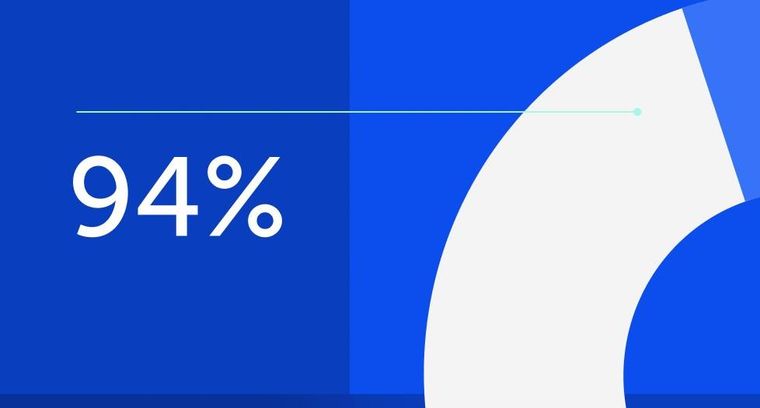
94% of researchers rate our articles as excellent or good
Learn more about the work of our research integrity team to safeguard the quality of each article we publish.
Find out more
ORIGINAL RESEARCH article
Front. Immunol., 04 May 2023
Sec. Multiple Sclerosis and Neuroimmunology
Volume 14 - 2023 | https://doi.org/10.3389/fimmu.2023.1110672
This article is part of the Research TopicBiomarkers in Autoimmune Diseases of the Central Nervous SystemView all 22 articles
Background: Increasing evidence indicates the importance of CD8+ T cells in autoimmune attack against CNS myelin and axon in multiple sclerosis (MS). Previous research has also discovered that myelin-reactive T cells have memory phenotype functions in MS patients. However, limited evidence is available regarding the role of CD8+ memory T cell subsets in MS. This study aimed to explore potential antigen-specific memory T cell-related biomarkers and their association with disease activity.
Methods: The myelin oligodendrocyte glycoprotein (MOG)-specific CD8+ memory T cell subsets and their related cytokines (perforin, granzyme B, interferon (IFN)-γ) and negative co-stimulatory molecules (programmed cell death protein 1 (PD-1), T- cell Ig and mucin domain 3 (Tim-3)) were analyzed by flow cytometry and real-time PCR in peripheral blood of patients with relapsing-remitting MS.
Results: We found that MS patients had elevated frequency of MOG-specific CD8+ T cells, MOG-specific central memory T cells (TCM), MOG-specific CD8+ effector memory T cells (TEM), and MOG-specific CD8+ terminally differentiated cells (TEMRA); elevated granzyme B expression on MOG-specific CD8+ TCM; and, on MOG-specific CD8+ TEM, elevated granzyme B and reduced PD-1 expression. The Expanded Disability Status Scale score (EDSS) in MS patients was correlated with the frequency of MOG-specific CD8+ TCM, granzyme B expression in CD8+ TCM, and granzyme B and perforin expression on CD8+ TEM, but with reduced PD-1 expression on CD8+ TEM.
Conclusion: The dysregulation of antigen-specific CD8+ memory T cell subsets, along with the abnormal expression of their related cytokines and negative co-stimulatory molecules, may reflect an excessive or persistent inflammatory response induced during early stages of the illness. Our findings strongly suggest positive regulatory roles for memory T cell populations in MS pathogenesis, probably via molecular mimicry to trigger or promote abnormal peripheral immune responses. Furthermore, downregulated PD-1 expression may stimulate a positive feedback effect, promoting MS-related inflammatory responses via the interaction of PD-1 ligands. Therefore, these parameters are potential serological biomarkers for predicting disease development in MS.
Multiple sclerosis (MS) is a chronic inflammatory demyelinating disease of the central nervous system (CNS). The disease frequently has a relapsing-remitting course and, in the later phases, tends to cause irreparable and severe neurological disability. Although its etiology remains uncertain, it is widely speculated that autoreactive T cell responses directed against CNS myelin are responsible for its pathogenesis (1). Importantly, several studies have found that MS patients have different memory phenotypes of myelin-reactive T cells.
Conventional CD4+ and CD8+ memory T cells can be divided into CCR7+CD45RA− central memory T cells (TCM), CCR7−CD45RA− effector memory T cells (TEM), and CD45RA+CCR7− terminally differentiated cells (TEMRA), with district homing and effector properties (2–4). TCM and naïve cells home to secondary lymphoid tissues in physiological circumstances, whereas TEM and TEMRA traffick to non-lymphoid organs that are inflamed and exert effects such as stimulating interferon (IFN)-γ secretion and causing potent cytotoxicities. TCM, in contrast, are longer-lived with a greater proliferative capacity (5, 6).
Mounting evidence indicates that CD8+ T cells have a significant role in autoimmune CNS attack in MS. CD8+ T cells are accumulated in white matter lesions. These cells frequently outnumber CD4+ T cells at this location, and are in a quiet neibourhood close to oligodendrocytes and demyelinated CNS axons (7–9), the latter of which are believed to produce early neurological symptoms (10). CD8+ T cells are present in immune cell infiltrates in the early phases of MS cortical demyelinating lesions (11). Strikingly, CD8+ T cells with an effector-memory phenotype have been found to accumulate in the MS lesions, exhibiting inflammatory and cytotoxic potential due to enhanced expression of granzyme B and interferon (IFN)-γ (12, 13). In parallel with these findings, we previously reported that MS patients display elevated CD8+CCR7+CD45RA− TCM, which tends to decrease after treatment with the immunomodulatory agent IFN-β1α (14). Together, these findings strongly suggest that a skewed distribution of autoreactive CD8+ memory T-cell subsets is involved in the disease pathogenesis, given that an increased frequency of circulating CD4+ TEM has been demonstrated in MS patients after specific antigen-driven stimulation. Notably, human leucocyte antigen (HLA)-A*03:01 is associated with about a two-fold increase in risk of developing MS—independent of HLA-DR2 (15–17).
However, further research is still required to understand the distribution of memory T-cell subsets in the illness and the potential contribution of abnormal T-cell homeostasis to the pathophysiology of inflammation. Thus, we analyzed circulating antigen-specific memory T-cell subsets using the pentamer, HLA-A*03:01-RVVHLYRNGK (myelin oligodendrocyte glycoprotein (MOG)46-55 peptide) and its related cytokines, to determine if these cell populations are correlated with disease activity.
Twenty-four patients with definite MS (five men and 19 women; mean age 35.2 ± 5.3 years) were included. All patients experienced relapsing-remitting MS (RRMS) and had never been using immunosuppressive medications, including glucocorticosteroids, for more than 6 months prior to the study. Five of these RRMS patients were chosen for serial examination while receiving treatment with teriflunomide (Aubagio®, Sanofi, Paris, France). Patients underwent clinical neurological examination including expanded disability status scale (EDSS), blood sampling before and after 2 and 4 weeks of treatment (18).
Twenty-three patients with atherothrombotic stroke (6 men and 17 women; mean age 36.7 ± 5.9 years) were recruited as “other neurological diseases” (OND) controls. Twenty-four healthy individuals participated as the healthy controls (“HC”, six men and 18 women; mean age 35.0 ± 6.5 years). All participating subjects were Han Chinese and tested positive for the A3 allele via HLA-A genotyping using polymerase chain reaction (PCR) sequence-specific primers (PCR-SSP). At the Department of Clinical Chemistry, whole blood cell counts and leucocyte differential analysis, were measured for patients and HC.
Blood samples that had been heparinized were gathered between 9 and 12 AM. Blood samples from teriflunomide-treated individuals were taken before, and after 2 and 4 weeks of therapy as part of a serial trial.
To examine the expression of multiple cytokines (perforin, granzyme B, IFN-γ) and checkpoint receptor members ((programmed cell death protein 1 (PD-1) and T-cell Ig and mucin domain 3 (Tim-3)) in the antigen-specific CD8+ memory T-cell subsets, PBMCs were isolated by density gradient centrifugation and resuspended in phosphate buffer saline (PBS) containing 2% fetal calf serum. Thereafter, CD8+ TCM, TEM, and TEMRA were isolated with allophycocyanin (APC)-labelled Pro5MHC Pentamer [HLA-A*03:01 MOG46-55 pentamers-RVVHLYRNGK] (Proimmune, Oxford, UK), phycoerythrin (PE)-cy7-labelled anti-CCR7 (eBioscience, San Diego, CA), APC-cy7-labelled anti-CD8 (BD Pharmingen, San Diego, CA, USA), and peridinin chlorophyll protein (PerCP)-Cy5.5-labelled-anti-CD45RA (Tonbo Biosciences, San Diego, CA) monoclonal antibody (MoAb) via a flow sorter (Becton Dickinson, San Jose, CA, USA).
Antigen-specific CD8+ memory T-cell subsets were characterized in peripheral blood mononuclear cells (PBMCs) of the patients and controls by assessing PD-1 and Tim-3 expression via four-color direct fluorescence staining and flow cytometry using a FACScan (BD Biosciences, San Jose, CA, USA). After washing with PBS (0.5% BSA, pH 7.2), the cells were resuspended in PBS, to a final concentration of 1 × 106 cells/mL. The following MoAb were added to 1 × 106 cells following the manufacturer’s instructions: APC-cy7-labelled CD8; APC-labelled Pro5 MHC Pentamer [HLA-A*03:01 MOG46-55 pentamers-RVVHLYRNGK]; PE-cy7-labelled anti-CCR7; PerCP-Cyanine5.5-labelled-anti-CD45RA, Super Bright (SB)645-labelled anti-PD-1 (Thermo Fisher Scientific, Waltham, MA, USA); SB600-labelled anti-Tim-3 (Thermo Fisher Scientific). After 30-min incubation at room temperature, the cells were washed with PBS, fixed with 1% paraformaldehyde, and finally analyzed using a FACScan.
To perform intracellular cytokine staining, 1 × 106 cells were incubated with the Cell Stimulation Cocktail (Tonbo Bioscience) for 4–5 h at 37°C in complete RPMI. Intracellular staining with eFluor 450-labelled anti-perforin (Thermo Fisher Scientific), Brilliant Violet (BV)510-labelled anti-granzyme B (BD Biosciences), and BV711-labelled anti-IFN-γ (BD Biosciences) MoAbs was completed following fixation/permeabilization according the manufacturer’s instructions (Cytofix/Cytoperm, BD Biosciences).
RNA isolation was performed using RNeasy® Mini Kit (Qiagen, Hilden, Germany), according to standard protocol. Reverse transcription was conducted with HiScript III 1st Strand cDNA Synthesis Kit (Nanjing Vazyme Biotech Co. Nanjing, China) using random hexamers and primer containing 50 µM oligio (dt). The process was carried out at 25°C for 10 min, 48°C for 30 min, and 95°C for 5 min on a T100 PCR system (Bio-Rad Laboratories, Hercules, CA, USA).
Perforin, granzyme B, IFN-γ, PD-1, and Tim-3 mRNA were quantified using cDNA-specific primers (Sangon Biotec, Shanghai, China) as described elsewhere (Table 1) (19, 20). Twenty-five nanograms of cDNA and 200 nM forward and reverse primers were added to the PCR reactions using the AceQ Universal SYBR qPCR Master Mix (Nanjing Vazyme Biotech, Nanjing, China). β-Actin was chosen as the endogenous control. Real-time PCR was conducted using an ABI PRISM 7500 sequencing detector (Applied Biosystems, Foster City, CA, USA). Perforin, granzyme B, IFN- γ, PD-1, Tim-3, and 18 S PCR conditions were: hold at 50°C for 90 s, then 95°C for 10 min, followed by 40 cycles at 95°C for 15 s, 60°C for 1 min, and 72°C for 45 s.
Age, disease course, EDSS, and blood leukocyte count data are shown as means ± standard deviation. Memory T-cell subsets, surface and intracellular expression of cytokines, PD-1, and Tim-3 mRNA data are shown as medians with range. Categorical variables (sex) are expressed as percentages. Normal distribution data were analyzed using one-way ANOVA or a Pearson correlation test. Non-normal distribution data were analyzed using Kruskal-Wallis analysis or a Spearman correlation test. Categorical variables were analyzed utilizing a chi-square test. Receiver operating characteristic (ROC) curve analysis was conducted for quantitative MOG-specific CD8+ TCM, MOG-specific CD8+granzyme-B+ TCM or TEM, MOG-specific CD8+perforin+ TEM, and MOG-specific CD8+ PD-1+ TEM frequency, followed by the calculation of area under the ROC curve (AUC). P < 0.05 was deemed statistically significant.
Table 2 shows the clinical and laboratory data gathered from patients during blood sampling. There were no remarkable differences among the three groups.
Table 2 Baseline characteristics of patients with multiple sclerosis (MS), other neurological disease (OND), and healthy controls (HC).
Table 3 shows the comparison of MOG-specific CD8+ T cells, naïve T cells and memory T cell subsets in peripheral blood between patients with MS,OND and HC.
Table 3 Comparison of MOG-specific CD8+ T cells and memory T cell subsets in peripheral blood between patients with multiple sclerosis (MS), other neurological disease (OND) and healthy controls (HC).
MS Patients had an increased proportion of MOG-specific CD8+ T cells, MOG-specific CD8+ TCM (HLA-A*03:01/MOG46-55 pentamers+CCR7+CD45RA−), MOG-specific CD8+ TEM (HLA-A*03:01/MOG46-55 pentamers+CCR7−CD45RA−), and MOG-specific CD8+ TEMRA (HLA-A*03:01/MOG46-55 pentamers+CCR7−CD45RA+) compared to OND patients and HC via FACScan (Figure 1). In contrast, MS patients had a lower proportion of MOG-specific naive CD8+ T cells either OND patients or HC. After 2 and 4 weeks of therapy, five MS patients showed a tendency for a gradual decrease in MOG-specific CD8+ TCM and TEM (Figures 2B, C), while the remaining cell subsets displayed minor or irregular alternations (Figures 2A, D).
Figure 1 Region 1 (R1) was selected to set the mononuclear cell gate according to the forward light scatter (FSC) and side light scatter (SSC) properties. Region 2 (R2) was used to set the second gate, to separate MOG-specific CD8+ T cells for analysis of memory T cell subsets. Regions 3, 4, and 5 (R3–R5) were selected to set the central memory T cell (TCM), effector memory T cell (TEM) and terminally differentiated cell (TEMRA) gates, respectively, for perforin, granzyme B, interferon (IFN)-γ, programmed cell death protein 1 (PD-1), and T- cell Ig and mucin domain 3 (Tim-3) analysis.
Figure 2 Serial study of the MOG-specific memory T-cell subsets (A–D) in the peripheral blood from five patients with multiple sclerosis (MS) before and after 14 d and 28 d of treatment with teriflunomide.
MS patients demonstrated elevated expression of granzyme B, and reduced expression of PD-1, on MOG-specific CD8+ TEM, compared to HC (Figures 3A, D), as well as increased expression of granzyme B on MOG-specific CD8+ TCM, when compared with HC (Figure 3F). Although there was a slight increase in perforin expression, no marked differences were found between these three groups (Figures 3C, H). OND patients demonstrated slightly higher expression of Tim-3 on MOG-specific CD8+ TEM and CD8+ TCM than HC, but did not reach statistical significance (Figures 3E, J). However, we did not measure the above cytokines or PD-1 and Tim-3 on CD8+ TEMRA, owing to the extremely low proportion of this cell population in peripheral blood. Five MS patients treated with teriflunomide exhibited a continuous increase in PD-1 expression on MOG-specific CD8+ TEM after 2- and 4-weeks therapy (Figure 4D), while the expression of the remaining cytokines or co-stimulatory molecules presented slight or irregular changes (Figures 4A-C, E-J).
Figure 3 Peripheral blood expression of perforin, granzyme B, interferon (IFN)-γ, programmed cell death protein 1 (PD-1), and T- cell Ig and mucin domain 3 (Tim-3) on MOG-specific CD8+ effector memory T cells (TEM) (A–E) and CD8+ central memory T cells (TCM) (F–J), in patients with multiple sclerosis (MS), those with other neurological disease (OND), and healthy controls (HC). Horizontal lines: medians. * P < 0.05, ** P < 0.01.
Figure 4 Serial analysis of perforin, granzyme B, interferon (IFN)-γ, programmed cell death protein 1 (PD-1), and T- cell Ig and mucin domain 3 (Tim-3) on MOG-specific CD8+ central memory T cells (TCM) and CD8+ effector memory T cells (TEM) in the peripheral blood of five patients with multiple sclerosis (MS) before and after 14 d and 28 d of treatment with teriflunomide (A–J).
Of the isolated CD8+ memory T cell subsets, MOG-specific CD8+ TEM exhibited lower PD-1 and Tim-3 mRNA expression in MS patients than in HC, while PD-1 expression did not differ significantly between MS and OND patients (Figures 5D, E). MS patients displayed significantly higher mRNA expression of granzyme B in MOG-specific CD8+ TCM than OND patients and HC (Figure 5F). However, no significant difference in above proinflammatory cytokines or PD-1 and Tim-3 mRNA expression in TEMRA were found between MS patients and control groups.
Figure 5 Peripheral blood mRNA expression of granzyme B, interferon (IFN)-γ, perforin, PD1, and TIM3 on CD8+ effector memory T cells (TEM) (A–E), CD8+ central memory T cells (TCM) (F–J), and terminally differentiated cells (TEMRA) (K–O), in patients with multiple sclerosis (MS), those with other neurological disease (OND), and healthy controls (HC). Horizontal lines: medians.
ROC curves were generated to calculate the AUC on the basis of the optimal cut-off value, as well as maximum sensitivity and specificity. For the MOG-specific CD8+ TCM proportion, AUC was 0.9089 (optimal threshold cutoff, 6.65%; sensitivity, 91.67%; and specificity, 75%); for MOG-specific CD8+granzyme-B+ TCM, AUC was 0.6528 (optimal threshold cutoff, 7.605%; sensitivity, 66.67%; specificity, 75%); for MOG-specific CD8+granzyme-B+ TEM, AUC was 0.691 (optimal threshold cut-off, 4.364%; sensitivity, 100%; specificity, 29.17%); for MOG-specific CD8+perforin+ TEM, AUC was 0.678% (optimal threshold cutoff, 0.5885%; sensitivity, 58.33%; specificity, 87.5%); for MOG-specific CD8+ PD-1+ TEM, AUC was 0.6918 (optimal threshold cutoff, 0.0415%, sensitivity 75%, specificity, 70.83%) (Figure 6).
Figure 6 Receiver operating characteristic (ROC) curve analysis, in patients with multiple sclerosis (MS), of the frequency of MOG-specific CD8+ central memory T cells (TCM), MOG-specific CD8+granzyme-B+ TCM or effector memory T cells (TEM), MOG-specific CD8+perforin+ TEM, and MOG-specific CD8+ PD-1+ TEM.
In MS patients, EDSS score before treatment was correlated with the frequency of MOG-specific CD8+ TCM (r = 0.421, P = 0.041); granzyme B expression in CD8+ TCM (r = 0.507, P = 0.012); and, for CD8+ TEM, the expression of granzyme B (r = 0.512, P = 0.01), perforin (r = 0.446, P = 0.029), and PD-1 (r = −0.520, P = 0.009). EDSS score after treatment of teriflunomide was correlated with the frequency of MOG-specific CD8+ TEM (r = 0.975, P = 0.033). Moreover, EDSS score after 4 weeks of treatment with teriflunomide was correlated with the granzyme B expression in MOG-specific CD8+ TEM (r = 0.975, P = 0.033).
To the best of our knowledge, this is the first human-based research utilizing MHC pentamers to identify myelin antigen-specific CD8+ T cells and their memory T cell subsets in MS patients. As a result, MS patients had higher frequency of MOG-specific CD8+ T cells, MOG-specific CD8+ TCM, MOG-specific CD8+ TEM, and MOG-specific CD8+ TEMRA, in contrast to a lower frequency of MOG-specific naïve CD8+ T cells; elevated granzyme B expression on MOG-specific CD8+ TCM; and, on MOG-specific CD8+ TEM, elevated granzyme B and reduced PD-1 expression. In MS patients, EDSS was correlated with the frequency of MOG-specific CD8+ TCM, granzyme B expression in CD8+ TCM, and granzyme-B and perforin expression on CD8+ TEM, but with reduced PD-1 expression on CD8+ TEM.
Our results indicate that MS patients have a skewed distribution of antigen-specific CD8+ memory T cell subsets, with more TCM, TEM, and TEMRA, and fewer naive CD8+ T cells, than HC. These findings validate the derangement of these cell populations during the inflammatory process in MS. Together with our previous report revealing elevated IL-15 release into circulation (14), our current findings suggest that the differentiation of naïve cells is what causes the rise in CD8+ TCM and TEM because IL-15 is generally considered a central regulator of primary and memory antigen-specific CD8+ T cell production (21, 22). Interestingly, patients with atherosclerotic stroke and HC do not differ in the frequency of antigen-specific CD8+ T cells and their memory T cell subsets, since the former category of patients exhibits CD4+ memory T cells and CD8+ T cells in the atherosclerotic tissue from their carotid arteries (23). Hence, further research is required to elucidate this.
Several sphingosine 1-phosphate (S1P1) receptor antagonists, such as fingolimod (FTY720), have been commonly used for the treatment of RRMS; these antagonists selectively retain CCR7+ naïve T cells and TCM, and particularly autoreactive Th17 cells, within the secondary lymphoid organs (24–26). Nonetheless, the exact role of CD8+ T cell subsets such as TCM and TEM in MS remains elusive. However, in our recently published pilot study, adoptive transfer of autoreactive CD8+ TCM into Rag-1−/− mice failed to induce EAE symptoms or EAE-related pathology (27). Notably, a lower proportion of memory CD8+ T cell subsets (particularly effector memory and TEMRA) has been observed in patients with untreated RRMS than in HC, probably due to inherent (i.e., genetically determined) defects rather than a pathophysiological effect of MS (28, 29). In our experiments, despite an irregular change in total MOG-specific CD8+ T cells in MS patients, MOG-specific CD8+ TCM and CD8+ TEM exhibited a decreasing trend at 14 and 28 d post-treatment with teriflunomide, a well-known immunosuppressant agent (30). Together with a recent MS study revealing markedly lower IFN-γ and tumor necrosis factor-α levels on TEMRA and TEM following 12 months of teriflunomide use (31), our results indicate that these cell populations play a positive regulatory role in disease pathogenesis, presumably via a mechanism of molecular mimicry to trigger or promote abnormal peripheral immune responses and, consequently, aggravate neuroinflammation, and myelin and axonal damage in the brain. The positive correlations that we observed here between disease severity and the frequencies of MOG-specific CD8+ TCM and MOG-specific CD8+ granzyme B+ TCM or TEM further support this view. More importantly, these markers are potentially valuable in defining RRMS and secondary progressive MS (SPMS), because growing evidence suggests the involvement of distinct memory T cell subsets in different forms of MS and/or at different disease stages (29, 32, 33).
As negative co-stimulatory molecules, Tim-3 and PD-1 are expressed on the cell surface and negatively modulate the immune response; their signaling impairs functional activities of CD8+ T cell, eventually leading to CD8+ T cell exhaustion, particularly in chronic viral infection and tumors (34, 35). Co-expression of Tim-3 and PD-1 are characteristic of the most severely exhausted CD8+ T cell subset (36, 37). Blockade of Tim-3 and PD-1 pathway can reverse this exhaustion and rescue the T-cell function (38, 39). However, it is difficult to determine their function on CD8 + T cells, since TIM-3 is implicated in both T cell exhaustion and activation (35, 40–44). Here, MS patients showed a significant reduction in PD-1 and slight reduction in Tim-3 surface expression on MOG-specific CD8+ TEM, compared to HC. This suggests that the molecule dysregulation in CD8+ memory T cells might be more prevalent in CD8+ TEM than in CD8+ TCM. However, these results should be interpreted with caution, because the expression of PD-1 and Tim-3 are insufficient to define the function of CD8+ T cells in the absence of their ligands. Nevertheless, more efforts are needed to explore their expression as well as the effects of these two costimulatory pathways on CD8+ T cells in our future study.
The reduced PD-1 and Tim-3 mRNA expression in MOG-specific CD8+ TEM that we observed in MS patients further substantiates our findings. Consistent with our results, a previous study (45) reported that CD4+ and CD8+ T cells stimulated by myelin basic protein demonstrated significantly higher PD-1-positive cell frequency in stable than in acute MS. Tim-3 activation, on the other hand, stimulates the formation of effector T cells as evidenced by the acquisition of an activated effector phenotype, elevated cytokine secretion, higher proliferative activities, and a transcription program linked with the differentiation of human antigen-specific CD8 T cells (42). Indeed, there was a trend towards increased expression of PD-1 on CD8+ TEM during 4 weeks of treatment with teriflunomide. Taken together, these findings for MS suggest aberrant PD-1 and Tim-3 co-stimulation in CD8+ TEM rather than in CD8+ TCM. PD-1 may reduce the inflammation caused by local cell–cell interactions, by halting co-stimulation of the host immune cells such as lymphocytes, or by suppressing apoptotic signaling via interaction with PD-1 ligands (40, 46). Tim-3 may exert complex regulatory effects on the immunoactivity of CD8+ memory T cell subsets in different stages of MS. Together with our ROC analysis of abnormal antigen-specific memory T cell subsets, our findings strongly suggest that dysregulated PD-1 and Tim-3 co-stimulation are implicated in MS pathogenesis, and may therefore be useful for assessing disease severity.
In conclusion, in MS patients, we observed remarkable upregulation of antigen-specific CD8+ TEM, TEMRA and TCM, with elevated intracellular expression of granzyme B and reduced expression of PD-1 in MOG-specific CD8+ TEM. This may implicate a persistent chronic CD8+ memory T cell-mediated inflammatory response, potentially induced in the early stages of this disorder. More strikingly, MS severity was at least partially reflected in the elevated MOG-specific CD8+ TCM frequency and granzyme B and perforin expression, and reduced PD-1 expression. These are therefore potential serological biomarkers for predicting the development of MS. Nevertheless, further studies, such as those with a prospective cohort design, are required. Given that other disorders (e.g., oncological malignancies) also demonstrate dysregulated CD8+ memory T cells, it is necessary to evaluate their specificity, sensitivity, cytotoxicity, and negative co-stimulation to demonstrate whether these markers might be specific for MS. Other issues should be addressed, such as their prognostic vs. non-prognostic value in predicting acute episodes in patients diagnosed as clinically isolated syndrome (CIS). Our findings strongly suggest positive regulatory roles for memory T cell populations in MS pathogenesis, probably via molecular mimicry to trigger or promote abnormal peripheral immune responses. Furthermore, downregulated PD-1 expression may stimulate a positive feedback effect, promoting MS-related inflammatory responses via the interaction of its ligands (PDL1), although the expression of PD-1 is not sufficient to define the function of CD8+ T cells in the absence of its ligand. Our discovery of antigen-specific CD8+ T cell subsets and PD-1 expression in MS paves the way for identifying potential MS biomarkers and, more importantly, for exploring a novel treatment approach against this disease through intervention in the PD-1–PDL1 pathway.
The raw data supporting the conclusions of this article will be made available by the authors, without undue reservation.
The studies involving human participants were reviewed and approved by Ethics Committee of Beijing Anzhen Hospital, Capital Medical University. The patients/participants provided their written informed consent to participate in this study.
P-JL and G-ZL wrote the article. G-ZL designed the research. P-JL and T-TY performed the research. Z-XF and Z-YW analyzed the data. G-BY, LM, W-LZ, J-FL, X-HZ, and B-YY contributed new reagents/analytical tools. All authors contributed to the article and approved the submitted version.
The National Natural Science Foundation secured assistance for this research (NSF 81870951 and 82071342).
The authors would like to express their tremendous gratitude to all participants.
The authors declare that the research was conducted in the absence of any commercial or financial relationships that could be construed as a potential conflict of interest.
All claims expressed in this article are solely those of the authors and do not necessarily represent those of their affiliated organizations, or those of the publisher, the editors and the reviewers. Any product that may be evaluated in this article, or claim that may be made by its manufacturer, is not guaranteed or endorsed by the publisher.
AUC, area under the receiver operating characteristic curve; CNS, central nervous system; EDSS, Expanded Disability Status Scale; HC, healthy controls; IFN, interferon; MOG, myelin oligodendrocyte glycoprotein; MS, multiple sclerosis; OND, other neurological disease; PBMC, peripheral blood mononuclear cells; PD-1, programmed cell death protein 1; PCR, polymerase chain reaction; ROC, receiver operating characteristic; RRMS, relapsing-remitting multiple sclerosis; TCM, central memory T cells; TEM, effector memory T cells; TEMRA, terminally differentiated cells; Tim-3, T- cell Ig and mucin domain 3.
1. Rodríguez Murúa S, Farez MF, Quintana FJ. The immune response in multiple sclerosis. Annu Rev Pathol (2022) 17:121–39. doi: 10.1146/annurev-pathol-052920-040318
2. Sallusto F, Lenig D, Förster R, Lipp M, Lanzavecchia A. Two subsets of memory T lymphocytes with distinct homing potentials and effector functions. Nature(1999) (6754) 401:708–12. doi: 10.1038/44385
3. Sani MM, Ashari NSM, Abdullah B, Wong KK, Musa KI, Mohamud R, et al. Reduced CD4+ terminally differentiated effector memory T cells in moderate-severe house dust mites sensitized allergic rhinitis patients. Asian Pac J Allergy Immunol (2019) 37(3):138–46. doi: 10.12932/AP-191217-0220
4. Delgobo M, Heinrichs M, Hapke N, Ashour D, Appel M, Srivastava M, et al. Terminally differentiated CD4+ T cells promote myocardial inflammaging. Front Immunol (2021) 12:584538. doi: 10.3389/fimmu.2021.584538
5. Mahnke YD, Brodie TM, Sallusto F, Roederer M, Lugli E. The who's who of T-cell differentiation: human memory T-cell subsets. Eur J Immunol (2013) 43(11):2797–809. doi: 10.1002/eji.201343751
6. Sallusto F, Geginat J, Lanzavecchia A. Central memory and effector memory T cell subsets: function, generation, and maintenance. Annu Rev Immunol (2004) 22:745–63. doi: 10.1146/annurev.immunol.22.012703.104702
7. Frischer JM, Bramow S, Dal-Bianco A, Lucchinetti CF, Rauschka H, Schmidbauer M, et al. The relation between inflammation and neurodegeneration in multiple sclerosis brains. Brain (2009) 132:1175–89. doi: 10.1093/brain/awp070
8. Liblau RS, Wong FS, Mars LT, Santamaria P. Autoreactive CD8 T cells in organ-specific autoimmunity: emerging targets for therapeutic intervention. Immunity (2002) 17:1–6. doi: 10.1016/s1074-7613(02)00338-2
9. Neumann H, Medana IM, Bauer J, Lassmann H. Cytotoxic T lymphocytes in autoimmune and degenerative CNS diseases. Trends Neurosci (2002) 25:313–9. doi: 10.1016/s0166-2236(02)02154-9
10. Kipp M, Victor M, Martino G, Franklin RJ. Endogeneous remyelination: findings in human studies. CNS Neurol Disord Drug Targets (2012) 11(5):598–609. doi: 10.2174/187152712801661257
11. Lucchinetti CF, Popescu BFG, Bunyan RF, Moll NM, Roemer SF, Lassmann H, et al. Inflammatory cortical demyelination in early multiple sclerosis. N Engl J Med (2011) 365:2188–97. doi: 10.1056/NEJMoa1100648
12. Ifergan I, Kebir H, Terouz S, Alvarez JI, Lécuyer M-A, Gendron S, et al. Role of ninjurin-1 in the migration of myeloid cells to central nervous system inflammatory lesions. Ann Neurol (2011) 70:751–63. doi: 10.1002/ana.22519
13. Van Nierop GP, Van Luijn MM, Michels SS, Melief M-J, Janssen M, Langerak AW, et al. Phenotypic and functional characterization of T cells in white matter lesions of multiple sclerosis patients. Acta Neuropathol (2017) 134:383–401. doi: 10.1007/s00401-017-1744-4
14. Liu G-Z, Fang L-B, Hjelmström P, Gao X-G. Increased CD8+ central memory T cells in patients with multiple sclerosis. Mult Scler (2007) 13(2):149–55. doi: 10.1177/1352458506069246
15. Burfoot RK, Jensen CJ, Field J, Stankovich J, Varney MD, Johnson LJ, et al. SNP mapping and candidate gene sequencing in the class I region of the HLA complex: searching for multiple sclerosis susceptibility genes in tasmanians. Tissue Antigens (2008) 71(1):42–50. doi: 10.1111/j.1399-0039.2007.00962.x
16. Fogdell-Hahn A, Ligers A, Grønning M, Hillert J, Olerup O. Multiple sclerosis: a modifying influence of HLA class I genes in an HLA class II associated autoimmune disease. Tissue Antigens (2000) 55(2):140–8. doi: 10.1034/j.1399-0039.2000.550205.x
17. Harbo HF, Lie BA, Sawcer S, Celius EG, Dai K-Z, Oturai A, et al. Genes in the HLA class I region may contribute to the HLA class II-associated genetic susceptibility to multiple sclerosis. Tissue Antigens (2004) 63(3):237–47. doi: 10.1111/j.0001-2815.2004.00173.x
18. Kurtzke JF. Rating neurologic impairment in multiple sclerosis: an expanded disability status scale (EDSS). Neurology (1983) 33:444–52. doi: 10.1212/wnl.33.11.1444
19. Sawayama T, Sakaguchi K, Senoh T, Ohta T, Nishimura M, Takaki A, et al. Effects of pulsing procedure of interleukin-12 in combination with interleukin-2 on the activation of peripheral blood lymphocytes derived from patients with hepatocellular carcinoma. Acta Med Okayama (2003) 57(6):285–92. doi: 10.18926/AMO/32813
20. Mahmoudian RA, Mozhgani S, Abbaszadegan MR, Mokhlessi L, Montazer M, Gholamin M. Correlation between the immune checkpoints and EMT genes proposes potential prognostic and therapeutic targets in ESCC. J Mol Histol. (2021) 52(3):597–609. doi: 10.1007/s10735-021-09971-3
21. Weninger W, Crowley MA, Manjunath N, von Andrian UH. Migratory properties of naive, effector,and memory CD8(+) T cells. J Exp Med (2001) 194:953–66. doi: 10.1084/jem.194.7.953
22. Schluns KS, Williams K, Ma A, Zheng XX, Lefrançois L. Cutting edge: requirement for IL-15 in the generation of primary and memory antigen-specific CD8 T cells. J Immunol (2002) 168(10):4827–31. doi: 10.4049/jimmunol.168.10.4827
23. Gao J, Shi L, Gu J, Zhang D, Wang W, Zhu X, et al. Difference of immune cell infiltration between stable and unstable carotid artery atherosclerosis. J Cell Mol Med (2021) 25(23):10973–9. doi: 10.1111/jcmm.17018
24. Gräler MH, Goetzl EJ. The immunosuppressant FTY720 down-regulates sphingosine 1-phosphate G-proteincoupled receptors. FASEB J (2004) 18(3):551–3. doi: 10.1096/fj.03-0910fje
25. Matloubian M, Lo CG, Cinamon G, Lesneski MJ, Xu Y, Brinkmann V, et al. Lymphocyte egress from thymus and peripheral lymphoid organs is dependent on S1P receptor 1. Nature (2004) 427(6972):355–60. doi: 10.1038/nature02284
26. Gholamnezhadjafari R, Falak R, Tajik N, Aflatoonian R, Ali Keshtkar A, Rezaei A. Effect of FTY720 (fingolimod) on graft survival in renal transplant recipients: a systematic review protocol. BMJ Open (2016) 6(4):e010114. doi: 10.1136/bmjopen-2015-010114
27. Ting-Ting Y, Hong J, Ya-Juan X, Yang H, Pen-Ju L, He Y, et al. Priliminary study on pathogenic role of autoreactive CD8+ central memory T cells in experimental autoimmune encephalomyelitis. Chin Neuroimmunol Neurol (2021) 28(2):93–102. doi: 10.3969/j.jssn.1006-2963
28. Pender MP, Csurhes PA, Pfluger CMM, Burrows SR. Deficiency of CD8+Effector memory T cells is an early and persistent feature of multiple sclerosis. Mult Scler J (2014) 20(14):1825–32. doi: 10.1177/1352458514536252
29. Canto-Gomes J, Silva CS, Rb-Silva R, Boleixa D, da Silva AM, Cheynier R, et al. Low memory T cells blood counts and high naïve regulatory T cells percentage at relapsing remitting multiple sclerosis diagnosis. Front Immunol (2022) 13:901165. doi: 10.3389/fimmu.2022.901165
30. Bar-Or A, Pachner A, Menguy-Vacheron F, Kaplan J, Wiendl H. Teriflunomide and its mechanism of action in multiple sclerosis. Drugs (2014) 74(6):659–74. doi: 10.1007/s40265-014-0212-x
31. Tilly G, Cadoux M, Garcia A, Morille J, Wiertlewski S, Pecqueur C, et al. Teriflunomide treatment of multiple sclerosis selectively modulates CD8 memory T cells. Front Immunol (2021) 12:730342. doi: 10.3389/fimmu.2021.730342
32. Nielsen BR, Ratzer R, Börnsen L, von Essen MR, Christensen JR, Sellebjerg F. Characterization of naïve, memory and effector T cells in progressive multiple sclerosis. J Neuroimmunol (2017) 310:17–25. doi: 10.1016/j.jneuroim.2017.06.001
33. Canto-Gomes J, Da Silva-Ferreira S, Silva CS, Boleixa D, Martins da Silva A, González-Suárez I, et al. People with primary progressive multiple sclerosis have a lower number of central memory T cells and HLA-DR+ tregs. Cells (2023) 12(3):439. doi: 10.3390/cells12030439
34. Takano S, Saito H, Ikeguchi M. An increased number of PD-1+ and Tim-3+ CD8+ T cells is involved in immune evasion in gastric cancer. Surg Today (2016) 46:1341–7. doi: 10.1007/s00595-016-1305-9
35. Fourcade J, Sun Z, Benallaoua M, Guillaume P, Luescher IF, Sander C, et al. Upregulation of Tim-3 and PD-1 expression is associated with tumor antigen-specifc CD8+ T cell dysfunction in melanoma patients. J Exp Med (2010) 207:2175–86. doi: 10.1084/jem.20100637
37. Xu YY, Wang SC, Lin YK, Li DJ, DU MR. Tim-3 and PD-1 regulate CD8+ T cell function to maintain early pregnancy in mice. J Reprod Dev (2017) 63(3):289–94. doi: 10.1262/jrd.2016-177
38. Kim JE, Patel MA, Mangraviti A, Kim ES, Theodros D, Velarde E, et al. Combination therapy with anti-PD-1, antiTIM-3, and focal radiation results in regression of murine gliomas. Clin Cancer Res (2017) 23:124–36. doi: 10.1158/1078-0432
39. Liu J, Zhang S, Hu Y, Yang Z, Li J, Liu X, et al. Targeting PD-1 and Tim-3 pathways to reverse CD8 T-cell exhaustion and enhance ex vivo T-cell responses to autologous Dendritic/Tumor vaccines. J Immunother (2016) 39:171–80. doi: 10.1097/CJI.0000000000000122
40. Verdon DJ, Mulazzani M, Jenkins MR. Cellular and molecular mechanisms of CD8+ T cell differentiation, dysfunction and exhaustion. Int J Mol Sci (2020) 21(19):7357. doi: 10.3390/ijms21197357
41. Gorman JV, Starbeck-Miller G, Pham NL, Traver GL, Rothman PB, Harty JT, et al. Tim-3 directly enhances CD8 T cell responses to acute listeria monocytogenes infection. J Immunol (2014) 192:3133–42. doi: 10.4049/jimmunol.1302290
42. Sabins NC, Harman BC, Barone LR, Shen S, Santulli-Marotto S. Differential expression of immune checkpoint modulators on in vitro primed CD4(+) and CD8(+) T cells. Front Immunol (2016) 7:221. doi: 10.3389/fimmu.2016.00221
43. Qiu Y, Chen J, Liao H, Zhang Y, Wang H, Li S, et al. Tim-3-expressing CD4+ and CD8+ T cells in human tuberculosis (TB) exhibit polarized effector memory phenotypes and stronger anti-TB effector functions. PloS Pathog (2012) 8(11):e1002984. doi: 10.1371/journal.ppat.1002984
44. Jin HT, Anderson AC, Tan WG, West EE, Ha SJ, Araki K, et al. Cooperation of Tim-3 and PD-1 in CD8 T-cell exhaustion during chronic viral infection. Proc Natl Acad Sci USA (2010) 107:14733–8. doi: 10.1073/pnas.1009731107
45. Trabattoni D, Saresella M, Pacei M, Marventano I, Mendozzi L, Rovaris M, et al. Costimulatory pathways in multiple sclerosis: distinctive expression of PD-1 and PD-L1 in patients with different patterns of disease. J Immunol (2009) 183(8):4984–93. doi: 10.4049/jimmunol.0901038
Keywords: multiple sclerosis, peripheral blood mononuclear cells, memory T cells, oligodendrocyte glycoprotein, pentamer
Citation: Liu P-J, Yang T-T, Fan Z-X, Yuan G-B, Ma L, Wang Z-Y, Lu J-F, Yuan B-Y, Zou W-L, Zhang X-H and Liu G-Z (2023) Characterization of antigen-specific CD8+ memory T cell subsets in peripheral blood of patients with multiple sclerosis. Front. Immunol. 14:1110672. doi: 10.3389/fimmu.2023.1110672
Received: 29 November 2022; Accepted: 24 April 2023;
Published: 04 May 2023.
Edited by:
Honghao Wang, Guangzhou First People’s Hospital, ChinaReviewed by:
Sheng Chen, Shanghai Jiao Tong University, ChinaCopyright © 2023 Liu, Yang, Fan, Yuan, Ma, Wang, Lu, Yuan, Zou, Zhang and Liu. This is an open-access article distributed under the terms of the Creative Commons Attribution License (CC BY). The use, distribution or reproduction in other forums is permitted, provided the original author(s) and the copyright owner(s) are credited and that the original publication in this journal is cited, in accordance with accepted academic practice. No use, distribution or reproduction is permitted which does not comply with these terms.
*Correspondence: Guang-Zhi Liu, Z3Vhbmd6aGkyMDAyQGhvdG1haWwuY29t
†These authors have contributed equally to this work
Disclaimer: All claims expressed in this article are solely those of the authors and do not necessarily represent those of their affiliated organizations, or those of the publisher, the editors and the reviewers. Any product that may be evaluated in this article or claim that may be made by its manufacturer is not guaranteed or endorsed by the publisher.
Research integrity at Frontiers
Learn more about the work of our research integrity team to safeguard the quality of each article we publish.