- Laboratory of Molecular Immunology, Department of Animal Resource Sciences, Graduate School of Agricultural and Life Sciences, The University of Tokyo, Tokyo, Japan
In today’s aging society, dementia is an urgent problem to be solved because no treatment or preventive methods have been established. This review focuses on oral administration of lipopolysaccharide (LPS), an outer membrane component of Gram-negative bacteria, as a novel preventive drug for dementia. LPS is also called endotoxin and is well known to induce inflammation when administered systemically. On the other hand, although we humans routinely ingest LPS derived from symbiotic bacteria of edible plants, the effect of oral administration of LPS has hardly been studied. Recently, oral administration of LPS was reported to prevent dementia by inducing neuroprotective microglia. Furthermore, it has been suggested that colony stimulating factor 1 (CSF1) is involved in the dementia prevention mechanism by oral administration of LPS. Thus, in this review, we summarized the previous studies of oral administration of LPS and discussed the predicted dementia prevention mechanism. In addition, we showed the potential of oral LPS administration as a preventive drug for dementia by highlighting research gaps and future issues for clinical application development.
Introduction
Currently, as the aging of the population progresses worldwide, dementia such as Alzheimer’s disease caused by aging is one of the global issues to be resolved. The WHO reports that there are currently over 55 million people with dementia, and this number is expected to reach 75 million in 2030 and 132 million in 2050 (1). However, there is still no effective preventive or therapeutic method for dementia, and urgent research and development is required. As research progresses, it has become clear that the activation of microglia, which are tissue-resident macrophages in the central nervous system (CNS), is important in the pathology of dementia. Microglia, which play a central role in the innate immune system, control neuronal activity through synaptic phagocytosis and cytokine production in the physiological condition and maintain CNS homeostasis. On the other hand, pathological microglia, which are activated and induced in pathological conditions, contribute to neural network damage through xenophagocytosis, inflammatory factors, and cytotoxic factors (2). Therefore, controlling microglial activation can lead to the prevention and treatment of dementia.
Lipopolysaccharide (LPS) is a glycolipid that constitutes the outer membrane of Gram-negative bacteria. LPS activates various cells including macrophages via toll-like receptor 4 (TLR4). LPS, also called endotoxin, is well known to cause fever, systemic inflammation, and shock when injected intravenously (The LD50 for intraperitoneally administered LPS is 27 mg/kg.) (3). In the field of neuroscience, a single systemic administration of LPS at a high dose (approximately 5 mg/kg) has been generally used as a Parkinson’s model or an encephalitis model to induce neuroinflammation (4, 5). It has been reported that a single high-dose LPS systemic administration promotes amyloid-β accumulation and exacerbates cognitive decline in Alzheimer’s disease models (6). The underlying mechanism of this neuropathy involves inflammatory microglia induced by a single high dose of LPS (7). On the other hand, repetitive low-dose systemic administration of LPS (0.2-0.9 mg/kg) contributes to the prevention of neurological dysfunctions such as Alzheimer’s disease and ischemic neuropathy by inducing neuroprotective microglia (8, 9). The neuroprotective effects of repetitive low-dose LPS administration have already been described in detail in our previous review (10).
However, even with repeated low doses of LPS, systemic administration of LPS causes body weight loss in experimental animals (8). Therefore, the problem is that systemic administration of LPS and subsequent elevation of blood LPS concentration (bioavailability), even in very small doses, carry the risk of systemic inflammation. In contrast, we have elucidated that oral administration of LPS (OAL) at a dose of 0.01-1 mg/kg contributes to the prevention and treatment of various diseases while maintaining high safety (11, 12). Furthermore, we have recently shown that OAL prevents dementia by inducing neuroprotective microglia (13, 14). In this review, we summarize previous studies on OAL and propose the potential of OAL as a new therapeutic strategy. The data sources for this review were comprehensively searched using the PubMed with keywords of “lipopolysaccharide” and “oral administration/treatment” (last searched February 16, 2023). Data published within the last ten years were prioritized, focusing on the novel and comprehensive reports.
Distinct mechanism between oral and intravenous administration of LPS
LPS is a glycolipid that constitutes the outer membrane of Gram-negative bacteria, and induces strong fever, systemic inflammation, and shock when administered intravenously (3). Therefore, LPS is called endotoxin, and intravenous administration of LPS has long been used as a model of sepsis and systemic inflammation. The maximum tolerated dose of LPS for intravenous administration in humans is 4 ng/kg, and the inclusion of LPS in injection drug is prohibited (15). Under these circumstances, LPS has been recognized as a dangerous substance that should be eliminated in the medical field. On the other hand, a minority research group including ours has found that LPS is contained in many edible plants and that human beings take LPS orally on a daily basis. Furthermore, it has been elucidated that orally administered LPS suppresses rather than promotes inflammation and contributes to disease prevention. In other words, LPS exhibits completely opposite effects when administered orally and when administered intravenously. Now, the “aufheben” of recognition for LPS is about to occur from endotoxin as an inflammatory inducer to disease preventive agent via oral route.
In fact, LPS is detected in many edible plants, including wheat, rice, and buckwheat (16). The LPS is derived from symbiotic bacteria of edible plants. Other Gram-negative bacteria found in edibles include Acetobacter spp. used to produce vinegar and Caspian Sea yogurt, Zymomonas spp. and Xanthomonas spp. used to produce tequila, nata de coco and xanthan gum, and Enterobacter spp. and Pantoea spp. used to ferment salapao in Thailand and rye bread in Finland (16). In other words, we humans have already unconsciously orally ingested LPS derived from food. In fact, animal safety studies have confirmed that LPS is not toxic when administered orally, even at doses of 500-4500 mg/kg, which are far higher than doses that are toxic when administered intravenously (16–18). Therefore, it is thought that the pharmacokinetics of LPS are completely different between intravenous and oral administration, resulting in different pharmacological effects.
Studies on disease prevention effect of OAL
Previous studies on OAL are summarized in Table 1. Although there are very few OAL studies compared to studies in the LPS intravenous model, most of them demonstrate health maintenance effects of OAL. Märklin et al. revealed that OAL regulates granulopoiesis by inducing hematopoietic stem cells and progenitor cells into the neutrophil lineage via TLR4 (19). This study indicates that OAL contributes to maintaining homeostasis.
It has also been reported that OAL has the effect of reducing the side effects of antibiotics. Antibiotics significantly reduce intestinal vancomycin-resistant killing by inducing downregulation of the C-type lectin RegIIIγ, whereas Brandl et al. show that OAL re-induces RegIIIγ to enhance innate immunity against vancomycin-resistant bacteria (20). In addition, although antibiotics reduce the TNF-induced effects of cancer immunotherapy, Iida et al. demonstrated that OAL restores TNF expression and TNF-producing leukocytes in tumors (21). Muller et al. demonstrated that antibiotics inhibit colony stimulating factor 1 (CSF1)-mediated gastrointestinal motility regulation by crosstalk between macrophages and intestinal neurons, while OAL prevents antibiotic-induced intestinal CSF1 downregulation and intestinal macrophage depletion (22).
Furthermore, disease preventive effects of OAL have also been reported. Khoury et al. showed that OAL in combination with myelin basic protein (MBP) suppressed experimental autoimmune encephalomyelitis (EAE) (23). Márquez-Velasco et al. revealed that OAL protects against sepsis induced by cecal ligation and puncture (24). Ametaj et al. reported that OAL to perinatal dairy cows modulates the postpartum plasma metabolite and mineral profiles and enhances the mucosal IgA (25–28). Kihl et al. showed that OAL induced suppression of inflammatory cytokine expression in pancreatic lymph nodes (29). These studies indicate that OAL is effective in maintaining homeostasis and in preventing or treating disease. Incidentally, all the LPS used in the above studies are derived from Escherichia coli.
Pantoea agglomerans is one of the plant symbiotic bacteria, and widely exists in edible plants such as wheat, rice, and root vegetables. P. agglomerans promotes the growth of symbiotic plants by suppressing fungal growth, fixing nitrogen, and dissolving inorganic phosphorus (38). In fact, the bacteria are also used as a food preservative (39, 40). In addition, the safety of oral administration of P. agglomerans-derived LPS is guaranteed by objective tests based on Organization for Economic Co-operation and Development (OECD) standards (17). Therefore, we focused on this P. agglomerans-derived LPS as a food-experienced LPS, and elucidated the preventive and therapeutic effects of OAL in various disease models. For example, OAL induces increases in blood IFN-γ/IL-12 levels and NK cell counts, and enhances the effects of anticancer drugs (11, 30). OAL also reduces blood IgE levels and improves atopic dermatitis (31). Furthermore, OAL suppresses atherosclerosis by inducing improvement of glucose tolerance and reduction of blood LDL and inflammatory cytokines (12). Importantly, human studies also show that OAL has the protective effect on hyperglycemia and hyperlipidemia (32), the inhibitory effect on bone density loss in middle-aged and elderly women (33), and the effect on improving blood flow (34). Because OAL promotes the phagocytic activity of peritoneal macrophages (41), it is suggested that macrophages are involved in the disease prevention mechanism by OAL.
Studies on disease exacerbation effect of OAL
On the other hand, there are a few reports that OAL exacerbates pathologies. For example, OAL has been reported to exacerbate collagen-induced arthritis (35) and autoimmune diseases (36). There is also a report that OAL to heat-stressed chickens increases enteritis (37). In addition, although it is not OAL, it has been reported that oral administration of Porphyromonas gingivalis, a periodontal disease bacterium, invades the blood from the periodontal lesion site, induces endotoxemia, and exacerbates Alzheimer’s disease (42, 43). In these reports of disease exacerbation by OAL, an increase in blood LPS was observed. In contrast, LPS was not detected in blood in OAL under normal conditions (14). Therefore, under physiological conditions, OAL is hypothesized to act on the immune system of the oral or upper gastrointestinal mucosa to induce disease-preventing effects without inducing elevation of blood LPS concentration (44). On the other hand, in exceptionally severe leaky gut and mucosal immune barrier breakdown due to periodontal disease or immunodeficiency, LPS may be absorbed into blood and cause inflammation. Thus, in the future, it is important to identify complications and medical history that require attention in OAL treatment.
Diversity of LPS activity depending on bacterial origin
Although there is diversity in the LPS structure depending on the bacterial origin, the differences in the LPS activation mechanisms are less clear. However, at least it is known that lipid A structure influences the difference in LPS activity. For example, LPS from Bacteroides dorei, a predominant Gram-negative bacterium in the human gut flora, is not only non-immunogenic per se, but also inhibits TLR4-dependent cytokine production (45). The lipid A of Escherichia-derived LPS has 6 acyl groups and the lipid A of Salmonella-derived LPS has 7 acyl groups, whereas the lipid A of B. dorei LPS has 4 acyl groups (46).
On the other hand, lipid A of P. agglomerans-derived LPS has 7 acyl groups, similar to Salmonella-derived LPS (47). The LD50 of P. agglomerans LPS is also almost the same as that of Escherichia- and Salmonella-derived LPS (48). In addition, P. agglomerans-derived LPS induces pro-inflammatory cytokine production through TLR4 signaling like Escherichia-derived LPS and Salmonella-derived LPS (49). Therefore, P. agglomerans-derived LPS is similar to Escherichia-derived LPS and Salmonella-derived LPS in terms of TLR4 activity and lipid A acyl group structure.
It is also interesting to explore the diversity of LPS activity by bacterial origin. However, considering the previous studies on OAL shown in Table 1, it can be expected that both Escherichia-derived LPS and Salmonella-derived LPS exhibit disease prevention effects equivalent to P. agglomerans-derived LPS when administered orally. This is because the contact of LPS with healthy mucous membranes is considered to be more important than the origin of LPS in the mechanism of disease prevention by OAL as described above.
However, what is important here is the fact that at least oral administration of LPS derived from P. agglomerans, a food symbiotic bacterium, has been verified for its safety and disease prevention effect. In the future, it is expected that P. agglomerans-derived LPS, whose safety has already been confirmed, will be developed for clinical application as the mainstay of dementia preventive drugs. At the same time, in order to establish a more effective dementia prevention method, the diversity of LPS derived from other Gram-negative bacteria and their OAL effects should be elucidated.
Preventive effect of OAL on dementia via neuroprotective microglia
We recently have demonstrated that OAL prevents dementia (13, 14). OAL inhibited amyloid-β deposition and tau phosphorylation by promoting microglial amyloid-β phagocytosis and prevented brain atrophy. On the other hand, microglia depletion by PLX3397 canceled the preventive effect of OAL on dementia. These results indicate that microglia play an important role in the prevention mechanism of dementia by OAL. Furthermore, comprehensive genetic analysis revealed that OAL-transformed microglia (OAL-microglia) highly expressed genes involved in inflammation control, tissue repair, and promotion of phagocytosis, such as CSF1 receptor (CSF1R), IL-10, and heat shock protein (HSP). The gene expression pattern of OAL-microglia was different from that of normal and dementia-induced microglia. These results indicate that OAL prevents dementia by inducing neuroprotective microglia.
CSF1R regulates survival and proliferation of macrophages, and CSF1R signaling contributes to neuroprotective microglia induction (50–54). In addition, peripheral CSF1 levels correlate with CSF1R levels in CNS (55, 56), and peripheral administration of CSF1R ligands ameliorates neurological disorders (57–59). Indeed, our in vitro analysis also showed that CSF1R activation of microglia increased the phagocytosis of amyloid-β, promoted IL-10 and HSP gene expression, and exhibited neuroprotective effects (14). CSF1R ligands include CSF1 and IL-34, and CSF1 further has isoforms of membranous CSF1 (m-CSF1) and secretory CSF1 (s-CSF1). We found that OAL promoted m-CSF1 expression on peripheral leukocytes (14). Therefore, it is strongly suggested that OAL contributes to the prevention of dementia by promoting m-CSF1 expression on peripheral leukocytes, activating CSF1R on microglia, and inducing transformation to neuroprotective microglia. As mentioned above, because CSF1 is also reported to be involved in the maintenance of gastrointestinal motility homeostasis by crosstalk between muscularis macrophages and enteric neurons by OAL (22), CSF1R signaling is thought to play an important role in the disease prevention mechanism of OAL.
OAL-microglia and CSF1R signaling
The choroid plexus functions as a site of crosstalk between CNS cells and the peripheral immune system (60). In the physiological state, peripheral leukocytes communicate with healthy CNS cells in the choroid plexus for homeostasis maintenance without entering the tissue parenchyma. In contrast, in pathological conditions, peripheral leukocytes sense CNS abnormalities such as alarmins in the choroid plexus and prevent neuropathy by crosstalking with CNS cells through neuroprotective molecules. Interestingly, the choroid plexus behaves like an active barrier under certain conditions. Thus, the choroid plexus selectively recruits immune cells into the CNS, facilitating close communication between peripheral leukocytes and CNS cells to suppress neuropathy.
By the way, in contrast to s-CSF1 which has an endocrine effect systemically, m-CSF1 shows local juxtacrine or paracrine effect (61). Major cells in brain parenchyma include microglia, astrocyte, oligodendrocyte, and neuron. Among these cells, only microglia express CSF1R. Therefore, it is considered that OAL-induced m-CSF1 on peripheral leukocytes crosstalk restrictively with microglia via CSF1R. Considering the functions of the choroid plexus described above, the choroid plexus is the predicted communication site for restricted signaling between m-CSF1 on peripheral leukocytes and CSF1R on microglia. Indeed, unlike the dementia model, OAL had little effect on expression of the neuroprotective genes in microglia of naïve mice (14). Therefore, only in pathological but not physiological conditions, the microglial CSF1R activation by m-CSF1 on peripheral leukocytes may induce transformation to neuroprotective microglia (Figure 1). In such an anomaly-sensing system, m-CSF1 is certainly more rational than s-CSF1, as it transmits signals only to the necessary target in a “just-in-time system” manner. It is likely that OAL exerts its disease-preventing effect in other tissues as well, through mechanisms similar to those in the brain. In other words, it is supposed that OAL-induced m-CSF1high leukocytes monitor the whole body while circulating, and when abnormalities are detected, they activate the CSF1R signal of tissue-resident macrophages, thereby inducing transformation to protective macrophages.
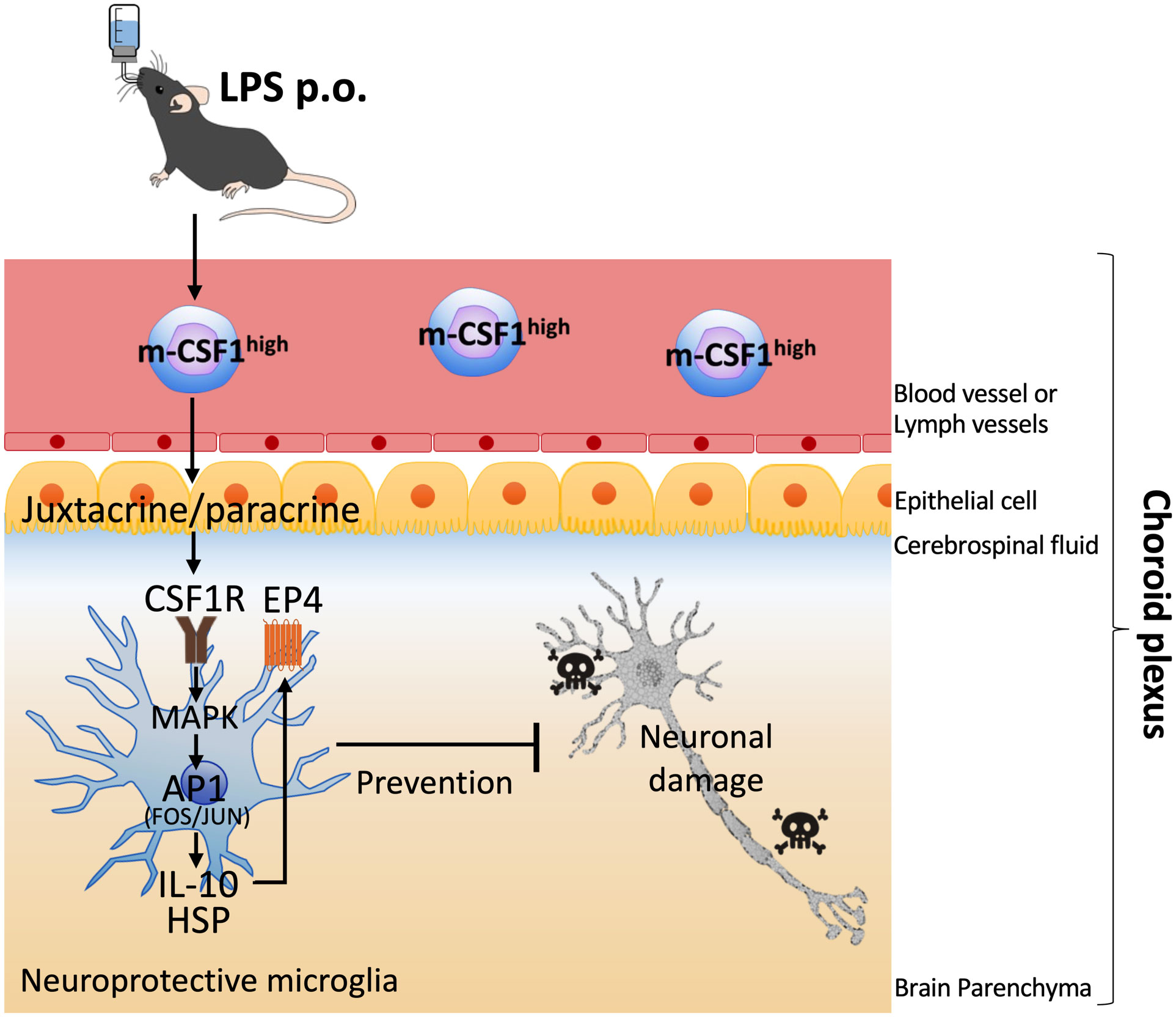
Figure 1 Hypothetical mechanism of induction of neuroprotective microglia by OAL. OAL promotes m-CSF1 expression on peripheral leukocytes. In the physiological state, peripheral leukocytes communicate with healthy CNS cells in the choroid plexus for homeostasis maintenance. In pathological conditions, the microglial CSF1R activation by m-CSF1 on peripheral leukocytes induces transformation to neuroprotective microglia, which contributes to the prevention of dementia. OAL, oral administration of LPS; m-CSF1, membranous colony stimulating factor 1; CNS, central nervous system; CSF1R, CSF1 receptor.
OAL-microglia in dementia models are characterized by high expression of the following genes (14): genes of CSF1R, IL-10, IL-12B, prostaglandin-E2 EP4 receptor (encoded by Ptger4 gene), c-Jun, and heat shock protein (HSP) family (14). IL-10 is an anti-inflammatory cytokine and interestingly synergizes with IL-12B to regulate inflammation in tumor models (62). Activation of EP4 receptor suppresses brain inflammation (63–65), and also synergizes with CSF1 signaling (66). c-Jun forms activator protein 1 (AP-1), a transcription factor, which is activated downstream of CSF1 signal involving in transformation to anti-inflammatory macrophage (51, 54). AP-1 is also involved in the induction of IL-10, IL-12, HSP (67–70). Exogenous HSP promotes Aβ phagocytosis of microglia and induces neuroprotection (71).
On the other hand, CSF1R activation promotes IL-10 and HSP expression in microglia (14), and MAPK and c-Jun activation are involved in the upstream signals (51, 52, 72, 73). In addition, it has also been reported that EP4 receptor and HSP expression are elevated downstream of IL-10 (74, 75). These facts indicate that the gene expression patterns of CSF1R-stimulated microglia and OAL-microglia are highly similar, suggesting that m-CSF1 and CSF1R are key molecules that mediate neuroprotective OAL-microglia induction. In the future, it is necessary to clarify the detailed mechanism of how CSF1R signaling is involved in the induction of OAL-microglia.
In addition to CSF1R signal, TLR4 is expected to be involved in the OAL mechanism because OAL promotes phagocytosis of peritoneal macrophages in a TLR4-dependent manner (41). However, other OAL mechanisms are still largely unknown. It should be elucidated how CSF1R, TLR4 and other signals are involved in the OAL mechanism.
Discussion
As one of the efforts to solve dementia, a dietary method called Mediterranean-DASH Intervention for Neurodegenerative Delay diet (MIND diet) has been proposed to prevent dementia (76, 77). The MIND diet includes vegetables and whole grains, and these edible plants contain high levels of LPS (16). Especially in grains, LPS is abundant on the outside because symbiotic bacteria exist on the surface of the plant. Thus, whole grains contain more LPS than refined grains (78). Interestingly, human studies have shown that consumption of brown rice with high LPS content contributes to the prevention of cognitive decline in the elderly (79). Because inactivation of LPS requires exposure to a temperature of 250°C for more than 30 min or 180°C for more than 3 h (80), LPS is hardly inactivated during the cooking process of brown rice. Therefore, it is considered that the activity of LPS does not change whether it is derived from viable or dead bacteria. Accordingly, it is suggested that one of the functional components of MIND diet including brown rice may be LPS.
Brown rice contains as much as 10 μg/g of LPS compared to 0.037 μg/g of LPS in white rice (78, 81). LPS in other cereals is 8.8 μg/g in wheat bran, 7.5 μg/g in wheat germ, and 2.95 μg/g in Barley sprouts (16). One serving of brown rice (65 g) provides 0.65 mg of LPS, and eating three servings of brown rice a day yields 1.95 mg of LPS per day. Assuming a body weight of 60 kg, it corresponds to an LPS intake of 32.5 μg/kg per day. Because LPS is also taken from organic foods other than brown rice, the total LPS intake is expected to be slightly higher than this dose.
As shown in Table 1, LPS intake dose in humans that is effective in suppressing blood sugar levels, preventing osteoporosis, and improving blood flow is about 0.5 mg/day, which is 8.3 μg/kg. On the other hand, LPS intake dose in mice that is effective in preventing arteriosclerosis and enhancing anticancer drugs is about 0.3~0.5 mg/kg, which is approximately 60 times higher than the human dose. This difference is probably due to differences in animal species. In mouse dementia model, not 0.3 mg/kg but 1 mg/kg LPS has been shown to prevent cognitive brain decline (13). A simple calculation suggests that the LPS intake dose that can prevent dementia in humans is about 8.3 x 3.3 = 27 μg/kg. In fact, LPS intake was 32.5 μg/kg in the brown rice study that confirmed the dementia preventive effect. Therefore, this assumed capacity can be said to be reasonable. Based on the above, the LPS intake dose that induces dementia-preventing effects in humans is considered to be around 32.5 μg/kg/day. However, assuming application to human clinical therapy in the future, one of the important issues is to strictly determine the optimal dose of OAL in humans.
However, it is difficult to immediately expect the function of OAL only with food because the quality and quantity of orally ingested LPS are uncertain. Although our previous research has revealed that LPS is certainly contained in food, it is still unknown from what source and to what extent LPS is ingested. In addition, plant cultivation using chemical fertilizers and pesticides kills LPS-expressing symbiotic bacteria, thereby reducing the LPS content in food. Therefore, modern humans are considered to have decreased oral LPS intake from diet. Furthermore, because LPS has structural differences such as Lipid A and sugar chains depending on the bacteria (82), it is thought that there is qualitative diversity in the biological activity of LPS. In order to utilize LPS as a functional material, it is necessary to clarify the quality of LPS, standardize the food content, and elucidate the dose-activity relationship regarding preventive effects, thereby optimizing the expression of OAL effects. For practical use, forms such as tablets and powdered supplements are considered desirable.
In addition to the decrease in dietary LPS intake, excessive sterilization, overuse of antibiotics, and a decrease in contact with soil due to asphalt pavement in urban areas further deprives modern humans of opportunities to contact LPS. In other words, it can be said that modern society is suffering from a lack of LPS. Insufficient LPS exposure during childhood suppresses the induction of immune tolerance, and as a result promotes the development of allergic diseases (83), suggesting that LPS plays an important role in establishing the hygiene hypothesis. Furthermore, as described above, because OAL exhibits preventive and therapeutic effects against various diseases, the modern LPS-deficient environment may also be related to the pathological background of diseases other than allergies.
In order to apply OAL clinically in the future, there are still problems to be solved (Figure 2). One of them is the elucidation of mechanism by which OAL exerts its effect. By what route does orally administered LPS induce enhanced m-CSF1 expression in peripheral leukocytes? How do m-CSF1-expressing leukocytes interact with microglia to regulate CSF1R signaling? How do microglia induced by CSF1R activation exert their neuroprotective effects? Do signals other than m-CSF1/CSF1R participate in OAL neuroprotective mechanisms? These questions need to be resolved. Besides, screening LPS with higher efficacy from various LPS from different bacteria origins will further expand the possibilities of OAL. Importantly, as mentioned above, immunodeficient patients with mucosal immune barrier disruption may have to be excluded from OAL treatment in consideration of the risk of LPS absorption into the blood (Figure 3). LPS has the risk of inducing inflammation and shock when it circulates systemically. Therefore, in the future, it will be necessary to stipulate prescription conditions for OAL, such as refraining from OAL treatment if patients have periodontal disease or gastrointestinal mucositis. In order to prevent the risk of LPS absorption into the blood, it may be effective to completely cure mucositis before OAL treatment and to take LPS with a mucosal protectant. Based on the fact, it is necessary to investigate the optimal dose of OAL in pharmacological, pharmacokinetic and clinical studies.
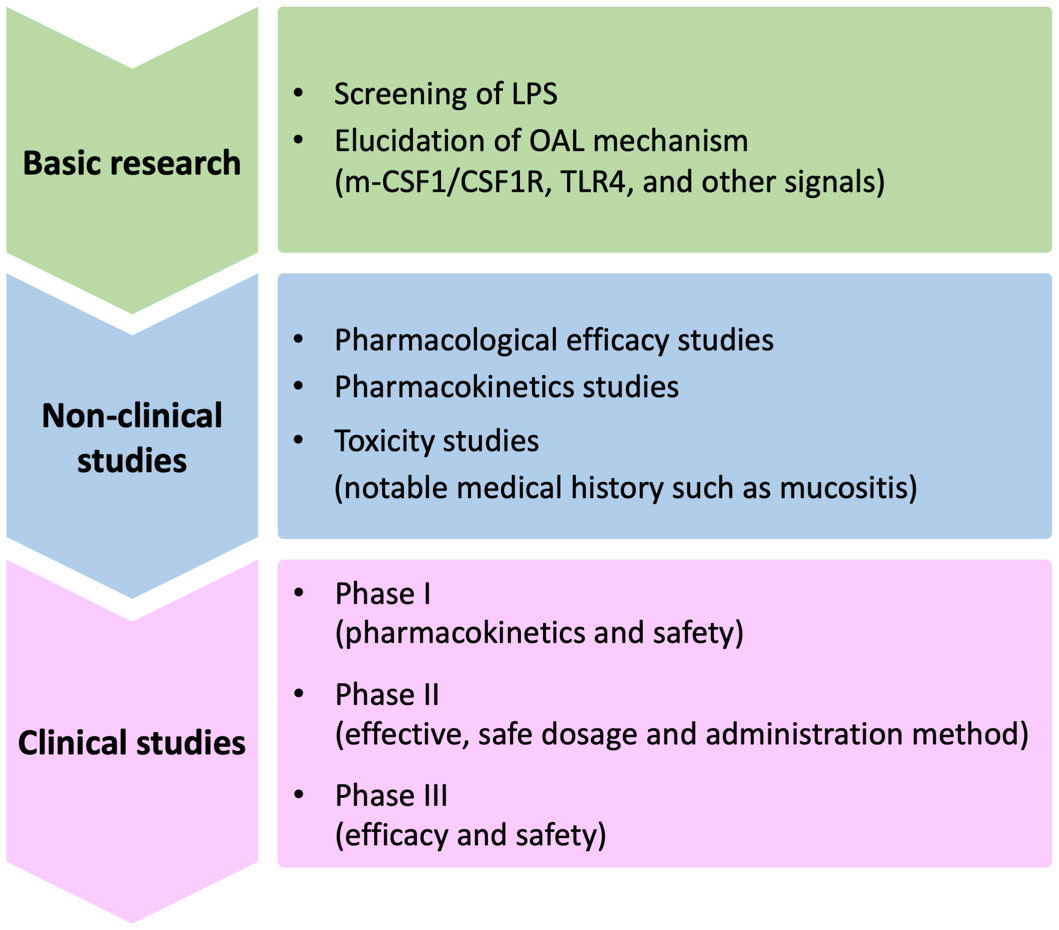
Figure 2 Issues to be solved for clinical application of OAL. Issues to be resolved in the future for clinical application of OAL can be broadly divided into basic research, non-clinical studies, and clinical studies. First, in basic research, the OAL mechanism involving CSF1R signaling must be elucidated. It will also be important to extract LPS with high efficacy through LPS screening. Next, in non-clinical studies, it is necessary to improve the safety and efficacy of OAL through pharmacological, pharmacokinetic, and toxicity studies. Safety in patients with specific and noteworthy medical histories such as mucositis must also be verified there. Based on the above studies, in the final clinical trial, it is necessary to confirm the safety and efficacy in humans and determine the optimal dosage. OAL, oral administration of LPS; m-CSF1, membranous colony stimulating factor 1; CSF1R, CSF1 receptor; TLR4, toll-like receptor 4.
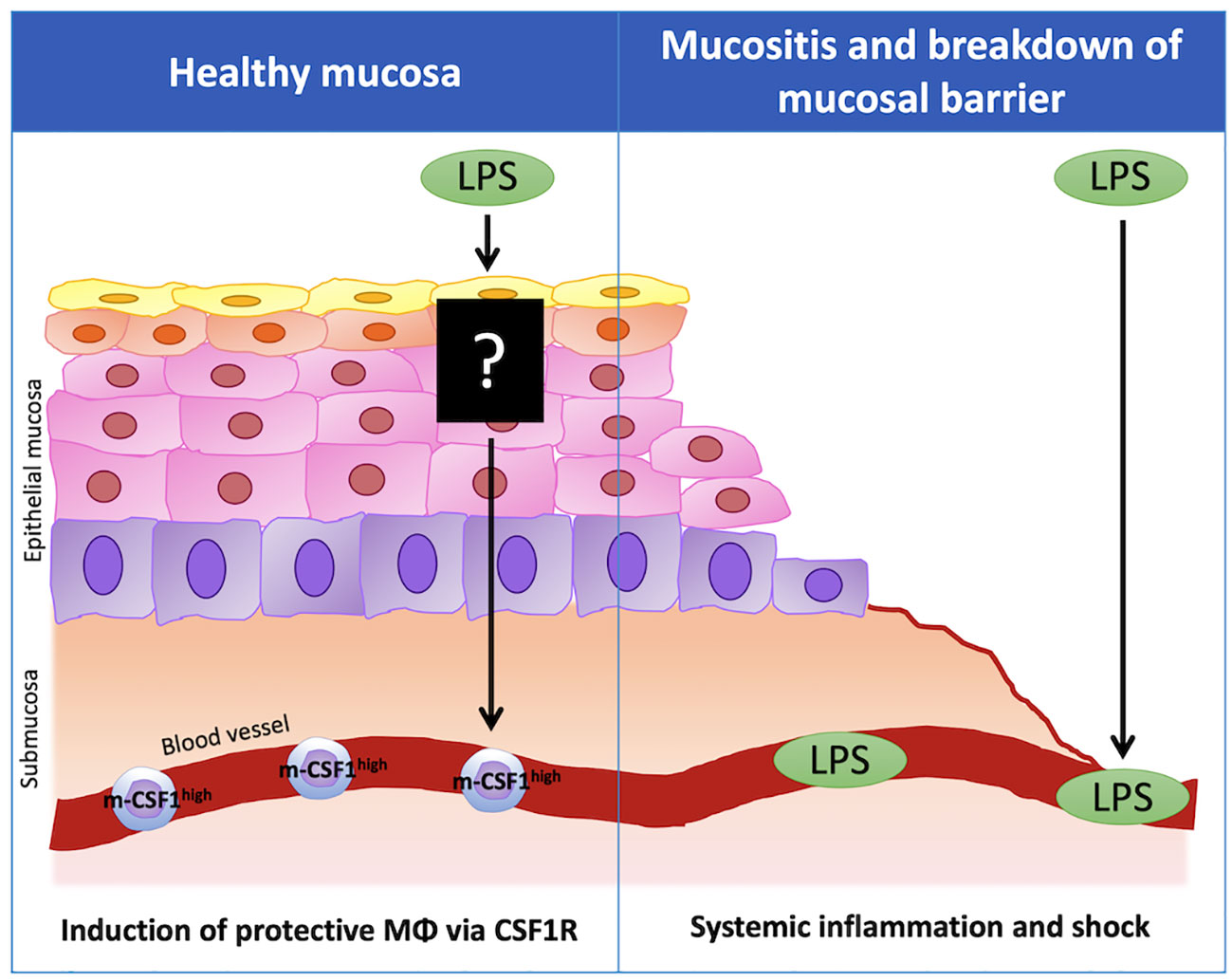
Figure 3 The importance of healthy mucosa for disease-preventing effect of OAL. In healthy mucosa, LPS taken by the oral route does not enter the blood, but acts on the immune system in the oral or upper gastrointestinal mucosa and induces the expression of m-CSF1 in peripheral leukocytes. The leukocytes that highly express m-CSF1 (m-CSF1high leukocytes) patrol the choroid plexus and maintain brain homeostasis. Upon sensing pathological conditions, m-CSF1high leukocytes activate microglial CSF1R signaling in the choroid plexus, inducing transformation to neuroprotective microglia to prevent neuropathy. On the other hand, when the mucosal barrier is disrupted due to mucositis, LPS may be absorbed into the blood and induce inflammation and shock when it circulates systemically. m-CSF1, membranous colony stimulating factor 1; CSF1R, CSF1 receptor.
In conclusion, this review showed that microglia regulation by OAL could be an innovative preventive method for dementia. One of the advantages of OAL is its simplicity. Because it can be easily taken at home, it is easy to disseminate in society, and it can sufficiently cover the estimated 50 to 100 million people at risk of dementia. The LPS purification method has already been established, and the production cost can be kept low. It is possible to reduce the dementia risk population in a short period by making OAL treatment available to a large number of people. In the future, solving the problems and filling the research gap is expected to lead to the realization of clinical application of OAL.
Author contributions
HM conceptualized and wrote the manuscript. All authors contributed to the article and approved the submitted version.
Funding
This study was funded by the Control of Innate Immunity Collaborative Innovation Partnership. This study was also supported by a grant from the Cross-ministerial Strategic Innovation Promotion Program (SIP-No. 14533073) of the Council for Science from Technology and Innovation (CSTI) in the Cabinet Office of the Japanese Government and the National Agriculture and Food Research Organization (NARO). CSTI and NARO had no role in the study design, data collection and analysis, decision to publish, or preparation of the manuscript.
Conflict of interest
HM was employed by the Control of Innate Immunity, Collaborative Innovation Partnership from 2018-2021. This does not affect my adherence to journal policies on sharing data and materials.
Publisher’s note
All claims expressed in this article are solely those of the authors and do not necessarily represent those of their affiliated organizations, or those of the publisher, the editors and the reviewers. Any product that may be evaluated in this article, or claim that may be made by its manufacturer, is not guaranteed or endorsed by the publisher.
References
1. World Health Organization. Global action plan on the public health response to dementia 2017 - 2025. (2017). Geneva, Switzerland: the WHO Document Production Services.
2. Leng F, Edison P. Neuroinflammation and microglial activation in Alzheimer disease: where do we go from here? Nat Rev Neurol (2021) 17:157–72. doi: 10.1038/s41582-020-00435-y
3. Li Q, Li L, Fei X, Zhang Y, Qi C, Hua S, et al. Inhibition of autophagy with 3-methyladenine is protective in a lethal model of murine endotoxemia and polymicrobial sepsis. Innate Immun (2018) 24:231–9. doi: 10.1177/1753425918771170
4. Batista CRA, Gomes GF, Candelario-Jalil E, Fiebich BL, de Oliveira ACP. Lipopolysaccharide-induced neuroinflammation as a bridge to understand neurodegeneration. Int J Mol Sci (2019) 20:2293. doi: 10.3390/ijms20092293
5. Deng I, Corrigan F, Zhai G, Zhou X-F, Bobrovskaya L. Lipopolysaccharide animal models of parkinson’s disease: Recent progress and relevance to clinical disease. Brain Behav Immun Health (2020) 4:100060. doi: 10.1016/j.bbih.2020.100060
6. Zhan X, Stamova B, Sharp FR. Lipopolysaccharide associates with amyloid plaques, neurons and oligodendrocytes in alzheimer’s disease brain: A review. Front Aging Neurosci (2018) 10:42. doi: 10.3389/fnagi.2018.00042
7. Ye X, Zhu M, Che X, Wang H, Liang XJ, Wu C, et al. Lipopolysaccharide induces neuroinflammation in microglia by activating the MTOR pathway and downregulating Vps34 to inhibit autophagosome formation. J Neuroinflamm (2020) 17:18. doi: 10.1186/s12974-019-1644-8
8. Wendeln AC, Degenhardt K, Kaurani L, Gertig M, Ulas T, Jain G, et al. Innate immune memory in the brain shapes neurological disease hallmarks. Nature (2018) 556:332–8. doi: 10.1038/s41586-018-0023-4
9. Neher JJ, Cunningham C. Priming microglia for innate immune memory in the brain. Trends Immunol (2019) 40:358–74. doi: 10.1016/j.it.2019.02.001
10. Mizobuchi H, Soma GI. Low-dose lipopolysaccharide as an immune regulator for homeostasis maintenance in the central nervous system through transformation to neuroprotective microglia. Neural Regener Res (2021) 16:1928–34. doi: 10.4103/1673-5374.308067
11. Hebishima T, Matsumoto Y, Watanabe G, Soma G, Kohchi C, Taya K, et al. Oral adminitsration of immunopotentiator from pantoea agglomerans 1 (IP-PA1) improves the survival of B16 melanoma-inoculated model mice. Exp Anim (2011) 60:101–9. doi: 10.1538/expanim.60.101
12. Kobayashi Y, Inagawa H, Kohchi C, Kazumura K, Tsuchiya H, Miwa T, et al. Oral administration of pantoea agglomeransderived lipopolysaccharide prevents development of atherosclerosis in high-fat diet-fed apoE-deficient mice via ameliorating hyperlipidemia, pro-inflammatory mediators and oxidative responses. PloS One (2018) 13:e0195008. doi: 10.1371/journal.pone.0195008
13. Kobayashi Y, Inagawa H, Kohchi C, Kazumura K, Tsuchiya H, Miwa T, et al. Oral administration of pantoea agglomerans-derived lipopolysaccharide prevents metabolic dysfunction and alzheimer’s disease-related memory loss in senescence-accelerated prone 8 (SAMP8) mice fed a high-fat diet. PloS One (2018) 13:e0198493. doi: 10.1371/journal.pone.0198493
14. Mizobuchi H, Yamamoto K, Yamashita M, Nakata Y, Inagawa H, Kohchi C, et al. Prevention of diabetes-associated cognitive dysfunction through oral administration of lipopolysaccharide derived from pantoea agglomerans. Front Immunol (2021) 12:650176. doi: 10.3389/fimmu.2021.650176
15. Engelhardt R, Mackensen A, Galanos C. Phase I trial of intravenously administered endotoxin (Salmonella abortus equi) in cancer patients. Cancer Res (1991) 51:2524–30.
16. Taniguchi Y, Yoshioka N, Nishizawa T, Inagawa H, Kohchi C, Soma GI. Utility and safety of LPS- based fermented flour extract as a macrophage activator. Anticancer Res (2009) 29:859–64.
17. Phipps KR, Sulaiman C, Simon R, Holalagoudar S, Kohchi C, Nakata Y. Subchronic (90-day) toxicity assessment of somacy-FP100, a lipopolysaccharide-containing fermented wheat flour extract from pantoea agglomerans. J Appl Toxicol (2020) 40:1342–52. doi: 10.1002/jat.3987
18. Samarasinghe MB, Sehested J, Larsen T, Hernández-Castellano LE. Oral administration of lipopolysaccharides from escherichia coli (serotype O111:B4) does not induce an effective systemic immune response in milk-fed Holstein calves. J Dairy Sci (2020) 103:5525–31. doi: 10.3168/jds.2019-17404
19. Märklin M, Bugl S, Wirths S, Frick JS, Müller MR, Kopp HG, et al. Oral intake of lipopolysaccharide regulates toll-like receptor 4-dependent granulopoiesis. Exp Biol Med (2020) 245:1254–9. doi: 10.1177/1535370220931043
20. Brandl K, Plitas G, Mihu CN, Ubeda C, Jia T, Fleisher M, et al. Vancomycin-resistant enterococci exploit antibiotic-induced innate immune deficits. Nature (2008) 455:804–7. doi: 10.1038/nature07250
21. Iida N, Dzutsev A, Stewart CA, Smith L, Bouladoux N, Weingarten RA, et al. Commensal bacteria control cancer response to therapy by modulating the tumor microenvironment. Sci (1979) (2013) 342:967–70. doi: 10.1126/science.1240527
22. Muller PA, Koscsó B, Rajani GM, Stevanovic K, Berres ML, Hashimoto D, et al. Crosstalk between muscularis macrophages and enteric neurons regulates gastrointestinal motility. Cell (2014) 158:300–13. doi: 10.1016/j.cell.2014.04.050
23. Khoury SJ, Lider O, Weiner HL. Suppression of experimental autoimmune encephalomyelitis by oral administration of myelin basic protein. III. synergistic effect of lipopolysaccharide’. Cell Immunol (1990) 131:302–10. doi: 10.1016/0008-8749(90)90256-q
24. Márquez-Velasco R, Massó F, Hernández-Pando R, Montaño LF, Springall R, Amezcua-Guerra LM, et al. LPS pretreatment by the oral route protects against sepsis induced by cecal ligation and puncture. regulation of proinflammatory response and IgM anti-LPS antibody production as associated mechanisms. Inflammation Res (2007) 56:385–90. doi: 10.1007/s00011-007-6116-4
25. Ametaj BN, Sivaraman S, Dunn SM, Zebeli Q. Repeated oral administration of lipopolysaccharide from escherichia coli 0111:B4 modulated humoral immune responses in periparturient dairy cows. Innate Immun (2012) 18:638–47. doi: 10.1177/1753425911434851
26. Zebeli Q, Mansmann D, Sivaraman S, Dunn SM, Ametaj BN. Oral challenge with increasing doses of LPS modulated the patterns of plasma metabolites and minerals in periparturient dairy cows. Innate Immun (2013) 19:298–314. doi: 10.1177/1753425912461287
27. Iqbal S, Zebeli Q, Mansmann DA, Dunn SM, Ametaj BN. Repeated oronasal exposure to lipopolysaccharide induced mucosal IgA responses in periparturient dairy cows. PloS One (2014) 9:e103504. doi: 10.1371/journal.pone.0103504
28. Iqbal S, Zebeli Q, Mansmann DA, Dunn SM, Ametaj BN. Oral administration of LPS and lipoteichoic acid prepartum modulated reactants of innate and humoral immunity in periparturient dairy cows. Innate Immun (2014) 20:390–400. doi: 10.1177/1753425913496125
29. Kihl P, Krych L, Deng L, Kildemoes AO, Laigaard A, Hansen LH, et al. Oral LPS dosing induces local immunological changes in the pancreatic lymph nodes in mice. J Diabetes Res (2019) 2019:1649279. doi: 10.1155/2019/1649279
30. Hebishima T, Matsumoto Y, Watanabe G, Soma GI, Kohch C, Taya K, et al. Recovery from immunosuppression-related disorders in humans and animals by IP-PA1, an edible lipopolysaccharide. Anticancer Res (2010) 30:3113–8.
31. Wakame K, Komatsu K-I, Inagawa H, Nishizawa T. Immunopotentiator from pantoea agglomerans prevents atopic dermatitis induced by dermatophagoides farinae extract in NC/Nga mouse. Anticancer Res (2015) 35:4501–8.
32. Nakata K, Taniguchi Y, Yoshioka N, Yoshida A, Inagawa H, Nakamoto T, et al. A mixture of salacia oblonga extract and IP-PA1 reduces fasting plasma glucose (FPG) and low-density lipoprotein (LDL) cholesterol levels. Nutr Res Pract (2011) 5:435–42. doi: 10.4162/nrp.2011.5.5.435
33. Nakata K, Nakata Y, Inagawa H, Nakamoto T, Yoshimura H, Soma GI. Pantoea agglomerans lipopolysaccharide maintains bone density in premenopausal women: A randomized, double-blind, placebo-controlled trial. Food Sci Nutr (2014) 2:638–46. doi: 10.1002/fsn3.145
34. Nakata Y, Kohchi C, Ogawa K, Nakamoto T, Yoshimura H, Soma GI. Effects of 3 months continuous intake of supplement containing pantoea agglomerans LPS to maintain normal bloodstream in adults: Parallel double-blind randomized controlled study. Food Sci Nutr (2018) 6:197–206. doi: 10.1002/fsn3.547
35. Yoshino S, Sasatomi E, Mori Y, Masaru S. Oral administration of lipopolysaccharide exacerbates collagen-induced arthritis in mice. J Immunol References (1999) 163:3417–22.
36. Murakami M, Tsubata T, Shinkura R, Nisitani S, Okamoto M, Yoshioka H, et al. Oral administration of lipopolysaccharides activates b-1 cells in the peritoneal cavity and lamina propria of the gut and induces autoimmune symptoms in an autoantibody transgenic mouse. J Exp Med (1994) 180:111–21. doi: 10.1084/jem.180.1.111
37. Reisinger N, Emsenhuber C, Doupovec B, Mayer E, Schatzmayr G, Nagl V, et al. Endotoxin translocation and gut inflammation are increased in broiler chickens receiving an oral lipopolysaccharide (LPS) bolus during heat stress. Toxins (Basel) (2020) 12:622. doi: 10.3390/toxins12100622
38. Dutkiewicz J, Mackiewicz B, Lemieszek MK, Golec M, Milanowski J. Pantoea agglomerans: A mysterious bacterium of evil and good. part IV. beneficial effects. Ann Agric Environ Med (2016) 23:206–22. doi: 10.5604/12321966.1203879
39. Nunes C, Usall J, Teixidó N, Fons E, Viñas I. Post-harvest biological control by pantoea agglomerans (CPA-2) on golden delicious apples. J Appl Microbiol (2002) 92:247–55. doi: 10.1046/j.1365-2672.2002.01524.x
40. Usall J, Smilanick J, Palou L, Denis-Arrue N, Teixido N, Torres R, et al. Postharvest biology and technology preventive and curative activity of combined treatments of sodium carbonates and pantoea agglomerans CPA-2 to control postharvest green mold of citrus fruit. Postharvest Biol Technol (2008) 50:1–7. doi: 10.1016/j.postharvbio.2008.03.001
41. Inagawa H, Kobayashi Y, Kohchi C, Zhang R, Shibasaki Y, Soma GI. Primed activation of macrophages by oral administration of lipopolysaccharide derived from pantoea agglomerans. In Vivo (Brooklyn) (2016) 30:205–11.
42. Zenobia C, Darveau RP. Does oral endotoxin contribute to systemic inflammation? Front Oral Health (2022) 3:911420. doi: 10.3389/froh.2022.911420
43. Ishida N, Ishihara Y, Ishida K, Tada H, Funaki-Kato Y, Hagiwara M, et al. Periodontitis induced by bacterial infection exacerbates features of alzheimer’s disease in transgenic mice. NPJ Aging Mech Dis (2017) 3:15. doi: 10.1038/s41514-017-0015-x
44. Inagawa H, Kohchi C, Soma GI. Oral administration of lipopolysaccharides for the prevention of various diseases: Benefit and usefulness. Anticancer Res (2011) 31:2431–6.
45. d’Hennezel E, Abubucker S, Murphy LO, Cullen TW. Total lipopolysaccharide from the human gut microbiome silences toll-like receptor signaling. mSystems (2017) 2:e00046–17. doi: 10.1128/msystems.00046-17
46. Vatanen T, Kostic AD, D’Hennezel E, Siljander H, Franzosa EA, Yassour M, et al. Variation in microbiome LPS immunogenicity contributes to autoimmunity in humans. Cell (2016) 165:842–53. doi: 10.1016/j.cell.2016.04.007
47. Tsukioka D, Nishizawa T, Miyase T, Achiwa K, Suda T, Soma G-I, et al. Structural characterization of lipid a obtained from pantoea agglomerans lipopolysaccharide. FEMS Microbiol Lett (1997) 149:239–44. doi: 10.1111/j.1574-6968.1997.tb10335.x
48. Inagawa H, Nishizawa T, Tsukioka D, Suda T, Chiba Y, Okutomi T, et al. Homeostasis as regulated by activated macrophage. II. LPS of plant origin other than wheat flour and their concomitant bacteria. Chem Pharm Bull (Tokyo) (1992) 40:994–7. doi: 10.1248/cpb.40.994
49. Fukasaka M, Asari D, Kiyotoh E, Okazaki A, Gomi Y, Tanimoto T, et al. A lipopolysaccharide from pantoea agglomerans is a promising adjuvant for sublingual vaccines to induce systemic and mucosal immune responses in mice via TLR4 pathway. PloS One (2015) 10:e0126849. doi: 10.1371/journal.pone.0126849
50. Mitrasinovic OM, Grattan A, Robinson CC, Lapustea NB, Poon C, Ryan H, et al. Microglia overexpressing the macrophage colony-stimulating factor receptor are neuroprotective in a microglial-hippocampal organotypic coculture system. J Neurosci (2005) 25:4442–51. doi: 10.1523/JNEUROSCI.0514-05.2005
51. Yang Y, Qin J, Lan L, Li N, Wang C, He P, et al. M-CSF cooperating with NFκB induces macrophage transformation from M1 to M2 by upregulating c-jun. Cancer Biol Ther (2014) 15:99–107. doi: 10.4161/cbt.26718
52. Chu CH, Wang S, Li CL, Chen SH, Hu CF, Chung YL, et al. Neurons and astroglia govern microglial endotoxin tolerance through macrophage colony-stimulating factor receptor-mediated ERK1/2 signals. Brain Behav Immun (2016) 55:260–72. doi: 10.1016/j.bbi.2016.04.015
53. Pepe G, de Maglie M, Minoli L, Villa A, Maggi A, Vegeto E. Selective proliferative response of microglia to alternative polarization signals. J Neuroinflamm (2017) 14:236. doi: 10.1186/s12974-017-1011-6
54. Casals-Casas C, Álvarez E, Serra M, de la Torre C, Farrera C, Sánchez-Tilló E, et al. CREB and AP-1 activation regulates MKP-1 induction by LPS or m-CSF and their kinetics correlate with macrophage activation versus proliferation. Eur J Immunol (2009) 39:1902–13. doi: 10.1002/eji.200839037
55. Guan Z, Kuhn JA, Wang X, Colquitt B, Solorzano C, Vaman S, et al. Injured sensory neuron-derived CSF1 induces microglia proliferation and DAP12-dependent pain. Nat Neurosci (2016) 19:94–101. doi: 10.1016/j.physbeh.2017.03.040
56. Knight AC, Brill SA, Queen SE, Tarwater PM, Mankowski JL. Increased microglial CSF1R expression in the SIV/macaque model of HIV CNS disease. J Neuropathol Exp Neurol (2018) 77:199–206. doi: 10.1093/jnen/nlx115
57. Boissonneault V, Filali M, Lessard M, Relton J, Wong G, Rivest S. Powerful beneficial effects of macrophage colony-stimulating factor on β-amyloid deposition and cognitive impairment in alzheimers disease. Brain (2009) 132:1078–92. doi: 10.1093/brain/awn331
58. Luo J, Elwood F, Britschgi M, Villeda S, Zhang H, Ding Z, et al. Colony-stimulating factor 1 receptor (CSF1R) signaling in injured neurons facilitates protection and survival. J Exp Med (2013) 210:157–72. doi: 10.1084/jem.20120412
59. Laflamme N, Cisbani G, Préfontaine P, Srour Y, Bernier J, St-Pierre MK, et al. mCSF-induced microglial activation prevents myelin loss and promotes its repair in a mouse model of multiple sclerosis. Front Cell Neurosci (2018) 12:178. doi: 10.3389/fncel.2018.00178
60. Shechter R, London A, Schwartz M. Orchestrated leukocyte recruitment to immune-privileged sites: Absolute barriers versus educational gates. Nat Rev Immunol (2013) 13:206–18. doi: 10.1038/nri3391
61. Douglass TG, Driggers L, Zhang JG, Hoa N, Delgado C, Williams CC, et al. Macrophage colony stimulating factor: Not just for macrophages anymore! a gateway into complex biologies. Int Immunopharmacol (2008) 8:1354–76. doi: 10.1016/j.intimp.2008.04.016
62. Lopez MV, Adris SK, Bravo AI, Chernajovsky Y, Podhajcer OL. IL-12 and IL-10 expression synergize to induce the immune-mediated eradication of established colon and mammary tumors and lung metastasis. J Immunol (2005) 175:5885–94. doi: 10.4049/jimmunol.175.9.5885
63. Woodling NS, Wang Q, Priyam PG, Larkin P, Shi J, Johansson JU, et al. Suppression of Alzheimer-associated inflammation by microglial prostaglandin-E2 EP4 receptor signaling. J Neurosci (2014) 34:5882–94. doi: 10.1523/JNEUROSCI.0410-14.2014
64. Pradhan SS, Salinas K, Garduno AC, Johansson JU, Wang Q, Manning-Bog A, et al. Anti-inflammatory and neuroprotective effects of PGE2 EP4 signaling in models of parkinson’s disease. J Neuroimmune Pharmacol (2017) 12:292–304. doi: 10.1016/j.physbeh.2017.03.040
65. Shi J, Johansson J, Woodling NS, Wang Q, Montine TJ, Andreasson K. The prostaglandin e 2 e-prostanoid 4 receptor exerts anti-inflammatory effects in brain innate immunity. J Immunol (2010) 184:7207–18. doi: 10.4049/jimmunol.0903487
66. Digiacomo G, Ziche M, dello SP, Donnini S, Rovida E. Prostaglandin E2 transactivates the colony-stimulating factor-1 receptor and synergizes with colony-stimulating factor-1 in the induction of macrophage migration via the mitogen-activated protein kinase ERK1/2. FASEB J (2015) 29:2545–54. doi: 10.1096/fj.14-258939
67. Saraiva M, O’Garra A. The regulation of IL-10 production by immune cells. Nat Rev Immunol (2010) 10:170–81. doi: 10.1038/nri2711
68. Zhu C, Gagnidze K, Gemberling JHM, Plevy SE. Characterization of an activation protein-1-binding site in the murine interleukin-12 p40 promoter: Demonstration of novel functional elements by a reductionist approach. J Biol Chem (2001) 276:18519–28. doi: 10.1074/jbc.M100440200
69. Assimakopoulou M, Varakis J. AP-1 and heat shock protein 27 expression in human astrocytomas. J Cancer Res Clin Oncol (2001) 127:727–32. doi: 10.1007/s004320100280
70. Souza V, Escobar MDC, Gómez-Quiroz L, Bucio L, Hernández E, Cossio EC, et al. Acute cadmium exposure enhances AP-1 DNA binding and induces cytokines expression and heat shock protein 70 in HepG2 cells. Toxicology (2004) 197:213–28. doi: 10.1016/j.tox.2004.01.006
71. Kakimura J-I, Kitamura Y, Takata K, Umeki M, Suzuki S, Shibagaki K, et al. Microglial activation and amyloid-β clearance induced by exogenous heat-shock proteins. FASEB J (2002) 16:601–3. doi: 10.1096/fj.01-0530fje
72. Gosse SG, Bourgin C, Liu WQ, Garbay C, Mouchiroud G. M-CSF stimulated differentiation requires persistent MEK activity and MAPK phosphorylation independent of Grb2-sos association and phosphatidylinositol 3-kinase activity. Cell Signal (2005) 17:1352–62. doi: 10.1016/j.cellsig.2005.02.002
73. Huang F, Cao J, Liu Q, Zou Y, Li H, Yin T. Original article MAPK/ERK signal pathway involved expression of COX-2 and VEGF by IL-1β induced in human endometriosis stromal cells in vitro. Int J Clin Exp Pathol (2013) 6:2129–36.
74. Samiea A, Yoon JSJ, Cheung ST, Chamberlain TC, Mui ALF. Interleukin-10 contributes to PGE2 signalling through upregulation of EP4 via SHIP1 and STAT3. PloS One (2020) 15:e0230427. doi: 10.1371/journal.pone.0230427
75. Ripley BJM, Stephanou A, Isenberg DA, Latchman DS. Interleukin-10 activates heat-shock protein 90β gene expression. Immunology (1999) 97:226–31. doi: 10.1046/j.1365-2567.1999.00773.x
76. Morris MC, Tangney CC, Wang Y, Sacks FM, Bennett DA, Aggarwal NT. MIND diet associated with reduced incidence of alzheimer’s disease. Alzheimer’s Dementia (2015) 11:1007–14. doi: 10.1016/j.jalz.2014.11.009
77. Hosking DE, Eramudugolla R, Cherbuin N, Anstey KJ. MIND not Mediterranean diet related to 12-year incidence of cognitive impairment in an Australian longitudinal cohort study. Alzheimer’s Dementia (2019) 15:581–9. doi: 10.1016/j.jalz.2018.12.011
78. Inagawa H, Saika T, Nisizawa T, Kohchi C, Uenobe M, Soma GI. Dewaxed brown rice contains a significant amount of lipopolysaccharide pointing to macrophage activation via TLRs. Anticancer Res (2016) 36:3599–605.
79. Uenobe M, Saika T, Waku N, Ohno M, Inagawa H. Efficacy of continuous ingestion of dewaxed brown rice on the cognitive functions of the residents of elderly welfare facilities: A pilot test using crossover trial. Food Sci Nutr (2019) 7:3520–6. doi: 10.1002/fsn3.1202
80. Miyamoto T, Okano S, Kasai N. Inactivation of escherichia coli endotoxin by soft hydrothermal processing. Appl Environ Microbiol (2009) 75:5058–63. doi: 10.1128/AEM.00122-09
81. Tamura Y, Inagawa H, Nakata Y, Kohchi C, Soma G-I. Effects of the subaleurone layer of rice on macrophage activation and protection of pollen allergy in a murine model. Anticancer Res (2015) 35:4467–72.
82. Michaud JP, Hallé M, Lampron A, Thériault P, Préfontaine P, Filali M, et al. Toll-like receptor 4 stimulation with the detoxified ligand monophosphoryl lipid a improves alzheimer’s disease-related pathology. Proc Natl Acad Sci U.S.A. (2013) 110:1941–6. doi: 10.1073/pnas.1215165110
Keywords: microglia, lipopolysaccharide, oral administration, neuroprotection, cognitive dysfunction, dementia
Citation: Mizobuchi H (2023) Oral route lipopolysaccharide as a potential dementia preventive agent inducing neuroprotective microglia. Front. Immunol. 14:1110583. doi: 10.3389/fimmu.2023.1110583
Received: 29 November 2022; Accepted: 27 February 2023;
Published: 09 March 2023.
Edited by:
Koji Wakame, Hokkaido University of Science, JapanCopyright © 2023 Mizobuchi. This is an open-access article distributed under the terms of the Creative Commons Attribution License (CC BY). The use, distribution or reproduction in other forums is permitted, provided the original author(s) and the copyright owner(s) are credited and that the original publication in this journal is cited, in accordance with accepted academic practice. No use, distribution or reproduction is permitted which does not comply with these terms.
*Correspondence: Haruka Mizobuchi, bWl6b2J1Y2hpQGcuZWNjLnUtdG9reW8uYWMuanA=
†ORCID: Haruka Mizobuchi, orcid.org/0000-0002-6667-4820