- 1Key Laboratory for Regenerative Medicine of Ministry of Education, Institute of Hematology, School of Medicine, Jinan University, Guangzhou, China
- 2Department of Pathology, School of Medicine, Jinan University, Guangzhou, China
Hematological malignancy develops and applies various mechanisms to induce immune escape, in part through an immunosuppressive microenvironment. Adenosine is an immunosuppressive metabolite produced at high levels within the tumor microenvironment (TME). Adenosine signaling through the A2A receptor expressed on immune cells, such as T cells, potently dampens immune responses. Extracellular adenosine generated by ectonucleoside triphosphate diphosphohydrolase-1 (CD39) and ecto-5’-nucleotidase (CD73) molecules is a newly recognized ‘immune checkpoint mediator’ and leads to the identification of immunosuppressive adenosine as an essential regulator in hematological malignancies. In this Review, we provide an overview of the detailed distribution and function of CD39 and CD73 ectoenzymes in the TME and the effects of CD39 and CD73 inhibition on preclinical hematological malignancy data, which provides insights into the potential clinical applications for immunotherapy.
1 Introduction
In the tumor microenvironment (TME), unusually high extracellular adenosine concentrations promote tumor proliferation through various immunosuppressive mechanisms. Adenosine triphosphate (ATP) represents the currency for energy metabolism inside the cell. By contrast, extracellular space usually derives from passive leakage from necrotic or injured cells, enhancing inflammation, hypoxia, and cancer (1, 2). High extracellular ATP (eATP) concentrations influence cell metabolism, adhesion, and migration in acute inflammation, in which the ectonucleotidases CD39 and CD73 take part in catabolizing nucleotides and producing immunosuppressant adenosine (ADO), which are devoted to restoring homeostasis. The ATP degradation pathway proceeds through CD39, which converts eATP or ADP to AMP, and CD73, which hydrolyzes and converts AMP to ADO (3) (Figure 1). Although ectonucleotidases help prevent excessive inflammation and tissue damage, their contribution to generating an immunosuppressive microenvironment in tumor biology is more worrying. In hematological malignancies, the overexpression of CD39 and CD73 has been linked to increased homing to protected niches, increased survival, proliferation, and modulation of immune responses toward tolerance (4, 5). In some instances, ectonucleotidases have become reliable markers for monitoring disease and stratifying patient subsets or molecular targets for novel treatment strategies.
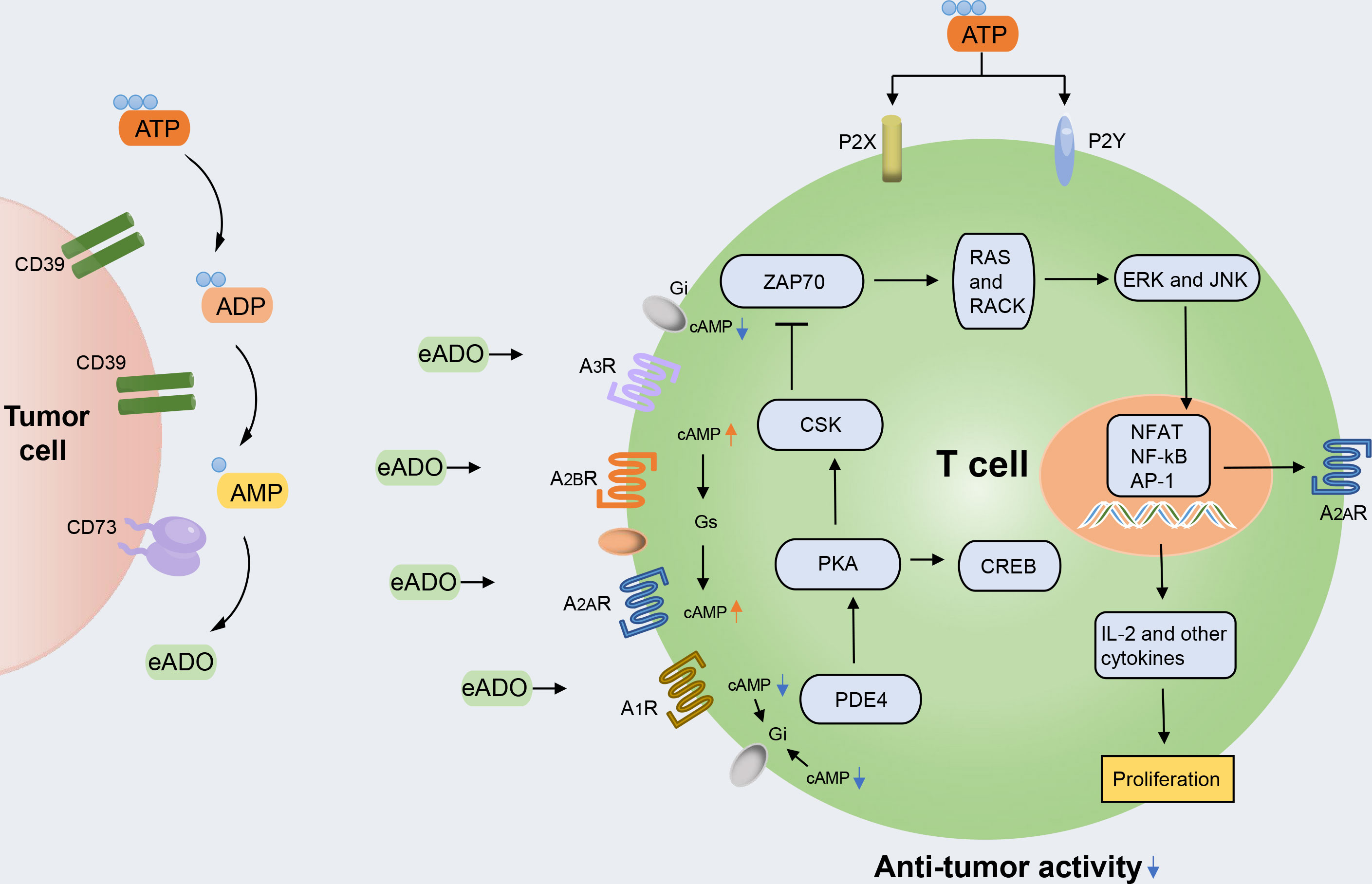
Figure 1 The ATP-adenosine pathway regulates immune response in the tumor microenvironment (TME). The accumulated extracellular ATP (eATP) can activate immune cell inflammation activity by stimulating type 2 purinergic receptors (P2XR and P2YR). The accumulated extracellular ADO (eADO) can bind to the downstream purinergic receptors (A1R, A2AR, A2BR, and A3R), resulting in the accumulation of cAMP. cAMP accumulation leads to protein kinase A (PKA) phosphorylation and the activation of C-terminal Src kinase (CSK), which reduces downstream LCK-dependent activation of ZAP70, extracellular signal-regulated kinase 1 (ERK1), and JNK and protein kinase C (PKC). PKA activation also activates cAMP-responsive element-binding protein 1 (CREB), which contributes to the inhibition of the major pro-inflammatory transcription factor nuclear factor-κB (NF-κB). Through this intracellular signaling pathway, the TCR-mediated activation of immune cells is counteracted.
In this review, we discuss the structure and function of CD39 and CD73 in physiological conditions and then focus on their expression and roles in the TME of several hematological malignancies. In addition, we illustrate their potential as new targets in hematological malignancies, and the experimental findings and clinical trials of CD39 or CD73 therapies are extensively discussed.
2 Classic features of CD39 and CD73
The cascade starting with ATP and leading to ADO production is governed by CD39 and CD73, which affect purinergic signaling by modulating ligand availability (6). CD39 is an extracellular enzyme known as ecto-nucleotide triphosphate diphosphohydrolase 1 (ENTPDase1), which belongs to the membrane-bound extracellular nucleoside triphosphate diphosphate hydrolase family. It is an integral membrane protein depending on Ca2+ and Mg2+. Human CD39, encoded by the ENTPD1 gene on the 10q24.1 chromosome, is a protein composed of 510 amino acids, and its molecular weight is approximately 78 kDa. CD39 contains seven heavily glycosylated N-linked glycosylation sites, 11 cysteine residues, and two transmembrane regions. These two transmembrane regions include a small cytoplasmic domain containing NH2- and COOH-terminal segments and a large extracellular hydrophobic domain containing five highly conserved domains known as apyrase conserved domains (ACRs) 1-5. This structure is significant for the catabolic activity of the enzyme and the maintenance of molecular structural integrity and contributes to nucleotide binding (7).
CD73, also known as ecto-5’-nucleotidase (ecto-5’-NT), is a membrane-bound glycoprotein connected by glycosylphosphatidylinositols (GPI) (8). Encoded by the NT5E gene located on human chromosome 6q14-21, CD73 is a protein molecule composed of 574 amino acids (according to its cDNA sequence), the molecular weight of which in its naturally purified form is 70 kDa (9). CD73 consists of three domains: the N-terminal domain with a metal binding site, the C-terminal domain in which the catalytic site is located, and the bridged α-helix domain (10). The non-covalent hydrophobic interaction at the C terminus and the binding of two zinc ions can stabilize the homodimerization of CD73 and achieve complete catalytic activity. CD73 homodimer can effectively hydrolyze AMP and convert it into ADO by opening and closing conformational cycles.
For this reason, it is also called the rate-limiting enzyme of the second step of purine nucleotide metabolism (11). ADO is a nucleoside molecule produced by the hydrolysis of ATP and is a critical signal molecule in the ATP-adenosine pathway. ADO can bind to four adenosine receptors belonging to the same G protein-coupled receptor (GPCR) family: A1R, A2AR, A2BR, and A3R. Among these, A1R and A3R are preferentially coupled to Gi protein to inhibit the action of adenylate cyclase and reduce the production of cyclic adenosine monophosphate (cAMP). However, A2AR and A2BR are generally Gs-coupled and trigger the action of adenylate cyclase and subsequently promote the production and accumulation of intracellular cAMP (12, 13). cAMP accumulation can activate both the canonical protein kinase A (PKA) and the non-canonical EPAC pathways (5). Additionally, all four adenosine receptors have been shown to induce the mitogen-activated protein kinase (MAPK) and JUN N-terminal kinase (JNK) pathways (14) (Figure 1).
3 CD39 and CD73 in the TME
3.1 CD39 and CD73 expressed on immune cells
Interactions between tumor cells and their immunological microenvironment are essential for the pathophysiology of lymphocytes, myeloid-derived suppressor cells (MDSCs), dendritic cells (DCs), and macrophages, which can co-express CD39 and CD73 (15) (Figure 2). Human B cells co-express CD39 and CD73 while the former was initially described as a B cell activation marker and expresses A1, A2, and A3 adenosine receptors (16, 17). It has been characterized that the phenotype and functionality of CD39+ human regulatory B cell (Breg) promotes Breg functions and shows high proliferative capacity while acting through adenosine generation and interleukin-10 (IL-10) secretion to immunosuppress T cells (18). CD73 is broadly expressed in human peripheral blood (PB) B cells and can also be expressed in memory B cells that develop outside of the germinal center, such as in the context of an extrafollicular reaction (19). Notably, adenosine-producing B cells produce significantly more interleukin-6 (IL-6) and IL-10, and activation of A1 and A2A receptors promote expansion and increase the differentiation of B cells toward class-switched B cells (20). Natural killer (NK) cells belonging to the innate immune subset are involved in anti-tumor immunity and contribute to the effects of ATP through type 2 purinergic receptors (P2XR and P2YR). CD39 and CD73 expression levels in NK cells are low but increase under specific conditions. CD39 can inhibit NK cell-mediated damage and decrease interferon-γ (IFN-γ) secretion (21). Additionally, CD73 expression is virtually absent in NK cells in healthy individuals but significant in tumor-infiltrating tissues, which suggests that NK cells can exert immunosuppressive function through the production of adenosine, environmental factors permitting (22).
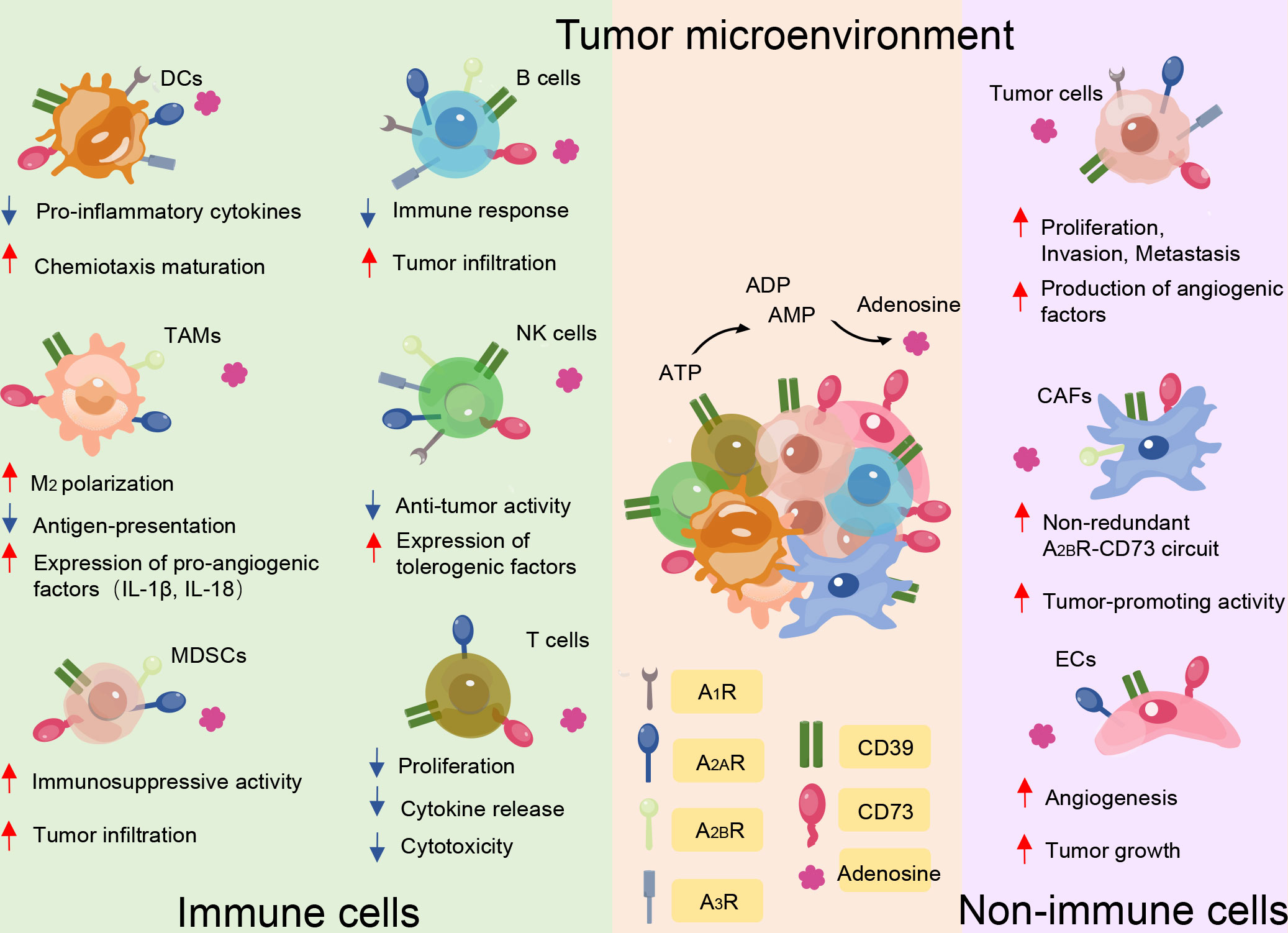
Figure 2 CD39 and CD73 serve as major immune suppressive mediators in the tumor microenvironment mainly through the generation of eADO. Besides the effect of ectonucleotidases on tumor cell proliferation, angiogenesis, infiltration, and metastasis, CD39 and CD73 expression by immune cells and non-immune cells impairs anti-tumor immunity by suppressing the function of protective immune cells, including T cells, B cells, natural killer (NK) cells, dendritic cells (DCs), myeloid derived suppressor cells (MDSCs), and tumor-associated macrophages, while maintaining the function of non-immune cells, including tumor cells, cancer-associated fibroblasts (CAFs), and endothelial cells (ECs). The red and blue arrows indicate whether functions are enhanced or reduced by adenosine binding to the different receptor subtypes.
CD39 and CD73 also exert their pro-tolerogenic effects on myeloid compartments. CD39 and CD73 levels of MDSCs are higher in tumor patients than in healthy controls (23). A positive correlation between intratumor CD39- and CD73-expressing MDSCs and tumor stage, node involvement, and metastasis status in non-small cell lung cancer has been reported (24). In further research, MDSCs expressing high levels of CD39 and CD73 increased immunosuppressive activity ex vivo compared with myeloid cells present in colorectal cancer (25). Thus, MDSCs that infiltrate tumors are probably an important source of extracellular adenosine, which contributes to tumor immune escape. eATP can activate the immune system through the stimulation of P2XR7 on DCs and promote an increase of interleukin-1β (IL-1β) and interleukin-18 (IL-18) secretion (26). Furthermore, IL-1β facilitates macrophage maturation and increases cytokine production (27). Additionally, CD39 is expressed on DCs, affecting DC-driven CD4+ T cell activation and differentiation through NLRP3 inflammasome, which is activated by the ATP-adenosine pathway (15, 28). NLRP3 is a prerequisite for IL-1β and IL-18 production (29). Furthermore, the accumulation of adenosine can impair the normal function of DCs, the so-called immune-suppressive regulatory DCs (30). Tumor-associated macrophages co-express CD39 and the eATP receptor. Inhibiting CD39 on macrophages significantly increases their production of tumor necrosis factor-α (TNF) and interleukin-12 (IL-12), while decreasing IL-10 secretion, thus inhibiting tumor growth (31, 32). It has been suggested that these macrophages that produce ADO suppress the activation of CD4+ T cells in vitro (33). In the context of a subgroup, the classification of immune cells based on CD39 and CD73 better reflects their function.
3.2 CD39 and CD73 are expressed on non-immune cells
Increasing evidence has also verified that CD39 and CD73 are the key regulatory molecules in tumor development, including tumor growth, metastasis, and angiogenesis, and their suppressive effects on the immune system in the TME (15). A high density of angiogenesis can support the sustenance of tumor cell growth, and angiogenesis is also an important pathway for the distant invasion of tumor cells.
CD39 is highly expressed on cancer-associated fibroblasts (CAFs) in ovarian cancer and pancreatic cancer (34). In a mouse model of chronic pancreatitis and fibrosis, it was shown that CD39-deficient mice develop significantly limited fibrosis. Additionally, tissue and plasma levels of anti-fibrotic IFN-γ significantly increased (35). These results suggest a role for CD39+ CAFs in promoting parenchymal fibrosis in pancreatic tissue (34). Elevated CD73 activity correlates strongly with high CAF abundance in colorectal cancer tissues (36). Furthermore, in a mouse model with ovarian cancer, a previous study demonstrated that CD73 on CAFs promotes tumor immune escape (37). ATP is well known to modulate a variety of processes linked to endothelial cell activation and increase the intracellular levels of Ca2+, which induces cytoskeletal rearrangements. In addition, ATP is released by endothelial cells during changes in flow or after exposure to hypoxic conditions, activating P2YR and promoting the release of vessel relaxation (38). In the TME, the expression of CD39 in the vascular system, especially endothelial cells, can promote tumor growth by scavenging eATP and promoting angiogenesis (39). In melanoma, lung carcinoma, and colon tumors, suppressed tumor growth in CD39-deficient mice has been associated with decreased angiogenesis; CD39 co-expression with CD73 in endothelial cells will ultimately generate adenosine, which promotes angiogenesis (34). Indeed, CD73-mediated adenosine and A2A signaling in endothelial cells have been shown to promote angiogenesis in a variety of experimental conditions, including during tumorigenesis (40) (Figure 2).
4 CD39 and CD73 are expressed on different T cell populations in the TME
The immortality of malignant cells demonstrates the host anti-tumor immune responses’ failure and induces an immunosuppressive microenvironment in which they can freely grow and expand. It has been shown that adenosine concentration is significantly increased in the TME, and a variety of immune cells, especially T cell subsets, are involved in the immunosuppression process (Figure 3). In effector T cells, after adenosine receptor activation, type I protein kinase A (PKA) and its C-terminal Src kinase (CSK) phosphorylation are activated to inhibit SRC family tyrosine kinases LCK and FYN. This attenuates the activation of transcription factors that are downstream of T cell receptor (TCR) activation, including NFAT, nuclear factor-κB (NF-κB), and AP-1. TCR activation increases A2ARs through NF-κB-dependent induction (41). The generation of high local concentrations of adenosine by CD39 and CD73 leads to potent immunosuppression via the impairment of T cell activation and function, with simultaneous enhancement of regulatory T cells (Tregs) (42). Hence, the ability to block adenosine generation by inhibiting the enzymatic activity of CD39 and CD73 provides a direct line of attack on adenosine-mediated immunosuppression, and the ATP-adenosine pathway functions as a critical modulator of innate and adaptive immunity with the TME.
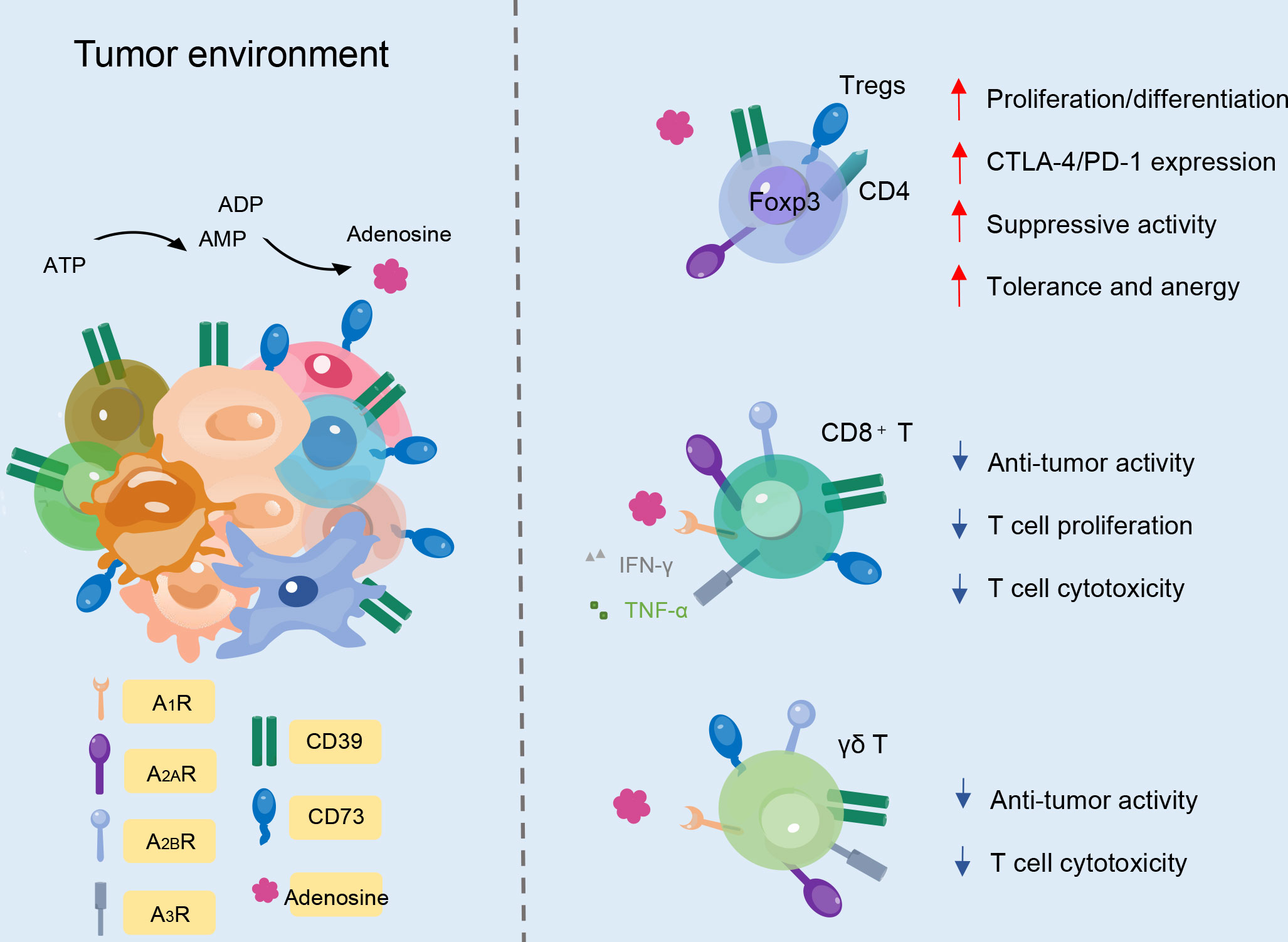
Figure 3 CD39 and CD73 expression on different T cell subtypes. In Foxp3+CD4+ Treg cells, CD39 and CD73 improve immunosuppressive activity. In CD8+ T cells, ectonucleotidases decrease cytotoxicity, proliferation, and anti-tumor activity. Likewise, CD39 and CD73 expression on γδ T cells can also decrease anti-tumor activity and T cell cytotoxicity.
4.1 CD39 and CD73 are expressed on Treg cells
In human PB, approximately one-third of CD4+ T cells and a small proportion of CD8+ T cells express CD39 (15). On the contrary, CD73 is expressed by less than 50% of CD8+ T cells and by less than 10% of CD4+ T cells (15). Adenosine in turn modulates Treg function. Tregs play an indispensable role in maintaining immunological unresponsiveness to self-antigens, and counteraction of the immunosuppressive features of the TME is an attractive strategy for cancer treatment. ADO produced by CD39 and CD73 through the ATP-adenosine pathway can regulate the function of Tregs, activate receptors on Tregs to promote proliferation, and increase the expression of immunosuppressive receptors to enhance immunosuppressive function (43). In the TME, the aggregation of Tregs is associated with high CD39 expression, which promotes adenosine accumulation, tumor growth, and angiogenesis (44, 45). Compared with traditional Tregs, CD39+ Tregs show more vital inhibitory ability (46). Studies also have shown that CD39+ Tregs specifically suppress Interleukin-17 (IL-17) production to some extent, preventing the transdifferentiation of Tregs into T helper 17 (Th17) cells or endowing already differentiated Th17 cells with an immunosuppressive phenotype. Additionally, inhibition of human CD73 can reduce immunosuppression mediated by Tregs (47).
4.2 CD39 and CD73 are expressed on CD8+ T cells
Some studies have suggested that CD8+ T cells expressing CD39 and CD73 also show regulatory characteristics. Meanwhile, CD39 is potentially involved in mediating the suppressive abilities of tumor-infiltrating CD8+ Tregs (48). The isolated CD39+CD8+ T cells from tumor-infiltrating lymphocytes (TILs) can inhibit T cell proliferation in vitro, which mediates tumor invasion, and display a gene signature of exhaustion (49). CD8+ T cells express a high frequency of CD39 in solid tumors and non-solid tumors, which affects their normal cytotoxicity and ability to secrete cytokines (50). The phenotypes of exhaustion mean that the production of TNF-α, IFN-γ, and interleukin-2 (IL-2) cytokines decreases, accompanied by the upregulation of co-inhibitory receptors, including programmed cell death 1 (PD-1), cytotoxic T-lymphocyte-associated protein 4 (CTLA4), lymphocyte-activation gene 3 (LAG3), T cell immunoreceptor with Ig and ITIM domains (TIGIT), and T-cell immunoglobulin mucin-3 (TIM-3) (48, 51, 52). IL-6 and transforming growth factor-β (TGF-β) are additional factors that contribute to the upregulation of CD39 on CD8+ T cells and subsequently potentiate the immunosuppressive activity in the TME. Beyond the TME, CD39+CD8+ T cells are also abundant in invaded lymph nodes and metastases and in the peripheral circulation lymphoid organs (51, 53). Interestingly, the expansion of the CD39+CD8+ T cell population in the blood is associated with clinical responses to anti-PD-1 therapy (53).
4.3 CD39 and CD73 are Expressed on γδ T Cells
Human γδ TCR-expressing cells constitute 1–5% of total T cells in the PB and play an indispensable role in the immune system. γδ T cells belong to the non-conventional lymphocyte family though they can produce many cytokines, such as IFN-γ, and act cytotoxically (54). γδ T cells are composed of different subpopulations with different functions. Recent research has shown that activated murine γδ T cells co-express CD73 and CD39 and display immunosuppressive functions, while most resting γδ τ cells do not constitutively express CD39 (55). CD39 has been identified as a marker of regulatory γδ T cells (15, 55). In murine lymph nodes, the CD25+CD39+ γδ T cell population can suppress the proliferation of αβ T cells in vitro (55). In the TME, CD39+ γδ T cells of invasive mouse pancreatic tumors are upregulated, together with other immunosuppressive factors, and support tumorigenesis by inhibiting αβ T cell proliferation (56). Vγ9Vδ2 T cells are a subset of γδ T cells in the peripheral circulation and function by detecting self and pathogen-associated phosphoantigens (pAgs). Normally, these cells do not express CD39 or CD73 but can upregulate CD39 upon TCR stimulation. Gruenbacher et al. proved that CD39 dephosphorylates pAgs, which specifically activate Vγ9Vδ2 T cells, rendering them inactivate at stimulating γδ T cells, and thus revealed a previously unrecognized immunoregulatory role of CD39 (57). CD73 is expressed in more than 90% of peripheral γδ T cells (58). In a study of CD73 deficient mice, CD73 proved essential for γδ T cell development and might participate in its regulatory function (58). γδ T cells express different levels of CD73 before and after their activation, and the level of CD73 expression correlates with the pro- and anti-inflammatory activities of γδ T cells in Th17 autoimmune responses (59). Researchers have found that CD73-expressing γδ T cells are much more potent at converting AMP to adenosine than all other CD73+ immune cell types (59).
5 The role of ectonucleotidases CD39 and CD73 in hematological malignancy
5.1 Acute myeloid leukemia
Acute myeloid leukemia (AML) is a progressive myeloproliferative malignant tumor, which is mainly characterized by abnormal proliferation of primitive and immature myeloid cells in the bone marrow (BM) and PB (60). It has been shown that there is an abnormally high CD39 expression in Treg cells in patients with AML (35). Nicolas Dulphy et al. found that compared with healthy people, the proportion of Tregs in the circulation of AML patients increased, and the frequency of CD39 decreased (36). However, the percentage of CD39+ Tregs did not decrease, which suggests that the function of CD39 in Tregs of AML patients could be maintained. At the same time, only a few patients and healthy people expressed CD73 in the Tregs, and the frequency was deficient. The increase of Treg subsets indicated that there is an overall immunosuppressive environment in tissues and BM in patients with AML (61, 62).
It has been suggested that CD39+CD8+ T cells can be used as a potential marker of exhaustion in patients with AML (63). In a study by Brauneck et al., TIGIT+CD73-CD8+ from AML patients showed a distinct characteristic, both in PB and BM. These cells were divided into PD1+TIGIT+CD73-CD8+ T and CD39+TIGIT+CD73-CD8+ T cell subsets. As the disease progressed, the proportion of PD1+TIGIT+CD73-CD8+ T cells gradually increased, and this was maintained in remission (63). The latest study suggested CD39 could be used as a marker of poor treatment response and prognosis in patients with AML. Aroua et al. graded the fold enrichment of the CD39 expression cells in AML patients after chemotherapy and found that the disease-free survival rate of the ‘high CD39 ratio’ group was significantly worse than that of the ‘low CD39 ratio’ group (64). Moreover, when the focus was on patients under 60, this survival disadvantage was more significant, indicating that CD39 could be used as a prognostic marker of adverse response to chemotherapy in AML (64). The drug blocking the inhibition of CD39 activity can not only block the mitochondrial metabolic reprogramming related to AraC resistance but also significantly enhance its cytotoxicity and sensitivity to AML cells in vivo and in vitro (64, 65). Additionally, Franziska Brauneck et al. found that γδ T cells in patients with AML expresses high levels of CD39 and PD-1, TIM-3, TIGIT, and other immunosuppressive receptors, which is similar to that of CD8+ T cells but higher than that of CD4+ T cells (66). In further analysis, the researchers found that CD39 expression on Vδ1 T cells is significantly increased and significantly co-expressed with PD-1, TIM-3, and TIGIT, which shows further depletion characteristics (66).
Similarly, CD73 is also closely related to T-cell depletion in patients with AML and can be used as an essential target (67). The frequency of CD73 expression in CD8+ T cells of newly diagnosed AML patients is significantly lower than that of healthy controls. This suggests that the downregulation of CD73 expression is phenotypically related to T cell depletion, and the expression of CD73 on CD8+ T cells is increased significantly after complete remission. Therefore, the low expression of CD73 on CD8+ T cells is associated with a high burden of leukemia (67). Contrary to the long-recognized negative immune regulation of ATP-adenosine signal in tumor tissue and the increase of CD73 associated with poor prognosis, the researchers found that the expression of CD73 on CD8+ T cells in patients with AML is related to the enhancement of immune response and has a higher function (67). On the other hand, CD73-CD8+ T cells express high levels of inhibitory receptors, such as PD-1, TIGIT, and immunosuppressive molecules, and have the ability to produce cytokines, including IL-2, TNF-α, and IFN-γ, is decreased, thus increasing the likelihood of apoptosis (67). Therefore, understanding the specific distribution pattern of CD73 in each cancer type or disease state is very important for the optimal design of clinical studies of cancer treatment of CD73 (67, 68).
5.2 Chronic lymphocytic leukemia
Chronic lymphocytic leukemia (CLL) is the most common type of leukemia in adults and is characterized by the proliferation and progressive accumulation of functionally deficient B cells in PB, BM, and lymphoid tissues (69). The clinical course of the disease is highly variable, and some patients have a good prognosis and a long survival time, while others can rapidly develop invasive lymphoma or leukemia (70). To better differentiate prognostic subsets, novel biological parameters have been added to clinical staging systems, and TME appears to play a critical role in genesis and progression. The expression level of CD39 on CLL cells is significantly higher than that of normal lymphocytes, and the levels of CD39+CD4+ T and CD39+CD8+ T cells in PB are also significantly higher (71). Compared with CD39low T cells, the time-to-first treatment of CLL patients with CD39high T cells is significantly shorter, which indicates that the expression of CD39 on CD4+ T cells is closely related to the more advanced stage of the disease and that CD39 plays a role in the invasion of the disease (71, 72). In addition, the number of CD39+CD4+ T cells increases in CLL patients with poor prognostic markers, which is associated with a shorter initial treatment time. In addition, the frequency of CD39+CD4+ T cells in CLL patients with cytogenetic abnormalities with poor prognosis is also similar to that in patients with normal- or low- or moderate-risk cytogenetic abnormalities (73). Above all, the data suggest that CD39+CD4+ T cells are associated with a poor prognosis in patients with CLL (73). In patients with CLL, the increase of Tregs has also been associated with disease progression, and the unique proportion of CD39+ Tregs subsets is related to the disease stage of CLL (74). However, compared with healthy controls, Foxp3+ and Foxp3-CD39+CD4+ T cells in CLL are increased, and the levels of these two subsets are related to the severity of CLL. This suggests that the expression of Foxp3 on CD39+CD4+ T cells has no additional predictive value for the prognosis of CLL patients (73). The results referred to above were obtained from a cross-sectional study, so it is not clear whether CD39 expression on T cells increases with the deterioration of the disease.
CD73 expression may also be related to the prognosis of CLL. M. Kicova et al. showed that high CD73 expression is related to the significant shortening of the overall survival time of CLL patients (75). This was the first time that researchers have directly proven the effect of CD73 expression on the survival of patients with CLL. In addition, CD73 expression has been found on B cells in CLL patients, and Serra et al. found that high CD73 expression is associated with more aggressive clinical behavior, which is characterized by large CLL clones and poor prognosis (75, 76). Therefore, further research is needed to determine the effect of CD73 expression in patients with progressive disease.
5.3 Multiple myeloma
Multiple myeloma (MM) is the second most common hematological malignancy and is characterized by abnormal proliferation of clonal and terminally differentiated B cells in the BM. Owing to the heterogeneity of its disease progression and the changes in the bone marrow microenvironment, most patients have a recurrence, and the prognosis of different patients is very different (77, 78). Therefore, individualized treatment of MM is critical. In patients with malignant MM, the number of CD39+ Tregs is increased and they participate in the inhibition of the Th17 response. Additionally, they are used as a myeloma cell promoter that produces IL-17, especially in myeloma-permitted BM environments (79, 80). The appearance of activated CD39+ Treg cells and BM resident CD39+ Tregs may represent the early changes caused by malignant MM cells, thus promoting the clinical progress of MM (79).
In addition, Rui Yang et al. detected the expression of CD39 on CD8+ T cells of MM patients. Interestingly, similar to CD8+ TIL cells related to antigen-specific depletion, these CD39+CD8+T cells can also co-express PD-1 (81). In addition, Arghya Ray et al. found that targeted CD73 therapy, alone or in combination with an immune stimulant TLR-7 agonist, can enhance the activity of MM-specific CD8+ cytotoxic T cells, which is a promising new strategy to restore patients’ anti-MM immunity (23, 82). In the BM of patients with MM, the expression of CD39 on γδ T cells is significantly increased, especially on Vδ1 T cell subsets (66). Moreover, CD39 is often co-expressed with inhibitory receptors, such as TIGIT, PD-1, and TIM-3 on γδ T cells, which suggests that γδ T cells may be in a state of depletion. Therefore, targeted CD39 has potential application value in activating and enhancing the cytotoxicity of γδ T cells.
5.4 Diffuse large B-cell lymphoma
Diffuse large B-cell lymphoma (DLBCL) is the most common subtype of aggressive non-Hodgkin’s lymphoma and can occur de novo or as a result of the transformation of indolent lymphoma (83). Owing to the heterogeneity of DLBCL, approximately one-third of patients still have a poor prognosis. The latest study found that the ATP-adenosine axis can inhibit the activity of CD8+ T cells, and the combination of PD-1 and CD73 can define more dysfunctional CD8+ T cell subsets (84). Targeting of the PD-1/PD-L1 (programmed cell death-ligand 1) immunosuppressive pathway combined with CD73 inhibitors may provide additional clinical benefits and partially overcome primary and secondary drug resistance to PD-1/PD-L1 blockade, as well as put forward a strong theoretical basis for precise immunotherapy and further the development of CD73 immunotherapy strategies for DLBCL patients.
6 Clinical study of the ectonucleotidases CD39 and CD73 in tumor immunity
The specific expression pattern of the ectonucleotidases CD39 and CD73 make them capable of serving as markers to selectively tag leukemia cells and deliver therapeutic agents while limiting off-targets. Additionally, as they operate in a coordinated cascade of events, the inhibition of one of them is sufficient to block the downstream processes. Hence, their intervention opens the possibility of modulating immunosuppression.
Recent studies have shown that blocking CD39 and CD73 can not only prevent the accumulation of adenosine but also restore anti-tumor immunity by stabilizing extracellular pro-inflammatory ATP (23). As a drug target for cancer, various drugs against CD39 have entered clinical trials (Table 1). CD39 inhibitors, including ARL67156 and POM-1, are effective in animal models of follicular lymphoma, sarcoma, or mouse melanoma, resulting in the partial overcoming of poor T cell response to stimulation, enhanced response to chemotherapeutic drugs, and inhibition of tumor growth, respectively (47). After administration of CD39 inhibitor ARL67156, eATP in tumors increases, which promotes the recruitment of dendritic cells and CD4+ and CD8+ cells producing IFN-γ and simultaneously promotes the immune control of autophagy-deficient tumors (85). Considering the delicate balance between eATP and extracellular adenosine in regulating the immune response in TME, CD39-guided therapy may affect tumor-immune interaction in other aspects (23). In addition to monotherapy, some preclinical studies have shown that there is a synergistic blocking effect between the release of immunosuppressive tumor microenvironment and anti-PD-1/PD-L1 resulting from targeting of the ATP-adenosine pathway (including CD39 and CD73) (85). Preclinical studies have also shown that the synergistic effect of targeted CD39 antagonist IPH5201 and PD-L1 checkpoint inhibitors have better complete regression and improved survival than PD-L1 inhibitors alone (86).
CD73 can also be expressed in normal cells, and for this reason, therapy targeting CD73 (such as an anti-CD73 monoclonal antibody) is often considered a non-specific therapy (87). Interestingly, studies have proved that CD73 is highly effective in targeted therapy for cancer (Table 2). Antagonists targeting CD73 often combine with other immune checkpoint blockers to improve the prognosis for cancer patients. Tests of CD73 small molecule inhibitor AB680 in pancreatic cancer patients have shown that it can effectively restore T cell proliferation, cytokine secretion, and suppressed cytotoxicity (88). Consistent with the previously reported anti-tumor effect of immune checkpoint blockers combined with CD73 targeted drugs, the use of AB680 combined with PD-1 blocking in vitro can overcome the inhibitory effect of adenosine on human T cells and enhance the anti-tumor activity of drugs and the anti-tumor effect in vivo (88). In addition, in the breast cancer model, anti-CD73 antibodies partially prevent lung metastasis in mice (89). Currently, a therapeutic anti-CD73 antibody MEDI9447 is also in clinical trials with patients with solid cancer (NCT02503774; NCT03611556) (90).
7 Conclusion
Although ectonucleotidases CD39 and CD73 represent promising targets for novel therapeutic strategies, most current therapeutic strategies come from solid tumors. They involve hematological malignancies in which they can act as disease and prognostic markers and, to some extent, directly contribute to leukemia progress and expansion. The proper design of clinical trials incorporating a comprehensive biomarker strategy will be paramount for robustly impacting tumor-immune interactions and regulating the suppressive TME. As discussed previously, there are additional combination regimens that can synergize with CD39 or CD73 blockade to provide potential benefits to patients. It is worth noting that some key issues remain unaddressed, including determining the consequences of targeting CD39, CD73, and adenosine receptors on extracellular ATP levels, evaluating the activity of the dual targeting of CD39 and CD73, and developing reliable methods to measure extracellular adenosine levels in the TME.
Author contributions
XLW and ZYJ contributed to the outline and revision of the manuscript; XJ wrote the initial manuscript draft; XFW prepared the figure; JMZ and YXX organized the literature for the manuscript; PLW and XJ revised the final draft. All authors read and approved the submitted manuscript.
Funding
This study was supported by grants from the Science and Technology Program of Guangzhou City (202201010164), the National Natural Science Foundation of China (81800143, 81770150, 81200388, and 82170220), the Natural Science Foundation of Guangdong Province (2020A1515010817 and 2022A1515010313), the National Innovation and Entrepreneurship Training Program for Undergraduates (202010559081), Guangdong’s Innovation and Entrepreneurship Training Program for Undergraduates (202010559081 and 202210559083), the “Challenge Cup” National Undergraduate curricular academic science and technology works by race (21112027), and Guangdong College Students’ Scientific and Technological Innovation (CX22438, CX22446, and CX21285).
Conflict of interest
The authors declare that the research was conducted in the absence of any commercial or financial relationships that could be construed as a potential conflict of interest.
Publisher’s note
All claims expressed in this article are solely those of the authors and do not necessarily represent those of their affiliated organizations, or those of the publisher, the editors and the reviewers. Any product that may be evaluated in this article, or claim that may be made by its manufacturer, is not guaranteed or endorsed by the publisher.
References
1. Kepp O, Loos F, Liu P, Kroemer G. Extracellular nucleosides and nucleotides as immunomodulators. Immunol Rev (2017) 280(1):83–92. doi: 10.1111/imr.12571
2. Dosch M, Gerber J, Jebbawi F, Beldi G. Mechanisms of Atp release by inflammatory cells. Int J Mol Sci (2018) 19(4):1–16. doi: 10.3390/ijms19041222
3. Longhi MS, Robson SC, Bernstein SH, Serra S, Deaglio S. Biological functions of ecto-enzymes in regulating extracellular adenosine levels in neoplastic and inflammatory disease states. J Mol Med (Berl) (2013) 91(2):165–72. doi: 10.1007/s00109-012-0991-z
4. Vaisitti T, Arruga F, Deaglio S. Targeting the adenosinergic axis in chronic lymphocytic leukemia: A way to disrupt the tumor niche? Int J Mol Sci (2018) 19(4):1–21. doi: 10.3390/ijms19041167
5. Antonioli L, Blandizzi C, Pacher P, Hasko G. Immunity, inflammation and cancer: A leading role for adenosine. Nat Rev Cancer (2013) 13(12):842–57. doi: 10.1038/nrc3613
6. Vaisitti T, Arruga F, Guerra G, Deaglio S. Ectonucleotidases in blood malignancies: A tale of surface markers and therapeutic targets. Front Immunol (2019) 10:2301. doi: 10.3389/fimmu.2019.02301
7. Heine P, Braun N, Sévigny J, Robson SC, Servos J, Zimmermann H. The c-terminal cysteine-rich region dictates specific catalytic properties in chimeras of the ectonucleotidases Ntpdase1 and Ntpdase2. Eur J Biochem (2001) 268(2):364–73. doi: 10.1046/j.1432-1033.2001.01896.x
8. Colgan SP, Eltzschig HK, Eckle T, Thompson LF. Physiological roles for ecto-5'-Nucleotidase (Cd73). Purinergic Signal (2006) 2(2):351–60. doi: 10.1007/s11302-005-5302-5
9. Resta R, Yamashita Y, Thompson LF. Ecto-enzyme and signaling functions of lymphocyte Cd73. Immunol Rev (1998) 161:95–109. doi: 10.1111/j.1600-065x.1998.tb01574.x
10. Fini C, Talamo F, Cherri S, Coli M, Floridi A, Ferrara L, et al. Biochemical and mass spectrometric characterization of soluble ecto-5'-Nucleotidase from bull seminal plasma. Biochem J (2003) 372(2):443–51. doi: 10.1042/bj20021687
11. Knapp K, Zebisch M, Pippel J, El-Tayeb A, Muller CE, Strater N. Crystal structure of the human ecto-5'-Nucleotidase (Cd73): Insights into the regulation of purinergic signaling. Structure (2012) 20(12):2161–73. doi: 10.1016/j.str.2012.10.001
12. Mosenden R, Tasken K. Cyclic amp-mediated immune regulation–overview of mechanisms of action in T cells. Cell Signal (2011) 23(6):1009–16. doi: 10.1016/j.cellsig.2010.11.018
13. Young A, Ngiow SF, Barkauskas DS, Sult E, Hay C, Blake SJ, et al. Co-Inhibition of Cd73 and A2ar adenosine signaling improves anti-tumor immune responses. Cancer Cell (2016) 30(3):391–403. doi: 10.1016/j.ccell.2016.06.025
14. Schulte G, Fredholm BB. Signalling from adenosine receptors to mitogen-activated protein kinases. Cell Signal (2003) 15(9):813–27. doi: 10.1016/s0898-6568(03)00058-5
15. Allard B, Longhi MS, Robson SC, Stagg J. The ectonucleotidases Cd39 and Cd73: Novel checkpoint inhibitor targets. Immunol Rev (2017) 276(1):121–44. doi: 10.1111/imr.12528
16. Zacca ER, Amezcua Vesely MC, Ferrero PV, Acosta CDV, Ponce NE, Bossio SN, et al. B Cells from patients with rheumatoid arthritis show conserved Cd39-mediated regulatory function and increased Cd39 expression after positive response to therapy. J Mol Biol (2021) 433(1):166687. doi: 10.1016/j.jmb.2020.10.021
17. Saze Z, Schuler PJ, Hong CS, Cheng D, Jackson EK, Whiteside TL. Adenosine production by human b cells and b cell-mediated suppression of activated T cells. Blood (2013) 122(1):9–18. doi: 10.1182/blood-2013-02-482406
18. Figueiro F, Muller L, Funk S, Jackson EK, Battastini AM, Whiteside TL. Phenotypic and functional characteristics of Cd39(High) human regulatory b cells (Breg). Oncoimmunology (2016) 5(2):e1082703. doi: 10.1080/2162402X.2015.1082703
19. Sweet RA, Cullen JL, Shlomchik MJ. Rheumatoid factor b cell memory leads to rapid, switched antibody-forming cell responses. J Immunol (2013) 190(5):1974–81. doi: 10.4049/jimmunol.1202816
20. Kaku H, Cheng KF, Al-Abed Y, Rothstein TL. A novel mechanism of b cell-mediated immune suppression through Cd73 expression and adenosine production. J Immunol (2014) 193(12):5904–13. doi: 10.4049/jimmunol.1400336
21. Hu G, Wu P, Cheng P, Zhang Z, Wang Z, Yu X, et al. Tumor-infiltrating Cd39(+)Gammadeltatregs are novel immunosuppressive T cells in human colorectal cancer. Oncoimmunology (2017) 6(2):e1277305. doi: 10.1080/2162402X.2016.1277305
22. Chatterjee D, Tufa DM, Baehre H, Hass R, Schmidt RE, Jacobs R. Natural killer cells acquire Cd73 expression upon exposure to mesenchymal stem cells. Blood (2014) 123(4):594–5. doi: 10.1182/blood-2013-09-524827
23. Moesta AK, Li XY, Smyth MJ. Targeting Cd39 in cancer. Nat Rev Immunol (2020) 20(12):739–55. doi: 10.1038/s41577-020-0376-4
24. Li J, Wang L, Chen X, Li L, Li Y, Ping Y, et al. Cd39/Cd73 upregulation on myeloid-derived suppressor cells Via tgf-Beta-Mtor-Hif-1 signaling in patients with non-small cell lung cancer. Oncoimmunology (2017) 6(6):e1320011. doi: 10.1080/2162402X.2017.1320011
25. Limagne E, Euvrard R, Thibaudin M, Rebe C, Derangere V, Chevriaux A, et al. Accumulation of mdsc and Th17 cells in patients with metastatic colorectal cancer predicts the efficacy of a folfox-bevacizumab drug treatment regimen. Cancer Res (2016) 76(18):5241–52. doi: 10.1158/0008-5472.CAN-15-3164
26. Mariathasan S, Weiss DS, Newton K, McBride J, O'Rourke K, Roose-Girma M, et al. Cryopyrin activates the inflammasome in response to toxins and atp. Nature (2006) 440(7081):228–32. doi: 10.1038/nature04515
27. Pan MH, Maresz K, Lee PS, Wu JC, Ho CT, Popko J, et al. Inhibition of tnf-alpha, il-1alpha, and il-1beta by pretreatment of human monocyte-derived macrophages with menaquinone-7 and cell activation with tlr agonists in vitro. J Med Food (2016) 19(7):663–9. doi: 10.1089/jmf.2016.0030
28. Mascanfroni ID, Yeste A, Vieira SM, Burns EJ, Patel B, Sloma I, et al. Il-27 acts on dcs to suppress the T cell response and autoimmunity by inducing expression of the immunoregulatory molecule Cd39. Nat Immunol (2013) 14(10):1054–63. doi: 10.1038/ni.2695
29. Zhao H, Bo C, Kang Y, Li H. What else can Cd39 tell us? Front Immunol (2017) 8:727. doi: 10.3389/fimmu.2017.00727
30. Veglia F, Gabrilovich DI. Dendritic cells in cancer: The role revisited. Curr Opin Immunol (2017) 45:43–51. doi: 10.1016/j.coi.2017.01.002
31. Savio LEB, de Andrade Mello P, Figliuolo VR, de Avelar Almeida TF, Santana PT, Oliveira SDS, et al. Cd39 limits P2x7 receptor inflammatory signaling and attenuates sepsis-induced liver injury. J Hepatol (2017) 67(4):716–26. doi: 10.1016/j.jhep.2017.05.021
32. Cohen HB, Ward A, Hamidzadeh K, Ravid K, Mosser DM. Ifn-gamma prevents adenosine receptor (A2br) upregulation to sustain the macrophage activation response. J Immunol (2015) 195(8):3828–37. doi: 10.4049/jimmunol.1501139
33. Montalban Del Barrio I, Penski C, Schlahsa L, Stein RG, Diessner J, Wockel A, et al. Adenosine-generating ovarian cancer cells attract myeloid cells which differentiate into adenosine-generating tumor associated macrophages - a self-amplifying, Cd39- and Cd73-dependent mechanism for tumor immune escape. J Immunother Cancer (2016) 4:49. doi: 10.1186/s40425-016-0154-9
34. Allard D, Allard B, Stagg J. On the mechanism of anti-Cd39 immune checkpoint therapy. J Immunother Cancer (2020) 8(1):1–11. doi: 10.1136/jitc-2019-000186
35. Kunzli BM, Nuhn P, Enjyoji K, Banz Y, Smith RN, Csizmadia E, et al. Disordered pancreatic inflammatory responses and inhibition of fibrosis in Cd39-null mice. Gastroenterology (2008) 134(1):292–305. doi: 10.1053/j.gastro.2007.10.030
36. Yu M, Guo G, Huang L, Deng L, Chang CS, Achyut BR, et al. Cd73 on cancer-associated fibroblasts enhanced by the a(2b)-mediated feedforward circuit enforces an immune checkpoint. Nat Commun (2020) 11(1):515. doi: 10.1038/s41467-019-14060-x
37. Turcotte M, Spring K, Pommey S, Chouinard G, Cousineau I, George J, et al. Cd73 is associated with poor prognosis in high-grade serous ovarian cancer. Cancer Res (2015) 75(21):4494–503. doi: 10.1158/0008-5472.CAN-14-3569
38. Lohman AW, Billaud M, Isakson BE. Mechanisms of atp release and signalling in the blood vessel wall. Cardiovasc Res (2012) 95(3):269–80. doi: 10.1093/cvr/cvs187
39. Kunzli BM, Bernlochner MI, Rath S, Kaser S, Csizmadia E, Enjyoji K, et al. Impact of Cd39 and purinergic signalling on the growth and metastasis of colorectal cancer. Purinergic Signal (2011) 7(2):231–41. doi: 10.1007/s11302-011-9228-9
40. Allard B, Turcotte M, Spring K, Pommey S, Royal I, Stagg J. Anti-Cd73 therapy impairs tumor angiogenesis. Int J Cancer (2014) 134(6):1466–73. doi: 10.1002/ijc.28456
41. Cekic C, Linden J. Purinergic regulation of the immune system. Nat Rev Immunol (2016) 16(3):177–92. doi: 10.1038/nri.2016.4
42. Verma NK, Wong BHS, Poh ZS, Udayakumar A, Verma R, Goh RKJ, et al. Obstacles for T-lymphocytes in the tumour microenvironment: Therapeutic challenges, advances and opportunities beyond immune checkpoint. EBioMedicine (2022) 83:104216. doi: 10.1016/j.ebiom.2022.104216
43. Ohta A, Kini R, Ohta A, Subramanian M, Madasu M, Sitkovsky M. The development and immunosuppressive functions of Cd4(+) Cd25(+) Foxp3(+) regulatory T cells are under influence of the adenosine-A2a adenosine receptor pathway. Front Immunol (2012) 3:190. doi: 10.3389/fimmu.2012.00190
44. Borsellino G, Kleinewietfeld M, Di Mitri D, Sternjak A, Diamantini A, Giometto R, et al. Expression of ectonucleotidase Cd39 by Foxp3+ treg cells: Hydrolysis of extracellular atp and immune suppression. Blood (2007) 110(4):1225–32. doi: 10.1182/blood-2006-12-064527
45. Peres RS, Donate PB, Talbot J, Cecilio NT, Lobo PR, Machado CC, et al. Tgf-beta signalling defect is linked to low Cd39 expression on regulatory T cells and methotrexate resistance in rheumatoid arthritis. J Autoimmun (2018) 90:49–58. doi: 10.1016/j.jaut.2018.01.004
46. Lu Y, Wang X, Gu J, Lu H, Zhang F, Li X, et al. Itreg induced from Cd39(+) naive T cells demonstrate enhanced proliferate and suppressive ability. Int Immunopharmacol (2015) 28(2):925–30. doi: 10.1016/j.intimp.2015.03.039
47. Mandapathil M, Hilldorfer B, Szczepanski MJ, Czystowska M, Szajnik M, Ren J, et al. Generation and accumulation of immunosuppressive adenosine by human Cd4+Cd25highfoxp3+ regulatory T cells. J Biol Chem (2010) 285(10):7176–86. doi: 10.1074/jbc.M109.047423
48. Vieyra-Lobato MR, Vela-Ojeda J, Montiel-Cervantes L, Lopez-Santiago R, Moreno-Lafont MC. Description of Cd8(+) regulatory T lymphocytes and their specific intervention in graft-Versus-Host and infectious diseases, autoimmunity, and cancer. J Immunol Res (2018) 2018:3758713. doi: 10.1155/2018/3758713
49. Gallerano D, Ciminati S, Grimaldi A, Piconese S, Cammarata I, Focaccetti C, et al. Genetically driven Cd39 expression shapes human tumor-infiltrating Cd8(+) T-cell functions. Int J Cancer (2020) 147(9):2597–610. doi: 10.1002/ijc.33131
50. Timperi E, Barnaba V. Cd39 regulation and functions in T cells. Int J Mol Sci (2021) 22(15):1–13. doi: 10.3390/ijms22158068
51. Canale FP, Ramello MC, Nunez N, Araujo Furlan CL, Bossio SN, Gorosito Serran M, et al. Cd39 expression defines cell exhaustion in tumor-infiltrating Cd8(+) T cells. Cancer Res (2018) 78(1):115–28. doi: 10.1158/0008-5472.CAN-16-2684
52. Noble A, Mehta H, Lovell A, Papaioannou E, Fairbanks L. Il-12 and il-4 activate a Cd39-dependent intrinsic peripheral tolerance mechanism in Cd8(+) T cells. Eur J Immunol (2016) 46(6):1438–48. doi: 10.1002/eji.201545939
53. Simoni Y, Becht E, Fehlings M, Loh CY, Koo SL, Teng KWW, et al. Bystander Cd8(+) T cells are abundant and phenotypically distinct in human tumour infiltrates. Nature (2018) 557(7706):575–9. doi: 10.1038/s41586-018-0130-2
54. Kalyan S, Kabelitz D. Defining the nature of human gammadelta T cells: A biographical sketch of the highly empathetic. Cell Mol Immunol (2013) 10(1):21–9. doi: 10.1038/cmi.2012.44
55. Otsuka A, Hanakawa S, Miyachi Y, Kabashima K. Cd39: A new surface marker of mouse regulatory gammadelta T cells. J Allergy Clin Immunol (2013) 132(6):1448–51. doi: 10.1016/j.jaci.2013.05.037
56. Daley D, Zambirinis CP, Seifert L, Akkad N, Mohan N, Werba G, et al. Gammadelta T cells support pancreatic oncogenesis by restraining alphabeta T cell activation. Cell (2016) 166(6):1485–99 e15. doi: 10.1016/j.cell.2016.07.046
57. Gruenbacher G, Gander H, Rahm A, Idzko M, Nussbaumer O, Thurnher M. Ecto-atpase Cd39 inactivates isoprenoid-derived Vgamma9vdelta2 T cell phosphoantigens. Cell Rep (2016) 16(2):444–56. doi: 10.1016/j.celrep.2016.06.009
58. Coffey F, Lee SY, Buus TB, Lauritsen JP, Wong GW, Joachims ML, et al. The tcr ligand-inducible expression of Cd73 marks gammadelta lineage commitment and a metastable intermediate in effector specification. J Exp Med (2014) 211(2):329–43. doi: 10.1084/jem.20131540
59. Li X, Liang D, Shao H, Born WK, Kaplan HJ, Sun D. Adenosine receptor activation in the Th17 autoimmune responses of experimental autoimmune uveitis. Cell Immunol (2019) 339:24–8. doi: 10.1016/j.cellimm.2018.09.004
60. Weigert N, Rowe JM, Lazarus HM, Salman MY. Consolidation in aml: Abundant opinion and much unknown. Blood Rev (2022) 51:100873. doi: 10.1016/j.blre.2021.100873
61. Schick J, Vogt V, Zerwes M, Kroell T, Kraemer D, Kohne CH, et al. Antileukemic T-cell responses can be predicted by the composition of specific regulatory T-cell subpopulations. J Immunother (2013) 36(4):223–37. doi: 10.1097/CJI.0b013e31829180e7
62. Dulphy N, Henry G, Hemon P, Khaznadar Z, Dombret H, Boissel N, et al. Contribution of Cd39 to the immunosuppressive microenvironment of acute myeloid leukaemia at diagnosis. Br J Haematol (2014) 165(5):722–5. doi: 10.1111/bjh.12774
63. Brauneck F, Haag F, Woost R, Wildner N, Tolosa E, Rissiek A, et al. Increased frequency of Tigit(+)Cd73-Cd8(+) T cells with a tox(+) tcf-1low profile in patients with newly diagnosed and relapsed aml. Oncoimmunology (2021) 10(1):1930391. doi: 10.1080/2162402X.2021.1930391
64. Aroua N, Boet E, Ghisi M, Nicolau-Travers ML, Saland E, Gwilliam R, et al. Extracellular atp and Cd39 activate camp-mediated mitochondrial stress response to promote cytarabine resistance in acute myeloid leukemia. Cancer Discovery (2020) 10(10):1544–65. doi: 10.1158/2159-8290.CD-19-1008
65. Luna-Yolba R, Marmoiton J, Gigo V, Marechal X, Boet E, Sahal A, et al. Disrupting mitochondrial electron transfer chain complex I decreases immune checkpoints in murine and human acute myeloid leukemic cells. Cancers (Basel) (2021) 13(14):1–18. doi: 10.3390/cancers13143499
66. Brauneck F, Weimer P, Schulze Zur Wiesch J, Weisel K, Leypoldt L, Vohwinkel G, et al. Bone marrow-resident Vdelta1 T cells Co-express tigit with pd-1, Tim-3 or Cd39 in aml and myeloma. Front Med (Lausanne) (2021) 8:763773. doi: 10.3389/fmed.2021.763773
67. Kong Y, Jia B, Zhao C, Claxton DF, Sharma A, Annageldiyev C, et al. Downregulation of Cd73 associates with T cell exhaustion in aml patients. J Hematol Oncol (2019) 12(1):40. doi: 10.1186/s13045-019-0728-3
68. Jia B, Zhao C, Rakszawski KL, Claxton DF, Ehmann WC, Rybka WB, et al. Eomes(+)T-Bet(Low) Cd8(+) T cells are functionally impaired and are associated with poor clinical outcome in patients with acute myeloid leukemia. Cancer Res (2019) 79(7):1635–45. doi: 10.1158/0008-5472.CAN-18-3107
69. Hallek M, Shanafelt TD, Eichhorst B. Chronic lymphocytic leukaemia. Lancet (2018) 391(10129):1524–37. doi: 10.1016/S0140-6736(18)30422-7
70. Ciszak L, Frydecka I, Wolowiec D, Szteblich A, Kosmaczewska A. Patients with chronic lymphocytic leukaemia (Cll) differ in the pattern of ctla-4 expression on cll cells: The possible implications for immunotherapy with ctla-4 blocking antibody. Tumour Biol (2016) 37(3):4143–57. doi: 10.1007/s13277-015-4217-1
71. Mosaad Zaki E, Mohamed Zahran A, Abdelazeem Metwaly A, Hafez R, Hussein S, Elaiw Mohammed A. Impact of Cd39 expression on Cd4+ T lymphocytes and 6q deletion on outcome of patients with chronic lymphocytic leukemia. Hematol/Oncol Stem Cell Ther (2019) 12(1):26–31. doi: 10.1016/j.hemonc.2018.09.002
72. Abousamra NK, Salah El-Din M, Hamza Elzahaf E, Esmael ME. Ectonucleoside triphosphate diphosphohydrolase-1 (E-Ntpdase1/Cd39) as a new prognostic marker in chronic lymphocytic leukemia. Leuk Lymphoma (2015) 56(1):113–9. doi: 10.3109/10428194.2014.907893
73. Perry C, Hazan-Halevy I, Kay S, Cipok M, Grisaru D, Deutsch V, et al. Increased Cd39 expression on Cd4(+) T lymphocytes has clinical and prognostic significance in chronic lymphocytic leukemia. Ann Hematol (2012) 91(8):1271–9. doi: 10.1007/s00277-012-1425-2
74. D'Arena G, Laurenti L, Minervini MM, Deaglio S, Bonello L, De Martino L, et al. Regulatory T-cell number is increased in chronic lymphocytic leukemia patients and correlates with progressive disease. Leuk Res (2011) 35(3):363–8. doi: 10.1016/j.leukres.2010.08.010
75. Kicova M, Michalova Z, Coma M, Gabzdilova J, Dedinska K, Guman T, et al. The expression of Cd73 on pathological b-cells is associated with shorter overall survival of patients with cll. Neoplasma (2020) 67(4):933–8. doi: 10.4149/neo_2020_190826N822
76. Serra S, Horenstein AL, Vaisitti T, Brusa D, Rossi D, Laurenti L, et al. Cd73-generated extracellular adenosine in chronic lymphocytic leukemia creates local conditions counteracting drug-induced cell death. Blood (2011) 118(23):6141–52. doi: 10.1182/blood-2011-08-374728
77. Premkumar V, Bhutani D, Lentzsch S. Modern treatments and future directions for Relapsed/Refractory multiple myeloma patients. Clin Lymphoma Myeloma Leuk (2020) 20(11):736–43. doi: 10.1016/j.clml.2020.06.023
78. Casey M, Nakamura K. The cancer-immunity cycle in multiple myeloma. Immunotargets Ther (2021) 10:247–60. doi: 10.2147/ITT.S305432
79. Marsh-Wakefield F, Kruzins A, McGuire HM, Yang S, Bryant C, Fazekas de St Groth B, et al. Mass cytometry discovers two discrete subsets of Cd39(-)Treg which discriminate mgus from multiple myeloma. Front Immunol (2019) 10:1596. doi: 10.3389/fimmu.2019.01596
80. Fletcher JM, Lonergan R, Costelloe L, Kinsella K, Moran B, O'Farrelly C, et al. Cd39+Foxp3+ regulatory T cells suppress pathogenic Th17 cells and are impaired in multiple sclerosis. J Immunol (2009) 183(11):7602–10. doi: 10.4049/jimmunol.0901881
81. Yang R, Elsaadi S, Misund K, Abdollahi P, Vandsemb EN, Moen SH, et al. Conversion of Atp to adenosine by Cd39 and Cd73 in multiple myeloma can be successfully targeted together with adenosine receptor A2a blockade. J Immunother Cancer (2020) 8(1):1–14. doi: 10.1136/jitc-2020-000610
82. Ray A, Song Y, Du T, Buon L, Tai YT, Chauhan D, et al. Identification and validation of ecto-5' nucleotidase as an immunotherapeutic target in multiple myeloma. Blood Cancer J (2022) 12(4):50. doi: 10.1038/s41408-022-00635-3
83. Wang X, Zhang T, Song Z, Li L, Zhang X, Liu J, et al. Tumor Cd73/A2ar adenosine immunosuppressive axis and tumor-infiltrating lymphocytes in diffuse Large b-cell lymphoma: Correlations with clinicopathological characteristics and clinical outcome. Int J Cancer (2019) 145(5):1414–22. doi: 10.1002/ijc.32144
84. Zhang T, Liu H, Jiao L, Zhang Z, He J, Li L, et al. Genetic characteristics involving the Pd-1/Pd-L1/L2 and Cd73/A2ar axes and the immunosuppressive microenvironment in Dlbcl. J Immunother Cancer (2022) 10(4):1–13. doi: 10.1136/jitc-2021-004114
85. Guo S, Han F, Zhu W. Cd39 - a bright target for cancer immunotherapy. BioMed Pharmacother (2022) 151:113066. doi: 10.1016/j.biopha.2022.113066
86. Perrot I, Michaud HA, Giraudon-Paoli M, Augier S, Docquier A, Gros L, et al. Blocking antibodies targeting the Cd39/Cd73 immunosuppressive pathway unleash immune responses in combination cancer therapies. Cell Rep (2019) 27(8):2411–25 e9. doi: 10.1016/j.celrep.2019.04.091
87. Ghalamfarsa G, Kazemi MH, Raoofi Mohseni S, Masjedi A, Hojjat-Farsangi M, Azizi G, et al. Cd73 as a potential opportunity for cancer immunotherapy. Expert Opin Ther Targets (2019) 23(2):127–42. doi: 10.1080/14728222.2019.1559829
88. Jeffrey JL, Lawson KV, Powers JP. Targeting metabolism of extracellular nucleotides Via inhibition of ectonucleotidases Cd73 and Cd39. J Med Chem (2020) 63(22):13444–65. doi: 10.1021/acs.jmedchem.0c01044
89. Stagg J, Divisekera U, McLaughlin N, Sharkey J, Pommey S, Denoyer D, et al. Anti-Cd73 antibody therapy inhibits breast tumor growth and metastasis. Proc Natl Acad Sci U.S.A. (2010) 107(4):1547–52. doi: 10.1073/pnas.0908801107
Keywords: CD39, CD73, T cells, hematological malignancy, immunotherapy
Citation: Jiang X, Wu X, Xiao Y, Wang P, Zheng J, Wu X and Jin Z (2023) The ectonucleotidases CD39 and CD73 on T cells: The new pillar of hematological malignancy. Front. Immunol. 14:1110325. doi: 10.3389/fimmu.2023.1110325
Received: 28 November 2022; Accepted: 12 January 2023;
Published: 27 January 2023.
Edited by:
Mazdak Ganjalikhani Hakemi, Isfahan University of Medical Sciences, Isfahan, IranReviewed by:
Yishan Ye, Zhejiang University, ChinaJean-René Pallandre, INSERM U1098 Interactions Hôte-Greffon-Tumeur & Ingénierie Cellulaire et Génique, France
Copyright © 2023 Jiang, Wu, Xiao, Wang, Zheng, Wu and Jin. This is an open-access article distributed under the terms of the Creative Commons Attribution License (CC BY). The use, distribution or reproduction in other forums is permitted, provided the original author(s) and the copyright owner(s) are credited and that the original publication in this journal is cited, in accordance with accepted academic practice. No use, distribution or reproduction is permitted which does not comply with these terms.
*Correspondence: Xiuli Wu, c2l1bGllckAxNjMuY29t; Zhenyi Jin, amluemhlbnlpam51QDE2My5jb20=
†ORCID: Xiuli Wu, orcid.org/0000-0002-5202-6515
Zhenyi Jin, orcid.org/0000-0002-3812-3626