- 1West African Centre for Cell Biology of Infectious Pathogens (WACCBIP), College of Basic and Applied Sciences, University of Ghana, Accra, Ghana
- 2Department of Biosciences and Biotechnology, University of Medical Sciences, Ondo, Nigeria
- 3Department of Biochemistry, Cell and Molecular Biology, College of Basic and Applied Sciences, University of Ghana, Accra, Ghana
- 4Medical Research Council Unit, The Gambia at the London School of Hygiene and Tropical Medicine, Banjul, Gambia
- 5Environmental and Public Health Microbiology, Department of Biological Science, Benson Idahosa University, Benin City, Edo State, Nigeria
- 6Department of Ophthalmology, Korle-bu Teaching Hospital, Accra, Ghana
- 7Virology Department, Noguchi Memorial Institute for Medical Research, University of Ghana, Accra, Ghana
In December 2019, a novel pneumonic condition, Coronavirus disease 2019 (COVID- 19) caused by severe acute respiratory syndrome coronavirus 2 (SARS-CoV-2), broke out in China and spread globally. The presentation of COVID-19 is more severe in persons with underlying medical conditions such as Tuberculosis (TB), Human Immunodeficiency Virus/Acquired Immunodeficiency Syndrome (HIV/AIDS) and other pneumonic conditions. All three diseases are of global concern and can significantly affect the lungs with characteristic cytokine storm, immunosuppression, and respiratory failure. Co-infections of SARS-CoV-2 with HIV and Mycobacterium tuberculosis (Mtb) have been reported, which may influence their pathogenesis and disease progression. Pulmonary TB and HIV/AIDS patients could be more susceptible to SARS-CoV-2 infection leading to lethal synergy and disease severity. Therefore, the biological and epidemiological interactions of COVID-19, HIV/AIDS, and TB need to be understood holistically. While data is needed to predict the impact of the COVID-19 pandemic on these existing diseases, it is necessary to review the implications of the evolving COVID-19 management on HIV/AIDS and TB control, including therapy and funding. Also, the impact of long COVID on patients, who may have this co-infection. Thus, this review highlights the implications of COVID-19, HIV/AIDS, and TB co-infection compares disease mechanisms, addresses growing concerns, and suggests a direction for improved diagnosis and general management.
1 Introduction
The novel coronavirus disease 2019 (COVID-19) was declared a pandemic by the World Health Organization (WHO) on March 11, 2020 (1). It is the third major viral pandemic after the Human immunodeficiency virus/acquired immunodeficiency syndrome (HIV/AIDS) and the Spanish flu (2, 3). The COVID-19 is caused by the novel Severe Acute Respiratory Syndrome Coronavirus 2 (SARS-CoV-2), which has infected more than 620 million people globally, including approximately nine million Africans (4). Transmission of SARS-CoV-2 occurs primarily through infected saliva and respiratory secretions or droplets (5). Symptoms of COVID-19 include fever, cough, general malaise, and dyspnea, while hypertension and diabetes as the most common underlying comorbidities (6–9). Also, some COVID-19 patients experience severe disease, which is characterized by acute respiratory distress syndrome (ARDS) and lung injury due to a damaged alveolar lumen (9–11). Others have cognitive and neurological problems due to post-COVID-19 conditions termed long COVID-19 conditions (12).
Secondary or co-infections, with bacteria (Mycobacterium tuberculosis (Mtb)), fungi, and viruses (influenza, coronavirus, HIV, metapneumovirus), have been observed in some COVID-19 patients (13–15) with varying incidences and about 50% secondary infections in non-survivors (15–22). Co-infections with SARS-CoV-2 contribute significantly to disease morbidity and mortality (22) and people living with HIV (PLWH) are four times more likely to experience post-COVID-19 conditions (23). Post-COVID-19 conditions known as the ‘‘Long-term effects of Coronavirus’ are persistent symptoms and consequences that developed after one month from COVID-19 diagnosis (12, 24). People of low socioeconomic status, those having pre-existing conditions and unvaccinated individuals have a higher risk of developing long COVID-19 (25, 26). This is of grave medical and socio-economic concern, especially where tuberculosis (TB) and HIV/AIDS burdens are high such as in Africa. Understanding host-pathogen biology and geographical variations of co-infection with TB and HIV are of utmost importance. Therefore, this review aims to highlight the effect of COVID-19, HIV/AIDS, and TB co-infections and address growing concerns. The information will contribute to COVID-19, HIV/AIDS and TB management, especially in Africa.
2 The burden of HIV/AIDS and TB co-infection in Africa
HIV is a comorbid infection in COVID-19 patients (27). It has infected about 74.9 million people globally, with more than 32 million deaths from AIDS-related illnesses (28). In 2021, about 38.4 million people were living with HIV (PLWH), with more than half in Africa (28). Tuberculosis, despite being preventable and treatable, is still one of the major causes of death, especially in PLWH - accounting for about one-third of global deaths (29). Globally, HIV/AIDS and TB are collectively the two most deadly infectious diseases and are tragically interconnected (30). As HIV suppresses the immune system, it predisposes patients to Mtb infection, hastens progression to active disease, and increases latent TB reactivation by 20-folds (31, 32). Alone, TB was the leading cause of death from a single infectious pathogen, before the COVID-19 pandemic (33), and the ninth leading cause of mortality globally (34). Africa has a high TB infection burden - 25% of the global new cases and deaths - but the lowest reduction rate worldwide (34). Twenty-two of the 30 high-burden HIV/TB countries are in Africa (35) (Figure 1).
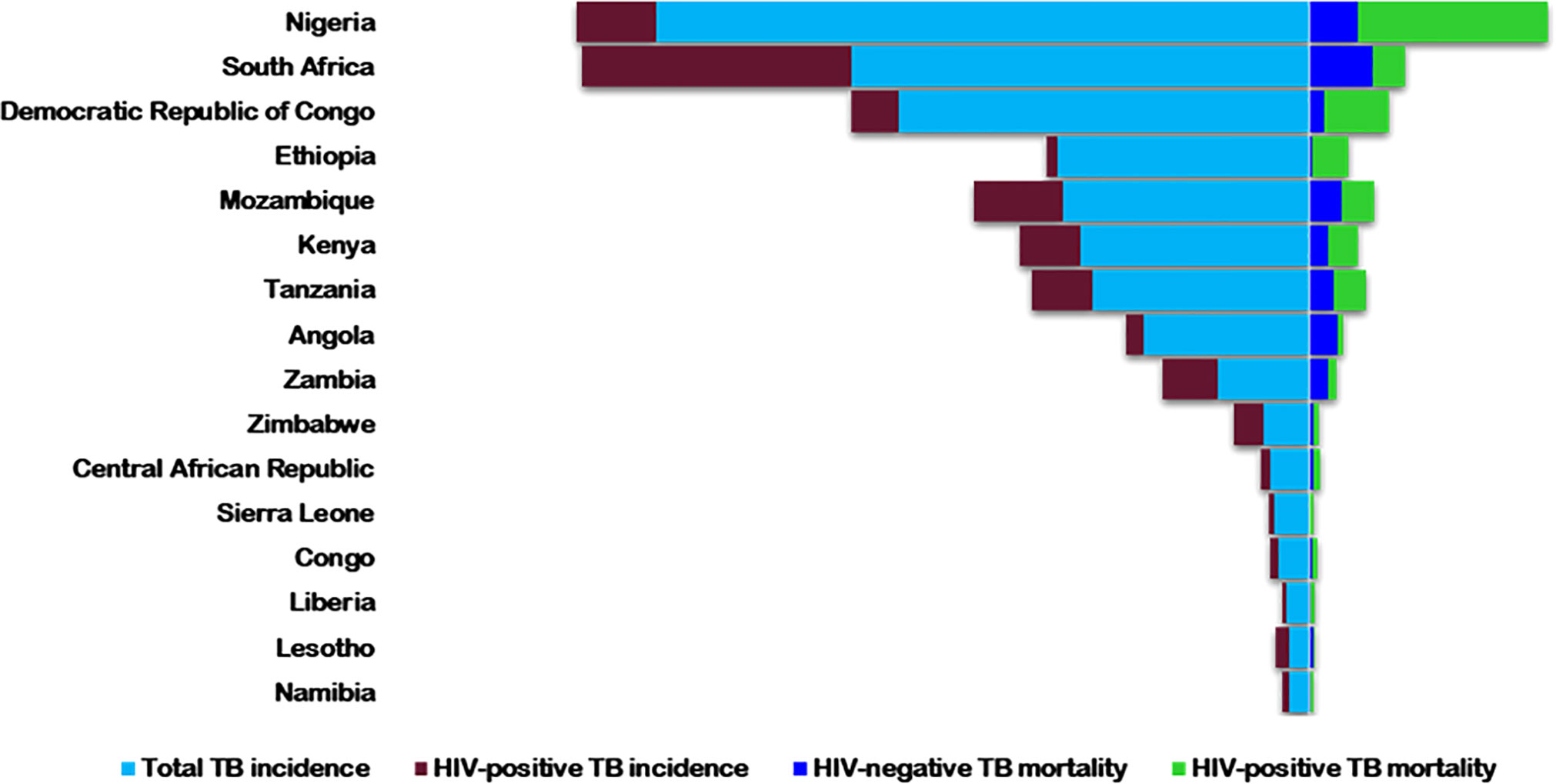
Figure 1 The 2018 estimated rates of HIV+/-TB incidence and mortality in the highest TB/HIV buden countries in Africa. Rates are per 100,000 populations [World Health Organization, Globa; Tuberculosis Report 2019(38)].
The interaction between HIV/AIDS and TB presents a “toxic negative synergistic effect” mostly impacting people within impoverished regions and causing high mortality (36). Therefore, triple infection with SARS-CoV-2, HIV, and Mtb is concerning as evidence and experience from other viruses and chronic illnesses show that HIV/Mtb co-infected patients have a greater risk of severity with secondary infections (37).
2.1 COVID-19, TB and HIV/AIDS: Cytokine storm syndromes and immunosuppression
Cytokine storm is a dysfunctional release of cytokines in response to infections or external stimuli, resulting in acute/severe inflammation and multiple organ damage (38). This complex mechanism involves loss of regulatory control of pro- and anti-inflammatory cytokine production, at local and systemic levels (39). Elevated levels of pro-inflammatory cytokines, including interleukin-1β (IL-1β), IL-8, IL-6, CXC-chemokine ligand 10 (CXCL10), and CC-chemokine ligand 2 (CCL2) have been observed in severe COVID-19 patients (40) and the profuse inflammatory responses during SARS-CoV-2 infection promote additional unrestrained pulmonary inflammation, likely a prime cause of case mortality (41). Rapid viral replication and cellular damage, virus-induced Angiotensin-Converting Enzyme 2 (ACE-2) downregulation and shedding, and antibody-dependent enhancement (ADE) are accountable for the hostile SARS-CoV-2-mediated inflammation (42). The debut rapid viral replication can lead to massive epithelial and endothelial cell death and vascular leakage, triggering excessive pro-inflammatory cytokines production (43). Additionally, increase Angiotensin II levels in SARS-CoV-2 infection secondary to ACE-2 down-regulation, triggers NF-κB and IL-6-STAT3 inflammatory pathways, particularly in non-immune cells, including endothelial and epithelial cells (44). This pathway forms the IL-6 amplifier positive feedback cycle, resulting in excessive activation and cytokine storm (44) leading to vascular permeability and ARDS.
The intricate interaction between Mtb and the immune system is associated with the release of cytokine array, including IL-6, IL-10, IL-12, IL-17, IL-18, IL-22, IL-23, IL-27, TNF-α, IFN-γ, IFN-β, IL-1β, and TGF-β, secreted by different cell types (45, 46). Excessive pro-inflammatory cytokines release, leading to cytokine storm, has been associated with lung damage and TB severity. However, Mtb-induced cytokine is a “circular model” that balances pro- and anti-inflammatory cytokines (46). Studies have suggested that pulmonary TB and COVID-19 co-infection can likely cause prolonged respiratory symptoms, fever and unresolved radiological abnormalities (47). Latent TB patients exposed to SARS-CoV-2 produce increased cytokines and chemokines levels associated with both resistance and susceptibility to active TB disease (48) suggesting induced activation of latent TB by COVID-19 when the balance between active TB resistance and susceptibility cytokines tips towards susceptibility.
HIV infection concords with immune activation, exhibited by the increase of several plasma cytokines (49–51), specifically seen at early infection. Acute HIV infection is distinguished by the onset of cytokine storm preceding peak viremia (50–52). At the viremia peak, the cytokine storm partially resolves with persistent elevation of some cytokines such as TNF, IFNɣ, IL-22, and CXCL10 above pre-infection physiologic levels and into the chronic phase in untreated infections (52). Although anti-retroviral therapy (ART) greatly lowers the plasma levels of inflammatory cytokines like CXCL10, residual immune activation prevails despite early ART initiation (53–55). While T cell activation during early infection is known to influence subsequent disease progression (56), plasma cytokine production during acute infection defines the disease prognosis (57).
Cytokine storm occurs early in HIV infection contributing to disease progression; however, it occurs late in SARS-CoV-2 infection constituting an aspect of disease progression. Therefore, the anti-inflammatory effects of immunosuppression could be protective to alleviate poor outcomes in cytokine storm-related COVID-19 patients (58). Hence, it will be interesting to know if HIV has a protective advantage against severe forms of COVID-19. Several studies claim an overall asymptomatic or mild COVID-19 course in immunocompromised patients, including children undergoing anticancer therapy (59), immunosuppressive chronic drugs users (60), transplant recipients (61), and poorly controlled HIV patients (62). In these studies, a handful of severely affected individuals recovered, and low fatality rates were recorded.
Given the high cytokine levels induced by SARS-CoV-2, inflammation-related lung damage treatment is critical. However, interventions to reduce inflammation may negatively affect viral clearance, which could prevail as sub-clinical infection in the host (63). Similarly, immune-suppressive conditions that reduce inflammatory response have been reported to enhance prolonged virus shedding even after symptoms have resolved (64). This effect of immune suppression on delayed viral clearance can allow for the accumulation of mutations driving the emergence of new SARS-CoV-2 variants. Therefore, the need for clinicians to be careful when assessing COVID-19 in immune-compromised persons, including HIV patients since they could likely experience prolonged shedding of infectious SARS-CoV-2. More clinical studies are needed to find a balance between immune activation and inflammation inhibition.
Although SARS-CoV-2, Mtb, and HIV are individually involved in lung pathologies, the kinetics of cytokine storm in each infection is different. It is imperative to understand these differences, especially in regions with the likelihood of co-infections. This will provide specific guidelines for the management of patients in communities where these infections co-exist.
2.2 Ménage à Trios: COVID, TB, and HIV/AIDS
Apart from the global economic burden, the COVID-19 pandemic has greatly impacted countries burdened with HIV/TB co-infection, reversing gains made within the past decade (33). Few cases of TB/HIV/COVID-19 triple infection have been reported with the need for hospitalization in several instances (65) while a case of triple infection with extra-pulmonary TB, HIV and COVID-19 was successfully treated without complications (66). SARS-CoV-2 is known to primarily enter cells by binding to the ACE-2 receptor using its envelope spike (S) glycoprotein (67). However, the CD147 (basigin) receptor is an alternative route of SARS-CoV-2 infection via Cyclophilin A (CyPA)/CD147 interaction (68). This interaction plays a vital role in the ability of SARS-CoV- 2 to enter the host cells via the binding of SARS-CoV-2 S-protein (CD147-S protein) to the CD147 surface molecule (69).
The CD147 similarly enhances HIV infection (69) while CyPA expressly incorporates into HIV-1 virions, significantly enhancing an early cellular infection. In HIV and SARS-CoV-2 infection, the viral protein binds to CD147 together with CyPA. The question here is, what does it mean for the host cell if they are infected with the two different types of viruses? Therefore, the need to investigate co-infections at the molecular level for more insight and understanding of the ‘ménage à trios.
Interestingly, studies have revealed that HIV infection in hospitalized COVID -19 patients is not an imperative comorbid factor (70) as admission rates, morbidity, and mortality in HIV/COVID-19 co-infections are similar to rates seen in the general population (71). Similarly, SARS-CoV infection was reported to cause momentary repression of cellular immunity, leading to further susceptibility and exacerbating reactivation of new Mtb infection (72). Therefore, the risk of severe COVID-19 or death in PLWH is lower than perceived and the prognosis better for people on ART (73, 74), while SARS-CoV-2-infected HIV patients without ART are more likely to be hospitalized and die (75). In a study assessing COVID-19 risk in PLWH, administration of nucleotide reverse transcriptase inhibitors, tenofovir disoproxil fumarate (TDF)/emtricitabine (FTC), tenofovir alafenamide (TAF)/FTC, lowered COVID-19 risk and related hospitalization in West and Central Africa, while HIV-positive men older than 70 years had a greater risk of contracting COVID-19 (76). This suggests that TDF/FTC prevents COVID-19 in PLWH by inhibiting RNA-dependent RNA polymerase (RNAdRNAp) (76). Infection with SARS-CoV-2 enhances T-cell exhaustion in PLWH, especially in those without proper ART, leading to severe outcomes compared to patients on ART (77). However, cases of non-fatality (78) imply that HIV infection regardless of ART may not be a risk factor for COVID-19 severity/fatality. Since HIV replication gradually destroys the naive and memory CD4+ T-lymphocytes, incapacitating the immune system (79), low CD4 counts due to COVID-19/HIV co-infections could result in reduced immune response contributing to less COVID-19 complications (73).
The majority of COVID-19 cases are asymptomatic; hence, a primary HIV infection could activate, that is, increase the severity of most asymptomatic COVID-19 cases. Also, the two HIV types, HIV-1 and HIV-2, with differential aggressiveness, could impact the severity or symptomatology of the disease. Nonetheless, the link between HIV and SARS-CoV-2 remains unclear (80), despite reports of significant COVID-19 deaths in immunosuppressed patients (81). More data tying the latter effects of this triplet are needed to understand the underlying impact of co-infection, especially with increased reports and complications of post-COVID-19 conditions.
Generally, the long-term pulmonary sequelae in post-TB patients are well described but not fully characterized in post-COVID-19 patients. Furthermore, the experience of SARS-CoV-2 infection in TB patients remains limited and poor treatment outcomes are predicted in people with both, especially if TB treatment is interrupted. A key feature of Mtb infection is the depletion of nicotinamide adenosine dinucleotide (NAD+), a central factor of metabolism, respiration, redox balance, and biosynthesis of molecular building blocks (82). This promotes Mtb pathogenesis and shaped drug design, as most TB drugs are NAD analogues or metabolized into NAD+ intermediates (83). NAD+ depletion is dependent on age and other conditions like obesity and type-2 diabetes, which are known risk factors for COVID-19 severity and mortality (84). Infection with SARS-CoV-2 also depletes cellular NAD+, which may be a primary determinant of the COVID-19 spectrum and a risk for mortality (85) suggesting that prior TB infection could enhance COVID-19 progression and vice versa.
Tuberculosis has been considered a risk factor for severe COVID-19 (86) with higher COVID-19 case fatality rates observed in areas like Peru with a high TB burden (87). Also, a case report of severe COVID-19 in an infant with disseminated TB has been reported (88). On a cellular level, the FCN1+/SPP1+ macrophage lineage is enriched in the lungs of severe COVID-19 patients. A study showed that some persons with active TB have increased levels of this macrophage lineage in circulation implying a risk of severe disease in TB/SARS-CoV-2 coinfection (89). In vitro, Mtb induces ACE2 and TMPRSS2 expression in macrophages leading to increased SARS-CoV-2 infection susceptibility, and consequently, Mtb-infected macrophages co-cultured with SARS-CoV-2 express a milieu of inflammatory response genes (89). Considered together, these factors suggest that there is a risk of severe disease in TB/SARS-CoV-2 co-infection. Contrarily, severe COVID-19 risk and mortality seem less in TB/COVID-19 co-infected patients in some studies (90, 91) indicating some protective effect of TB against severe COVID-19, but this needs to be specifically investigated. However, co-morbidities such as old age, respiratory diseases and diabetes contribute to increased severity and mortality in patients with COVID-19/TB co-infection (92, 93). A higher percentage of TB patients with COVID-19 have active pulmonary TB (91, 92, 94, 95) probably due to damage to the lungs making them more susceptible, nonetheless most patients present with mild symptoms with few reports of severe disease and death (66, 93). TB/COVID-19 co-infection leads to reduced SARS-CoV-2-specific immune response but increased TB-specific immunity without severe COVID-19 (96) meaning co-infection reduces COVID-19 immunity. The effect of COVID-19 on TB and vice versa is still unclear but it is important to tease out confounding factors that most likely contribute to the different outcomes in COVID/TB coinfections for better prognosis.
Immunity may be temporarily inhibited with the use of immunosuppressive drugs on SARS-CoV-2, resulting in latent Mtb activation and exacerbating the clinical outcomes (89). Primary TB protects the host by producing effective systemic immunity that prevents disseminated infection, while post-primary TB evades and distorts systemic immunity. As COVID-19 pathogenesis also depends on immune response, its severity may correlate with the phase of TB infection leading to an increase in TB burden as recorded in 2021 (33). This would necessitate a better understanding of these interactions to develop management strategies that will prevent a further upsurge of Mtb infection. The disease outcome and severity in patients with all three infections will far surpass that in an individual infected with either HIV or Mtb. Suffice to say that the above are predictions considering untreated patients. With treatment, COVID severity in TB and/or HIV-infected individuals may be significantly low.
2.3 Potential implications of COVID-19 on the control of TB and HIV/AIDS
The COVID-19 pandemic has caused an unprecedented interruption to health services, impeding the management of diseases like HIV/AIDS and TB. The urgent COVID-19 response posed competition in healthcare services, disrupted drugs and diagnostic kits supply chain, hindered access to healthcare and increased the burden on health practitioners leading to reduced clinical care because of the high influx of patients (97). This interruption has led to the reversal of global gains in combating HIV/AIDS and TB, especially in Africa (33). Also, the use of different drugs in patients with co-morbidities of SARS-CoV-2 with TB and/or HIV/AIDS could lead to drug-drug interactions, of which the immediate implications may not be seen. It could result in reduced or increased drug absorption, thereby modulating the action of drugs with adverse effects, reducing efficiency and increasing toxicity (98). Antivirals such as lopinavir, ritonavir, and remdesivir, used in COVID-19 therapy may interact with TB and/or HIV/AIDS treatments (99). Drug-drug interactions between protease inhibitors and rifampicin have been observed in HIV/AIDS and TB co-infection, reducing the efficiency of protease inhibitors (99), which may compromise COVID-19 treatment. New COVID-19 therapeutic approaches in HIV/AIDS or TB co-morbidities are needed to help tackle these diseases.
High-income or developed countries that mostly fund TB and HIV/AIDS control interventions have been severely affected by the COVID-19 pandemic and thus consigned their resources inward to alleviate the burden within their healthcare sectors. This jettisoned resources for research, and TB and HIV/AIDS control efforts in Africa. The Global Fund, to fight HIV/AIDS, TB, and other diseases, was also diverted to the COVID-19 response (100). Although the continuity of the fight against HIV/AIDS and TB has continued, the increase in SARS-CoV-2 transmission has reduced HIV/AIDS control in Africa, with high dependence on external partners for funding. Likewise, the enormous funds made available to combat COVID-19 in domains like research, therapeutics, diagnostics, personal protective equipment, construction, and hospital renovations have negatively impacted TB and HIV/AIDS funding in developing countries like Africa with a high HIV/TB co-infection burden. The question is, is the future somewhat bleak for the fight to eliminate TB and maintain a low HIV viral load in patients?
2.4 Management of TB and HIV amidst COVID-19 and stigmatization
Several antivirals have been incorporated into COVID-19 treatment and suppression (101, 102). Administering antivirals shortly after the onset of COVID-19 symptoms can reduce viral shedding, and prophylactic treatment of contacts could reduce infection risk (103). As high antivirals are needed for COVID-19 treatment with adequate stock of prophylaxis, two things are likely to happen: firstly, drug resistance and drug shortage due to increased usage. A few proposed COVID-19 drugs have antiviral resistance (104, 105) and overusing them could escalate this resistance, thus, rendering them useless. Drug resistance could result in increased morbidity and mortality, especially in viral and bacterial co-infected patients, including PLWH and TB. Secondly, drug overuse could aggravate both COVID-19 and AIDS conditions leading to complications. Continuous focus on COVID-19 at the expense of TB and HIV/AIDS might increase incidence and mortality rates in sub-Saharan Africa, already grappling with the burden of the earlier two epidemics. There is a significant risk of disruption of HIV/AIDS and TB treatments due to the diversion of efforts in combating COVID-19 (106). The continuous spread of COVID-19 in Africa stretches an already overwhelmed and inadequately resourced healthcare system (107) as TB incidence increases.
Thus, necessary measures need to be taken to ensure that combating COVID-19 is not at the expense of the gains made so far in HIV/AIDS and TB control. Ensuring a sufficient global supply of tests and treatment amidst the pandemic will be invaluable in sustaining TB and HIV/AIDS control interventions. Moreover, concurrent efforts in the management of these diseases are paramount to avoid exacerbating their morbidities and mortalities. Bulk distribution of HIV/AIDS and TB standard course medications could prevent treatment interruption due to efforts in combating the COVID-19 pandemic. Furthermore, proper and regular maintenance of testing equipment and facilities will help in sustaining efforts in the control of the three diseases. The provision of more diagnostic machines will equally be helpful to facilitate the workload.
Stigmatization is a discrediting social label that changes an individual’s self-image disqualifying them from full social acceptance (108). Stigma has been associated with HIV/AIDS and TB, worsening in HIV/TB co-infection (109). While ongoing efforts are made to tackle HIV/TB-associated stigma, there might be a shift to triple stigmatization with COVID-19. The stigma associated with COVID-19 is because of its novelty with many uncertainties and most infected individuals may not present for treatment, thereby continuing the spread of the pathogen (110). To reduce this triple stigma, modifications of policies and practices might be required while efforts are continuously made to reduce the spread of all three pathogens.
3 Conclusion
The COVID-19 response is unprecedented and has impacted TB and HIV/AIDS testing. Due to inadequate guidelines and patterns for SARS-CoV-2 co-infection, gleaning from past experiences with similar pathogens like SARS-CoV and MERS is of utmost benefit in tackling SARS-CoV-2 co-infections (111–116). Considering the inevitability of combined infections with HIV/AIDS and TB in Africa, comprehensive microbiologic surveys using syndromic multiplex panels and clinical evaluations are necessary. Also, research progress on HIV/AIDS and TB has been impeded, and clinical trials suspended in most countries as several researchers are redirected their efforts to COVID-19. However, increased collaboration with COVID-19 research has established new approaches to augment testing, and therapeutic and vaccine development, with potential long-term medical research advantages beyond the COVID-19 pandemic. Although COVID-19 vaccination is significantly high in many Western and Asian countries (117, 118), markedly changing the dynamics of the pandemic, repeated outbreaks and long-COVID-19 conditions are still being reported in several of these countries/regions. Thus, eradicating the disease seems to be hampered by inadequate knowledge and understanding of the SARS-CoV-2 biology, host immune response to the virus, COVID-19 treatment options, and vaccine efficacies and hesitancy, especially in Africa. Past investments in infectious disease training and research have produced remarkable gains in the COVID-19 response, indicating the need to retain them. Since several African countries have well-developed HIV and Mtb clinical trial infrastructure, it will be beneficial to incorporate SARS-CoV-2 in these collaborative efforts for a sustainable outcome to avoid losing ongoing gains, due to consequences of co-infections. Finally, adopting holistic medical care for COVID-19, HIV/AIDS, and TB co-infected patients is vital, with regular communications between TB/HIV programs and public health laboratories.
Author contributions
AU conceptualized the study; AU, AD, KT, NN, FO, CA, and STE wrote the first draft of the manuscript; IAO and PQ edited and LP, GAA, and PKQ critically reviewed the manuscript. All authors contributed to the article and approved the submitted version.
Funding
This work was supported by the Crick African Network which receives its funding from the UK’s Global Challenges Research Fund (MR/P028071/1), and by the Francis Crick Institute which receives its core funding from Cancer Research UK (FC1001647), the UK Medical Research Council (FC1001647), and the Wellcome Trust (FC1001647). For the purpose of Open Access, the author has applied a CC BY public copyright licence to any Author Accepted Manuscript version arising from this submission.
Conflict of interest
The authors declare that the research was conducted in the absence of any commercial or financial relationships that could be construed as a potential conflict of interest.
Publisher’s note
All claims expressed in this article are solely those of the authors and do not necessarily represent those of their affiliated organizations, or those of the publisher, the editors and the reviewers. Any product that may be evaluated in this article, or claim that may be made by its manufacturer, is not guaranteed or endorsed by the publisher.
References
1. World Health Organization. WHO director-general’s opening remarks at the media briefing on COVID-19 - 11 march 2020 (2020). Available at: https://www.who.int/director-general/speeches/detail/who-director-general-s-opening-remarks-at-the-media-briefing-on-covid-19—11-march-2020.
2. Johnson NPAS, Mueller J. Updating the accounts: global mortality of the 1918-1920 “Spanish” influenza pandemic. Bull Hist Med (2002) 76(1):105–15. doi: 10.1353/bhm.2002.0022
3. Patterson KD, Pyle GF. The geography and mortality of the 1918 influenza pandemic. In: Bulletin of the history of medicine (United States: Johns Hopkins University Press) (1991) 65(1):4–21. Available at: https://pubmed.ncbi.nlm.nih.gov/2021692/.
4. World Health Organization. WHO coronavirus (COVID-19) dashboard | WHO coronavirus (COVID-19) dashboard with vaccination data (2022). Available at: https://covid19.who.int/.
5. World Health Organization. Naming the coronavirus disease (COVID-19) and the virus that causes it (2020). Available at: https://www.who.int/emergencies/diseases/novel-coronavirus-2019/technical-guidance/naming-the-coronavirus-disease-(covid-2019)-and-the-virus-that-causes-it.
6. Huang C, Wang Y, Li X, Ren L, Zhao J, Hu Y, et al. Clinical features of patients infected with 2019 novel coronavirus in wuhan, China. Lancet (2020) 395(10223):497–506. doi: 10.1016/S0140-6736(20)30183-5
7. Moein ST, Hashemian SM, Mansourafshar B, Khorram-Tousi A, Tabarsi P, Doty RL. Smell dysfunction: A biomarker for COVID-19. Int Forum Allergy Rhinol (2020) 10(8):944–50. doi: 10.1002/alr.22587
8. Mao L, Jin H, Wang M, Hu Y, Chen S, He Q, et al. Neurologic manifestations of hospitalized patients with coronavirus disease 2019 in wuhan, China. JAMA Neurol (2020) 77(6):683–90. doi: 10.1001/jamaneurol.2020.1127
9. Xu J, Chu M, Zhong F, Tan X, Tang G, Mai J, et al. Digestive symptoms of COVID-19 and expression of ACE2 in digestive tract organs. Cell Death Discovery (2020) 6(1):76. doi: 10.1038/s41420-020-00307-w
10. Fang Z, Yi F, Wu K, Lai K, Sun X, Zhong N, et al. Clinical characteristics of coronavirus disease 2019 (COVID-19): An updated systematic review. medRxiv (2020) doi: 10.1101/2020.03.07.20032573
11. Wölfel R, Corman VM, Guggemos W, Seilmaier M, Zange S, Müller MA, et al. Virological assessment of hospitalized patients with COVID-2019. Nature (2020) 581(7809):465–9. doi: 10.1038/s41586-020-2196-x
12. Center for Disease Control and Prevention. Long COVID or post-COVID conditions. CDC (2022) United States: U.S. Department of Health & Human Services. Available at: https://www.cdc.gov/coronavirus/2019-ncov/long-term-effects/index.html.
13. Bhaskaran K, Rentsch CT, MacKenna B, Schultze A, Mehrkar A, Bates CJ, et al. HIV Infection and COVID-19 death: A population-based cohort analysis of UK primary care data and linked national death registrations within the OpenSAFELY platform. Lancet HIV (2021) 8(1):e24–32. doi: 10.1016/S2352-3018(20)30305-2
14. Tadolini M, Codecasa LR, García-García JM, Blanc FX, Borisov S, Alffenaar JW, et al. Froissart sequelae and c-19 co-infection: First cohort of 49 cases. active tuberculosis, sequelae and COVID-19 co-infection: first cohort of 49 cases. Eur Respir J (2020) 56(1):2001398. doi: 10.1183/13993003.01398-2020
15. Lai CC, Wang CY, Hsueh PR. Co-Infections among patients with COVID-19: The need for combination therapy with non-anti-SARS-CoV-2 agents? J Microbiol Immunol Infection (2020) 53(4):505–512. doi: 10.1016/j.jmii.2020.05.013
16. Arentz M, Yim E, Klaff L, Lokhandwala S, Riedo FX, Chong M, et al. Characteristics and outcomes of 21 critically ill patients with COVID-19 in Washington state. JAMA - J Am Med Assoc (2020) 323(16):1612–14. doi: 10.1001/jama.2020.4326
17. Ding Q, Lu P, Fan Y, Xia Y, Liu M. The clinical characteristics of pneumonia patients coinfected with 2019 novel coronavirus and influenza virus in wuhan, China. J Med Virol (2020) 92(9):1549–55. doi: 10.1002/jmv.25781
18. Nowak MD, Sordillo EM, Gitman MR, Paniz Mondolfi AE. Co-Infection in SARS-CoV-2 infected patients: Where are influenza virus and Rhinovirus/Enterovirus? J Med Virol (2020) 92(10):1699–700. doi: 10.1002/jmv.25953
19. Pongpirul WA, Mott JA, Woodring JV, Uyeki TM, MacArthur JR, Vachiraphan A, et al. Clinical characteristics of patients hospitalized with coronavirus disease, Thailand. Emerg Infect Dis (2020) 26(7):1580–5. doi: 10.3201/eid2607.200598
20. Touzard-Romo F, Tapé C, Lonks JR. Co-Infection with SARS-CoV-2 and human metapneumovirus. R I Med J (2013) 103(2):75–6.
21. Wu X, Cai Y, Huang X, Yu X, Zhao L, Wang F, et al. Co-Infection with SARS-CoV-2 and influenza a virus in patient with pneumonia, China. Emerg Infect Dis (2020) 26(6):1324–6. doi: 10.3201/eid2606.200299
22. Lv Z, Cheng S, Le J, Huang J, Feng L, Zhang B, et al. Clinical characteristics and co-infections of 354 hospitalized patients with COVID-19 in wuhan, China: a retrospective cohort study. Microbes Infect (2020) 22(4-5):195–9. doi: 10.1016/j.micinf.2020.05.007
23. Long COVID more common in people with HIV | aidsmap (2022). Available at: https://www.aidsmap.com/news/mar-2022/long-covid-more-common-people-hiv.
24. Samannodi M, Alwafi H, Naser AY, Qurashi AAAL, Qedair JT, Salawati E, et al. Determinants of post-COVID-19 conditions among SARS-CoV-2-Infected patients in Saudi Arabia: A web-based cross-sectional study. Diseases (2022) 10(3):55. doi: 10.3390/diseases10030055
25. Office for National Statistics. Technical article: Updated estimates of the prevalence of post-acute symptoms among people with coronavirus (COVID-19) in the UK - office for national statistics (2021). Available at: https://www.ons.gov.uk/peoplepopulationandcommunity/healthandsocialcare/conditionsanddiseases/articles/technicalarticleupdatedestimatesoftheprevalenceofpostacutesymptomsamongpeoplewithcoronaviruscovid19intheuk/26april2020to1august2021.
26. Natinal Institute of Health. HIV | COVID-19 treatment guidelines (2022). Available at: https://www.covid19treatmentguidelines.nih.gov/special-populations/hiv/.
27. Mirzaei H, McFarland W, Karamouzian M, Sharifi H. COVID-19 among people living with HIV: A systematic review. United States of America: Springer (2020). doi: 10.1007/s10461-020-02983-2
28. United Nations Programme on HIV and AIDS. Global HIV & AIDS statistics — fact sheet. Geneva, Switzerland: UNAIDS (2022). Available at: https://www.unaids.org/en/resources/fact-sheet.
29. United Nations Programme on HIV and AIDS. Fact sheet - world tuberculosis day 2022 (2022). Available at: https://www.unaids.org/sites/default/files/media_asset/20220324_TB_FactSheet_en.pdf.
30. Riou J, Althaus CL. Pattern of early human-to-human transmission of wuhan 2019 novel coronavirus (2019-nCoV), December 2019 to January 2020. vol. 25, eurosurveillance. Stockholm, Sweden: European Centre for Disease Prevention and Control (ECDC (2020).
31. Valdez H, Carlson NL, Post AB, Asaad R, Heeger PS, Lederman MM, et al. HIV Long-term non-progressors maintain brisk CD8 T cell responses to other viral antigens. Aids (2002) 16(8):1113–8. doi: 10.1097/00002030-200205240-00004
32. Pawlowski A, Jansson M, Sköld M, Rottenberg ME, Källenius G. Tuberculosis and HIV Co-infection. PloS Pathog (2012) 8(2):e1002464. doi: 10.1371/journal.ppat.1002464
33. Geneva: World Health Organization. Global tuberculosis report (Geneva, Switzerland: World Health Organization), Vol. 2021. (2021).
34. World Health Organization. Tuberculosis (TB). Brazzaville, Republic of Congo: WHO | Regional Office for Africa (2022). Available at: https://www.afro.who.int/health-topics/tuberculosis-tb.
35. World Health Organization. Global tuberculosis report 2019. Geneva: World Health Organization (2019).
36. Bell LCK, Noursadeghi M. Pathogenesis of HIV-1 and mycobacterium tuberculosis co-infection. Nat Rev Microbiol (2018) 16(2):80–90. doi: 10.1038/nrmicro.2017.128
37. Ramkissoon S, Mwambi HG, Matthews AP. Modelling HIV and MTB Co-infection including combined treatment strategies. PloS One (2012) 7(11):e49492. doi: 10.1371/journal.pone.0049492
38. Gu Y, Hsu ACY, Pang Z, Pan H, Zuo X, Wang G, et al. Role of the innate cytokine storm induced by the influenza a virus. Viral Immunol (2019) 32(6):244–51. doi: 10.1089/vim.2019.0032
39. Ye Q, Wang B, Mao J. The pathogenesis and treatment of the “Cytokine storm” in COVID-19. J Infect (2020) 80(6):607–13. doi: 10.1016/j.jinf.2020.03.037
40. Rothan HA, Byrareddy SN. The epidemiology and pathogenesis of coronavirus disease (COVID-19) outbreak. J Autoimmun (2020) 109:102433. doi: 10.1016/j.jaut.2020.102433
41. Jin Y, Yang H, Ji W, Wu W, Chen S, Zhang W, et al. Virology, epidemiology, pathogenesis, and control of COVID-19. Viruses (2020) 12(4):372. doi: 10.3390/v12040372
42. Cao X. COVID-19: immunopathology and its implications for therapy. Nat Rev Immunol (2020) 20(5):269–70. doi: 10.1038/s41577-020-0308-3
43. Yang P, Wang X. COVID-19 : a new challenge for human beings. Cell Mol Immunol (2020) 17:555–557, 19–21. doi: 10.1038/s41423-020-0407-x
44. Hirano T, Immunity M--. U. COVID-19: A new virus, but a familiar receptor and cytokine release syndrome. Immunity (2020) 52(5):731–3. doi: 10.1016/j.immuni.2020.04.003
45. de Martino M, Lodi L, Galli L, Chiappini E. Immune response to mycobacterium tuberculosis: A narrative review. Front Pediatr (2019) 7:350. doi: 10.3389/fped.2019.00350
46. Etna MP, Giacomini E, Severa M, Coccia EM. Pro-and anti-inflammatory cytokines in tuberculosis: A two-edged sword in TB pathogenesis. Semin Immunol (2014) 26:543–51. doi: 10.1016/j.smim.2014.09.011
47. Nyanti LE, Wong ZH, Sachdev Manjit Singh B, Chang AKW, Jobli AT, Chua HH. Pulmonary tuberculosis and COVID-19 coinfection: Hickam’s dictum revisited. Respir Med Case Rep (2022) 37:101653. doi: 10.1016/j.rmcr.2022.101653
48. Rajamanickam A, Pavan Kumar N, Chandrasekaran P, Nancy A, Bhavani PK, Selvaraj N, et al. Effect of SARS-CoV-2 seropositivity on antigen – specific cytokine and chemokine responses in latent tuberculosis. Cytokine (2022) 150(155785). doi: 10.1016/j.cyto.2021.155785
49. Pastor L, Casellas A, Carrillo J, Alonso S, Parker E. IP-10 levels as an accurate screening tool to detect acute HIV infection in resource-limited settings. Sci Rep (2017) 7:1–9. doi: 10.1038/s41598-017-08218-0
50. Pastor L, Parker E, Carrillo J, Urrea V, Fuente-Soro L, Respeito D, et al. A cytokine pattern that differentiates preseroconversion from postseroconversion phases of primary HIV infection. J Acquir Immune Defic Syndr (2017) 74(4):459–66. doi: 10.1097/QAI.0000000000001272
51. Stacey AR, Norris PJ, Qin L, Haygreen EA, Taylor E, Heitman J, et al. Induction of a striking systemic cytokine cascade prior to peak viremia in acute human immunodeficiency virus type 1 infection, in contrast to more modest and delayed responses in acute hepatitis b and c virus infections. J Virol (2009) 83(8):3719–33. doi: 10.1128/JVI.01844-08
52. McMichael AJ, Borrow P, Tomaras GD, Goonetilleke N, Haynes BF. The immune response during acute HIV-1 infection: Clues for vaccine development. Nat Rev Immunol (2010) 10(1):11–23. doi: 10.1038/nri2674
53. French M, King M, Tschampa J. Serum immune activation markers are persistently increased in patients with HIV infection after 6 years of antiretroviral therapy despite suppression of viral replication. J Infect Dis (2009) 200(8):1212–5. doi: 10.1086/605890
54. Guihot A, Dentone C, Assoumou L, Parizot C, Calin R. Residual immune activation in combined antiretroviral therapy-treated patients with maximally suppressed viremia. AIDS (2016) 30(2):327–30. doi: 10.1097/QAD.0000000000000815
55. Teigler JE, Leyre L, Chomont N, Slike B, Jian N, Eller MA, et al. Distinct biomarker signatures in HIV acute infection associate with viral dynamics and reservoir size. Am Soc Clin Investig (2018) 3(10):1–12. doi: 10.1172/jci.insight.98420
56. Deeks SG, Kitchen CMR, Liu L, Guo H, Gascon R, Narvá Ez AB, et al. Immune activation set point during early HIV infection predicts subsequent CD4 T-cell changes independent of viral load. Blood (2004) 104:942–7. doi: 10.1182/blood-2003-09-3333
57. Roberts L, Passmore JAS, Williamson C, Little F, Bebell LM, Mlisana K, et al. Plasma cytokine levels during acute HIV-1 infection predict HIV disease progression. AIDS (2010) 24(6):819–31. doi: 10.1097/QAD.0b013e3283367836
58. Antonio R, Silvia M. Immunosuppression drug-related and clinical manifestation of coronavirus disease 2019: a therapeutical hypothesis. Am J Transplant (2020) 20(7):1947–8. doi: 10.1111/ajt.15905
59. Hrusak O, Kalina T, Wolf J, Balduzzi A, Provenzi M, Rizzari C, et al. Flash survey on severe acute respiratory syndrome coronavirus-2 infections in paediatric patients on anticancer treatment. Eur J Cancer (2020) 132:11–6. doi: 10.1016/j.ejca.2020.03.021
60. Han Y, Jiang M, Xia D, He L, Lv X, Liao X, et al. COVID-19 in a patient with long-term use of glucocorticoids: A study of a familial cluster. Clin Immunol (2020) 214:1–4. doi: 10.1016/j.clim.2020.108413
61. Bussalino E, De Maria A, Russo R, Paoletti E. Immunosuppressive therapy maintenance in a kidney transplant recipient SARS-CoV-2 pneumonia: a case report. Am J Transplant (2020) 20(7):1922–4. doi: 10.1111/ajt.15920
62. Calza L, Bon I, Tadolini M, Borderi M, Colangeli V, Badia L, et al. COVID-19 in patients with HIV-1 infection: a single-centre experience in northern Italy. Infection (2020) 49(2):333–7. doi: 10.1007/s15010-020-01492-7
63. Rouse BT, Sehrawat S. Immunity and immunopathology to viruses: What decides the outcome? Nat Rev Immunol (2010) 10(7):514–26. doi: 10.1038/nri2802
64. Nakajima Y, Ogai A, Furukawa K, Arai R, Anan R, Nakano Y, et al. Prolonged viral shedding of SARS-CoV-2 in an immunocompromised patient. J Infect Chemother (2021) 27(2):387–9. doi: 10.1016/j.jiac.2020.12.001
65. Lagrutta L, Sotelo CA, Estecho BR, Beorda WJ, Francos JL, González Montaner P. La unidad febril de urgencias del hospital muñiz frente a COVID-19, HIV y tuberculosis. Med (2021) (Spanish: B Aires) 81(2):143–8.
66. Kozińska M, Augustynowicz-Kopeć E. COVID-19 in patients with active tuberculosis. Diagnostics (2021) 11(10). doi: 10.3390/diagnostics11101768
67. Yan T, Xiao R, Lin G. Angiotensin-converting enzyme 2 in severe acute respiratory syndrome coronavirus and SARS-CoV-2: A double-edged sword? FASEB J (2020) 34(5):6017–26. doi: 10.1096/fj.202000782
68. Liu C, Zhou Q, Li Y, Garner LV, Watkins SP, Carter LJ, et al. Research and development on therapeutic agents and vaccines for COVID-19 and related human coronavirus diseases. ACS Cent Science (2020) 6:315–31. doi: 10.1021/acscentsci.0c00272
69. Gubernatorova EO, Gorshkova EA, Polinova AI, Drutskaya MS. IL-6: Relevance for immunopathology of SARS-CoV-2. Cytokine Growth Factor Rev (2020) 53:13–24. doi: 10.1016/j.cytogfr.2020.05.009
70. Richardson S, Hirsch JS, Narasimhan M, Crawford JM, McGinn T, Davidson KW, et al. Presenting characteristics, comorbidities, and outcomes among 5700 patients hospitalized with COVID-19 in the new York city area. JAMA 323(20):2052–9. doi: 10.1001/jama.2020.6775
71. Masukume G, Mapanga W, Grinberg S, van Zyl DS. COVID-19 and HIV co-infection an emerging consensus. J Med Virol 93(1):170–1. doi: 10.1002/jmv.26270
72. Riou C, du Bruyn E, Stek C, Daroowala R, Goliath RT, Abrahams F, et al. Relationship of SARS-CoV-2–specific CD4 response to COVID-19 severity and impact of HIV-1 and tuberculosis coinfection. J Clin Invest (2021) 131(12):e149125. doi: 10.1172/JCI149125
73. Qasim A, Mansour M, Kousa O, Awad D, Abuhazeem B, Millner P, et al. A case of coronavirus disease 2019 in acquired immunodeficiency syndrome patient: A case report and review of the literature. Intractable Rare Dis Res (2020) 9(4):256–9. doi: 10.5582/irdr.2020.03081
74. Mondi A, Cimini E, Colavita F, Cicalini S, Pinnetti C, Matusali G, et al. COVID-19 in people living with HIV: Clinical implications of dynamics of the immune response to SARS-CoV-2. J Med Virol (2021) 93(3):1796–804. doi: 10.1002/jmv.26556
75. Jassat W, Cohen C, Tempia S, Masha M, Goldstein S, Kufa T, et al. Risk factors for COVID-19-related in-hospital mortality in a high HIV and tuberculosis prevalence setting in south Africa: a cohort study. (2021) 8(9):554–67. doi: 10.1016/S2352-3018(21)00151-X
76. del Amo J, Polo R, Moreno S, Díaz A, Martínez E, Arribas JR, et al. Incidence and severity of COVID-19 in HIV-positive persons receiving antiretroviral Therapy : A cohort study. Ann Intern Med (2020) 173(7):536–41. doi: 10.7326/M20-3689
77. Sharov KS. HIV/SARS-CoV-2 co-infection: T cell profile, cytokine dynamics and role of exhausted lymphocytes konstantin. Int J Infect Dis (2020) 102(2021):163–9. doi: 10.1016/j.ijid.2020.10.049
78. Calza L, Bon I, Borderi M, Colangeli V, Borioni A, Re MC, et al. COVID-19 outcomes in uncontrolled HIV infection. (2021) 86(1):2020–2. doi: 10.1097/QAI.0000000000002537
79. Mattapallil JJ, Douek DC, Hill B, Nishimura Y, Martin M, Roederer M. Massive infection and loss of memory CD4+ T cells in multiple tissues during acute SIV infection. Nature (2005) 434(7037):1093–7. doi: 10.1038/nature03501
80. Blanco JL, Ambrosioni J, Garcia F, Martínez E, Soriano A, Mallolas J, et al. COVID-19 in patients with HIV: clinical case series. Lancet HIV (2020) 7:e314–6. doi: 10.1016/S2352-3018(20)30111-9
81. Wang Y, Wang Y, Chen Y, Qin Q. Unique epidemiological and clinical features of the emerging 2019 novel coronavirus pneumonia (COVID-19) implicate special control measures. J Med Virol (2020) 92(6):568–76. doi: 10.1002/jmv.25748
82. Rodionova IA, Schuster BM, Guinn KM, Sorci L, Scott DA, Li X, et al. Metabolic and bactericidal effects of targeted suppression of NadD and NadE enzymes in mycobacteria. MBio (2014) 5(1):747–60. doi: 10.1128/mBio.00747-13
83. Depaix A, Kowalska J. NAD analogs in aid of chemical biology and medicinal chemistry. Molecules (2019) 24(22):4187. doi: 10.3390/molecules24224187
84. Drucker DJ. Diabetes, obesity, metabolism, and SARS-CoV-2 infection: the end of the beginning. Cell Metab (2021) 33(3):479–98. doi: 10.1016/j.cmet.2021.01.016
85. Miller R, Wentzel AR, Richards GA. COVID-19: NAD+ deficiency may predispose the aged, obese and type2 diabetics to mortality through its effect on SIRT1 activity. Med Hypotheses (2020) 144:110044. doi: 10.1016/j.mehy.2020.110044
86. Migliori GB, Casco N, Jorge AL, Palmero DJ, Alffenaar JW, Denholm J, et al. Tuberculosis and COVID-19 co-infection: description of the global cohort. Eur Respir J (2022) 59(3):1–15. doi: 10.1183/13993003.02538-2021).
87. Rojas-Bolivar D, Intimayta-Escalante C, Cardenas-Jara A, Jandarov R, Huaman MA. COVID-19 case fatality rate and tuberculosis in a metropolitan setting. J Med Virol (2021) 93(5):3273–6. doi: 10.1002/jmv.26868
88. Fraser S, Ellsworth M, Perez N, Hamilton H, Fletcher S, Brown D, et al. Cerebral infarctions in an infant with COVID-19 delta variant infection and disseminated tuberculosis to. Pediatr Neurol (2022) 126:112–3. doi: 10.1016/j.pediatrneurol.2021.10.014
89. Sheerin D, Abhimanyu, Peton N, Vo W, Allison CC, Wang X, et al. Immunopathogenic overlap between COVID-19 and tuberculosis identified from transcriptomic meta-analysis and human macrophage infection. iScience (2022) 25(6):104464. doi: 10.1016/j.isci.2022.104464
90. Parolina L, Pshenichnaya N, Vasilyeva I, Lizinfed I, Urushadze N, Guseva V, et al. Clinical characteristics of COVID-19 in TB patients and factors associated with the disease severity. Int J Infect Dis (2022) 124(Suppl 1):S82–9. doi: 10.1016/j.ijid.2022.04.041
91. Gül Ş, Akalın Karaca ES, Özgün Niksarlıoğlu EY, Çınarka H, Uysal MA. Coexistence of tuberculosis and COVID-19 pneumonia: A presentation of 16 patients from Turkey with their clinical features. Tuberk Toraks. (2022) 70(1):8–14. doi: 10.5578/tt.20229902
92. He G, Wu J, Shi J, Dai J, Gamber M, Jiang X, et al. COVID-19 in tuberculosis patients: a report of three cases. J Med Virol (2020) 92(10):1802–6. doi: 10.1002/jmv.25943
93. Modi P, Khanna R, Reddy N, Patankar A, Patel S, Nair G, et al. COVID-19 and tuberculosis co-infection in pregnancy - a case series and review. J Mother Child. (2021) 25(2):127–34. doi: 10.34763/jmotherandchild.20212502.d-21-00002
94. Zaini J, Fadhillah MR, Reisa T, Isbaniah F, Handayani RRD. Tuberculosis and COVID-19 coinfection: A report of two cases at a tertiary referral in Indonesia. J Infect Dev Ctries. (2022) 16(3):478–83. doi: 10.3855/jidc.15481
95. Gortaire EV, Sívori M, Jajati M, Trullás MF. ARTÍCULO ORIGINAL COINFECCIÓN DE COVID-19 y TUBERCULOSIS EXTRAPULMONAR. Materiales y métodos (2022) 82(2):167–71.
96. Petrone L, Petruccioli E, Vanini V, Cuzzi G, Gualano G, Vittozzi P, et al. Coinfection of tuberculosis and COVID-19 limits the ability to in vitro respond to SARS-CoV-2. Int J Infect Dis (2021) 1135:582–7. doi: 10.1016/j.ijid.2021.02.090
97. Amimo F, Lambert B, Magit A. What does the COVID-19 pandemic mean for HIV, tuberculosis, and malaria control? Trop Med Health (2020) 48(1):32. doi: 10.1186/s41182-020-00219-6
98. Campbell JE, Cohall D. Pharmacodynamics-a pharmacognosy perspective. pharmacognosy: Fundamentals, applications and strategy. (2017), 513–25. doi: 10.1016/B978-0-12-802104-0.00026-3
99. Tamuzi JL, Birhanu AT, Shumba CS, Adetokunboh O, Uwimana-nico J, Haile ZT, et al. Implications of COVID-19 in high burden HIV/TB countries : A systematic review of evidence. BMC Infect Dis (2020) 20(1):744. doi: 10.1186/s12879-020-05450-4
100. The global fund. global fund survey: Majority of HIV, TB and malaria programs face disruptions as a result of COVID-19 - COVID-19 - the global fund to fight AIDS, tuberculosis and malaria (2022). Available at: https://www.theglobalfund.org/en/covid-19/news/2020-06-17-global-fund-survey-majority-of-hiv-tb-and-malaria-programs-face-disruptions-as-a-result-of-covid-19/.
101. Coomes EA, Haghbayan H. Favipiravir, an antiviral for COVID-19? J Antimicrob Chemother (2020) 75(7):2013–4. doi: 10.1093/jac/dkaa171
102. Martinez MA. Compounds with therapeutic potential against novel respiratory 2019 coronavirus. Antimicrob Agents Chemother (2020) 64(5). doi: 10.1128/AAC.00399-20
103. Mitjà O, Clotet B. Use of antiviral drugs to reduce COVID-19 transmission. Lancet Glob Heal (2020) 8(5):e639–40. doi: 10.1016/S2214-109X(20)30114-5
104. Goldhill DH, Te Velthuis AJW, Fletcher RA, Langat P, Zambon M, Lackenby A, et al. The mechanism of resistance to favipiravir in influenza. Proc Natl Acad Sci U S A. (2018) 115(45):11613–8. doi: 10.1073/pnas.1811345115
105. Pennings PS. HIV Drug resistance : problems and perspectives. Infect Dis Rep (2013) 5(1):21–5. doi: 10.4081/idr.2013.s1.e5
106. Togun T, Kampmann B, Stoker NG, Lipman M. Anticipating the impact of the COVID-19 pandemic on TB patients and TB control programmes. Ann Clin Microbiol Antimicrob (2020) 19(1):1–6. doi: 10.1186/s12941-020-00363-1
107. Wolters F, Van De Bovenkamp J, Van Den Bosch B, Van Den Brink S, Broeders M, Hoa N, et al. Multi-center evaluation of cepheid xpert® xpress SARS-CoV-2 point-of-care test during the SARS-CoV-2 pandemic. J Clin Virol J (2020) 128(2020):104426. doi: 10.1016/j.jcv.2020.104426
108. Erving G. Stigma : notes on the management of spoiled identity Vol. p. Englewood Cliffs NJ, editor. (Touchstone Publisher, New York United States of America: Prentice-Hall) (1963) p. 1–147.
109. Tornheim JA, Dooley KE. Tuberculosis associated with HIV infection. Microbiol Spectr. (2017) 5(1):1–16. doi: 10.1128/9781555819866.ch34
110. World Health Organization. . social stigma associated with COVID-19 (2020). Available at: https://www.who.int/publications/i/item/social-stigma-associated-with-covid-19.
111. Dolatian M, Ghiasi N, Valizadeh R, Kellner SJ, Mahmoodi Z, Nanbakhsh M. SARS, MERS and COVID-19 status in HIV-positive patients: A systematic review. J Cell Mol Anesth (2021) 6(1):75–80. doi: 10.22037/jcma.v6i1.32203
112. Wong CY, Wong KY, Law TSG, Shum TT, Li YK, Pang WK. Tuberculosis in a SARS outbreak. J Chin Med Assoc (2004) 67(11):579–82.
113. Liu W, Fontanet A, Zhang P-H, Zhan L, Xin Z-T, Tang F, et al. Pulmonary tuberculosis and SARS, China (2006). Available at: https://stacks.cdc.gov/view/cdc/15869.
114. Low JG, Lee CC, Leo YS, Low JGH, Lee CC, Leo YS. Severe acute respiratory syndrome and pulmonary tuberculosis. Clin Infect Dis (2004) 38(12):e123-5. doi: 10.1086/421396
115. Alfaraj SH, Al-Tawfiq JA, Altuwaijri TA, Memish ZA. Middle East respiratory syndrome coronavirus and pulmonary tuberculosis coinfection: Implications for infection control. Intervirology (2017) 60(1–2):53–5. doi: 10.1159/000477908
116. Perlman DC, El-Helou P, Salomon N. Tuberculosis in patients with human immunodeficiency virus infection. Semin Respir Infect (1999) 14(4):344–52.
117. Vicente P, Suleman A. COVID-19 in Europe: from outbreak to vaccination. BMC Public Heal (2022) 22(1):1–17. doi: 10.1186/s12889-022-14454-5
118. Watson OJ, Barnsley G, Toor J, Hogan AB, Winskill P, Ghani AC. Global impact of the first year of COVID-19 vaccination: a mathematical modelling study. Lancet Infect Dis (2022) 22(9):1293–302. http://www.thelancet.com/article/S1473309922003206/fulltext. doi: 10.1016/S1473-3099(22)00320-6
Keywords: COVID-19, HIV/AIDS, tuberculosis, co-infection, pandemic, susceptibility, immunosuppression
Citation: Udoakang AJ, Djomkam Zune AL, Tapela K, Nganyewo NN, Olisaka FN, Anyigba CA, Tawiah-Eshun S, Owusu IA, Paemka L, Awandare GA and Quashie PK (2023) The COVID-19, tuberculosis and HIV/AIDS: Ménage à Trois. Front. Immunol. 14:1104828. doi: 10.3389/fimmu.2023.1104828
Received: 22 November 2022; Accepted: 11 January 2023;
Published: 27 January 2023.
Edited by:
Alexander Yaw Debrah, Kwame Nkrumah University of Science and Technology, GhanaReviewed by:
Antonino Di Caro, Saint Camillus International University of Health and Medical Sciences, ItalyCopyright © 2023 Udoakang, Djomkam Zune, Tapela, Nganyewo, Olisaka, Anyigba, Tawiah-Eshun, Owusu, Paemka, Awandare and Quashie. This is an open-access article distributed under the terms of the Creative Commons Attribution License (CC BY). The use, distribution or reproduction in other forums is permitted, provided the original author(s) and the copyright owner(s) are credited and that the original publication in this journal is cited, in accordance with accepted academic practice. No use, distribution or reproduction is permitted which does not comply with these terms.
*Correspondence: Aniefiok John Udoakang, YXVkb2FrYW5nQHVuaW1lZC5lZHUubmc=; bWFuZGl0aDIwMDRAeWFob28uY29t; Alexandra Lindsey Djomkam Zune, ZGpvbWthbWxpbmRzZXlAeWFob28uY29t; Peter Kojo Quashie, cHF1YXNoaWVAdWcuZWR1Lmdo
†These authors have contributed equally to this work