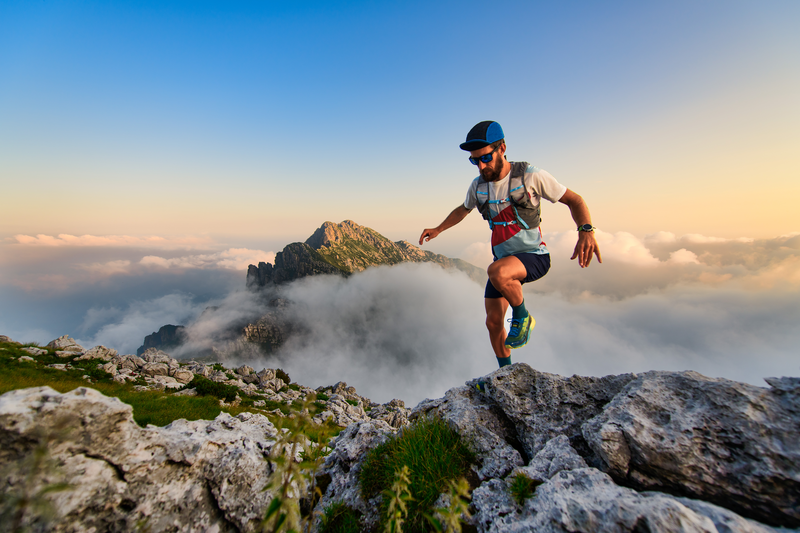
94% of researchers rate our articles as excellent or good
Learn more about the work of our research integrity team to safeguard the quality of each article we publish.
Find out more
REVIEW article
Front. Immunol. , 16 March 2023
Sec. Cancer Immunity and Immunotherapy
Volume 14 - 2023 | https://doi.org/10.3389/fimmu.2023.1103617
Colitis-associated colorectal cancer is the most serious complication of ulcerative colitis. Long-term chronic inflammation increases the incidence of CAC in UC patients. Compared with sporadic colorectal cancer, CAC means multiple lesions, worse pathological type and worse prognosis. Macrophage is a kind of innate immune cell, which play an important role both in inflammatory response and tumor immunity. Macrophages are polarized into two phenotypes under different conditions: M1 and M2. In UC, enhanced macrophage infiltration produces a large number of inflammatory cytokines, which promote tumorigenesis of UC. M1 polarization has an anti-tumor effect after CAC formation, whereas M2 polarization promotes tumor growth. M2 polarization plays a tumor-promoting role. Some drugs have been shown to that prevent and treat CAC effectively by targeting macrophages.
Ulcerative colitis (UC) is a chronic nonspecific intestinal inflammation caused by a variety of factors including heredity, immunity and environment exposure. Hemorrhagic diarrhea, abdominal spastic pain, and weight loss are the most common symptoms (1). In the past few decades, the incidence trend of UC is on the rise (2). Most ulcerative colitis patients only need medication to control their symptoms. When severe bleeding, obstruction or tumorigenesis occur, surgical treatment is required. Colitis-associated colorectal cancer (CAC) is the most serious complication of UC. The risk of tumorigenesis of UC includes long course of disease, wide range of lesions, and primary sclerosing cholangitis (3). Previous studies have shown that the incidence of colorectal cancer in patients with ulcerative colitis in Asia is 0.02%, 4.81% and 13.91% in 10 years, 20 years and 30 years, respectively (4). CAC is distinguished from sporadic colorectal cancer by the presence of multiple lesions, more serious pathological types, and a poor prognosis (5, 6). CAC and sporadic colorectal cancer(S-CRC) have different pathogenesis. S-CRC develops according to the sequence of adenoma–dysplasia–carcinoma, while CAC follows the sequence of inflammation–dysplasia–carcinoma. DNA damage caused by inflammation and reactive oxygen species promotes the occurrence of mutations. The mutations that occur in the development of CAC are similar to those of S-CRC, but the order of mutations is opposite. For example, in the development of CAC, the mutation of TP53 occurs in the early stage and the mutation of APC occurs in the late stage. In the process of sporadic colorectal cancer, TP53 mutation occurs in the late stage and APC mutation exists in the early stage (7). Despite accounting for a small proportion of colorectal cancer, CAC is still a leading cause of mortality and the reason for colectomy in UC patients (8).
The cause of UC tumorigenesis is not clearly understood. However, now most physician believe that it is related to the inflammatory microenvironment caused by intestinal inflammation (9). Inflammatory factors act on the intestinal mucosa, promoting the occurrence of tumor. Macrophages, as innate immune cells, contribute to the UC’s inflammatory response. Furthermore, macrophages play an important role in tumors. Therefore, it is critical to comprehend the role of macrophages in UC carcinogenesis and CAC progression. This article reviews the role of macrophages in the occurrence and progression of CAC.
Macrophage is an innate immune cell, most of which originate from monocytes (10). Recent research suggests that have monocytes may not be the only source of macrophages, and intestinal macrophages are derived from resident macrophages in the intestine. Macrophages secrete cytokines (11), remove cell fragments (12), kill pathogens (13), and are involved in inflammation (14), tissue repair (15), angiogenesis (16) and so on. Macrophages exist in many tissues of the body and play different roles in different tissues: alveolar macrophages in the lungs can maintain the stability of the environment in the alveoli (17), osteoclasts in bones have the ability of bone remodeling (18), and a large number of macrophages in the liver are called Kupffer cells which participate in immune response in liver (19, 20).
Macrophages are white blood cells that exist in tissue. It is generally believed that macrophages are derived from monocytes, while monocytes are derived from the granulocyte-macrophage colony (GM-CFUc) forming unit in bone marrow. Monocytes migrate from blood to different tissues and form groups of cells with different functions, such as “inflammatory” monocytes and “resident” monocytes distinguished by the expression of cell surface marker CX3CR1 (21). Inflammatory monocytes have a short half-life and can differentiate into inflammatory macrophages and dendritic cells. Inflammatory monocytes may also be one of the sources of resident monocytes. Resident macrophages are usually produced by resident monocytes and sometimes by inflammatory monocytes. Resident macrophages and Foxp3+T cells play an important role in maintaining the stability of intestinal environment through the mechanism of IL-10 and TGF-β dependence. When intestinal inflammation occurs, inflammatory monocytes migrate to the intestinal tract and differentiate into dendritic cells and inflammatory macrophages, which can produce a variety of cytokines involved in inflammatory response (22). Inflammatory macrophages are usually activated as M1 phenotype, while resident macrophages are usually activated as M2 phenotype. This process, which is affected by a variety of complex factors, is known as polarization. The polarization of macrophages is dynamic and could be reversed under certain conditions. The polarized macrophages mainly include M1 activated by classical pathway and M2 activated by alternative pathway (23). M2 can be divided into four subtypes in response to various stimuli: M2a (24), M2b (25), M2c (26) and M2d (27).
Inflammatory cytokines, such as IFN- γ, TNF-α, and the microbial product LPS stimulate M1 polarization of macrophages (28). TLR2, TLR4, CD80, CD86, and CCR7 are among the surface receptors expressed by M1. And M1 secretes a variety of inflammatory cytokines and chemokines, including TNF-α, IL-6, IL-1, IL-12, ROS, CXCL9, CXCL10, CXCL11, CCL2, CCL3, CCL4 and CCL5 (29). Polarized M1 macrophages have increased antigen presentation ability (30). As a result, M1 shows strong inflammation, antibacterial and anti-tumor performance. Th-2 cytokines IL-4, IL-10 and IL-13 can stimulate M2 polarization of macrophages (31). Dectin-1, mannose receptor, scavenger receptor A/B, DC-SIGN (CD209) and CD163, CCR2, CXCR1, and CXCR2 expression in M2 macrophages. As the main member of tumor-associated macrophages TAMs, M2 has strong phagocytic ability, removes fragments and apoptotic cells, promote tissue repair, angiogenesis, suppresses immunity, and promotes tumor progression and metastasis (32).
The immune state of the body is maintained by the coordination of a variety of immune cells. The interaction between macrophages and other immune cells is complex and diverse, which can regulate the direction and intensity of immune response through a variety of ways and mechanisms, thus having different effects on the body.
T cells are important immune cells, which participate in adaptive immunity. In tumor immunity, T cells can directly kill tumor cells and coordinate different anti-tumor immunity. As antigen presenting cells, macrophages affect the immune response of CD8+T cells by phagocytosis and degradation of antigens such as pathogens and tumor cells, or by regulating the expression of costimulatory molecules (33). In addition, macrophages can regulate the immune response of CD8+T cells by secreting cytokines and chemokines. M1 macrophages can promote the activation and proliferation of CD8+T cells and enhance their killing ability by releasing cytokines such as IL-12 and IL-18, thus clearing pathogens and tumor cells. M2 macrophages may inhibit the activation and proliferation of CD8+T cells and weaken their killing ability by releasing immunosuppressive factors such as IL-10, which may lead to immune tolerance or immune escape (34). M1 macrophages can secrete chemokines such as CXCL9/10 to attract CD8+T cells to the infection site and promote inflammatory response, while M2 macrophages can secrete chemokines such as CCL17 to attract Treg to the inflammatory site, thus inhibiting the inflammatory response (35, 36). In addition, Th1 cells secrete cytokines such as IFN- γ, which can stimulate M1 polarization of macrophages. And M1 macrophages can also secrete some chemokines, such as CXCL10 and CXCL11, which can attract and activate Th1 cells and enhance cellular immune response. Th2 cytokines such as IL-4 and IL-13 can stimulate M2 macrophage differentiation and activation which in turn can promote sustained Th2 T cell activation and survival (12). M1 macrophages activate signal transduction pathways such as NF- κB pathway and STAT3 pathway by secreting cytokines such as IL-1 β, IL-6 and IL-23, and then promote the differentiation and function of Th17 cells, thereby enhancing inflammatory response. M2 macrophages inhibit the differentiation and function of Th17 cells by producing anti-inflammatory factors such as IL-10 and TGF- β, thus reducing inflammatory response (37). Some studies have shown that IL-17 produced by Th17 can promote the differentiation and function of M1 macrophages, while inhibit the differentiation and function of M2 macrophages, thus enhancing the inflammatory response (38). However, other studies have also found that IL-17A can induce the differentiation and function of M2 macrophages, thus reducing the inflammatory response (39).
B cells can secrete antibodies to participate in adaptive immunity. Macrophages can recognize and absorb antigens such as pathogens and present them to B cells, thus activating the immune response of B cells and promoting the production of antibodies (40). And M1 macrophages can activate B cells by secreting TNF- α, IL-6 and other cytokines, promote the proliferation and differentiation of B cells, and induce B cells to secrete antibodies such as IgM and IgG. On the other hand, M2 macrophages can secrete immunosuppressive factors such as IL-10 and TGF- β to inhibit the activation and differentiation of B cells, thus reducing the level of humoral immunity (41).
The interaction between M1 macrophages and NK cells usually helps to enhance cytotoxicity, while the interaction between M2 macrophages and NK cells may lead to immunosuppression. On the one hand, M1 macrophages can activate NK cells by secreting cytokines such as IL-12, IL-15, IL-18 and IFN-γ. These cytokines can promote the proliferation and differentiation of NK cells and enhance their cytotoxicity. On the other hand, the interaction between M2 macrophages and NK cells is relatively complex. Some studies have shown that M2 macrophages can inhibit the activity of NK cells by secreting cytokines such as IL-10. In addition, M2 macrophages can also inhibit the function of NK cells by expressing costimulatory molecules such as PD-L1 (42).
Unlike other lymphocytes, ILCs does not have T cell receptor or B cell receptor, so it does not participate in specific immune response. On the contrary, they play an important role in innate immunity. ILC is usually divided into three different subtypes: ILC1,ILC2 and ILC3, which play an important role in regulating immune response. M1 macrophages can secrete IL-12 and IL-18 to promote the differentiation and function of ILC1. ILC1 mainly produces IFN- γ and participates in antiviral and cytotoxic effects. M2 macrophages can secrete IL-33 to promote the differentiation and function of ILC2. ILC2 mainly produces cytokines such as IL-4, IL-5, IL-9 and IL-13, which are involved in anti-parasite infection, allergic reaction and tissue repair. Macrophages can secrete IL-23 to regulate the differentiation and function of ILC3. ILC3 mainly produces cytokines such as IL-17 and IL-22, and participates in antifungal and antibacterial effects (43, 44).
Skin and mucosa are the first line of immune defense of the body. In intestinal mucosa, macrophages and dendritic cells play an innate immune role, while B cells and T cells participate in adaptive immune response. Macrophages are important cells in chronic inflammation and the pathological processes. And macrophage infiltration is a sign of chronic inflammation. The polarization of macrophages is associated with some autoimmune diseases, such as UC (45) and systemic lupus erythematosus (46). Under physiological conditions, macrophages in the intestine phagocytize microorganisms and present antigens to activate T cells. Excessive activation of macrophages transforms physiological inflammation into pathological damage of intestinal mucosa. The number of macrophages increased significantly in active ulcerative colitis, suggesting that macrophages were involved in the occurrence and development of UC (47). And during the active phase of UC, most of the macrophages in the lamina propria of the intestinal wall are M1 phenotype. M1 breaks down tight junction proteins, destroys the epithelial barrier, induces apoptosis of epithelial cells, and leads to excessive inflammation (48). M1 plays a major role in intestinal inflammation, while M2 plays an antagonistic role which eliminates inflammation and promotes tissue healing. Previous studies have confirmed that increasing the proportion of M2 could reduce the symptoms of colitis in mice (49).
A variety of inflammatory cytokines secreted by macrophages play an important role in UC. IL-6 is an important mediator in inflammatory response, which directly participates in inflammatory response and corresponding injury process. IL-6 increases the permeability of epithelial cells, which promotes the infiltration of macrophages and aggravate the development of ulcerative colitis. Previous studies have shown that the level of IL-6 is positively correlated with the severity of intestinal inflammation (50). IL-18 is also highly expressed in serum and colon tissues of patients with ulcerative colitis. The application of IL-18 inhibitor relieves the symptoms of mouse colitis model and reduce the expression of many inflammatory factors (51). TNF-α regulates the NF-κB pathway by promoting IκBα degradation, NF-κB p65 phosphorylation and NF-κB nuclear transfer, which aggravates the injury of ulcerative colitis (52). Infliximab has been used as a TNF-α antagonist in the treatment of ulcerative colitis (53).
These cytokines not only play a role in the inflammatory response, but also participate in the tumorigenesis and progression of tumors. TNF- α is a kind of inflammatory cytokine with strong anti-tumor effect (54). Many kinds of immune cells, including macrophages, can produce TNF- α. It has been shown to promote tumor growth in chronic inflammatory diseases, although TNF- α has anti-tumor property. In chronic inflammation, by production of RONS, TNF- α promotes DNA damage caused by oxidative stress and promotes the tumorigenesis of CAC (55). And targeting TNF- α may prevent or reduce the tumorigenesis of CAC (56). Similarly, IL-6 can also promote early CAC tumorigenesis by inducing oxidative stress (57).
Interestingly, although TNF- α has anti-tumor effect, even some studies have shown that TNF- α can promote tumor cell apoptosis and inhibit tumor liver metastasis in colorectal cancer (58). However, more studies have found that TNF- α plays an important role in tumor progression. By stimulating epithelial-mesenchymal transformation, TNF- α can enhance the ability of invasion and metastasis of colon cancer cells (59). And TNF- α can promote angiogenesis by inducing human fibroblasts to secrete VEGF (60). TNF- α can also promote tumor lymphangiogenesis and lymphatic metastasis (61). In addition, TNF- α promote the progression and metastasis of CRC through NF- κ B pathway and induction of MACC1 (62, 63). By blocking TNF- α, the promotion of TNF- α on tumor can be reduced (64, 65).
Macrophages have the dual functions of anti-tumor and promoting tumor in cancer. In view of the fact that M2 plays a promoting role in tumor development and metastasis, reducing the proportion of M2 polarization could be used as a way to treat tumor (66). On the other hand, M1 has the anti-tumor effect of promoting tumor immunity (67). The polarization of macrophages is affected by tumor microenvironment, which indicates that macrophage has the ability to become potential target for tumor therapy. In CAC, macrophages not only play a role in tumor immunity, but also play a role in the inflammatory environment before tumor formation. Overactivated macrophages not only aggravate inflammatory damage, but also promote tumorigenesis.
Inflammation is an important cause of carcinogenesis of UC. Macrophage infiltration is one of the characteristics of inflammation in UC. A large number of infiltrating macrophages aggravate the mucosal damage caused by inflammation and promote the transformation from inflammation to tumor. The infiltration of macrophages and the release of pro-inflammatory factors promote the carcinogenesis of epithelial cells.
CCL2 and CX3CL1 are important cytokines that induce macrophage infiltration. By promoting the expression of these cytokines, macrophage infiltration is enhanced and the occurrence and development of CAC is promoted. MUC1 is a kind of cell surface mucin which exists in intestinal epithelial cells and some leukocytes. MUC1 promotes the expression of CCL2 and mediates the recruitment and activation of macrophages. Activated macrophages secrete IL-6 and promote the occurrence and development of CAC through IL-6/STAT3 pathway (68). Glucocorticoid is a drug for the treatment of active UC. In an acute experimental UC mouse study, it was found that glucocorticoid increased the expression of CCL2 and CX3CL1, promoted macrophage infiltration and promoted the occurrence of UC and CAC by targeting mTOR signal of intestinal epithelial cells (69). Corticotrophin-releasing hormone receptor (CRHR1) belongs to the CRH family and is highly expressed in inflammatory tissues adjacent to CAC tumor tissue. CRHR1 promotes the recruitment of macrophages, secretes more inflammatory factors such as IL-1b, IL-6 and TNF- α, and promotes the early occurrence of CAC (70).
And inhibition of macrophage infiltration reduces the occurrence and development of inflammation and CAC. By inhibiting the expression of CCL2, GDC-0575, a CHK1 inhibitor, reduces macrophage infiltration in the mouse colon, which may inhibit the occurrence of CAC and colitis (71). Suppressor of AP‐1 (SAR1), a tumor suppressor, down-regulates the expression of p-STAT1 and STAT1 in CAC cells, inhibits the activation of MCP-1/CCR2 axis, reduces macrophage infiltration, which may inhibit the tumorigenesis of CAC (72). Serum amyloid A (SAA) is an evolutionarily conserved protein family associated with inflammation. It was found that in the CAC mouse model, SAA deficient mice showed a decrease in macrophage infiltration, which may inhibits tumorigenesis of CAC (73). Nerve injury inducer protein 1 (ninjurin1) is a homogenous cell surface adhesion molecule, which plays an important role in cell migration. Overexpression of Ninjurin1 in macrophages weakens the infiltration of macrophages by targeting FAK signaling pathway, thus may inhibiting the tumor progression of CAC (74). Diphenyleneiodonium (DPI) is an inhibitor of NADPH oxidase, which inhibits a variety of inflammatory responses. Low-dose DPI inhibits macrophage infiltration and migration, reduce the production of pro-inflammatory cytokines such as TNF- α and IL-6, reduce inflammatory response and may inhibit tumorigenesis (75). Estrogen receptor β (Erβ) reduce the occurrence and development of tumor by reducing macrophage infiltration and reducing the production of TNF-α (76). As a non-coding RNA, miRNA cannot encode proteins, but it can still exert its biological functions in a variety of physiological or pathological processes. A large number of previous studies have confirmed that miRNA plays an important role in inflammation and tumor (77, 78). Abdullah et al. found that overexpression of miR-132 inhibits macrophage infiltration and the production of proinflammatory cytokines, which may inhibit CAC (79).
These factors that affect macrophage infiltration are quite pleiotropic and have numerous downstream targeting effects, so the impact on CAC may not be direct, and may not all be caused by macrophage infiltration. And the effect of macrophage infiltration on CAC mainly occurred in the early stage. The underlying mechanism may be the large amount of macrophage infiltration leads to more severe intestinal inflammation. A large number of cytokines produced by macrophages promote DNA damage and promote the process of inflammation-dysplasia-tumor. The regulation of intestinal macrophage infiltration affects the tumorigenesis of CAC (Figure 1). Therefore, early inhibition of macrophage infiltration may be of significance to not only reduce the inflammation of ulcerative colitis but also prevent the occurrence of CAC.
Figure 1 The regulation of intestinal macrophage infiltration affects the occurrence of CAC. MUC1, Glu, CRHR1 and SAA enhance macrophage infiltration to promote the tumorigenesis. SARI, GDC-0575, Ninjurin1, DPI, ER-β, miR-132 reduce macrophage infiltration to inhibit the tumorigenesis.
Macrophages express different phenotypes under the influence of different environments, which is called macrophage polarization (80). The polarized macrophages mainly include M1 activated by classical pathway and M2 activated by alternative pathway. M1 and M2 have different functions. M1 secretes inflammatory cytokines and enhances the inflammatory response, but it has the anticancer effect of enhancing tumor immunity in tumor tissues. M2 promotes tissue repair and has anti-inflammatory effect, but it is considered to inhibit tumor immunity in tumor tissue. This means that macrophage polarization can not only affect the occurrence of CAC by regulating inflammation, but also directly affect CAC by regulating tumor microenvironment.
Excessive M1 polarization aggravates the inflammatory symptoms of the UC, promotes mutation involvement and provides an inflammatory environment suitable for tumorigenesis. M2 antagonize the pro-inflammatory effect of M1 and inhibit tumorigenesis. Low-dose DPI inhibited the classical activation pathway of macrophages and the level of M1 by down-regulating the pathways of STAT3, NF-κB and ERK, and inhibited the tumorigenesis of CAC in the early stage (75). MiR-146b targets MyD88 and IRAK1, regulates TLR4 pathway, inhibits M1 activation, and then reduces inflammation and inhibits the tumorigenesis of CAC (81). In the early stage of CAC, xanthine oxidoreductase (XOR) mediated M1 macrophage polarization, which promoted the tumorigenesis of CAC (82). In macrophage, Fibrinogen-like protein 2 (Fgl2) deficiency promotes M1 polarization and inhibit M2 polarization, which promote the tumorigenesis of CAC (83).
However, inflammation is not the only factor affecting the tumorigenesis of UC. M2 macrophages in tumor microenvironment also have the effect of pro-tumor. In myofibroblasts, MyD88 secretes OPN through TLR pathway, binds to CD44 and αvβ3 on macrophages, promotes M2 polarization through STAT3 and PPARγ pathway, which promotes the tumorigenesis of CAC (84). And a study found that the loss of SLC7A2 enhanced M2 polarization to promote the tumorigenesis of CAC (85).
After tumor formation, M1 plays an anti-tumor role through direct tumoricidal mechanisms or by promoting CD8 cytotoxic T cell and NK cell killing of tumor cells in later phases of CAC progression. M2 promotes tumor immune escape and enhanced tumor progression, especially invasion and metastasis. In a study on vitexin in CAC mice, vitexin inhibited M1 polarization in inflammatory tissue, but promoted M1 polarization in tumor tissue, which reduces tumorigenesis, inhibited tumor progression, and reduced tumor load (86). Transient receptor potential vanilloid 1 (TRPV1) is an ion channel expressed in a variety of immune cells. In the early stage of CAC, TRPV1 directly stimulates M1 polarization and promotes tumorigenesis. After that, TRPV1 regulates the Calcineurin/NFATc2 pathway to increase the phenotype of Th2, enhance M2 polarization and promote tumor progression (87). IL-28B inhibits M2 polarization of macrophages through STAT3 and JNK signaling pathways, which reduces the expression of M2 cytokines Arg-1 and TGF-β, to inhibit the tumor progression of CAC (88). Hematopoietic cell kinase (HCK) is one of nine non-receptor tyrosine kinases in the SRC family, which has the function of regulating innate immunity. HCK promotes M2 polarization which promotes the tumor progression of CAC (89) (Figure 2).
Figure 2 In the early stage, DPI, miR146b and Fgl2 inhibit M1 polarization, and Fgl2 promote M2 polarization, which both inhibit the tumorigenesis of CAC. XOR promotes M1 polarization and enhance the tumor progression of CAC. In the later stage, vitexin promotes M1 polarization, IL-28B and Embelin inhibit M2 polarization, which both inhibit CAC progress. TRPV1, HCK and OPN promote M2 polarization and enhance the tumor progression of CAC.
Tumor immunity is an important factor affecting tumor progression. The main reason for the effect of macrophage polarization on CAC is that polarized M1 and M2 play different functions in tumor immunity.
M1 produces ROS, which can directly kill tumor cells and inhibit tumor growth. M1 macrophages can produce a series of pro-inflammatory cytokines, including TNF- α, IL-1 β, IL-6, IL-12. These cytokines can stimulate the activity of immune cells such as NK cells and CD8+T cells and enhance their ability to attack tumor cells (90). In addition, M1 macrophages can activate tumor-specific T cells through antigen presentation and mature DC cells, thus promoting anti-tumor immune response (91). Recent studies have shown that M1 macrophages can also enhance anti-tumor immune response by inhibiting immunosuppressive cells in tumor microenvironment, including Tregs and MDSCs (92).M2 cells play an important role in different stages of tumor progression. In the early stage of tumor, M2 macrophages are mainly involved in tumor growth and angiogenesis. They secrete a variety of growth factors and cytokines, including VEGF, TGF- β, EGF, which promote tumor cell proliferation and neovascularization. In the middle stage of the tumor, M2 macrophages mainly play an immunosuppressive role. They can inhibit the activity of tumor immune cells and reduce the immune recognition and killing of tumor cells by secreting a variety of cytokines and surface molecules, including IL-10, TGF- β, PD-L1. In the late stage of tumor, M2 macrophages are mainly involved in tumor invasion and metastasis. By secreting a variety of proteases and lysosomal enzymes, they can promote tumor cell infiltration and invasion of surrounding tissues, and promote tumor cell metastasis and distant metastasis (36, 93–95). In general, M2 macrophages have complex mechanisms in the process of tumor growth and development, which can not only promote tumor growth and angiogenesis, but also inhibit the activity of tumor immune cells and promote tumor invasion and metastasis. Tumor cells also produce cytokines that affect the differentiation and function of immune cells. Some studies have shown that G-CSF released by tumor cells can activate MDSCs, thus forming an immune microenvironment suitable for tumor progression. In mechanism, MDSCs expresses high levels of inhibitory receptors and ligands, such as PD-L1, B7-H1 and CTLA-4, which can bind to T cell activation signal molecules (such as CD28), thus inhibiting T cell activation and proliferation. MDSCs can produce a variety of immunosuppressive molecules, including ROS, TGF- β, IL-10. These molecules can directly or indirectly inhibit the function and proliferation of T cells. MDSCs can promote T cell apoptosis through a variety of mechanisms, including secreting apoptosis-inducing molecules, expressing apoptosis-inducing receptors, inhibiting survival signals (96–98). These production of factors by MDSC and M2 macrophages provide a productive microenvironment tumor invasion and metastasis during tumor progression to malignant carcinoma stage tumors, including CAC.
In addition to infiltration of a large number of macrophage and break of the balance of macrophage polarization, macrophages also affect CAC through some other mechanisms such as regulating the secretion of inflammatory cytokines or tumor-promoting factors and targeting NF-κB pathway in macrophages.
Pou3f1 is a member of POU family and participates in cell apoptosis and immune response (99, 100). Nuclear factor of activated T cell 3 (Nfatc3) targets Pou3f1 to increase its expression, while Pou3f1 can promote inflammation in macrophages. Knockout of Pou3f1 decreased the levels of pro-inflammatory cytokines such as IL-1β, IL-6 and TNF-α, and inhibited the tumorigenesis of CAC (101). G protein-coupled receptor 35 (GPR35) is a soliton G protein-coupled receptor that interacts with sodium-potassium pumps. In macrophages, GPR35 promotes macrophages to release angiogenic factors and promote angiogenesis and tumor metastasis through the activation of Na/k-ATPase and non-receptor tyrosine kinase SRC (102). Dana et al. found that in macrophages, EGFR signal activates M2 polarization through STAT6, which promotes angiogenesis and CAC progression. Interestingly, in this study, M1 polarization is also activated by EGFR signals via NF-κB. The promotion of angiogenesis and tumor metastasis is due to the joint action of M1 and M2 to produce angiogenic factors CXCL1 and VEGF (103). Macrophage scavenger receptor A1 (SR-A1) is a pattern recognition receptor, which is mainly expressed in macrophages. In macrophages, SR-A1 inhibits the classical and non-classical activation of NF-κB signal through TRAF6 and TRAF3, respectively, thus inhibiting the occurrence of colitis and CAC (104). Elongation factor Tu GTP binding domain containing 2 (EFTUD2) is a mRNA splicing protein that could promote the occurrence of CAC by regulating the inflammatory response of macrophages. In macrophages, EFTUD2 deletion reduces the secretion of inflammatory factors and inhibits the occurrence of CAC by inhibiting the activation of TLR4-NF-κB pathway (105). MiRNA26a is overexpressed in macrophages, targeting IL-6, TLR3 and PKCσ, inhibiting the activation of NF-κB/STAT3 pathway and inhibiting the occurrence and development of CAC (106). M1 promotes the expression of TNF-related apoptosis-inducing ligands (TRAIL) in adipose tissue-derived stem cells (ASCs), while ASCs induce apoptosis of CD133+ tumor cells and reduce the number of M2 through TRAIL, which inhibits the progress of CAC (107).
The discovery of these mechanisms not only gives us a deep understanding of the role of macrophages in CAC, but also provides a new direction for the treatment of CAC by targeting these molecular pathways. At present, some potential drugs for the treatment of CAC, especially traditional Chinese medicine, play a role by affecting macrophage infiltration or polarization. Some drugs that directly target these molecular pathways are highly anticipated.
5-ASA is thought to reduce the risk of CAC in patients with UC, which may be related to its effect on controlling inflammation (108). In view of the important role of macrophages in CAC, some drugs can prevent and treat CAC by targeting macrophages (Table 1).
Dimethyl itaconate (DI) inhibits the secretion of IL-1 β, thus reduces the production of CCL2, restricts the recruitment of macrophages by the combination of CCL2 and CCR2, which inhibits CAC (109). EGFR signaling pathway plays an important role in the growth and metastasis of colorectal cancer. However, most of the studies focused on the colon epithelial cells. It has been found that EGFR in macrophages can regulate macrophage polarization and promote the tumorigenesis and angiogenesis of CAC. Targeting EGFR with cetuximab eliminates the cancer promoting effect of macrophages (103, 110). Ultra-low dose of DPI reduces the M1 polarization and macrophage infiltration of macrophages, and then inhibit the tumorigenesis and tumor progression of CAC. But it cannot kill CAC tumor cells directly (75). OTSSP167 inhibits macrophage infiltration and M1 polarization by targeting Maternal embryonic leucine zipper kinase (MELK), thereby inhibiting the tumorigenesis of CAC (111). Mannose inhibits M2 polarization to inhibit tumorigenesis of CAC (112). Embelin is a small molecule inhibitor of X-junction apoptotic protein inhibitor with anti-tumor effect. In the late stage of CAC, embelin reduce M2 polarization to reduce angiogenesis and MMP expression, which inhibits tumor metastasis (122).
Many traditional Chinese medicines have definite therapeutic effects on UC. Some traditional Chinese medicines or their active components regulate the occurrence and progression of CAC by targeting macrophages. Yi-Yi-Fu-Zi-Bai-Jiang-San (YYFZBJS) is a traditional Chinese medicine, and its extract inhibits the imbalance of enterotoxigenic bacteroid fragilis (ETBF) in the intestinal tract. The imbalance of ETBF stimulates STAT3-mediated polarization of M2 macrophages to promote malignant transformation of adenomas, thus promoting the occurrence and development of CAC. By inhibiting ETBF imbalance, YYFZBJS inhibits M2 polarization and plays an antitumor role in CAC (113). Ganoderma lucidum polysaccharide (GLP) is a component of Ganoderma lucidum, which reduce intestinal macrophage infiltration, down-regulate the expression of inflammatory factors such as IL-1 β, and attenuate the tumorigenesis of CAC (114). Shaoyao decoction (SYD), as a traditional Chinese medicine, also inhibit the proliferation of macrophages, reduce the expression of NF-κB and cytokines IL-1 β, IL-6, TNF-α, which inhibits the tumorigenesis of CAC. In addition, SYD down-regulates EMT and inhibits CAC by inhibiting the expression of cytokines (115). YTE17 is the active fraction of Garcinia yunnanensis. In CAC mouse model, YTE17 suppresses M2 polarization by down-regulating JNK, STAT3 and ERK signal pathways, thus inhibiting the tumorigenesis of CAC (116). Triptolide is the active ingredient of Tripterygium wilfordii, which has the effects of suppressing immunity and anti-inflammation. Triptolide inhibits M2 polarization of macrophages and reduce the secretion of anti-inflammatory cytokines. By inhibiting the promoting effect of M2 on the growth of CAC tumor, it plays an anti-tumor effect (117, 118). Isoliquiritigenin (ISL) is a flavonoid extracted from licorice and has anti-inflammatory effects. In CAC mouse model, ISL inhibits M2 polarization through cox-2/PGE2 pathway and IL-6/STAT3 pathway, and then prevents against tumorigenesis of CAC (119). Vitexin is the active ingredient of many traditional Chinese herbal medicines, which is found in hawthorn, mung bean and other traditional Chinese medicines. It has antioxidant, anti-inflammatory and antitumor effects. In the CAC mice, the M1 in the inflammatory tissues adjacent to the tumor of the mice treated with vitexin decreased and M2 increased. In tumor tissues, vitexin enhanced M1 polarization of macrophages. The reduction of inflammation of inflammatory tissue inhibits the occurrence of tumor. M1 polarization in tumor tissue promotes tumor immunity and reduces tumor burden (86). Dihydroartemisinin (DHA) is an active metabolite of artemisinin compounds, which could inhibit inflammation and tumor progression. At the early stage of CAC formation, DHA inhibits macrophage activation, reduces macrophage infiltration which inhibits the occurrence of CAC by inhibiting TLR4 signal. And in the late stage of CAC, DHA inhibited tumor growth by enhancing cell cycle arrest and apoptosis in tumor cells (120). Corylin is a natural polyphenol compound, which has antioxidant effect. It was found that corylin inhibits M1 polarization so that inhibits the tumorigenesis of CAC (121).
It is worth noting that these drugs mentioned above are in the preclinical animal model stage. Further human clinical trials are needed to determine whether it can be transferred to humans. In spite of this, we still have great expectations for targeted macrophage therapy for CAC.
In addition to drugs, diet also affects CAC by regulating macrophages. β-carotene (BC) has the ability of antioxidation. Previous study have found that drinking carrot juice rich in β-carotene reduces cell DNA damage (123). In addition, BC inhibits the growth of human leukemia and colon cancer by regulating NF-κB signal pathway (124). In CAC, BC inhibits tumor growth by inhibiting M2 polarization of macrophages (125).
Obesity is a risk factor for colorectal cancer. In obese mice model, IL-6 induces the expression of chemokine CCL-20 in M2, recruits immune cells expressing CCR-6, and promotes the progress of CAC (126). A study on western diet found that the western diet with high fat and low fiber promoted the infiltration of macrophages in colon tissue of mice, aggravated intestinal inflammation and promoted the development of CAC (127). Metformin combined with probiotics seems to inhibit the infiltration of macrophages and the destruction of intestinal epithelial barrier caused by western diet, thus inhibiting the occurrence of CAC (128).
The development from UC to CAC includes two processes: inflammation and tumor. Macrophages are also involved in both inflammation and tumor. Obviously, in the inflammatory stage of UC, excessive macrophage infiltration aggravates the damage of inflammation and promotes the occurrence of CAC. However, macrophages polarize into M1 macrophages and M2 macrophages, which play different roles in inflammation and tumor. M1 macrophages have the effects of promoting inflammation and antitumor, while M2 macrophages have the effects of anti-inflammation and promoting cancer. Before the tumor forms, M1 plays a pro-inflammatory role in promoting the tumorigenesis. After the formation of CAC, M1 polarization inhibits the progression of CAC by tumor immunity, while M2 promotes tumor progression and metastasis. Interestingly, some studies have found that M2 not only promotes tumor progression, but also increases the incidence of CAC (84, 85). This may be because M2 play a tumor-promoting role in the early stage of tumor formation.
Some drugs, especially traditional Chinese medicine, have been confirmed that play a role in the prevention and treatment of CAC by targeting macrophages. In the future, there is still great potential and possibility to prevent the occurrence of CAC and improve the prognosis of CAC patients by targeting macrophages.
GL provided direction and guidance throughout the preparation of this manuscript. MZ and XL wrote and edited the manuscript. QZ reviewed and made significant revisions to the manuscript. JY collected and prepared the related papers. All authors contributed to the article and approved the submitted version.
The authors declare that the research was conducted in the absence of any commercial or financial relationships that could be construed as a potential conflict of interest.
All claims expressed in this article are solely those of the authors and do not necessarily represent those of their affiliated organizations, or those of the publisher, the editors and the reviewers. Any product that may be evaluated in this article, or claim that may be made by its manufacturer, is not guaranteed or endorsed by the publisher.
1. Ungaro R, Mehandru S, Allen PB, Peyrin-Biroulet L, Colombel JF. Ulcerative colitis. Lancet Lond Engl (2017) 389(10080):1756–70. doi: 10.1016/S0140-6736(16)32126-2
2. Ng SC, Shi HY, Hamidi N, Underwood FE, Tang W, Benchimol EI, et al. Worldwide incidence and prevalence of inflammatory bowel disease in the 21st century: A systematic review of population-based studies. Lancet Lond Engl (2017) 390(10114):2769–78. doi: 10.1016/S0140-6736(17)32448-0
3. Lakatos L, Mester G, Erdélyi Z, David G, Pandúr T, Balogh M, et al. [Risk factors for ulcerative colitis associated colorectal cancers in a Hungarian cohort of ulcerative colitis patients]. Orv Hetil (2006) 147(4):175–81.
4. Bopanna S, Ananthakrishnan AN, Kedia S, Yajnik V, Ahuja V. Risk of colorectal cancer in Asian patients with ulcerative colitis: A systematic review and meta-analysis. Lancet Gastroenterol Hepatol (2017) 2(4):269–76. doi: 10.1016/S2468-1253(17)30004-3
5. Watanabe T, Konishi T, Kishimoto J, Kotake K, Muto T, Sugihara K, et al. Ulcerative colitis-associated colorectal cancer shows a poorer survival than sporadic colorectal cancer: A nationwide Japanese study. Inflammation Bowel Dis (2011) 17(3):802–8. doi: 10.1002/ibd.21365
6. Leowardi C, Schneider ML, Hinz U, Harnoss JM, Tarantino I, Lasitschka F, et al. Prognosis of ulcerative colitis-associated colorectal carcinoma compared to sporadic colorectal carcinoma: A matched pair analysis. Ann Surg Oncol (2016) 23(3):870–6. doi: 10.1245/s10434-015-4915-3
7. Porter RJ, Arends MJ, Churchhouse AMD, Din S. Inflammatory bowel disease-associated colorectal cancer: Translational risks from mechanisms to medicines. J Crohns Colitis (2021) 15(12):2131–41. doi: 10.1093/ecco-jcc/jjab102
8. Mattar MC, Lough D, Pishvaian MJ, Charabaty A. Current management of inflammatory bowel disease and colorectal cancer. Gastrointest Cancer Res GCR (2011) 4(2):53–61.
9. Itzkowitz SH, Yio X. Inflammation and cancer IV. colorectal cancer in inflammatory bowel disease: the role of inflammation. Am J Physiol Gastrointest Liver Physiol (2004) 287(1):G7–17. doi: 10.1152/ajpgi.00079.2004
10. Güç E, Pollard JW. Redefining macrophage and neutrophil biology in the metastatic cascade. Immunity (2021) 54(5):885–902. doi: 10.1016/j.immuni.2021.03.022
11. Park SH, Kang K, Giannopoulou E, Qiao Y, Kang K, Kim G, et al. Type I interferons and the cytokine TNF cooperatively reprogram the macrophage epigenome to promote inflammatory activation. Nat Immunol (2017) 18(10):1104–16. doi: 10.1038/ni.3818
12. Martinez FO, Helming L, Gordon S. Alternative activation of macrophages: An immunologic functional perspective. Annu Rev Immunol (2009) 27:451–83. doi: 10.1146/annurev.immunol.021908.132532
13. Subramanian Vignesh K, Landero Figueroa JA, Porollo A, Caruso JA, Deepe GS. Granulocyte macrophage-colony stimulating factor induced zn sequestration enhances macrophage superoxide and limits intracellular pathogen survival. Immunity (2013) 39(4):697–710. doi: 10.1016/j.immuni.2013.09.006
14. Virga F, Cappellesso F, Stijlemans B, Henze AT, Trotta R, Van Audenaerde J, et al. Macrophage miR-210 induction and metabolic reprogramming in response to pathogen interaction boost life-threatening inflammation. Sci Adv (2021) 7(19):eabf0466. doi: 10.1126/sciadv.abf0466
15. Hoeffel G, Debroas G, Roger A, Rossignol R, Gouilly J, Laprie C, et al. Sensory neuron-derived TAFA4 promotes macrophage tissue repair functions. Nature (2021) 594(7861):94–9. doi: 10.1038/s41586-021-03563-7
16. Qiu S, Xie L, Lu C, Gu C, Xia Y, Lv J, et al. Gastric cancer-derived exosomal miR-519a-3p promotes liver metastasis by inducing intrahepatic M2-like macrophage-mediated angiogenesis. J Exp Clin Cancer Res CR (2022) 41(1):296. doi: 10.1186/s13046-022-02499-8
17. Liang Y, Yang N, Pan G, Jin B, Wang S, Ji W. Elevated IL-33 promotes expression of MMP2 and MMP9 via activating STAT3 in alveolar macrophages during LPS-induced acute lung injury. Cell Mol Biol Lett (2018) 23:52. doi: 10.1186/s11658-018-0117-x
18. Xie J, Huang Z, Yu X, Zhou L, Pei F. Clinical implications of macrophage dysfunction in the development of osteoarthritis of the knee. Cytokine Growth Factor Rev (2019) 46:36–44. doi: 10.1016/j.cytogfr.2019.03.004
19. Bonnardel J, T’Jonck W, Gaublomme D, Browaeys R, Scott CL, Martens L, et al. Stellate cells, hepatocytes, and endothelial cells imprint the kupffer cell identity on monocytes colonizing the liver macrophage niche. Immunity (2019) 51(4):638–654.e9. doi: 10.1016/j.immuni.2019.08.017
20. De Simone G, Andreata F, Bleriot C, Fumagalli V, Laura C, Garcia-Manteiga JM, et al. Identification of a kupffer cell subset capable of reverting the T cell dysfunction induced by hepatocellular priming. Immunity (2021) 54(9):2089–2100.e8. doi: 10.1016/j.immuni.2021.05.005
21. Strauss-Ayali D, Conrad SM, Mosser DM. Monocyte subpopulations and their differentiation patterns during infection. J Leukoc Biol (2007) 82(2):244–52. doi: 10.1189/jlb.0307191
22. Mowat AM, Bain CC. Mucosal macrophages in intestinal homeostasis and inflammation. J Innate Immun (2011) 3(6):550–64. doi: 10.1159/000329099
23. Li B, Liu F, Ye J, Cai X, Qian R, Zhang K, et al. Regulation of macrophage polarization through periodic photo-thermal treatment to facilitate osteogenesis. Small Weinh Bergstr Ger (2022) 18(38):e2202691. doi: 10.1002/smll.202202691
24. Kubota T, Inoue M, Kubota N, Takamoto I, Mineyama T, Iwayama K, et al. Downregulation of macrophage Irs2 by hyperinsulinemia impairs IL-4-indeuced M2a-subtype macrophage activation in obesity. Nat Commun (2018) 9(1):4863. doi: 10.1038/s41467-018-07358-9
25. Chen MM, Xiao X, Lao XM, Wei Y, Liu RX, Zeng QH, et al. Polarization of tissue-resident TFH-like cells in human hepatoma bridges innate monocyte inflammation and M2b macrophage polarization. Cancer Discov (2016) 6(10):1182–95. doi: 10.1158/2159-8290.CD-16-0329
26. Tian L, Yu Q, Liu D, Chen Z, Zhang Y, Lu J, et al. Epithelial-mesenchymal transition of peritoneal mesothelial cells is enhanced by M2c macrophage polarization. Immunol Invest (2022) 51(2):301–15. doi: 10.1080/08820139.2020.1828911
27. Nikovics K, Morin H, Riccobono D, Bendahmane A, Favier AL. Hybridization-chain-reaction is a relevant method for in situ detection of M2d-like macrophages in a mini-pig model. FASEB J Off Publ Fed Am Soc Exp Biol (2020) 34(12):15675–86. doi: 10.1096/fj.202001496R
28. Su X, Yu Y, Zhong Y, Giannopoulou EG, Hu X, Liu H, et al. Interferon-γ regulates cellular metabolism and mRNA translation to potentiate macrophage activation. Nat Immunol (2015) 16(8):838–49. doi: 10.1038/ni.3205
29. Wu K, Yuan Y, Yu H, Dai X, Wang S, Sun Z, et al. The gut microbial metabolite trimethylamine n-oxide aggravates GVHD by inducing M1 macrophage polarization in mice. Blood (2020) 136(4):501–15. doi: 10.1182/blood.2019003990
30. Klichinsky M, Ruella M, Shestova O, Lu XM, Best A, Zeeman M, et al. Human chimeric antigen receptor macrophages for cancer immunotherapy. Nat Biotechnol (2020) 38(8):947–53. doi: 10.1038/s41587-020-0462-y
31. Verreck FAW, de Boer T, Langenberg DML, Hoeve MA, Kramer M, Vaisberg E, et al. Human IL-23-producing type 1 macrophages promote but IL-10-producing type 2 macrophages subvert immunity to (myco)bacteria. Proc Natl Acad Sci USA (2004) 101(13):4560–5. doi: 10.1073/pnas.0400983101
32. Rao X, Zhou X, Wang G, Jie X, Xing B, Xu Y, et al. NLRP6 is required for cancer-derived exosome-modified macrophage M2 polarization and promotes metastasis in small cell lung cancer. Cell Death Dis (2022) 13(10):891. doi: 10.1038/s41419-022-05336-0
33. Gordon S, Taylor PR. Monocyte and macrophage heterogeneity. Nat Rev Immunol (2005) 5(12):953–64. doi: 10.1038/nri1733
34. Vermare A, Guérin MV, Peranzoni E, Bercovici N. Dynamic CD8+ T cell cooperation with macrophages and monocytes for successful cancer immunotherapy. Cancers (2022) 14(14):3546. doi: 10.3390/cancers14143546
35. Arango Duque G, Descoteaux A. Macrophage cytokines: involvement in immunity and infectious diseases. Front Immunol (2014) 5:491. doi: 10.3389/fimmu.2014.00491
36. Cendrowicz E, Sas Z, Bremer E, Rygiel Tp. The role of macrophages in cancer development and therapy. Cancers (2021) 13(8). doi: 10.3390/cancers13081946
37. Liu T, Zhang L, Joo D, Sun Sc. NF-κB signaling in inflammation. Signal Transduct Target Ther (2017) 2. doi: 10.1038/sigtrans.2017.2
38. de la Paz Sánchez-Martínez M, Blanco-Favela F, Mora-Ruiz MD, Chávez-Rueda AK, Bernabe-García M, Chávez-Sánchez L. IL-17-differentiated macrophages secrete pro-inflammatory cytokines in response to oxidized low-density lipoprotein. Lipids Health Dis (2017) 16(1):196. doi: 10.1186/s12944-017-0588-1
39. Nishikawa K, Seo N, Torii M, Ma N, Muraoka D, Tawara I, et al. Interleukin-17 induces an atypical M2-like macrophage subpopulation that regulates intestinal inflammation. PloS One (2014) 9(9):e108494. doi: 10.1371/journal.pone.0108494
40. Chaplin DD. Overview of the immune response. J Allergy Clin Immunol (2010) 125(2 Suppl 2):S3–23. doi: 10.1016/j.jaci.2009.12.980
41. Martinez FO, Gordon S. The M1 and M2 paradigm of macrophage activation: Time for reassessment. F1000prime Rep (2014) 6:13. doi: 10.12703/P6-13
42. Zhou J, Zhang S, Guo C. Crosstalk between macrophages and natural killer cells in the tumor microenvironment. Int Immunopharmacol (2021) 101(Pt B):108374. doi: 10.1016/j.intimp.2021.108374
43. Yin G, Zhao C, Pei W. Crosstalk between macrophages and innate lymphoid cells (ILCs) in diseases. Int Immunopharmacol (2022) 110:108937. doi: 10.1136/gutjnl-2012-303063
44. Vivier E, Artis D, Colonna M, Diefenbach A, Di Santo JP, Eberl G, et al. Innate lymphoid cells: 10 years on. Cell (2018) 174(5):1054–66. doi: 10.1016/j.cell.2018.07.017
45. Uo M, Hisamatsu T, Miyoshi J, Kaito D, Yoneno K, Kitazume MT, et al. Mucosal CXCR4+ IgG plasma cells contribute to the pathogenesis of human ulcerative colitis through FcγR-mediated CD14 macrophage activation. Gut (2013) 62(12):1734–44. doi: 10.1136/gutjnl-2012-303063
46. Barrera-Vargas A, Gómez-Martín D, Carmona-Rivera C, Merayo-Chalico J, Torres-Ruiz J, Manna Z, et al. Differential ubiquitination in NETs regulates macrophage responses in systemic lupus erythematosus. Ann Rheum Dis (2018) 77(6):944–50. doi: 10.1136/annrheumdis-2017-212617
47. Na YR, Stakenborg M, Seok SH, Matteoli G. Macrophages in intestinal inflammation and resolution: A potential therapeutic target in IBD. Nat Rev Gastroenterol Hepatol (2019) 16(9):531–43. doi: 10.1038/s41575-019-0172-4
48. Lissner D, Schumann M, Batra A, Kredel LI, Kühl AA, Erben U, et al. Monocyte and M1 macrophage-induced barrier defect contributes to chronic intestinal inflammation in IBD. Inflamm Bowel Dis (2015) 21(6):1297–305. doi: 10.1097/MIB.0000000000000384
49. Kim W, Jang JH, Zhong X, Seo H, Surh YJ. 15-Deoxy-△12,14-Prostaglandin J2 promotes resolution of experimentally induced colitis. Front Immunol (2021) 12:615803. doi: 10.3389/fimmu.2021.615803
50. Takac B, Mihaljević S, Stefanić M, Glavas-Obrovac L, Kibel A, Samardzija M. Importance of interleukin 6 in pathogenesis of inflammatory bowel disease. Coll Antropol (2014) 38(2):659–64.
51. Sivakumar PV, Westrich GM, Kanaly S, Garka K, Born TL, Derry JMJ, et al. Interleukin 18 is a primary mediator of the inflammation associated with dextran sulphate sodium induced colitis: blocking interleukin 18 attenuates intestinal damage. Gut (2002) 50(6):812–20. doi: 10.1136/gut.50.6.812
52. Dou W, Zhang J, Ren G, Ding L, Sun A, Deng C, et al. Mangiferin attenuates the symptoms of dextran sulfate sodium-induced colitis in mice via NF-κB and MAPK signaling inactivation. Int Immunopharmacol (2014) 23(1):170–8. doi: 10.1016/j.intimp.2014.08.025
53. Danese S, Colombel JF, Lukas M, Gisbert JP, D’Haens G, Hayee B, et al. Etrolizumab versus infliximab for the treatment of moderately to severely active ulcerative colitis (GARDENIA): A randomised, double-blind, double-dummy, phase 3 study. Lancet Gastroenterol Hepatol (2022) 7(2):118–27. doi: 10.1016/S2468-1253(21)00294-6
54. Balkwill F. Tumour necrosis factor and cancer. Nat Rev Cancer (2009) 9(5):361–71. doi: 10.1038/nrc2628
55. Yu H, Dai C, Zhu W, Jin Y, Wang C. PFKFB3 increases IL-1β and TNF-α in intestinal epithelial cells to promote tumorigenesis in colitis-associated colorectal cancer. J Oncol (2022) 2022:6367437. doi: 10.1155/2022/6367437
56. Onizawa M, Nagaishi T, Kanai T, Oshima S, Nemoto Y, Nemoto Y, et al. Signaling pathway via TNF-alpha/NF-kappaB in intestinal epithelial cells may be directly involved in colitis-associated carcinogenesis. Am J Physiol Gastrointest Liver Physiol (2009) 296(4):G850–859. doi: 10.1152/ajpgi.00071.2008
57. Landskron G, de la Fuente M, Thuwajit P, Thuwajit C, Hermoso MA. Chronic inflammation and cytokines in the tumor microenvironment. J Immunol Res (2014) 2014:149185. doi: 10.1155/2014/149185
58. Kee JY, Ito A, Hojo S, Hashimoto I, Igarashi Y, Tsuneyama K, et al. CXCL16 suppresses liver metastasis of colorectal cancer by promoting TNF-α-induced apoptosis by tumor-associated macrophages. BMC Cancer (2014) 14:949. doi: 10.1186/1471-2407-14-949
59. Wang H, Wang HS, Zhou BH, Li CL, Zhang F, Wang XF, et al. Epithelial-mesenchymal transition (EMT) induced by TNF-α requires AKT/GSK-3β-mediated stabilization of snail in colorectal cancer. PloS One (2013) 8(2):e56664. doi: 10.1371/journal.pone.0056664
60. Danese S, Sans M, de la Motte C, Graziani C, West G, Phillips MH, et al. Angiogenesis as a novel component of inflammatory bowel disease pathogenesis. Gastroenterology (2006) 130(7):2060–73. doi: 10.1053/j.gastro.2006.03.054
61. Ji H, Cao R, Yang Y, Zhang Y, Iwamoto H, Lim S, et al. TNFR1 mediates TNF-α-induced tumour lymphangiogenesis and metastasis by modulating VEGF-C-VEGFR3 signalling. Nat Commun (2014), 5:4944. doi: 10.1038/ncomms5944
62. Zhang J, Zhao Y, Hou T, Zeng H, Kalambhe D, Wang B, et al. Macrophage-based nanotherapeutic strategies in ulcerative colitis. J Control Release Off J Control Release Soc (2020) 320:363–80. doi: 10.1016/j.jconrel.2020.01.047
63. Kobelt D, Zhang C, Clayton-Lucey IA, Glauben R, Voss C, Siegmund B, et al. Pro-inflammatory TNF-α and IFN-γ promote tumor growth and metastasis via induction of MACC1. Front Immunol (2020) 11:980. doi: 10.3389/fimmu.2020.00980
64. Popivanova BK, Kitamura K, Wu Y, Kondo T, Kagaya T, Kaneko S, et al. Blocking TNF-alpha in mice reduces colorectal carcinogenesis associated with chronic colitis. J Clin Invest (2008) 118(2):560–70. doi: 10.1172/JCI32453
65. Grimm M, Lazariotou M, Kircher S, Höfelmayr A, Germer CT, von Rahden BHA, et al. Tumor necrosis factor-α is associated with positive lymph node status in patients with recurrence of colorectal cancer–indications for anti-TNF-α agents in cancer treatment. Anal Cell Pathol Amst (2010) 33(3):151–63. doi: 10.1155/2010/891869
66. Xiao H, Guo Y, Li B, Li X, Wang Y, Han S, et al. M2-like tumor-associated macrophage-targeted codelivery of STAT6 inhibitor and IKKβ siRNA induces M2-to-M1 repolarization for cancer immunotherapy with low immune side effects. ACS Cent Sci (2020) 6(7):1208–22. doi: 10.1021/acscentsci.9b01235
67. Natoli M, Herzig P, Pishali Bejestani E, Buchi M, Ritschard R, Lloyd GK, et al. Plinabulin, a distinct microtubule-targeting chemotherapy, promotes M1-like macrophage polarization and anti-tumor immunity. Front Oncol (2021) 11:644608. doi: 10.3389/fonc.2021.644608
68. Sheng YH, Davies JM, Wang R, Wong KY, Giri R, Yang Y, et al. MUC1-mediated macrophage activation promotes colitis-associated colorectal cancer via activating the interleukin-6/Signal transducer and activator of transcription 3 axis. Cell Mol Gastroenterol Hepatol (2022) 14(4):789–811. doi: 10.1016/j.jcmgh.2022.06.010
69. Zhang Z, Dong L, Jia A, Chen X, Yang Q, Wang Y, et al. Glucocorticoids promote the onset of acute experimental colitis and cancer by upregulating mTOR signaling in intestinal epithelial cells. Cancers (2020) 12(4):E945. doi: 10.3390/cancers12040945
70. Liu Y, Fang X, Yuan J, Sun Z, Li C, Li R, et al. The role of corticotropin-releasing hormone receptor 1 in the development of colitis-associated cancer in mouse model. Endocr Relat Cancer (2014) 21(4):639–51. doi: 10.1530/ERC-14-0239
71. Li M, Huang T, Li X, Shi Z, Sheng Y, Hu M, et al. GDC-0575, a CHK1 inhibitor, impairs the development of colitis and colitis-associated cancer by inhibiting CCR2+ macrophage infiltration in mice. OncoTargets Ther (2021) 14:2661–72. doi: 10.2147/OTT.S297132
72. Zong G, Zhu Y, Zhang Y, Wang Y, Bai H, Yang Q, et al. SR-A1 suppresses colon inflammation and tumorigenesis through negative regulation of NF-κB signaling. Biochem Pharmacol (2018) 154:335–43. doi: 10.1016/j.bcp.2018.05.017
73. Davis TA, Conradie D, Shridas P, de Beer FC, Engelbrecht AM, de Villiers WJS. Serum amyloid a promotes inflammation-associated damage and tumorigenesis in a mouse model of colitis-associated cancer. Cell Mol Gastroenterol Hepatol (2021) 12(4):1329–41. doi: 10.1016/j.jcmgh.2021.06.016
74. Woo JK, Jang YS, Kang JH, Hwang JI, Seong JK, Lee SJ, et al. Ninjurin1 inhibits colitis-mediated colon cancer development and growth by suppression of macrophage infiltration through repression of FAK signaling. Oncotarget (2016) 7(20):29592–604. doi: 10.18632/oncotarget.9020
75. Kuai Y, Liu H, Liu D, Liu Y, Sun Y, Xie J, et al. An ultralow dose of the NADPH oxidase inhibitor diphenyleneiodonium (DPI) is an economical and effective therapeutic agent for the treatment of colitis-associated colorectal cancer. Theranostics (2020) 10(15):6743–57. doi: 10.7150/thno.43938
76. Hases L, Archer A, Williams C. ERβ and inflammation. Adv Exp Med Biol (2022) 1390:213–25. doi: 10.1007/978-3-031-11836-4_12
77. Emmi G, Bagni G, Lastraioli E, Di Patti F, Bettiol A, Fiorillo C, et al. A unique circulating miRNA profile highlights thrombo-inflammation in behçet’s syndrome. Ann Rheum Dis (2022) 81(3):386–97. doi: 10.1136/annrheumdis-2021-220859
78. Sun Z, Shao B, Liu Z, Dang Q, Guo Y, Chen C, et al. LINC01296/miR-141-3p/ZEB1-ZEB2 axis promotes tumor metastasis via enhancing epithelial-mesenchymal transition process. J Cancer (2021) 12(9):2723–34. doi: 10.7150/jca.55626
79. Alzahrani AM, Hanieh H, Ibrahim HIM, Mohafez O, Shehata T, Bani Ismail M, et al. Enhancing miR-132 expression by aryl hydrocarbon receptor attenuates tumorigenesis associated with chronic colitis. Int Immunopharmacol (2017) 52:342–51. doi: 10.1016/j.intimp.2017.09.015
80. Mantovani A, Sica A, Sozzani S, Allavena P, Vecchi A, Locati M. The chemokine system in diverse forms of macrophage activation and polarization. Trends Immunol (2004) 25(12):677–86. doi: 10.1016/j.it.2004.09.015
81. Deng F, He S, Cui S, Shi Y, Tan Y, Li Z, et al. A molecular targeted immunotherapeutic strategy for ulcerative colitis via dual-targeting nanoparticles delivering miR-146b to intestinal macrophages. J Crohns Colitis (2019) 13(4):482–94. doi: 10.1093/ecco-jcc/jjy181
82. Li H, Zhang C, Zhang H, Li H. Xanthine oxidoreductase promotes the progression of colitis-associated colorectal cancer by causing DNA damage and mediating macrophage M1 polarization. Eur J Pharmacol (2021), 906:174270. doi: 10.1016/j.ejphar.2021.174270
83. Zhu Y, Zhou J, Feng Y, Chen L, Zhang L, Yang F, et al. Control of intestinal inflammation, colitis-associated tumorigenesis, and macrophage polarization by fibrinogen-like protein 2. Front Immunol (2018) 9:87. doi: 10.3389/fimmu.2018.00087
84. Yuan Q, Gu J, Zhang J, Liu S, Wang Q, Tian T, et al. MyD88 in myofibroblasts enhances colitis-associated tumorigenesis via promoting macrophage M2 polarization. Cell Rep (2021) 34(5):108724. doi: 10.1016/j.celrep.2021.108724
85. Coburn LA, Singh K, Asim M, Barry DP, Allaman MM, Al-Greene NT, et al. Loss of solute carrier family 7 member 2 exacerbates inflammation-associated colon tumorigenesis. Oncogene (2019) 38(7):1067–79. doi: 10.1038/s41388-018-0492-9
86. Chen Y, Wang B, Yuan X, Lu Y, Hu J, Gao J, et al. Vitexin prevents colitis-associated carcinogenesis in mice through regulating macrophage polarization. Phytomedicine Int J Phytother Phytopharm (2021) 83:153489. doi: 10.1016/j.phymed.2021.153489
87. Jiang X, Wang C, Ke Z, Duo L, Wu T, Wang W, et al. The ion channel TRPV1 gain-of-function reprograms the immune microenvironment to facilitate colorectal tumorigenesis. Cancer Lett (2022) 527:95–106. doi: 10.1016/j.canlet.2021.12.012
88. Cheng D, Wang H, Cheng H, Zhang H, Dong G, Yan F, et al. IL-28B reprograms tumor-associated macrophages to promote anti-tumor effects in colon cancer. Int Immunopharmacol (2022) 109:108799. doi: 10.1016/j.intimp.2022.108799
89. Poh AR, Love CG, Masson F, Preaudet A, Tsui C, Whitehead L, et al. Inhibition of hematopoietic cell kinase activity suppresses myeloid cell-mediated colon cancer progression. Cancer Cell (2017) 31(4):563–575.e5. doi: 10.1016/j.ccell.2017.03.006
90. Aminin D, Wang YM. Macrophages as a “weapon” in anticancer cellular immunotherapy. Kaohsiung J Med Sci (2021) 37(9):749–58. doi: 10.1002/kjm2.12405
91. Muntjewerff EM, Meesters LD, van den Bogaart G. Antigen cross-presentation by macrophages. Front Immunol (2020) 11:1276. doi: 10.3389/fimmu.2020.01276
92. Qiu Y, Chen T, Hu R, Zhu R, Li C, Ruan Y, et al. Next frontier in tumor immunotherapy: macrophage-mediated immune evasion. biomark Res (2021) 9(1):72. doi: 10.1186/s40364-021-00327-3
93. Noy R, Pollard JW. Tumor-associated macrophages: from mechanisms to therapy. Immunity (2014) 41(1):49–61. doi: 10.1016/j.immuni.2014.06.010
94. Cheruku S, Rao V, Pandey R, Rao Chamallamudi M, Velayutham R, Kumar N. Tumor-associated macrophages employ immunoediting mechanisms in colorectal tumor progression: Current research in macrophage repolarization immunotherapy. Int Immunopharmacol (2023) 116:109569. doi: 10.1016/j.intimp.2022.109569
95. Larionova I, Cherdyntseva N, Liu T, Patysheva M, Rakina M, Kzhyshkowska J. Interaction of tumor-associated macrophages and cancer chemotherapy. Oncoimmunology (2019) 8(7):1596004. doi: 10.1080/2162402X.2019.1596004
96. Li W, Zhang X, Chen Y, Xie Y, Liu J, Feng Q, et al. G-CSF is a key modulator of MDSC and could be a potential therapeutic target in colitis-associated colorectal cancers. Protein Cell (2016) 7(2):130–40. doi: 10.1007/s13238-015-0237-2
97. Sun HW, Wu WC, Chen HT, Xu YT, Yang YY, Chen J, et al. Glutamine deprivation promotes the generation and mobilization of MDSCs by enhancing expression of G-CSF and GM-CSF. Front Immunol (2020) 11:616367. doi: 10.3389/fimmu.2020.616367
98. Lee YS, Saxena V, Bromberg JS, Scalea JR. G-CSF promotes alloregulatory function of MDSCs through a c-kit dependent mechanism. Cell Immunol (2021) 364:104346. doi: 10.1016/j.cellimm.2021.104346
99. Fionda C, Di Bona D, Kosta A, Stabile H, Santoni A, Cippitelli M. The POU-domain transcription factor Oct-6/POU3F1 as a regulator of cellular response to genotoxic stress. Cancers (2019) 11(6):E810. doi: 10.3390/cancers11060810
100. Hofmann E, Reichart U, Gausterer C, Guelly C, Meijer D, Müller M, et al. Octamer-binding factor 6 (Oct-6/Pou3f1) is induced by interferon and contributes to dsRNA-mediated transcriptional responses. BMC Cell Biol (2010) 11:61. doi: 10.1186/1471-2121-11-61
101. Lin Y, Wang D, Zhao H, Li D, Li X, Lin L. Pou3f1 mediates the effect of Nfatc3 on ulcerative colitis-associated colorectal cancer by regulating inflammation. Cell Mol Biol Lett (2022) 27(1):75. doi: 10.1186/s11658-022-00374-0
102. Pagano E, Elias JE, Schneditz G, Saveljeva S, Holland LM, Borrelli F, et al. Activation of the GPR35 pathway drives angiogenesis in the tumour microenvironment. Gut (2022) 71(3):509–20. doi: 10.1136/gutjnl-2020-323363
103. Hardbower DM, Coburn LA, Asim M, Singh K, Sierra JC, Barry DP, et al. EGFR-mediated macrophage activation promotes colitis-associated tumorigenesis. Oncogene (2017) 36(27):3807–19. doi: 10.1038/onc.2017.23
104. Dai L, Liu Y, Yin Y, Li J, Dong Z, Chen N, et al. SARI suppresses colitis-associated cancer development by maintaining MCP-1-mediated tumour-associated macrophage recruitment. J Cell Mol Med (2020) 24(1):189–201. doi: 10.1111/jcmm.14699
105. Lv Z, Wang Z, Luo L, Chen Y, Han G, Wang R, et al. Spliceosome protein Eftud2 promotes colitis-associated tumorigenesis by modulating inflammatory response of macrophage. Mucosal Immunol (2019) 12(5):1164–73. doi: 10.1038/s41385-019-0184-y
106. Zhang W, Fu X, Xie J, Pan H, Han W, Huang W. miR-26a attenuates colitis and colitis-associated cancer by targeting the multiple intestinal inflammatory pathways. Mol Ther Nucleic Acids (2021) 24:264–73. doi: 10.1016/j.omtn.2021.02.029
107. Eom YW, Akter R, Li W, Lee S, Hwang S, Kim J, et al. M1 macrophages promote TRAIL expression in adipose tissue-derived stem cells, which suppresses colitis-associated colon cancer by increasing apoptosis of CD133+ cancer stem cells and decreasing M2 macrophage population. Int J Mol Sci (2020) 21(11):E3887. doi: 10.3390/ijms21113887
108. Zhao LN, Li JY, Yu T, Chen GC, Yuan YH, Chen QK. 5-aminosalicylates reduce the risk of colorectal neoplasia in patients with ulcerative colitis: An updated meta-analysis. PloS One (2014) 9(4):e94208. doi: 10.1371/journal.pone.0094208
109. Wang Q, Li XL, Mei Y, Ye JC, Fan W, Cheng GH, et al. The anti-inflammatory drug dimethyl itaconate protects against colitis-associated colorectal cancer. J Mol Med Berl Ger (2020) 98(10):1457–66. doi: 10.1007/s00109-020-01963-2
110. Zhang W, Chen L, Ma K, Zhao Y, Liu X, Wang Y, et al. Polarization of macrophages in the tumor microenvironment is influenced by EGFR signaling within colon cancer cells. Oncotarget (2016) 7(46):75366–78. doi: 10.18632/oncotarget.12207
111. Tang B, Zhu J, Fang S, Wang Y, Vinothkumar R, Li M, et al. Pharmacological inhibition of MELK restricts ferroptosis and the inflammatory response in colitis and colitis-propelled carcinogenesis. Free Radic Biol Med (2021) 172:312–29. doi: 10.1016/j.freeradbiomed.2021.06.012
112. Liu Q, Li X, Zhang H, Li H. Mannose attenuates colitis-associated colorectal tumorigenesis by targeting tumor-associated macrophages. J Cancer Prev (2022) 27(1):31–41. doi: 10.15430/JCP.2022.27.1.31
113. Chai N, Xiong Y, Zhang Y, Cheng Y, Shi W, Yao Y, et al. YYFZBJS inhibits colorectal tumorigenesis by remodeling gut microbiota and influence on M2 macrophage polarization in vivo and in vitro. Am J Cancer Res (2021) 11(11):5338–57. doi: 10.2139/ssrn.3837225
114. Guo C, Guo D, Fang L, Sang T, Wu J, Guo C, et al. Ganoderma lucidum polysaccharide modulates gut microbiota and immune cell function to inhibit inflammation and tumorigenesis in colon. Carbohydr Polym (2021), 267:118231. doi: 10.1016/j.carbpol.2021.118231
115. Lin X, Yi Z, Diao J, Shao M, Zhao L, Cai H, et al. ShaoYao decoction ameliorates colitis-associated colorectal cancer by downregulating proinflammatory cytokines and promoting epithelial-mesenchymal transition. J Transl Med (2014) 12:105. doi: 10.1186/1479-5876-12-105
116. Sui H, Tan H, Fu J, Song Q, Jia R, Han L, et al. The active fraction of garcinia yunnanensis suppresses the progression of colorectal carcinoma by interfering with tumorassociated macrophage-associated M2 macrophage polarization in vivo and in vitro. FASEB J Off Publ Fed Am Soc Exp Biol (2020) 34(6):7387–403. doi: 10.1096/fj.201903011R
117. Li H, Xing X, Zhang X, Li L, Jiang Z, Wang T, et al. Effects of triptolide on the sphingosine kinase - sphingosine-1-phosphate signaling pathway in colitis-associated colon cancer. Int Immunopharmacol (2020) 88:106892. doi: 10.1016/j.intimp.2020.106892
118. Li H, Li L, Mei H, Pan G, Wang X, Huang X, et al. Antitumor properties of triptolide: phenotype regulation of macrophage differentiation. Cancer Biol Ther (2020) 21(2):178–88. doi: 10.1080/15384047.2019.1679555
119. Zhao H, Zhang X, Chen X, Li Y, Ke Z, Tang T, et al. Isoliquiritigenin, a flavonoid from licorice, blocks M2 macrophage polarization in colitis-associated tumorigenesis through downregulating PGE2 and IL-6. Toxicol Appl Pharmacol (2014) 279(3):311–21. doi: 10.1016/j.taap.2014.07.001
120. Bai B, Wu F, Ying K, Xu Y, Shan L, Lv Y, et al. Therapeutic effects of dihydroartemisinin in multiple stages of colitis-associated colorectal cancer. Theranostics (2021) 11(13):6225–39. doi: 10.7150/thno.55939
121. Chang ZY, Liu HM, Leu YL, Hsu CH, Lee TY. Modulation of gut microbiota combined with upregulation of intestinal tight junction explains anti-inflammatory effect of corylin on colitis-associated cancer in mice. Int J Mol Sci (2022) 23(5):2667. doi: 10.3390/ijms23052667
122. Wu T, Dai Y, Wang W, Teng G, Jiao H, Shuai X, et al. Macrophage targeting contributes to the inhibitory effects of embelin on colitis-associated cancer. Oncotarget (2016) 7(15):19548–58. doi: 10.18632/oncotarget.6969
123. Lee HJ, Park YK, Kang MH. The effect of carrot juice, β-carotene supplementation on lymphocyte DNA damage, erythrocyte antioxidant enzymes and plasma lipid profiles in Korean smoker. Nutr Res Pract (2011) 5(6):540–7. doi: 10.4162/nrp.2011.5.6.540
124. Palozza P, Serini S, Torsello A, Di Nicuolo F, Piccioni E, Ubaldi V, et al. Beta-carotene regulates NF-kappaB DNA-binding activity by a redox mechanism in human leukemia and colon adenocarcinoma cells. J Nutr (2003) 133(2):381–8. doi: 10.1093/jn/133.2.381
125. Lee NY, Kim Y, Kim YS, Shin JH, Rubin LP, Kim Y. β-carotene exerts anti-colon cancer effects by regulating M2 macrophages and activated fibroblasts. J Nutr Biochem (2020) 82:108402. doi: 10.1016/j.jnutbio.2020.108402
126. Wunderlich CM, Ackermann PJ, Ostermann AL, Adams-Quack P, Vogt MC, Tran ML, et al. Obesity exacerbates colitis-associated cancer via IL-6-regulated macrophage polarisation and CCL-20/CCR-6-mediated lymphocyte recruitment. Nat Commun (2018) 9(1):1646. doi: 10.1038/s41467-018-03773-0
127. Kim IW, Myung SJ, Do MY, Ryu YM, Kim MJ, Do EJ, et al. Western-Style diets induce macrophage infiltration and contribute to colitis-associated carcinogenesis. J Gastroenterol Hepatol (2010) 25(11):1785–94. doi: 10.1111/j.1440-1746.2010.06332.x
Keywords: ulcerative colitis, colitis-associated colorectal cancer, macrophage infiltration, macrophage polarization, macrophage
Citation: Zhang M, Li X, Zhang Q, Yang J and Liu G (2023) Roles of macrophages on ulcerative colitis and colitis-associated colorectal cancer. Front. Immunol. 14:1103617. doi: 10.3389/fimmu.2023.1103617
Received: 20 November 2022; Accepted: 06 March 2023;
Published: 16 March 2023.
Edited by:
Hiroshi Nakase, Sapporo Medical University, JapanCopyright © 2023 Zhang, Li, Zhang, Yang and Liu. This is an open-access article distributed under the terms of the Creative Commons Attribution License (CC BY). The use, distribution or reproduction in other forums is permitted, provided the original author(s) and the copyright owner(s) are credited and that the original publication in this journal is cited, in accordance with accepted academic practice. No use, distribution or reproduction is permitted which does not comply with these terms.
*Correspondence: Gang Liu, bGcxMDU5QHRtdS5lZHUuY24=
†These authors have contributed equally to this work and share first authorship
Disclaimer: All claims expressed in this article are solely those of the authors and do not necessarily represent those of their affiliated organizations, or those of the publisher, the editors and the reviewers. Any product that may be evaluated in this article or claim that may be made by its manufacturer is not guaranteed or endorsed by the publisher.
Research integrity at Frontiers
Learn more about the work of our research integrity team to safeguard the quality of each article we publish.