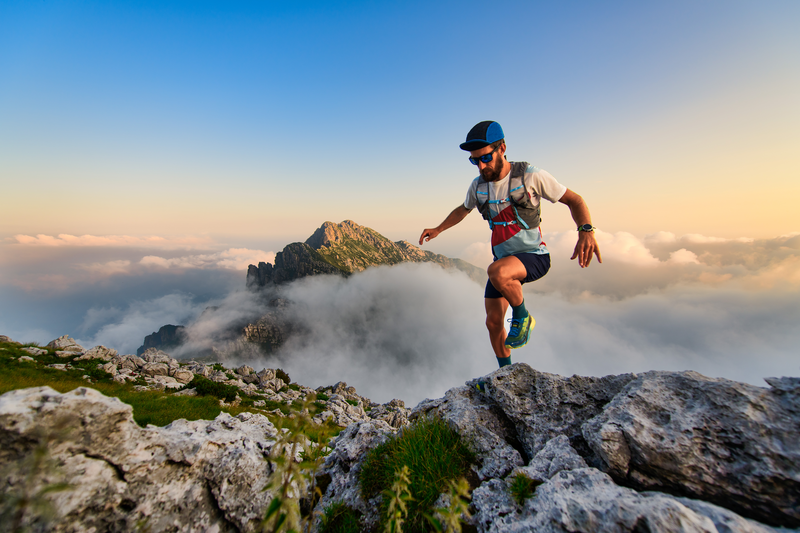
95% of researchers rate our articles as excellent or good
Learn more about the work of our research integrity team to safeguard the quality of each article we publish.
Find out more
REVIEW article
Front. Immunol. , 13 February 2023
Sec. Cytokines and Soluble Mediators in Immunity
Volume 14 - 2023 | https://doi.org/10.3389/fimmu.2023.1100869
This article is part of the Research Topic Progress and challenges with therapeutic targeting of chemokine receptors View all 10 articles
Rheumatoid arthritis (RA) is an autoimmune disease that commonly causes inflammation and bone destruction in multiple joints. Inflammatory cytokines, such as IL-6 and TNF-α, play important roles in RA development and pathogenesis. Biological therapies targeting these cytokines have revolutionized RA therapy. However, approximately 50% of the patients are non-responders to these therapies. Therefore, there is an ongoing need to identify new therapeutic targets and therapies for patients with RA. In this review, we focus on the pathogenic roles of chemokines and their G-protein-coupled receptors (GPCRs) in RA. Inflamed tissues in RA, such as the synovium, highly express various chemokines to promote leukocyte migration, tightly controlled by chemokine ligand-receptor interactions. Because the inhibition of these signaling pathways results in inflammatory response regulation, chemokines and their receptors could be promising targets for RA therapy. The blockade of various chemokines and/or their receptors has yielded prospective results in preclinical trials using animal models of inflammatory arthritis. However, some of these strategies have failed in clinical trials. Nonetheless, some blockades showed promising results in early-phase clinical trials, suggesting that chemokine ligand-receptor interactions remain a promising therapeutic target for RA and other autoimmune diseases.
Chemokines are a family of small chemotactic cytokines (approximately 8-15 kDa). Chemokine ligand-receptor interactions control leukocyte migration during inflammation, promoting migration from the circulation into the extravascular space in inflamed tissues (1, 2). Nearly 50 chemokines have been identified in mammals (3), commonly formed by four conserved cysteine residues—the first and third and the second and fourth forming disulfide bridges. Chemokines are divided into four subclasses according to the position of the first two conserved N-terminal cysteine residues: CC-chemokines (β-chemokines), having adjacent cysteine residues; CXC-chemokines (α-chemokines), having two cysteine residues separated by another amino acid; CX3C-chemokines (δ-chemokines), having two cysteine residues separated by three other amino acids; and C-chemokines (γ-chemokines), with only the second and fourth cysteine residues (4, 5). The glutamate-leucine-arginine (ELR)-positive (CXCL1, CXCL2, CXCL3, CXCL5, CXCL6, CXCL7, and CXCL8) but not ELR-negative CXC chemokines (CXCL4, CXCL4L1, CXCL9, CXCL10, and CXCL11) have three amino acid residues (Glu-Leu-Arg) before the first conserved cysteine residue. The ELR motif is important for angiogenesis (6, 7). Some chemokines are activated by matrix metalloproteinase-mediated (MMPs)-mediated proteolysis (8).
Chemokine receptors are expressed on the surface of immune cells. “Classical” chemokine receptors are G-protein-coupled transmembrane receptors (GPCRs) and induce cell migration, whereas “atypical” chemokine receptors (ACKRs) are not coupled to G proteins and regulate cell migration (9, 10). ACKRs scavenge chemokines to regulate chemokine gradients and dampen inflammation in a G protein-independent manner (3, 11, 12). Chemokine ligand-receptor interactions are presented in Table 1 (13).
The chemokine system may play a central role in rheumatoid arthritis (RA) pathogenesis. Several chemokines are highly expressed in the blood and inflammatory tissues, such as arthritic joints, of patients with RA. Furthermore, some genes encoding chemokine ligands and receptors have been reported as risk factors for RA development (14–42), and their expression is associated with clinical disease activity and severity (43–69). The regulation of immune cell recruitment into joints represents a major hallmark for therapeutic intervention, as the inhibition of the chemokine system can suppress the characteristic inflammation of RA, thereby halting its pathogenesis.
In this review, we summarize the pathogenic roles of chemokines and their receptors in RA. In addition, we provide evidence from recent human clinical trials using inhibitors of the chemokine system in RA and discuss the potential clinical benefits of chemokine blockade in patients with RA.
RA is an autoimmune disease characterized by autoantibody production, leading to the settlement of inflammatory processes with cytokine and chemokine production. This results in synovial inflammation, hyperplasia and swelling, cartilage and bone destruction and deformity, and systemic features, such as cardiovascular, pulmonary, and skeletal disorders (70).
Inflammatory cytokines, including interleukin (IL)-1, IL-6, and tumor necrosis factor (TNF)-α, play important roles in RA development. Biological agents, such as TNF-α and IL-6 inhibitors, have revolutionized RA therapies (71). However, approximately 50% of patients with RA are non-responders to these therapeutic approaches (72). Therefore, there is an ongoing need to identify novel targets and treatment strategies for RA.
Animal models of inflammatory arthritis have provided determinant information for the understanding of RA pathogenesis and development of RA therapeutics. Models such as type II collagen-induced arthritis (CIA) (73), collagen antibody-induced arthritis (CAIA) (74), K/BxN arthritogenic serum transfer model of arthritis (K/BxN) (75), and adjuvant-induced arthritis (AIA) (76) show RA-like arthritic phenotypes, including synovial hyperplasia with leukocyte infiltration and bone destruction. Furthermore, models of inflammatory arthritis and RA also show upregulated expression of chemokine ligands and their receptors in the serum, immune cells, and synovium (77–84). Thus, these animal models are useful for elucidating the pathogenic role of chemokines in RA.
Various chemokines are highly expressed in the serum, synovial fluids (SFs), and synovial tissues (STs) of patients with RA compared with those of healthy donors (HD) (Table 2). For instance, the CC-chemokines CCL2, CCL5, CCL11, CCL13, CCL18, CCL19, CCL20, CCL22, CXC-chemokine CXCL2, CXCL5, CXCL8, CXCL9, CXCL10, CXCL11, CXCL12, CXCL13, and CXCL16 were increased in the serum and/or plasma of patients with RA compared with those of HD (43, 44, 46, 47, 54, 57, 85–90).
Peripheral blood mononuclear cells (PBMCs) derived from patients with RA highly express CCL2, CCL3, CXCL2, and CX3CL1 compared to those derived from HD (91–93). These chemokines are differentially produced by different immune cells in patients with RA: T cells produce CCL3, CCL4, CCL5, and CXCL13 (93–96); B cells express CXCL9/10 (97); monocytes generate CCL2, CCL18, CCL19, and CX3CL1 (93, 98, 99); macrophages express CCL25, CXCL4, CXCL7, and CX3CL1 (93, 100, 101); dendritic cells (DCs) produce CCL17, CCL18, and CCL19 (102–104); and neutrophils generate CCL3 and CCL18 (103, 105, 106).
CC-chemokines are expressed in RA synovial endothelial cells (ECs) in different concentrations (high-abundance: CCL7, CCL8, CCL14, CCL16, CCL19, and CCL22; low-abundance: CCL1-3, CCL5, CCL10, CCL11, CCL12, CCL13, CCL15, CCL17, CCL18, CCL20, CCL21, CCL23, CCL24, CCL25, CCL26, CCL27, and CCL28 (107), whereas ELR+ CXC-chemokines (CXCL1, CXCL2, CXCL3, CXCL5, and CXCL6) are expressed in the SFs of patients with RA (108). Additionally, various CC-chemokines (CCL1, CCL2, CCL3, CCL4, CCL5, CCL7, CCL11, CCL13, CCL15, CCL17, CCL18, CCL19, CCL20, CCL21, CCL25, and CCL28), CXCL8, CXCL9, and CXCL10 are also expressed in SFs, STs, and/or fibroblast-like synoviocytes (FLSs) derived from patients with RA (86, 91, 100, 102, 109–121).
Cartilage and chondrocytes from patients with RA express CCL2, CCL5, CCL13, CCL18, CCL25, CXCL1, CXCL8, CXCL10, and XCL1 (109, 118, 122, 123). In addition, osteoclasts (OCs) and OC progenitors (OCPs) from patients with RA produce CCL2, CCL3, CCL4, CCL5, CXCL9, CXCL10, and CX3CL1 (124, 125).
Several chemokines (CCL3, CCL4, CCL5, CCL3L1, CCL21, CCL26, CXCL8, CXCL9, CXCL10, CXCL12, and CXCL13) have been reported as risk factors for RA development (11–25). Certain chemokines (CCL2, CCL5, CCL20, CCL23, CCL25, CXCL2, CXCL5, CXCL7, CXCL8, CXCL9, CXCL11, CXCL12, and CXCL13) are associated with disease activity and/or severity (40–58). Moreover, CCL23, CXCL9, CXCL10, CXCL11, and CXCL13 may be potential biomarkers for RA (48, 56).
Multiple chemokine receptors as well as chemokines contribute to RA pathogenesis (Table 3). Polymorphisms in CCR2, CCR5, CCR6, and CCR7-encoding genes are considered risk factors for RA development (29–42). CD4+ cells expressing CCR5 are increased in the blood of patients with active RA compared with that of patients with inactive RA patients and HD. Furthermore, CD4+ cells expressing CX3CR1 are decreased in patients with RA, and the CD4+ CD95+ T cell subset expressing CCR7 is associated with disease activity (63). In addition, CXCR4 and CXCL12 show higher expression in the serum and joints of patients with active RA than in those of HD and patients with RA remission. Moreover, the expression of these chemokines in the synovium has been correlated with disease score in patients with RA treated with TNF-α inhibitors (54, 55).
Chemokine receptors on T cells [CCR2, CCR4, CCR5, CCR6, CCR7, CXCR3, CXCR4, CXCR6, and CX3CR1 (111, 126–129)], B cells [CCR5, CCR6, CCR7, CXCR3, CXCR4, and CXCR5 (130–132)], monocytes [CCR1, CCR2, CCR5, CCR9, CXCR4, and CX3CR1 (33, 100, 133–137)], macrophages [CCR7, CCR9, and CXCR3 (100, 138)], and neutrophils [CCR1, CCR5, CXCR1, and CXCR2 (79, 106, 139)] were more highly expressed in patients with RA than in HD.
Stromal cells of patients with RA also express chemokine receptors. For instance, ECs express CCR7, CCR10, CXCR2, CXCR4, CXCR5, CXCR6, CXCR7, and ACKR1 (6, 140–147), whereas FLSs express CCR2, CCR3, CCR5, CCR6, CCR9, CXCR2, CXCR4, CXCR6, and ACKR6 (86, 100, 115, 148–150). OCs and OCPs express CCR1, CCR2, CCR3, CCR4, CCR7, CCR9, CXCR2, CXCR3, CXCR4, and CX3CR1 (124, 125).
Chemokines and their receptors control lymphocyte recruitment to inflamed joints in RA patients and animal models (Figure 1). In RA patients, the recruitment of T cells into the synovium is controlled by CCR4, CCR5, CXCR3, CXCR4, and CXCR6 (95, 97, 102, 126, 127, 129, 151–154). Inhibition of CCL2, CCL5, or CXCL12 suppresses Th1 cell migration in vitro, suggesting that these chemokines might promote Th1 cell recruitment to the RA synovium (129). CD4+ T cells of patients with RA treated in vitro with anti-CCL22 antibodies differentiate into regulatory T cells (Tregs) via STAT5 activation (85). In SCID mice implanted with human RA synovium, recruitment of CD4+ CD28-T cells, resembling effector memory T cells, is controlled by CCL5 and CXCL12 (127). CCR6 promotes Th17 cell recruitment into the inflamed joint in SKG arthritic mice (155), myostatin-deficient (KO) mice, TNF-α transgenic (Tg) arthritic mice (156), and chemotactic ability of Th17 cells derived from patients with RA in vitro model (155, 157). In addition, the CCR4 blockade suppresses Th17 cell migration to the arthritic joints in CIA mice (84). The CIA model also shows joint infiltration of CCR6+ type 3 innate lymphoid cells (iLC3s), which highly express IL-17A and IL-22. Furthermore, the number of CCR6+ iLC3s in the SF of patients with RA is correlated with disease activity (158).
Figure 1 The major contribution of chemokine ligand-receptor interactions in RA patients. I. T cell recruitment: FLSs generate CCL2, CCL5, CCL20, CXCL13, and CXCL16; OCs produce CXCL10; ECs release CCL22; monocytes, macrophages, and OCs produce CX3CL1, promoting T cell recruitment into the arthritic joints through the indicated chemokine receptors. II. B cell recruitment: FLSs generate CCL19-21, CXCL12, and CXCL13, enhancing B cell recruitment into arthritic joints through the respective chemokine receptors. III. Neutrophil recruitment: FLSs generate CCL3-5, CXCL1, CXCL2, CXCL5, and CXCL8, leading to neutrophil recruitment into arthritic joints through the indicated chemokine receptors. IV. Monocyte recruitment: FLSs generate CCL2, CCL5, CCL25, and CXCL12; synovial macrophages produce CCL25 and CX3CL1; OCs produce CCL2, CCL5, and CX3CL1, promoting monocyte recruitment into the arthritic joints through chemokine receptor signaling. V. Synovial macrophage development: FLSs and macrophages generate CCL25, which promotes monocyte differentiation into macrophages. VI. Osteoclast progenitor recruitment: FLSs generate CCL11, CCL19, and CCL21, while ECs generate CCL19, leading to OCP recruitment into arthritic joints through the indicated chemokine receptors. VII. Osteoclast differentiation: FLSs and macrophages generate CCL25, and synovial macrophages and OCs generate CX3CL1, promoting osteoclast differentiation through chemokine receptors. VIII. Osteoclastogenesis: FLSs, T cells, and neutrophils generate CCL3; FLSs generate CXCL2; and OCs generate CXCL10, ensuring osteoclastogenesis through the indicated chemokine receptors. IX. Endothelial cell recruitment: FLSs generate CCL28, CXCL13, and CXCL16, stimulating endothelial cell recruitment into arthritic joints through the indicated chemokine receptors. X. Angiogenesis: FLSs generate CCL21, CCL28, CXCL8, CXCL12, CXCL13, and CXCL16, supporting angiogenesis through indicated chemokine receptors. Black arrow indicates chemokine production, and red bar shows chemokine ligand-receptor interaction.
Follicular helper T (Tfh) cells contribute to the formation and maintenance of germinal centers (GC). CXCR5+ Tfh cells are increased in the blood of patients with RA and CIA mice. Furthermore, the number of these cells correlates with the levels of clinical RA markers, such as C-reactive protein, rheumatoid factor, and erythrocyte sedimentation rate (159). In transwell experiments, the CXCL13/CXCR5 axis showed chemotactic activity in B cells of patients with RA (130). CXCR5 KO mice are resistant to CIA development; B cell-specific CXCR5 deficiency leads to mild arthritis with impaired germinal center (GC) response and antibody production, whereas T cell-specific CXCR5 deficiency promotes resistance to arthritis development by impaired GC response, antibody production, and inflammatory cytokine response (160).
CCL19, CXCL12, and CXCL13 levels in the serum of patients with RA are associated with the clinical response to rituximab (89). In addition, these chemokine levels in the SFs of patients are also correlated with both the number of CD19+ CD24hi CD27+ B cells and disease activity and severity (161). The CCL20/CCR6, CXCL12/CXCR4, and CXCL13/CXCR5 axes regulate B cell migration into RA SFs (130, 161, 162), whereas the CCL19/CCR7, CCL20/CCR6, CCL21/CCR7, and CXCL12/CXCR4 axes regulate B cell recruitment into the RA synovium (130, 131).
CCL2 and CXCL8 enhance neutrophil chemotactic ability in cells from patients with RA, and CCR2 KO mice are resistant to AIA model through the suppression of CCL2/CCR2-induced neutrophil recruitment (163). CCL3 expression is associated with the neutrophil number in the SFs from patients with RA (106). Furthermore, the chemotactic activities of CCL4 and CCL5 are also correlated with the number of neutrophils in the SFs from patients with RA (94). An amino-terminal-modified methionylated form of CCL5 (Met-RANTES) antagonized the binding of CCL3 and CCL5 to their receptors CCR1 and CCR5, respectively, and the blockade inhibited arthritis in AIA rats via the suppression of neutrophil and macrophage migration into the joints (164).
ELR-positive CXC chemokines (CXCL1, CXCL2, CXCL3, CXCL5, CXCL6, CXCL7, and CXCL8) regulate neutrophil migration and angiogenesis via the receptor CXCR2 (6). CXCL5 expressed in RA SFs promotes neutrophil recruitment to EC in vitro (165). CXCL1 and CXCL5 induce neutrophil migration into the articular cavity of AIA mice, and chemotaxis is inhibited by the blockade of CXCR1/CXCR2 with repertaxin (79). CXCL1 and CXCL8 induce neutrophil chemotaxis in vitro, which is also inhibited by the blockade of CXCR1/CXCR2 and DF 2162, the later inhibiting neutrophil recruitment in zymosan-induced arthritis in mice and AIA in rats (166, 167). Furthermore, in vitro, the ligand for CXCR1, CXCR2, CXCL2 enhances murine neutrophil migration, and the CXCL2-neutralizing antibody inhibits migration (139). Both CXCR2 and CCR1 are expressed in mice neutrophils, and their abrogation attenuates inflammatory arthritis in K/BxN mice (168). Recent in vivo imaging of joints showed that CCR1 promotes neutrophil crawling on the joint endothelium, whereas CXCR2 amplifies late neutrophil recruitment and survival in the joint (169). CXCL9 blocking peptide decreases neutrophil recruitment into the joints of AIA mice (170).
In vitro approaches further clarified the role of some of these chemokines in RA samples. CCL2/CCR2 and CCL5/CCR5 enhance monocyte chemotaxis (171). CCL3, highly expressed in RA SFs, enhances macrophage chemotaxis (172). CCL25 induces the chemotactic activity of monocytes and differentiation into macrophages (100). CCR9 abrogation suppressed CD11b+ cell migration into joints in a CIA model (82). The CXCL12/CXCR4 axis promotes monocyte migration into the joints of RA ST-transplanted SCID mice (173). Furthermore, increased CX3CL1 expression in SFs of RA patients induced monocyte chemotaxis via CX3CR1 in vitro (93).
Increased OC differentiation and activity lead to bone loss and joint destruction in patients with RA. CCL3 enhanced osteoclastogenesis via OC migration and activation in the AIA rat model (174). CCL11/CCR3 induced OCP migration and bone resorption in vitro (175). CCL19 and CCL21, increased in RA SFs and serum, and their receptor CCR7, expressed in murine OCPs. These chemokines did not affect OC differentiation but promoted OC migration and increased OC resorption activity in vitro and in vivo (176). The CCL25/CCR9 axis initiates the transformation of OCPs into mature OCs in vitro (100). CXCL2 promotes monocyte recruitment and osteoclastogenesis in RA samples in vitro, as well as in mouse bone marrow-derived macrophages (90, 177). CXCL10 KO and CXCR3 KO in mice ameliorated arthritis in CAIA model by suppressing macrophage and T cell accumulation in arthritic joints. In addition, CXCL10 and CXCR3 inhibition decreased osteoclastogenic cytokine levels in the serum and spleen of CAIA (154). Furthermore, in vitro, CX3CL1/CX3CR1 regulates monocyte, DC, and OCP differentiation into osteoclasts (125, 178).
Several chemokines contribute to cartilage damage in arthritic joints. For instance, interferon-γ (IFN-γ) enhances CCL13 expression, inducing RA FLS proliferation in the cartilage of patients with RA in vitro (123). CXCL12, which induces MMP-3 production in chondrocytes in vitro, is also highly expressed in the SFs of patients with RA (179). CCL5 induces both MMP-1- and MMP-13-mediated collagen degradation in the SFs of patients with RA (180). In addition, CXCR4-CXCL12 signaling increased both MMP-9 and MMP-13 production in human chondrocytes in vitro (181).
Chemokine receptors are also expressed in stromal cells, although their functions remain unknown. Angiogenesis is determinant for RA pathogenesis, namely for synovial proliferation and pannus formation (182). CCL21, in vitro, induces human microvascular ECs angiogenesis and migration via CCR7, suggesting that the CCL21/CCR7 axis may contribute to angiogenesis in RA (140). The CXCL12/CXCR4 axis also showed angiogenic activity in RA SFs in Matrigel in vivo (145) and the CXCL13/CXCR5 axis facilitated EC migration and angiogenesis in CIA mice (142). CCL28 and CCR10, highly expressed in RA synovium, regulate angiogenesis by EC recruitment, and CCL28 blockade inhibits EC migration and capillary formation (141). The CXCL16/CXCR6 axis promoted chemotactic and angiogenic activity in human umbilical vein ECs (HUVEC), which is a cell line (147). The CXCL12/CXCR4 and CXCL12/CXCR7 axes promote angiogenic activity in HUVEC, contributing to RA angiogenesis. CXCR7 is also expressed on ECs in the RA synovium. Furthermore, CXCR7 blockade ameliorated arthritis in CIA mice by suppressing angiogenesis (183).
FLS-producing inflammatory cytokines and degenerative enzymes initiate synovial inflammation and joint damage in RA (184). Several chemokines (CCL11, CCL25, CXCL4, CXCL7, CXCL10, and CX3CL1) mediate the FLS chemotactic activity in RA in vitro models (82, 86, 100, 185, 186). In addition to this chemotactic activity, some chemokines (CCL2, CCL5, CCL18, CCL20, and CXCL12) increase the production of IL-6, CCL2, CXCL8, MMP-3, and COX-2 from FLS of patients with RA in vitro models (100, 115, 149, 150). The CX3CL1/CX3CR1 axis enhances MHVEC migration in vitro and angiogenesis in Matrigel in vivo (187). The CCL21/CCR7 axis induces VEGF and angiotensin 1 (Ang1) production in RA fibroblasts and CXCL8 and Ang1 production in macrophages (119).
In general, the signaling of “classical” G protein-coupled chemokine receptors is mediated by activating pertussis toxin-sensitive Gi-type G proteins. Activated G proteins regulate multiple downstream signaling cascades, such as the JAK/STAT pathway and PI3K phosphorylation (188). In contrast, signaling of “atypical” chemokine receptors is independent of G proteins and remains somewhat unclear. In this section, we provide an update on arthritis animal models and clinical trials using drugs targeting chemokines and their receptors while discussing their potential as therapeutic targets.
Animal experiments are useful in the testing and development of new therapeutic agents and treatment approaches. Some chemokine ligands and receptors in KO, Tg, and naturally mutant mice are used as arthritic models (Table 4). For instance, CCL3 KO mice showed milder clinical and histopathological scores in the CAIA model (189), whereas plt/plt mice, a naturally occurring CCL19 and CCL20 mutant strain, also showed mild arthritis in CIA model (190). CXCL10 KO mice showed mild arthritis in CAIA model through the inhibition of macrophage and T-cell migration into the synovium (154). CXCL14 Tg mice showed exacerbated autoimmune arthritis in a CIA model, caused by an excessive immune response against type II collagen (191).
CCR2 KO in the DBA/1J background exacerbated the CIA model because of the enhanced Th17 cell response and increased autoantibody production (192, 193). CCR2 deficiency in IL-1Ra KO mice enhanced neutrophil migration (194). Furthermore, CCR2 deficiency in DBA/1J caused severe arthritis in CIA with cutaneous M. avium infection (195). In contrast, CCR2 KO in C57BL/6 mice showed decreased neutrophil infiltration into arthritic joints in AIA model (163). CCR4, CCR6, CCR7, CCR9, CXCR5, and CXCR6 deficiency ameliorated arthritis in CIA mice by suppressing the migration of Th17 cells (CCR4), DC (CCR7), and CD11b+ splenocytes (CCR9) (82, 84, 160, 190, 196, 197). CCR5 KO mice showed conflicting results, with a reduced clinical score in CIA model in one study (198) and no changes in others (193). Although CCR6 KO mice were resistant to CIA model, the deficient CCR6 did not improve in an animal model of K/BxN and TNF-α Tg mice (196). In addition, CCR7 inhibition decreased autoantibody production and T cell proliferation in AIA mice (199). CXCR3 KO mice showed mild arthritis in CAIA model via the inhibition of both macrophage and T cell migration into the synovium (154). CXCR4-conditional KO in T cells reduced arthritic symptoms in CIA mice by inhibiting T cell migration (200). T cell- or B cell-specific CXCR5 KO mice, as well as fully CXCR5 KO mice, were resistant to both CIA and AIA models (160). CXCR6 KO mice showed resistance to K/BxN serum-induced arthritis and CIA model (147).
The blockade of a single chemokine (CCL2, CCL5, CCL24, CXCL8, CXCL9, CXCL10, and CXCL16) or chemokine receptor (CCR2, CCR5, CCR9, CXCR1, CXCR2, CXCR3, and CXCR4) demonstrated preventive and/or therapeutic effects in distinct animal models (Table 5). For instance, monomeric mutant CCL2, but not CCL5 mutant (44AANA47), ameliorated arthritis in AIA rats (201). Met-RANTES, which antagonizes the binding of CCL5 to CCR1 and CCR5, reduced the arthritic score and decreased macrophage infiltration into STs in CIA mice and AIA rats (83, 164). The anti-CCL5 antibody, but not the anti-CCL3 antibody, reduced the arthritic score in AIA rats (202). CCL24 blockade ameliorated arthritic symptoms in rats with AIA model (203). Anti-CXCL5 antibody ameliorated arthritis in the AIA rat model by inhibiting neutrophil migration (204). CXCL8-based decoy proteins prevented CXCR1 and CXCR2 signaling in neutrophils and ameliorated arthritis in AIA mice (205). The CXCL9 blocking peptide, which competes with CCL3 and CXCL6 binding, reduced neutrophil migration in AIA mice (170). Monoclonal bispecific antibodies against TNF-α and CXCL10 attenuated arthritis symptoms in mice by inhibiting CXCL10-mediated CD8+ T cell migration (206). Anti-CXCL16 antibody attenuated arthritis in CIA mice by suppressing T cell recruitment (126). Anti-CX3CL1 antibody decreased arthritic symptoms by inhibiting osteoclast migration into the synovium of CIA mice (207).
Regarding chemokine receptors, CCR1 antagonist J-113863 decreased the arthritic score but did not affect auto-antibody production in CIA mice (208). Small-molecule inhibitors of CCR2 combined with Methotrexate (MTX) treatment reduced both the arthritic score and bone destruction via the suppression of OC activity in CIA mice (209). Compound 22, a CCR4 inhibitor, ameliorated arthritis by reducing Th17 cell migration into the joints of CIA mice (84). A CCR5 antagonist (maraviroc) decreased the arthritic score and CD8+ T cell activation in CIA mice (210); however, other CCR5 antagonists (MCC22) did not change the arthritic score in K/B.g7 arthritic mice (211). In CIA monkeys, a CCR5 antagonist (SCH-X) reduced arthritic score but did not change biomarker expression (212). CCR9 antagonist (CCX8037) reduced the arthritic score by inhibiting CD11b+ splenocyte recruitment into joints in CIA mice (82). The CXCR1/CXCR2 antagonist (SCH563705), but not the CCR2 antagonist (MK0812), reduced the arthritic score in CAIA mice (213). Furthermore, the blockade of CXCR1 and CXCR2 (DF 2162) ameliorated arthritis by inhibiting neutrophil migration in AIA rats (167). Anti-CXCR3 antibody reduced the arthritic scores and T cell influx into joints in adaptive transfer-induced arthritic rats (214). The CXCR3 antagonist (AMG487) contributed to the modulation of the Th17/Treg cell balance in CIA mice (215). Other CXCR3 antagonists, such as SCH 546738 and JN-2, also treated arthritis in CIA mice (216, 217). A CXCR4 antagonist (T140) reduced the arthritic score and auto-antibody production in CIA mice (218).
Based on valuable animal research, various therapeutic agents against chemokine ligands or their receptors have been developed and tested in patients with RA (219). However, several chemokines or chemokine receptor inhibitors have failed to show positive results in clinical trials (Table 6). For instance, the CCL2-blocking antibody (ABN912) did not promote clinical improvements in patients with RA (220). In addition, the CCR2 antibody (MLN1202) failed at phase IIa of the clinical trial due to the reduction of monocyte levels and no changes in synovial biomarkers (221).
Animal experiments have suggested CCR5 as a good RA therapeutic candidate (198, 210–212, 222). However, reports showed that CCR5 is not determinant for RA development (223–225), and all clinical trials using CCR5 antagonists failed (226–228).
A phase II clinical trial with a CCR1 antagonist (CCX354-C) showed good efficacy in the ACR20 response in patients with abundant CCX354-C in plasma but not in those with poor CCX354-C plasma concentration. However, ACR responses did not significantly vary between placebo- and CCX354-C-treated patients (229). CCR1 antagonist (MLN3897, 10 mg, once, daily) combined with MTX had no discernible effects on the disease, despite high MLN3897 plasma concentrations and receptor occupancy of the therapeutic target (230). Another trial using a CCR1 antagonist (CP-481,715) and MTX also failed in phase II (231). CCR1 ligands, CCL3 and CCL5, can bind to other chemokine receptors, CCR3, CCR4, and CCR5 (Table 1). Therefore, even though CCR1 on leukocytes might be inhibited, other chemokine receptors can still promote leukocyte recruitment into inflamed joints in RA. This could explain the failures in the use of CCR1 as a therapeutic target.
In contrast, the combination of CXCL10 blocking antibody (MDX-1100) and MTX showed a mild therapeutic effect on the ACR20 response; however, ACR50, ACR70, and EULAR were not significantly different between the treatment and placebo groups. The frequency of adverse events (AEs) in MDX-1100-treated patients was higher than that in placebo-treated patients; but any MDX-1100-treated patients experienced serious AEs (232). Phase III of the clinical trial using MDX-1100 has not yet been launched.
The clinical trial using CX3CL1 blocking antibody (E6011, 200-400 mg) was effective for ACR20, ACR50 and ACR70 responses in RA patients with an inadequate response to MTX. The incidence of AEs and treatment-related AEs (TEAEs) were similar across the four treatment groups (placebo, E6011 100 mg, 200 mg, and 400/200 mg groups). Nonetheless, the incidence of serious AEs was similar between E6011- and placebo-treated patients. AEs such as nasopharyngitis, upper respiratory tract infections, bronchitis, pharyngitis, stomatitis, and back pain occurred in over 5% of the overall patients (233). However E6011 was no clear benefit in the ACR20 response rate was observed in RA patients with an inadequate response to biological DMARDs (234).
Chemokine-targeted therapy encompassed several AEs; however, the overall incidence of AEs was 40-50%, and the incidence of serious AE was 0-5% in chemokine-targeted therapies (Table 6). These numbers increased to an AE incidence of 60-80% and serious AEs of 5-25% in patients treated with anti-IL-6R antibody, tocilizumab (235–237). Furthermore, AE incidence was 50-70%, and serious AE incidence was 5-10% in trials using anti-TNF-α antibody, infliximab (238–240). These clinical findings suggest that chemokine-targeted therapy is safer for patients with RA than cytokine-targeted therapy.
In addition to the above-mentioned blockade agents, other inhibitors of chemokine ligands or their receptors have demonstrated therapeutic effects on arthritis in RA models. Thus, these chemokine ligands and respective receptors may be promising targets for new RA therapies.
In this review, we summarize the functional roles of chemokine ligand–receptor interactions in arthritic joints of animal models and RA patients. Although several inhibitors of chemokines and/or their receptors have shown therapeutic effects in animal models of arthritis and clinical trials of patients with RA, limited therapeutic effects have been reported, suggesting that chemokine-targeted therapy still requires improvement. In targeting chemokine receptors, the choice of the most relevant receptor and ensuring high receptor occupancy at all times might be the key to therapeutic effects. In addition, inhibition of a single chemokine alone may not be sufficient to completely suppress leukocyte migration due to the functional overlap between chemokine systems. Therefore, the combined targeting of multiple chemokines and/or their receptors may be a more effective approach for human RA. Our previous study in animal models demonstrated that broadly cross-reactive chemokine-blocking antibodies for CXCR2 ligands dramatically ameliorated inflammatory arthritis compared with inhibition with antibodies against a single chemokine (241).
Further understanding of the importance of different chemokines at different stages of RA is required for the development of drugs that effectively target the system. We have previously developed an in vivo imaging technique to fully dissect the functional roles of chemokines and their receptors in inflamed joints in animal models (242). Interestingly, CXCR2 and ACKR1 are required for neutrophil apoptosis in the joint space, whereas the classical C5aR1 and atypical C5a and C5aR2 receptors are required for neutrophil apoptosis in the joint (146, 169). Altogether, the development of effective inhibitors of chemokines and their receptors has untapped therapeutic potential in RA.
MM and YM conceived the manuscript; MM prepared the draft manuscript; MM, JS, CM, KY and YM approved the published version of the manuscript.
The work of MM is supported by JSPS KAKENHI (JP21K06955, JP21K07379, JP21H02394), SRF foundation (2022Y003), Kansai Medical University alumni association (Katano Prize) and Kansai Medical University Molecular Imaging Center of Diseases. The work of JS and MM is supported by JSPS KAKENHI (JP22K08532). YM is supported by the Japanese Society for the Promotion of Science (JSPS) KAKENHI grant number JP22K08531, AMED under Grant Number 22jm0210069h0004 and 22jm0610070h0001, Takeda Science Foundation, The Uehara Memorial Foundation and The Naito Foundation.
The authors declare that the research was conducted in the absence of any commercial or financial relationships that could be construed as a potential conflict of interest.
All claims expressed in this article are solely those of the authors and do not necessarily represent those of their affiliated organizations, or those of the publisher, the editors and the reviewers. Any product that may be evaluated in this article, or claim that may be made by its manufacturer, is not guaranteed or endorsed by the publisher.
1. David BA, Kubes P. Exploring the complex role of chemokines and chemoattractants in vivo on leukocyte dynamics. Immunol Rev (2019) 289(1):9–30. doi: 10.1111/imr.12757
2. Rossi B, Angiari S, Zenaro E, Budui SL, Constantin G. Vascular inflammation in central nervous system diseases: adhesion receptors controlling leukocyte-endothelial interactions. J Leukoc Biol (2011) 89(4):539–56. doi: 10.1189/jlb.0710432
3. Griffith JW, Sokol CL, Luster AD. Chemokines and chemokine receptors: positioning cells for host defense and immunity. Annu Rev Immunol (2014) 32:659–702. doi: 10.1146/annurev-immunol-032713-120145
4. Palomino DC, Marti LC. Chemokines and immunity. Einstein (Sao Paulo) (2015) 13(3):469–73. doi: 10.1590/S1679-45082015RB3438
5. Koch AE. Chemokines and their receptors in rheumatoid arthritis: future targets? Arthritis Rheum (2005) 52(3):710–21. doi: 10.1002/art.20932
6. Strieter RM, Burdick MD, Gomperts BN, Belperio JA, Keane MP. CXC chemokines in angiogenesis. Cytokine Growth Factor Rev (2005) 16(6):593–609. doi: 10.1016/j.cytogfr.2005.04.007
7. Strieter RM, Polverini PJ, Kunkel SL, Arenberg DA, Burdick MD, Kasper J, et al. The functional role of the ELR motif in CXC chemokine-mediated angiogenesis. J Biol Chem (1995) 270(45):27348–57. doi: 10.1074/jbc.270.45.27348
8. Van Lint P, Libert C. Chemokine and cytokine processing by matrix metalloproteinases and its effect on leukocyte migration and inflammation. J Leukoc Biol (2007) 82(6):1375–81. doi: 10.1189/jlb.0607338
9. Zhao J, Wei K, Jiang P, Chang C, Xu L, Xu L, et al. G-Protein-Coupled receptors in rheumatoid arthritis: Recent insights into mechanisms and functional roles. Front Immunol (2022) 13:907733. doi: 10.3389/fimmu.2022.907733
10. Torphy RJ, Yee EJ, Schulick RD, Zhu Y. Atypical chemokine receptors: emerging therapeutic targets in cancer. Trends Pharmacol Sci (2022) 43(12):1085–97. doi: 10.1016/j.tips.2022.09.009
11. Bonecchi R, Graham GJ. Atypical chemokine receptors and their roles in the resolution of the inflammatory response. Front Immunol (2016) 7:224. doi: 10.3389/fimmu.2016.00224
12. Nibbs RJ, Graham GJ. Immune regulation by atypical chemokine receptors. Nat Rev Immunol (2013) 13(11):815–29. doi: 10.1038/nri3544
13. Miyabe Y, Miyabe C, Iwai Y, Luster AD. Targeting the chemokine system in rheumatoid arthritis and vasculitis. JMA J (2020) 3(3):182–92. doi: 10.31662/jmaj.2020-0019
14. Ben Kilani MS, Achour Y, Perea J, Cornelis F, Bardin T, Chaudru V, et al. Characterization of copy number variants for CCL3L1 gene in rheumatoid arthritis for French trio families and Tunisian cases and controls. Clin Rheumatol (2016) 35(8):1917–22. doi: 10.1007/s10067-015-3156-y
15. McKinney C, Merriman ME, Chapman PT, Gow PJ, Harrison AA, Highton J, et al. Evidence for an influence of chemokine ligand 3-like 1 (CCL3L1) gene copy number on susceptibility to rheumatoid arthritis. Ann Rheum Dis (2008) 67(3):409–13. doi: 10.1136/ard.2007.075028
16. Kuo SJ, Huang CC, Tsai CH, Hsu HC, Su CM, Tang CH. Chemokine c-c motif ligand 4 gene polymorphisms associated with susceptibility to rheumatoid arthritis. BioMed Res Int (2018) 2018:9181647. doi: 10.1155/2018/9181647
17. Luterek-Puszynska K, Malinowski D, Paradowska-Gorycka A, Safranow K, Pawlik A. CD28, CTLA-4 and CCL5 gene polymorphisms in patients with rheumatoid arthritis. Clin Rheumatol (2017) 36(5):1129–35. doi: 10.1007/s10067-016-3496-2
18. Yao TC, Tsai YC, Huang JL. Association of RANTES promoter polymorphism with juvenile rheumatoid arthritis. Arthritis Rheum (2009) 60(4):1173–8. doi: 10.1002/art.24422
19. Wang CR, Guo HR, Liu MF. RANTES promoter polymorphism as a genetic risk factor for rheumatoid arthritis in the Chinese. Clin Exp Rheumatol (2005) 23(3):379–84.
20. Li G, Zhao J, Li B, Ma J, Zhao Q, Wang X, et al. Associations between CCL21 gene polymorphisms and susceptibility to rheumatoid arthritis: a meta-analysis. Rheumatol Int (2017) 37(10):1673–81. doi: 10.1007/s00296-017-3784-4
21. Chae SC, Park YR, Shim SC, Lee IK, Chung HT. Eotaxin-3 gene polymorphisms are associated with rheumatoid arthritis in a Korean population. Hum Immunol (2005) 66(3):314–20. doi: 10.1016/j.humimm.2005.01.002
22. Dieguez-Gonzalez R, Akar S, Calaza M, Gonzalez-Alvaro I, Fernandez-Gutierrez B, Lamas JR, et al. Lack of association with rheumatoid arthritis of selected polymorphisms in 4 candidate genes: CFH, CD209, eotaxin-3, and MHC2TA. J Rheumatol (2009) 36(8):1590–5. doi: 10.3899/jrheum.090022
23. Zhang R, Sun P, Jiang Y, Chen Z, Huang C, Zhang X, et al. Genome-wide haplotype association analysis and gene prioritization identify CCL3 as a risk locus for rheumatoid arthritis. Int J Immunogenet (2010) 37(4):273–8. doi: 10.1111/j.1744-313X.2010.00920.x
24. Lo SF, Huang CM, Lin HC, Chen WC, Tsai CH, Tsai FJ. Cytokine (IL-6) and chemokine (IL-8) gene polymorphisms among rheumatoid arthritis patients in Taiwan. Clin Exp Rheumatol (2008) 26(4):632–7.
25. Kotrych D, Dziedziejko V, Safranow K, Drozdzik M, Pawlik A. CXCL9 and CXCL10 gene polymorphisms in patients with rheumatoid arthritis. Rheumatol Int (2015) 35(8):1319–23. doi: 10.1007/s00296-015-3234-0
26. Ibrahim I, Humphreys J, Mokhtar I, Marshall T, Verstappen S, Symmons D, et al. Association of chemokine CXC ligand 12 gene polymorphism (rs1746048) with cardiovascular mortality in patients with rheumatoid arthritis: results from the Norfolk arthritis register. Ann Rheum Dis (2015) 74(11):2099–102. doi: 10.1136/annrheumdis-2015-207851
27. Joven B, Gonzalez N, Aguilar F, Santiago B, Galindo M, Alcami J, et al. Association between stromal cell-derived factor 1 chemokine gene variant and radiographic progression of rheumatoid arthritis. Arthritis Rheum (2005) 52(1):354–6. doi: 10.1002/art.20743
28. Kwon YC, Lim J, Bang SY, Ha E, Hwang MY, Yoon K, et al. Genome-wide association study in a Korean population identifies six novel susceptibility loci for rheumatoid arthritis. Ann Rheum Dis (2020) 79(11):1438–45. doi: 10.1136/annrheumdis-2020-217663
29. Leng RX, Di DS, Ni J, Wu XX, Zhang LL, Wang XF, et al. Identification of new susceptibility loci associated with rheumatoid arthritis. Ann Rheum Dis (2020) 79(12):1565–71. doi: 10.1136/annrheumdis-2020-217351
30. Caliz R, Canet LM, Lupianez CB, Canhao H, Escudero A, Filipescu I, et al. Gender-specific effects of genetic variants within Th1 and Th17 cell-mediated immune response genes on the risk of developing rheumatoid arthritis. PloS One (2013) 8(8):e72732. doi: 10.1371/journal.pone.0072732
31. Toson B, Dos Santos EJ, Adelino JE, Sandrin-Garcia P, Crovella S, Louzada-Junior P, et al. CCR5Delta32 and the genetic susceptibility to rheumatoid arthritis in admixed populations: a multicentre study. Rheumatol (Oxford) (2017) 56(3):495–7. doi: 10.1093/rheumatology/kew398
32. Han SW, Sa KH, Kim SI, Lee SI, Park YW, Lee SS, et al. CCR5 gene polymorphism is a genetic risk factor for radiographic severity of rheumatoid arthritis. Tissue Antigens (2012) 80(5):416–23. doi: 10.1111/j.1399-0039.2012.01955.x
33. Kohem CL, Brenol JC, Xavier RM, Bredemeier M, Brenol CV, Dedavid e Silva TL, et al. The chemokine receptor CCR5 genetic polymorphism and expression in rheumatoid arthritis patients. Scand J Rheumatol (2007) 36(5):359–64. doi: 10.1080/03009740701393999
34. Akhtar M, Khan S, Ali Y, Haider S, Ud Din J, Islam ZU, et al. Association study of CCR6 rs3093024 with rheumatoid arthritis in a Pakistani cohort. Saudi J Biol Sci (2020) 27(12):3354–8. doi: 10.1016/j.sjbs.2020.08.045
35. Mo XB, Sun YH, Zhang YH, Lei SF. Integrative analysis highlighted susceptibility genes for rheumatoid arthritis. Int Immunopharmacol (2020) 86:106716. doi: 10.1016/j.intimp.2020.106716
36. Li G, Cunin P, Wu D, Diogo D, Yang Y, Okada Y, et al. The rheumatoid arthritis risk variant CCR6DNP regulates CCR6 via PARP-1. PloS Genet (2016) 12(9):e1006292. doi: 10.1371/journal.pgen.1006292
37. Jiang L, Yin J, Ye L, Yang J, Hemani G, Liu AJ, et al. Novel risk loci for rheumatoid arthritis in han Chinese and congruence with risk variants in europeans. Arthritis Rheumatol (2014) 66(5):1121–32. doi: 10.1002/art.38353
38. Chang WC, Woon PY, Wei JC, Chang CM, Hsu YW, Guo YC, et al. A single-nucleotide polymorphism of CCR6 (rs3093024) is associated with susceptibility to rheumatoid arthritis but not ankylosing spondylitis, in a Taiwanese population. J Rheumatol (2012) 39(8):1765–6. doi: 10.3899/jrheum.120040
39. Perkins EA, Landis D, Causey ZL, Edberg Y, Reynolds RJ, Hughes LB, et al. Association of single-nucleotide polymorphisms in CCR6, TAGAP, and TNFAIP3 with rheumatoid arthritis in African americans. Arthritis Rheum (2012) 64(5):1355–8. doi: 10.1002/art.33464
40. Teng E, Leong KP, Li HH, Thong B, Koh ET, Loi PL, et al. Analysis of a genome-wide association study-linked locus (CCR6) in Asian rheumatoid arthritis. DNA Cell Biol (2012) 31(4):607–10. doi: 10.1089/dna.2011.1350
41. Stahl EA, Raychaudhuri S, Remmers EF, Xie G, Eyre S, Thomson BP, et al. Genome-wide association study meta-analysis identifies seven new rheumatoid arthritis risk loci. Nat Genet (2010) 42(6):508–14. doi: 10.1038/ng.582
42. Yu F, Hu G, Li L, Yu B, Liu R. Identification of key candidate genes and biological pathways in the synovial tissue of patients with rheumatoid arthritis. Exp Ther Med (2022) 23(6):368. doi: 10.3892/etm.2022.11295
43. Pandya JM, Lundell AC, Andersson K, Nordstrom I, Theander E, Rudin A. Blood chemokine profile in untreated early rheumatoid arthritis: CXCL10 as a disease activity marker. Arthritis Res Ther (2017) 19(1):20. doi: 10.1186/s13075-017-1224-1
44. Aldridge J, Lundell AC, Andersson K, Mark L, Lund Hetland M, Ostergaard M, et al. Blood chemokine levels are markers of disease activity but not predictors of remission in early rheumatoid arthritis. Clin Exp Rheumatol (2022) 40(7):1393–402. doi: 10.55563/clinexprheumatol/idogmj
45. Huang Y, Zheng S, Wang R, Tang C, Zhu J, Li J. CCL5 and related genes might be the potential diagnostic biomarkers for the therapeutic strategies of rheumatoid arthritis. Clin Rheumatol (2019) 38(9):2629–35. doi: 10.1007/s10067-019-04533-1
46. Yang MH, Wu FX, Xie CM, Qing YF, Wang GR, Guo XL, et al. Expression of CC chemokine ligand 5 in patients with rheumatoid arthritis and its correlation with disease activity and medication. Chin Med Sci J (2009) 24(1):50–4. doi: 10.1016/s1001-9294(09)60059-6
47. Pournazari M, Feizollahi P, Roghani SA, Assar S, Soufivand P, Soleymani B, et al. Increased plasma levels of CCL20 in peripheral blood of rheumatoid arthritis patients and its association with clinical and laboratory parameters. Clin Rheumatol (2022) 41(1):265–70. doi: 10.1007/s10067-021-05899-x
48. Ren C, Li M, Zheng Y, Wu F, Du W, Quan R. Identification of diagnostic genes and vital microRNAs involved in rheumatoid arthritis: based on data mining and experimental verification. PeerJ (2021) 9:e11427. doi: 10.7717/peerj.11427
49. Kuan WP, Tam LS, Wong CK, Ko FW, Li T, Zhu T, et al. CXCL 9 and CXCL 10 as sensitive markers of disease activity in patients with rheumatoid arthritis. J Rheumatol (2010) 37(2):257–64. doi: 10.3899/jrheum.090769
50. Kraan MC, Patel DD, Haringman JJ, Smith MD, Weedon H, Ahern MJ, et al. The development of clinical signs of rheumatoid synovial inflammation is associated with increased synthesis of the chemokine CXCL8 (interleukin-8). Arthritis Res (2001) 3(1):65–71. doi: 10.1186/ar141
51. Chen Y, Liao R, Yao Y, Wang Q, Fu L. Machine learning to identify immune-related biomarkers of rheumatoid arthritis based on WGCNA network. Clin Rheumatol (2022) 41(4):1057–68. doi: 10.1007/s10067-021-05960-9
52. Guerrero S, Sanchez-Tirado E, Agui L, Gonzalez-Cortes A, Yanez-Sedeno P, Pingarron JM. Simultaneous determination of CXCL7 chemokine and MMP3 metalloproteinase as biomarkers for rheumatoid arthritis. Talanta (2021) 234:122705. doi: 10.1016/j.talanta.2021.122705
53. Imam AM, Hamed AM, Nasef SI, Hassan AM, Omar HH. Biochemical analysis of c-X-C motif chemokine ligand 10 (CXCL10) as a biomarker in patients with rheumatoid arthritis. Egypt J Immunol (2019) 26(2):79–86.
54. Peng L, Zhu N, Mao J, Huang L, Yang Y, Zhou Z, et al. Expression levels of CXCR4 and CXCL12 in patients with rheumatoid arthritis and its correlation with disease activity. Exp Ther Med (2020) 20(3):1925–34. doi: 10.3892/etm.2020.8950
55. Kanbe K, Chiba J, Inoue Y, Taguchi M, Yabuki A. SDF-1 and CXCR4 in synovium are associated with disease activity and bone and joint destruction in patients with rheumatoid arthritis treated with golimumab. Mod Rheumatol (2016) 26(1):46–50. doi: 10.3109/14397595.2015.1054088
56. Li Z, Chen YE, Zulipikaer M, Xu C, Fu J, Deng T, et al. Identification of PSMB9 and CXCL13 as immune-related diagnostic markers for rheumatoid arthritis by machine learning. Curr Pharm Des (2022) 28(34):2842–54. doi: 10.2174/1381612828666220831085608
57. Greisen SR, Mikkelsen C, Hetland ML, Ostergaard M, Horslev-Petersen K, Junker P, et al. CXCL13 predicts long-term radiographic status in early rheumatoid arthritis. Rheumatol (Oxford) (2022) 61(6):2590–5. doi: 10.1093/rheumatology/keab763
58. Tuckwell K, Gabay C, Sornasse T, Laubender RP, Wang J, Townsend MJ. Levels of CXCL13 and sICAM-1 correlate with disease activity score in patients with rheumatoid arthritis treated with tocilizumab. Adv Rheumatol (2019) 59(1):54. doi: 10.1186/s42358-019-0097-1
59. Greisen SR, Schelde KK, Rasmussen TK, Kragstrup TW, Stengaard-Pedersen K, Hetland ML, et al. CXCL13 predicts disease activity in early rheumatoid arthritis and could be an indicator of the therapeutic ‘window of opportunity’. Arthritis Res Ther (2014) 16(5):434. doi: 10.1186/s13075-014-0434-z
60. Jones JD, Hamilton BJ, Challener GJ, de Brum-Fernandes AJ, Cossette P, Liang P, et al. Serum c-X-C motif chemokine 13 is elevated in early and established rheumatoid arthritis and correlates with rheumatoid factor levels. Arthritis Res Ther (2014) 16(2):R103. doi: 10.1186/ar4552
61. Rioja I, Hughes FJ, Sharp CH, Warnock LC, Montgomery DS, Akil M, et al. Potential novel biomarkers of disease activity in rheumatoid arthritis patients: CXCL13, CCL23, transforming growth factor alpha, tumor necrosis factor receptor superfamily member 9, and macrophage colony-stimulating factor. Arthritis Rheum (2008) 58(8):2257–67. doi: 10.1002/art.23667
62. Wu R, Long L, Zhou Q, Su J, Su W, Zhu J. Identification of hub genes in rheumatoid arthritis through an integrated bioinformatics approach. J Orthop Surg Res (2021) 16(1):458. doi: 10.1186/s13018-021-02583-3
63. Aldahlawi AM, Elshal MF, Ashgan FT, Bahlas S. Chemokine receptors expression on peripheral CD4-lymphocytes in rheumatoid arthritis: Coexpression of CCR7 and CD95 is associated with disease activity. Saudi J Biol Sci (2015) 22(4):453–8. doi: 10.1016/j.sjbs.2015.02.011
64. Sanchez-Zuno GA, Bucala R, Hernandez-Bello J, Roman-Fernandez IV, Garcia-Chagollan M, Nicoletti F, et al. Canonical (CD74/CD44) and non-canonical (CXCR2, 4 and 7) MIF receptors are differentially expressed in rheumatoid arthritis patients evaluated by DAS28-ESR. J Clin Med (2021) 11(1):120. doi: 10.3390/jcm11010120
65. Burska AN, Thu A, Parmar R, Bzoma I, Samans B, Raschke E, et al. Quantifying circulating Th17 cells by qPCR: potential as diagnostic biomarker for rheumatoid arthritis. Rheumatol (Oxford) (2019) 58(11):2015–24. doi: 10.1093/rheumatology/kez162
66. Luo Q, Fu P, Guo Y, Fu B, Guo Y, Huang Q, et al. Increased TIGIT(+)PD1(+)CXCR5()CD4(+)T cells are associated with disease activity in rheumatoid arthritis. Exp Ther Med (2022) 24(4):642. doi: 10.3892/etm.2022.11579
67. Anaparti V, Tanner S, Zhang C, O’Neil L, Smolik I, Meng X, et al. Increased frequency of TIGIT+ CD4 T cell subset in autoantibody-positive first-degree relatives of patients with rheumatoid arthritis. Front Immunol (2022) 13:932627. doi: 10.3389/fimmu.2022.932627
68. Xu T, Yan T, Li F, Li B, Li P. The role of different circulating T follicular helper cell markers in rheumatoid arthritis. J Interferon Cytokine Res (2022) 42(3):108–17. doi: 10.1089/jir.2021.0168
69. Zhao L, Li Z, Zeng X, Xia C, Xu L, Xu Q, et al. Circulating CD4(+) FoxP3(-) CXCR5(-) CXCR3(+) PD-1(hi) cells are elevated in active rheumatoid arthritis and reflect the severity of the disease. Int J Rheum Dis (2021) 24(8):1032–9. doi: 10.1111/1756-185X.14170
70. McInnes IB, Schett G. The pathogenesis of rheumatoid arthritis. N Engl J Med (2011) 365(23):2205–19. doi: 10.1056/NEJMra1004965
71. Feldmann M, Maini SR. Role of cytokines in rheumatoid arthritis: an education in pathophysiology and therapeutics. Immunol Rev (2008) 223:7–19. doi: 10.1111/j.1600-065X.2008.00626.x
72. Bystrom J, Clanchy FI, Taher TE, Al-Bogami MM, Muhammad HA, Alzabin S, et al. Response to treatment with TNFalpha inhibitors in rheumatoid arthritis is associated with high levels of GM-CSF and GM-CSF(+) T lymphocytes. Clin Rev Allergy Immunol (2017) 53(2):265–76. doi: 10.1007/s12016-017-8610-y
73. Brand DD, Latham KA, Rosloniec EF. Collagen-induced arthritis. Nat Protoc (2007) 2(5):1269–75. doi: 10.1038/nprot.2007.173
74. Khachigian LM. Collagen antibody-induced arthritis. Nat Protoc (2006) 1(5):2512–6. doi: 10.1038/nprot.2006.393
75. Monach PA, Mathis D, Benoist C. The K/BxN arthritis model. Curr Protoc Immunol (2008) 15:15.22.1–15.22.12. doi: 10.1002/0471142735.im1522s81. Chapter 15: Unit 15 22.
76. van Eden W, Wagenaar-Hilbers JP, Wauben MH. Adjuvant arthritis in the rat. Curr Protoc Immunol (2001) 15:15.4.1–15.4.8. doi: 10.1002/0471142735.im1504s19. Chapter 15: Unit 15 14.
77. Herrera-Aco DR, Medina-Campos ON, Pedraza-Chaverri J, Sciutto-Conde E, Rosas-Salgado G, Fragoso-Gonzalez G. Alpha-mangostin: Anti-inflammatory and antioxidant effects on established collagen-induced arthritis in DBA/1J mice. Food Chem Toxicol (2019) 124:300–15. doi: 10.1016/j.fct.2018.12.018
78. Booth G, Newham P, Barlow R, Raines S, Zheng B, Han S. Gene expression profiles at different stages of collagen-induced arthritis. Autoimmunity (2008) 41(7):512–21. doi: 10.1080/08916930802095210
79. Grespan R, Fukada SY, Lemos HP, Vieira SM, Napimoga MH, Teixeira MM, et al. CXCR2-specific chemokines mediate leukotriene B4-dependent recruitment of neutrophils to inflamed joints in mice with antigen-induced arthritis. Arthritis Rheum (2008) 58(7):2030–40. doi: 10.1002/art.23597
80. Wang Y, Wei D, Lai Z, Le Y. Triptolide inhibits CC chemokines expressed in rat adjuvant-induced arthritis. Int Immunopharmacol (2006) 6(12):1825–32. doi: 10.1016/j.intimp.2006.07.029
81. Haas CS, Martinez RJ, Attia N, Haines GK 3rd, Campbell PL, Koch AE. Chemokine receptor expression in rat adjuvant-induced arthritis. Arthritis Rheum (2005) 52(12):3718–30. doi: 10.1002/art.21476
82. Yokoyama W, Kohsaka H, Kaneko K, Walters M, Takayasu A, Fukuda S, et al. Abrogation of CC chemokine receptor 9 ameliorates collagen-induced arthritis of mice. Arthritis Res Ther (2014) 16(5):445. doi: 10.1186/s13075-014-0445-9
83. Plater-Zyberk C, Hoogewerf AJ, Proudfoot AE, Power CA, Wells TN. Effect of a CC chemokine receptor antagonist on collagen induced arthritis in DBA/1 mice. Immunol Lett (1997) 57(1-3):117–20. doi: 10.1016/s0165-2478(97)00075-8
84. Honzawa T, Matsuo K, Hosokawa S, Kamimura M, Kaibori Y, Hara Y, et al. CCR4 plays a pivotal role in Th17 cell recruitment and expansion in a mouse model of rheumatoid arthritis. Int Immunol (2022) 34(12):635–42. doi: 10.1093/intimm/dxac041
85. Wang L, Wang L, Hao P, Cao Q, Zhang Z. Anti-CCL22 increases regulatory T cells in CD4(+) T cells of rheumatoid arthritis patients via STAT5 pathway. Exp Ther Med (2020) 19(3):2127–32. doi: 10.3892/etm.2019.8404
86. Wakabayashi K, Isozaki T, Tsubokura Y, Fukuse S, Kasama T. Eotaxin-1/CCL11 is involved in cell migration in rheumatoid arthritis. Sci Rep (2021) 11(1):7937. doi: 10.1038/s41598-021-87199-7
87. Yamaguchi A, Nozawa K, Fujishiro M, Kawasaki M, Suzuki F, Takamori K, et al. CC motif chemokine ligand 13 is associated with rheumatoid arthritis pathogenesis. Mod Rheumatol (2013) 23(5):856–63. doi: 10.1007/s10165-012-0752-4
88. van Lieshout AW, Fransen J, Flendrie M, Eijsbouts AM, van den Hoogen FH, van Riel PL, et al. Circulating levels of the chemokine CCL18 but not CXCL16 are elevated and correlate with disease activity in rheumatoid arthritis. Ann Rheum Dis (2007) 66(10):1334–8. doi: 10.1136/ard.2006.066084
89. Sellam J, Rouanet S, Hendel-Chavez H, Miceli-Richard C, Combe B, Sibilia J, et al. CCL19, a b cell chemokine, is related to the decrease of blood memory b cells and predicts the clinical response to rituximab in patients with rheumatoid arthritis. Arthritis Rheum (2013) 65(9):2253–61. doi: 10.1002/art.38023
90. Wang X, Sun L, He N, An Z, Yu R, Li C, et al. Increased expression of CXCL2 in ACPA-positive rheumatoid arthritis and its role in osteoclastogenesis. Clin Exp Immunol (2021) 203(2):194–208. doi: 10.1111/cei.13527
91. Zhang L, Yu M, Deng J, Lv X, Liu J, Xiao Y, et al. Chemokine signaling pathway involved in CCL2 expression in patients with rheumatoid arthritis. Yonsei Med J (2015) 56(4):1134–42. doi: 10.3349/ymj.2015.56.4.1134
92. Zhang G, Liu HB, Zhou L, Cui XQ, Fan XH. CCL3 participates in the development of rheumatoid arthritis by activating AKT. Eur Rev Med Pharmacol Sci (2018) 22(20):6625–32. doi: 10.26355/eurrev_201810_16137
93. Ruth JH, Volin MV, Haines GK 3rd, Woodruff DC, Katschke KJ Jr., Woods JM, et al. Fractalkine, a novel chemokine in rheumatoid arthritis and in rat adjuvant-induced arthritis. Arthritis Rheum (2001) 44(7):1568–81. doi: 10.1002/1529-0131(200107)44:7<1568::AID-ART280>3.0.CO;2-1
94. Stanczyk J, Kowalski ML, Grzegorczyk J, Szkudlinska B, Jarzebska M, Marciniak M, et al. RANTES and chemotactic activity in synovial fluids from patients with rheumatoid arthritis and osteoarthritis. Mediators Inflammation (2005) 2005(6):343–8. doi: 10.1155/MI.2005.343
95. Wang CR, Liu MF. Regulation of CCR5 expression and MIP-1alpha production in CD4+ T cells from patients with rheumatoid arthritis. Clin Exp Immunol (2003) 132(2):371–8. doi: 10.1046/j.1365-2249.2003.02126.x
96. Kobayashi S, Watanabe T, Suzuki R, Furu M, Ito H, Ito J, et al. TGF-beta induces the differentiation of human CXCL13-producing CD4(+) T cells. Eur J Immunol (2016) 46(2):360–71. doi: 10.1002/eji.201546043
97. Nakayama T, Yoshimura M, Higashioka K, Miyawaki K, Ota Y, Ayano M, et al. Type 1 helper T cells generate CXCL9/10-producing T-bet(+) effector b cells potentially involved in the pathogenesis of rheumatoid arthritis. Cell Immunol (2021) 360:104263. doi: 10.1016/j.cellimm.2020.104263
98. Lin YC, Lin YC, Huang MY, Kuo PL, Wu CC, Lee MS, et al. Tumor necrosis factor-alpha inhibitors suppress CCL2 chemokine in monocytes via epigenetic modification. Mol Immunol (2017) 83:82–91. doi: 10.1016/j.molimm.2017.01.009
99. Ellingsen T, Hansen I, Thorsen J, Moller BK, Tarp U, Lottenburger T, et al. Upregulated baseline plasma CCL19 and CCR7 cell-surface expression on monocytes in early rheumatoid arthritis normalized during treatment and CCL19 correlated with radiographic progression. Scand J Rheumatol (2014) 43(2):91–100. doi: 10.3109/03009742.2013.803149
100. Umar S, Palasiewicz K, Van Raemdonck K, Volin MV, Romay B, Ahmad I, et al. CCL25 and CCR9 is a unique pathway that potentiates pannus formation by remodeling RA macrophages into mature osteoclasts. Eur J Immunol (2021) 51(4):903–14. doi: 10.1002/eji.202048681
101. Yeo L, Adlard N, Biehl M, Juarez M, Smallie T, Snow M, et al. Expression of chemokines CXCL4 and CXCL7 by synovial macrophages defines an early stage of rheumatoid arthritis. Ann Rheum Dis (2016) 75(4):763–71. doi: 10.1136/annrheumdis-2014-206921
102. Hillen MR, Moret FM, van der Wurff-Jacobs K, Radstake T, Hack CE, Lafeber F, et al. Targeting CD1c-expressing classical dendritic cells to prevent thymus and activation-regulated chemokine (TARC)-mediated T-cell chemotaxis in rheumatoid arthritis. Scand J Rheumatol (2017) 46(1):11–6. doi: 10.3109/03009742.2016.1158311
103. van Lieshout AW, van der Voort R, le Blanc LM, Roelofs MF, Schreurs BW, van Riel PL, et al. Novel insights in the regulation of CCL18 secretion by monocytes and dendritic cells via cytokines, toll-like receptors and rheumatoid synovial fluid. BMC Immunol (2006) 7:23. doi: 10.1186/1471-2172-7-23
104. Radstake TR, van der Voort R, ten Brummelhuis M, de Waal Malefijt M, Looman M, Figdor CG, et al. Increased expression of CCL18, CCL19, and CCL17 by dendritic cells from patients with rheumatoid arthritis, and regulation by fc gamma receptors. Ann Rheum Dis (2005) 64(3):359–67. doi: 10.1136/ard.2003.017566
105. Auer J, Blass M, Schulze-Koops H, Russwurm S, Nagel T, Kalden JR, et al. Expression and regulation of CCL18 in synovial fluid neutrophils of patients with rheumatoid arthritis. Arthritis Res Ther (2007) 9(5):R94. doi: 10.1186/ar2294
106. Hatano Y, Kasama T, Iwabuchi H, Hanaoka R, Takeuchi HT, Jing L, et al. Macrophage inflammatory protein 1 alpha expression by synovial fluid neutrophils in rheumatoid arthritis. Ann Rheum Dis (1999) 58(5):297–302. doi: 10.1136/ard.58.5.297
107. Rump L, Mattey DL, Kehoe O, Middleton J. An initial investigation into endothelial CC chemokine expression in the human rheumatoid synovium. Cytokine (2017) 97:133–40. doi: 10.1016/j.cyto.2017.05.023
108. Reich N, Beyer C, Gelse K, Akhmetshina A, Dees C, Zwerina J, et al. Microparticles stimulate angiogenesis by inducing ELR(+) CXC-chemokines in synovial fibroblasts. J Cell Mol Med (2011) 15(4):756–62. doi: 10.1111/j.1582-4934.2010.01051.x
109. Momohara S, Okamoto H, Iwamoto T, Mizumura T, Ikari K, Kawaguchi Y, et al. High CCL18/PARC expression in articular cartilage and synovial tissue of patients with rheumatoid arthritis. J Rheumatol (2007) 34(2):266–71.
110. Wong PK, Cuello C, Bertouch JV, Roberts-Thomson PJ, Ahern MJ, Smith MD, et al. Effects of pulse methylprednisolone on macrophage chemotactic protein-1 and macrophage inflammatory protein-1alpha in rheumatoid synovium. J Rheumatol (2001) 28(12):2634–6.
111. Patel DD, Zachariah JP, Whichard LP. CXCR3 and CCR5 ligands in rheumatoid arthritis synovium. Clin Immunol (2001) 98(1):39–45. doi: 10.1006/clim.2000.4957
112. Yu X, Song Z, Rao L, Tu Q, Zhou J, Yin Y, et al. Synergistic induction of CCL5, CXCL9 and CXCL10 by IFN-gamma and NLRs ligands on human fibroblast-like synoviocytes-a potential immunopathological mechanism for joint inflammation in rheumatoid arthritis. Int Immunopharmacol (2020) 82:106356. doi: 10.1016/j.intimp.2020.106356
113. Klimiuk PA, Sierakowski S, Latosiewicz R, Skowronski J, Cylwik JP, Cylwik B, et al. Histological patterns of synovitis and serum chemokines in patients with rheumatoid arthritis. J Rheumatol (2005) 32(9):1666–72.
114. Haringman JJ, Smeets TJ, Reinders-Blankert P, Tak PP. Chemokine and chemokine receptor expression in paired peripheral blood mononuclear cells and synovial tissue of patients with rheumatoid arthritis, osteoarthritis, and reactive arthritis. Ann Rheum Dis (2006) 65(3):294–300. doi: 10.1136/ard.2005.037176
115. Nanki T, Nagasaka K, Hayashida K, Saita Y, Miyasaka N. Chemokines regulate IL-6 and IL-8 production by fibroblast-like synoviocytes from patients with rheumatoid arthritis. J Immunol (2001) 167(9):5381–5. doi: 10.4049/jimmunol.167.9.5381
116. Miyazaki Y, Nakayamada S, Kubo S, Nakano K, Iwata S, Miyagawa I, et al. Th22 cells promote osteoclast differentiation via production of IL-22 in rheumatoid arthritis. Front Immunol (2018) 9:2901. doi: 10.3389/fimmu.2018.02901
117. Chen CY, Fuh LJ, Huang CC, Hsu CJ, Su CM, Liu SC, et al. Enhancement of CCL2 expression and monocyte migration by CCN1 in osteoblasts through inhibiting miR-518a-5p: implication of rheumatoid arthritis therapy. Sci Rep (2017) 7(1):421. doi: 10.1038/s41598-017-00513-0
118. Endres M, Andreas K, Kalwitz G, Freymann U, Neumann K, Ringe J, et al. Chemokine profile of synovial fluid from normal, osteoarthritis and rheumatoid arthritis patients: CCL25, CXCL10 and XCL1 recruit human subchondral mesenchymal progenitor cells. Osteoarthritis Cartilage (2010) 18(11):1458–66. doi: 10.1016/j.joca.2010.08.003
119. Pickens SR, Chamberlain ND, Volin MV, Pope RM, Mandelin AM 2nd, Shahrara S. Characterization of CCL19 and CCL21 in rheumatoid arthritis. Arthritis Rheum (2011) 63(4):914–22. doi: 10.1002/art.30232
120. Aldridge J, Ekwall AH, Mark L, Bergstrom B, Andersson K, Gjertsson I, et al. T Helper cells in synovial fluid of patients with rheumatoid arthritis primarily have a Th1 and a CXCR3(+)Th2 phenotype. Arthritis Res Ther (2020) 22(1):245. doi: 10.1186/s13075-020-02349-y
121. Lindberg J, Klint E, Catrina AI, Nilsson P, Klareskog L, Ulfgren AK, et al. Effect of infliximab on mRNA expression profiles in synovial tissue of rheumatoid arthritis patients. Arthritis Res Ther (2006) 8(6):R179. doi: 10.1186/ar2090
122. Borzi RM, Mazzetti I, Macor S, Silvestri T, Bassi A, Cattini L, et al. Flow cytometric analysis of intracellular chemokines in chondrocytes in vivo: constitutive expression and enhancement in osteoarthritis and rheumatoid arthritis. FEBS Lett (1999) 455(3):238–42. doi: 10.1016/s0014-5793(99)00886-8
123. Iwamoto T, Okamoto H, Kobayashi S, Ikari K, Toyama Y, Tomatsu T, et al. A role of monocyte chemoattractant protein-4 (MCP-4)/CCL13 from chondrocytes in rheumatoid arthritis. FEBS J (2007) 274(18):4904–12. doi: 10.1111/j.1742-4658.2007.06013.x
124. Sucur A, Jajic Z, Artukovic M, Matijasevic MI, Anic B, Flegar D, et al. Chemokine signals are crucial for enhanced homing and differentiation of circulating osteoclast progenitor cells. Arthritis Res Ther (2017) 19(1):142. doi: 10.1186/s13075-017-1337-6
125. Muraoka S, Kaneko K, Motomura K, Nishio J, Nanki T. CX3CL1/fractalkine regulates the differentiation of human peripheral blood monocytes and monocyte-derived dendritic cells into osteoclasts. Cytokine (2021) 146:155652. doi: 10.1016/j.cyto.2021.155652
126. Nanki T, Shimaoka T, Hayashida K, Taniguchi K, Yonehara S, Miyasaka N. Pathogenic role of the CXCL16-CXCR6 pathway in rheumatoid arthritis. Arthritis Rheum (2005) 52(10):3004–14. doi: 10.1002/art.21301
127. Zhang X, Nakajima T, Goronzy JJ, Weyand CM. Tissue trafficking patterns of effector memory CD4+ T cells in rheumatoid arthritis. Arthritis Rheum (2005) 52(12):3839–49. doi: 10.1002/art.21482
128. Sawai H, Park YW, He X, Goronzy JJ, Weyand CM. Fractalkine mediates T cell-dependent proliferation of synovial fibroblasts in rheumatoid arthritis. Arthritis Rheum (2007) 56(10):3215–25. doi: 10.1002/art.22919
129. Shadidi KR, Aarvak T, Henriksen JE, Natvig JB, Thompson KM. The chemokines CCL5, CCL2 and CXCL12 play significant roles in the migration of Th1 cells into rheumatoid synovial tissue. Scand J Immunol (2003) 57(2):192–8. doi: 10.1046/j.1365-3083.2003.01214.x
130. Armas-Gonzalez E, Dominguez-Luis MJ, Diaz-Martin A, Arce-Franco M, Castro-Hernandez J, Danelon G, et al. Role of CXCL13 and CCL20 in the recruitment of b cells to inflammatory foci in chronic arthritis. Arthritis Res Ther (2018) 20(1):114. doi: 10.1186/s13075-018-1611-2
131. Nanki T, Takada K, Komano Y, Morio T, Kanegane H, Nakajima A, et al. Chemokine receptor expression and functional effects of chemokines on b cells: implication in the pathogenesis of rheumatoid arthritis. Arthritis Res Ther (2009) 11(5):R149. doi: 10.1186/ar2823
132. Henneken M, Dorner T, Burmester GR, Berek C. Differential expression of chemokine receptors on peripheral blood b cells from patients with rheumatoid arthritis and systemic lupus erythematosus. Arthritis Res Ther (2005) 7(5):R1001–1013. doi: 10.1186/ar1776
133. Katschke KJ Jr., Rottman JB, Ruth JH, Qin S, Wu L, LaRosa G, et al. Differential expression of chemokine receptors on peripheral blood, synovial fluid, and synovial tissue monocytes/macrophages in rheumatoid arthritis. Arthritis Rheum (2001) 44(5):1022–32. doi: 10.1002/1529-0131(200105)44:5<1022::AID-ANR181>3.0.CO;2-N
134. Schmutz C, Cartwright A, Williams H, Haworth O, Williams JH, Filer A, et al. Monocytes/macrophages express chemokine receptor CCR9 in rheumatoid arthritis and CCL25 stimulates their differentiation. Arthritis Res Ther (2010) 12(4):R161. doi: 10.1186/ar3120
135. Cecchinato V, D’Agostino G, Raeli L, Nerviani A, Schiraldi M, Danelon G, et al. Redox-mediated mechanisms fuel monocyte responses to CXCL12/HMGB1 in active rheumatoid arthritis. Front Immunol (2018) 9:2118. doi: 10.3389/fimmu.2018.02118
136. Wang B, Zinselmeyer BH, Runnels HA, LaBranche TP, Morton PA, Kreisel D, et al. In vivo imaging implicates CCR2(+) monocytes as regulators of neutrophil recruitment during arthritis. Cell Immunol (2012) 278(1-2):103–12. doi: 10.1016/j.cellimm.2012.07.005
137. Chara L, Sanchez-Atrio A, Perez A, Cuende E, Albarran F, Turrion A, et al. Monocyte populations as markers of response to adalimumab plus MTX in rheumatoid arthritis. Arthritis Res Ther (2012) 14(4):R175. doi: 10.1186/ar3928
138. Van Raemdonck K, Umar S, Palasiewicz K, Volkov S, Volin MV, Arami S, et al. CCL21/CCR7 signaling in macrophages promotes joint inflammation and Th17-mediated osteoclast formation in rheumatoid arthritis. Cell Mol Life Sci (2020) 77(7):1387–99. doi: 10.1007/s00018-019-03235-w
139. Inoue A, Matsumoto I, Tanaka Y, Umeda N, Takai C, Kawaguchi H, et al. TIARP attenuates autoantibody-mediated arthritis via the suppression of neutrophil migration by reducing CXCL2/CXCR2 and IL-6 expression. Sci Rep (2016) 6:38684. doi: 10.1038/srep38684
140. Pickens SR, Chamberlain ND, Volin MV, Pope RM, Talarico NE, Mandelin AM 2nd, et al. Role of the CCL21 and CCR7 pathways in rheumatoid arthritis angiogenesis. Arthritis Rheum (2012) 64(8):2471–81. doi: 10.1002/art.34452
141. Chen Z, Kim SJ, Essani AB, Volin MV, Vila OM, Swedler W, et al. Characterising the expression and function of CCL28 and its corresponding receptor, CCR10, in RA pathogenesis. Ann Rheum Dis (2015) 74(10):1898–906. doi: 10.1136/annrheumdis-2013-204530
142. Tsai CH, Chen CJ, Gong CL, Liu SC, Chen PC, Huang CC, et al. CXCL13/CXCR5 axis facilitates endothelial progenitor cell homing and angiogenesis during rheumatoid arthritis progression. Cell Death Dis (2021) 12(9):846. doi: 10.1038/s41419-021-04136-2
143. Flytlie HA, Hvid M, Lindgreen E, Kofod-Olsen E, Petersen EL, Jorgensen A, et al. Expression of MDC/CCL22 and its receptor CCR4 in rheumatoid arthritis, psoriatic arthritis and osteoarthritis. Cytokine (2010) 49(1):24–9. doi: 10.1016/j.cyto.2009.10.005
144. Koch AE, Volin MV, Woods JM, Kunkel SL, Connors MA, Harlow LA, et al. Regulation of angiogenesis by the c-X-C chemokines interleukin-8 and epithelial neutrophil activating peptide 78 in the rheumatoid joint. Arthritis Rheum (2001) 44(1):31–40. doi: 10.1002/1529-0131(200101)44:1<31::AID-ANR5>3.0.CO;2-4
145. Pablos JL, Santiago B, Galindo M, Torres C, Brehmer MT, Blanco FJ, et al. Synoviocyte-derived CXCL12 is displayed on endothelium and induces angiogenesis in rheumatoid arthritis. J Immunol (2003) 170(4):2147–52. doi: 10.4049/jimmunol.170.4.2147
146. Miyabe Y, Miyabe C, Mani V, Mempel TR, Luster AD. Atypical complement receptor C5aR2 transports C5a to initiate neutrophil adhesion and inflammation. Sci Immunol (2019) 4(35):eaav5951. doi: 10.1126/sciimmunol.aav5951
147. Isozaki T, Arbab AS, Haas CS, Amin MA, Arendt MD, Koch AE, et al. Evidence that CXCL16 is a potent mediator of angiogenesis and is involved in endothelial progenitor cell chemotaxis: studies in mice with K/BxN serum-induced arthritis. Arthritis Rheum (2013) 65(7):1736–46. doi: 10.1002/art.37981
148. Hou SM, Chen PC, Lin CM, Fang ML, Chi MC, Liu JF. CXCL1 contributes to IL-6 expression in osteoarthritis and rheumatoid arthritis synovial fibroblasts by CXCR2, c-raf, MAPK, and AP-1 pathway. Arthritis Res Ther (2020) 22(1):251. doi: 10.1186/s13075-020-02331-8
149. Takayasu A, Miyabe Y, Yokoyama W, Kaneko K, Fukuda S, Miyasaka N, et al. CCL18 activates fibroblast-like synoviocytes in patients with rheumatoid arthritis. J Rheumatol (2013) 40(6):1026–8. doi: 10.3899/jrheum.121412
150. Alaaeddine N, Hilal G, Baddoura R, Antoniou J, Di Battista JA. CCL20 stimulates proinflammatory mediator synthesis in human fibroblast-like synoviocytes through a MAP kinase-dependent process with transcriptional and posttranscriptional control. J Rheumatol (2011) 38(9):1858–65. doi: 10.3899/jrheum.110049
151. Yang PT, Kasai H, Zhao LJ, Xiao WG, Tanabe F, Ito M. Increased CCR4 expression on circulating CD4(+) T cells in ankylosing spondylitis, rheumatoid arthritis and systemic lupus erythematosus. Clin Exp Immunol (2004) 138(2):342–7. doi: 10.1111/j.1365-2249.2004.02617.x
152. Nanki T, Hayashida K, El-Gabalawy HS, Suson S, Shi K, Girschick HJ, et al. Stromal cell-derived factor-1-CXC chemokine receptor 4 interactions play a central role in CD4+ T cell accumulation in rheumatoid arthritis synovium. J Immunol (2000) 165(11):6590–8. doi: 10.4049/jimmunol.165.11.6590
153. Buckley CD, Amft N, Bradfield PF, Pilling D, Ross E, Arenzana-Seisdedos F, et al. Persistent induction of the chemokine receptor CXCR4 by TGF-beta 1 on synovial T cells contributes to their accumulation within the rheumatoid synovium. J Immunol (2000) 165(6):3423–9. doi: 10.4049/jimmunol.165.6.3423
154. Lee JH, Kim B, Jin WJ, Kim HH, Ha H, Lee ZH. Pathogenic roles of CXCL10 signaling through CXCR3 and TLR4 in macrophages and T cells: relevance for arthritis. Arthritis Res Ther (2017) 19(1):163. doi: 10.1186/s13075-017-1353-6
155. Hirota K, Yoshitomi H, Hashimoto M, Maeda S, Teradaira S, Sugimoto N, et al. Preferential recruitment of CCR6-expressing Th17 cells to inflamed joints via CCL20 in rheumatoid arthritis and its animal model. J Exp Med (2007) 204(12):2803–12. doi: 10.1084/jem.20071397
156. Fennen M, Weinhage T, Kracke V, Intemann J, Varga G, Wehmeyer C, et al. A myostatin-CCL20-CCR6 axis regulates Th17 cell recruitment to inflamed joints in experimental arthritis. Sci Rep (2021) 11(1):14145. doi: 10.1038/s41598-021-93599-6
157. Kaneko S, Kondo Y, Yokosawa M, Furuyama K, Segawa S, Tsuboi H, et al. The RORgammat-CCR6-CCL20 axis augments Th17 cells invasion into the synovia of rheumatoid arthritis patients. Mod Rheumatol (2018) 28(5):814–25. doi: 10.1080/14397595.2017.1416923
158. Takaki-Kuwahara A, Arinobu Y, Miyawaki K, Yamada H, Tsuzuki H, Irino K, et al. CCR6+ group 3 innate lymphoid cells accumulate in inflamed joints in rheumatoid arthritis and produce Th17 cytokines. Arthritis Res Ther (2019) 21(1):198. doi: 10.1186/s13075-019-1984-x
159. Cao G, Chi S, Wang X, Sun J, Zhang Y. CD4+CXCR5+PD-1+ T follicular helper cells play a pivotal role in the development of rheumatoid arthritis. Med Sci Monit (2019) 25:3032–40. doi: 10.12659/MSM.914868
160. Moschovakis GL, Bubke A, Friedrichsen M, Falk CS, Feederle R, Forster R. T Cell specific Cxcr5 deficiency prevents rheumatoid arthritis. Sci Rep (2017) 7(1):8933. doi: 10.1038/s41598-017-08935-6
161. Guo X, Xu T, Zheng J, Cui X, Li M, Wang K, et al. Accumulation of synovial fluid CD19(+)CD24(hi)CD27(+) b cells was associated with bone destruction in rheumatoid arthritis. Sci Rep (2020) 10(1):14386. doi: 10.1038/s41598-020-71362-7
162. Rempenault C, Mielle J, Schreiber K, Corbeau P, Macia L, Combe B, et al. CXCR5/CXCL13 pathway, a key driver for migration of regulatory B10 cells, is defective in patients with rheumatoid arthritis. Rheumatol (Oxford) (2022) 61(5):2185–96. doi: 10.1093/rheumatology/keab639
163. Talbot J, Bianchini FJ, Nascimento DC, Oliveira RD, Souto FO, Pinto LG, et al. CCR2 expression in neutrophils plays a critical role in their migration into the joints in rheumatoid arthritis. Arthritis Rheumatol (2015) 67(7):1751–9. doi: 10.1002/art.39117
164. Shahrara S, Proudfoot AE, Woods JM, Ruth JH, Amin MA, Park CC, et al. Amelioration of rat adjuvant-induced arthritis by met-RANTES. Arthritis Rheum (2005) 52(6):1907–19. doi: 10.1002/art.21033
165. Smith E, McGettrick HM, Stone MA, Shaw JS, Middleton J, Nash GB, et al. Duffy Antigen receptor for chemokines and CXCL5 are essential for the recruitment of neutrophils in a multicellular model of rheumatoid arthritis synovium. Arthritis Rheum (2008) 58(7):1968–73. doi: 10.1002/art.23545
166. Cunha TM, Barsante MM, Guerrero AT, Verri WA Jr., Ferreira SH, Coelho FM, et al. Treatment with DF 2162, a non-competitive allosteric inhibitor of CXCR1/2, diminishes neutrophil influx and inflammatory hypernociception in mice. Br J Pharmacol (2008) 154(2):460–70. doi: 10.1038/bjp.2008.94
167. Barsante MM, Cunha TM, Allegretti M, Cattani F, Policani F, Bizzarri C, et al. Blockade of the chemokine receptor CXCR2 ameliorates adjuvant-induced arthritis in rats. Br J Pharmacol (2008) 153(5):992–1002. doi: 10.1038/sj.bjp.0707462
168. Chou RC, Kim ND, Sadik CD, Seung E, Lan Y, Byrne MH, et al. Lipid-cytokine-chemokine cascade drives neutrophil recruitment in a murine model of inflammatory arthritis. Immunity (2010) 33(2):266–78. doi: 10.1016/j.immuni.2010.07.018
169. Miyabe Y, Miyabe C, Murooka TT, Kim EY, Newton GA, Kim ND, et al. Complement C5a receptor is the key initiator of neutrophil adhesion igniting immune complex-induced arthritis. Sci Immunol (2017) 2(7):eaaj2195. doi: 10.1126/sciimmunol.aaj2195
170. Boff D, Crijns H, Janssens R, Vanheule V, Menezes GB, Macari S, et al. The chemokine fragment CXCL9(74-103) diminishes neutrophil recruitment and joint inflammation in antigen-induced arthritis. J Leukoc Biol (2018) 104(2):413–22. doi: 10.1002/JLB.3MA1217-502R
171. Lebre MC, Vergunst CE, Choi IY, Aarrass S, Oliveira AS, Wyant T, et al. Why CCR2 and CCR5 blockade failed and why CCR1 blockade might still be effective in the treatment of rheumatoid arthritis. PloS One (2011) 6(7):e21772. doi: 10.1371/journal.pone.0021772
172. Koch AE, Kunkel SL, Harlow LA, Mazarakis DD, Haines GK, Burdick MD, et al. Macrophage inflammatory protein-1 alpha. a novel chemotactic cytokine for macrophages in rheumatoid arthritis. J Clin Invest (1994) 93(3):921–8. doi: 10.1172/JCI117097
173. Blades MC, Ingegnoli F, Wheller SK, Manzo A, Wahid S, Panayi GS, et al. Stromal cell-derived factor 1 (CXCL12) induces monocyte migration into human synovium transplanted onto SCID mice. Arthritis Rheum (2002) 46(3):824–36. doi: 10.1002/art.10102
174. Toh K, Kukita T, Wu Z, Kukita A, Sandra F, Tang QY, et al. Possible involvement of MIP-1alpha in the recruitment of osteoclast progenitors to the distal tibia in rats with adjuvant-induced arthritis. Lab Invest (2004) 84(9):1092–102. doi: 10.1038/labinvest.3700132
175. Kindstedt E, Holm CK, Sulniute R, Martinez-Carrasco I, Lundmark R, Lundberg P. CCL11, a novel mediator of inflammatory bone resorption. Sci Rep (2017) 7(1):5334. doi: 10.1038/s41598-017-05654-w
176. Lee J, Park C, Kim HJ, Lee YD, Lee ZH, Song YW, et al. Stimulation of osteoclast migration and bone resorption by c-c chemokine ligands 19 and 21. Exp Mol Med (2017) 49(7):e358. doi: 10.1038/emm.2017.100
177. Ha J, Choi HS, Lee Y, Kwon HJ, Song YW, Kim HH. CXC chemokine ligand 2 induced by receptor activator of NF-kappa b ligand enhances osteoclastogenesis. J Immunol (2010) 184(9):4717–24. doi: 10.4049/jimmunol.0902444
178. Matsuura T, Ichinose S, Akiyama M, Kasahara Y, Tachikawa N, Nakahama KI. Involvement of CX3CL1 in the migration of osteoclast precursors across osteoblast layer stimulated by interleukin-1ss. J Cell Physiol (2017) 232(7):1739–45. doi: 10.1002/jcp.25577
179. Kanbe K, Takagishi K, Chen Q. Stimulation of matrix metalloprotease 3 release from human chondrocytes by the interaction of stromal cell-derived factor 1 and CXC chemokine receptor 4. Arthritis Rheum (2002) 46(1):130–7. doi: 10.1002/1529-0131(200201)46:1<130::aid-art10020>3.0.co;2-d
180. Agere SA, Akhtar N, Watson JM, Ahmed S. RANTES/CCL5 induces collagen degradation by activating MMP-1 and MMP-13 expression in human rheumatoid arthritis synovial fibroblasts. Front Immunol (2017) 8:1341. doi: 10.3389/fimmu.2017.01341
181. Kanbe K, Takemura T, Takeuchi K, Chen Q, Takagishi K, Inoue K. Synovectomy reduces stromal-cell-derived factor-1 (SDF-1) which is involved in the destruction of cartilage in osteoarthritis and rheumatoid arthritis. J Bone Joint Surg Br (2004) 86(2):296–300. doi: 10.1302/0301-620x.86b2.14474
182. Wang Y, Wu H, Deng R. Angiogenesis as a potential treatment strategy for rheumatoid arthritis. Eur J Pharmacol (2021) 910:174500. doi: 10.1016/j.ejphar.2021.174500
183. Watanabe K, Penfold ME, Matsuda A, Ohyanagi N, Kaneko K, Miyabe Y, et al. Pathogenic role of CXCR7 in rheumatoid arthritis. Arthritis Rheum (2010) 62(11):3211–20. doi: 10.1002/art.27650
184. Tsaltskan V, Firestein GS. Targeting fibroblast-like synoviocytes in rheumatoid arthritis. Curr Opin Pharmacol (2022) 67:102304. doi: 10.1016/j.coph.2022.102304
185. Wang W, Deng Z, Liu G, Yang J, Zhou W, Zhang C, et al. Platelet-derived extracellular vesicles promote the migration and invasion of rheumatoid arthritis fibroblast-like synoviocytes via CXCR2 signaling. Exp Ther Med (2021) 22(4):1120. doi: 10.3892/etm.2021.10554
186. Volin MV, Huynh N, Klosowska K, Chong KK, Woods JM. Fractalkine is a novel chemoattractant for rheumatoid arthritis fibroblast-like synoviocyte signaling through MAP kinases and akt. Arthritis Rheum (2007) 56(8):2512–22. doi: 10.1002/art.22806
187. Volin MV, Woods JM, Amin MA, Connors MA, Harlow LA, Koch AE. Fractalkine: a novel angiogenic chemokine in rheumatoid arthritis. Am J Pathol (2001) 159(4):1521–30. doi: 10.1016/S0002-9440(10)62537-0
188. Tripathi DK, Poluri KM. Molecular insights into kinase mediated signaling pathways of chemokines and their cognate G protein coupled receptors. Front Biosci (Landmark Ed) (2020) 25(7):1361–85. doi: 10.2741/4860
189. Chintalacharuvu SR, Wang JX, Giaconia JM, Venkataraman C. An essential role for CCL3 in the development of collagen antibody-induced arthritis. Immunol Lett (2005) 100(2):202–4. doi: 10.1016/j.imlet.2005.03.012
190. Moschovakis GL, Bubke A, Friedrichsen M, Ristenpart J, Back JW, Falk CS, et al. The chemokine receptor CCR7 is a promising target for rheumatoid arthritis therapy. Cell Mol Immunol (2019) 16(10):791–9. doi: 10.1038/s41423-018-0056-5
191. Chen L, Guo L, Tian J, He H, Marinova E, Zhang P, et al. Overexpression of CXC chemokine ligand 14 exacerbates collagen-induced arthritis. J Immunol (2010) 184(8):4455–9. doi: 10.4049/jimmunol.0900525
192. Rampersad RR, Tarrant TK, Vallanat CT, Quintero-Matthews T, Weeks MF, Esserman DA, et al. Enhanced Th17-cell responses render CCR2-deficient mice more susceptible for autoimmune arthritis. PloS One (2011) 6(10):e25833. doi: 10.1371/journal.pone.0025833
193. Quinones MP, Ahuja SK, Jimenez F, Schaefer J, Garavito E, Rao A, et al. Experimental arthritis in CC chemokine receptor 2-null mice closely mimics severe human rheumatoid arthritis. J Clin Invest (2004) 113(6):856–66. doi: 10.1172/JCI20126
194. Fujii H, Baba T, Ishida Y, Kondo T, Yamagishi M, Kawano M, et al. Ablation of the Ccr2 gene exacerbates polyarthritis in interleukin-1 receptor antagonist-deficient mice. Arthritis Rheum (2011) 63(1):96–106. doi: 10.1002/art.30106
195. Quinones MP, Jimenez F, Martinez H, Estrada CA, Willmon O, Dudley M, et al. CC chemokine receptor (CCR)-2 prevents arthritis development following infection by mycobacterium avium. J Mol Med (Berl) (2006) 84(6):503–12. doi: 10.1007/s00109-006-0039-3
196. Bonelli M, Puchner A, Goschl L, Hayer S, Niederreiter B, Steiner G, et al. CCR6 controls autoimmune but not innate immunity-driven experimental arthritis. J Cell Mol Med (2018) 22(11):5278–85. doi: 10.1111/jcmm.13783
197. Slauenwhite D, Gebremeskel S, Doucette CD, Hoskin DW, Johnston B. Regulation of cytokine polarization and T cell recruitment to inflamed paws in mouse collagen-induced arthritis by the chemokine receptor CXCR6. Arthritis Rheumatol (2014) 66(11):3001–12. doi: 10.1002/art.38816
198. Bao L, Zhu Y, Zhu J, Lindgren JU. Decreased IgG production but increased MIP-1beta expression in collagen-induced arthritis in c-c chemokine receptor 5-deficient mice. Cytokine (2005) 31(1):64–71. doi: 10.1016/j.cyto.2005.03.003
199. Wengner AM, Hopken UE, Petrow PK, Hartmann S, Schurigt U, Brauer R, et al. CXCR5- and CCR7-dependent lymphoid neogenesis in a murine model of chronic antigen-induced arthritis. Arthritis Rheum (2007) 56(10):3271–83. doi: 10.1002/art.22939
200. Chung SH, Seki K, Choi BI, Kimura KB, Ito A, Fujikado N, et al. CXC chemokine receptor 4 expressed in T cells plays an important role in the development of collagen-induced arthritis. Arthritis Res Ther (2010) 12(5):R188. doi: 10.1186/ar3158
201. Shahrara S, Proudfoot AE, Park CC, Volin MV, Haines GK, Woods JM, et al. Inhibition of monocyte chemoattractant protein-1 ameliorates rat adjuvant-induced arthritis. J Immunol (2008) 180(5):3447–56. doi: 10.4049/jimmunol.180.5.3447
202. Barnes DA, Tse J, Kaufhold M, Owen M, Hesselgesser J, Strieter R, et al. Polyclonal antibody directed against human RANTES ameliorates disease in the Lewis rat adjuvant-induced arthritis model. J Clin Invest (1998) 101(12):2910–9. doi: 10.1172/JCI2172
203. Ablin JN, Entin-Meer M, Aloush V, Oren S, Elkayam O, George J, et al. Protective effect of eotaxin-2 inhibition in adjuvant-induced arthritis. Clin Exp Immunol (2010) 161(2):276–83. doi: 10.1111/j.1365-2249.2010.04172.x
204. Halloran MM, Woods JM, Strieter RM, Szekanecz Z, Volin MV, Hosaka S, et al. The role of an epithelial neutrophil-activating peptide-78-like protein in rat adjuvant-induced arthritis. J Immunol (1999) 162(12):7492–500.
205. Falsone A, Wabitsch V, Geretti E, Potzinger H, Gerlza T, Robinson J, et al. Designing CXCL8-based decoy proteins with strong anti-inflammatory activity in vivo. Biosci Rep (2013) 33(5):e00068. doi: 10.1042/BSR20130069
206. Kang SE, Park JK, Yoo HJ, Kang HS, Park YW, Park BC, et al. Efficacy of novel bispecific antibody targeting TNF-alpha/CXCL10 in the treatment of experimental arthritis. Transl Res (2021) 232:75–87. doi: 10.1016/j.trsl.2021.01.004
207. Hoshino-Negishi K, Ohkuro M, Nakatani T, Kuboi Y, Nishimura M, Ida Y, et al. Role of anti-fractalkine antibody in suppression of joint destruction by inhibiting migration of osteoclast precursors to the synovium in experimental arthritis. Arthritis Rheumatol (2019) 71(2):222–31. doi: 10.1002/art.40688
208. Amat M, Benjamim CF, Williams LM, Prats N, Terricabras E, Beleta J, et al. Pharmacological blockade of CCR1 ameliorates murine arthritis and alters cytokine networks. vivo. Br J Pharmacol (2006) 149(6):666–75. doi: 10.1038/sj.bjp.0706912
209. Flegar D, Filipovic M, Sucur A, Markotic A, Lukac N, Sisl D, et al. Preventive CCL2/CCR2 axis blockade suppresses osteoclast activity in a mouse model of rheumatoid arthritis by reducing homing of CCR2(hi) osteoclast progenitors to the affected bone. Front Immunol (2021) 12:767231. doi: 10.3389/fimmu.2021.767231
210. Ansari MA, Nadeem A, Bakheet SA, Attia SM, Shahid M, Alyousef FS, et al. Chemokine receptor 5 antagonism causes reduction in joint inflammation in a collagen-induced arthritis mouse model. Molecules (2021) 26(7):1839. doi: 10.3390/molecules26071839
211. Dutta R, Lunzer MM, Auger JL, Akgun E, Portoghese PS, Binstadt BA. A bivalent compound targeting CCR5 and the mu opioid receptor treats inflammatory arthritis pain in mice without inducing pharmacologic tolerance. Arthritis Res Ther (2018) 20(1):154. doi: 10.1186/s13075-018-1661-5
212. Vierboom MP, Zavodny PJ, Chou CC, Tagat JR, Pugliese-Sivo C, Strizki J, et al. Inhibition of the development of collagen-induced arthritis in rhesus monkeys by a small molecular weight antagonist of CCR5. Arthritis Rheum (2005) 52(2):627–36. doi: 10.1002/art.20850
213. Min SH, Wang Y, Gonsiorek W, Anilkumar G, Kozlowski J, Lundell D, et al. Pharmacological targeting reveals distinct roles for CXCR2/CXCR1 and CCR2 in a mouse model of arthritis. Biochem Biophys Res Commun (2010) 391(1):1080–6. doi: 10.1016/j.bbrc.2009.12.025
214. Mohan K, Issekutz TB. Blockade of chemokine receptor CXCR3 inhibits T cell recruitment to inflamed joints and decreases the severity of adjuvant arthritis. J Immunol (2007) 179(12):8463–9. doi: 10.4049/jimmunol.179.12.8463
215. Bakheet SA, Ansari MA, Nadeem A, Attia SM, Alhoshani AR, Gul G, et al. CXCR3 antagonist AMG487 suppresses rheumatoid arthritis pathogenesis and progression by shifting the Th17/Treg cell balance. Cell Signal (2019) 64:109395. doi: 10.1016/j.cellsig.2019.109395
216. Jenh CH, Cox MA, Cui L, Reich EP, Sullivan L, Chen SC, et al. A selective and potent CXCR3 antagonist SCH 546738 attenuates the development of autoimmune diseases and delays graft rejection. BMC Immunol (2012) 13:2. doi: 10.1186/1471-2172-13-2
217. Kim B, Lee JH, Jin WJ, Kim HH, Ha H, Lee ZH. JN-2, a c-X-C motif chemokine receptor 3 antagonist, ameliorates arthritis progression in an animal model. Eur J Pharmacol (2018) 823:1–10. doi: 10.1016/j.ejphar.2018.01.037
218. Tamamura H, Fujisawa M, Hiramatsu K, Mizumoto M, Nakashima H, Yamamoto N, et al. Identification of a CXCR4 antagonist, a T140 analog, as an anti-rheumatoid arthritis agent. FEBS Lett (2004) 569(1-3):99–104. doi: 10.1016/j.febslet.2004.05.056
219. Miyabe Y, Lian J, Miyabe C, Luster AD. Chemokines in rheumatic diseases: pathogenic role and therapeutic implications. Nat Rev Rheumatol (2019) 15(12):731–46. doi: 10.1038/s41584-019-0323-6
220. Haringman JJ, Gerlag DM, Smeets TJ, Baeten D, van den Bosch F, Bresnihan B, et al. A randomized controlled trial with an anti-CCL2 (anti-monocyte chemotactic protein 1) monoclonal antibody in patients with rheumatoid arthritis. Arthritis Rheum (2006) 54(8):2387–92. doi: 10.1002/art.21975
221. Vergunst CE, Gerlag DM, Lopatinskaya L, Klareskog L, Smith MD, van den Bosch F, et al. Modulation of CCR2 in rheumatoid arthritis: a double-blind, randomized, placebo-controlled clinical trial. Arthritis Rheum (2008) 58(7):1931–9. doi: 10.1002/art.23591
222. Duan H, Yang P, Fang F, Ding S, Xiao W. CCR5 small interfering RNA ameliorated joint inflammation in rats with adjuvant-induced arthritis. Immunol Lett (2014) 162(2 Pt B):258–63. doi: 10.1016/j.imlet.2014.09.018
223. Pokorny V, McQueen F, Yeoman S, Merriman M, Merriman A, Harrison A, et al. Evidence for negative association of the chemokine receptor CCR5 d32 polymorphism with rheumatoid arthritis. Ann Rheum Dis (2005) 64(3):487–90. doi: 10.1136/ard.2004.023333
224. John S, Smith S, Morrison JF, Symmons D, Worthington J, Silman A, et al. Genetic variation in CCR5 does not predict clinical outcome in inflammatory arthritis. Arthritis Rheum (2003) 48(12):3615–6. doi: 10.1002/art.11360
225. Zuniga JA, Villarreal-Garza C, Flores E, Barquera R, Perez-Hernandez N, Montes de Oca JV, et al. Biological relevance of the polymorphism in the CCR5 gene in refractory and non-refractory rheumatoid arthritis in mexicans. Clin Exp Rheumatol (2003) 21(3):351–4.
226. van Kuijk AW, Vergunst CE, Gerlag DM, Bresnihan B, Gomez-Reino JJ, Rouzier R, et al. CCR5 blockade in rheumatoid arthritis: a randomised, double-blind, placebo-controlled clinical trial. Ann Rheum Dis (2010) 69(11):2013–6. doi: 10.1136/ard.2010.131235
227. Gerlag DM, Hollis S, Layton M, Vencovsky J, Szekanecz Z, Braddock M, et al. Preclinical and clinical investigation of a CCR5 antagonist, AZD5672, in patients with rheumatoid arthritis receiving methotrexate. Arthritis Rheum (2010) 62(11):3154–60. doi: 10.1002/art.27652
228. Fleishaker DL, Garcia Meijide JA, Petrov A, Kohen MD, Wang X, Menon S, et al. Maraviroc, a chemokine receptor-5 antagonist, fails to demonstrate efficacy in the treatment of patients with rheumatoid arthritis in a randomized, double-blind placebo-controlled trial. Arthritis Res Ther (2012) 14(1):R11. doi: 10.1186/ar3685
229. Tak PP, Balanescu A, Tseluyko V, Bojin S, Drescher E, Dairaghi D, et al. Chemokine receptor CCR1 antagonist CCX354-C treatment for rheumatoid arthritis: CARAT-2, a randomised, placebo controlled clinical trial. Ann Rheum Dis (2013) 72(3):337–44. doi: 10.1136/annrheumdis-2011-201605
230. Vergunst CE, Gerlag DM, von Moltke L, Karol M, Wyant T, Chi X, et al. MLN3897 plus methotrexate in patients with rheumatoid arthritis: safety, efficacy, pharmacokinetics, and pharmacodynamics of an oral CCR1 antagonist in a phase IIa, double-blind, placebo-controlled, randomized, proof-of-concept study. Arthritis Rheum (2009) 60(12):3572–81. doi: 10.1002/art.24978
231. Gladue RP, Brown MF, Zwillich SH. CCR1 antagonists: what have we learned from clinical trials. Curr Top Med Chem (2010) 10(13):1268–77. doi: 10.2174/156802610791561237
232. Yellin M, Paliienko I, Balanescu A, Ter-Vartanian S, Tseluyko V, Xu LA, et al. Randomized, double-blind, placebo-controlled study evaluating the efficacy and safety of MDX-1100, a fully human anti-CXCL10 monoclonal antibody, in combination with methotrexate in patients with rheumatoid arthritis. Arthritis Rheum (2012) 64(6):1730–9. doi: 10.1002/art.34330
233. Tanaka Y, Takeuchi T, Yamanaka H, Nanki T, Umehara H, Yasuda N, et al. Long-term safety and efficacy of e6011, an anti-fractalkine monoclonal antibody, in patients with rheumatoid arthritis inadequately responding to methotrexate. Mod Rheumatol (2023) 21:road004. doi: 10.1093/mr/road004
234. Tanaka Y, Takeuchi T, Yamanaka H, Nanki T, Umehara H, Yasuda N, et al. Long-term evaluation of e6011, an anti-fractalkine monoclonal antibody, in patients with rheumatoid arthritis inadequately responding to biological disease-modifying antirheumatic drugs. Mod Rheumatol (2023) 21:road005. doi: 10.1093/mr/road005
235. Baek HJ, Lim MJ, Park W, Park SH, Shim SC, Yoo DH, et al. Efficacy and safety of tocilizumab in Korean patients with active rheumatoid arthritis. Korean J Intern Med (2019) 34(4):917–31. doi: 10.3904/kjim.2017.159
236. Elmedany SH, Mohamed AE, Galil SMA. Efficacy and safety profile of intravenous tocilizumab versus intravenous abatacept in treating female Saudi Arabian patients with active moderate-to-severe rheumatoid arthritis. Clin Rheumatol (2019) 38(8):2109–17. doi: 10.1007/s10067-019-04508-2
237. Bouajina E, Zakraoui L, Kchir M, Kochbati S, Baklouti S. Safety and efficacy of tocilizumab as monotherapy or in combination with methotrexate in Tunisian patients with active rheumatoid arthritis and inadequate response to disease-modifying anti-rheumatic drugs in conditions close to clinical practice. Clin Rheumatol (2020) 39(5):1449–55. doi: 10.1007/s10067-019-04815-8
238. Choe JY, Prodanovic N, Niebrzydowski J, Staykov I, Dokoupilova E, Baranauskaite A, et al. A randomised, double-blind, phase III study comparing SB2, an infliximab biosimilar, to the infliximab reference product remicade in patients with moderate to severe rheumatoid arthritis despite methotrexate therapy. Ann Rheum Dis (2017) 76(1):58–64. doi: 10.1136/annrheumdis-2015-207764
239. Matsuno H, Matsubara T. A randomized double-blind parallel-group phase III study to compare the efficacy and safety of NI-071 and infliximab reference product in Japanese patients with active rheumatoid arthritis refractory to methotrexate. Mod Rheumatol (2019) 29(6):919–27. doi: 10.1080/14397595.2018.1533063
240. Genovese MC, Sanchez-Burson J, Oh M, Balazs E, Neal J, Everding A, et al. Comparative clinical efficacy and safety of the proposed biosimilar ABP 710 with infliximab reference product in patients with rheumatoid arthritis. Arthritis Res Ther (2020) 22(1):60. doi: 10.1186/s13075-020-2142-1
241. Angelini A, Miyabe Y, Newsted D, Kwan BH, Miyabe C, Kelly RL, et al. Directed evolution of broadly crossreactive chemokine-blocking antibodies efficacious in arthritis. Nat Commun (2018) 9(1):1461. doi: 10.1038/s41467-018-03687-x
Keywords: rheumatoid arthritis, chemokine, chemokine receptor, migration, leukocyte, blockade
Citation: Murayama MA, Shimizu J, Miyabe C, Yudo K and Miyabe Y (2023) Chemokines and chemokine receptors as promising targets in rheumatoid arthritis. Front. Immunol. 14:1100869. doi: 10.3389/fimmu.2023.1100869
Received: 17 November 2022; Accepted: 31 January 2023;
Published: 13 February 2023.
Edited by:
Elias J. Lolis, Yale University, United StatesReviewed by:
Chayan Munshi, Berlin School of Business and Innovation (BSBI), GermanyCopyright © 2023 Murayama, Shimizu, Miyabe, Yudo and Miyabe. This is an open-access article distributed under the terms of the Creative Commons Attribution License (CC BY). The use, distribution or reproduction in other forums is permitted, provided the original author(s) and the copyright owner(s) are credited and that the original publication in this journal is cited, in accordance with accepted academic practice. No use, distribution or reproduction is permitted which does not comply with these terms.
*Correspondence: Yoshishige Miyabe, eW9zaGlzaGlnZS5taXlhYmVAbWFyaWFubmEtdS5hYy5qcA==
Disclaimer: All claims expressed in this article are solely those of the authors and do not necessarily represent those of their affiliated organizations, or those of the publisher, the editors and the reviewers. Any product that may be evaluated in this article or claim that may be made by its manufacturer is not guaranteed or endorsed by the publisher.
Research integrity at Frontiers
Learn more about the work of our research integrity team to safeguard the quality of each article we publish.