- 1Institute of Chinese Medical Sciences, State Key Laboratory of Quality Research in Chinese Medicine, University of Macau, Macao, Macao SAR, China
- 2Department of Pharmceutical Sciences, Faculty of Health Sciences, University of Macau, Macao, Macao SAR, China
- 3MoE Frontiers Science Center for Precision Oncology, University of Macau, Macao, Macao SAR, China
- 4Guangdong-Hong Kong-Macau Joint Lab on Chinese Medicine and Immune Disease Research, Macao, Macao SAR, China
Immune checkpoint inhibitors (ICIs) by targeting PD-1/PD-L1 or CTLA-4 have markedly improved the outcome of cancer patients. However, most solid tumor patients can’t benefit from such therapy. Identification of novel biomarkers to predict the responses of ICIs is crucial to enhance their therapeutic efficacy. TNFR2 is highly expressed by the maximally immunosuppressive subset of CD4+Foxp3+ regulatory T cells (Tregs), especially those present in tumor microenvironment (TME). Since Tregs represent a major cellular mechanism in tumor immune evasion, TNFR2 may be a useful biomarker to predict the responses to ICIs therapy. This notion is supported by our analysis of the computational tumor immune dysfunction and exclusion (TIDE) framework from published single-cell RNA-seq data of pan-cancer databases. The results show that, as expected, TNFR2 is highly expressed by tumor-infiltrating Tregs. Interestingly, TNFR2 is also expressed by the exhausted CD8 T cells in breast cancer (BRCA), hepatocellular carcinoma (HCC), lung squamous cell carcinoma (LUSC), and melanoma (MELA). Importantly, high expression of TNFR2 is associated with poor responses to the treatment with ICIs in BRCA, HCC, LUSC, and MELA. In conclusion, the expression of TNFR2 in TME may be a reliable biomarker for the precision of ICIs treatment of cancer patients, and this idea merits further research.
Introduction
The development of immune checkpoint inhibitors (ICIs) by targeting CTLA-4, PD-1 or PD-L1 has now revolutionized cancer treatment (1–3). ICIs interrupt co-inhibitory signaling pathways and relieve or overcome tumor-induced immunosuppression, thereby reinvigorating anti-tumor immune responses (2, 4). Despite the outcomes being promising, the majority of patients cannot derive the benefits from PD-1/PD-L1 and CTLA-4 inhibitors therapy, due to low response rates and immune-related adverse events (irAEs) (5–8). The onset of irAEs is highly unpredictable, severe, and sometimes even fatal. The identification of novel biomarkers to predict the responses of ICIs is crucial to enhance the efficacy of their treatments. Recently, a substantial number of studies have been performed to explore the potential irAE biomarkers, including cytokines/chemokines, autoantibodies, immunogenetics, and microbial biomarkers (9–13). To date, no single biomarker has been identified to precisely predict the responses in patients receiving ICIs treatment.
CD4+Foxp3+ regulatory T cells (Tregs) are central regulators of anti-tumor immune responses (14) and they represent a major cellular mechanism of tumor immune evasion (15, 16). Tumor necrosis factor receptor 2 (TNFR2), one of two receptors that mediate the biological function of TNF, is predominantly expressed by highly immunosuppressive subset of Tregs in mice and humans (17–20). There is now compelling evidence indicate that TNFR2 plays a decisive role in the activation, proliferative expansion, immunosuppressive function, and phenotypic stability of Tregs (17, 19, 21). Furthermore, TNFR2 is also expressed by other types of immunosuppressive cells such as myeloid-derived suppressor cells (MDSCs), mesenchymal stem cells (MSCs), and some tumor cells (16, 22, 23). We have proposed that TNFR2 behaves as an immune checkpoint stimulator and oncoprotein (15). Interestingly, it was reported that TNF could upregulate PD-L1 expression in pancreatic cancer cells through TNFR2 signaling, and consequently induced PD-1/PD-L1-mediated immune evasion (24). Targeting TNFR2 was able to significantly improve the anti-tumor efficacy in multiple tumor models (25–27). We further hypothesize that TNFR2 may be a useful biomarker for the prediction of the responses to ICIs therapy.
TNFR2 is preferentially expressed by tumor-infiltrating Tregs and exhausted CD8 T cells
To test the hypothesis, we first examined the expression of TNFR2 in different types of tumor-infiltrating T cells, by re-analyzing and integrating the pan-cancer single-cell landscape with UMAP visualization from the Zemin Zhang group’s study (28), based on their framework of scDVA (short for single-cell RNA-seq data visualization and analysis) among those various tumor types. It was reported by us and others that TNFR2 could be expressed by mouse antigen-experienced CD4+Foxp3- conventional T cells, including those in the tumor environment (29, 30), we thus firstly analyzed TNFR2 expression by subsets of cells in CD4 T cell metaclusters. As expected, the expression of TNFR2 by CD4+FoxP3+ Tregs was much higher than that expressed by conventional CD4 T cells in human cancers (Figures 1A, B). Therefore, high expression of TNFR2 remained to be a trustful marker of Tregs in human tumors.
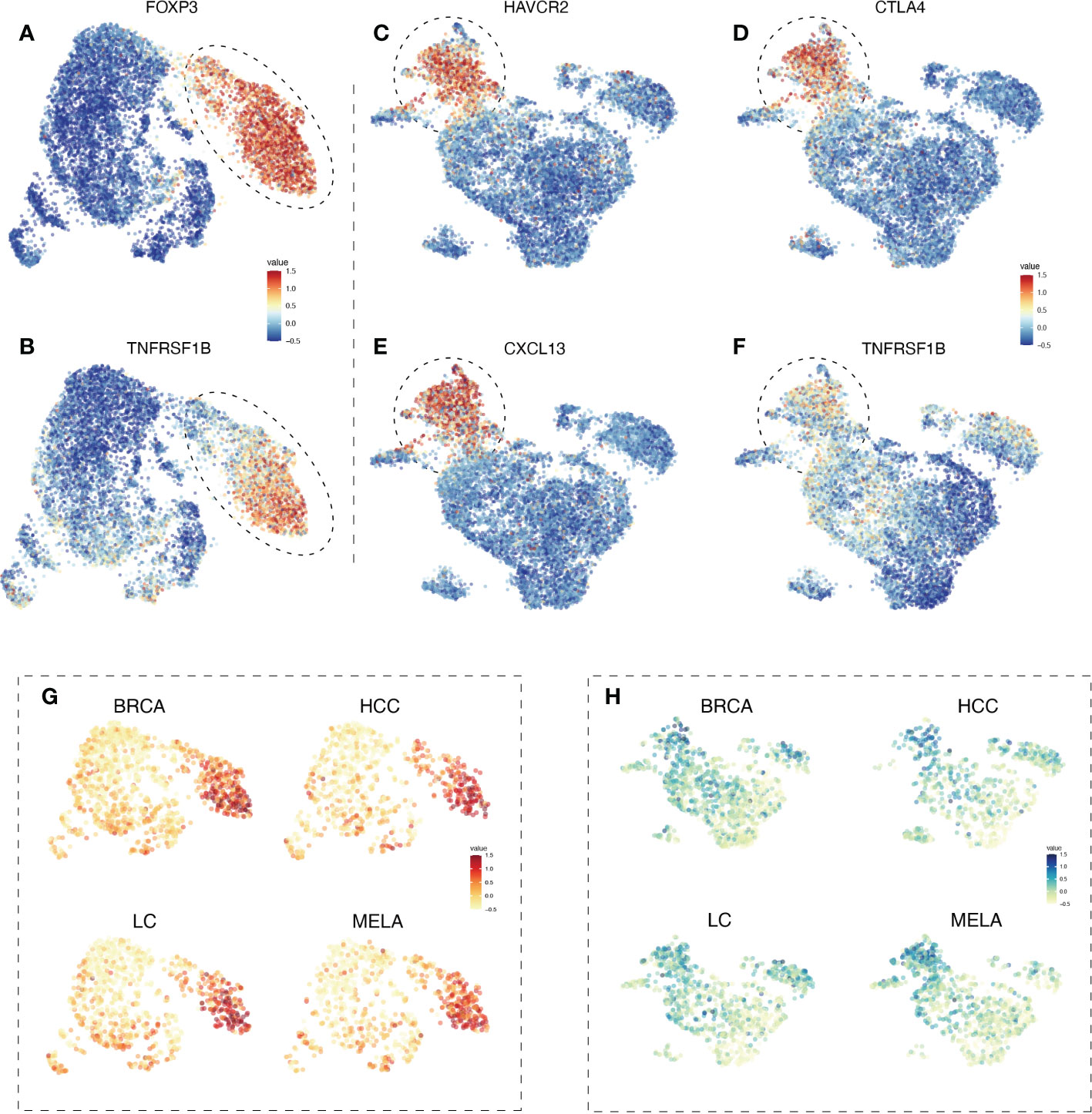
Figure 1 The expression profile of TNFR2 in tumor-infiltrating Tregs and exhausted CD8 T cells in BRCA, HCC, LC, and MELA. To estimate the differentiation of TNFR2 expression among tumor-infiltrating immune cells, we re-analyzed and integrated the pan-cancer single-cell landscape from the Zemin Zhang group’s study, based on their framework of scDVA (short for single-cell RNA-seq data visualization and analysis). UMAP visualization shows the expression of FOXP3 (A) in CD4 T cell metaclusters. The dotted line represents regulatory T cells. (B) TNFR2 expression in CD4 T cell metaclusters. In CD8 T cell metaclusters, the expression of selected marker genes, such as HAVCR2 (C), CTLA4 (D), and CXCL13 (E) are shown. Dotted line represents terminal exhausted CD8 T cells. (F) TNFR2 expression in CD8 T cell metaclusters. UMAP visualization shows the expression of TNFR2 in CD4 T cell metaclusters (G) and CD8 T cell metaclusters (H) across four cancer types (BRCA, HCC, LC, and MELA).
CD8 T cells could also express TNFR2 and the signal of TNFR2 in CD8 cytotoxic T lymphocytes (CTLs) was purportedly mediated by the anti-tumor effect of agonistic antibodies against TNFR2 (27). We analyzed TNFR2 expression on tumor-infiltrating CD8 T cells in human cancers. The results showed that CD8 T cells indeed expressed high levels of TNFR2. Interestingly, CD8 cells with high TNFR2 expression appeared to have a terminal exhausted phenotype, as defined by the expression of CTLA4, HAVCR2, and CXCL13 (Figures 1C–F). Furthermore, we analyzed the expression of selected marker genes across several cancer types. UMAP visualization demonstrated that high expression of TNFR2 was presented in tumor-infiltrating Tregs and exhausted CD8 T cells across human breast cancer (BRCA), hepatocellular carcinoma (HCC), lung cancer (LC), and melanoma (MELA) (Figures 1G, H). TNFR2 expression by CD4 T and CD8 T cell metaclusters in other 17 human cancer types were analyzed as well, and results consistently showed that TNFR2-expressing cells are mostly concentrated by Tregs in CD4 T cells, while distribution of TNFR2-expressing cells are less homogenous in the CD8 T cells in these cancer types (Supplementary Figure 1). Besides, the expression profile of TNF in tumor-infiltrating T cells was also analyzed. The results showed that there was no clear pattern of TNF expression in CD4 and CD8 T cells (Supplementary Figure 2).
Association of TNFR2 with responsiveness to ICIs therapy
To further assess the potential relationship of TNFR2 signaling on the state of the immune-suppressive tumor environment, and to predict the response of patients to the treatment with ICIs, we evaluated the expression of immune-checkpoint–relevant gene markers of SIGLEC15, TIGIT, CD274 (PD-L1), HAVCR2, PDCD1 (PD-1), CTLA4, LAG3, and PDCD1LG2 (PD-L2) (31, 32). The results showed that TNFR2 expression was positively correlated with the expression of these checkpoint markers in BRCA, HCC, LUSC, and MELA, respectively (Figures 2A–D). Next, we assessed the correlation between TNFR2 expression and the ICIs response by Tumor Immune Dysfunction and Exclusion (TIDE) score (33). The results showed that higher TNFR2 expression was associated with higher TIDE scores, complying with poorer responses to ICIs therapy, in patients with BRCA, HCC, LUSC, and MELA (Figures 2E–H). Therefore, the results of our analysis clearly indicate that TNFR2 expression is associated with a general immunosuppressive state in tumor environments. Thus, high levels of TNFR2 expression are associated with poor responses of patients to ICIs treatment.
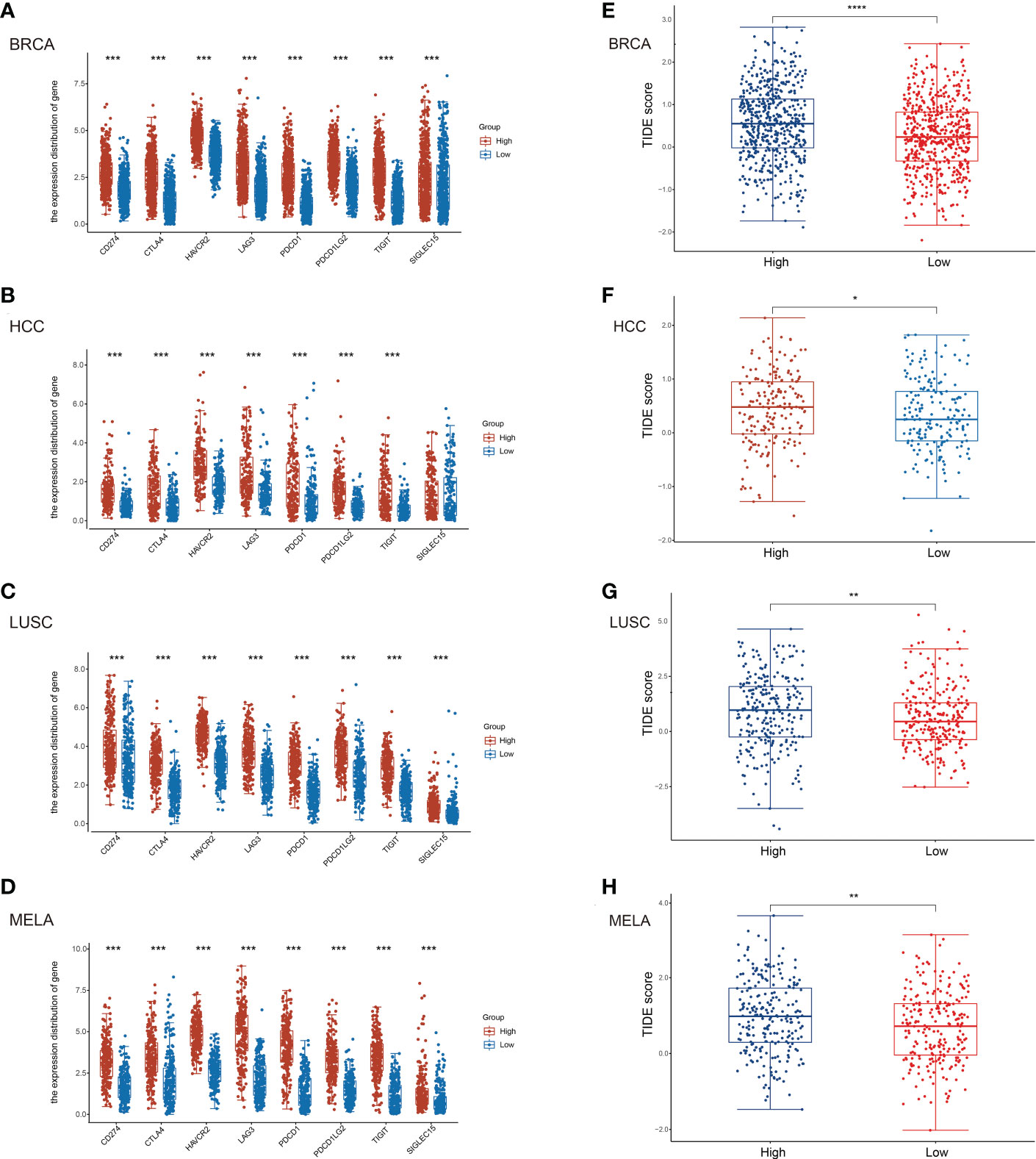
Figure 2 Correlation of TNFR2 expression and immune checkpoint gene markers. To predict the correlation between TNFR2 expression and the responses to immune checkpoint inhibitor therapy, (TIDE) score was evaluated. According to the median value of TNFR2 expression, tabulated tumor samples and types (BRCA, HCC, LUSC, and MELA) were divided into TNFR2high and TNFR2low groups. Then, TIDE score was used to predict the correlation between TNFR2 expression and immune checkpoint inhibitor responses. The correlation between TNFR2 and representative immune checkpoints gene markers, such as CD274 (PD-L1), CTLA4, HAVCR2, LAG3, PDCD1 (PD-1), PDCD1LG2 (PD-L2), TIGIT, and SIGLEC15 were shown in BRCA (A), HCC (B), LUSC (C) and MELA (D). TIDE score was used to predict the correlation between TNFR2 expression and ICIs responses in BRCA (E), HCC (F), LUSC (G), and MELA (H). *P < 0.05, **P < 0.01, ***P < 0.001, ****P < 0.0001.
Discussion and perspective
We and others have shown that targeting TNFR2 with antagonistic antibodies could mobilize anti-tumor immune responses by eliminating Treg activity, yielding a potent anti-tumor effect both in mice and humans (25–27, 34–36). Fcγ-binding could result in the conversion of antagonistic TNFRSF receptor-specific antibodies into strong agonists (37), therefore, further investigation is needed to clarity if the anti-tumor effect of these antibodies is truly caused by the inhibition of the TNFR2 signal. In fact, it was shown that TNFR2 agonistic antibody Y9 could inhibit tumor growth by acting directly on CD8 CTLs in tumors and stimulating their activation and expansion in murine cancer models (27). Based on the above contradictory results, pharmacological companies are currently developing both agonistic and antagonistic anti-TNFR2 antibodies simultaneously (38), in chance one of them may eventually be proved useful in the clinic as a cancer immunotherapeutic agent. Therefore, further understanding TNFR2 expression by T cell subsets in tumor microenvironment is important to clarify the primary cellular target of both agonistic and antagonistic TNFR2 antibodies. In this study, we found that among tumor-infiltrating CD4 T cells, TNFR2 is quite selectively expressed by Foxp3+ Tregs. Notably, although Foxp3 expression is a standard marker for murine Tregs, caution should be taken to use Foxp3 as a marker for human Tregs, since it can also be expressed by the activated human T cells upon TCR triggering (39). Thus, further study is needed to verify if all Foxp3-expressing cells in the metacluster are bona-fide Tregs or not. The expression of TNFR2 by tumor-infiltrating CD8 T cells appeared to be less homogeneous and relatively discrete, as compared with its expression in CD4 T cells. Nevertheless, it is clear that TNFR2 is mainly expressed by CD8 T cells with an exhaustive phenotype. Our results are consistent with a number of previous studies that suggest both Tregs and exhausted CD8 CTLs are potential targets of TNFR2 antibody treatments (25, 27, 34, 35, 40). Based on our results, it is possible to propose that antagonistic antibodies may dampen the immunosuppressive function of Tregs, while the exhaustive phenotype of CD8 CTLs may be reversed simultaneously.
Our results also indicate that TNFR2 expression levels are associated with the responsiveness of ICIs treatment. The expression of TNF, an endogenous ligand of TNFR2 with the capacity to up-regulate TNFR2 expression by Tregs (41), in tumor tissue could be markedly upregulated after ICIs treatment (42). It was shown that TNF derived from CD8+ T cells and CD8- T cells represent a crucial effector mechanism in ICI-responsive tumors (43). Nevertheless, it was also reported that blockage of TNF overcome the resistance to anti-PD-1 treatment in experimental melanoma (44), and the treatment with TNF inhibitors enhanced the anti-tumor effect of combined CTLA-4 and PD-1 immunotherapy (45). However, a preclinical study revealed that the treatment with anti-mouse TNFR2 surrogate antibody (suAb) abrogated TNF-induced expansion of Tregs in vitro and decreased expression of PD-1 on CD8 tumor-infiltrating lymphocytes (TILs) in vivo (46). Thus, it is highly possible that TNF-TNFR2 axis is attributable to the resistance to ICIs therapy. This notion is supported by the evidence from us and others that blockade of TNFR2 with antagonistic antibodies could markedly enhance the efficacy of ICIs treatment in mouse tumor models (25, 35, 47). For example, in a cohort of metastatic melanoma patients treated with CTLA4 and PD-1 blockade, a deep immune analysis of tumor samples found that TNF was markedly upregulated after PD-1 treatment (42).
Taken together, our analysis clearly indicates that the expression of TNFR2 in TME may be a reliable biomarker for the precision ICIs treatment of cancer patients. Our results also support the notion that both tumor-infiltrating Tregs and exhausted CD8 CTLs are major cellular targets of antagonistic TNFR2 antibody treatment, for the reason that both subsets of tumor-infiltrating lymphocytes express high levels of TNFR2. These possibilities merit further clinical research.
Data availability statement
The original contributions presented in the study are included in the article/Supplementary Material. Further inquiries can be directed to the corresponding author.
Author contributions
PL and MJ designed the study, PL, MJ, YW and MI analyzed and interpreted the data, and drafted the manuscript. XC, the corresponding author, was involved in designing the work and approving the final version to be published. All authors agree to submit the manuscript.
Funding
This project has been funded by The Science and Technology Development Fund, Macau SAR (FDCT, File No. 0056/2019/AFJ, 0099/2021/A2, and SKL-QRCM(UM)-2020-2022), University of Macau (File No. CPG2023-00025-ICMS, MYRG2022-00260-ICMS), and the 2020 Guangdong Provincial Science and Technology Innovation Strategy Special Fund (Guangdong-Hong Kong-Macau Joint Lab), No: 2020B1212030006.
Conflict of interest
The authors declare that the research was conducted in the absence of any commercial or financial relationships that could be construed as a potential conflict of interest.
Publisher’s note
All claims expressed in this article are solely those of the authors and do not necessarily represent those of their affiliated organizations, or those of the publisher, the editors and the reviewers. Any product that may be evaluated in this article, or claim that may be made by its manufacturer, is not guaranteed or endorsed by the publisher.
Supplementary material
The Supplementary Material for this article can be found online at: https://www.frontiersin.org/articles/10.3389/fimmu.2023.1097090/full#supplementary-material
Abbreviations
BRCA, breast cancer; CTLs, cytotoxic T lymphocytes; HCC, hepatocellular carcinoma; ICIs, immune checkpoint inhibitors; irAEs, immune-related adverse events; LUSC, lung squamous cell carcinoma; LC, Lung cancer; MELA, melanoma; MSCs, mesenchymal stem cells; MDSCs, myeloid-derived suppressor cells; Tregs, regulatory T cells; scDVA, single-cell RNA-seq data visualization and analysis; TIDE, tumor immune dysfunction and exclusion; TME, tumor microenvironment; TNF, tumor necrosis factor; TNFR2, tumor necrosis factor receptor 2.
References
1. Twomey JD, Zhang B. Cancer immunotherapy update: Fda-approved checkpoint inhibitors and companion diagnostics. AAPS J (2021) 23(2):39. doi: 10.1208/s12248-021-00574-0
2. Bagchi S, Yuan R, Engleman EG. Immune checkpoint inhibitors for the treatment of cancer: Clinical impact and mechanisms of response and resistance. Annu Rev Pathol (2021) 16:223–49. doi: 10.1146/annurev-pathol-042020-042741
3. Robert C. A decade of immune-checkpoint inhibitors in cancer therapy. Nat Commun (2020) 11(1):3801. doi: 10.1038/s41467-020-17670-y
4. O'Donnell JS, Teng MWL, Smyth MJ. Cancer immunoediting and resistance to T cell-based immunotherapy. Nat Rev Clin Oncol (2019) 16(3):151–67. doi: 10.1038/s41571-018-0142-8
5. Borghaei H, Paz-Ares L, Horn L, Spigel DR, Steins M, Ready NE, et al. Nivolumab versus docetaxel in advanced nonsquamous non-Small-Cell lung cancer. N Engl J Med (2015) 373(17):1627–39. doi: 10.1056/NEJMoa1507643
6. Garon EB, Rizvi NA, Hui R, Leighl N, Balmanoukian AS, Eder JP, et al. Pembrolizumab for the treatment of non-Small-Cell lung cancer. N Engl J Med (2015) 372(21):2018–28. doi: 10.1056/NEJMoa1501824
7. Ansell SM, Lesokhin AM, Borrello I, Halwani A, Scott EC, Gutierrez M, et al. Pd-1 blockade with nivolumab in relapsed or refractory hodgkin's lymphoma. N Engl J Med (2015) 372(4):311–9. doi: 10.1056/NEJMoa1411087
8. Polk A, Svane IM, Andersson M, Nielsen D. Checkpoint inhibitors in breast cancer - current status. Cancer Treat Rev (2018) 63:122–34. doi: 10.1016/j.ctrv.2017.12.008
9. Cabrita R, Lauss M, Sanna A, Donia M, Skaarup Larsen M, Mitra S, et al. Tertiary lymphoid structures improve immunotherapy and survival in melanoma. Nature (2020) 577(7791):561–5. doi: 10.1038/s41586-019-1914-8
10. Hasan Ali O, Berner F, Bomze D, Fassler M, Diem S, Cozzio A, et al. Human leukocyte antigen variation is associated with adverse events of checkpoint inhibitors. Eur J Cancer (2019) 107:8–14. doi: 10.1016/j.ejca.2018.11.009
11. Jia XH, Geng LY, Jiang PP, Xu H, Nan KJ, Yao Y, et al. The biomarkers related to immune related adverse events caused by immune checkpoint inhibitors. J Exp Clin Cancer Res (2020) 39(1):284. doi: 10.1186/s13046-020-01749-x
12. Osorio JC, Ni A, Chaft JE, Pollina R, Kasler MK, Stephens D, et al. Antibody-mediated thyroid dysfunction during T-cell checkpoint blockade in patients with non-Small-Cell lung cancer. Ann Oncol (2017) 28(3):583–9. doi: 10.1093/annonc/mdw640
13. Routy B, Le Chatelier E, Derosa L, Duong CPM, Alou MT, Daillere R, et al. Gut microbiome influences efficacy of pd-1-Based immunotherapy against epithelial tumors. Science (2018) 359(6371):91–7. doi: 10.1126/science.aan3706
14. Tanaka A, Sakaguchi S. Regulatory T cells in cancer immunotherapy. Cell Res (2017) 27(1):109–18. doi: 10.1038/cr.2016.151
15. Chen X, Oppenheim JJ. Targeting Tnfr2, an immune checkpoint stimulator and oncoprotein, is a promising treatment for cancer. Sci Signal (2017) 10(462):eaal2328. doi: 10.1126/scisignal.aal2328
16. Yang Y, Islam MS, Hu Y, Chen X. Tnfr2: Role in cancer immunology and immunotherapy. Immunotargets Ther (2021) 10:103–22. doi: 10.2147/ITT.S255224
17. Chen X, Baumel M, Mannel DN, Howard OM, Oppenheim JJ. Interaction of tnf with tnf receptor type 2 promotes expansion and function of mouse Cd4+Cd25+ T regulatory cells. J Immunol (Baltimore Md 1950) (2007) 179(1):154–61. doi: 10.4049/jimmunol.179.1.154
18. Chen X, Subleski JJ, Kopf H, Howard OM, Mannel DN, Oppenheim JJ. Cutting edge: Expression of Tnfr2 defines a maximally suppressive subset of mouse Cd4+Cd25+Foxp3+ T regulatory cells: Applicability to tumor-infiltrating T regulatory cells. J Immunol (Baltimore Md 1950) (2008) 180(10):6467–71. doi: 10.4049/jimmunol.180.10.6467
19. Chen X, Wu X, Zhou Q, Howard OM, Netea MG, Oppenheim JJ. Tnfr2 is critical for the stabilization of the Cd4+Foxp3+ regulatory t. cell phenotype in the inflammatory environment. J Immunol (Baltimore Md 1950) (2013) 190(3):1076–84. doi: 10.4049/jimmunol.1202659
20. Govindaraj C, Scalzo-Inguanti K, Madondo M, Hallo J, Flanagan K, Quinn M, et al. Impaired Th1 immunity in ovarian cancer patients is mediated by Tnfr2+ tregs within the tumor microenvironment. Clin Immunol (2013) 149(1):97–110. doi: 10.1016/j.clim.2013.07.003
21. Nguyen DX, Ehrenstein MR. Anti-tnf drives regulatory T cell expansion by paradoxically promoting membrane tnf-Tnf-Rii binding in rheumatoid arthritis. J Exp Med (2016) 213(7):1241–53. doi: 10.1084/jem.20151255
22. Zhao X, Rong L, Zhao X, Li X, Liu X, Deng J, et al. Tnf signaling drives myeloid-derived suppressor cell accumulation. J Clin Invest (2012) 122(11):4094–104. doi: 10.1172/JCI64115
23. Kelly ML, Wang M, Crisostomo PR, Abarbanell AM, Herrmann JL, Weil BR, et al. Tnf receptor 2, not tnf receptor 1, enhances mesenchymal stem cell-mediated cardiac protection following acute ischemia. Shock (2010) 33(6):602–7. doi: 10.1097/SHK.0b013e3181cc0913
24. Zhang X, Lao M, Xu J, Duan Y, Yang H, Li M, et al. Combination cancer immunotherapy targeting Tnfr2 and pd-1/Pd-L1 signaling reduces immunosuppressive effects in the microenvironment of pancreatic tumors. J Immunother Cancer (2022) 10:e003982. doi: 10.1136/jitc-2021-003982
25. Case K, Tran L, Yang M, Zheng H, Kuhtreiber WM, Faustman DL. Tnfr2 blockade alone or in combination with pd-1 blockade shows therapeutic efficacy in murine cancer models. J Leukoc Biol (2020) 107(6):981–91. doi: 10.1002/JLB.5MA0420-375RRRRR
26. Fu Q, Shen Q, Tong J, Huang L, Cheng Y, Zhong W. Anti-tumor necrosis factor receptor 2 antibody combined with anti-Pd-L1 therapy exerts robust antitumor effects in breast cancer. Front Cell Dev Biol (2021) 9:720472. doi: 10.3389/fcell.2021.720472
27. Tam EM, Fulton RB, Sampson JF, Muda M, Camblin A, Richards J, et al. Antibody-mediated targeting of Tnfr2 activates Cd8(+) T cells in mice and promotes antitumor immunity. Sci Transl Med (2019) 11(512):eaax0720. doi: 10.1126/scitranslmed.aax0720
28. Zheng L, Qin S, Si W, Wang A, Xing B, Gao R, et al. Pan-cancer single-cell landscape of tumor-infiltrating T cells. Science (2021) 374(6574):abe6474. doi: 10.1126/science.abe6474
29. Chen X, Hamano R, Subleski JJ, Hurwitz AA, Howard OM, Oppenheim JJ. Expression of costimulatory Tnfr2 induces resistance of Cd4+Foxp3- conventional T cells to suppression by Cd4+Foxp3+ regulatory T cells. J Immunol (Baltimore Md 1950) (2010) 185(1):174–82. doi: 10.4049/jimmunol.0903548
30. Alam MS, Otsuka S, Wong N, Abbasi A, Gaida MM, Fan Y, et al. Tnf plays a crucial role in inflammation by signaling Via T cell Tnfr2. Proc Natl Acad Sci USA (2021) 118(50):e2109972118. doi: 10.1073/pnas.2109972118
31. Wang J, Sun J, Liu LN, Flies DB, Nie X, Toki M, et al. Siglec-15 as an immune suppressor and potential target for normalization cancer immunotherapy. Nat Med (2019) 25(4):656–66. doi: 10.1038/s41591-019-0374-x
32. Kitsou M, Ayiomamitis GD, Zaravinos A. High expression of immune checkpoints is associated with the til load, mutation rate and patient survival in colorectal cancer. Int J Oncol (2020) 57(1):237–48. doi: 10.3892/ijo.2020.5062
33. Jiang P, Gu S, Pan D, Fu J, Sahu A, Hu X, et al. Signatures of T cell dysfunction and exclusion predict cancer immunotherapy response. Nat Med (2018) 24(10):1550–8. doi: 10.1038/s41591-018-0136-1
34. Nie Y, He J, Shirota H, Trivett AL, Yang D, Klinman DM, et al. Blockade of Tnfr2 signaling enhances the immunotherapeutic effect of cpg odn in a mouse model of colon cancer. Sci Signaling (2018) 11(511):eaan0790. doi: 10.1126/scisignal.aan0790
35. Jiang M, Liu J, Yang D, Tross D, Li P, Chen F, et al. A Tnfr2 antibody by countering immunosuppression cooperates with Hmgn1 and R848 immune stimulants to inhibit murine colon cancer. Int Immunopharmacol (2021) 101(Pt A):108345. doi: 10.1016/j.intimp.2021.108345
36. Yang M, Tran L, Torrey H, Song Y, Perkins H, Case K, et al. Optimizing Tnfr2 antagonism for immunotherapy with tumor microenvironment specificity. J Leukoc Biol (2020) 107(6):971–80. doi: 10.1002/jlb.5ab0320-415rrrrr
37. Medler J, Nelke J, Weisenberger D, Steinfatt T, Rothaug M, Berr S, et al. Tnfrsf receptor-specific antibody fusion proteins with targeting controlled fcgammar-independent agonistic activity. Cell Death Dis (2019) 10(3):224. doi: 10.1038/s41419-019-1456-x
38. Bai J, Ding B, Li H. Targeting Tnfr2 in cancer: All roads lead to Rome. (2022) 13:844931. doi: 10.3389/fimmu.2022.844931
39. Kmieciak M, Gowda M, Graham L, Godder K, Bear HD, Marincola FM, et al. Human T cells express Cd25 and Foxp3 upon activation and exhibit Effector/Memory phenotypes without any Regulatory/Suppressor function. J Transl Med (2009) 7:89. doi: 10.1186/1479-5876-7-89
40. Sum CS, Danton M, hu Q, Pritsker A, Lin R, Yu R, et al. Abstract 1869: Novel Tnfr2 antibodies to overcome T cell exhaustion and suppressive tumor microenvironment. Cancer Res (2021) 81(13_Supplement):1869. doi: 10.1158/1538-7445.Am2021-1869
41. Hamano R, Huang J, Yoshimura T, Oppenheim JJ, Chen X. Tnf optimally activatives regulatory T cells by inducing tnf receptor superfamily members Tnfr2, 4-1bb and Ox40. Eur J Immunol (2011) 41(7):2010–20. doi: 10.1002/eji.201041205
42. Chen PL, Roh W, Reuben A, Cooper ZA, Spencer CN, Prieto PA, et al. Analysis of immune signatures in longitudinal tumor samples yields insight into biomarkers of response and mechanisms of resistance to immune checkpoint blockade. Cancer Discovery (2016) 6(8):827–37. doi: 10.1158/2159-8290.CD-15-1545
43. Vredevoogd DW, Kuilman T, Ligtenberg MA, Boshuizen J, Stecker KE, de Bruijn B, et al. Augmenting immunotherapy impact by lowering tumor tnf cytotoxicity threshold. Cell (2019) 178(3):585–99.e15. doi: 10.1016/j.cell.2019.06.014
44. Bertrand F, Montfort A, Marcheteau E, Imbert C, Gilhodes J, Filleron T, et al. Tnfalpha blockade overcomes resistance to anti-Pd-1 in experimental melanoma. Nat Commun (2017) 8(1):2256. doi: 10.1038/s41467-017-02358-7
45. Perez-Ruiz E, Minute L, Otano I, Alvarez M, Ochoa MC, Belsue V, et al. Prophylactic tnf blockade uncouples efficacy and toxicity in dual ctla-4 and pd-1 immunotherapy. Nature (2019) 569(7756):428–32. doi: 10.1038/s41586-019-1162-y
46. Zhao X, Liu X, Gao Z, Wang M, Li W, Pang R, et al. Abstract 1029: Dissecting the mechanism of action targeting Tnfr2 with a lignd blocking anti-Tnfr2 antibody that elicits potent anti-tumor activity. Cancer Res (2021) 81(13 Supplement):1029. doi: 10.1158/1538-7445.Am2021-1029
Keywords: TNFR2, immune checkpoint inhibitors, tumor microenvironment, CD4 + Foxp3 + regulatory T cells, exhausted CD8 T cells, biomarker
Citation: Liao P, Jiang M, Islam MS, Wang Y and Chen X (2023) TNFR2 expression predicts the responses to immune checkpoint inhibitor treatments. Front. Immunol. 14:1097090. doi: 10.3389/fimmu.2023.1097090
Received: 13 November 2022; Accepted: 02 February 2023;
Published: 14 February 2023.
Edited by:
Tobias R. Kollmann, University of Western Australia, AustraliaReviewed by:
Harald Wajant, University Hospital Würzburg, GermanyCopyright © 2023 Liao, Jiang, Islam, Wang and Chen. This is an open-access article distributed under the terms of the Creative Commons Attribution License (CC BY). The use, distribution or reproduction in other forums is permitted, provided the original author(s) and the copyright owner(s) are credited and that the original publication in this journal is cited, in accordance with accepted academic practice. No use, distribution or reproduction is permitted which does not comply with these terms.
*Correspondence: Xin Chen, eGNoZW5AdW0uZWR1Lm1v
†These authors have contributed equally to this work