- 1Stomatological Hospital, Southern Medical University, Guangzhou, China
- 2Department of General Surgery, Nanfang Hospital, The First School of Clinical Medicine, Southern Medical University, Guangzhou, China
Digestive tract-related cancers account for four of the top ten high-risk cancers worldwide. In recent years, cancer immunotherapy, which exploits the innate immune system to attack tumors, has led to a paradigm shifts in cancer treatment. Gut microbiota modification has been widely used to regulate cancer immunotherapy. Dietary compounds and traditional Chinese medicine (TCM) can alter the gut microbiota and its influence on toxic metabolite production, such as the effect of iprindole on lipopolysaccharide (LPS), and involvement in various metabolic pathways that are closely associated with immune reactions. Therefore, it is an effective strategy to explore new immunotherapies for gastrointestinal cancer to clarify the immunoregulatory effects of different dietary compounds/TCMs on intestinal microbiota. In this review, we have summarized recent progress regarding the effects of dietary compounds/TCMs on gut microbiota and their metabolites, as well as the relationship between digestive cancer immunotherapy and gut microbiota. We hope that this review will act as reference, providing a theoretical basis for the clinical immunotherapy of digestive cancer via gut microbiota modulation.
Introduction
Gastrointestinal cancers, including colorectal and gastric cancer, are among the top five cancer types with the highest mortality rates according to data published by the World Health Organization in 2021 (1). Although significant progress has been made in cancer treatment, improving cancer survival and life expectancy remains a challenge worldwide (2). In the past decade, immune checkpoint blockade (ICB) therapy, which interferes with the interaction between immune checkpoints and receptors, has demonstrated promising therapeutic effects in cancer treatments (3). Antibodies targeting programmed cell death 1 (PD-1), programmed cell death ligand 1 (PD-L1), and cytotoxic T lymphocyte-associated antigen-4 (CTLA-4) have achieved early success in clinical trials, inducing durable remission in various tumor types (4–6). Unfortunately, effective immunotherapy is limited in most patients owing to the immunosuppressive tumor environment, benefitting only 10–40% of patients. In particular, driver gene mutations (7), low tumor-infiltrating lymphocytes (TIL) (8), defects in the antigen presentation process (9), and T cell function loss and failure inhibit immunotherapy effects. Therefore, the efficacy of immunotherapy in tumor treatment requires further improvement.
Hundreds of trillions of microbes make up the gut microbiome, which is the largest microbial community in the human body. Gut microbiota maintain the physiochemical conditions of the gut and aid host digestion, nutrient metabolism, toxin neutralization, and resistance to parasites (10, 11). Actinobacteria, Bacteroidetes, Firmicutes, and Fusobacteria constitute the majority of the human gut microbiota (12). Fungi such as Aspergillus and Candida are also present in the gut microbiome (13). In addition to genetic factors and maternal status during pregnancy, acquired environmental factors affecting gut microbiota include diet (14, 15), lifestyle choices (16), and emotions (17). Previous studies have demonstrated that gut microbiota perturbations influence digestive cancer immunotherapy development. For example, microbiota can ameliorate immunosuppression by altering the tumor microenvironment (18, 19). Lactobacillus and Bifidobacterium reduce the polarization of invasive monocytes to M2 macrophages and increase M1 phenotype development by upregulating immune factors such as interleukin (IL)-10, thereby inhibiting immune escape and further reducing digestive tumor growth and metastasis (20). Furthermore, products metabolized by microbiota are also involved in immune regulation. Short-chain fatty acids (SCFAs) restrain specific enzymes involved in the transmission of genetic materials that alter the metabolism and gene regulation of immune cells, contributing to a positive impact on digestive tumor therapy. Moreover, inosine from B. longum promotes T helper (TH)1 cell differentiation and enhances the therapeutic effect of ICB, mediated by the T cell-specific adenosine A2A receptor (A2AR) (21).
Dietary compounds such as dietary fiber, flavonoids, alkaloids, and polysaccharides are bioactive metabolites which play a crucial role in maintaining health and adjusting physiological functions (Food and Drug Administration and HSS, 2016). They can transform intestinal microbial components and produce intestinal metabolites, such as hydrogen, methane, SCFAs and B vitamins after microflora fermentation in the large intestine (22). Traditional Chinese medicine (TCM) has been used therapeutically for several millennia. Nearly 100 species of Chinese herbal crude drugs and their preparations are widely used in the medical industry and are included in the European Pharmacopoeia and the United States Pharmacopoeia (23). The chemical composition of TCMs is complex including sugars, amino acids, proteins, vitamins, and dietary compounds originating from plant cell walls (24). Most TCMs are administered orally and interact with the microflora, which affects immune function and influences cancer immunotherapy (25). Recent studies have shown that dietary compounds/TCMs can modulate intestinal microbiota structure and metabolic pathways, improve the composition of the tumor immune microenvironment, and show potential in turning a cold tumor (i.e. immune desert) into a hot tumor (i.e. immune-infiltrated) to enhance immunotherapy efficacy (26–28). Some dietary compounds/TCMs can be fermented or converted by the gut microbiota to form bioactive components. For example, puerarin and isoflavone glycosides can be metabolized by gut flora into daidzein and pistil isoflavones that are more effective than their precursors (29).
Although ICB remains one of the most commonly used techniques to clinically treat cancer, several studies have used dietary compounds/TCMs as a supplementary treatment choice to explore new immunotherapies with high efficiency and few adverse reactions, and improve the survivability and quality of life of patients with tumors (30–34). We have summarized these dietary compounds/TCMs currently under preclinical or clinical research and critically evaluated their future development potential to provide new ideas for tumor immunotherapy through the intervention of microflora. Figure 1 illustrates the links between digestive cancer immunotherapy, dietary compounds/TCMs, and the intestinal microbiota.
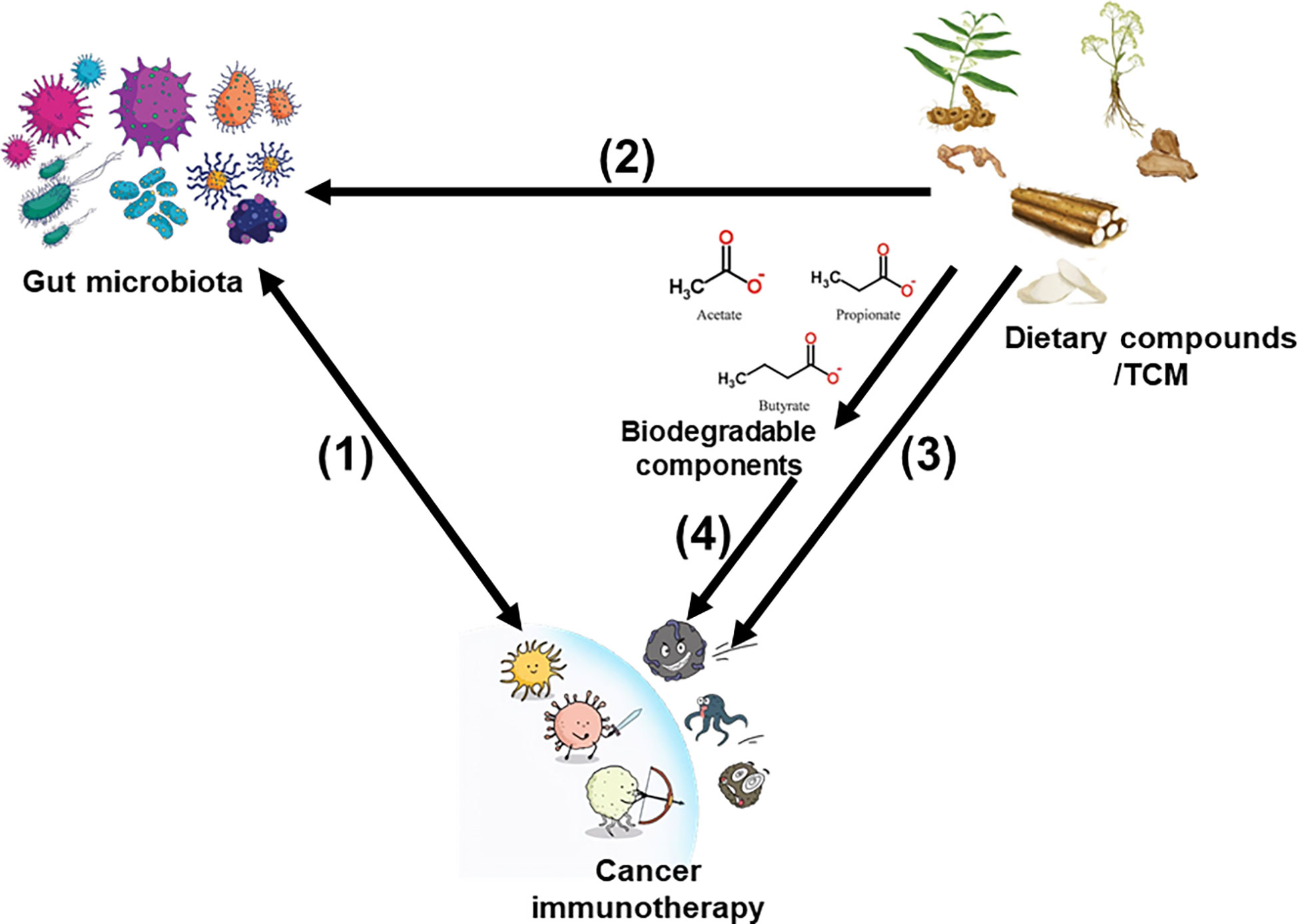
Figure 1 Links betweencancer immunotherapy, dietary compounds/TCM, and gut microbiota. (1) The relationship between gut microbiota and cancer immunotherapy is bidirectional. (2) Dietary compounds/TCM modulate gut microbiota composition and metabolism. (3) Dietary compounds/TCM can directly improve the immune system. (4) Biodegradable components produced by intestinal microbiota derived from dietary compounds/TCM also improve cancer immunotherapy effects.
Gut microbiota involved in digestive tract tumorigenesis
Many studies have demonstrated the alteration of gut microbiota in digestive cancer patients. The efforts to identify the specific processes by which the gut microbiota contributes to the development of cancer have received a lot of attention.
Gastric cancer
Numerous virulence factors are produced by Helicobacter pylori, including the oncoproteins cytotoxin-associated gene A (CagA) and vacuolating cytotoxin A (VacA), which have been identified as the primary virulence factors involved in the pathogenesis of gastric cancer (35). Additionally, Helicobacter pylori causes gastric atrophy and gastric acid shortage, which promote excessive microbial growth in the stomach, resulting in more nitrogen derivatives in the diet that can be converted into carcinogens (36).
Colon cancer
It was reported that Fusobacterium are abundant in patients with colorectal cancer (CRC) (37). Surface adhesion protein (FadA), the primary factor controlling the adherence and invasion of Fusobacterium, can bind to β-catenin and lead to its activation, which in turn triggers inflammation and tumor growth (38). Additionally, many bacterial metabolites may also cause genomic instability, leading to tumorigenesis. For instance, Enterobacteriaceae produce colibactin, which stimulates the overexpansion of intestinal epithelial cells and aids in the development of CRC by generating DNA damage, mutation, and genomic instability (39). Enterococcus faecalis can induce double-stranded DNA breaks and promote the development of CRC in mice by producing superoxide free radicals (40). Similarly, secondary bile acids produced by gut bacteria can affect the mitotic process of intestinal epithelial cells, induce DNA damage, and increase the risk of CRC (41). The metabolism of gut bacteria also produces other cancer-promoting substances such as glucuronidase that can transform the precarcinogens in food or drugs into carcinogens (42); or produce carcinogenic chemicals such as N-nitroso compounds (NOCs) (43) and hydrogen sulfide (H2S) (44).
Hepatocellular carcinoma
A growing body of literature points to an increased abundance of lipopolysaccharide (LPS)- producing bacteria (Neisseria, Enterobacteriaceae, and Vermicella) in patients with hepatocellular carcinoma (HCC) (45–47). It is reported that LPS can activate the toll-like receptor (TLR) 4 and NF-κB pathways and trigger the production of cytokines such as TNF-α and IL-6 that promote cancer progression (48). Secondary bile acids can make the intestinal mucosa more permeable, allowing intestinal pathogens to translocate to the liver and cause HCC (49, 50). Through the portal venous system, the liver is connected to intestinal bacterial components and their metabolites, which may cause inflammatory alterations, hepatotoxicity, and finally, HCC. For instance, alterations in the gut microbiota raised the levels of hepatobiliary acid, which caused hepatocarcinogenesis in an obesity-induced human liver cancer xenograft mice model (51).
Esophageal cancer
Fusobacteria is one of the most common microorganisms found in the esophagus (52). Matrix metalloproteinases (MMPs) can be secreted by intestinal epithelial cells when Fusobacterium is present (53). Activated MMPs can encourage the growth of tumor cells by increasing the breakdown of the esophageal extracellular matrix, stimulating tumor angiogenesis, and controlling cell adhesion and motility (54). Fusobacterium simultaneously triggers the IL-6/p-STAT3/c-MYC signaling pathway and encourages M2-type differentiation of macrophages through a TLR4-dependent mechanism, promoting tumor growth (55). Therefore, Fusobacterium is a potential target for the treatment of esophageal cancer.
Digestive cancer immunotherapy via gut microbiota modulation
Due to the resistance of a large number of patients to chemotherapy drugs, gut microbiota- mediated immunotherapy has become one of the most promising methods in cancer research in recent years (56). The gut microbiota plays a key role in the occurrence and development of digestive cancer by regulating metabolism, immune response and inflammation (57). The relationship between gut microbiota and digestive cancer immunotherapy is shown in Figure 2.
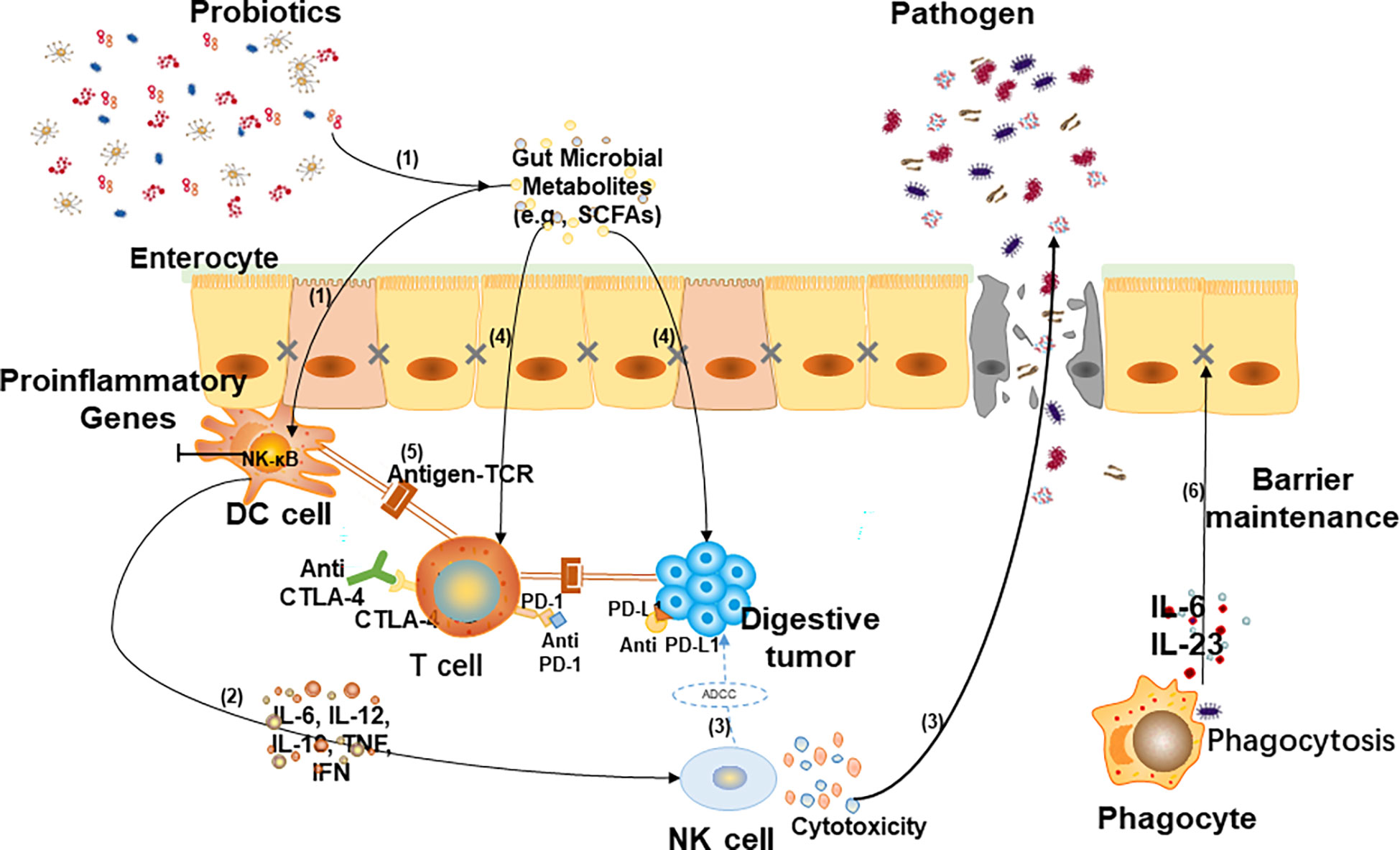
Figure 2 Relationship between gut microbiota and digestive cancer immunotherapy. (1) When tumors arise, gut flora and its metabolites (such as short-chain fatty acids) activate dendritic (DC) cells. (2) Cytokines are involved in activating NK cells and promoting the migration of NK cells to tumor sites. (3) Killer (NK) cells can directly kill tumor cells via antibody-dependent cellular cytotoxicity (ADCC) and inhibit pathogenicity through cytotoxicity. (4) Some metabolites shape the immune system by regulating T cell differentiation and also participate in tumor-killing by interacting with host cell surface receptors. (5) Anti-cytotoxic T lymphocyte-associated antigen-4 (CTLA-4) enhance the interaction between DC cells and T cells, and anti-programmed cell death ligand 1 (PD-L1) stimulate T cell immune response to promote immunotherapy. (6) Phagocytes eliminate pathogens by phagocytosis and secret cytokines to recover intestinal barrier dysfunction.
Gastric cancer
Bifidobacterium augmented anti-CTLA-4 checkpoint blockade in mouse models (58), whereas antibiotic-treated or sterile mice showed no response to this blockade. More abundant bacterial species such as Enterococcus faecium enhance anti-PD-L1 treatment in mouse models (18). Recent research has shown that the use of probiotics as adjunctive therapy for Helicobacter pylori infection can effectively inhibit the progression of gastric cancer (59, 60). Probiotics administered to patients with gastric cancer following total gastrectomy were found to improve immune function and reduce inflammation (61).
Colon cancer
Eubacterium, Lactobacillus, and Streptococcus can release multiple metabolites that influence the immune system, such as SCFAs, which are positively connected to the anti-PD-1/PD-L1 response (62). SCFAs alter inflammation, including the activation, proliferation, and differentiation of anti-inflammatory T regulatory (Treg) cells or pro-inflammatory Th1 and Th17 cells, and affect the polarization of pro-inflammatory M1 and anti-inflammatory M2 macrophages (63). Furthermore, SCFAs cause tumor growth by activating mitogen-activated protein kinase and PI3K (phosphatidylinositol-3-kinases) signaling by increasing somatomedin C (IGF-1) levels. In addition, virulence factors are important components of gut flora that affect CRC immunotherapy (64). TcdB produced by Clostridium difficile inhibits TH and memory B-cell differentiation (65). Therefore, modulating the Clostridium difficile count is beneficial for immune recovery. Interestingly, in some microflora such as Bacteroides fragilis, the cross-reaction between bacterial antigen and tumor neoantigen can activate antitumor T cells (66). In contrast, the enterotoxins BFT (Bacteroides fragilis enterotoxin) and IL-17 produced by Bacteroides fragilis induce the differentiation of monocytic myeloid-derived suppressor cells into intestinal epithelial cells, which can selectively upregulate arginase 1 (Arg1) and type 2 NO synthase (NOS2) to produce NO, inhibit T cell proliferation, and promote CRC generation (67).
Hepatocellular carcinoma
The function of dendritic cells can be enhanced by oral Bifidobacterium administration, which increases CD8+ T cell accumulation in HCC tissue (68). Similarly, SagA, an enzyme expressed by Enterococcus faecium, enhances the effect of immunotherapy in HCC (69). Microbial metabolites, including amino acid derivatives and secondary BAs, are also involved in immunoregulation. Lactobacillus is able to convert tryptophan into indole and its derivatives, which are major aromatic hydrocarbon receptors (AHRs) that play a pivotal role in intestinal immunobarrier function (70). In addition, the bacterial metabolites lactic acid and pyruvate, enhance the immune response by inducing GPR31-mediated dendritic cell differentiation (71).
Esophageal cancer
Immune checkpoint inhibitor (ICI) therapies have been evaluated for their effect on specific microbes in esophageal cancer, including cytotoxic T lymphocyte-associated protein 4 (CTLA-4) and programmed cell death 1 (PD-1)/PD-1 ligand (PD-L1) inhibitors (72). Vetizou et al. found that anti-CTLA-4 therapy was effective only when B. fragilis and/or B. thetaiotaomicron and Burkholderiales populations were present and are therapeutic when T cells are specific for B. fragilis and B. thetaiotamicron (58). In addition, the reintroduction of B. fragilis cells and/or polysaccharides or adoptive transfer of B. fragilis-specific T cells restored therapeutic efficacy and reduced immune-mediated colitis through activation of Th1 cells with cross-reactivity to bacterial antigens and tumour neoantigens. These results indicate that reconstruction of intestinal flora is beneficial for esophageal cancer treatment through immune regulation.
Interactions between dietary compounds and gut microbiota
Dietary compounds affect microbiota metabolites
SCFAs
Several epidemiological studies have shown that in inflammatory disease and digestive cancer, particularly gastric and colon cancer, the incidence rate is related to SCFA shortage in the diet (73). SCFAs, mainly composed of acetate, propionate, and butyrate, inhibit histone deacetylase (HDAC) and G protein-coupled receptor (GPCR) activation pathways to induce phagocytes to secrete chemokines and anti-inflammatory factors, block phagocytes from releasing tumor necrosis factor (TNF), and promote T lymphocyte proliferation and differentiation for the treatment of tumors (74, 75). Notably, when treating intestinal inflammation with dietary fiber, high-dose butyrate caused by continuous inulin intake may cause stagnation of colonic epithelial stem cell proliferation and even inflammation and obstruction of the urinary system, thus damaging the immune system (76). This indicates that the dietary compound dose also affects the metabolic function of microbiota.
Tryptophan
After treatment with active ginseng polysaccharides, indoleamine 2,3-dioxygenase (IDO) activity was substantially reduced, causing the microflora to produce more L-tryptophan and less L-kynurenine. Therefore, dietary compounds that lower IDO activity to modulate microfloral metabolites are a potential route of anti-PD-1 therapy resistance (76). Furthermore, intestinal microbes can break down TRP to produce indole-containing metabolites, which modulate the host immune system by activating the ligand-gated transcription factor AHR.
Secondary bile acids
The intestinal microbes convert primary bile acids into secondary bile acids (lithocholic and deoxycholic acids) in the large intestine. Two primary bile acid receptors, farnesoid X receptor (FXR) and G-protein coupled bile acid receptor (TGR), modulate the synthesis, metabolism, and redistribution of bile acids through interactions with the gut microbiota (77). The development of Romboutsia following treatment with Tremella fuciformis polysaccharides can increase the production of deoxycholic acid in the intestine and alter the metabolism of bile acids, which has a considerable influence on the treatment of colitis (78). Kaempferol has been used to increase the expression of sterol 27-hydroxylase (CYP27A1) and FXR to counteract the declining trend of deoxycholic acid (79). This decreased the tumor burden in ApcMin/+ mice and repaired the intestinal barrier. Moreover, the increase in secondary bile acids was closely related to the gut microbiota as demonstrated by the greater number of species with anticancer capabilities in the kaempferol therapy group (80).
Pyruvate and lactic acid
The gut microorganisms can ferment dietary fiber to produce pyruvate and lactic acid. When the expression of GPR81 and Wnt3 lactic acid-specific receptors in Paneth cells and stromal cells is increased, lactic acid stimulates the proliferation of intestinal epithelial stem cells and prevents intestinal damage. Lactic acid also affects the expression of CX3CR1, a phagocyte in the lamina propria that modulates intestinal immune function (80). Codium fragile extract boosted the percentage of beneficial bacteria and decreased the degree of pyruvate fermentation and glycolysis, reducing the inflammatory reaction induced by a high-fat diet (81). Additionally, to reduce intestinal inflammation and treat metabolic disorders of the microbiota, polysaccharides extracted from Rosa Roxburghii Tratt (RTFP) can decrease the Firmicutes/Bacteroides ratio, lower the levels of d-lactic acid and LPS, and suppress the TLR4/NF-κB signaling pathway. It has been reported that RTFP can be administered as a natural anti-inflammatory agent to minimize colitis caused by chronic obesity (82).
Dietary compounds affect microbiota composition
According to their natural properties, intestinal microbiota can be divided into nine phyla, of which Firmicutes (64%), Bacteroides (28%), Proteus (8%), and Actinomycetes (3%) account for 98% of the flora (83). Dietary compounds indirectly affect immune responses by regulating the microbiota composition. For instance, insoluble dietary fiber extracted from barley leaves increased Parasutterella and Alistipes abundance to a certain extent and decreased Akkermansia abundance, as well as markedly relieved acute colitis symptoms and decreased levels of inflammatory factors such as IL-6, TNF-α, and IL-1β in colitis mice. In addition, short-term rice bran consumption reduces the Firmicutes: Bacteroidetes ratio in humans but may increase it in the long run (84). Therefore, there may be a time difference in the impact of dietary compounds in reducing CRC risk (85). Additionally, genetically modified mice with the same epigenome but two different gut microbes were fed four equal-calorie diets with the same dietary fiber composition. Integrated transcriptomic and metabolomic analyses showed that the metabolic results of the final amino acids and lipids in each group were different, indicating that gut microbiota structures also affected metabolism of dietary compounds (86).
Metabolic regulation of intestinal flora on dietary compounds
The metabolism of dietary compounds by gut microbiota is mainly catabolic, which reduces the molecular weight of drugs, weakens polarity, and increases fat solubility and the efficacy. According to research on ginseng metabolism, microbiota can metabolize the ginsenosides Rb1 and RD into compound K, which has a potent anticancer effect (87). The oral bioavailability of food components can also be improved by gut flora. Curcumin can prevent tumor growth in vivo by increasing the chemical sensitivity of HCC cells to 5-FU via blocking the G2/M phase of the cell cycle, and reducing the activation of downstream protein kinases in the PI3K/AKT/mTOR signaling pathway (88). Glycyrrhizin is a flavonoid food component that can be metabolized by gut flora to yield three potential metabolites: pantothenic acid (M3), resorcinol (M4), and M5 to achieve antitumor activity (89, 90).
Interactions between TCM and gut microbiota
TCM is mostly used in decoctions. The main components of TCM ingested by the human body from such decoctions include polysaccharides, peptides, flavonoids, alkaloids, polyphenols and anthocyanins. As specific components are more complex than those of dietary compounds, TCM has multi- component, level, and target effects. The herbal medicine WangShiBoChiWan (WSBCW) increases the number of Bifidobacterium and Desulfovibrio, restrains Bacteroides fragillis in the gut, upregulates intestinal junction proteins, increases long villi length, and reduces the levels of inflammatory factor (91). XiaoChaiHuTang (XCHT) partially reverses gut dysbiosis associated with CRC progression inhibition, and the mechanism may be related to the TLR4/MyD88/nuclear factor (NF)-κB downregulation of signaling pathways (92). Fecal microbiota transplantation from GeGen QinLian decoction (FMT-GQD) treatment inhibits nucleotide-binding and regulates important pathways, including the oligomerization domain (NOD), receptor-interacting-serine, threonine-protein kinase 2 (RIP2), and NF-κB signaling pathways, which could influence the expression of related downstream inflammatory factors and inhibit the activation and differentiation of CD4+ T cells to influence the immune system (93).
Gut bacteria can produce a large number of enzyme systems, mainly including glucuronidase β-glucose enzyme, nitroreductase, and protease to degrade and release a variety of active ingredients that are convertible, directly altering the toxicity of TCM (94). By diacylation, esterification, and elimination of methyl hydroxyl, the intestinal microbiota can transform aconitine, the primary poisonous component of aconite, into monoester diterpene alkaloids that act as anti-inflammatories (95). However, the negative effects of gut flora that increase TCM toxicity are also significant. The hepatotoxic and carcinogenic effects of cycasin, an azo-glucoside found in the plants of the Cycadaceae family, may be caused by the microflora modulation via converting it into three different carcinogens, diazomethane cycasin, diazomethane cycasin, and cycasin (96). Consequently, comprehensive research into the dual effects of gut flora metabolism on TCM is necessary for digestive cancer immunotherapy. The relationships between dietary compounds/TCMs and intestinal microflora have been demonstrated in Figure 3.
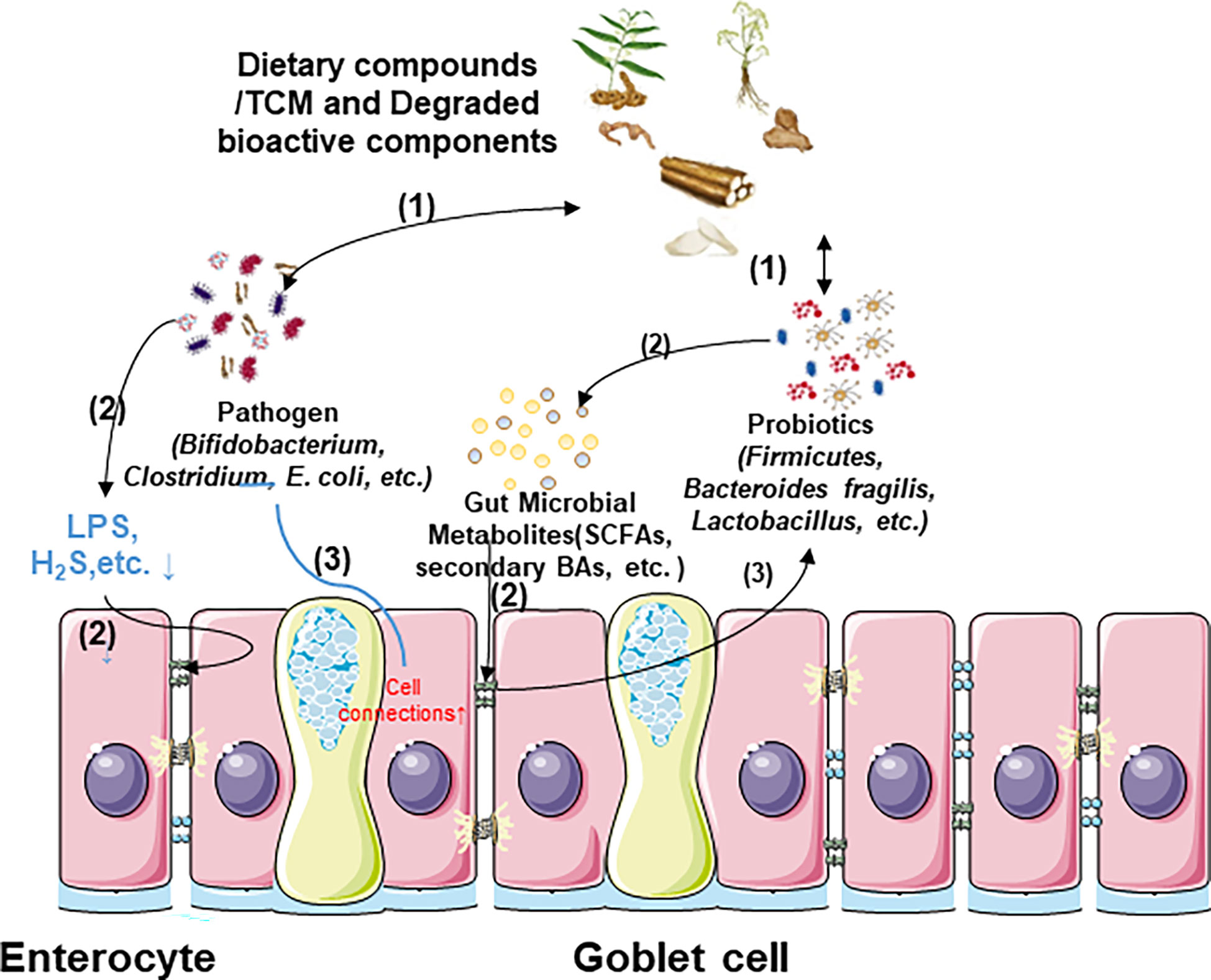
Figure 3 Relationship between dietary compounds/TCM and gut microbiota. (1) Dietary compounds/TCM and degraded bioactive components can modulate the microflora structure, and the different composition of gut flora among species also influences their curative effect. (2) Some gut microbial metabolites produced by probiotics restore intestinal epithelial cell barrier, and the reduction of harmful metabolites produced by pathogens also decreases intestinal barrier damage. (3) Cell barrier restoration is also conducive to gut flora stability.
Dietary compounds/TCM regulate immunotherapy through interstinal microbiota intervention
Many active components of dietary compounds/TCM participate in regulating cancer immunotherapy, which may be linked to improved intestinal health and microbial metabolites. The mechanisms have been detailed in Tables 1, 2.
Dietary fiber
Pectin, a soluble fiber extracted from plant cell walls, can be used to block the proliferation cycle of tumor cells, thereby inhibiting CRC (97, 119). The gut flora of CRC subjects in tumor-bearing mice was dosed orally with pectin, which significantly improved the anti-PD-1 monoclonal antibody effect (82). Moreover, mice treated with gut flora from patients resistant to anti-PD-1 antibody showed a similar effect. Inulin is a plant-derived polysaccharide fiber present in Jerusalem artichoke and chicory tubers. It can be adsorbed into intestinal mucosa folds through the thickening effect, which is conducive to its interaction with gut flora. Akkermansia, Lactobacillus, and Rosebacilli are the main SCFA-producing bacteria, and their abundance increased significantly after inulin gel administration. Notably, high-dose inulin intake for 14 days (approximately 450 mg per day) slows tumor growth in mice before tumor modeling but does not work in synergy with anti-PD-1 (98). This may be related to a reduction in gut flora diversity caused by a single diet. Approximately 90% CRC tumors exhibit abnormal Wnt/β-catenin pathway activation (120). Albuca Bracteate Polysaccharides (ABP) treatment inhibits β-catenin expression. Furthermore, ABP and 5-FU (first-line drugs for CRC treatment) synergistically affected CRC tumor cell growth, migration, and invasion, and the antitumor effect of their combination was better than that of 5-FU or ABP alone. Fucoidan significantly increased Lactobacillus levels and decreased Fusobacterium levels in CRC mouse models, which alleviated macrophage and T cell infiltration, and reduced colonic inflammation (100). Moreover, fucoidan also inhibited the Wnt/β-catenin pathway (121). Dietary fibers with similar effects also contain cellulose and apple and jujube polysaccharides, which reduce the abundance of differential bacteria correlated with IL-6, IL-1β, and TNF-α concentrations to inhibit colon tumor formation (101, 102, 122).
Flavonoids
Corylin is one kind of flavonoids isolated from the fruit and seed of Psoralea corylifolia (104). Corylin improved intestinal homeostasis to further reduce tumor cell-induced inflammation by inhibiting the TLR4/p38/AP-1 pathway, inflammatory factors, and the contact between bacteria and epithelial cells in CRC mice. Apigenin has been reported to prevent atrophic gastritis and subsequent gastric cancer caused by Helicobacter pylori. Apigenin also treats tumors by regulating gut flora associated with SCFA production, such as Bifidobacterium and Lactobacillus (103, 123). Bound polyphenol of the inner shell (BPIS) sharply restores Lactobacillus and Bifidobacterium abundance in the intestine of CRC mouse models, with an increase in various lymphoid subgroups such as CD4+, CD8+T cells, and NKT cells in the blood (105). BPIS also increases the number of microbiota products, including SCFAs and indole derivatives, which boost intestinal junction recovery and alleviate inflammation in tumor-bearing mice (124). The above result shows that BPIS can regulate oncogenic inflammation. The initial response to harmful stimuli is acute inflammation, with chronic inflammation potentially resulting from the persistence of inflammatory factors (125). Inflammatory cells and cytokines act as tumor promoters during chronic inflammation, affecting cell survival, proliferation, invasion, as well as angiogenesis. Moreover, the effect of inflammation on most cancers is double-edged, since cancer also affects inflammation. Inflammation has a close relationship with tumors, making inflammation an important target for anticancer treatment (126). Activating anti-cancer immunity cells can improve the cancer-killing ability of the immune system (127, 128). These results showed that BPIS may become a new cancer drug through microbial restoration and immune regulation. Other flavonoid compounds with anti-tumor effects include carnosic acid (106) and baicalin (129).
Glycosides
An early study showed that Ginsenoside Rk3 exerts antitumor effects in vitro, without toxic effects on normal cells and lymphocytes (105). Later, it was found that a crucial factor in avoiding HCC is the LPS-TLR4 signaling pathway that Ginsenoside Rk3 inhibits by enhancing gut microbial imbalance (107). The mechanism of Rk3 against esophageal cancer is also related to this pathway. Neohesperidin (NHP), found in citrus fruits, upregulates Firmicutes and Proteobacteria and downregulates Bacteroides abundance. Furthermore, NHP treatment significantly increases IFN-γ expression and CD4+ and CD8+ T cell infiltration in mouse tumor cells, whereas the antibiotic cocktail (ABX) suppresses this effect (108). Consequently, NHP, a microecological regulator, induces antitumor immunity by improving immune checkpoint efficacy as a glycoside.
Biological pigments
Anthocyanins are polyphenolic compounds widely present in plants. Bilberry anthocyanin extracts (BAE) assist in systemic nutritional status and immunity improvement by increasing the levels of Lachnospiraceae johnsonii in Firmicutes (109). Meanwhile, BAE induces T cell responses and increases SCFA production by upregulating Clostridia to ferment resistant starch and nonstarch polysaccharides. Simultaneously, the ratio of aerobes decreased remarkably, and the proportion of anaerobes increased with BAE, showing that oxygen content reduction may help BAE improve the microflora environment. Recombinant phycoerythrin (RPE) is a light-harvesting pigment that greatly reduces tumor weight, increases the incubation period of tumor cells, and inhibits cancer growth in H22-bearing mice. Moreover, RPE improved the probiotic level and sharply reduced the pathogen level compared to the cyclophosphamide group (110). Safflower yellow (SY), the main active ingredient from Carthamus tinctorius, modulates microbiota composition in BC mice, including increased Bacteroides fragilis and Clostridium counts, which could suppress pro-inflammatory factor activity and induce CD8+T cell and butyric acid production. This finding provides evidence that SY improves the immune microenvironment by affecting the immune cell components of the liver and modulating the abundance of inflammation-related gut microbiota (111).
Furthermore, combined treatment with multiple dietary compounds requires more attention. Combined GLP and GPS treatment significantly improved the intestinal barrier by blocking colonic polyp growth, transforming M1 to M2, effectively adjusting epithelial–mesenchymal transition markers and cutting carcinogenic signaling molecules in ApcMin/+ mice. Their combination also greatly promotes SCFA-producing bacteria and inhibits sulfate-reducing bacteria (130). However, some highly disconcerted effects were also observed. Soluble fermentable fiber inulin may lead to gut flora imbalance and icteric hepatic cellular cancer (131). Excessive inulin increases SCFA production and stimulates immune cells to produce inflammatory factors, including IL-1α, IL-1β, IL-6, and IL-10, which cause acute lamellar inflammation and laminitis. Therefore, the application of dietary compounds in immunotherapy requires more comprehensive investigations.
TCM
GQD is a classical TCM formula, and its active compounds, including baicalin, Glaxo, and berberine, greatly reduce the inflammatory response and oxidative stress both in vivo and in vitro, which have been used in ulcerative colitis therapy (132, 133). Additionally, GQD and anti-PD-1 combination therapy downregulates PD-1 and increases IL-2, indicating that the combined treatment restores T-cell function to a certain extent by suppressing the checkpoint blockade. Combination therapy also increased Bacteroides groups, which reduces the pro-inflammatory activity of the mouse small intestine and exerts immune regulation by releasing extracellular bacterial DNA. Notably, combination therapy changes glycerophospholipid and sphingolipid metabolism, which could be used as biomarkers for monitoring patients with CRC (134).
Experimental results have indicated that sporoderm-broken Ganoderma reverses the tumor xenotransplantation-mediated microbiota structural shift (135) by increasing the immunoactivity-related genera, and by reducing microbiota, such as Bacteroides, which cause immunologic suppression and carcinogenic effects (136). The transformation of microbiota leads to changes in a series of key metabolites, including several amide acids necessary to form SCFAs. The Yiyi Fuzi Baijiang decoction (YFBD), composed of Coix seed and Patrinia villosa, is a TCM used to treat gastrointestinal disorders. Coix seeds and Patrinia villosa demonstrated antitumor hyperplasia effects in several carcinoma cell lines (137, 138). YFBD inhibited CRC cell proliferation and development in ApcMin/+ mice without significant weight changes or immune recovery. YFBD also changes ApcMin/+ mice intestinal bacteria, such as Bacteroides fragilis and Trichospiroideae, which regulate the Treg/Th17 ratio to control carcinogenesis (139).
Siwu-Yin inhibits esophageal precancerous lesion occurrence by increasing Turicibacter abundance, regulating bile acid synthesis and secretion metabolic pathways, and improving macrophage polarization (117). Accordingly, TCM has great potential in preventing digestion cancer progression in addition to its application in tumor immunotherapy. Furthermore, Danggui Buxue decoction (DBD) significantly improves bone marrow suppression-mediated anemia after CRC chemotherapy, while treating CRC by increasing butyric acid-producing bacterial abundance (118). This suggests a novel use of TCM for treating the postoperative side effects of digestive cancer.
Conclusions
With the development of 16S rRNA high-throughput sequencing, TCM microencapsulation, and CAR-T technology, we have reached a new level of understanding regarding the modulation of gut flora by dietary compounds/TCM for digestive cancer immunotherapy. Dietary compounds/TCM are primarily metabolized in the intestine. Long-lasting effects on the gut microbiota from dietary compounds/TCM can strengthen the biological function via their conversion into bioactive metabolites. The studies mentioned above provide prospective methods to arrest tumor progression by enhancing the intestinal barrier, inhibiting pathogens, restraining inflammatory reactions, provoking tumor cell apoptosis and metabolism, and controlling SCFA secretion. However, research must overcome some obstacles.
Firstly, most research focused on the correlation analysis of dietary compounds/TCM on the structure and composition of gut flora, intestinal immune inflammatory reaction, intestinal barrier function and bacterial metabolites, but further multi-channel research has been neglected. In addition, the vast majority of experimental designs only stay in one stage, such as in precancerous lesions of digestive tract tumors, or in the tumor development or pre-late stages. We believe time series analysis is required to conduct a longitudinal survey on the tumor immune methods of dietary compounds/TCM modulating gut microbiota, which may provide more information. Notably, most digestive tumor models were conducted on only one strain of mice, resulting in a lack of comparisons and inductions between different strains of mice. Furthermore, the therapeutic effects of dietary compounds/TCM in different species (such as rabbits, monkeys, etc.) should also be investigated.
We recommend that future research should focus on the following aspects. Firstly, some dietary compounds/TCMs have shortcomings such as low bioavailability and bioactivity, toxic or side effects, and insufficient supply, which remain the main obstacles to clinical transformation. Secondly, exactly which specific species of gut microbiota, or even which specific enzymes in the microbiota can metabolize dietary compounds/TCM remains to be elucidated. Moreover, the specific components of dietary compounds/TCM that play an immunomodulatory role after being metabolized should also be investigated. It is necessary to identify more precise targets to reduce the increased toxicity of dietary compounds/TCM after metabolism. Thirdly, regarding some TCM with complex prescriptions, it may be difficult to standardize research materials, because factors such as different processing methods or different raw material origins can affect the quality. Fourthly, since the therapeutic response of dietary compounds/TCM is affected by different digestive cancer heterogeneity, more information is needed to select the proper treatment and mode of natural compound administration. Therefore, more studies are required focusing on the effect of one specific type of digestive tumor or a single component of dietary compounds/TCM on tumor immunotherapy. Fifthly, the modification of dietary supplements/TCM, in comparison to western drugs, is still poorly understood, which make it more difficult to achieve selective targeted drug delivery, leading to many uncertainties about the efficacy of this method. We suggest that interdisciplinary methods, such as nanomedical technology and precision medicine, are required to improve the safety of dietary compounds/TCMs and achieve better therapeutic effects. These efforts will pave the way for the use of dietary compounds/TCMs in clinical researches. In summary, we hope this review will help to understand the specific mechanisms by which dietary components/TCM improve immunotherapy based on microbiota and provide a theoretical basis for the development of new drugs for treating malignant digestive tumor growth, recurrence, and complications.
Author contributions
XF, WG and YH contributed to conception and design of the study. ZL organized the database. XF and ZL wrote the first draft of the manuscript. All authors contributed to manuscript revision, read, and approved the submitted version.
Funding
This work was supported by grants from Natural Science Foundation of China (82272062; 82001948; 82001298; 81971746) and Guangdong Basic and Applied Basic Research Foundation (2019A1515110635; 2022A1515010462), Outstanding Youths Development Scheme of Nanfang Hospital, Southern Medical University (2022J004) and GuangZhou Basic and Applied Basic Research Foundation (SL2022A04J02053).
Acknowledgments
I would like to thank all the tutors and fellow students who have encouraged and helped in the completion of this article. Thanks for their contributions.
Conflict of interest
The authors declare that the research was conducted in the absence of any commercial or financial relationships that could be construed as a potential conflict of interest.
Publisher’s note
All claims expressed in this article are solely those of the authors and do not necessarily represent those of their affiliated organizations, or those of the publisher, the editors and the reviewers. Any product that may be evaluated in this article, or claim that may be made by its manufacturer, is not guaranteed or endorsed by the publisher.
References
1. Sung H, Ferlay J, Siegel RL, Laversanne M, Soerjomataram I, Jemal A, et al. Global cancer statistics 2020: GLOBOCAN estimates of incidence and mortality worldwide for 36 cancers in 185 countries. CA Cancer J Clin (2021) 71:209–49. doi: 10.3322/caac.21660
2. Bray F, Laversanne M, Weiderpass E, Soerjomataram I. The ever-increasing importance of cancer as a leading cause of premature death worldwide. CANCER-AM Cancer Soc (2021) 127:3029–30. doi: 10.1002/cncr.33587
3. Kalbasi A, Ribas A. Tumour-intrinsic resistance to immune checkpoint blockade. Nat Rev Immunol (2020) 20:25–39. doi: 10.1038/s41577-019-0218-4
4. Postow MA, Callahan MK, Wolchok JD. Immune checkpoint blockade in cancer therapy. J Clin Oncol (2015) 33:1974–82. doi: 10.1200/JCO.2014.59.4358
5. Sharma P, Pachynski RK, Narayan V, Flechon A, Gravis G, Galsky MD, et al. Nivolumab plus ipilimumab for metastatic castration-resistant prostate cancer: Preliminary analysis of patients in the CheckMate 650 trial. Cancer Cell (2020) 38:489–99. doi: 10.1016/j.ccell.2020.08.007
6. Antonia S, Goldberg SB, Balmanoukian A, Chaft JE, Sanborn RE, Gupta A, et al. Safety and antitumour activity of durvalumab plus tremelimumab in non-small cell lung cancer: a multicentre, phase 1b study. Lancet Oncol (2016) 17:299–308. doi: 10.1016/S1470-2045(15)00544-6
7. Li L, Rao X, Wen Z, Ding X, Wang X, Xu W, et al. Implications of driver genes associated with a high tumor mutation burden identified using next-generation sequencing on immunotherapy in hepatocellular carcinoma. Oncol Lett (2020) 19:2739–48. doi: 10.3892/ol.2020.11372
8. Wan H, Liu Z, Tan X, Wang G, Xu Y, Xie L, et al. Application of immune cell infiltration in the diagnosis and prognosis of non-small cell lung cancer. Sheng Wu Gong Cheng Xue Bao (2020) 36:740–9. doi: 10.13345/j.cjb.190232
9. Schumacher TN, Schreiber RD. Neoantigens in cancer immunotherapy. SCIENCE (2015) 348:69–74. doi: 10.1126/science.aaa4971
10. Chen F, Dai X, Zhou CC, Li KX, Zhang YJ, Lou XY, et al. Integrated analysis of the faecal metagenome and serum metabolome reveals the role of gut microbiome-associated metabolites in the detection of colorectal cancer and adenoma. GUT (2022) 71:1315–25. doi: 10.1136/gutjnl-2020-323476
11. Minton K. Microbiome effects on immune surveillance of intestinal tumours. Nat Rev Immunol (2021) 21:692–3. doi: 10.1038/s41577-021-00629-5
12. Mariat D, Firmesse O, Levenez F, Guimaraes V, Sokol H, Dore J, et al. The Firmicutes/Bacteroidetes ratio of the human microbiota changes with age. BMC Microbiol (2009) 9:123. doi: 10.1186/1471-2180-9-123
13. Raimondi S, Amaretti A, Gozzoli C, Simone M, Righini L, Candeliere F, et al. Longitudinal survey of fungi in the human gut: ITS profiling, phenotyping, and colonization. Front Microbiol (2019) 10:1575. doi: 10.3389/fmicb.2019.01575
14. Beam A, Clinger E, Hao L. Effect of diet and dietary components on the composition of the gut microbiota. NUTRIENTS (2021) 13(8):2795. doi: 10.3390/nu13082795
15. Bibbo S, Ianiro G, Giorgio V, Scaldaferri F, Masucci L, Gasbarrini A, et al. The role of diet on gut microbiota composition. Eur Rev Med Pharmacol Sci (2016) 20:4742–9.
16. Sepp E, Smidt I, Rööp T, Štšepetova J, Kõljalg S, Mikelsaar M, et al. Comparative analysis of gut microbiota in centenarians and young people: Impact of eating habits and childhood living environment. Front Cell Infect MI (2022) 12:851404. doi: 10.3389/fcimb.2022.851404
17. Roth W, Zadeh K, Vekariya R, Ge Y, Mohamadzadeh M. Tryptophan metabolism and gut-brain homeostasis. Int J Mol Sci (2021) 22(6):2973. doi: 10.3390/ijms22062973
18. Matson V, Fessler J, Bao R, Chongsuwat T, Zha Y, Alegre ML, et al. The commensal microbiome is associated with anti-PD-1 efficacy in metastatic melanoma patients. SCIENCE (2018) 359:104–8. doi: 10.1126/science.aao3290
19. Routy B, Le Chatelier E, Derosa L, Duong C, Alou MT, Daillere R, et al. Gut microbiome influences efficacy of PD-1-based immunotherapy against epithelial tumors. SCIENCE (2018) 359:91–7. doi: 10.1126/science.aan3706
20. Kim SY, Shin JS, Chung KS, Han HS, Lee HH, Lee JH, et al. Immunostimulatory effects of live lactobacillus sakei K040706 on the CYP-induced immunosuppression mouse model. NUTRIENTS (2020) 12(11):3573. doi: 10.3390/nu12113573
21. Mager LF, Burkhard R, Pett N, Cooke N, Brown K, Ramay H, et al. Microbiome-derived inosine modulates response to checkpoint inhibitor immunotherapy. SCIENCE (2020) 369:1481–9. doi: 10.1126/science.abc3421
22. Kim MS, Hwang SS, Park EJ, Bae JW. Strict vegetarian diet improves the risk factors associated with metabolic diseases by modulating gut microbiota and reducing intestinal inflammation. Environ Microbiol Rep (2013) 5:765–75. doi: 10.1111/1758-2229.12079
23. Leong F, Hua X, Wang M, Chen T, Song Y, Tu P, et al. Quality standard of traditional Chinese medicines: comparison between European pharmacopoeia and Chinese pharmacopoeia and recent advances. Chin Med (2020) 15:76. doi: 10.1186/s13020-020-00357-3
24. Xu HY, Zhang YQ, Liu ZM, Chen T, Lv CY, Tang SH, et al. ETCM: an encyclopaedia of traditional Chinese medicine. Nucleic Acids Res (2019) 47:D976–82. doi: 10.1093/nar/gky987
25. Gopalakrishnan V, Helmink BA, Spencer CN, Reuben A, Wargo JA. The influence of the gut microbiome on cancer, immunity, and cancer immunotherapy. Cancer Cell (2018) 33:570–80. doi: 10.1016/j.ccell.2018.03.015
26. Han X, Wei Q, Lv Y, Weng L, Huang H, Wei Q, et al. Ginseng-derived nanoparticles potentiate immune checkpoint antibody efficacy by reprogramming the cold tumor microenvironment. Mol Ther (2022) 30:327–40. doi: 10.1016/j.ymthe.2021.08.028
27. Chen DS, Mellman I. Elements of cancer immunity and the cancer–immune set point. NATURE (2017) 541:321–30. doi: 10.1038/nature21349
28. Gerard CL, Delyon J, Wicky A, Homicsko K, Cuendet MA, Michielin O. Turning tumors from cold to inflamed to improve immunotherapy response. Cancer Treat Rev (2021) 101:102227. doi: 10.1016/j.ctrv.2021.102227
29. Lin TL, Lu CC, Lai WF, Wu TS, Lu JJ, Chen YM, et al. Role of gut microbiota in identification of novel TCM-derived active metabolites. Protein Cell (2021) 12:394–410. doi: 10.1007/s13238-020-00784-w
30. Su XL, Wang JW, Che H, Wang CF, Jiang H, Lei X, et al. Clinical application and mechanism of traditional Chinese medicine in treatment of lung cancer. Chin Med J (Engl) (2020) 133:2987–97. doi: 10.1097/CM9.0000000000001141
31. Spencer CN, McQuade JL, Gopalakrishnan V, McCulloch JA, Vetizou M, Cogdill AP, et al. Dietary fiber and probiotics influence the gut microbiome and melanoma immunotherapy response. SCIENCE (2021) 374:1632–40. doi: 10.1126/science.aaz7015
32. Zitvogel L, Derosa L, Kroemer G. Modulation of cancer immunotherapy by dietary fibers and over-the-counter probiotics. Cell Metab (2022) 34:350–2. doi: 10.1016/j.cmet.2022.02.004
33. Unger WWJ, Laban S, Kleijwegt FS, van der Slik AR, Roep BO. Induction of treg by monocyte-derived DC modulated by vitamin D3 or dexamethasone: Differential role for PD-L1. Eur J Immunol (2009) 39:3147–59. doi: 10.1002/eji.200839103
34. Turbitt WJ, Demark-Wahnefried W, Peterson CM, Norian LA. Targeting glucose metabolism to enhance immunotherapy: Emerging evidence on intermittent fasting and calorie restriction mimetics. Front Immunol (2019) 10:1402. doi: 10.3389/fimmu.2019.01402
35. Capurro MI, Greenfield LK, Prashar A, Xia S, Abdullah M, Wong H, et al. VacA generates a protective intracellular reservoir for helicobacter pylori that is eliminated by activation of the lysosomal calcium channel TRPML1. Nat Microbiol (2019) 4:1411–23. doi: 10.1038/s41564-019-0441-6
36. Sung J, Coker OO, Chu E, Szeto CH, Luk S, Lau H, et al. Gastric microbes associated with gastric inflammation, atrophy and intestinal metaplasia 1 year after helicobacter pylori eradication. GUT (2020) 69:1572–80. doi: 10.1136/gutjnl-2019-319826
37. Garrett WS. The gut microbiota and colon cancer. SCIENCE (2019) 364:1133–5. doi: 10.1126/science.aaw2367
38. Mima K, Nishihara R, Qian ZR, Cao Y, Sukawa Y, Nowak JA, et al. Fusobacterium nucleatum in colorectal carcinoma tissue and patient prognosis. GUT (2016) 65:1973–80. doi: 10.1136/gutjnl-2015-310101
39. Wilson MR, Jiang Y, Villalta PW, Stornetta A, Boudreau PD, Carra A, et al. The human gut bacterial genotoxin colibactin alkylates DNA. SCIENCE (2019) 363(6428):eaar7785. doi: 10.1126/science.aar7785
40. Huycke MM, Abrams V, Moore DR. Enterococcus faecalis produces extracellular superoxide and hydrogen peroxide that damages colonic epithelial cell DNA. CARCINOGENESIS (2002) 23:529–36. doi: 10.1093/carcin/23.3.529
41. Marquet P, Duncan SH, Chassard C, Bernalier-Donadille A, Flint HJ. Lactate has the potential to promote hydrogen sulphide formation in the human colon. FEMS Microbiol Lett (2009) 299:128–34. doi: 10.1111/j.1574-6968.2009.01750.x
42. Cheng KW, Tseng CH, Chen IJ, Huang BC, Liu HJ, Ho KW, et al. Inhibition of gut microbial beta-glucuronidase effectively prevents carcinogen-induced microbial dysbiosis and intestinal tumorigenesis. Pharmacol Res (2022) 177:106115. doi: 10.1016/j.phrs.2022.106115
43. Engemann A, Focke C, Humpf HU. Intestinal formation of n-nitroso compounds in the pig cecum model. J Agric Food Chem (2013) 61:998–1005. doi: 10.1021/jf305040e
44. Hanson BT, Dimitri Kits K, Löffler J, Burrichter AG, Fiedler A, Denger K, et al. Sulfoquinovose is a select nutrient of prominent bacteria and a source of hydrogen sulfide in the human gut. ISME J (2021) 15:2779–91. doi: 10.1038/s41396-021-00968-0
45. Schneider KM, Mohs A, Gui W, Galvez E, Candels LS, Hoenicke L, et al. Imbalanced gut microbiota fuels hepatocellular carcinoma development by shaping the hepatic inflammatory microenvironment. Nat Commun (2022) 13:3964. doi: 10.1038/s41467-022-31312-5
46. Fedirko V, Tran HQ, Gewirtz AT, Stepien M, Trichopoulou A, Aleksandrova K, et al. Exposure to bacterial products lipopolysaccharide and flagellin and hepatocellular carcinoma: a nested case-control study. BMC Med (2017) 15:72. doi: 10.1186/s12916-017-0830-8
47. Gola A, Dorrington MG, Speranza E, Sala C, Shih RM, Radtke AJ, et al. Commensal-driven immune zonation of the liver promotes host defence. NATURE (2021) 589:131–6. doi: 10.1038/s41586-020-2977-2
48. Ryu JK, Kim SJ, Rah SH, Kang JI, Jung HE, Lee D, et al. Reconstruction of LPS transfer cascade reveals structural determinants within LBP, CD14, and TLR4-MD2 for efficient LPS recognition and transfer. IMMUNITY (2017) 46:38–50. doi: 10.1016/j.immuni.2016.11.007
49. Jia W, Xie G, Jia W. Bile acid-microbiota crosstalk in gastrointestinal inflammation and carcinogenesis. Nat Rev Gastroenterol Hepatol (2018) 15:111–28. doi: 10.1038/nrgastro.2017.119
50. Banales JM, Inarrairaegui M, Arbelaiz A, Milkiewicz P, Muntane J, Munoz-Bellvis L, et al. Serum metabolites as diagnostic biomarkers for cholangiocarcinoma, hepatocellular carcinoma, and primary sclerosing cholangitis. HEPATOLOGY (2019) 70:547–62. doi: 10.1002/hep.30319
51. Jia X, Lu S, Zeng Z, Liu Q, Dong Z, Chen Y, et al. Characterization of gut microbiota, bile acid metabolism, and cytokines in intrahepatic cholangiocarcinoma. HEPATOLOGY (2020) 71:893–906. doi: 10.1002/hep.30852
52. Dong L, Yin J, Zhao J, Ma SR, Wang HR, Wang M, et al. Microbial similarity and preference for specific sites in healthy oral cavity and esophagus. Front Microbiol (2018) 9:1603. doi: 10.3389/fmicb.2018.01603
53. Gursoy UK, Kononen E, Uitto VJ. Stimulation of epithelial cell matrix metalloproteinase (MMP-2, -9, -13) and interleukin-8 secretion by fusobacteria. Oral Microbiol Immunol (2008) 23:432–4. doi: 10.1111/j.1399-302X.2008.00453.x
54. Yu T, Guo F, Yu Y, Sun T, Ma D, Han J, et al. Fusobacterium nucleatum promotes chemoresistance to colorectal cancer by modulating autophagy. CELL (2017) 170:548–63. doi: 10.1016/j.cell.2017.07.008
55. Hu L, Liu Y, Kong X, Wu R, Peng Q, Zhang Y, et al. Fusobacterium nucleatum facilitates M2 macrophage polarization and colorectal carcinoma progression by activating TLR4/NF-kappaB/S100A9 cascade. Front Immunol (2021) 12:658681. doi: 10.3389/fimmu.2021.658681
56. Kim J, Manspeaker MP, Thomas SN. Augmenting the synergies of chemotherapy and immunotherapy through drug delivery. Acta BIOMATER (2019) 88:1–14. doi: 10.1016/j.actbio.2019.02.012
57. Lloyd-Price J, Mahurkar A, Rahnavard G, Crabtree J, Orvis J, Hall AB, et al. Erratum: Strains, functions and dynamics in the expanded human microbiome project. NATURE (2017) 551:256. doi: 10.1038/nature24485
58. Vetizou M, Pitt JM, Daillere R, Lepage P, Waldschmitt N, Flament C, et al. Anticancer immunotherapy by CTLA-4 blockade relies on the gut microbiota. SCIENCE (2015) 350:1079–84. doi: 10.1126/science.aad1329
59. Liou JM, Lee YC, El-Omar EM, Wu MS. Efficacy and long-term safety of h. pylori eradication for gastric cancer prevention. Cancers (Basel) (2019) 11(5):593. doi: 10.3390/cancers11050593
60. He C, Peng C, Xu X, Li N, Ouyang Y, Zhu Y, et al. Probiotics mitigate helicobacter pylori-induced gastric inflammation and premalignant lesions in INS-GAS mice with the modulation of gastrointestinal microbiota. HELICOBACTER (2022) 27:e12898. doi: 10.1111/hel.12898
61. Zheng C, Chen T, Wang Y, Gao Y, Kong Y, Liu Z, et al. A randomised trial of probiotics to reduce severity of physiological and microbial disorders induced by partial gastrectomy for patients with gastric cancer. J Cancer (2019) 10:568–76. doi: 10.7150/jca.29072
62. Peng Z, Cheng S, Kou Y, Wang Z, Jin R, Hu H, et al. The gut microbiome is associated with clinical response to anti-PD-1/PD-L1 immunotherapy in gastrointestinal cancer. Cancer Immunol Res (2020) 8:1251–61. doi: 10.1158/2326-6066.CIR-19-1014
63. Bachem A, Makhlouf C, Binger KJ, de Souza DP, Tull D, Hochheiser K, et al. Microbiota-derived short-chain fatty acids promote the memory potential of antigen-activated CD8(+) T cells. IMMUNITY (2019) 51:285–97. doi: 10.1016/j.immuni.2019.06.002
64. Matsushita M, Fujita K, Hayashi T, Kayama H, Motooka D, Hase H, et al. Gut microbiota-derived short-chain fatty acids promote prostate cancer growth via IGF1 signaling. Cancer Res (2021) 81:4014–26. doi: 10.1158/0008-5472.CAN-20-4090
65. Amadou AS, Shadid T, Ballard JD, Lang ML. Clostridioides difficile infection induces an inferior IgG response to that induced by immunization and is associated with a lack of T follicular helper cell and memory b cell expansion. Infect Immun (2020) 88(3):e00829-19. doi: 10.1128/IAI.00829-19
66. Chang J, Liu X, Ren H, Lu S, Li M, Zhang S, et al. Pseudomonas exotoxin a-based immunotherapy targeting CCK2R-expressing colorectal malignancies: An In vitro and In vivo evaluation. Mol Pharm (2021) 18:2285–97. doi: 10.1021/acs.molpharmaceut.1c00095
67. Thiele OE, Fan H, Tam AJ, Dejea CM, Destefano SC, Wu S, et al. The myeloid immune signature of enterotoxigenic bacteroides fragilis-induced murine colon tumorigenesis. Mucosal Immunol (2017) 10:421–33. doi: 10.1038/mi.2016.53
68. Sivan A, Corrales L, Hubert N, Williams JB, Aquino-Michaels K, Earley ZM, et al. Commensal bifidobacterium promotes antitumor immunity and facilitates anti-PD-L1 efficacy. SCIENCE (2015) 350:1084–9. doi: 10.1126/science.aac4255
69. Griffin ME, Espinosa J, Becker JL, Luo JD, Carroll TS, Jha JK, et al. Enterococcus peptidoglycan remodeling promotes checkpoint inhibitor cancer immunotherapy. SCIENCE (2021) 373:1040–6. doi: 10.1126/science.abc9113
70. Song X, Sun X, Oh SF, Wu M, Zhang Y, Zheng W, et al. Microbial bile acid metabolites modulate gut RORgamma(+) regulatory T cell homeostasis. NATURE (2020) 577:410–5. doi: 10.1038/s41586-019-1865-0
71. Tanoue T, Morita S, Plichta DR, Skelly AN, Suda W, Sugiura Y, et al. A defined commensal consortium elicits CD8 T cells and anti-cancer immunity. NATURE (2019) 565:600–5. doi: 10.1038/s41586-019-0878-z
72. Cramer P, Bresalier RS. Gastrointestinal and hepatic complications of immune checkpoint inhibitors. Curr Gastroenterol Rep (2017) 19:3. doi: 10.1007/s11894-017-0540-6
73. Liang W, Yang Y, Wang H, Wang H, Yu X, Lu Y, et al. Gut microbiota shifts in patients with gastric cancer in perioperative period. MEDICINE (2019) 98:e16626. doi: 10.1097/MD.0000000000016626
74. Gasaly N, de Vos P, Hermoso MA. Impact of bacterial metabolites on gut barrier function and host immunity: A focus on bacterial metabolism and its relevance for intestinal inflammation. Front Immunol (2021) 12:658354. doi: 10.3389/fimmu.2021.658354
75. Moniri NH, Farah Q. Short-chain free-fatty acid G protein-coupled receptors in colon cancer. Biochem Pharmacol (2021) 186:114483. doi: 10.1016/j.bcp.2021.114483
76. Singh V, Yeoh BS, Walker RE, Xiao X, Saha P, Golonka RM, et al. Microbiota fermentation-NLRP3 axis shapes the impact of dietary fibres on intestinal inflammation. GUT (2019) 68:1801–12. doi: 10.1136/gutjnl-2018-316250
77. Huang J, Liu D, Wang Y, Liu L, Li J, Yuan J, et al. Ginseng polysaccharides alter the gut microbiota and kynurenine/tryptophan ratio, potentiating the antitumour effect of antiprogrammed cell death 1/programmed cell death ligand 1 (anti-PD-1/PD-L1) immunotherapy. GUT (2022) 71:734–45. doi: 10.1136/gutjnl-2020-321031
78. Xu Y, Xie L, Zhang Z, Zhang W, Tang J, He X, et al. Tremella fuciformis polysaccharides inhibited colonic inflammation in dextran sulfate sodium-treated mice via Foxp3+ T cells, gut microbiota, and bacterial metabolites. Front Immunol (2021) 12:648162. doi: 10.3389/fimmu.2021.648162
79. Li X, Khan I, Huang G, Lu Y, Wang L, Liu Y, et al. Kaempferol acts on bile acid signaling and gut microbiota to attenuate the tumor burden in ApcMin/+ mice. Eur J Pharmacol (2022) 918:174773. doi: 10.1016/j.ejphar.2022.174773
80. Hu T, Wu H, Yu Y, Xu Y, Li E, Liao S, et al. Preparation, structural characterization and prebiotic potential of mulberry leaf oligosaccharides. Food Funct (2022) 13:5287–98. doi: 10.1039/D1FO04048K
81. Kim J, Choi JH, Oh T, Ahn B, Unno T. Codium fragile ameliorates high-fat diet-induced metabolism by modulating the gut microbiota in mice. NUTRIENTS (2020) 12:1848. doi: 10.3390/nu12061848
82. Ferreira-Lazarte A, Fernández J, Gallego-Lobillo P, Villar CJ, Lombó F, Moreno FJ, et al. Behaviour of citrus pectin and modified citrus pectin in an azoxymethane/dextran sodium sulfate (AOM/DSS)-induced rat colorectal carcinogenesis model. Int J Biol MACROMOL (2021) 167:1349–60. doi: 10.1016/j.ijbiomac.2020.11.089
83. Beatrice L PAJS. Overview of experimental data on reduction of colorectal cancer risk by inulin-type fructans. J Nutr (2018) 137(11 Suppl):2580S–4S. doi: 10.1093/jn/137.11.2580S
84. Tian M, Li D, Ma C, Feng Y, Hu X, Chen F. Barley leaf insoluble dietary fiber alleviated dextran sulfate sodium-induced mice colitis by modulating gut microbiota. NUTRIENTS (2021) 13(3):846. doi: 10.3390/nu13030846
85. So WKW, Chan JYW, Law BMH, Choi KC, Ching JYL, Chan KL, et al. Effects of a rice bran dietary intervention on the composition of the intestinal microbiota of adults with a high risk of colorectal cancer: A pilot randomised-controlled trial. NUTRIENTS (2021) 13:526. doi: 10.3390/nu13020526
86. Murga-Garrido SM, Hong Q, Cross TL, Hutchison ER, Han J, Thomas SP, et al. Gut microbiome variation modulates the effects of dietary fiber on host metabolism. MICROBIOME (2021) 9(1):117. doi: 10.1186/s40168-021-01061-6
87. Zheng F, Zhang MY, Wu YX, Wang YZ, Li FT, Han MX, et al. Biotransformation of ginsenosides (Rb1, Rb2, Rb3, rc) in human intestinal bacteria and its effect on intestinal flora. Chem BIODIVERS (2021) 18(12):e2100296. doi: 10.1002/cbdv.202100296
88. Jin M, Kong L, Han Y, Zhang S. Gut microbiota enhances the chemosensitivity of hepatocellular carcinoma to 5-fluorouracil in vivo by increasing curcumin bioavailability. Phytother Res (2021) 35:5823–37. doi: 10.1002/ptr.7240
89. Márquez J, Mena J, Hernandez-Unzueta I, Benedicto A, Sanz E, Arteta B, et al. Ocoxin® oral solution slows down tumor growth in an experimental model of colorectal cancer metastasis to the liver in balb/c mice. Oncol Rep (2016) 35:1265–72. doi: 10.3892/or.2015.4486
90. Keranmu A, Pan L, Fu J, Han P, Yu H, Zhang Z, et al. Biotransformation of liquiritigenin into characteristic metabolites by the gut microbiota. MOLECULES (2022) 27:3057. doi: 10.3390/molecules27103057
91. Yin S, Sun C, Ji Y, Abdolmaleky H, Zhou J. Herbal medicine WangShiBaoChiWan improves gastrointestinal health in mice via modulation of intestinal tight junctions and gut microbiota and inhibition of inflammation. BioMed Pharmacother (2021) 138:111426. doi: 10.1016/j.biopha.2021.111426
92. Shao S, Jia R, Zhao L, Zhang Y, Guan Y, Wen H, et al. Xiao-Chai-Hu-Tang ameliorates tumor growth in cancer comorbid depressive symptoms via modulating gut microbiota-mediated TLR4/MyD88/NF-κB signaling pathway. PHYTOMEDICINE (2021) 88:153606. doi: 10.1016/j.phymed.2021.153606
93. Deng L, Shi Y, Liu P, Wu S, Lv Y, Xu H, et al. GeGen QinLian decoction alleviate influenza virus infectious pneumonia through intestinal flora. BioMed Pharmacother (2021) 141:111896. doi: 10.1016/j.biopha.2021.111896
94. Lin L, Luo L, Zhong M, Xie T, Liu Y, Li H, et al. Gut microbiota: a new angle for traditional herbal medicine research. RSC Adv (2019) 9:17457–72. doi: 10.1039/C9RA01838G
95. Wangchuk P, Navarro S, Shepherd C, Keller PA, Pyne SG, Loukas A. Diterpenoid alkaloids of aconitum laciniatum and mitigation of inflammation by 14-o-acetylneoline in a murine model of ulcerative colitis. Sci REP-UK (2015) 5:12845. doi: 10.1038/srep12845
96. Nicolas GR, Chang PV. Deciphering the chemical lexicon of host–gut microbiota interactions. Trends Pharmacol Sci (2019) 40:430–45. doi: 10.1016/j.tips.2019.04.006
97. Zhang SL, Mao YQ, Zhang ZY, Li ZM, Kong CY, Chen HL, et al. Pectin supplement significantly enhanced the anti-PD-1 efficacy in tumor-bearing mice humanized with gut microbiota from patients with colorectal cancer. THERANOSTICS (2021) 11:4155–70. doi: 10.7150/thno.54476
98. Han K, Nam J, Xu J, Sun X, Huang X, Animasahun O, et al. Generation of systemic antitumour immunity via the in situ modulation of the gut microbiome by an orally administered inulin gel. Nat BioMed Eng (2021) 5:1377–88. doi: 10.1038/s41551-021-00749-2
99. Yuan X, Xue J, Tan Y, Yang Q, Qin Z, Bao X, et alAlbuca Bracteate Polysaccharides Synergistically Enhance the Anti-Tumor Efficacy of 5-Fluorouracil Against Colorectal Cancer by Modulating β-Catenin Signaling and Intestinal Flora. Front Pharmacology (2021) 12:736627. 10.3389/fphar.2021.736627
100. Li X, Xin S, Zheng X, Lou L, Ye S, Li S, et al. Inhibition of the occurrence and development of inflammation-related colorectal cancer by fucoidan extracted from sargassum fusiforme. J Agric Food Chem (2022) 70:9463–76. doi: 10.1021/acs.jafc.2c02357
101. Li Q, Chen C, Liu C, Sun W, Liu X, Ci Y, et al. The effects of cellulose on AOM/DSS-treated C57BL/6 colorectal cancer mice by changing intestinal flora composition and inflammatory factors. Nutr Cancer (2021) 73:502–13. doi: 10.1080/01635581.2020.1756355
102. Li Y, Wang S, Sun Y, Xu W, Zheng H, Wang Y, et al. Apple polysaccharide protects ICR mice against colitis associated colorectal cancer through the regulation of microbial dysbiosis. Carbohydr Polym (2020) 230:115726. doi: 10.1016/j.carbpol.2019.115726
103. Bian S, Wan H, Liao X, Wang W. Inhibitory effects of apigenin on tumor carcinogenesis by altering the gut microbiota. Mediators Inflammation (2020) 2020:7141970. doi: 10.1155/2020/7141970
104. Wang T, Tseng W, Leu Y, Chen C, Lee W, Chi Y, et al. The flavonoid corylin exhibits lifespan extension properties in mouse. Nat Commun (2022) 13:1238. doi: 10.1038/s41467-022-28908-2
105. Yang R, Shan S, Zhang C, Shi J, Li H, Li Z. Inhibitory effects of bound polyphenol from foxtail millet bran on colitis-associated carcinogenesis by the restoration of gut microbiota in a mice model. J AGR Food Chem (2020) 68:3506–17. doi: 10.1021/acs.jafc.0c00370
106. Li S, Yang H, Li L, Wang W, Tan HY, Qu Y, et al. The involvement of gut microbiota in the anti-tumor effect of carnosic acid via IL-17 suppression in colorectal cancer. Chem Biol Interact (2022) 365:110080. doi: 10.1016/j.cbi.2022.110080
107. Qu L, Ma X, Fan D. Ginsenoside Rk3 suppresses hepatocellular carcinoma development through targeting the gut-liver axis. J AGR Food Chem (2021) 69:10121–37. doi: 10.1021/acs.jafc.1c03279
108. Gong Y, Dong R, Gao X, Li J, Jiang L, Zheng J, et al. Neohesperidin prevents colorectal tumorigenesis by altering the gut microbiota. Pharmacol Res (2019) 148:104460. doi: 10.1016/j.phrs.2019.104460
109. Wang L, Jiang G, Jing N, Liu X, Li Q, Liang W, et al. Bilberry anthocyanin extracts enhance anti-PD-L1 efficiency by modulating gut microbiota. Food Funct (2020) 11:3180–90. doi: 10.1039/D0FO00255K
110. Qi H, Liu Y, Qi X, Liang H, Chen H, Jiang P, et al. Dietary recombinant phycoerythrin modulates the gut microbiota of H22 tumor-bearing mice. Mar Drugs (2019) 17:665. doi: 10.3390/md17120665
111. Fu H, Liu X, Jin L, Lang J, Hu Z, Mao W, et al. Safflower yellow reduces DEN-induced hepatocellular carcinoma by enhancing liver immune infiltration through promotion of collagen degradation and modulation of gut microbiota. Food Funct (2021) 12:10632–43. doi: 10.1039/D1FO01321A
112. Zhang H, Hui D, Li Y, Xiong G, Fu X. Canmei formula reduces colitis-associated colorectal carcinogenesis in mice by modulating the composition of gut microbiota. Front Oncol (2019) 9:1149. doi: 10.3389/fonc.2019.01149
113. Yang H, Li S, Qu Y, Li L, Li Y, Wang D. Anti-colorectal cancer effects of inonotus hispidus (Bull.: Fr.) p. karst. spore powder through regulation of gut microbiota-mediated JAK/STAT signaling. NUTRIENTS (2022) 14(16):3299. doi: 10.3390/nu14163299
114. Zhang MM, Yin DK, Rui XL, Shao FP, Li JC, Xu L, et al. Protective effect of pai-Nong-San against AOM/DSS-induced CAC in mice through inhibiting the wnt signaling pathway. Chin J Nat Med (2021) 19:912–20. doi: 10.1016/S1875-5364(22)60143-2
115. Jiang F, Liu M, Wang H, Shi G, Chen B, Chen T, et al. Wu Mei wan attenuates CAC by regulating gut microbiota and the NF-kB/IL6-STAT3 signaling pathway. BioMed Pharmacother (2020) 125:109982. doi: 10.1016/j.biopha.2020.109982
116. Liu Y, Jiang Z, Yang H, Yuan J, Zeng J, Wu J, et al. Gui shao tea extracts inhibit gastric cancer growth in vitro and in vivo and prolong survival in nude mice. Front BIOSCI-LANDMRK (2022) 27:250. doi: 10.31083/j.fbl2708250
117. Shi HJ, Chen XY, Chen XR, Wu ZB, Li JY, Sun YQ, et al. Chinese Medicine formula siwu-yin inhibits esophageal precancerous lesions by improving intestinal flora and macrophage polarization. Front Pharmacol (2022) 13:812386. doi: 10.3389/fphar.2022.812386
118. Shi X, Zhu Z, Yue S, Tang Y, Chen Y, Pu Z, et al. Studies on blood enrichment and anti-tumor effects of combined danggui buxue decoction, fe and rhEPO based on colon cancer-related anemia model and gut microbiota modulation. Chin J Nat Medicines (2021) 19:422–31. doi: 10.1016/S1875-5364(21)60041-9
119. Annunziata G, Maisto M, Schisano C, Ciampaglia R, Narciso V, Hassan S, et al. Effect of grape pomace polyphenols with or without pectin on TMAO serum levels assessed by LC/MS-based assay: A preliminary clinical study on Overweight/Obese subjects. Front Pharmacol (2019) 10:575. doi: 10.3389/fphar.2019.00575
120. Gupta R, Bhatt LK, Johnston TP, Prabhavalkar KS. Colon cancer stem cells: Potential target for the treatment of colorectal cancer. Cancer Biol Ther (2019) 20:1068–82. doi: 10.1080/15384047.2019.1599660
121. Xue M, Ge Y, Zhang J, Liu Y, Wang Q, Hou L, et al. Fucoidan inhibited 4T1 mouse breast cancer cell growth in vivo and in vitro via downregulation of wnt/beta-catenin signaling. Nutr Cancer (2013) 65:460–8. doi: 10.1080/01635581.2013.757628
122. Ji X, Peng Q, Yuan Y, Shen J, Xie X, Wang M. Isolation, structures and bioactivities of the polysaccharides from jujube fruit (Ziziphus jujuba mill.): A review. Food Chem (2017) 227:349–57. doi: 10.1016/j.foodchem.2017.01.074
123. Kuo C, Weng B, Wu C, Yang S, Wu D, Wang Y. Apigenin has anti-atrophic gastritis and anti-gastric cancer progression effects in helicobacter pylori -infected Mongolian gerbils. J ETHNOPHARMACOL (2014) 151:1031–9. doi: 10.1016/j.jep.2013.11.040
124. Kaburagi T, Yamano T, Fukushima Y, Yoshino H, Mito N, Sato K. Effect of lactobacillus johnsonii La1 on immune function and serum albumin in aged and malnourished aged mice. NUTRITION (2007) 23:342–50. doi: 10.1016/j.nut.2007.02.001
125. Boyle M, Chun C, Strojny C, Narayanan R, Bartholomew A, Sundivakkam P, et al. Chronic inflammation and angiogenic signaling axis impairs differentiation of dental-pulp stem cells. PloS One (2014) 9:e113419. doi: 10.1371/journal.pone.0113419
126. Schreiber RD, Old LJ, Smyth MJ. Cancer immunoediting: integrating immunity's roles in cancer suppression and promotion. SCIENCE (2011) 331:1565–70. doi: 10.1126/science.1203486
127. Mohamed E, Sierra RA, Trillo-Tinoco J, Cao Y, Innamarato P, Payne KK, et al. The unfolded protein response mediator PERK governs myeloid cell-driven immunosuppression in tumors through inhibition of STING signaling. IMMUNITY (2020) 52:668–82. doi: 10.1016/j.immuni.2020.03.004
128. Hokey DA, Larregina AT, Erdos G, Watkins SC, Falo LJ. Tumor cell loaded type-1 polarized dendritic cells induce Th1-mediated tumor immunity. Cancer Res (2005) 65:10059–67. doi: 10.1158/0008-5472.CAN-05-1692
129. Chen ME, Su CH, Yang JS, Lu CC, Hou YC, Wu JB, et al. Baicalin, baicalein, and lactobacillus rhamnosus JB3 alleviated helicobacter pylori infections in vitro and in vivo. J Food Sci (2018) 83:3118–25. doi: 10.1111/1750-3841.14372
130. Khan I, Huang G, Li X, Liao W, Leong WK, Xia W, et al. Mushroom polysaccharides and jiaogulan saponins exert cancer preventive effects by shaping the gut microbiota and microenvironment in apc mice. Pharmacol Res (2019) 148:104448. doi: 10.1016/j.phrs.2019.104448
131. Singh V, Yeoh BS, Chassaing B, Xiao X, Saha P, Aguilera Olvera R, et al. Dysregulated microbial fermentation of soluble fiber induces cholestatic liver cancer. CELL (2018) 175:679–94. doi: 10.1016/j.cell.2018.09.004
132. Lee I, Hyun Y, Kim D. Berberine ameliorates TNBS-induced colitis by inhibiting lipid peroxidation, enterobacterial growth and NF-κB activation. Eur J Pharmacol (2010) 648:162–70. doi: 10.1016/j.ejphar.2010.08.046
133. Kwon H, Oh S, Kim J. Glabridin, a functional compound of liquorice, attenuates colonic inflammation in mice with dextran sulphate sodium-induced colitis. Clin Exp Immunol (2008) 151:165–73. doi: 10.1111/j.1365-2249.2007.03539.x
134. Junior AGDF, Serafim PVPP, de Melo AAAA, Felipe AVAV, Turco EGLE, Da Silva IDCG, et al. Analysis of the lipid profile in patients with colorectal cancer in advanced stages. Asian Pacific J Cancer prevention: APJCP (2018) 19:1287–93. doi: 10.22034/APJCP.2018.19.5.1287
135. Wu X, Cao J, Li M, Yao P, Li H, Xu W, et al. An integrated microbiome and metabolomic analysis identifies immunoenhancing features of ganoderma lucidum spores oil in mice. Pharmacol Res (2020) 158:104937. doi: 10.1016/j.phrs.2020.104937
136. Zhang Z, Liu L, Tang H, Jiao W, Zeng S, Xu Y, et al. Immunosuppressive effect of the gut microbiome altered by high-dose tacrolimus in mice. Am J Transplant (2018) 18:1646–56. doi: 10.1111/ajt.14661
137. Guo M, Ding S, Zhao C, Gu X, He X, Huang K, et al. Red ginseng and semen coicis can improve the structure of gut microbiota and relieve the symptoms of ulcerative colitis. J ETHNOPHARMACOL (2015) 162:7–13. doi: 10.1016/j.jep.2014.12.029
138. Zakharzhevskaya NB, Vanyushkina AA, Altukhov IA, Shavarda AL, Butenko IO, Rakitina DV, et al. Outer membrane vesicles secreted by pathogenic and nonpathogenic bacteroides fragilis represent different metabolic activities. Sci REP-UK (2017) 7(1):5008. doi: 10.1038/s41598-017-05264-6
139. Cervantes-Barragan L, Chai JN, Tianero MD, Di Luccia B, Ahern PP, Merriman J, et al. Lactobacillus reuteri induces gut intraepithelial CD4 + CD8αα + T cells. SCIENCE (2017) 357:806–10. doi: 10.1126/science.aah5825
Glossary
Keywords: digestive cancer, immunotherapy, traditional Chinese medicine, dietary compounds, microbiota, inflammatory factor, SCFAs
Citation: Feng X, Li Z, Guo W and Hu Y (2023) The effects of traditional Chinese medicine and dietary compounds on digestive cancer immunotherapy and gut microbiota modulation: A review. Front. Immunol. 14:1087755. doi: 10.3389/fimmu.2023.1087755
Received: 02 November 2022; Accepted: 17 January 2023;
Published: 06 February 2023.
Edited by:
Chuanzhao Zhang, Guangdong Provincial People’s Hospital, ChinaReviewed by:
H. Atakan Ekiz, Izmir Institute of Technology, TürkiyeYuqin Chen, First Affiliated Hospital of Guangzhou Medical University, China
Copyright © 2023 Feng, Li, Guo and Hu. This is an open-access article distributed under the terms of the Creative Commons Attribution License (CC BY). The use, distribution or reproduction in other forums is permitted, provided the original author(s) and the copyright owner(s) are credited and that the original publication in this journal is cited, in accordance with accepted academic practice. No use, distribution or reproduction is permitted which does not comply with these terms.
*Correspondence: Weihong Guo, 307014735@qq.com; Yanfeng Hu, yfenghu@qq.com
†These authors have contributed equally to this work