- 1Department of Radiation Oncology, Affiliated Cancer Hospital and Institute of Guangzhou Medical University, Guangzhou, China
- 2State Key Laboratory of Respiratory Diseases, Guangzhou Institute of Respiratory Disease, Guangzhou Medical University, Guangzhou, China
Background: Inhibitors of programmed cell death 1 (PD-1)/programmed cell death ligand 1(PD-L1) checkpoint have been approved for metastatic triple negative breast cancer (mTNBC) in patients positive for PD-L1 expression. Negative results from the recent phase III trials (IMPassion131 and IMPassion132) have raises questions on the efficacy of PD-1/PD-L1 checkpoint inhibitors and the predictive value of PD-L1 expression. Here we attempt to systematically analyze the biomarker value of PD-L1 expression for predicting the response of PD-1/PD-L1 checkpoint inhibitors in mTNBC.
Materials and methods: PubMed database was searched until Dec 2021 for studies evaluating PD-1/PD-L1 checkpoint inhibitors plus/minus chemotherapy in mTNBC. Outcome of interest included objective response rate (ORR), progression-free survival (PFS), and overall survival (OS). Review Manager (RevMan) version 5.4. was used for data-analysis.
Results: In total, 20 clinical trials comprising 3962 mTNBC patients (ICT: 2665 (67%); CT: 1297 (33%) were included in this study. Overall ORR was 22% (95%CI, 14-30%) and significant improvement was observed for PD-L1+ patients (ORR 1.78 [95%CI, 1.45-2.19], p<0.00001) as compared to PD-L1- cohort. Pooled outcome also indicated a significant 1-year PFS and 2-year OS advantage for patients with PD-L1 expression (1-year PFS: ORR 1.39 [95%CI, 1.04-1.85], p=0.02; I2 = 0%; 2-year OS: (ORR 2.47 [95%CI, 1.30-4.69], p=0.006; I2 = 63%). Subgroup analysis indicated that PD-L1 expression can successfully predict tumor response and 2-year OS benefit in mTNBC patients regardless of the type of investigating agent, line of treatment administration, and to some extent the type of treatment. Biomarker ability of PD-L1 expression to predict 1-year PFS was slightly better with pembrolizumab (p=0.09) than atezolizumab (p=0.18), and significantly better when treatment was administered in the first-line setting (OR 1.38 [95%CI, 1.02-1.87], p=0.04) and chemotherapy was added (OR 1.38 [95%CI, 1.02-1.86], p=0.03). Immune-related toxicity of any grade and grade≥3 was 39% (95%CI, 26%-52%) and 10% (95%CI, 8%-13%), respectively.
Conclusions: PD-L1 expression can predict objective response rate and 2-year OS in mTNBC patients receiving PD-1/PD-L1 checkpoint inhibitors. One-year PFS is also predicted in selected patients. PD-L1 expression can be a useful biomarker of efficacy of PD-1/PD-L1 checkpoint inhibitors in mTNBC.
Introduction
Triple negative breast cancer (TNBC), which constitutes about 15 to 20% of the breast cancer cases, is characterized by the lack of expression for hormone receptors (estrogen receptor [ER-] and progesterone receptor [PR-]) and human epidermal growth factor receptor 2 (HER2) (1–3). Multi-omics studies have further identified that majority of TNBC (about 55%-81%) express highly proliferative basal-like genes which can describe its aggressive nature (3). Prognosis is poor and 3-year recurrence is high despite a greater response to chemotherapy as compared to other breast cancer subgroups (3–5). Metastasis is common and is the major cause of death (2). 5-year survival rate is less than 30% for metastatic TNBC (2).
Immunotherapy in the form of immune checkpoint inhibition (ICI) was considered as an alternative and complementary cancer treatment option for TNBC due to its high genomic instability, infiltration of tumor-infiltrating lymphocytes (TILs) and elevated expression of programmed cell death protein ligand 1 (PD-L1) (6–8). Programmed cell death protein expressed on the T cells engages its ligand PD-L1 expressed on tumor cells thereby mediating tumor immune escape via suppression of antigen-specific T cell immune responses (8). Interruption of this PD-1/PD-L1 interaction with either anti-PD-1 monoclonal antibody (mAb) or anti-PD-L1 mAb results in activation of anti-tumor immune response (8) (Figure 1A). Additionally, PD-L1 is also expressed on other tumor-infiltrating immune cells mainly the antigen presenting cells (APCs) such as dendritic cells (DCs) and macrophages among others (9). Studies have identified the indispensable role of PD-L1 expression on such immune cells for the therapeutic efficacy of PD-1/PD-L1 blockade therapy (9, 10). As such, PD-L1 expression on tumor cells as well as host immune cells is evaluated for their biomarker efficacy.
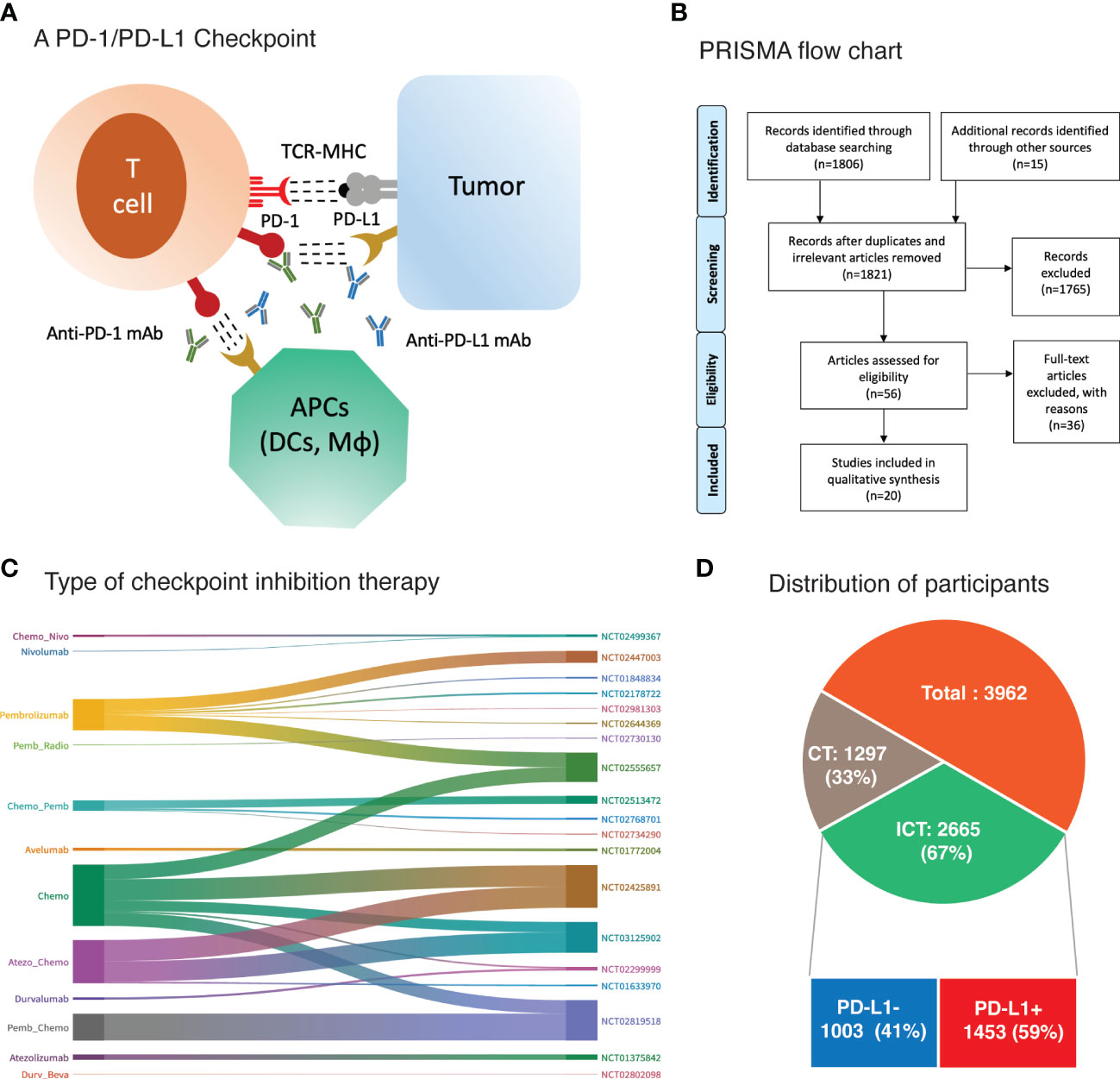
Figure 1 (A) Binding of checkpoint proteins, such as PD-1 on T cells and PD-L1 on tumor cells and antigen presenting cells (APCs), keeps T cells from killing tumor cells in the body. Blocking this interaction of PD-1 and PD-L1 with an immune checkpoint inhibitor (anti-PD-L1 or anti-PD-1) allows the T cells to kill tumor cells. DCs, dendritic cells; Mϕ, macrophages (B) PRISMA flow diagram of research strategy and study selection. (C) Sankey diagram depicting treatment regimen and corresponding trial registration number. (D) Main cohorts and number of participants.
Durable responses were observed in the earlier TNBC trials for anti-PD-1 (pembrolizumab) and anti-PD-L1 (atezolizumab) monoclonal antibodies alone or in combination with chemotherapy (11–15). Results of subsequent phase III trials (IMPassion130 and KEYNOTE-355) had led to approval of these agents in combination with chemotherapy for advanced metastatic TNBC patients (16, 17). The approval was based on the progression free survival benefit observed for TNBC patients receiving the combined treatment particularly in patients positive for PD-L1 expression. However, long term benefit is still unclear and OS results for KEYNOTE-355 is yet to come (17). Moreover, IMPassion131 phase III trial of atezolizumab showed no PFS advantage with combined treatment for PD-L1+ patients (18). These outcomes not only question the long-term benefit of PD-1 inhibitors but also cast doubt on the biomarker value of PD-L1 expression which had formed the basis for FDA approval.
In this study, we have gathered the efficacy data from various TNBC immunotherapy trials to evaluate the status of immunotherapy in advanced TNBC with a focus on PD-L1 expression as biomarker.
Methods and materials
This systematic-review and meta-analysis was carried out following the updated version 2020 of PRISMA (Preferred Reporting Items for Systematic Reviews and Meta-Analyses) guidelines (19).
Eligibility criteria
The PICOT (Population, Index prognostic factors, Comparator prognostic factors, Outcomes, Timings, and Settings) system was utilized for identifying the key elements of this review.
◼ Target population and treatment: Advanced metastatic triple negative breast cancer (TNBC) patients receiving immunotherapy, mainly PD-1/PD-L1 checkpoint inhibitors, with or without chemotherapy.
◼ Index Prognostic factors: PD-L1 expression was the only main index prognostic factor.
◼ Comparator prognostic factors: not applicable for this review.
◼ Outcome of interest: Main efficacy outcomes of interest included objective response rate (ORR), progression-free survival (PFS) and overall survival (OS). Definition of ORR and PFS were based on Response Evaluation Criteria in Solid Tumors (RECIST) version 1.1. Frequencies of adverse events graded according to the National Cancer Institute Common Terminology Criteria for Adverse Events (CTCAE), version 4.0. was also evaluated between the treatment cohorts.
◼ Timing: PD-L1 expression was assessed before immunotherapy
◼ Setting: Cancer hospitals and treatment centers.
Study design and language restrictions
Single arm studies that provided treatment comparison based on PD-L1 status and comparative clinical trials (CTs) that compared PD-1/PD-L1 checkpoint inhibitors +/- chemotherapy to chemotherapy alone were pursued with English language restrictions.
Research strategy and study selection
PubMed was formally searched with several key terms until Dec, 2021. Further potential studies were identified through screening references of relevant articles. A step-wise procedure comprising retrieving, organizing, and screening was followed by two reviewers to select studies meeting the eligibility criteria. Disagreements were solved after consulting the third author.
Data extraction
Modified form “The Cochrane Collaboration Data Collection form for RCTs” was used for data extraction that is available from Cochrane website. Characteristics of the included studies and attributes of participants were extracted that included first author, publication year, trail designation, national clinical trial (NCT) registration number, trial design, number and type of participants, and treatment type. Participants’ attributes included age, PD-L1 expression status, PD-L1 assessment assay, and the lines of previous therapy for metastatic disease. Relevant outcome data was also extracted for performing meta-analysis.
Quality assessment
The Cochrane Collaboration Tool were used to assess the quality of included randomized controlled trials (20). Assessments included sequence generation, allocation of sequence concealment, blinding of participants and personnel, blinding of outcomes and assessments, incomplete outcome data, selective outcome reporting, and other biases. Non-randomized clinical trials were assessed with the methodological index for non-randomized studies (MINORS), which contains eight items of assessment and is recognized as the most appropriate guideline to evaluate the methodological quality of non-randomized trials (21). As a meta-analysis of biomarker assessment, the risk of bias was also assessed by “The Quality In Prognosis Studies (QUIPS) tool” for studies reporting biomarker analysis (22, 23). The QUIPS tool used six important domains (1): study participation (2), study attrition (3), prognostic factor measurement (4), outcome measurement (5) study confounding, and (6) statistical analysis and reporting. Quality of evidence assessment was carried out with The Grading of Recommendations Assessment, Development and Evaluation (GRADE) (24). Major outcomes were graded as high, moderate, low, or very low depending upon the following elements: study design, risk of bias, inconsistencies, imprecision of the results, indirectness, and publication bias.
Measurement of treatment effect and data synthesis
Number of events for objective response rates (ORR: Complete Responses + Partial Responses) and adverse events were pooled using Mantel-Haenszel method (19, 25). Pooling of number of events for single-arm studies was carried out with the help of a step-by-step guide for meta-analysis of descriptive data analysis developed by Neyeloff, et al. (26). Hazard ratios for PFS and OS were recorded directly from the study or extracted from the K-M curves using the Digital Equalizer and methods for incorporating summary time-to-event data into the meta-analysis according to Tierney et al. (27, 28). Pooling of HRs was done by applying inverse variance method (19, 25). Heterogeneity was assessed using Chi2 test and I2 value and graded as low (I2 = 25%), moderate (I2 = 50%), and high (I2 = 75%) according to I2 values (29). Random effects model was adopted when heterogeneity exceeded 50% (I2 ≥50%). Significance level was set at p<0.05.
Results
The research strategy and study selection are illustrated in Figure 1B. A total of 1806 published studies were identified upon initial database search. Title and abstract screening excluded 1765 studies for various reasons including duplicates, irrelevant, lacking data of main outcomes, and not target agent/s, etcetera. Further scrutiny for eligibility and full text reading yielded 20 studies that were included in this meta-analysis (11–18, 30–41). General characteristics of the included studies and participants are presented in Table 1. The detailed treatment regimen is highlighted in Figure 1C, along with the national clinical trial number for each study. Overall, 3962 patients with mTNBC were available for analysis as depicted in Figure 1D. A total of 2665 (67%) participants had received PD-1 (nivolumab and pembrolizumab) or PD-L1 inhibitor (avelumab, atezolizumab, and durvalumab) as monotherapy or in combination with chemotherapy. This cohort was described as ICT cohort. While the remaining (33%) were administered with chemotherapy alone (CT cohort). Patients with PD-L1+ expression were 1453 (59%) and 1003 (41%) patients were negative for PD-L1 expression in the ICT cohort. Pembrolizumab was investigated in 12 studies; atezolizumab in 4; durvalumab in 2; and avelumab in only one study (Figure 1C and Table 1). Pembrolizumab was also administered with induction chemotherapy in few trials (31, 32) and was followed by radiotherapy in a single trial (33). Likewise, three studies had evaluated immune modulating agents along with pembrolizumab (34–36). However, these studies had only reported response rates in mTNBC and were not incorporated into the biomarker analysis due to lack of relevant data (33–36). PD-L1 expression was assessed with PD-L1 IHC 22C3 pharmDx assay in studies involving pembrolizumab measuring the PD-L1 expression on tumor cells as well as immune cells which was termed as combined positive score (CPS) (11, 12, 17, 30, 41). Atezolizumab studies (Phase III RCTs) utilized VENTANA PD-L1 (SP142) assay assessing the PD-L1 expression on immune cells (IC) alone as the phase I trial had revealed a significant improvement based on the PD-L1 expression on immune cells and not tumor cells (TC) (15, 16, 18). Durvalumab also showed better predictive value of PD-L1 expression on immune cells in a phase II trial after an initial pilot study demonstrated no significant predictive value of PD-L1 expression assessed on tumor cells (38, 39). Details are highlighted in Table 1.
Quality assessment of the studies and evidence
Quality assessment was carried out using The Cochrane Collaboration Tool. Only three RCTs were double-blinded exhibiting a low risk of bias (16–18). Detail assessment is highlighted in Supplementary Table 1. Quality assessment of the included studies based on the MINORS and QUIPS tools are detailed in Supplementary Tables 2, 3. MINORS demonstrated that about 80% of the studies (11–18, 30, 32, 33, 36–41) had low risk of bias (score 10-15) and 20% exhibited a moderate risk of bias (score <10) (31, 34, 35). QUIPS tool, which assess the risk of bias in the studies involving assessment of prognostic factors, showed 66% of the studies had low risk of bias. Nonetheless, 33% of the studies demonstrated high risk of bias in terms of participants (30), study attrition (30), prognostic factor (15, 30), and confounding (40, 41). Quality of evidence assessment based on the GRADE quality tool is presented in Supplementary Table 4, which indicated that main three outcomes carried low risk of bias and were considered of critical importance with moderate to low certainty.
Objective response rate
Objective response rates for the entire mTNBC cohort were available from 19 studies involving 2617 patients. Pooled analysis revealed an ORR of 22% (95%CI, 14-30%) (Supplementary Figure 1). When compared to CT alone, the ORR was higher in ICT cohort (OR 1.32 [95%CI, 1.13-1.55], p=0.0007) (Supplementary Figure 2A).
Overall, nine studies (n=2277) reported ORR for PD-L1 expression difference. A direct comparison of PD-L1+ cohort (n=1330) to PD-L1- cohort (n=947) showed significantly improved ORR in patients with PD-L1 expression (OR 1.78 [95%CI, 1.45-2.19], p<0.00001) (Figure 2A). There was no heterogeneity (Chi² = 8.67, df = 9 (P = 0.47); I² = 0%) and publication bias observed for this outcome as shown in Figure 2C. The ORR was even higher in patients with ≥10% PD-L1 expression (OR 2.30 [95%CI, 1.38-3.81], p=0.001). Moreover, there was evidence of an increasing probability of objective response with increasing PD-L1 expression in the KEYNOTE-012 trial (p=0.028) (14). Four studies (n=1701) have reported ORR for treatment difference (ICT versus CT) in PD-L1+ (n=3019) and PD-L1- cohorts (n=1318). Pooled analysis showed significantly improved ORR for PD-L1+ positive patients in the ICT cohort (ORR 1.55 [95%CI, 1.25-1.92], p<0.0001) without any heterogeneity (I² = 0%) (Figure 2B). However, PD-L1- TNBC cohort showed no significant change in ORR for the treatment difference (ORR 0.95 [95%CI, 0.59-1.52], p=0.82). Difference between these two subgroups was close to significance (Chi² = 3.41, df = 1 (P = 0.06), I² = 70.7%).
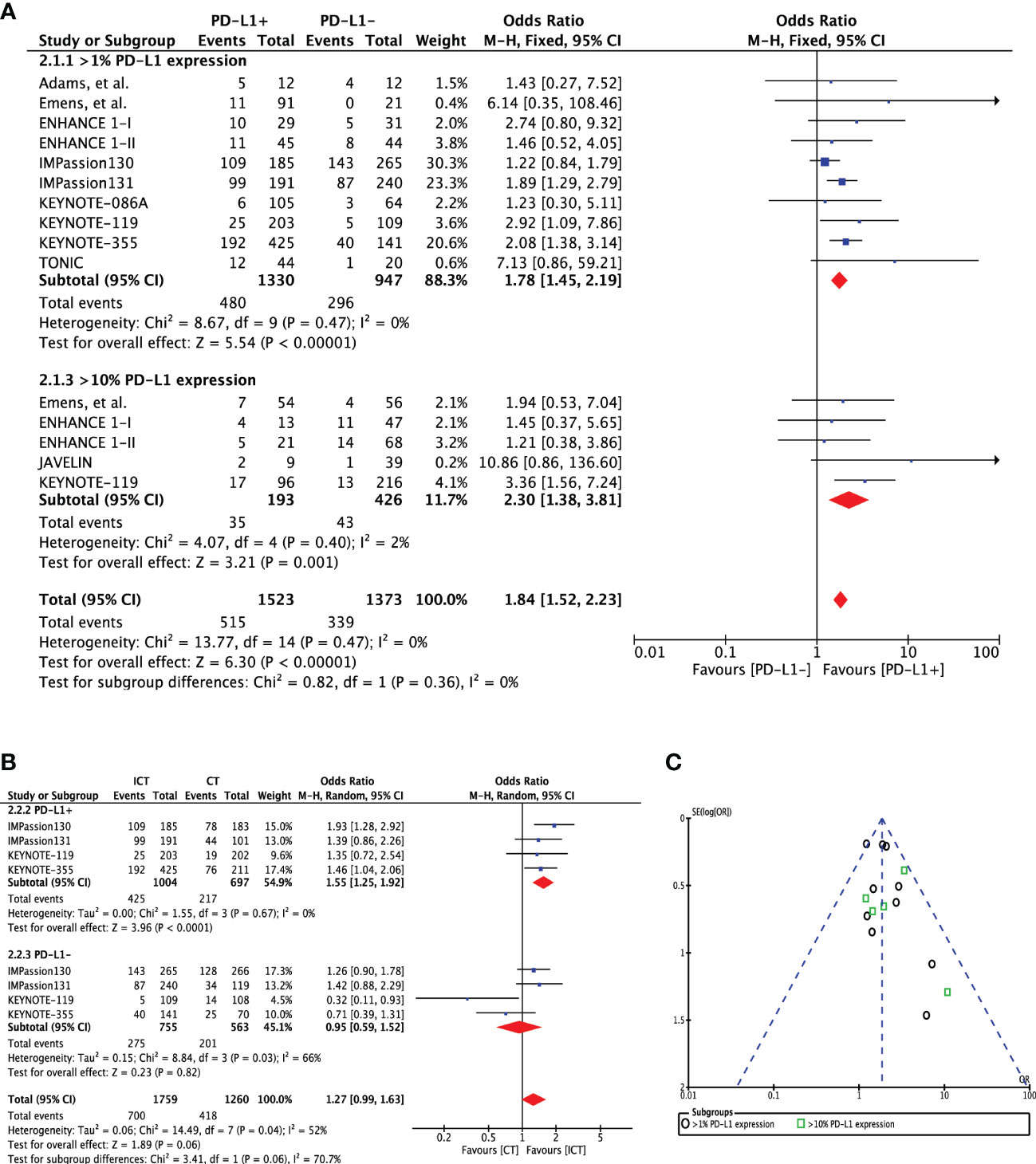
Figure 2 (A) Comparison of objective response rate (ORR) in advanced metastatic TNBC (mTNBC) patients treated with PD-1 checkpoint inhibition therapy based on PD-L1 expression. (B) Comparison of objective response rate (ORR) in advanced metastatic TNBC (mTNBC) patients (PD-L1+ & PD-L1-) between patients receiving PD-1 checkpoint inhibitors plus chemotherapy (ICT) and CT alone. (C) Funnel plot of publication bias assessment in objective response rate (ORR) analysis.
Progression-free survival
Progression-free survival was assessed using 1-year and overall PFS outcomes. One-year PFS event rates for PD-L1 expression cohorts were available in eight studies (n=1967). Pooled outcome indicated a significant 1-year PFS advantage for patients with PD-L1 expression (OR 1.39 [95%CI, 1.04-1.85], p=0.02; I2 = 0%) (Figure 3A). There was no heterogeneity (Chi² = 4.85, df = 8 (P = 0.77); I² = 0%) and publication bias observed for this outcome as shown in Figure 3D. A direct comparison of overall PFS in these cohorts was available in 5 studies. Extracted hazard ratios were pooled which showed no difference between the cohorts (HR 0.88 [95%CI, 0.72-1.09], p=0.24; I2 = 0%) (Figure 3B). In KEYNOTE-012 trial, significant reduction in the hazard to progression or death was shown with increasing PD-L1 expression (p=0.012) (14).
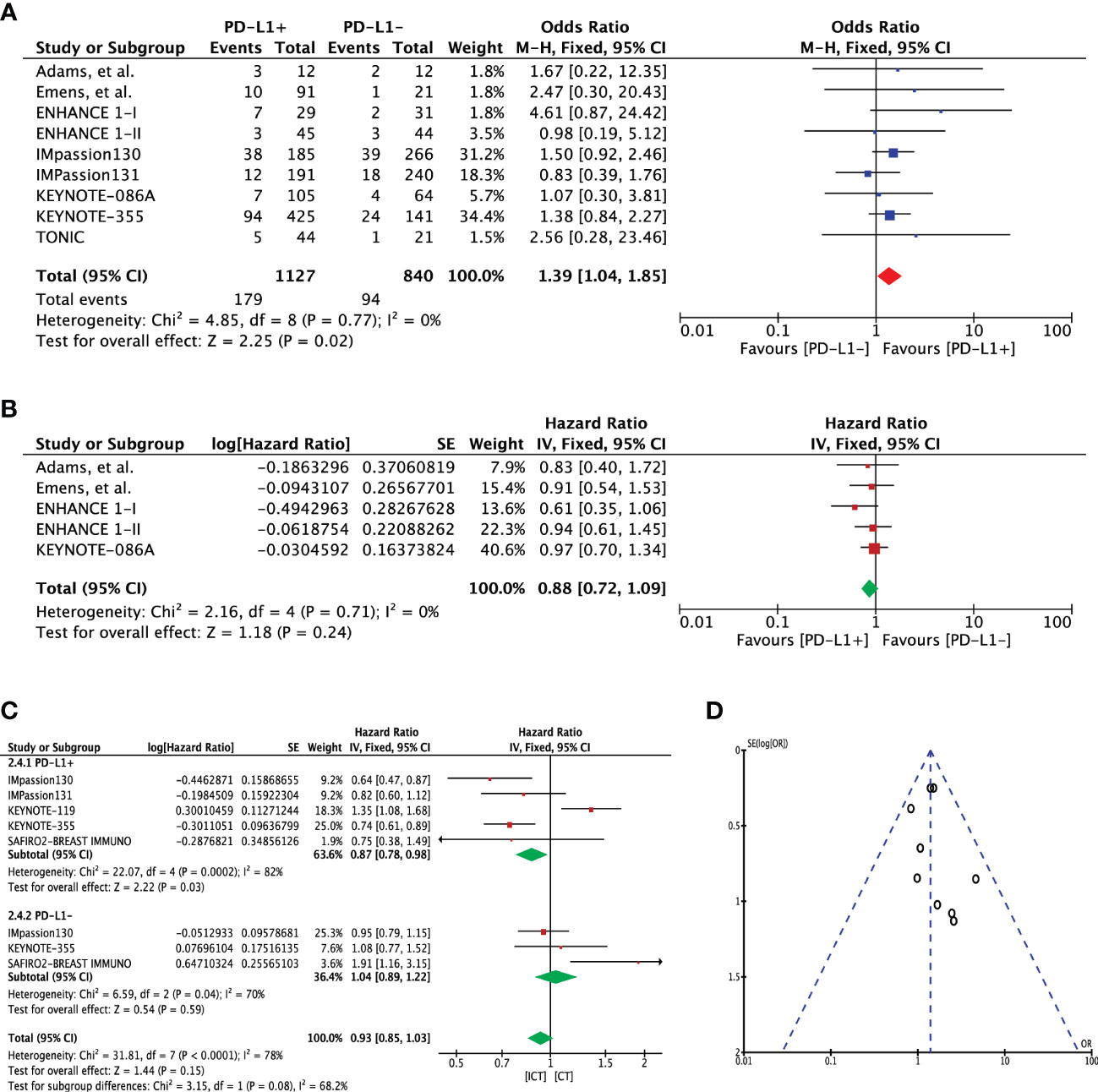
Figure 3 (A) Comparison of 1-year progression-free survival (PFS) in advanced metastatic TNBC (mTNBC) patients treated with PD-1 checkpoint inhibition therapy based on PD-L1 expression. (B) Comparison of progression-free survival (PFS) in advanced metastatic TNBC (mTNBC) patients treated with PD-1 checkpoint inhibition therapy based on PD-L1 expression. (C) Comparison of progression-free survival (PFS) in PD-L1+ and PD-L1- metastatic TNBC (mTNBC) patients between patients receiving PD-1 checkpoint inhibitors plus chemotherapy (ICT) and CT alone. (D) Funnel plot of publication bias assessment in 1-year progression-free survival (PFS) analysis.
Five studies (n=3104) reported PFS for treatment difference (ICT=1807 versus CT=1297). Pooled analysis showed significantly improved PFS for PD-L1+ positive patients in the ICT (PFS 0.87 [95%CI, 0.78-0.98], p=0.03); however, heterogeneity was high (I² = 82%) (Figure 3C). Application of random effects model resulted in loss of significance difference (HR 0.84 [95%CI, 0.62-1.15], p=0.28) (Supplementary Figure 3A). On the other hand, PD-L1- TNBC cohort showed no significant change in PFS for the treatment difference (HR 1.04 [95%CI, 0.89-1.22], p=0.59). Difference between these two subgroups was close to significance (Chi² = 3.15, df = 1 (P = 0.08), I² = 68.2%) (Figure 3C). In an attempt to address the heterogeneity, sensitivity analysis was carried out with excluding the KEYNOTE-119 trial which included patients whom had received more than two lines of treatments for metastatic disease. Upon exclusion, pooled analysis showed significant improvement in PFS with no heterogeneity (HR 0.73 [95%CI, 0.64-0.84], p<0.0001, I² = 0%), which also resulted in significant subgroup difference (Chi² = 10.82, df = 1 (P = 0.001), I² = 90.8%) (Supplementary Figure 3B). A similar outcome was demonstrated when the entire mTNBC cohort (regardless of PD-L1 expression status) was considered for the treatment difference (Supplementary Figures 2B, C).
Overall survival
Overall survival was assessed using 2-year and overall survival outcomes. Two-year OS event rates for PD-L1 expression cohorts were available in eight studies (n=1683). Pooled outcome indicated a significant 2-year OS advantage for patients with PD-L1 expression (OR 2.47 [95%CI, 1.30-4.69], p=0.006; I2 = 63%) (Figure 4A). Heterogeneity was high; hence, random effects model was adopted. No publication bias was observed for this outcome as shown in Figure 4D. A direct comparison of overall survival for these two cohorts was available in 5 studies. Extracted hazard ratios were pooled which also indicated a significant difference between the cohorts (HR 0.75 [95%CI, 0.61-0.94], p=0.01; I2 = 0%) (Figure 4B). Four studies (n=2257) reported OS for treatment difference (ICT=1241 versus CT=1061). Pooled analysis showed significantly improved OS for PD-L1+ positive patients in the ICT (HR 0.83 [95%CI, 0.71-0.6], p=0.02); however, heterogeneity was high (I² = 82%) (Figure 4C). On the other hand, PD-L1- TNBC cohort showed no significant change in OS for the treatment difference (HR 0.99 [95%CI, 0.81-1.22], p=0.95). Difference between these two subgroups showed no significance (Chi² = 1.96, df = 1 (P = 0.16), I² = 48.9%). Comparison of overall survival when the entire mTNBC cohort (regardless of PD-L1 expression status) was considered showed no survival advantage for ICT (Supplementary Figure 2D).
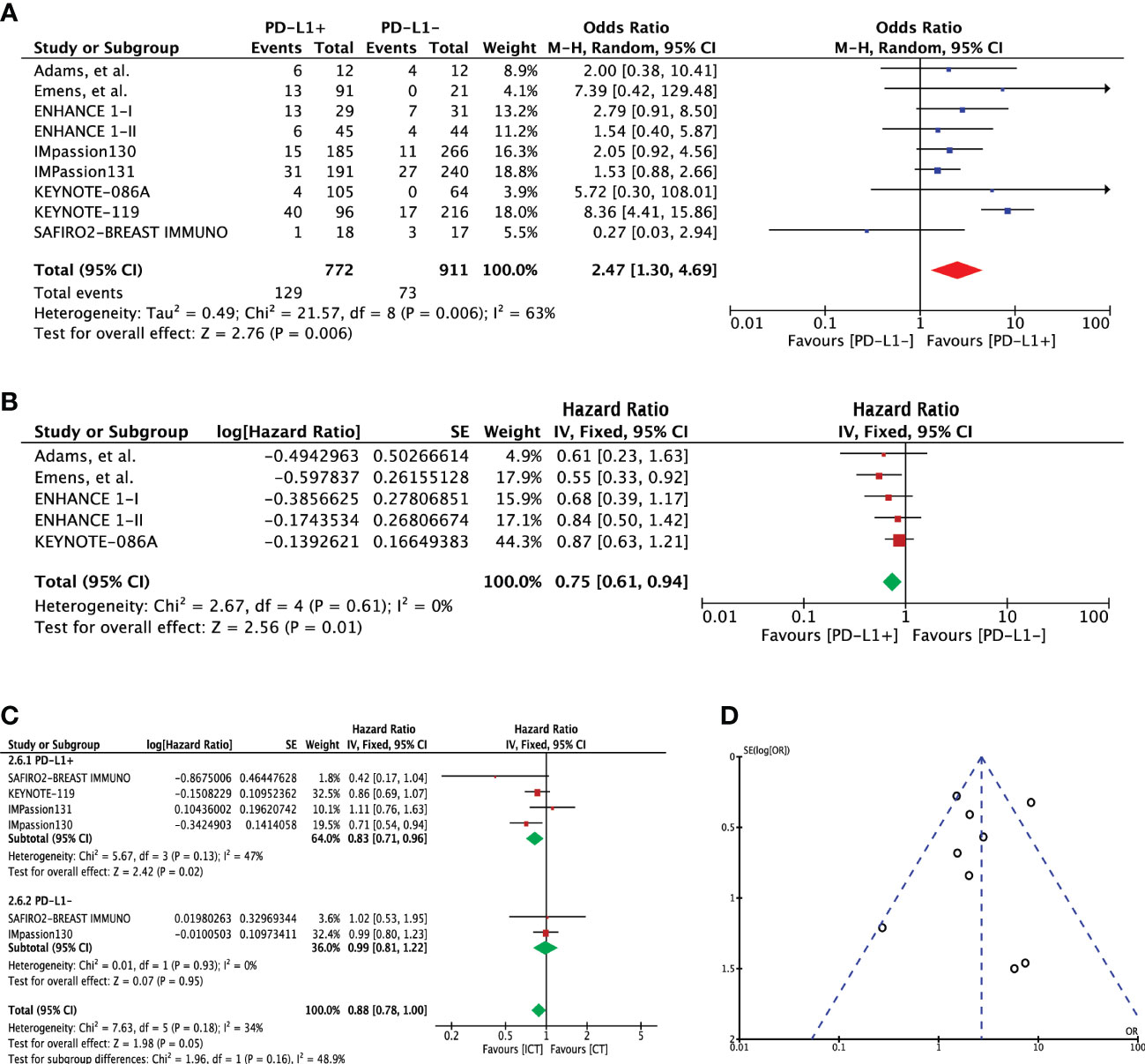
Figure 4 (A) Comparison of 2-year overall survival (OS) in advanced metastatic TNBC (mTNBC) patients treated with PD-1 checkpoint inhibition therapy based on PD-L1 expression. (B) Comparison of overall survival (OS) in advanced metastatic TNBC (mTNBC) patients treated with PD-1 checkpoint inhibition therapy based on PD-L1 expression. (C) Comparison of overall survival (OS) in PD-L1+ and PD-L1- metastatic TNBC (mTNBC) patients between patients receiving PD-1 checkpoint inhibitors plus chemotherapy (ICT) and CT alone. (D) Funnel plot of publication bias assessment in 2-year overall survival (OS) analysis.
Subgroup analysis
As the included studies were heterogenous according to the type of therapeutic agents, line of treatment and the addition of chemotherapy, we further carried out subgroup analysis to investigate the predictive significance of PD-L1 expression (Table 2). First of all, we considered the studies that either included pembrolizumab or atezolizumab as the investigating agent. The response was slightly higher in pembrolizumab cohort (OR 2.08 [95%CI, 1.49-2.90], p<0.0001) than atezolizumab (OR 1.55 [95%CI, 1.19-2.02], p=0.001) with no significant difference between the two agents (subgroup differences: Chi² = 1.86, df = 1 (P = 0.17), I² = 46.3%) (Figure 5A). Pembrolizumab showed to improve the 1-year PFS (OR 1.44 [95%CI, 0.94-2.21], p=0.09) as compared to atezolizumab (p=0.18) but still no significant differences were observed for subgroups (p=0.74) (Supplementary Figure 4A). While 2-year OS was significantly increased with PD-L1 expression in both subgroups (Pembrolizumab: OR 3.97 [95%CI, 1.61-9.80], p=0.003; Atezolizumab: OR 1.76 [95%CI, 1.14-2.72], p=0.01; subgroup difference: p=0.11) (Supplementary Figure 5A). This comparison also the rules out the impact of different PD-L1 expression assays (SP142 & 222C3) and scoring algorithms (CPS vs IC) on the overall significance of PD-L1 expression.
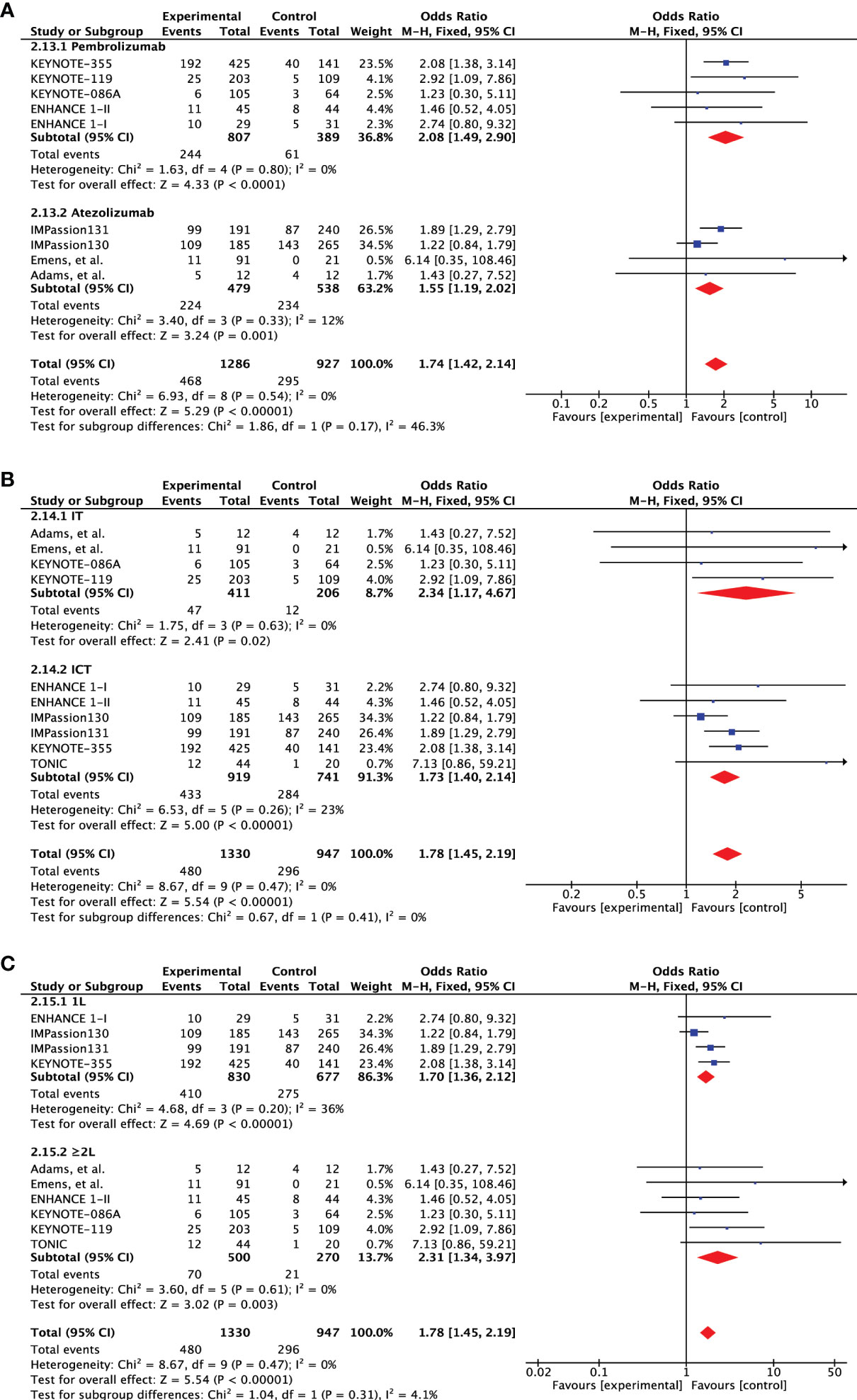
Figure 5 Subgroup analysis based on objective response rate (ORR). (A) Forest plot of comparison of objective response rate (ORR) between PD-L1+ and PD-L1- metastatic triple-negative breast cancer treated with pembrolizumab and atezolizumab, (B) PD-1 checkpoint inhibitors alone (IT) and PD-1 checkpoint inhibitors plus chemotherapy (ICT), and (C) treatment administered in first- and second-line setting.
Second, we also evaluated the effect of chemotherapy addition to PD-1 inhibitors by considering studies evaluating single agent (IT) and combined therapy (ICT). The response was almost double for both treatment strategies (IT: OR 2.34 [95%CI, 1.17-4.67], p=0.02; ICT: OR 1.73 [95%CI, 1.40-2.14], p<0.00001) with no statistical difference (subgroup differences: Chi² = 0.67, df = 1 (P = 0.41), I² = 0%) (Figure 5B). IT failed to improve 1-year PFS and was significantly enhanced by ICT (OR 1.38 [95%CI, 1.02-1.86], p=0.03) but still no significant differences were identified (p=0.92) (Supplementary Figure 4B). A similar outlook was demonstrated for 2-year OS but the difference in IT cohort was close to significance (p=0.07) (Supplementary Figure 5B).
Lastly, we also evaluated if the significance of PD-L1 expression is maintained when therapy is administered in the second line setting? Predictive ability of PD-L1 expression was slightly better in first line setting (OR 1.70 [95%CI, 1.36-2.12], p<0.00001) as compared to the second line administration (OR 2.31 [95%CI, 1.34-3.97], p=0.003) (Figure 5C). However, the effect showed no statistical difference. On the other hand, improvement in 1-year PFS was only seen in the first-line setting (OR 1.38 [95%CI, 1.02-1.87], p=0.04) (Supplementary Figure 4C). Although both subgroups showed significant 2-year OS advantage, the subgroups difference tended towards significance (p=0.08) (Supplementary Figure 5C).
Safety concerns
Toxicity data were reported in the form of treatment-related adverse events (TRAEs) and immunotherapy-related adverse events (IRAEs).
General toxicity
Based on the results from 7 studies (n=1401), frequency of any grade treatment-related adverse events was 71% (95%CI, 49%-92%) as shown in Supplementary Figure 6A. Frequency of any grade≥3 was 30% (95%CI, 17%-44%) (Supplementary Figure 6B). Additionally, general toxicity of ICT to CT alone was also evaluated. No difference in TRAEs (OR 1.58 [0.99, 2.52], p=0.06) or any grade ≥3 TRAEs (OR 0.90 [0.47, 1.73], p=0.75) was demonstrated (Supplementary Figure 7).
Toxicity of special interest
Toxicity of special or clinical interest mainly included adverse events associated with immunotherapy. Based on the results from 10 studies (n=2290), frequency of any grade treatment-related adverse events was 39% (95%CI, 26%-52%) (Supplementary Figure 8A). Frequency of any grade≥3 was 10% (95%CI, 8%-13%) (Supplementary Figure 8B). Compared to CT alone, toxicity of special interest was significantly more common in the IT group (OR 2.89 [1.56, 5.38], p=0.0008) (Supplementary Figure 7). A significant rise was also observed in grade ≥ 3 irAEs as well (OR 2.53 [1.66, 5.53], p=0.02).
Discussion
Immune checkpoint inhibition (ICI) therapy has been extensively evaluated in the management of triple negative breast cancer patients. ICI as monotherapy or in combination with chemotherapy have demonstrated anti-cancer activity in early-stage as well as metastatic TNBC (11–18, 42–46). Our study result indicates a 22% response rate for PD-1/PD-L1 checkpoint inhibitors as monotherapy or in combination with chemotherapy in mTNBC. The later approach was also shown superior to chemotherapy alone in terms of ORR and PFS. However, no overall survival advantage was demonstrated for the combined approach.
PD-L1 expression has been regarded as prognostic factor in various cancers (47–50). Its biomarker value to predict response to PD-1/PD-L1 checkpoint inhibition has also been acknowledged across cancers (50–55). Among breast cancer subtypes, TNBC exhibit higher tumor mutational burden (TMB), tumor infiltrates of lymphocytes (TILs) and PD-L1 expression, indicating its candidacy for immune checkpoint inhibition therapy (6–8). In TNBC, PD-L1 expression as biomarker have failed to predict pathological response rate in the neoadjuvant setting and long-term OS benefit in the metastatic setting (56). Our study systematically accumulated data to analyze the biomarker significance of PD-L1 expression in the metastatic TNBC patients. The outcome revealed that PD-L1 expression can significantly predict objective response, 1-year PFS, 2-year OS and overall survival. However, overall PFS was not significantly different between these two groups based on the direct comparison analysis. Nonetheless, the studies that provided the direct PFS comparison between PD-L1+ and PD-L1- patients were comprised of smaller number of participants (12, 13, 15, 30). While major phase III trials reported treatment difference in each subgroup (PD-L1+/- cohort) separately with no direct comparison between the PD-L1+ and PD-L1- patients (16–18). Besides, a much larger population cohort would be required to detect a PFS difference of any significant value in a population with such a heterogenous and extensive disease burden as mTNBC (57). On the other hand, OS is affected by several other patient factors other than the treatment as compared to PFS which is a direct measure of clinical performance (58). In the treatment comparative analysis, the response rate, PFS and OS was significantly higher with ICT in PD-L1 positive population. While PD-L1 negative patients failed to derive such benefit. Overall, these outcomes strongly underline biomarker value of PD-L1 expression in mTNBC.
PD-L1 expression was assessed on the tumor cells and/or the tumor infiltrating lymphocytes and macrophages (11–18, 30, 32, 38, 39, 41). Studies involving pembrolizumab had reported the combined positive score (CPS), defined as the ratio of PD-L1–positive cells (tumor cells, lymphocytes, and macrophages) out of the total number of tumor cells × 100 (11, 12, 17, 30, 41). The prognostic and predictive efficacy of atezolizumab was initially assessed with PD-L1 expression on the tumor cells (TC) and immune cells (IC) (13, 15). However, the phase Ib trial conducted by Emens, et al. indicated a significant predictive value for PD-L1 expression on immune cells only (15). Therefore, subsequent phase III trials had only evaluated the predictive efficacy of atezolizumab based on the IC-derived PD-L1 which was defined as a percentage of tumor area (consisting of TC and associated intra-tumoral and continuous peri-tumoral stroma) occupied by IC with discernible PD-L1 staining of any intensity (16, 18). Subgroup analysis revealed that predictive value of PD-L1 expression was maintained regardless of the scoring algorithm used. PD-L1 expression was able to predict ORR and 2-year OS to both of these agents. Although a near to significant improvement in 1-year PFS was observed for pembrolizumab in PD-L1+ patients, PD-L1 expression failed to predict 1-year PFS for these two subgroups. This subgroup analysis also established that the type of detection assay (SP142 used for atezolizumab & 222C3 for pembrolizumab) also had no impact on the clinical activity. This outcome is in concordance with the observations made in comparative analysis of these immunohistochemistry assays in IMPassion130 study, which indicated significant prevalence differences (prevalence rates for PD-L1 IC ≥1% cutoff for SP142, SP263, and 22C3 were 46.4%, 74.9%, and 73.1%, respectively. And for PD-L1 22C3 CPS ≥1 was 80.9%) but similar clinical activity with A+nP vs P+nP for all four subgroups (SP142 IC ≥1%, SP263 IC ≥1%, 22C3 IC ≥1%, and 22C3 CPS ≥1; PFS HR = 0.60 to 0.68; OS HR = 0.74 to 0.79) (59). These observations indicate that the lack of analytical equivalency among PD-L1 expression detection assays exert no impact on the biomarker ability of PD-L1 expression in predicting clinical activity. PD-L1 expression also varies between primary tumor and metastatic sites as previously been reported for mTNBC (60, 61). The specific site of PD-L1 assessment was not clearly reported in these trials but a post hoc analysis of IMPassion130 revealed a difference in PD-L1 expression between primary tumor and metastatic sites (44% vs 36%, p=0.014) of mTNBC patients with no apparent influence on the predictive value of PD-L1 expression (59). A negative impact has been reported for number of previous lines of therapy on the response to PD-1 checkpoint inhibitors in lung cancer and melanoma (62–64). Likewise, overall response was higher in mTNBC patients receiving PD-1/PD-L1 checkpoint inhibitors as first line treatment compared to the patients previously treated for metastatic disease (12–16). However, previous therapy had no effect on the biomarker efficacy of PD-L1 expression according to the outcomes of our study. Nonetheless, 1-year PFS could only be predicted by PD-L1 expression when the treatment was administered in the first line setting.
Moreover, use of PD-1/PD-L1 checkpoint inhibitors in mTNBC have shown durable responses as monotherapy but with high heterogeneity from study to study and its supremacy over chemotherapy alone is controversial (11–18). In fact, combined approach has also yield contradictory results in phase III trials in terms of PFS and OS (16, 18). Although PD-L1 expression was able to predict clinical response when analysis was restricted to single-agent and combined approach separately, 1-year PFS and 2-year OS was only predicted when immunotherapy was combined with chemotherapy. It may indicate that the two treatments are applied in combination may induce strong and long-term responses which also result in survival benefits. In addition, preclinical evidence also indicates a synergistic interplay between these two treatments. Chemotherapeutic agents have shown augment mutational load and neoantigen presentation, suppress the immune-suppressive cells, sensitize tumor cells to effector cytokines produced by T cells, induce the expression of major histocompatibility complex class I (MHC-I) and PD-L1 on tumor cells (65–73). These outcomes firmly support the use of PD-L1 expression as prognostic and predictive of response to immune checkpoint inhibition in mTNBC. As expected, the combination of two treatments has led to added toxicity mainly observed in the form of immune-related adverse events. Toxicity associated with immune checkpoint inhibitors has been recognized as a separate entity of special consideration and management needs (74). Nonetheless, no late-onset or long-term safety concerns were reported with addition of PD-1 checkpoint inhibitors.
Our study is limited by several factors. First, a number of non-randomized clinical trials were included which may impart certain degree of inevitable heterogeneity. Second, certain studies involved small number of patients which contributed high heterogeneity to the pooled outcomes of ORR and adverse events of single-arm studies. Certain dissimilarities were noticed in these studies in reference to the type of agent (PD-1 or PD-L1 inhibitor), chemotherapy regimen, PD-L1 expression assessment assays, treatment combination (IT versus ICT) and line of therapy. Subgroup analysis were undertaken to address these issues; nonetheless, these differences may still impact pooled analysis.
Conclusion
In summary, the results indicate that PD-L1 expression can successfully predict objective response rate and 2-year OS in mTNBC patients receiving PD-1/PD-L1 checkpoint inhibitors plus/minus chemotherapy. Short-term progression-free survival (1-year) is only predicted by PD-L1 expression when treatment is administered in the first-line setting and with chemotherapy. It can also predict the ORR, PFS and OS for PD-L1+ mTNBC patients receiving PD-1 checkpoint inhibitors plus chemotherapy as compared to chemotherapy alone. Overall, PD-L1 expression can be a useful biomarker of efficacy of PD-1/PD-L1 checkpoint inhibitors in mTNBC.
Data availability statement
The original contributions presented in the study are included in the article/Supplementary Material. Further inquiries can be directed to the corresponding authors.
Author contributions
MK, KD, MA, and BW designed the project, performed data extraction, and statistical analysis. MK wrote the initial manuscript. JL, AR, CC, ZH, WQ, YT, and YY provided critical assessment and supervision. All authors contributed to the article and approved the submitted version.
Funding
This study was supported by the National Natural Science Foundation of China (Nos. 82002858 and 81872195), Guangzhou Key Medical Discipline Construction Project Fund (Radiation Oncology) and Key Clinical Technology of Guangzhou (2019ZD17).
Conflict of interest
The authors declare that the research was conducted in the absence of any commercial or financial relationships that could be construed as a potential conflict of interest.
Publisher’s note
All claims expressed in this article are solely those of the authors and do not necessarily represent those of their affiliated organizations, or those of the publisher, the editors and the reviewers. Any product that may be evaluated in this article, or claim that may be made by its manufacturer, is not guaranteed or endorsed by the publisher.
Supplementary material
The Supplementary Material for this article can be found online at: https://www.frontiersin.org/articles/10.3389/fimmu.2023.1060308/full#supplementary-material
References
1. Chacón RD, Costanzo MV. Triple-negative breast cancer. Breast Cancer Res (2010) 12(2):S3. doi: 10.1186/bcr2574
2. Bianchini G, Balko JM, Mayer IA, Sanders ME, Gianni L. Triple-negative breast cancer: challenges and opportunities of a heterogeneous disease. Nat Rev Clin Oncol (2016) 13(11):674–90. doi: 10.1038/nrclinonc.2016.66
3. Denkert C, Liedtke C, Tutt A, von Minckwitz G. Molecular alterations in triple-negative breast cancer-the road to new treatment strategies. Lancet (2017) 389(10087):2430–42. doi: 10.1016/s0140-6736(16)32454-0
4. Early Breast Cancer Trialists' Collaborative G, Peto R, Davies C, Godwin J, Gray R, Pan HC, et al. Comparisons between different polychemotherapy regimens for early breast cancer: meta-analyses of long-term outcome among 100,000 women in 123 randomised trials. Lancet (London England) (2012) 379(9814):432–44. doi: 10.1016/S0140-6736(11)61625-5
5. Carey LA, Dees EC, Sawyer L, Gatti L, Moore DT, Collichio F, et al. The triple negative paradox: Primary tumor chemosensitivity of breast cancer subtypes. Clin Cancer Res (2007) 13(8):2329. doi: 10.1158/1078-0432.CCR-06-1109
6. Adams S, Gray RJ, Demaria S, Goldstein L, Perez EA, Shulman LN, et al. Prognostic value of tumor-infiltrating lymphocytes in triple-negative breast cancers from two phase III randomized adjuvant breast cancer trials: ECOG 2197 and ECOG 1199. J Clin Oncol (2014) 32(27):2959–66. doi: 10.1200/jco.2013.55.0491
7. Vogelstein B, Papadopoulos N, Velculescu VE, Zhou S, Diaz LA, Kinzler KW. Cancer genome landscapes. Science (2013) 339(6127):1546. doi: 10.1126/science.1235122
8. Mittendorf EA, Philips AV, Meric-Bernstam F, Qiao N, Wu Y, Harrington S, et al. PD-L1 expression in triple-negative breast cancer. Cancer Immunol Res (2014) 2(4):361–70. doi: 10.1158/2326-6066.Cir-13-0127
9. Tang F, Zheng P. Tumor cells versus host immune cells: whose PD-L1 contributes to PD-1/PD-L1 blockade mediated cancer immunotherapy? Cell Bioscience (2018) 8(1):34. doi: 10.1186/s13578-018-0232-4
10. Tang H, Liang Y, Anders RA, Taube JM, Qiu X, Mulgaonkar A, et al. PD-L1 on host cells is essential for PD-L1 blockade-mediated tumor regression. J Clin Invest (2018) 128(2):580–8. doi: 10.1172/JCI96061
11. Adams S, Loi S, Toppmeyer D, Cescon DW, De Laurentiis M, Nanda R, et al. Pembrolizumab monotherapy for previously untreated, PD-L1-positive, metastatic triple-negative breast cancer: cohort b of the phase II KEYNOTE-086 study. Ann Oncol (2019) 30(3):405–11. doi: 10.1093/annonc/mdy518
12. Adams S, Schmid P, Rugo HS, Winer EP, Loirat D, Awada A, et al. Pembrolizumab monotherapy for previously treated metastatic triple-negative breast cancer: cohort a of the phase II KEYNOTE-086 study. Ann Oncol (2019) 30(3):397–404. doi: 10.1093/annonc/mdy517
13. Adams S, Diamond JR, Hamilton E, Pohlmann PR, Tolaney SM, Chang CW, et al. Atezolizumab plus nab-paclitaxel in the treatment of metastatic triple-negative breast cancer with 2-year survival follow-up: A phase 1b clinical trial. JAMA Oncol (2019) 5(3):334–42. doi: 10.1001/jamaoncol.2018.5152
14. Nanda R, Chow LQ, Dees EC, Berger R, Gupta S, Geva R, et al. Pembrolizumab in patients with advanced triple-negative breast cancer: Phase ib KEYNOTE-012 study. J Clin Oncol (2016) 34(21):2460–7. doi: 10.1200/JCO.2015.64.8931
15. Emens LA, Cruz C, Eder JP, Braiteh F, Chung C, Tolaney SM, et al. Long-term clinical outcomes and biomarker analyses of atezolizumab therapy for patients with metastatic triple-negative breast cancer: A phase 1 study. JAMA Oncol (2019) 5(1):74–82. doi: 10.1001/jamaoncol.2018.4224
16. Schmid P, Rugo HS, Adams S, Schneeweiss A, Barrios CH, Iwata H, et al. Atezolizumab plus nab-paclitaxel as first-line treatment for unresectable, locally advanced or metastatic triple-negative breast cancer (IMpassion130): Updated efficacy results from a randomised, double-blind, placebo-controlled, phase 3 trial. Lancet Oncol (2020) 21(1):44–59. doi: 10.1016/S1470-2045(19)30689-8
17. Cortes J, Cescon DW, Rugo HS, Nowecki Z, Im S-A, Yusof MM, et al. Pembrolizumab plus chemotherapy versus placebo plus chemotherapy for previously untreated locally recurrent inoperable or metastatic triple-negative breast cancer (KEYNOTE-355): A randomised, placebo-controlled, double-blind, phase 3 clinical trial. Lancet (2020) 396(10265):1817–28. doi: 10.1016/S0140-6736(20)32531-9
18. Miles D, Gligorov J, André F, Cameron D, Schneeweiss A, Barrios C, et al. Primary results from IMpassion131, a double-blind, placebo-controlled, randomised phase III trial of first-line paclitaxel with or without atezolizumab for unresectable locally advanced/metastatic triple-negative breast cancer. Ann Oncol (2021) 32(8):994–1004. doi: 10.1016/j.annonc.2021.05.801
19. Review manager web (RevMan web). the cochrane collaboration (2019). Available at: https://revman.cochrane.org.
20. Page MJ, McKenzie JE, Bossuyt PM, Boutron I, Hoffmann TC, Mulrow CD, et al. The PRISMA 2020 statement: An updated guideline for reporting systematic reviews. PloS Med (2021) 18(3):e1003583. doi: 10.1371/journal.pmed.1003583
21. Slim K, Nini E, Forestier D, Kwiatkowski F, Panis Y, Chipponi J. Methodological index for non-randomized studies (minors): Development and validation of a new instrument. ANZ J Surg (2003) 73(9):712–6. doi: 10.1046/j.1445-2197.2003.02748.x
22. Riley RD, Moons KGM, Snell KIE, Ensor J, Hooft L, Altman DG, et al. A guide to systematic review and meta-analysis of prognostic factor studies. BMJ (2019) 364:k4597. doi: 10.1136/bmj.k4597
23. Hayden JA, van der Windt DA, Cartwright JL, Côté P, Bombardier C. Assessing bias in studies of prognostic factors. Ann Intern Med (2013) 158(4):280–6. doi: 10.7326/0003-4819-158-4-201302190-00009
24. Guyatt GH, Oxman AD, Vist GE, Kunz R, Falck-Ytter Y, Alonso-Coello P, et al. GRADE: an emerging consensus on rating quality of evidence and strength of recommendations. BMJ (2008) 336(7650):924. doi: 10.1136/bmj.39489.470347.AD
25. DerSimonian R, Laird N. Meta-analysis in clinical trials. Control Clin Trials. (1986) 7(3):177–88. doi: 10.1016/0197-2456(86)90046-2
26. Neyeloff JL, Fuchs SC, Moreira LB. Meta-analyses and forest plots using a microsoft excel spreadsheet: Step-by-step guide focusing on descriptive data analysis. BMC Res Notes (2012) 5(1):52. doi: 10.1186/1756-0500-5-52
27. Automeris. WebPlotDigitizer. Available at: https://automeris.io/WebPlotDigitizer (Accessed March 15, 2021).
28. Tierney JF, Stewart LA, Ghersi D, Burdett S, Sydes MR. Practical methods for incorporating summary time-to-event data into meta-analysis. Trials. (2007) 8(1):16. doi: 10.1186/1745-6215-8-16
29. Higgins JPT, Thompson SG, Deeks JJ, Altman DG. Measuring inconsistency in meta-analyses. BMJ (Clinical Res ed) (2003) 327(7414):557–60. doi: 10.1136/bmj.327.7414.5
30. Tolaney SM, Kalinsky K, Kaklamani VG, Adamo DR, Aktan G, Tsai ML, et al. Eribulin plus pembrolizumab in patients with metastatic triple-negative breast cancer (ENHANCE 1): A phase Ib/II study. Clin Cancer Res (2021) 27(11):3061. doi: 10.1158/1078-0432.CCR-20-4726
31. Anders CK, Moore D, Sambade M, Cuaboy L, Garrett A, Woodcock M, et al. Abstract P2-09-05: LCCC 1525: A phase II study of a priming dose of cyclophosphamide prior to pembrolizumab to treat metastatic triple negative breast cancer (mTNBC). Cancer Res (2019) 79(4_Supplement):P2-09-5-P2–5. doi: 10.1158/1538-7445.SABCS18-P2-09-05
32. Voorwerk L, Slagter M, Horlings HM, Sikorska K, van de Vijver KK, de Maaker M, et al. Immune induction strategies in metastatic triple-negative breast cancer to enhance the sensitivity to PD-1 blockade: The TONIC trial. Nat Med (2019) 25(6):920–8. doi: 10.1038/s41591-019-0432-4
33. McArthur HL, Barker CA, Gucalp A, Lebron-Zapata L, Wen YH, Phung A, et al. A single-arm, phase II study assessing the efficacy of pembrolizumab (pembro) plus radiotherapy (RT) in metastatic triple negative breast cancer (mTNBC). J Clin Oncol (2018) 36(5_suppl):14–14. doi: 10.1200/JCO.2018.36.5_suppl.14
34. O'Day S, Borges V, Chmielowski B, Rao R, Abu-Khalaf M, Stopeck A, et al. Abstract P2-09-08: Imprime PGG, a novel innate immune modulator, combined with pembrolizumab in a phase 2 multicenter, open label study in chemotherapy-resistant metastatic triple negative breast cancer (TNBC). Cancer Res (2019) 79(4_Supplement):P2-09-8-P2–8. doi: 10.1158/1538-7445.SABCS18-P2-09-08
35. Spira AI, Hamid O, Bauer TM, Borges VF, Wasser JS, Smith DC, et al. Efficacy/safety of epacadostat plus pembrolizumab in triple-negative breast cancer and ovarian cancer: Phase I/II ECHO-202 study. J Clin Oncol (2017) 35(15_suppl):1103–. doi: 10.1200/JCO.2017.35.15_suppl.1103
36. Veitch ZWN, Cescon DW, Elston S, Lien S, Yang C, Wang BX, et al. (INSPIRE) trial of pembrolizumab (pembro) with serial immune and genomic profiling in patients (pts) with metastatic triple negative breast cancer (mTNBC). J Clin Oncol (2018) 36(15_suppl):1094–. doi: 10.1200/JCO.2018.36.15_suppl.1094
37. Page DB, Pucilowska J, Bennetts L, Kim I, Sanchez K, Martel M, et al. Abstract P2-09-03: Updated efficacy of first or second-line pembrolizumab (pembro) plus capecitabine (cape) in metastatic triple negative breast cancer (mTNBC) and correlations with baseline lymphocyte and naïve CD4+ T-cell count. Cancer Res (2019) 79(4_Supplement):P2–09-3-P2—3. doi: 10.1158/1538-7445.SABCS18-P2-09-03
38. Bachelot T, Filleron T, Bieche I, Arnedos M, Campone M, Dalenc F, et al. Durvalumab compared to maintenance chemotherapy in metastatic breast cancer: the randomized phase II SAFIR02-BREAST IMMUNO trial. Nat Med (2021) 27(2):250–5. doi: 10.1038/s41591-020-01189-2
39. Quintela-Fandino M, Holgado E, Manso L, Morales S, Bermejo B, Colomer R, et al. Immuno-priming durvalumab with bevacizumab in HER2-negative advanced breast cancer: a pilot clinical trial. Breast Cancer Res BCR. (2020) 22(1):124–. doi: 10.1186/s13058-020-01362-y
40. Dirix LY, Takacs I, Jerusalem G, Nikolinakos P, Arkenau HT, Forero-Torres A, et al. Avelumab, an anti-PD-L1 antibody, in patients with locally advanced or metastatic breast cancer: a phase 1b JAVELIN solid tumor study. Breast Cancer Res Treat (2018) 167(3):671–86. doi: 10.1007/s10549-017-4537-5
41. Winer EP, Lipatov O, Im SA, Goncalves A, Muñoz-Couselo E, Lee KS, et al. Pembrolizumab versus investigator-choice chemotherapy for metastatic triple-negative breast cancer (KEYNOTE-119): a randomised, open-label, phase 3 trial. Lancet Oncol (2021) 22(4):499–511. doi: 10.1016/S1470-2045(20)30754-3
42. Loibl S, Untch M, Burchardi N, Huober J, Sinn BV, Blohmer JU, et al. A randomised phase II study investigating durvalumab in addition to an anthracycline taxane-based neoadjuvant therapy in early triple-negative breast cancer: clinical results and biomarker analysis of GeparNuevo study. Ann Oncol (2019) 30(8):1279–88. doi: 10.1093/annonc/mdz158
43. Gianni L, Huang C-S, Egle D, Bermejo B, Zamagni C, Thill M, et al. Abstract GS3-04: Pathologic complete response (pCR) to neoadjuvant treatment with or without atezolizumab in triple negative, early high-risk and locally advanced breast cancer. NeoTRIPaPDL1 Michelangelo randomized study. Cancer Res (2020) 80(4 Supplement):GS3–04. doi: 10.1158/1538-7445.SABCS19-GS3-04
44. Mittendorf EA, Zhang H, Barrios CH, Saji S, Jung KH, Hegg R, et al. Neoadjuvant atezolizumab in combination with sequential nab-paclitaxel and anthracycline-based chemotherapy versus placebo and chemotherapy in patients with early-stage triple-negative breast cancer (IMpassion031): A randomised, double-blind, phase 3 trial. Lancet (2020) 396(10257):1090–100. doi: 10.1016/s0140-6736(20)31953-x
45. Nanda R, Liu MC, Yau C, Shatsky R, Pusztai L, Wallace A, et al. Effect of pembrolizumab plus neoadjuvant chemotherapy on pathologic complete response in women with early-stage breast cancer: An analysis of the ongoing phase 2 adaptively randomized I-SPY2 trial. JAMA Oncol (2020) 6(5):676–84. doi: 10.1001/jamaoncol.2019.6650
46. Schmid P, Cortes J, Pusztai L, McArthur H, Kümmel S, Bergh J, et al. Pembrolizumab for early triple-negative breast cancer. New Engl J Med (2020) 382(9):810–21. doi: 10.1056/NEJMoa1910549
47. Matikas A, Zerdes I, Lövrot J, Richard F, Sotiriou C, Bergh J, et al. Prognostic implications of PD-L1 expression in breast cancer: Systematic review and meta-analysis of immunohistochemistry and pooled analysis of transcriptomic data. Clin Cancer Res (2019) 25(18):5717–26. doi: 10.1158/1078-0432.CCR-19-1131
48. Wang G, Fu X, Chang Y, Li X, Wu X, Li L, et al. B7-CD28 gene family expression is associated with prognostic and immunological characteristics of diffuse large b-cell lymphoma. Aging (Albany NY). (2019) 11(12):3939–57. doi: 10.18632/aging.102025
49. Zheng S, Luo X, Dong C, Zheng D, Xie J, Zhuge L, et al. A B7-CD28 family based signature demonstrates significantly different prognoses and tumor immune landscapes in lung adenocarcinoma. Int J Cancer (2018) 143(10):2592–601. doi: 10.1002/ijc.31764
50. Khan M, Lin J, Liao G, Tian Y, Liang Y, Li R, et al. Comparative analysis of immune checkpoint inhibitors and chemotherapy in the treatment of advanced non-small cell lung cancer: A meta-analysis of randomized controlled trials. Med (Baltimore). (2018) 97(33):e11936–e. doi: 10.1097/MD.0000000000011936
51. Lu S, Stein JE, Rimm DL, Wang DW, Bell JM, Johnson DB, et al. Comparison of biomarker modalities for predicting response to PD-1/PD-L1 checkpoint blockade: A systematic review and meta-analysis. JAMA Oncol (2019) 5(8):1195–204. doi: 10.1001/jamaoncol.2019.1549
52. Incorvaia L, Fanale D, Badalamenti G, Barraco N, Bono M, Corsini LR, et al. Programmed death ligand 1 (PD-L1) as a predictive biomarker for pembrolizumab therapy in patients with advanced non-Small-Cell lung cancer (NSCLC). Adv Ther (2019) 36(10):2600–17. doi: 10.1007/s12325-019-01057-7
53. Ghate K, Amir E, Kuksis M, Hernandez-Barajas D, Rodriguez-Romo L, Booth CM, et al. PD-L1 expression and clinical outcomes in patients with advanced urothelial carcinoma treated with checkpoint inhibitors: A meta-analysis. Cancer Treat Rev (2019) 76:51–6. doi: 10.1016/j.ctrv.2019.05.002
54. Roviello G, Corona SP, Nesi G, Mini E. Results from a meta-analysis of immune checkpoint inhibitors in first-line renal cancer patients: Does PD-L1 matter? Ther Adv Med Oncol (2019) 11:1758835919861905. doi: 10.1177/1758835919861905
55. Kitano S, Nakayama T, Yamashita M. Biomarkers for immune checkpoint inhibitors in melanoma. Front Oncol (2018) 8:270–. doi: 10.3389/fonc.2018.00270
56. Sternschuss M, Yerushalmi R, Saleh RR, Amir E, Goldvaser H. Efficacy and safety of neoadjuvant immune checkpoint inhibitors in early-stage triple-negative breast cancer: A systematic review and meta-analysis. J Cancer Res Clin Oncol (2021) 147(11):3369–79. doi: 10.1007/s00432-021-03591-w
57. Broglio KR, Berry DA. Detecting an overall survival benefit that is derived from progression-free survival. J Natl Cancer Inst (2009) 101(23):1642–9. doi: 10.1093/jnci/djp369
58. Mushti SL, Mulkey F, Sridhara R. Evaluation of overall response rate and progression-free survival as potential surrogate endpoints for overall survival in immunotherapy trials. Clin Cancer Res (2018) 24(10):2268–75. doi: 10.1158/1078-0432.CCR-17-1902
59. Rugo HS, Loi S, Adams S, Schmid P, Schneeweiss A, Barrios CH, et al. PD-L1 immunohistochemistry assay comparison in atezolizumab plus nab-Paclitaxel-Treated advanced triple-negative breast cancer. J Natl Cancer Inst (2021) 113(12):1733–43. doi: 10.1093/jnci/djab108
60. Boman C, Zerdes I, Mårtensson K, Bergh J, Foukakis T, Valachis A, et al. Discordance of PD-L1 status between primary and metastatic breast cancer: A systematic review and meta-analysis. Cancer Treat Rev (2021) 99:102257. doi: 10.1016/j.ctrv.2021.102257
61. Rozenblit M, Huang R, Danziger N, Hegde P, Alexander B, Ramkissoon S, et al. Comparison of PD-L1 protein expression between primary tumors and metastatic lesions in triple negative breast cancers. J Immunother Cancer (2020) 8(2):e001558. doi: 10.1136/jitc-2020-001558
62. Rittmeyer A, Barlesi F, Waterkamp D, Park K, Ciardiello F, von Pawel J, et al. Atezolizumab versus docetaxel in patients with previously treated non-small-cell lung cancer (OAK): a phase 3, open-label, multicentre randomised controlled trial. Lancet (London England). (2017) 389(10066):255–65. doi: 10.1016/s0140-6736(16)32517-x
63. Long GV, Trefzer U, Davies MA, Kefford RF, Ascierto PA, Chapman PB, et al. Dabrafenib in patients with Val600Glu or Val600Lys BRAF-mutant melanoma metastatic to the brain (BREAK-MB): A multicentre, open-label, phase 2 trial. Lancet Oncol (2012) 13(11):1087–95. doi: 10.1016/S1470-2045(12)70431-X
64. Davies MA, Saiag P, Robert C, Grob JJ, Flaherty KT, Arance A, et al. Dabrafenib plus trametinib in patients with BRAF(V600)-mutant melanoma brain metastases (COMBI-MB): A multicentre, multicohort, open-label, phase 2 trial. Lancet Oncol (2017) 18(7):863–73. doi: 10.1016/S1470-2045(17)30429-1
65. de Biasi AR, Villena-Vargas J, Adusumilli PS. Cisplatin-induced antitumor immunomodulation: A review of preclinical and clinical evidence. Clin Cancer Res (2014) 20(21):5384–91. doi: 10.1158/1078-0432.CCR-14-1298
66. Suzuki E, Kapoor V, Jassar AS, Kaiser LR, Albelda SM. Gemcitabine selectively eliminates splenic gr-1+/CD11b+ myeloid suppressor cells in tumor-bearing animals and enhances antitumor immune activity. Clin Cancer Res (2005) 11(18):6713–21. doi: 10.1158/1078-0432.CCR-05-0883
67. Apetoh L, Ladoire S, Coukos G, Ghiringhelli F. Combining immunotherapy and anticancer agents: The right path to achieve cancer cure? Ann Oncol (2015) 26(9):1813–23. doi: 10.1093/annonc/mdv209
68. Szikriszt B, Póti Á, Pipek O, Krzystanek M, Kanu N, Molnár J, et al. A comprehensive survey of the mutagenic impact of common cancer cytotoxics. Genome Biol (2016) 17:99. doi: 10.1186/s13059-016-0963-7
69. Gravett AM, Trautwein N, Stevanović S, Dalgleish AG, Copier J. Gemcitabine alters the proteasome composition and immunopeptidome of tumour cells. Oncoimmunology. (2018) 7(6):e1438107. doi: 10.1080/2162402X.2018.1438107
70. Kodumudi KN, Woan K, Gilvary DL, Sahakian E, Wei S, Djeu JY. A novel chemoimmunomodulating property of docetaxel: Suppression of myeloid-derived suppressor cells in tumor bearers. Clin Cancer Res (2010) 16(18):4583–94. doi: 10.1158/1078-0432.CCR-10-0733
71. Hato SV, Khong A, de Vries IJ, Lesterhuis WJ. Molecular pathways: the immunogenic effects of platinum-based chemotherapeutics. Clin Cancer Res (2014) 20(11):2831–7. doi: 10.1158/1078-0432.CCR-13-3141
72. Song Z, Yu X, Zhang Y. Altered expression of programmed death-ligand 1 after neo-adjuvant chemotherapy in patients with lung squamous cell carcinoma. Lung Cancer. (2016) 99:166–71. doi: 10.1016/j.lungcan.2016.07.013
73. Peng J, Hamanishi J, Matsumura N, Abiko K, Murat K, Baba T, et al. Chemotherapy induces programmed cell death-ligand 1 overexpression via the nuclear factor-κB to foster an immunosuppressive tumor microenvironment in ovarian cancer. Cancer Res (2015) 75(23):5034–45. doi: 10.1158/0008-5472.CAN-14-3098
74. Brahmer JR, Lacchetti C, Schneider BJ, Atkins MB, Brassil KJ, Caterino JM, et al. Management of immune-related adverse events in patients treated with immune checkpoint inhibitor therapy: American society of clinical oncology clinical practice guideline. J Clin Oncol (2018) 36(17):1714–68. doi: 10.1200/jco.2017.77.6385
Keywords: breast cancer, immunotherapy, immune checkpoint inhibitors (ICI), pembrolizumab, atezolizumab, survival, immune-related toxicity
Citation: Khan M, Du K, Ai M, Wang B, Lin J, Ren A, Chen C, Huang Z, Qiu W, Yuan Y and Tian Y (2023) PD-L1 expression as biomarker of efficacy of PD-1/PD-L1 checkpoint inhibitors in metastatic triple negative breast cancer: A systematic review and meta-analysis. Front. Immunol. 14:1060308. doi: 10.3389/fimmu.2023.1060308
Received: 03 October 2022; Accepted: 13 February 2023;
Published: 06 March 2023.
Edited by:
Arne Östman, Karolinska Institutet (KI), SwedenReviewed by:
Judith Ramage, University of Nottingham, United KingdomHuiping Li, Beijing Cancer Hospital, Peking University, China
Copyright © 2023 Khan, Du, Ai, Wang, Lin, Ren, Chen, Huang, Qiu, Yuan and Tian. This is an open-access article distributed under the terms of the Creative Commons Attribution License (CC BY). The use, distribution or reproduction in other forums is permitted, provided the original author(s) and the copyright owner(s) are credited and that the original publication in this journal is cited, in accordance with accepted academic practice. No use, distribution or reproduction is permitted which does not comply with these terms.
*Correspondence: Yunhong Tian, dGlhbnl1bmhvbmcyMDIwQGd6aG11LmVkdS5jbg==; Yawei Yuan, eXVhbnlhd2VpQGd6aG11LmVkdS5jbg==
†These authors have contributed equally to this work