- 1Department of Medicine, Division of Rheumatology, University of Washington, Seattle, WA, United States
- 2Department of Microbiology, University of Washington, Seattle, WA, United States
- 3Department of Immunology, University of Washington, Seattle, WA, United States
Purpose and methods: B cell-activating factor (BAFF) contributes to the pathogenesis of autoimmune diseases including systemic lupus erythematosus (SLE). Although several anti-BAFF Abs and derivatives have been developed for the treatment of SLE, the specific sources of BAFF that sustain autoantibody (auto-Ab) producing cells have not been definitively identified. Using BAFF-RFP reporter mice, we identified major changes in BAFF-producing cells in two mouse spontaneous lupus models (Tlr7 Tg mice and Sle1), and in a pristane-induced lupus (PIL) model.
Results: First, we confirmed that similar to their wildtype Tlr7 Tg and Sle1 mice counterparts, BAFF-RFP Tlr7 Tg mice and BAFF-RFP Sle1 mice had increased BAFF serum levels, which correlated with increases in plasma cells and auto-Ab production. Next, using the RFP reporter, we defined which cells had dysregulated BAFF production. BAFF-producing neutrophils (Nphs), monocytes (MOs), cDCs, T cells and B cells were all expanded in the spleens of BAFF-RFP Tlr7 Tg mice and BAFF-RFP Sle1 mice compared to controls. Furthermore, Ly6Chi inflammatory MOs and T cells had significantly increased BAFF expression per cell in both spontaneous lupus models, while CD8- DCs up-regulated BAFF expression only in the Tlr7 Tg mice. Similarly, pristane injection of BAFF-RFP mice induced increases in serum BAFF levels, auto-Abs, and the expansion of BAFF-producing Nphs, MOs, and DCs in both the spleen and peritoneal cavity. BAFF expression in MOs and DCs, in contrast to BAFF from Nphs, was required to maintain homeostatic and pristane-induced systemic BAFF levels and to sustain mature B cell pools in spleens and BMs. Although acting through different mechanisms, Nph, MO and DC sources of BAFF were each required for the development of auto-Abs in PIL mice.
Conclusions: Our findings underscore the importance of considering the relative roles of specific myeloid BAFF sources and B cell niches when developing treatments for SLE and other BAFF-associated autoimmune diseases.
Introduction
B-cell-activating factor (BAFF) (also known as BLyS or Tnfsf13b) is a member of the TNF family of ligands that plays an essential role for mature B cells to develop, survive and respond to Ag (1–4). BAFF binds to three different TNF receptor family members: BAFFR, TACI and BCMA; signaling through these receptors functions as a key survival factor for maintaining mature B cell homeostasis (5–7). Baff-/- and Baffr-/- mice develop few or no mature B cells due to a block in B cell development from the newly formed transitional 1 (T1) to the T2 B cell stage onwards (5–7). We and others have shown that BAFF plays a key protective role against infectious diseases, including West Nile virus, HIV and Salmonella typhimurium (8–12).
On the other hand, BAFF overexpression contributes to the pathogenesis of a number of autoimmune diseases, including IgA nephropathy, type I diabetes, systemic sclerosis, Sjögren’s syndrome, rheumatoid arthritis, and systemic lupus erythematosus (SLE) (13–19). Mice overexpressing BAFF develop an SLE-like disease, and blocking BAFF receptors in these animals inhibits the development of kidney disease and prolongs survival (20, 21). Furthermore, mouse strains that develop spontaneous SLE have increased serum BAFF levels (14, 15, 20). Elevated serum BAFF levels and increased BAFF gene expression in peripheral blood cells are associated with disease activity in SLE patients (22–24). More recently, a genotype carrying a variant of the gene encoding BAFF was associated with increased disease severity in SLE (25).
The anti-BAFF Ab, belimumab, was the first BAFF-blocking drug approved for the treatment of SLE (15, 26–30). Other BAFF-targeting agents and belimumab in combination with other biologics are being assessed as treatments for lupus patients (29). The heterogeneity in clinical responses to belimumab and other BAFF-blocking drugs underscores the need to understand more fully the complexity of the BAFF system (3, 27, 28). While the downstream effects of blocking BAFF receptors have been examined extensively, the upstream regulation of BAFF production and how it affects the development of autoimmunity in vivo has not been investigated in detail.
In this study, we used BAFF-red fluorescent protein (RFP) reporter (BAFF-RFP) mice and BAFF conditional knockout (cKO) lines we previously developed (10) to identify BAFF sources implicated in the development of auto-Abs in lupus. We crossed BAFF-RFP reporter mice with two spontaneous mouse lupus models: Tlr7 Tg mice (31–33) and Sle1 mice (34, 35). Mice overexpressing TLR7 develop pathogenic auto-Abs and an SLE-like disease (33). Tlr7 Tg mice also exhibit peripheral myeloid expansion, dependent on Type I IFN (IFN-I) signaling, a major BAFF inducer (10, 36, 37). Sle1 (B6.NZMc1) mice contain an SLE-susceptibility locus that mediates the loss of tolerance to nuclear Ags and generates a robust, spontaneous anti-chromatin auto-Ab response that peaks at 7-9 mo of age (35, 38). In addition, we used a pristane-induced lupus (PIL) model (39) as an inducible lupus system readily suitable for studies using our BAFF cKO lines. The hydrocarbon oil pristane induces an SLE-like renal disease, ectopic lymphoid tissues and auto-Abs by 6-8 mo p.i (39). In the PIL model, similarly to Tlr7 Tg mice, the SLE-like autoimmune phenotype is dependent on robust TLR7-mediated IFN-I production by inflammatory MOs (40, 41). In all three models, BAFF-RFP Tlr7 Tg mice, BAFF-RFP Sle1 mice, and pristane-treated BAFF-RFP mice, we observed increased BAFF serum levels, which correlated with increases in plasma cells (PCs) and auto-Ab production. BAFF-producing DCs, MOs and Nphs each expanded upon induction of autoimmunity, and each of these cellular sources of BAFF was required for the optimal induction of auto-Abs. However, DCs, MOs and Nphs regulated B cells and auto-Ab production through different mechanisms. DCs and MOs, but not Nphs, were required for the systemic production of BAFF and the maintenance of mature B cells in pristane-induce lupus. Our findings highlight how distinct BAFF sources may play different roles in the development of autoreactive B cells in lupus. The identification of specific BAFF-producing cells and niches implicated in SLE autoimmunity may be useful for designing new effective B cell targeted therapies.
Materials and methods
Mice
57BL/6J (WT) mice were purchased from Jackson Labs (Bar Harbor, ME). BAFF-RFP mice and Bafffl/fl mice (on the B6 background) were described previously (10). Briefly, BAFF-RFP reporter mice were generated by replacing a Tnfsf13b/Baff allele with a targeting construct expressing IRES-RFP. The Tnfsf13b/Baff is functionally knocked-out where the endogenous reporter expresses the RFP protein translated under the control of an IRES site. Thus, the BAFF-RFP signal is a measurement of BAFF expression and correlates with both Baff mRNA and BAFF protein expression (10). These heterozygous BAFF-RFP+/- (BAFF-RFP) mice express the RFP protein on one Baff allele and the WT Baff on the other. Tlr7.1 Tg mice and Sle1 mice (31–36) (provided by KBE). zDCCre, Cx3cr1Cre and Mrp8Cre mice were purchased from Jackson Labs (Bar Harbor, ME, USA). BAFF cKO mice where Baff is selectively deleted in either Nphs (Bafffl/fl Mrp8Cre), cDCs (Bafffl/fl zDCCre) or MOs (Bafffl/fl Cx3cr1Cre) were described previously (10). Briefly, a ~50% BAFF mRNA reduction was restricted to cDCs in Bafffl/fl zDCCre, and an ~85% reduction of BAFF mRNA was restricted to CX3CR1hi MOs in Bafffl/fl Cx3cr1Cre. In Bafffl/fl Mrp8Cre BAFF mRNA was selectively, but not specifically reduced in Nphs by 85-90%, as some reduction was observed in MOs too. However, our previous studies do not show any overlap in the phenotype of Bafffl/fl Mrp8Cre vs. Bafffl/fl Cx3cr1Cre mice (10, 11). All mice were age- and sex-matched for experiments and used at 8-12 wks of age. Mice were housed in a specific pathogen free environment; all procedures were approved by the University of Washington Institutional Animal Care and Use Committee.
Spontaneous lupus models
To generate BAFF-RFP expressing spontaneous lupus models, BAFF-RFP mice were crossed with Tlr7.1 Tg mice or Sle1 mice. Blood samples, spleens and bone marrows (BMs) were obtained from 6-8 months (mo) old BAFF-RFP Tlr7.1 mice and Tlr7.1 mice, and 7-9 mo BAFF-RFP Sle1 mice and Sle1 mice. WT mice and heterozygous BAFF-RFP controls were also included in the experiments.
Pristane induced lupus
To induce a lupus-like disease, 0.5 ml or 0.4 ml of pristane (2,6,10,14-tetramethylpentadecane, TMPD) (Sigma-Aldrich, St. Louis, MO, USA) was administered by i.p. injection into 2-3 mo old mice (40). Controls mice were left untreated (naïve). Blood samples were obtained before pristane treatment and after treatment at day 7, day 14, day 28 and monthly thereafter. For short-term experiments with BAFF-RFP mice, spleens and peritoneal exudate cells (PECs) were harvested 2 wks after treatment. For long-term experiments with BAFF cKO mice, spleens and BMs were harvested 6 mo after pristane injections; untreated (naïve) and pristane treated mice were 8-9 mo old at the time of tissue harvest. Spleens and BMs from 2-3 mo old BAFF cKO mice and Baff fl/fl mice were also harvested as controls at the time of injection.
Tissue harvest and cell isolation
Spleens, BM cells and PECs were harvested and cell suspension obtained as described previously (9, 10, 42). Briefly, spleens were removed and dissociated by enzymatic digestion at 37°CC with liberase TL and Dnase I (Roche, Indianapolis, IN, USA), followed by mincing the tissue between the ends of two frosted microscope glass slides to obtain a single cell suspension. BM cells were isolated by cutting one end of the femur and flushing BM cells out of the bone by centrifugation. After erythrocytes were lysed, splenocytes, BM cells and PECs from peritoneal lavage were filtered and processed for staining for flow cytometry.
Flow cytometry
Single cell suspensions obtained from spleens, BMs and PECs were incubated with Aqua Live-Dead fixable viability dye (Molecular Probes, Life Technologies, Waltham, MA, USA) in the absence of FBS, to discriminate dead cells. Cells were then blocked using an anti-FC receptor Ab (anti-CD16/CD32) (2.4G2) (BioLegend, (San Diego, CA, USA) and stained for surface markers and then fixed in 1-2% paraformaldehyde. Cells were stained with mAbs conjugated to FITC, allophycocyanin, eFluor450, allophycocyanin-eFluor780, PerCPCy5.5, PE-Cy7, AlexaFluor647, BUV395, BV605, BV421, BV711, BV650 and BUV395. For the analyses of splenic, PEC- and BM cell-subsets, nine- to twelve-colors flow cytometry was performed using combinations of mAbs against: CD19 (1D3), CD11b (M1/70), IgM (II/41), CD5 (53-7.3), and CD11c (N418) from eBioscience (San Diego, CA, USA); B220 (RA3-6B2), CD93 (AA4.1), CD38 (90), GL7 (GL7), CD138 (281-2) and Ly6C (AL-21) from BD Horizon/Biosciences (San Jose, CA, USA); CD19 (1D3), B220 (RA3-6B2), CD3 (17A2), NK1.1 (PK136), CD8α (53-6.7), Ly6G (1A8), SiglecH (440c), CD21/35 (7E9), CD23 (B3B4), IgD (11-26c.2a), CD93 (AA4.1) and CD24 (M1/69) from BioLegend (San Diego, CA, USA). The BAFF-RFP signal was detected in the PE channel. Cells were processed with an LSRII flow cytometer (Becton Dickinson, Franklin Lakes, NJ, USA) using a FACSDiva software and data were analyzed using FlowJo (v.10, Tree Star). The gating strategy used for splenic, PEC and BM myeloid cell populations and T cells was described previously (9, 10, 42). For splenic myeloid cells, B cells (CD19+CD3-) and T cells (CD19-CD3+) were gated out; non-B cells and non-T cells (CD19- CD3- gate) populations were defined as follows: Nphs, CD11bhiLy6GhiLy6CintSSCint-NK1.1-; MOs, CD11bhiSSC-Ly6G-NK1.1-; Ly6Clo MOs, CD11bhiLy6CloCD11c-SSC-Ly6G-NK1.1-; Ly6Chi MOs, CD11bhiLy6ChiCD11c-SSC-Ly6G-NK1.1-; Ly6Chi DCs, CD11bhiLy6ChiCD11chi SSC-Ly6G-NK1.1-; CD8+ cDCs, CD11chiCD8+B220-Ly6G-NK1.1-; CD8- cDCs, CD11chiCD8-B220-Ly6G-NK1.1-. In BM, myeloid cells were defined in B220-CD19-NK1.1-SiglecH-CD11b+ gate as follows: Nphs, Ly6GhiLy6CintSSCint-; Ly6Chi MOs, CD11c-CD115+CX3CR1+CCR2hi MHCII-Ly6G-SSC-; preDCs, CD11c+Ly6C-CD115+CX3CR1hiCCR2+MHCII+Ly6G-SSC-. BM T cells were defined as CD3+ CD19- in the NK1.1-SiglecH-CD11b- gate. In PECs Nphs were defined as CD19-B220-CD11bhiLy6GhiLy6CintSSCint-; other myeloid cells in the gate CD19-B220-CD11b+Ly6Glo-SSClo were defined as follows: Ly6Chi MO, Ly6Chi CD11c-; Ly6Chi Mph/DC, Ly6Chi CD11c+; Mph, Ly6Cint CD11clo; DC, Ly6Clo CD11chi.
Splenic B cells subsets were defined as described previously (9, 10, 43). After gating out debris, doublets and dead cells, B cell subsets in the CD19+ B220+ gate were defined as follows: Follicular (FO) B cells, CD24midCD21/35midCD93-CD23-; Marginal zone (MZ) B cells, CD24hiCD21/35hiCD93-CD23-; MZ B cell precursors (MZP), CD24hiCD21/35hiCD93loCD23+; Newly-formed transitional 2 (T2) B cells, CD24hiCD21/35int/hiCD93+CD23+; Newly-formed transitional 1 (T1) B cells CD24hiCD21/35loCD93+CD23-. Germinal center (GC) B cells were defined as CD19+ B220+CD38-GL7+. BM B cell precursors and PCs were gated as previously described (10). NK cells, pDCs and CD11b+ cells were gated out and in the NK1.1-SiglecH-CD11b- gate we defined PreProB cells as B220-CD43+CD19-; ProB cells as B220loCD43+CD19+; PreB cells as B220+CD43-CD19+IgM-IgD-; newly formed B cells (NFB) as B220+CD43-CD19+IgM+IgD-; mature B cells (Mature B) as B220+CD43-CD19+IgM+IgD+; PEC B cells were defined as B220+CD19+ (CD5-CD23+IgMloIgDhi) B cells and B1 B cells B220lo-CD19hiCD23-IgMhiIgDlo, subsequently subdivided in CD5+ B1a B cells and CD5- B1b cells. Splenic PCs, PEC PCs and long-lived PCs in the BM were defined as B220loCD138+.
Auto-Ab and BAFF ELISAs
Sera were isolated from blood, collected via the retro-orbital route. Serum levels of BAFF were determined using the Mouse BAFF/BLyS/TNFSF13B DuoSet ELISA kit (R&D systems, Inc., Minneapolis, MN) according to the manufacturer’s instructions. For specific auto-Ab ELISAs (44), 96 well Immuno plates (Nunc) were coated with: Sm/RNP (5μg/ml; Arotec Diagnostic Limited ATR01-10); yeast RNA (5μg/ml; Sigma R6750); calf thymus dsDNA (25μg/ml; Sigma-Aldrich D4522) or calf thymus histone (10μg/ml; Sigma-Aldrich H9250) diluted in 1X PBS and incubated overnight at 4°C. Prior to coating with yeast RNA and calf thymus histone, the plates were coated with Poly L Lysine (25μg/ml; Sigma P6516) for 2 h at RT or overnight at 4°C. Plates were then blocked for 2 h at RT with 1% BSA/PBS prior to addition of diluted serum for 2 h at RT. For ssRNA ELISA plates were blocked with 5% Goat serum/PBS for 2 h at RT and diluted serum were incubated overnight at 4°C. Specific Ab were detected using goat anti-mouse IgG Ab coupled with HRP (Southern Biotech, Birmingham, AL) and allowed to incubate for 2 h at RT. HRP activity was visualized using tetramethylbenzidine peroxidase (Bio-Rad Laboratories, Hercules, CA). Absorbance for all ELISA assays were measured at 450 nm with a Synergy plate reader (BioTek). For the standard curve, goat anti-mouse IgG (Southern Biotech, Birmingham, AL) was used for coating, and serial dilutions of recombinant IgG (Southern Biotech, Birmingham, AL) were used to calculate the absolute quantities of anti-SmRNP IgG and anti-ssRNA IgG. Serial dilutions of sera were used to determine the relative amount of anti-dsDNA and anti-Histone in different groups, measured by the OD at 450nm.
Statistical analyses
Timeline data were analyzed using a 2-way ANOVA with Tukey’s multiple comparison test. Analyses between more than two groups were performed using 1-way ANOVA with Holm-Sidak method for multiple comparisons. Analyses between two groups were performed using unpaired Student t test or Mann Whitney test. GraphPad Prism was used for statistical analyses. Differences of p<0.05 were considered significant.
Results
Induction of BAFF, autoantibodies and plasma cells in BAFF-RFP Tlr7.1 Tg mice and BAFF-RFP Sle1 mice
To investigate BAFF regulation and BAFF-producing cells in lupus prone mice, we crossed our BAFF-RFP reporter mice with Tlr7.1 Tg mice and Sle1 mice. Heterozygous BAFF-RFP+/- (BAFF-RFP) mice express lower BAFF levels, as one Baff allele expresses the RFP protein as a measurement of BAFF expression and the other allele expresses wild-type (WT) Baff (10, 11). However, BAFF-RFP still retain a reduced population of mature B cells, and thus can be used for functional studies of B cells and Ab responses (10, 11). We first assessed how either the Tlr7.1 Tg or the Sle1 background affected serum BAFF levels. Both 6-8 mo old Tlr7.1 Tg mice and the 7-9 mo old Sle1 mice had increased serum BAFF levels compared to age-matched WT control B6 mice, although the Tlr7.1 Tg mice had more pronounced increases in serum BAFF compared to the Sle1 mice (Figure 1A, upper panel). Similarly, BAFF levels in the sera of BAFF-RFP Tlr7.1 Tg mice and BAFF-RFP Sle1 mice were significantly up-regulated compared to BAFF-RFP control mice (Figure 1A, lower panel). As expected, since the heterozygous BAFF-RFP lines express half the normal levels of BAFF protein, they had about half the BAFF serum levels of the parental lines (Figure 1A).
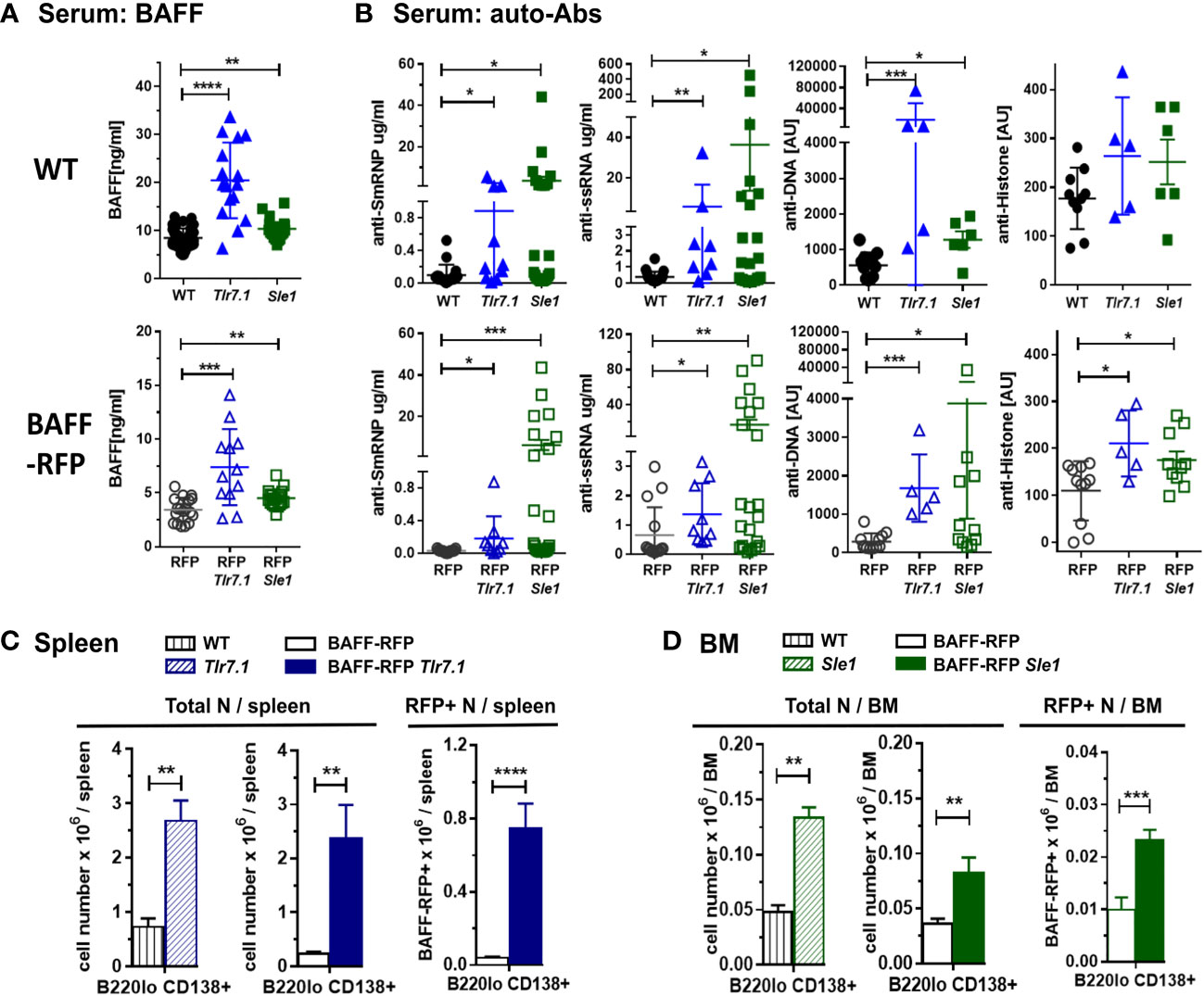
Figure 1 Induction of BAFF, auto-antibodies and PCs in BAFF-RFP Tlr7.1 mice and BAFF-RFP Sle1 mice. BAFF-RFP (BAFF-RFP+/-) mice were crossed with Tlr7.1 mice and Sle1 mice, 6-8 mo old Tlr7.1 genotypes and 7-9 mo old Sle1 genotypes were analyzed (A, B) Serum from WT, Tlr7.1 and Sle1 mice (upper panels) and BAFF-RFP, BAFF-RFP Tlr7.1 and BAFF-RFP Sle1 mice (lower panels) were collected and analyzed by ELISA for BAFF titers (A) and anti-SmRNP, anti-ssRNA, anti-DNA and anti-Histone auto-Abs (B). (A) graphs summarize BAFF data from more than three independent experiments (WT, Tlr7.1 and Sle1 mice: N=16-43; BAFF-RFP, BAFF-RFP Tlr7.1 and BAFF-RFP Sle1: N=12-19). (B) graphs summarize auto-Ab data from more than two independent experiments (WT, Tlr7.1 and Sle1 mice: N=5-20; BAFF-RFP, BAFF-RFP Tlr7.1 and BAFF-RFP Sle1: N=5-22). (C, D), Spleens from BAFF-RFP Tlr7.1, BAFF-RFP, Tlr7.1 and WT mice (C) and BM from BAFF-RFP Sle1, BAFF-RFP, Sle1 and WT mice (D) were harvested and analyzed by flow cytometry for B220lo CD138+ PC numbers. Total PC numbers in (C, D) left panels, and BAFF-RFP+ PC numbers in (C, D) right panels, are shown as bar graphs (mean ± SEM) and summarize data from three independent experiments (WT, Tlr7.1 and Sle1 mice: N=7-9; BAFF-RFP, BAFF-RFP Tlr7.1 and BAFF-RFP Sle1: N=9-13). Statistics were performed using one-way ANOVA with Holm-Sidak method for multiple comparisons tests (A, B), or two-tailed unpaired Student t test (C, D); *p<0.05, **p<0.01, ***p<0.001, ****p<0.0001.
Next, we tested whether the Tlr7.1 Tg or Sle1 background was sufficient to induce an autoimmune phenotype in the BAFF-RFP mice, even in the context of reduced mature B cells. As expected, Tlr7.1 Tg mice and Sle1 control mice had elevated titers of anti-SmRNP, -ssRNA and -dsDNA IgG auto-Abs compared to WT mice (Figure 1B, upper panels). Similarly, BAFF-RFP Tlr7.1 Tg mice and BAFF-RFP Sle1 mice had substantially increased serum auto-Ab levels compared to BAFF-RFP controls (Figure 1B, lower panels). Surprisingly, the mean levels of serum IgG auto-Abs in BAFF-RFP Sle1 mice were comparable to those in Sle1 mice. Furthermore, the fold increase in anti -SmRNP and -dsDNA IgG auto-Abs in BAFF-RFP Sle1 mice compared to BAFF-RFP mice was greater than in Sle1 mice compared to WT controls (SmRNP: 226 -fold in RFP Sle1/RFP in vs 37 -fold in Sle1/WT; dsDNA: 13.4-fold in RFP Sle1/RFP vs 2.3 -fold in Sle1/WT). Anti-histone IgG Abs were also up-regulated to a similar extent in BAFF-RFP Tlr7.1 Tg mice and Tlr7.1 Tg mice (Figure 1B).
Consistent with the increased auto-Ab levels, spleens of BAFF-RFP Tlr7.1 Tg mice had increased PC numbers compared to BAFF-RFP mice and were comparable to those of Tlr7.1 Tg mice (Figure 1C and Supplementary Figure S1A). PCs were not upregulated in the BM of either Tlr7.1 Tg mice or BAFF-RFP Tlr7.1 Tg mice (data not shown). Sle1 mice had expanded levels of PCs in the BM (Figure 1D and Supplementary Figure S1B), and to a lesser extent, in the spleen (Supplementary Figure S1C). We previously showed that PCs are also BAFF producers (10). BAFF-RFP PCs were also substantially expanded in the spleens of BAFF-RFP Tlr7.1 Tg mice (Figure 1C, right panel) and in the BM of BAFF-RFP Sle1 mice (Figure 1D, right panel). In summary, BAFF-RFP mice with either the Tlr7.1 Tg or Sle1 lupus genotype developed an autoimmune phenotype comparable to their parental line including higher systemic BAFF levels, expanded PCs in spleens and BMs, and increased anti-chromatin auto-Abs.
Regulation of BAFF-RFP expression in splenic and BM myeloid and lymphoid cells from BAFF-RFP Tlr7.1 and Sle1 mice
Next, we investigated how a Tlr7 or Sle1 background affected BAFF expression in myeloid and lymphoid subsets. Compared to controls, BAFF-RFP Tlr7.1 mice had increased BAFF expression in all splenic MO subsets and in CD8- DCs (Figures 2A, B) as well as in BM preDCs (Figure 2C). Interestingly, TLR7 overexpression did not affect BAFF expression in either splenic or BM Nphs, which constitutively express high BAFF levels (Figures 2A–C). Surprisingly, TLR7 overexpression also upregulated BAFF in splenic and BM T cells (Figures 2A–C) as well as in several splenic B cell subsets including B1 B cells (Figures 2D, E). The mature FO and MZ B cells and T2 B cells from BAFF-RFP Tlr7.1 mice had increased BAFF expression, while T1 B cells were not affected (Figure 2E). TLR7 overexpression, in addition to up-regulating BAFF expression on a per cell basis, induced the expansion of several BAFF-producing myeloid and lymphoid splenic cell populations (Figure 2F).
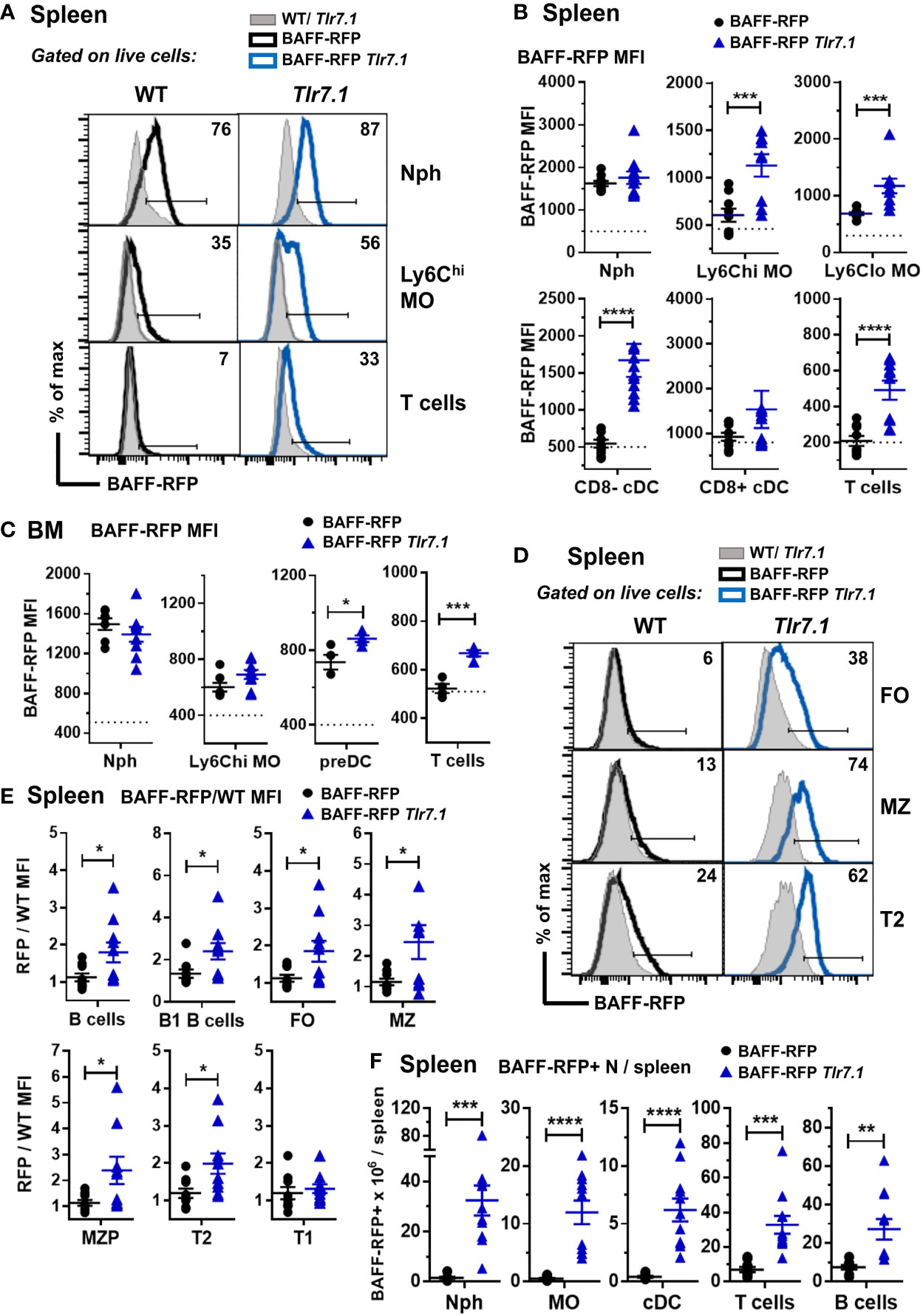
Figure 2 BAFF-RFP expression is upregulated in splenic and BM myeloid cells and in splenic T and B cells from BAFF-RFP Tlr7.1 mice. Spleens (A, B, D-F) and BM (C) from 6-8 mo old BAFF-RFP Tlr7.1, BAFF-RFP, Tlr7.1 and WT mice were harvested, and cell populations were analyzed by flow cytometry. For the gating strategy used for myeloid cells, B and T cells see Methods. (A-C, F), Nph, Ly6chi and Ly6Clo MOs, CD8- and CD8+ cDCs, DC precursors (preDC), T cells. (D–F), total CD19+ B220+ B cells, B1 B cells, FO B cells, MZ B cells, MZ precursors (MZP), T2 and T1 B cells. Data shown are representative histograms (A, D), or dot plot graphs showing mean ± SEM of RFP MFI (Median Fluorescence Intensity) (B, C, E), and numbers of BAFF-RFP+ cell populations per spleen (F), from three independent experiments (WT and Tlr7.1 mice: N=7-8; BAFF-RFP and BAFF-RFP Tlr7.1: N=9-11). In A and D numbers indicate % of BAFF-RFP+ cells. In B and C dotted lines show RFP MFI background in WT control. In E, data are shown as ratio of RFP/WT MFI due to the increased RFP signal background in Tlr7.1 vs WT B cells. Statistics were performed by one-way ANOVA with Holm-Sidak method for multiple comparisons; *p<0.05, **p<0.01, ***p<0.001, ****p<0.0001.
Myeloid and lymphoid cells were not as profoundly expanded in the Sle1 mice as in the Tlr7.1 Tg mice (45). However, similar to the BAFF-RFP Tlr7.1 mice, BAFF was up-regulated in splenic inflammatory Ly6Chi MOs and to a lower extent in T cells from BAFF-RFP Sle1 mice (Figures 3A, B). In contrast to the TLR7 Tg mice, splenic Nphs from BAFF-RFP Sle1 mice had lower BAFF expression per cell than Nphs from BAFF-RFP controls (Figures 3A, B), possibly due to Nph activation. Indeed, we and others have shown that upon activation, Nphs not only release BAFF from the intracellular stores but also down-regulate BAFF expression (11). cDC- and B cell-BAFF expression per cell did not change in the spleens of Sle1 autoimmune mice (Figure 3B). However, similar to TLR7 overexpression, Sle1 induced the expansion of several BAFF-producing lymphoid and myeloid cell populations, including Nphs (Figure 3C). Thus, in both Tlr7.1 Tg and Sle1 spontaneous SLE models, increased systemic BAFF levels and the development of autoimmunity were associated with a significant expansion of all the major BAFF-producing myeloid cell populations: Nphs, MOs, and cDCs.
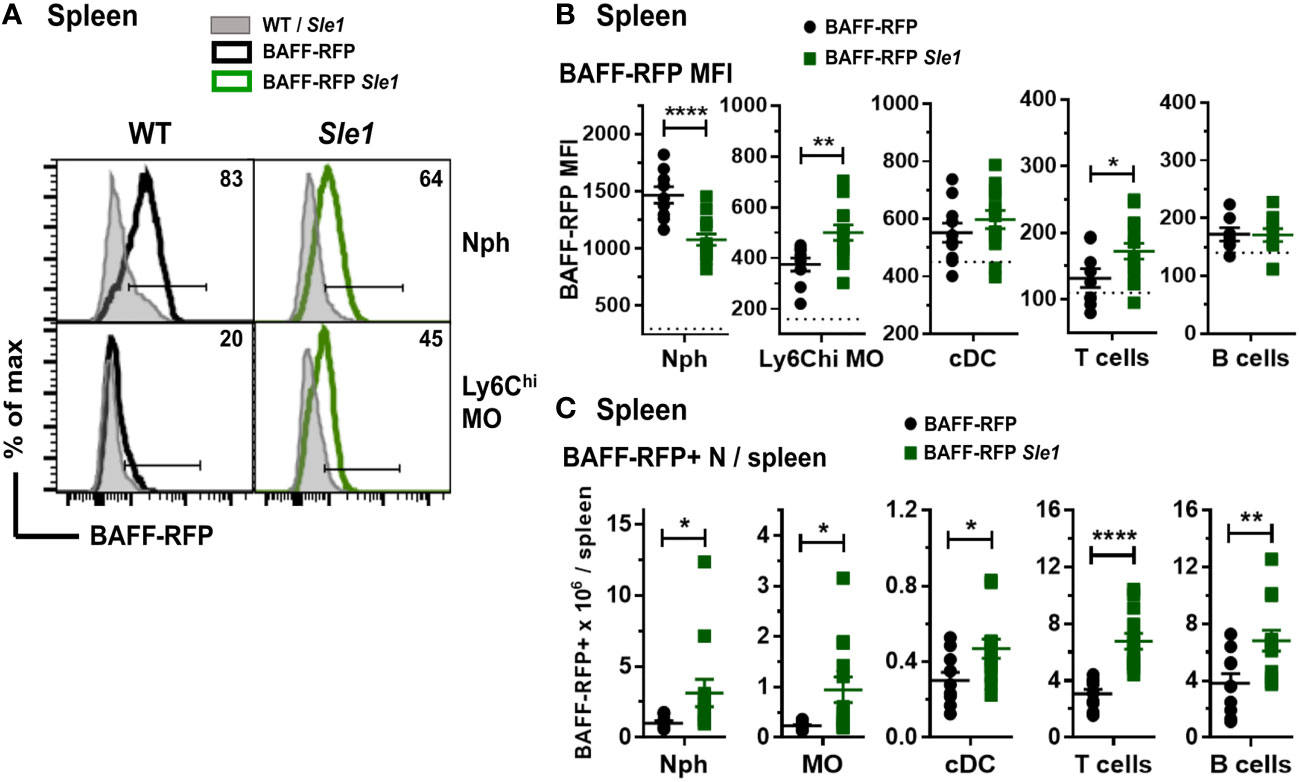
Figure 3 Changes in BAFF-RFP expression in splenic myeloid cells from BAFF-RFP Sle1 mice. Spleens from 7-9 mo old BAFF-RFP Sle1, BAFF-RFP, Sle1, WT mice were harvested, and cell populations were analyzed by flow cytometry. For gating strategy of splenic myeloid cells, B and T cells see Methods. Data shown are representative histograms (A), or dot plot graphs showing mean ± SEM of RFP MFI (B), and numbers of BAFF-RFP+ cell populations per spleen (C), from three independent experiments (WT and Sle1 mice: N=9; BAFF-RFP and BAFF-RFP Sle1: N=9-13). In A numbers indicate % of BAFF-RFP+ cells. In (B) dotted lines show RFP MFI background in WT control. Statistics were performed by one-way ANOVA with Holm-Sidak method for multiple comparisons; *p<0.05, **p<0.01, ****p<0.0001.
Increased serum BAFF and expansion of BAFF-RFP+ myeloid cells in the spleen and peritoneal cavity of pristane treated lupus-prone mice
In order to explore the effects of depletion of BAFF in specific immune cell types, we used a pristane-induced mouse model of lupus (10, 11). As with Tlr7Tg mice, the SLE-like autoimmune phenotype of PIL mice is dependent on IFN-I production and expansion of inflammatory myeloid cells (39). Pristane treatment of WT B6 mice induced an increase in serum BAFF levels that peaked at 30 days post-injection (Figure 4A, left panel). As with WT mice, BAFF-RFP mice up-regulated serum BAFF that peaked and doubled 30 days after pristane injection (Figure 4A, right panel). Since the BAFF-RFP mice express only one wild-type BAFF allele, constitutive and induced serum BAFF levels were lower in these mice than in WT mice (Figure 4A). As expected from previous studies in B6 mice (46), WT mice had a modest up-regulation of anti-SmRNP by 3-4 mo post pristane injection (Figure 4B). In pristane-treated BAFF-RFP mice, auto-Ab responses were delayed and lower than in WT mice, but still detectable and significantly different from BAFF-RFP untreated mice (Figure 4B). As previously reported (38), pristane did not induce anti-DNA or anti-histone auto-Abs in the B6 strain (data not shown). Reeves et al. showed that pristane injection induces recruitment of Nphs and inflammatory MOs to the peritoneal cavity and spleen, peaking two weeks post-injection (41). At this time point, BAFF-RFP expression was up-regulated in splenic MO subsets, but decreased in Nphs from BAFF-RFP mice, similar to what we detected in BAFF-RFP Sle1 mice (Figures 4C, D). Also, as in the spontaneous lupus models, BAFF+ Nphs and BAFF+ inflammatory MOs/DCs were expanded in the spleens of pristane-treated BAFF-RFP mice (Figure 4E). As expected, two weeks after pristane treatment Ly6Chi inflammatory MO and mature CD11c+ Mph/DCs were also expanded in the peritoneal cavity of BAFF-RFP mice, as well as in WT mice (Figure 5A). These inflammatory MOs and Mph/DCs also up-regulated BAFF-RFP expression on a per cell basis (Figures 5B, C), and together with BAFF-RFP+ Nphs, were substantially increased in numbers after pristane injection (Figure 5D).
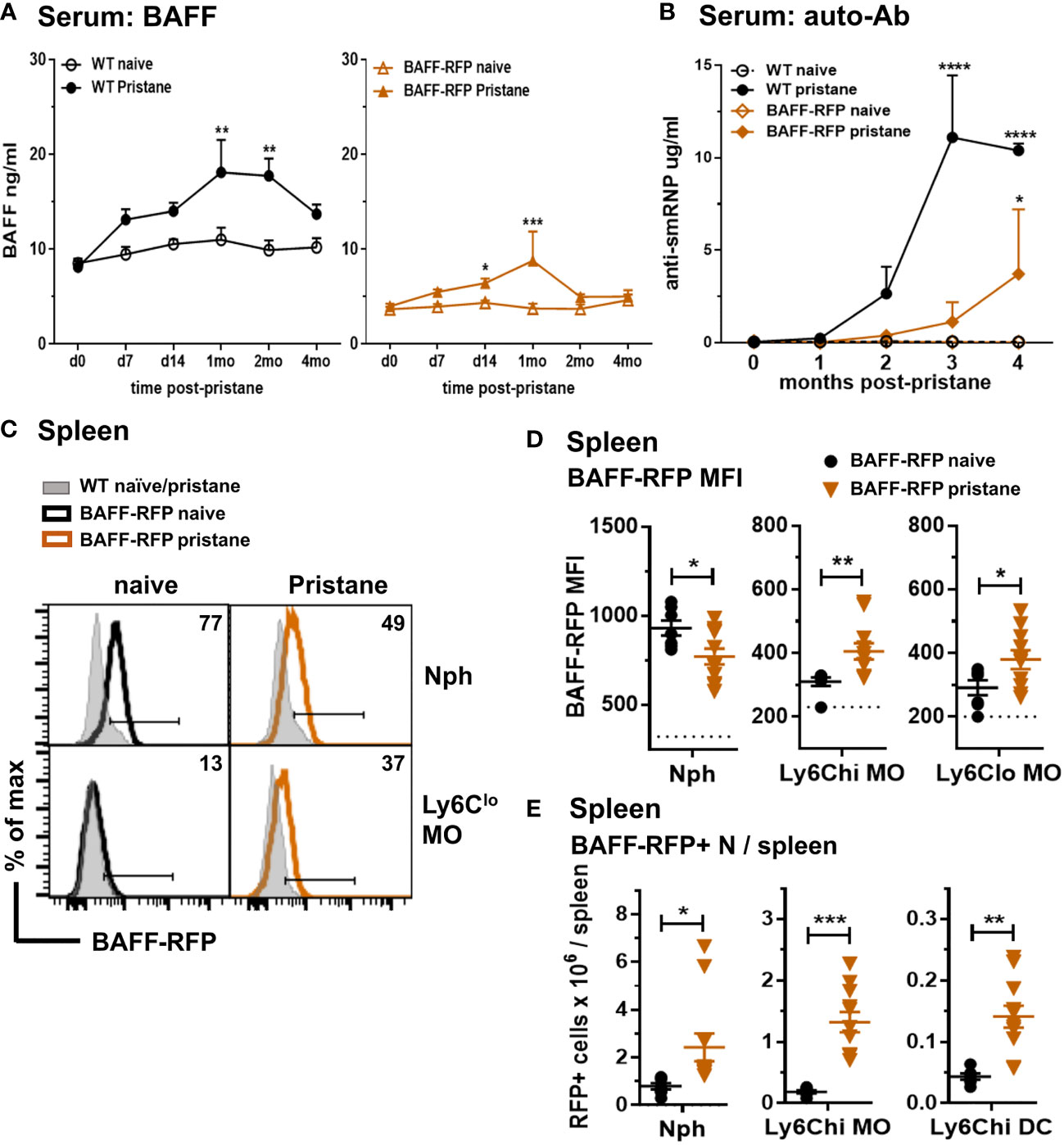
Figure 4 Increase in serum BAFF and auto-Abs and expansion of BAFF-RFP+ myeloid cells in spleen after pristane treatment. WT and BAFF-RFP mice were injected or not with 500µl pristane i.p. Serum was collected at the indicated time points, BAFF (A) and auto-Abs (B) were measured by ELISA. (A), BAFF titers in naïve and pristane-treated WT mice (left panel) and BAFF-RFP mice (right panel). (B), Anti-SmRNP IgG in pristane-treated WT and BAFF-RFP mice, naïve mice are shown as controls. In (A, B) graphs show representative data from one of two independent experiments with at least three mice per group. Statistics were performed using 2-way ANOVA with Tukey’s multiple comparison test; **p<0.01, ****p<0.0001. In (A, B) statistics shown are the comparison between pristane-treated and naïve mice in each group (WT pristane vs WT naïve and BAFF-RFP Pristane vs. BAFF-RFP naïve). In (C–E), two weeks after pristane injection spleens were harvested, and splenic cell populations were analyzed by flow cytometry. For gating strategy see Methods. Data are shown as representative BAFF-RFP histograms (C), or dot plots graphs showing mean ± SEM of RFP MFI (D), and numbers of BAFF-RFP+ cell populations per spleen (E) summarizing data from two independent experiments (naïve and pristane treated WT mice: N=4-6; naïve and pristane treated BAFF-RFP mice: N=7-11). In (C) numbers indicate % of BAFF-RFP+ cells. In (D) dotted lines show RFP MFI background in WT control. Statistics were performed by one-way ANOVA with Holm-Sidak method for multiple comparisons; *p<0.05, **p<0.01, ***p<0.001.
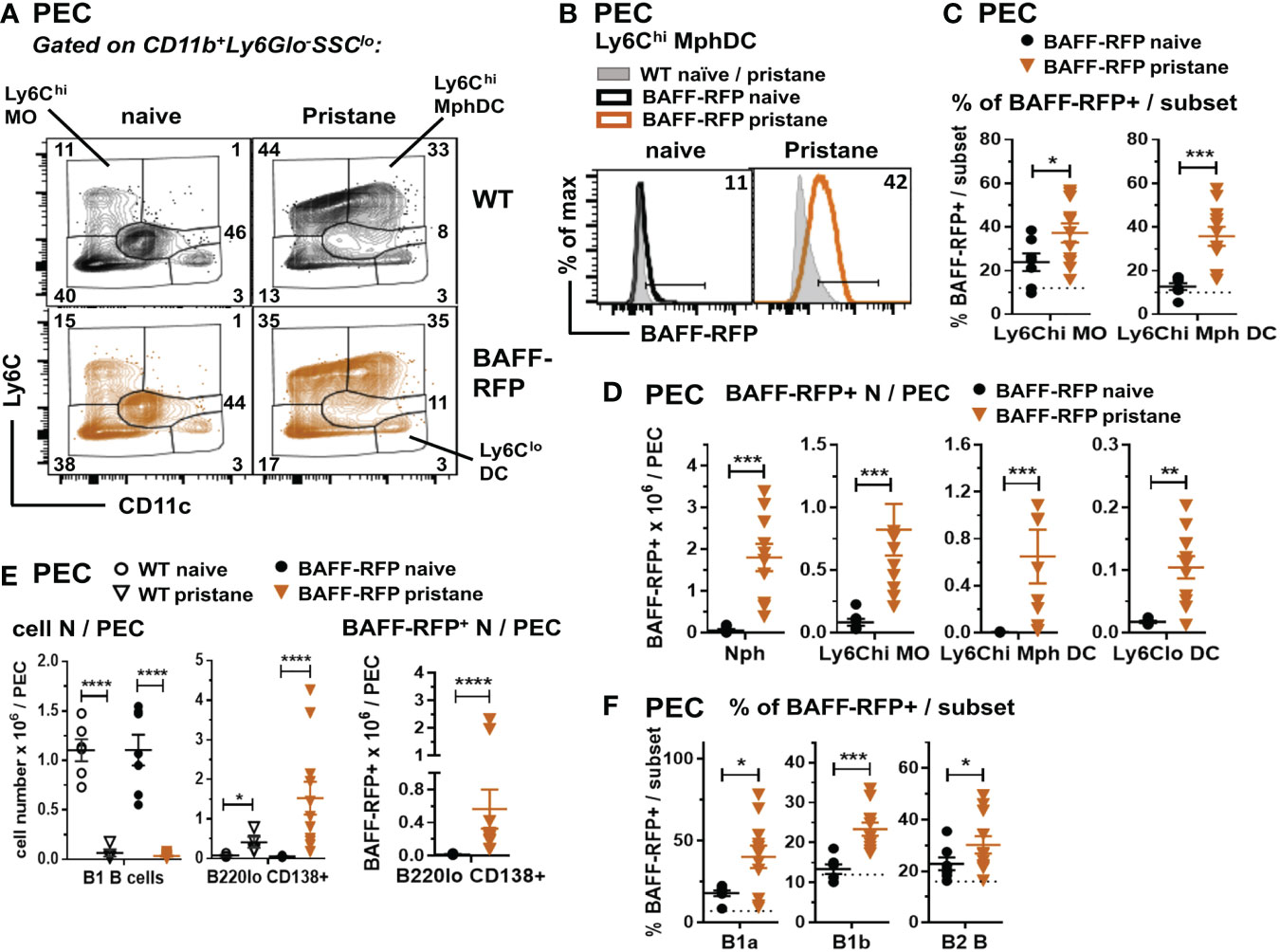
Figure 5 Expansion of BAFF-RFP+ Nphs and inflammatory MO/Mph/DCs and PCs in the peritoneal cavity after pristane injection. WT and BAFF-RFP mice were injected or not with 500µl pristane i.p.; two weeks later peritoneal cavity exudate cells (PEC) were harvested, and cell populations were analyzed by flow cytometry. For gating strategy of myeloid cells (A–D) and B cells (E, F) see Methods. Data are shown as representative dot plots (A), BAFF-RFP histograms (B), or dot plot graphs showing mean ± SEM of the % of BAFF-RFP+ cells per subset (C, F), numbers of BAFF-RFP+ cell populations per spleen (D, E left panel), and total numbers cell subsets per spleen (E left and central panel) summarizing data from two independent experiments (naïve and pristane-treated WT mice: N=4-6; naïve and pristane-treated BAFF-RFP mice: N=7-11). In (B) numbers indicate % of BAFF-RFP+ cells. In (C, F) dotted lines show RFP background in WT control. Statistics were performed by one-way ANOVA with Holm-Sidak method for multiple comparisons; *p<0.05, **p<0.01, ***p<0.001, ****p<0.0001.
Together with the significant expansion of myeloid populations, peritoneal B2 and B1 B cells are substantially reduced by pristane treatment (41). Interestingly, although B1 B cells were reduced in the peritoneal cavity of either WT or BAFF-RFP mice, PC numbers and BAFF-RFP+ PCs were expanded after pristane injection (Figure 5E). Furthermore, the remaining B1a and B1b B cells, and to a lesser extent B2 B cells, normally expressing low BAFF levels, upregulated BAFF-RFP expression upon pristane injection (Figure 5F). Thus, our data suggest that in PIL, as well as in the spontaneous lupus models (Figures 1C, D; 2D–F; 3C), autocrine and paracrine BAFF from PCs and other B cell subsets could also contribute to sustain B cells.
In summary, similar to the spontaneous lupus models, PIL induced the expansion of splenic and peritoneal BAFF producing -Nphs and -inflammatory MOs/DCs and an increase in systemic BAFF levels, together with the expansion of PCs and induction of auto-Abs.
BAFF from MOs and cDCs is required for homeostatic and pristane–induced systemic BAFF levels
Since myeloid cells have been implicated as the major producers of BAFF (10), and were expanded in spontaneous lupus models as well as in PIL, we asked whether deletion of BAFF in one of the major subpopulations of myeloid cells would influence BAFF levels and downstream effects in PIL. Surprisingly, already at the time of pristane injection (day 0) 2-3 mo old naïve Bafffl/fl zDCCre+ (BAFF cDC cKO) mice and Bafffl/fl Cx3cr1Cre+ (BAFF MO cKO) mice, but not Bafffl/fl Mrp8Cre+ (BAFF Nph cKO) mice had lower BAFF levels in sera compared to naive Baff fl/fl mice (Figures 6A–C and Supplementary Figure S2A). Over a period of 6 mo, only BAFF cDC cKO mice had significantly reduced BAFF levels compared to naive Baff fl/fl mice (Supplementary Figure S2A). Furthermore, while pristane treatment of Baff fl/fl control mice induced a sustained increase in serum BAFF levels, it did not up-regulate systemic BAFF levels in BAFF cDC cKO mice for up to 6 mo post-injection (Figure 6A and Supplementary Figure S2B). In mice lacking BAFF in MOs, serum BAFF slightly increased in the first few months post-pristane injection but was significantly lower than in Baff fl/fl control mice over the course of the experiment (Figure 6B and Supplementary Figure S2C). In contrast, BAFF Nph cKO mice initially up-regulated systemic BAFF levels to a similar extent as Baff fl/fl control mice, but at 3 and 6 mo post-pristane injection BAFF was significantly lower in these mice than in the controls (Figure 6C and Supplementary Figure S2C). Serum BAFF levels in zDCCre+, Cx3cr1Cre+ and Mrp8Cre+ control mice were similar to Baff fl/fl mice (Supplementary Figure S2D). Therefore, cDC-BAFF is required to sustain homeostatic levels of BAFF in the serum, while BAFF from either MOs or cDCs is required for pristane-induced systemic BAFF levels. Although Nphs are major BAFF producers (10), Nph-BAFF is not required to sustain homeostatic BAFF levels in the serum, but does contribute to the upregulation of serum BAFF at later stages of pristane-induced autoimmunity.
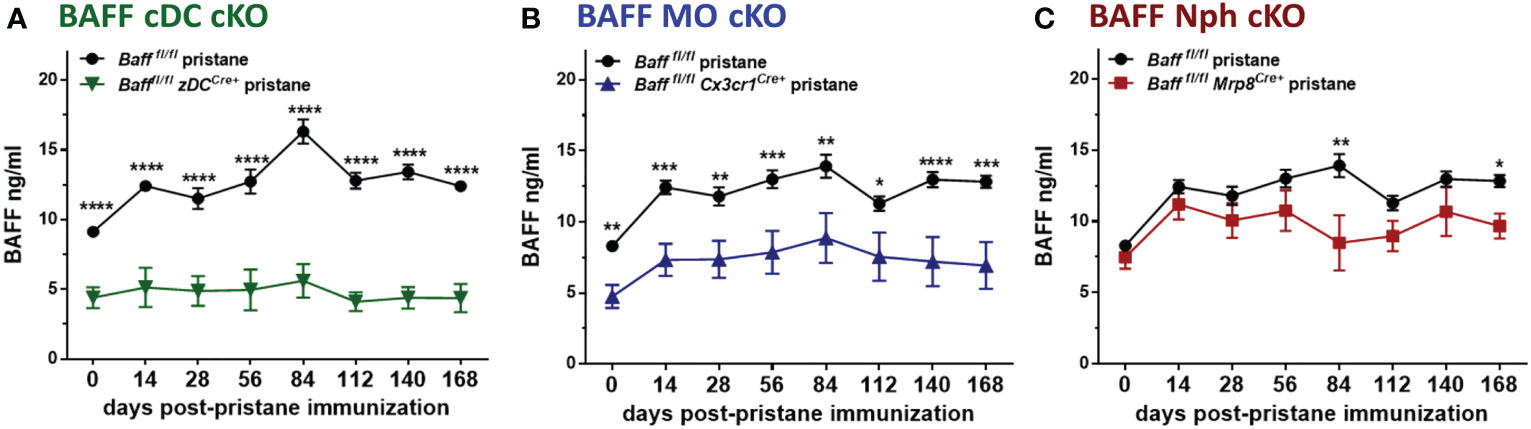
Figure 6 BAFF from MOs and cDCs is required to maintain homeostatic and pristane–induced systemic levels of BAFF. (A-C), Bafffl/fl, (A) Bafffl/fl zDCCre+ mice (BAFF cDC cKO), (B) Bafffl/fl Cx3cr1Cre+ mice (BAFF MO cKO) and (C) Bafffl/fl Mrp8Cre+ mice (BAFF Nph cKO) were treated for 6 mo with 400µl pristane. At the indicated time points sera were collected and BAFF levels were measured by ELISA. Graphs summarize data from two to three independent experiments with three or more mice per group (Bafffl/fl, N=6-21; Bafffl/fl zDCCre+, N=6-14; Bafffl/fl Cx3cr1Cre+, N=13-18; Bafffl/fl Mrp8Cre+, N=9-10). Statistics were performed using 2-way ANOVA with Tukey’s multiple comparison test. *p<0.05, **p<0.01, ***p<0.001, ****p<0.0001.
BAFF from DCs and MOs skews MO differentiation toward a more inflammatory Ly6Chi MO during long-term pristane treatment
We next analyzed whether selective depletion of BAFF from DCs, MOs or Nphs altered splenic myeloid and B cell populations. Unexpectedly, we found that BAFF depletion from cDCs or MOs, but not Nphs, affected the phenotype of inflammatory Ly6Chi MO in the spleen (47–49). Specifically, splenic Ly6Cint/hi MOs from either 8-9 mo-old untreated or pristane-treated BAFF cDC cKO mice had lower Ly6C MFI levels compared to those from Baff fl/fl mice (Figures 7A–D). The same phenotype was observed in mice lacking BAFF in MOs, but only after pristane injection (Figures 7A–D). In contrast to older mice, Ly6Cint/hi MOs from 2-3 mo old mice were not significantly different in BAFF cDC cKO mice, BAFF MO cKO mice and Baff fl/fl mice (data not shown). No changes in Ly6C MFI occurred in Ly6Cint/hi DCs (CD11c+) from BAFF cDC cKO and BAFF MO cKO mice (Figures 7B, D), suggesting that lack of BAFF production by cDCs or MOs, skewed MO differentiation toward a Ly6Cint MO/Mph subset rather than MO-derived DCs. Thus, in an autoimmune setting, DC-BAFF and MO-BAFF were required to maintain high expression of Ly6C in inflammatory MOs.
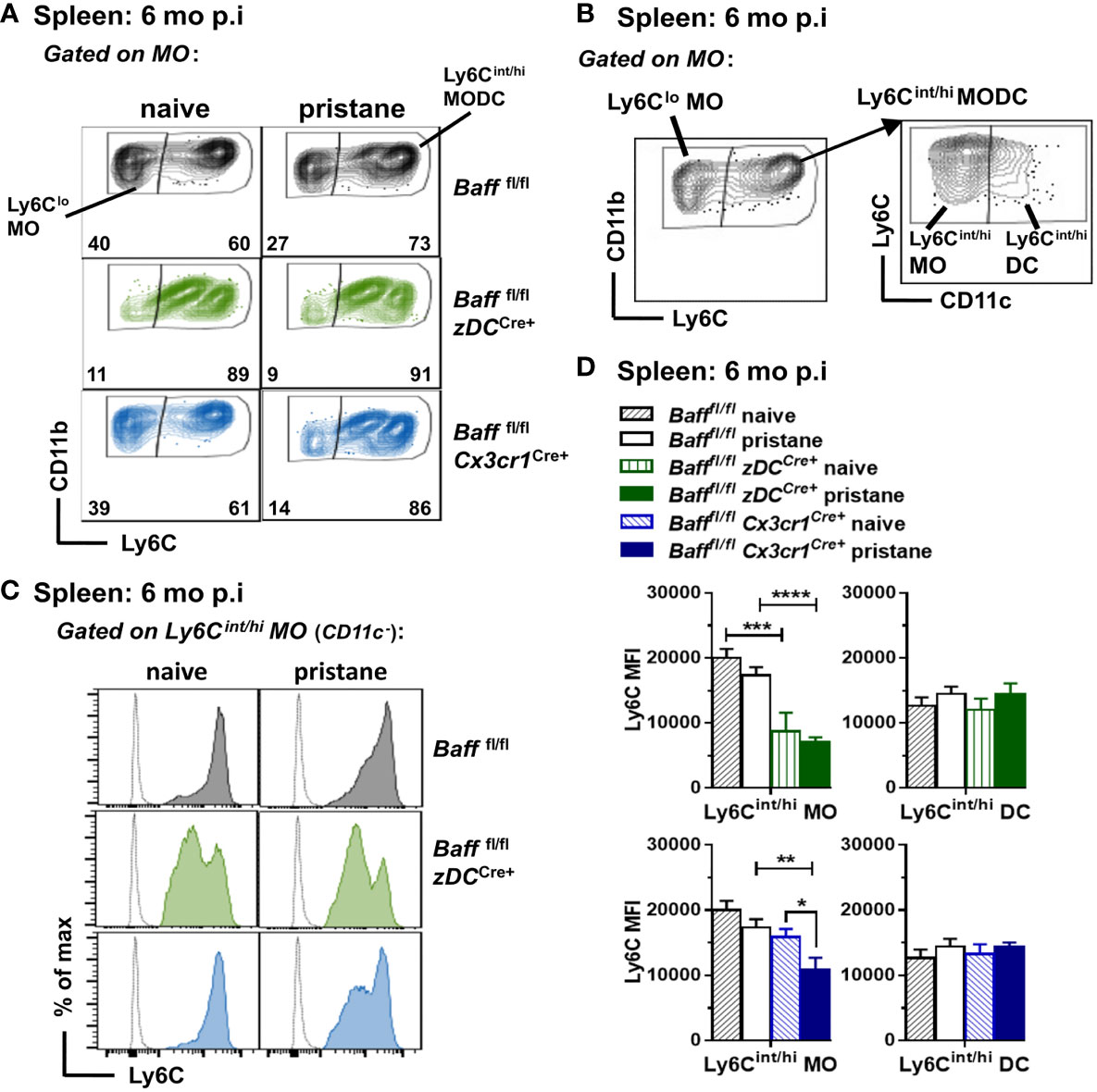
Figure 7 BAFF from DCs and MOs after pristane injection skews MO differentiation toward Ly6Chi inflammatory MOs. Bafffl/fl, Bafffl/fl zDCCre+ mice (BAFF cDC cKO) and Bafffl/fl Cx3cr1Cre+ mice (BAFF MO cKO) were injected or not with 400µl pristane; 6 mo later splenic MO subsets were analyzed by flow cytometry (see Methods for gating strategy). (A), Representative dot plots of MO subsets in all the groups. (B), Representative dot plots for MO subsets gating strategy to identify Ly6Cint/hi MO and MO-derived DCs. (B), left panel shows Ly6Clo MO and Ly6Cint/hi MODC in the MO gate (CD19- CD3- NK1.1- CD11bhi Ly6G- SSC-Hlo); right panel shows Ly6Cin/hi MOs (CD11c-) and Ly6Cint/hi DCs (CD11c+) in the Ly6Cint/hi MODC population. (C), shows representative Ly6C histograms of Ly6Cin/hi MOs (CD11c-). (D), shows bar graphs of Ly6C MFI in Ly6Cin/hi MO and Ly6Cint/hi DC subsets, summarizing data from two to three independent experiments (Bafffl/fl naive, N=8; Bafffl/fl pristane, N=18; Bafffl/fl zDCCre+ naive, N=3; Bafffl/fl zDCCre+ pristane, N=10; Bafffl/fl Cx3cr1Cre+ naive, N=4; Bafffl/fl Cx3cr1Cre+ pristane, N=12). In (A) numbers indicate % of cells in Ly6Clo MO and Ly6Chint/hi MODC subsets. Statistics were performed by one-way ANOVA with Holm-Sidak method for multiple comparisons; *p<0.05, **p<0.01, ***p<0.001, ****p<0.0001.
BAFF from MOs and cDCs is required to sustain mature B cell pools in spleen and BM in pristane-treated mice
We then investigated whether the absence of BAFF-producing MOs, DCs or Nphs affected splenic B cell levels in pristane-induced lupus-prone mice. Before pristane injection, B cell subsets in spleens of 2-3 mo old mice lacking BAFF from DCs, MOs and Nphs were not significantly different from control Baff fl/fl mice, although a trend toward a decrease in FO and MZ B cells was observed in BAFF cDC cKO mice (Supplementary Figure S3A and data not shown). Thus, the lower BAFF serum levels observed in BAFF cDC cKO mice and BAFF MO cKO mice before pristane injection (Figure 6) did not affect mature splenic B cells homeostasis, confirming previous findings using BM chimeras (50). In the long-term (6 mo) pristane experiments we left some mice untreated (naïve) as controls. In contrast to the younger mice, these 8-9 mo old naïve mice lacking BAFF in cDCs had lower numbers of mature B cells and PCs than untreated Baff fl/fl mice (Figure 8A). Specifically, when BAFF was missing in cDCs, spleens had lower numbers of FO, MZ and MZP B cells, while newly formed T2 and T1 B cells were unchanged (Figure 8A, lower panels). Like older naive mice, pristane-treated BAFF cDC cKO mice had lower mature B cell and PC numbers compared to Baff fl/fl mice (Figure 8A and Supplementary Figure S3B). However, in addition to mature B cells, pristane-treated BAFF cDC cKO mice had fewer T2 B cells and CD11c+ B cells than Baff fl/fl mice, indicating that in pristane-treated mice the lack of BAFF derived from cDCs more broadly affected B cell numbers.
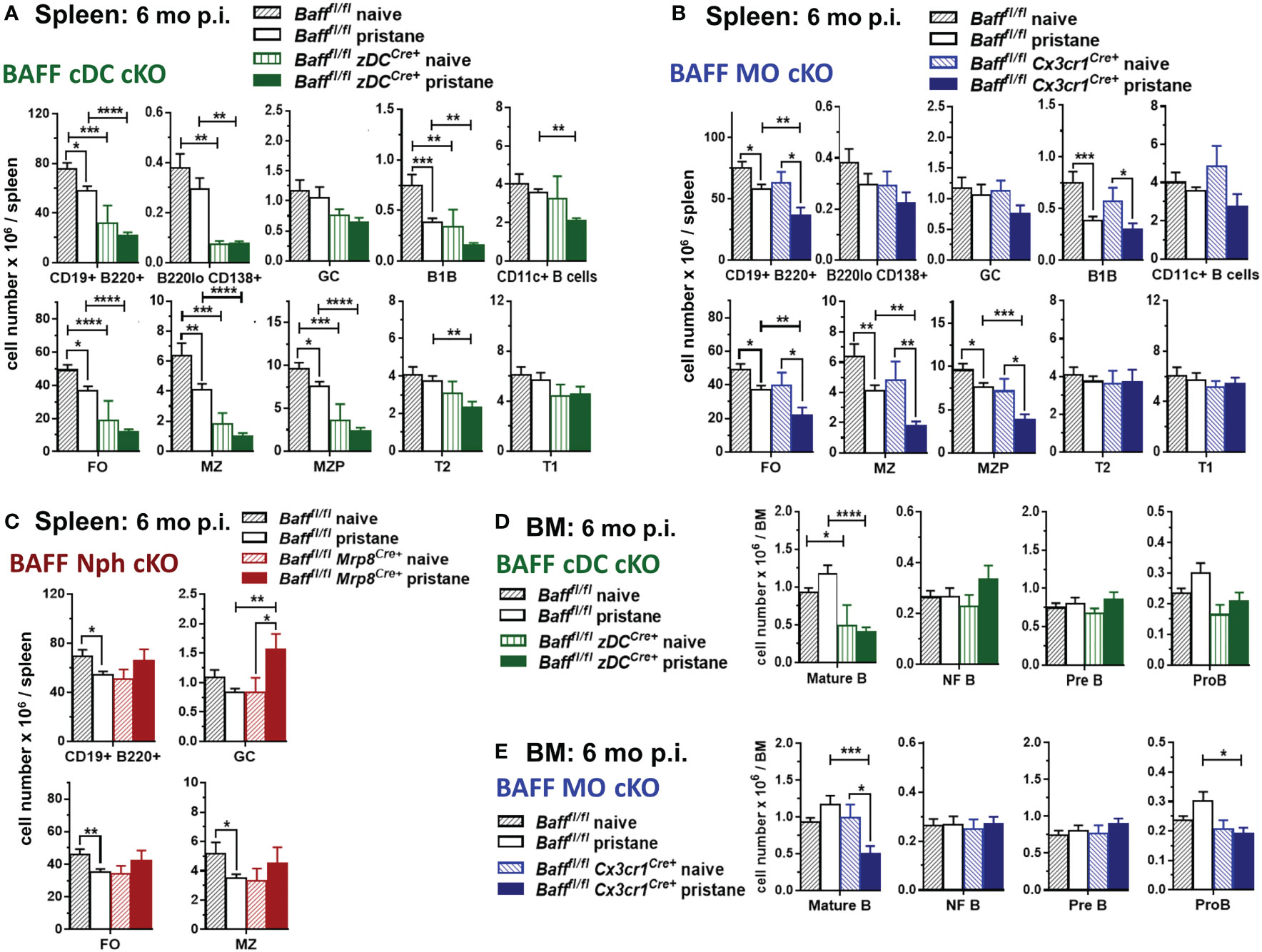
Figure 8 BAFF from cDCs and MOs is required for long-term maintenance of mature splenic and BM B cells after pristane treatment. Bafffl/fl mice, Bafffl/fl zDCCre+ mice (BAFF cDC cKO) (A, D), Bafffl/fl Cx3cr1Cre+ mice (BAFF MO cKO) (B, E) and Bafffl/fl Mrp8Cre+ mice (BAFF Nph cKO) (C) were injected or not with 400µl pristane; 6 mo later splenic B cell subsets (A-C) and BM B cell precursors (D, E) were analyzed by flow cytometry. For gating strategy of B cell populations see Methods. Graphs summarize data from two to three independent experiments, Bafffl/fl naive, N=8; Bafffl/fl pristane, N=18; Bafffl/fl zDCCre+ naive, N=3; Bafffl/fl zDCCre+ pristane, N=10; Bafffl/fl Cx3cr1Cre+ naive, N=4; Bafffl/fl Cx3cr1Cre+ pristane, N=12; Bafffl/fl Mrp8Cre+naive, N=2; Bafffl/fl Mrp8Cre+pristane, N=9. Statistics were performed by one-way ANOVA with Holm-Sidak method for multiple comparisons; *p<0.05, **p<0.01, ***p<0.001, ****p<0.0001.
In contrast to mice lacking BAFF in cDCs, naïve 8-9 mo old BAFF MO cKO mice had similar numbers of splenic B cells as naïve Baff fl/fl mice (Figure 8B). However, BAFF from MOs was required to maintain normal mature B cells numbers after pristane injection (Figure 8B and Supplementary Figure S3B). Lack of BAFF from Nphs did not significantly affect FO or MZ B cells in either 8-9 mo naïve mice or pristane-treated mice (Figure 8C and Supplementary Figure S3B). Interestingly, pristane-treated BAFF Nph cKO mice had increased numbers of GC B cells compared to Baff fl/fl mice, suggesting that Nph-derived BAFF may affect the generation of GC B cells, induced by pristane (Figure 8C).
Since cDC-derived BAFF contributed to systemic BAFF levels in naive mice independently of age, and it was required to maintain splenic mature B cells only in older mice, we examined whether BAFF produced by cDCs played a role in mature B cell homeostasis in the BM. BMs from either younger or older, as well as pristane-treated, BAFF cDC KO mice had lower numbers of mature B cells, but similar numbers of B cell precursors, compared to Baff fl/fl mice (Figure 8D and Supplementary Figure S4). MO-derived BAFF also contributed to mature B cell homeostasis in the BM of 2-3 mo old mice (Supplementary Figure S4). In long-term experiments, the absence of BAFF-producing MOs affected BM mature B cells only after pristane treatment, similarly to what we observed in the spleen (Figure 8E). BMs from either younger naïve or pristane-treated BAFF MO cKO mice had lower numbers of Pro-B cells, suggesting a possible role for MO-BAFF, but not cDC-BAFF, in earlier stages of B cell development (Figure 8E and Supplementary Figure S4). In contrast, Nph-derived BAFF did not contribute to mature B cell homeostasis in the BM of pristane treated mice (data not shown).
Taken together our data from Figures 6, 8 highlight how different myeloid BAFF sources play overlapping, but also, specific roles in supporting systemic BAFF and B cell homeostasis in an inducible lupus-like setting. First, in younger mice cDC-derived and MO-derived BAFF are required to maintain systemic BAFF levels and mature B cell homeostasis in the BM. Second, BAFF produced by cDCs is also required to sustain systemic BAFF and mature B cell homeostasis in the spleen and BM of older mice. Third, after induction of autoimmunity in PIL, cDCs and MOs are major cellular BAFF sources driving the up-regulation of systemic BAFF and sustaining mature B cells in the spleen and BM. Finally, Nph-derived BAFF does not play a major role in splenic mature B cell homeostasis but contributes to the increase in pristane-induced systemic BAFF and regulates GC B cell numbers during PIL.
Nphs, MOs and cDCs are all BAFF sources required for the induction of anti-SmRNP IgG autoantibodies in pristane–induced lupus mice
Finally, we tested whether the decrease in systemic BAFF levels in BAFF cDC cKO mice and BAFF MO cKO mice affected the auto-Ab responses induced by pristane. Anti-SmRNP IgG auto-Abs were induced in Baff fl/fl mice and peaked 4-6 mo after pristane injection; however, when BAFF was absent from either cDCs or MOs, the auto-Ab responses were substantially reduced (Figures 9A, B). Surprisingly, even though systemic levels of BAFF, mature B cells and PCs were normal in mice lacking Nph-BAFF, pristane-induced anti-SmRNP IgG was significantly lower in these mice compared to Baff fl/fl control mice (Figure 9C). Serum levels of auto-Abs were not reduced in all the corresponding control Cre mice (data not shown). These data suggest that although BAFF-producing Nphs may have a more localized effect on B cells, they still play a significant role in promoting auto-Ab production in this model. In conclusion, BAFF produced from three myeloid subsets- cDCs, MOs. and Nphs- is required for the induction of auto-Abs in a lupus-like autoimmune model.
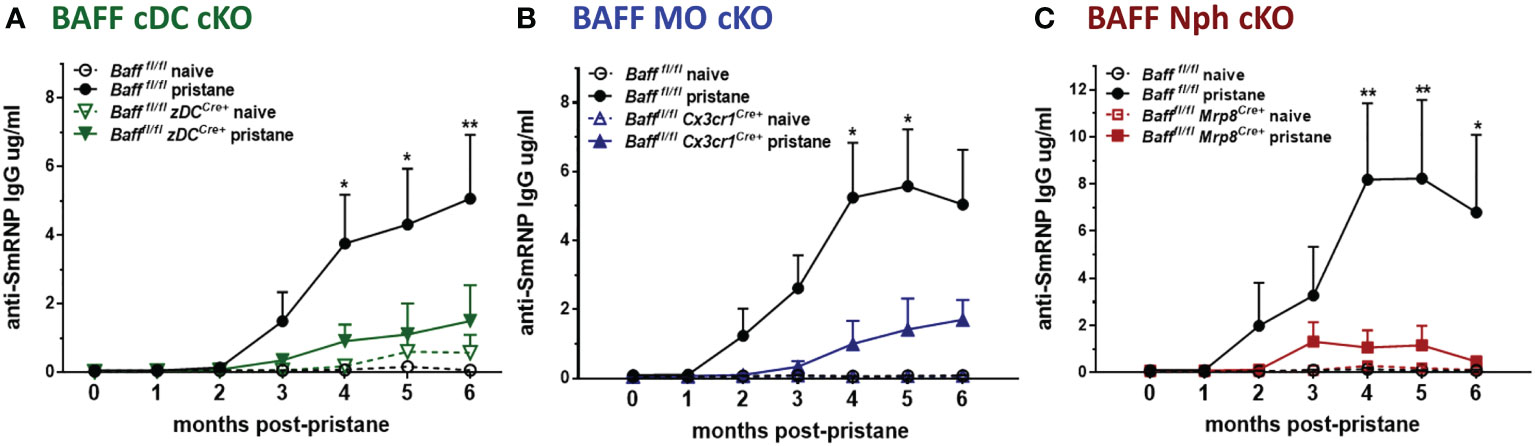
Figure 9 BAFF from Nphs, MOs and cDCs is required for the induction of pristane–induced anti-smRNP antibodies. Bafffl/fl, Bafffl/fl zDCCre+ mice (BAFF cDC cKO) (A), Bafffl/fl Cx3cr1Cre+ mice (BAFF MO cKO) (B) and Bafffl/fl Mrp8Cre+ mice (BAFF Nph cKO) (C) were treated or not for 6 mo with 400µl pristane. At the indicated time points sera were collected and anti-SmRNP Abs were measured by ELISA. Graphs summarize data from two to three independent experiments with three or more mice per group (Bafffl/fl naïve, N=4-6; Bafffl/fl pristane, N=10-19; Bafffl/fl zDCCre+ naïve, N=3; Bafffl/fl zDCCre+ pristane, N=9; Bafffl/fl Cx3cr1Cre+naïve, N=4; Bafffl/fl Cx3cr1Cre+ pristane, N=10; Bafffl/fl Mrp8Cre+ naïve, N=2; Bafffl/fl Mrp8Cre+pristane, N=9). Statistics were performed using 2-way ANOVA with Tukey’s multiple comparison test; *p<0.05, **p<0.01. Statistics shown are comparison between pristane treated Bafffl/fl mice and pristane treated BAFF cKO mice.
Discussion
BAFF plays an essential role in the pathogenesis of SLE and other autoimmune diseases. Since the anti-BAFF Ab, Belimumab, was approved for the treatment of SLE, several new drugs targeting different forms of BAFF or the BAFF/APRIL axis are being developed (26, 29, 30). However, still little is known about how, when, and where BAFF regulates the development of autoreactive B cells (15, 27–29, 51). In the current study, using novel BAFF-RFP reporter mice, BAFF cKO mice and both spontaneous as well an inducible mouse lupus-models, we defined cDC-, MO- and Nph-BAFF as major BAFF sources regulating specific B cell niches. Each of these cellular sources was required for the full development of SLE auto-Ab responses in an inducible lupus-model.
We and others have identified BAFF produced by cDCs and MO-derived DCs as essential for B cell and Ab responses in secondary lymphoid organs (10, 11, 42, 52, 53).
Previous studies described peripheral myeloid cells expansion in Tlr7 Tg mice but did not address BAFF expression (36, 45). Utilizing heterozygous BAFF-RFP reporter mice, which when crossed with Tlr7.1 Tg or Sle1 mice spontaneously developed a lupus-like phenotype, we found that both mouse models had expanded splenic BAFF+-Nph, -MOs and -cDCs. Furthermore, BAFF expression was up-regulated on a per cells basis in inflammatory MOs in both Tlr7 Tg and Sle1 mice, while CD8- DCs increased BAFF expression only in the Tlr7 Tg model. In addition to myeloid cells, T cells upregulated BAFF expression and BAFF+ T cells were expanded in both models. Although BAFF expression in T cells has been suggested in CD4 T cells in human SLE (54–56), our results are the first clear demonstration that BAFF expression in T cells may be as high as in myeloid cells in mouse lupus. BAFF+ B cells were expanded in the TLR7 Tg mice, and BAFF expression per cell increased in mature B cell subsets. BAFF+ PCs and other BAFF+ B cells in the peritoneal cavity were expanded in PIL These findings agree with other studies linking the elevated expression of BAFF in B cells in mouse lupus-models and SLE patients and the production of auto-Abs (57, 58). The ability of B cells to produce autocrine BAFF and sustain their own survival could play an important role in Type-I IFN and TLR7-driven autoimmunity. The exact contribution of BAFF+ T or B lymphocyte populations in the development or exacerbation of autoimmunity needs to be further explored.
Very few studies have investigated the production of tissue or serum BAFF in the PIL model (59–62). Here, we determined that serum BAFF is up-regulated and peaks at one month post-pristane injection. In PIL BAFF was elevated in multiple myeloid cell populations similar to the two spontaneous models. The reduced transcription of BAFF in splenic Nphs from pristane-treated BAFF-RFP mice, as well as BAFF-RFP Sle1 mice may be due to Nph activation as in a Salmonella infection model (11). Nphs constitutively store high levels of BAFF that is released upon activation and drives PC responses (63, 64). Pristane-induced expansion of BAFF-expressing Nphs, MOs and DCs, similar to the spontaneous lupus models, was associated with increased BAFF serum levels, PC’s expansion, and induction of auto-Abs. MO recruitment, IFN-I induction and auto-Ab production in PIL all require TLR7/Myd88 signaling (40, 65). The inhibition of TLR7-mediated activation and BAFF production by DCs protects from PIL (60). Thus, in a lupus model where the TLR7/IFN-I axis is implicated in the development of autoimmunity, BAFF-producing DCs, MOs and Nphs each contribute to the induction of auto-Abs.
All pristane-treated mice in this study developed lipogranulomas (not shown), indicating disease development (65). However, we could not conclusively determine quantitative differences between the groups on lipogranuloma formation. The PIL model on the B6 background has some limitations (39); pristane-treated B6 mice do not develop robust anti-chromatin auto-Ab responses and develop very mild glomerulonephritis (60, 62). Even though renal function was not examined in this study, previous reports have suggested that all three myeloid BAFF sources studied here may be involved in the regulation of IgG deposits in the kidney (60, 62, 66). While BAFF overexpression can efficiently amplify underlying predisposition to clinical disease, it may not be sufficient to drive the development of severe glomerular pathology in mice (1, 67, 68). Therefore, it will be of future interest to test whether the deletion of any of these specific myeloid BAFF sources could improve renal disease in other more robust lupus-prone mice models.
While all three myeloid BAFF sources were required for the induction of auto-Abs, each subset affected systemic BAFF levels and mature B cells pools differently. For example, while either MO-BAFF or cDC-BAFF was required to maintain systemic BAFF levels and mature B cell homeostasis in the BM of younger mice, only BAFF produced by cDCs was required for mature B cell homeostasis in older mice. DC-BAFF was required to sustain mature splenic B cells only in older untreated or pristane treated mice, while MO-BAFF affected the B cell pool only after the induction of autoimmunity. Further studies are needed to assess the involvement of myeloid-derived BAFF in regulating B cell homeostasis during aging, which could also play a role in the development of autoimmunity (69).
Nph-BAFF, in contrast to cDC-BAFF and MO-BAFF, did not play a major role in determining systemic BAFF levels or FO and MZ B cell homeostasis. Using long-term neutrophil-depletion, Coquery et al. reported that Nphs contribute to excess serum BAFF levels and B cell responses in a congenic lupus-prone mouse model (70). In contrast, in PIL mice we found that Nph-BAFF was required for auto-Ab production, but only partially supported normal BAFF serum levels. This difference could be due to differences in the two models used or could reflect the possibility that Nph-depletion independently affects other BAFF-sources. Surprisingly, conditional BAFF depletion from Nphs increased GC B cell numbers in PIL, implying that Nph-BAFF may play a role in regulating GC formation. Consistent with our finding, Nph depletion early in lupus resulted in a striking acceleration in the onset of lupus renal disease and GC formation in secondary lymphoid organs (71). Given the complex role of Nphs in lupus (66, 72), the effect of Nph-BAFF on GC, serum BAFF and auto-Abs in PIL mice can be interpreted in the context of different disease kinetics and the localized effects of Nph-BAFF in specific B cell niches. For example, although Nphs play a major role in extrafollicular (EF) humoral responses, they can also localize in T-cell zones and interact with GC B cells in lupus-prone mice (70, 73). Both EF and GC-dependent B cell activation pathways contribute to pathogenic auto-Ab production in SLE, and clinical data suggest that these two pathways maybe responsible for some heterogeneity in human SLE patients (28). Thus, a possible explanation for our findings is that Nph-derived BAFF may skew toward EF B cell responses, away from GC formation. Our findings are consistent with a growing number of studies suggesting that a BAFF-producing Ly6Glo Nph subset supports Ag-specific humoral responses in lupus as well as after microbial infections (74–76). Thus, our data suggest that Nphs and Nph-BAFF may play a more pathogenic role in the development of autoreactive B cells in lupus and highlight the importance of defining the specific context where Nphs interact with B cells.
Reeves et al. found that in PIL mice, Ly6Chi inflammatory MOs are the primary source of IFN-I (41, 77). IFN-I sustains the chronic inflammatory response that leads to autoimmunity, by promoting Ly6Chi inflammatory MOs continuous recruitment to the peritoneum and lymphoid tissues, and blocking their ability to differentiate into Ly6Clo/int MO/Mphs (41, 77). Ly6Clo/int MO-derived Mphs are mature stages of MO differentiation, more phagocytic and less inflammatory than Ly6Chi MO, they play a major role in the resolution of inflammation, tissue repair and homeostasis (47–49). Here we found that DC-BAFF and MO-BAFF, but not Nph-BAFF, were required to maintain high expression levels of the inflammatory marker Ly6C on MOs in PIL mice. Consistent with our results, BAFF receptors on human MOs are associated with the release of inflammatory mediators, while suppressing phagocytosis and migration in vitro (78–80). Furthermore, lupus and Sjogren’s syndrome patients have abnormally elevated expression of BAFF receptors on MOs (54, 81). Thus, an interesting possibility is that DC-BAFF and MO-BAFF contribute to the amplification of the inflammatory cascade by promoting inflammatory Ly6Chi MOs and restraining their differentiation into more mature MOs (47–49). Future research could test whether BAFF directly induces production of IFN-I or other cytokines in MOs creating a positive feedback circuit, and whether BAFF regulation of MOs may play a role in the exacerbation of lupus autoimmunity induced by chronic inflammatory responses (77, 82).
As in the PIL model where IFN-I drives the autoimmune response, the high serum BAFF levels we observed in Tlr7.1 Tg mice was likely due to the substantial increase in IFN-I induced by TLR7 overexpression (31, 32, 36). A similar correlation between up-regulation of TLR7 and high BAFF serum levels occurs in immune thrombocytopenia, another autoimmune disorder (83). Both TLR7 and IFN-I signaling, particularly in myeloid populations, are strong drivers of lupus-like pathology and auto-Ab production in Sle mice (84, 85). However, previous studies only found higher BAFF levels in the serum when crossing Sle1 mice with an autoimmune accelerator that overexpresses TLR7, resulting in severe systemic autoimmunity and kidney disease (38, 86). Thus, the increase in systemic BAFF in Sle1 mice, which has been previously overlooked, further supports a role for BAFF in the induction of autoreactive B cells and auto-Abs in spontaneous lupus-like disease models (14, 15). Increases in systemic BAFF levels correlate with disease severity and auto-Ab responses in other lupus-prone mice like MRLlpr/lpr mice and NZBWF1 (20, 70, 87), as well as in SLE patients (22–24, 88–90).
Humoral autoimmune responses in our parental lines were consistent with those described by us and others in Tlr7.1 Tg mice (31–33, 45), and Sle1 mice (35, 38). Notably, the induction of auto-Abs and the increase in PC numbers in BAFF-RFP Tlr7.1 Tg mice and BAFF-RFP Sle1 mice, which had only one functional BAFF allele and reduced mature B cells, was comparable to that of their parental lines. Similarly, high PC numbers and some auto-Abs were induced in BAFF-RFP mice also after pristane-induced autoimmunity. In autoimmune settings transitional/newly formed (NF) B cells may become more responsive to BAFF and play a role in the development of autoimmunity including SLE (28, 33, 44, 91–94). Since, NF B cells can differentiate in PCs and produce IgG Abs (9, 28, 33, 91, 92), it is possible that in the BAFF-RFP mice NF B cells contributed to auto-Abs responses in the lupus-prone models.
Despite the redundancy in the BAFF system, depletion of BAFF in anyone of the three myeloid cell types, DCs, MOs or Nphs was sufficient to ameliorate humoral autoimmunity. However, each BAFF source has a different role in regulating BAFF serum levels and seems to sustain mature B cells acting through different mechanisms. Consistent with our results, a few studies have pointed out a possible involvement of MO- and DC-BAFF in human SLE (95, 96). IFNα and SLE-immune complexes (IC) induce BAFF production and mobilization by blood MOs and DCs in SLE-patients with active disease, but not in healthy individuals or SLE patients with non-active disease (95). Serum from SLE patients via IFN-I instructs MOs to differentiate into DCs, which in turn mediate IgG-plasmablast differentiation via BAFF and IL-10 (96). Also, recent studies in mice and humans have highlighted how inflammatory MO-derived DCs and the plasticity of MOs play a critical role in mediating SLE autoimmunity and pathogenesis (77, 82, 97, 98). In addition, specific monocyte/DC signatures related to clinical disease, type I IFN signatures and responsiveness to treatments have been identified in Lupus patients (99, 100). Thus, it would be interesting to see whether BAFF expression correlates with different myeloid cell-signatures in pathological conditions and the ability to respond to BAFF-neutralizing agents. It is possible that some of the BAFF heterogeneity in SLE patients may reflect variable responses to inflammatory signals that induce BAFF in different subsets/locations (27, 28, 101). Our data, suggest that specific BAFF sources and B cell niches, implicated in the development of autoreactive B cells, may be important to consider when designing new lupus treatments (3, 19, 28, 51). In a recent study, BAFF gene silencing has effectively improved disease outcome in experimental SLE (102). DC- and MO-targeting and new technologies are starting to show promising results in autoimmune diseases (103, 104). Thus, targeting upstream signals of BAFF production by myeloid cell populations, or targeting BAFF in specific myeloid cells or lymphoid compartments, could be helpful in future lupus therapies (105).
Data availability statement
The original contributions presented in the study are included in the article/Supplementary Material. Further inquiries can be directed to the corresponding author.
Ethics statement
The animal study was reviewed and approved by University of Washington Institutional Animal Care and Use Committee.
Author contributions
Conceptualization, DG, EC, NG. Data curation, DG, RK, KD. Formal analysis, DG, RK. Funding acquisition, EC, NG, DG, KE. Investigation, DG, RK, KD. Methodology, DG, RK. Project Administration, DG, NG, EC. Resources, EC, NG. Supervision, DG, NG, EC. Visualization, DG. Writing – original draft, DG. Writing – review and editing, DG, EC, KE, RK. All authors contributed to the article and approved the submitted version.
Funding
This work was supported by a grant from the National Institutes of Health (R01 AI44257, EC/NG).
Acknowledgments
We thank Dr. Tomas Mustelin for helpful insights and discussion. We thank William D. Selke for technical support. We thank Michele Black and the Cell and Analysis Flow Cytometry and Imaging Core in the Department of Immunology at the University of Washington for their support.
Conflict of interest
The authors declare that the research was conducted in the absence of any commercial or financial relationships that could be construed as a potential conflict of interest.
Publisher’s note
All claims expressed in this article are solely those of the authors and do not necessarily represent those of their affiliated organizations, or those of the publisher, the editors and the reviewers. Any product that may be evaluated in this article, or claim that may be made by its manufacturer, is not guaranteed or endorsed by the publisher.
Supplementary material
The Supplementary Material for this article can be found online at: https://www.frontiersin.org/articles/10.3389/fimmu.2023.1050528/full#supplementary-material
Abbreviations
Ab, antibody; B6, C57BL/6.; BAFF, B cell activating factor; BM, bone marrow; cKO, conditional knockout; cDC, conventional DC; DC, dendritic cell; FO, follicular; GC, germinal center; IFN-I, Type I IFN; KO, knockout; MO, monocyte; Mph, macrophage; MZ, marginal zone; Nph, neutrophil; PC, plasma cell; PECs, peritoneal exudate cells; PIL, pristine-induced lupus; RFP, red fluorescent protein; SLE, systemic lupus erythematosus; Tg, transgenic.
References
1. Mackay F, Schneider P. Cracking the BAFF code. Nat Rev Immunol (2009) 9:491–502. doi: 10.1038/nri2572
2. Vincent FB, Saulep-Easton D, Figgett WA, Fairfax KA, Mackay F. The BAFF/APRIL system: Emerging functions beyond b cell biology and autoimmunity. Cytokine Growth Factor Rev (2013) 24:203–15. doi: 10.1016/j.cytogfr.2013.04.003
3. Goenka R, Scholz JL, Sindhava VJ, Cancro MP. New roles for the BLyS/BAFF family in antigen-experienced b cell niches. Cytokine Growth Factor Rev (2014) 25:107–13. doi: 10.1016/j.cytogfr.2014.01.001
4. Eslami M, Schneider P. Function, occurrence and inhibition of different forms of BAFF. Curr Opin Immunol (2021) 71:75–80. doi: 10.1016/j.coi.2021.06.009
5. Mackay F, Figgett WA, Saulep D, Lepage M, Hibbs ML. B-cell stage and context-dependent requirements for survival signals from BAFF and the b-cell receptor. Immunol Rev (2010) 237:205–25. doi: 10.1111/j.1600-065X.2010.00944.x
6. Smulski CR, Eibel H. BAFF and BAFF-receptor in b cell selection and survival. Front Immunol (2018) 9:2285. doi: 10.3389/fimmu.2018.02285
7. Schweighoffer E, Tybulewicz VL. BAFF signaling in health and disease. Curr Opin Immunol (2021) 71:124–31. doi: 10.1016/j.coi.2021.06.014
8. Borhis G, Trovato M, Chaoul N, Ibrahim HM, Richard Y. B-Cell-Activating factor and the b-cell compartment in HIV/SIV infection. Front Immunol (2017) 8:1338. doi: 10.3389/fimmu.2017.01338
9. Giordano D, Draves KE, Young LB, Roe K, Bryan MA, Dresch C, et al. Protection of mice deficient in mature b cells from West Nile virus infection by passive and active immunization. PloS Pathog (2017) 13:e1006743. doi: 10.1371/journal.ppat.1006743
10. Giordano D, Kuley R, Draves KE, Roe K, Holder U, Giltiay NV, et al. BAFF produced by neutrophils and dendritic cells is regulated differently and has distinct roles in antibody responses and protective immunity against West Nile virus. J Immunol (2020) 204:1508–20. doi: 10.4049/jimmunol.1901120
11. Kuley R, Draves KE, Fuller DH, Giltiay NV, Clark EA, Giordano D. B cell activating factor (BAFF) from neutrophils and dendritic cells is required for protective b cell responses against salmonella typhimurium infection. PloS One (2021) 16:e0259158. doi: 10.1371/journal.pone.0259158
12. Sakai J, Akkoyunlu M. The role of BAFF system molecules in host response to pathogens. Clin Microbiol Rev (2017) 30:991–1014. doi: 10.1128/CMR.00046-17
13. Boneparth A, Davidson A. B-cell activating factor targeted therapy and lupus. Arthritis Res Ther (2012) 14:1–8. doi: 10.1186/ar3920
14. Vincent FB, Morand EF, Schneider P, Mackay F. The BAFF/APRIL system in SLE pathogenesis. Nat Rev Rheumatol (2014) 10:365–73. doi: 10.1038/nrrheum.2014.33
15. Samy E, Wax S, Huard B, Hess H, Schneider P. Targeting BAFF and APRIL in systemic lupus erythematosus and other antibody-associated diseases. Int Rev Immunol (2017) 36:3–19. doi: 10.1080/08830185.2016.1276903
16. Shabgah AG, Shariati-Sarabi Z, Tavakkol-Afshari J, Mohammadi M. The role of BAFF and APRIL in rheumatoid arthritis. J Cell Physiol (2019) 234:17050–63. doi: 10.1002/jcp.28445
17. Nocturne G, Mariette X. B cells in the pathogenesis of primary sjögren syndrome. Nat Rev Rheumatol (2018) 14:133–45. doi: 10.1038/nrrheum.2018.1
18. Sanges S, Guerrier T, Launay D, Lefèvre G, Labalette M, Forestier A, et al. Role of b cells in the pathogenesis of systemic sclerosis. Rev Médecine Interne (2017) 38:113–24. doi: 10.1016/j.revmed.2016.02.016
19. Möckel T, Basta F, Weinmann-Menke J, Schwarting A. B cell activating factor (BAFF): Structure, functions, autoimmunity and clinical implications in systemic lupus erythematosus (SLE). Autoimmun Rev (2021) 20:102736. doi: 10.1016/j.autrev.2020.102736
20. Gross JA, Johnston J, Mudri S, Enselman R, Dillon SR, Madden K, et al. TACI and BCMA are receptors for a TNF homologue implicated in b-cell autoimmune disease. Nature (2000) 404:995–9. doi: 10.1038/35010115
21. Groom JR, Fletcher CA, Walters SN, Grey ST, Watt SV, Sweet MJ, et al. BAFF and MyD88 signals promote a lupuslike disease independent of T cells. J Exp Med (2007) 204:1959–71. doi: 10.1084/jem.20062567
22. Petri M, Stohl W, Chatham W, McCune WJ, Chevrier M, Ryel J, et al. Association of plasma b lymphocyte stimulator levels and disease activity in systemic lupus erythematosus. Arthritis Rheum (2008) 58:2453–9. doi: 10.1002/art.23678
23. Salazar-Camarena DC, Ortiz-Lazareno PC, Cruz A, Oregon-Romero E, Machado-Contreras JR, Muñoz-Valle JF, et al. Association of BAFF, APRIL serum levels, BAFF-r, TACI and BCMA expression on peripheral b-cell subsets with clinical manifestations in systemic lupus erythematosus. Lupus (2016) 25:582–92. doi: 10.1177/0961203315608254
24. Zollars E, Bienkowska J, Czerkowicz J, Allaire N, Ranger AM, Magder L, et al. BAFF (B cell activating factor) transcript level in peripheral blood of patients with SLE is associated with same-day disease activity as well as global activity over the next year. Lupus Sci Med (2015) 2:e000063. doi: 10.1136/lupus-2014-000063
25. Friebus-Kardash J, Trendelenburg M, Eisenberger U, Ribi C, Chizzolini C, Huynh-Do U, et al. Susceptibility of BAFF-var allele carriers to severe SLE with occurrence of lupus nephritis. BMC Nephrol (2019) 20:1–10. doi: 10.1186/s12882-019-1623-4
26. Vincent FB, Morand EF, Mackay F. BAFF and innate immunity: new therapeutic targets for systemic lupus erythematosus. Immunol Cell Biol (2012) 90:293–303. doi: 10.1038/icb.2011.111
27. Stohl W. Inhibition of b cell activating factor (BAFF) in the management of systemic lupus erythematosus (SLE). Expert Rev Clin Immunol (2017) 13:623–33. doi: 10.1080/1744666X.2017.1291343
28. Jackson SW, Davidson A. BAFF inhibition in SLE–is tolerance restored? Immunol Rev (2019) 292:102–19. doi: 10.1111/imr.12810
29. Lee WS, Amengual O. B cells targeting therapy in the management of systemic lupus erythematosus. Immunol Med (2020) 43:16–35. doi: 10.1080/25785826.2019.1698929
30. Shin W, Lee HT, Lim H, Lee SH, Son JY, Lee JU, et al. BAFF-neutralizing interaction of belimumab related to its therapeutic efficacy for treating systemic lupus erythematosus. Nat Commun (2018) 9:1200. doi: 10.1038/s41467-018-03620-2
31. Deane JA, Pisitkun P, Barrett RS, Feigenbaum L, Town T, Ward JM, et al. Control of toll-like receptor 7 expression is essential to restrict autoimmunity and dendritic cell proliferation. Immunity (2007) 27:801–10. doi: 10.1016/j.immuni.2007.09.009
32. Hwang S-H, Lee H, Yamamoto M, Jones LA, Dayalan J, Hopkins R, et al. B cell TLR7 expression drives anti-RNA autoantibody production and exacerbates disease in systemic lupus erythematosus–prone mice. J Immunol (2012) 189:5786–96. doi: 10.4049/jimmunol.1202195
33. Giltiay NV, Chappell CP, Sun X, Kolhatkar N, Teal TH, Wiedeman AE, et al. Overexpression of TLR7 promotes cell-intrinsic expansion and autoantibody production by transitional T1 b cells. J Exp Med (2013) 210:2773–89. doi: 10.1084/jem.20122798
34. Morel L, Mohan C, Yu Y, Croker BP, Tian N, Deng A, et al. Functional dissection of systemic lupus erythematosus using congenic mouse strains. J Immunol (1997) 158:6019–28. doi: 10.4049/jimmunol.158.12.6019
35. Mohan C, Alas E, Morel L, Yang P, Wakeland EK. Genetic dissection of SLE pathogenesis. Sle1 on murine chromosome 1 leads to a selective loss of tolerance to H2A/H2B/DNA subnucleosomes. J Clin Invest (1998) 101:1362–72. doi: 10.1172/JCI728
36. Buechler MB, Teal TH, Elkon KB, Hamerman JA. Cutting edge: Type I IFN drives emergency myelopoiesis and peripheral myeloid expansion during chronic TLR7 signaling. J Immunol (2013) 190:886–91. doi: 10.4049/jimmunol.1202739
37. Sjöstrand M, Johansson A, Aqrawi L, Olsson T, Wahren-Herlenius M, Espinosa A. The expression of BAFF is controlled by IRF transcription factors. J Immunol (2016) 196:91–6. doi: 10.4049/jimmunol.1501061
38. Morel L, Croker BP, Blenman KR, Mohan C, Huang G, Gilkeson G, et al. Genetic reconstitution of systemic lupus erythematosus immunopathology with polycongenic murine strains. Proc Natl Acad Sci (2000) 97:6670–5. doi: 10.1073/pnas.97.12.6670
39. Reeves WH, Lee PY, Weinstein JS, Satoh M, Lu L. Induction of autoimmunity by pristane and other naturally occurring hydrocarbons. Trends Immunol (2009) 30:455–64. doi: 10.1016/j.it.2009.06.003
40. Lee PY, Kumagai Y, Li Y, Takeuchi O, Yoshida H, Weinstein J, et al. TLR7-dependent and FcγR-independent production of type I interferon in experimental mouse lupus. J Exp Med (2008) 205:2995–3006. doi: 10.1084/jem.20080462
41. Lee PY, Li Y, Kumagai Y, Xu Y, Weinstein JS, Kellner ES, et al. Type I interferon modulates monocyte recruitment and maturation in chronic inflammation. Am J Pathol (2009) 175:2023–33. doi: 10.2353/ajpath.2009.090328
42. Giordano D, Draves KE, Li C, Hohl TM, Clark EA. Nitric oxide regulates BAFF expression and T cell–independent antibody responses. J Immunol (2014) 193:1110–20. doi: 10.4049/jimmunol.1303158
43. Roe K, Shu GL, Draves KE, Giordano D, Pepper M, Clark EA. Targeting antigens to CD180 but not CD40 programs immature and mature b cell subsets to become efficient APCs. J Immunol (2019) 203:1715–29. doi: 10.4049/jimmunol.1900549
44. Du SW, Jacobs HM, Arkatkar T, Rawlings DJ, Jackson SW. Integrated b cell, toll-like, and BAFF receptor signals promote autoantibody production by transitional b cells. J Immunol (2018) 201:3258–68. doi: 10.4049/jimmunol.1800393
45. Sun X, Wiedeman A, Agrawal N, Teal TH, Tanaka L, Hudkins KL, et al. Increased ribonuclease expression reduces inflammation and prolongs survival in TLR7 transgenic mice. J Immunol (2013) 190:2536–43. doi: 10.4049/jimmunol.1202689
46. Satoh M, Richards HB, Shaheen VM, Yoshida H, Shaw M, Naim JO, et al. Widespread susceptibility among inbred mouse strains to the induction of lupus autoantibodies by pristane. Clin Exp Immunol (2000) 121:399–405. doi: 10.1046/j.1365-2249.2000.01276.x
47. Ginhoux F, Jung S. Monocytes and macrophages: developmental pathways and tissue homeostasis. Nat Rev Immunol (2014) 14:392–404. doi: 10.1038/nri3671
48. Jakubzick CV, Randolph GJ, Henson PM. Monocyte differentiation and antigen-presenting functions. Nat Rev Immunol (2017) 17:349–62. doi: 10.1038/nri.2017.28
49. Li Y, Zhang Y, Pan G, Xiang L, Luo D, Shao J. Occurrences and functions of Ly6Chi and Ly6Clo macrophages in health and disease(2022) (Accessed November 7, 2022).
50. Gorelik L, Gilbride K, Dobles M, Kalled SL, Zandman D, Scott ML. Normal b cell homeostasis requires b cell activation factor production by radiation-resistant cells. J Exp Med (2003) 198:937–45. doi: 10.1084/jem.20030789
51. Murphy G, Isenberg DA. New therapies for systemic lupus erythematosus — past imperfect, future tense. Nat Rev Rheumatol (2019) 15:403. doi: 10.1038/s41584-019-0235-5
52. Bergamin F, Vincent IE, Summerfield A, McCullough KC. Essential role of antigen-presenting cell-derived BAFF for antibody responses. Eur J Immunol (2007) 37:3122–30. doi: 10.1002/eji.200636791
53. Litinskiy MB, Nardelli B, Hilbert DM, He B, Schaffer A, Casali P, et al. DCs induce CD40-independent immunoglobulin class switching through BLyS and APRIL. Nat Immunol (2002) 3:822. doi: 10.1038/ni829
54. Chen Y, Yang M, Long D, Li Q, Zhao M, Wu H, et al. Abnormal expression of BAFF and its receptors in peripheral blood and skin lesions from systemic lupus erythematosus patients. Autoimmunity (2020) 53:192–200. doi: 10.1080/08916934.2020.1736049
55. Yoshimoto K, Takahashi Y, Ogasawara M, Setoyama Y, Suzuki K, Tsuzaka K, et al. Aberrant expression of BAFF in T cells of systemic lupus erythematosus, which is recapitulated by a human T cell line, loucy. Int Immunol (2006) 18:1189–96. doi: 10.1093/intimm/dxl053
56. Morimoto S, Nakano S, Watanabe T, Tamayama Y, Mitsuo A, Nakiri Y, et al. Expression of b-cell activating factor of the tumour necrosis factor family (BAFF) in T cells in active systemic lupus erythematosus: the role of BAFF in T cell-dependent b cell pathogenic autoantibody production. Rheumatol Oxf Engl (2007) 46:1083–6. doi: 10.1093/rheumatology/kem097
57. Chu VT, Enghard P, Riemekasten G, Berek C. In vitro and In vivo activation induces BAFF and APRIL expression in b cells. J Immunol (2007) 179:5947–57. doi: 10.4049/jimmunol.179.9.5947
58. Chu VT, Enghard P, Schürer S, Steinhauser G, Rudolph B, Riemekasten G, et al. Systemic activation of the immune system induces aberrant BAFF and APRIL expression in b cells in patients with systemic lupus erythematosus. Arthritis Rheum (2009) 60:2083–93. doi: 10.1002/art.24628
59. Coquery CM, Loo WM, Wade NS, Bederman AG, Tung KS, Lewis JE, et al. BAFF regulates follicular helper T cells and affects their accumulation and interferon-γ production in autoimmunity. Arthritis Rheumatol (2015) 67:773–84. doi: 10.1002/art.38950
60. Lech M, Skuginna V, Kulkarni OP, Gong J, Wei T, Stark RW, et al. Lack of SIGIRR/TIR8 aggravates hydrocarbon oil-induced lupus nephritis. J Pathol (2010) 220:596–607. doi: 10.1002/path.2678
61. Liu X, Jiao Y, Cui B, Gao X, Xu J, Zhao Y. The potential protective role of hepatitis b virus infection in pristane-induced lupus in mice. Lupus (2016) 25:1180–9. doi: 10.1177/0961203316631637
62. Lu A, Li H, Niu J, Wu S, Xue G, Yao X, et al. Hyperactivation of the NLRP3 inflammasome in myeloid cells leads to severe organ damage in experimental lupus. J Immunol (2017) 198:1119–29. doi: 10.4049/jimmunol.1600659
63. Parsa R, Lund H, Georgoudaki A-M, Zhang X-M, Guerreiro-Cacais AO, Grommisch D, et al. BAFF-secreting neutrophils drive plasma cell responses during emergency granulopoiesis. J Exp Med (2016) 213:1537–53. doi: 10.1084/jem.20150577
64. Scapini P, Carletto A, Nardelli B, Calzetti F, Roschke V, Merigo F, et al. Proinflammatory mediators elicit secretion of the intracellular b-lymphocyte stimulator pool (BLyS) that is stored in activated neutrophils: implications for inflammatory diseases. Blood (2005) 105:830–7. doi: 10.1182/blood-2004-02-0564
65. Nacionales DC, Kelly-Scumpia KM, Lee PY, Weinstein JS, Lyons R, Sobel E, et al. Deficiency of the type I interferon receptor protects mice from experimental lupus. Arthritis Rheum (2007) 56:3770–83. doi: 10.1002/art.23023
66. Smith C, Kaplan M. The role of neutrophils in the pathogenesis of systemic lupus erythematosus. Curr Opin Rheumatol (2015) 27:448–53. doi: 10.1097/BOR.0000000000000197
67. Stohl W, Jacob N, Guo S, Morel L. Constitutive overexpression of BAFF in autoimmune-resistant mice drives only some aspects of systemic lupus erythematosus–like autoimmunity. Arthritis Rheum (2010) 62:2432–42. doi: 10.1002/art.27502
68. Stohl W, Xu D, Kim KS, Koss MN, Jorgensen TN, Deocharan B, et al. BAFF overexpression and accelerated glomerular disease in mice with an incomplete genetic predisposition to systemic lupus erythematosus. Arthritis Rheum (2005) 52:2080–91. doi: 10.1002/art.21138
69. Huang Z, Chen B, Liu X, Li H, Xie L, Gao Y, et al. Effects of sex and aging on the immune cell landscape as assessed by single-cell transcriptomic analysis. Proc Natl Acad Sci (2021) 118:e2023216118. doi: 10.1073/pnas.2023216118
70. Coquery CM, Wade NS, Loo WM, Kinchen JM, Cox KM, Jiang C, et al. Neutrophils contribute to excess serum BAFF levels and promote CD4+ T cell and b cell responses in lupus-prone mice. PloS One (2014) 9:e102284. doi: 10.1371/journal.pone.0102284
71. Bird AK, Chang M, Barnard J, Goldman BI, Meednu N, Rangel-Moreno J, et al. Neutrophils slow disease progression in murine lupus via modulation of autoreactive germinal centers. J Immunol (2017) 199:458–66. doi: 10.4049/jimmunol.1700354
72. Fresneda Alarcon M, McLaren Z, Wright HL. Neutrophils in the pathogenesis of rheumatoid arthritis and systemic lupus erythematosus: Same foe different M.O(2021) (Accessed February 2, 2022).
73. Wang X, Liu X, Zhang Y, Wang Z, Zhu G, Han G, et al. Interleukin (IL)-39 [IL-23p19/Epstein–Barr virus-induced 3 (Ebi3)] induces differentiation/expansion of neutrophils in lupus-prone mice. Clin Exp Immunol (2016) 186:144–56. doi: 10.1111/cei.12840
74. Puga I, Cols M, Barra CM, He B, Cassis L, Gentile M, et al. B cell–helper neutrophils stimulate the diversification and production of immunoglobulin in the marginal zone of the spleen. Nat Immunol (2012) 13:170–80. doi: 10.1038/ni.2194
75. Deniset JF, Surewaard BG, Lee W-Y, Kubes P. Splenic Ly6Ghigh mature and Ly6Gint immature neutrophils contribute to eradication of s. pneumoniae. J Exp Med (2017) 214:1333–50. doi: 10.1084/jem.20161621
76. Jeremic I, Djuric O, Nikolic M, Vlajnic M, Nikolic A, Radojkovic D, et al. Neutrophil extracellular traps-associated markers are elevated in patients with systemic lupus erythematosus. Rheumatol Int (2019) 39:1849–57. doi: 10.1007/s00296-019-04426-1
77. Lee PY, Weinstein JS, Nacionales DC, Scumpia PO, Li Y, Butfiloski E, et al. A novel type I IFN-producing cell subset in murine lupus. J Immunol (2008) 180:5101–8. doi: 10.4049/jimmunol.180.7.5101
78. Chang SK, Arendt BK, Darce JR, Wu X, Jelinek DF. A role for BLyS in the activation of innate immune cells. Blood (2006) 108:2687–94. doi: 10.1182/blood-2005-12-017319
79. Jeon S-T, Kim W-J, Lee S-M, Lee M-Y, Park S-B, Lee S-H, et al. Reverse signaling through BAFF differentially regulates the expression of inflammatory mediators and cytoskeletal movements in THP-1 cells. Immunol Cell Biol (2010) 88:148–56. doi: 10.1038/icb.2009.75
80. Chen J, He D, Chen Q, Guo X, Yang L, Lin X, et al. BAFF is involved in macrophage-induced bortezomib resistance in myeloma. Cell Death Dis (2017) 8:e3161–1. doi: 10.1038/cddis.2017.533
81. Yoshimoto K, Suzuki K, Takei E, Ikeda Y, Takeuchi T. Elevated expression of BAFF receptor, BR3, on monocytes correlates with b cell activation and clinical features of patients with primary sjögren’s syndrome. Arthritis Res Ther (2020) 22:1–10. doi: 10.1186/s13075-020-02249-1
82. Porat A, Giat E, Kowal C, He M, Son M, Latz E, et al. DNA-Mediated interferon signature induction by SLE serum occurs in monocytes through two pathways: A mechanism to inhibit both pathways(2018) (Accessed November 2, 2022).
83. Yang Q, Xu S, Li X, Wang B, Wang X, Ma D, et al. Pathway of toll-like receptor 7/B cell activating Factor/B cell activating factor receptor plays a role in immune thrombocytopenia. In Vivo. PloS One (2011) 6:e22708. doi: 10.1371/journal.pone.0022708
84. Celhar T, Lu HK, Benso L, Rakhilina L, Lee HY, Tripathi S, et al. TLR7 protein expression in mild and severe lupus-prone models is regulated in a leukocyte, genetic, and IRAK4 dependent manner(2019) (Accessed January 27, 2022).
85. Sriram U, Varghese L, Bennett HL, Jog NR, Shivers DK, Ning Y, et al. Myeloid dendritic cells from B6.NZM Sle1/Sle2/Sle3 lupus-prone mice express an IFN signature that precedes disease onset. J Immunol (2012) 189:80–91. doi: 10.4049/jimmunol.1101686
86. Wu Y-Y, Kumar R, Iida R, Bagavant H, Alarcón-Riquelme ME. BANK1 regulates IgG production in a lupus model by controlling TLR7-dependent STAT1 activation. PloS One (2016) 11:e0156302. doi: 10.1371/journal.pone.0156302
87. Chang N-H, Cheung Y-H, Loh C, Pau E, Roy V, Cai Y-C, et al. B cell activating factor (BAFF) and T cells cooperate to breach b cell tolerance in lupus-prone new Zealand black (NZB) mice. PloS One (2010) 5:e11691. doi: 10.1371/journal.pone.0011691
88. Zhang J, Roschke V, Baker KP, Wang Z, Alarcón GS, Fessler BJ, et al. Cutting edge: A role for b lymphocyte stimulator in systemic lupus erythematosus. J Immunol (2001) 166:6–10. doi: 10.4049/jimmunol.166.1.6
89. McCarthy EM, Lee RZ, Ní Gabhann J, Smith S, Cunnane G, Doran MF, et al. Elevated b lymphocyte stimulator levels are associated with increased damage in an Irish systemic lupus erythematosus cohort. Rheumatology (2013) 52:1279–84. doi: 10.1093/rheumatology/ket120
90. Duan JH, Jiang Y, Mu H, Tang ZQ. Expression of BAFF and BR3 in patients with systemic lupus erythematosus. Braz J Med Biol Res Rev Bras Pesqui Medicas E Biol (2016) 49. doi: 10.1590/1414-431X20154853
91. Giltiay NV, Giordano D, Clark EA. The plasticity of newly formed b cells. J Immunol (2019) 203:3095–104. doi: 10.4049/jimmunol.1900928
92. Jacobs HM, Thouvenel CD, Leach S, Arkatkar T, Metzler G, Scharping NE, et al. Cutting edge: BAFF promotes autoantibody production via TACI-dependent activation of transitional b cells. J Immunol (2016) 196:3525–31. doi: 10.4049/jimmunol.1600017
93. Vossenkämper A, Lutalo PMK, Spencer J. Translational mini-review series on b cell subsets in disease. transitional b cells in systemic lupus erythematosus and sjögren’s syndrome: clinical implications and effects of b cell-targeted therapies. Clin Exp Immunol (2012) 167:7–14. doi: 10.1111/j.1365-2249.2011.04460.x
94. Rawlings DJ, Metzler G, Wray-Dutra M, Jackson SW. Altered b cell signalling in autoimmunity. Nat Rev Immunol (2017) 17:421–36. doi: 10.1038/nri.2017.24
95. López P, Scheel-Toellner D, Rodríguez-Carrio J, Caminal-Montero L, Gordon C, Suárez A. Interferon-α-induced b-lymphocyte stimulator expression and mobilization in healthy and systemic lupus erthymatosus monocytes. Rheumatology (2014) 53:2249–58. doi: 10.1093/rheumatology/keu249
96. Joo H, Coquery C, Xue Y, Gayet I, Dillon SR, Punaro M, et al. Serum from patients with SLE instructs monocytes to promote IgG and IgA plasmablast differentiation. J Exp Med (2012) 209:1335–48. doi: 10.1084/jem.20111644
97. Miyagawa F, Tagaya Y, Ozato K, Horie K, Asada H. Inflammatory monocyte-derived dendritic cells mediate autoimmunity in murine model of systemic lupus erythematosus. J Transl Autoimmun (2020) 3:100060. doi: 10.1016/j.jtauto.2020.100060
98. Parikh SV, Malvar A, Shapiro J, Turman JM, Song H, Alberton V, et al. A novel inflammatory dendritic cell that is abundant and contiguous to T cells in the kidneys of patients with lupus nephritis(2021) (Accessed November 2, 2022).
99. Jin Z, Fan W, Jensen MA, Dorschner JM, Bonadurer GF, Vsetecka DM, et al. Single-cell gene expression patterns in lupus monocytes independently indicate disease activity, interferon and therapy. Lupus Sci Med (2017) 4:e000202. doi: 10.1136/lupus-2016-000202
100. Zhang Z, Maurer K, Perin JC, Song L, Sullivan KE. Cytokine-induced monocyte characteristics in SLE. BioMed Res Int (2010) 2010:e507475. doi: 10.1155/2010/507475
101. Gupta S, Kaplan MJ. Bite of the wolf: innate immune responses propagate autoimmunity in lupus. J Clin Invest (2021) 131:e144918. doi: 10.1172/JCI144918
102. Guiteras J, Ripoll É, Bolaños N, De Ramon L, Fontova P, Lloberas N, et al. The gene silencing of IRF5 and BLYSS effectively modulates the outcome of experimental lupus nephritis. Mol Ther - Nucleic Acids (2021) 24:807–21. doi: 10.1016/j.omtn.2021.03.019
103. Castenmiller C, Keumatio-Doungtsop B-C, van Ree R, de Jong EC, van Kooyk Y. Tolerogenic immunotherapy: Targeting DC surface receptors to induce antigen-specific tolerance(2021) (Accessed November 30, 2022).
104. Cifuentes-Rius A, Desai A, Yuen D, Johnston APR, Voelcker NH. Inducing immune tolerance with dendritic cell-targeting nanomedicines. Nat Nanotechnol (2021) 16:37–46. doi: 10.1038/s41565-020-00810-2
Keywords: BAFF, autoimmunity, SLE, B cells, autoantibodies, dendritic cells, monocytes, neutrophils
Citation: Giordano D, Kuley R, Draves KE, Elkon KB, Giltiay NV and Clark EA (2023) B cell-activating factor (BAFF) from dendritic cells, monocytes and neutrophils is required for B cell maturation and autoantibody production in SLE-like autoimmune disease. Front. Immunol. 14:1050528. doi: 10.3389/fimmu.2023.1050528
Received: 21 September 2022; Accepted: 09 February 2023;
Published: 27 February 2023.
Edited by:
Betty Diamond, Feinstein Institute for Medical Research, New York, United StatesReviewed by:
Anja Kerstein-Staehle, University of Lübeck, GermanyCharles S. Via, Uniformed Services University, United States
Copyright © 2023 Giordano, Kuley, Draves, Elkon, Giltiay and Clark. This is an open-access article distributed under the terms of the Creative Commons Attribution License (CC BY). The use, distribution or reproduction in other forums is permitted, provided the original author(s) and the copyright owner(s) are credited and that the original publication in this journal is cited, in accordance with accepted academic practice. No use, distribution or reproduction is permitted which does not comply with these terms.
*Correspondence: Daniela Giordano, giordano@uw.edu
†Present address: Runa Kuley, Center for Life Sciences, Mahindra University, Hyderabad, India
Natalia V. Giltiay, Kyverna Therapeutics, Emeryville, CA, United States
‡These authors have contributed equally to this work