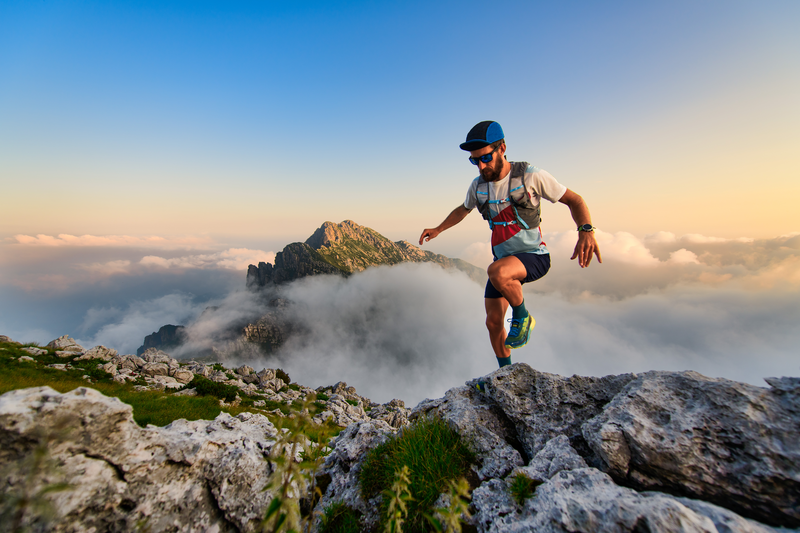
95% of researchers rate our articles as excellent or good
Learn more about the work of our research integrity team to safeguard the quality of each article we publish.
Find out more
REVIEW article
Front. Immunol. , 01 March 2023
Sec. Cancer Immunity and Immunotherapy
Volume 14 - 2023 | https://doi.org/10.3389/fimmu.2023.1046771
This article is part of the Research Topic Advances of Immunosuppression Reversal Strategies in Engineered Adoptive Cell Therapies Against Solid Tumors: from Basic Research to Clinical Trials View all 7 articles
Hepatocellular carcinoma (HCC) is a high-incidence malignant tumor worldwide and lacks effective treatment options. Targeted drugs are the preferred recommendations for the systemic treatment of hepatocellular carcinoma. Immunotherapy is a breakthrough in the systemic treatment of malignant tumors, including HCC. However, either targeted therapy or immunotherapy alone is inefficient and has limited survival benefits on part of HCC patients. Investigations have proved that tyrosine kinase inhibitors (TKIs) have regulatory effects on the tumor microenvironment and immune response, which are potential sensitizers for immunotherapy. Herein, a combination therapy using TKIs and immunotherapy has been explored and demonstrated to improve the effectiveness of treatment. As an effective immunotherapy, adoptive T cell therapy in solid tumors is required to improve tumor infiltration and killing activity which can be possibly achieved by combination with TKIs.
According to GLOBOCAN2020 (a global cancer statistic online database), primary liver cancer, a common malignant tumor with high morbidity and mortality, ranks 6th in tumor incidence and 3rd in tumor-related mortality globally. Hepatocellular carcinoma (HCC) is the most common type of primary liver cancer (accounts for 75%-85%) (1). As the greatest etiological risk factor for HCC, viral infections including hepatitis B virus (HBV), hepatitis C virus (HCV), and hepatitis delta virus (HDV) are considered the major cause of HCC cases (2). Liver fibrosis and cirrhosis, caused by chronic hepatitis infections, will further affect the patients’ liver function and the anti-tumor therapy, reducing the survival rate of patients. The high mortality rate of HCC is attributed to its insidious nature and lack of effective treatment options. Early-stage HCC patients with hepatic resection and transplantation have a 5-year survival of 70–80% (3). However, the majority of HCC patients are diagnosed in the intermediate stages or advanced stages resulting in a significant decrease in survival rate due to missing the best treatment opportunities and lack of effective therapies (4, 5).
Tyrosine kinase inhibitors (TKIs) with antiangiogenic properties are used as first-line systemic therapy in advanced HCC. Although TKIs have certain survival benefits for HCC patients, the inevitable problem of primary or acquired resistance has become the bottleneck that interferes the long-term response and limits the improvement of prognosis. In addition to resistance issues, the lack of identified driver mutations, underlying liver cirrhosis, and impaired functional reserves are also difficulties in treating advanced HCC patients.
Adoptive T cell therapy is an emerging research topic in tumor immunotherapy that progress rapidly from basic research to clinical application. Adoptive T cell therapy refers to manipulating and expanding autologous or allogeneic T cells in vitro followed by infusing into tumor patients to generate a robust immune-mediated antitumor response (6). Previous research has demonstrated the promising efficacy of adoptive T cell therapy in the treatment of hematologic tumors. There are growing investigations conducted to evaluate adoptive T cell therapy in solid tumors, including HCC. However, several barriers are identified, including the immunosuppression of the tumor microenvironment (TME) (7, 8) and target antigen heterogeneity (9). To improve the survival time and living quality of HCC patients, researchers are engaged in overcoming these issues mentioned above.
Recently, it has been discovered that TKIs also have immunomodulatory effects besides their well-known antiangiogenic properties (10). This finding obtained great attention to the combination therapy of TKIs with other immune therapies. In this review, we discuss this new combination therapy with a focus on TKIs as potential sensitizers of adoptive T cells for HCC. In the beginning, an introduction to diagnostic methods and current treatment strategies will be given. The discovery of immunomodulatory effects of TKIs and the underlying mechanisms will then be presented. Finally, current adoptive T cell therapies for HCC together with their combination therapies with different TKIs will be discussed.
Diagnostic imaging and Alpha-fetoprotein (AFP) testing are the most common ways for HCC screening and diagnosis (3). However, imaging methods, including ultrasound, CT, and MRI, are less effective for HCC diagnosis at early stage (11, 12). As to AFP testing, a serum biomarker for HCC normally used in conjunction with imaging, it is not sensitive or specific for HCC that about 30% of HCC patients with confirmed liver cancer have no significant increase in AFP (13). Since more curative approaches are available to early-stage HCC patients resulting in a higher survival rate, advanced screening techniques and diagnostic methods capable of detecting HCC at early stage are required to gain the optimal therapeutic outcome (3).
Recently, new screening techniques have been studied and shown advantages in early screening and early diagnosis of liver cancer such as the liquid biopsy method which is based on circulating tumor cells (CTC), circulating tumor DNA (ctDNA), extracellular vehicles (EVs), circulating cell-free RNA (cfRNA) (14, 15). It has been reported that ctDNA is superior to serum AFP in sensitivity and specificity for early screening of HCC (16). In addition, the development of molecular biology technology has discovered the important role of non-coding RNA (ncRNA) in tumorigenesis and progression, providing alternative ways for early-stage HCC detection. ncRNAs, taking up to 60% of the transcriptional output in human cells, are untranslated transcripts and can be classified based on their length, such as microRNAs (22-25 nucleotides) and long-ncRNAs (>200 nucleotides) (17, 18). Researchers have found abnormal expression of miRNA in liver cancer, such as miRNA-502c-3p, miRNA-342-3p, and miRNA-21 (19–22). The accuracy of using a miRNA panel (consisting of 7 miRNAs) can improve the diagnosis of HCC to reach a detection rate close to 90% (23). LncRNA is also associated with cancer progression and can function as HCC diagnostic markers such as TUG1, ZFAS1, and SCARNA10 (21, 24–26). Compared with traditional tumor markers, ncRNA expression abnormalities may appear earlier and can potentially become a category of more convenient, accurate, and non-invasive detection markers.
Tumors can be characterized by The Barcelona Clinic Liver Cancer (BCLC) staging system via performance status, the size and number of tumors, vascular invasion, extrahepatic metastases, and liver function (27). Treatment strategies for tumors in each stage are varied. Liver transplantation, hepatic radical resection, and ablation are preferred and applicable to early-stage HCC. Surgery, including hepatic resection and liver transplantation, has been considered as curative therapy, which is currently the most important way to help patients achieve long-term survival (28–31). Hepatic resection is recommended for patients with excellent performance status, good liver function, and no clinically significant portal hypertension (32). Ablation delivers heat directly to tumors to induce tumor necrosis. It is also a potentially curative procedure for HCC at early stages, with similar survival rates to surgical resection (33–35). As to intermediate-stage HCC patients, transarterial chemoembolization (TACE) is the first candidate. The current treatments for advanced HCC are systemic therapies, including chemotherapy, targeted therapy, and immunotherapy (36–38). Though curative and locoregional therapies showed promising prognoses, more than 60% of HCC patients were diagnosed at the middle and advanced stages, eventually receiving systemic therapy. Systemic therapy thus plays a vital role in liver cancer treatment.
Since chemotherapy showed unsatisfactory outcomes and related to severe toxicities, molecularly targeted therapy and immunotherapy have played a critical role in the systemic treatment of advanced HCC (39–41). Several molecular targeted therapy drugs with effects on angiogenesis, cell proliferation, and metastasis have been shown to improve overall survival (OS) in advanced HCC. The main molecular targeted drugs in use are TKIs and monoclonal antibodies.
TKIs belong to a category of tumor angiogenesis inhibitors. Sorafenib is the first multikinase inhibitor approved for the treatment of HCC based on its benefit in two multicenter clinical trials (42–44). Sorafenib works through the inhibition of the receptor tyrosine kinases of vascular endothelial growth factor receptor (VEGFR), platelet-derived growth factor receptor (PDGFR), fibroblast growth factor receptor(FGFR)-β, and c-KIT, blocking the RAF/MEK/ERK pathway (45, 46). Lenvatinib showed a non-inferiority in overall survival and superiority in progression-free survival, time to progression and objective response rate (ORR) compared to sorafenib in a randomized phase 3 non-inferiority trial and has been approved as another first-line treatment of HCC (47). The second-line TKIs are cabozantinib and regrafinib. These agents commonly have effects targeting various receptors including VEGFR, PDGFR, and FGFR (48).
For monoclonal antibodies, bevacizumab and ramucirumab are currently used in clinics based on the promising results obtained from previous clinical studies. The combination of bevacizumab (a monoclonal antibody that targets VEGF, inhibiting angiogenesis) and atezolizumab (an immune checkpoint inhibitor that targets programmed cell death protein-1 and reverses T-cell suppression) has become one of the first-line treatment because it showed better overall and improved survival with unresectable HCC compared with sorafenib in IMbrave150, a global phase III randomized trial (49). Ramucirumab is a human immunoglobulin G1 monoclonal antibody and a VEGFR2 inhibitor. It is now a second-line therapy because, in the REACH-2 trial, ramucirumab showed a significant improvement compared with placebo in median OS(mOS) for advanced HCC patients who had prior sorafenib treatment (50).
In the last two decades, significant breakthroughs have been made in immunotherapy for tumors, including monoclonal antibodies, tumor vaccines, adoptive transfer of immune cells, and immunomodulatory agents (39, 51). Immune checkpoints are key members of immunoregulatory pathways regulated by ligand/receptor interactions, playing an important role oftenly in preventing overactive immune responses. Immune checkpoint inhibitors (ICIs) are monoclonal antibodies that regulate T cell activity through a series of targets, such as programmed cell death protein-1 and its ligand (PD-1/PD-L1), cytotoxic T lymphocyte protein 4 (CTLA-4), mucin domain-containing molecule-3 (Tim-3), and lymphocyte activation gene 3 protein (LAG-3), to exert anti-tumor effects (40). Including atezolizumab, pembrolizumab, ipilimumab, and nivolumab, ICIs and their combination regimens have been used as first-line and second-line treatments for hepatocellular carcinoma in clinical practices (52). In addition to the above commonly used treatment strategies, antiviral therapy is also an optional method considering that chronic HBV/HCV infection is a major cause of HCC. Studies have shown antiviral therapy benefits the survival of HBV-associated HCC patients (53, 54). For example, anti-HBV drugs have been reported to suppress the growth of HBV-expressing hepatoma cells via down-regulation of the hepatitis B virus X protein (55).
Although the HCC treatment options and their efficiency have been developed in recent years, and the application of multidisciplinary treatment strategies shows better outcomes compared with monotherapies, the current objective remission rate and the prognosis are still unsatisfactory (56). The obstacles, such as the recurrence rate of surgery, inevitable drug resistance of targeted drugs, and a low response to systemic cancer therapy, are at urgent need to be overcome.
Recently, new treatments and optimization of current treatment regimens through combination therapies have been studied to improve prognosis. Several clinical trials have been conducted and showed promising outcomes. For example, several new molecular kinase inhibitors like palbociclib, ribociclib, and tivantinib have been under investigation and have shown their effectiveness (57, 58). As to combination therapies, an interesting finding has been reported that TKIs possess synergistic antitumor effects with immunotherapies in the clinical trials of combination therapy (58). In this section, we will introduce the discovery of TKIs’ immunomodulatory effect first and then discuss the related mechanisms.
The immunomodulatory effect of TKIs was discovered in combination therapies. Both preclinical and clinical trials of combinations of anti-PD-1 and TKIs have shown promising outcomes for HCC treatments (59). In a phase Ib open-label multicentre study, 100 HCC patients were enrolled, who were diagnosed as BCLC stage B (n=29) or C (n=71) diseases. The result showed that the ORR of lenvatinib plus pembrolizumab treatment was 46.0% (95% CI, 36.0% to 56.3%) by modified Response Evaluation Criteria in Solid Tumors (mRECIST), without new or unexpected toxicities resulting from the combination therapy (60). Notably, 11 patients (11%) were evaluated to have a complete response (CR). At the data cut-off date, median progression-free survival (mPFS) was 9.3 months (95% CI, 5.6 to 9.7 months) and mOS was 22.0 months (95% CI, 20.4 months to NE), showing a promising antitumor activity observed in unresectable HCC with lenvatinib plus pembrolizumab treatment. Immunomodulatory effects of TKIs were also observed in mouse models of liver cancer. Kenichi Nomoto et al. found that lenvatinib had greater antitumor activity in immunocompetent mice than in immunodeficient mice, which implies that part of the antitumor effect of lenvatinib originates from the immune responses (61). It was further demonstrated that lenvatinib plus anti-PD-1 antibody enhanced tumor regression and increased treatment response rate. Both studies showed an increased percentage of CD8+ T cells, confirming the synergistic effect of TKIs and immunotherapy (61, 62).
Targeting on the varied functional mechanisms of the therapeutic targets, TKIs induce immunomodulatory effects with increased antitumor effects and decreased immunosuppression based on the following main categories of mechanisms: (a) normalize tumor capillaries, (b) enhance immune cell infiltration and activation in tumors, (c) reverse immunosuppression of TME, (d) promote the release and presentation of tumor antigens (Figure 1).
Figure 1 The underlying immunomodulatory mechanisms of TKIs. The immunomodulatory effects of TKIs are based on the following: (A) normalize tumor capillaries, (B) enhance immune cell infiltration and activation in tumors, (C) reverse immunosuppression of TME, (D) promote the release and presentation of tumor antigens. TKIs, tyrosine kinase inhibitors; TAMs, tumor-associated macrophages; Tregs, regulatory T cells; MDSCs, myeloid-derived suppressor cells; TME, tumor microenvironment; DCs, dendritic cells.
Different from normal vessels, pathological angiogenesis in tumors shows abnormal structures and functional defects (63). Pathologic neovascularization is the critical manner of tumor blood supply, which further induced hypoperfusion, hypoxia, acidosis, and tumor metastasis (64, 65). Neovascularization is regulated by more than 40 molecules acting proangiogenicly or antiangiogenicly, respectively (66–68). Angiogenesis inhibitors including TKIs are first applied to decrease tumor vessel formation, resulting in blocking nutrient supply and inducing tumor cell dormancy (63). Unexpectedly, the alleviation of hypoxia and acidosis in tumor tissue has been found in clinical application when using lower doses of antiangiogenic agents (69), which may synergize with radiotherapy, chemotherapy, and immune therapy. Rakesh K. Jain first proposed the rationale that antiangiogenic therapy normalizes tumor vasculature networks before their destruction and hence improves the delivery of oxygen (70). This rationale based on the antiangiogenic therapy rectifies the imbalance of proangiogenic and antiangiogenic factors, which results in the elimination of the excess endothelial cells and the immature and inefficient blood vessels. Subsequent pre-clinical studies verified this hypothesis and revealed that inhibition of multiple tumor-promoting effects of VEGF is the key factor (63). However, sustained antiangiogenic therapy cannot maintain its effect in normalizing the pathogenic tumor vessels. Instead, sustained antiangiogenic results in the reduction rather than normalization of pathogenic tumor vessels. Thus, extensive experiments are still needed to explore an optimized strategy of vascular normalization which is potentially applicable in clinical practice.
Besides the effect of normalizing tumor vasculature, TKIs can also improve the infiltration and activation of antitumor immune cells to interfere with tumor progression. CD8+ T cells are the most important type of tumor-killing cells, and TKIs significantly increase the proportion of anti-tumor effector T cells (61, 71). Another investigation showed that TKIs enhanced the effector functions of human NK cells by activation of the RAS/RAF/ERK pathway in a dose- and time-dependent manner (72). TKIs have been reported to induce increased expression of recognizing and activating ligands for NK cells, T cells, NKT cells, NKT-like cells, and γσT cells, promoting chemotaxis and tumor killing (73–76). Down-regulation of major histocompatibility complex class I (MHC-I) expression of tumor cells by TKIs is also a major underlying mechanism to increase NK cell responses (77). Moreover, TKIs can induce tumor cells to release neutrophil chemotactic factors, resulting in a rapid neutrophil infiltration into the tumor and enhanced tumor eradication (78).
In addition to insufficient immune infiltration, immunosuppressive factors in TME also prevent immune cells from exerting anti-tumor effects. TKIs can reverse the immunosuppression of TME by reducing the infiltration and immunosuppressing effect of tumor-associated macrophages (TAMs), regulatory T cells (Tregs), and myeloid-derived suppressor cells (MDSCs) (79). TAM is involved in the formation of the immunosuppressive microenvironment and the inhibition of anticancer immune responses by secreting immunosuppressive factors, inhibiting the proliferation and activation of T cells, and promoting tumor invasion and metastasis (80). Ulrike Protzer et al. investigated the influence of sorafenib on TAM in vitro and in vivo. The results revealed that sorafenib stimulated macrophages to produce cytokines such as IL-6, TNF-a, and IL-12, and subsequently induced antitumor NK cell responses via the NF-κB pathway (81). Other studies validated sorafenib’s modulatory effect on TAM by decreasing the percentage of TAMs and restoring the classical activation of macrophages, thus decreasing the tumor burden (77, 81, 82).
Interactions of TKIs and cancer cells are considered to mediate immunomodulatory activity by promoting tumor antigen presentation. T cells are known to specifically recognize tumour antigens and become activated to execute their anti-tumor functions, while insufficient presentation of tumour antigens is an important reason for the lack of T cell responsiveness in tumor patients (83). TKIs induce cytotoxicity through the inhibition of multiple tyrosine kinases to block the VEGFR and PDGFR pathways. Subsequent cell death can expose tumour antigens and activate antigen presentation by dendritic cells (DCs). This cross-presentation of antigens activates T cells, completing a so-called “immune sensitization” (83, 84). On the other hand, VEGF inhibits the adhesion of lymphocytes to endothelial cells through intercellular adhesion molecule-1 (ICAM-1), vascular cell adhesion molecule-1 (VCAM-1), and Fas Ligand (FasL), thereby affecting the infiltration of T cells into the tumor tissue (85, 86). In addition, VEGF inhibits the maturation of DCs, and the development and activation of T cells (87, 88). Negative regulation of VEGF functions on DCs and T cells is the potential mechanism of TKIs promoting antigen presentation.
Showing a promising antitumor activity, the landscape of treatment strategy for HCC may change dramatically with the advance of immunotherapy in the near future. Since the immunomodulation effect of TKIs has been discovered, combination therapy of TKIs with other immunotherapies has also been under evaluation, such as ICIs and immune cells (89). Adoptive T cell therapy is one of the immunotherapies and has shown great value for clinical translation. Currently, several adoptive T cell therapies have been approved by Food and Drug Administration (FDA) for lymphoma, myeloma, and leukemia (90). In recent years, there are many attempts in applying adoptive T cell therapies for other tumors. In this section, we will first summarize the current state of applying adoptive T cell therapy for HCC and discuss the related challenges. The combination therapy of TKIs with adoptive T cells will also be discussed.
T cells are important effector cells in the specific immune response of the somatic machinery to tumor cells and are currently the most widely studied effector cells in pericyte therapy. T cell-based pericyte therapies include autologous T cells, allogeneic T cells, tumor-infiltrating lymphocytes (TILs), chimeric antigen receptor T cells (CAR-T cells), T cell receptor engineered T cells (TCR-T cells) and other engineered T cells (9). Up to date, 63 researches of T cell-based therapies for HCC have been registered at ClinicalTrials (https://clinicaltrials.gov/ ), with CAR-T cells account for the largest proportion among all T cell transfer approaches (Table 1). CAR-T cells originally showed their remarkable efficacy in treating hematological malignancies (91). The chimeric antigen receptors (CARs) contain an extracellular antigen-recognition domain, a transmembrane domain, and an intracellular signaling domain, which recognize tumor-specific antigens expressed and achieve non-MHC-restricted activations (92). Glypian-3 (GPC-3) has been demonstrated to relate with immunoreactivity towards tumor cells and is highly expressed in HCC, becoming a notable candidate target in HCC. CAR-T cells targeting GPC-3 have been shown effective in animal models with orthotopic xenografts and patient-derived xenografts highly expressing GPC-3 (93, 94).
Table 1 Clinical Trials of Adoptive T Cell Therapy for HCC Registered at ClinicalTrials from 2008 to 2022.
Adoptive T cell therapy can specifically and efficiently kill target cells. However, there are barriers and challenges for the clinical application of adoptive T cells. Theoretically, adoptive T cells can enhance cellular immune responses to eliminate cancer cells, but adoptive T cell therapy has demonstrated low clinical efficacy in the treatment of solid tumors, including HCC, due to the limited expansion, poor persistence, terminal differentiation and dysfunction or exhaustion of T cells (95, 96). Target antigen heterogeneity is also a barrier for adoptive T cell therapy application in solid tumors (9). Contrary to hematologic malignancies, solid tumors usually lack consistently expressed specific antigens for T cells to identify and become activated, consequently causing adoptive T cells fail to exert their anti-tumor effects. Off-target effects and cytokine storm also hinder the clinical performance of adoptive T cell therapy. In addition, the immunosuppression effect of the TME is a major barrier for the recruitment of adoptive T cells into tumors, fulfilling their antitumor activity (7, 8). TME is a complex circumstance including blood vessels, immune cells, fibroblasts, various signaling molecules, and extracellular matrix (97). Accumulation of immune cells, such as TAMs, MDSCs, and Tregs, as well as cytokines, including transforming growth factor-β, interleukin-6, and interleukin-1, are known to induce immunosuppression. As a result of interactions between cytokines, immune cells, and tumor cells, TME participates in the regulation of metastasis and anticancer drug sensitivity (97). In HCC, the immune-suppressing liver environments and chronic inflammation caused by liver diseases interfere with the effects of immunotherapy (98). There is an urgent need to more comprehensively understand adoptive T cell therapy effects and mechanisms, and optimize its application strategies.
As discussed in section 3, TKIs have been found having immunomodulatory effects and have shown improved patient outcomes by combination therapies. Together with the obstacles faced by current adoptive T cell therapy for HCC, it is rational to consider the possibility of combining TKIs with adoptive T cell therapy to achieve better performance. We here summarize the immunomodulatory effects of commonly used TKIs and discuss their potential applications as sensitizers in combination therapies with adoptive T cells.
The immunoregulatory effects of sorafenib have been observed recently. Sorafenib may enhance the infiltration and activation of T cells in the tumor microenvironment for various types of tumors by increasing the production of T cell-recruiting cytokines and chemokines (99–101). Treatment of serial low doses of sorafenib enhanced the activation, cytotoxicity, and migration of CD8+ T cells (102). Sorafenib inhibits the expression of multiple immunosuppressive factors, such as indoleamine 2,3-dioxygenase(IDO), transforming growth factor-beta (TGF-beta), VEGF, interleukin-10 (IL-10), monocyte chemoattractant protein-1 (MCP-1), by down-regulation of the STAT3 signaling pathway (102). In HCC, several studies have proven sorafenib can enhance antitumor responses through increasing the proportion of tumor-specific effector CD8+ T cells and reducing the proportion of exhausted or immunosuppressive immune cells such as PD-1-expressing CD8+ T cells and Foxp3+ Tregs (103, 104). The enhancement of antitumor immunity of sorafenib implies its potential as a sensitizer for adoptive T cell therapy. A recent study has confirmed this theory that combined treatment of GPC3-specific CAR-T cell therapy with subpharmacologic doses of sorafenib demonstrated an enhanced antitumor effect both in vitro and in vivo (105). Lenvatinib showed better antitumor activity than that of sorafenib in immunocompetent mice but not in immunodeficient mice (61). Further investigation revealed that lenvatinib modulates antitumor immune responses by reducing tumor-associated macrophages and increasing activated CD8+ T cells secreting interferon-γ and granzyme B (62). Lenvatinib prevents Treg differentiation and infiltration, and reverses T cell suppression, by reducing tumor PD-L1 level (106, 107).
As second-line TKIs, regorafenib and cabozantinib also exhibit immunomodulatory effects and antiangiogenetic functions. Regorafenib promoted T cell activation, M1 macrophage polarization, and proliferation/activation of cocultured T cells via p38 kinase/Creb1/Klf4 axis, therefore enhancing antitumor immunity independently from its antiangiogenic effects (108). The antitumor effect of T cells is a human leukocyte antigen class I (HLA-I)-dependent immune response. HLA-I is essential for tumor antigen presentation and subsequent antitumor immunity. Tumor cells evade immune detection by acquiring deficiencies in HLA antigen processing and presentation pathways (109). It has been shown that regorafenib can increase the expression of cell surface HLA-I, and upregulate various genes associated with the HLA-I antigen processing pathway, as well as its transcriptional regulators (110). Cabozantinib showed its immunomodulatory activity making murine colon tumor cells more sensitive to immune-mediated killing by altering the phenotype of tumor cells, the proportions of immune cell subpopulations in the peripheral circulation, and the tumor microenvironment (71). Subsequent research revealed that cabozantinib significantly increased the infiltration of neutrophils and reduced the proportions of intratumor CD8+PD1+ T cells in HCC (111).
With the progress of molecular biological research, the complex and diverse phenotypes, mechanisms, and therapeutic responses of cancer have been gradually uncovered, which are translated to the clinical treatment of cancer. Reprogramming of cellular metabolism replaces the metabolic processes that operate in most normal tissue cells, maintaining their rapid proliferation, invasion, migration, and metastasis (112). In HCC, for instance, abnormal lipid metabolism triggered by SPIN1/SREBP1/FASN axis has been reported to enhance tumor growth (113). Recently, TKIs have been reported to have effects on the metabolic balance of multiple endogenous metabolic pathways, including lipid metabolism (114). More research on the functioning mechanism of TKIs in tumor treatment is needed to guide the clinical application of TKIs in cancer treatment.
Early-stage clinical studies combining targeted therapy with immunotherapy such as ICIs are ongoing and have shown a synergistic effect, suggesting combined treatment for HCC in the future (115, 116). Investigations have proved the immunoregulatory effects of targeted therapy on tumor microenvironment and immune responses (117), making it a potential sensitizer for adoptive T cell therapy. However, the regulatory mechanism of targeted therapy on immune responses, TME, and adoptive T cells have not yet been elucidated. There is also a need for more direct evidence to confirm the clinical benefits of the combination of targeted therapy and adoptive T cell therapy. Further optimization for the combination therapy is still needed for the selection of targeted therapy and the corresponding immune cells to be affected. Therefore, a comprehensive understanding of combination therapy is necessary to optimize the management of HCC and to bring survival benefits for the patients.
It remains a challenge to assess the effects of combination therapies. For HCC patients treated with targeted therapy or immune therapy, mRECIST and immune RECIST (iRECIST) can be applied (118, 119). Tumor antigens, for example AFP and GPC-3, can provide reference value for efficacy evaluation (13, 120). However, current evaluation methods all showed inevitable low sensitivity and hysteresis. Liquid biopsy can dynamically monitor and reflect the treatment efficacy and can be used as a supplement to imaging, but standardization of the evaluation criteria is still needed (121, 122). ncRNA has been trialed as a novel biomarker in clinical diagnosis and efficacy monitoring, but still needs to be extensively validated (123). The development of specific and sensitive molecular markers for the monitoring of the cancer prognosis and treatment efficacy of HCC is still an urgent issue to be settled.
LJL, PZ, YC, and LL devised the study, wrote and revised the manuscript. PZ, XW, SH, YWC, YC, LL, and LJL contributed to the literature search and gave insightful suggestions in revising this work. All authors contributed to the article and approved the submitted version.
This study was supported by the National Natural Science Foundation of China (82070096).
BioRender was used to generate Figure 1.
The authors declare that the research was conducted in the absence of any commercial or financial relationships that could be construed as a potential conflict of interest.
All claims expressed in this article are solely those of the authors and do not necessarily represent those of their affiliated organizations, or those of the publisher, the editors and the reviewers. Any product that may be evaluated in this article, or claim that may be made by its manufacturer, is not guaranteed or endorsed by the publisher.
1. Sung H, Ferlay J, Siegel RL, Laversanne M, Soerjomataram I, Jemal A, et al. Global cancer statistics 2020: GLOBOCAN estimates of incidence and mortality worldwide for 36 cancers in 185 countries. CA Cancer J Clin (2021) 71(3):209–49. doi: 10.3322/caac.21660
2. D’Souza S, Lau KC, Coffin CS, Patel TR. Molecular mechanisms of viral hepatitis induced hepatocellular carcinoma. World J Gastroenterol (2020) 26(38):5759–83. doi: 10.3748/wjg.v26.i38.5759
3. Llovet JM, Kelley RK, Villanueva A, Singal AG, Pikarsky E, Roayaie S, et alHepatocellular carcinoma. Nat Rev Dis Primers (2021) 7(1):7. doi: 10.1038/s41572-021-00245-6
4. Yang JD, Hainaut P, Gores GJ, Amadou A, Plymoth A, Roberts LR. A global view of hepatocellular carcinoma: Trends, risk, prevention and management. Nat Rev Gastroenterol Hepatol (2019) 16(10):589–604. doi: 10.1038/s41575-019-0186-y
5. Zeng H, Chen W, Zheng R, Zhang S, Ji JS, Zou X, et al. Changing cancer survival in China during 2003–15: a pooled analysis of 17 population-based cancer registries. Lancet Global Health (2018) 6(5):e555–e67. doi: 10.1016/s2214-109x(18)30127-x
6. Met O, Jensen KM, Chamberlain CA, Donia M, Svane IM. Principles of adoptive T cell therapy in cancer. Semin Immunopathol (2019) 41(1):49–58. doi: 10.1007/s00281-018-0703-z
7. Terrén I, Orrantia A, Vitallé J, Zenarruzabeitia O, Borrego F. And tumor microenvironment. Front Immunol (2019) 10:2278. doi: 10.3389/fimmu.2019.02278
8. Nakamura K, Smyth MJ. Myeloid immunosuppression and immune checkpoints in the tumor microenvironment. Cell Mol Immunol (2020) 17(1):1–12. doi: 10.1038/s41423-019-0306-1
9. Kirtane K, Elmariah H, Chung CH, Abate-Daga D. Adoptive cellular therapy in solid tumor malignancies: Review of the literature and challenges ahead. J Immunother Cancer (2021) 9(7):1–11. doi: 10.1136/jitc-2021-002723
10. Lin Y-Y, Tan C-T, Chen C-W, Ou D-L, Cheng A-L, Hsu C. Immunomodulatory effects of current targeted therapies on hepatocellular carcinoma: Implication for the future of immunotherapy. Semin In Liver Dis (2018) 38(4):379–88. doi: 10.1055/s-0038-1673621
11. Tzartzeva K, Obi J, Rich NE, Parikh ND, Marrero JA, Yopp A, et al. Surveillance imaging and alpha fetoprotein for early detection of hepatocellular carcinoma in patients with cirrhosis: A meta-analysis. Gastroenterology (2018) 154(6):1706–18 e1. doi: 10.1053/j.gastro.2018.01.064
12. Yang JD. Detect or not to detect very early stage hepatocellular carcinoma? the western perspective. Clin Mol Hepatol (2019) 25(4):335–43. doi: 10.3350/cmh.2019.0010
13. Hu X, Chen R, Wei Q, Xu X. The landscape of alpha fetoprotein in hepatocellular carcinoma: Where are we? Int J Biol Sci (2022) 18(2):536–51. doi: 10.7150/ijbs.64537
14. Maravelia P, Silva DN, Rovesti G, Chrobok M, Stal P, Lu YC, et al. Liquid biopsy in hepatocellular carcinoma: Opportunities and challenges for immunotherapy. Cancers (Basel) (2021) 13(17):4334. doi: 10.3390/cancers13174334
15. Roskams-Hieter B, Kim HJ, Anur P, Wagner JT, Callahan R, Spiliotopoulos E, et al. Plasma cell-free RNA profiling distinguishes cancers from pre-malignant conditions in solid and hematologic malignancies. NPJ Precis Oncol (2022) 6(1):28. doi: 10.1038/s41698-022-00270-y
16. Xu R-H, Wei W, Krawczyk M, Wang W, Luo H, Flagg K, et al. Circulating tumour DNA methylation markers for diagnosis and prognosis of hepatocellular carcinoma. Nat Mater (2017) 16(11):1155–61. doi: 10.1038/nmat4997
17. Grillone K, Riillo C, Scionti F, Rocca R, Tradigo G, Guzzi PH, et al. Non-coding RNAs in cancer: platforms and strategies for investigating the genomic “dark matter”. J Exp Clin Cancer Research: CR (2020) 39(1):117. doi: 10.1186/s13046-020-01622-x
18. Anastasiadou E, Jacob LS, Slack FJ. Non-coding RNA networks in cancer. Nat Rev Cancer (2018) 18(1):5-18. doi: 10.1038/nrc.2017.99
19. Komoll R-M, Hu Q, Olarewaju O, von Döhlen L, Yuan Q, Xie Y, et al. MicroRNA-342-3p is a potent tumour suppressor in hepatocellular carcinoma. J Hepatol (2021) 74(1):122–34. doi: 10.1016/j.jhep.2020.07.039
20. Xiong D-D, Dang Y-W, Lin P, Wen D-Y, He R-Q, Luo D-Z, et al. A circRNA-miRNA-mRNA network identification for exploring underlying pathogenesis and therapy strategy of hepatocellular carcinoma. J Transl Med (2018) 16(1):220. doi: 10.1186/s12967-018-1593-5
21. Han TS, Hur K, Cho HS, Ban HS. Epigenetic associations between lncRNA/circRNA and miRNA in hepatocellular carcinoma. Cancers (Basel) (2020) 12(9):1–31. doi: 10.3390/cancers12092622
22. Oura K, Morishita A, Masaki T. Molecular and functional roles of MicroRNAs in the progression of hepatocellular carcinoma-a review. Int J Mol Sci (2020) 21(21):8362. doi: 10.3390/ijms21218362
23. Zhou J, Yu L, Gao X, Hu J, Wang J, Dai Z, et al. Plasma microRNA panel to diagnose hepatitis b virus-related hepatocellular carcinoma. J Clin Oncol (2011) 29(36):4781–8. doi: 10.1200/JCO.2011.38.2697
24. Lin YH, Wu MH, Huang YH, Yeh CT, Cheng ML, Chi HC, et al. Taurine up-regulated gene 1 functions as a master regulator to coordinate glycolysis and metastasis in hepatocellular carcinoma. Hepatology (2018) 67(1):188–203. doi: 10.1002/hep.29462
25. Huang Z, Zhou JK, Peng Y, He W, Huang C. The role of long noncoding RNAs in hepatocellular carcinoma. Mol Cancer (2020) 19(1):77. doi: 10.1186/s12943-020-01188-4
26. Han Y, Jiang W, Wang Y, Zhao M, Li Y, Ren L. Serum long non-coding RNA SCARNA10 serves as a potential diagnostic biomarker for hepatocellular carcinoma. BMC Cancer (2022) 22(1):431. doi: 10.1186/s12885-022-09530-3
27. Benson AB, D’Angelica MI, Abbott DE, Anaya DA, Anders R, Are C, et al. Hepatobiliary cancers, version 2.2021, NCCN clinical practice guidelines in oncology. J Natl Compr Canc Netw (2021) 19(5):541–65. doi: 10.6004/jnccn.2021.0022
28. Mazzaferro V, Regalia E, Doci R, Andreola S, Pulvirenti A, Bozzetti F, et al. Liver transplantation for the treatment of small hepatocellular carcinomas in patients with cirrhosis. N Engl J Med (1996) 334(11):693–9. doi: 10.1056/NEJM199603143341104
29. Ko SE, Lee MW, Ahn S, Rhim H, Kang TW, Song KD, et al. Laparoscopic hepatic resection versus laparoscopic radiofrequency ablation for subcapsular hepatocellular carcinomas smaller than 3 cm: Analysis of treatment outcomes using propensity score matching. Korean J Radiol (2022) 23(6):615–24. doi: 10.3348/kjr.2021.0786
30. Zhou XD, Tang ZY, Yang BH, Lin ZY, Ma ZC, Ye SL, et al. Experience of 1000 patients who underwent hepatectomy for small hepatocellular carcinoma. Cancer (2001) 91(8):1479–86. doi: 10.1002/1097-0142(20010415)91:8<1479::aid-cncr1155>3.0.co;2-0
31. Kardashian A, Florman SS, Haydel B, Ruiz RM, Klintmalm GB, Lee DD, et al. Liver transplantation outcomes in a U.S. multicenter cohort of 789 patients with hepatocellular carcinoma presenting beyond Milan criteria. Hepatology (2020) 72(6):2014–28. doi: 10.1002/hep.31210
32. Roayaie S, Jibara G, Tabrizian P, Park J-W, Yang J, Yan L, et al. The role of hepatic resection in the treatment of hepatocellular cancer. Hepatology (2015) 62(2):440–51. doi: 10.1002/hep.27745
33. Xia Y, Li J, Liu G, Wang K, Qian G, Lu Z, et al. Long-term effects of repeat hepatectomy vs percutaneous radiofrequency ablation among patients with recurrent hepatocellular carcinoma: A randomized clinical trial. JAMA Oncol (2020) 6(2):255–63. doi: 10.1001/jamaoncol.2019.4477
34. Ng KKC, Chok KSH, Chan ACY, Cheung TT, Wong TCL, Fung JYY, et al. Randomized clinical trial of hepatic resection versus radiofrequency ablation for early-stage hepatocellular carcinoma. Br J Surg (2017) 104(13):1775–84. doi: 10.1002/bjs.10677
35. Izumi N, Hasegawa K, Nishioka Y, Takayama T, Yamanaka N, Kudo M, et al. A multicenter randomized controlled trial to evaluate the efficacy of surgery vs. radiofrequency ablation for small hepatocellular carcinoma (SURF trial). J Clin Oncol (2019) 37(15_suppl):4002. doi: 10.1200/JCO.2019.37.15_suppl.4002
36. Llovet JM, Kelley RK, Villanueva A, Singal AG, Pikarsky E, Roayaie S, et al. Hepatocellular carcinoma. Nat Rev Dis Primers (2021) 7(1):6. doi: 10.1038/s41572-020-00240-3
37. Bruix J, Chan SL, Galle PR, Rimassa L, Sangro B. Systemic treatment of hepatocellular carcinoma: An EASL position paper. J Hepatol (2021) 75(4):960–74. doi: 10.1016/j.jhep.2021.07.004
38. Gordan JD, Kennedy EB, Abou-Alfa GK, Beg MS, Brower ST, Gade TP, et al. Systemic therapy for advanced hepatocellular carcinoma: ASCO guideline. J Clin Oncol (2020) 38(36):4317–45. doi: 10.1200/JCO.20.02672
39. Tagliamonte M, Mauriello A, Cavalluzzo B, Ragone C, Manolio C, Petrizzo A, et al. Tackling hepatocellular carcinoma with individual or combinatorial immunotherapy approaches. Cancer Lett (2020) 473:25–32. doi: 10.1016/j.canlet.2019.12.029
40. Khan AA, Liu ZK, Xu X. Recent advances in immunotherapy for hepatocellular carcinoma. Hepatobiliary Pancreat Dis Int (2021) 20(6):511–20. doi: 10.1016/j.hbpd.2021.06.010
41. Llovet JM, Pinyol R, Kelley RK, El-Khoueiry A, Reeves HL, Wang XW, et al. Molecular pathogenesis and systemic therapies for hepatocellular carcinoma. Nat Cancer (2022) 3(4):386–401. doi: 10.1038/s43018-022-00357-2
42. Cheng A-L, Kang Y-K, Chen Z, Tsao C-J, Qin S, Kim JS, et al. Efficacy and safety of sorafenib in patients in the Asia-pacific region with advanced hepatocellular carcinoma: A phase III randomised, double-blind, placebo-controlled trial. Lancet Oncol (2009) 10(1):25–34. doi: 10.1016/s1470-2045(08)70285-7
43. Rimassa L, Santoro A. Sorafenib therapy in advanced hepatocellular carcinoma: The SHARP trial. Expert Rev Anticancer Ther (2009) 9(6):739–45. doi: 10.1586/era.09.41
44. Llovet JM, Ricci S, Mazzaferro V, Hilgard P, Gane E, Blanc J-F, et al. Sorafenib in advanced hepatocellular carcinoma. N Engl J Med (2008) 359(4):378–90. doi: 10.1056/NEJMoa0708857
45. Wilhelm SM, Carter C, Tang L, Wilkie D, McNabola A, Rong H, et al. BAY 43-9006 exhibits broad spectrum oral antitumor activity and targets the RAF/MEK/ERK pathway and receptor tyrosine kinases involved in tumor progression and angiogenesis. Cancer Res (2004) 64(19):7099–109. doi: 10.1158/0008-5472.CAN-04-1443
46. Liu L, Cao Y, Chen C, Zhang X, McNabola A, Wilkie D, et al. Sorafenib blocks the RAF/MEK/ERK pathway, inhibits tumor angiogenesis, and induces tumor cell apoptosis in hepatocellular carcinoma model PLC/PRF/5. Cancer Res (2006) 66(24):11851–8. doi: 10.1158/0008-5472.CAN-06-1377
47. Kudo M, Finn RS, Qin S, Han K-H, Ikeda K, Piscaglia F, et al. Lenvatinib versus sorafenib in first-line treatment of patients with unresectable hepatocellular carcinoma: A randomised phase 3 non-inferiority trial. Lancet (2018) 391(10126):1163–73. doi: 10.1016/S0140-6736(18)30207-1
48. Wong KM, King GG, Harris WP. The treatment landscape of advanced hepatocellular carcinoma. Curr Oncol Rep (2022) 24(7):917–27. doi: 10.1007/s11912-022-01247-7
49. Finn RS, Qin S, Ikeda M, Galle PR, Ducreux M, Kim T-Y, et al. Atezolizumab plus bevacizumab in unresectable hepatocellular carcinoma. N Engl J Med (2020) 382(20):1894–905. doi: 10.1056/NEJMoa1915745
50. Zhu AX, Kang Y-K, Yen C-J, Finn RS, Galle PR, Llovet JM, et al. Ramucirumab after sorafenib in patients with advanced hepatocellular carcinoma and increased α-fetoprotein concentrations (REACH-2): a randomised, double-blind, placebo-controlled, phase 3 trial. Lancet Oncol (2019) 20(2):282–96. doi: 10.1016/S1470-2045(18)30937-9
51. Johnston MP, Khakoo SI. Immunotherapy for hepatocellular carcinoma: Current and future. World J Gastroenterol (2019) 25(24):2977–89. doi: 10.3748/wjg.v25.i24.2977
52. Parikh ND, Pillai A. Recent advances in hepatocellular carcinoma treatment. Clin Gastroenterol Hepatol (2021) 19(10):2020–4. doi: 10.1016/j.cgh.2021.05.045
53. Gao Q, Zhu H, Dong L, Shi W, Chen R, Song Z, et al. Integrated proteogenomic characterization of HBV-related hepatocellular carcinoma. Cell (2019) 179(2):561–77. doi: 10.1016/j.cell.2019.08.052
54. Yeh M-L, Hung C-H, Tseng K-C, Lai H-C, Chen C-Y, Kuo H-T, et al. Long-term outcome of liver complications in patients with chronic HBV/HCV co-infection after antiviral therapy: A real-world nationwide study on Taiwanese chronic hepatitis c cohort (T-COACH). Hepatol Int (2021) 15(5):1109–21. doi: 10.1007/s12072-021-10220-8
55. Zhang S, Gao S, Zhao M, Liu Y, Bu Y, Jiang Q, et al. Anti-HBV drugs suppress the growth of HBV-related hepatoma cells via down-regulation of hepatitis b virus X protein. Cancer Lett (2017) 392:94–104. doi: 10.1016/j.canlet.2017.02.003
56. Serper M, Taddei TH, Mehta R, D’Addeo K, Dai F, Aytaman A, et al. Association of provider specialty and multidisciplinary care with hepatocellular carcinoma treatment and mortality. Gastroenterology (2017) 152(8):1954–64. doi: 10.1053/j.gastro.2017.02.040
57. De Mattia E, Cecchin E, Guardascione M, Foltran L, Di Raimo T, Angelini F, et al. Pharmacogenetics of the systemic treatment in advanced hepatocellular carcinoma. World J Gastroenterol (2019) 25(29):3870–96. doi: 10.3748/wjg.v25.i29.3870
58. Llovet JM, Montal R, Sia D, Finn RS. Molecular therapies and precision medicine for hepatocellular carcinoma. Nat Rev Clin Oncol (2018) 15(10):599–616. doi: 10.1038/s41571-018-0073-4
59. Lin YY, Tan CT, Chen CW, Ou DL, Cheng AL, Hsu C. Immunomodulatory effects of current targeted therapies on hepatocellular carcinoma: Implication for the future of immunotherapy. Semin Liver Dis (2018) 38(4):379–88. doi: 10.1055/s-0038-1673621
60. Finn RS, Ikeda M, Zhu AX, Sung MW, Baron AD, Kudo M, et al. Phase ib study of lenvatinib plus pembrolizumab in patients with unresectable hepatocellular carcinoma. J Clin Oncol (2020) 38(26):2960–70. doi: 10.1200/JCO.20.00808
61. Kimura T, Kato Y, Ozawa Y, Kodama K, Ito J, Ichikawa K, et al. Immunomodulatory activity of lenvatinib contributes to antitumor activity in the Hepa1-6 hepatocellular carcinoma model. Cancer Sci (2018) 109(12):3993–4002. doi: 10.1111/cas.13806
62. Kato Y, Tabata K, Kimura T, Yachie-Kinoshita A, Ozawa Y, Yamada K, et al. Lenvatinib plus anti-PD-1 antibody combination treatment activates CD8+ T cells through reduction of tumor-associated macrophage and activation of the interferon pathway. PloS One (2019) 14(2):e0212513. doi: 10.1371/journal.pone.0212513
63. Jain RK. Antiangiogenesis strategies revisited: From starving tumors to alleviating hypoxia. Cancer Cell (2014) 26(5):605–22. doi: 10.1016/j.ccell.2014.10.006
64. Folkman J. Tumor angiogenesis: Therapeutic implications. N Engl J Med (1971) 285(21):1182–6. doi: 10.1056/NEJM197111182852108
65. Siemann DW, Horsman MR. Modulation of the tumor vasculature and oxygenation to improve therapy. Pharmacol Ther (2015) 153:107–24. doi: 10.1016/j.pharmthera.2015.06.006
66. Huang Y, Kim BYS, Chan CK, Hahn SM, Weissman IL, Jiang W. Improving immune-vascular crosstalk for cancer immunotherapy. Nat Rev Immunol (2018) 18(3):195–203. doi: 10.1038/nri.2017.145
67. Katayama Y, Uchino J, Chihara Y, Tamiya N, Kaneko Y, Yamada T, et al. Tumor neovascularization and developments in therapeutics. Cancers (Basel) (2019) 11(3):316. doi: 10.3390/cancers11030316
68. Jaszai J, Schmidt MHH. Trends and challenges in tumor anti-angiogenic therapies. Cells (2019) 8(9):1102. doi: 10.3390/cells8091102
69. Fukumura D, Kloepper J, Amoozgar Z, Duda DG, Jain RK. Enhancing cancer immunotherapy using antiangiogenics: opportunities and challenges. Nat Rev Clin Oncol (2018) 15(5):325–40. doi: 10.1038/nrclinonc.2018.29
70. Jain RK. Normalizing tumor vasculature with anti-angiogenic therapy: A new paradigm for combination therapy. Nat Med (2001) 7(9):987–9. doi: 10.1038/nm0901-987
71. Kwilas AR, Ardiani A, Donahue RN, Aftab DT, Hodge JW. Dual effects of a targeted small-molecule inhibitor (cabozantinib) on immune-mediated killing of tumor cells and immune tumor microenvironment permissiveness when combined with a cancer vaccine. J Transl Med (2014) 12:294. doi: 10.1186/s12967-014-0294-y
72. Lohmeyer J, Nerreter T, Dotterweich J, Einsele H, Seggewiss-Bernhardt R. Sorafenib paradoxically activates the RAS/RAF/ERK pathway in polyclonal human NK cells during expansion and thereby enhances effector functions in a dose- and time-dependent manner. Clin Exp Immunol (2018) 193(1):64–72. doi: 10.1111/cei.13128
73. Zhang Q, Liu H, Wang H, Lu M, Miao Y, Ding J, et al. Lenvatinib promotes antitumor immunity by enhancing the tumor infiltration and activation of NK cells. Am J Cancer Res (2019) 9(7):1382–95.
74. Lanier LL. NKG2D receptor and its ligands in host defense. Cancer Immunol Res (2015) 3(6):575–82. doi: 10.1158/2326-6066.CIR-15-0098
75. Almeida JS, Couceiro P, Lopez-Sejas N, Alves V, Ruzickova L, Tarazona R, et al. NKT-like (CD3+CD56+) cells in chronic myeloid leukemia patients treated with tyrosine kinase inhibitors. Front Immunol (2019) 10:2493. doi: 10.3389/fimmu.2019.02493
76. Arai J, Goto K, Stephanou A, Tanoue Y, Ito S, Muroyama R, et al. Predominance of regorafenib over sorafenib: Restoration of membrane-bound MICA in hepatocellular carcinoma cells. J Gastroenterol Hepatol (2018) 33(5):1075–81. doi: 10.1111/jgh.14029
77. Hage C, Hoves S, Strauss L, Bissinger S, Prinz Y, Poschinger T, et al. Sorafenib induces pyroptosis in macrophages and triggers natural killer cell-mediated cytotoxicity against hepatocellular carcinoma. Hepatology (2019) 70(4):1280–97. doi: 10.1002/hep.30666
78. Patnaik A, Swanson KD, Csizmadia E, Solanki A, Landon-Brace N, Gehring MP, et al. Cabozantinib eradicates advanced murine prostate cancer by activating antitumor innate immunity. Cancer Discov (2017) 7(7):750–65. doi: 10.1158/2159-8290.CD-16-0778
79. Zhou S-L, Zhou Z-J, Hu Z-Q, Huang X-W, Wang Z, Chen E-B, et al. Tumor-associated neutrophils recruit macrophages and T-regulatory cells to promote progression of hepatocellular carcinoma and resistance to sorafenib. Gastroenterology (2016) 150(7):1646–58. doi: 10.1053/j.gastro.2016.02.040
80. Fu LQ, Du WL, Cai MH, Yao JY, Zhao YY, Mou XZ. The roles of tumor-associated macrophages in tumor angiogenesis and metastasis. Cell Immunol (2020) 353:104119. doi: 10.1016/j.cellimm.2020.104119
81. Sprinzl MF, Reisinger F, Puschnik A, Ringelhan M, Ackermann K, Hartmann D, et al. Sorafenib perpetuates cellular anticancer effector functions by modulating the crosstalk between macrophages and natural killer cells. Hepatology (2013) 57(6):2358–68. doi: 10.1002/hep.26328
82. Farsaci B, Donahue RN, Coplin MA, Grenga I, Lepone LM, Molinolo AA, et al. Immune consequences of decreasing tumor vasculature with antiangiogenic tyrosine kinase inhibitors in combination with therapeutic vaccines. Cancer Immunol Res (2014) 2(11):1090–102. doi: 10.1158/2326-6066.CIR-14-0076
83. Jhunjhunwala S, Hammer C, Delamarre L. Antigen presentation in cancer: Insights into tumour immunogenicity and immune evasion. Nat Rev Cancer (2021) 21(5):298–312. doi: 10.1038/s41568-021-00339-z
84. Fu C, Jiang A. Dendritic cells and CD8 T cell immunity in tumor microenvironment. Front Immunol (2018) 9:3059. doi: 10.3389/fimmu.2018.03059
85. Ramjiawan RR, Griffioen AW, Duda DG. Anti-angiogenesis for cancer revisited: Is there a role for combinations with immunotherapy? Angiogenesis (2017) 20(2):185–204. doi: 10.1007/s10456-017-9552-y
86. Motz GT, Santoro SP, Wang L-P, Garrabrant T, Lastra RR, Hagemann IS, et al. Tumor endothelium FasL establishes a selective immune barrier promoting tolerance in tumors. Nat Med (2014) 20(6):607–15. doi: 10.1038/nm.3541
87. Gabrilovich D, Ishida T, Oyama T, Ran S, Kravtsov V, Nadaf S, et al. Vascular endothelial growth factor inhibits the development of dendritic cells and dramatically affects the differentiation of multiple hematopoietic lineages vivo. Blood (1998) 92(11):4150–66.
88. Palazon A, Tyrakis PA, Macias D, Veliça P, Rundqvist H, Fitzpatrick S, et al. An HIF-1α/VEGF-A axis in cytotoxic T cells regulates tumor progression. Cancer Cell (2017) 32(5):669–83. doi: 10.1016/j.ccell.2017.10.003
89. Pan C, Liu H, Robins E, Song W, Liu D, Li Z, et al. Next-generation immuno-oncology agents: Current momentum shifts in cancer immunotherapy. J Hematol Oncol (2020) 13(1):29. doi: 10.1186/s13045-020-00862-w
90. Amini L, Silbert SK, Maude SL, Nastoupil LJ, Ramos CA, Brentjens RJ, et al. Preparing for CAR T cell therapy: Patient selection, bridging therapies and lymphodepletion. Nat Rev Clin Oncol (2022) 19(5):342–55. doi: 10.1038/s41571-022-00607-3
91. Haslauer T, Greil R, Zaborsky N, Geisberger R. CAR T-cell therapy in hematological malignancies. Int J Mol Sci (2021) 22(16):8996. doi: 10.3390/ijms22168996
92. Labanieh L, Majzner RG, Mackall CL. Programming CAR-T cells to kill cancer. Nat BioMed Eng (2018) 2(6):377–91. doi: 10.1038/s41551-018-0235-9
93. Gao H, Li K, Tu H, Pan X, Jiang H, Shi B, et al. Development of T cells redirected to glypican-3 for the treatment of hepatocellular carcinoma. Clin Cancer Res (2014) 20(24):6418–28. doi: 10.1158/1078-0432.CCR-14-1170
94. Jiang Z, Jiang X, Chen S, Lai Y, Wei X, Li B, et al. Anti-GPC3-CAR T cells suppress the growth of tumor cells in patient-derived xenografts of hepatocellular carcinoma. Front Immunol (2016) 7:690. doi: 10.3389/fimmu.2016.00690
95. Chan JD, Lai J, Slaney CY, Kallies A, Beavis PA, Darcy PK. Cellular networks controlling T cell persistence in adoptive cell therapy. Nat Rev Immunol (2021) 21(12):769–84. doi: 10.1038/s41577-021-00539-6
96. Petersen CT, Hassan M, Morris AB, Jeffery J, Lee K, Jagirdar N, et al. Improving T-cell expansion and function for adoptive T-cell therapy using ex vivo treatment with PI3Kδ inhibitors and VIP antagonists. Blood Adv (2018) 2(3):210–23. doi: 10.1182/bloodadvances.2017011254
97. Li L, Yu R, Cai T, Chen Z, Lan M, Zou T, et al. Effects of immune cells and cytokines on inflammation and immunosuppression in the tumor microenvironment. Int Immunopharmacol (2020) 88:106939. doi: 10.1016/j.intimp.2020.106939
98. Ringelhan M, Pfister D, O’Connor T, Pikarsky E, Heikenwalder M. The immunology of hepatocellular carcinoma. Nat Immunol (2018) 19(3):222–32. doi: 10.1038/s41590-018-0044-z
99. Sunay MME, Foote JB, Leatherman JM, Edwards JP, Armstrong TD, Nirschl CJ, et al. Sorafenib combined with HER-2 targeted vaccination can promote effective T cell immunity. vivo. Int Immunopharmacol (2017) 46:112–23. doi: 10.1016/j.intimp.2017.02.028
100. Mathew NR, Baumgartner F, Braun L, O’Sullivan D, Thomas S, Waterhouse M, et al. Sorafenib promotes graft-versus-leukemia activity in mice and humans through IL-15 production in FLT3-ITD-mutant leukemia cells. Nat Med (2018) 24(3):282–91. doi: 10.1038/nm.4484
101. Romero AI, Chaput N, Poirier-Colame V, Rusakiewicz S, Jacquelot N, Chaba K, et al. Regulation of CD4(+)NKG2D(+) Th1 cells in patients with metastatic melanoma treated with sorafenib: Role of IL-15Rα and NKG2D triggering. Cancer Res (2014) 74(1):68–80. doi: 10.1158/0008-5472.CAN-13-1186
102. Chuang HY, Chang YF, Liu RS, Hwang JJ. Serial low doses of sorafenib enhance therapeutic efficacy of adoptive T cell therapy in a murine model by improving tumor microenvironment. PloS One (2014) 9(10):e109992. doi: 10.1371/journal.pone.0109992
103. Chen ML, Yan BS, Lu WC, Chen MH, Yu SL, Yang PC, et al. Sorafenib relieves cell-intrinsic and cell-extrinsic inhibitions of effector T cells in tumor microenvironment to augment antitumor immunity. Int J Cancer (2014) 134(2):319–31. doi: 10.1002/ijc.28362
104. Kalathil SG, Lugade AA, Miller A, Iyer R, Thanavala Y. PD-1(+) and Foxp3(+) T cell reduction correlates with survival of HCC patients after sorafenib therapy. JCI Insight (2016) 1(11). doi: 10.1172/jci.insight.86182
105. Wu X, Luo H, Shi B, Di S, Sun R, Su J, et al. Combined antitumor effects of sorafenib and GPC3-CAR T cells in mouse models of hepatocellular carcinoma. Mol Ther (2019) 27(8):1483–94. doi: 10.1016/j.ymthe.2019.04.020
106. Yi C, Chen L, Lin Z, Liu L, Shao W, Zhang R, et al. Lenvatinib targets FGF receptor 4 to enhance antitumor immune response of anti-programmed cell death-1 in HCC. Hepatology (2021) 74(5):2544–60. doi: 10.1002/hep.31921
107. Torrens L, Montironi C, Puigvehí M, Mesropian A, Leslie J, Haber PK, et al. Immunomodulatory effects of lenvatinib plus anti-programmed cell death protein 1 in mice and rationale for patient enrichment in hepatocellular carcinoma. Hepatology (2021) 74(5):2652–69. doi: 10.1002/hep.32023
108. Ou D-L, Chen C-W, Hsu C-L, Chung C-H, Feng Z-R, Lee B-S, et al. Regorafenib enhances antitumor immunity via inhibition of p38 kinase/Creb1/Klf4 axis in tumor-associated macrophages. J For Immunotherapy Cancer (2021) 9(3):1–23. doi: 10.1136/jitc-2020-001657
109. Hazini A, Fisher K, Seymour L. Deregulation of HLA-I in cancer and its central importance for immunotherapy. J Immunother Cancer (2021) 9(8):1–45. doi: 10.1136/jitc-2021-002899
110. Takahashi A, Umemura A, Yano K, Okishio S, Kataoka S, Okuda K, et al. Tyrosine kinase inhibitors stimulate HLA class I expression by augmenting the IFNγ/STAT1 signaling in hepatocellular carcinoma cells. Front Oncol (2021) 11:707473. doi: 10.3389/fonc.2021.707473
111. Esteban-Fabro R, Willoughby CE, Pique-Gili M, Montironi C, Abril-Fornaguera J, Peix J, et al. Cabozantinib enhances anti-PD1 activity and elicits a neutrophil-based immune response in hepatocellular carcinoma. Clin Cancer Res (2022) 28(11):2449–60. doi: 10.1158/1078-0432.CCR-21-2517
112. Hanahan D, Weinberg RA. Hallmarks of cancer: The next generation. Cell (2011) 144(5):646–74. doi: 10.1016/j.cell.2011.02.013
113. Zhao M, Bu Y, Feng J, Zhang H, Chen Y, Yang G, et al. SPIN1 triggers abnormal lipid metabolism and enhances tumor growth in liver cancer. Cancer Lett (2020) 470:54–63. doi: 10.1016/j.canlet.2019.11.032
114. Zhao Q, Wu ZE, Li B, Li F. Recent advances in metabolism and toxicity of tyrosine kinase inhibitors. Pharmacol Ther (2022) 237:108256. doi: 10.1016/j.pharmthera.2022.108256
115. Xing R, Gao J, Cui Q, Wang Q. Strategies to improve the antitumor effect of immunotherapy for hepatocellular carcinoma. Front Immunol (2021) 12:783236. doi: 10.3389/fimmu.2021.783236
116. Llovet JM, De Baere T, Kulik L, Haber PK, Greten TF, Meyer T, et al. Locoregional therapies in the era of molecular and immune treatments for hepatocellular carcinoma. Nat Rev Gastroenterol Hepatol (2021) 18(5):293–313. doi: 10.1038/s41575-020-00395-0
117. Tan HY, Wang N, Lam W, Guo W, Feng Y, Cheng YC. Targeting tumour microenvironment by tyrosine kinase inhibitor. Mol Cancer (2018) 17(1):43. doi: 10.1186/s12943-018-0800-6
118. Seymour L, Bogaerts J, Perrone A, Ford R, Schwartz LH, Mandrekar S, et al. iRECIST: Guidelines for response criteria for use in trials testing immunotherapeutics. Lancet Oncol (2017) 18(3):e14–e52. doi: 10.1016/s1470-2045(17)30074-8
119. Llovet JM, Lencioni R. mRECIST for HCC: Performance and novel refinements. J Hepatol (2020) 72(2):288–306. doi: 10.1016/j.jhep.2019.09.026
120. Zhu AX, Abbas AR, de Galarreta MR, Guan Y, Lu S, Koeppen H, et al. Molecular correlates of clinical response and resistance to atezolizumab in combination with bevacizumab in advanced hepatocellular carcinoma. Nat Med (2022) 28(8):1599–611. doi: 10.1038/s41591-022-01868-2
121. Muraoka M, Maekawa S, Katoh R, Komiyama Y, Nakakuki N, Takada H, et al. Usefulness of cell-free human telomerase reverse transcriptase mutant DNA quantification in blood for predicting hepatocellular carcinoma treatment efficacy. Hepatol Commun (2021) 5(11):1927–38. doi: 10.1002/hep4.1762
122. Ao H, Xin Z, Jian Z. Liquid biopsy to identify biomarkers for immunotherapy in hepatocellular carcinoma. Biomark Res (2021) 9(1):91. doi: 10.1186/s40364-021-00348-y
Keywords: hepatocellular carcinoma, tyrosine kinase inhibitors, adoptive T cell therapy, immunotherapy, combination therapy
Citation: Liang L, Wang X, Huang S, Chen Y, Zhang P, Li L and Cui Y (2023) Tyrosine kinase inhibitors as potential sensitizers of adoptive T cell therapy for hepatocellular carcinoma. Front. Immunol. 14:1046771. doi: 10.3389/fimmu.2023.1046771
Received: 17 September 2022; Accepted: 13 February 2023;
Published: 01 March 2023.
Edited by:
Zhaohua Hou, Memorial Sloan Kettering Cancer Center, United StatesReviewed by:
Hui Chen, University of California, San Diego, United StatesCopyright © 2023 Liang, Wang, Huang, Chen, Zhang, Li and Cui. This is an open-access article distributed under the terms of the Creative Commons Attribution License (CC BY). The use, distribution or reproduction in other forums is permitted, provided the original author(s) and the copyright owner(s) are credited and that the original publication in this journal is cited, in accordance with accepted academic practice. No use, distribution or reproduction is permitted which does not comply with these terms.
*Correspondence: Peng Zhang, cGVuZy56aGFuZ0BzaWF0LmFjLmNu; Liang Li, bGlsQHN1c3RlY2guZWR1LmNu; Yong Cui, b3Q5OEBzaW5hLmNvbQ==
Disclaimer: All claims expressed in this article are solely those of the authors and do not necessarily represent those of their affiliated organizations, or those of the publisher, the editors and the reviewers. Any product that may be evaluated in this article or claim that may be made by its manufacturer is not guaranteed or endorsed by the publisher.
Research integrity at Frontiers
Learn more about the work of our research integrity team to safeguard the quality of each article we publish.