- 1Medical Scientist Training Program, School of Medicine, Johns Hopkins University, Baltimore, MD, United States
- 2Ghost Lab, Division of Intramural Research, National Institute of Allergy and Infectious Diseases, National Institutes of Health, Bethesda, MD, United States
The Danger Model predicts that there are some molecules that no immune system can ever be fully tolerant of, namely proteins that are only transiently expressed during times of stress, infection, or injury. Among these are the danger/alarm signals themselves. Accordingly, a fleeting autoantibody response to danger signals is expected during times when they are released. Depending on context, these autoantibodies may serve beneficial “housekeeping” functions by removing surplus danger signals from the circulation or, conversely, create an immunodeficiency. Here, we will focus on the Type 1 Interferons as examples of foreseeable targets for a transient autoantibody response, but the principles outlined should hold for other danger-associated molecules as well.
1 Introduction
Danger signals (such as DNA, RNA, ATP, heat shock proteins, hyaluron breakdown products, inflammatory cytokines, HMGB1, interferons, etc.) are molecules that are fleetingly released as a result of infection, injury, toxins or other forms of non-physiological cell stress or death (1). They also represent a unique set of self-proteins that are predicted to be targets for an autoimmune response. To explain why, we start with two primary assumptions of the Danger Model (2, 3), namely that,
1. Antigen presenting cells (APCs) are primarily activated to induce immune responses by danger signals that have been released from stressed, damaged, or infected tissues (Figure 1A).
2. Tolerance is induced to any molecule (foreign or self) that is present and persists in the absence of activated APCs (Figure 1B).
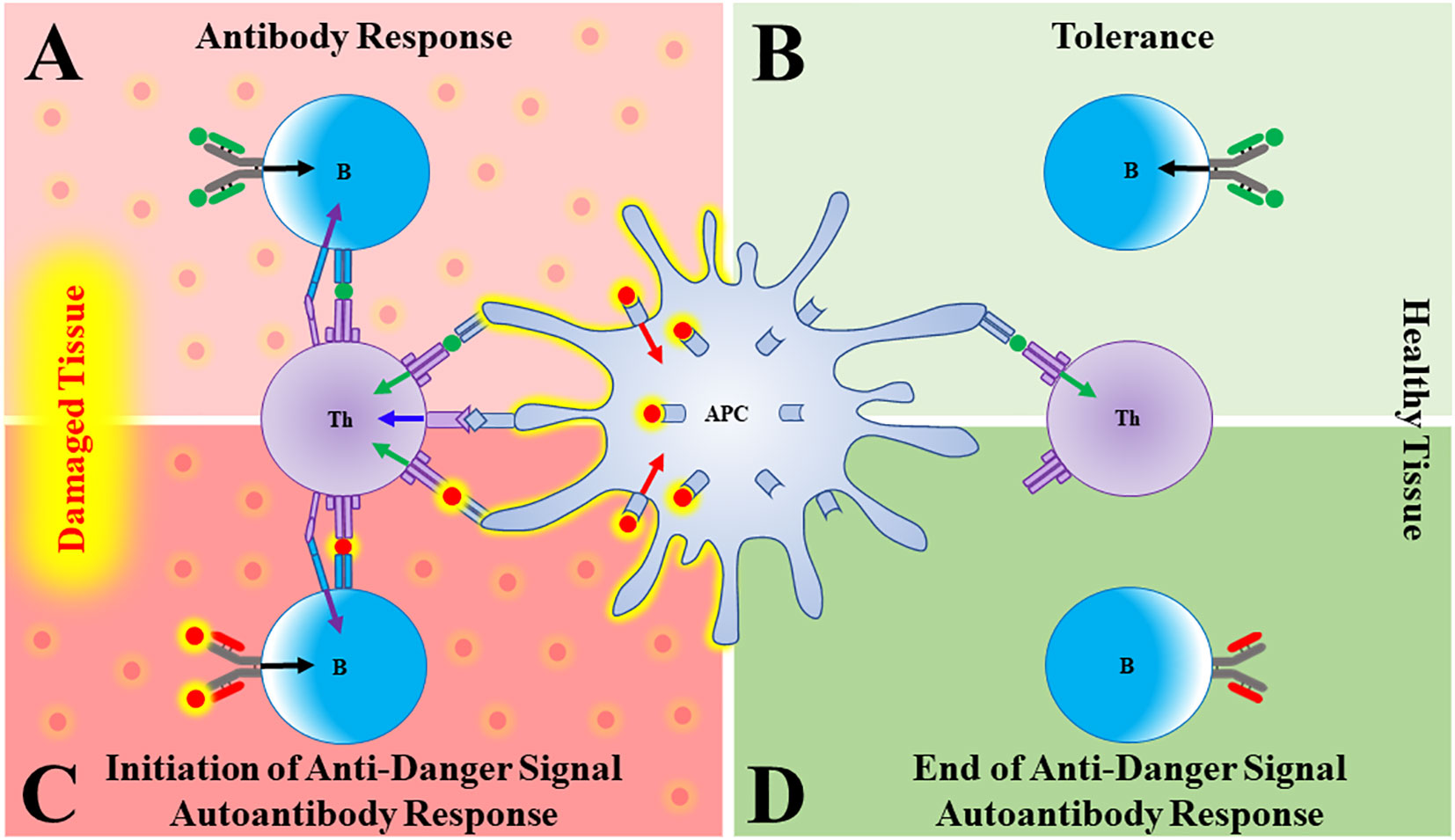
Figure 1 Autoantibodies to danger signals. (A) In damaged/distressed tissue, an antigen presenting cell (APC) that has been activated by a danger signal (red sphere, red arrow) presents antigen (green sphere) to a T cell, providing T cell Signal 1 (green arrow) and co-stimulation (blue arrow). The T cell can now give a “help” signal (purple arrow) to a B cell that had bound its cognate antigen (green sphere, black arrow), leading to a productive antibody response. (B) In the absence of danger signals (such as in healthy tissue), APCs are not activated and both T and B cells that bind antigen go on to die, thus generating tolerance. (C) As danger signals are elaborated in response to damage, a B cell bearing a B cell receptor (BCR) that binds the danger signal itself will become activated and produce antibody. (D) As the tissue recovers, danger signals wane. A B cell specific for danger signals will therefore go unstimulated and the anti-danger signal autoantibody response will fade.
Most forms of clinically overt autoimmunity are uncommon because activation of self-directed immune responses requires simultaneous failures of central and peripheral tolerance. While the thymus promotes central tolerance to self-proteins through clonal deletion of immature autoreactive T cells, the thymus cannot induce tolerance to antigens that are not found there (4–6). Thus, peripheral tolerance mechanisms exist to eliminate autoreactive B and T cells that bind peripheral self-antigens in the absence of danger-activated APCs (Figure 1B).
We propose that an autoimmune response to danger signals is expected because 1) it should be difficult to induce central tolerance to self-molecules that are produced transiently and 2) peripheral tolerance mechanisms will necessarily fail to generate tolerance towards danger signals because danger signals themselves can activate APCs.
2 Prediction
Let’s use the Type 1 interferons (T1IFNs) as an example. These molecules are produced only transiently (often in response to infection), and so are not normally present. Therefore, just like lymphocytes specific for pathogens, newly produced T1IFN-reactive T or B cells in a healthy individual would circulate as unactivated naïve cells due to lack of Signal 1. We would predict, however, that during an episode where T1IFNs are released/elaborated in response to danger, these naïve cells would encounter both T1IFN (Signal 1) and activated APCs (Signal 2), resulting in the production of anti-T1IFN autoantibodies (Figure 1C). Once the damage instigating agent has been cleared, T1IFNs stop being produced, activated APCs die, and the autoimmune response should wane as T1IFN-reactive T and B cells die or revert to a memory state, ready to respond to the next insult (Figure 1D).
3 Evidence
When central tolerance is disturbed, as it is in patients with thymic malignancies or with genetic or acquired deficiencies in autoimmune regulator (AIRE), neutralizing anti-T1IFN autoantibodies are common (7–11). Similar IgG autoantibodies that neutralize T1IFNs can also result from infections. For example, they were recently identified in 10-20% of patients with critical COVID-19 (12). Studying the temporal dynamics of anti-T1IFN autoantibodies, we found that autoantibody levels remained stable in patients with AIRE deficiency and thymic malignancies, but were highly dynamic in patients with acute COVID-19 (13). In the COVID-19 patients, both the levels and the neutralizing activity of autoantibodies peaked shortly after disease onset and then rapidly declined to undetectable levels during convalescence. Our data suggested that the increase in T1IFN production during the early stages of viral infection triggered a short-lived response of high titer neutralizing autoantibodies in these patients.
4 Perhaps a purpose
We started to wonder: If complete central and peripheral tolerance to transiently expressed danger signals would be nearly impossible using the logic of the Danger Model, could there be a function for these autoreactive cells and antibodies? Decades ago, Mel Cohn suggested that germline-encoded autoreactive “housekeeping antibodies” served the important role of clearing autogenously generated waste products from the extracellular space (14). One could envisage that the continued presence of danger signals long after an infection is cleared could lead to prolonged and unnecessary immune responses – both wasteful and potentially dangerous. Thus, transient autoantibodies directed against danger signals could be an evolutionarily selected characteristic. Indeed, binding (non-neutralizing) anti-IFN-γ autoantibodies that wax and wane in response to viral disease have been observed since 1990 (15) and transient anti-HMGB1 autoantibodies emerge in some patients with septic shock and are highly predictive of survival (16). In patients with systemic lupus erythematosus, neutralizing anti-IFN-α autoantibodies were found to have positive effects akin to those of therapeutic anti-IFN monoclonals (17). Similarly, endogenous disease-suppressing autoantibodies that neutralize TNF-α have been found in some patients with rheumatoid arthritis (18). These findings support the idea that the immune system has evolved to generate autoantibodies for the purpose of restraining immunopathology.
5 Thoughts: Anti-T1IFN autoantibodies in viral disease
It has become popular to consider that neutralizing anti-T1IFN autoantibodies underlie susceptibility to severe viral diseases (11, 12, 19–22). However, recent data with COVID-19 pokes a hole in this view. Specifically, Meisel and colleagues identified several patients with AIRE deficiency who experienced mild COVID-19 disease despite having high levels of potently neutralizing anti-T1IFN autoantibodies (23). An alternative view could be that these autoantibodies instead may be helpful disease modifiers, for example, by neutralizing or encouraging phagocytosis of excess cytokine to prevent hyperinflammation during the later stages of COVID-19. Supporting this idea is a study on COVID-19 patients admitted to the ICU where Abers et al. found that 10-week survival was higher in patients with autoantibodies (5 out of 26 = 19.2%) than in patients without (11 out of 192 = 5.7%) (24). Thus, “housekeeping” anti-cytokine autoAbs may play an important and underexplored role in the host response to viral disease. In other cases, they may simply be epiphenomena caused by a perfect storm of high levels of T1IFNs, activated APCs and rare(ish)? autoreactive lymphocytes.
6 Conclusions
The Danger Model predicts that transient cytokines that can activate APCs are particularly logical targets for an autoantibody response, and that a short-lived autoantibody response to danger signals might occur during any infection/injury in otherwise healthy individuals. Depending on context and neutralization activity, anti-danger signal autoantibodies may benefit the host by restraining immunopathology or, conversely, provoke an immunodeficiency (25, 26) or autoimmune disease. Further study of anti-danger signal autoantibodies may provide novel insights into how the immune system regulates itself and how derangements in this process may lead to disease.
Data availability statement
The original contributions presented in the study are included in the article/Supplementary Material. Further inquiries can be directed to the corresponding author.
Author contributions
Writing – original draft, ES. Writing – review, suggestions, and editing, ES and PM. All authors contributed to the article and approved the submitted version.
Funding
This research was supported [in part] by the Intramural Research Program of the NIH, National Institute of Allergy and Infectious Diseases, and in part by the Medical Scientist Training Program at the Johns Hopkins School of Medicine.
Acknowledgments
We thank Lindsey B. Rosen for her mentorship and support. We thank Steven Holland for funding and support. We thank Maximilian König for helpful discussions and for editing an early draft of the manuscript. We thank Hector Ibáñez for creative and insightful discussions.
Conflict of interest
The authors declare that the research was conducted in the absence of any commercial or financial relationships that could be construed as a potential conflict of interest.
Publisher’s note
All claims expressed in this article are solely those of the authors and do not necessarily represent those of their affiliated organizations, or those of the publisher, the editors and the reviewers. Any product that may be evaluated in this article, or claim that may be made by its manufacturer, is not guaranteed or endorsed by the publisher.
References
1. O'Reilly S. Pound the alarm: Danger signals in rheumatic diseases. Clin Sci (London Engl 1979) (2015) 128(5):297–305. doi: 10.1042/CS20140467
2. Matzinger P. The danger model: A renewed sense of self. Sci (New York N.Y.) (2002) 296(5566):301–5. doi: 10.1126/science.1071059
3. Matzinger P. Tolerance, danger, and the extended family. Annu Rev Immunol (1994) 12:991–1045. doi: 10.1146/annurev.iy.12.040194.005015
4. Bonomo A, Matzinger P. Thymus epithelium induces tissue-specific tolerance. J Exp Med (1993) 177(4):1153–64. doi: 10.1084/jem.177.4.1153
5. Coutinho A. The le douarin phenomenon: a shift in the paradigm of developmental self-tolerance. Int J Dev Biol (2005) 49(2-3):131–6. doi: 10.1387/ijdb.041965ac
6. Goldman KP, Park CS, Kim M, Matzinger P, Anderson CC. Thymic cortical epithelium induces self tolerance. Eur J Immunol (2005) 35(3):709–17. doi: 10.1002/eji.200425675
7. Meager A, Visvalingam K, Peterson P, Möll K, Murumägi A, Krohn K, et al. Anti-interferon autoantibodies in autoimmune polyendocrinopathy syndrome type 1. PLoS Med (2006) 3(7):e289. doi: 10.1371/journal.pmed.0030289
8. Chen K, Wu W, Mathew D, Zhang Y, Browne SK, Rosen LB, et al. Autoimmunity due to RAG deficiency and estimated disease incidence in RAG1/2 mutations. J Allergy Clin Immunol (2014) 133(3):880–2.e10. doi: 10.1016/j.jaci.2013.11.038
9. Walter JE, Rosen LB, Csomos K, Rosenberg JM, Mathew D, Keszei M, et al. Broad-spectrum antibodies against self-antigens and cytokines in RAG deficiency. J Clin Invest (2015) 125(11):4135–48. doi: 10.1172/JCI80477
10. Ströbel P, Murumägi A, Klein R, Luster M, Lahti M, Krohn K, et al. Deficiency of the autoimmune regulator AIRE in thymomas is insufficient to elicit autoimmune polyendocrinopathy syndrome type 1 (APS-1). J Pathol (2007) 211(5):563–71. doi: 10.1002/path.2141
11. Bastard P, Orlova E, Sozaeva L, Lévy R, James A, Schmitt MM, et al. Preexisting autoantibodies to type I IFNs underlie critical COVID-19 pneumonia in patients with APS-1. J Exp Med (2021) 218(7):e20210554. doi: 10.1084/jem.20210554
12. Bastard P, Rosen LB, Zhang Q, Michailidis E, Hoffmann HH, Zhang Y, et al. Autoantibodies against type I IFNs in patients with life-threatening COVID-19. Sci (New York N.Y.) (2020) 370(6515):eabd4585. doi: 10.1126/science.abd4585
13. Shaw ER, Rosen LB, Cheng A, Dobbs K, Delmonte OM, Ferré EMN, et al. Temporal dynamics of anti-type 1 interferon autoantibodies in patients with coronavirus disease 2019. Clin Infect Dis an Off Publ Infect Dis Soc America (2022) 75(1):e1192–4. doi: 10.1093/cid/ciab1002
14. Cohn M. The self/nonself discrimination: Reconstructing a cabbage from sauerkraut. Res Immunol (1992) 143(3):323–34. doi: 10.1016/s0923-2494(92)80132-5
15. Caruso A, Bonfanti C, Colombrita D, De Francesco M, De Rango C, Foresti I, et al. Natural antibodies to IFN-gamma in man and their increase during viral infection. J Immunol (Baltimore Md. 1950) (1990) 144(2):685–90.
16. Barnay-Verdier S, Fattoum L, Borde C, Kaveri S, Gibot S, Maréchal V. Emergence of autoantibodies to HMGB1 is associated with survival in patients with septic shock. Intensive Care Med (2011) 37(6):957–62. doi: 10.1007/s00134-011-2192-6
17. Gupta S, Tatouli IP, Rosen LB, Hasni S, Alevizos I, Manna ZG, et al. Distinct functions of autoantibodies against interferon in systemic lupus erythematosus: A comprehensive analysis of anticytokine autoantibodies in common rheumatic diseases. Arthritis Rheumatol (Hoboken N.J.) (2016) 68(7):1677–87. doi: 10.1002/art.39607
18. Wildbaum G, Nahir MA, Karin N. Beneficial autoimmunity to proinflammatory mediators restrains the consequences of self-destructive immunity. Immunity (2003) 19(5):679–88. doi: 10.1016/s1074-7613(03)00291-7
19. Puel A, Bastard P, Bustamante J, Casanova JL. Human autoantibodies underlying infectious diseases. J Exp Med (2022) 219(4):e20211387. doi: 10.1084/jem.20211387
20. Bastard P, Gervais A, Le Voyer T, Rosain J, Philippot Q, Manry J, et al. Autoantibodies neutralizing type I IFNs are present in ~4% of uninfected individuals over 70 years old and account for ~20% of COVID-19 deaths. Sci Immunol (2021) 6(62):eabl4340. doi: 10.1126/sciimmunol.abl4340
21. Zhang Q, Pizzorno A, Miorin L, Bastard P, Gervais A, Le Voyer T, et al. Autoantibodies against type I IFNs in patients with critical influenza pneumonia. J Exp Med (2022) 219(11):e20220514. doi: 10.1084/jem.20220514
22. Bastard P. Why do people die from COVID-19? Sci (New York N.Y.) (2022) 375(6583):829–30. doi: 10.1126/science.abn9649
23. Meisel C, Akbil B, Meyer T, Lankes E, Corman VM, Staudacher O, et al. Mild COVID-19 despite autoantibodies against type I IFNs in autoimmune polyendocrine syndrome type 1. J Clin Invest (2021) 131(14):e150867. doi: 10.1172/JCI150867
24. Abers MS, Rosen LB, Delmonte OM, Shaw E, Bastard P, Imberti L, et al. Neutralizing type-I interferon autoantibodies are associated with delayed viral clearance and intensive care unit admission in patients with COVID-19. Immunol Cell Biol (2021) 99(9):917–21. doi: 10.1111/imcb.12495
25. Browne SK. Anticytokine autoantibody-associated immunodeficiency. Annu Rev Immunol (2014) 32:635–57. doi: 10.1146/annurev-immunol-032713-120222
Keywords: type 1 interferon, autoantibodies, danger model, danger signal, autoimmunity, virus
Citation: Shaw ER and Matzinger P (2023) Transient autoantibodies to danger signals. Front. Immunol. 14:1046300. doi: 10.3389/fimmu.2023.1046300
Received: 16 September 2022; Accepted: 02 January 2023;
Published: 18 January 2023.
Edited by:
Adnane Achour, Karolinska Institutet (KI), SwedenReviewed by:
Steven O’Reilly, STipe Therapeutics, DenmarkAbdulraouf Ramadan, LAPIX Therapeutics, United States
Copyright © 2023 Shaw and Matzinger. This is an open-access article distributed under the terms of the Creative Commons Attribution License (CC BY). The use, distribution or reproduction in other forums is permitted, provided the original author(s) and the copyright owner(s) are credited and that the original publication in this journal is cited, in accordance with accepted academic practice. No use, distribution or reproduction is permitted which does not comply with these terms.
*Correspondence: Elana R. Shaw, eshaw12@jhmi.edu