- 1Cleveland Clinic Lerner College of Medicine, Case Western Reserve University, Cleveland, OH, United States
- 2Department of Inflammation and Immunity, Lerner Research Institute, Cleveland Clinic, Cleveland, OH, United States
- 3Medical Intensive Care Unit, Cleveland Clinic, Cleveland, OH, United States
- 4Department of Quantitative Health Sciences, Cleveland Clinic, Cleveland, OH, United States
- 5Department of Dermatology, The University of Oklahoma College of Medicine, Oklahoma City, OK, United States
- 6Department of Infectious Disease, Cleveland Clinic, Cleveland, OH, United States
- 7Department of Dermatology, University Hospitals Cleveland Medical Center, Cleveland, OH, United States
- 8Department of Dermatology, Dermatology and Plastic Surgery Institute, Cleveland Clinic, Cleveland, OH, United States
- 9Department of Anatomic Pathology, Pathology and Lab Medicine, Cleveland Clinic, Cleveland, OH, United States
- 10Department of Molecular Medicine, Cleveland Clinic Lerner College of Medicine, Case Western Reserve University, Cleveland, OH, United States
Hospitalized patients have an increased risk of developing hospital-acquired sacral pressure injury (HASPI). However, it is unknown whether SARS-CoV-2 infection affects HASPI development. To explore the role of SARS-CoV-2 infection in HASPI development, we conducted a single institution, multi-hospital, retrospective study of all patients hospitalized for ≥5 days from March 1, 2020 to December 31, 2020. Patient demographics, hospitalization information, ulcer characteristics, and 30-day-related morbidity were collected for all patients with HASPIs, and intact skin was collected from HASPI borders in a patient subset. We determined the incidence, disease course, and short-term morbidity of HASPIs in COVID-19(+) patients, and characterized the skin histopathology and tissue gene signatures associated with HASPIs in COVID-19 disease. COVID-19(+) patients had a 63% increased HASPI incidence rate, HASPIs of more severe ulcer stage (OR 2.0, p<0.001), and HASPIs more likely to require debridement (OR 3.1, p=0.04) compared to COVID-19(-) patients. Furthermore, COVID-19(+) patients with HASPIs had 2.2x increased odds of a more severe hospitalization course compared to COVID-19(+) patients without HASPIs. HASPI skin histology from COVID-19(+) patients predominantly showed thrombotic vasculopathy, with the number of thrombosed vessels being significantly greater than HASPIs from COVID-19(-) patients. Transcriptional signatures of a COVID-19(+) sample subset were enriched for innate immune responses, thrombosis, and neutrophil activation genes. Overall, our results suggest that immunologic dysregulation secondary to SARS-CoV-2 infection, including neutrophil dysfunction and abnormal thrombosis, may play a pathogenic role in development of HASPIs in patients with severe COVID-19.
Introduction
Patients with coronavirus disease 2019 (COVID-19) develop multi-organ manifestations and tissue damage secondary to both intrinsic properties of the SARS-CoV-2 virus and the resultant host immune response. The skin is among the organs affected by COVID-19, with manifestations including various localized or diffuse exanthems and retiform or acral purpura associated with thrombotic vasculopathy (1–5). Skin manifestations in COVID-19 disease may contribute to adverse symptoms and exacerbate patient morbidity, but significant skin necrosis leading to permanent damage in the form of scarring is rarely reported. Although several groups have reported challenges detecting SARS-CoV-2 virus in the skin; some studies have demonstrated the presence of viral Spike protein via immunohistochemistry (6–8).
Sacral ulcers are a leading cause of cutaneous-related patient morbidity in hospitalized patients (9, 10). The most common cause of sacral ulcers in hospitalized patients are hospital-acquired sacral pressure injuries (HASPIs), which occur secondary to unremitting pressure on the sacral and/or buttocks region (11, 12). Given the significant risk of HASPIs in hospitalized patients, most hospitals have developed protocols involving monitoring for early pressure-induced changes and routine off-loading measures in attempts to prevent them. Risk factors for HASPI formation include prolonged periods of immobility, decreased sensory perception, decreased mobility, increased moisture, and poor nutrition, many of which are typically present in hospitalized COVID-19 patients (11). Although small case series have reported the development of sacral ulcers in hospitalized COVID-19 patients, detailed analyses concerning incidence and prevalence of such ulcers, as well as risk factors associated with their development, are lacking (13, 14).
Despite the frequent presence of common HASPI risk factors, our clinical experience is that some COVID-19 patients acquire HASPIs that develop and progress more rapidly than usual, which may be associated with retiform purpura (13, 15). Based on these observations, we hypothesized that factors inherent to SARS-CoV-2 infection contribute to formation of HASPIs in some COVID-19 patients. This study explored the pathogenesis and determined the risk and associated morbidity of HASPIs in hospitalized COVID-19 patients. In addition to characterizing the clinical features of COVID-19(+) patients with HASPIs, histologic features of sacral skin biopsies acquired from COVID-19(+) patients with HASPIs were compared to sacral skin samples from pressure ulcers (PU) in COVID-19(-) patients. Furthermore, the transcriptional landscapes of sacral skin samples from COVID-19(+) and COVID-19(-) patients were analyzed to identify SARS-CoV-2-associated contributions.
We found that COVID-19(+) patients are at an increased risk of developing HASPIs and HASPI-associated morbidity compared to COVID-19(-) patients, even when controlling for baseline risk factors for ulceration. A large percentage of sacral skin biopsies from the borders of HASPIs of COVID-19(+) patients displayed thrombotic vasculopathy (TV), with significantly more thrombosed vessels per tissue section compared to sacral skin samples from PU in COVID-19(-) patients. Furthermore, the transcriptional profile differences in sacral biopsies from COVID-19(+) patients with TV support abnormal thrombosis and enhanced neutrophil extracellular trap (NET)-driven inflammation in sacral skin of COVID-19(+) patients compared to sacral skin specimens from PU in COVID-19(-) patients. These findings implicate thrombotic and inflammatory responses to SARS-CoV-2 infection in the promotion of cutaneous vascular thromboses, tissue ischemia, and eventual cutaneous ulceration.
Results
Risk of HASPI development and severity is increased in hospitalized COVID-19 patients
To understand the risk of HASPI development in COVID-19 disease, the incidence of sacral ulcers was compared between COVID-19(+) and COVID-19(-) patients in our study cohort (Supplementary Figure 1). Clinical and demographic characteristics of hospitalized patients during the study period were compared based on COVID-19 status, regardless of HASPI status. On average, COVID-19(+) patients were significantly older (p<0.001), with an increased percentage of males (p=0.003) and non-whites (p<0.001) compared to COVID-19(-) patients (Supplementary Table 1). COVID-19(+) patients had a significantly higher baseline risk of ulcer formation, longer length of hospitalization, higher rate of intensive care unit (ICU) admission, and a higher rate of mechanical ventilation compared to COVID-19(-) patients (Supplementary Table 1; p<0.001 for all 3 outcomes). Of 293 patients with HASPIs, COVID-19(+) patients had a HASPI occurrence of 7.5 per 10,000 days (49 patients; 16.7%), while HASPIs occurred in COVID-19(-) patients at 4.6 per 10,000 days (244 patients; 83.3%) (Supplementary Table 1). This translated to a 63% increased incidence and a 2-fold higher relative risk for HASPIs in COVID-19(+) patients compared to COVID-19(-) patients.
COVID-19 severity is associated with HASPI risk and morbidity in relation to co-morbidities
Next, the hospitalization course of COVID-19(+) patients with and without HASPIs were compared in relation to their comorbidities (Supplementary Table 2). COVID-19(+) patients with HASPIs had a higher risk of ulcer formation at admission (Braden score of 14 vs. 19, p<0.001), longer hospitalization length (29 vs. 8 days, p<0.001), and more severe COVID-19 disease course, including increased requirement of mechanical ventilation or death (p<0.001) as compared to COVID-19(+) patients without HASPIs (Supplementary Table 2).
Multivariable regression analysis controlling for age at admission and patient co-morbidities (hypertension, chronic obstructive pulmonary disease (COPD), congestive heart failure and diabetes) was performed to assess impact of co-morbidities on HASPI development and hospitalization course (Supplementary Table 3). When controlling for age and comorbidities, COVID-19(+) patients who developed HASPIs were 2.2-fold more likely to have a severe COVID-19 hospitalization course compared to COVID-19(+) patients without HASPIs.
In patients that developed HASPIs, COVID-19(+) patients are more likely to develop severe HASPIs that require debridement
Clinical and demographic characteristics in the subset of COVID-19(+) (49 patients) and COVID-19(-) (244 patients) hospitalized patients who developed HASPIs were compared to examine the impact of COVID-19 on disease course and ulcer phenotypes (Table 1). Amongst patients who developed HASPIs, COVID-19(+) patients had a higher baseline risk of ulcer formation at hospital admission (Braden score of 14 vs. 18, p<0.001) and a higher frequency of requiring intubation (20.4% vs 3.3%, p<0.001) compared to COVID-19(-) patients (Table 1). On the other hand, gender, hospitalization length, and ICU admission rate did not differ between the two groups. A larger percentage of COVID-19(+) patients with HASPIs were black (p=0.03) (Table 1). Time to HASPI and HASPI size did not differ between COVID-19(+) and COVID-19(-) patients; however, COVID-19(+) patients were more likely to have a severe HASPI stage compared to COVID-19(-) patients (24.9% vs. 9.0% Stage 3 or 4 ulcer p=0.003).
We also compared laboratory parameters for patients who developed HASPIs to determine if there were differences among COVID-19(+) and COVID-19(-) patients. COVID-19(+) patients had significantly higher maximum ferritin concentrations compared to COVID-19(-) patients (1,303 vs. 813 ng/mL, p=0.007). Other laboratory parameters, including maximum D-dimer concentration, maximum white blood cell (WBC) count, and neutrophil-to-lymphocyte ratio did not significantly differ between the two groups (Table 1).
We performed multivariable regression modeling, controlling for Braden ulcer risk at admission, hypertension, congestive heart failure, chronic obstructive pulmonary disease, diabetes mellitus, age at admission, hospitalization length, requirement for ICU admission, and requirement for intubation to assess the impact of SARS-CoV-2 infection on HASPI development and characteristics (Table 2). Within our cohort, COVID-19 positivity was associated with 40% increased odds of developing a HASPI (p=0.03) even after controlling for Braden ulcer risk score at admission and other variables. Additionally, COVID-19(+) patients were 2.1-fold more likely to develop a HASPI of more severe stage (p<0.001) and 3.1-fold more likely to require debridement (p=0.045) compared to COVID-19(-) patients. Time to HASPI development and wound size were not significantly associated with COVID-19 status based on multivariable regression (Table 2). Overall, 30-day morbidity did not differ amongst the two groups (Supplementary Table 4). However, a greater percentage of COVID-19(+) patients required surgical debridement compared to COVID-19(-) patients (14.3% vs. 5.7%, p=0.047; Supplementary Table 4).
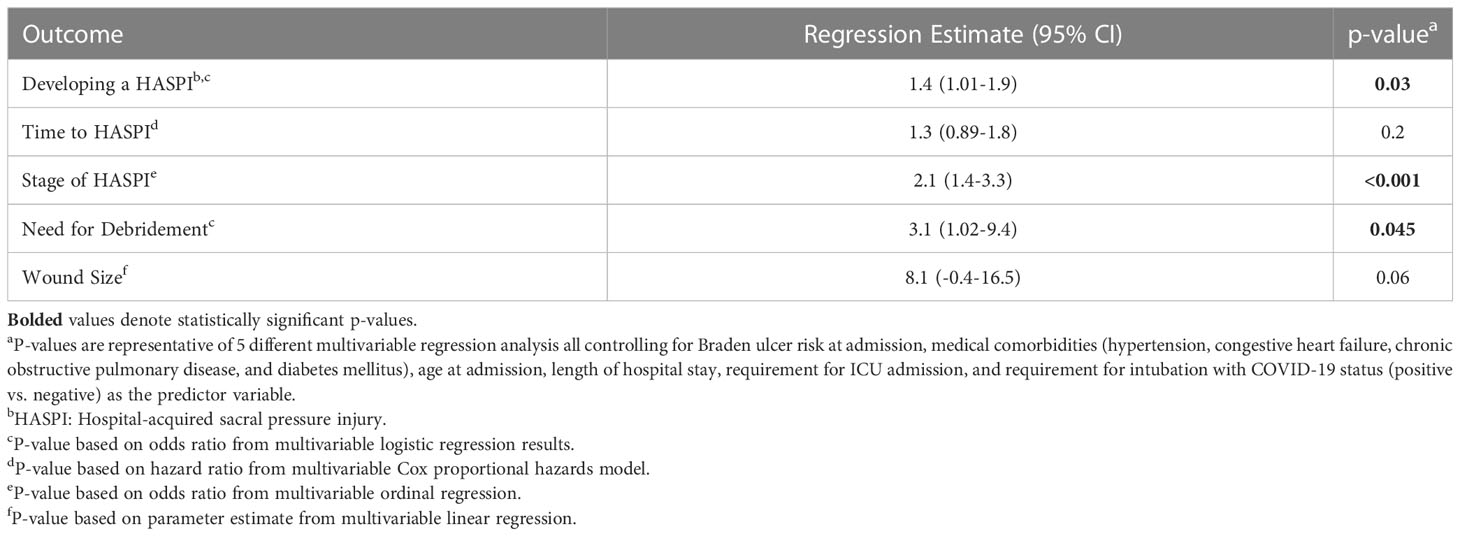
Table 2 Multivariable logistic regression model assessing the impact of COVID-19 status (positive vs. negative) on HASPI development and characteristics.
Thrombotic vasculopathy is a prominent histopathologic feature in a significant percentage of COVID-19(+) patient HASPIs
The histopathological features of skin biopsies from the edges of HASPIs in COVID-19(+) patients were compared to similar tissue specimens from COVID-19(-) patients with PUs. Qualitative assessments revealed that biopsies of COVID-19(+) patients with HASPIs could be broadly categorized into 2 groups: histologic features consistent with thrombotic vasculopathy (TV; 67%) or histologic features consistent with PUs (33%); (Figure 1; Table 3). In our study, PU is defined to be a cutaneous ulceration secondary to tissue ischemia caused entirely by unremitting pressure on the involved anatomic location, without important contributions from other pathologic processes. Whereas specimens with a TV pattern displayed diffuse fibrin thrombi within superficial and deep dermal and subcutaneous blood vessels, in addition to dermal neutrophils with occasional leukocytoclasis, specimens with a PU pattern displayed only occasional vascular thromboses with a sparse superficial dermal inflammatory infiltrate, but also displayed dermal neutrophils with occasional leukocytoclasis (Figures 1A, B; Supplementary Figures 2A, B, 3A, B, 4A, B; Table 3). Specimens in the COVID-19(-) PU control group typically displayed mixed dermal inflammation and dilated vascular spaces with congestion and only occasional fibrin thrombi (Figure 1C; Supplementary Figures 2C, 3C, 4C; Table 3). Most specimens had an intact epidermis, but two COVID-19(-) PU control specimens had at least partial epidermal ulceration (20%). Additionally, COVID-19(-) PU specimens lacked leukocytoclasis and typically had eosinophils within the dermal inflammatory infiltrate or within vascular lumina (Figure 1C; Supplementary Figure 2C).
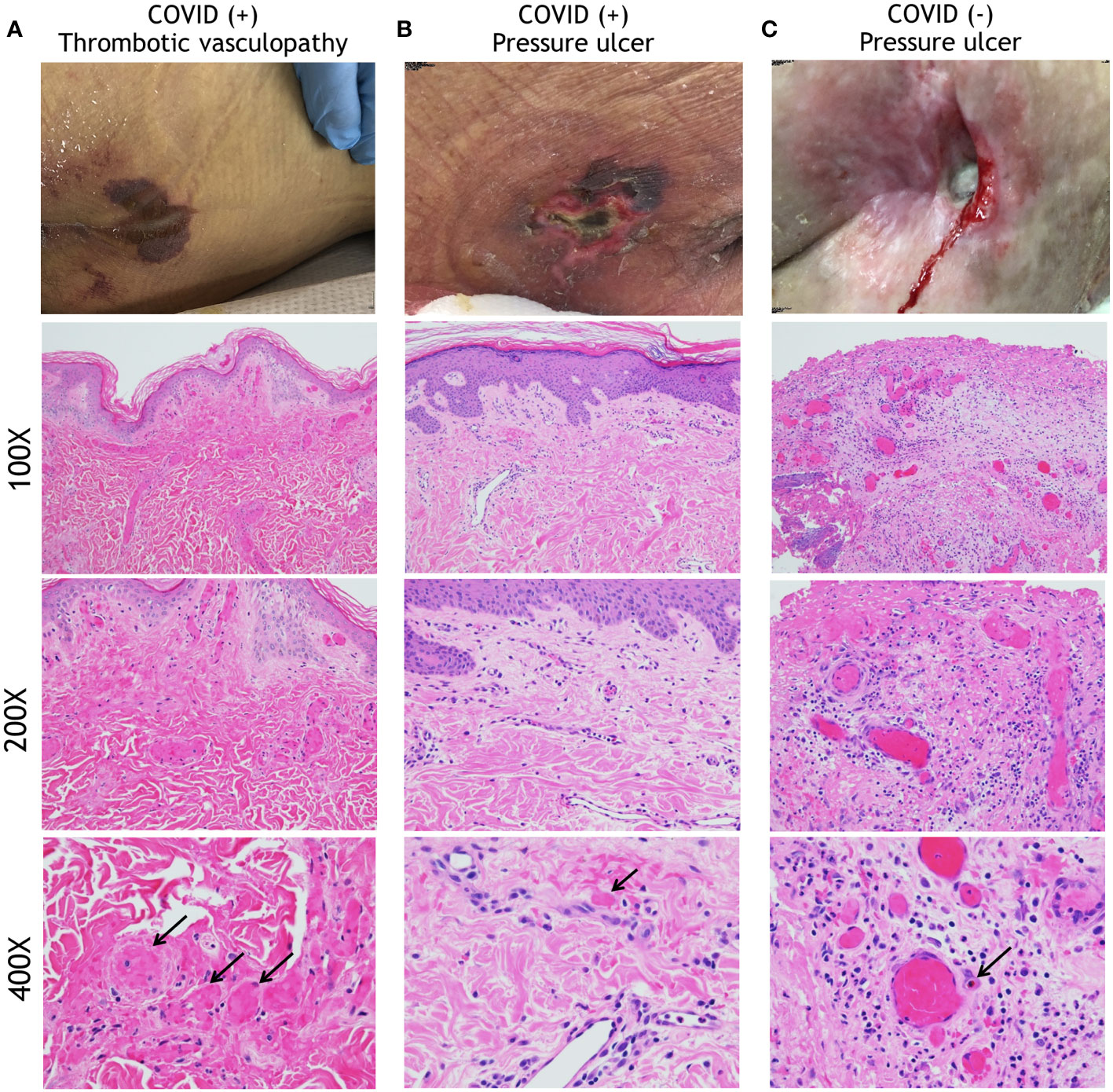
Figure 1 Histopathology analysis of HASPIs. (A) COVID-19-related Thrombotic Vasculopathy. A man in his 30s was intubated in the ICU due to severe COVID-19 with an acute purpuric rash on the buttocks that subsequently ulcerated. A low-power photomicrograph (100X) reveals a relatively normal and intact epidermis with diffuse fibrin thrombi involving superficial and deep dermal vessels. A higher power image (200X) further highlights diffuse fibrin thrombi and occasional inflammatory cells within the dermis. At even higher power (400X) occasional neutrophils and leukocytoclasis can be seen. (B) A woman in her 80s who developed a sacral ulcer with surrounding purpura in the setting of COVID-19 after 6 weeks of intubation. At low power (100X), a photomicrograph displays an intact epidermis with mostly patent vascular spaces in the dermis. At higher power (200X), a sparse superficial dermal inflammatory infiltrate with occasional neutrophils is noted. A 400X image reveals a rare vascular thrombosis. Other vessels display intact neutrophils within their lumens. (C) A pressure ulcer in a COVID-19(-), wheelchair-bound man in his 70s. A low-power (100X) image reveals an ulcer with superficial fibrin necrosis, mixed inflammation, and dilated vascular spaces with congestion and occasional fibrin thrombi. A higher power (200X) image reveals centrally located vessels with congestion and peripheral vessels with fibrin thrombi. At 400X magnification, a mixed inflammatory infiltrate is seen surrounding vessels that are either congested or with contained fibrin thrombi. Neutrophils are intact, without obvious leukocytoclasis. An eosinophil is present within a vascular lumen (arrow, (400X)). Eosinophils were often seen in COVID-19(-)-associated ulcer specimens, either within vascular lumina or in the dermis.
The clinical and histological characteristics of biopsied patients were further analyzed in a quantitative manner (Table 3). Age and sex of patients with sacral tissue specimens did not differ, however average hospitalization length and severity of disease course of biopsied COVID-19(+) patients (both TV and PU) was significantly greater than in COVID(-) patients (p=0.02 and p<0.001, respectively). COVID-19(-) patients with tissue specimens available had ulcers of more severe stage compared to the two COVID-19(+) groups. Additionally, due to frequent consultation to evaluate unusual purpuric sacral rashes in COVID-19(+) patients, skin tissue specimens were taken from COVID-19(+) patients at time points closer to onset of ulceration compared to the COVID-19(-) patients (19.5 vs. 59.5 days). There were no significant differences observed in the maximum D-dimer levels and the absolute neutrophil counts between the three groups; however, not all subjects had D-dimer levels reported, especially in COVID-19(-) patients. Histologically, COVID-19(+) TV biopsies had a significantly greater number of vascular thromboses in the superficial and deep dermis, but not in the subcutis, and significantly more leukocytoclasis in the upper dermis, compared to COVID-19(+) PU and COVID-19(-) PU histologic sections (Table 3).
Genes involved in interferon-related viral responses and neutrophil extracellular trap formation are uniquely upregulated in COVID-19(+) TV skin
The combination of clinical retiform purpura and histologic thrombotic vasculopathy in the majority of the COVID-19(+) patients with HASPIs led us to hypothesize that immunologic dysregulation due to SARS-CoV-2 infection may contribute to the pathogenesis of HASPIs in these patients. To explore this, the differentially expressed gene (DEG) profile of the tissue specimens from the TV and PU COVID-19(+) groups were compared to the COVID-19(-) PU controls. Dimensionality reduction using principal component analysis (PCA) revealed that the COVID-19(+) TV and PU groups segregated into separate clusters that were distinct from the COVID-19(-) PU control group (Figure 2A).
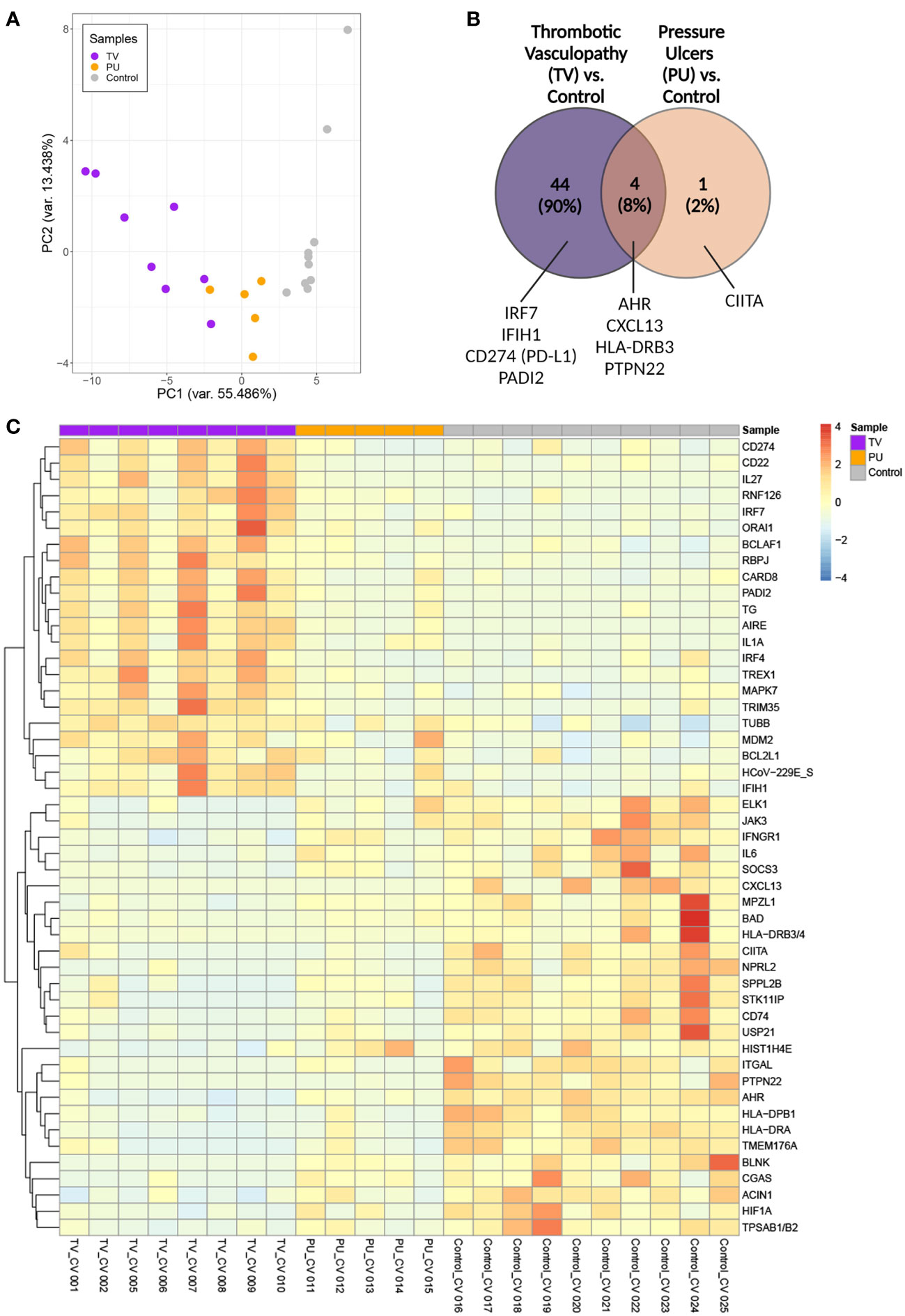
Figure 2 Transcriptomic analysis of sacral ulcer biopsies from COVID-19(+) patients reveals distinct signatures between TV and PU groups relative to COVID-19(-) PU control ulcers. (A) Principal component analysis of all patient samples using normalized counts from the differentially expressed genes with FDR adjusted p-value of < 0.05 indicates the distance between COVID-19(+) TV (TV), COVID-19(+) PU (PU), and COVID-19(-) PU (Control) samples. (B) Venn diagram showing differentially expressed genes (FDR adjusted p-value of < 0.05) in COVID-19(+) patients with TV and PU compared with COVID-19(-) PU control. Representative genes are indicated. (C) Supervised two-dimensional Hierarchical clustering of the 49 genes whose average normalized counts were significantly different in COVID-19(+) TV and PU groups compared to COVID-19(-) PU control samples (FDR adjusted p-value of < 0.05).
Among 44 DEGs that were unique to the COVID-19(+) TV group (Figure 2B), genes involved in responses to viral infection, such as the interferon regulatory factor 7 (IRF7) transcription factor and interferon-induced with helicase C domain 1 (IFIH1) that encodes the cytosolic nucleic acid sensor MDA-5, were identified among the top TV-specific up-regulated genes compared to COVID-19(+) PU and COVID-19(-) PU groups. CD274 (PD-L1), which has been implicated in dysregulated neutrophil responses in severe COVID-19, and PADI2, an enzyme involved in the citrullination of arginine residues and neutrophil extracellular trap (NET) formation, were also highly expressed in the TV group compared to the other two groups (16).
HLA-DRB3, CXCL13, PTPN22 and AHR were identified as genes that were commonly down-regulated in both COVID-19(+) TV and COVID-19(+) PU groups compared to the COVID-19(-) PU control group. These genes are key players in antigen processing and T and B cell homeostasis. Only CIITA, a master regulator of MHC class II genes, was differentially expressed and down-regulated in the COVID-19(+) PU group compared to the COVID-19(-) PU controls. Taken together, this data suggests that innate immune responses are primarily dysregulated in COVID-19(+) TV pathology in comparison to COVID-19(-) PUs. In contrast, antigen processing and T and B cell signaling mechanisms are potentially downregulated in both COVID-19(+) TV and PU groups.
Hierarchical clustering of differentially-expressed genes reveals unique immunologic pathway dysregulation in COVID-19(+) TV skin compared to COVID-19(+) and (-) PU skin
To better characterize the transcriptional signatures in skin tissue from the three ulcer groups, hierarchical clustering of the top up- and down-regulated genes in each disease category was performed (Figure 2C). The signatures associated with the COVID-19(+) TV and COVID-19(-) PU group were distinct from one another, supporting unique biological mechanisms of disease pathogenesis in sacral skin as a result of severe SARS-CoV-2 infection. In comparison to the COVID-19(-) PU controls, genes highly expressed in the TV group fell into categories that included neutrophil dysfunction (CD274, PADI2), inhibition of B and T cell responses (CD22, IRF4, ORAI1), and upregulation of pro-inflammatory response pathways (CARD8, MAPK7, IRF7, IFIH1). In contrast to COVID-19(+) TV, highly expressed in the COVID-19(-) PU control group included MHC class II antigen processing (HLA-DRB3/4, HLA-DPB1, HLA-DRA, CIITA, and CD74), mast cell degranulation (TPSAB1/B2), B cell activation (CXCL13, BLNK, IL-6) and histone modification (USP21, HIST1H4E). An apoptotic gene signature was found in both COVID-19(+) TV and COVID-19(-) PU control groups but not in the COVID-19(+) PU samples, consistent with the level of tissue necrosis in these tissue specimens. However, these apoptotic gene signatures were characterized by distinct DEGs expressed in the COVID-19(+) TV (BCLAF1, BCL2L1) and COVID-19(-) PU control groups (BAD, ACIN1, HIF1A) (Figure 2C).
SARS-CoV-2-specific transcripts were also measured to determine whether transcriptional profiles and immune responses in the skin of COVID-19(+) patients may be a direct, local response to viral infection or a result of systemic immune responses. Although a majority of the COVID-19(+) TV tissue samples were positive for the presence of a limited set of SARS-CoV-2 transcripts (Supplementary Figure 5), these differences in transcript level do not persist as significant when false discovery rate (FDR) testing was performed on the entire Nanostring panel. Therefore, it is unclear from this limited sample set whether local SARS-CoV-2 infection contributes to HASPI pathology.
Taken together, these results indicate distinct transcriptional differences in HASPI skin of COVID-19(+) TV patients compared to COVID-19(+) PU and COVID-19(-) PU controls. Genes that were highly upregulated in COVID-19(+) TV were downregulated in both PU groups indicative of similar mechanisms of pathogenesis in PU. Upregulation of genes involved in neutrophil dysfunction and pro-inflammatory response mechanisms, specifically genes involved in response to viral infection, suggests that transcriptional differences observed in the COVID-19(+) TV group might be a result of SARS-CoV-2 infection (either locally or systemically) and account for the unique histopathological findings of thrombotic vasculopathy (Figure 1A).
COVID-19(+) TV skin displays upregulation of unique biologic pathways compared to COVID-19(-) PU control skin
To understand the effect of infection and thrombotic vasculopathy in the COVID-19(+) TV group compared to the COVID-19(-) PU control group, the top 10 enriched Gene Ontology (GO) biological pathways in the COVID-19(+) TV and COVID-19(-) PU control groups were identified, followed by a sub-analysis to determine the significantly altered transcripts within these pathways (Figure 3). Both distinct and common biological mechanisms were identified in each set of analyses (Figures 3A, B). Top biological pathways in the COVID-19(+) TV group up-regulated in comparison to COVID-19(-) PU controls included positive regulation of pro-inflammatory cytokine production, defense response to virus, and the innate immune response pathway (Figure 3A). Hematopoietic or lymphoid organ development and T cell activation involved in immune response represented top down-regulated pathways in the COVID-19(+) TV group compared to the COVID-19(-) PU control group (Figure 3B). Antigen processing and presentation of exogenous peptide antigen via MHC class II biological pathway was also down-regulated in the COVID-19(+) TV group compared to the COVID-19(-) PU control group, indicating hampered adaptive immune responses in the COVID-19(+) TV group (Figure 3B). Pathway analysis did not reveal major pathways that were distinctly up- or down-regulated in the COVID-19(+) PU group compared to the COVID-19(-) PU group, suggesting similar biological mechanisms contribute to disease pathology of PU regardless of infection status.
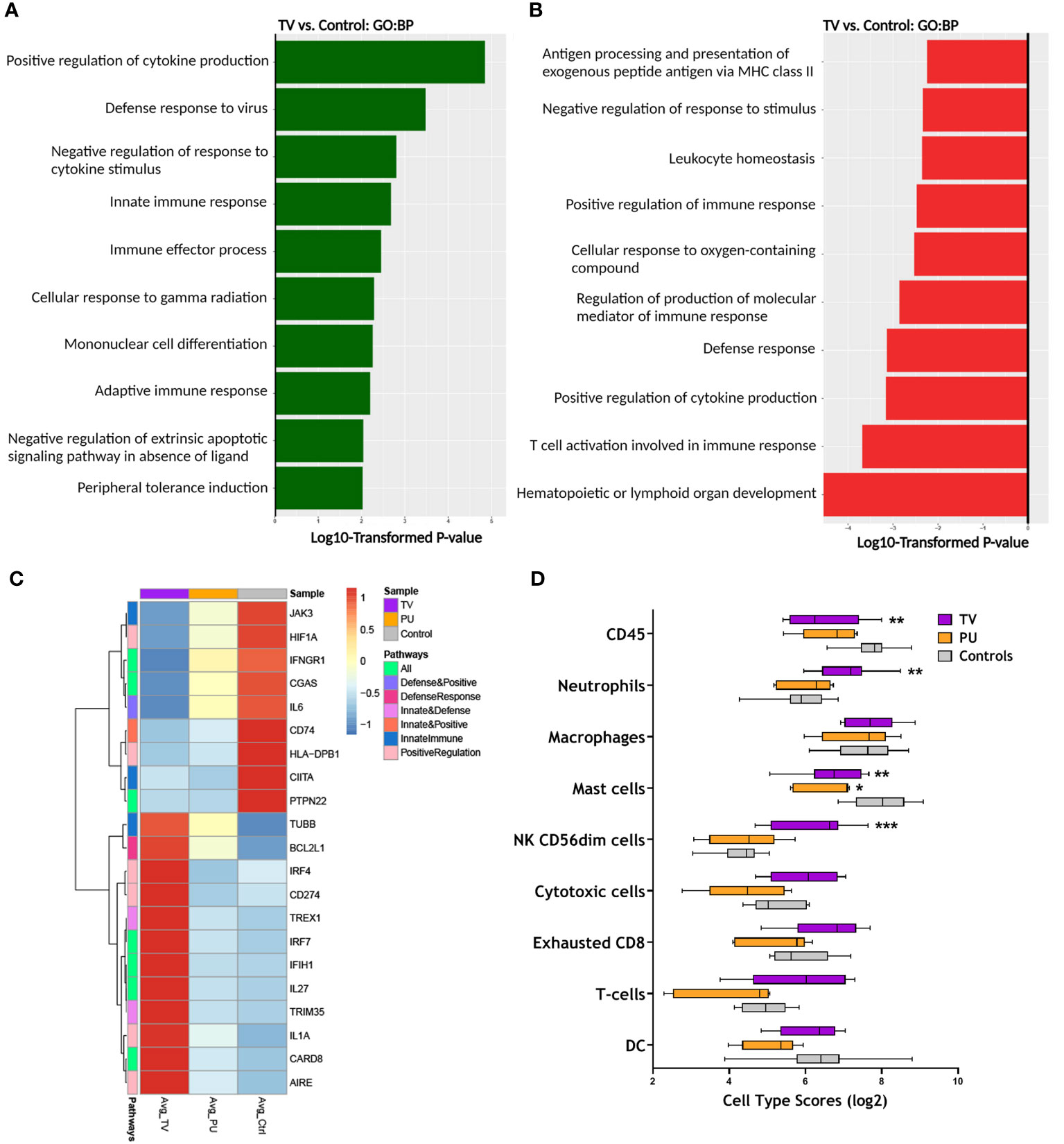
Figure 3 RNA expression reveals distinct immune responses in TV ulcers. (A) Top enriched GO biological pathways (N=10) in COVID-19(+) TV (TV) samples compared with COVID-19(-) PU (Control). (B) Top GO biological pathways (N=10) downregulated in TV samples relative to controls. (C) Clustered heatmap comparing average normalized counts of COVID-19(+) TV and COVID-19(+) PU (PU) groups with COVID-19(-) PU Control samples depicts differentially expressed genes (FDR adjusted p-value of <0.05) involved in positive regulation of cytokine production, defense response to virus, and innate immune response pathways. Genes involved in pathways were identified from GO biological pathways (D) Box and whisker plot shows the relative immune cell type scores of TV, PU, and Control samples. Cell type scores were determined using NanoString Advanced Analysis.
The analysis was expanded to uncover transcriptional signatures in the above three biological pathways up-regulated in COVID-19(+) TV specimens compared to COVID-19(-) PU control specimens (Figure 3C). Select genes that were highly expressed in COVID-19(+) TV specimens compared to COVID-19(+) PU and COVID-19(-) PU control groups play a central role in regulation of innate immune responses and defense to viral infection, including CARD8, IL1A, CD274, TRIM35, IRF7, and IFIH1 (Figure 3C). Both PU groups displayed similar trends in gene expression in the subset of genes that were upregulated in the COVID-19(+) TV group.
As several of the GO pathways altered in COVID-19(+) TV samples relate to regulation of immune cell populations, cell type scores were calculated to identify differences in the skin immune cell landscape in the tissue specimens from the three HASPI groups. Neutrophils and CD56+dim natural killer (NK) cells were significantly higher in the COVID-19(+) TV group compared to the other disease groups (Figure 3D). The identification of a neutrophil gene signature in the COVID-19(+) TV samples is consistent with results of our DEG and GO biological pathway analyses supporting involvement of innate immune response (CD274, PADI2), as well as histologic differences in the inflammatory cell infiltrates observed in the disease groups (Figure 3D and Figure 2, Table 3). Taken together, these results demonstrate that HASPI tissue from the COVID-19(+) TV group displays upregulation of unique biologic pathways, including defense response to virus and innate immune responses, and a differential immune cell signature, compared to the COVID-19(+) PU and COVID-19(-) PU groups, suggesting differing HASPI pathogeneses. It also reinforces the histologic observations that neutrophils might be key players in COVID-19(+) TV pathology.
Genes associated with thrombosis, PADI2-associated NET formation, and exacerbated inflammatory responses are specifically up-regulated in COVID-19(+) TV skin
Neutrophils have been implicated as key players in mechanisms contributing to immunothrombosis in COVID-19 disease (17–19). Given this and our above results, gene-set enrichment analysis (GSEA) was utilized to more completely characterize transcriptional signatures associated with thrombosis, immune cell chemotaxis, and neutrophil-specific biological functions in our HASPI groups. First, examined were genes involved in thrombosis and complement genes that may contribute to enhanced thrombosis. Notably, CXCL10, a cytokine that is implicated among the three key players contributing to COVID-19 cytokine storm and increased fatal COVID-19 outcomes (20), was highly expressed in the COVID-19(+) TV group compared to the other disease groups. Additionally, genes associated with thrombotic mechanisms, including MEFV, STAT5B, CD55, CRP, CTLA4, TLR4, CCR1, CFP, C4A/B and IL23R, were only upregulated in the COVID-19(+) TV group (Figure 4A). Alternatively, complement activation genes were similarly expressed in all three ulcer groups (Figure 4A). These findings suggest that COVID-19(+) TV HASPIs may have abnormal thrombosis without enhanced complement activation due to upregulation of genes that mediate thrombosis.
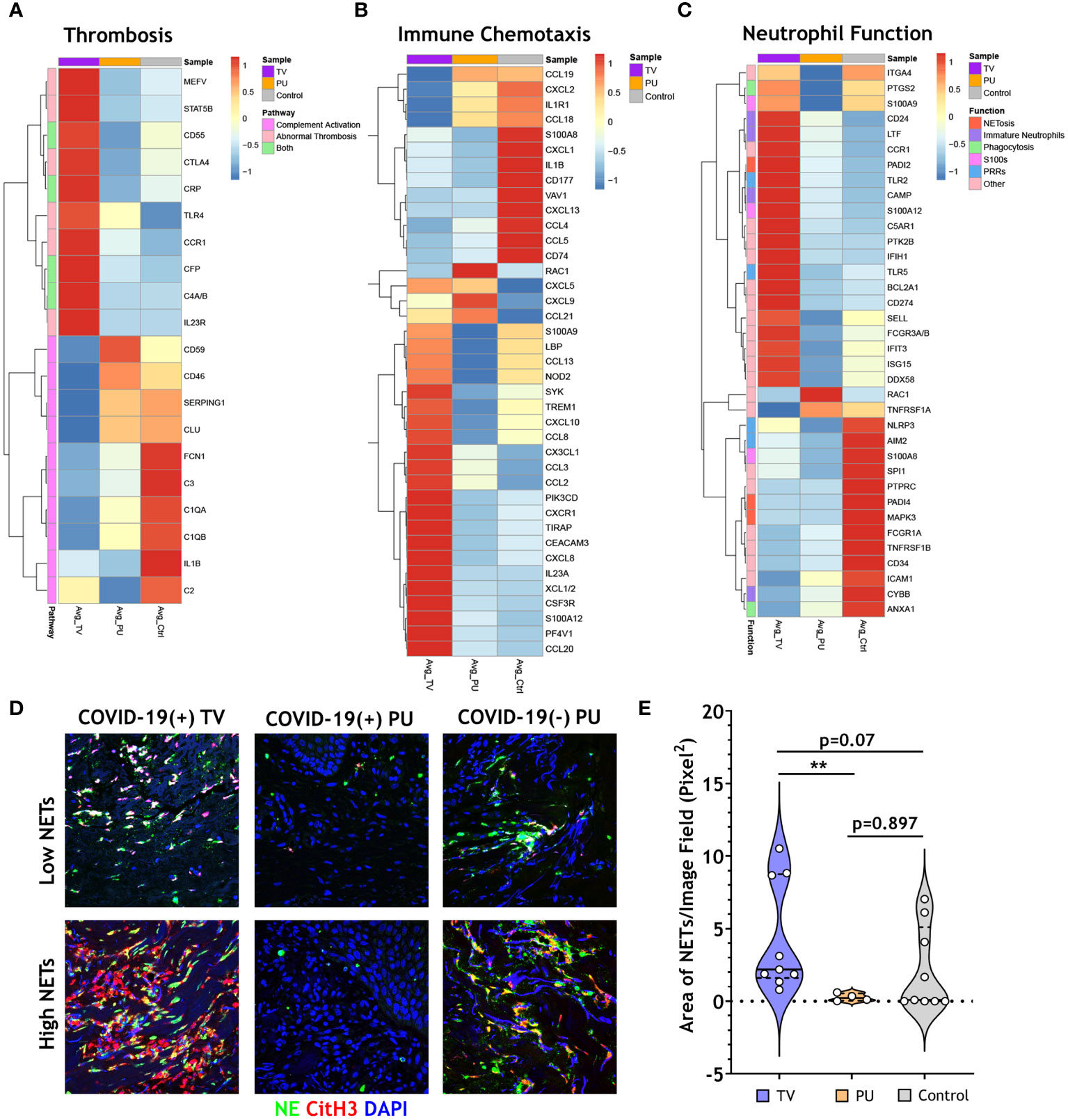
Figure 4 COVID-19 thrombotic vasculopathy is characterized by abnormal thrombosis and neutrophil extracellular trap formation. (A) Clustered heatmap shows the average normalized counts of genes involved in thrombosis and complement activation for COVID-19(+) TV (TV) and COVID-19(+) PU (PU) samples compared to COVID-19(-) PU (Control). Pathway specific genes were selected based on GO biological pathways. (B) Clustered heatmap illustrates the average normalized counts of genes involved in immune cell chemotaxis and extravasation for COVID-19(+)TV and PU samples compared to COVID-19(-) PU Controls. Genes were selected based on GO biological pathways. (C) Neutrophil specific genes arranged by function (NETosis, Immature Neutrophils, Phagocytosis, S100s, PRRs, or Other) represented in a clustered heatmap comparing the average normalized counts of DEGs in COVID-19(+) TV and PU groups relative to COVID-19(-) PU Controls. (D) Immunofluorescence staining of NETs in skin tissue sections. Markers of NETs used include NE (green), CitH3 (red) and nuclei (blue). Images acquired using confocal microscopy, 40X oil objective (E) Quantification of tissue NETs in the skin using Image J analysis.
Next, the dataset was surveyed for all genes that are involved in immune cell chemotaxis and extravasation (Figure 4B). In both COVID-19(+) TV and COVID-19(-) PU control groups a gene expression signature associated with neutrophil activation and chemotaxis was expressed; however, these signatures were characterized by unique subsets of genes in COVID-19(+) TV (TREM1, CXCR1, CXCL8, and S100A12) and COVID-19(-) PU control (CXCL1, CXCL2, and CD177) groups (Figure 4B). A small subset of genes involved in neutrophil and T cell activation (CXCL5, CXCL9, CCL21) were expressed in both COVID-19(+) TV and COVID-19(-) PU groups. Additionally, genes associated with antimicrobial defense mechanisms, including alarmins (S100A9, S100A12) and pattern recognition receptors (LBP, and NOD2), were up-regulated in the COVID-19(+) TV group compared to the COVID-19(-) PU control group, while suppressed in the COVID-19(+) PU group. While some genes involved in monocyte and lymphocyte migration and function were expressed in the COVID-19(+) TV group (CCL13, CCL8, and CCL20), a greater number of these genes were expressed in the COVID-19(-) PU control group (CCL19, CCL18, CXCL13, CCL4, CCL5, CXCL9). Only two genes (RAC1, CXCL9) were upregulated in COVID-19(+) PU compared to the other two disease groups. These genes are involved in the control of cell growth and lymphocyte chemoattraction, respectively. These findings support the histologic findings of different immune cell populations present in the HASPI groups. Taken together, these results demonstrate that while neutrophil activation and dysfunction may play a role in HASPI skin of both COVID-19(+) TV and COVID-19(-) PU groups, abnormal thrombosis and exacerbated inflammatory responses appear to be unique to HASPI skin in the COVID-19(+) TV group.
NET formation and upregulation of genes associated with the immature neutrophil subset are significantly elevated in COVID-19(+) TV skin
Schulte-Schrepping et al., utilized single-cell RNA sequencing of whole blood and peripheral blood mononuclear cells from COVID-19(+) patients to study the role of mature and immature neutrophil subsets in COVID-19 disease pathogenesis (16). We utilized this published dataset of neutrophil function-related genes that contribute to systemic inflammation in COVID-19 disease and compared them to the transcriptional signatures in our HASPI skin tissue dataset. Genes contributing to dysregulated NET formation were highly expressed in both COVID-19(+) TV and COVID-19(-) PU groups, but not in the COVID-19(+) PU group (Figure 4C). However, genes related to distinct mechanisms of NET induction were differentially expressed in the COVID-19(+) TV and COVID-19(-) PU control groups, with COVID-19(+) TV samples expressing PADI2 versus PADI4 expression in COVID-19(-) PU controls.
Immature neutrophils, or low-density granulocytes, have been shown to undergo uncontrolled NET formation in several autoinflammatory and autoimmune diseases, contributing to disease pathology (21). Genes associated with the immature neutrophil subset (CD24, LTF, CAMP) were up-regulated in COVID-19(+) TV skin, but not in COVID-19(+) PU or COVID-19(-) PU skin tissue (Figure 4C). Several other neutrophil function-related genes were also uniquely expressed in the COVID-19(+) TV group, including those related to antiviral response (IFIT3, DDX58, PTGS2, and IFIH1) and Toll-like receptor (TLR)-mediated signaling (TLR2, TLR5). Finally, CD274 (PD-L1), a known inhibitor of T cell activation, was highly expressed in COVID-19(+) TV skin tissue compared to the other 2 groups. These results reveal that while certain neutrophil chemotactic genes are expressed in both COVID-19(+) TV and COVID-19(-) PU control groups, expression of genes associated with the immature neutrophil subset is a distinct characteristic of the COVID-19(+) TV group, suggesting this neutrophil subset might contribute to enhanced vascular thrombosis at the local skin tissue level.
Finally, NETs in skin tissue from all three HASPI groups were visualized by immunofluorescent staining. Rare NETs were detected in the COVID-19(+) PU group (Figure 4D), which is consistent with the low NET-specific DEGs detected in this group (Figure 4C). This is in contrast to the visualization of NETs mainly in the dermis in all of the COVID-19(+) TV samples, which ranged from relatively low to extensive NETosis (Figure 4D). NETs were also variably detected in COVID-19(-) PU control tissue specimens, with five of nine COVID-19(-) PU skin specimens (~55%) demonstrating a lack of detectable NETs (Figure 4D). This was supported by image analysis to quantify the area of NETs within the tissue, which demonstrated the median total NET area in the COVID-19(+) TV group was modestly elevated compared to COVID-19(-) PU controls (p=0.07) (Figure 4E). Total area of NETs in the COVID-19(+) TV tissue was significantly higher than in the COVID-19(+) PU group (p<0.01), indicating a potential role for neutrophils in abnormal thrombosis-mediated biological mechanisms. These results demonstrate that while NETs may contribute to pathology in both COVID-19(+) TV and COVID-19(-) PU groups, mechanisms of NET induction (PADI2 vs. PADI4) and neutrophil subsets that potentially contribute to disease pathology (immature vs. mature neutrophil subsets) differ, possibly due to local SARS-CoV-2 infection.
Discussion
Although the hallmark of COVID-19 disease is acute respiratory distress syndrome and respiratory failure, manifestations are known to occur in many organ systems (22). Inflammation related to COVID-19 can cause transient or permanent organ damage, adding considerable morbidity to patients who survive their COVID-19 disease course. Numerous cutaneous eruptions have been described in the setting of COVID-19, but the peer-reviewed literature has focused mainly on outbreaks that resolve without significant tissue damage. Alternatively, HASPIs represent localized areas of significant and often permanent skin damage. HASPIs occur mainly in hospitalized patients and are associated with substantial patient morbidity and healthcare costs, with estimates ranging from $10 billion to >$26 billion annually (23). Given the significant impact of HASPIs and requirement for hospitalization in a substantial percentage of patients with COVID-19, we explored the incidence of HASPIs and risk factors for their development in a large cohort at a single tertiary care, multi-hospital institution.
This study revealed a number of important findings surrounding HASPIs in COVID-19(+) patients. Overall, COVID-19(+) patients had a 63% increased incidence of developing a HASPI compared to COVID-19(-) patients (Supplementary Table 1). Additionally, COVID-19(+) hospitalized patients have a 40% increased odds of developing HASPIs compared to COVID-19(-) patients, even when controlling for baseline ulceration risk at admission, ICU admission, and other variables (Table 2). Although the overall etiology of HASPIs in COVID-19 patients is likely multifactorial, there are numerous possible explanations for the increased risk we found in these patients. One obvious possibility is that COVID-19(+) patients have increased risk of ulceration at time of admission. However, the elevated risk of HASPIs in COVID-19(+) patients persisted compared to COVID-19(-) patients when controlling for risk of ulceration in a multivariable model. Another possibility is that meticulous routine care for these patients was compromised to protect caregivers from excess exposure to COVID-19(+) patients. However, protocols for frequent skin checks for HASPIs were not changed at our institution during the COVID-19 pandemic. This makes lack of adequate care an unlikely cause of increased HASPIs in our COVID-19(+) patient population and suggests other factors contribute to this increased risk, potentially including factors inherently related to SARS-CoV-2 infection.
This study identified that COVID-19(+) patients are at risk of more severe HASPIs and more often require surgical debridement compared to COVID-19(-) patients (Tables 1, 2). In particular, the finding that almost 25% of the COVID-19(+) HASPIs were Stage 3 or Stage 4 (compared to <10% in the COVID-19(-) cohort) is important, as these stages of pressure injuries are considered hospital ‘never events’. These ‘never events’ were defined by the National Quality Forum as events that are measurable and preventable, and that may lead to severe patient morbidity and mortality. The increased incidence of these severe ulcerations, despite continuing standard clinical monitoring protocols during COVID-19 to prevent them, is concerning.
Importantly, when controlling for age, co-morbidities, and other variables COVID-19(+) patients with HASPIs were more likely to have a severe hospitalization course compared to COVID-19(+) patients without HASPI. These results support that development of a HASPI is a poor prognostic sign in the setting of COVID-19. However, it is unclear whether a severe hospitalization course increases risk of HASPI development, or vice versa. Regardless, this finding aligns with the hypothesis that HASPIs in COVID-19(+) patients likely arise as a result of both intrinsic and extrinsic disease factors.
A comparison of laboratory parameters revealed COVID-19(+) HASPI patients had significantly higher maximum levels of serum ferritin compared to COVID-19(-) HASPI patients (Table 1). Hyperferritinemia has been associated with poor prognosis in COVID-19 patients and has been implicated to have a pathogenic role in cytokine storm (24). In the setting of an inflammatory state, ferritin has also been hypothesized to be a marker of cellular damage. Thus, COVID-19-associated inflammation may result in more significant cellular damage compared to other medical conditions leading to hospitalization, resulting in an overall catabolic state that promotes HASPI ulceration.
Surprisingly, maximum D-dimer levels were not significantly higher in COVID-19(+) patients with HASPIs compared to COVID-19(-) patients with HASPIs in our cohort. However, a high percentage of COVID-19(-) patients had no D-dimer levels drawn, suggesting a selection bias towards evaluating D-dimer levels in those patients who may have had clinical features supporting a thrombotic state (Table 1). Elevated D-dimer levels strongly correlate with an increased risk of thrombotic events in COVID-19 patients and, importantly, all COVID-19(+) HASPI patients in our cohort had elevated D-dimer levels (25). Factors other than elevated D-dimer levels, such as elevated expression of thrombotic genes or heightened triggering of complement pathway activation, may also contribute to thrombotic pathology in COVID-19 patients.
SARS-CoV-2-induced thrombotic events in the skin microcirculation represent another possible contributor to increased HASPI risk in COVID-19 patients. Thrombotic events in the skin and other organs of patients with COVID-19 are well-described (2, 3). Although these events typically do not appear to result in significant skin damage, they may promote eventual ulceration in areas like the sacrum that are additionally affected by pressure. In support of this, we have previously seen and reported several patients with severe COVID-19 who developed unusual retiform purpura on the buttocks associated with histologic thrombotic vasculopathy and subsequent development of sacral ulcers (13, 15).
In our cohort, 25 patients had skin specimens of their HASPIs available, 15 of which were biopsies performed on COVID-19(+) patients (TV and PU). We compared the histologic, cellular and molecular characteristics of these specimens in detail to each other and to those in COVID-19(-) PU control skin tissue. Significantly higher numbers of vascular thromboses and leukocytoclasis were observed in skin tissue from the COVID-19(+) TV group compared to the other groups, supporting the role of thrombotic vasculopathy as a potential contributor to subsequent ulceration in some COVID-19(+) patients.
Interestingly, vascular thromboses were detected occasionally in our COVID-19(-) PU control specimens. In fact, vascular thromboses have been described in skin biopsies of HASPIs in COVID-19(-) patients, and vascular occlusion has long been hypothesized to play an important pathogenic role in the development of PUs (26, 27). However, vascular thromboses were far more numerous and prominent in COVID-19(+) TV tissue compared to what we found in COVID-19(-) PU skin and compared to what has been described in prior histologic studies of PUs, suggesting additional pathogenic contributors to thrombotic vasculopathy in the COVID-19(+) TV group, such as factors inherent to COVID-19 (17, 28, 29). Additionally, some prior histologic studies of PUs describe vascular thromboses underlying ulcerated skin, suggesting they may represent secondary phenomena related to inflammation in areas of ulcer, whereas our COVID-19(+) TV tissue contained diffuse vascular thromboses in skin with intact epidermis, prior to ulceration (30, 31).
Although the majority of skin specimens from COVID-19(+) patients showed a TV pattern, one-third showed a PU histologic pattern with far less vascular thromboses compared to TV skin tissue. Although not statistically significant, mean maximum D-dimer levels were higher in the COVID-19(+) TV group compared to the COVID-19(+) PU group. This raises the possibility that there may also be a threshold D-dimer level, above which there is increased risk of vascular thrombosis in cutaneous vessels under pressure during viral infection, which might contribute to the unique histologic observations in this study.
Transcriptional analyses on skin specimens from the sites of sacral ulcer formation evaluated the inflammatory landscape in skin tissue during SARS-CoV-2 infection in comparison to sacral ulcers obtained from uninfected patients. This type of analysis has not been previously performed. Our results showed that COVID-19(+) TV skin is associated with up-regulation of genes related to innate immune responses and viral defense responses, and down-regulation of T-cell mediated immune responses (Figures 3A, B). Importantly, biological pathways and DEGs upregulated in COVID-19(+) TV skin were mainly categorized as genes that would potentially contribute to abnormal thromboses and immunothrombosis, consistent with our histopathology results (Figure 4). Analysis of DEGs showed that while interferon response genes and neutrophil-associated genes were highly expressed in COVID-19(+) TV skin, genes associated with MHC class II antigen presentation were down-regulated, indicative of stunted adaptive immune responses in COVID-19(+) TV (Figure 2C). Consistent with results of histopathological observations (Table 3), we also observed that genes associated with mechanisms of dysregulated immature neutrophil responses were highly expressed in COVID-19(+) TV skin compared to COVID-19(-) PU skin (Figures 4B, C). Given the known association between SARS-CoV-2 infection and immature neutrophil responses, this result further implicates SARS-CoV-2 infection itself with augmentation of ischemia in these areas and contribution to eventual tissue necrosis and ulceration. Although trending higher, we were unable to detect significant presence of SARS-CoV-2 transcripts in the COVID-19(+) TV samples in our limited dataset, so are unable to conclusively determine whether the immune dysregulation and enhanced thrombosis observed is a direct, local response to virus present in the skin or a more systemic response to SARS-CoV-2 infection. Interestingly, genes related to complement activation were upregulated in all disease groups, indicating that the alternative complement activation pathway was triggered in infected as well as uninfected skin tissue. Overall, our results suggest SARS-CoV-2 infection may amplify ischemia and eventual tissue necrosis and ulceration in skin under pressure by increasing thrombosis and neutrophil dysregulation, and not complement activation.
Recently, Yang et al. (32) examined the importance of CXCL10 plasma levels (interferon γ-inducible protein 10; IP-10) in COVID-19 disease severity and progression of COVID-19 disease. They reported that 5 cytokines, including CXCL10, were associated with fatal outcomes during infection (32). Several other studies have also shown the prognostic value of CXCL10 in determining COVID-19 disease course (33, 34). CXCL10 was found to be up-regulated in COVID-19(+) TV skin in comparison to COVID-19(+) PU and COVID-19(-) PU skin in our study (Figure 4A). These results suggest COVID-19 severity may be important for precipitating cutaneous vasculopathy in skin under pressure, which would further support a threshold is needed for SARS-CoV-2-associated contributions to cutaneous thrombosis.
Cytokines, such as IL1A and IL27, which have been shown to be higher in patients with more severe COVID-19 disease (35–37), were highly expressed in COVID-19(+) TV skin in comparison to the other groups. We also found that alarmins (S100A9, S100A12) and pattern-recognition receptors (PRRs) including TLR2, TLR4, TLR5, IFIH1, and NOD2 had higher expression in COVID-19(+) TV skin. It has been shown that SARS-CoV-2 spike protein binds TLR4 to increase the cell surface expression of ACE2 and facilitate viral entry (38, 39) exacerbating innate immune responses during infection. Expression of SARS-CoV-2-specific genes and higher transcript levels of PRRs in COVID-19(+) TV skin could potentially explain the enhanced innate immune responses observed with examination of GO biological pathways, and a contribution of neutrophil dysfunction in promoting cutaneous vascular thromboses.
Cell type scoring demonstrated that neutrophils were enriched in COVID-19(+) TV skin compared to COVID-19(+) PU and COVID-19(-) PU skin. While we also observed changes in CD56+dim NK cell, CD45+ cell, and mast cell signatures, we focused our subsequent efforts on specifically understanding the role of neutrophils in the ulcer phenotypes with and without SARS-CoV-2 infection since neutrophils have been shown to play an important role in COVID-19 disease pathogenesis, particularly in relation to thromboses and multi-organ damage (19, 40, 41). A study of dysregulated myeloid cell responses in COVID-19 revealed that presence of CD274 and ARG1 expressing dysfunctional mature neutrophils contributed to severe COVID-19 disease (16). In our dataset, the expression of CD274 was significantly higher in TV skin compared to skin in the other groups. Given that T cell mediated immune responses were down-regulated in COVID-19(+) TV skin, it is possible that presence of CD274high (PD-L1+) mature neutrophils in TV skin tissue suppress T cell responses due to the inhibitory interaction between PD-L1 and PD-1, resulting in stunted adaptive immune responses. In support of this, mature CD274 (PD-L1+) neutrophils have been shown to exert suppressive effects on T cell function in various other diseases like cancer and HIV infection (42, 43).
The presence of NETs in skin tissue was directly evaluated to understand whether exacerbated NET formation contributed to inflammation in the skin. Results showed that NETs were significantly higher in COVID-19(+) TV vs. PU skin, indicating that neutrophils and NETs potentially contribute to cutaneous thrombotic vasculopathy during severe COVID-19 infection. While NETs were also observed in COVID-19(-) PU (controls), the baseline levels in 5 patients were nearly zero, indicating that exacerbated NET formation might be a consistent feature of TV in COVID-19(+) patients. In addition to significantly higher NETs in COVID-19(+) TV vs. COVID-19(+) PU skin, COVID-19(+) TV skin also had more intense neutrophil elastase and CD61 staining than COVID-19(+) PU skin, correlating with the known ability of NETs to propagate inflammation and thrombosis (Figure 4D and Supplementary Figure 3). The reason for the difference in NETs in COVID-19(+) TV and PU skin is not entirely clear. However, previous work has supported that COVID-19 is a pro-NETotic state that is influenced by disease severity and levels of certain clinical biomarkers (44). The degree of NETosis is likely influenced by many factors in a given COVID-19 patient, including genetic factors, amount of viral-damaged epithelial cells, levels of various inflammatory cytokines, extent of platelet and endothelial cell activation and others. Although details concerning how NETosis is triggered in the setting of severe COVID-19 requires additional work, our results support that presence of significant cutaneous NETosis correlates with increased cutaneous thromboses, and likely contributes to development of HASPIs in this particular population of severe COVID-19 patients.
Our study has several advantages and limitations. Advantages include the establishment of a comprehensive COVID-19 registry at our institution that allowed identification of all COVID-19(+) patients admitted to our institutional hospitals, as well as a relatively large cohort utilized for analysis. Additionally, the standard protocol to photograph ulcers allowed objective confirmation that patients were accurately labeled as having HASPIs. More importantly, our results correlate clinical findings with histopathologic and molecular results. The comparison of COVID-19(+) patients with HASPIs to both COVID-19(+) patients without HASPIs and COVID-19(-) patients with HASPIs has not previously been done, especially with quantification of genes in sacral skin under pressure in all 3 groups. We were also able to corroborate the contribution of NETs to local tissue inflammation indicated by our transcript analyses using immunofluorescence imaging.
Limitations include the retrospective nature of our study and possibility that electronic medical records did not always contain accurate documentation of data points (i.e. date of ulcer onset). One important data point that we could not completely characterize was skin color due to absence of images and/or incomplete disclosure of ethnicity for several patients in our cohort. However, we believe it is notable that our COVID-19(+) HASPI cohort had a large number of black patients. As black patients have been found to be at increased risk for severe COVID-19 compared to other ethnic groups, our ethnicity data further supports a link between severe COVID-19 and HASPI development, and suggests clinicians should be particularly meticulous in monitoring black COVID-19(+) patients for HASPI development (45, 46). Additionally, laboratory data points and skin tissue specimens were not always captured on similar days during hospitalizations or in all patients to allow precise comparison. The number of biopsies evaluated in each cohort was relatively small with only limited amounts of tissue available for research. However, given HASPIs are typically diagnosed clinically and patients with severe COVID-19 can be difficult to biopsy for various reasons, we believe our biopsy sample numbers are adequate to gain insights into central pathways altered in these patients. These limitations impacted our ability to conclusively determine whether these dysregulated pathways are altered in response to local or systemic SARS-CoV-2 infection and will need to be explored further in a larger study cohort with a secondary method to confirm presence of virus in cutaneous tissue. We were unable to utilize fresh biopsies for transcript-level analyses and corroborate with systemic markers due to the unavailability of these resources in severely ill patients during the initial months of the outbreak. Therefore, the transcriptional analyses were limited to bulk tissue digests of paraffin-embedded tissue, precluding single cell transcriptomic analyses. Furthermore, as this study consists of a cohort from a single institution, our results are not necessarily generalizable. This study also focused specifically on the role of neutrophils and NETs in disease pathology; however, future studies will explore the role of other cells types, including platelets that were significantly altered in the three disease groups.
In conclusion, we show that COVID-19(+) patients are at an increased risk of HASPIs compared to COVID-19(-) patients, and COVID-19(+) patients with HASPIs develop ulcerations of more severe stage that more often require debridement than COVID-19(-) patients. Furthermore, we show that thrombotic vasculopathy was prominent in sacral skin of COVID-19(+) patients with HASPIs compared to controls, and TV tissue displayed increased expression of DEGs known to contribute to abnormal thrombosis and ischemia. Overall, our results implicate SARS-CoV-2 infection as a contributing factor to HASPIs in at least some COVID-19 patients.
Unlike other reports of skin manifestations in COVID-19 patients, our overall findings support the skin is yet another organ system that may sustain significant damage secondary to COVID-19. Recognizing risk of HASPIs and the potential role of SARS-CoV-2 infection in COVID-19 patients is important, as they may contribute to significant future morbidity, healthcare expenditure, and even mortality in patients who recover from COVID-19. Further research is needed to uncover detailed pathogenic factors leading to increased HASPI risk in COVID-19(+) patients, as well as how to optimally prevent them from occurring. For now, healthcare workers should be especially attentive to potential early signs of HASPI development in patients hospitalized with COVID-19, especially those with serious disease, and be meticulous with currently proven preventative clinical interventions.
Materials and methods
Patient identification
Patients with HASPIs were identified as having been hospitalized for greater than 5 days within our institution between March 1 and December 31, 2020, with an ICD-10 diagnosis of sacral ulcer or photographic documentation of sacral ulceration at some point during hospitalization (Supplementary Table 5). We defined a sacral ulcer as any new onset ulceration involving the sacral and/or buttocks areas acquired during an inpatient hospital admission at one of our facilities within the above time period. The decision to utilize an image search was made because nursing staff at our institution are instructed to image all potential HASPIs for documentation and clinical improvement purposes, and because photographs provide objective proof of ulceration. Based on our search criteria, we assumed all sacral ulcers that developed during hospitalization represented HASPIs unless otherwise stated in clinical notes and will refer to them as such from this point forward.
Patients that did not undergo a polymerase chain reaction (PCR)-based COVID-19 test at admission or during hospitalization, those hospitalized for <5 days, and those with sacral ulcers already present at hospital admission were excluded from our study. Additionally, stage 1 ulcer patients were excluded as these types of ulcers are difficult to reliably detect and are not routinely documented by our wound care teams. Some patients were hospitalized more than once during our defined study period. However, only the hospitalization associated with an initial development of a HASPI was included for our study purposes.
Among the 143,223 patients hospitalized at our institution during our defined study period (March 2020-December 2020), 58,766 patients met our inclusion/exclusion criteria and comprised our study cohort (Supplementary Figure 1). These patients were hospitalized for a total 591,763 days. Of our 58,766 patients, 5,531 (9.4%) were COVID-19(+) patients (total hospitalization days = 64,980; average 11.8 days) and 53,528 (89.6%) were COVID-19(-) patients (total hospitalization days=526,783; average 9.8 days). A total of 3,352 patients had a diagnosis of sacral ulcer during their hospitalization with or without an image of their sacral region in their electronic medical records. Of these patients, 2,992 had their sacral ulcers documented prior to or upon admission and 67 were seen at an outside hospital and were excluded from our study. Thus, our final study cohort consisted of 293 patients with HASPIs (Supplementary Figure 1).
Patient and ulcer characteristics
For all patients who met our inclusion/exclusion criteria, we thoroughly reviewed electronic medical records to obtain detailed clinical information. Clinical data acquired included demographic information (age, gender, race), HASPI ulceration risk (based on Braden risk assessment at hospital admission), presence of specific medical comorbidities (obesity, hypertension, diabetes mellitus, and chronic obstructive pulmonary disease (COPD)) index hospitalization information (COVID-19 status, length of stay, intensive care unit (ICU) admission, intubation/mechanical ventilation requirements), laboratory results (including maximum D-dimer concentration, maximum ferritin concentration, and maximum white blood cell count (WBC)), HASPI characteristics (time from admission to ulcer formation, ulcer stage, and ulcer size), and 30-day HASPI-related morbidity information (defined as either need for surgical debridement or subsequent ulcer-related infection within 30-days of discharge).
The Braden risk assessment score is a validated clinical tool calculated by nursing staff throughout our institution as part of routine patient skin assessments at admission and every 12 hours thereafter. This standardized risk scale assigns numerical ratings (ranging from 1-4) to six domains, including sensory perception, moisture, activity, mobility, nutrition, and friction/shear forces (47). The sum of these domains is used to risk stratify patients for HASPI formation; a lower score is correlated with increased risk of HASPI development (48). The primary outcome of our study was development of HASPI during the indexed hospitalization. Our secondary outcome was severity of hospitalization course, which was an ordinal categorical variable defined as either (1) hospitalization only, (2) hospitalization requiring ICU admission, (3) hospitalization requiring ICU admission and need for mechanical ventilation, and (4) hospitalization leading to death.
COVID-19 status was determined by nasal swab and PCR-based diagnostic testing. If a patient tested positive for COVID-19 at least once during the index hospitalization, they were classified as COVID-19(+). Time to ulcer formation was measured in days from admission to first mention and/or photographic documentation of HAPSI in the patient’s EMR, whichever occurred first. Ulcer size was defined as the largest recorded area (measured in cm2) of sacral ulceration during the index hospitalization. Ulcer stage was defined as most severe stage of ulcer (2, 3, 4, or unstageable) recorded during hospitalization according to National Pressure Injury Advisory Panel (NPIAP) classifications. Severe stage of HASPI was defined as either Stage 3 or Stage 4 HASPI according to NPIAP staging criteria. These specific stages were selected as they are considered hospital ‘never events’ by the NPIAP due to their associated, independent risk of increased morbidity and mortality (49).
Histopathology analysis of tissue specimens
Histopathologic characteristics of biopsied patients were qualitatively and quantitatively assessed using hematoxylin and eosin (H&E) stained tissue specimens by a board-certified dermatopathologists (APF and JDM). For COVID-19(+) patients, 4mm biopsies from (typically) areas of sacral purpura were obtained. For control COVID-19(-) specimens, which were typically submitted to pathology during debridement, a search of Cleveland Clinic pathology archives was performed to find specimens from PUs. H&E sections of all available specimens were reviewed to identify those appropriate to use as control specimens. Any specimens with histologic features suggestive of secondary infection, extensive epidermal ulceration, or prominent fibrosis (supporting significant chronicity) were excluded. Ten specimens were found to have histologic features suggestive of inherent pressure-induced changes. For these, a 4mm wide area was demarcated and examined to be consistent with the area examined in COVID-19(+) tissue. When possible, areas with intact epidermis were chosen in COVID-19(-) specimens to also reduce variability with tissue examined from COVID-19(+) patients. Histopathologic features that were evaluated included neutrophil infiltrate, vascular thrombosis, total number of thromboses, intraluminal neutrophils and leukocytoclasis (Table 3). These features were scored for their presence or absence, in addition to quantitating them if present. Phosphotungstic Acid Hematoxylin (PTAH/fibrin) and CD61 staining to evaluate microthrombi formation was performed on biopsied tissues samples obtained from the cases highlighted in Figure 1.
NanoString analysis
RNA was extracted from Formalin-Fixed and Paraffin Embedded (FFPE) tissue biopsies, using the RNeasy FFPE kit (Qiagen) with deparaffinization solution (Qiagen). Total RNA concentrations were determined using a Nanodrop ND-1000 spectrophotometer (Thermo Fisher Scientific). One-hundred nanograms of RNA was then hybridized with the NanoString Human Autoimmune Profiling Panel and spike in Coronavirus Panel Plus probe sets (NanoString Technologies). Transcriptional analysis of immune related genes and SARS-CoV-2 genes in tissue biopsies was conducted using the NanoString nCounter Pro Analysis System (NanoString Technologies). Raw data was initially processed using the nSolver Analysis Software (V.4.0). Quality control and normalization was performed using the 6 positive control, 8 negative control, and 20 housekeeping probes. Samples flagged for failing quality control were removed. Remaining data was assessed for cell type scoring using nSolver Advanced Analysis (V.2.0) and exported for subsequent analysis in R software.
Bioinformatics analysis
Analysis was performed using R (version 4.1.0). Venn diagram was generated utilizing DEGs from TV vs Control results against PU vs Control. Resulting residuals for principal component analysis (PC1 and PC2) were plotted using ‘ggplot2’ package (version 3.3.5). For pathway analysis, DEGs were separated into up- and down-regulated gene lists (fold change > 0 and fold change < 0 respectively). Package ‘gprofiler2’ (version 0.2.1) to find up- and down-regulated pathways. GO pathways were filtered to remove redundant pathways to generate the data by GO’s hierarchically structured database. Using ordered p-values from pathway analysis, the top 10 up-regulated and down-regulated pathways were obtained and p-values were log10-transformed for Figures 3A, B. Heatmaps were generated using normalized counts for specific gene sets (pheatmap version 1.0.12). Gene set enrichment analysis (GSEA) was utilized to identify genes involved in specific biological functions like neutrophil activation, abnormal thrombosis and complement activation. COVID-19-specific neutrophil genes (Figure 4C) were analyzed using published single-cell sequencing data. The p-value cutoffs that were used to determine significant genes from the NanoString data was FDR adjusted p-values < 0.05.
Neutrophil extracellular traps immunofluorescence staining and confocal imaging
Paraffin embedded 5 μm tissue sections were sectioned by the Cleveland Clinic Histology Core. Sections were deparaffinized and blocked using blocking buffer (Hank’s balanced salt solution containing 2% bovine serum albumin and 2% goat serum) for 1 hour at room temperature. Qualitative evaluation of presence of NETs in the tissue was performed using antibodies for anti-neutrophil elastase (NE/ELA2; R&D Systems, Catalogue# MAB91671-100; monoclonal mouse IgG; RRID : AB_2923234) and Anti-Histone H3 (citrulline R2 + R8 + R17; Abcam, Catalogue# ab5103; Rabbit polyclonal IgG; RRID : AB_304752). Primary antibody dilutions were made in the blocking buffer (NE, CitH3; used at 1:100 dilution) and added to the sections for an overnight incubation at 4°CC. Next day, slides were washed in 1X PBS (3X) and secondary antibodies were added to the sections for 1 hour at room temperature (1:1000 dilution in blocking buffer, Goat anti-Mouse 633 and Goat anti-Rabbit 568). After the 1 hour incubation, slides were washed in 1X PBS (3X) and mounted with DAPI (ProLong™ Gold Antifade Mountant with DAPI, ThermoFisher Scientific, Catalogue# P36931). Images were obtained using an inverted Leica SP8 confocal microscope using objective lens 40X oil/1X zoom factor. Five fields/tissue section were imaged for the purpose of quantitative assessment of presence of tissue NETs in the epidermis as well as the dermis. Images obtained from various disease groups were qualitatively assessed for presence of high, medium-low and no tissue NETs. Area of NETs was quantified using Image J software (version 1.53r 21).
Statistical analysis
Categorical variables are presented as count (percentage) and continuous variables as median (interquartile range). Wilcoxon rank-sum was utilized for two-sample continuous variable comparisons. Chi-square and Fisher’s Exact tests were utilized to compare nominal categorical data across groups. Cochrane-Armitage exact trend test was utilized to compare univariate ordinal categorical data. Multivariable logistic and multivariable Cox proportional hazards regression were used to model binary and time-to-event outcomes, respectively. Multivariable linear regression was used to model wound size. Potential for multicollinearity was explored using variance inflation factor analysis. One-way ANOVA was utilized for analysis of tissue NETs with post-hoc Tukey analysis. An a level of 5% (two-sided) was utilized for all comparisons. Statistical analysis was performed using SAS JMP Pro (version 15) and Prism (version 9.3.1).
Data availability statement
The datasets presented in this study can be found in online repositories. The names of the repository/repositories and accession number(s) can be found below: www.ncbi.nlm.nih.gov/geo/, GSE214647.
Ethics statement
The studies involving human participants were reviewed and approved by Cleveland Clinic Institutional Review Board. The patients/participants provided their written informed consent to participate in this study.
Author contributions
JN, SJ, AF and CM conceptualized and designed the study. JN, SJ, KC, AF and CM acquired funding for the study. JN, SJ and AP carried out laboratory-based investigations. JN, SJ, AP, RM, JG, AW, SS, JJ, LK, AN, JM, EM-C, SG, KC, AF, CM carried out clinical-based investigations and analysis of data including statistical analysis. AF and CM supervised the study. JN, SJ, AP and RM created the original figures and tables. AP and RM performed analysis of the Nanostring data. JN and SJ wrote the original draft of the manuscript. JN, SJ, AP, AF and CM wrote, reviewed and edited the manuscript. The order of authors was based on their sequential roles in the study. All authors contributed to the article and approved the submitted version.
Funding
This publication was made possible by a COVID-19 Themed Pilot Grant Award (to AF and CM) from the Clinical and Translational Science Collaborative (CTSC) of Cleveland from the National Center for Advancing Translational Sciences (NCATS) component of the National Institutes of Health and NIH roadmap for Medical Research (UL1TR002548). This work was also funded by the Office of the Assistant Secretary of Defense for Health Affairs through the Congressionally Directed Medical Research Programs Peer Reviewed Medical Research Program (W81XWH-16-0439/PR150299 to CM). This study was also supported by the Crohn’s & Colitis Foundation (#662997 to SJ). JN was supported by the Case Investigative and Translational Dermatology Training Program funded by the National Institute of Arthritis and Musculoskeletal and Skin Diseases of the National Institutes of Health (T32AR007569 to KC). This work utilized the Leica SP8 confocal microscope that was purchased with funding from the National Institutes of Health Shared Instrumentation Grant 1S10OD019972-01. Opinions, interpretations, conclusions, and recommendations are those of the authors and are not necessarily endorsed by the funders.
Acknowledgments
We would like to thank Emily Jiang from the Cleveland Clinic Department of Business Intelligence; Greg Strnad from the Cleveland Clinic COVID-19 Registry; and Janine Sot from the Cleveland Clinic Department of Dermatology.
Conflict of interest
The authors declare that the research was conducted in the absence of any commercial or financial relationships that could be construed as a potential conflict of interest.
Publisher’s note
All claims expressed in this article are solely those of the authors and do not necessarily represent those of their affiliated organizations, or those of the publisher, the editors and the reviewers. Any product that may be evaluated in this article, or claim that may be made by its manufacturer, is not guaranteed or endorsed by the publisher.
Supplementary material
The Supplementary Material for this article can be found online at: https://www.frontiersin.org/articles/10.3389/fimmu.2023.1031336/full#supplementary-material
References
1. Galvan Casas C, Catala A, Carretero Hernandez G, Rodriguez-Jimenez P, Fernandez-Nieto D, Rodriguez-Villa Lario A, et al. Classification of the cutaneous manifestations of COVID-19: A rapid prospective nationwide consensus study in Spain with 375 cases. Br J Dermatol (2020) 183(1):71–7. doi: 10.1111/bjd.19163
2. Magro C, Mulvey JJ, Berlin D, Nuovo G, Salvatore S, Harp J, et al. Complement associated microvascular injury and thrombosis in the pathogenesis of severe COVID-19 infection: A report of five cases. Transl Res (2020) 220:1–13. doi: 10.1016/j.trsl.2020.04.007
3. Magro CM, Mulvey JJ, Laurence J, Sanders S, Crowson AN, Grossman M, et al. The differing pathophysiologies that underlie COVID-19-associated perniosis and thrombotic retiform purpura: A case series. Br J Dermatol (2021) 184(1):141–50. doi: 10.1111/bjd.19415
4. Droesch C, Do MH, DeSancho M, Lee EJ, Magro C, Harp J. Livedoid and purpuric skin eruptions associated with coagulopathy in severe COVID-19. JAMA Dermatol (2020) 156(9):1–3. doi: 10.1001/jamadermatol.2020.2800
5. Freeman EE, McMahon DE, Lipoff JB, Rosenbach M, Kovarik C, Desai SR, et al. The spectrum of COVID-19-associated dermatologic manifestations: An international registry of 716 patients from 31 countries. J Am Acad Dermatol (2020) 83(4):1118–29. doi: 10.1016/j.jaad.2020.06.1016
6. Gehlhausen JR, Little AJ, Ko CJ, Emmenegger M, Lucas C, Wong P, et al. Lack of association between pandemic chilblains and SARS-CoV-2 infection. Proc Natl Acad Sci USA (2022) 119(9):e2122090119. doi: 10.1073/pnas.2122090119
7. Liu J, Li Y, Liu L, Hu X, Wang X, Hu H, et al. Infection of human sweat glands by SARS-CoV-2. Cell Discov (2020) 6(1):84. doi: 10.1038/s41421-020-00229-y
8. Magro C, Nuovo G, Mulvey JJ, Laurence J, Harp J, Crowson AN. The skin as a critical window in unveiling the pathophysiologic principles of COVID-19. Clin Dermatol (2021) 39(6):934–65. doi: 10.1016/j.clindermatol.2021.07.001
9. Avsar P, Moore Z, Patton D, O'Connor T, Budri AM, Nugent L. Repositioning for preventing pressure ulcers: a systematic review and meta-analysis. J Wound Care (2020) 29(9):496–508. doi: 10.12968/jowc.2020.29.9.496
10. Reddy M, Gill SS, Rochon PA. Preventing pressure ulcers: A systematic review. JAMA (2006) 296(8):974–84. doi: 10.1001/jama.296.8.974
11. Lyder CH. Pressure ulcer prevention and management. JAMA (2003) 289(2):223–6. doi: 10.1001/jama.289.2.223
12. Thomas DR. Does pressure cause pressure ulcers? An inquiry into the etiology of pressure ulcers. J Am Med Dir Assoc (2010) 11(6):397–405. doi: 10.1016/j.jamda.2010.03.007
13. Young S, Narang J, Kumar S, Kwizera E, Malik P, Billings SD, et al. Large Sacral/buttocks ulcerations in the setting of coagulopathy: A case series establishing the skin as a target organ of significant damage and potential morbidity in patients with severe COVID-19. Int Wound J (2020) 17(6):2033–7. doi: 10.1111/iwj.13457
14. Rrapi R, Chand S, Lo JA, Gabel CK, Song S, Holcomb Z, et al. The significance of pressure injuries and purpura in COVID-19 patients hospitalized at a large urban academic medical center: A retrospective cohort study. J Am Acad Dermatol (2021) 85(2):462–4. doi: 10.1016/j.jaad.2021.03.051
15. McBride JD, Narang J, Simonds R, Agrawal S, Rodriguez ER, Tan CD, et al. Development of sacral/buttock retiform purpura as an ominous presenting sign of COVID-19 and clinical and histopathologic evolution during severe disease course. J Cutan Pathol (2021) 48(9):1166–72. doi: 10.1111/cup.14038
16. Schulte-Schrepping J, Reusch N, Paclik D, Bassler K, Schlickeiser S, Zhang B, et al. Severe COVID-19 is marked by a dysregulated myeloid cell compartment. Cell (2020) 182(6):1419–40 e23. doi: 10.1016/j.cell.2020.08.001
17. Bonaventura A, Vecchie A, Dagna L, Martinod K, Dixon DL, Van Tassell BW, et al. Endothelial dysfunction and immunothrombosis as key pathogenic mechanisms in COVID-19. Nat Rev Immunol (2021) 21(5):319–29. doi: 10.1038/s41577-021-00536-9
18. Reusch N, De Domenico E, Bonaguro L, Schulte-Schrepping J, Bassler K, Schultze JL, et al. Neutrophils in COVID-19. Front Immunol (2021) 12:652470. doi: 10.3389/fimmu.2021.652470
19. Ackermann M, Anders HJ, Bilyy R, Bowlin GL, Daniel C, De Lorenzo R, et al. Patients with COVID-19: in the dark-NETs of neutrophils. Cell Death Differ (2021) 28(11):3125–39. doi: 10.1038/s41418-021-00805-z
20. Vaninov N. In the eye of the COVID-19 cytokine storm. Nat Rev Immunol (2020) 20(5):277. doi: 10.1038/s41577-020-0305-6
21. Papayannopoulos V. Neutrophil extracellular traps in immunity and disease. Nat Rev Immunol (2018) 18(2):134–47. doi: 10.1038/nri.2017.105
22. Gupta A, Madhavan MV, Sehgal K, Nair N, Mahajan S, Sehrawat TS, et al. Extrapulmonary manifestations of COVID-19. Nat Med (2020) 26(7):1017–32. doi: 10.1038/s41591-020-0968-3
23. Wassel CL, Delhougne G, Gayle JA, Dreyfus J, Larson B. Risk of readmissions, mortality, and hospital-acquired conditions across hospital-acquired pressure injury (HAPI) stages in a US national hospital discharge database. Int Wound J (2020) 17(6):1924–34. doi: 10.1111/iwj.13482
24. Terpos E, Ntanasis-Stathopoulos I, Elalamy I, Kastritis E, Sergentanis TN, Politou M, et al. Hematological findings and complications of COVID-19. Am J Hematol (2020) 95(7):834–47. doi: 10.1002/ajh.25829
25. Zhan H, Chen H, Liu C, Cheng L, Yan S, Li H, et al. Diagnostic value of d-dimer in COVID-19: A meta-analysis and meta-regression. Clin Appl Thromb Hemost (2021) 27:10760296211010976. doi: 10.1177/10760296211010976
26. Parish LC, Lowthian P, Witkowski JA. The decubitus ulcer: many questions but few definitive answers. Clin Dermatol (2007) 25(1):101–8. doi: 10.1016/j.clindermatol.2006.09.013
27. Lowthian PT. Trauma and thrombosis in the pathogenesis of pressure ulcers. Clin Dermatol (2005) 23(1):116–23. doi: 10.1016/j.clindermatol.2004.10.001
28. Witkowski JA, Parish LC. Histopathology of the decubitus ulcer. J Am Acad Dermatol (1982) 6(6):1014–21. doi: 10.1016/S0190-9622(82)70085-4
29. Salcido R, Donofrio JC, Fisher SB, LeGrand EK, Dickey K, Carney JM, et al. Histopathology of pressure ulcers as a result of sequential computer-controlled pressure sessions in a fuzzy rat model. Adv Wound Care (1994) 7(5):23–4,26,28 passim.
30. Vande Berg JS, Rudolph R. Pressure (decubitus) ulcer: variation in histopathology–a light and electron microscope study. Hum Pathol (1995) 26(2):195–200. doi: 10.1016/0046-8177(95)90037-3
31. Kitagawa HS A, Nakatani T, Sugama J, Konya C, Okuwa M, Nagakawa T. Histological examination of pressure ulcer tissue in terminally ill cancer patients. Japan J Nurs Sci (2004) 1:35–46. doi: 10.1111/j.1742-7924.2004.00008.x
32. Yang Y, Shen C, Li J, Yuan J, Wei J, Huang F, et al. Plasma IP-10 and MCP-3 levels are highly associated with disease severity and predict the progression of COVID-19. J Allergy Clin Immunol (2020) 146(1):119–27 e4. doi: 10.1016/j.jaci.2020.04.027
33. Chen Y, Wang J, Liu C, Su L, Zhang D, Fan J, et al. IP-10 and MCP-1 as biomarkers associated with disease severity of COVID-19. Mol Med (2020) 26(1):97. doi: 10.1186/s10020-020-00230-x
34. Lev S, Gottesman T, Sahaf Levin G, Lederfein D, Berkov E, Diker D, et al. Observational cohort study of IP-10's potential as a biomarker to aid in inflammation regulation within a clinical decision support protocol for patients with severe COVID-19. PloS One (2021) 16(1):e0245296. doi: 10.1371/journal.pone.0245296
35. Angioni R, Sanchez-Rodriguez R, Munari F, Bertoldi N, Arcidiacono D, Cavinato S, et al. Age-severity matched cytokine profiling reveals specific signatures in covid-19 patients. Cell Death Dis (2020) 11(11):957. doi: 10.1038/s41419-020-03151-z
36. Tamayo-Velasco A, Martinez-Paz P, Penarrubia-Ponce MJ, de la Fuente I, Perez-Gonzalez S, Fernandez I, et al. HGF, IL-1alpha, and IL-27 are robust biomarkers in early severity stratification of COVID-19 patients. J Clin Med (2021) 10(9):2017. doi: 10.3390/jcm10092017
37. Kleymenov DA, Bykonia EN, Popova LI, Mazunina EP, Gushchin VA, Kolobukhina LV, et al. A deep look into COVID-19 severity through dynamic changes in blood cytokine levels. Front Immunol (2021) 12:771609. doi: 10.3389/fimmu.2021.771609
38. Aboudounya MM, Heads RJ. COVID-19 and toll-like receptor 4 (TLR4): SARS-CoV-2 may bind and activate TLR4 to increase ACE2 expression, facilitating entry and causing hyperinflammation. Med Inflamm (2021) 2021:8874339. doi: 10.1155/2021/8874339
39. Zhao Y, Kuang M, Li J, Zhu L, Jia Z, Guo X, et al. SARS-CoV-2 spike protein interacts with and activates TLR41. Cell Res (2021) 31(7):818–20. doi: 10.1038/s41422-021-00495-9
40. Meizlish ML, Pine AB, Bishai JD, Goshua G, Nadelmann ER, Simonov M, et al. A neutrophil activation signature predicts critical illness and mortality in COVID-19. Blood Adv (2021) 5(5):1164–77. doi: 10.1182/bloodadvances.2020003568
41. Szturmowicz M, Demkow U. Neutrophil extracellular traps (NETs) in severe SARS-CoV-2 lung disease. Int J Mol Sci (2021) 22(16):8854. doi: 10.3390/ijms22168854
42. Wang TT, Zhao YL, Peng LS, Chen N, Chen W, Lv YP, et al. Tumour-activated neutrophils in gastric cancer foster immune suppression and disease progression through GM-CSF-PD-L1 pathway. Gut (2017) 66(11):1900–11. doi: 10.1136/gutjnl-2016-313075
43. Bowers NL, Helton ES, Huijbregts RP, Goepfert PA, Heath SL, Hel Z. Immune suppression by neutrophils in HIV-1 infection: role of PD-L1/PD-1 pathway. PloS Pathog (2014) 10(3):e1003993. doi: 10.1371/journal.ppat.1003993
44. Zuo Y, Yalavarthi S, Shi H, Gockman K, Zuo M, Madison JA, et al. Neutrophil extracellular traps in COVID-19. JCI Insight (2020) 5(11):e138999. doi: 10.1172/jci.insight.138999
45. Yancy CW. COVID-19 and African americans. JAMA (2020) 323(19):1891–2. doi: 10.1001/jama.2020.6548
46. Mackey K, Ayers CK, Kondo KK, Saha S, Advani SM, Young S, et al. Racial and ethnic disparities in COVID-19-Related infections, hospitalizations, and deaths : A systematic review. Ann Intern Med (2021) 174(3):362–73. doi: 10.7326/M20-6306
47. Braden BJ. The braden scale for predicting pressure sore risk: reflections after 25 years. Adv Skin Wound Care (2012) 25(2):61. doi: 10.1097/01.ASW.0000411403.11392.10
48. Moore ZE, Patton D. Risk assessment tools for the prevention of pressure ulcers. Cochrane Database Syst Rev (2019) 1:CD006471. doi: 10.1002/14651858.CD006471.pub4
Keywords: COVID-19, SARS-CoV-2, sacral ulcer, pressure ulcer, thrombotic vasculopathy, thrombosis, immune activation, neutrophil extracellular trap
Citation: Narang J, Jatana S, Ponti AK, Musich R, Gallop J, Wei AH, Seck S, Johnson J, Kokoczka L, Nowacki AS, McBride JD, Mireles-Cabodevila E, Gordon S, Cooper K, Fernandez AP and McDonald C (2023) Abnormal thrombosis and neutrophil activation increase hospital-acquired sacral pressure injuries and morbidity in COVID-19 patients. Front. Immunol. 14:1031336. doi: 10.3389/fimmu.2023.1031336
Received: 29 August 2022; Accepted: 08 March 2023;
Published: 21 March 2023.
Edited by:
Martijn van Griensven, Maastricht University, NetherlandsReviewed by:
Minhui Guan, University of Missouri, United StatesMeihong Deng, Feinstein Institute for Medical Research, United States
Copyright © 2023 Narang, Jatana, Ponti, Musich, Gallop, Wei, Seck, Johnson, Kokoczka, Nowacki, McBride, Mireles-Cabodevila, Gordon, Cooper, Fernandez and McDonald. This is an open-access article distributed under the terms of the Creative Commons Attribution License (CC BY). The use, distribution or reproduction in other forums is permitted, provided the original author(s) and the copyright owner(s) are credited and that the original publication in this journal is cited, in accordance with accepted academic practice. No use, distribution or reproduction is permitted which does not comply with these terms.
*Correspondence: Christine McDonald, mcdonac2@ccf.org
†These authors have contributed equally to this work and share first authorship
‡ORCID: Anthony P.Fernandez, orcid.org/0000-0003-4490-8427
‡These authors have contributed equally to this work and share senior authorship