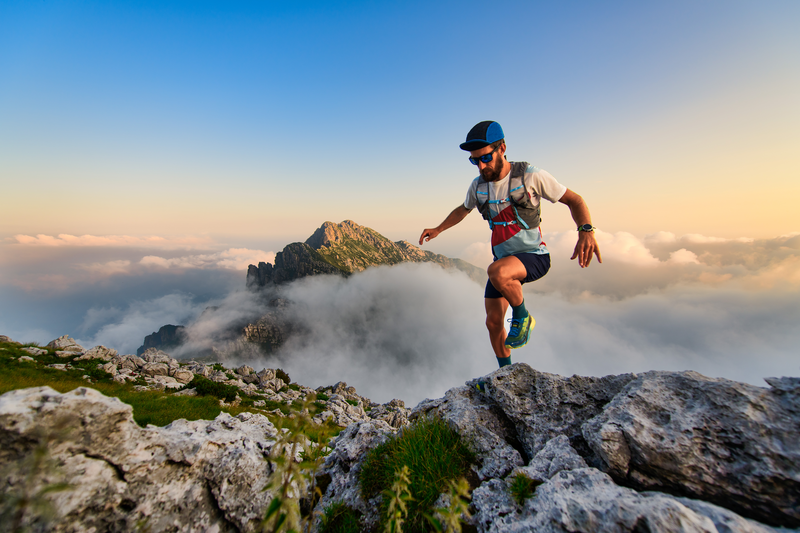
95% of researchers rate our articles as excellent or good
Learn more about the work of our research integrity team to safeguard the quality of each article we publish.
Find out more
REVIEW article
Front. Immunol. , 14 February 2023
Sec. NK and Innate Lymphoid Cell Biology
Volume 14 - 2023 | https://doi.org/10.3389/fimmu.2023.1009867
Preeclampsia (PE) is a disease that is unique to pregnancy and affects multiple systems. It can lead to maternal and perinatal mortality. The precise etiology of PE is unclear. Patients with PE may have systemic or local immune abnormalities. A group of researchers has proposed that the immune communication between the fetus and mother is primarily moderated by natural killer (NK) cells as opposed to T cells, since NK cells are the most abundant immune cells in the uterus. This review examines the immunological roles of NK cells in the pathogenesis of PE. Our aim is to provide obstetricians with a comprehensive and updated research progress report on NK cells in PE patients. It has been reported that decidual NK (dNK) cells contribute to the process of uterine spiral artery remodeling and can modulate trophoblast invasion. Additionally, dNK cells can stimulate fetal growth and regulate delivery. It appears that the count or proportion of circulating NK cells is elevated in patients with or at risk for PE. Changes in the number or function of dNK cells may be the cause of PE. The Th1/Th2 equilibrium in PE has gradually shifted to an NK1/NK2 equilibrium based on cytokine production. An improper combination of killer cell immunoglobulin-like receptor (KIR) and human leukocyte antigen (HLA)-C may lead to insufficient activation of dNK cells, thereby causing PE. In the etiology of PE, NK cells appear to exert a central effect in both peripheral blood and the maternal-fetal interface. To maintain immune equilibrium both locally and systemically, it is necessary to take therapeutic measures directed at NK cells.
Preeclampsia (PE) is a disease that is unique to pregnancy and affects multiple systems. It can lead to maternal and perinatal mortality (1). Its incidence rate is approximately 2–8% (2). PE is referred to as a “Great Obstetrical Syndrome” (3). Approximately 50,000–60,000 pregnant women worldwide die annually from PE (4, 5). PE is defined as hypertension (systolic blood pressure ≥ 140mmHg and/or diastolic blood pressure ≥ 90mmHg) that occurs for the first time after twenty weeks of gestation and may include one or more of the following manifestations: proteinuria (≥ 300mg/day), maternal organ damage (liver, kidney, or nervous system), blood system involvement (such as thrombocytopenia), or evidence of placental dysfunction (such as fetal growth restriction and/or abnormal uterine artery Doppler) (6–8). Severe PE is characterized by significantly elevated blood pressure (≥160/110mmHg), proteinuria, pulmonary edema, severe neurological symptoms, liver and kidney dysfunction, or low platelets (9). PE can gradually progress to eclampsia, accelerated hypertension, acute renal failure, liver subcapsular hematoma, liver rupture, and heart failure, and can also result in placental abruption and intrauterine fetal death (10). Furthermore, the risk of developing cardiovascular illness is twelve times higher in females who have experienced PE, suggesting a link between PE and the development of cardiovascular disease (11). Early-onset PE (occurring prior to 34 weeks) and late-onset PE (occurring after 34 weeks) are subtypes of PE. For many years, the most effective treatment for PE has been termination of pregnancy. However, since it is often necessary to prematurely deliver the fetus and placenta, the prognosis for newborns is poor (12). Therefore, it is essential to investigate the pathogenesis of PE and implement effective treatments to improve the prognosis of mothers and infants.
PE can be caused by genetic factors, vascular endothelial cell damage, angiogenesis disorders, decreased invasion of trophoblasts, and insufficient recasting of uterine spiral arteries (13, 14). However, the precise etiology of PE is unclear. Research has demonstrated that patients with PE may have systemic or local immune abnormalities (15–17). Both the innate and the adaptive immune systems, such as neutrophils, monocytes, natural killer (NK) cells and T cells, contribute to the onset and progression of PE (18, 19). The two-stage theory of PE is gradually gaining acceptance. The first stage is characterized by poor placental formation in the first trimester, while the second stage is characterized by placental oxidative stress in late pregnancy. The first stage typically occurs prior to the onset of clinical symptoms and is characterized by impaired spiral artery remodeling, insufficient blood flow at the maternal-fetal interface and placental ischemia. The second stage typically manifests as a systemic inflammatory response in leukocytes and endothelial cells of the blood vessels.
Some researchers have hypothesized that the mother must acquire immune tolerance to the semi-allogeneic fetus (20). However, recently, this theory’s validity has been called into question. It is now believed that the maternal-fetal immune interaction is in an activated state and that the immune system at the implantation site should be activated rather than inhibited. Additionally, the immune homeostasis at the materno-fetal interface is functional and finely regulated in healthy pregnancies (21). A new theory regarding the immunological etiology of PE has been proposed by a few researchers. They believe that the primary mode of immune communication between the fetus and mother is moderated by NK cells rather than T cells, as NK cells are the most abundant immune cells in the uterus (22). Furthermore, CD56 cells in maternal peripheral blood begin to increase from the 12th week of pregnancy, while T cells begin to change until late pregnancy (22). Fragments of syncytiotrophoblast also support the theory that NK cells, rather than T cells, are the predominant cell type in PE. These fragments are released into the maternal blood circulation during the normal replacement and renewal of the placental surface (22). In PE patients, these debris are found in increased quantities (23). It is also worth noting that in human placental tissues, there is no major histocompatibility complex (MHC) on the surface of syncytiotrophoblast, except for the soluble form of human leukocyte antigen (HLA)-G (24). This means that it cannot stimulate the antigen response of maternal T cells (22). On the other hand, NK cells can regulate the inflammatory response by both promoting and inhibiting inflammation (22). As a result, NK cells play a crucial role in PE. Our aim is to provide obstetricians with a comprehensive and updated research progress report on NK cells in PE patients.
NK cells have cytotoxic effects on both tumor and infected cells and are important to the innate immune system. NK cells are classified as CD3-CD56+ lymphocytes in humans. They account for about 10% of the total peripheral blood lymphocytes and can be distributed into two subtypes according to CD56 and CD16 expression. Approximately 90% of all peripheral blood NK (pNK) cells are CD56dimCD16+, mainly playing a cytotoxic role. In contrast, CD56brightCD16- NK cells have little cytotoxic nature. They secrete a group of cytokines, such as granulocyte macrophage colony stimulating factor (GM-CSF), interferon-γ (IFN-γ), transforming growth factor-β (TGF-β), interleukin-10 (IL-10) and IL-13 (25–28). CD16 has the ability to stimulate antibody-dependent cell-mediated cytotoxicity (ADCC). Therefore, the decreased CD16 expression confers greater regulatory functions and diminished cytotoxic properties to uterine NK (uNK) and decidual NK (dNK) cells. The cytotoxicity of NK cells is modulated by three factors. Firstly, NK cells go through an education procedure, and only self-identified NK cells are cytotoxic (29). Moreover, these cytotoxic NK cells are influenced by a complex network that includes intricate communication between target cells and activating or inhibiting receptors on the surface of NK cells. Lastly, NK cytotoxicity is lower in the resting state than in the “primed” state. In contrast to the phenotype of pNK cells (CD56dimCD16+), uNK and dNK cells have a higher CD56 density and less CD16 expression, existing as CD56brightCD16- NK cells, which show a phenotype of tissue resident NK (trNK) cells (30).
Because of the presence of diverse cell receptors on NK cells, the cytotoxic effect and cytokine production of these cells varies (31). NK cell receptors include killer cell immunoglobulin-like receptors (KIRs), the C-type lectin heterodimer family (CD94/NKG2), the Ly49 homodimers, the NK cytotoxicity receptors (NCRs), and the immunoglobulin-like transcripts (ILTs) (32, 33). The NK receptors repertoire can also be divided into two classes, activating and inhibitory receptors. The activating receptors consist of KIRs (KIR2DS1, KIR2DS2, KIR2DS4, KIR2DS5, KIR3DS1), C type lectin receptors (NKG2D, CD94/NKG2C, CD94/NKG2E/H), NCRs (NKp30, NKp44, NKp46), and immunoglobulin (Ig)-like receptors (2B4) (18). On the other hand, the inhibitory receptors include KIRs (KIR2DL1, KIR2DL2/3, KIR2DL5, KIR3DL1, KIR3DL2, KIR3DL7), C-type lectin receptors (CD94/NKG2A, CD161), Ig-like receptors (ILT-2), and leukocyte inhibitory receptors (LAIR-1) (18). CD56dim NK cells express KIRs. However, the CD56bright subgroup lacks KIRs but expresses a greater number of CD94/NKG2A receptors. The CD94/NKG2 receptors are MHC class I specific receptors and contain inhibitory CD94/NKG2A and activating CD94/NKG2C receptors. The NCRs include NKp30, NKp44, and NKp46. NKp46 and NKp30 are simultaneously expressed. Moreover, the NKp44 expression rises when NK cells are activated. NK cells interact with MHC antigens via a vast array of distinct receptors. HLA-E on extravillous cytotrophoblast (EVT) inhibits the trophoblast killing by interacting with CD94/NKG2A (34). HLA-G combines with KIR2DL4 receptors on NK cells to produce cytokines, chemicals, and angiogenic elements that stimulate trophoblast invasion and vascular development during embryo implantation. The communication between dNK cells and trophoblast HLA-C is of particular interest. Paternity partially affects the binding between trophoblast cells and dNK cells due to the polymorphism of HLA-C and KIR2D receptors.
Programmed death 1 (PD-1) is an immune checkpoint that limits immune activity by transmitting an inhibiting signal to T cells, thus participating in immunologic suppression in maternal-fetal tolerance (35). The expressions of PD-1 ligands (PD-L1 and PD-L2) have been confirmed in human decidual tissues (36). A recent study showed that PD-1 was also expressed by NK cells (37). The expression of PD-L1 from decidual CD56+ NK cells of normal pregnant women in the first trimester was significantly higher than that in peripheral blood (38). This indicates that PD-1/PD-L1 pathway is involved in maintaining immune homeostasis at the maternal-fetal interface in the first trimester of human pregnancy. In another mice experiment, researchers detected the expression of PD-1 by dNK cells from pregnant BALB-c mice at two weeks of gestation (39). Their findings showed that the expression level of PD-1 by dNK cells was significantly increased compared with that by pNK cells (39). Since the study was carried out on 14.5 days of pregnancy in mice, it is similar to the third trimester in human pregnancy. Indeed, PD-1 receptor was recently identified on dNK cells in the third trimester of human pregnancy (40). Moreover, with the development of pregnancy, the expression of PD-1 on dNK cells increases gradually (40). Therefore, this NK inhibitory receptor may play a crucial role in pregnancy, which needs further investigation.
The origin of dNK cells is not entirely established. Currently, there are three theories. Some authors hypothesized that the recruitment of pNK cells into the uterus causes dNK cell aggregation (41). Since pNK cells and dNK cells exhibited similar chemokine receptor models, including extremely low levels of CCR1 and CCR5 when they contacted decidual stromal cells (41). The second theory proposes that certain precursor dNK cells develop and mature gradually from endometrial NK (eNK) cells in response to pregnancy-related elements including IL-15 and progesterone (42, 43). According to the third theory, dNK cells could differentiate gradually from the hematopoietic precursor cells in decidua under the influence of unique stromal elements in the decidual microenvironment (44). Therefore, the dNK cell origin is complex, and the aforementioned three theories could coexist, indicating that additional research is required.
In the menstrual cycle, the number of eNK cells fluctuates in non-pregnant females. uNK cells make up approximately 20% of endometrial leucocytes in the proliferative stage and 40-50% in the secretory stage in the endometrium of non-pregnant females (45). A small number of agranular NK cells can be observed in the endometrium before ovulation and in the proliferative (follicular stage) of the menstrual cycle (46). Under the influence of progesterone, the proportion of these cells increased significantly after ovulation. However, when progesterone levels decrease, these cells die two days ahead of menstruation (28). During the mid-to-late secretory (luteal) phase, they rapidly increase and become granular, encircling the spiral arteries and endometrial glands (47). When uNK cells disappear prior to menstruation, the expression of soluble factors that maintain vascular integrity decreases, resulting in the monthly shedding of endometrium that initiates the menstrual cycle, indicating that uNK cells are included in endometrial renewal, differentiation, and decomposition (48).
During a normal pregnancy, the proportion of pNK cells increases in early pregnancy, decreases in middle pregnancy, and then further decreases in late pregnancy (49). The same pattern of NK cytotoxicity is observed in pregnancy (50). In early pregnancy, dNK cells constitute 70-80% of decidual leukocytes with the highest expression level (51). The focus is on whether the phenotype and importance of dNK cells vary in pregnancy. It is widely known that decidual CD16+ NK cells are lower in late pregnancy than in early pregnancy (52). However, the expression level of CD16+ dNK cells at term was greatly higher than that in early pregnancy (53). In the third trimester of near-term pregnancy, 40% of decidual lymphocytes are CD56+ NK cells (54). It was not different between the first and second trimesters in the number of CD56+ cells in the decidua basalis (placental bed containing EVTs), same as the decidua parietalis (non-placental bed without trophoblast cells) (55). However, the number of CD56+ NK cells decreased greatly in the third trimester (55). The percentage of CD56+ NK cells expressing perforin and granzyme B in placental bed biopsies at 16 to 20 weeks of gestation was significantly lower than at 8 to 10 and 12 to 14 weeks of gestation (56). In addition, there was no difference between the first and second trimesters in the number and expression level of cytokines secreted by dNK cells (57). Compared to the first trimester, dNK cells in the second trimester had more activating receptors, such as NKG2D and NKp80, but their degranulation capacity decreased significantly (57). In addition, other researchers discovered that the dNK cell number in CD45+ cells during full-term pregnancy was significantly lower, but its degranulation ability was significantly higher than in early pregnancy (58). The same team also discovered that dNK cells had fewer HLA-C-binding receptors, including KIR2DS1, KIR2DL2/3, and KIR2DL1, but more HLA-E-binding NKG2A receptors than those in the first trimester (58). In full-term pregnancy, dNK cells have distinct receptors and gene expression profiles, indicating that they are a special kind of NK cells. However, the dNK cell function in full-term pregnancy is unknown. Previous research has demonstrated that dNK cells gradually decrease beginning in the second trimester and almost completely disappear in term pregnancy (28). The diversity of results may be attributable to the various detection methods. Previous experiments determined the presence of NK cells through coloring the cytoplasmic granules. Granular leukocytes are only a few in decidua after 20 weeks of gestation (56). In subsequent tests, however, the distribution of NK cells was analyzed using CD56 immunostaining. In the third trimester, there are still a small number of agranular CD56+ dNK cells that are usually ignored. Consequently, dNK cells may undergo both functional and phenotypic changes at various stages of pregnancy.
Reproduction-related dNK cell functions can be widely found in the existing studies (Figure 1). The process of spiral artery remodeling was divided into two stages. In the initial phase, the myometrium loses its elasticity and the endothelial cell layer ruptures (59). In the second stage, endovascular trophoblast cells enter the spiral arteries and take the place of the decidual endothelium and a portion of the myometrium (59). dNK cells contribute to the two phases of spiral artery remodeling (59–61). How dNK cells influence the spiral artery remodeling is unclear. Nevertheless, dNK cells secrete some factors, including placental growth factor (PlGF), TGF-β1, vascular endothelial growth factor-C (VEGF-C), angiopoietin 1 (Ang1), Ang2, matrix metalloproteinases-2 (MMP-2), and MMP-9 (61–63). These factors all participate in spiral artery remodeling (61, 62, 64). Moreover, dNK cells can interact with specific HLA ligands on trophoblast cells and indirectly contribute to the trophoblast-mediated recasting of spiral arteries (28).
Figure 1 The schema of roles of dNK cells in reproduction. dNK cells contribute to the spiral artery remodeling by producing PlGF, TGF-β1, VEGF-C, Ang1, Ang2, MMP-2, and MMP-9. Besides, dNK cells secrete IFN-γ, IL-8, and IP-10, which inhibit trophoblast invasion. CXCR1 and CXCR3 are the corresponding receptors of IL-8 and IP-10, respectively. dNK cells stimulate fetal development by secreting pleiotrophin and osteoglycin. PBX1 raises the mRNA expression levels of pleiotrophin and osteoglycin. The activation of NKT cells induces a systemic inflammatory response, leading to the onset of parturition.
Experiments with mice yielded an abundance of knowledge about the function of dNK cells in the spiral artery recasting in a healthy pregnancy. Similar to humans, the dNK cells in mice are stimulated (65) and produce numerous factors, such as IFN-γ, IL-22 (66, 67), PlGF (67) and VEGF (68). dNK cells in mice can express Ly49 receptors similar to human KIRs (69). In NK cell-deficient mice, the remodeling of spiral arteries is defective, and the structure of the decidua and myometrium is abnormal in the second trimester (70). Spiral artery remodeling was enhanced when NK cells were restored to NK cell-deficient mice (71). Similarly, IFN-γ administration to NK cell-deficient mice restored spiral artery remodeling, indicating that IFN-γ is important to spiral artery remodeling (66). Besides, IFN-γ may act on the initial stage of spiral artery remodeling (66). In addition, other factors are involved in the remodeling of spiral arteries in mice, including nitric oxide (72) and VEGF (73).
Maternal dNK cells surround trophoblasts and regulate trophoblast invasion in a dose-dependent pattern (74). In the first trimester, dNK cells are a primary source of IFN-γ in the decidua, and IFN-γ may inhibit the invasion of EVT (62). It has been discovered that in human purified infiltrating trophoblasts, dNK cells could secrete IL-8, IFN-inducible protein (IP-10), and their corresponding receptors CXCR1 and CXCR3 (46). Experiments conducted in vitro revealed that after the addition of neutralizing antibodies IL-8 and IP-10, the capacity of dNK cells to induce trophoblast migration was significantly less than that of pNK cells (46). In brief, these experiments showed that IL-8 and IP-10 produced by dNK cells will inhibit trophoblast invasion (75).
Since mice are not ideal models for deep trophoblast incursion, rats are often used to explore the relationship between dNK cells and trophoblast invasion (76, 77). On the 15th, 17th and 20th day of pregnancy in rats, serial sections of the mesometrial triangle (similar to the human placental bed) were made, and the dNK cells were stained (43). It was discovered that dNK cells disappeared from the myometrium following EVT invasion, indicating that dNK cells modulate EVT invasion (43). dNK cells can also control the invasion of endovascular trophoblasts, as the invasion of endovascular trophoblasts increased on day 13.5 of pregnancy following the removal of NK cells in the first trimester (78). It is noteworthy that different species have distinct NK cell types. Methodologically, there is currently no model that can eliminate dNK cells only or eliminate this cell population by targeting specific antibodies.
dNK cells could stimulate fetal growth and development. Prior to successful placentation in humans and mice, the CD94a+ subtype of dNK cells stimulated fetal growth by producing development-improving elements such as osteoglycin and pleiotrophin (79). The lack of these factors may lead to fetal bone dysplasia and growth restriction in mice (79). It has been revealed that the transcription factor PBX homeobox 1 (PBX1) can increase the mRNA expression levels of growth-promoting elements in dNK cells, thereby promoting fetal development in murine pregnancy models (80).
In the third trimester, dNK cells become agranular in the cytoplasm, suggesting that their function changes and they gradually prepare for delivery (55). dNK cells have been reported to regulate delivery. Using single cell RNA-sequencing to compare the basal plate, placental villous tissues, and chorioamniotic membranes of full-term and preterm pregnant women, researchers discovered that these tissues contained many lymphocytes, including T cells and NK cells (81). Moreover, compared to full-term pregnant women without labor, the single-cell signatures of NK cells were significantly elevated in full-term pregnant females with spontaneous delivery (81).
NKT cells are a small subpopulation of T cells that express certain surface markers on the NK cell surface. NKT cells can activate NK cells by producing IFN-γ. Even though only 0.01%- 0.1% of peripheral blood lymphocytes are NKT cells, their number in decidua is 10 times that of peripheral blood (82). Experiments in vivo revealed a remarkable rise in the activated NKT cell number in the decidua of preterm pregnant mice, indicating that the stimulation of NKT cells caused preterm birth by promoting systemic inflammatory response (83). However, the specific mechanism by which dNK cells cause delivery initiation is still unclear.
Collectively, dNK cells perform many important functions including modulating spiral artery remodeling, regulating trophoblast invasion, promoting fetal development and the initiation of labor in pregnancy. However, it has been revealed that dNK cells vanish by the end of the pregnancy (55, 58), therefore, dNK cells that play a role may have specific phenotypes or dNK cells in early pregnancy.
Changes in pNK cells may occur in patients with PE, but the direction and magnitude of these changes are controversial (Table 1). According to the scientific literature, the cytotoxicity of pNK cells in PE patients may be higher (18, 85, 91, 92, 94–96), lower (97), or equal (84) to that of normal controls. The level of CD56+/NKp44+ cells in females with PE was significantly higher than that of healthy pregnant women at 12-20 weeks of gestation (18). The increased percentage of pNK cells may indicate the correspondingly elevated dNK cells, which may aid in identifying asymptomatic PE patients in advance. In comparison to women with healthy pregnancies, females who later developed PE had a higher proportion of pNK cells during early pregnancy (90). However, another assay revealed that the increased proportions of pNK cells were critical regulators of postpartum PE, not PE during pregnancy (98). Before delivery in the third trimester, the proportions of CD56+ NK cells in the peripheral blood of PE patients were remarkably higher than those of healthy mothers (93). Moreover, the increase in pNK cells in the early-onset PE population was more than that in the late-onset PE population (92). Consequently, it appears that the count or proportion of circulating NK cells is elevated in patients with or at risk for PE.
Similarly, several studies have demonstrated that the function of pNK cells is enhanced in PE. In comparison to normal pregnancies, the IFN-γ level secreted by pNK cells was significantly higher in PE patients (99), as was the expression of NKG2A and NKG2C (88, 89). The ratio of type 1 to type 2 NK cells was enhanced in the peripheral blood of patients with PE (86). However, the pregnancy-promoting factors produced by NK cells, such as VEGF (100) and galectin-1 (101), were clearly reduced (Figure 2). Mucin-16, or CA-125, is a glycoprotein produced by decidual cells (102, 103). The binding between Mucin-16 and circulating CD16-/CD56bright NK cells was stronger in PE patients than in full-term normal pregnant women (104). The greater combination of Mucin-16 and CD16-/CD56bright NK cells may reduce these cells’ ability to secrete angiogenic cytokines, resulting in poor remodeling of spiral arteries and insufficient invasion of trophoblasts, leading to PE. In summary, these results show that circulating NK cells in patients with PE are dysfunctional and activated.
Figure 2 Changes of pNK cells in PE. In PE patients, increased circulating pro-inflammatory cytokines IL-12 and IL-18 induce IFN-γ production by pNK cells, which leads to the Th1 immune bias of PE. In addition, elevated circulating IL-15 induces the systemic inflammatory response by activating pNK cells. pNK cells from PE cases produce less VEGF and more galectin. Mucin-16 has a stronger binding with pNK cells in PE. The inhibitory NKG2A, activating NKG2C and NKp44 in pNK cells of PE patients are increased. Besides, KIR2DL1+ NK cells are decreased, whereas KIR2DL2/3+ NK cells are increased in PE. In females with PE, there is a lower expression of NKp46 on pNK cells, which causes the increased expression of TNF-α produced by pNK cells. Higher production of TNF-α results in an NK1 shift of NK cells, which is one of the etiologies of PE.
Although the immune cell mechanism in decidua has been studied for many years, the function of dNK cells in PE remains unknown (Table 2). A few experiments have demonstrated a rise in the NK cell proportion in the gestational tissues of PE patients (92, 114, 116). During delivery, the number of CD56+ and CD94+ cells in PE placentas was remarkably higher, while placental IL-12 expression was significantly lower compared to healthy pregnancies (108). Researchers examined the composition of leukocytes in the decidua of PE patients and discovered that dNK cells were greatly increased in PE relative to those from normal pregnant women (54). Similarly, the CD16+/CD56+ NK cell proportion was remarkably enhanced in the decidua of PE patients (106). Patients with PE had abundant dNK cells with increased CD56 density in their decidua, showing that most dNK cells in PE were in a resting immature state (114). The increased production of CD56+ dNK cells in PE creates a decidual microenvironment that captures dNK cells by distorting their conversion and trafficking. The increased number of dNK cells, cytolytic enzymes, and intracellular inflammatory cytokines may inhibit trophoblast migration and invasion, constrain spiral artery remodeling, kill trophoblast cells, and ultimately initiate PE.
Nonetheless, other studies discovered that the count of CD56+ NK cells in the decidua of patients with PE was greatly lower than in normal pregnancy (110, 112, 113). In PE patients, for instance, the CD16+ dNK cell number greatly decreased, indicating that these cells had a protective effect on normal pregnancy (115). According to an assay, the CD56+ NK cell number in the decidua had no connection with the disease severity (113). As the etiology of PE involves systemic vascular endothelial cell response and multiple other factors, the clinical manifestations of PE are not solely related to the dNK cell number. In one study, placental bed biopsies were taken from twelve normal pregnancies, eight intrauterine growth retardation (IUGR) patients without hypertension, and twelve PE patients (109). They discovered that the CD56+ dNK cell number in people with PE and IUGR was greatly lower than in healthy pregnant women (109). In another separate experiment, the number of CD56+ dNK cells was less in PE with IUGR than in healthy pregnancy (107). These outcomes are unexpected given that PE is typically considered as an inflammatory response (117, 118). Oncology studies have demonstrated, however, that pro-inflammatory status is a necessary condition for cancer cells to invade healthy tissues (119, 120). Since trophoblast cells share many similarities with cancer cells, the decreased invasion of trophoblasts in the placenta may be related to the compromise of these inflammatory cells. In addition, the decreased number of dNK cells may reduce the production of angiogenic factors like VEGF, Ang2 and PlGF, resulting in inadequate spiral artery remodeling (62, 121).
Alternatively, many authors have hypothesized the dNK cell function in PE has shifted rather than their absolute number (122). dNK cells separated from pregnant women who had poor spiral artery recasting were less capable of chemically attracting trophoblasts (122). This phenomenon may be attributable to the decreased cytokines produced by dNK cells, which affected the spiral artery development and contributed to hypertension in pregnant women (123). The function of dNK cells is distinct from their number, and the former appears to be more essential because it determines the biological response.
A study also revealed that it was not significantly different between PE patients and normal pregnancies in the number of CD16-/CD56+ dNK cells (110). Similarly, another study collected decidual tissues from ten normal pregnant women and nine women who had PE (111). Their results indicated that dNK cells continued to exist during pregnancy, while showing no statistical difference between the two groups in the dNK cell number (111). Moreover, the increase in the proportion of dNK cells during the implantation window did not increase the risk of PE in the index pregnancy (124).
The variation in experimental results may be attributed to altered experimental methods, the use of PE samples with varying disease severity (with or without IUGR), or the distinct position of the collected tissues in the placenta (dNK cells near the spiral arteries or total dNK cells in the placenta). Some studies obtained placental tissues via cesarean delivery (106), whereas others obtained placental bed biopsies (54). Due to the invasive nature of placental bed biopsy, the probe may become contaminated with peripheral blood, resulting in changes in the dNK cell number. In one study, researchers obtained decidual tissues from the placental surface after placental delivery or performed vaginal curettage (110). This approach offers minimal contamination from maternal peripheral blood and may represent the true in vivo state. Even though the debris on the probe was meticulously cleaned, it is not possible to rule out the possibility of peripheral blood contamination. Besides, the detection method of dNK cells by fluorescence activated cell sorting (FACS) (110) or immunohistochemistry (IHC) (113) was another factor that affected the results. Both approaches have advantages and disadvantages: FACS has more advantages in the precision of quantitative detection, and it can detect a greater number of cells with less interobserver bias; IHC reveals the distribution of various cell types in tissues and can reveal the interaction between cells. Specifically, the percentages of decidual CD56+ NKp46+ cells were elevated in PE, whereas the percentages of NK cells labeled with other receptors (NKp44, NKp80, or NKG2D) were not increased (114) (Figure 3). Due to the different etiology of early-onset and late-onset PE, the increase in pNK and dNK cells in early-onset PE was more pronounced than in late-onset PE when compared to healthy full-term pregnancies (92). The count of dNK cells in IUGR patients (with or without PE) was remarkably lower than in healthy pregnancies (107, 109) and was associated with defective spiral artery remodeling (107).
Figure 3 Hypothetical model of alternations of dNK cells in PE. The incidence of PE is significantly increased if the mother has KIR AA genotype and the trophoblast has HLA-C2. The expressions of activating KIR2DS2, 3, and 5 are decreased on dNK cells from PE patients. Besides, the expressions of HLA-G on trophoblast cells and its receptor LILRB1 on dNK cells are diminished in PE. The expression of NKp46 on dNK cells is elevated in PE. The increased expression of TGF-β1 in decidua of females with PE suppresses the activation of specific subpopulations of dNK cell, thus resulting in PE. Lower levels of IFN-γ secreted by dNK cells result in defective spiral artery remodeling and abnormal trophoblast invasion, leading to impaired placentation and ultimately causing PE.
Both rats and mice are the most popular animal models for examining the dNK cell function in PE because they can be used to examine dNK cells at various pregnancy stages. In an in vitro experiment, it was determined that dNK cells in the mesometrial triangle of the PE mouse model decreased significantly during the second trimester, and this decrease was associated with the malfunctioning spiral artery recasting (76, 125). Another PE rat model revealed a correlation between decreased trophoblast invasion and spiral artery recasting and the lower dNK cell count (76). In a separate experiment using BPH/5 mice as PE models, researchers found that the proportion of dNK cells was reduced (126). It has been confirmed that an augment in cytolytic NK (cNK) cells in the placenta of PE rats results in hypertension and growth retardation in their offspring (127). This study demonstrated that Reduced Uterine Perfusion Pressure (RUPP) promoted the activation and proliferation of cNK cells, and that NK cell removal ameliorated hypertension, fetal growth restriction, and inflammation (127). Adoptive transfer of NK cells from RUPP to normal pregnant rats resulted in NK cell stimulation, increased uterine artery resistance index (UARI) and oxidative stress reaction in the placenta, which ultimately caused PE and IUGR (128). RUPP acceptors of NK cells from normal pregnant rats exhibited normal NK activation, plasma soluble fms-like tyrosine kinase receptor-1 (sFlt1), plasma and decidual VEGF, and normal UARI, thereby compromising hypertension and fetal growth restriction (128). The studies point out that cNK cells matter in the pathogenesis of PE, while normal NK cells alleviate hypertension and promote fetal development (128). Compared to removing NK cells from healthy pregnant rats, removing NK cells from PE rats decreased trophoblast cell invasion, but did not affect spiral artery recasting (129). Consequently, similar to the findings in humans, the number of dNK cells in animal models of PE decreased, and their functions may have been altered. More PE model experiments on dNK cells are necessary.
The Th1/Th2 equilibrium in PE has gradually shifted to an NK1/NK2 equilibrium based on cytokine production (130, 131). Compared with healthy pregnant females, the ratio of NK1/NK2 cells in the peripheral blood of patients with PE was significantly higher (86). NK cells from PE patients were more cytotoxic than those from normal pregnancies and produced more tumor necrosis factor (TNF)-α and IFN-γ (132). Both cytokines were greatly activated in patients with PE and rats with RUPP (99, 127, 133). The administration of TNF-α to pregnant rats increased mean arterial pressure and vascular resistance in the kidney (134). In rats with placental ischemia, the removal of NK cells reduces blood pressure by inhibiting TNF-α signaling. In addition, IFN-γ is an essential contributor to the etiology of PE caused by cNK cells and may be used as a therapeutic target to alleviate PE symptoms (135). Significantly elevated IFN-γ was secreted by NK cells in the peripheral blood of women with severe PE, and this elevation was associated with syncytiotrophoblast microparticles from the placenta (136). Nevertheless, the level of IFN-γ secreted by dNK cells in PE patients was remarkably less than in regular pregnancies, indicating that the dNK cell function was markedly diminished (114). In the third trimester, IFN-γ activity was greatly enhanced in the decidua of patients with PE, which altered the local immune response (106).
Increased production of IL-12 and IL-18 causes a systemic inflammatory response, which in turn induces NK cell stimulation and increases IFN-γ expression, thereby altering the favorable Th2 immune response into the Th1 response prevalent in PE (86). Consistently, the serum expression of IL-12 was higher in pre-eclamptic patients than in healthy mothers (108, 137). Nonetheless, the reduced expression of IL-12 in the placentas of PE patients indicated that the regulatory function of NK cells was compromised (105, 108). In addition, decidual macrophages produce IL-15, which is essential for the differentiation, proliferation, cytotoxicity, surface receptor expression, and cytokine secretion of dNK cells (138, 139). The elevated IL-15 expression in the peripheral blood of PE women suggests that PE is in a pro-inflammatory state (108). However, the placental expression of IL-15 in PE did not differ significantly from that in healthy pregnancy (108). Moreover, the NK cell depletion decreased the expression of IL-17 in peripheral blood, indicating that placental ischemia may stimulate cNKs to produce IL-17 (140). This result is similar to an earlier study indicating that NK cells in patients with PE are capable of producing IL-17 (140).
TGF-β1 can transform CD56dim CD16+ NK cells into CD56bright CD16- NK cells, completing the NK cell transformation (141, 142). Moreover, TGF-β1 was reported to impair the function of CD56+ dNK cells (114). dNK cells with low cytotoxicity modulate vascular remodeling by secreting TGF-β1 (62, 143, 144). An experiment with rodents demonstrated that inhibiting the TGF-β1 signal slowed the maturation of NK cells (145). Consequently, TGF-β1 is a primary factor in modulating the function of dNK cells and promoting maternal-fetal immune tolerance. The TGF-β1 expression in the decidua of females with PE was higher than that of females with normal pregnancies, and the increased TGF-β1 expression inhibited the stimulation of certain subsets of dNK cells, thereby causing PE (114). TGF-β1 levels in the circulation and cord blood of late-onset PE patients were greatly lower than those of normal controls, but they were similar to those in early-onset PE cases (146). In addition, one study found that the TGF-β1 expression level in placental tissue of PE cases was remarkably higher than that of healthy pregnant females (147), whereas another research discovered that the TGF-β1 expression level in serum did not differ greatly between PE and fit mothers (148). These discrepancies may be attributed to the unique experimental samples, the racial and ethnic diversity of the subjects, and the limited number of patients in some assays. Additionally, it is barely specified whether the active TGF-β1 was tested in these experiments. Consequently, these results must be evaluated with caution. It is necessary to establish a unified detection standard for TGF-β1 and conduct a large-scale study to determine whether TGF-β1 is associated with the occurrence of PE. In addition, angiogenic factors secreted by circulating NK cells also changed in PE patients. Significantly fewer VEGF-producing pNK cells were found in PE patients than in fit mothers (100). In response to stimulation, pNK cells changed their phenotype and ceased to produce VEGF, but began to secrete inflammatory factors.
During the proliferative and secretory phases of the menstrual cycle, eNK cells in the uterus express NKp46, while NKp30 and NKp44 are either negative or weakly positive, respectively (42). However, another study found that eNK cells in the endometrium in the secretory stage were positive for NKp46, NKp30, and NKp44 (132). Few studies have been conducted regarding NCRs in the field of reproduction. Females with poor pregnancy outcomes may have an aberrant repertoire of NCRs, indicating that NK cells in this population are dysfunctional. NCR-positive cells are more cytotoxic, and women with reproductive failure may have higher cytotoxic activity. Studies have analyzed the expression of NCRs in circulating NK cells of PE patients. PE patients had significantly fewer CD56+/NKp46+ cells and CD56bright/NKp46+ cells than normotensive pregnant females (132). Notably, the decrease of CD56+/NKp46+ cells occurred three to four months before the onset of PE (132). Consequently, NKp46 can be used as an index to predict PE, similar to sFlt1 and PlGF (149, 150). Besides, the declined expression of NKp46 on NK cells in PE patients increased the production of TNF-α and shifted NK cells to the NK1 phenotype (18).
Various activating or inhibiting receptors are present on the NK cell surface. The bias between activating and inhibitory receptors determines the cytotoxicity of NK cells. In addition to NCRs, there are also CD94/NKG2 receptors that play a role in the pathogenesis of PE. The CD94/NKG2 receptors include the activating receptor CD94/NKG2C and the inhibitory receptor CD94/NKG2A. NKG2D is another activating receptor unrelated to CD94 and owns low homology with NKG2A and NKG2C (151). Combined with HLA-E, the CD94/NKG2A receptor on the surface of dNK cells generates inhibiting signals to prevent cell lysis. Experiments on mice demonstrated that depletion of NKG2A during pregnancy led to defective vascular recasting (152). By sequencing the entire genomes of 7,219 PE patients, researchers discovered a 7% increase in the risk of maternal HLA-B failing to educate NKG2A+ NK cells (152). HLA-B could bind with activating KIR2DS1, 2, 3, 5, and KIR3DS1 receptors. Patients with PE had significantly higher levels of inhibitory NKG2A and activating NKG2C in their circulating NK cells than normal expectant mothers or healthy women (88). It appears that NK cells attempt to keep a balanced level of NK-cell receptor expression, even though PE patients are about to experience a systemic inflammatory response and have activated pNK cells. In severe PE cases without HELLP (hemolysis, elevated liver enzyme levels, and a low platelet count) syndrome, the proportion of NK cells containing NKG2A or NKG2C was significantly elevated (89). This indicates that patients with severe PE without HELLP have elevated NK cytotoxicity due to the NKG2 receptor (89). The mRNA expression levels of NKG2D receptor and its ligands were remarkably higher in the placentas of PE patients in comparison to those of normal controls (91). The impairment of chorionic villi detected in PE may be due to the enhanced local cytotoxicity caused by the NKG2D receptor-ligand pathway (91). Alternatively, a strong inflammatory reaction may stimulate this pathway, but the signal cannot be neutralized by regulatory cytokines (91).
KIR is a predominant receptor expressed on NK cells, combing with HLA-C on trophoblast cells. Trophoblast cells express HLA-C, HLA-G, and HLA-E. Maternal KIR genes are polymorphic and can be either AA or Bx (153). The KIR AA haplotype lacks the majority of activating receptors, except for KIR2DS4. The KIR Bx haplotype owns most activating KIRs (154). As ligands for KIRs, HLA-C can be categorized into two distinct kinds. HLA-C1 binds to KIR2DL2 and KIR2DL3, whereas HLA-C2 combines with KIR2DL1 and KIR2DS1. C2 is superior to C1 in its ability to combine with KIRs (155).
It has been reported that the incidence of PE is increased if the mother has KIR AA genotype and the fetus inherits the father’s HLA-C2 (156, 157). The combination of KIR AA and HLA-C2 may result in insufficient stimulation of dNK cells, affecting the remodeling of spiral arteries and ultimately leading to PE. In patients with PE, the binding of maternal Bx and fetal HLA-C2 increases the risk of atherosclerosis (158). Having additional HLA-C2 from the father significantly increases the PE risk (159). Through high-resolution KIR phenotyping and genotyping of the single NK cell, researchers discovered that women with KIR2DL1A were more susceptible to PE than those with KIR2DL1B (160). A study conducted in Mexico collected decidua tissues from 10 normal pregnancies and 9 patients with PE (111). They discovered a greater number of inhibitory KIRs in the decidua of PE patients (111). In comparison to normal pregnancies, CD158a+ (KIR2DL1) NK cells in the peripheral blood of PE sufferers were lower, whereas CD158b+ (KIR2DL2/3) NK cells were higher (161). Moreover, the ratio of CD158a+/CD158a+ NK cells is linked with a rise in blood pressure (161).
In patients with PE, activating KIRs were found to be reduced (162, 163). The activating KIR2DS1 binding to HLA-C2 protects European and Ethiopian women from PE (156, 157, 164). KIR2DS1 stimulates the activation of dNK cells by enhancing angiogenesis and immune response, thereby promoting a healthy pregnancy (27). However, in Caucasians and Africans, KIR2DS4 and KIR2DS5 play a protective role in PE (165–167). Besides, the expressions of activating KIR2DS2, 3, and 5 were lower in Chinese females with PE relative to those with normal pregnancies (163). The whole number of activating receptors in PE sufferers was significantly lower than in the control group (163).
Since Caucasians tend to carry more HLA-C2 molecules than Japanese, Japanese females who marry or have children with Caucasian men tend to have PE than those with Japanese men. Intriguingly, the findings revealed that the incidence of PE in Japanese women with Caucasian husbands was similar to those with Japanese partners (1.54% vs. 2.67%) (162). The peripheral blood of pregnant females and newborns from 259 patients with severe PE or eclampsia and 259 women with healthy pregnancies was collected in a separate study (168). They found that KIR/HLA-C combination and severe PE risk were not associated (168). Accordingly, the association between KIR/HLA-C and PE requires further investigation.
By combining with KIR2DL4 on NK cells, HLA-G inhibits NK cell cytotoxicity and regulates cytokine production (169). Additionally, soluble HLA-G stimulated the production of IFN-γ by both pNK and dNK cells (170). Since soluble HLA-G stimulated the IFN-γ production by dNK cells, it promoted the reformation of spiral arteries and normal implantation (170). Significantly lower HLA-G expression was detected in EVT of PE patients (171–173). In pre-eclamptic placentas, the expressions of HLA-G and its receptor leukocyte immunoglobulin-like receptor subfamily B1 (LILRB1) were significantly lower than in those of normotensive pregnant females (174). Additionally, the expressions of HLA-G and soluble HLA-G in PE sufferers’ peripheral blood were lower than those of women with healthy pregnancies, indicating that insufficient immune tolerance may be the cause of PE (173). The decreased HLA-G expression increases the propensity of NK cells to damage trophoblast cells. Therefore, the reduction of HLA-G on EVT and the NK cell elimination may restrict the invasion of trophoblasts at the maternal-fetal interface, resulting in the constriction and failed conversion of spiral arteries. Notably, another test revealed that the HLA-G expression in PE decidual trophoblasts was similar to that of normal pregnancy (175). Some researchers believed that placental necrosis in PE was responsible for the decrease of HLA-G (176).
Since the outcome of a pregnancy cannot be predicted in advance, it is difficult to obtain dNK cells during the first trimester. To address this issue, specialists sort pregnancies using Doppler ultrasound of the uterine artery. During a healthy pregnancy, the uterine spiral artery undergoes recasting, resulting in increased maternal blood circulation that supplies the placental bed with low pressure. If spiral artery recasting is defective, the resistance index (RI) of the uterine artery will increase correspondingly. RI of the uterine artery can indirectly represent the degree of spiral artery recasting in early pregnancy (177, 178). Some researchers segregated human pNK cells and transformed them into idNK cells, which resemble dNK cells in function and phenotype (179). After administering idNK cells to pregnant mice with elevated uterine artery RI, the number of RI was reduced and placental perfusion was improved (179). dNK cells from expectant mothers with a normal uterine artery RI induced trophoblast invasion more effectively than those from pregnant females with a high uterine artery RI (180). Additionally, dNK cells from high uterine artery RI induced greater endothelial cell activation and TNF-α production than those from normal RI, indicating that the function of these cells has changed (181). In early pregnancy, the expressions of KIR2DL/S1, 3, 5 and LILRB1 were significantly lower in dNK cells from increased uterine artery RI than in those from normal RI (182). This may result in abnormal binding between trophoblasts and dNK cells (122, 123), as well as changes in the production of mediators by dNK cells (123). Pregnant females with PE may have different variations in the uterine artery Doppler parameters during pregnancy (183). Consequently, the monitoring of uterine artery Doppler can be used to evaluate changes in the number or function of dNK cells in PE patients.
Regarding the treatment of PE, several studies have demonstrated that anti-inflammatory compounds may alter the NK cell number in peripheral blood or decidua, resulting in effective PE treatment. The addition of anti-inflammatory IL-4 to RUPP rat models cut down the number of NK cells in the placenta (184), as did treatment with 17-hydroxyprogesterone caproate (17-OHPC) (185). In addition, the superoxide dismutase mimic tempol decreased the percentages of total NK and cNK cells in peripheral blood (186). Consequently, these specific anti-inflammatory drugs may be used to improve the symptoms of PE due to their ability to regulate NK cells.
In comparison to normal pregnancies, PE patients have changes in the NK cell number affected by progesterone (18, 28, 132). Indirectly or directly, progesterone can decrease the number, activation, and cytotoxicity of circulating NK cells (51). Moreover, progesterone participates in the dNK cell proliferation and differentiation, influencing placentation and fetal development (51, 187–189). However, there are not much research to support the use of progesterone or 17-OHPC to prevent PE (190). Moreover, 17-OHPC did not reduce the risk of recurrent preterm birth (191). Therefore, the therapeutic role of 17-OHPC in patients with PE is unclear.
Intravenous immunoglobulin (IVIg) reduces NK cell cytotoxicity via direct antibody action or immunomodulation (192). First of all, IVIg decreased the number of pNK cells in recurrent pregnancy loss (RPL) and achieved reproductive success (193). In addition, IVIg increased the expression of CD94 on NK cells, thereby inhibiting their activity (194). In another study, 31 patients with RPL or repeated implantation failure (RIF) and 15 fertile women participated (195). Patients in the experimental group had more CD56+ CD3+ NKT cells than those in the control group (195). IVIg therapy greatly reduced the NKT cell number in the study group (195). It can be concluded that IVIg could reduce the number of NKT cells in RPL or RIF patients, thus promoting a healthy pregnancy. IVIg treatment was effective for patients with RIF (196). Before embryo transfer, researchers measured the number of circulating CD16+/CD56+ NK cells. Then, seven days before embryo transfer, IVIg was administered to women with elevated CD16+/CD56+ NK cells (196). Compared to placebo, IVIg infusion significantly improved pregnancy outcomes (196). Therefore, IVIg may be an acceptable treatment for PE patients with abnormal NK cell number or activity.
In recent years, it has been illustrated that intralipid can be used to maintain pregnancy (197). Intralipid is primarily made up of purified soybean oil and egg phospholipids. Clinically, intralipid infusion is frequently used to provide malnourished patients with energy and essential fatty acids. Whether intralipid has an immunoregulatory effect remains controversial (198). Several studies point out that intralipid possesses immune activity (199–201). Similar to IVIg, intralipid was reported to suppress the cytotoxicity of NK cells (200, 201). However, future research is necessary to examine the possibility of intralipid in the treatment of PE patients.
The maternal immune mechanism of PE is well studied, but there is rarely any research about the effect of PE on neonatal immune system. PE patients exhibit an increased inflammatory response, which may impair the immune function of newborns. Alternatively, because maternal-fetal immune tolerance is interactive, the fetal immune system may contribute to maternal pregnancy complications. The NKp30 expression in cord blood NK cells of PE patients was remarkably elevated, whereas that of NKG2D was marginally decreased compared to normal pregnancies (202). The innate immune system of a pre-eclamptic fetus initiates an inflammatory response in the uterus, which is crucial for the function of neonatal NK cells (202). After binding with their respective ligands, aberrant expressions of NKp30 and NKG2D impair the fine regulation of neonatal NK cells. Another study examined the phenotype of NK cells in the umbilical cord blood of both PE sufferers and fit expectant mothers during the third trimester (203). In PE patients’ cord blood, the ratio of CD56hi CD16- non-activated/regulatory NK cells to CD56lo CD16+ activated/effector NK cells was significantly decreased (203). This indicates that the pre-eclamptic fetus has an abnormal immune system (203). In addition, the increased inflammatory response and the stimulation of the alternative complement pathway in the cord blood of PE patients indicate that PE affects the neonatal immune compartment (204). The number of CD3-/CD56+16+ NK cells in PE sufferers’ cord blood was significantly higher than that of normotensive pregnant women (205), which was consistent with another study (206). The increase of NK cells transported from the mother to the fetus during PE can explain the rich NK cells in the cord blood of pre-eclamptic females (205). It has been reported that the mother-to-fetus cell shift is elevated in PE (207). Similarly, there is an increase in fetus-to-mother cell transfer in PE (208–211).
In this review, we summarize the research progress of NK cells in the etiology of PE. We speculate that the innate immune system, as opposed to the adaptive one, plays an important role in immune regulation in pregnancy. NK cells appear to exert a central effect in both peripheral blood and maternal-fetal interface in the etiology of PE. Even though a previous perception believes that dNK cells are “hazardous” cells, current studies suggest that they are necessary for normal implantation, placentation and fetal growth. Accordingly, dNK cells are considered as “peaceful” cells in pregnancy complications, and they collaborate with other members of the innate immune system to construct a healthy reproductive system. Changes in the number or function of dNK cells may be the cause of PE. Genetic studies based on animal models have shown that dNK cells are involved in spiral artery remodeling and trophoblast invasion at the maternal–fetal interface. The abnormal interaction between dNK cells and trophoblasts or maternal cells may contribute to the progression of PE, but the evidence is insufficient.
Even though significant progress has been made in knowing the function of NK cells, the difficulty of obtaining human samples in the first trimester and the limited extrapolation from animal experiments impede the research of NK cells. In addition, the signal pathway governing the dNK cell function during pregnancy, which can predict the occurrence of PE, is unclear. To maintain immune equilibrium both locally and systemically, it is necessary to take therapeutic measures directed at NK cells. Before developing treatments that target these immune cells, it is important to understand the complex mechanism of interaction between decidual cells, as well as the normal function of dNK cells during the first versus third pregnancies and the contrast between these alternations in PE. To determine the reference interval of cytotoxicity and angiogenesis of dNK cells between healthy pregnancies and PE cases, clinical trials are required. To clarify the mechanism of PE, in vitro experiments and animal models of PE may mimic the phenotypic alternations of NK cells observed in clinical patients. In addition, basic research aids in the exploration of new NK cell targets and the development of novel technologies to improve maternal and fetal outcomes of PE. In order to improve the prediction, diagnosis, and treatment of PE, therefore, clinically applicable research results are required. We postulate that a more comprehensive understanding of dNK functions and dNK receptor–regulated communications can make a difference in the beneficial role of these cells in PE. The prospective experiments in other pregnancy complications caused by placental abnormalities are requisite to expand on these observations to initiate a latent NK cell-based method to prevent or treat PE and other relevant diseases of impaired placentation.
XW and XY contributed to the writing of this review. XY designed and revised the manuscript. All authors contributed to the article and approved the submitted version.
The authors declare that the research was conducted in the absence of any commercial or financial relationships that could be construed as a potential conflict of interest.
All claims expressed in this article are solely those of the authors and do not necessarily represent those of their affiliated organizations, or those of the publisher, the editors and the reviewers. Any product that may be evaluated in this article, or claim that may be made by its manufacturer, is not guaranteed or endorsed by the publisher.
1. Belmonte B, Mangogna A, Gulino A, Cancila V, Morello G, Agostinis C, et al. Distinct roles of classical and lectin pathways of complement in preeclamptic placentae. Front Immunol (2022) 13:882298. doi: 10.3389/fimmu.2022.882298
2. Abalos E, Cuesta C, Grosso AL, Chou D, Say L. Global and regional estimates of preeclampsia and eclampsia: A systematic review. Eur J Obstet Gynecol Reprod Biol (2013) 170(1):1–7. doi: 10.1016/j.ejogrb.2013.05.005
3. Brosens I, Puttemans P, Benagiano G. Placental bed research: I. the placental bed: From spiral arteries remodeling to the great obstetrical syndromes. Am J Obstet Gynecol (2019) 221(5):437–56. doi: 10.1016/j.ajog.2019.05.044
4. Högberg U. The world health report 2005: "Make every mother and child count" - including africans. Scand J Public Health (2005) 33(6):409–11. doi: 10.1080/14034940500217037
5. Guedes-Martins L. Chronic hypertension and pregnancy. Adv Exp Med Biol (2017) 956:395–407. doi: 10.1007/5584_2016_81
6. Mol BWJ, Roberts CT, Thangaratinam S, Magee LA, de Groot CJM, Hofmeyr GJ. Pre-eclampsia. Lancet (2016) 387(10022):999–1011. doi: 10.1016/S0140-6736(15)00070-7
7. Brown MA, Magee LA, Kenny LC, Karumanchi SA, McCarthy FP, Saito S, et al. Hypertensive disorders of pregnancy: Isshp classification, diagnosis, and management recommendations for international practice. Hypertension (2018) 72(1):24–43. doi: 10.1161/HYPERTENSIONAHA.117.10803
8. Burton GJ, Redman CW, Roberts JM, Moffett A. Pre-eclampsia: Pathophysiology and clinical implications. BMJ (2019) 366. doi: 10.1136/bmj.l2381
9. Mihu D, Razvan C, Malutan A, Mihaela C. Evaluation of maternal systemic inflammatory response in preeclampsia. Taiwan J Obstet Gynecol (2015) 54(2):160–6. doi: 10.1016/j.tjog.2014.03.006
10. Magee LA, Pels A, Helewa M, Rey E, von Dadelszen P. Diagnosis, evaluation, and management of the hypertensive disorders of pregnancy. Pregnancy Hypertens (2014) 4(2):105–45. doi: 10.1016/j.preghy.2014.01.003
11. Ahmed R, Dunford J, Mehran R, Robson S, Kunadian V. Pre-eclampsia and future cardiovascular risk among women: A review. J Am Coll Cardiol (2014) 63(18):1815–22. doi: 10.1016/j.jacc.2014.02.529
12. Hladunewich M, Karumanchi SA, Lafayette R. Pathophysiology of the clinical manifestations of preeclampsia. Clin J Am Soc Nephrol (2007) 2(3):543–9. doi: 10.2215/CJN.03761106
13. Kanasaki K, Kalluri R. The biology of preeclampsia. Kidney Int (2009) 76(8):831–7. doi: 10.1038/ki.2009.284
14. Yang X, Meng T. Mir-215-5p decreases migration and invasion of trophoblast cells through regulating Cdc6 in preeclampsia. Cell Biochem Funct (2020) 38(4):472–9. doi: 10.1002/cbf.3492
15. Ahn H, Park J, Gilman-Sachs A, Kwak-Kim J. Immunologic characteristics of preeclampsia, a comprehensive review. Am J Reprod Immunol (2011) 65(4):377–94. doi: 10.1111/j.1600-0897.2010.00913.x
16. Zeng B, Kwak-Kim J, Liu Y, Liao A-H. Treg cells are negatively correlated with increased memory b cells in pre-eclampsia while maintaining suppressive function on autologous b-cell proliferation. Am J Reprod Immunol (2013) 70(6):454–63. doi: 10.1111/aji.12154
17. Yang X, Yang Y, Yuan Y, Liu L, Meng T. The roles of uterine natural killer (Nk) cells and Kir/Hla-c combination in the development of preeclampsia: A systematic review. BioMed Res Int (2020) 2020:4808072. doi: 10.1155/2020/4808072
18. Fukui A, Yokota M, Funamizu A, Nakamua R, Fukuhara R, Yamada K, et al. Changes of nk cells in preeclampsia. Am J Reprod Immunol (2012) 67(4):278–86. doi: 10.1111/j.1600-0897.2012.01120.x
19. Zhao Y, Zhang X, Du N, Sun H, Chen L, Bao H, et al. Immune checkpoint molecules on T cell subsets of pregnancies with preeclampsia and gestational diabetes mellitus. J Reprod Immunol (2020) 142:103208. doi: 10.1016/j.jri.2020.103208
20. Billingham RE, Brent L, Medawar PB. Actively acquired tolerance of foreign cells. Nature (1953) 172(4379):603–6. doi: 10.1038/172603a0
21. Mor G, Cardenas I, Abrahams V, Guller S. Inflammation and pregnancy: The role of the immune system at the implantation site. Ann N Y Acad Sci (2011) 1221:80–7. doi: 10.1111/j.1749-6632.2010.05938.x
22. Sargent IL, Borzychowski AM, Redman CWG. Nk cells and human pregnancy–an inflammatory view. Trends Immunol (2006) 27(9):399–404. doi: 10.1016/j.it.2006.06.009
23. Hahn S, Huppertz B, Holzgreve W. Fetal cells and cell free fetal nucleic acids in maternal blood: New tools to study abnormal placentation? Placenta (2005) 26(7):515–26. doi: 10.1016/j.placenta.2004.10.017
24. Solier C, Aguerre-Girr M, Lenfant F, Campan A, Berrebi A, Rebmann V, et al. Secretion of pro-apoptotic intron 4-retaining soluble hla-G1 by human villous trophoblast. Eur J Immunol (2002) 32(12):3576–86. doi: 10.1002/1521-4141(200212)32:12<3576::Aid-immu3576>3.0.Co;2-m
25. Laresgoiti-Servitje E, Gómez-López N, Olson DM. An immunological insight into the origins of pre-eclampsia. Hum Reprod Update (2010) 16(5):510–24. doi: 10.1093/humupd/dmq007
26. Fu B, Li X, Sun R, Tong X, Ling B, Tian Z, et al. Natural killer cells promote immune tolerance by regulating inflammatory Th17 cells at the human maternal-fetal interface. Proc Natl Acad Sci U.S.A. (2013) 110(3):E231–40. doi: 10.1073/pnas.1206322110
27. Higuma-Myojo S, Sasaki Y, Miyazaki S, Sakai M, Siozaki A, Miwa N, et al. Cytokine profile of natural killer cells in early human pregnancy. Am J Reprod Immunol (2005) 54(1):21–9. doi: 10.1111/j.1600-0897.2005.00279.x
28. Moffett-King A. Natural killer cells and pregnancy. Nat Rev Immunol (2002) 2(9):656–63. doi: 10.1038/nri886
29. Orr MT, Lanier LL. Natural killer cell education and tolerance. Cell (2010) 142(6):847–56. doi: 10.1016/j.cell.2010.08.031
30. Jabrane-Ferrat N. Features of human decidual nk cells in healthy pregnancy and during viral infection. Front Immunol (2019) 10:1397. doi: 10.3389/fimmu.2019.01397
31. Moretta L, Moretta A. Unravelling natural killer cell function: Triggering and inhibitory human nk receptors. EMBO J (2004) 23(2):255–9. doi: 10.1038/sj.emboj.7600019
32. Lanier LL. Nk cell receptors. Annu Rev Immunol (1998) 16:359–93. doi: 10.1146/annurev.immunol.16.1.359
33. Raulet DH, Vance RE, McMahon CW. Regulation of the natural killer cell receptor repertoire. Annu Rev Immunol (2001) 19:291–330. doi: 10.1146/annurev.immunol.19.1.291
34. Ishitani A, Sageshima N, Hatake K. The involvement of hla-e and -f in pregnancy. J Reprod Immunol (2006) 69(2):101–13. doi: 10.1016/j.jri.2005.10.004
35. Zhang Y, Liu Z, Tian M, Hu X, Wang L, Ji J, et al. The altered pd-1/Pd-L1 pathway delivers the 'One-two punch' effects to promote the Treg/Th17 imbalance in pre-eclampsia. Cell Mol Immunol (2018) 15(7):710–23. doi: 10.1038/cmi.2017.70
36. Guleria I, Khosroshahi A, Ansari MJ, Habicht A, Azuma M, Yagita H, et al. A critical role for the programmed death ligand 1 in fetomaternal tolerance. J Exp Med (2005) 202(2):231–7. doi: 10.1084/jem.20050019
37. Pesce S, Greppi M, Tabellini G, Rampinelli F, Parolini S, Olive D, et al. Identification of a subset of human natural killer cells expressing high levels of programmed death 1: A phenotypic and functional characterization. J Allergy Clin Immunol (2017) 139(1):335–46.e3. doi: 10.1016/j.jaci.2016.04.025
38. Meggyes M, Miko E, Szigeti B, Farkas N, Szereday L. The importance of the pd-1/Pd-L1 pathway at the maternal-fetal interface. BMC Pregnancy Childbirth (2019) 19(1):74. doi: 10.1186/s12884-019-2218-6
39. Meggyes M, Lajko A, Palkovics T, Totsimon A, Illes Z, Szereday L, et al. Feto-maternal immune regulation by Tim-3/Galectin-9 pathway and pd-1 molecule in mice at day 14.5 of pregnancy. Placenta (2015) 36(10):1153–60. doi: 10.1016/j.placenta.2015.07.124
40. Vacca P, Pesce S, Greppi M, Fulcheri E, Munari E, Olive D, et al. Pd-1 is expressed by and regulates human group 3 innate lymphoid cells in human decidua. Mucosal Immunol (2019) 12(3):624–31. doi: 10.1038/s41385-019-0141-9
41. Carlino C, Stabile H, Morrone S, Bulla R, Soriani A, Agostinis C, et al. Recruitment of circulating nk cells through decidual tissues: A possible mechanism controlling nk cell accumulation in the uterus during early pregnancy. Blood (2008) 111(6):3108–15. doi: 10.1182/blood-2007-08-105965
42. Manaster I, Mizrahi S, Goldman-Wohl D, Sela HY, Stern-Ginossar N, Lankry D, et al. Endometrial nk cells are special immature cells that await pregnancy. J Immunol (2008) 181(3):1869–76. doi: 10.4049/jimmunol.181.3.1869
43. Faas MM, de Vos P. Uterine nk cells and macrophages in pregnancy. Placenta (2017) 56:44–52. doi: 10.1016/j.placenta.2017.03.001
44. Vacca P, Vitale C, Montaldo E, Conte R, Cantoni C, Fulcheri E, et al. Cd34+ hematopoietic precursors are present in human decidua and differentiate into natural killer cells upon interaction with stromal cells. Proc Natl Acad Sci U.S.A. (2011) 108(6):2402–7. doi: 10.1073/pnas.1016257108
45. Yang X, Gilman-Sachs A, Kwak-Kim J. Ovarian and endometrial immunity during the ovarian cycle. J Reprod Immunol (2019) 133:7–14. doi: 10.1016/j.jri.2019.04.001
46. Mahajan D, Sharma NR, Kancharla S, Kolli P, Tripathy A, Sharma AK, et al. Role of natural killer cells during pregnancy and related complications. Biomolecules (2022) 12(1). doi: 10.3390/biom12010068
47. Le Bouteiller P, Piccinni M-P. Human nk cells in pregnant uterus: Why there? Am J Reprod Immunol (2008) 59(5):401–6. doi: 10.1111/j.1600-0897.2008.00597.x
48. Igarashi T, Konno R, Okamoto S, Moriya T, Satoh S, Yajima A. Involvement of granule-mediated apoptosis in the cyclic changes of the normal human endometrium. Tohoku J Exp Med (2001) 193(1):13–25. doi: 10.1620/tjem.193.13
49. De Carolis C, Perricone C, Perricone R. Nk cells, autoantibodies, and immunologic infertility: A complex interplay. Clin Rev Allergy Immunol (2010) 39(3):166–75. doi: 10.1007/s12016-009-8184-4
50. Hidaka Y, Amino N, Iwatani Y, Kaneda T, Mitsuda N, Morimoto Y, et al. Changes in natural killer cell activity in normal pregnant and postpartum women: Increases in the first trimester and postpartum period and decrease in late pregnancy. J Reprod Immunol (1991) 20(1):73–83. doi: 10.1016/0165-0378(91)90024-k
51. Dosiou C, Giudice LC. Natural killer cells in pregnancy and recurrent pregnancy loss: Endocrine and immunologic perspectives. Endocr Rev (2005) 26(1):44–62. doi: 10.1210/er.2003-0021
52. Haller H, Radillo O, Rukavina D, Tedesco F, Candussi G, Petrović O, et al. An immunohistochemical study of leucocytes in human endometrium, first and third trimester basal decidua. J Reprod Immunol (1993) 23(1):41–9. doi: 10.1016/0165-0378(93)90025-d
53. Vargas ML, Sántos JL, Ruiz C, Montes MJ, Alemán P, García-Tortosa C, et al. Comparison of the proportions of leukocytes in early and term human decidua. Am J Reprod Immunol (1993) 29(3):135–40. doi: 10.1111/j.1600-0897.1993.tb00578.x
54. Stallmach T, Hebisch G, Orban P, Lü X. Aberrant positioning of trophoblast and lymphocytes in the feto-maternal interface with pre-eclampsia. Virchows Arch (1999) 434(3):207–11. doi: 10.1007/s004280050329
55. Williams PJ, Searle RF, Robson SC, Innes BA, Bulmer JN. Decidual leucocyte populations in early to late gestation normal human pregnancy. J Reprod Immunol (2009) 82(1):24–31. doi: 10.1016/j.jri.2009.08.001
56. Bulmer JN, Williams PJ, Lash GE. Immune cells in the placental bed. Int J Dev Biol (2010) 54(2-3):281–94. doi: 10.1387/ijdb.082763jb
57. Zhang J, Dunk CE, Kwan M, Jones RL, Harris LK, Keating S, et al. Human dnk cell function is differentially regulated by extrinsic cellular engagement and intrinsic activating receptors in first and second trimester pregnancy. Cell Mol Immunol (2017) 14(2):203–13. doi: 10.1038/cmi.2015.66
58. de Mendonça Vieira R, Meagher A, Crespo ÂC, Kshirsagar SK, Iyer V, Norwitz ER, et al. Human term pregnancy decidual nk cells generate distinct cytotoxic responses. J Immunol (2020) 204(12):3149–59. doi: 10.4049/jimmunol.1901435
59. Smith SD, Dunk CE, Aplin JD, Harris LK, Jones RL. Evidence for immune cell involvement in decidual spiral arteriole remodeling in early human pregnancy. Am J Pathol (2009) 174(5):1959–71. doi: 10.2353/ajpath.2009.080995
60. Harris LK. Review: Trophoblast-vascular cell interactions in early pregnancy: How to remodel a vessel. Placenta (2010) 31 Suppl:S93–S8. doi: 10.1016/j.placenta.2009.12.012
61. Robson A, Harris LK, Innes BA, Lash GE, Aljunaidy MM, Aplin JD, et al. Uterine natural killer cells initiate spiral artery remodeling in human pregnancy. FASEB J (2012) 26(12):4876–85. doi: 10.1096/fj.12-210310
62. Lash GE, Schiessl B, Kirkley M, Innes BA, Cooper A, Searle RF, et al. Expression of angiogenic growth factors by uterine natural killer cells during early pregnancy. J Leukoc Biol (2006) 80(3):572–80. doi: 10.1189/jlb.0406250
63. Naruse K, Lash GE, Innes BA, Otun HA, Searle RF, Robson SC, et al. Localization of matrix metalloproteinase (Mmp)-2, mmp-9 and tissue inhibitors for mmps (Timps) in uterine natural killer cells in early human pregnancy. Hum Reprod (2009) 24(3):553–61. doi: 10.1093/humrep/den408
64. Moses MA. The regulation of neovascularization of matrix metalloproteinases and their inhibitors. Stem Cells (1997) 15(3):180–9. doi: 10.1002/stem.150180
65. Croy BA, Chen Z, Hofmann AP, Lord EM, Sedlacek AL, Gerber SA. Imaging of vascular development in early mouse decidua and its association with leukocytes and trophoblasts. Biol Reprod (2012) 87(5):125. doi: 10.1095/biolreprod.112.102830
66. Ashkar AA, Di Santo JP, Croy BA. Interferon gamma contributes to initiation of uterine vascular modification, decidual integrity, and uterine natural killer cell maturation during normal murine pregnancy. J Exp Med (2000) 192(2):259–70. doi: 10.1084/jem.192.2.259
67. Chen Z, Zhang J, Hatta K, Lima PDA, Yadi H, Colucci F, et al. Dba-lectin reactivity defines mouse uterine natural killer cell subsets with biased gene expression. Biol Reprod (2012) 87(4):81. doi: 10.1095/biolreprod.112.102293
68. Tayade C, Hilchie D, He H, Fang Y, Moons L, Carmeliet P, et al. Genetic deletion of placenta growth factor in mice alters uterine nk cells. J Immunol (2007) 178(7):4267–75. doi: 10.4049/jimmunol.178.7.4267
69. Yadi H, Burke S, Madeja Z, Hemberger M, Moffett A, Colucci F. Unique receptor repertoire in mouse uterine nk cells. J Immunol (2008) 181(9):6140–7. doi: 10.4049/jimmunol.181.9.6140
70. Guimond MJ, Luross JA, Wang B, Terhorst C, Danial S, Croy BA. Absence of natural killer cells during murine pregnancy is associated with reproductive compromise in Tge26 mice. Biol Reprod (1997) 56(1):169–79. doi: 10.1095/biolreprod56.1.169
71. Guimond MJ, Wang B, Croy BA. Engraftment of bone marrow from severe combined immunodeficient (Scid) mice reverses the reproductive deficits in natural killer cell-deficient tg epsilon 26 mice. J Exp Med (1998) 187(2):217–23. doi: 10.1084/jem.187.2.217
72. Hunt JS, Miller L, Vassmer D, Croy BA. Expression of the inducible nitric oxide synthase gene in mouse uterine leukocytes and potential relationships with uterine function during pregnancy. Biol Reprod (1997) 57(4):827–36. doi: 10.1095/biolreprod57.4.827
73. Wang C, Umesaki N, Nakamura H, Tanaka T, Nakatani K, Sakaguchi I, et al. Expression of vascular endothelial growth factor by granulated metrial gland cells in pregnant murine uteri. Cell Tissue Res (2000) 300(2):285–93. doi: 10.1007/s004410000198
74. Hu Y, Dutz JP, MacCalman CD, Yong P, Tan R, von Dadelszen P. Decidual nk cells alter in vitro first trimester extravillous cytotrophoblast migration: A role for ifn-gamma. J Immunol (2006) 177(12):8522–30. doi: 10.4049/jimmunol.177.12.8522
75. Hanna J, Goldman-Wohl D, Hamani Y, Avraham I, Greenfield C, Natanson-Yaron S, et al. Decidual nk cells regulate key developmental processes at the human fetal-maternal interface. Nat Med (2006) 12(9):1065–74. doi: 10.1038/nm1452
76. Spaans F, Melgert BN, Chiang C, Borghuis T, Klok PA, de Vos P, et al. Extracellular atp decreases trophoblast invasion, spiral artery remodeling and immune cells in the mesometrial triangle in pregnant rats. Placenta (2014) 35(8):587–95. doi: 10.1016/j.placenta.2014.05.013
77. Soares MJ, Chakraborty D, Karim Rumi MA, Konno T, Renaud SJ. Rat placentation: An experimental model for investigating the hemochorial maternal-fetal interface. Placenta (2012) 33(4):233–43. doi: 10.1016/j.placenta.2011.11.026
78. Chakraborty D, Rumi MAK, Konno T, Soares MJ. Natural killer cells direct hemochorial placentation by regulating hypoxia-inducible factor dependent trophoblast lineage decisions. Proc Natl Acad Sci U.S.A. (2011) 108(39):16295–300. doi: 10.1073/pnas.1109478108
79. Fu B, Zhou Y, Ni X, Tong X, Xu X, Dong Z, et al. Natural killer cells promote fetal development through the secretion of growth-promoting factors. Immunity (2017) 47(6):1100–13. doi: 10.1016/j.immuni.2017.11.018
80. Zhou Y, Fu B, Xu X, Zhang J, Tong X, Wang Y, et al. Pbx1 expression in uterine natural killer cells drives fetal growth. Sci Transl Med (2020) 12(537). doi: 10.1126/scitranslmed.aax1798
81. Pique-Regi R, Romero R, Tarca AL, Sendler ED, Xu Y, Garcia-Flores V, et al. Single cell transcriptional signatures of the human placenta in term and preterm parturition. Elife (2019) 8. doi: 10.7554/eLife.52004
82. Tsuda H, Sakai M, Michimata T, Tanebe K, Hayakawa S, Saito S. Characterization of nkt cells in human peripheral blood and decidual lymphocytes. Am J Reprod Immunol (2001) 45(5):295–302. doi: 10.1111/j.8755-8920.2001.450505.x
83. St Louis D, Romero R, Plazyo O, Arenas-Hernandez M, Panaitescu B, Xu Y, et al. Invariant nkt cell activation induces late preterm birth that is attenuated by rosiglitazone. J Immunol (2016) 196(3):1044–59. doi: 10.4049/jimmunol.1501962
84. Hill JA, Hsia S, Doran DM, Bryans CI. Natural killer cell activity and antibody dependent cell-mediated cytotoxicity in preeclampsia. J Reprod Immunol (1986) 9(3):205–12. doi: 10.1016/0165-0378(86)90014-8
85. Matthiesen L, Berg G, Ernerudh J, Håkansson L. Lymphocyte subsets and mitogen stimulation of blood lymphocytes in preeclampsia. Am J Reprod Immunol (1999) 41(3):192–203. doi: 10.1111/j.1600-0897.1999.tb00532.x
86. Borzychowski AM, Croy BA, Chan WL, Redman CWG, Sargent IL. Changes in systemic type 1 and type 2 immunity in normal pregnancy and pre-eclampsia may be mediated by natural killer cells. Eur J Immunol (2005) 35(10):3054–63. doi: 10.1002/eji.200425929
87. van Nieuwenhoven ALV, Moes H, Heineman MJ, Santema J, Faas MM. Cytokine production by monocytes, nk cells, and lymphocytes is different in preeclamptic patients as compared with normal pregnant women. Hypertens Pregnancy (2008) 27(3):207–24. doi: 10.1080/10641950701885006
88. Bachmayer N, Sohlberg E, Sundström Y, Hamad RR, Berg L, Bremme K, et al. Women with pre-eclampsia have an altered Nkg2a and Nkg2c receptor expression on peripheral blood natural killer cells. Am J Reprod Immunol (2009) 62(3):147–57. doi: 10.1111/j.1600-0897.2009.00724.x
89. Bueno-Sánchez JC, Agudelo-Jaramillo B, Escobar-Aguilerae LF, Lopera A, Cadavid-Jaramillo AP, Chaouat G, et al. Cytokine production by non-stimulated peripheral blood nk cells and lymphocytes in early-onset severe pre-eclampsia without hellp. J Reprod Immunol (2013) 97(2):223–31. doi: 10.1016/j.jri.2012.11.007
90. Laganà AS, Giordano D, Loddo S, Zoccali G, Vitale SG, Santamaria A, et al. Decreased endothelial progenitor cells (Epcs) and increased natural killer (Nk) cells in peripheral blood as possible early markers of preeclampsia: A case-control analysis. Arch Gynecol Obstet (2017) 295(4):867–72. doi: 10.1007/s00404-017-4296-x
91. Vinnars M-T, Björk E, Nagaev I, Ottander U, Bremme K, Holmlund U, et al. Enhanced Th1 and inflammatory mrna responses upregulate nk cell cytotoxicity and Nkg2d ligand expression in human pre-eclamptic placenta and target it for nk cell attack. Am J Reprod Immunol (2018) 80(1):e12969. doi: 10.1111/aji.12969
92. Du M, Wang W, Huang L, Guan X, Lin W, Yao J, et al. Natural killer cells in the pathogenesis of preeclampsia: A double-edged sword. J Matern Fetal Neona (2022) 35(6):1028–35. doi: 10.1080/14767058.2020.1740675
93. Seamon K, Kurlak LO, Warthan M, Stratikos E, Strauss JF, Mistry HD, et al. The differential expression of Erap1/Erap2 and immune cell activation in pre-eclampsia. Front Immunol (2020) 11:396. doi: 10.3389/fimmu.2020.00396
94. Toder V, Blank M, Gleicher N, Voljovich I, Mashiah S, Nebel L. Activity of natural killer cells in normal pregnancy and edema-Proteinuria-Hypertension gestosis. Am J Obstet Gynecol (1983) 145(1):7–10. doi: 10.1016/0002-9378(83)90332-0
95. Marinoni E, De Pità O, Bresadola M, Ippoliti F, Di Iorio R. Cell-mediated immunity imbalance in pregnancy-induced hypertension. Gynecol Obstet Invest (1994) 38(4):236–40. doi: 10.1159/000292488
96. Minagawa M, Narita J, Tada T, Maruyama S, Shimizu T, Bannai M, et al. Mechanisms underlying immunologic states during pregnancy: Possible association of the sympathetic nervous system. Cell Immunol (1999) 196(1):1–13. doi: 10.1006/cimm.1999.1541
97. Alanen A, Lassila O. Deficient natural killer cell function in preeclampsia. Obstet Gynecol (1982) 60(5):631–4.
98. Brien M-E, Boufaied I, Soglio DD, Rey E, Leduc L, Girard S. Distinct inflammatory profile in preeclampsia and postpartum preeclampsia reveal unique mechanisms. Biol Reprod (2019) 100(1):187–94. doi: 10.1093/biolre/ioy164
99. Darmochwal-Kolarz D, Rolinski J, Leszczynska-Goarzelak B, Oleszczuk J. The expressions of intracellular cytokines in the lymphocytes of preeclamptic patients. Am J Reprod Immunol (2002) 48(6):381–6. doi: 10.1034/j.1600-0897.2002.01089.x
100. Molvarec A, Ito M, Shima T, Yoneda S, Toldi G, Stenczer B, et al. Decreased proportion of peripheral blood vascular endothelial growth factor-expressing T and natural killer cells in preeclampsia. Am J Obstet Gynecol (2010) 203(6):567. doi: 10.1016/j.ajog.2010.07.019
101. Molvarec A, Blois SM, Stenczer B, Toldi G, Tirado-Gonzalez I, Ito M, et al. Peripheral blood galectin-1-Expressing T and natural killer cells in normal pregnancy and preeclampsia. Clin Immunol (2011) 139(1):48–56. doi: 10.1016/j.clim.2010.12.018
102. O'Brien TJ, Hardin JW, Bannon GA, Norris JS, Quirk JG. Ca 125 antigen in human amniotic fluid and fetal membranes. Am J Obstet Gynecol (1986) 155(1):50–5. doi: 10.1016/0002-9378(86)90076-1
103. Quirk JG, Brunson GL, Long CA, Bannon GA, Sanders MM, O'Brien TJ. Ca 125 in tissues and amniotic fluid during pregnancy. Am J Obstet Gynecol (1988) 159(3):644–9. doi: 10.1016/s0002-9378(88)80026-7
104. Tyler C, Kapur A, Felder M, Belisle JA, Trautman C, Gubbels JAA, et al. The mucin Muc16 (Ca125) binds to nk cells and monocytes from peripheral blood of women with healthy pregnancy and preeclampsia. Am J Reprod Immunol (2012) 68(1):28–37. doi: 10.1111/j.1600-0897.2012.01113.x
105. Wilczyński JR, Tchórzewski H, Głowacka E, Banasik M, Lewkowicz P, Szpakowski M, et al. Cytokine secretion by decidual lymphocytes in transient hypertension of pregnancy and pre-eclampsia. Mediators Inflammation (2002) 11(2):105–11. doi: 10.1080/09629350220131962
106. Wilczyński JR, Tchórzewski H, Banasik M, Głowacka E, Wieczorek A, Lewkowicz P, et al. Lymphocyte subset distribution and cytokine secretion in third trimester decidua in normal pregnancy and preeclampsia. Eur J Obstet Gynecol Reprod Biol (2003) 109(1):8–15. doi: 10.1016/s0301-2115(02)00350-0
107. Eide IP, Rolfseng T, Isaksen CV, Mecsei R, Roald B, Lydersen S, et al. Serious foetal growth restriction is associated with reduced proportions of natural killer cells in decidua basalis. Virchows Arch (2006) 448(3):269–76. doi: 10.1007/s00428-005-0107-z
108. Bachmayer N, Rafik Hamad R, Liszka L, Bremme K, Sverremark-Ekström E. Aberrant uterine natural killer (Nk)-cell expression and altered placental and serum levels of the nk-cell promoting cytokine interleukin-12 in pre-eclampsia. Am J Reprod Immunol (2006) 56(5-6):292–301. doi: 10.1111/j.1600-0897.2006.00429.x
109. Williams PJ, Bulmer JN, Searle RF, Innes BA, Robson SC. Altered decidual leucocyte populations in the placental bed in pre-eclampsia and foetal growth restriction: A comparison with late normal pregnancy. Reproduction (2009) 138(1):177–84. doi: 10.1530/REP-09-0007
110. Rieger L, Segerer S, Bernar T, Kapp M, Majic M, Morr A-K, et al. Specific subsets of immune cells in human decidua differ between normal pregnancy and preeclampsia–a prospective observational study. Reprod Biol Endocrinol (2009) 7:132. doi: 10.1186/1477-7827-7-132
111. Sánchez-Rodríguez EN, Nava-Salazar S, Mendoza-Rodríguez CA, Moran C, Romero-Arauz JF, Ortega E, et al. Persistence of decidual nk cells and kir genotypes in healthy pregnant and preeclamptic women: A case-control study in the third trimester of gestation. Reprod Biol Endocrinol (2011) 9:8. doi: 10.1186/1477-7827-9-8
112. Lockwood CJ, Huang SJ, Chen C-P, Huang Y, Xu J, Faramarzi S, et al. Decidual cell regulation of natural killer cell-recruiting chemokines: Implications for the pathogenesis and prediction of preeclampsia. Am J Pathol (2013) 183(3):841–56. doi: 10.1016/j.ajpath.2013.05.029
113. Milosevic-Stevanovic J, Krstic M, Radovic-Janosevic D, Popovic J, Tasic M, Stojnev S. Number of decidual natural killer cells & macrophages in pre-eclampsia. Indian J Med Res (2016) 144(6):823–30. doi: 10.4103/ijmr.IJMR_776_15
114. Zhang J, Dunk CE, Shynlova O, Caniggia I, Lye SJ. Tgfb1 suppresses the activation of distinct dnk subpopulations in preeclampsia. EBioMedicine (2019) 39:531–9. doi: 10.1016/j.ebiom.2018.12.015
115. Zhang J, Lye SJ. The immune potential of decidua-resident Cd16cd56 nk cells in human pregnancy. Hum Immunol (2021) 82(5):332–9. doi: 10.1016/j.humimm.2021.01.014
116. Akhlaq M, Nagi AH, Yousaf AW. Placental morphology in pre-eclampsia and eclampsia and the likely role of nk cells. Indian J Pathol Microbiol (2012) 55(1):17–21. doi: 10.4103/0377-4929.94848
117. Saito S, Sakai M. Th1/Th2 balance in preeclampsia. J Reprod Immunol (2003) 59(2):161–73. doi: 10.1016/s0165-0378(03)00045-7
118. Redman CWG, Sargent IL. Placental stress and pre-eclampsia: A revised view. Placenta (2009) 30 Suppl A:S38–42. doi: 10.1016/j.placenta.2008.11.021
119. Mantovani A, Allavena P, Sica A, Balkwill F. Cancer-related inflammation. Nature (2008) 454(7203):436–44. doi: 10.1038/nature07205
120. Whiteside TL. The tumor microenvironment and its role in promoting tumor growth. Oncogene (2008) 27(45):5904–12. doi: 10.1038/onc.2008.271
121. Li XF, Charnock-Jones DS, Zhang E, Hiby S, Malik S, Day K, et al. Angiogenic growth factor messenger ribonucleic acids in uterine natural killer cells. J Clin Endocrinol Metab (2001) 86(4):1823–34. doi: 10.1210/jcem.86.4.7418
122. Wallace AE, Host AJ, Whitley GS, Cartwright JE. Decidual natural killer cell interactions with trophoblasts are impaired in pregnancies at increased risk of preeclampsia. Am J Pathol (2013) 183(6):1853–61. doi: 10.1016/j.ajpath.2013.08.023
123. Wallace AE, Fraser R, Gurung S, Goulwara SS, Whitley GS, Johnstone AP, et al. Increased angiogenic factor secretion by decidual natural killer cells from pregnancies with high uterine artery resistance alters trophoblast function. Hum Reprod (2014) 29(4):652–60. doi: 10.1093/humrep/deu017
124. Wong AWY, Archer B, Mariee N, Li TC, Laird SM. Do uterine natural killer cell numbers in peri-implantation endometrium predict hypertensive disorder in pregnancy in women with a history of reproductive failure? J Reprod Immunol (2014) 106:34–40. doi: 10.1016/j.jri.2014.04.005
125. Winship AL, Koga K, Menkhorst E, Van Sinderen M, Rainczuk K, Nagai M, et al. Interleukin-11 alters placentation and causes preeclampsia features in mice. Proc Natl Acad Sci U.S.A. (2015) 112(52):15928–33. doi: 10.1073/pnas.1515076112
126. Sones JL, Cha J, Woods AK, Bartos A, Heyward CY, Lob HE, et al. Decidual Cox2 inhibition improves fetal and maternal outcomes in a preeclampsia-like mouse model. JCI Insight (2016) 1(3). doi: 10.1172/jci.insight.75351
127. Elfarra J, Amaral LM, McCalmon M, Scott JD, Cunningham MW, Gnam A, et al. Natural killer cells mediate pathophysiology in response to reduced uterine perfusion pressure. Clin Sci (Lond) (2017) 131(23):2753–62. doi: 10.1042/CS20171118
128. Travis OK, Baik C, Tardo GA, Amaral L, Jackson C, Greer M, et al. Adoptive transfer of placental ischemia-stimulated natural killer cells causes a preeclampsia-like phenotype in pregnant rats. Am J Reprod Immunol (2021) 85(6):e13386. doi: 10.1111/aji.13386
129. Golic M, Haase N, Herse F, Wehner A, Vercruysse L, Pijnenborg R, et al. Natural killer cell reduction and uteroplacental vasculopathy. Hypertension (2016) 68(4):964–73. doi: 10.1161/HYPERTENSIONAHA.116.07800
130. Carter LL, Dutton RW. Relative perforin- and fas-mediated lysis in T1 and T2 Cd8 effector populations. J Immunol (1995) 155(3):1028–31. doi: 10.4049/jimmunol.155.3.1028
131. Peritt D, Robertson S, Gri G, Showe L, Aste-Amezaga M, Trinchieri G. Differentiation of human nk cells into Nk1 and Nk2 subsets. J Immunol (1998) 161(11):5821–4. doi: 10.4049/jimmunol.161.11.5821
132. Fukui A, Funamizu A, Yokota M, Yamada K, Nakamua R, Fukuhara R, et al. Uterine and circulating natural killer cells and their roles in women with recurrent pregnancy loss, implantation failure and preeclampsia. J Reprod Immunol (2011) 90(1):105–10. doi: 10.1016/j.jri.2011.04.006
133. LaMarca B, Speed J, Fournier L, Babcock SA, Berry H, Cockrell K, et al. Hypertension in response to chronic reductions in uterine perfusion in pregnant rats: Effect of tumor necrosis factor-alpha blockade. Hypertension (2008) 52(6):1161–7. doi: 10.1161/HYPERTENSIONAHA.108.120881
134. LaMarca BBD, Bennett WA, Alexander BT, Cockrell K, Granger JP. Hypertension produced by reductions in uterine perfusion in the pregnant rat: Role of tumor necrosis factor-alpha. Hypertension (2005) 46(4):1022–5. doi: 10.1161/01.HYP.0000175476.26719.36
135. Travis OK, Tardo GA, Giachelli C, Siddiq S, Nguyen HT, Crosby MT, et al. Interferon Γ neutralization reduces blood pressure, uterine artery resistance index, and placental oxidative stress in placental ischemic rats. Am J Physiol Regul Integr Comp Physiol (2021) 321(2):R112–R24. doi: 10.1152/ajpregu.00349.2020
136. Germain SJ, Sacks GP, Sooranna SR, Soorana SR, Sargent IL, Redman CW. Systemic inflammatory priming in normal pregnancy and preeclampsia: The role of circulating syncytiotrophoblast microparticles. J Immunol (2007) 178(9):5949–56. doi: 10.4049/jimmunol.178.9.5949
137. Daniel Y, Kupferminc MJ, Baram A, Jaffa AJ, Fait G, Wolman I, et al. Plasma interleukin-12 is elevated in patients with preeclampsia. Am J Reprod Immunol (1998) 39(6):376–80. doi: 10.1111/j.1600-0897.1998.tb00372.x
138. Verma S, Hiby SE, Loke YW, King A. Human decidual natural killer cells express the receptor for and respond to the cytokine interleukin 15. Biol Reprod (2000) 62(4):959–68. doi: 10.1095/biolreprod62.4.959
139. Ashkar AA, Black GP, Wei Q, He H, Liang L, Head JR, et al. Assessment of requirements for il-15 and ifn regulatory factors in uterine nk cell differentiation and function during pregnancy. J Immunol (2003) 171(6):2937–44. doi: 10.4049/jimmunol.171.6.2937
140. Toldi G, Rigó J, Stenczer B, Vásárhelyi B, Molvarec A. Increased prevalence of il-17-Producing peripheral blood lymphocytes in pre-eclampsia. Am J Reprod Immunol (2011) 66(3):223–9. doi: 10.1111/j.1600-0897.2011.00987.x
141. Keskin DB, Allan DSJ, Rybalov B, Andzelm MM, Stern JNH, Kopcow HD, et al. Tgfbeta promotes conversion of Cd16+ peripheral blood nk cells into Cd16- nk cells with similarities to decidual nk cells. Proc Natl Acad Sci U.S.A. (2007) 104(9):3378–83. doi: 10.1073/pnas.0611098104
142. Sanjabi S, Oh SA, Li MO. Regulation of the immune response by tgf-B: From conception to autoimmunity and infection. Cold Spring Harb Perspect Biol (2017) 9(6). doi: 10.1101/cshperspect.a022236
143. Jokhi PP, King A, Sharkey AM, Smith SK, Loke YW. Screening for cytokine messenger ribonucleic acids in purified human decidual lymphocyte populations by the reverse-transcriptase polymerase chain reaction. J Immunol (1994) 153(10):4427–35. doi: 10.4049/jimmunol.153.10.4427
144. Clark DA, Vince G, Flanders KC, Hirte H, Starkey P. Cd56+ lymphoid cells in human first trimester pregnancy decidua as a source of novel transforming growth factor-beta 2-related immunosuppressive factors. Hum Reprod (1994) 9(12):2270–7. doi: 10.1093/oxfordjournals.humrep.a138436
145. Marcoe JP, Lim JR, Schaubert KL, Fodil-Cornu N, Matka M, McCubbrey AL, et al. Tgf-B: is responsible for nk cell immaturity during ontogeny and increased susceptibility to infection during mouse infancy. Nat Immunol (2012) 13(9):843–50. doi: 10.1038/ni.2388
146. Vinayagam V, Bobby Z, Habeebullah S, Chaturvedula L, Bharadwaj SK. Maternal and cord blood plasma seng and tgf-B1 in patients with hypertensive disorders of pregnancy: A pilot study in a south Indian population. J Clin Diagn Res (2017) 11(3):QC32–QC4. doi: 10.7860/JCDR/2017/22790.9600
147. Yu L, Kuang L-Y, He F, Du L-L, Li Q-L, Sun W, et al. The role and molecular mechanism of long nocoding rna-Meg3 in the pathogenesis of preeclampsia. Reprod Sci (2018) 25(12):1619–28. doi: 10.1177/1933719117749753
148. Molvarec A, Szarka A, Walentin S, Beko G, Karádi I, Prohászka Z, et al. Serum leptin levels in relation to circulating cytokines, chemokines, adhesion molecules and angiogenic factors in normal pregnancy and preeclampsia. Reprod Biol Endocrinol (2011) 9:124. doi: 10.1186/1477-7827-9-124
149. Moore Simas TA, Crawford SL, Solitro MJ, Frost SC, Meyer BA, Maynard SE. Angiogenic factors for the prediction of preeclampsia in high-risk women. Am J Obstet Gynecol (2007) 197(3):244.e1–.e8. doi: 10.1016/j.ajog.2007.06.030
150. Poon LCY, Kametas NA, Maiz N, Akolekar R, Nicolaides KH. First-trimester prediction of hypertensive disorders in pregnancy. Hypertension (2009) 53(5):812–8. doi: 10.1161/HYPERTENSIONAHA.108.127977
151. Raulet DH, Vance RE. Self-tolerance of natural killer cells. Nat Rev Immunol (2006) 6(7):520–31. doi: 10.1038/nri1863
152. Shreeve N, Depierreux D, Hawkes D, Traherne JA, Sovio U, Huhn O, et al. The Cd94/Nkg2a inhibitory receptor educates uterine nk cells to optimize pregnancy outcomes in humans and mice. Immunity (2021) 54(6):1231–44. doi: 10.1016/j.immuni.2021.03.021
153. Hsu KC, Chida S, Geraghty DE, Dupont B. The killer cell immunoglobulin-like receptor (Kir) genomic region: Gene-order, haplotypes and allelic polymorphism. Immunol Rev (2002) 190:40–52. doi: 10.1034/j.1600-065x.2002.19004.x
154. Bashirova AA, Martin MP, McVicar DW, Carrington M. The killer immunoglobulin-like receptor gene cluster: Tuning the genome for defense. Annu Rev Genomics Hum Genet (2006) 7:277–300. doi: 10.1146/annurev.genom.7.080505.115726
155. Winter CC, Gumperz JE, Parham P, Long EO, Wagtmann N. Direct binding and functional transfer of nk cell inhibitory receptors reveal novel patterns of hla-c allotype recognition. J Immunol (1998) 161(2):571–7. doi: 10.4049/jimmunol.161.2.571
156. Hiby SE, Walker JJ, O'Shaughnessy KM, Redman CWG, Carrington M, Trowsdale J, et al. Combinations of maternal kir and fetal hla-c genes influence the risk of preeclampsia and reproductive success. J Exp Med (2004) 200(8):957–65. doi: 10.1084/jem.20041214
157. Kelemu T, Erlandsson L, Seifu D, Hansson E, Abebe M, Teklu S, et al. Polymorphism in killer cell immunoglobulin-like receptors and human leukocyte antigen-c and predisposition to preeclampsia in Ethiopian pregnant women population. J Reprod Immunol (2020) 141:103169. doi: 10.1016/j.jri.2020.103169
158. Johnsen GM, Størvold GL, Drabbels JJM, Haasnoot GW, Eikmans M, Spruyt-Gerritse MJ, et al. The combination of maternal kir-b and fetal hla-C2 is associated with decidua basalis acute atherosis in pregnancies with preeclampsia. J Reprod Immunol (2018) 129:23–9. doi: 10.1016/j.jri.2018.07.005
159. Hiby SE, Apps R, Sharkey AM, Farrell LE, Gardner L, Mulder A, et al. Maternal activating kirs protect against human reproductive failure mediated by fetal hla-C2. J Clin Invest (2010) 120(11):4102–10. doi: 10.1172/JCI43998
160. Huhn O, Chazara O, Ivarsson MA, Retière C, Venkatesan TC, Norman PJ, et al. High-resolution genetic and phenotypic analysis of Kir2dl1 alleles and their association with pre-eclampsia. J Immunol (2018) 201(9):2593–601. doi: 10.4049/jimmunol.1800860
161. Xu X, Zhao X, Chen L, Liu M, Hu Z, Ke J, et al. Cd158a /Cd158b nk cell imbalance correlates with hypertension in patients with pre-eclampsia. Am J Reprod Immunol (2022) 87(5):e13532. doi: 10.1111/aji.13532
162. Saito S, Takeda Y, Sakai M, Nakabayahi M, Hayakawa S. The incidence of pre-eclampsia among couples consisting of Japanese women and Caucasian men. J Reprod Immunol (2006) 70(1-2):93–8. doi: 10.1016/j.jri.2005.12.005
163. Long W, Shi Z, Fan S, Liu L, Lu Y, Guo X, et al. Association of maternal kir and fetal hla-c genes with the risk of preeclampsia in the Chinese han population. Placenta (2015) 36(4):433–7. doi: 10.1016/j.placenta.2014.05.008
164. Gentle NL, Loubser S, Paximadis M, Puren A, Tiemessen CT. Killer-cell immunoglobulin-like receptor (Kir) and human leukocyte antigen (Hla) class I genetic diversity in four south African populations. Hum Immunol (2017) 78(7-8):503–9. doi: 10.1016/j.humimm.2017.05.006
165. Nakimuli A, Chazara O, Hiby SE, Farrell L, Tukwasibwe S, Jayaraman J, et al. A kir b centromeric region present in africans but not europeans protects pregnant women from pre-eclampsia. Proc Natl Acad Sci U.S.A. (2015) 112(3):845–50. doi: 10.1073/pnas.1413453112
166. Kennedy PR, Chazara O, Gardner L, Ivarsson MA, Farrell LE, Xiong S, et al. Activating Kir2ds4 is expressed by uterine nk cells and contributes to successful pregnancy. J Immunol (2016) 197(11):4292–300. doi: 10.4049/jimmunol.1601279
167. Nakimuli A, Chazara O, Byamugisha J, Elliott AM, Kaleebu P, Mirembe F, et al. Pregnancy, parturition and preeclampsia in women of African ancestry. Am J Obstet Gynecol (2014) 210(6):510–20. doi: 10.1016/j.ajog.2013.10.879
168. Larsen TG, Hackmon R, Geraghty DE, Hviid TVF. Fetal human leukocyte antigen-c and maternal killer-cell immunoglobulin-like receptors in cases of severe preeclampsia. Placenta (2019) 75:27–33. doi: 10.1016/j.placenta.2018.11.008
169. Lu H-Q, Hu R. The role of immunity in the pathogenesis and development of pre-eclampsia. Scand J Immunol (2019) 90(5):e12756. doi: 10.1111/sji.12756
170. van der Meer A, Lukassen HGM, van Cranenbroek B, Weiss EH, Braat DDM, van Lierop MJ, et al. Soluble hla-G promotes Th1-type cytokine production by cytokine-activated uterine and peripheral natural killer cells. Mol Hum Reprod (2007) 13(2):123–33. doi: 10.1093/molehr/gal100
171. Hara N, Fujii T, Yamashita T, Kozuma S, Okai T, Taketani Y. Altered expression of human leukocyte antigen G (Hla-G) on extravillous trophoblasts in preeclampsia: Immunohistological demonstration with anti-Hla-G specific antibody "87g" and anti-cytokeratin antibody "Cam5.2". Am J Reprod Immunol (1996) 36(6):349–58. doi: 10.1111/j.1600-0897.1996.tb00185.x
172. Goldman-Wohl DS, Ariel I, Greenfield C, Hochner-Celnikier D, Cross J, Fisher S, et al. Lack of human leukocyte antigen-G expression in extravillous trophoblasts is associated with pre-eclampsia. Mol Hum Reprod (2000) 6(1):88–95. doi: 10.1093/molehr/6.1.88
173. Yie S-m, Li L-h, Li Y-m, Librach C. Hla-G protein concentrations in maternal serum and placental tissue are decreased in preeclampsia. Am J Obstet Gynecol (2004) 191(2):525–9. doi: 10.1016/j.ajog.2004.01.033
174. Wedenoja S, Yoshihara M, Teder H, Sariola H, Gissler M, Katayama S, et al. Fetal hla-G mediated immune tolerance and interferon response in preeclampsia. EBioMedicine (2020) 59:102872. doi: 10.1016/j.ebiom.2020.102872
175. Shobu T, Sageshima N, Tokui H, Omura M, Saito K, Nagatsuka Y, et al. The surface expression of hla-f on decidual trophoblasts increases from mid to term gestation. J Reprod Immunol (2006) 72(1-2):18–32. doi: 10.1016/j.jri.2006.02.001
176. Sageshima N, Ishitani A, Omura M, Akasaki M, Umekage H, Katabuchi H, et al. Necrotic feature of the trophoblasts lacking hla-G expression in normal and pre-eclamptic placentas. Am J Reprod Immunol (2003) 49(3):174–82. doi: 10.1034/j.1600-0897.2003.00010.x
177. Melchiorre K, Wormald B, Leslie K, Bhide A, Thilaganathan B. First-trimester uterine artery Doppler indices in term and preterm pre-eclampsia. Ultrasound Obstet Gynecol (2008) 32(2):133–7. doi: 10.1002/uog.5400
178. Prefumo F, Sebire NJ, Thilaganathan B. Decreased endovascular trophoblast invasion in first trimester pregnancies with high-resistance uterine artery Doppler indices. Hum Reprod (2004) 19(1):206–9. doi: 10.1093/humrep/deh037
179. Cavalli RC, Cerdeira AS, Pernicone E, Korkes HA, Burke SD, Rajakumar A, et al. Induced human decidual nk-like cells improve utero-placental perfusion in mice. PloS One (2016) 11(10):e0164353. doi: 10.1371/journal.pone.0164353
180. Fraser R, Whitley GS, Johnstone AP, Host AJ, Sebire NJ, Thilaganathan B, et al. Impaired decidual natural killer cell regulation of vascular remodelling in early human pregnancies with high uterine artery resistance. J Pathol (2012) 228(3):322–32. doi: 10.1002/path.4057
181. Fraser R, Whitley GSJ, Thilaganathan B, Cartwright JE. Decidual natural killer cells regulate vessel stability: Implications for impaired spiral artery remodelling. J Reprod Immunol (2015) 110:54–60. doi: 10.1016/j.jri.2015.04.003
182. Wallace AE, Whitley GS, Thilaganathan B, Cartwright JE. Decidual natural killer cell receptor expression is altered in pregnancies with impaired vascular remodeling and a higher risk of pre-eclampsia. J Leukoc Biol (2015) 97(1):79–86. doi: 10.1189/jlb.2A0614-282R
183. Tian Y, Yang X. A review of roles of uterine artery Doppler in pregnancy complications. Front Med (Lausanne) (2022) 9:813343. doi: 10.3389/fmed.2022.813343
184. Cottrell JN, Amaral LM, Harmon A, Cornelius DC, Cunningham MW, Vaka VR, et al. Interleukin-4 supplementation improves the pathophysiology of hypertension in response to placental ischemia in rupp rats. Am J Physiol Regul Integr Comp Physiol (2019) 316(2):R165–R71. doi: 10.1152/ajpregu.00167.2018
185. Elfarra JT, Cottrell JN, Cornelius DC, Cunningham MW, Faulkner JL, Ibrahim T, et al. 17-hydroxyprogesterone caproate improves T cells and nk cells in response to placental ischemia; new mechanisms of action for an old drug. Pregnancy Hypertens (2020) 19:226–32. doi: 10.1016/j.preghy.2019.11.005
186. Shields CA, McCalmon M, Ibrahim T, White DL, Williams JM, LaMarca B, et al. Placental ischemia-stimulated T-helper 17 cells induce preeclampsia-associated cytolytic natural killer cells during pregnancy. Am J Physiol Regul Integr Comp Physiol (2018) 315(2):R336–R43. doi: 10.1152/ajpregu.00061.2018
187. Schumacher A, Costa S-D, Zenclussen AC. Endocrine factors modulating immune responses in pregnancy. Front Immunol (2014) 5:196. doi: 10.3389/fimmu.2014.00196
188. Borzychowski AM, Chantakru S, Minhas K, Paffaro VA, Yamada AT, He H, et al. Functional analysis of murine uterine natural killer cells genetically devoid of oestrogen receptors. Placenta (2003) 24(4):403–11. doi: 10.1053/plac.2002.0924
189. van den Heuvel MJ, Xie X, Tayade C, Peralta C, Fang Y, Leonard S, et al. A review of trafficking and activation of uterine natural killer cells. Am J Reprod Immunol (2005) 54(6):322–31. doi: 10.1111/j.1600-0897.2005.00336.x
190. Meher S, Duley L. Progesterone for preventing pre-eclampsia and its complications. Cochrane Database Syst Rev (2006) 4):CD006175. doi: 10.1002/14651858.CD006175
191. Blackwell SC, Gyamfi-Bannerman C, Biggio JR, Chauhan SP, Hughes BL, Louis JM, et al. 17-ohpc to prevent recurrent preterm birth in singleton gestations (Prolong study): A multicenter, international, randomized double-blind trial. Am J Perinatol (2020) 37(2):127–36. doi: 10.1055/s-0039-3400227
192. Yang X, Meng T. Is there a role of intravenous immunoglobulin in immunologic recurrent pregnancy loss? J Immunol Res (2020) 2020:6672865. doi: 10.1155/2020/6672865
193. Kwak JY, Kwak FM, Ainbinder SW, Ruiz AM, Beer AE. Elevated peripheral blood natural killer cells are effectively downregulated by immunoglobulin G infusion in women with recurrent spontaneous abortions. Am J Reprod Immunol (1996) 35(4):363–9. doi: 10.1111/j.1600-0897.1996.tb00495.x
194. Shimada S, Takeda M, Nishihira J, Kaneuchi M, Sakuragi N, Minakami H, et al. A high dose of intravenous immunoglobulin increases Cd94 expression on natural killer cells in women with recurrent spontaneous abortion. Am J Reprod Immunol (2009) 62(5):301–7. doi: 10.1111/j.1600-0897.2009.00739.x
195. van den Heuvel MJ, Peralta CG, Hatta K, Han VK, Clark DA. Decline in number of elevated blood Cd3(+) Cd56(+) nkt cells in response to intravenous immunoglobulin treatment correlates with successful pregnancy. Am J Reprod Immunol (2007) 58(5):447–59. doi: 10.1111/j.1600-0897.2007.00529.x
196. Heilmann L, Schorsch M, Hahn T. Cd3-Cd56+Cd16+ natural killer cells and improvement of pregnancy outcome in Ivf/Icsi failure after additional ivig-treatment. Am J Reprod Immunol (2010) 63(3):263–5. doi: 10.1111/j.1600-0897.2009.00790.x
197. Dakhly DMR, Bayoumi YA, Sharkawy M, Gad Allah SH, Hassan MA, Gouda HM, et al. Intralipid supplementation in women with recurrent spontaneous abortion and elevated levels of natural killer cells. Int J Gynaecol Obstet (2016) 135(3):324–7. doi: 10.1016/j.ijgo.2016.06.026
198. Shreeve N, Sadek K. Intralipid therapy for recurrent implantation failure: New hope or false dawn? J Reprod Immunol (2012) 93(1):38–40. doi: 10.1016/j.jri.2011.11.003
199. Granato D, Blum S, Rössle C, Le Boucher J, Malnoë A, Dutot G. Effects of parenteral lipid emulsions with different fatty acid composition on immune cell functions in vitro. JPEN J Parenter Enteral Nutr (2000) 24(2):113–8. doi: 10.1177/0148607100024002113
200. Roussev RG, Acacio B, Ng SC, Coulam CB. Duration of intralipid's suppressive effect on nk cell's functional activity. Am J Reprod Immunol (2008) 60(3):258–63. doi: 10.1111/j.1600-0897.2008.00621.x
201. Roussev RG, Ng SC, Coulam CB. Natural killer cell functional activity suppression by intravenous immunoglobulin, intralipid and soluble human leukocyte antigen-G. Am J Reprod Immunol (2007) 57(4):262–9. doi: 10.1111/j.1600-0897.2007.00473.x
202. Sohlberg E, Saghafian-Hedengren S, Bachmayer N, Hamad RR, Bremme K, Holmlund U. Pre-eclampsia affects cord blood nk cell expression of activation receptors and serum cytokine levels but not cb monocyte characteristics. Am J Reprod Immunol (2014) 71(2):178–88. doi: 10.1111/aji.12169
203. Loewendorf AI, Nguyen TA, Yesayan MN, Kahn DA. Preeclampsia is characterized by fetal nk cell activation and a reduction in regulatory T cells. Am J Reprod Immunol (2015) 74(3):258–67. doi: 10.1111/aji.12393
204. Hoffman MC, Rumer KK, Kramer A, Lynch AM, Winn VD. Maternal and fetal alternative complement pathway activation in early severe preeclampsia. Am J Reprod Immunol (2014) 71(1):55–60. doi: 10.1111/aji.12162
205. Bujold E, Chaiworapongsa T, Romero R, Gervasi MT, Espinoza J, Goncalves LF, et al. Neonates born to pre-eclamptic mothers have a higher percentage of natural killer cells (Cd3-/Cd56+16+) in umbilical cord blood than those without pre-eclampsia. J Matern Fetal Neona (2003) 14(5):305–12. doi: 10.1080/jmf.14.5.305.312
206. Darmochwal-Kolarz D, Leszczynska-Gorzelak B, Rolinski J, Oleszczuk J. Pre-eclampsia affects the immunophenotype of neonates. Immunol Lett (2001) 77(2):67–71. doi: 10.1016/s0165-2478(01)00205-x
207. Brune T, Koch HG, Plümpe U, Coenen-Worch V, Harms E, Louwen F. Effect of pathological perinatal conditions on the maternofetal transfer of mononuclear cells. Fetal Diagn Ther (2002) 17(2):110–4. doi: 10.1159/000048019
208. Holzgreve W, Ghezzi F, Di Naro E, Gänshirt D, Maymon E, Hahn S. Disturbed feto-maternal cell traffic in preeclampsia. Obstet Gynecol (1998) 91(5 Pt 1):669–72. doi: 10.1016/s0029-7844(98)00068-4
209. Al-Mufti R, Hambley H, Albaiges G, Lees C, Nicolaides KH. Increased fetal erythroblasts in women who subsequently develop pre-eclampsia. Hum Reprod (2000) 15(7):1624–8. doi: 10.1093/humrep/15.7.1624
210. Jansen MW, Korver-Hakkennes K, van Leenen D, Visser W, in 't Veld PA, de Groot CJ, et al. Significantly higher number of fetal cells in the maternal circulation of women with pre-eclampsia. Prenat Diagn (2001) 21(12):1022–6. doi: 10.1002/pd.176
Keywords: preeclampsia, NK cells, pregnancy, immune, placenta
Citation: Wei X and Yang X (2023) The central role of natural killer cells in preeclampsia. Front. Immunol. 14:1009867. doi: 10.3389/fimmu.2023.1009867
Received: 02 August 2022; Accepted: 31 January 2023;
Published: 14 February 2023.
Edited by:
Cristina Bottino, University of Genoa, ItalyCopyright © 2023 Wei and Yang. This is an open-access article distributed under the terms of the Creative Commons Attribution License (CC BY). The use, distribution or reproduction in other forums is permitted, provided the original author(s) and the copyright owner(s) are credited and that the original publication in this journal is cited, in accordance with accepted academic practice. No use, distribution or reproduction is permitted which does not comply with these terms.
*Correspondence: Xiuhua Yang, eWFuZ3hpdWh1YWppbmdAaG90bWFpbC5jb20=
Disclaimer: All claims expressed in this article are solely those of the authors and do not necessarily represent those of their affiliated organizations, or those of the publisher, the editors and the reviewers. Any product that may be evaluated in this article or claim that may be made by its manufacturer is not guaranteed or endorsed by the publisher.
Research integrity at Frontiers
Learn more about the work of our research integrity team to safeguard the quality of each article we publish.