- 1Barcelona Institute for Global Health, Hospital Clínic - Universitat de Barcelona, Barcelona, Spain
- 2Barcelona Institute for Global Health, Spanish Consortium for Research on Epidemiology and Public Health (CIBERESP), Center for Genomic Regulation (CRG), Barcelona Institute of Science and Technology (BIST), Barcelona, Spain
- 3Barcelona Center for Maternal-Fetal and Neonatal Medicine (BCNatal), Hospital Sant Joan de Déu and Hospital Clínic, Institut de Recerca Sant Joan de Déu (IR-SJD), Barcelona, Spain
- 4Biomolecular screening and Protein Technologies Unit, Centre for Genomic Regulation (CRG), The Barcelona Institute of Science and Technology, Barcelona, Spain
- 5Pathogenesis and treatment of autoimmunity department, Institut D'Investigacions Biomèdiques August Pi i Sunyer (IDIBAPS), Barcelona, Spain
- 6Julia McFarlane Diabetes Research Centre (JMDRC), and Department of Microbiology, Immunology and Infectious Diseases, Snyder Institute for Chronic Diseases, Cumming School of Medicine, University of Calgary, Calgary, AB, Canada
- 7Barcelona Institute for Global Health, CIBER de Enfermedades Infecciosas (CIBERINFEC), Barcelona, Spain
SARS-CoV-2 infected pregnant women are at increased risk of severe COVID-19 than non-pregnant women and have a higher risk of adverse pregnancy outcomes like intrauterine/fetal distress and preterm birth. However, little is known about the impact of SARS-CoV-2 infection on maternal and neonatal immunological profiles. In this study, we investigated the inflammatory and humoral responses to SARS-CoV-2 in maternal and cord blood paired samples. Thirty-six pregnant women were recruited at delivery at Hospital Sant Joan de Déu, Barcelona, Spain, between April-August 2020, before having COVID-19 available vaccines. Maternal and pregnancy variables, as well as perinatal outcomes, were recorded in questionnaires. Nasopharyngeal swabs and maternal and cord blood samples were collected for SARS-CoV-2 detection by rRT-PCR and serology, respectively. We measured IgM, IgG and IgA levels to 6 SARS-CoV-2 antigens (spike [S], S1, S2, receptor-binding domain [RBD], nucleocapsid [N] full-length and C-terminus), IgG to N from 4 human coronaviruses (OC43, HKU1, 229E and NL63), and the concentrations of 30 cytokines, chemokines and growth factors by Luminex. Mothers were classified as infected or non-infected based on the rRT-PCR and serology results. Sixty-four % of pregnant women were infected with SARS-CoV-2 (positive by rRT-PCR during the third trimester and/or serology just after delivery). None of the newborns tested positive for rRT-PCR. SARS-CoV-2 infected mothers had increased levels of virus-specific antibodies and several cytokines. Those with symptoms had higher cytokine levels. IFN-α was increased in cord blood from infected mothers, and in cord blood of symptomatic mothers, EGF, FGF, IL-17 and IL-15 were increased, whereas RANTES was decreased. Maternal IgG and cytokine levels showed positive correlations with their counterparts in cord blood. rRT-PCR positive mothers showed lower transfer of SARS-CoV-2-specific IgGs, with a stronger effect when infection was closer to delivery. SARS-CoV-2 infected mothers carrying a male fetus had higher antibody levels and higher EGF, IL-15 and IL-7 concentrations. Our results show that SARS-CoV-2 infection during the third trimester of pregnancy induces a robust antibody and cytokine response at delivery and causes a significant reduction of the SARS-CoV-2-specific IgGs transplacental transfer, with a stronger negative effect when the infection is closer to delivery.
Introduction
Coronavirus 2019 (COVID-19) pandemic caused by the severe acute respiratory syndrome coronavirus 2 (SARS-CoV-2), has resulted in more than six million deaths and has infected over 500 million people as of July 19, 2022 (1). The most severe outcomes of COVID-19 have been documented in geriatric individuals and pregnant women with chronic diseases, including hypertension, diabetes, and cardiopulmonary problems, or with some other respiratory viral infections (2).
The immune status of pregnant women adapts to ensure tolerance to the fetus by changing the cellular composition and the functions of immune cells. T cell-mediated immunity and humoral responses are suppressed particularly during the third trimester (3), production of IL-4 and IL-10 are increased while IL-2 and IFN-γ are reduced in peripheral blood mononuclear cells (4, 5). As a consequence, pregnant women are at increased risk of morbidity and mortality from respiratory viral infections (6–9). Several studies show that pregnant women with COVID-19 are more likely to be hospitalized and have increased rates of ICU admissions and mechanical ventilation compared with non-pregnant women with COVID-19, resulting in a higher risk of mortality (10–15).
The maternal inflammatory response induced by SARS-CoV-2 infection may also have deleterious effects on the offspring. Although vertical transmission has been hardly observed in SARS-CoV-2 to date (16), with a rate of around 3% (17), pregnant women with COVID-19 are at increased risk of adverse pregnancy outcomes like intrauterine/fetal distress and preterm birth (11, 18). The pro-inflammatory cytokines induced during infection may enter the amniotic cavity and interfere with normal fetal development (19–21) causing short- and long-term damage (22–24) such as miscarriage, restricted fetal growth, or still-birth (25) and neurodevelopmental affection as seen in other viral infections ranging from autism spectrum disorder, attention deficit hyperactivity disorder, and cognitive dysfunction, to anxiety, depression, and schizophrenia (26). Recent studies evidence that SARS-CoV-2 infection during pregnancy results in offspring neurodevelopmental morbidity (26). Maternal infection may also alter the ability of antibodies to transfer across the placenta as has been shown for other infections like HIV (27), malaria (28), dengue (29) or Zika (30) and recently in COVID-19 (31–34). Vaccination of pregnant women with an mRNA COVID-19 vaccine resulted in a significantly greater antibody persistence in infants than infection at 20 to 32 weeks gestation. At 6 months, 57% of infants born to vaccinated mothers had detectable antibodies compared with 8% of infants born to infected mothers (35).
However, our knowledge is limited regarding the immune response in pregnant women infected by SARS-CoV-2 and the immunopathological mechanisms in maternal and neonatal outcomes. Studying the maternal immune response after infection and the in-utero antibody transfer can help understand neonatal vulnerability to infection and protection.
In this study, we investigated the inflammatory and humoral responses to SARS-CoV-2 in maternal and cord blood samples collected from pregnant women who had tested positive during the third trimester of pregnancy, and their neonates, by measuring antibody levels against SARS-CoV-2 antigens, their IgG transplacental transfer, and cytokine concentrations.
Materials and methods
Study design, participants and sample collection
This is a prospective case-control study in which pregnant women were recruited at delivery at Hospital Sant Joan de Déu (HSJD), Barcelona (Spain) between April and August 2020, before having COVID-19 available vaccines. Women approached were those visited at the hospital, or who arrived in labor or with a programmed induction of labor. To capture the maximum number of SARS-CoV-2 infected mothers inclusion criteria were: i) women with a positive SARS-CoV-2 rRT-PCR; and/or ii) symptoms consistent with SARS-CoV-2 infection (high temperature, fatigue, muscular pain, diarrhea, taste and smell loss, cough, difficulty breathing, pneumonia); and/or iii) direct contact (more than 15 min and less than 2 m distance) with someone diagnosed with COVID-19 by rRT-PCR during the third trimester. Pregnant women who did not meet any of the inclusion criteria were recruited for the control group. Women with multiple gestations, aged <18 or >45 years, with a fetus affected by abnormal karyotype, structural abnormalities, congenital infections, or who did not communicate in Catalan, Spanish or English, were excluded from the study. Thirty-six mother-newborn pairs were enrolled in the study. The study protocol was approved by the Ethics Committee for Clinical Research of the HSJD with the approval code number PIC 48-20, and informed consent was obtained from each participant.
Maternal (age, parity, ethnic group and pathologies) and pregnancy (high blood pressure, gestational diabetes, fetal growth retardation, preterm delivery or other pregnancy complications) variables as well as perinatal outcomes (type of delivery or any newborn complications), were recorded in a questionnaire or obtained from medical records.
Maternal and neonatal nasopharyngeal swabs, and maternal peripheral and cord blood samples, were collected just after delivery. Nasopharyngeal swabs were used for SARS-CoV-2 detection by rRT-PCR. Blood samples were processed to isolate serum that was stored at -80˚C until the analysis of antibody levels and cytokines. Data from the rRT-PCR and serology tests performed with the samples collected just after delivery was used to reallocate mothers to the case (infected) or control (not infected) groups, for the subsequent analyses.
Antibody luminex assays
IgM, IgG and IgA antibodies to six SARS-CoV-2 antigens were measured in mother and cord serum samples by Luminex as previously described (36). SARS-CoV-2 antigens included in the panel were: the spike full-length protein (S), its subunits S1 and S2, the receptor-binding domain (RBD), the nucleocapsid full-length protein (N FL) and its C-terminus region (N CT) (37). The full-length N proteins from the four HuCoVs OC43, HKU1, 229E and NL63 (37) were also included in the IgG panel. A positive control curve and four blanks were included in each assay plate for QA/QC purposes, and 129 pre-pandemic samples as negative controls to estimate the seropositivity cutoffs. To quantify IgM, test samples and controls were pre-treated with anti-human IgG (Gullsorb) to avoid IgG interferences. Paired mother-cord samples were tested in the same assay plate. At least 50 microspheres per analyte/well were acquired, and the median fluorescence intensity (MFI) was reported for each analyte. Assay positivity cutoffs specific for each isotype and antigen were calculated as 10 to the mean plus 3 standard deviations (SD) of log10-transformed MFI of the 129 pre-pandemic controls. A more detailed description of the antigens and the assay are included in the Supplementary Material.
Cytokine luminex assay
The Cytokine Human Magnetic 30-Plex Panel from Invitrogen™ was used to measure the concentrations of 30 analytes in serum from mothers and cord blood samples. Samples were tested using half of the reagents following a modification of the manufacturer’s protocol (38–40). Paired mother-cord samples were tested in the same assay plate. Each plate included 16 serial dilutions (2-fold) of a standard curve, two blank controls and three positive controls of high, medium and low concentrations for QA/QC purposes. Samples were acquired on a Luminex® 100/200. The interpolation of concentrations and imputation of missing data was done using the R package drLumi (41). A more detailed description of the analytes and the assay are included in the Supplementary Material.
Groups definitions and statistical analysis
SARS-CoV-2 infected mother groups were reallocated using serological data from the antibody Luminex assay. Thus, mothers were classified as infected when having a SARS-CoV-2 positive rRT-PCR during the third trimester and/or being seropositive just after delivery, and non-infected when not having reported any SARS-CoV-2 rRT-PCR positive during the third trimester and being seronegative just after delivery. Pregnant women were considered seropositive if positive for one or more isotype-antigen pairs. Time since the infection was estimated as the time since positive rRT-PCR or symptoms onset to delivery. This variable was not available for three mothers who were seropositive just after delivery but did not report any positive rRT-PCR or symptoms.
A comparison of clinical and demographic characteristics between infected and non-infected mothers was performed by the Wilcoxon-rank-sum-test for age (continuous) and by Fisher’s exact test for the rest of the variables (categorical). Antibody and cytokine data were log10-transformed to perform the statistical analysis and non-parametric tests were used. The IgG transplacental transfer was calculated as the log10-transformed ratio of antibody levels (MFI) in cord vs. mother in paired samples. Comparisons of antibody and cytokine levels between the study groups were performed by the Wilcoxon-rank-sum-test. Correlations between analytes were assessed with Spearman’s rank correlation coefficient ρ (rho) and p-values were computed via the asymptotic t approximation. Univariable linear regression models were performed to assess the associations between IgG transplacental transfer (ratio MFI Cord/MFI Mother) as the outcome variable and SARS-CoV-2 infection, infection during the third trimester or just after delivery, time since infection, gestational age, and birth weight as predictor variables. Nominal p-values (not adjusted for multiple testing due to small sample size) of <0.05 were considered statistically significant. All data processing and statistical analyses were performed using the R software version 4.1.1.
Results
Pregnancy outcomes in SARS-CoV-2 infected mothers
Our study included 36 pregnant women, 23 SARS-CoV-2 infected and 13 non-infected. No differences were observed between these two groups regarding parity, maternal age at delivery, ethnicity or pathologies (Table 1). Among the non-infected women, two reported COVID-19 compatible symptoms probably from another viral infection. Although having symptoms consistent with COVID-19 was an inclusion criterion for those infected or possibly infected with SARS-CoV-2, we included these two cases in the non-infected group because they were neither rRT-PCR positive nor seropositive. Thirty-nine % (9/23) of the infected women showed pregnancy complications vs. 15% (2/13) of the non-infected, although differences were not statistically significant (p=0.086). Pregnancy complications were of different etiologies without a predominant one (Table 1). Twenty-two % (5/23) of the newborns from infected women had complications vs. none of the 13 born from non-infected mothers, although differences were not statistically significant (p=0.089). Newborn complications consisted of three premature, one with hyperbilirubinemia and one with lymphopenia. None of the newborns tested positive for SARS-CoV-2 rRT-PCR just after delivery, and neither showed detectable levels of IgM or IgA in cord blood, which are indicative of fetal infection (data not shown).
Antibody response in SARS-CoV-2 infected mothers
From the infected group (N=23), 11 were rRT-PCR positive in the third trimester and seropositive just after delivery; eight did not report any rRT-PCR positive during pregnancy but were seropositive just after delivery (5 reported symptoms during the third trimester and 3 were asymptomatic), and 4 were rRT-PCR positive but seronegative just after delivery, denoting recent infections or deficiency in producing antibodies. Anti-SARS-CoV-2 antibody levels did not differ between infected rRT-PCR positive (N=15) and negative (N=8) mothers during the third trimester (Figure 1). Infected mothers showed higher antibody levels compared to non-infected mothers (p≤0.01) regardless of the rRT-PCR status, except for IgM against N antigen (Figure 1). Among the infected mothers, those with symptoms showed an overall trend of higher antibody levels compared to asymptomatic mothers although not statistically significant except for IgA to RBD (p=0.049) (Figure S1).
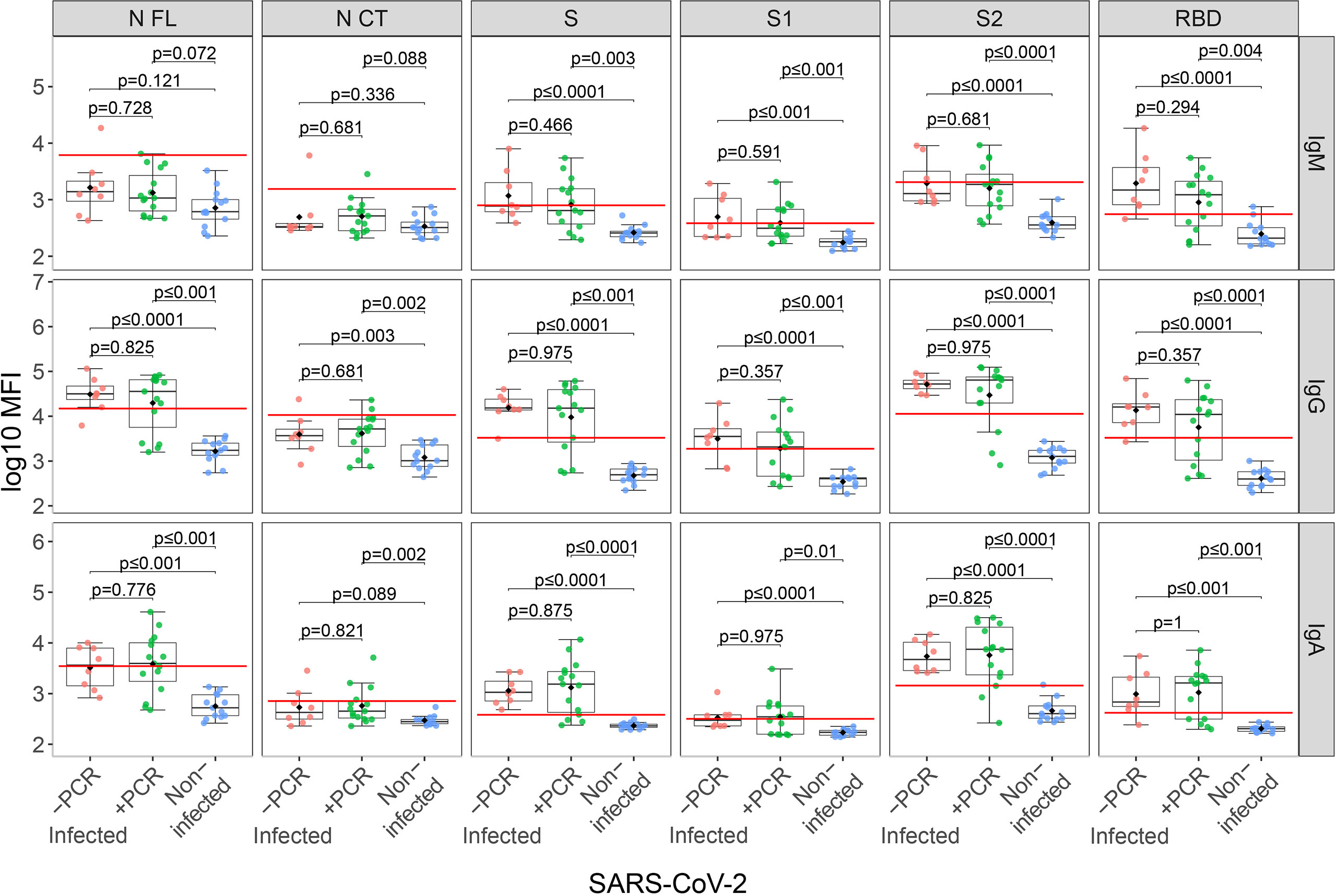
Figure 1 Anti-SARS-CoV-2 antibody levels in mothers stratified by infection and rRT-PCR (PCR) results. Comparison of anti-SARS-CoV-2 IgM, IgG and IgA serum levels (log10 median fluorescence intensity, MFI) between infected mothers who tested COVID-19 positive by rRT-PCR during the third trimester (+PCR, N=15, in green), those that tested negative (-PCR, N=8, in red) and non-infected mothers (N=13, in blue). The boxplots represent the median (bold line), the mean (black diamond), the 1st and 3rd quartiles (box) and the largest and smallest values within 1.5 times the inter-quartile range (whiskers). Groups were compared by the Wilcoxon-rank-sum-test. The red line indicates the seropositivity cutoff calculated as 10 to the mean plus 3 standard deviations (SD) of log10-transformed MFI of 129 pre-pandemic controls. Antigens: nucleocapsid full-length (N FL) and C-terminus (N CT), spike full-length (S), S1 and S2 subunits, and receptor-binding domain (RBD).
Effect of time since infection on SARS-CoV-2 antibody levels
We evaluated the association of antibody levels with time since infection in paired infected mother-cord samples. Anti-SARS-CoV-2 IgG levels in cord blood showed moderate positive associations with time since infection (ρ=0.3-0.54) for all antigens, being lower when the infection was closer to delivery, as expected, and the associations were statistically significant for S, S1 and RBD (Figure 2). However, only IgG levels to S1 and RBD showed moderate positive associations with time since infection in maternal samples (ρ=0.3) and were not statistically significant (Figure 2).
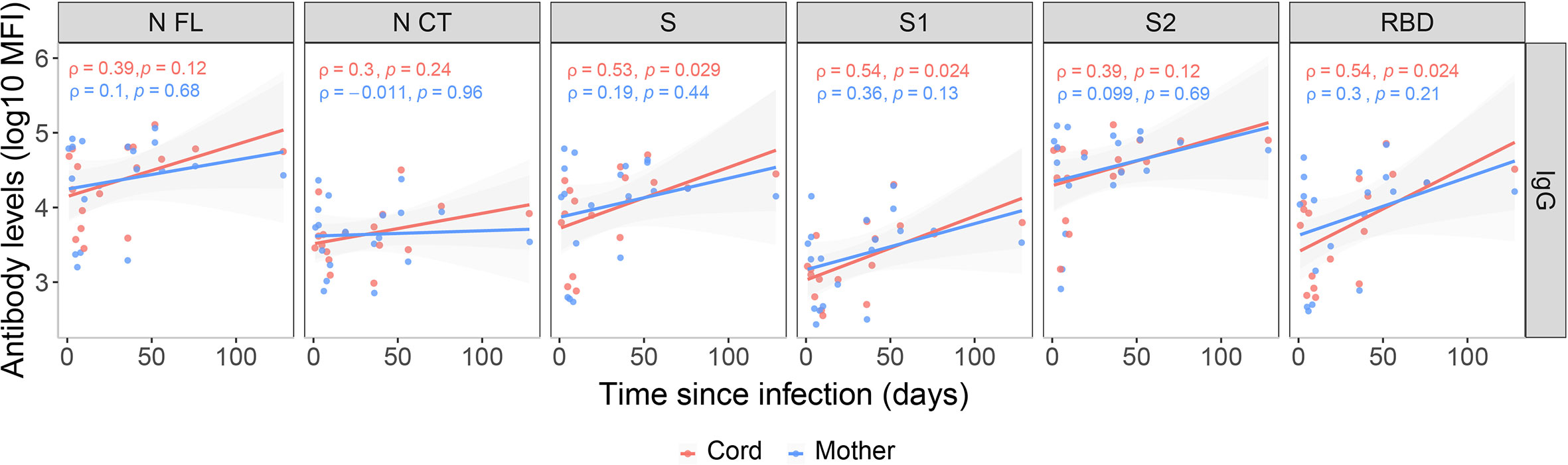
Figure 2 Correlations between anti-SARS-CoV-2 IgG levels and time since infection. Correlations of anti-SARS-CoV-2 IgG levels (log10 median fluorescence intensity, MFI) in infected mothers (blue) and cord blood (red) with time since infection (days since positive rRT-PCR or symptoms onset), represented as a linear model with standard error as confidence interval (shaded areas) and assessed by the Spearman test, showing the rho (ρ) and p-values. Only data from SARS-CoV-2 infected mothers and their newborns are included (N=20). Antigens: nucleocapsid full-length (N FL) and C-terminus (N CT), spike full-length (S), S1 and S2 subunits, and receptor-binding domain (RBD).
IgG transplacental transfer in SARS-CoV-2 infected mothers
Among infected mothers, antigen-specific IgG levels in serum showed moderate to strong positive correlations with levels in cord blood (ρ=0.54-0.8, p<0.05, Figure 3A). Contrary to what was observed in mother samples, IgG levels to S antigens in cord blood showed significant differences depending on the the maternal rRT-PCR status during the third trimester (p<0.05), being higher in the infected but rRT-PCR negative (Figure 3B), suggesting a negative effect of infection during the third trimester in the IgG transfer. To confirm this observation, we assessed the effect of the SARS-CoV-2 infection on the IgG transplacental transfer by linear regression models in paired seropositive infected mothers and cord blood. The rRT-PCR positive mothers just after the delivery or during the third trimester transferred fewer anti-SARS-CoV-2 IgGs to cord blood compared to rRT-PCR negative, except for N FL antigen when tested positive during the third trimester (Figures 4A and S2A, p<0.01). Then, we investigated if this IgG transplacental transfer reduction was extensible to HuCoVs antigens by linear regression models in all study population. Being infected with SARS-CoV-2 reduced the transference of IgG to 229E N antigen (p<0.05) and having a positive rRT-PCR, either during the third trimester or just after delivery, to OC43 N antigen (p<0.05) (Figures 4B and S2B). This suggests a most noticeable negative effect for anti-SARS-CoV-2 antibodies. Gestational age and birth weight, two variables strongly associated with antibody transfer, did not affect the SARS-CoV-2 IgG transplacental transfer in seropositive infected mothers (Figure S3).
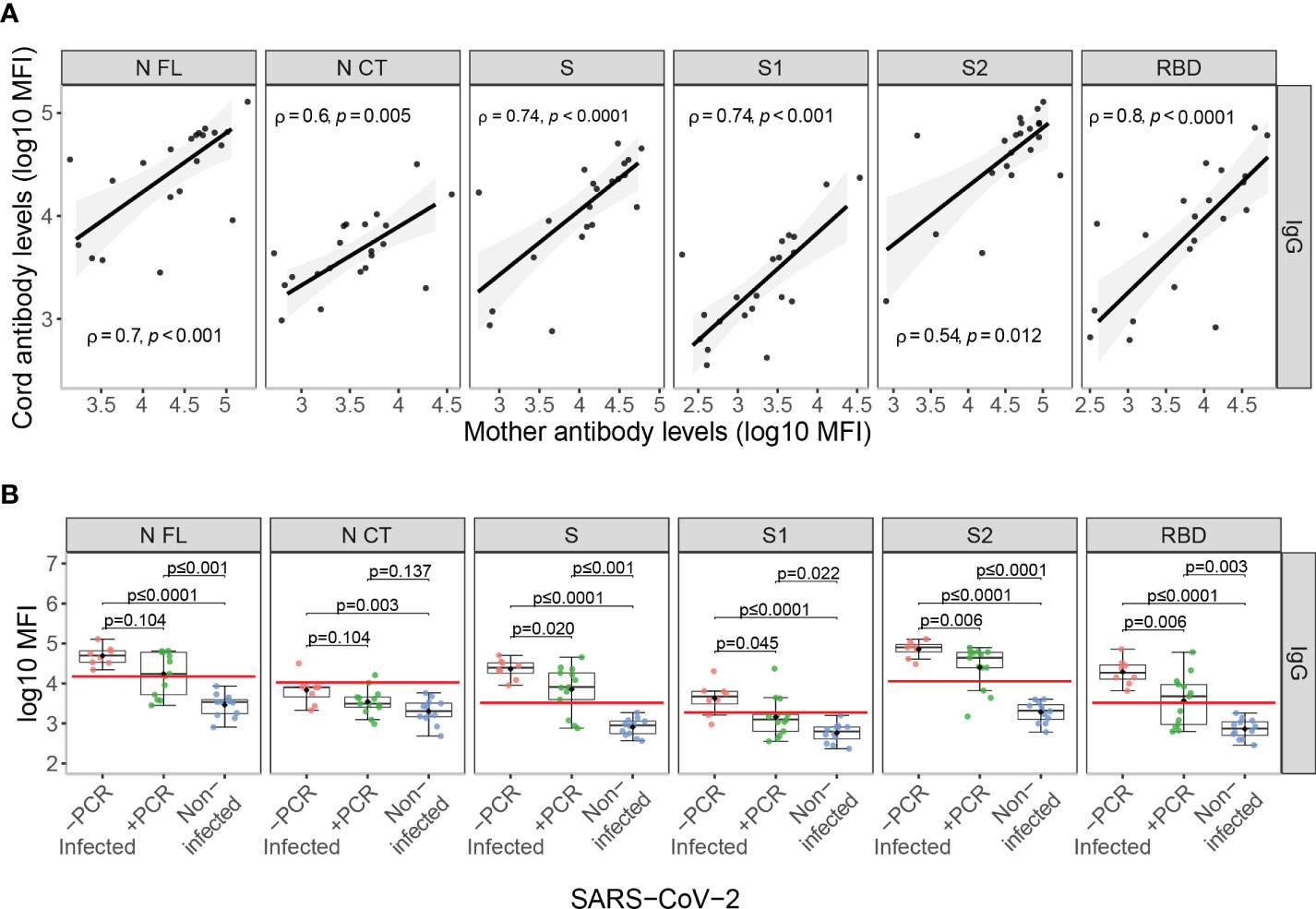
Figure 3 Anti-SARS-CoV-2 antibody levels in cord blood. (A) Correlations of anti-SARS-CoV-2 IgG levels (log10 median fluorescence intensity, MFI) in paired infected mothers and cord blood samples represented as a linear model with standard error as confidence interval (shaded areas) and assessed by the Spearman test, showing the rho (ρ) and p-values. (B) Comparison of anti-SARS-CoV-2 IgG cord blood levels (log10 MFI) between infected mothers who tested COVID-19 rRT-PCR positive (+PCR, N=13, in green) during the third trimester, those that tested rRT-PCR negative (-PCR, N=8, in red) and from non-infected mothers (N=12, in blue). The boxplots represent the median (bold line), the mean (black diamond), the 1st and 3rd quartiles (box) and the largest and smallest values within 1.5 times the inter-quartile range (whiskers). Groups were compared by the Wilcoxon-rank-sum-test. The red line indicates the seropositivity cutoff calculated as 10 to the mean plus 3 standard deviations (SD) of log10-transformed MFI of 129 pre-pandemic controls. Antigens: nucleocapsid full-length (N FL) and C-terminus (N CT), spike full-length (S), S1 and S2 subunits, and receptor-binding domain (RBD).
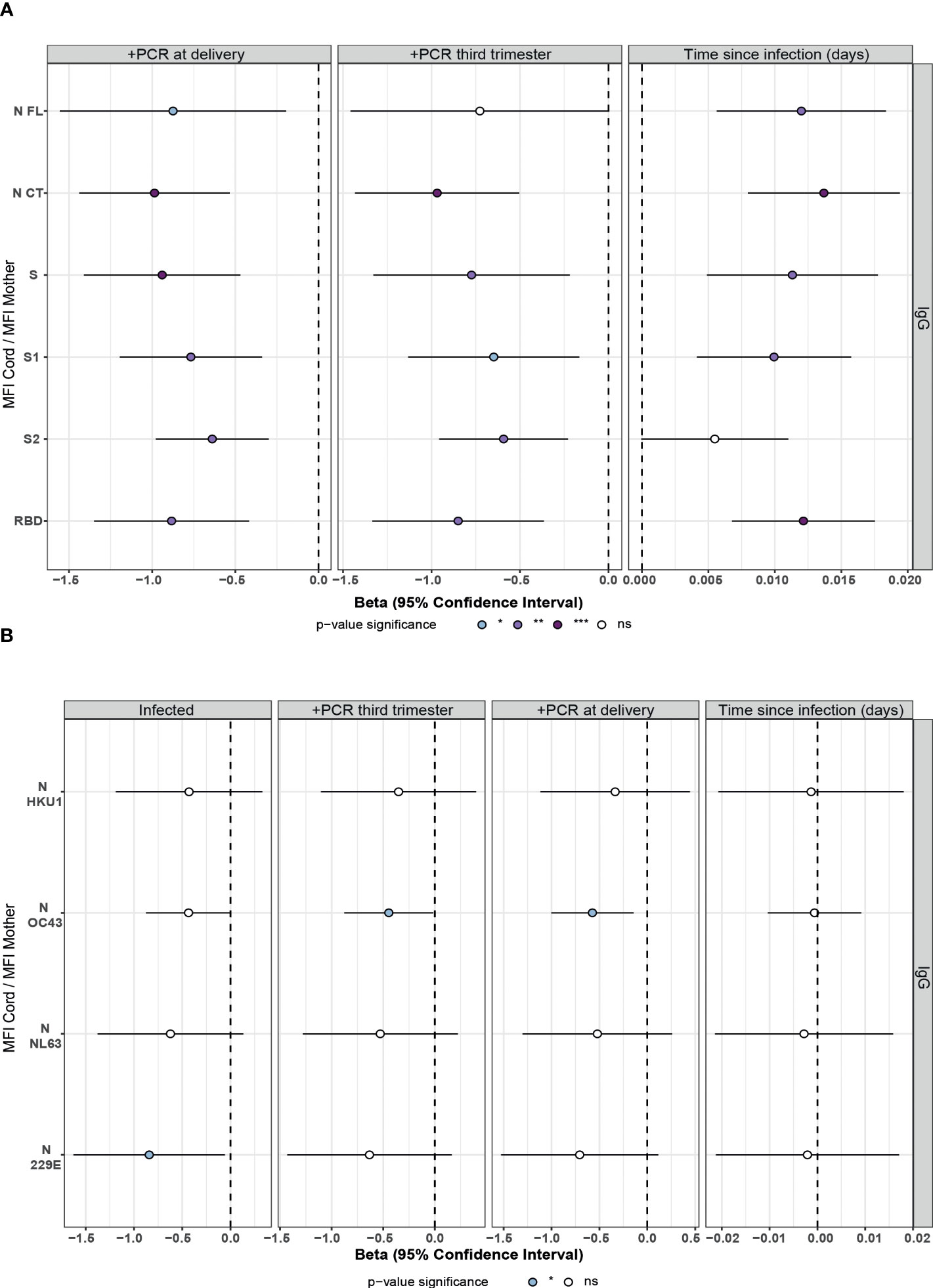
Figure 4 Association of SARS-CoV-2 infection and time since infection on IgG transfer from mother to the newborn in univariable linear regression models. Forest plots show the effect of SARS-CoV-2 infection, positive rRT-PCR (+PCR) during the third trimester or at delivery, and the time since infection (days since positive rRT-PCR or symptoms onset) on IgG transfer from mother to the newborn (MFI Cord/MFI Mother) for (A) SARS-CoV-2 antigens in seropositive paired infected mothers and cord blood and (B) HuCoVs N proteins in all study population. Univariable linear regression models were fitted to calculate the betas (dots) and 95% confidence intervals (CI) (lines). The color of the dots represents the p-value significance, where *p-value ≤ 0.05, **p-value ≤ 0.01 and ***p-value ≤ 0.001, ns, not significant. Antigens: nucleocapsid full-length (N FL) and C-terminus (N CT), spike full-length (S), S1 and S2 subunits, and receptor-binding domain (RBD).
According to the lower anti-SARS-CoV-2 IgG levels observed in cord blood in recent compared to past infections (Figure 2), there was a positive effect of time since infection on anti-SARS-CoV-2 IgG transplacental transfer (Figures 4A and S4). This observation together with the lack of correlation of maternal antibody levels with time since infection, confirms the impact of SARS-CoV-2 infection on IgG transplacental transfer and suggests a stronger negative effect when the infection is closer to delivery. Time since infection did not affect the HuCoVs IgG transference (Figure 4B).
Fetus sex effect on SARS-CoV-2 antibody response
SARS-CoV-2 infected mothers with a male fetus had higher SARS-CoV-2 antibody levels in serum compared to those with a female fetus (Figure 5A), specifically IgM against S, S1 and RBD (p<0.05); IgA against N antigens and S1 (p<0.05); and IgG to all antigens (p<0.05). However, no differences were found in cord blood samples (data not shown). Also, no differences were found by fetus sex in antibody levels against the N antigen of the HuCoVs, neither in the mother nor cord blood (data not shown). Interestingly, there was a trend of lower anti-SARS-CoV-2 IgG transplacental transfer in mothers carrying a male fetus compared to mothers carrying a female (Figure 5B), but not statistically significant. The same trend, not statistically significant, by fetus sex was observed for the transfer of IgG to N of the HuCoVs in SARS-CoV-2 infected mothers (Figure S5).
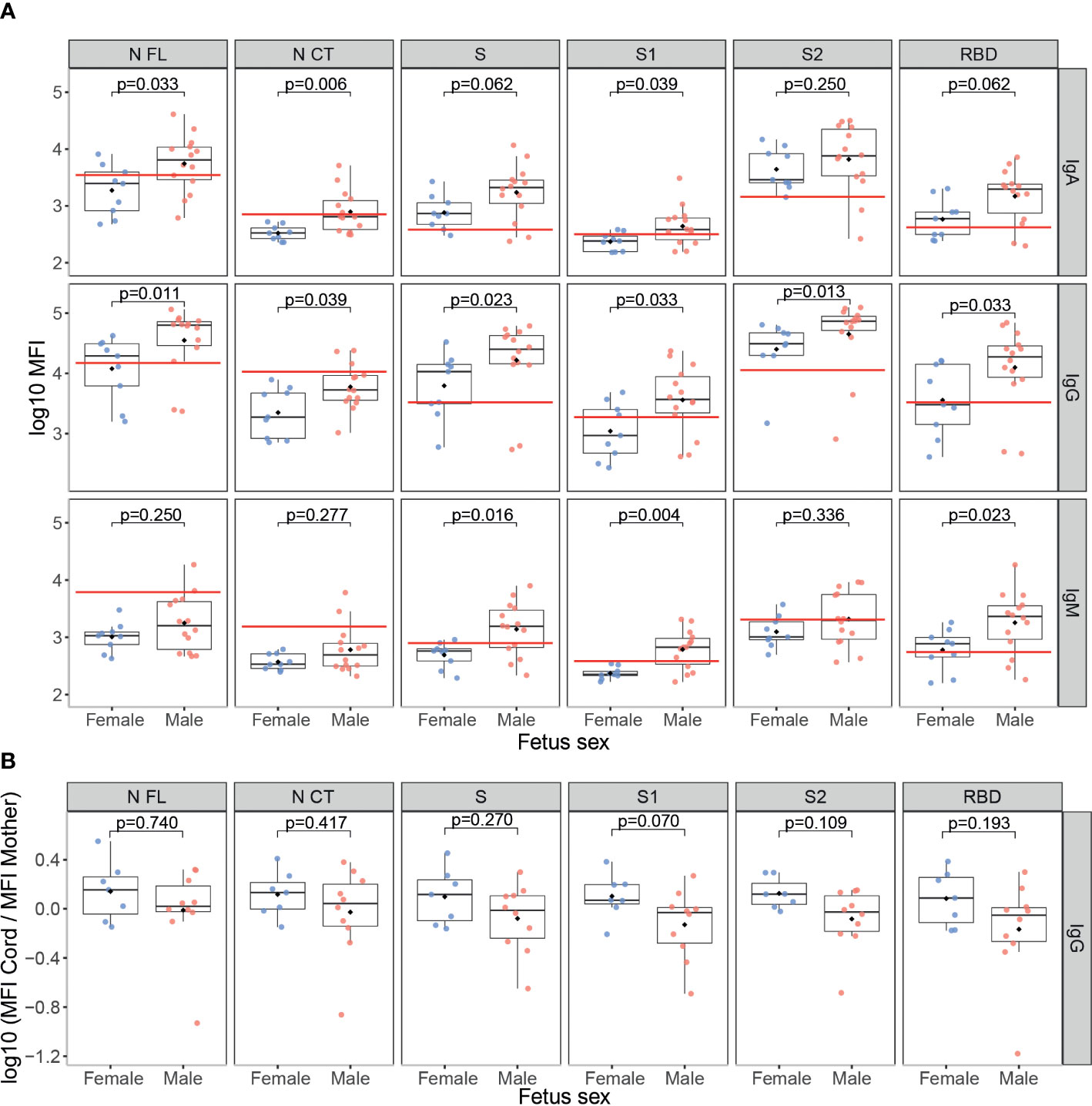
Figure 5 Anti-SARS-CoV-2 antibody levels by fetus sex. (A) Comparison of anti-SARS-CoV-2 levels (log10 median fluorescence intensity, MFI) in infected mothers between those who had a male fetus (N=14, in red) and those who had a female fetus (N=9, in blue). (B) Comparison of the ratios of IgG levels (log10 MFI) to SARS-CoV-2 antigens in cord blood vs. mother peripheral blood from seropositive mothers who had a male fetus (N=10, in red) and those that had a female fetus (N=7, in blue). The boxplots represent the median (bold line), the mean (black diamond), the 1st and 3rd quartiles (box) and the largest and smallest values within 1.5 times the inter-quartile range (whiskers). Groups were compared by the Wilcoxon-rank-sum-test. The red line indicates the seropositivity cutoff calculated as 10 to the mean plus 3 standard deviations (SD) of log10-transformed MFI of 129 pre-pandemic controls. Antigens: nucleocapsid full-length (N FL) and C-terminus (N CT), spike full-length (S), S1 and S2 subunits, and receptor-binding domain (RBD).
Cytokine profile in SARS-CoV-2 infected mothers
SARS-CoV-2 infected mothers had higher serum concentrations of the growth factor EGF, the Th2 cytokine IL-13, the pro-inflammatory marker IL-2R, the anti-inflammatory cytokine IL-17, and the chemokines IP-10, MIG and MIP-1β (p<0.05) compared to non-infected mothers (Figures 6A and S6). Those with symptoms at any time during the third trimester showed higher levels of growth factors EGF, G-CSF and HGF, the pro-inflammatory cytokines IL-1β and IL-6, and the chemokines IP-10, MCP-1 and MIG (p<0.05) compared to the asymptomatic ones (Figures 6B and S7); and when the symptoms were at delivery, FGF, the anti-inflammatory cytokine IL-15, the Th1 markers IL-2, IL-2R, MIP-1α, MIP-1β and the pro-inflammatory cytokine TNF-α (p<0.05) were also increased (Figures 6C and S8).
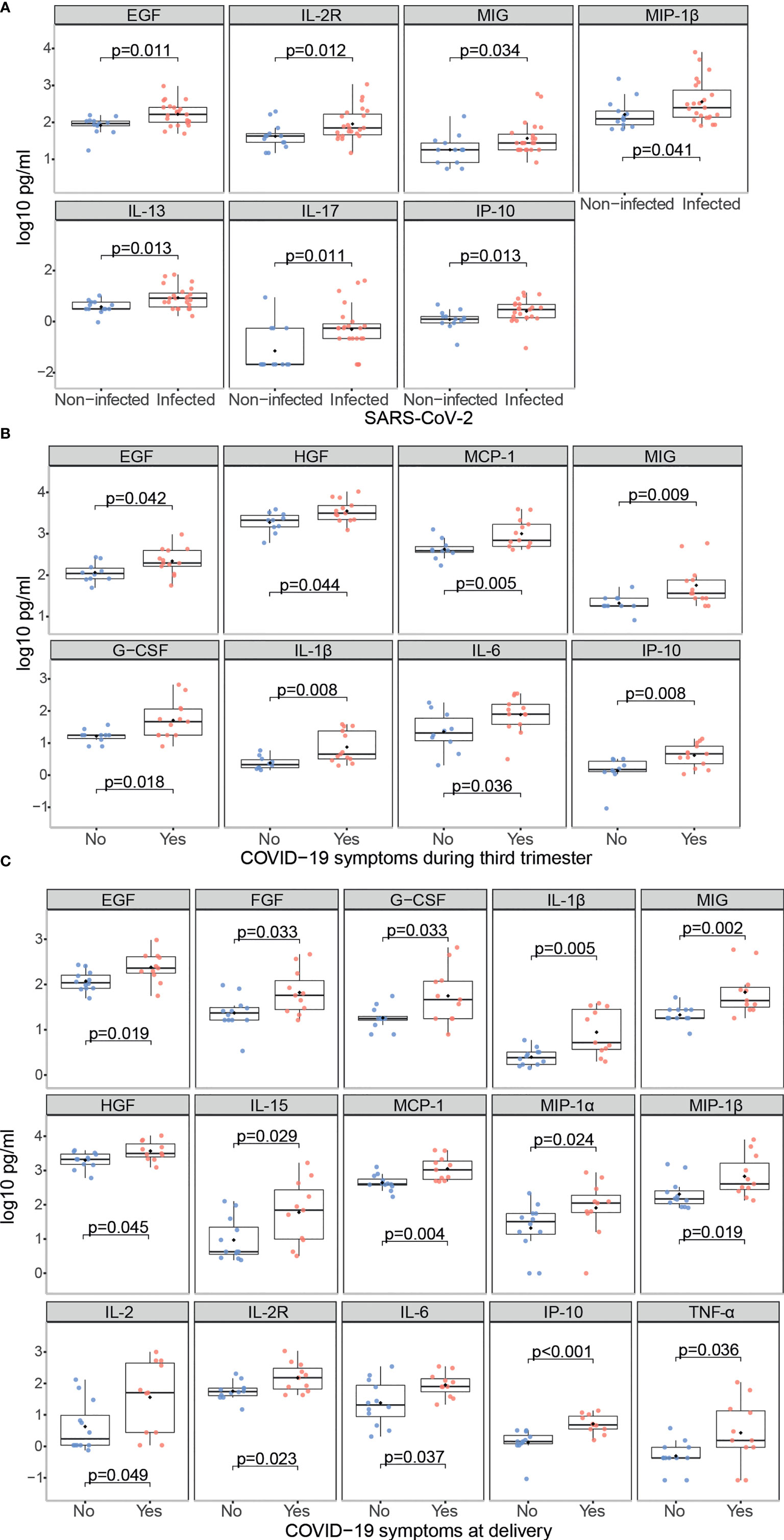
Figure 6 Cytokine, chemokine and growth factor concentrations in SARS-CoV-2 infected mothers. Comparison of inflammatory markers concentrations (log10 pg/ml) in serum between (A) Infected (N=23, in red) vs. non-infected (N=13, in blue) mothers; (B) Infected symptomatic (N=13, in red) vs. asymptomatic (N=10, in blue) mothers during the third trimester; (C) Infected symptomatic (N=11, in red) vs. asymptomatic (N=12, in blue) mothers at delivery. Groups were compared by the Wilcoxon-rank-sum-test. Only significant comparisons are shown, the remaining are in Figures S6–8.
Newborns from infected mothers showed higher concentrations of the pro-inflammatory cytokine IFN-α (p=0.044) in cord blood than those from non-infected mothers (Figures 7A and S9). Newborns from mothers with symptoms at any time during the third trimester presented higher levels of EGF, FGF and IL-17 (p<0.05) in cord blood compared to those from asymptomatic ones (Figures 7B and S10); and when symptoms were at delivery IL-15 was also increased while the chemokine RANTES was decreased (p<0.05) (Figures 7C and S11). Infected mothers showed positive significant correlations for EGF, HGF, IL-13, IL-17, IL-1β, IL-1RA, IL-5, IL-7 and IL-8, and MIP-1β (ρ=0.44-0.77, p<0.05) concentrations in serum and cord blood (Figures 8 and S12).
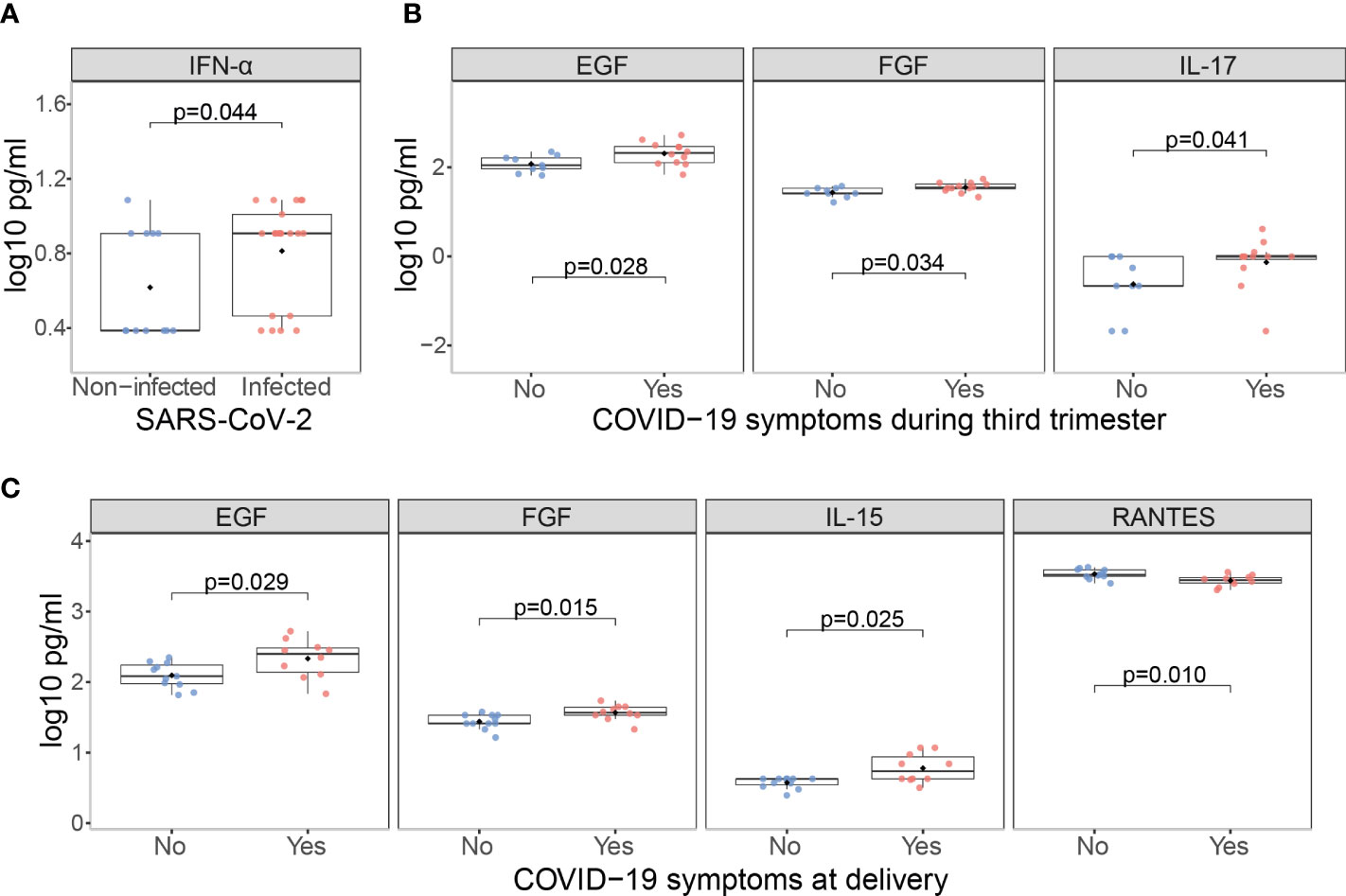
Figure 7 Cytokine, chemokine and growth factor concentrations in cord blood from children born from SARS-CoV-2 infected mothers. Comparison of inflammatory markers concentrations (log10 pg/ml) in cord blood between (A) Infected (N=21, in red) vs. non-infected (N=12, in blue) mothers; (B) Infected symptomatic (N=12, in red) vs. asymptomatic (N=9, in blue) mothers during the third trimester; (C) Infected symptomatic (N=11, in red) vs. asymptomatic (N=12, in blue) mothers at delivery. Groups were compared by the Wilcoxon-rank-sum-test. Only significant comparisons are shown, the remaining are in Figures S9–11.
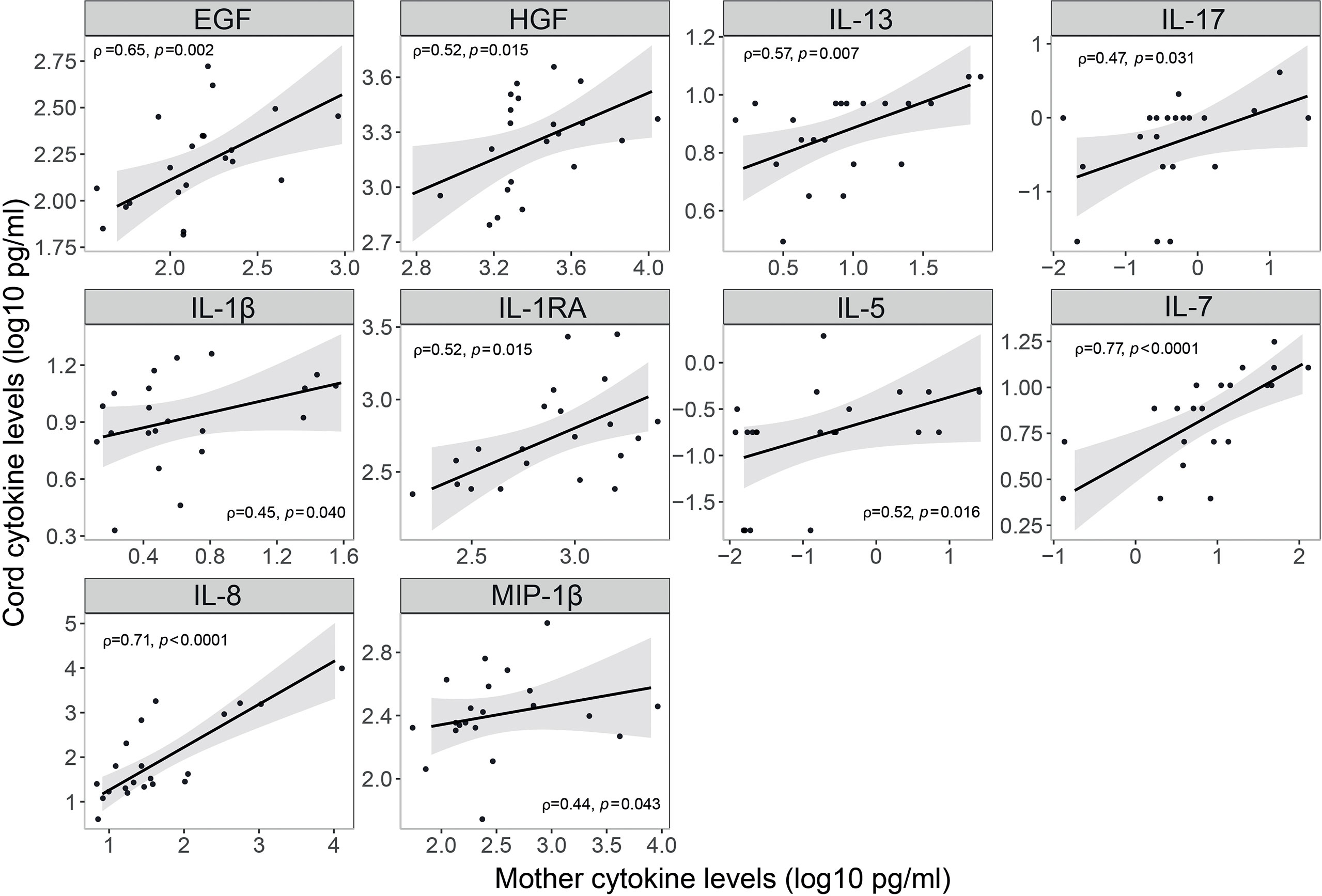
Figure 8 Correlations of cytokine, chemokine and growth factor concentrations in serum and cord blood from SARS-CoV-2 infected mothers. Correlations of inflammatory markers concentrations (log10 pg/ml) in serum blood and cord blood from SARS-CoV-2 infected mothers are represented as a linear model with standard error as a confidence interval (shaded areas). Correlations were assessed by the Spearman test, and the rho (ρ) and p-values are shown. Only significant correlations are shown, the remaining are in Figure S12.
Fetus sex effect on SARS-CoV-2 cytokine response
SARS-CoV-2 infected women carrying a male fetus had higher concentrations of EGF, IL-15 and the regulatory cytokine IL-7 in serum compared to women carrying a female fetus (Figure S13, p<0.05). There were no differences in any of the analytes tested by the sex of the fetus in cord samples (data not shown).
Discussion
The SARS-CoV-2 infection during the third trimester induced a robust antibody and cytokine response detected at delivery in the mother and cord blood. Symptomatic mothers induced higher levels of cytokines and SARS-CoV-2 specific antibodies than asymptomatic women, and maternal levels correlated with cord blood levels. However, the infection during the third trimester caused an impairment of the IgG transplacental transfer that was stronger when the infection was closer to delivery. Furthermore, we observed that mothers carrying a male fetus induced higher anti-SARS-CoV-2 antibody levels and had higher EGF, IL-15 and IL-7 concentrations than mothers carrying a female fetus.
Among the infected pregnant women, those with symptoms had higher anti-SARS-CoV-2 antibody levels, especially IgA and IgM to RBD. Higher RBD-IgA levels in symptomatic infections in non-pregnant adults have been previously reported (42–44). Two recent studies have also reported higher RBD-IgG levels at delivery in pregnant patients with symptomatic infections compared to the asymptomatic ones (31, 45), and it probably impacts the passive transfer of protective IgA through breast milk.
SARS-CoV-2 infected mothers with symptoms presented increased levels of several cytokines compared to the asymptomatic ones, and cytokine serum levels correlated with cord blood levels. In a SARS-CoV-2 infection, inflammatory cytokine storms are an important cause of severe manifestations of COVID-19. This is critically important in the case of pregnant women because of the consequences for the fetus. We observed higher levels of IL-1β, IL-6 and TNF in symptomatic mothers, and of IFN-α in cord blood from SARS-CoV-2 infected mothers. Elevated levels of these cytokines may affect fetal development, increase the risk of neurological diseases, and lead to abortion in the first and second trimesters and preterm birth in the third trimester (46). We also observed increased levels of IL-17 in cord blood from infected symptomatic mothers. High levels of IL-17 can lead to cortical dysplasia and behavioral abnormalities in fetuses and increase the probability that offspring develop mental illness in adulthood (46). However, cytokine data have to be considered with caution due to the dramatic fluctuation of cytokines intrapartum. Normal delivery is pro-inflammatory and women with pregnancy complications, such as preterm delivery or fetal growth retardation, have an ever more pro-inflammatory profile (47), making it difficult to disentangle what is due to COVID-19 or to inflammatory changes in the labor process.
We observed that maternal antibody levels highly correlated to cord blood levels, similar to previous studies (31, 45). However, rRT-PCR positive mothers during the third trimester of pregnancy had a reduction in the IgG transplacental transfer, also observed in previous studies (31–34). We also found that the negative effect of the infection on the IgG transfer was stronger as the infection was closer to delivery. Previous studies have reported that the IgG transfer ratio at birth is significantly lower for third trimester as compared to second-trimester infections (48–50). This could be because the mother has been seropositive for a shorter period, therefore maternal antibody levels may have not reached the peak response and there has been less time for transfer of antibodies. However, we did not detect an effect of antibody levels in the mother by time since infection. Moreover, in the transfer ratio, the IgG levels in cord blood we corrected by the IgG levels in the mother. In addition, although it has been previously documented that preterm newborns have reduced IgG transplacental transfer (51, 52), prematurity was ruled out as the cause of the reduction of SARS-CoV-2 IgG transfer because neither gestational age nor birth weight were associated. All together suggest that SARS-CoV-2 infections closer to term have a stronger negative impact on the IgG transplacental transfer. Interestingly, the reduction in IgG transfer was more marked for the anti-SARS-CoV-2 IgGs than for the anti-HuCoV IgGs. This stronger effect on the transfer of anti-SARS-CoV-2 IgGs vs. other IgGs has also been observed in the studies by Edlow et al. (34) and Atyeo et al. (49). They suggest a potential alteration in the Fc region glycosylation of SARS-CoV-2–specific antibodies probably mediated by the inflammation (34, 49), which may result in a compromised transfer by the Fc receptors (53). Neonatal transferred anti-SARS-CoV-2 antibodies may also be of lower activity (49) and short-lived (54), which raises further concern.
Mothers carrying a male fetus had higher concentrations of EGF, IL-15 and IL-7 cytokines, and induced higher antibody levels against SARS-CoV-2 than those carrying a female, but IgG transfer to the fetus was less efficient in the first ones. Previous studies have shown that fetal sex influences maternal serum cytokine and antibody profiles (55–57). In the context of SARS-CoV-2 infection, a recent study has reported similar results to ours, and a more pronounced SARS-CoV-2-specific antibody glycosylation in mothers carrying a male fetus, which would explain the reduced transplacental transfer (58).
In line with previous studies, SARS-CoV-2 infected pregnant women and their newborns showed more gestational complications than the non-infected women, although differences did not reach statistical significance. An increased risk of adverse birth outcomes, preterm birth, preeclampsia, cesarean section, and even stillbirth, neonatal death and maternal mortality has been reported in COVID-19 positive pregnant women (59–63). A surveillance study by the CDC also showed that pregnant women suffer a greater clinical burden due to COVID-19 than their non-pregnant counterparts (10). A meta-analysis of 86 studies reported a high proportion of pregnant women with COVID-19 who had preterm birth (22%) and cesarean delivery (48%), and higher estimated rates of admission to the ICU (7% vs. 4% of non-pregnant women) (64).
One of the main strengths of the study is the broad panel of antigen-specific antibodies of three isotypes, including SARS-CoV-2 and HuCoVs, and cytokines measured, which allows having a very comprehensive picture of the humoral immune response in SARS-CoV-2 infected pregnant women and their newborns. However, the study has some limitations. The main one is the small sample size. Also, we did not adjust the p-values for multiple comparisons due to the small sample size, and therefore results should be taken with caution and require further validation. Larger sample size would have increased the statistical power to detect additional differences. It would have also been interesting to have histological data on placental samples to assess associations with the humoral response, the antibody transfer, and the delivery outcomes.
Results from this study indicate that we should pay greater attention to women infected with SARS-CoV-2 and their newborns. Children born to mothers with SARS-CoV-2 during pregnancy should be longitudinally observed to assess long-term outcomes. In addition, the vaccination of pregnant women should be highly recommended. Safety data on COVID-19 vaccines in pregnancy are rapidly accumulating without safety concerns being detected (65–68). However, additional longitudinal studies of vaccinated pregnant women at different trimesters and information on vaccine breakthroughs are necessary to inform maternally, pregnancy, and infant outcomes.
Future studies should further address the impact of SARS-CoV-2 infection in vaccinated and unvaccinated mothers along with gestational age on immune responses, how those affect fetal development, antibody mammary transfer, and the mechanisms underlying them. This knowledge may provide insights for vaccination strategies to assure the greatest protection for both mother and neonate.
Data availability statement
The raw data supporting the conclusions of this article will be made available by the authors upon request.
Ethics statement
The study was reviewed and approved by the Ethics Committee for Clinical Research of the Hospital Sant Joan de Déu. The patients/participants provided their written informed consent to participate in this study.
Author contributions
EMa, GM, and CD designed the study. EMu processed the samples at HSJD. MB managed the database. RR and RA developed and performed the serological and cytokines assays, the analysis and interpretation of the results. MV-S, RS, and MV gave support with the assays and analysis. LI, CC, PSa, PSe, DP, and NM produced the antigens. GM and CD supervised the assays and data analyses. RA and RR wrote the draft of the manuscript and GM, CD, MG-R, MB, and EMa reviewed the manuscript. All authors contributed to the article and approved the submitted version.
Funding
This work was supported by the Fundació Privada Daniel Bravo Andreu. RR had the support of the Health Department, Catalan Government (PERIS SLT017/20/000224). LI work was supported by the PID2019-110810RB-I00 grant from the Spanish Ministry of Science & Innovation. We acknowledge support from the Spanish Ministry of Science and Innovation and State Research Agency through the “Centro de Excelencia Severo Ochoa 2019-2023” Program (CEX2018-000806-S), and support from the Generalitat de Catalunya through the CERCA Program.
Acknowledgments
We are grateful to the participants of the study. Special thanks to the team members from ISGlobal, specifically Laura Puyol for the organization and coordination of shipment of samples and materials, Diana Barrios and Alfons Jiménez for the technical support in the lab, Gemma Ruiz-Olalla for the statistical support and Jordi Chi for the production of N antigens.
Conflict of interest
The authors declare that the research was conducted in the absence of any commercial or financial relationships that could be construed as a potential conflict of interest.
Publisher’s note
All claims expressed in this article are solely those of the authors and do not necessarily represent those of their affiliated organizations, or those of the publisher, the editors and the reviewers. Any product that may be evaluated in this article, or claim that may be made by its manufacturer, is not guaranteed or endorsed by the publisher.
Supplementary material
The Supplementary Material for this article can be found online at: https://www.frontiersin.org/articles/10.3389/fimmu.2022.999136/full#supplementary-material
References
1. WHO. WHO coronavirus disease (COVID-19) dashboard . Available at: https://covid19whoint/ (Accessed 11 April 2021).
2. Kumar R, Yeni CM, Utami NA, Masand R, Asrani RK, Patel SK, et al. SARS-CoV-2 infection during pregnancy and pregnancy-related conditions: Concerns, challenges, management and mitigation strategies-a narrative review. J Infect Public Health [Internet] (2021) 14(7):863–75. doi: 10.1016/j.jiph.2021.04.005
3. Aghaeepour N, Ganio EA, Mcilwain D, Tsai AS, Tingle M, van Gassen S, et al. An immune clock of human pregnancy. Sci Immunol (2017) 2(15):eaan2946. doi: 10.1126/sciimmunol.aan2946
4. Marzi M, Vigano A, Trabattoni D, Villa ML, Salvaggio A, Clerici E, et al. Characterization of type 1 and type 2 cytokine production profile in physiologic and pathologic human pregnancy. Clin Exp Immunol (1996) 106(1):127–33. doi: 10.1046/j.1365-2249.1996.d01-809.x
5. Robinson DP, Klein SL. Pregnancy and pregnancy-associated hormones alter immune responses and disease pathogenesis. Horm Behav (2012) 62(3):263–71. doi: 10.1016/j.yhbeh.2012.02.023
6. Jamieson DJ, Honein MA, Rasmussen SA, Williams JL, Swerdlow DL, Biggerstaff MS, et al. H1N1 2009 influenza virus infection during pregnancy in the USA. Lancet. (2009) 374(9688):451–8. doi: 10.1016/S0140-6736(09)61304-0
7. Rasmussen SA, Jamieson DJ, Uyeki TM. Effects of influenza on pregnant women and infants. Am J Obstet Gynecol (2012) 207(3 Suppl):S3–8. doi: 10.1016/j.ajog.2012.06.068
8. Liu H, Wang LL, Zhao SJ, Kwak-Kim J, Mor G, Liao AH. Why are pregnant women susceptible to COVID-19? an immunological viewpoint. J Reprod Immunol (2020) 139:103122. doi: 10.1016/j.jri.2020.103122
9. Mullins E, Evans D, Viner RM, O’Brien P, Morris E. Coronavirus in pregnancy and delivery: rapid review. Ultrasound obstetrics gynecology (2020) 55(5):586–92. doi: 10.1002/uog.22014
10. Ellington S, Strid P, Tong VT, Woodworth K, Galang RR, Zambrano LD, et al. Characteristics of women of reproductive age with laboratory-confirmed SARS-CoV-2 infection by pregnancy status - united states, January 22-June 7, 2020. MMWR Morb Mortal Wkly Rep (2020) 69(25):769–75. doi: 10.15585/mmwr.mm6925a1
11. CDC. Investigating the impact of COVID-19 during pregnancy. (2021). Available at: https://www.cdc.gov/coronavirus/2019-ncov/cases-updates/special-populations/pregnancy-data-on-covid-19/what-cdc-is-doing.html (Accessed 8 July 2022)
12. Lokken EM, Huebner EM, Taylor GG, Hendrickson S, Vanderhoeven J, Kachikis A, et al. Disease severity, pregnancy outcomes, and maternal deaths among pregnant patients with severe acute respiratory syndrome coronavirus 2 infection in Washington state. Am J Obstet Gynecol (2021) 225(1):77.e1–77.e14. doi: 10.1016/j.ajog.2020.12.1221
13. Lokken EM, Taylor GG, Huebner EM, Vanderhoeven J, Hendrickson S, Coler B, et al. Higher severe acute respiratory syndrome coronavirus 2 infection rate in pregnant patients. Am J Obstetrics Gynecol (2021) 225(1):75.e1–75.e16. doi: 10.1016/j.ajog.2021.02.011
14. Zambrano LD, Ellington S, Strid P, Galang RR, Oduyebo T, Tong VT, et al. Update: Characteristics of symptomatic women of reproductive age with laboratory-confirmed SARS-CoV-2 infection by pregnancy status - united states, January 22-October 3, 2020. MMWR Morb Mortal Wkly Rep (2020) 69(44):1641–7. doi: 10.15585/mmwr.mm6944e3
15. WAPM. Maternal and perinatal outcomes of pregnant women with SARS-CoV-2 infection. Ultrasound Obstet Gynecol (2021) 57(2):232–41. doi: 10.1002/uog.23107
16. Perlman JM, Salvatore C. Coronavirus disease 2019 infection in newborns. Clin Perinatol (2022) 49(1):73–92. doi: 10.1016/j.clp.2021.11.005
17. Yuan J, Qian H, Cao S, Dong B, Yan X, Luo S, et al. Is there possibility of vertical transmission of COVID-19: a systematic review. Transl Pediatr (2021) 10(2):423–34. doi: 10.21037/tp-20-144
18. Akhtar H, Patel C, Abuelgasim E, Harky A. COVID-19 (SARS-CoV-2) infection in pregnancy: A systematic review. Vol 85 Gynecologic obstetric Invest (2020) p:295–306. doi: 10.1159/000509290
19. Yockey LJ, Iwasaki A. Interferons and proinflammatory cytokines in pregnancy and fetal development. (2018) 49(3):397–412. doi: 10.1016/j.immuni.2018.07.017
20. Racicot K, Mor G. Risks associated with viral infections during pregnancy. J Clin Invest (2017) 127(5):1591–9. doi: 10.1172/JCI87490
21. Chudnovets A, Liu J, Narasimhan H, Liu Y, Burd I. Role of inflammation in virus pathogenesis during pregnancy. J Virol (2020) 95(2):e01381–19. doi: 10.1128/JVI.01381-19
22. Prochaska E, Jang M, Burd I. COVID-19 in pregnancy: Placental and neonatal involvement. Am J Reprod Immunol (2020) 84(5):e13306. doi: 10.1111/aji.13306
23. Estes ML, McAllister AK. Maternal immune activation: Implications for neuropsychiatric disorders. Science. (2016) 353(6301):772–7. doi: 10.1126/science.aag3194
24. Mor G, Cardenas I. The immune system in pregnancy: a unique complexity. Am J Reprod Immunol (2010) 63(6):425–33. doi: 10.1111/j.1600-0897.2010.00836.x
25. Rad HS, Röhl J, Stylianou N, Allenby MC, Bazaz SR, Warkiani ME, et al. The effects of COVID-19 on the placenta during pregnancy. Front Immunol (2021) 12:743022. doi: 10.3389/fimmu.2021.743022
26. Shook LL, Sullivan EL, Lo JO, Perlis RH, Edlow AG. COVID-19 in pregnancy: implications for fetal brain development. Trends Mol Med (2022) 28(4):319–30. doi: 10.1016/j.molmed.2022.02.004
27. Cumberland P, Shulman CE, Maple PAC, Bulmer JN, Dorman EK, Kawuondo K, et al. Maternal HIV infection and placental malaria reduce transplacental antibody transfer and tetanus antibody levels in newborns in Kenya. J Infect Dis (2007) 196(4):550–7. doi: 10.1086/519845
28. Ogolla S, Daud II, Asito AS, Sumba OP, Ouma C, Vulule J, et al. Reduced transplacental transfer of a subset of Epstein-Barr virus-specific antibodies to neonates of mothers infected with plasmodium falciparum malaria during pregnancy. Clin Vaccine Immunol (2015) 22(11):1197–205. doi: 10.1128/CVI.00270-15
29. Perret C, Chanthavanich P, Pengsaa K, Limkittikul K, Hutajaroen P, Bunn JEG, et al. Dengue infection during pregnancy and transplacental antibody transfer in Thai mothers. J Infect (2005) 51(4):287–93. doi: 10.1016/j.jinf.2004.10.003
30. Castanha PMS, Souza W v, Braga C, de Araújo TVB, Ximenes RAA, Albuquerque MdeFPM, et al. Perinatal analyses of zika- and dengue virus-specific neutralizing antibodies: A microcephaly case-control study in an area of high dengue endemicity in Brazil. PLoS Negl Trop Dis (2019) 13(3):e0007246. doi: 10.1371/journal.pntd.0007246
31. Kubiak JM, Murphy EA, Yee J, Cagino KA, Friedlander RL, Glynn SM, et al. Severe acute respiratory syndrome coronavirus 2 serology levels in pregnant women and their neonates. Am J Obstet Gynecol (2021) 225(1):73.e1–7. doi: 10.1016/j.ajog.2021.01.016
32. Sherer ML, Lei J, Creisher PS, Jang M, Reddy R, Voegtline K, et al. Pregnancy alters interleukin-1 beta expression and antiviral antibody responses during severe acute respiratory syndrome coronavirus 2 infection. Am J Obstet Gynecol (2021) 225(3):301.e1–301.e14. doi: 10.1016/j.ajog.2021.03.028
33. Flannery DD, Gouma S, Dhudasia MB, Mukhopadhyay S, Pfeifer MR, Woodford EC, et al. Assessment of maternal and neonatal cord blood SARS-CoV-2 antibodies and placental transfer ratios. JAMA Pediatr (2021) 175(6):594–600. doi: 10.1001/jamapediatrics.2021.0038
34. Edlow AG, Li JZ, Collier ARY, Atyeo C, James KE, Boatin AA, et al. Assessment of maternal and neonatal SARS-CoV-2 viral load, transplacental antibody transfer, and placental pathology in pregnancies during the COVID-19 pandemic. JAMA Netw Open (2020) 3(12):e2030455. doi: 10.1001/jamanetworkopen.2020.30455
35. Shook LL, Atyeo CG, Yonker LM, Fasano A, Gray KJ, Alter G, et al. Durability of anti-spike antibodies in infants after maternal COVID-19 vaccination or natural infection. JAMA (2022) 327(11):1087–1089. doi: 10.1001/jama.2022.1206
36. Dobaño C, Ramírez-Morros A, Alonso S, Vidal-Alaball J, Ruiz-Olalla G, Vidal M, et al. Persistence and baseline determinants of seropositivity and reinfection rates in health care workers up to 12.5 months after COVID-19. BMC Med (2021) 19(1):155. doi: 10.1186/s12916-021-02032-2
37. Dobaño C, Santano R, Jiménez A, Vidal M, Chi J, Rodrigo Melero N, et al. Immunogenicity and crossreactivity of antibodies to the nucleocapsid protein of SARS-CoV-2: utility and limitations in seroprevalence and immunity studies. Trans Res (2021) 232:60–74. doi: 10.1016/j.trsl.2021.02.006
38. Aguilar R, Campo JJ, Chicuecue S, Cisteró P, Català A, Luis L, et al. Changing plasma cytokine, chemokine and growth factor profiles upon differing malaria transmission intensities. Malaria J (2019) 18(1):406. doi: 10.1186/s12936-019-3038-x
39. Ballart C, Torrico MC, Vidal G, Torrico F, Lozano D, Gállego M, et al. Clinical and immunological characteristics of tegumentary leishmaniasis cases in Bolivia. PLoS Negl Trop Dis (2021) 15(3):e0009223. doi: 10.1371/journal.pntd.0009223
40. Natama HM, Moncunill G, Rovira-Vallbona E, Sanz H, Sorgho H, Aguilar R, et al. Modulation of innate immune responses at birth by prenatal malaria exposure and association with malaria risk during the first year of life. BMC Med (2018) 16(1):198. doi: 10.1186/s12916-018-1187-3
41. Sanz H, Aponte JJ, Harezlak J, Dong Y, Ayestaran A, Nhabomba A, et al. drLumi: An open-source package to manage data, calibrate, and conduct quality control of multiplex bead-based immunoassays data analysis. PLoS One (2017) 12(11):1:e018790. doi: 10.1371/journal.pone.0187901
42. Garcia-Basteiro AL, Moncunill G, Tortajada M, Vidal M, Guinovart C, Jiménez A, et al. Seroprevalence of antibodies against SARS-CoV-2 among health care workers in a large Spanish reference hospital. Nat Commun (2020) 11(1):3500. doi: 10.1038/s41467-020-17318-x
43. Moncunill G, Mayor A, Santano R, Jiménez A, Vidal M, Tortajada M, et al. SARS-CoV-2 seroprevalence and antibody kinetics among health care workers in a Spanish hospital after three months of follow-up. J Infect Dis (2020) 223(1):62–71. doi: 10.1093/infdis/jiaa696
44. Shields AM, Faustini SE, Perez-Toledo M, Jossi S, Allen JD, Al-Taei S, et al. Serological responses to SARS-CoV-2 following non-hospitalised infection: clinical and ethnodemographic features associated with the magnitude of the antibody response. BMJ Open Respir Res (2021) 8(1):e000872. doi: 10.1136/bmjresp-2020-000872
45. Joseph NT, Dude CM, Verkerke HP, Irby LS, Dunlop AL, Patel RM, et al. Maternal antibody response, neutralizing potency, and placental antibody transfer after severe acute respiratory syndrome coronavirus 2 (SARS-CoV-2) infection. Obstet Gynecol (2021) 138(2):189–97. doi: 10.1097/AOG.0000000000004440
46. Dang D, Wang L, Zhang C, Li Z, Wu H. Potential effects of SARS-CoV-2 infection during pregnancy on fetuses and newborns are worthy of attention. J Obstet Gynaecol Res (2020) 46(10):1951–7. doi: 10.1111/jog.14406
47. Denney JM, Nelson E, Wadhwa P, Waters T, Mathew L, Goldenberg RL, et al. Cytokine profiling: Variation in immune modulation with preterm birth vs. uncomplicated term birth identifies pivotal signals in pathogenesis of preterm birth. J Perinatal Med (2021) 49(3):299–309. doi: 10.1515/jpm-2020-0025/html
48. Beharier O, Plitman Mayo R, Raz T, Nahum Sacks K, Schreiber L, Suissa-Cohen Y, et al. Efficient maternal to neonatal transfer of antibodies against SARS-CoV-2 and BNT162b2 mRNA COVID-19 vaccine. J Clin Invest (2021) 131(13):e154834. doi: 10.1172/JCI150319
49. Atyeo C, Pullen KM, Bordt EA, Fischinger S, Burke J, Michell A, et al. Compromised SARS-CoV-2-specific placental antibody transfer. Cell. (2021) 184(3):628–42.e10. doi: 10.1016/j.cell.2020.12.027
50. Palmeira P, Quinello C, Silveira-Lessa AL, Zago CA, Carneiro-Sampaio M. IgG placental transfer in healthy and pathological pregnancies. Salumets A editor Clin Dev Immunol (2012) 2012:985646. doi: 10.1155/2012/985646
51. Okoko BJ, Wesumperuma HL, Fern J, Yamuah LK, Hart CA. The transplacental transfer of IgG subclasses: influence of prematurity and low birthweight in the Gambian population. Ann Trop Paediatr (2002) 22(4):325–32. doi: 10.1179/027249302125001985
52. Costa-Carvalho BT, Vieria HM, Dimantas RB, Arslanian C, Naspitz CK, Solé D, et al. Transfer of IgG subclasses across placenta in term and preterm newborns. razilian J Med Biol Res = Rev Bras pesquisas medicas e biologicas (1996) 29(2):201–4.
53. Jennewein MF, Abu-Raya B, Jiang Y, Alter G, Marchant A. Transfer of maternal immunity and programming of the newborn immune system. Semin Immunopathol (2017) 39(6):605–13. doi: 10.1007/s00281-017-0653-x
54. Gao J, Li W, Hu X, Wei Y, Wu J, Luo X, et al. Disappearance of SARS-CoV-2 antibodies in infants born to women with COVID-19, wuhan, China. Emerging Infect diseases (2020) Vol. 26:2491–4. doi: 10.3201/eid2610.202328
55. Ramiro-Cortijo D, de la Calle M, Böger R, Hannemann J, Lüneburg N, López-Giménez MR, et al. Male Fetal sex is associated with low maternal plasma anti-inflammatory cytokine profile in the first trimester of healthy pregnancies. Cytokine. (2020) 136:155290. doi: 10.1016/j.cyto.2020.155290
56. Mitchell AM, Palettas M, Christian LM. Fetal sex is associated with maternal stimulated cytokine production, but not serum cytokine levels, in human pregnancy. Brain Behav Immun (2017) 60:32–7. doi: 10.1016/j.bbi.2016.06.015
57. Moncunill G, Dobaño C, González R, Smolen KK, Manaca MN, Balcells R, et al. Association of maternal factors and HIV infection with innate cytokine responses of delivering mothers and newborns in Mozambique. Front Microbiol (2020) 11:1452. doi: 10.3389/fmicb.2020.01452
58. Bordt EA, Shook LL, Atyeo C, Pullen KM, de Guzman RM, Meinsohn MC, et al. Maternal SARS-CoV-2 infection elicits sexually dimorphic placental immune responses. Sci Transl Med (2021) 13(617):eabi7428. doi: 10.1126/scitranslmed.abi7428
59. Yang R, Mei H, Zheng T, Fu Q, Zhang Y, Buka S, et al. Pregnant women with COVID-19 and risk of adverse birth outcomes and maternal-fetal vertical transmission: a population-based cohort study in wuhan, China. BMC Med (2020) 18(1):330. doi: 10.1186/s12916-020-01798-1
60. Carrasco I, Muñoz-Chapuli M, Vigil-Vázquez S, Aguilera-Alonso D, Hernández C, Sánchez-Sánchez C, et al. SARS-COV-2 infection in pregnant women and newborns in a Spanish cohort (GESNEO-COVID) during the first wave. BMC Pregnancy Childbirth (2021) 21(1):326. doi: 10.1186/s12884-021-03784-8
61. Villar J, Ariff S, Gunier RB, Thiruvengadam R, Rauch S, Kholin A, et al. Maternal and neonatal morbidity and mortality among pregnant women with and without COVID-19 infection: The INTERCOVID multinational cohort study. JAMA Pediatr [Internet] (2021) 175(8):817–26. doi: 10.1001/jamapediatrics.2021.1050
62. Laresgoiti-Servitje E, Cardona-Pérez JA, Hernández-Cruz RG, Helguera-Repetto AC, Valdespino-Vázquez MY, Moreno-Verduzco ER, et al. COVID-19 infection in pregnancy: PCR cycle thresholds, placental pathology, and perinatal outcomes. Viruses (2021) 13(9):1884. doi: 10.3390/v13091884
63. Chamseddine RS, Wahbeh F, Chervenak F, Salomon LJ, Ahmed B, Rafii A. Pregnancy and neonatal outcomes in SARS-CoV-2 infection: A systematic review. Marozio L editor J Pregnancy (2020) 2020:4592450. doi: 10.1155/2020/4592450
64. Khalil A, Kalafat E, Benlioglu C, O’Brien P, Morris E, Draycott T, et al. SARS-CoV-2 infection in pregnancy: A systematic review and meta-analysis of clinical features and pregnancy outcomes. EClinicalMedicine. (2020) 25:100446. doi: 10.1016/j.eclinm.2020.100446
65. Goldshtein I, Nevo D, Steinberg DM, Rotem RS, Gorfine M, Chodick G, et al. Association between BNT162b2 vaccination and incidence of SARS-CoV-2 infection in pregnant women. JAMA [Internet] (2021) 326(8):728–35. doi: 10.1001/jama.2021.11035
66. Adhikari EH, Spong CY. COVID-19 vaccination in pregnant and lactating women. JAMA. (2021) 325(11):1039–40. doi: 10.1001/jama.2021.1658
67. Theiler RN, Wick M, Mehta R, Weaver AL, Virk A, Swift M. Pregnancy and birth outcomes after SARS-CoV-2 vaccination in pregnancy. Am J Obstet Gynecol MFM (2021) 3(6):100467. doi: 10.1016/j.ajogmf.2021.100467
Keywords: SARS-CoV-2, maternal and neonatal immunity, antibodies, cytokines, transplacental transfer
Citation: Rubio R, Aguilar R, Bustamante M, Muñoz E, Vázquez-Santiago M, Santano R, Vidal M, Melero NR, Parras D, Serra P, Santamaria P, Carolis C, Izquierdo L, Gómez-Roig MD, Dobaño C, Moncunill G and Mazarico E (2022) Maternal and neonatal immune response to SARS-CoV-2, IgG transplacental transfer and cytokine profile. Front. Immunol. 13:999136. doi: 10.3389/fimmu.2022.999136
Received: 20 July 2022; Accepted: 05 September 2022;
Published: 27 September 2022.
Edited by:
E. Marion Schneider, University of Ulm, GermanyReviewed by:
Miguel de Mulder Rougvie, Cornell University, United StatesRakesh Kumar, Askham Bryan College, United Kingdom
Copyright © 2022 Rubio, Aguilar, Bustamante, Muñoz, Vázquez-Santiago, Santano, Vidal, Melero, Parras, Serra, Santamaria, Carolis, Izquierdo, Gómez-Roig, Dobaño, Moncunill and Mazarico. This is an open-access article distributed under the terms of the Creative Commons Attribution License (CC BY). The use, distribution or reproduction in other forums is permitted, provided the original author(s) and the copyright owner(s) are credited and that the original publication in this journal is cited, in accordance with accepted academic practice. No use, distribution or reproduction is permitted which does not comply with these terms.
*Correspondence: Gemma Moncunill, Z2VtbWEubW9uY3VuaWxsQGlzZ2xvYmFsLm9yZw==; Carlota Dobaño, Y2FybG90YS5kb2Jhbm9AaXNnbG9iYWwub3Jn
†These authors have contributed equally to this work and share first authorship
‡These authors have contributed equally to this work and share senior authorship