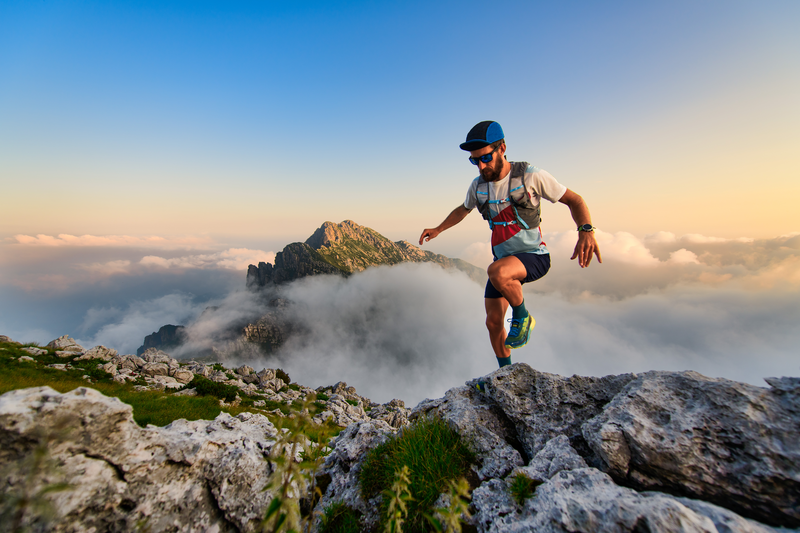
95% of researchers rate our articles as excellent or good
Learn more about the work of our research integrity team to safeguard the quality of each article we publish.
Find out more
MINI REVIEW article
Front. Immunol. , 15 September 2022
Sec. NK and Innate Lymphoid Cell Biology
Volume 13 - 2022 | https://doi.org/10.3389/fimmu.2022.998378
This article is part of the Research Topic Therapeutic Potential of Innate and Innate-like Effector Lymphocytes in Autoimmune and Inflammatory Diseases View all 14 articles
Invariant natural killer T (iNKT) cells are innate T cells that are recognized for their potent immune modulatory functions. Over the last three decades, research in murine models and human observational studies have revealed that iNKT cells can act to limit inflammatory pathology in a variety of settings. Since iNKT cells are multi-functional and can promote inflammation in some contexts, understanding the mechanistic basis for their anti-inflammatory effects is critical for effectively harnessing them for clinical use. Two contrasting mechanisms have emerged to explain the anti-inflammatory activity of iNKT cells: that they drive suppressive pathways mediated by other regulatory cells, and that they may cytolytically eliminate antigen presenting cells that promote excessive inflammatory responses. How these activities are controlled and separated from their pro-inflammatory functions remains a central question. Murine iNKT cells can be divided into four functional lineages that have either pro-inflammatory (NKT1, NKT17) or anti-inflammatory (NKT2, NKT10) cytokine profiles. However, in humans these subsets are not clearly evident, and instead most iNKT cells that are CD4+ appear oriented towards polyfunctional (TH0) cytokine production, while CD4- iNKT cells appear more predisposed towards cytolytic activity. Additionally, structurally distinct antigens have been shown to induce TH1- or TH2-biased responses by iNKT cells in murine models, but human iNKT cells may respond to differing levels of TCR stimulation in a way that does not neatly separate TH1 and TH2 cytokine production. We discuss the implications of these differences for translational efforts focused on the anti-inflammatory activity of iNKT cells.
iNKT cells are innate T lymphocytes that are present in all individuals and use a unique “semi-invariant” TCR, comprised of a canonically rearranged TCRα chain (TRAV10-TRAJ18) paired with TCRβ chains utilizing TRBV25-1 in diverse rearrangements (1–3). The TCRs of iNKT cells are specific for CD1d, a non-classical antigen presenting molecule that has minimal polymorphism at the amino acid level in human populations (4). CD1d molecules are constitutively expressed by professional APCs, including B cells, monocytes, macrophages, and DCs (5), and also by non-hematopoietic cells (particularly epithelial cells) in a variety of tissues (6). CD1d molecules are specialized for presenting lipidic antigens, which are structurally conserved molecules that are not highly mutable (7). Antigens recognized by iNKT cells derive from both self and microbial sources (8). Self-lipids recognized by iNKT cells are constitutively presented by CD1d+ APCs, and may also be up-regulated during inflammation or cellular stress (9). Hence, because of their status as ‘donor-unrestricted’ T cells that recognize conserved antigens and do not mediate alloreactivity, iNKT cells are ideal candidates for allogeneic cellular immunotherapies. Due to their self-lipid recognition iNKT cells can be used for adoptive cellular immunotherapies without added antigens. Alternatively, they can be specifically activated by synthetic mimetics of their lipid antigens.
Extensive studies have demonstrated remarkable potency of iNKT cells in limiting TH1-driven pathology in multiple settings, including autoimmune diseases, inflammation associated with obesity, and graft versus host disease (GVHD) [reviewed in (10–12)]. However, a central conundrum about iNKT cells is that they can also potently promote TH1 responses. Their TH1-promoting functions have been associated with enhanced defense against infections and cancer (reviewed in (13, 14)), but also appear to play pathological roles in certain contexts, including atherosclerosis, sickle cell disease, and endotoxic shock (reviewed in (15–17)). Thus, in order to successfully exploit the potential of iNKT cells to treat inflammatory disease, it may be important to selectively engage their anti-inflammatory pathways.
Two distinct mechanistic processes have been identified that may explain how iNKT cells limit TH1-driven inflammation. The first is a regulatory axis characterized by iNKT cell production of TH2 (IL-4, IL-13) or regulatory (IL-10, TGFβ) cytokines, and by activation of anti-inflammatory cells including M2-polarized macrophages, myeloid-derived suppressor cells (MDSCs), and Tregs (Figure 1A). The second is a cytolytic pathway involving iNKT-mediated killing of inflammatory antigen presenting cells (APCs) that activate TH1 effectors (Figure 1B).
Figure 1 iNKT cell anti-inflammatory mechanisms. (A) iNKT cells interact with myeloid cell types to initiate the activation of regulatory pathways. Recognition of antigens presented by CD1d molecules expressed by myeloid cells induces iNKT cells to produce cytokines like IL-4, IL-10, or IL-13, that in turn act on the APCs. IL-4 and IL-10 promote macrophage differentiation into an M2 phenotype. IL-13 promotes monocyte differentiation into APCs that express suppressive cytokines such as IL-10 and TGF-β. Secretion of ATP by iNKT cells leads to upregulation of the checkpoint inhibitors PD-L1 and PD-L2, and iNKT interaction with monocytes induces secretion of PGE2 by mechanisms that have not yet been determined. Additionally, IL-2 produced by iNKT cells helps to drive the expansion of Tregs. (B) iNKT cells can lyse pro-inflammatory APCs, leading to reduced T cell activation. In this case, recognition of antigens presented by CD1d molecules activates iNKT cells to release cytolytic granules that induce apoptosis of pro-inflammatory APCs.
Studies investigating insulitis in non-obese diabetic (NOD) mice were amongst the first to elucidate the regulatory activity of iNKT cells, with early work revealing a critical link to IL-4 and IL-10 production (18–21), and further analysis showing that they promote the differentiation of tolerogenic APCs that limit the activation of autoreactive T cells (22–25). A similar axis has been observed in murine models of diet-induced obesity, where adipose-resident iNKT cells play a powerful role in glucose tolerance by promoting macrophage polarization into a non-inflammatory M2 phenotype through secretion of IL-4 and IL-10 (26, 27), and by transactivating regulatory T cells via secretion of IL-2 (28). iNKT cells also contribute to the resolution phase of sterile inflammation in the liver by promoting monocyte transition into an anti-inflammatory phenotype through secretion of IL-4 (29, 30). In murine models of allogeneic hematopoietic transplantation, iNKT cells protect against GVHD through IL-4 dependent mechanisms (31–33), and by promoting the regulatory functions of myeloid-derived suppressor cells (MDSCs) while driving Treg expansion via secretion of IL-2 (34–36).
Analyses of human iNKT cells have suggested that they may participate in similar regulatory processes. IL-10 producing iNKT cells recently identified in the intestinal lamina propria of Crohn’s Disease patients showed suppressive activity towards pathogenic CD4+ T cells, and the frequency of IL-10 producing iNKT cells in colon tissue of these patients correlated inversely with TH1 and TH17 cell frequency, and was associated with reduced disease severity, higher TGFB gene expression, and lower levels of inflammatory proteins (37). Moreover, co-culture of human Tregs with iNKT cells led to increased Treg FOXP3 expression, enhanced IL-10 secretion, and more profound inhibition of conventional T cell proliferation (38).
Human iNKT cells can also mediate potent suppression of T cell IFN-γ production by modulating the functions of monocytic cells. Our research group showed that GM-CSF and IL-13 secretion by human iNKT cells induced monocytes to differentiate into tolerogenic APCs that produced high levels of IL-10, expressed the checkpoint inhibitors PDL-1 and PDL-2, and potently suppressed T cell proliferation and IFN-γ secretion (39, 40). The regulatory phenotype of the APCs was due to iNKT cell release of extracellular ATP, which signaled through the P2X7 receptor on the monocytes to induce upregulation of PD-L1 and PD-L2 (41). This iNKT-monocyte interaction resembles a pathway observed in a murine model in which IL-13 secreted by CD1d-restricted T cells promoted monocyte expression of TGFβ, which led to suppression of T cell effector responses (42, 43), although the role of TGFβ in the human iNKT-monocyte pathway remains unclear.
We also used a xenotransplantation model of hematopoietic engraftment to investigate the impact of the human iNKT-monocyte pathway in vivo. The addition of allogeneic adult iNKT cells to human cord blood mononuclear cell grafts resulted in dramatically improved engraftment, which was due to iNKT cells inducing cord blood monocytes to secrete prostaglandin E2, which potently suppressed T cell IFN-γ production (44). Since hematopoietic engraftment is suppressed by excessive IFN-γ (45), this analysis shows that human iNKT cells can engage powerful regulatory pathways that limit adverse effects of human TH1 activation in vivo.
A number of studies have suggested that iNKT cells may also control inflammation by eliminating pro-inflammatory APCs through a mechanism involving CD1d-dependent activation of the iNKT cells and lysis of APCs by cytotoxic granule deposition (46–50). Human iNKT cells were found to kill monocyte-derived DCs and blood DCs, but did not kill monocytes or plasmacytoid DCs, suggesting they specifically target certain types of APCs (46, 49). In another analysis, human iNKT cells preferentially eliminated monocyte-derived DCs that produced high levels of IL-12 while those that produced mainly IL-10 were spared, resulting in a DC population that limited TH1 activation (48). Together these studies suggest that this cytolytic pathway selectively targets pro-inflammatory APCs, and might thereby limit pathological inflammation. Consistent with this, in mice infected with a highly pathogenic strain of influenza A virus, iNKT cells were associated with reduced accumulation of inflammatory monocytes in the lungs (50). iNKT cell activity in this model was associated with reduced levels of MCP-1 (a chemokine that recruits monocytes and CD4+ T cells), reduced damage to lung tissue, and improved survival even though viral loads were not affected (50). The effect of iNKT cells was thought to be due to their cytolytic activity against influenza-infected monocytes, suggesting that iNKT cells may limit pathological inflammation during viral infections by eliminating inflammatory APCs. However, an important note is that in all of these studies the iNKT cells were experimentally exposed to strong TCR stimulation prior to analysis of their cytolytic activity. Therefore, the physiological conditions that might lead to APC-targeted cytolytic activity by iNKT cells remain unclear.
iNKT cells can be activated in two ways: either through TCR-mediated recognition of antigen presented by CD1d, or through TCR-independent pathways such as exposure to the cytokines IL-12 or IL-18, or LFA-1 ligation by high-density ICAM-1 (51–54). These TCR-independent pathways selectively induce iNKT cells to produce IFN-γ and not TH2 or regulatory cytokines (52, 54). Additionally, iNKT cells require a TCR signal for cytolysis of target cells (55–57). Thus, the anti-inflammatory activities of iNKT cells are probably highly dependent on TCR-recognition of antigens presented by CD1d molecules. Since it is clear that iNKT cells can mediate regulatory effects in the absence of infectious challenges, the antigens required for their anti-inflammatory pathways must be constitutively or chronically present. However, the sources and nature of the antigens that physiologically activate iNKT cells, and correspondingly the processes that contribute to their increased or decreased activation in different contexts, remain an ongoing area of inquiry.
Due to their shared use of a canonical TCRα chain, all iNKT cells recognize an unusual type of glycolipid in which the sugar head group is present in an α-anomeric configuration. Certain microbes produce glycolipids of this type that are potent antigens for iNKT cells (reviewed in (8)). Recent studies indicate that bacterial species that can be found within the normal gut microbiota can produce similar antigenic lipids (58, 59), although these may be counter-regulated by related forms produced by other bacteria that are antagonists (60). These studies suggest that, particularly at mucosal sites, TCR-dependent activation of iNKT cells may fluctuate according to the composition of the microbial community.
iNKT cells can also recognize self-lipids as antigens. Mammalian cells do not directly synthesize the α-linked glycolipids recognized by iNKT cells, but the β-linked forms they produce may be converted at low frequencies to α-linked forms that are strongly antigenic (61, 62). Additionally, iNKT cells can recognize mammalian β-linked glycolipids as weak agonists (63). Some antigenic self-lipids, including lysophospholipids, glycosylated sphingolipids, and neutral lipids, are specifically upregulated during inflammation or cellular stress (64–70). Conversely, some non-antigenic self-lipids, such as sphingomyelin, can inhibit presentation of antigenic species (66). Together, the available data suggest that antigenic self-lipids are constitutively present, but are maintained in a manner that is only weakly agonistic for iNKT cells, and that during inflammation or cellular stress the abundance or nature of the antigenic self-lipids changes in a way that provides stronger TCR signals to iNKT cells. Additionally, as discussed below, activation by self antigens can be markedly enhanced by TCR-independent signals (71, 72).
Exposure to inflammatory cytokines (IL-12, IL-18) or elevated levels of the adhesion ligand ICAM-1 selectively promotes iNKT cell IFN-γ secretion (51, 52, 54, 71, 72). Thus, when these signals are present, such as during inflammation, iNKT cells probably predominantly promote inflammatory responses. In contrast, the TCR-dependent activation pathway can promote either pro-inflammatory or anti-inflammatory outcomes (reviewed in (73)), and it has been of considerable interest to understand how TCR-mediated activation of iNKT cells leads to these contrasting effects. Two central factors have emerged: first, that the iNKT cell population contains multiple functionally distinct subsets; and second, that iNKT cell functional responses vary according to antigen characteristics.
In contrast to conventional T cells that become polarized into different effector phenotypes by priming in the periphery, iNKT cells are already cytokine competent as they exit the thymus (74). Murine iNKT cells are segregated into four functionally distinct subsets based on their expression of master-regulator transcription factors that govern cytokine production (Tbet, GATA3, RORγT, E4BP4) and on differences in expression levels of PLZF (promyelocytic leukemia zinc finger), a transcription factor that promotes cellular characteristics associated with innate lymphocytes (28, 75–78). NKT1 cells have a TH1 cytokine profile, often express a cytotoxic effector program, and are PLZFloTbethi; NKT2 cells are characterized by high levels of IL-4 secretion and are PLZFhiGATA3+; NKT17 cells produce IL-17 and express RORγT with intermediate levels of PLZF; NKT10 cells produce IL-10, are preferentially found within adipose tissues, and are negative for PLZF but express E4BP4 (Figure 2A). NKT1, NKT2, and NKT17 lineages are generated during thymic selection, and are thought to home to distinct tissues (79). In contrast, NKT10 cells may originate from other subsets and differentiate into a regulatory phenotype as a result of exposure to factors in adipose tissues (27). The identification of these iNKT sub-lineages has led to the paradigm that the anti-inflammatory effects of iNKT cells are due to NKT2 or NKT10 cells, which become activated in different situations than NKT1 and NKT17 subsets as a result of differences in tissue localization.
Figure 2 Determinants of the nature of the functional response mediated by iNKT cells. In both mice and humans the nature of the response mediated by iNKT cells may depend on the subset of iNKT cells activated or on the characteristics of the antigenic stimulation leading to activation. However, there are important differences between mice and humans in each of these parameters. (A, C) Murine iNKT cells can be classified into four lineages with functionally segregated cytokine profiles; whereas the two major subsets of human iNKT cells are characterized by comparatively polyfunctional cytokine production (CD4+) or a more TH1/cytotoxic profile (CD4-). (B, D) Structural features of lipid antigens can bias murine iNKT cell responses towards either a TH1 or TH2 output, whereas human iNKT cell cytokine production proceeds in a hierarchical manner depending on the strength of the TCR signal. Antigens that stimulate a TH1-biased response in mice typically also produce a strong secondary wave of IFN-γ production by NK cells, whereas strong agonists produce this effect from human iNKT cells.
In contrast, it has thus far not been straightforward to categorize human iNKT cells into NKT1, NKT2, and NKT17 lineages matching those in mice. Similar to their murine counterparts, most human iNKT cells express PLZF (80–82), and are characterized by an innate-like transcriptional profile that results in a “poised-effector” status allowing them to rapidly mediate functional responses (83). Multi-parameter flow cytometric analyses and gene expression studies have revealed human iNKT cells to express a diverse selection of cytokines and chemokines (84–88). Human iNKT cells can be segregated into two major subsets according to CD4 expression (84, 85). Those that express CD4 often appear to co-produce GM-CSF, IL-13, TNF-α, IFN-γ, IL-4, and IL-2, while those lacking CD4 appear more specialized for cytolysis (Figure 2C). These two major populations are sub-divided into further subsets characterized by additional markers (e.g. CD8α, CD161, CD62L) with distinctions in functional characteristics, but it is not clear that these subsets equate to the NKT1, NKT2, or NKT17 lineages observed in mice (89, 90). It is also not clear whether anti-inflammatory activity segregates according to CD4+ or CD4- status of human iNKT cells, although CD4+ iNKT cells are the ones that have been found to induce regulatory functions in monocytic cells, and the CD4- subset has appeared more likely to kill DCs.
The prototypical iNKT antigen is called α-galactosylceramide (α-GalCer) (91), and synthetic forms of this lipid have proved extremely valuable as pharmacological agents that activate iNKT cells in a highly specific manner (92). Observations that structural variants of α-GalCer can produce substantially different immunological outcomes in vivo have led to interest in using these agents to selectively tune iNKT responses towards pro- or anti-inflammatory functions (92). Administration of α-GalCer to mice potently stimulates iNKT cells, and induces a mixed response where TH1, TH2, and regulatory cytokines are all produced, although with different kinetics (93). In contrast, certain analogues of α-GalCer have been shown to produce a TH2-biased cytokine response (94, 95), while other variants produce a highly TH1-biased response (96) (Figure 2B). The mechanisms underlying these differential responses appear complex. One component may be that certain variants induce biased cytokine production from iNKT cells themselves (97), while another important element likely relates to whether or not antigen-driven interactions between iNKT cells and APCs result in release of cytokines (e.g. IL-12) that activate a secondary IFN-γ response by NK cells (96, 98). A key factor may be the relative duration of antigen presentation by CD1d molecules, with more durable antigens being associated with TH1-biased responses (99). Additionally, TH1-biasing forms of α-GalCer may be selectively presented by APCs that produce IL-12, whereas TH2-biasing forms may be more promiscuously presented and thus less likely to produce a secondary wave of IFN-γ production by NK cells (100). It is not clear whether antigen variants selectively activate different iNKT cell subsets, or bias the cytokine profile produced within a given subset (for example, by inducing higher IL-4 production by NKT1 cells, or increased IFN-γ by NKT2 or NKT17 cells), or whether any structural variants selectively promote IL-10 production. Interestingly, repeated administration of α-GalCer results in selective loss of its TH1-promoting features, but under such “anergizing” conditions α-GalCer retains the ability to induce IL-4 secretion and to promote control of EAE pathology (101).
Whether human iNKT cell responses can be modulated similarly using α-GalCer structural variants remains an open question. It has become clear that TCR differences between murine and human iNKT cells result in significant discrepancies in TCR-signaling strength induced by lipid variants (102). Perhaps more importantly, polyfunctional human iNKT cells show a hierarchy of cytokine production in response to TCR stimulation that does not neatly segregate into clear TH1 or TH2 patterns. Weak TCR stimulation of human iNKT cells preferentially induces production of IL-3, GM-CSF, and IL-13, with increasing stimulation leading first to IFN-γ, then IL-4, then IL-2 (44, 103, 104) (Figure 2D). Secondary induction of NK cell IFN-γ secretion was associated with activation of human iNKT cells by strong TCR agonists (104). It is therefore not clear that it will be feasible to selectively polarize human immune responses towards IL-4 production through the use of specific lipid antigen variants, although it may be possible to drive IL-13 production through administration of weak agonists.
The potential of engaging iNKT cells therapeutically to treat TH1-inflammatory pathology is well supported by pre-clinical studies in murine models, in vitro experiments using human cells, and ex vivo analyses of human subjects, but clinical data have been limited. Recently, however, a pilot clinical trial using allogeneic iNKT cells as a cellular immunotherapy to treat patients who were intubated with acute respiratory distress syndrome (ARDS) secondary to SARS-CoV-2 infection has shown highly promising results, with 77% survival of treated patients compared to a national average of 40% survival for other intubated SARS-CoV-2 patients during the same period of enrollment (105). Understanding whether such iNKT cell therapies work through one of the regulatory pathways shown in Figure 1A, or through elimination of inflammatory cells via cytolysis as depicted in Figure 1B, has important implications. For example, if APC killing is a key component it may be necessary to deliver a strong TCR signal to the iNKT cells to prime their cytolytic activity. Alternatively, if a regulatory pathway is involved it may be beneficial to generate iNKT cells that are biased towards production of TH2 cytokines or IL-10, depending on the pathway.
Also critical to developing effective iNKT cell therapies is to determine whether human iNKT cells include stable regulatory subsets, or whether polyfunctional iNKT cells are converted into a regulatory phenotype through particular signals. If a stable NKT10 lineage exists in humans, an attractive option might be to specifically engage these cells for immunotherapy. Alternatively, if human iNKT cells generally retain functional plasticity, it may be important to identify methods to specifically promote their regulatory functions. To this end, a recent study found that the presence of IL-7 during in vitro expansion of human iNKT cells resulted in a CD4+ population with enhanced TH2 cytokine production (106), while exposure to short chain fatty acids, palmitate, or the mTOR inhibitor rapamycin may induce a regulatory phenotype (27, 37, 107). Another important consideration is that iNKT immunotherapy that engages TH2 pathways would likely be contraindicated in certain inflammatory diseases, including asthma, chronic obstructive pulmonary disease, and ulcerative colitis, where TH2 cytokine production by iNKT cells has been associated with disease-exacerbating effects (reviewed in (108–110)).
Overall, studies of human and murine iNKT cells over the last three decades clearly support the potential of this unique population to be utilized clinically to control inflammatory pathology. Key areas of further investigation will be to better understand the antigens that physiologically or pharmacologically activate human iNKT cells, and to determine the impact of iNKT cell antigenic activation in different tissues or by distinct APCs. For example, since lipid antigens can be retained locally at the site of administration (111), or distributed to distal sites through binding to lipid transport proteins (112, 113), it may be possible to control the location of iNKT cell activation. Additionally, since iNKT cells promote anti-inflammatory outcomes through interactions with multiple distinct APC populations, it may be possible to direct specific effects through engaging particular APC types, such as the regulatory B cells that ameliorate arthritic pathology (114). It will also be of importance to understand roles of non-invariant populations of CD1d-restricted T cells (often called type II NKT cells, reviewed in reference (115)), and to determine whether these other T cell populations promote or counter-regulate anti-inflammatory outcomes mediated by the “type I” iNKT cells discussed here. Finally, given the likely importance of TCR and CD1d structural differences, the difference in abundance between murine and human iNKT cells (common experimental mouse strains have ~100-fold higher frequencies of iNKT cells than humans), and of additional CD1 molecules (CD1a,b, c, and e) expressed in humans that may impact antigen availability or T cell responses (7, 116, 117), an important step for translating iNKT-based immunotherapies to the clinic may be the development of new animal models that better capture determinants that affect human iNKT cell functions.
NSB and JEG wrote the manuscript and generated the figures. All authors listed have made a substantial, direct, and intellectual contribution to the work and approved it for publication.
Major support provided by NIH R01 AI136500 to JG; NB also supported by funding from the University of Wisconsin-Madison’s Office of the Vice Chancellor for Research and Graduate Education through the Fall Competition Program.
Author JG is a member of the Scientific Advisory Board of MiNK Therapeutics.
The remaining author declares that the research was conducted in the absence of any commercial or financial relationships that could be construed as a potential conflict of interest.
All claims expressed in this article are solely those of the authors and do not necessarily represent those of their affiliated organizations, or those of the publisher, the editors and the reviewers. Any product that may be evaluated in this article, or claim that may be made by its manufacturer, is not guaranteed or endorsed by the publisher.
1. Gumperz JE. Antigen specificity of semi-invariant CD1d-restricted T cell receptors: the best of both worlds? Immunol Cell Biol (2004) 82(3):285–94. doi: 10.1111/j.0818-9641.2004.01257.x
2. Zajonc DM, Kronenberg M. Carbohydrate specificity of the recognition of diverse glycolipids by natural killer T cells. Immunol Rev (2009) 230(1):188–200. doi: 10.1111/j.1600-065X.2009.00802.x
3. Van Rhijn I, Godfrey DI, Rossjohn J, Moody DB. Lipid and small-molecule display by CD1 and MR1. Nat Rev Immunol (2015) 15(10):643–54. doi: 10.1038/nri3889
4. Oteo M, Parra JF, Mirones I, Gimenez LI, Setien F, Martinez-Naves E. Single strand conformational polymorphism analysis of human CD1 genes in different ethnic groups. Tissue Antigens (1999) 53(6):545–50. doi: 10.1034/j.1399-0039.1999.530604.x
5. Exley M, Garcia J, Wilson SB, Spada F, Gerdes D, Tahir SM, et al. CD1d structure and regulation on human thymocytes, peripheral blood T cells, b cells and monocytes. Immunology (2000) 100(1):37–47. doi: 10.1046/j.1365-2567.2000.00001.x
6. Canchis PW, Bhan AK, Landau SB, Yang L, Balk SP, Blumberg RS. Tissue distribution of the non-polymorphic major histocompatibility complex class I-like molecule, CD1d. Immunology (1993) 80(4):561–5.
7. Moody DB, Cotton RN. Four pathways of CD1 antigen presentation to T cells. Curr Opin Immunol (2017) 46:127–33. doi: 10.1016/j.coi.2017.07.013
8. McEwen-Smith RM, Salio M, Cerundolo V. CD1d-dependent endogenous and exogenous lipid antigen presentation. Curr Opin Immunol (2015) 34:116–25. doi: 10.1016/j.coi.2015.03.004
9. Salio M, Cerundolo V. Linking inflammation to natural killer T cell activation. PloS Biol (2009) 7(10):e1000226. doi: 10.1371/journal.pbio.1000226
10. Van Kaer L, Wu L. Therapeutic potential of invariant natural killer T cells in autoimmunity. Front Immunol (2018) 9:519. doi: 10.3389/fimmu.2018.00519
11. Rakhshandehroo M, Kalkhoven E, Boes M. Invariant natural killer T cells in adipose tissue: novel regulators of immune-mediated metabolic disease. Cell Mol Life Sci (2013) 70(24):4711–27. doi: 10.1007/s00018-013-1414-1
12. Negrin RS. Immune regulation in hematopoietic cell transplantation. Bone Marrow Transplant (2019) 54(Suppl 2):765–8. doi: 10.1038/s41409-019-0600-7
13. Kinjo Y, Takatsuka S, Kitano N, Kawakubo S, Abe M, Ueno K, et al. Functions of CD1d-restricted invariant natural killer T cells in antimicrobial immunity and potential applications for infection control. Front Immunol (2018) 9:1266. doi: 10.3389/fimmu.2018.01266
14. Shimizu K, Iyoda T, Yamasaki S, Kadowaki N, Tojo A, Fujii AS. NK and NKT cell-mediated immune surveillance against hematological malignancies. Cancers (Basel) (2020) 12(4):817. doi: 10.3390/cancers12040817
15. Felley L, Gumperz JE. Are human iNKT cells keeping tabs on lipidome perturbations triggered by oxidative stress in the blood? Immunogenetics (2016) 68(8):611–22. doi: 10.1007/s00251-016-0936-8
16. Field JJ, Nathan DG, Linden J. Targeting iNKT cells for the treatment of sickle cell disease. Clin Immunol (2011) 140(2):177–83. doi: 10.1016/j.clim.2011.03.002
17. Leung B, Harris HW. NKT cells: the culprits of sepsis? J Surg Res (2011) 167(1):87–95. doi: 10.1016/j.jss.2010.09.038
18. Hammond KJ, Poulton LD, Palmisano LJ, Silveira PA, Godfrey DI, Baxter AG. Alpha/beta-T cell receptor (TCR)+CD4-CD8- (NKT) thymocytes prevent insulin-dependent diabetes mellitus in nonobese diabetic (NOD)/Lt mice by the influence of interleukin (IL)-4 and/or IL-10. J Exp Med (1998) 187(7):1047–56. doi: 10.1084/jem.187.7.1047
19. Lehuen A, Lantz O, Beaudoin L, Laloux V, Carnaud C, Bendelac A, et al. Overexpression of natural killer T cells protects Valpha14- Jalpha281 transgenic nonobese diabetic mice against diabetes. J Exp Med (1998) 188(10):1831–9. doi: 10.1084/jem.188.10.1831
20. Hong S, Wilson MT, Serizawa I, Wu L, Singh N, Naidenko OV, et al. The natural killer T-cell ligand alpha-galactosylceramide prevents autoimmune diabetes in non-obese diabetic mice. Nat Med (2001) 7(9):1052–6. doi: 10.1038/nm0901-1052
21. Falcone M, Facciotti F, Ghidoli N, Monti P, Olivieri S, Zaccagnino L, et al. Up-regulation of CD1d expression restores the immunoregulatory function of NKT cells and prevents autoimmune diabetes in nonobese diabetic mice. J Immunol (2004) 172(10):5908–16. doi: 10.4049/jimmunol.172.10.5908
22. Naumov YN, Bahjat KS, Gausling R, Abraham R, Exley MA, Koezuka Y, et al. Activation of CD1d-restricted T cells protects NOD mice from developing diabetes by regulating dendritic cell subsets. Proc Natl Acad Sci U S A (2001) 98(24):13838–43. doi: 10.1073/pnas.251531798
23. Beaudoin L, Laloux V, Novak J, Lucas B, Lehuen A. NKT cells inhibit the onset of diabetes by impairing the development of pathogenic T cells specific for pancreatic beta cells. Immunity (2002) 17(6):725–36. doi: 10.1016/S1074-7613(02)00473-9
24. Chen YG, Choisy-Rossi CM, Holl TM, Chapman HD, Besra GS, Porcelli SA, et al. Activated NKT cells inhibit autoimmune diabetes through tolerogenic recruitment of dendritic cells to pancreatic lymph nodes. J Immunol (2005) 174(3):1196–204. doi: 10.4049/jimmunol.174.3.1196
25. Wang J, Cho S, Ueno A, Cheng L, Xu BY, Desrosiers MD, et al. Ligand-dependent induction of noninflammatory dendritic cells by anergic invariant NKT cells minimizes autoimmune inflammation. J Immunol (2008) 181(4):2438–45. doi: 10.4049/jimmunol.181.4.2438
26. Lynch L, Nowak M, Varghese B, Clark J, Hogan AE, Toxavidis V, et al. Adipose tissue invariant NKT cells protect against diet-induced obesity and metabolic disorder through regulatory cytokine production. Immunity (2012) 37(3):574–87. doi: 10.1016/j.immuni.2012.06.016
27. LaMarche NM, Kane H, Kohlgruber AC, Dong H, Lynch L, Brenner MB. Distinct iNKT cell populations use IFNgamma or ER stress-induced IL-10 to control adipose tissue homeostasis. Cell Metab (2020) 32(2):243–58.e6. doi: 10.1016/j.cmet.2020.05.017
28. Lynch L, Michelet X, Zhang S, Brennan PJ, Moseman A, Lester C, et al. Regulatory iNKT cells lack expression of the transcription factor PLZF and control the homeostasis of t(reg) cells and macrophages in adipose tissue. Nat Immunol (2015) 16(1):85–95. doi: 10.1038/ni.3047
29. Liew PX, Lee WY, Kubes P. iNKT cells orchestrate a switch from inflammation to resolution of sterile liver injury. Immunity (2017) 47(4):752–65.e5. doi: 10.1016/j.immuni.2017.09.016
30. Goto T, Ito Y, Satoh M, Nakamoto S, Nishizawa N, Hosono K, et al. Activation of iNKT cells facilitates liver repair after hepatic ischemia reperfusion injury through acceleration of macrophage polarization. Front Immunol (2021) 12:754106. doi: 10.3389/fimmu.2021.754106
31. Hashimoto D, Asakura S, Miyake S, Yamamura T, Van Kaer L, Liu C, et al. Stimulation of host NKT cells by synthetic glycolipid regulates acute graft-versus-host disease by inducing Th2 polarization of donor T cells. J Immunol (2005) 174(1):551–6. doi: 10.4049/jimmunol.174.1.551
32. Pillai AB, George TI, Dutt S, Strober S. Host natural killer T cells induce an interleukin-4-dependent expansion of donor CD4+CD25+Foxp3+ T regulatory cells that protects against graft-versus-host disease. Blood (2009) 113(18):4458–67. doi: 10.1182/blood-2008-06-165506
33. Leveson-Gower DB, Olson JA, Sega EI, Luong RH, Baker J, Zeiser R, et al. Low doses of natural killer T cells provide protection from acute graft-versus-host disease via an IL-4-dependent mechanism. Blood (2011) 117(11):3220–9. doi: 10.1182/blood-2010-08-303008
34. Du J, Paz K, Thangavelu G, Schneidawind D, Baker J, Flynn R, et al. Invariant natural killer T cells ameliorate murine chronic GVHD by expanding donor regulatory T cells. Blood (2017) 129(23):3121–5. doi: 10.1182/blood-2016-11-752444
35. Schneidawind D, Baker J, Pierini A, Buechele C, Luong RH, Meyer EH, et al. Third-party CD4+ invariant natural killer T cells protect from murine GVHD lethality. Blood (2015) 125(22):3491–500. doi: 10.1182/blood-2014-11-612762
36. Schneidawind D, Pierini A, Alvarez M, Pan Y, Baker J, Buechele C, et al. CD4+ invariant natural killer T cells protect from murine GVHD lethality through expansion of donor CD4+CD25+FoxP3+ regulatory T cells. Blood (2014) 124(22):3320–8. doi: 10.1182/blood-2014-05-576017
37. Burrello C, Strati F, Lattanzi G, Diaz-Basabe A, Mileti E, Giuffre MR, et al. IL10 secretion endows intestinal human iNKT cells with regulatory functions towards pathogenic T lymphocytes. J Crohns Colitis (2022). doi: 10.1093/ecco-jcc/jjac049
38. Venken K, Decruy T, Aspeslagh S, Van Calenbergh S, Lambrecht BN, Elewaut D. Bacterial CD1d-restricted glycolipids induce IL-10 production by human regulatory T cells upon cross-talk with invariant NKT cells. J Immunol (2013) 191(5):2174–83. doi: 10.4049/jimmunol.1300562
39. Hegde S, Chen X, Keaton JM, Reddington F, Besra GS, Gumperz JE. NKT cells direct monocytes into a DC differentiation pathway. J Leukoc Biol (2007) 81(5):1224–35. doi: 10.1189/jlb.1206718
40. Hegde S, Jankowska-Gan E, Roenneburg DA, Torrealba J, Burlingham WJ, Gumperz JE. Human NKT cells promote monocyte differentiation into suppressive myeloid antigen-presenting cells. J Leukoc Biol (2009) 86(4):757–68. doi: 10.1189/jlb.0209059
41. Hegde S, Lockridge JL, Becker YA, Ma S, Kenney SC, Gumperz JE. Human NKT cells direct the differentiation of myeloid APCs that regulate T cell responses via expression of programmed cell death ligands. J Autoimmun (2011) 37(1):28–38. doi: 10.1016/j.jaut.2011.03.001
42. Terabe M, Matsui S, Noben-Trauth N, Chen H, Watson C, Donaldson DD, et al. NKT cell-mediated repression of tumor immunosurveillance by IL-13 and the IL-4R-STAT6 pathway. Nat Immunol (2000) 1(6):515–20. doi: 10.1038/82771
43. Terabe M, Matsui S, Park JM, Mamura M, Noben-Trauth N, Donaldson DD, et al. Transforming growth factor-beta production and myeloid cells are an effector mechanism through which CD1d-restricted T cells block cytotoxic T lymphocyte-mediated tumor immunosurveillance: abrogation prevents tumor recurrence. J Exp Med (2003) 198(11):1741–52. doi: 10.1084/jem.20022227
44. Hess NJ, SB N, Bobeck EA, McDougal CE, Ma S, Sauer JD, et al. iNKT cells coordinate immune pathways to enable engraftment in nonconditioned hosts. Life Sci Alliance (2021) 4(7). doi: 10.26508/lsa.202000999
45. Muller AM, Florek M, Kohrt HE, Kupper NJ, Filatenkov A, Linderman JA, et al. Blood stem cell activity is arrested by Th1-mediated injury preventing engraftment following nonmyeloablative conditioning. J Immunol (2016) 197(10):4151–62. doi: 10.4049/jimmunol.1500715
46. Nicol A, Nieda M, Koezuka Y, Porcelli S, Suzuki K, Tadokoro K, et al. Dendritic cells are targets for human invariant Valpha24+ natural killer T-cell cytotoxic activity: an important immune regulatory function. Exp Hematol (2000) 28(3):276–82. doi: 10.1016/S0301-472X(99)00149-6
47. Yang OO, Racke FK, Nguyen PT, Gausling R, Severino ME, Horton HF, et al. CD1d on myeloid dendritic cells stimulates cytokine secretion from and cytolytic activity of V alpha 24J alpha q T cells: a feedback mechanism for immune regulation. J Immunol (2000) 165(7):3756–62. doi: 10.4049/jimmunol.165.7.3756
48. Liu TY, Uemura Y, Suzuki M, Narita Y, Hirata S, Ohyama H, et al. Distinct subsets of human invariant NKT cells differentially regulate T helper responses via dendritic cells. Eur J Immunol (2008) 38(4):1012–23. doi: 10.1002/eji.200737838
49. Schmid H, Ribeiro EM, Secker KA, Duerr-Stoerzer S, Keppeler H, Dong R, et al. Human invariant natural killer T cells promote tolerance by preferential apoptosis induction of conventional dendritic cells. Haematologica (2022) 107(2):427–36. doi: 10.3324/haematol.2020.267583
50. Kok WL, Denney L, Benam K, Cole S, Clelland C, McMichael AJ, et al. Pivotal advance: Invariant NKT cells reduce accumulation of inflammatory monocytes in the lungs and decrease immune-pathology during severe influenza a virus infection. J Leukoc Biol (2012) 91(3):357–68. doi: 10.1189/jlb.0411184
51. Nagarajan NA, Kronenberg M. Invariant NKT cells amplify the innate immune response to lipopolysaccharide. J Immunol (2007) 178(5):2706–13. doi: 10.4049/jimmunol.178.5.2706
52. Wang X, Bishop KA, Hegde S, Rodenkirch LA, Pike JW, Gumperz JE. Human invariant natural killer T cells acquire transient innate responsiveness via histone H4 acetylation induced by weak TCR stimulation. J Exp Med (2012) 209(5):987–1000. doi: 10.1084/jem.20111024
53. Holzapfel KL, Tyznik AJ, Kronenberg M, Hogquist KA. Antigen-dependent versus -independent activation of invariant NKT cells during infection. J Immunol (2014) 192(12):5490–8. doi: 10.4049/jimmunol.1400722
54. Sharma A, Lawry SM, Klein BS, Wang X, Sherer NM, Zumwalde NA, et al. LFA-1 ligation by high-density ICAM-1 is sufficient to activate IFN-gamma release by innate T lymphocytes. J Immunol (2018) 201(8):2452–61. doi: 10.4049/jimmunol.1800537
55. Metelitsa LS, Naidenko OV, Kant A, Wu HW, Loza MJ, Perussia B, et al. Human NKT cells mediate antitumor cytotoxicity directly by recognizing target cell CD1d with bound ligand or indirectly by producing IL-2 to activate NK cells. J Immunol (2001) 167(6):3114–22. doi: 10.4049/jimmunol.167.6.3114
56. Metelitsa LS, Weinberg KI, Emanuel PD, Seeger RC. Expression of CD1d by myelomonocytic leukemias provides a target for cytotoxic NKT cells. Leukemia (2003) 17(6):1068–77. doi: 10.1038/sj.leu.2402943
57. Chen X, Wang X, Besra GS, Gumperz JE. Modulation of CD1d-restricted NKT cell responses by CD4. J Leukoc Biol (2007) 82(6):1455–65. doi: 10.1189/jlb.0307163
58. von Gerichten J, Lamprecht D, Opalka L, Soulard D, Marsching C, Pilz R, et al. Bacterial immunogenic alpha-galactosylceramide identified in the murine large intestine: dependency on diet and inflammation. J Lipid Res (2019) 60(11):1892–904. doi: 10.1194/jlr.RA119000236
59. Wieland Brown LC, Penaranda C, Kashyap PC, Williams BB, Clardy J, Kronenberg M, et al. Production of alpha-galactosylceramide by a prominent member of the human gut microbiota. PloS Biol (2013) 11(7):e1001610. doi: 10.1371/journal.pbio.1001610
60. An D, Oh SF, Olszak T, Neves JF, Avci FY, Erturk-Hasdemir D, et al. Sphingolipids from a symbiotic microbe regulate homeostasis of host intestinal natural killer T cells. Cell (2014) 156(1-2):123–33. doi: 10.1016/j.cell.2013.11.042
61. Kain L, Webb B, Anderson BL, Deng S, Holt M, Costanzo A, et al. The identification of the endogenous ligands of natural killer T cells reveals the presence of mammalian alpha-linked glycosylceramides. Immunity (2014) 41(4):543–54. doi: 10.1016/j.immuni.2014.08.017
62. Kain L, Costanzo A, Webb B, Holt M, Bendelac A, Savage PB, et al. Endogenous ligands of natural killer T cells are alpha-linked glycosylceramides. Molec Immunol (2015) 68(2 Pt A):94–7. doi: 10.1016/j.molimm.2015.06.009
63. Ortaldo JR, Young HA, Winkler-Pickett RT, Bere EW Jr., Murphy WJ, Wiltrout RH. Dissociation of NKT stimulation, cytokine induction, and NK activation in vivo by the use of distinct TCR-binding ceramides. J Immunol (2004) 172(2):943–53. doi: 10.4049/jimmunol.172.2.943
64. Paget C, Mallevaey T, Speak AO, Torres D, Fontaine J, Sheehan KC, et al. Activation of invariant NKT cells by toll-like receptor 9-stimulated dendritic cells requires type I interferon and charged glycosphingolipids. Immunity (2007) 27(4):597–609. doi: 10.1016/j.immuni.2007.08.017
65. Salio M, Speak AO, Shepherd D, Polzella P, Illarionov PA, Veerapen N, et al. Modulation of human natural killer T cell ligands on TLR-mediated antigen-presenting cell activation. Proc Natl Acad Sci U S A (2007) 104(51):20490–5. doi: 10.1073/pnas.0710145104
66. Fox LM, Cox DG, Lockridge JL, Wang X, Chen X, Scharf L, et al. Recognition of lyso-phospholipids by human natural killer T lymphocytes. PloS Biol (2009) 7(10):e1000228. doi: 10.1371/journal.pbio.1000228
67. Facciotti F, Ramanjaneyulu GS, Lepore M, Sansano S, Cavallari M, Kistowska M, et al. Peroxisome-derived lipids are self antigens that stimulate invariant natural killer T cells in the thymus. Nat Immunol (2012) 13(5):474–80. doi: 10.1038/ni.2245
68. Gately CM, Podbielska M, Counihan T, Hennessy M, Leahy T, Moran AP, et al. Invariant natural killer T-cell anergy to endogenous myelin acetyl-glycolipids in multiple sclerosis. J Neuroimmunol (2013) 259(1-2):1–7. doi: 10.1016/j.jneuroim.2013.02.020
69. Bedard M, Shrestha D, Priestman DA, Wang Y, Schneider F, Matute JD, et al. Sterile activation of invariant natural killer T cells by ER-stressed antigen-presenting cells. Proc Natl Acad Sci USA (2019) 116(47):23671–81. doi: 10.1073/pnas.1910097116
70. Govindarajan S, Verheugen E, Venken K, Gaublomme D, Maelegheer M, Cloots E, et al. ER stress in antigen-presenting cells promotes NKT cell activation through endogenous neutral lipids. EMBO Rep (2020) 21(6):e48927. doi: 10.15252/embr.201948927
71. Brigl M, Bry L, Kent SC, Gumperz JE, Brenner MB. Mechanism of CD1d-restricted natural killer T cell activation during microbial infection. Nat Immunol (2003) 4(12):1230–7. doi: 10.1038/ni1002
72. Brigl M, Tatituri RV, Watts GF, Bhowruth V, Leadbetter EA, Barton N, et al. Innate and cytokine-driven signals, rather than microbial antigens, dominate in natural killer T cell activation during microbial infection. J Exp Med (2011) 208(6):1163–77. doi: 10.1084/jem.20102555
73. Godfrey DI, Kronenberg M. Going both ways: immune regulation via CD1d-dependent NKT cells. J Clin Invest (2004) 114(10):1379–88. doi: 10.1172/JCI200423594
74. Stetson DB, Mohrs M, Reinhardt RL, Baron JL, Wang ZE, Gapin L, et al. Constitutive cytokine mRNAs mark natural killer (NK) and NK T cells poised for rapid effector function. J Exp Med (2003) 198(7):1069–76. doi: 10.1084/jem.20030630
75. Watarai H, Sekine-Kondo E, Shigeura T, Motomura Y, Yasuda T, Satoh R, et al. Development and function of invariant natural killer T cells producing T(h)2- and T(h)17-cytokines. PloS Biol (2012) 10(2):e1001255. doi: 10.1371/journal.pbio.1001255
76. Constantinides MG, Bendelac A. Transcriptional regulation of the NKT cell lineage. Cur Opin Immunol (2013) 25(2):161–7. doi: 10.1016/j.coi.2013.01.003
77. Lee YJ, Holzapfel KL, Zhu J, Jameson SC, Hogquist KA. Steady-state production of IL-4 modulates immunity in mouse strains and is determined by lineage diversity of iNKT cells. Nat Immunol (2013) 14(11):1146–54. doi: 10.1038/ni.2731
78. Wang H, Hogquist KA. How lipid-specific T cells become effectors: The differentiation of iNKT subsets. Front Immunol (2018) 9:1450. doi: 10.3389/fimmu.2018.01450
79. Lee YJ, Wang H, Starrett GJ, Phuong V, Jameson SC, Hogquist KA. Tissue-specific distribution of iNKT cells impacts their cytokine response. Immunity (2015) 43(3):566–78. doi: 10.1016/j.immuni.2015.06.025
80. Savage AK, Constantinides MG, Han J, Picard D, Martin E, Li B, et al. The transcription factor PLZF directs the effector program of the NKT cell lineage. Immunity (2008) 29(3):391–403. doi: 10.1016/j.immuni.2008.07.011
81. Eidson M, Wahlstrom J, Beaulieu AM, Zaidi B, Carsons SE, Crow PK, et al. Altered development of NKT cells, gammadelta T cells, CD8 T cells and NK cells in a PLZF deficient patient. PloS One (2011) 6(9):e24441. doi: 10.1371/journal.pone.0024441
82. Constantinides MG, Picard D, Savage AK, Bendelac A. A naive-like population of human CD1d-restricted T cells expressing intermediate levels of promyelocytic leukemia zinc finger. J Immunol (2011) 187(1):309–15. doi: 10.4049/jimmunol.1100761
83. Gutierrez-Arcelus M, Teslovich N, Mola AR, Polidoro RB, Nathan A, Kim H, et al. Lymphocyte innateness defined by transcriptional states reflects a balance between proliferation and effector functions. Nat Commun (2019) 10(1):687. doi: 10.1038/s41467-019-08604-4
84. Gumperz JE, Miyake S, Yamamura T, Brenner MB. Functionally distinct subsets of CD1d-restricted natural killer T cells revealed by CD1d tetramer staining. J Exp Med (2002) 195(5):625–36. doi: 10.1084/jem.20011786
85. Lee PT, Benlagha K, Teyton L, Bendelac A. Distinct functional lineages of human Valpha24 natural killer T cells. J Exp Med (2002) 195(5):637–41. doi: 10.1084/jem.20011908
86. Montoya CJ, Pollard D, Martinson J, Kumari K, Wasserfall C, Mulder CB, et al. Characterization of human invariant natural killer T subsets in health and disease using a novel invariant natural killer T cell-clonotypic monoclonal antibody, 6B11. Immunology (2007) 122(1):1–14. doi: 10.1111/j.1365-2567.2007.02647.x
87. Zhou L, Adrianto I, Wang J, Wu X, Datta I, Mi QS. Single-cell RNA-seq analysis uncovers distinct functional human NKT cell Sub-populations in peripheral blood. Front Cell Dev Biol (2020) 8:384. doi: 10.3389/fcell.2020.00384
88. Erkers T, Xie BJ, Kenyon LJ, Smith B, Rieck M, Jensen KP, et al. High-parametric evaluation of human invariant natural killer T cells to delineate heterogeneity in allo- and autoimmunity. Blood (2020) 135(11):814–25. doi: 10.1182/blood.2019001903
89. O’Reilly V, Zeng SG, Bricard G, Atzberger A, Hogan AE, Jackson J, et al. Distinct and overlapping effector functions of expanded human CD4+, CD8alpha+ and CD4-CD8alpha- invariant natural killer T cells. PloS One (2011) 6(12):e28648. doi: 10.1371/journal.pone.0028648
90. Chan AC, Leeansyah E, Cochrane A, d’Udekem d’Acoz Y, Mittag D, Harrison LC, et al. Ex-vivo analysis of human natural killer T cells demonstrates heterogeneity between tissues and within established CD4(+) and CD4(-) subsets. Clin Exp Immunol (2013) 172(1):129–37. doi: 10.1111/cei.12045
91. Kawano T, Cui J, Koezuka Y, Toura I, Kaneko Y, Motoki K, et al. CD1d-restricted and TCR-mediated activation of valpha14 NKT cells by glycosylceramides. Science (1997) 278(5343):1626–9. doi: 10.1126/science.278.5343.1626
92. Carreno LJ, Saavedra-Avila NA, Porcelli SA. Synthetic glycolipid activators of natural killer T cells as immunotherapeutic agents. Clin Transl Immunol (2016) 5(4):e69. doi: 10.1038/cti.2016.14
93. Burdin N, Brossay L, Kronenberg M. Immunization with alpha-galactosylceramide polarizes CD1-reactive NK T cells towards Th2 cytokine synthesis. Eur J Immunol (1999) 29(6):2014–25. doi: 10.1002/(SICI)1521-4141(199906)29:06<2014::AID-IMMU2014>3.0.CO;2-G
94. Miyamoto K, Miyake S, Yamamura T. A synthetic glycolipid prevents autoimmune encephalomyelitis by inducing TH2 bias of natural killer T cells. Nature (2001) 413(6855):531–4. doi: 10.1038/35097097
95. Yu KO, Im JS, Molano A, Dutronc Y, Illarionov PA, Forestier C, et al. Modulation of CD1d-restricted NKT cell responses by using n-acyl variants of alpha-galactosylceramides. Proc Natl Acad Sci U S A (2005) 102(9):3383–8. doi: 10.1073/pnas.0407488102
96. Schmieg J, Yang G, Franck RW, Tsuji M. Superior protection against malaria and melanoma metastases by a c-glycoside analogue of the natural killer T cell ligand alpha-galactosylceramide. J Exp Med (2003) 198(11):1631–41. doi: 10.1084/jem.20031192
97. Goff RD, Gao Y, Mattner J, Zhou D, Yin N, Cantu C, et al. Effects of lipid chain lengths in alpha-galactosylceramides on cytokine release by natural killer T cells. J Am Chem Soc (2004) 126(42):13602–3. doi: 10.1021/ja045385q
98. Arora P, Baena A, Yu KO, Saini NK, Kharkwal SS, Goldberg MF, et al. A single subset of dendritic cells controls the cytokine bias of natural killer T cell responses to diverse glycolipid antigens. Immunity (2014) 40(1):105–16. doi: 10.1016/j.immuni.2013.12.004
99. Sullivan BA, Nagarajan NA, Wingender G, Wang J, Scott I, Tsuji M, et al. Mechanisms for glycolipid antigen-driven cytokine polarization by Valpha14i NKT cells. J Immunol (2010) 184(1):141–53. doi: 10.4049/jimmunol.0902880
100. Bai L, Constantinides MG, Thomas SY, Reboulet R, Meng F, Koentgen F, et al. Distinct APCs explain the cytokine bias of alpha-galactosylceramide variants in vivo. J Immunol (2012) 188(7):3053–61. doi: 10.4049/jimmunol.1102414
101. Parekh VV, Wilson MT, Olivares-Villagomez D, Singh AK, Wu L, Wang CR, et al. Glycolipid antigen induces long-term natural killer T cell anergy in mice. J Clin Invest (2005) 115(9):2572–83. doi: 10.1172/JCI24762
102. Dangerfield EM, Cheng JM, Knight DA, Weinkove R, Dunbar PR, Hermans IF, et al. Species-specific activity of glycolipid ligands for invariant NKT cells. Chembiochem (2012) 13(9):1349–56. doi: 10.1002/cbic.201200095
103. Wang X, Chen X, Rodenkirch L, Simonson W, Wernimont S, Ndonye RM, et al. Natural killer T-cell autoreactivity leads to a specialized activation state. Blood (2008) 112(10):4128–38. doi: 10.1182/blood-2008-05-157529
104. Bricard G, Venkataswamy MM, Yu KO, Im JS, Ndonye RM, Howell AR, et al. Alpha-galactosylceramide analogs with weak agonist activity for human iNKT cells define new candidate anti-inflammatory agents. PloS One (2010) 5(12):e14374. doi: 10.1371/journal.pone.0014374
105. Purbhoo M, Yigit B, Moskowitz D, Lim M, Shapiro I, Alsaraby A, et al. 400 persistence and tissue distribution of agent-797 – a native allogeneic iNKT cell-therapy drug product. J Immunother Cancer (2021) 9(Suppl 2):A432–A. doi: 10.1136/jitc-2021-SITC2021.400
106. Trujillo-Ocampo A, Cho HW, Clowers M, Pareek S, Ruiz-Vazquez W, Lee SE, et al. IL-7 during antigenic stimulation using allogeneic dendritic cells promotes expansion of CD45RA(-)CD62L(+)CD4(+) invariant NKT cells with Th-2 biased cytokine production profile. Front Immunol (2020) 11:567406. doi: 10.3389/fimmu.2020.567406
107. Huijts CM, Schneiders FL, Garcia-Vallejo JJ, Verheul HM, de Gruijl TD, van der Vliet HJ, et al. mTOR inhibition per Se induces nuclear localization of FOXP3 and conversion ofof Invariant NKT (iNKT) cells into immunosuppressive regulatory iNKT cells. J Immunol (2015) 195(5):2038–45. doi: 10.4049/jimmunol.1402710
108. Iwamura C, Nakayama T. Role of CD1d- and MR1-restricted T cells in asthma. Front Immunol (2018) 9:1942. doi: 10.3389/fimmu.2018.01942
109. Paget C, Trottein F. Role of type 1 natural killer T cells in pulmonary immunity. Mucosal Immunol (2013) 6(6):1054–67. doi: 10.1038/mi.2013.59
110. van Dieren JM, van der Woude CJ, Kuipers EJ, Escher JC, Samsom JN, Blumberg RS, et al. Roles of CD1d-restricted NKT cells in the intestine. Inflammation Bowel Dis (2007) 13(9):1146–52. doi: 10.1002/ibd.20164
111. Li X, Kawamura A, Andrews CD, Miller JL, Wu D, Tsao T, et al. Colocalization of a CD1d-binding glycolipid with a radiation-attenuated sporozoite vaccine in lymph node-resident dendritic cells for a robust adjuvant effect. J Immunol (2015) 195(6):2710–21. doi: 10.4049/jimmunol.1403017
112. Freigang S, Kain L, Teyton L. Transport and uptake of immunogenic lipids. Mol Immunol (2013) 55(2):179–81. doi: 10.1016/j.molimm.2012.10.016
113. Major AS, Joyce S, Van Kaer L. Lipid metabolism, atherogenesis and CD1-restricted antigen presentation. Trends Mol Med (2006) 12(6):270–8. doi: 10.1016/j.molmed.2006.04.004
114. Oleinika K, Rosser EC, Matei DE, Nistala K, Bosma A, Drozdov I, et al. CD1d-dependent immune suppression mediated by regulatory b cells through modulations of iNKT cells. Nat Commun (2018) 9(1):684. doi: 10.1038/s41467-018-02911-y
115. Singh AK, Tripathi P, Cardell SL. Type II NKT cells: An elusive population with immunoregulatory properties. Front Immunol (2018) 9:1969. doi: 10.3389/fimmu.2018.01969
116. Fox LM, Miksanek J, May NA, Scharf L, Lockridge JL, Veerapen N, et al. Expression of CD1c enhances human invariant NKT cell activation by alpha-GalCer. Cancer Immun (2013) 13:9. doi: 10.1158/1424-9634.DCL-9.13.2
Keywords: iNKT cell, CD1d, anti-inflammatory, immunotherapy, immuno-regulatory, immunomodulatory
Citation: Bharadwaj NS and Gumperz JE (2022) Harnessing invariant natural killer T cells to control pathological inflammation. Front. Immunol. 13:998378. doi: 10.3389/fimmu.2022.998378
Received: 19 July 2022; Accepted: 25 August 2022;
Published: 15 September 2022.
Edited by:
Luc Van Kaer, Vanderbilt University Medical Center, United StatesReviewed by:
Mariolina Salio, University of Oxford, United KingdomCopyright © 2022 Bharadwaj and Gumperz. This is an open-access article distributed under the terms of the Creative Commons Attribution License (CC BY). The use, distribution or reproduction in other forums is permitted, provided the original author(s) and the copyright owner(s) are credited and that the original publication in this journal is cited, in accordance with accepted academic practice. No use, distribution or reproduction is permitted which does not comply with these terms.
*Correspondence: Jenny E. Gumperz, amVndW1wZXJ6QHdpc2MuZWR1
Disclaimer: All claims expressed in this article are solely those of the authors and do not necessarily represent those of their affiliated organizations, or those of the publisher, the editors and the reviewers. Any product that may be evaluated in this article or claim that may be made by its manufacturer is not guaranteed or endorsed by the publisher.
Research integrity at Frontiers
Learn more about the work of our research integrity team to safeguard the quality of each article we publish.