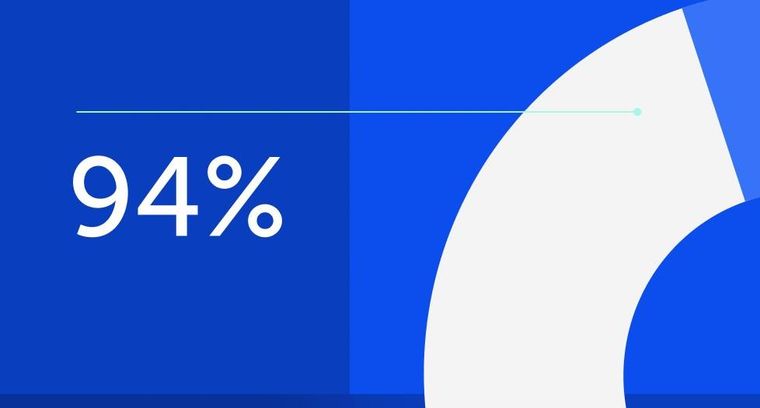
94% of researchers rate our articles as excellent or good
Learn more about the work of our research integrity team to safeguard the quality of each article we publish.
Find out more
ORIGINAL RESEARCH article
Front. Immunol., 20 October 2022
Sec. NK and Innate Lymphoid Cell Biology
Volume 13 - 2022 | https://doi.org/10.3389/fimmu.2022.997806
This article is part of the Research TopicWomen in NK and Innate Lymphoid Cell BiologyView all 9 articles
Natural killer (NK) cells are emerging as unique players in the immune response against cancer; however, only limited data are available on tumor infiltrating NK cells in head and neck squamous cell carcinoma (HNSCC), one of the most common cancer. Occurrence of HNSCC is closely related to the immune microenvironment, and immunotherapy is increasingly being applied to this setting. However, the limited success of this type of treatment in this tumor calls for further investigation in the field.
Surgical HNSSC specimens of 32 consecutive patients were mechanically and enzymatically dissociated. Tumor cells were separated from infiltrating cells by short centrifugation and infiltrating NK cells were phenotypically and functionally characterized by multiple antibody staining and flow cytometry. Tumor infiltrating NK cells in HNSCC showed a peculiar phenotype predominantly characterized by increased NKG2A and reduced Siglec-7, NKG2D, NKp30 and CD16 expression. This phenotype was associated with a decreased ability to perform antibody-dependent cellular cytotoxicity (ADCC). However, NK, CD4 and CD8 shared an increment of glucocorticoid-induced tumor necrosis factor-related (GITR) costimulatory receptor which could be exploited for immunotherapy with agonistic anti-GITR antibodies combined with checkpoint inhibitors.
Head and neck squamous cell carcinoma (HNSCC) is one of the most common cancer with 878348 new cases and 444347 deaths in 2020 worldwide (1). HNSCC includes carcinoma originating from oral cavity, nasopharynx, hypopharynx, oropharynx and larynx, is closely associated to alcohol abuse and tobacco smoke and it may be associated with infection with oncogenic strains of human papillomavirus (HPV), such as HPV-16 and HPV-18. HPV positive HNSCC have a better prognosis than HPV-negative (2, 3). Treatment depends on the stage and the site of the disease, primary treatment options are surgical resection followed by chemotherapy and radiotherapy. The monoclonal antibody Cetuximab directed against epidermal growth factor receptor (EGFR) is used alone or in combination with chemotherapy, for the treatment of patients with recurrent or metastatic disease (4). Recently, the immune checkpoint inhibitors Nivolumab and Pembrolizumab have also been approved for the treatment of cisplatin-refractory recurrent or metastatic HNSCC (5, 6). Most patients have an advanced stage disease at diagnosis, because of the lack of important symptoms or reduced symptom awareness (7) and a local recurrence and lymphnode metastasis are frequently observed.
Unfortunately, five year survival rate is quite low, (56%-62%) and it has modestly improved over the years (8–10). A better survival is associated with the presence of tumor immune infiltrate (11–13), as shown by patients with a tumor microenvironment enriched in tumor infiltrating lymphocytes (TIL). Interestingly, most HPV positive HNSCC tumors are highly infiltrated by lymphocytes and have the best survival rates (13, 14), suggesting that the number of TIL plays an important role in the control of tumor growth. A recent study has shown that tumor immune microenvironment (TIME) in HNSCC is associated with immune checkpoint therapy response and better prognosis (15). Furthermore, the presence of defective NK genes related with NK cell cytotoxicity pathway and phenotypes, were associated with higher risk of developing cancer (15). Increasing data have shown that NK cells are not merely innate effector cells, but they are fundamental in the recruitment and activation of adaptive immunity, through the secretion of IFNγ which induces activation of T helper cells (15, 16). Moreover, in recent years different studies supported an increasing potential of NK cells in the control of different types of cancers, including HNSCC, where a favorable prognosis has been associated with NK cell infiltration (17–21).
However, a number of immune escape mechanisms are exploited by tumors to avoid NK cell recognition, thus a better knowledge of peripheral and intratumoral NK cell profile in patients with HNSCC is needed to identify possible therapeutic targets.
The activity of NK cells is finely tuned by several membrane receptors able to switch the NK cell function towards activation or inhibition after ligand recognition and interaction (22). The low affinity FcγRIIIA (CD16) is one of the most potent activating receptors of NK cells that mediates antibody-dependent cell-mediated cytotoxicity (ADCC) (23). It is associated with ITAM-containing
CD3 ζ-chain and its activation allows NK cells to recognize and kill antibody-coated target cells via ADCC and to release immunoregulatory cytokines.
Spontaneous NK cell lytic activity is mediated by several activating receptor-ligand axes, including the natural killer group 2, member D (NKG2D) activating receptor, and its ligands. NKG2D is a dimeric, type II membrane protein constitutively expressed by NK cells in humans, as well as by almost all CD8+ αβ T cells and γδ T cells (24). NKG2D receptors recognize several molecules, such as MHC class I chain-related molecules (MIC)A/B and the cytomegalovirus UL-16 protein (ULBP1-6), the expression of which is induced on the cell surface as a result of cellular stress conditions (25). Therefore, NKG2D plays a pivotal role in immune surveillance and antitumor immune responses (26–28). NKp30 is a natural cytotoxicity receptor constitutively expressed by NK cells whose engagement by its ligand transduces a strong activation signal to the cell (29). Siglec-7 is an inhibitory NK receptor whose reduced frequency is associated with decreased cell function in physiologic (30) and pathologic conditions (31–34).
PD-1 and NKG2A are checkpoint molecules that have been shown to harness NK and T cell immune responses in cancer (35). NKG2A forms a heterodimer with CD94. The NKG2A/CD94 complex binds to the non-classical MHC I molecule HLA-E in humans and transduces inhibitory signals which suppress NK and CD8+ T cell activity (36). Interestingly, NK cells are endowed by innate and adaptive properties, being able of long-term ‘memory-like’ immune responses (37, 38). Individual repertoire of NK cells may be shaped by environmental exposure, such as CMV infection, which is able to lead to a stable expansion of adaptive NK cell population characterized by the expression of NKG2C activating receptor (39–44). Sequence variations in CMV UL40-encoded peptides control the activation and expansion of adaptive NK cells (40–42), and the peptide variant VMAPRTLFL induces increased proliferation of NKG2C population compared with other CMV peptides (40, 41). Such expanded NK cells are identified by the lack of FcϵR1γ adaptor protein and display an altered receptor expression pattern characterized by reduced expression of NKp46, NKp30, CD16, Siglec-7 and NKG2A compared to conventional, FcεR1γ positive NK cells. Importantly, they have increased ability to perform ADCC and to produce IFN gamma (44–48).
In this study we explore immune infiltrating NK cell phenotype and function in intratumor, peritumor and peripheral blood of HNSCC patients and controls and evaluate NK cell function after CMV peptide-driven expansion of adaptive NK cells.
Thirty-two individuals undergoing curative resection of HNSCC at the Fondazione IRCCS Policlinico San Matteo, Pavia, Italy were enrolled in the study. For each patient peripheral blood, tumor tissue, and, whenever possible, surrounding non-tumor tissue, were collected. Peripheral blood mononuclear cells collected from 19 healthy volunteers were used as control. Table 1 shows the demographic and clinical features of patients and controls. The study protocol conformed to the ethical guidelines of the 1975 Declaration of Helsinki and was approved by the Institutional Review Board and Ethical Committee of Fondazione IRCCS Policlinico San Matteo, document number 20190063538.
Peripheral blood mononuclear cell (PBMC) isolation was performed with Lympholyte (Cedarlane) density gradient (d=1.0770) centrifugation following the manufacturer’s instructions. To isolate tumor- (TIL) and non-tumor infiltrating lymphocytes (NIL) freshly dissected tissues were enzymatically and mechanically dissociated with the Tumor Dissociation Kit and gentle MACS Dissociator (Miltenyi Biotec), according to the manufacturer’s instructions. The resulting cell suspension was then filtered over a 70 µm cell strainer (Miltenyi Biotec) and centrifuged at 50xg for 1 min. The supernatant containing lymphocytes was washed, counted and resuspended in complete RPMI-1640 medium supplemented with 10% fetal bovine serum (FBS), 2mM L-glutamine and antibiotic antimycotic solution (Sigma-Aldrich) (RPMI complete). Cells were immediately used for immunophenotype staining or stimulated for functional analysis. Left-over PBMC, TIL and NIL were frozen in 90% FBS and 10% dimethylsulphoxide and stored in liquid nitrogen.
The K562 erythroleukemic and SW-480 colorectal carcinoma cell lines (ATCC) were grown in RPMI complete. The UM-SCC-22A squamous carcinoma cell line (Sigma-Aldrich) was maintained in complete Dulbecco’s Modified Eagle Medium (DMEM, ThermoFisher Scientific) supplemented with 10% FCS, 1 mM Sodium Pyruvate (Sigma-Aldrich), 1% antibiotic antimycotic solution, 1% L-glutamine and 1X non-essential amino acid (Sigma-Aldrich) (DMEM complete).
To establish in vitro primary HNSCC cell cultures, the cell pellet obtained after dissociation of resected tumor tissue was resuspended in CellGro SCGM (Cell Genix, Freiburg, Germany) supplemented with 10% fetal bovine serum (FBS; HyClone, GE Healthcare, South Logan, Utah, USA) and 1% antibiotic antimycotic solution (CellGro complete) and plated in 6-well plate. Viable tumor cells attached to the flask within 24 hours. Cultures at 75% to 100% confluence were selected for subculture by trypsinization with 0.05% trypsin/0.53 mM EDTA solution (Corning). The neoplastic origin of cultured cells was confirmed by an experienced histopathologist. Briefly, 50,000 formalin-fixed cells were stained with hematoxylin-eosin to evaluate in detail their morphologic features.
2x106 freshly isolated PBMC, TIL and, when available, NIL were used for flow-cytometric immunophenotypic analysis using a 12-color FACSCelesta (BD Biosciences). LIVE/DEAD® Fixable Near-IR Dead Cell Stain Kit (Thermo Fisher Scientific) was used to determine cell viability. The immunophenotyping panel used to characterize the main lymphocyte subsets is given in Supplementary Table 1. Staining of nuclear trascription factor FcϵRIγ was performed using the Foxp3/Transcription Factor Staining Buffer Set (eBioscience) according to the manufacturer’s instructions.
Freshly isolated PBMC and TIL were stimulated overnight with IL12 (10 ng/ml), IL-15 (1 ng/ml) (Peprotech) and IL-18 (50 ng/ml, R&D System) in complete RPMI medium. After incubation, cells were harvested and co-cultured with K562 target cells at a 5:1 effector:target cell ratio for 5h at 37°C in the presence of anti-CD107a BV786 and BD GolgiPlug™ Protein Transport Inhibitor (BD). To assess the cytokine production, cells were fixed with BD Cytofix/Cytoperm (BD) and permeabilized with the BD Perm/Wash buffer (BD) and subsequently stained with anti-IFNγ PE (BD) and analyzed by FACSCelesta.
Antibody-dependent cell-mediated cytotoxicity (ADCC) was performed using SW480 colon cancer cells as targets in the presence or absence of 10 μg/ml Cetuximab. Briefly, IL-12/15/18 pre-activated PBMC were incubated for 5 h with target cells at an E:T ratio of 5:1 in the presence of brefeldin A (GolgiPlug, BD). IFNγ production in NK cells was detected by intracellular staining and analyzed by FACSCelesta.
Cryopreserved PBMC from 5 CMV+ HNSCC patients and 6 CMV+ control subjects were thawed, washed and rested for 2h in complete RPMI medium at 37°C. Subsequently, PBMC were seeded in 48 -well plates (106 cells/well) and cultured for 12 days in complete medium supplemented with IL-2 250 IU/ml in the presence or absence of 400 μM synthetic peptides (HCMV-VMAPRTLFL or –VMAPRTLLL, peptides&elephants). IL-2-containing medium (250 IU/ml) was half-replaced at day 3, 6 and 9. At day 12, expanded NK cells were harvested and co-cultured with SW480 or UM-SCC-22A and/or autologous primary tumor cells at a ratio of 5:1 to assess their ADCC function. One ng/ml of IL-15 was added to culture on the day before functional assays
HCMV IgG serology was determined with DRG IgG CMV ELISA kit (DRG International).
Statistical analysis was performed using the GraphPad Prism 8.4.3 software (GraphPad, La Jolla, CA, USA). Data distribution was examined by the D’Agostino & Pearson normality test. The Mann-Whitney U test was used to compare control subjects and HNSCC patients, more data sets were compared by the non-parametric Kruskal-Wallis followed by Dunn’s multiple test. The non-parametric Wilcoxon signed-rank test or the one-way Friedman followed by Dunn’s multiple comparison tests were used, as appropriate, to compare data within the same group. Correlations between variables were analyzed by Pearson’s rank correlation coefficient. Differences were deemed statistically significant when p ≤ 0.05.
We explored the quality of NK cell phenotype and function in peripheral blood, tumor and non tumor infiltrating lymphocytes (PB-NK, TIL-NK and NIL-NK respectively) in HNSCC tumor specimens. Tumor and non tumor tissues were scarcely infiltrated by NK cells, whereas T cells were largely predominant. Unfortunately, the number of cells retrieved from tissue was occasionally insufficient to allow performance of all the experiments planned. Specifically, the surrounding non-tumor tissue was often very scarcely infiltrated by immune cells. To allow clear data reporting, we showed paired data comparing PB-, TIL- and NIL- NK cell phenotype of HNSCC patients. Comparison between HNSCC patients and controls, showed that the proportion of circulating NK cells was similar, whereas these cells were strongly reduced in the non tumor and, particularly, in the tumor compartment compared with paired PB-NK (Figure 1A). The peripheral NK cell compartment was characterized by a reduced proportion of CD56bright and Siglec-7-expressing NK cells in HNSCC subjects (Figures 1B, C). While no differences were observed in the CD56bright subset distribution in tissues, the frequency of Siglec-7 positive NK cells was significantly reduced in TIL compared with paired PB and NIL (Figure 1C, G).
Figure 1 (A) Frequency of CD3-CD56 + NK cells and (B) CD56bright NK cells in PBMC from control subjects (CTRL PB), and from HNSCC patients (HNSCC-PB) as matched tumor-infiltrating (TIL) and non- tumor-infiltrating lymphocytes (NIL). Proportion of (C) Siglec-7-, (D) NKG2A-, (E) TIM-3- and (F) NKG2C- positive NK cells. (G) Representative concatenated flow cytometry plots showing the proportion of NK cells expressing the indicated phenotypic markers in 6 representative CTRL PB, 6 HNSCC-PB, 6 HNSCC-TIL and 6 HNSCC-NIL. The Mann–Whitney U test was used to compare CTRL and HNSCC subjects. Paired data were analyzed by the Wilcoxon signed rank test. All plots include observations from 25th to 75th percentile. The horizontal line represents the median value. *p < 0.05, **p < 0.01, ****p < 0.0001.
The frequency of circulating NK cells expressing NKG2A and TIM-3 was increased compared with controls (Figures 1D, E, G). NKG2A positive NK cells were further augmented inside the tumor tissue compared with PB and NIL NK cells (Figure 1D). The proportion of NK cells expressing NKG2C receptor was also increased in the peripheral blood of patients compared with control PB and in tumor tissue when compared with the non tumor compartment (Figure 1F, G).
No differences in the frequency of circulating FcεRIγ negative adaptive/memory NK cell population were found in the two groups, despite there being a small, though statistically significant, increase in the tumor compartment compared with the periphery in HNSCC patients (Figure 2A). Other immune regulatory receptors were not differentially expressed in peripheral blood NK cells from patients and controls, but some of these molecules (CD69, PD-1, GITR and CXCR6) were significantly upregulated (Figures 2B–E, G) and other significantly reduced (NKG2D, NKP30, CD16 and CD57) (Figures 3A–E) in TIL-NK compared with circulating NK cells. Interestingly, the frequency of NK cells coexpressing PD-1 and TIGIT exhaustion markers was significantly increased in tumor compared with peripheral blood compartment (Figure 2F). TIGIT+ and NKp46+ NK cell frequencies did not differ between the compartments analyzed (Supplementary Figure 1). Thus, there were increased frequencies of NK cells coexpressing PD-1 and TIGIT in the tumor microenvironment. Significantly lower frequencies of NK cells expressing NKG2D, NKp30 and CD16 activating receptors and higher frequency of NK cells expressing the NKG2A inhibitory molecule, were found in TIL. Moreover, Siglec-7, a NK receptor whose reduced frequency is associated with decreased cell function in physiologic (30) and pathologic conditions (31–34), was also reduced.
Figure 2 (A) Frequency of FcϵRIγ negative CD56+/CD57+ adaptive NK cells in PBMC from control subjects (CTRL PB), and from HNSCC patients (HNSCC-PB) as well as matched tumor-infiltrating lymphocytes (TIL) and surrounding non-tumor-infiltrating lymphocytes (NIL). Percentage of NK cells expressing (B) CD69, (C) PD-1 (D) GITR and (E) CXCR6. (F) Frequency of PD-1+TIGIT+ NK cells. (G) Representative concatenated flow cytometry plots showing the percentage of expression of the indicated phenotypic markers on CD3-CD56+ NK cells. The Mann–Whitney U test was used to compare CTRL and HNSCC subjects. Paired data were analyzed by the Wilcoxon signed rank test. *p < 0.05, **p < 0.01, ***p < 0.001, ****p < 0.0001.
Figure 3 Frequences of NK cells expressing (A) NKG2D, (B) NKp30, (C) CD16 and (D) CD57 were determined in PBMC from control subjects (CTRL PB), and from HNSCC patients (HNSCC-PB) as well as matched tumor- (TIL) and surrounding non-tumor infiltrating lymphocytes (NIL). (E) Representative concatenated flow cytometry plots showing the percentage of expression of the indicated phenotypic markers on CD3-CD56+ NK cells. The Mann–Whitney U test was used to compare CTRL and HNSCC subjects. Paired data were analyzed by the Wilcoxon signed rank test. *p < 0.05, **p < 0.01, ***p < 0.001, ****p < 0.0001.
A decreased frequency of three strong activating receptors and the increase of NKG2A would support the concept that intratumoral NK cells are dysfunctional. To address this hypothesis, we compared TIL and paired PBMC function, by analyzing NK cell IFNγ production and degranulation activity in the presence of the MHC class I non-expressing K562 cells or with EGFR+ SW480 colon carcinoma target cells in the presence of Cetuximab in an ADCC assay. A cytokine combo including IL12+IL18+IL15 was employed as a stimulus because of their established ability to synergize and induce high levels of IFNγ (49). No changes in CD107a expression or IFNγ secretion was observed in NK cells from controls and patients in the presence of K562 target cells (Supplementary Figure 2). As shown if Figures 4A, B, spontaneous cytotoxicity or ADCC measured either as IFNγ secretion or as degranulation as a readout was significantly decreased in intratumoral NK cells compared with paired HNSSC peripheral and control NK cells. Cetuximab induced a significant increased activity in controls and patients, both in circulating and intratumor NK cells. IFNγ secretion and CD107a expression of circulating NK cells were inversely correlated to the frequency of TIGIT expressing NK cells (Figures 4C, D). IFNγ secretion by TIL-NK cells was inversely correlated to the frequency of TIGIT expressing TIL-NK cels (Figure 4E).
Figure 4 (A) IFNγ production and (B) degranulation ability by IL12+15+18 pre-activated NK cells following stimulation with SW480 with or without Cetuximab. Paired data were analyzed by the Wilcoxon signed rank test. The non-parametric Kruskal-Wallis followed by Dunn’s multiple test were used to compare different datasets. *p < 0.05, **p < 0.01, ***p < 0.001. (C) IFNγ and (D) CD107a expression negatively correlated with TIGIT expression on peripheral NK cells. (E) Intratumoral IFNγ expression negatively correlate with TIGIT expression on tumor infiltrating NK cells. The Pearson test was used to examine correlations.
NK cell effector properties can be exploited in treating certain tumors due to their ability to elicit ADCC. Adaptive NK cells have unique features, being characterized by increased ADCC activity and by resistance to myeloid-derived suppressor cell and Treg-mediated suppression (50, 51). To evaluate the potential of these cells in HNSCC, we expanded this population from PBMC from CMV positive patients by culturing cells in the presence of CMV UL-40 peptide VMAPRTLFL or a control peptide (VMAPRTLLL), and compared the ability of NK cells to secrete IFNγ and CD107a as surrogates of cytotoxicity. We used one commercial EGFR expressing cell lines as target cells, the UM-SCC-2A squamous cell carcinoma of the oral cavity and autologous primary tumor cells. Increased IFNγ secretion by NK cells primed with VMAPRTLFL was observed in controls (Figure 5A) and patients (Figures 5B, C) compared with unstimulated and control peptide-stimulated cells in the presence of UM-SCC-2A target cells (Figures 5A, B) or of primary tumor cells (Figure 5C). In contrast, degranulation of CD107a was similar in peptide-primed and unprimed NK cells (Supplementary Figure 3). Supplementary Figure 4 shows EGFR expression in primary tumor cells
Figure 5 (A) IFNγ secretion by control and (B, C) HNSCC patient NK cells after 10-day cell culture with 400μm VMAPRTLLL (LLL) or VMAPRTLFL(LFL) UL-40 CMV peptide in HCMV+ individuals. (A, B) UM-SCC-22A or (C) autologous primary tumor cells were used as targets in an ADCC assay in the presence of Cetuximab or a control antibody. Paired data were analyzed by the Wilcoxon signed rank test. The one-way Friedman followed by Dunn’s multiple comparison tests were used, as appropriate, to compare data within the same group. *p < 0.05.
As a corollary of this study, we also compared peripheral and intratumoral T cell phenotype in controls and HNSCC patients. Frequencies of circulating CD4 and CD8 T cells were not different in the two groups, while there was a significant reduction in the proportion of CD4 T helper cells, and an enrichment in the frequency of CD8+ T cells in TIL (Figures 6A, B, D). A significantly increased frequency of the regulatory T cell population (Treg) was also observed in the peripheral blood of HNSCC patients compared with controls, and a further significant increment was found in the intratumoral tissue when compared with paired circulating T cells and with non tumoral tissue (Figure 6C, D). Moreover, frequencies of circulating CD4 and CD8 T cells expressing the immunostimulatory checkpoint GITR (Figures 7A, B, E) or the inhibitory receptor TIGIT (Figures 7C, D, F) were significantly increased in HNSCC patients compared with controls. Interestingly, their relative proportions were further increased in TIL compared with PBMC and NIL (Figure 7). Moreover, the proportions of intratumoral CD4 and CD8 T cells expressing the CD69 homing marker, as well as PD-1, TIM-3 inhibitory co-stimulatory molecules and CXCR6 were increased compared with the peripheral compartment (Figure 8). TIGIT (Figures 7C, D) and TIM-3 (Figures 8C, G, I) inhibitory receptors were specifically increased in TIL and not in NIL CD4 and CD8 T cells, suggesting that the tumor microenvironment plays a crucial role in inhibiting tumor-specific T-cell responses. Circulating PD-1+,TIM-3+, CD69+ and CXCR6+ CD4 and CD8 T cells frequencies did not differ between the two groups (Figure 8).
Figure 6 (A) Frequencies of CD4+ T cells, (B) CD8+ T cells, (C) Treg cells in PBMC from healthy donors (CTRL PB) and HNSCC patients (HNSCC-PB) and in matched tumor-infiltrating (TIL) and surrounding non-tumor-infiltrating lymphocytes (NIL). The Mann–Whitney U test was used to compare CTRL and HNSCC subjects. Paired data were analyzed by the Wilcoxon signed rank test. *p < 0.05, **p < 0.01, ***p < 0.001. (D) UMAP (Uniform Manifold Approximation and Projection for Dimension Reduction) representation of manually gated CD4, CD8 and Treg cells.
Figure 7 Expression of (A, B) the GITR costimulatory molecule and (C, D) of the TIGIT checkpoint molecule on CD4+ and CD8+ T cells. The Mann–Whitney U test was used to compare CTRL and HNSCC subjects. Paired data were analyzed by the Wilcoxon signed rank test. *p < 0.05, **p < 0.01, ***p < 0.001, ****p < 0.0001. (E) Representative concatenated flow cytometry plots showing the percentage of GITR-expressing CD4+ and CD8+ T cells. (F) Representative concatenated flow cytometry plots showing the percentage of TIGIT expression on CD4+ and CD8+ T cells.
Figure 8 Proportions of (A) CD69, (B) PD-1-, (C) TIM-3-, and (D) CXCR6- positive CD4 T cells in PBMC from control subjects (CTRL PB), from HNSCC patients (HNSCC-PB) as well as matched tumor-infiltrating (TIL) and non-tumor-infiltrating lymphocytes (NIL). Frequencies of CD8 T cells expressing (E) CD69, (F) PD-1-, (G) TIM-3-, and (H) CXCR6. The Mann–Whitney U test was used to compare CTRL and HNSCC subjects. Paired data were analyzed by the Wilcoxon signed rank test. **p < 0.01, ***p < 0.001. (I) Representative concatenated flow cytometry plots showing the percentage of CD4+ and CD8+ T cells expressing the indicated phenotypic markers.
In this study we have examined the NK and T cell immunophenotype in peripheral blood and in tumor tissue of patients with HNSCC undergoing surgery. Other studies had previously characterized HNSCC circulating immune cells; however most were limited to assessing the distribution of the principal lymphocyte populations (20, 52–54) and only few analyzed NK cell inhibitory and activating receptors (55–57). In agreement with others, we observed reduced frequencies of the immunoregulatory CD56bright NK cell population (58) and increased frequencies of TIM-3+ and NKG2A+ NK cells (56, 59) in the peripheral blood of patients with HNSCC compared with healthy donors. Moreover, the proportion of Siglec-7+ NK cells was significantly reduced in patients. Interestingly, a recent study showed that reduced Siglec-7 expression predicts NK cell dysfunction in hepatocellular carcinoma (60), confirming the role of this receptor in the modulation of NK cell activity, as already observed in physiological and pathological conditions (30–34).
Several recent studies pointed to the relevance of the immune infiltrate in HNSCC, showing the prognostic role of TIL specific markers, including CD56 and CD57 (20, 61, 62), CD3, CD4 and CD8 (63–68). A meta-analysis showed that M2 macrophages and CD57+ natural killer cells were the most promising predictors of survival in oral cancer patients (69).
On this basis, it has been proposed that incorporation of TIL evaluation in the TNM system could improve the prognostic performance, and help physicians in clinical decisions. NK cells are potentially very effective against tumor growth, being able to kill tumor cells when activating signals prevail on inhibitory signals. However, the tumor immunosuppressive microenvironment may inhibit NK cell function by tipping the balance toward inhibition. Our study shows that intratumoral NK cells are characterized by an inhibitory phenotypic pattern, with reduced frequencies of cells expressing the major activating receptors NKG2D, NKp30 and CD16. The importance of NKG2D in tumor control is highlighted by the fact that tumors release NKG2D ligands MICA/B and ULBP1/2/3 by proteolytic cleavage to escape NKG2D-mediated NK cell cytotoxicity (70) and that antibody targeting the site of proteolytic cleavage prevent release of MICA/B and reduce melanoma metastasis in a humanized mouse model (71). This mechanism of immune evasion has also been observed in HNSCC in an interesting study showing that increased levels of soluble NKG2D ligands are present in the plasma of HNSCC patients (72) and that depletion of these ligands from patients’ plasma restores the ability to kill target cells in vitro (73). In agreement with others (57, 74) we have observed increased frequencies of NKG2A positive intratumoral NK cells compared with matched PBMC. Different types of tumors overexpress NKG2A and its ligand HLA-E (75, 76), and blocking NKG2A is an effective strategy to enhance antitumor activity by NK and CD8 T cells (77). We observed high level of HLA-E in primary tumor cells obtained from 4 patients with HNSCC (Supplementary Figure 5).
Not surprisingly, the functional activity of intratumoral NK cells was reduced, reflecting the prevalent inhibitory phenotype, which was also accompanied by an enrichment in PD-1+ TIGIT+ NK cells
and a reduction in CD16, a receptor capable to bind the antibody Fc fragment, thus enabling NK cells to perform ADCC. Downregulation of CD16 is induced in different tumors and viral infections, through matrix metalloproteinases (MMPs) induced shedding (78, 79), as an escape mechanism to avoid ADCC. Among MMPs, ADAM-17 mediated cleavage has been recognized as responsible of CD16 shedding in breast cancer (78, 80). ADCC process is triggered by Cetuximab, an anti EGFR antibody, which is widely used in cancer immunotherapy, including locally advanced- or recurrent/metastatic HNSCC (4, 81). Cetuximab engagement of CD16 activates NK cells and induces IFNγ secretion (82), an important soluble factor which promotes dendritic cell maturation leading to increased tumor antigen presentation and expansion of EGFR specific T cells (83). Our data show that intratumoral NK cells have reduced ability to secrete IFNγ in the presence of cetuximab after stimulation with a potent combination of cytokines, while peripheral NK cells from patients produced IFNγ similarly to controls. Interestingly, patients with higher frequencies of TIGIT expressing NK cells produced less IFNγ in the peripheral blood and intratumor compartments. TIGIT is a co-inhibitory receptor on the immune cell surface that binds ligands CD155 and CD112 (84). Different strategies are employed to increase NK cell activity against solid tumors in a number of clinical and pre- clinical studies (85). Blocking TIGIT interaction with its ligands reduces NK cell exhaustion in vitro and in a murine model (86, 87). Moreover, several anti-TIGIT monoclonal antibodies are under investigation in phase I/II studies (88).
Similarly to NK cells, intratumoral T cells were characterized by an exhausted phenotype, with increased proportions of TIM-3, PD-1 and TIGIT expressing CD4 and CD8 T cells. Moreover, patients’ Treg cells were increased in both the circulating and intratumoral compartments, further supporting the inhibitory role of the TME.
Increased frequencies of GITR+ NK cells were found in TIL and, in agreement with others (89, 90) we also observed a significant enrichment of GITR+ CD4 and CD8 T cells. GITR is a costimulatory receptor which has a dual positive effect supporting the survival and expansion of activated T cells and at the same time deactivating the Treg population within tumors (91). For this reason, GITR represents an ideal targetable immunomodulatory receptor that could provide a powerful tool to diminish the suppressive potential of intratumoral Treg and enhance anti-tumor immunity. Of note, different clinical trials are testing GITR agonist antibodies in combination with immune checkpoint inhibitors in advanced tumors (92–95). On this basis, considering the increased frequencies of NK and T cells expressing GITR, the use of agonistic GITR combined with blocking PD-1 and/or TIGIT antibodies could be beneficial in patients with HNSCC.
Increased proportions of CXCR6 were found in intratumoral NK and T cells, probably caused by ligand-receptor chemotaxis of CXCL16/CXCR6. Interestingly, a recent study in mice showed that CXCR6 is highly expressed in intratumoral CD8+ T cells and that these cells are more immunocompetent and could enhance the effect of anti-PD-1 blockade to delay tumor progression (96). CXCR6 has been recognized as a marker for liver resident NK cells (97) and has been associated to murine CMV virus specific adaptive-memory NK cells (98, 99). Adaptive NK cells have peculiar characteristics that have been exploited in adoptive anticancer therapy, being characterized by increased ADCC activity and by resistance to myeloid-derived suppressor cells and to Treg-mediated suppression (50, 51). Interestingly, a lower leukemia relapse rate and a superior disease-free survival at 1 year has been associated with CMV reactivation and this protective effect strongly correlated with adaptive NK-cell expansion (100). We have observed an increased IFNγ secretion, but not CD107a expression, by NK cells after 12 days expansion of adaptive NK cells in CMV+ patients, confirming that these cells are specialized in IFNγ secretion after Fc binding of therapeutic antibodies and that NK cell expansion by UL40 peptide stimulation combined with IL2 is a feasible way to obtain the expansion of highly functional NK cells in CMV positive subjects.
In conclusion our data show that the HNSCC tumor microenvironment affects NK and T cells, inducing an inhibitory/exhausted phenotype which is reflected in reduced NK cell ability to produce IFNγ after cetuximab engagement. The concomitant increase of costimulatory receptor GITR in intratumoral NK and T cells suggests that agonist engagement of GITR could be an effective treatment in HNSCC patients, particularly if combined with immune checkpoint blocking antibodies anti-PD-1 and anti-TIGIT.
The raw data supporting the conclusions of this article will be made available by the authors, without undue reservation.
The studies involving human participants were reviewed and approved by Institutional Review Board and Ethical Committee of Fondazione IRCCS Policlinico San Matteo, Pavia, Italiy. Document number 20190063538. The patients/participants provided their written informed consent to participate in this study.
DM, SV, and MM provided substantial contribution to the conception and design of the study, acquisition, analysis and interpretation of data, and revised the manuscript critically for important intellectual content. SV and MM supervised the team, obtained funding and had leadership responsibility for the research activity planning and execution. BO, SM, and GP contributed to acquisition, analysis, validation and interpretation of data. GT, AL and MB were responsible for data curation and were in charge of patient care. PM performed the histopathologic analysis. All authors critically read, edited and approved the final version of the manuscript.
This work was funded by Ricerca Corrente, Ministero della Salute, Nr. 08071419 to SV.
We thank patients and donors included in the study.
The authors declare that the research was conducted in the absence of any commercial or financial relationships that could be construed as a potential conflict of interest
All claims expressed in this article are solely those of the authors and do not necessarily represent those of their affiliated organizations, or those of the publisher, the editors and the reviewers. Any product that may be evaluated in this article, or claim that may be made by its manufacturer, is not guaranteed or endorsed by the publisher.
The Supplementary Material for this article can be found online at: https://www.frontiersin.org/articles/10.3389/fimmu.2022.997806/full#supplementary-material
1. Available at: https://gco.iarc.fr/today/online-analysistable?v=2020&mode=cancer&mode_population=continents&population=900&populations=900&key=asr&sex=0&cancer=39&type=0&statistic=5&prevalence=0&population_group=0&ages_group%5B%5D=0&ages_group%5B%5D=17&group_cancer=1&include_nmsc=0&include_nmsc_other=1 (Accessed 5 June 2022).
2. Gillison ML, Koch WM, Capone RB, Spafford M, Westra WH, Wu L, et al. Evidence for a causal association between human papillomavirus and a subset of head and neck cancers. J Natl Cancer Inst (2000) 92(9):709–20. doi: 10.1093/jnci/92.9.709
3. Ritchie JM, Smith EM, Summersgill KF, Hoffman HT, Wang D, Klussmann JP, et al. Human papillomavirus infection as a prognostic factor in carcinomas of the oral cavity and oropharynx. Int J Cancer (2003) 104(3):336–44. doi: 10.1002/ijc.10960
4. Bonner JA, Harari PM, Giralt J, Azarnia N, Shin DM, Cohen RB, et al. Radiotherapy plus cetuximab for squamous-cell carcinoma of the head and neck. N Engl J Med (2006) 354:567–78. doi: 10.1056/NEJMoa053422
5. Ferris RL, Blumenschein G Jr, Fayette J, Guigay J, Colevas AD, Licitra L, et al. Nivolumab for recurrent squamous-cell carcinoma of the head and neck. N Engl J Med (2016) 375:1856–67. doi: 10.1056/NEJMoa1602252
6. Seiwert TY, Burtness B, Mehra R, Weiss J, Berger R, Eder JP, et al. Safety and clinical activity of pembrolizumab for treatment of recurrent or metastatic squamous cell carcinoma of the head and neck (KEYNOTE-012): an open-label, multicentre, phase 1b trial. Lancet Oncol (2016) 17:956–65. doi: 10.1016/S1470-2045(16)30066-3
7. van Harten MC, de Ridder M, Hamming-Vrieze O, Smeele LE, Balm AJ, van den Brekel MW. The association of treatment delay and prognosis in head and neck squamous cell carcinoma (HNSCC) patients in a dutch comprehensive cancer center. Oral Oncol (2014) 50(4):282–90. doi: 10.1016/j.oraloncology.2013.12.018
8. Matta A, Ralhan R. Overview of current and future biologically based targeted therapies in head and neck squamous cell carcinoma. Head Neck Oncol (2009) 1(1):6. doi: 10.1186/1758-3284-1-6
9. Reyes-Gibby CC, Anderson KO, Merriman KW, Todd KH, Shete SS, Hanna EY. Survival patterns in squamous cell carcinoma of the head and neck: pain as an independent prognostic factor for survival. J Pain (2014) 15(10):1015–22. doi: 10.1016/jpain.2014.07.003
10. Pulte D, Brenner H. Changes in survival in head and neck cancers in the late 20th and early 21st century: a period analysis. Oncologist (2010) 15:994–1001. doi: 10.1634/theoncologist.2009-0289
11. Laban S, Hoffmann TK. Human papillomavirus immunity in oropharyngeal cancer: Time to change the game? Clin Cancer Res (2018) 24:505–7. doi: 10.1158/1078-0432.CCR-17-2991
12. Mann JE, Smith JD, Birkeland AC, Bellile E, Swiecicki P, Mierzwa M, et al. Analysis of tumor-infiltrating CD103 resident memory T-cell content in recurrent laryngeal squamous cell carcinoma. Cancer Immunol Immunother (2019) 68:213–20. doi: 10.1007/s00262-018-2256-3
13. Ward MJ, Thirdborough SM, Mellows T, Riley C, Harris S, Suchak K, et al. Tumour-infiltrating lymphocytes predict for outcome in HPV-positive oropharyngeal cancer. Br J Cancer (2014) 110:489–500. doi: 10.1038/bjc.2013.639
14. Wood O, Clarke J, Woo J, Mirza AH, Woelk CH, Thomas GJ, et al. Head and neck squamous cell carcinomas are characterized by a stable immune signature within the primary tumor over time and space. Clin Cancer Res (2017) 23:7641–9. doi: 10.1158/1078-0432.CCR-17-0373
15. Xu X, Li J, Zou J, Feng X, Zhang C, Zheng R, et al. Association of germline variants in natural killer cells with tumor immune microenvironment subtypes, tumor-infiltrating lymphocytes, immunotherapy response, clinical outcomes, and cancer risk. JAMA Netw Open (2019) 2(9):e199292. doi: 10.1001/jamanetworkopen.2019.9292
16. Vivier E, Raulet DH, Moretta A, Caligiuri MA, Zitvogel L, Lanier LL, et al. Innate or adaptive immunity? the example of natural killer cells. Science (2011) 331(6013):44–9. doi: 10.1126/science.1198687
17. Mandal R, Şenbabaoğlu Y, Desrichard A, Havel JJ, Dalin MG, Riaz N, et al. The head and neck cancer immune landscape and its immunotherapeutic implications. JCI Insight (2016) 1(17):e89829. doi: 10.1172/jci.insight.89829
18. Wagner S, Wittekindt C, Reuschenbach M, Hennig B, Thevarajah M, Würdemann N, et al. CD56-positive lymphocyte infiltration in relation to human papillomavirus association and prognostic significance in oropharyngeal squamous cell carcinoma. Int J Cancer (2016) 138:2263–73. doi: 10.1002/ijc.29962
19. Lu J, Chen XM, Huang HR, Zhao FP, Wang F, Liu X, et al. Detailed analysis of inflammatory cell infiltration and the prognostic impact on nasopharyngeal carcinoma. Head Neck (2018) 40:1245–53. doi: 10.1002/hed.25104
20. Caruntu A, Moraru L, Lupu M, Vasilescu F, Dumitrescu M, Cioplea M, et al. Prognostic potential of tumor-infiltrating immune cells in resectable oral squamous cell carcinoma. Cancers (Basel) (2021) 13(9):2268. doi: 10.3390/cancers13092268
21. Santos EM, Rodrigues de Matos F, Freitas de Morais E, Galvão HC, de Almeida Freitas R. Evaluation of CD8+ and natural killer cells defense in oral and oropharyngeal squamous cell carcinoma. J Craniomaxillofac Surg (2019) 47:676–81. doi: 10.1016/j.jcms.2019.01.036
22. Lanier LL. Up on the tightrope: Natural killer cell activation and inhibition. Nat Immunol (2008) 9:495–502. doi: 10.1038/ni1581
23. Nimmerjahn F, Ravetch JV. Fcgamma receptors as regulators of immune responses. Nat Rev Immunol (2008) 8:34–7. doi: 10.1038/nri2206
24. Raulet DH. Roles of the NKG2D immunoreceptor and its ligands. Nat Rev Immunol (2003) 3:81–790. doi: 10.1038/nri1199
25. Gassser S, Orsulic S, EJ B, Raulet DH. The DNA damage pathway regulates innate immune system ligands of the NKG2D receptor. Nature (2005) 436:1186–90. doi: 10.1038/nature03884
26. Diefenbach A, Jensen ER, Jamieson AM, Raulet DH. Rae1 and H60 ligands of the NKG2D receptor stimulate tumour immunity. Nature (2001) 413:165–7. doi: 10.1038/35093109
27. Cerwenka A, Baron JL, Lanier LL. Ectopic expression of retinoic acid early inducible-1 gene (RAE-1) permits natural killer cell-mediated rejection of a MHC class I-bearing tumor in vivo. Proc Natl Acad Sci USA (2001) 98:1521–11526. doi: 10.1073/pnas.201238598
28. Smyth MJ, Swann J, Cretney E, Zerafa N, Yokoyama WM, Hayakawa Y. NKG2D function protects the host from tumor initiation. J Exp Med (2005) 202:583–8. doi: 10.1084/jem.20050994
29. Kruse PH, Matta J, Ugolini S, Vivier E. Natural cytotoxicity receptors and their ligands. Immunol Cell Biol (2014) 92:221–9. doi: 10.1038/icb.2013.98
30. Shao JY, Yin WW, Zhang QF, Liu Q, Peng ML, Hu HD, et al. Siglec-7 defines a highly functional natural killer cell subset and inhibits cell-mediated activities. Scand J Immunol (2016) 84(3):182–90. doi: 10.1111/sji.12455
31. Zulu MZ, Naidoo KK, Mncube Z, Jaggernath M, Goulder PJR, Ndung'u T, et al. Reduced expression of siglec-7, NKG2A, and CD57 on terminally differentiated CD56-CD16+ natural killer cell subset is associated with natural killer cell dysfunction in chronic HIV-1 clade c infection. AIDS Res Hum Retroviruses (2017) 33(12):1205–13. doi: 10.1089/AID.2017.0095
32. Yang L, Feng Y, Wang S, Jiang S, Tao L, Li J, et al. Siglec-7 is an indicator of natural killer cell function in acute myeloid leukemia. Int Immunopharmacol (2021) 99:107965. doi: 10.1016/j.intimp.2021.107965
33. Varchetta S, Mele D, Oliviero B, Mantovani S, Ludovisi S, Cerino A, et al. Unique immunological profile in patients with COVID-19. Cell Mol Immunol (2021) 18(3):604–12. doi: 10.1038/s41423-020-00557-9
34. Varchetta S, Mele D, Lombardi A, Oliviero B, Mantovani S, Tinelli C, et al. Lack of siglec-7 expression identifies a dysfunctional natural killer cell subset associated with liver inflammation and fibrosis in chronic HCV infection. Gut (2016) 65(12):1998–2006. doi: 10.1136/gutjnl-2015-310327
35. Demaria O, Cornen S, Daëron M, Morel Y, Medzhitov R, Vivier E. Harnessing innate immunity in cancer therapy. Nature (2019) 574:45–56. doi: 10.1038/s41586-019-1593-5
36. Braud VM, Allan DS, O'Callaghan CA, Söderström K, D'Andrea A, Ogg GS, et al. HLA-e binds to natural killer cell receptors CD94/ NKG2A, b and c. Nature (1998) 391:795–9. doi: 10.1038/35869
37. Morvan MG, Lanier LL. NK cells and cancer: you can teach innate cells new tricks. Nat Rev Cancer (2016) 16(1):7–19. doi: 10.1038/nrc.2015.5
38. Fehniger TA, Cooper MA. Harnessing NK cell memory for cancer immunotherapy. Trends Immunol (2016) 37:877–88. doi: 10.1016/j.it.2016.09.005
39. Gumá M, Angulo A, Vilches C, Gómez-Lozano N, Malats N, López-Botet M. Imprint of human cytomegalovirus infection on the NK cell receptor repertoire. Blood (2004) 104:3664–71. doi: 10.1182/blood-2004-05-2058
40. Hammer Q, Rückert T, Borst EM, Dunst J, Haubner A, Durek P, et al. Peptide-specific recognition of human cytomegalovirus strains controls adaptive natural killer cells. Nat Immunol (2018) 19:453–63. doi: 10.1038/s41590-018-0082-6
41. Rölle A, Meyer M, Calderazzo S, Jäger D, Momburg F. Distinct HLA-e peptide complexes modify antibody-driven effector functions of adaptive NK cells. Cell Rep (2018) 24(8):1967–1976.e4. doi: 10.1016/j.celrep.2018.07.069
42. Béziat V, Dalgard O, Asselah T, Halfon P, Bedossa P, Boudifa A, et al. CMV drives clonal expansion of NKG2C+ NK cells expressing self-specific KIRs in chronic hepatitis patients. Eur J Immunol (2012) 42(2):447–57. doi: 10.1002/eji.201141826
43. Gumá M, Budt M, Sáez A, Brckalo T, Hengel H, Angulo A, et al. Expansion of CD94/NKG2C+ NK cells in response to human cytomegalovirus-infected fibroblasts. Blood (2006) 107:3624–31. doi: 10.1182/blood-2005-09-3682
44. Rölle A, Pollmann J, Ewen EM, Le VT, Halenius A, Hengel H, et al. IL-12-producing monocytes and HLA-e control HCMV-driven NKG2C+ NK cell expansion. J Clin Invest (2014) 124:5305–16. doi: 10.1172/JCI77440
45. Schlums H, Cichocki F, Tesi B, Theorell J, Beziat V, Holmes TD, et al. Cytomegalovirus infection drives adaptive epigenetic diversification of NK cells with altered signaling and effector function. Immunity (2015) 42:443–56. doi: 10.1016/j.immuni.2015.02.008
46. Lee J, Zhang T, Hwang I, Kim A, Nitschke L, Kim M, et al. Epigenetic modification and antibody-dependent expansion of memory-like NK cells in human cytomegalovirus-infected individuals. Immunity (2015) 42(3):431–42. doi: 10.1016/j.immuni.2015.02.013
47. Darboe A, Danso E, Clarke E, Umesi A, Touray E, Wegmuller R, et al. Enhancement of cytokine-driven NK cell IFN-γ production after vaccination of HCMV infected africans. Eur J Immunol (2017) 47:1040–50. doi: 10.1002/eji.201746974
48. Capuano C, Pighi C, Battella S, Santoni A, Palmieri G, Galandrini R. Memory NK cell features exploitable in anticancer immunotherapy. J Immunol Res (2019) 2019:8795673. doi: 10.1155/2019/8795673
49. Lusty E, Poznanski SM, Kwofie K, Mandur TS, Lee DA, Richards CD, et al. IL-18/IL-15/IL-12 synergy induces elevated and prolonged IFN-γ production by ex vivo expanded NK cells which is not due to enhanced STAT4 activation. Mol Immunol (2017) 88:138–47. doi: 10.1016/j.molimm.2017.06.025
50. Sarhan D, Hippen KL, Lemire A, Hying S, Luo X, Lenvik T, et al. Adaptive NK cells resist regulatory T-cell suppression driven by IL37. Cancer Immunol Res (2018) 6(7):766–75. doi: 10.1158/2326-6066.CIR-17-0498
51. Sarhan D, Cichocki F, Zhang B, Yingst A, Spellman SR, Cooley S, et al. Adaptive NK cells with low TIGIT expression are inherently resistant to myeloid-derived suppressor cells. Cancer Res (2016) 76(19):5696–706. doi: 10.1158/0008-5472.CAN-16-0839
52. Caruntu A, Moraru L, Surcel M, Munteanu A, Tanase C, Constantin C, et al. Assessment of immune cell populations in tumor tissue and peripheral blood samples from head and neck squamous cell carcinoma patients. Anal Cell Pathol (Amst) (2021) 2021:2328218. doi: 10.1155/2021/2328218
53. Dutta A, Banerjee A, Saikia N, Phookan J, Baruah MN, Baruah S. Negative regulation of natural killer cell in tumor tissue and peripheral blood of oral squamous cell carcinoma. Cytokine (2015) 76(2):123–30. doi: 10.1016/j.cyto.2015.09.006
54. Grimm M, Feyen O, Hofmann H, Teriete P, Biegner T, Munz A, et al. Immunophenotyping of patients with oral squamous cell carcinoma in peripheral blood and associated tumor tissue. Tumour Biol (2016) 37(3):3807–16. doi: 10.1007/s13277-015-4224-2
55. Niu M, Combs SE, Linge A, Krause M, Baumann M, Lohaus F, et al. Comparison of the composition of lymphocyte subpopulations in non-relapse and relapse patients with squamous cell carcinoma of the head and neck before, during radiochemotherapy and in the follow-up period: a multicenter prospective study of the German cancer consortium radiation oncology group (DKTK-ROG). Radiat Oncol (2021) 16(1):141. doi: 10.1186/s13014-021-01868-5
56. Schilling B, Halstead ES, Schuler P, Harasymczuk M, Egan JE, Whiteside TL. IRX-2, a novel immunotherapeutic, enhances and protects NK-cell functions in cancer patients. Cancer Immunol Immunother (2012) 61(9):1395–405. doi: 10.1007/s00262-011-1197-x
57. Habif G, Crinier A, André P, Vivier E, Narni-Mancinelli E. Targeting natural killer cells in solid tumors. Cell Mol Immunol (2019) 16(5):415–22. doi: 10.1038/s41423-019-0224-2
58. Wulff S, Pries R, Börngen K, Trenkle T, Wollenberg B. Decreased levels of circulating regulatory NK cells in patients with head and neck cancer throughout all tumor stages. Anticancer Res (2009) 29(8):3053–7.
59. Concha-Benavente F, Kansy B, Moskovitz J, Moy J, Chandran U, Ferris RL. PD-L1 mediates dysfunction in activated PD-1+ NK cells in head and neck cancer patients. Cancer Immunol Res (2018) 6(12):1548–60. doi: 10.1158/2326-6066.CIR-18-0062
60. Tao L, Wang S, Yang L, Jiang L, Li J, Wang X. Reduced siglec-7 expression on NK cells predicts NK cell dysfunction in primary hepatocellular carcinoma. Clin Exp Immunol (2020) 201(2):161–70. doi: 10.1111/cei.13444
61. Taghavi N, Bagheri S, Akbarzadeh A. Prognostic implication of CD57, CD16, and TGF-β expression in oral squamous cell carcinoma. J Oral Pathol Med (2016) 45(1):58–62. doi: 10.1111/jop.12320
62. Bisheshar SK, De Ruiter EJ, Devriese LA, Willems SM. The prognostic role of NK cells and their ligands in squamous cell carcinoma of the head and neck: a systematic review and meta-analysis. Oncoimmunology (2020) 9(1):1747345. doi: 10.1080/2162402X.2020.1747345
63. Zhang D, Tang D, Heng Y, Zhu XK, Zhou L, Tao L, et al. Prognostic impact of tumor-infiltrating lymphocytes in laryngeal squamous cell carcinoma patients. Laryngoscope (2021) 131(4):E1249–55. doi: 10.1002/lary.29196
64. Wang J, Tian S, Sun J, Zhang J, Lin L, Hu C. The presence of tumour-infiltrating lymphocytes (TILs) and the ratios between different subsets serve as prognostic factors in advanced hypopharyngeal squamous cell carcinoma. BMC Cancer (2020) 20(1):731. doi: 10.1186/s12885-020-07234-0
65. Huang Y, Lin C, Kao HK, Hung SY, Ko HJ, Huang YC, et al. Digital image analysis of CD8+ and CD3+ tumor-infiltrating lymphocytes in tongue squamous cell carcinoma. Cancer Manag Res (2020) 12:8275–85. doi: 10.2147/CMAR.S255816
66. Almangush A, De Keukeleire S, Rottey S, Ferdinande L, Vermassen T, Leivo I, et al. Tumor-infiltrating lymphocytes in head and neck cancer: Ready for prime time? Cancers (Basel) (2022) 14(6):1558. doi: 10.3390/cancers14061558
67. Spector ME, Bellile E, Amlani L, Zarins K, Smith J, Brenner JC, et al. Prognostic value of tumor-infiltrating lymphocytes in head and neck squamous cell carcinoma. JAMA Otolaryngol Head Neck Surg (2019) 145(11):1012–9. doi: 10.1001/jamaoto.2019.2427
68. Borsetto D, Tomasoni M, Payne K, Polesel J, Deganello A, Bossi P, et al. Prognostic significance of CD4+ and CD8+ tumor-infiltrating lymphocytes in head and neck squamous cell carcinoma: A meta-analysis. Cancers (Basel) (2021) 13(4):781. doi: 10.3390/cancers13040781
69. Hadler-Olsen E, Wirsing AM. Tissue-infiltrating immune cells as prognostic markers in oral squamous cell carcinoma: a systematic review and meta-analysis. Br J Cancer (2019) 120(7):714–27. doi: 10.1038/s41416-019-0409-6
70. Kaiser BK, Yim D, Chow IT, Gonzalez S, Dai Z, Mann HH, et al. Disulphide-isomerase-enabled shedding of tumour-associated NKG2D ligands. Nature (2007) 447(7143):482–6. doi: 10.1038/nature05768
71. Ferrari de Andrade L, Tay RE, Pan D, Luoma AM, Ito Y, Badrinath S, et al. Antibody-mediated inhibition of MICA and MICB shedding promotes NK cell-driven tumor immunity. Science (2018) 359(6383):1537–42. doi: 10.1126/science.aao0505
72. Klöß S, Chambron N, Gardlowski T, Arseniev L, Koch J, Esser R, et al. Increased sMICA and TGFβ1 levels in HNSCC patients impair NKG2D-dependent functionality of activated NK cells. Oncoimmunology (2015) 4(11):e1055993. doi: 10.1080/2162402X.2015.1055993
73. Weil S, Memmer S, Lechner A, Huppert V, Giannattasio A, Becker T, et al. Natural killer group 2D ligand depletion reconstitutes natural killer cell immunosurveillance of head and neck squamous cell carcinoma. Front Immunol (2017) 8:387. doi: 10.3389/fimmu.2017.00387
74. van Montfoort N, Borst L, Korrer MJ, Sluijter M, Marijt KA, Santegoets SJ, et al. NKG2A blockade potentiates CD8 T cell immunity induced by cancer vaccines. Cell. (2018) 175(7):1744–1755.e15. doi: 10.1016/j.cell.2018.10.028
75. Sun C, Xu J, Huang Q, Huang M, Wen H, Zhang C, et al. High NKG2A expression contributes to NK cell exhaustion and predicts a poor prognosis of patients with liver cancer. Oncoimmunology (2017) 6(1):e1264562. doi: 10.1080/2162402X.2016.1264562
76. Zhen ZJ, Ling JY, Cai Y, Luo WB, He YJ. Impact of HLA-e gene polymorphism on HLA-e expression in tumor cells and prognosis in patients with stage III colorectal cancer. Med Oncol (2013) 30(1):482. doi: 10.1007/s12032-013-0482-2
77. André P, Denis C, Soulas C, Bourbon-Caillet C, Lopez J, Arnoux T, et al. Anti-NKG2A mAb is a checkpoint inhibitor that promotes anti-tumor immunity by unleashing both T and NK cells. Cell (2018) 175(7):1731–1743.e13. doi: 10.1016/j.cell.2018.10.014
78. Romee R, Foley B, Lenvik T, Wang Y, Zhang B, Ankarlo D, et al. NK cell CD16 surface expression and function is regulated by a disintegrin and metalloprotease-17 (ADAM17). Blood (2013) 121(18):3599–608. doi: 10.1182/blood-2012-04-425397
79. Oliviero B, Mantovani S, Varchetta S, Mele D, Grossi G, Ludovisi S, et al. Hepatitis c virus-induced NK cell activation causes metzincin-mediated CD16 cleavage and impaired antibody-dependent cytotoxicity. J Hepatol (2017) 66(6):1130–7. doi: 10.1016/j.jhep.2017.01.032
80. Pham DH, Kim JS, Kim SK, Shin DJ, Uong NT, Hyun H, et al. Effects of ADAM10 and ADAM17 inhibitors on natural killer cell expansion and antibody-dependent cellular cytotoxicity against breast cancer cells in vitro. Anticancer Res (2017) 37(10):5507–13. doi: 10.21873/anticanres.11981
81. Vermorken JB, Remenar E, Hitt R, Kawecki A, Rottey S, Knierim L, et al. Platinum-based chemotherapy (CT) plus cetuximab in recurrent or metastatic squamous cell carcinoma of the head and neck cancer (R/M-SCCHN): 5-year follow-up data for the extreme trial. J Clin Onco (2014) 32(15_suppl):6021–1. doi: 10.1200/jco.2014.32.15_suppl.6021
82. García-Foncillas J, Sunakawa Y, Aderka D, Wainberg Z, Ronga P, Witzler P, et al. Distinguishing features of cetuximab and panitumumab in colorectal cancer and other solid tumors. Front Oncol (2019) 9:849. doi: 10.3389/fonc.2019.00849
83. Srivastava RM, Lee SC. Cetuximab-activated natural killer and dendritic cells collaborate to trigger tumor antigen–specific T-cell immunity in head and neck cancer patients. Clin Cancer Res (2013) 19(7):1858–72. doi: 10.1158/1078-0432.CCR-12-2426
84. Anderson AC, Joller N, Kuchroo VK. Lag-3, Tim-3, and TIGIT: Co-inhibitory receptors with specialized functions in immune regulation. Immunity (2016) 44(5):989–1004. doi: 10.1016/j.immuni.2016.05.001
85. Lian G, Mak TS, Yu X, Lan HY. Challenges and recent advances in NK cell-targeted immunotherapies in solid tumors. Int J Mol Sci2 (2021) 23(1):164. doi: 10.3390/ijms23010164
86. Xu F, Sunderland A, Zhou Y, Schulick RD, Edil BH, Zhu Y. Blockade of CD112R and TIGIT signaling sensitizes human natural killer cell functions. Cancer Immunol Immunother (2017) 66(10):1367–75. doi: 10.1007/s00262-017-2031-x
87. Zhang Q, Bi J, Zheng X, Chen Y, Wang H, Wu W, et al. Blockade of the checkpoint receptor TIGIT prevents NK cell exhaustion and elicits potent anti-tumor immunity. Nat Immunol (2018) 19(7):723–32. doi: 10.1038/s41590-018-0132-0
88. Harjunpää H, Guillerey C. TIGIT as an emerging immune checkpoint. Clin Exp Immunol (2020) 200(2):108–19. doi: 10.1111/cei.13407
89. Puntigam LK, Jeske SS, Götz M, Greiner J, Laban S, Theodoraki MN, et al. Immune checkpoint expression on immune cells of HNSCC patients and modulation by chemo- and immunotherapy. Int J Mol Sci (2020) 21(15):5181. doi: 10.3390/ijms21155181
90. Vence L, Bucktrout SL, Fernandez Curbelo I, Blando J, Smith BM, Mahne AE, et al. Characterization and comparison of GITR expression in solid tumors. Clin Cancer Res (2019) 25(21):6501–10. doi: 10.1158/1078-0432.CCR-19-0289
91. Shevach EM, Stephens GL. The GITR-GITRL interaction: co-stimulation or contrasuppression of regulatory activity? Nat Rev Immunol (2006) 6(8):613–8. doi: 10.1038/nri1867
92. Zappasodi R, Sirard C, Li Y, Budhu S, Abu-Akeel M, Liu C, et al. Rational design of anti-GITR-based combination immunotherapy. Nat Med (2019) 25(5):759–66. doi: 10.1038/s41591-019-0420-8
93. Davar D, Zappasodi R, Wang H, Naik GS, Sato T, Bauer T, et al. Phase IB study of GITR agonist antibody TRX518 singly and in combination with gemcitabine, pembrolizumab or nivolumab in patients with advanced solid tumors. Clin Cancer Res (2022) 28(18):3990-4002. doi: 10.1158/1078-0432.CCR-22-0339. 0339.2022.
94. Heinhuis KM, Carlino M, Joerger M, Di Nicola M, Meniawy T, Rottey S, et al. Safety, tolerability, and potential clinical activity of a glucocorticoid-induced TNF receptor-related protein agonist alone or in combination with nivolumab for patients with advanced solid tumors: A phase 1/2a dose-escalation and cohort-expansion clinical trial. JAMA Oncol (2020) 6(1):100–7. doi: 10.1001/jamaoncol.2019.3848
95. Balmanoukian AS, Infante JR, Aljumaily R, Naing A, Chintakuntlawar AV, Rizvi NA, et al. Safety and clinical activity of MEDI1873, a novel GITR agonist, in advanced solid tumors. Clin Cancer Res (2020) 26(23):6196–203. doi: 10.1158/1078-0432.CCR-20-0452
96. Wang B, Wang Y, Sun X, Deng G, Huang W, Wu X, et al. CXCR6 is required for antitumor efficacy of intratumoral CD8+ T cell. J Immunother Cancer. (2021) 9(8):e003100. doi: 10.1136/jitc-2021-003100
97. Stegmann KA, Robertson F, Hansi N, Gill U, Pallant C, Christophides T, et al. CXCR6 marks a novel subset of T-bet(lo)Eomes(hi) natural killer cells residing in human liver. Sci Rep (2016) 6:26157. doi: 10.1038/srep26157
98. Paust S, Gill HS, Wang BZ, Flynn MP, Moseman EA, Senman B, et al. Critical role for the chemokine receptor CXCR6 in NK cell-mediated antigen specific memory of haptens and viruses. Nat Immunol (2010) 11(12):1127–35. doi: 10.1038/ni.1953
99. Sun JC, Beilke JN, Lanier LL. Adaptive immune features of natural killer cells. Nature (2009) 457(7229):557–61. doi: 10.1038/nature07665
Keywords: tumor microenvironment, HNSCC, natural killer cells, GITR, PD-1, NKG2C, adaptive NK cells
Citation: Mele D, Pessino G, Trisolini G, Luchena A, Benazzo M, Morbini P, Mantovani S, Oliviero B, Mondelli MU and Varchetta S (2022) Impaired intratumoral natural killer cell function in head and neck carcinoma. Front. Immunol. 13:997806. doi: 10.3389/fimmu.2022.997806
Received: 19 July 2022; Accepted: 06 October 2022;
Published: 20 October 2022.
Edited by:
Alessandra Zingoni, Sapienza University of Rome, ItalyReviewed by:
Norberto Walter Zwirner, Consejo Nacional de Investigaciones Científicas y Técnicas (CONICET), ArgentinaCopyright © 2022 Mele, Pessino, Trisolini, Luchena, Benazzo, Morbini, Mantovani, Oliviero, Mondelli and Varchetta. This is an open-access article distributed under the terms of the Creative Commons Attribution License (CC BY). The use, distribution or reproduction in other forums is permitted, provided the original author(s) and the copyright owner(s) are credited and that the original publication in this journal is cited, in accordance with accepted academic practice. No use, distribution or reproduction is permitted which does not comply with these terms.
*Correspondence: Stefania Varchetta, cy52YXJjaGV0dGFAc21hdHRlby5wdi5pdA==; Mario U. Mondelli, bWFyaW8ubW9uZGVsbGlAdW5pcHYuaXQ=
Disclaimer: All claims expressed in this article are solely those of the authors and do not necessarily represent those of their affiliated organizations, or those of the publisher, the editors and the reviewers. Any product that may be evaluated in this article or claim that may be made by its manufacturer is not guaranteed or endorsed by the publisher.
Research integrity at Frontiers
Learn more about the work of our research integrity team to safeguard the quality of each article we publish.