- 1Department of Pathophysiology, College of Basic Medical Sciences, Jilin University, Changchun, China
- 2Department of Pathology, Affiliated Hospital to Changchun University of Chinese Medicine, Changchun, Jilin, China
There is increasing evidence for the key role of the immune microenvironment in the occurrence and development of hepatocellular carcinoma. As an important component of the immune microenvironment, the polarization state and function of macrophages determine the maintenance of the immunosuppressive tumor microenvironment. Hepatocellular carcinoma tumor-derived exosomes, as information carriers, regulate the physiological state of cells in the microenvironment and control cancer progression. In this review, we focus on the role of the exosome content in disease outcomes at different stages in the progression of hepatitis B virus/hepatitis C virus-induced hepatocellular carcinoma. We also explore the mechanism by which macrophages contribute to the formation of hepatocellular carcinoma and summarize the regulation of macrophage functions by the heterogeneity of exosome loading in liver cancer. Finally, with the rise of exosome modification in immunotherapy research on hepatocellular carcinoma, we summarize the application prospects of exosome-based targeted drug delivery.
Introduction
Liver cancer has a 5-year relative survival rate of only 20% (1). Liver cancer caused by chronic infection with hepatitis B virus (HBV) and hepatitis C virus (HCV) accounts for approximately 50%–80% of cases (2). Other risk factors include aflatoxin exposure, tobacco and alcohol use, non-alcoholic fatty liver disease, obesity, and diabetes. The distribution of these risk factors varies according to the population, time, and region (1, 3). Therefore, as liver cancer is a chronic inflammation-related cancer, it is crucial to study the role of exosomes during diseaseprogression and in the immune microenvironment.
Macrophages are abundant in the liver and are essential cells in the tumor microenvironment (TME) in liver cancer. During the initial stages of liver cancer at the time of niche formation, hepatic macrophages display an inflammatory phenotype, namely, the M1 type; these cells damage neighboring cells by continuous secretion of reactive oxygen species. In solid tumors that successfully escape immune surveillance, macrophages disproportionately differentiate into the M2 phenotype with anti-inflammatory activity, that is, tumor-associated macrophages (TAMs), which have proangiogenic, matrix remodeling, distal metastasis, and immunosuppressive effects (4). The recruitment of hepatic macrophages in human liver cancer is correlated with disease progression and a poor prognosis (5). Hepatoma cells also play crucial regulatory roles in macrophage proliferation and differentiation during tumor progression (6).
Hypoxia has become one of the most intensively studied features of the TME (7). In this context, in addition to directly secreting cytokines (8), exosome-mediated communication between tumor cells and the stroma is considered an important step in remodeling the TME (9). Multiple studies have demonstrated the adaptive tuning of extracellular vesicle (EV) secretion and contents of liver cancer cells during progression, providing a basis for subsequent remodeling of the surrounding niche (10, 11) and, specifically, for altering TAMs.
In this review, we summarize the roles of exosomes and macrophages during the progression of viral hepatitis to liver cancer, including but not limited to the effects of exosomal contents on macrophages and exosome-based therapeutic prospects for liver cancer.
Involvement of exosomes in hepatocarcinogenesis
Analyses of liver cancer progression associated with chronic inflammation are still needed. Hepatocyte exosomes function as messengers in the formation and evolution of the liver cancer niche (12). Exosomes are released into the intercellular space or enter the hepatic microvasculature to participate in intercellular signal communication or material transport, thereby regulating the pathophysiological state of the liver (13).
In the physiological state, liver parenchymal cells, which make up approximately 80% of the liver volume, secrete exosomes loaded with neutral ceramidase and sphingosine kinase 2 (SK2), which are recognized by recipient hepatocytes and upregulate sphingosine-1-phosphate (S1P) production by target cells, thereby promoting hepatocyte repair and regeneration (14).
In some pathological conditions, liver parenchymal cells, hepatic stellate cells (HSCs), and Kupffer cells (KCs) are the main donor and recipient cells of exosomes and are associated with hepatitis, cirrhosis, and liver cancer (15). The hepatocyte exosomal cargo plays diverse roles in the microenvironment during liver cancer progression (Figure 1).
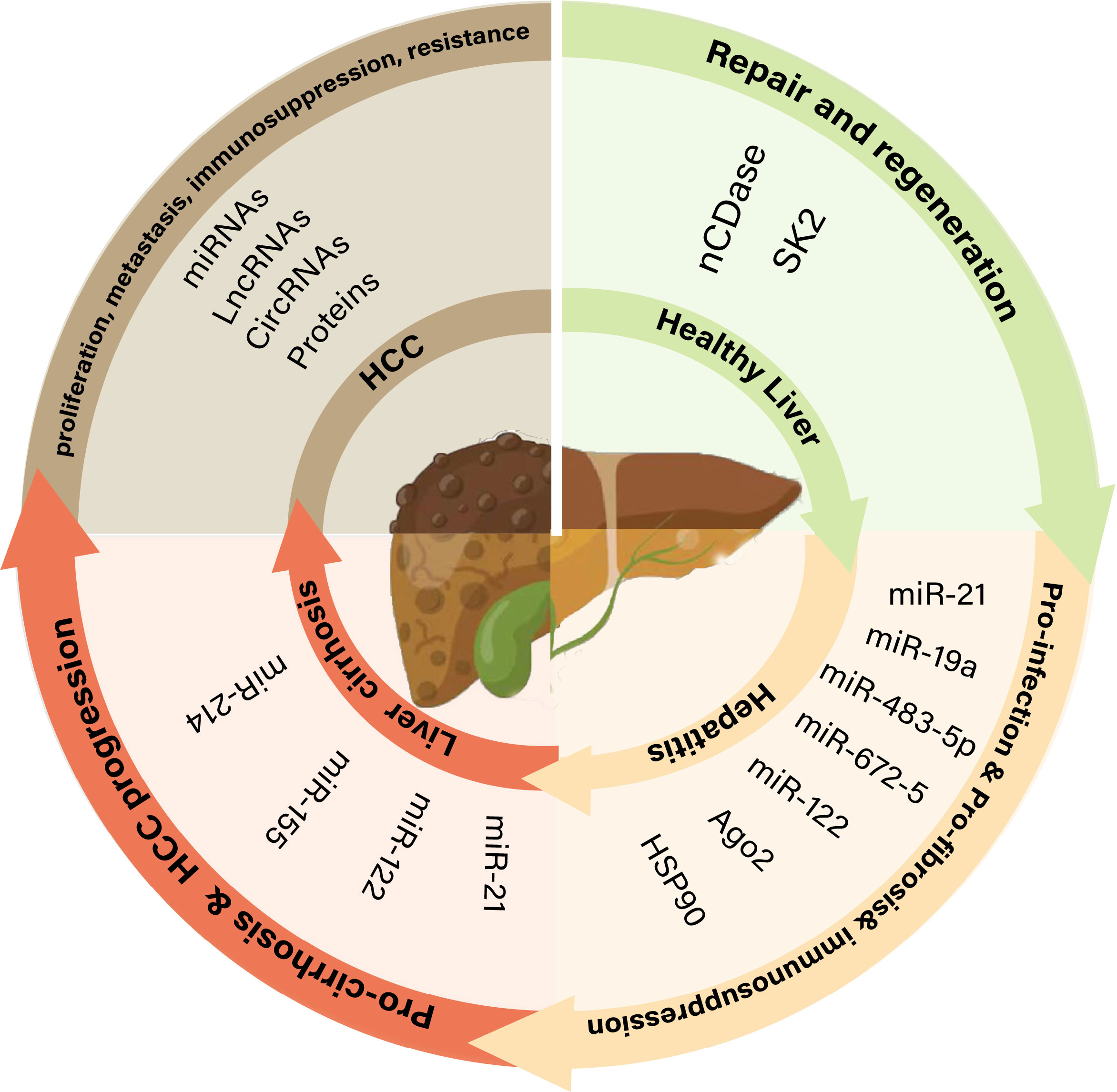
Figure 1 The role of exosomes in different pathophysiological states of the liver. nCDase, neutral ceramidase; SK2, sphingosine kinase 2; Ago2, argonaute-2; Hsp90, heat shock protein 90; lncRNAs, long non-coding RNAs; miRNAs, MicroRNAs; circRNAs, Circular RNAs.
Viral Hepatitis
Hepatitis caused by HBV/HCV infection is one of the main causes of hepatocellular carcinoma (HCC) (2). Exosomes secreted from hepatocytes infected with HCV carry virus-derived Ago2, heat shock protein 90 (Hsp90), and miR-122, which mediate the stable transmission of HCV in the liver (16–18). Exosome-mediated viral transport helps the virus evade immune system surveillance. MicroRNAs (miRNAs) released from virus-infected hepatocytes inhibit natural killer (NK) cell proliferation and survival and facilitate the evasion of host innate immunity (19). Exosomes containing HCV RNA reduce Toll-like receptor 3 (TLR3) activation and interfere with antiviral interferon-stimulated gene activation (20). T-cell immunoglobulin and mucin domain-containing molecule 3 (TIM-3)/galectin 9 (Gal-9) in exosomes increase HCV-infected hepatocytes, affect monocyte differentiation, and inhibit the immune response (21).
Cirrhosis
During liver cirrhosis, HSCs along with other cells of the liver parenchyma (liver sinusoidal endothelial cells and KCs) play important roles in the development and progression of liver fibrosis (22). Exosomes released by injured hepatocytes are internalized by stellate cells, leading to phenotypic switching of quiescent stellate cells. HSC activation is a major driver of the initiation, progression, and resolution of liver fibrosis (23).
Exosomes released from injured hepatic stellate structures contain abundant fibrotic components that promote fibrosis via multiple pathways, such as by stimulating fibroblasts and myofibroblasts to produce collagen from the bone marrow and portal fibrocytes. Connective tissue growth factor (CTGF), a multifunctional heparin-binding glycoprotein, contributes to the promotion of multiple fibrotic processes (24). CTGF, which is widely expressed in activated HSC-derived exosomes, regulates the activation and migration of HSCs and immune responses, whereas exosomes produced by quiescent HSCs are enriched in miR-214 and twist, attenuating the profibrotic function of activated HSCs (25, 26). Exosomes derived from liver sinusoidal endothelial cells regulate the migratory capacity of HSCs via adhesion.
Hepatocellular Carcinoma
HCC is a common malignancy with poor overall survival. The main risk factors for HCC include viral hepatitis, excessive alcohol consumption, and smoking. However, the pathogenesis of HCC is complicated and difficult to determine. Extensive evidence suggests that exosomes derived from cells carry tumor-specific markers, which mediate intercellular communication between cancer cell populations and promote the migration and invasion of recipient cells (27). For non-immune cells, HCC exosomes regulate tumor niche formation by promoting tumor-associated fibroblast transformation and angiogenesis by altering the endothelial vascular phenotype (28, 29). Liver cancer exosomes mainly mediate tumor cell immune escape by inhibiting their maintenance and proliferation, promoting phenotypic transformation, and blocking functional activation (30).
These effects promoting HCC progression depend on proteins and non-coding RNAs (ncRNAs) in exosomes. They are transferred by exosomes and participate in the communication between HCC cells and targeted cells in the TME, thereby affecting tumor angiogenesis, metastasis, and drug and radiotherapy resistance. Therefore, we summarized the current research status of proteins and ncRNAs in HCC exosomes to further emphasize the potential value of these abnormally expressed exosome molecules in HCC as biomarkers for the diagnosis, prognosis, and treatment of HCC (Table 1).
Hypoxia promotes the production and release of exosomes
HCC is a hypermetabolic tumor of the digestive system. Based on the high rate of cell proliferation, the altered blood supply system participates in the exchange of substances within the tumor (66). Therefore, hypoxic signals contribute to liver cancer formation, proliferation, and metastasis (67). In addition to the adaptive changes in cellular components within the TME in response to hypoxia, hepatoma cells transmit post-hypoxic regulatory signals to other cells by secreting EVs (68). Cancer cells with different phenotypes communicate via exosomes to complete the phenotypic transformation and promote the progression of liver cancer (69). For example, exosomes from highly metastatic MHCC97H cells can communicate with less metastatic HCC cells, increasing their migration, chemotaxis, and invasion (70). Similarly, the EVs of cisplatin-resistant non-small-cell lung cancer cell lines secreted pyruvate kinase M2 (PKM2) under hypoxic conditions. The phagocytosis of these EVs by cisplatin-sensitive non-small-cell lung cancer cell lines induced decreased sensitivity to cisplatin (71).
The adaptive response of tumor cells to hypoxia is mostly regulated by hypoxia-inducible factor 1 (HIF1). HIF1α/2α is also highly expressed in liver cancer (72). Under normoxia, the two proline residues of the HIF-1/2α subunit are hydroxylated by prolyl hydroxylase domain (PHD) enzymes, promoting binding to von Hippel–Lindau (VHL), which mediates the degradation of the hydroxylated HIF-1/2α subunit via the ubiquitin-proteasome pathway. However, under hypoxic conditions, the generation and release of EVs are regulated by HIF. During EV biogenesis, RAS superfamily proteins (RABs) are involved in the formation and fusion of membrane buds, and HIF can directly affect the RAS. That is, under hypoxic conditions, HIF is activated to promote the transcription of RABs and finally promote the generation and secretion of exosomes (73, 74). HIF can promote the expression and activation of a series of cell surface receptors, such as epidermal growth factor receptor, glucose transporter receptor, and transferrin receptor, and promote cell internalization and endocytosis (75).
The mechanism by which EV contents (nucleic acids, proteins, etc.) are specifically sorted under hypoxia remains unclear. This process is related to endosomal sorting complex required for transport (ESCRT) complexes and ceramides and may be related to posttranslational processes (76). This modified protein complex is closely related to ubiquitin-like 3 (UBL3)/membrane-anchored Ub-fold protein (MUB). In models of lung injury, proteins and peptides in vesicles were more ubiquitinated under hypoxia (77). This indicates that ubiquitination regulates the loading process of exosome contents under hypoxia.
In addition, HIF1-independent regulation of adaptive responses to hypoxia has been reported, such as phosphoinositide 3-kinase (PI3K), serine-threonine kinase (AKT), mammalian target of rapamycin (mTOR), Nuclear factor kappa-light-chain-enhancer of activated B cells (NF-κB) Rab-GTPase, Wnt/β-catenin, mitogen-activated protein kinases, and oxidative stress (78).
Hepatocellular Carcinoma (HCC)-associated macrophages
Origin and function of macrophages
Under physiological conditions, the liver has a rich blood supply and abundant innate immune cells (such as KCs, NK cells, and T cells). Resident macrophages in the liver are mainly composed of KCs and monocyte-derived macrophages (79). In healthy liver, KCs are the main resident hepatic macrophages. KCs are generally believed to have originated from yolk sac-derived colony-stimulating factor 1 receptor (CSF1R) + erythroid/myeloid progenitors (EMPs), which are present in the fetal liver during embryogenesis. They can maintain liver homeostasis by removing metabolic waste and cell debris, regulating cholesterol homeostasis, maintaining iron homeostasis and iron cycling, mediating immune responses, and promoting immune tolerance (80). Some circulation-derived monocyte-macrophage populations recognize liver-invading bacteria and recruit neutrophils. In the human liver, hepatic macrophages consist of CD68+macrophage receptor with collagenous structure (MARCO)+KCs, CD68+MARCO-macrophages, and CD14+monocytes. CD68+MARCO+-KCs usually overexpress immune tolerance-related genes and have anti-inflammatory effects, and CD68+MARCO-macrophages and CD14+monocytes have pro-inflammatory effects (5).
From the progression of chronic hepatitis to fully developed tumors, there is a high degree of heterogeneity in the intratumoral microenvironment, with a highly invasive anterior and middle hypoxic and necrotic areas and tumor cells with high and low proliferation (81). There are also different TAM phenotypes in liver cancer, and research on the classification of TAMs and their heterogeneity is still in its infancy (82). In short, TAMs are collections of macrophages, including infiltrating and resident macrophages, originating from various cellular sources. TAM polarization shows plasticity, and cells can exhibit either phenotype. Several studies have provided evidence that the acquisition of an M2-like polarized macrophage phenotype by TAMs promotes tumor progression by promoting angiogenesis, immunosuppression, and growth factor secretion, ultimately leading to metastasis (83).
TAMs secrete excessive proangiogenic factors [e.g., vascular endothelial growth factor (VEGF), platelet-derived growth factor, and transforming growth factor beta (TGFB)] and cell proliferation-stimulating factors (e.g., Interleukin(IL)-1β, IL-6, chemokine (C-C motif) ligand 2 (CCL2), tumor necrosis factor, and VEGF), which strongly promote tumor growth and development (84, 85).
Mechanisms underlying macrophage uptake of exosomes
When exosomal vesicles come in contact with the surface of macrophages, they trigger a functional response (e.g., proliferation and differentiation) via membrane surface ligand–receptor recognition signals and/or transport of their contents into the cell, antigen presentation, etc. (86).
Macrophages are initially recognized by protein receptors and adhesion molecules (e.g., tetraspanins, integrins, proteoglycans, and lectins) on the exosome surface. Exosomes are then taken up by activating cell membrane-expressed receptors, fusion with the macrophage plasma membrane, or endocytosis (87). The final contents are delivered to macrophages to exert biological functions. An increasing number of studies have evaluated the mechanism underlying the uptake. For example, exosomes derived from pancreatic cancer preferentially bind to F4/80+ and CD11b+ KCs in the liver, which is promoted by intercellular adhesion molecules and CD11b ligands (88). A study of the liver metastasis of pancreatic cancer cells suggested that endoplasmic reticulum aminopeptidase 1 (ERAP1)-secreting exosomes enhance the phagocytic capacity and NO synthesis activity of macrophages (89). However, some circulating exosomes can protect against phagocytosis by macrophage CD47 enrichment.
The effect of exosomes from non-metastatic K7 and Dunn osteosarcoma cells and the metastatic sublines K7M3 and DLM8 on macrophage phagocytosis was evaluated in a study of osteosarcoma lung metastasis (85). Exosomes secreted by the highly metastatic K7M3 and DLM8 cell lines were incubated with MHS mouse alveolar macrophages, which induced the mRNA expression of IL-10, TGFB2, and CCL22 (markers of M2 macrophages). Reduced macrophage phagocytosis, exocytosis, and macrophage-mediated tumor cell killing were also observed. By contrast, exosomes from non-metastatic K7 or Dunn cells failed to inhibit macrophage phagocytosis, exocytosis, and cytotoxicity and did not induce increases in the mRNA expression of IL10, TGFB2, or CCL22.
The uptake of exosomes by macrophages is inseparable from clathrin-dependent endocytosis in which caveolin-1 is essential for the formation of pits (membrane depressions) and accumulates in membrane depressions (90). Clathrin protein heavy chain 1 (Cltc) is encoded by the cltc gene and is highly expressed in macrophages (91). When Cltc1 is knocked out, phagocytosis by monocyte-macrophages is inhibited.
HCC Tumor-Derived exosomes are involved in the regulation of the polarization and function of macrophages
Liver macrophages can be activated to M1 and IL-13 via the classical activation pathway (bacterial lipopolysaccharide and interferon-gamma secreted by Th1 cells) and alternative activation pathways (cytokines IL-4, IL-10, and IL-13 secreted by Th2 cells). There are two subtypes of M2 macrophages, and these can be further subdivided into M2a, M2b, M2c, and M2d. M1-type macrophages mainly secrete pro-inflammatory factors, such as IL-12, IL-6, IL-18, IL-23, and tumor necrosis factor, and increase the expression of nitric oxide synthase, which is responsible for the defense against pathogen infection. The M2 type expresses high levels of IL-10, IL-1a/b inhibitor, mannose receptor (MRC1), arginase 1 (Arg1), and other anti-inflammatory factors. These two polarization modes are classic models for studies of macrophages (92, 93).
Immunity and metabolism are highly integrated and coordinated. In the initial stage of tissue hypoxia, the anaerobic glycolysis and pentose phosphate pathways of M1 macrophages are activated, whereas M2 macrophages mainly use oxidative phosphorylation and aerobic glycolysis to meet the energy requirements for tissue repair and remodeling. M1 macrophages are considered the most likely precursors of tumor-infiltrating macrophages, and TAMs are frequently M2 macrophages (94).
The long non-coding RNA (lncRNA) TUC339 is highly expressed in HCC-derived exosomes, which can be transferred across HCC cells to promote tumor growth and metastasis (55, 95). Furthermore, the exosomal long non-coding RNA (lncRNA) TUC339 can be transferred to neighboring macrophages to modulate M1/M2 polarization and suppress antitumor immune responses in vitro. Microarray studies have demonstrated that exosomal TUC339 downregulated TLR signaling and Fcγ receptor (FcγR)-mediated phagocytosis pathways in macrophages, and TUC339 knockdown increased the phagocytic activity of macrophages. TUC339 is also involved in cytokine and chemokine receptor signaling, although the exact mechanism is unclear. Tumor cell-derived exosomes also carry miRNAs that regulate the expression of immune response-related genes. miR150 is highly expressed in the plasma of patients with HCC and in HCC-derived exosomes and promotes the growth of vascular endothelial cells by secreting the TAM-derived cytokine factor VEGF (40). VEGF levels are reduced in the plasma and tumor tissues of tumor-bearing mice treated with miR150 inhibitors. HCC exosomal miR-23a-3p upregulates the programmed cell death ligand 1 (PD-L1) expression in macrophages via Signal transducer and activator of transcription 3 (STAT3) signaling, which significantly attenuates melatonin-treated HCC cell-derived exosomes (41). PD-L1 expression in phagocytes has been demonstrated in vivo. HCC-derived exosomes significantly increased CD11b+F4/80+CD206+ macrophages, accompanied by upregulation of M2-specific markers, including C-C chemokine ligand 17 (ccl17), C-C chemokine ligand 22 (ccl22), and arg-1. M2 polarization in vitro and in HCC-bearing mouse models is driven by miR146a, which is directly regulated by the zinc finger transcription factor Sal-like protein-4 (SALL4) in HCC cells (96, 97). The exosomal lncRNA HMMR-AS1 mediates macrophage polarization via the miR-147a/ARID3A axis under hypoxia and affects the progression of HCC (98) (Figure 2).
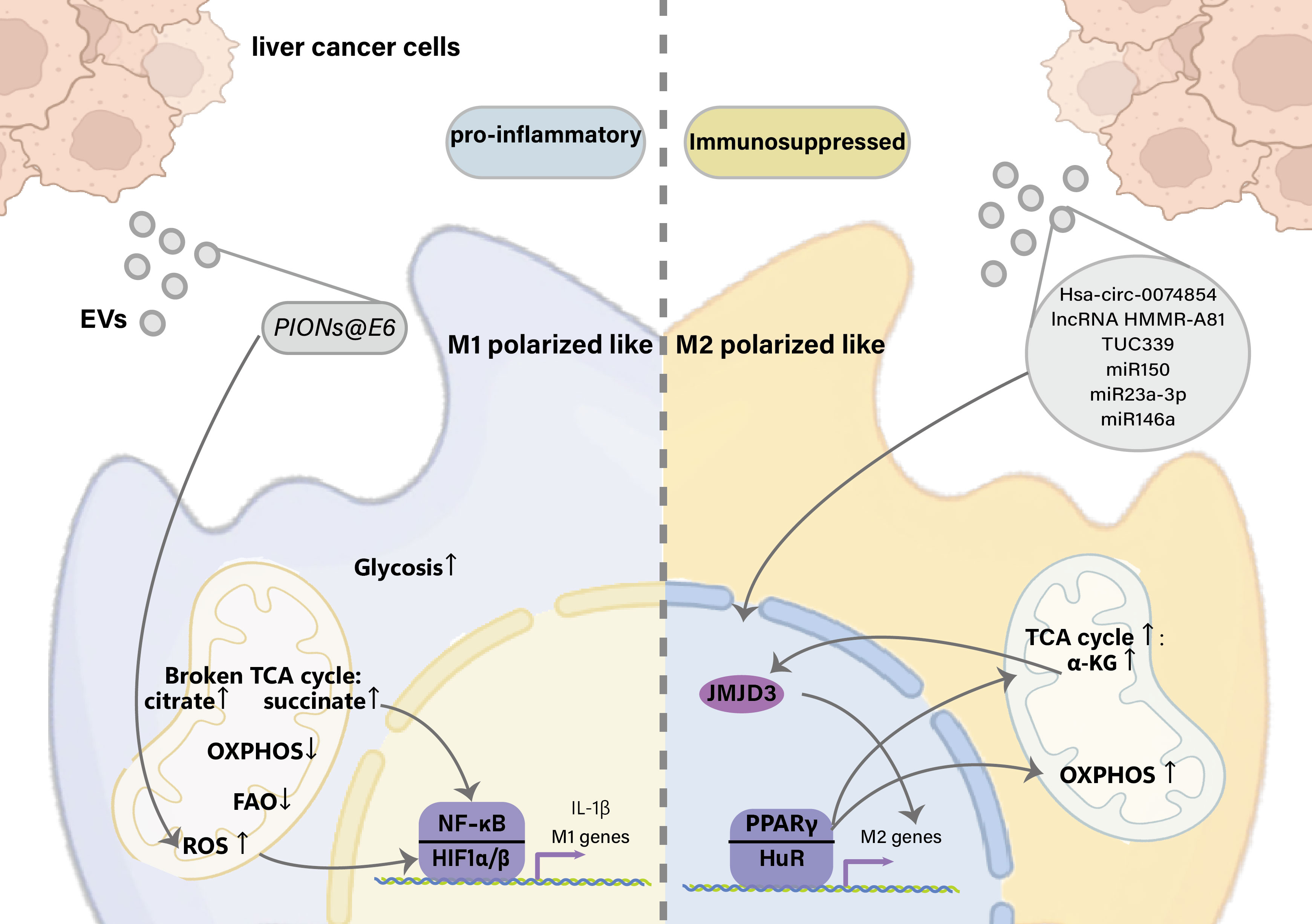
Figure 2 Effects of liver cancer-derived exosomes on macrophage polarization. EVs, Extracellular vesicles; TCA, tricarboxylic acid cycle; OXPHOS, Oxidative phosphorylation; FAO, Fatty Acid Oxidation; HIF1, hypoxia-inducible factor 1; NF-κB, Nuclear factor kappa-light-chain-enhancer of activated B cells; IL-1β, Interleukin 1 beta; α-KG, α-Ketoglutaric acid; JMJD3, Jumonji domain-containing protein-3; PPARγ, Peroxisome proliferator-activated receptor γ; HuR, Hu antigen R.
Therapeutic prospects related to exosomes in liver cancer cells
Role of H-TEXs in liver cancer drug resistance
In the TME, exosomes act as key regulators of the effects of chemotherapeutics by modulating drug efflux, epithelial–mesenchymal transition (EMT), autophagy phenotype, and immunosuppression (Table 2).
Exosome-related drug delivery system based on liver cancer therapy
Additionally, exosomes can assist in the early diagnosis of tumors, monitoring, and prognostic analyses. Because exosomes are endogenous vesicles, they benefit from low immunogenicity, good biodegradability, low toxicity, and the ability to cross the blood–brain barrier, making them good carriers for drug delivery (106). For example, using doxorubicin, Yong et al. (107) took advantage of the ability of tumor cells to efflux chemotherapeutic drugs through EVs to achieve chemoresistance and encapsulated porous silicon nanoparticles loaded with Adriamycin into tumor cell-derived exosomes. Liang et al. (108) demonstrated that tumor-repopulating cell (TRC)-derived three-dimensional (3D) extracellular microparticles (MPs), which benefit from their softness, accumulate substantially and readily penetrate the liver tumor parenchyma for efficient delivery of chemotherapeutic drugs into TRCs. This results in effective suicide-like TRC killing and favorable therapeutic outcomes. Cytospin-A-related softness of 3D-MPs plays an important role in regulating the in vivo transport process. These findings reveal a new aspect of MP biology and provide potentially effective strategies for drug delivery in cancer therapy. This Trojan horse-like nanodrug delivery system using tumor exosomes as a carrier has been shown to have a higher inhibitory effect on tumor cells in vivo, and the tail vein injection of tumor-bearing mice is comparable to that of doxorubicin alone. Greater enrichment was observed in the exosome-encapsulated doxorubicin-treated group than in the doxorubicin group. Similarly, the exosome chemotherapeutic drug loading method has been evaluated in research on glioma. However, the liver, the largest solid organ in the human body, contains the most tissue-resident macrophages, and KCs, which account for 80%–90% of all tissue macrophages in the body, are responsible for capturing and removing foreign bodies. Therefore, avoiding the capture of the mononuclear phagocytic cell system and ensuring the delivery of sufficient doses of drugs to the liver tumor area after entering the blood circulation are major issues that need to be resolved in cancer-targeted drug delivery research (109). Belhadj et al. (110) described an “eat/don’t-eat” decision switch for macrophages to evade phagocytosis by modifying CD47 outside of EVs. The effectiveness of this switch was verified by Du et al. (111). They engineered an exosome armed with three moieties, surface functionalization with CD47, membrane loading with ferroptosis inducer erastin, and core with photosensitizer RB. The exosomes displayed high delivery efficiency to tumors. Upon irradiation with a 532-nm laser in the tumor region, Erastin (Er) and Rose Bengal (RB) synergistically induced cell death.
Exosome-based immunotherapy in HCC
Cancer immunotherapy reverses the immunosuppressive TME (112). Exosome-targeted immunotherapy of HCC is often associated with dendritic cell (DC)-derived exosomes (DEXs), which have great potential for immunotherapy applications (113, 114).
Lu et al. (115) infected a DC cell line (DC2.4), which was established by transfecting Granulocyte-macrophage colony-stimulating factor (GM-CSF) (Csf2), Myc, and Raf genes into C57BL/6 mice, with a lentivirus-expressing murine α-fetoprotein (AFP). They found that DC-AFP-derived exosomes (DEX-AFP) elicited strong antigen-specific immune responses, resulting in significantly delayed tumor growth and prolonged survival in various HCC mouse models (115). Zuo et al. (116) also used DEX as a carrier for a liver cancer vaccine to initiate a specific immune response against HCC. They decorated DEX with an HCC-targeting peptide (P47-P), an AFP epitope (AFP212-A2), and a functional domain of high-mobility group nucleosome-binding protein 1 (N1ND-N) and demonstrated its potential for the individualized treatment of HCC via universal DEX vaccines (116). Zhong et al. (117) enhanced the antitumor efficacy of a DEX vaccine for HCC using microwave ablation. Zuo et al. (118) demonstrated that alarmin-coated exosomes elicited durable large-scale antitumor immunity in mouse liver tumors. Shi et al. (102) showed that the combination of DC-TEX and a programmed cell death protein 1 (PD-1) antibody (Ab) enhances the efficacy of sorafenib.
However, immunotherapy clinical trials have shown that substantial work is still needed before these findings can be applied to the treatment of cancer in clinical settings. Despite the challenges, DEX remains a promising immunotherapeutic strategy. DEX acts as a stable vesicle with a long shelf life, and its immunostimulatory properties are easily manipulated (through donor DCs). Research advancements have expanded the use of DEX-based cancer treatments in clinical settings (119).
Discussion
In this review, we evaluated the important role of exosomes in HCC progression and immunotherapy. Given that TAMs are a key component of the microenvironment, we summarize the regulatory mechanism by which liver cancer-derived exosomes regulate macrophage polarization, demonstrating that exosomes are a promising tool to target macrophages for HCC immunotherapy.
It should be emphasized that the interaction between the tumor and immunity is dynamic, heterogeneous, and bidirectional, including the immune response to drugs or external stimuli. Furthermore, the tumor cell state and even the genome are altered (120). Even so, the regulatory function of exosomes as messengers cannot be ignored, especially in the treatment of HCC. Chemotherapy resistance has become a major obstacle in improving the prognosis of patients. Targeting exosomes could be a promising strategy for reversing drug tolerance. In addition, the improvement of the efficacy of chemotherapy in patients with HCC by exosome anticancer drug delivery provides a new perspective for clinical treatment.
Author contributions
Conceptualization, SY and LZ. Writing original manuscript, SY. Visualization, SY and JF. Writing review and editing, SY, JF,LX, YZ, JW, BL, JS and XY. All authors contributed to the article and approved the submitted version.
Funding
National Natural Science Foundation of China (81672948); Jilin Provincial Research Foundation for Health Technology Innovation (2021JC034).
Conflict of interest
The authors declare that the research was conducted in the absence of any commercial or financial relationships that could be construed as a potential conflict of interest.
Publisher’s note
All claims expressed in this article are solely those of the authors and do not necessarily represent those of their affiliated organizations, or those of the publisher, the editors and the reviewers. Any product that may be evaluated in this article, or claim that may be made by its manufacturer, is not guaranteed or endorsed by the publisher.
References
1. Siegel RL, Miller KD, Fuchs HE, Jemal A. Cancer statistics, 2022. CA Cancer J Clin (2022) 72(1):7–33. doi: 10.3322/caac.21708
2. Chen Y, Tian Z. HBV-induced immune imbalance in the development of HCC. Front Immunol (2019) 10:2048. doi: 10.3389/fimmu.2019.02048
3. Pandyarajan V, Govalan R, Yang JD. Risk factors and biomarkers for chronic hepatitis b associated hepatocellular carcinoma. Int J Mol Sci (2021) 22(2):479. doi: 10.3390/ijms22020479
4. Tian Z, Hou X, Liu W, Han Z, Wei L. Macrophages and hepatocellular carcinoma. Cell Biosci (2019) 9:79. doi: 10.1186/s13578-019-0342-7
5. Wen Y, Lambrecht J, Ju C, Tacke F. Hepatic macrophages in liver homeostasis and diseases-diversity, plasticity and therapeutic opportunities. Cell Mol Immunol (2021) 18(1):45–56. doi: 10.1038/s41423-020-00558-8
6. Wu J, Gao W, Tang Q, Yu Y, You W, Wu Z, et al. M2 macrophage-derived exosomes facilitate HCC metastasis by transferring alphaM beta2 integrin to tumor cells. Hepatology (2021) 73(4):1365–80. doi: 10.1002/hep.31432
7. Guo Y, Xiao Z, Yang L, Gao Y, Zhu Q, Hu L, et al. Hypoxiainducible factors in hepatocellular carcinoma (Review). Oncol Rep (2020) 43(1):3–15. doi: 10.3892/or.2019.7397
8. Xu J, Zhang J, Zhang Z, Gao Z, Qi Y, Qiu W, et al. Hypoxic glioma-derived exosomes promote M2-like macrophage polarization by enhancing autophagy induction. Cell Death Dis (2021) 12(4):373. doi: 10.1038/s41419-021-03664-1
9. Bister N, Pistono C, Huremagic B, Jolkkonen J, Giugno R, Malm T. Hypoxia and extracellular vesicles: A review on methods, vesicular cargo and functions. J Extracell Vesicles (2020) 10(1):e12002. doi: 10.1002/jev2.12002
10. Reinfeld BI, Madden MZ, Wolf MM, Chytil A, Bader JE, Patterson AR, et al. Cell programmed nutrient partitioning in the tumor microenvironment. Nature(2021) 593(7858):282–88. doi: 10.1038/s41586-021-03442-1
11. Milane L, Singh A, Mattheolabakis G, Suresh M, Amiji MM. Exosome mediated communication within the tumor microenvironment. J Control Release (2015) 219:278–94. doi: 10.1016/j.jconrel.2015.06.029
12. Sung S, Kim J, Jung Y. Liver-derived exosomes and their implications in liver pathobiology. Int J Mol Sci (2018) 19(12):3715. doi: 10.3390/ijms19123715
13. Jiao Y, Xu P, Shi H, Chen D, Shi H. Advances on liver cell-derived exosomes in liver diseases. J Cell Mol Med (2021) 25(1):15–26. doi: 10.1111/jcmm.16123
14. Nojima H, Freeman CM, Schuster RM, Japtok L, Kleuser B, Edwards MJ, et al. Hepatocyte exosomes mediate liver repair and regeneration via sphingosine-1-phosphate. J Hepatol (2016) 64(1):60–8. doi: 10.1016/j.jhep.2015.07.030
15. Conde-Vancells J, Rodriguez-Suarez E, Embade N, Gil D, Matthiesen R, Valle M, et al. Characterization and comprehensive proteome profiling of exosomes secreted by hepatocytes. J Proteome Res (2008) 7(12):5157–66. doi: 10.1021/pr8004887
16. Bukong TN, Momen-Heravi F, Kodys K, Bala S, Szabo G. Exosomes from hepatitis c infected patients transmit HCV infection and contain replication competent viral RNA in complex with Ago2-miR122-HSP90. PLos Pathog (2014) 10(10):e1004424. doi: 10.1371/journal.ppat.1004424
17. Chahal J, Gebert LFR, Gan HH, Camacho E, Gunsalus KC, MacRae IJ, et al. miR-122 and ago interactions with the HCV genome alter the structure of the viral 5' terminus. Nucleic Acids Res (2019) 47(10):5307–24. doi: 10.1093/nar/gkz194
18. Masaki T, Arend KC, Li Y, Yamane D, McGivern DR, Kato T, et al. miR-122 stimulates hepatitis c virus RNA synthesis by altering the balance of viral RNAs engaged in replication versus translation. Cell Host Microbe (2015) 17(2):217–28. doi: 10.1016/j.chom.2014.12.014
19. Yang Y, Han Q, Hou Z, Zhang C, Tian Z, Zhang J. Exosomes mediate hepatitis b virus (HBV) transmission and NK-cell dysfunction. Cell Mol Immunol (2017) 14(5):465–75. doi: 10.1038/cmi.2016.24
20. Grunvogel O, Colasanti O, Lee JY, Kloss V, Belouzard S, Reustle A, et al. Secretion of hepatitis c virus replication intermediates reduces activation of toll-like receptor 3 in hepatocytes. Gastroenterology (2018) 154(8):2237–51 e16. doi: 10.1053/j.gastro.2018.03.020
21. Harwood NM, Golden-Mason L, Cheng L, Rosen HR, Mengshol JA. HCV-infected cells and differentiation increase monocyte immunoregulatory galectin-9 production. J Leukoc Biol (2016) 99(3):495–503. doi: 10.1189/jlb.5A1214-582R
22. Zhang CY, Yuan WG, He P, Lei JH, Wang CX. Liver fibrosis and hepatic stellate cells: Etiology, pathological hallmarks and therapeutic targets. World J Gastroenterol (2016) 22(48):10512–22. doi: 10.3748/wjg.v22.i48.10512
23. Benbow JH, Marrero E, McGee RM, Brandon-Warner E, Attal N, Feilen NA, et al. Hepatic stellate cell-derived exosomes modulate macrophage inflammatory response. Exp Cell Res (2021) 405(1):112663. doi: 10.1016/j.yexcr.2021.112663
24. Li X, Chen R, Kemper S, Brigstock DR. Extracellular vesicles from hepatocytes are therapeutic for toxin-mediated fibrosis and gene expression in the liver. Front Cell Dev Biol (2019) 7:368. doi: 10.3389/fcell.2019.00368
25. Chen L, Charrier A, Zhou Y, Chen R, Yu B, Agarwal K, et al. Epigenetic regulation of connective tissue growth factor by MicroRNA-214 delivery in exosomes from mouse or human hepatic stellate cells. Hepatology (2014) 59(3):1118–29. doi: 10.1002/hep.26768
26. Chen L, Chen R, Kemper S, Charrier A, Brigstock DR. Suppression of fibrogenic signaling in hepatic stellate cells by Twist1-dependent microRNA-214 expression: Role of exosomes in horizontal transfer of Twist1. Am J Physiol Gastrointest Liver Physiol (2015) 309(6):G491–9. doi: 10.1152/ajpgi.00140.2015
27. Wang H, Lu Z, Zhao X. Tumorigenesis, diagnosis, and therapeutic potential of exosomes in liver cancer. J Hematol Oncol (2019) 12(1):133. doi: 10.1186/s13045-019-0806-6
28. Conigliaro A, Costa V, Lo Dico A, Saieva L, Buccheri S, Dieli F, et al. CD90+ liver cancer cells modulate endothelial cell phenotype through the release of exosomes containing H19 lncRNA. Mol Cancer (2015) 14:155. doi: 10.1186/s12943-015-0426-x
29. Zeng Z, Li Y, Pan Y, Lan X, Song F, Sun J, et al. Cancer-derived exosomal miR-25-3p promotes pre-metastatic niche formation by inducing vascular permeability and angiogenesis. Nat Commun (2018) 9(1):5395. doi: 10.1038/s41467-018-07810-w
30. Han Q, Zhao H, Jiang Y, Yin C, Zhang J. HCC-derived exosomes: Critical player and target for cancer immune escape. Cells (2019) 8(6):558. doi: 10.3390/cells8060558
31. Li R, Wang Y, Zhang X, Feng M, Ma J, Li J, et al. Exosome-mediated secretion of LOXL4 promotes hepatocellular carcinoma cell invasion and metastasis. Mol Cancer (2019) 18(1):18. doi: 10.1186/s12943-019-0948-8
32. Gai X, Tang B, Liu F, Wu Y, Wang F, Jing Y, et al. mTOR/miR-145-regulated exosomal GOLM1 promotes hepatocellular carcinoma through augmented GSK-3beta/MMPs. J Genet Genomics (2019) 46(5):235–45. doi: 10.1016/j.jgg.2019.03.013
33. Sun H, Wang C, Hu B, Gao X, Zou T, Luo Q, et al. Exosomal S100A4 derived from highly metastatic hepatocellular carcinoma cells promotes metastasis by activating STAT3. Signal Transduct Target Ther (2021) 6(1):187. doi: 10.1038/s41392-021-00579-3
34. Ye L, Zhang Q, Cheng Y, Chen X, Wang G, Shi M, et al. Tumor-derived exosomal HMGB1 fosters hepatocellular carcinoma immune evasion by promoting TIM-1(+) regulatory b cell expansion. J Immunother Cancer (2018) 6(1):145. doi: 10.1186/s40425-018-0451-6
35. Fu Q, Zhang Q, Lou Y, Yang J, Nie G, Chen Q, et al. Primary tumor-derived exosomes facilitate metastasis by regulating adhesion of circulating tumor cells via SMAD3 in liver cancer. Oncogene (2018) 37(47):6105–18. doi: 10.1038/s41388-018-0391-0
36. Jiang K, Dong C, Yin Z, Li R, Mao J, Wang C, et al. Exosome-derived ENO1 regulates integrin alpha6beta4 expression and promotes hepatocellular carcinoma growth and metastasis. Cell Death Dis (2020) 11(11):972. doi: 10.1038/s41419-020-03179-1
37. Dai W, Wang Y, Yang T, Wang J, Wu W, Gu J. Downregulation of exosomal CLEC3B in hepatocellular carcinoma promotes metastasis and angiogenesis via AMPK and VEGF signals. Cell Commun Signal (2019) 17(1):113. doi: 10.1186/s12964-019-0423-6
38. Qiu QC, Wang L, Jin SS, Liu GF, Liu J, Ma L, et al. CHI3L1 promotes tumor progression by activating TGF-beta signaling pathway in hepatocellular carcinoma. Sci Rep (2018) 8(1):15029. doi: 10.1038/s41598-018-33239-8
39. Lee H-Y, Chen C-K, Ho C-M, Lee S-S, Chang C-Y, Chen K-J, et al. EIF3C-enhanced exosome secretion promotes angiogenesis and tumorigenesis of human hepatocellular carcinoma. Oncotarget (2018) 9(17):13193. doi: 10.18632/oncotarget.24149
40. Liu Y, Zhao L, Li D, Yin Y, Zhang CY, Li J, et al. Microvesicle-delivery miR-150 promotes tumorigenesis by up-regulating VEGF, and the neutralization of miR-150 attenuate tumor development. Protein Cell (2013) 4(12):932–41. doi: 10.1007/s13238-013-3092-z
41. Liu J, Fan L, Yu H, Zhang J, He Y, Feng D, et al. Endoplasmic reticulum stress causes liver cancer cells to release exosomal miR-23a-3p and up-regulate programmed death ligand 1 expression in macrophages. Hepatology (2019) 70(1):241–58. doi: 10.1002/hep.30607
42. Fu X, Liu M, Qu S, Ma J, Zhang Y, Shi T, et al. Exosomal microRNA-32-5p induces multidrug resistance in hepatocellular carcinoma via the PI3K/Akt pathway. J Exp Clin Cancer Res (2018) 37(1):52. doi: 10.1186/s13046-018-0677-7
43. Fang T, Lv H, Lv G, Li T, Wang C, Han Q, et al. Tumor-derived exosomal miR-1247-3p induces cancer-associated fibroblast activation to foster lung metastasis of liver cancer. Nat Commun (2018) 9(1):191. doi: 10.1038/s41467-017-02583-0
44. Yokota Y, Noda T, Okumura Y, Kobayashi S, Iwagami Y, Yamada D, et al. Serum exosomal miR-638 is a prognostic marker of HCC via downregulation of VE-cadherin and ZO-1 of endothelial cells. Cancer Sci (2021) 112(3):1275–88. doi: 10.1111/cas.14807
45. Li W, Xin X, Li X, Geng J, Sun Y. Exosomes secreted by M2 macrophages promote cancer stemness of hepatocellular carcinoma via the miR-27a-3p/TXNIP pathways. Int Immunopharmacol (2021) 101(Pt A):107585. doi: 10.1016/j.intimp.2021.107585
46. Kim HS, Kim JS, Park NR, Nam H, Sung PS, Bae SH, et al. Exosomal miR-125b exerts anti-metastatic properties and predicts early metastasis of hepatocellular carcinoma. Front Oncol (2021) 11:637247. doi: 10.3389/fonc.2021.637247
47. Zhang HY, Liang HX, Wu SH, Jiang HQ, Wang Q, Yu ZJ. Overexpressed tumor suppressor exosomal miR-15a-5p in cancer cells inhibits PD1 expression in CD8+T cells and suppresses the hepatocellular carcinoma progression. Front Oncol (2021) 11:622263. doi: 10.3389/fonc.2021.622263
48. Lin XJ, Fang JH, Yang XJ, Zhang C, Yuan Y, Zheng L, et al. Hepatocellular carcinoma cell-secreted exosomal MicroRNA-210 promotes angiogenesis In vitro and in vivo. Mol Ther Nucleic Acids (2018) 11:243–52. doi: 10.1016/j.omtn.2018.02.014
49. Xue X, Wang X, Zhao Y, Hu R, Qin L. Exosomal miR-93 promotes proliferation and invasion in hepatocellular carcinoma by directly inhibiting TIMP2/TP53INP1/CDKN1A. Biochem Biophys Res Commun (2018) 502(4):515–21. doi: 10.1016/j.bbrc.2018.05.208
50. Lin Q, Zhou C-R, Bai M-J, Zhu D, Chen J-W, Wang H-F, et al. Exosome-mediated miRNA delivery promotes liver cancer EMT and metastasis. Am J Trans Res (2020) 12(3):1080.
51. Yang B, Feng X, Liu H, Tong R, Wu J, Li C, et al. High-metastatic cancer cells derived exosomal miR92a-3p promotes epithelial-mesenchymal transition and metastasis of low-metastatic cancer cells by regulating PTEN/Akt pathway in hepatocellular carcinoma. Oncogene (2020) 39(42):6529–43. doi: 10.1038/s41388-020-01450-5
52. Zhang Z, Li X, Sun W, Yue S, Yang J, Li J, et al. Loss of exosomal miR-320a from cancer-associated fibroblasts contributes to HCC proliferation and metastasis. Cancer Lett (2017) 397:33–42. doi: 10.1016/j.canlet.2017.03.004
53. Zhou Y, Ren H, Dai B, Li J, Shang L, Huang J, et al. Hepatocellular carcinoma-derived exosomal miRNA-21 contributes to tumor progression by converting hepatocyte stellate cells to cancer-associated fibroblasts. J Exp Clin Cancer Res (2018) 37(1):324. doi: 10.1186/s13046-018-0965-2
54. Zhao S, Li J, Zhang G, Wang Q, Wu C, Zhang Q, et al. Exosomal miR-451a functions as a tumor suppressor in hepatocellular carcinoma by targeting LPIN1. Cell Physiol Biochem (2019) 53(1):19–35. doi: 10.33594/000000118
55. Li X, Lei Y, Wu M, Li N. Regulation of macrophage activation and polarization by HCC-derived exosomal lncRNA TUC339. Int J Mol Sci (2018) 19(10):2958. doi: 10.3390/ijms19102958
56. Wang D, Xing N, Yang T, Liu J, Zhao H, He J, et al. Exosomal lncRNA H19 promotes the progression of hepatocellular carcinoma treated with propofol via miR-520a-3p/LIMK1 axis. Cancer Med (2020) 9(19):7218–30. doi: 10.1002/cam4.3313
57. Wang J, Pu J, Zhang Y, Yao T, Luo Z, Li W, et al. Exosome-transmitted long non-coding RNA SENP3-EIF4A1 suppresses the progression of hepatocellular carcinoma. Aging (Albany NY) (2020) 12(12):11550. doi: 10.18632/aging.103302
58. Li B, Mao R, Liu C, Zhang W, Tang Y, Guo Z. LncRNA FAL1 promotes cell proliferation and migration by acting as a CeRNA of miR-1236 in hepatocellular carcinoma cells. Life Sci (2018) 197:122–9. doi: 10.1016/j.lfs.2018.02.006
59. Ma D, Gao X, Liu Z, Lu X, Ju H, Zhang N. Exosome-transferred long non-coding RNA ASMTL-AS1 contributes to malignant phenotypes in residual hepatocellular carcinoma after insufficient radiofrequency ablation. Cell Prolif (2020) 53(9):e12795. doi: 10.1111/cpr.12795
60. Zhang H, Deng T, Ge S, Liu Y, Bai M, Zhu K, et al. Exosome circRNA secreted from adipocytes promotes the growth of hepatocellular carcinoma by targeting deubiquitination-related USP7. Oncogene (2019) 38(15):2844–59. doi: 10.1038/s41388-018-0619-z
61. Wang G, Liu W, Zou Y, Wang G, Deng Y, Luo J, et al. Three isoforms of exosomal circPTGR1 promote hepatocellular carcinoma metastasis via the miR449a-MET pathway. EBioMedicine (2019) 40:432–45. doi: 10.1016/j.ebiom.2018.12.062
62. Zhang PF, Gao C, Huang XY, Lu JC, Guo XJ, Shi GM, et al. Cancer cell-derived exosomal circUHRF1 induces natural killer cell exhaustion and may cause resistance to anti-PD1 therapy in hepatocellular carcinoma. Mol Cancer (2020) 19(1):110. doi: 10.1186/s12943-020-01222-5
63. Chen W, Quan Y, Fan S, Wang H, Liang J, Huang L, et al. Exosome-transmitted circular RNA hsa_circ_0051443 suppresses hepatocellular carcinoma progression. Cancer Lett (2020) 475:119–28. doi: 10.1016/j.canlet.2020.01.022
64. Hu K, Li NF, Li JR, Chen ZG, Wang JH, Sheng LQ. Exosome circCMTM3 promotes angiogenesis and tumorigenesis of hepatocellular carcinoma through miR-3619-5p/SOX9. Hepatol Res (2021) 51(11):1139–52. doi: 10.1111/hepr.13692
65. Zhang T, Jing B, Bai Y, Zhang Y, Yu H. Circular RNA circTMEM45A acts as the sponge of MicroRNA-665 to promote hepatocellular carcinoma progression. Mol Ther Nucleic Acids (2020) 22:285–97. doi: 10.1016/j.omtn.2020.08.011
66. Mo Z, Liu D, Rong D, Zhang S. Hypoxic characteristic in the immunosuppressive microenvironment of hepatocellular carcinoma. Front Immunol (2021) 12:611058. doi: 10.3389/fimmu.2021.611058
67. Zhang B, Tang B, Gao J, Li J, Kong L, Qin L. A hypoxia-related signature for clinically predicting diagnosis, prognosis and immune microenvironment of hepatocellular carcinoma patients. J Transl Med (2020) 18(1):342. doi: 10.1186/s12967-020-02492-9
68. Yaghoubi S, Najminejad H, Dabaghian M, Karimi MH, Abdollahpour-Alitappeh M, Rad F, et al. How hypoxia regulate exosomes in ischemic diseases and cancer microenvironment? IUBMB Life (2020) 72(7):1286–305. doi: 10.1002/iub.2275
69. Yuen VW, Wong CC. Hypoxia-inducible factors and innate immunity in liver cancer. J Clin Invest (2020) 130(10):5052–62. doi: 10.1172/JCI137553
70. Chen L, Guo P, He Y, Chen Z, Chen L, Luo Y, et al. HCC-derived exosomes elicit HCC progression and recurrence by epithelial-mesenchymal transition through MAPK/ERK signalling pathway. Cell Death Dis (2018) 9(5):513. doi: 10.1038/s41419-018-0534-9
71. Wang D, Zhao C, Xu F, Zhang A, Jin M, Zhang K, et al. Cisplatin-resistant NSCLC cells induced by hypoxia transmit resistance to sensitive cells through exosomal PKM2. Theranostics (2021) 11(6):2860–75. doi: 10.7150/thno.51797
72. Ju C, Colgan SP, Eltzschig HK. Hypoxia-inducible factors as molecular targets for liver diseases. J Mol Med (Berl) (2016) 94(6):613–27. doi: 10.1007/s00109-016-1408-1
73. Jafari R, Rahbarghazi R, Ahmadi M, Hassanpour M, Rezaie J. Hypoxic exosomes orchestrate tumorigenesis: molecular mechanisms and therapeutic implications. J Transl Med (2020) 18(1):474. doi: 10.1186/s12967-020-02662-9
74. Zhong L, Liao D, Li J, Liu W, Wang J, Zeng C, et al. Rab22a-NeoF1 fusion protein promotes osteosarcoma lung metastasis through its secretion into exosomes. Signal Transduct Target Ther (2021) 6(1):59. doi: 10.1038/s41392-020-00414-1
75. Bao MH, Wong CC. Hypoxia, metabolic reprogramming, and drug resistance in liver cancer. Cells (2021) 10(7):1715. doi: 10.3390/cells10071715
76. Ageta H, Tsuchida K. Post-translational modification and protein sorting to small extracellular vesicles including exosomes by ubiquitin and UBLs. Cell Mol Life Sci (2019) 76(24):4829–48. doi: 10.1007/s00018-019-03246-7
77. Wong CC, Kai AK, Ng IO. The impact of hypoxia in hepatocellular carcinoma metastasis. Front Med (2014) 8(1):33–41. doi: 10.1007/s11684-013-0301-3
78. Lee JW, Ko J, Ju C, Eltzschig HK. Hypoxia signaling in human diseases and therapeutic targets. Exp Mol Med (2019) 51(6):1–13. doi: 10.1038/s12276-019-0235-1
79. Anthony BJ, Ramm GA, McManus DP. Role of resident liver cells in the pathogenesis of schistosomiasis. Trends Parasitol (2012) 28(12):572–9. doi: 10.1016/j.pt.2012.09.005
80. Davies LC, Taylor PR. Tissue-resident macrophages: then and now. Immunology (2015) 144(4):541–8. doi: 10.1111/imm.12451
81. Craig AJ, von Felden J, Garcia-Lezana T, Sarcognato S, Villanueva A. Tumour evolution in hepatocellular carcinoma. Nat Rev Gastroenterol Hepatol (2020) 17(3):139–52. doi: 10.1038/s41575-019-0229-4
82. Tacke F, Zimmermann HW. Macrophage heterogeneity in liver injury and fibrosis. J Hepatol (2014) 60(5):1090–6. doi: 10.1016/j.jhep.2013.12.025
83. Wang C, Ma C, Gong L, Guo Y, Fu K, Zhang Y, et al. Macrophage polarization and its role in liver disease. Front Immunol (2021) 12:803037. doi: 10.3389/fimmu.2021.803037
84. Shao J, Li S, Liu Y, Zheng M. Extracellular vesicles participate in macrophage-involved immune responses under liver diseases. Life Sci (2020) 240:117094. doi: 10.1016/j.lfs.2019.117094
85. Wolf-Dennen K, Gordon N, Kleinerman ES. Exosomal communication by metastatic osteosarcoma cells modulates alveolar macrophages to an M2 tumor-promoting phenotype and inhibits tumoricidal functions. Oncoimmunology (2020) 9(1):1747677. doi: 10.1080/2162402X.2020.1747677
86. Parada N, Romero-Trujillo A, Georges N, Alcayaga-Miranda F. Camouflage strategies for therapeutic exosomes evasion from phagocytosis. J Adv Res (2021) 31:61–74. doi: 10.1016/j.jare.2021.01.001
87. Kanno S, Hirano S, Sakamoto T, Furuyama A, Takase H, Kato H, et al. Scavenger receptor MARCO contributes to cellular internalization of exosomes by dynamin-dependent endocytosis and macropinocytosis. Sci Rep (2020) 10(1):21795. doi: 10.1038/s41598-020-78464-2
88. Costa-Silva B, Aiello NM, Ocean AJ, Singh S, Zhang H, Thakur BK, et al. Pancreatic cancer exosomes initiate pre-metastatic niche formation in the liver. Nat Cell Biol (2015) 17(6):816–26. doi: 10.1038/ncb3169
89. Goto Y, Ogawa Y, Tsumoto H, Miura Y, Nakamura TJ, Ogawa K, et al. Contribution of the exosome-associated form of secreted endoplasmic reticulum aminopeptidase 1 to exosome-mediated macrophage activation. Biochim Biophys Acta Mol Cell Res (2018) 1865(6):874–88. doi: 10.1016/j.bbamcr.2018.03.009
90. Simon L, Campos A, Leyton L, Quest AFG. Caveolin-1 function at the plasma membrane and in intracellular compartments in cancer. Cancer Metastasis Rev (2020) 39(2):435–53. doi: 10.1007/s10555-020-09890-x
91. Zhou X, Liu X, Yang X, Wang L, Hong Y, Lian K, et al. Tumor progress intercept by intervening in caveolin-1 related intercellular communication via ROS-sensitive c-myc targeting therapy. Biomaterials (2021) 275:120958. doi: 10.1016/j.biomaterials.2021.120958
92. Saha S, Shalova IN, Biswas SK. Metabolic regulation of macrophage phenotype and function. Immunol Rev (2017) 280(1):102–11. doi: 10.1111/imr.12603
93. Shapouri-Moghaddam A, Mohammadian S, Vazini H, Taghadosi M, Esmaeili SA, Mardani F, et al. Macrophage plasticity, polarization, and function in health and disease. J Cell Physiol (2018) 233(9):6425–40. doi: 10.1002/jcp.26429
94. Viola A, Munari F, Sanchez-Rodriguez R, Scolaro T, Castegna A. The metabolic signature of macrophage responses. Front Immunol (2019) 10:1462. doi: 10.3389/fimmu.2019.01462
95. Alzahrani FA, El-Magd MA, Abdelfattah-Hassan A, Saleh AA, Saadeldin IM, El-Shetry ES, et al. Potential effect of exosomes derived from cancer stem cells and MSCs on progression of DEN-induced HCC in rats. Stem Cells Int (2018) 2018:8058979. doi: 10.1155/2018/8058979
96. Yin C, Han Q, Xu D, Zheng B, Zhao X, Zhang J. SALL4-mediated upregulation of exosomal miR-146a-5p drives T-cell exhaustion by M2 tumor-associated macrophages in HCC. Oncoimmunology (2019) 8(7):1601479. doi: 10.1080/2162402X.2019.1601479
97. Frundt T, Krause L, Hussey E, Steinbach B, Kohler D, von Felden J, et al. Diagnostic and prognostic value of miR-16, miR-146a, miR-192 and miR-221 in exosomes of hepatocellular carcinoma and liver cirrhosis patients. Cancers (Basel) (2021) 13(10):2484. doi: 10.3390/cancers13102484
98. Wang X, Zhou Y, Dong K, Zhang H, Gong J, Wang S. Exosomal lncRNA HMMR-AS1 mediates macrophage polarization through miR-147a/ARID3A axis under hypoxia and affects the progression of hepatocellular carcinoma. Environ Toxicol (2022) 37(6):1357–72. doi: 10.1002/tox.23489
99. Qu Z, Wu J, Wu J, Luo D, Jiang C, Ding Y. Exosomes derived from HCC cells induce sorafenib resistance in hepatocellular carcinoma both in vivo and in vitro. J Exp Clin Cancer Res (2016) 35(1):159. doi: 10.1186/s13046-016-0430-z
100. Takahashi K, Yan IK, Kogure T, Haga H, Patel T. Extracellular vesicle-mediated transfer of long non-coding RNA ROR modulates chemosensitivity in human hepatocellular cancer. FEBS Open Bio (2014) 4:458–67. doi: 10.1016/j.fob.2014.04.007
101. Takahashi K, Yan IK, Wood J, Haga H, Patel T. Involvement of extracellular vesicle long noncoding RNA (linc-VLDLR) in tumor cell responses to chemotherapy. Mol Cancer Res (2014) 12(10):1377–87. doi: 10.1158/1541-7786.MCR-13-0636
102. Shi S, Rao Q, Zhang C, Zhang X, Qin Y, Niu Z. Dendritic cells pulsed with exosomes in combination with PD-1 antibody increase the efficacy of sorafenib in hepatocellular carcinoma model. Transl Oncol (2018) 11(2):250–8. doi: 10.1016/j.tranon.2018.01.001
103. Liu DX, Li PP, Guo JP, Li LL, Guo B, Jiao HB, et al. Exosomes derived from HBV-associated liver cancer promote chemoresistance by upregulating chaperone-mediated autophagy. Oncol Lett (2019) 17(1):323–31. doi: 10.3892/ol.2018.9584
104. Lou G, Chen L, Xia C, Wang W, Qi J, Li A, et al. MiR-199a-modified exosomes from adipose tissue-derived mesenchymal stem cells improve hepatocellular carcinoma chemosensitivity through mTOR pathway. J Exp Clin Cancer Res (2020) 39(1):4. doi: 10.1186/s13046-019-1512-5
105. Xu J, Ji L, Liang Y, Wan Z, Zheng W, Song X, et al. CircRNA-SORE mediates sorafenib resistance in hepatocellular carcinoma by stabilizing YBX1. Signal Transduct Target Ther (2020) 5(1):298. doi: 10.1038/s41392-020-00375-5
106. Slomka A, Mocan T, Wang B, Nenu I, Urban SK, Gonzales-Carmona M, et al. EVs as potential new therapeutic Tool/Target in gastrointestinal cancer and HCC. Cancers (Basel) (2020) 12(10):3019. doi: 10.3390/cancers12103019
107. Yong T, Zhang X, Bie N, Zhang H, Zhang X, Li F, et al. Tumor exosome-based nanoparticles are efficient drug carriers for chemotherapy. Nat Commun (2019) 10(1):3838. doi: 10.1038/s41467-019-11718-4
108. Liang Q, Bie N, Yong T, Tang K, Shi X, Wei Z, et al. The softness of tumour-cell-derived microparticles regulates their drug-delivery efficiency. Nat BioMed Eng (2019) 3(9):729–40. doi: 10.1038/s41551-019-0405-4
109. Yong T, Wei Z, Gan L, Yang X. Extracellular vesicle-based drug delivery systems for enhanced anti-tumor therapies through modulating cancer-immunity cycle. Adv Mater (2022), e2201054. doi: 10.1002/adma.202201054
110. Belhadj Z, He B, Deng H, Song S, Zhang H, Wang X, et al. A combined "eat me/don't eat me" strategy based on extracellular vesicles for anticancer nanomedicine. J Extracell Vesicles (2020) 9(1):1806444. doi: 10.1080/20013078.2020.1806444
111. Du J, Wan Z, Wang C, Lu F, Wei M, Wang D, et al. Designer exosomes for targeted and efficient ferroptosis induction in cancer via chemo-photodynamic therapy. Theranostics (2021) 11(17):8185–96. doi: 10.7150/thno.59121
112. Riley RS, June CH, Langer R, Mitchell MJ. Delivery technologies for cancer immunotherapy. Nat Rev Drug Discovery (2019) 18(3):175–96. doi: 10.1038/s41573-018-0006-z
113. Xu Z, Zeng S, Gong Z, Yan Y. Exosome-based immunotherapy: a promising approach for cancer treatment. Mol Cancer (2020) 19(1):160. doi: 10.1186/s12943-020-01278-3
114. Pitt JM, Andre F, Amigorena S, Soria JC, Eggermont A, Kroemer G, et al. Dendritic cell-derived exosomes for cancer therapy. J Clin Invest (2016) 126(4):1224–32. doi: 10.1172/JCI81137
115. Lu Z, Zuo B, Jing R, Gao X, Rao Q, Liu Z, et al. Dendritic cell-derived exosomes elicit tumor regression in autochthonous hepatocellular carcinoma mouse models. J Hepatol (2017) 67(4):739–48. doi: 10.1016/j.jhep.2017.05.019
116. Zuo B, Zhang Y, Zhao K, Wu L, Qi H, Yang R, et al. Universal immunotherapeutic strategy for hepatocellular carcinoma with exosome vaccines that engage adaptive and innate immune responses. J Hematol Oncol (2022) 15(1):46. doi: 10.1186/s13045-022-01266-8
117. Zhong X, Zhou Y, Cao Y, Ding J, Wang P, Luo Y, et al. Enhanced antitumor efficacy through microwave ablation combined with a dendritic cell-derived exosome vaccine in hepatocellular carcinoma. Int J Hyperthermia (2020) 37(1):1210–8. doi: 10.1080/02656736.2020.1836406
118. Zuo B, Qi H, Lu Z, Chen L, Sun B, Yang R, et al. Alarmin-painted exosomes elicit persistent antitumor immunity in large established tumors in mice. Nat Commun (2020) 11(1):1790. doi: 10.1038/s41467-020-15569-2
119. Viaud S, Thery C, Ploix S, Tursz T, Lapierre V, Lantz O, et al. Dendritic cell-derived exosomes for cancer immunotherapy: What's next? Cancer Res (2010) 70(4):1281–5. doi: 10.1158/0008-5472.CAN-09-3276
Keywords: exosomes, hepatocellular carcinoma, liver cancer, hypoxia, TAM, macrophage, therapy, drug resistance
Citation: Yu S, Zhou L, Fu J, Xu L, Liu B, Zhao Y, Wang J, Yan X and Su J (2022) H-TEX-mediated signaling between hepatocellular carcinoma cells and macrophages and exosome-targeted therapy for hepatocellular carcinoma. Front. Immunol. 13:997726. doi: 10.3389/fimmu.2022.997726
Received: 19 July 2022; Accepted: 21 September 2022;
Published: 13 October 2022.
Edited by:
Jinyan Wang, China Medical University, ChinaReviewed by:
Soumya Basu, Dr. D. Y. Patil Biotechnology and Bioinformatics Institute, IndiaXinyi Tang, Mayo Clinic, United States
Copyright © 2022 Yu, Zhou, Fu, Xu, Liu, Zhao, Wang, Yan and Su. This is an open-access article distributed under the terms of the Creative Commons Attribution License (CC BY). The use, distribution or reproduction in other forums is permitted, provided the original author(s) and the copyright owner(s) are credited and that the original publication in this journal is cited, in accordance with accepted academic practice. No use, distribution or reproduction is permitted which does not comply with these terms.
*Correspondence: Xiaoyu Yan, yanxy@jlu.edu.cn; Jing Su, jingsu@jlu.edu.cn
†These authors share senior authorship