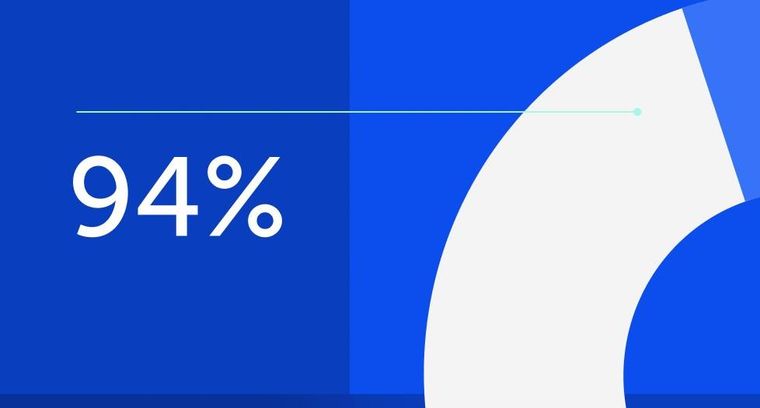
94% of researchers rate our articles as excellent or good
Learn more about the work of our research integrity team to safeguard the quality of each article we publish.
Find out more
ORIGINAL RESEARCH article
Front. Immunol., 06 September 2022
Sec. Microbial Immunology
Volume 13 - 2022 | https://doi.org/10.3389/fimmu.2022.996446
This article is part of the Research TopicA year in review: Discussions in Microbial ImmunologyView all 7 articles
Adenosine shows a significant immunosuppressive effect in sepsis via binding to the adenosine 2a receptor (A2aR). Both genetic deletion and pharmacological inhibition of the A2aR may improve survival in sepsis. However, available research on this protective mechanism is quite limited. We used an A2aR antagonist (ZM241385) to treat a cecal ligation and puncture model of normal mice or regulatory T-cell (Treg)-depletion mice and found that the protective effect of ZM241385 is dependent on Tregs. Mechanically, A2aR inactivation was associated with decreased frequencies and reduced function of Foxp3+ Tregs, as evidenced by Foxp3 and CTLA-4 expression and classical effector T-cell proliferative assays, suggesting Treg modulation is a potential protective mechanism against sepsis. Simultaneously, the function and quantity of abdominal neutrophils were improved with ZM241385 treatment. To see if a link exists between them, Tregs and neutrophils were co-cultured, and it was found that ZM241385 blocked the inhibitory effect of Tregs on neutrophils. According to our research, Tregs play a key role in how A2aR antagonists improve sepsis prognosis and bacterial clearance.
Sepsis is a complicated condition that emerges as an abnormal host response to an infection and is linked to immediate organ failure and a high mortality risk (1). Due to the lack of specific drug therapy, sepsis mortality remains high (2, 3). More research on sepsis is required in the future.
Recently, researchers found that immunosuppression is a general immune condition in sepsis (4–6). In patients who die from sepsis, immunosuppression has been characterized as an increased number of regulatory T-cells (Tregs) (7). The majority of researches have concluded that the depletion of Tregs enhances bacterial clearance but does not benefit sepsis survival (8, 9). Many studies have indicated that the elimination of Tregs can induce a fatal autoimmune illness (10, 11), and this would be the reason why the depletion of Tregs enhances bacterial clearance but causes significant tissue damage in sepsis. Other studies suggest that modulating the suppressive functions of Tregs by silencing Foxp3 would benefit the sepsis model (12). However, there is still no effective method to moderately regulate Treg function in sepsis.
Recent research indicates that adenosine induces potent immunosuppression in sepsis via the adenosine 2a receptor (A2aR) (13, 14). Genetic depletion of the A2aR improved sepsis survival via bacterial clearance in several studies with an accompanying increase in bacterial clearance (15, 16). An A2aR antagonist (ZM241385) has been tested before and showed a similar protective effect (15); however, the mechanism of said A2aR antagonist has not yet been described. Previous research indicated that A2aR agonists could enhance Treg Foxp3, CTLA-4, CD39, and CD73 expression levels (17, 18). As described above, the immunosuppression activity of Tregs plays a critical role in sepsis. Thus, we hypothesize that the use of an A2aR antagonist is a proper method to inhibit the immunosuppression activity of Tregs, which could be a possible mechanism to improve sepsis survival.
A previous study described the influence of an A2aR antagonist on macrophages (15). As is known, enhancing macrophage function by granulocyte-macrophage colony-stimulating factor did not improve the survival rate of sepsis patients (19, 20). Here, we pay more attention to the effect of an A2aR antagonist on Treg function in a model of polymicrobial sepsis caused by cecal ligation and puncture (CLP). First, the A2aR antagonist ZM241385 was administrated to normal mice and Treg-depletion mice treated by PC61, an antibody of CD25. Then, co-culturing of Tregs and neutrophils was performed to explain the mechanism by which Tregs influence bacterial clearance. Our data indicated that ZM241385 improved sepsis survival by inhibiting Treg function, which inhibits bacterial clearance function of neutrophils.
Male wild-type C57BL/6J mice aged 8–10 weeks (Beijing Vital River Laboratory Animal Technology Co., Beijing, China) were fed in a specific pathogen-free environment in Tianjin Medical University General Hospital Animal Laboratory (Tianjin, China). The average weight of the mice was 22-28g. All procedures involving the use of animals in research were authorized by the ethics committee of Tianjin Medical University General Hospital (approval no. IRB2022-DWFL-44). The mice were kept in an environment with a day/night cycle of 12 hours and had access to food and water at all times.
The CLP mouse model was prepared as previously described (21). Mice were rendered unconscious using a gas anesthetic equipment and isoflurane. For the purpose of inducing sepsis, a 1-cm midline laparotomy incision was performed in the abdomen after disinfection; the cecum was then exteriorized, ligated in the center with 4-0 silk suture, and punctured with a 22-gauge needle at a spot 0.5cm from the cecal tip. The cecum was restored to the peritoneal cavity after a little drop of cecal content was expelled. Two layers of silk sutures were then used to seal the abdominal wound. After being revived with a subcutaneous injection of 1 mL of sterile saline (0.9% solution) into the scruff of the mice’s necks, the animals were kept warm on a thermal blanket and released back into their cages with unrestricted access to food and drink. The survival of one group of mice was examined every day for fourteen days. A second set of mice was re-anesthetized with isoflurane 24 hours following surgery, and their blood, peritoneal lavage fluid (PLF), and other organs were taken as shown below.
A lipopolysaccharide (LPS)-induced sepsis model was established via a single intravenous injection of LPS (1 mg/kg, O26:B6; Sigma-Aldrich, St. Louis, MO, USA) (22). In order to deplete Tregs in vivo, anti-CD25 antibody (100 μg, Clone PC61; BioLegend, San Diego, CA, USA) was injected intravenously 1 day before the CLP model was created (23). Separately, rat immunoglobulin (Ig)G (BioLegend) was injected into the control mice. The effect of Treg depletion was confirmed by flow cytometry. To test the A2aR antagonist effect, the mice were injected both immediately after the operation and 24 h thereafter with ZM241385 (5 mg/kg delivered subcutaneously) (MedChem Express, Shanghai, China) or normal saline.
After opening the chest, blood samples were taken aseptically by cardiac puncture, then placed on ice until further processing. The whole blood needed to be mixed with ethylenediaminetetraacetic acid EDTA (final volume, 4 mM) immediately to prevent coagulation. After completing successive dilutions for bacteriological examination (see below), the blood was centrifuged at 2000 ×g for 15 minutes, and the plasma obtained was kept at -80°C until further use.
The abdominal skin was cleansed with 75% ethanol before being opened to reveal the abdominal wall for peritoneal lavage. The peritoneal cavity was then filled with four milliliters of sterile physiological saline using a 22-gauge needle. After gently massaging the belly for one minute with the needle tip in the peritoneum, peritoneal fluid was extracted using the needle. When the retrieved PLF was ready to be processed for a bacteriological analysis, it was put on ice. The PLF was centrifuged at 1000 ×g for 10 min. after being serially diluted to determine colony-forming unit (CFU) counts (see below), and the supernatant was kept at -80°C until further analysis.
All samples of PLF and blood were prepared at 4°C environment and diluted with sterile phosphate-buffered saline (PBS), and 100 μL of each sample was spread on 5% sheep blood Trypticase™ soy agar (BD Bioscience, San Diego, CA, USA) plates, which were subsequently cultivated for 24 hours at 37°C in a damp chamber. The number of colonies was calculated and represented as CFUs per milliliter.
We utilized commercial ELISA kits in accordance with the manufacturer’s recommendations (Dakewe Biotech Co., Ltd., Shenzhen, China) to measure the concentration of interleukin (IL)-10, IL-6, and tumor necrosis factor (TNF)-a in the peripheral blood and the peritoneal cavity or the concentration of IL-10 and transforming growth factor (TGF)-β in the supernatant of Treg culture liquid, respectively.
As previously described, peritoneal cells were collected from PLF (21). To keep peritoneal cells active, ice-cold PBS is installed into the peritoneal cavity instead of sterile physiological saline. Then, PLF was centrifugation at 300 ×g for 10 min at 4°C and washed twice with PBS. To prepare for flow cytometry, the pellet was suspended in 1 ml of cold PBS.
Anti-mouse CD16/32 antibody was used to block Fc receptors on PLF or spleen cells by treating them with cold for 20 minutes. Single-cell suspensions were stained with a combination of the following antibodies: PerCP-anti-CD11b (M1/70), FITC-anti-Ly6G (1A8), PerCP-anti-CD4 (GK1.5), PE-anti-CD25 (PC61), FITC-anti-Foxp3 (FJK-16s), and APC-anti-CTLA-4 (UC10-4B9) (all from BioLegend). Neutrophils were recognized as CD11b+Ly6G+, and Tregs as CD4+CD25+Foxp3+. The cells were fixed and permeabilized following the kit’s instructions (BioLegend) before being stained for Foxp3. PerCP-conjugated rat IgG2b, PE-conjugated rat IgG2a, FITC-conjugated rat IgG2b, and APC-conjugated rat IgG1 were all used as isotype controls (all from BioLegend). Fluorescence-activated cell sorting (FACS) analysis was performed using an Accuir C6 Plus cell analyzer (BD Bioscience). Flow cytometry data were analyzed with FlowJo (Tree Star, Inc., Ashland, OR, USA). For all samples, ≥1×105 cells were collected to generate scatter plots.
The splenocytes were isolated by passing spleen tissue through a cell strainer with a mesh size of 70 nm using the back of a sterile 3 mL syringe plunger. With the aid of red blood cell lysis buffer, RBCs were successfully removed. Single splenocytes were centrifugated at 300 ×g for 10 min at 4°C and washed twice with PBS. One milliliter of cold PBS containing 2% fetal bovine serum was used to suspend the pellet. Then, the cell suspension was incubated with a CD16/CD32 antibody to block the Fc receptors, and stained with CD4-PerCP and CD25-PE antibodies. For cell co-culture experiments, CD4+CD25+ Tregs were isolated by FACS using a FACSAria IIu instrument (BD Bioscience). The purity of Treg populations assessed by 2-color flow cytometry was >95%. As previously described (24), Tregs (5×104-per well) were plated in 48-well plates precoated with CD3/CD28 antibodies (BD Bioscience) in RPMI 1640 supplemented with 10% fetal bovine serum, 1% penicillin-streptomycin (Sigma-Aldrich), and 2 mM of l-glutamine (Invitrogen, Carlsbad, CA, USA). Treg supernatant was collected after 24 h and cultured for TGF-β and IL-10 examination.
Similar to a previous study, we used the proliferation of carboxyfluorescein succinimidyl ester (CFSE) (Molecular Probes, Eugene, OR, USA)-labeled CD4+CD25- T-cells (Tconvs) to measure the regulatory activity of Tregs (18). Briefly, cells were rinsed with PBS and treated with 1 μM of CFSE for 8 min to be labeled with CFSE at 37°C. Then, to remove any remaining CFSE, the cells were washed twice with fetal bovine serum. Tregs were isolated as described above from sepsis mice. Inhibition of effector T-cell proliferation was used to assess regulatory activity of Tregs. Tconvs were isolated from the spleens of C57BL/6 mice with the FACSAria IIu instrument and labeled with CFSE. Tconvs (1×105 cells) were co-cultured with Tregs in a 1:1 ratio. Tconv cell proliferation was induced with anti-CD3 monoclonal antibody (1 μg/mL; BD Biosciences), anti-CD28 (1 μg/mL; BD Biosciences), and IL-2 (5 μg/mL; BD Biosciences) for 4 days in a 48-well plate, and after CD4+CD25- gating, the degree of Tconv proliferation was examined (25). In another set of experiments, ZM241385 (1 μM; MedChem Express) was added.
Male mice aged 8 to 12 weeks had their femurs and tibias dissected. Before flushing the bone marrow cells from the tibias and femurs with ice-cold PBS containing 10% fetal bovine serum, we first cut the ends of each tibia and femur using dissecting scissors to expose the marrow. For tibias, we used two 1-mL quantities of buffer, and for femurs, we used three 1-mL volumes of buffer. By gently pipetting the combined bone marrow eluates back into suspension, we next carried out filtering using a 70-m nylon cell strainer (Becton, Dickinson, and Co., Franklin Lakes, NJ, USA) to remove cell clumps and bone particles. Using a centrifuge set at 300 g for 6 minutes at 4°C, the cell suspension was twice washed with PBS, and the pellet was then suspended in 1 mL of ice-cold PBS containing 4% fetal bovine serum. Then, the cell suspension was incubated with a CD16/CD32 antibody and stained with CD11b-PerCP and Ly6G-FITC antibodies. For cell co-culture experiments, CD11b+Ly6G+ neutrophils were isolated by FACS using the FACSAria IIu instrument. The purity of neutrophil cell populations assessed by 2-color flow cytometry was >95%.
As previously described (24), Tregs (5×104 per well) were plated in 48-well plates pre-coated with CD3/CD28 antibodies (BD Bioscience) in RPMI 1640 supplemented with 10% fetal bovine serum, 2 mM of l-glutamine (Invitrogen), and 1% penicillin-streptomycin (Sigma-Aldrich). Then, neutrophils (5×105 per well) were added to the co-culture at 37°C. After 5h, neutrophil phagocytosis and oxidative bursts were measured by flow cytometry analysis (see below). In another set of experiments, ZM241385 (1μM; MedChem Express) was added as described in previous research (26).
Using a modified procedure, phagocytosis was evaluated by flow cytometry as previously mentioned (27). Briefly, 24 hours after CLP, peritoneal cells were collected, the red blood cells were lysed, and the cells were stained for neutrophil-specific surface markers. The stained cells were then incubated with opsonized PE-labeled Escherichia coli (K-12 strain) bioparticles (catalog no. E2861; Thermo Fisher Scientific, Waltham, MA, USA) for 10 min at 37°C, and analyses using flow cytometry were then carried out soon after.
As previously described, a modified procedure was used to measure the generation of reactive oxygen species (ROS) using flow cytometry (21). Briefly, at 24 hours post-CLP, peritoneal cells were collected. Following RBC lysis, peritoneal cells were loaded in the dark for 30 minutes at 37°C with 5 μM/mL of ROS Brite™ (10 µM; AAT Bioquest, Sunnyvale, CA, USA), which is a general oxidative stress indicator. As soon as the cells were washed and labeled for neutrophil-specific surface markers, flow cytometry analysis was done.
All statistical comparisons were analyzed by the GraphPad Prism software program (GraphPad Software, San Diego, CA, USA). The log-rank test was used to examine the survival curves. In order to compare cytokine levels, CFUs, and other lab variables, we employed two-tailed t testing. For all experiments, p < 0.05 was accepted as statistically significant. Data are shown as mean ± standard error of the mean values unless otherwise noted.
A clinically relevant model of polymicrobial peritonitis caused by CLP that has been widely used to study sepsis was employed to establish severe sepsis in mice (28, 29). We tested different kinds of administration of ZM241385 and found the proper method to improve sepsis survival (Figure 1A). Bacterial clearance has been wildly used to evaluate sepsis and corelates with survival (13, 15). We found that the ZM241385-treated sepsis group experienced higher bacterial clearance from both the blood and peritoneal cavity (Figure 1B). Cytokine storm is another important indicator for sepsis survival (4). We found that IL-6, IL-10 and TNF-α levels of the ZM241385-treated sepsis group were decreased in the blood and peritoneal cavity (Figure 1C).
Figure 1 ZM241385 protects against CLP-induced mortality with reduced cytokine storm and improved bacterial clearance. Mice were subjected to CLP or ZM241385+CLP (n = 30 per group). Following CLP, the survival rates were assessed for a continuous 7 days. (A) Administration of 5 mg/kg of ZM241385 for 2 days led to a better effect than other doses in C57BL/6J mice. Statistical differences were determined by the log-rank test. (B) PLF and peripheral blood were taken 24 hours after CLP (n = 8-11 per group). Bacterial CFUs were calculated using 100 μL of each sample after dilution on Trypticase™ soy agar blood plates. ZM241385-treated mice exhibited a lower bacterial burden. (C) Mice treated with the selective A2aR antagonist ZM241385 had lower levels of IL-10 and IL-6 in their plasma and peritoneum (5 mg/kg, delivered subcutaneously once daily). Comparing animals treated with ZM241385 to mice treated with a vehicle, TNF-α levels in the blood were reduced. Levels of IL-6, IL-10, and TNF-α were measured by ELISA in plasma and PLF obtained 24h after CLP. Data are presented as mean ± standard error of the mean values of n = 7-10 mice per group. These findings are typical of three independent trials. The individual mice are represented by symbols. *p < 0.05, **p < 0.01.
In order to test whether an A2aR antagonist could improve sepsis survival by decreasing system inflammation, LPS was used to establish endotoxemia in mice (30). Even using several different administrations of ZM241385, survival from LPS-induced endotoxemia did not improve (Figure 2A). In several studies, Tregs show abilities to inhibit bacterial clearance (31–33). PC61 was used to achieve short-term depletion of Tregs (23, 34), and the result of depletion was confirmed by flow cytometry (Figure 2C). Then, CLP model creation and administration of ZM241385 proceeded in the Treg-deleted mice. Ultimately, we found that ZM241385 did not benefit sepsis survival (Figure 2B).
Figure 2 ZM241385 (5 mg/kg) did not improve LPS-induced sepsis survival and CLP-induced sepsis survival in Tregs deleted mice. (A) The LPS induced sepsis model was administrated by a single intravenous injection of LPS (1 mg/kg). Mice were subjected to LPS or ZM241385+LPS (n = 10 per group). The survival rates were evaluated for a consecutive 7 days after CLP. (B) To deplete Tregs in vivo, 100 μg of anti-CD25 antibody (Clone PC61) was injected intravenously one day before the CLP model. Rat IgG was injected into the control mice. The effect of deletion is confirmed by Flow Cytometry. Septic mice were subjected to normal saline (NS) or ZM241385 (n = 10 per group). (C) The survival rates were evaluated for a consecutive 7 days after CLP. A statistical difference was determined by the log-rank test.
In sepsis, Treg activity is enhanced, which is characterized by increased expression levels of CTLA-4 (35, 36) and Foxp3, higher IL-10 and TGF-β production, and greater suppression of T-cell proliferation (37). We found that the A2aR antagonist ZM241385 inhibited Treg expansion, Foxp3 and CTLA-4 (Figure 3A) expression, and IL-10 or TGF-β secretion (Figure 3B). The inhibition of T-cell proliferation has been tested to describe Treg inhibition activity in several studies (38, 39). We found that the A2aR antagonist ZM241385 inhibited Treg inhibitory activity on T-cell proliferation (Figures 3C, D). Reduced neutrophil antimicrobial activity has been linked to increased mortality in sepsis (40, 41). We found that neutrophils in PLF increased in ZM241385-treated sepsis mice (Figure 4A), and neutrophil phagocytosis (Figure 4B) and ROS production (Figure 4C) were similarly enhanced in this group.
Figure 3 A2aR blockade treatment modulates the frequency and function of Tregs in CLP mice. Tregs were collected for testing from the spleens of normal, CLP and ZM241385-treated CLP mice. Tregs were identified by CD4+CD25+Foxp3+ to elevate percentages of CD4+ cells. (A) Percentages of Tregs from ZM241385-treated CLP mice were decreased compared to those from the CLP group. Expression levels of Foxp3 and CTLA-4 in Tregs were measured by flow cytometry. Representative histograms and the mean fluorescence intensity (MFI) of Foxp3 and CTLA-4 expression are represented. (B) Tregs from the CLP group secreted more cytokines than those from the ZM241385-treated group and naïve group. (C, D) Treg suppression of T-cell proliferation might be reduced if the A2aR was blocked. The individual mice are represented by symbols. *p < 0.05, **p < 0.01.
Figure 4 ZM241385 improves neutrophil phagocytosis and ROS generation in CLP-induced sepsis. Mice treated with vehicle or ZM241385 were collected for peritoneal cells 24 hours after CLP. Neutrophils were identified by Ly6G+CD11b+ in elevated numbers and percentages (A) and were assessed for phagocytic capacity (B) or ROS production (C). In Fig. 3B and Fig. 3C panels, CD11b+ and Ly6G+ cells were gated first, as in Fig. 3A. After that, all the cells in Fig. 3B and Fig. 3C were Ly6G+ and considered neutrophils. The Y axis of these panels is used to describe the function of neutrophils. The function of neutrophils is normal distribution. We make the same mistake in each test by drawing the rectangular box as the positive groups. Meanwhile, the fluorescence curve was used to show the difference between each group. The individual mice are represented by symbols. *p < 0.05, **p < 0.01.
Previous research has indicated that Tregs inhibit neutrophil functions in several mechanisms (24, 42). Co-culture of Tregs and neutrophils has been wildly used to reveal the inhibitory effect of Tregs on neutrophils (43, 44). In order to prove whether the A2aR antagonist ZM241385 enhanced neutrophil function via a Treg-dependent mechanism in sepsis, we performed a co-culture of Tregs and neutrophils (Figure 5A) in different conditions and found the following results (Figure 5B). First, ZM241385 did not enhance neutrophil phagocytosis or ROS generation function. Then, we found that Tregs from sepsis mice greatly inhibited neutrophil phagocytosis and ROS generation function. Finally, ZM241385 enhanced neutrophil phagocytosis and ROS generation function in the co-culture of Tregs and neutrophils. In summary, the inhibition of Treg activity by an A2aR antagonist is the mechanism for enhanced neutrophil function in sepsis.
Figure 5 ZM241385 influenced Treg-mediated inhibition of neutrophil phagocytosis and ROS generation in vitro. CD4+CD25+ Tregs and CD11b+Ly6G+ neutrophils were isolated by FACS using the FACSAria IIu instrument. The purity of Treg and neutrophil populations assessed by 2-color flow cytometry was >95%. (A) Neutrophils were co-cultured with septic CD4+CD25+ Tregs and their phagocytic capacity or ROS generation ability was evaluated as previously described. (B) We found that neutrophil phagocytosis and ROS generation could be inhibited by septic Tregs and restored by ZM241385. *p < 0.05, **p < 0.01.
Adenosine is one of the oldest organic molecules on Earth, having appeared > 100 million years before the birth of the first living form (45, 46). Pharmacology intervention of A2aRs has been used clinically in several conditions; for example, an A2aR agonist has been used in myocardial perfusion imaging (47), and an A2aR antagonist has been used in Parkinson’s disease (48, 49). In this study, we used an A2aR antagonist for the treatment of polymicrobial sepsis and found that the survival of our CLP mouse model was improved by ZM241385. In contrast, ZM241385 showed no benefit in LPS-induced endotoxemia. It is the associated enhanced bacterial clearance but light tissue damage that is the major beneficial effect of an A2aR antagonist to improve sepsis survival. Consistently, an A2aR agonist paired with antibiotics protected against sepsis created by E. coli injection by inhibiting the excessive inflammatory reaction caused by the rapid drug-mediated death of vast numbers of bacteria (50).
Immunosuppression in sepsis has been linked to Tregs and myeloid-derived suppressor cells (51). Here, we paid more attention to Tregs because prior research has shown that both adenosine and Treg levels rise in the blood of sepsis patients (51, 52). Some studies have indicated that A2aR agonists can enhance the expression levels of Foxp3, CTLA-4, CD39, and CD73 in Tregs (17, 18). Whether or not an A2aR antagonist inhibits Treg function has not been studied. We found in sepsis that the A2aR antagonist ZM241385 inhibited Treg expansion, CTLA-4 and Foxp3 expression, secretion of IL-10 and TGF-β and inhibitory activity on T-cell proliferation. In detail, ZM241385 seems to mostly target CTLA-4 expression, whereas its effect on Treg numbers or FoxP3 expression is moderate. This is consistent with what was found in earlier studies, which showed that the sepsis model would be helped by adjusting the suppressive functions of Tregs (12) but not by getting rid of them (8, 9). In addition, we cautiously found that ZM241385 did not improve sepsis survival without Tregs. In summary, an A2aR antagonist improved sepsis survival by blocking the Treg-induced inhibition of bacterial clearance.
Both neutrophils and macrophiles can clear bacteria in sepsis (53, 54). A previous study indicated that an A2aR antagonist enhanced macrophage functions (15). In this study, we found that neutrophil count and the activities of phagocytosis and ROS production in PLF were enhanced by the A2aR antagonist ZM241385. As neutrophils hold an essential role of pathogen control (55, 56) and neutropenia is a major reason for fatal infection (50), we did not examine the role of neutrophils in bacterial clearance by depletion of neutrophils.
Previous research has demonstrated that Tregs may inhibit neutrophil ROS generation and promote neutrophil apoptosis and death through many mechanisms, including the contact-dependent CTLA-4/B7-1 route and the IL-10 impact (24, 42). Here, we validated the idea that Tregs from sepsis mice strongly inhibited neutrophil phagocytosis and ROS production. Differently, we found that the A2aR antagonist ZM241385 could reduce the inhibitory activity of Tregs on neutrophils. As it could not affect neutrophil function alone, our data indicate that the A2aR antagonist ZM241385 just interfered with the inhibitory activity of Tregs in sepsis.
There are also some imperfections in our study. First, the fact that PC61 was used to delete Tregs may influence some CD25+ effector T-cells (57). However, PC61 has been used in several studies to delete Tregs in the short term (23, 34). Importantly, we preferred to find the effect of an A2aR antagonist in a model with a normal gene background, which is more similar to the typical clinical condition. Second, we found that the A2aR antagonist ZM241385 could inhibit Treg function in sepsis, but we did not test the molecule mechanisms of A2aR signal enhancement of Treg activity. These mechanisms must be investigated in future studies.
Collectively, our data suggest that an A2aR antagonist would be a proper treatment to inhibit Treg activity in sepsis and consequently enhance neutrophil phagocytosis and ROS generation function to enhance bacterial clearance and improve the survival rate. More research is needed to describe the intracellular pathway of Tregs to gain a better understanding of immunosuppression in sepsis.
The original contributions presented in the study are included in the article/supplementary material. Further inquiries can be directed to the corresponding author.
The animal study was reviewed and approved by the ethics committee of Tianjin Medical University General Hospital.
TM and TZ contributed to the conception and design of the study. TM organized the database. TZ performed the statistical analysis. TZ and JZ wrote the first draft of the manuscript. JZ, JF, GC, and TZ wrote sections of the manuscript. TZ, JZ, JF, and GC performed experiments. All authors contributed to manuscript revision and have read and approved the submitted version.
This work was supported by grants from the National Natural Science Foundation of China (82172122 to TM), and Tianjin Municipal Health Commission (RC20145 to TZ). This work was also funded by the Tianjin Key Medical Discipline (Specialty) Construction Project.
We thank Liu Ye (Department of Neurology, Tianjin Medical University General Hospital, Tianjin, China) and Li Hong-Yue (Department of General Surgery, Tianjin Medical University General Hospital, Tianjin, China) for their technical assistance.
The authors declare that the research was conducted in the absence of any commercial or financial relationships that could be construed as a potential conflict of interest.
All claims expressed in this article are solely those of the authors and do not necessarily represent those of their affiliated organizations, or those of the publisher, the editors and the reviewers. Any product that may be evaluated in this article, or claim that may be made by its manufacturer, is not guaranteed or endorsed by the publisher.
1. Singer M, Deutschman CS, Seymour CW, Shankar-Hari M, Annane D, Bauer M, et al. The third international consensus definitions for sepsis and septic shock (Sepsis-3). JAMA (2016) 315(8):801–10. doi: 10.1001/jama.2016.0287
2. Kheterpal S, Singh K, Topol EJ. Digitising the prediction and management of sepsis. Lancet (2022) 399(10334):1459. doi: 10.1016/S0140-6736(22)00658-4
3. Mushtaq A, Kazi F. Updates in sepsis management. Lancet Infect Dis (2022) 22(1):24. doi: 10.1016/S1473-3099(21)00773-8
4. Chousterman BG, Swirski FK, Weber GF. Cytokine storm and sepsis disease pathogenesis. Semin Immunopathol (2017) 39(5):517–28. doi: 10.1007/s00281-017-0639-8
5. Ayala A, Perl M, Venet F, Lomas-Neira J, Swan R, Chung CS. Apoptosis in sepsis: Mechanisms, clinical impact and potential therapeutic targets. Curr Pharm Des (2008) 14(19):1853–9. doi: 10.2174/138161208784980617
6. Hotchkiss RS, Coopersmith CM, McDunn JE, Ferguson TA. The sepsis seesaw: Tilting toward immunosuppression. Nat Med (2009) 15(5):496–7. doi: 10.1038/nm0509-496
7. Venet F, Lepape A, Monneret G. Clinical review: Flow cytometry perspectives in the icu - from diagnosis of infection to monitoring of injury-induced immune dysfunctions. Crit Care (2011) 15(5):231. doi: 10.1186/cc10333
8. Okeke EB, Okwor I, Mou Z, Jia P, Uzonna JE. Cd4+Cd25+ regulatory T cells attenuate lipopolysaccharide-induced systemic inflammatory responses and promotes survival in murine escherichia coli infection. Shock (2013) 40(1):65–73. doi: 10.1097/SHK.0b013e318296e65b
9. Ono S, Kimura A, Hiraki S, Takahata R, Tsujimoto H, Kinoshita M, et al. Removal of increased circulating Cd4+Cd25+Foxp3+ regulatory T cells in patients with septic shock using hemoperfusion with polymyxin b-immobilized fibers. Surgery (2013) 153(2):262–71. doi: 10.1016/j.surg.2012.06.023
10. Sakaguchi S, Mikami N, Wing JB, Tanaka A, Ichiyama K, Ohkura N. Regulatory T cells and human disease. Annu Rev Immunol (2020) 38:541–66. doi: 10.1146/annurev-immunol-042718-041717
11. Mukhatayev Z, Ostapchuk YO, Fang D, Le Poole IC. Engineered antigen-specific regulatory T cells for autoimmune skin conditions. Autoimmun Rev (2021) 20(3):102761. doi: 10.1016/j.autrev.2021.102761
12. Venet F, Chung CS, Kherouf H, Geeraert A, Malcus C, Poitevin F, et al. Increased circulating regulatory T cells (Cd4(+)Cd25 (+)Cd127 (-)) contribute to lymphocyte anergy in septic shock patients. Intensive Care Med (2009) 35(4):678–86. doi: 10.1007/s00134-008-1337-8
13. Nascimento DC, Viacava PR, Ferreira RG, Damaceno MA, Pineros AR, Melo PH, et al. Sepsis expands a Cd39(+) plasmablast population that promotes immunosuppression Via adenosine-mediated inhibition of macrophage antimicrobial activity. Immunity (2021) 54(9):2024–41.e8. doi: 10.1016/j.immuni.2021.08.005
14. Fredholm BB, IJzerman AP, Jacobson KA, Klotz KN, Linden J. International union of pharmacology. xxv. nomenclature and classification of adenosine receptors. Pharmacol Rev (2001) 53(4):527–52.
15. Nemeth ZH, Csoka B, Wilmanski J, Xu D, Lu Q, Ledent C, et al. Adenosine A2a receptor inactivation increases survival in polymicrobial sepsis. J Immunol (2006) 176(9):5616–26. doi: 10.4049/jimmunol.176.9.5616
16. Belikoff B, Hatfield S, Sitkovsky M, Remick DG. Adenosine negative feedback on A2a adenosine receptors mediates hyporesponsiveness in chronically septic mice. Shock (2011) 35(4):382–7. doi: 10.1097/SHK.0b013e3182085f12
17. Sitkovsky M, Lukashev D, Deaglio S, Dwyer K, Robson SC, Ohta A. Adenosine A2a receptor antagonists: Blockade of adenosinergic effects and T regulatory cells. Br J Pharmacol (2008) 153 Suppl 1:S457–64. doi: 10.1038/bjp.2008.23
18. Ohta A, Kini R, Ohta A, Subramanian M, Madasu M, Sitkovsky M. The development and immunosuppressive functions of Cd4(+) Cd25(+) Foxp3(+) regulatory T cells are under influence of the adenosine-A2a adenosine receptor pathway. Front Immunol (2012) 3:190. doi: 10.3389/fimmu.2012.00190
19. Presneill JJ, Harris T, Stewart AG, Cade JF, Wilson JW. A randomized phase ii trial of granulocyte-macrophage colony-stimulating factor therapy in severe sepsis with respiratory dysfunction. Am J Respir Crit Care Med (2002) 166(2):138–43. doi: 10.1164/rccm.2009005
20. Meisel C, Schefold JC, Pschowski R, Baumann T, Hetzger K, Gregor J, et al. Granulocyte-macrophage colony-stimulating factor to reverse sepsis-associated immunosuppression: A double-blind, randomized, placebo-controlled multicenter trial. Am J Respir Crit Care Med (2009) 180(7):640–8. doi: 10.1164/rccm.200903-0363OC
21. Liu Y, Hu JN, Luo N, Zhao J, Liu SC, Ma T, et al. The essential involvement of the omentum in the peritoneal defensive mechanisms during intra-abdominal sepsis. Front Immunol (2021) 12:631609:631609. doi: 10.3389/fimmu.2021.631609
22. Hong C, Lu H, Huang X, Chen M, Jin R, Dai X, et al. Neutrophils as regulators of macrophage-induced inflammation in a setting of allogeneic bone marrow transplantation. Stem Cell Rep (2022) 17(7):1561–75. doi: 10.1016/j.stemcr.2022.05.021
23. Bai M, Zhang L, Fu B, Bai J, Zhang Y, Cai G, et al. Il-17a improves the efficacy of mesenchymal stem cells in ischemic-reperfusion renal injury by increasing treg percentages by the cox-2/Pge2 pathway. Kidney Int (2018) 93(4):814–25. doi: 10.1016/j.kint.2017.08.030
24. Lewkowicz P, Lewkowicz N, Sasiak A, Tchorzewski H. Lipopolysaccharide-activated Cd4+Cd25+ T regulatory cells inhibit neutrophil function and promote their apoptosis and death. J Immunol (2006) 177(10):7155–63. doi: 10.4049/jimmunol.177.10.7155
25. Dikiy S, Li J, Bai L, Jiang M, Janke L, Zong X, et al. A distal Foxp3 enhancer enables interleukin-2 dependent thymic treg cell lineage commitment for robust immune tolerance. Immunity (2021) 54(5):931–46.e11. doi: 10.1016/j.immuni.2021.03.020
26. Bauza G, Moitra R, Remick D. Adenosine receptor antagonists effect on plasma-enhanced killing. Shock (2014) 41(1):62–6. doi: 10.1097/SHK.0000000000000055
27. Piliponsky AM, Shubin NJ, Lahiri AK, Truong P, Clauson M, Niino K, et al. Basophil-derived tumor necrosis factor can enhance survival in a sepsis model in mice. Nat. Immunol (2019) 20(2):129–40. doi: 10.1038/s41590-018-0288-7
28. Nascimento DC, Alves-Filho JC, Sonego F, Fukada SY, Pereira MS, Benjamim C, et al. Role of regulatory T cells in long-term immune dysfunction associated with severe sepsis. Crit Care Med (2010) 38(8):1718–25. doi: 10.1097/CCM.0b013e3181e78ad0
29. Rocheteau P, Chatre L, Briand D, Mebarki M, Jouvion G, Bardon J, et al. Sepsis induces long-term metabolic and mitochondrial muscle stem cell dysfunction amenable by mesenchymal stem cell therapy. Nat Commun (2015) 6:10145. doi: 10.1038/ncomms10145
30. Gomez G, Sitkovsky MV. Differential requirement for A2a and A3 adenosine receptors for the protective effect of inosine in vivo. Blood (2003) 102(13):4472–8. doi: 10.1182/blood-2002-11-3624
31. Pacheco Y, Lim CX, Weichhart T, Valeyre D, Bentaher A, Calender A. Sarcoidosis and the mtor, Rac1, and autophagy triad. Trends Immunol (2020) 41(4):286–99. doi: 10.1016/j.it.2020.01.007
32. Teh CE, Preston SP, Robbins AK, Stutz MD, Cooney J, Clark MP, et al. Caspase-8 has dual roles in regulatory T cell homeostasis balancing immunity to infection and collateral inflammatory damage. Sci Immunol (2022) 7(69):eabn8041. doi: 10.1126/sciimmunol.abn8041
33. Manangeeswaran M, Jacques J, Tami C, Konduru K, Amharref N, Perrella O, et al. Binding of hepatitis a virus to its cellular receptor 1 inhibits T-regulatory cell functions in humans. Gastroenterology (2012) 142(7):1516–25.e3. doi: 10.1053/j.gastro.2012.02.039
34. Setiady YY, Coccia JA, Park PU. In vivo depletion of Cd4+Foxp3+ treg cells by the Pc61 anti-Cd25 monoclonal antibody is mediated by fcgammariii+ phagocytes. Eur J Immunol (2010) 40(3):780–6. doi: 10.1002/eji.200939613
35. Hou TZ, Qureshi OS, Wang CJ, Baker J, Young SP, Walker LS, et al. A transendocytosis model of ctla-4 function predicts its suppressive behavior on regulatory T cells. J Immunol (2015) 194(5):2148–59. doi: 10.4049/jimmunol.1401876
36. Hou TZ, Verma N, Wanders J, Kennedy A, Soskic B, Janman D, et al. Identifying functional defects in patients with immune dysregulation due to lrba and ctla-4 mutations. Blood (2017) 129(11):1458–68. doi: 10.1182/blood-2016-10-745174
37. Scumpia PO, Delano MJ, Kelly KM, O’Malley KA, Efron PA, McAuliffe PF, et al. Increased natural Cd4+Cd25+ regulatory T cells and their suppressor activity do not contribute to mortality in murine polymicrobial sepsis. J Immunol (2006) 177(11):7943–9. doi: 10.4049/jimmunol.177.11.7943
38. Corthesy B, Lassus A, Terrettaz J, Tranquart F, Bioley G. Efficacy of a therapeutic treatment using gas-filled microbubble-associated phospholipase A2 in a mouse model of honeybee venom allergy. Allergy (2016) 71(7):957–66. doi: 10.1111/all.12859
39. Canavan JB, Afzali B, Scotta C, Fazekasova H, Edozie FC, Macdonald TT, et al. A rapid diagnostic test for human regulatory T-cell function to enable regulatory T-cell therapy. Blood (2012) 119(8):e57–66. doi: 10.1182/blood-2011-09-380048
40. Shen XF, Cao K, Jiang JP, Guan WX, Du JF. Neutrophil dysregulation during sepsis: An overview and update. J Cell Mol Med (2017) 21(9):1687–97. doi: 10.1111/jcmm.13112
41. Alves-Filho JC, Sonego F, Souto FO, Freitas A, Verri WA Jr., Auxiliadora-Martins M, et al. Interleukin-33 attenuates sepsis by enhancing neutrophil influx to the site of infection. Nat Med (2010) 16(6):708–12. doi: 10.1038/nm.2156
42. Montagnoli C, Fallarino F, Gaziano R, Bozza S, Bellocchio S, Zelante T, et al. Immunity and tolerance to aspergillus involve functionally distinct regulatory T cells and tryptophan catabolism. J Immunol (2006) 176(3):1712–23. doi: 10.4049/jimmunol.176.3.1712
43. Lewkowicz N, Mycko MP, Przygodzka P, Cwiklinska H, Cichalewska M, Matysiak M, et al. Induction of human il-10-Producing neutrophils by lps-stimulated treg cells and il-10. Mucosal Immunol (2016) 9(2):364–78. doi: 10.1038/mi.2015.66
44. Li P, Mao L, Liu X, Gan Y, Zheng J, Thomson AW, et al. Essential role of program death 1-ligand 1 in regulatory T-Cell-Afforded protection against blood-brain barrier damage after stroke. Stroke (2014) 45(3):857–64. doi: 10.1161/STROKEAHA.113.004100
45. Miller SL, Urey HC. Organic compound synthesis on the primitive earth. Science (1959) 130(3370):245–51. doi: 10.1126/science.130.3370.245
46. Oro J, Kimball AP. Synthesis of purines under possible primitive earth conditions. i. adenine from hydrogen cyanide. Arch Biochem Biophys (1961) 9:217–27. doi: 10.1016/0003-9861(61)90033-9
48. Ren X, Chen JF. Caffeine and parkinson’s disease: Multiple benefits and emerging mechanisms. Front Neurosci (2020) 14:602697:602697. doi: 10.3389/fnins.2020.602697
49. Byeon JJ, Park MH, Shin SH, Park Y, Lee BI, Choi JM, et al. In vitro, in silico, and in vivo assessments of pharmacokinetic properties of Zm241385. Molecules (2020) 25(5):1106. doi: 10.3390/molecules25051106
50. Segal BH, Freifeld AG. Antibacterial prophylaxis in patients with neutropenia. J Natl Compr Canc Netw (2007) 5(2):235–42. doi: 10.6004/jnccn.2007.0023
51. Venet F, Monneret G. Advances in the understanding and treatment of sepsis-induced immunosuppression. Nat Rev Nephrol (2018) 14(2):121–37. doi: 10.1038/nrneph.2017.165
52. Burn GL, Foti A, Marsman G, Patel DF, Zychlinsky A. The neutrophil. Immunity (2021) 54(7):1377–91. doi: 10.1016/j.immuni.2021.06.006
53. Margraf A, Lowell CA, Zarbock A. Neutrophils in acute inflammation: Current concepts and translational implications. Blood (2022) 139(14):2130–44. doi: 10.1182/blood.2021012295
54. Rowe SE, Wagner NJ, Li L, Beam JE, Wilkinson AD, Radlinski LC, et al. Reactive oxygen species induce antibiotic tolerance during systemic staphylococcus aureus infection. Nat Microbiol (2020) 5(2):282–90. doi: 10.1038/s41564-019-0627-y
55. Liew PX, Kubes P. The neutrophil’s role during health and disease. Physiol Rev (2019) 99(2):1223–48. doi: 10.1152/physrev.00012.2018
56. Swain SD, Rohn TT, Quinn MT. Neutrophil priming in host defense: Role of oxidants as priming agents. Antioxid Redox Signal (2002) 4(1):69–83. doi: 10.1089/152308602753625870
Keywords: septic peritonitis, adenosine 2A receptor, regulatory T-cells, neutrophils, bacterial clearance
Citation: Zhang T, Zhao J, Fu J, Chen G and Ma T (2022) Improvement of the sepsis survival rate by adenosine 2a receptor antagonists depends on immune regulatory functions of regulatory T-cells. Front. Immunol. 13:996446. doi: 10.3389/fimmu.2022.996446
Received: 17 July 2022; Accepted: 18 August 2022;
Published: 06 September 2022.
Edited by:
Ian Marriott, University of North Carolina at Charlotte, United StatesReviewed by:
Tiezheng Hou, University College London, United KingdomCopyright © 2022 Zhang, Zhao, Fu, Chen and Ma. This is an open-access article distributed under the terms of the Creative Commons Attribution License (CC BY). The use, distribution or reproduction in other forums is permitted, provided the original author(s) and the copyright owner(s) are credited and that the original publication in this journal is cited, in accordance with accepted academic practice. No use, distribution or reproduction is permitted which does not comply with these terms.
*Correspondence: Tao Ma, dGFvbWFAdG11LmVkdS5jbg==
Disclaimer: All claims expressed in this article are solely those of the authors and do not necessarily represent those of their affiliated organizations, or those of the publisher, the editors and the reviewers. Any product that may be evaluated in this article or claim that may be made by its manufacturer is not guaranteed or endorsed by the publisher.
Research integrity at Frontiers
Learn more about the work of our research integrity team to safeguard the quality of each article we publish.