- Third Hospital of Shanxi Medical University, Shanxi Bethune Hospital, Shanxi Academy of Medical Sciences, Tongji Shanxi Hospital, Taiyuan, China
Sjögrens syndrome (SS) is caused by autoantibodies that attack proprioceptive salivary and lacrimal gland tissues. Damage to the glands leads to dry mouth and eyes and affects multiple systems and organs. In severe cases, SS is life-threatening because it can lead to interstitial lung disease, renal insufficiency, and lymphoma. Histological examination of the labial minor salivary glands of patients with SS reveals focal lymphocyte aggregation of T and B cells. More studies have been conducted on the role of B cells in the pathogenesis of SS, whereas the role of T cells has only recently attracted the attention of researchers. This review focusses on the role of various populations of T cells in the pathogenesis of SS and the progress made in research to therapeutically targeting T cells for the treatment of patients with SS.
Introduction
T cell development, activation and differentiation
T cells originate from hematopoietic stem cells in the bone marrow and develop in the thymus, T cell receptor (TCR) diversity is generated through TCR gene rearrangement and then, T cells undergo positive selection to obtain major histocompatibility complex (MHC) restriction, expressing CD4 or CD8. The initial T cells then undergo negative selection to gain autoimmune tolerance. Gene mutations most likely occur in the variable region of the TCR and antigen recognition can only be achieved by binding to the MHC. If gene mutations occur in the TCR, negative and positive selection screening fails and some TCRs with different affinities to antigen peptides are preserved and differentiate into atypical and highly reactive T cells, which may cause autoimmune diseases (1–3).
The initial T cells enter the peripheral immune organs through the lymphatic and blood systems, and after TCR binds to the antigen (first signal) and co-stimulatory molecule (second signal), thereafter T cells become activated and differentiate into effector and memory T cells. The co-stimulator achieves the proinflammatory effect by activating T and B cells, whereas co-inhibitors inhibit activation. The immune checkpoint is mainly composed of co-stimulatory and co-inhibitory receptors or ligands. When external viruses and foreign bodies invade the body, immune cells become activated to produce an immune response, and immune checkpoints prevents excessive immunization (4).
T cells are divided into CD4+ and CD8+ T cells according to surface CD molecule expression. CD4+ T cells differentiate into specific subsets, depending on the presence of different cytokines, such as Th1, Th2, Th17, Treg, Tfh, and Tfr cells. CD8+ T cells differentiate into Tc1 and Tc2 cells and release interferon (IFN)-γ or IFN-α to regulate the immune response. Natural killer cell (NK)-like T cells directly kill cells and regulate immunity by secreting cytokines and chemokines that activate T and B cells. Memory T cells are mainly retained and can be quickly activated and differentiated into effector T cells when exposed to the same antigen: exerting an immune response. T cells play an important role in the occurrence and development of autoimmune diseases. This study reviews the role of T cells in the pathogenesis of SS and discusses SS therapies targeting T cells.
Role of CD4+ T cells in SS
There are fewer CD4+ T cells in the peripheral blood of individuals with SS than in healthy individuals: mainly due to aging and decreased proliferative capacity of naive CD4+ T cells (5). Additionally, an increase in the number of CD4+ T cells was observed in the labial glands of patients in the early stage of SS. However, as the disease developed, the CD4+ T cell count in the labial glands gradually decreased, whereas the B cell count increased in the middle and late stages of SS (6). This increase in CD4+ T may be caused by CD4+ T cell migration from the peripheral blood to exocrine glands (7). In mouse SS models, the CD4+ T cell count increased in the spleen, lymph nodes, and exocrine glands, whereas the CD4+ T cell count in the peripheral blood has rarely been studied and the evidence is inconclusive (8). The following sections summarize the roles of different T cell subsets in SS (Table 1 and Figure 1 ).
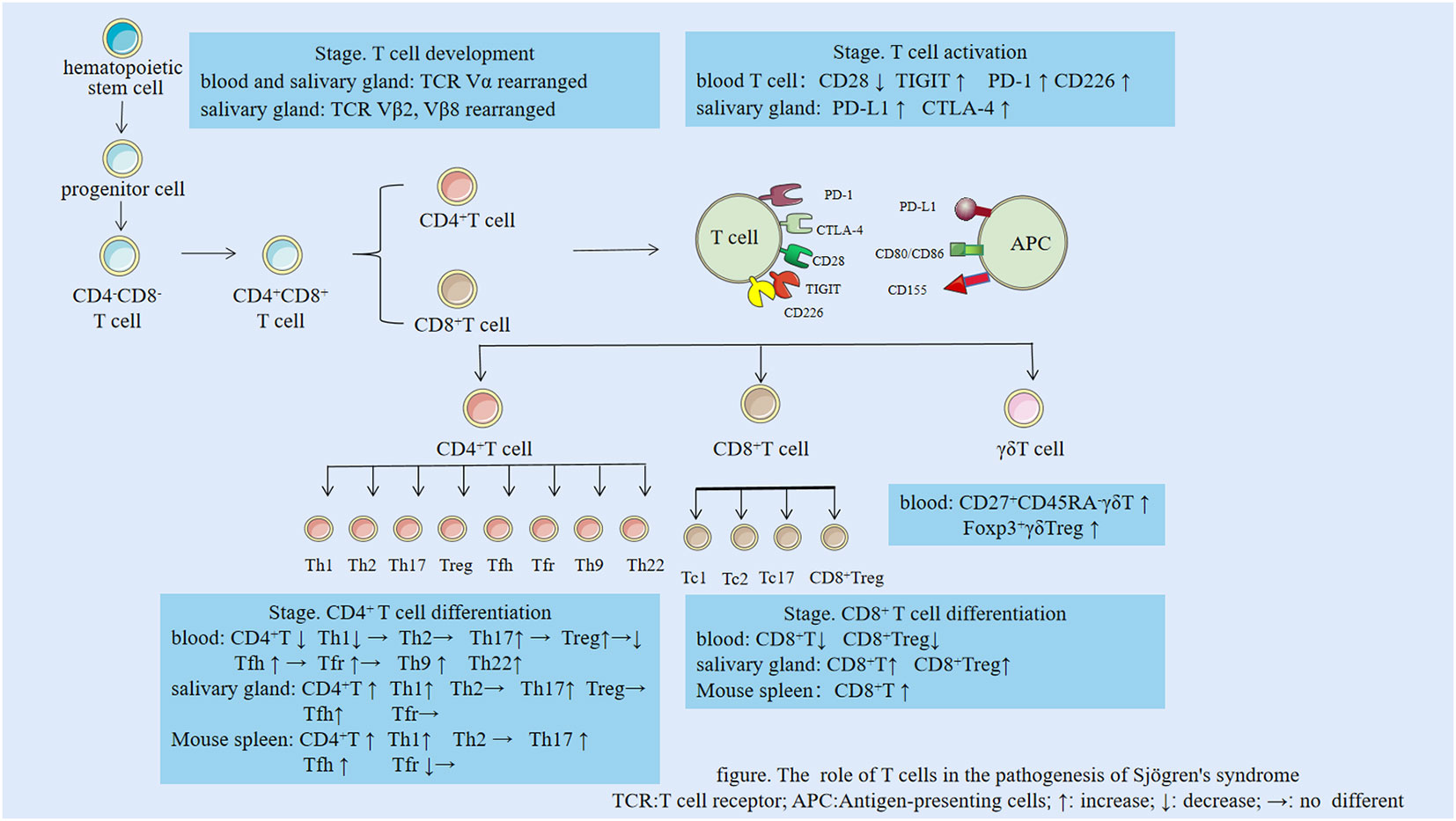
Figure 1 The role of T cells in the pathogenesis of Sjögren’s syndrome. TCR, T cell receptor; APC, Antigen-presenting cells; ↑: increase; ↓: decrease; →: no difference.
Th1 and Th2 cells
Changes in the number of Th1 and Th2 cells with disease progression were the focus of early research on SS. Immunohistochemical staining of salivary gland samples from patients with SS shows an increased number of local Th1 cells, but no significant change in Th2 cells, indicating an increased Th1/Th2 ratio (25). In contrast, the number of Th1 cells in the peripheral blood of patients with SS is significantly lower than that in healthy individuals, without significant changes in Th2 cells. Thus, in the peripheral blood, the Th1/Th2 ratio is decreased (9). This conclusion was confirmed in studies that used enzyme-linked immunosorbent assays to test the peripheral blood of patients with SS (10). Yet, Sudzius et al. found that the numbers of Th1 and Th2 cells in the peripheral blood of patients with SS were not significantly different from those in the control group (11). The observed opposite changes in the number of Th1 cells in the local salivary glands and peripheral blood may be explained by Th1 cell migration from the peripheral blood to glands, but there is no definitive correlation between the numbers of Th1 cells in these two locations. Some researchers believe that these changes reflect a systemic immunity reaction. Th1 cells mainly accumulate in the early stage of SS, before germinal center formation, whereas Th2 cells mainly appear during the infiltration stage of SS, after germinal center formation, when B cells in the salivary glands proliferate extensively. Therefore, it is possible that the studied patients were in the early-stage SS which explains the increase in the number of Th1 cells in the salivary glands and the lack of change in the number of Th2 cells (25, 35, 36).
Th1 and Th2 cells were also analyzed in the spleen and salivary glands of a mouse SS model. The spleen weight was increased because of the immune response. Enzyme-linked immunosorbent assays showed that Th1 cells in the spleen were increased, whereas Th2 cells were not detected. The number of Th1 cells was also increased in the enlarged mouse salivary glands, whereas Th2 cells were either undetectable or present only in small amounts. These findings might be explained by the generally small number of Th2 cells in mouse peripheral glands or the Th1/Th2 ratio imbalance; an increase in Th1 cells could inhibit Th2 cell differentiation (30, 31).
Th1 cells mainly secrete IFN-γ, interleukin (IL)-2, IFN-α, and C-X-C motif chemokine receptor 3 (CXCR3), of which INF-γ is the most important inflammatory factor (7). Early analysis of the saliva in patients with SS using the reference microsphere method that used probe labeling and immunohistochemistry showed that IFN-γ, TNF-α, and TNF-α/IL-4 levels were significantly increased, indicating that an increase in Th1 cell-related inflammatory factors may trigger SS (35, 37, 38). Interaction with IL-12, secreted by B cells and macrophages, prompts Th1 cells to induce an immune response. A small dose of IL-12 promoted CD4+ T cell differentiation into Th1 cells via the STAT4 phosphorylation pathway. IL-12 induces IFN-γ production, which stimulates IL-12 production, promoting Th1 cell differentiation. The lack of IL-6 normalizes IL-12 levels, alleviating SS manifestations in a mouse model (39). IL-27, a member of the IL-12 family, induces T, B, NK, and dendritic cell differentiation. Notably, IL-27 is elevated in the peripheral blood of patients with SS, which may promote Th1 cell differentiation via the STAT1 pathway, change the Th1/Treg cell ratio, and stimulate T cells to cause SS manifestations. IL-27 mediates inflammatory effects in autoimmune diseases such as SS and rheumatoid arthritis. Compared with normal non-obese diabetic (NOD) mice, IL-27-negative NOD mice had less inflammation in the lacrimal and salivary glands. Furthermore, T cells extracted from the spleen of NOD.Il27ra−/− mice and transferred to NOD. Rag1−/− mice failed to induce type 1 diabetes, which confirmed that the lack of IL-27 could prevent the occurrence of autoimmune diseases (40).
Th2 cells mainly secrete IL-4 and IL-13 and are involved in antibody formation and humoral immunity. Th2 cells are increased in the blood of patients with SS (41). Genotype investigation of cytokines regulating Th1/Th2 differentiation showed that their frequencies did not differ in disease activity between patients with SS and healthy controls (42). Thus, SS is primarily a Th1 cell-mediated condition, although Th2 cells may appear in mild cases (42). Mikulicz’s disease is a kind of IgG4-related diseases. Compared with primary SS, both diseases can cause lymphocyte infiltration in lacrimal gland and salivary gland. A comparison of patients with SS with a patient with Mikulicz’s disease showed that the two conditions had similar histological features in the lacrimal and salivary glands (43). The Mikulicz’s disease case was characterized by a prominent infiltration of IgG4-producing plasma cells, higher infiltration of IL-4 and IL-13, and lack of IFN-γ effect, whereas patients with SS had anti-SSA and anti-SSB antibodies, indicating that both conditions have a Th2 tendency. However, the sample size was too small to draw definitive conclusions (43).
Th17 and Treg cells
Flow cytometry single cell analysis and immunohistochemistry revealed a significant increase in Th17 cells in the peripheral blood and labial gland tissues of patients with SS compared to that in normal healthy controls (12–14). However, one study found no such difference (11). The increased Th17 cell frequency was also noted in the peripheral blood, spleen, and salivary glands of NOD mice (29).
Th17 cells secrete IL-17A and participate in inflammatory responses in many autoimmune diseases. While IL-17A levels are significantly increased in the peripheral blood of patients with SS, it does not significantly correlate with an increase in disease activity or decrease after immunosuppressant treatment. However, immunosuppressant treatment significantly decreased IL-17 mRNA levels in patients with SS (44). The lack of significant differences in IL-17A levels in the peripheral blood of patients with SS and healthy controls may be due to the fact that IL-17A is produced by Th17 cells upon gland infiltration (45).
In a mouse SS model, IL-17A increased in the blood and salivary glands in a sex-depended manner. Inflammation in female mice occurs faster than in male mice, and the number of migrated lymphocytes is increased. Additionally, in the absence of IL-17, symptoms improve faster in female mice than in male mice (46).
Th17 cells exhibit strong plasticity and can be converted into Th1 cells. Th17 cells secrete IL-17A without producing IFN-γ. However, after stimulation with IL-12, Th17 cells start secreting IFN-γ. IL-17A+IFN-γ+ Th1 cells differ substantially from classical CD4+ Th1 cells in the submandibular and lymph nodes; therefore, Th1 cells were hypothesized to be transformed by Th17 cells (47). Th1 cell subtypes transformed from Th17 cells may be more pathogenic than normal Th17 cells (13, 48). Mice injected with IL-17A into their salivary glands gradually developed dryness and had increased levels of serum antibodies. IL-17A promotes IL-8 participation in neutrophil chemotaxis at the injured site, induces the formation of germinal centers, and activates B cells (49). Il17a−/− mice immunized with the salivary gland proteins did not show SS signs, however they rapidly developed SS upon implantation of Th17 cells generated in vitro (50). Furthermore, the number of Th1 cells increased, indicating that Th17 cells infiltrated the gland via the Th1 pathway, which might be because of the plasticity of Th17 cells (50).
Regulatory T cells (Tregs) are immunomodulatory CD25+FOXP3+cells that suppress effector T cell activation. Fewer CD25+ Tregs were detected in the peripheral blood of patients with SS, whereas their number did not change in the bilateral parotid glands and the parotid gland cells were not damaged (15). The number of CD25+FOXP3+ Tregs in the peripheral blood of patients with SS was the same as or higher than that in the healthy control group, whereas during the inactive stage of SS, the number of CD25+FOXOP3+ Tregs was relatively low (16). Immunohistochemical staining showed no significant changes in CD25+ Tregs in the labial glands of patients with SS and normal individuals (15, 26, 27). However, the differences in these results may be related to the different surface markers used in the experiments (51).
T and B lymphocytes increase with an increase in Tregs upon ageing. However, Tregs lose their immunosuppressive function with age. Tregs are plastic, and are converted into Th1, Th2, and Th17-like cells to secrete related inflammatory factors. In NOD mice, the lacrimal gland inflammation and the number of Tregs increased with age, but no inhibition of Treg function was observed. Whether Tregs are converted to other cells with age cause inflammation, or become less inhibitory remains to be established (52).
Furthermore, sex is an important factor in SS. Estrogens promote Treg cell immunosuppression, reducing lacrimal gland inflammation, whereas androgens inhibit Treg cell function. Previous studies discovered that males with SS were primarily affected by lacrimal gland inflammation, whereas females with SS were affected by salivary gland inflammation. Lacrimal gland inflammation occurs with or without Treg cells in lymphocyte transfer in male NOD mice, which suggest that androgens prevent Treg cell function. Androgen’s involvement in the development of lacrimal gland inflammation is suspected as female NOD mice rarely trigger lacrimal gland inflammation, whereas lymphocyte transfer from female to male mice causes lacrimal gland inflammation (53).
Tfh and Tfr cells
The median number of T follicular helper (Tfh) cells (CXCR5+PD-1+) in the peripheral blood of patients with SS is significantly higher than in individuals without SS, and Tfh cells stimulated proliferation of B cells unrelated to disease activity (17, 18). The frequency of Tfh cells in the peripheral blood of SS patients is not significantly increased compared to that in healthy controls, but there were more activated Tfh cells. Furthermore, the number of CCR7lowPD-1highTfh cells in the peripheral blood of patients with SS is significantly increased, indicating that CCR7lowPD-1highTfh cells may be involved in glandular inflammation and disease activity (21). There are many subtypes of Tfh cells, such as Tfh1, Tfh2, and Tfh17-like cells, the latter are significantly increased in the peripheral blood of patients with SS (22). Additionally, some experiments showed that CXCR5+PD-1+ Tfh cells in the blood of patients with SS were unchanged compared with those in individuals without SS, but the effects of Tfh1-like cells were more significant than those of Tfh2 and Tfh17-like cells (19, 20). An increase in Tfh (CD4+CXCR5+) cells was detected in the labial glands of patients with SS compared to that in normal controls. Furthermore, Tfh cells facilitated B-cell differentiation and their presence was associated with anti-nuclear antibodies (28).
In a mouse SS model, Tfh (CD4+CXCR5+PD1+) cells were elevated in the spleen and salivary glands, and there was no difference in the number of Tfh1, Tfh2, and Tfh17 cells in the spleen when compared to those in controls. IL-21 inhibited Tfh activation by spleen DNA binding inhibitor 3 (ID3) in NOD mice in vivo, whereas an IL-21 blocking agent injection in NOD mice decreased the number of Tfh cells and reduced the inflammatory reaction. ID3 attenuates mouse salivary and lacrimal gland inflammation and may act as part of the PAX3-ID3 signaling pathway, as IL-21R-Fc administration augmented the number of PAX3- and ID3-positive cells in the salivary glands of NOD mice, while reducing the pathologically increased proportion of Tfh and IL-17-producing T cells (32). Stimulation of this pathway may be beneficial for the treatment of SS and other autoimmune diseases (32). Germinal center enlargement in the spleen was also observed in a mouse SS model, and the number of Tfh (CD4+CXCR5+) cells positively correlated with disease status, confirming the importance of Tfh cells in SS (54).
Tfh cells expressing CXCR5, PD-1, and IL-21, interact with B and T cells and secrete related cytokines to promote germinal center formation. However, CCR9+ Tfh cells in the peripheral blood are more closely related to SS than CCR5+ Tfh cells. CCR5+ Tfh cells promote high CCL5 expression and cause inflammatory cell accumulation by secreting IL-7 (55, 56). Tfh cells can be present in the germinal centers in patients with late-stage SS, affecting the secretion of antibodies by B cells. Tfh cells in the peripheral circulation differ from Tfh cells in the germinal center in that they express little or no BCL-6. Circulating Tfh1 cells secrete IL-21, IFN-γ, and IL-10; Tfh2 cells secrete IL-21, IL-4, and IL-13; whereas Tfh17 cells secrete IL-17, IL-21, IL-22, and other cytokines (57, 58). Therefore, if different Tfh surface markers are selected in the peripheral blood and tissues, the expression of different populations of Tfh cells in SS may vary.
The number of T follicular regulatory (Tfr) (FOXP3+) cells was significantly increased in the peripheral blood of patients with SS, and the Tfr/Tfh ratio increase was positively correlated with the degree of salivary gland infiltration. The Tfr/Tfh ratio can also show the presence of ectopic lymphoid tissue (23, 24). No changes in the number of Tfr (FOXP3+) cells in the peripheral blood of patients with SS were observed in other studies. However, the number of Tfr cells in autoantibody-positive patients was higher than that in autoantibody-negative patients. Additionally, the number of Tfr cells in the labial gland of patients with SS did not significantly change compared to that in healthy controls (24). In a mouse SS model, no changes in the number of Tfr (CXCR5+PD1+CD4+FOXP3+) cells in the spleen were observed (34). Tfh and Tfr cells were found in the cervical lymph nodes of NOD mice, but no changes in the number of Tfr cells in the salivary glands were noted. Tfr cells share the same CXCR5 recognition signal as Tfh cells, and express FOXP3 and PRDM (33). The FOXP3, PD-1, and ICOS expression levels in Tfr cells from tissues and blood vary greatly. There are more Tfr cells in peripheral blood than in tissues, indicating that Tfr does not completely inhibit B cells and enters the blood after secondary lymphoid tissue production and before the action of B cells (59, 60).
Th9 cells
Th9 cells, first discovered in 2008, are a new subtype of CD4+ T cells. TGF-β and IL-4 induce differentiation of CD4+ T cells into Th9 cells, which secrete IL-9. Th2 cells can be converted into Th9 cells, which are induced only by TGF-β. Additionally, IL-21, IL-10, TNF-α, and TLR2 promote Th9 cell differentiation, whereas IFN-γ and PRDM inhibit their differentiation (61). IL-9 inhibits Tregs, promotes Th17 cell differentiation, and participates in B-lymphocytic tumor development (62, 63). Th9 cells are significantly elevated in the peripheral blood and synovial tissue of patients with RA compared to that in healthy controls (64). There was no significant decrease in Th9 cells in the peripheral blood after infliximab treatment (65). Similarly, the proportion of Th9 cells in the peripheral blood of patients with systemic lupus erythematosus was also increased. The number of Th9 cells in the spleen and kidneys of lupus mice was higher than that in normal mice and correlated with germinal center formation (66). Flow cytometry showed an increased number of Th9 cells and no significant changes in IL-9-producing Th2 cells in the peripheral blood of patients with systemic sclerosis (67). Although there has been no relevant research on Th9 cells in SS, the above-mentioned evidence indicates the importance of Th9 cells in various autoimmune diseases.
Th22 cells
In addition to secreting IL-22, IL-13, and TNF-α, Th22 can be converted into Th1 and Th2 cells under certain conditions. IL-6 and TNF-α promote Th22 differentiation, whereas high doses of TGF-β inhibit Th22 cell differentiation (68). A peripheral blood flow assay using CCR4+, CCR6+, and CCR10+ as Th22 cell surface markers showed that Th22 cells of patients with RA were significantly increased compared with that in the healthy control group; after methotrexate and leflunomide treatment, the peripheral Th22 cell levels were decreased in patients with RA (69). Additionally, both IL-22 levels and Th22 cell counts were significantly higher in the peripheral blood of patients with SLE than that in the normal controls and correlated with disease activity (70, 71). However, one trial found lower peripheral blood IL-22 and higher IL-17 and IL-23 levels in patients with SLE compared to that in healthy controls, suggesting independence to disease activity. This could possibly be because of the specific subset of IL-22-producing CD4+ T cells in human peripheral blood (72). In a study where the salivary glands of 19 patients with primary SS and 16 patients with non-specific sialadenitis were biopsied by immunohistochemistry, IL-22 expression of patients with SS and the control group was increased (73). The comparison between SS patients and the healthy control group showed that the serum IL-22 level of SS patients was significantly increased, and the serum IL-22 level was significantly positively correlated with anti-SSA, anti-SSB, and rheumatoid factor (RF) (74). Although studies on Th22 in SS are lacking, Th22 cells have shown a relative advantage in various autoimmune diseases.
The comparison between SS patients and the healthy control group showed that the serum IL-22 level of SS patients was significantly increased, and the serum IL-22 level was significantly positively correlated with anti-SSA, anti-SSB, and RF. Although studies on Th22 in sjogren’s syndrome are lacking, Th22 cells have shown a relative advantage in various autoimmune diseases.
Role of CD8+ T lymphocytes in SS
The decreased number of CD8+ T cells in the peripheral blood of patients with SS may be due to persistent viral infection. CD8+ T cells secrete perforin and kill cells infected by viruses. Therefore, the participation in the elimination of viral infections continuously depletes CD8+ T cells from the peripheral blood (75). The decrease in circulating CD8+ T cells may also be due to their migration to and accumulation in the glands (76). CD8+ T cells accumulate in the exocrine glands of patients with SS. CD8+ T cells can differentiate into Tc1 cells that secrete IFN-γ and TNF-α and have strong killing activity. They can also differentiate into Tc2 cells that secrete IL-4 and IL-13 that enhance inflammation. Tc17 cells secrete IL-17, and CD8+ Tregs secrete IL-21 (76). CD8+ Tregs, that inhibit lymphocyte function and prevent the continuous development of immune responses, are significantly reduced in the peripheral blood of patients with SS. In contrast, in the late stage of SS, the number of CD8+ Tregs may increase (77). Generally, the number of CD8+ Tregs cells are undetectable or slightly reduced in the peripheral blood of SS, suggesting that it is inversely proportional to disease activity, and there are also large numbers of IFN-γ and CD8+ Tregs in the lacrimal gland, that inactivate dendritic cells and thereby alleviate SS manifestations (76). In NOD mice with CD8+ Treg depletion, the activation of dendritic cells and stimulation of the generation of CD4+ T cells that secrete IL-17A increased the number of Th17 cells, leading to corneal damage and more severe SS pathology. A decrease in CD8+ Treg cells and an increase in Th17 cells are important factors involved in SS aggravation (78). The CD4+CD8+ T cells were significantly increased in the peripheral blood of patients with SS compared to that in healthy controls. The number of these cells positively correlated with IL-10 levels and negatively correlated with disease activity, possibly indicating a protective role of CD4+CD8+ T cells in SS (79, 80). CD8+ T cells in the labial glands of patients with SS are excessively active and characterized by abnormal proliferation, therefore the tissue accumulates memory CD8+ T cells, which secrete high levels of IFN-γ, leading to SS manifestations (76). Immunofluorescence detection experiments also revealed an increased number of tissue-resident memory CD8+ T cells in the salivary gland epithelial cells of patients with SS (81).
CD8+ T cells were increased in both the spleen and salivary glands of NOD mice (78, 81), especially in the late stage of SS. CD8+ T cells reside in glandular tissues and cause apoptosis of salivary gland cells through the FASL and CTL pathways. Knockout of IFN-γ in mice alleviated salivary acinus atrophy, restored the secretory function, and reduced the germinal center size (81). It is possible that IFN-γ released by CD8+ T cells in the submandibular glands and acting on CXCR3 caused the destruction of the acinus. The same study also found that depletion of CD8+ T cells normalized the antibody secretion of salivary glands and blood levels in mice. CD8+ T cells could be used as a target to treat SS (81)(Table 2).
Role of NK-like cells in SS
Natural killer (NK) cells are natural immune cells. The total number of NK cells in the peripheral blood of patients with SS is decreased compared to that in healthy controls. NK cell activity and expression levels of the NK cell activation receptors CD2, NKG2D (C-type lectin-like NK receptor), and NKP62 (natural cytotoxic receptor), as well as serum IL-18 and TNF-α levels are elevated in patients with SS (82–85). The proportion of CD56+ NK cells in patients with SS was also found to be higher than in healthy controls. The ratio of CD56 strongly positive cells to CD56 weakly positive cells positively correlated with IgG levels and was directly proportional to disease activity, indicating that this ratio could be used as a specific indicator of SS. In healthy people, the number of CD56dim cells is 10 times higher than that in CD56bright cells, whereas in SS, the number of CD56bright cells increases, while the number of CD56dim cells decreases, leading to an overall increase in the CD56bright/CD56dim ratio. Secreted cytokines tend to migrate to the glands, causing inflammation. Upon the treatment of patients with SS with hormones and immunosuppressants, the number of CD56dim NK cells increased, but the CD56bright/CD56dim ratio and number of CD56bright NK cells did not change (86). NKP30 is an NK cell-specific activation receptor that regulates the interaction between NK and dendritic cells and stimulates IFN-γ secretion to promote salivary gland inflammation. The number of NKP30+ (CD56+CD16+) NK cells in the peripheral blood of patients with SS is increased, and this parameter is sensitive to formal immunotherapy (87, 88).
Additionally, there is another type of NK-like T cells, which is divided into type I NK-like T cells (iNKT) and type II NK-like T cells. iNKT cells, which produce IL-17 and IFN-γ, are elevated in the peripheral blood of patients with SS, but are not detected in the labial glands, possibly because they are difficult to identify in the labial glands using the existing technology (89, 90). NK-like T cells have the same functions as both CD3+ T and CD56+ NK cells. The number of NK-like T cells in the peripheral blood of SS patients was decreased compared to that in the healthy group, which positively correlated with the numbers of CD4+ T, CD8+ T, and NK cells, but not with that of B cells (91, 92). As SS developed, NK-like T cells gradually decreased, and the number of NK-like T cells in treated patients gradually returned to normal (93). In other studies, the number of NK-like T cells in the peripheral blood of SS patients was increased, which may be due to insufficient sample size, or different course of disease and treatment between patients (94–96). It has also been reported that the numbers of CD3+CD161+ NK-like T cells in the peripheral blood of patients with SS and healthy controls were not significantly different. This discrepancy may be due to the detection of different surface markers of NK-like T-cells (82). The numbers of NK cells in the labial glands of patients with SS and salivary glands of NOD mice were increased compared to the respective numbers in unaffected individuals, presumably due to the migration of NK cells from the peripheral blood to the salivary gland tissue (97).
Role of memory γδT cells in SS
γδT cells, a type of T cell present in the skin, colon, and reproductive tract, with a limited number detected in peripheral blood and lymphocytes, play specific biological roles. γδT cells are separated into CD4+ γδT, CD8+ γδT, and γδTreg cells. CD4+γδT cells express Th1-, Th2-, and Th17-related cytokines, CD8+γδT cells are primarily connected with viral infections, and γδTreg cells’ physiological activities are still being researched (98). Human peripheral blood γδT cells can be classified according to their expression of CD27 and CD45 as follows: naive (CD27 + CD45 +), effector memory (CD27+CD45-), central memory (CD27+CD45-), and terminally differentiated (CD27-CD45+), patients with SLE have more CD27+CD45-γδT cells in their peripheral blood and fewer Foxp3+γδTreg cells that express Foxp3 compared to healthy controls, as well as a substantial increase in Vδ1 cells after glucocorticoid and cyclophosphamide treatment (99). After treatment with biologic agents, CCR6+ Vγ9+Vδ2+ T cell counts were significantly reduced and returned to normal in the peripheral blood of patients with psoriasis compared to healthy controls (100). Therefore, γδT cells play an important role in autoimmune diseases, but the function of γδT cells in dry syndrome still needs further investigation.
Role of memory T cells in SS
CD4+ memory T cells were increased in the bilateral parotid glands of patients with SS compared to healthy controls, whereas there were fewer CD8+ memory T cells. However, both cell types could lead to glandular fibrosis. The CD4+CD45+ memory T cells in the parotid gland are very similar to Tfh signals that help B cell recruitment and form germinal centers, therefore induce SS (101, 102). Large numbers of tissue-resident CD8+ and CD4+ memory T cells were detected in patients and in a mouse SS model: the number of CD8+ memory T cells was greater than that of CD4+ memory T cells, and CD69+CD103+ memory CD8+ T cells were predominant. When these CD8+ memory T cells were ablated in mouse experiments, the pathological manifestations of SS in mouse salivary glands were alleviated (81).
Role of T cell checkpoint in the pathogenesis of SS
T-cell checkpoints, which include co-stimulatory and co-inhibitory signals, are secondary signals for T-cell activation. T-cell checkpoint receptor dysfunction is associated with autoimmune disease development. CD28, PD-1, CTLA-4, CD226, and TIGIT are expressed on T cells and CD80/CD86, PD-L1, and CD155 are expressed on antigen-presenting cells. CD28, CD226 are co-stimulatory signals; CD28 binds to CD80/CD86 and CD226 binds to CD155, decreasing the TCR threshold and promoting T cell proliferation, survival, and cytokine production. CTLA-4, PD-1, TIGIT are co-inhibitory signals; CTLA-4 binds to CD80/CD86, PD-1 to PD-L1, and TIGIT to CD155, inducing cell survival, preventing apoptosis, and downregulating or terminating T cell activation (103).
Soluble CD28 expression was elevated in the peripheral blood of patients with SS with deficiency of T cells. Whereas CTLA-4 was elevated in the salivary glands of patients with SS, which was associated with disease susceptibility and the presence of autoantibodies (104–106). PD-1 and its ligand PD-L1 play an important role in autoimmune diseases. PD-1 is expressed on T cells, and its interaction with PD-L1 inhibits TCR signal transduction and induces immature CD4+ T cells differentiation into Tregs, thereby suppressing immune reactions. PD-1 expression on T lymphocytes in the peripheral blood of patients with SS was increased compared to that in healthy controls, but PD-1 did not correlate with the levels of anti-nuclear antibodies, immunoglobulins, or the erythrocyte sedimentation rate. Patients with SS have higher PD-L1 expression in the salivary gland epithelium, likely because of stimulation by IFN-γ. PD-1/PD-L1 expression changes may contribute to the pathogenesis of SS. The role of the PD-1/PD-L1 interaction in SS has not been thoroughly investigated and further research is required (107, 108). Recently, it was discovered that CD226 and TIGIT proteins, which are competitively expressed on T and NK cells as novel immune checkpoint proteins, were significantly elevated in the peripheral blood of patients with SS. Further, TIGIT+CD4+ T cells in patients with SS showed greater activity than those in healthy individuals. CD226 is a co-stimulatory molecule, whereas TIGIT is a co-inhibitory molecule that binds to its ligand CD155, competing with CD226, to exert its inhibitory function. The disrupted balance between the co-stimulatory and co-inhibitory molecules is an important factor that causes an immune response. Anti-CD226 and anti-TIGIT treatments maintain the immune balance by regulating Tregs, which is important for rheumatic disease treatment (109). Notably, dryness symptoms caused by the treatment of tumors with immune checkpoint inhibitors are different from those caused by SS. The former mainly occur in older males, tend to have less ocular involvement, and are accompanied by different antibody and histopathological features (110, 111). In conclusion, the application of modulator immune checkpoints as novel therapeutic targets for the treatment of SS remains to be investigated.
Role of TCR in the pathogenesis of SS
TCR is composed of an α-chain and a β-chain, and each chain includes a variable, easily mutated region, and a constant (C) region. In patients with SS and in mouse models of SS, the TCR is rearranged. The variable region of TCR in T cells from salivary gland cells in patients with SS does not play a corresponding role in inflammation, and the preferential amplification of Vβ transcripts, especially Vβ2 and Vβ8, is likely to be closely related to the stimulated immunity (112). Vα was also found to be rearranged in T cells from salivary glands, and antigen-specific and clonal expansion of T cells in the peripheral blood and salivary glands of patients with SS was studied using single-cell analysis. The salivary gland expansion clones and TCRα sequence frequencies were increased in patients, and salivary glands became hypofunctional. The TCRα sequence starts simultaneously in two gene sequences, which may lead to increased levels of gene expression and elevated self-reactivity. These phenomena may provide an important basis for SS development (113, 114). The expression levels of the first 50 differential genes in the minor salivary glands of patients with primary SS and normal control groups were compared by quantitative reverse transcription polymerase chain reaction, and it was found that TCR played an important role in T lymphocyte activation (115). Peripheral blood samples from patients with primary SS and healthy control groups were studied using single-cell RNA sequencing technology and TCR variable genes CD4+ T cells were found to be amplified in patients with primary SS (116). Single-cell RNA sequencing analysis of the submandibular glands of mice with Sjogren’s syndrome revealed that TCR signals were enriched in the mice with SS (117).
Development of T cell therapy in SS
There are no well-established treatments targeting T cells and their secreted inflammatory factors in SS, but it is an actively researched topic (Table 3 and Figure 2).
Abatacept inhibits T cell proliferation by blocking the co-stimulatory signals of CD86/CD80 on antigen-presenting cells and CD28 on T cells, thereby inhibiting the immune response, reducing inflammation, and increasing the secretion of the salivary glands. Tfh cells are also reduced by this treatment. In a 1-year multicenter study of 68 patients with SS treated with intravenous abatacept, saliva flow and tear volume were significantly increased, serum IgG levels were decreased, and dry symptoms were improved (118). The effect was more significant at early treatment intervention. In 15 patients, adverse reactions, mainly infections, were observed and most of these were transient (118). In another randomized, double-blind, controlled trial of 187 patients with SS, abatacept treatment did not significantly improve gland characteristics in individuals with SS. Twenty patients experienced severe adverse reactions, such as septic shock and drug hypersensitivity. Current clinical trials are single-center phase III trials (119).
Applying Tofacitinib application, an inhibitor of Janus kinase (JAK) 1/3, to dry eyes alleviated ocular inflammation and was well tolerated, so the drug is currently in phase I/II of clinical trials (120). Baricitinib, a selective JAK1 and JAK2 inhibitor, is used to treat RA. In a recently published clinical trial involving 11 patients with SS, baricitinib improved arthritis and rash symptoms, and significantly alleviated the active stage of pulmonary interstitial disease. It is currently in the initial stages of clinical trials (121). JAK 1 inhibitors, spleen tyrosine kinase, and selective Bruton tyrosine kinase filgotinib, lanraplenib, and irabrutinib, respectively, block corresponding signal transduction pathways, which might be beneficial for the treatment of SS. In a multicenter, double-blind, randomized study of 150 patients with primary or secondary SS treated with filgotinib, lanraplenib, tirabrutinib, and placebo, filgotinib-treated patients had reduced type I IFN activity, increased number of memory B cells, and decreased levels of RF, IgM, IgG, and IgA, respectively, compared with that in patients that received the placebo. IFN activity was significantly increased in the lanraplenib group, and the numbers of B cells and precursor plasma cells in the peripheral blood of patients treated with tirabrutinib were significantly decreased, as was the ESSDAI score (122).
Alefacept, originally developed for psoriasis, inhibits T cell activation and proliferation by binding to CD2 expressed on the T cell surface, causing a decrease in the numbers of CD4+ and CD8+ T cells. To the best of our knowledge, there has been no relevant research on the treatment of SS with alefacept. However, this drug was used in type I diabetes clinical trials, and treatment reduced insulin use and decreased the frequency of hypoglycemic events (123). It is currently in phase II of clinical trials for diabetes (132).
Mesenchymal stem cells (MSCs) that can be isolated from the bone marrow, fat, umbilical cord, and gums are characterized by their self-renewal ability and differentiation potential. MSCs also play an anti-inflammatory and immunomodulatory role. IL-10 induces CD4+ T cells to differentiate into Tregs, thereby weakening the immune response and alleviating SS (133, 134). Umbilical cord mesenchymal stem cells (UCMSCs) inhibit T cell proliferation in patients with SS, increasing the number of Tregs and lowering the number of Th17 and Th1 cells. In a clinical experiment involving 24 patients with SS, intravenous injections of UCMSCs led to the recovery of salivary gland function, improvement of dry mouth symptoms, and suppression of inflammatory reactions. No adverse reactions were observed and this treatment is currently in the final stage of a phase II trial (121, 124, 125).
The M3 acetylcholine receptor (M3R) is a G protein-coupled receptor that plays an important role in the secretory function of salivary and lacrimal glands. Autoantibodies against M3R and an increase in M3R reactive CD4+ T cells were detected in the peripheral blood of patients with SS. In an experimental mouse SS model, the M3R-reactive CD4+ T cells secreted IL-17 and IFN-γ. M3R-reactive CD4+ T cell peptide ligands inhibited IFN-γ production in vitro and alleviated salivary gland inflammation in vivo, thus the M3R-reactive CD4+ T cells may become a potential target for the treatment of SS (135). Other biological therapies targeting T cells, such as anti-CD40 and anti-ICOSL, are still under investigation.
Targeting cytokines in SS
IFN-α kinoid is an anti-IFN-α vaccine that stimulates generation of an anti-IFN-α antibody in the peripheral blood of MRL/lpr mice. The reduced level of IFN-α improved dry symptoms of SS without adverse reactions. This treatment is still at the preclinical stage, and it requires many tests to assess its efficacy and suitability for clinical application (126). Interestingly, oral mucosal administration of a low IFN-α dose to 497 patients with SS in a phase III clinical trial significantly increased saliva flow without causing significant adverse events (136).
Infliximab and etanercept, which both target TNF-α, have been shown to improve ocular fatigue symptoms, but were ineffective in patients with dry eyes and dry mouth. Currently, a multicenter phase II clinical trial of infliximab is underway, but so far, xerosis symptoms and the degree of salivary gland infiltration were not improved and there were serious adverse reactions, such as transfusion reaction, autoimmune liver disease, and pneumococcal septicemia (127). In a clinical trial of subcutaneously applied etanercept in 15 SS patients, the salivary and lacrimal gland functions were not significantly improved, and some individuals had local side effects (128).
IL-6 is involved in most autoimmune reactions, and elevated IL-6 levels are observed in the peripheral blood and salivary glands of patients with SS. Therefore, IL-6-targeting treatment for SS may be promising. The recombinant humanized anti-human IL-6 receptor monoclonal antibody tocilizumab did not improve the systemic involvement and dry symptoms in a cohort of 55 patients with SS, compared with the manifestations in the placebo group (129). The treatment group is currently involved in a stage II/III clinical trial.
Low IL-2 doses increase the number of CD4+ Tregs and normalize the Th17 cell/Treg ratio, suppressing immune reactions. Administration of a low IL-2 dose to a cohort of 190 patients with SS restored the number of Tregs in the peripheral blood, which was significantly lower in patients, whereas the proportion of Th17 cells was not significantly changed in comparison to the healthy control group. Thus, therapy with low-dose IL-2 can restore the Th17/Treg balance in the peripheral blood of patients with primary SS, and this treatment has now completed its phase II clinical trial (130).
Blocking IL-7 and CXCR3 reduces the secretion of various cytokines by T cells. Compared to the control group, patients with SS had increased serum IL-7R levels and elevated IL-7 and IL-7R expression in the labial glands, and IL-7 was related to increased inflammation and lymphoma (137). Therefore, targeting the IL-7/IL-7R pathway may be an effective therapeutic strategy. IL-1 levels were significantly increased in the salivary glands of patients with SS, and this cytokine is an important target for autoimmune diseases. In IL-1 knockout autoimmune deficient mice, although there was no reduction in lymphocyte infiltration of the lacrimal gland or eye, ocular surface keratosis and epithelial lesions were significantly reduced, implying that the IL-1 receptor antagonist anakinra could be used for the treatment of ocular surface fatigue (131). IL-22 was significantly increased in the salivary glands and serum of patients with SS, indicating that IL-22 may also be an important therapeutic target for SS (138).
Conclusion
Various T-cell subsets are essential for the development of SS. However, there are many T cell classifications and subtypes, and further research is needed to elucidate their mechanisms of action. This review summarized the current understanding of the roles of various T cell subsets in SS and discussed attempts to therapeutically target T cells. We hope that our review will be a useful primer for everyone interested in the development of SS therapies.
Author contributions
All authors contributed to the study conception and design. DM, LZ and KX initiated the project. QA and DM drafted and wrote the manuscript. JZ, ZW, YS, BY, and XZ collected the references. All authors contributed to the article and approved the submitted version.
Funding
This work was supported by the National Natural Science Foundation of China [grant number 82001741] and the Applied Basic Research Project of the Shanxi Science and Technology Department [grant number 201801D221380]
Conflict of interest
The authors declare that the research was conducted in the absence of any commercial or financial relationships that could be construed as a potential conflict of interest.
Publisher’s note
All claims expressed in this article are solely those of the authors and do not necessarily represent those of their affiliated organizations, or those of the publisher, the editors and the reviewers. Any product that may be evaluated in this article, or claim that may be made by its manufacturer, is not guaranteed or endorsed by the publisher.
References
1. Kumar BV, Connors TJ, Farber DL. Human T cell development, localization, and function throughout life. Immunity (2018) 48(2):202–13. doi: 10.1016/j.immuni.2018.01.007
2. Geltink RIK, Kyle RL, Pearce EL. Unraveling the complex interplay between T cell metabolism and function. Annu Rev Immunol (2018) 36:461–88. doi: 10.1146/annurev-immunol-042617-053019
3. Germain RN. Ligand-dependent regulation of T cell development and activation. Immunol Res (2003) 27(2-3):277–86. doi: 10.1385/ir:27:2-3:277
4. Gibney GT, Weiner LM, Atkins MB. Predictive biomarkers for checkpoint inhibitor-based immunotherapy. Lancet Oncol (2016) 17(12):e542–e51. doi: 10.1016/s1470-2045(16)30406-5
5. Fessler J, Fasching P, Raicht A, Hammerl S, Weber J, Lackner A, et al. Lymphopenia in primary sjögren’s syndrome is associated with premature aging of naïve Cd4+ T cells. Rheumatol (Oxford) (2021) 60(2):588–97. doi: 10.1093/rheumatology/keaa105
6. Wang Q, Che N, Lu C, Sun X, Wang Y, Wang Q, et al. Correlation of peripheral Cd4+Granzb+Ctls with disease severity in patients with primary sjögren’s syndrome. Arthritis Res Ther (2021) 23(1):257. doi: 10.1186/s13075-021-02632-6
7. Yao Y, Ma JF, Chang C, Xu T, Gao CY, Gershwin ME, et al. Immunobiology of T cells in sjogren’s syndrome. Clin Rev Allergy Immunol (2021) 60(1):111–31. doi: 10.1007/s12016-020-08793-7
8. Iizuka M, Wakamatsu E, Tsuboi H, Nakamura Y, Hayashi T, Matsui M, et al. Pathogenic role of immune response to M3 muscarinic acetylcholine receptor in sjögren’s syndrome-like sialoadenitis. J Autoimmun (2010) 35(4):383–9. doi: 10.1016/j.jaut.2010.08.004
9. van Woerkom JM, Kruize AA, Wenting-van Wijk MJ, Knol E, Bihari IC, Jacobs JW, et al. Salivary gland and peripheral blood T helper 1 and 2 cell activity in sjogren’s syndrome compared with non-sjogren’s sicca syndrome. Ann Rheum Dis (2005) 64(10):1474–9. doi: 10.1136/ard.2004.031781
10. Kohriyama K, Katayama Y. Disproportion of helper T cell subsets in peripheral blood of patients with primary sjögren’s syndrome. Autoimmunity (2000) 32(1):67–72. doi: 10.3109/08916930008995989
11. Sudzius G, Mieliauskaite D, Butrimiene I, Siaurys A, Mackiewicz Z, Dumalakiene I. Activity of T-helper cells in patients with primary sjogren’s syndrome. In Vivo (2013) 27(2):263–8.
12. Voigt A, Bohn K, Sukumaran S, Stewart CM, Bhattacharya I, Nguyen CQ. Unique glandular ex-vivo Th1 and Th17 receptor motifs in sjogren’s syndrome patients using single-cell analysis. Clin Immunol (2018) 192:58–67. doi: 10.1016/j.clim.2018.04.009
13. Verstappen GM, Corneth OBJ, Bootsma H, Kroese FGM. Th17 cells in primary sjogren’s syndrome: Pathogenicity and plasticity. J Autoimmun (2018) 87:16–25. doi: 10.1016/j.jaut.2017.11.003
14. Kwok SK, Cho ML, Her YM, Oh HJ, Park MK, Lee SY, et al. Tlr2 ligation induces the production of il-23/Il-17 Via il-6, Stat3 and nf-kb pathway in patients with primary sjogren’s syndrome. Arthritis Res Ther (2012) 14(2):R64. doi: 10.1186/ar3780
15. Liu MF, Lin LH, Weng CT, Weng MY. Decreased Cd4+Cd25+Bright T cells in peripheral blood of patients with primary sjogren’s syndrome. Lupus (2008) 17(1):34–9. doi: 10.1177/0961203307085248
16. Furuzawa-Carballeda J, Hernández-Molina G, Lima G, Rivera-Vicencio Y, Férez-Blando K, Llorente L. Peripheral regulatory cells immunophenotyping in primary sjögren’s syndrome: A cross-sectional study. Arthritis Res Ther (2013) 15(3):R68. doi: 10.1186/ar4245
17. Rios-Rios WJ, Sosa-Luis SA, Torres-Aguilar H. T Cells subsets in the immunopathology and treatment of sjogren’s syndrome. Biomolecules (2020) 10(11). doi: 10.3390/biom10111539
18. Verstappen GM, Kroese FGM, Bootsma H. T Cells in primary sjogren’s syndrome: Targets for early intervention. Rheumatol (Oxford) (2019) 60(7):3088–98. doi: 10.1093/rheumatology/kez004
19. Szabo K, Jambor I, Szanto A, Horvath IF, Tarr T, Nakken B, et al. The imbalance of circulating follicular T helper cell subsets in primary sjogren’s syndrome associates with serological alterations and abnormal b-cell distribution. Front Immunol (2021) 12:639975. doi: 10.3389/fimmu.2021.639975
20. Ivanchenko M, Aqrawi LA, Björk A, Wahren-Herlenius M, Chemin K. Foxp3(+) Cxcr5(+) Cd4(+) T cell frequencies are increased in peripheral blood of patients with primary sjögren’s syndrome. Clin Exp Immunol (2019) 195(3):305–9. doi: 10.1111/cei.13244
21. Kim JW, Lee J, Hong SM, Lee J, Cho ML, Park SH. Circulating Ccr7(Lo)Pd-1(Hi) follicular helper T cells indicate disease activity and glandular inflammation in patients with primary sjogren’s syndrome. Immune Netw (2019) 19(4):e26. doi: 10.4110/in.2019.19.e26
22. Li XY, Wu ZB, Ding J, Zheng ZH, Li XY, Chen LN, et al. Role of the frequency of blood Cd4(+) Cxcr5(+) Ccr6(+) T cells in autoimmunity in patients with sjogren’s syndrome. Biochem Biophys Res Commun (2012) 422(2):238–44. doi: 10.1016/j.bbrc.2012.04.133
23. Kasashima S, Kawashima A, Kurose N, Ozaki S, Ikeda H, Harada KI. Regional disturbance of the distribution of T regulatory cells and T helper cells associated with irregular-shaped germinal centers in immunoglobulin G4-related sialadenitis. Virchows Arch (2021) 479(6):1221–32. doi: 10.1007/s00428-021-03187-2
24. Fonseca VR, Graca L. Contribution of Foxp3(+) tfr cells to overall human blood Cxcr5(+) T cells. Clin Exp Immunol (2019) 195(3):302–4. doi: 10.1111/cei.13245
25. Mitsias DI, Tzioufas AG, Veiopoulou C, Zintzaras E, Tassios IK, Kogopoulou O, et al. The Th1/Th2 cytokine balance changes with the progress of the immunopathological lesion of sjogren’s syndrome. Clin Exp Immunol (2002) 128(3):562–8. doi: 10.1046/j.1365-2249.2002.01869.x
26. Saito M, Otsuka K, Ushio A, Yamada A, Arakaki R, Kudo Y, et al. Unique phenotypes and functions of follicular helper T cells and regulatory T cells in sjogren’s syndrome. Curr Rheumatol Rev (2018) 14(3):239–45. doi: 10.2174/1573397113666170125122858
27. Alunno A, Nocentini G, Bistoni O, Petrillo MG, Bartoloni Bocci E, Ronchetti S, et al. Expansion of Cd4+Cd25-gitr+ regulatory T-cell subset in the peripheral blood of patients with primary sjögren’s syndrome: Correlation with disease activity. Reumatismo (2012) 64(5):293–8. doi: 10.4081/reumatismo.2012.293
28. Jin L, Yu D, Li X, Yu N, Li X, Wang Y, et al. Cd4+Cxcr5+ follicular helper T cells in salivary gland promote b cells maturation in patients with primary sjogren’s syndrome. Int J Clin Exp Pathol (2014) 7(5):1988–96. doi: 10.1002/(SICI)1521-4141(199810)28:10<3336::AID-IMMU3336>3.0.CO;2-R
29. Hwang SH, Woo JS, Moon J, Yang S, Park JS, Lee J, et al. Il-17 and Ccr9(+)A4β7(-) Th17 cells promote salivary gland inflammation, dysfunction, and cell death in sjögren’s syndrome. Front Immunol (2021) 12:721453. doi: 10.3389/fimmu.2021.721453
30. Yanagi K, Ishimaru N, Haneji N, Saegusa K, Saito I, Hayashi Y. Anti-120-Kda alpha-fodrin immune response with Th1-cytokine profile in the nod mouse model of sjögren’s syndrome. Eur J Immunol (1998) 28(10):3336–45. doi: 10.1002/(sici)1521-4141(199810)28:10<3336::Aid-immu3336>3.0.Co;2-r
31. Hayashi T, Shimoyama N, Mizuno T. Destruction of salivary and lacrimal glands by Th1-polarized reaction in a model of secondary sjogren’s syndrome in lupus-prone female nzb X Nzwf(1) mice. Inflammation (2012) 35(2):638–46. doi: 10.1007/s10753-011-9356-y
32. Park JS, Kim SM, Choi J, Jung KA, Hwang SH, Yang S, et al. Interleukin-21-Mediated suppression of the Pax3-Id3 pathway exacerbates the development of sjogren’s syndrome Via follicular helper T cells. Cytokine (2020) 125:154834. doi: 10.1016/j.cyto.2019.154834
33. Fonseca VR, Romao VC, Agua-Doce A, Santos M, Lopez-Presa D, Ferreira AC, et al. The ratio of blood T follicular regulatory cells to T follicular helper cells marks ectopic lymphoid structure formation while activated follicular helper T cells indicate disease activity in primary sjogren’s syndrome. Arthritis Rheumatol (2018) 70(5):774–84. doi: 10.1002/art.40424
34. Fu W, Liu X, Lin X, Feng H, Sun L, Li S, et al. Deficiency in T follicular regulatory cells promotes autoimmunity. J Exp Med (2018) 215(3):815–25. doi: 10.1084/jem.20170901
35. Konttinen YT, Kemppinen P, Koski H, Li TF, Jumppanen M, Hietanen J, et al. T(H)1 cytokines are produced in labial salivary glands in sjögren’s syndrome, but also in healthy individuals. Scand J Rheumatol (1999) 28(2):106–12. doi: 10.1080/030097499442577
36. de Souza TR, de Albuquerque Tavares Carvalho A, Duarte AP, Porter SR, Leao JC, Gueiros LA. Th1 and Th2 polymorphisms in sjogren’s syndrome and rheumatoid arthritis. J Oral Pathol Med (2014) 43(6):418–26. doi: 10.1111/jop.12149
37. Singh N, Cohen PL. The T cell in sjogren’s syndrome: Force majeure, not spectateur. J Autoimmun (2012) 39(3):229–33. doi: 10.1016/j.jaut.2012.05.019
38. Mustafa W, Zhu J, Deng G, Diab A, Link H, Frithiof L, et al. Augmented levels of macrophage and Th1 cell-related cytokine mrna in submandibular glands of Mrl/Lpr mice with autoimmune sialoadenitis. Clin Exp Immunol (1998) 112(3):389–96. doi: 10.1046/j.1365-2249.1998.00609.x
39. Maier-Moore JS, Horton CG, Mathews SA, Confer AW, Lawrence C, Pan Z, et al. Interleukin-6 deficiency corrects nephritis, lymphocyte abnormalities, and secondary sjogren’s syndrome features in lupus-prone Sle1.Yaa mice. Arthritis Rheumatol (2014) 66(9):2521–31. doi: 10.1002/art.38716
40. Ciecko AE, Foda B, Barr JY, Ramanathan S, Atkinson MA, Serreze DV, et al. Interleukin-27 is essential for type 1 diabetes development and sjogren syndrome-like inflammation. Cell Rep (2019) 29(10):3073–86 e5. doi: 10.1016/j.celrep.2019.11.010
41. Wu GL, Li TY, Fan YS, Yu GY, Chen J. Effect of Chinese herbal medicine for nourishing yin, supplementing qi, and activating blood on the Th1/Th2 immune balance in peripheral blood in patients with primary sjogren’s syndrome. Chin J Integr Med (2013) 19(9):696–700. doi: 10.1007/s11655-013-1565-9
42. Pertovaara M, Antonen J, Hurme M. Th2 cytokine genotypes are associated with a milder form of primary sjogren’s syndrome. Ann Rheum Dis (2006) 65(5):666–70. doi: 10.1136/ard.2005.040956
43. Kudo-Tanaka E, Nakatsuka S, Hirano T, Kawai M, Katada Y, Matsushita M, et al. A case of mikulicz’s disease with Th2-biased cytokine profile: Possible feature discriminable from sjogren’s syndrome. Mod Rheumatol (2009) 19(6):691–5. doi: 10.1007/s10165-009-0214-9
44. Fei Y, Zhang W, Lin D, Wu C, Li M, Zhao Y, et al. Clinical parameter and Th17 related to lymphocytes infiltrating degree of labial salivary gland in primary sjogren’s syndrome. Clin Rheumatol (2014) 33(4):523–9. doi: 10.1007/s10067-013-2476-z
45. Verstappen GM, Kroese FGM, Meiners PM, Corneth OB, Huitema MG, Haacke EA, et al. B cell depletion therapy normalizes circulating follicular Th cells in primary sjögren syndrome. J Rheumatol (2017) 44(1):49–58. doi: 10.3899/jrheum.160313
46. Voigt A, Esfandiary L, Wanchoo A, Glenton P, Donate A, Craft WF, et al. Sexual dimorphic function of il-17 in salivary gland dysfunction of the C57bl/6.Nod-Aec1aec2 model of sjögren’s syndrome. Sci Rep (2016) 6:38717. doi: 10.1038/srep38717
47. Nanke Y, Kobashigawa T, Yago T, Kawamoto M, Yamanaka H, Kotake S. Detection of ifn-Γ+Il-17+ cells in salivary glands of patients with sjögren’s syndrome and mikulicz’s disease: Potential role of Th17•Th1 in the pathogenesis of autoimmune diseases. Nihon Rinsho Meneki Gakkai Kaishi (2016) 39(5):473–7. doi: 10.2177/jsci.39.473
48. Alunno A, Carubbi F, Bistoni O, Caterbi S, Bartoloni E, Mirabelli G, et al. T Regulatory and T helper 17 cells in primary sjögren’s syndrome: Facts and perspectives. Mediators Inflammation (2015) 2015:243723. doi: 10.1155/2015/243723
49. Nguyen CQ, Yin H, Lee BH, Carcamo WC, Chiorini JA, Peck AB. Pathogenic effect of interleukin-17a in induction of sjögren’s syndrome-like disease using adenovirus-mediated gene transfer. Arthritis Res Ther (2010) 12(6):R220. doi: 10.1186/ar3207
50. Lin X, Rui K, Deng J, Tian J, Wang X, Wang S, et al. Th17 cells play a critical role in the development of experimental sjogren’s syndrome. Ann Rheum Dis (2015) 74(6):1302–10. doi: 10.1136/annrheumdis-2013-204584
51. Alunno A, Petrillo MG, Nocentini G, Bistoni O, Bartoloni E, Caterbi S, et al. Characterization of a new regulatory Cd4+ T cell subset in primary sjogren’s syndrome. Rheumatol (Oxford) (2013) 52(8):1387–96. doi: 10.1093/rheumatology/ket179
52. Coursey TG, Bian F, Zaheer M, Pflugfelder SC, Volpe EA, de Paiva CS. Age-related spontaneous lacrimal keratoconjunctivitis is accompanied by dysfunctional T regulatory cells. Mucosal Immunol (2017) 10(3):743–56. doi: 10.1038/mi.2016.83
53. Lieberman SM, Kreiger PA, Koretzky GA. Reversible lacrimal gland-protective regulatory T-cell dysfunction underlies Male-specific autoimmune dacryoadenitis in the non-obese diabetic mouse model of sjogren syndrome. Immunology (2015) 145(2):232–41. doi: 10.1111/imm.12439
54. Otsuka K, Yamada A, Saito M, Ushio A, Sato M, Kisoda S, et al. Achaete-scute homologue 2-regulated follicular helper T cells promote autoimmunity in a murine model for sjogren syndrome. Am J Pathol (2019) 189(12):2414–27. doi: 10.1016/j.ajpath.2019.08.008
55. Hinrichs AC, Blokland SLM, Lopes AP, Wichers CGK, Kruize AA, Pandit A, et al. Transcriptome analysis of Ccr9+ T helper cells from primary sjogren’s syndrome patients identifies Ccl5 as a novel effector molecule. Front Immunol (2021) 12:702733. doi: 10.3389/fimmu.2021.702733
56. Ji LS, Sun XH, Zhang X, Zhou ZH, Yu Z, Zhu XJ, et al. Mechanism of follicular helper T cell differentiation regulated by transcription factors. J Immunol Res (2020) 2020:1826587. doi: 10.1155/2020/1826587
57. Chen W, Yang F, Xu G, Ma J, Lin J. Follicular helper T cells and follicular regulatory T cells in the immunopathology of primary sjogren’s syndrome. J Leukoc Biol (2021) 109(2):437–47. doi: 10.1002/JLB.5MR1020-057RR
58. Kurata I, Matsumoto I, Sumida T. T Follicular helper cell subsets: A potential key player in autoimmunity. Immunol Med (2021) 44(1):1–9. doi: 10.1080/25785826.2020.1776079
59. Fonseca VR, Agua-Doce A, Maceiras AR, Pierson W, Ribeiro F, Romão VC, et al. Human blood T(Fr) cells are indicators of ongoing humoral activity not fully licensed with suppressive function. Sci Immunol (2017) 2(14). doi: 10.1126/sciimmunol.aan1487
60. Verstappen GM, Nakshbandi U, Mossel E, Haacke EA, van der Vegt B, Vissink A, et al. Is the T follicular regulatory : Follicular helper T cell ratio in blood a biomarker for ectopic lymphoid structure formation in sjogren’s syndrome? comment on the article by fonseca et al. Arthritis Rheumatol (2018) 70(8):1354–5. doi: 10.1002/art.40488
61. Chen J, Guan L, Tang L, Liu S, Zhou Y, Chen C, et al. T Helper 9 cells: A new player in immune-related diseases. DNA Cell Biol (2019) 38(10):1040–7. doi: 10.1089/dna.2019.4729
62. Heim J, Almanzar G, Schmalzing M, Gernert M, Tony HP, Prelog M. Induction of il-9 in peripheral lymphocytes of rheumatoid arthritis patients and healthy donors by Th17-inducing cytokine conditions. Front Immunol (2021) 12:668095. doi: 10.3389/fimmu.2021.668095
63. Chowdhury K, Kumar U, Das S, Chaudhuri J, Kumar P, Kanjilal M, et al. Synovial il-9 facilitates neutrophil survival, function and differentiation of Th17 cells in rheumatoid arthritis. Arthritis Res Ther (2018) 20(1):18. doi: 10.1186/s13075-017-1505-8
64. Talotta R, Berzi A, Doria A, Batticciotto A, Ditto MC, Atzeni F, et al. The immunogenicity of branded and biosimilar infliximab in rheumatoid arthritis according to Th9-related responses. Int J Mol Sci (2017) 18(10). doi: 10.3390/ijms18102127
65. Talotta R, Berzi A, Atzeni F, Dell’Acqua D, Sarzi Puttini P, Trabattoni D. Evaluation of Th9 lymphocytes in peripheral blood of rheumatoid arthritis patients and correlation with anti-tumor necrosis factor therapy: Results from an in vitro pivotal study. Reumatismo (2016) 68(2):83–9. doi: 10.4081/reumatismo.2016.875
66. Ciccia F, Guggino G, Ferrante A, Cipriani P, Giacomelli R, Triolo G. Interleukin-9 and T helper type 9 cells in rheumatic diseases. Clin Exp Immunol (2016) 185(2):125–32. doi: 10.1111/cei.12807
67. Guggino G, Lo Pizzo M, Di Liberto D, Rizzo A, Cipriani P, Ruscitti P, et al. Interleukin-9 over-expression and T helper 9 polarization in systemic sclerosis patients. Clin Exp Immunol (2017) 190(2):208–16. doi: 10.1111/cei.13009
68. Jiang Q, Yang G, Xiao F, Xie J, Wang S, Lu L, et al. Role of Th22 cells in the pathogenesis of autoimmune diseases. Front Immunol (2021) 12:688066. doi: 10.3389/fimmu.2021.688066
69. Miyazaki Y, Nakayamada S, Kubo S, Nakano K, Iwata S, Miyagawa I, et al. Th22 cells promote osteoclast differentiation Via production of il-22 in rheumatoid arthritis. Front Immunol (2018) 9:2901. doi: 10.3389/fimmu.2018.02901
70. Hossein-Khannazer N, Zian Z, Bakkach J, Kamali AN, Hosseinzadeh R, Anka AU, et al. Features and roles of T helper 22 cells in immunological diseases and malignancies. Scand J Immunol (2021) 93(5):e13030. doi: 10.1111/sji.13030
71. Ye Z, Zhao L, Gao Q, Jiang Y, Jiang Z, Chu CQ. Analysis of il-22 and Th22 cells by flow cytometry in systemic lupus erythematosus. Methods Mol Biol (2020) 2108:29–42. doi: 10.1007/978-1-0716-0247-8_3
72. Lee V, Zayat A, Wakefield RJ. The effect of joint position on Doppler flow in finger synovitis. Ann Rheum Dis (2009) 68(4):603–4. doi: 10.1136/ard.2008.089755
73. Ciccia F, Guggino G, Rizzo A, Ferrante A, Raimondo S, Giardina A, et al. Correction: Potential involvement of il-22 and il-22-Producing cells in the inflamed salivary glands of patients with sjogren’s syndrome. Ann Rheum Dis (2021) 80(5):e81. doi: 10.1136/ard.2011.154013.corr1
74. Lavoie TN, Stewart CM, Berg KM, Li Y, Nguyen CQ. Expression of interleukin-22 in sjögren’s syndrome: Significant correlation with disease parameters. Scand J Immunol (2011) 74(4):377–82. doi: 10.1111/j.1365-3083.2011.02583.x
75. Narkeviciute I, Sudzius G, Mieliauskaite D, Mackiewicz Z, Butrimiene I, Viliene R, et al. Are cytotoxic effector cells changes in peripheral blood of patients with sjögren’s syndrome related to persistent virus infection: Suggestions and conundrums. Cell Immunol (2016) 310:123–30. doi: 10.1016/j.cellimm.2016.08.013
76. Zhou H, Yang J, Tian J, Wang S. Cd8(+) T lymphocytes: Crucial players in sjögren’s syndrome. Front Immunol (2020) 11:602823. doi: 10.3389/fimmu.2020.602823
77. Zhai X, Wang Y, Guo H, Liang Z, Feng M, Wu Y, et al. Altered levels of circulating Cd8(+)Cxcr5(+)Pd-1(+)T follicular cytotoxic cells in primary sjögren’s syndrome. Clin Rheumatol (2022) 41(6):1697–708. doi: 10.1007/s10067-022-06098-y
78. Barr JY, Wang X, Meyerholz DK, Lieberman SM. Cd8 T cells contribute to lacrimal gland pathology in the nonobese diabetic mouse model of sjögren syndrome. Immunol Cell Biol (2017) 95(8):684–94. doi: 10.1038/icb.2017.38
79. Wang S, Shen H, Bai B, Wu J, Wang J. Increased Cd4(+)Cd8(+) double-positive T cell in patients with primary sjögren’s syndrome correlated with disease activity. J Immunol Res (2021) 2021:6658324. doi: 10.1155/2021/6658324
80. Ferraccioli GF, Tonutti E, Casatta L, Pegoraro I, De Vita S, Sala P, et al. Cd4 cytopenia and occasional expansion of Cd4+Cd8+Lymphocytes in sjögren’s syndrome. Clin Exp Rheumatol (1996) 14(2):125–30.
81. Gao CY, Yao Y, Li L, Yang SH, Chu H, Tsuneyama K, et al. Tissue-resident memory Cd8+ T cells acting as mediators of salivary gland damage in a murine model of sjögren’s syndrome. Arthritis Rheumatol (2019) 71(1):121–32. doi: 10.1002/art.40676
82. Izumi Y, Ida H, Huang M, Iwanaga N, Tanaka F, Aratake K, et al. Characterization of peripheral natural killer cells in primary sjögren’s syndrome: Impaired nk cell activity and low nk cell number. J Lab Clin Med (2006) 147(5):242–9. doi: 10.1016/j.lab.2006.01.001
83. Deshmukh US, Bagavant H. When killers become helpers. Sci Transl Med (2013) 5(195):195fs29. doi: 10.1126/scitranslmed.3006850
84. Gianchecchi E, Delfino DV, Fierabracci A. Natural killer cells: Potential biomarkers and therapeutic target in autoimmune diseases? Front Immunol (2021) 12:616853. doi: 10.3389/fimmu.2021.616853
85. Miyasaka N, Seaman W, Bakshi A, Sauvezie B, Strand V, Pope R, et al. Natural killing activity in sjögren’s syndrome. Anal Defective Mech Arthritis Rheum (1983) 26(8):954–60. doi: 10.1002/art.1780260803
86. Ming B, Wu T, Cai S, Hu P, Tang J, Zheng F, et al. The increased ratio of blood Cd56(Bright) nk to Cd56(Dim) nk is a distinguishing feature of primary sjogren’s syndrome. J Immunol Res (2020) 2020:7523914. doi: 10.1155/2020/7523914
87. Wenink MH, Leijten EFA, Cupedo T, Radstake T. Review: Innate lymphoid cells: Sparking inflammatory rheumatic disease? Arthritis Rheumatol (2017) 69(5):885–97. doi: 10.1002/art.40068
88. Rusakiewicz S, Nocturne G, Lazure T, Semeraro M, Flament C, Caillat-Zucman S, et al. Ncr3/Nkp30 contributes to pathogenesis in primary sjogren’s syndrome. Sci Transl Med (2013) 5(195):195ra96. doi: 10.1126/scitranslmed.3005727
89. Rizzo C, La Barbera L, Lo Pizzo M, Ciccia F, Sireci G, Guggino G. Invariant nkt cells and rheumatic disease: Focus on primary sjogren syndrome. Int J Mol Sci (2019) 20(21). doi: 10.3390/ijms20215435
90. Guggino G, Ciccia F, Raimondo S, Giardina G, Alessandro R, Dieli F, et al. Invariant nkt cells are expanded in peripheral blood but are undetectable in salivary glands of patients with primary sjögren’s syndrome. Clin Exp Rheumatol (2016) 34(1):25–31.
91. Sudzius G, Mieliauskaite D, Siaurys A, Viliene R, Butrimiene I, Characiejus D, et al. Distribution of peripheral lymphocyte populations in primary sjögren’s syndrome patients. J Immunol Res (2015) 2015:854706. doi: 10.1155/2015/854706
92. Davies R, Hammenfors D, Bergum B, Jakobsen K, Solheim M, Vogelsang P, et al. Patients with primary sjögren’s syndrome have alterations in absolute quantities of specific peripheral leucocyte populations. Scand J Immunol (2017) 86(6):491–502. doi: 10.1111/sji.12622
93. Zhou X, Li Q, Li Y, Fu J, Sun F, Li Y, et al. Diminished natural killer T-like cells correlates with aggravated primary sjögren’s syndrome. Clin Rheumatol (2022) 41(4):1163–8. doi: 10.1007/s10067-021-06011-z
94. Pontarini E, Sciacca E, Grigoriadou S, Rivellese F, Lucchesi D, Fossati-Jimack L, et al. Nkp30 receptor upregulation in salivary glands of sjögren’s syndrome characterizes ectopic lymphoid structures and is restricted by rituximab treatment. Front Immunol (2021) 12:706737. doi: 10.3389/fimmu.2021.706737
95. Szodoray P, Papp G, Horvath IF, Barath S, Sipka S, Nakken B, et al. Cells with regulatory function of the innate and adaptive immune system in primary sjögren’s syndrome. Clin Exp Immunol (2009) 157(3):343–9. doi: 10.1111/j.1365-2249.2009.03966.x
96. Szodoray P, Gal I, Barath S, Aleksza M, Horvath IF, Gergely P Jr., et al. Immunological alterations in newly diagnosed primary sjögren’s syndrome characterized by skewed peripheral T-cell subsets and inflammatory cytokines. Scand J Rheumatol (2008) 37(3):205–12. doi: 10.1080/03009740801910361
97. Roescher N, Lodde BM, Vosters JL, Tak PP, Catalan MA, Illei GG, et al. Temporal changes in salivary glands of non-obese diabetic mice as a model for sjögren’s syndrome. Oral Dis (2012) 18(1):96–106. doi: 10.1111/j.1601-0825.2011.01852.x
98. Lu H, Li DJ, Jin LP. Γδt cells and related diseases. Am J Reprod Immunol (2016) 75(6):609–18. doi: 10.1111/aji.12495
99. Wu YL, Ding YP, Tanaka Y, Shen LW, Wei CH, Minato N, et al. Γδ T cells and their potential for immunotherapy. Int J Biol Sci (2014) 10(2):119–35. doi: 10.7150/ijbs.7823
100. Laggner U, Di Meglio P, Perera GK, Hundhausen C, Lacy KE, Ali N, et al. Identification of a novel proinflammatory human skin-homing Vγ9vδ2 T cell subset with a potential role in psoriasis. J Immunol (2011) 187(5):2783–93. doi: 10.4049/jimmunol.1100804
101. Joachims ML, Leehan KM, Dozmorov MG, Georgescu C, Pan Z, Lawrence C, et al. Sjogren’s syndrome minor salivary gland Cd4(+) memory T cells associate with glandular disease features and have a germinal center T follicular helper transcriptional profile. J Clin Med (2020) 9(7). doi: 10.3390/jcm9072164
102. Brito-Zeron P, Baldini C, Bootsma H, Bowman SJ, Jonsson R, Mariette X, et al. Sjogren syndrome. Nat Rev Dis Primers (2016) 2:16047. doi: 10.1038/nrdp.2016.47
103. Edner NM, Carlesso G, Rush JS, Walker LSK. Targeting Co-stimulatory molecules in autoimmune disease. Nat Rev Drug Discovery (2020) 19(12):860–83. doi: 10.1038/s41573-020-0081-9
104. Ceeraz S, Nowak EC, Burns CM, Noelle RJ. Immune checkpoint receptors in regulating immune reactivity in rheumatic disease. Arthritis Res Ther (2014) 16(5):469. doi: 10.1186/s13075-014-0469-1
105. Warner BM, Baer AN, Lipson EJ, Allen C, Hinrichs C, Rajan A, et al. Sicca syndrome associated with immune checkpoint inhibitor therapy. Oncologist (2019) 24(9):1259–69. doi: 10.1634/theoncologist.2018-0823
106. Hebbar M, Jeannin P, Magistrelli G, Hatron PY, Hachulla E, Devulder B, et al. Detection of circulating soluble Cd28 in patients with systemic lupus erythematosus, primary sjögren’s syndrome and systemic sclerosis. Clin Exp Immunol (2004) 136(2):388–92. doi: 10.1111/j.1365-2249.2004.02427.x
107. Zamani MR, Aslani S, Salmaninejad A, Javan MR, Rezaei N. Pd-1/Pd-L and autoimmunity: A growing relationship. Cell Immunol (2016) 310:27–41. doi: 10.1016/j.cellimm.2016.09.009
108. Ren Y, Cui G, Gao Y. Research progress on inflammatory mechanism of primary sjögren syndrome. Zhejiang Da Xue Xue Bao Yi Xue Ban (2021) 50(6):783–94. doi: 10.3724/zdxbyxb-2021-0072
109. Deng C, Chen Y, Li W, Peng L, Luo X, Peng Y, et al. Alteration of Cd226/Tigit immune checkpoint on T cells in the pathogenesis of primary sjogren’s syndrome. J Autoimmun (2020) 113:102485. doi: 10.1016/j.jaut.2020.102485
110. Mavragani CP, Moutsopoulos HM. Sicca syndrome following immune checkpoint inhibition. Clin Immunol (2020) 217:108497. doi: 10.1016/j.clim.2020.108497
111. Pringle S, van der Vegt B, Wang X, van Bakelen N, Hiltermann TJN, Spijkervet FKL, et al. Lack of conventional acinar cells in parotid salivary gland of patient taking an anti-Pd-L1 immune checkpoint inhibitor. Front Oncol (2020) 10:420. doi: 10.3389/fonc.2020.00420
112. Sumida T, Matsumoto I, Maeda T, Nishioka K. T-Cell receptor in sjögren’s syndrome. Br J Rheumatol (1997) 36(6):622–9. doi: 10.1093/rheumatology/36.6.622
113. Joachims ML, Leehan KM, Lawrence C, Pelikan RC, Moore JS, Pan Z, et al. Single-cell analysis of glandular T cell receptors in sjogren’s syndrome. JCI Insight (2016) 1(8). doi: 10.1172/jci.insight.85609
114. Karabiyik A, Peck AB, Nguyen CQ. The important role of T cells and receptor expression in sjogren’s syndrome. Scand J Immunol (2013) 78(2):157–66. doi: 10.1111/sji.12079
115. Hjelmervik TO, Petersen K, Jonassen I, Jonsson R, Bolstad AI. Gene expression profiling of minor salivary glands clearly distinguishes primary sjögren’s syndrome patients from healthy control subjects. Arthritis Rheum (2005) 52(5):1534–44. doi: 10.1002/art.21006
116. Hong X, Meng S, Tang D, Wang T, Ding L, Yu H, et al. Single-cell rna sequencing reveals the expansion of cytotoxic Cd4(+) T lymphocytes and a landscape of immune cells in primary sjögren’s syndrome. Front Immunol (2020) 11:594658. doi: 10.3389/fimmu.2020.594658
117. Horeth E, Oyelakin A, Song EC, Che M, Bard J, Min S, et al. Transcriptomic and single-cell analysis reveals regulatory networks and cellular heterogeneity in mouse primary sjögren’s syndrome salivary glands. Front Immunol (2021) 12:729040. doi: 10.3389/fimmu.2021.729040
118. Tsuboi H, Toko H, Honda F, Abe S, Takahashi H, Yagishita M, et al. Abatacept ameliorates both glandular and extraglandular involvements in patients with sjögren’s syndrome associated with rheumatoid arthritis: Findings from an open-label, multicenter, 1-year, prospective study: The rose (Rheumatoid arthritis with orencia trial toward sjögren’s syndrome endocrinopathy) and rose ii trials. Mod Rheumatol (2022). doi: 10.1093/mr/roac011
119. Baer AN, Gottenberg JE, St Clair EW, Sumida T, Takeuchi T, Seror R, et al. Efficacy and safety of abatacept in active primary sjögren’s syndrome: Results of a phase iii, randomised, placebo-controlled trial. Ann Rheum Dis (2020) 80(3):339–48. doi: 10.1136/annrheumdis-2020-218599
120. Liew SH, Nichols KK, Klamerus KJ, Li JZ, Zhang M, Foulks GN. Tofacitinib (Cp-690,550), a janus kinase inhibitor for dry eye disease: Results from a phase 1/2 trial. Ophthalmology (2012) 119(7):1328–35. doi: 10.1016/j.ophtha.2012.01.028
121. Liu Y, Li C, Wang S, Guo J, Guo J, Fu J, et al. Human umbilical cord mesenchymal stem cells confer potent immunosuppressive effects in sjögren’s syndrome by inducing regulatory T cells. Mod Rheumatol (2021) 31(1):186–96. doi: 10.1080/14397595.2019.1707996
122. Price E, Bombardieri M, Kivitz A, Matzkies F, Gurtovaya O, Pechonkina A, et al. Safety and efficacy of filgotinib, lanraplenib, and tirabrutinib in Sjögren’s syndrome: Randomised, phase 2, double-blind, placebo-controlled study. Rheumatol (Oxford) (2022). doi: 10.1093/rheumatology/keac167
123. Rigby MR, DiMeglio LA, Rendell MS, Felner EI, Dostou JM, Gitelman SE, et al. Targeting of memory T cells with alefacept in new-onset type 1 diabetes (T1dal study): 12 month results of a randomised, double-blind, placebo-controlled phase 2 trial. Lancet Diabetes Endocrinol (2013) 1(4):284–94. doi: 10.1016/s2213-8587(13)70111-6
124. Cortes-Troncoso J, Jang SI, Perez P, Hidalgo J, Ikeuchi T, Greenwell-Wild T, et al. T Cell exosome-derived mir-142-3p impairs glandular cell function in sjögren’s syndrome. JCI Insight (2020) 5(9). doi: 10.1172/jci.insight.133497
125. Xu J, Wang D, Liu D, Fan Z, Zhang H, Liu O, et al. Allogeneic mesenchymal stem cell treatment alleviates experimental and clinical sjögren syndrome. Blood (2012) 120(15):3142–51. doi: 10.1182/blood-2011-11-391144
126. Killian M, Colaone F, Haumont P, Nicco C, Cerles O, Chouzenoux S, et al. Therapeutic potential of anti-interferon A vaccination on sjs-related features in the Mrl/Lpr autoimmune mouse model. Front Immunol (2021) 12:666134. doi: 10.3389/fimmu.2021.666134
127. Betül Türkoğlu E, Tuna S, Alan S, İhsan Arman M, Tuna Y, Ünal M. Effect of systemic infliximab therapy in patients with sjögren’s syndrome. Turk J Ophthalmol (2015) 45(4):138–41. doi: 10.4274/tjo.48379
128. Zandbelt MM, de Wilde P, van Damme P, Hoyng CB, van de Putte L, van den Hoogen F. Etanercept in the treatment of patients with primary sjögren’s syndrome: A pilot study. J Rheumatol (2004) 31(1):96–101.
129. Felten R, Devauchelle-Pensec V, Seror R, Duffau P, Saadoun D, Hachulla E, et al. Interleukin 6 receptor inhibition in primary sjögren syndrome: A multicentre double-blind randomised placebo-controlled trial. Ann Rheum Dis (2020) 80(3):329–38. doi: 10.1136/annrheumdis-2020-218467
130. Miao M, Hao Z, Guo Y, Zhang X, Zhang S, Luo J, et al. Short-term and low-dose il-2 therapy restores the Th17/Treg balance in the peripheral blood of patients with primary sjögren’s syndrome. Ann Rheum Dis (2018) 77(12):1838–40. doi: 10.1136/annrheumdis-2018-213036
131. Chen YT, Nikulina K, Lazarev S, Bahrami AF, Noble LB, Gallup M, et al. Interleukin-1 as a phenotypic immunomodulator in keratinizing squamous metaplasia of the ocular surface in sjögren’s syndrome. Am J Pathol (2010) 177(3):1333–43. doi: 10.2353/ajpath.2010.100227
132. Felten R, Scher F, Sibilia J, Gottenberg JE, Arnaud L. The pipeline of targeted therapies under clinical development for primary sjögren’s syndrome: A systematic review of trials. Autoimmun Rev (2019) 18(6):576–82. doi: 10.1016/j.autrev.2018.12.008
133. Mirzaei R, Zamani F, Hajibaba M, Rasouli-Saravani A, Noroozbeygi M, Gorgani M, et al. The pathogenic, therapeutic and diagnostic role of exosomal microrna in the autoimmune diseases. J Neuroimmunol (2021) 358:577640. doi: 10.1016/j.jneuroim.2021.577640
134. Chihaby N, Orliaguet M, Le Pottier L, Pers JO, Boisramé S. Treatment of sjögren’s syndrome with mesenchymal stem cells: A systematic review. Int J Mol Sci (2021) 22(19). doi: 10.3390/ijms221910474
135. Asashima H, Tsuboi H, Takahashi H, Hirota T, Iizuka M, Kondo Y, et al. The anergy induction of M3 muscarinic acetylcholine receptor-reactive Cd4+ T cells suppresses experimental sialadenitis-like sjögren’s syndrome. Arthritis Rheumatol (2015) 67(8):2213–25. doi: 10.1002/art.39163
136. Cummins MJ, Papas A, Kammer GM, Fox PC. Treatment of primary sjögren’s syndrome with low-dose human interferon Alfa administered by the oromucosal route: Combined phase iii results. Arthritis Rheum (2003) 49(4):585–93. doi: 10.1002/art.11199
137. Hillen MR, Blokland SL, Risselada AP, Bikker A, Lauwerys BR, Kruize AA, et al. High soluble il-7 receptor expression in sjögren’s syndrome identifies patients with increased immunopathology and dryness. Ann Rheum Dis (2016) 75(9):1735–6. doi: 10.1136/annrheumdis-2016-209236
Keywords: T cells, Sjögren’s syndrome, autoimmune, cytokines, T-cell treatment
Citation: An Q, Zhao J, Zhu X, Yang B, Wu Z, Su Y, Zhang L, Xu K and Ma D (2022) Exploiting the role of T cells in the pathogenesis of Sjögren’s syndrome for therapeutic treatment. Front. Immunol. 13:995895. doi: 10.3389/fimmu.2022.995895
Received: 16 July 2022; Accepted: 17 October 2022;
Published: 28 October 2022.
Edited by:
Cuong Nguyen, University of Florida, United StatesReviewed by:
Alexandria Voigt, University of Florida, United StatesMuhammad Shahnawaz Soyfoo, Université libre de Bruxelles, Belgium
Copyright © 2022 An, Zhao, Zhu, Yang, Wu, Su, Zhang, Xu and Ma. This is an open-access article distributed under the terms of the Creative Commons Attribution License (CC BY). The use, distribution or reproduction in other forums is permitted, provided the original author(s) and the copyright owner(s) are credited and that the original publication in this journal is cited, in accordance with accepted academic practice. No use, distribution or reproduction is permitted which does not comply with these terms.
*Correspondence: Dan Ma, ZGFuZGFuODQwNTA5QDE2My5jb20=
†These authors have contributed equally to this work