- 1Institute for Medical Microbiology, Immunology and Parasitology, University Hospital Bonn, Bonn, Germany
- 2German Center for Infection Research (DZIF), Partner Site Bonn-Cologne, Bonn, Germany
Filariae are parasitic roundworms, which can cause debilitating diseases such as lymphatic filariasis and onchocerciasis. Lymphatic filariasis, also known as elephantiasis, and onchocerciasis, commonly referred to as river blindness, can lead to stigmatizing pathologies and present a socio-economic burden for affected people and their endemic countries. Filariae typically induce a type 2 immune response, which is characterized by cytokines, i.e., IL-4, IL-5 and IL-13 as well as type 2 immune cells including alternatively activated macrophages, innate lymphoid cells and Th2 cells. However, the hallmark characteristic of filarial infections is a profound eosinophilia. Eosinophils are innate immune cells and pivotal in controlling helminth infections in general and filarial infections in particular. By modulating the function of other leukocytes, eosinophils support and drive type 2 immune responses. Moreover, as primary effector cells, eosinophils can directly attack filariae through the release of granules containing toxic cationic proteins with or without extracellular DNA traps. At the same time, eosinophils can be a driving force for filarial pathology as observed during tropical pulmonary eosinophilia in lymphatic filariasis, in dermatitis in onchocerciasis patients as well as adverse events after treatment of onchocerciasis patients with diethylcarbamazine. This review summarizes the latest findings of the importance of eosinophil effector functions including the role of eosinophil-derived proteins in controlling filarial infections and their impact on filarial pathology analyzing both human and experimental animal studies.
1 Filariae
Filariasis are vector-borne diseases caused by filarial nematodes. Infection occurs via blood-feeding insects (e.g. mosquitoes) that transmit the infective third stage larvae (L3) during their blood meal. Following two moltings, adult filariae develop in the definite mammalian host, mate and start to release their progeny, the microfilariae (MF). Dependent on the filarial species, MF are mainly found in the peripheral blood or skin. Upon ingestion of the MF (L1 stage) during another blood meal of the appropriate insect vector, MF develop via two moltings into the L3 stage to complete the life-cycle (Figure 1) (1). Filariae cause several tropical diseases including onchocerciasis, lymphatic filariasis (LF), loiasis and mansonellosis. While some filariae, such as the pathogenic agents for onchocerciasis and LF, can cause severe pathology in infected patients, others like the filariae inducing loiasis and mansonellosis, lead mainly to asymptomatic infections and only occasionally cause clinical symptoms (2–4). A summary of the causative agents, the geographical distribution and estimated number of infected individuals, the associated clinical symptoms and the vectors transmitting the diseases is given in Table 1. In short, onchocerciasis, is caused by Onchocerca volvulus, and an estimated 21 million people are infected, which can lead to vision impairment or blindness (1.15 million people) as well as severe dermatitis (15 million people) (1, 5). LF is caused by Brugia malayi, Brugia timori and Wuchereria bancrofti with 51 million people infected. 17 million LF patients suffer from lymphedema in the lower extremities and 20 million men from lymphedema in the scrotum (hydrocele). Few LF patients develop tropical pulmonary eosinophilia (TPE), which is characterized by asthma-like symptoms (1, 6). Loiasis, the eye-worm, is caused by Loa loa, which can lead to edema (calabar swellings) and temporary painful sensations in the eye, which are both a results of the migrating adult filariae (4). Mansonellosis is caused by Mansonella perstans, Mansonella ozzardi and Mansonella streptocerca. M. perstans is most common with an estimated 120 million infections, although it represents one of the most neglected filarial diseases, as there are no specific symptoms associated, but unspecific symptoms such as abdominal pain, headache and subcutaneous swellings are repeatedly reported (7) (Table 1). Importantly, following treatment with MF-killing (microfilaridical) drugs, most severe adverse events can occur, such as life-threatening encephalitis in loiasis patients or severe dermatitis and blindness in onchocerciasis patients treated with diethylcarbamazine (DEC) (14, 15).
To study filariae, animal models have been extensively used and contributed to our current understanding about the protective immune responses, filarial immunomodulation and allowed to identify new drugs against filariae (16–18). Given that mice are not susceptible to human-pathogenic filariae, surrogate filarial nematode species are often used. Onchocerca ochengi is a natural parasite of cattle and is closely related to the human filarial nematode O. volvulus. Similarly to the human infection, adult worms reside in subcutaneous nodules, while MF can be found in the skin (Table 2) (21). It is regularly used to test antifilarial drugs and vaccines (22–24, 33, 34). Gerbils and immunodeficient mice also maintain O. ochengi or B. malayi filariae after intraperitoneal implantation, which allows preclinical testing of drug candidates in a small rodent model (35–37). To study LF, the B. malayi ferret or mouse model can be used, where B. malayi L3 are injected into the footpath of ferrets or hind limb of mice, leading to adult worm development in the lymphatics and lymphedema in the limb (Table 2) (27, 28, 38). Onchocerciasis-induced keratitis on the other hand is investigated in mice injected with O. volvulus antigen or MF, which leads to opacification and keratitis (25, 26, 39). To study the immune response to filariae in immunocompetent mice, the filarial Litomosoides sigmodontis model is an excellent tool, since the induced immune responses resemble those observed in human filarial infections (Table 2) (40, 41). L. sigmodontis L3 larvae are transmitted through the bite of the tropical rat mite Ornithonyssus bacoti during a blood meal; the L3 larvae migrate through the skin via the lymphatics to the pleural cavity. Gravid females start to release MF into the peripheral blood, which can be taken up again by the mites. Within the mites, the MF develop into the L3 stage (19).
Studying model organisms has advanced our knowledge on protective immunity and pathology development during filarial infections. Thus, it was demonstrated that filarial infections provoke a type 2 immune response in the host with eosinophilia being a hallmark of filarial infections. Eosinophils are the predominant cell type during filarial infection and they contribute to protection and the development of pathogenesis. Several reviews published so far, described the role of eosinophils and other granulocytes during helminth infections in general (42–44). However, they focused almost exclusively on intestinal helminths and blood flukes, while the present review summarizes the current state-of-the-art knowledge on eosinophil-mediated protection and pathology during filarial infections.
2 Eosinophils and their effector functions
2.1 Eosinophil development and activation
Filarial infections provoke a type 2 immune response in the host, which is initiated by epithelial cell-derived alarmins such as thymic stromal lymphopoietin (TSLP), Interleukin (IL)-25 and IL-33 as a response to the tissue damage caused by the multicellular parasites (45). In response, type 2-related cytokines including IL-4, IL-5, IL-9, IL-10, and IL-13 are produced (46, 47), which support the induction and expansion of innate lymphoid type 2 cells (ILC2s), eosinophils, alternatively activated macrophages (AAMs) and T helper 2 cells, as well as antibody isotypes IgG1 (mouse), IgG4 (human) and IgE (48). A hallmark of filarial infections is a significant increase of blood eosinophils (from 120/mm3 under homeostatic conditions to >450/mm3) (49, 50).
Under homeostatic conditions, eosinophils are derived from the bone marrow and migrate quickly into the tissue, primarily the gastrointestinal tract, the lung, the uterus, mammary gland tissue, as well as adipose tissue and thymus (51–54). During allergy and filarial infection eosinophil numbers significantly increase and eosinophils are recruited in high numbers to the sites of tissue repair and inflammation (53, 55). While eosinophils have been shown to be involved during bacterial and viral infections, eosinophils contribute to control helminth infections as well (51). Tissue damage caused by the migrating filariae triggers the production of the alarmin IL-33, mainly by dying epithelial and endothelial cells, adipocytes and fibroblasts (51, 56). Furthermore, the alarmin IL-25 is produced by Th2 cells, mast cells and eosinophils. Both alarmins induce IL-5 release by Th2 cells and ILC2s. IL-5, together with IL-3 and granulocyte-macrophage colony-stimulating factor (GM-CSF), drives the development of eosinophils in the bone marrow. Moreover, IL-5 is a key cytokine not only involved in the development of eosinophils but also in the priming and activation of eosinophils (57). IL-4 and IL-13, produced by Th2 cells and ILC2s, impact eosinophil recruitment as well. Both cytokines signal via the IL-4R, which is expressed among others on fibroblasts and epithelial cells, leading to the release of chemokines, which stimulate eosinophil migration. These chemokines include the eotaxins CCL11 (eotaxin 1), CCL24 (eotaxin 2) and CCL5 (RANTES) (58). Other sources of eotaxins include eosinophils, monocytes, lymphocytes, dermal fibroblasts, epithelial cells and macrophages (59–62). IL-4 and IL-13 also induce the upregulation of the adhesion molecule VCAM-1 on endothelia cells at the site of infection and thus enhance the adhesion of eosinophils and their local accumulation (57, 63, 64).
2.2 Eosinophil effector functions
Eosinophils are equipped with Fc receptors as well as pattern-recognition-receptors (PRR), which enables them to recognize pathogen-associated molecular patterns (PAMPs) and damage-associated molecular patterns (DAMPs) (51). As response to PAMPs and DAMPs, eosinophils can interact with other cells either through the expression of MHCII molecules, by releasing cytokines and chemokines or by mediating the release of their intracellular granules containing toxic proteins (51). Thus, eosinophils can interact with T cells through MHCII expression and drive their proliferation and cytokine production; they can also mediate Th2 cell recruitment through release of the chemoattractant molecules CCL22 and CCL17 (65, 66). Moreover, eosinophils drive the maturation of AAMs through the release of IL-4 and IL-13, supporting tissue repair through fibroblast recruitment and tissue remodeling (67).
A pivotal eosinophil effector mechanism is the release of their cytotoxic granules, which contain anti-microbial peptides and inflammatory mediators that support the elimination of invading pathogens and enhance the ongoing inflammation. Eosinophils store different types of secretory organelles in the cytosol including the most abundant form, the crystalloid granules, as well as primary granules, small granules and secretory vesicles. The crystalloid granules mainly contain four highly basic proteins, namely major basic protein (MBP), eosinophil cationic protein (ECP), eosinophil-derived neurotoxin (EDN) and eosinophil peroxidase (EPO), while the primary granules contain Charcot-Leyden crystal (CLC) forming proteins (51). MBP is the most highly cationic protein in eosinophil granules (68). Furthermore, the granules contain two ribonucleases A: EDN, which shows strong antiviral activity, and ECP, which has been described to form pores rather than have ribonuclease activity (69). Equivalent to the myeloid peroxidase found in neutrophils, EPO is a haloperoxidase and is associated with bacterial killing through the production of reactive oxygen species (ROS) (53). Lastly, CLC-forming proteins can be found in primary granules of human, but not murine eosinophils (70). CLC are formed upon the release of galectin-10 proteins, which accumulate and form hexagonal and bipyramidal-shaped crystals (71–73) (Box 1). CLC were described for tape worm infections, filarial diseases and other eosinophil-associated diseases such as allergies and asthma (71, 72, 80, 81).
Box 1. Eosinophil granule proteins
Crystalloid granules
Major basic protein (MBP)
• Reduces worm burden in L. sigmodontis-infected mice (74)
• Is deposited on O. volvulus MF during Mazzotti reaction (75)
• Deposit on B. malayi MF in TPE (76)
• Kills B. malayi and B. pahangi MF in vitro (77)
Eosinophil cationic protein (ECP)
• Deposit on O. volvulus MF in lymph node after diethylcarbamazine (DEC) treatment (78)
• Kills B. malayi and B. pahangi MF in vitro (77)
Eosinophil-derived neurotoxin (EDN)
• Kills B. malayi and B. pahangi MF in vitro (77)
Eosinophil peroxidase (EPO)
• Reduces worm burden in L. sigmodontis-infected mice (74)
• Is deposited on O. volvulus MF in lymph node after DEC treatment (78)
• Kills B. malayi and B. pahangi MF in vitro in combination with ROS (77)
Primary granules
Charcot-Leyden crystal (CLC) proteins
• Found in tape worm infections and filarial diseases (71)
• Contributes to eosinophil ETosis (79)
In general, the release of eosinophil granules can be mediated through several mechanisms. Following crosslinking of Fc receptors such as FcγRII, FcγRIII, FcαRI and FcϵRI by IgG1, IgG3, IgG2, IgA and IgE, antibody-dependent cellular cytotoxicity (ADCC) occurs, leading to cell degranulation, activation and/or phagocytosis. Especially during secondary filarial infections and after vaccination, eosinophil-mediated ADCC plays an important role in the killing of the filariae (29, 82, 83). Furthermore, PAMPs and DAMPs can be recognized through PRR expressed on eosinophils such as toll-like receptors (TLRs) (TLR1, 2, 3, 4, 5, 6, 7, 9 and 10) or C-type lectin receptors (CLR) (51). TLR2 and TLR6 are of particular importance, as filariae including the causative agents of LF, onchocerciasis and mansonellosis, but not loiasis, contain endosymbiotic Wolbachia bacteria, which trigger an inflammatory response through TLR2 and TLR6 recognition (8, 84). Wolbachia can also be recognized by the nucleotide-binding oligomerization domain-containing protein 2 (NOD2), which supports neutrophil recruitment to the skin and subsequent L3 larval elimination in murine filariasis (85). The binding of TLR and CLR ligands leads to the release of granules containing cytotoxic cationic proteins as well as cytokines and chemokines through exocytosis (granules fuse with the plasma membrane), piecemeal degranulation (shuttling of the granular content via secretory vesicles from the granules to the plasma membrane) and cytolysis (rupture of the plasma membrane). The latter can be associated with or without extracellular DNA traps (extracellular DNA trap cell death (ETosis)). ETosis is a form of cell death, where intracellular DNA is explosively released into the surrounding. It is distinct from apoptosis and necrosis, as DNA remains condensed and compact (86).
3 Eosinophils as protective immune cells against filariae
3.1 Direct effects of eosinophils against filariae
Eosinophilia is a hallmark of filarial infections and it was shown in humans as well as in mice that eosinophils can contribute to the protective effect against filariae (16). Elevated serum ECP and EDN levels have been observed in different helminth infections, including onchocerciasis, LF as well as schistosomiasis and are indicative of an ongoing infection (87). Furthermore, studies have shown that eosinophils respond in particular to MF and that increased eosinophil counts as well as high levels of eosinophil-derived proteins were associated with the presence of MF in onchocerciasis patients (88). Accordingly, onchocercomata, which are the nodules containing adult O. volvulus filariae, contain abundant eosinophils in the presence of MF, while nodules with male worms, dead worms and female worms without MF present significantly lower eosinophil counts. Analysis of onchocercomata with and without MF from the same individual support that this is independent of the host’s immune response (89). In contrast to onchocerciasis patients, in L. loa-infected persons, individuals native to endemic regions generally have high MF counts and low eosinophil numbers, while temporary residents show significantly higher eosinophil counts and eosinophil granule protein levels but lower MF counts (90).
Using the L. sigmodontis rodent model it was shown that eosinophil numbers increase in the pleural cavity as early as 11 days post infection and thus before adult worms and MF have developed and peak together with the highest MF counts (41, 91). The importance of eosinophils as mediator of protective immunity was demonstrated through several knockout (KO) mouse strains. Indirect evidence for the importance of eosinophils in protective immunity against filariae was obtained by experiments using CCL11/eotaxin-1 and IL-5 KO mice. While IL-5 is the most crucial factor for the development and survival of eosinophils, CCL11 is a chemokine responsible for eosinophil recruitment and activation (51). Even though wild type and eotaxin-1 KO mice had similar larval worm burdens, during patency (adult worms and MF are present) CCL11 KO mice had a significantly higher worm burden in comparison to wild type mice (92). This was accompanied with comparable eosinophil infiltration suggesting that eosinophil recruitment is still mediated in the absence of CCL11. However, CCL11 KO mice harbored eosinophils with reduced activation as shown by CD80 and CD86 expression. Thus, eosinophil activation appears to be crucial for adult worm elimination. Interestingly, only the adult worm burden and not MF numbers were affected by the lack of CCL11 (92). In contrast, mice lacking IL-5 showed reduced eosinophil counts at the site of infection as well as a higher adult worm and MF burden (16). Moreover, these mice presented prolonged microfilaremia and delayed adult worm clearance. Additionally, IL-5 appeared to be crucial for worm containment since IL-5 KO mice presented less nodule formation (93). Accordingly, IL-5 transgenic mice, which overexpress IL-5, exhibited enhanced macrophage and eosinophil attachment to the larvae as early as 10 days post infection. This resulted in increased nodule formation during patency as well as lower adult worm numbers (94). Interestingly, IL-5 overexpression accelerated larval growth during the early infection, suggesting that eosinophil-mediated protection increases developmental pressure on the larvae (94, 95). However, studies have shown that IL-5 signaling has not only an impact on eosinophils but may affect neutrophils as well (96). Thus, protection mediated by IL-5 may not be exclusively caused by eosinophils.
Direct evidence for the importance of eosinophils in mediating protection against filariae was demonstrated in studies using infections in dblGATA mice, which have IL-5 signaling but lack eosinophils. Similar to the IL-5 KO mice, dblGATA mice showed a higher worm and MF burden with an earlier onset of microfilaremia and prolonged maintenance of the infection compared to wild type controls (16). While only 50% of wild type mice became MF positive, all dblGATA mice developed peripheral blood microfilaremia.
Moreover, another study showed that eosinophils control adult B. malayi infections. While IL-4Rα KO mice were still able to generate eosinophilia and controlled the infection, mice became susceptible after depleting eosinophils through CCR3. Thus, the adult worm control was apparent even in the absence of IL-4Rα signaling, highlighting the importance of eosinophils and not AAMs in controlling adult worm burden (30).
Thus, IL-5 and eosinophils appear to significantly contribute to the clearance of chronic infections; attacking adult worms and MF.
Filariae are master modulators of the host immune response and there is indirect evidence that filariae also modulate eosinophil-mediated protection against filariae. The release of Wolbachia bacteria from adult O. volvulus attracts and initiates a strong neutrophil response. Onchocercomata of untreated cattle infected with O. ochengi showed mostly neutrophil accumulation, while eosinophils were rarely seen around the adult worms. However, oxytetracycline treatment of O. ochengi depleted Wolbachia resulting in an increase in eosinophils and eosinophil degranulation around the filariae. This however, required prolonged antifilarial treatment to permanently deplete Wolbachia otherwise neutrophils returned to the onchocercomata (97). Thus, antibiotic treatment, leading to the depletion of Wolbachia bacteria, replaced neutrophils with eosinophils, which degranulated at the cuticle of the worms (98). On the other hand, direct macrofilaricidal drugs, which did not deplete Wolbachia, failed to induce eosinophil accumulation and degranulation (10). Thus, it has been suggested that the release of Wolbachia may confer the longevity of the filariae through a defensive mutualism, leading to neutrophil accumulation and preventing effective eosinophil defense (10). This masking may be driven through neutrophil trap formation, which was shown in O. volvulus onchocercomata. Wolbachia triggered the release of neutrophil DNA traps and thereby prevented eosinophil accumulation (8, 99). Thus, an evolutionary adaptation of the filariae may has developed to reduce eosinophil-mediated protection and increase its survival in the host.
3.2 Eosinophil proteins and eosinophil traps against filariae
Protective effects of eosinophils against filariae are mainly mediated through toxic proteins released by the eosinophils. In general, granulocyte-related proteins such as ECP and EDN are increased in patients with onchocerciasis and bancroftian filariasis and the levels of those proteins exceeded by far the levels described for other eosinophil-related diseases, e.g. bronchial asthma and atopic dermatitis. Thus, ECP and EDN serum levels are biomarkers reflecting an ongoing filarial disease (87, 100).
The protective effect of eosinophil granular proteins was shown in mice lacking EPO and MBP, which rendered mice more susceptible to a L. sigmodontis infection (74). Shortly before adult worm development, a higher worm burden was recovered from the pleural cavity of these mice compared to control mice. This may be due to the lack of the direct effect of the eosinophil-derived proteins in attacking the filariae, but also indirectly through modulating the subsequent immune response. Lack of MBP for example resulted in increased IL-10 expression in macrophages, while EPO deficiency increased the IL-5 production by CD4+ T cells. Thus, eosinophils and their mediators modulate type 2 immune responses, which affects filarial elimination (74, 101). Deposition of eosinophil proteins on the surface of filariae is a common feature of filariae and direct cytotoxic effects of eosinophil proteins on filariae are supported by several in vitro studies. MBP was detected on the surface of O. volvulus MF in the skin during the Mazzotti reaction, which is an inflammatory response caused by dying MF following topical administration of DEC in onchocerciasis patients (75). Similarly, ECP and EPO were found on the surface of O. volvulus MF in the lymph node after oral treatment with ivermectin (78). MBP deposition was also detected on B. malayi MF in a model of TPE (76). In vitro, these proteins showed marked anthelminthic activity. MBP, EDN and ECP killed Brugia pahangi and B. malayi MF in a concentration dependent manner. EDN was the least effective, while EPO, in the presence of ROS, was extremely potent even at low concentrations (77) (Box 1).
Another important mechanism of granulocytes is the release of DNA traps. While smaller pathogens such as viruses and bacteria can be phagocytosed, multicellular pathogens like filariae need to be contained through a different mechanism. Especially for neutrophils, DNA trap formation has been shown in various studies as a response to helminths and other pathogens (44, 102–105). Similarly, eosinophils are able to release DNA traps (106). However, even though eosinophils are important players in the defense against helminths, little is known about eosinophil ETosis (EETosis) in response to parasites in general and filariae in particular. So far, EETosis has only been shown in response to the nematodes Haemonchus contortus (105) and Strongyloides ratti larvae (103). Regarding EETosis in response to filariae, we showed for the first time that eosinophils released DNA traps not only in response to MF of L. sigmodontis but also in response to MF of the canine heartworm Dirofilaria immitis, suggesting a conserved mechanism. In vitro blocking studies demonstrated that the DNA release during EETosis was mediated by the dectin-1 receptor. In vitro, the DNA traps entrapped the MF and reduced their motility. In vivo, L. sigmodontis MF coated with eosinophil DNA traps were cleared faster from the circulation, suggesting that these traps may be an essential mechanism in MF clearance (102).
Of note, for human eosinophils it was shown that CLCs form during the process of EETosis. As murine eosinophils lack galectin-10, the protein responsible for CLC formation (79), additional studies using human eosinophils have to be performed to elucidate the specific role of CLCs in EETosis and their effect on filariae.
3.3 Eosinophils and vaccine efficacy against filariae
Next to mass drug administrations and the development of new drug candidates (2), current attempts to eliminate filarial diseases include the development of a potent anti-filarial vaccine, which would help to control the transmission of the disease (107). Several animal studies investigated the protective effect using irradiation-attenuated L3 larvae (irr. L3), which results in protective effects ranging from 30 to 100% (108–113). The immune reaction towards the irr. L3 appears to be dependent on larval-specific antibodies as well as eosinophils. In jirds vaccinated with irr. Acanthocheilonema viteae- or B. malayi L3, eosinophil-rich granuloma were observed after challenge infection and strong eosinophil degranulation was associated with the destruction of the worm cuticles (114, 115). O. volvulus-vaccinated and subsequently challenged mice were also able to kill the in diffusion chambers intraperitoneally implanted adult filariae. Eosinophils were the only cells that accumulated in the diffusion chambers of the immunized mice and depletion of IL-5 prevented the protective effect (29). Similarly, vaccination efficacy in L. sigmodontis-infected mice was dependent on eosinophils and IL-5. After challenge infection of mice vaccinated with irr. L3, IL-5 and eosinophils accumulated in the skin and incoming L3 larvae were eliminated within the first 2 days post infection (82, 83). In accordance, anti-IL-5 antibody treatment, suppressed tissue eosinophilia and prevented the protection (82).
Vaccination-mediated protection was also dependent on B cells and antibodies. Lack of B cells and antibodies in µMT mice abolished the protective effect even though eosinophil infiltration occurred at the site of infection (116). In this context, degranulation of eosinophils was significantly reduced in immunized and challenged µMT mice compared to wild type mice, suggesting that antibody-complexes are required for eosinophil effector function through ADCC (116). In accordance, in vitro motility inhibition of intestinal nematode S. ratti L3 larvae by eosinophils was dependent on antibodies from infected animals. Only in the presence of antibody-containing plasma from infected but not naïve animals, S. ratti L3-induced DNA release by eosinophils inhibited the larval motility (103).
In summary, eosinophils not only contribute to protection during primary infection in response to adult worms and MF, but are crucial for mediating protection during vaccinations (Figure 2).
4 Eosinophils and filarial pathology
By successfully inducing an immunosuppressive milieu, filariae hamper protective immunity and prevent at the same time the development of pathology that, in filariasis patients, is a result of an unbalanced immune response towards the filariae (117). Thus, a balanced host response includes sufficient parasite control and maintenance of immune homeostasis without excessive tissue damage. Failure of this balance results in excessive immune-mediated inflammation and thus, in pathology. Clinical manifestations observed in the different filarial diseases are a result of inflammation towards different filarial stages. While pathology in LF patients (lymphedema, hydrocele and elephantiasis) and Calabar swelling in loiasis patients is a result of the immune reaction to the adult worms, dermatitis and ocular lesions in onchocerciasis patients and TPE in LF is caused by the response to dead MF.
4.1 Eosinophils and pathology: Adult worms
Lymphedema, hydrocele and elephantiasis in LF patients is primarily a result of excessive immune responses towards the adult worms residing in the lymphatic vessels. However, the impact of eosinophils on pathology development is less well understood. The pathology development is primarily due to a failure to induce T cell hyporesponsiveness (118). Vascular endothelial growth factor released by endothelial cells and the secretion of pro-inflammatory cytokines have been implicated in LF pathology by enhancing leucocyte adhesion, increasing vascular permeability and promoting lymphangiogenesis. Increased frequencies of Th1, Th9 and Th17 cells and reduced numbers of Th2 cells are associated with filarial pathology (118, 119). In addition, elevated levels of innate pro-inflammatory cytokines such as C-reactive protein, IL-6, IL-8 and TNF in the peripheral blood have been associated with disease pathology (118), while only indirect evidence for the contribution of eosinophils exist. Locally, dysregulation of matrix metalloproteinases (MMPs) and their inhibitors (TIMPs) (120), which are released by a variety of cells including macrophages, epidermal cells, fibroblasts and granulocytes, are frequently linked to filarial pathology as well as elevated levels of type 2 cytokines, e.g., IL-5, IL-13 and TGFβ (121). This was further studied using a B. malayi infection model in ferrets. There, infective L3 larvae were injected into the hind-footpad of ferrets inducing LF pathology, including parasitological, immunological and histological changes remarkably similar to human filarial infections. Using this model, it was shown that perivascular and subcutaneous inflammation was associated with increased infiltration of plasma cells and histiocytes and also with increased neutrophil and eosinophil numbers (28). Thus, eosinophils and eosinophil-associated IL-5 may be involved during LF pathology development. However, in W. bancrofti patients, the pathology was rather connected to decreased serum fibrosis markers and increased levels of hyaluronan, while serum ECP and EPO concentrations were not altered among patients with lymphatic pathology compared to asymptomatic patients. An increase in ECP levels at the limit of significance was observed in patients with elephantiasis (100).
An additional example of the importance of unregulated immune responses is seen in loiasis pathology development. Temporary residents, with less exposure to the parasites, show a significantly higher prevalence for Calabar swellings compared to persons native to the region. Calabar swellings are edema in the subcutaneous tissue, caused by the migrating adult filariae. The prevalence of Calabar swellings in temporary residents was associated with elevated levels of eosinophil numbers, eosinophil-associated proteins IL-5 and GM-CSF, as well as filarial-specific IgG. On the other hand people native to endemic countries showed immune tolerance with reduced eosinophil numbers and eosinophil-associated responses, higher MF loads and lower frequencies of pathology (90). Thus, eosinophil presence and responses are related to disease pathology in loiasis patients.
In summary, while many animal studies underline the importance of eosinophil-mediated protection against adult worms, evidence for eosinophils contributing to pathology based on inflammatory responses towards the adult worms is only indirect.
4.2 Eosinophils and pathology: Microfilariae
4.2.1 Lung pathology and tropical pulmonary eosinophilia (TPE)
A severe pathology observed in some LF patients is TPE caused by MF of W. bancrofti and B. malayi. It is characterized by increased peripheral eosinophil numbers and asthma-like symptoms, i.e., cough, dyspnoea and wheezing. The symptoms result from an immunological hyperresponsiveness to the MF and patients show high levels of serum IgE and filarial-specific antibodies (11, 122, 123). Thereby, MF are trapped and cleared in the pulmonary circulation. As a result, microfilaremia is rarely observed in TPE patients. MF trapping leads to the release of antigens triggering chronic inflammation with peribronchial and perivascular exudates as well as acute eosinophil infiltration. Within the lower airway, severe eosinophilic inflammation occurs and eosinophils massively release their granules into the lung parenchyma. EDN has been found to be increased in bronchoalveolar lavage (BAL) of patients with TPE. MBP-2, which is only found in eosinophils, is also increased and may be a suitable biomarker for TPE. Especially MBP has been associated with airway hyperreactivity leading to fibrosis. Thus, eosinophils and their granules serve a dual role by eliminating MF and by inducing lung pathology (11, 124).
Indirect evidence that eosinophils are responsible for TPE pathology is given by studies demonstrating that individuals treated with DEC, which removes MF, leads to a marked decrease in lung eosinophilia and an improvement of the clinical symptoms (125). Patients treated with DEC continue to show a mild persistent inflammation with sustained eosinophilic alveolitis as well as higher free oxygen radicals in the bronchoalveolar fluid (126). Thus, the immune response by eosinophils towards the MF rather than the MF themselves are responsible for the immunopathology.
Direct evidence of eosinophil-mediated lung damage during TPE was demonstrated in a TPE-mouse model, where freeze-thawed B. malayi MF are used to sensitize mice followed by a challenge with viable MF (127). Neutralization of the α4 and β7 integrins, which mediates eosinophil migration, prevented pathology development, reduced peripheral eosinophilia, reduced lung eosinophil infiltration, and subsequently minimized lung damage (32). However, it has to be mentioned that neutralization of α4 and β7 integrin also impairs the migration of several T helper cell subsets to the lung. Thus, the results may not exclusively demonstrate the impact of eosinophils on lung damage.
Skewing the type 2 immune response towards a more pronounced type 1 response by administering IL-12 significantly reduced IgG1 and IgE levels, eosinophilia as well as MBP levels and lessened lung pathology in the TPE mouse model (76). Further evidence for eosinophil-mediated pathology during TPE was shown in mice lacking IL-5, which prevented peripheral and pulmonary eosinophilia as well as airway hyperresponsiveness (128). Similarly, our own unpublished results show that TPE induction using L. sigmodontis MF in dblGATA mice, which lack eosinophils, resulted in reduced lung pathology.
Even in asymptomatic LF patients, eosinophils have been shown to induce clinical symptoms after treatment. Following DEC treatment, patients often develop symptoms such as fever, headache and lethargy and this is accompanied by a significant increase in IL-5 levels followed by an increase in eosinophil counts (129, 130). One study conducted by Gopinath et al. closely followed the cytokine and chemokine response as well as eosinophil blood counts after treatment (129). Four hours following treatment, patients developed clinical symptoms, which peaked 24h after treatment. This was accompanied by a drop in peripheral eosinophil counts reflecting the immediate migration of eosinophils into the tissue or adhesion to dying MF followed by a significant eosinophil increase within 3-4 days post treatment. The eosinophil-mediated response was also reflected in the increase in eosinophil-associated cytokines and chemokines such as IL-5 and CCL5/RANTES as well as the increase in the eosinophil-associated proteins MBP and EDN in the peripheral blood upon treatment. Moreover, DEC treatment increased the expression of the integrin markers VLA-4, α4 and β7, which mediate eosinophil adhesion to endothelia or to dying MF (129).
During natural infection with L. sigmodontis, eosinophil-mediated lung pathology is also observed. Adult L. sigmodontis worms, residing in the pleural cavity, release MF that are thought to enter the peripheral blood via the lung capillaries, inducing lung injury. It was shown that MF in L. sigmodontis-infected jirds induced fibrotic polyps on the visceral mesothelium with high infiltrates of macrophages, lymphocytes and eosinophils (131). In mice, hypertrophic mesothelium was seen in infected wild type as well as in eosinophil-deficient mice regardless of the presence of MF. However, hyperplasia was mainly seen in MF-positive wild type mice and was absent in MF-negative wild type mice and mice lacking eosinophils (131). Therefore, eosinophils not only contribute to protective immunity against MF but also to pathology development during LF and murine filariasis.
4.2.2 Skin and eye pathology
Pathology in onchocerciasis patients is induced by the immune response towards the dying MF in the skin leading to dermatitis with the most severe form of chronic hyperreactive onchocerciasis called sowda, and in the cornea leading to vision impairment and even blindness (132). Most individuals living in endemic areas have acquired a parasite-specific anergy with reduced cellular responses and modulated inflammatory responses. Hyperreactive response to the parasite antigen on the other hand results in pathology, which is associated with high parasite-specific IgE and IgG levels as well as absence of skin MF (133). Moreover, in ocular lesions, MF migration itself is not responsible for eliciting pathology, but clinical manifestations result from the inflammation in response to the dead/dying MF; in the eye this leads to antigen release that triggers a local inflammatory response, resulting in corneal inflammation characterized by an opaque area called punctate keratitis. Heavily infected individuals with high MF numbers in the cornea develop sclerosing keratitis with scaring of the cornea and in visual impairment (134).
To study the immune response in the cornea of onchocerciasis patients, an O. volvulus keratitis mouse model helped to decipher the mechanism of immunopathology. To induce ocular lesions, mice are treated with intraconjunctival or intrastromal injection of viable MF, or with intrastromal injections of O. volvulus antigen after immunization with filarial antigen. This drives a Th2 response with local production of IL-4 and IL-5 (26). As a response, a biphasic accumulation of granulocytes occurs, with neutrophils being the first cells to arrive at the site of inflammation followed by eosinophils, inducing inflammation in the cornea leading to ocular lesions (25, 26). So far, it is known that CD4+ T cells and B cells play an essential role during the development of keratitis by driving granulocyte recruitment. Depletion of CD4+ T cells reduced pathology, which was associated with significantly reduced recruitment of eosinophils (135). Similarly, B cell-deficient mice failed to develop corneal disease through impaired recruitment of eosinophils and neutrophils, which was mediated through circulating immune complexes (136). Thus, the presence of eosinophils and neutrophils is associated with disease development. However, the exact mechanism and the contribution of both cell types to the development of keratitis is less well understood. It is assumed that eosinophils and neutrophils both contribute to the inflammation. Thereby, their granular content, which is released into the cornea, is suggested to mediate pathology. For example, in vitro it was shown that MBP is highly toxic to corneal cells (137). However, the essential role of eosinophils and neutrophils on disease outcome has still to be demonstrated. In this regard, type 2 and type 1 cytokines modulated severity of keratitis, which was associated with eosinophil numbers. IL-4 KO mice presented reduced keratitis that correlated with impaired eosinophil recruitment to the cornea (138), while IL-12-treated mice experienced enhanced eosinophil recruitment and excessive pathology (139). Thus, eosinophil presence appears to be essential for pathology development. However, IL-5 KO mice develop keratitis in the absence of eosinophils. The biphasic response of neutrophils followed by eosinophils is ablated in these mice resulting in a constitutive presence of neutrophils in the cornea at early and late time points (140). These data suggest, that neutrophils rather than eosinophils mediate corneal opacification. Moreover, neutrophil- but not eosinophil-mediated keratitis was demonstrated in response to Wolbachia. Injection of Wolbachia-containing worm extract into the cornea of mice induced keratitis, while injection of Wolbachia-depleted extract and extracts from the rodent filaria A. viteae, which lack Wolbachia bacteria, resulted in reduced ocular lesions with lower neutrophil recruitment. While neutrophil recruitment was ablated in the absence of Wolbachia, eosinophil accumulation was independent of Wolbachia (39). Thus, neutrophils seem to be pivotal for the induction of ocular lesions in onchocerciasis patients, while the exact contribution of eosinophils has still to be determined.
Interestingly, during experimental-induced dermatitis of onchocerciasis, eosinophils and neutrophils follow a similar recruitment pattern as in the cornea. Injection of O. volvulus antigen into the ear of immunized mice resulted in dermatitis with intense infiltration of neutrophils followed by eosinophils (141). Thus, a similar mechanism during skin manifestations and keratitis may be in place. However, while the role of eosinophils in ocular lesion may be questionable, a more prominent role of eosinophils during onchocerciasis-related skin manifestations has been observed. Sowda patients show significantly higher serum levels of ECP in comparison to hyporesponsive generalized onchocerciasis patients. Especially MF density is low or absent in sowda patients; thus, increased ECP levels could reflect a higher degree of eosinophil activation and MF killing (87).
Since dying MF elucidate an eosinophil-mediated response, pathology is observed after DEC treatment in onchocerciasis patients due to the rapid death of MF in the skin and the cornea. It was already described in 1984 that onchocerciasis patients treated with DEC showed marked inflammation in the skin characterized by extensive eosinophil infiltration into the dermis and epidermis with intradermal abscesses containing dead MF and eosinophils (75), which was mediated through eotaxin release (60). Interestingly, only dead and dying MF were coated by MBP, while viable MF did not stain for MBP suggesting that eosinophils damage the MF of O. volvulus through the release of toxic proteins such as MBP (75). Similarly, dead MF elucidated higher DNA release by eosinophils in comparison to viable MF in vitro (102). The eosinophil-associated inflammation in the skin of DEC-treated onchocerciasis patients is referred to as Mazzotti reaction. After oral treatment with DEC, peripheral eosinophil counts first decrease followed by a significant increase in peripheral blood eosinophil counts as well as an increase in eosinophil-associated proteins (EDN, MBP), which coincide with the infiltration and degranulation of eosinophils in the papillary dermis (142). In addition, neutrophils and their proteins are found around the dying MF in the skin and probably work together with the eosinophils to kill MF after DEC treatment (143). Therefore, onchocerciasis patients should not be treated with DEC to prevent the strong immune reaction towards the MF and thus development of ophthalmological and dermal pathology (2).
Similarly, ivermectin treatment in onchocerciasis patients induced a significant increase in CCL5/RANTES and eotaxin expression in the skin post treatment, which resulted in an increase of skin eosinophils 24h after treatment (144). Moreover, eosinophils, together with macrophages, mast cells and some T cells, encircled degenerated MF after ivermectin treatment of hyperreactive onchocerciasis patients (145). Skin MF were markedly reduced after treatment and primarily found in the regional lymph nodes, where they were surrounded by eosinophils and eosinophil-derived toxic deposits such as ECP, EPO and cationic leukocyte antigen (CLA) (78, 146, 147). This clearance of MF within lymph nodes of ivermectin-treated patients renders this treatment safe for use of onchocerciasis patients, preventing the risk of permanent visual impairment.
DEC or ivermectin treatment, which causes a rapid decline in MF, can also result in post-treatment adverse events in loiasis patients with high MF counts. For DEC treatment clinical manifestations were associated with eosinophil infiltration into the skin and subsequent migration to peripheral lymph nodes (148). A study investigating loiasis in temporary residents showed that DEC treatment resulted in severe adverse events in 7 out of 20 patients with endomyocardial fibrosis and renal disease, which was associated with increased eosinophil numbers, IgE and filarial-specific antibodies (149). On the other hand, treatment of loiasis patients with reslizumab, an anti-IL-5 antibody, decreased absolute eosinophil counts in the peripheral blood, but had no effect on MF clearance nor did it lead to an improvement of adverse events suggesting that eosinophil depletion has less impact on post-DEC adverse events in loiasis patients than assumed (150).
These data indicate that eosinophils may contribute to skin and eye pathology in onchocerciasis patients. Especially after treatment with DEC, eosinophil responses coincided with the clinical symptoms in onchocerciasis patients. Based on the data received from animal experiments, it can be assumed that eosinophils promote MF killing and may support microfilaricidal drug action (Figure 3). However, some of the studies are contradictory for the role of eosinophils and further research is required to define the specific role of eosinophils and neutrophils in the development of filarial pathology.
5 Gaps in understanding and future work
Eosinophils serve several roles during filarial infection by promoting protection on the one hand and contributing to pathology development on the other hand. The great task protective immunity has to achieve is a balanced host response, which includes sufficient parasite control and maintenance of immune homeostasis without excessive tissue damage.
Even though eosinophil function during protective immunity and disease pathology in filarial infection has been studied, several questions remain unanswered.
5.1 Direct effects of eosinophils on protection
While granular proteins appear to be involved in protective immunity against filariae, the role of DNA traps in filarial containment has still to be elucidated. While smaller pathogens can be phagocytosed, larger multicellular organisms such as filariae need to be contained through a different mechanism. Thus, it can be assumed that this may be mediated through extracellular DNA traps to prevent parasite escape and to concentrate and focus granular proteins on the surface of the parasite and thus limit excessive systemic inflammation. Such a response was observed for the fungi Aspergillus fumigatus that was entrapped in the lung by neutrophil DNA traps that contributed to fungal killing (151). Since eosinophils can inhibit MF motility in vitro and DNA traps support MF removal from the peripheral circulation (102), the question remains where those entrapped MF are removed (role of spleen, lung and other organs), if eosinophils also contribute to the containment of MF after their release from the adult worms, and if ETosis supports drug-induced MF removal. Furthermore, with Dectin-1 the receptor inducing EETosis was identified (102), however, the structure on the MF that induces EETosis is not yet known. Given that dead MF lead to a stronger induction of EETosis, PAMPs and DAMPs from dead and viable MF may differ or use different pathways.
Moreover, the role of complement-mediated toxicity and ADCC for eosinophils during primary filarial infection is not completely known. Antibodies and ADCC appear to be an important mechanism during secondary infections and after vaccination, however, during primary infection little is known. It is known that IgG4 from MF+, MF- LF patients and endemic normal but not from LF patients with pathology can inhibit the activation of granulocytes, suggesting that antibody-mediated toxicity by granulocytes may contribute to pathology in LF patients (152). However, the contribution of ADCC by eosinophils for protection against filariae is not known. For other helminth infections, we know that antibodies and complement contribute only marginally to the protective effect by eosinophils during primary infection. Eosinophil-mediated immunity against Nippostrongylus brasiliensis is independent of complement (153) and antibody-dependent killing of Schistosoma mansoni requires additional eosinophil activation or pre-exposure of the eosinophils to the parasite (154). Similarly, S. ratti L3 larvae motility was only inhibited by immune serum and not in the presence of naïve serum suggesting that helminth-specific antibodies are required to attack the worm (103). However, the exact role of complement and antibodies during primary filarial infection needs to be elucidated yet.
5.2 Interaction of eosinophils with other immune cells during filarial infections
Depletion experiments and usage of KO mice suggest specific roles of eosinophils and neutrophils during filarial infection, however kinetics on granulocyte recruitment indicate a close interplay of eosinophils and neutrophils during protective immunity as well as pathology development (155). However, the exact interplay between eosinophils and neutrophils during filarial infection remains unclear. This includes the recruitment and activation of each other, their interaction during the containment and elimination of the filariae, as well as the development of pathology, e.g. ocular lesions and skin manifestations in onchocerciasis patients. The current results suggest that eosinophils are recruited after neutrophil accumulation in the skin and cornea of onchocerciasis patients. The question remains if neutrophils directly induce eosinophil recruitment or if this is mediated through T cells. Further, it has to be clarified which cell type is responsible for pathology development in onchocerciasis patients and if both granulocyte populations have redundant roles.
Moreover, eosinophils seem to interact with macrophages during filarial infection (30, 96), but more experiments analyzing the exact mechanism on how eosinophils impact macrophage plasticity locally and systemically during filarial infection are required.
In addition, in diseases such as allergies (156), eosinophils affect T cell responses by promoting T cell proliferation, activation and polarization through type 1 and type 2 cytokine release (157). Such an unbalanced T cell hyperresponsiveness was also associated with the occurrence of lymphedema in LF patients, but the exact role of eosinophils in this context is not known. The interaction of eosinophils and T cells was suggested in patients suffering of two eosinophil-driven diseases, allergy and filarial infection. Concomitant filarial infection and allergy increased parasite antigen-driven Th2, Th9 and regulatory T cell-related cytokine expression in comparison to filaria-infected and non-allergic patients. This correlated with IgE levels, eosinophil numbers and their degranulation products (158). The authors suggested that this particular immune response could affect parasite control and associated pathology.
5.3 Direct effects of eosinophils on pathology
While the role of eosinophils in hyper- and hyporesponsive onchocerciasis patients has been shown, the causative effect remains unclear. Hyperresponsive patients present marked eosinophil responses, while hyporesponsive patients are characterized by reduced eosinophil numbers and responses. Thus, the differences in immune status among patients appear to be a result of an unbalanced immune responses towards a more pronounced Th17 and Th1 shift in hyperreactive onchocerciasis patients rather than a regulatory response in hyporesponsive patients. Eosinophils are able to produce type 1 and type 2 cytokines and different subtypes of eosinophils have been described including resident eosinophils that rather show an anti-inflammatory phenotype, responsible for tissue homeostasis, and inducible eosinophils with a marked pro-inflammatory phenotype (159). Thus, eosinophils and their different phenotypes could contribute to the different disease outcomes among onchocerciasis patients and explain why some patients develop pathology, while others remain asymptomatic and maintain a high parasite burden. Differences in the composition of resident and inducible eosinophils could be also the case for patients suffering from TPE. Similarly, it can be hypothesized that differences in the ETosis capacity may contribute to disease pathology, which, when balanced, limits inflammation and clears filariae, while excessive ETosis may drive pathology. Thus, future studies should analyze the composition of eosinophil phenotypes in the different filarial disease settings and determine whether filarial immunomodulation is involved.
Even though eosinophil function during the Mazzotti reaction in onchocerciasis patients has been investigated, several questions on the eosinophil-mediated pathology remain unanswered. The exact interplay of eosinophils and DEC has not be elucidated yet and the question remains how and whether the drug impacts eosinophil function directly. Identifying the exact mechanism on drug and eosinophil-responses may open up new and improved treatment strategies for filariasis, which will not only include macro- or microfilaricidal activity but also lessen associated adverse events.
5.4 Targeting eosinophils as treatment option
Monoclonal antibodies targeting eosinophil-development are used for other eosinophil-associated pathologies, such as allergic asthma. Mepolizumab and reslizumab, monoclonal anti-IL-5 antibodies, and benralizumab, which targets IL-5Rα, are approved for the treatment of asthma and have shown to improve allergic asthma (160, 161). Thus, similar treatments in filariasis patients could lessen symptoms. However, one study using reslizumab prior DEC treatment in loiasis patients failed to reduce adverse events (150). Moreover, monoclonal antibody treatment for the treatment of filarial pathology faces several limitations. The therapy is extremely cost intensive, requires intense patient care and often requires parental administration. However, most countries affected by filarial infections lack an advanced health care system making monoclonal antibody therapies impossible. Furthermore, drugs currently used for mass drug administration do not kill the adult filariae. Thus, impairing protective immune responses by eosinophils, may facilitate filarial survival and transmission of the diseases. The latter does not only refer to filarial diseases, but also other helminth infections, such as intestinal helminths (162).
6 Summary
Eosinophilia and increases in eosinophil protein levels are often associated with an ongoing helminth infection and can serve as first diagnostic indication. Eosinophils provide essential effector functions to control filarial infections but also induce filarial pathology. Tables 3, 4 summaries the findings on eosinophil-mediated protection and pathology in human filariasis and in filarial animal models.
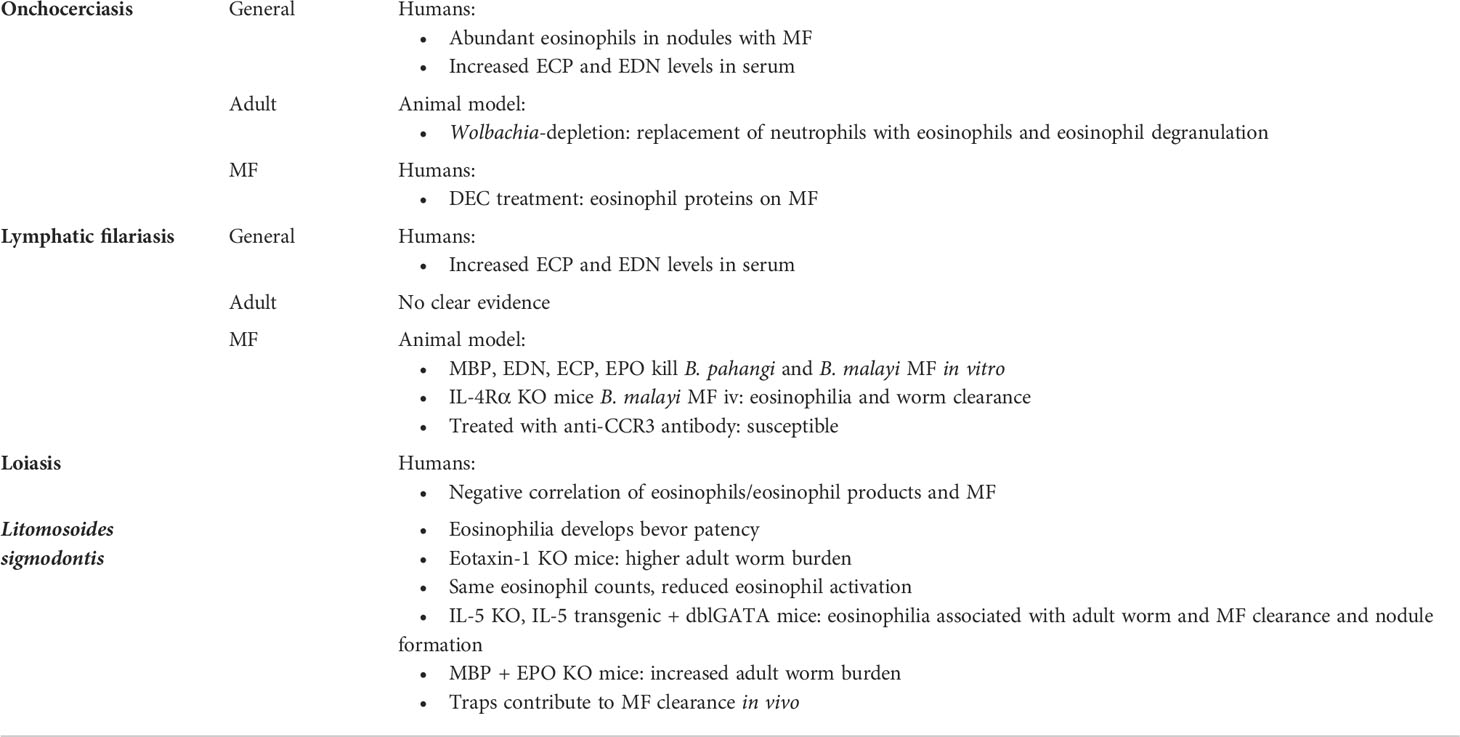
Table 3 Summary of eosinophil involvement in protective immune responses during filarial infections.
Although a direct evidence for the role of eosinophils is not always given, animal experiments convincingly demonstrate that eosinophils are important to control microfilaremia and adult worm burden. Moreover, eosinophils are shown to be of particular importance for vaccination efficacy in animal models. With regard to filarial pathology, eosinophil-mediated inflammatory responses against MF are likely supporting the development of filarial pathology, as discussed for the development of keratitis and dermatitis in onchocerciasis patients or lung inflammation during TPE in LF patients. Similarly, following DEC treatment, eosinophil- rather than neutrophil-mediated responses seem to trigger skin lesions in onchocerciasis patients. Future studies will have to further elucidate the exact role of the different eosinophil phenotypes during filarial infection and distinguish them from the role of neutrophils. Further research on eosinophil function, eosinophil plasticity and interaction with other immune cells is required.
Author contributions
Writing original draft: AE and MH. Writing review and editing: AE, AH, and MH. All authors contributed to the article and approved the submitted version.
Funding
MH and AE are funded by the German Center for Infection Research (DZIF Translational Thematic Unit: Novel Antibiotics grant #09.701) and by the Deutsche Forschungsgesellschaft (DFG grant HU2144/3-1). AH received support from the DZIF (#09.807, #09.806, #09.822 and #09.914), the Deutsche Forschungsgesellschaft (DFG grant PF673/3-1) and the Federal Ministry of Education and Research (BMBF, grant 16GW0227K).
Conflict of interest
The authors declare that the research was conducted in the absence of any commercial or financial relationships that could be construed as a potential conflict of interest.
Publisher’s note
All claims expressed in this article are solely those of the authors and do not necessarily represent those of their affiliated organizations, or those of the publisher, the editors and the reviewers. Any product that may be evaluated in this article, or claim that may be made by its manufacturer, is not guaranteed or endorsed by the publisher.
References
2. Ehrens A, Hoerauf A, Hubner MP. Current perspective of new anti-wolbachial and direct-acting macrofilaricidal drugs as treatment strategies for human filariasis. GMS Infect Dis (2022) 10:Doc02. doi: 10.3205/id000079
3. Ta-Tang TH, Crainey JL, Post RJ, Luz SL, Rubio JM. Mansonellosis: Current perspectives. Res Rep Trop Med (2018) 9:9–24. doi: 10.2147/RRTM.S125750
5. Taylor MJ, Hoerauf A, Bockarie M. Lymphatic filariasis and onchocerciasis. Lancet (2010) 376(9747):1175–85. doi: 10.1016/S0140-6736(10)60586-7
6. WHO. Global programme to eliminate lymphatic filariasis: Progress report, 2019. Weekly epidemiological record, Vol. 95. (2020), 509–24.
7. Mediannikov O, Ranque S. Mansonellosis, the most neglected human filariasis. New Microbes New Infect (2018) 26:S19–22. doi: 10.1016/j.nmni.2018.08.016
8. Tamarozzi F, Turner JD, Pionnier N, Midgley A, Guimaraes AF, Johnston KL, et al. Wolbachia endosymbionts induce neutrophil extracellular trap formation in human onchocerciasis. Sci Rep (2016) 6:35559. doi: 10.1038/srep35559
9. WHO. Progress in eliminating onchocerciasis in the who region of the americas: Doxycycline treatment as an end-game strategy. Weekly epidemiological Rep (2019) 37(94):415–9.
10. Hansen RD, Trees AJ, Bah GS, Hetzel U, Martin C, Bain O, et al. A worm's best friend: Recruitment of neutrophils by wolbachia confounds eosinophil degranulation against the filarial nematode onchocerca ochengi. Proc Biol Sci (2011) 278(1716):2293–302. doi: 10.1098/rspb.2010.2367
11. Vijayan VK. Tropical pulmonary eosinophilia: Pathogenesis, diagnosis and management. Curr Opin Pulm Med (2007) 13(5):428–33. doi: 10.1097/MCP.0b013e3281eb8ec9
12. Padgett JJ, Jacobsen KH. Loiasis: African eye worm. Trans R Soc Trop Med Hyg (2008) 102(10):983–9. doi: 10.1016/j.trstmh.2008.03.022
13. Nutman TB, Reese W, Poindexter RW, Ottesen EA. Immunologic correlates of the hyperresponsive syndrome of loiasis. J Infect Dis (1988) 157(3):544–50. doi: 10.1093/infdis/157.3.544
14. Awadzi K. Clinical picture and outcome of serious adverse events in the treatment of onchocerciasis. Filaria J (2003) 2 Suppl 1:S6. doi: 10.1186/1475-2883-2-s1-s6
15. Gardon J, Gardon-Wendel N, Demanga N, Kamgno J, Chippaux JP, Boussinesq M. Serious reactions after mass treatment of onchocerciasis with ivermectin in an area endemic for loa loa infection. Lancet (1997) 350(9070):18–22. doi: 10.1016/S0140-6736(96)11094-1
16. Frohberger SJ, Ajendra J, Surendar J, Stamminger W, Ehrens A, Buerfent BC, et al. Susceptibility to l. sigmodontis infection is highest in animals lacking il-4r/Il-5 compared to single knockouts of il-4r, il-5 or eosinophils. Parasit Vectors (2019) 12(1):248. doi: 10.1186/s13071-019-3502-z
17. Gondorf F, Berbudi A, Buerfent BC, Ajendra J, Bloemker D, Specht S, et al. Chronic filarial infection provides protection against bacterial sepsis by functionally reprogramming macrophages. PLoS Pathog (2015) 11(1):e1004616. doi: 110.1371/journal.ppat.1004616
18. Hübner MP, Martin C, Specht S, Koschel M, Dubben B, Frohberger SJ, et al. Oxfendazole mediates macrofilaricidal efficacy against the filarial nematode litomosoides sigmodontis in vivo and inhibits onchocerca spec. motility in vitro. PloS Negl Trop Dis (2020) 14(7):e0008427. doi: 10.1371/journal.pntd.0008427
19. Hübner MP, Torrero MN, McCall JW, Mitre E. Litomosoides sigmodontis: A simple method to infect mice with L3 larvae obtained from the pleural space of recently infected jirds (Meriones unguiculatus). Exp Parasitol (2009) 123(1):95–8. doi: 10.1016/j.exppara.2009.05.009
20. Fulton A, Babayan SA, Taylor MD. Use of the litomosoides sigmodontis infection model of filariasis to study type 2 immunity. Methods Mol Biol (2018) 1799:11–26. doi: 10.1007/978-1-4939-7896-0_2
21. Trees AJ, Graham SP, Renz A, Bianco AE, Tanya V. Onchocerca ochengi infections in cattle as a model for human onchocerciasis: Recent developments. Parasitology (2000) 120 Suppl:S133–42. doi: 10.1017/s0031182099005788
22. Zhan B, Bottazzi ME, Hotez PJ, Lustigman S. Advancing a human onchocerciasis vaccine from antigen discovery to efficacy studies against natural infection of cattle with onchocerca ochengi. Front Cell Infect Microbiol (2022) 12:869039. doi: 10.3389/fcimb.2022.869039
23. Allen JE, Adjei O, Bain O, Hoerauf A, Hoffmann WH, Makepeace BL, et al. Of mice, cattle, and humans: The immunology and treatment of river blindness. PloS Negl Trop Dis (2008) 2(4):e217. doi: 10.1371/journal.pntd.0000217
24. Tchakoute VL, Bronsvoort M, Tanya V, Renz A, Trees AJ. Chemoprophylaxis of onchocerca infections: In a controlled, prospective study ivermectin prevents calves becoming infected with o. ochengi. Parasitology (1999) 118(Pt 2):195–9. doi: 10.1017/s0031182098003680
25. Chakravarti B, Lass JH, Bardenstein DS, Diaconu E, Roy CE, Herring TA, et al. Immune-mediated onchocerca volvulus sclerosing keratitis in the mouse. Exp Eye Res (1993) 57(1):21–7. doi: 10.1006/exer.1993.1094
26. Pearlman E, Lass JH, Bardenstein DS, Kopf M, Hazlett FE Jr., Diaconu E, et al. Interleukin 4 and T helper type 2 cells are required for development of experimental onchocercal keratitis (River blindness). J Exp Med (1995) 182(4):931–40. doi: 10.1084/jem.182.4.931
27. Crandall RB, Thompson JP, Connor DH, McGreevy PB, Crandall CA. Pathology of experimental infection with brugia malayi in ferrets: Comparison with occult filariasis in man. Acta Trop (1984) 41(4):373–81.
28. Jackson-Thompson BM, Kim SY, Jaiswal S, Scott JR, Jones SR, Morris CP, et al. Brugia malayi infection in ferrets - a small mammal model of lymphatic filariasis. PloS Negl Trop Dis (2018) 12(3):e0006334. doi: 10.1371/journal.pntd.0006334
29. Lange AM, Yutanawiboonchai W, Scott P, Abraham D. Il-4- and il-5-Dependent protective immunity to onchocerca volvulus infective larvae in Balb/Cbyj mice. J Immunol (1994) 153(1):205–11.
30. Pionnier N, Sjoberg H, Furlong-Silva J, Marriott A, Halliday A, Archer J, et al. Eosinophil-mediated immune control of adult filarial nematode infection can proceed in the absence of il-4 receptor signaling. J Immunol (2020) 205(3):731–40. doi: 10.4049/jimmunol.1901244
31. Abraham D, Grieve RB, Holy JM, Christensen BM. Immunity to larval brugia malayi in Balb/C mice: Protective immunity and inhibition of larval development. Am J Trop Med Hyg (1989) 40(6):598–604. doi: 10.4269/ajtmh.1989.40.598
32. Sharma P, Sharma A, Srivastava M. In vivo neutralization of Alpha4 and Beta7 integrins inhibits eosinophil trafficking and prevents lung injury during tropical pulmonary eosinophilia in mice. Eur J Immunol (2017) 47(9):1501–12. doi: 10.1002/eji.201747086
33. Luu L, Bah GS, Okah-Nnane NH, Hartley CS, Glover AF, Walsh TR, et al. Co-Administration of adjuvanted recombinant ov-103 and ov-Ral-2 vaccines confer protection against natural challenge in a bovine onchocerca ochengi infection model of human onchocerciasis. Vaccines (Basel) (2022) 10(6):861. doi: 10.3390/vaccines10060861
34. Bah GS, Schneckener S, Hahnel SR, Bayang NH, Fieseler H, Schmuck GM, et al. Emodepside targets slo-1 channels of onchocerca ochengi and induces broad anthelmintic effects in a bovine model of onchocerciasis. PloS Pathog (2021) 17(6):e1009601. doi: 10.1371/journal.ppat.1009601
35. Halliday A, Guimaraes AF, Tyrer HE, Metuge HM, Patrick CN, Arnaud KO, et al. A murine macrofilaricide pre-clinical screening model for onchocerciasis and lymphatic filariasis. Parasit Vectors (2014) 7:472. doi: 10.1186/s13071-014-0472-z
36. Akama T, Freund YR, Berry PW, Carter DS, Easom EE, Jarnagin K, et al. Macrofilaricidal benzimidazole-benzoxaborole hybrids as an approach to the treatment of river blindness: Part 1. amide linked analogs. ACS Infect Dis (2020) 6(2):173–9. doi: 10.1021/acsinfecdis.9b00396
37. Sjoberg HT, Pionnier N, Aljayyoussi G, Metuge HM, Njouendou AJ, Chunda VC, et al. Short-course, oral flubendazole does not mediate significant efficacy against onchocerca adult Male worms or brugia microfilariae in murine infection models. PloS Negl Trop Dis (2019) 13(1):e0006356. doi: 10.1371/journal.pntd.0006356
38. Furlong-Silva J, Cross SD, Marriott AE, Pionnier N, Archer J, Steven A, et al. Tetracyclines improve experimental lymphatic filariasis pathology by disrupting interleukin-4 receptor-mediated lymphangiogenesis. J Clin Invest (2021) 131(5):e140853. doi: 10.1172/jci140853
39. Saint Andre A, Blackwell NM, Hall LR, Hoerauf A, Brattig NW, Volkmann L, et al. The role of endosymbiotic wolbachia bacteria in the pathogenesis of river blindness. Science (2002) 295(5561):1892–5. doi: 10.1126/science.1068732
40. Risch F, Ritter M, Hoerauf A, Hübner MP. Human filariasis-contributions of the litomosoides sigmodontis and acanthocheilonema viteae animal model. Parasitol Res (2021) 120(12):4125–43. doi: 10.1007/s00436-020-07026-2
41. Finlay CM, Allen JE. The immune response of inbred laboratory mice to litomosoides sigmodontis: A route to discovery in myeloid cell biology. Parasite Immunol (2020) 42(7):e12708. doi: 10.1111/pim.12708
42. Mitre E, Klion AD. Eosinophils and helminth infection: Protective or pathogenic? Semin Immunopathol (2021) 43(3):363–81. doi: 10.1007/s00281-021-00870-z
43. Obata-Ninomiya K, Domeier PP, Ziegler SF. Basophils and eosinophils in nematode infections. Front Immunol (2020) 11:583824. doi: 10.3389/fimmu.2020.583824
44. Ajendra J. Lessons in type 2 immunity: Neutrophils in helminth infections. Semin Immunol (2021) 53:101531. doi: 10.1016/j.smim.2021.101531
45. Roan F, Obata-Ninomiya K, Ziegler SF. Epithelial cell-derived cytokines: More than just signaling the alarm. J Clin Invest (2019) 129(4):1441–51. doi: 10.1172/JCI124606
46. Allen JE, Maizels RM. Diversity and dialogue in immunity to helminths. Nat Rev Immunol (2011) 11(6):375–88. doi: 10.1038/nri2992
47. Maizels RM, Yazdanbakhsh M. Immune regulation by helminth parasites: Cellular and molecular mechanisms. Nat Rev Immunol (2003) 3(9):733–44. doi: 10.1038/nri1183
48. Hoerauf A, Satoguina J, Saeftel M, Specht S. Immunomodulation by filarial nematodes. Parasite Immunol (2005) 27(10-11):417–29. doi: 10.1111/j.1365-3024.2005.00792.x
49. Noordin R, Arifin N, Balachandra D, Ahmad H. Chapter 8 - serodiagnosis of strongyloides stercoralis infection. In: Methods in microbiolog. Academic press (2020).
50. Hartl S, Breyer MK, Burghuber OC, Ofenheimer A, Schrott A, Urban MH, et al. Blood eosinophil count in the general population: Typical values and potential confounders. Eur Respir J (2020) 55(5):1901874. doi: 10.1183/13993003.01874-2019
51. Rosenberg HF, Dyer KD, Foster PS. Eosinophils: Changing perspectives in health and disease. Nat Rev Immunol (2013) 13(1):9–22. doi: 10.1038/nri3341
52. Lombardi C, Berti A, Cottini M. The emerging roles of eosinophils: Implications for the targeted treatment of eosinophilic-associated inflammatory conditions. Curr Res Immunol (2022) 3:42–53. doi: 10.1016/j.crimmu.2022.03.002
53. Rothenberg ME, Hogan SP. The eosinophil. Annu Rev Immunol (2006) 24:147–74. doi: 10.1146/annurev.immunol.24.021605.090720
54. Weller PF, Spencer LA. Functions of tissue-resident eosinophils. Nat Rev Immunol (2017) 17(12):746–60. doi: 10.1038/nri.2017.95
55. Fulkerson PC, Rothenberg ME. Targeting eosinophils in allergy, inflammation and beyond. Nat Rev Drug Discov (2013) 12(2):117–29. doi: 10.1038/nrd3838
56. Meiners J, Reitz M, Rudiger N, Turner JE, Heepmann L, Rudolf L, et al. Il-33 facilitates rapid expulsion of the parasitic nematode strongyloides ratti from the intestine Via Ilc2- and il-9-Driven mast cell activation. PloS Pathog (2020) 16(12):e1009121. doi: 10.1371/journal.ppat.1009121
57. Rankin SM, Conroy DM, Williams TJ. Eotaxin and eosinophil recruitment: Implications for human disease. Mol Med Today (2000) 6(1):20–7. doi: 10.1016/s1357-4310(99)01635-4
58. Doran E, Cai F, Holweg CTJ, Wong K, Brumm J, Arron JR. Interleukin-13 in asthma and other eosinophilic disorders. Front Med (Lausanne) (2017) 4:139. doi: 10.3389/fmed.2017.00139
59. Makepeace BL, Martin C, Turner JD, Specht S. Granulocytes in helminth infection - who is calling the shots? Curr Med Chem (2012) 19(10):1567–86. doi: 10.2174/092986712799828337
60. Pearlman E, Toe L, Boatin BA, Gilles AA, Higgins AW, Unnasch TR. Eotaxin expression in onchocerca volvulus-induced dermatitis after topical application of diethylcarbamazine. J Infect Dis (1999) 180(4):1394–7. doi: 10.1086/315041
61. Mochizuki M, Bartels J, Mallet AI, Christophers E, Schroder JM. Il-4 induces eotaxin: A possible mechanism of selective eosinophil recruitment in helminth infection and atopy. J Immunol (1998) 160(1):60–8.
62. Rothenberg ME, MacLean JA, Pearlman E, Luster AD, Leder P. Targeted disruption of the chemokine eotaxin partially reduces antigen-induced tissue eosinophilia. J Exp Med (1997) 185(4):785–90. doi: 10.1084/jem.185.4.785
63. Bochner BS, Klunk DA, Sterbinsky SA, Coffman RL, Schleimer RP. Il-13 selectively induces vascular cell adhesion molecule-1 expression in human endothelial cells. J Immunol (1995) 154(2):799–803.
64. Fukuda T, Fukushima Y, Numao T, Ando N, Arima M, Nakajima H, et al. Role of interleukin-4 and vascular cell adhesion molecule-1 in selective eosinophil migration into the airways in allergic asthma. Am J Respir Cell Mol Biol (1996) 14(1):84–94. doi: 10.1165/ajrcmb.14.1.8534490
65. Jacobsen EA, Ochkur SI, Pero RS, Taranova AG, Protheroe CA, Colbert DC, et al. Allergic pulmonary inflammation in mice is dependent on eosinophil-induced recruitment of effector T cells. J Exp Med (2008) 205(3):699–710. doi: 10.1084/jem.20071840
66. Wang HB, Ghiran I, Matthaei K, Weller PF. Airway eosinophils: Allergic inflammation recruited professional antigen-presenting cells. J Immunol (2007) 179(11):7585–92. doi: 10.4049/jimmunol.179.11.7585
67. Spencer LA, Szela CT, Perez SA, Kirchhoffer CL, Neves JS, Radke AL, et al. Human eosinophils constitutively express multiple Th1, Th2, and immunoregulatory cytokines that are secreted rapidly and differentially. J Leukoc Biol (2009) 85(1):117–23. doi: 10.1189/jlb.0108058
68. Gleich GJ, Frigas E, Loegering DA, Wassom DL, Steinmuller D. Cytotoxic properties of the eosinophil major basic protein. J Immunol (1979) 123(6):2925–7.
69. Slifman NR, Loegering DA, McKean DJ, Gleich GJ. Ribonuclease activity associated with human eosinophil-derived neurotoxin and eosinophil cationic protein. J Immunol (1986) 137(9):2913–7.
70. Weller PF, Goetzl EJ, Austen KF. Identification of human eosinophil lysophospholipase as the constituent of charcot-Leyden crystals. Proc Natl Acad Sci U.S.A. (1980) 77(12):7440–3. doi: 10.1073/pnas.77.12.7440
71. Arora VK, Singh N, Bhatia A. Charcot-Leyden crystals in fine needle aspiration cytology. Acta Cytol (1997) 41(2):409–12. doi: 10.1159/000332532
72. Ghafouri B, Irander K, Lindbom J, Tagesson C, Lindahl M. Comparative proteomics of nasal fluid in seasonal allergic rhinitis. J Proteome Res (2006) 5(2):330–8. doi: 10.1021/pr050341h
74. Specht S, Saeftel M, Arndt M, Endl E, Dubben B, Lee NA, et al. Lack of eosinophil peroxidase or major basic protein impairs defense against murine filarial infection. Infect Immun (2006) 74(9):5236–43. doi: 10.1051/parasite/2006132165
75. Kephart GM, Gleich GJ, Connor DH, Gibson DW, Ackerman SJ. Deposition of eosinophil granule major basic protein onto microfilariae of onchocerca volvulus in the skin of patients treated with diethylcarbamazine. Lab Invest (1984) 50(1):51–61.
76. Mehlotra RK, Hall LR, Higgins AW, Dreshaj IA, Haxhiu MA, Kazura JW, et al. Interleukin-12 suppresses filaria-induced pulmonary eosinophilia, deposition of major basic protein and airway hyperresponsiveness. Parasite Immunol (1998) 20(10):455–62. doi: 10.1046/j.1365-3024.1998.00174.x
77. Hamann KJ, Gleich GJ, Checkel JL, Loegering DA, McCall JW, Barker RL. In vitro killing of microfilariae of brugia pahangi and brugia malayi by eosinophil granule proteins. J Immunol (1990) 144(8):3166–73.
78. Wildenburg G, Darge K, Knab J, Tischendorf FW, Bonow I, Buttner DW. Lymph nodes of onchocerciasis patients after treatment with ivermectin: Reaction of eosinophil granulocytes and their cationic granule proteins. Trop Med Parasitol (1994) 45(2):87–96.
79. Ueki S, Tokunaga T, Melo RCN, Saito H, Honda K, Fukuchi M, et al. Charcot-Leyden crystal formation is closely associated with eosinophil extracellular trap cell death. Blood (2018) 132(20):2183–7. doi: 10.1182/blood-2018-04-842260
80. Ackerman SJ, Gleich GJ, Weller PF, Ottesen EA. Eosinophilia and elevated serum levels of eosinophil major basic protein and charcot-Leyden crystal protein (Lysophospholipase) after treatment of patients with bancroft's filariasis. J Immunol (1981) 127(3):1093–8.
81. Bhatia V, Sarin SK. Hepatic visceral larva migrans: Evolution of the lesion, diagnosis, and role of high-dose albendazole therapy. Am J Gastroenterol (1994) 89(4):624–7.
82. Martin C, Al-Qaoud KM, Ungeheuer MN, Paehle K, Vuong PN, Bain O, et al. Il-5 is essential for vaccine-induced protection and for resolution of primary infection in murine filariasis. Med Microbiol Immunol (2000) 189(2):67–74. doi: 10.1007/pl00008258
83. Le Goff L, Loke P, Ali HF, Taylor DW, Allen JE. Interleukin-5 is essential for vaccine-mediated immunity but not innate resistance to a filarial parasite. Infect Immun (2000) 68(5):2513–7. doi: 10.1128/IAI.68.5.2513-2517.2000
84. Hise AG, Daehnel K, Gillette-Ferguson I, Cho E, McGarry HF, Taylor MJ, et al. Innate immune responses to endosymbiotic wolbachia bacteria in brugia malayi and onchocerca volvulus are dependent on Tlr2, Tlr6, Myd88, and mal, but not Tlr4, trif, or tram. J Immunol (2007) 178(2):1068–76. doi: 10.4049/jimmunol.178.2.1068
85. Ajendra J, Specht S, Ziewer S, Schiefer A, Pfarr K, Parcina M, et al. Nod2 dependent neutrophil recruitment is required for early protective immune responses against infectious litomosoides sigmodontis L3 larvae. Sci Rep (2016) 6:39648. doi: 10.1038/srep39648
86. Spencer LA, Bonjour K, Melo RC, Weller PF. Eosinophil secretion of granule-derived cytokines. Front Immunol (2014) 5:496. doi: 10.3389/fimmu.2014.00496
87. Tischendorf FW, Brattig NW, Buttner DW, Pieper A, Lintzel M. Serum levels of eosinophil cationic protein, eosinophil-derived neurotoxin and myeloperoxidase in infections with filariae and schistosomes. Acta Trop (1996) 62(3):171–82. doi: 10.1016/s0001-706x(96)00038-1
88. Arndts K, Specht S, Debrah AY, Tamarozzi F, Klarmann Schulz U, Mand S, et al. Immunoepidemiological profiling of onchocerciasis patients reveals associations with microfilaria loads and ivermectin intake on both individual and community levels. PloS Negl Trop Dis (2014) 8(2):e2679. doi: 10.1371/journal.pntd.0002679
89. Wildenburg G, Kromer M, Buttner DW. Dependence of eosinophil granulocyte infiltration into nodules on the presence of microfilariae producing onchocerca volvulus. Parasitol Res (1996) 82(2):117–24. doi: 10.1007/s004360050081
90. Herrick JA, Metenou S, Makiya MA, Taylar-Williams CA, Law MA, Klion AD, et al. Eosinophil-associated processes underlie differences in clinical presentation of loiasis between temporary residents and those indigenous to loa-endemic areas. Clin Infect Dis (2015) 60(1):55–63. doi: 10.1093/cid/ciu723
91. Torrero MN, Hübner MP, Larson D, Karasuyama H, Mitre E. Basophils amplify type 2 immune responses, but do not serve a protective role, during chronic infection of mice with the filarial nematode litomosoides sigmodontis. J Immunol (2010) 185(12):7426–34. doi: 10.4049/jimmunol.0903864
92. Gentil K, Lentz CS, Rai R, Muhsin M, Kamath AD, Mutluer O, et al. Eotaxin-1 is involved in parasite clearance during chronic filarial infection. Parasite Immunol (2014) 36(2):60–77. doi: 10.1111/pim.12079
93. Volkmann L, Bain O, Saeftel M, Specht S, Fischer K, Brombacher F, et al. Murine filariasis: Interleukin 4 and interleukin 5 lead to containment of different worm developmental stages. Med Microbiol Immunol (Berl) (2003) 192(1):23–31. doi: 10.1007/s00430-002-0155-9
94. Martin C, Le Goff L, Ungeheuer MN, Vuong PN, Bain O. Drastic reduction of a filarial infection in eosinophilic interleukin-5 transgenic mice. Infect Immun (2000) 68(6):3651–6. doi: 10.1128/IAI.68.6.3651-3656.2000
95. Babayan SA, Read AF, Lawrence RA, Bain O, Allen JE. Filarial parasites develop faster and reproduce earlier in response to host immune effectors that determine filarial life expectancy. PloS Biol (2010) 8(10):e1000525. doi: 10.1371/journal.pbio.1000525
96. Al-Qaoud KM, Pearlman E, Hartung T, Klukowski J, Fleischer B, Hoerauf A. A new mechanism for il-5-Dependent helminth control: Neutrophil accumulation and neutrophil-mediated worm encapsulation in murine filariasis are abolished in the absence of il-5. Int Immunol (2000) 12(6):899–908. doi: 10.1093/intimm/12.6.899
97. Nfon CK, Makepeace BL, Njongmeta LM, Tanya VN, Bain O, Trees AJ. Eosinophils contribute to killing of adult onchocerca ochengi within onchocercomata following elimination of wolbachia. Microbes Infect (2006) 8(12-13):2698–705. doi: 10.1016/j.micinf.2006.07.017
98. Brattig NW, Buttner DW, Hoerauf A. Neutrophil accumulation around onchocerca worms and chemotaxis of neutrophils are dependent on wolbachia endobacteria. Microbes Infect (2001) 3(6):439–46. doi: 10.1016/s1286-4579(01)01399-5
99. Tamarozzi F, Turner JD, Pionnier N, Midgley A, Guimaraes AF, Johnston KL, et al. Wolbachia endosymbionts induce neutrophil extracellular trap formation in human onchocerciasis. Sci Rep (2016) 6:35559. doi: 10.1038/srep35559
100. Esterre P, Plichart C, Huin-Blondey MO, Nguyen LN, Hartmann D, Guerret S, et al. Circulating fibrosis markers, eosinophil cationic protein and eosinophil protein X in patients with wuchereria bancrofti infection: Association with clinical status. Parasite (2006) 13(2):165–70. doi: 10.1051/parasite/2006132165
101. McSorley HJ, Maizels RM. Helminth infections and host immune regulation. Clin Microbiol Rev (2012) 25(4):585–608. doi: 10.1128/CMR.05040-11
102. Ehrens A, Lenz B, Neumann AL, Giarrizzo S, Reichwald JJ, Frohberger SJ, et al. Microfilariae trigger eosinophil extracellular DNA traps in a dectin-1-Dependent manner. Cell Rep (2021) 34(2):108621. doi: 10.1016/j.celrep.2020.108621
103. Ehrens A, Rudiger N, Heepmann L, Linnemann L, Hartmann W, Hubner MP, et al. Eosinophils and neutrophils eliminate migrating strongyloides ratti larvae at the site of infection in the context of extracellular DNA trap formation. Front Immunol (2021) 12:715766. doi: 10.3389/fimmu.2021.715766
104. Munoz-Caro T, Conejeros I, Zhou E, Pikhovych A, Gartner U, Hermosilla C, et al. Dirofilaria immitis microfilariae and third-stage larvae induce canine netosis resulting in different types of neutrophil extracellular traps. Front Immunol (2018) 9:968. doi: 10.3389/fimmu.2018.00968
105. Munoz-Caro T, Rubio RM, Silva LM, Magdowski G, Gartner U, McNeilly TN, et al. Leucocyte-derived extracellular trap formation significantly contributes to haemonchus contortus larval entrapment. Parasit Vectors (2015) 8:607. doi: 10.1186/s13071-015-1219-1
106. Mukherjee M, Lacy P, Ueki S. Eosinophil extracellular traps and inflammatory pathologies-untangling the web! Front Immunol (2018) 9:2763. doi: 10.3389/fimmu.2018.02763
107. Abraham D, Graham-Brown J, Carter D, Gray SA, Hess JA, Makepeace BL, et al. Development of a recombinant vaccine against human onchocerciasis. Expert Rev Vaccines (2021) 20(11):1459–70. doi: 10.1080/14760584.2021.1977125
108. Babayan SA, Allen JE, Taylor DW. Future prospects and challenges of vaccines against filariasis. Parasite Immunol (2012) 34(5):243–53. doi: 10.1111/j.1365-3024.2011.01350.x
109. Babayan SA, Attout T, Harris A, Taylor MD, Le Goff L, Vuong PN, et al. Vaccination against filarial nematodes with irradiated larvae provides long-term protection against the third larval stage but not against subsequent life cycle stages. Int J Parasitol (2006) 36(8):903–14.
110. Babayan SA, Attout T, Vuong PN, Le Goff L, Gantier JC, Bain O. The subcutaneous movements of filarial infective larvae are impaired in vaccinated hosts in comparison to primary infected hosts. Filaria J (2005) 4:3.
111. Card C, Wilson DS, Hirosue S, Rincon-Restrepo M, de Titta A, Guc E, et al. Adjuvant-free immunization with infective filarial larvae as lymphatic homing antigen carriers. Sci Rep (2020) 10(1):1055. doi: 10.1038/s41598-020-57995-8
112. Fujiwara RT, Loukas A, Mendez S, Williamson AL, Bueno LL, Wang Y, et al. Vaccination with irradiated ancylostoma caninum third stage larvae induces a Th2 protective response in dogs. Vaccine (2006) 24(4):501–9. doi: 10.1016/j.vaccine.2005.07.091
113. Hübner MP, Torrero MN, Mitre E. Type 2 immune-inducing helminth vaccination maintains protective efficacy in the setting of repeated parasite exposures. Vaccine (2010) 28(7):1746–57. doi: 10.1016/j.vaccine.2009.12.016
114. Yates JA, Higashi GI. Ultrastructural observations on the fate of brugia malayi in jirds previously vaccinated with irradiated infective stage larvae. Am J Trop Med Hyg (1986) 35(5):982–7. doi: 10.4269/ajtmh.1986.35.982
115. Bleiss W, Oberlander U, Hartmann S, Adam R, Marko A, Schonemeyer A, et al. Protective immunity induced by irradiated third-stage larvae of the filaria acanthocheilonema viteae is directed against challenge third-stage larvae before molting. J Parasitol (2002) 88(2):264–70. doi: 10.1645/0022-3395(2002)088[0264:PIIBIT]2.0.CO;2
116. Martin C, Saeftel M, Vuong PN, Babayan S, Fischer K, Bain O, et al. B-cell deficiency suppresses vaccine-induced protection against murine filariasis but does not increase the recovery rate for primary infection. Infect Immun (2001) 69(11):7067–73. doi: 10.1128/IAI.69.11.7067-7073.2001
117. Katawa G, Layland LE, Debrah AY, von Horn C, Batsa L, Kwarteng A, et al. Hyperreactive onchocerciasis is characterized by a combination of Th17-Th2 immune responses and reduced regulatory T cells. PloS Negl Trop Dis (2015) 9(1):e3414. doi: 10.1371/journal.pntd.0003414
118. Babu S, Bhat SQ, Pavan Kumar N, Lipira AB, Kumar S, Karthik C, et al. Filarial lymphedema is characterized by antigen-specific Th1 and Th17 proinflammatory responses and a lack of regulatory T cells. PloS Negl Trop Dis (2009) 3(4):e420. doi: 10.1371/journal.pntd.0000420
119. Debrah AY, Mand S, Specht S, Marfo-Debrekyei Y, Batsa L, Pfarr K, et al. Doxycycline reduces plasma vegf-C/Svegfr-3 and improves pathology in lymphatic filariasis. PloS Pathog (2006) 2(9):e92. doi: 10.1371/journal.ppat.0020092
120. Anuradha R, George JP, Pavankumar N, Kumaraswami V, Nutman TB, Babu S. Altered circulating levels of matrix metalloproteinases and inhibitors associated with elevated type 2 cytokines in lymphatic filarial disease. PloS Negl Trop Dis (2012) 6(6):e1681. doi: 10.1371/journal.pntd.0001681
121. Bennuru S, Maldarelli G, Kumaraswami V, Klion AD, Nutman TB. Elevated levels of plasma angiogenic factors are associated with human lymphatic filarial infections. Am J Trop Med Hyg (2010) 83(4):884–90. doi: 10.4269/ajtmh.2010.10-0039
122. Ottesen EA, Nutman TB. Tropical pulmonary eosinophilia. Annu Rev Med (1992) 43:417–24. doi: 10.1146/annurev.me.43.020192.002221
123. Ong RK, Doyle RL. Tropical pulmonary eosinophilia. Chest (1998) 113(6):1673–9. doi: 10.1378/chest.113.6.1673
124. Mullerpattan JB, Udwadia ZF, Udwadia FE. Tropical pulmonary eosinophilia–a review. Indian J Med Res (2013) 138(3):295–302.
125. Tsanglao WR, Nandan D, Chandelia S, Arya NK, Sharma A. Filarial tropical pulmonary eosinophilia: A condition masquerading asthma, a series of 12 cases. J Asthma (2019) 56(7):791–8. doi: 10.1080/02770903.2018.1490748
126. Rom WN, Vijayan VK, Cornelius MJ, Kumaraswami V, Prabhakar R, Ottesen EA, et al. Persistent lower respiratory tract inflammation associated with interstitial lung disease in patients with tropical pulmonary eosinophilia following conventional treatment with diethylcarbamazine. Am Rev Respir Dis (1990) 142(5):1088–92. doi: 10.1164/ajrccm/142.5.1088
127. Egwang TG, Kazura JW. The Balb/C mouse as a model for immunological studies of microfilariae-induced pulmonary eosinophilia. Am J Trop Med Hyg (1990) 43(1):61–6. doi: 10.4269/ajtmh.1990.43.61
128. Hall LR, Mehlotra RK, Higgins AW, Haxhiu MA, Pearlman E. An essential role for interleukin-5 and eosinophils in helminth-induced airway hyperresponsiveness. Infect Immun (1998) 66(9):4425–30. doi: 10.1128/IAI.66.9.4425-4430.1998
129. Gopinath R, Hanna LE, Kumaraswami V, Perumal V, Kavitha V, Vijayasekaran V, et al. Perturbations in eosinophil homeostasis following treatment of lymphatic filariasis. Infect Immun (2000) 68(1):93–9. doi: 10.1128/IAI.68.1.93-99.2000
130. Limaye AP, Ottesen EA, Kumaraswami V, Abrams JS, Regunathan J, Vijayasekaran V, et al. Kinetics of serum and cellular interleukin-5 in posttreatment eosinophilia of patients with lymphatic filariasis. J Infect Dis (1993) 167(6):1396–400. doi: 10.1093/infdis/167.6.1396
131. Fercoq F, Remion E, Vallarino-Lhermitte N, Alonso J, Raveendran L, Nixon C, et al. Microfilaria-dependent thoracic pathology associated with eosinophilic and fibrotic polyps in filaria-infected rodents. Parasit Vectors (2020) 13(1):551. doi: 10.1186/s13071-020-04428-0
132. Tamarozzi F, Halliday A, Gentil K, Hoerauf A, Pearlman E, Taylor MJ. Onchocerciasis: The role of wolbachia bacterial endosymbionts in parasite biology, disease pathogenesis, and treatment. Clin Microbiol Rev (2011) 24(3):459–68. doi: 10.1128/CMR.00057-10
133. Brattig NW. Pathogenesis and host responses in human onchocerciasis: Impact of onchocerca filariae and wolbachia endobacteria. Microbes Infect (2004) 6(1):113–28. doi: 10.1016/j.micinf.2003.11.003
134. Hall LR, Pearlman E. Pathogenesis of onchocercal keratitis (River blindness). Clin Microbiol Rev (1999) 12(3):445–53. doi: 10.1128/CMR.12.3.445
135. Hall LR, Kaifi JT, Diaconu E, Pearlman E. Cd4(+) depletion selectively inhibits eosinophil recruitment to the cornea and abrogates onchocerca volvulus keratitis (River blindness). Infect Immun (2000) 68(9):5459–61. doi: 10.1128/IAI.68.9.5459-5461.2000
136. Hall LR, Lass JH, Diaconu E, Strine ER, Pearlman E. An essential role for antibody in neutrophil and eosinophil recruitment to the cornea: B cell-deficient (Micromt) mice fail to develop Th2-dependent, helminth-mediated keratitis. J Immunol (1999) 163(9):4970–5.
137. Trocme SD, Hallberg CK, Gill KS, Gleich GJ, Tyring SK, Brysk MM. Effects of eosinophil granule proteins on human corneal epithelial cell viability and morphology. Invest Ophthalmol Vis Sci (1997) 38(3):593–9.
138. Pearlman E, Lass JH, Bardenstein DS, Diaconu E, Hazlett FE Jr., Albright J, et al. Onchocerca volvulus-mediated keratitis: Cytokine production by il-4-Deficient mice. Exp Parasitol (1996) 84(2):274–81. doi: 10.1006/expr.1996.0113
139. Pearlman E, Lass JH, Bardenstein DS, Diaconu E, Hazlett FE Jr., Albright J, et al. Il-12 exacerbates helminth-mediated corneal pathology by augmenting inflammatory cell recruitment and chemokine expression. J Immunol (1997) 158(2):827–33.
140. Pearlman E, Hall LR, Higgins AW, Bardenstein DS, Diaconu E, Hazlett FE, et al. The role of eosinophils and neutrophils in helminth-induced keratitis. Invest Ophthalmol Vis Sci (1998) 39(7):1176–82.
141. Pearlman E, Garhart CA, Grand DJ, Diaconu E, Strine ER, Hall LR. Temporal recruitment of neutrophils and eosinophils to the skin in a murine model for onchocercal dermatitis. Am J Trop Med Hyg (1999) 61(1):14–8. doi: 10.4269/ajtmh.1999.61.14
142. Ackerman SJ, Kephart GM, Francis H, Awadzi K, Gleich GJ, Ottesen EA. Eosinophil degranulation. an immunologic determinant in the pathogenesis of the mazzotti reaction in human onchocerciasis. J Immunol (1990) 144(10):3961–9.
143. Gutierrez-Pena EJ, Knab J, Buttner DW. Neutrophil granule proteins: Evidence for the participation in the host reaction to skin microfilariae of onchocerca volvulus after diethylcarbamazine administration. Parasitology (1996) 113(Pt 4):403–14. doi: 10.1017/s0031182000066543
144. Cooper PJ, Beck LA, Espinel I, Deyampert NM, Hartnell A, Jose PJ, et al. Eotaxin and rantes expression by the dermal endothelium is associated with eosinophil infiltration after ivermectin treatment of onchocerciasis. Clin Immunol (2000) 95(1 Pt 1):51–61. doi: 10.1006/clim.1999.4829
145. Korten S, Hoerauf A, Kaifi JT, Buttner DW. Low levels of transforming growth factor-beta (Tgf-beta) and reduced suppression of Th2-mediated inflammation in hyperreactive human onchocerciasis. Parasitology (2011) 138(1):35–45. doi: 10.1017/S0031182010000922
146. Darge K, Lucius R, Monson MH, Behrendsen J, Buttner DW. Immunohistological and electron microscopic studies of microfilariae in skin and lymph nodes from onchocerciasis patients after ivermectin treatment. Trop Med Parasitol (1991) 42(4):361–7.
147. Duke BO, Soula G, Zea-Flores G, Bratthauer GL, Doumbo O. Migration and death of skin-dwelling onchocerca volvulus microfilariae after treatment with ivermectin. Trop Med Parasitol (1991) 42(1):25–30.
148. Herrick JA, Legrand F, Gounoue R, Nchinda G, Montavon C, Bopda J, et al. Posttreatment reactions after single-dose diethylcarbamazine or ivermectin in subjects with loa loa infection. Clin Infect Dis (2017) 64(8):1017–25. doi: 10.1093/cid/cix016
149. Nutman TB, Miller KD, Mulligan M, Ottesen EA. Loa loa infection in temporary residents of endemic regions: Recognition of a hyperresponsive syndrome with characteristic clinical manifestations. J Infect Dis (1986) 154(1):10–8. doi: 10.1093/infdis/154.1.10
150. Legrand F, Herrick J, Makiya M, Ramanathan R, Thompson R, Rampertaap S, et al. A randomized, placebo-controlled, double-blind pilot study of single-dose humanized anti-Il5 antibody (Reslizumab) for the reduction of eosinophilia following diethylcarbamazine treatment of loa loa infection. Clin Infect Dis (2021) 73(7):e1624–e31. doi: 10.1093/cid/ciaa1365
151. Bruns S, Kniemeyer O, Hasenberg M, Aimanianda V, Nietzsche S, Thywissen A, et al. Production of extracellular traps against aspergillus fumigatus in vitro and in infected lung tissue is dependent on invading neutrophils and influenced by hydrophobin roda. PloS Pathog (2010) 6(4):e1000873. doi: 10.1371/journal.ppat.1000873
152. Prodjinotho UF, Hoerauf A, Adjobimey T. Igg4 antibodies from patients with asymptomatic bancroftian filariasis inhibit the binding of Igg1 and Igg2 to C1q in a fc-Fc-Dependent mechanism. Parasitol Res (2019) 118(10):2957–2968. doi: 10.1007/s00436-019-06451-2
153. Giacomin PR, Gordon DL, Botto M, Daha MR, Sanderson SD, Taylor SM, et al. The role of complement in innate, adaptive and eosinophil-dependent immunity to the nematode nippostrongylus brasiliensis. Mol Immunol (2008) 45(2):446–55. doi: 10.1016/j.molimm.2007.05.029
154. Khalife J, Dunne DW, Richardson BA, Mazza G, Thorne KJ, Capron A, et al. Functional role of human igg subclasses in eosinophil-mediated killing of schistosomula of schistosoma mansoni. J Immunol (1989) 142(12):4422–7.
155. Ajendra J, Chenery AL, Parkinson JE, Chan BHK, Pearson S, Colombo SAP, et al. Il-17a both initiates, Via ifngamma suppression, and limits the pulmonary type-2 immune response to nematode infection. Mucosal Immunol (2020) 13(6):958–68. doi: 10.1038/s41385-020-0318-2
156. MacKenzie JR, Mattes J, Dent LA, Foster PS. Eosinophils promote allergic disease of the lung by regulating Cd4(+) Th2 lymphocyte function. J Immunol (2001) 167(6):3146–55. doi: 10.4049/jimmunol.167.6.3146
157. Blanchard C, Rothenberg ME. Biology of the eosinophil. Adv Immunol (2009) 101:81–121. doi: 10.1016/S0065-2776(08)01003-1
158. Gazzinelli-Guimaraes PH, Bonne-Annee S, Fujiwara RT, Santiago HC, Nutman TB. Allergic sensitization underlies hyperreactive antigen-specific Cd4+ T cell responses in coincident filarial infection. J Immunol (2016) 197(7):2772–9. doi: 10.4049/jimmunol.1600829
159. Kanda A, Yun Y, Van Bui D, Nguyen LM, Kobayashi Y, Suzuki K, et al. Corrigendum to "the multiple functions and subpopulations of eosinophils in tissues under steady-state and pathological conditions" [Allergol int 70 (2021) 9-18]. Allergol Int (2021) 70(2):277. doi: 10.1016/j.alit.2021.01.002
160. Ridolo E, Pucciarini F, Nizi MC, Makri E, Kihlgren P, Panella L, et al. Mabs for treating asthma: Omalizumab, mepolizumab, reslizumab, benralizumab, dupilumab. Hum Vaccin Immunother (2020) 16(10):2349–56. doi: 10.1080/21645515.2020.1753440
161. Agache I, Beltran J, Akdis C, Akdis M, Canelo-Aybar C, Canonica GW, et al. Efficacy and safety of treatment with biologicals (Benralizumab, dupilumab, mepolizumab, omalizumab and reslizumab) for severe eosinophilic asthma. a systematic review for the eaaci guidelines - recommendations on the use of biologicals in severe asthma. Allergy (2020) 75(5):1023–42. doi: 10.1111/all.14221
Keywords: filaria, eosinophil, lymphatic filariasis, onchocerciasis, granulocyte, ETosis, EPO, TPE
Citation: Ehrens A, Hoerauf A and Hübner MP (2022) Eosinophils in filarial infections: Inducers of protection or pathology? Front. Immunol. 13:983812. doi: 10.3389/fimmu.2022.983812
Received: 01 July 2022; Accepted: 05 October 2022;
Published: 31 October 2022.
Edited by:
Dario S. Zamboni, University of São Paulo, BrazilReviewed by:
Alan L. Scott, Johns Hopkins University, United StatesPedro H. Gazzinelli-Guimaraes, National Institute of Allergy and Infectious Diseases (NIH), United States
Copyright © 2022 Ehrens, Hoerauf and Hübner. This is an open-access article distributed under the terms of the Creative Commons Attribution License (CC BY). The use, distribution or reproduction in other forums is permitted, provided the original author(s) and the copyright owner(s) are credited and that the original publication in this journal is cited, in accordance with accepted academic practice. No use, distribution or reproduction is permitted which does not comply with these terms.
*Correspondence: Alexandra Ehrens, YWVocmVuc0B1bmktYm9ubi5kZQ==