- 1IFOM ETS- The AIRC Institute of Molecular Oncology Joint Research Laboratory, Centre for Inflammation and Tissue Homeostasis, Institute for Stem Cell Science and Regenerative Medicine (inStem), Bangalore, Karnataka, India
- 2School of Chemical and Biotechnology, Shanmugha Arts, Science, Technology and Research Academy (SASTRA) University, Thanjavur, Tamil Nadu, India
- 3Unilever Research & Development Shanghai, Shanghai, China
- 4Unilever Research & Development Bangalore, Bangalore, India
As the interface between the body and the environment, the skin functions as the physical barrier against external pathogens and toxic agents. In addition, the skin is an immunologically active organ with a plethora of resident adaptive and innate immune cells, as well as effector molecules that provide another layer of protection in the form of an immune barrier. A major subpopulation of these immune cells are the Foxp3 expressing CD4 T cells or regulatory T cells (T-regs). The canonical function of T-regs is to keep other immune cells in check during homeostasis or to dissipate a robust inflammatory response following pathogen clearance or wound healing. Interestingly, recent data has uncovered unconventional roles that vary between different tissues and we will highlight the emerging non-lymphoid functions of cutaneous T-regs. In light of the novel functions of other immune cells that are routinely being discovered in the skin, their regulation by T-regs implies that T-regs have executive control over a broad swath of biological activities in both homeostasis and disease. The blossoming list of non-inflammatory functions, whether direct or indirect, suggests that the role of T-regs in a regenerative organ such as the skin will be a field ripe for discovery for decades to come.
Skin as an immune organ
The skin is an immunologically active organ that harbors multiple immune effector molecules and cells which belong to both innate and adaptive branches of immunity. Apart from its role as a physical barrier protecting the body from the external environment, the skin through the activity of immune cells and molecules, forms the first line of natural immunological defence against infection (1).
The skin is a multi-layered structure, and each layer contains cells and effector molecules that contributes towards the mounting of an immune response and hence infection protection. The epidermis for example contains Langerhans cells and T cells, which, can be found in the stratum basale and stratum spinosum. Apart from this, the dermis harbours dendritic cell (DC) subsets, including dermal DCs and plasmacytoid DCs (pDCs), and T cell subsets, including CD4+ T helper 1 (TH1), TH2 and TH17 cells, γδ T cells natural killer T (NKT) cells and Tregs.
In addition to the immune cells linked to an adaptive immune response, innate immunity activators are also present in the skin. Key innate immune activators present in the skin layers are the TLRs, NLRs, cytokines, chemokines, antimicrobial peptides, and lipids. In addition, macrophages, mast cells and fibroblasts and keratinocytes are also present in the skin tissue. All these acts in a concerted fashion and they co-ordinate a strong immune response against invading pathogens. Skin keratinocytes also produce antimicrobial peptides and lipids as a part of its natural innate immune response against pathogens. The production of things like antimicrobial peptides (AMPs) and lipids (AMLs) forms a part of a natural and evolutionarily conserved defence mechanism of eukaryotic cells against bacteria and viruses. AMPs and AMLs prevent microbial infection of skin by direct killing of the pathogen, recruitment of host immune cells and modulation of cytokine production (2).
The optimal regulation of this skin resident immune system and its effectors molecules (AMPs, AMLs) is thus important for skin health and hygiene and maintenance of such an activity is driven by the efficient crosstalk between the skin cells, immune cells and effectors and the microbes. In the following sections of this review, we will cover in the key role played by the immune cells in maintaining this with a focus on the role of the Tregs.
Cyclical regulation of cutaneous immune cells under homeostatic conditions
Most of the T regulatory cells isolated from human skin expresses CCR4. It is also shown that a good percentage of peripheral blood CD4+ CD25hi FOXP3+ T-regs express cutaneous lymphocyte antigen (CLA). These cells were shown to be capable of immunosuppressing activated CD3+ T cells suggesting that they might be recruited to maintain immune tolerance in human skin in homeostatic conditions (3). FACS analysis of healthy human skin have shown that a major portion of T-regs in human skin are memory T-regs as they express CD45RO. These cells were at one point activated due to the expression of CTLA-4. There are reduced number of T-regs in fetal skin compared to adult skin. Immunofluorescence analysis have shown that in human skin, T-regs reside close to follicular epithelium compared to interfollicular dermis whereas conventional T cells are distributed throughout the dermis. More T-regs are present in the areas where there is high density of hair like the scalp. Memory T-regs are non-migratory as they lacked CCR7 (4).
Under homeostatic conditions, the hair follicles undergo cycles of growth (anagen), regression (catagen) and quiescence (telogen) throughout life (Figure 1). In rodents, hair growth commences in the embryo and continues postnatally followed by catagen and telogen phases. The early growth phase is called morphogenesis and it starts from the anterior and progresses to the tail in rodents. Interestingly, unlike rodent hair follicles that cycle synchronously for the first two cycles (5, 6), the hair follicles in human skin cycle independently from the adjacent hair follicle. The stem cells in various niches of the hair follicle proliferate and differentiate to maintain the hair follicle unit, and requires communication between the epithelial compartment and the mesenchymal compartment (17). The hair follicles and the dermal white adipose tissue (dWAT) expand during anagen and shrink during the catagen and telogen stages.
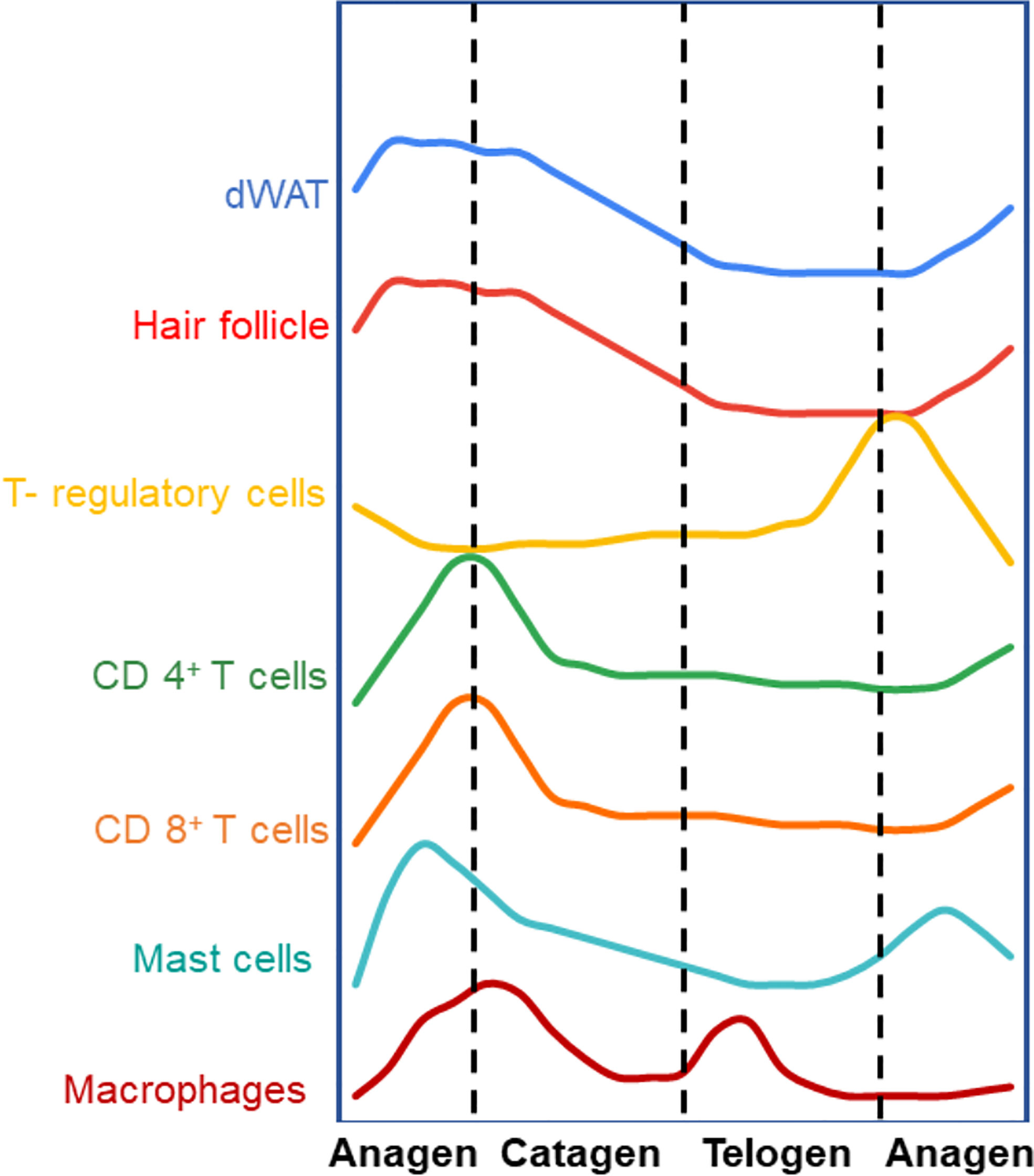
Figure 1 Different compartments of the skin cycle under homeostatic conditions (Arbitrary levels). The hair follicle is well known to undergo regenerative cycles of anagen (growth), catagen (regression) and telogen (resting phase). Immune cells and dermal white adipose tissue (dWAT) cycle synchronously with respect to different stages of hair follicle. Most of the immune cells follow a similar trend where they increase in number during anagen and decline with catagen and telogen. Interestingly, T-regs cycle in the opposite fashion – T-regs are more in number in the telogen phase than anagen or catagen. This could signify a possible regulatory relationship between these different cell types though mechanisms governing this cyclical behavior are yet to be defined. (5–16).
dWAT is layer of white adipose tissue which resides in the dermal layer skin under the fibroblasts and above the muscle layer panniculus carnosus (18). Well know function of dWAT is the thermoregulation but recent studies have unearthed importance of dWAT in antimicrobial peptide secretion (19), wound healing, and hair follicle cycling (7, 20). Similar to the dynamics of the hair follicle and dWAT, many resident immune cells cycle in both numbers and activity in the skin (Figure 1). This concurrent cycling between immune cells with the different cutaneous structures suggests that there may be a regulatory and functional link between them. For instance, macrophages cycle in sync with the hair follicle. By IF and FACS analysis of murine back skin, it was shown that macrophages gradually increase in number after the commencement of the anagen phase and attains a peak at mid telogen, followed by a significant decrease in the late telogen phase due to apoptosis and migration (8). Interestingly, these changes in macrophage number along with their release of Wnt7b and Wnt10a during apoptosis are necessary for induction of the anagen phase of the hair follicle (8). During the late anagen phase, an increased number of a subset of macrophage-like cells expressing FGF5 was observed (9, 10). These cells then migrate to the lower dermal region where they interact with the FGFR1 on dermal papillae to induce the regression of hair follicle marking the beginning of catagen phase.
Similar to macrophages, mast cells numbers and degranulation of its stored cytokines also in sync with the hair follicle cycle (Figure 1). The mast cell population accumulates towards the end of anagen and just before catagen and decrease in numbers during telogen. Around 70% of mast cells at very early anagen are degranulated whereas only 5.8% are degranulated in telogen skin (11, 12). It has been shown that mast cell degranulation is essential for HF cycling, primarily in at the anagen phase (12) through its secretion of factors such as adrenocorticotropic hormone, and Substance P (13).
In addition to the innate immune cells, some adaptive immune cells also cycle under homeostatic conditions in the skin. CD4+ and CD8+ T cells, expressing the αβ TCR, spontaneously appear in the early neonatal skin (6). In the epidermis of murine dorsal skin, the highest number of CD4+ T-cells are observed at postnatal day 3 followed by a gradual decline in number. CD8+ T cells are the prime T cell population in the epidermis, whereas the CD4+ T cell population dominates the dermis (6). Analysis of murine skin by immunofluorescence staining over different stages of the hair cycle has shown that CD4+ T cells and CD8+ T cells are higher in number in the anagen skin when compared to telogen skin. In both depilation and cyclosporin induced hair cycling, the higher number of these T-cells in anagen vs telogen is exaggerated. As with innate immune cells, this trend is opposite with respect to T-regs in skin (6, 14–16). Interestingly, despite the cyclic fluctuations in the number of these cells, the ratio of CD4+/CD8+ T-cells remains constant in steady state (6).
Recently new roles of T-regs in regulating the homeostasis of various skin compartments that undergo cyclical rounds of regeneration have been reported. For instance, T-regs themselves undergo cyclic changes in numbers as well as in activation markers (such as; CD25, ICOS, Ki67, CTLA-4, and GITR) with respect to the cyclic changes in hair follicle, dWAT and other immune cells (Figure 1) (16). Consistent with their immune suppressive activities, cutaneous T-regs are generally higher when innate and adaptive immune cells numbers and activity are low and vice versa. It is also interesting to note that only the T-regs in close proximity to the hair follicle stem cell region showed an active (amoeboid) morphology. On the other hand, T-regs further away from the hair follicle showed a more spherical morphology indicative of a lack of activity. This could indicate spatial differences in T-reg activity and function with respect to other cells within the skin (16). We expect that the increasing use of single cell analysis will facilitate the further understanding of novel roles of T-regs during cyclic regeneration of the skin.
Introduction to cutaneous T-regs
Given its role as a master regulator of other immune cells, T-regs may play an executive role in the cellular dynamics of other immune cells thereby contributing to the cyclic changes in the skin directly or indirectly. The discovery of T-regs was reported in the mid-90’s as a subpopulation within the pool of CD4+CD25+ T-cells. CD25 was established as a marker of T-regs (21), with a lot of skepticism, since activated T effector (T-eff) cells highly express CD25. However, the skepticism started to dissipate with the discovery of CD45RB expression on T-regs, which helped to distinguish the T-regs from the T-eff cells (22). Finally, the discovery of a new specific marker forkhead box transcription factor FOXP3 in 2003 settled the debate over the unambiguous existence of T-regs (CD4+ CD25+ FOXP3+ T-regs). The expression of FOXP3 is both necessary and sufficient for T-reg immune suppressive activity (23–26) and its absence leads to development of severe lymphoproliferative autoimmune disease in mice (Scurfy mouse) and in humans (IPEX syndrome) (27, 28). On a cautionary note, recent studies show that the FOXP3 can be expressed in various mammalian cell types during embryonic stages and only becomes restricted to T-regs in adults. Thus, it is difficult to perform lineage tracing experiments in embryogenesis using FOXP3 alone to understand T-reg development in the skin (29).
These T-regs, referred to as natural T-regs (nT-regs), arise from the thymus where they acquire the ability to distinguish self from non-self-antigens (30, 31). Studies from thymectomized mice, which develop lymphoproliferative disease, demonstrated that the transfer of healthy CD4+ cells (which includes both T-eff and T-regs) prevented the development of this phenotype. This study also revealed that IL2 is important in the maturation of T-regs by stabilizing FOXP3 expression. Later it was found that CD4 T cells can be induced to become T-regs (induced T-regs or iT-regs) in the peripheral tissues as well as in vitro cultures by secreted factors such as retinoic acid and TGF beta (32–34).
T-regs are a heterogenous group of cells both in lymphoid and non-lymphoid tissues. Single cell analysis of mouse T-regs from these two groups of tissues suggested that skin T-regs resembled colonic T-regs (35), though interpretations should be predicated on the low number of cells captured. Nevertheless, the sequencing data revealed that the chemokine receptors Ccr4, Ccr8, and Cxcr4 were upregulated in both colon and skin T-regs, while Ccr6 was specific to the skin T-regs. They also found shared expression of genes in the skin and colonic T-regs such as Gata3, Il1rl1, Tnfrsf4, Rora (35). More than 95% of T-regs in adult skin express CD45RO, which indicates that the cells have previously encountered an antigen outside the thymus (36). Skin T-regs have higher levels of the T-reg activation markers such as CTLA4, CD25, ICOS and Foxp3 compared to T-regs in peripheral blood. A switch in expression (either up or down) of 812 genes was discovered along the brachial lymph node to skin migration. Initially, T cell migration and glycolytic process help in the adaptation of T-regs in skin followed by cell proliferation; cytokine production and fatty acid homeostasis. The adaptation of T-regs in mouse skin was conserved in human skin T-regs as well.
There is evidence that suggest that T-regs express the transcription factors of the target cells they are attempting to suppress. For example, M.A. Koch et al., showed in 2009 that in response to interferon-γ, mouse T-regs upregulated the T helper 1 (Th1)-specifying transcription factor T-bet, which is required for the homeostasis and function of T-reg cells during Th1-mediated inflammation (37). Similarly, it was observed that interferon regulatory factor-4 (IRF4), a transcription factor essential for T helper-2 effector cell differentiation, endows mouse T-regs with the ability to suppress T helper-2 responses (38). Moreover, deletion of the transcription factor STAT3 (transcription factor required for T helper-17 differentiation) from mouse T-regs resulted in the development of fatal intestinal inflammation (39). Single cell RNA sequencing of skin T-regs revealed that mouse T-regs preferentially expressed high levels T helper-2 associated transcription factor aka GATA3 during homeostasis. GATA3 deletion from mouse T-regs resulted in exacerbation of T helper-2 cytokine secretion and fibrosis in the skin (40). Fibrosis is the manifestation of chronically activated fibroblasts that secrete excessive amounts of ECM and leads to an increase in the dermal layer. Moreover, in mouse models of atopic dermatitis, retinoid-related orphan receptor α (RORα) in skin T-regs is important for restraining allergic skin inflammation (41).
More recently, single cell RNA-sequencing on isolated T-regs from the skin and skin draining lymph nodes (SDLN) of mice revealed a transcriptionally distinct feature of skin T-regs (42). As compared to the SDLN T-regs, skin T-regs are transcriptionally enriched in pathways associated with TGFβ and integrin signalling. In addition, there was a significant increase in the expression of receptor-ligand pairs that link to T-reg-epithelial cell crosstalk. The distinct transcriptomic signature implies a preferential interaction of skin T-regs with the skin epithelium to exert tissue specific functions. Further stratifying T-reg populations into those derived from the dermis and the epidermis revealed strong transcriptomic homogeny between the two T-reg populations. However, there was a notable preferential expression of Itgb8 gene, which form the TGFβ activating integrin αvβ8, in the epidermal T-regs. Epidermal T-regs also showed increased SMAD2/3 phosphorylation in response to TGFβ stimulation as compared to those of the dermis and SDLN. The TGFβ – integrin αvβ8 axis plays a major role in tissue repair/remodelling in the context of injury and skin pathology (43). Interestingly, while skin T-reg was shown to facilitate epidermal barrier repair (44), activation of latent TGF-β in T-regs via the TGF-β/αvβ8 pathway was shown to delay epithelial repair efficiency. Instead, the TGF-β/αvβ8 pathway elicited innate immune protection against Staphylococcus aureus infection. This implies the functional heterogeneity of tissue T-regs and the potential effect of tissue cytokine milieu on non-lymphoid T-reg responses. The exact mechanism of how skin T-regs balance the kinetics of tissue repair and pathogen defense in anatomically distinct areas of the skin remain to be elucidated.
Though similar to colonic T-regs, skin T-regs have unique features which may imply tissue specific functions. For example, the mice skin exclusively consists of T-regs that are lacking Id3 (the inhibitors of DNA binding 3) when compared to lymphoid T-regs. The Id3 negative cutaneous T-regs express inhibitory markers and probably more suppressive (45). Another molecule, arginase 2, has been shown to be preferentially expressed in healthy human skin T-regs compared to the skin T-effs or circulating T-regs. Arginase 2 increases in human T-regs in metastatic melanoma, and reduces in T-regs from psoriatic skin. Decreased levels of arginase 2 renders T-regs dysfunctional to suppress T-eff cells and vice vera. Arginase 2 helps T-regs to maintain tissue T-reg signatures, to regulate inflammation and enhance metabolic fitness (46). ARG2 expression in Tregs was shown to attenuate mTOR activity with time after Treg activation.
In 2011, the Abbas lab unraveled how chronic or repeated exposure to self-antigen within tissues leads to an attenuation of pathological autoimmune responses. Using a model of cutaneous self-antigen expression, it was found that self-antigen specific T-regs migrate out of the thymus. Further T-regs activate, proliferate, and differentiate into more potent suppressors in the SDLN and then migrate to the skin to mitigate autoimmune and skin inflammation. A subset of T-regs also confer a memory phenotype and are maintained for long periods with enhanced suppression capacity (47). These results are consistent with reported differences between the skin resident T-regs and those that have migrated into the tissue. For example, half of the T-regs in an inflamed mice ear are migratory and have been shown to have higher levels of CTLA4 and Nrp1 and lower levels of CD25 and CD39 when compared to the resident T-regs (48). The diversity in gene expression and function points to the varying potential of T-regs in suppressing inflammation. Important questions arise from these observations: 1. Does the diversity of T-reg subsets suggest that they perform multiple non-overlapping functions in the skin? and 2. Are some of those cutaneous functions associated with the non-immune cells?
Trafficking of cutaneous T-regs
As an example of its role as an immunological barrier, the human skin contains more T cells than the bloodstream. It is estimated that there are around 1 million T cells per square centimeter and 10 billion T cells in the entire skin (49). T-regs represent around 20% of the tissue resident CD4+ T cells in human skin and 20-60 percent in the mouse skin (4, 16, 41). Where do these T cells come from and how do they migrate to the skin?
The cutaneous migration of T-regs is important as the inability of T-regs to home to the skin is permissive of dermatitis and cutaneous inflammation (50). T-regs enter the skin is after post-natal day 6 (51), indicating that these cells are not required for the embryonic and early development this organ. However, there is a peak in the number of T-regs at post-natal day 13 when roughly 80 percent of CD4+ T cells are T-regs, which have migrated from the thymus (51). The significance of this peak is linked to the tolerance of commensal microbes (51). Following this peak there is a dip in the number of T-regs and a second peak at post-natal day 23. The importance of this second wave of T-regs is its association with the proliferation of hair follicle stem cells and subsequent growth of the hair follicle (16). Further, in steady state human skin resident memory T-regs reside in the epidermis and in the follicular epithelium in proximity to dermal dendritic cell (DDC) (52). It would thus be important to determine if there is an increase in a specific type of T-regs (nT-regs vs. iT-reg) given their differential representation in other organs in physiology and disease. Moreover, whether the cyclical changes observed in T-regs and T-cells in the skin is due to interconversion between natural and induced subtypes is yet to be addressed.
The migration of T-regs into the skin from the thymus and lymph nodes is guided by signals arising from the tissue resident cells and the corresponding expression of their cognate receptors on the T-regs. One class of migratory signals is the chemokine and chemokine receptor. The CCR4 chemokine receptor is robustly expressed by skin-homing T cells and the CCR4 ligand, TARC (Thymus and activation-regulated chemokine) is expressed by the endothelial cells of the cutaneous vasculature (53). Upon genetic ablation of CCR4 expression in T cells, homing of T cells to the skin is prevented (54). Cutaneous T-regs have also been shown to express CCR4, thus loss of CCR4 in T-regs, confers to them a competitive disadvantage with other CCR4 expressing T-cells to migrate into the skin and lungs. This leads to lymphocytic infiltration and severe inflammation in the skin (55).
Upon reaching the skin through the vasculature, cell adhesion molecules on the T-regs aid in binding the cell to the endothelium and promote extravasation. Surface expression of the ligands for E and P selectins found on endothelial cells is required for the exit of the T-regs from the circulation (3, 56). In a model of contact hypersensitivity, rolling of endogenous T-regs in dermal postcapillary venules was dependent on overlapping contributions of P- and E-selectin. However, after a repeated challenge, T-regs but not conventional CD4+ T cells became P-selectin independent. This was also supported by the reduced capacity of T-regs to bind P-selectin. Inhibition of E-selectin during a repeated challenge resulted in exacerbation of inflammation. This report suggested that there is a dynamic molecular shift in T-regs: the initial migration requires both P- and E-selectin whereas repeated challenge requires only E-selectin (57). The α-1,3-fucosyltransferase VII (FuT7) enzyme is responsible for catalyzing the formation of the carbohydrate determinants of E- and P-selectin. Consistent with this, the loss of FuT7 also reduced T-reg cell accumulation in the mouse skin, resulting in selective onset of severe cutaneous inflammation (50). Additionally, layilin (a C-type lectin-like receptor) is preferentially and highly expressed on a subset of activated Tregs in human skin (58). Layilin is induced by TCR-mediated activation (by IL-2 or TGF-β) and facilitates Treg adhesion in skin. Moreover, Layilin expression on T-regs limits their suppressive capacity (58).
Under homeostatic conditions, microbial-epithelial interactions also contribute to the migration of T-regs into the skin. The commensal bacteria of the skin microbiome play important roles in maintaining homeostasis (59). The tolerance of the skin commensal microbes is maintained by the cutaneous T-regs as they accumulate in the skin at postnatal days 6–13 (51). In the absence of this microbial interaction (such as the case of germ-free mice), a 20 percent reduction in the number of neonatal skin T-regs was reported (60). Furthermore, the microbiome induces the expression of CCL20 in the hair follicle cells and T-regs possess the receptor for CCL20 (i.e., CCR6) which aids in their retention near the hair follicle (60). CCR6 is a G protein-coupled receptor expressed on immature DCs, innate lymphoid cells, T-regs, Th17 cells and B cells (61). In human oral squamous cell carcinoma, CCR6 expressing T-reg were more suppressive with higher FOXP3 expression compared to CCR6 negative T-regs (62). Human skin T-regs express high levels of the memory markers such as CD27 and BCL-2, suggesting that they are effector memory T-regs (4). The expression memory markers can be a result of the interaction of T-regs with the skin microbiome.
Unconventional roles of cutaneous T-regs: Barrier function and wound healing
The functional importance of cutaneous T-regs in supporting skin barrier integrity was shown in mouse models where T-regs were selectively impaired in their ability to migrate to the skin. These mutant mice spontaneously developed visible dermatitis accompanied by a dense dermal infiltration of leukocytes and thickening of the epidermis (50, 55). Even in the absence of immunogenic foreign antigens, the lack of constitutive cutaneous T-reg migration can results in progressive immune responses. This can result from the mounting of an immune response from self-antigens, or from the skin’s own microbiome or the environment, which normally do not elicit a strong inflammatory response.
It is increasingly recognized that the skin microbiome is an integral part of the skin barrier that influence many aspects of skin health (63) and shape the cutaneous innate and adaptive immunity (64). An important method by which the skin microbiome contributes to homeostatic immunity is via regulating the immigration of T-regs into the skin to establish tolerance (51). While early skin colonization of commensal is critical for establishing commensal-specific immune tolerance (51), skin T-regs are also involved in regulating commensal-specific T cell responses in relation to local immunity and tissue remodeling (65). Harrison et al. reported that Foxp3+ T-regs are localized in closed proximity to S. epidermidis-induced CD8+ T cells in murine epidermis. Reduced level of skin T-regs through conditional deletion of GATA-3 in Treg cells were shown to selectively unleash type-2 cytokines by commensal-specific, RORγt-committed type-17 cells. which may be licensed by alarmins associated with tissue damages (65). This data suggests a potential role of skin T-reg in regulating commensal-specific T cell plasticity, allowing the cells to exert pleiotropic immunity and tissue repair functions, while preventing tissue predisposition to inflammation.
In addition, recent insights have revealed the unconventional roles of immune cells, in not only protecting the wound site from infection but actively promoting restoration of tissue homeostasis. Upon breaching of the physical barrier of the skin the wound healing program is initiated and is comprised of three sequential and overlapping phases: inflammation, proliferation, and remodeling. The early phase of the wound repair process is the inflammatory phase, which is rapidly launched by damage-associated molecular patterns (DAMPs) and chemokines released by the cells in wound areas that recruit and activate innate immune cells and later adaptive immune cells. These immune cells are critical to fending off infections but they must be tightly regulated to avoid secondary damage to the tissue.
Though regulated by different chemokines produced from numerous cell sources in the skin, it is tempting to speculate that different T-reg sub-populations may also contribute to the sequential appearance and activity of the innate and adaptive immune cells during the wound healing process. This is based on the correlation with the sequential recruitment of T-regs in the wound healing program. For instance, CD25+ T-regs are increased at an early-stage post wounding (day 3) while ICOS+ and CTLA4+ T-regs are increased at later stages (Day 7) (66). Interestingly, early ablation of T-regs (2 day prior to wounding up to 5 days post wounding) leads to significantly slower wound closure rates. However, depletion of these cells at later stages (post wounding day 5 to 10) had no effect on wound closure kinetics (66). The early ablation of T-regs in the wounded skin leads to exacerbated production of IFNγ (66), which increases monocyte differentiation to M1 (pro-inflammatory) macrophages as well as their accumulation (67). A functional consequence of accumulating the M1 macrophage is a delay in the wound healing program (68). There are additional instances in which T-regs impact the wound healing program. For example, upon depletion of T-regs in the wounded mice, the suppression of the immune responses is absent which leads to impaired barrier restoration (44). T-reg cells were shown to preferentially suppress early accumulation of IL-17A CD4+ T cells at the site of injury. In these settings, the T-reg mediated control of IL-17-CXCL5-neutrophil axis of inflammation is absent which in turn prevents the differentiation of hair follicle stem cells into the epithelial keratinocytes to aid in reepithelialization (44). Notably, in their model, absence of Treg cells compromised epidermal barrier repair upon injury but did not alter skin barrier integrity under homeostatic condition.
Differentiation of dermal fibroblast into myofibroblasts (ECM producing activated fibroblast) is another key event in tissue regeneration which requires tight regulation. In this context, T-regs prevent Th2 responses in the skin to suppress myofibroblast activation and thus chronic reduction of T-regs leads to increased TH2 cytokine production in skin, leading to persistent fibroblast activation and fibrosis (40). In a recent study in 2021, the number of IL-10+ and TGF-β+ T-regs were shown to increase after the topical application of 4% sodium dodecyl sulfate mediated skin barrier disruption in the mice (69). IL-10+ and TGF-β+ T-regs might play roles in suppressing inflammation and/or facilitate the barrier repair. Altogether, these observations highlight the importance of cutaneous T-regs as an integral component in maintaining skin immune homeostasis, thereby sustaining a healthy skin barrier function and support/regulating tissue regeneration (Figure 2)
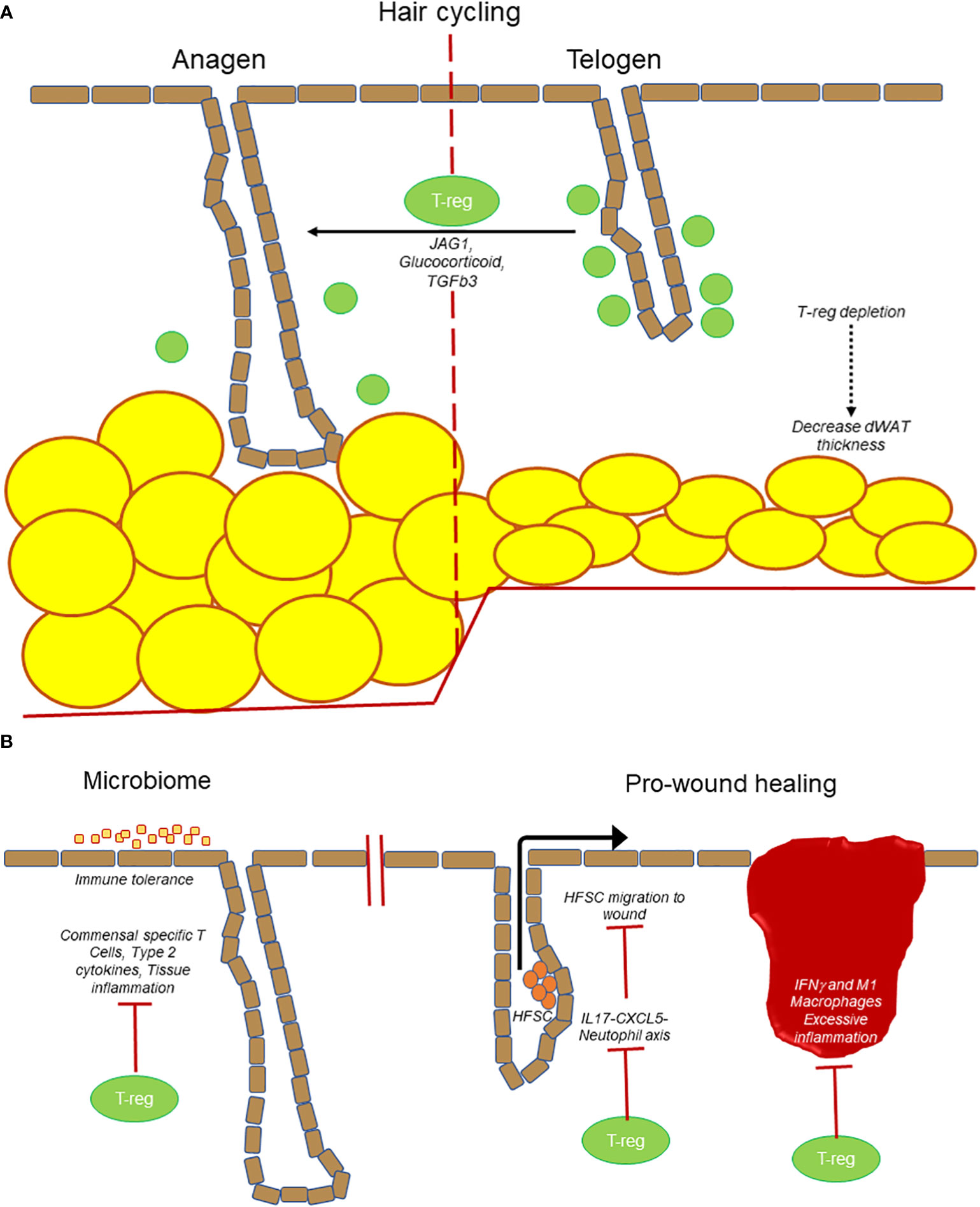
Figure 2 T-regs are at the center of many processes in the skin. (A) T-regs play a direct role in hair follicle cycling by promoting transition from telogen to anagen stage. Loss of T-regs also results in a decrease in dWAT thickness. (B) T-regs establish immune tolerance and prevent microbiome specific inflammation. T-regs also promote wound healing by suppressing IL17-CXCL5-neutophil axis to promote hair follicle stem cell (HFSC) migration. T-regs prevent excessive inflammation (IFNγ and M1 macrophages) to prevent delayed wound healing.
The reparative roles of T-regs are not only limited to the skin as it has also been reported to promote healing in the lungs and muscle (70, 71). A distinct population of T-regs can help in muscle repair by promoting satellite cell function and muscle repair by amphiregulin. A similar mechanism was reported in lung tissue repair by amphiregulin belonging to the same family of EGFR (70). Although there are very few T-regs in the brain, recent work (72) suggests that these cells play a role in restoring neurological homeostasis after ischemic stroke. These anecdotes suggest that we have just scratched the surface of understanding the full extent the role of T-regs in tissue repair throughout the body.
Hair follicle cycling
Hair follicles are “mini-organs” that undergo continuous cycles of regeneration. Interestingly, an emerging area of interest is the regulation of hair follicles by immune cells. At telogen stage, T-regs localize near the hair follicle stem cell niche known as the bulge region. In the well-established model of depilation-induced hair follicle regeneration, it was found that T-regs preferentially express the Notch ligand, Jagged1, which activates the Notch target genes transcripts on the hair follicle stem cells to initiate the transition from telogen to anagen (growth) stage. Subcutaneous administration of microbeads coated with Jag1-Fc was able to partially rescue HFSC activation and induction of anagen in the absence of Tregs. The result of Notch and Jagged1 interaction results in the proliferation and differentiation of hair follicle stem cells (16). More recently, it was reported that glucocorticoid signaling in T-regs is important in stimulating TGFβ3 production that can signal to the nearby hair follicle stem cells to enter anagen (73). Altogether these findings suggest that T-regs have direct unconventional functions in the skin to regulate the regeneration of the hair follicles (Figure 2).
Impact of T-regs via its regulation of other immune cells with unconventional functions in the skin
Other components of the skin undergo cyclic regeneration such as the dermis, adipose tissue, and epidermis, and it is tempting to propose that the cyclical nature of T-regs likewise regulates these processes either directly or through their modulation of other immune cell activities. It is well established that T-regs suppress various immune cells from generating an autoimmune response or ameliorating the inflammatory activity after infection or injury or antibody response. Thus, T-regs can function at a secondary level to control adaptive immune cells that in turn have novel functions in the skin (Figure 2).
Non-classical functions of adaptive immune cells
CD4+ and CD8+ T-cells are present in the skin at lower frequencies than macrophages, but during wound healing, infection, or autoimmunity, the numbers of T cells skyrocket. T cells have been found to affect numerous cells of the skin including dermal fibroblasts, keratinocytes, and hair follicles during pathological conditions (74, 75). The interaction of T cells with different compartments and cell types in the skin can be through the secretion of various cytokines. For example, some cytokines mediate interactions between the T-cells and fibroblasts- thereby affecting the activation status of the former:
● IL22 is a cytokine produced by subsets of T cells and has been linked to fibroblast function during wound healing. Both in vivo and in vitro evidence has revealed that IL22 in wounds displays severe defects in the dermal compartment and inhibits the ability of fibroblasts to produce ECM and differentiate into myofibroblasts (76).
● Similarly, in the GVHD-SSc mice that exhibit fibrotic skin, it was found that ICOS+ TFH-like (Inducible T-cell co-stimulator+ T Follicular Helper-like) cells produce IL21 and MMP 12, which cause activation of fibroblasts that lead to the pathology. Upon administration of anti-ICOS antibody or IL21 neutralization in GVHD-SSc, skin fibrosis was reduced (77).
● IL13 secreted by CD8 T cells has been shown to increase the ECM production from dermal fibroblasts (78). Similarly, when conditioned media from CD8+ CD28- T cells isolated from patients with the skin fibrotic disease scleroderma was added to dermal fibroblasts, there was an increase in collagen and fibronectin production. This effect was inhibited by the pre-treatment of IL13 antibody suggesting IL13 production from CD8 T cells causes activation of fibroblasts (79).
● TGFβ is a well-known cytokine that causes fibroblast activation and can be produced by various cell types in the skin. Conditioned media collected from the CD4 T cells from the skin of burn wound patients had significantly higher TGFβ levels compared to the normal subjects. Upon treatment of this conditioned media on fibroblasts, an increase in cell proliferation, collagen synthesis, smooth muscle actin levels, and collagen contraction was observed (80).
Interestingly, depletion of T-regs leads to an increase in Th2 cytokine production in the skin and the spontaneous activation of fibroblasts, which results in dermal fibrosis (40). T-reg depletion in the bleomycin-induced fibrosis model likewise exacerbated the fibrotic phenotype. Specific deletion of the transcription factor GATA3 in T-regs phenocopied the depletion of T-regs in terms of Th2 cytokine production and enhanced fibrosis. Moreover, antibody neutralization of IL4, the primary cytokine associated with Th2 type inflammation, augmented the fibrotic phenotype.
A major question in fibrogenesis is the source of these activated fibroblasts. Using a lineage tracing technique in bleomycin-treated mice, John Varga’s group demonstrated that the dermal adipocytes undergo an adipose to mesenchymal transition (AMT) (81). The resulting adipose-derived fibroblasts were actively producing collagens and contributing to fibrogenesis. This is consistent with observations in the fibrotic skin of both mouse and patients with scleroderma where an increase in the dermal layer is accompanied by a decrease in the dWAT layer (81–86). Interestingly, the study mentioned above by Rosenblum’s group (40), also noticed a decrease in the dWAT layer upon depletion of T-regs and was more pronounced upon bleomycin treatment. These reports imply a possible role of T-regs in the regulation of the dWAT layer. The histologic images of the skin section of the scurfy mice, which has complete loss of immunosuppressive activity of T-regs – display loss of dWAT, a phenotype that is often overlooked (87–91). In addition, the transient loss of T-regs also results in a decrease in the dWAT layer and an increase in the thickness of the fascia layer (92). In summary these data suggest that the T-regs in the skin prevent spontaneous inflammation, development of fibrosis, loss of dWAT layer and thickness of fascia layer. Nevertheless, questions remain about the role(s) that T-regs or Th2 inflammation play in the regulation of dWAT, whether these signals mediate an AMT in vivo, and if there more direct roles that T-regs can play, as reported in the case of hair follicle stem cells (16).
In addition to T-cell-derived cytokines, B cells have been shown to activate fibroblast in a contact-dependent manner. Co-culturing experiments revealed that B cells are capable of stimulating collagen secretion by dermal fibroblasts which is enhanced by B-cell activating factor (BAFF) (93). Likewise, a co-culture of circulating B cells and dermal fibroblasts isolated from SSc patients induced IL-6, TGF-β1, CCL2, and collagen secretion, as well as alpha-SMA, TIMP1, and MMP9 expression in dermal fibroblasts (93). Similarly, upon antibody mediated depletion of B cells in the Tight Skin model of systemic sclerosis, there was a significant reduction of fibrosis (94). Altogether, these results suggest that CD4 T+, and CD8 T+ can activate fibroblast via secreted factors and B cells achieve a similar response in a contact-dependent manner.
The thickening of the epidermal layer (epidermal hyperplasia) because of increased proliferation of the keratinocytes is a hallmark of the wound healing program as well as one of the major signs of diseased skin during pathological inflammation. The coincident observation of epidermal hyperplasia and inflammation suggest causal relationship. In fact, in vitro studies on epithelial keratinocytes suggests that IL22, which is often sourced from T-cells, can enhance the proliferative and migratory capacity of epidermal keratinocytes while their differentiation is repressed (95, 96). Further, it was reported that a subpopulation of lesional psoriatic T lymphocytes can enhance the proliferation of keratinocytes in vitro (97). Apart from the proliferation of keratinocytes apoptosis is associated with the pathogenesis of eczematous disorders. T cell infiltration leads to keratinocyte apoptosis by Fas ligand and Fas receptor interaction and increases susceptibility to INF-g mediated apoptosis (98). Another mode of inducing keratinocyte apoptosis is through the secretion of granzyme B from CD8 T cells (99). These results suggest that T cells can cause both proliferation and apoptosis of keratinocytes in different settings.
These findings suggest that T-regs have non-classical roles owing to their regulation of T and B-cells that are themselves increasingly recognized for unconventional activities (Figure 2). Given the heterogeneity of each of these cell types, it is likely that they will exhibit diverse activities, and many more novel cutaneous functions will be uncovered in both physiological and pathological scenarios.
Non-classical functions of Innate immune cells
In addition to the adaptive immune cells, the innate immune cells likewise impact homeostasis of various compartments of the skin. These innate immune cells are also regulated by T-regs and form another indirect link between T-regs and skin homeostasis (Figure 2). Langerhans cells (LCs) perform barrier functions by forming tight junctions with keratinocytes in the epidermis and scout the surroundings for potential pathogens (100). They are also involved in scavenging apoptotic keratinocytes, a process necessary for the maintenance of immune tolerance in the epidermis (101) Under stress, specific regions of the HF secrete different cytokines that differentially influence LC activity. The S1P1 + bulge cells secrete CCL8 to prevent the influx of LC during stress thereby protecting the stem cell niche. The isthmus and infundibular regions of the hair follicle recruit LCs by the production of CCL2 and CCL20. In fact, it was shown that in the absence of HF stem cells, Langerhans cells do not repopulate the epidermis after LC depletion signifying the cross-talk between HFs and the LC population (102). Studies on human skin have shown that skin resident memory T-regs residing in the epidermis and in the follicular epithelium are in close proximity to CD1a+ LC and CD1c/BDCA 1+ dermal dendritic cell (DDC) population in steady-state (52). As T-regs are also in close proximity to the hair follicle stem cell region, it would be interesting to ascertain whether they also influence LC indirectly via hair follicles or directly by cell-cell contact.
T-regs regulate macrophages mainly through IL10, which is crucial for immune tolerance (103, 104). T-regs are also capable of inducing macrophage apoptosis by the Fas/Fas ligand pathway during septic shock (105). Therefore, its effect on macrophages endows T-regs with the ability to influence hair follicle cycling since macrophages have been shown to be responsible for the induction of hair follicle anagen phase and regression phase by producing different cell signaling molecules such as Wnt and FGF (9, 106). As mentioned previously, apoptosis of macrophages releases Wnt7b and 10a and induces anagen phase of the hair cycle. Consistent with this, inhibition of macrophage apoptosis or Wnt signaling, results in a delay in the entry of the hair follicle into anagen (8). Additionally, oncostatin M (OSMR), released by a subset of TREM2+ macrophages during the telogen phase, contributes to the maintenance of hair follicle stem cell quiescence during the telogen phase (107). Consequently, ablation of OSMR beta or STAT5 can lead to early anagen induction. Interestingly, there is an inverse correlation of T-regs and macrophages during the hair cycle suggesting that T-regs indirectly regulates this follicular regenerative process through its control of macrophages. However, the direct link between T-regs and macrophages in the skin and their effect on hair follicle cycling remains to be established.
Another interaction that has thus far been reported only in inflammatory conditions is the crosstalk between T-regs and mast cells. In these scenarios T-regs have a dual effect on mast cells. T-regs can inhibit mast cell degranulation and also stimulate the production of IL-6, a cytokine with pleiotropic effects ranging from mediating local and systemic inflammation, tumorigenesis and autoimmune diseases (108). Role of Mast cells (MC) in hair follicle homeostasis came to light from a study in lesional alopecia areata (109). It was reported that MCs in alopecia areata switch from immune-inhibitory to pro-inflammatory phenotype suggested by the decreased TGFβ1, IL-10 and increased Tryptase immunoreactivity. Further, upon cyclosporine stimulated and depilation induced anagen, MC degranulation was observed during the early stages of anagen. The secretome of mast cells which contain numerous cytokines such as ACTH (adrenocorticotrophic hormone), substance 48/80, and the neuropeptide substance P were all able to induce anagen in mice skin (110). Similarly, induction of anagen was significantly delayed by MC degranulation inhibitors. However, the direct connection between T-regs and mast cells in the skin and their effect on hair follicle cycling remains to be established. In a mouse skin transplantation model where anti-CD154 and donor-specific transfusion (DST) was used to induce tolerance, a marked link between MCs and T-regs was observed through IL-9. IL 9 is a mast cell growth factor that can enhance the survival of primary MC, and induce the production of inflammatory cytokines, mast cell proteases, and high-affinity IgE receptors (FceR1 alpha). Upon activation, T-regs up-regulated IL9 production and a higher level of IL 9 was observed in tolerant grafts as opposed to rejected grafts. This tolerance could be due to the suppression of alloreactive CD8 T cells via the T-reg-IL9-MC axis (111, 112).
Future prospective
As discussed above, perturbations in T-regs or its downstream effectors often results in skin phenotypes in mice. This correlates with many human skin diseases (Figure 3) that are accompanied by changes in T-reg numbers or activity such as scleroderma (113–116), alopecia (117–119), psoriasis (120–123), atopic dermatitis (27, 124, 125) and vitiligo (126–128). Likewise, diabetic patients have compromised T-regs (129–131) which likely contributes to their chronic non-healing wounds. The status of T-regs in inflammatory conditions and their utility in combating scenarios such chronic wounds is largely unknown and an area that is ripe for investigation. As the major node of regulation of the immune responses in various tissues including skin, it is not too surprising that many cutaneous diseases are marked by perturbations in T-regs or its downstream effectors, thereby suggesting a causal role in pathogenesis. The role of T-regs in human diseases has been extensively covered elsewhere (132, 133), so we will only give a brief overview of this topic.
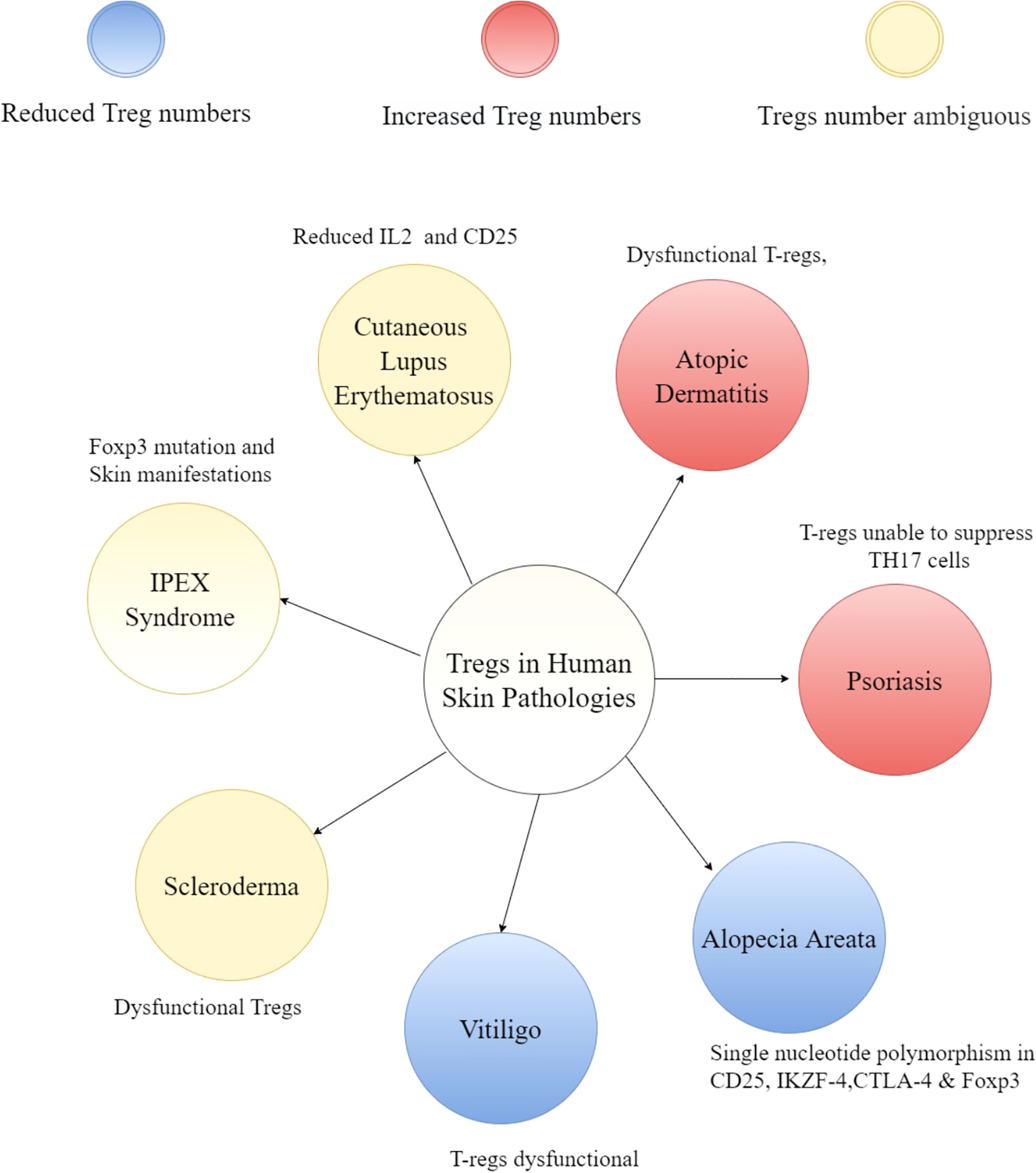
Figure 3 Overview of T-regs in human skin pathologies. A hub and spoke diagram summarizing the potential impact of T-regs and cytokines influencing the niche disease state.
Though there is a debate about whether the number of T-regs are altered in the fibrotic skin disease scleroderma, it is clear that these cells are dysfunctional (134, 135). In the case of alopecia areata, in which the hair follicles lose their immune privilege and are targeted by CD8+ T-cells, the number of T-regs are reduced (118) and unable to perform suppressive functions in-vitro (136). Genome-wide association studies (GWAS) in alopecia revealed single-nucleotide polymorphisms (SNPs) in regions encoding Treg signature genes such as CD25, the ikaros family member Eos (IKZF4), cytotoxic T-lymphocyte antigen 4 (CTLA-4) and Foxp3 (117, 119). These SNPs can possibly render T-regs dysfunctional. Dysfunctional T-regs have also been reported in psoriatic plaques which are unable to suppress TH17 responses (122). Further studies have revealed that T-regs in psoriasis can differentiate into IL-17 producing cells (137, 138). Similarly, T-regs can produce TH2 cytokines and contribute to the progression of atopic dermatitis (139). Tregs have also been shown to attenuate skin inflammation in several mouse models of atopic dermatitis (41, 140, 141). These results strongly suggest role of T-regs in various skin pathologies and likelihood of therapeutic window for many inflammatory skin diseases. For example, IL-2 treatment to boost T-regs have shown promising results of hair regeneration in patients with alopecia areata (142). Similarly, IL-2 mediated augmentation of T-regs reduces skin fibrosis in some patients suffering from graft-versus-host disease (GVHD) (143, 144). Interestingly, autologous hematopoietic stem cell transplantation which led to increase in the number of T-regs (along with higher expression of CTLA-4 and GITR on Tregs) resulted in clinical improvement in systemic sclerosis patients (145).
In the last two decades the unconventional roles of T-regs in skin and other tissues have been unraveled in both physiology and disease. Given the phenotypic diversity of T-regs and the ability to control both the innate and adaptive arms of immunity, we expect in the next few years a plethora of new and exciting functions of T-regs would be uncovered. The increased understanding of the diverse activities of T-regs will also open novel avenues for therapeutic intervention of many common inflammatory skin diseases. Lastly, development of T-reg based immunotherapies could potentially improve the quality of life in patients suffering from skin inflammatory pathologies.
Author contributions
EH, PP, C-CC, HJ, AM, and CJ wrote different sections of the review article. Order of authorship was determined based on the amount written. EH and CJ organized the review, assigned authors for each section, and revised the manuscript. All authors contributed to the article and approved the submitted version.
Funding
Research in the Jamora Laboratory is supported by core funds from the Institute for Stem Cell Science and Regenerative Medicine (inStem), Bellary Road, Bangalore, India and grants from the Department of Biotechnology of the Government of India (BT/PR8738/AGR/36/770/2013) and (BT/PR32539/BRB/10/1814/2019). EH was supported by an Indian Council for Medical Research (ICMR) Senior Research Fellowship. Animal studies in the Jamora lab is partially supported by the National Mouse Research Resource (NaMoR) grant BT/PR5981/MED/31/181/2012;2013-2016;2018 and 102/IFD/SAN/5003/2017-2018 from the Department of Biotechnology.
Conflict of interest
Authors CC and AM were employed by company Unilever.
The remaining authors declare that the research was conducted in the absence of any commercial or financial relationships that could be construed as a potential conflict of interest.
Publisher’s note
All claims expressed in this article are solely those of the authors and do not necessarily represent those of their affiliated organizations, or those of the publisher, the editors and the reviewers. Any product that may be evaluated in this article, or claim that may be made by its manufacturer, is not guaranteed or endorsed by the publisher.
References
1. Nestle FO, di Meglio P, Qin JZ, Nickoloff BJ. Skin immune sentinels in health and disease. Nat Rev Immunol (2009) 9:679–91. doi: 10.1038/NRI2622
2. Lai Y, Gallo RL. AMPed up immunity: how antimicrobial peptides have multiple roles in immune defense. Trends Immunol (2009) 30:131–41. doi: 10.1016/J.IT.2008.12.003
3. Hirahara K, Liu L, Clark RA, Yamanaka K, Fuhlbrigge RC, Kupper TS. The majority of human peripheral blood CD4+CD25highFoxp3+ regulatory T cells bear functional skin-homing receptors. J Immunol (2006) 177:4488–94. doi: 10.4049/JIMMUNOL.177.7.4488
4. Rodriguez RS, Pauli ML, Neuhaus IM, Yu SS, Arron ST, Harris HW, et al. Memory regulatory T cells reside in human skin. J Clin Invest (2014) 124:1027–36. doi: 10.1172/JCI72932
5. Müller-Röver S, Handjiski B, van der Veen C, Eichmüller S, Foitzik K, McKay IA, et al. A comprehensive guide for the accurate classification of murine hair follicles in distinct hair cycle stages. J Invest Dermatol (2001) 117:3–15. doi: 10.1046/J.0022-202X.2001.01377.X
6. Paus R, van der Veen C, Eichmüller S, Kopp T, Hagen E, Müller-Röver S, et al. Generation and cyclic remodeling of the hair follicle immune system in mice. J Invest Dermatol (1998) 111:7–18. doi: 10.1046/J.1523-1747.1998.00243.X
7. Rivera-Gonzalez G, Shook B, Horsley V. Adipocytes in skin health and disease. Cold Spring Harb Perspect Med (2014) 4:1–18. doi: 10.1101/CSHPERSPECT.A015271
8. Castellana D, Paus R, Perez-Moreno M. Macrophages contribute to the cyclic activation of adult hair follicle stem cells. PloS Biol (2014) 12:1–16. doi: 10.1371/JOURNAL.PBIO.1002002
9. Suzuki S, Kato T, Takimoto H, Masui S, Oshima H, Ozawa K, et al. Localization of rat FGF-5 protein in skin macrophage-like cells and FGF-5S protein in hair follicle: possible involvement of two fgf-5 gene products in hair growth cycle regulation. J Invest Dermatol (1998) 111:963–72. doi: 10.1046/J.1523-1747.1998.00427.X
10. Suzuki S, Ota Y, Ozawa K, Imamura T. Dual-mode regulation of hair growth cycle by two fgf-5 gene products. J Invest Dermatol (2000) 114:456–63. doi: 10.1046/J.1523-1747.2000.00912.X
11. Paus R, Maurer M, Slominski A, Czarnetzki BM. Mast cell involvement in murine hair growth. Dev Biol (1994) 163:230–40. doi: 10.1006/DBIO.1994.1139
12. Maurer M, Paus R, Czarnetzki BM. Mast cells as modulators of hair follicle cycling. Exp Dermatol (1995) 4:266–71. doi: 10.1111/J.1600-0625.1995.TB00256.X
13. Paus R, Heinzelmann T, Schultz KD, Furkert J, Fechner K, Czarnetzki BM. Hair growth induction by substance p. Lab Invest (1994) 71:134–40.
14. Paus R, Christoph T, Müller-Röver S. Immunology of the hair follicle: a short journey into terra incognita. J Investig Dermatol Symp Proc (1999) 4:226–34. doi: 10.1038/SJ.JIDSP.5640217
15. Rahmani W, Sinha S, Biernaskie J. Immune modulation of hair follicle regeneration. NPJ Regener Med (2020) 5:1–13. doi: 10.1038/S41536-020-0095-2
16. Ali N, Zirak B, Rodriguez RS, Pauli ML, Truong HA, Lai K, et al. Regulatory T cells in skin facilitate epithelial stem cell differentiation. Cell (2017) 169:1119–1129.e11. doi: 10.1016/J.CELL.2017.05.002
17. Morgan BA. The dermal papilla: an instructive niche for epithelial stem and progenitor cells in development and regeneration of the hair follicle. Cold Spring Harb Perspect Med (2014) 4:1–15. doi: 10.1101/CSHPERSPECT.A015180
18. Driskell RR, Jahoda CAB, Chuong CM, Watt FM, Horsley V. Defining dermal adipose tissue. Exp Dermatol (2014) 23:629–31. doi: 10.1111/EXD.12450
19. Zhang Lj, Chen SX, Guerrero-Juarez CF, Li F, Tong Y, Liang Y, et al. Age-related loss of innate immune antimicrobial function of dermal fat is mediated by transforming growth factor beta. Immunity (2019) 50:121–136.e5. doi: 10.1016/J.IMMUNI.2018.11.003
20. Alexander CM, Kasza I, Yen CLE, Reeder SB, Hernando D, Gallo RL, et al. Dermal white adipose tissue: a new component of the thermogenic response. J Lipid Res (2015) 56:2061–9. doi: 10.1194/JLR.R062893
21. Sakaguchi S, Sakaguchi N, Asano M, Itoh M, Toda M. Immunologic self-tolerance maintained by activated T cells expressing IL-2 receptor alpha-chains (CD25). breakdown of a single mechanism of self-tolerance causes various autoimmune diseases. J Immunol (1995) 155:1151–64.
22. Powrie F, Mason D. OX-22high CD4+ T cells induce wasting disease with multiple organ pathology: prevention by the OX-22low subset. J Exp Med (1990) 172:1701–8. doi: 10.1084/JEM.172.6.1701
23. Fontenot JD, Gavin MA, Rudensky AY. Foxp3 programs the development and function of CD4+CD25+ regulatory T cells. Nat Immunol (2003) 4:986–92. doi: 10.1038/NI904
24. Hori S, Nomura T, Sakaguchi S. Control of regulatory T cell development by the transcription factor Foxp3. Science (2003) 299:981–5. doi: 10.1126/SCIENCE.1079490
25. Khattri R, Cox T, Yasayko SA, Ramsdell F. An essential role for scurfin in CD4+CD25+ T regulatory cells. Nat Immunol (2003) 4:993–8. doi: 10.1038/NI909
26. Fontenot JD, Rasmussen JP, Williams LM, Dooley JL, Farr AG, Rudensky AY. Regulatory T cell lineage specification by the forkhead transcription factor foxp3. Immunity (2005) 22:329–41. doi: 10.1016/J.IMMUNI.2005.01.016
27. Bennett CL, Christie J, Ramsdell F, Brunkow ME, Ferguson PJ, Whitesell L, et al. The immune dysregulation, polyendocrinopathy, enteropathy, X-linked syndrome (IPEX) is caused by mutations of FOXP3. Nat Genet (2001) 27:20–1. doi: 10.1038/83713
28. Brunkow ME, Jeffery EW, Hjerrild KA, Paeper B, Clark LB, Yasayko SA, et al. Disruption of a new forkhead/winged-helix protein, scurfin, results in the fatal lymphoproliferative disorder of the scurfy mouse. Nat Genet (2001) 27:68–73. doi: 10.1038/83784
29. Devaud C, Darcy PK, Kershaw MH. Foxp3 expression in T regulatory cells and other cell lineages. Cancer Immunol Immunother (2014) 63:869–76. doi: 10.1007/S00262-014-1581-4
30. Setoguchi R, Hori S, Takahashi T, Sakaguchi S. Homeostatic maintenance of natural Foxp3(+) CD25(+) CD4(+) regulatory T cells by interleukin (IL)-2 and induction of autoimmune disease by IL-2 neutralization. J Exp Med (2005) 201:723–35. doi: 10.1084/JEM.20041982
31. Fontenot JD, Rasmussen JP, Gavin MA, Rudensky AY. A function for interleukin 2 in Foxp3-expressing regulatory T cells. Nat Immunol (2005) 6:1142–51. doi: 10.1038/NI1263
32. Schmitt EG, Williams CB. Generation and function of induced regulatory T cells. Front Immunol (2013) 4:152. doi: 10.3389/FIMMU.2013.00152
33. Chen WJ, Jin W, Hardegen N, Lei KJ, Li L, Marinos N, et al. Conversion of peripheral CD4+CD25- naive T cells to CD4+CD25+ regulatory T cells by TGF-beta induction of transcription factor Foxp3. J Exp Med (2003) 198:1875–86. doi: 10.1084/JEM.20030152
34. Floess S, Freyer J, Siewert C, Baron U, Olek S, Polansky J, et al. Epigenetic control of the foxp3 locus in regulatory T cells. PloS Biol (2007) 5:0169–78. doi: 10.1371/JOURNAL.PBIO.0050038
35. Miragaia RJ, Gomes T, Chomka A, Jardine L, Riedel A, Hegazy AN, et al. Single-cell transcriptomics of regulatory T cells reveals trajectories of tissue adaptation. Immunity (2019) 50:493–504.e7. doi: 10.1016/J.IMMUNI.2019.01.001
36. Chen X, Oppenheim JJ. Resolving the identity myth: key markers of functional CD4+FoxP3+ regulatory T cells. Int Immunopharmacol (2011) 11:1489–96. doi: 10.1016/J.INTIMP.2011.05.018
37. Koch MA, Tucker-Heard G, Perdue NR, Killebrew JR, Urdahl KB, Campbell DJ. The transcription factor T-bet controls regulatory T cell homeostasis and function during type 1 inflammation. Nat Immunol (2009) 10:595–602. doi: 10.1038/NI.1731
38. Zheng Y, Chaudhry A, Kas A, DeRoos P, Kim JM, Chu TT, et al. Regulatory T-cell suppressor program co-opts transcription factor IRF4 to control T(H)2 responses. Nature (2009) 458:351–6. doi: 10.1038/NATURE07674
39. Chaudhry A, Rudra D, Treuting P, Samstein RM, Liang Y, Kas A, et al. CD4+ regulatory T cells control TH17 responses in a Stat3-dependent manner. Science (2009) 326:986–91. doi: 10.1126/SCIENCE.1172702
40. Kalekar LA, Cohen JN, Prevel N, Sandoval PM, Mathur AN, Moreau JM, et al. Regulatory T cells in skin are uniquely poised to suppress profibrotic immune responses. Sci Immunol (2019) 4:1–26. doi: 10.1126/SCIIMMUNOL.AAW2910
41. Malhotra N, Leyva-Castillo JM, Jadhav U, Barreiro O, Kam C, O’Neill NK, et al. RORα-expressing T regulatory cells restrain allergic skin inflammation. Sci Immunol (2018) 3:1–25. doi: 10.1126/SCIIMMUNOL.AAO6923
42. Moreau JM, Dhariwala MO, Gouirand V, Boda DP, Boothby IC, Lowe MM, et al. Regulatory T cells promote innate inflammation after skin barrier breach via TGF-β activation. Sci Immunol (2021) 6:1–21. doi: 10.1126/SCIIMMUNOL.ABG2329
43. Konkel JE, Zhang D, Zanvit P, Chia C, Zangarle-Murray T, Jin W, et al. Transforming growth factor-β signaling in regulatory T cells controls T helper-17 cells and tissue-specific immune responses. Immunity (2017) 46:660–74. doi: 10.1016/J.IMMUNI.2017.03.015/ATTACHMENT/74822171-D378-4460-B0AE-CF1E37ABFB97/MMC1.PDF
44. Mathur AN, Zirak B, Boothby IC, Tan M, Cohen JN, Mauro TM, et al. Treg-cell control of a CXCL5-IL-17 inflammatory axis promotes hair-Follicle-Stem-Cell differentiation during skin-barrier repair. Immunity (2019) 50:655–667.e4. doi: 10.1016/J.IMMUNI.2019.02.013
45. Sullivan JM, Höllbacher B, Campbell DJ. Cutting edge: Dynamic expression of Id3 defines the stepwise differentiation of tissue-resident regulatory T cells. J Immunol (2019) 202:31–6. doi: 10.4049/JIMMUNOL.1800917
46. Lowe MM, Boothby I, Clancy S, Ahn RS, Liao W, Nguyen DN, et al. Regulatory T cells use arginase 2 to enhance their metabolic fitness in tissues. JCI Insight (2019) 4:1–18. doi: 10.1172/JCI.INSIGHT.129756
47. Rosenblum MD, Gratz IK, Paw JS, Lee K, Marshak-Rothstein A, Abbas AK. Response to self antigen imprints regulatory memory in tissues. Nature (2011) 480:538–42. doi: 10.1038/NATURE10664
48. Ikebuchi R, Fujimoto M, Nakanishi Y, Okuyama H, Moriya T, Kusumoto Y, et al. Functional phenotypic diversity of regulatory T cells remaining in inflamed skin. Front Immunol (2019) 10:1098. doi: 10.3389/FIMMU.2019.01098
49. Clark RA, Chong B, Mirchandani N, Brinster NK, Yamanaka K, Dowgiert RK, et al. The vast majority of CLA+ T cells are resident in normal skin. J Immunol (2006) 176:4431–9. doi: 10.4049/JIMMUNOL.176.7.4431
50. Dudda JC, Perdue N, Bachtanian E, Campbell DJ. Foxp3+ regulatory T cells maintain immune homeostasis in the skin. J Exp Med (2008) 205:1559–85. doi: 10.1084/JEM.20072594
51. Scharschmidt TC, Vasquez KS, Truong HA, Gearty SV, Pauli ML, Nosbaum A, et al. A wave of regulatory T cells into neonatal skin mediates tolerance to commensal microbes. Immunity (2015) 43:1011–21. doi: 10.1016/J.IMMUNI.2015.10.016
52. Seneschal J, Clark RA, Gehad A, Baecher-Allan CM, Kupper TS. Human epidermal langerhans cells maintain immune homeostasis in skin by activating skin resident regulatory T cells. Immunity (2012) 36:873–84. doi: 10.1016/J.IMMUNI.2012.03.018
53. Campbell JJ, Haraldsen G, Pan J, Rottman J, Qin S, Ponath P, et al. The chemokine receptor CCR4 in vascular recognition by cutaneous but not intestinal memory T cells. Nature (1999) 400:776–80. doi: 10.1038/23495
54. Tubo NJ, McLachlan JB, Campbell JJ. Chemokine receptor requirements for epidermal T-cell trafficking. Am J Pathol (2011) 178:2496–503. doi: 10.1016/J.AJPATH.2011.02.031
55. Sather BD, Treuting P, Perdue N, Miazgowicz M, Fontenot JD, Rudensky AY, et al. Altering the distribution of Foxp3(+) regulatory T cells results in tissue-specific inflammatory disease. J Exp Med (2007) 204:1335–47. doi: 10.1084/JEM.20070081
56. Tietz W, Allemand Y, Borges E, von Laer D, Hallmann R, Vestweber D, et al. CD4+ T cells migrate into inflamed skin only if they express ligands for e- and p-selectin. J Immunol (1998) 161:963–70.
57. Abeynaike LD, Deane JA, Westhorpe CLV, Chow Z, Alikhan MA, Kitching AR, et al. Regulatory T cells dynamically regulate selectin ligand function during multiple challenge contact hypersensitivity. J Immunol (2014) 193:4934–44. doi: 10.4049/JIMMUNOL.1400641
58. Mehta P, Gouirand V, Boda DP, Zhang J, Gearty SV, Zirak B, et al. Layilin anchors regulatory T cells in skin. J Immunol (2021) 207:1763–75. doi: 10.4049/JIMMUNOL.2000970
59. Byrd AL, Belkaid Y, Segre JA. The human skin microbiome. Nat Rev Microbiol (2018) 16:143–55. doi: 10.1038/NRMICRO.2017.157
60. Scharschmidt TC, Vasquez KS, Pauli ML, Leitner EG, Chu K, Truong HA, et al. Commensal microbes and hair follicle morphogenesis coordinately drive treg migration into neonatal skin. Cell Host Microbe (2017) 21:467–477.e5. doi: 10.1016/J.CHOM.2017.03.001
61. Meitei HT, Jadhav N, Lal G. CCR6-CCL20 axis as a therapeutic target for autoimmune diseases. Autoimmun Rev (2021) 20:1–7. doi: 10.1016/J.AUTREV.2021.102846
62. Lee J-J, Kao K-C, Chiu Y-L, Jung C-J, Liu C-J, Cheng S-J, et al. Enrichment of human CCR6 + regulatory T cells with superior suppressive activity in oral cancer. J Immunol (2017) 199:467–76. doi: 10.4049/JIMMUNOL.1601815
63. Eyerich S, Eyerich K, Traidl-Hoffmann C, Biedermann T. Cutaneous barriers and skin immunity: Differentiating a connected network. Trends Immunol (2018) 39:315–27. doi: 10.1016/J.IT.2018.02.004
64. Belkaid Y, Tamoutounour S. The influence of skin microorganisms on cutaneous immunity. Nat Rev Immunol (2016) 16:353–66. doi: 10.1038/NRI.2016.48
65. Harrison OJ, Linehan JL, Shih HY, Bouladoux N, Han SJ, Smelkinson M, et al. Commensal-specific T cell plasticity promotes rapid tissue adaptation to injury. Sci (1979) (2019) 363:1–27. doi: 10.1126/SCIENCE.AAT6280/SUPPL_FILE/AAT6280_HARRISON_SM.PDF
66. Nosbaum A, Prevel N, Truong H-A, Mehta P, Ettinger M, Scharschmidt TC, et al. Cutting edge: Regulatory T cells facilitate cutaneous wound healing. J Immunol (2016) 196:2010–4. doi: 10.4049/JIMMUNOL.1502139
67. Wang N, Liang H, Zen K. Molecular mechanisms that influence the macrophage m1-m2 polarization balance. Front Immunol (2014) 5:614. doi: 10.3389/FIMMU.2014.00614
68. Sindrilaru A, Peters T, Wieschalka S, Baican C, Baican A, Peter H, et al. An unrestrained proinflammatory M1 macrophage population induced by iron impairs wound healing in humans and mice. J Clin Invest (2011) 121:985–97. doi: 10.1172/JCI44490
69. Toyama S, Moniaga CS, Nakae S, Kurosawa M, Ogawa H, Tominaga M, et al. Regulatory t cells exhibit interleukin-33-dependent migratory behavior during skin barrier disruption. Int J Mol Sci (2021) 22:1–13. doi: 10.3390/IJMS22147443/S1
70. Arpaia N, Green JA, Moltedo B, Arvey A, Hemmers S, Yuan S, et al. A distinct function of regulatory T cells in tissue protection. Cell (2015) 162:1078–89. doi: 10.1016/J.CELL.2015.08.021
71. Burzyn D, Kuswanto W, Kolodin D, Shadrach JL, Cerletti M, Jang Y, et al. A special population of regulatory T cells potentiates muscle repair. Cell (2013) 155:1–24. doi: 10.1016/J.CELL.2013.10.054
72. Ito M, Komai K, Nakamura T, Srirat T, Yoshimura A. Tissue regulatory T cells and neural repair. Int Immunol (2019) 31:361–9. doi: 10.1093/INTIMM/DXZ031
73. Liu Z, Hu X, Liang Y, Yu J, Li H, Shokhirev MN, et al. Glucocorticoid signaling and regulatory T cells cooperate to maintain the hair-follicle stem-cell niche. Nat Immunol (2022) 23:1086–1097. doi: 10.1038/S41590-022-01244-9
74. Ho AW, Kupper TS. T Cells and the skin: from protective immunity to inflammatory skin disorders. Nat Rev Immunol (2019) 19:490–502. doi: 10.1038/S41577-019-0162-3
75. Bertolini M, McElwee K, Gilhar A, Bulfone-Paus S, Paus R. Hair follicle immune privilege and its collapse in alopecia areata. Exp Dermatol (2020) 29:703–25. doi: 10.1111/EXD.14155
76. McGee HM, Schmidt BA, Booth CJ, Yancopoulos GD, Valenzuela DM, Murphy AJ, et al. IL-22 promotes fibroblast-mediated wound repair in the skin. J Invest Dermatol (2013) 133:1321–9. doi: 10.1038/JID.2012.463
77. Taylor DK, Mittereder N, Kuta E, Delaney T, Burwell T, Dacosta K, et al. T Follicular helper-like cells contribute to skin fibrosis. Sci Transl Med (2018) 10:1–15. doi: 10.1126/SCITRANSLMED.AAF5307
78. Fuschiotti P, Larregina AT, Ho J, Feghali-Bostwick C, Medsger TA. Interleukin-13-producing CD8+ T cells mediate dermal fibrosis in patients with systemic sclerosis. Arthritis Rheum (2013) 65:236–46. doi: 10.1002/ART.37706
79. Li G, Larregina AT, Domsic RT, Stolz DB, Medsger TA, Lafyatis R, et al. Skin-resident effector memory CD8 + CD28 - T cells exhibit a profibrotic phenotype in patients with systemic sclerosis. J Invest Dermatol (2017) 137:1042–50. doi: 10.1016/J.JID.2016.11.037
80. Wang JF, Jiao H, Stewart TL, Shankowsky HA, Scott PG, Tredget EE. Increased TGF-beta-producing CD4+ T lymphocytes in postburn patients and their potential interaction with dermal fibroblasts in hypertrophic scarring. Wound Repair Regener (2007) 15:530–9. doi: 10.1111/J.1524-475X.2007.00261.X
81. Marangoni RG, Korman BD, Wei J, Wood TA, Graham LV, Whitfield ML, et al. Myofibroblasts in murine cutaneous fibrosis originate from adiponectin-positive intradermal progenitors. Arthritis Rheumatol (2015) 67:1062–73. doi: 10.1002/ART.38990
82. Marangoni RG, Lu TT. The roles of dermal white adipose tissue loss in scleroderma skin fibrosis. Curr Opin Rheumatol (2017) 29:585–90. doi: 10.1097/BOR.0000000000000437
83. Liu SY, Wu JJ, Chen ZH, Zou ML, Teng YY, Zhang KW, et al. Insight into the role of dermal white adipose tissue loss in dermal fibrosis. J Cell Physiol (2022) 237:169–77. doi: 10.1002/JCP.30552
84. Marangoni RG, Korman B, Varga J. Adipocytic progenitor cells give rise to pathogenic myofibroblasts: Adipocyte-to-Mesenchymal transition and its emerging role in fibrosis in multiple organs. Curr Rheumatol Rep (2020) 22:1–8. doi: 10.1007/S11926-020-00957-W
85. Varga J, Marangoni RG. Systemic sclerosis in 2016: Dermal white adipose tissue implicated in SSc pathogenesis. Nat Rev Rheumatol (2017) 13:71–2. doi: 10.1038/NRRHEUM.2016.223
86. Chia JJ, Zhu T, Chyou S, Dasoveanu DC, Carballo C, Tian S, et al. Dendritic cells maintain dermal adipose-derived stromal cells in skin fibrosis. J Clin Invest (2016) 126:4331–45. doi: 10.1172/JCI85740
87. Hadaschik EN, Wei X, Leiss H, Heckmann B, Niederreiter B, Steiner G, et al. Regulatory T cell-deficient scurfy mice develop systemic autoimmune features resembling lupus-like disease. Arthritis Res Ther (2015) 17:1–12. doi: 10.1186/S13075-015-0538-0
88. Sharma R, Sung SSJ, Fu SM, Ju S. Regulation of multi-organ inflammation in the regulatory T cell-deficient scurfy mice. J BioMed Sci (2009) 16:1–8. doi: 10.1186/1423-0127-16-20
89. Aschermann S, Lehmann CHK, Mihai S, Schett G, Dudziak D, Nimmerjahn F. B cells are critical for autoimmune pathology in scurfy mice. Proc Natl Acad Sci U.S.A. (2013) 110:19042–7. doi: 10.1073/PNAS.1313547110
90. Muramatsu K, Ujiie H, Kobayashi I, Nishie W, Izumi K, Ito T, et al. Regulatory T-cell dysfunction induces autoantibodies to bullous pemphigoid antigens in mice and human subjects. J Allergy Clin Immunol (2018) 142:1818–1830.e6. doi: 10.1016/j.jaci.2018.03.014
91. Lahl K, Mayer CT, Bopp T, Huehn J, Loddenkemper C, Eberl G, et al. Nonfunctional regulatory T cells and defective control of Th2 cytokine production in natural scurfy mutant mice. J Immunol (2009) 183:5662–72. doi: 10.4049/jimmunol.0803762
92. Boothby IC, Kinet MJ, Boda DP, Kwan EY, Clancy S, Cohen JN, et al. Early-life inflammation primes a T helper 2 cell-fibroblast niche in skin. Nature (2021) 599:667–72. doi: 10.1038/s41586-021-04044-7
93. François A, Chatelus E, Wachsmann D, Sibilia J, Bahram S, Alsaleh G, et al. B lymphocytes and b-cell activating factor promote collagen and profibrotic markers expression by dermal fibroblasts in systemic sclerosis. Arthritis Res Ther (2013) 15:1–9. doi: 10.1186/AR4352
94. Hasegawa M, Hamaguchi Y, Yanaba K, Bouaziz JD, Uchida J, Fujimoto M, et al. B-lymphocyte depletion reduces skin fibrosis and autoimmunity in the tight-skin mouse model for systemic sclerosis. Am J Pathol (2006) 169:954–66. doi: 10.2353/AJPATH.2006.060205
95. Wolk K, Witte E, Wallace E, Döcke WD, Kunz S, Asadullah K, et al. IL-22 regulates the expression of genes responsible for antimicrobial defense, cellular differentiation, and mobility in keratinocytes: a potential role in psoriasis. Eur J Immunol (2006) 36:1309–23. doi: 10.1002/EJI.200535503
96. Boniface K, Bernard F-X, Garcia M, Gurney AL, Lecron J-C, Morel F. IL-22 inhibits epidermal differentiation and induces proinflammatory gene expression and migration of human keratinocytes. J Immunol (2005) 174:3695–702. doi: 10.4049/jimmunol.174.6.3695
97. Prinz JC, Groß B, Vollmer S, Trommler P, Strobel I, Meurer M, et al. T Cell clones from psoriasis skin lesions can promote keratinocyte proliferation in vitro via secreted products. Eur J Immunol (1994) 24:593–8. doi: 10.1002/EJI.1830240315
98. Trautmann A, Akdis M, Kleemann D, Altznauer F, Simon HU, Graeve T, et al. T Cell-mediated fas-induced keratinocyte apoptosis plays a key pathogenetic role in eczematous dermatitis. J Clin Invest (2000) 106:25–35. doi: 10.1172/JCI9199
99. Shimizu M, Higaki Y, Higaki M, Kawashima M. The role of granzyme b-expressing CD8-positive T cells in apoptosis of keratinocytes in lichen planus. Arch Dermatol Res (1997) 289:527–32. doi: 10.1007/S004030050234
100. Kubo A, Nagao K, Yokouchi M, Sasaki H, Amagai M. External antigen uptake by langerhans cells with reorganization of epidermal tight junction barriers. J Exp Med (2009) 206:2937–46. doi: 10.1084/JEM.20091527/VIDEO-4
101. Hatakeyama M, Fukunaga A, Washio K, Taguchi K, Oda Y, Ogura K, et al. Anti-inflammatory role of langerhans cells and apoptotic keratinocytes in ultraviolet-B-Induced cutaneous inflammation. J Immunol (2017) 199:2937–47. doi: 10.4049/JIMMUNOL.1601681
102. Nagao K, Kobayashi T, Moro K, Ohyama M, Adachi T, Kitashima DY, et al. Stress-induced production of chemokines by hair follicles regulates the trafficking of dendritic cells in skin. Nat Immunol (2012) 13:744–52. doi: 10.1038/NI.2353
103. Ehrchen J, Steinmüller L, Barczyk K, Tenbrock K, Nacken W, Eisenacher M, et al. Glucocorticoids induce differentiation of a specifically activated, anti-inflammatory subtype of human monocytes. Blood (2007) 109:1265–74. doi: 10.1182/BLOOD-2006-02-001115
104. Zigmond E, Bernshtein B, Friedlander G, Walker CR, Yona S, Kim KW, et al. Macrophage-restricted interleukin-10 receptor deficiency, but not IL-10 deficiency, causes severe spontaneous colitis. Immunity (2014) 40:720–33. doi: 10.1016/J.IMMUNI.2014.03.012
105. Venet F, Pachot A, Debard A-L, Bohe J, Bienvenu J, Lepape A, et al. Human CD4+CD25+ regulatory T lymphocytes inhibit lipopolysaccharide-induced monocyte survival through a Fas/Fas ligand-dependent mechanism. J Immunol (2006) 177:6540–7. doi: 10.4049/JIMMUNOL.177.9.6540
106. Qiu W, Lei M, Zhou L, Bai X, Lai X, Yu Y, et al. Hair follicle stem cell proliferation, akt and wnt signaling activation in TPA-induced hair regeneration. Histochem Cell Biol (2017) 147:749–58. doi: 10.1007/S00418-017-1540-1
107. Wang ECE, Dai Z, Ferrante AW, Drake CG, Christiano AM. A subset of TREM2 + dermal macrophages secretes oncostatin m to maintain hair follicle stem cell quiescence and inhibit hair growth. Cell Stem Cell (2019) 24:654–669.e6. doi: 10.1016/J.STEM.2019.01.011
108. Ganeshan K, Bryce PJ. Regulatory T cells enhance mast cell production of IL-6 via surface-bound TGF-β. J Immunol (2012) 188:594–603. doi: 10.4049/jimmunol.1102389
109. Bertolini M, Zilio F, Rossi A, Kleditzsch P, Emelianov VE, Gilhar A, et al. Abnormal interactions between perifollicular mast cells and CD8+ T-cells may contribute to the pathogenesis of alopecia areata. PloS One (2014) 9:1–16. doi: 10.1371/JOURNAL.PONE.0094260
110. Paus R, Heinzelmann T, Robicsek S, Czarnetzki BM, Maurer M. Substance p stimulates murine epidermal keratinocyte proliferation and dermal mast cell degranulation in situ. Arch Dermatol Res (1995) 287:500–2. doi: 10.1007/BF00373436
111. Sayed BA, Brown MA. Mast cells as modulators of T-cell responses. Immunol Rev (2007) 217:53–64. doi: 10.1111/J.1600-065X.2007.00524.X
112. Lu LF, Lind EF, Gondek DC, Bennett KA, Gleeson MW, Pino-Lagos K, et al. Mast cells are essential intermediaries in regulatory T-cell tolerance. Nature (2006) 442:997–1002. doi: 10.1038/NATURE05010
113. Antiga E, Quaglino P, Bellandi S, Volpi W, del Bianco E, Comessatti A, et al. Regulatory T cells in the skin lesions and blood of patients with systemic sclerosis and morphoea. Br J Dermatol (2010) 162:1056–63. doi: 10.1111/J.1365-2133.2010.09633.X
114. Lee CG, Homer RJ, Zhu Z, Lanone S, Wang X, Koteliansky V, et al. Interleukin-13 induces tissue fibrosis by selectively stimulating and activating transforming growth factor beta(1). J Exp Med (2001) 194:809–21. doi: 10.1084/JEM.194.6.809
115. Chiaramonte MG, Mentink-Kane M, Jacobson BA, Cheever AW, Whitters MJ, Goad MEP, et al. Regulation and function of the interleukin 13 receptor alpha 2 during a T helper cell type 2-dominant immune response. J Exp Med (2003) 197:687–701. doi: 10.1084/JEM.20020903
116. Yang X, Yang J, Xing X, Wan L, Li M. Increased frequency of Th17 cells in systemic sclerosis is related to disease activity and collagen overproduction. Arthritis Res Ther (2014) 16:R4. doi: 10.1186/ar4430
117. Petukhova L, Duvic M, Hordinsky M, Norris D, Price V, Shimomura Y, et al. Genome-wide association study in alopecia areata implicates both innate and adaptive immunity. Nature (2010) 466:113–7. doi: 10.1038/NATURE09114
118. Han YM, Sheng YY, Xu F, Qi SS, Liu XJ, Hu RM, et al. Imbalance of T-helper 17 and regulatory T cells in patients with alopecia areata. J Dermatol (2015) 42:981–8. doi: 10.1111/1346-8138.12978
119. Conteduca G, Rossi A, Megiorni F, Parodi A, Ferrera F, Tardito S, et al. Single nucleotide polymorphisms in the promoter regions of Foxp3 and ICOSLG genes are associated with alopecia areata. Clin Exp Med (2014) 14:91–7. doi: 10.1007/S10238-012-0224-3
120. di Meglio P, Villanova F, Nestle FO. Psoriasis. Cold Spring Harb Perspect Med (2014) 4:1–30. doi: 10.1101/CSHPERSPECT.A015354
121. Sugiyama H, Gyulai R, Toichi E, Garaczi E, Shimada S, Stevens SR, et al. Dysfunctional blood and target tissue CD4+CD25high regulatory T cells in psoriasis: mechanism underlying unrestrained pathogenic effector T cell proliferation. J Immunol (2005) 174:164–73. doi: 10.4049/JIMMUNOL.174.1.164
122. Goodman WA, Levine AD, Massari JV, Sugiyama H, McCormick TS, Cooper KD. IL-6 signaling in psoriasis prevents immune suppression by regulatory T cells. J Immunol (2009) 183:3170–6. doi: 10.4049/JIMMUNOL.0803721
123. Ahn R, Yan D, Chang HW, Lee K, Bhattarai S, Huang ZM, et al. RNA-Seq and flow-cytometry of conventional, scalp, and palmoplantar psoriasis reveal shared and distinct molecular pathways. Sci Rep (2018) 8:1–12. doi: 10.1038/S41598-018-29472-W
124. van Gool F, Nguyen MLT, Mumbach MR, Satpathy AT, Rosenthal WL, Giacometti S, et al. A mutation in the transcription factor Foxp3 drives T helper 2 effector function in regulatory T cells. Immunity (2019) 50:362–377.e6. doi: 10.1016/J.IMMUNI.2018.12.016
125. Ito Y, Adachi Y, Makino T, Higashiyama H, Fuchizawa T, Shimizu T, et al. Expansion of FOXP3-positive CD4+CD25+ T cells associated with disease activity in atopic dermatitis. Ann Allergy Asthma Immunol (2009) 103:160–5. doi: 10.1016/S1081-1206(10)60170-6
126. Lili Y, Yi W, Ji Y, Yue S, Weimin S, Ming L. Global activation of CD8+ cytotoxic T lymphocytes correlates with an impairment in regulatory T cells in patients with generalized vitiligo. PloS One (2012) 7:1–10. doi: 10.1371/JOURNAL.PONE.0037513
127. Hegab DS, Attia MAS. Decreased circulating T regulatory cells in Egyptian patients with nonsegmental vitiligo: Correlation with disease activity. Dermatol Res Pract (2015) 2015:1–7. doi: 10.1155/2015/145409
128. Tembhre MK, Parihar AS, Sharma VK, Sharma A, Chattopadhyay P, Gupta S. Alteration in regulatory T cells and programmed cell death 1-expressing regulatory T cells in active generalized vitiligo and their clinical correlation. Br J Dermatol (2015) 172:940–50. doi: 10.1111/BJD.13511
129. Kukreja A, Cost G, Marker J, Zhang C, Sun Z, Lin-Su K, et al. Multiple immuno-regulatory defects in type-1 diabetes. J Clin Invest (2002) 109:131–40. doi: 10.1172/JCI13605
130. Visperas A, Vignali DAA. Are regulatory T cells defective in type 1 diabetes and can we fix them? J Immunol (2016) 197:3762–70. doi: 10.4049/JIMMUNOL.1601118
131. Hull CM, Peakman M, Tree TIM. Regulatory T cell dysfunction in type 1 diabetes: what’s broken and how can we fix it? Diabetologia (2017) 60:1839–50. doi: 10.1007/S00125-017-4377-1
132. Kalekar LA, Rosenblum MD. Regulatory T cells in inflammatory skin disease: from mice to humans. Int Immunol (2019) 31:457–63. doi: 10.1093/INTIMM/DXZ020
133. Ujiie H. Regulatory T cells in autoimmune skin diseases. Exp Dermatol (2019) 28:642–6. doi: 10.1111/EXD.13535
134. Ugor E, Simon D, Almanzar G, Pap R, Najbauer J, Németh P, et al. Increased proportions of functionally impaired regulatory T cell subsets in systemic sclerosis. Clin Immunol (2017) 184:54–62. doi: 10.1016/J.CLIM.2017.05.013
135. Macdonald KG, Dawson NAJ, Huang Q, Dunne JV, Levings MK, Broady R. Regulatory T cells produce profibrotic cytokines in the skin of patients with systemic sclerosis. J Allergy Clin Immunol (2015) 135:946–955.e9. doi: 10.1016/J.JACI.2014.12.1932
136. Shin BS, Furuhashi T, Nakamura M, Torii K, Morita A. Impaired inhibitory function of circulating CD4+CD25+ regulatory T cells in alopecia areata. J Dermatol Sci (2013) 70:141–3. doi: 10.1016/J.JDERMSCI.2013.01.006
137. Bovenschen HJ, van de Kerkhof PC, van Erp PE, Woestenenk R, Joosten I, Koenen HJPM. Foxp3+ regulatory T cells of psoriasis patients easily differentiate into IL-17A-producing cells and are found in lesional skin. J Invest Dermatol (2011) 131:1853–60. doi: 10.1038/JID.2011.139
138. Koenen HJPM, Smeets RL, Vink PM, van Rijssen E, Boots AMH, Joosten I. Human CD25highFoxp3pos regulatory T cells differentiate into IL-17-producing cells. Blood (2008) 112:2340–52. doi: 10.1182/BLOOD-2008-01-133967
139. Reefer AJ, Satinover SM, Solga MD, Lannigan JA, Nguyen JT, Wilson BB, et al. Analysis of CD25hiCD4+ “regulatory” T-cell subtypes in atopic dermatitis reveals a novel TH2-like population. J Allergy Clin Immunol (2008) 121:415–422.e3. doi: 10.1016/J.JACI.2007.11.003
140. Fyhrquist N, Lehtimäki S, Lahl K, Savinko T, Lappeteläinen AM, Sparwasser T, et al. Foxp3+ cells control Th2 responses in a murine model of atopic dermatitis. J Invest Dermatol (2012) 132:1672–80. doi: 10.1038/JID.2012.40
141. Shin JU, Kim SH, Noh JY, Kim JH, Kim HR, Jeong KY, et al. Allergen-specific immunotherapy induces regulatory T cells in an atopic dermatitis mouse model. Allergy (2018) 73:1801–11. doi: 10.1111/ALL.13465
142. Castela E, le Duff F, Butori C, Ticchioni M, Hofman P, Bahadoran P, et al. Effects of low-dose recombinant interleukin 2 to promote T-regulatory cells in alopecia areata. JAMA Dermatol (2014) 150:748–51. doi: 10.1001/JAMADERMATOL.2014.504
143. Koreth J, Matsuoka K, Kim HT, McDonough SM, Bindra B, Alyea EP, et al. Interleukin-2 and regulatory T cells in graft-versus-host disease. N Engl J Med (2011) 365:2055–66. doi: 10.1056/NEJMOA1108188
144. Matsuoka KI, Koreth J, Kim HT, Bascug G, McDonough S, Kawano Y, et al. Low-dose interleukin-2 therapy restores regulatory T cell homeostasis in patients with chronic graft-versus-host disease. Sci Transl Med (2013) 5:1–25. doi: 10.1126/SCITRANSLMED.3005265
Keywords: skin, skin immunology, skin disease, regulatory T (Treg) cells, inflammation, innate immunity, adaptive immunity
Citation: Hajam EY, Panikulam P, Chu C-C, Jayaprakash H, Majumdar A and Jamora C (2022) The expanding impact of T-regs in the skin. Front. Immunol. 13:983700. doi: 10.3389/fimmu.2022.983700
Received: 01 July 2022; Accepted: 26 August 2022;
Published: 15 September 2022.
Edited by:
Dipayan Rudra, ShanghaiTech University, ChinaReviewed by:
Tatiana Akimova, University of Pennsylvania, United StatesNiwa Ali, King’s College London, United Kingdom
Copyright © 2022 Hajam, Panikulam, Chu, Jayaprakash, Majumdar and Jamora. This is an open-access article distributed under the terms of the Creative Commons Attribution License (CC BY). The use, distribution or reproduction in other forums is permitted, provided the original author(s) and the copyright owner(s) are credited and that the original publication in this journal is cited, in accordance with accepted academic practice. No use, distribution or reproduction is permitted which does not comply with these terms.
*Correspondence: Colin Jamora, colinj@instem.res.in