- 1Department of Orthopaedics and Traumatology, Faculty of Medicine, Prince of Wales Hospital, The Chinese University of Hong Kong, Hong Kong, China
- 2Li Ka Shing Institute of Health Sciences, The Chinese University of Hong Kong, Hong Kong, China
- 3SH Ho Scoliosis Research Laboratory, Joint Scoliosis Research Center of the Chinese University of Hong Kong and Nanjing University, The Chinese University of Hong Kong, Hong Kong, China
Osteoarthritis (OA) is a prevalent joint disease, which is associated with progressive articular cartilage loss, synovial inflammation, subchondral sclerosis and meniscus injury. The molecular mechanism underlying OA pathogenesis is multifactorial. Long non-coding RNAs (lncRNAs) are non-protein coding RNAs with length more than 200 nucleotides. They have various functions such as modulating transcription and protein activity, as well as forming endogenous small interfering RNAs (siRNAs) and microRNA (miRNA) sponges. Emerging evidence suggests that lncRNAs might be involved in the pathogenesis of OA which opens up a new avenue for the development of new biomarkers and therapeutic strategies. The purpose of this review is to summarize the current clinical and basic experiments related to lncRNAs and OA with a focus on the extensively studied H19, GAS5, MALAT1, XIST and HOTAIR. The potential translational value of these lncRNAs as therapeutic targets for OA is also discussed.
Introduction
Osteoarthritis (OA) is a prevalent joint disease in aging and obese populations, resulting in joint pain, stiffness, and movement limitation (1). It has been estimated that OA affects more than 240 million people all over the world which is projected to double in the next 20 years (2). OA is regarded as one of the leading causes of major health and socioeconomic burdens in many countries (3). OA was once considered as a disease of articular cartilage alone. However, it is now generally believed that OA pathogenesis is associated with pathological changes of other joint tissues, such as synovial inflammation, subchondral bone remodeling and meniscal degeneration (4, 5). Risk factors, such as aging, obesity, trauma, genetic predisposition, and bone density, have been implicated in the onset and development of OA (6). Despite these well documented factors and other routinely used clinical parameters such as patient history and radiographic examination, there is still a lack of sensitive approach to detect OA at its reversible stage (7, 8). In the clinics, multiple conservative treatments are available at the early stage of OA, such as physical measures or pharmacological anti-inflammatory and analgesic drugs (6). Surgical interventions, such as osteotomies and total replacement surgeries are served as the ultimate therapeutic options to rehabilitate the persistent pain and functional limitations of patients suffering from severe OA (9, 10). Obviously, these approaches are not able to halt or the progressive degeneration in the joints. Collectively, a better understanding of the molecular mechanism underlying this complex pathogenesis will provide an insight into the development of more specific and sensitive biomarkers as well as disease-modifying drugs for OA prevention and treatment (11).
In human genome, approximately 2% of genome is made up of protein-coding genes. The remaining 98% genome was thought to be nonfunctional evolutionary leftovers. It is now evidenced that these widely distributed non-coding genomes can be classified into two groups, namely short (< 200 nucleotides) and lncRNAs (> 200 nucleotides) which have diverse biological functions in various human diseases (12). In general, lncRNAs modulate the expression of target genes or the activity of downstream pathways by direct binding to DNA, RNA and proteins (13). Increasing evidence reveals that there are differential expressions of lncRNAs at cellular and tissue levels in human OA condition (14), suggesting the undefined roles of lncRNAs in OA development and progression (15), and potentially a new class of biomarkers for OA (16).
To supplement our current understanding as summarized in previous reviews and to update the landscape of lncRNAs research in OA (17, 18), this review takes a more comprehensive approach to critically review the current findings about the role of lncRNAs in OA pathobiology and diagnosis with emphasis on those extensively studied lncRNAs, including lncRNA H19, GAS5, MALAT1, XIST and HOTAIR and their effects on various joint tissues, and to propose novel treatment strategies via targeting lncRNAs.
This review on clinical and basic studies was conducted to provide a current understanding about the lncRNAs research on multiple joint tissues of OA pathogenesis through searching published articles on the PubMed, Google Scholar, and ScienceDirect databases from February 2003 to August 2022. The searching keywords include (“long non-coding RNA” OR “lncRNA”) AND (“osteoarthritis” OR “arthritis” OR “osteoarthritis treatment”) AND (“plasma” OR “synovial fluid” OR “body fluid” OR “cartilage” OR “synovium” OR “subchondral bone” OR “meniscus” OR “chondrocyte” OR “synoviocyte” OR “osteoblast” OR “exosome” OR “nanoparticle” OR “siRNA” OR “Gene-editing”).
Classification and function of lncRNAs
One common classification of lncRNAs is based on their positions to protein-coding genes: (i) Sense lncRNAs and (ii) antisense IncRNAs are those overlap with the same and opposite strand of coding genes, respectively; (iii) Intronic lncRNAs are those locate in the same intronic region of protein-coding genes. While (iv) bidirectional lncRNAs are transcribed from the same promoter as the protein-coding genes, but in the opposite direction and (v) long intergenic noncoding RNAs (lincRNAs) locate in the genomic interval between two genes (19) (Figure 1). In addition, lncRNAs can be further classified by their interactions with targets, including decoy lncRNAs, guide lncRNAs, scaffold lncRNAs, stabilizing lncRNAs and competitive endogenous-lncRNAs. Decoy lncRNAs sequester DNA-binding proteins to limit their bindings to DNA recognition elements. Guide lncRNAs recruit chromatin remodeling agents to impart specificity to genomic locations through either DNA-protein or RNA-DNA recognition. While scaffold lncRNAs join several proteins together in a complex, and stabilizing lncRNAs bind to target mRNA transcripts, stabilize and promote their translations. Competitive endogenous-lncRNAs (ceRNAs) or ‘RNA sponges’ compete with miRNAs to limit their effects on protein-coding mRNA targets (20).
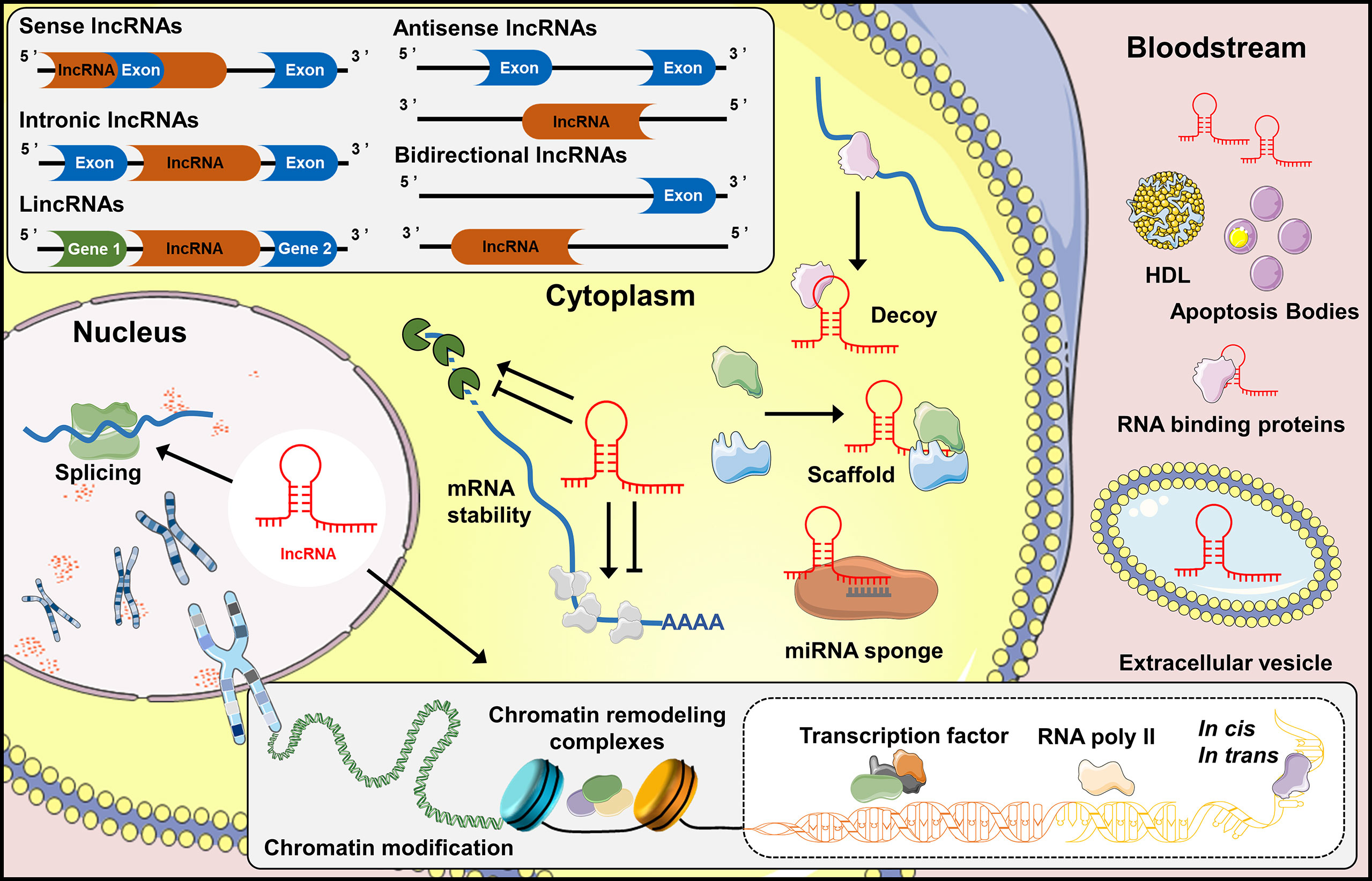
Figure 1 Biogenesis and function of lncRNAs. Classification of lncRNAs into five classes: sense lncRNAs, intronic lncRNAs, lincRNAs, antisense lncRNAs and bidirectional lncRNAs, based upon their genomic locations and transcription. LncRNAs regulate the expression of genes in the cytoplasm by interacting directly with microRNAs (miRNAs) or proteins, and stabilizing mRNA transcripts. Noncoding transcripts in the nucleus are known to regulate gene expression at the level of chromatin modification, transcription and post-transcriptional processing. In addition, lncRNAs are considered as biomarkers or participant in tissue crosstalk by entering the bloodstream directly, or bound to carrier proteins, even incorporated into extracellular vesicles which can be further released into bloodstream.
Extensive research over the past decade has deciphered various biological functions of lncRNAs (21). In general, lncRNAs regulate gene expression via chromatin modification, transcription and post-transcriptional processes (22). During chromatin modification, lncRNAs recruit chromatin remodeling complexes to specific chromatin loci (23). Transcriptional regulation is the core role of lncRNAs in which they serve as pervasive enhancers or promoters of transcription. In addition, lncRNAs also behave as RNA binding proteins, transcription factors and RNA polymerase (RNAP) II in regulating the initiation of transcription (21). During post-transcriptional regulation, lncRNAs mediate mRNA dynamics in both cis- and trans-targets (24). Overall, lncRNAs serve as master regulators of gene expression, and it is not surprising that the value of lncRNAs in key aspects of OA progression has attracted considerable attention.
Overview of lncRNAs in OA pathogenesis
Currently, most of the studies focused on the lncRNAs functions in OA cartilage/chondrocyte. Given that OA is a disease of the whole joint (25), it is of clinical value to provide an overview regarding the lncRNAs expression in different joint tissues. The section summarizes some recent key findings about the dysregulation of lncRNAs expression and their potential biological roles in cartilage degradation, synovial inflammation, dysfunction of subchondral bone homeostasis and meniscus injury. The full list of literature search can be referred to Table 1.
lncRNAs in Cartilage
Cartilage is an integral part of the skeletal system and is mostly composed of chondrocytes. Chondrocytes can secrete cartilage matrix and maintain joint activity (124), making this cell type indispensable to the dynamic and continuous processes of extracellular matrix (ECM) deposition and remodeling to maintain homeostasis of cartilage (125). However, such balance is disrupted in OA, and finally resulting in degeneration of cartilage matrix (notably type II collagen, COL2), production of fibrous ECM, aberrant proliferation, senescence and hypertrophy of chondrocytes, as well as secretion of inflammatory cytokines (126). Previous studies described the abnormal expression of lncRNAs in OA cartilages or chondrocytes, indicating the probable link between lncRNAs and the aberrant chondrocyte function (127, 128). Liu and colleagues are one of the pioneer groups to profile lncRNA in human OA cartilage tissues, providing a new insight into the mechanism of cartilage injury and the progression of ECM degradation (52). Similarly, Hoolwerff and colleagues reported the differential expression of lncRNAs with OA pathophysiology in cartilage, and they discussed the potential of antisense lncRNA P3H2-AS1 on collagen chain assembly in lesioned OA cartilage via the regulation of P3H2 expression (129). On the other hand, Pearson et al. identified 125 lncRNAs were differentially expressed upon IL-1β stimulation in primary human OA chondrocytes. Amongst, two novel lncRNAs, namely ClLinc01 and ClLinc02, were found to mediate the secretion of proinflammatory cytokines in IL-1 stimulated human chondrocytes, suggesting that some lncRNAs might mediate the response of chondrocytes to inflammation and inflammation-driven cartilage degeneration within the OA joint (92). Of note, different types of cellular model, such as cartilage derived primary cell culture or immortalized cell line with or without prior stimulation, were used in previous studies to delineate the effects of various lncRNAs on chondrocytes (130). Whether these effects are associated with or even causative factors in OA development or progression requires further investigation with appropriate transgenic animal models.
lncRNAs in Synovium
Synovium is a specialized connective membrane lining the inner surface of synovial joint capsules, and almost 75% of cells in the synovium are fibroblast-like synoviocytes (FLS) (131). Increasing evidence shows that FLS secretes proinflammatory cytokines which mediate the degradation of cartilage during OA progression (132), which has been speculated to be associated with disease progression (133). Till now, the effects of lncRNAs on OA synovium remains elusive. Early work by Xiang and colleagues identified the differential expressions of 17 lncRNAs in OA synovium of aged patients undergoing total knee replacement surgery, in which some of these lncRNAs were found to be related to immune response. The recruitment of younger control subjects requiring arthroscopic meniscectomy in this case-control study is ethically sound but not ideal to exclude the influences of the acute injury of meniscus on the lncRNAs in the synovial microenvironment (134). Li and colleagues focused on a hepatocellular carcinoma associated lncRNA (ANRIL) and found a higher level of ANRIL in the OA cartilage tissue when compared with that of normal cartilage tissue obtained from subjects requiring traumatic emergency amputation without OA or rheumatic arthritis. Then primary chondrocytes isolated from the collected cartilage tissues, and commercially available normal and OA synoviocytes were used to show differentially upregulated ANRIL expression in OA synoviocytes but not in OA chondrocytes. It appears that ANRIL dysregulation in OA is cell-type specific, affecting the proliferation of synoviocytes via binding to miR-122-5p (117). However, it should be noted that the information of the subjects where those chondrocytes and synoviocytes derived from (such as age and sex) were not provided, which should be taken into consideration.
lncRNAs in Subchondral bone
Impaired mineralization is a pathological feature of osteoarthritic subchondral bone. Such distinct microstructural alterations, including sclerotic changes and osteophyte formation, are both believed to arise from elevated bone turnover with an increase in osteoblastic over osteoclastic activities (135). In addition, the subchondral bone is also considered as a major site of OA pain, likely due to the innervation with sensory neurons and vascular channels (136). From bone remodeling perspective, it is evidenced that several lncRNAs could regulate osteoblast and osteoclast activities, and there are attempts to modulate lncRNAs expression in vivo via various strategies (137). Therefore, it is of interest to ask whether aberrant subchondral bone remodeling in OA is associated with lncRNAs dysregulation. By comparing subchondral bone samples collected from hip and knee, Tuerlings and colleagues identified 21 lncRNAs differentially expressed between preserved and lesioned OA subchondral bone significantly. It is interesting to note that a further stratified analysis identified 15 lncRNAs were differentially expressed in knee samples but none in hip samples (122). These findings prompt to further research questions. 1) Whether lncRNAs differential expression in OA subchondral bone is site-specific and associated with aberrant mechanical loading? 2) What are the biological functions of these lncRNAs in OA subchondral bone remodeling? Further investigation on the effects of lncRNAs on osteoblasts, osteoclasts and osteocytes functions related to subchondral bone mineralization and remodeling is warranted to develop a more comprehensive understanding of the lncRNAs and their roles and therapeutic values in OA.
lncRNAs in Meniscus
Meniscus is a crucial tissue for supporting the structure, stability, and biomechanical function of the knee joint (138). During OA progression, it undergoes various histopathological changes, including tears, calcification, and atypical cell arrangement (139). Till now, there is limited studies exploring the mechanism of meniscal pathogenesis in OA, and only two studies were found to investigate the expression level of lncRNAs in OA meniscus tissues. The work by Brophy and colleagues depicted the transcriptome profile in the meniscus between end stage OA patients and patients undergoing arthroscopic partial meniscectomy with no evidence of OA. The subjects in the OA group were older and had higher BMI. Twenty-six and 10 lncRNAs were found up- and down-regulated in the OA group, respectively. Lnc-RPL19-1 and lnc-ICOSLG-5 were highlighted because of their correlations with some cartilage disease related genes. qPCR was performed to validate the microarray results (140). Recently, Jiang and colleagues performed a whole-transcriptome profile of lncRNAs dysregulation using isolated meniscus cells from OA patients with and without IL-1β, suggesting a potential crosslink between menisci and cartilage during OA. Of note, LCN2 and RAB27B were consistently upregulated in both OA meniscus and IL-1β treated primary meniscus cells derived from three OA meniscus samples, and appears to be associated with OA severity (123). Although different samples were used in the analysis, these two works both illustrated the potential link between inflammatory phenotype in meniscus and lncRNAs, which is subjected to further investigation to confirm the molecular mechanisms and biological functions of these lncRNAs in OA meniscus injury.
Clinical biomarkers of lncRNAs for OA diagnosis
In general, the secretion and transport of lncRNAs into extracellular environment are mediated by three manners (1): Direct release of extracellular RNAs by joint tissues and cells (2). Encapsulated in high density lipoprotein (HDL) or apoptosis bodies or associated with protein complexes (3). Packed in membrane vesicles, such as exosomes and micro-vesicles (141). In clinical research, serum and synovial fluid are often the preferred biological fluid samples for OA biomarker discovery (142). Recent detections of the extracellular lncRNAs in these biological fluids of OA subjects implicate that they might serve as alternative indicators for OA onset and progression (Table 2).
Circulation
Previous studies have shown that there is a relationship between the blood level of lncRNAs and OA progression (Table 2). For instance, lncRNA DILC (145), and lncRNA FER1L4 (146) were also found to be closely associated with OA inflammatory condition in plasma. As ANCR is known to regulate TGF-β signaling, Li and colleagues proposed that the plasma levels of TGF-β1 and ANCR could differentiate OA patients from healthy control subjects. They found a higher TGF-β1 and a lower ANCR level in OA plasma (N=62) when compared with that of healthy controls (N=46), which was inversely correlated. The mean area under curve (AUC) for OA plasma TGF-β1 and ANCR were 0.8929 and 0.8845, respectively (147). However, it is not shown if combination of plasma TGF-β1 and ANCR could enhance the sensitivity and specificity. Zhou et al. indicated that the expression of lncRNA H19 was negatively correlated with bone metabolic index of OA patients, such as Procollagen I N-Terminal Propeptide (PINP), N-MID-Osteocalcin, bone Gla protein (BGP), and bone alkaline phosphatase (BALP). Particularly, lncRNA H19 is highly correlated with K-L grading, VAS, WOMAC and Lysholm scores, suggesting H19 was associated with disease severity in OA patients (144). These two biomarkers discovery studies show encouraging AUC value, however, discussion on confounding factors and validation with separate cohort were missing.
Synovial fluid
Based on current findings, it is reasonable to speculate that the expression of lncRNAs is cell and tissue specific in OA joint. Therefore, the information from research on synovial fluid is likely to provide additional clues on the clinical values of lncRNAs as OA biomarkers. Qi and colleagues showed lower levels of CAIF in the synovial fluid collected from the hip and knee of OA patients, and CAIF was inversely and significantly correlated with IL-6 expression level (150). Meanwhile, Xiao and colleagues reported lower levels of lncRNA MIR4436-2HG in both plasma and synovial fluid of OA patients. The mean AUC for CAIF and MIR-4435-2HG were found to be 0.89 and 0.96, respectively. It is interesting to note that 1 or 3 months treatment including exercise, prescription of non-steroidal anti-inflammatory drugs (NSAIDs) and joint burden reduction seems to increase the plasma level of MIR-4435-2HG (148). Although the study design, the details of these treatment and the compliance were not mentioned, this preliminary result suggests that lncRNAs level in circulation could be modulated. In these studies, healthy volunteers were recruited as control group for the collection of synovial fluid. If the collections of synovial fluid from mild to moderate stages are also ethically feasible, it will be of clinical interest to determine the correlations between lncRNAs level in synovial fluid and OA severity and progression in order to explore the prognostic value of those selected lncRNAs.
Others
LncRNAs in cells/extracellular carriers within the blood and synovial fluid are another sources of biomarker candidates (160). The expression profile of lncRNAs in peripheral blood leukocytes of OA patients showed that LINC00167 may serve as a potential early diagnosis marker for OA in clinical practice (157). In addition, lncRNA GAS5 in the peripheral blood mononuclear cells isolated from the knee of OA patients was also lower than that of healthy subjects, indicating a novel marker for occurrence and progression of OA (156). The first study of IncRNA profiles in human OA synovial exosomes by Wu et al. found that exosomal lncRNA PCGEM1 is a potential indicator to distinguish the early stage of OA from the late-stage. Moreover, the expression of lncRNA PCGEM1 in synovial exosome rather than that in plasma was found to be closely associated with the WOMAC Index (159).
Biological functions of lncRNAs in OA pathogenesis
lncRNA H19
H19 lncRNA is located on chromosome 11p15.5, and its transcription product, H19 RNA, primarily resides in cytoplasm (161). It is the first reported mammalian lncRNA (162), which is highly expressed during fetal stage but markedly down-regulated after birth. H19 was found to be upregulated in OA cartilage, and appears to be associated with the disease progression (26, 163, 164). In primary human chondrocytes, H19 and H19-derived miR675 increased the matrix production of differentiated chondrocytes via activating COL2 transcription (165). Furthermore, H19 could regulate the proliferation and apoptosis of chondrocytes treated by IL-1b via sponging miR106a-5p (27). Meanwhile, lncRNA H19 upregulated IL-38, which is bound to IL- 36R and brought about suppression of knee joint inflammation in mouse chondrocytes (28). Inconsistent outcomes were observed in different in vitro models and upon different stimulations. Knockdown of lncRNA H19 could alleviate apoptosis and inflammatory response via sponging miR130a in LPS-stimulated human C28/I2 chondrocytea (166). Furthermore, the effect of H19 silencing suppressed the expression of matrix metalloproteinases (MMPs) family (MMP1 and MMP3) via targeting miR-140-5p in human HC-A chondrocyte cells , suggesting a protective role of H19 on the degradation of the chondrocyte extracellular matrix (29). Besides OA chondrocyte, H19 RNA level in OA synovial tissue was also found to be significantly higher those that in synovium of normal and trauma joint (30). However, there is a lack of strong evidence supporting that H19 RNA upregulation is a sign of inflammation of synovial FLSs nor polarization of synovial macrophages (167). Notably, rats FLS-derived exosomal lncRNA H19 was found to promote chondrocyte viability and migration, as well as inhibit ECM degradation in IL-1β-induced chondrocytes by targeting miR106b-5p expression (168). Altogether, these studies suggest that lncRNA H19 may play an essential role in the crosstalk between synovium and cartilage during OA progression, and H19-targeted therapy is expected to open new perspectives for OA management.
lncRNA GAS5
The growth arrest-specific 5 (GAS5) lncRNA is located on chromosome 1q25.1 and consists of 12 exons with a short open reading frame (ORF) (169). Its name reflects its nature and predominant expression in growth-arrested cells (170). As such, GAS5 is mainly responsible for suppressing multiple anti-apoptotic genes, thereby enhancing the vulnerability of cells to pro-apoptotic signals (171). In OA cartilage, GAS5 was found to be upregulated with positive correlation pattern to the disease stages (172, 173). Overexpression of GAS5 was reported to increase the activity level of chondrocyte catabolism (several MMPs), and apoptosis (31). Meanwhile, GAS5 can serve as negative regulators for miR21 (31), miR34a (32), miR137 (33), miR144 (173) and miR27a (174). It is also evidenced that GAS5 could directly target KLF2 to alleviate LPS-induced inflammatory damage in murine chondrocytic ATDC5 cell line (175). On the contrary, the expression levels of GAS5 in synovial fluid and tissues were significantly lower in OA (34, 155), which possibly implicate different functions of GAS5 in OA synovium. Considering the small sample size (N=45) and a lack of in vivo functional analysis, future study is required to evaluate the function of GAS5 in OA synovium by including a clinical study with a larger sample size and experiments with appropriate animal models.
lncRNA MALAT1
Metastasis-associated lung adenocarcinoma transcript 1 (MALAT1), also known as NEAT2 for nuclear-enriched abundant transcript 2, is transcribed by RNA polymerase II at human chromosome 11q13 (176). It is a highly abundant nuclear transcript localized to the nuclear speckles and have a longer half-life (9–12 h) than other lncRNAs owing to bipartite triple helix structure (177, 178). MALAT1 is upregulated in human OA cartilage and IL-1β-induced chondrocyte cells (35). Overexpression of MALAT1 in human chondrocytes inhibited cells viability and promoted cartilage ECM degradation through targeting miR145 (35). Also, lncRNA MALAT1 overexpression in human C28/I2 chondrocyte cells was proved to promote chondrocyte migration, inflammation suppression, and ECM degradation (179). Besides, MALAT1 could act as sponges for other miRNAs, like miR127-5p (36), miR150-5p (180) and miR146a (181), thus likely to play some regulatory roles in OA cartilage. It should be noted that lower level of MALAT1 was also reported in IL-1b stimulated rat chondrocytes, which enhanced cell proliferation and type II collagen (Col II) expression by blocking JNK signaling activation (182). In synovium, the synovial fibroblasts isolated from OA patients had a higher expression of MALAT1 compared with that of normal subjects, which could be owing to proinflammatory challenge in synoviocytes especially to IL-6 and CXCL-8 (37). It is worth mentioning that MALAT1 is the first lncRNA to be investigated in OA subchondral bone. Higher expression level of MALAT1 was reported in both knee and hip subchondral bone of patients with OA, and its expression in the osteoblasts appears to be associated with the production of inflammatory prostacyclins. Since the subchondral bone is considered to be an important site of OA pain, MALAT1 may play an important role in the development of OA bone pain and inflammation (38). Based on current evidence, it appears that MALTA1 plays more pro-inflammatory role in OA synovial and subchondral bone, which represents a potential candidate for research on OA pathogenesis and therapeutic target.
lncRNA XIST
X-inactive specific transcript (XIST) encodes a 17-kb lncRNA which, despite being capped, spliced and polyadenylated, it is retained in the nucleus (183). lncRNA XIST and its associated chromatin modifying complex play vital roles in the regulation of the X-chromosome inactivation process (184). Emerging evidence indicates that it is correlated with the modification of ECM component of OA (185). XIST was upregulated in OA cartilage and promoted MMP-13 and ADAMTS-5 expression in human chondrocytes, indicating its role in ECM degradation through functioning as a ceRNA of miR1277-5p (186). Notably, the consistency results could be seen in the studies of XIST in terms of repressing the development of OA as indicated by different models. For instance, in IL-1b induced human C28/I2 chondrocyte cells, the knockdown of XIST expression suppressed the production of IL-6, TNF-α, PGE2 and NO through the interaction with miR130a (187). XIST regulated IL-1β-induced chondrocyte growth, apoptosis and ECM synthesis through sponging with miR-142-5p in human chondrosarcoma cell line SW1353 (188). Moreover, the silencing of XIST could promote cell viability but inhibit cell apoptosis through acting as a sponge for miR149-5p in human CHON-001 chondrocyte cell line (39). In addition, XIST expression was significantly upregulated in the OA synovium compared with that in normal synovium. More importantly, XIST/miR376c‐5p/OPN axis has been proven to modulate the inflammatory microenvironment in OA synovial macrophage, subsequently affecting chondrocyte apoptosis and ECM degradation (42).
lncRNA HOTAIR
HOX transcript antisense RNA (HOTAIR) resides within the intergenic region in HOXC cluster on chromosome 12, and acts as a crucial modulator of chromatin re-modeling and transcriptional silencing (189). As an epigenetic agent, HOTAIR can interact with various factors, leading to genomic stability, proliferation, survival, invasion, migration, metastasis, and drug resistance (190). In OA cartilage, HORAIR was upregulated than that of normal samples (164). HOTAIR was reported as a promising promoter for ADAMTS-5 expression and ECM degradation in human OA articular chondrocytes (47). HOTAIR silencing reduced cartilage tissue damage in OA mice, and promoted the expression of collagen II and aggrecan in cartilage tissue, while inhibited the expression of MMP-13 and ADAMTS-5 by targeting miR-20b/PTEN axis in mouse primary chondrocytes (191). Interestingly, cumulative evidence shows that Wnt/β-catenin pathway might play a certain role in the pathogenesis of cartilage damage, and lncRNA HOTAIR could directly bind to miR17-5p and indirectly regulate FUT2/β-catenin axis in connection with OA progression, such as ECM degradation and cell apoptosis (48). Wnt inhibitory factor 1 (WIF-1), a key inhibitor of the Wnt/β-catenin pathway, could be directly modulated by HOTAIR and interfered with the activation of downstream pathway and relative genes expression on cartilage degradation in human chondrosarcoma cell line SW1353 (49). Overexpression of HOTAIR in human CHON-001 chondrocyte cell line could aggravate LPS-induced cell apoptosis and inflammatory cytokines influx, including IL-1β, IL-6, IL-8 and TNF-α. While blocking HOTAIR could suppress cleavage of caspase-3 and p62 proteins and elevated secretion of IL-6 and TNF-α via suppression of miR222-3p (43). Meanwhile, HOTAIR inhibited chondrocytes proliferation via sponging with other miRNAs, including miR130a-3p (44), miR1277-5p (45), miR107 (46), and miR221 (192). Therefore, all HOTAIR-related factors form a comprehensive regulatory network, suggesting the central role of HOTAIR in the physiology of chondrocytes during OA (130).
Collectively, the identification of disease-specific lncRNAs for OA pathophysiology, including H19, GAS5, MALAT1, XIST, HOTAIR and future identified lncRNAs, emphasized the general consistency of lncRNAs functions in various tissues, which might be further developed as lncRNAs-targeted therapies for OA treatment in the future.
Targeting lncRNAs: A novel treatment strategy for OA?
Based on current evidence, it is worthwhile to explore if targeting lncRNAs could be a novel strategy for preventing and/or treating OA. Till now, according to clinical trials registries (clincialtrials.gov), there is only one registered clinical trial studying the role of lncRNAs as biomarkers for OA articular microenvironment. Without relevant clinical studies can be included for discussion, we attempted to propose strategies developed for lncRNA delivery and targeting with reference to published animal studies (Figure 2).
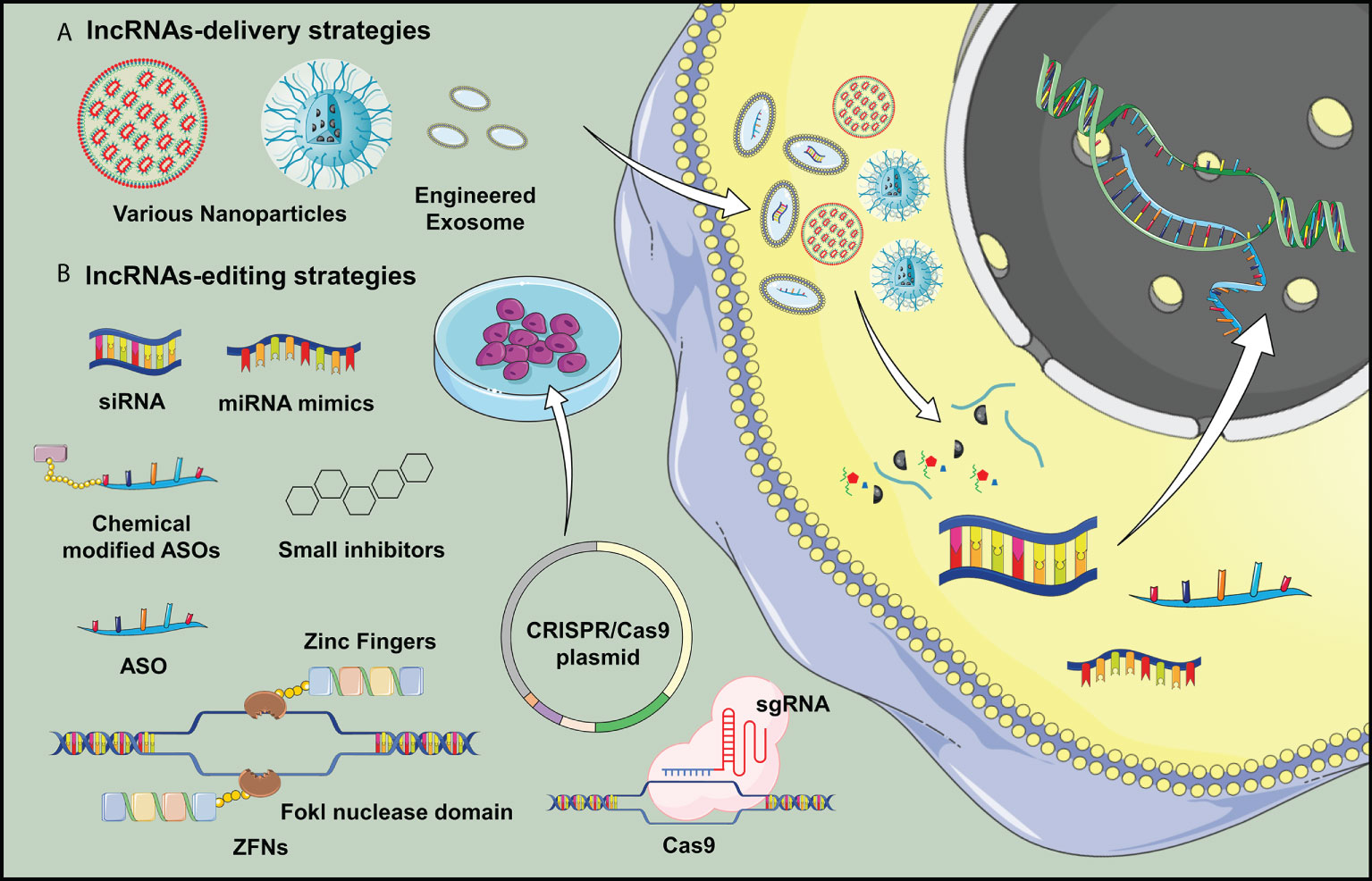
Figure 2 Potential delivery strategies for lncRNA H19 is proposed in OA treatment. (A) Nanotechnology and lncRNA-loaded exosomes could overcome the low efficiency of in vivo transgene lncRNA transfection, which would be applicable for widespread clinical application of gene therapy targeting lncRNAs. (B) Various transgene technologies may benefit lncRNA overexpression or downregulations in vivo studies, which opened a new door in studying the delivery of genetic material for OA treatment.
Extracellular vesicles hold some promise to be a vehicle for selective delivery of target genes into tissues of interest (193). In animal study, intra-articular injection of exosomes with overexpressed lncRNA H19 is found to promote cartilage repair and restore OA joint homeostasis (194). Liu and colleagues highlighted the possible mechanism for OA therapy by cellular delivery of exosomal lncRNA KLF3-AS1, which could facilitate cartilage repair by promoting chondrocyte proliferation and migration and inhibiting apoptosis (195). Zhang and colleagues also reported that targeting lncRNA NEAT1 through artificial exosomes could be one of the options to elevate chondrocyte proliferation for OA treatment (196). Pan and colleagues confirmed the effect of MALAT1 on chondrocytes, which exhibited a slight cartilage damage and a smooth surface after intra-articular injection of LAMAT1 extracellular vesicles in OA animal model (179). In addition, the use of nanoparticles as an effective delivery vehicle for targeting lncRNAs provides a new therapeutic strategy owing to improved stability, biocompatibility, and high-dose therapeutic payloads (197). Recent advancement in lipid nanoparticles, polymeric nanocarrier and metal-based delivery system provides novel approaches for delivering of nucleic acids and lncRNAs-based therapeutic agents (198–200). At the time of writing, although nanoparticle delivery strategies for targeting lncRNAs in OA field has not been reported, therapeutic carriers, exosomes and nanomaterials pose enormous potential as vehicles loading gene-editing systems for OA treatment.
Considering upregulation of lncRNAs in OA pathogenesis appears to be the most common aberrant change, it is reasonable to propose approaches which can inhibit their expression or activity. Short interfering RNAs (siRNAs) is currently one of the in vivo feasible methods that has been shown to alleviate joint inflammation and decrease the expression of pro-inflammatory mediators by targeting lncRNA PVT1 in OA mice (201). Other in vivo approaches to regulate lncRNAs expression, such as locked nucleic acids (LNA) and ASOs have been shown to be effective to inhibit cancer progression (202, 203), which is pending for testing in OA animal models. Gene-editing enzymatic systems, such as zinc finger nucleases (ZFNs) and clustered regularly interspaced short palindromic repeats (CRISPR), are known far superior to RNAi technique for lncRNAs knockdown (204). Recently, some small molecule inhibitors are identified to systematically target lncRNA expression by masking the binding sites or disrupting the RNA structure (205).
Conclusions and future direction
Increasing evidence indicates that lncRNAs are playing certain important roles associated with the pathological changes of OA joints through diverse actions on various joint components, which is exemplified by lncRNAs H19, GAS5, MALAT1, XIST and HOTAIR in this review.
The roles of lncRNAs have been mainly investigated with OA cartilage tissues and chondrocytes, and found to participate in the regulation of cartilage metabolism and chondrocyte function as a miRNA sponge regulating target genes expression. However, this kind of action and post-transcriptional regulation on target genes/proteins might not represent the whole picture of lncRNAs function in the context of OA. In addition, it should be admitted that the diverse methods employed in previous studies for lncRNA expression and functional analyses, such as the source of the testing cells, experimental procedures and even stimulation approaches, might lead to inconsistent findings.
In addition, the following questions remain elusive (1): the cause of lncRNAs dysregulation in the onset, development and progression of OA is still unclear. Whether the inflammation, hypoxia (26) or mechanical stress (206) are the major upstream factors leading to the aberrant expression of lncRNAs (2). Numerous miRNAs or proteins are reported to be downstream targets of lncRNAs, but their roles in line with lncRNAs dysfunction in OA pathogenesis remains largely unclear (3). In view of the diverse biological functions of lncRNAs, it is uncertain whether the effect of lncRNAs on the development and progression of OA is tissue- and/or cell-specific.
In view of the association with OA phenotypes, the clinical value of lncRNAs as biomarkers for disease severity and prognostication also draws much attention. However, it should be admitted that this kind of preliminary findings need to be validated further. It will be desired to (1) develop a standardized lncRNAs testing system, including sample preparation, extraction, selection of appropriate endogenous controls (2); other statistical approaches such as predictive value, likelihood ratio, odd ratio and so on subjecting to the purpose of the biomarkers under investigation (3); conduct a multi-center study with a larger sample size to eliminate discrepancy such as ethnicity and sampling bias (4); perform a longitudinal study to validate lncRNAs as biomarkers for OA.
It appears that the modulation of the expression and activity of IncRNAs might be a novel strategy for OA management. Despite therapeutic nuclei acids hav been reported in OA treatment, several technical concerns including mechanism of action and an effective and specific delivery approach are not fully understood nor developed for OA application. Furthermore, the clinical application of lncRNAs-based therapy requires more stringent and robust investigation particularly safety issues including immunogenicity, cytotoxicity and long-term safety profile (207). In addition, the specificity of targeting lncRNAs is very important, and further studies are needed to avoid off-target side effects. Last but not least, a suitable target lncRNAs would lead to a more effective approach for OA treatment, and the focus of disease-specific lncRNAs described herein might draw some attention collaterally as the fields of gene-delivery and editing therapy develop.
Author contributions
RL prepared the draft of the manuscript, which was revised by HS and WL. All authors have read and approved the final version of the manuscript.
Funding
This work was partly supported by the General Research Fund (Ref. No. 24121622), Area of Excellence (Ref. No. AoE/M-402/20) and Research Matching Grant Scheme, University Grants Committee, Hong Kong; Start-up Fund, The Chinese University of Hong Kong, Hong Kong; Innovation and Technology Fund (Ref. No. PRP/090/20FX), Hong Kong; 2020 Rising Star Award provided by American Society for Bone and Mineral Research.
Conflict of interest
The authors declare that the research was conducted in the absence of any commercial or financial relationships that could be construed as a potential conflict of interest.
Publisher’s note
All claims expressed in this article are solely those of the authors and do not necessarily represent those of their affiliated organizations, or those of the publisher, the editors and the reviewers. Any product that may be evaluated in this article, or claim that may be made by its manufacturer, is not guaranteed or endorsed by the publisher.
References
1. Katz JN, Arant KR, Loeser RF. Diagnosis and treatment of hip and knee osteoarthritis: A review. JAMA (2021) 325(6):568–78. doi: 10.1001/jama.2020.22171
2. Hunter DJ, Bierma-Zeinstra S. Osteoarthritis. Lancet (2019) 393(10182):1745–59. doi: 10.1016/S0140-6736(19)30417-9
3. Hunter DJ, Schofield D, Callander E. The individual and socioeconomic impact of osteoarthritis. Nat Rev Rheumatol (2014) 10(7):437–41. doi: 10.1038/nrrheum.2014.44
4. Hu Y, Chen X, Wang S, Jing Y, Su J. Subchondral bone microenvironment in osteoarthritis and pain. Bone Res (2021) 9(1):20. doi: 10.1038/s41413-021-00147-z
5. Mobasheri A, Rayman MP, Gualillo O, Sellam J, van der Kraan P, Fearon U. The Role of Metabolism in the Pathogenesis of Osteoarthritis. Nat Rev Rheumatol (2017) 13(5):302–11. doi: 10.1038/nrrheum.2017.50
6. Zhang Y, Liu T, Yang H, He F, Zhu X. Melatonin: A novel candidate for the treatment of osteoarthritis. Ageing Res Rev (2022) 78:101635. doi: 10.1016/j.arr.2022.101635
7. Okuyan HM, Begen MA. LncRNAs in osteoarthritis. Clin Chim Acta (2022) 532:145–63. doi: 10.1016/j.cca.2022.05.030
8. Budd E, Nalesso G, Mobasheri A. Extracellular genomic biomarkers of osteoarthritis. Expert Rev Mol Diagn (2018) 18(1):55–74. doi: 10.1080/14737159.2018.1415757
9. Chen T, Weng W, Liu Y, Aspera-Werz RH, Nussler AK, Xu J. Update on novel non-operative treatment for osteoarthritis: Current status and future trends. Front Pharmacol (2021) 12:755230. doi: 10.3389/fphar.2021.755230
10. Madry H. Surgical therapy in osteoarthritis. Osteoarthritis Cartilage (2022) 30(8):1019–34. doi: 10.1016/j.joca.2022.01.012
11. Zhu X, Chen F, Lu K, Wei A, Jiang Q, Cao W. PPARgamma preservation via promoter demethylation alleviates osteoarthritis in mice. Ann Rheum Dis (2019) 78(10):1420–9. doi: 10.1136/annrheumdis-2018-214940
12. Kapranov P, Cheng J, Dike S, Nix DA, Duttagupta R, Willingham AT, et al. RNA Maps reveal new RNA classes and a possible function for pervasive transcription. Science (2007) 316(5830):1484–8. doi: 10.1126/science.1138341
13. Bartel DP. MicroRNAs: target recognition and regulatory functions. Cell (2009) 136(2):215–33. doi: 10.1016/j.cell.2009.01.002
14. Abbasifard M, Kamiab Z, Bagheri-Hosseinabadi Z, Sadeghi I. The role and function of long non-coding RNAs in osteoarthritis. Exp Mol Pathol (2020) 114:104407. doi: 10.1016/j.yexmp.2020.104407
15. Ali SA, Peffers MJ, Ormseth MJ, Jurisica I, Kapoor M. The non-coding RNA interactome in joint health and disease. Nat Rev Rheumatol (2021) 17(11):692–705. doi: 10.1038/s41584-021-00687-y
16. Xie F, Liu YL, Chen XY, Li Q, Zhong J, Dai BY, et al. Role of MicroRNA, LncRNA, and exosomes in the progression of osteoarthritis: A review of recent literature. Orthop Surg (2020) 12(3):708–16. doi: 10.1111/os.12690
17. Wang J, Sun Y, Liu J, Yang B, Wang T, Zhang Z, et al. Roles of long noncoding RNA in osteoarthritis (Review). Int J Mol Med (2021) 48(1):133–40. doi: 10.3892/ijmm.2021.4966
18. Ghafouri-Fard S, Poulet C, Malaise M, Abak A, Mahmud Hussen B, Taheriazam A, et al. The emerging role of non-coding RNAs in osteoarthritis. Front Immunol (2021) 12:773171. doi: 10.3389/fimmu.2021.773171
19. McCabe EM, Rasmussen TP. lncRNA involvement in cancer stem cell function and epithelial-mesenchymal transitions. Semin Cancer Biol (2020) 75:38–48. doi: 10.1016/j.semcancer.2020.12.012
20. Thomson DW, Dinger ME. Endogenous microRNA sponges: Evidence and controversy. Nat Rev Genet (2016) 17(5):272–83. doi: 10.1038/nrg.2016.20
21. Long Y, Wang X, Youmans DT, Cech TR. How do lncRNAs regulate transcription? Sci Adv (2017) 3(9):eaao2110. doi: 10.1126/sciadv.aao2110
22. Mercer TR, Dinger ME, Mattick JS. Long non-coding RNAs: Insights into functions. Nat Rev Genet (2009) 10(3):155–9. doi: 10.1038/nrg2521
23. Qian X, Yang J, Qiu Q, Li X, Jiang C, Li J, et al. LCAT3, a novel m6A-regulated long non-coding RNA, plays an oncogenic role in lung cancer via binding with FUBP1 to activate c-MYC. J Hematol Oncol (2021) 14(1):112. doi: 10.1186/s13045-021-01123-0
24. Chen LL. Linking long noncoding RNA localization and function. Trends Biochem Sci (2016) 41(9):761–72. doi: 10.1016/j.tibs.2016.07.003
25. Kraus VB, Blanco FJ, Englund M, Karsdal MA, Lohmander LS. Call for standardized definitions of osteoarthritis and risk stratification for clinical trials and clinical use. Osteoarthritis Cartilage (2015) 23(8):1233–41. doi: 10.1016/j.joca.2015.03.036
26. Steck E, Boeuf S, Gabler J, Werth N, Schnatzer P, Diederichs S, et al. Regulation of H19 and its encoded microRNA-675 in osteoarthritis and under anabolic and catabolic in vitro conditions. J Mol Med (Berl) (2012) 90(10):1185–95. doi: 10.1007/s00109-012-0895-y
27. Zhang X, Liu X, Ni X, Feng P, Wang YU. Long non-coding RNA H19 modulates proliferation and apoptosis in osteoarthritis via regulating miR-106a-5p. J Biosci (2019) 44(6):128–37. doi: 10.1007/s12038-019-9943-x
28. Zhou Y, Li J, Xu F, Ji E, Wang C, Pan Z. Long noncoding RNA H19 alleviates inflammation in osteoarthritis through interactions between TP53, IL-38, and IL-36 receptor. Bone Joint Res (2022) 11(8):594–607. doi: 10.1302/2046-3758.118.BJR-2021-0188.R1
29. Yang B, Xu L, Wang S. Regulation of lncRNA-H19/miR-140-5p in cartilage matrix degradation and calcification in osteoarthritis. Ann Palliat Med (2020) 9(4):1896–904. doi: 10.21037/apm-20-929
30. Stuhlmuller B, Kunisch E, Franz J, Martinez-Gamboa L, Hernandez MM, Pruss A, et al. Detection of oncofetal h19 RNA in rheumatoid arthritis synovial tissue. Am J Pathol (2003) 163(3):901–11. doi: 10.1016/S0002-9440(10)63450-5
31. Song J, Ahn C, Chun CH, Jin EJ. A long non-coding RNA, GAS5, plays a critical role in the regulation of miR-21 during osteoarthritis. J Orthop Res (2014) 32(12):1628–35. doi: 10.1002/jor.22718
32. Ji Q, Qiao X, Liu Y, Wang D, Yan J. Silencing of longchain noncoding RNA GAS5 in osteoarthritic chondrocytes is mediated by targeting the miR34a/Bcl2 axis. Mol Med Rep (2020) 21(3):1310–9. doi: 10.3892/mmr.2019.10900. doi: 10.26355/eurrev_202011_23582.
33. Gao ST, Yu YM, Wan LP, Liu ZM, Lin JX. LncRNA GAS5 induces chondrocyte apoptosis by down-regulating miR-137. Eur Rev Med Pharmacol Sci (2020) 24(21):10984–91. doi: 10.26355/eurrev_202011_23582
34. Cai L, Huang N, Zhang X, Wu S, Wang L, Ke Q. Long non-coding RNA plasmacytoma variant translocation 1 and growth arrest specific 5 regulate each other in osteoarthritis to regulate the apoptosis of chondrocytes. Bioengineered (2022) 13(5):13680–8. doi: 10.1080/21655979.2022.2063653
35. Liu C, Ren S, Zhao S, Wang Y. LncRNA MALAT1/MiR-145 adjusts IL-1beta-Induced chondrocytes viability and cartilage matrix degradation by regulating ADAMTS5 in human osteoarthritis. Yonsei Med J (2019) 60(11):1081–92. doi: 10.3349/ymj.2019.60.11.1081
36. Liang J, Xu L, Zhou F, Liu AM, Ge HX, Chen YY, et al. MALAT1/miR-127-5p regulates osteopontin (OPN)-mediated proliferation of human chondrocytes through PI3K/Akt pathway. J Cell Biochem (2018) 119(1):431–9. doi: 10.1002/jcb.26200
37. Nanus DE, Wijesinghe SN, Pearson MJ, Hadjicharalambous MR, Rosser A, Davis ET, et al. Regulation of the inflammatory synovial fibroblast phenotype by metastasis-associated lung adenocarcinoma transcript 1 long noncoding RNA in obese patients with osteoarthritis. Arthritis Rheumatol (2020) 72(4):609–19. doi: 10.1002/art.41158
38. Alnajjar FA, Sharma-Oates A, Wijesinghe SN, Farah H, Nanus DE, Nicholson T, et al. The expression and function of metastases associated lung adenocarcinoma transcript-1 long non-coding RNA in subchondral bone and osteoblasts from patients with osteoarthritis. Cells (2021) 10(4):786–92. doi: 10.3390/cells10040786
39. Liu Y, Liu K, Tang C, Shi Z, Jing K, Zheng J. Long non-coding RNA XIST contributes to osteoarthritis progression via miR-149-5p/DNMT3A axis. BioMed Pharmacother (2020) 128:110349. doi: 10.1016/j.biopha.2020.110349
40. Shen XF, Cheng Y, Dong QR, Zheng MQ. MicroRNA-675-3p regulates IL-1beta-stimulated human chondrocyte apoptosis and cartilage degradation by targeting GNG5. Biochem Biophys Res Commun (2020) 527(2):458–65. doi: 10.1016/j.bbrc.2020.04.044
41. Li L, Lv G, Wang B, Kuang L. The role of lncRNA XIST/miR-211 axis in modulating the proliferation and apoptosis of osteoarthritis chondrocytes through CXCR4 and MAPK signaling. Biochem Biophys Res Commun (2018) 503(4):2555–62. doi: 10.1016/j.bbrc.2018.07.015
42. Li L, Lv G, Wang B, Kuang L. XIST/miR-376c-5p/OPN axis modulates the influence of proinflammatory M1 macrophages on osteoarthritis chondrocyte apoptosis. J Cell Physiol (2020) 235(1):281–93. doi: 10.1002/jcp.28968
43. Wang J, Luo X, Cai S, Sun J, Wang S, Wei X. Blocking HOTAIR protects human chondrocytes against IL-1beta-induced cell apoptosis, ECM degradation, inflammatory response and oxidative stress via regulating miR-222-3p/ADAM10 axis. Int Immunopharmacol (2021) 98:107903. doi: 10.1016/j.intimp.2021.107903
44. He B, Jiang D. HOTAIR-induced apoptosis is mediated by sponging miR-130a-3p to repress chondrocyte autophagy in knee osteoarthritis. Cell Biol Int (2020) 44(2):524–35. doi: 10.1002/cbin.11253
45. Wang B, Sun Y, Liu N, Liu H. LncRNA HOTAIR modulates chondrocyte apoptosis and inflammation in osteoarthritis via regulating miR-1277-5p/SGTB axis. Wound Repair Regen (2021) 29(3):495–504. doi: 10.1111/wrr.12908
46. Lu J, Wu Z, Xiong Y. Knockdown of long noncoding RNA HOTAIR inhibits osteoarthritis chondrocyte injury by miR-107/CXCL12 axis. J Orthop Surg Res (2021) 16(1):410. doi: 10.1186/s13018-021-02547-7
47. Dou P, Hu R, Zhu W, Tang Q, Li D, Li H, et al. Long non-coding RNA HOTAIR promotes expression of ADAMTS-5 in human osteoarthritic articular chondrocytes. Pharmazie (2017) 72(2):113–7. doi: 10.1691/ph.2017.6649
48. Hu J, Wang Z, Shan Y, Pan Y, Ma J, Jia L. Long non-coding RNA HOTAIR promotes osteoarthritis progression via miR-17-5p/FUT2/beta-catenin axis. Cell Death Dis (2018) 9(7):711. doi: 10.1038/s41419-018-0746-z
49. Yang Y, Xing D, Wang Y, Jia H, Li B, Li JJ. A long non-coding RNA, HOTAIR, promotes cartilage degradation in osteoarthritis by inhibiting WIF-1 expression and activating wnt pathway. BMC Mol Cell Biol (2020) 21(1):53. doi: 10.1186/s12860-020-00299-6
50. Kim D, Song J, Han J, Kim Y, Chun CH, Jin EJ. Two non-coding RNAs, MicroRNA-101 and HOTTIP contribute cartilage integrity by epigenetic and homeotic regulation of integrin-alpha1. Cell Signal (2013) 25(12):2878–87. doi: 10.1016/j.cellsig.2013.08.034
51. He X, Gao K, Lu S, Wu R. LncRNA HOTTIP leads to osteoarthritis progression via regulating miR-663a/Fyn-related kinase axis. BMC Musculoskelet Disord (2021) 22(1):67. doi: 10.1186/s12891-020-03861-7
52. Liu Q, Zhang X, Dai L, Hu X, Zhu J, Li L, et al. Long noncoding RNA related to cartilage injury promotes chondrocyte extracellular matrix degradation in osteoarthritis. Arthritis Rheumatol (2014) 66(4):969–78. doi: 10.1002/art.38309
53. Li YF, Li SH, Liu Y, Luo YT. Long noncoding RNA CIR promotes chondrocyte extracellular matrix degradation in osteoarthritis by acting as a sponge for mir-27b. Cell Physiol Biochem (2017) 43(2):602–10. doi: 10.1159/000480532
54. Wang CL, Peng JP, Chen XD. LncRNA-CIR promotes articular cartilage degeneration in osteoarthritis by regulating autophagy. Biochem Biophys Res Commun (2018) 505(3):692–8. doi: 10.1016/j.bbrc.2018.09.163
55. Liu Q, Hu X, Zhang X, Dai L, Duan X, Zhou C, et al. The TMSB4 pseudogene LncRNA functions as a competing endogenous RNA to promote cartilage degradation in human osteoarthritis. Mol Ther (2016) 24(10):1726–33. doi: 10.1038/mt.2016.151
56. Zhao Y, Zhao J, Guo X, She J, Liu Y. Long non-coding RNA PVT1, a molecular sponge for miR-149, contributes aberrant metabolic dysfunction and inflammation in IL-1beta-simulated osteoarthritic chondrocytes. Biosci Rep (2018) 38(5):1–11. doi: 10.1042/BSR20180576
57. Lu X, Yu Y, Yin F, Yang C, Li B, Lin J, et al. Knockdown of PVT1 inhibits IL-1beta-induced injury in chondrocytes by regulating miR-27b-3p/TRAF3 axis. Int Immunopharmacol (2020) 79:106052. doi: 10.1016/j.intimp.2019.106052
58. Park S, Lee M, Chun CH, Jin EJ. The lncRNA, nespas, is associated with osteoarthritis progression and serves as a potential new prognostic biomarker. Cartilage (2019) 10(2):148–56. doi: 10.1177/1947603517725566
59. Wang G, Bu X, Zhang Y, Zhao X, Kong Y, Ma L, et al. LncRNA-UCA1 enhances MMP-13 expression by inhibiting miR-204-5p in human chondrocytes. Oncotarget (2017) 8(53):91281–90. doi: 10.18632/oncotarget.20108
60. Fan X, Yuan J, Xie J, Pan Z, Yao X, Sun X, et al. Long non-protein coding RNA DANCR functions as a competing endogenous RNA to regulate osteoarthritis progression via miR-577/SphK2 axis. Biochem Biophys Res Commun (2018) 500(3):658–64. doi: 10.1016/j.bbrc.2018.04.130
61. Zhang L, Zhang P, Sun X, Zhou L, Zhao J. Long non-coding RNA DANCR regulates proliferation and apoptosis of chondrocytes in osteoarthritis via miR-216a-5p-JAK2-STAT3 axis. Biosci Rep (2018) 38(6)1–11. doi: 10.1042/BSR20181228
62. Li XP, Wei X, Wang SQ, Sun G, Zhao YC, Yin H, et al. Differentiation antagonizing non-protein coding RNA knockdown alleviates lipopolysaccharide-induced inflammatory injury and apoptosis in human chondrocyte primary chondrocyte cells through upregulating miRNA-19a-3p. Orthop Surg (2021) 13(1):276–84. doi: 10.1111/os.12845
63. Sun J, Song X, Su L, Cao S. Long non-coding RNA LncHIFCAR promotes osteoarthritis development via positively regulating HIF-1alpha and activating the PI3K/AKT/mTOR pathway. Int J Clin Exp Pathol (2018) 11(6):3000–9.
64. Zhu JK, He TD, Wei ZX, Wang YM. LncRNA FAS-AS1 promotes the degradation of extracellular matrix of cartilage in osteoarthritis. Eur Rev Med Pharmacol Sci (2018) 22(10):2966–72. doi: 10.26355/eurrev_201805_15051
65. Cao L, Wang Y, Wang Q, Huang J. LncRNA FOXD2-AS1 regulates chondrocyte proliferation in osteoarthritis by acting as a sponge of miR-206 to modulate CCND1 expression. BioMed Pharmacother (2018) 106:1220–6. doi: 10.1016/j.biopha.2018.07.048
66. Tang L, Ding J, Zhou G, Liu Z. LncRNAp21 promotes chondrocyte apoptosis in osteoarthritis by acting as a sponge for miR451. Mol Med Rep (2018) 18(6):5295–301. doi: 10.3892/mmr.2018.9506.
67. Li Y, Li Z, Li C, Zeng Y, Liu Y. Long noncoding RNA TM1P3 is involved in osteoarthritis by mediating chondrocyte extracellular matrix degradation. J Cell Biochem (2019) 120(8):12702–12. doi: 10.1002/jcb.28539
68. Huang B, Yu H, Li Y, Zhang W, Liu X. Upregulation of long noncoding TNFSF10 contributes to osteoarthritis progression through the miR-376-3p/FGFR1 axis. J Cell Biochem (2019) 120(12):19610–20. doi: 10.1002/jcb.29267
69. Wei W, He S, Wang Z, Dong J, Xiang D, Li Y, et al. LINC01534 promotes the aberrant metabolic dysfunction and inflammation in IL-1beta-Simulated osteoarthritic chondrocytes by targeting miR-140-5p. Cartilage (2021) 13(2_suppl):898S–907S. doi: 10.1177/1947603519888787
70. Xue H, Yu P, Wang WZ, Niu YY, Li X. The reduced lncRNA NKILA inhibited proliferation and promoted apoptosis of chondrocytes via miR-145/SP1/NF-kappaB signaling in human osteoarthritis. Eur Rev Med Pharmacol Sci (2020) 24(2):535–48. doi: 10.26355/eurrev_202001_20030
71. Zhang Y, Ma L, Wang C, Wang L, Guo Y, Wang G. Long noncoding RNA LINC00461 induced osteoarthritis progression by inhibiting miR-30a-5p. Aging (Albany NY) (2020) 12(5):4111–23. doi: 10.18632/aging.102839
72. Chen K, Fang H, Xu N. LncRNA LOXL1-AS1 is transcriptionally activated by JUND and contributes to osteoarthritis progression via targeting the miR-423-5p/KDM5C axis. Life Sci (2020) 258:118095. doi: 10.1016/j.lfs.2020.118095
73. Zhou L, Gu M, Ma X, Wen L, Zhang B, Lin Y, et al. Long non-coding RNA PCAT-1 regulates apoptosis of chondrocytes in osteoarthritis by sponging miR-27b-3p. J Bone Miner Metab (2021) 39(2):139–47. doi: 10.1007/s00774-020-01128-8
74. Zhang G, Zhang Q, Zhu J, Tang J, Nie M. LncRNA ARFRP1 knockdown inhibits LPS-induced the injury of chondrocytes by regulation of NF-kappaB pathway through modulating miR-15a-5p/TLR4 axis. Life Sci (2020) 261:118429. doi: 10.1016/j.lfs.2020.118429
75. Li Z, Wang J, Yang J. TUG1 knockdown promoted viability and inhibited apoptosis and cartilage ECM degradation in chondrocytes via the miR-17-5p/FUT1 pathway in osteoarthritis. Exp Ther Med (2020) 20(6):154. doi: 10.3892/etm.2020.9283
76. Chen C, Xu Y. Long noncoding RNA LINC00671 exacerbates osteoarthritis by promoting ONECUT2-mediated Smurf2 expression and extracellular matrix degradation. Int Immunopharmacol (2021) 90:106846. doi: 10.1016/j.intimp.2020.106846
77. Lu JF, Qi LG, Zhu XB, Shen YX. LncRNA RMRP knockdown promotes proliferation and inhibits apoptosis in osteoarthritis chondrocytes by miR-206/CDK9 axis. Pharmazie (2020) 75(10):500–4. doi: 10.1691/ph.2020.0591
78. Aili D, Wu T, Gu Y, Chen Z, Wang W. Knockdown of long non-coding RNA KCNQ1OT1 suppresses the progression of osteoarthritis by mediating the miR-211-5p/TCF4 axis in vitro. Exp Ther Med (2021) 21(5):455. doi: 10.3892/etm.2021.9886
79. Wang B, Liu X. Long non-coding RNA KCNQ1OT1 promotes cell viability and migration as well as inhibiting degradation of CHON-001 cells by regulating miR-126-5p/TRPS1 axis. Adv Rheumatol (2021) 61(1):31. doi: 10.1186/s42358-021-00187-3
80. Li X, Li Y, Yang X, Liao R, Chen L, Guo Q, et al. PR11-364P22.2/ATF3 protein interaction mediates IL-1beta-induced catabolic effects in cartilage tissue and chondrocytes. J Cell Mol Med (2021) 25(13):6188–202. doi: 10.1111/jcmm.16561
81. Jiang M, Xu K, Ren H, Wang M, Hou X, Cao J. Role of lincRNA-Cox2 targeting miR-150 in regulating the viability of chondrocytes in osteoarthritis. Exp Ther Med (2021) 22(2):800. doi: 10.3892/etm.2021.10232
82. Zhou C, He T, Chen L. LncRNA CASC19 accelerates chondrocytes apoptosis and proinflammatory cytokine production to exacerbate osteoarthritis development through regulating the miR-152-3p/DDX6 axis. J Orthop Surg Res (2021) 16(1):399. doi: 10.1186/s13018-021-02543-x
83. Long H, Li Q, Xiao Z, Yang B. LncRNA MIR22HG promotes osteoarthritis progression via regulating miR-9-3p/ADAMTS5 pathway. Bioengineered (2021) 12(1):3148–58. doi: 10.1080/21655979.2021.1945362
84. Wang Z, Huang C, Zhao C, Zhang H, Zhen Z, Xu D. Knockdown of LINC01385 inhibits osteoarthritis progression by modulating the microRNA-140-3p/TLR4 axis. Exp Ther Med (2021) 22(5):1244. doi: 10.3892/etm.2021.10679
85. Xu Y, Duan L, Liu S, Yang Y, Qiao Z, Shi L. Long intergenic non-protein coding RNA 00707 regulates chondrocyte apoptosis and proliferation in osteoarthritis by serving as a sponge for microRNA-199-3p. Bioengineered (2022) 13(4):11137–45. doi: 10.1080/21655979.2022.2061287
86. Qian M, Shi Y, Lu W. LINC00707 knockdown inhibits IL-1beta-induced apoptosis and extracellular matrix degradation of osteoarthritis chondrocytes by the miR-330-5p/FSHR axis. Immunopharmacol Immunotoxicol (2022) 44(4):1–11. doi: 10.1080/08923973.2022.2076241
87. Ren J, Li Y, Wuermanbieke S, Hu S, Huang G. N(6)-methyladenosine (m(6)A) methyltransferase METTL3-mediated LINC00680 accelerates osteoarthritis through m(6)A/SIRT1 manner. Cell Death Discovery (2022) 8(1):240. doi: 10.1038/s41420-022-00890-0
88. Tang S, Cao Y, Cai Z, Nie X, Ruan J, Zhou Z, et al. The lncRNA PILA promotes NF-kappaB signaling in osteoarthritis by stimulating the activity of the protein arginine methyltransferase PRMT1. Sci Signal (2022) 15(735):eabm6265. doi: 10.1126/scisignal.abm6265
89. Wu X, Yin S, Yan L, Liu Y, Shang L, Liu J. lncRNA DLEU1 modulates proliferation, inflammation, and extracellular matrix degradation of chondrocytes through regulating miR-671-5p. J Immunol Res (2022) 2022:1816217. doi: 10.1155/2022/1816217
90. Su W, Xie W, Shang Q, Su B. The long noncoding RNA MEG3 is downregulated and inversely associated with VEGF levels in osteoarthritis. BioMed Res Int (2015) 2015:356893. doi: 10.1155/2015/356893
91. Wang A, Hu N, Zhang Y, Chen Y, Su C, Lv Y, et al. MEG3 promotes proliferation and inhibits apoptosis in osteoarthritis chondrocytes by miR-361-5p/FOXO1 axis. BMC Med Genomics (2019) 12(1):201. doi: 10.1186/s12920-019-0649-6
92. Pearson MJ, Philp AM, Heward JA, Roux BT, Walsh DA, Davis ET, et al. Long intergenic noncoding RNAs mediate the human chondrocyte inflammatory response and are differentially expressed in osteoarthritis cartilage. Arthritis Rheumatol (2016) 68(4):845–56. doi: 10.1002/art.39520
93. Zheng J, Li Q. Methylene blue regulates inflammatory response in osteoarthritis by noncoding long chain RNA CILinc02. J Cell Biochem (2019) 120(3):3331–8. doi: 10.1002/jcb.27602
94. Zhang G, Wu Y, Xu D, Yan X. Long noncoding RNA UFC1 promotes proliferation of chondrocyte in osteoarthritis by acting as a sponge for miR-34a. DNA Cell Biol (2016) 35(11):691–5. doi: 10.1089/dna.2016.3397
95. Shen H, Wang Y, Shi W, Sun G, Hong L, Zhang Y. LncRNA SNHG5/miR-26a/SOX2 signal axis enhances proliferation of chondrocyte in osteoarthritis. Acta Biochim Biophys Sin (Shanghai) (2018) 50(2):191–8. doi: 10.1093/abbs/gmx141
96. Zhang X, Huang CR, Pan S, Pang Y, Chen YS, Zha GC, et al. Long non-coding RNA SNHG15 is a competing endogenous RNA of miR-141-3p that prevents osteoarthritis progression by upregulating BCL2L13 expression. Int Immunopharmacol (2020) 83:106425. doi: 10.1016/j.intimp.2020.106425
97. Jiang H, Pang H, Wu P, Cao Z, Li Z, Yang X. LncRNA SNHG5 promotes chondrocyte proliferation and inhibits apoptosis in osteoarthritis by regulating miR-10a-5p/H3F3B axis. Connect Tissue Res (2021) 62(6):605–14. doi: 10.1080/03008207.2020.1825701
98. Yue Y, Zhibo S, Feng L, Yuanzhang B, Fei W. SNHG5 protects chondrocytes in interleukin-1beta-stimulated osteoarthritis via regulating miR-181a-5p/TGFBR3 axis. J Biochem Mol Toxicol (2021) 35(10):e22866. doi: 10.1002/jbt.22866
99. Xiao Y, Yan X, Yang Y, Ma X. Downregulation of long noncoding RNA HOTAIRM1 variant 1 contributes to osteoarthritis via regulating miR-125b/BMPR2 axis and activating JNK/MAPK/ERK pathway. BioMed Pharmacother (2019) 109:1569–77. doi: 10.1016/j.biopha.2018.10.181
100. Yang Q, Li X, Zhou Y, Fu W, Wang J, Wei Q. A LINC00341-mediated regulatory pathway supports chondrocyte survival and may prevent osteoarthritis progression. J Cell Biochem (2019) 120(6):10812–20. doi: 10.1002/jcb.28372
101. Ai D, Yu F. LncRNA DNM3OS promotes proliferation and inhibits apoptosis through modulating IGF1 expression by sponging MiR-126 in CHON-001 cells. Diagn Pathol (2019) 14(1):106. doi: 10.1186/s13000-019-0877-2
102. Lu C, Li Z, Hu S, Cai Y, Peng K. LncRNA PART-1 targets TGFBR2/Smad3 to regulate cell viability and apoptosis of chondrocytes via acting as miR-590-3p sponge in osteoarthritis. J Cell Mol Med (2019) 23(12):8196–205. doi: 10.1111/jcmm.14690
103. Zhu YJ, Jiang DM. LncRNA PART1 modulates chondrocyte proliferation, apoptosis, and extracellular matrix degradation in osteoarthritis via regulating miR-373-3p/SOX4 axis. Eur Rev Med Pharmacol Sci (2019) 23(19):8175–85. doi: 10.26355/eurrev_201910_19124
104. Liu F, Liu X, Yang Y, Sun Z, Deng S, Jiang Z, et al. NEAT1/miR-193a-3p/SOX5 axis regulates cartilage matrix degradation in human osteoarthritis. Cell Biol Int (2020) 44(4):947–57. doi: 10.1002/cbin.11291
105. Xiao P, Zhu X, Sun J, Zhang Y, Qiu W, Li J, et al. LncRNA NEAT1 regulates chondrocyte proliferation and apoptosis via targeting miR-543/PLA2G4A axis. Hum Cell (2021) 34(1):60–75. doi: 10.1007/s13577-020-00433-8
106. Lu M, Zhou E. Long noncoding RNA LINC00662-miR-15b-5p mediated GPR120 dysregulation contributes to osteoarthritis. Pathol Int (2020) 70(3):155–65. doi: 10.1111/pin.12875
107. Zhi L, Zhao J, Zhao H, Qing Z, Liu H, Ma J. Downregulation of LncRNA OIP5-AS1 induced by IL-1beta aggravates osteoarthritis via regulating miR-29b-3p/PGRN. Cartilage (2021) 13(2_suppl):1345S–55S. doi: 10.1177/1947603519900801
108. Lu G, Li L, Wang B, Kuang L. LINC00623/miR-101/HRAS axis modulates IL-1beta-mediated ECM degradation, apoptosis and senescence of osteoarthritis chondrocytes. Aging (Albany NY) (2020) 12(4):3218–37. doi: 10.18632/aging.102801
109. Xu J, Pei Y, Lu J, Liang X, Li Y, Wang J, et al. LncRNA SNHG7 alleviates IL-1beta-induced osteoarthritis by inhibiting miR-214-5p-mediated PPARGC1B signaling pathways. Int Immunopharmacol (2021) 90:107150. doi: 10.1016/j.intimp.2020.107150
110. Feng L, Yang ZM, Li YC, Wang HX, Lo JHT, Zhang XT, et al. Linc-ROR promotes mesenchymal stem cells chondrogenesis and cartilage formation via regulating SOX9 expression. Osteoarthritis Cartilage (2021) 29(4):568–78. doi: 10.1016/j.joca.2020.12.020
111. Qin GH, Yang WC, Yao JN, Zhao Y, Wu XJ. LncRNA OIP5-AS1 affects the biological behaviors of chondrocytes of patients with osteoarthritis by regulating micro-30a-5p. Eur Rev Med Pharmacol Sci (2021) 25(3):1215–24. doi: 10.26355/eurrev_202102_24825
112. Yang Y, Sun Z, Liu F, Bai Y, Wu F. FGD5-AS1 inhibits osteoarthritis development by modulating miR-302d-3p/TGFBR2 axis. Cartilage (2021) 13(2_suppl):1412S–20S. doi: 10.1177/19476035211003324
113. Shi J, Cao F, Chang Y, Xin C, Jiang X, Xu J, et al. Long non-coding RNA MCM3AP-AS1 protects chondrocytes ATDC5 and CHON-001 from IL-1beta-induced inflammation via regulating miR-138-5p/SIRT1. Bioengineered (2021) 12(1):1445–56. doi: 10.1080/21655979.2021.1905247
114. Xie W, Jiang L, Huang X, Shang H, Gao M, You W, et al. lncRNA MEG8 is downregulated in osteoarthritis and regulates chondrocyte cell proliferation, apoptosis and inflammation. Exp Ther Med (2021) 22(4):1153. doi: 10.3892/etm.2021.10587
115. Gu Y, Wang G, Xu H. Long non-coding RNA ZNFX1 antisense 1 (ZFAS1) suppresses anti-oxidative stress in chondrocytes during osteoarthritis by sponging microRNA-1323. Bioengineered (2022) 13(5):13188–200. doi: 10.1080/21655979.2022.2074770
116. Li X, Ren W, Xiao ZY, Wu LF, Wang H, Guo PY. GACAT3 promoted proliferation of osteoarthritis synoviocytes by IL-6/STAT3 signaling pathway. Eur Rev Med Pharmacol Sci (2018) 22(16):5114–20. doi: 10.26355/eurrev_201808_15705
117. Li X, Huang TL, Zhang GD, Jiang JT, Guo PY. LncRNA ANRIL impacts the progress of osteoarthritis via regulating proliferation and apoptosis of osteoarthritis synoviocytes. Eur Rev Med Pharmacol Sci (2019) 23(22):9729–37. doi: 10.26355/eurrev_201911_19535
118. Kang Y, Song J, Kim D, Ahn C, Park S, Chun CH, et al. PCGEM1 stimulates proliferation of osteoarthritic synoviocytes by acting as a sponge for miR-770. J Orthop Res (2016) 34(3):412–8. doi: 10.1002/jor.23046
119. Zeng G, Deng G, Xiao S, Li F. Fibroblast-like synoviocytes-derived exosomal PCGEM1 accelerates IL-1beta-induced apoptosis and cartilage matrix degradation by miR-142-5p/RUNX2 in chondrocytes. Immunol Invest (2022) 51(5):1284–301. doi: 10.1080/08820139.2021.1936010
120. Jia J, Sun J, Liao W, Qin L, Su K, He Y, et al. Knockdown of long noncoding RNA AK094629 attenuates the interleukin1beta induced expression of interleukin6 in synoviumderived mesenchymal stem cells from the temporomandibular joint. Mol Med Rep (2020) 22(2):1195–204. doi: 10.3892/mmr.2020.11193
121. Zhang P, Sun J, Liang C, Gu B, Xu Y, Lu H, et al. lncRNA IGHCgamma1 acts as a ceRNA to regulate macrophage inflammation via the miR-6891-3p/TLR4 axis in osteoarthritis. Mediators Inflamm (2020) 2020:9743037. doi: 10.1155/2020/9743037
122. Tuerlings M, van Hoolwerff M, van Bokkum JM, Suchiman HED, Lakenberg N, Broekhuis D, et al. Long non-coding RNA expression profiling of subchondral bone reveals AC005165.1 modifying FRZB expression during osteoarthritis. Rheumatol (Oxford) (2021) 61(7):3023–32. doi: 10.1093/rheumatology/keab826
123. Jiang Z, Du X, Wen X, Li H, Zeng A, Sun H, et al. Whole-transcriptome sequence of degenerative meniscus cells unveiling diagnostic markers and therapeutic targets for osteoarthritis. Front Genet (2021) 12:754421. doi: 10.3389/fgene.2021.754421
124. Chen S, Fu P, Wu H, Pei M. Meniscus, articular cartilage and nucleus pulposus: a comparative review of cartilage-like tissues in anatomy, development and function. Cell Tissue Res (2017) 370(1):53–70. doi: 10.1007/s00441-017-2613-0
125. Goldring MB, Marcu KB. Cartilage homeostasis in health and rheumatic diseases. Arthritis Res Ther (2009) 11(3):224. doi: 10.1186/ar2592
126. Hodgkinson T, Kelly DC, Curtin CM, O’Brien FJ. Mechanosignalling in cartilage: An emerging target for the treatment of osteoarthritis. Nat Rev Rheumatol (2021) 18:67–84. doi: 10.1038/s41584-021-00724-w
127. Fu M, Huang G, Zhang Z, Liu J, Zhang Z, Huang Z, et al. Expression profile of long noncoding RNAs in cartilage from knee osteoarthritis patients. Osteoarthritis Cartilage (2015) 23(3):423–32. doi: 10.1016/j.joca.2014.12.001
128. Li X, Liao Z, Deng Z, Chen N, Zhao L. Combining bulk and single-cell RNA-sequencing data to reveal gene expression pattern of chondrocytes in the osteoarthritic knee. Bioengineered (2021) 12(1):997–1007. doi: 10.1080/21655979.2021.1903207
129. van Hoolwerff M, Metselaar PI, Tuerlings M, Suchiman HED, Lakenberg N, Ramos YFM, et al. Elucidating epigenetic regulation by identifying functional cis-acting long noncoding RNAs and their targets in osteoarthritic articular cartilage. Arthritis Rheumatol (2020) 72(11):1845–54. doi: 10.1002/art.41396
130. Tu J, Huang W, Zhang W, Mei J, Zhu C. The emerging role of lncRNAs in chondrocytes from osteoarthritis patients. BioMed Pharmacother (2020) 131:110642. doi: 10.1016/j.biopha.2020.110642
131. Firestein GS, McInnes IB. Immunopathogenesis of rheumatoid arthritis. Immunity (2017) 46(2):183–96. doi: 10.1016/j.immuni.2017.02.006
132. Doherty M. Synovial inflammation and osteoarthritis progression: effects of nonsteroidal antiinflammatory drugs. Osteoarthritis Cartilage (1999) 7(3):319–20. doi: 10.1053/joca.1998.0179
133. Wenham CY, Conaghan PG. The role of synovitis in osteoarthritis. Ther Adv Musculoskelet Dis (2010) 2(6):349–59. doi: 10.1177/1759720X10378373
134. Xiang S, Li Z, Bian Y, Weng X. Identification of changed expression of mRNAs and lncRNAs in osteoarthritic synovium by RNA-sequencing. Gene (2019) 685:55–61. doi: 10.1016/j.gene.2018.10.076
135. Dai G, Xiao H, Liao J, Zhou N, Zhao C, Xu W, et al. Osteocyte TGFbeta1Smad2/3 is positively associated with bone turnover parameters in subchondral bone of advanced osteoarthritis. Int J Mol Med (2020) 46(1):167–78. doi: 10.3892/ijmm.2020.4576
136. Suri S, Gill SE, Massena de Camin S, Wilson D, McWilliams DF, Walsh DA. Neurovascular invasion at the osteochondral junction and in osteophytes in osteoarthritis. Ann Rheum Dis (2007) 66(11):1423–8. doi: 10.1136/ard.2006.063354
137. Silva AM, Moura SR, Teixeira JH, Barbosa MA, Santos SG, Almeida MI. Long noncoding RNAs: a missing link in osteoporosis. Bone Res (2019) 7:10. doi: 10.1038/s41413-019-0048-9
138. Makris EA, Hadidi P, Athanasiou KA. The knee meniscus: structure-function, pathophysiology, current repair techniques, and prospects for regeneration. Biomaterials (2011) 32(30):7411–31. doi: 10.1016/j.biomaterials.2011.06.037
139. Murphy CA, Garg AK, Silva-Correia J, Reis RL, Oliveira JM, Collins MN. The meniscus in normal and osteoarthritic tissues: Facing the structure property challenges and current treatment trends. Annu Rev BioMed Eng (2019) 21:495–521. doi: 10.1146/annurev-bioeng-060418-052547
140. Brophy RH, Zhang B, Cai L, Wright RW, Sandell LJ, Rai MF. Transcriptome comparison of meniscus from patients with and without osteoarthritis. Osteoarthritis Cartilage (2018) 26(3):422–32. doi: 10.1016/j.joca.2017.12.004
141. Shi T, Gao G, Cao Y. Long noncoding RNAs as novel biomarkers have a promising future in cancer diagnostics. Dis Markers (2016) 2016:9085195. doi: 10.1155/2016/9085195
142. De Ceuninck F, Sabatini M, Pastoureau P. Recent progress toward biomarker identification in osteoarthritis. Drug Discovery Today (2011) 16(9-10):443–9. doi: 10.1016/j.drudis.2011.01.004
143. Dang X, Lian L, Wu D. The diagnostic value and pathogenetic role of lncRNA-ATB in patients with osteoarthritis. Cell Mol Biol Lett (2018) 23:55. doi: 10.1186/s11658-018-0118-9
144. Zhou L, Wan Y, Cheng Q, Shi B, Zhang L, Chen S. The expression and diagnostic value of LncRNA H19 in the blood of patients with osteoarthritis. Iran J Public Health (2020) 49(8):1494–501. doi: 10.18502/ijph.v49i8.3893
145. Huang J, Liu L, Yang J, Ding J, Xu X. lncRNA DILC is downregulated in osteoarthritis and regulates IL-6 expression in chondrocytes. J Cell Biochem (2019) 120(9):16019–24. doi: 10.1002/jcb.28880
146. He J, Wang L, Ding Y, Liu H, Zou G. lncRNA FER1L4 is dysregulated in osteoarthritis and regulates IL-6 expression in human chondrocyte cells. Sci Rep (2021) 11(1):13032. doi: 10.1038/s41598-021-92474-8
147. Li Q, Zhang Z, Guo S, Tang G, Lu W, Qi X. LncRNA ANCR is positively correlated with transforming growth factor-beta1 in patients with osteoarthritis. J Cell Biochem (2019) 120(9):14226–32. doi: 10.1002/jcb.28881
148. Xiao Y, Bao Y, Tang L, Wang L. LncRNA MIR4435-2HG is downregulated in osteoarthritis and regulates chondrocyte cell proliferation and apoptosis. J Orthop Surg Res (2019) 14(1):247. doi: 10.1186/s13018-019-1278-7
149. Ni S, Xu C, Zhuang C, Zhao G, Li C, Wang Y, et al. LncRNA LUADT1 regulates miR-34a/SIRT1 to participate in chondrocyte apoptosis. J Cell Biochem (2020) 122(9):1003–8. doi: 10.1002/jcb.29637
150. Qi K, Lin R, Xue C, Liu T, Wang Y, Zhang Y, et al. Long non-coding RNA (LncRNA) CAIF is downregulated in osteoarthritis and inhibits LPS-induced interleukin 6 (IL-6) upregulation by downregulation of MiR-1246. Med Sci Monit (2019) 25:8019–24. doi: 10.12659/MSM.917135
151. Yang F, Zhao M, Sang Q, Yan C, Wang Z. Long non-coding RNA PMS2L2 is down-regulated in osteoarthritis and inhibits chondrocyte proliferation by up-regulating miR-34a. J Immunotoxicol (2022) 19(1):74–80. doi: 10.1080/1547691X.2022.2049664
152. Zhang C, Wang P, Jiang P, Lv Y, Dong C, Dai X, et al. Upregulation of lncRNA HOTAIR contributes to IL-1beta-induced MMP overexpression and chondrocytes apoptosis in temporomandibular joint osteoarthritis. Gene (2016) 586(2):248–53. doi: 10.1016/j.gene.2016.04.016
153. Sun Y, Kang S, Pei S, Sang C, Huang Y, et al. Mir93-5p Inhibits Chondrocyte Apoptosis in Osteoarthritis by Targeting Lncrna Casc2. BMC Musculoskelet Disord (2020) 21(1):26–32. doi: 10.1186/s12891-019-3025-y
154. Zhang H, Li J, Shao W, Shen N. LncRNA CTBP1-AS2 is upregulated in osteoarthritis and increases the methylation of miR-130a gene to inhibit chondrocyte proliferation. Clin Rheumatol (2020) 39(11):3473–8. doi: 10.1007/s10067-020-05113-4
155. Zhang D, Qiu S. LncRNA GAS5 upregulates Smad4 to suppress the apoptosis of chondrocytes induced by lipopolysaccharide. Arch Gerontol Geriatr (2021) 97:104478. doi: 10.1016/j.archger.2021.104478
156. Zhang H, Wu Y, Li W, Chen H. Clinical significance and mechanism of LncRNA GAS-5 in osteoarthritis. Am J Transl Res (2021) 13(7):8465–70.
157. Jiang L, Zhou Y, Shen J, Chen Y, Ma Z, Yu Y, et al. RNA Sequencing reveals LINC00167 as a potential diagnosis biomarker for primary osteoarthritis: A multi-stage study. Front Genet (2020) 11:539489. doi: 10.3389/fgene.2020.539489
158. Meng Y, Qiu S, Sun L, Zuo J. Knockdown of exosomemediated lncPVT1 alleviates lipopolysaccharideinduced osteoarthritis progression by mediating the HMGB1/TLR4/NFkappaB pathway via miR935p. Mol Med Rep (2020) 22(6):5313–25. doi: 10.3892/mmr.2020.11594
159. Zhao Y, Xu J. Synovial fluid-derived exosomal lncRNA PCGEM1 as biomarker for the different stages of osteoarthritis. Int Orthop (2018) 42(12):2865–72. doi: 10.1007/s00264-018-4093-6
160. Wu X, Bian B, Lin Z, Wu C, Sun Y, Pan Y, et al. Identification of exosomal mRNA, lncRNA and circRNA signatures in an osteoarthritis synovial fluid-exosomal study. Exp Cell Res (2022) 410(1):112881. doi: 10.1016/j.yexcr.2021.112881
161. Wang J, Sun J, Yang F. The role of long non-coding RNA H19 in breast cancer. Oncol Lett (2020) 19(1):7–16. doi: 10.3892/ol.2019.11093
162. Pachnis V, Belayew A, Tilghman SM. Locus unlinked to alpha-fetoprotein under the control of the murine raf and rif genes. Proc Natl Acad Sci U S A (1984) 81(17):5523–7. doi: 10.1073/pnas.81.17.5523
163. Zhou X, Cao H, Wang M, Zou J, Wu W. Moderate-intensity treadmill running relieves motion-induced post-traumatic osteoarthritis mice by up-regulating the expression of lncRNA H19. BioMed Eng Online (2021) 20(1):111. doi: 10.1186/s12938-021-00949-6
164. Xing D, Liang JQ, Li Y, Lu J, Jia HB, Xu LY, et al. Identification of long noncoding RNA associated with osteoarthritis in humans. Orthop Surg (2014) 6(4):288–93. doi: 10.1111/os.12147
165. Dudek KA, Lafont JE, Martinez-Sanchez A, Murphy CL. Type II collagen expression is regulated by tissue-specific miR-675 in human articular chondrocytes. J Biol Chem (2010) 285(32):24381–7. doi: 10.1074/jbc.M110.111328
166. Hu Y, Li S, Zou Y. Knockdown of LncRNA H19 relieves LPS-induced damage by modulating miR-130a in osteoarthritis. Yonsei Med J (2019) 60(4):381–8. doi: 10.3349/ymj.2019.60.4.381
167. Wang Y, Yu D, Liu Z, Zhou F, Dai J, Wu B, et al. Exosomes from embryonic mesenchymal stem cells alleviate osteoarthritis through balancing synthesis and degradation of cartilage extracellular matrix. Stem Cell Res Ther (2017) 8(1):189. doi: 10.1186/s13287-017-0632-0
168. Tan F, Wang D, Yuan Z. The fibroblast-like synoviocyte derived exosomal long non-coding RNA H19 alleviates osteoarthritis progression through the miR-106b-5p/TIMP2 axis. Inflammation (2020) 43(4):1498–509. doi: 10.1007/s10753-020-01227-8
169. Smith CM, Steitz JA. Classification of gas5 as a multi-small-nucleolar-RNA (snoRNA) host gene and a member of the 5’-terminal oligopyrimidine gene family reveals common features of snoRNA host genes. Mol Cell Biol (1998) 18(12):6897–909. doi: 10.1128/MCB.18.12.6897
170. Filippova EA, Fridman MV, Burdennyy AM, Loginov VI, Pronina IV, Lukina SS, et al. Long noncoding RNA GAS5 in breast cancer: Epigenetic mechanisms and biological functions. Int J Mol Sci (2021) 22(13):6810–26. doi: 10.3390/ijms22136810
171. Zhou Z, Chen J, Huang Y, Liu D, Chen S, Qin S. Long noncoding RNA GAS5: A new factor involved in bone diseases. Front Cell Dev Biol (2021) 9:807419. doi: 10.3389/fcell.2021.807419
172. Xiao K, Yang Y, Bian Y, Feng B, Li Z, Wu Z, et al. Identification of differentially expressed long noncoding RNAs in human knee osteoarthritis. J Cell Biochem (2019) 120(3):4620–33. doi: 10.1002/jcb.27750
173. Ji Q, Qiao X, Liu Y, Wang D. Expression of long-chain noncoding RNA GAS5 in osteoarthritis and its effect on apoptosis and autophagy of osteoarthritis chondrocytes. Histol Histopathol (2021) 36(4):475–84. doi: 10.14670/HH-18-312
174. Zheng T, Zhou Q, Huang J, Lai J, Ji G, Kong D. Xanthohumol inhibited mechanical stimulation-induced articular ECM degradation by mediating lncRNA GAS5/miR-27a axis. Front Pharmacol (2021) 12:737552. doi: 10.3389/fphar.2021.737552
175. Li F, Sun J, Huang S, Su G, Pi G. LncRNA GAS5 overexpression reverses LPS-induced inflammatory injury and apoptosis through up-regulating KLF2 expression in ATDC5 chondrocytes. Cell Physiol Biochem (2018) 45(3):1241–51. doi: 10.1159/000487455
176. Muller-Tidow C, Diederichs S, Thomas M, Serve H. Genome-wide screening for prognosis-predicting genes in early-stage non-small-cell lung cancer. Lung Cancer (2004) 45(Suppl 2):S145–50. doi: 10.1016/j.lungcan.2004.07.979
177. Yoshimoto R, Mayeda A, Yoshida M, Nakagawa S. MALAT1 long non-coding RNA in cancer. Biochim Biophys Acta (2016) 1859(1):192–9. doi: 10.1016/j.bbagrm.2015.09.012
178. Brown JA, Bulkley D, Wang J, Valenstein ML, Yario TA, Steitz TA, et al. Structural insights into the stabilization of MALAT1 noncoding RNA by a bipartite triple helix. Nat Struct Mol Biol (2014) 21(7):633–40. doi: 10.1038/nsmb.2844
179. Pan C, Huang W, Chen Q, Xu J, Yao G, Li B, et al. LncRNA malat-1 from MSCs-derived extracellular vesicles suppresses inflammation and cartilage degradation in osteoarthritis. Front Bioeng Biotechnol (2021) 9:772002. doi: 10.3389/fbioe.2021.772002
180. Zhang Y, Wang F, Chen G, He R, Yang L. LncRNA MALAT1 promotes osteoarthritis by modulating miR-150-5p/AKT3 axis. Cell Biosci (2019) 9:54. doi: 10.1186/s13578-019-0302-2
181. Li H, Xie S, Li H, Zhang R, Zhang H. LncRNA MALAT1 mediates proliferation of LPS treated-articular chondrocytes by targeting the miR-146a-PI3K/Akt/mTOR axis. Life Sci (2020) 254:116801. doi: 10.1016/j.lfs.2019.116801
182. Gao GC, Cheng XG, Wei QQ, Chen WC, Huang WZ. Long noncoding RNA MALAT-1 inhibits apoptosis and matrix metabolism disorder in interleukin-1beta-induced inflammation in articular chondrocytes via the JNK signaling pathway. J Cell Biochem (2019) 120(10):17167–79. doi: 10.1002/jcb.28977
183. Cerase A, Pintacuda G, Tattermusch A, Avner P. Xist localization and function: new insights from multiple levels. Genome Biol (2015) 16:166. doi: 10.1186/s13059-015-0733-y
184. da Rocha ST, Heard E. Novel players in X inactivation: insights into xist-mediated gene silencing and chromosome conformation. Nat Struct Mol Biol (2017) 24(3):197–204. doi: 10.1038/nsmb.3370
185. Akbari Dilmaghnai N, Shoorei H, Sharifi G, Mohaqiq M, Majidpoor J, Dinger ME, et al. Non-coding RNAs modulate function of extracellular matrix proteins. BioMed Pharmacother (2021) 136:111240. doi: 10.1016/j.biopha.2021.111240
186. Wang T, Liu Y, Wang Y, Huang X, Zhao W, Zhao Z. Long non-coding RNA XIST promotes extracellular matrix degradation by functioning as a competing endogenous RNA of miR-1277-5p in osteoarthritis. Int J Mol Med (2019) 44(2):630–42. doi: 10.3892/ijmm.2019.4240
187. Xiao Y, Liu L, Zheng Y, Liu W, Xu Y. Kaempferol attenuates the effects of XIST/miR-130a/STAT3 on inflammation and extracellular matrix degradation in osteoarthritis. Future Med Chem (2021) 13(17):1451–64. doi: 10.4155/fmc-2021-0127
188. Sun P, Wu Y, Li X, Jia Y. miR-142-5p protects against osteoarthritis through competing with lncRNA XIST. J Gene Med (2020) 22(4):e3158. doi: 10.1002/jgm.3158
189. Rinn JL, Kertesz M, Wang JK, Squazzo SL, Xu X, Brugmann SA, et al. Functional demarcation of active and silent chromatin domains in human HOX loci by noncoding RNAs. Cell (2007) 129(7):1311–23. doi: 10.1016/j.cell.2007.05.022
190. Kong W, Yin G, Zheng S, Liu X, Zhu A, Yu P, et al. Long noncoding RNA (lncRNA) HOTAIR: Pathogenic roles and therapeutic opportunities in gastric cancer. Genes Dis (2022) 9(5):1269–80. doi: 10.1016/j.gendis.2021.07.006
191. Chen Y, Zhang L, Li E, Zhang G, Hou Y, Yuan W, et al. Long-chain non-coding RNA HOTAIR promotes the progression of osteoarthritis via sponging miR-20b/PTEN axis. Life Sci (2020) 253:117685. doi: 10.1016/j.lfs.2020.117685
192. Zheng T, Huang J, Lai J, Zhou Q, Liu T, Xu Q, et al. Long non-coding RNA HOTAIRincreased mechanical stimulation-induced apoptosis by regulating microRNA-221/BBC3 axis in C28/I2 cells. Bioengineered (2021) 12(2):10734–44. doi: 10.1080/21655979.2021.2003129
193. Herrmann IK, Wood MJA, Fuhrmann G. Extracellular vesicles as a next-generation drug delivery platform. Nat Nanotechnol (2021) 16(7):748–59. doi: 10.1038/s41565-021-00931-2
194. Yan L, Liu G, Wu X, et al. The Umbilical Cord Mesenchymal Stem Cell-Derived Exosomal Lncrna H19 Improves Osteochondral Activity through Mir-29b-3p/Foxo3 Axis. Clin Transl Med (2021) 11(1):e255–70. doi: 10.1002/ctm2.255
195. Liu Y, Zou R, Wang Z, Wen C, Zhang F, Lin F. Exosomal KLF3-AS1 from hMSCs promoted cartilage repair and chondrocyte proliferation in osteoarthritis. Biochem J (2018) 475(22):3629–38. doi: 10.1042/BCJ20180675
196. Zhang S, Jin Z. Bone mesenchymal stem cell-derived extracellular vesicles containing long noncoding RNA NEAT1 relieve osteoarthritis. Oxid Med Cell Longev (2022) 2022:5517648. doi: 10.1155/2022/5517648
197. Pi YN, Qi WC, Xia BR, Lou G, Jin WL. Long non-coding RNAs in the tumor immune microenvironment: Biological properties and therapeutic potential. Front Immunol (2021) 12:697083. doi: 10.3389/fimmu.2021.697083
198. Vaidya AM, Sun Z, Ayat N, Schilb A, Liu X, Jiang H, et al. Systemic delivery of tumor-targeting siRNA nanoparticles against an oncogenic LncRNA facilitates effective triple-negative breast cancer therapy. Bioconjug Chem (2019) 30(3):907–19. doi: 10.1021/acs.bioconjchem.9b00028
199. Watanabe S, Hayashi K, Toh K, Kim HJ, Liu X, Chaya H, et al. In vivo rendezvous of small nucleic acid drugs with charge-matched block catiomers to target cancers. Nat Commun (2019) 10(1):1894. doi: 10.1038/s41467-019-09856-w
200. Lin B, Lu L, Wang Y, Zhang Q, Wang Z, Cheng G, et al. Nanomedicine directs neuronal differentiation of neural stem cells via silencing long noncoding RNA for stroke therapy. Nano Lett (2021) 21(1):806–15. doi: 10.1021/acs.nanolett.0c04560
201. Wang YZ, Yao L, Liang SK, Ding LB, Feng L, Guan J, et al. LncPVT1 promotes cartilage degradation in diabetic OA mice by downregulating miR-146a and activating TGF-beta/SMAD4 signaling. J Bone Miner Metab (2021) 39(4):534–46. doi: 10.1007/s00774-020-01199-7
202. Shermane Lim YW, Xiang X, Garg M, Le MT, Li-Ann Wong A, Wang L, et al. The double-edged sword of H19 lncRNA: Insights into cancer therapy. Cancer Lett (2021) 500:253–62. doi: 10.1016/j.canlet.2020.11.006
203. Liu SJ, Dang HX, Lim DA, Feng FY, Maher CA. Long noncoding RNAs in cancer metastasis. Nat Rev Cancer (2021) 21(7):446–60. doi: 10.1038/s41568-021-00353-1
204. Gutschner T, Baas M, Diederichs S. Noncoding RNA gene silencing through genomic integration of RNA destabilizing elements using zinc finger nucleases. Genome Res (2011) 21(11):1944–54. doi: 10.1101/gr.122358.111
205. Aguilar R, Spencer KB, Kesner B, Rizvi NF, Badmalia MD, Mrozowich T, et al. Targeting xist with compounds that disrupt RNA structure and X inactivation. Nature (2022) 604:160–6. doi: 10.1038/s41586-022-04537-z
206. Wang CL, Zuo B, Li, Zhu JF, Xiao F, Zhang XL, et al. The long noncoding RNA H19 attenuates force-driven cartilage degeneration via miR-483-5p/Dusp5. Biochem Biophys Res Commun (2020) 529(2):210–7. doi: 10.1016/j.bbrc.2020.05.180
Keywords: long non-coding RNA, osteoarthritis, pathogenesis, biomarkers, therapeutic strategies
Citation: Wang R, Shiu HT and Lee WYW (2022) Emerging role of lncRNAs in osteoarthritis: An updated review. Front. Immunol. 13:982773. doi: 10.3389/fimmu.2022.982773
Received: 30 June 2022; Accepted: 08 September 2022;
Published: 11 October 2022.
Edited by:
Shanshan Hu, Anhui Medical University, ChinaReviewed by:
Jin Lin, Zhejiang University, ChinaYolande Ramos, Leiden University Medical Center (LUMC), Netherlands
Copyright © 2022 Wang, Shiu and Lee. This is an open-access article distributed under the terms of the Creative Commons Attribution License (CC BY). The use, distribution or reproduction in other forums is permitted, provided the original author(s) and the copyright owner(s) are credited and that the original publication in this journal is cited, in accordance with accepted academic practice. No use, distribution or reproduction is permitted which does not comply with these terms.
*Correspondence: Wayne Yuk Wai Lee, d2F5bmVsZWVAY3Voay5lZHUuaGs=