- 1Department of Anesthesiology and Intensive Care Medicine, University Hospital, Ulm, Germany
- 2Institute for Anesthesiologic Pathophysiology and Process Engineering, Ulm University, Ulm, Germany
- 3Institute of Comparative Molecular Endocrinology (CME), Ulm University, Ulm, Germany
- 4Immunology and Respiratory, Boehringer Ingelheim Pharma GmbH & Co KG, Biberach, Germany
Introduction: We previously showed that attenuated glucocorticoid receptor (GR) function in mice (GRdim/dim) aggravates systemic hypotension and impairs organ function during endotoxic shock. Hemorrhagic shock (HS) causes impaired organ perfusion, which leads to tissue hypoxia and inflammation with risk of organ failure. Lung co-morbidities like chronic obstructive pulmonary disease (COPD) can aggravate tissue hypoxia via alveolar hypoxia. The most common cause for COPD is cigarette smoke (CS) exposure. Therefore, we hypothesized that affecting GR function in mice (GRdim/dim) and pre-traumatic CS exposure would further impair hemodynamic stability and organ function after HS.
Methods: After 3 weeks of CS exposure, anesthetized and mechanically ventilated GRdim/dim and GR+/+ mice underwent pressure-controlled HS for 1h via blood withdrawal (mean arterial pressure (MAP) 35mmHg), followed by 4h of resuscitation with re-transfusion of shed blood, colloid fluid infusion and, if necessary, continuous intravenous norepinephrine. Acid–base status and organ function were assessed together with metabolic pathways. Blood and organs were collected at the end of the experiment for analysis of cytokines, corticosterone level, and mitochondrial respiratory capacity. Data is presented as median and interquartile range.
Results: Nor CS exposure neither attenuated GR function affected survival. Non-CS GRdim/dim mice had a higher need of norepinephrine to keep target hemodynamics compared to GR+/+ mice. In contrast, after CS exposure norepinephrine need did not differ significantly between GRdim/dim and GR+/+ mice. Non-CS GRdim/dim mice presented with a lower pH and increased blood lactate levels compared to GR+/+ mice, but not CS exposed mice. Also, higher plasma concentrations of some pro-inflammatory cytokines were observed in non-CS GRdim/dim compared to GR+/+ mice, but not in the CS group. With regards to metabolic measurements, CS exposure led to an increased lipolysis in GRdim/dim compared to GR+/+ mice, but not in non-CS exposed animals.
Conclusion: Whether less metabolic acidosis or increased lipolysis is the reason or the consequence for the trend towards lower catecholamine need in CS exposed GRdim/dim mice warrants further investigation.
Introduction
Hemorrhagic shock (HS) is one of the major mediators of trauma-related mortality (1). During HS as well as during resuscitation from HS impaired organ blood flow may lead to tissue hypoxia, resulting in risk of organ damage (2). Tissue hypoxia itself induces inflammation (3), which in turn aggravates the risk of organ damage. Both, tissue hypoxia and inflammation may lead to enhanced radical formation and mitochondrial dysfunction, altogether leading to multiorgan failure (MOF (1, 2, 4),). During resuscitation after HS, it is important to maintain mean arterial pressure (MAP) to ensure perfusion of the most important organs as heart, kidney, liver, lung, or brain. At best, this hemodynamic state enables microcirculatory perfusion of these important organs to prevent longer impairment of O2-availability and, thus irreversible organ dysfunction or damage (5). On a cellular level, impaired O2-availability may lead to mitochondrial dysfunction with uncoupling of mitochondrial respiration and a lack of mitochondrial substrates, resulting in reduced production of adenosine triphosphate (ATP) and increased oxidative stress (5). Besides this mitochondrial dysfunction due to a reduced O2-availability, during and after shock a hypermetabolic condition with insulin resistance and increased oxygen demands often occurs (6). This, in turn, causes impaired glucose and oxygen availability on a cellular level and, ultimately, lead to metabolic failure. Together with the mitochondrial dysfunction this metabolic failure further impairs organ function after shock (7).
Our group showed already that pre-traumatic cigarette-smoke (CS) exposure enhanced post-traumatic inflammation and radical stress, thereby aggravating lung dysfunction (8). CS is the major risk factor for chronic obstructive pulmonary disease (COPD) (9) and COPD as a lung co-morbidity increases pulmonary and systemic inflammation and might increase tissue hypoxia via alveolar hypoxia (10). Furthermore, CS exposure-induced COPD aggravated acute lung injury (ALI) in a murine model of blunt chest trauma (8, 11), causing the strongest inflammatory response in mice after HS and resuscitation under intensive care treatment (12).
Glucocorticoids (GCs) mediate their effects through the glucocorticoid receptor (GR) (13). The GR is an intracellular, ligand-activated transcription factor, which regulates gene transcription by several mechanisms: as a protein dimer it can bind palindromic DNA sequences (glucocorticoid response elements – GREs) or DNA half sites as a monomeric protein (GR monomer (14)). In a non-resuscitated mouse model of endotoxemia, we previously showed that the GR is crucial for the resolution of systemic inflammation (15). In a more recent study, we demonstrated that intact GR dimer also mediates hemodynamic stability in a resuscitated mouse model of endotoxemia, indicated by a higher need of catecholamines in mice with a impaired GR dimerization function (GRdim/dim) to keep hemodynamic targets (16). In addition to the mouse model of endotoxemia, we already showed that HS led to impaired lung mechanics and aggravated lung inflammation in GRdim/dim mice compared to littermate control wildtype mice (GR+/+) (17). This previous study showed that functional GR signaling plays a crucial role to attenuate HS-induced lung damage. Based on this study, we hypothesized that an attenuation of GR function together with CS exposure as a lung co-morbidity may lead to organ dysfunctions due to metabolic disturbances and an increased hemodynamic instability during resuscitation from HS with the effects on lung function already published (17). To ensure clinical transferability of the results, we used our murine model of HS with resuscitation similar to intensive care management (infusion of crystalloids and norepinephrine (NE) to achieve hemodynamic targets, lung-protective mechanical ventilation, measurements of gas exchange, and control of body temperature (17–19)) together with a detailed analysis of the metabolic state (18, 20) and mitochondrial function (16, 21) in CS exposed animals (21).
Material and methods
This study was approved by the federal authorities for animal research of the Regierungspräsidium Tübingen, Baden-Wuerttemberg, Germany (approved animal experimentation number: 1359, August 17th, 2017), and performed in adherence with the National Institutes of Health Guidelines on the Use of Laboratory Animals and the European Union ‘‘Directive 2010/63 EU on the protection of animals used for scientific purposes.’’ GRdim/dim mice (Nr3c1tm3Gsc) (22) were bred in a mixed background (129/SvEv x C57BL/6) and housed in the animal facility at University Ulm or in the housing facility at Boehringer Ingelheim (Biberach). GR+/+ littermate controls were used as wild-type mice. Animals always had free access to food and water, were kept under standardized conditions, and were equally distributed in terms of age (16-26 weeks) and body weight (23-34 g). Groups comprised 7 animals (GR+/+ non-CS), 9 animals (GRdim/dim non-CS), 8 animals (GR+/+ CS exposure), and 9 animals (GRdim/dim CS exposure) of both gender. Due to the complicated surgery 2 animals in the GR+/+ non-CS group and 1 animal in the GR+/+ CS group deceased during surgery.
Implementation of general anesthesia and surgery
Anesthesia was induced via sevoflurane (2.5%; sevoflurane, Abbott, Wiesbaden, HE, Germany) as described previously (19, 21), followed by intraperitoneal injection (ip) of ketamine (120µg·g-1; Ketanest-S, Pfizer, New York City, NY), midazolam (1.25µg·g-1; Midazolam-ratiopharm, Ratiopharm, Ulm, BW, Germany) and fentanyl (0.25µg·g-1; Fentanyl-hameln, Hameln Pharma Plus GmbH, Hameln, NI, Germany). Afterwards, animals were placed on a closed-loop-system for body temperature control (19, 21). Lung-protective mechanical ventilation using a small animal ventilator (FlexiVent, Scireq, MO, Canada) was performed via a tracheostomy (19, 21). Surgical instrumentation comprised catheters in the jugular vein for fluid administration, the carotid artery for hemodynamic measurements, the femoral artery for blood removal, and the bladder to collect urine (19, 21). General anesthesia was titrated to guarantee complete tolerance against noxious stimuli and was sustained by continuous intravenous (iv) administration of ketamine, midazolam, and fentanyl to reach deep sedation (0.85 [0.79; 0.88] μl·g-1·min.-1 for all animals). Animals were mechanically ventilated with ventilator settings being FiO2 0.21%, respiratory rate 150·min-1, tidal volume of 6 mL·kg-1, and inspiratory/expiratory time ratio 1:2. Ventilation was modified to maintain an arterial PaCO2 between 30 mmHg and 40 mmHg throughout the experiment as described previously (21). Therefore, blood gas analyses were performed before HS, 2h after re-transfusion of shed blood, and at the end of the experiment. However, PaCO2 values reported in Table 1 were measured at the end of experiment where adaptation of ventilation was no longer practicable. Therefore, PaCO2 values may vary and appear out of that range at the end of the experiment. Positive end-expiratory pressure (PEEP) was adjusted according to the arterial PaO2 (PaO2/FiO2-ratio>300mmHg: PEEP=3cmH2O; PaO2/FiO2-ratio<300mmHg: PEEP=5cmH2O; PaO2/FiO2-ratio<200mmHg: PEEP=8cmH2O) (19, 21). Recruitment maneuvers (5s hold at 18 cmH2O) were repeated hourly to avoid any impairment of thoraco-pulmonary compliance due to anesthesia- and/or supine position-induced atelectasis. A detailed description of the intensive care measures in mice performed in the present study (mouse intensive care unit, MICU) can be found elsewhere (23).
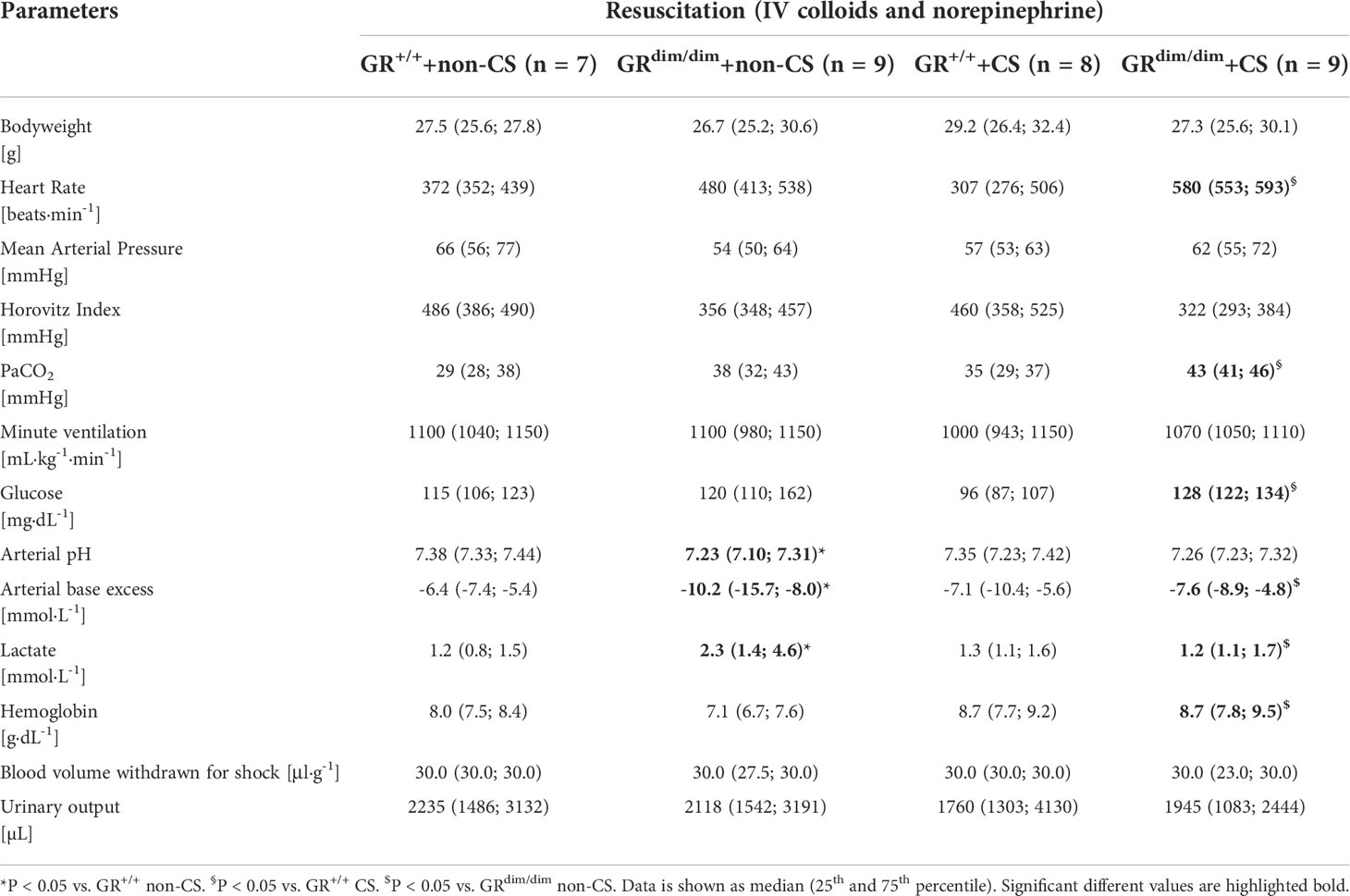
Table 1 Hemodynamic and metabolic measurements as well as ventilation parameters in GRdim/dim and GR+/+ mice with or without cigarette-smoke (CS) exposure and after hemorrhagic shock(HS) with subsequent resuscitation (4h) at the end of the experiment.
Cigarette smoke inhalation procedure
CS exposure was performed for 5 days per week over a period of 3 weeks using a standardized protocol as described previously (8, 11, 24). Particle concentration was monitored by a real time ambient particle monitor (MicroDust Pro, Casella, Amherst, NH, USA). In pilot experiments, this CS-exposure procedure had not caused any effect on the behavior, body weight, or respiratory pattern. Control animals (non-CS) were exposed to room air. After CS exposure, mice were transported from Boehringer Ingelheim (Biberach) to Ulm University and there were allowed to recover for 1 week to avoid acute stress effects induced by the CS procedure per se (11, 25).
Induction of hemorrhagic shock
After the initiation of lung-protective mechanical ventilation and instrumentation for vascular access, mice underwent 1h of HS. HS was performed by removing blood out of the femoral catheter with citrate-coated syringes (about 30µL·g-1) to titrate mean arterial pressure (MAP) to 35 mmHg. Blood was further removed or re-transfused to keep MAP at 35 mmHg for 1h. Besides, fluid administration was temporarily stopped. At the start of the resuscitation phase, shed blood was re-transfused, together with the administration of hydroxyethyl starch 6% (10μl·g-1 h-1) and NE dissolved in balanced electrolyte solution (≤0.5 ml·h-1 for all animals) via the jugular vein catheter titrated to maintain MAP≥55 mmHg. The bladder was punctured to collect urine during the experiment. After 4h of resuscitation, animals were exsanguinated, blood and tissue samples were taken immediately thereafter, and prepared for further analyses (19, 21).
Analysis of metabolic pathways and kidney function
Thirty minutes before induction of hemorrhagic shock, mice received a primed continuous infusion of stable, non-radioactive-labeled 13C6-glucose, 15N2-urea, 2H5-glycerol, and 5,5,5–2H3-leucine. Expiratory breath gas was sampled 0.5, 1.5, 2.5, 3.5, 4.5, and 5.5 h (end of experiment) after start of the stable isotope infusion. Arterial blood was sampled at time points 0.5, 2.5, 4.5, and 5.5 h. Together with urine output, gas chromatography/mass spectrometry measurement of plasma und urinary creatinine concentrations using 2H3-creatinine as internal standard allowed for calculating creatinine clearance (18). Expiratory gas was analyzed immediately after collection by gas chromatography/mass spectrometry (GC/MS) for total expiratory CO2 concentration and 13CO2 enrichment to assess glucose oxidation rate. Plasma and urine samples were stored at −80°C until sample workup. For metabolic analysis, we only analyzed animals that survived the whole observation period (4 h) in order to guarantee a steady state of isotopes (n=7 for GRdim/dim and GR+/+ non-CS, n=8 for GR+/+ CS, and n=9 for GRdim/dim CS). For further details see supplemental material.
Parameters of hemodynamics, gas exchange, and metabolism
Systemic hemodynamics and body temperature were recorded hourly. Blood gas tensions, acid-base status, glycaemia, and lactatemia were assessed at the end of the resuscitation period via arterial blood gas analysis (ABL800 Felx; Radiometer, Krefeld, Germany) (19, 21).
Mitochondrial respiration
Mitochondrial respiratory capacity was determined via high-resolution respirometry with a clark-electrode-based system (Oxygraph 2k, OROBOROS Instruments Corp., Innsbruck, Austria) as described previously (19). Post-mortem muscle, heart, liver, and brain biopsies were mechanically homogenized in Mir05 (respiration medium). Mir05 is composed of 0.5mM EGTA, 3mM MgCl2·6H2O, 60mM Lactobionic acid, 20mM Taurine, 10mM KH2PO4, 20mM HEPES, 110mM Sucrose, 1g·L-1 bovine serum albumin). 1.5-2 mg of tissue (1.5mg: heart, 2mg tissue: muscle, liver, and brain) were added to the Oxygraph chamber. By the addition of a defined sequence of substrates and inhibitors, various states of mitochondrial function could be assessed. Complex I activity was determined after addition of 10mM pyruvate, 10mM glutamate, 5mM malate and 5mM ADP. 10µM cytochrome c was added to check for mitochondrial integrity. Maximum oxidative phosphorylation (OxPhos) was evaluated after subsequent addition of 1mM octanoyl-carnitine and 10mM succinate. Leak compensation was assessed after inhibition of the ATP-synthase by 2.5µM oligomycin, followed by stepwise titration of the uncoupling agent Carbonyl cyanide-4-(trifluoromethoxy)-phenylhydrazone (FCCP, final concentration 1.5µM) to reach maximum respiratory activity of the electron transfer system in the uncoupled state (ETS).
Measurements of cytokine, chemokine, and corticosterone concentrations in plasma
Cytokines, chemokines, and growth factors were measured using Bio-Plex Pro Mouse Cytokine 23-plex Assay (Group I) (Biorad) simultaneously in the plasma according to the manufacturer´s protocol. The multiplex-assay was performed with Bio-Plex 200 machine (Biorad) and the Bio-Plex Manager TM 6.1 software (Biorad). Plasma corticosterone levels were determined with an enzyme linked immunosorbent assay (ELISA) kit (TECAN, IBL international GmbH, RE52211) according to the manufactures protocol. In short, plasma samples were incubated in the anti-corticosterone antibody coated wells alongside with a horse-reddish peroxide (HRP) conjugated corticosterone competitor for one hour at room temperature. After five subsequent washing steps, HRP substrate was added for 15 min followed by stop solution and immediate measure of color intensity at 450 nm using Fluostar OPTIMA plate reader. Corticosterone concentrations were calculated according to the provided standards using a 4-parametric fit.
Statistical analysis
Unless stated otherwise, all data are presented as median (25th and 75th percentile). Data sets were analyzed using non-parametric statistics, i.e. Mann-Whitney U-test (one factor, two independent samples) or Kruskal-Wallis test with post hoc Dunn’s comparison testing (one factor, four independent samples). P-values <0.05 were considered statistically significant. Quantitative graphical presentations and statistical analyses were accomplished by using GraphPad Prism 9 (GraphPad Software Inc, La Jolla, Calif).
Results
Survival
All CS exposed animals survived to the end of the observation period during resuscitation from GRdim/dim HS (4h). Only two animals with an attenuation of GR function and non-CS exposure did not reach the end of the observation period, however, the survival rates did not show any significant intergroup differences and have been reported previously in the supplementary materials of a publication of our group (17).
Hemodynamics, acid–base balance, parameters of organ (dys)function as well as mitochondrial respiration
To examine the effects of an attenuated GR function as well as exposure of CS on organ function during resuscitation from 1h of HS, hemodynamics, metabolic parameters, and mitochondrial respiration were investigated. Table 1 summarizes the parameters of hemodynamics and acid–base status. Heart rate was significantly higher in GRdim/dim compared to GR+/+ mice with CS-exposure, whereas mean arterial pressure (MAP) did not differ between groups. In non-CS exposed mice, GRdim/dim mice had a significantly higher need of catecholamines to keep hemodynamic stability compared to GR+/+ mice (Figure 1). In contrast, need of catecholamines did not differ in CS-exposed GRdim/dim and GR+/+ mice (Figure 1). After CS exposure, arterial partial pressure of carbon dioxide (PaCO2) was significantly higher in GRdim/dim compared to GR+/+ mice, whereas minute ventilation did not differ between groups (Table 1). In non-CS exposed mice, pH as well as base excess (BE) were lower and lactate levels were higher in GRdim/dim compared to GR+/+ mice (Table 1). Because increased lactate levels may be linked to disturbances in mitochondrial respiration (6, 26), important metabolic organs as muscle, heart, liver and brain were investigated for mitochondrial respiration in the current study. However, mitochondrial respiration (Figure 2) in tissue from muscle, heart, liver, and brain in GRdim/dim and GR+/+ mice with or without CS exposure revealed no intergroup differences in all four organs analyzed in the current study. In summary, after resuscitation from HS, the attenuated function of the GR resulted in a higher need of catecholamines to keep hemodynamic stability and a lower pH together with increased lactate levels. These differences were not present in CS exposed mice.
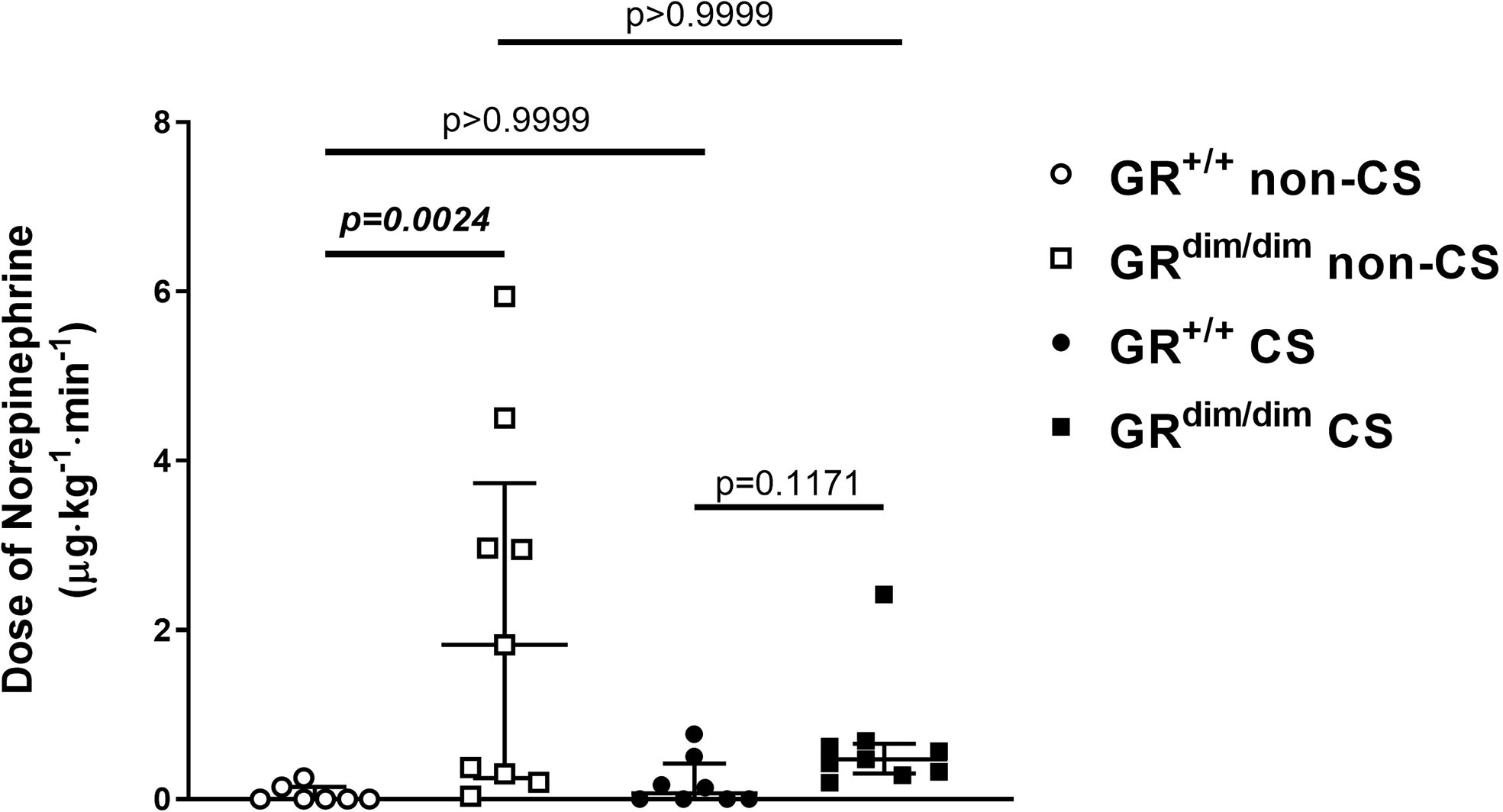
Figure 1 Doses of norepinephrine(NE) in mechanically ventilated GRdim/dim and GR+/+ mice with or without cigarette-smoke (CS) exposure and after 1 h of hemorrhagic shock (HS, mean arterial pressure (MAP) 35 mmHg) and subsequent resuscitation (colloids, NE) for 4 h. NE was titrated intravenously during resuscitation to keep MAP ≥55mmHg. GR+/+ mice non-CS: n = 7, GRdim/dim mice non-CS: n = 9, GR+/+ mice CS: n=8, GRdim/dim mice CS: n = 9. Data is presented as median (25th and 75th percentile and minimum/maximum).
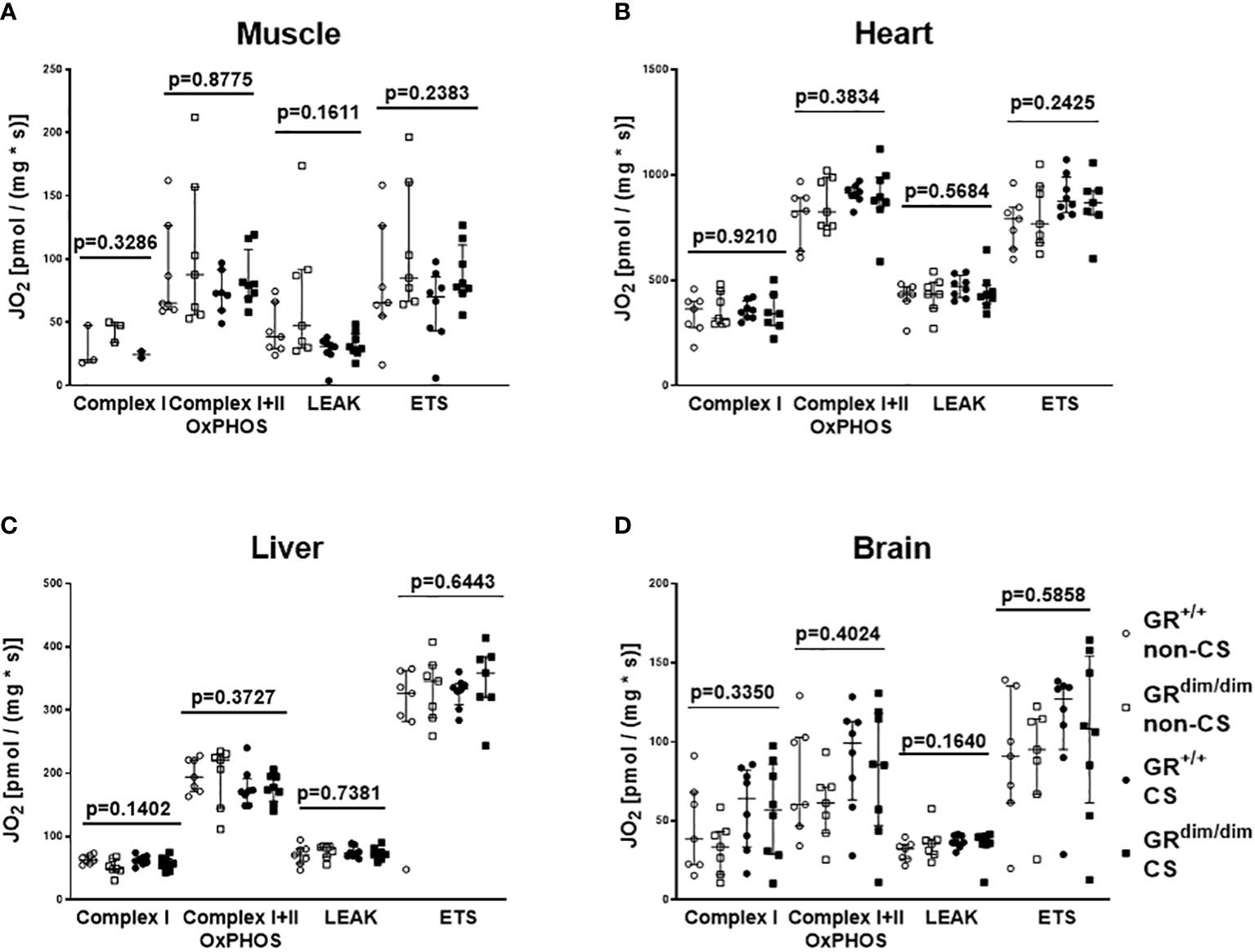
Figure 2 Mitochondrial respiration (JO2) in tissue from muscle (A), heart (B), liver (C), and brain (D) in GRdim/dim and GR+/+ mice with or without cigarette-smoke (CS) exposure and after 1 h of hemorrhagic shock (HS, MAP 35 mmHg) and subsequent resuscitation (colloids, NE) for 4 h. Overall p-values from Kruskal-Wallis-Test are shown above. GR+/+ mice non-CS: n = 3-7, GRdim/dim mice non-CS: n = 3-7, GR+/+ mice CS: n = 2-8, GRdim/dim mice CS: n=8. For the group GRdim/dim CS in muscle tissue there were no measurements available due to a measurement problem. Data is presented as median (25th and 75th percentile and minimum/maximum).
Measurements of plasma cytokine levels at the end of 4 hours of resuscitation after hemorrhagic shock
To determine effects of an attenuation of GR function and CS exposure on inflammatory parameters, plasma concentration of cytokines and chemokines were determined. In the non-CS group, concentration of interleukine-6 (IL-6), interleukine-12 p40 (IL-12 p40), and Eotaxin were significantly higher in plasma of GRdim/dim mice compared to GR+/+ mice (Table 2). Interestingly, there were no intergroup differences for the measured plasma cytokines when animals were exposed to CS before HS. Therefore, only the attenuation of GR function led to an increase in some plasma cytokines, whereas this increase was prevented by CS pre-exposure.
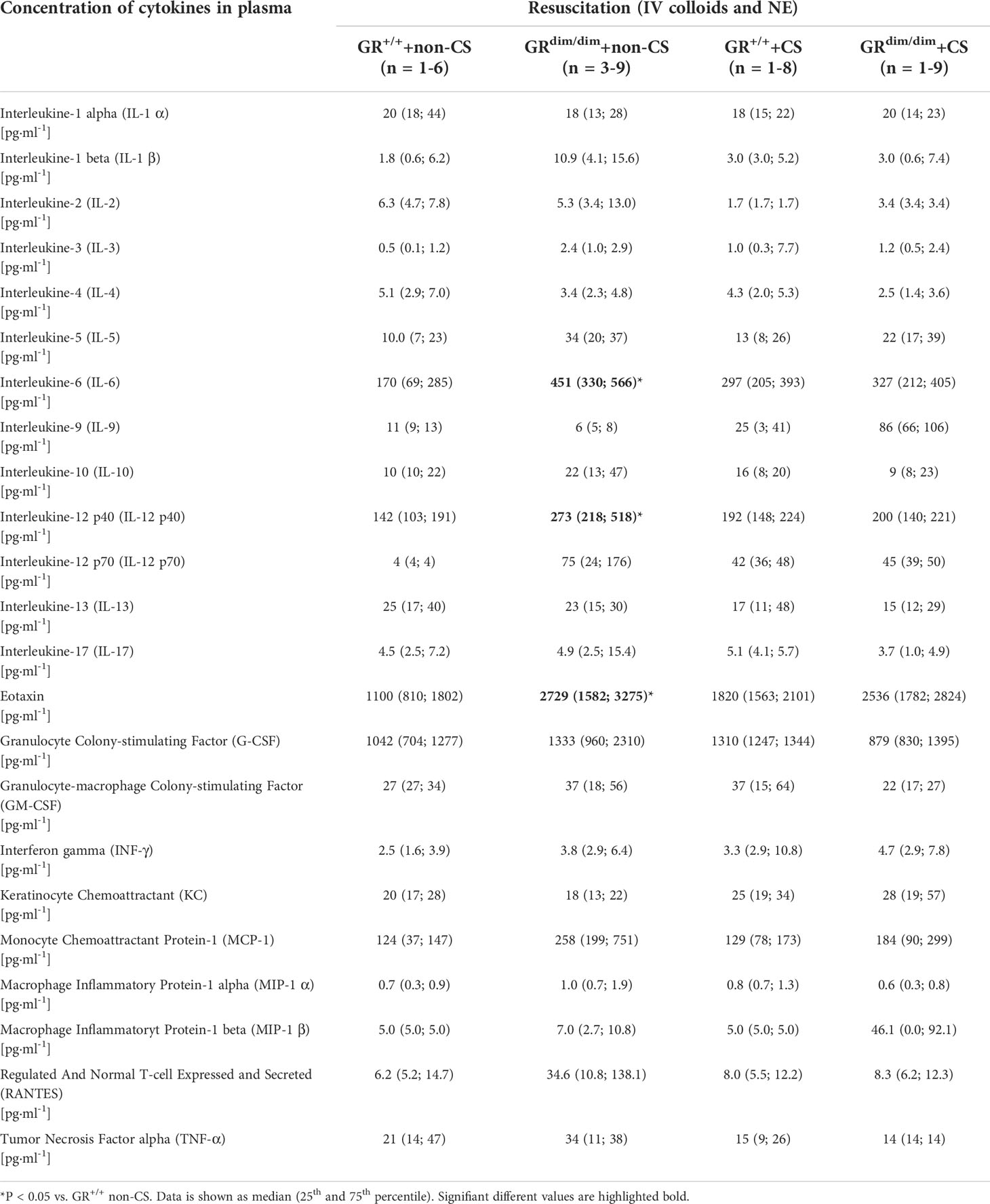
Table 2 Concentration of cytokines in plasma in GRdim/dim and GR+/+ mice with or without cigarette-smoke (CS) exposure and after HS with subsequent resuscitation (4 h) at the end of the experiment.
Effects of an attenuation of GR function and CS exposure on metabolic parameters
Despite no differences in minute ventilation during resuscitation (Table 1), GRdim/dim mice showed higher CO2 values in the exhaled air compared to GR+/+ mice, both after CS exposure during the observation period (Figure 3A, together with increased arterial partial pressure of CO2, see Table 1). The same genotype dependent difference appeared for the concentration of glucose in the plasma, whereas the rate of endogenous glucose production as well as glucose oxidation rate showed no inter-group differences (Figures 3C, D). After CS exposure, rate of appearance for urea (marker for hepatic metabolic capacity as well as kidney function) was significantly lower in GRdim/dim mice compared to GRdim/dim mice with no CS exposure (Figure 4A). Measures of lipolysis (measured via rate of appearance for glycerol), protein degradation (rate of appearance for leucine), and kidney function (creatinine clearance, urine output [Table 1]) showed no intergroup differences (Figures 4C, D). Taken together, during resuscitation after HS, CS exposed GRdim/dim mice presented with an increased glucose concentration in plasma (Figure 3B), which does not come from an increase in gluconeogenesis or decrease in glucose oxidation (Figures 3C, D). Furthermore, due to no differences in kidney function (creatinine clearance, Figure 4B) as well as protein breakdown (rate of appearance for leucine, Figure 4D), the reduced rate of appearance of urea in CS exposed GRdim/dim mice (Figure 4A) is most likely a result of a reduced hepatic synthesis.
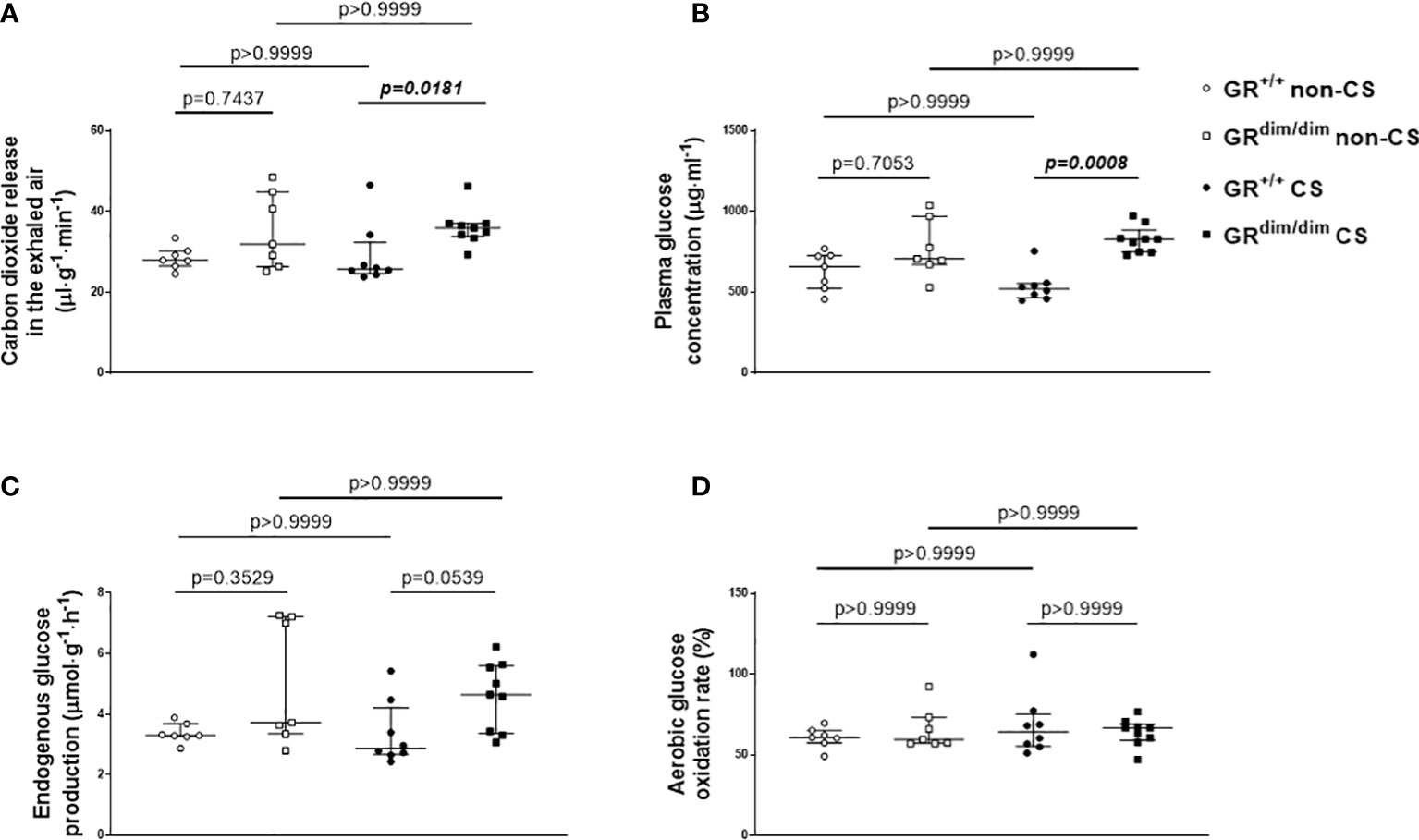
Figure 3 Glucose metabolism. (A) Carbon dioxide release via the exhaled air, (B) plasma glucose concentration, (C) endogenous glucose production rate, and (D) glucose oxidation as percentage of oxidation of infused tracer. GR+/+ mice non-CS: n=7, GRdim/dim mice non-CS: n = 7, GR+/+ mice CS: n = 8, GRdim/dim mice CS: n = 9. Data is presented as median (25th and 75th percentile and minimum/maximum).
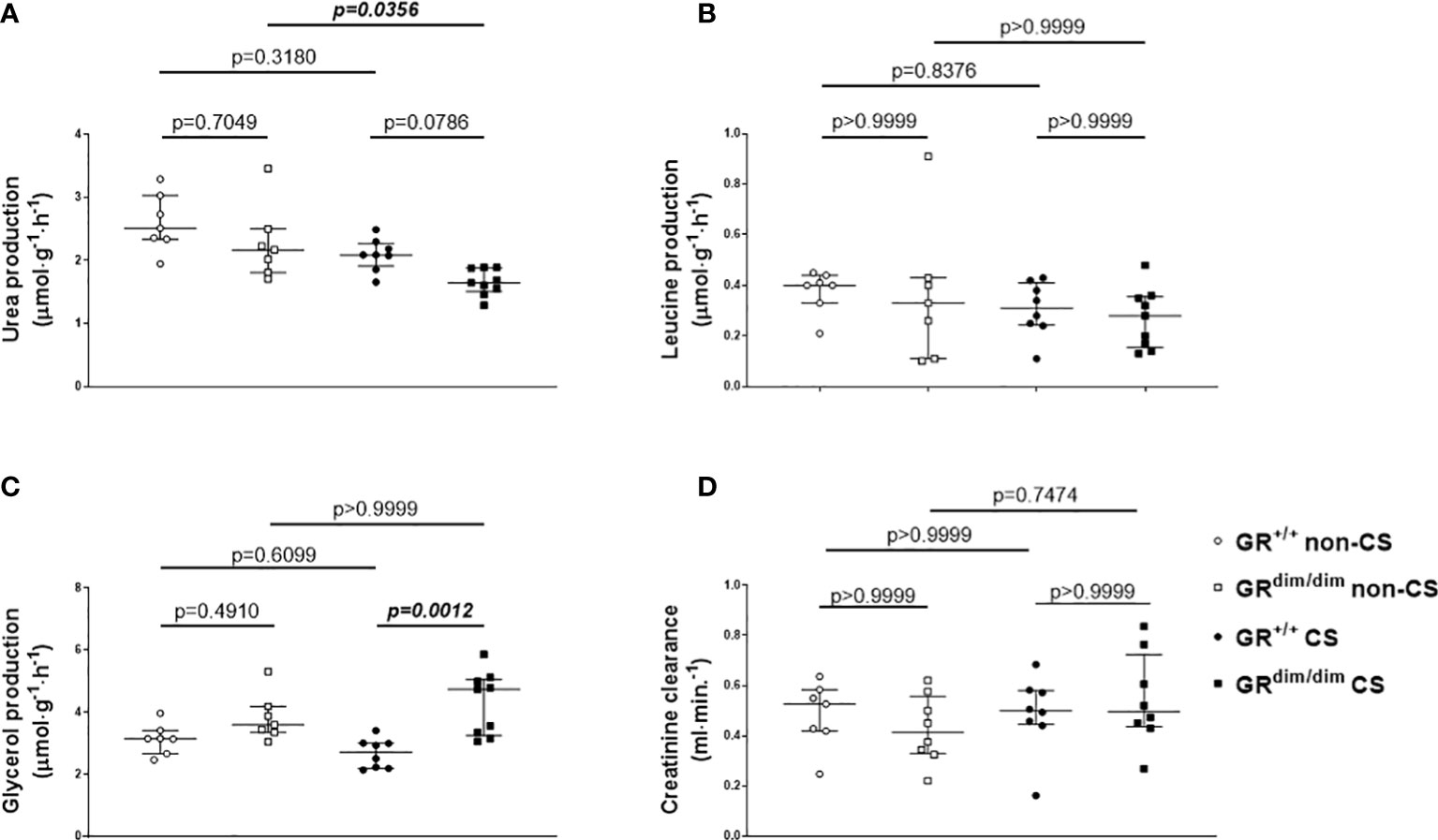
Figure 4 Parameters of metabolic and kidney function. Rates of appearance of (A) urea, (B) leucine, (C) glycerol as well as (D) creatinine clearance, as measures for hepatic metabolic capacity, protein degradation, lipolysis, and kidney function, respectively. *P < 0.05. #P < 0.01. GR+/+ mice non-CS: n = 7, GRdim/dim mice non-CS: n = 7-8, GR+/+ mice CS: n=8, GRdim/dim mice CS: n = 8-9. Data is presented as median (25th and 75th percentile and minimum/maximum).
Measurements of corticosterone concentration in plasma
As the above measured parameters are well known to be influenced by corticosteroids, we determined the concentration of corticosterone in plasma. Neither an attenuation of GR function nor CS exposure had any effects on plasma corticosterone levels in mice in the present study under intensive care conditions (non-CS GR+/+: 112 (78;141), non-CS GRdim/dim: 85 (35; 156); CS GR+/+: 93 (65; 147), CS GRdim/dim: 82 (55; 119), P=0.6852).
Discussion
In the present study, we examined the effects of a dysfunction in the glucocorticoid receptor (GRdim/dim) on hemodynamics, metabolic parameters, mitochondrial function, and inflammation during resuscitation from hemorrhagic shock (HS) in mice with pre-traumatic cigarette smoke (CS) exposure with the effects on lung function already published in a previous paper (17).
Neither an attenuation of GR function (GRdim/dim) nor CS exposure prior to resuscitation from HS showed an effect on survival rates. However, we found that non-CS exposed GRdim/dim mice needed significantly higher doses of norepinephrine (NE) to keep hemodynamic stability compared to GR+/+ mice. Furthermore, lower pH and higher lactate levels as well as an increase in systemic cytokines was present in non-CS GRdim/dim compared to GR+/+ mice. Surprisingly, these genotype dependent differences disappeared in CS exposed animals.
Compared to a mouse model based on lipopolysaccharide (LPS)-induced systemic inflammation, we previously reported a significantly increased mortality in GRdim/dim mice compared to GR+/+ mice (16). In that LPS model, GRdim/dim mice presented with significant higher need for NE to keep hemodynamic stability, like in the present study the non-CS exposed GRdim/dim mice during resuscitation from HS. However, in the present study there was no effect on survival. Although resuscitation manners (except for colloids) as well as definition for hemodynamic stability (MAP≥55mmHg) were similar in both studies, with regards on survival rates it must be considered that the observation time in the LPS study was 6 h and in the present study 4 hours. If the observation time would have been 6 h in the present study, there may would have been more animals not surviving the whole observation period after HS in the non-CS GRdim/dim group with a probably significant effect on survival rates. Interestingly, CS exposure led to a higher hemodynamic stability in GRdim/dim mice following HS shown by a trend towards a decreased dose of NE in the present study. We can only speculate for possible reasons for this effect of CS exposure on hemodynamics. A possible increased sympathetic activity with reduced demand of additional catecholamine support in CS exposed mice during resuscitation from HS has been reported before (21). This may have been the reason for the absolute lower catecholamine need in CS exposed GRdim/dim mice during resuscitation from HS compared to non-CS exposed animals. An interaction of CS exposure and glucocorticoid receptor (GR) function might also be a possible explanation for the lower NE need in CS exposed animals, because one study reported that maternal tobacco smoke exposure increased serum corticosterone levels in adult male rat offspring (27). Another study reported that CS exposure during breastfeeding in rats showed changes in serum corticosterone level in in obese adult offspring (28). However, with the results in the present study, we can only speculate about possible effects of CS exposure on GR function. This needs further investigation.
In a previous study from our group with a similar murine model of resuscitation from HS, subsequent CS exposure per se had the strongest impact on inflammatory responses, shown by an increased systemic inflammation (21). Furthermore, in a murine model of blunt chest trauma (without HS), pre-traumatic CS exposure also enhanced post-traumatic inflammation (8). In the present study, an increased systemic inflammation could only be seen in part due to the attenuation of GR function in non-CS mice (increased plasma IL-6, IL-12 p40, and Eotaxin in non-CS GRdim/dim compared to non-CS GR+/+ mice, Table 2), whereas there were no differences in systemic cytokines in CS exposed mice. Although anti-inflammatory effects via CS exposure have been reported in a murine model of allergic asthma (29), such protective effects are more unlikely in the present study, because our CS exposure model has, so far, only shown pro-inflammatory effects in our murine trauma models (8, 21). As mentioned above, the short observation time of 4h also might be a reason for the missing inflammatory effect of CS exposure in the present murine trauma model.
With regards to metabolic parameters, in the present study only GRdim/dim mice without a CS exposure presented with a lower pH, a lower base excess (BE), and higher lactate levels compared to GR+/+ mice (Table 1). The higher lactate levels are in agreement with the study by Vandewalle and colleagues showing an impaired lactate clearance upon LPS-exposure in GRdim/dim mice even in the absence of resuscitation (30). With regards to the lower pH and lower BE in non-CS GRdim/dim mice shown in the present study it must be considered that the lactate levels alone cannot be the reason for this metabolic acidosis. A metabolic acidosis in mice due to other fixed acids than lactic acid has been reported before (11, 24). Furthermore, in the present study, pH, BE, and lactate levels were similar in non-CS exposed GR+/+ and CS exposed GR+/+ and GRdim/dim mice with a trend towards a slightly lower pH in the latter group, which might have been induced by the higher PaCO2 values (Table 1). Therefore, CS exposure had no effects on pH, BE, and lactate levels on GR+/+ mice in the present study. This is in line with previous studies also showing no effect of CS exposure on pH or lactate levels in a more recent mouse model of HS (21).
Hemodynamic instability coincides with an increased need of catecholamines. It is well known that both, endogenously produced as well as externally administered catecholamines exert non-hemodynamic effects and impact metabolism (31). Vital organs such as heart, brain, and kidney may receive energy under stress situations like HS through release of lactate from skeletal muscle as well as by breaking down amino acids, glycogen, and triglycerides to generate glucose, fatty acids, and ketone bodies, all mediated via an increased release of endogenous catecholamines (32, 33). Therefore, the increased glucose level in CS exposed GRdim/dim mice in the present study could have been induced via increased doses of catecholamines. However, the need of catecholamines to keep hemodynamic stability was higher in non-CS exposed GRdim/dim mice with no effect on glucose levels compared to GR+/+ mice in this group. Furthermore, we found no differences in endogenous glucose production or aerobic glucose oxidation between all groups of mice (Figure 3). Therefore, the increased glucose concentration in CS exposed GRdim/dim mice does not come from an increased dose of catecholamines or an increase in gluconeogenesis or decrease in glucose oxidation. Moreover, it is most likely an effect of a reduced peripheral uptake of glucose in tissue which utilizes glucose in a non-oxidative way in this group of mice. We can only speculate for possible reasons for this effect. On one hand, in our subsequent studies in LPS-challenged GRdim/dim and GR+/+ mice we found no differences for glucose concentrations between GRdim/dim and GR+/+ mice (16). On the other hand, in previous studies CS exposure in wildtype mice lead to a trend towards lower glucose levels (21, 24). Therefore, the increased glucose level in CS exposed GRdim/dim mice in the present study are more likely related to both, the combination of CS exposure and attenuation of GR function, which warrants further investigation.
Besides a catecholamine-induced energy production via increased carbohydrate, catecholamines also mediate energy production via a ketotic effect through an increase in fat and protein oxidation (32, 34). The ketotic impacts of both epinephrine and NE are similar at physiologically low concentrations, whereas the ketotic effect of NE predominates at pathophysiological concentrations, possibly due to an antiketogenic effect resulting from the epinephrine-induced increase of glucose and subsequent insulin concentrations (31), with the latter one may be missing in the present study. With measurements of the rate of appearance of urea in the present study, we were able to determine hepatic metabolic capacity as well as in part kidney function. After CS exposure, GRdim/dim mice produced significantly less urea compared to non-CS GRdim/dim mice and had a trend towards a lower urea production compared to CS exposed GR+/+ mice (Figure 4A). With no differences between the groups for kidney function measured via plasma creatinine concentration, creatinine clearance, and urine output in the present study we can exclude differences in urea excretion via the kidney (Figures 4A, D). With no differences in rate of appearance for leucine (protein degradation, Figure 4B), we can also exclude differences in urea metabolized to amino acids. Therefore, primarily the reduced urea in CS exposed GRdim/dim mice after resuscitation from HS in the present study is most likely a result of a reduced hepatic synthesis. However, this is in contrast with the results of the rate of appearance for glucose, since both, urea and glucose are metabolized in the periportal cells and therefore we should have seen effects on both, rate of appearance in urea and glucose (35).
Last, in CS exposed GRdim/dim mice the rate of appearance for glycerol was higher compared to CS exposed GR+/+ mice, which indicates an increase in lipolysis. However, with no differences in plasma corticosterone concentrations in CS exposed GRdim/dim and GR+/+ mice in the present study, it is at least less likely that differences in corticosterone concentrations due to an attenuation of GR function might have altered glucose or lipid metabolism in GRdim/dim mice in the present study.
Conclusion
Cigarette smoke (CS) exposure before hemorrhagic shock (HS) led to an increased hemodynamic stability in mice with an attenuated GR function (GRdim/dim), indicated by a trend towards an absolute lower norepinephrine (NE) need. CS exposed GRdim/dim mice also presented with less metabolic changes (pH, base excess, and lactate levels), and showed an increase in lipid oxidation compared to non-CS GRdim/dim mice. If the more stable pH and BE values, the lower lactate levels, or the switch to lipid oxidation to possibly increase the efficiency of energy production to may maintain organ function were the reason or the consequence for the lower NE need in these mice warrants further investigation.
Data availability statement
The raw data supporting the conclusions of this article will be made available by the authors, without undue reservation.
Ethics statement
The animal study was reviewed and approved by Regierungspraesidium Tuebingen, Baden-Wuerttemberg, Germany.
Author contributions
MW, JP, PR, JT, and SV conceived and designed the study. MW, JP, TM, UW, CT, MK, JV, SK, MG, MF, EC, UB, PR, and SV performed the experiments and organ analysis. MW, JP, TM, UW, JV, EC, PR, JT, and SV analyzed the data and interpreted the results. MW, JP, and SV prepared the figures. MW, JP, and SV wrote the manuscript. PR and JT revised the manuscript. All authors contributed to the article and approved the submitted version.
Funding
Publication costs are funded by the Deutsche Forschungsgemeinschaft (DFG, German Research Foundation)—Project ID INST 40/600-1—Collaborative Research Center (CRC) Projektnummer 251293561 - SFB 1149 to MW and SV. SV received funding from Deutsche Forschungsgemeinschaft (DFG, German Research Foundation), GRK 2203 and DFG VE 994/2-1. PR and JT received funding from the Deutsche Forschungsgemeinschaft (DFG, German Research Foundation)—Collaborative Research Center (CRC) Projektnummer 251293561 - SFB 1149 and DFG Tu 220/13-1 to JT.
Conflict of interest
Authors CT, MK, and JB are employed by Boehringer Ingelheim Pharma GmbH & Co KG.
The remaining authors declare that the research was conducted in the absence of any commercial or financial relationships that could be construed as a potential conflict of interest.
Publisher’s note
All claims expressed in this article are solely those of the authors and do not necessarily represent those of their affiliated organizations, or those of the publisher, the editors and the reviewers. Any product that may be evaluated in this article, or claim that may be made by its manufacturer, is not guaranteed or endorsed by the publisher.
Supplementary material
The Supplementary Material for this article can be found online at: https://www.frontiersin.org/articles/10.3389/fimmu.2022.980707/full#supplementary-material
References
1. Angele MK, Schneider CP, Chaudry IH. Bench-to-bedside review: Latest results in hemorrhagic shock. Crit Care (2008) 12(4):218. doi: 10.1186/cc6919
2. Denk S, Weckbach S, Eisele P, Braun CK, Wiegner R, Ohmann JJ, et al. Role of hemorrhagic shock in experimental polytrauma. Shock (2017) 49(2):154–63. doi: 10.1097/SHK.0000000000000925
3. Eltzschig HK, Carmeliet P. Hypoxia and inflammation. N Engl J Med (2011) 364(7):656–65. doi: 10.1056/NEJMra0910283
4. Douzinas EE, Livaditi O, Tasoulis M-K, Prigouris P, Bakos D, Goutas N, et al. Nitrosative and oxidative stresses contribute to post-ischemic liver injury following severe hemorrhagic shock: The role of hypoxemic resuscitation. PLoS One (2012) 7(3):e32968. doi: 10.1371/journal.pone.0032968
5. Merz T, Denoix N, Huber-Lang M, Singer M, Radermacher P, McCook O. Microcirculation vs. mitochondria-what to target? Front Med (Lausanne) (2020) 7:416. doi: 10.3389/fmed.2020.00416
6. Barth E, Albuszies G, Baumgart K, Matejovic M, Wachter U, Vogt J, et al. Glucose metabolism and catecholamines. Crit Care Med (2007) 35(9 Suppl):S508–18. doi: 10.1097/01.CCM.0000278047.06965.20
7. Vanhorebeek I, Gunst J, Ellger B, Boussemaere M, Lerut E, Debaveye Y, et al. Hyperglycemic kidney damage in an animal model of prolonged critical illness. Kidney Int (2009) 76(5):512–20. doi: 10.1038/ki.2009.217
8. Wagner K, Gröger M, McCook O, Scheuerle A, Asfar P, Stahl B, et al. Blunt chest trauma in mice after cigarette smoke-exposure: Effects of mechanical ventilation with 100% O2. PloS One (2015) 10(7):e0132810. doi: 10.1371/journal.pone.0132810
9. Geldmacher H, Biller H, Herbst A, Urbanski K, Allison M, Buist AS, et al. Die prävalenz der chronisch obstruktiven lungenerkrankung (COPD) in deutschland. Ergebnisse der BOLD-Studie Dtsch Med Wochenschr (2008) 133(50):2609–14. doi: 10.1055/s-0028-1105858
10. Sinden NJ, Stockley RA. Systemic inflammation and comorbidity in COPD: a result of 'overspill' of inflammatory mediators from the lungs? Review of the evidence. Thorax (2010) 65(10):930–6. doi: 10.1136/thx.2009.130260
11. Hartmann C, Hafner S, Scheuerle A, Möller P, Huber-Lang M, Jung B, et al. The role of cystathionine-γ-Lyase in blunt chest trauma in cigarette smoke exposed mice. Shock (2017) 47(4):491–9. doi: 10.1097/SHK.0000000000000746
12. Minei JP, Cuschieri J, Sperry J, Moore EE, West MA, Harbrecht BG, et al. The changing pattern and implications of multiple organ failure after blunt injury with hemorrhagic shock. Crit Care Med (2012) 40(4):1129–35. doi: 10.1097/CCM.0b013e3182376e9f
13. Vettorazzi S, Nalbantoglu D, Gebhardt JCM, Tuckermann J. A guide to changing paradigms of glucocorticoid receptor function-a model system for genome regulation and physiology. FEBS J (2021). doi: 10.1111/febs.16100
14. Lim H-W, Uhlenhaut NH, Rauch A, Weiner J, Hübner S, Hübner N, et al. Genomic redistribution of GR monomers and dimers mediates transcriptional response to exogenous glucocorticoid in vivo. Genome Res (2015) 25(6):836–44. doi: 10.1101/gr.188581.114
15. Vettorazzi S, Bode C, Dejager L, Frappart L, Shelest E, Klaßen C, et al. Glucocorticoids limit acute lung inflammation in concert with inflammatory stimuli by induction of SphK1. Nat Commun (2015) 6:7796. doi: 10.1038/ncomms8796
16. Wepler M, Preuss JM, Merz T, Hartmann C, Wachter U, McCook O, et al. Impaired glucocorticoid receptor dimerization aggravates LPS-induced circulatory and pulmonary dysfunction. Front Immunol (2019) 10:3152. doi: 10.3389/fimmu.2019.03152
17. Preuss JM, Burret U, Gröger M, Kress S, Scheuerle A, Möller P, et al. Impaired glucocorticoid receptor signaling aggravates lung injury after hemorrhagic shock. Cells (2021) 11(1):112. doi: 10.3390/cells11010112
18. Langgartner D, Wachter U, Hartmann C, Gröger M, Vogt J, Merz T, et al. Effects of psychosocial stress on subsequent hemorrhagic shock and resuscitation in Male mice. Shock (2019) 51(6):725–30. doi: 10.1097/SHK.0000000000001204
19. Gröger M, Wepler M, Wachter U, Merz T, McCook O, Kress S, et al. The effects of genetic 3-mercaptopyruvate sulfurtransferase deficiency in murine traumatic-hemorrhagic shock. Shock (2018) 51(4):472–478. doi: 10.1097/SHK.0000000000001165
20. Gröger M, Hogg M, Abdelsalam E, Kress S, Hoffmann A, Stahl B, et al. Effects of sodium thiosulfate during resuscitation from trauma-and-Hemorrhage in cystathionine gamma lyase (CSE) knockout mice. Shock (2022) 57(1):131–9. doi: 10.1097/SHK.0000000000001828
21. Hartmann C, Gröger M, Noirhomme J-P, Scheuerle A, Möller P, Wachter U, et al. In-depth characterization of the effects of cigarette smoke exposure on the acute trauma response and hemorrhage in mice. Shock (2018) 51(1):68–77. doi: 10.1097/SHK.0000000000001115
22. Reichardt HM, Kaestner KH, Tuckermann J, Kretz O, Wessely O, Bock R, et al. DNA Binding of the glucocorticoid receptor is not essential for survival. Cell (1998) 93(4):531–41. doi: 10.1016/s0092-8674(00)81183-6
23. Merz T, Kress S, Gröger M, Radermacher P, McCook O. Mouse intensive care unit (MICU). Methods Mol Biol (2021) 2321:121–35. doi: 10.1007/978-1-0716-1488-4_11
24. Hafner S, Wagner K, Weber S, Gröger M, Wepler M, McCook O, et al. Role of the purinergic receptor P2XR4 after blunt chest trauma in cigarette smoke-exposed mice. Shock (2017) 47(2):193–9. doi: 10.1097/SHK.0000000000000726
25. Wollin L, Pieper MP. Tiotropium bromide exerts anti-inflammatory activity in a cigarette smoke mouse model of COPD. Pulm Pharmacol Ther (2010) 23(4):345–54. doi: 10.1016/j.pupt.2010.03.008
26. Vogt JA, Wachter U, Wagner K, Calzia E, Gröger M, Weber S, et al. Effects of glycemic control on glucose utilization and mitochondrial respiration during resuscitated murine septic shock. Intensive Care Med Exp (2014) 2(1):19. doi: 10.1186/2197-425X-2-19
27. Zinkhan EK, Lang BY, Yu B, Wang Y, Jiang C, Fitzhugh M, et al. Maternal tobacco smoke increased visceral adiposity and serum corticosterone levels in adult male rat offspring. Pediatr Res (2014) 76(1):17–23. doi: 10.1038/pr.2014.58
28. Novaes Soares P, Silva Tavares Rodrigues V, Cherem Peixoto T, Calvino C, Aparecida Miranda R, Pereira Lopes B, et al. Cigarette smoke during breastfeeding in rats changes glucocorticoid and vitamin d status in obese adult offspring. Int J Mol Sci (2018) 19(10):3084. doi: 10.3390/ijms19103084
29. Tilp C, Bucher H, Haas H, Duechs MJ, Wex E, Erb KJ. Effects of conventional tobacco smoke and nicotine-free cigarette smoke on airway inflammation, airway remodelling and lung function in a triple allergen model of severe asthma. Clin Exp Allergy (2016) 46(7):957–72. doi: 10.1111/cea.12665
30. Vandewalle J, Timmermans S, Paakinaho V, Vancraeynest L, Dewyse L, Vanderhaeghen T, et al. Combined glucocorticoid resistance and hyperlactatemia contributes to lethal shock in sepsis. Cell Metab (2021) 33(9):1763–76.e5. doi: 10.1016/j.cmet.2021.07.002
31. Hartmann C, Radermacher P, Wepler M, Nußbaum B. Non-hemodynamic effects of catecholamines. Shock (2017) 48(4):390–400. doi: 10.1097/SHK.0000000000000879
32. Gjedsted J, Buhl M, Nielsen S, Schmitz O, Vestergaard ET, Tønnesen E, et al. Effects of adrenaline on lactate, glucose, lipid and protein metabolism in the placebo controlled bilaterally perfused human leg. Acta Physiol (Oxf) (2011) 202(4):641–8. doi: 10.1111/j.1748-1716.2011.02316.x
33. Steinberg D, Nestel PJ, Buskirk ER, Thompson RH. Calorigenic effect of norepinephrine correlated with plasma free fatty acid turnover and oxidation. J Clin Invest (1964) 43:167–76. doi: 10.1172/JCI104901
34. Bearn AG, Billing B, Sherlock S. The effect of adrenaline and noradrenaline on hepatic blood flow and splanchnic carbohydrate metabolism in man. J Physiol (1951) 115(4):430–41. doi: 10.1113/jphysiol.1951.sp004679
Keywords: catecholamines, cigarettes smoke exposure, glucocorticoid receptor function, hemodynamic function, hemorrhagic shock, metabolic function, inflammation
Citation: Wepler M, Preuss JM, Tilp C, Keck M, Blender J, Wachter U, Merz T, Vogt J, Kress S, Gröger M, Hoffmann A, Fink M, Calzia E, Burret U, Radermacher P, Tuckermann JP and Vettorazzi S (2022) Cigarette smoke exposure reduces hemorrhagic shock induced circulatory dysfunction in mice with attenuated glucocorticoid receptor function. Front. Immunol. 13:980707. doi: 10.3389/fimmu.2022.980707
Received: 28 June 2022; Accepted: 23 August 2022;
Published: 12 September 2022.
Edited by:
Tom E. Mollnes, University of Oslo, NorwayReviewed by:
Nikola Cesarovic, ETH Zürich, SwitzerlandPatrick Geraghty, Downstate Health Sciences University, United States
Copyright © 2022 Wepler, Preuss, Tilp, Keck, Blender, Wachter, Merz, Vogt, Kress, Gröger, Hoffmann, Fink, Calzia, Burret, Radermacher, Tuckermann and Vettorazzi. This is an open-access article distributed under the terms of the Creative Commons Attribution License (CC BY). The use, distribution or reproduction in other forums is permitted, provided the original author(s) and the copyright owner(s) are credited and that the original publication in this journal is cited, in accordance with accepted academic practice. No use, distribution or reproduction is permitted which does not comply with these terms.
*Correspondence: Martin Wepler, bWFydGluLndlcGxlckB1bmktdWxtLmRl; Sabine Vettorazzi, c2FiaW5lLnZldHRvcmF6emlAdW5pLXVsbS5kZQ==