- School of Pharmacy, Second Military Medical University, Shanghai, China
Fungal infections are a growing health care challenge. Neutrophils play a key role in defense against fungal infections. There are many effective ways for neutrophils to eliminate fungal invaders, such as phagocytosis, oxidative bursts, and the formation of extracellular traps. This process has received considerable attention and has made rapid progress since neutrophil extracellular traps (NETs) formation was described. Here, we describe the formation, induction, and function of NETs, as well as fungal strategies against NETs hunting. We highlight the effects of NETs on common fungal pathogens and how these pathogens survive.
Introduction
Fungal infections have long been a public health challenge. It mainly affects immunocompromised populations, such as solid organ transplant recipients and AIDS patients (1). Fungal co-infection has also been reported in COVID-19 patients during these years of pandemics (2). Among COVID-19 patients on mechanical ventilation in the ICU, fungal co-infection rates have been reported as high as 26.7% (3). Innate immune system plays an important role in defense against fungal infections. Mucosal barriers and chemicals work with natural killer cells and phagocytes. It is reviewed that Aspergillus species can interact with the innate immune system including macrophages, neutrophils, dendritic cells, and the complement system (4). These cells and proteins recognize and kill fungal pathogens, protecting our bodies from infection. Neutrophil (polymorphonuclear leukocyte), which is one kind of the phagocytes, plays a decisive role in this process (5). Invasive fungal diseases occur in up to 24% of patients with leukemia (6). Neutrophils can kill fungal pathogens by phagocytosis, production of reactive oxygen species (ROS) and formation of extracellular traps. Neutrophil extracellular traps (NETs) were first described as an antibacterial mechanism of innate immunity in 2004 when Volker Brinkmann et al. discovered that NETs could kill bacteria (7). In recent years, much progress has been made in the study of NETs, and the fungicidal effects of NETs have been described, such as the fungicidal effects of NETs on Candida spp. and Aspergillus spp. (8, 9). Rather than waiting to be killed, fungi have their own ways of fighting back. For example, Aspergillus spp. can invade the innate immune system by interfering with complement system and phagocytes [reviewed in (4)]. In this article, we focus on the interactions between NETs and several common fungal pathogens. The killing process of NETs against fungal pathogens and the strategies of pathogen resistance were reviewed.
Formation of NETs
NETs are fibrous three-dimensional network structures composed of nucleic acids and various granular proteins that neutrophils can release out of the cell in response to various stimuli. This structure traps pathogens such as bacteria and fungi, limits their spread through the body and kills them with high concentrations of toxic proteins. Other immune cells, such as eosinophils and mast cells, can also form similar structures, killing a variety of microorganisms and enhancing inflammatory immune responses (10, 11). NETs help eliminate pathogens, but excess NETs can cause damage to surrounding tissues either by themselves or by increasing the pro-inflammatory response. The generation of NETs is closely related to the occurrence and development of various diseases (12–14).
Since 2004, two major ways of releasing NETs have been identified, a) NETosis and b) rapid release of live neutrophils. NETosis is a classic way of releasing NETs, usually neutrophils release NETs by decondensation of chromatin resulting in cell death (15). Later, a novel way was discovered to form NETs by releasing mitochondrial DNA. This novel way of NETs formation does not require neutrophil death and therefore does not limit the lifespan of these cells (16).
NETosis
NETosis begins with oxidative burst and activation of peptidyl arginine deiminase 4 (PAD4), which catalyzes the citrullination of arginine residues. The process leads to disassembly of nuclear envelope and chromatin decondensation (17). Chromatin then combine with neutrophil elastase (NE) and other cytoplasmic enzymes to form NETs. And NETs release upon plasma membrane rupture. The whole process takes about 4 hours and results in neutrophil death (15, 18–20). A recent study showed that intact F-actin dynamics and myosin II function are essential for the formation of NETs in response to different stimuli including Candida albicans. Neutrophils in patients with actin polymerization defects also failed to exhibit NETs, confirming this conclusion (21).
Rapid release of live neutrophils
Live neutrophils can generate NETs under some stimuli. Interestingly, these NETs contain mitochondrial DNA instead of nuclear DNA (16). The process can be very quick since neutrophils expel mitochondrial DNA and assemble NETs outside the cell. Both this approach and the NETosis approach rely on ROS (22). There is also a ROS-independent fast-release mechanism without neutrophil death. In some Gram-positive bacterial infections, the nuclear membranes of neutrophils are separated and nuclear DNA is extruded out of the cell through vesicles. The anuclear neutrophils are still capable of migration and phagocytosis (23, 24).
Induction of NETs in fungal infections
The induction of NETs is affected by a variety of inducing factors and the number of NETs produced is different. ROS (25), IL-8 (7), lipopolysaccharide (LPS) (7), complementary 5a (C5a) (16), phorbol-12-myristate-13-acetate (PMA) (7, 18), and glucose oxidase (GO) (25) are all inducers that can trigger the formation of NETs. And among them, ROS is one of the key factors that trigger the formation of NETs.
Fungal pathogens can also trigger the release of NETs. C. albicans is the most widely discussed fungal pathogen in the NETs release field (26). In addition, other Candida spp. (27, 28), Aspergillus fumigatus (8), Histoplasma capsulatum (29), Phialophora verrucose (30), Paracoccidioides brasiliensis (31), and Scedosporium apiospermum (32), have all been described as NETs release inducers. The common dermatophyte Trichophyton rubrum is also found to be a NETs inducer. Both conidia and hyphae of T. rubrum can induce NETs formation in a dose-dependent manner (33). Cryptococcus neoformans itself is not an inducing factor, but the capsular polysaccharide glucuronoxylomannogalactan (GXMGal) can induce the formation of NETs (34). Unfortunately, Candida auris, a recent emerging global public health threat, cannot induce the formation of NETs and is not effectively killed by neutrophils (35). Fungi come in a variety of forms, from small yeast to large hyphae and biofilms, which require neutrophils to respond in different ways to eliminate them. Both yeast and hyphal forms of C. albicans can activate NETs formation (26). However, the extracellular matrix of C. albicans biofilms does not trigger NETs, but instead impairs the NETs formation (36). Interestingly, recent studies have shown that the nucleic acids in the extracellular matrix of C. albicans biofilms can stimulate the release of NETs (37). A number of components in C. albicans have also been shown to stimulate NETs release, including dectin-2 (38), aspartic proteases, mannans, β-glucans (39), and farnesol (40).
Function of NETs in fungal infections
NETs are mixtures of nucleic acids, histones, granular proteins, and cytoplasmic proteins, including NE, myelperoxidase (MPO), lysozyme C, and gelatinase (20). These components lead to the release of chemokines, the production of cytokines, the promotion of inflammatory disease and, of course, the killing of microorganisms (20). The mixture can trap fungi inside its 3D network structure and cause damage to fungi through the components it releases. In recent years, studies on the effects of NETs on C. albicans, Aspergillus spp., and C. neoformans have made progress (Table 1).
C. albicans
Opportunistic fungal pathogen C. albicans is a component of intestinal commensal microbiota that colonizes the intestines, skin, and oral mucosa of healthy humans (44). In immunocompromised populations, such as neutropenia patients, it can shift from colonization to invasion and spread in the body, causing systemic infection (45). Candida spp. are the most common pathogens of invasive fungal diseases (44, 46), in which C. albicans is the major cause for candidiasis (47). As a model organism, C. albicans is the first fungus to be shown to induce NETs in vitro (26).
On the one hand, the sensitivity of different morphologies of C. albicans to NETs immunoclearance is different. NETs have been shown to kill both yeast and hyphal forms of C. albicans (26). However, NETs cannot be produced upon C. albicans biofilms. Time-lapse imaging showed that neutrophils adhered only to hyphae and migrated on the biofilms (36). Another study showed that sub-inhibitory concentrations of echinocandins, an effective antibiofilm drug, promote the formation of NETs in C. albicans biofilms, including structures of DNA, histones, and antimicrobial proteins with antifungal activity (48). On the other hand, different isolates of C. albicans also modulate the function of NETs. By using a panel of clinical C. albicans strains, Madhu Shankar et al. found that the prototype strain SC5314 induced the most potent accumulation of ROS and NETs by neutrophils from all the isolates tested (49).
In response to microbial infections, neutrophils initiate NETosis via protein kinase C (PKC) and activate the nicotinamide adenine dinucleotide phosphate (NADPH) oxidase signaling cascade, leading to the accumulation of ROS (39, 50). Studies have shown that C. albicans-induced NETs production requires PKC, and PKC inhibitor Gö6976 can block this process (51). Another important component of NETs that kills fungi is calprotectin. Lack of calprotectin in NETs resulted in a complete loss of antifungal activity in vitro (52).
NETs also unmask C. albicans and make it expose immunogenic epitopes to the host. NETs trigger fungal cell wall remodeling and enhance immune recognition by Dectin-1 β-glucan receptors. This process involves fungal MAPK pathways, which dynamically relocalize cell wall remodeling machinery including Chs3, Phr1 and Sur7 (53).
Aspergillus spp.
Aspergillus spp. are common spore-releasing environmental fungi. However, for immunocompromised individuals who are unable to adequately clear the spores from their lungs, they may develop invasive pulmonary aspergillosis (IPA), which is life-threatening (54). Two high-risk groups were patients with neutropenia or hematologic malignancy and patients with chronic granulomatosis (CGD) (55, 56).
Unlike C. albicans, Aspergillus spp. may be less susceptible to NETs. NETs did not kill either A. fumigatus or A. nidulans conidia (41, 57). They are more inclined to be engulfed by living neutrophils (8). Another study proves that adding DNase to neutrophils do not affect the killing efficiency of Aspergillus hyphae, which indicates that NETs formation does not contribute to this fungal killing process (42). NETs are more robust towards A. fumigatus hyphae than conidia, which is confirmed by both in vitro and in vivo experiments (8, 41).
Patients with CGDs usually exhibit deficient phagocyte NADPH oxidase function, which is essential in the formation of NETs (15). In a case report, CGD patients reconstructed the generation of NETs through gene therapy and restored neutrophil clearance of A. nidulans conidia and hyphae, which is associated with rapid cure of IPA (58). The authors soon verified this connection experimentally. Restoring NADPH function through gene complementation can restore the production of NETs in vitro (43). Further studies have shown that calprotectin plays a key role in human innate immunity against Aspergillus infection (43).
Some studies came to somewhat an opposite conclusion. On one hand, it is proved by confocal imaging that neutrophils from CGD patients can still form NETs under the stimulation of Aspergillus hyphae, although these cells with genetic immunodeficiencies have antifungal deficiency (42). The researchers also find that neutrophils from CGD patients cannot initiate NETs formation in response to PMA, which suggests different mechanisms between PMA and Aspergillus hyphae in inducing NETs formation (42). A relevant study shows that Aspergillus and β-glucan-induced NETs formation is regulated by PAD4 and CR3. The hyphae killing process, however, is only dependent on CR3 (59). On the other hand, it suggested that inhibition of NETs release might contribute to the treatment of patients with IPA (60). They found that in the IPA model, mice lacking PAD4 had a lower fungal burden in their lungs and less acute lung injury. This indicates that NETs release causes tissue damage and impairs fungal clearance in IPA mouse models (60).
C. neoformans
C. neoformans is also an opportunistic fungal pathogen with a small-size yeast form and a unique polysaccharide capsule. It is one of the most common pathogens for meningitis (61). Polysaccharide capsules are considered to be a key virulence factor (62). It comprises approximately 88% glucuronoxylomannan (GXM),10% GXMGal, and 2% mannoproteins (63, 64). Although wild-type C. neoformans and its GXM do not induce NETs, NET-enriched supernatants induced by a mutant acapsular strain exhibit fungicidal activity against wild-type strains. (34) (Figure 1)
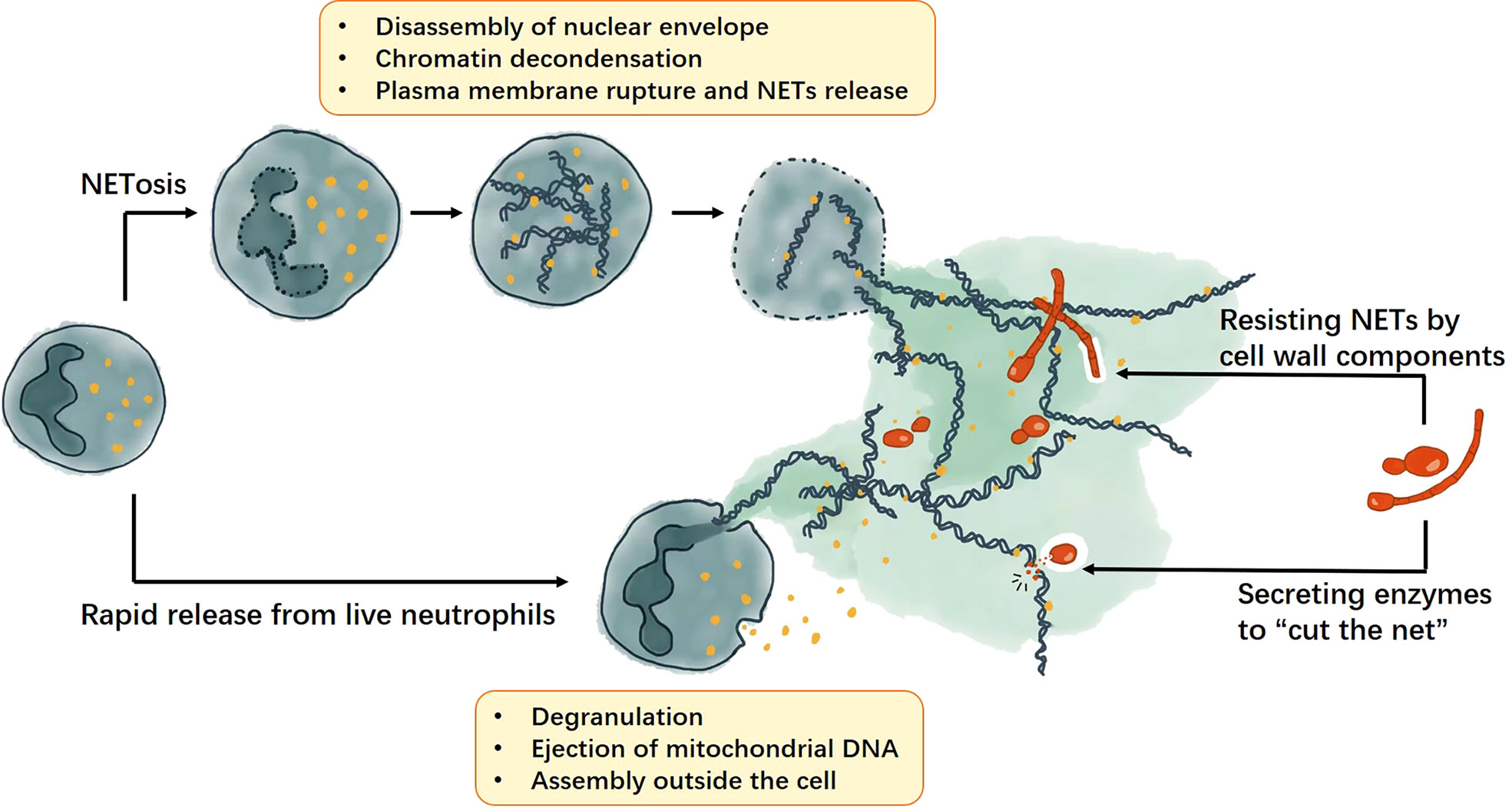
Figure 1 The formation of NETs and the fungal strategies against NETs. NETs can be produced though NETosis or rapid release from live cells. The NETosis process includes disassembly of nuclear envelope, chromatin decondensation, plasma membrane rupture and NETs release; whereas rapid release from live cells require cell degranulation and ejection of mitochondrial DNA, then the NETs will be assembled outside the cell. For fungi, they can resist NETs by cell wall components and secrete enzymes to help them escape the NETs.
Fungal strategies against NETs
Hosts can kill fungi by producing NETs, but the fungi won’t stand still. Fungal defense strategies against NETs are varied and can be generally divided into two categories: modulation of NETs formation and escape from NETs. On the one hand, fungi can modulate the formation of NETs through their own components, thus resisting or even inhibiting the fungicidal effects of NETs. This phenomenon is common in Aspergillus spp., C. albicans, and C. neoformans. On the other hand, fungi can release active proteins, usually enzymes that target nuclear acids, to help themselves escape NETs.
Modulation of NETs formation
Aspergillus spp. can use galactosaminogalactan (GAG) to enhance resistance to NETs. GAG is an exopolysaccharide produced by A. fumigatus and is associated with adherence and complete virulence. Enhancing GAG in less pathogenic A. nidulans at the genetic level can increase its virulence and resistance to NADPH oxidase-dependent NETs in vitro. It indicates that cell wall-bound GAG enhances virulence through mediating resistance to NETs (65). CcpA is another important protein to reduce recognition by the innate immune system. Lacking of CcpA causes higher activation of neutrophils and speeds up the oxidative burst progress, and A. fumigatus ΔccpA conidia shows highly attenuated virulence even in immunosuppressed mice (66).
C. albicans also has its unique ways to resist NETs. C. albicans biofilms of clinical isolates uniformly impair NETs release at different depths and architectures (67). Another way for C. albicans to modulate NETs formation is by arresting proteinous components of NETs, including elastase, myeloperoxidase, lactotransferrin, and histones. These NETs components are involved in cell surface contact with C. albicans. Adhesins on the surface of C. albicans, such as the agglutinin-like sequence protein family Als3, can adsorb NETs proteins and increase the pathogen’s potency in host cell destruction, suggesting that the efficiency of fungal entrapment might be altered (68).
C. neoformans appears to be quite “invisible” to NETs. The fungus itself and its major capsular polysaccharide glucuronoxylomannan (GXM) do not trigger NETs formation. Moreover, both inhibit the production of PMA-induced NETs. In addition, both GXM and GXMGal block the production of ROS through PMA-activated neutrophils (34).
Escape from NETs
C. albicans and C. glabrata can escape from being trapped in NETs through their 3’-nucleotidase/nuclease (3’NT/NU) activity. 3’NT/NU is an ectonucleotidase that hydrolyze AMP and nucleic acids. When NETs trap Candida cells, the cells promote NETs disruption and this process can be blocked by 3’NT/NU inhibitor ammonium tetrathiomolybdate (69).
Besides, C. albicans can escape NETs by secreting DNase. Strains that secrete more DNase showed greater resistance to neutrophil killing. And the antifungal activity of neutrophils decreases significantly after NETs being degraded by exogenous DNase I or catalase (70).
Outlook
It is like a seesaw battle between NETs and pathogenic fungi. NETs are activated when fungi invade the host, trapping the fungi and killing them. The fungi, in turn, find their ways to resist NETs’ fungicidal effect or escape traps.
Research on NETs has been a hotspot in recent years. NETs protect the host from infections by killing pathogens including bacteria, fungi, viruses, and parasites. However, in addition to antimicrobial effects, excess NETs increase pro-inflammatory responses and cause damage to surrounding tissues, which has negative effects in many infectious and non-infectious diseases. For example, dysfunction of NETs can damage host tissues, promote the development of autoimmunity and thrombosis (71). NETs are also involved in nearly all the inflammation-related diseases, including systemic lupus erythematosus (72), rheumatoid arthritis (72), atherosclerosis (73), diabetes (74), asthma (75), tumors (75), and wound healing (76). Since its discovery, people have been enthusiastic about this field, and there are still many unknowns to explore. Taking the antifungal activity as an example, the role of NETs in fungal infections is still unclear. What are the molecular mechanisms underlying the induction, formation, and antifungal processes of NETs? Why do different fungi, or even different strains of the same species induce NETs differently?
As our understanding of NETs’ underlying mechanisms increases, it may provide a useful tool for diagnosis and treatment of related diseases. On the one hand, we can identify new targets and design drugs that enhance the antifungal ability of NETs without causing tissue damage. On the other hand, ideal NETs release blockers may be discovered and used to avoid tissue damage without compromising antimicrobial effects. We can also use synergists to reduce immune escape or resistance of fungi to NETs. Another interesting idea is that, according to a recent study (77), extracellular traps can be trained as a memory response. There may one day be a vaccine to help increase the antimicrobial function of NETs in high-risk populations.
Author contributions
All authors listed have made direct and intellectual contribution to the work and approved it for publication.
Funding
This study was supported by the National Natural Science Foundation of China (81772124), the Shanghai Science and Technology Commission “Science and Technology Innovation Action Plan” biomedical science and technology support special project (20S11902900) and the biosafety program (20SWAQX29-1-6).
Conflict of interest
The authors declare that the research was conducted in the absence of any commercial or financial relationships that could be construed as a potential conflict of interest.
Publisher’s note
All claims expressed in this article are solely those of the authors and do not necessarily represent those of their affiliated organizations, or those of the publisher, the editors and the reviewers. Any product that may be evaluated in this article, or claim that may be made by its manufacturer, is not guaranteed or endorsed by the publisher.
References
1. Azoulay E, Russell L, Van de Louw A, Metaxa V, Bauer P, Povoa P, et al. Diagnosis of severe respiratory infections in immunocompromised patients. Intensive Care Med (2020) 46(2):298–314. doi: 10.1007/s00134-019-05906-5
2. Rawson TM, Wilson RC, Holmes A. Understanding the role of bacterial and fungal infection in COVID-19. Clin Microbiol Infect (2021) 27(1):9–11. doi: 10.1016/j.cmi.2020.09.025
3. White PL, Dhillon R, Cordey A, Hughes H, Faggian F, Soni S, et al. A national strategy to diagnose coronavirus disease 2019-associated invasive fungal disease in the intensive care unit. Clin Infect Dis (2021) 73(7):e1634–44. doi: 10.1093/cid/ciaa1298
4. Brakhage AA, Bruns S, Thywissen A, Zipfel PF, Behnsen J. Interaction of phagocytes with filamentous fungi. Curr Opin Microbiol (2010) 13(4):409–15. doi: 10.1016/j.mib.2010.04.009
5. Urban CF, Nett JE. Neutrophil extracellular traps in fungal infection. Semin Cell Dev Biol (2019) 89:47–57. doi: 10.1016/j.semcdb.2018.03.020
6. Cornely OA, Maertens J, Winston DJ, Perfect J, Ullmann AJ, Walsh TJ, et al. Posaconazole vs. fluconazole or itraconazole prophylaxis in patients with neutropenia. N Engl J Med (2007) 356(4):348–59. doi: 10.1056/NEJMoa061094
7. Brinkmann V, Reichard U, Goosmann C, Fauler B, Uhlemann Y, Weiss DS, et al. Neutrophil extracellular traps kill bacteria. Science (2004) 303(5663):1532–5. doi: 10.1126/science.1092385
8. Bruns S, Kniemeyer O, Hasenberg M, Aimanianda V, Nietzsche S, Thywissen A, et al. Production of extracellular traps against aspergillus fumigatus in vitro and in infected lung tissue is dependent on invading neutrophils and influenced by hydrophobin RodA. PloS Pathog (2010) 6(4):e1000873. doi: 10.1371/journal.ppat.1000873
9. Branzk N, Lubojemska A, Hardison SE, Wang Q, Gutierrez MG, Brown GD, et al. Neutrophils sense microbe size and selectively release neutrophil extracellular traps in response to large pathogens. Nat Immunol (2014) 15(11):1017–25. doi: 10.1038/ni.2987
10. von Köckritz-Blickwede M, Goldmann O, Thulin P, Heinemann K, Norrby-Teglund A, Rohde M, et al. Phagocytosis-independent antimicrobial activity of mast cells by means of extracellular trap formation. Blood (2008) 111(6):3070–80. doi: 10.1182/blood-2007-07-104018
11. Kim HJ, Sim MS, Lee DH, Kim C, Choi Y, Park HS, et al. Lysophosphatidylserine induces eosinophil extracellular trap formation and degranulation: Implications in severe asthma. Allergy (2020) 75(12):3159–70. doi: 10.1111/all.14450
12. Carestia A, Kaufman T, Rivadeneyra L, Landoni VI, Pozner RG, Negrotto S, et al. Mediators and molecular pathways involved in the regulation of neutrophil extracellular trap formation mediated by activated platelets. J Leukoc Biol (2016) 99(1):153–62. doi: 10.1189/jlb.3A0415-161R
13. Fadini GP, Menegazzo L, Scattolini V, Gintoli M, Albiero M, Avogaro A. A perspective on NETosis in diabetes and cardiometabolic disorders. Nutr Metab Cardiovasc Dis (2016) 26(1):1–8. doi: 10.1016/j.numecd.2015.11.008
14. Masucci MT, Minopoli M, Del Vecchio S, Carriero MV. The emerging role of neutrophil extracellular traps (NETs) in tumor progression and metastasis. Front Immunol (2020) 11:1749. doi: 10.3389/fimmu.2020.01749
15. Fuchs TA, Abed U, Goosmann C, Hurwitz R, Schulze I, Wahn V, et al. Novel cell death program leads to neutrophil extracellular traps. J Cell Biol (2007) 176(2):231–41. doi: 10.1083/jcb.200606027
16. Yousefi S, Mihalache C, Kozlowski E, Schmid I, Simon HU. Viable neutrophils release mitochondrial DNA to form neutrophil extracellular traps. Cell Death Differ. (2009) 16(11):1438–44. doi: 10.1038/cdd.2009.96
17. Thiam HR, Wong SL, Qiu R, Kittisopikul M, Vahabikashi A, Goldman AE, et al. NETosis proceeds by cytoskeleton and endomembrane disassembly and PAD4-mediated chromatin decondensation and nuclear envelope rupture. Proc Natl Acad Sci U.S.A. (2020) 117(13):7326–37. doi: 10.1073/pnas.1909546117
18. Remijsen Q, Vanden Berghe T, Wirawan E, Asselbergh B, Parthoens E, De Rycke R, et al. Neutrophil extracellular trap cell death requires both autophagy and superoxide generation. Cell Res (2011) 21(2):290–304. doi: 10.1038/cr.2010.150
19. Rohrbach AS, Slade DJ, Thompson PR, Mowen KA. Activation of PAD4 in NET formation. Front Immunol (2012) 3:360. doi: 10.3389/fimmu.2012.00360
20. Nija RJ, Sanju S, Sidharthan N, Mony U. Extracellular trap by blood cells: Clinical implications. Tissue Eng Regener Med (2020) 17(2):141–53. doi: 10.1007/s13770-020-00241-z
21. Sprenkeler EGG, Tool ATJ, Henriet SSV, van Bruggen R, Kuijpers TW. Formation of neutrophil extracellular traps requires actin cytoskeleton rearrangements. Blood (2022) 139(21):3166–80. doi: 10.1182/blood.2021013565
22. Yousefi S, Gold JA, Andina N, Lee JJ, Kelly AM, Kozlowski E, et al. Catapult-like release of mitochondrial DNA by eosinophils contributes to antibacterial defense. Nat Med (2008) 14(9):949–53. doi: 10.1038/nm.1855
23. Pilsczek FH, Salina D, Poon KK, Fahey C, Yipp BG, Sibley CD, et al. A novel mechanism of rapid nuclear neutrophil extracellular trap formation in response to staphylococcus aureus. J Immunol (2010) 185(12):7413–25. doi: 10.4049/jimmunol.1000675
24. Yipp BG, Petri B, Salina D, Jenne CN, Scott BN, Zbytnuik LD, et al. Infection-induced NETosis is a dynamic process involving neutrophil multitasking in vivo. Nat Med (2012) 18(9):1386–93. doi: 10.1038/nm.2847
25. Cheng ML, Ho HY, Lin HY, Lai YC, Chiu DT. Effective NET formation in neutrophils from individuals with G6PD Taiwan-hakka is associated with enhanced NADP(+) biosynthesis. Free Radic Res (2013) 47(9):699–709. doi: 10.3109/10715762.2013.816420
26. Urban CF, Reichard U, Brinkmann V, Zychlinsky A. Neutrophil extracellular traps capture and kill candida albicans yeast and hyphal forms. Cell Microbiol (2006) 8(4):668–76. doi: 10.1111/j.1462-5822.2005.00659.x
27. Svobodová E, Staib P, Losse J, Hennicke F, Barz D, Józsi M. Differential interaction of the two related fungal species candida albicans and candida dubliniensis with human neutrophils. J Immunol (2012) 189(5):2502–11. doi: 10.4049/jimmunol.1200185
28. Campos-Garcia L, Jimenez-Valdes RJ, Hernandez-Bello R, Palma-Nicolas J, Gonzalez GM, Sanchez-Gonzalez A. Candida albicans and non-albicans isolates from bloodstream have different capacities to induce neutrophil extracellular traps. J Fungi. (Basel) (2019) 5(2):28-43. doi: 10.3390/jof5020028
29. Thompson-Souza GA, Santos GMP, Silva JC, Muniz VS, Braga YAV, Figueiredo RT, et al. Histoplasma capsulatum-induced extracellular DNA trap release in human neutrophils. Cell Microbiol (2020) 22(7):e13195. doi: 10.1111/cmi.13195
30. Liu Q, Yi W, Jiang S, Song J, Liang P. Neutrophil extracellular traps serve as key effector molecules in the protection against phialophora verrucosa. Mycopathologia (2021) 186(3):367–75. doi: 10.1007/s11046-021-00554-0
31. Mejía SP, Cano LE, López JA, Hernandez O, González Á. Human neutrophils produce extracellular traps against paracoccidioides brasiliensis. Microbiol (Reading) (2015) 161(Pt 5):1008–17. doi: 10.1099/mic.0.000059
32. Luna-Rodríguez CE, González GM, Montoya AM, Treviño-Rangel RJ, Sánchez-González A. Production of neutrophil extracellular traps (NETs) in response to scedosporium apiospermum in a murine model of pulmonary infection. Microb Pathog (2020) 149:104349. doi: 10.1016/j.micpath.2020.104349
33. Reis APC, Celestrino GA, Igoa MVB, Jesus TM, França TT, Moreira DVS, et al. The dermatophyte trichophyton rubrum induces neutrophil extracellular traps release by human neutrophils. J Fungi. (Basel) (2022) 8(2):147–55. doi: 10.3390/jof8020147
34. Rocha JD, Nascimento MT, Decote-Ricardo D, Côrte-Real S, Morrot A, Heise N, et al. Capsular polysaccharides from cryptococcus neoformans modulate production of neutrophil extracellular traps (NETs) by human neutrophils. Sci Rep (2015) 5:8008. doi: 10.1038/srep08008
35. Johnson CJ, Davis JM, Huttenlocher A, Kernien JF, Nett JE. Emerging fungal pathogen candida auris evades neutrophil attack. mBio (2018) 9(4):e01403–18. doi: 10.1128/mBio.01403-18
36. Johnson CJ, Cabezas-Olcoz J, Kernien JF, Wang SX, Beebe DJ, Huttenlocher A, et al. The extracellular matrix of candida albicans biofilms impairs formation of neutrophil extracellular traps. PloS Pathog (2016) 12(9):e1005884. doi: 10.1371/journal.ppat.1005884
37. Smolarz M, Zawrotniak M, Satala D, Rapala-Kozik M. Extracellular nucleic acids present in the candida albicans biofilm trigger the release of neutrophil extracellular traps. Front Cell Infect Microbiol (2021) 11:681030. doi: 10.3389/fcimb.2021.681030
38. Wu SY, Weng CL, Jheng MJ, Kan HW, Hsieh ST, Liu FT, et al. Candida albicans triggers NADPH oxidase-independent neutrophil extracellular traps through dectin-2. PloS Pathog (2019) 15(11):e1008096. doi: 10.1371/journal.ppat.1008096
39. Zawrotniak M, Bochenska O, Karkowska-Kuleta J, Seweryn-Ozog K, Aoki W, Ueda M, et al. Aspartic proteases and major cell wall components in candida albicans trigger the release of neutrophil extracellular traps. Front Cell Infect Microbiol (2017) 7:414. doi: 10.3389/fcimb.2017.00414
40. Zawrotniak M, Wojtalik K, Rapala-Kozik M. Farnesol, a quorum-sensing molecule of candida albicans triggers the release of neutrophil extracellular traps. Cells (2019) 8(12):1611–27. doi: 10.3390/cells8121611
41. McCormick A, Heesemann L, Wagener J, Marcos V, Hartl D, Loeffler J, et al. NETs formed by human neutrophils inhibit growth of the pathogenic mold aspergillus fumigatus. Microbes Infect (2010) 12(12-13):928–36. doi: 10.1016/j.micinf.2010.06.009
42. Gazendam RP, van Hamme JL, Tool AT, Hoogenboezem M, van den Berg JM, Prins JM, et al. Human neutrophils use different mechanisms to kill aspergillus fumigatus conidia and hyphae: Evidence from phagocyte defects. J Immunol (2016) 196(3):1272–83. doi: 10.4049/jimmunol.1501811
43. Bianchi M, Niemiec MJ, Siler U, Urban CF, Reichenbach J. Restoration of anti-aspergillus defense by neutrophil extracellular traps in human chronic granulomatous disease after gene therapy is calprotectin-dependent. J Allergy Clin Immunol (2011) 127(5):1243–1252.e1247. doi: 10.1016/j.jaci.2011.01.021
44. Kullberg. BJ, Arendrup MC. Invasive candidiasis. New Engl J Med (2015) 373(15):1445–56. doi: 10.1056/NEJMra1315399
46. Azie N, Neofytos D, Pfaller M, Meier-Kriesche HU, Quan SP, Horn D, et al. (Prospective antifungal therapy) Alliance(R) registry and invasive fungal infections: update 2012. Diagn Microbiol Infect Dis (2012) 73(4):293–300. doi: 10.1016/j.diagmicrobio.2012.06.012
48. Hoyer AR, Johnson CJ, Hoyer MR, Kernien JF, Nett JE. Echinocandin treatment of candida albicans biofilms enhances neutrophil extracellular trap formation. Antimicrob Agents Chemother (2018) 62(9):e00797–18. doi: 10.1128/aac.00797-18
49. Shankar M, Lo TL, Traven A. Natural variation in clinical isolates of candida albicans modulates neutrophil responses. mSphere (2020) 5(4):e00501–20. doi: 10.1128/mSphere.00501-20
50. Kaplan MJ, Radic M. Neutrophil extracellular traps: double-edged swords of innate immunity. J Immunol (2012) 189(6):2689–95. doi: 10.4049/jimmunol.1201719
51. Kenny EF, Herzig A, Krüger R, Muth A, Mondal S, Thompson PR, et al. Diverse stimuli engage different neutrophil extracellular trap pathways. Elife (2017) 6:e24437. doi: 10.7554/eLife.24437
52. Urban CF, Ermert D, Schmid M, Abu-Abed U, Goosmann C, Nacken W, et al. Neutrophil extracellular traps contain calprotectin, a cytosolic protein complex involved in host defense against candida albicans. PloS Pathog (2009) 5(10):e1000639. doi: 10.1371/journal.ppat.1000639
53. Hopke A, Nicke N, Hidu EE, Degani G, Popolo L, Wheeler RT. Neutrophil attack triggers extracellular trap-dependent candida cell wall remodeling and altered immune recognition. PloS Pathog (2016) 12(5):e1005644. doi: 10.1371/journal.ppat.1005644
54. Latgé JP, Chamilos G. Aspergillus fumigatus and aspergillosis in 2019. Clin Microbiol Rev (2019) 33(1):e00140–18. doi: 10.1128/cmr.00140-18
55. Kosmidis C, Denning DW. The clinical spectrum of pulmonary aspergillosis. Thorax (2015) 70(3):270–7. doi: 10.1136/thoraxjnl-2014-206291
56. King J, Henriet SSV, Warris A. Aspergillosis in chronic granulomatous disease. J Fungi. (Basel) (2016) 2(2):15–30. doi: 10.3390/jof2020015
57. Silva JDC, Thompson-Souza GA, Barroso MV, Neves JS, Figueiredo RT. Neutrophil and eosinophil DNA extracellular trap formation: Lessons from pathogenic fungi. Front Microbiol (2021) 12:634043. doi: 10.3389/fmicb.2021.634043
58. Bianchi M, Hakkim A, Brinkmann V, Siler U, Seger RA, Zychlinsky A, et al. Restoration of NET formation by gene therapy in CGD controls aspergillosis. Blood (2009) 114(13):2619–22. doi: 10.1182/blood-2009-05-221606
59. Clark HL, Abbondante S, Minns MS, Greenberg EN, Sun Y, Pearlman E. Protein deiminase 4 and CR3 regulate aspergillus fumigatus and β-Glucan-Induced neutrophil extracellular trap formation, but hyphal killing is dependent only on CR3. Front Immunol (2018) 9:1182. doi: 10.3389/fimmu.2018.01182
60. Alflen A, Aranda Lopez P, Hartmann AK, Maxeiner J, Bosmann M, Sharma A, et al. Neutrophil extracellular traps impair fungal clearance in a mouse model of invasive pulmonary aspergillosis. Immunobiology (2020) 225(1):151867. doi: 10.1016/j.imbio.2019.11.002
61. Xiao M, Chen SC, Kong F, Fan X, Cheng JW, Hou X, et al. Five-year China hospital invasive fungal surveillance net (CHIF-NET) study of invasive fungal infections caused by noncandidal yeasts: species distribution and azole susceptibility. Infect Drug Resist (2018) 11:1659–67. doi: 10.2147/idr.s173805
62. McClelland EE, Bernhardt P, Casadevall A. Estimating the relative contributions of virulence factors for pathogenic microbes. Infect Immun (2006) 74(3):1500–4. doi: 10.1128/iai.74.3.1500-1504.2006
63. Cherniak R, Valafar H, Morris LC, Valafar F. Cryptococcus neoformans chemotyping by quantitative analysis of 1H nuclear magnetic resonance spectra of glucuronoxylomannans with a computer-simulated artificial neural network. Clin Diagn Lab Immunol (1998) 5(2):146–59. doi: 10.1128/cdli.5.2.146-159.1998
64. Heiss C, Klutts JS, Wang Z, Doering TL, Azadi P. The structure of cryptococcus neoformans galactoxylomannan contains beta-d-glucuronic acid. Carbohydr. Res (2009) 344(7):915–20. doi: 10.1016/j.carres.2009.03.003
65. Lee MJ, Liu H, Barker BM, Snarr BD, Gravelat FN, Al Abdallah Q, et al. The fungal exopolysaccharide galactosaminogalactan mediates virulence by enhancing resistance to neutrophil extracellular traps. PloS Pathog (2015) 11(10):e1005187. doi: 10.1371/journal.ppat.1005187
66. Voltersen V, Blango MG, Herrmann S, Schmidt F, Heinekamp T, Strassburger M, et al. Proteome analysis reveals the conidial surface protein CcpA essential for virulence of the pathogenic fungus aspergillus fumigatus. mBio (2018) 9(5):e01557–18. doi: 10.1128/mBio.01557-18
67. Kernien JF, Johnson CJ, Nett JE. Conserved inhibition of neutrophil extracellular trap release by clinical candida albicans biofilms. J Fungi. (Basel) (2017) 3(3):49–59. doi: 10.3390/jof3030049
68. Karkowska-Kuleta J, Smolarz M, Seweryn-Ozog K, Satala D, Zawrotniak M, Wronowska E, et al. Proteinous components of neutrophil extracellular traps are arrested by the cell wall proteins of candida albicans during fungal infection, and can be used in the host invasion. Cells (2021) 10(10):2736–63. doi: 10.3390/cells10102736
69. Afonso M, Mestre AR, Silva G, Almeida AC, Cunha RA, Meyer-Fernandes JR, et al. Candida extracellular nucleotide metabolism promotes neutrophils extracellular traps escape. Front Cell Infect Microbiol (2021) 11:678568. doi: 10.3389/fcimb.2021.678568
70. Zhang X, Zhao S, Sun L, Li W, Wei Q, Ashman RB, et al. Different virulence of candida albicans is attributed to the ability of escape from neutrophil extracellular traps by secretion of DNase. Am J Transl Res (2017) 9(1):50–62.
71. Jorch SK, Kubes P. An emerging role for neutrophil extracellular traps in noninfectious disease. Nat Med (2017) 23(3):279–87. doi: 10.1038/nm.4294
72. Chapman EA, Lyon M, Simpson D, Mason D, Beynon RJ, Moots RJ, et al. Caught in a trap? proteomic analysis of neutrophil extracellular traps in rheumatoid arthritis and systemic lupus erythematosus. Front Immunol (2019) 10:423. doi: 10.3389/fimmu.2019.00423
73. An Z, Li J, Yu J, Wang X, Gao H, Zhang W, et al. Neutrophil extracellular traps induced by IL-8 aggravate atherosclerosis via activation NF-κB signaling in macrophages. Cell Cycle (2019) 18(21):2928–38. doi: 10.1080/15384101.2019.1662678
74. Berezin A. Neutrophil extracellular traps: The core player in vascular complications of diabetes mellitus. Diabetes Metab Syndr (2019) 13(5):3017–23. doi: 10.1016/j.dsx.2018.07.010
75. Radermecker C, Sabatel C, Vanwinge C, Ruscitti C, Maréchal P, Perin F, et al. Locally instructed CXCR4(hi) neutrophils trigger environment-driven allergic asthma through the release of neutrophil extracellular traps. Nat Immunol (2019) 20(11):1444–55. doi: 10.1038/s41590-019-0496-9
76. Heuer A, Stiel C, Elrod J, Königs I, Vincent D, Schlegel P, et al. Therapeutic targeting of neutrophil extracellular traps improves primary and secondary intention wound healing in mice. Front Immunol (2021) 12:614347. doi: 10.3389/fimmu.2021.614347
Keywords: neutrophil, neutrophil extracellular traps, fungal infection, immunology, Candida, Aspergillus
Citation: Zhong H, Lu R-Y and Wang Y (2022) Neutrophil extracellular traps in fungal infections: A seesaw battle in hosts. Front. Immunol. 13:977493. doi: 10.3389/fimmu.2022.977493
Received: 24 June 2022; Accepted: 24 August 2022;
Published: 14 September 2022.
Edited by:
Wenjie Fang, Shanghai Changzheng Hospital, ChinaReviewed by:
Kerstin Voigt, Friedrich Schiller University Jena, GermanyLei Zhang, Shaanxi Provincial People’s Hospital, China
Copyright © 2022 Zhong, Lu and Wang. This is an open-access article distributed under the terms of the Creative Commons Attribution License (CC BY). The use, distribution or reproduction in other forums is permitted, provided the original author(s) and the copyright owner(s) are credited and that the original publication in this journal is cited, in accordance with accepted academic practice. No use, distribution or reproduction is permitted which does not comply with these terms.
*Correspondence: Yan Wang, d2FuZ3lhbnNtbXVAMTI2LmNvbQ==