- 1Department of Clinical and Toxicological Analyses, School of Pharmaceutical Sciences, University of São Paulo, SP, São Paulo, Brazil
- 2Núcleo de Pesquisa em Ciências Médicas, Fundação Universidade para o Desenvolvimento do Alto Vale do Itajaí – UNIDAVI, Rio do Sul, SC, Brazil
- 3Center for Stem Cells and Regenerative Medicine, King’s College London, London, United Kingdom
- 4Department of Morphology and Genetics, Federal University of São Paulo, São Paulo, SP, Brazil
Maternal neutrophils cells are players in gestational tolerance and fetus delivery. Nonetheless, their actions in each phase of the pregnancy are unknown. We here investigated the role of maternal neutrophil depletion before the blastocyst implantation phase and outcomes in the pregnancy index, placenta, and fetus development. Neutrophils were pharmacologically depleted by i.p. injection of anti-Gr1 (anti-neutrophils; 200 µg) 24 hours after plug visualization in allogeneic-mated C57BL/6/BALB/c mice. Depletion of peripheral neutrophils lasted until 48 hours after anti-Gr1 injection (gestational day 1.5-3.5). On gestational day 5.5, neutrophil depletion impaired the blastocyst implantation, as 50% of pregnant mice presented reduced implantation sites. On gestational day 18.5, neutrophil depletion reduced the pregnancy rate and index, altered the placenta disposition in the uterine horns, and modified the structure of the placenta, detected by reduced junctional zone, associated with decreased numbers of giant trophoblast cells, spongiotrophoblast. Reduced number of placenta cells labeled for vascular endothelial growth factor (VEGF), platelet-endothelial cell adhesion molecule (PECAM-1), and intercellular cell adhesion molecule (ICAM-1), important markers of angiogenesis and adhesiveness, were detected in neutrophil depleted mice. Furthermore, neutrophil depletion promoted a higher frequency of monocytes, natural killers, and T regulatory cells, and lower frequency of cytotoxic T cells in the blood, and abnormal development of offspring. Associated data obtained herein highlight the pivotal role of neutrophils actions in the early stages of pregnancy, and address further investigations on the imbricating signaling evoked by neutrophils in the trophoblastic interaction with uterine epithelium.
Introduction
Mammalian reproduction is a complex phenomenon, which generally leads to inefficient outcomes. The majority of conceptions do not evolve, especially by losses before the blastocyst implantation (1, 2), which takes place in a very short period of gestation named window of implantation (WOI). During WOI occurs the synchronization of embryonic development and uterine differentiation into a receptive status (3). WOI is characterized by pre-receptive and receptive periods followed by refractory no receptive phases. In humans and rodents, implantation of blastocysts begins with apposition, followed by attachment to trophoblast outgrowth and decidualization, which are spatiotemporally regulated by many players, such as endocrine/growth factors/immune mediators of cell-cell and cell-matrix interactions (3, 4). Disruptions to the implantation process are also considered causes of pregnancy-associated complications, such as preeclampsia, intrauterine growth restriction, and preterm birth (5, 6).
The maternal immune response in the endometrium is pivotal to the establishment of WOI. The fetal antigen contact with the maternal endometrium modifies the local repertoire of immune cells, which display a fundamental role in trophoblast invasion, vascular remodeling by extravillous trophoblast, embryo invasion, and maternal tolerance (7, 8). The innate immune response in the blastocyst implantation period is not characterized as classical inflammation, as it occurs to enhance the adhesiveness of the uterine epithelium and the formation of new vessels for the blood supply. Therefore, innate-mediated inflammation must be local and adjustable to the process, as both impaired and exacerbated innate response leads to failure in the blastocyst-endometrium interactions (9).
Neutrophils are the predominant circulating innate immune cells in humans prompted to migrate into the site of inflammation to eliminate insulting agents. More recently, the role of neutrophils in host defense and homeostasis has been outspread by identifying neutrophil phenotypes, which display pro or anti-inflammatory properties and influence the resolution of inflammation and tissue repair (10). Neutrophil switching to distinct subtypes is dependent on the phase of the inflammatory process and modulated by the chemical and cellular composition of the surrounding inflammatory microenvironment (11).
In this context, it has been highlighted that neutrophils acquire different phenotypes during pregnancy, with fundamental roles in each phase. Studies describing neutrophils phenotype in the placental microenvironment are scarce. However, all studies corroborate that neutrophils display a proangiogenic phenotype. In the first trimester, neutrophils are found in the human decidua, in an area mainly involved in tissue remodeling and physiological decidual implant reaction, displaying high resistance to apoptosis, do not exerting a suppressive activity, and expressing fibro/angiogenic factors (12) In the second trimester, the number of neutrophils at decidua basalis is increased and high levels of activation markers and angiogenesis-related proteins, such as vascular endothelial growth factor-A (VEGF) are detected (13). In pregnant mice, the augmented neutrophils numbers were observed on gestational days 8 – 10, which coincides with the period of spiral arterial angiogenesis (13).
Several chemical mediators in the uterine microenvironment modulate neutrophil recruitment and functional phenotypes in normal pregnancy (14–16). Studies have demonstrated that placental cell debris and IL-8 are involved in the neutrophil migration to decidua (17). During coitus, inflammatory neutrophils are recruited for the elimination of excessive sperm through phagocytosis; nevertheless, in the following phases, they are inhibited to allow the healthy motile sperm to reach the oviduct (18). Moreover, neutrophils are found in the uterine stroma at the early phase of WOI and proangiogenic neutrophils were identified in the second‐trimester decidua of normal pregnancy, displaying the ability to perform transendothelial invasion and induce endothelial tube formation in vitro in comparison to resting circulating neutrophil (13, 15). During maternal tolerance mediated by T cells, mice neutrophils in the pregnant uterus acquire an anti-inflammatory phenotype, undergo apoptosis, and release intracellular granules into T cells, favoring the Treg maternal tolerance, and placenta angiogenesis; the frequency of anti-inflammatory neutrophils is reduced in the blood of pre-eclampsia mothers (19). During childbirth, local neutrophils are classical pro-inflammatory cells, which contribute to fetus expulsion (20). Moreover, it is evident that pro-inflammatory activated neutrophils are involved in the pre-eclampsia pathogenesis, and a higher neutrophil-lymphocyte ratio is related to preterm labor (7, 18, 19, 21).
Some studies using antibody-mediated circulating neutrophil depletion approaches have highlighted the role of these cells in the placenta and fetus development. In this context, Higashisaka and collaborators showed that neutrophil depletion in mice on gestational day 15 just exacerbated the pregnancy complications induced by the silica nanoparticles, being that neutrophil depletion per se was not able to impair the pregnancy outcomes (22). On the other hand, Nadkarni and collaborators showed that neutrophil depletion on gestational days 5 and 8 promoted abnormal placentation and fetus alterations (19). These studies suggest that the effects of neutrophil depletion can be associated with the earlier stages of placentation. Indeed, we show that circulating neutrophils are required for efficient placentation and fetal development.
Material and methods
Animals and allogenic pregnancy
Mice were maintained and reproduced at Animal House at the School of Pharmaceutical Sciences, University of Sao Paulo (Brazil) with chow and water ad libitum in a temperature-controlled room (22–25°C and 70% humidity) with a 12-hr light-dark cycle. Allogeneic pregnancy was performed by mating male Balb/C with female C57Bl/6 mice (both 5–6 weeks old). Females were caged overnight with males (3:1), and successful mating was verified the following morning by the presence of a vaginal plug. All procedures were performed according to the Brazilian Society for the Science of Laboratory Animals (SBCAL) and approved by the Institutional Animal Care and Use Committee from the Faculty of Pharmaceutical Sciences of the University of Sao Paulo (Protocol number 557).
Peripheral maternal neutrophil depletion
Neutrophil depletion was achieved by intraperitoneal injection of 200 µg anti-Gr1 antibody (clone RB6-8C5; e-Bioscience/Invitrogen, Waltham, Massachusetts, USA), isotype-matched control antibody (clone eB149/10H5; Rt IgG2b k; e-Bioscience/Invitrogen), or phosphate-buffered saline (Control) into C57Bl/6 pregnant at 24 h after vaginal plug visualization (reported as gestational day 0.5). Maternal blood was collected from the abdominal aorta and used to perform leukogram and flow cytometry.
Peripheral leukocyte profile and immunophenotyping by flow cytometry
The effectiveness of maternal peripheral neutrophil depletion and peripheral leukocyte profile was evaluated by total and differential leukogram, and flow cytometry. For the evaluation of maternal peripheral neutrophil depletion, peripheral blood was collected by aortic puncture in tubes containing heparin on gestational day 3.5. The leukogram was performed under optical microscopy by manual counting in the Neubauer chamber using Turk’s solution. The morphological evaluation was performed using Panotico staining smears (peripheral blood mononuclear cells - MNs and peripheral polymorphonuclear cells - PMNs).
Flow cytometry was employed to confirm the efficacy of anti-Gr1 injection using peripheral blood collected by aortic puncture in tubes containing heparin. Leukocyte population, PMNs and MNs were isolated using forward versus side scatter channels.
Peripheral leukocyte profile was investigated by flow cytometry. Leukocytes were obtained from abdominal aorta on gestational day 18.5. Whole blood was lysed with an ammonium lysis buffer. Isolated leukocytes were incubated with PE-anti-mouse CD3; FITC anti-mouse CD4, APC anti-mouse CD8, APC anti-mouse FoxP3, PE anti-mouse B220; FITC anti-mouse F4/80; PerCP Cy5 anti-mouse-Gr1, and FITC anti-NK1.1 (BD-Bioscience or Invitrogen) for 50 minutes at room temperature and in the dark. In sequence, the labeling cells were acquired in an Accuri C6 flow cytometer (BD Biosciences), and 10,000 events were considered for analysis. The data were expressed as the frequency of positive cells.
Cesarean section procedure
C-sections were performed under aseptic conditions on gestational days 5.5 to count the number of implantation sites and on 18.5 to investigate the reproductive and pregnancy outcomes and to collect the fetus and placentas for posterior analysis. C-sections were performed after mice were anesthetized with xylazine and ketamine (7 mg/kg and 77 mg/kg, respectively; i.p; Vetbrands, Jacarei, SP, Brazil). In addition, on gestational day 18.5, maternal blood was collected from the abdominal aorta and used to identify the leukocyte profile by flow cytometry.
Reproductive and pregnancy outcomes calculation
On gestational day 5.5, the uterus was surgically removed for implantation points count. The pregnancy index and outcomes were performed as previously described (23, 24). Briefly, the successful pregnancy index was calculated considering the ratio of mice that presented vaginal plugs and the presence of live fetuses at C-section performed on gestational day 18.5. Also, the uterus and ovary were removed followed by the count of fetuses and resorption points. The uterus, placenta, and fetus were also weighted, and a placenta index was calculated by considering the ratio between the weight of the fetus and the weight of their respective placentas.
Histological procedures and analyses
Placentas were fixed in 4% buffered paraformaldehyde and histologically processed for inclusion in paraffin. Sections of 4 μm were stained according to analyses performed. The photomicrographs were obtained by optical microscopy using high-power objectives on the Imager.A2 Zeiss microscope (Zeiss, Oberkochen, Germany). The quantification of all the parameters cited below was carried out using ImageJ software by analyzing 5 fields of each section.
Placenta morphometric analysis
Placenta morphometry (total size, decidua, junctional zone, and labyrinth area) was measured in hematoxylin and eosin-stained (H&E) sections using the Axiovision software (Zeiss, Oberkochen, Germany). Periodic Acid Schiff (PAS) staining was performed using a commercial kit and following the manufacturer’s instructions (EasyPath; São Paulo, Brazil) to count the glycogen-positive cells. Also, the giant trophoblast cells and spongiotrophoblast were counted based on morphologic characteristics. The cell count was performed using the Cell counter tool from Image J software (National Institutes of Health, Bethesda, USA). Five fields were analyzed in the junctional zone from each placenta section by two independent observers.
Immunohistochemistry
After placenta section permeabilization (0.01% Triton X; Sigma) (Sigma-Aldrich, Burlington, USA) and antigen retrieval (sodium citrate buffer, 10 mM, pH 6.0, 30 minutes in 96°C), endogenous peroxidase was blocked with three rounds of 3% hydrogen peroxide. Slides were incubated with blocking buffer (5% Tris-buffered saline–bovine serum albumin; 1 h) and incubated overnight with purified rabbit anti-VEGF (1:200; Invitrogen) goat anti-ICAM, or anti-PECAM antibodies (both 1:25; Santa Cruz Biotechnology, Dallas, USA). Thus, the slides were washed with tris-buffered saline three times and then incubated with anti-rabbit (1:250; Abcam, Cambridge, UK) or anti-goat (1:100; R&D System, Minneapolis, USA) horseradish peroxidase (HRP) antibody, followed by 3,3-diaminobenzidine (DAB; DAKO, Glostrup, DEN), and hematoxylin counterstaining. As negative control of reactions, slides were incubated only with anti-rabbit or anti-goat HRP antibodies.
Statistical analysis
Data were analyzed using Student’s t-test or one-factor analysis of variance (One-Way ANOVA) followed by Tukey post-hoc test using GraphPad software version 7. Differences between assessed means were considered statistically significant assuming p < 0.05. All results are shown as mean ± standard error of the mean. When needed, nominal data were statistically evaluated using the Fischer Exact Test and p < 0.05 was considered statistically significant.
Results
Neutrophils depletion before the implantation phase reduced the pregnancy index
Neutrophil depletion at the pre-receptive phase of implantation, was carried out by i.p. of anti-Gr1 24 hours after vaginal plug visualization (Figure 1A). In this experimental approach, a decrease in the circulating neutrophils number was observed up to 48 hours after anti-Gr1 i.p injection, while the mononuclear cells population was not affected (Figures 1B and b’). The depletion of circulating neutrophils abrogated implantation points in 50% of the pregnant mice, as observed by laparotomy performed on gestational day 5.5. Differently, all control animals (100%) presented around ten points of implantation. It is important to mention that the remaining 50% of mice treated with anti-Gr1, presented around eight and just one had almost twenty points of implantation (Figures 1C, D). On gestational day 18.5, pregnant mice were submitted for cesarean section and reproductive parameters were analyzed. Herein, it was observed that the number of resorption sites in neutrophil-depleted mice was similar to those found in the control/isotype groups (Figure 1E) Ilustrative image of the absence of resorption sites is exhibited in the Figure 1F - f'. Only one anti-Gr1 animal presented higher resorption sites as shown in Figure 1 - f''. Nonetheless, the pregnancy failure rate was around 60% in the neutrophil-depleted group. On the other hand, the control/isotype group showed a rate of pregnancy success of around 86% (Supplementary Table 1). Furthermore, in the neutrophil-depleted mice that had a successful pregnancy, the number of the fetuses was similar to the control group (Figure 1G), and the weight gain of pregnant mice and the weight of the pregnancy uterus were not altered (Supplementary Figures 1A, B). The gross analysis of the uterus showed that neutrophil depletion altered the placenta’s disposition inside of uterine horns. As shown in Figure 1H, the control/isotype mice showed the same number of placenta on both uterine horns (four fetuses on each horn - left/right). On the other hand, neutrophil-depleted mice presented three and five placentas on the right and left horns, respectively, showing no equidistant pattern as observed for the control/isotype group. Moreover, the placental weight and index were not changed (Supplementary Figures 1C, D).
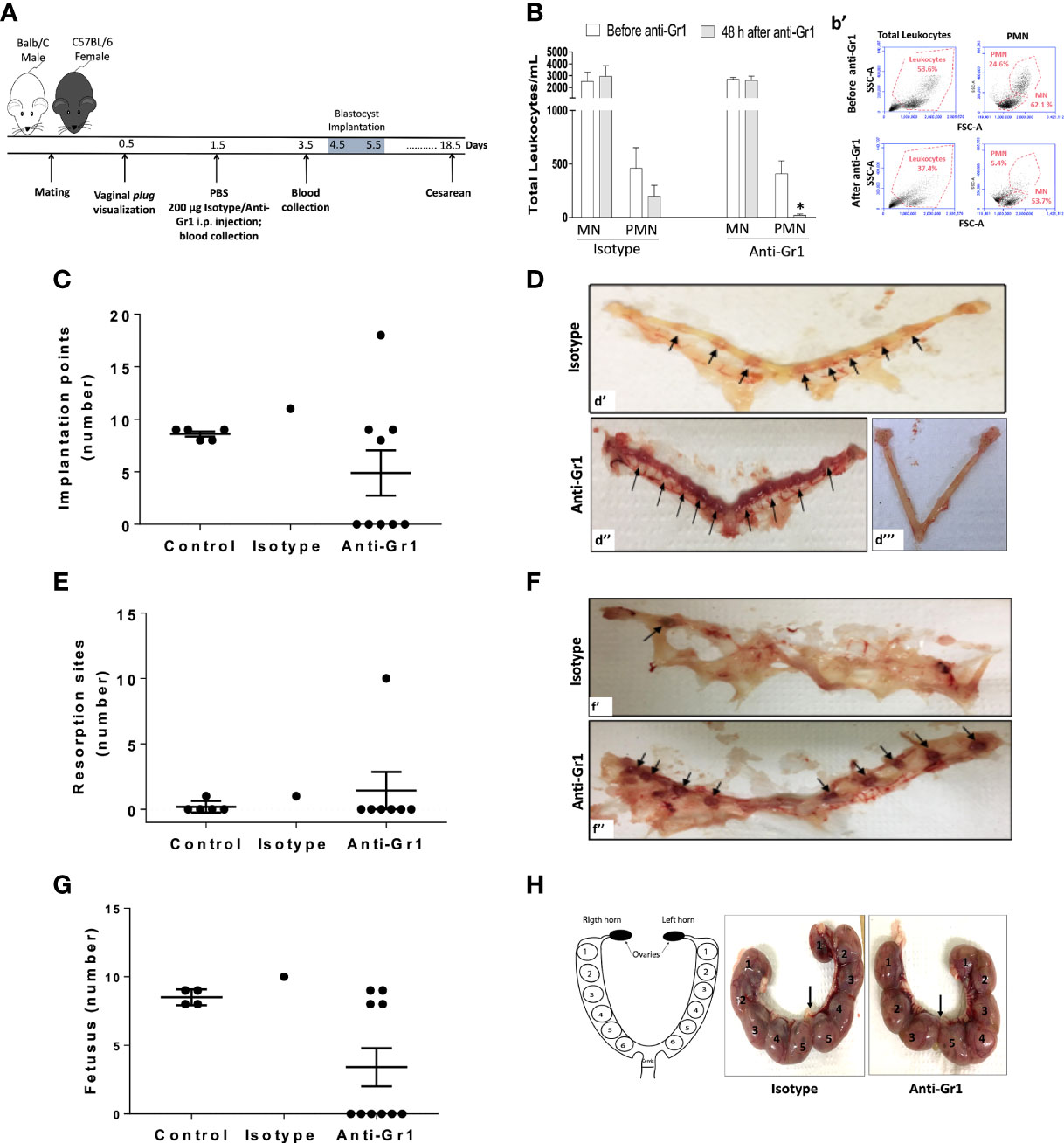
Figure 1 Neutrophil depletion before the implantation phase of pregnancy reduces pregnancy index and reproductive outcomes. Anti-Gr1/isotype injection and the subsequent endpoints were illustrated in the experimental workflow (A). The total number of mononuclear cells (MN) and polymorphonuclear (PMN) was monitored before and after 48h of anti-Gr1/isotype i.p. injection by manual count (B) and flow cytometry (b’), respectively. On gestational day 5.5, mice were euthanized for implantation points count (C). Representative images of implantation points (D - arrows) in the isotype (d’), and in the Anti-Gr1 (d’’) groups are shown. The lack of implantation points observed in anti-Gr1 treated mice is also depicted (d’’’). On gestational day 18.5, pregnant mice were submitted for cesarean and the number of resorption points (E), fetuses (G), and disposition of fetuses in the uterine horns (H) was analyzed. Representative images of resorption sites (F) in the isotype (f’) and Anti-Gr1 (f’’) are shown. Schematic diagram, isotype, and Anti-Gr1 placenta distribution into the uterine horns are demonstrated (H). Each number indicates a fetus inside of the placenta. Arrow indicates cervix location. Data were statistically analyzed using One-Way ANOVA followed by Tukey’s post-test (n= 5 – 9 animals/group) and p < 0.05 was considered statistically significant. * p < 0.05 versus respective control before Anti-Gr1 injection (B). No significant difference was observed (C, E, G).
Neutrophils depletion impaired the development of the junctional zone
Macroscopic analysis of the placenta from neutrophil-depleted mice showed a reduced size and altered macrostructure compared to the control/isotype group (Figures 2A–C). Thus, morphometric analyses were performed. Placenta sections stained with H&E were used to measure the total area and the three main placental layers: the labyrinth (Lb), the junctional zone (JZ), and the maternal decidua (Dc) (Figures 2D–F). As shown in Figure 2G, the neutrophil-depleted mice showed a reduced total area in comparison to control/isotype groups. The labyrinth and decidua areas were not altered by neutrophil depletion (Figures 2H, I). However, it was verified that anti-Gr1 treatment decreased the junctional zone area (Figure 2J). Considering the role of the junctional zone as an endocrine compartment and potential energy source (25, 26), we evaluated whether the number of cell types that comprise this layer (glycogen trophoblast cells - GlyTCs; spongiotrophoblast - SpT; and trophoblast giant cells - TGCs) was altered. As shown in Figures 2K–M, at the junctional zone, it was verified the presence of GlyTC (arrow) exhibiting vacuoles containing particles of glycogen (PAS positive); TGCs (*) characterized by the largest cytoplasm and located at the boundary between JZ and Dc zones; and SpTs (arrowhead) identified as clusters of tightly packed polygonal cells that comprise the middle layer of the placenta sandwiched between the outer secondary TGCs and the Lb layer. Further, we observed that the number of GlyTC was not significantly altered by neutrophil depletion (Figure 2N). However, we verified that the counting of TGCs and SpT was decreased in neutrophil-depleted mice (Figures 2O, P).
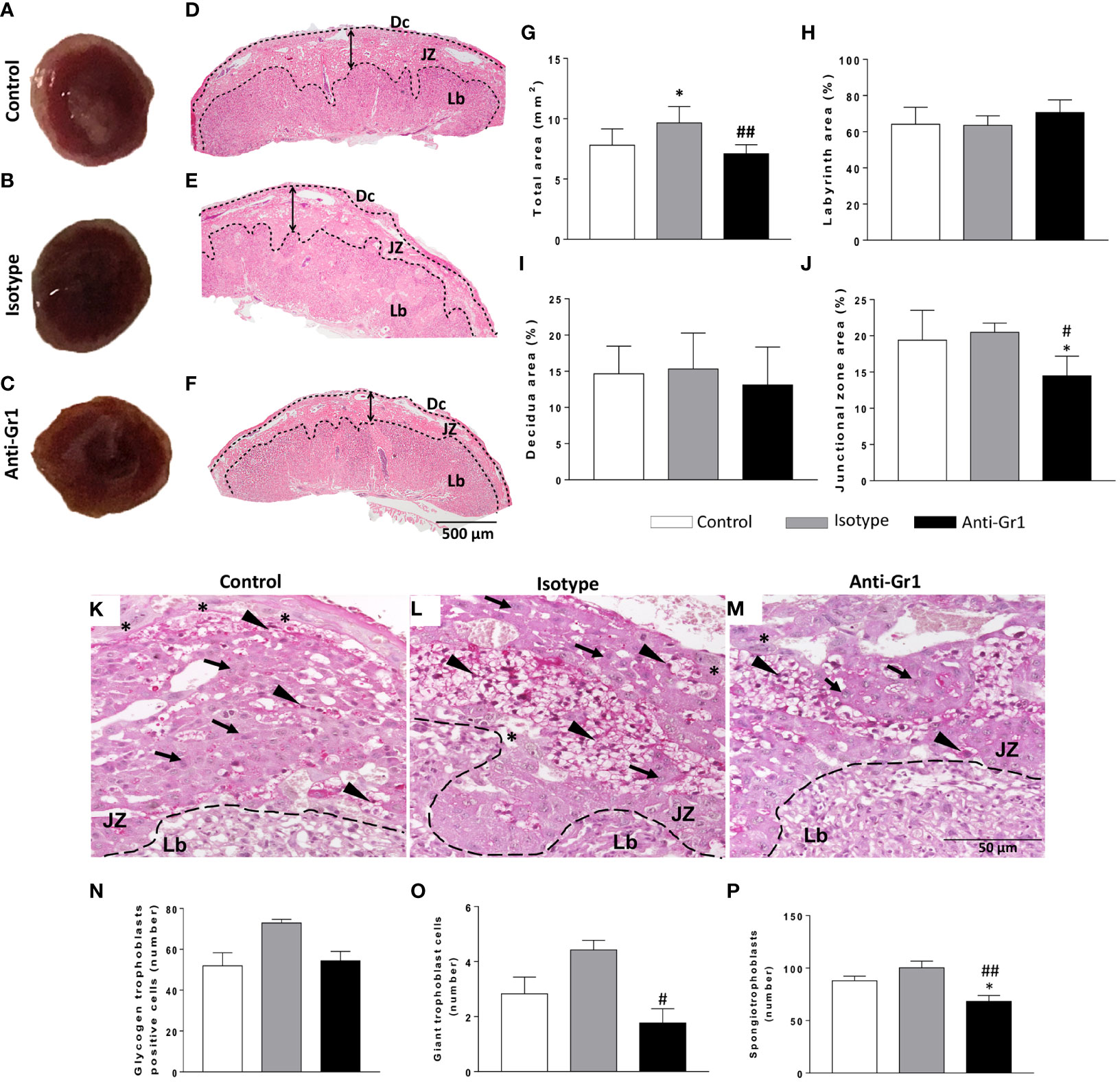
Figure 2 Neutrophil depletion altered placenta morphology and reduced the number of specialized cells in the junctional zone. On gestational day 18.5, placentas were removed for gross analyses (A-C) and then submitted for histology evaluation with H&E staining (D-F). Morphometrical analysis was employed to measure the total area (G), the Lb- labyrinth (H), Dc - decidua (I), and JZ - junctional zone (J) using PAS staining. The count of the types of cells in the junctional zone and the presence of glycogen trophoblast-positive cells was performed by analyzing five fields (M). The trophoblast subtypes were quantified and indicated in the sections as glycogen-trophoblast cells (N), giant trophoblast cells (O), and spongiotrophoblast (P). * < 0.05 in comparison to control group; #p < 0.05; ##p < 0.01 in comparison to isotype control. Data were statistically analyzed using One-Way ANOVA followed by Tukey’s post-test (n = 6 – 9). Results were expressed as mean ± SEM. Arrowheads in PAS staining (K-M) indicate spongiotrophoblast (SpTs); arrows indicate glycogen trophoblasts (GlyTCs), and asterisks indicate giant trophoblast cells (TGCs). Embedding paraffin; sections thickness 4 µm. Bars 50 µm.
The angiogenesis and adhesiveness markers in the junctional zone were altered by neutrophils depletion
Since VEGF, PECAM-1, and ICAM-1 are important for angiogenesis and placentation, their expression was assessed in the junctional zone by immunohistochemistry. We verified that VEGF protein was expressed by TGCs and SpTs (Figures 3A–C). However, the neutrophil depletion reduced the number of VEGF-positive cells when compared to control/isotype groups (Figure 3L). The expression of PECAM- 1 (Figures 3D–F) and ICAM-1 (Figures 3G–I) was located especially in cells next to maternal vessels, being this pattern was observed for all studied groups (Figures 3J, K show the negative control). Nonetheless, the number of positive cells for the adhesion markers decreased in the neutrophil-depleted compared to the control/isotype groups (Figures 3M, N).
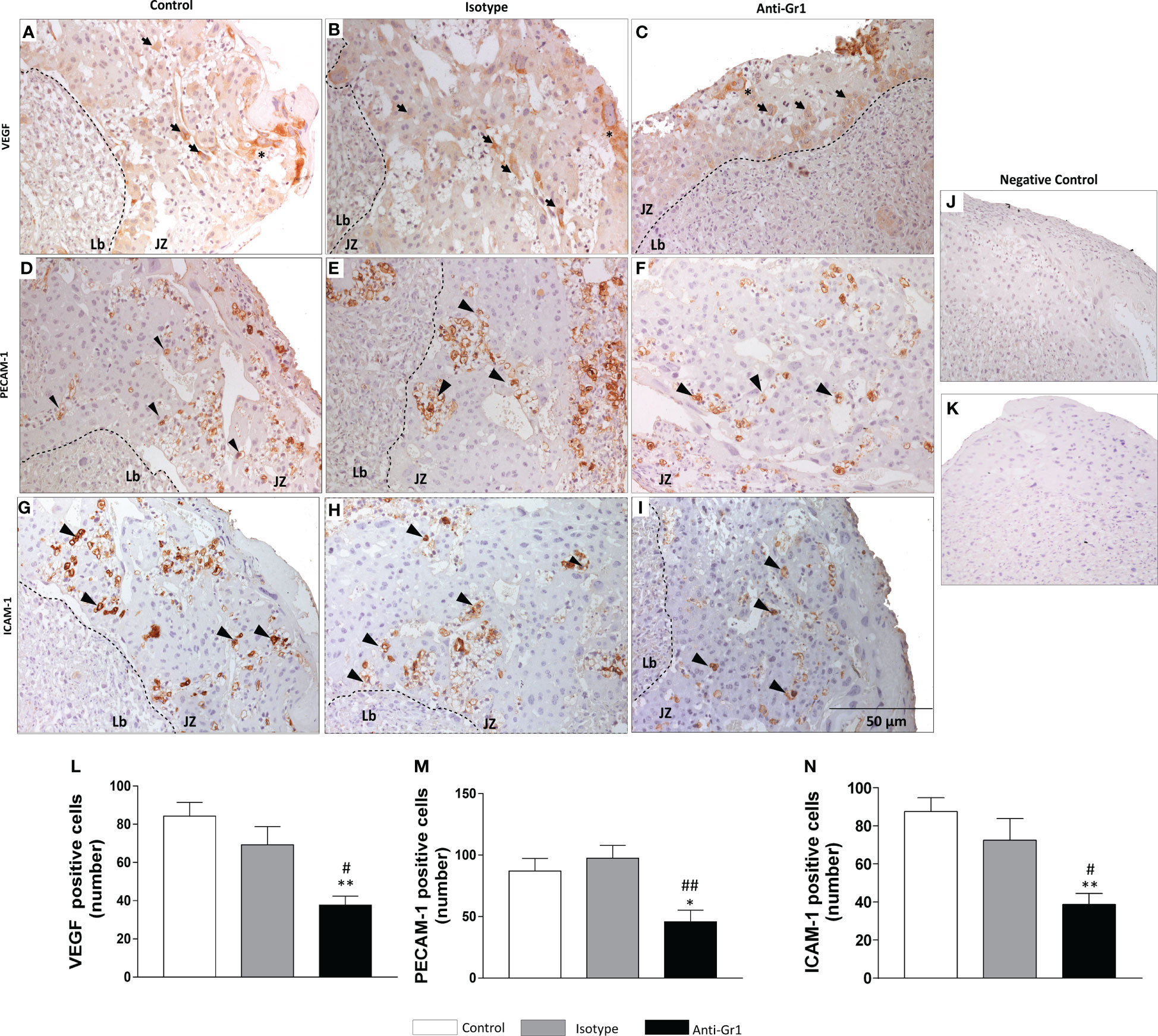
Figure 3 Neutrophil depletion altered angiogenesis and adhesiveness markers in the placenta. Expression and distribution of VEGF (A-C), PECAM-1 (D-F), and ICAM-1 (G-I) in the junctional zone of placenta were evaluated by immunohistochemistry. Negative control sections labeled with anti-rabbit (J) and anti-goat (K) HRP-conjugated antibodies. Count of VEGF (L), PECAM-1 (M) and ICAM-1 (N) positive cells. *, **p < 0.05; 0.01 in comparison to control group; #, ##p <0.05, 0.01 in comparison to isotype. Data were statistically analyzed using One-Way ANOVA followed by Tukey’s post-test (n= 4 – 6 placentas). Results were expressed as mean ± SEM. Immunoreactive cells for adhesiveness markers are indicated as arrowheads. Asterisks - giant trophoblast cells; TGCs. Arrows - spongiotrophoblast – SpTs. Counterstaining with hematoxylin; embedding paraffin; sections thickness 4 µm. Bars 50 µm.
Neutrophils depletion modified the circulating leukocytes profile and promoted morphologic alterations in the offspring
The analysis of circulating leukocytes performed at the end of pregnancy showed the frequency of monocytes (F4/80+) and natural killers’ cells (NK.1+), which were higher in neutrophil-depleted mice in comparison to control/isotype groups. The frequency of neutrophils (Ly6G+) and B lymphocytes (B220+) was similar for both groups (Figure 4A). Furthermore, the circulating CD4+ and CD8+ cells were reduced in the neutrophil-depleted group (Figure 4B). However, in the CD4+ population, the frequency of T regulatory cells (FoxP3+) was increased in the neutrophil-depleted group (Figure 4C).
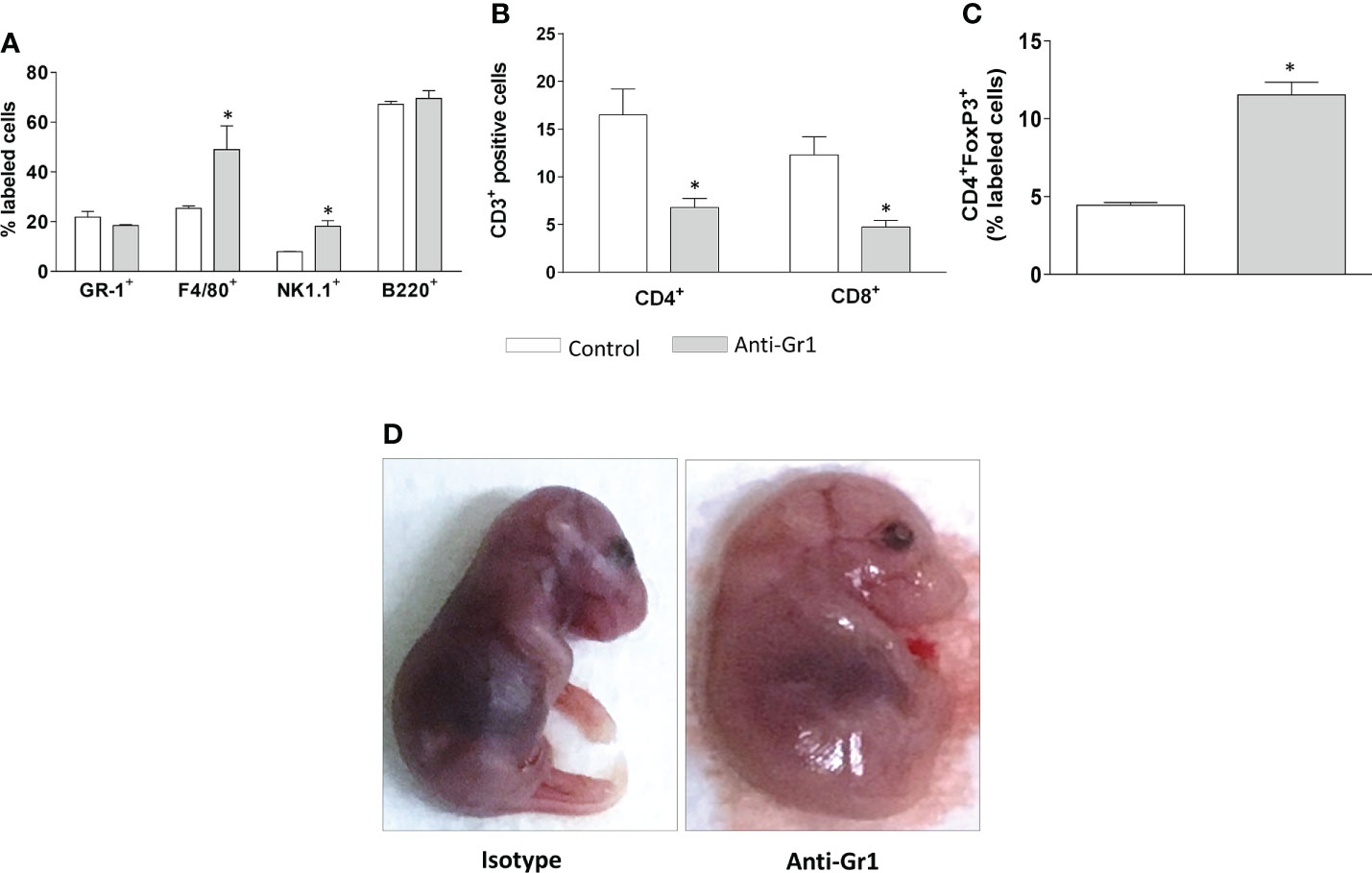
Figure 4 Neutrophil depletion altered the peripheral leukocyte profile and offspring development. On gestational day 18.5, peripheral leukocytes from mice were collected for immunophenotyping of granulocytes (Gr-1), monocytes (F4/80), natural killers cells (NK1.1), and mature B cells (B220) (A). T lymphocyte subtypes were divided into CD3+ CD4/CD8 positive cells (B) and T regulatory cells, characterized as CD3+CD4+FoxP3+ positive cells (C). The fetuses were carefully removed for gross analyses (D). *p < 0.05 in comparison to the control group. Data were statistically analyzed using One-Way ANOVA followed by Tukey’s post-test (n= 3 – 4 animals/group). Results were expressed as mean ± SEM.
Considering that neutrophil depletion affected the placenta structure, we performed a gross evaluation of the offspring. Indeed, neutrophil depletion promoted significant abnormalities, as offspring presented an overall swollen appearance, indicative of generalized edema, and the limbs remained attached to the body (Figure 4D). It was also observed that the alterations displayed a pattern among the fetuses (Supplementary Figure 2A). The fetal weight was not changed (Supplementary Figure 2B). Altogether the gross analyses may indicate that neutrophil depletion evokes delayed fetal development.
Discussion
Pre-implantation embryos are highly sensitive to environmental conditions during the early development process (27), which includes embryonic epigenome remodeling, and the first cell differentiation. In this phase, blastocyst differentiates into inner cell mass which gives rise to the embryo, and trophectoderm will form the placenta (28).
It is well known that the implantation site resembles an inflammatory response, with a profusion of recruited immune cells (29) into the endometrial stroma and lumen from the blood (30–32). Innate immune cells are the significant population of leukocytes in the uterus at the time of blastocyst implantation. Uterine NK (uNK) cells are the most abundant population, although DCs, macrophages, neutrophils, and mast cells are also present (33). Even though the role of immune cells in pregnancy has been extensively investigated, many questions remain open. Thus, approaches such as immune cell depletion have been applied to better understand the role of the immune system at different phases of pregnancy. In this context, it has been shown the depletion of T regulatory cells in the early phase of pregnancy causes increased fetal resorption, maternal systemic inflammation, enhanced uterine artery resistance to flow and increased nitric oxide modulation of blood pressure (34). Furthermore, macrophages depletion evokes blastocyst implantation failure due to disruption of the luteal microvascular network associated with the decreased production of progesterone, which is essential for establishing pregnancy (35). Recently, the neutrophil’s role in the placenta has been also discussed, as they address their role in the earliest phases of gestation (19, 22). Indeed, we here observed the depletion of neutrophils sustained at the beginning of WOI promoted a reduction of implantation points and compromised the placenta architecture and fetal development, reinforcing the signaling induced by neutrophils is necessary to the implantation of the blastocyst.
Implantation depends on a genetically healthy blastocyst and uterine receptivity (36). However, the immune cells’ repertoire decides whether the uterine endometrium will be receptive or refractive. An immune-competent and receptive endometrium will tolerate and support the trophoblast invasion to initiate the placentation (33). Mouse placenta is organized in the maternal decidua, junctional zone, and labyrinth. The trophoblast giant cells (TGC) and spongiotrophoblast (SpT) are found in the junctional zone and the syncytiotrophoblast in the labyrinth (37). The TGCs help the embryo invade the uterine epithelium and implant it into the endometrium (38). These cells also acquire an invasive phenotype, penetrating deeply into the endometrial stroma and contacting maternal arteries, promoting vascular remodeling, the crucial step that supports the anatomical foundations for placenta development (39). While SpT probably has a structural function and produces several layer-specific secreted factors that may prevent the growth of maternal blood vessels into the fetal placenta (40). Here, morphometric analyses showed the lack of maternal peripheral neutrophils decreased the placenta area due to junctional zone reduction, which presented a decreased TGC and SpT cells number. The reduced junctional zone observed in different experimental conditions has been associated with changing the endocrine environment in the placenta locally and with systemic effects on both fetus and mother (41–43). Also, the control of invasion of trophoblast cells depends on their interaction with immune cells (44, 45). Herein, the neutrophils depletion at the beginning of pregnancy seems to alter the invasion of trophoblast, and consequently impairs the proper placenta development.
Besides morphometric analyses, angiogenic and adhesiveness markers were assessed in the junctional zone. The VEGF-induced signaling controls the angiogenesis-vascularization during placentation. It is expressed in arteriolar smooth muscle, endothelium, trophoblast, uNKs, and decidual cells (46–49). The VEGF expression in the placenta microenvironment has been associated with the modulation of the TGC development and differentiation, and consequent maintenance of the homeostasis of the maternal vascular space in the mouse placenta (50). Additionally, placental VEGF has related to the modulation of metalloproteinase 2 and 9, which play a role in the endometrial tissue remodeling at implantation (51), decidualization (52), and trophoblast invasiveness (53, 54). The imbalance in the VEGF expression is associated with the abnormal placenta and its pathologies such as pre-eclampsia (55). Furthermore, it has been demonstrated that neutrophils at decidua basalis adopt a unique phenotype, expressing high levels of activation markers and angiogenesis-related proteins such as VEGF (13). Although immunohistochemical staining is not the most accurate for quantitative determination, here, we found a reduced number of VEGF-positive trophoblast cells associated with neutrophil depletion during the pre-receptive phase of embryo implantation.
Homotypic and heterotypic cell adhesions are pivotal to blastocyst implantation, and deficiencies in cell-cell interactions impair this process. Immunoglobulins cell adhesions, such as ICAM-1 and PECAM-1, display pivotal roles in immune response and tissue structure, as they are adhesion membrane proteins that cross-link with actin filaments. Also, they are relevant players in intracellular signaling during cell adhesions (56, 57). In pregnancy, ICAM-1 expression on the apical membrane of uterine epithelial cells and on the inner cells of trophoblast formation is enhanced at the time of implantation, which seems to be induced by progesterone secretion (58, 59). Furthermore, ICAM-1 is involved in maternal tolerance maintenance by controlling the secretion of Th2 cytokines (60). By being expressed on endothelial cells, PECAM-1 is highly involved in the angiogenesis process and its up-regulation on the placenta is expected, as novel vessels are required to irrigate the growing fetus. Several pieces of evidence support the role of PECAM-1 on pregnancy failures, as in preeclampsia, cytotrophoblasts fail to express PECAM-1 (54, 61). Mothers down expressing PECAM-1 suffer recurrent implantation failures, in a mechanism depending on the secretion of transforming growth factor β1 induced by PECAM-1 (62), and the impairment of PECAM-1 expression on blastocyst has been pointed out as an emerging marker to successfully assisted transfer embryo (63). Furthermore, PECAM-1 is detected early in the pluripotent inner cell mass of mouse blastocyst, which contains no vascular cells (64) (61), suggesting that it may exert additional roles on blastocyst implantation beyond angiogenesis. Despite the mechanisms displayed by neutrophils needing to be further evaluated, it is possible to infer that the impairment of VEGF, ICAM-1, and PECAM-1 positive cells associated with neutrophil depletion at the earlier phase of pregnancy contributes to placenta and fetus deficiencies shown here.
Many factors are involved in fetal malformation that includes specific genetic defects or congenital abnormalities in the embryo itself, intrauterine infections, and defects in placentation (39, 65). The association between placental dysfunction and fetus alterations has been explored in different contexts. It was shown that the majority of animal lethality found during genetic manipulation was associated with placental dysmorphologies, which were correlated with abnormalities, such as forebrain development, heart chamber, and septum morphology, subcutaneous edema, and overall artery or vein topology (66). Furthermore, alterations in the placenta during preeclampsia cause defective trophoblast invasion, spiral arterial impairment, and maternal decidua remodeling, leading to maternal and fetal complications or diseases (67, 68). Moreover, impairment in the development of the junctional, zone promotes endocrine changes in the placenta environment leading to reduced fetal growth (41, 43). Hence, the placental alterations promoted by neutrophil depletion during the pre-receptive phase of embryo implantation may be associated with the morphological alterations found in the offspring.
Altogether, the data obtained here highlight the relevance of maternal neutrophils in the earliest phase of pregnancy and to sustain placentation and fetus development. The reduced number of neutrophils in the pre-receptive phase led to fetal morphological alterations and an imbalance in the maternal peripheral leukocyte profile. The present data shed light on the intrinsic communication between neutrophils and pregnancy successful; further investigations will provide data to elucidate the fine-tuning mechanisms and signaling triggered by neutrophils during blastocyst implantation.
Data availability statement
The raw data supporting the conclusions of this article will be made available by the authors, without undue reservation.
Ethics statement
The animal study was reviewed and approved by Institutional Animal Care and Use Committee from the Faculty of Pharmaceutical Sciences of the University of Sao Paulo.
Author contributions
Conceptualization, CH, SF, and SS; Data curation, PS and SS; Funding acquisition, SF; Investigation, CH, PS, CG, and SS; Experiments, CH, AS, PS, and SS; Statistical analysis, CH, and SS; Supervision, SF, and SS; Validation, SF, and SS; Writing—original draft, CH, PS, SF, and SS; Writing—review and editing, CH, PS, SF, and SS. All authors contributed to the article and approved the submitted version.
Funding
This work was supported by FAPESP (Fundação de Amparo á Pesquisa do Estado de São Paulo), grant number 2014/07328-4; SF is a fellow researcher of CNPq (Conselho Nacional de Pesquisa); CH and SS were post-doctoral fellows of CAPES (Coordenação de Aperfeiçoamento de Pessoal de Níel Superior); PS is a PhD fellow of the FAPESP, grant number 2020/14368-3. AS is a Msc fellow of the CAPES.
Acknowledgments
The authors thank Franciani Rodrigues da Rocha from Núcleo de Pesquisa em Ciências Médicas, Fundação Universidade para o Desenvolvimento do Alto Vale do Itajaí – UNIDAVI; Rio do Sul, SC, Brazil for the statistical analysis support.
Conflict of interest
The authors declare that the research was conducted in the absence of any commercial or financial relationships that could be construed as a potential conflict of interest.
Publisher’s note
All claims expressed in this article are solely those of the authors and do not necessarily represent those of their affiliated organizations, or those of the publisher, the editors and the reviewers. Any product that may be evaluated in this article, or claim that may be made by its manufacturer, is not guaranteed or endorsed by the publisher.
Supplementary material
The Supplementary Material for this article can be found online at: https://www.frontiersin.org/articles/10.3389/fimmu.2022.969336/full#supplementary-material
Supplementary Figure 1 | Effects of neutrophil depletion on maternal weight gain, fetuses, and placental weight and index. Pregnant mice were weight on gestational day 1 and before euthanasia on day 18.5 (A). After the cesarean, placenta (B) and pregnant utero (C) were weighted. The placenta index was based on the ratio of fetus weight in relation to its respective placenta (D). Data were statistically analyzed using test “t” (n = 3 - 6). No significant difference was observed.
Supplementary Figure 2 | Effects of neutrophil depletion on the offspring development. Representative image of offspring from control/isotype and anti-Gr1 groups (A). Fetus weight (B).
Supplementary Table 1 | Pregnancy index (PI) was calculated considering the of number of females delivering live pups/number of females with evidence of pregnancy*100. Data was statistically evaluated using Fischer Exact Test and results obtained showed there was no statistical association between Isotype/PBS and anti-Gr1 (0.13) as p < 0.05 was considered statistically significant.
References
1. Norwitz ER, Schust DJ, Fisher SJ. Implantation and the survival of early pregnancy. N Engl J Med (2001) 345: 1400–8. doi: 10.1056/nejmra000763
2. Ng SW, Norwitz GA, Pavlicev M, Tilburgs T, Simón C, Norwitz ER. Endometrial decidualization: The primary driver of pregnancy health. Int J Mol Sci (2020) 21: 4092. doi: 10.3390/ijms21114092
3. Governini L, Luongo FP, Haxhiu A, Piomboni P, Luddi A. Main actors behind the endometrial receptivity and successful implantation. Tissue Cell (2021) 73:101656. doi: 10.1016/j.tice.2021.101656
4. Guo X, Yi H, Li TC, Wang Y, Wang H, Chen X. Role of vascular endothelial growth factor (Vegf) in human embryo implantation: Clinical implications. Biomolecules (2021) 11: 253. doi: 10.3390/biom11020253
5. Boeldt DS, Bird IM. Vascular adaptation in pregnancy and endothelial dysfunction in preeclampsia. J Endocrinol (2017) 232: R27–R44. doi: 10.1530/JOE-16-0340
6. Ball E, Bulmer JN, Ayis S, Lyall F, Robson SC. Late sporadic miscarriage is associated with abnormalities in spiral artery transformation and trophoblast invasion. J Pathol (2006) 208: 535–42. doi: 10.1002/path.1927
7. Bert S, Ward EJ, Nadkarni S. Neutrophils in pregnancy: New insights into innate and adaptive immune regulation. Immunology (2021) 164: 665–676. doi: 10.1111/imm.13392
8. Liu Y, Gao S, Zhao Y, Wang H, Pan Q, Shao Q. Decidual natural killer cells: A good nanny at the maternal-fetal interface during early pregnancy. Front Immunol (2021) 12:663660. doi: 10.3389/fimmu.2021.663660
9. Schjenken JE, Green ES, Overduin TS, Mah CY, Russell DL, Robertson SA. Endocrine disruptor compounds–a cause of impaired immune tolerance driving inflammatory disorders of pregnancy? Front Endocrinol (Lausanne) (2021) 12:607539. doi: 10.3389/fendo.2021.607539
10. Hellebrekers P, Vrisekoop N, Koenderman L. Neutrophil phenotypes in health and disease. Eur J Clin Invest (2018) 48: e12943. doi: 10.1111/eci.12943
11. Kim HR, Kim YS, Yoon JA, Yang SC, Park M, Seol DW, et al. Estrogen induces EGR1 to fine-tune its actions on uterine epithelium by controlling PR signaling for successful embryo implantation. FASEB J (2018) 32:1184–95. doi: 10.1096/fj.201700854RR
12. Croxatto D, Micheletti A, Montaldo E, Orecchia P, Loiacono F, Canegallo F, et al. Group 3 innate lymphoid cells regulate neutrophil migration and function in human decidua. Mucosal Immunol (2016) 9: 1372–1383. doi: 10.1038/mi.2016.10
13. Amsalem H, Kwan M, Hazan A, Zhang J, Jones RL, Whittle W, et al. Identification of a novel neutrophil population: Proangiogenic granulocytes in second-trimester human decidua. J Immunol (2014) 193:3070–9. doi: 10.4049/jimmunol.1303117
14. Giaglis S, Stoikou M, Grimolizzi F, Subramanian BY, van Breda SV, Hoesli I, et al. Neutrophil migration into the placenta: Good, bad or deadly? Cell Adhes Migr (2016) 10:208–25. doi: 10.1080/19336918.2016.1148866
15. Giaglis S, Stoikou M, Chowdhury CS, Schaefer G, Grimolizzi F, Rossi SW, et al. Multimodal regulation of NET formation in pregnancy: Progesterone antagonizes the pro-NETotic effect of estrogen and G-CSF. Front Immunol (2016) 7:565. doi: 10.3389/fimmu.2016.00565
16. Calo G, Sabbione F, Vota D, Paparini D, Ramhorst R, Trevani A, et al. Trophoblast cells inhibit neutrophil extracellular trap formation and enhance apoptosis through vasoactive intestinal peptide-mediated pathways. Hum Reprod (2017) 32:55–64. doi: 10.1093/humrep/dew292
17. Gupta AK, Hasler P, Holzgreve W, Gebhardt S, Hahn S. Induction of neutrophil extracellular DNA lattices by placental microparticles and IL-8 and their presence in preeclampsia. Hum Immunol (2005) 66:1146–54. doi: 10.1016/j.humimm.2005.11.003
18. Hahn S, Giaglis S, Hoesli I, Hasler P. Neutrophil NETs in reproduction: From infertility to preeclampsia and the possibility of fetal loss. Front Immunol (2012) 3:362. doi: 10.3389/fimmu.2012.00362
19. Nadkarni S, Smith J, Sferruzzi-Perri AN, Ledwozyw A, Kishore M, Haas R, et al. Neutrophils induce proangiogenic T cells with a regulatory phenotype in pregnancy. Proc Natl Acad Sci U.S.A. (2016) 113:E8415–E8424. doi: 10.1073/pnas.1611944114
20. Gomez-Lopez N, StLouis D, Lehr MA, Sanchez-Rodriguez EN, Arenas-Hernandez M. Immune cells in term and preterm labor. Cell Mol Immunol (2014) 11:571–81. doi: 10.1038/cmi.2014.46
21. Vakili S, Torabinavid P, Tabrizi R, Shojazadeh A, Asadi N, Hessami K. The association of inflammatory biomarker of neutrophil-to-Lymphocyte ratio with spontaneous preterm delivery: A systematic review and meta-analysis. Mediators Inflammation (2021) 2021:6668381. doi: 10.1155/2021/6668381
22. Higashisaka K, Nakashima A, Iwahara Y, Aoki A, Nakayama M, Yanagihara I, et al. Neutrophil depletion exacerbates pregnancy complications, including placental damage, induced by silica nanoparticles in mice. Front Immunol (2018) 9:1850. doi: 10.3389/fimmu.2018.01850
23. Hurt B, Schulick R, Edil B, El KC, Barnett C. The American journal of surgery cancer-promoting mechanisms of tumor-associated neutrophils. Am J Surg (2017); 214: 1–7. doi: 10.1016/j.amjsurg.2017.08.003
24. Hebeda CB, Machado ID, Reif-Silva I, Moreli JB, Oliani SM, Nadkarni S, et al. Endogenous annexin A1 (AnxA1) modulates early-phase gestation and offspring sex-ratio skewing. J Cell Physiol (2018) 233:6591–603. doi: 10.1002/jcp.26258
25. Coan PM, Conroy N, Burton GJ, Ferguson-Smith AC. Origin and characteristics of glycogen cells in the developing murine placenta. Dev Dyn (2006) 235:3280–94. doi: 10.1002/dvdy.20981
26. Simmons DG, Cross JC. Determinants of trophoblast lineage and cell subtype specification in the mouse placenta. Dev Biol (2005) 284:12–24. doi: 10.1016/j.ydbio.2005.05.010
27. Monk D, Mackay DJG, Eggermann T, Maher ER, Riccio A. Genomic imprinting disorders: lessons on how genome, epigenome and environment interact. Nat Rev Genet (2019) 20: 235–248. doi: 10.1038/s41576-018-0092-0
28. Marikawa Y, Alarcón VB. Establishment of trophectoderm and inner cell mass lineages in the mouse embryo. Mol Reprod Dev (2009) 76: 1019–32. doi: 10.1002/mrd.21057
29. Griffith OW, Chavan AR, Protopapas S, Maziarz J, Romero R, Wagner GP. Embryo implantation evolved from an ancestral inflammatory attachment reaction. Proc Natl Acad Sci U.S.A. (2017) 114:E6566–E6575. doi: 10.1073/pnas.1701129114
30. Schjenken JE, Glynn DJ, Sharkey DJ, Robertson SA. TLR4 signaling is a major mediator of the female tract response to seminal fluid in mice. Biol Reprod (2015) 93:68. doi: 10.1095/biolreprod.114.125740
31. De M, Choudhuri R, Wood GW. Determination of the number and distribution of macrophages, lymphocytes, and granulocytes in the mouse uterus from mating through implantation. J Leukoc Biol (1991) 50: 252–62. doi: 10.1002/jlb.50.3.252
32. Robertson SA, Mau VJ, Tremellen KP, Seamark RF. Role of high molecular weight seminal vesicle proteins in eliciting the uterine inflammatory response to semen in mice. J Reprod Fertil (1996) 107: 265–77. doi: 10.1530/jrf.0.1070265
33. Schumacher A, Sharkey DJ, Robertson SA, Zenclussen AC. Immune cells at the fetomaternal interface: How the microenvironment modulates immune cells to foster fetal development. J Immunol (2018) 201:325–334. doi: 10.4049/jimmunol.1800058
34. Care AS, Bourque SL, Morton JS, Hjartarson EP, Robertson SA, Davidge ST. Reduction in regulatory t cells in early pregnancy causes uterine artery dysfunction in mice. Hypertension (2018) 72: 177–187. doi: 10.1161/HYPERTENSIONAHA.118.10858
35. Care AS, Diener KR, Jasper MJ, Brown HM, Ingman WV, Robertson SA. Macrophages regulate corpus luteum development during embryo implantation in mice. J Clin Invest (2013) 123:3472–87. doi: 10.1172/JCI60561
36. Paria BC, Reese J, Das SK, Dey SK. Deciphering the cross-talk of implantation: Advances and challenges. Science (2002) 296:2185–8. doi: 10.1126/science.1071601
37. Watson ED, Cross JC. Development of structures and transport functions in the mouse placenta. Physiology (2005) 20:180–93. doi: 10.1152/physiol.00001.2005
38. Latos PA, Hemberger M. From the stem of the placental tree: Trophoblast stem cells and their progeny. Dev (2016) 143:3650–66. doi: 10.1242/dev.133462
39. Woods L, Perez-Garcia V, Hemberger M. Regulation of placental development and its impact on fetal growth–new insights from mouse models. Front Endocrinol (Lausanne) (2018) 9:570. doi: 10.3389/fendo.2018.00570
40. Cross JC, Hemberger M, Lu Y, Nozaki T, Whiteley K, Masutani M, et al. Trophoblast functions, angiogenesis and remodeling of the maternal vasculature in the placenta. Mol Cell Endocrinol (2002); 187: 207–12. doi: 10.1016/S0303-7207(01)00703-1
41. Salas M, John R, Saxena A, Barton S, Frank D, Fitzpatrick G, et al. Placental growth retardation due to loss of imprinting of Phlda2. Mech Dev (2004) 121:1199–210. doi: 10.1016/j.mod.2004.05.017
42. Tunster SJ, Van De Pette M, John RM. Impact of genetic background on placental glycogen storage in mice. Placenta (2012) 33:124–7. doi: 10.1016/j.placenta.2011.11.011
43. Fowden AL, Moore T. Maternal-fetal resource allocation: Co-operation and conflict. Placenta (2012) 33:e11-5. doi: 10.1016/j.placenta.2012.05.002
44. Stieglitz F, Celik AA, von Kaisenberg C, Camps MA, Blasczyk R, Bade-Döding C. The microstructure in the placenta is influenced by the functional diversity of HLA-G allelic variants. Immunogenetics (2019) 71:455–46. doi: 10.1007/s00251-019-01121-0
45. Fu B, Zhou Y, Ni X, Tong X, Xu X, Dong Z, et al. Natural killer cells promote fetal development through the secretion of growth-promoting factors. Immunity (2017) 47:1100–13. doi: 10.1016/j.immuni.2017.11.018
46. Hoffmann P, Feige JJ, Alfaidy N. Placental expression of EG-VEGF and its receptors PKR1 (Prokineticin receptor-1) and PKR2 throughout mouse gestation. Placenta (2007) 28:1049–58. doi: 10.1016/j.placenta.2007.03.008
47. Zhang J, Chen Z, Smith GN, Croy BA. Natural killer cell-triggered vascular transformation: Maternal care before birth? Cell Mol Immunol (2011) 8:1–11. doi: 10.1038/cmi.2010.38
48. Hofmann AP, Gerber SA, Croy BA. Uterine natural killer cells pace early development of mouse decidua basalis. Mol Hum Reprod (2014) 20:66–76. doi: 10.1093/molehr/gat060
49. Ventureira MR, Sobarzo C, Argandoña F, Palomino WA, Barbeito C, Cebral E. Decidual vascularization during organogenesis after perigestational alcohol ingestion. Reproduction (2019) 158:109–22. doi: 10.1530/REP-18-0230
50. Fan X, Muruganandan S, Shallie PD, Dhal S, Petitt M, Nayak NR. Vegf maintains maternal vascular space homeostasis in the mouse placenta through modulation of trophoblast giant cell functions. Biomolecules (2021) 11:1062. doi: 10.3390/biom11071062
51. Novaro V, Pustovrh C, Colman-Lerner A, Radisky D, Lo Nostro F, Paz D, et al. Nitric oxide induces gelatinase a (matrix metalloproteinase 2) during rat embryo implantation. Fertil Steril (2002) 78:487–96. doi: 10.1016/S0015-0282(02)04240-1
52. Fontana V, Coll TA, Sobarzo CMA, Tito LP, Calvo JC, Cebral E. Matrix metalloproteinase expression and activity in trophoblastdecidual tissues at organogenesis in CF-1 mouse. J Mol Histol (2012) 78:487–96. doi: 10.1007/s10735-012-9429-8
53. Staun-Ram E, Goldman S, Gabarin D, Shalev E. Expression and importance of matrix metalloproteinase 2 and 9 (MMP-2 and -9) in human trophoblast invasion. Reprod Biol Endocrinol (2004) 2:59. doi: 10.1186/1477-7827-2-59
54. Gualdoni G, Gomez Castro G, Hernández R, Barbeito C, Cebral E. Comparative matrix metalloproteinase-2 and -9 expression and activity during endotheliochorial and hemochorial trophoblastic invasiveness. Tissue Cell (2022) 74:101698. doi: 10.1016/j.tice.2021.101698
55. Cerdeira AS, Karumanchi SA. Angiogenic factors in preeclampsia and related disorders. Cold Spring Harb Perspect Med (2012) 2:a006585. doi: 10.1101/cshperspect.a006585
56. Yonemura S, Tsukita S, Tsukita S. Direct involvement of ezrin/radixin/moesin (ERM)-binding membrane proteins in the organization of microvilli in collaboration with activated ERM proteins. J Cell Biol (1999) 145:1497–509. doi: 10.1083/jcb.145.7.1497
57. Ley K, Laudanna C, Cybulsky MI, Nourshargh S. Getting to the site of inflammation: The leukocyte adhesion cascade updated. Nat Rev Immunol (2007) 7:678–89. doi: 10.1038/nri2156
58. Lecce L, Kaneko Y, Madawala RJ, Murphy CR. ICAM1 and fibrinogen-γ are increased in uterine epithelial cells at the time of implantation in rats. Mol Reprod Dev (2011) 78:318–27. doi: 10.1002/mrd.21307
59. Matsumoto H, Daikoku T, Wang H, Sato E, Dey SK. Differential expression of Ezrin/Radixin/Moesin (ERM) and ERM-associated adhesion molecules in the blastocyst and uterus suggests their functions during implantation. Biol Reprod (2004) 70:729–36. doi: 10.1095/biolreprod.103.022764
60. Takeshita T, Satomi M, Akira S, Nakagawa Y, Takahashi H, Araki T. Preventive effect of monoclonal antibodies to intercellular adhesion molecule-1 and leukocyte function-associate antigen-1 on murine spontaneous fetal resorption. Am J Reprod Immunol (2000) 43:80–5. doi: 10.1111/j.8755-8920.2000.430308.x
61. Cho S, Sohn YD, Kim S, Rajakumar A, Badell ML, Sidell N, et al. Reduced angiovasculogenic and increased inflammatory profiles of cord blood cells in severe but not mild preeclampsia. Sci Rep (2021) 11:3630. doi: 10.1038/s41598-021-83146-8
62. Guo F, Si C, Zhou M, Wang J, Zhang D, Leung PCK, et al. Decreased PECAM1-mediated TGF-β1 expression in the mid-secretory endometrium in women with recurrent implantation failure. Hum Reprod (2018) 33:832–843. doi: 10.1093/humrep/dey022
63. Freis A, Roesner S, Marshall A, Rehnitz J, von Horn K, Capp E, et al. Non-invasive embryo assessment: Altered individual protein profile in spent culture media from embryos transferred at day 5. Reprod Sci (2021) 28:1866–1873. doi: 10.1007/s43032-020-00362-9
64. Robson P, Stein P, Zhou B, Schultz RM, Baldwin HS. Inner cell mass-specific expression of a cell adhesion molecule (PECAM-1/CD31) in the mouse blastocyst. Dev Biol (2001) 234:317–29. doi: 10.1006/dbio.2001.0274
65. Sharma D, Shastri S, Sharma P. Intrauterine growth restriction: Antenatal and postnatal aspects. Clin Med Insights Pediatr (2016) 10:67–83. doi: 10.4137/cmped.s40070
66. Perez-Garcia V, Fineberg E, Wilson R, Murray A, Mazzeo CI, Tudor C, et al. Placentation defects are highly prevalent in embryonic lethal mouse mutants. Nature (2018) 555:463–8. doi: 10.1038/nature26002
67. Roberts JM, Escudero C. The placenta in preeclampsia. Pregnancy Hypertens (2012) 2:72–83. doi: 10.1016/j.preghy.2012.01.001
Keywords: anti-granulocytes, pregnancy index, abnormal fetal development, maternal immune system, placenta morphology, window of implantation
Citation: Hebeda CB, Savioli AC, Scharf P, de Paula-Silva M, Gil CD, Farsky SHP and Sandri S (2022) Neutrophil depletion in the pre-implantation phase impairs pregnancy index, placenta and fetus development. Front. Immunol. 13:969336. doi: 10.3389/fimmu.2022.969336
Received: 14 June 2022; Accepted: 29 August 2022;
Published: 29 September 2022.
Edited by:
Kenneth Beaman, Rosalind Franklin University of Medicine and Science, United StatesReviewed by:
Shanmuga Priyaa Madhukaran, University of Texas Southwestern Medical Center, United StatesAgnieszka Waclawik, Institute of Animal Reproduction and Food Research (PAS), Poland
Copyright © 2022 Hebeda, Savioli, Scharf, de Paula-Silva, Gil, Farsky and Sandri. This is an open-access article distributed under the terms of the Creative Commons Attribution License (CC BY). The use, distribution or reproduction in other forums is permitted, provided the original author(s) and the copyright owner(s) are credited and that the original publication in this journal is cited, in accordance with accepted academic practice. No use, distribution or reproduction is permitted which does not comply with these terms.
*Correspondence: Silvana Sandri, c3NhbmRyaUB1c3AuYnI=