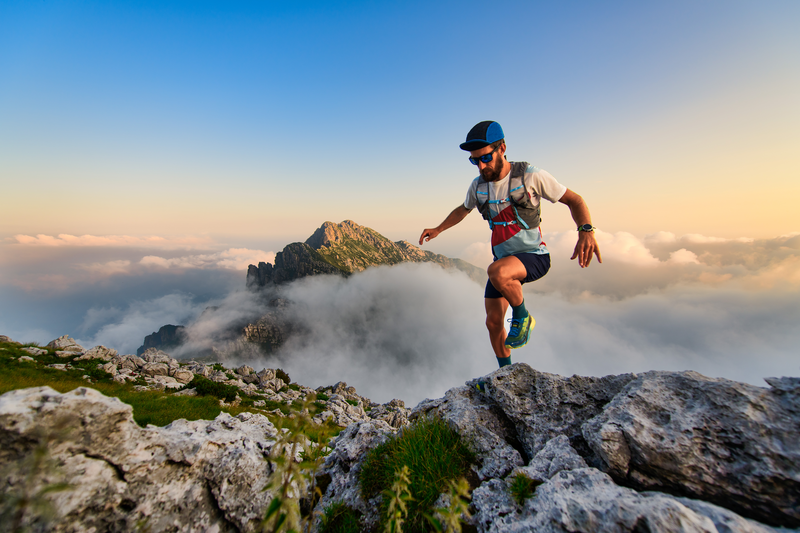
95% of researchers rate our articles as excellent or good
Learn more about the work of our research integrity team to safeguard the quality of each article we publish.
Find out more
MINI REVIEW article
Front. Immunol. , 29 July 2022
Sec. Viral Immunology
Volume 13 - 2022 | https://doi.org/10.3389/fimmu.2022.965018
This article is part of the Research Topic How do innate host responses shape antiviral immunity? View all 10 articles
Chronic hepatitis B virus (HBV) infection remains to be a substantial global burden, especially for end-stage liver diseases. It is well accepted that HBV-specific T and B cells are essential for controlling HBV infection. Toll-like receptors (TLRs) represent one of the major first-line antiviral defenses through intracellular signaling pathways that induce antiviral inflammatory cytokines and interferons, thereby shaping adaptive immunity. However, HBV has evolved strategies to counter TLR responses by suppressing the expression of TLRs and blocking the downstream signaling pathways, thus limiting HBV-specific adaptive immunity and facilitating viral persistence. Recent studies have stated that stimulation of the TLR signaling pathway by different TLR agonists strengthens host innate immune responses and results in suppression of HBV replication. In this review, we will discuss how TLR-mediated responses shape HBV-specific adaptive immunity as demonstrated in different experimental models. This information may provide important insight for HBV functional cure based on TLR agonists as immunomodulators.
Hepatitis B virus (HBV) infection affects approximately 3.5% of the world’s population and remains a major cause of end-stage liver disease, such as cirrhosis and hepatocellular carcinoma (HCC) (1). HBV-specific adaptive immune responses play an essential role in HBV clearance. During acute HBV infections, vigorous HBV-specific T-cell responses contribute to viral clearance (2). However, HBV-specific T cells exhibit quantitative and functional defects accompanied by an exhausted phenotype with upregulation of several coinhibitory molecules during chronic HBV infection (3). This is considered as a major contributing factor to HBV persistence. Currently available treatment regimens, including pegylated interferon alpha (Peg-IFN-α) and nucleos(t)ide analogs (NAs), can effectively suppress HBV replication but rarely achieve a functional cure. In recent years, various immunotherapeutic agents that aim to restore HBV-specific immune responses have been investigated, including anti-PD1 antibodies (4), CTLA4 inhibitors and pattern recognition receptor (PRR) agonists (5). However, these novel treatment options are still under preclinical or early clinical evaluation.
Toll-like receptors (TLRs), members of the evolutionarily ancient family of PRRs, play a central role in responses to microbial pathogens by recognizing pathogen-associated molecular patterns (PAMPs) (6). Twelve and 10 functional TLRs have been identified in mice and humans, respectively (7). Based on the cellular localization and the respective PAMP ligands, TLRs are largely divided into two groups. TLR3, TLR7, TLR8, and TLR9 are localized in intracellular vesicles, such as endosomes, endoplasmic reticulum (ER), and lysosomes, and recognize viral DNA (TLR9) or RNA [double-stranded RNA (TLR3), single-stranded RNA (TLR7 and TLR8)] (8, 9). TLR1, TLR2, TLR4, TLR5, and TLR6 are localized in the cell surface and recognize extracellular bacterial and fungal cell wall components and some viral proteins, including lipoproteins (recognized by TLR1, TLR2, and TLR6), lipopolysaccharide (LPS) (TLR4), and flagellin (TLR5) (8). Upon recognizing respective PAMPs, TLRs selectively recruit distinct adaptor molecules, such as TRIF and MyD88, and initiate downstream signaling events that result in the secretion of type I interferon (IFN), inflammatory cytokines, and chemokines (9, 10). In this review, we will discuss the interaction between TLRs and HBV and how different TLR ligands regulate HBV-specific T-cell responses.
The recognition of HBV by the innate immune system involves three types of host cells: hepatocytes, innate immune cells, such as dendritic cells (DCs) and macrophages, and hepatic non-parenchymal cells (11). Previously, acute HBV infection has been reported to only weakly induce the expression of type I IFN and innate immune genes within the liver of infected animals (12) and patients (13–15). However, Hösel et al. found that HBV could be recognized by Kupffer cells (KCs), present in primary human hepatocytes (PHHs) culture in vitro. This recognition leads to the activation of the NF-κB signaling pathway and the subsequent release of proinflammatory cytokines but does not induce an interferon response in KCs (16). Moreover, a recent study also showed that infection of PHHs with HBV induces the secretion of proinflammatory cytokines through TLR2 signaling but not IFNs (17). Consistent with these findings, another study showed that mouse B cells could be activated by HBV particles through the TLR2–MyD88–mTOR axis (18). Furthermore, HBsAg has been reported to be recognized by TLR4 on monocytes or myeloid DCs via CD14 and increase the production of IL-10 (19, 20). These data suggest that host innate immunity could indeed sense HBV infection, although it may be weak.
Interestingly, the genetic single-nucleotide polymorphisms (SNPs) of TLRs have potential effects on the outcome of HBV infection. The TLR3 (rs3775290, rs3775291) and TLR4 (rs4986790) SNP variants link to a higher risk of chronic HBV infection and HCC (21, 22), while mutations of rs3804099 and rs4696480 in TLRs correlate with HBsAg reduction and liver function improvement (23).
During chronic infection, HBV modulates TLR response (24). The impaired expression of TLRs in immune cells from patients with chronic hepatitis B infection (CHB) has been reported in several studies. Peripheral blood mononuclear cells (PBMCs) from CHB patients displayed a significantly reduced expression of TLR transcripts, including TLR1, 2, 4, and 6 (25). Similarly, PBMCs from CHB patients showed a reduced expression of TLR3 (26), TLR8 (27), TLR7, and TLR9 (28, 29) as well as the TLR signaling molecules IRAK4, TRAF3, and IRF7 (30). Additionally, patients who achieved a complete response sustained higher TLR8 mRNA levels in PBMCs than non-responders at week 12 after Peg-IFN-α therapy (27). Consistent with this finding, partial restoration of TLR2 and TLR3 expression in PBMCs has been observed in patients with virological response after treatment (26, 31).
In addition to downregulating TLR expression, HBV infection also impairs the functional response of TLR signaling. PBMCs from CHB patients exhibit impaired cytokine secretion after challenging with TLR2, TLR4 (25, 32), and TLR 8 ligand (27). Consistent with this finding, PBMCs and plasmacytoid DCs (pDCs) from CHB patients have shown significantly decreased IFN-α production in response to TLR7 and TLR9 ligands (33, 34). Indeed, HBV components have been reported to interrupt the intracellular signaling pathways of TLRs. HBsAg inhibits IRF7 expression and nuclear translocation in pDCs (34) and also interferes with the NF-κB pathway by interacting with the TAK1–TAB2 complex (35). Moreover, HBeAg has been reported to disrupt homotypic TIR : TIR interactions and thus suppress TIR-mediated activation of the NF-κB and IFN-α promoters (36). Furthermore, HBV polymerase inhibits the activity of IKKs and thereby suppresses TLR3- and TLR4-induced NF-κB signaling (37). Wu et al. showed that supernatant from TLR3-stimulated liver sinusoidal endothelial cells (LSECs) suppressed HBV replication in hepatocytes (38). However, HBV components, such as HBsAg, HBeAg, and HBV virions, suppressed the activation of IRF-3, NF-κB, MAPK, and ERK 1/2 and abrogated TLR-induced antiviral activity in LSECs (39, 40). Taken together, these studies suggest that various HBV components can interrupt the TLR signaling pathway, which might explain the impaired function of the TLR signaling pathway during HBV infection.
Of note, persistent inflammation in CHB patients is also partly responsible for the impairment of TLR response. For instance, IFN-α production by pDCs in response to TLR7 or 9 ligands is negatively correlated with alanine aminotransferase (ALT) levels (41, 42).
TLR agonists may directly inhibit HBV replication in hepatocytes or indirectly suppress HBV replication by antiviral cytokines produced by other innate immune cells (43, 44). On the other hand, HBV-specific T- and B-cell responses ultimately determine the outcomes of HBV infections. In chronic HBV infections, HBV-specific T cells display quantitative and functional defects, a state referred to as T-cell exhaustion (45). Moreover, exhausted T cells are more susceptible to tumor necrosis factor-alpha (TNF-α)-related apoptosis-inducing ligand (TRAIL)-dependent NK-cell-mediated lysis due to the upregulation of the TRAIL death receptor (46). Therefore, restoring HBV-specific T-cell responses in CHB patients represents a promising strategy to achieve HBV functional cure.
Several studies have investigated the effects of TLR agonists to restore HBV-specific CD8+ T-cell responses. The liver is a specialized immunological organ with a unique composition of innate immune cells, including liver resident cells, such as parenchymal cells, and non-parenchymal cells, that is, LSECs, KCs, and hepatic stellate cells, and recruited immune cells, such as DCs, macrophages, and T and B cells. Activation of TLR signaling in these hepatic cells can induce the production of type I IFN and a variety of proinflammatory cytokines, such as TNF, IL-6, IL-12, and IL-18 (47), which play essential roles in controlling HBV replication (38, 48) but also modulate specific immune responses (Figure 1). For instance, IL-6 could control the expression of HBx and suppress HBV replication through modulating the activity of HBV enhancer I, and it also participates in the activation of NK cells and cytotoxic T cells (CTLs) (49). IL-12 and IL-18 could rescue the exhausted CD8 T-cell responses (50) or promote the secretion of IFN-γ by T cells (51).
Figure 1 TLRs regulate HBV-specific T-cell responses. TLRs are expressed in T cells, hepatocytes, and hepatic non-parenchymal cells, including LSECs, KCs, and DCs. TLRs may directly shape the T-cell response as costimulatory molecules. Stimulation of TLRs by their ligands leads to the activation of the downstream MyD88/TRIF-dependent signaling pathway in hepatic non-parenchymal cells and promotes the maturation of these cells, thus promoting antigen presentation to T cells and the production of IFNs, proinflammatory cytokines, and chemokines. IFNs exert antiviral effects against HBV in infected hepatocytes. Chemokines and inflammatory cytokines recruit DCs, macrophages, and specific T cells into the liver and promote HBV-specific T-cell activation and proliferation. Activated CLTs then kill infected hepatocytes. CTL, cytotoxic T cell; DC, dendritic cell; HBV, hepatitis B virus; IFN, interferon; LSEC, liver sinusoidal endothelial cell; KC, Kupffer cell; TLR, Toll-like receptor.
Recent studies have stated that TLRs may serve as costimulatory molecules on T cells (52, 53). The expression of TLR2 is detected in activated CD8 T cells on their surface, and TLR2 stimulation reduces the requirement of costimulatory signals delivered by antigen-presenting cells (APCs) and directly promotes their differentiation, proliferation, and effector function (53–55). A recent study also demonstrated that the TLR2 agonist P3C could directly enhance TCR-dependent CD8 T-cell activation by increasing cellular glycolysis and glutaminolysis (52). Similarly, the TLR7 ligand also enhanced the effector functions of TCR-dependent CD8 T cells primed by CD3, and the effects were accompanied by upregulation of glucose uptake and glycolysis (56). Moreover, human effector CD8+ T cells constitutively express TLR3 and TLR9. Stimulation of these TLRs by respective ligands directly promotes IFN-γ production by T cells (57, 58). Overall, these data suggest that activation of TLR signaling in T cells directly promotes T-cell activation, proliferation, and effector functions. However, the direct effect of TLR ligands on T cells in the treatment of CHB requires further investigation.
As professional APCs, DCs exhibit a potent capacity to prime naïve T cells and induce them to develop into different subtypes of T cells based on the origin of the maturation signals. There are two major DC subsets identified in humans: myeloid DCs (mDCs) and pDCs (59). These cells express different sets of TLRs. Specifically, pDCs predominantly express TLR7 and TLR9, while mDCs express a wider range of TLRs, including TLR1, TLR2, TLR3, TLR4, TLR5, TLR6, and TLR8 (60). Therefore, pDCs can sense the nucleic acids of viral pathogens through TLR7 and TLR9 and induce the secretion of type I IFN. pDCs exhibit a dysfunctional phenotype during chronic HBV infection, indicated by downregulation of TLR9 (29, 61) and reduction in antigen-presenting and migration capacity (62) and decreased production of IFN-α and inflammatory cytokines in the response to TLR7 and 9 ligands (29, 33, 34, 41, 63). The functional deficit of DCs might participate in T-cell dysfunction given the impaired interaction between DCs and T cells, including failure of DCs to increase HLA-II and costimulatory molecules and defective induction of antigen-specific CLT proliferation and cytokine production (64). However, two studies stated that the ability of pDCs in PBMCs to stimulate T-cell proliferation was similar between CHB patients and healthy control (42, 65). Despite these discrepancies, therapeutic vaccines targeting DC function might represent an opportunity to improve HBV-specific T-cell responses.
Some studies have investigated TLR agonists as vaccine adjuvants to improve DC function and thus induce HBV-specific T-cell responses. Synthetic long peptides (SLPs) are linear amino acid sequences that are most efficiently presented by DCs (66). Dou et al. found that the presence of the TLR2 ligand promoted the effects of HBV core protein-derived SLP to boost CD4 and CD8+ T-cell responses in CHB patients ex vivo (67). Later, the same group identified that HBV-core SLP that conjugates with the TLR2 ligand also triggered a functional T-cell response, but it reduced the cross-presentation efficiency of the SLP-containing epitope by DC subsets (68). However, the cross-presentation can be improved by either placing a valine–citrulline linker between the TLR2 ligand and the long SLP or by shortening the SLP (68).
Murata et al. demonstrated that antigen presentation by hepatocytes is more efficient than by hematopoietic cells for inducing HBV-specific CD8+ T-cell responses in the liver (69). Yan et al. reported that the TLR5 ligand flagellin (SF) could modulate the intrahepatic CD8+ T-cell response by regulating the responses of hepatocytes (70). Coculture of SF-treated primary mouse hepatocytes with splenocytes results in the activation of CD8+ T cells in the coculture system during anti-CD3 stimulation or antigen-specific activation (70).
LSECs are liver-resident APCs that have competent capacity in antigen cross-presentation to CD8+ T cells (71). However, the antigen-specific interaction of LSECs with CD8+ T cells does not induce T-cell activation under physiological conditions (72). Nevertheless, T-cell tolerance induced by LSECs can be regulated by several factors, such as NOD1 ligand, DAP (73), TLR2 agonist (74), and combinatorial stimulation of CD28 and IL-12 (75). Liu et al. illustrated that stimulation of LSECs with the TLR1/2 ligand promoted the maturation of LSECs and enabled them to further activate virus-specific CD8+ T cells in mice (74). Mechanistically, IL-12 produced by LSECs was an essential mediator of LSEC-mediated CD8+ T-cell immunity (74).
KCs are resident macrophages in the sinusoids of the liver that express TLRs and secrete large amounts of inflammatory mediators that regulate antiviral immunity during HBV infection (76). Human KCs express TLR2–4, whereas KCs from rats and mice express TLR1-9 (26). Previous data demonstrated that the TLR2 ligand P3C further strengthens the tolerogenic and suppressive properties of intrahepatic myeloid-derived cells (iMDCs) in mice. Mechanistically, the enhanced inhibition of T-cell activation was mainly induced by KCs via secreting IL-10 (77). Consistent with this finding, another group identified that KCs support HBV-mediated CD8 T-cell exhaustion via the HBV core antigen–TLR2 interaction in mice (78). On the other hand, KCs produce CXCL8 upon HBV exposure (16), which potentially attracts NK and NKT cells into the liver during the early phase of HBV infection. Uwatoku et al. found that KCs are crucial for DC recruitment to the liver through N-acetyl galactosamine-specific C-type lectin interactions (79). The increased DC recruitment may promote HBV antigen presentation and thus enhance HBV-specific T-cell responses. As antigen-presenting cells, mouse KCs also present antigens to T cells, thus inducing T-cell proliferation and the production of IFN-γ (80, 81). However, the antigen-presenting function of KCs is much weaker than that of DCs. A recent study identified a subset of KCs (KC2) that could cross-present hepatocellular antigens to CD8 T cells upon IL-2 administration and thus improve the antiviral functions of T cells (82).
In a persistent HBV replication mouse model utilizing hydrodynamic injection (HI) of the pAAV/HBV 1.2 plasmid, HBV-specific immune responses are primed but do not clear HBV from the liver (83). Therefore, this model was used to test the effects of TLR agonists on HBV-specific immune responses. Previously, our group indicated that intrahepatic application of TLR3 ligand poly(I:C) after establishment of persistent HBV replication efficiently recruited CD8+ T cells into the liver, enhanced HBV-specific T-cell responses, and cleared HBV in an IFN-γ- and CXCR3-dependent manner (84, 85). Later, we constructed calcium phosphate nanoparticles carrying poly(I:C) conjugated with F4/80, which promoted liver targeting by conventional intravenous injection. These nanoparticles exerted a similar enhancing effect on HBV-specific T-cell responses like HI of poly(I:C) (86). However, simultaneous or prior activation of TLR3 signaling by HI of poly(I:C) results in expansion of Tregs, KCs, and myeloid-derived suppressor cells (MDSCs), all of which impair the HBV-specific T-cell response and thus inhibit HBV clearance (85). Consistent with this finding, another study investigated the HBV-specific T-cell immunity and anti-HBV effect by TLR2 activation (P3C) in the same model at different time points, which found that only TLR2 pre-activation could enhance the intrahepatic HBV-specific T-cell response (87). In detail, pre-activation of TLR2 reduced the number of hepatic F4/80+ macrophages but increased the number of CD11c+ DCs, which is helpful for the initiation of the HBV-specific T-cell responses in the following time period (76). Huang et al. reported that TLR signaling induced intrahepatic aggregates of myeloid cells that enabled the population expansion of CTLs during chronic viral liver infection (88). Hepatic CTL proliferation was restricted to myeloid-cell aggregates for T-cell population expansion (iMATES) that were composed of inflammatory monocyte-derived CD11b+ cells (88). These findings suggest that the application of TLR ligands at the right time and in the right location can enhance virus-specific T-cell responses by recruiting immune cells into the liver. Of note, the HI mouse model is quite different from human beings. Therefore, the findings in mouse studies should be carefully evaluated and verified in human research.
TLR agonists are potential antiviral agents in chronic HBV infection. Indeed, several studies have investigated the anti-HBV effects of TLR ligands in clinical studies (Table 1). An earlier study tested the efficacy of polyadenylic.polyuridylic acid [poly(A).poly (U), TLR3 ligand] in CHB patients and found that normalization of ALT levels and HBeAg seroconversion were noted in approximately 57.9% of treated patients, suggesting that poly(A).poly (U) may be effective in the treatment of CHB patients (89). The safety and efficacy of the TLR7 agonist GS9620 have been assessed in CHB patients (90–92). GS9620 promoted ISG-15 expression, HBV-specific T-cell responses, and NK-cell activation and function and reduced the ability of NK cells to lysis T cells. However, HBsAg levels are not significantly reduced in the treated patients (90, 91, 93). Another study used different TLR agonists to stimulate mononuclear cells derived from chronic HBV- or HCV-infected livers and found that the TLR8 ligand ssRNA40 could induce the production of IFN-γ in chronic HBV- or HCV-infected livers by mucosal-associated invariant T cells (MAIT) and NK cells (94). Media from PBMCs that were stimulated with the TLR8 agonist GS-9688 reduced HBV replication in HBV-infected PHHs (95). A recent study found that GS-9688 could activate antiviral effector function in PBMCs from CHB patients by multiple immune mediators (HBV-specific CD8+ T cells, CD4+ follicular helper T cells, NK cells, and MAIT) (96). Similarly, TLR8 agonists enhance HBV-specific B-cell responses via improving monocyte-mediated follicular helper T-cell function in CHB patients (97). Taken together, the agonists of TLR3, 7, and 8 may serve as potential antiviral agents against chronic HBV infections, but further investigation is needed to evaluate their toxicity, tolerated range, and efficacy when used alone or applied together with current antiviral drugs.
Despite the availability of an effective prophylactic vaccine, HBV infection remains a major challenge worldwide. During chronic HBV infection, HBV suppresses the expression of TLRs and downstream cytokines through various HBV components and thus limits HBV-specific adaptive immunity and suppresses virus clearance. Thus, restoration of HBV-specific immune responses may be essential for sustained viral control. Accumulated studies suggest that TLR-mediated innate immune responses could enhance HBV-specific responses and thus suppress HBV replication and expression. To achieve a functional cure of CHB, a combined strategy with current antiviral treatment, activation of TLR-mediated immunity, and restoration of HBV adaptive immunity should be investigated in future studies in both animal models and clinical trials. It will be useful to understand the various underlying mechanisms how TLRs mediate immune activations and identify those contributing to HBV-specific immune control. It is also important to find a way to direct immune cells to the liver and to let those to exert antiviral functions.
YD and ML conceptualized and drafted this review. JW, JL, XZ, and DY edited the review. All authors contributed to the article and approved the submitted version.
This work was contributed by a scholarship from the Medical Faculty of University of Duisburg-Essen, a grant from Deutsche Forschungsgemeinschaft (RTG 1949/2), and grants of National Natural Science Foundation of China (81672022 and 82170636).
The authors declare that the research was conducted in the absence of any commercial or financial relationships that could be construed as a potential conflict of interest.
All claims expressed in this article are solely those of the authors and do not necessarily represent those of their affiliated organizations, or those of the publisher, the editors and the reviewers. Any product that may be evaluated in this article, or claim that may be made by its manufacturer, is not guaranteed or endorsed by the publisher.
1. Hutin Y, Nasrullah M, Easterbrook P, Nguimfack BD, Burrone E, Averhoff F, et al. Access to treatment for hepatitis b virus infection - worldwide, 2016. MMWR Morb Mortal Wkly Rep (2018) 67:773–7. doi: 10.15585/mmwr.mm6728a2
2. Thimme R, Wieland S, Steiger C, Ghrayeb J, Reimann KA, Purcell RH, et al. CD8(+) T cells mediate viral clearance and disease pathogenesis during acute hepatitis b virus infection. J Virol (2003) 77:68–76. doi: 10.1128/JVI.77.1.68-76.2003
3. Bertoletti A, Ferrari C. Adaptive immunity in HBV infection. J Hepatol (2016) 64:S71–83. doi: 10.1016/j.jhep.2016.01.026
4. Salimzadeh L, Le Bert N, Dutertre CA, Gill US, Newell EW, Frey C, et al. PD-1 blockade partially recovers dysfunctional virus-specific b cells in chronic hepatitis b infection. J Clin Invest (2018) 128:4573–87. doi: 10.1172/JCI121957
5. Bertoletti A, Bert NL. Immunotherapy for chronic hepatitis b virus infection. Gut Liver (2018) 12:497–507. doi: 10.5009/gnl17233
6. Fitzgerald KA, Kagan JC. Toll-like receptors and the control of immunity. Cell (2020) 180:1044–66. doi: 10.1016/j.cell.2020.02.041
7. Kawai T, Akira S. Toll-like receptors and their crosstalk with other innate receptors in infection and immunity. Immunity (2011) 34:637–50. doi: 10.1016/j.immuni.2011.05.006
8. Akira S, Uematsu S, Takeuchi O. Pathogen recognition and innate immunity. Cell (2006) 124:783–801. doi: 10.1016/j.cell.2006.02.015
9. Kawai T, Akira S. The role of pattern-recognition receptors in innate immunity: update on toll-like receptors. Nat Immunol (2010) 11:373–84. doi: 10.1038/ni.1863
10. Zhang E, Lu M. Toll-like receptor (TLR)-mediated innate immune responses in the control of hepatitis b virus (HBV) infection. Med Microbiol Immunol (2015) 204:11–20. doi: 10.1007/s00430-014-0370-1
11. Ma Z, Zhang E, Yang D, Lu M. Contribution of toll-like receptors to the control of hepatitis b virus infection by initiating antiviral innate responses and promoting specific adaptive immune responses. Cell Mol Immunol (2015) 12:273–82. doi: 10.1038/cmi.2014.112
12. Wieland S, Thimme R, Purcell RH, Chisari FV. Genomic analysis of the host response to hepatitis b virus infection. Proc Natl Acad Sci U.S.A. (2004) 101:6669–74. doi: 10.1073/pnas.0401771101
13. Fisicaro P, Valdatta C, Boni C, Massari M, Mori C, Zerbini A, et al. Early kinetics of innate and adaptive immune responses during hepatitis b virus infection. GUT (2009) 58:974–82. doi: 10.1136/gut.2008.163600
14. Dunn C, Peppa D, Khanna P, Nebbia G, Jones M, Brendish N, et al. Temporal analysis of early immune responses in patients with acute hepatitis b virus infection. Gastroenterology (2009) 137:1289–300. doi: 10.1053/j.gastro.2009.06.054
15. Suslov A, Boldanova T, Wang X, Wieland S, Heim MH. Hepatitis b virus does not interfere with innate immune responses in the human liver. Gastroenterology (2018) 154:1778–90. doi: 10.1053/j.gastro.2018.01.034
16. Hosel M, Quasdorff M, Wiegmann K, Webb D, Zedler U, Broxtermann M, et al. Not interferon, but interleukin-6 controls early gene expression in hepatitis b virus infection. Hepatology (2009) 50:1773–82. doi: 10.1002/hep.23226
17. Zhang Z, Trippler M, Real CI, Werner M, Luo X, Schefczyk S, et al. Hepatitis b virus particles activate toll-like receptor 2 signaling initially upon infection of primary human hepatocytes. Hepatology (2020) 72:829–44. doi: 10.1002/hep.31112
18. Li Q, Wang J, Islam H, Kirschning C, Lu H, Hoffmann D, et al. Hepatitis b virus particles activate b cells through the TLR2-MyD88-mTOR axis. Cell Death Dis (2021) 12:34. doi: 10.1038/s41419-020-03284-1
19. Vanlandschoot P, Roobrouck A, Van Houtte F, Leroux-Roels G. Recombinant HBsAg, an apoptotic-like lipoprotein, interferes with the LPS-induced activation of ERK-1/2 and JNK-1/2 in monocytes. Biochem Biophys Res Commun (2002) 297:486–91. doi: 10.1016/S0006-291X(02)02243-X
20. van Montfoort N, van der Aa E, van den Bosch A, Brouwers H, Vanwolleghem T, Janssen H, et al. Hepatitis b virus surface antigen activates myeloid dendritic cells via a soluble CD14-dependent mechanism. J Virol (2016) 90:6187–99. doi: 10.1128/JVI.02903-15
21. Sghaier I, Zidi S, Mouelhi L, Ghazoueni E, Brochot E, Almawi WY, et al. TLR3 and TLR4 SNP variants in the liver disease resulting from hepatitis b virus and hepatitis c virus infection. Br J BioMed Sci (2019) 76:35–41. doi: 10.1080/09674845.2018.1547179
22. Fischer J, Koukoulioti E, Schott E, Fulop B, Heyne R, Berg T, et al. Polymorphisms in the toll-like receptor 3 (TLR3) gene are associated with the natural course of hepatitis b virus infection in Caucasian population. Sci Rep (2018) 8:12737. doi: 10.1038/s41598-018-31065-6
23. Lin Y, Gao ZX, Shen X, Chen MJ, Li YT, Li SL, et al. Correlation between polymorphisms in toll-like receptor genes and the activity of hepatitis b virus among treatment-naive patients: a case-control study in a han Chinese population. BMC Infect Dis (2018) 18:28. doi: 10.1186/s12879-018-2943-x
24. Ma Z, Cao Q, Xiong Y, Zhang E, Lu M. Interaction between hepatitis b virus and toll-like receptors: Current status and potential therapeutic use for chronic hepatitis b. Vaccines (Basel) (2018) 6(1):6. doi: 10.3390/vaccines6010006
25. Chen Z, Cheng Y, Xu Y, Liao J, Zhang X, Hu Y, et al. Expression profiles and function of toll-like receptors 2 and 4 in peripheral blood mononuclear cells of chronic hepatitis b patients. Clin Immunol (2008) 128:400–8. doi: 10.1016/j.clim.2008.04.006
26. Huang YW, Lin SC, Wei SC, Hu JT, Chang HY, Huang SH, et al. Reduced toll-like receptor 3 expression in chronic hepatitis b patients and its restoration by interferon therapy. Antivir Ther (2013) 18:877–84. doi: 10.3851/IMP2630
27. Deng G, Ge J, Liu C, Pang J, Huang Z, Peng J, et al. Impaired expression and function of TLR8 in chronic HBV infection and its association with treatment responses during peg-IFN-alpha-2a antiviral therapy. Clin Res Hepatol Gastroenterol (2017) 41:386–98. doi: 10.1016/j.clinre.2016.12.006
28. Xu N, Yao HP, Sun Z, Chen Z. Toll-like receptor 7 and 9 expression in peripheral blood mononuclear cells from patients with chronic hepatitis b and related hepatocellular carcinoma. Acta Pharmacol Sin (2008) 29:239–44. doi: 10.1111/j.1745-7254.2008.00711.x
29. Vincent IE, Zannetti C, Lucifora J, Norder H, Protzer U, Hainaut P, et al. Hepatitis b virus impairs TLR9 expression and function in plasmacytoid dendritic cells. PloS One (2011) 6:e26315. doi: 10.1371/journal.pone.0026315
30. Momeni M, Zainodini N, Bidaki R, Hassanshahi G, Daneshvar H, Khaleghinia M, et al. Decreased expression of toll like receptor signaling molecules in chronic HBV infected patients. Hum Immunol (2014) 75:15–9. doi: 10.1016/j.humimm.2013.09.015
31. Visvanathan K, Lang T, Ryan K, Wilson R, Skinner NA, Thompson AJ, et al. Toll-IL1 receptor-mediated innate immune responses vary across HBV genotype and predict treatment response to pegylated-IFN in HBeAg-positive CHB patients. J Viral Hepat (2016) 23:170–9. doi: 10.1111/jvh.12477
32. Heiberg IL, Winther TN, Paludan SR, Hogh B. Pattern recognition receptor responses in children with chronic hepatitis b virus infection. J Clin Virol (2012) 54:229–34. doi: 10.1016/j.jcv.2012.04.013
33. Xu N, Yao HP, Lv GC, Chen Z. Downregulation of TLR7/9 leads to deficient production of IFN-alpha from plasmacytoid dendritic cells in chronic hepatitis b. Inflammation Res (2012) 61:997–1004. doi: 10.1007/s00011-012-0493-z
34. Xu Y, Hu Y, Shi B, Zhang X, Wang J, Zhang Z, et al. HBsAg inhibits TLR9-mediated activation and IFN-alpha production in plasmacytoid dendritic cells. Mol Immunol (2009) 46:2640–6. doi: 10.1016/j.molimm.2009.04.031
35. Deng F, Xu G, Cheng Z, Huang Y, Ma C, Luo C, et al. Hepatitis b surface antigen suppresses the activation of nuclear factor kappa b pathway via interaction with the TAK1-TAB2 complex. Front Immunol (2021) 12:618196. doi: 10.3389/fimmu.2021.618196
36. Lang T, Lo C, Skinner N, Locarnini S, Visvanathan K, Mansell A. The hepatitis b e antigen (HBeAg) targets and suppresses activation of the toll-like receptor signaling pathway. J Hepatol (2011) 55:762–9. doi: 10.1016/j.jhep.2010.12.042
37. Liu D, Wu A, Cui L, Hao R, Wang Y, He J, et al. Hepatitis b virus polymerase suppresses NF-kappaB signaling by inhibiting the activity of IKKs via interaction with Hsp90beta. PloS One (2014) 9:e91658. doi: 10.1371/journal.pone.0091658
38. Wu J, Lu M, Meng Z, Trippler M, Broering R, Szczeponek A, et al. Toll-like receptor-mediated control of HBV replication by nonparenchymal liver cells in mice. Hepatology (2007) 46:1769–78. doi: 10.1002/hep.21897
39. Wu J, Meng Z, Jiang M, Pei R, Trippler M, Broering R, et al. Hepatitis b virus suppresses toll-like receptor-mediated innate immune responses in murine parenchymal and nonparenchymal liver cells. Hepatology (2009) 49:1132–40. doi: 10.1002/hep.22751
40. Jiang M, Broering R, Trippler M, Poggenpohl L, Fiedler M, Gerken G, et al. Toll-like receptor-mediated immune responses are attenuated in the presence of high levels of hepatitis b virus surface antigen. J Viral Hepatitis (2014) 21:860–72. doi: 10.1111/jvh.12216
41. Woltman AM, Op DBM, Biesta PJ, Shi CC, Janssen HL. Hepatitis b virus lacks immune activating capacity, but actively inhibits plasmacytoid dendritic cell function. PloS One (2011) 6:e15324. doi: 10.1371/journal.pone.0015324
42. van der Molen RG, Sprengers D, Binda RS, de Jong EC, Niesters HG, Kusters JG, et al. Functional impairment of myeloid and plasmacytoid dendritic cells of patients with chronic hepatitis b. Hepatology (2004) 40:738–46. doi: 10.1002/hep.20366
43. Asadi-Asadabad S, Sarvnaz H, Amiri MM, Mobini M, Khoshnoodi J, Hojjat-Farsangi M, et al. Influence of pattern recognition receptor ligands on induction of innate immunity and control of hepatitis b virus infection. Viral Immunol (2021) 34:531–41. doi: 10.1089/vim.2021.0040
44. Lucifora J, Bonnin M, Aillot L, Fusil F, Maadadi S, Dimier L, et al. Direct antiviral properties of TLR ligands against HBV replication in immune-competent hepatocytes. Sci Rep (2018) 8:5390. doi: 10.1038/s41598-018-23525-w
45. Boni C, Fisicaro P, Valdatta C, Amadei B, Di Vincenzo P, Giuberti T, et al. Characterization of hepatitis b virus (HBV)-specific T-cell dysfunction in chronic HBV infection. J Virol (2007) 81:4215–25. doi: 10.1128/JVI.02844-06
46. Peppa D, Gill US, Reynolds G, Easom NJW, Pallett LJ, Schurich A, et al. Up-regulation of a death receptor renders antiviral T cells susceptible to NK cell–mediated deletion. J Exp Med (2013) 210:99–114. doi: 10.1084/jem.20121172
47. Wu J, Meng Z, Jiang M, Zhang E, Trippler M, Broering R, et al. Toll-like receptor-induced innate immune responses in non-parenchymal liver cells are cell type-specific. Immunology (2010) 129:363–74. doi: 10.1111/j.1365-2567.2009.03179.x
48. Isogawa M, Robek MD, Furuichi Y, Chisari FV. Toll-like receptor signaling inhibits hepatitis b virus replication in vivo. J Virol (2005) 79:7269–72. doi: 10.1128/JVI.79.11.7269-7272.2005
49. Lan T, Chang L, Wu L, Yuan YF. IL-6 plays a crucial role in HBV infection. J Clin Transl Hepatol (2015) 3:271–6. doi: 10.14218/JCTH.2015.00024
50. Schurich A, Pallett LJ, Lubowiecki M, Singh HD, Gill US, Kennedy PT, et al. The third signal cytokine IL-12 rescues the anti-viral function of exhausted HBV-specific CD8 T cells. PloS Pathog (2013) 9:e1003208. doi: 10.1371/journal.ppat.1003208
51. Kimura K, Kakimi K, Wieland S, Guidotti LG, Chisari FV. Interleukin-18 inhibits hepatitis b virus replication in the livers of transgenic mice. J Virol (2002) 76:10702–7. doi: 10.1128/JVI.76.21.10702-10707.2002
52. Zhang E, Ma Z, Li Q, Yan H, Liu J, Wu W, et al. TLR2 stimulation increases cellular metabolism in CD8(+) T cells and thereby enhances CD8(+) T cell activation, function, and antiviral activity. J Immunol (2019) 203:2872–86. doi: 10.4049/jimmunol.1900065
53. Komai-Koma M, Jones L, Ogg GS, Xu D, Liew FY. TLR2 is expressed on activated T cells as a costimulatory receptor. Proc Natl Acad Sci U.S.A. (2004) 101:3029–34. doi: 10.1073/pnas.0400171101
54. Cottalorda A, Verschelde C, Marcais A, Tomkowiak M, Musette P, Uematsu S, et al. TLR2 engagement on CD8 T cells lowers the threshold for optimal antigen-induced T cell activation. Eur J Immunol (2006) 36:1684–93. doi: 10.1002/eji.200636181
55. Geng D, Zheng L, Srivastava R, Asprodites N, Velasco-Gonzalez C, Davila E. When toll-like receptor and T-cell receptor signals collide: a mechanism for enhanced CD8 T-cell effector function. Blood (2010) 116:3494–504. doi: 10.1182/blood-2010-02-268169
56. Li Q, Yan Y, Liu J, Huang X, Zhang X, Kirschning C, et al. Toll-like receptor 7 activation enhances CD8+ T cell effector functions by promoting cellular glycolysis. Front Immunol (2019) 10:2191. doi: 10.3389/fimmu.2019.02191
57. Tabiasco J, Devevre E, Rufer N, Salaun B, Cerottini JC, Speiser D, et al. Human effector CD8+ T lymphocytes express TLR3 as a functional coreceptor. J Immunol (2006) 177:8708–13. doi: 10.4049/jimmunol.177.12.8708
58. Bendigs S, Salzer U, Lipford GB, Wagner H, Heeg K. CpG-oligodeoxynucleotides co-stimulate primary T cells in the absence of antigen-presenting cells. Eur J Immunol (1999) 29:1209–18. doi: 10.1002/(SICI)1521-4141(199904)29:04<1209::AID-IMMU1209>3.0.CO;2-J
59. Kadowaki N. The divergence and interplay between pDC and mDC in humans. Front Biosci (Landmark Ed) (2009) 14:808–17. doi: 10.2741/3279
60. Golsaz-Shirazi F, Amiri MM, Shokri F. Immune function of plasmacytoid dendritic cells, natural killer cells, and their crosstalk in HBV infection. Rev Med Virol (2018) 28:e2007. doi: 10.1002/rmv.2007
61. Xie Q, Shen HC, Jia NN, Wang H, Lin LY, An BY, et al. Patients with chronic hepatitis b infection display deficiency of plasmacytoid dendritic cells with reduced expression of TLR9. Microbes Infect (2009) 11:515–23. doi: 10.1016/j.micinf.2009.02.008
62. Yonejima A, Mizukoshi E, Tamai T, Nakagawa H, Kitahara M, Yamashita T, et al. Characteristics of impaired dendritic cell function in patients with hepatitis b virus infection. Hepatology (2019) 70:25–39. doi: 10.1002/hep.30637
63. Sukriti S, Trehanpati N, Kumar M, Pande C, Hissar SS, Sarin SK. Functionally aberrant dendritic cell subsets and expression of DC-SIGN differentiate acute from chronic HBV infection. Hepatol Int (2016) 10:916–23. doi: 10.1007/s12072-016-9763-0
64. Zheng BJ, Zhou J, Qu D, Siu KL, Lam TW, Lo HY, et al. Selective functional deficit in dendritic cell–T cell interaction is a crucial mechanism in chronic hepatitis b virus infection. J Viral Hepat (2004) 11:217–24. doi: 10.1111/j.1365-2893.2004.00497.x
65. Tavakoli S, Mederacke I, Herzog-Hauff S, Glebe D, Grun S, Strand D, et al. Peripheral blood dendritic cells are phenotypically and functionally intact in chronic hepatitis b virus (HBV) infection. Clin Exp Immunol (2008) 151:61–70. doi: 10.1111/j.1365-2249.2007.03547.x
66. Bijker MS, van den Eeden SJ, Franken KL, Melief CJ, van der Burg SH, Offringa R. Superior induction of anti-tumor CTL immunity by extended peptide vaccines involves prolonged, DC-focused antigen presentation. Eur J Immunol (2008) 38:1033–42. doi: 10.1002/eji.200737995
67. Dou Y, van Montfoort N, van den Bosch A, de Man RA, Zom GG, Krebber WJ, et al. HBV-derived synthetic long peptide can boost CD4+ and CD8+ T-cell responses in chronic HBV patients ex vivo. J Infect Dis (2018) 217:827–39. doi: 10.1093/infdis/jix614
68. Dou Y, Jansen D, van den Bosch A, de Man RA, van Montfoort N, Araman C, et al. Design o TLR2-ligand-synthetic long peptide conjugates for therapeutic vaccination of chronic HBV patients. Antiviral Res (2020) 178:104746. doi: 10.1016/j.antiviral.2020.104746
69. Murata Y, Kawashima K, Sheikh K, Tanaka Y, Isogawa M. Intrahepatic cross-presentation and hepatocellular antigen presentation play distinct roles in the induction of hepatitis b virus-specific CD8(+) T cell responses. J Virol (2018) 92(21):e00920–18. doi: 10.1128/JVI.00920-18
70. Yan H, Zhong M, Yang J, Guo J, Yu J, Yang Y, et al. TLR5 activation in hepatocytes alleviates the functional suppression of intrahepatic CD8(+) T cells. Immunology (2020) 161:325–44. doi: 10.1111/imm.13251
71. Knolle PA, Bottcher J, Huang LR. The role of hepatic immune regulation in systemic immunity to viral infection. Med Microbiol Immunol (2015) 204:21–7. doi: 10.1007/s00430-014-0371-0
72. Bottcher JP, Knolle PA, Stabenow D. Mechanisms balancing tolerance and immunity in the liver. Dig Dis (2011) 29:384–90. doi: 10.1159/000329801
73. Huang S, Zou S, Chen M, Gao X, Chen L, Yang X, et al. Local stimulation of liver sinusoidal endothelial cells with a NOD1 agonist activates T cells and suppresses hepatitis b virus replication in mice. J Immunol (2018) 200:3170–9. doi: 10.4049/jimmunol.1700921
74. Liu J, Jiang M, Ma Z, Dietze KK, Zelinskyy G, Yang D, et al. TLR1/2 ligand-stimulated mouse liver endothelial cells secrete IL-12 and trigger CD8+ T cell immunity in vitro. J Immunol (2013) 191:6178–90. doi: 10.4049/jimmunol.1301262
75. Bottcher JP, Schanz O, Wohlleber D, Abdullah Z, Debey-Pascher S, Staratschek-Jox A, et al. Liver-primed memory T cells generated under noninflammatory conditions provide anti-infectious immunity. Cell Rep (2013) 3:779–95. doi: 10.1016/j.celrep.2013.02.008
76. Yuan F, Zhang W, Mu D, Gong J. Kupffer cells in immune activation and tolerance toward HBV/HCV infection. Adv Clin Exp Med (2017) 26:739–45. doi: 10.17219/acem/62759
77. Liu J, Yu Q, Wu W, Huang X, Broering R, Werner M, et al. TLR2 stimulation strengthens intrahepatic myeloid-derived cell-mediated T cell tolerance through inducing kupffer cell expansion and IL-10 production. J Immunol (2018) 200:2341–51. doi: 10.4049/jimmunol.1700540
78. Li M, Sun R, Xu L, Yin W, Chen Y, Zheng X, et al. Kupffer cells support hepatitis b virus-mediated CD8+ T cell exhaustion via hepatitis b core antigen-TLR2 interactions in mice. J Immunol (2015) 195:3100–9. doi: 10.4049/jimmunol.1500839
79. Uwatoku R, Suematsu M, Ezaki T, Saiki T, Tsuiji M, Irimura T, et al. Kupffer cell-mediated recruitment of rat dendritic cells to the liver: roles of n-acetylgalactosamine-specific sugar receptors. Gastroenterology (2001) 121:1460–72. doi: 10.1053/gast.2001.29594
80. Ebrahimkhani MR, Mohar I, Crispe IN. Cross-presentation of antigen by diverse subsets of murine liver cells. Hepatology (2011) 54:1379–87. doi: 10.1002/hep.24508
81. You Q, Cheng L, Kedl RM, Ju C. Mechanism of T cell tolerance induction by murine hepatic kupffer cells. Hepatology (2008) 48:978–90. doi: 10.1002/hep.22395
82. De Simone G, Andreata F, Bleriot C, Fumagalli V, Laura C, Garcia-Manteiga JM, et al. Identification of a kupffer cell subset capable of reverting the T cell dysfunction induced by hepatocellular priming. Immunity (2021) 54:2089–100. doi: 10.1016/j.immuni.2021.05.005
83. Du Y, Broering R, Li X, Zhang X, Liu J, Yang D, et al. In vivo mouse models for hepatitis b virus infection and their application. Front Immunol (2021) 12:766534. doi: 10.3389/fimmu.2021.766534
84. Wu J, Huang S, Zhao X, Chen M, Lin Y, Xia Y, et al. Poly(I:C) treatment leads to interferon-dependent clearance of hepatitis b virus in a hydrodynamic injection mouse model. J Virol (2014) 88:10421–31. doi: 10.1128/JVI.00996-14
85. Zou S, Du Y, Huang S, Chen M, Yang X, Li S, et al. Simultaneous or prior activation of intrahepatic type I interferon signaling leads to hepatitis b virus persistence in a mouse model. J Virol (2021) 95:e3421. doi: 10.1128/JVI.00034-21
86. Du Y, Yang X, Li J, Sokolova V, Zou S, Han M, et al. Delivery of toll-like receptor 3 ligand poly(I:C) to the liver by calcium phosphate nanoparticles conjugated with an F4/80 antibody exerts an anti-hepatitis b virus effect in a mouse model. Acta Biomater (2021) 133:297–307. doi: 10.1016/j.actbio.2021.01.045
87. Lin Y, Huang X, Wu J, Liu J, Chen M, Ma Z, et al. Pre-activation of toll-like receptor 2 enhances CD8(+) T-cell responses and accelerates hepatitis b virus clearance in the mouse models. Front Immunol (2018) 9:1495. doi: 10.3389/fimmu.2018.01495
88. Huang LR, Wohlleber D, Reisinger F, Jenne CN, Cheng RL, Abdullah Z, et al. Intrahepatic myeloid-cell aggregates enable local proliferation of CD8(+) T cells and successful immunotherapy against chronic viral liver infection. Nat Immunol (2013) 14:574–83. doi: 10.1038/ni.2573
89. Hahm KB, Han KY, Kim WH, Yim DS, Chon CY, Moon YM, et al. Efficacy of polyadenylic.polyuridylic acid in the treatment of chronic active hepatitis b. Int J Immunopharmacol (1994) 16:217–25. doi: 10.1016/0192-0561(94)90015-9
90. Agarwal K, Ahn SH, Elkhashab M, Lau AH, Gaggar A, Bulusu A, et al. Safety and efficacy of vesatolimod (GS-9620) in patients with chronic hepatitis b who are not currently on antiviral treatment. J Viral Hepat (2018) 25:1331–40. doi: 10.1111/jvh.12942
91. Gane EJ, Lim YS, Gordon SC, Visvanathan K, Sicard E, Fedorak RN, et al. The oral toll-like receptor-7 agonist GS-9620 in patients with chronic hepatitis b virus infection. J Hepatol (2015) 63:320–8. doi: 10.1016/j.jhep.2015.02.037
92. Janssen H, Brunetto MR, Kim YJ, Ferrari C, Massetto B, Nguyen AH, et al. Safety, efficacy and pharmacodynamics of vesatolimod (GS-9620) in virally suppressed patients with chronic hepatitis b. J Hepatol (2018) 68:431–40. doi: 10.1016/j.jhep.2017.10.027
93. Boni C, Vecchi A, Rossi M, Laccabue D, Giuberti T, Alfieri A, et al. TLR7 agonist increases responses of hepatitis b virus-specific T cells and natural killer cells in patients with chronic hepatitis b treated with Nucleos(T)Ide analogues. Gastroenterology (2018) 154:1764–77. doi: 10.1053/j.gastro.2018.01.030
94. Jo J, Tan AT, Ussher JE, Sandalova E, Tang XZ, Tan-Garcia A, et al. Toll-like receptor 8 agonist and bacteria trigger potent activation of innate immune cells in human liver. PloS Pathog (2014) 10:e1004210. doi: 10.1371/journal.ppat.1004210
95. Mackman RL, Mish M, Chin G, Perry JK, Appleby T, Aktoudianakis V, et al. Discovery of GS-9688 (Selgantolimod) as a potent and selective oral toll-like receptor 8 agonist for the treatment of chronic hepatitis b. J Med Chem (2020) 63:10188–203. doi: 10.1021/acs.jmedchem.0c00100
96. Amin OE, Colbeck EJ, Daffis S, Khan S, Ramakrishnan D, Pattabiraman D, et al. Therapeutic potential of TLR8 agonist GS-9688 (Selgantolimod) in chronic hepatitis b: Remodeling of antiviral and regulatory mediators. Hepatology (2021) 74:55–71. doi: 10.1002/hep.31695
Keywords: Toll-like receptor, hepatitis B virus, adaptive immunity, innate immunity, T cell response
Citation: Du Y, Wu J, Liu J, Zheng X, Yang D and Lu M (2022) Toll-like receptor-mediated innate immunity orchestrates adaptive immune responses in HBV infection. Front. Immunol. 13:965018. doi: 10.3389/fimmu.2022.965018
Received: 09 June 2022; Accepted: 30 June 2022;
Published: 29 July 2022.
Edited by:
Laure Perrin-Cocon, Institut National de la Santé et de la Recherche Médicale (INSERM), FranceReviewed by:
Sonja I. Buschow, Erasmus Medical Center, NetherlandsCopyright © 2022 Du, Wu, Liu, Zheng, Yang and Lu. This is an open-access article distributed under the terms of the Creative Commons Attribution License (CC BY). The use, distribution or reproduction in other forums is permitted, provided the original author(s) and the copyright owner(s) are credited and that the original publication in this journal is cited, in accordance with accepted academic practice. No use, distribution or reproduction is permitted which does not comply with these terms.
*Correspondence: Mengji Lu, bWVuZ2ppLmx1QHVuaS1kdWUuZGU=
Disclaimer: All claims expressed in this article are solely those of the authors and do not necessarily represent those of their affiliated organizations, or those of the publisher, the editors and the reviewers. Any product that may be evaluated in this article or claim that may be made by its manufacturer is not guaranteed or endorsed by the publisher.
Research integrity at Frontiers
Learn more about the work of our research integrity team to safeguard the quality of each article we publish.