- 1Guangdong-Hong Kong-Macau Joint Lab on Chinese Medicine and Immune Disease Research, Clinical and Basic Research Team of Traditional Chinese Medicine (TCM) Prevention and Treatment of Non small cell lung cancer (NSCLC), Department of Oncology, The Second Clinical College of Guangzhou University of Chinese Medicine, Guangdong Provincial Hospital of Chinese Medicine, Guangdong Provincial Key Laboratory of Clinical Research on Traditional Chinese Medicine Syndrome, Guangzhou University of Chinese Medicine, Guangzhou, China
- 2Department of Organ Transplantation, Second Affiliated Hospital of Guangzhou Medical University, Guangzhou, China
- 3Institute of Rehabilitation Medicine, Shanghai University of Traditional Chinese Medicine, Shanghai, China
- 4Department of Cerebrovascular Disease, Guangdong Provincial Hospital of Chinese Medicine, The Second Clinical College of Guangzhou University of Chinese Medicine, Guangzhou, China
- 5Department of Oncology, The Second Affiliated Hospital of Guangzhou University of Chinese Medicine, Guangdong Provincial Hospital of Chinese Medicine, Guangzhou, China
- 6State Key Laboratory of Dampness Syndrome of Chinese Medicine, The Second Affiliated Hospital of Guangzhou University of Chinese Medicine, Guangdong Provincial Hospital of Chinese Medicine, Guangzhou, China
- 7Guangdong Provincial Key Laboratory of Clinical Research on Traditional Chinese Medicine Syndrome, The Second Affiliated Hospital of Guangzhou University of Chinese Medicine, Guangzhou, China
Programmed cell death protein-1 (PD-1) is a checkpoint receptor expressed on the surface of various immune cells. PD-L1, the natural receptor for PD-1, is mainly expressed in tumor cells. Studies have indicated that PD-1 and PD-L1 are closely associated with the progression of human cancers and are promising biomarkers for cancer therapy. Moreover, the interaction of PD-1 and PD-L1 is one of the important mechanism by which human tumors generate immune escape. This article provides a review on the role of PD-L1/PD-1, mechanisms of immune response and resistance, as well as immune-related adverse events in the treatment of anti-PD-1/PD-L1 immunotherapy in human cancers. Moreover, we summarized a large number of clinical trials to successfully reveal that PD-1/PD-L1 Immune-checkpoint inhibitors have manifested promising therapeutic effects, which have been evaluated from different perspectives, including overall survival, objective effective rate and medium progression-free survival. Finally, we pointed out the current problems faced by PD-1/PD-L1 Immune-checkpoint inhibitors and its future prospects. Although PD-1/PD-L1 immune checkpoint inhibitors have been widely used in the treatment of human cancers, tough challenges still remain. Combination therapy and predictive models based on integrated biomarker determination theory may be the future directions for the application of PD-1/PD-L1 Immune-checkpoint inhibitors in treating human cancers.
Introduction
PD-1 is a representative immunosuppressive checkpoint and mainly expressed in macrophages, B lymphocytes, dendritic cells (DCs), monocytes, tumor-specific activated T cells, myeloid cells and natural killer (NK) cells under conditions of chronic antigen exposure (1–3). PD-L1 is one of the PD-1 ligands. PD-L1 expression has been shown to be a valuable biomarker for the prognosis and prediction of the sensitivity of PD-1/PD-L1 inhibitors. The expression of PD-L1 is mainly expressed in tumor cells, tumor-infiltrating cells and antigen-presenting cells (APCs) in many cancers (1, 4). In recent years, a number of studies have confirmed the clinical significance of PD-1/PD-L1 antibodies and their prognostic impact on human cancers (5, 6). However, the relationship between this biomarker and its clinical significance is imperfect and varies in different types of human cancers (7).
In general, PD-1/PD-L1 inhibitory checkpoints suppress T cell receptor-mediated cytotoxicity and CD8+ T cell proliferation by interacting with the ligand PD-L1, thus avoiding the killing effect of the autoimmune system on tumor cells and immune surveillance (8–10). Immune checkpoint antibodies as promising cancer therapeutic strategies are based on their natural role acting as T cell-activated co-inhibitory receptors. Undoubtedly, the co-stimulatory and co-inhibitory receptors of T cells play an important role in the treatment of PD-1/PD-L1 immune checkpoint inhibitors (11). Expression of PD-L1 in tumor cells or tumor-associated stromal cells is a potential predictive marker for response and outcome of anti-PD-1/PD-L1 immunotherapy (1, 12).
Despite the remarkable efficacy of PD-1/PD-L1 immunocheckpoint inhibitors in the treatment of tumors, some problems also remain, such as drug resistance and adverse events. The presence of drug resistance significantly reduces the efficacy of anti-PD-1/PD-L1 immunotherapy. Exploring the mechanisms of PD-1/PD-L1 immunocheckpoint inhibitors resistance will contribute to the discovery of new immunotherapeutic strategies to control disease progression and provide a more sustainable survival benefit for patients (13). In addition, PD-1/PD-L1 immunocheckpoint inhibitors acting as immunomodulatory drugs can significantly enhance the natural defense of the immune system against cancers, while inevitably leading to some immune-related adverse events, the erroneous stimulation of the immune system leads to immune injuries to the normal tissues of the body (14). Therefore, in order to further improve the treatment outcome and reduce the risk of patients, it is necessary to learn more about the immune-related adverse events of PD-1/PD-L1 immunocheckpoint inhibitors in the treatment of human cancers.
In any case, we believe that tumor immunotherapy based on PD-1/PD-L1 inhibitors will become a promising strategy for human cancers. This article will focus on the role of PD-1/PD-L1 and the application of PD-1/PD-L1 inhibitors in human cancers.
Mechanisms of tumor immunotherapy based on PD-1/PD-L1
At present, the immunotherapy mechanisms of anti-PD-1/PD-L1 antibody have been relatively clear. The activation of T cells relies mainly on dual signals. The first signal consists of the binding of MHC-presenting antigen to T cell receptor (TCR). The second signal constitute by co-stimulatory and co-inhibitory signals (15). The interaction between PD-1 on T cells and PD-L1 on tumor cells or APCs can effectively inhibit T cell activation and even cause T cell apoptosis, decreased cytokine production, t-cell lysis and induction of tolerance to antigen, thus making the tumor escape from the immune surveillance (16). PD-1/PD-L1 inhibitors respectively bind to PD-1 or PD-L1 to prevent the interaction between PD-1 and PD-L1, then the recognition and killing effect of immune cells is restored and the immune escape of tumor cells is avoided (Figure 1).
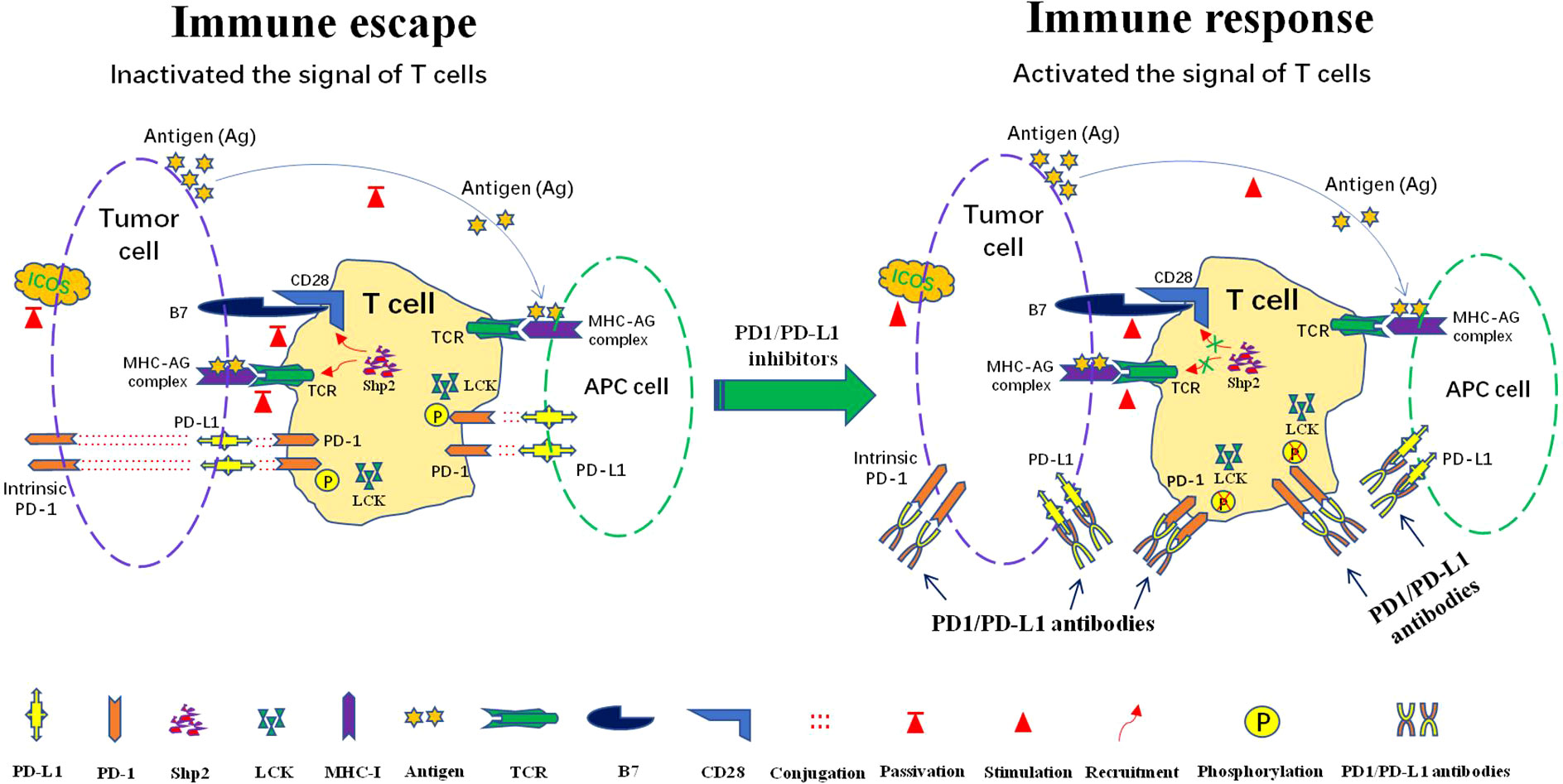
Figure 1 Mechanisms of the response to anti-PD1/PD-L1 immunotherapy: PD-L1 is expressed in tumor cells and antigen presenting cells (APCs). PD-1 is mainly expressed in T cells, some tumor cells also express intrinsic PD-1. Immune escape occur after interaction of PD-1 and PD-L1. PD-1 can be phosphorylated by LCK to recruit tyrosine phosphatase Shp2, consequently inactivating CD28 and T cell receptor (TCR) function and signaling pathway, thus attenuating the activation signal of T cells and causing immune escape. Lck kinase activity is required to maintain PD-1/Shp2-mediated inhibitory signaling. The intervene of PD-1/PD-L1 immunocheckpoint inhibitors can effectively block the interaction between PD-L1 and PD-1, which in turn blocks the recruitment of SHP-2 and reactivates T cells signal for immune function.
PD-1 activation significantly inhibits TCR signaling, CD28 co-stimulatory signaling and inducible T cell co-stimulator(ICOS) signaling (17–19). Recent studies have suggested that after activation by its ligand PD-L1, PD-1 is phosphorylated by protein tyrosine kinase Lck to recruit tyrosine phosphatase Shp2 (Src homologous phosphatase 2), followed by dephosphorylation of TCR and CD28, and subsequent inhibition of T cell-associated signaling (20–23)(Figure 1). When PD-1/PD-L1 immune checkpoint inhibitors intervene, the intramembrane motif of PD-1 cannot be phosphorylated by lymphocyte-specific protein tyrosine kinase (LCK), resulting in the failure of cell recruitment to SHP-2. TCR and CD28 dephosphorylation is blocked, which leads to efficient delivery of activation signals to downstream proteins and signaling pathways, ultimately stimulating T cell proliferation and differentiation. Eventually, the immune function of T cells is effectively performed (24) (Figure 1). Interestingly, some tumor cells also express intrinsic PD-1 to promote the occurrence and development of tumors independent of adaptive immunity. PD-1 checkpoint inhibitors can also block the binding of intrinsic PD-1 and PD-L1 to inhibit tumor growth (25, 26) (Figure 1).
Tumor cells evolve themselves to lose the ability to present tumor antigens in order to avoid recognition by cytotoxic T cells and APCs (27). Recent studies showed that major histocompatibility complex class-I and -II (MHC-I and MHC-II) were required for tumor antigen presentation and immunosurveillance (28–30). In many malignancies, downregulation of MHC-I/II is associated with immunosuppression, metastatic progression and poor prognosis, as well as predict anti-PD-1/PD-L1 therapy response (31). Researchers have attempted to find ways to upregulate MCH-II expression in tumor cells with a view to improve the response rate to PD-1/PD-L1 immunotherapy. They found that epigenetic and ERK signaling cascades were effective in suppressing the expression of intrinsic MHC II in non-small cell lung cancer (32). Therefore, the combined blocking strategy for these pathways may generate a novel positive response to PD-1/PD-L1 immune checkpoint therapy in human cancers. In addition, lung epithelial MHC-II was needed for surface expression of PD-L1 (33). The results of a clinical study showed that recurrent or metastatic nasopharyngeal carcinoma with high expression of both MHC-II and PD-L1 responded better to treatment with camrelizumab (anti-PD-1) (34). In conclusion, the above results suggest that MHC-II and PD-L1 influence each other not only in expression but also in function for the treatment of PD-1/PD-L1 Immune-checkpoint inhibitors in human cancer.
Mechanisms of PD-1/PD-L1 inhibitors resistance
In recent years, immune checkpoints blockade therapy targeting the PD-1/PD-L1 axis has pushed tumor immunotherapy to a new revolutionary-like milestone and achieved surprising therapeutic effects in a variety of malignancies. However, most patients have developed resistance to PD-1/PD-L1 inhibitors, which severely limits its application and becomes a serious clinical problem that cannot be ignored in this field. Therefore, it is urgent to deeply reveal the molecular mechanism of immune checkpoint inhibitor resistance and improve the response rate of patients to PD-1/PD-L1 immunotherapy. Herein, we have summarized the molecular mechanisms of resistance to common PD-1/PD-L1 immune checkpoint inhibitors (Figure 2).
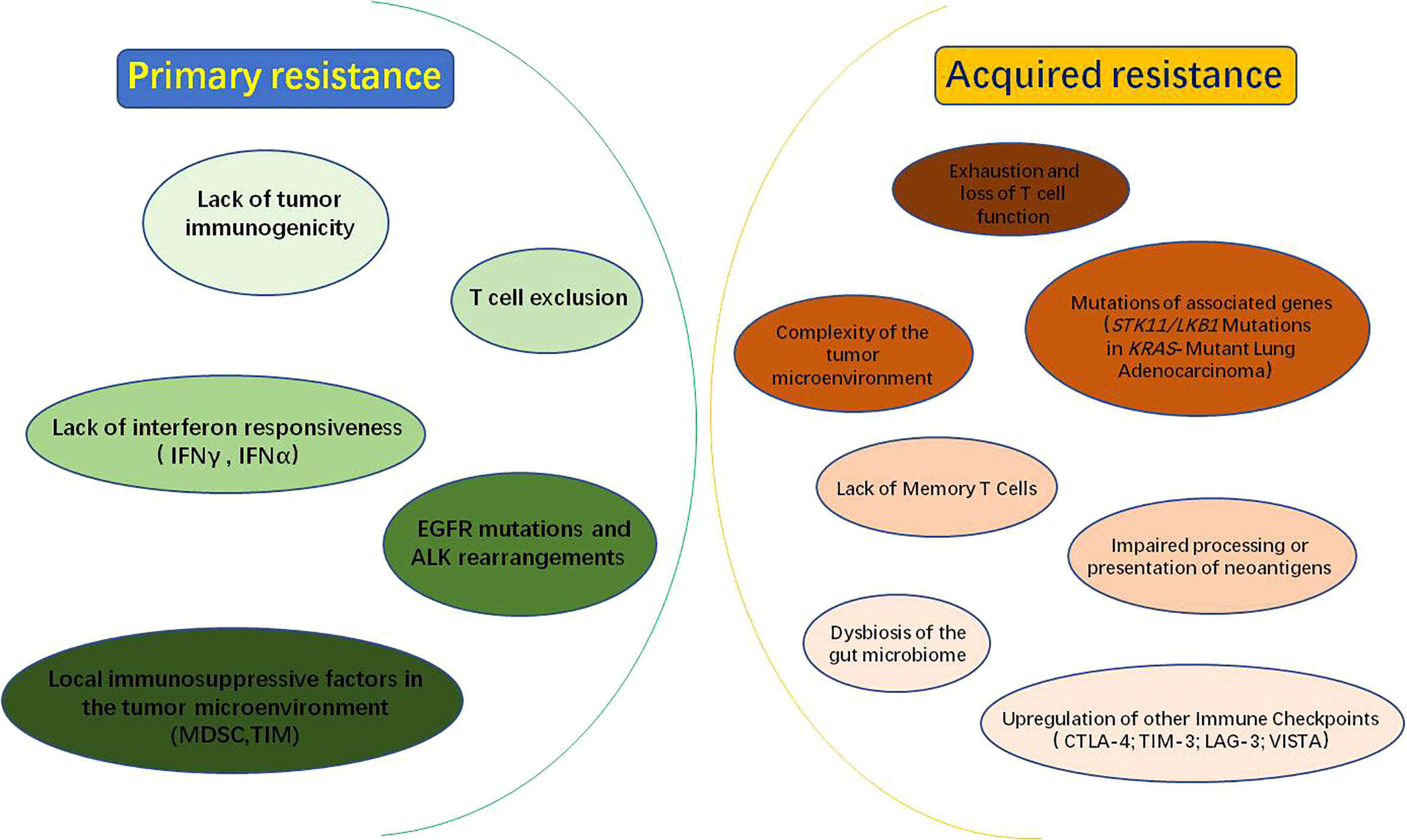
Figure 2 Mechanisms of PD-1/PD-L1 inhibitors resistance: PD-1/PD-L1 inhibitor resistance is divided into primary resistance and acquired resistance. Mechanisms of primary resistance include lack of tumor immunogenicity; T-cell rejection; lack of interferon responsiveness, such as IFNγ (interferon Gamma) and IFNα (interferon alpha); EGFR (epidermal growth factor receptor) mutations and ALK (anaplastic lymphoma kinase) rearrangements; local immunosuppressive factors within the tumor microenvironment, such as MDSC (myeloid-derived suppressor cell) and TIM (tumor-infiltrating myeloid cell). While, the mechanisms of acquired resistance may be related to the following factors: exhaustion and loss of T cell function; impaired processing or presentation of neoantigens; complexity of the tumor microenvironment; mutations in associated genes, such as STK11/LKB1; dysbiosis of the gut microbiome; lack of Memory T Cells and upregulation of other Immune Checkpoints, such as CTLA-4(cytotoxic T-lymphocyte antigen-4), TIM-3(T cell immunoglobulin and mucin domain-containing molecule-3), LAG-3(lymphocyte activation gene-3) and VISTA(V-domain Ig suppressor of T cell activation).
The resistance to PD-1/PD-L1 inhibitors includes primary resistance and acquired resistance. Primary resistance is defined as patients who have never shown clinical response or stable disease when using PD-1/PD-L1 blockade. The mechanism of primary resistance includes lack of tumor immunogenicity (35), T cell exclusion (36), lack of interferon responsiveness (37), epidermal growth factor receptor (EGFR) mutations and anaplastic lymphoma kinase(ALK) rearrangements (38), local immunosuppressive factors in tumor microenvironment (39) and other factors (Figure 2). While acquired drug resistance means that PD-1/PD-L1 inhibitors show a durable and effective response at the beginning of treatment, but therapeutic effect of inhibitors is significantly diminished or non-responsive after a period of treatment for some patients (40). The mechanisms may be closely associated with exhaustion and loss of T cell function (41–43), impaired processing or presentation of neoantigens (44), complexity of the tumor microenvironment (45), mutations in associated genes (46), dysbiosis of the gut microbiome (47), lack of Memory T Cells (48) and upregulation of others immune checkpoints (49) (Figure 2).
Tumor cells may interact closely with stromal cells, immune cells, other suppressive immune checkpoints and cytokines in the surrounding environment, thus protecting them from detection and elimination by immune surveillance (45). In general, T lymphocytes accomplish immune clearance of tumors by recognizing specific antigens on the surface of tumor cell membranes, thereby killing tumor cells. Therefore, effective tumor-specific antigen is an important factor for the efficacy of immune response. If the structure of the specific antigen is similar to the immune tolerance antigen or autoantigen, resulting in the inability of APCs to recognize and initiate T-cell activation, thus acquired resistance may developed (50, 51). In addition, some tumors cause a decrease of normal mature dendritic cells (DCs) and an increase in the number of immature DCs by secreting certain suppressors, such as IL-10 and VEGF. When tumors recruit these immature DCs, effector T cells are not effectively activated during antigen presentation (24, 52). These patients will fail to generate an effective immune response with PD-1/PD-L1 blockers, resulting in drug resistance and immune escape.
Main clinical trials and outcomes for PD-1/PD-L1 inhibitors in the treatment of human cancers
In recent years, a large number of clinical trials have been conducted with PD-1/PDL1 Immune-checkpoint inhibitors, and their therapeutic effects have been evaluated from different perspectives, including overall survival (OS), objective response rate (ORR) and medium progression-free survival (PFS), respectively (53–55). Herein, we reviewed some clinical trials of PD-1/PDL1 immunocheckpoint inhibitors (Table 1). We found that the same inhibitor has completely different therapeutic effects and responsiveness against different cancers (Table 1). This will provide better reference for the selection of PD1/PD-L1 inhibitors for different cancers in future clinical practice.
Immune-related adverse events caused by PD-1/PD-L1 inhibitors in the treatment of human cancers
Over the past few decades, cancer immunotherapies represented by PD-1/PD-L1 immune checkpoint inhibitors have changed the landscape of cancer treatment. However, this has also inevitably led to some immune-related adverse events (irAEs), and these irAEs are usually characterized by long duration and delayed onset (58, 78–86). In this study, we have reviewed some of the common immune-related adverse events associated with PD-1/PD-L1 immune checkpoint inhibitors for cancer treatment (Table 2).
PD-1/PD-L1 and inhibitors in human solid cancers
Lung cancer
Lung cancer is the most common cancer and the leading cause of cancer death worldwide. Fortunately, the advent of immune checkpoint inhibitors has improved the outlook for patients with advanced lung cancers. Tumor immunotherapy targeting PD-1/PD-L1 have revolutionized the treatment of lung cancer (70, 72, 90).
Elevated PD-L1 expression correlates with higher efficacy of immunotherapy, implying that PD-L1 has high predictive value as a cancer biomarker (62, 91). The anticancer efficacy of PD-L1 inhibitors is significantly better than that of chemotherapy in advanced non-small cell lung cancer (NSCLC) patients with high PD-L1 expression (58),as well as in patients with previously untreated metastatic squamous NSCLC (60). Remarkably, PD-L1 expression may also be induced by chemotherapy or targeted therapies (92). Therefore, if PD-L1 protein expression is to be used as a biomarker to guide immunotherapy, fresh specimens may need to be collected after other treatments and before the start of immunotherapy to assess PD-L1 expression. Moreover, tumor microenvironment plays an important role in the anticancer effect of PD-1/PD-L1 immunocheckpoint inhibitors. Recent studies have shown that low-dose apatinib (VEGFR2-TKI) significantly improves the therapeutic effect of PD-1/PD-L1 inhibitors by modulating the tumor microenvironment, delaying tumor growth, reducing the number of metastases and prolonging survival in mouse models (93).
In patients with lung cancer, PD-1 and PD-L1 can be detected not only in tissues but also in the serum and plasma of patients (94–96). The clinical diagnosis and prevention of soluble PD-1 and PD-L1 lung cancer in blood is of great importance because blood samples are easily available and easily detectable (96). By detecting the expression levels of PD-1 and PD-L1 in the blood of lung cancer patients, the drug treatment regimen of PD-1 and PD-L1 can be formulated based on the test results, and the patients’ response to immunotherapy can be further assessed. The purpose of individualized treatment of lung cancer is absolutely achieved.
Unfortunately, although many patients have achieved long-term survival benefits with PD-1/PD-L1 inhibitors, some patients have experienced rapid tumor progression after immunotherapy, known as hyperprogressive disease (HPD) (97, 98). In pretreated NSCLC patients, HPD is more common with PD-1/PD-L1 inhibitors compared to chemotherapy, and patients treated with PD-1/PD-L1 inhibitors are also associated with a high metastatic burden and poor prognosis (73). Currently, the combination of PD-1/PD-L1 immune checkpoint inhibitors with other antitumor agents has become an important treatment strategy. For example, the combination of pembrolizumab antibody (anti-PD-1) with carboplatin plus paclitaxel reduced the risk of death in advanced NSCLC. Atezolizumab antibody(anti-PD-L1) combined with carboplatin plus paclitaxel also improved the treatment outcome in advanced NSCLC (99).
The rapid development of anti-PD-1/PD-L1 inhibitors for advanced NSCLC has greatly improved patient prognosis. However, the vast majority of NSCLC patients are ineffective to PD-(L)1 blockade. Therefore, more clinical trials are required to explore immunomodulatory pathways in an effort to enhance non-responders or hyposensitive individuals to achieve desired therapeutic outcomes. In addition, understanding the mechanisms of resistance to immune checkpoint inhibitors will help determine combination therapy strategies for advanced lung cancer.
Breast cancer
In the past few years, anti-PD-1/PD-L1 antibodies have shown promising therapeutic effects, and great anti-tumor effects have been observed when used alone or in combination with conventional treatment. However, immunotherapy is still rarely used in the treatment of breast cancer. In fact, breast cancer is generally thought to have a weaker immunogenicity than other types of tumors (100).
Encouragingly, specific PD-1/PD-L1 antibodies can effectively block PD-1 or PD-L1 in breast cancer. Especially, metastatic triple negative breast cancer (mTNBC) shows a potential response to PD-1/PD-L1inhibitors. For example, pembrolizumab antibody(anti-PD-1) has significant antitumor activity and safety in patients with PD-L1-positive mTNBC (16, 59). Moreover, atezolizumab antibody (anti-PD-L1) effectively prolong progression-free survival in patients with mTNBC, and paclitaxel enhanced the therapeutic effect of atezolizumab (69). In addition, patients with metastatic breast cancer (MBC) were treated with avelumab (anti-PD-L1) for 2-50 weeks and followed up for 6-15 months. The results showed that the objective response rate (ORR) was significantly increased in patients with PD-L1 positive tumor-associated immune cells, which suggested that PD-L1 is associated with higher response rates to avelumab in patients with MBC (74).
However, it has also been suggested that PD-1 inhibitors are less effective in mTNBC and that better strategies should be adopted to make the tumor microenvironment more sensitive to PD-L1 inhibitors. For example, short-term adriamycin and cisplatin may induce a more favorable tumor microenvironment in mTNBC and increase the anticancer effect of PD-1 blockers (101). In the past, chemotherapy was the standard first-line treatment for mTNBC, but the efficacy was not satisfactory. Recent study have found that the combination of PD-1/PD-L1 Immune-checkpoint inhibitors and chemotherapy may be a new promising clinical paradigm for the treatment of triple-negative breast cancer (102).
Moreover, PD-L1 expression is associated with high-risk clinicopathological parameters and poor prognosis in patients with primary breast cancer (PBC). A meta-analysis, including 47 studies with a total of 14,367 PBC patients, suggested that PD-L1 high expression associates with large tumor size, histologic grade, Ki-67 high level, ER and PR negative, TNBC subtype and shorter survival time (103). In addition, the expression of PD-L1 in breast cancer stem cells has attracted interest in recent years, and studies have found a significant increase in PD-L1 protein in breast cancer stem cells; therefore, targeting PD-L1 in stem cells may become a new promising therapeutic strategy for breast cancer (104). In any case, PD-1 and PD-L1 have been increasingly studied in breast cancer in recent years, and PD-1/PD-L1 immunocheckpoint inhibitors have shown promising applications in the treatment of breast cancer.
Gastric cancer
Gastric cancer (GC) is a common malignancy and the third leading cause of cancer death worldwide. The 5-year survival rate of patients with advanced gastric cancer was only 5% and 20%, and the median overall survival rate was 10 months. Advanced gastric cancer has a poor prognosis and limited therapeutic options (89, 105, 106). Therefore, it is urgent to explore some new molecular targets and treatments.
Clinical studies have confirmed the efficacy of programmed cell death 1 (PD-1)-targeted therapy for patients with metastatic GC. For example, the anti-PD-1 antibody pembrolizumab has promising anti-tumor activity and manageable toxicity in the treatment of patients with recurrent or metastatic GC (61). Moreover, anticancer effect and responsiveness of pembrolizumab are closely related to PD-L1 expression. Pembrolizumab has a significantly higher ORR in PD-L1-positive GC than in PD-L1-negative GC (56).
It is well known that autophagy is a highly conserved homeostasis process that plays a key role in tumor formation, cell survival, cell metabolism, immune response and tumorigenesis (107–109). Recent studies have shown that autophagy is highly associated with the expression level of PD-L1. Autophagy inhibition increases the expression of PD-L1 in GC, autophagy-related protein LC3 expression is also positively correlated with PD-L1 in primary GC (110). Therefore, autophagy may be closely related to anti-PD-1/PD-L1 immunotherapy in human GC.
Although anti-PD-1/PD-L1 immunotherapy is widely recognized in the clinical practice of gastric cancer, some studies have questioned that single PD-1/PD-L1 inhibitor do not result in relative improvements in OS and PFS compared with chemotherapy in patients with advanced GC or gastroesophageal junction cancer. However, they also determined that PD-1/PD-L1 inhibitors appear to enhance antitumor activity in patients with advanced gastric junction cancers (111). Therefore, further randomized clinical trials are needed to confirm those findings.
Angiosarcoma
Angiosarcoma (AS) is rare malignant endothelial-cell tumors of vascular or lymphatic origin, and is among the most aggressive subtypes of soft-tissue sarcomas (112, 113). In recent years, immunotherapy targeting PD-1/PD-L1 has become a hotspot in the treatment of AS (114, 115). A recent study analyzed PD-L1 expression levels in angiosarcomas at different sites in humans and showed that PD-L1 was abnormally expressed in about 66% of the samples (116). In addition, a 63-year-old male patient with nasal AS that received pembrolizumab 2 mg/kg every 21 days for 13 cycles had no new tumor progression during the 8 months after therapy, which suggested that PD-1/PD-L1 immune checkpoint inhibitors have significant efficacy against angiosarcomas (117).
Cutaneous angiosarcoma (CAS) is the most common form of AS. Positive PD-L1 expression predicts worse outcome in CAS (118). Malignant progression and prognosis of CAS are closely associated not only with high expression of PD-L1, but also with the presence of tumor-infiltrating lymphocytes (TILs) (114). Since high PD-L1 expression is closely related to the progression of CAS, it is also critical to explore upstream regulators that can increase PD-L1 expression. Recent study revealed that PD-L1 expression was closely associated with atypical protein kinase C lambda/iota (aPKCλ). Inhibition of aPKCλ expression in HUVECs significantly reduced the expression of PD-L1 (119). Therefore, the combination of immune checkpoint inhibitors and aPKC inhibitors may be a potential therapeutic strategy for patients with CAS.
With the development of genomic sequencing technology, treatment strategies for advanced diseases have advanced significantly. Whole genome sequencing (WGS) can provide valuable information for treatment of PD-1/PD-L1 Immune-checkpoint inhibitors in the treatment of AS. For example, patients with metastatic AS who underwent WGS analysis were found to have hypermutated tumor characteristics associated with a positive response to PD-1 Immune-checkpoint inhibitors (120). Subsequently, corresponding scheme was established for the patient to receive the anti-PD-1 antibody pembrolizumab, and the metastases almost completely disappeared after 4 weeks therapy (120). Taken together, PD-1/PD-L1 expression is related to AS progression, and growing evidence suggests that the treatment with PD-1/PD-L1 immunocheckpoint inhibitors may be a promising strategy for AS patients.
Prostate cancer
Prostate cancer (PC) remains the most commonly diagnosed malignant disease in men worldwide (121). At present, PD-1/PD-L1 immunocheckpoint inhibitors have brought significant clinical benefits to some patients with PC. Further study will help guide the development of immunotherapy for advanced PC (122, 123). Patients with high density of PD-1+ lymphocytes were at significantly higher risk of clinical failure, and it was positive association between a high density of PD-1+ lymphocytes and worse clinical failure-free survival (124). Pembrolizumab(anti-PD-1) achieve durable objective responses in a group of severely pretreated patients with advanced PD-L1 positive PC (125). Moreover, recent study suggested that the combination of PD-1/PD-L1 checkpoint inhibitors and radiotherapy was a promising strategy for treating PC (126). Interestingly, PD-1/PD-L1 inhibitors significantly enhanced the efficacy of SA-GM-CSF surface-modified tumor vaccines against PC, which may be a new application for PD-1/PD-L1 inhibitors in the treatment of PC (127). In addition, PD-1/PD-L1 inhibitors had potential of lasting response to microsatellite instability-high (MSI-H) or defective mismatch repair (dMMR) molecular phenotype of prostate cancer (128). Nevertheless, more relevant studies may be needed to confirm this issue.
However, it was reported that PD-L1/PD-1 blockade had a poor effect in PC, due to the low immunogenicity of PC. Early clinical trials confirmed that patients with metastatic castration-resistant prostate cancer (mCRPC) did not significantly respond to PD-1 inhibitors, and they believed that loss of PTEN is responsible for upregulation of PD-L1, followed by constituting innate immune resistance (129). However, subsequent studies revealed that high PD-L1 expression is not significantly related to the loss of PTEN, but rather to the regulation of inflammatory cytokines (130).
All in all, although PD-1/PD-L1 Immune-checkpoint inhibitors have made revolutionary breakthroughs in the treatment of a wide range of human cancers, only a small percentage of prostate cancer patients have achieved significant clinical benefit. However, many experts support that we should still encourage clinical trials with PD-1/PD-L1 inhibitors in PC patients and exploring the mechanisms of PD-1/PD-L1 inhibitors resistance will optimize treatment options and guide the next steps in immunotherapy for PC.
Colorectal carcinoma
Colorectal cancer (CRC) is one of the most common neoplasms accompanied by a high rate of morbidity and mortality, immune checkpoint molecules have been identified as a novel treatment for CRC, such as PD-1 and PD-L1 (131, 132).
Currently, several clinical studies gave the clinical conclusions for PD-1 inhibitors in CRC with dMMR and MSI-H (133, 134). In a non-randomized phase II clinical trial enrolling 74 metastatic CRC(mCRC) patients with dMMR/MSI-H, patients were treated with nivolumab antibody (anti-PD-1) with relatively satisfactory clinical results. The results showed that ORR is 31.1% was achieved, disease control longer than 12 weeks was achieved in 69% of patients, twelve months PFS was 50.4% and 12 months OS was 73.4% (135). Immune checkpoint inhibitors have achieved clearer therapeutic effect in mCRC with dMMR or high microsatellite instability (MSI-H) (133, 136). However, patients with proficient mismatch repair(pMMR) or microsatellite stable (MSS) tumors have not gained enough benefit from immunotherapy (137). This may be related to the higher expression of PD-1 and PD-L1 in dMMR tumors compared to pMMR tumors (138). For pMMR CRC patients with poor responsiveness to immunotherapy, recent study has demonstrated a significant synergistic inhibitory effect of pembrolizumab(anti-PD-1) combined with ibrutinib (139). The combination of pembrolizumab and azacitidine for chemotherapy-refractory mCRC has also achieved a safe, tolerable and positive clinical efficacy (140). Moreover, the chemotherapy agent FOLFOXIRI plus bevacizumab is known to increase the immunogenicity of pMMR or MSS tumors. Importantly, the addition of atezolizumab(anti-PD-L1) to the first-line FOLFOXIRI plus bevacizumab significantly improve progression-free survival in patients with previously untreated metastatic colorectal cancer (141). Taken together, the application of PD-1/PD-L1 inhibitors in combination with other antitumor agents will bring light to the treatment of refractory or metastatic CRC.
It is generally believed that PD-1/PD-L1 cause immune escape from tumors by inhibiting tumor immune processes. Reduction of T cell cytotoxicity may be one of the key mechanisms of tumor PD-L1-induced inhibition of antitumor immunity. A recent study suggested that PD-L1 expressed in CRC significantly inhibited the cytotoxicity of CD8+ T cells, which led to tumor immune escape (142). In addition, tumor immune escape may be caused by upregulation of tumor cell infiltrating immune cells (TIICs) or their ligands at suppressive immune checkpoint in CRC, such as PD-1, PD-L1 and CTLA-4. Changes in DNA methylation patterns and enrichment of methylated histone markers in promoter regions may be the main reasons for upregulation of immune checkpoint (ICs) in CRC (143). Furthermore, a study aimed at analyzing the prognostic value of PD-L1 in CRC cells and tumor cell infiltrates (TILs) revealed that high expression of PD-1 and PD-L1 was associated with a better prognosis in colorectal cancer patients and TILs-PD-1 may be an independent prognostic factor for OS and disease-free survival (DFS) in CRC patients (144).
Anyway, PD-L1 inhibitor-based immunotherapy is considered a promising approach for targeting colorectal cancer. The binding of PD-L1 and PD-1 in tumor cells or tumor microenvironment induces immunosuppressive signals that reduce T cell proliferation and lead to tumor immune escape.
Hepatocelullar carcinoma
Hepatocelullar carcinoma (HCC) is the third leading cause of cancer death and the sixth most common malignancy worldwide (145). Recent study showed that PD-1/PD-L1 expression played an important role and interacted with CD8+ T-cell immune responses to regulate the immune homeostasis and prognosis of HCC patients (146). Recent studies revealed that amplification or high expression of PD-L1 was significantly and independently associated with poor survival in HCC patients, which confirmed that the PD-1/PD-L1 axis is a promising potential target for HCC immunotherapy (147).
Recent studies have shown that immunocheckpoint inhibitor therapy significantly improves the overall survival of HCC patients (148, 149). For example, some PD-1 inhibitors, such as pembrolizumab and nivolumab, were effective and well tolerated in patients with advanced HCC (63, 88). PD-L1 mediated the growth inhibition of herbal medicines baicalin and flavonol on HCC by decreasing STAT3 activity, thereby restoring the anti-cancer sensitivity of T cells (150). More importantly, anti-PD-1/PD-L1 antibodies combined with other therapies was considered an effective strategy for the treatment of HCC (151). In addition to binding to cell membranes, PD-1/PD-L1 could also dissociate in the blood of patients and is called as soluble PD-1/PD-L1 (94, 152). Recent studies have shown that high expression of soluble PD-L1 was significantly associated with an increased risk of death (153, 154). Furthermore, soluble PD-1 and PD-L1 were independent prognostic factors with opposite roles in predicting disease-free survival (DFS) and overall survival (OS) in HCC patients (155).
Currently, clinical trials about the application of PD-1/PD-L1 checkpoint inhibitors in HCC are underway or completed, some of which have shown promising therapeutic expectations. Nonetheless, the clinical benefits for other patients are not satisfactory. Comprehensive predictive biomarkers are necessary to identify HCC patients who are more likely to respond to immunosuppression and thus guide clinical therapy strategies.
Bladder cancer
Bladder cancer (BC) is the most common cancer of the human urinary system, with poor prognosis and high recurrence rate (156, 157). Immunotherapy based on PD-1/PD-L1 immunecheckpoints has been approved and successfully performed in the treatment of BC (158–160). Recent studies have identified significant differences in the expression levels of PD-1 and PD-L1 between higher-grade BC and lower-grade BC. The expressions of PD-1 and PD-L1 in higher-grade BC are higher than those in lower-grade BC. Therefore, PD-1 and PD-L1 may be important biomarkers related to the pathological grading of BC and play a mediating role in the progression of BC (161). Moreover, PD-L1 may be novel combined biomarkers for predicting tumor invasitivity and immune checkpoint response in BC (162). Taken together, PD-1/PD-L1 are closely associated with tumorigenesis, treatment and prognosis of human BC.
PD-L1 positive BC patients with heavily pretreated have shown a manageable safety profile and meaningful clinical outcomes after treatment of durvalumab antibody (Anti-PD-L1), ORR was 46.4% and responses were ongoing in 12 of 13 responding patients (163). Another study evaluated the safety and antitumor activity of avelumab (Anti-PD-L1) in patients with metastatic urothelial BC. Results suggested that patients achieved a median progression-free survival of 11.6 weeks, median OS of 13.7 months and 12-months OS rate of 54.3% (164). There were many other similar clinical studies that demonstrate that PD-1/PD-L1 Immune-checkpoint inhibitors are well associated with durable responses and prolonged survival in metastatic urothelial BC. Recent studies revealed that the expression and function of PD-L1 in BC were closely related to autophagy. PD-L1 could be upregulated by autophagy-related proteins (e.g ATG7), ultimately enhancing the stem cell-like properties and invasive capacity of BC cells (165). Therefore, PD-1/PD-L1 Immune-checkpoint inhibitors combined with autophagy inhibitors may be a promising therapeutic approach for human BC. In addition, anti-PD-1/PD-L1 immunetherapy combined with radiotherapy has significant local and distal synergistic anticancer effects (166).
Overall, PD-1/PD-L1 Immune-checkpoint inhibitors have shown promising results in terms of clinical efficacy in patients with advanced and metastatic BC. However, more additional data is urgently needed for the evaluation of reliability and safety of anti-PD-1/PD-L1 treatment. These exciting advances will bring more benefit and hope for BC patients.
Ovarian cancer
Ovarian cancer(OC) is the seventh most common cancer and the eighth leading cause of cancer death in women worldwide (167). Recent studies have shown that the application of PD-1/PD-L1 inhibitors in the treatment of OC has attracted extensive attention of researchers (168–170). PD-1 and its ligand were significantly expressed in tumor cells and immune system cells of OC patients (171).
Recent studies claimed that PD-1/PD-L1 immune checkpoint inhibitors do not perform well in the treatment of recurrent epithelial OC (172, 173). However, well efficacy was achieved by the combination of PD-1/PD-L1 inhibitor (Nivolumab) and anti-angiogenic drug (Bevacizumab). Their interaction may exert synergistic effects by modulating the microenvironment (66). In addition, combination therapy with PARP and immune checkpoint inhibition has yielded encouraging results in ovarian cancer (174, 175). Taken together, combination therapy may be an effective strategy for the treatment of OC, offering a potential therapeutic opportunity for OC.
Although combined immunotherapy have evolved rapidly in the treatment of OC and have been successfully applied, some emerging issues need to be addressed in clinical practice, such as the dose and sequence of optimal synergy, differences in immunotherapy response across OC subtypes and possible side effects of the interaction of two medicines. Therefore, we appeal that more clinical trials of combination immunotherapy should be performed to obtain relevant clinical data, including efficacy, stability and immune-related adverse effects. so that the combined immunotherapy will be more widely applied in the treatment of OC in the near future.
Pancreatic cancer
Pancreatic cancer (PC) is one of the leading causes of cancer death worldwide. In the last two decades, the number of pancreatic cancer patients diagnosed each year has doubled worldwide (176). PD-L1 was identified as a novel maker of prognosis in patients with PC, and the up-regulation of PD-L1 was found in human PC tissues (177). PD-L1 is involved in the regulation of PC stemness, epigenetic mechanisms and metastasis. Blocking PD-1 significantly inhibited the PC growth by enhancing INF-γ production and decreasing IL-10 production in a mouse model (178). Therefore, PD-1/PD-L1 expression and related signaling may play an important role in the progression of PC.
In recent years, although PD-1/PD-L1 immunecheckpoint inhibitors have been rapidly developed in the treatment of various cancers. However, the outcomes of PD-1/PD-L1 immunecheckpoint inhibitors monotherapy are not satisfactory in PC (179). Currently, two main reasons are believed to be responsible for such failures. First, pancreatic cancer is inherently non-immunogenic. Second, immunosuppression due to high tumor burden is another reason for which PC cannot be treated by PD-1/PD-L1 blockade alone, immune escape of pancreatic tumors is closely related to the excessive development of immunosuppressive T cells (177). Therefore, the combination therapeutic strategies may bring new hope for the treatment of PC with PD-1/PD-L1 inhibitors. For example, the combination of anti-PD-1 inhibitory antibodies and anti-ox40 agonist antibodies decreased the proportion of T regulatory, and increased the number of memory CD4+ and CD8+ T cells, thereby attenuating the immune escape response and enhancing the anticancer effects of anti-PD-1 in PC (180). In addition, a study suggested that Anti-TNFR2 and anti-PD-L1 combination therapy significantly inhibited the growth of PC through relieving tumor immunosuppression and generating robust memory recall (181). Moreover, Anti-PD-1 antibody immunotherapy combined with gemcitabine significantly inhibited PC and liver metastasis by enhancing the immune response mediated by Th1 lymphocytes and M1 macrophages (182).
All in all, although anti-PD-1/PD-L1 inhibitors have rapidly developed as a priority of immunotherapy strategy for various cancers. However, the poor therapeutic outcomes were observed in the treatment of PC because of the particularity of pancreatic cancer, such as a high tumor burden, non-immunogenicity and immunosuppressive tumor microenvironment. The combination of anti-PD-1/PD-L1 immunotherapy with other anti-tumor agents that overcome these specific properties will significantly improve the therapeutic effect of anti-PD-1/PD-L1 immunotherapy in PC.
PD-1/PD-L1 and inhibitors in human hematological malignancies
Leukemia
Leukemia is the common name for several malignant diseases with an increasing number of white blood cells in the blood and/or bone marrow. Leukemia includes acute myeloid leukemia, chronic myeloid leukemia, acute lymphoblastic leukemia, chronic lymphocytic leukemia, and so on (183). Acute myeloid leukemia (AML) is the most common form of leukemia and hematological malignancy with a poor clinical prognosis and characterized by uncontrolled proliferation of hematopoietic stem cells in the bone marrow (184, 185).
Recent results has shown that high expression of PD-1 and PD-L1 was associated with poorer overall survival (OS) and clinical outcome in AML patients (186, 187). In addition, a clinical trial has shown encouraging response and overall survival rates for patients with relapsed/refractory (R/R) acute myeloid leukemia (AML) treated with nivolumab(anti-PD-1) and azacitidine, which suggested that nivolumab in combination with azacitidine appears to be a safe and effective treatment for AML (188).
However, the clinical response to PD-1/PD-L1 blockade varied in different AML patients (189). A recent study revealed that the majority of immune-checkpoint receptor genes were downregulated in bone marrow (BM)-infiltrating CD8+ T cells and partially in CD4+ T cells due to pathological chromatin remodeling via histone deacetylation. Therefore, the dysfunction of CD8+ T cells in AML was mainly due to pathological epigenetic silencing of activated IC receptors rather than due to signaling by immune inhibitory IC receptors (190). This may explain the limited role of PD-1/PD-L1 antibodies in AML patients. In conclusion, anti-PD-1/PD-L1 therapy may be a new immunotherapeutic strategy for AML. However, further studies are still necessary.
Multiple myeloma
Multiple myeloma (MM) is a genetically heterogeneous clonal plasma cell disorder, which is the second most common malignancy in the hematological system (191, 192). The immune dysfunction is critical for the genesis of MM. The interaction of PD-L1 and PD-1 inhibited the body’s immune function and promoted immune escape by preventing tumor-reactive T cells from being activated and functioning (193). PD-L1 and PD-1 were higher on their tumor cells and T-cells in MM patients, respectively. MM cells with high PD-L1 expression effectively protected themselves against MM-specific t-cell killing, which could be reversed by anti-pd-1 or PD-L1 antibodies (194). In addition, PD-L1 expression on malignant myeloma plasma cells was related to an increased risk of MM (195).
However, the role of PD-L1/PD-1 axis in MM is still debated, the clinical outcomes of PD-1/PD-L1 inhibitors alone for MM are not very encouraging, the combination of PD-1/PD-L1 inhibitors with other drugs for multiple myeloma appears to be promising (196, 197). Recent study showed that pembrolizumab (anti-PD-1) in combination with belapectin (Galectin-3 Inhibitor) significantly enhanced the activation of effector memory T cells and the percentage of effector memory T cell proliferation in MM patients. Moreover, pembrolizumab in combination with belapectin was associated with fewer immune-related adverse events compared to pembrolizumab monotherapy (198)). Moreover,PD-1inhibitor in combination with CD38 monoclonal antibody was also a promising strategy for the treatment of CD38-positive MM (194). In fact, in vitro experiments have also demonstrated that PD-1/PD-L1 inhibitors directly enhance NK cell- and T cell-mediated immune responses against MM, and lenalidomide (immunomodulator) significantly enhanced such immune responses (199). Overall, PD-1/PD-L1 expressions in MM have shown an important clinical significance and its inhibitors have a certain potential in the treatment of MM, but the conclusions of their effectiveness are inconsistent and more rigorous clinical and basic studies are required to confirm that.
Lymphoma
Lymphoma is a kind of heterogeneous lymph-like malignancy (200).The World Health Organization classifies lymphomas into more than 80 subtypes in 2017 year based on their morphology, immunophenotype, genetic lesion, molecular profile, clinical features and cell type of origin, such as B cell lymphoma, T cell lymphoma, Hodgkin’s lymphoma and so on (200, 201). Preliminary clinical data suggested that checkpoint inhibitors were a promising therapeutic strategy for certain lymphoid malignancies. However, the expression level and role of PD-1/PD-L1 in lymphoma cells and tumor microenvironment varied depending on the subtype (202). For example, increased infiltration of PD-1+ tumor-infiltrating lymphocytes (TILs) was a positive prognostic predictor in diffuse large B-cell lymphoma (DLBCL) but not in Hodgkin’s lymphoma (HL) (202). The NK cell-associated and monocyte/macrophage-associated immune escape due to the PD-1/PD-L1 pathway was more prominent in HL than DLBCL (203).
Anyway, PD-1/PD-L1 inhibitors have still made some promising achievements in the research and treatment of lymphoma. For instance, a clinical trial suggested that PD-L1 may be the most promising soluble biomarker for classical Hodgkin lymphoma (CHL) (204). The objective response rate(ORR) to nivolumab was 66.3% (53/80) in a multicenter, multicohort, single-arm phase 2 trial for classical Hodgkin’s lymphoma after failure of autologous stem cell transplantation and brentuximab vedotin (65). Moreover, GLS-010, a recombinant human anti-programmed death-1 monoclonal antibody, has demonstrated favorable response and safety in clinical trials for the treatment of advanced solid tumors or lymphomas (77). In a recent phase 2 study, pembrolumab significantly improved the PFS and OS in patients with relapsed/refractory (R/R) CHL after autologous stem cell transplantation (ASCT) and achieved 82% PFS at 18 months and 100% OS at 18 months, which suggested that pembrolumab is a promising approach for post-ASCT consolidation in patients with R/R CHL (205). Furthermore, the results of a small phase 1b study showed that the ORR was 36% in patients with R/R diffuse large B-cell lymphoma (DLBCL) treated with nivolumab (67). However, in a subsequent larger phase 2 study, the ORR to nivolumab treatment was only 10% and 3% respectively, median response time was 11 and 8 months respectively in patients with R/R DLBCL who are ineligible for autologous hematopoietic cell transplantation (AHCT) or experienced failure with AHCT (87). Taken together, considering the diversity and complexity of lymphomas, more precise and individual clinical trials are necessary to elucidate the role of PD-1/PD-L1 inhibitors for the treatment of lymphomas in the future.
Problems and prospects
Currently, there is an increasing number of studies targeting PD-1/PD-L1 immune checkpoint inhibitors in human cancers including solid tumors and hematological malignancies. PD-1 and PD-L1 are expressed in tumor-infiltrating immune cells and most solid tumors, and they are closely associated with tumor development and prognosis (206–209). PD-L1-positive patients have a significantly lower 5-year survival rate than patients with non-PD-L1-positive tumors, and PD-L1 expression is an independent prognostic indicator (210). PD-L1 expression is associated with many factors, such as age, tumor size, depth of infiltration, lymph node metastasis, lymphovascular infiltration, venous infiltration and disease stage. Recent studies have shown that PD-L1 and PD-1 expressions are often closely linked. In patients with high PD-L1 expression, PD-1 levels in T cells are also high, which may be an intrinsic factor for the immune escape of tumors (210). Activated T cells play a key role in tumor suppression. PD-1 is mainly expressed in activated T cells and inhibits T cell function through binding to PD-L1, thereby promoting immune escape (22).Therefore, blockade of interaction between PD-1 and PD-L1 can significantly enhance immune function and inhibit tumor growth. A multicenter phase 1 trial showed that intravenous anti-PD-L1 antibody significantly inhibited tumor progression (objective remission rate of 6-17%) and prolonged disease stability (12-41% at 24 weeks) (211).
Although PD-1/PD-L1 expression is closely associated with tumor progression and treatment, using PD-1/PD-L1 as the only predictive biomarker for cancer immunotherapy still remains problematic. For example, the low accuracy of PD-L1 detection brings unnecessary obstacles for examination and anti-PD-L1 treatment in patients. The main reasons are considered as follows: Firstly, different studies may use antibodies of varying sensitivity. Secondly, the criteria for positive PD-L1 staining were inconsistent across studies. Thirdly, the expression level of PD-L1 in different sites of tumor tissues is variable, and even if the same sites are sampled at different times, the results of PD-L1 detection can be affected (212). Fortunately, some studies have found that some other factors can work together with PD-L1 as biomarkers to better predict the responsiveness of anti-PD-1/PD-L1 therapy. For example, high expression of TMB (tumor mutational burden), T-cell-inflamed gene-expression profile(GEP) and PD-L1 together reflect the potential for higher response of pembrolizumab in various types of cancers (57).
Taken together, PD-1/PD-L1 immune checkpoint inhibitors have been widely used in the treatment of a variety of cancers (64, 65, 68, 71, 75, 76). However, serious challenges still remain, such as the small number of beneficiary populations, primary and acquired drug resistance, lack of predictive and prognostic biomarkers, and treatment-related adverse effects (22). In addition, there are few predictive biomarkers that can identify the type of patients who will benefit from treatment (213, 214). Moreover, PD-L1 expression is heterogeneous and dynamic in tests with different antibodies and different scoring criteria complicate the interpretation of the test results (215–217). Therefore, considering the multifactorial characteristics of tumor immune crosstalk, prediction models based on comprehensive biomarker determination theory may be more feasible for future applications. Finally, in response to the treatment resistance of PD-1/PD-L1 blockers, some investigators believe that combined therapy, nanoimmunotherapy and intestinal microbial therapy may be promising therapeutic strategy (218). In any case, PD-1/PD-L1 Immune-checkpoint inhibitors definitely have wildly application prospects and clinical value in the treatment of human cancers.
Author contributions
QT, YC, and XL drafted the manuscript and were involved in data analysis in the whole manuscript. SL, YS, and YY were involved in technical support and revised the manuscript. WW and LH contributed to funding and writing suggestions. SW revised the manuscript and contributed to the conception and design of the work. All authors approve it for publication and agree to be accountable for all aspects of the work.
Funding
This work was supported by the grants from the Natural Science Foundation of China (81974543, 81903991, 81703551, 81871863), the Natural Science Foundation of Guangdong Province (2019A1515011362, 2021A1515410007, 2021A1515220023), the Guangzhou science and technology plan project (202002030155, 202102010160), the Scientific Research Project in Universities of Guangdong Provincial Department of Education (2020KTSCX029), the Chinese medicine science and technology research project of Guangdong Provincial Hospital of Chinese Medicine (YN2019MJ09, YN2019QJ06), the Guangdong Provincial Key Laboratory of Clinical Research on Traditional Chinese Medicine Syndrome (ZH2020KF03), the Key project of State Key Laboratory of dampness syndrome of Chinese medicine (SZ2021ZZ38, SZ2021ZZ29), the Science and Technology Planning Project of Guangdong Province (2017B030314166) and the Research Fund for Bajian Talents of Guangdong Provincial Hospital of Chinese Medicine(BJ2022KY13).
Conflict of interest
The authors declare that the research was conducted in the absence of any commercial or financial relationships that could be construed as a potential conflict of interest.
Publisher’s note
All claims expressed in this article are solely those of the authors and do not necessarily represent those of their affiliated organizations, or those of the publisher, the editors and the reviewers. Any product that may be evaluated in this article, or claim that may be made by its manufacturer, is not guaranteed or endorsed by the publisher.
References
1. Daud AI, Wolchok JD, Robert C, Hwu WJ, Weber JS, Ribas A, et al. Programmed death-ligand 1 expression and response to the anti-programmed death 1 antibody pembrolizumab in melanoma. J Clin Oncol (2016) 34(34):4102–9. doi: 10.1200/jco.2016.67.2477
2. Jalili-Nik M, Soltani A, Mashkani B, Rafatpanah H, Hashemy SI. PD-1 and PD-L1 inhibitors foster the progression of adult T-cell Leukemia/Lymphoma. Int Immunopharmacol (2021) 98:107870. doi: 10.1016/j.intimp.2021.107870
3. Nakamura T, Sato T, Endo R, Sasaki S, Takahashi N, Sato Y, et al. STING agonist loaded lipid nanoparticles overcome anti-PD-1 resistance in melanoma lung metastasis via NK cell activation. J Immunother Cancer (2021) 9(7). doi: 10.1136/jitc-2021-002852
4. Zuo H, Wan Y. Inhibition of myeloid PD-L1 suppresses osteoclastogenesis and cancer bone metastasis. Cancer Gene Ther (2022). doi: 10.1038/s41417-022-00446-5
5. Chen L, Mo DC, Hu M, Zhao SJ, Yang QW, Huang ZL. PD-1/PD-L1 inhibitor monotherapy in recurrent or metastatic squamous cell carcinoma of the head and neck: A meta-analysis. Am J Otolaryngol (2022) 43(2):103324. doi: 10.1016/j.amjoto.2021.103324
6. Luke JJ, Rutkowski P, Queirolo P, Del Vecchio M, Mackiewicz J, Chiarion-Sileni V, et al. Pembrolizumab versus placebo as adjuvant therapy in completely resected stage IIB or IIC melanoma (KEYNOTE-716): A randomised, double-blind, phase 3 trial. Lancet (2022) 399(10336):1718–29. doi: 10.1016/s0140-6736(22)00562-1
7. Saito Y, Fujiwara Y, Shinchi Y, Mito R, Miura Y, Yamaguchi T, et al. Classification of PD-L1 expression in various cancers and macrophages based on immunohistocytological analysis. Cancer Sci (2022). doi: 10.1111/cas.15442
8. Banchereau R, Chitre AS, Scherl A, Wu TD, Patil NS, De Almeida P, et al. Intratumoral CD103+ CD8+ T cells predict response to PD-L1 blockade. J Immunother Cancer (2021) 9(4). doi: 10.1136/jitc-2020-002231
9. Peña-Asensio J, Calvo H, Torralba M, Miquel J, Sanz-De-Villalobos E, Larrubia JR. Anti-PD-1/PD-L1 based combination immunotherapy to boost antigen-specific CD8(+) T cell response in hepatocellular carcinoma. Cancers (Basel) (2021) 13(8). doi: 10.3390/cancers13081922
10. Song D, Powles T, Shi L, Zhang L, Ingersoll MA, Lu YJ. Bladder cancer, a unique model to understand cancer immunity and develop immunotherapy approaches. J Pathol (2019) 249(2):151–65. doi: 10.1002/path.5306
11. Zhu HH, Feng Y, Hu XS. Emerging immunotherapy targets in lung cancer. Chin Med J (Engl) (2020) 133(20):2456–65. doi: 10.1097/cm9.0000000000001082
12. Zandberg DP, Algazi AP, Jimeno A, Good JS, Fayette J, Bouganim N, et al. Durvalumab for recurrent or metastatic head and neck squamous cell carcinoma: Results from a single-arm, phase II study in patients with ≥25% tumour cell PD-L1 expression who have progressed on platinum-based chemotherapy. Eur J Cancer (2019) 107:142–52. doi: 10.1016/j.ejca.2018.11.015
13. Zhuang Y, Liu C, Liu J, Li G. Resistance mechanism of PD-1/PD-L1 blockade in the cancer-immunity cycle. Onco Targets Ther (2020) 13:83–94. doi: 10.2147/ott.S239398
14. Baxi S, Yang A, Gennarelli RL, Khan N, Wang Z, Boyce L, et al. Immune-related adverse events for anti-PD-1 and anti-PD-L1 drugs: Systematic review and meta-analysis. Bmj (2018) 360:k793. doi: 10.1136/bmj.k793
15. O'neill RE, Cao X. Co-Stimulatory and co-inhibitory pathways in cancer immunotherapy. Adv Cancer Res (2019) 143:145–94. doi: 10.1016/bs.acr.2019.03.003
16. Schütz F, Stefanovic S, Mayer L, Von Au A, Domschke C, Sohn C. PD-1/PD-L1 pathway in breast cancer. Oncol Res Treat (2017) 40(5):294–7. doi: 10.1159/000464353
17. Ai L, Xu A, Xu J. Roles of PD-1/PD-L1 pathway: Signaling, cancer, and beyond. Adv Exp Med Biol (2020) 1248:33–59. doi: 10.1007/978-981-15-3266-5_3
18. Sun L, Li CW, Chung EM, Yang R, Kim YS, Park AH, et al. Targeting glycosylated PD-1 induces potent antitumor immunity. Cancer Res (2020) 80(11):2298–310. doi: 10.1158/0008-5472.Can-19-3133
19. Duhen R, Fesneau O, Samson KA, Frye AK, Beymer M, Rajamanickam V, et al. PD-1 and ICOS co-expression identifies tumor-reactive CD4 T cells in human solid tumors. J Clin Invest (2022). doi: 10.1172/jci156821
20. Hui E, Cheung J, Zhu J, Su X, Taylor MJ, Wallweber HA, et al. T Cell costimulatory receptor CD28 is a primary target for PD-1-mediated inhibition. Science (2017) 355(6332):1428–33. doi: 10.1126/science.aaf1292
21. Kamphorst AO, Wieland A, Nasti T, Yang S, Zhang R, Barber DL, et al. Rescue of exhausted CD8 T cells by PD-1-targeted therapies is CD28-dependent. Science (2017) 355(6332):1423–7. doi: 10.1126/science.aaf0683
22. Xia L, Liu Y, Wang Y. PD-1/PD-L1 blockade therapy in advanced non-Small-Cell lung cancer: Current status and future directions. Oncologist (2019) 24(Suppl 1):S31–41. doi: 10.1634/theoncologist.2019-IO-S1-s05
23. Liu Y, Wu L, Tong R, Yang F, Yin L, Li M, et al. PD-1/PD-L1 inhibitors in cervical cancer. Front Pharmacol (2019) 10:65. doi: 10.3389/fphar.2019.00065
24. Wang Z, Wu X. Study and analysis of antitumor resistance mechanism of PD1/PD-L1 immune checkpoint blocker. Cancer Med (2020) 9(21):8086–121. doi: 10.1002/cam4.3410
25. Cao Z, Kon N, Liu Y, Xu W, Wen J, Yao H, et al. An unexpected role for p53 in regulating cancer cell-intrinsic PD-1 by acetylation. Sci Adv (2021) 7(14). doi: 10.1126/sciadv.abf4148
26. Yao H, Wang H, Li C, Fang JY, Xu J. Cancer cell-intrinsic PD-1 and implications in combinatorial immunotherapy. Front Immunol (2018) 9:1774. doi: 10.3389/fimmu.2018.01774
27. Leko V, Rosenberg SA. Identifying and targeting human tumor antigens for T cell-based immunotherapy of solid tumors. Cancer Cell (2020) 38(4):454–72. doi: 10.1016/j.ccell.2020.07.013
28. Dersh D, Phelan JD, Gumina ME, Wang B, Arbuckle JH, Holly J, et al. Genome-wide screens identify lineage- and tumor-specific genes modulating MHC-i- and MHC-II-Restricted immunosurveillance of human lymphomas. Immunity (2021) 54(1):116–131.e10. doi: 10.1016/j.immuni.2020.11.002
29. Ghasemi F, Tessier TM, Gameiro SF, Maciver AH, Cecchini MJ, Mymryk JS. High MHC-II expression in Epstein-Barr virus-associated gastric cancers suggests that tumor cells serve an important role in antigen presentation. Sci Rep (2020) 10(1):14786. doi: 10.1038/s41598-020-71775-4
30. Burr ML, Sparbier CE, Chan KL, Chan YC, Kersbergen A, Lam EYN, et al. An evolutionarily conserved function of polycomb silences the MHC class I antigen presentation pathway and enables immune evasion in cancer. Cancer Cell (2019) 36(4):385–401.e8. doi: 10.1016/j.ccell.2019.08.008
31. Tu K, Yu Y, Wang Y, Yang T, Hu Q, Qin X, et al. Combination of chidamide-mediated epigenetic modulation with immunotherapy: Boosting tumor immunogenicity and response to PD-1/PD-L1 blockade. ACS Appl Mater Interfaces (2021) 13(33):39003–17. doi: 10.1021/acsami.1c08290
32. Neuwelt AJ, Kimball AK, Johnson AM, Arnold BW, Bullock BL, Kaspar RE, et al. Cancer cell-intrinsic expression of MHC II in lung cancer cell lines is actively restricted by MEK/ERK signaling and epigenetic mechanisms. J Immunother Cancer (2020) 8(1). doi: 10.1136/jitc-2019-000441
33. Shenoy AT, Lyon De Ana C, Arafa EI, Salwig I, Barker KA, Korkmaz FT, et al. Antigen presentation by lung epithelial cells directs CD4(+) T(RM) cell function and regulates barrier immunity. Nat Commun (2021) 12(1):5834. doi: 10.1038/s41467-021-26045-w
34. Yang Y, Zhou T, Chen X, Li J, Pan J, He X, et al. Efficacy, safety, and biomarker analysis of camrelizumab in previously treated recurrent or metastatic nasopharyngeal carcinoma (CAPTAIN study). J Immunother Cancer (2021) 9(12). doi: 10.1136/jitc-2021-003790
35. Kawakami Y, Ohta S, Sayem MA, Tsukamoto N, Yaguchi T. Immune-resistant mechanisms in cancer immunotherapy. Int J Clin Oncol (2020) 25(5):810–7. doi: 10.1007/s10147-019-01611-x
36. Mariathasan S, Turley SJ, Nickles D, Castiglioni A, Yuen K, Wang Y, et al. TGFβ attenuates tumour response to PD-L1 blockade by contributing to exclusion of T cells. Nature (2018) 554(7693):544–8. doi: 10.1038/nature25501
37. Bullock BL, Kimball AK, Poczobutt JM, Neuwelt AJ, Li HY, Johnson AM, et al. Tumor-intrinsic response to IFNγ shapes the tumor microenvironment and anti-PD-1 response in NSCLC. Life Sci Alliance (2019) 2(3). doi: 10.26508/lsa.201900328
38. Gainor JF, Shaw AT, Sequist LV, Fu X, Azzoli CG, Piotrowska Z, et al. EGFR mutations and ALK rearrangements are associated with low response rates to PD-1 pathway blockade in non-small cell lung cancer: A retrospective analysis. Clin Cancer Res (2016) 22(18):4585–93. doi: 10.1158/1078-0432.Ccr-15-3101
39. Antonios JP, Soto H, Everson RG, Moughon D, Orpilla JR, Shin NP, et al. Immunosuppressive tumor-infiltrating myeloid cells mediate adaptive immune resistance via a PD-1/PD-L1 mechanism in glioblastoma. Neuro Oncol (2017) 19(6):796–807. doi: 10.1093/neuonc/now287
40. Nowicki TS, Hu-Lieskovan S, Ribas A. Mechanisms of resistance to PD-1 and PD-L1 blockade. Cancer J (2018) 24(1):47–53. doi: 10.1097/ppo.0000000000000303
41. Huang AC, Postow MA, Orlowski RJ, Mick R, Bengsch B, Manne S, et al. T-Cell invigoration to tumour burden ratio associated with anti-PD-1 response. Nature (2017) 545(7652):60–5. doi: 10.1038/nature22079
42. Koyama S, Akbay EA, Li YY, Herter-Sprie GS, Buczkowski KA, Richards WG, et al. Adaptive resistance to therapeutic PD-1 blockade is associated with upregulation of alternative immune checkpoints. Nat Commun (2016) 7:10501. doi: 10.1038/ncomms10501
43. Peng DH, Rodriguez BL, Diao L, Chen L, Wang J, Byers LA, et al. Collagen promotes anti-PD-1/PD-L1 resistance in cancer through LAIR1-dependent CD8(+) T cell exhaustion. Nat Commun (2020) 11(1):4520. doi: 10.1038/s41467-020-18298-8
44. Veldman J, Visser L, Berg AVD, Diepstra A. Primary and acquired resistance mechanisms to immune checkpoint inhibition in Hodgkin lymphoma. Cancer Treat Rev (2020) 82:101931. doi: 10.1016/j.ctrv.2019.101931
45. Bai J, Gao Z, Li X, Dong L, Han W, Nie J. Regulation of PD-1/PD-L1 pathway and resistance to PD-1/PD-L1 blockade. Oncotarget (2017) 8(66):110693–707. doi: 10.18632/oncotarget.22690
46. Skoulidis F, Goldberg ME, Greenawalt DM, Hellmann MD, Awad MM, Gainor JF, et al. STK11/LKB1 mutations and PD-1 inhibitor resistance in KRAS-mutant lung adenocarcinoma. Cancer Discovery (2018) 8(7):822–35. doi: 10.1158/2159-8290.Cd-18-0099
47. Routy B, Le Chatelier E, Derosa L, Duong CPM, Alou MT, Daillère R, et al. Gut microbiome influences efficacy of PD-1-based immunotherapy against epithelial tumors. Science (2018) 359(6371):91–7. doi: 10.1126/science.aan3706
48. Ribas A, Shin DS, Zaretsky J, Frederiksen J, Cornish A, Avramis E, et al. PD-1 blockade expands intratumoral memory T cells. Cancer Immunol Res (2016) 4(3):194–203. doi: 10.1158/2326-6066.Cir-15-0210
49. Pathak R, Pharaon RR, Mohanty A, Villaflor VM, Salgia R, Massarelli E. Acquired resistance to PD-1/PD-L1 blockade in lung cancer: Mechanisms and patterns of failure. Cancers (Basel) (2020) 12(12). doi: 10.3390/cancers12123851
50. Patel SA, Minn AJ. Combination cancer therapy with immune checkpoint blockade: Mechanisms and strategies. Immunity (2018) 48(3):417–33. doi: 10.1016/j.immuni.2018.03.007
51. Rizvi NA, Hellmann MD, Snyder A, Kvistborg P, Makarov V, Havel JJ, et al. Cancer immunology. mutational landscape determines sensitivity to PD-1 blockade in non-small cell lung cancer. Science (2015) 348(6230):124–8. doi: 10.1126/science.aaa1348
52. Martín-Gayo E, Sierra-Filardi E, Corbí AL, Toribio ML. Plasmacytoid dendritic cells resident in human thymus drive natural treg cell development. Blood (2010) 115(26):5366–75. doi: 10.1182/blood-2009-10-248260
53. Seto T, Nosaki K, Shimokawa M, Toyozawa R, Sugawara S, Hayashi H, et al. Phase II study of atezolizumab with bevacizumab for non-squamous non-small cell lung cancer with high PD-L1 expression (@Be study). J Immunother Cancer (2022) 10(2). doi: 10.1136/jitc-2021-004025
54. Schoenfeld JD, Giobbie-Hurder A, Ranasinghe S, Kao KZ, Lako A, Tsuji J, et al. Durvalumab plus tremelimumab alone or in combination with low-dose or hypofractionated radiotherapy in metastatic non-small-cell lung cancer refractory to previous PD(L)-1 therapy: An open-label, multicentre, randomised, phase 2 trial. Lancet Oncol (2022) 23(2):279–91. doi: 10.1016/s1470-2045(21)00658-6
55. Paz-Ares LG, Ramalingam SS, Ciuleanu TE, Lee JS, Urban L, Caro RB, et al. First-line nivolumab plus ipilimumab in advanced NSCLC: 4-year outcomes from the randomized, open-label, phase 3 CheckMate 227 part 1 trial. J Thorac Oncol (2022) 17(2):289–308. doi: 10.1016/j.jtho.2021.09.010
56. Kim ST, Cristescu R, Bass AJ, Kim KM, Odegaard JI, Kim K, et al. Comprehensive molecular characterization of clinical responses to PD-1 inhibition in metastatic gastric cancer. Nat Med (2018) 24(9):1449–58. doi: 10.1038/s41591-018-0101-z
57. Ott PA, Bang YJ, Piha-Paul SA, Razak ARA, Bennouna J, Soria JC, et al. T-Cell-Inflamed gene-expression profile, programmed death ligand 1 expression, and tumor mutational burden predict efficacy in patients treated with pembrolizumab across 20 cancers: KEYNOTE-028. J Clin Oncol (2019) 37(4):318–27. doi: 10.1200/jco.2018.78.2276
58. Reck M, Rodriguez-Abreu D, Robinson AG, Hui R, Csoszi T, Fulop A, et al. Pembrolizumab versus chemotherapy for PD-L1-Positive non-Small-Cell lung cancer. N Engl J Med (2016) 375(19):1823–33. doi: 10.1056/NEJMoa1606774
59. Adams S, Loi S, Toppmeyer D, Cescon DW, De Laurentiis M, Nanda R, et al. Pembrolizumab monotherapy for previously untreated, PD-L1-positive, metastatic triple-negative breast cancer: Cohort b of the phase II KEYNOTE-086 study. Ann Oncol (2019) 30(3):405–11. doi: 10.1093/annonc/mdy518
60. Paz-Ares L, Luft A, Vicente D, Tafreshi A, Gümüş M, Mazières J, et al. Pembrolizumab plus chemotherapy for squamous non-Small-Cell lung cancer. N Engl J Med (2018) 379(21):2040–51. doi: 10.1056/NEJMoa1810865
61. Muro K, Chung HC, Shankaran V, Geva R, Catenacci D, Gupta S, et al. Pembrolizumab for patients with PD-L1-positive advanced gastric cancer (KEYNOTE-012): a multicentre, open-label, phase 1b trial. Lancet Oncol (2016) 17(6):717–26. doi: 10.1016/s1470-2045(16)00175-3
62. Garon EB, Rizvi NA, Hui R, Leighl N, Balmanoukian AS, Eder JP, et al. Pembrolizumab for the treatment of non-small-cell lung cancer. N Engl J Med (2015) 372(21):2018–28. doi: 10.1056/NEJMoa1501824
63. El-Khoueiry AB, Sangro B, Yau T, Crocenzi TS, Kudo M, Hsu C, et al. Nivolumab in patients with advanced hepatocellular carcinoma (CheckMate 040): an open-label, non-comparative, phase 1/2 dose escalation and expansion trial. Lancet (2017) 389(10088):2492–502. doi: 10.1016/s0140-6736(17)31046-2
64. Alley EW, Lopez J, Santoro A, Morosky A, Saraf S, Piperdi B, et al. Clinical safety and activity of pembrolizumab in patients with malignant pleural mesothelioma (KEYNOTE-028): preliminary results from a non-randomised, open-label, phase 1b trial. Lancet Oncol (2017) 18(5):623–30. doi: 10.1016/s1470-2045(17)30169-9
65. Younes A, Santoro A, Shipp M, Zinzani PL, Timmerman JM, Ansell S, et al. Nivolumab for classical hodgkin's lymphoma after failure of both autologous stem-cell transplantation and brentuximab vedotin: A multicentre, multicohort, single-arm phase 2 trial. Lancet Oncol (2016) 17(9):1283–94. doi: 10.1016/s1470-2045(16)30167-x
66. Liu JF, Herold C, Gray KP, Penson RT, Horowitz N, Konstantinopoulos PA, et al. Assessment of combined nivolumab and bevacizumab in relapsed ovarian cancer: A phase 2 clinical trial. JAMA Oncol (2019). doi: 10.1001/jamaoncol.2019.3343
67. Lesokhin AM, Ansell SM, Armand P, Scott EC, Halwani A, Gutierrez M, et al. Nivolumab in patients with relapsed or refractory hematologic malignancy: Preliminary results of a phase ib study. J Clin Oncol (2016) 34(23):2698–704. doi: 10.1200/jco.2015.65.9789
68. Hodi FS, Chiarion-Sileni V, Gonzalez R, Grob JJ, Rutkowski P, Cowey CL, et al. Nivolumab plus ipilimumab or nivolumab alone versus ipilimumab alone in advanced melanoma (CheckMate 067): 4-year outcomes of a multicentre, randomised, phase 3 trial. Lancet Oncol (2018) 19(11):1480–92. doi: 10.1016/s1470-2045(18)30700-9
69. Schmid P, Adams S, Rugo HS, Schneeweiss A, Barrios CH, Iwata H, et al. Atezolizumab and nab-paclitaxel in advanced triple-negative breast cancer. N Engl J Med (2018) 379(22):2108–21. doi: 10.1056/NEJMoa1809615
70. Rittmeyer A, Barlesi F, Waterkamp D, Park K, Ciardiello F, Von Pawel J, et al. Atezolizumab versus docetaxel in patients with previously treated non-small-cell lung cancer (OAK): A phase 3, open-label, multicentre randomised controlled trial. Lancet (2017) 389(10066):255–65. doi: 10.1016/s0140-6736(16)32517-x
71. Yang J, Dong L, Yang S, Han X, Han Y, Jiang S, et al. Safety and clinical efficacy of toripalimab, a PD-1 mAb, in patients with advanced or recurrent malignancies in a phase I study. Eur J Cancer (2020) 130:182–92. doi: 10.1016/j.ejca.2020.01.028
72. Wang Z, Ying J, Xu J, Yuan P, Duan J, Bai H, et al. Safety, antitumor activity, and pharmacokinetics of toripalimab, a programmed cell death 1 inhibitor, in patients with advanced non-small cell lung cancer: A phase 1 trial. JAMA Netw Open (2020) 3(10):e2013770. doi: 10.1001/jamanetworkopen.2020.13770
73. Ferrara R, Mezquita L, Texier M, Lahmar J, Audigier-Valette C, Tessonnier L, et al. Hyperprogressive disease in patients with advanced non-small cell lung cancer treated with PD-1/PD-L1 inhibitors or with single-agent chemotherapy. JAMA Oncol (2018) 4(11):1543–52. doi: 10.1001/jamaoncol.2018.3676
74. Dirix LY, Takacs I, Jerusalem G, Nikolinakos P, Arkenau HT, Forero-Torres A, et al. Avelumab, an anti-PD-L1 antibody, in patients with locally advanced or metastatic breast cancer: a phase 1b JAVELIN solid tumor study. Breast Cancer Res Treat (2018) 167(3):671–86. doi: 10.1007/s10549-017-4537-5
75. Song Y, Gao Q, Zhang H, Fan L, Zhou J, Zou D, et al. Treatment of relapsed or refractory classical Hodgkin lymphoma with the anti-PD-1, tislelizumab: results of a phase 2, single-arm, multicenter study. Leukemia (2020) 34(2):533–42. doi: 10.1038/s41375-019-0545-2
76. Song Y, Wu J, Chen X, Lin T, Cao J, Liu Y, et al. A single-arm, multicenter, phase II study of camrelizumab in relapsed or refractory classical Hodgkin lymphoma. Clin Cancer Res (2019) 25(24):7363–9. doi: 10.1158/1078-0432.Ccr-19-1680
77. Liu D, Ma C, Lu P, Gong J, Ye D, Wang S, et al. Dose escalation and expansion (phase Ia/Ib) study of GLS-010, a recombinant fully human antiprogrammed death-1 monoclonal antibody for advanced solid tumors or lymphoma. Eur J Cancer (2021) 148:1–13. doi: 10.1016/j.ejca.2021.01.020
78. De Velasco G, Je Y, Bossé D, Awad MM, Ott PA, Moreira RB, et al. Comprehensive meta-analysis of key immune-related adverse events from CTLA-4 and PD-1/PD-L1 inhibitors in cancer patients. Cancer Immunol Res (2017) 5(4):312–8. doi: 10.1158/2326-6066.Cir-16-0237
79. Ramos-Casals M, Brahmer JR, Callahan MK, Flores-Chávez A, Keegan N, Khamashta MA, et al. Immune-related adverse events of checkpoint inhibitors. Nat Rev Dis Primers (2020) 6(1):38. doi: 10.1038/s41572-020-0160-6
80. Ferris RL, Blumenschein G Jr., Fayette J, Guigay J, Colevas AD, Licitra L, et al. Nivolumab for recurrent squamous-cell carcinoma of the head and neck. N Engl J Med (2016) 375(19):1856–67. doi: 10.1056/NEJMoa1602252
81. Herbst RS, Baas P, Kim DW, Felip E, Pérez-Gracia JL, Han JY, et al. Pembrolizumab versus docetaxel for previously treated, PD-L1-positive, advanced non-small-cell lung cancer (KEYNOTE-010): A randomised controlled trial. Lancet (2016) 387(10027):1540–50. doi: 10.1016/s0140-6736(15)01281-7
82. Fradet Y, Bellmunt J, Vaughn DJ, Lee JL, Fong L, Vogelzang NJ, et al. Randomized phase III KEYNOTE-045 trial of pembrolizumab versus paclitaxel, docetaxel, or vinflunine in recurrent advanced urothelial cancer: results of >2 years of follow-up. Ann Oncol (2019) 30(6):970–6. doi: 10.1093/annonc/mdz127
83. Fehrenbacher L, Spira A, Ballinger M, Kowanetz M, Vansteenkiste J, Mazieres J, et al. Atezolizumab versus docetaxel for patients with previously treated non-small-cell lung cancer (POPLAR): A multicentre, open-label, phase 2 randomised controlled trial. Lancet (2016) 387(10030):1837–46. doi: 10.1016/s0140-6736(16)00587-0
84. Lee MS, Ryoo BY, Hsu CH, Numata K, Stein S, Verret W, et al. Atezolizumab with or without bevacizumab in unresectable hepatocellular carcinoma (GO30140): An open-label, multicentre, phase 1b study. Lancet Oncol (2020) 21(6):808–20. doi: 10.1016/s1470-2045(20)30156-x
85. Balar AV, Galsky MD, Rosenberg JE, Powles T, Petrylak DP, Bellmunt J, et al. Atezolizumab as first-line treatment in cisplatin-ineligible patients with locally advanced and metastatic urothelial carcinoma: A single-arm, multicentre, phase 2 trial. Lancet (2017) 389(10064):67–76. doi: 10.1016/s0140-6736(16)32455-2
86. Desai J, Deva S, Lee JS, Lin CC, Yen CJ, Chao Y, et al. Phase IA/IB study of single-agent tislelizumab, an investigational anti-PD-1 antibody, in solid tumors. J Immunother Cancer (2020) 8(1). doi: 10.1136/jitc-2019-000453
87. Ansell SM, Minnema MC, Johnson P, Timmerman JM, Armand P, Shipp MA, et al. Nivolumab for Relapsed/Refractory diffuse Large b-cell lymphoma in patients ineligible for or having failed autologous transplantation: A single-arm, phase II study. J Clin Oncol (2019) 37(6):481–9. doi: 10.1200/jco.18.00766
88. Zhu AX, Finn RS, Edeline J, Cattan S, Ogasawara S, Palmer D, et al. Pembrolizumab in patients with advanced hepatocellular carcinoma previously treated with sorafenib (KEYNOTE-224): A non-randomised, open-label phase 2 trial. Lancet Oncol (2018) 19(7):940–52. doi: 10.1016/s1470-2045(18)30351-6
89. Wang F, Wei XL, Wang FH, Xu N, Shen L, Dai GH, et al. Safety, efficacy and tumor mutational burden as a biomarker of overall survival benefit in chemo-refractory gastric cancer treated with toripalimab, a PD-1 antibody in phase Ib/II clinical trial NCT02915432. Ann Oncol (2019) 30(9):1479–86. doi: 10.1093/annonc/mdz197
90. De Ruysscher D, Ramalingam S, Urbanic J, Gerber DE, Tan DSW, Cai J, et al. CheckMate 73L: A phase 3 study comparing nivolumab plus concurrent chemoradiotherapy followed by nivolumab with or without ipilimumab versus concurrent chemoradiotherapy followed by durvalumab for previously untreated, locally advanced stage III non-Small-Cell lung cancer. Clin Lung Cancer (2022) 23(3):e264–8. doi: 10.1016/j.cllc.2021.07.005
91. Tsoukalas N, Kiakou M, Tsapakidis K, Tolia M, Aravantinou-Fatorou E, Baxevanos P, et al. PD-1 and PD-L1 as immunotherapy targets and biomarkers in non-small cell lung cancer. J BUON (2019) 24(3):883–8.
92. Yu H, Boyle TA, Zhou C, Rimm DL, Hirsch FR. PD-L1 expression in lung cancer. J Thorac Oncol (2016) 11(7):964–75. doi: 10.1016/j.jtho.2016.04.014
93. Zhao S, Ren S, Jiang T, Zhu B, Li X, Zhao C, et al. Low-dose apatinib optimizes tumor microenvironment and potentiates antitumor effect of PD-1/PD-L1 blockade in lung cancer. Cancer Immunol Res (2019) 7(4):630–43. doi: 10.1158/2326-6066.CIR-17-0640
94. Takeuchi M, Doi T, Obayashi K, Hirai A, Yoneda K, Tanaka F, et al. Soluble PD-L1 with PD-1-binding capacity exists in the plasma of patients with non-small cell lung cancer. Immunol Lett (2018) 196:155–60. doi: 10.1016/j.imlet.2018.01.007
95. Tiako Meyo M, Jouinot A, Giroux-Leprieur E, Fabre E, Wislez M, Alifano M, et al. Predictive value of soluble PD-1, PD-L1, VEGFA, CD40 ligand and CD44 for nivolumab therapy in advanced non-small cell lung cancer: A case-control study. Cancers (Basel) (2020) 12(2). doi: 10.3390/cancers12020473
96. Abu Hejleh T, Furqan M, Ballas Z, Clamon G. The clinical significance of soluble PD-1 and PD-L1 in lung cancer. Crit Rev Oncol Hematol (2019) 143:148–52. doi: 10.1016/j.critrevonc.2019.08.009
97. Wang X, Wang F, Zhong M, Yarden Y, Fu L. The biomarkers of hyperprogressive disease in PD-1/PD-L1 blockage therapy. Mol Cancer (2020) 19(1):81. doi: 10.1186/s12943-020-01200-x
98. Kim CG, Kim KH, Pyo KH, Xin CF, Hong MH, Ahn BC, et al. Hyperprogressive disease during PD-1/PD-L1 blockade in patients with non-small-cell lung cancer. Ann Oncol (2019) 30(7):1104–13. doi: 10.1093/annonc/mdz123
99. Zhang Y, Zhou H, Zhang L. Which is the optimal immunotherapy for advanced squamous non-small-cell lung cancer in combination with chemotherapy: anti-PD-1 or anti-PD-L1? J Immunother Cancer (2018) 6(1):135. doi: 10.1186/s40425-018-0427-6
100. Planes-Laine G, Rochigneux P, Bertucci F, Chretien AS, Viens P, Sabatier R, et al. PD-1/PD-L1 targeting in breast cancer: The first clinical evidences are emerging. A literature review. Cancers (Basel) (2019) 11(7). doi: 10.3390/cancers11071033
101. Voorwerk L, Slagter M, Horlings HM, Sikorska K, Van De Vijver KK, De Maaker M, et al. Immune induction strategies in metastatic triple-negative breast cancer to enhance the sensitivity to PD-1 blockade: The TONIC trial. Nat Med (2019) 25(6):920–8. doi: 10.1038/s41591-019-0432-4
102. Cyprian FS, Akhtar S, Gatalica Z, Vranic S. Targeted immunotherapy with a checkpoint inhibitor in combination with chemotherapy: A new clinical paradigm in the treatment of triple-negative breast cancer. Bosn J Basic Med Sci (2019) 19(3):227–33. doi: 10.17305/bjbms.2019.4204
103. Huang W, Ran R, Shao B, Li H. Prognostic and clinicopathological value of PD-L1 expression in primary breast cancer: A meta-analysis. Breast Cancer Res Treat (2019) 178(1):17–33. doi: 10.1007/s10549-019-05371-0
104. Wu Y, Chen M, Wu P, Chen C, Xu ZP, Gu W. Increased PD-L1 expression in breast and colon cancer stem cells. Clin Exp Pharmacol Physiol (2017) 44(5):602–4. doi: 10.1111/1440-1681.12732
105. Janjigian YY, Shitara K, Moehler M, Garrido M, Salman P, Shen L, et al. First-line nivolumab plus chemotherapy versus chemotherapy alone for advanced gastric, gastro-oesophageal junction, and oesophageal adenocarcinoma (CheckMate 649): A randomised, open-label, phase 3 trial. Lancet (2021) 398(10294):27–40. doi: 10.1016/s0140-6736(21)00797-2
106. Kim JH, Ryu MH, Park YS, Ma J, Lee SY, Kim D, et al. Predictive biomarkers for the efficacy of nivolumab as ≥ 3(rd)-line therapy in patients with advanced gastric cancer: A subset analysis of ATTRACTION-2 phase III trial. BMC Cancer (2022) 22(1):378. doi: 10.1186/s12885-022-09488-2
107. Hernandez GA, Perera RM. Autophagy in cancer cell remodeling and quality control. Mol Cell (2022) 82(8):1514–27. doi: 10.1016/j.molcel.2022.03.023
108. Wang J, Gong M, Fan X, Huang D, Zhang J, Huang C. Autophagy-related signaling pathways in non-small cell lung cancer. Mol Cell Biochem (2022) 477(2):385–93. doi: 10.1007/s11010-021-04280-5
109. Rakesh R, Priyadharshini LC, Sakthivel KM, Rasmi RR. Role and regulation of autophagy in cancer. Biochim Biophys Acta Mol Basis Dis (2022) 1868(7):166400. doi: 10.1016/j.bbadis.2022.166400
110. Wang X, Wu WKK, Gao J, Li Z, Dong B, Lin X, et al. Autophagy inhibition enhances PD-L1 expression in gastric cancer. J Exp Clin Cancer Res (2019) 38(1):140. doi: 10.1186/s13046-019-1148-5
111. Wang BC, Zhang ZJ, Fu C, Wang C. Efficacy and safety of anti-PD-1/PD-L1 agents vs chemotherapy in patients with gastric or gastroesophageal junction cancer: A systematic review and meta-analysis. Med (Baltimore) (2019) 98(47):e18054. doi: 10.1097/MD.0000000000018054
112. Spiker AM, Mangla A, Ramsey ML. Angiosarcoma. Treasure Island (FL): StatPearls Publishing Copyright © 2022, StatPearls Publishing LLC (2022).
113. Digrazia GN, Zinzuwadia S, Aragao AP, Camren GP, Hibbeln JF, Gerena M, et al. Rare primary pulmonary angiosarcoma with aberrant neuroendocrine differentiation. Clin Imaging (2022) 82:88–93. doi: 10.1016/j.clinimag.2021.11.002
114. Googe PB, Flores K, Jenkins F, Merritt B, Moschos SJ, Grilley-Olson JE. Immune checkpoint markers in superficial angiosarcomas: PD-L1, PD-1, CD8, LAG-3, and tumor-infiltrating lymphocytes. Am J Dermatopathol (2021) 43(8):556–9. doi: 10.1097/dad.0000000000001843
115. Xu W, Wang K, Gu W, Nie X, Zhang H, Tang C, et al. Case report: Complete remission with anti-PD-1 and anti-VEGF combined therapy of a patient with metastatic primary splenic angiosarcoma. Front Oncol (2022) 12:809068. doi: 10.3389/fonc.2022.809068
116. Botti G, Scognamiglio G, Marra L, Pizzolorusso A, Di Bonito M, De Cecio R, et al. Programmed death ligand 1 (PD-L1) expression in primary angiosarcoma. J Cancer (2017) 8(16):3166–72. doi: 10.7150/jca.19060
117. Sindhu S, Gimber LH, Cranmer L, Mcbride A, Kraft AS. Angiosarcoma treated successfully with anti-PD-1 therapy - a case report. J Immunother Cancer (2017) 5(1):58. doi: 10.1186/s40425-017-0263-0
118. Shimizu A, Kaira K, Okubo Y, Utsumi D, Yasuda M, Asao T, et al. Positive PD-L1 expression predicts worse outcome in cutaneous angiosarcoma. J Glob Oncol (2017) 3(4):360–9. doi: 10.1200/jgo.2016.005843
119. Kawamura A, Kawamura T, Riddell M, Hikita T, Yanagi T, Umemura H, et al. Regulation of programmed cell death ligand 1 expression by atypical protein kinase c lambda/iota in cutaneous angiosarcoma. Cancer Sci (2019) 110(5):1780–9. doi: 10.1111/cas.13981
120. Momen S, Fassihi H, Davies HR, Nikolaou C, Degasperi A, Stefanato CM, et al. Dramatic response of metastatic cutaneous angiosarcoma to an immune checkpoint inhibitor in a patient with xeroderma pigmentosum: whole-genome sequencing aids treatment decision in end-stage disease. Cold Spring Harb Mol Case Stud (2019) 5(5). doi: 10.1101/mcs.a004408
121. Álvarez Múgica M, Jalón Monzón A. [Tissue biomarkers in prostate cancer.]. Arch Esp Urol (2022) 75(2):185–94.
122. Katongole P, Sande OJ, Reynolds SJ, Joloba M, Kajumbula H, Kalungi S, et al. Soluble programmed death-ligand 1 (sPD-L1) is elevated in aggressive prostate cancer disease among African men. Oncol Ther (2022) 10(1):185–93. doi: 10.1007/s40487-022-00184-6
123. Zhou X, Zou L, Liao H, Luo J, Yang T, Wu J, et al. Abrogation of HnRNP l enhances anti-PD-1 therapy efficacy via diminishing PD-L1 and promoting CD8(+) T cell-mediated ferroptosis in castration-resistant prostate cancer. Acta Pharm Sin B (2022) 12(2):692–707. doi: 10.1016/j.apsb.2021.07.016
124. Ness N, Andersen S, Khanehkenari MR, Nordbakken CV, Valkov A, Paulsen EE, et al. The prognostic role of immune checkpoint markers programmed cell death protein 1 (PD-1) and programmed death ligand 1 (PD-L1) in a large, multicenter prostate cancer cohort. Oncotarget (2017) 8(16):26789–801. doi: 10.18632/oncotarget.15817
125. Hansen AR, Massard C, Ott PA, Haas NB, Lopez JS, Ejadi S, et al. Pembrolizumab for advanced prostate adenocarcinoma: Findings of the KEYNOTE-028 study. Ann Oncol (2018) 29(8):1807–13. doi: 10.1093/annonc/mdy232
126. Han HJ, Li YR, Roach M 3rd, Aggarwal R. Dramatic response to combination pembrolizumab and radiation in metastatic castration resistant prostate cancer. Ther Adv Med Oncol (2020) 12:1758835920936084. doi: 10.1177/1758835920936084
127. Shi X, Zhang X, Li J, Zhao H, Mo L, Shi X, et al. PD-1/PD-L1 blockade enhances the efficacy of SA-GM-CSF surface-modified tumor vaccine in prostate cancer. Cancer Lett (2017) 406:27–35. doi: 10.1016/j.canlet.2017.07.029
128. Abida W, Cheng ML, Armenia J, Middha S, Autio KA, Vargas HA, et al. Analysis of the prevalence of microsatellite instability in prostate cancer and response to immune checkpoint blockade. JAMA Oncol (2019) 5(4):471–8. doi: 10.1001/jamaoncol.2018.5801
129. Crane CA, Panner A, Murray JC, Wilson SP, Xu H, Chen L, et al. PI(3) kinase is associated with a mechanism of immunoresistance in breast and prostate cancer. Oncogene (2009) 28(2):306–12. doi: 10.1038/onc.2008.384
130. Martin AM, Nirschl TR, Nirschl CJ, Francica BJ, Kochel CM, Van Bokhoven A, et al. Paucity of PD-L1 expression in prostate cancer: Innate and adaptive immune resistance. Prostate Cancer Prostatic Dis (2015) 18(4):325–32. doi: 10.1038/pcan.2015.39
131. Jin C, Zhu X, Huang X, Gong T, Wei Z, You J. Efficacy and safety of PD-1/PD-L1 and CTLA-4 immune checkpoint inhibitors in colorectal cancer: A meta-analysis. J Comp Eff Res (2022) 11(3):203–12. doi: 10.2217/cer-2021-0134
132. Yaghoubi N, Soltani A, Ghazvini K, Hassanian SM, Hashemy SI. PD-1/ PD-L1 blockade as a novel treatment for colorectal cancer. BioMed Pharmacother (2019) 110:312–8. doi: 10.1016/j.biopha.2018.11.105
133. Morse MA, Overman MJ, Hartman L, Khoukaz T, Brutcher E, Lenz HJ, et al. Safety of nivolumab plus low-dose ipilimumab in previously treated microsatellite instability-High/Mismatch repair-deficient metastatic colorectal cancer. Oncologist (2019) 24(11):1453–61. doi: 10.1634/theoncologist.2019-0129
134. Overman MJ, Lonardi S, Wong KYM, Lenz HJ, Gelsomino F, Aglietta M, et al. Durable clinical benefit with nivolumab plus ipilimumab in DNA mismatch repair-Deficient/Microsatellite instability-high metastatic colorectal cancer. J Clin Oncol (2018) 36(8):773–9. doi: 10.1200/jco.2017.76.9901
135. Overman MJ, Mcdermott R, Leach JL, Lonardi S, Lenz HJ, Morse MA, et al. Nivolumab in patients with metastatic DNA mismatch repair-deficient or microsatellite instability-high colorectal cancer (CheckMate 142): An open-label, multicentre, phase 2 study. Lancet Oncol (2017) 18(9):1182–91. doi: 10.1016/s1470-2045(17)30422-9
136. Lenz HJ, Van Cutsem E, Luisa Limon M, Wong KYM, Hendlisz A, Aglietta M, et al. First-line nivolumab plus low-dose ipilimumab for microsatellite instability-High/Mismatch repair-deficient metastatic colorectal cancer: The phase II CheckMate 142 study. J Clin Oncol (2022) 40(2):161–70. doi: 10.1200/jco.21.01015
137. Ciardiello D, Vitiello PP, Cardone C, Martini G, Troiani T, Martinelli E, et al. Immunotherapy of colorectal cancer: Challenges for therapeutic efficacy. Cancer Treat Rev (2019) 76:22–32. doi: 10.1016/j.ctrv.2019.04.003
138. Möller K, Blessin NC, Höflmayer D, Büscheck F, Luebke AM, Kluth M, et al. High density of cytotoxic T-lymphocytes is linked to tumoral PD-L1 expression regardless of the mismatch repair status in colorectal cancer. Acta Oncol (2021) 60(9):1210–7. doi: 10.1080/0284186x.2021.1933585
139. Kim DW, Tan E, Zhou JM, Schell MJ, Martinez M, Yu J, et al. A phase 1/2 trial of ibrutinib in combination with pembrolizumab in patients with mismatch repair proficient metastatic colorectal cancer. Br J Cancer (2021) 124(11):1803–8. doi: 10.1038/s41416-021-01368-z
140. Kuang C, Park Y, Augustin RC, Lin Y, Hartman DJ, Seigh L, et al. Pembrolizumab plus azacitidine in patients with chemotherapy refractory metastatic colorectal cancer: A single-arm phase 2 trial and correlative biomarker analysis. Clin Epigenet (2022) 14(1):3. doi: 10.1186/s13148-021-01226-y
141. Antoniotti C, Rossini D, Pietrantonio F, Catteau A, Salvatore L, Lonardi S, et al. Upfront FOLFOXIRI plus bevacizumab with or without atezolizumab in the treatment of patients with metastatic colorectal cancer (AtezoTRIBE): a multicentre, open-label, randomised, controlled, phase 2 trial. Lancet Oncol (2022) 23(7):876–87. doi: 10.1016/s1470-2045(22)00274-1
142. Juneja VR, Mcguire KA, Manguso RT, Lafleur MW, Collins N, Haining WN, et al. PD-L1 on tumor cells is sufficient for immune evasion in immunogenic tumors and inhibits CD8 T cell cytotoxicity. J Exp Med (2017) 214(4):895–904. doi: 10.1084/jem.20160801
143. Sasidharan Nair V, Toor SM, Taha RZ, Shaath H, Elkord E. DNA Methylation and repressive histones in the promoters of PD-1, CTLA-4, TIM-3, LAG-3, TIGIT, PD-L1, and galectin-9 genes in human colorectal cancer. Clin Epigenet (2018) 10(1):104. doi: 10.1186/s13148-018-0539-3
144. Li Y, Liang L, Dai W, Cai G, Xu Y, Li X, et al. Prognostic impact of programed cell death-1 (PD-1) and PD-ligand 1 (PD-L1) expression in cancer cells and tumor infiltrating lymphocytes in colorectal cancer. Mol Cancer (2016) 15(1):55. doi: 10.1186/s12943-016-0539-x
145. Gong XQ, Tao YY, Wu YK, Liu N, Yu X, Wang R, et al. Progress of MRI radiomics in hepatocellular carcinoma. Front Oncol (2021) 11:698373. doi: 10.3389/fonc.2021.698373
146. Huang CY, Wang Y, Luo GY, Han F, Li YQ, Zhou ZG, et al. Relationship between PD-L1 expression and CD8+ T-cell immune responses in hepatocellular carcinoma. J Immunother (2017) 40(9):323–33. doi: 10.1097/CJI.0000000000000187
147. Du SS, Chen GW, Yang P, Chen YX, Hu Y, Zhao QQ, et al. Radiation therapy promotes hepatocellular carcinoma immune cloaking via PD-L1 upregulation induced by cGAS-STING activation. Int J Radiat Oncol Biol Phys (2022) 112(5):1243–55. doi: 10.1016/j.ijrobp.2021.12.162
148. Cheng H, Sun G, Chen H, Li Y, Han Z, Li Y, et al. Trends in the treatment of advanced hepatocellular carcinoma: Immune checkpoint blockade immunotherapy and related combination therapies. Am J Cancer Res (2019) 9(8):1536–45.
149. Pinato DJ, Mauri FA, Spina P, Cain O, Siddique A, Goldin R, et al. Clinical implications of heterogeneity in PD-L1 immunohistochemical detection in hepatocellular carcinoma: The blueprint-HCC study. Br J Cancer (2019) 120(11):1033–6. doi: 10.1038/s41416-019-0466-x
150. Ke M, Zhang Z, Xu B, Zhao S, Ding Y, Wu X, et al. Baicalein and baicalin promote antitumor immunity by suppressing PD-L1 expression in hepatocellular carcinoma cells. Int Immunopharmacol (2019) 75:105824. doi: 10.1016/j.intimp.2019.105824
151. Xu F, Jin T, Zhu Y, Dai C. Immune checkpoint therapy in liver cancer. J Exp Clin Cancer Res (2018) 37(1):110. doi: 10.1186/s13046-018-0777-4
152. Zhu X, Lang J. Soluble PD-1 and PD-L1: predictive and prognostic significance in cancer. Oncotarget (2017) 8(57):97671–82. doi: 10.18632/oncotarget.18311
153. Li XS, Li JW, Li H, Jiang T. Prognostic value of programmed cell death ligand 1 (PD-L1) for hepatocellular carcinoma: A meta-analysis. Biosci Rep (2020) 40(4). doi: 10.1042/bsr20200459
154. Mocan T, Ilies M, Nenu I, Craciun R, Horhat A, Susa R, et al. Serum levels of soluble programmed death-ligand 1 (sPD-L1): A possible biomarker in predicting post-treatment outcomes in patients with early hepatocellular carcinoma. Int Immunopharmacol (2021) 94:107467. doi: 10.1016/j.intimp.2021.107467
155. Chang B, Huang T, Wei H, Shen L, Zhu D, He W, et al. The correlation and prognostic value of serum levels of soluble programmed death protein 1 (sPD-1) and soluble programmed death-ligand 1 (sPD-L1) in patients with hepatocellular carcinoma. Cancer Immunol Immunother (2019) 68(3):353–63. doi: 10.1007/s00262-018-2271-4
156. Lin Y, Shen Z, Tao T, Xu C, Xia K, Xuan Q, et al. Study on the effect of polymer nano-loaded drug system on bladder cancer perfusion. J Nanosci Nanotechnol (2021) 21(2):955–61. doi: 10.1166/jnn.2021.18638
157. Macgregor M, Safizadeh Shirazi H, Chan KM, Ostrikov K, Mcnicholas K, Jay A, et al. Cancer cell detection device for the diagnosis of bladder cancer from urine. Biosens Bioelectron (2021) 171:112699. doi: 10.1016/j.bios.2020.112699
158. Chen R, Zhou X, Liu J, Huang G. Relationship between the expression of PD-1/PD-L1 and (18)F-FDG uptake in bladder cancer. Eur J Nucl Med Mol Imaging (2019) 46(4):848–54. doi: 10.1007/s00259-018-4208-8
159. Inman BA, Longo TA, Ramalingam S, Harrison MR. Atezolizumab: A PD-L1-Blocking antibody for bladder cancer. Clin Cancer Res (2017) 23(8):1886–90. doi: 10.1158/1078-0432.CCR-16-1417
160. Bellmunt J, Powles T, Vogelzang NJ. A review on the evolution of PD-1/PD-L1 immunotherapy for bladder cancer: The future is now. Cancer Treat Rev (2017) 54:58–67. doi: 10.1016/j.ctrv.2017.01.007
161. Kawahara T, Ishiguro Y, Ohtake S, Kato I, Ito Y, Ito H, et al. PD-1 and PD-L1 are more highly expressed in high-grade bladder cancer than in low-grade cases: PD-L1 might function as a mediator of stage progression in bladder cancer. BMC Urol (2018) 18(1):97. doi: 10.1186/s12894-018-0414-8
162. Flores-Martin JF, Perea F, Exposito-Ruiz M, Carretero FJ, Rodriguez T, Villamediana M, et al. A combination of positive tumor HLA-I and negative PD-L1 expression provides an immune rejection mechanism in bladder cancer. Ann Surg Oncol (2019) 26(8):2631–9. doi: 10.1245/s10434-019-07371-2
163. Massard C, Gordon MS, Sharma S, Rafii S, Wainberg ZA, Luke J, et al. Safety and efficacy of durvalumab (MEDI4736), an anti-programmed cell death ligand-1 immune checkpoint inhibitor, in patients with advanced urothelial bladder cancer. J Clin Oncol (2016) 34(26):3119–25. doi: 10.1200/jco.2016.67.9761
164. Apolo AB, Infante JR, Balmanoukian A, Patel MR, Wang D, Kelly K, et al. Avelumab, an anti-programmed death-ligand 1 antibody, in patients with refractory metastatic urothelial carcinoma: Results from a multicenter, phase ib study. J Clin Oncol (2017) 35(19):2117–24. doi: 10.1200/jco.2016.71.6795
165. Zhu J, Li Y, Luo Y, Xu J, Liufu H, Tian Z, et al. A feedback loop formed by ATG7/Autophagy, FOXO3a/miR-145 and PD-L1 regulates stem-like properties and invasion in human bladder cancer. Cancers (Basel) (2019) 11(3). doi: 10.3390/cancers11030349
166. Rompre-Brodeur A, Shinde-Jadhav S, Ayoub M, Piccirillo CA, Seuntjens J, Brimo F, et al. PD-1/PD-L1 immune checkpoint inhibition with radiation in bladder cancer: In situ and abscopal effects. Mol Cancer Ther (2020) 19(1):211–20. doi: 10.1158/1535-7163.MCT-18-0986
167. Asante DB, Calapre L, Ziman M, Meniawy TM, Gray ES. Liquid biopsy in ovarian cancer using circulating tumor DNA and cells: Ready for prime time? Cancer Lett (2020) 468:59–71. doi: 10.1016/j.canlet.2019.10.014
168. Pawłowska A, Suszczyk D, Okła K, Barczyński B, Kotarski J, Wertel I. Immunotherapies based on PD-1/PD-L1 pathway inhibitors in ovarian cancer treatment. Clin Exp Immunol (2019) 195(3):334–44. doi: 10.1111/cei.13255
169. Eggold JT, Chow S, Melemenidis S, Wang J, Natarajan S, Loo PE, et al. Abdominopelvic FLASH irradiation improves PD-1 immune checkpoint inhibition in preclinical models of ovarian cancer. Mol Cancer Ther (2022) 21(2):371–81. doi: 10.1158/1535-7163.Mct-21-0358
170. Zhu J, Yan L, Wang Q. Efficacy of PD-1/PD-L1 inhibitors in ovarian cancer: a single-arm meta-analysis. J Ovarian Res (2021) 14(1):112. doi: 10.1186/s13048-021-00862-5
171. Pietak P, Pietrzyk N, Pawlowska A, Suszczyk D, Bednarek W, Kotarski J, et al. [The meaning of PD-1/PD-L1 pathway in ovarian cancer pathogenesis]. Wiad Lek (2018) 71(5):1089–94.
172. Moore KN, Pignata S. Trials in progress: IMagyn050/GOG 3015/ENGOT-OV39. a phase III, multicenter, randomized study of atezolizumab versus placebo administered in combination with paclitaxel, carboplatin, and bevacizumab to patients with newly-diagnosed stage III or stage IV ovarian, fallopian tube, or primary peritoneal cancer. Int J Gynecol Cancer (2019). doi: 10.1136/ijgc-2018-000071
173. Liu Z, Ravindranathan R, Kalinski P, Guo ZS, Bartlett DL. Rational combination of oncolytic vaccinia virus and PD-L1 blockade works synergistically to enhance therapeutic efficacy. Nat Commun (2017) 8:14754. doi: 10.1038/ncomms14754
174. Farkkila A, Gulhan DC, Casado J, Jacobson CA, Nguyen H, Kochupurakkal B, et al. Immunogenomic profiling determines responses to combined PARP and PD-1 inhibition in ovarian cancer. Nat Commun (2020) 11(1):1459. doi: 10.1038/s41467-020-15315-8
175. Boussios S, Karihtala P, Moschetta M, Karathanasi A, Sadauskaite A, Rassy E, et al. Combined strategies with poly (ADP-ribose) polymerase (PARP) inhibitors for the treatment of ovarian cancer: A literature review. Diagnostics (Basel) (2019) 9(3). doi: 10.3390/diagnostics9030087
176. Klein AP. Pancreatic cancer epidemiology: understanding the role of lifestyle and inherited risk factors. Nat Rev Gastroenterol Hepatol (2021) 18(7):493–502. doi: 10.1038/s41575-021-00457-x
177. Feng M, Xiong G, Cao Z, Yang G, Zheng S, Song X, et al. PD-1/PD-L1 and immunotherapy for pancreatic cancer. Cancer Lett (2017) 407:57–65. doi: 10.1016/j.canlet.2017.08.006
178. Nagaraju GP, Malla RR, Basha R, Motofei IG. Contemporary clinical trials in pancreatic cancer immunotherapy targeting PD-1 and PD-L1. Semin Cancer Biol (2021). doi: 10.1016/j.semcancer.2021.11.003
179. Karamitopoulou E, Andreou A, Pahud De Mortanges A, Tinguely M, Gloor B, Perren A. PD-1/PD-L1-Associated immunoarchitectural patterns stratify pancreatic cancer patients into Prognostic/Predictive subgroups. Cancer Immunol Res (2021) 9(12):1439–50. doi: 10.1158/2326-6066.Cir-21-0144
180. Ma Y, Li J, Wang H, Chiu Y, Kingsley CV, Fry D, et al. Combination of PD-1 inhibitor and OX40 agonist induces tumor rejection and immune memory in mouse models of pancreatic cancer. Gastroenterology (2020) 159(1):306–319.e12. doi: 10.1053/j.gastro.2020.03.018
181. Zhang X, Lao M, Xu J, Duan Y, Yang H, Li M, et al. Combination cancer immunotherapy targeting TNFR2 and PD-1/PD-L1 signaling reduces immunosuppressive effects in the microenvironment of pancreatic tumors. J Immunother Cancer (2022) 10(3). doi: 10.1136/jitc-2021-003982
182. Ho TTB, Nasti A, Seki A, Komura T, Inui H, Kozaka T, et al. Combination of gemcitabine and anti-PD-1 antibody enhances the anticancer effect of M1 macrophages and the Th1 response in a murine model of pancreatic cancer liver metastasis. J Immunother Cancer (2020) 8(2). doi: 10.1136/jitc-2020-001367
183. Chennamadhavuni A, Lyengar V, Shimanovsky A. Leukemia. Treasure Island (FL: StatPearls Publishing Copyright © 2022, StatPearls Publishing LLC (2022).
184. Nepstad I, Hatfield KJ, Grønningsæter IS, Reikvam H. The PI3K-Akt-mTOR signaling pathway in human acute myeloid leukemia (AML) cells. Int J Mol Sci (2020) 21(8). doi: 10.3390/ijms21082907
185. Meleveedu KS, Chen D, Nadiminti K, Sidiqi H, Khan S, Alkhateeb H, et al. PD-1/PD-L1 expression in extramedullary lesions of acute myeloid leukemia. Leuk Lymphoma (2021) 62(3):764–7. doi: 10.1080/10428194.2019.1675880
186. Chen C, Liang C, Wang S, Chio CL, Zhang Y, Zeng C, et al. Expression patterns of immune checkpoints in acute myeloid leukemia. J Hematol Oncol (2020) 13(1):28. doi: 10.1186/s13045-020-00853-x
187. Huang J, Tan J, Chen Y, Huang S, Xu L, Zhang Y, et al. A skewed distribution and increased PD-1+Vβ+CD4+/CD8+ T cells in patients with acute myeloid leukemia. J Leukoc Biol (2019) 106(3):725–32. doi: 10.1002/jlb.Ma0119-021r
188. Daver N, Garcia-Manero G, Basu S, Boddu PC, Alfayez M, Cortes JE, et al. Efficacy, safety, and biomarkers of response to azacitidine and nivolumab in Relapsed/Refractory acute myeloid leukemia: A nonrandomized, open-label, phase II study. Cancer Discovery (2019) 9(3):370–83. doi: 10.1158/2159-8290.Cd-18-0774
189. Chen C, Xu L, Gao R, Wang S, Zhang Y, Wang C, et al. Transcriptome-based Co-expression of BRD4 and PD-1/PD-L1 predicts poor overall survival in patients with acute myeloid leukemia. Front Pharmacol (2020) 11:582955. doi: 10.3389/fphar.2020.582955
190. Radpour R, Stucki M, Riether C, Ochsenbein AF. Epigenetic silencing of immune-checkpoint receptors in bone marrow- infiltrating T cells in acute myeloid leukemia. Front Oncol (2021) 11:663406. doi: 10.3389/fonc.2021.663406
191. Jelinek T, Paiva B, Hajek R. Update on PD-1/PD-L1 inhibitors in multiple myeloma. Front Immunol (2018) 9:2431. doi: 10.3389/fimmu.2018.02431
192. Ye W, Wu X, Liu X, Zheng X, Deng J, Gong Y. Comparison of monoclonal antibodies targeting CD38, SLAMF7 and PD-1/PD-L1 in combination with Bortezomib/Immunomodulators plus dexamethasone/prednisone for the treatment of multiple myeloma: An indirect-comparison meta-analysis of randomised controlled trials. BMC Cancer (2021) 21(1):994. doi: 10.1186/s12885-021-08588-9
193. Oliva S, Troia R, D'agostino M, Boccadoro M, Gay F. Promises and pitfalls in the use of PD-1/PD-L1 inhibitors in multiple myeloma. Front Immunol (2018) 9:2749. doi: 10.3389/fimmu.2018.02749
194. Verkleij CPM, Jhatakia A, Broekmans MEC, Frerichs KA, Zweegman S, Mutis T, et al. Preclinical rationale for targeting the PD-1/PD-L1 axis in combination with a CD38 antibody in multiple myeloma and other CD38-positive malignancies. Cancers (Basel) (2020) 12(12). doi: 10.3390/cancers12123713
195. Dhodapkar MV, Sexton R, Das R, Dhodapkar KM, Zhang L, Sundaram R, et al. Prospective analysis of antigen-specific immunity, stem-cell antigens, and immune checkpoints in monoclonal gammopathy. Blood (2015) 126(22):2475–8. doi: 10.1182/blood-2015-03-632919
196. Tremblay-Lemay R, Rastgoo N, Chang H. Modulating PD-L1 expression in multiple myeloma: an alternative strategy to target the PD-1/PD-L1 pathway. J Hematol Oncol (2018) 11(1):46. doi: 10.1186/s13045-018-0589-1
197. Costa F, Marchica V, Storti P, Malavasi F, Giuliani N. PD-L1/PD-1 axis in multiple myeloma microenvironment and a possible link with CD38-mediated immune-suppression. Cancers (Basel) (2021) 13(2). doi: 10.3390/cancers13020164
198. Curti BD, Koguchi Y, Leidner RS, Rolig AS, Sturgill ER, Sun Z, et al. Enhancing clinical and immunological effects of anti-PD-1 with belapectin, a galectin-3 inhibitor. J Immunother Cancer (2021) 9(4). doi: 10.1136/jitc-2021-002371
199. Görgün G, Samur MK, Cowens KB, Paula S, Bianchi G, Anderson JE, et al. Lenalidomide enhances immune checkpoint blockade-induced immune response in multiple myeloma. Clin Cancer Res (2015) 21(20):4607–18. doi: 10.1158/1078-0432.Ccr-15-0200
200. Jiang M, Bennani NN, Feldman AL. Lymphoma classification update: T-cell lymphomas, Hodgkin lymphomas, and histiocytic/dendritic cell neoplasms. Expert Rev Hematol (2017) 10(3):239–49. doi: 10.1080/17474086.2017.1281122
201. De Leval L, Jaffe ES. Lymphoma classification. Cancer J (2020) 26(3):176–85. doi: 10.1097/ppo.0000000000000451
202. Xie M, Huang X, Ye X, Qian W. Prognostic and clinicopathological significance of PD-1/PD-L1 expression in the tumor microenvironment and neoplastic cells for lymphoma. Int Immunopharmacol (2019) 77:105999. doi: 10.1016/j.intimp.2019.105999
203. Vari F, Arpon D, Keane C, Hertzberg MS, Talaulikar D, Jain S, et al. Immune evasion via PD-1/PD-L1 on NK cells and monocyte/macrophages is more prominent in Hodgkin lymphoma than DLBCL. Blood (2018) 131(16):1809–19. doi: 10.1182/blood-2017-07-796342
204. Veldman J, Alsada ZND, Van Den Berg A, Plattel WJ, Diepstra A, Visser L. Soluble PD-L1 is a promising disease biomarker but does not reflect tissue expression in classic Hodgkin lymphoma. Br J Haematol (2021) 193(3):506–14. doi: 10.1111/bjh.17362
205. Armand P, Chen YB, Redd RA, Joyce RM, Bsat J, Jeter E, et al. PD-1 blockade with pembrolizumab for classical Hodgkin lymphoma after autologous stem cell transplantation. Blood (2019) 134(1):22–9. doi: 10.1182/blood.2019000215
206. Yi M, Zheng X, Niu M, Zhu S, Ge H, Wu K. Combination strategies with PD-1/PD-L1 blockade: current advances and future directions. Mol Cancer (2022) 21(1):28. doi: 10.1186/s12943-021-01489-2
207. Yin J, Wu Y, Yang X, Gan L, Xue J. Checkpoint inhibitor pneumonitis induced by anti-PD-1/PD-L1 therapy in non-Small-Cell lung cancer: Occurrence and mechanism. Front Immunol (2022) 13:830631. doi: 10.3389/fimmu.2022.830631
208. Zhang H, Tan S, Fang C, Zhang Q, Cao X, Liu Y. PD-1/PD-L1 correlates with the efficacy of the treatment of concurrent chemoradiotherapy in cervical cancer. Front Oncol (2022) 12:858164. doi: 10.3389/fonc.2022.858164
209. Pinard CJ, Hocker SE, Poon AC, Inkol JM, Matsuyama A, Wood RD, et al. Evaluation of PD-1 and PD-L1 expression in canine urothelial carcinoma cell lines. Vet Immunol Immunopathol (2022) 243:110367. doi: 10.1016/j.vetimm.2021.110367
210. Saito H, Kono Y, Murakami Y, Shishido Y, Kuroda H, Matsunaga T, et al. Highly activated PD-1/PD-L1 pathway in gastric cancer with PD-L1 expression. Anticancer Res (2018) 38(1):107–12. doi: 10.21873/anticanres.12197
211. Brahmer JR, Tykodi SS, Chow LQ, Hwu WJ, Topalian SL, Hwu P, et al. Safety and activity of anti-PD-L1 antibody in patients with advanced cancer. N Engl J Med (2012) 366(26):2455–65. doi: 10.1056/NEJMoa1200694
212. Wang X, Teng F, Kong L, Yu J. PD-L1 expression in human cancers and its association with clinical outcomes. Onco Targets Ther (2016) 9:5023–39. doi: 10.2147/OTT.S105862
213. Gandini S, Massi D, Mandala M. PD-L1 expression in cancer patients receiving anti PD-1/PD-L1 antibodies: A systematic review and meta-analysis. Crit Rev Oncol Hematol (2016) 100:88–98. doi: 10.1016/j.critrevonc.2016.02.001
214. Aguiar PN Jr., De Mello RA, Hall P, Tadokoro H, Lima Lopes G. PD-L1 expression as a predictive biomarker in advanced non-small-cell lung cancer: updated survival data. Immunotherapy (2017) 9(6):499–506. doi: 10.2217/imt-2016-0150
215. Scognamiglio G, De Chiara A, Di Bonito M, Tatangelo F, Losito NS, Anniciello A, et al. Variability in immunohistochemical detection of programmed death ligand 1 (PD-L1) in cancer tissue types. Int J Mol Sci (2016) 17(5). doi: 10.3390/ijms17050790
216. Pinato DJ, Shiner RJ, White SD, Black JR, Trivedi P, Stebbing J, et al. Intra-tumoral heterogeneity in the expression of programmed-death (PD) ligands in isogeneic primary and metastatic lung cancer: Implications for immunotherapy. Oncoimmunology (2016) 5(9):e1213934. doi: 10.1080/2162402X.2016.1213934
217. Hofman P. PD-L1 immunohistochemistry for non-small cell lung carcinoma: which strategy should be adopted? Expert Rev Mol Diagn (2017) 17(12):1097–108. doi: 10.1080/14737159.2017.1398083
Keywords: PD-1/PD-L1, immunecheckpoint inhibitor, clinical application, biomarker, human cancers
Citation: Tang Q, Chen Y, Li X, Long S, Shi Y, Yu Y, Wu W, Han L and Wang S (2022) The role of PD-1/PD-L1 and application of immune-checkpoint inhibitors in human cancers. Front. Immunol. 13:964442. doi: 10.3389/fimmu.2022.964442
Received: 08 June 2022; Accepted: 23 August 2022;
Published: 13 September 2022.
Edited by:
Lekh N. Dahal, University of Liverpool, United KingdomReviewed by:
Francesca Romana Mariotti, Bambino Gesù Children’s Hospital, (IRCCS), ItalyChunwan Lu, Tianjin University, China
Copyright © 2022 Tang, Chen, Li, Long, Shi, Yu, Wu, Han and Wang. This is an open-access article distributed under the terms of the Creative Commons Attribution License (CC BY). The use, distribution or reproduction in other forums is permitted, provided the original author(s) and the copyright owner(s) are credited and that the original publication in this journal is cited, in accordance with accepted academic practice. No use, distribution or reproduction is permitted which does not comply with these terms.
*Correspondence: Wanyin Wu, wwanyin@126.com; Ling Han, linghan99@gzucm.edu.cn; Sumei Wang, wangsumei198708@163.com
†These authors have contributed equally to this work