- 1Department of Radiology, Shengjing Hospital of China Medical University, Shenyang, China
- 2Department of Urology, Shengjing Hospital of China Medical University, Shenyang, China
The high recurrence rate of non-muscle invasive bladder cancer (BC) and poor prognosis of advanced BC are therapeutic challenges that need to be solved. Bacillus Calmette-Guerin (BCG) perfusion was the pioneer immunotherapy for early BC, and the discovery of immune checkpoint inhibitors has created a new chapter in the treatment of advanced BC. The benefit of immunotherapy is highly anticipated, but its effectiveness still needs to be improved. In this review, we collated and analysed the currently available information and explored the mechaisms by which the internal immune imbalance of BC leads to tumour progression. The relationship between immunity and progression and the prognosis of BC has been explored through tests using body fluids such as blood and urine. These analytical tests have attempted to identify specific immuyne cells and cytokines to predict treatment outcomes and recurrence. The diversity and proportion of immune and matrix cells in BC determine the heterogeneity and immune status of tumours. The role and classification of immune cells have also been redefined, e.g., CD4 cells having recognised cytotoxicity in BC. Type 2 immunity, including that mediated by M2 macrophages, Th2 cells, and interleukin (IL)-13, plays an important role in the recurrence and progression of BC. Pathological fibrosis, activated by type 2 immunity and cancer cells, enhances the rate of cancer progression and irreversibility. Elucidating the immune status of BC and clarifying the mechanisms of action of different cells in the tumour microenvironment is the research direction to be explored in the future.
Introduction
There are an estimated 500,000 new cases of bladder cancer (BC) and 200,000 related deaths worldwide each year. In the US alone, > 80,000 new cases of BC and 17,000 deaths occur each year (1–3). In recent decades, the treatment of BC has improved gradually (4, 5) and the latest major development started in 1977 with the introduction of Bacillus Calmette-Guerin (BCG) in therapeutic management (6). In the past few years, tumour molecular profiling and immune checkpoint blockade have led to unprecedented progress in the treatment of BC (7–11). However, immune checkpoint inhibitors (ICIs) only provide survival benefits to 20–30% of BC patients (12–15). However, this limited effectiveness has not prevented ICIs from replacing previous chemotherapy regimens as the first and second-line treatment of BC (16–18). Immune-related therapies have featured prominently in the history of BC treatment. Researchers have focused more attention on human immune surveillance and elimination mechanisms, with the goal of eliminating BC cells in the body. However, the effectiveness of immunotherapy for BC still needs to be improved.
The function of the cellular components in the BC microenvironment is related to their molecular subtypes; precise tumour status markers need to be further explored. The accumulation of high-throughput data has provided a strategy to elucidate the mechanisms underlying cancer development (19–21). The most representative data hosted on The Cancer Genome Atlas (TCGA), comprising chromatin landscape, transcriptome, epigenetic alterations, and clinical data (22–25). Based on multi-omics data analysis, muscle invasive BC (MIBC) has been divided into six molecular subtypes (26, 27), including the stroma-rich and basal/squamous subtypes, which have lower tumour purity.
In addition, the luminal-infiltrated subtype shows high expression of epithelial-mesenchymal transition (EMT) markers and high resistance to cisplatin-based chemotherapy (26, 28). EMT markers, such as Twist, Snail and Zeb1, also induce chemoresistance in cancer (29). In various malignant tumours, including urothelial carcinoma (UC), there is a positive correlation between T cell infiltration and expression of EMT-related genes (30). T cell infiltration has been revealed to not be directly involved in the effect of ICIs (31). Furthermore, the infiltration of immune and stromal cells, which decreases tumour purity, is related to BC progression and poor prognosis (32).
The immune status of BC is not only associated with its staging and progression but also closely related to the outcome of BCG treatment and chemotherapy. Changes in the immune status of the microenvironment of BC affect the recruitment of a variety of cells and cause fluctuations in immune cells in the blood and urine. Type 2 immunity, which mediates wound healing fibrosis, is related to the progression, recurrence, and refractory nature of BC. In this review, we have collated and analysed the above information and explored the reasons for tumour progression caused by the internal immune imbalance in BC.
Miscellaneous cells similar to those involved in wound repair appear in the BC tissue
The urothelium, submucosa, and muscle layers are part of the bladder wall, bladder tumours mostly originate in the urothelium layer (33, 34). The innate immune system shows anti-tumour activity based on the detection of tumour-associated antigens (TAAs) and damage-related molecular patterns (DAMPs). TAAs and DAMPs are presented by antigen-presenting cells (APCs), such as dendritic cells (DCs) and macrophages, to cytotoxic lymphocytes, causing activation and subsequent tumour infiltration (35–37). A tumour is an incurable wound that is closely associated with traumatic inflammation (38). Cancer cells likely gain survival advantages by reducing the expression of the MHCI-like molecules, HLA-A, -B, and -C to evade cytotoxic lymphocytes (39). Nectin-4 is a TAA that is expressed on the surface of 97% of the UC. Currently, combinatorial treatment with pembrolizumab and enfortumab vedotin—an antibody-drug conjugate targeting nectin-4—has been approved by the FDA as a first-line treatment for cisplatin-ineligible patients with locally advanced or metastatic UC (40). Identifying and amplifying TAA signals and fully mobilising immune cells to attack tumour cells may be a future solution for BC.
The development of tumours and trauma involves the same cell types, including platelets (41). In trauma, the fascia acts as an external reservoir for the formation of a temporary stroma for scarring and provides numerous fibroblasts for rapid sealing of large open wounds (42). The submucosa of the bladder is similar to the fascia in trauma and stores numerous fibroblasts. The inflammatory infiltration in early BC spreads to the submucosa, which permanently activates fibroblasts and increases their conversion into cancer-associated fibroblasts (CAFs).
CAFs are stimulated by surrounding cells or activated by signal crosstalk in the tumour microenvironment (TME), similar to wound repair, and are mainly derived from resident fibroblasts (43–47). The downregulation of major histocompatibility (MHC) class I antigen of cancer cells and upregulation of programmed cell death 1 ligand 1 (PDL1) in the TME are the mechanisms underlying the evasion of cytotoxic T cells by cancer cells (48, 49).
The proliferation of tumour cells and their inability to be eliminated leads to long-term immune cell infiltration and further recruitment of fibroblasts in the stroma. This is completely different from established fibrosis where the monocyte-derived cell population is dismantled and degraded with the disappearance of inflammation after wound repair (50). In the progression of BC, the innate advantages of the different bladder wall layers and the infiltration of immune cells facilitate the recruitment of more stromal cells to enhance tumour heterogeneity and enrich the signal exchange in the TME (Figure 1).
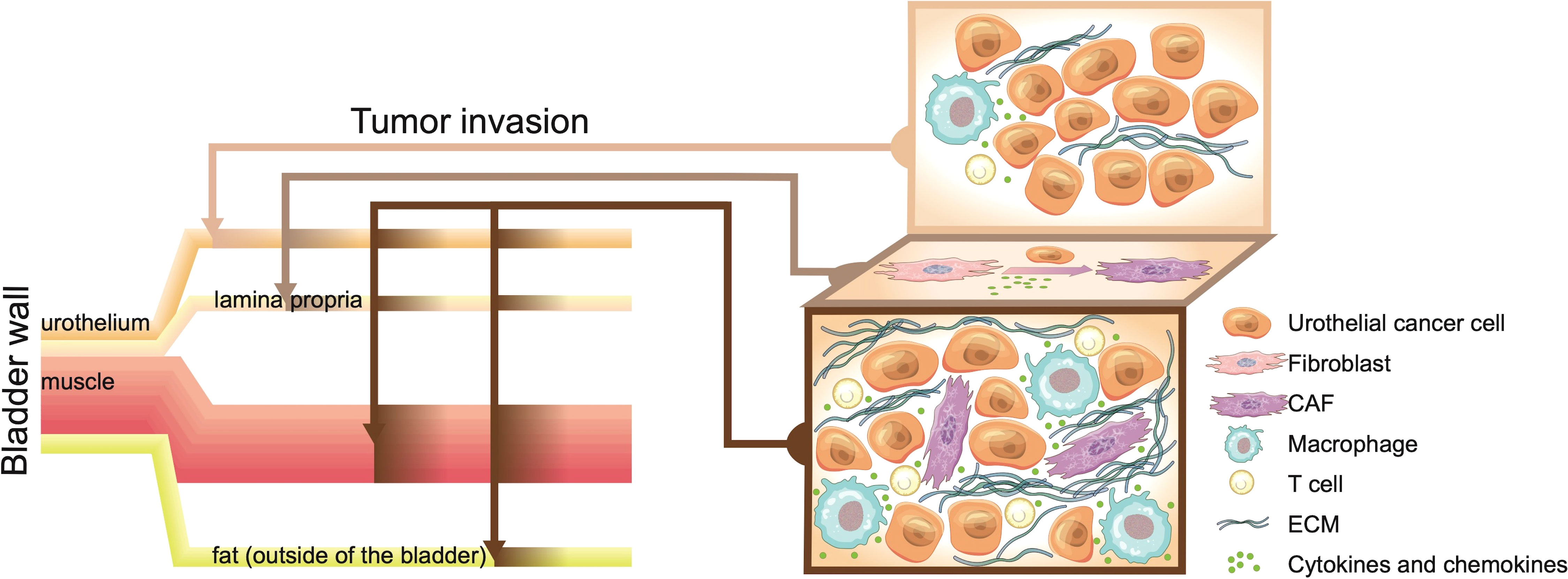
Figure 1 The bladder wall contains the urothelial layer, fibroblast-rich lamina propria, and muscle layer. Once bladder cancer (BC) tumours break through the bladder, they invade the external fat and adjacent tissues. Early BC is limited to the urothelial layer, with low immune cell infiltration and the highest tumour purity. Continued inflammatory infiltration and proliferation of cancer cells recruit and activate fibroblasts. The lamina propria, which is rich in fibroblasts, provides numerous cancer-associated fibroblasts (CAFs) for cancer progression and increases the heterogeneity of cancer tissues. The invasion or breakthrough of BC tumours through the lamina propria layer is similar to the repair process in the early stage of trauma, with infiltration of immune cells and fibroblasts. However, the immune infiltration and disordered stroma in BC continue to increase. Cancer cells that cannot be eliminated by the immune system exchange numerous signals with the complex cell environment, which makes the incurable “bladder scar” continue to recruit immune cells and fibroblasts.
Overactive CAFs thrive in BC
CAFs are the main stromal cell type and are involved in the occurrence and development of various cancers (51). CAFs have multiple functions, including matrix deposition and remodelling, extensive interaction with cancer cells, and signal crosstalk with infiltrating immune cells (52, 53). In the immune-excluded phenotype, immune and tumour cells are separated by tumour stroma, preventing immune cells from killing tumour cells (54–56). Common markers of CAFs are vimentin, α-smooth muscle actin (SMA), fibroblast activation protein (FAP), S100 calcium-binding protein A4 (S100A4), and platelet-derived growth factor receptor-β (PDGFRβ) (57). The specific markers of CAFs in BC were identified using biocomputing; these included PDGFRβ (32).
Highly infiltrating CAFs and their markers are associated with the progression and poor prognosis of BC (58–61) and, along with high EMT/stromal-related gene expression, induce significant resistance to PD-1 blockade in UC (62). CAFs increased the resistance of BC cells to cisplatin through oestrogen receptor β (ERβ)/BCL2 signalling (63).
CAFs in BC can be divided into two subtypes, i.e., inflammatory and myo-CAFs (iCAFs and mCAFs, respectively) (64). iCAFs (PDGFRA+) strongly express various cytokines and chemokines, including CXCL12, IL-6, CXCL14, CXCL1, and CXCL2, which are very similar to the iCAFs described in pancreatic cancer (65). CXCL2—majorly from iCAFs—interacted with CXCR4 to induce tumour-associated macrophage (TAM) aggregation (66). Moreover, iCAFs—which are more closely related to the poor prognosis of BC than mCAFs (RGS5+)—might degrade and remodel the extracellular matrix, promote the proliferation of tumour and stromal cells, and recruit immune cells to tumours (64). However, mCAFs are considered the most abundant cell subtype in the stroma in many other types of tumours, which support the growth, survival, and metastasis of tumour cells (41, 67). Research on CAFs is still in its infancy, and the accurate classification of CAFs is crucial for the in-depth elucidation of their mechanism of action. Numerous experiments are still needed to verify this in the future.
Cytotoxic immune cells
Immune surveillance mediates tumour clearance mainly through the action of cytotoxic CD8+ T cells (68–70). A high density of CD8+ T cell infiltration is associated with a good prognosis in BC (71, 72). However, T cell immunoreceptors with Ig and ITIM domain (TIGIT)-positive CD8+ T cells are related factors for the poor prognosis of MIBC and poor response to adjuvant chemotherapy (73). TIGIT, a new co-inhibitory receptor, downregulates the cytotoxicity and activation of T cells (74). Single-cell sequencing analysis has allowed for the comparison of CD8+ T cells in BC tumour tissue with normal tissues, the cell status and composition are not significantly different. In contrast, single-cell analysis of CD4+ T cells showed several tumour-specific states, including various states of Tregs, which also included clonally expanded cytotoxic CD4+ T cell (75). These CD4+ T cells kill autologous tumours in a major histocompatibility complex class II (MHCII)-dependent manner and are suppressed by Tregs (75). The previous concept defines the role of CD4+ T cells in anti-tumour immunity as indirect, mainly through Th cells to support CD8+ T cell-mediated tumour killing or Tregs to limit this class response (76, 77).
However, accumulating evidence has shown that some cytotoxic CD4+ T cells directly kill tumour cells and may play a key role in anti-tumour immunity (75). Mature CD4+ Th cells have also been shown to have phenotypic plasticity, inhibit CD8+ lineage genes under certain conditions, and exhibit MHCII-limited cytotoxicity (78). Because of its direct cytotoxicity, CD4+ T cell-mediated tumour killing has emerged as a unique mechanism of anti-tumour immunity.
In BC, two cytotoxic CD4+ T cell subpopulations have been identified, one expressing granzyme K (GZMK) and the other granzyme B (GZMB). CD4 GZMB subgroups express high levels of cytotoxic molecules GZMB, perforin, granulysin (Gnly), and natural killer (NK) cell granule protein 7 (NKG7), whereas CD4 GZMK expresses high levels of GZMK and lower levels of NKG7. Both the subsets produced high levels of the anti-tumour cytokine interferon (IFN)-γ and tumour necrosis factor (TNF)-α (75).
The unique biological role of cytotoxic CD4+ T cells still needs considerable investigation, especially the mechanism underlying cell population development and regulation and the mechanism by which cell populations contribute to tumour cell death. Uncovering the subpopulations of T cells and their mechanism of action would help improve the understanding of the response and drug resistance to ICIs, and the identification of novel targets for immunotherapy.
Immunosuppressive cells
The role of immunosuppressive cells such as Tregs and macrophages in BC has not been fully elucidated. Tregs inhibit CD8+ T cell function by releasing immunosuppressive cytokines (including IL-10 and IL-35) (79, 80). Tregs in tissues play an important role in promoting tissue homeostasis and regeneration. Infiltration of FOXP3+ Tregs, which are involved in regression of inflammation, is associated with a better prognosis in BC (81, 82). However, high levels of C-C motif chemokine receptor 8 (CCR8)+ Tregs are associated with immunotolerance, low survival rates, and low chemotherapy response rates in MIBC (83).
CCR8, which mediates the immunosuppressive function, is an important chemokine receptor expressed in Tregs (84, 85). In BC, CCR8 maintains the stability of Tregs and enhances their inhibitory function by upregulating the expression of transcription factors FOXO1 and c-MAF (83). Blocking CCR8 decreases the stability of Tregs and enhances the therapeutic effect of PD-1 inhibitors (83). Human basic leucine zipper ATF-like transcription factor (BATF)+ CCR8+ Tregs from normal skin and adipose tissue have the same characteristics as non-lymphoid T follicular helper-like (Tfh-like) cells, with tissue regeneration and wound healing properties.
BATF+ CCR8+ Tregs also have the same characteristics as tumour-resident Tregs (86). BATF+ CCR8+ Tregs destroy the caspase recruitment domain family member 11- B-cell lymphoma 10- MALT1 paracaspase (CBM) signal body complex to modulate the function of Tregs and promote their production of IFN-γ. This not only inhibits tumour growth but also prepares the TME for successful immune checkpoint therapy (87). However, the exact role of Tregs in BC has not yet been clarified. Highly plastic macrophages, which usually exhibit an immunomodulatory M2 phenotype in tumours, are called TAMs.
Macrophages are polarised to the M2 phenotype and inhibit CD8+ T cell function after being manipulated by tumour-derived signals, including angiopoietin-2, macrophage-colony-stimulating factor (M-CSF), CCL2, and vascular endothelial growth factor (VEGF) (88, 89). Although the role of TAM in BC has not been confirmed, its infiltration may affect angiogenesis, tumour grade, and prognosis (90, 91). The TAM subgroups of BC that express dendritic cell-specific C-type lectin (DC-SIGN) significantly activate multiple M2-like signalling pathways, which are associated with poor prognosis and chemotherapy resistance. Moreover, blocking DC-SIGN has been shown to reduce the secretion of inflammatory cytokines in TAM and enhance the cytotoxicity of CD8+ T cells to MIBC cells mediated by PD-1 inhibition (92).
Immune escape or suppression: Antigen presentation, recognition, and monitoring
To elicit an effective anti-tumour response, antigen presentation must be successful in the following two aspects. First, cancer neoantigens must be absorbed by specific APCs, mainly DCs, and cross-presented to activate naive CD8+ T cells (93). Second, neoantigens must be directly presented by tumour cells to enable triggered CD8+ T cells to recognise and kill them (94). Tumours have developed a variety of mechanisms to reduce these steps of antigen presentation and evade immune recognition by inhibiting DC function and downregulating tumour cell MHC expression.
DCs are a group of immune cells that play a central role in antigen presentation and effective anti-tumour T cell responses. UC has been found to induce high expression of the inhibitory receptors (IR, B, and T lymphocyte associated) BTLA and T cell immunoglobulin and mucin-domain containing-3 (TIM-3) in DCs in blood and tumours and mediates the decline and dysfunction of cytokine secretion in DCs (95). The MHCI and MHCII antigen presentation pathways play an important role in controlling the immune response (96).
Cytotoxic immune cells in BC, namely CD4+ T and CD8+ T cells, killed tumour cells by recognising MHCII and MHCI, respectively (75, 97). Single-cell-sequencing results showed that the downregulation of MHCII in BC cells contributes to the formation of an immunosuppressive TME (64). The downregulation or absence of MHCI in the TME leads to the dysfunction of CD8+ T cells (95). Although complete downregulation of MHC presentation appears to be an attractive escape mechanism for tumours to evade immune recognition, the immune system has an important checkpoint to monitor the loss of MHC presentation.
NK cells detect the loss of MHC surface expression as a stress signal and target stressed cells (94). Therefore, tumours have also evolved more subtle immune evasion strategies to deplete NK cells without eliminating the surface expression of MHC. Increasing the concentration of IL-21 in the TME could reverse the failure of NK cells by activating the phosphoinositide 3-kinase (PI3K)-AKT-FoxO1 and signal transducer and activator of transcription 1 (STAT1) signalling pathways to improve the prognosis of advanced tumours (98).
Liquid biopsy and BC
Liquid biopsy is an easily accessible, non-invasive technique that provides real-time information about cancer (99). Blood-perfusing cancer tumours are key carriers of related substances that can be used to detect the presence and progression of cancer (100–104). In BC, in addition to blood, urine also directly makes contact with the tumour and exfoliates tumour cells, which can be used in the diagnosis of UC (105). Extracellular vesicles (EVs) and cell-free DNA (cfDNA) in the blood and urine can be used to monitor the progression, relapse, and treatment response of BC (106–108).
EVs including exosomes serve as a medium for signal communication between cells in cancer tissues (109–113). Both EVs and cfDNA penetrate adjacent body fluid compartments (109, 114) and abnormal changes in their levels in liquid biopsy are related to the heterogeneity of cells in cancer tissues. In peripheral blood, the percentage of CD3+CD25+ T lymphocytes in BC patients is significantly higher than that in healthy controls (115). However, the phenotypic overlap between peripheral blood mononuclear cells (PBMCs) and tumour-infiltrating lymphocytes (TILs) is the lowest.
The T cell checkpoint and T cell receptor (TCR) composition is similar between urine-derived lymphocytes (UDLs) and TILs, suggesting that UDLs are derived from the bladder tumour tissue. Viable CD3+ T lymphocytes, including CD8+, CD4+ FoxP3- (CD4eff), and CD4+ FOXP3+ (T regulatory cells, Tregs), have been detected in the urine of MIBC patients (116). However, the mechanism by which lymphocytes enter the urine is still unclear. UDLs can be used to accurately determine the immune status and lymphocyte composition in the TME of BC patients (Figure 2). In other words, the immune content in UDLs is closer to that in the tumour, while the immune content in blood does not decrease, but is greatly different from that in the tumour. This phenomenon remains to be clarified.
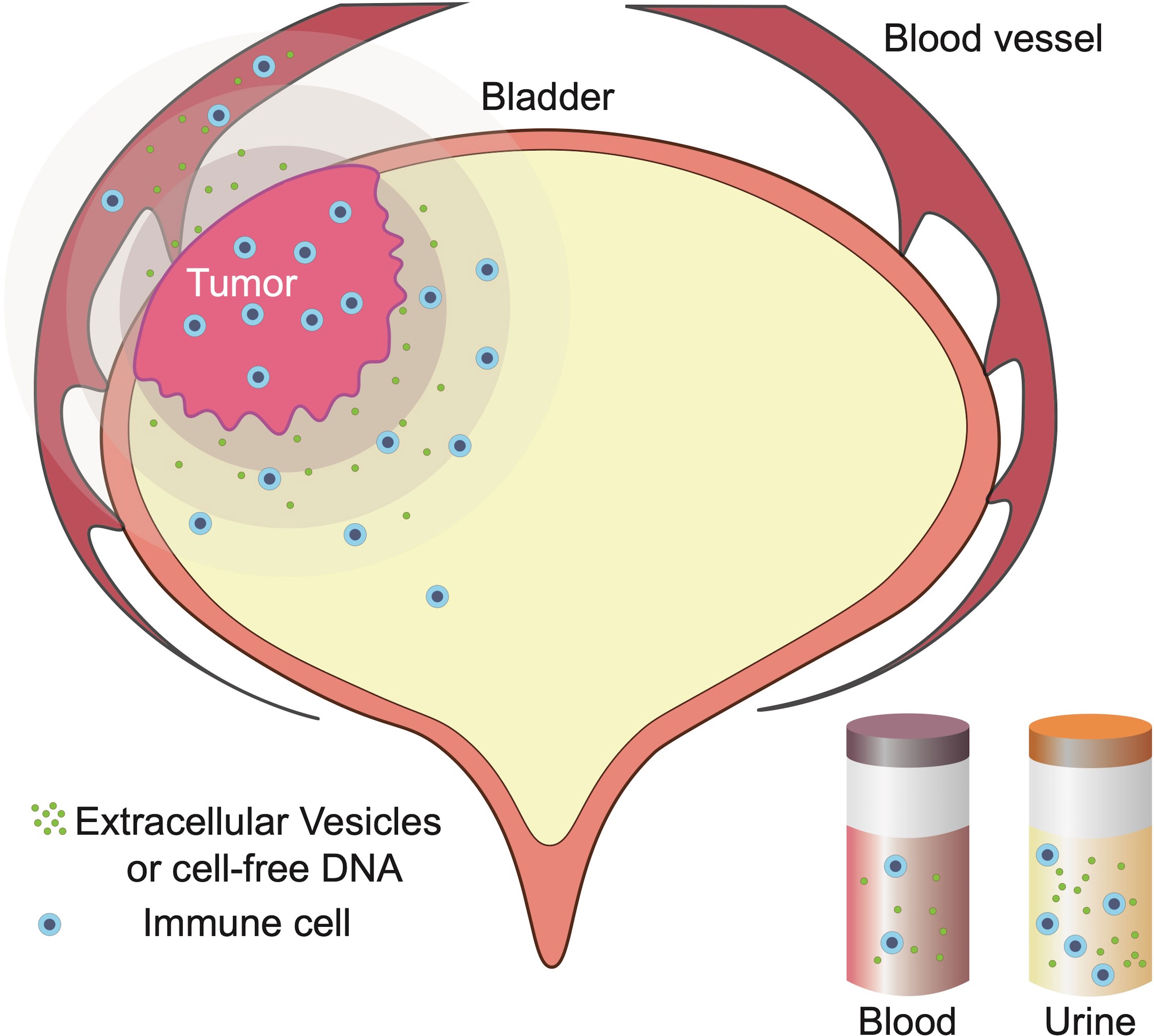
Figure 2 Blood flowing through bladder cancer (BC) tissue and urine that comes in contact with BC tissue reflects changes to the tumour microenvironment (TME). Currently, free cells and molecules in the urine may be shed from the tumour, similar to infused lymphocytes and molecules in BC.
Immunotherapy and BC
The mechanisms of action of BCG in non-muscle invasive bladder cancer (NMIBC) have not been completely elucidated (36). The intense inflammation induced by BCG involves a variety of chemokines and cytokines, which lead to infiltration of innate and adaptive immune cells, mainly neutrophils, T cells, and monocytes (117, 118). BCG Connaught and Tice are the two most widely used BCG strains in North America and Europe (119); different strains induce different immune responses.
Patients treated with BCG Connaught have significantly better recurrence-free survival rates than those treated with BCG Tice, which may be because its induction of T-helper 1 (Th1) and initiation of CD8+ T cells is superior to that of BCG Tice. The difference in treatment results may be attributable to the single nucleotide polymorphism (SNP) of the BCG strain (120). In addition, relapse after BCG treatment is associated with a lower ratio of T cells to monocytic myeloid-derived suppressor cells (M-MDSCs), which are known to inhibit immune function in tumours (121, 122).
M-MDSCs and the innate counterpart of Th2 cells, group 2 innate lymphocytes (ILC2), are detectable in urine after BCG treatment and may be recruited and induced by ILC2 to secrete IL-13. The ILC2/IL-13 axis mediates a mechanism that drives the immunosuppressive microenvironment, which is closely involved in the failure of BCG immunotherapy (123). Previous studies reported that the imbalance of Th1/Th2 cytokine IL-2/IL-10, that is, overproduction of IL-10, may contribute to the poor prognosis of NMIBC.
Controlling and reversing the production of IL-10 and promoting the overproduction of IL-2 would help eliminate tumours (124). In NMIBC patients who failed to respond to BCG treatment, the immunosuppressive cell subsets of traditional Tregs have a higher level of infiltration than other cells (125, 126). There is a significant difference in the expression of PD-L1 in Tregs expressing Forkhead box A1 (FoxA1) (127, 128).
The enrichment of PD-L1-positive Tregs that may be induced by IFN-β during BCG treatment increases, leading to a decrease in the therapeutic effect of BCG (127, 128). Compared with the expression of PD-L1 on tumour cells, T cells may be a more important non-classical source of PD-L1. Blocking the PD-1/PD-L1 interaction may increase immune-related BCG activity (128–130). Overall, the effect of BCG treatment mainly depends on the immune status of the local microenvironment and the detection of cytokines and immune cells in urine could predict the outcome of BCG treatment.
Because of the higher mutation spectrum, MIBC has a better response rate to ICIs and lower mutation rate than melanoma and non-small cell lung cancer (131–137). ICIs, such as PD-1 and PD-L1 inhibitors, have also become first-line treatment strategies for patients with advanced urothelial cancer who are not suitable candidates for platinum chemotherapy (57, 138). Nevertheless, the response rate to ICIs still needs to be improved and the sum of the objective and complete response rates in urothelial cancer is < 30% (3, 139, 140). The mechanism by which the immune checkpoint is blocked in BC remains to be further elucidated.
PD-L1 is not only expressed in cancer cells but also on the surface of a variety of immune cells, especially in lysosomal associated membrane protein 3 (LAMP3)+ DCs. The DC subgroup may directly inhibit CD8+ T cells (64). The high expression of PD-L1 in BC patients is favourable for treatment with PD-L1 inhibitors (8, 9, 141, 142). However, the type 2 immunity associated with tumour progression is also closely related to the expression of PD-L1. Group 2 innate lymphoid cells (ILC2s) expressing PD-L1 stimulate Th2 cells to increase GATA binding protein 3 (GATA3) expression and IL-13 production. As important participants in type 2 immunity, stromal cells were significantly associated with a lower response rate to PD-1 inhibitors (62).
The loss of PD-L1 on ILC2s can impair early Th2 polarisation and cytokine production (143). Surprisingly, the interaction between PD-L1 on ILC2s and PD-1 on CD4+ T cells did not inhibit the T cell response (143). However, we can confirm that type 2 immunity is detrimental to immunotherapy, including BCG and ICIs. The mechanism of action of immune checkpoints needs to be further elucidated, especially the markers of immune checkpoints on different cells in the immune microenvironment. In addition, the effect of the interaction among immune checkpoints on various functions between cells needs to be further investigated.
Chemotherapy and BC
Although chemotherapy is still the first-line treatment for BC, its effects are limited (17). Three or four cycles of neoadjuvant chemotherapy (NAC) have been established as the standard adjuvant treatment for eligible MIBC patients (17, 144, 145). A meta-analysis of 11 clinical trials with a total of 3,000 patients showed the benefits of NAC with respect to improving the 5-year overall survival (OS) and reducing the risk of death (146). However, the correlation between the therapeutic effect of chemotherapy and the TME of BC patients has not been clarified. The gene expression profile was used to evaluate the role of the immune-related gene expression of BC patients in chemotherapy activity, and it was found not to be related to the effect of chemotherapy (147).
It may be that the expression of immune-related genes does not reflect the proportion of immune cell subsets, and therefore, the association between chemotherapy and immune status could not be identified. Chemotherapy can affect the internal TME in BC patients. For patients with urothelial cancer who have not received chemotherapy, the prognosis of failure of ICI therapy is often worse than for patients who received chemotherapy (13). FoxA1, which is a specific expression factor of Tregs induced by IFN-β, is related to the chemotherapy resistance of BC (127, 148).
Chemotherapy can enhance anti-tumour immunity, including stimulation of CD4+ effector T (Teff) cells and CD8+ Teff cells (149). NAC treatment decreases the expression of the depletion marker PD-1 in CD8+ and CD4+ Teff cells and increases that of the activation markers T-bet and the cytotoxic molecules granzyme B and perforin in the sentinel lymph nodes of BC patients (150). However, the phenotype and function of T cells in the sentinel lymph node in patients whose BC is not downgraded did not show these positive immune effects (150).
Long-term cancer control and complete remission may involve CD8+ T cell immune responses. The C-X-C motif chemokine receptor 3 alt (CXCR3alt)-CXCL11 chemokine system, which has stimulatory effects on CD8+ T cells, can predict the responsiveness of NAC in MIBC (151). CAFs, which play a role in the development of drug resistance in a variety of tumours, increase the resistance of BC cells to cisplatin by enhancing the transmission of ER β/Bcl-2 signals (63, 152). Therefore, the success of a refined NAC regimen to improve immunogenicity—perhaps by enhancing cytotoxicity or selecting low stroma infiltration—could be achieved by maximising the effectiveness of chemotherapy and immune promotion.
Inflammation and BC
Inflammation has been identified as a driving factor for many cancers (153). Inflammation markers such as C-reactive protein (CRP) and IL-6 promote the progression of MIBC (154–156). Both CRP and IL-6 increase during the acute inflammatory phase and their levels reflect their severity (157–159). Active CRP increases the secretion of cytokine IL-6 (160). IL-6 is a cytokine with various physiological functions, including regulation of immune cell proliferation and differentiation (161). IL-6 not only amplifies cancer-causing chronic inflammation but also mediates the internal mechanism of tumour cells that drive cancer progression (162). IL-6 induces PI3K-AKT, mitogen-activated protein kinase (MAPK)/extracellular signal-regulated kinase (ERK), nuclear factor (NF)-κB, and STAT3 signalling (162).
CRP also has prognostic significance with respect to NMIBC. However, relapses confined to the bladder mucosa layer have a less effect on systemic inflammation and CRP is not ideal for predicting disease recurrence (163). Higher CRP levels are associated not only with poor prognosis for radical cystectomy, but also with poor prognosis after chemotherapy and radiotherapy in metastatic BC (164–168). However, CRP has been shown to have a tumour-killing activity in both in vivo and in vitro (160). Such results revealed the complexity of the role of CRP in BC. In tumours, CRP is involved in the recruitment of monocytes/macrophages, a phenomenon that increases the complexity of the TME (169–171). Perhaps CRP does not directly kill camouflaged cancer cells, as it is non-toxic to normal cells (160).
A gradual increase in inflammatory infiltration increases the number and various types of immune cells that accumulate in the TME. The progression of tumours is similar to wound healing. Following the accumulation of a considerable amount of inflammatory factors, the inflammatory phase subsides and the tissue repair process commences (172). Similar to the inflammation subsidence and tissue reconstruction phase, which enhance wound healing, the complex and multi-source signals present in the TME promote cancer progression.
Type 2 immunity and BC
Typically, Th1 induces M1 macrophages and cytotoxic T cells to kill tumour cells in the pro-inflammatory type 1 immune response (173). Type 2 immunity is usually involved in the development of tolerance in the tumour environment, where Th2/M2-related cells are involved in immunosuppression and tumour progression (174, 175).
M2 macrophages
M2 macrophages, often referred to as immunosuppressive cells, are also called TAMs (176). The M2 phenotype has been found to promote the progression of BC by suppressing inflammation (177). Squamous cell carcinoma-like BC, which dominates in MIBC, has greater M2 macrophage infiltration (178). Studies revealing the molecular mechanisms between BC and M2 macrophages are limited. Bone morphogenetic protein 4 (BMP4) secreted by BC cells induces M2 macrophage polarisation (179). Moreover, lysine-specific demethylase 6A (KDM6A), which is frequently mutated in BC, leads to activation of cytokine and chemokine pathways, which promotes the polarisation of M2 macrophages (180).
Th2
Th2 is a predominant class of CD4+ Th cells characterised by the production of IL-4/IL-13 cytokines. There are few high-quality studies related to Th2 in BC. However, it is known that M2 macrophages recruit Th2 cells through the actions of CCL11/CCL22 (181–184). Th2 differentiation could be induced by IL-4 secreted by B cells, NK cells, naive CD4+ T cells, and mast cells (185). Furthermore, IL-4/IL-13 has been shown to contribute to cancer growth and metastasis (186, 187).
IL-13
IL-13, which plays an important role in type 2 immunity, was found to be abnormally elevated in the urine of BC patients (188, 189). IL-13, described as an inhibiting inflammatory cytokine (190, 191), participates in immune surveillance in cancer, inhibits cancer cell apoptosis, and promotes tumour growth (192–194).
CAFs
After BC tumours break through the muscle layer of the bladder or metastasise to distant sites, the CAFs and extracellular matrix (ECM) in the TME increases significantly (32, 64). However, excessive deposition of fibroblasts and ECM during wound healing can lead to scarring or fibrosis (195–198). The study of the wound healing process has considerably facilitated the understanding of cancer progression. Excessive type 2 immune activation involves Th2 cells and IL−4- and IL−13−activated M2 macrophages, which recruit more fibroblasts and cause excessive ECM deposition by IL-4/IL-13 and fibroblast growth factor (FGF)/PDGF, respectively. The progression of BC reduces the purity of the tumour and increases the proportion of stroma in the tumour (26, 199). The proliferation rate of CAFs, the main component of the stroma, has been shown to be faster in BC than under normal conditions, which contributes greatly to the growth and increased heterogeneity of the tumour.
In addition to the activation of type 2 immunity, the proliferation of CAFs is also related to the signals provided by cancer cells in the TME. BC cells trigger the differentiation of fibroblasts into CAFs through exosome-mediated transforming growth factor (TGF)-β transport and activation of the SMAD pathway (200). In oral squamous cell carcinoma, gastric cancer, and liver cancer, CAFs promote the proliferation and metastasis of cancer cells through exosomes (201–203). The signals produced by cancer cells disrupt the immune microenvironment and convert the type 2 immune response, which is targeted at repairing and maintaining homeostasis, into a persistent source of malignant tumours (Figure 3).
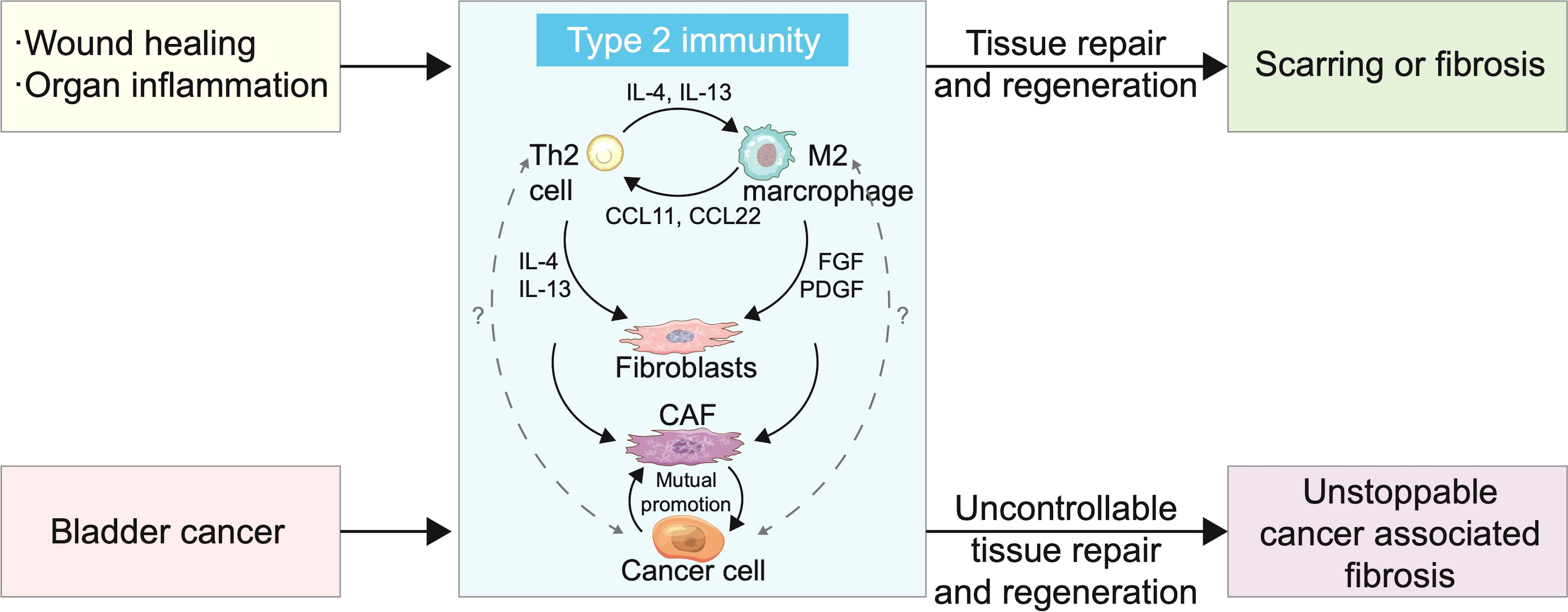
Figure 3 Type 2 immunity and bladder cancer (BC) cells cause rapid and persistent progression of cancer-associated fibrosis. Scarring or fibrosis caused by wound healing or inflammation of organs is associated with overactivation of type 2 immunity. T helper 2 (Th2) cells and M2 polarized macrophages promote mutual differentiation and jointly recruit fibroblasts. The proportion of M2 cells and cancer-associated fibroblasts (CAFs) in BC increased with tumour progression and interleukin (IL)-13 level increased significantly in tumours, which suggests that BC was closely related to type 2 immunity. CAFs have shown signal crosstalk with cancer cells in a variety of cancers, promoting differentiation, proliferation, and metastasis. Cancer cell involvement makes type 2 immunity persistent and uncontrollable, resulting in tumour metastasis.
Next generation therapeutics
The use of ICIs has impacted the treatment of BC. Antibody-drug conjugates (ADCs), such as enfortumab vedotin, are approved for patients locally advanced or metastatic UC who were unresponsive to PD-1/PD-L1 therapy (204). ADCs—agents targeting Trop-2, HER2, and EpCAM—are undergoing clinical evaluation for UC (205) and have the potential to emerge as next generation UC treatments. Similarly, some bispecific antibodies and fusion proteins in the research and development phase, such as T cell engagers, Treg depleting ATOR-1015, and super agonistic cytokine traps ALT-803, are likely to introduce a change in the manner UC is treated (206–210). The next generation of treatments, based on activation of the immune system and recruitment of effector cells to kill cancer cells while minimising the side effects, and a variety of other treatments, are undergoing clinical trials for BC (211).
Conclusions and future directions of BC research
Immune status is the main factor determining prognosis in BC and is evaluated using relatively invasive body fluid testing (99, 106). The significance of fluctuations in immune cells and cytokines in blood and urine in patients with BC has not been fully demonstrated. In the future, it may be possible to predict the prognosis and guide treatment of BC patients by analysing the immune state of bodily fluids, which are reflective. The role of type 2 immunity and the related cytokines in the host immune system and inflammatory diseases is to suppress type 1 and Th17-driven inflammation and participate in the repair and regeneration of damaged tissue (181, 182, 212).
Overreaction of type 2 immunity leads to pathological fibrosis, such as liver and pulmonary fibrosis (213–216). The mechanism underlying the development of fibrotic disorders mediated by type 2 immunity is unclear, but signals that continuously activate tissue repair are known to contribute to this condition. The involvement of cancer cells further complicates the imbalance of type 2 immunity in the TME. Studies have shown that blocking type 2 immune cytokines, such as IL-4 and IL-13, can interfere with the inhibition of type 1 and Th17-driven inflammation, which can induce considerable neutrophilic inflammation.
In the experimental myeloid schistosomiasis and pulmonary cell tumour model, blocking IL-13 significantly reduced fibrosis, but type 1 and Th17-driven inflammation simultaneously exacerbated liver and lung damage (217). Cancer-related fibrosis of the TME, likely induced by the blockade of type 2 cytokines, slows down the pathological fibrosis associated with the uncontrollable nature of tumour and active cytotoxicity of type 1 immunity (Figure 4). However, there may also be excessive accumulation of type 1 and Th17-related inflammatory molecules, with considerable inflammatory fluids providing nutrition for BC cells, which promotes their proliferation and metastasis.
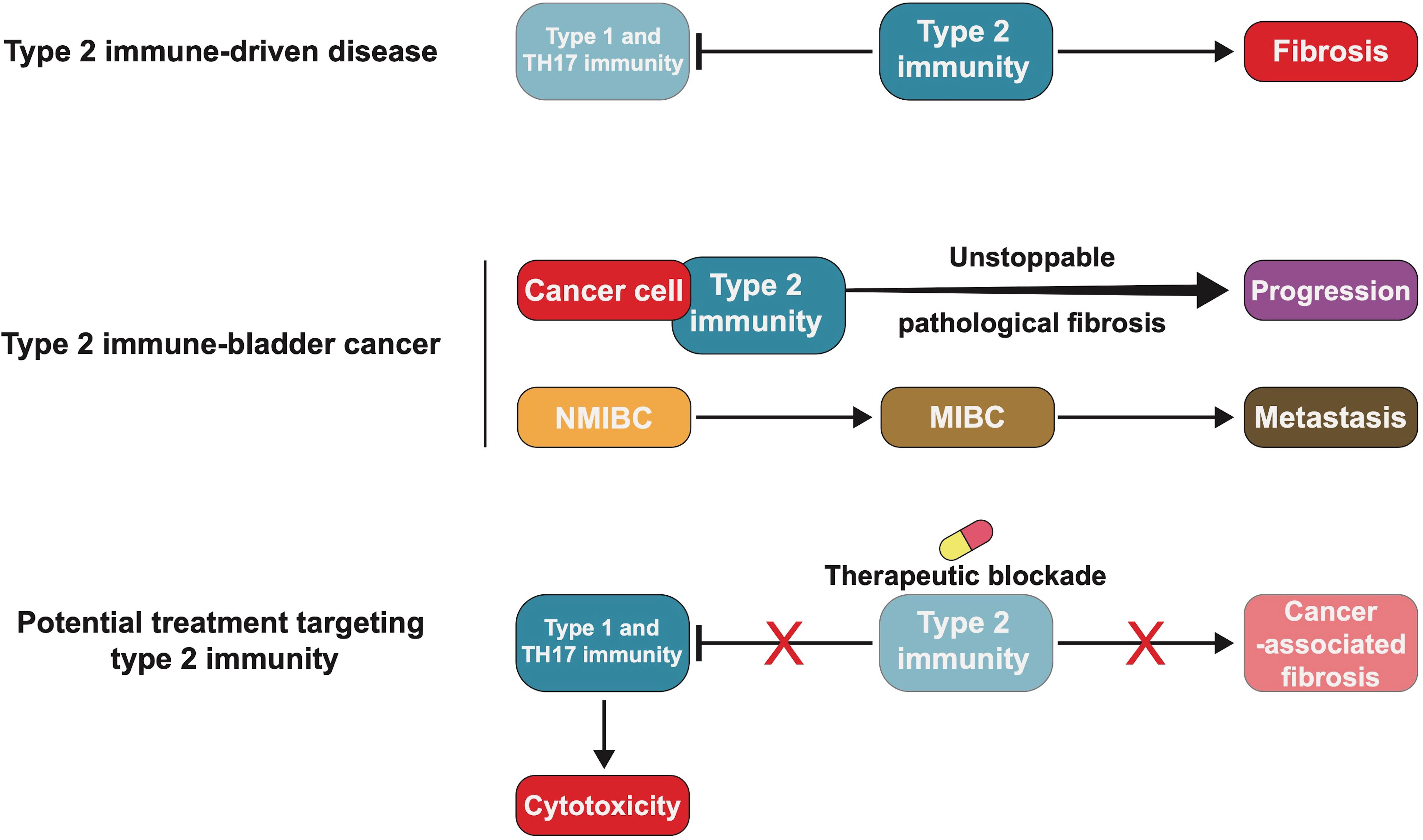
Figure 4 Blocking type 2 immunity may be an effective treatment for bladder cancer (BC). Type 2 immune-driven diseases, such as scarring and organ fibrosis, are caused by excessive recruitment of fibroblasts and inhibition of the cytotoxicity of type 1 and T helper 17 (TH17) immunity. BC cells interact with type 2 immunity-related molecules, making pathological fibrosis persistent and causing tumour progression or metastasis. Blocking type 2 immunity could prevent pathological fibrosis and in BC may be worth future exploration and study.
In the allergenic mouse model, the dual blockade of IL-13 and IL-17A protected mice from acidophilic and neutrophilic inflammation and the damage caused by rebound inflammation (217, 218). This also prompted us to attempt to treat BC using dual blockade (217, 219). The study of the immune status and mechanism of action of related molecules in BC is still in its infancy and identification of the immune stage and control of immune status should be the primary focus of future research. The correlations among multiple immune, matrix, and cancer cells need to be studied and elucidated in future studies.
Author contributions
BL, SP, and SL conceptualised and designed the study and wrote the article. ZL, XL, and BW collected the documents and reviewed the manuscript. YZ, MS and XC revised the manuscript. All authors contributed to the article and approved the submitted version.
Funding
The present study was supported by 345 Talent Project from Shengjing Hospital of China Medical University (Grant Nos. M0312 and M0340), and the Doctoral Scientific Research Foundation of Liaoning Province (Grant No. 2020-BS-120).
Conflict of interest
The authors declare that the research was conducted in the absence of any commercial or financial relationships that could be construed as a potential conflict of interest.
Publisher’s note
All claims expressed in this article are solely those of the authors and do not necessarily represent those of their affiliated organizations, or those of the publisher, the editors and the reviewers. Any product that may be evaluated in this article, or claim that may be made by its manufacturer, is not guaranteed or endorsed by the publisher.
Glossary
References
1. Richters A, Aben KKH, Kiemeney L. The global burden of urinary bladder cancer: An update. World J Urol (2020) 38(8):1895–904. doi: 10.1007/s00345-019-02984-4
2. Siegel RL, Miller KD, Jemal A. Cancer statistics, 2019. CA Cancer J Clin (2019) 69(1):7–34. doi: 10.3322/caac.21551
3. Lenis AT, Lec PM, Chamie K, Mshs MD. Bladder cancer: A review. JAMA (2020) 324(19):1980–91. doi: 10.1001/jama.2020.17598
5. Kamat AM, Hahn NM, Efstathiou JA, Lerner SP, Malmström PU, Choi W, et al. Bladder cancer. Lancet (2016) 388(10061):2796–810. doi: 10.1016/s0140-6736(16)30512-8
6. Kulkarni GS. Nadofaragene firadenovec: a new gold standard for BCG-unresponsive bladder cancer? Lancet Oncol (2021) 22(1):8–9. doi: 10.1016/s1470-2045(20)30586-6
7. Hurst C, Rosenberg J, Knowles M. SnapShot: Bladder cancer. Cancer Cell (2018) 34(2):350–.e351. doi: 10.1016/j.ccell.2018.07.013
8. Brower V. Anti-PD-L1 antibody active in metastatic bladder cancer. Lancet Oncol (2015) 16(1):e11. doi: 10.1016/s1470-2045(14)71167-2
9. Brower V. Anti-PD-L1 inhibitor durvalumab in bladder cancer. Lancet Oncol (2016) 17(7):e275. doi: 10.1016/s1470-2045(16)30242-x
10. Gourd E. EMA restricts use of anti-PD-1 drugs for bladder cancer. Lancet Oncol (2018) 19(7):e341. doi: 10.1016/s1470-2045(18)30433-9
11. Ott PA, Hu-Lieskovan S, Chmielowski B, Govindan R, Naing A, Bhardwaj N, et al. A phase ib trial of personalized neoantigen therapy plus anti-PD-1 in patients with advanced melanoma, non-small cell lung cancer, or bladder cancer. Cell (2020) 183(2):347–62.e324. doi: 10.1016/j.cell.2020.08.053
12. Bandini M, Ross JS, Raggi D, Gallina A, Colecchia M, Lucianò R, et al. Predicting the pathologic complete response after neoadjuvant pembrolizumab in muscle-invasive bladder cancer. J Natl Cancer Inst (2021) 113(1):48–53. doi: 10.1093/jnci/djaa076
13. Gómez de Liaño Lista A, van Dijk N, de Velasco Oria de Rueda G, Necchi A, Lavaud P, Morales-Barrera R, et al. Clinical outcome after progressing to frontline and second-line anti-PD-1/PD-L1 in advanced urothelial cancer. Eur Urol (2020) 77(2):269–76. doi: 10.1016/j.eururo.2019.10.004
14. Niglio SA, Jia R, Ji J, Ruder S, Patel VG, Martini A, et al. Programmed death-1 or programmed death ligand-1 blockade in patients with platinum-resistant metastatic urothelial cancer: A systematic review and meta-analysis. Eur Urol (2019) 76(6):782–9. doi: 10.1016/j.eururo.2019.05.037
15. Witjes JA, Bruins HM, Cathomas R, Compérat EM, Cowan NC, Gakis G, et al. European Association of urology guidelines on muscle-invasive and metastatic bladder cancer: Summary of the 2020 guidelines. Eur Urol (2021) 79(1):82–104. doi: 10.1016/j.eururo.2020.03.055
16. Parikh RB, Adamson BJS, Khozin S, Galsky MD, Baxi SS, Cohen A, et al. Association between FDA label restriction and immunotherapy and chemotherapy use in bladder cancer. JAMA (2019) 322(12):1209–11. doi: 10.1001/jama.2019.10650
17. Patel VG, Oh WK, Galsky MD. Treatment of muscle-invasive and advanced bladder cancer in 2020. CA Cancer J Clin (2020) 70(5):404–23. doi: 10.3322/caac.21631
18. Voelker R. Immunotherapy for bladder cancer. JAMA (2017) 317(23):2363. doi: 10.1001/jama.2017.6976
19. Consortium. ITP-CAoWG. Pan-cancer analysis of whole genomes. Nature (2020) 578(7793):82–93. doi: 10.1038/s41586-020-1969-6
20. Abeler-Dörner L, Laing AG, Lorenc A, Ushakov DS, Clare S, Speak AO, et al. High-throughput phenotyping reveals expansive genetic and structural underpinnings of immune variation. Nat Immunol (2020) 21(1):86–100. doi: 10.1038/s41590-019-0549-0
21. Gröbner SN, Worst BC, Weischenfeldt J, Buchhalter I, Kleinheinz K, Rudneva VA, et al. The landscape of genomic alterations across childhood cancers. Nature (2018) 555(7696):321–7. doi: 10.1038/nature25480
23. Hoadley KA, Yau C, Hinoue T, Wolf DM, Lazar AJ, Drill E, et al. Cell-of-Origin patterns dominate the molecular classification of 10,000 tumors from 33 types of cancer. Cell (2018) 173(2):291–304.e296. doi: 10.1016/j.cell.2018.03.022
24. Hutter C, Zenklusen JC. The cancer genome atlas: Creating lasting value beyond its data. Cell (2018) 173(2):283–5. doi: 10.1016/j.cell.2018.03.042
25. Weinstein JN, Collisson EA, Mills GB, Shaw KR, Ozenberger BA, Ellrott K, et al. The cancer genome atlas pan-cancer analysis project. Nat Genet (2013) 45(10):1113–20. doi: 10.1038/ng.2764
26. Robertson AG, Kim J, Al-Ahmadie H, Bellmunt J, Guo G, Cherniack AD, et al. Comprehensive molecular characterization of muscle-invasive bladder cancer. Cell (2017) 171(3):540–56.e525. doi: 10.1016/j.cell.2017.09.007
27. Kamoun A, de Reyniès A, Allory Y, Sjödahl G, Robertson AG, Seiler R, et al. A consensus molecular classification of muscle-invasive bladder cancer. Eur Urol (2020) 77(4):420–33. doi: 10.1016/j.eururo.2019.09.006
28. Seiler R, Ashab HAD, Erho N, van Rhijn BWG, Winters B, Douglas J, et al. Impact of molecular subtypes in muscle-invasive bladder cancer on predicting response and survival after neoadjuvant chemotherapy. Eur Urol (2017) 72(4):544–54. doi: 10.1016/j.eururo.2017.03.030
29. Zheng X, Carstens JL, Kim J, Scheible M, Kaye J, Sugimoto H, et al. Epithelial-to-mesenchymal transition is dispensable for metastasis but induces chemoresistance in pancreatic cancer. Nature (2015) 527(7579):525–30. doi: 10.1038/nature16064
30. Robinson DR, Wu YM, Lonigro RJ, Vats P, Cobain E, Everett J, et al. Integrative clinical genomics of metastatic cancer. Nature (2017) 548(7667):297–303. doi: 10.1038/nature23306
31. Pan S, Zhan Y, Chen X, Wu B, Liu B. Bladder cancer exhibiting high immune infiltration shows the lowest response rate to immune checkpoint inhibitors. Front Oncol (2019) 91101:1101. doi: 10.3389/fonc.2019.01101
32. Liu B, Zhan Y, Chen X, Hu X, Wu B, Pan S. Weighted gene co-expression network analysis can sort cancer-associated fibroblast-specific markers promoting bladder cancer progression. J Cell Physiol (2021) 236(2):1321–31. doi: 10.1002/jcp.29939
33. Elsen S, Lerut E, Van Cleynenbreugel B, van der Aa F, van Poppel H, de Witte PA. Biodistribution of Evans blue in an orthotopic AY-27 rat bladder urothelial cell carcinoma model: Implication for the improved diagnosis of non-muscle-invasive bladder cancer (NMIBC) using dye-guided white-light cystoscopy. BJU Int (2015) 116(3):468–77. doi: 10.1111/bju.13113
34. Lepiarczyk E, Bossowska A, Kaleczyc J, Skowrońska A, Majewska M, Majewski M, et al. The influence of resiniferatoxin (RTX) and tetrodotoxin (TTX) on the distribution, relative frequency, and chemical coding of noradrenergic and cholinergic nerve fibers supplying the porcine urinary bladder wall. Toxins (Basel) (2017) 9(10):310. doi: 10.3390/toxins9100310
35. Kroemer G, Galluzzi L, Kepp O, Zitvogel L. Immunogenic cell death in cancer therapy. Annu Rev Immunol (2013) 31:3151–72. doi: 10.1146/annurev-immunol-032712-100008
36. Daza J, Charap A, Wiklund PN, Sfakianos JP. Role of the innate immune system in the development, progression, and therapeutic response of bladder cancer. Eur Urol Focus (2020) 6(4):650–2. doi: 10.1016/j.euf.2020.02.013
37. Woo SR, Corrales L, Gajewski TF. Innate immune recognition of cancer. Annu Rev Immunol (2015) 33:454–74. doi: 10.1146/annurev-immunol-032414-112043
38. Balkwill F, Mantovani A. Inflammation and cancer: Back to virchow? Lancet (2001) 357(9255):539–45. doi: 10.1016/s0140-6736(00)04046-0
39. Morrison BJ, Steel JC, Morris JC. Reduction of MHC-I expression limits T-lymphocyte-mediated killing of cancer-initiating cells. BMC Cancer (2018) 18(1):469. doi: 10.1186/s12885-018-4389-3
40. Heath EI, Rosenberg JE. The biology and rationale of targeting nectin-4 in urothelial carcinoma. Nat Rev Urol (2021) 18(2):93–103. doi: 10.1038/s41585-020-00394-5
41. Schwörer S, Vardhana SA, Thompson CB. Cancer metabolism drives a stromal regenerative response. Cell Metab (2019) 29(3):576–91. doi: 10.1016/j.cmet.2019.01.015
42. Correa-Gallegos D, Jiang D, Christ S, Ramesh P, Ye H, Wannemacher J, et al. Patch repair of deep wounds by mobilized fascia. Nature (2019) 576(7786):287–92. doi: 10.1038/s41586-019-1794-y
43. Yoon H, Tang CM, Banerjee S, Delgado AL, Yebra M, Davis J, et al. TGF-β1-mediated transition of resident fibroblasts to cancer-associated fibroblasts promotes cancer metastasis in gastrointestinal stromal tumor. Oncogenesis (2021) 10(2):13. doi: 10.1038/s41389-021-00302-5
44. Butti R, Nimma R, Kundu G, Bulbule A, Kumar TVS, Gunasekaran VP, et al. Tumor-derived osteopontin drives the resident fibroblast to myofibroblast differentiation through Twist1 to promote breast cancer progression. Oncogene (2021) 40(11):2002–17. doi: 10.1038/s41388-021-01663-2
45. Melissari MT, Chalkidi N, Sarris ME, Koliaraki V. Fibroblast reprogramming in gastrointestinal cancer. Front Cell Dev Biol (2020) 8630:630. doi: 10.3389/fcell.2020.00630
46. Wang Y, Jing Y, Ding L, Zhang X, Song Y, Chen S, et al. Epiregulin reprograms cancer-associated fibroblasts and facilitates oral squamous cell carcinoma invasion via JAK2-STAT3 pathway. J Exp Clin Cancer Res (2019) 38(1):274. doi: 10.1186/s13046-019-1277-x
47. Nayar S, Campos J, Smith CG, Iannizzotto V, Gardner DH, Mourcin F, et al. Immunofibroblasts are pivotal drivers of tertiary lymphoid structure formation and local pathology. Proc Natl Acad Sci U S A (2019) 116(27):13490–7. doi: 10.1073/pnas.1905301116
48. Burr ML, Sparbier CE, Chan KL, Chan YC, Kersbergen A, Lam EYN, et al. An evolutionarily conserved function of polycomb silences the MHC class I antigen presentation pathway and enables immune evasion in cancer. Cancer Cell (2019) 36(4):385–401.e388. doi: 10.1016/j.ccell.2019.08.008
49. Daassi D, Mahoney KM, Freeman GJ. The importance of exosomal PDL1 in tumour immune evasion. Nat Rev Immunol (2020) 20(4):209–15. doi: 10.1038/s41577-019-0264-y
50. Duffield JS, Lupher M, Thannickal VJ, Wynn TA. Host responses in tissue repair and fibrosis. Annu Rev Pathol (2013) 8:241–276. doi: 10.1146/annurev-pathol-020712-163930
51. Su S, Chen J, Yao H, Liu J, Yu S, Lao L, et al. CD10(+)GPR77(+) cancer-associated fibroblasts promote cancer formation and chemoresistance by sustaining cancer stemness. Cell (2018) 172(4):841–56.e816. doi: 10.1016/j.cell.2018.01.009
52. Sahai E, Astsaturov I, Cukierman E, DeNardo DG, Egeblad M, Evans RM, et al. A framework for advancing our understanding of cancer-associated fibroblasts. Nat Rev Cancer (2020) 20(3):174–86. doi: 10.1038/s41568-019-0238-1
53. Park D, Sahai E, Rullan A. SnapShot: Cancer-associated fibroblasts. Cell (2020) 181(2):486–.e481. doi: 10.1016/j.cell.2020.03.013
54. Chen DS, Mellman I. Elements of cancer immunity and the cancer-immune set point. Nature (2017) 541(7637):321–30. doi: 10.1038/nature21349
55. Joyce JA, Fearon DT. T Cell exclusion, immune privilege, and the tumor microenvironment. Science (2015) 348(6230):74–80. doi: 10.1126/science.aaa6204
56. Salmon H, Franciszkiewicz K, Damotte D, Dieu-Nosjean MC, Validire P, Trautmann A, et al. Matrix architecture defines the preferential localization and migration of T cells into the stroma of human lung tumors. J Clin Invest (2012) 122(3):899–910. doi: 10.1172/jci45817
57. Tran L, Xiao JF, Agarwal N, Duex JE, Theodorescu D. Advances in bladder cancer biology and therapy. Nat Rev Cancer (2021) 21(2):104–21. doi: 10.1038/s41568-020-00313-1
58. Liu B, Pan S, Liu J, Kong C. Cancer-associated fibroblasts and the related runt-related transcription factor 2 (RUNX2) promote bladder cancer progression. Gene (2021) 775:145451. doi: 10.1016/j.gene.2021.145451
59. Du Y, Jiang X, Wang B, Cao J, Wang Y, Yu J, et al. The cancer-associated fibroblasts related gene CALD1 is a prognostic biomarker and correlated with immune infiltration in bladder cancer. Cancer Cell Int (2021) 21(1):283. doi: 10.1186/s12935-021-01896-x
60. Mezheyeuski A, Segersten U, Leiss LW, Malmström PU, Hatina J, Östman A, et al. Fibroblasts in urothelial bladder cancer define stroma phenotypes that are associated with clinical outcome. Sci Rep (2020) 10(1):281. doi: 10.1038/s41598-019-55013-0
61. Calvete J, Larrinaga G, Errarte P, Martín AM, Dotor A, Esquinas C, et al. The coexpression of fibroblast activation protein (FAP) and basal-type markers (CK 5/6 and CD44) predicts prognosis in high-grade invasive urothelial carcinoma of the bladder. Hum Pathol (2019) 91:61–8. doi: 10.1016/j.humpath.2019.07.002
62. Wang L, Saci A, Szabo PM, Chasalow SD, Castillo-Martin M, Domingo-Domenech J, et al. EMT- and stroma-related gene expression and resistance to PD-1 blockade in urothelial cancer. Nat Commun (2018) 9(1):3503. doi: 10.1038/s41467-018-05992-x
63. Long X, Xiong W, Zeng X, Qi L, Cai Y, Mo M, et al. Cancer-associated fibroblasts promote cisplatin resistance in bladder cancer cells by increasing IGF-1/ERβ/Bcl-2 signalling. Cell Death Dis (2019) 10(5):375. doi: 10.1038/s41419-019-1581-6
64. Chen Z, Zhou L, Liu L, Hou Y, Xiong M, Yang Y, et al. Single-cell RNA sequencing highlights the role of inflammatory cancer-associated fibroblasts in bladder urothelial carcinoma. Nat Commun (2020) 11(1):5077. doi: 10.1038/s41467-020-18916-5
65. Öhlund D, Handly-Santana A, Biffi G, Elyada E, Almeida AS, Ponz-Sarvise M, et al. Distinct populations of inflammatory fibroblasts and myofibroblasts in pancreatic cancer. J Exp Med (2017) 214(3):579–96. doi: 10.1084/jem.20162024
66. Mota JM, Leite CA, Souza LE, Melo PH, Nascimento DC, de-Deus-Wagatsuma VM, et al. Post-sepsis state induces tumor-associated macrophage accumulation through CXCR4/CXCL12 and favors tumor progression in mice. Cancer Immunol Res (2016) 4(4):312–22. doi: 10.1158/2326-6066.Cir-15-0170
67. Kalluri R. The biology and function of fibroblasts in cancer. Nat Rev Cancer (2016) 16(9):582–98. doi: 10.1038/nrc.2016.73
68. Collier JL, Weiss SA, Pauken KE, Sen DR, Sharpe AH. Not-so-opposite ends of the spectrum: CD8(+) T cell dysfunction across chronic infection, cancer and autoimmunity. Nat Immunol (2021) 22(7):809–19. doi: 10.1038/s41590-021-00949-7
69. Kallies A, Zehn D, Utzschneider DT. Precursor exhausted T cells: key to successful immunotherapy? Nat Rev Immunol (2020) 20(2):128–36. doi: 10.1038/s41577-019-0223-7
70. Reina-Campos M, Scharping NE, Goldrath AW. CD8(+) T cell metabolism in infection and cancer. Nat Rev Immunol (2021) 21(11):718–38. doi: 10.1038/s41577-021-00537-8
71. van Wilpe S, Gerretsen ECF, van der Heijden AG, de Vries IJM, Gerritsen WR, Mehra N. Prognostic and predictive value of tumor-infiltrating immune cells in urothelial cancer of the bladder. Cancers (Basel) (2020) 12(9):2692. doi: 10.3390/cancers12092692
72. Sharma P, Shen Y, Wen S, Yamada S, Jungbluth AA, Gnjatic S, et al. CD8 tumor-infiltrating lymphocytes are predictive of survival in muscle-invasive urothelial carcinoma. Proc Natl Acad Sci USA (2007) 104(10):3967–72. doi: 10.1073/pnas.0611618104
73. Liu Z, Zhou Q, Wang Z, Zhang H, Zeng H, Huang Q, et al. Intratumoral TIGIT(+) CD8(+) T-cell infiltration determines poor prognosis and immune evasion in patients with muscle-invasive bladder cancer. J Immunother Cancer (2020) 8(2):e000978. doi: 10.1136/jitc-2020-000978
74. Wu L, Mao L, Liu JF, Chen L, Yu GT, Yang LL, et al. Blockade of TIGIT/CD155 signaling reverses T-cell exhaustion and enhances antitumor capability in head and neck squamous cell carcinoma. Cancer Immunol Res (2019) 7(10):1700–13. doi: 10.1158/2326-6066.Cir-18-0725
75. Oh DY, Kwek SS, Raju SS, Li T, McCarthy E, Chow E, et al. Intratumoral CD4(+) T cells mediate anti-tumor cytotoxicity in human bladder cancer. Cell (2020) 181(7):1612–25.e1613. doi: 10.1016/j.cell.2020.05.017
76. Borst J, Ahrends T, Bąbała N, Melief CJM, Kastenmüller W. CD4(+) T cell help in cancer immunology and immunotherapy. Nat Rev Immunol (2018) 18(10):635–47. doi: 10.1038/s41577-018-0044-0
77. Pellicci DG, Koay HF, Berzins SP. Thymic development of unconventional T cells: how NKT cells, MAIT cells and γδ T cells emerge. Nat Rev Immunol (2020) 20(12):756–70. doi: 10.1038/s41577-020-0345-y
78. Mucida D, Husain MM, Muroi S, van Wijk F, Shinnakasu R, Naoe Y, et al. Transcriptional reprogramming of mature CD4+ helper T cells generates distinct MHC class II-restricted cytotoxic T lymphocytes. Nat Immunol (2013) 14(3):281–9. doi: 10.1038/ni.2523
79. Sundstedt A, O’Neill EJ, Nicolson KS, Wraith DC. Role for IL-10 in suppression mediated by peptide-induced regulatory T cells. vivo. J Immunol (2003) 170(3):1240–8. doi: 10.4049/jimmunol.170.3.1240
80. Sawant DV, Yano H, Chikina M, Zhang Q, Liao M, Liu C, et al. Adaptive plasticity of IL-10(+) and IL-35(+) t(reg) cells cooperatively promotes tumor T cell exhaustion. Nat Immunol (2019) 20(6):724–35. doi: 10.1038/s41590-019-0346-9
81. Winerdal ME, Marits P, Winerdal M, Hasan M, Rosenblatt R, Tolf A, et al. FOXP3 and survival in urinary bladder cancer. BJU Int (2011) 108(10):1672–8. doi: 10.1111/j.1464-410X.2010.10020.x
82. Hori S, Nomura T, Sakaguchi S. Control of regulatory T cell development by the transcription factor Foxp3. Science (2003) 299(5609):1057–61. doi: 10.1126/science.1079490
83. Wang T, Zhou Q, Zeng H, Zhang H, Liu Z, Shao J, et al. CCR8 blockade primes anti-tumor immunity through intratumoral regulatory T cells destabilization in muscle-invasive bladder cancer. Cancer Immunol Immunother (2020) 69(9):1855–67. doi: 10.1007/s00262-020-02583-y
84. Barsheshet Y, Wildbaum G, Levy E, Vitenshtein A, Akinseye C, Griggs J, et al. CCR8(+)FOXp3(+) t(reg) cells as master drivers of immune regulation. Proc Natl Acad Sci USA (2017) 114(23):6086–91. doi: 10.1073/pnas.1621280114
85. Karin N. Chemokines and cancer: new immune checkpoints for cancer therapy. Curr Opin Immunol (2018) 51:140–5. doi: 10.1016/j.coi.2018.03.004
86. Delacher M, Simon M, Sanderink L, Hotz-Wagenblatt A, Wuttke M, Schambeck K, et al. Single-cell chromatin accessibility landscape identifies tissue repair program in human regulatory T cells. Immunity (2021) 54(4):702–20.e717. doi: 10.1016/j.immuni.2021.03.007
87. Di Pilato M, Kim EY, Cadilha BL, Prüßmann JN, Nasrallah MN, Seruggia D, et al. Targeting the CBM complex causes t(reg) cells to prime tumours for immune checkpoint therapy. Nature (2019) 570(7759):112–6. doi: 10.1038/s41586-019-1215-2
88. Mantovani A, Biswas SK, Galdiero MR, Sica A, Locati M. Macrophage plasticity and polarization in tissue repair and remodelling. J Pathol (2013) 229(2):176–85. doi: 10.1002/path.4133
89. Murdoch C, Tazzyman S, Webster S, Lewis CE. Expression of tie-2 by human monocytes and their responses to angiopoietin-2. J Immunol (2007) 178(11):7405–11. doi: 10.4049/jimmunol.178.11.7405
90. Takeuchi H, Tanaka M, Tanaka A, Tsunemi A, Yamamoto H. Predominance of M2-polarized macrophages in bladder cancer affects angiogenesis, tumor grade and invasiveness. Oncol Lett (2016) 11(5):3403–8. doi: 10.3892/ol.2016.4392
91. Wang Y, Yan K, Wang J, Lin J, Bi J. M2 macrophage Co-expression factors correlate with immune phenotype and predict prognosis of bladder cancer. Front Oncol (2021) 11609334:609334. doi: 10.3389/fonc.2021.609334
92. Hu B, Wang Z, Zeng H, Qi Y, Chen Y, Wang T, et al. Blockade of DC-SIGN(+) tumor-associated macrophages reactivates antitumor immunity and improves immunotherapy in muscle-invasive bladder cancer. Cancer Res (2020) 80(8):1707–19. doi: 10.1158/0008-5472.Can-19-2254
93. Wculek SK, Cueto FJ, Mujal AM, Melero I, Krummel MF, Sancho D. Dendritic cells in cancer immunology and immunotherapy. Nat Rev Immunol (2020) 20(1):7–24. doi: 10.1038/s41577-019-0210-z
94. Jhunjhunwala S, Hammer C, Delamarre L. Antigen presentation in cancer: insights into tumour immunogenicity and immune evasion. Nat Rev Cancer (2021) 21(5):298–312. doi: 10.1038/s41568-021-00339-z
95. Chevalier MF, Bohner P, Pieraerts C, Lhermitte B, Gourmaud J, Nobile A, et al. Immunoregulation of dendritic cell subsets by inhibitory receptors in urothelial cancer. Eur Urol (2017) 71(6):854–7. doi: 10.1016/j.eururo.2016.10.009
96. Neefjes J, Jongsma ML, Paul P, Bakke O. Towards a systems understanding of MHC class I and MHC class II antigen presentation. Nat Rev Immunol (2011) 11(12):823–36. doi: 10.1038/nri3084
97. Philip M, Schietinger A. CD8(+) T cell differentiation and dysfunction in cancer. Nat Rev Immunol (2021) 22(4):209–23. doi: 10.1038/s41577-021-00574-3
98. Seo H, Jeon I, Kim BS, Park M, Bae EA, Song B, et al. IL-21-mediated reversal of NK cell exhaustion facilitates anti-tumour immunity in MHC class I-deficient tumours. Nat Commun (2017) 8:15776. doi: 10.1038/ncomms15776
99. Ghosh RK, Pandey T, Dey P. Liquid biopsy: A new avenue in pathology. Cytopathology (2019) 30(2):138–43. doi: 10.1111/cyt.12661
100. Abbasi J. Blood test flags multiple cancers in Large study. Jama (2020) 323(22):2239. doi: 10.1001/jama.2020.9266
101. Garber K. Blood test may predict cancer immunotherapy benefit. Science (2018) 360(6396):1387. doi: 10.1126/science.360.6396.1387
102. Kaiser J. ‘Liquid biopsy’ for cancer promises early detection. Science (2018) 359(6373):259. doi: 10.1126/science.359.6373.259
103. Kaiser J. Cancer DNA blood test gets real-world trial. Science (2020) 368(6490):461. doi: 10.1126/science.368.6490.461
104. Lennon AM, Buchanan AH, Kinde I, Warren A, Honushefsky A, Cohain AT, et al. Feasibility of blood testing combined with PET-CT to screen for cancer and guide intervention. Science (2020) 369(6499):eabb9601. doi: 10.1126/science.abb9601
105. Kinoshita H, Ogawa O, Kakehi Y, Mishina M, Mitsumori K, Itoh N, et al. Detection of telomerase activity in exfoliated cells in urine from patients with bladder cancer. J Natl Cancer Inst (1997) 89(10):724–30. doi: 10.1093/jnci/89.10.724
106. Birkenkamp-Demtröder K, Christensen E, Nordentoft I, Knudsen M, Taber A, Høyer S, et al. Monitoring treatment response and metastatic relapse in advanced bladder cancer by liquid biopsy analysis. Eur Urol (2018) 73(4):535–40. doi: 10.1016/j.eururo.2017.09.011
107. Junker K, Heinzelmann J, Beckham C, Ochiya T, Jenster G. Extracellular vesicles and their role in urologic malignancies. Eur Urol (2016) 70(2):323–31. doi: 10.1016/j.eururo.2016.02.046
108. Togneri FS, Ward DG, Foster JM, Devall AJ, Wojtowicz P, Alyas S, et al. Genomic complexity of urothelial bladder cancer revealed in urinary cfDNA. Eur J Hum Genet (2016) 24(8):1167–74. doi: 10.1038/ejhg.2015.281
109. Marar C, Starich B, Wirtz D. Extracellular vesicles in immunomodulation and tumor progression. Nat Immunol (2021) 22(5):560–70. doi: 10.1038/s41590-021-00899-0
110. Möller A, Lobb RJ. The evolving translational potential of small extracellular vesicles in cancer. Nat Rev Cancer (2020) 20(12):697–709. doi: 10.1038/s41568-020-00299-w
111. Pegtel DM, Gould SJ. Exosomes. Annu Rev Biochem (2019) 88:487–514. doi: 10.1146/annurev-biochem-013118-111902
112. Puno MR, Weick EM, Das M, Lima CD. SnapShot: The RNA exosome. Cell (2019) 179(1):282–282.e281. doi: 10.1016/j.cell.2019.09.005
113. Shah R, Patel T, Freedman JE. Circulating extracellular vesicles in human disease. N Engl J Med (2018) 379(10):958–66. doi: 10.1056/NEJMra1704286
114. Cheng ML, Pectasides E, Hanna GJ, Parsons HA, Choudhury AD, Oxnard GR. Circulating tumor DNA in advanced solid tumors: Clinical relevance and future directions. CA Cancer J Clin (2021) 71(2):176–90. doi: 10.3322/caac.21650
115. Reyes E, Carballido J, Prieto A, Molto L, Manzano L, Alvarez-Mon M. T Lymphocytes infiltrating the bladder wall of patients with carcinoma of urinary bladder are in vivo activated. Eur Urol (1997) 31(4):472–7. doi: 10.1159/000474509
116. Wong YNS, Joshi K, Khetrapal P, Ismail M, Reading JL, Sunderland MW, et al. Urine-derived lymphocytes as a non-invasive measure of the bladder tumor immune microenvironment. J Exp Med (2018) 215(11):2748–59. doi: 10.1084/jem.20181003
117. Redelman-Sidi G, Glickman MS, Bochner BH. The mechanism of action of BCG therapy for bladder cancer–a current perspective. Nat Rev Urol (2014) 11(3):153–62. doi: 10.1038/nrurol.2014.15
118. Bisiaux A, Thiounn N, Timsit MO, Eladaoui A, Chang HH, Mapes J, et al. Molecular analyte profiling of the early events and tissue conditioning following intravesical bacillus calmette-guerin therapy in patients with superficial bladder cancer. J Urol (2009) 181(4):1571–80. doi: 10.1016/j.juro.2008.11.124
119. Herr HW, Morales A. History of bacillus calmette-guerin and bladder cancer: an immunotherapy success story. J Urol (2008) 179(1):53–6. doi: 10.1016/j.juro.2007.08.122
120. Rentsch CA, Birkhäuser FD, Biot C, Gsponer JR, Bisiaux A, Wetterauer C, et al. Bacillus calmette-guérin strain differences have an impact on clinical outcome in bladder cancer immunotherapy. Eur Urol (2014) 66(4):677–88. doi: 10.1016/j.eururo.2014.02.061
121. Marvel D, Gabrilovich DI. Myeloid-derived suppressor cells in the tumor microenvironment: expect the unexpected. J Clin Invest (2015) 125(9):3356–64. doi: 10.1172/jci80005
122. Ugel S, De Sanctis F, Mandruzzato S, Bronte V. Tumor-induced myeloid deviation: when myeloid-derived suppressor cells meet tumor-associated macrophages. J Clin Invest (2015) 125(9):3365–76. doi: 10.1172/jci80006
123. Chevalier MF, Trabanelli S, Racle J, Salomé B, Cesson V, Gharbi D, et al. ILC2-modulated T cell-to-MDSC balance is associated with bladder cancer recurrence. J Clin Invest (2017) 127(8):2916–29. doi: 10.1172/jci89717
124. Saint F, Kurth N, Maille P, Vordos D, Hoznek A, Soyeux P, et al. Urinary IL-2 assay for monitoring intravesical bacillus calmette-guérin response of superficial bladder cancer during induction course and maintenance therapy. Int J Cancer (2003) 107(3):434–40. doi: 10.1002/ijc.11352
125. Pichler R, Fritz J, Zavadil C, Schäfer G, Culig Z, Brunner A. Tumor-infiltrating immune cell subpopulations influence the oncologic outcome after intravesical bacillus calmette-guérin therapy in bladder cancer. Oncotarget (2016) 7(26):39916–30. doi: 10.18632/oncotarget.9537
126. Miyake M, Tatsumi Y, Gotoh D, Ohnishi S, Owari T, Iida K, et al. Regulatory T cells and tumor-associated macrophages in the tumor microenvironment in non-muscle invasive bladder cancer treated with intravesical bacille calmette-guérin: A long-term follow-up study of a Japanese cohort. Int J Mol Sci (2017) 18(10):2186. doi: 10.3390/ijms18102186
127. Liu Y, Carlsson R, Comabella M, Wang J, Kosicki M, Carrion B, et al. FoxA1 directs the lineage and immunosuppressive properties of a novel regulatory T cell population in EAE and MS. Nat Med (2014) 20(3):272–82. doi: 10.1038/nm.3485
128. Chevalier MF, Schneider AK, Cesson V, Dartiguenave F, Lucca I, Jichlinski P, et al. Conventional and PD-L1-expressing regulatory T cells are enriched during BCG therapy and may limit its efficacy. Eur Urol (2018) 74(5):540–4. doi: 10.1016/j.eururo.2018.06.045
129. Inman BA, Sebo TJ, Frigola X, Dong H, Bergstralh EJ, Frank I, et al. PD-L1 (B7-H1) expression by urothelial carcinoma of the bladder and BCG-induced granulomata: associations with localized stage progression. Cancer (2007) 109(8):1499–505. doi: 10.1002/cncr.22588
130. Wang Y, Liu J, Yang X, Liu Y, Liu Y, Li Y, et al. Bacillus calmette-guérin and anti-PD-L1 combination therapy boosts immune response against bladder cancer. Onco Targets Ther (2018) 11:2891–9. doi: 10.2147/ott.S165840
131. Samstein RM, Lee CH, Shoushtari AN, Hellmann MD, Shen R, Janjigian YY, et al. Tumor mutational load predicts survival after immunotherapy across multiple cancer types. Nat Genet (2019) 51(2):202–6. doi: 10.1038/s41588-018-0312-8
132. Cristescu R, Mogg R, Ayers M, Albright A, Murphy E, Yearley J, et al. Pan-tumor genomic biomarkers for PD-1 checkpoint blockade-based immunotherapy. Science (2018) 362(6411):eaar3593. doi: 10.1126/science.aar3593
133. Goodman AM, Kato S, Bazhenova L, Patel SP, Frampton GM, Miller V, et al. Tumor mutational burden as an independent predictor of response to immunotherapy in diverse cancers. Mol Cancer Ther (2017) 16(11):2598–608. doi: 10.1158/1535-7163.Mct-17-0386
134. Hodi FS, O’Day SJ, McDermott DF, Weber RW, Sosman JA, Haanen JB, et al. Improved survival with ipilimumab in patients with metastatic melanoma. N Engl J Med (2010) 363(8):711–23. doi: 10.1056/NEJMoa1003466
135. Borghaei H, Paz-Ares L, Horn L, Spigel DR, Steins M, Ready NE, et al. Nivolumab versus docetaxel in advanced nonsquamous non-small-cell lung cancer. N Engl J Med (2015) 373(17):1627–39. doi: 10.1056/NEJMoa1507643
136. Herbst RS, Baas P, Kim DW, Felip E, Pérez-Gracia JL, Han JY, et al. Pembrolizumab versus docetaxel for previously treated, PD-L1-positive, advanced non-small-cell lung cancer (KEYNOTE-010): A randomised controlled trial. Lancet (2016) 387(10027):1540–50. doi: 10.1016/s0140-6736(15)01281-7
137. Fehrenbacher L, Spira A, Ballinger M, Kowanetz M, Vansteenkiste J, Mazieres J, et al. Atezolizumab versus docetaxel for patients with previously treated non-small-cell lung cancer (POPLAR): a multicentre, open-label, phase 2 randomised controlled trial. Lancet (2016) 387(10030):1837–46. doi: 10.1016/s0140-6736(16)00587-0
138. Suzman DL, Agrawal S, Ning YM, Maher VE, Fernandes LL, Karuri S, et al. FDA Approval summary: Atezolizumab or pembrolizumab for the treatment of patients with advanced urothelial carcinoma ineligible for cisplatin-containing chemotherapy. Oncologist (2019) 24(4):563–9. doi: 10.1634/theoncologist.2018-0084
139. Bellmunt J, de Wit R, Vaughn DJ, Fradet Y, Lee JL, Fong L, et al. Pembrolizumab as second-line therapy for advanced urothelial carcinoma. N Engl J Med (2017) 376(11):1015–26. doi: 10.1056/NEJMoa1613683
140. Balar AV, Castellano D, O’Donnell PH, Grivas P, Vuky J, Powles T, et al. First-line pembrolizumab in cisplatin-ineligible patients with locally advanced and unresectable or metastatic urothelial cancer (KEYNOTE-052): A multicentre, single-arm, phase 2 study. Lancet Oncol (2017) 18(11):1483–92. doi: 10.1016/s1470-2045(17)30616-2
141. Bajorin DF, Witjes JA, Gschwend JE, Schenker M, Valderrama BP, Tomita Y, et al. Adjuvant nivolumab versus placebo in muscle-invasive urothelial carcinoma. N Engl J Med (2021) 384(22):2102–14. doi: 10.1056/NEJMoa2034442
142. Powles T, Park SH, Voog E, Caserta C, Valderrama BP, Gurney H, et al. Avelumab maintenance therapy for advanced or metastatic urothelial carcinoma. N Engl J Med (2020) 383(13):1218–30. doi: 10.1056/NEJMoa2002788
143. Schwartz C, Khan AR, Floudas A, Saunders SP, Hams E, Rodewald HR, et al. ILC2s regulate adaptive Th2 cell functions via PD-L1 checkpoint control. J Exp Med (2017) 214(9):2507–21. doi: 10.1084/jem.20170051
144. Ferro M, de Cobelli O, Musi G, Lucarelli G, Terracciano D, Pacella D, et al. Three vs. four cycles of neoadjuvant chemotherapy for localized muscle invasive bladder cancer undergoing radical cystectomy: A retrospective multi-institutional analysis. Front Oncol (2021) 11651745:651745. doi: 10.3389/fonc.2021.651745
145. D’Andrea D, Black PC, Zargar H, Dinney CP, Soria F, Cookson MS, et al. Identifying the optimal number of neoadjuvant chemotherapy cycles in patients with muscle invasive bladder cancer. J Urol (2022) 207(1):70–6 . doi: 10.1097/ju.0000000000002190
146. Advanced Bladder Cancer (ABC) Meta-analysis Collaboration.Neoadjuvant chemotherapy in invasive bladder cancer: update of a systematic review and meta-analysis of individual patient data advanced bladder cancer (ABC) meta-analysis collaboration. Eur Urol (2005) 48(2):202–5. doi: 10.1016/j.eururo.2005.04.006
147. Necchi A, Raggi D, Gallina A, Ross JS, Farè E, Giannatempo P, et al. Impact of molecular subtyping and immune infiltration on pathological response and outcome following neoadjuvant pembrolizumab in muscle-invasive bladder cancer. Eur Urol (2020) 77(6):701–10. doi: 10.1016/j.eururo.2020.02.028
148. He Y, Wang L, Wei T, Xiao YT, Sheng H, Su H, et al. FOXA1 overexpression suppresses interferon signaling and immune response in cancer. J Clin Invest (2021) 131(14):e147025. doi: 10.1172/jci147025
149. Zitvogel L, Galluzzi L, Smyth MJ, Kroemer G. Mechanism of action of conventional and targeted anticancer therapies: reinstating immunosurveillance. Immunity (2013) 39(1):74–88. doi: 10.1016/j.immuni.2013.06.014
150. Krantz D, Hartana CA, Winerdal ME, Johansson M, Alamdari F, Jakubczyk T, et al. Neoadjuvant chemotherapy reinforces antitumour T cell response in urothelial urinary bladder cancer. Eur Urol (2018) 74(6):688–92. doi: 10.1016/j.eururo.2018.06.048
151. Vollmer T, Schlickeiser S, Amini L, Schulenberg S, Wendering DJ, Banday V, et al. The intratumoral CXCR3 chemokine system is predictive of chemotherapy response in human bladder cancer. Sci Transl Med (2021) 13(576):eabb3735. doi: 10.1126/scitranslmed.abb3735
152. Meads MB, Gatenby RA, Dalton WS. Environment-mediated drug resistance: a major contributor to minimal residual disease. Nat Rev Cancer (2009) 9(9):665–74. doi: 10.1038/nrc2714
153. Bruni D, Angell HK, Galon J. The immune contexture and immunoscore in cancer prognosis and therapeutic efficacy. Nat Rev Cancer (2020) 20(11):662–80. doi: 10.1038/s41568-020-0285-7
154. Masson-Lecomte A, Rava M, Real FX, Hartmann A, Allory Y, Malats N. Inflammatory biomarkers and bladder cancer prognosis: a systematic review. Eur Urol (2014) 66(6):1078–91. doi: 10.1016/j.eururo.2014.07.033
155. Tamalunas A, Buchner A, Kretschmer A, Jokisch F, Schulz G, Eismann L, et al. Impact of routine laboratory parameters in patients undergoing radical cystectomy for urothelial carcinoma of the bladder: A long-term follow-up. Urol Int (2020) 104(7-8):551–8. doi: 10.1159/000506263
156. Rodler S, Buchner A, Ledderose ST, Eismann L, Volz Y, Pfitzinger P, et al. Prognostic value of pretreatment inflammatory markers in variant histologies of the bladder: is inflammation linked to survival after radical cystectomy? World J Urol (2021) 39(7):2537–43. doi: 10.1007/s00345-020-03482-8
157. Pepys MB, Baltz ML. Acute phase proteins with special reference to c-reactive protein and related proteins (pentaxins) and serum amyloid a protein. Adv Immunol (1983) 34:141–212. doi: 10.1016/s0065-2776(08)60379-x
158. Ganapathi MK, May LT, Schultz D, Brabenec A, Weinstein J, Sehgal PB, et al. Role of interleukin-6 in regulating synthesis of c-reactive protein and serum amyloid a in human hepatoma cell lines. Biochem Biophys Res Commun (1988) 157(1):271–7. doi: 10.1016/s0006-291x(88)80043-3
159. Jones SA, Novick D, Horiuchi S, Yamamoto N, Szalai AJ, Fuller GM. C-reactive protein: a physiological activator of interleukin 6 receptor shedding. J Exp Med (1999) 189(3):599–604. doi: 10.1084/jem.189.3.599
160. Potempa LA, Rajab IM, Olson ME, Hart PC. C-reactive protein and cancer: Interpreting the differential bioactivities of its pentameric and monomeric, modified isoforms. Front Immunol (2021) 12744129:744129. doi: 10.3389/fimmu.2021.744129
161. Garbers C, Heink S, Korn T, Rose-John S. Interleukin-6: designing specific therapeutics for a complex cytokine. Nat Rev Drug Discovery (2018) 17(6):395–412. doi: 10.1038/nrd.2018.45
162. Briukhovetska D, Dörr J, Endres S, Libby P, Dinarello CA, Kobold S. Interleukins in cancer: from biology to therapy. Nat Rev Cancer (2021) 21(8):481–99. doi: 10.1038/s41568-021-00363-z
163. Mbeutcha A, Shariat SF, Rieken M, Rink M, Xylinas E, Seitz C, et al. Prognostic significance of markers of systemic inflammatory response in patients with non-muscle-invasive bladder cancer. Urol Oncol (2016) 34(11):483.e417–483.e424. doi: 10.1016/j.urolonc.2016.05.013
164. Grimm T, Buchner A, Schneevoigt B, Kretschmer A, Apfelbeck M, Grabbert M, et al. Impact of preoperative hemoglobin and CRP levels on cancer-specific survival in patients undergoing radical cystectomy for transitional cell carcinoma of the bladder: results of a single-center study. World J Urol (2016) 34(5):703–8. doi: 10.1007/s00345-015-1680-7
165. Gakis G, Todenhöfer T, Renninger M, Schilling D, Sievert KD, Schwentner C, et al. Development of a new outcome prediction model in carcinoma invading the bladder based on preoperative serum c-reactive protein and standard pathological risk factors: the TNR-c score. BJU Int (2011) 108(11):1800–5. doi: 10.1111/j.1464-410X.2011.10234.x
166. Hwang EC, Hwang IS, Yu HS, Kim SO, Jung SI, Hwang JE, et al. Utility of inflammation-based prognostic scoring in patients given systemic chemotherapy first-line for advanced inoperable bladder cancer. Jpn J Clin Oncol (2012) 42(10):955–60. doi: 10.1093/jjco/hys124
167. Yoshida S, Saito K, Koga F, Yokoyama M, Kageyama Y, Masuda H, et al. C-reactive protein level predicts prognosis in patients with muscle-invasive bladder cancer treated with chemoradiotherapy. BJU Int (2008) 101(8):978–81. doi: 10.1111/j.1464-410X.2007.07408.x
168. Saito K, Urakami S, Komai Y, Yasuda Y, Kubo Y, Kitsukawa S, et al. Impact of c-reactive protein kinetics on survival of patients with advanced urothelial carcinoma treated by second-line chemotherapy with gemcitabine, etoposide and cisplatin. BJU Int (2012) 110(10):1478–84. doi: 10.1111/j.1464-410X.2012.11153.x
169. Barna BP, James K, Deodhar SD. Activation of human monocyte tumoricidal activity by c-reactive protein. Cancer Res (1987) 47(15):3959–63.
170. Zahedi K, Mortensen RF. Macrophage tumoricidal activity induced by human c-reactive protein. Cancer Res (1986) 46(10):5077–83.
171. Barna BP, Deodhar SD, Gautam S, Yen-Lieberman B, Roberts D. Macrophage activation and generation of tumoricidal activity by liposome-associated human c-reactive protein. Cancer Res (1984) 44(1):305–10.
172. Schäfer M, Werner S. Cancer as an overhealing wound: an old hypothesis revisited. Nat Rev Mol Cell Biol (2008) 9(8):628–38. doi: 10.1038/nrm2455
173. Mellman I, Coukos G, Dranoff G. Cancer immunotherapy comes of age. Nature (2011) 480(7378):480–9. doi: 10.1038/nature10673
174. Xue J, Schmidt SV, Sander J, Draffehn A, Krebs W, Quester I, et al. Transcriptome-based network analysis reveals a spectrum model of human macrophage activation. Immunity (2014) 40(2):274–88. doi: 10.1016/j.immuni.2014.01.006
175. DeNardo DG, Barreto JB, Andreu P, Vasquez L, Tawfik D, Kolhatkar N, et al. CD4(+) T cells regulate pulmonary metastasis of mammary carcinomas by enhancing protumor properties of macrophages. Cancer Cell (2009) 16(2):91–102. doi: 10.1016/j.ccr.2009.06.018
176. Sharifi L, Nowroozi MR, Amini E, Arami MK, Ayati M, Mohsenzadegan M. A review on the role of M2 macrophages in bladder cancer; pathophysiology and targeting. Int Immunopharmacol (2019) 76:105880. doi: 10.1016/j.intimp.2019.105880
177. van der Horst G, Bos L, van der Pluijm G. Epithelial plasticity, cancer stem cells, and the tumor-supportive stroma in bladder carcinoma. Mol Cancer Res (2012) 10(8):995–1009. doi: 10.1158/1541-7786.Mcr-12-0274
178. Tan TZ, Rouanne M, Tan KT, Huang RY, Thiery JP. Molecular subtypes of urothelial bladder cancer: Results from a meta-cohort analysis of 2411 tumors. Eur Urol (2019) 75(3):423–32. doi: 10.1016/j.eururo.2018.08.027
179. Martínez VG, Rubio C, Martínez-Fernández M, Segovia C, López-Calderón F, Garín MI, et al. BMP4 induces M2 macrophage polarization and favors tumor progression in bladder cancer. Clin Cancer Res (2017) 23(23):7388–99. doi: 10.1158/1078-0432.Ccr-17-1004
180. Kobatake K, Ikeda KI, Nakata Y, Yamasaki N, Ueda T, Kanai A, et al. Kdm6a deficiency activates inflammatory pathways, promotes M2 macrophage polarization, and causes bladder cancer in cooperation with p53 dysfunction. Clin Cancer Res (2020) 26(8):2065–79. doi: 10.1158/1078-0432.Ccr-19-2230
181. Minutti CM, Knipper JA, Allen JE, Zaiss DM. Tissue-specific contribution of macrophages to wound healing. Semin Cell Dev Biol (2017) 61:3–11. doi: 10.1016/j.semcdb.2016.08.006
182. Van Dyken SJ, Locksley RM. Interleukin-4- and interleukin-13-mediated alternatively activated macrophages: Roles in homeostasis and disease. Annu Rev Immunol (2013) 31:317–343. doi: 10.1146/annurev-immunol-032712-095906
183. Wynn TA, Vannella KM. Macrophages in tissue repair, regeneration, and fibrosis. Immunity (2016) 44(3):450–62. doi: 10.1016/j.immuni.2016.02.015
184. Nakayama T, Hirahara K, Onodera A, Endo Y, Hosokawa H, Shinoda K, et al. Th2 cells in health and disease. Annu Rev Immunol (2017) 35:53–84. doi: 10.1146/annurev-immunol-051116-052350
185. Kumar S, Jeong Y, Ashraf MU, Bae YS. Dendritic cell-mediated Th2 immunity and immune disorders. Int J Mol Sci (2019) 20(9):2159. doi: 10.3390/ijms20092159
186. Li Z, Jiang J, Wang Z, Zhang J, Xiao M, Wang C, et al. Endogenous interleukin-4 promotes tumor development by increasing tumor cell resistance to apoptosis. Cancer Res (2008) 68(21):8687–94. doi: 10.1158/0008-5472.Can-08-0449
187. Nappo G, Handle F, Santer FR, McNeill RV, Seed RI, Collins AT, et al. The immunosuppressive cytokine interleukin-4 increases the clonogenic potential of prostate stem-like cells by activation of STAT6 signalling. Oncogenesis (2017) 6(5):e342. doi: 10.1038/oncsis.2017.23
188. Gieseck RL 3rd, Wilson MS, Wynn TA. Type 2 immunity in tissue repair and fibrosis. Nat Rev Immunol (2018) 18(1):62–76. doi: 10.1038/nri.2017.90
189. Margel D, Pevsner-Fischer M, Baniel J, Yossepowitch O, Cohen IR. Stress proteins and cytokines are urinary biomarkers for diagnosis and staging of bladder cancer. Eur Urol (2011) 59(1):113–9. doi: 10.1016/j.eururo.2010.10.008
190. Schmid-Grendelmeier P, Altznauer F, Fischer B, Bizer C, Straumann A, Menz G, et al. Eosinophils express functional IL-13 in eosinophilic inflammatory diseases. J Immunol (2002) 169(2):1021–7. doi: 10.4049/jimmunol.169.2.1021
191. Brown KD, Zurawski SM, Mosmann TR, Zurawski G. A family of small inducible proteins secreted by leukocytes are members of a new superfamily that includes leukocyte and fibroblast-derived inflammatory agents, growth factors, and indicators of various activation processes. J Immunol (1989) 142(2):679–87.
192. Terabe M, Matsui S, Noben-Trauth N, Chen H, Watson C, Donaldson DD, et al. NKT cell-mediated repression of tumor immunosurveillance by IL-13 and the IL-4R-STAT6 pathway. Nat Immunol (2000) 1(6):515–20. doi: 10.1038/82771
193. Skinnider BF, Elia AJ, Gascoyne RD, Trümper LH, von Bonin F, Kapp U, et al. Interleukin 13 and interleukin 13 receptor are frequently expressed by Hodgkin and reed-sternberg cells of Hodgkin lymphoma. Blood (2001) 97(1):250–5. doi: 10.1182/blood.v97.1.250
194. Kapp U, Yeh WC, Patterson B, Elia AJ, Kägi D, Ho A, et al. Interleukin 13 is secreted by and stimulates the growth of Hodgkin and reed-sternberg cells. J Exp Med (1999) 189(12):1939–46. doi: 10.1084/jem.189.12.1939
195. Mascharak S, desJardins-Park HE, Davitt MF, Griffin M, Borrelli MR, Moore AL, et al. Preventing engrailed-1 activation in fibroblasts yields wound regeneration without scarring. Science (2021) 372(6540):eaba2374. doi: 10.1126/science.aba2374
196. Abbasi S, Sinha S, Labit E, Rosin NL, Yoon G, Rahmani W, et al. Distinct regulatory programs control the latent regenerative potential of dermal fibroblasts during wound healing. Cell Stem Cell (2021) 28(3):581–3. doi: 10.1016/j.stem.2021.02.004
197. Yokota T, McCourt J, Ma F, Ren S, Li S, Kim TH, et al. Type V collagen in scar tissue regulates the size of scar after heart injury. Cell (2020) 182(3):545–62.e523. doi: 10.1016/j.cell.2020.06.030
198. Ramos R, Plikus MV. Moving on after trauma: Fibroblasts thrive in the right environment. Cell Stem Cell (2020) 27(3):349–51. doi: 10.1016/j.stem.2020.08.007
199. Meeks JJ, Lerner SP. Molecular landscape of non-muscle invasive bladder cancer. Cancer Cell (2017) 32(5):550–1. doi: 10.1016/j.ccell.2017.08.015
200. Ringuette Goulet C, Bernard G, Tremblay S, Chabaud S, Bolduc S, Pouliot F. Exosomes induce fibroblast differentiation into cancer-associated fibroblasts through TGFβ signaling. Mol Cancer Res (2018) 16(7):1196–204. doi: 10.1158/1541-7786.Mcr-17-0784
201. Li YY, Tao YW, Gao S, Li P, Zheng JM, Zhang SE, et al. Cancer-associated fibroblasts contribute to oral cancer cells proliferation and metastasis via exosome-mediated paracrine miR-34a-5p. EBioMedicine (2018) 36:209–20. doi: 10.1016/j.ebiom.2018.09.006
202. Xu G, Zhang B, Ye J, Cao S, Shi J, Zhao Y, et al. Exosomal miRNA-139 in cancer-associated fibroblasts inhibits gastric cancer progression by repressing MMP11 expression. Int J Biol Sci (2019) 15(11):2320–9. doi: 10.7150/ijbs.33750
203. Zhang Z, Li X, Sun W, Yue S, Yang J, Li J, et al. Loss of exosomal miR-320a from cancer-associated fibroblasts contributes to HCC proliferation and metastasis. Cancer Lett (2017) 397:33–42. doi: 10.1016/j.canlet.2017.03.004
204. Chang E, Weinstock C, Zhang L, Charlab R, Dorff SE, Gong Y, et al. FDA Approval summary: Enfortumab vedotin for locally advanced or metastatic urothelial carcinoma. Clin Cancer Res (2021) 27(4):922–7. doi: 10.1158/1078-0432.Ccr-20-2275
205. Lattanzi M, Rosenberg JE. The emerging role of antibody-drug conjugates in urothelial carcinoma. Expert Rev Anticancer Ther (2020) 20(7):551–61. doi: 10.1080/14737140.2020.1782201
206. Vafa O, Trinklein ND. Perspective: Designing T-cell engagers with better therapeutic windows. Front Oncol (2020) 10446:446. doi: 10.3389/fonc.2020.00446
207. Ma W, Ma J, Lei T, Zhao M, Zhang M. Targeting immunotherapy for bladder cancer by using anti-CD3 × CD155 bispecific antibody. J Cancer (2019) 10(21):5153–61. doi: 10.7150/jca.29937
208. Kvarnhammar AM, Veitonmäki N, Hägerbrand K, Dahlman A, Smith KE, Fritzell S, et al. The CTLA-4 x OX40 bispecific antibody ATOR-1015 induces anti-tumor effects through tumor-directed immune activation. J Immunother Cancer (2019) 7(1):103. doi: 10.1186/s40425-019-0570-8
209. Furuya H, Chan OTM, Pagano I, Zhu C, Kim N, Peres R, et al. Effectiveness of two different dose administration regimens of an IL-15 superagonist complex (ALT-803) in an orthotopic bladder cancer mouse model. J Transl Med (2019) 17(1):29. doi: 10.1186/s12967-019-1778-6
210. Knudson KM, Hicks KC, Alter S, Schlom J, Gameiro SR. Mechanisms involved in IL-15 superagonist enhancement of anti-PD-L1 therapy. J Immunother Cancer (2019) 7(1):82. doi: 10.1186/s40425-019-0551-y
211. Bogen JP, Grzeschik J, Jakobsen J, Bähre A, Hock B, Kolmar H. Treating bladder cancer: Engineering of current and next generation antibody-, fusion protein-, mRNA-, cell- and viral-based therapeutics. Front Oncol (2021) 11672262:672262. doi: 10.3389/fonc.2021.672262
212. Wynn TA. Type 2 cytokines: mechanisms and therapeutic strategies. Nat Rev Immunol (2015) 15(5):271–82. doi: 10.1038/nri3831
213. McSorley HJ, Maizels RM. Helminth infections and host immune regulation. Clin Microbiol Rev (2012) 25(4):585–608. doi: 10.1128/cmr.05040-11
214. Fairfax K, Nascimento M, Huang SC, Everts B, Pearce EJ. Th2 responses in schistosomiasis. Semin Immunopathol (2012) 34(6):863–71. doi: 10.1007/s00281-012-0354-4
215. Aceves SS, Broide DH. Airway fibrosis and angiogenesis due to eosinophil trafficking in chronic asthma. Curr Mol Med (2008) 8(5):350–8. doi: 10.2174/156652408785161023
216. Churg A, Müller NL, Wright JL. Respiratory bronchiolitis/interstitial lung disease: fibrosis, pulmonary function, and evolving concepts. Arch Pathol Lab Med (2010) 134(1):27–32. doi: 10.5858/134.1.27
217. Ramalingam TR, Gieseck RL, Acciani TH, MH K, Cheever AW, Mentink-Kane MM, et al. Enhanced protection from fibrosis and inflammation in the combined absence of IL-13 and IFN-γ. J Pathol (2016) 239(3):344–54. doi: 10.1002/path.4733
218. Choy DF, Hart KM, Borthwick LA, Shikotra A, Nagarkar DR, Siddiqui S, et al. TH2 and TH17 inflammatory pathways are reciprocally regulated in asthma. Sci Transl Med (2015) 7(301):301ra129. doi: 10.1126/scitranslmed.aab3142
Keywords: bladder cancer, BCG, immunotherapy, immune cells, type 2 immunity
Citation: Pan S, Li S, Zhan Y, Chen X, Sun M, Liu X, Wu B, Li Z and Liu B (2022) Immune status for monitoring and treatment of bladder cancer. Front. Immunol. 13:963877. doi: 10.3389/fimmu.2022.963877
Received: 08 June 2022; Accepted: 22 August 2022;
Published: 08 September 2022.
Edited by:
Ja Hyeon Ku, Seoul National University, South KoreaReviewed by:
Sujit S. Nair, Icahn School of Medicine at Mount Sinai, United StatesChuanyu Sun, Fudan University, China
Haitao Liu, Shanghai General Hospital, China
Copyright © 2022 Pan, Li, Zhan, Chen, Sun, Liu, Wu, Li and Liu. This is an open-access article distributed under the terms of the Creative Commons Attribution License (CC BY). The use, distribution or reproduction in other forums is permitted, provided the original author(s) and the copyright owner(s) are credited and that the original publication in this journal is cited, in accordance with accepted academic practice. No use, distribution or reproduction is permitted which does not comply with these terms.
*Correspondence: Bitian Liu, liu_bitian@163.com; btliu@cmu.edu.cn
†These authors contributed equally to this work