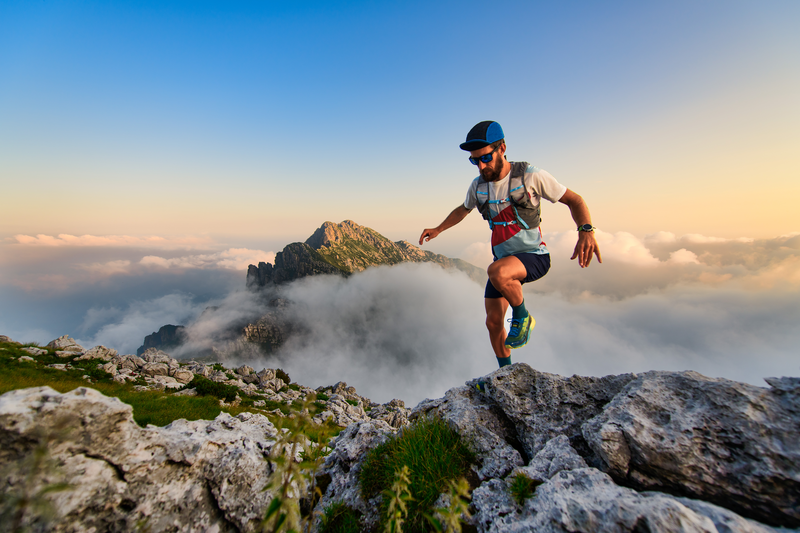
95% of researchers rate our articles as excellent or good
Learn more about the work of our research integrity team to safeguard the quality of each article we publish.
Find out more
REVIEW article
Front. Immunol. , 23 August 2022
Sec. Microbial Immunology
Volume 13 - 2022 | https://doi.org/10.3389/fimmu.2022.953354
This article is part of the Research Topic Modulation of the immune system by bacteria: from evasion to therapy View all 17 articles
Urinary tract infection (UTI) caused by uropathogens is the most common infectious disease and significantly affects all aspects of the quality of life of the patients. However, uropathogens are increasingly becoming antibiotic-resistant, which threatens the only effective treatment option available-antibiotic, resulting in higher medical costs, prolonged hospital stays, and increased mortality. Currently, people are turning their attention to the immune responses, hoping to find effective immunotherapeutic interventions which can be alternatives to the overuse of antibiotic drugs. Bladder infections are caused by the main nine uropathogens and the bladder executes different immune responses depending on the type of uropathogens. It is essential to understand the immune responses to diverse uropathogens in bladder infection for guiding the design and development of immunotherapeutic interventions. This review firstly sorts out and comparatively analyzes the immune responses to the main nine uropathogens in bladder infection, and summarizes their similarities and differences. Based on these immune responses, we innovatively propose that different microbial bladder infections should adopt corresponding immunomodulatory interventions, and the same immunomodulatory intervention can also be applied to diverse microbial infections if they share the same effective therapeutic targets.
Urinary tract infection (UTI) is the most common infectious disease of the urinary system caused by diverse uropathogens, affecting females and males of all ages (1). In 2019, the overall global incident cases of UTI were 4046.12 ✕ 105, with 871.90 ✕ 105 for males and 3174.22 ✕ 105 for females (2). Notably, the incident cases of UTI increased by 60.40% in the past thirty decades. UTI results in dysuria, frequency, urgency, suprapubic pain, hematuria, and serious sequelae including frequent recurrences, pyelonephritis with sepsis, renal damage, and pre-term birth and significantly affects all aspects of the quality of life of the patients (3, 4). In addition, UTI ranges in severity from mild self-limitation to severe sepsis, with 20-40% mortality (2). UTI has been causing a huge burden on human health, medical resources, and financial expenditure (2). In the United States alone, UTI results in >10 million outpatient visits and $3.5 billion in societal costs per year (2, 5).
UTI is caused by main nine pathogens, epidemiologically covering almost 100% of UTI confirmed cases (1). These pathogens include uropathogen escherichia coli (UPEC), Klebsiella pneumoniae (K. pneumoniae), Staphylococcus saprophyticus (S. saprophyticus), Enterococcus faecalis (E. faecalis), Group B Streptococcus (GBS), Proteus mirabilis (P. mirabilis), Pseudomonas aeruginosa (P. aeruginosa), Staphylococcus aureus (S. aureus), and Candida spp. (Candida.) (1). Antibiotics are the first-line treatment options for UTI but the effectiveness is being increasingly limited due to the rise of bacterial resistance (6, 7) (Table 1). More than 80% resistance of Escherichia coli (E. coli) isolated from UTI to amoxicillin-clavulanic acid, ciprofloxacin, and trimethoprim-sulfamethoxazole has been observed in developing countries (39). In developed countries such as the United States, the resistance of Enterobacteria to some antibiotics for UTI has exceeded 30% (39, 40). Both the World Health Organization (WHO) and the Infectious Disease Society of America (IDSA) claimed the lack of antibiotics for the main pathogens of UTI and urged countries around the world to develop new drugs and therapies that can replace the overuse of antibiotics (41, 42). Thus, people move their sights on the immune responses hoping to find some effective therapeutic targets to combat the infection (4, 43–45).
The bladder possesses a wide range of immune responses against diverse uropathogens, including inhibitors of adhesion and antimicrobial protein production (4, 43–45). The bladder immune responses to invading uropathogens have some in common but also show differences depending on the type of uropathogens. For example, both UPEC and GBS stimulate bladder epithelial cells (BECs) to produce the antimicrobial peptide LL-37, and it is surprising that LL-37 has antibacterial effects on UPEC, but promotes GBS infection in the bladder (46–48). As such, individual immunomodulatory intervention options for UTI should be taken based on immune responses to the specific uropathogen in the bladder. Improved understanding of the bladder immune responses to diverse uropathogens is crucial for our ability to design immunomodulatory interventions and target them properly.
In this Review, we comparatively analyzed the similar and different immune responses triggered by the main nine uropathogens in the bladder. Based on the immune responses, we discussed the immune therapeutic targets with great prospects in-depth and innovatively proposed that when the bladder infection is treated through the modulation of immune responses, different uropathogens should adopt corresponding modulation options to improve the therapeutic effects.
Since the differences in virulence factors of the nine uropathogens (Table 1), the immune responses against the nine uropathogens are diverse in the bladder. In this section, we summarize the characteristics and research status of immune responses to the major nine uropathogens in bladder infection.
UPEC is the most common uropathogen of bladder infection (49). When UPEC ascends to the bladder along the urinary tract, it adheres to the mannose receptors of BECs through type I fimbriae (50). Tamm-Horsfall glycoprotein (THP), the most abundant urine protein, plays a key role to prevent the adhesion of UPEC to the BECs (51, 52). THP has a high-mannose structure among its disaccharides, which binds to the type I fimbriae and competes with the mannose receptors of BECs, thereby reducing the adhesion and colonization of UPEC to the bladder, and leading to the elimination of UPEC through urination (53, 54). In addition, the THP can prevent excessive inflammation in bladder infection via inhibition of the chemotaxis and reactive oxygen species (ROS) production by binding to sialic acid-binding Ig-like lectin-9 (Siglec-9) receptor of the neutrophils (55). Once UPEC successfully adheres to BECs, extracellular immune responses will be activated by lipopolysaccharide (LPS) and type I fimbriae of UPEC via binding to toll-like receptor 4 (TLR4) on BECs (56). The activation of TLR4 stimulates BECs to secrete stromal-cell derived factor 1 (SDF-1), and interleukin- 6 (IL-6) (57, 58). SDF-1 can bind to the CXC-motif chemokine receptor 4 (CXCR4) on neutrophils and recruit them to accumulate to the infection site (57). The aggregated neutrophils have the ability to engulf UPEC and can be significantly enhanced by BECs-secreted pentraxins (PTX3) (59). Cytokine IL-6 upon activation of TLR4 promotes the expression of C-X3-C motif chemokine 1 (CX3CL1) and recruits macrophages to the epithelium, which kill UPEC by phagocytosis and lipocalin-2 (LCN2) (60). LCN2 can restrict access of UPEC to iron, one of the key nutrients for the growth of UPEC, and starve them to death (61). Besides, IL-6 can enhance the expression of antimicrobial peptides (AMPs), such as ribonuclease 7 (RNase 7) and LL-37, which exert antibacterial effects by disrupting the microbial membrane (47, 58, 62–64). In the bladder of mice lacking RNase 7 and LL-37, the UPEC communities are significantly increased (47, 63). (Figure 1)
Figure 1 Extracellular immune responses to UPEC in the bladder. At the beginning of infection, THP reduces the adhesion of UPEC to the BECs. In addition, the THP can prevent excessive inflammation and ROS production of neutrophils. Once adhesion, BECs secrete SDF-1, PTX3, and IL-6. SDF-1 recruit neutrophils, T-cells, and NK cells to the site of infection. PTX3 promotes neutrophils to engulf UPEC, and IL-6 promotes the expression of CX3CL1 to recruit macrophages which kill UPEC by phagocytosis and LCN2. IL-6 also enhances the release of AMPs through phosphorylation of Stat3.AMPs, antimicrobial peptides; BEC, bladder epithelial cells; CXCR4, CXC-motif chemokine receptor 4; CX3CL1, C-X3-C motif chemokine 1; LCN2, lipocalin-2; IL-6, interleukin 6; NK cells, natural killer cells; PTX3, Pentraxins; SDF-1, stromal cell-derived factor1; Siglec-9, sialic acid-binding Ig-like lectin-9; Stat3, signal transducers and activators of transcription 3; THP, Tamm-Horsfall protein; UPEC, Uropathogenic Escherichia coli.
Some UPEC survives from the extracellular immune responses and invades BECs, which then initiate the intracellular efflux immune responses (65, 66). Once BECs are invaded, two waves of UPEC expulsion in an innate immune signaling-orchestrated process occur (67). The first wave is mediated by the activation of TLR4 between 4 and 6h after infection followed by the second mucolipin transient receptor potential 3 (TRPML3)-activated wave occurring around 8h after infection (67). In the first wave of UPEC expulsion, UPEC is encapsulated within RAB27b+ vesicle and activates TLR4 by type I fimbriae (67, 68). Activation of TLR4 signaling advances the K33-linked polyubiquitination of TNF receptor associated factors (TRAF3), which is then sensed by the RalGDS-activating exocyst complex to locate and tether vesicles (68). After that, Sec 6 and Sec 15, two submit of the activated exocyst complex, stimulate collaboration between Rab11a/Rab11FIP3/Dynein and Rab27b/MyRIP/MyosinVIIa to transport UPEC-containing vesicles (67, 69). In addition, the activation of TLR4 can lead to the increase of cyclic adenosine monophosphate (cAMP) which subsequently stimulates the caveolin-1/Rab27b/PKA/MyRIP complex formation, and as a consequence, expels UPEC from infected BECs (70). Once UPEC escapes the first wave of efflux immune response by destroying the RAB27b+ vesicle, the second wave is initiated by lysosomal autophagy (71). After the lysosome engulfed UPEC, the pH of the lysosome will change from acid to neutral, and TRPML3 is able to sense the UPEC-mediated lysosome neutralization of pH and release calcium ions, which leads to the efflux of UPEC (71). (Figure 2)
Figure 2 Intracellular immune responses to UPEC in the bladder. After invading BECs, TLR4 is activated by UPEC to promote the K33-linked polyubiquitination of TRAF3, which is sensed by the RalGDS-activating exocyst complex to locate and tether vesicles. Then, the Sec 6 and Sec 15 of the exocyst complex stimulate collaboration between Rab11a/Rab11FIP3/Dynein and Rab27b/MyRIP/MyosinVIIa to transport UPEC-containing vesicles. Once the lysosome engulfs UPEC, TRPML3 senses the pH neutralization and then releases calcium ions, leading to the efflux of UPEC. BEC, bladder epithelial cells; TLR4, toll-like receptor 4; TRPML3, transient receptor potential 3; UPEC, uropathogenic Escherichia coli.
BECs can adopt more intense immune responses against UPEC by secretion of IL-6, IL-17, tumor necrosis factor-α (TNF-α), C-X-C motif chemokine ligand 1 (CXCL1), CXCL2, and CXCL5, which result in extensive neutrophil recruitment to induction of BECs’ death and exfoliation (72–75). BECs’ death and exfoliation carry a large amount of UPEC into the urine and then excretes UPEC by urination (72–75). In addition, in response to α-hemolysin, which is a virulence factor expressed by UPEC, human BECs induce the production of IL-1β and IL-18 through p38/ERK/ROS/NLRP3/caspase-1 signaling to recruit mast cells, which can produce tryptase to promote the exfoliation of BECs (76, 77). A point worthy of attention is that ROS and inflammation associated with NOD-like receptor thermal protein domain associated protein 3 (NLRP3) or cyclooxygenase-2 (COX-2) also contribute to BECs’ exfoliation (76–79). However, excessive ROS and inflammation are believed to do more harm than good to the host, since the bladder infection gradually intensifies with the increase of ROS and inflammation (80, 81). Although the exfoliation of BECs promotes the excretion of UPEC into the urine, it also exposes deep immature epithelium, thus allowing UPEC to invade them and form quiescent intracellular reservoirs (QIRs), which can avoid immune responses and antibiotics (82). In order to prevent the formation of QIRs caused by shedding, the proliferation ability of the epithelial layer after shedding is enhanced (83). This ability is mainly related to Th2 cells, as Th2 cells have an ability to secret epidermal growth factor (EGF), transforming growth factor-α (TGFα), and insulin-like growth factors-1 (IGF-1), which contribute to epithelial regeneration (84). The differentiation of Th2 cells in the bladder mainly depends on dendritic cells (DCs) presenting UPEC antigen to CD4+ T cells after infection (84). In addition, sonic hedgehog (SHH) expressed by basal stem cells and peroxisome proliferator-activated receptor-γ (Pparg) expressed by BECs also contribute to the regeneration and proliferation of BECs (85–87). (Figure 3)
Figure 3 The exfoliation and regeneration of BECs in UPEC bladder infection. Cytokines from BECs are released to recruit neutrophils to induce cell death and exfoliation. Besides, Type 1 fimbriated UPEC activates TLR4 and causes the expression of COX-2, which promotes inflammation and exfoliation of BECs. Moreover, α-hemolysin produced by UPEC recruits mast cells through the ROS/NLRP3/caspase-1/IL-1β, which produces tryptase to mediate the BECs exfoliation. To repair shed BECs, transitional BECs will regenerate under the influence of EGF, TGF-α, IGF-1, SHH, and Pparg. BECs, bladder epithelial cells; EGF, epidermal growth factor; ERK, extracellular signal-related kinase; IGF-1, insulin-like growth factors-1; IL-1β, interleukin 1β; JNK, c-Jun-NH2-terminal kinase; NLRP3, NOD-like receptor thermal protein domain associated protein 3; PAR2, Protease-activated receptor 2; Pparg, peroxisome proliferator-activated receptor-γ; ROS, reactive oxygen species; SHH, sonic hedgehog; TGF-α, transforming growth factor-α; UPEC, uropathogenic Escherichia coli.
K. pneumoniae, one of the most common pathogens of intensive care unit infections, is the second leading cause of UTI from community or hospital sources (1, 88–90). Similar to the effects of THP on UPEC, THP exerts anti-adhesion and anti-inflammation effects on K. pneumoniae (91). In the THP-deficient mouse models, K. pneumoniae load in the urine and bladder significantly increased, as well as the number of inflammatory cells (91, 92). Once K.pneumoniae adheres to and invades BECs, intracellular immune defense mechanisms are initiated to inhibit the internalization of K.pneumoniae and promote its efflux. The first mechanism is initiated by TLR4, which down-regulates Rho through the expression of cAMP, and ultimately achieves the goal of inhibiting the invasion of K.pneumoniae (92). The second mechanism is mediated by high-mobility group protein N2 (HMGN2), which plays a key role in the inhibition of K.pneumoniae internalization by reduction of bacteria-induced activation of extracellular signal-regulated kinase (ERK1/2) and the polymerization of actin (93, 94). The last mechanism is that the invasion of K.pneumoniae promotes the synthesis of dual oxidase 2, which has the ability to inhibit bacterial internalization by the production of intracellular ROS (95, 96). The proper concentration of ROS has antibacterial against invading pathogenic bacteria (95, 97–99). (Figure 4A)
Figure 4 Immune responses to K. pneumoniae and S.saprophyticus in the bladder. (A) In the urine, THP exerts anti-adhesion and anti-inflammation effects on K. pneumoniae. Once adhered, K. pneumoniae lipopolysaccharide activates TLR4 to initiate AC-3/cAMP/PKA signaling pathway, then down-regulates Rac-1 and abrogates the endocytic lipid raft. HMGN2 also can inhibit K. pneumoniae internalization by inhibiting the attachment of bacteria and reducing bacterial-induced ERK1/2 activation and actin polymerization. In addition, the ROS promoted by oxidase 2 can inhibit endocytosis. (B) Before adhesion, RegIIIγ, RNase 7, and THP have anti-adhesion and sterilization abilities to S. saprophyticus. The acidic urine environment suppresses S. saprophyticu uptake and utilization of iron thus limiting its growth. After the adhesion, BECs produce TNF-α, MIP-1, IL-1, IL-6, and IL-12 to recruit macrophages. Upon the activation of TLR4 by PAMP, macrophages phagocytize S.saprophyticus depending on genes associated with GRIM-19. AC-3, adenylyl cyclase-3; cAMP, cyclic adenosine monophosphate; ERK1/2, extracellular-regulated kinase 1/2; GRIM-19, genes associated with retinoid-IFN-induced mortality-19; HMGN2, high-mobility group protein N2; IL-1, interleukin-1; INF-γ, interferon-γ; K.pneumoniae, Klebsiella pneumoniae; MIP-1, macrophage inflammatory protein-1; PAMP, pathogen-associated molecular pattern; PKA, protein kinase A; RegIIIγ, regenerating islet-derived 3γ; RNase 7, ribonuclease 7; ROS, reactive oxygen species; S.saprophyticus, Staphylococcus saprophytes; THP, Tamm-Horsfall protein; TLR4, toll-like receptor 4; TNF-α, tumor necrosis factor-α.
The type I fimbriae of K.pneumoniae is involved in the triggering of multiple immune responses in the bladder, which are very similar to UPEC type I fimbriae-induced immune responses (91, 92). Both UPEC and K.pneumoniae can be inhibited by the effect of THP against type I fimbriae, and they can both increase cAMP through type I fimbriae to regulate actin and ultimately promote bacterial efflux (53, 70, 91, 92). In addition, the UPEC and K. pneumoniae type I fimbriae play similar roles in the pathogenic process of bladder infection, as both of them rely on type I fimbriae to attach, invade, and form intracellular bacterial communities (1). By comparing the nucleic acid sequences of UPEC and K.pneumoniae type I fimbriae, they are highly homologous, which can explain why UPEC and K. pneumoniae type I fimbriae play similar roles in the pathogenicity and stimulate resembling immune responses of bladder infection (100, 101). However, K.pneumoniae carries the gene fimK but lacks the gene fimX, leading to reduce expression of type I fimbriae, which may explain K. pneumoniae form fewer intracellular bacterial communities (IBCs) and have lower titers in the bladder than UPEC and are more easily cleared by host defense response during infection (102).
Bladder infection caused by S.saprophyticus is most likely to occur in sexually active, non-pregnant women (103). Generally speaking, when S.saprophyticus contaminates the vaginal area, it ascends through the urinary tract (103). In the ascending process, S.saprophyticus uses citrate in urine to synthesize carboxylate siderophores and obtain iron ions in urine to supply its nutrition and growth (104). In order to limit the growth of S.saprophyticus, the bladder maintains a weakly acidic urine environment to reduce the activity of citrate synthase and thereby reduce the synthesis of citrate, ultimately achieving the goal of limiting S.saprophyticus from obtaining iron and starving them to death (104, 105). In addition, THP in urine has the ability to inhibit the adhesion of S.saprophyticus to BECs, which is similar to the effects on UPEC (53, 91). However, the antibacterial ability of urine is limited, as some S.saprophyticus still survive from THP and the acidic environment and adhere to BECs, stimulating BECs to increase the expression of AMPs including regenerating islet-derived 3γ (RegIIIγ) and RNase 7 (106, 107). RegIIIγ is able to promote the proliferation and repair of the injury epithelial cells (108, 109). RNase 7 mainly binds to the negatively charged bacterial cell membrane through cationic residues on its surface, destroys the physical and physiological functions of the bacteria, and ultimately kills the bacteria (62). In addition to AMPs, BECs mediate the production of cytokines, such as TNF-α, macrophage inflammatory protein-1 (MIP-1), IL-1, IL-6, and IL-12, to recruit the macrophages (14). Macrophages depend on genes associated with retinoid-IFN-induced mortality-19 (GRIM-19), a component of the mitochondrial respiratory chain, to phagocytize S.saprophyticus (110, 111). In GRIM-19-deficient macrophages, the expression of IL-1, IL-6, IL-12, interferon-γ (INF-γ) cytokines, and phagocytic ability are significantly reduced (110). (Figure 4B)
The immune responses to S.saprophyticus in bladder infection have differences from these to other uropathogens, as the urine pH and GRIM-19 have abilities to inhibit the growth of S.saprophyticus (104, 110). Acidic urine reduces the synthesis of citrate, consequently resulting in inhibition of S.saprophyticus growth, and GRIM-19 molecule exerts immune defense effects by regulating the phagocytic ability of macrophages in bladder infection (104, 110). Therefore, modulating urine pH and GRIM-19 is a promising target for S.saprophyticus UTI.
E.faecalis is one of the most resistant gram-positive bacteria in UTI, which has caused great trouble for clinical treatment (112). Current research on the immune responses to E.faecalis bladder infection are more about the responses of macrophages, DCs, and Natural killer (NK) cells (113, 114).
Under normal circumstances, activation of TLR2-Toll/interleukin-1 receptor (TIR) on macrophages can trigger the production of chemokines dependent on the NF-κB signaling pathway, and recruit immune cells in the bladder (115, 116). However, E.faecalis has a TIR domain-containing protein structure, which is similar to the TIR domain of TLR2 on macrophages (113, 117). Hence, the TIR domain-containing protein of E.faecalis (TcpF) has an ability to compete with the TIR domain of human TLR2 to form TLR dimers, thereby further eliminating downstream signals and ultimately inhibiting the immune responses of macrophages in the bladder (113, 117). Therefore, immune responses of macrophages to E.faecalis and UPEC co-infected in the bladder are significantly inhibited compared to the infection of UPEC alone, consequently promoting UPEC virulence during a mixed-species bladder infection (113, 118).
Different from immunosuppressive effects on macrophages, E.faecalis has the ability to intensify the proliferation and activation of NK cells, which in turn promote the maturation and differentiation of DCs (114). In addition, NK cells also can be activated by E.faecalis-induced DC-derived effectors signals. E. faecalis specific DC/NK interaction is necessary for the killing of transformed or infected cells in E.faecalis bladder infection (114). (Figure 5A) The adaptive immune responses in the bladder are limited, widely assumed to the restricted ability of mature DCs to capture and present antigens in the bladder (119, 120). Exogenously regulating the DC/NK interaction may be one of the effective strategies to enhance bladder adaptive immune responses.
Figure 5 Immune responses to E. faecalis and GBS in the bladder. (A) Initially, RNase 7 in the urine binds to the E. faecalis and plays a bactericidal effect. Once E. faecalis adheres to BECs, TcpF of which binds to the TIR on macrophages, eliminating downstream MyD88 and NF-kB signals and suppressing the immune responses. However, the proliferation and activation of NK cells are intensified, which promote the maturation and differentiation of DCs. In turn, NK cells can be specifically activated to kill E. faecalis through derived effectors signals from infected DCs. (B) In the urine, LL-37 sticks to GBS and promotes its growth and adhesion. After adhesion, GBS induces the expression of IL-8, IL-1β, IL-1α, IL-6, TNF-α, GM-CSF to recruit immune cells and mediate inflammation. Macrophages and DCs secrete IL-1β and IL-18 against the GBS infection under the activation of the NLRP3 inflammasome by β-hemolysin/cytolysin of GBS. Neutrophils engulf GBS to play an antibacterial effect. DCs, dendritic cells; E. faecalis, Enterococcus faecalis; GBS, Group B Streptococcus; GM-CSF, granulocyte-macrophage colony-stimulating factor; IL-8, interleukin-8; MyD88, myeloiddifferentiationfactor88; NF-kB, Nuclear factor kappa beta; NK cells, natural killer cells; NLRP3, NOD-like receptor thermal protein domain associated protein 3; RNase 7, ribonuclease 7; TcpF, TLR2-Toll/Interleukin-1 receptor domain-containing protein of E. Faecalis.
GBS is a common commensal of the human genitourinary tract in healthy people (121). Nevertheless, this bacterium can cause life-threatening hazards to pregnant women, the elderly, and immunocompromised individuals (122–124).
When the immune function of the body is compromised, GBS in the urethra will express a variety of virulence factors to damage and adhere to the bladder tissue (122–124). AMPs in the urine are the first line of defense, however, LL-37, one of the AMPs, has no antibacterial effect on GBS (46). On the contrary, the load of GBS increases with the rise of LL-37 (46). Under the action of LL-37, GBS further adheres to the BECs, and this adherence promotes the expression of many cytokines, including IL-8, IL-1β, IL-1α, IL-6, TNF-α, granulocyte-macrophage colony-stimulating factor (GM-CSF) to mediate the occurrence of inflammation and recruit the immune cells including neutrophils, macrophages, and DCs to the infected sites (22, 125, 126). Neutrophils reach the focal point of infection producing anti-infective effects through various biological effects such as phagocytosis and cytokine production (125–128). Macrophages and DCs also make significant contributions to host defenses by secretion of IL-1β and IL-18 through the activation of the NLRP3 inflammasome, deficiency of which has GBS communities increased (129, 130). (Figure 5B) However, immune responses of neutrophils and macrophages can be inhibited by GBS virulence factors, as the cytokines production of macrophages and neutrophils increased when the bladder was infected by the virulence factor capsule sialic acid-deficient GBS (23, 131).
Compared with the anti-bacterial effects of LL-37 on UPEC infection, LL-37 plays an opposite role in GBS infection, which promotes GBS growth and proliferation (46, 47, 132). The role of NLRP3 may also differ between GBS and UPEC infection, as NLRP3-deficient mice were more susceptible to GBS infection and have GBS load increased. Whereas UPEC burden was significantly reduced in NLRP3-deficient BECs (76, 129). As these colonization differences between GBS and UPEC were observed based on the different NLRP3-deficient cells but have not been validated in the same cells and in vivo yet, which needs to be further explored (76, 129). Due to the differences in immune responses of the bladder between UPEC and GBS infection, when treating bladder infection caused by GBS, we should adopt different immunomodulation options from that of UPEC.
P.mirabilis, which showed high resistance rates to ampicillin, nitrofurantoin, and amoxicillin-clavulanate, is the sixth most common pathogen of uncomplicated UTI (1, 25). When the P.mirabilis reaches the mouth of the urethra, it moves up the urethra through the swing of the flagella and reaches the bladder (133). During the ascending process, many immune mediators in the urine including complement (C1q and C3), LL-37, and human β-defensin (hBD) are hydrolyzed by ZapA (Mirabilysin), which is a 54-kDa extracellular proteolytic enzyme with broad-spectrum degradation activity encoded by P.mirabilis (26). Similar to the effects on UPEC and K.pneumoniae, THP and RNase 7 in the urine resist the adhesion and invasion of P.mirabilis to BECs (106, 134). Some P.mirabilis survive from THP and RNase 7 and adhere to BECs through fimbriae (135). Once the P.mirabilis successfully adhere, a number of leukocytes migrate to the epithelium mediated by the production of c-c chemokine ligand 20 (CCL20), CXCL2, and CCL2 under the stimulation of flagella (136). However, the migration of leukocytes is demonstrated ineffective in clearing P.mirabilis (136). (Figure 6A)
Figure 6 Immune responses to P. mirabilis, Paeruginosa and Candida spp. in the bladder. (A) Before adhesion, THP and RNase 7 resist the adhesion and invasion of P.mirabilis to the bladder, and P.mirabilis has countermeasures by expressing extracellular metalloprotease ZapA, which has hydrolytic activity. In addition, ZapA can hydrolyze complement (C1q and C3), LL-37, and human hBD in the urine. Notably, BECs can produce CCL20, CXCL2, and CCL2, and then promote numbers of leukocytes migrate to the epithelium, the specific role of which is not determined. (B) Under the iron restriction, P.aeruginosa has a stronger colonization ability on BECs. Once P.aeruginosa adheres to the BECs, the BECs increase the expression of MIP-1α to recruit neutrophils, which against the bladder infection of P.aeruginosa. (C) In the urine, THP and LL-37 respectively bind to the Als3 and Xog1p glycoprotein of Candida to inhibit adhesion. After Candida adhesion, BECs express COX-2 through EGFR-ERK/p38-RSK-CREB-1 pathway, leading to the synthesis of prostaglandins, which mediate the occurrence of inflammation. BECs, bladder epithelial cells; Candida, Candida spp; CCL20, c-c chemokine ligand 20; COX-2, cyclooxygenase-2; CREB-1, cAMP-response element-binding protein-1; CXCL2, C-X-C motif chemokine ligand 2; EGFR, epidermal growth factor receptor; ERK, extracellular regulated protein kinases; hBD, β-defensin; MIP-1α, macrophage inflammatory protein-1α; P.aeruginosa, Pseudomonas aeruginosa; P. mirabilis, Proteus mirabilis; RNase 7, ribonuclease 7; RSK, ribosomal s6 kinase; THP, tamm-horsfall protein.
There are very few reports on the immune responses to the effective inhibition of P.mirabilis in bladder infection. Two broad-spectrum antibacterial mediators, THP and RNase 7, in the urine have been reported to inhibit the growth of P.mirabilis (106, 134). However, many immune responses and immune mediators in the urine are suppressed by ZapA (26). In addition, it has been reported that the anti-MrpA (structural subunit of MR/P fimbriae) antibodies in urine and serum can be neutralized by P.mirabilis (137). Therefore, the antibacterial immune responses to P.mirabilis in bladder infection remain lacking and need more to be explored in the future.
Of all uropathogens in bladder infection, P.aeruginosa is a relatively small pathogenic bacterium in UTI, but it has caused great trouble for clinical treatment, as many antibiotics such as topiperacillin-tazobactam, ceftazidime, and cefepime, which are effective against other uropathogens, hardly have effects on P.aeruginosa (32). The current research on the immune responses to P.aeruginosa in the bladder is extremely limited. Before P.aeruginosa adhere to the bladder, the growth of P.aeruginosa is firstly affected by iron restriction and THP (34, 138, 139). Surprisingly, the burden of P.aeruginosa and histopathological conditions in the bladder and kidney increase under iron-restricted conditions. Consistently, in vitro experiments showed that iron-restricted media increases the adhesion of P.aeruginosa to the BECs and inhibits macrophage to phagocytose P.aeruginosa (138). The reason why iron restriction can aggravate the P.aeruginosa bladder infection may be attributed to the enhancement of quorum sensing (QS) signaling molecules under iron deficiency conditions (140, 141). Furthermore, when mice are infected with THP-coated P.aeruginosa, the bacterial burden and pathological changes in the kidney are significantly enhanced (139). Therefore, THP and iron restriction have beneficial effects on P.aeruginosa colonization (34, 138, 139). Once the bladder is colonized by P.aeruginosa, it will increase the expression of MIP-1α to recruit neutrophils, which can effectively decrease the burden of P.aeruginosa in the bladder (142). (Figure 6B)
Many immune responses that have spectral antibacterial effects on other uropathogens have no effects on P.aeruginosa, or may even aggravate the infection of P.aeruginosa. In addition, many antibiotics, which are effective against other uropathogens, do not affect P.aeruginosa bladder infection (32). Hence, it is pretty urgent to continue to explore the effective immune defenses for P.aeruginosa in bladder infection so that propose some feasible immunomodulatory interventions.
Candida. is a common uropathogenic fungus in UTI, especially in immunocompromised patients (143). Generally speaking, Candida. mainly causes disease through its hyphae, Candida. adheres to the BECs through the agglutin-like sequence (Als3) glycoprotein structure on the hyphae in the bladder (144, 145). To combat this adhesion process, the THP already present in the bladder urine binds to Als3, thereby inhibiting the adhesion of Candida. to the BECs (144). In addition to THP, LL-37 binds to the Xog1p glycoprotein of the Candida. cell wall to reduce adhesion to BECs (146, 147). However, once Candida. adheres to the BECs, COX-2 will be induced in BECs through the EGFR-ERK/p38-RSK-CREB-1 pathway, the upregulation of which leads to the synthesis of prostaglandins, triggering inflammation (148, 149). (Figure 6C)
Candida. is the only fungus among the nine major uropathogens and the bladder executes different mechanisms of immune responses to Candida. from those to bacteria. For example, THP and LL-37 exert an anti-adhesion effect on both Candida. and other bacteria, THP targets the hyphae to inhibit the adhesion of Candida (144–146).. In bacterial infection, THP targets the fimbriae (52, 91, 134). LL-37 reduces adhesion of the Candida. by binding to its glycoprotein, in bacterial infection, LL-37 exerts anti-adhesion by disrupting the bacterial membrane (47, 146).
S.aureus is the most common gram-positive bacteria in hospital-acquired infections, which mainly occur in catheter-induced UTI (150, 151). The immune responses of the bladder to S.aureus are blank. However, there are many patients with cystitis caused by S.aureus, which is highly resistant to antibiotics (1, 18, 152). It is necessary to carry out research work on the immune responses to S.aureus in bladder infection.
Based on the above summarized immune responses to diverse uropathogens in bladder infection, we deemed that maybe an immune target has antibacterial effects on a variety of uropathogens in bladder infection, and on the other side, some immune mediators play opposite roles in bladder infection (Table 2). In this section, we discuss the potential immunomodulatory interventions for bladder infection caused by different uropathogens.
THP, a broad-spectrum anti-infective protein in bladder infection, has the ability to against many uropathogens, inclusive of UPEC, K.pneumoniae, P.mirabilis, S.saprophyticus, and Candida (91, 134, 144, 145, 153, 154). It plays the antibacterial effect mainly by reducing the colonization of uropathogens on BECs, as THP can occupy the binding sites of uropathogens to BECs (91, 134, 144, 145, 153, 154). Therefore, the upregulation of THP may be an excellent intervention option for the bladder infection caused by UPEC, K.pneumoniae, P.mirabilis, S.saprophyticus, and Candida. Clinical experiments showed that the level of THP in patients who take cranberry extract orally increases, and the urine from these patients has a stronger inhibitory effect on the adhesion of UPEC (52, 155). However, whether this intervention is effective for bladder infection caused by P.aeruginosa is not determined, as the THP can lead to an increase in P.aeruginosa load (139). In conclusion, the upregulation of THP is an excellent way to combat the bladder infection of UPEC, K.pneumoniae, S.saprophyticus, P.mirabilis, and Candida.
Over accumulated ROS is involved in the induction of BECs injury and death in bladder infection, but the proper concentration of ROS has antibacterial effects (76, 77, 95, 97–99). Uropathogens including UPEC, K.pneumoniae, S.saprophyticus, P.mirabilis, P.aeruginosa, and Candida. induce an increase in ROS level in bladder infection (156). Reducing the expression of ROS seems to have a therapeutic effect on UTI (157, 158). The results of a systematic review showed that vitamin C, a drug candidate with antioxidant capacity, has the ability to prevent the occurrence of UTI, and anthocyanins can inhibit ROS to treat UTI caused by K.pneumoniae and P.aeruginosa (157, 158). Among the anthocyanin extracts of all plants, blueberry is an excellent candidate because of its very rich anthocyanin content (159, 160). We conclude that reducing the content of ROS through the use of antioxidant drugs is a promising intervention for bladder infection.
Iron restriction, as another broad-spectrum antibacterial method, inhibits the growth of a variety of uropathogens in bladder infection, including UPEC, K.pneumoniae, S.saprophyticus, and P.mirabilis (61, 104, 161–163). Exogenous regulation of iron content in urine is an excellent immune regulation target for the treatment of bladder infection. Animal experiments showed that the dietary restriction of iron significantly reduces the iron content, followed by bacterial burden, bacteriuria, as well as inflammatory responses decreasing in UPEC bladder infection, and the exogenous injection of lactoferrin, an iron-binding glycoprotein, also significantly reduces the UPEC load and the infiltration of neutrophils (164, 165). However, the intervention effects of iron restriction on UTI caused by P.aeruginosa are not verified, because iron restriction does not inhibit the growth of P.aeruginosa, but increases the bacterial load in the bladder (138). In conclusion, restricting access to iron is a promising intervention for bladder infection caused by UPEC, K.pneumoniae, S.saprophyticus, and P.mirabilis, which may not apply to P.aeruginosa.
AMPs are a large class of compounds that participate in a variety of innate immune responses and are considered to be promising compounds to deal with antimicrobial resistance (166). RNase 7 has antibacterial effects on UPEC, S.saprophyticus, and P.mirabilis, RegIIIγ has antibacterial effects on S.saprophyticus, LCN2 has antibacterial effects on UPEC, and LL-37 has antibacterial effects on UPEC and Candida (47, 58, 61, 106, 107, 147). Therefore, RNase 7, RegIIIγ, LCN2, and LL-37 may have therapeutic effects against the above uropathogens in bladder infection. Notably, different AMPs and even different segments of the same AMP have different antimicrobial effects. Taking RNase 7 as an example, fragments of RNase 7 have different antibacterial effects on uropathogens, the F:1-97 fragment has the most antibacterial activity against UPEC and S.saprophyticus, while all N-terminal fragments except the F:1-45 fragment have the most antibacterial activity against P.mirabilis (106). Notably, LL-37 does not have a killing effect on GBS, on the contrary, it will promote GBS bladder infection (46).
Among hormones, insulin has the ability to promote the secretion of RNase 7, RNase4, and LCN2, which are proven to be against bladder infection caused by a variety of uropathogens (167, 168). In addition, insulin reduces the risk factor of blood sugar, thereby reducing the susceptibility of diabetic patients to bladder infection of UPEC, K.pneumoniae, E.faecalis, GBS, S.aureus, and Candida (124, 169–173).. However, a prospective study showed that diabetic patients who used insulin for a long time had a higher risk of UTI than diabetic patients who did not use insulin. The reason for the inconsistency may be that the blood and urine sugar of patients taking insulin is higher than that of patients without taking insulin (174). Insulin may not be suitable for people with low blood sugar, because it can cause hypoglycemia and lead to undesirable consequences such as coma (175). Except for insulin, estrogen also changes the bacterial burden in bladder infection (176–178). Female, compared with male, had lower bacterial burdens and stronger immune responses (178). This may be because of the increase of IL-17 mediated by estrogen, as IL-17 initiates many anti-bacterial pathways, including antimicrobial peptide and chemokine expression and the direct killing effects on bacteria (178–181). Differently, exogenous androgen can increase the burden of UPEC and mediate the development of cystitis into pyelonephritis (176, 177).
cAMP plays an important role in the efflux of UPEC and K.pneumoniae from BECs in bladder infection (70, 92). Many drugs, that are proven by US-Food and Drug Administration certification (like Liraglutide, Terbutaline, and so on) can increase the production of cAMP. Liraglutide, a glucagon-like peptide-1 (GLP-1) receptor agonist, is shown to increase cAMP to inhibit the replication of the hepatitis C virus (182). Terbutaline can reduce LPS-induced human pulmonary microvascular endothelial cell damage by increasing cAMP (183). cAMP is proven to be a potential immunomodulatory target for bacterial bladder infection, but there is a lack of research to prove their therapeutic effects, further research is needed (70).
BECs play important roles as the first line of defense in bladder infection, because it produces many immune factors to mediate the immune responses, and meanwhile, it prevents the invasion of bacteria into the deep immature epithelium to form QIRs (184). Hyaluronic acid (HA), a high molecular weight glycosaminoglycan, not only induces the production of LCN2 and IL-8 in HA/flagellin-challenged epithelial cells but is also involved in the enhancement of the physical barrier of BECs (185). As clinical data showed that intravesical injection of HA can indeed achieve the purpose of treatment for infected humans (186–188). Similar to HA, clinical trials showed that 25-hydroxyvitamin D3 also has the role of protecting the bladder epithelial integrity in postmenopausal women, as 25-hydroxyvitamin D3 induces expression of occludin and claudin-14, which are the tight junction proteins in the urinary tract (189). In addition to protecting mature epithelial integrity, the measures to promote the regeneration of immature epithelium should be taken into consideration. Briefly, HA, 25-hydroxyvitamin D3 and so on which can repair urothelium are excellent targets to combat the infection of UPEC.
COX-2 and NLRP-3 were shown to favor infections by exacerbating inflammation (76–79, 148, 149, 190). Inhibiting the synthesis of COX-2 or NLRP-3 can protect mice from cystitis induced by uropathogens, but except GBS-induced cystitis, because GBS colonized more in NLRP-3-deficient mice compared with wild type mice (76–79, 129, 148, 149, 190). Therefore, inhibiting inflammation by targeting COX-2 or NLRP-3 theoretically has a certain therapeutic value against uropathogens except for GBS (129). However, a randomized controlled trial with a sample size of 253 showed that targeting COX-2 by using NSAIDs is less effective than antibiotics and may even promote the progression of cystitis to pyelonephritis (191). Another randomized controlled trial with a sample size of 383 also showed that NSAIDs are less effective than antibiotics in the treatment of bladder infections, and may even lead to pyelonephritis and serious adverse events (192). To sum up, although the basic experiments confirmed the value of anti-inflammatory in the intervention of bladder infection, it should be cautious in clinical application for UTI.
Vaccination holds a promising approach against different microbial bladder infections. Many vaccines designed against individual-specific uropathogens are currently in the stage of basic or clinical trials (193–195). For UPEC bladder infection, there are vaccines targeting type 1 fimbriae, hemolysins, siderophore receptors, cytotoxic necrotizing factor 1 (CNF1), and LPS (194, 196–198). For P.mirabilis bladder infection, there are vaccines targeting MR/P fimbriae and hemolysins (199, 200). For E. faecalis bladder infection, there is endocarditis- and biofilm-associated (Ebp) fimbriae vaccine (201). To make the vaccines against the diversity of uropathogens, the vaccines can be extracted from a range of uropathogens to form a multivalent vaccine. For example, Urovac (Solco Basel Ltd, Basel, Switzerland) consists of 10 heat-killed uropathogens, including 6 serotypes of UPEC, P.vulgaris, K.pneumoniae, and E.faecalis (202). Although most vaccines have been demonstrated highly efficacious in reducing the incidence and severity of UTI in animal models, there is a lack of large-scale clinical trials to prove their efficacy and safety. As the purpose of vaccination is to induce immune memory of the specific pathogens, the vaccines are effective on the corresponding uropathogens but not on others. If a broad anti-infective effect is desired in the treatment of bladder infection, then a multivalent vaccine is an option.
Probiotics can inhibit the adherence, growth, and colonization of uropathogens and reduce inflammation in the urinary tract by producing antibacterial substances such as lactic acid and hydrogen peroxide, or by directly competing for the adhesion sites between UPEC and the BECs (203–205). The efficacy and safety of probiotics in the treatment of bladder infection have been confirmed by extensive clinical trials, which include Lactobacillus rhamnosus, Lactobacillus acidophilus, Lactobacillus fermentum, Lactobacillus reuteri, Bifidobacterium bifidum, and Bifidobacterium lactis (206–209). However, different probiotics were demonstrated to have diverse antibacterial effects. Lactobacillus acidophilus has an average inhibition zone of 16 mm for UPEC but for E.faecalis was 12mm (210). Lactobacillus salivarius UCM572 had anti-adhesion effects against UPEC, however, the anti-adhesion effect on other uropathogens was not demonstrated (211). Furthermore, the anti-adhesion effects of different Lactobacillus strains against Candida, K.pneumoniae, P.aeruginosa, and Proteus were reported to be different (212). Therefore, when probiotics are used to treat different microbial bladder infections, appropriate probiotic strains should be selected according to the specific uropathogens in bladder infection.
Because of the diverse effects of immunomodulatory interventions on different uropathogens, corresponding immunotherapies should be taken for different uropathogenic bladder infections for better therapeutic effects. However, compared with great advances in the understanding of bladder immune responses trigged by UPEC, understanding of the bladder immune responses caused by other uropathogens remains relatively limited, which results in relatively few individual immunomodulatory options for other uropathogens which we came up with. Further research needs to pay more attention to the immune responses to other uropathogens besides UPEC. In addition, most of the immunomodulatory interventions were proven efficacious in animal models, further clinical research needs to demonstrate the consistency of the effects, and then which will achieve better therapeutic effects in the future.
Antibiotic therapy is the only option for UTI treatment but in recent years it is becoming more limited due to the increasing resistance of UTIs to routinely applied antibiotics. Immunomodulatory interventions have been suggested to be alternatives. However, the bladder executes different immune responses depending on the type of uropathogens, thus one immunomodulatory target has diverse effects on different uropathogens. The similarities and differences in immune responses to the main nine uropathogens in bladder infection were sorted out and comparably analyzed in this Review. To improve the effects of immunomodulatory interventions on different microbial bladder infections, specific uropathogenic bladder infections should adopt corresponding immunomodulatory targets to intervene, and one immunomodulatory intervention can be applied to diverse microbial infections, under the condition that they share the same effective therapeutic targets. Only through individual treatments in different uropathogenic bladder infection by immunomodulatory interventions can achieve better therapeutic results as alternatives for antibiotics in the future.
LL, YL, and HC researched data for the article and wrote the manuscript. HC and XX made substantial contributions to discussions of content, and reviewed and edited the manuscript. All authors contributed to multiple parts of the paper, as well as the final style. All authors contributed to the article and approved the submitted version.
The authors acknowledge funding received from the Department of Science and Technology of Sichuan Province and Southwest Medical School (2021ZYD0084, 2022NSFSC1381, 2021ZKZD006, and 2021ZKZD004).
The authors declare that the research was conducted in the absence of any commercial or financial relationships that could be construed as a potential conflict of interest.
All claims expressed in this article are solely those of the authors and do not necessarily represent those of their affiliated organizations, or those of the publisher, the editors and the reviewers. Any product that may be evaluated in this article, or claim that may be made by its manufacturer, is not guaranteed or endorsed by the publisher.
1. Flores-Mireles AL, Walker JN, Caparon M, Hultgren SJ. Urinary tract infections: Epidemiology, mechanisms of infection and treatment options. Nat Rev Microbiol (2015) 13(5):269–84. doi: 10.1038/nrmicro3432
2. Zhu C, Wang DQ, Zi H, Huang Q, Gu JM, Li LY, et al. Epidemiological trends of urinary tract infections, urolithiasis and benign prostatic hyperplasia in 203 countries and territories from 1990 to 2019. Mil Med Res (2021) 8(1):64. doi: 10.1186/s40779-021-00359-8
3. Sureshbabu RP, Aramthottil P, Anil N, Sumathy S, Varughese SA, Sreedevi A, et al. Risk factors associated with preterm delivery in singleton pregnancy in a tertiary care hospital in south India: A case control study. Int J Womens Health (2021) 13:369–77. doi: 10.2147/IJWH.S282251
4. Lacerda Mariano L, Ingersoll MA. The immune response to infection in the bladder. Nat Rev Urol (2020) 17(8):439–58. doi: 10.1038/s41585-020-0350-8
5. Bruxvoort KJ, Bider-Canfield Z, Casey JA, Qian L, Pressman A, Liang AS, et al. Outpatient urinary tract infections in an era of virtual healthcare: Trends from 2008 to 2017. Clin Infect Dis (2020) 71(1):100–8. doi: 10.1093/cid/ciz764
6. Sihra N, Goodman A, Zakri R, Sahai A, Malde S. Nonantibiotic prevention and management of recurrent urinary tract infection. Nat Rev Urol (2018) 15(12):750–76. doi: 10.1038/s41585-018-0106-x
7. Gajdacs M, Urban E. Comparative epidemiology and resistance trends of proteae in urinary tract infections of inpatients and outpatients: A 10-year retrospective study. Antibiotics (Basel) (2019) 8(3):91. doi: 10.3390/antibiotics8030091
8. Gu J, Song P, Chen X, Yang Z, Zhang X, Bai Y. Comparative study of the bacterial distribution and antimicrobial susceptibility of uropathogens in older and younger patients with urinary stones. BMC Geriatr (2022) 22(1):195. doi: 10.1186/s12877-022-02886-y
9. Govindarajan DK, Kandaswamy K. Virulence factors of uropathogens and their role in host pathogen interactions. Cell Surf (2022) 8:100075. doi: 10.1016/j.tcsw.2022.100075
10. Subashchandrabose S, Mobley HLT. Virulence and fitness determinants of uropathogenic escherichia coli. Microbiol Spectr (2015) 3(4):UTI-0015-2012. doi: 10.1128/microbiolspec.UTI-0015-2012
11. Chathley U, Sharma S, Chhibber S. Lipopolysaccharide-induced resistance in mice against ascending urinary tract infection with klebsiella pneumoniae. Folia Microbiol (Praha) (1996) 41(4):373–6. doi: 10.1007/bf02814718
12. Tarkkanen A, Allen B, Williams P, Kauppi M, Haahtela K, Siitonen A, et al. Fimbriation, capsulation, and iron-scavenging systems of klebsiella strains associated with human urinary tract infection. Infect Immun (1992) 60(3):1187–92. doi: 10.1128/iai.60.3.1187-1192.1992
13. Li G, Sun S, Zhao ZY, Sun Y. The pathogenicity of rmpa or aerobactin-positive klebsiella pneumoniae in infected mice. J Int Med Res (2019) 47(9):4344–52. doi: 10.1177/0300060519863544
14. Kline KA, Ingersoll MA, Nielsen HV, Sakinc T, Henriques-Normark B, Gatermann S, et al. Characterization of a novel murine model of staphylococcus saprophyticus urinary tract infection reveals roles for ssp and sdri in virulence. Infect Immun (2010) 78(5):1943–51. doi: 10.1128/IAI.01235-09
15. Ekwealor PA, Ugwu MC, Ezeobi I, Amalukwe G, Ugwu BC, Okezie U, et al. Antimicrobial evaluation of bacterial isolates from urine specimen of patients with complaints of urinary tract infections in awka, Nigeria. Int J Microbiol (2016) 2016:9740273. doi: 10.1155/2016/9740273
16. Mortimer TD, Annis DS, O'Neill MB, Bohr LL, Smith TM, Poinar HN, et al. Adaptation in a fibronectin binding autolysin of staphylococcus saprophyticus. mSphere (2017) 2(6):e00511-17. doi: 10.1128/mSphere.00511-17
17. Deutch CE. Limited effectiveness of over-the-Counter plant preparations used for the treatment of urinary tract infections as inhibitors of the urease activity from staphylococcus saprophyticus. J Appl Microbiol (2017) 122(5):1380–8. doi: 10.1111/jam.13430
18. Juralowicz E, Bartoszko-Tyczkowska A, Tyczkowska-Sieron E, Kurnatowska I. Etiology and bacterial susceptibility to antibiotics in patients with recurrent lower urinary tract infections. Pol Arch Intern Med (2020) 130(5):373–81. doi: 10.20452/pamw.15284
19. Nallapareddy SR, Singh KV, Sillanpaa J, Zhao M, Murray BE. Relative contributions of ebp pili and the collagen adhesin ace to host extracellular matrix protein adherence and experimental urinary tract infection by enterococcus faecalis Og1rf. Infect Immun (2011) 79(7):2901–10. doi: 10.1128/IAI.00038-11
20. Xu W, Flores-Mireles AL, Cusumano ZT, Takagi E, Hultgren SJ, Caparon MG. Host and bacterial proteases influence biofilm formation and virulence in a murine model of enterococcal catheter-associated urinary tract infection. NPJ Biofilms Microbiomes (2017) 3:28. doi: 10.1038/s41522-017-0036-z
21. Ahmed N, Le Jeune A, Torelli R, Sanguinetti M, Giard J-C, Hartke A, et al. The extracytoplasmic function sigma factor sigv plays a key role in the original model of lysozyme resistance and virulence of enterococcus faecalis. PloS One (2010) 5(3):e9658. doi: 10.1371/journal.pone.0009658
22. Kulkarni R, Randis TM, Antala S, Wang A, Amaral FE, Ratner AJ. Beta-Hemolysin/Cytolysin of group b streptococcus enhances host inflammation but is dispensable for establishment of urinary tract infection. PloS One (2013) 8(3):e59091. doi: 10.1371/journal.pone.0059091
23. Kline KA, Schwartz DJ, Gilbert NM, Hultgren SJ, Lewis AL. Immune modulation by group b streptococcus influences host susceptibility to urinary tract infection by uropathogenic escherichia coli. Infect Immun (2012) 80(12):4186–94. doi: 10.1128/IAI.00684-12
24. Guo Y, Deng X, Liang Y, Zhang L, Zhao GP, Zhou Y. The draft genomes and investigation of serotype distribution, antimicrobial resistance of group b streptococcus strains isolated from urine in suzhou, China. Ann Clin Microbiol Antimicrob (2018) 17(1):28. doi: 10.1186/s12941-018-0280-y
25. Shrestha LB, Baral R, Poudel P, Khanal B. Clinical, etiological and antimicrobial susceptibility profile of pediatric urinary tract infections in a tertiary care hospital of Nepal. BMC Pediatr (2019) 19(1):36. doi: 10.1186/s12887-019-1410-1
26. Belas R, Manos J, Suvanasuthi R. Proteus Mirabilis zapa metalloprotease degrades a broad spectrum of substrates, including antimicrobial peptides. Infect Immun (2004) 72(9):5159–67. doi: 10.1128/IAI.72.9.5159-5167.2004
27. Scavone P, Villar S, Umpierrez A, Zunino P. Role of Proteus mirabilis Mr/P fimbriae and flagella in adhesion, cytotoxicity and genotoxicity induction in T24 and vero cells. Pathog Dis (2015) 73(4):ftv017. doi: 10.1093/femspd/ftv017
28. Nielubowicz GR, Mobley HL. Host-pathogen interactions in urinary tract infection. Nat Rev Urol (2010) 7(8):430–41. doi: 10.1038/nrurol.2010.101
29. Dumanski A, Hedelin H, Edin-Liljegren A, Beauchemin D, McLean RJ. Unique ability of the Proteus mirabilis capsule to enhance mineral growth in infectious urinary calculi. Infect Immun (1994) 62(7):2998–3003. doi: 10.1128/iai.62.7.2998-3003.1994
30. Himpsl SD, Pearson MM, Arewang CJ, Nusca TD, Sherman DH, Mobley HL. Proteobactin and a yersiniabactin-related siderophore mediate iron acquisition in Proteus mirabilis. Mol Microbiol (2010) 78(1):138–57. doi: 10.1111/j.1365-2958.2010.07317.x
31. Yuan F, Huang Z, Yang T, Wang G, Li P, Yang B, et al. Pathogenesis of Proteus mirabilis in catheter-associated urinary tract infections. Urol Int (2021) 105(5-6):354–61. doi: 10.1159/000514097
32. Al-Orphaly M, Hadi HA, Eltayeb FK, Al-Hail H, Samuel BG, Sultan AA, et al. Epidemiology of multidrug-resistant pseudomonas aeruginosa in the middle East and north Africa region. mSphere (2021) 6(3):e00202–21. doi: 10.1128/mSphere.00202-21
33. Kroken A, Chen C, Evans D, Yahr T, Fleiszig SJ. Pseudomonas aeruginosathe impact of exos on internalization by epithelial cells is independent of and correlates with bistability of type three secretion system gene expression. mBio (2018) 9(3):e00668-18. doi: 10.1128/mBio.00668-18
34. Newman J, Floyd R, Fothergill JL. The contribution of pseudomonas aeruginosa virulence factors and host factors in the establishment of urinary tract infections. FEMS Microbiol Lett (2017) 364(15):fnx124. doi: 10.1093/femsle/fnx124
35. Montagut E, Marco M. Biological and clinical significance of quorum sensing alkylquinolones: Current analytical and bioanalytical methods for their quantification. Anal Bioanal Chem (2021) 413(18):4599–618. doi: 10.1007/s00216-021-03356-x
36. Gharaghani M, Rezaei-Matehkolaei A, Hardani AK, Zarei Mahmoudabadi A. Genotypic diversity and antifungal susceptibility pattern of candida albicans species isolated from hospitalized paediatric patients with urinary tract infection in Iran. J Appl Microbiol (2021) 131(2):1017–27. doi: 10.1111/jam.15006
37. Kauffman C. Diagnosis and management of fungal urinary tract infection. Infect Dis Clin North Am (2014) 28(1):61–74. doi: 10.1016/j.idc.2013.09.004
38. Walker JN, Flores-Mireles AL, Pinkner CL, Schreiber H, Joens MS, Park AM, et al. Catheterization alters bladder ecology to potentiate staphylococcus aureus infection of the urinary tract. Proc Natl Acad Sci U.S.A. (2017) 114(41):E8721–E30. doi: 10.1073/pnas.1707572114
39. Kot B. Antibiotic resistance among uropathogenic escherichia coli. Pol J Microbiol (2019) 68(4):403–15. doi: 10.33073/pjm-2019-048
40. Talan DA, Takhar SS, Krishnadasan A, Mower WR, Pallin DJ, Garg M, et al. Emergence of extended-spectrum beta-lactamase urinary tract infections among hospitalized emergency department patients in the united states. Ann Emerg Med (2021) 77(1):32–43. doi: 10.1016/j.annemergmed.2020.08.022
41. Boucher HW, Ambrose PG, Chambers HF, Ebright RH, Jezek A, Murray BE, et al. White paper: Developing antimicrobial drugs for resistant pathogens, narrow-spectrum indications, and unmet needs. J Infect Dis (2017) 216(2):228–36. doi: 10.1093/infdis/jix211
42. WHO. Global priority list of antibiotic-resistant bacteria to guide research, discovery, and development of new antibiotics. In: World health organization (2017). World Health Organization (Switzerland). Available at: https://www.who.int/news/item/27-02-2017-who-publishes-list-of-bacteria-for-which-new-antibiotics-are-urgently-needed.
43. Abraham SN, Miao Y. The nature of immune responses to urinary tract infections. Nat Rev Immunol (2015) 15(10):655–63. doi: 10.1038/nri3887
44. Schwab S, Jobin K, Kurts C. Urinary tract infection: Recent insight into the evolutionary arms race between uropathogenic escherichia coli and our immune system. Nephrol Dial Transplant (2017) 32(12):1977–83. doi: 10.1093/ndt/gfx022
45. Ortega Martell JA. Immunology of urinary tract infections. GMS Infect Dis (2020) 8:Doc21. doi: 10.3205/id000065
46. Patras KA, Coady A, Babu P, Shing SR, Ha AD, Rooholfada E, et al. Host cathelicidin exacerbates group b streptococcus urinary tract infection. mSphere (2020) 5(2):e00932–19. doi: 10.1128/mSphere.00932-19
47. Chromek M, Slamová Z, Bergman P, Kovács L, Podracká L, Ehrén I, et al. The antimicrobial peptide cathelicidin protects the urinary tract against invasive bacterial infection. Nat Med (2006) 12(6):636–41. doi: 10.1038/nm1407
48. Wnorowska U, Piktel E, Durnas B, Fiedoruk K, Savage PB, Bucki R. Use of ceragenins as a potential treatment for urinary tract infections. BMC Infect Dis (2019) 19(1):369. doi: 10.1186/s12879-019-3994-3
49. Tamadonfar KO, Omattage NS, Spaulding CN, Hultgren SJ. Reaching the end of the line: Urinary tract infections. Microbiol Spectr (2019) 7(3):BAI-0014-2019. doi: 10.1128/microbiolspec.BAI-0014-2019
50. Hatton NE, Baumann CG, Fascione MA. Developments in mannose-based treatments for uropathogenic escherichia coli-induced urinary tract infections. Chembiochem (2021) 22(4):613–29. doi: 10.1002/cbic.202000406
51. Devuyst O, Olinger E, Rampoldi L. Uromodulin: From physiology to rare and complex kidney disorders. Nat Rev Nephrol (2017) 13(9):525–44. doi: 10.1038/nrneph.2017.101
52. Scharf B, Sendker J, Dobrindt U, Hensel A. Influence of cranberry extract on tamm-horsfall protein in human urine and its antiadhesive activity against uropathogenic escherichia coli. Planta Med (2019) 85(2):126–38. doi: 10.1055/a-0755-7801
53. Weiss G, Stanisich J, Sauer M, Lin C, Eras J, Zyla D, et al. Architecture and function of human uromodulin filaments in urinary tract infections. Science (2020) 369(6506):1005–10. doi: 10.1126/science.aaz9866
54. Pak J, Pu Y, Zhang ZT, Hasty DL, Wu XR. Tamm-horsfall protein binds to type 1 fimbriated escherichia coli and prevents e. coli from binding to uroplakin ia and ib receptors. J Biol Chem (2001) 276(13):9924–30. doi: 10.1074/jbc.M008610200
55. Patras KA, Coady A, Olson J, Ali SR, RamachandraRao SP, Kumar S, et al. Tamm-horsfall glycoprotein engages human siglec-9 to modulate neutrophil activation in the urinary tract. Immunol Cell Biol (2017) 95(10):960–5. doi: 10.1038/icb.2017.63
56. Behzadi E, Behzadi P. The role of toll-like receptors (Tlrs) in urinary tract infections (Utis). Cent Eur J Urol (2016) 69(4):404–10. doi: 10.5173/ceju.2016.871
57. Isaacson B, Hadad T, Glasner A, Gur C, Granot Z, Bachrach G, et al. Stromal cell-derived factor 1 mediates immune cell attraction upon urinary tract infection. Cell Rep (2017) 20(1):40–7. doi: 10.1016/j.celrep.2017.06.034
58. Ching CB, Gupta S, Li B, Cortado H, Mayne N, Jackson AR, et al. Interleukin-6/Stat3 signaling has an essential role in the host antimicrobial response to urinary tract infection. Kidney Int (2018) 93(6):1320–9. doi: 10.1016/j.kint.2017.12.006
59. Jaillon S, Moalli F, Ragnarsdottir B, Bonavita E, Puthia M, Riva F, et al. The humoral pattern recognition molecule Ptx3 is a key component of innate immunity against urinary tract infection. Immunity (2014) 40(4):621–32. doi: 10.1016/j.immuni.2014.02.015
60. Bottek J, Soun C, Lill JK, Dixit A, Thiebes S, Beerlage A-L, et al. Spatial proteomics revealed a Cx3cl1-dependent crosstalk between the urothelium and relocated macrophages through il-6 during an acute bacterial infection in the urinary bladder. Mucosal Immunol (2020) 13(4):702–14. doi: 10.1038/s41385-020-0269-7
61. Owusu-Boaitey N, Bauckman KA, Zhang T, Mysorekar IU. Macrophagic control of the response to uropathogenic e. coli infection by regulation of iron retention in an il-6-Dependent manner. Immun Inflammation Dis (2016) 4(4):413–26. doi: 10.1002/iid3.123
62. Spencer JD, Schwaderer AL, Wang H, Bartz J, Kline J, Eichler T, et al. Ribonuclease 7, an antimicrobial peptide upregulated during infection, contributes to microbial defense of the human urinary tract. Kidney Int (2013) 83(4):615–25. doi: 10.1038/ki.2012.410
63. Eichler T, Bender K, Murtha MJ, Schwartz L, Metheny J, Solden L, et al. Ribonuclease 7 shields the kidney and bladder from invasive uropathogenic escherichia coli infection. J Am Soc Nephrol (2019) 30(8):1385–97. doi: 10.1681/ASN.2018090929
64. Pierce K, Eichler T, Mosquera Vasquez C, Schwaderer A, Simoni A, Creacy S, et al. Ribonuclease 7 polymorphism Rs1263872 reduces antimicrobial activity and associates with pediatric urinary tract infections. J Clin Invest (2021) 131(22):e149807. doi: 10.1172/jci149807
65. Hirakawa H, Suzue K, Kurabayashi K, Tomita H. The tol-pal system of uropathogenic escherichia coli is responsible for optimal internalization into and aggregation within bladder epithelial cells, colonization of the urinary tract of mice, and bacterial motility. Front Microbiol (2019) 10:1827. doi: 10.3389/fmicb.2019.01827
66. Kim W-J, Shea AE, Kim J-H, Daaka Y. Uropathogenic escherichia coli invades bladder epithelial cells by activating kinase networks in host cells. J Biol Chem (2018) 293(42):16518–27. doi: 10.1074/jbc.RA118.003499
67. Miao Y, Bist P, Wu J, Zhao Q, Li Q-j, Wan Y, et al. Collaboration between distinct rab small gtpase trafficking circuits mediates bacterial clearance from the bladder epithelium. Cell Host Microbe (2017) 22(3):330–42.e4. doi: 10.1016/j.chom.2017.08.002
68. Miao Y, Wu J, Abraham SN. Ubiquitination of innate immune regulator Traf3 orchestrates expulsion of intracellular bacteria by exocyst complex. Immunity (2016) 45(1):94–105. doi: 10.1016/j.immuni.2016.06.023
69. Wang C, Bauckman KA, Ross ASB, Symington JW, Ligon MM, Scholtes G, et al. A non-canonical autophagy-dependent role of the Atg16l1(T300a) variant in urothelial vesicular trafficking and uropathogenic escherichia coli persistence. Autophagy (2019) 15(3):527–42. doi: 10.1080/15548627.2018.1535290
70. Song J, Bishop B, Li G, Grady R, Stapleton A, Abraham SN. Tlr4-mediated expulsion of bacteria from infected bladder epithelial cells. Proc Natl Acad Sci U.S.A. (2009) 106(35):14966–71. doi: 10.1073/pnas.0900527106
71. Miao Y, Li G, Zhang X, Xu H, Abraham SN. A trp channel senses lysosome neutralization by pathogens to trigger their expulsion. Cell (2015) 161(6):1306–19. doi: 10.1016/j.cell.2015.05.009
72. Duell BL, Carey AJ, Tan CK, Cui X, Webb RI, Totsika M, et al. Innate transcriptional networks activated in bladder in response to uropathogenic escherichia coli drive diverse biological pathways and rapid synthesis of il-10 for defense against bacterial urinary tract infection. J Immunol (2012) 188(2):781–92. doi: 10.4049/jimmunol.1101231
73. Ingersoll MA, Kline KA, Nielsen HV, Hultgren SJ. G-Csf induction early in uropathogenic escherichia coli infection of the urinary tract modulates host immunity. Cell Microbiol (2008) 10(12):2568–78. doi: 10.1111/j.1462-5822.2008.01230.x
74. Sivick KE, Schaller MA, Smith SN, Mobley HL. The innate immune response to uropathogenic escherichia coli involves il-17a in a murine model of urinary tract infection. J Immunol (2010) 184(4):2065–75. doi: 10.4049/jimmunol.0902386
75. Schiwon M, Weisheit C, Franken L, Gutweiler S, Dixit A, Meyer-Schwesinger C, et al. Crosstalk between sentinel and helper macrophages permits neutrophil migration into infected uroepithelium. Cell (2014) 156(3):456–68. doi: 10.1016/j.cell.2014.01.006
76. Demirel I, Persson A, Brauner A, Sarndahl E, Kruse R, Persson K. Activation of the Nlrp3 inflammasome pathway by uropathogenic escherichia coli is virulence factor-dependent and influences colonization of bladder epithelial cells. Front Cell Infect Microbiol (2018) 8:81. doi: 10.3389/fcimb.2018.00081
77. Wu Z, Li Y, Liu Q, Liu Y, Chen L, Zhao H, et al. Pyroptosis engagement and bladder urothelial cell-derived exosomes recruit mast cells and induce barrier dysfunction of bladder urothelium after uropathogenic E.Coli infection. Am J Physiol Cell Physiol (2019) 317(3):C544–C55. doi: 10.1152/ajpcell.00102.2019
78. Hannan TJ, Roberts PL, Riehl TE, van der Post S, Binkley JM, Schwartz DJ, et al. Inhibition of cyclooxygenase-2 prevents chronic and recurrent cystitis. EBioMedicine (2014) 1(1):46–57. doi: 10.1016/j.ebiom.2014.10.011
79. Chen T, Tsai J, Huang H, Teng C, Chien S, Kuo H, et al. Regulation of cyclooxygenase-2 expression in human bladder epithelial cells infected with type I fimbriated uropathogenic e. coli. Cell Microbiol (2011) 13(11):1703–13. doi: 10.1111/j.1462-5822.2011.01650.x
80. Himpsl SD, Shea AE, Zora J, Stocki JA, Foreman D, Alteri CJ, et al. The oxidative fumarase fumc is a key contributor for e. coli fitness under iron-limitation and during uti. PloS Pathog (2020) 16(2):e1008382. doi: 10.1371/journal.ppat.1008382
81. Bessaiah H, Pokharel P, Habouria H, Houle S, Dozois CM. Yqhg contributes to oxidative stress resistance and virulence of uropathogenic escherichia coli and identification of other genes altering expression of type 1 fimbriae. Front Cell Infect Microbiol (2019) 9:312. doi: 10.3389/fcimb.2019.00312
82. Sharma K, Thacker VV, Dhar N, Clapes Cabrer M, Dubois A, Signorino-Gelo F, et al. Early invasion of the bladder wall by solitary bacteria protects upec from antibiotics and neutrophil swarms in an organoid model. Cell Rep (2021) 36(3):109351. doi: 10.1016/j.celrep.2021.109351
83. Hicks RM. The mammalian urinary bladder: An accommodating organ. Biol Rev Camb Philos Soc (1975) 50(2):215–46. doi: 10.1111/j.1469-185x.1975.tb01057.x
84. Wu J, Hayes BW, Phoenix C, Macias GS, Miao Y, Choi HW, et al. A highly polarized Th2 bladder response to infection promotes epithelial repair at the expense of preventing new infections. Nat Immunol (2020) 21(6):671–83. doi: 10.1038/s41590-020-0688-3
85. Liu C, Tate T, Batourina E, Truschel ST, Potter S, Adam M, et al. Pparg promotes differentiation and regulates mitochondrial gene expression in bladder epithelial cells. Nat Commun (2019) 10(1):4589. doi: 10.1038/s41467-019-12332-0
86. Shin K, Lee J, Guo N, Kim J, Lim A, Qu L, et al. Hedgehog/Wnt feedback supports regenerative proliferation of epithelial stem cells in bladder. Nature (2011) 472(7341):110–4. doi: 10.1038/nature09851
87. Bohnenpoll T, Wittern AB, Mamo TM, Weiss AC, Rudat C, Kleppa MJ, et al. A shh-Foxf1-Bmp4 signaling axis regulating growth and differentiation of epithelial and mesenchymal tissues in ureter development. PloS Genet (2017) 13(8):e1006951. doi: 10.1371/journal.pgen.1006951
88. Alfouzan W, Dhar R, Abdo NM, Alali WQ, Rabaan AA. Epidemiology and microbiological profile of common healthcare associated infections among patients in the intensive care unit of a general hospital in Kuwait: A retrospective observational study. J Epidemiol Glob Health (2021) 3:302–9. doi: 10.2991/jegh.k.210524.001
89. Chang Y, Jeon K, Lee SM, Cho YJ, Kim YS, Chong YP, et al. The distribution of multidrug-resistant microorganisms and treatment status of hospital-acquired Pneumonia/Ventilator-associated pneumonia in adult intensive care units: A prospective cohort observational study. J Korean Med Sci (2021) 36(41):e251. doi: 10.3346/jkms.2021.36.e251
90. Wang G, Zhao G, Chao X, Xie L, Wang H. The characteristic of virulence, biofilm and antibiotic resistance of klebsiella pneumoniae. Int J Environ Res Public Health (2020) 17(17):6278. doi: 10.3390/ijerph17176278
91. Raffi HS, Bates JM Jr., Laszik Z, Kumar S. Tamm-horsfall protein acts as a general host-defense factor against bacterial cystitis. Am J Nephrol (2005) 25(6):570–8. doi: 10.1159/000088990
92. Song J, Bishop B, Li G, Duncan M, Abraham SN. Tlr4-initiated and camp-mediated abrogation of bacterial invasion of the bladder. Proc Natl Acad Sci U.S.A. (2007) 1(4):287–98. doi: 10.1016/j.chom.2007.05.007
93. Nanduri R, Furusawa T, Bustin M. Biological functions of hmgn chromosomal proteins. Int J Mol Sci (2020) 21(2):449. doi: 10.3390/ijms21020449
94. Wu G, Cao Y, Fan B, Zheng F, Gao X, Liu N, et al. High-mobility group protein N2 (Hmgn2) inhibited the internalization of klebsiella pneumoniae into cultured bladder epithelial cells. Acta Biochim Biophys Sin (Shanghai) (2011) 43(9):680–7. doi: 10.1093/abbs/gmr064
95. Lu H, Wu Q, Yang H. Duox2 promotes the elimination of the klebsiella pneumoniae strain K5 from T24 cells through the reactive oxygen species pathway. Int J Mol Med (2015) 36(2):551–8. doi: 10.3892/ijmm.2015.2234
96. Dang PM, Rolas L, El-Benna J. The dual role of reactive oxygen species-generating nicotinamide adenine dinucleotide phosphate oxidases in gastrointestinal inflammation and therapeutic perspectives. Antioxid Redox Signal (2020) 33(5):354–73. doi: 10.1089/ars.2020.8018
97. Aviello G, Knaus UG. Nadph oxidases and ros signaling in the gastrointestinal tract. Mucosal Immunol (2018) 11(4):1011–23. doi: 10.1038/s41385-018-0021-8
98. Van Acker H, Coenye T. The role of reactive oxygen species in antibiotic-mediated killing of bacteria. Trends Microbiol (2017) 25(6):456–66. doi: 10.1016/j.tim.2016.12.008
99. Pinegin B, Vorobjeva N, Pashenkov M, Chernyak B. The role of mitochondrial ros in antibacterial immunity. J Cell Physiol (2018) 233(5):3745–54. doi: 10.1002/jcp.26117
100. Gerlach G, Clegg S, Allen BL. Identification and characterization of the genes encoding the type 3 and type 1 fimbrial adhesins of klebsiella pneumoniae. J Bacteriol (1989) 171(3):1262–70. doi: 10.1128/jb.171.3.1262-1270.1989
101. Rosen DA, Pinkner JS, Walker JN, Elam JS, Jones JM, Hultgren SJ. Molecular variations in klebsiella pneumoniae and escherichia coli fimh affect function and pathogenesis in the urinary tract. Infect Immun (2008) 76(7):3346–56. doi: 10.1128/IAI.00340-08
102. Rosen DA, Pinkner JS, Jones JM, Walker JN, Clegg S, Hultgren SJ. Utilization of an intracellular bacterial community pathway in klebsiella pneumoniae urinary tract infection and the effects of fimk on type 1 pilus expression. Infection Immun (2008) 76(7):3337–45. doi: 10.1128/iai.00090-08
103. Ehlers S, Merrill SA. Staphylococcus saprophyticus. In: Statpearls. Treasure Island (FL: StatPearls Publishing Copyright © 2022, StatPearls Publishing LLC (2022).
104. Souza BSV, Silva KCS, Parente AFA, Borges CL, Paccez JD, Pereira M, et al. The influence of ph on staphylococcus saprophyticus iron metabolism and the production of siderophores. Microbes Infection (2019) 21(10):456–63. doi: 10.1016/j.micinf.2019.04.008
105. Böswald L, Matzek D, Kienzle E, Popper B. Influence of strain and diet on urinary ph in laboratory mice. Anim (Basel) (2021) 11(3):702. doi: 10.3390/ani11030702
106. Wang H, Schwaderer AL, Kline J, Spencer JD, Kline D, Hains DS. Contribution of structural domains to the activity of ribonuclease 7 against uropathogenic bacteria. Antimicrobial Agents Chemother (2012) 57(2):766–74. doi: 10.1128/aac.01378-12
107. Spencer JD, Jackson AR, Li B, Ching CB, Vonau M, Easterling RS, et al. Expression and significance of the Hip/Pap and regiiigamma antimicrobial peptides during mammalian urinary tract infection. PloS One (2015) 10(12):e0144024. doi: 10.1371/journal.pone.0144024
108. Fan H, Wang Y, Zhang X, Chen J, Zhou Q, Yu Z, et al. Ginsenoside compound K ameliorates imiquimod-induced psoriasis-like dermatitis through inhibiting Reg3a/Regiiigamma expression in keratinocytes. Biochem Biophys Res Commun (2019) 515(4):665–71. doi: 10.1016/j.bbrc.2019.06.007
109. Lai Y, Li D, Li C, Muehleisen B, Radek KA, Park HJ, et al. The antimicrobial protein Reg3a regulates keratinocyte proliferation and differentiation after skin injury. Immunity (2012) 37(1):74–84. doi: 10.1016/j.immuni.2012.04.010
110. Chen Y, Lu H, Liu Q, Huang G, Lim CP, Zhang L, et al. Function of grim-19, a mitochondrial respiratory chain complex I protein, in innate immunity. J Biol Chem (2012) 287(32):27227–35. doi: 10.1074/jbc.M112.340315
111. Nallar SC, Kalvakolanu DV. Grim-19: A master regulator of cytokine induced tumor suppression, metastasis and energy metabolism. Cytokine Growth Factor Rev (2017) 33:1–18. doi: 10.1016/j.cytogfr.2016.09.001
112. Bhola P, Mvelase NR, Balakrishna Y, Mlisana KP, Swe Swe-Han K. Antimicrobial susceptibility patterns of uropathogens isolated from pregnant women in kwazulu-natal province: 2011 - 2016. S Afr Med J (2020) 110(9):872–6. doi: 10.7196/SAMJ.2020.v110i9.14468
113. Kraemer TD, Quintanar Haro OD, Domann E, Chakraborty T, Tchatalbachev S. The tir domain containing locus ofenterococcus faecalisis predominant among urinary tract infection isolates and downregulates host inflammatory response. Int J Microbiol (2014) 2014:1–9. doi: 10.1155/2014/918143
114. Kathirvel S, Mani M, Gopala Krishnan GK, Sethumadhavan A, Vijayalakshmi T, Ponnan SM, et al. Molecular characterization of enterococcus faecalis isolates from urinary tract infection and interaction between enterococcus faecalis encountered dendritic and natural killer cells. Microb Pathog (2020) 140:103944. doi: 10.1016/j.micpath.2019.103944
115. Cai S, Zhu G, Cen X, Bi J, Zhang J, Tang X, et al. Synthesis, structure-activity relationships and preliminary mechanism study of n-benzylideneaniline derivatives as potential Tlr2 inhibitors. Bioorg Med Chem (2018) 26(8):2041–50. doi: 10.1016/j.bmc.2018.03.001
116. Munoz MD, Gutierrez LJ, Delignat S, Russick J, Gomez Mejiba SE, Lacroix-Desmazes S, et al. The nitrone spin trap 5,5dimethyl1pyrroline noxide binds to toll-like receptor-2-Tir-Bb-Loop domain and dampens downstream inflammatory signaling. Biochim Biophys Acta Mol Basis Dis (2019) 1865(6):1152–9. doi: 10.1016/j.bbadis.2019.01.005
117. Zou J, Baghdayan AS, Payne SJ, Shankar N. A tir domain protein from e. faecalis attenuates Myd88-mediated signaling and nf-kappab activation. PloS One (2014) 9(11):e112010. doi: 10.1371/journal.pone.0112010
118. Tien BYQ, Goh HMS, Chong KKL, Bhaduri-Tagore S, Holec S, Dress R, et al. Enterococcus faecalis promotes innate immune suppression and polymicrobial catheter-associated urinary tract infection. Infection Immun (2017) 85(12):e00378–17. doi: 10.1128/iai.00378-17
119. Gause WC, Mora-Bau G, Platt AM, van Rooijen N, Randolph GJ, Albert ML, et al. Macrophages subvert adaptive immunity to urinary tract infection. PloS Pathog (2015) 11(7):e1005044. doi: 10.1371/journal.ppat.1005044
120. Chan Cheryl Y, St. John Ashley L, Abraham Soman N. Mast cell interleukin-10 drives localized tolerance in chronic bladder infection. Immunity (2013) 38(2):349–59. doi: 10.1016/j.immuni.2012.10.019
121. Jalalifar S, Havaei SA, Motallebirad T, Moghim S, Fazeli H, Esfahani BN. Determination of surface proteins profile, capsular genotyping, and antibiotic susceptibility patterns of group b streptococcus isolated from urinary tract infection of Iranian patients. BMC Res Notes (2019) 12(1):437. doi: 10.1186/s13104-019-4428-4
122. Ulett KB, Benjamin WH Jr., Zhuo F, Xiao M, Kong F, Gilbert GL, et al. Diversity of group b streptococcus serotypes causing urinary tract infection in adults. J Clin Microbiol (2009) 47(7):2055–60. doi: 10.1128/JCM.00154-09
123. Girma W, Yimer N, Kassa T, Yesuf E. Group b streptococcus recto-vaginal colonization in near-term pregnant women, southwest Ethiopia. Ethiop J Health Sci (2020) 30(5):687–96. doi: 10.4314/ejhs.v30i5.7
124. John PP, Baker BC, Paudel S, Nassour L, Cagle H, Kulkarni R. Exposure to moderate glycosuria induces virulence of group b streptococcus. J Infect Dis (2021) 223(5):843–7. doi: 10.1093/infdis/jiaa443
125. Leclercq SY, Sullivan MJ, Ipe DS, Smith JP, Cripps AW, Ulett GC. Pathogenesis of streptococcus urinary tract infection depends on bacterial strain and β-Hemolysin/Cytolysin that mediates cytotoxicity, cytokine synthesis, inflammation and virulence. Sci Rep (2016) 6(1):29000. doi: 10.1038/srep29000
126. Ulett GC, Webb RI, Ulett KB, Cui X, Benjamin WH, Crowley M, et al. Group b streptococcus (Gbs) urinary tract infection involves binding of gbs to bladder uroepithelium and potent but gbs-specific induction of interleukin 1alpha. J Infect Dis (2010) 201(6):866–70. doi: 10.1086/650696
127. Burgener SS, Schroder K. Neutrophil extracellular traps in host defense. Cold Spring Harb Perspect Biol (2020) 12(7):a037028. doi: 10.1101/cshperspect.a037028
128. Tsai CY, Hsieh SC, Liu CW, Lu CS, Wu CH, Liao HT, et al. Cross-talk among polymorphonuclear neutrophils, immune, and non-immune cells Via released cytokines, granule proteins, microvesicles, and neutrophil extracellular trap formation: A novel concept of biology and pathobiology for neutrophils. Int J Mol Sci (2021) 22(6):3119. doi: 10.3390/ijms22063119
129. Costa A, Gupta R, Signorino G, Malara A, Cardile F, Biondo C, et al. Activation of the Nlrp3 inflammasome by group b streptococci. J Immunol (2012) 188(4):1953–60. doi: 10.4049/jimmunol.1102543
130. Tsai CM, Riestra AM, Ali SR, Fong JJ, Liu JZ, Hughes G, et al. Siglec-14 enhances Nlrp3-inflammasome activation in macrophages. J Innate Immun (2020) 12(4):333–43. doi: 10.1159/000504323
131. Kline KA, Schwartz DJ, Lewis WG, Hultgren SJ, Lewis AL, Camilli A. Immune activation and suppression by group b streptococcus in a murine model of urinary tract infection. Infection Immun (2011) 79(9):3588–95. doi: 10.1128/iai.00122-11
132. Babikir IH, Abugroun EA, Bilal NE, Alghasham AA, Abdalla EE, Adam I. The impact of cathelicidin, the human antimicrobial peptide ll-37 in urinary tract infections. BMC Infect Dis (2018) 18(1):17. doi: 10.1186/s12879-017-2901-z
133. Pearson MM. Methods for studying swarming and swimming motility. Methods Mol Biol (2019) 2021:15–25. doi: 10.1007/978-1-4939-9601-8_3
134. Raffi HS, Bates JM Jr., Laszik Z, Kumar S. Tamm-horsfall protein protects against urinary tract infection by Proteus mirabilis. J Urol (2009) 181(5):2332–8. doi: 10.1016/j.juro.2009.01.014
135. Armbruster CE, Mobley HLT, Pearson MM. Pathogenesis of Proteus mirabilis infection. EcoSal Plus (2018) 8(1):ESP-0009-2017. doi: 10.1128/ecosalplus.ESP-0009-2017
136. Umpiérrez A, Scavone P, Romanin D, Marqués JM, Chabalgoity JA, Rumbo M, et al. Innate immune responses to Proteus mirabilis flagellin in the urinary tract. Microbes Infection (2013) 15(10-11):688–96. doi: 10.1016/j.micinf.2013.06.007
137. Scavone P, Umpierrez A, Rial A, Chabalgoity JA, Zunino P. Native flagellin does not protect mice against an experimental Proteus mirabilis ascending urinary tract infection and neutralizes the protective effect of mrpa fimbrial protein. Antonie Van Leeuwenhoek (2014) 105(6):1139–48. doi: 10.1007/s10482-014-0175-7
138. Mittal R, Sharma S, Chhibber S, Harjai K. Iron dictates the virulence of pseudomonas aeruginosa in urinary tract infections. J Biomed Sci (2008) 15(6):731–41. doi: 10.1007/s11373-008-9274-7
139. Harjai K, Mittal R, Chhibber S, Sharma S. Contribution of tamm–horsfall protein to virulence of pseudomonas aeruginosa in urinary tract infection. Microbes Infection (2005) 7(1):132–7. doi: 10.1016/j.micinf.2004.09.005
140. Sun F, Li N, Wang L, Feng H, Shen D, Wang M. Iron interferes with quorum sensing-mediated cooperation in pseudomonas aeruginosa by affecting the expression of ppyr and mext, in addition to rhlr. J Microbiol (2020) 58(11):938–44. doi: 10.1007/s12275-020-0264-4
141. Montagut EJ, Marco MP. Biological and clinical significance of quorum sensing alkylquinolones: Current analytical and bioanalytical methods for their quantification. Anal Bioanal Chem (2021) 18:4599–618. doi: 10.1007/s00216-021-03356-x
142. Mittal R, Chhibber S, Sharma S, Harjai KJM. Macrophage inflammatory protein-2, neutrophil recruitment and bacterial persistence in an experimental mouse model of urinary tract infection. Microbes Infect (2004) 6(14):1326–32. doi: 10.1016/j.micinf.2004.08.008
143. Poloni JAT, Rotta LN. Urine sediment findings and the immune response to pathologies in fungal urinary tract infections caused by candida spp. J Fungi (Basel) (2020) 6(4):245. doi: 10.3390/jof6040245
144. Coady A, Ramos AR, Olson J, Nizet V, Patras KA, Deepe GS. Tamm-horsfall protein protects the urinary tract againstcandida albicans. Infection Immun (2018) 86(12):e00451–18. doi: 10.1128/iai.00451-18
145. Chen H, Zhou X, Ren B, Cheng L. The regulation of hyphae growth in candida albicans. Virulence (2020) 11(1):337–48. doi: 10.1080/21505594.2020.1748930
146. Tsai PW, Yang CY, Chang HT, Lan CY. Human antimicrobial peptide ll-37 inhibits adhesion of candida albicans by interacting with yeast cell-wall carbohydrates. PloS One (2011) 6(3):e17755. doi: 10.1371/journal.pone.0017755
147. Arkowitz RA, Tsai P-W, Yang C-Y, Chang H-T, Lan C-Y. Characterizing the role of cell-wall β-1,3-Exoglucanase Xog1p in candida albicans adhesion by the human antimicrobial peptide ll-37. PloS One (2011) 6(6):e21394. doi: 10.1371/journal.pone.0021394
148. Wang S-H, Wang S-C, Chen P-C, Wang S-T, Liu Y-W. Induction of cyclooxygenase-2 gene bycandida albicansthrough egfr, erk, and P38 pathways in human urinary epithelium. Med Mycol (2016) 3:314–22. doi: 10.1093/mmy/myw082
149. Mahesh G, Anil Kumar K, Reddanna P. Overview on the discovery and development of anti-inflammatory drugs: Should the focus be on synthesis or degradation of Pge2? J Inflammation Res (2021) 14:253–63. doi: 10.2147/JIR.S278514
150. Rafa E, Walaszek MZ, Walaszek MJ, Domanski A, Rozanska A. The incidence of healthcare-associated infections, their clinical forms, and microbiological agents in intensive care units in southern Poland in a multicentre study from 2016 to 2019. Int J Environ Res Public Health (2021) 18(5):2238. doi: 10.3390/ijerph18052238
151. Litwin A, Fedorowicz O, Duszynska W. Characteristics of microbial factors of healthcare-associated infections including multidrug-resistant pathogens and antibiotic consumption at the university intensive care unit in Poland in the years 2011-2018. Int J Environ Res Public Health (2020) 17(19):6943. doi: 10.3390/ijerph17196943
152. Bazaid AS, Saeed A, Alrashidi A, Alrashidi A, Alshaghdali K, AH S, et al. Antimicrobial surveillance for bacterial uropathogens in ha'il, Saudi Arabia: A five-year multicenter retrospective study. Infect Drug Resist (2021) 14:1455–65. doi: 10.2147/IDR.S299846
153. Orskov I, Ferencz A, Orskov FJL. Tamm-horsfall protein or uromucoid is the normal urinary slime that traps type 1 fimbriated escherichia coli. Lancet (1980) 1(8173):887. doi: 10.1016/s0140-6736(80)91396-3
154. Schaeffer C, Devuyst O, Rampoldi L. Uromodulin: Roles in health and disease. Annu Rev Physiol (2021) 83:477–501. doi: 10.1146/annurev-physiol-031620-092817
155. Scharf B, Schmidt TJ, Rabbani S, Stork C, Dobrindt U, Sendker J, et al. Antiadhesive natural products against uropathogenic e. coli: What can we learn from cranberry extract? J Ethnopharmacol (2020) 257:112889. doi: 10.1016/j.jep.2020.112889
156. Kurutas EB, Ciragil P, Gul M, Kilinc M. The effects of oxidative stress in urinary tract infection. Mediators Inflammation (2005) 2005(4):242–4. doi: 10.1155/MI.2005.242
157. Cerezo AB, Cătunescu GM, González MM-P, Hornedo-Ortega R, Pop CR, Rusu CC, et al. Anthocyanins in blueberries grown in hot climate exert strong antioxidant activity and may be effective against urinary tract bacteria. Antioxidants (2020) 9(6):478. doi: 10.3390/antiox9060478
158. Ghouri F, Hollywood A, Ryan K. A systematic review of non-antibiotic measures for the prevention of urinary tract infections in pregnancy. BMC Pregnancy Childbirth (2018) 18(1):99. doi: 10.1186/s12884-018-1732-2
159. Kalt W, Cassidy A, Howard LR, Krikorian R, Stull AJ, Tremblay F, et al. Recent research on the health benefits of blueberries and their anthocyanins. Adv Nutr (2020) 11(2):224–36. doi: 10.1093/advances/nmz065
160. Wu X, Beecher G, Holden J, Haytowitz D, Gebhardt S, Prior RL, et al. Concentrations of anthocyanins in common foods in the united states and estimation of normal consumption. J Agric Food Chem (2006) 54(11):4069–75. doi: 10.1021/jf060300l
161. Lima A, Zunino P, D'Alessandro B, Piccini C. An iron-regulated outer-membrane protein of Proteus mirabilis is a haem receptor that plays an important role in urinary tract infection and in in vivo growth. J Med Microbiol (2007) 56(12):1600–7. doi: 10.1099/jmm.0.47320-0
162. Robinson AE, Lowe JE, Koh E-I, Henderson JP. Uropathogenic enterobacteria use the yersiniabactin metallophore system to acquire nickel. J Biol Chem (2018) 293(39):14953–61. doi: 10.1074/jbc.RA118.004483
163. Dogan O, Vatansever C, Atac N, Albayrak O, Karahuseyinoglu S, Sahin OE, et al. Virulence determinants of colistin-resistant k. pneumoniae high-risk clones. Biol (Basel) (2021) 10(5):436. doi: 10.3390/biology10050436
164. Patras KA, Ha AD, Rooholfada E, Olson J, Ramachandra Rao SP, Lin AE, et al. Augmentation of urinary lactoferrin enhances host innate immune clearance of uropathogenic escherichia coli. J Innate Immun (2019) 11(6):481–95. doi: 10.1159/000499342
165. Bauckman K, Matsuda R, Higgins C, DeBosch B, Wang C, Mysorekar IU. Dietary restriction of iron availability attenuates upec pathogenesis in a mouse model of urinary tract infection. Am J Physiol Renal Physiol (2019) 316(5):F814–F22. doi: 10.1152/ajprenal.00133.2018
166. Annunziato G, Costantino G. Antimicrobial peptides (Amps): A patent review (2015-2020). Expert Opin Ther Pat (2020) 30(12):931–47. doi: 10.1080/13543776.2020.1851679
167. Eichler TE, Becknell B, Easterling RS, Ingraham SE, Cohen DM, Schwaderer AL, et al. Insulin and the phosphatidylinositol 3-kinase signaling pathway regulate ribonuclease 7 expression in the human urinary tract. Kidney Int (2016) 90(3):568–79. doi: 10.1016/j.kint.2016.04.025
168. Murtha M, Eichler T, Bender K, Metheny J, Li B, Schwaderer A, et al. Insulin receptor signaling regulates renal collecting duct and intercalated cell antibacterial defenses. J Clin Invest (2018) 128(12):5634–46. doi: 10.1172/jci98595
169. Ho C-H, Fan C-K, Wu C-C, Yu H-J, Liu H-T, Chen K-C, et al. Enhanced uropathogenic escherichia coli-induced infection in uroepithelial cells by sugar through tlr-4 and Jak/Stat1 signaling pathways. J Microbiol Immunol Infection (2019) 2:193–205. doi: 10.1016/j.jmii.2019.05.008
170. Esmailzadeh A, Zarrinfar H, Fata A, Sen T. High prevalence of candiduria due to non-albicans candida species among diabetic patients: A matter of concern? J Clin Lab Anal (2018) 32(4):e22343. doi: 10.1002/jcla.22343
171. Saenkham P, Jennings-Gee J, Hanson B, Kock ND, Adams LG, Subashchandrabose S. Hyperglucosuria induced by dapagliflozin augments bacterial colonization in the murine urinary tract. Diabetes Obes Metab (2020) 22(9):1548–55. doi: 10.1111/dom.14064
172. Mama M, Manilal A, Gezmu T, Kidanewold A, Gosa F, Gebresilasie A. Prevalence and associated factors of urinary tract infections among diabetic patients in arba minch hospital, arba minch province, south Ethiopia. Turk J Urol (2019) 45(1):56–62. doi: 10.5152/tud.2018.32855
173. Giannakopoulos X, Sakkas H, Ragos V, Tsiambas E, Bozidis P, M Evangelou A, et al. Impact of enterococcal urinary tract infections in immunocompromised - neoplastic patients. J BUON (2019) 24(5):1768–75.
174. Al-Rubeaan KA, Moharram O, Al-Naqeb D, Hassan A, Rafiullah MR. Prevalence of urinary tract infection and risk factors among Saudi patients with diabetes. World J Urol (2013) 31(3):573–8. doi: 10.1007/s00345-012-0934-x
175. Amiel SA. The consequences of hypoglycaemia. Diabetologia (2021) 64(5):963–70. doi: 10.1007/s00125-020-05366-3
176. Olson PD, Hruska KA, Hunstad DA. Androgens enhance Male urinary tract infection severity in a new model. J Am Soc Nephrol (2016) 27(6):1625–34. doi: 10.1681/ASN.2015030327
177. Olson PD, McLellan LK, Hreha TN, Liu A, Briden KE, Hruska KA, et al. Androgen exposure potentiates formation of intratubular communities and renal abscesses by escherichia coli. Kidney Int (2018) 94(3):502–13. doi: 10.1016/j.kint.2018.04.023
178. Zychlinsky Scharff A, Rousseau M, Lacerda Mariano L, Canton T, Consiglio C, Albert M, et al. Sex differences in il-17 contribute to chronicity in Male versus female urinary tract infection. JCI Insight (2019) 5:e122998. doi: 10.1172/jci.insight.122998
179. Papotto PH, Ribot JC, Silva-Santos B. Il-17+ Γδ T cells as kick-starters of inflammation. Nat Immunol (2017) 18(6):604–11. doi: 10.1038/ni.3726
180. Veldhoen M. Interleukin 17 is a Chief orchestrator of immunity. Nat Immunol (2017) 18(6):612–21. doi: 10.1038/ni.3742
181. Baldwin CL, Yirsaw A, Gillespie A, Le Page L, Zhang F, Damani-Yokota P, et al. Gammadelta T cells in livestock: Responses to pathogens and vaccine potential. Transbound Emerg Dis (2020) 67 Suppl 2:119–28. doi: 10.1111/tbed.13328
182. Lee MY, Chen WC, Hsu WH, Chen SC, Lee JC. Liraglutide inhibits hepatitis c virus replication through an amp activated protein kinase dependent mechanism. Int J Mol Sci (2019) 20(18):4569. doi: 10.3390/ijms20184569
183. Duan X, Yang Y, Yang A, Zhao Y, Fan F, Niu L, et al. Terbutaline attenuates lps-induced injury of pulmonary microvascular endothelial cells by Camp/Epac signaling. Drug Dev Res (2021) 83(3):699–707. doi: 10.1002/ddr.21901
184. Wu J, Miao Y, Abraham SN. The multiple antibacterial activities of the bladder epithelium. Ann Transl Med (2017) 5(2):35. doi: 10.21037/atm.2016.12.71
185. Mowbray C, Shams S, Chung G, Stanton A, Aldridge P, Suchenko A, et al. High molecular weight hyaluronic acid: A two-pronged protectant against infection of the urogenital tract? Clin Trans Immunol (2018) 7(6):e1021. doi: 10.1002/cti2.1021
186. Damiano R, Quarto G, Bava I, Ucciero G, De Domenico R, Palumbo MI, et al. Prevention of recurrent urinary tract infections by intravesical administration of hyaluronic acid and chondroitin sulphate: A placebo-controlled randomised trial. Eur Urol (2011) 59(4):645–51. doi: 10.1016/j.eururo.2010.12.039
187. Cicione A, Cantiello F, Ucciero G, Salonia A, Torella M, De Sio M, et al. Intravesical treatment with highly-concentrated hyaluronic acid and chondroitin sulphate in patients with recurrent urinary tract infections: Results from a multicentre survey. Can Urological Assoc J (2014) 8(9-10):E721–7. doi: 10.5489/cuaj.1989
188. De Vita D, Giordano S. Effectiveness of intravesical hyaluronic Acid/Chondroitin sulfate in recurrent bacterial cystitis: A randomized study. Int Urogynecol J (2012) 23(12):1707–13. doi: 10.1007/s00192-012-1794-z
189. Mohanty S, Kamolvit W, Hertting O, Brauner A. Vitamin d strengthens the bladder epithelial barrier by inducing tight junction proteins during e. coli urinary tract infection. Cell Tissue Res (2020) 380(3):669–73. doi: 10.1007/s00441-019-03162-z
190. Ambite I, Puthia M, Nagy K, Cafaro C, Nadeem A, Butler DS, et al. Molecular basis of acute cystitis reveals susceptibility genes and immunotherapeutic targets. PloS Pathog (2016) 12(10):e1005848. doi: 10.1371/journal.ppat.1005848
191. Kronenberg A, Butikofer L, Odutayo A, Muhlemann K, da Costa BR, Battaglia M, et al. Symptomatic treatment of uncomplicated lower urinary tract infections in the ambulatory setting: Randomised, double blind trial. BMJ (2017) 359:j4784. doi: 10.1136/bmj.j4784
192. Vik I, Bollestad M, Grude N, Baerheim A, Damsgaard E, Neumark T, et al. Ibuprofen versus pivmecillinam for uncomplicated urinary tract infection in women-a double-blind, randomized non-inferiority trial. PloS Med (2018) 15(5):e1002569. doi: 10.1371/journal.pmed.1002569
193. Poolman JT, Wacker M. Extraintestinal pathogenic escherichia coli, a common human pathogen: Challenges for vaccine development and progress in the field. J Infect Dis (2016) 213(1):6–13. doi: 10.1093/infdis/jiv429
194. O'Brien VP, Hannan TJ, Nielsen HV, Hultgren SJ. Drug and vaccine development for the treatment and prevention of urinary tract infections. Microbiol Spectr (2016) 4(1):UTI-0013-2012. doi: 10.1128/microbiolspec.UTI-0013-2012
195. Aziminia N, Hadjipavlou M, Philippou Y, Pandian SS, Malde S, Hammadeh MY. Vaccines for the prevention of recurrent urinary tract infections: A systematic review. BJU Int (2019) 123(5):753–68. doi: 10.1111/bju.14606
196. Hasanzadeh S, Farokhi M, Habibi M, Shokrgozar MA, Ahangari Cohan R, Rezaei F, et al. Silk fibroin nanoadjuvant as a promising vaccine carrier to deliver the FimH-IutA antigen for urinary tract infection. ACS Biomater Sci Eng (2020) 6(8):4573–82. doi: 10.1021/acsbiomaterials.0c00736
197. Frick-Cheng AE, Sintsova A, Smith SN, Pirani A, Snitkin ES, Mobley HLT. Ferric citrate uptake is a virulence factor in uropathogenic escherichia coli. mBio (2022) 13(3):e0103522. doi: 10.1128/mbio.01035-22
198. Huttner A, Hatz C, van den Dobbelsteen G, Abbanat D, Hornacek A, Frölich R, et al. Safety, immunogenicity, and preliminary clinical efficacy of a vaccine against extraintestinal pathogenic escherichia coli in women with a history of recurrent urinary tract infection: A randomised, single-blind, placebo-controlled phase 1b trial. Lancet Infect Dis (2017) 17(5):528–37. doi: 10.1016/S1473-3099(17)30108-1
199. Habibi M, Asadi Karam MR, Bouzari S. Construction and evaluation of the immune protection of a recombinant divalent protein composed of the MrpA from MR/P fimbriae and flagellin of Proteus mirabilis strain against urinary tract infection. Microb Pathog (2018) 117:348–55. doi: 10.1016/j.micpath.2018.02.023
200. Alamuri P, Eaton KA, Himpsl SD, Smith SN, Mobley HL. Vaccination with proteus toxic agglutinin, a hemolysin-independent cytotoxin in vivo, protects against Proteus mirabilis urinary tract infection. Infect Immun (2009) 77(2):632–41. doi: 10.1128/IAI.01050-08
201. Flores-Mireles AL, Pinkner JS, Caparon MG, Hultgren SJ. EbpA vaccine antibodies block binding of enterococcus faecalis to fibrinogen to prevent catheter-associated bladder infection in mice. Sci Transl Med (2014) 6(254):254ra127. doi: 10.1126/scitranslmed.3009384
202. Grischke EM, Rüttgers H. Treatment of bacterial infections of the female urinary tract by immunization of the patients. Urol Int (1987) 42(5):338–41. doi: 10.1159/000281988
203. Osset J, Bartolomé RM, García E, Andreu A. Assessment of the capacity of lactobacillus to inhibit the growth of uropathogens and block their adhesion to vaginal epithelial cells. J Infect Dis (2001) 183(3):485–91. doi: 10.1086/318070
204. Mastromarino P, Brigidi P, Macchia S, Maggi L, Pirovano F, Trinchieri V, et al. Characterization and selection of vaginal lactobacillus strains for the preparation of vaginal tablets. J Appl Microbiol (2002) 93(5):884–93. doi: 10.1046/j.1365-2672.2002.01759.x
205. Barrons R, Tassone D. Use of lactobacillus probiotics for bacterial genitourinary infections in women: A review. Clin Ther (2008) 30(3):453–68. doi: 10.1016/j.clinthera.2008.03.013
206. Sadeghi-Bojd S, Naghshizadian R, Mazaheri M, Ghane Sharbaf F, Assadi F. Efficacy of probiotic prophylaxis after the first febrile urinary tract infection in children with normal urinary tracts. J Pediatr Infect Dis Soc (2020) 9(3):305–10. doi: 10.1093/jpids/piz025
207. Stapleton AE, Au-Yeung M, Hooton TM, Fredricks DN, Roberts PL, Czaja CA, et al. Randomized, placebo-controlled phase 2 trial of a lactobacillus crispatus probiotic given intravaginally for prevention of recurrent urinary tract infection. Clin Infect Dis (2011) 52(10):1212–7. doi: 10.1093/cid/cir183
208. Forster CS, Hsieh MH, Cabana MD. Perspectives from the society for pediatric research: Probiotic use in urinary tract infections, atopic dermatitis, and antibiotic-associated diarrhea: An overview. Pediatr Res (2021) 90(2):315–27. doi: 10.1038/s41390-020-01298-1
209. Aragón IM, Herrera-Imbroda B, Queipo-Ortuño MI, Castillo E, Del Moral JS, Gómez-Millán J, et al. The urinary tract microbiome in health and disease. Eur Urol Focus (2018) 4(1):128–38. doi: 10.1016/j.euf.2016.11.001
210. Shim YH, Lee SJ, Lee JW. Antimicrobial activity of lactobacillus strains against uropathogens. Pediatr Int (2016) 58(10):1009–13. doi: 10.1111/ped.12949
211. de Llano DG, Arroyo A, Cárdenas N, Rodríguez JM, Moreno-Arribas MV, Bartolomé B. Strain-specific inhibition of the adherence of uropathogenic bacteria to bladder cells by probiotic lactobacillus spp. Pathog Dis (2017) 75(4):ftx043. doi: 10.1093/femspd/ftx043
Keywords: bladder infection, uropathogens, immune responses, individual intervention, uropathogen escherichia coli
Citation: Li L, Li Y, Yang J, Xie X and Chen H (2022) The immune responses to different Uropathogens call individual interventions for bladder infection. Front. Immunol. 13:953354. doi: 10.3389/fimmu.2022.953354
Received: 26 May 2022; Accepted: 05 August 2022;
Published: 23 August 2022.
Edited by:
Maria Kaparakis-Liaskos, La Trobe University, AustraliaReviewed by:
Razvan-Cosmin Petca, Carol Davila University of Medicine and Pharmacy, RomaniaCopyright © 2022 Li, Li, Yang, Xie and Chen. This is an open-access article distributed under the terms of the Creative Commons Attribution License (CC BY). The use, distribution or reproduction in other forums is permitted, provided the original author(s) and the copyright owner(s) are credited and that the original publication in this journal is cited, in accordance with accepted academic practice. No use, distribution or reproduction is permitted which does not comply with these terms.
*Correspondence: Xiang Xie, eGlhbmd4aWVAc3dtdS5lZHUuY24=; Huan Chen, aHVhbmNoZW5Ac3dtdS5lZHUuY24=
†These authors have contributed equally to this work
Disclaimer: All claims expressed in this article are solely those of the authors and do not necessarily represent those of their affiliated organizations, or those of the publisher, the editors and the reviewers. Any product that may be evaluated in this article or claim that may be made by its manufacturer is not guaranteed or endorsed by the publisher.
Research integrity at Frontiers
Learn more about the work of our research integrity team to safeguard the quality of each article we publish.