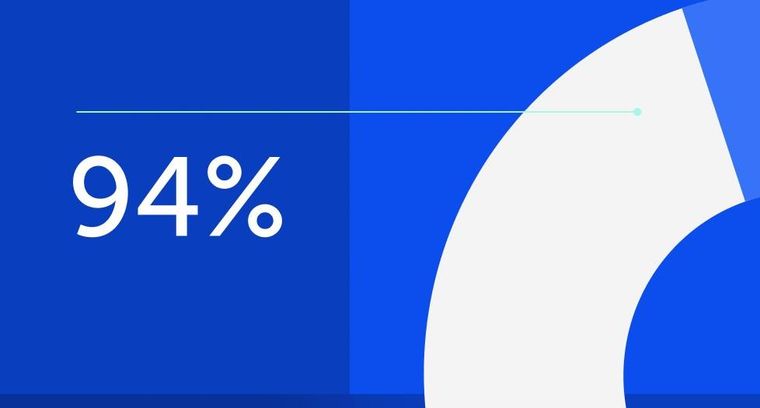
94% of researchers rate our articles as excellent or good
Learn more about the work of our research integrity team to safeguard the quality of each article we publish.
Find out more
ORIGINAL RESEARCH article
Front. Immunol., 29 July 2022
Sec. Inflammation
Volume 13 - 2022 | https://doi.org/10.3389/fimmu.2022.939106
Aspirin eugenol ester (AEE) was a novel drug compound with aspirin and eugenol esterified. AEE had various pharmacological activities, such as anti-inflammatory, antipyretic, analgesic, anti-oxidative stress and so on. In this study, it was aimed to investigate the effect of AEE on the acute lung injury (ALI) induced by lipopolysaccharide (LPS) in rats. In vitro experiments evaluated the protective effect of AEE on the LPS-induced A549 cells. The tumor necrosis factor-α (TNF-α), interleukin-6 (IL-6), and interleukin-1β (IL-1β) were measured in the cell supernatant. The Wistar rats were randomly divided into five groups (n = 8): control group, model group (LPS group), LPS + AEE group (AEE, 54 mg·kg−1), LPS + AEE group (AEE, 108 mg·kg−1), LPS + AEE group (AEE, 216 mg·kg−1). The lung wet-to-dry weight (W/D) ratio and immune organ index were calculated. WBCs were counted in bronchoalveolar lavage fluid (BALF) and total protein concentration was measured. Hematoxylin-Eosin (HE) staining of lung tissue was performed. Glutathione (GSH), glutathione peroxidase (GPx), catalase (CAT), antioxidant superoxide dismutase (SOD), total antioxidant capacity (T-AOC), lactate dehydrogenase (LDH), C-reactive protein (CRP), myeloperoxidase (MPO), malondialdehyde (MDA), macrophage mobility inhibitory factor (MIF), TNF-α, IL-6, and IL-1β activity were measured. The metabolomic analysis of rat serum was performed by UPLC-QTOF-MS/MS. From the results, compared with LPS group, AEE improved histopathological changes, reduced MDA, CRP, MPO, MDA, and MIF production, decreased WBC count and total protein content in BALF, pro-inflammatory cytokine levels, immune organ index and lung wet-dry weight (W/D), increased antioxidant enzyme activity, in a dose-dependent manner. The results of serum metabolomic analysis showed that the LPS-induced ALI caused metabolic disorders and oxidative stress in rats, while AEE could ameliorate it to some extent. Therefore, AEE could alleviate LPS-induced ALI in rats by regulating abnormal inflammatory responses, slowing down oxidative stress, and modulating energy metabolism.
Acute lung injury (ALI) is a type of acute inflammatory disease that is prone to develop into acute respiratory distress syndrome (ARDS), which has a high morbidity and mortality (1–3). The development of ALI can be caused by many factors, such as pneumonia, shock, trauma, burns, severe septic infection, and other non-cardiac diseases (4, 5). Until now, there is still a lack of effective drugs in the treatment of ALI (6, 7). ALI is characterized by pulmonary edema, production of pro-inflammatory mediators, uncontrolled oxidative stress, and inflammatory response, finally leading to impaired lung function (8, 9). The potential pathogenesis of ALI is the imbalance between the inflammatory and anti-inflammatory responses of the body, which leads to the development of various diseases (10). Studies suggest that there may be some preventive effect on the development of ALI by modulating the relationship between inflammation and anti-inflammation (11).
LPS is one of the important components of the cell wall of gram-negative bacteria, which consists of lipids and polysaccharides (12, 13). LPS can induce inflammatory cell infiltration and inflammatory factor release in the organism, causing strong immune responses in the body (14, 15). Therefore, LPS has been widely used to simulate inflammatory responses, including ALI (16, 17). Studies have shown that LPS could induce lung injury through several inflammatory mechanisms. When animal organisms were injected with LPS, the immune system was activated and released large amounts of pro-inflammatory cytokines, which disrupted the inflammatory and anti-inflammatory balance, leading to the development of lung inflammation (18, 19).
AEE, which is a novel medicinal compound, is synthesized by esterification of aspirin with eugenol using the prodrug principle. AEE had pharmacological activities against inflammatory (20), oxidative stress (21–24), hypolipidemia (25, 26), atherosclerosis (27, 28), thrombosis (29, 30), and anti-acute liver injury (31). In previous studies, aspirin had been found to effectively inhibit paraquat-induced oxidative stress injury, inflammatory response, and pulmonary edema, thereby alleviating paraquat-induced ALI (32). Aspirin pretreatment decreased NF-κB expression, showing a good protective effect against hyperoxia-induced ALI (33). Aspirin provides excellent protection against severe bleeding-induced ALI (34, 35). Therefore, it is speculated that AEE may also have some preventive and palliative value in ALI.
In this study, the protective effect of AEE against LPS-induced inflammation in A549 cells was firstly investigated, and then AEE was investigated against LPS-induced ALI among rats. It was aimed to explore the possibility of AEE as a novel drug for the treatment of ALI patients and promote the comprehensive utilization of AEE.
Human alveolar epithelial A549 cells was obtained from American Type Culture Collection (ATCC, Manassas, VA, USA). AEE (purity 99.5%) was prepared in Lanzhou Institute of Husbandry and Pharmaceutical Sciences of CAAS (Lanzhou, China). LPS from Escherichia coli 055: B5 was obtained from Solarbio (Beijing, China). Ham’s F-12K medium, 0.05% Trypsin-EDTA, and fetal bovine serum were from Gibco (Grand Island, NY, USA). The enzyme-linked immunosorbent assay (ELISA) kits for determinations of IL-6, IL-1β, TNF-α, GSH, GPx, CAT, SOD, T-AOC, LDH, CRP, MPO, MDA and MIF were produced by Shanghai Mlbio (Shanghai, China). Cell counting kit-8 was purchased from Beyotime (Shanghai, China). BCA protein assay kit was supplied by Solarbio (Beijing, China). Cell culture plate was purchased from Corning (Beijing, China). Carboxymethylcellulose sodium (CMC-Na) was supplied by Tianjin Chemical Reagent Company (Tianjin, China). The other reagents with analytical grade were purchased from Sinopharm group (Shanghai, China).
A549 cells were cultured in 10% fetal bovine serum (FBS), 90% Ham’s F-12K medium media at 37°C under humidified atmospheric conditions containing 5% CO2. When reaching 90% ~ 95% confluency by observation under an inverted microscope, they were subcultured with 0.05% trypsin-EDTA. Then the groups were randomly divided into three groups (control, LPS and AEE pretreatment group).
To test the viability effects of AEE and LPS on A549 cells, the cell counting kit-8 (CCK-8) assay was performed. Briefly, 1×105 cells were seeded in 96-well plates. After treatment, 10 µL CCK-8 reagent was added to each well. Finally, the number of viable cells were assessed by measurement of the absorbance at 450 nm (Multiskan™ FC; Thermo Scientific™, USA).
A549 cells (1 × 105 cells) were seeded in 6-well plates, and incubated in a humidified atmosphere at 37°C with 5% CO2 for 24 h until fully attached. The cells were treated with different concentrations of AEE (4, 8 and 32 μM) for 24 h, which were selected according to the cytotoxicity, and then stimulated with LPS (10 μg·mL-1) for 24 h. The cell-free supernatants were collected, and stored at -80°C for cytokine assays.
First, 0.5% CMC-Na solution was made up. AEE was dissolved in 0.5% CMC-Na solution to form the storage suspension solution of 40 mg·mL-1. LPS was dissolved in saline to form the storage solution of 5 mg·mL-1. The amount of drug administered to the rats were calculated based on the recorded body weight of the rats as AEE (54, 108 and 216 mg·kg-1 body weight) and LPS (5 mg·kg-1 body weight).
Sixty male specific pathogen-free Wistar rats (7 weeks old) weighing 160 ~ 180 g were bought from the Lanzhou Veterinary Research Institute, Chinese Academy of Agricultural Sciences (Lanzhou, China). All animals were housed in groups in SPF-rated laboratory with controlled relative humidity (55-65%), 12-hour light/dark cycle and temperature (24 ± 2°C), and food and water were offered ad libitum. The rats were randomly divided into five groups (n = 8), such as control, model (LPS group), LPS + AEE low (AEE, 54 mg·kg-1), LPS + AEE middle (AEE, 108 mg·kg-1) and LPS + AEE group high (AEE, 216 mg·kg-1). In vivo models of ALI in Wistar rats were established using intraperitoneal injection (i.p.) of LPS (5 mg·kg-1). Briefly, Wistar rats were given either the vehicle alone (0.5% CMC-Na) or the vehicle combination containing AEE (54, 108 and 216 mg·kg-1·d-1) for 14 d. On the 14th day of the experiment, all rats were fasted for 12 h and then dosed with 0.5% CMC-Na and different doses of AEE by gavage. After 60 min, LPS (5 mg·kg-1) were injected as i.p. into each group, except for the control group. After 6 h of LPS treatment, animals were anesthetized as i.p. with 80 mg·kg-1 sodium pentobarbital. The blood samples were collected by cardiac puncture for further analysis. Then the lung tissue was carefully harvested, quick-freezed in liquid nitrogen, and then stored at -80°C. All experimental protocols and procedures were approved by the Institutional Animal Care and Use Committee of Lanzhou Institute of Husbandry and Pharmaceutical Science of Chinese Academy of Agricultural Sciences (Approval No. NKMYD20201123; Approval Date: 23 November 2020). Animal welfare and experimental procedures were performed strictly in accordance with the Guidelines for the Care and Use of Laboratory Animals issued by the US National Institutes of Health.
After blood samples were collected by cardiac puncture, their chest cavities were opened, and then the cannula was inserted into the trachea and the lung lobes were flushed three times, each time with 1 mL saline. The bronchoalveolar lavage fluid (BALF) were centrifuged at 2500 rpm for 10 min at 4°C, and the supernatants were collected and stored at -80°C for subsequent testing. The BALF protein concentrations were analyzed by the bicinchoninic acid (BCA) method using the BCA kit (Solarbio, Shanghai, China) according to the manufacturer’s instructions. The absorbance was measured at 562 nm and the protein content was calculated. The BALF was performed the white blood cell count.
The wet/dry weight ratio of lung tissue was measured. Briefly, wet weight was determined by excision of the left lower lung lobe, and dry weight was determined through putting the lung lobe in an oven at 65°C for 72 h to remove all moisture, and then weighed the dried lung.
The spleen tissues were collected to calculate the immune organ index. Immune organ index = spleen index (mg)/rat body weight (g).
The left lung lobe was fixed in 10% formaldehyde. After fixation, the lung tissue was embedded in paraffin, sectioned to 5 μm thickness, and stained with hematoxylin-eosin stain (H&E). Then they were pathologically observed.
The levels of IL-6, IL-1β and TNF-α in the serum and the cell supernatant were measured using the appropriate kits according to the manufacturer’s instructions.
The levels of GSH, GPx, CAT, SOD, T-AOC, LDH, CRP, MPO, MDA and MIF in the ALI rats were measured using the appropriate kits according to the manufacturer’s instructions.
Based on the above experimental results, control group, model group (LPS group), LPS + AEE high group (AEE, 216 mg·kg−1) were selected for metabolomic analysis. The serum was thawed at ambient temperature, acetonitrile (3:1, V/V) was added for precipitating the protein, then vortexed and mixed for 1 min and stood for 10 min. The samples were centrifuged at 14000 rpm for 10 min at 4°C. The supernatant was filtered through the 0.22 μm filter membrane and subsequently analyzed by UPLC-QTOF-MS/MS.
Liquid chromatography was performed on a 1290 UPLC system (Agilent Technologies Inc., California, USA). The LC conditions was as followed. The column was an Agilent ZORBAX SB C18 (2.1 × 150 mm, 1.8 μm) and the column temperature was 35°C. The injection volume was 2 μL and the autosampler temperature was set at 4°C. Mobile phase: 0.1% formic acid in water (A) and 0.1% formic acid in acetonitrile (B) at a flow rate of 0.3 mL·min-1. The gradient elution of A was as followed: 95% A from 0 to 3 min, 95 - 35% A from 3 to 4 min, 35 - 30% A from 4 to 7 min, 30 - 15% A from 7 to 10 min, 15 - 10% A from 10 to 15 min, 10 - 5% A from 15 to 18 min, and 18 to 20 min kept at 95% A.
Agilent 6530 Q-TOF (Agilent Technologies, USA) was used to carry out mass spectrometry of serum samples with a dual electrospray ionization source (ESI) operating in positive and negative ion modes. The fragment voltage was set to 135 V and the skimmer voltage was set to 65 V. In positive ionization mode, the capillary voltage was 4000 V while in negative ionization mode, it was 3500 V. The dry gas temperature was 350°C, and the flow rate was 10 L·min-1. The nebulizer pressure was set to 45 psi. The ions were scanned in the region of 50-1000 m/z.
The samples were tested by the UPLC/Q-TOF-MS system and the original data were obtained. The original data were processed using MS-DIAL V 4.38 to filter noise, baseline comparison, peak identification, data reduction, and normalization. Finally, the two-dimensional data matrix including mass-to-charge ratio, peak area and retention time were obtained. The obtained data were subjected to multivariate statistical analysis using SIMCA-P (version 13.0, Umetrics AB, Umea, Sweden) software, and the results were presented as principal component analysis (PCA) score plots and partial least squares discriminant analysis (OPLS-DA) score plots, respectively. The OPLS-DA model was also evaluated using the R2X, R2Y and Q2 parameters, and the permutation test was used to prevent overfitting of the model. R2Y can indicate the fit of the model and Q2 can indicate the predictability of the model, and the magnitude of these two parameters can reflect the reliability and accuracy of the model. Finally, the metabolites with VIP > 1 and P < 0.05 were screened for metabolite variability analysis in different groups. The screened differential metabolites were analyzed by targeted MS/MS using a UPLC/Q-TOF-MS analysis system, which combined their mass/charge (m/z) and retention time to obtain their accurate fragment ion information. Then, the information on the differential metabolites of concern was finalized by performing the spectral library comparison in METLIN and Human Metabolome Database (HMDB). According to the information on the differential metabolites, MetaboAnalyst 4.0 and KEGG database were used for the analysis of relevant metabolic pathways.
All data are presented as means ± SD. The differences among different treatment groups were analyzed with one-way ANOVA followed with Duncan’s multiple comparisons. Statistical significance was considered at p<0.05.
The results showed that AEE (4 ~ 32 μM) had no cytotoxicity to A549 cells for 24 h. When the concentration was greater than 32 μM, the cell viability started to decrease (Figure 1A). Therefore, the concentrations of AEE below 32 μM were selected for subsequent experiments. It is known from Figure 1B that LPS had no cytotoxicity to A549 cells in the range of 3 to 12 μM for 24 h. When the concentration of LPS was 25 μM, the viability of A549 cells decreased slightly, therefore, the LPS concentration should be less than 25 μM for subsequent tests.
Figure 1 The effects of AEE and LPS with different concentrations on A549 cell viability, respectively. (A) AEE, (B) LPS. Values are presented as the means ± SD where applicable (n = 6). *P < 0.05 compared to control group.
The results (Figure 2) showed that the levels of IL-6, IL-1β, and TNF-α were higher in the LPS group than in the control group. The levels of IL-6, IL-1β, and TNF-α were decreased in the AEE-treated group in a dose-dependent manner compared with the LPS group. These data indicated that AEE could improve the inflammatory response to LPS via inhibiting the release of cytokines IL-6, IL-1β and TNF-α.
Figure 2 Effect of AEE on LPS-induced inflammatory cytokines. (A) TNF-α, (B) IL-6 and (C) IL-1β in the supernatant of A549 cells. (D) TNF-α, (E) IL-6, and (F) IL-1β in the serum of LPS-induced ALI rats. Values are presented as the means ± SD where applicable (n = 6). *P < 0.05 compared to control group, #P < 0.05 compared to LPS group.
The results (Figures 3A, B) indicated that the WBC count and total protein content in the BALF of the LPS group were higher than those of the control group. Compared with the LPS group, the WBC count and total protein content in the BALF of the AEE-treated group were significantly decreased in the dose-dependence manner.
Figure 3 Effect of AEE on the number of WBC in BALF and total protein level in BALF of LPS-induced ALI rats. (A) WBC in BALF, (B) Total protein in BALF. Values are presented as the means ± SD where applicable (n = 8). *P < 0.05 compared to control group, #P < 0.05 compared to LPS group.
The effect of AEE on the splenic index of LPS-induced ALI in rats was investigated. The results (Figure 4) showed that the splenic index of the LPS group was higher than the control group. However, after AEE treatment, the spleen index of rats decreased significantly compared with the LPS group. Moreover, the decrease in spleen index was related to the dose of AEE.
Figure 4 Effect of AEE on splenic index in LPS-induced ALI rats. Values are presented as the means ± SD where applicable (n = 8). *P < 0.05 compared to control group, #P < 0.05 compared to LPS group.
To evaluate the degree of edema in rat lungs, the wet to dry (W/D) weight ratio of lung tissue was calculated. As shown in Figure 5, compared with the control group, the W/D ratio of the LPS group was significantly increased, which indicated that LPS treatment significantly increased the W/D ratio. However, the W/D ratio of lung tissue was significantly decreased in a dose-dependent manner after AEE treatment compared with the LPS group.
Figure 5 Effect of AEE on lung wet to dry weight ratio (W/D). Values are presented as the means ± SD where applicable (n = 8). *P < 0.05 compared to control group, #P < 0.05 compared to LPS group.
The results (Figures 6A–C) showed that the levels of CRP, MPO and MIF in the serum of rats in the LPS group were higher than in the control group. Compared with the LPS group, there were significant decreases in the levels of CRP, MPO, and MIF in the serum of rats in the AEE-treated group. All these data indicated that AEE could prevent lung inflammation during ALI in rats by decreasing CRP, MPO, and MIF in a dose-dependent manner.
Figure 6 Effect of AEE on concentrations of CRP (A), MIF (B) in serum, MPO (C) in lung tissue. Values are presented as the means ± SD where applicable (n = 8). *P < 0.05 compared to control group, #P < 0.05 compared to LPS group.
To explore the anti-oxidative stress effect of AEE on LPS-induced ALI in rats, the following oxidative stress indicators were selected: GSH, GPx, CAT, T-AOC, SOD, LDH, and MDA. As shown in Figures 7A–G, compared with the control group, GSH, GPx, CAT, T-AOC, and SOD levels were significantly decreased in the LPS group, while LDH and MDA levels were significantly increased. Compared with the LPS group, the levels of GSH, GPx, CAT, T-AOC, and SOD in the AEE-treated group increased significantly in a dose-dependent manner, while the levels of LDH and MDA decreased significantly.
Figure 7 Effect of AEE on the level of oxidative stress in LPS-induced ALI rats. (A) GSH, (B) GPx, (C) CAT, (D) T-AOC, (E) SOD, (F) LDH, (G) MDA. Values are presented as the means ± SD where applicable (n = 8). *P < 0.05 compared to control group, #P < 0.05 compared to LPS group.
After HE staining of lung tissue, histopathology was observed. As shown in Figure 8, compared with the control, the LPS group showed necrosis of alveolar epithelial cells, the disintegration of nuclei, proliferation of alveolar epithelial cells, and infiltration of inflammatory cells, mainly neutrophils, which were seen in the necrotic area and in the interstitial space. Compared with the LPS group, AEE-treated groups all had some degree of reducing LPS-induced lung histopathological damage in a dose-dependent manner.
Figure 8 Effect of AEE on histopathological changes in the lungs of LPS-induced ALI rats. (A) Control group, (B) LPS group, (C) LPS + AEE group (AEE, 54 mg kg-1), (D) LPS + AEE group (AEE, 108 mg kg-1), (E) LPS + AEE group (AEE, 216 mg kg-1), (F) Inflammatory change score chart. *P < 0.05 compared to control group, #P < 0.05 compared to LPS group.
Metabolite data of serum samples obtained from three experimental groups were further analyzed by principal component analysis (PCA). As shown in Figures 9A, B, G, H, there were significant changes in the metabolite profiles of the serum samples after drug administration. Under the positive and negative modes, the first two principal components explained 87.8% and 82.9% of the total variance, respectively, which showed a significant separation. To further decrease the effect of irrelevant factors on the analysis results and improve the separation and identification of metabolites, the effects of AEE on metabolites in LPS-induced AIL rats were investigated, and three experimental groups were modeled and analyzed using orthogonal partial least squares discriminant analysis (OPLS-DA). Moreover, the OPLS-DA models of each group were validated with the permutation tests. The parameters of the permutation tests for the OPLS-DA model for each group were shown in Figures 9C, E, I, K. The OPLS-DA models were analyzed between the LPS group and the other groups. Results of the OPLS-DA score plot showed a clear separation between the LPS group and the other groups and there were no overlaps in either the positive or negative mode. the R2X, R2Y and Q2 values of the OPLS-DA model indicated that the model was not overfitted, which matched the sample reality and had predictive power (Figures 9D, F, J, L).
Figure 9 Metabolomic analysis of the effect of AEE on LPS-induced ALI in rats. (A, G) PCA score plots based on rat sera obtained from control, LPS and AEE groups in positive and negative modes, ESI+: R2 = 0.878, ESI-: R2 = 0.829. (B, H) The loading plots of AEE and LPS groups in positive and negative modes. (C, I) OPLS-DA score plots for AEE and LPS groups in positive and negative models, ESI+: R2 X = 0.807, R2 Y = 0.926, Q2 = 0.768; ESI-: R2 X = 0.646, R2 Y = 0.998, Q2 = 0.958. (D, J) Permutation test for the OPLS-DA model, ESI+: intercepts of R2 = 0.626 and Q2 = -0.502 and ESI-: intercepts of R2 = 0.946 and Q2 = -0.245. (E, K) OPLS-DA score plots for control and LPS groups in positive and negative modes, ESI+: R2 X = 0.977, R2 Y = 1, Q2 = 0.992; ESI-: R2 X = 0.887, R2 Y = 1, Q2 = 0.951. (F, L) Permutation test for the OPLS-DA model, ESI+: intercepts of R2 = 0.999 and Q2 = -0.16, ESI-: intercepts of R2 = 0.991 and Q2 = -0.243. Values are presented as the means ± SD where applicable (n = 6).
The potential differential metabolites were selected by VIP (VIP > l) and t-test P < 0.05 in the OPLS-DA model, and the differential metabolites were analyzed by Target MS/MS scan. To obtain secondary characteristic ion fragments of the differential metabolites for comparison in relevant databases. As shown in Table 1, 18 differential metabolites were finally screened in the serum sample analysis, including 2-Methylglutaric acid, Suberylglycine, Spermidine, Doxylamine, Delphinidin, D-Alanine, Gamma-glutamylcysteine, 2’-Deoxyguanosine-5’-monophosphate, Pterin, N-Acetyl-L-tyrosine, Benzyl benzoate, Flavone, L-Histidinol, Mannitol 1-phosphate, m-Coumaric acid, 5-Aminopentanoic acid, Benzocaine, Ferulic acid. After AEE treatment, the levels of these differential metabolites were normalized due to up- or down-regulation, which indicated that AEE could improve the metabolic bias caused by LPS in rats.
To more deeply investigate the effect of AEE on the metabolic pathways in the organism of LPS-induced ALI rats, the metabolomic pathways were analyzed using MetaboAnalyst 5.0. Figure 10 showed an overview of the metabolic pathway analysis in the form of bar and bubble charts. There were 6 main metabolic pathways: Spermidine and Spermine Biosynthesis, Glutathione Metabolism, Cysteine Metabolism, Methionine Metabolism, Glutamate Metabolism, and Purine Metabolism. The effects of pathways were mainly focused on Glutathione metabolism, beta-Alanine metabolism, Arginine and proline metabolism, and Purine metabolism. The LPS-induced ALI in rats was mainly reflected in oxidative stress and energy metabolism. The results indicated that LPS-induced ALI caused metabolic disorders and oxidative stress in rats and AEE could alleviate it to some extent.
With the development of society, the problem of environmental pollution is becoming more and more serious, especially air pollution (36). According to the statistics of relevant organizations, the number of deaths caused by air pollution is up to 7 million per year worldwide (37). The fine particles in the air and other harmful substances can enter the organism with breathing, which leads to the activation of the body’s immune system and further induces oxidative stress, inflammatory reactions, and local tissue damage (38). This increases the occurrence of various respiratory diseases, including asthma, respiratory tract infections, decreased lung function, and acute lung injury, which seriously affects people’s health (39–43). Therefore, this experiment investigated the protective effect of AEE on LPS-induced inflammation in A549 cells and the protective effect of AEE on LPS-induced ALI in rats. The effects of AEE on ALI were investigated from both in vitro assays and in vivo experiments to provide the theoretical basis for the comprehensive and rational use of AEE.
In this study, the LPS concentration of 10 μg·mL-1 was chosen to induce the inflammation model in A549 cells based on the results of the cytotoxicity assay. Previous studies also showed that LPS worked at a concentration of 10 μg·mL-1 on A549 cells (44), and the results of this assay were also consistent with them. According to the toxicity of AEE on A549 cells, three concentrations of the low, medium, and high (4,8,32 μM) were chosen for the follow-up experiments. IL-6, IL-1β, and TNF-α act as pro-inflammatory factors, which are involved in the inflammatory response when the cells are attacked by LPS (45). Compared with the control, the levels of IL-6, IL-1β, and TNF-α in the supernatant of cells in the LPS group were significantly increased, indicating that A549 cells occurred an inflammatory response. Compared with the LPS group, the levels of IL-6, IL-1β and TNF-α were significantly decreased in the AEE-treated group, which showed a dose-dependent effect. This indicated that AEE had a protective effect on inflammation in A549 cells.
According to the preliminary results of in vitro experiments, in vivo experiments were also conducted. Experimental animals were selected as Wistar rats, and the modeling drug was chosen as LPS with the concentration of 5 mg·kg-1 (46, 47).
The results in this study showed that AEE could reduce LPS-induced lung inflammation and injury in rats. Compared with the LPS group, AEE significantly decreased the serum levels of IL-6, IL-1β, and TNF-α, which indicated that AEE could reduce the release of inflammatory mediators in the blood to some extent and alleviate the damage to lung tissue caused by LPS.
C-reactive protein (CRP) is a protein that rises sharply in response to infection or tissue damage in the body (48). In this study, the serum CRP level was higher in the LPS group than the control group, indicating the presence of acute inflammation in the rats. Compared with the LPS group, the AEE group showed a significant decrease in CRP content, which indicated the therapeutic effect of AEE on acute inflammation. Myeloperoxidase (MPO) is a hemoglobin peroxidase expressed most richly in neutrophils (49, 50). As essential mediators of the innate immune system, neutrophils were rapidly recruited to sites of inflammation, and recognized, phagocytosed, inactivated foreign microorganisms, playing a pivotal role in host defense (51, 52). The level of MPO was positively correlated with the number of neutrophils (53). During infection, MPO is involved in the inflammatory response, but it can cause oxidative reactions and the formation of large amounts of oxides, which may cause damage to the tissues (54). The MPO level in the LPS group was higher than in the control group. However, the level of MPO in the AEE group was lower than in the LPS group, which suggested that AEE could reduce the harmful effects of inflammation on the lungs. Neutrophil aggregation is a key character of inflammation in the lung. MPO is a marker of neutrophil function and activation and its activity level can implicitly reflect the extent of neutrophil aggregation at the site of inflammation (46). Therefore, neutrophils were not tested in this study, but the changes in neutrophils could be inferred from the changes in MPO. Macrophage migration inhibitory factor (MIF) is a pro-inflammatory cytokine that is expressed in a variety of cells (55). MIF plays a pathogenic role in many inflammatory diseases, including acute and chronic inflammation, sepsis (56). AEE reduced MIF levels, which further confirmed the protective effect of AEE against inflammation. Certainly, if a number of macrophages and neutrophils were tested and analyzed with the levels of MPO and MIF, the results were more definite and perfect.
One of the main characteristics of ALI is pulmonary edema (57). The primary cause of pulmonary edema is endothelial damage due to inflammatory response, resulting in microvascular leakage (58, 59). The lung W/D ratio is an important indicator of pulmonary edema (60). The increase in BALF protein concentration due to protein extravasation is another important indicator of pulmonary edema (61). Therefore, to evaluate the therapeutic effect of AEE on pulmonary edema, the following experiments were conducted. The result showed that AEE group significantly decreased the lung W/D ratio compared with the LPS group, which indicated that AEE inhibited the accumulation of fluid in the lung tissue. The detection of protein concentration in BALF showed that AEE group significantly reduced the protein concentration in BALF, which indicated that AEE inhibited protein extravasation in lung tissue. Assaying the WBC count in BALF showed that the WBC count in BALF decreased in AEE group, which further suggested a therapeutic effect of AEE on inflammation. In addition, morphological changes in lung tissue were observed by H&E staining. The results showed that AEE group alleviated the pathological changes such as alveolar epithelial cell proliferation, inflammatory cell infiltration, and alveolar epithelial cell necrosis to some extent.
The non-normal immune response of the organism is an essential factor in triggering ALI (62). The spleen is the most important immune organ of the body, and its relative proportion is an essential indicator of nonspecific immunity, they play an active role in non-specific immunity in immune defense (63, 64). As the results showed, compared with the LPS group, the spleen index of rats in AEE group was significantly lower, which indicated that AEE had a protective effect on the spleen.
According to studies, the inflammatory response may trigger oxidative stress, and oxidative stress can exacerbate the inflammatory response, which can interact with each other in multiple ways (65–67). Several studies showed that oxidative stress and the inflammatory response were essential factors in triggering lung injury (68, 69). MDA is a product of lipid oxidation under oxidative stress conditions, which has a damaging effect on cells (70). SOD is the most important free radical scavenger in the body, maintaining the metabolic homeostasis of the body (71). LDH is a crucial oxidoreductase in organisms that is an indicator of oxidative stress. CAT and GSH are essential non-protein antioxidants that clears lipid peroxide radicals. GPx clear oxidation products and is associated with the transport of GSH. T-AOC is the total antioxidant level consisting of various antioxidant substances and antioxidant enzymes (72–74). Some results showed that in LPS-induced ALI, MDA levels increased while antioxidant activity decreased (19, 75, 76). In the study, the above indices were chosen to evaluate the protective effect of AEE on oxidative stress in rats. Compared with the LPS group, the levels of MDA and LDH were significantly decreased and the levels of SOD, CAT, GSH, GPx, and T-AOC were somewhat restored in the AEE group. It indicated that the protective effect of AEE on LPS-induced ALI in rats might be its antioxidant capacity.
The action mechanism of AEE on the serum of ALI rats were investigated by an untargeted metabolomics approach using UPLC-Q-TOF/MS. The results showed that the mechanism of AEE on ALI may mainly involve Spermidine and Spermine Biosynthesis, Glutathione Metabolism, Cysteine Metabolism, Methionine Metabolism, Glutamate Metabolism, and Purine Metabolism. Spermidine and spermine biosynthesis may be one of the metabolic pathways through which AEE plays a protective role in LPS-induced ALI. Spermidine and spermine can alleviate oxidative stress, and spermidine is involved in protecting cells from oxidative stress by clearing ROS (77–79). The biosynthetic pathway of spermidine was inhibited in the LPS group, while the production of spermidine was increased in the AEE group, which suggested that AEE might alleviate LPS-induced ALI in rats by clearing excess ROS. Cysteine metabolism, methionine metabolism, and glutathione metabolic pathways are closely related to oxidative stress (80). Methionine residues can also act as endogenous antioxidants, which can be used as a marker that the body is in a state of oxidative stress (81). Glutathione (γ-glutamyl-cysteinyl-glycine, GSH) plays an important role in the antioxidant process because of its ability to effectively clean up free radicals and other reactive oxygen species (82). The changes in GSH levels indicate changes in the oxidative status of the body, and when the body is stimulated by oxidation, GSH synthesis increases (83). The metabolomic results showed that the biosynthetic pathway of Gamma-glutamylcysteine was inhibited in the LPS group, while the production of Gamma-glutamylcysteine was increased in the AEE group. Purine metabolism is an important response to oxidative stress, and abnormal purine metabolism can generate damaging reactive oxygen species (ROS) (84, 85). 2’-Deoxyguanosine 5’-monophosphate production was increased in the LPS group, indicating oxidative stress in rats. In contrast, 2’-Deoxyguanosine 5’-monophosphate production was decreased in the AEE group, which suggested an antioxidant effect of AEE. In the subsequent study, we will further explore the action mechanism of AEE on ALI in rats and elucidate the signaling pathway of AEE action on ALI in rats.
AEE had a protective effect against LPS-induced ALI in rats. In vitro and in vivo experiments demonstrated that AEE can reduce inflammation in rat lung by decreasing the release of pro-inflammatory factors as IL-6, IL-1β, and TNF-α. AEE alleviated pulmonary edema in rats by inhibiting lung tissue protein exudation, decreasing lung wet-to-dry (W/D) weight ratio, decreasing immune organ index, and improving lung histopathology. In addition, AEE showed an alleviating effect on inflammation-induced oxidative stress, which suggested an anti-oxidative stress effect of AEE. From metabolomics, AEE potential mechanism was related to the regulation of oxidative stress and metabolic pathway disorders of energy metabolism. Therefore, AEE could be used as a potential drug to improve ALI.
The original contributions presented in the study are included in the article/supplementary material. Further inquiries can be directed to the corresponding authors.
The animal study was reviewed and approved by Institutional Animal Care and Use Committee of Lanzhou Institute of Husbandry and Pharmaceutical Science of Chinese Academy of AgriculturalSciences.
J-YL and Y-JY designed the experiments and revised the manuscript. QT and Z-DZ designed and performed the experiments, and wrote the manuscript. X-WL synthesized and purified AEE. L-XB, S-HL, W-BG and ZQ supplied reagents. All authors contributed to the article and approved the submitted version.
This study was supported by the Special Fund for National natural science foundation of China (Grant/Award Numbers: 31872518).
The authors declare that the research was conducted in the absence of any commercial or financial relationships that could be construed as a potential conflict of interest.
All claims expressed in this article are solely those of the authors and do not necessarily represent those of their affiliated organizations, or those of the publisher, the editors and the reviewers. Any product that may be evaluated in this article, or claim that may be made by its manufacturer, is not guaranteed or endorsed by the publisher.
AEE, Aspirin eugenol ester; LPS, Lipopolysaccharide; ALI, Acute lung injury; TNF-α, Tumor necrosis factor-α; IL-6, Interleukin-6; IL-1β, Interleukin-1β; WBC, White blood cell; W/D, Lung wet-to-dry weight; BALF, Bronchoalveolar lavage fluid; HE, Hematoxylin-Eosin; FBS, fetal bovine serum; NEAA, Non-essential amino acids; CCK-8, Cell counting kit-8; BCA, Bicinchoninic acid; GSH, Glutathione; GPx, Glutathione peroxidase; CAT, Catalase; SOD, Superoxide dismutase; T-AOC, Total antioxidant capacity; LDH, Lactate dehydrogenase; CRP, C-reactive protein; MPO, Myeloperoxidase; MDA, Malondialdehyde; MIF, Macrophage mobility inhibitory factor; CMC-Na, Carboxymethylcellulose.
1. Kim YY, Lee S, Kim MJ, Kang BC, Dhakal H, Choi YA, et al. Tyrosol attenuates lipopolysaccharide-induced acute lung injury by inhibiting the inflammatory response and maintaining the alveolar capillary barrier. Food Chem Toxicol (2017) 109:526–33. doi: 10.1016/j.fct.2017.09.053
2. Niu X, Zang L, Li W, Xiao X, Yu J, Yao Q, et al. Anti-inflammatory effect of yam glycoprotein on lipopolysaccharide-induced acute lung injury via the NLRP3 and NF-κB/TLR4 signaling pathway. Int Immunopharmacol (2020) 81:106024. doi: 10.1016/j.intimp.2019.106024
3. Aggarwal NR, King LS, D'Alessio FR. Diverse macrophage populations mediate acute lung inflammation and resolution. Am J Physiol Lung Cell Mol Physiol (2014) 306(8):L709–25. doi: 10.1152/ajplung.00341.2013
4. Zhu T, Zhang W, Wang D-X. Insulin up-regulates epithelial sodium channel in LPS-induced acute lung injury model in rats by SGK1 activation. Injury (2012) 43(8):1277–83. doi: 10.1016/j.injury.2012.04.004
5. Wang Y, Huang C, Bian E, Lei T, Lv X, Li J. NLRC5 negatively regulates inflammatory responses in LPS-induced acute lung injury through NF-κB and p38 MAPK signal pathways. Toxicol Appl Pharmacol (2020) 403:115150. doi: 10.1016/j.taap.2020.115150
6. Sahu B, Narota A, Naura AS. Pharmacological inhibition of poly (ADP-ribose) polymerase by olaparib, prevents acute lung injury associated cognitive deficits potentially through suppression of inflammatory response. Eur J Pharmacol (2020) 877:173091. doi: 10.1016/j.ejphar.2020.173091
7. Kuo M-Y, Liao M-F, Chen F-L, Li Y-C, Yang M-L, Lin R-H, et al. Luteolin attenuates the pulmonary inflammatory response involves abilities of antioxidation and inhibition of MAPK and NFκB pathways in mice with endotoxin-induced acute lung injury. Food Chem Toxicol (2011) 49(10):2660–6. doi: 10.1016/j.fct.2011.07.012
8. Chu P-Y, Chien S-P, Hsu D-Z, Liu M-Y. Protective effect of sesamol on the pulmonary inflammatory response and lung injury in endotoxemic rats. Food Chem Toxicol (2010) 48(7):1821–6. doi: 10.1016/j.fct.2010.04.014
9. Elicker BM, Jones KT, Naeger DM, JA. Imaging of acute lung injury. Radiol Clin North Am (2016) 54(6):1119–32. doi: 10.1016/j.rcl.2016.05.006
10. Wan M, Yao Y-F, Wu W, Fu W-W, Wu R-T, Li W-J. Chimonanthus nitens oliv. essential oil mitigates lipopolysaccharide-induced acute lung injury in rats. Food Chem Toxicol (2021) 156:112445. doi: 10.1016/j.fct.2021.112445
11. Tang J, Xu L, Zeng Y, Gong F. Effect of gut microbiota on LPS-induced acute lung injury by regulating the TLR4/NF-kB signaling pathway. Int Immunopharmacol (2021) 91:107272. doi: 10.1016/j.intimp.2020.107272
12. Chen H, Bai C, Wang X. The value of the lipopolysaccharide-induced acute lung injury model in respiratory medicine. Expert Rev Respir Med (2010) 4(6):773–83. doi: 10.1586/ers.10.71
13. Wang M, Zhong H, Zhang X, Huang X, Wang J, Li Z, et al. EGCG promotes PRKCA expression to alleviate LPS-induced acute lung injury and inflammatory response. Sci Rep (2021) 11(1):11014. doi: 10.1038/s41598-021-90398-x
14. de Souza Xavier Costa N, Ribeiro Júnior G, Dos Santos Alemany AA, Belotti L, Zati DH, Frota Cavalcante M, et al. Early and late pulmonary effects of nebulized LPS in mice: An acute lung injury model. PloS One (2017) 12(9):e0185474. doi: 10.1371/journal.pone.0185474
15. Zhang B, Liu Z-Y, Li Y-Y, Luo Y, Liu M-L, Dong H-Y, et al. Antiinflammatory effects of matrine in LPS-induced acute lung injury in mice. Eur J Pharm Sci (2011) 44(5):573–9. doi: 10.1016/j.ejps.2011.09.020
16. Yuan C-B, Tian L, Yang B, Zhou H-Y. Isoalantolactone protects LPS-induced acute lung injury through Nrf2 activation. Microb Pathog (2018) 123:213–8. doi: 10.1016/j.micpath.2018.07.010
17. Shaaban AA, El-Kashef DH, Hamed MF, El-Agamy DS. Protective effect of pristimerin against LPS-induced acute lung injury in mice. Int Immunopharmacol (2018) 59:31–9. doi: 10.1016/j.intimp.2018.03.033
18. Zhang X-P, Zhang J, Ma M-L, Cai Y, Xu R-J, Xie Q, et al. Pathological changes at early stage of multiple organ injury in a rat model of severe acute pancreatitis. Hepatobil Pancreat Dis Int (2010) 9(1):83–7.
19. Mokhtari-Zaer A, Norouzi F, Askari VR, Khazdair MR, Roshan NM, Boskabady M, et al. The protective effect of nigella sativa extract on lung inflammation and oxidative stress induced by lipopolysaccharide in rats. J Ethnopharmacol (2020) 253:112653. doi: 10.1016/j.jep.2020.112653
20. Ma N, Yang G-Z, Liu X-W, Yang Y-J, Mohamed I, Liu G-R, et al. Impact of aspirin eugenol ester on cyclooxygenase-1, cyclooxygenase-2, c-reactive protein, prothrombin and arachidonate 5-lipoxygenase in healthy rats. Iranian J Pharm Res (2017) 16(4):1443–51.
21. Huang M-Z, Yang Y-J, Liu X-W, Qin Z, Li J-Y. Aspirin eugenol ester reduces HO-induced oxidative stress of HUVECs via mitochondria-lysosome axis. Oxid Med Cell Longev (2019) 2019:8098135. doi: 10.1155/2019/8098135
22. Huang M-Z, Yang Y-J, Liu X-W, Qin Z, Li J-Y. Aspirin eugenol ester attenuates oxidative injury of vascular endothelial cells by regulating NOS and Nrf2 signalling pathways. Br J Pharmacol (2019) 176(7):906–18. doi: 10.1111/bph.14592
23. Zhang Z-D, Yang Y-J, Liu X-W, Qin Z, Li S-H, Li J-Y. Corrigendum to "Aspirin eugenol ester ameliorates paraquat-induced oxidative damage through ROS/p38-MAPK-mediated mitochondrial apoptosis pathway" [Toxicology 453 (2021) 152721]. Toxicology (2021) 454:152763. doi: 10.1016/j.tox.2021.152721
24. Zhang Z-D, Huang M-Z, Yang Y-J, Liu X-W, Qin Z, Li S-H, et al. Aspirin eugenol ester attenuates paraquat-induced hepatotoxicity by inhibiting oxidative stress. Front Physiol (2020) 11:582801. doi: 10.3389/fphys.2020.582801
25. Karam I, Ma N, Liu X-W, Kong X-J, Zhao X-L, Yang Y-J, et al. Lowering effects of aspirin eugenol ester on blood lipids in rats with high fat diet. Lipids Health Dis (2016) 15(1):196. doi: 10.1186/s12944-016-0369-2
26. Karam I, Ma N, Liu X-W, Li S-H, Kong X-J, Li J-Y, et al. Regulation effect of aspirin eugenol ester on blood lipids in wistar rats with hyperlipidemia. BMC Vet Res (2015) 11:217. doi: 10.1186/s12917-015-0523-5
27. Ma N, Yang Y, Liu X, Kong X, Li S, Qin Z, et al. UPLC-Q-TOF/MS-based metabonomic studies on the intervention effects of aspirin eugenol ester in atherosclerosis hamsters. Sci Rep (2017) 7(1):10544. doi: 10.1038/s41598-017-11422-7
28. Ma N, Liu X, Kong X, Li S, Jiao Z, Qin Z, et al. Feces and liver tissue metabonomics studies on the regulatory effect of aspirin eugenol eater in hyperlipidemic rats. Lipids Health Dis (2017) 16(1):240. doi: 10.1186/s12944-017-0633-0
29. Shen D-S, Yang Y-J, Kong X-J, Ma N, Liu X-W, Li S-H, et al. Aspirin eugenol ester inhibits agonist-induced platelet aggregation in vitro by regulating PI3K/Akt, MAPK and sirt 1/CD40L pathways. Eur J Pharmacol (2019) 852:1–13. doi: 10.1016/j.ejphar.2019.02.032
30. Ma N, Liu X-W, Yang Y-J, Shen D-S, Zhao X-L, Mohamed I, et al. Evaluation on antithrombotic effect of aspirin eugenol ester from the view of platelet aggregation, hemorheology, TXB2/6-keto-PGF1α and blood biochemistry in rat model. BMC Vet Res (2016) 12(1):108.
31. Zhang Z-D, Yang Y-J, Liu X-W, Qin Z, Li S-H, Li J-Y. The protective effect of aspirin eugenol ester on paraquat-induced acute liver injury rats. Front Med (Lausanne) (2020) 7:589011. doi: 10.3389/fmed.2020.589011
32. Hu X, Shen H, Wang Y, Zhang L, Zhao M. Aspirin-triggered resolvin D1 alleviates paraquat-induced acute lung injury in mice. Life Sci (2019) 218:38–46. doi: 10.1016/j.lfs.2018.12.028
33. Chen C-M, Tung Y-T, Wei C-H, Lee P-Y, Chen W. Anti-inflammatory and reactive oxygen species suppression through aspirin pretreatment to treat hyperoxia-induced acute lung injury in NF-κB-Luciferase inducible transgenic mice. Antioxid (Basel) (2020) 9(5). doi: 10.3390/antiox9050429
34. Tuinman PR, Vlaar AP, Binnenkade JM, Juffermans NP. The effect of aspirin in transfusion-related acute lung injury in critically ill patients. Anaesthesia (2012) 67(6):594–9. doi: 10.1111/j.1365-2044.2011.07054.x
35. Looney MR, Nguyen JX, Hu Y, Van Ziffle JA, Lowell CA, Matthay MA. Platelet depletion and aspirin treatment protect mice in a two-event model of transfusion-related acute lung injury. J Clin Invest (2009) 119(11):3450–61. doi: 10.1172/JCI38432
36. Grigg J. Air pollution and suppression of lung function growth: A triumph for epidemiology. Am J Respir Crit Care Med (2020) 201(4):400–1. doi: 10.1164/rccm.201911-2219ED
37. Shaddick G, Thomas ML, Amini H, Broday D, Cohen A, Frostad J, et al. Data integration for the assessment of population exposure to ambient air pollution for global burden of disease assessment. Environ Sci Technol (2018) 52(16):9069–78. doi: 10.1021/acs.est.8b02864
38. Garshick E, Grady ST, Hart JE, Coull BA, Schwartz JD, Laden F, et al. Indoor black carbon and biomarkers of systemic inflammation and endothelial activation in COPD patients. Environ Res (2018) 165:358–64. doi: 10.1016/j.envres.2018.05.010
39. Tiotiu AI, Novakova P, Nedeva D, Chong-Neto HJ, Novakova S, Steiropoulos P, et al. Impact of air pollution on asthma outcomes. Int J Environ Res Public Health (2020) 17(17):6212. doi: 10.3390/ijerph17176212
40. Hoek G, Pattenden S, Willers S, Antova T, Fabianova E, Braun-Fahrländer C, et al. PM10, and children's respiratory symptoms and lung function in the PATY study. Eur Respir J (2012) 40(3):538–47. doi: 10.1183/09031936.00002611
41. Kurt OK, Zhang J, Pinkerton KE. Pulmonary health effects of air pollution. Curr Opin Pulm Med (2016) 22(2):138–43. doi: 10.1097/MCP.0000000000000248
42. Khaniabadi YO, Daryanoosh M, Sicard P, Takdastan A, Hopke PK, Esmaeili S, et al. Chronic obstructive pulmonary diseases related to outdoor PM, O, SO, and NO in a heavily polluted megacity of Iran. Environ Sci pollut Res Int (2018) 25(18):17726–34. doi: 10.1007/s11356-018-1902-9
43. Hansel NN, McCormack MC, Kim V. The effects of air pollution and temperature on COPD. COPD (2016) 13(3):372–9. doi: 10.3109/15412555.2015.1089846
44. Zhou J, Qi C, Fang X, Wang Z, Zhang S, Li D, et al. DJ-1 modulates Nrf2-mediated MRP1 expression by activating Wnt3a/β-catenin signalling in A549 cells exposed to cigarette smoke extract and LPS. Life Sci (2021) 276:119089. doi: 10.1016/j.lfs.2021.119089
45. Hu X, Li H, Fu L, Liu F, Wang H, Li M, et al. The protective effect of hyperin on LPS-induced acute lung injury in mice. Microb Pathog (2019) 127:116–20. doi: 10.1016/j.micpath.2018.11.048
46. Zhao Y, Zhang Y, Kong H, Cheng G, Qu H, Zhao Y. Protective effects of carbon dots derived from carbonisata against acute lung injury induced by lipopolysaccharides in rats. Int J Nanomedicine (2022) 17:1–14. doi: 10.2147/IJN.S338886
47. Hu J-T, Lai J, Zhou W, Chen XF, Zhang C, Pan YP, et al. Hypothermia alleviated LPS-induced acute lung injury in rat models through TLR2/MyD88 pathway. Exp Lung Res (2018) 44(8-9):397–404. doi: 10.1080/01902148.2018.1557299
48. Jimenez RV, Szalai AJ. Therapeutic lowering of c-reactive protein. Front Immunol (2020) 11:619564. doi: 10.3389/fimmu.2020.619564
49. Kargapolova Y, Geißen S, Zheng R, Baldus S, Winkels H, Adam M. The enzymatic and non-enzymatic function of myeloperoxidase (MPO) in inflammatory communication. Antioxid (Basel) (2021) 10(4):562. doi: 10.3390/antiox10040562
50. Arnhold J. The dual role of myeloperoxidase in immune response. Int J Mol Sci (2020) 21(21):8057. doi: 10.3390/ijms21218057
51. Ndrepepa G. Myeloperoxidase - a bridge linking inflammation and oxidative stress with cardiovascular disease. Clin Chim Acta (2019) 493:36–51. doi: 10.1016/j.cca.2019.02.022
52. Nyssen P, Maho A, Malempré R, Matagne A, Mouithys-Mickalad A, Hoebeke M. Propofol inhibits the myeloperoxidase activity by acting as substrate through a redox process. Biochim Biophys Acta Gen Subj (2022) 1866(5):130100. doi: 10.1016/j.bbagen.2022.130100
53. de Oliveira MTP, Coutinho D, Guterres SS, Pohlmann AR, Silva PMRE, Martins MA, et al. Resveratrol-loaded lipid-core nanocapsules modulate acute lung inflammation and oxidative imbalance induced by LPS in mice. Pharmaceutics (2021) 13(5):683. doi: 10.3390/pharmaceutics13050683
54. Jiang W, Li M, He F, Yao W, Bian Z, Wang X, et al. Protective effects of Asiatic acid against spinal cord injury-induced acute lung injury in rats. Inflammation (2016) 39(6):1853–61. doi: 10.1007/s10753-016-0414-3
55. Lee JPW, Foote A, Fan H, Peral de Castro C, Lang T, Jones SA, et al. Loss of autophagy enhances MIF/macrophage migration inhibitory factor release by macrophages. Autophagy (2016) 12(6):907–16. doi: 10.1080/15548627.2016.1164358
56. Bruchfeld A, Wendt M, Miller EJ. Macrophage migration inhibitory factor in clinical kidney disease. Front Immunol (2016) 7:8. doi: 10.3389/fimmu.2016.00008
57. Hu X, Tian Y, Qu S, Cao Y, Li S, Zhang W, et al. Protective effect of TM6 on LPS-induced acute lung injury in mice. Sci Rep (2017) 7(1):572. doi: 10.1038/s41598-017-00551-8
58. Grommes J, Soehnlein O. Contribution of neutrophils to acute lung injury. Mol Med (2011) 17(3-4):293–307. doi: 10.2119/molmed.2010.00138
59. Di A, Mehta D, Malik AB. ROS-activated calcium signaling mechanisms regulating endothelial barrier function. Cell Calcium (2016) 60(3):163–71. doi: 10.1016/j.ceca.2016.02.002
60. Zhu R, Zhao Y, Li X, Bai T, Wang S, Wang W, et al. Effects of penehyclidine hydrochloride on severe acute pancreatitis-associated acute lung injury in rats. BioMed Pharmacother (2018) 97:1689–93. doi: 10.1016/j.biopha.2017.12.025
61. Müller-Redetzky HC, Suttorp N, Witzenrath M. Dynamics of pulmonary endothelial barrier function in acute inflammation: mechanisms and therapeutic perspectives. Cell Tissue Res (2014) 355(3):657–73. doi: 10.1007/s00441-014-1821-0
62. Lee K-Y, Rhim J-W, Kang J-H. Hyperactive immune cells (T cells) may be responsible for acute lung injury in influenza virus infections: a need for early immune-modulators for severe cases. Med Hypotheses (2011) 76(1):64–9. doi: 10.1016/j.mehy.2010.08.032
63. Fan H, Zhang S, Li N, Fan P, Hu X, Liang K, et al. Stable expression ratios of five pyroptosis-inducing cytokines in the spleen and thymus of mice showed potential immune regulation at the organ level. Lupus (2020) 29(3):290–302. doi: 10.1177/0961203320903802
64. Cooper GN, Stuart AE. Non-specific immunity in pneumococcal infection of mice[J]. Nature (1961) 191:295–6. doi: 10.1038/191295a0
65. Mittal M, Siddiqui MR, Tran K, Reddy SP. Reactive oxygen species in inflammation and tissue injury. Antioxid Redox Signal (2014) 20(7):1126–67. doi: 10.1089/ars.2012.5149
66. Mateen S, Shahzad S, Ahmad S, Naeem SS, Khalid S, Akhtar K, et al. Cinnamaldehyde and eugenol attenuates collagen induced arthritis via reduction of free radicals and pro-inflammatory cytokines. Phytomedicine (2019) 53:70–8. doi: 10.1016/j.phymed.2018.09.004
67. Biswas SK. Does the interdependence between oxidative stress and inflammation explain the antioxidant paradox? Oxid Med Cell Longev (2016) 2016:5698931. doi: 10.1155/2016/5698931
68. Zhou Z, Su Y, Fa X-E. Isorhynchophylline exerts anti-inflammatory and anti-oxidative activities in LPS-stimulated murine alveolar macrophages. Life Sci (2019) 223:137–45. doi: 10.1016/j.lfs.2019.03.017
69. Guo R-F, Ward PA. Role of oxidants in lung injury during sepsis. Antioxid Redox Signal (2007) 9(11):1991–2002. doi: 10.1089/ars.2007.1785
70. Barrera G, Pizzimenti S, Daga M, Dianzani C, Arcaro A, Cetrangolo GP, et al. Lipid peroxidation-derived aldehydes, 4-hydroxynonenal and malondialdehyde in aging-related disorders. Antioxid (Basel) (2018) 7(8). doi: 10.3390/antiox7080102
71. Hu Q, Wei B, Wei L, Hua K, Yu X, Li H, et al. Sodium tanshinone IIA sulfonate ameliorates ischemia-induced myocardial inflammation and lipid accumulation in beagle dogs through NLRP3 inflammasome. Int J Cardiol (2015) 196:183–92. doi: 10.1016/j.ijcard.2015.05.152
72. Wu X, Cao W, Jia G, Zhao H, Chen X, Wu C, et al. New insights into the role of spermine in enhancing the antioxidant capacity of rat spleen and liver under oxidative stress. Anim Nutr (2017) 3(1):85–90. doi: 10.1016/j.aninu.2016.11.005
73. Khazri O, Charradi K, Limam F, El May MV, Aouani E. Grape seed and skin extract protects against bleomycin-induced oxidative stress in rat lung. BioMed Pharmacother (2016) 81:242–9. doi: 10.1016/j.biopha.2016.04.004
74. Abdel-Halim KY, Osman SR, Abdou GY. In vivo evaluation of oxidative stress and biochemical alteration as biomarkers in glass clover snail, monacha cartusiana exposed to zinc oxide nanoparticles. Environ pollut (2020) 257:113120. doi: 10.1016/j.envpol.2019.113120
75. Dikmen N, Cellat M, Etyemez M, İşler CT, Uyar A, Aydın T, et al. Ameliorative effects of oleuropein on lipopolysaccharide-induced acute lung injury model in rats. Inflammation (2021) 44(6):2246–59. doi: 10.1007/s10753-021-01496-x
76. Baradaran Rahimi V, Rakhshandeh H, Raucci F, Buono B, Shirazinia R, Samzadeh Kermani A, et al. Anti-inflammatory and anti-oxidant activity of extract on LPS-induced rat lung injury. Molecules (2019) 24(1):139. doi: 10.3390/molecules24010139
77. Ha HC, Sirisoma NS, Kuppusamy P, Zweier JL, Woster PM, Casero RA. The natural polyamine spermine functions directly as a free radical scavenger. Proc Natl Acad Sci U.S.A. (1998) 95(19):11140–5. doi: 10.1073/pnas.95.19.11140
78. Rider JE, Hacker A, Mackintosh CA, Pegg AE, Woster PM. Spermine and spermidine mediate protection against oxidative damage caused by hydrogen peroxide. Amino Acids (2007) 33(2):231–40. doi: 10.1007/s00726-007-0513-4
79. Smirnova OA, Isaguliants MG, Hyvonen MT, Keinanen TA, Tunitskaya VL, Vepsalainen J, et al. Chemically induced oxidative stress increases polyamine levels by activating the transcription of ornithine decarboxylase and spermidine/spermine-N1-acetyltransferase in human hepatoma HUH7 cells. Biochimie (2012) 94(9):1876–83. doi: 10.1016/j.biochi.2012.04.023
80. Gao H, Yang T, Chen X, Song Y. Changes of lipopolysaccharide-induced acute kidney and liver injuries in rats based on metabolomics analysis. J Inflammation Res (2021) 14:1807–25. doi: 10.2147/JIR.S306789
81. Levine RL, Mosoni L, Berlett BS, Stadtman ER. Methionine residues as endogenous antioxidants in proteins. Proc Natl Acad Sci USA (1996) 93(26):15036–40. doi: 10.1073/pnas.93.26.15036
82. Fang Y-Z, Yang S, Wu G. Free radicals, antioxidants, and nutrition. Nutrition (2002) 18(10):872–9. doi: 10.1016/S0899-9007(02)00916-4
83. Ercal N, Gurer-Orhan H, Aykin-Burns N. Toxic metals and oxidative stress part I: mechanisms involved in metal-induced oxidative damage. Curr Top Med Chem (2001) 1(6):529–39. doi: 10.2174/1568026013394831
84. Tian R, Yang C, Chai S-M, Guo H, Seim I, Yang G. Evolutionary impacts of purine metabolism genes on mammalian oxidative stress adaptation. Zool Res (2022) 43(2):241–54. doi: 10.24272/j.issn.2095-8137.2021.420
Keywords: aspirin eugenol ester (AEE), acute lung injury, inflammation, oxidative stress, metabolites
Citation: Tao Q, Zhang Z-D, Qin Z, Liu X-W, Li S-H, Bai L-X, Ge W-B, Li J-Y and Yang Y-J (2022) Aspirin eugenol ester alleviates lipopolysaccharide-induced acute lung injury in rats while stabilizing serum metabolites levels. Front. Immunol. 13:939106. doi: 10.3389/fimmu.2022.939106
Received: 08 May 2022; Accepted: 06 July 2022;
Published: 29 July 2022.
Edited by:
Yongqing Li, University of Michigan, United StatesReviewed by:
Emanuel Kennedy-Feitosa, Federal University Rural Semi-Arid, BrazilCopyright © 2022 Tao, Zhang, Qin, Liu, Li, Bai, Ge, Li and Yang. This is an open-access article distributed under the terms of the Creative Commons Attribution License (CC BY). The use, distribution or reproduction in other forums is permitted, provided the original author(s) and the copyright owner(s) are credited and that the original publication in this journal is cited, in accordance with accepted academic practice. No use, distribution or reproduction is permitted which does not comply with these terms.
*Correspondence: Jian-Yong Li, bGlqeTE5NzFAMTYzLmNvbQ==; Ya-Jun Yang, eWFueXVlMTAyMjRAMTYzLmNvbQ==
†These authors have contributed equally to this work
Disclaimer: All claims expressed in this article are solely those of the authors and do not necessarily represent those of their affiliated organizations, or those of the publisher, the editors and the reviewers. Any product that may be evaluated in this article or claim that may be made by its manufacturer is not guaranteed or endorsed by the publisher.
Research integrity at Frontiers
Learn more about the work of our research integrity team to safeguard the quality of each article we publish.