- 1School and Hospital of Stomatology, Shanxi Medical University, Taiyuan, China
- 2Shanxi Province Key Laboratory of Oral Diseases Prevention and New Materials, Taiyuan, China
- 3Science and Technology Information and Strategy Research Center of Shanxi, Taiyuan, China
The therapeutic outcomes of exosome-based therapies have greatly exceeded initial expectations in many clinically intractable diseases due to the safety, low toxicity, and immunogenicity of exosomes, but the production of the exosomes is a bottleneck for wide use. To increase the yield of the exosomes, various solutions have been tried, such as hypoxia, extracellular acidic pH, etc. With a limited number of cells or exosomes, an alternative approach has been developed to improve the efficacy of exosomes through cell pretreatment recently. Melatonin is synthesized from tryptophan and secreted in the pineal gland, presenting a protective effect in pathological conditions. As a new pretreatment method, melatonin can effectively enhance the antioxidant, anti-inflammatory, and anti-apoptotic function of exosomes in chronic kidney disease, diabetic wound healing, and ischemia-reperfusion treatments. However, the current use of melatonin pretreatment varies widely. Here, we discuss the effects of melatonin pretreatment on the heterogeneity of exosomes based on the role of melatonin and further speculate on the possible mechanisms. Finally, the therapeutic use of exosomes and the usage of melatonin pretreatment are described.
Introduction
The therapeutic outcomes of exosome-based therapies have greatly exceeded initial expectations in clinical refractory diseases (1), such as cardiovascular diseases, neurodegenerative diseases, and cancer progression (2). Compared with cell therapy, the exosome therapeutic platform has the advantages of safety, low toxicity, and immunogenicity (3), which can be administered repeatedly (4). As a new therapeutic platform, there are still many technical problems during application. The low yield of exosomes remains a bottleneck in the large-scale implementation (5).
Isolation of exosomes is the first step. Standard exosome isolation techniques are based on differential ultracentrifugation, size, bead, and polymer (6). All these methods are considered tedious and challenging because they require large sample volumes, multi-step operations, and several days. The low production of exosomes greatly hinders their wide application (5, 7). In the clinic, a dose of 10 (8) exosomes is typically needed per treatment for a patient (9), which requires more than a week and several hundred milliliters of cell supernatant (10, 11). Thus, current productivity is insufficient (12).
Various solutions have been tried to increase the production of exosomes. For example, Ban et al. found that exosome production was increased through changes in environmental pH values (13), but pH affected the stability of the exosomes. Wang et al. reported that toward microfluidic-based exosome isolation, it affects the biological functions of exosomes, such as the targeting of exosomes (6). Hao et al. suggested that knockdown or overexpression of certain genes promotes the release of exosomes but destroys the natural structure of exosomes (8). Up to now, how to regulate exosomes release without impacting the native structure or functions remains unsolved (14).
With a limited number of cells or exosomes, an alternative approach has been developed to improve the efficacy of exosomes through cell pretreatment recently. Melatonin (N-acetyl-5-methoxytryptamine) can effectively enhance the antioxidant, anti-inflammatory, and anti-apoptotic functions of exosomes. Exosomes extracted from cells pretreated with Melatonin are named MT-exosomes (15, 16). Some studies revealed that the therapeutic potency of exosomes can be improved by melatonin pretreatment in chronic kidney disease (CKD), ischemia-reperfusion (IR) treatments, and wound healing in diabetes (15–18). It is considered a booster to break through the barrier of exosome application quickly. However, there is great variability in the effect on MT-exosomes and how to use the melatonin pretreatment.
Here, we discuss the effects of melatonin pretreatment on the heterogeneity of exosomes based on the role of melatonin. Given the application of melatonin pretreatment on exosomes is immature, we further speculate on the possible mechanisms. Finally, the therapeutic use of exosomes and the usage of melatonin pretreatment are summarized. Encouragingly, melatonin pretreatment provides a unique and new approach to the exosomes, which gives a new way to overcome the “low yield bottlenecks” of exosomes in the therapeutic platform.
Melatonin and melatonin pretreatment
Melatonin, an indole derivative discovered in 1958, is a natural neurotransmitter (19). Controlled by the master clock of the suprachiasmatic nucleus (SCN) of the hypothalamus, melatonin is secreted by the pineal gland according to the circadian rhythm (20) (Figure 1). Melatonin has been known for decades as an animal hormone. However, later in the 1990s, it was also found in plants and is now widely considered a common parental molecule that permeates and influences the viability of all cells (21).
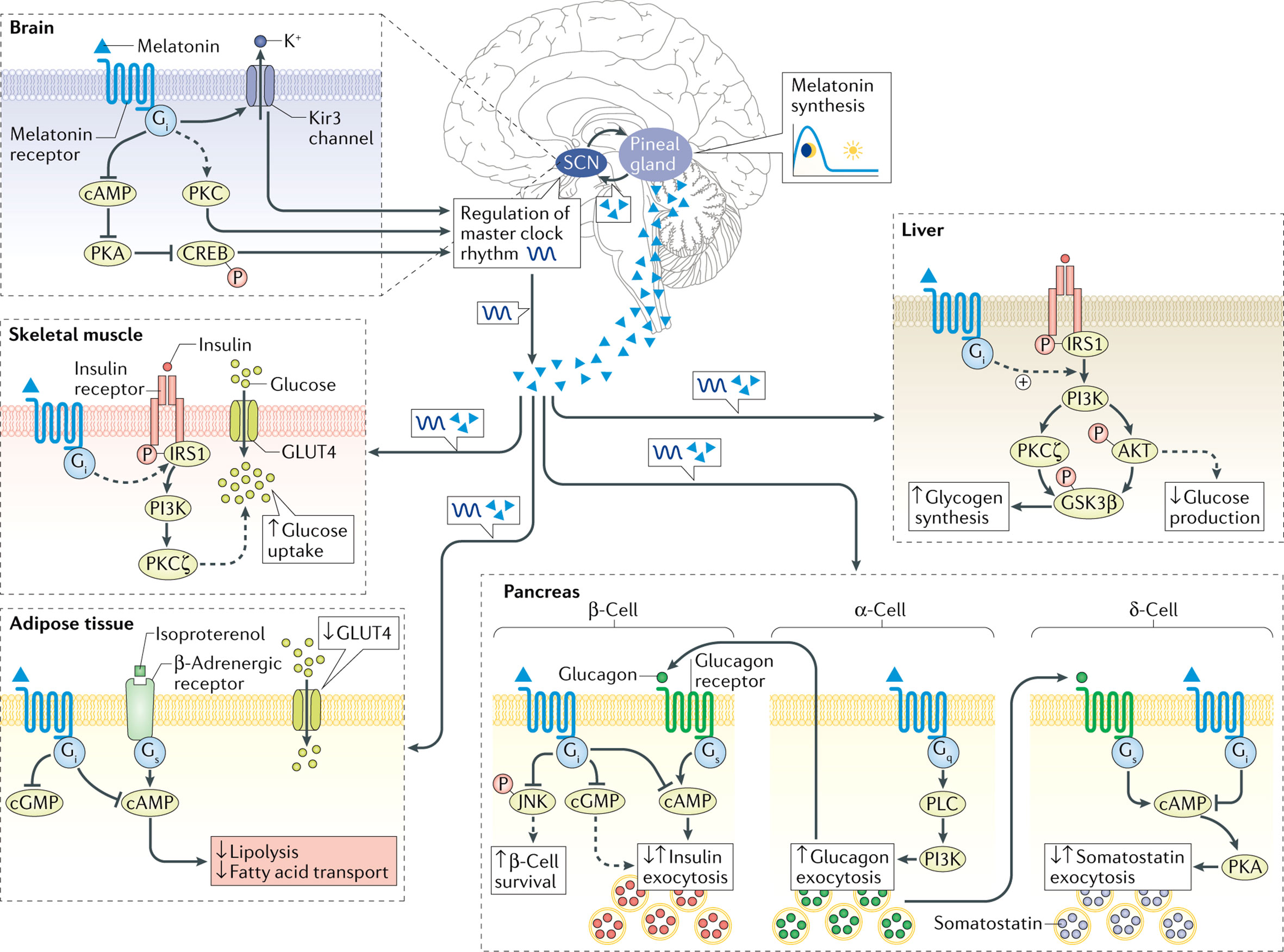
Figure 1 Metabolic processes influenced by melatonin signaling in peripheral and central tissues. Broken lines correspond to the function of melatonin, which exact pathways remain to be elucidated. The dark blue wave-formed line illustrates the circadian clock oscillation. Reprinted by permission from Copyright clearance center: Springer Nature, Nature Reviews Endocrinology, Melatonin in type 2 diabetes mellitus and obesity, Angeliki Karamitri et al, COPYRIGHT (2018).
Melatonin may be a cornucopia, with various therapeutic effects such as antioxidant, anti-inflammatory, anti-infective, and anti-tumor, assisting to treat fertility disorders, osteoporosis, cardiovascular disease, Alzheimer’s disease, obesity, influenza, gastrointestinal tumors, and non–small cell lung cancer (22, 23). In many countries in Europe and the United States, melatonin is widely used both as a prescription drug and as a non-prescription supplement (24).
Melatonin can also be used for pretreatment, meaning that it is added in advance of treatment or injury. Melatonin pretreatment not only improves the therapeutic effect of some diseases (25–27), such as blast injury and kidney IR injury, but also alleviates the toxicity of drugs (28) and plants (Table 1) (29–33). Studies have found that melatonin pretreatment improves the survival rate and angiogenesis of cells (34), as well as the therapeutic effect on solid organ cells (35). Pan et al. found that melatonin inhibited the increase in the ameloblast-lineage cell line (ALC) number in a time-dependent and dose-dependent manner (0, 10–10, 10–8, 10–6, 10–4, and 10−3 M). With the increase in melatonin concentration, the alkaline phosphatase activity of ALCs gradually increased (36). Cells from different sources respond differently to melatonin concentrations. Cucielo et al. found that melatonin alters the mitochondrial membrane and the size of human ovarian carcinoma cells (SKOV-3 cells). After melatonin treatment, SKOV-3 cells showed a statistically significant reduction in mitochondrial metabolism at all concentrations (1.6, 3.2, and 4 mM) (37). Wang et al. found that P21 (cell cycle–associated gene) expression was decreased in granulosa cells treated with the high-concentration (10−5 M) melatonin and increased in that treated with the low-concentration (10−9 M) melatonin (38). Moreover, melatonin pretreatment also affects the exosome release from the donor cells and significantly enhances the therapeutic effects of MT-exosomes (39, 40), which are discussed in the following sections.
Effects of melatonin pretreatment on exosome heterogeneity
The heterogeneity of exosomes mainly includes the size and content of exosomes (41), functional effects on recipient cells, and cell origin; different combinations lead to complex heterogeneity of exosomes (2). Whether melatonin pretreatment affects exosome heterogeneity or not is controversial. In this section, we mainly discuss the heterogeneity of MT-exosomes including size, production, microRNA (miRNA), and protein (Figure 2). The effects of melatonin pretreatment on exosomes, among others, may vary with specific cell types and conditions.
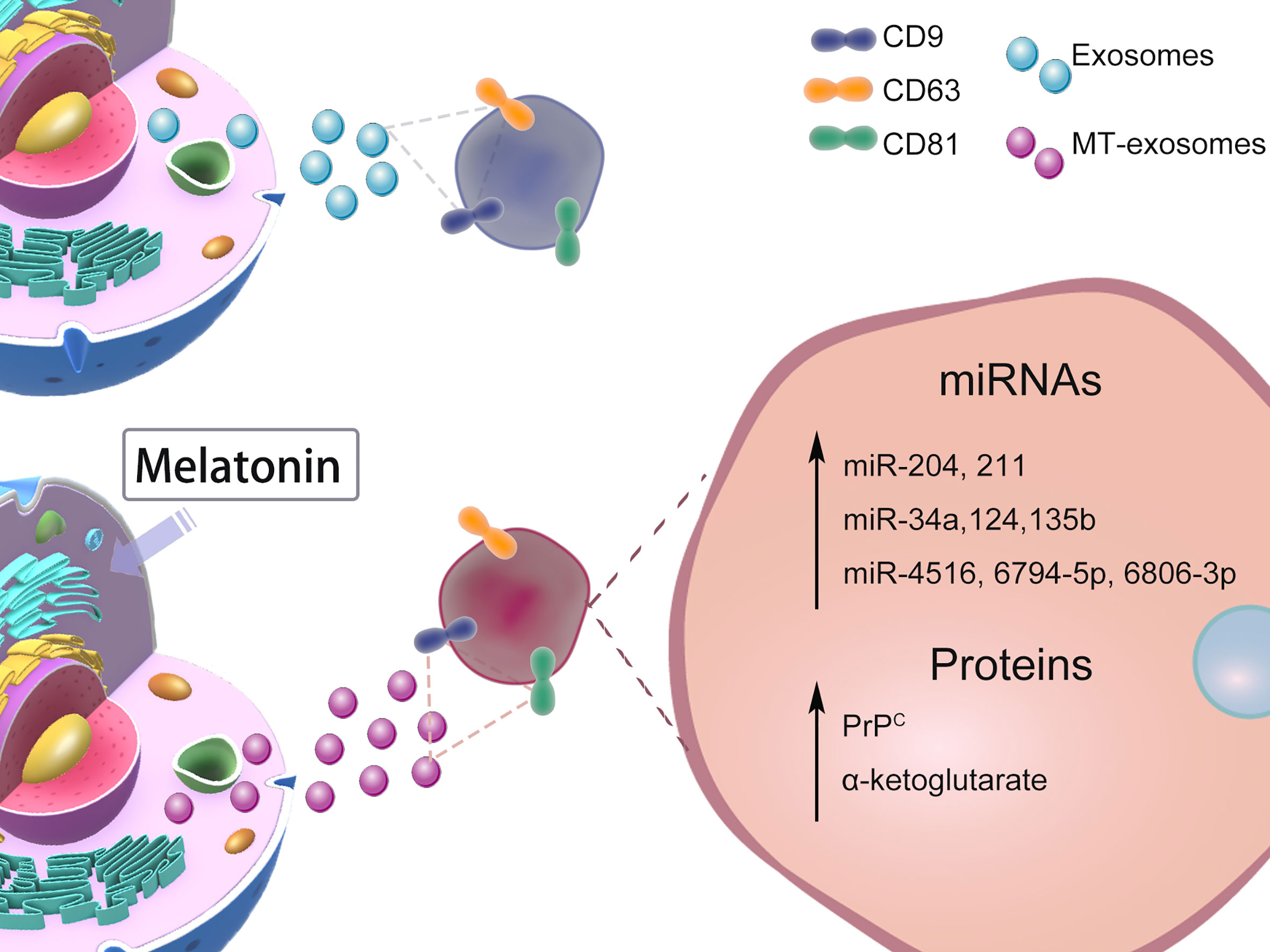
Figure 2 Effects of Melatonin Pretreatment on Exosome heterogeneity: size, production, miRNAs, proteins. The heterogeneity of the effect of melatonin pretreatment on exosomes is still controversial. We mainly list the changes in size, production, and content of exosomes reported by current related studies.
Size and production of MT-exosomes
Melatonin pretreatment affects the exosome size and production with variable effects. On the one hand, some studies suggest that melatonin pretreatment has no significant effect on the size and production of exosomes. Liu et al. observed that both MT-exosomes and exosomes were oval bilayer lipid membrane vesicles with a diameter of about 120 nm and had no significant difference in production (approximately 7.0 × 108 and 7.5 × 108, respectively) (15). Cheng et al. also demonstrated that melatonin pretreatment does not affect exosome production in hepatocellular carcinoma (HCC) (42).
On the other hand, some researchers hold different views (43). Abd-Elhafeez et al. found melatonin pretreatment significantly increases the size and production of exosomes in telocytes (44). Pournaghi et al. reported that melatonin pretreatment significantly reduced the exosome size but increased the production in bovine granulosa cells (45). Ozansoy et al. found that the production of exosomes in SH-SY5Y human neuroblastoma cells decreased by 36.23% after pretreatment with melatonin (46).
Given the current evidence, melatonin may affect the exosomes from various donor cells through different biogenesis mechanisms (45). Heterogeneity can endow unique properties on exosomes based on the organ and tissue from which they originate (2). In addition, considering the difference in experimental conditions, we believe that the concentration of melatonin is another significant factor, which is discussed in the following section.
miRNAs change in MT-exosomes
The heterogeneity of exosomes includes size and yield, as well as content (miRNAs and proteins). In CKD-MSCs, Yoon et al. found that miR-4516, miR-6806-3p, and miR-6794-5p were increased in MT-exosomes (16). In smooth muscle, Xu et al. reported that melatonin pretreatment significantly increased the expression of miR-204 and miR-211 in exosomes from smooth muscle cells (47). In bone, it is considered that melatonin pretreatment increases the content of miR-181 in exosomes and further improves the osteogenesis effect of exosomes (48).
In chronic inflammatory diseases or autoimmune diseases, Heo et al. found the specific miR-34a, miR-124, and miR-135b of anti-inflammatory macrophages (M2) expressed significantly higher in MT-exosomes than in exosomes (49). MT-exosomes can additively attenuate inflammation, which may provide a therapeutic target for immunoregulation and anti-inflammatory responses (50).
Proteins change in MT-exosomes
Specific molecules on the surface of exosomes determine the internalization, immune escape, and targeting of the delivery of exosomes (51), which mediate signaling and help them escape the internalization and into target cells (52). Heo et al. suggested that the exosome marker CD9 was not affected by melatonin pretreatment (49). The same results were found in the CD9, CD81, CD63, apoptosis-linked gene 2-interacting protein X (Alix), and tumor susceptibility gene 101 (TSG101) (2), which demonstrates that exosomes and MT-exosomes have similar surface proteins (15, 16, 42, 49).
After melatonin pretreatment, the internal proteins carried by exosomes change differently. In the murine hindlimb ischemia model, the expression of the cellular prion protein (PrPC) significantly increases in MT-exosomes of healthy MSCs and CKD-MSCs (16), enhancing the proliferation and release of angiogenic cytokines. Liu et al. found that the α-ketoglutarate (metabolite contents) level from MT-exosomes was increased to alleviate inflammatory response (53).
Interestingly, Ozansoy et al. found that the secretion of tau in exosomes of the amyloid-beta (Aβ) toxicity model varied with the time sequence of melatonin treatment. The amount of total tau was not impacted by melatonin pretreatment but significantly reduced by post-treatment of melatonin in exosomes (46). They suggested that the amyloid-beta and melatonin play an important role in the mechanism of exosomes, and the effect of exosomal tau content was time-sensitive in the 8-hour application cycle.
As the product of cells, exosomes are heterogeneous, and it is difficult to isolate and to pool identical exosomes in exosome studies. Currently, there are many methods for analyzing exosome heterogeneity, such as flow cytometer, immunoaffinity capture, and asymmetric flow-field flow fractionation (54). Super-resolution microscopy enables us to accurately quantify multiple characteristics of exosome secretion by single human macrophages (55). This may be a crucial way to resolve and understand the heterogeneity of MT-exosomes.
Possible mechanism of melatonin pretreatment on exosomes
It is inconclusive that the heterogeneity of exosomes is more or less affected by melatonin pretreatment. Alterations in exosome heterogeneity may contribute to the complexity of exosomes, the mechanism of which remains unknown. In this section, we further speculate on the mechanism by which exosomes are pretreated with melatonin as follows (Figure 3). Notably, the mechanisms involved may vary with specific cell types and different conditions.
Generally, exosomes are produced through the endocytic pathway and released through exocytosis (41). Exosomes originate from the terminal endosomes formed by the inward budding of the multivesicular bodies (MVBs) membrane (56, 57). After MVBs dock with the plasma membrane, exosomes are released to the extracellular through exocytosis (58), and midbody remnants are produced by cytokine abscission of interconnecting bridges between dividing cells (59). Otherwise, a part of the MVBs is transported to be merged with the lysosomes to degrade the cargo, and the autophagosome encapsulating the protein and/or organelles can fuse with the endosomes forming an amphisome, which then undergoes lysosomal breakdown (60). Exosomes are regulated in different steps, including their biogenesis, cargo selection, and cell-specific uptake. Among them, exosome biogenesis is a multi-step biological process, which represents the complexity of exosome composition and is also the focus of exosome-related research (61).
Melatonin pretreatment regulates the biogenesis of exosomes by modulating the exocytosis of donor cells. Melatonin pretreatment enhances cell exocytosis to release exosomes via the phosphatidylinositol 3-kinase/protein kinase B (PI3K/Akt) axis inhibiting the activity of glycogen synthase kinase 3 (GSK-3) (62). Melatonin pretreatment also increases the flexibility and fluidity of donor cell membranes and promotes cell exocytosis (63). Moreover, melatonin on fatty acid metabolism may be a molecular mechanism affecting the production of exosomes from donor cells (43, 45).
Melatonin pretreatment affects the biogenesis of exosomes by triggering cell exocytosis and autophagy (64, 65). Autophagy is a regulated self-degrading process that modulates changes in exosome biogenesis in response to changes in external stimuli (66). The melatonin pretreatment in the donor cell causes cell autophagy, which likely changes the biogenesis of exosomes (67). Different autophagy proteins have been proven to regulate exosome biogenesis (68, 69). Autophagy-related proteins (ATGs) are essential regulators of cytosol and membrane autophagy (70). Guo et al. found that ATG5 and ATG16L1 have common signaling pathways between autophagy and exosome biogenesis (69). Melatonin directly stimulates autophagy by activating the ATG4, 5, 7, 10, 12, and 16, increasing the microtubule-associated protein 1A/1B light chain 3 (LC3) II/I rate (71). Melatonin can enhance the autophagy mechanism of cells (67), increasing MVB autophagosome fusion and generating amphisomes (72). Then, amphisomes use specific GTPases (including Rab8a and Rab27a) to release exosomes and autophagic contents (68). Thus, the exosome production and the contents they carry are altered.
To further explore the possible effects of melatonin pretreatment on exosome biogenesis, Amini et al. conducted a bioinformatics analysis based on common genes associated with the melatonin signaling pathway, exosomes biogenesis, and the Wnt cascade (43). Results showed that Pax2 and the transducin-like enhancer of split 4 (TLE4) had interactions between melatonin and exosome biogenesis. It is almost certain that the biogenesis of exosomes was regulated by melatonin pretreatment. Host cells receive external or internal stimuli by inducing specific intracellular signals that then regulate exosome biogenesis (73). The detailed mechanism regarding the effect of melatonin pretreatment on exosome biogenesis still needs to be further clarified in the future.
In addition to regulating exosome biogenesis, we should also consider whether exosomes are qualified to transfer melatonin between cells. Exosomes are a carrier that reveals the nature of the donor cells and the influence of external factors on the donor cells. Direct passive diffusion of melatonin through the cell membrane is acceptable given its lipophilicity and rapid passive diffusion. Researchers continue to explore the mechanism of the transfer of the melatonin receptor MT1 through internalization and endocytic transport (43). When melatonin binds to MT1, the vacuolar sorting machinery uses Rab5 to transfer the internalized MT1 to early endosomes. Endosomes carrying MT1 can cycle to the plasma membrane due to the activity of other GTPases.
Therapeutic potential of MT-exosomes
Currently, the heterogeneity and mechanism of melatonin pretreatment affecting exosomes are still controversial, but it is undeniable that MT-exosomes have greater therapeutic potential. The use of melatonin pretreatment to enhance the therapeutic effect of exosomes is summarized in Table 2.
Anti-inflammatory effects of MT-exosomes
Studies have shown that MT-exosomes significantly improve the polarization of macrophages from proinflammatory macrophages (M1) to anti-inflammatory macrophages (M2) (49). Wang et al. found that melatonin enhances the anti-inflammatory potential of the exosomes through the Toll-like receptors/nuclear factor kappa-B (TLR4/NF-κB) pathway to combat post-stroke inflammation (74). MT-exosomes promote the transformation of macrophages to M2 type by a phosphatase and tensin homolog deleted on the chromosome 10 (PTEN)/AKT signaling pathway (15). MT-exosomes reduce inflammatory cytokines, including interleukin-1β (IL-1β), IL-18, IL-6, and tumor necrosis factor–alpha (TNF-α), increasing the release of anti-inflammatory factors IL-10 and transforming growth factor–β (TGF-β) (Table 3).
Liu et al. suggested that melatonin mediates the inflammatory response by increasing α-ketoglutarate (α-KG) level and transferring to macrophages through exosomes in adipose tissue (Figure 4) (53). Melatonin pretreatment promotes the production of α-KG, which increases the DNA demethylation of adipocytes. Melatonin drives the circadian amplitude of mitochondrial isocitrate dehydrogenase 2 (Idh2) in adipose inflammation, and the gene clock regulates Idh2 at the transcription level. Sirtuin 1 (Sirt1) and Idh2 formed a complex to increase α-KG levels that exosomes inactivate signal transducer and the transcriptional activator 3 (STAT3)/NF-kappaB pathways. Exosomes α-KG transports to macrophages, which promote their transformation to M2 and reduce inflammation (TNFα, IL-6, and IL-1β).
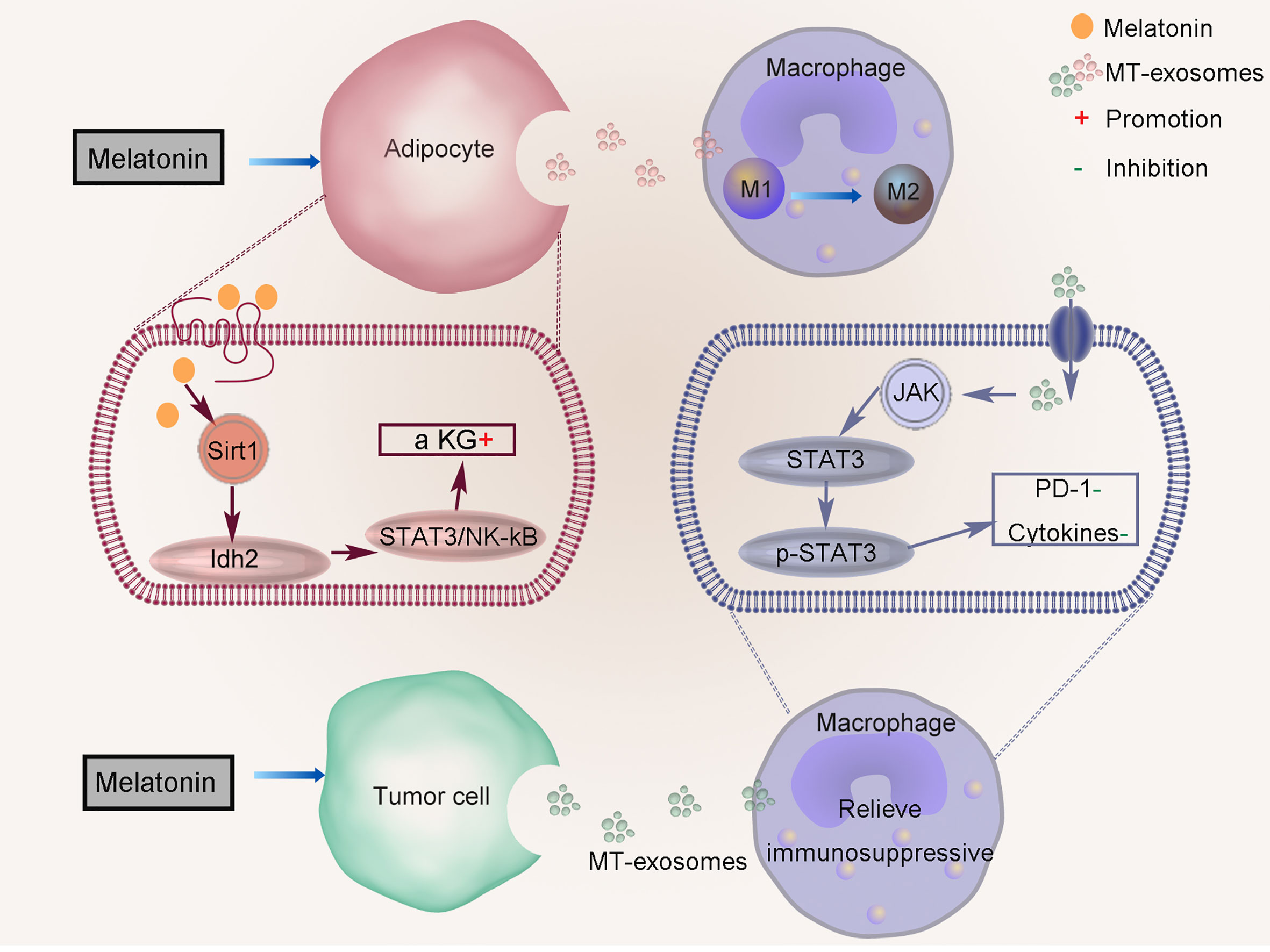
Figure 4 Regulation of anti-inflammatory effect of MT-exosomes. Melatonin mediates the inflammatory response by increasing α-ketoglutarate (α KG) level and transferring to macrophages through exosomes in adipose tissue. MT-exosomes from HCC cells reverse this effect by down-regulating the expression of PD-1 and altering the secretion of macrophage cytokines through inactivating the STAT3 signaling pathway.
The role of MT-exosome is to improve its efficacy based on the therapy of exosomes, which can better alleviate the progression of cell pathology. MT-exosomes can alleviate the pathogenic effects of pathological exosomes on cells, such as exosomes secreted by HCCs. Tumor cells can change the immune status of the tumor microenvironment by upregulating programmed death-ligand 1 (PD-1), and related inflammatory factors such as TNF-a, IL-6, and IL-1β increase significantly. Cheng et al. found that MT-exosomes from HCC cells reverse this effect by downregulating the expression of PD-1 and altering the secretion of macrophage cytokines by inactivating the STAT3 signaling pathway (Figure 4) (42).
Antioxidant and anti-apoptotic effects of MT-exosomes
Melatonin is a powerful antioxidant that scavenges different types of free radicals in body fluids and cells (77, 78). Chen et al. reported that melatonin exerts the protective effect of cellular oxidative stress, also through its anti-apoptosis effect (79). Compared with exosomes, MT-exosomes could play a neuroprotective role by increasing the expression of the anti-apoptotic protein B-cell lymphoma 2 gene (Bcl2) (75).
Inflammation, oxidation, and apoptosis are closely associated with each other. IR injury is a complex, sterile inflammatory cascade (80) and a major cause of cell death and organ damage in many diseases such as myocardial infarction, stroke, and acute kidney injury (81). MT-exosomes have been found to suppress inflammation, oxidative stress, and apoptosis in protecting the liver against IR injury (76). Alzahrani et al. found that treatment with MT-exosomes provided the best protective effect against renal IR injury, compared to the therapy by MSCs or exosomes (75). MT-exosomes decline oxidative stress status (malondialdehyde level and NADPH oxidase 2 protein) and increase antioxidant status (heme oxygenase-1 gene and superoxide dismutase, catalase, glutathione, and peroxidase activities). Moreover, MT-exosomes decline apoptosis (B-cell lymphoma 2–associated X protein genes and caspase 3 activity) and induce an anti-apoptotic effect (Bcl-2) (Table 3). Through the expression of antioxidant and anti-apoptotic markers, it is not difficult to find the enhancement of the antioxidant and anti-apoptotic ability of MT-exosomes.
Usage of melatonin pretreatment
The full use of melatonin pretreatment is firstly summarized in this review. Some studies do not mention whether the concentration of melatonin pretreatment could cause damage to other tissues or any cells. Therefore, we should also pay attention to the potential risks of melatonin pretreatment.
Route of administration
There are two main methods of pretreatment with melatonin. The former is that melatonin is injected into the body through intravenous injection, and then the blood is collected to extract exosomes (74). The latter is to collect melatonin-pretreated cells and then collect the exosomes secreted by the cells (15).
Concentration
Based on Amini et al. and Pournagi et al., et al., recent findings about melatonin pretreatment alter exosome size and production in bovine granulosa cells in a dose-dependent manner in which higher-melatonin concentrations (0.1 nM~0.1 mM) contribute to the elevated release of small-sized exosomes (43, 45).
There is no uniform standard for the concentration of melatonin pretreatment in vivo and in vitro (Table 2) (82, 83). Further consideration needs to be given to the variation of optimal concentration of melatonin pretreatment from individual to individual. Through dissolving melatonin in 0.9% saline (with 5% DMSO), Wang et al. injected intraperitoneally (10 mg/kg) the solution for 7 consecutive days and found reduced brain damage in the stroke rats (84).
Indications
Melatonin can enhance the therapeutic effect of exosomes, mainly including anti-inflammatory and antioxidant effects. Current studies have focused on IR (brain, liver, and kidney), liver cancer, CKD, and wound healing in diabetes (Figure 5). As described above, the MT-exosomes were significantly superior to the exosomes, increasing the therapeutic efficiency by about 0.5 to 3 times (42, 74, 75). However, there is currently no unified standard for quantifying the efficiency of exosome treatment. The quantification of exosome therapeutic efficiency should be further confirmed by further studies.
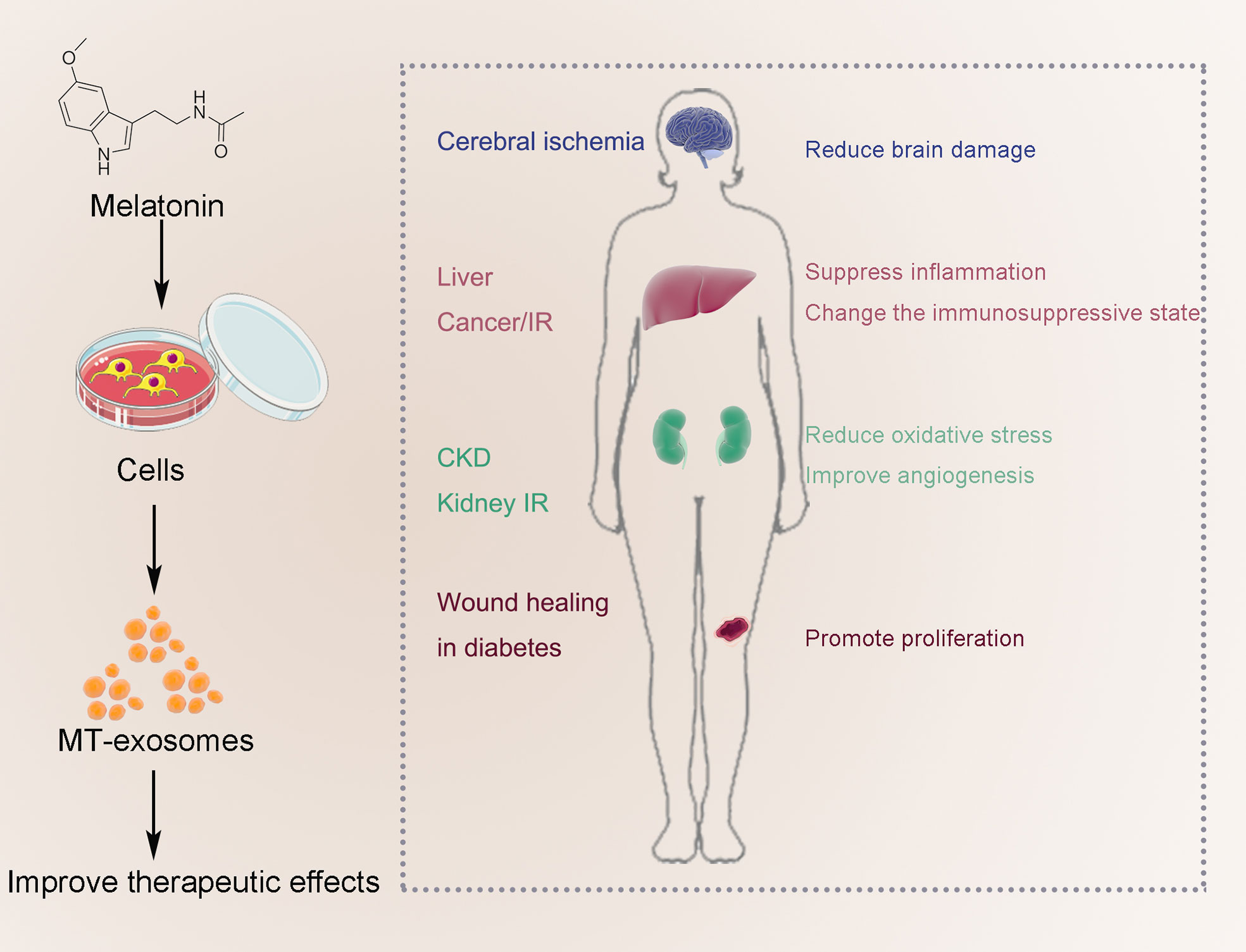
Figure 5 Improve the therapeutic effect of MT-exosomes, mainly including anti-inflammatory and antioxidant effects. Current studies have focused on IR (brain, liver and kidney), liver cancer, AIC, CKD, traumatic spinal cord injury, embryonic development and wound healing in diabetes.
Potential risks
Melatonin is widely used in medicine and food (24). As observed in animal and human studies, the acute toxicity of melatonin is not lethal even at 800 mg/kg (85). Therefore, the potential risks of melatonin were often overlooked. However, there are different responses to melatonin in clinical trials: mild side effects of melatonin in the short and medium terms, such as irritability, headache, and drowsiness (86, 87). Severe or clinically significant adverse events are rare, including excitement, nightmares, mood swings, fatigue, and skin irritation. Most of these effects either subside spontaneously within a few days without adjusting the dose or subside immediately after discontinuation of the treatment (88, 89). There is no evidence that people develop tolerance to melatonin (85). The risk of melatonin pretreatment has not been clearly reported so far, and we speculate its potential risk through the side effects of melatonin.
Melatonin pretreatment can repair abnormal cells to play its anti-inflammatory and antioxidant role. It is worth considering whether melatonin pretreatment can cause damage to normal cells (90). Melatonin pretreatment is relatively nontoxic but has side effects at high concentrations. The cell survival rate began to decrease when the concentration was higher than 0.1 mM (45). Whether the melatonin pretreatment makes the same effect on the secreted exosomes or not is still unclear. Therefore, we should pay more attention to its potential risks in the future, and more preclinical studies are needed to explore the impact, focusing on possible complications.
Concluding remarks and perspectives
As nanoscale biological vesicles, exosomes protect their contents from degradation and facilitate their intercellular transfer, and their natural origin and biological properties facilitate the application of exosomes, making them safe and effective for drug delivery. How to better use exosomes as a therapeutic drug has become a focus of attention.
Melatonin is a potent-free radical scavenger and metal chelator with the ability to relieve oxidative stress and inflammatory responses and stabilize cell membranes. Melatonin pretreatment has emerged as a potentially favored alternative approach to enhance exosome function. There are differences in the pretreatment methods of melatonin, including the way, concentration, and time of pretreatment. The melatonin pretreatment can improve the therapeutic potential of exosomes, such as anti-inflammatory, antioxidant, and anti-apoptotic, by changing the proteins and miRNAs carried in exosomes. Related research has focused on cancer, IR, CKD, and wound healing in diabetes. Melatonin is considered one of the most promising approaches to breaking through the dilemma of exosomes.
However, the application of melatonin pretreatment on exosomes is inchoate, and the complexity of exosome heterogeneity makes the exosomes secreted by cells varied after melatonin pretreatment. There are unknown features of the interaction between exosomes with melatonin pretreatment, which needed to be explored in the future: (1) More specific molecular mechanism of melatonin pretreatment on exosomes. We should always remember that mechanisms may vary with specific cell types and conditions. (2) Cross-talk between melatonin pretreatment with different extracellular vesicles subtypes (exosomes are a subtype of extracellular vesicles). (3) The effects of melatonin pretreatment on exosomes from different cell sources and different disease states are likely to be different. (4) Assessing possible risks and application barriers of melatonin pretreatment on exosomes when there is a reproducible effect of melatonin pretreatment on exosomes. (5) Exploring the long-term effects on cells and the exosomes they secrete with melatonin pretreatment, and the possibility of producing exosomes in bulk.
Author contributions
ZZ and RW contributed equally. ZZ wrote the manuscript. RW was involved in drafting the manuscript. JW, YH, and QX searched the references. XW and LW were responsible for the design and critical revision of the manuscript. All authors read and approved the final manuscript.
Funding
This work was supported by the National Natural Science Foundation of China (grant numbers 81801004 and 82071155), Shanxi Province Key Research and Development Project (grant numbers 201903D321148), and the Open Project of Shanxi Province Key Laboratory of Oral Diseases Prevention and New Materials (grant numbers KF2020-07).
Conflict of interest
The authors declare that the research was conducted in the absence of any commercial or financial relationships that could be construed as a potential conflict of interest.
Publisher’s note
All claims expressed in this article are solely those of the authors and do not necessarily represent those of their affiliated organizations, or those of the publisher, the editors and the reviewers. Any product that may be evaluated in this article, or claim that may be made by its manufacturer, is not guaranteed or endorsed by the publisher.
References
1. Wang X, Xiang Z, Liu Y, Huang C, Pei Y, Wang X, et al. Exosomes derived from Vδ2-T cells control Epstein-Barr virus-associated tumors and induce T cell antitumor immunity. Sci Trans Med (2020) 12(563):eaaz3426. doi: 10.1126/scitranslmed.aaz3426”
2. Kalluri R, LeBleu VS. The biology, function, and biomedical applications of exosomes. Sci (New York N.Y.) (2020) 367(6478):eaau6977. doi: 10.1126/science.aau6977”
3. Su G, Lei X, Wang Z, Xie W, Wen D, Wu Y. Mesenchymal stem cell-derived exosomes affect macrophage phenotype: A cell-free strategy for the treatment of skeletal muscle disorders. Curr Mol Med (2022). doi: 10.2174/1566524022666220511123625”
4. Yang J, Zhang L. The roles and therapeutic approaches of MSC-derived exosomes in colorectal cancer. Clin Trans oncol: Off Publ Fed Spanish Oncol Soci Natl Cancer Inst Mexico (2022) 24(6):959–67. doi: 10.1007/s12094-021-02750-2”
5. Haraszti RA, Miller R, Stoppato M, Sere YY, Coles A, Didiot MC, et al. Exosomes produced from 3D cultures of MSCs by tangential flow filtration show higher yield and improved activity. Mol ther: J Am Soc Gene Ther (2018) 26(12):2838–47. doi: 10.1016/j.ymthe.2018.09.015”
6. Wang J, Ma P, Kim DH, Liu BF, Demirci U. Towards microfluidic-based exosome isolation and detection for tumor therapy. Nano Today (2021) 37:101066. doi: 10.1016/j.nantod.2020.101066”
7. Fan J, Lee CS, Kim S, Chen C, Aghaloo T, Lee M. Generation of small RNA-modulated exosome mimetics for bone regeneration. ACS nano (2020) 14(9):11973–84. doi: 10.1021/acsnano.0c05122
8. Hao Y, Song H, Zhou Z, Chen X, Li H, Zhang Y, et al. Promotion or inhibition of extracellular vesicle release: Emerging therapeutic opportunities. J Controlled release: Off J Controlled Release Soc (2021), 340: 136–148. doi: 10.1016/j.jconrel.2021.10.019
9. Cully M. Exosome-based candidates move into the clinic. Nat Rev Drug Discovery (2021) 20(1):6–7. doi: 10.1038/d41573-020-00220-y
10. Su T, Xiao Y, Xiao Y, Guo Q, Li C, Huang Y, et al. Bone marrow mesenchymal stem cells-derived exosomal MiR-29b-3p regulates aging-associated insulin resistance. ACS nano (2019) 13(2):2450–62. doi: 10.1021/acsnano.8b09375
11. Patel GK, Khan MA, Zubair H, Srivastava SK, Khushman M, Singh S, et al. Comparative analysis of exosome isolation methods using culture supernatant for optimum yield, purity and downstream applications. Sci Rep (2019) 9(1):5335. doi: 10.1038/s41598-019-41800-2
12. Mitchell JP, Court J, Mason MD, Tabi Z, Clayton A. Increased exosome production from tumour cell cultures using the integra CELLine culture system. J Immunol Methods (2008) 335(1-2):98–105. doi: 10.1016/j.jim.2008.03.001
13. Ban JJ, Lee M, Im W, Kim M. Low pH increases the yield of exosome isolation. Biochem Biophys Res Commun (2015) 461(1):76–9. doi: 10.1016/j.bbrc.2015.03.172
14. Yang D, Zhang W, Zhang H, Zhang F, Chen L, Ma L, et al. Progress, opportunity, and perspective on exosome isolation - efforts for efficient exosome-based theranostics. Theranostics (2020) 10(8):3684–707. doi: 10.7150/thno.41580
15. Liu W, Yu M, Xie D, Wang L, Ye C, Zhu Q, et al. Melatonin-stimulated MSC-derived exosomes improve diabetic wound healing through regulating macrophage M1 and M2 polarization by targeting the PTEN/AKT pathway. Stem Cell Res Ther (2020) 11(1):259. doi: 10.1186/s13287-020-01756-x
16. Yoon YM, Lee JH, Song KH, Noh H, Lee SH. Melatonin-stimulated exosomes enhance the regenerative potential of chronic kidney disease-derived mesenchymal stem/stromal cells via cellular prion proteins. J pineal Res (2020) 68(3):e12632. doi: 10.1111/jpi.12632
17. Novais AA, Chuffa LGA, Zuccari D, Reiter RJ. Exosomes and melatonin: Where their destinies intersect. Front Immunol (2021) 12:692022. doi: 10.3389/fimmu.2021.692022
18. Yea JH, Yoon YM, Lee JH, Yun CW, Lee SH. Exosomes isolated from melatonin-stimulated mesenchymal stem cells improve kidney function by regulating inflammation and fibrosis in a chronic kidney disease mouse model. J Tissue Eng (2021) 12:20417314211059624. doi: 10.1177/20417314211059624
19. Xia Y, Chen S, Zeng S, Zhao Y, Zhu C, Deng B, et al. Melatonin in macrophage biology: Current understanding and future perspectives. J pineal Res (2019) 66(2):e12547. doi: 10.1111/jpi.12547
20. Pevet P, Challet E. Melatonin: both master clock output and internal time-giver in the circadian clocks network. J Physiol (2011) 105(4-6):170–82. doi: 10.1016/j.jphysparis.2011.07.001
21. Socaciu AI, Ionuţ R, Socaciu MA, Ungur AP, Bârsan M, Chiorean A, et al. Melatonin, an ubiquitous metabolic regulator: functions, mechanisms and effects on circadian disruption and degenerative diseases. Rev Endocrine Metab Disord (2020) 21(4):465–78. doi: 10.1007/s11154-020-09570-9
22. Lynch HJ, Wurtman RJ, Moskowitz MA, Archer MC, Ho MH. Daily rhythm in human urinary melatonin. Sci (New York N.Y.) (1975) 187(4172):169–71. doi: 10.1126/science.1167425
23. Ferlazzo N, Andolina G, Cannata A, Costanzo MG, Rizzo V, Currò M, et al. Is melatonin the cornucopia of the 21st century? Antioxidants (Basel Switzerland) (2020) 9(11):1088. doi: 10.3390/antiox9111088
24. Bandyopadhyay D, Chattopadhyay A. Reactive oxygen species-induced gastric ulceration: protection by melatonin. Curr Med Chem (2006) 13(10):1187–202. doi: 10.2174/092986706776360842
25. de Farias ACS, de Pieri Pickler K, Bernardo HT, Baldin SL, Dondossola ER, Rico EP. Melatonin pretreatment protects against status epilepticus, glutamate transport, and oxidative stress induced by kainic acid in zebrafish. Mol Neurobiol (2022) 59(1):266–75. doi: 10.1007/s12035-021-02579-4
26. Zhang Y, Cong P, Tong C, Jin H, Liu Y, Hou M. Melatonin pretreatment alleviates blast-induced oxidative stress in the hypothalamic-pituitary-gonadal axis by activating the Nrf2/HO-1 signaling pathway. Life Sci (2021) 280:119722. doi: 10.1016/j.lfs.2021.119722
27. Yang J, Liu H, Han S, Fu Z, Wang J, Chen Y, et al. Melatonin pretreatment alleviates renal ischemia-reperfusion injury by promoting autophagic flux via TLR4/MyD88/MEK/ERK/mTORC1 signaling. FASEB journal: Off Publ Fed Am Soci Exp Biol (2020) 34(9):12324–37. doi: 10.1096/fj.202001252R
28. Liang L, Zeng T, Zhao Y, Lu R, Guo B, Xie R, et al. Melatonin pretreatment alleviates the long-term synaptic toxicity and dysmyelination induced by neonatal sevoflurane exposure via MT1 receptor-mediated wnt signaling modulation. J Pineal Res (2021) 71(4):e12771. doi: 10.1111/jpi.12771
29. Jahan MS, Shu S, Wang Y, Hasan MM, El-Yazied AA, Alabdallah NM, et al. Melatonin pretreatment confers heat tolerance and repression of heat-induced senescence in tomato through the modulation of ABA- and GA-mediated pathways. Front Plant Sci (2021) 12:650955. doi: 10.3389/fpls.2021.650955
30. Tousi S, Zoufan P, Ghahfarrokhie AR. Alleviation of cadmium-induced phytotoxicity and growth improvement by exogenous melatonin pretreatment in mallow (Malva parviflora) plants. Ecotoxicol Environ Saf (2020) 206:111403. doi: 10.1016/j.ecoenv.2020.111403
31. De Butte M, Gieseking B. Efficacy of a low-dose melatonin pretreatment in protecting against the neurobehavioral consequences of chronic hypoperfusion in middle-aged female rats. Behav Brain Res (2020) 377:112257. doi: 10.1016/j.bbr.2019.112257
32. Wang Y, Wang X, Chen J, Li S, Zhai H, Wang Z. Melatonin pretreatment attenuates acute methamphetamine-induced aggression in male ICR mice. Brain Res (2019) 1715:196–202. doi: 10.1016/j.brainres.2019.04.002
33. Nawaz MA, Jiao Y, Chen C, Shireen F, Zheng Z, Imtiaz M, et al. Melatonin pretreatment improves vanadium stress tolerance of watermelon seedlings by reducing vanadium concentration in the leaves and regulating melatonin biosynthesis and antioxidant-related gene expression. J Plant Physiol (2018) 220:115–27. doi: 10.1016/j.jplph.2017.11.003
34. Mias C, Trouche E, Seguelas MH, Calcagno F, Dignat-George F, Sabatier F, et al. Ex vivo pretreatment with melatonin improves survival, proangiogenic/mitogenic activity, and efficiency of mesenchymal stem cells injected into ischemic kidney. Stem Cells (Dayton Ohio) (2008) 26(7):1749–57. doi: 10.1634/stemcells.2007-1000
35. Zhao J, Young YK, Fradette J, Eliopoulos N. Melatonin pretreatment of human adipose tissue-derived mesenchymal stromal cells enhances their prosurvival and protective effects on human kidney cells. Am J Physiol Renal Physiol (2015) 308(12):F1474–83. doi: 10.1152/ajprenal.00512.2014
36. Pan J, Ren Q, Yang Z, Guo Y, Kwon K, Shen C, et al. The effect of melatonin on the mouse ameloblast-lineage cell line ALCs. Sci Rep (2022) 12(1):8225. doi: 10.1038/s41598-022-11912-3
37. Cucielo MS, Cesário RC, Silveira HS, Gaiotte LB, Dos Santos SAA, de Campos Zuccari DAP, et al. Melatonin reverses the warburg-type metabolism and reduces mitochondrial membrane potential of ovarian cancer cells independent of MT1 receptor activation. Molecules (2022) 27(14):4350. doi: 10.3390/molecules27144350
38. Wang S, Liu W, Wen A, Yang B, Pang X. Luzindole and 4P-PDOT block the effect of melatonin on bovine granulosa cell apoptosis and cell cycle depending on its concentration. PeerJ (2021) 9:e10627. doi: 10.7717/peerj.10627
39. Tang Y, Cai B, Yuan F, He X, Lin X, Wang J, et al. Melatonin pretreatment improves the survival and function of transplanted mesenchymal stem cells after focal cerebral ischemia. Cell Transplant (2014) 23(10):1279–91. doi: 10.3727/096368913x667510
40. Zhang Y, Yang N, Huang X, Zhu Y, Gao S, Liu Z, et al. Melatonin engineered adipose-derived biomimetic nanovesicles regulate mitochondrial functions and promote myocardial repair in myocardial infarction. Front Cardiovasc Med (2022) 9:789203. doi: 10.3389/fcvm.2022.789203
41. Gurung S, Perocheau D, Touramanidou L, Baruteau J. The exosome journey: from biogenesis to uptake and intracellular signalling. Cell Comm Signaling: CCS (2021) 19(1):47. doi: 10.1186/s12964-021-00730-1
42. Cheng L, Liu J, Liu Q, Liu Y, Fan L, Wang F, et al. Exosomes from melatonin treated hepatocellularcarcinoma cells alter the immunosupression status through STAT3 pathway in macrophages. Int J Biol Sci (2017) 13(6):723–34. doi: 10.7150/ijbs.19642
43. Amini H, Rezabakhsh A, Heidarzadeh M, Hassanpour M, Hashemzadeh S, Ghaderi S, et al. An examination of the putative role of melatonin in exosome biogenesis. Front Cell Dev Biol (2021) 9:686551. doi: 10.3389/fcell.2021.686551
44. Abd-Elhafeez HH, Mokhtar DM, Hassan AH. Effect of melatonin on telocytes in the seminal vesicle of the soay ram: An immunohistochemical, ultrastructural and morphometrical study. Cells tissues organs (2017) 203(1):29–54. doi: 10.1159/000449500
45. Pournaghi M, Khodavirdilou R, Saadatlou MAE, Nasimi FS, Yousefi S, Mobarak H, et al. Effect of melatonin on exosomal dynamics in bovine cumulus cells. Process Biochem (2021) 106:78–87. doi: 10.1016/j.procbio.2021.03.008
46. Ozansoy M, Ozansoy MB, Yulug B, Cankaya S, Kilic E, Goktekin S, et al. Melatonin affects the release of exosomes and tau-content in in vitro amyloid-beta toxicity model. J Clin Neurosci (2020) 73:237–44. doi: 10.1016/j.jocn.2019.11.046
47. Xu F, Zhong JY, Lin X, Shan SK, Guo B, Zheng MH, et al. Melatonin alleviates vascular calcification and ageing through exosomal miR-204/miR-211 cluster in a paracrine manner. J pineal Res (2020) 68(3):e12631. doi: 10.1111/jpi.12631
48. Liu W, Yu M, Chen F, Wang L, Ye C, Chen Q, et al. A novel delivery nanobiotechnology: engineered miR-181b exosomes improved osteointegration by regulating macrophage polarization. J nanobiotechnol (2021) 19(1):269. doi: 10.1186/s12951-021-01015-y
49. Heo JS, Lim JY, Yoon DW, Pyo S, Kim J. Exosome and melatonin additively attenuates inflammation by transferring miR-34a, miR-124, and miR-135b. BioMed Res Int (2020) 2020:1621394. doi: 10.1155/2020/1621394
50. Mauriz JL, Collado PS, Veneroso C, Reiter RJ, González-Gallego J. A review of the molecular aspects of melatonin’s anti-inflammatory actions: recent insights and new perspectives. J pineal Res (2013) 54(1):1–14. doi: 10.1111/j.1600-079X.2012.01014.x
51. Hessvik NP, Llorente A. Current knowledge on exosome biogenesis and release. Cell Mol Life sciences: CMLS (2018) 75(2):193–208. doi: 10.1007/s00018-017-2595-9
52. Kamerkar S, LeBleu VS, Sugimoto H, Yang S, Ruivo CF, Melo SA, et al. Exosomes facilitate therapeutic targeting of oncogenic KRAS in pancreatic cancer. Nature (2017) 546(7659):498–503. doi: 10.1038/nature22341
53. Liu Z, Gan L, Zhang T, Ren Q, Sun C. Melatonin alleviates adipose inflammation through elevating alpha-ketoglutarate and diverting adipose-derived exosomes to macrophages in mice. J pineal Res (2018) 64(1). doi: 10.1111/jpi.12455
54. Huang G, Lin G, Zhu Y, Duan W, Jin D. Emerging technologies for profiling extracellular vesicle heterogeneity. Lab chip (2020) 20(14):2423–37. doi: 10.1039/d0lc00431f
55. Dechantsreiter S, Ambrose AR, Worboys JD, Lim JME, Liu S, Shah R, et al. Heterogeneity in extracellular vesicle secretion by single human macrophages revealed by super-resolution microscopy. J extracellular vesicles (2022) 11(4):e12215. doi: 10.1002/jev2.12215
56. Lai RC, Arslan F, Lee MM, Sze NS, Choo A, Chen TS, et al. Exosome secreted by MSC reduces myocardial ischemia/reperfusion injury. Stem Cell Res (2010) 4(3):214–22. doi: 10.1016/j.scr.2009.12.003
57. Toh WS, Lai RC, Zhang B, Lim SK. MSC exosome works through a protein-based mechanism of action. Biochem Soc Trans (2018) 46(4):843–53. doi: 10.1042/bst20180079
58. Hu SQ, Zhang QC, Meng QB, Hu AN, Zou JP, Li XL. Autophagy regulates exosome secretion in rat nucleus pulposus cells via the RhoC/ROCK2 pathway. Exp Cell Res (2020) 395(2):112239. doi: 10.1016/j.yexcr.2020.112239
59. Rai A, Greening DW, Xu R, Chen M, Suwakulsiri W, Simpson RJ. Secreted midbody remnants are a class of extracellular vesicles molecularly distinct from exosomes and microparticles. Commun Biol (2021) 4(1):400. doi: 10.1038/s42003-021-01882-z
60. Fader CM, Colombo MI. Autophagy and multivesicular bodies: two closely related partners. Cell Death Diff (2009) 16(1):70–8. doi: 10.1038/cdd.2008.168
61. Jadli AS, Ballasy N, Edalat P, Patel VB. Inside(sight) of tiny communicator: exosome biogenesis, secretion, and uptake. Mol Cell Biochem (2020) 467(1-2):77–94. doi: 10.1007/s11010-020-03703-z
62. Liu D, Wei N, Man HY, Lu Y, Zhu LQ, Wang JZ. The MT2 receptor stimulates axonogenesis and enhances synaptic transmission by activating akt signaling. Cell Death Diff (2015) 22(4):583–96. doi: 10.1038/cdd.2014.195
63. Sunyer-Figueres M, Vázquez J, Mas A, Torija MJ, Beltran G. Transcriptomic insights into the effect of melatonin in saccharomyces cerevisiae in the presence and absence of oxidative stress. Antioxidants (Basel Switzerland) (2020) 9(10):947. doi: 10.3390/antiox9100947
64. Shrivastava S, Devhare P, Sujijantarat N, Steele R, Kwon YC, Ray R, et al. Knockdown of autophagy inhibits infectious hepatitis c virus release by the exosomal pathway. J Virol (2016) 90(3):1387–96. doi: 10.1128/jvi.02383-15
65. Baixauli F, López-Otín C, Mittelbrunn M. Exosomes and autophagy: coordinated mechanisms for the maintenance of cellular fitness. Front Immunol (2014) 5:403. doi: 10.3389/fimmu.2014.00403
66. Joshi BS, de Beer MA, Giepmans BNG, Zuhorn IS. Endocytosis of extracellular vesicles and release of their cargo from endosomes. ACS nano (2020) 14(4):4444–55. doi: 10.1021/acsnano.9b10033
67. Xu J, Camfield R, Gorski SM. The interplay between exosomes and autophagy - partners in crime. J Cell Sci (2018) 131(15):jcs215210. doi: 10.1242/jcs.215210
68. Murrow L, Malhotra R, Debnath J. ATG12-ATG3 interacts with alix to promote basal autophagic flux and late endosome function. Nat Cell Biol (2015) 17(3):300–10. doi: 10.1038/ncb3112
69. Guo H, Chitiprolu M, Roncevic L, Javalet C, Hemming FJ, Trung MT, et al. Atg5 disassociates the V(1)V(0)-ATPase to promote exosome production and tumor metastasis independent of canonical macroautophagy. Dev Cell (2017) 43(6):716–730.e7. doi: 10.1016/j.devcel.2017.11.018
70. Hassanpour M, Rezabakhsh A, Pezeshkian M, Rahbarghazi R, Nouri M. Distinct role of autophagy on angiogenesis: highlights on the effect of autophagy in endothelial lineage and progenitor cells. Stem Cell Res Ther. (2018) 9(1):305. doi: 10.1186/s13287-018-1060-5
71. Jin H, Zhang Z, Wang C, Tang Q, Wang J, Bai X, et al. Melatonin protects endothelial progenitor cells against AGE-induced apoptosis via autophagy flux stimulation and promotes wound healing in diabetic mice. Exp Mol Med (2018) 50(11):1–15. doi: 10.1038/s12276-018-0177-z
72. Villarroya-Beltri C, Baixauli F, Mittelbrunn M, Fernández-Delgado I, Torralba D, Moreno-Gonzalo O, et al. ISGylation controls exosome secretion by promoting lysosomal degradation of MVB proteins. Nat Commun (2016) 7:13588. doi: 10.1038/ncomms13588
73. Palmulli R, van Niel G. To be or not to be … secreted as exosomes, a balance finely tuned by the mechanisms of biogenesis. Essays Biochem (2018) 62(2):177–91. doi: 10.1042/ebc20170076
74. Wang K, Ru J, Zhang H, Chen J, Lin X, Lin Z, et al. Melatonin enhances the therapeutic effect of plasma exosomes against cerebral ischemia-induced pyroptosis through the TLR4/NF-kappaB pathway. Front Neurosci (2020) 14:848. doi: 10.3389/fnins.2020.00848
75. Alzahrani FA. Melatonin improves therapeutic potential of mesenchymal stem cells-derived exosomes against renal ischemia-reperfusion injury in rats. Am J Trans Res (2019) 11(5):2887–907.
76. Sun CK, Chen CH, Chang CL, Chiang HJ, Sung PH, Chen KH, et al. Melatonin treatment enhances therapeutic effects of exosomes against acute liver ischemia-reperfusion injury. Am J Trans Res (2017) 9(4):1543–60.
77. du Plessis SS, Hagenaar K, Lampiao F. The in vitro effects of melatonin on human sperm function and its scavenging activities on NO and ROS. Andrologia (2010) 42(2):112–6. doi: 10.1111/j.1439-0272.2009.00964.x
78. Zavodnik IB, Domanski AV, Lapshina EA, Bryszewska M, Reiter RJ. Melatonin directly scavenges free radicals generated in red blood cells and a cell-free system: chemiluminescence measurements and theoretical calculations. Life Sci (2006) 79(4):391–400. doi: 10.1016/j.lfs.2006.01.030
79. Chen Z, Zhao C, Liu P, Huang H, Zhang S, Wang X. Anti-apoptosis and autophagy effects of melatonin protect rat chondrocytes against oxidative stress via regulation of AMPK/Foxo3 pathways. Cartilage (2021), 13(2_suppl):1041S–1053S. doi: 10.1177/19476035211038748
80. Iske J, Hinze CA, Salman J, Haverich A, Tullius SG, Ius F. The potential of ex vivo lung perfusion on improving organ quality and ameliorating ischemia reperfusion injury. Am J transplantation: Off J Am Soc Transplant Am Soc Transplant Surgeons (2021), 21(12):3831–3839. doi: 10.1111/ajt.16784
81. Chen Y, Fan H, Wang S, Tang G, Zhai C, Shen L. Ferroptosis: A novel therapeutic target for ischemia-reperfusion injury. Front Cell Dev Biol (2021) 9:688605. doi: 10.3389/fcell.2021.688605
82. Talib WH, Alsayed AR, Abuawad A, Daoud S, Mahmod AI. Melatonin in cancer treatment: Current knowledge and future opportunities. Molecules (2021) 26(9):2506. doi: 10.3390/molecules26092506
83. Majidinia M, Sadeghpour A, Mehrzadi S, Reiter RJ, Khatami N, Yousefi B. Melatonin: A pleiotropic molecule that modulates DNA damage response and repair pathways. J Pineal Res (2017) 63(1). doi: 10.1111/jpi.12416
84. Wang K, Ru J, Zhang H, Chen J, Lin X, Lin Z, et al. Melatonin enhances the therapeutic effect of plasma exosomes against cerebral ischemia-induced pyroptosis through the TLR4/NF-κB pathway. Front Neurosci (2020) 14:848. doi: 10.3389/fnins.2020.00848
85. Savage RA, Zafar N, Yohannan S, Miller JMM. Melatonin. in StatPearls. Treasure Island (FL: StatPearls Publishing Copyright (2021).
86. Andersen LP, Gögenur I, Rosenberg J, Reiter RJ. The safety of melatonin in humans. Clin Drug Invest (2016) 36(3):169–75. doi: 10.1007/s40261-015-0368-5
87. Wasdell MB, Jan JE, Bomben MM, Freeman RD, Rietveld WJ, Tai J, et al. A randomized, placebo-controlled trial of controlled release melatonin treatment of delayed sleep phase syndrome and impaired sleep maintenance in children with neurodevelopmental disabilities. J Pineal Res (2008) 44(1):57–64. doi: 10.1111/j.1600-079X.2007.00528.x
88. Seabra ML, Bignotto M, Pinto LR Jr., Tufik S. Randomized, double-blind clinical trial, controlled with placebo, of the toxicology of chronic melatonin treatment. J Pineal Res (2000) 29(4):193–200. doi: 10.1034/j.1600-0633.2002.290401.x
89. Besag FMC, Vasey MJ, Lao KSJ, Wong ICK. Adverse events associated with melatonin for the treatment of primary or secondary sleep disorders: A systematic review. CNS Drugs (2019) 33(12):1167–86. doi: 10.1007/s40263-019-00680-w
Keywords: exosomes, melatonin, pretreatment, heterogeneity, therapeutic
Citation: Zhou Z, Wang R, Wang J, Hao Y, Xie Q, Wang L and Wang X (2022) Melatonin pretreatment on exosomes: Heterogeneity, therapeutic effects, and usage. Front. Immunol. 13:933736. doi: 10.3389/fimmu.2022.933736
Received: 01 May 2022; Accepted: 29 August 2022;
Published: 16 September 2022.
Edited by:
Rosa M. Sainz, University of Oviedo, SpainReviewed by:
Yen-Yi Zhen, Kaohsiung Medical University, TaiwanReza Rahbarghazi, Tabriz University of Medical Sciences, Iran
Luiz Gustavo Chuffa, Universidade Estadual Paulista, Brazil
Copyright © 2022 Zhou, Wang, Wang, Hao, Xie, Wang and Wang. This is an open-access article distributed under the terms of the Creative Commons Attribution License (CC BY). The use, distribution or reproduction in other forums is permitted, provided the original author(s) and the copyright owner(s) are credited and that the original publication in this journal is cited, in accordance with accepted academic practice. No use, distribution or reproduction is permitted which does not comply with these terms.
*Correspondence: Xing Wang, kqwx100@163.com; Lu Wang, wdl02011@126.com
†These authors have contributed equally to this work