- 1Department of Medicine, Baylor College of Medicine, Houston TX, United States
- 2Dan L Duncan Comprehensive Cancer Center, Baylor College of Medicine, Houston, TX, United States
- 3Institute for Clinical and Translational Research, Baylor College of Medicine, Houston, TX, United States
- 4Institute of Super Cell, BGI-Shenzhen, Shenzhen, China
- 5Department of Pipeline Development, Biomap, Inc, San Francisco, CA, United States
Peptide-based cancer vaccines have been shown to boost immune systems to kill tumor cells in cancer patients. However, designing an effective T cell epitope peptide-based cancer vaccine still remains a challenge and is a major hurdle for the application of cancer vaccines. In this study, we constructed for the first time a library of peptide-based cancer vaccines and their clinical attributes, named CancerVaccine (https://peptidecancervaccine.weebly.com/). To investigate the association factors that influence the effectiveness of cancer vaccines, these peptide-based cancer vaccines were classified into high (HCR) and low (LCR) clinical responses based on their clinical efficacy. Our study highlights that modified peptides derived from artificially modified proteins are suitable as cancer vaccines, especially for melanoma. It may be possible to advance cancer vaccines by screening for HLA class II affinity peptides may be an effective therapeutic strategy. In addition, the treatment regimen has the potential to influence the clinical response of a cancer vaccine, and Montanide ISA-51 might be an effective adjuvant. Finally, we constructed a high sensitivity and specificity machine learning model to assist in designing peptide-based cancer vaccines capable of providing high clinical responses. Together, our findings illustrate that a high clinical response following peptide-based cancer vaccination is correlated with the right type of peptide, the appropriate adjuvant, and a matched HLA allele, as well as an appropriate treatment regimen. This study would allow for enhanced development of cancer vaccines.
Introduction
Cancer is a heterogeneous disease with mixed clinical outcomes (1). Conventional cancer treatments tend to non-specifically kill tumor cells. Some of these tumor cells survive due to resistance to therapy and drug toxicity, eventually leading to tumor relapse and metastasis (2, 3). Cancer immunotherapy is a treatment strategy that uses a patient’s own immune system to fight the cancerous cells (4, 5). Immunotherapy has become a promising alternative cancer treatment after surgery, radiotherapy, and chemotherapy in recent years because of its mild side effects and significant therapeutic benefits (6–8). T cell epitope peptide-based cancer vaccine is one of the representative strategies of cancer immunotherapy, relying on short peptide fragments to engineer the induction of highly targeted immune responses (9–11).
Previous studies (12–17) have demonstrated the effectiveness of peptide-based cancer vaccines in treating several common types of cancer, such as breast cancer, melanoma, colorectal and lung cancer. This strategy exploits the fact that the surfaces of cancer cells have various peptide epitopes (i.e., peptides of usually 8-17 residues in length), which bind to major histocompatibility complex (MHC) proteins. T cells can attack these cancer cells after recognizing the peptide/MHC complex (18). T cells aimed to induce immune recognition of tumor cells are then able to eradicate them by generating a sustained and potent anti-tumor immune response. Therefore, a key determinant for an anti-tumor immune response to lead to the effective killing of cancer cells is the selection of immunogenic peptide epitopes as the target (19). Many peptide epitopes have been identified and molecularly characterized in experiments (12–17). While there are many options in selecting immunogenic antigens, it is not clear which selected epitopes can induce the dominant immune system mediated by T cells. Many clinical studies in cancer vaccines have been initiated to assess the therapeutic potential of active immunization or vaccination with peptide epitopes in cancer patients. However, it is still unclear what the ideal characteristics of selected peptide epitopes should be and which could induce stronger anti-tumor responses. Therefore, it remains highly challenging to design an effective cancer vaccine that can achieve a meaningful clinical benefit in patients.
There have been many breakthroughs in prior studies that investigated the optimal conditions for designing a peptide-based cancer vaccine (20–25). Thomas et al, Zhang et al, and Liu et al found differences in the therapeutic efficacy of peptide-based cancer vaccines prepared from different sources of peptides (26–28). Furthermore, patients with certain HLA alleles might be more sensitive to respond to cancer vaccines (29, 30). The same cancer vaccine with different adjuvants might also have an impact on the outcome of treatment (28, 31). In addition, different treatment strategies could also affect the efficacy and side effects of cancer vaccines, such as the dose of vaccine used, injection interval, number of injections, and injection methods (28, 32). However, due to the limited number of clinical trials available, combined with the difference in cancer types and patient conditions, it is difficult to improve a cancer vaccine design by directly referring to the design of other cancer vaccines. Machine learning (ML) options such as Random forest (RF) (33)could improve cancer vaccine design by utilizing large data repositories to identify novel features and more complex interactions among these features.
In this study, a library of peptide-based cancer vaccines used in clinical studies from public and private sources was established from multiple sources, such as PubMed, ClinicalTrials.gov, and Web of Science, using databases up to January 1, 2022. The statistical analysis of types of peptides, adjuvants, treatment regimen, human leukocyte antigen (HLA) alleles of peptides, and other features in vaccine therapy was obtained from the results in high clinical response (HCR) and low clinical response (LCR) in the database to find the associations which influence the treatment effect of cancer immunotherapy. Finally, we built a random forest model to help distinguish which kinds of cancer vaccines in patients are most likely to achieve a high clinical response.
Material and methods
Data utilized in this study screening and extraction
We screen and extracted all the peptide-based cancer vaccine relevant studies, retrieved from the PubMed, ClinicalTrials.gov, and Web of Science, using databases up to January 1, 2022. All studies were browsed, searched, prioritized, and filtered by the investigators based on the keywords: peptide*, vaccine*, cancer/tumor*, human, HLA, clinical. These extracted studies were then reviewed according to the inclusion and exclusion criteria. In cases in which the results obtained were different, the case was discussed further to obtain consensus. Further details are provided in the following sections. Finally, A total of 705 peptides resulting from 152 clinical studies containing peptide-based cancer vaccine features and clinical treatment information were registered in our library, which has been posted to our web-accessible library, CancerVaccine (https://peptidecancervaccine.weebly.com/).
Inclusion criteria
The inclusion criteria were as follows: 1. The study focused on human cancer research. 2. The study used the peptide as the vaccine to treat cancer patients. 3. They are not review reports; there are cancer detail descriptions and treatment information about the clinical trials.
Exclusion criteria
The exclusion criteria were as follows: 1. The peptide-based cancer vaccine was not associated with humans. 2. There was no associated peptide information. 3. There was no treatment information for the peptide-based cancer vaccine.
Feature selection procedure
Exploratory data analysis: First, we checked the types of variables. There were no missing values in the data (Figure S3A). Next, we created a bar graph for the categorical variables; if the levels of all categorical variables looked correct, we kept the original levels for these variables (Figure S3B). Finally, four features were recommended for the model: injection interval and injection time, adjuvants, and HLA alleles; the blue dot represents the optimal solution, as shown in Figure S3C.
Classifier
We use a random forest model (random Forest package in R) (34) to construct a feature-based classifier. The prediction performance (estimated by 10-fold cross-validation) is best when the top 4 features with the most differentiation are included (injection interval, injection times, adjuvant types, and HLA alleles), indicating that these 4 features have the greatest differentiation power. Using these 4 features as predictors, the area under the receiver operating characteristic (ROC) curve (AUC) was 0.97. The ROC curves were plotted using the pROC R package.
The area under the precision-recall curve
For computing the AUPRC, we used the function metrics.precision_recall_curve and metrics.auc from the R package, ROCR 1.0-11 version (35). We first randomly divided the library cohort with known high or low clinical response into a training set (50% randomly selected samples) and a test set (50% randomly selected samples) based on cancer type. Then, the merged training set was used as the training set and the merged test set was used as the test set. Finally, we logit-transformed AUPRC values before testing (using two-tailed Welch’s t-test). We carried out 1,000 replications of 5-fold cross-validation; within each replication, across the 5-folds, we obtained prediction scores for each cancer type from the fold in which the cancer type was in the test set, enabling us to compute an overall AUPRC within each replication.
Statistical analysis
The R statistical package was used for all data processing and statistical analysis (R package: stats v3.6.2). All details of the statistical tests are specified in the associated text or figure legends. For the statistical analyses, P-values were calculated by using the “Wilcox_test” function from the R package: stats v3.6.2, which applies the two-sided Wilcoxon rank-sum test and corrected multiple testing using the Holm–Bonferroni method. A statistically significant difference was assumed when P ≤ 0.05.
Results
Data filtering and features of selected trials
To investigate what classes of peptide-based cancer vaccine can help achieve satisfactory results from clinical treatment, we reviewed a total of 302 relevant studies, retrieved from the PubMed, ClinicalTrials.gov, and Web of Science, using databases up to January 1, 2022. PubMed.gov is a free search engine that accesses the MEDLINE database on life sciences and biomedical topics primarily at the U.S. National Institutes of Health’s National Library of Medicine. The database of ClinicalTrials.gov is a service of the U.S. National Institute of Health. After removing duplicates, we screened 206 potentially relevant articles by scanning the titles and abstracts. We reviewed the full text and screened the candidate studies according to the inclusion criteria, and 43 studies were excluded. Of the remaining 163 studies, 11 were excluded due to describing the same repeated clinical trials. A total of 705 peptides resulting from 152 clinical studies containing peptide-based cancer vaccine features and clinical treatment information were registered in our library (12–14, 36–163). Details of the study identification process can be found in Figure 1A. The final study population included 6,713 participants. All studies were retrospective studies published between January 1, 1997 and January 1, 2022, and involved various tumor types. The details of the library in this study have been posted to our web-accessible library, CancerVaccine (https://peptidecancervaccine.weebly.com/).
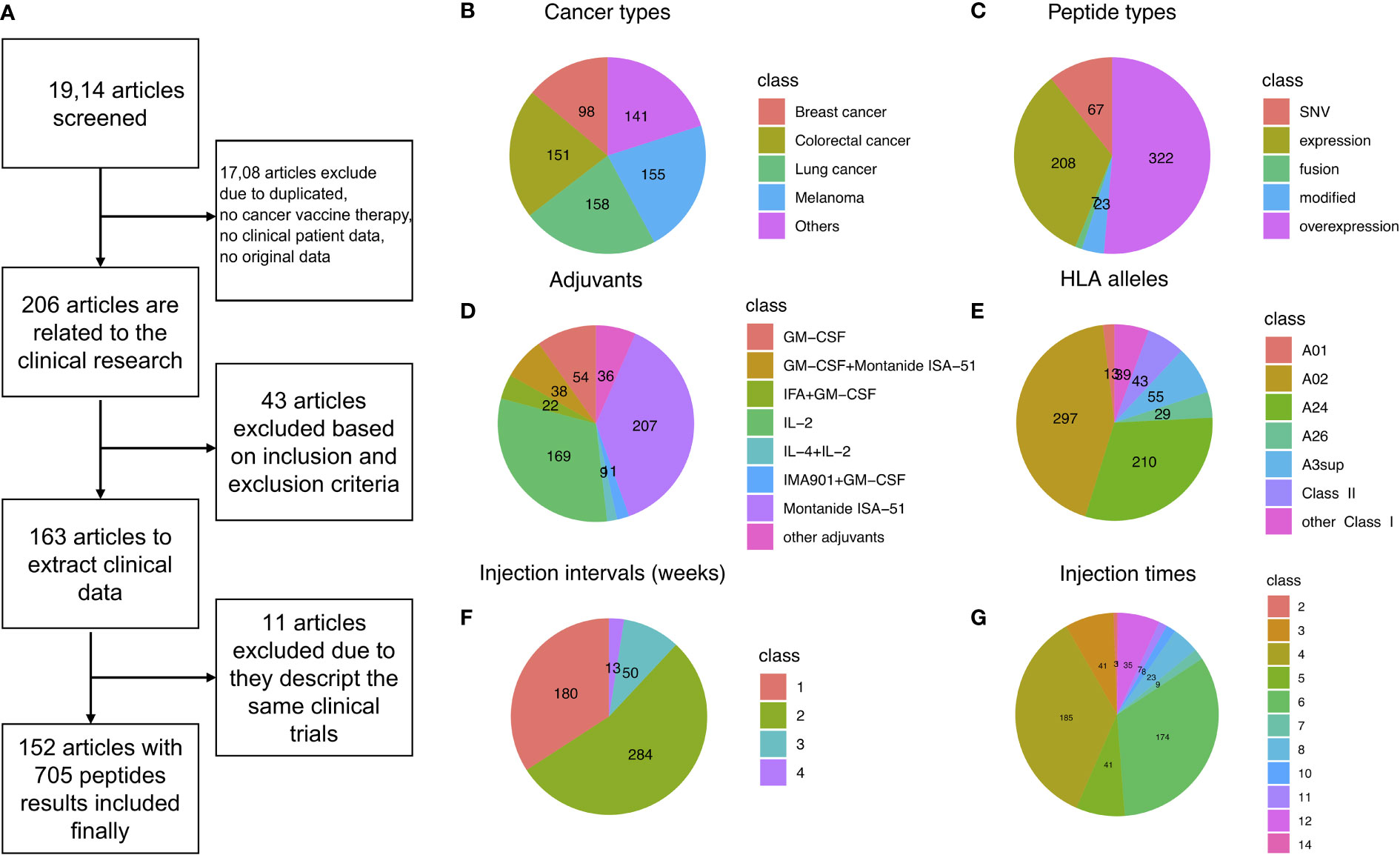
Figure 1 Data filtering summary landscape of the library, CancerVaccine (https://peptidecancervaccine.weebly.com/). (A) Data filtering process. (B) The landscape of cancer types. (C) The landscape of peptide types. (D) The landscape of adjuvants. (E) The landscape of HLA alleles. (F) The landscape of treatment regimen (injection interval). (G) The landscape of treatment regimen (injection times).
In order to analyze this library in a comprehensive and in-depth manner, we mapped the types of cancer and peptide, adjuvant, HLA allele, and treatment regimen (injection interval and injection times) landscapes of the library, as shown in Figures 1B-G, respectively. We found that melanoma, colorectal cancer, and breast cancer are the most common cancer types investigated in cancer vaccine therapy (Figure 1B). The peptides were divided into five categories based on the origin of peptides: tumor expressed peptides, tumor overexpressed peptides, fusion peptides, modified peptides, and single-nucleotide variant (SNV) peptides. For tumor expressed peptides, the genes in which the peptides were co-expressed are found in both cancer and normal tissues (144, 164–166). For tumor overexpressed peptides, the genes in which the peptides were located are found overexpressed in the tumor tissue only (129, 167, 168). For fusion peptides, the peptides were derived from gene fusion (57, 122, 130, 136, 155). For modified peptides, the amino acids (AA) had been artificially modified (15, 65, 67, 89, 99, 107, 110, 118, 134, 138, 147, 169–171). The SNV derived peptides were neoantigen, or a new AA sequence that forms on cancer cells when somatic mutations occur in tumor DNA sequences (40, 44, 58, 62, 67, 70, 80, 83, 84, 106, 114, 117, 123, 131, 143, 152, 156, 159, 161, 163, 165).
We found that more than half the peptides (51.4%) used in cancer vaccine preparation were overexpressed in the targeted tumor cells (Figure 1C). We also found that Montanide ISA-51 was the most widely used adjuvant in cancer vaccines. IL-2 was the most widely used cytokine as a vaccine adjuvant in cancer vaccines (Figure 1D). Interestingly, most clinical phase peptides are focused on the HLA class I alleles, especially the A02 and A24, as shown in Figure 1E. In addition, we noted that more than half of peptide-based cancer vaccines were injected weekly (53.9%, Figure 1F) and more than half of the patients had greater than four vaccine injections (Figure 1G).
The prognostic evaluation of anti-tumor effect in clinical trials
After building this library, we wanted to further explore the causes which influence the effectiveness of peptide-based cancer vaccine results. We divided these peptide-based cancer vaccine results into high clinical response (HCR) and low clinical response (LCR) results based on their clinical efficacy and safety (172).
The specific classification criteria and basis of a high clinical response were presented in the form of an evolutionary tree, as shown in Figure 2. The prerequisite criteria was whether there have been any vaccine-related deaths; if there were vaccine-related deaths, it was excluded from this study. A total of 78 peptides which involved 673 patients were excluded from this study. We next examined whether patients in the best objective response (complete or partial response, according to modified World Health Organization criteria) had been reported (172–174). Due to the complex tumor microenvironment and vaccine technology limitations, it is difficult to achieve a complete response with vaccine therapy; therefore, the best objective response indicates that the clinical response of the vaccine therapy is high (175). We also looked at cases where there were no best objective response patients, investigating whether more than 50% of patients achieved stable disease (SD) or progression-free survival (PFS) if the previous conditions were not met. If more than half of the patients achieved SD or PFS, we took this as an indication that the cancer vaccine was effective (174, 176). Finally, we compared whether the overall survival time was significantly longer in the vaccine group than in the control group; a significantly longer overall survival time in the vaccine group could also indicate that the vaccine worked well (177, 178). If none of these progressive conditions were met, then the clinical result was classified as a low clinical response result. The clinical efficacy evaluation was performed using the immune-related response criteria (irRC) (179) and response evaluation criteria in solid tumors (RECIST 1.1) standard criteria (180). Toxicities were reported using the World Health Organization grading system (181). In total, 273 high clinical response results (3,233 patients involved) and 354 low clinical response results (2,807 patients involved) were included in this study (Figure 2).
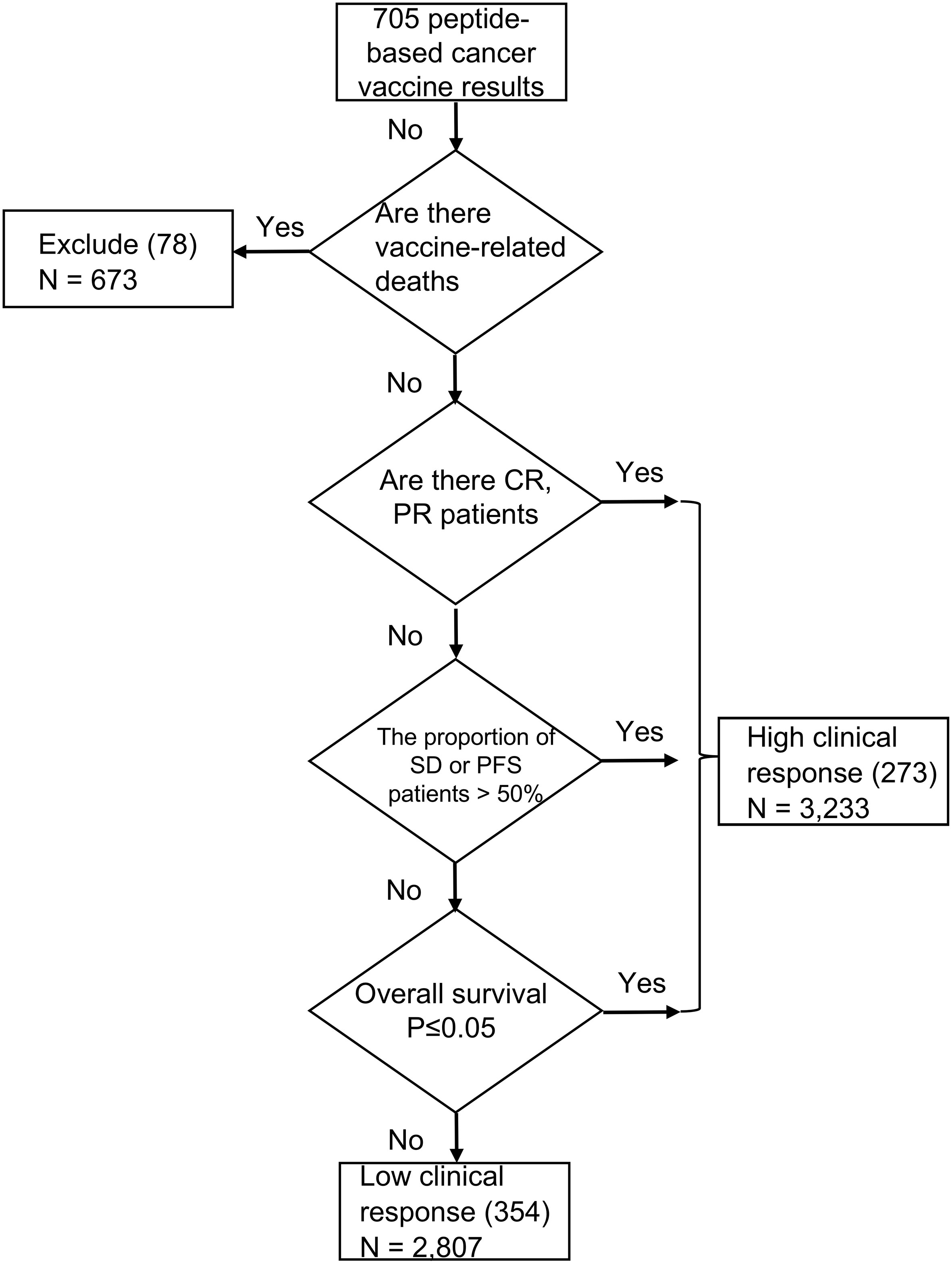
Figure 2 Cancer vaccine criteria and feature comparison. The specific criteria of a clinical treatment response. A total of 78 peptides resulting which involved 673 patients were excluded. 273 high clinical response results (3,233 patients involved) and 354 low clinical response results (2,807 patients involved) were finally included in this study.
To further investigate which factors influence the efficacy of cancer vaccines, we specifically explored the types of peptides, HLA alleles, adjuvants, and treatment regimens (injection interval and injection times) in the high and low clinical response groups. Further details are provided in the following sections.
Modified and tumor overexpressed peptides could be suitably selected for cancer vaccines
Cancer vaccines face a number of challenges, including finding suitable sources of peptides that work best in vivo. The peptides were divided into five categories based on the origin of peptides (182, 183): the tumor expressed peptides, tumor overexpressed peptides, fusion peptides, modified peptides, and single-nucleotide variant (SNV) peptides. We summarized their distribution in the results from high and low clinical responses (Figure 3). As shown in Figure 3A, most of the peptides in the library are expressed or overexpressed in cancer. The overexpressed peptides achieved many of the high clinical response results (41.3%), especially in colorectal cancer (59.6% vs. 40.4% in high vs. low clinical response results, Figures 3A, C). The fusion cancer vaccines were not as efficacious in clinical trials, as they did not lead to any high clinical responses (Figure 3A). However, modified peptides, in which the amino acids (AA) were artificially modified, appeared to be the most suitable method for cancer vaccine (69.6% of modified peptides achieved high clinical response results), especially for melanoma immunotherapy (84.2%, Figures 3B, C). In this study, we also listed the top 8 most frequent peptide gene names and the top 18 most commonly used peptides, as shown in Figure S1.
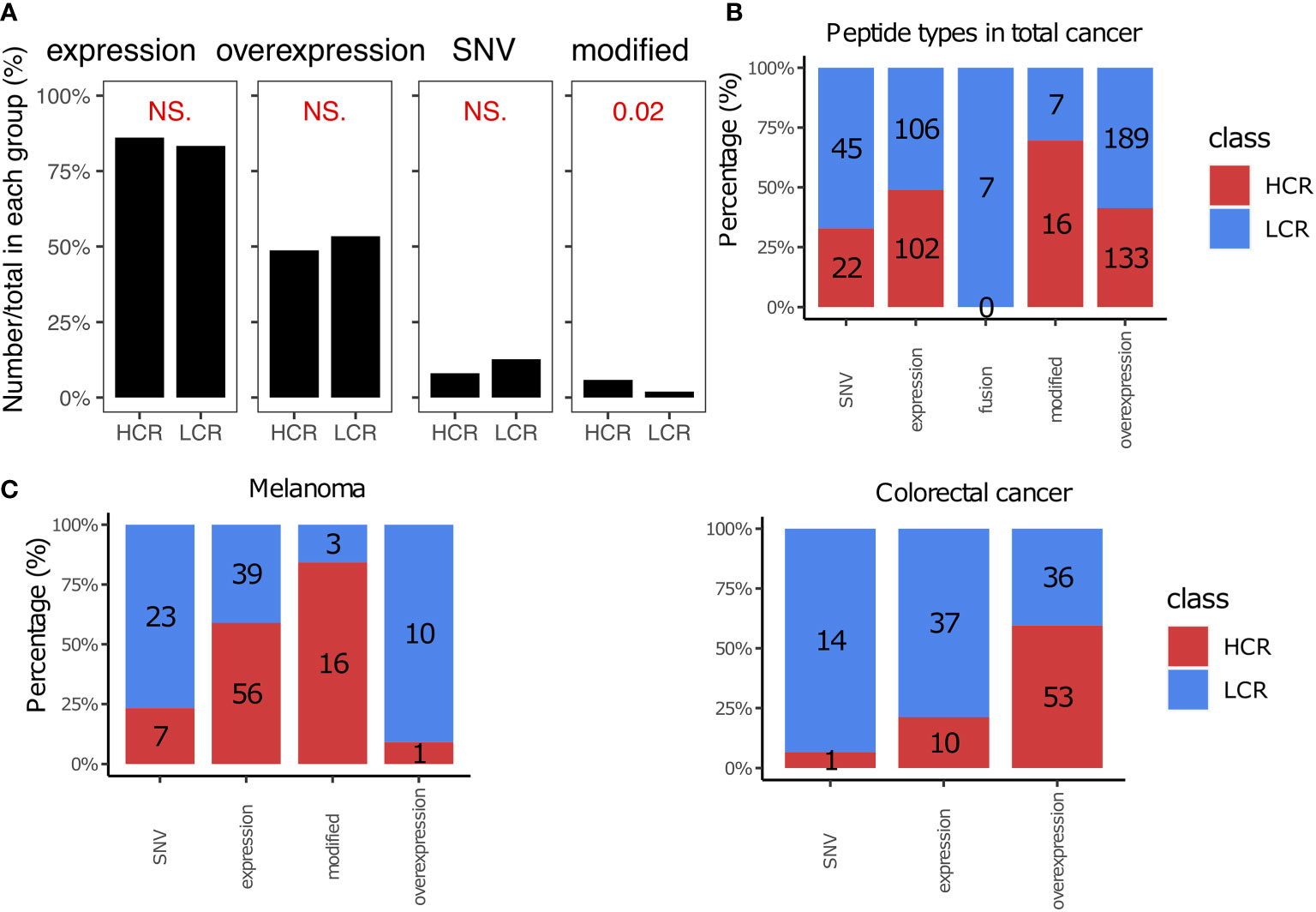
Figure 3 Peptide types in high and low clinical response results. (A) Comparison of peptide types between high clinical response (HCR) results and low clinical response (LCR) results. The distribution of peptide types in HCR and LCR results. (B) The distribution of peptide types in HCR and LCR results. (C) The distribution of peptide types in HCR and LCR results in melanoma and colorectal cancer, respectively. P-values were calculated using two-sided Wilcoxon rank-sum tests. NS., not significant.
HLA class II peptide-based cancer vaccines could achieve high clinical response results
The cytotoxic T cell (CTL) epitope peptides were restricted with HLA alleles when they were prepared as cancer vaccines (102, 184–186). Previous studies have reported that an accurate HLA allele is a key factor in successful cancer vaccinations (186, 187). More than 90% of cancer vaccines in our library are typed for HLA Class I alleles (Figure 4A), with the most common being HLA- A02, A24, A3sup, A26, and A01. This is generally consistent with the proportional rank of these alleles in the population (188, 189). We noticed that patients with melanoma achieved more high clinical response results when using peptides typed for HLA-A01 and HLA Class II. For example, all peptides with HLA-A01 alleles achieved high clinical response results in melanoma (13 vs. 0, Figures 4B, C), although this is limited by the sample size and we may need more data to verify whether a similar trend exists in other cancer types (Figure S4). The peptides with HLA Class II alleles also achieved more high clinical response results (28 vs. 15, P = 0.0049, Figures 4A, B), especially for melanoma and lung cancer patients (Figure 4C). HLA Class II alleles are highly associated with the CD4+ T cells; CD4+ T cells primarily mediate anti-tumor immunity by providing help for CD8+ CTL and antibody responses, as well as via secretion of effector cytokines such as interferon-γ (IFNγ) and tumor necrosis factor-α (TNFα). Under specific contexts, they can also mediate anti-tumor immunity via direct cytotoxicity against tumor cells (190–193). Therefore, peptide epitopes targeting HLA Class II could be more likely to achieve high clinical response results.
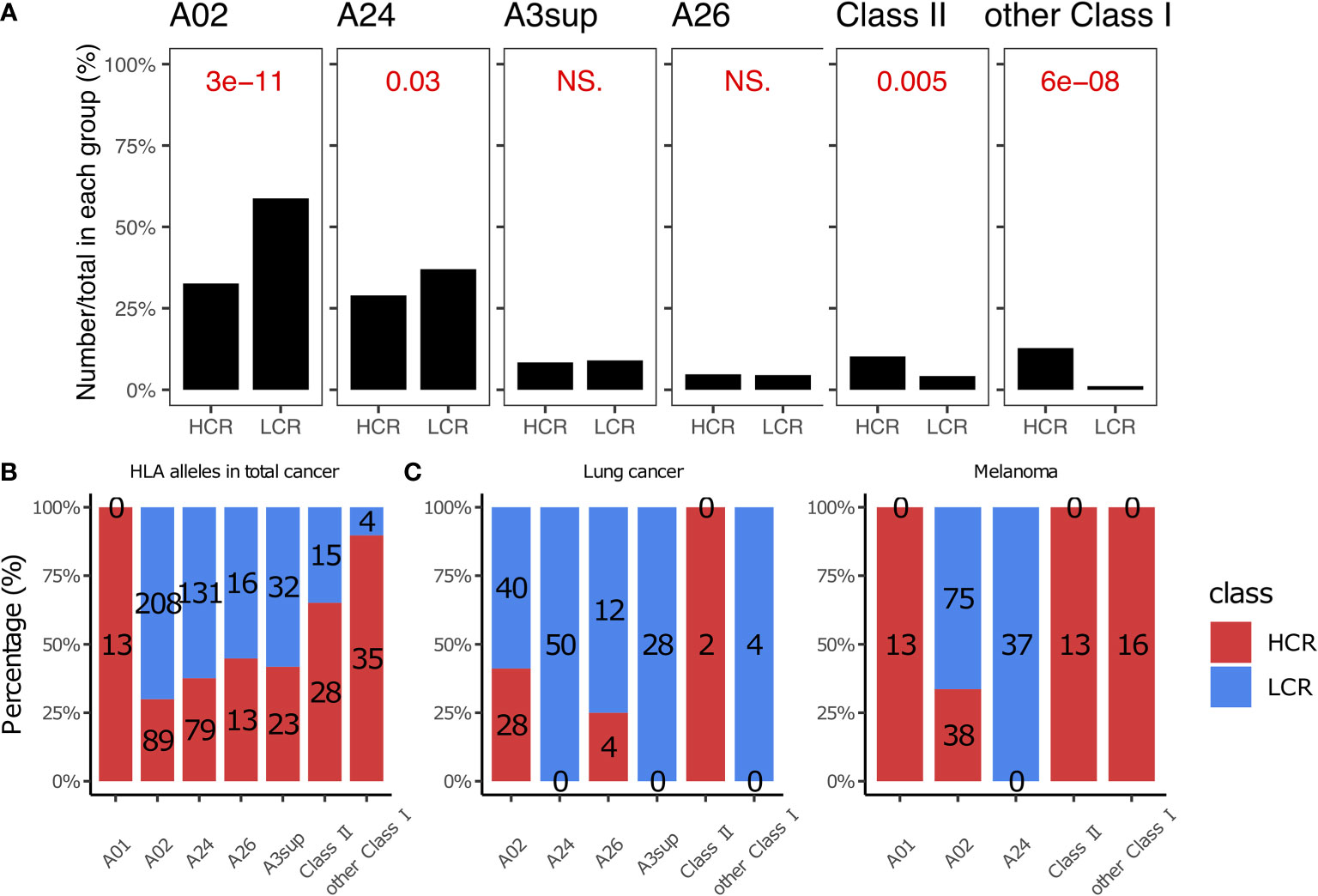
Figure 4 HLA alleles in high and low clinical response results. (A) Comparison of HLA alleles between HCR and LCR results. (B) The distribution of HLA alleles in HCR and LCR results. (C) The distribution of HLA alleles in HCR and LCR results in melanoma and lung cancer, respectively. P-values were calculated using two-sided Wilcoxon rank-sum tests. NS, not significant.
Montanide ISA-51 was identified as an effective adjuvant in the treatment of cancer vaccines, especially for breast and colorectal cancers
An adjuvant is an ingredient that can help create a stronger immune response in patients receiving the vaccine (194, 195). Many cancer vaccines use adjuvants to enhance therapeutic efficacy.
We found that Montanide ISA-51 and Granulocyte-macrophage colony-stimulating factor (GM-CSF) were the two most widely used adjuvants in cancer vaccines (Figure 5A), and they also appeared most frequently in high clinical response results (P = 6.83e-19 and 0.045, respectively, Figures 5A, B). Montanide ISA-51, in particular, was the most frequently used adjuvant with high clinical response results (59.4%, Figure 5B), especially in breast cancer and colorectal cancer (92.1% and 95.6%, respectively, Figure 5C). Cytokines in cancer immunity and immunotherapy, cytokine modulation is necessary for efficacious treatment of experimental neuropathic pain (196). IL-2, IL-4, and IL-12 were the most widely used cytokines as vaccine adjuvants, especially IL-2, which was used as an immune adjuvant in many kinds of cancer types, such as lung cancer, colorectal cancer, and melanoma (Figure 5C). However, IL-2, IL-4, and IL-12 are not very effective when used alone in peptide-based cancer vaccines (Figures 5B, C).
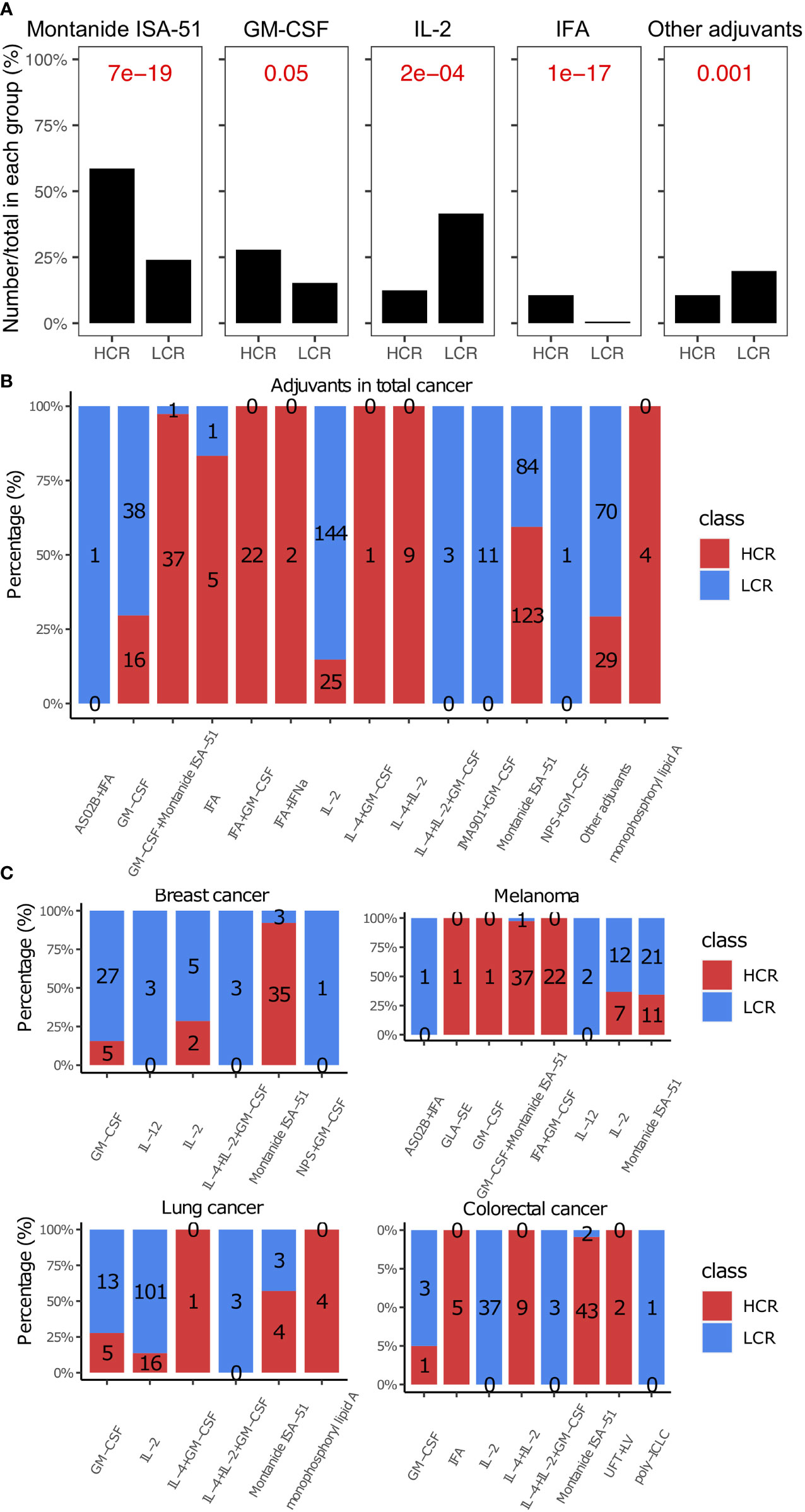
Figure 5 Adjuvants in high and low clinical response results. (A) Comparison of adjuvants between HCR and LCR results. (B) The distribution of adjuvants in HCR and LCR results. (C) The distribution of adjuvants in HCR and LCR results in four main cancer types (breast cancer, melanoma, lung cancer, and colorectal cancer). P-values were calculated using two-sided Wilcoxon rank-sum tests.
Treatment regimens with weekly intervals and greater than four injections could be more likely to achieve a better clinical response
The type of treatment regimen is also one of the major challenges affecting the effectiveness of cancer vaccine therapy (32, 197, 198). We evaluated the influences of treatment regimens on cancer vaccine efficacy.
Our study found a significant difference in the vaccine injection intervals between the HCR and LCR results (Figure 6A, left). Treatment regimens with shorter vaccine injection intervals yielded more high clinical response results. The number of high clinical response results decreases from 141 to 4 with the increase in vaccine injection intervals, implying that shorter vaccine injection intervals (weeks) may be more favorable for patients to achieve high clinical response results, as shown in Figure 6A. We also found that the number of vaccine injections associated with high clinical response results was significantly higher than that of the low clinical response results (Figure 6A, right). Patients with greater than four vaccination injections achieved more high clinical response results (Figure 6C). We also found similar results in main cancer types, such as breast cancer, melanoma, colorectal cancer, and lung cancer. The details are shown in the supplement materials [injection interval (Figure S2A) and injection times (Figure S2B), respectively].
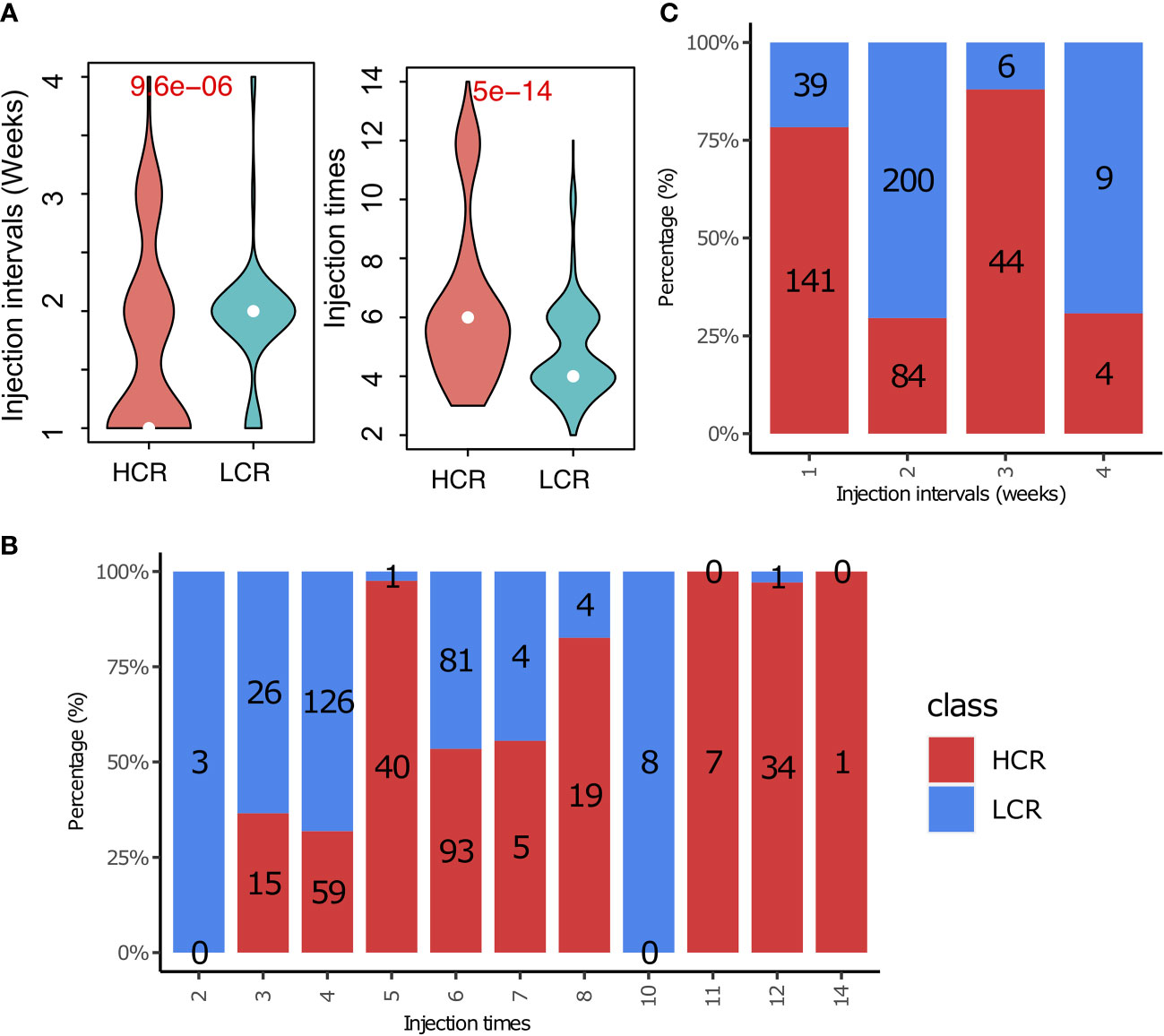
Figure 6 Treatment regimens play an important role in cancer immunotherapy. (A) The distribution of treatment regimens (injection interval) in HCR and LCR results. (B) The distribution of treatment regimens (injection times) in HCR and LCR results. (C) Comparison of treatment regimens (injection interval and injection times) between HCR and LCR results. P-values were calculated using two-sided Wilcoxon rank-sum tests.
Generation of a random forest model from clinical responses for cancer vaccines
Given that some redundant or irrelevant features in the new data set may exert an influence on the classifying effects of a machine learning model, the importance value of cancer vaccine features was first calculated by means of a Random Forest algorithm, followed by the selection of the optimal features based on each feature’s importance (Figure 7A and Supplemental Figure S3). From the methodological perspective of feature selection, the random forest is a kind of embedded feature selector that can automatically produce the relative importance of features during the model training process. We investigated the relative importance of multiple features, such as the peptide types, adjuvant, HLA alleles, tumor stages, chemotherapy, and treatment regimens, in cancer vaccines. Four features were chosen for the random forest-based modeling in this study: injection interval, injection times, adjuvant types, and HLA alleles (Figure 7A).
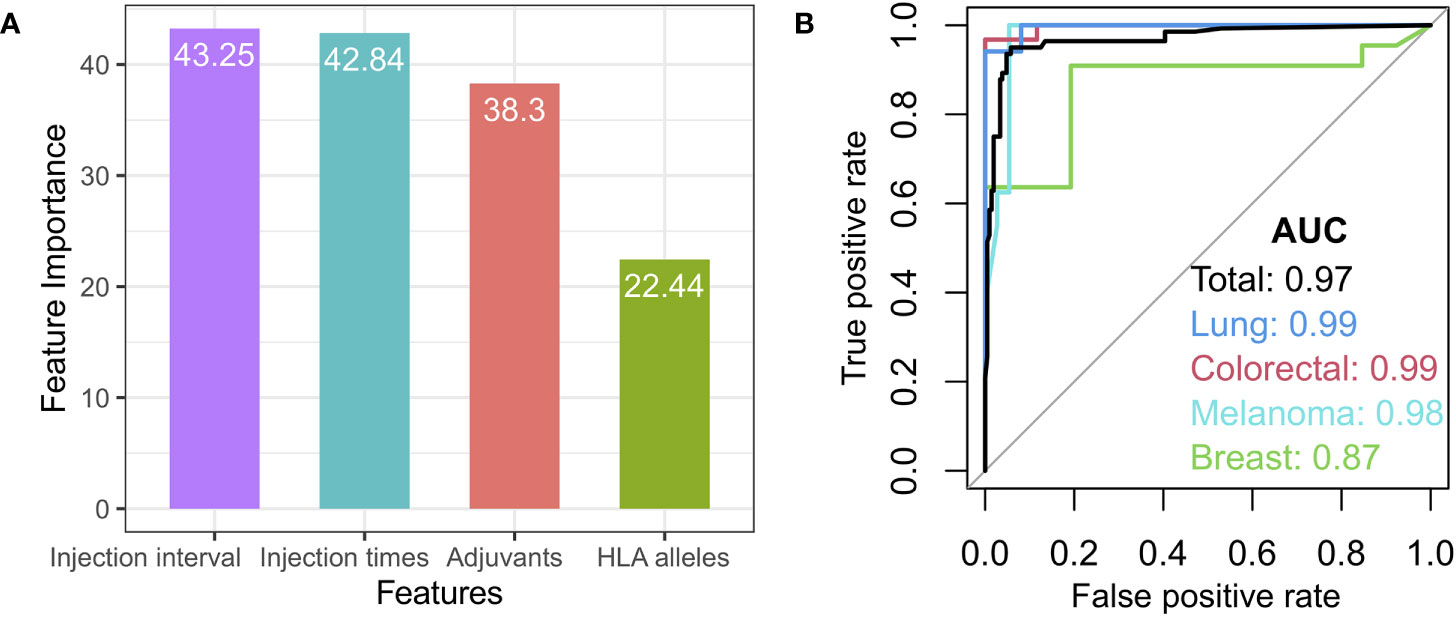
Figure 7 Features selection and model. (A) The variable importance for the selected features, such as injection interval, injection times, adjuvant types, and HLA alleles. (B) Receiver Operating Characteristic (ROC) curve for the total test set (black) and independent breast cancer (green), melanoma (light blue), lung cancer (blue), and colorectal cancer datasets (red).
For the random forest-based modeling, the library cohort with known high or low clinical response was randomly split into a training set (50% randomly selected samples) and a test set (50% randomly selected samples) according to the cancer types. The merged training set was used as the training set and the merged test set as the test set. The model was trained on the training set and tested on the test set, with 1,000 repeated nested 5-fold cross-validation. The model achieved a high area under the curve (AUC) of 0.97 sensitivity on the test set (Figure 7B, black curve). The prediction model’s performance was first assessed in four independent cancer type cohorts (breast cancer, melanoma, lung cancer, and colorectal cancer) with the equilibrium of class distribution and balanced data. Our model also achieved AUCs of 0.87, 0.99, 0.99, and 0.98 for independent breast cancer, melanoma, lung cancer, and colorectal cancer datasets, respectively, demonstrating that our model could predict their vaccine responses from the features we selected (Figure 7B). In addition, the prediction model was also evaluated from the perspectives of the average precision score and precision-recall (AUPRC) (Figure S3D).
Random forest yields high discriminative performance in cancer vaccine clinical response prediction. Thus, the model could be helpful in identifying cancer vaccines with high clinical responses.
Discussion
Immunotherapies such as peptide-based cancer vaccines have proven to be effective in enhancing the immune response in cancer patients to fight cancer cells. Cancer vaccines that specifically target high expression of gp100 in melanoma have already been approved (199). However, one of the key factors limiting the application of immunotherapy is how to rationally design a peptide-based cancer vaccine that generates an anti-tumor immune response leading to the effective killing of tumor cells (200–202). The goal of our study is to determine the key criteria for cancer vaccines that may lead to better clinical outcomes.
We collected T cell epitopes from several databases that had been applied to clinical studies to construct in silico a library of peptide-based cancer vaccines. These candidate T cell epitopes could activate CD8+ or CD4+ T cells to induce cytotoxicity for tumor cells. The selected peptide epitopes could be used as cancer vaccines or as target antigens for adoptive cell therapy of DC, CTL, TCR-T, and CAR-T cells. To find the associations which influence treatment effectiveness of cancer vaccines, several critical factors, including types of peptides, HLA alleles, adjuvants, and treatment regimens, were analyzed in patients with high and low clinical responses.
We found that studies often chose tumor expressed or overexpressed peptides to prepare cancer vaccines (Figures 1, 3). However, modified peptides in which the amino acids (AAs) always were artificially modified, could be more suitable for cancer vaccines, especially for melanoma immunotherapy (65, 67, 79, 99, 110, 118, 147) (Figure 3). The reason modified peptides are more effective may be because the AAs of modified peptides are altered and these modified peptides will be treated as exogenous peptides, which are more easily recognized by T cells and therefore more immunogenic (12, 203–205). Alternatively, the genes of these modified peptides are always expressed or overexpressed in tumor cells (Figure S1), and their comparative wild-type peptides are usually known to have binding affinities for certain HLA alleles (206–208). Based on this information, we could make targeted modifications to the modified peptides, further enhancing the affinity of modified peptides for pMHC, potentially making modified peptides more immunogenic and therapeutically effective. However, it is possible that modified peptides are also expressed in normal cells, which could lead to uncertain side effects (205, 208).
Based on our study, Montanide ISA-51, in particular, was the most frequently used adjuvant with high clinical response results (59.4%, P = 1.3e-17, Figure 5), especially in breast cancer and colorectal cancer (92.1% and 95.6%, respectively, as shown in Figure 5A, C). Montanide ISA-51 can enhance antigen-specific antibody titers and cytotoxic T-lymphocyte responses. Doorn et al. reported that a proper mixture of peptide epitopes and Montanide ISA-51 could help effectively avoid or mitigate adverse events (209, 210). Because cytokine modulation is necessary for efficacious treatment of experimental neuropathic pain (196). Many cytokines, such as IL-2, IL-4, and IL-12, were widely used cytokines as vaccine adjuvants. IL-2 in particular was used as an immune adjuvant in many cancer types, such as lung cancer, colorectal cancer, and melanoma. However, as vaccination adjuvants, these cytokines are less efficient than Montanide ISA-51 or GM-CSF (Figure 5). There are several possible explanations for this. One explanation is that cytokines are susceptible to degradation due to their short half-life (211, 212). Another explanation could be that the main role of adjuvants is to activate the immune system and activate effector T cells (194). If we use IL-2, IL-4, and IL-12 as the adjuvant in cancer vaccine therapy, we need them to activate effector T cells. However, IL-2 has a higher affinity and stimulatory effect on regulatory T cells (Tregs). Therefore, IL-2 will activate Treg cells along with effector T cells. Tregs are a specialized subpopulation of T cells that act to suppress the immune response. Thus, it is difficult to achieve a high clinical response with IL-2 alone. IL-4 is a cytokine that induces the differentiation of naive helper T cells (Th0 cells) to Th2 cells, thereby inducing immunosuppression (213). Therefore, IL-4 generally does not have a good antitumor effect in cancer vaccines as an adjuvant alone. IL-12 is an interleukin that is naturally produced by dendritic cells (214). It has a strong anti-tumor therapeutic effect, but IL-12 is difficult to use in molecular therapy alone (215). Thus, IL-2, IL-4, and IL-12 are not very effective when used alone in peptide-based cancer vaccines. Antigen-specific specificity is important in cancer vaccinations. However, antigen-specific cytokines do not exist; cytokines can activate many non-specific or tumor growth promoting pathways, which is ineffective and unhelpful for the specificity of a cancer vaccine (216, 217). IL-2, IL-4, and IL-12 may need to be modified or combined with other adjuvants, such as Montanide ISA-51 or GM-CSF in peptide-based cancer vaccine therapies to increase effectiveness.
In addition to these cancer vaccines that utilized various adjuvants, we also observed that many cases did not use any adjuvants in cancer vaccine preparation, which could be a factor leading to the poor clinical outcomes of many peptide vaccinations in clinical trials (38, 41, 49, 53, 68, 72, 74, 81, 82, 86, 95, 98, 110, 117, 127, 131, 143, 151, 160, 218–221)
The treatment regimen also plays an important role in the therapeutic efficacy of cancer vaccines. The treatment regimens with weekly injection intervals and greater than four vaccination injections were more likely to achieve a high clinical response (Figure 6). Short vaccine injection intervals and multiple vaccinations could continually activate the immune system, ensuring that there are enough cytotoxic T cells to kill tumor cells, enhancing the tumor-killing effect and making it easier to get a high clinical response (222). However, many of the available treatment regimens did not use shorter vaccine intervals and more frequent injections. This could be because many of the patients who participated in the cancer vaccine clinical trials had gone through various conventional treatments, and many of them also had stage III or stage IV tumors. As a result, they were already in a weakened condition. Shortened vaccination intervals and an increased frequency could lead to stronger side effects that would be difficult for these weaker patients to tolerate. Therefore, shorter intervals and more frequent injections may be more appropriate for patients with early-stage tumors, while relatively frail patients could have longer injection intervals, but no less than four injections are recommended.
Finally, we investigated the correlation between multiple features, such as the peptide types, adjuvant, HLA alleles, and treatment regimens, in cancer vaccines, and we built a random forest model to distinguish the peptide-based cancer vaccines with high clinical responses (Figures 7, S3). In addition, we also investigated the effect of the tumor stages and chemotherapy, which could reflect the patient’s health condition and medical treatment prior to vaccination on the model prediction. We summarized their distribution in the results from the high and low clinical responses (Figures S4A, B). The relative importance of the tumor stages and chemotherapy are weaker than the peptide types, adjuvant, HLA alleles, and treatment regimens in this study (Figure S4C). The prediction accuracy of our model was also not improved by introducing them (Figure S4D). Possible causes for this are that many clinical trials included patients with different tumor stages, and some others omitted patients’ tumor stages information. There is no significant difference between the chemotherapy group and the no or unknown chemotherapy group (Figure S4B). The cause could be that many of the cancer vaccine trials included patients who had undergone conventional medical treatments, which included but are not limited to chemotherapy. Moreover, some clinical trials excluded patients who had received chemotherapy (41, 45) or required patients to wait a period of time after chemotherapy before participating in cancer vaccine therapy to eliminate and minimize the impact of chemotherapy on vaccine therapy (49, 64, 74, 133, 220). Therefore, we think that the tumor stage and chemotherapy may have less impact on the improvement of prediction accuracy (Figure S4D).
We found a combination of the modified peptides, Montanide ISA-51, a short interval between vaccine injections, and multiple vaccinations could be helpful in effectively activating the immune system to kill tumors, enhancing the tumor-killing effect, and resulting in high clinical responses.
The severe acute respiratory syndrome coronavirus 2 (SARS-CoV-2) virus is prone to specific mutations that alter viral surface peptide epitopes, making the virus more susceptible to immune escape (223). Peptide-based tumor vaccine research has also contributed to the development of COVID-19 vaccines targeting COVID-19-specific peptide epitopes (224, 225).
There are several limitations noted in this study. First, there are no prior studies that quantitatively distinguish between high or low results in cancer vaccine clinical trials; it is possible that we missed information in classifying a high or low response result. Next, due to lack of sufficient data for a single tumor type, we could not directly explore the difference in each cancer type between the high- and low- clinical response groups.
In addition, the effect of the tumor mutation burden, the dose of the cancer vaccine, and the specific method of injection, such as subcutaneous, intranasal, intravenous, and transdermal, may also need to be further explored in the future studies. Lastly, the effect of coupling multiple features on cancer vaccine efficacy is complex and was not investigated in depth in this study. Thus, future studies can explore further the effects of coupling multiple features on cancer vaccine efficacy based on a larger cohort.
Altogether, we presented CancerVaccine, a peptide-based cancer vaccine library that stored and aggregated the results of peptide-based cancer vaccines and their clinical attributes. CancerVaccine can be accessed at https://peptidecancervaccine.weebly.com/. We demonstrated that CancerVaccine is a versatile resource that can be used to screen for useful peptides epitopes and aid in the design of new cancer vaccines. Our study describes a design strategy in peptide vaccination treatment relating to the appropriate types of peptides, suitable adjuvants, matched HLA alleles, and suitable treatment regimens. Furthermore, we developed a high AUC machine learning model, which could be helpful in identifying peptide-based cancer vaccines with high clinical responses. The results of this study could impact future exploration of vaccine designs, taking into consideration identifying suitable peptide antigens and treatment conditions for cancer and personalized immunotherapy.
Data availability statement
The original contributions presented in the study are included in the article/Supplementary Material. Further inquiries can be directed to the corresponding author/s.
Author contributions
C-CC and CJ conceived the project. CJ, JL, WZ, ZZ, and GL prepared and analyzed the database. CJ and C-CC, evaluated the conclusions, wrote the manuscript. CJ, C-CC, WH, BL, and XZ reviewed and revised the content. All authors read and approved the final manuscript. All authors contributed to the article and approved the submitted version.
Funding
This work is supported by the Cancer Prevention Research Institute of Texas (CPRIT) (RR180061).
Acknowledgments
We would like to give special thanks to Chao Cheng and other members of the Chao Cheng lab for their valuable discussions and critical feedback. We especially thank Aude Angelini, Xiaoshi Zhang, Si Qiu, Wenhui Li, and Xiuying Li for their valuable suggestions.
Conflict of interest
Author C-CC was employed by the company Biomap, Inc. Authors WZ, ZZ, GL, XZ and BL are employed by the company BGI-Shenzhen.
The remaining authors declare that the research was conducted in the absence of any commercial or financial relationships that could be construed as a potential conflict of interest.
Publisher’s note
All claims expressed in this article are solely those of the authors and do not necessarily represent those of their affiliated organizations, or those of the publisher, the editors and the reviewers. Any product that may be evaluated in this article, or claim that may be made by its manufacturer, is not guaranteed or endorsed by the publisher.
Supplementary material
The Supplementary Material for this article can be found online at: https://www.frontiersin.org/articles/10.3389/fimmu.2022.931612/full#supplementary-material
References
1. Arora A, Olshen AB, Seshan VE, Shen R. Pan-cancer identification of clinically relevant genomic subtypes using outcome-weighted integrative clustering. Genome Med (2020) 12:1–13. doi: 10.1186/s13073-020-00804-8
2. Chang L, Graham P, Hao J, Ni J, Deng J, Bucci J, et al. Cancer stem cells and signaling pathways in radioresistance. Oncotarget (2016) 7:11002–17. doi: 10.18632/oncotarget.6760
3. Lyakhovich A, Lleonart ME. Bypassing mechanisms of mitochondria-mediated cancer stem cells resistance to chemo- and radiotherapy. Oxid Med Cell Longev (2016) 2016:10. doi: 10.1155/2016/1716341
4. Wang R-F, Wang HY. Immune targets and neoantigens for cancer immunotherapy and precision medicine. Cell Res (2017) 27:11–37. doi: 10.1038/cr.2016.155
5. Fischbach MA, Bluestone JA, Lim WA. Cell-based therapeutics: the next pillar of medicine. Sci Transl Med (2013) 5:179ps7. doi: 10.1126/scitranslmed.3005568
6. Rosenberg SA. Progress in human tumour immunology and immunotherapy. Nature (2001) 411:380–4. doi: 10.1038/35077246
7. Rosenberg SA, Yang JC, Restifo NP. Cancer immunotherapy: moving beyond current vaccines. Nat Med (2004) 10:909–15. doi: 10.1038/nm1100
8. Rook AH, Raphael BA. Progress in immunotherapy of cancer. N Engl J Med (2012) 367:1168. doi: 10.1056/NEJMc1208946
9. Li W, Joshi MD, Singhania S, Ramsey KH, Murthy AK. Peptide vaccine: Progress and challenges. Vaccines (2014) 2:515–36. doi: 10.3390/vaccines2030515
10. Stephens AJ, Burgess-Brown NA, Jiang S. Beyond just peptide antigens: The complex world of peptide-based cancer vaccines. Front Immunol (2021) 12:696791. doi: 10.3389/fimmu.2021.696791
11. Chukwudozie OS, Gray CM, Fagbayi TA, Chukwuanukwu RC, Oyebanji VO, Bankole TT, et al. Immuno-informatics design of a multimeric epitope peptide based vaccine targeting SARS-CoV-2 spike glycoprotein. PloS One (2021) 16:1–25. doi: 10.1371/journal.pone.0248061
12. Carreno BM, Magrini V, Becker-Hapak M, Kaabinejadian S, Hundal J, Petti AA, et al. Cancer immunotherapy. a dendritic cell vaccine increases the breadth and diversity of melanoma neoantigen-specific T cells. Science (2015) 348:803–8. doi: 10.1126/science.aaa3828
13. Peoples GE, Holmes JP, Hueman MT, Mittendorf EA, Amin A, Khoo S, et al. Combined clinical trial results of a HER2/neu (E75) vaccine for the prevention of recurrence in high-risk breast cancer patients: U.S. military cancer institute clinical trials group study I-01 and I-02. Clin Cancer Res (2008) 14:797–803. doi: 10.1158/1078-0432.CCR-07-1448
14. Walter S, Weinschenk T, Stenzl A, Zdrojowy R, Pluzanska A, Szczylik C, et al. Multipeptide immune response to cancer vaccine IMA901 after single-dose cyclophosphamide associates with longer patient survival. Nat Med (2012) 18:1254–61. doi: 10.1038/nm.2883
15. Weber JS, Dummer R, de Pril V, Lebbé C, Hodi FS. Patterns of onset and resolution of immune-related adverse events of special interest with ipilimumab: detailed safety analysis from a phase 3 trial in patients with advanced melanoma. Cancer (2013) 119:1675–82. doi: 10.1002/cncr.27969
16. Wu YL, Park K, Soo RA, Sun Y, Tyroller K, Wages D, et al. INSPIRE: A phase III study of the BLP25 liposome vaccine (L-BLP25) in Asian patients with unresectable stage III non-small cell lung cancer. BMC Cancer (2011) 11:430. doi: 10.1186/1471-2407-11-430
17. Yadav M, Jhunjhunwala S, Phung QT, Lupardus P, Tanguay J, Bumbaca S, et al. Predicting immunogenic tumour mutations by combining mass spectrometry and exome sequencing. Nature (2014) 515:572–6. doi: 10.1038/nature14001
18. Hundal J, Carreno BM, Petti AA, Linette GP, Griffith OL, Mardis ER, et al. pVAC-seq: A genome-guided in silico approach to identifying tumor neoantigens. Genome Med (2016) 8:11. doi: 10.1186/s13073-016-0264-5
19. Chen DS, Mellman I. Oncology meets immunology: The cancer-immunity cycle. Immunity (2013) 39:1–10. doi: 10.1016/j.immuni.2013.07.012
20. Sultan H, Fesenkova VI, Addis D, Fan AE, Kumai T, Wu J, et al. Designing therapeutic cancer vaccines by mimicking viral infections. Cancer Immunol Immunother (2017) 66:203–13. doi: 10.1007/s00262-016-1834-5
21. Sultan H, Kumai T, Nagato T, Wu J, Salazar AM, Celis E. The route of administration dictates the immunogenicity of peptide-based cancer vaccines in mice. Cancer Immunol Immunother (2019) 68:455–66. doi: 10.1007/s00262-018-02294-5
22. Kumai T, Lee S, Cho H-I, Sultan H, Kobayashi H, Harabuchi Y, et al. Optimization of peptide vaccines to induce robust antitumor CD4 T-cell responses. Cancer Immunol Res (2017) 5:72–83. doi: 10.1158/2326-6066.CIR-16-0194
23. Noguchi M, Matsumoto K, Uemura H, Arai G, Eto M, Naito S, et al. An open-label, randomized phase II trial of personalized peptide vaccination in patients with bladder cancer that progressed after platinum-based chemotherapy. Clin Cancer Res (2016) 22:54–60. doi: 10.1158/1078-0432.CCR-15-1265
24. Jiang C, Chao C, Cheng C. Abstract 6618: A database of potential T cell epitopes for cancer immunotherapy. Immunology (2020) 80:6618–8. doi: 10.1158/1538-7445.AM2020-6618
25. Jiang C, Liu G, Zhang W, Zhuang Z, Li W, Qiu S, et al. Abstract LB051: The landscape of peptide vaccinein clinical trials for cancer. Cancer Res (2021) 81:LB051–1. doi: 10.1158/1538-7445.AM2021-LB051
26. Zhang L, Huang Y, Lindstrom AR, Lin TY, Lam KS, Li Y. Peptide-based materials for cancer immunotherapy. Theranostics (2019) 9:7807–25. doi: 10.7150/thno.37194
27. Liu J, Fu M, Wang M, Wan D, Wei Y, Wei X. Cancer vaccines as promising immuno-therapeutics: platforms and current progress. J Hematol Oncol (2022) 15:1–26. doi: 10.1186/s13045-022-01247-x
28. Thomas S, Prendergast GC. Cancer vaccines: A brief overview. Methods Mol Biol (2016) 1403:755–61. doi: 10.1007/978-1-4939-3387-7_43
29. Sakamoto S, Matsueda S, Takamori S, Toh U, Noguchi M, Yutani S, et al. Immunological evaluation of peptide vaccination for cancer patients with the HLA -A11+ or -A33+ allele. Cancer Sci (2017) 108:598–603. doi: 10.1111/cas.13189
30. Sayad A, Akbari MT, Mehdizadeh M, Taheri M, Hajifathali A. HLA-A*26 and susceptility of iranian patients with non-Hodgkin lymphoma. Iran J Immunol (2014) 11:269–74. doi: IJIv11i4A5
31. Malonis RJ, Lai JR, Vergnolle O. Peptide-based vaccines: Current progress and future challenges. Chem Rev (2020) 120:3210–29. doi: 10.1021/acs.chemrev.9b00472
32. Liu W, Tang H, Li L, Wang X, Yu Z, Li J. Peptide-based therapeutic cancer vaccine: Current trends in clinical application. Cell Prolif (2021) 54:1–16. doi: 10.1111/cpr.13025
33. Fernández-Delgado M, Sirsat MS, Cernadas E, Alawadi S, Barro S, Febrero-Bande M. An extensive experimental survey of regression methods. Neural Netw (2019) 111:11–34. doi: 10.1016/j.neunet.2018.12.010
34. Liaw A, Wiener M. Classification and regression by randomForest. R News (2002) 2:18–22. doi: 10.1177/154405910408300516
35. Sing T, Sander O, Beerenwinkel N, Lengauer T. ROCR: Visualizing classifier performance in r. Bioinformatics (2005) 21:3940–1. doi: 10.1093/bioinformatics/bti623
36. Salazar LG, Fikes J, Southwood S, Ishioka G, Knutson KL, Gooley TA, et al. Immunization of cancer patients with HER-2/neu-derived peptides demonstrating high-affinity binding to multiple class II alleles. Clin Cancer Res (2003) 9:5559–65.
37. Mittendorf EA, Clifton GT, Holmes JP, Schneble E, van Echo D, Ponniah S, et al. Final report of the phase I/II clinical trial of the E75 (nelipepimut-s) vaccine with booster inoculations to prevent disease recurrence in high-risk breast cancer patients. Ann Oncol (2014) 25:1735–42. doi: 10.1093/annonc/mdu211
38. Wada S, Tsunoda T, Baba T, Primus FJ, Kuwano H, Shibuya M, et al. Rationale for antiangiogenic cancer therapy with vaccination using epitope peptides derived from human vascular endothelial growth factor receptor 2. Cancer Res (2005) 65:4939–46. doi: 10.1158/0008-5472.CAN-04-3759
39. Yajima N, Yamanaka R, Mine T, Tsuchiya N, Homma J, Sano M, et al. Immunologic evaluation of personalized peptide vaccination for patients with advanced malignant glioma. Clin Cancer Res (2005) 11:5900–11. doi: 10.1158/1078-0432.CCR-05-0559
40. Nishida S, Koido S, Takeda Y, Homma S, Komita H, Takahara A, et al. Wilms tumor gene (WT1) peptide-based cancer vaccine combined with gemcitabine for patients with advanced pancreatic cancer. J Immunother (2014) 37:105–14. doi: 10.1097/CJI.0000000000000020
41. Sadanaga N, Nagashima H, Mashino K, Tahara K, Yamaguchi H, Ohta M, et al. Dendritic cell vaccination with MAGE peptide is a novel therapeutic approach for gastrointestinal carcinomas. Clin Cancer Res (2001) 7:2277–84.
42. Okuno K, Sugiura F, Inoue K, Sukegawa Y. Clinical trial of a 7-peptide cocktail vaccine with oral chemotherapy for patients with metastatic colorectal cancer. Anticancer Res (2014) 34:3045–52.
43. Liénard D, Rimoldi D, Marchand M, Dietrich P-Y, van Baren N, Geldhof C, et al. Ex vivo detectable activation of melan-a-specific T cells correlating with inflammatory skin reactions in melanoma patients vaccinated with peptides in IFA. Cancer Immun (2004) 4:4.
44. Babatz J, Rollig C, Lobel B, Folprecht G, Haack M, Gunther H, et al. Induction of cellular immune responses against carcinoembryonic antigen in patients with metastatic tumors after vaccination with altered peptide ligand-loaded dendritic cells. Cancer Immunol Immunother (2006) 55:268–76. doi: 10.1007/s00262-005-0021-x
45. Creelan BC, Antonia S, Noyes D, Hunter TB, Simon GR, Bepler G, et al. Phase II trial of a GM-CSF-producing and CD40L-expressing bystander cell line combined with an allogeneic tumor cell-based vaccine for refractory lung adenocarcinoma. J Immunother (2013) 36:442–50. doi: 10.1097/CJI.0b013e3182a80237
46. Yoshiyama K, Terazaki Y, Matsueda S, Shichijo S, Noguchi M, Yamada A, et al. Personalized peptide vaccination in patients with refractory non-small cell lung cancer. Int J Oncol (2012) 40:1492–500. doi: 10.3892/ijo.2012.1351
47. Baxevanis CN, Voutsas IF, Gritzapis AD, Perez SA, Papamichail M. HER-2/neu as a target for cancer vaccines. Immunotherapy (2010) 2:213–26. doi: 10.2217/imt.09.89
48. Imai N, Harashima N, Ito M, Miyagi Y, Harada M, Yamada A, et al. Identification of lck-derived peptides capable of inducing HLA-A2-restricted and tumor-specific CTLs in cancer patients with distant metastases. Int J Cancer (2001) 94:237–42. doi: 10.1002/ijc.1461
49. Fay JW, Palucka AK, Paczesny S, Dhodapkar M, Johnston DA, Burkeholder S, et al. Long-term outcomes in patients with metastatic melanoma vaccinated with melanoma peptide-pulsed CD34+ progenitor-derived dendritic cells. Cancer Immunol Immunother (2006) 55:1209–18. doi: 10.1007/s00262-005-0106-6
50. Takahashi R, Ishibashi Y, Hiraoka K, Matsueda S, Kawano K, Kawahara A, et al. Phase II study of personalized peptide vaccination for refractory bone and soft tissue sarcoma patients. Cancer Sci (2013) 104:1285–94. doi: 10.1111/cas.12226
51. Sangha R, Butts C. L-BLP25: a peptide vaccine strategy in non small cell lung cancer. Clin Cancer Res (2007) 13:s4652–4. doi: 10.1158/1078-0432.CCR-07-0213
52. Yoshikawa T, Nakatsugawa M, Suzuki S, Shirakawa H, Nobuoka D, Sakemura N, et al. HLA-A2-restricted glypican-3 peptide-specific CTL clones induced by peptide vaccine show high avidity and antigen-specific killing activity against tumor cells. Cancer Sci (2011) 102:918–25. doi: 10.1111/j.1349-7006.2011.01896.x
53. Disis ML, Wallace DR, Gooley TA, Dang Y, Slota M, Lu H, et al. Concurrent trastuzumab and HER2/neu-specific vaccination in patients with metastatic breast cancer. J Clin Oncol (2009) 27:4685–92. doi: 10.1200/JCO.2008.20.6789
54. Miyatake T, Ueda Y, Morimoto A, Enomoto T, Nishida S, Shirakata T, et al. WT1 peptide immunotherapy for gynecologic malignancies resistant to conventional therapies: a phase II trial. J Cancer Res Clin Oncol (2013) 139:457–63. doi: 10.1007/s00432-012-1348-2
55. Hazama S, Nakamura Y, Takenouchi H, Suzuki N, Tsunedomi R, Inoue Y, et al. A phase I study of combination vaccine treatment of five therapeutic epitope-peptides for metastatic colorectal cancer; safety, immunological response, and clinical outcome. J Transl Med (2014) 12:63. doi: 10.1186/1479-5876-12-63
56. Harashima N, Tanaka K, Sasatomi T, Shimizu K, Miyagi Y, Yamada A, et al. Recognition of the lck tyrosine kinase as a tumor antigen by cytotoxic T lymphocytes of cancer patients with distant metastases. Eur J Immunol (2001) 31:323–32. doi: 10.1002/1521-4141(200102)31:2<323::AID-IMMU323>3.0.CO;2-0
57. Tada F, Abe M, Hirooka M, Ikeda Y, Hiasa Y, Lee Y, et al. Phase I/II study of immunotherapy using tumor antigen-pulsed dendritic cells in patients with hepatocellular carcinoma. Int J Oncol (2012) 41:1601–9. doi: 10.3892/ijo.2012.1626
58. Khleif SN, Abrams SI, Hamilton JM, Bergmann-Leitner E, Chen A, Bastian A, et al. A phase I vaccine trial with peptides reflecting ras oncogene mutations of solid tumors. J Immunother (1999) 22:155–65. doi: 10.1097/00002371-199903000-00007
59. Tanaka F, Haraguchi N, Isikawa K, Inoue H, Mori M. Potential role of dendritic cell vaccination with MAGE peptides in gastrointestinal carcinomas. Oncol Rep (2008) 20:1111–6.
60. Miyagi Y, Imai N, Sasatomi T, Yamada A, Mine T, Katagiri K, et al. Induction of cellular immune responses to tumor cells and peptides in colorectal cancer patients by vaccination with SART3 peptides. Clin Cancer Res (2001) 7:3950–62.
61. Ogata R, Matsueda S, Yao A, Noguchi M, Itoh K, Harada M. Identification of polycomb group protein enhancer of zeste homolog 2 (EZH2)-derived peptides immunogenic in HLA-A24+ prostate cancer patients. Prostate (2004) 60:273–81. doi: 10.1002/pros.20078
62. Hamid O, Solomon JC, Scotland R, Garcia M, Sian S, Ye W, et al. Alum with interleukin-12 augments immunity to a melanoma peptide vaccine: Correlation with time to relapse in patients with resected high-risk disease. Clin Cancer Res (2007) 13:215–22. doi: 10.1158/1078-0432.CCR-06-1450
63. Butterfield LH, Ribas A, Meng WS, Dissette VB, Amarnani S, Vu HT, et al. T-Cell responses to HLA-A*0201 immunodominant peptides derived from alpha-fetoprotein in patients with hepatocellular cancer. Clin Cancer Res (2003) 9:5902–8.
64. Brunsvig PF, Aamdal S, Gjertsen MK, Kvalheim G, Markowski-Grimsrud CJ, Sve I, et al. Telomerase peptide vaccination: a phase I/II study in patients with non-small cell lung cancer. Cancer Immunol Immunother (2006) 55:1553–64. doi: 10.1007/s00262-006-0145-7
65. Becker JC, Andersen MH, Hofmeister-Muller V, Wobser M, Frey L, Sandig C, et al. Survivin-specific T-cell reactivity correlates with tumor response and patient survival: a phase-II peptide vaccination trial in metastatic melanoma. Cancer Immunol Immunother (2012) 61:2091–103. doi: 10.1007/s00262-012-1266-9
66. Scardino A, Alves P, Gross DA, Tourdot S, Graff-Dubois S, Angevin E, et al. Identification of HER-2/neu immunogenic epitopes presented by renal cell carcinoma and other human epithelial tumors. Eur J Immunol (2001) 31:3261–70. doi: 10.1002/1521-4141(200111)31:11<3261::AID-IMMU3261>3.0.CO;2-4
67. Slingluff CL, Petroni GR, Chianese-Bullock KA, Smolkin ME, Hibbitts S, Murphy C, et al. Immunologic and clinical outcomes of a randomized phase II trial of two multipeptide vaccines for melanoma in the adjuvant setting. Clin Cancer Res (2007) 13:6386–95. doi: 10.1158/1078-0432.CCR-07-0486
68. Liu KJ, Wang CC, Chen LT, Cheng AL, Lin DT, Wu YC, et al. Generation of carcinoembryonic antigen (CEA)-specific T-cell responses in HLA-A*0201 and HLA-A*2402 late-stage colorectal cancer patients after vaccination with dendritic cells loaded with CEA peptides. Clin Cancer Res (2004) 10:2645–51. doi: 10.1158/1078-0432.CCR-03-0430
69. Shomura H, Shichijo S, Komatsu N, Matsueda S, Mine T, Rikimaru T, et al. Identification of epidermal growth factor receptor-derived peptides recognised by both cellular and humoral immune responses in HLA-A24+ non-small cell lung cancer patients. Eur J Cancer (2004) 40:1776–86. doi: 10.1016/j.ejca.2004.04.003
70. Kabaker K, Shell K, Kaufman HL. Vaccines for colorectal cancer and renal cell carcinoma. Cancer J (2011) 17:283–93. doi: 10.1097/PPO.0b013e318232ff44
71. Rapoport AP, Aqui NA, Stadtmauer EA, Vogl DT, Fang HB, Cai L, et al. Combination immunotherapy using adoptive T-cell transfer and tumor antigen vaccination on the basis of hTERT and survivin after ASCT for myeloma. Blood (2011) 117:788–97. doi: 10.1182/blood-2010-08-299396
72. Morse MA, Deng Y, Coleman D, Hull S, Kitrell-Fisher E, Nair S, et al. A phase I study of active immunotherapy with carcinoembryonic antigen peptide (CAP-1)-pulsed, autologous human cultured dendritic cells in patients with metastatic malignancies expressing carcinoembryonic antigen. Clin Cancer Res (1999) 5:1331–8.
73. Cormier JN, Salgaller ML, Prevette T, Barracchini KC, Rivoltini L, Restifo NP, et al. Enhancement of cellular immunity in melanoma patients immunized with a peptide from MART-1/Melan a. Cancer J Sci Am (1997) 3:37–44.
74. Butts C, Murray N, Maksymiuk A, Goss G, Marshall E, Soulières D, et al. Randomized phase IIB trial of BLP25 liposome vaccine in stage IIIB and IV non-small-cell lung cancer. J Clin Oncol (2005) 23:6674–81. doi: 10.1200/JCO.2005.13.011
75. Nakao M, Shichijo S, Imaizumi T, Inoue Y, Matsunaga K, Yamada A, et al. Identification of a gene coding for a new squamous cell carcinoma antigen recognized by the CTL. J Immunol (2000) 164:2565–74. doi: 10.4049/jimmunol.164.5.2565
76. Ito M, Shichijo S, Miyagi Y, Kobayashi T, Tsuda N, Yamada A, et al. Identification of SART3-derived peptides capable of inducing HLA-A2-restricted and tumor-specific CTLs in cancer patients with different HLA-A2 subtypes. Int J Cancer (2000) 88:633–9. doi: 10.1002/1097-0215(20001115)88:4<633::AID-IJC18>3.0.CO;2-N
77. Kobayashi K, Noguchi M, Itoh K, Harada M. Identification of a prostate-specific membrane antigen-derived peptide capable of eliciting both cellular and humoral immune responses in HLA-A24+ prostate cancer patients. Cancer Sci (2003) 94:622–7. doi: 10.1111/j.1349-7006.2003.tb01493.x
78. Choi C, Witzens M, Bucur M, Feuerer M, Sommerfeldt N, Trojan A, et al. Enrichment of functional CD8 memory T cells specific for MUC1 in bone marrow of patients with multiple myeloma. Blood (2005) 105:2132–4. doi: 10.1182/blood-2004-01-0366
79. Sarnaik AA, Yu B, Yu D, Morelli D, Hall M, Bogle D, et al. Extended dose ipilimumab with a peptide vaccine: immune correlates associated with clinical benefit in patients with resected high-risk stage IIIc/IV melanoma. Clin Cancer Res (2011) 17:896–906. doi: 10.1158/1078-0432.CCR-10-2463
80. Bolonaki I, Kotsakis A, Papadimitraki E, Aggouraki D, Konsolakis G, Vagia A, et al. Vaccination of patients with advanced non – small-cell lung cancer with an optimized cryptic human telomerase reverse transcriptase peptide. J Clin Oncol (2007) 25:2727–34. doi: 10.1200/JCO.2006.10.3465
81. Barnea E, Beer I, Patoka R, Ziv T, Kessler O, Tzehoval E, et al. Analysis of endogenous peptides bound by soluble MHC class I molecules: A novel approach for identifying tumor-specific antigens. Eur J Immunol (2002) 32:213–22. doi: 10.1002/1521-4141(200201)32:1<213::AID-IMMU213>3.0.CO;2-8
82. Yang D, Nakao M, Shichijo S, Sasatomi T, Takasu H, Matsumoto H, et al. Identification of a gene coding for a protein possessing shared tumor epitopes capable of inducing HLA-A24-restricted cytotoxic T lymphocytes in cancer patients. Cancer Res (1999) 59:4056–63. doi: 10.1002/1521-4141(200201)32:1<213::AID-IMMU213>3.0.CO;2-8
83. Butler MO, Lee JS, Ansén S, Neuberg D, Hodi FS, Murray AP, et al. Long-lived antitumor CD8+ lymphocytes for adoptive therapy generated using an artificial antigen-presenting cell. Clin Cancer Res (2007) 13:1857–67. doi: 10.1158/1078-0432.CCR-06-1905
84. Lee P, Wang F, Kuniyoshi J, Rubio V, Stuges T, Groshen S, et al. Effects of interleukin-12 on the immune response to a multipeptide vaccine for resected metastatic melanoma. J Clin Oncol (2001) 19:3836–47. doi: 10.1200/JCO.2001.19.18.3836
85. Matsushita N, Aruga A, Inoue Y, Kotera Y, Takeda K, Yamamoto M. Phase I clinical trial of a peptide vaccine combined with tegafur-uracil plus leucovorin for treatment of advanced or recurrent colorectal cancer. Oncol Rep (2013) 29:951–9. doi: 10.3892/or.2013.2231
86. Yamada A, Kawano K, Koga M, Matsumoto T, Itoh K. Multidrug resistance-associated protein 3 is a tumor rejection antigen recognized by HLA-A2402-restricted cytotoxic T lymphocytes. Cancer Res (2001) 61:6459–66.
87. Kibe S, Yutani S, Motoyama S, Nomura T, Tanaka N, Kawahara A, et al. Phase II study of personalized peptide vaccination for previously treated advanced colorectal cancer. Cancer Immunol Res (2014) 2:1154–62. doi: 10.1158/2326-6066.CIR-14-0035
88. Tanaka T, Kitamura H, Inoue R, Nishida S, Takahashi-Takaya A, Kawami S, et al. Potential survival benefit of anti-apoptosis protein: survivin-derived peptide vaccine with and without interferon alpha therapy for patients with advanced or recurrent urothelial cancer–results from phase I clinical trials. Clin Dev Immunol (2013) 2013:262967. doi: 10.1155/2013/262967
89. Svane IM, Pedersen AE, Johansen JS, Johnsen HE, Nielsen D, Kamby C, et al. Vaccination with p53 peptide-pulsed dendritic cells is associated with disease stabilization in patients with p53 expressing advanced breast cancer; monitoring of serum YKL-40 and IL-6 as response biomarkers. Cancer Immunol Immunother (2007) 56:1485–99. doi: 10.1007/s00262-007-0293-4
90. Zeestraten ECM, Speetjens FM, Welters MJP, Saadatmand S, Stynenbosch LFM, Jongen R, et al. Addition of interferon-α to the p53-SLP® vaccine results in increased production of interferon-γ in vaccinated colorectal cancer patients: a phase I/II clinical trial. Int J Cancer (2013) 132:1581–91. doi: 10.1002/ijc.27819
91. Dai S, Zhou X, Wang B, Wang Q, Fu Y, Chen T, et al. Enhanced induction of dendritic cell maturation and HLA-A*0201-restricted CEA-specific CD8+ CTL response by exosomes derived from IL-18 gene-modified CEA-positive tumor cells. J Mol Med (2006) 84:1067–76. doi: 10.1007/s00109-006-0102-0
92. Noguchi M, Sasada T, Itoh K. Personalized peptide vaccination: A new approach for advanced cancer as therapeutic cancer vaccine. Cancer Immunol Immunother (2013) 62:919–29. doi: 10.1007/s00262-012-1379-1
93. Sato Y, Maeda Y, Shomura H, Sasatomi T, Takahashi M, Une Y, et al. A phase I trial of cytotoxic T-lymphocyte precursor-oriented peptide vaccines for colorectal carcinoma patients. Br J Cancer (2004) 90:1334–42. doi: 10.1038/sj.bjc.6601711
94. Lekka E, Gritzapis AD, Perez SA, Tsavaris N, Missitzis I, Mamalaki A, et al. Identification and characterization of a HER-2/neu epitope as a potential target for cancer immunotherapy. Cancer Immunol Immunother (2010) 59:715–27. doi: 10.1007/s00262-009-0791-7
95. Thorn M, Wang M, Kloverpris H, Schmidt EG, Fomsgaard A, Wenandy L, et al. Identification of a new hTERT-derived HLA-A*0201 restricted, naturally processed CTL epitope. Cancer Immunol Immunother (2007) 56:1755–63. doi: 10.1007/s00262-007-0319-y
96. Harada M, Kobayashi K, Matsueda S, Nakagawa M, Noguchi M, Itoh K. Prostate-specific antigen-derived epitopes capable of inducing cellular and humoral responses in HLA-A24+ prostate cancer patients. Prostate (2003) 57:152–9. doi: 10.1002/pros.10280
97. Karanikas V, Hwang LA, Pearson J, Ong CS, Apostolopoulos V, Vaughan H, et al. Antibody and T cell responses of patients with adenocarcinoma immunized with mannan-MUC1 fusion protein. J Clin Invest (1997) 100:2783–92. doi: 10.1172/JCI119825
98. Wobser M, Keikavoussi P, Kunzmann V, Weininger M, Andersen MH, Becker JC. Complete remission of liver metastasis of pancreatic cancer under vaccination with a HLA-A2 restricted peptide derived from the universal tumor antigen survivin. Cancer Immunol Immunother (2006) 55:1294–8. doi: 10.1007/s00262-005-0102-x
99. Filipazzi P, Pilla L, Mariani L, Patuzzo R, Castelli C, Camisaschi C, et al. Limited induction of tumor cross-reactive T cells without a measurable clinical benefit in early melanoma patients vaccinated with human leukocyte antigen class I-modified peptides. Clin Cancer Res (2012) 18:6485–96. doi: 10.1158/1078-0432.CCR-12-1516
100. Matsueda S, Takedatsu H, Yao A, Tanaka M, Noguchi M, Itoh K, et al. Identification of peptide vaccine candidates for prostate cancer patients with HLA-A3 supertype alleles. Clin Cancer Res (2005) 11:6933–43. doi: 10.1158/1078-0432.CCR-05-0682
101. Holmes JP, Gates JD, Benavides LC, Hueman MT, Carmichael MG, Patil R, et al. Optimal dose and schedule of an HER-2/neu (E75) peptide vaccine to prevent breast cancer recurrence: from US military cancer institute clinical trials group study I-01 and I-02. Cancer (2008) 113:1666–75. doi: 10.1002/cncr.23772
102. Sakamoto S, Matsueda S, Takamori S, Toh U, Noguchi M, Yutani S, et al. Immunological evaluation of peptide vaccination for cancer patients with the HLA-A26 allele. Cancer Sci (2015) 106:1257–63. doi: 10.1111/cas.12757
103. Kimura T, McKolanis JR, Dzubinski LA, Islam K, Potter DM, Salazar AM, et al. MUC1 vaccine for individuals with advanced adenoma of the colon: A cancer immunoprevention feasibility study. Cancer Prev Res (2013) 6:18–26. doi: 10.1158/1940-6207.CAPR-12-0275
104. Naito M, Komohara Y, Ishihara Y, Noguchi M, Yamashita Y, Shirakusa T, et al. Identification of lck-derived peptides applicable to anti-cancer vaccine for patients with human leukocyte antigen-A3 supertype alleles. Br J Cancer (2007) 97:1648–54. doi: 10.1038/sj.bjc.6604071
105. Staff C, Mozaffari F, Frödin J-E, Mellstedt H, Liljefors M. Telomerase (GV1001) vaccination together with gemcitabine in advanced pancreatic cancer patients. Int J Oncol (2014) 45:1293–303. doi: 10.3892/ijo.2014.2496
106. Gjertsen MK, Buanes T, Rosseland AR, Bakka A, Gladhaug I, Søreide O, et al. Intradermal ras peptide vaccination with granulocyte-macrophage colony-stimulating factor as adjuvant: Clinical and immunological responses in patients with pancreatic adenocarcinoma. Int J Cancer (2001) 92:441–50. doi: 10.1002/ijc.1205
107. Morita S, Oka Y, Tsuboi A, Kawakami M, Maruno M, Izumoto S, et al. A phase I/II trial of a WT1 (Wilms’ tumor gene) peptide vaccine in patients with solid malignancy: safety assessment based on the phase I data. Jpn J Clin Oncol (2006) 36:231–6. doi: 10.1093/jjco/hyl005
108. Speiser DE, Schwarz K, Baumgaertner P, Manolova V, Devevre E, Sterry W, et al. Memory and effector CD8 T-cell responses after nanoparticle vaccination of melanoma patients. J Immunother (2010) 33:848–58. doi: 10.1097/CJI.0b013e3181f1d614
109. Suzuki N, Hazama S, Ueno T, Matsui H, Shindo Y, Iida M, et al. A phase I clinical trial of vaccination with KIF20A-derived peptide in combination with gemcitabine for patients with advanced pancreatic cancer. J Immunother (2014) 37:36–42. doi: 10.1097/CJI.0000000000000012
110. McDermott D, Haanen J, Chen T-T, Lorigan P, O’Day S. Efficacy and safety of ipilimumab in metastatic melanoma patients surviving more than 2 years following treatment in a phase III trial (MDX010-20). Ann Oncol (2013) 24:2694–8. doi: 10.1093/annonc/mdt291
111. Karbach J, Gnjatic S, Bender A, Neumann A, Weidmann E, Yuan J, et al. Tumor-reactive CD8+ T-cell responses after vaccination with NY-ESO-1 peptide, CpG 7909 and montanide® ISA-51: Association with survival. Int J Cancer (2010) 126:909–18. doi: 10.1002/ijc.24850
112. Tsuruma T, Hata F, Torigoe T, Furuhata T, Idenoue S, Kurotaki T, et al. Phase I clinical study of anti-apoptosis protein, survivin-derived peptide vaccine therapy for patients with advanced or recurrent colorectal cancer. J Transl Med (2004) 2:19. doi: 10.1186/1479-5876-2-19
113. Benavides LC, Gates JD, Carmichael MG, Patil R, Patel R, Holmes JP, et al. The impact of HER2/neu expression level on response to the E75 vaccine: from U.S. military cancer institute clinical trials group study I-01 and I-02. Clin Cancer Res (2009) 15:2895–904. doi: 10.1158/1078-0432.CCR-08-1126
114. Barve M, Bender J, Senzer N, Cunningham C, Greco FA, McCune D, et al. Induction of immune responses and clinical efficacy in a phase II trial of IDM-2101, a 10-epitope cytotoxic T-lymphocyte vaccine, in metastatic non-small-cell lung cancer. J Clin Oncol (2008) 26:4418–25. doi: 10.1200/JCO.2008.16.6462
115. Asahara S, Takeda K, Yamao K, Maguchi H, Yamaue H. Phase I/II clinical trial using HLA-A24-restricted peptide vaccine derived from KIF20A for patients with advanced pancreatic cancer. J Transl Med (2013) 11:291. doi: 10.1186/1479-5876-11-291
116. Takahashi R, Toh U, Iwakuma N, Takenaka M, Otsuka H, Furukawa M, et al. Feasibility study of personalized peptide vaccination for metastatic recurrent triple-negative breast cancer patients. Breast Cancer Res (2014) 16:R70. doi: 10.1186/bcr3685
117. Fong L, Hou Y, Rivas A, Benike C, Yuen A, Fisher GA, et al. Altered peptide ligand vaccination with Flt3 ligand expanded dendritic cells for tumor immunotherapy. Proc Natl Acad Sci U S A (2001) 98:8809–14. doi: 10.1073/pnas.141226398
118. Slingluff CL, Lee S, Zhao F, Chianese-Bullock KA, Olson WC, Butterfield LH, et al. A randomized phase II trial of multiepitope vaccination with melanoma peptides for cytotoxic T cells and helper T cells for patients with metastatic melanoma (E1602). Clin Cancer Res (2013) 19:4228–38. doi: 10.1158/1078-0432.CCR-13-0002
119. Terazaki Y, Yoshiyama K, Matsueda S, Watanabe N, Kawahara A, Naito Y, et al. Immunological evaluation of personalized peptide vaccination in refractory small cell lung cancer. Cancer Sci (2012) 103:638–44. doi: 10.1111/j.1349-7006.2012.02202.x
120. Inoue Y, Takaue Y, Takei M, Kato K, Kanai S, Harada Y, et al. Induction of tumor specific cytotoxic T lymphocytes in prostate cancer using prostatic acid phosphatase derived HLA-A2402 binding peptide. J Urol (2001) 166:1508–13. doi: 10.1016/S0022-5347(05)65821-1
121. Morse MA, Niedzwiecki D, Marshall JL, Garrett C, Chang DZ, Aklilu M, et al. A randomized phase II study of immunization with dendritic cells modified with poxvectors encoding CEA and MUC1 compared with the same poxvectors plus GM-CSF for resected metastatic colorectal cancer. Ann Surg (2013) 258:879–86. doi: 10.1097/SLA.0b013e318292919e
122. Holmes JP, Benavides LC, Gates JD, Carmichael MG, Hueman MT, Mittendorf EA, et al. Results of the first phase I clinical trial of the novel II-key hybrid preventive HER-2/neu peptide (AE37) vaccine. J Clin Oncol (2008) 26:3426–33. doi: 10.1200/JCO.2007.15.7842
123. Toubaji A, Achtar M, Provenzano M, Herrin VE, Behrens R, Hamilton M, et al. Pilot study of mutant ras peptide-based vaccine as an adjuvant treatment in pancreatic and colorectal cancers. Cancer Immunol Immunother (2008) 57:1413–20. doi: 10.1007/s00262-008-0477-6
124. Mittendorf EA, Holmes JP, Murray JL, von Hofe E, Peoples GE. CD4+ T cells in antitumor immunity: utility of an li-key HER2/neu hybrid peptide vaccine (AE37). Expert Opin Biol Ther (2009) 9:71–8. doi: 10.1517/14712590802614538
125. Domchek SM, Recio A, Mick R, Clark CE, Carpenter EL, Fox KR, et al. Telomerase-specific T-cell immunity in breast cancer: effect of vaccination on tumor immunosurveillance. Cancer Res (2007) 67:10546–55. doi: 10.1158/0008-5472.CAN-07-2765
126. Mittendorf EA, Clifton GT, Holmes JP, Clive KS, Patil R, Benavides LC, et al. Clinical trial results of the HER-2/neu (E75) vaccine to prevent breast cancer recurrence in high-risk patients: From US military cancer institute clinical trials group study I-01 and I-02. Cancer (2012) 118:2594–602. doi: 10.1002/cncr.26574
127. Harrop R, Drury N, Shingler W, Chikoti P, Redchenko I, Carroll MW, et al. Vaccination of colorectal cancer patients with TroVax given alongside chemotherapy (5-fluorouracil, leukovorin and irinotecan) is safe and induces potent immune responses. Cancer Immunol Immunother (2008) 57:977–86. doi: 10.1007/s00262-007-0428-7
128. Kameshima H, Tsuruma T, Kutomi G, Shima H, Iwayama Y, Kimura Y, et al. Immunotherapeutic benefit of α-interferon (IFNα) in survivin2B-derived peptide vaccination for advanced pancreatic cancer patients. Cancer Sci (2013) 104:124–9. doi: 10.1111/cas.12046
129. Czerniecki BJ, Koski GK, Koldovsky U, Xu S, Cohen PA, Mick R, et al. Targeting HER-2/neu in early breast cancer development using dendritic cells with staged interleukin-12 burst secretion. Cancer Res (2007) 67:1842–52. doi: 10.1158/0008-5472.CAN-06-4038
130. Sato N, Hirohashi Y, Tsukahara T, Kikuchi T, Sahara H, Kamiguchi K, et al. Molecular pathological approaches to human tumor immunology. Pathol Int (2009) 59:205–17. doi: 10.1111/j.1440-1827.2009.02353.x
131. Bernhardt SL, Gjertsen MK, Trachsel S, Moller M, Eriksen JA, Meo M, et al. Telomerase peptide vaccination of patients with non-resectable pancreatic cancer: A dose escalating phase I/II study. Br J Cancer (2006) 95:1474–82. doi: 10.1038/sj.bjc.6603437
132. Slingluff CL, Petroni GR, Olson W, Czarkowski A, Grosh WW, Smolkin M, et al. Helper T-cell responses and clinical activity of a melanoma vaccine with multiple peptides from MAGE and melanocytic differentiation antigens. J Clin Oncol (2008) 26:4973–80. doi: 10.1200/JCO.2008.17.3161
133. Masuzawa T, Fujiwara Y, Okada K, Nakamura A, Takiguchi S, Nakajima K, et al. Phase I/II study of s-1 plus cisplatin combined with peptide vaccines for human vascular endothelial growth factor receptor 1 and 2 in patients with advanced gastric cancer. Int J Oncol (2012) 41:1297–304. doi: 10.3892/ijo.2012.1573
134. Svane IM, Pedersen AE, Nikolajsen K, Zocca M-B. Alterations in p53-specific T cells and other lymphocyte subsets in breast cancer patients during vaccination with p53-peptide loaded dendritic cells and low-dose interleukin-2. Vaccine (2008) 26:4716–24. doi: 10.1016/j.vaccine.2008.06.085
135. Gritzapis AD, Voutsas IF, Lekka E, Tsavaris N, Missitzis I, Sotiropoulou P, et al. Identification of a novel immunogenic HLA-A*0201-binding epitope of HER-2/neu with potent antitumor properties. J Immunol (2008) 181:146–54. doi: 10.4049/jimmunol.181.1.146
136. Dakappagari NK, Pyles J, Parihar R, Carson WE, Young DC, Kaumaya PTP. A chimeric multi-human epidermal growth factor receptor-2 b cell epitope peptide vaccine mediates superior antitumor responses. J Immunol (2003) 170:4242–53. doi: 10.4049/jimmunol.170.8.4242
137. Slingluff CLJ, Petroni GR, Yamshchikov GV, Barnd DL, Eastham S, Galavotti H, et al. Clinical and immunologic results of a randomized phase II trial of vaccination using four melanoma peptides either administered in granulocyte-macrophage colony-stimulating factor in adjuvant or pulsed on dendritic cells. J Clin Oncol (2003) 21:4016–26. doi: 10.1200/JCO.2003.10.005
138. Iiyama T, Udaka K, Takeda S, Takeuchi T, Adachi YC, Ohtsuki Y, et al. WT1 (Wilms’ tumor 1) peptide immunotherapy for renal cell carcinoma. Microbiol Immunol (2007) 51:519–30. doi: 10.1111/j.1348-0421.2007.tb03940.x
139. Slingluff CLJ, Petroni GR, Yamshchikov GV, Hibbitts S, Grosh WW, Chianese-Bullock KA, et al. Immunologic and clinical outcomes of vaccination with a multiepitope melanoma peptide vaccine plus low-dose interleukin-2 administered either concurrently or on a delayed schedule. J Clin Oncol (2004) 22:4474–85. doi: 10.1200/JCO.2004.10.212
140. Ito M, Shichijo S, Tsuda N, Ochi M, Harashima N, Saito N, et al. Molecular basis of T cell-mediated recognition of pancreatic cancer cells. Cancer Res (2001) 61:2038–46.
141. Speetjens FM, Kuppen PJK, Welters MJP, Essahsah F, Van Den Brink AMEGV, Lantrua MGK, et al. Induction of p53-specific immunity by a p53 synthetic long peptide vaccine in patients treated for metastatic colorectal cancer. Clin Cancer Res (2009) 15:1086–95. doi: 10.1158/1078-0432.CCR-08-2227
142. Miles D, Papazisis K. Rationale for the clinical development of STn-KLH (Theratope) and anti-MUC-1 vaccines in breast cancer. Clin Breast Cancer (2003) 3 Suppl 4:S134–8. doi: 10.3816/CBC.2003.s.002
143. Kotsakis A, Papadimitraki E, Vetsika EK, Aggouraki D, Dermitzaki EK, Hatzidaki D, et al. A phase II trial evaluating the clinical and immunologic response of HLA-A2(+) non-small cell lung cancer patients vaccinated with an hTERT cryptic peptide. Lung Cancer (2014) 86:59–66. doi: 10.1016/j.lungcan.2014.07.018
144. Sawada Y, Yoshikawa T, Nobuoka D, Shirakawa H, Kuronuma T, Motomura Y, et al. Phase I trial of a glypican-3-derived peptide vaccine for advanced hepatocellular carcinoma: Immunologic evidence and potential for improving overall survival. Clin Cancer Res (2012) 18:3686–96. doi: 10.1158/1078-0432.CCR-11-3044
145. Brunsvig PF, Kyte JA, Kersten C, Sundstrøm S, Møller M, Nyakas M, et al. Telomerase peptide vaccination in NSCLC: a phase II trial in stage III patients vaccinated after chemoradiotherapy and an 8-year update on a phase I/II trial. Clin Cancer Res (2011) 17:6847–57. doi: 10.1158/1078-0432.CCR-11-1385
146. Yao A, Harada M, Matsueda S, Ishihara Y, Shomura H, Noguchi M, et al. Identification of parathyroid hormone-related protein-derived peptides immunogenic in human histocompatibility leukocyte antigen-A24+ prostate cancer patients. Br J Cancer (2004) 91:287–96. doi: 10.1038/sj.bjc.6601960
147. Schaefer C, Butterfield LH, Lee S, Kim GG, Visus C, Albers A, et al. Function but not phenotype of melanoma peptide-specific CD8(+) T cells correlate with survival in a multiepitope peptide vaccine trial (ECOG 1696). Int J Cancer (2012) 131:874–84. doi: 10.1002/ijc.26481
148. Rongcun Y, Salazar-Onfray F, Charo J, Malmberg KJ, Evrin K, Maes H, et al. Identification of new HER2/neu-derived peptide epitopes that can elicit specific CTL against autologous and allogeneic carcinomas and melanomas. J Immunol (1999) 163:1037–44.
149. Ishikawa H, Imano M, Shiraishi O, Yasuda A, Peng YF, Shinkai M, et al. Phase i clinical trial of vaccination with LY6K-derived peptide in patients with advanced gastric cancer. Gastric Cancer (2014) 17:173–80. doi: 10.1007/s10120-013-0258-6
150. Markovic SN, Suman VJ, Ingle JN, Kaur JS, Pitot HC, Loprinzi CL, et al. Peptide vaccination of patients with metastatic melanoma: improved clinical outcome in patients demonstrating effective immunization. Am J Clin Oncol (2006) 29:352–60. doi: 10.1097/01.coc.0000217877.78473.a4
151. Neumann F, Sturm C, Hülsmeyer M, Dauth N, Guillaume P, Luescher IF, et al. Fab antibodies capable of blocking T cells by competitive binding have the identical specificity but a higher affinity to the MHC-peptide-complex than the T cell receptor. Immunol Lett (2009) 125:86–92. doi: 10.1016/j.imlet.2009.06.002
152. Alves PM, Viatte S, Fagerberg T, Michielin O, Bricard G, Bouzourene H, et al. Immunogenicity of the carcinoembryonic antigen derived peptide 694 in HLA-A2 healthy donors and colorectal carcinoma patients. Cancer Immunol Immunother (2007) 56:1795–805. doi: 10.1007/s00262-007-0323-2
153. Tamura M, Nishizaka S, Maeda Y, Ito M, Harashima N, Harada M, et al. Identification of cyclophilin b-derived peptides capable of inducing histocompatibility leukocyte antigen-A2-restricted and tumor-specific cytotoxic T lymphocytes. Jpn J Cancer Res (2001) 92:762–7. doi: 10.1111/j.1349-7006.2001.tb01159.x
154. Amin A, Benavides LC, Holmes JP, Gates JD, Carmichael MG, Hueman MT, et al. Assessment of immunologic response and recurrence patterns among patients with clinical recurrence after vaccination with a preventive HER2/neu peptide vaccine: from US military cancer institute clinical trials group study I-01 and I-02. Cancer Immunol Immunother (2008) 57:1817–25. doi: 10.1007/s00262-008-0509-2
155. Kawaguchi S, Wada T, Ida K, Sato Y, Nagoya S, Tsukahara T, et al. Phase I vaccination trial of SYT-SSX junction peptide in patients with disseminated synovial sarcoma. J Transl Med (2005) 3:1. doi: 10.1186/1479-5876-3-1
156. de Vries IJM, Bernsen MR, Lesterhuis WJ, Scharenborg NM, Strijk SP, Gerritsen M-JP, et al. Immunomonitoring tumor-specific T cells in delayed-type hypersensitivity skin biopsies after dendritic cell vaccination correlates with clinical outcome. J Clin Oncol (2005) 23:5779–87. doi: 10.1200/JCO.2005.06.478
157. Clive KS, Tyler JA, Clifton GT, Holmes JP, Ponniah S, Peoples GE, et al. The GP2 peptide: a HER2/neu-based breast cancer vaccine. J Surg Oncol (2012) 105:452–8. doi: 10.1002/jso.21723
158. Ullenhag GJ, Frödin J-E, Mosolits S, Kiaii S, Hassan M, Bonnet MC, et al. Immunization of colorectal carcinoma patients with a recombinant canarypox virus expressing the tumor antigen ep-CAM/KSA (ALVAC-KSA) and granulocyte macrophage colony- stimulating factor induced a tumor-specific cellular immune response. Clin Cancer Res (2003) 9:2447–56.
159. Oka Y, Tsuboi A, Taguchi T, Osaki T, Kyo T, Nakajima H, et al. Induction of WT1 (Wilms’ tumor gene)-specific cytotoxic T lymphocytes by WT1 peptide vaccine and the resultant cancer regression. Proc Natl Acad Sci U S A (2004) 101:13885–90. doi: 10.1073/pnas.0405884101
160. Svane IM, Pedersen AE, Johnsen HE, Nielsen D, Kamby C, Gaarsdal E, et al. Vaccination with p53-peptide-pulsed dendritic cells, of patients with advanced breast cancer: report from a phase I study. Cancer Immunol Immunother (2004) 53:633–41. doi: 10.1007/s00262-003-0493-5
161. Schwartzentruber DJ, Lawson DH, Richards JM, Conry RM, Miller DM, Treisman J, et al. Gp100 peptide vaccine and interleukin-2 in patients with advanced melanoma. N Engl J Med (2011) 364:2119–27. doi: 10.1056/NEJMoa1012863
162. Hazama S, Nakamura Y, Tanaka H, Hirakawa K, Tahara K, Shimizu R, et al. A phase II study of five peptides combination with oxaliplatin-based chemotherapy as a first-line therapy for advanced colorectal cancer (FXV study). J Transl Med (2014) 12:108. doi: 10.1186/1479-5876-12-108
163. Meyer RG, Korn S, Micke P, Becker K, Huber C, Wölfel T, et al. An open-label, prospective phase I/II study evaluating the immunogenicity and safety of a ras peptide vaccine plus GM-CSF in patients with non-small cell lung cancer. Lung Cancer (2007) 58:88–94. doi: 10.1016/j.lungcan.2007.05.003
164. Bolhassani A, Safaiyan S, Rafati S. Improvement of different vaccine delivery systems for cancer therapy. Mol Cancer (2011) 10:3. doi: 10.1186/1476-4598-10-3
165. Carreno BM, Magrini V, Becker-Hapak M, Kaabinejadian S, Hundal J, Petti AA, et al. A dendritic cell vaccine increases the breadth and diversity of melanoma neoantigen-specific T cells. Science (2015) 348:1–9. doi: 10.1126/science.aaa3828
166. Brichard VG, Lejeune D. Cancer immunotherapy targeting tumour-specific antigens: Towards a new therapy for minimal residual disease. Expert Opin Biol Ther (2008) 8:951–68. doi: 10.1517/14712598.8.7.951
167. Zaremba S, Barzaga E, Zhu M, Soares N, Tsang KY, Schlom J. Identification of an enhancer agonist cytotoxic T lymphocyte peptide from human carcinoembryonic antigen. Cancer Res (1997) 57:4570–7.
168. Goldman B, DeFrancesco L. The cancer vaccine roller coaster. Nat Biotechnol (2009) 27:129–39. doi: 10.1038/nbt0209-129
169. Robert C, Schadendorf D, Messina M, Hodi FS, O’Day S. Efficacy and safety of retreatment with ipilimumab in patients with pretreated advanced melanoma who progressed after initially achieving disease control. Clin Cancer Res (2013) 19:2232–9. doi: 10.1158/1078-0432.CCR-12-3080
170. Hodi FS, O’Day SJS, McDermott DF, Weber RW, Sosman JA, Haanen JB, et al. Improved survival with ipilimumab in patients with metastatic melanoma. N Engl J Med (2010) 363:711–23. doi: 10.1056/NEJMoa1003466
171. Revicki DA, van den Eertwegh AJM, Lorigan P, Lebbe C, Linette G, Ottensmeier CH, et al. Health related quality of life outcomes for unresectable stage III or IV melanoma patients receiving ipilimumab treatment. Health Qual Life Outcomes (2012) 10:66. doi: 10.1186/1477-7525-10-66
172. Weissman JP, Samlowski W, Meoz R. Hedgehog inhibitor induction with addition of concurrent superficial radiotherapy in patients with locally advanced basal cell carcinoma: A case series. Oncologist (2021) 26:e2247–53. doi: 10.1002/onco.13959
173. Raphael BA, Shin DB, Suchin KR, Morrissey KA, Vittorio CC, Kim EJ, et al. High clinical response rate of sézary syndrome to immunomodulatory therapies: Prognostic markers of response. Arch Dermatol (2011) 147:1410–5. doi: 10.1001/archdermatol.2011.232
174. Mamounas EP, Fisher B. Preoperative chemotherapy for operable breast cancer. Cancer Treat Res (2000) 103:137–55. doi: 10.1007/978-1-4757-3147-7_7
175. Boussios S, Sheriff M, Rassy E, Moschetta M, Samartzis EP, Hallit R, et al. Immuno-oncology: a narrative review of gastrointestinal and hepatic toxicities. Ann Transl Med (2021) 9:423. doi: 10.21037/atm-20-7361
176. Dimopoulos MA, Terpos E. Lenalidomide: An update on evidence from clinical trials. Blood Rev (2010) 24:S21–6. doi: 10.1016/S0268-960X(10)70005-9
177. Moore DH. Treatment of stage IB2 (bulky) cervical carcinoma. Cancer Treat Rev (2003) 29:401–6. doi: 10.1016/S0305-7372(03)00075-6
178. Wistuba-Hamprecht K, Martens A, Heubach F, Romano E, Geukes Foppen M, Yuan J, et al. Peripheral CD8 effector-memory type 1 T-cells correlate with outcome in ipilimumab-treated stage IV melanoma patients. Eur J Cancer (2017) 73:61–70. doi: 10.1016/j.ejca.2016.12.011
179. Wolchok JD, Hoos A, O’Day S, Weber JS, Hamid O, Lebbé C, et al. Guidelines for the evaluation of immune therapy activity in solid tumors: Immune-related response criteria. Clin Cancer Res (2009) 15:7412–20. doi: 10.1158/1078-0432.CCR-09-1624
180. Duffaud F, Therasse P. [New guidelines to evaluate the response to treatment in solid tumors]. Bull Cancer (2000) 87:881–6.
181. Louis DN, Ohgaki H, Wiestler OD, Cavenee WK, Burger PC, Jouvet A, et al. The 2007 WHO classification of tumours of the central nervous system. Acta Neuropathol (2007) 114:97–109. doi: 10.1007/s00401-007-0243-4
182. Löwik DWPM, van Hest JCM. Peptide based amphiphiles. Chem Soc Rev (2004) 33:234–45. doi: 10.1039/B212638A
183. Yamada A, Sasada T, Noguchi M, Itoh K. Next-generation peptide vaccines for advanced cancer. Cancer Sci (2013) 104:15–21. doi: 10.1111/cas.12050
184. Takedatsu H, Shichijo S, Katagiri K, Sawamizu H, Sata M, Itoh K. Identification of peptide vaccine candidates sharing among HLA-A3 +, -A11+, -A31+, and -A33+ cancer patients. Clin Cancer Res (2004) 10:1112–20. doi: 10.1158/1078-0432.CCR-0797-3
185. Abelin JG, Harjanto D, Malloy M, Suri P, Colson T, Goulding SP, et al. Defining HLA-II ligand processing and binding rules with mass spectrometry enhances cancer epitope prediction. Immunity (2019) 51:1–14. doi: 10.1016/j.immuni.2019.08.012
186. Hu Z, Ott PA, Wu CJ. Towards personalized, tumour-specific, therapeutic vaccines for cancer. Nat Rev Immunol (2018) 18:168–82. doi: 10.1038/nri.2017.131
187. Nagorsen D, Thiel E. HLA typing demands for peptide-based anti-cancer vaccine. Cancer Immunol Immunother (2008) 57:1903–10. doi: 10.1007/s00262-008-0493-6
188. González-Galarza FF, Takeshita LYC, Santos EJM, Kempson F, Maia MHT, Da Silva ALS, et al. Allele frequency net 2015 update: New features for HLA epitopes, KIR and disease and HLA adverse drug reaction associations. Nucleic Acids Res (2015) 43:D784–8. doi: 10.1093/nar/gku1166
189. Jiang C, Schaafsma E, Hong W, Zhao Y, Zhu K, Chao CC, et al. Influence of T cell-mediated immune surveillance on somatic mutation occurrences in melanoma. Front Immunol (2022) 12:703821. doi: 10.3389/fimmu.2021.703821
190. Matsuzaki J, Qian F, Luescher I, Lele S, Ritter G, Shrikant PA, et al. Recognition of naturally processed and ovarian cancer reactive CD8 + T cell epitopes within a promiscuous HLA class II T-helper region of NY-ESO-1. Cancer Immunol Immunother (2008) 57:1185–95. doi: 10.1007/s00262-008-0450-4
191. Baxevanis CN, Voutsas IF, Tsitsilonis OE, Gritzapis AD, Sotiriadou R, Papamichail M. Tumor-specific CD4 + T lymphocytes from cancer patients are required for optimal induction of cytotoxic T cells against the autologous tumor. J Immunol (2000) 164:3902–12. doi: 10.4049/jimmunol.164.7.3902
192. Tay RE, Richardson EK, Toh HC. Revisiting the role of CD4+ T cells in cancer immunotherapy–new insights into old paradigms. Cancer Gene Ther (2021) 28:5–17. doi: 10.1038/s41417-020-0183-x
193. Borst J, Ahrends T, Bąbała N, Melief CJM, Kastenmüller W. CD4+ T cell help in cancer immunology and immunotherapy. Nat Rev Immunol (2018) 18:635–47. doi: 10.1038/s41577-018-0044-0
194. Coffman RL, Sher A, Seder RA. Vaccine adjuvants: Putting innate immunity to work. Immunity (2010) 33:492–503. doi: 10.1016/j.immuni.2010.10.002
195. De Gregorio E, Caproni E, Ulmer JB. Vaccine adjuvants: mode of action. Front Immunol (2013) 4:214. doi: 10.3389/fimmu.2013.00214
196. Sacerdote P, Franchi S, Moretti S, Castelli M, Procacci P, Magnaghi V, et al. Cytokine modulation is necessary for efficacious treatment of experimental neuropathic pain. J Neuroimmune Pharmacol (2013) 8:202–11. doi: 10.1007/s11481-012-9428-2
197. Hollingsworth RE, Jansen K. Turning the corner on therapeutic cancer vaccines. NPJ Vaccines (2019) 4:7. doi: 10.1038/s41541-019-0103-y
198. Carretero-Iglesia L, Couturaud B, Baumgaertner P, Schmidt J, Maby-El Hajjami H, Speiser DE, et al. High peptide dose vaccination promotes the early selection of tumor antigen-specific CD8 T-cells of enhanced functional competence. Front Immunol (2020) 10:3016. doi: 10.3389/fimmu.2019.03016
199. Nathan P, Hassel JC, Rutkowski P, Baurain J-F, Butler MO, Schlaak M, et al. Overall survival benefit with tebentafusp in metastatic uveal melanoma. N Engl J Med (2021) 385:1196–206. doi: 10.1056/NEJMoa2103485
200. Purcell AW, McCluskey J, Rossjohn J. More than one reason to rethink the use of peptides in vaccine design. Nat Rev Drug Discov (2007) 6:404–14. doi: 10.1038/nrd2224
201. Yu Z, Restifo NP. Cancer vaccines: Progress reveals new complexities. J Clin Invest (2002) 110:289–94. doi: 10.1172/JCI0216216
202. Cuzzubbo S, Mangsbo S, Nagarajan D, Habra K, Pockley AG, McArdle SEB. Cancer vaccines: Adjuvant potency, importance of age, lifestyle, and treatments. Front Immunol (2021) 11. doi: 10.3389/fimmu.2020.615240
203. McGranahan N, Furness AJS, Rosenthal R, Ramskov S, Lyngaa R, Saini SK, et al. Clonal neoantigens elicit T cell immunoreactivity and sensitivity to immune checkpoint blockade. Science (80- ) (2016) 354:1–11. doi: 10.1126/science.aaf1490
204. Iero M, Filipazzi P, Castelli C, Belli F, Valdagni R, Parmiani G, et al. Modified peptides in anti-cancer vaccines: Are we eventually improving anti-tumour immunity? Cancer Immunol Immunother (2009) 58:1159–67. doi: 10.1007/s00262-008-0610-6
205. Xu M, Liu X, Li P, Yang Y, Zhang W, Zhao S, et al. Modified natriuretic peptides and their potential role in cancer treatment. BioMed J (2021) 45:1–14. doi: 10.1016/j.bj.2021.06.007
206. Sun W, Shi J, Wu J, Zhang J, Chen H, Li Y, et al. A modified HLA-A*0201-restricted CTL epitope from human oncoprotein (hPEBP4) induces more efficient antitumor responses. Cell Mol Immunol (2018) 15:768–81. doi: 10.1038/cmi.2017.155
207. Chiangjong W, Chutipongtanate S, Hongeng S. Anticancer peptide: Physicochemical property, functional aspect and trend in clinical application (Review). Int J Oncol (2020) 57:678–96. doi: 10.3892/ijo.2020.5099
208. Xie M, Liu D, Yang Y. Anti-cancer peptides: Classification, mechanism of action, reconstruction and modification: Anticancer peptides. Open Biol (2020) 10::200004.
209. Van Doorn E, Liu H, Huckriede A, Hak E. Safety and tolerability evaluation of the use of montanide ISATM51 as vaccine adjuvant: A systematic review. Hum Vaccines Immunother (2016) 12:159–69. doi: 10.1080/21645515.2015.1071455
210. Aucouturier J, Dupuis L, Deville S, Ascarateil S, Ganne V. Montanide ISA 720 and 51: A new generation of water in oil emulsions as adjuvants for human vaccines. Expert Rev Vaccines (2002) 1:111–8. doi: 10.1586/14760584.1.1.111
211. Donnelly RP, Young HA, Rosenberg AS. An overview of cytokines and cytokine antagonists as therapeutic agents. Ann N Y Acad Sci (2009) 1182:1–13. doi: 10.1111/j.1749-6632.2009.05382.x
212. Liu C, Chu D, Kalantar-Zadeh K, George J, Young HA, Liu G. Cytokines: From clinical significance to quantification. Adv Sci (2021) 8:e2004433. doi: 10.1002/advs.202004433
213. Gadani SP, Cronk JC, Norris GT, Kipnis J. IL-4 in the brain: A cytokine to remember. J Immunol (2012) 189:4213–9. doi: 10.4049/jimmunol.1202246
214. Tatsumi T, Takehara T, Kanto T, Hori M, Miyagi T, Kuzushita N, et al. Administration of interleukin-12 enhances the therapeutic efficacy of dendritic cell-based tumor vaccines in mouse hepatocellular carcinoma. Cancer Res (2001) 61:7563–7.
215. Chmielewski M, Kopecky C, Hombach AA, Abken H. IL-12 release by engineered T cells expressing chimeric antigen receptors can effectively muster an antigen-independent macrophage response on tumor cells that have shut down tumor antigen expression. Cancer Res (2011) 71:5697–706. doi: 10.1158/0008-5472.CAN-11-0103
216. Setrerrahmane S, Xu H. Tumor-related interleukins: Old validated targets for new anti-cancer drug development. Mol Cancer (2017) 16:1–17. doi: 10.1186/s12943-017-0721-9
217. Zhao H, Wu L, Yan G, Chen Y, Zhou M, Wu Y, et al. Inflammation and tumor progression: signaling pathways and targeted intervention. Signal Transduct Target Ther (2021) 6:263. doi: 10.1038/s41392-021-00658-5
218. Bolonaki I, Kotsakis A, Papadimitraki E, Aggouraki D, Konsolakis G, Vagia A, et al. Vaccination of patients with advanced non-small-cell lung cancer with an optimized cryptic human telomerase reverse transcriptase peptide. J Clin Oncol (2007) 25:2727–34. doi: 10.1200/JCO.2006.10.3465
219. Zhang W, Lu X, Cui P, Piao C, Xiao M, Liu X, et al. Phase I/II clinical trial of a wilms’ tumor 1-targeted dendritic cell vaccination-based immunotherapy in patients with advanced cancer. Cancer Immunol Immunother (2019) 68:121–30. doi: 10.1007/s00262-018-2257-2
220. Wen PY, Reardon DA, Armstrong TS, Phuphanich S, Aiken RD, Landolfi JC, et al. A randomized double-blind placebo-controlled phase II trial of dendritic cell vaccine ICT-107 in newly diagnosed patients with glioblastoma. Clin Cancer Res (2019) 25:5799–807. doi: 10.1158/1078-0432.CCR-19-0261
221. Rosenbaum P, Artaud C, Bay S, Ganneau C, Campone M, Delaloge S, et al. The fully synthetic glycopeptide MAG-Tn3 therapeutic vaccine induces tumor-specific cytotoxic antibodies in breast cancer patients. Cancer Immunol Immunother (2020) 69:703–16. doi: 10.1007/s00262-020-02503-0
222. Pollard AJ, Bijker EM. A guide to vaccinology: from basic principles to new developments. Nat Rev Immunol (2021) 21:83–100. doi: 10.1038/s41577-020-00479-7
223. Harvey WT, Carabelli AM, Jackson B, Gupta RK, Thomson EC, Harrison EM, et al. SARS-CoV-2 variants, spike mutations and immune escape. Nat Rev Microbiol (2021) 19:409–24. doi: 10.1038/s41579-021-00573-0
224. Garrett ME, Galloway JG, Wolf C, Logue JK, Franko N, Chu HY, et al. Comprehensive characterization of the antibody responses to SARS-CoV-2 spike protein finds additional vaccine-induced epitopes beyond those for mild infection. Elife (2022) 11:1–20. doi: 10.7554/eLife.73490
Keywords: cancer vaccine, peptide, clinical response, machine learning, potential factors
Citation: Jiang C, Li J, Zhang W, Zhuang Z, Liu G, Hong W, Li B, Zhang X and Chao C-C (2022) Potential association factors for developing effective peptide-based cancer vaccines. Front. Immunol. 13:931612. doi: 10.3389/fimmu.2022.931612
Received: 29 April 2022; Accepted: 29 June 2022;
Published: 27 July 2022.
Edited by:
Yubin Li, University of Pennsylvania, United StatesReviewed by:
Xi Lou, University of Alabama at Birmingham, United StatesQiaosi Tang, University of Pennsylvania, United States
Copyright © 2022 Jiang, Li, Zhang, Zhuang, Liu, Hong, Li, Zhang and Chao. This is an open-access article distributed under the terms of the Creative Commons Attribution License (CC BY). The use, distribution or reproduction in other forums is permitted, provided the original author(s) and the copyright owner(s) are credited and that the original publication in this journal is cited, in accordance with accepted academic practice. No use, distribution or reproduction is permitted which does not comply with these terms.
*Correspondence: Chongming Jiang, chongming.jiang@bcm.edu; Cheng-Chi Chao, Chengchi@biomap.com