- 1Department of Laboratory Medicine and Pathobiology, University of Toronto, Toronto, ON, Canada
- 2Sandra A. Rotman (SAR) Laboratories, Sandra Rotman Centre for Global Health, University Health Network-Toronto General Hospital, Toronto, ON, Canada
- 3Department of Experimental Therapeutics, University Health Network-Toronto General Hospital, Toronto, ON, Canada
- 4Faculty of Medicine, University of Toronto, Toronto, ON, Canada
- 5Tropical Disease Unit, Division of Infectious Diseases, Department of Medicine, University of Toronto, Toronto, ON, Canada
Severe malaria (SM) is a leading cause of global morbidity and mortality, particularly in children in sub-Saharan Africa. However, existing malaria diagnostic tests do not reliably identify children at risk of severe and fatal outcomes. Dysregulated host immune and endothelial activation contributes to the pathogenesis of SM. Current research suggests that measuring markers of these pathways at presentation may have clinical utility as prognostic indicators of disease progression and risk of death. In this review, we focus on the available evidence implicating soluble urokinase-type plasminogen activator receptor (suPAR) as a novel and early predictor of severe and fatal malaria and discuss its potential utility for malaria triage and management.
1 Introduction
Malaria remains a primary cause of childhood illness and death in sub-Saharan Africa (1). Malaria rapid diagnostic tests (RDTs) are overwhelmingly the diagnostic tool used in malaria-endemic countries to confirm suspected cases. Although RDTs are less sensitive than microscopy or nucleic acid amplification techniques, they are fast, inexpensive, and a low-expertise method to quickly diagnose Plasmodium falciparum and Plasmodium vivax infections. Regardless of the method used to detect malaria parasites, no current diagnostic test can reliably predict which children will progress to severe or fatal disease. Dysregulated host immune and endothelial activation contributes to the pathogenesis of severe malaria (SM) (2). Host-based prognostic biomarkers, especially if incorporated into RDTs, have the potential to improve risk stratification and outcome of children with malaria (2–6).
Soluble urokinase-type plasminogen activator receptor (suPAR) is an indicator of immune and endothelial activation and plays an important role in processes including cell migration, adhesion, and chemotaxis. Elevated suPAR levels have been linked to poor prognosis in various infections including HIV-1, tuberculosis, and sepsis. Emerging evidence also suggests that increased circulating levels of suPAR are associated with disease severity and mortality in children with malaria. Here, we report an overview of urokinase/urokinase-type plasminogen activator receptor (uPA/uPAR) biology, review what is known about suPAR in pediatric malaria, and discuss the ability of suPAR to predict disease outcome and its potential as a druggable target. We summarize putative mechanisms through which suPAR may contribute to the pathogenesis of malaria. Lastly, we outline the need for research investigating the causal role of suPAR in malaria and the evaluation of a currently available suPAR point-of-care test as a potential triage tool for severe malaria in prospective trials.
2 Structure and Function of suPAR
uPAR is a glycosylphosphatydilinositol (GPI)-anchored protein that contains three homologous domains (D1, D2, and D3) and is expressed on the surface of various cell types including immune, endothelial, epithelial, and smooth muscle cells (7, 8). suPAR is the soluble form of the membrane-bound receptor uPAR that is released into circulation (9) (Figure 1). Three soluble forms of uPAR are produced by cleavage at the GPI anchor and/or linker region connecting D1 and D2: suPAR D1–D3 (full-length suPAR), suPAR D2D3 (cleaved suPAR), and suPAR D1. Full-length suPAR is involved in various cellular processes including proteolysis, migration, adhesion, and proliferation since it can interact with other extracellular matrix (ECM) proteins and receptors (e.g., integrins and vitronectins) (10, 11). Also, this soluble form of uPAR is a scavenger of urokinase-type plasminogen activator (uPA; also known as urokinase), the ligand for membrane-associated uPAR, in the ECM; thus, full-length suPAR can competitively inhibit uPAR (12). In contrast, cleaved suPAR is primarily involved in the chemotaxis of immune cells (e.g., monocytes and neutrophils) (13). Unlike these active forms of suPAR, suPAR D1 has no known biological functions and is rapidly cleared from circulation (14).
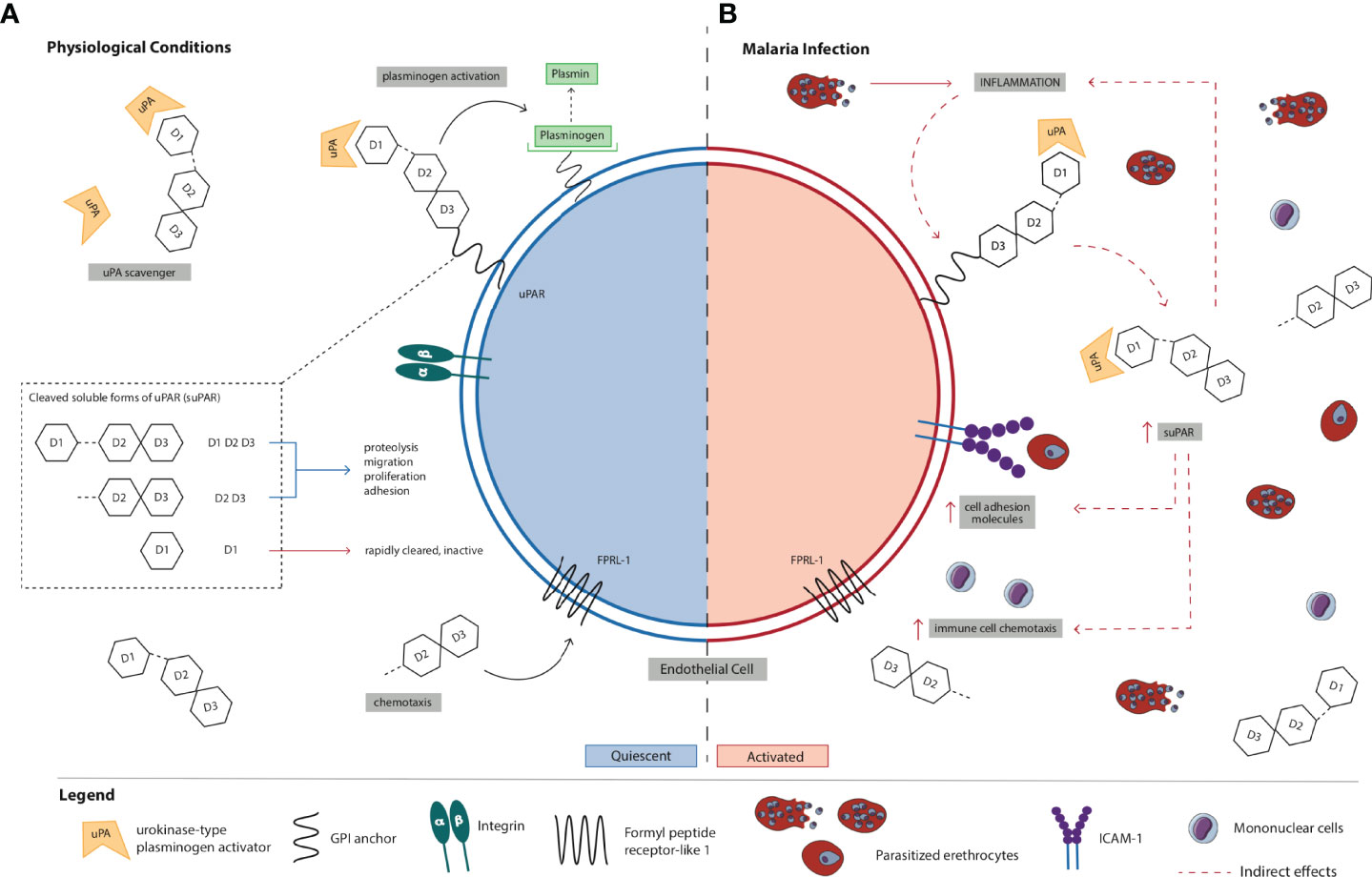
Figure 1 Schematic representation of the (A) normal physiological role of suPAR in inflammation and immune activation vs. (B) proposed mechanism of action of suPAR in the pathogenesis of severe malaria. (A) uPAR is a three-domain (D1, D2, and D3) GPI-anchored protein expressed on the cell surface of immune, endothelial, epithelial, and smooth muscle cells. Full-length suPAR is involved in a variety of cellular processes including proteolysis, migration, proliferation, and adhesion through its interactions with ECM proteins such as integrins and vitronectins as well as other receptors. Also, suPAR is a regulator of the plasminogen activation system since it can scavenge and bind the serine protease uPA in the ECM thereby competitively inhibiting uPAR. Cleaved suPAR interacts with FPRL-1 to induce chemotaxis of immune cells (e.g., monocytes, neutrophils). suPAR D1 is rapidly cleared from circulation and is biologically inactive. suPAR is released into circulation during infection, and therefore, the circulating concentrations of suPAR reflect the extent of immune activation and inflammation in an individual. Under normal physiological conditions, low levels of circulating suPAR are detected in the healthy population. (B) During P. falciparum infection, PEs bind to and sequester in microvascular endothelial cells. Recognition and binding of parasite products (e.g., PfGPI) to Toll-like receptors on monocytes and ECs activates them to produce and secrete pro-inflammatory cytokines (e.g., TNF-α) and chemokines, and there is upregulation of the expression of cell-adhesion molecules on endothelial cells (e.g., ICAM-1) to which PEs bind. Increased production of pro-inflammatory cytokines/chemokines and immune activation stimulate uPAR-expressing cells (e.g., monocytes) to produce and secrete suPAR. Initially, increased local levels of suPAR in children with malaria may promote protective innate immune responses in the host defense by promoting the recruitment of immune cells (e.g., neutrophils and monocytes) to acute sites of infection via its chemotactic effector functions. Through its interactions with other ECM proteins and receptors (e.g., integrins), suPAR can also trigger downstream signaling resulting in the upregulation and expression of adhesion molecules (e.g., ICAM-1). In the absence of early treatment, parasite numbers continue to increase and PEs further accumulate and sequester in microvascular ECs due to the upregulated expression of adhesion molecules. As a result, the production and secretion of pro-inflammatory cytokines/chemokines are exacerbated, ultimately resulting in a systemic increase in inflammation and immune activation. Elevated circulating suPAR may amplify the release of cytokines/chemokines and the recruitment of immune cells, which contributes to sustained systemic inflammation and immune activation. Excessive inflammation in malaria could also trigger biological processes leading to marked increases in circulating suPAR. Although further studies are needed to elucidate its role, suPAR may contribute to enhanced chemokine and cytokine secretion that culminates in endothelial dysfunction, multiorgan failure, and death in children with malaria. High circulating concentrations of suPAR in children with malaria may therefore reflect excessive activation of immune cells, adhesion of PEs at sites of inflammation, disturbances in hemostasis, or a combination of these. Collectively, suPAR may be involved in a positive feedback loop that results in high levels of local and systemic immune activation and inflammation through its own pro-inflammatory properties and by activating and recruiting other chemokines and cytokines via chemotaxis. Also, the pathobiology of severe falciparum malaria is associated with upregulation of coagulation pathways. Elevated suPAR levels may indirectly exert pro-coagulant effects by enhanced binding to uPA and competitive inhibition of membrane-bound uPAR thereby indirectly inhibiting uPA-dependent fibrinolysis or by stimulating activation of coagulation mechanisms via intrinsic and extrinsic pathways. Elevated levels of suPAR in children with malaria may reflect the degree to which some or all of these processes occur. EC, endothelial cell; ECM, extracellular matrix; P. falciparum, Plasmodium falciparum; PfGPI, GPI, Plasmodium falciparum glycosylphosphatidylinositol; GPI, glycosylphosphatidylinositol; ICAM-1, intercellular adhesion molecule-1; PE, parasitized erythrocyte; SM, severe malaria; suPAR, soluble urokinase-type plasminogen activator receptor; uPA, urokinase or urokinase-type plasminogen activator; uPAR, urokinase-type plasminogen activator receptor.
2.1 The uPA/uPAR System at the Intersection of Inflammation, Fibrinolysis, and Coagulation
uPA, a serine protease, and its cellular receptor uPAR exhibit pleiotropic functions during both physiological and pathological processes. The uPA/uPAR system mediates numerous critical cellular functions including extracellular proteolysis, chemotaxis, cell adhesion, vascular homeostasis, and tissue remodeling and repair (10, 15–17).
The uPA/uPAR system has a central role in fibrinolysis and the modulation of host inflammatory and immune responses (18, 19). Binding of uPA to membrane-bound uPAR catalyzes the activation of plasminogen to plasmin, an enzyme critical for the degradation of fibrin (20). Notably, uPA-mediated plasminogen activation is implicated in various processes requiring cell migration, which is an essential event in both physiological and pathological processes (e.g., cell recruitment, wound healing, and angiogenesis) (21–24).
The uPA molecule is directly involved in mechanisms of migration, adhesion, and chemotaxis (16). Importantly, uPA can regulate many of these processes independent of uPAR binding. Unlike uPA, which can directly mediate fibrinolysis by activating plasminogen, uPAR is thought to indirectly participate in local fibrinolysis through its chemotactic activity (25). Full-length suPAR, which contains the D1 uPA-binding domain, can bind many of the same ligands (e.g., uPA, integrins, and vitronectin) as membrane-bound uPAR (12, 26). Therefore, suPAR–integrin interactions may trigger downstream signaling events. In addition, increased production of suPAR may disrupt uPA/uPAR-dependent proteolysis and/or alter cell signaling pathways since suPAR competes with membrane-bound uPAR for uPA in the ECM (27). In effect, suPAR may be a negative regulator of uPA/uPAR-dependent plasminogen activation and contribute to inhibited fibrinolysis, i.e., suPAR may indirectly exert pro-coagulant functions. Thus, suPAR may induce and modulate uPAR-dependent cell signaling responses and the processes catalyzed by these molecules and their interaction with membrane-associated uPAR (16). In contrast, cleaved suPAR (suPAR D2D3), which lacks the D1 binding domain, is mainly involved in chemotaxis since it can activate formyl peptide receptor-like 1 (FPRL-1) thereby promoting the immune response. The production of this form of suPAR by activated neutrophils in sites of acute inflammation may contribute to the recruitment of monocytes to these sites during infection (28).
2.1.1 The uPA/uPAR System Promotes Inflammation and Immune Activation
In addition to its role in fibrinolysis, the uPA/uPAR system participates in inflammatory and immune activation pathways (Figure 1A). uPAR anchors uPA at the cell surface which favors ECM degradation and regulates cell migration, adhesion, and proliferation, thereby influencing the development of inflammatory and immune responses (15, 21). Since membrane-tethered uPAR lacks transmembrane and intracellular domains, it requires interactions with other coreceptors and proteins to induce cell signaling and mediate cytoskeletal reorganization (10, 29). uPAR on the cell surface interacts with integrin β1, β2, and β3 family members and G-protein-coupled receptors (GPRs) including FPRL-1 to modulate cell adhesion and migration (10, 16, 30). Membrane-bound uPAR is involved in the recruitment of leukocytes to acute sites of inflammation via integrated mechanisms including proteolysis, adhesion, migration, and mitogenesis (21, 22). The functional interaction between uPAR and integrins is believed to be critical for leukocyte recruitment and activation (21). In addition, membrane-bound uPAR interacts with FPRL-1 to exert chemotactic effector functions, which promotes the recruitment and activation of immune cells (e.g., monocytes, neutrophils, macrophages) during immune and inflammatory responses (16). Vitronectin, an extracellular protein, is another important uPAR ligand that promotes cell adhesion and migration (8). Vitronectin is at the intersection of the uPA/uPAR and integrin systems since it can bind both uPAR and integrins, and integrin-bound vitronectin is required for uPAR (31, 32). In addition, uPAR regulates integrin activity and uPAR-bound uPA enhances uPAR binding to vitronectin, which highlights the importance of the uPA/uPAR system in coordinating cell–cell and cell–ECM interactions (21, 29). uPAR and its partners cross-regulate each other and there is considerable cross-talk between these pathways (32).
2.2 uPAR/suPAR and Endothelial Dysfunction
Dysregulated host endothelial activation is a key pathophysiological feature in P. falciparum infection (2). uPAR and suPAR have previously been linked with endothelial dysfunction (33–35). uPAR is expressed on endothelial cells and upregulated during endothelial activation (36, 37). Post-mortem tissues of patients with cerebral malaria (CM) showed upregulated expression of uPAR on endothelial cells limited to CM-associated lesions, implicating uPAR in blood–brain barrier disruption and immunologic injury during CM (38). The authors also suggested that uPAR may be an additional adhesion molecule for parasitized erythrocytes (PEs) on activated endothelial cells since uPAR reactivity exclusively co-localized to lesions with PEs sequestered in cerebral microvasculature. suPAR levels also positively correlate with circulating markers of endothelial dysfunction (39, 40). The uPA/uPAR system has been suggested to contribute to the pathogenesis of SM by influencing platelet sequestration and/or activating the endothelium (37, 38, 41, 42). Upregulated expression of cellular adhesion molecules (e.g., ICAM-1) on endothelial cells promotes sequestration of PEs to the vascular endothelium in vivo (43, 44). Interactions between endothelial cells and immune cells stimulate the release of suPAR, suggesting a potential link between elevated levels of suPAR and inflammatory processes in the microvascular endothelium (45). Given the role of suPAR in modulating immune responses and cell adhesion, it is possible that suPAR may contribute to endothelial dysfunction in SM by inducing intracellular signaling cascades that lead to upregulated expression of cellular adhesion molecules on the surface of microvascular endothelial cells and/or promoting pro-inflammatory cytokine production, which may also contribute to the upregulation of host adhesion receptors.
2.3 uPA, uPAR, and suPAR Are Upregulated During Infection
The importance of the uPA/uPAR system in modulating immune and inflammatory responses during infection has been well documented. uPAR expression is upregulated on endothelial and hematopoietic cells during bacterial infection and in response to pro-inflammatory cytokines [e.g., tumor necrosis factor alpha (TNF-α), interferon gamma (IFN-γ), and interleukin 2 (IL-2)] (25). For example, patients with melioidosis have upregulated uPAR expression, and in the experimental model of Burkholderia pseudomallei melioidosis, uPAR knockout mice had reduced neutrophil migration to the primary site of infection and increased bacterial growth and organ inflammation (46). These findings indicate that uPAR deficiency may result in impaired host innate immune responses to infection. In vitro, uPAR-deficient macrophages and granulocytes had impaired phagocytosis of B. pseudomallei. Similar findings from other animal models show that uPAR deficiency in mice impairs the recruitment of neutrophils and leukocytes (22, 47). In vitro, antisense blockade of uPAR leads to impaired leukocyte migration (48). uPA-knockout mice have impaired recruitment of mononuclear cells during infection in vivo (49). Furthermore, the uPA/uPAR system is involved in the activation and differentiation of T cells; uPA and uPAR are upregulated during T-cell activation (19). In response to Pseudomonas aeruginosa infection, uPA- and uPAR-knockout mice have impaired lymphocyte recruitment to the lung, which suggests that uPA/uPAR may promote T-cell effector functions at the sites of infection (22). Collectively, these findings show that uPA and uPAR modulate host defense in response to infection and are important for protective immunity. The role of suPAR in innate immune responses in vivo is relatively unexplored. Evidence suggests that suPAR may elicit the recruitment and activation of pro-inflammatory cells (i.e., monocytes and neutrophils) (28). However, as with other innate immune responses, dysregulated suPAR production during infection has the potential to mediate disease pathobiology.
2.3.1 Dysregulation of the uPA/uPAR System Is Linked to Pathology
Upregulated uPA, uPAR, and suPAR are linked with various pathological conditions (50–55). In patients with systemic inflammation and cirrhosis, circulating suPAR levels are related to immune activation and function as a marker of poor clinical outcomes (56, 57). In vivo, suPAR may stimulate the recruitment and activation of leukocytes, which promotes inflammation and immune activation (28). Excessive inflammation is an established mechanism contributing to tissue damage and organ dysfunction in multiple infections (58–60). Thus, it is not unexpected that dysregulation of the uPA/uPAR system and suPAR, which promotes inflammation and immune activation, may mediate host pathobiology. Evidence from a preclinical study of HIV-1 supports this notion. In cell culture, suPAR expression and release was upregulated in lymphoid organs during HIV-1 infection (53). Cleaved suPAR in HIV-infected cells inhibited chemotaxis and induced virus expression, suggesting that suPAR contributes to dysregulated immune activation and pathogenesis in HIV infection. Considerable evidence from clinical studies also supports a link between suPAR and the pathophysiology and clinical outcomes of various life-threatening infections, including malaria (40, 61, 62).
3 suPAR Is a Marker of Disease Progression and Poor Prognosis in Infectious Diseases
The prognostic value of suPAR has previously been documented in infectious diseases of variable origin (bacterial, viral, and parasitic) including tuberculosis, bacteremia and/or sepsis, meningitis, hepatitis B and C, HIV-1, hemorrhagic fever, hantavirus, malaria, and most recently coronavirus disease 2019 (COVID-19) (63–72). Across multiple infectious diseases, suPAR levels are elevated and predict the severity of illness and poor clinical outcomes.
In patients with HIV-1 infection, suPAR is associated with disease severity, mortality risk, and ineffective immune recovery (54, 68). Elevated plasma suPAR levels (both intact and cleaved suPAR) have been reported to reflect immune activation and independently predict mortality outcome in HIV-1-infected patients (68). In a study of critically ill children with sepsis, circulating suPAR levels were significantly higher in non-survivors compared with survivors, and suPAR was a reliable indicator of ICU mortality (73). Consistent with these findings, suPAR was an excellent predictor of mortality outcome in patients with hemorrhagic fever (69). Similarly, patients with meningitis have elevated cerebrospinal fluid suPAR levels, and increased suPAR is a strong predictor of a fatal outcome (61). suPAR is also associated with clinical severity and case fatality in COVID-19 (40, 72).
In a study of patients with symptoms of COVID-19, suPAR levels were significantly higher in patients who developed severe and critical illness compared with those who were moderately ill, and it was shown that suPAR cutoffs could be used to risk-stratify patients (74, 75). In hospitalized COVID-19 patients, suPAR was predictive of in-hospital acute kidney injury (AKI) and the need for dialysis (76). At least in COVID-19, suPAR has been shown to be an early indicator of endogenous alarmins such as IL-1a, and its levels increase earlier than other biomarkers of disease progression, such as CRP, IL-6, and D-dimers (76, 77). It is this early warning, sentinel-like property of suPAR that positions it as a useful tool for patient triage and has been applied as such in at least three studies of COVID-19. suPAR point-of-care (POC) testing was used to triage and discharge patients with COVID-19 symptoms early from the emergency department (78).
Another set of studies used suPAR POC testing to quantify suPAR levels, risk-stratify patients to identify those at higher risk of respiratory failure or death, and provide early targeted treatment for these individuals with a drug intervention (anakinra) (79, 80).
4 The Prognostic Role of suPAR in Malaria
4.1 suPAR in Children With Malaria
Only a few studies to date have examined the prognostic role of suPAR in malaria (Supplementary Table 1). Limited data have linked elevated suPAR levels with disease severity and outcome in pediatric malaria. In a study of African children with acute P. falciparum malaria, Perch et al. found that serum suPAR levels at study inclusion were associated with parasite density and children with the highest parasitemia had significantly higher suPAR levels than children who had lower parasitemia or had a negative blood film (81). Of note, suPAR levels were significantly reduced in all malaria-positive children 7 days following initiation of antimalarial treatment. The most marked reduction in suPAR levels following treatment was in the group with the highest parasitemia where suPAR decreased to almost half its level at inclusion; however, this level was still significantly higher than the suPAR levels reported in all other groups on day 7. The authors suggest that elevated suPAR levels in children with high parasitemia may normalize over a longer treatment period. Similarly, Ostrowski et al. reported significantly higher plasma suPAR concentrations in malaria-positive children compared with those who were malaria-negative and healthy controls (82). Among the malaria-positive children, the highest suPAR levels were in children who had complicated diseases and in those who died. suPAR positively correlated with parasitemia and increased plasma suPAR concentration at admission was associated with a fatal outcome.
Contrary to the findings of Ostrowski et al., elevated suPAR levels were not associated with poor outcome in Cameroonian children with falciparum malaria (83). Instead, a gradual trend in increasing plasma suPAR levels was observed between children with asymptomatic malaria (AM) and those with uncomplicated malaria (UM) as well as between children with SM and those with CM. suPAR was excellent at discriminating between children with AM and those with UM with an area under the receiver operating characteristic curve (AUROC) of 0.958. However, a strong association with SM was not observed. suPAR levels were not informative in differentiating between severe (non-cerebral) and cerebral malaria. The lack of relationship between suPAR and SM reported in this study is unknown but may reflect, at least in part, the absence of fatal cases in the study population. In a study of Beninese children with P. falciparum infection, plasma suPAR levels were associated with malaria severity, coma, and mortality (84). suPAR was significantly higher in children with SM [CM/severe non-cerebral malaria (SNCM)] than in children with UM. Elevated suPAR levels were also associated with the presence of coma. suPAR was significantly higher in children with SM (CM/SNCM) who died compared with those who survived. However, suPAR was not a strong discriminator between SM subtypes (CM vs. SNCM). When compared with other inflammatory, angiogenic, and vascular markers, suPAR had relatively low prognostic accuracy in discriminating between UM vs. CM/SNCM, coma vs. no coma, and fatal vs. non-fatal cases with AUROC <0.70 for each analysis.
In summary, the available studies were largely underpowered to assess mortality outcomes. Therefore, the current evidence base is inadequate to determine whether suPAR levels are clinically useful as prognostic predictors in pediatric malaria. Additional larger prospective studies that enroll consecutive children with a broad disease severity spectrum are required to determine the utility of suPAR to risk-stratify children with malaria.
4.2 suPAR in Adults With Severe Malaria
Evidence for the prognostic role of suPAR in adults with malaria infection is limited. In a study of Bangladeshi adults with P. falciparum infection and AKI, increased suPAR levels were associated with increasing AKI severity, and levels at admission predicted a later requirement for renal replacement therapy (62). However, suPAR concentrations were not significantly different between survivors and non-survivors with AKI in SM. The authors posited that the lack of association between suPAR levels and mortality in this study may reflect the limited spectrum of malaria severity in their cohort (i.e., all patients had severe illness) and that including patients with UM might confirm the potential link between suPAR levels and mortality outcome in adults with SM. Alternatively, it is possible that elevated suPAR levels in adults with SM may not predict an increased risk of death or do so, albeit, to a lesser extent than in children. This could be expected since age is a known risk factor for fatal outcome in patients with SM and the risk of mortality is disproportionately higher in children under 5 years old (85).
4.3 suPAR in Malaria in Pregnancy
To date, there is only one study that has evaluated the prognostic role of suPAR in malaria in pregnancy (71). In their study, Ostrowski et al. investigated the relationship between suPAR levels and fetal outcome in pregnant African women. Plasma suPAR concentrations were measured in maternal (at enrolment) and cord (at delivery) samples in women with histology-confirmed placental malaria (active or past infection) and women with no history of malaria infection. They found that maternal suPAR levels were significantly higher in women with active malaria infection compared with women with past infection or non-infected. Cord suPAR levels did not differ across placental histology groups. Maternal suPAR was also positively correlated with maternal peripheral parasitemia. Importantly, in women with active infection, elevated maternal suPAR independently predicted low birth weight. Both maternal and cord suPAR levels were not associated with stillbirth in any of the histology groups. These findings suggest that maternal suPAR levels are potentially associated with adverse birth outcomes in women with malaria. However, cord suPAR levels were not associated with birth weight after adjusting for gestational age, suggesting that cord suPAR is less influenced by maternal malaria infection. The role of suPAR in malaria in pregnancy needs to be further evaluated in prospective studies with longitudinal sampling across pregnancy and assessment of additional adverse birth outcomes (e.g., preterm birth).
5 A Mechanistic Role for suPAR in Malaria Pathology?
Given that dysregulated endothelial and immune activation contributes to the pathogenesis of severe falciparum malaria, we propose a model of how suPAR may be a mediator of these pathways (Figure 1B). The limited preclinical data that exist suggest a role of the uPA/uPAR pathway in the pathogenesis of malaria. uPAR-deficient mice infected with Plasmodium berghei have reduced mortality, attenuated thrombocytopenia, absent platelet trapping, enhanced leukocytosis, and reduced apoptosis compared with wild-type mice (41). Evidence that uPA/uPAR deficiency in P. berghei-infected mice attenuates disease severity and delays mortality in vivo suggests that attenuation of this pathway (including suPAR) might be beneficial. In cell culture experiments, uPA binds to human erythrocytes infected with mature forms of P. falciparum and is required for the rupture of erythrocytes and the release of merozoites, which suggests that uPA may be an additional adhesion molecule for PEs (86). The only evidence to date that implicates the uPA/uPAR pathway to human malaria pathogenesis comes from a post-mortem study of patients with CM that reported increased expression of uPAR on macrophages, microglial cells, astrocytes, and endothelial cells in CM-associated lesions, suggesting that uPAR may contribute to blood–brain barrier disruptions and immunopathology in CM (38).
Additional studies are required to determine whether the uPA/uPAR pathway plays a mechanistic role in the pathogenesis of malaria. Antibodies or small molecular inhibitors that prevent uPAR cleavage or that specifically bind and sequester suPAR from circulation could provide further insights (55, 87). Experimental evidence from a murine model of focal segmental glomerulosclerosis (FSG) indicates that circulating levels of suPAR can contribute to the pathogenesis of FSG and that administration of blocking uPAR antibodies improves suPAR-induced kidney damage (55). If suPAR directly contributes to the pathogenesis of SM, this pathway could represent a potential therapeutic target.
6 Conclusions
Prognostic/severity markers could facilitate the early recognition and treatment of children with impending severe malaria. Integrating these markers into POC RDT platforms, or added to current malaria RDTs, could transform community-based triage of pediatric malaria cases, especially in low-resource settings (3). This could inform individualized management and potentially reduce malaria deaths. The few available studies investigating the prognostic role of suPAR in children with malaria have been underpowered to robustly assess clinical outcomes (most have few or no fatal cases) and/or use limited measures of disease severity. Discrepancies in suPAR levels reported across studies in patients with malaria may reflect differences in the assay used to quantify suPAR (Supplementary Table 1) and may limit the comparability of suPAR findings. Alternatively, they could be explained by varying baseline circulating suPAR concentrations in different patient populations; differences in age, malaria exposure, and immunity status; other coinfections or different etiologies inducing changes in suPAR; and/or underlying nutritional deficiencies which may affect the levels of inflammation and immune activation and, thus, circulating suPAR concentrations (63, 68, 88–91). Future studies controlling for these factors and using standardized commercial kits to quantify suPAR would help to elucidate the potential relationship between suPAR levels and clinical outcomes (e.g., mortality) in patients with malaria. In addition, large prospective studies including children across a broad disease severity spectrum with adequate power to assess mortality outcomes are required to confirm the potential value of suPAR to risk-stratify children with malaria. These studies would need to determine and validate quantitative cutoffs that can accurately identify patients needing urgent care and parenteral artesunate treatment. Several testing platforms are available to quantify suPAR concentrations including turbidimetric immunoassays, sandwich ELISAs, and magnetic bead-based multiplex assays. Given that a POC test for suPAR already exists, prospective trials can be designed to assess its clinical utility and establish cutoffs to guide triage and management decisions (74). The validation of a POC test based on suPAR levels that accurately risk-stratifies children with malaria could have a direct impact for enhanced triage and management and improved health outcomes for children in resource-limited settings.
Author Contributions
VS and VC conceived the ideas for preparing this review. AW created the figure and VS created the table. VS wrote the first draft of the manuscript. VC, AW, and KK wrote sections of the manuscript. All authors contributed to manuscript revision and read and approved the submitted version.
Funding
This work was supported by the Canadian Institutes of Research (CIHR) Foundation grant (FDN-148439 to KK) and the Canada Research Chair Program (KK). This work was also supported by a Collaborative Research Agreement Grant from Intellectual Ventures/Global Good (KK), the Bill and Melinda Gates Foundation Trust through Intellectual Ventures/Global Good, and donations from Kim Kertland and the Tesari Foundation.
Conflict of Interest
The authors declare that the research was conducted in the absence of any commercial or financial relationships that could be construed as a potential conflict of interest.
Publisher’s Note
All claims expressed in this article are solely those of the authors and do not necessarily represent those of their affiliated organizations, or those of the publisher, the editors and the reviewers. Any product that may be evaluated in this article, or claim that may be made by its manufacturer, is not guaranteed or endorsed by the publisher.
Supplementary Material
The Supplementary Material for this article can be found online at: https://www.frontiersin.org/articles/10.3389/fimmu.2022.931321/full#supplementary-material
Abbreviations
AKI, acute kidney injury; CI, confidence interval; CM, cerebral malaria; ECM, extracellular matrix; ELISA; enzyme-linked immunosorbent assay; FPRL-1, formyl peptide receptor-like 1; FSG, focal segmental glomerulosclerosis; GPI, glycosylphosphatidylinositol; ICAM-1, intercellular adhesion molecule 1; IFN-γ, interferon gamma; IL-2, interleukin 2; RDT, rapid diagnostic test; SM, severe malaria; suPAR, soluble urokinase-type plasminogen activator receptor; TLR, Toll-like receptor; TNF-α, tumor necrosis factor alpha; UM, uncomplicated malaria; uPA, urokinase; uPAR, urokinase-type plasminogen activator receptor.
References
2. Leligdowicz A, Richard-Greenblatt M, Wright J, Crowley VM, Kain KC. Endothelial Activation: The Ang/Tie Axis in Sepsis. Front Immunol (2018) 249:838. doi: 10.3389/fimmu.2018.00838
3. McDonald CR, Weckman A, Richard-Greenblatt M, Leligdowicz A, Kain KC. Integrated Fever Management: Disease Severity Markers to Triage Children With Malaria and non-Malarial Febrile Illness. Malar J (2018) 17:1–7. doi: 10.1186/s12936-018-2488-x
4. Erdman LK, Dhabangi A, Musoke C, Conroy AL, Hawkes M, Higgins S, et al. Combinations of Host Biomarkers Predict Mortality Among Ugandan Children With Severe Malaria: A Retrospective Case-Control Study. PloS One (2011) 6:e17440. doi: 10.1371/journal.pone.0017440
5. Erdman LK, Petes C, Lu Z, Dhabangi A, Musoke C, Cserti-Gazdewich CM, et al. Chitinase 3-Like 1 is Induced by Plasmodium Falciparum Malaria and Predicts Outcome of Cerebral Malaria and Severe Malarial Anaemia in a Case–Control Study of African Children. Malar J (2014) 13:1 –1. doi: 10.1186/1475-2875-13-279
6. Richard-Greenblatt M, Boillat-Blanco N, Zhong K, Mbarack Z, Samaka J, Mlaganile T, et al. Prognostic Accuracy of Soluble Triggering Receptor Expressed on Myeloid Cells (sTREM-1)-Based Algorithms in Febrile Adults Presenting to Tanzanian Outpatient Clinics. Clin Infect Dis (2020) 70:1304–12. doi: 10.1093/cid/ciz419
7. Barinka C, Parry G, Callahan J, Shaw DE, Kuo A, Bdeir K, et al. Structural Basis of Interaction Between Urokinase-Type Plasminogen Activator and its Receptor. J Mol Biol (2006) 363:482–95. doi: 10.1016/j.jmb.2006.08.063
8. Kanse SM, Kost C, Wilhelm OG, Andreasen PA, Preissner KT. The Urokinase Receptor is a Major Vitronectin-Binding Protein on Endothelial Cells. Exp Cell Res (1996) 224:344–53. doi: 10.1006/excr.1996.0144
9. Beaufort N, Leduc D, Rousselle JC, Magdolen V, Luther T, Namane A, et al. Proteolytic Regulation of the Urokinase Receptor/CD87 on Monocytic Cells by Neutrophil Elastase and Cathepsin G. J Immunol (2004) 172:540–9. doi: 10.4049/jimmunol.172.1.540
10. Wei Y, Lukashev M, Simon DI, Bodary SC, Rosenberg S, Doyle MV, et al. Regulation of Integrin Function by the Urokinase Receptor. Science (1996) 273:1551–5. doi: 10.1126/science.273.5281.1551
11. Masucci MT, Pedersen N, Blasi F. A Soluble, Ligand Binding Mutant of the Human Urokinase Plasminogen Activator Receptor. J Biol Chem (1991) 266:8655–8. doi: 10.1016/S0021-9258(18)31492-3
12. Mizukami IF, Todd RF III. A Soluble Form of the Urokinase Plasminogen Activator Receptor (suPAR) can Bind to Hematopoietic Cells. J Leukoc Biol (1998) 64:203–13. doi: 10.1002/jlb.64.2.203
13. Resnati M, Guttinger M, Valcamonica S, Sidenius N, Blasi F, Fazioli F. Proteolytic Cleavage of the Urokinase Receptor Substitutes for the Agonist-Induced Chemotactic Effect. EMBO J (1996) 15:1572–82. doi: 10.1002/j.1460-2075.1996.tb00502.x
14. Sidenius N, Sier CF, Blasi F. Shedding and Cleavage of the Urokinase Receptor (uPAR): Identification and Haracterization of uPAR Fragments In Vitro and In Vivo. FEBS Lett (2000) 475:52–6. doi: 10.1016/S0014-5793(00)01624-0
15. Estreicher A, Mühlhauser J, Carpentier JL, Orci L, Vassalli JD. The Receptor for Urokinase Type Plasminogen Activator Polarizes Expression of the Protease to the Leading Edge of Migrating Monocytes and Promotes Degradation of Enzyme Inhibitor Complexes. J Cell Biol (1990) 111:783–92. doi: 10.1083/jcb.111.2.783
16. Resnati M, Pallavicini I, Wang JM, Oppenheim J, Serhan CN, Romano M, et al. The Fibrinolytic Receptor for Urokinase Activates the G Protein-Coupled Chemotactic Receptor FPRL1/LXA4R. Proc Natl Acad Sci (2002) 99:1359–64. doi: 10.1073/pnas.022652999
17. Eming SA, Brachvogel B, Odorisio T, Koch M. Regulation of Angiogenesis: Wound Healing as a Model. Prog Histochem Cytochem (2007) 42:115–70. doi: 10.1016/j.proghi.2007.06.001
18. Plesner T, Ralfkiær E, Wittrup M, Johnsen H, Pyke C, Pedersen TL, et al. Expression of the Receptor for Urokinasetype Plasminogen Activator in Normal and Neoplastic Blood Cells and Hematopoietic Tissue. Am J Clin Pathol (1994) 102:835–41. doi: 10.1093/ajcp/102.6.835
19. Nykjaer A, Møller B, Todd RF, Christensen T, Andreasen PA, Gliemann J, et al. Urokinase Receptor. Activation Antigen Hum T Lymphocytes J Immunol (1994) 152:505–16. doi: 10.1016/0198-8859(94)90249-6
20. Choong PF, Nadesapillai AP. Urokinase Plasminogen Activator System: A Multifunctional Role in Tumor Progression and Metastasis. Clin Orthop Relat Res (2003) 415:S46–58. doi: 10.1097/01.blo0000093845.72468.bd
21. May AE, Kanse SM, Lund LR, Gisler RH, Imhof BA, Preissner KT. Urokinase Receptor (CD87) Regulates Leukocyte Recruitment via β2 Integrins In Vivo. J Exp Med (1998) 188:1029–37. doi: 10.1084/jem.188.6.1029
22. Gyetko MR, Sud S, Kendall T, Fuller JA, Newstead MW, Standiford TJ. Urokinase Receptor-Deficient Mice Have Impaired Neutrophil Recruitment in Response to Pulmonary Pseudomonas Aeruginosa Infection. J Immunol (2000) 165:1513–9. doi: 10.4049/jimmunol.165.3.1513
23. Heymans S, Luttun A, Nuyens D, Theilmeier G, Creemers E, Moons L, et al. Inhibition of Plasminogen Activators or Matrix Metalloproteinases Prevents Cardiac Rupture But Impairs Therapeutic Angiogenesis and Causes Cardiac Failure. Nat Med (1999) 5:1135–42. doi: 10.1038/13459
24. Stewart CE, Nijmeh HS, Brightling CE, Sayers I. uPAR Regulates Bronchial Epithelial Repair In Vitro and is Elevated in Asthmatic Epithelium. Thorax (2012) 67:477–87. doi: 10.1136/thoraxjnl-2011-200508
25. Mondino A, Blasi F. uPA and uPAR in Fibrinolysis, Immunity and Pathology. Trends Immunol (2004) 25:450–5. doi: 10.1016/j.it.2004.06.004
26. Tarui T, Mazar AP, Cines DB, Takada Y. Urokinase-Type Plasminogen Activator Receptor (CD87) is a Ligand for Integrins and Mediates Cell-Cell Interaction. J Biol Chem (2001) 276:3983–90. doi: 10.1074/jbc.M008220200
27. Simon DI, Wei Y, Zhang L, Rao NK, Xu H, Chen Z, et al. Identification of a Urokinase Receptor-Integrin Interaction Site: Promiscuous Regulator of Integrin Function. J Biol Chem (2000) 275:10228–34. doi: 10.1074/jbc.275.14.10228
28. Pliyev BK. Activated Human Neutrophils Rapidly Release the Chemotactically Active D2D3 Form of the Urokinase-Type Plasminogen Activator Receptor (uPAR/Cd87). Mol Cell Biochem (2009) 321:111–22. doi: 10.1007/s11010-008-9925-z
29. Wei Y, Waltz DA, Rao N, Drummond RJ, Rosenberg S, Chapman HA. Identification of the Urokinase Receptor as an Adhesion Receptor for Vitronectin. J Biol Chem (1994) 269:32380–8. doi: 10.1016/S0021-9258(18)31646-6
30. Yebra M, Goretzki L, Pfeifer M, Mueller BM. Urokinase-Type Plasminogen Activator Binding to its Receptor Stimulates Tumor Cell Migration by Enhancing Integrin-Mediated Signal Transduction. Exp Cell Res (1999) 250:231–40. doi: 10.1006/excr.1999.4510
31. Waltz DA, Chapman HA. Reversible Cellular Adhesion to Vitronectin Linked to Urokinase Receptor Occupancy. J Biol Chem (1994) 269:14746–50. doi: 10.1016/S0021-9258(17)36688-7
32. Ferraris GM, Schulte C, Buttiglione V, De Lorenzi V, Piontini A, Galluzzi M, et al. The Interaction Between uPAR and Vitronectin Triggers Ligand-Independent Adhesion Signalling by Integrins. EMBO J (2014) 33:2458–72. doi: 10.15252/embj.201387611
33. Beschorner R, Schluesener HJ, Nguyen TD, Magdolen V, Luther T, Pedal I, et al. Lesion-Associated Accumulation of uPAR/CD87-Expressing Infiltrating Granulocytes, Activated Microglial Cells/Macrophages and Upregulation by Endothelial Cells Following TBI and FCI in Humans. Neuropathol Appl Neurobiol (2000) 26:522–7. doi: 10.1046/j.0305-1846.2000.287.x
34. Lyngbæk S, Sehestedt T, Marott JL, Hansen TW, Olsen MH, Andersen O, et al. CRP and suPAR are Differently Related to Anthropometry and Subclinical Organ Damage. Int J Cardiol (2013) 167:781–5. doi: 10.1016/j.ijcard.2012.03.040
35. Raptis V, Loutradis C, Boutou AK, Faitatzidou D, Sioulis A, Ferro CJ, et al. Serum Copeptin, NLPR3, and suPAR Levels Among Patients With Autosomal-Dominant Polycystic Kidney Disease With and Without Impaired Renal Function. Cardiorenal Med (2020) 10:440–51. doi: 10.1159/000510834
36. Unseld M, Chilla A, Pausz C, Mawas R, Breuss J, Zielinski C, et al. PTEN Expression in Endothelial Cells is Down-Regulated by uPAR to Promote Angiogenesis. Thromb Haemost (2015) 114:379–89. doi: 10.1160/TH15-01-0016
37. Piguet PF, Vesin C, Donati Y, Tacchini-Cottier F, Belin D, Barazzone C. Urokinase Receptor (uPAR, CD87) is a Platelet Receptor Important for Kinetics and TNF-Induced Endothelial Adhesion in Mice. Circulation (1999) 99:3315–21. doi: 10.1161/01.CIR.99.25.3315
38. Fauser S, Deininger MH, Kremsner PG, Magdolen V, Luther T, Meyermann R, et al. Lesion Associated Expression of Urokinase-Type Plasminogen Activator Receptor (uPAR, CD87) in Human Cerebral Malaria. J Neuroimmunol (2000) 111:234–40. doi: 10.1016/S0165-5728(00)00368-4
39. Roca N, Jatem E, Martín ML, Munoz M, Molina M, Martínez C, et al. Relationship Between Soluble Urokinase-Type Plasminogen Activator Receptor and Serum Biomarkers of Endothelial Activation in Patients With Idiopathic Nephrotic Syndrome. Clin Kidney J (2021) 14:543–9. doi: 10.1093/ckj/sfz173
40. Keskinidou C, Vassiliou AG, Zacharis A, Jahaj E, Gallos P, Dimopoulou I, et al. Endothelial, Immunothrombotic, and Inflammatory Biomarkers in the Risk of Mortality in Critically Ill COVID-19 Patients: The Role of Dexamethasone. Diagnostics (2021) 11:1249. doi: 10.3390/diagnostics11071249
41. Piguet PF, Da Laperrousaz C, Vesin C, Tacchini-Cottier F, Senaldi G, Grau GE. Delayed Mortality and Attenuated Thrombocytopenia Associated With Severe Malaria in Urokinase-and Urokinase Receptor-Deficient Mice. Infect Immun (2000) 68:3822–9. doi: 10.1128/IAI.68.7.3822-3829.2000
42. Wassmer SC, Combes V, Grau GE. Pathophysiology of Cerebral Malaria: Role of Host Cells in the Modulation of Cytoadhesion. Ann N Y Acad Sci (2003) 992:30–8. doi: 10.1111/j.1749-6632.2003.tb03135.x
43. Willimann K, Matile H, Weiss NA, Imhof BA. In Vivo Sequestration of Plasmodium Falciparum-Infected Human Erythrocytes: A Severe Combined Immunodeficiency Mouse Model for Cerebral Malaria. J Exp Med (1995) 182:643–53. doi: 10.1084/jem.182.3.643
44. Dobbie MS, Hurst RD, Klein NJ, Surtees RA. Upregulation of Intercellular Adhesion Molecule-1 Expression on Human Endothelial Cells by Tumour Necrosis Factor-α in an In Vitro Model of the Blood–Brain Barrier. Brain Res (1999) 830:330–6. doi: 10.1016/S0006-8993(99)01436-5
45. Mustjoki S, Sidenius N, Vaheri A. Enhanced Release of Soluble Urokinase Receptor by Endothelial Cells in Contact With Peripheral Blood Cells. FEBS Lett (2000) 486:237–42. doi: 10.1016/S0014-5793(00)02312-7
46. Wiersinga WJ, Kager LM, Hovius JW, van der Windt GJ, de Vos AF, Meijers JC, et al. Urokinase Receptor is Necessary for Bacterial Defense Against Pneumonia-Derived Septic Melioidosis by Facilitating Phagocytosis. J Immunol (2010) 184:3079–86. doi: 10.4049/jimmunol.0901008
47. Rijneveld AW, Levi M, Florquin S, Speelman P, Carmeliet P, van der Poll T. Urokinase Receptor is Necessary for Adequate Host Defense Against Pneumococcal Pneumonia. J Immunol (2002) 168:3507–11. doi: 10.4049/jimmunol.168.7.3507
48. Sitrin RG, Todd R, Albrecht E, Gyetko MR. The Urokinase Receptor (CD87) Facilitates CD11b/CD18-Mediated Adhesion of Human Monocytes. J Clin Invest (1996) 97:1942–51. doi: 10.1172/JCI118626
49. Beck JM, Preston AM, Gyetko MR. Urokinase-Type Plasminogen Activator in Inflammatory Cell Recruitment and Host Defense Against Pneumocystis Carinii in Mice. Infect Immun (1999) 67:879–84. doi: 10.1128/IAI.67.2.879-884.1999
50. Winkler F, Kastenbauer S, Koedel U, Pfister HW. Role of the Urokinase Plasminogen Activator System in Patients With Bacterial Meningitis. Neurology (2002) 59:1350–5. doi: 10.1212/01.WNL.0000031427.81898.96
51. Sanderson-Smith ML, Zhang Y, Ly D, Donahue D, Hollands A, Nizet V, et al. A Key Role for the Urokinase Plasminogen Activator (uPA) in Invasive Group A Streptococcal Infection. PloS Pathog (2013) 9:e1003469. doi: 10.1371/journal.ppat.1003469
52. Bondu V, Bitting C, Poland VL, Hanson JA, Harkins MS, Lathrop S, et al. Upregulation of P2Y2R, Active uPA, and PAI-1 are Essential Components of Hantavirus Cardiopulmonary Syndrome. Front Cell Infect Microbiol (2018) 8:169. doi: 10.3389/fcimb.2018.00169
53. Aguirre-Ghiso JA, Liu D, Mignatti A, Kovalski K, Ossowski L. Urokinase Receptor and Fibronectin Regulate the ERKMAPK to P38mapk Activity Ratios That Determine Carcinoma Cell Proliferation or Dormancy. Vivo Mol Biol Cell (2001) 12:863–79. doi: 10.1091/mbc.12.4.863
54. Nebuloni M, Zawada L, Ferri A, Tosoni A, Zerbi P, Resnati M, et al. HIV-1 Infected Lymphoid Organs Upregulate Expression and Release of the Cleaved Form of uPAR That Modulates Chemotaxis and Virus Expression. PloS One (2013) 8:e70606. doi: 10.1371/journal.pone.0070606
55. Wei C, El Hindi S, Li J, Fornoni A, Goes N, Sageshima J, et al. Circulating Urokinase Receptor as a Cause of Focal Segmental Glomerulosclerosis. Nat Med (2011) 17:952–60. doi: 10.1038/nm.2411
56. Zimmermann HW, Reuken PA, Koch A, Bartneck M, Adams DH, Trautwein C, et al. Soluble Urokinase Plasminogen Activator Receptor is Compartmentally Regulated in Decompensated Cirrhosis and Indicates Immune Activation and Short-Term Mortality. J Intern Med (2013) 274:86–100. doi: 10.1111/joim.12054
57. Savva A, Raftogiannis M, Baziaka F, Routsi C, Antonopoulou A, Koutoukas P, et al. Soluble Urokinase Plasminogen Activator Receptor (suPAR) for Assessment of Disease Severity in Ventilator-Associated Pneumonia and Sepsis. J Infect (2011) 63:344–50. doi: 10.1016/j.jinf.2011.07.016
58. Karki R, Sharma BR, Tuladhar S, Williams EP, Zalduondo L, Samir P, et al. Synergism of TNF-α and IFN-γ Triggers Inflammatory Cell Death, Tissue Damage, and Mortality in SARS-CoV-2 Infection and Cytokine Shock Syndromes. Cell (2021) 184:149–68. doi: 10.1016/j.cell.2020.11.025
59. Loures FV, Pina A, Felonato M, Calich VL. TLR2 is a Negative Regulator of Th17 Cells and Tissue Pathology in a Pulmonary Model of Fungal Infection. J Immunol (2009) 183:1279–90. doi: 10.4049/jimmunol.0801599
60. Couper KN, Barnes T, Hafalla JC, Combes V, Ryffel B, Secher T, et al. Parasite-Derived Plasma Microparticles Contribute Significantly to Malaria Infection-Induced Inflammation Through Potent Macrophage Stimulation. PloS Pathog (2010) 6:e1000744. doi: 10.1371/journal.ppat.1000744
61. Østergaard C, Benfield T, Lundgren JD, Eugen-Olsen J. Soluble Urokinase Receptor is Elevated in Cerebrospinal Fluid From Patients With Purulent Meningitis and is Associated With Fatal Outcome. Scand J Infect Dis (2004) 36:14–9. doi: 10.1080/00365540310017366
62. Plewes K, Royakkers AA, Hanson J, Hasan MM, Alam S, Ghose A, et al. Correlation of Biomarkers for Parasite Burden and Immune Activation With Acute Kidney Injury in Severe Falciparum Malaria. Malar J (2014) 13:1–10. doi: 10.1186/1475-2875-13-91
63. Eugen-Olsen J, Gustafson P, Sidenius N, Fischer TK, Parner J, Aaby P, et al. The Serum Level of Soluble Urokinase Receptor is Elevated in Tuberculosis Patients and Predicts Mortality During Treatment: A Community Study From Guinea-Bissau. Int J Tuberc Lung Dis (2002) 6:686–92.
64. Mölkänen T, Ruotsalainen E, Thorball CW, Järvinen A. Elevated Soluble Urokinase Plasminogen Activator Receptor (suPAR) Predicts Mortality in Staphylococcus Aureus Bacteremia. Eur J Clin Microbiol Infect Dis (2011) 30:1417–24. doi: 10.1007/s10096-011-1236-8
65. Tzanakaki G, Paparoupa M, Kyprianou M, Barbouni A, Eugen-Olsen J, Kourea-Kremastinou J. Elevated Soluble Urokinase Receptor Values in CSF, Age and Bacterial Meningitis Infection are Independent and Additive Risk Factors of Fatal Outcome. Eur J Clin Microbiol Infect Dis (2012) 31:1157–62. doi: 10.1007/s10096-011-1423-7
66. Huang Z, Wang N, Huang S, Chen Y, Yang S, Gan Q, et al. Increased Serum Soluble Urokinase Plasminogen Activator Receptor Predicts Short-Term Outcome in Patients With Hepatitis B-Related Acute-on-Chronic Liver Failure. Gastroenterol Res Pract (2019) 2019: 1–10. doi: 10.1155/2019/3467690
67. Berres ML, Schlosser B, Berg T, Trautwein C, Wasmuth HE. Soluble Urokinase Plasminogen Activator Receptor is Associated With Progressive Liver Fibrosis in Hepatitis C Infection. J Clin Gastroenterol (2012) 46:334–8. doi: 10.1097/MCG.0b013e31822da19d
68. Ostrowski SR, Piironen T, Høyer-Hansen G, Gerstoft J, Pedersen BK, Ullum H. High Plasma Levels of Intact and Cleaved Soluble Urokinase Receptor Reflect Immune Activation and are Independent Predictors of Mortality in HIV-1-Infected Patients. J Acquir Immune Defic Syndr (2005) 39:23–31. doi: 10.1097/01.qai.0000157950.02076.a6
69. Yilmaz G, Mentese A, Kaya S, Uzun A, Karahan SC, Koksal I. The Diagnostic and Prognostic Significance of Soluble Urokinase Plasminogen Activator Receptor in Crimean-Congo Hemorrhagic Fever. J Clin Virol (2011) 50:209–11. doi: 10.1016/j.jcv.2010.11.014
70. Outinen TK, Tervo L, Mäkelä S, Huttunen R, Mäenpää N, Huhtala H, et al. Plasma Levels of Soluble Urokinase-Type Plasminogen Activator Receptor Associate With the Clinical Severity of Acute Puumala Hantavirus Infection. PloS One (2013) 8:e71335. doi: 10.1371/journal.pone.0071335
71. Ostrowski SR, Shulman CE, Peshu N, Staalsøe T, HØyer-Hansen G, Pedersen BK, et al. Elevated Plasma Urokinase Receptor Predicts Low Birth Weight Maternal Malaria. Parasite Immunol (2007) 29:37–46. doi: 10.1111/j.1365-3024.2006.00916.x
72. Huang M, Li L, Shen J, Wang Y, Wang R, Yuan C, et al. Plasma Levels of the Active Form of suPAR are Associated With COVID-19 Severity. Crit Care (2020) 24:1–3. doi: 10.1186/s13054-020-03336-0
73. Koch A, Voigt S, Kruschinski C, Sanson E, Dückers H, Horn A, et al. Circulating Soluble Urokinase Plasminogen Activator Receptor is Stably Elevated During the First Week of Treatment in the Intensive Care Unit and Predicts Mortality in Critically Ill Patients. Crit Care (2011) 15:1–4. doi: 10.1186/cc10037
74. Altintas I, Eugen-Olsen J, Seppälä S, Tingleff J, Stauning MA, El Caidi NO, et al. suPAR Cut-Offs for Risk Stratification in Patients With Symptoms of COVID-19. biomark Insights (2021) 16:11772719211034685. doi: 10.1177/11772719211034685
75. Enocsson H, Idoff C, Gustafsson A, Govender M, Hopkins F, Larsson M, et al. Soluble Urokinase Plasminogen Activator Receptor (suPAR) Independently Predicts Severity and Length of Hospitalisation in Patients With COVID-19. Front Med (2021) 8:1–12. doi: 10.3389/fmed.2021.791716
76. Azam TU, Shadid HR, Blakely P, O’Hayer P, Berlin H, Pan M, et al. Soluble Urokinase Receptor (SuPAR) in COVID-19–Related AKI. J Am Soc Nephrol (2020) 31:2725–35. doi: 10.1681/ASN.2020060829
77. Rovina N, Akinosoglou K, Eugen-Olsen J, Hayek S, Reiser J, Giamarellos-Bourboulis EJ. Soluble Urokinase Plasminogen Activator Receptor (suPAR) as an Early Predictor of Severe Respiratory Failure in Patients With COVID-19 Pneumonia. Crit Care (2020) 24:1–3. doi: 10.1186/s13054-020-02897-4
78. Stauning MA, Altintas I, Kallemose T, Eugen-Olsen J, Lindstrøm MB, Rasmussen LJ, et al. Soluble Urokinase Plasminogen Activator Receptor as a Decision Marker for Early Discharge of Patients With COVID-19 Symptoms in the Emergency Department. J Emerg Med (2021) 61:298–313. doi: 10.1016/j.jemermed.2021.03.012
79. Kyriazopoulou E, Panagopoulos P, Metallidis S, Dalekos GN, Poulakou G, Gatselis N, et al. An Open Label Trial of Anakinra to Prevent Respiratory Failure in COVID-19. Elife (2021) 10:e66125. doi: 10.7554/eLife.66125
80. Kyriazopoulou E, Poulakou G, Milionis H, Metallidis S, Adamis G, Tsiakos K, et al. Early Treatment of COVID-19 With Anakinra Guided by Soluble Urokinase Plasminogen Receptor Plasma Levels: A Double-Blind, Randomized Controlled Phase 3 Trial. Nat Med (2021) 27:1752–60. doi: 10.1038/s41591-021-01499-z
81. Perch M, Kofoed PE, Fischer TK, Co F, Rombo L, Aaby P, et al. Serum Levels of Soluble Urokinase Plasminogen Activator Receptor is Associated With Parasitemia in Children With Acute Plasmodium Falciparum Malaria Infection. Parasite Immunol (2004) 26:207–11. doi: 10.1111/j.0141-9838.2004.00695.x
82. Ostrowski SR, Ullum H, Goka BQ, Høyer-Hansen G, Obeng-Adjei G, Pedersen BK, et al. Plasma Concentrations of Soluble Urokinase-Type Plasminogen Activator Receptor are Increased in Patients With Malaria and are Associated With a Poor Clinical or a Fatal Outcome. J Infect Dis (2005) 191:1331–41. doi: 10.1086/428854
83. Tahar R, Albergaria C, Zeghidour N, Ngane VF, Basco LK, Roussilhon C. Plasma Levels of Eight Different Mediators and Their Potential as Biomarkers of Various Clinical Malaria Conditions in African Children. Malar J (2016) 15:1–5. doi: 10.1186/s12936-016-1378-3
84. Tornyigah B, Blankson SO, Adamou R, Moussiliou A, Rietmeyer L, Tettey P, et al. Specific Combinations of Inflammatory, Angiogenesis and Vascular Integrity Biomarkers Are Associated With Clinical Severity, Coma and Mortality in Beninese Children With Plasmodium Falciparum Malaria. Diagnostics (2022) 12:524. doi: 10.3390/diagnostics12020524
85. Dondorp AM, Lee SJ, Faiz MA, Mishra S, Price R, Tjitra E, et al. The Relationship Between Age and the Manifestations of and Mortality Associated With Severe Malaria. Clin Infect Dis (2008) 47:151–7. doi: 10.1086/589287
86. Roggwiller E, Fricaud AC, Blisnick T, Braun-Breton C. Host Urokinase-Type Plasminogen Activator Participates in the Release of Malaria Merozoites From Infected Erythrocytes. Mol Biochem Parasitol (1997) 86:49–59. doi: 10.1016/S0166-6851(97)02848-X
87. Kim EY, Dryer SE. RAGE and αvβ3-Integrin are Essential for suPAR Signaling in Podocytes. Biochim Biophys Acta Mol Basis Dis (2021) 1867:166186. doi: 10.1016/j.bbadis.2021.166186
88. Haupt TH, Rasmussen LJH, Kallemose T, Ladelund S, Andersen O, Pisinger C, et al. Healthy Lifestyles Reduce suPAR and Mortality in a Danish General Population Study. Immun Ageing (2019) 16:1–12. doi: 10.1186/s12979-018-0141-8
89. Farrington L, Vance H, Rek J, Prahl M, Jagannathan P, Katureebe A, et al. Both Inflammatory and Regulatory Cytokine Responses to Malaria are Blunted With Increasing Age in Highly Exposed Children. Malar J (2017) 16:1–11. doi: 10.1186/s12936-017-2148-6
90. Moncunill G, Mayor A, Jiménez A, Nhabomba A, Puyol L, Manaca MN, et al. Cytokine and Antibody Responses to Plasmodium Falciparum in Naïve Individuals During a First Malaria Episode: Effect of Age and Malaria Exposure. PloS One (2013) 8:e55756. doi: 10.1371/journal.pone.0055756
Keywords: soluble urokinase-type plasminogen activator receptor (suPAR), prognostic marker, triage, risk stratification, malaria
Citation: Stefanova V, Crowley VM, Weckman AM and Kain KC (2022) suPAR to Risk-Stratify Patients With Malaria. Front. Immunol. 13:931321. doi: 10.3389/fimmu.2022.931321
Received: 28 April 2022; Accepted: 13 May 2022;
Published: 10 June 2022.
Edited by:
Silvia Beatriz Boscardin, University of São Paulo, BrazilReviewed by:
Barna Vasarhelyi, Semmelweis University, HungaryCopyright © 2022 Stefanova, Crowley, Weckman and Kain. This is an open-access article distributed under the terms of the Creative Commons Attribution License (CC BY). The use, distribution or reproduction in other forums is permitted, provided the original author(s) and the copyright owner(s) are credited and that the original publication in this journal is cited, in accordance with accepted academic practice. No use, distribution or reproduction is permitted which does not comply with these terms.
*Correspondence: Kevin C. Kain, kkain8888@gmail.com