- 1Aix-Marseille Univ, Intitut Recherche pour le Développement (IRT), Assistance Publique Hôpitaux de Marseille (APHM), Microbe, Evolution, Phylogeny, Infection (MEPHI), Marseille, France
- 2Immunology Department, IHU-Méditerranée Infection, Marseille, France
- 3ImCheck Therapeutics, Marseille, France
- 4Aix-Marseille Univ, APHM, Hôpital de la Conception, Laboratoire d’Immunologie, Marseille, France
- 5Centre pour la Recherche sur le Cancer de Marseille (CRCM), Inserm UMR1068, Centre national de la recherche scientifique (CNRS) UMR7258, Institut Paoli Calmettes, Marseille, France
The T cell receptor Vγ9Vδ2 T cells bridge innate and adaptive antimicrobial immunity in primates. These Vγ9Vδ2 T cells respond to phosphoantigens (pAgs) present in microbial or eukaryotic cells in a butyrophilin 3A1 (BTN3) and butyrophilin 2A1 (BTN2A1) dependent manner. In humans, the rapid expansion of circulating Vγ9Vδ2 T lymphocytes during several infections as well as their localization at the site of active disease demonstrates their important role in the immune response to infection. However, Vγ9Vδ2 T cell deficiencies have been observed in some infectious diseases such as active tuberculosis and chronic viral infections. In this review, we are providing an overview of the mechanisms of Vγ9Vδ2 T cell-mediated antimicrobial immunity. These cells kill infected cells mainly by releasing lytic mediators and pro-inflammatory cytokines and inducing target cell apoptosis. In addition, the release of chemokines and cytokines allows the recruitment and activation of immune cells, promoting the initiation of the adaptive immune response. Finaly, we also describe potential new therapeutic tools of Vγ9Vδ2 T cell-based immunotherapy that could be applied to emerging infections.
Introduction
Gamma-delta (γδ) T cells are « unconventional » T lymphocytes that do not require major histocompatibility complex (MHC) presentation of antigen (1). Human γδ T cells are classified into two main subsets according to the expression of T cell receptor (TCR) δ chain (2). Vδ1 T cells are more common in mucosal tissues and are involved in the first line of the immune defense against solid tumors and infections. The Vδ2 T cells, that is a subset uniquely associated with Vγ9 chain (called Vγ9Vδ2), are abundant in the peripheral blood and play a role of immune effector in tumor surveillance and also in antimicrobial defense (2). Indeed, Vγ9Vδ2 T cells can directly kill infected cells through different mechanisms, and also prime and modulate functions of other innate and adaptive immune cells via cytokines, antigen presentation and cell contact to develop antimicrobial immunity (3).
Human Vγ9Vδ2 T cells, typically represent 2 to 5% of peripheral blood T cells, are expanded following infection with a wide range of microbial agents and can represent up to 50% of the peripheral T cell pool (3, 4). This subset of T cells is enriched in the circulation of patients with bacterial infections, including mycobacterial diseases, listeriosis, salmonellosis, brucellosis, tularemia, legionellosis and Q fever (5–11), and with protozoal parasite infections such as malaria, toxoplasmosis and leishmaniasis (12–14). Vγ9Vδ2 T cells are also increased in the bronchoalveolar lavage fluid of patients with active pulmonary tuberculosis or psittacosis (15), and in cerebral spinal fluid from patients with bacterial meningitis (M. tuberculosis, H. influenzae, S. pneumoniae, and N. meningitidis); such pattern is corrected by successful antibacterial therapy (16, 17). Bacterial vaginosis is also associated with an increase of Vγ9Vδ2 T cells in the female reproductive tract in women (18, 19). Furthermore, in patients with P. falciparum malaria, an increase in Vγ9Vδ2 T lymphocytes in human spleens during infection has also been observed (20). Globally, the rapid expansion of circulating Vγ9Vδ2 T lymphocytes during acute infections as well as their localization at the site of active disease indicate that Vγ9Vδ2 T cells may play an important role in the immune response to infection.
In contrast, it seems that the number of Vγ9Vδ2 T cells in the blood is reduced in patients with a viral infection (21–24). In patients with chronic hepatitis B, the frequency of peripheral and hepatic Vγ9Vδ2 T cells decreases with disease progression. Similarly, the frequency of Vγ9Vδ2 T cells is markedly reduced in the blood and the mucosal tissues of HIV patients, and interestingly is restored with highly active antiretroviral therapy (HAART) (25–27). These observations indicate that Vγ9Vδ2 T cells are activated early after infection but are lost if infection is not controlled. Recently, a decrease in the number of circulating Vγ9Vδ2 T cells has been reported in patients with coronavirus, especially SARS-CoV-2, which was followed by a return to normal levels in recovered patients (22, 23). The aim is to review the mechanisms of Vγ9Vδ2 T cell-mediated antimicrobial immunity and to report the potential therapeutic application of Vγ9Vδ2 T cell immunotherapy to infectious diseases.
Vγ9vδ2 T cell recruitment to the site of inflammation and their implication in tissue repair
The traffic of leukocytes to tissues is an essential step for the development of an immune response that is mainly controlled by the interactions between chemokines and their specific receptors (28). During infection the onset of local inflammation is associated with an increased chemokine production that plays a role in transendothelial migration of Vγ9Vδ2 T cells into the tissues. The majority of circulating Vγ9Vδ2 T cells have the potential to be rapidly recruited in tissues during the course of infection, due to their expression of inflammatory homing chemokine receptor CCR5 and CXCR3 (Figure 1) (28, 29). Indeed, CCR5 expressed on activated Vγ9Vδ2 T cells mediates their migration to influenza virus-infected sites (30). Similarly, high levels of CCR5 and CXCR3 receptors on Vγ9Vδ2 T cells are responsible of transendothelial migration of cells to the lungs in monkeys infected with M. tuberculosis or Bacille Calmette-Guerin (BCG) (31). A macaque model showed that Vγ9Vδ2 T cells exhibit trans-endothelial migration, interstitial localization, and granuloma infiltration in response to M. tuberculosis infections (32).
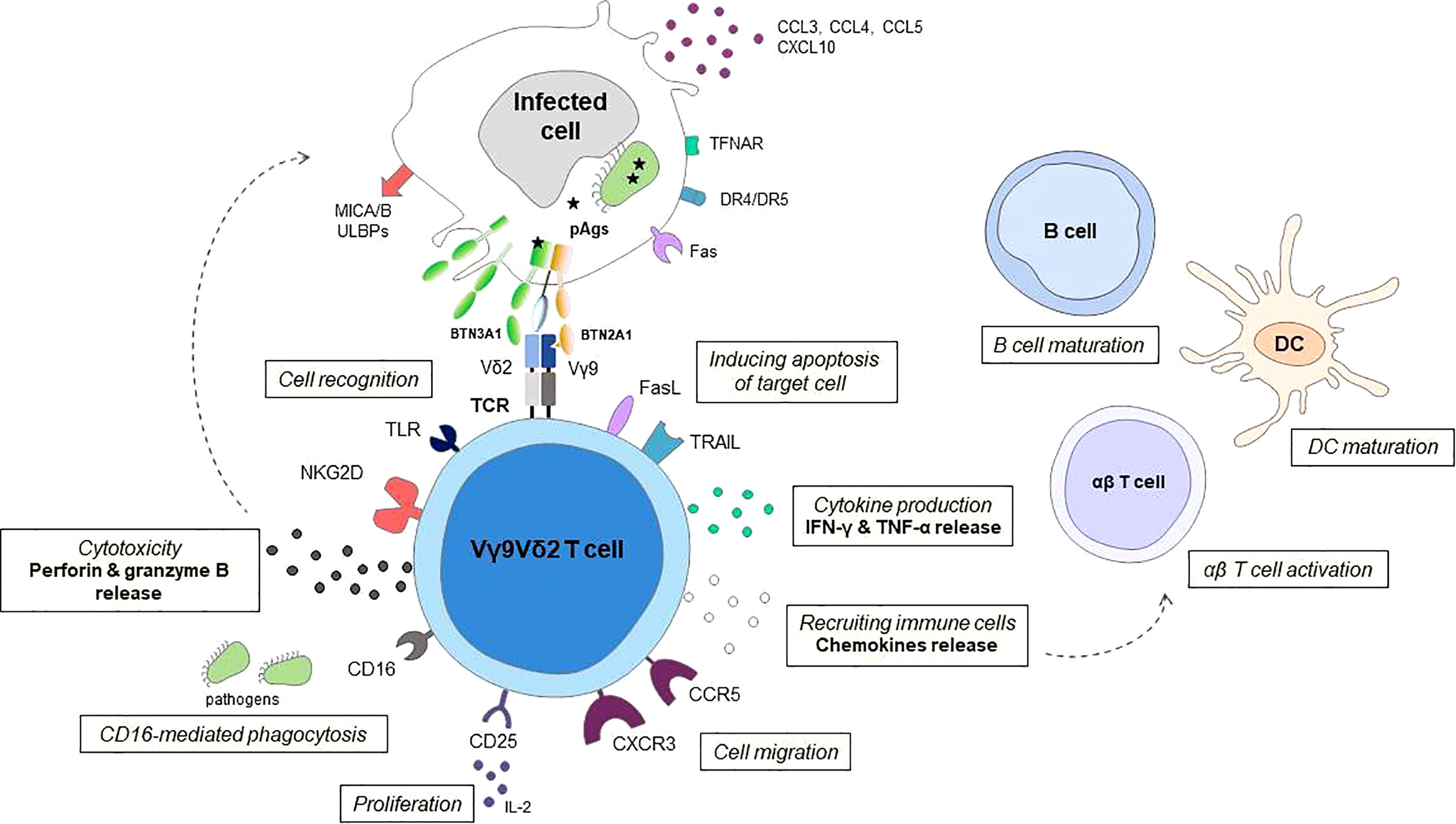
Figure 1 Schematic representation of effector mechanisms of Vγ9Vδ2 T cells in response to infection Vγ9Vδ2 T cells can distinguish between infected cells and normal cells using T cell receptor (TCR) and other cellular receptors especially natural killer group 2 member D receptor the (NKG2D) to sense isopentenyl pyrophosphate (IPP) levels and stress signals (such as MICA/B, ULBPs) displayed on target cells. The butyrophilin receptors BTN3A1 and BTN2A1 on target cells act to detect (pAgs) such as HMBPP and as a direct ligand for the Vγ9Vδ2 T cell receptor. Human Vγ9Vδ2 T cells can also recognize danger signals directly from pathogens through Toll-like receptors (TLRs). Following activation, Vγ9Vδ2 T cells kill infected cells by releasing lytic mediators (perforin, granzyme B), and pro-inflammatory cytokines, inducing target cell apoptosis via Fas/FasL, TNF-related apoptosis-inducing ligand (TRAIL) and TNF-α pathways, and antibody-dependent cell-mediated cytotoxicity (ADCC) through CD16 expression. In a CD16-dependent manner, Vγ9Vδ2 T cells may also have phagocytic functions. The chemokine receptors, including CCR5, control the ability of Vγ9Vδ2 T cell to migrate to the site of infection. The release of chemokines and cytokines allows recruitment of immune cells, enhance antigen priming of dendritic cells (DCs) and maturation of B cells. Vγ9Vδ2 T cells can display an APC-like phenotype and are able to present Ags and provide costimulatory signals sufficient for strong induction of αβ T cells, promoting the initiation of the adaptive immune response. The survival and proliferation of Vγ9Vδ2 T cells are mostly modulated by different cytokines, such as IL-2.
In addition to be anti-microbial effectors, Vγ9Vδ2 T cells, once activated locally or recruited to tissue compartments, might also participate to tissue repair or wound healing after post-infectious tissue damage. In acute bacterial peritonitis, Vγ9Vδ2 T cells accumulate rapidly at the site of infection and likely contribute to scarring in the peritoneal cavity, both directly via the local release of IFN-γ, and indirectly via induction of IL-6 production by mesothelial cells and peritoneal fibroblasts (33, 34). In addition, migrating Vγ9Vδ2 T cells can locally produce fibroblast growth factor-7 (FGF-7), a homeostatic mediator against tissue damages induced by bacterial infections (35). In a macaque model, induced expansion of Vγ9Vδ2 T cells by treatment with 4-hydroxy-3-methyl-but-2-enyl pyrophosphate (HMBPP) and IL-2 led to the apparent attenuation of plague lesions in lungs (35). These Vγ9Vδ2 T cells may therefore contribute to immune responses or tissue homeostasis against bacterial infections.
Recognition of infected cells by Vγ9vδ2 T cells
Recognition by phosphoantigens
In humans, Vγ9Vδ2 T cells recognise small pyrophosphate-containing molecules called phosphoantigens (pAgs) present in the malignant target cell or in the infected cells (Figure 1) (29). These small molecules are isopentenyl pyrophosphate (IPP) produced by infected cells or HMBPP produced by certain bacteria (Mycobacterium tuberculosis, Listeria monocytogenes) and parasites (Plasmodium falciparum, Toxoplasma gondii). It is important to note that the naturally occurring pAg HMBPP stimulates Vγ9Vδ2 T cells about 10,000-fold more efficiently than IPP (15, 36, 37), this recognition provides a formal basis for the role of Vγ9Vδ2 T cells in anti-infective immunity (38–40). A recent study showed that Vγ9Vδ2 T cell activation can occur independently of HMBPP produced by the bacteria but via the regulation of host cholesterol biosynthesis (41). Indeed, infection of human dendritic cells (DCs) with HMBPP-negative L. monocytogenes results in an upregulation of cholesterol metabolism in these cells, leading to increased intracellular IPP levels and direct activation of Vγ9Vδ2 T cells. On the other hand, Vγ9Vδ2 T cells can recognize a mycobacterial glycolipid component, 6-O-methylglucose lipopolysaccharide, which promotes TCR-dependent effector functions of Vγ9Vδ2 T cells against M. tuberculosis in vitro (42).
The recognition mechanisms of pAgs by Vγ9Vδ2 T cells involve the butyrophilin (BTN) protein family. The butyrophilin 3A1 (BTN3A1, CD277), expressed by both immune cells and somatic cells (43), directly binds pAg intracellularly through its B30.2 cytoplasmic domain leading to a conformational change in its ectodomain that is sensed by Vγ9Vδ2 T cells (44–46). BTN3A1 interacts at the plasma membrane with another member of the BTN family, BTN2A1 which is a direct ligand for the Vγ9 TCR chain, thus ensuring the synapse between Vγ9Vδ2 T cells and target cells (47–49). Several studies have confirmed that Vγ9Vδ2 T cell activation is dependent on BTN3A during infections. Indeed, the BTN3A blocking antibody (103.2 mAb) was able to inhibit the degranulation of Vγ9Vδ2 T cells when they were co-cultured with cells infected with M. tuberculosis, L. monocytogenes, P. falciparum or Epstein-Barr virus (38, 41, 42, 44, 50).
The expression of these butyrophilins can be modulated by infection in some cases. Indeed, the plasma membrane expression of BTN3A and BTN2A was induced on P. falciparum infected red blood cells (iRBCs) (38). In addition, we recently showed that intracellular bacteria, M. tuberculosis and C. burnetii increased BTN3A and BTN2A expression on monocytes, concomitantly to Vγ9Vδ2 T cell activation (manuscript submitted). In contrast, human immunodeficiency virus (HIV) infection did not appear to enhance BTN3A expression on DCs (51), indicating that basal BTN3A expression maybe sufficient for translating pAgs signal in HIV-infected cells.
Recognition via Nkg2d (natural killer group 2 member D) receptor
Other transmembrane activatory receptors, notably the NKG2D receptor, have been implicated in the effective triggering of antimicrobial responses by Vγ9Vδ2 T cells. Indeed, NKG2D can bind to its ligands including MICA/B (MHC class I-related chain proteins A and B) and UL16-binding proteins (ULBP1-4). Besides their expression on tumor cells, these ligands are upregulated on cells infected by Zika virus and EBV (52–55). This is also the case during infection with intracellular bacteria, for instance MICA is upregulated by DCs infected with M. tuberculosis (56) and ULBP1 by macrophages infected with M. tuberculosis and Brucella (57, 58).
Recognition via toll-like receptors
Human γδ T cells also recognise danger signals from pathogens via TLRs. Vγ9Vδ2 T cells can be activated by TLR3 and TLR4 ligands and exhibit enhanced antibacterial responses (59). On the other hand, TLR8 ligands were shown to inhibit the expansion of Vγ9Vδ2 T cells in vitro, while these can be potent co-stimuli for Vγ9Vδ2 T cell activation in a monocyte-dependent manner (60). Hence, Vγ9Vδ2 T cells may recognize infected cells through several different receptors involved in innate immune responses.
Antimicrobial responses of vγ9vδ2 T cells
Vγ9vδ2 T cells Kill infected cells in an innate immune manner
Human Vγ9Vδ2 T cells exert both a direct cytotoxic activity against pathogen-infected cells as well as a cell-mediated non-cytolytic activity based on cytokine production (Figure 1) (Table 1). Regarding direct cytotoxicity, Vγ9Vδ2 T cells have been shown to kill cells infected by M. tuberculosis, Brucella suis, Listeria monocytogenes, P. falciparum and influenza virus in vitro, through the secretion of cytolytic molecules such granzymes, granulysin and perforin (64–66, 68, 71, 74, 75), similar to their responses to malignat cells. In addition, apoptosis triggered by death inducible receptors, including Fas and tumor necrosis factor-related apoptosis-inducing ligand receptors (TRAIL), is a major mechanism of Vγ9Vδ2 T cells involved in the elimination of cells infected by Epstein-Barr and influenza virus (78, 79, 84, 93). Furthermore, engagement of NKG2D is sufficient to induce cytokine production and release of lytic granules; it increases TCR-dependent effector functions of Vγ9Vδ2 T cells in M. tuberculosis and Brucella infections (56, 58). In contrast, in other studies on M. tuberculosis or L. monocytogenes, NKG2D was not involved (41, 94). These discrepancies may be due to the different expression of NKG2D ligands between infections and between cell populations. On the other hand, NKG2D activation is required for Vγ9Vδ2 T cell cytotoxicity in viral infections with Epstein-Barr, influenza and Zika viruses (82).
Vγ9Vδ2 T cells have also been shown to be able of antibody dependent cell-mediated cytotoxicity (ADCC). Indeed, upon stimulation by pAgs, Vγ9Vδ2 T cells express CD16 (FcγRIIIa), an activatory Fcγ receptor that is constitutively expressed on NK cells and mediates ADCC (95, 96). Although total numbers of Vγ9Vδ2 T cells are decreased during HIV infection, resilient activated CD16+ Vγ9Vδ2 T cells were shown to retain the ability to induce ADCC and exert their antiviral functions in HIV disease (89). Moreover, Vγ9Vδ2 T cell expression of CD16 is increased in children in malaria-endemic regions, suggesting a potential role for Vγ9Vδ2 T cells in inciting antibody-mediated parasite killing (97). Besides ADCC, a recent study showed that Vγ9Vδ2 T cells destroy P. falciparum infected red blood cells (iRBCs) by a CD16-dependent phagocytosis mechanism (38). As a matter of fact, there are data suggesting that Vγ9Vδ2 T cells can phagocytose particles and act as professional antigen-presenting cells (pAPCs). In response to E. coli, peripheral human Vγ9Vδ2 T cells transitioned from cytokine-producing bacterial effectors to professional phagocytic killers in a CD16-dependent manner (98, 99). A recent study also showed that Vγ9Vδ2 T cells suppress P. falciparum by direct killing and phagocytosis (38).
Regarding cell-mediated non-cytolytic activity, there are abundant data documenting the pivotal role of IFN-γ and TNF-α secretion on Vγ9Vδ2 T cell responses during infection. In Vγ9Vδ2 T cell-depleted humanized mice, decreased resistance to acute lethal infections with Staphylococcus aureus, Escherichia coli, and Morganella morganii correlated with decreased serum IFN-γ titers, a cytokine known to control numerous bacterial infections (100). The release of IFN-γ is part of the effector mechanisms of Vγ9Vδ2 T cells in M. tuberculosis, B. suis and P. falciparum infection (64, 71, 101), and also inhibits influenza virus, HCV and SARS-CoV-1 replication (21, 30, 88, 102). Early in HSV-induced inflammation, activated Vγ9Vδ2 T cells secrete IFN-γ and TNF-α, and chemokines, that may affect the course of inflammation (19). Finally, the production of chemokines MIP-1α, MIP-1β and RANTES by Vγ9Vδ2 T cells has also been shown to block HIV replication in vitro by inhibiting the CCR5 co-receptor that is required for HIV entry (90).
Vγ9vδ2 T cells cooperation with immune cells
Vγ9Vδ2 T cells contribute to responses against pathogen infection by modulating indirectly the function of other immune cells. Activated Vγ9Vδ2 T cells can induce recruitment of immune cells by secreting chemokines and stimulating monocytes, neutrophils, DCs, B lymphocytes, and different subtypes of T cells through cytokine secretion, notably IFN-γ (Figure 1) (103–106). In patients with acute bacterial peritonitis, Vγ9Vδ2 T cells that accumulate at the site of infection favor the recruitment of monocytes, neutrophils, and lymphocytes and produce inflammatory cytokines that are controlled by BTN3A, as demonstrated by the inhibitory effect of BTN3A antagonist mAb 103.2 in this process (33). Vγ9Vδ2 T cells may impact DC function during infection. Indeed, Vγ9Vδ2 T cells may enhance DC activation through IFN-γ secretion and CD4+ cell responses to S. aureus (106). Several intracellular bacterial pathogens including M. tuberculosis, B. suis, C. burnetii, interfere with DC maturation, which results in poor priming of the adaptive immune response (107, 108). Brucella-infected DCs trigger Vγ9Vδ2 T cells activation that required cell-to-cell contact. In turn, co-culture with activated Vγ9Vδ2 T cells resulted in maturation of Brucella-infected DCs with increased expression of co-stimulatory CD80 and CD86, and enhanced IFN-γ and IL-12 secretion (72). In ten HIV patients naive of antiretroviral therapy, treatment with zoledronate and recombinant IL-2 achieved not only Vγ9Vδ2 T cells expansion and activation but also DC maturation and HIV-specific CD8+ T cell responses, although the eventual interaction between these immune compartments was not explored in the study (91).
Vγ9Vδ2 T cells were shown to induce differentiation and migration of neutrophils through the production of IL-17 during M. tuberculosis, L. monocytogenes infections and in bacterial meningitis (17, 109). Moreover, Vγ9Vδ2 T cells respond rapidly to neutrophils after phagocytosis of a broad range of bacteria at the site of infection, and in turn mediate the local differentiation of neighbouring neutrophils into APCs for both CD4+ and CD8+ T cells in vitro (110).
Vγ9Vδ2 T cells can also promote adaptive-like responses by sharing functions with APCs (111). Indeed, Vγ9Vδ2 T cells promote efficient adaptive immunity through processing and presenting influenza virus-derived peptides to CD4+ and CD8+ T cells (80, 81). In malaria patients, Vγ9Vδ2 T cells presented increased plasma membrane expression of APC markers HLA-DR and CD86. Similarly, in response to infected red blood cells in vitro, Vγ9Vδ2 T cells show an APC-like phenotype and are able of Ag presentation and αβT cell activation in vitro (112). Vγ9Vδ2 T cells may therefore promote the initiation of the adaptive response despite a possible impairment of conventional APCs. In response to E. coli and L. monocytogenes, human Vγ9Vδ2 T cells also display APC functions (99, 113). Futhermore, phosphoantigen-activated Vγ9Vδ2 T cells can inhibit IL-2-induced expansion of Tregs and reverse subsequent suppression of mycobacterium-specific T-cell immune responses (114).
Finally, it is well known that γδ T cells have a strong impact on humoral immunity. A subset of human Vγ9Vδ2 T cells isolated from peripheral blood expresses the CXC chemokine receptor type 5 (CXCR5) like T follicular helper cells, and, upon antigen stimulation, they are able to express the costimulatory molecules ICOS and CD40L, to produce cytokines such as IL-2, IL-4, and IL-10, and to help B cells for antibody production (Figure 1) (115, 116). In addition, Vγ9Vδ2 T cells activated with the phosphoantigen HMBPP and in presence of IL-21 can also influence the localization of B cell inside the germinal center, positioning them into the light zone thanks to the production of CXC motif chemokine 13 (CXCL13) (116). Surprisingly, during chronic Simian-Human Immunodeficiency Virus (SHIV) infection, Vγ9Vδ2 T cell activation boosted HIV Env-specific Ab titres (92). It has also been reported that human Vγ9Vδ2 T cells facilitated H9N2 influenza virus specific IgG production (81), and that the higher number of circulating Vγ9Vδ2 T cells was associated with higher anti-SARS-CoV-1 specific IgG titers (21).
Vγ9vδ2 T cell “memory” responses
The Vγ9Vδ2 T cells may acquire a memory effector phenotype (TEM cells) following several infections, as shown by the expression of the memory and activation markers CD27 and CD45RA. This phenotype has been reported in bacterial (31), parasitic (76), and viral infections (21, 22, 117).
In macaques, a clear memory-type response of Vγ9Vδ2 T cells was detected as early as four days after BCG re-infection and the magnitude of this expansion was 2-9-fold greater than that seen during primary BCG infection (67). A recall expansion of Vγ9Vδ2 T cells was also observed in macaques infected with L. monocytogenes or challenged with Salmonella and smallpox vaccines (69, 70, 118). In addition, studies in cattle and pigs showed similar responses to those found in macaques with a rapid γδ T cell proliferation after BCG vaccination (119–123). These observations demonstrate the essential role of γδ T cells in developing a long-term immunity against pathogens.
It is difficult to determine in humans whether a Vγ9Vδ2 T cell expansion observed during an infection represents a primary or recall response. Interestingly, Vγ9Vδ2 T cells induced by BCG or influenza vaccination develop memory responses (83, 124), and the numbers of memory Vγ9Vδ2 T cells correlates with protection in an P. falciparum sporozoite vaccine trial in a malaria endemic region (77). These data suggest that immunotherapy based on Vγ9Vδ2 T cells, which contribute to adaptive immunity, represents a great potential for the treatment of infections.
Overall, Vγ9Vδ2 T cells may act as an antimicrobial defense through different molecular mechanisms and also constitute a memory cell population that provides protection against subsequent infection. Hence, human Vγ9Vδ2 T cells may affect the progression and outcome of infectious diseases.
Vγ9vδ2 T cell deficiencies in infectious disease
Alterations of Vγ9Vδ2 T cell phenotype and/or functions have been reported in several infections usually due to intracellular pathogens. Hence, a loss of CD27 expression on circulating Vγ9Vδ2 T cells was reported in patients with active tuberculosis, suggesting an impairment of effector functions (61, 62). Indeed, Vγ9Vδ2 T cell expansion was accompanied by the dramatic reduction of the Vγ9Vδ2 T cells effectors (TEM and TEMRA cells), with decreased IFN-γ production and granulysin expression. This deficiency was restored by successful antimycobacterial therapy. A loss of cytotoxic activity is also observed in lung Vγ9Vδ2 T cells (63). These results suggest that a high bacterial burden leads to chronic stimulation of effector Vγ9Vδ2 T cells that may result in their loss or exhaustion. As a matter of fact, The progressive loss of reactive Vγ9Vδ2 T cells from the blood and bronchoalveolar fluid in pulmonary tuberculosis patients paralleles upregulation of FasL expression on Vγ9Vδ2 T cells resulting in fratricidal killing (1, 125). A progressive attenuation of the Vγ9Vδ2 response was also observed in children with high parasitaemia in malaria (73). Similarly, prophylaxis with antimalarial drug dihydroartemisinin-piperaquine (DHA-P) during early childhood prevents the development of dysfunctional Vγ9Vδ2 T cells (12, 73).
Patients with chronic HBV infection are usually characterized by a population of exhausted T cells, similarly the ability of Vγ9Vδ2 T cells to proliferate and to respond to a chemotactic signal is diminished, which may explain the reduced frequency of Vγ9Vδ2 T cells in the liver of these patients (85). In HIV and chronic HCV patients, peripheral Vγ9Vδ2 T cells are unable to proliferate and specifically to expand the cytotoxic subset (27, 61, 86, 87). In addition, it has been demonstrated that, during HIV infection, myeloid-derived suppressor cells (MDSC) are expanded and their frequency is inversely correlated with the capacity of Vγ9Vδ2 T cells to produce IFN-γ. However, in vitro MDSC depletion did not completely restore IFN-γ production by Vγ9Vδ2 T cells from HIV patients (126), suggesting that during HIV infection MDSC are not the unique player in dampening Vγ9Vδ2 T cell response. Finally, in chronic HCV infection and in HIV/HCV co-infection, direct acting antivirals (DAA) fail to restore Vγ9Vδ2-induced IFN-γ production. In contrast to other T cell subsets, Vγ9Vδ2 T cell dysfunction may persist in liver despite a successful HCV treatment for a reason that remains to be elucidated (87).
Overall, these data support a crucial role for Vγ2Vδ2 T cells in infectious diseases, since functional alterations of these cells can have a significant impact on the outcome of the infectious pathology.
Vγ9vδ2 T cell-based emerging therapeutic approaches
Overall, the data summarized above indicate that triggering Vγ9Vδ2 T cell cytotoxicity may be a promising strategy for the treatment of infectious diseases caused by intracellular pathogens. Specifically, proliferative, cytotoxic, and cytokine responses of human Vγ9Vδ2 T cell subset are induced by bisphosphonates, such as pamidronate (PAM) and zoledronic acid (Zol), through the intracellular accumulation of IPP and its metabolites. The administration of PAM, a common treatment for osteoporosis and Paget’s disease, to humanized mice decreases the disease severity and mortality caused by human influenza virus infection and EBV-induced lymphoproliferative disease by enhancing Vγ9Vδ2 T cells immunity (84, 93). On the other hand, Zol, a treatment for bone disease, is broadly used in vitro and ex vivo to stimulate effector Vγ9Vδ2 T cells (127). Zoledronate affects HCV, HCMV and West Nile virus replication by expanding IFN-γ-producing Vγ9Vδ2 T cells (88, 128, 129). As mentioned previously, low-dose IL-2 synergizes with bisphosphonates and hence, is an effective method to activate and expand Vγ9Vδ2 T cells both in vitro and in vivo. In HIV patients, Zol along with IL-2 allowed the rapid expansion of CD16-expressing T Vγ9Vδ2 cells in vitro, associated with enhanced ADCC cytotoxicity (130). In macaques, HMBPP/IL-2 administration induced remarkable Vγ9Vδ2 T cell expansion and resulted in apparent attenuation of plague lesions in lung tissues caused by Yersinia pestis infection (35). Similarly, Picostim (similar to HMBPP except one carbon difference)/IL-2 administration induced activation and expansion of effector Vγ9Vδ2 T cells during both the acute and chronic phases of SHIV infection and also increased resistance to tuberculosis in macaques (131), supporting a rationale to explore Vγ9Vδ2 T cell-targeting as treatment of drug-resistant tuberculosis or HIV-associated tuberculosis. Furthermore, IL-12 and also IL-15 enhance the proliferation and expansion of HMBPP-activated Vγ9Vδ2 T cells with effector functions capable of inhibiting intracellular mycobacterial growth (108, 132). On the other hand, IL-18 enhances the proliferative, cytotoxic and recall response of Vγ9Vδ2 T cells from HIV-1-infected individuals (133). In HIV seropositive individuals, where Vγ9Vδ2 T cells are typically reduced even after effective antiretroviral therapy and CD4 T-cell reconstitution, therapies directed at restoring the antiviral activity of Vγ9Vδ2 T cells represent an appealing potential treatment. This raises questions about the therapeutic use of these cells, including the minimal requirement for eliciting a response and the cytokines required for the boost of immune response. A new strategy for treating influenza virus infection has been suggested using the combination of PAM and CD137 agonist. Indeed, activation of the CD137/CD137L pathway could maintain the survival of Vγ9Vδ2 T cells, this may provide a new solution to avoid Vγ9Vδ2 T cell exhaustion and to increase the efficacy of γδ T cell-based immunotherapy (134). However, the clinical use of bisphosphonates as an anti-infective agent has certain limitations. Indeed, it has been reported that repeated pAg treatment may lead effector cells to a senescent or exhausted phenotype, and even lead to their death (135). Better antigens should be sought to help stimulating Vγ9Vδ2 T cells in vitro.
Besides pAg-induced activation of Vγ9Vδ2 T cells, a recently novel approach involved the development of a new class of molecules called immunoantibiotics, notably the IspH inhibitor, has been described as also inducing the expansion and activation of human Vγ9Vδ2 T cells (136). IspH, an enzyme in the isoprenoid synthesis pathway, is essential for the survival of most Gram-negative bacteria and the lack of IspH causes an accumulation of its substrate HMBPP, thus allowing the activation of cytotoxic Vγ9Vδ2 T cells. In a humanized mice model of E. coli infection, these prodrugs resulted in Vγ9Vδ2 T cell expansion and a lower bacterial load in the tissues (136). This strategy synergises direct antibiotic action with rapid immune response. In addition, these prodrugs allow the targeting of existing multi-resistant microbes (136), as well as decrease the chances of resistance emerging. Unlike antibiotics derived from natural sources, no IspH inhibitors have been discovered in microorganisms, which justify their therapeutical use (137).
Another approach would be to target specifically the ligands expressed on the plasma membrane of stressed cells, such as BTN3A, which are responsible for activation and effector functions of Vγ9Vδ2 T cells. Indeed, an important tool generated in BTN3A research are activating mAbs including the anti-BTN3A agonist 20.1, that mimics the pAg-induced Vγ9Vδ2 T cell activation (43, 138, 139). After successfully showing proof-of-concept of preclinical efficacy (140), another BTN3A agonist mAb, ICT01, is currently under evaluation in the EVICTION phase I/II clinical trial (NCT04243499) sponsored by ImCheck Therapeutics in patients with solid tumors and hematological malignancies (141, 142).
The activating anti-BTN3A mAb could represent important therapeutic tools in infections to overcome the imbalances in immune responses observed in some patients. In this context, we are currently testing the ability of the agonist anti-BTN3A 20.1 to modulate viral/bacterial replication in vitro in co-cultures of infected cells with Vγ9Vδ2 T cells (143). By enhancing Vγ9Vδ2 T cell cytotoxicity against infected cells, anti-BTN3A agonist antibodies could offer an alternative treatment strategy for infectious diseases. Combinations of newly emerging therapy with established treatments could minimize the potential side effects of immune reconstitution in the future.
Conclusion and perspectives
The unique features of Vγ9Vδ2 T cells make these cells ideal candidates that could be targeted to induce protective and durable immunity in the context of infectious diseases. Therapies must be developed to enhance the effector functions of these cells at the site of infection, which would be relevant especially in chronic infections such as HIV infection or tuberculosis where the effector Vγ9Vδ2 T cells are impaired. For the preparation of large number of cells for adoptive cell transfer, it is necessary to identify and develop better antigens, which stimulate the Vγ9Vδ2 T cells expansion in vitro. Targeting key receptors such as the BTN3A and BTN2A involved in activation and recognition of Vγ9Vδ2 T cells emerge as potential therapeutic strategies in infectious diseases. Therefore, further research might shed more light on the in-depth understanding of the underlying mechanisms of the antigen recognition and key factors influencing the Vγ9Vδ2 T cell activation during infectious diseases, which will be pivotal for developping effective Vγ9Vδ2 T cell-based therapies against pathogen infections.
Author contributions
LG, SM, and CC wrote/revised the manuscript. J-LM and DO supervised/revised the manuscript. All authors reviewed the manuscript and contributed to the work.
Funding
LG was supported by a Cifre fellowship from ImCheck Therapeutics.
Conflict of interest
DO is cofounder and shareholder of Imcheck Therapeutics, Emergence Therapeutics, Alderaan Biotechnology and Stealth. IO. CC, PF and LM are employees and shareholders of Imcheck Therapeutics.
The remaining authors declare that the research was conducted in the absence of any commercial or financial relationships that could be constructed as a potential conflict of interest.
Publisher’s note
All claims expressed in this article are solely those of the authors and do not necessarily represent those of their affiliated organizations, or those of the publisher, the editors and the reviewers. Any product that may be evaluated in this article, or claim that may be made by its manufacturer, is not guaranteed or endorsed by the publisher.
References
1. Carding SR, Egan PJ. γδ T cells: functional plasticity and heterogeneity. Nat Rev Immunol (2002) 2(5):336–45. doi: 10.1038/nri797
2. Zhou QH, Wu FT, Pang LT, Zhang TB, Chen Z. Role of γδ t cells in liver diseases and its relationship with intestinal microbiota. World J Gastroenterol (2020) 26(20):2559–69. doi: 10.3748/wjg.v26.i20.2559
3. Chen ZW. Immune biology of ag-specific γδ t cells in infections. Cell Mol Life Sci (2011) 68(14):2409–17. doi: 10.1007/s00018-011-0703-9
4. Morita CT, Jin C, Sarikonda G, Wang H. Nonpeptide antigens, presentation mechanisms, and immunological memory of human vγ2vδ2 t cells: discriminating friend from foe through the recognition of prenyl pyrophosphate antigens. Immunol Rev (2007) 215:59–76. doi: 10.1111/j.1600-065X.2006.00479.x
5. Balbi B, Valle MT, Oddera S, Giunti O, Manca F, Rossi GA, et al. T-lymphocytes with γδ+ vδ2+ antigen receptors are present in increased proportions in a fraction of patients with tuberculosis or with sarcoidosis. Am Rev Respir Dis (1993) 148:1685–90. doi: 10.1164/ajrccm/148.6_Pt_1.1685
6. Jouen-Beades F, Paris E, Dieulois C, Lemeland JF, Barre-Dezelus V, Marret S, et al. In vivo and in vitro activation and expansion of γδ t cells during listeria monocytogenes infection in humans. Infect Immun (1997) 65(10):4267–72. doi: 10.1128/iai.65.10.4267-4272.1997
7. Hara T, Mizuno Y, Takaki K, Takada H, Akeda H, Aoki T, et al. Predominant activation and expansion of v gamma 9-bearing gamma delta t cellsin vivo as well as in vitro in Salmonella Infection. J Clin Invest. (1992) 90(1):204–10. doi: 10.1172/JCI115837
8. Bertotto A, Gerli R, Spinozzi F, Muscat C, Scalise F, Castellueei G, et al. Lymphocytes bearing the γδ t cell receptor in acute Brucella melitensis infection. Eur J Immunol (1993) 23(5):1177–80. doi: 10.1002/eji.1830230531
9. Poquet Y, Kroca M, Halary F, Stenmark S, Peyrat MA, Bonneville M, et al. Expansion of vγ9vδ2 t cells is triggered byFrancisella tularensis-derived phosphoantigens in tularemia but not after tularemia vaccination. Infect Immun (1998) 66(5):2107–14. doi: 10.1128/IAI.66.5.2107-2114.1998
10. Kroca M, Johansson A, Sjöstedt A, Tärnvik A. Vγ9vδ2 t cells in human legionellosis. Clin Diagn Lab Immunol (2001) 8(5):949–54. doi: 10.1128/CDLI.8.5.949-954.2001
11. Schneider T, Jahn HU, Liesenfeld O, Steinhoff D, Riecken EO, Zeitz M, et al. The number and proportion of vυ9vδ2 t cells rise significantly in the peripheral blood of patients after the onset of acute Coxiella burnetii infection. Clin Infect Dis (1997) 24(2):261–4. doi: 10.1093/clinids/24.2.261
12. Jagannathan P, Lutwama F, Boyle MJ, Nankya F, Farrington LA, McIntyre TI, et al. Vδ2+ t cell response to malaria correlates with protection from infection but is attenuated with repeated exposure. Sci Rep (2017) 7(1):11487. doi: 10.1038/s41598-017-10624-3
13. Raziuddin S, Telmasani AW, El-Awad MEH, Al-Amari O, Al-Janadi M. γδ T cells and the immune response in visceral leishmaniasis. Eur J Immunol (1992) 22(5):1143–8. doi: 10.1002/eji.1830220506
14. Scalise F, Gerli R, Castellucci G, Spinozzi F, Fabietti GM, Crupi S, et al. lymphocytes bearing the γδ t-cell receptor in acute toxoplasmosis. Immunology. (1992) 76(4):668–70.
15. Chen ZW, Letvin NL. Vγ2vδ2+ t cells and anti-microbial immune responses. Microbes Infect (2003) 5(6):491–8. doi: 10.1016/S1286-4579(03)00074-1
16. Dieli F, Sireci G, Di Sano C, Champagne E, Fourniè JJ, Ivanyi J, et al. Predominance of vγ9/vδ2 t lymphocytes in the cerebrospinal fluid of children with tuberculous meningitis: reversal after chemotherapy. Mol Med (1999) 5(5):301–12. doi: 10.1007/BF03402066
17. Caccamo N, La Mendola C, Orlando V, Meraviglia S, Todaro M, Stassi G, et al. Differentiation, phenotype, and function of interleukin-17-producing human vγ9vδ2 t cells. Blood. (2011) 118(1):129–38. doi: 10.1182/blood-2011-01-331298
18. Alcaide ML, Strbo N, Romero L, Jones DL, Rodriguez VJ, Arheart K, et al. Bacterial vaginosis is associated with loss of gamma delta t cells in the female reproductive tract in women in the miami women interagency hiv study (wihs): a cross sectional study. PLoS One (2016) 11(4):e0153045. doi: 10.1371/journal.pone.0153045
19. Verjans GMGM, Roest RW, van der Kooi A, Osterhaus ADME. isopentenyl pyrophosphate-reactive vγ9vδ2 t helper 1-like cells are the major γδ t cell subset recovered from lesions of patients with genital herpes. J Infect Dis (2004) 190(3):489–93. doi: 10.1086/422393
20. Howard J, Zaidi I, Loizon S, Mercereau-Puijalon O, Déchanet-Merville J, Mamani-Matsuda M. Human vγ9vδ2 t lymphocytes in the immune response to p. falciparum infection. Front Immunol (2018) 9. doi: 10.3389/fimmu.2018.02760
21. Poccia F, Agrati C, Castilletti C, Bordi L, Gioia C, Horejsh D, et al. Anti-severe acute respiratory syndrome coronavirus immune responses: the role played by vγ9vδ2 t cells. J Infect Dis (2006) 193(9):1244–9. doi: 10.1086/502975
22. Rijkers G, Vervenne T, van der Pol P. More bricks in the wall against sars-cov-2 infection: involvement of γ9δ2 t cells. Cell Mol Immunol (2020) 17(7):771–2. doi: 10.1038/s41423-020-0473-0
23. Odak I, Barros-Martins J, Bošnjak B, Stahl K, David S, Wiesner O, et al. Reappearance of effector t cells is associated with recovery from covid-19. EBioMedicine. (2020) 57:102885. doi: 10.1016/j.ebiom.2020.102885
24. Rajoriya N, Fergusson JR, Leithead JA, Klenerman P. γδ T-lymphocytes in hepatitis c and chronic liver disease. Front Immunol (2014) 5. doi: 10.3389/fimmu.2014.00400
25. Wallace M, Scharko AM, Pauza CD, Fisch P, Imaoka K, Kawabata S, et al. Functional γδ t lymphocyte defect associated with human immunodeficiency virus infections. Mol Med (1997) 3(1):60–71. doi: 10.1007/BF03401668
26. Martini F, Poccia F, Goletti D, Carrara S, Vincenti D, D’Offizi G, et al. Acute human immunodeficiency virus replication causes a rapid and persistent impairment of vγ9vδ2 t cells in chronically infected patients undergoing structured treatment interruption. J Infect Dis (2002) 186(6):847–50. doi: 10.1086/342410
27. Li H, Peng H, Ma P, Ruan Y, Su B, Ding X, et al. Association between vγ2vδ2 t cells and disease progression after infection with closely related strains of hiv in china. Clin Infect Dis (2008) 46(9):1466–72. doi: 10.1086/587107
28. Brandes M, Willimann K, Lang AB, Nam KH, Jin C, Brenner MB, et al. Flexible migration program regulates γδ t-cell involvement in humoral immunity. Blood. (2003) 102(10):3693–701. doi: 10.1182/blood-2003-04-1016
29. Bonneville M, Scotet E. Human vγ9vδ2 t cells: promising new leads for immunotherapy of infections and tumors. Curr Opin Immunol (2006) 18(5):539–46. doi: 10.1016/j.coi.2006.07.002
30. Qin G, Liu Y, Zheng J, Ng IHY, Xiang Z, Lam KT, et al. Type 1 responses of human vγ9vδ2 t cells to influenza a viruses. J Virol (2011) 85(19):10109–16. doi: 10.1128/JVI.05341-11
31. Chen ZW, Letvin NL. Adaptive immune response of vγ2vδ2 t cells: a new paradigm. Trends Immunol (2003) 24(4):213–9. doi: 10.1016/S1471-4906(03)00032-2
32. Huang D, Shen Y, Qiu L, Chen CY, Shen L, Estep J, et al. Immune distribution and localization of phosphoantigen-specific vγ2vδ2 t cells in lymphoid and nonlymphoid tissues in Mycobacterium Tuberculosis Infection. Infect Immun (2008) 76(1):426–36. doi: 10.1128/IAI.01008-07
33. Liuzzi AR, Kift-Morgan A, Lopez-Anton M, Friberg IM, Zhang J, Brook AC, et al. Unconventional human t cells accumulate at the site of infection in response to microbial ligands and induce local tissue remodeling. J Immunol (2016) 197(6):2195–207. doi: 10.4049/jimmunol.1600990
34. Davey MS, Lin CY, Roberts GW, Heuston S, Brown AC, Chess JA, et al. Human neutrophil clearance of bacterial pathogens triggers anti-microbial γδ t cell responses in early infection. PLoS Pathog (2011) 7(5):e1002040. doi: 10.1371/journal.ppat.1002040
35. Huang D, Chen CY, Ali Z, Shao L, Shen L, Lockman HA, et al. Antigen-specific vγ2vδ2 t effector cells confer homeostatic protection against pneumonic plaque lesions. Proc Natl Acad Sci (2009) 106(18):7553–8. doi: 10.1073/pnas.0811250106
36. Eberl M, Hintz M, Reichenberg A, Kollas AK, Wiesner J, Jomaa H. Microbial isoprenoid biosynthesis and human γδ t cell activation. FEBS Lett (2003) 544(1):4–10. doi: 10.1016/S0014-5793(03)00483-6
37. Bonneville M, Fournié JJ. Sensing cell stress and transformation through vγ9vδ2 t cell-mediated recognition of the isoprenoid pathway metabolites. Microbes Infect (2005) 7(3):503–9. doi: 10.1016/j.micinf.2005.03.004
38. Junqueira C, Polidoro RB, Castro G, Absalon S, Liang Z, Sen Santara S, et al. γδ T cells suppress plasmodium falciparum Blood-Stage Infection by Direct Killing and Phagocytosis. Nat Immunol (2021) 22(3):347–57. doi: 10.1038/s41590-020-00847-4
39. Frencher JT, Shen H, Yan L, Wilson JO, Freitag NE, Rizzo AN, et al. Hmbpp-deficient listeria mutant immunization alters pulmonary/systemic responses, effector functions, and memory polarization of vγ2vδ2 t cells. J Leukoc Biol (2014) 96(6):957–67. doi: 10.1189/jlb.6HI1213-632R
40. Shen L, Frencher J, Huang D, Wang W, Yang E, Chen CY, et al. Immunization of vγ2vδ2 t cells programs sustained effector memory responses that control tuberculosis in nonhuman primates. Proc Natl Acad Sci (2019) 116(13):6371–8. doi: 10.1073/pnas.1811380116
41. Alice AF, Kramer G, Bambina S, Bahjat KS, Gough MJ, Crittenden MR. listeria monocytogenes-infected human monocytic derived dendritic cells activate vγ9vδ2 t cells independently of hmbpp production. Sci Rep (2021) 11(1):16347. doi: 10.1038/s41598-021-95908-5
42. Xia M, Hesser DC, De P, Sakala IG, Spencer CT, Kirkwood JS, et al. A subset of protective γ9δ2 t cells is activated by novel mycobacterial glycolipid components. Infect Immun (2016) 84(9):2449–62. doi: 10.1128/IAI.01322-15
43. Nerdal PT, Peters C, Oberg HH, Zlatev H, Lettau M, Quabius ES, et al. Butyrophilin 3a/cd277–dependent activation of human γδ t cells: accessory cell capacity of distinct leukocyte populations. J Immunol (2016) 197(8):3059–68. doi: 10.4049/jimmunol.1600913
44. Harly C, Guillaume Y, Nedellec S, Peigné CM, Mönkkönen H, Mönkkönen J, et al. Key implication of cd277/butyrophilin-3 (btn3a) in cellular stress sensing by a major human γδ t-cell subset. Blood. (2012) 120(11):2269–79. doi: 10.1182/blood-2012-05-430470
45. Sandstrom A, Peigné CM, Léger A, Crooks JE, Konczak F, Gesnel MC, et al. The intracellular b30.2 domain of butyrophilin 3a1 binds phosphoantigens to mediate activation of human vγ9vδ2 t cells. Immunity (2014) 40(4):490–500. dopi: 10.1016/j.immuni.2014.03.003
46. Gu S, Sachleben JR, Boughter CT, Nawrocka WI, Borowska MT, Tarrasch JT, et al. Phosphoantigen-induced conformational change of butyrophilin 3a1 (btn3a1) and its implication on vγ9vδ2 t cell activation. Proc Natl Acad Sci U S A. (2017) 114(35):E7311–20. doi: 10.1073/pnas.1707547114
47. Cano CE, Pasero C, De Gassart A, Kerneur C, Gabriac M, Fullana M, et al. Btn2a1, an immune checkpoint targeting vγ9vδ2 t cell cytotoxicity against malignant cells. Cell Rep (2021) 36(2):109359. doi: 10.1016/j.celrep.2021.109359
48. Rigau M, Ostrouska S, Fulford TS, Johnson DN, Woods K, Ruan Z, et al. Butyrophilin 2a1 is essential for phosphoantigen reactivity by γδ t cells. Science. (2020) 367(6478):eaay5516. doi: 10.1126/science.aay5516
49. Uldrich AP, Rigau M, Godfrey DI. Immune recognition of phosphoantigen-butyrophilin molecular complexes by γδ t cells. Immunol Rev (2020) 298(1):74–83. doi: 10.1111/imr.12923
50. Djaoud Z, Guethlein LA, Horowitz A, Azzi T, Nemat-Gorgani N, Olive D, et al. Two alternate strategies for innate immunity to epstein-barr virus: one using nk cells and the other nk cells and γδ t cells. J Exp Med (2017) 214(6):1827–41. doi: 10.1084/jem.20161017
51. Sacchi A, Rinaldi A, Tumino N, Casetti R, Agrati C, Turchi F, et al. HIV infection of monocytes-derived dendritic cells inhibits vγ9vδ2 t cells functions. PLoS One (2014) 9(10):e111095. doi: 10.1371/journal.pone.0111095
52. Nedellec S, Bonneville M, Scotet E. Human vγ9vδ2 t cells: from signals to functions. Semin Immunol (2010) 22(4):199–206. doi: 10.1016/j.smim.2010.04.004
53. Cimini E, Sacchi A, De Minicis S, Bordoni V, Casetti R, Grassi G, et al. Vδ2 T-cells kill zikv-infected cells by nkg2d-mediated cytotoxicity. Microorganisms. (2019) 7(9):350. doi; 10.3390/microorganisms7090350
54. Champsaur M, Lanier LL. Effect of nkg2d ligand expression on host immune responses. Immunol Rev (2010) 235(1):267–85. doi: 10.1111/j.0105-2896.2010.00893.x
55. Rincon-Orozco B, Kunzmann V, Wrobel P, Kabelitz D, Steinle A, Herrmann T. Activation of vγ9vδ2 t cells by nkg2d. J Immunol (2005) 175(4):2144–51. doi: 10.4049/jimmunol.175.4.2144
56. Das H, Groh V, Kuijl C, Sugita M, Morita CT, Spies T, et al. MICA engagement by human vγ2vδ2 t cells enhances their antigen-dependent effector function. Immunity. (2001) 15(1):83–93. doi: 10.1016/S1074-7613(01)00168-6
57. Vankayalapati R, Garg A, Porgador A, Griffith DE, Klucar P, Safi H, et al. Role of nk cell-activating receptors and their ligands in the lysis of mononuclear phagocytes infected with an intracellular bacterium. J Immunol (2005) 175(7):4611–7. doi: 10.4049/jimmunol.175.7.4611
58. Bessoles S, Ni M, Garcia-Jimenez S, Sanchez F, Lafont V. Role of NKG2D and its ligands in the anti-infectious activity of Vγ9vδ2 t cells against intracellular bacteria. Eur J Immunol (2011) 41(6):1619–28. doi: 10.1002/eji.201041230
59. Devilder MC, Allain S, Dousset C, Bonneville M, Scotet E. Early triggering of exclusive IFN-γ responses of human Vγ9vδ2 t cells by TLR-activated myeloid and plasmacytoid dendritic cells. J Immunol (2009) 183(6):3625–33. doi: 10.4049/jimmunol.0901571
60. Serrano R, Wesch D, Kabelitz D. Activation of human γδ t cells: modulation by toll-like receptor 8 ligands and role of monocytes. Cells. (2020) 9(3):713. doi: 10.3390/cells9030713
61. Gioia C, Agrati C, Casetti R, Cairo C, Borsellino G, Battistini L, et al. Lack of CD27–CD45RA–Vγ9vδ2+ t cell effectors in immunocompromised hosts and during active pulmonary tuberculosis. J Immunol (2002) 168(3):1484–9. doi: 10.4049/jimmunol.168.3.1484
62. Dieli F, Poccia F, Lipp M, Sireci G, Caccamo N, Di Sano C, et al. Differentiation of effector/memory Vδ2 t cells and migratory routes in lymph nodes or inflammatory sites. J Exp Med (2003) 198(3):391–7. doi: 10.1084/jem.20030235
63. El Daker S, Sacchi A, Montesano C, Altieri AM, Galluccio G, Colizzi V, et al. An abnormal phenotype of lung Vγ9vδ2 t cells impairs their responsiveness in tuberculosis patients. Cell Immunol (2013) 282(2):106–12. doi: 10.1016/j.cellimm.2013.05.001
64. La Manna MP, Orlando V, Tamburini B, Badami GD, Dieli F, Caccamo N. Harnessing unconventional t cells for immunotherapy of tuberculosis. Front Immunol (2020) 11. doi: 10.3389/fimmu.2020.02107
65. Spencer CT, Abate G, Sakala IG, Xia M, Truscott SM, Eickhoff CS, et al. Granzyme a produced by γ9δ2 t cells induces human macrophages to inhibit growth of an intracellular pathogen. PLoS Pathog (2013) 9(1):e1003119. doi: 10.1371/journal.ppat.1003119
66. Dieli F, Troye-Blomberg M, Ivanyi J, Fournié JJ, Krensky AM, Bonneville M, et al. Granulysin-dependent killing of intracellular and extracellularMycobacterium tuberculosis by Vγ9/Vδ2 t lymphocytes. J Infect Dis (2001) 184(8):1082–5. doi: 10.1086/323600
67. Shen Y, Zhou D, Qiu L, Lai X, Simon M, Shen L, et al. Adaptive immune response of Vγ2vδ2+ t cells during mycobacterial infections. Science. (2002) 295(5563):2255–8. doi: 10.1126/science.1068819
68. Ryan-Payseur B, Frencher J, Shen L, Chen CY, Huang D, Chen ZW. Multieffector-functional immune responses of HMBPP-specific Vγ2vδ2 T Cells in nonhuman primates inoculated with Listeria monocytogenes Δacta Prfa*. J Immunol (2012) 189(3):1285–93. doi: 10.4049/jimmunol.1200641
69. Khairallah C, Chu TH, Sheridan BS. Tissue adaptations of memory and tissue-resident gamma delta t cells. Front Immunol (2018) 9. doi: 10.3389/fimmu.2018.02636
70. Comeau K, Paradis P, Schiffrin EL. Human and murine memory γδ t cells: evidence for acquired immune memory in bacterial and viral infections and autoimmunity. Cell Immunol (2020) 357:104217. doi: 10.1016/j.cellimm.2020.104217
71. Oliaro J, Dudal S, Liautard J, Andrault JB, Liautard JP, Lafont V. Vγ9vδ2 t cells use a combination of mechanisms to limit the spread of the pathogenic bacteria brucella. J Leukoc Biol (2005) 77(5):652–60. doi: 10.1189/jlb.0704433
72. Ni M, Martire D, Scotet E, Bonneville M, Sanchez F, Lafont V. Full restoration of Brucella-infected dendritic cell functionality through Vγ9vδ2 t helper type 1 crosstalk. PLoS One (2012) 7(8):e43613. doi: 10.1371/journal.pone.0043613
73. Farrington LA, Jagannathan P, McIntyre TI, Vance HM, Bowen K, Boyle MJ, et al. Frequent malaria drives progressive Vδ2 t-cell loss, dysfunction, and CD16 up-regulation during early childhood. J Infect Dis (2016) 213(9):1483–90. doi: 10.1093/infdis/jiv600
74. Hernández-Castañeda MA, Happ K, Cattalani F, Wallimann A, Blanchard M, Fellay I, et al. γδ T cells kill Plasmodium falciparum in a granzyme- and granulysin-dependent mechanism during the late blood stage. J Immunol (2020) 204(7):1798–809. doi: 10.4049/jimmunol.1900725
75. Costa G, Loizon S, Guenot M, Mocan I, Halary F, de Saint-Basile G, et al. Control of Plasmodium palciparum erythrocytic cycle: γδ T cells target the red blood cell-invasive merozoites. Blood. (2011) 118(26):6952–62. doi: 10.1182/blood-2011-08-376111
76. Teirlinck AC, McCall MBB, Roestenberg M, Scholzen A, Woestenenk R, de MQ, et al. Longevity and composition of cellular immune responses following experimentalPlasmodium falciparum malaria infection in humans. PLoS Pathog (2011) 7(12):e1002389. doi: 10.1371/journal.ppat.1002389
77. Zaidi I, Diallo H, Conteh S, Robbins Y, Kolasny J, Orr-Gonzalez S, et al. γδ T cells are required for the induction of sterile immunity during irradiated sporozoite vaccinations. J Immunol (2017) 199(11):3781–8. doi: 10.4049/jimmunol.1700314
78. Qin G, Mao H, Zheng J, Sia SF, Liu Y, Chan PL, et al. Phosphoantigen-expanded human γδ t cells display potent cytotoxicity against monocyte-derived macrophages infected with human and avian influenza viruses. J Infect Dis (2009) 200(6):858–65. doi: 10.1086/605413
79. Li H, Xiang Z, Feng T, Li J, Liu Y, Fan Y, et al. Human vγ9vδ2-t cells efficiently kill influenza virus-infected lung alveolar epithelial cells. Cell Mol Immunol (2013) 10(2):159–64. doi: 10.1038/cmi.2012.70
80. Meuter S, Eberl M, Moser B. Prolonged antigen survival and cytosolic export in cross-presenting human γδ t cells. Proc Natl Acad Sci (2010) 107(19):8730–5. doi: 10.1073/pnas.1002769107
81. Chen Q, Wen K, Lv A, Liu M, Ni K, Xiang Z, et al. Human vγ9vδ2-t cells synergize cd4+ t follicular helper cells to produce influenza virus-specific antibody. Front Immunol (2018) 9. doi: 10.3389/fimmu.2018.00599
82. Sabbaghi A, Miri SM, Keshavarz M, Mahooti M, Zebardast A, Ghaemi A. Role of γδ t cells in controlling viral infections with a focus on influenza virus: implications for designing novel therapeutic approaches. Virol J (2020) 17(1):174. doi: 10.1186/s12985-020-01449-0
83. Hoft DF, Babusis E, Worku S, Spencer CT, Lottenbach K, Truscott SM, et al. Live and inactivated influenza vaccines induce similar humoral responses, but only live vaccines induce diverse t-cell responses in young children. J Infect Dis (2011) 204(6):845–53. doi: 10.1093/infdis/jir436
84. Xiang Z, Liu Y, Zheng J, Liu M, Lv A, Gao Y, et al. Targeted activation of human vγ9vδ2-t cells controls epstein-barr virus-induced b cell lymphoproliferative disease. Cancer Cell (2014) 26(4):565–76. doi: 10.1016/j.ccr.2014.07.026
85. Wu X, Zhang JY, Huang A, Li YY, Zhang S, Wei J, et al. Decreased vδ2 γδ t cells associated with liver damage by regulation of th17 response in patients with chronic hepatitis b. J Infect Dis (2013) 208(8):1294–304. doi: 10.1093/infdis/jit312
86. Yin W, Tong S, Zhang Q, Shao J, Liu Q, Peng H, et al. Functional dichotomy of vδ2 γδ t cells in chronic hepatitis c virus infections: role in cytotoxicity but not for ifn-γ production. Sci Rep (2016) 6(1):26296. doi: 10.1038/srep26296
87. Ghosh A, Mondal RK, Romani S, Bagchi S, Cairo C, Pauza CD, et al. Persistent gamma delta t-cell dysfunction in chronic hcv infection despite direct-acting antiviral therapy induced cure. J Viral Hepat. (2019) 26(9):1105–16. doi: 10.1111/jvh.13121
88. Agrati C, Alonzi T, De Santis R, Castilletti C, Abbate I, Capobianchi MR, et al. Activation of vγ9vδ2 t cells by non-peptidic antigens induces the inhibition of subgenomic hcv replication. Int Immunol (2006) 18(1):11–8. doi: 10.1093/intimm/dxh337
89. He X, Liang H, Hong K, Li H, Peng H, Zhao Y, et al. The potential role of cd16+ vγ2vδ2 t cell-mediated antibody-dependent cell-mediated cytotoxicity in control of hiv type 1 disease. AIDS Res Hum Retroviruses (2013) 29(12):1562–70. doi: 10.1089/aid.2013.0111
90. Poccia F, Battistini L, Cipriani B, Mancino G, Martini F, Gougeon ML, et al. Phosphoantigen-reactive vγ9vδ2 t lymphocytes suppress In Vitro human immunodeficiency virus type 1 replication by cell-released antiviral factors including cc chemokines. J Infect Dis (1999) 180(3):858–61. doi: 10.1086/314925
91. Poccia F, Gioia C, Martini F, Sacchi A, Piacentini P, Tempestilli M, et al. Zoledronic acid and interleukin-2 treatment improves immunocompetence in hiv-infected persons by activating vγ9vδ2 t cells. AIDS. (2009) 23(5):555–65. doi: 10.1097/QAD.0b013e3283244619
92. Chen ZW. Multifunctional immune responses of hmbpp-specific vγ2vδ2 t cells in m. tuberculosis and other infections. Cell Mol Immunol (2013) 10(1):58–64. doi: 10.1038/cmi.2012.46
93. Tu W, Zheng J, Liu Y, Sia SF, Liu M, Qin G, et al. The aminobisphosphonate pamidronate controls influenza pathogenesis by expanding a γδ t cell population in humanized mice. J Exp Med (2011) 208(7):1511–22. doi: 10.1084/jem.20110226
94. Martino A, Casetti R, Sacchi A, Poccia F. Central memory vγ9vδ2 t lymphocytes primed and expanded by bacillus calmette-guérin-infected dendritic cells kill mycobacterial-infected monocytes. J Immunol (2007) 179(5):3057–64. doi: 10.4049/jimmunol.179.5.3057
95. Lafont V, Liautard J, Liautard JP, Favero J. Production of tnf-α by human vγ9vδ2 t cells via Engagement of Fcγriiia, the Low affinity type 3 receptor for the fc portion of igg, expressed upon tcr activation by nonpeptidic antigen. J Immunol (2001) 166(12):7190–9. doi: 10.4049/jimmunol.166.12.7190
96. Couzi L, Pitard V, Sicard X, Garrigue I, Hawchar O, Merville P, et al. Antibody-dependent anti-cytomegalovirus activity of human γδ t cells expressing cd16 (fcγriiia). Blood. (2012) 119(6):1418–27. doi: 10.1182/blood-2011-06-363655
97. Dantzler KW, Jagannathan P. γδ T cells in antimalarial immunity: new insights into their diverse functions in protection and tolerance. Front Immunol (2018) 9:2445. doi: 10.3389/fimmu.2018.02445
98. Wu Y, Wu W, Wong WM, Ward E, Thrasher AJ, Goldblatt D, et al. Human γδ t cells: a lymphoid lineage cell capable of professional phagocytosis. J Immunol (2009) 183(9):5622–9. doi: 10.4049/jimmunol.0901772
99. Barisa M, Kramer AM, Majani Y, Moulding D, Saraiva L, Bajaj-Elliott M, et al. E. coli promotes human vγ9vδ2 t cell transition from cytokine-producing bactericidal effectors to professional phagocytic killers in a tcr-dependent manner. Sci Rep (2017) 7(1):2805. doi: 10.1038/s41598-017-02886-8
100. Wang L, Kamath A, Das H, Li L, Bukowski JF. Antibacterial effect of human vγ2vδ2 t cells in vivo. J Clin Invest (2001) 108(9):1349–57. doi: 10.1172/JCI200113584
101. Deroost K, Langhorne J. Gamma/delta t cells and their role in protection against malaria. Front Immunol (2018) 9. doi: 10.3389/fimmu.2018.02973
102. Jameson JM, Cruz J, Costanzo A, Terajima M, Ennis FA. A role for the mevalonate pathway in the induction of subtype cross-reactive immunity to influenza a virus by human γδ t lymphocytes. Cell Immunol (2010) 264(1):71–7. doi: 10.1016/j.cellimm.2010.04.013
103. Vantourout P, Hayday A. Six-of-the-best: unique contributions of γδ t cells to immunology. Nat Rev Immunol (2013) 13(2):88–100. doi: 10.1038/nri3384
104. Paul S, Lal G. Regulatory and effector functions of gamma-delta (γδ) t cells and their therapeutic potential in adoptive cellular therapy for cancer. Int J Cancer. (2016) 139(5):976–85. doi: 10.1002/ijc.30109
105. Eberl M, Roberts GW, Meuter S, Williams JD, Topley N, Moser B. A rapid crosstalk of human γδ t cells and monocytes drives the acute inflammation in bacterial infections. PLoS Pathog (2009) 5(2):e1000308. doi: 10.1371/journal.ppat.1000308
106. Cooper AJR, Lalor SJ, McLoughlin RM. Activation of human vδ2+ γδ t cells byStaphylococcus Aureus Promotes Enhanced Anti-Staphylococcal Adaptive Immunity. J Immunol (2020) 205(4):1039–49. doi: 10.4049/jimmunol.2000143
107. Gorvel L, Textoris J, Banchereau R, Amara AB, Tantibhedhyangkul W, von BK, et al. Intracellular bacteria interfere with dendritic cell functions: role of the type i interferon pathway. PLoS One (2014) 9(6):e99420. doi: 10.1371/journal.pone.0099420
108. Meraviglia S, Caccamo N, Salerno A, Sireci G, Dieli F. Partial and ineffective activation of vγ9vδ2 t cells byMycobacterium tuberculosis-Infected Dendritic Cells. J Immunol (2010) 185(3):1770–6. doi: 10.4049/jimmunol.1000966
109. Poggi A, Catellani S, Musso A, Zocchi MR. Gamma delta t lymphocytes producing ifnγ and il-17 in response toCandida albicans or mycobacterial antigens: possible implications for acute and chronic inflammation. Curr Med Chem (2009) 16(35):4743–9. doi: 10.2174/092986709789878238
110. Davey MS, Morgan MP, Liuzzi AR, Tyler CJ, Khan MWA, Szakmany T, et al. Microbe-specific unconventional t cells induce human neutrophil differentiation into antigen cross-presenting cells. J Immunol (2014) 193(7):3704–16. doi: 10.4049/jimmunol.1401018
111. Brandes M, Willimann K, Moser B. Professional antigen-presentation function by human γδ t cells. Science. (2005) 309(5732):264–8. doi: 10.1126/science.1110267
112. Howard J, Loizon S, Tyler CJ, Duluc D, Moser B, Mechain M, et al. The antigen-presenting potential of vγ9vδ2 t cells duringPlasmodium falciparum blood-stage infection. J Infect Dis (2017) 215(10):1569–79. doi: 10.1093/infdis/jix149
113. Zhu Y, Wang H, Xu Y, Hu Y, Chen H, Cui L, et al. Human γδ t cells augment antigen presentation inlisteria monocytogenes infection. Mol Med (2016) 22(1):737–46. doi: 10.2119/molmed.2015.00214
114. Gong G, Shao L, Wang Y, Chen CY, Huang D, Yao S, et al. Phosphoantigen-activated vγ2vδ2 t cells antagonize il-2-induced cd4+cd25+foxp3+ t regulatory cells in mycobacterial infection. Blood. (2009) 113(4):837–45. doi: 10.1182/blood-2008-06-162792
115. Caccamo N, Battistini L, Bonneville M, Poccia F, Fournié JJ, Meraviglia S, et al. CXCR5 Identifies a subset of vγ9vδ2 t cells which secrete il-4 and il-10 and help b cells for antibody production. J Immunol (2006) 177(8):5290–5. doi: 10.4049/jimmunol.177.8.5290
116. Rampoldi F, Ullrich L, Prinz I. Revisiting the interaction of γδ t-cells and b. Cells. (2020) 9(3):743. doi: 10.3390/cells9030743
117. Qin G, Liu Y, Zheng J, Xiang Z, Ng IHY, Malik Peiris JS, et al. Phenotypic and functional characterization of human γδ t-cell subsets in response to influenza a viruses. J Infect Dis (2012) 205(11):1646–53. doi: 10.1093/infdis/jis253
118. Workalemahu G, Wang H, Puan KJ, Nada MH, Kuzuyama T, Jones BD, et al. Metabolic engineering of salmonella vaccine bacteria to boost human vγ2vδ2 t cell immunity. J Immunol (2014) 193(2):708–21. doi: 10.4049/jimmunol.1302746
119. Blumerman SL, Herzig CTA, Baldwin CL. WC1+ γδ T cell memory population is induced by killed bacterial vaccine. Eur J Immunol (2007) 37(5):1204–16. doi: 10.1002/eji.200636216
120. Buza J, Kiros T, Zerihun A, Abraham I, Ameni G. Vaccination of calves with mycobacteria bovis bacilli calmete guerin (bcg) induced rapid increase in the proportion of peripheral blood γδ t cells. Vet Immunol Immunopathol (2009) 130(3):251–5. doi: 10.1016/j.vetimm.2008.12.021
121. Price S, Davies M, Villarreal-Ramos B, Hope J. Differential distribution of wc1+ γδ tcr+ t lymphocyte subsets within lymphoid tissues of the head and respiratory tract and effects of intranasal m. bovis bcg vaccination. Vet Immunol Immunopathol (2010) 136(1):133–7. doi: 10.1016/j.vetimm.2010.02.010
122. Lee J, Choi K, Olin MR, Cho SN, Molitor TW. γδ T cells in immunity induced by mycobacterium bovis bacillus calmette-guérin vaccination. Infect Immun (2004) 72(3):1504–11. doi: 10.1128/IAI.72.3.1504-1511.2004
123. Baldwin CL, Telfer JC. The bovine model for elucidating the role of γδ t cells in controlling infectious diseases of importance to cattle and humans. Mol Immunol (2015) 66(1):35–47. doi: 10.1016/j.molimm.2014.10.024
124. Hoft DF, Brown RM, Roodman ST. Bacille calmette-guérin vaccination enhances human γδ t cell responsiveness to mycobacteria suggestive of a memory-like phenotype. J Immunol (1998) 161(2):1045–54.
125. Yan L, Cui H, Xiao H, Zhang Q. Anergic pulmonary tuberculosis is associated with contraction of the vδ2+t cell population, apoptosis and enhanced inhibitory cytokine production. PLoS One (2013) 8(8):e71245. doi: 10.1371/journal.pone.0071245
126. Sacchi A, Tumino N, Sabatini A, Cimini E, Casetti R, Bordoni V, et al. Myeloid-derived suppressor cells specifically suppress ifn-γ production and antitumor cytotoxic activity of vδ2 t cells. Front Immunol (2018) 9. doi: 10.3389/fimmu.2018.01271
127. Yazdanifar M, Barbarito G, Bertaina A, Airoldi I. γδ T cells: the ideal tool for cancer immunotherapy. Cells. (2020) 9(5):1305. doi: 10.3390/cells9051305
128. Agrati C, Castilletti C, Cimini E, Romanelli A, Lapa D, Quartu S, et al. Antiviral activity of human vδ2 t-cells against wnv includes both cytolytic and non-cytolytic mechanisms. New Microbiol (2016) 39(2):139–42.
129. Daguzan C, Moulin M, Kulyk-Barbier H, Davrinche C, Peyrottes S, Champagne E. Aminobisphosphonates synergize with human cytomegalovirus to activate the antiviral activity of vγ9vδ2 cells. J Immunol (2016) 196(5):2219–29. doi: 10.4049/jimmunol.1501661
130. Poonia B, Pauza CD. Gamma delta t cells from hiv+ donors can be expanded in vitro by zoledronate/interleukin-2 to become cytotoxic effectors for antibody-dependent cellular cytotoxicity. Cytotherapy. (2012) 14(2):173–81. doi: 10.3109/14653249.2011.623693
131. Chen CY, Yao S, Huang D, Wei H, Sicard H, Zeng G, et al. Phosphoantigen/il-2 expansion and differentiation of vγ2vδ2 t cells increase resistance to tuberculosis in nonhuman primates. PLoS Pathog (2013) 9(8):e1003501. doi: 10.1371/journal.ppat.1003501
132. Yang R, Yao L, Shen L, Sha W, Modlin RL, Shen H, et al. IL-12 Expands and differentiates human vγ2vδ2 t effector cells producing antimicrobial cytokines and inhibiting intracellular mycobacterial growth. Front Immunol (2019) 0. doi: 10.3389/fimmu.2019.00913
133. Murday AS, Chaudhry S, Pauza CD. Interleukin-18 activates vγ9vδ2+ t cells from hiv-positive individuals: recovering the response to phosphoantigen. Immunology. (2017) 151(4):385–94. doi: 10.1111/imm.12735
134. Pei Y, Wen K, Xiang Z, Huang C, Wang X, Mu X, et al. CD137 Costimulation enhances the antiviral activity of vγ9vδ2-t cells against influenza virus. Signal Transduct Target Ther (2020) 5(1):1–10. doi: 10.1038/s41392-020-0174-2
135. Zou C, Zhao P, Xiao Z, Han X, Fu F, Fu L. γδ T cells in cancer immunotherapy. Oncotarget. (2016) 8(5):8900–9. doi; 10.18632/oncotarget.13051
136. Singh KS, Sharma R, Reddy PAN, Vonteddu P, Good M, Sundarrajan A, et al. Isph inhibitors kill gram-negative bacteria and mobilize immune clearance. Nature. (2021) 589(7843):597–602. doi: 10.1038/s41586-020-03074-x
137. de Weerdt I, Lameris R, Ruben JM, de Boer R, Kloosterman J, King LA, et al. A bispecific single-domain antibody boosts autologous vγ9vδ2-t cell responses toward cd1d in chronic lymphocytic leukemia. Clin Cancer Res (2021) 27(6):1744–55. doi: 10.1158/1078-0432.CCR-20-4576
138. Palakodeti A, Sandstrom A, Sundaresan L, Harly C, Nedellec S, Olive D, et al. The molecular basis for modulation of human vγ9vδ2 t cell responses by cd277/butyrophilin-3 (btn3a)-specific antibodies. J Biol Chem (2012) 287(39):32780–90. doi: 10.1074/jbc.M112.384354
139. Benyamine A, Loncle C, Foucher E, Blazquez JL, Castanier C, Chrétien AS, et al. BTN3A is a prognosis marker and a promising target for vγ9vδ2 t cells based-immunotherapy in pancreatic ductal adenocarcinoma(PDAC). OncoImmunology. (2018) 7(1):e1372080. doi: 10.1080/2162402X.2017.1372080
140. De Gassart A, Le KS, Brune P, Agaugué S, Sims J, Goubard A, et al. Development of ict01, a first-in-class, anti-btn3a antibody for activating vγ9vδ2 t cell-mediated antitumor immune response. Sci Transl Med (2021) 13(616):eabj0835. doi: 10.1126/scitranslmed.abj0835
141. Marabelle A, Jungels C, De Bono J, Vey N, Wermke M, Garralda E, et al. Abstract ct034: activation of the antitumor immune response of gamma9delta2 t cells in patients with solid or hematologic malignancies with ict01, a first-in-class, monoclonal antibody targeting butyrophilin 3a: the eviction study. Cancer Res (2021) 81(13_Supplement):CT034. doi: 10.1158/1538-7445.AM2021-CT034
142. Marabelle A, Jungels C, Bono JD, Vey N, Wermke M, Garralda E, et al. 316 Eviction study: preliminary results in solid tumor patients with ict01, a first-in-class, gamma9 delta2 t cell activating antibody targeting butyrophilin-3a. J Immunother Cancer. (2020) 8(Suppl 3):A194. doi: 10.1136/jitc-2020-SITC2020.0316
Keywords: Vγ9Vδ2 T cell, antimicrobial immunity, infectious diseases, butyrophilin, therapeutic approaches
Citation: Gay L, Mezouar S, Cano C, Frohna P, Madakamutil L, Mège J-L and Olive D (2022) Role of Vγ9vδ2 T lymphocytes in infectious diseases. Front. Immunol. 13:928441. doi: 10.3389/fimmu.2022.928441
Received: 25 April 2022; Accepted: 27 June 2022;
Published: 18 July 2022.
Edited by:
Xiaoli Wu, Tianjin University, ChinaReviewed by:
Massimo Massaia, University of Turin, ItalyOlga Vinogradova, University of Connecticut, United States
C. David Pauza, Viriom, Inc., United States
Copyright © 2022 Gay, Mezouar, Cano, Frohna, Madakamutil, Mège and Olive. This is an open-access article distributed under the terms of the Creative Commons Attribution License (CC BY). The use, distribution or reproduction in other forums is permitted, provided the original author(s) and the copyright owner(s) are credited and that the original publication in this journal is cited, in accordance with accepted academic practice. No use, distribution or reproduction is permitted which does not comply with these terms.
*Correspondence: Daniel Olive, daniel.olive@inserm.fr