- 1Division of Rheumatology, Department of Medicine, University of Virginia, Charlottesville, VA, United States
- 2Center for Immunity, Inflammation and Regenerative Medicine, Division of Nephrology, Department of Medicine, University of Virginia, Charlottesville, VA, United States
- 3Benaroya Research Institute, Virginia Mason, Seattle, WA, United States
- 4Department of Psychiatry and Neurobehavioral Sciences, University of Virginia, Charlottesville, VA, United States
HLA-DR3 (DR3) is one of the dominant HLA-DR alleles associated with systemic lupus erythematosus (SLE) susceptibility. Our previous studies showed multiple intramolecular DR3 restricted T cell epitopes in the Smith D (SmD) protein, from which we generated a non-homologous, bacterial epitope mimics library. From this library we identified ABC247-261 Mimic as one new DR3 restricted bacterial T cell epitope from the ABC transporter ATP-binding protein in Clostridium tetani. It activated and induced autoreactive SmD66-80-specific T cells and induced autoantibodies to lupus-related autoantigens in vivo. Compared to healthy donors, SLE patients have a greater percentage of cross-reactive T cells to ABC247-261 Mimic and SmD66-80. In addition, we analyzed the ability of single DR3 restricted Tetanus toxoid (TT) T cell epitopes to induce autoimmune T cells. We found that the immunodominant TT epitope TT826-845 stimulated SmD66-80 reactive T cells but failed to induce persistent anti-SmD autoantibodies compared to the ABC247-261 Mimic. Thus, exposure to the ABC247-261 Mimic epitope may contribute to autoimmunity in susceptible DR3 individuals.
Introduction
Systemic lupus erythematosus (SLE) is a prototypical, systemic autoimmune disease that occurs when the body’s immune system begins to attack its own healthy tissues (loss of self-tolerance) (1). Autoantibodies are one of the clinical markers of lupus and can be detected prior to symptoms and diagnosis (2). There is no cure for SLE and the exact mechanisms underlying disease development have yet to be elucidated.
The HLA-DR region is the dominant lupus susceptibility locus, particularly the HLA-DR3 (DR3) allele. This highlights the importance of T cells in lupus because the genes within the HLA-DR locus are important for antigen presentation to T cells (3–5). It is known that environmental antigens that share T cell epitopes with self-antigens can initiate adaptive immune responses that continue to be propagated by self-antigens, leading to disease in genetically susceptible individuals (6). Our laboratory has shown that in DR3 transgenic mice (DR3 mice), T cells specific for SLE-related autoantigens responded to bacterial mimics of self-peptides. For example, DR3 restricted T cells specific for SmD (7), and Ro60 (8) responded to several epitopes from environmental bacteria. We also identified numerous potential bacterial mimics that were able to induce the production of SLE-related autoantibodies in DR3 mice (9). However, the existence of cross-reactive T cells able to recognize self and non-homologous bacterial epitopes in humans remains to be demonstrated. Furthermore, if these cross-reactive T cells are present in humans, it is of interest whether they are activated by bacterial epitopes to induce lupus-related autoantibodies.
In this study, we examined the epitopes from SmD and the bacterium Clostridium tetani for the presence of shared T cell epitopes. To do this, we utilized MHC class II DR3 tetramers carrying different epitopes. Our results show that one bacterial epitope from the ABC transporter ATP-binding protein (ABC247-261 Mimic) activated SmD66-80 reactive T cells in both DR3 mice and humans. The percentages of cross-reactive T cells to ABC247-261 Mimic and SmD66-80 were significantly increased in DR3 positive SLE patients (DR3 SLE patients). Additionally, ABC247-261 Mimic induced persistent anti-SmD autoantibodies in DR3 mice. Our results suggest that environmental mimics of self-epitopes may be important for activating cross-reactive T cells, thus potentially leading to the initiation of autoimmune responses and SLE development in susceptible individuals.
Materials and methods
Human subjects
Patients from the University of Virginia Hospital fulfilling the American College of Rheumatology (ACR) criteria for systemic lupus erythematosus (SLE) (10) and healthy donors provided blood samples for HLA-DR typing. Patients’ and donors’ samples were archived and deidentified. Only HLA-DR3 (DR3) individuals were selected for this study. The three selected patients are two African American females and one Caucasian female over the age of 18. The three patients were all ANA and anti-SmD positive with leukopenia, oral mucosal and joint involvement. The patients were also anti-Tetanus toxoid (TT) positive [Mean, 0.81 ± 0.20] at 1 to 800 dilution. Seven of eight healthy donors were anti-TT positive [Mean, 0.96 ± 0.65] at 1 to 800 dilution (p=0.57).
Isolation of human peripheral blood mononuclear cells from whole blood
Peripheral blood mononuclear cells (PBMCs) were isolated by standard Ficoll-Paque PLUS (GE Healthcare, Chicago, IL) gradient centrifugation, resuspended in freezing media (10% DMSO in FCS), and stored in liquid nitrogen.
HLA-DR typing
DNA was extracted from blood clots and HLA-DR typing was done by the PCR method described by Bunce (11).
Stimulation and DR3 tetramer staining of mouse spleen cells and human PBMCs
This procedure was performed using modified versions of protocols described previously (12, 13). Briefly, human PBMCs were stimulated with 17.7 μg/ml of TT lysate (MassBiologics, University of Massachusetts Medical School, MA) for 5-7 days. Spleen cells from DR3 mice immunized with protein or peptide were harvested 10-14 days post immunization. Human PBMCs were stained with 1 μg of DR3 tetramer for 3-4 hours at 37°C; and mouse spleen cells were stained for 2 hours. After tetramer staining, the cells were washed in PBS containing 2% FCS (FACS buffer) and surface markers were detected by anti-CD4-FITC (Biolegend, San Diego, CA) and anti-CD3-APCeFluor™780 (Invitrogen, Waltham, MA) for human cells and anti-CD4-FITC and anti-CD3-APC/Fire™750 (Biolegend, San Diego, CA) for mouse cells on ice for 30 minutes after Fc blocking. Cells were analyzed by the BD LSR Fortessa™ Cell Analyzer (BD, Franklin Lakes, NJ). When determining the percentage of single epitope reactive T cells from dual tetramer staining as seen in Figure 6, for SmD66-80, the percentage of single SmD66-80 tetramer positive T cells was the sum of cells in Q1 and Q2; for the ABC247-261 Mimic and TT peptides, the percentage of single Clostridium tetani epitope tetramer positive T cells was the sum of cells in Q2 and Q3. Cross-reactive T cells between SmD66-80 and Clostridium tetani epitopes were detected in Q2.
Peptides and recombinant proteins
DR3 restricted TT peptides TT92-111, TT279-296, TT826-845, TT986-1005, TT1058-1077, TT1114-1133, and TT1272-1284, as well as the bacterial ABC247-261 Mimic peptide and peptides TT31-45 and TT506-525 were HPLC purified with ≥95% purity and obtained from GenScript (Piscataway, New Jersey). The overlapping, 30 amino acid length SmD peptides spanning the entire protein (119 amino acids), as well as 15mers SmD1-15 and SmD66-80 were also HPLC purified with ≥95% purity and obtained from ChinaPeptides (Shanghai, China). The recombinant SLE-related autoantigens used were described previously (14, 15). TT lysate was obtained from MassBiologics at the University of Massachusetts Medical School (UMMS). It was prepared from inactivated bacterial cultures followed by filtration and does not contain adjuvant.
Mouse strains and immunizations
The HLA-DRB1*0301, HLA-DRA1*0101 (DR3.A0/0E0/0) transgenic mice lacking endogenous class II molecules have been previously described (16). The HLA class II transgenic mice used in the present work were backcrossed to B10 mice for 10 generations, and thus carry B10 genetic backgrounds. All mice used in this study were bred and housed in specific pathogen-free conditions at the University of Virginia vivarium. Mouse experiments were approved by the Animal Care and Use Committees at the University of Virginia. For experiments analyzing T cell IFN-γ responses, two mice were immunized in the left footpad and base of tail with 100 μg of peptide or protein in IFA. For experiments analyzing antibody production, 4-5 mice were immunized in the left footpad and base of tail with 100 μg of peptide in CFA. On days 14 and 28 post immunization, mice received injections of 50 μg of peptide in IFA intraperitoneally. Sera were collected monthly and stored at -20°C.
T cell peptide-specific IFN-γ responses
For determining T cell specific IFN-γ responses to SmD66-80, ABC247-261 Mimic, and other TT peptides, two mice were immunized with each single peptide in the footpad and base of the tail, respectively and 10-14 days later spleen cells were pooled and analyzed. Cells were plated in 96-well round bottom plates at 2 x 106 cells per well with 20 µg/ml peptide at 37°C in 5% CO2 for 2 days. Culture supernatants were used to determine IFN-γ concentrations by ELISA (BD, Franklin Lakes, NJ), following the manufacturer’s instructions.
T-T hybridoma production and reactivity
SmD66-80 reactive T-T hybridomas were produced from the fusion of lymph node cells from DR3.A0/0E0/0 mice immunized with SmD in IFA with BW5147TCR-/- as described by Kruisbeek (17). For each fusion, cells were suspended in ten 96-well plates. Hybrid cells surviving hypoxanthine/aminopterin/thymidine (HAT) selection were expanded in 24-well plates. 105 T-T hybridoma cells were incubated with 2.5×105 syngeneic splenic cells in the presence of SmD66-80 for 14 hours. Interleukin (IL)-2 in culture supernatants was estimated by ELISA (BD, Franklin Lakes, NJ) following manufacturer’s instructions. Reactive hybridomas were cloned by limiting dilution. To determine the ability of the T-T hybridoma to respond to TT, hybridoma cells were incubated with irradiated syngeneic spleen cells in the presence of TT and IL-2 in culture supernatants was measured by ELISA.
Enzyme-linked immunosorbent assay
The experiments were performed using a modified version of the protocol described previously (14). Immulon 4HBX microtiter plates (ThermoFisher Scientific, Waltham, MA) were coated with 2 µg/ml of peptides overnight at 4°C and blocked with 1x PBS containing 0.1% Tween 20 with 3% BSA (blocking buffer) for 1 hour at room temperature. Mouse sera diluted 1 to 100 in blocking buffer were added to the plate and incubated for 2 hours. Secondary anti-mouse IgG in blocking buffer was added and incubated for 1hour. Then the substrate o-phenylenediamine (0.05%) (Sigma-Aldrich, St. Louis, MO) and 0.06% hydrogen peroxide in citrate-phosphate buffer, pH 5.0 (100 µl/well) was applied. Reaction was stopped after 15 minutes by the addition of 50 µl/well of 2.5 N sulfuric acid. Absorbance was read at 490 nm with a reference wavelength of 560 nm.
Antibody absorption
Peptide SmD66-80 was coupled to CNBr-activated Sepharose 4B beads (GE Healthcare, Chicago, IL) following the manufacturer’s instructions. The beads were incubated overnight with PBS containing 3% BSA at 4°C. Sera were diluted in 1x PBS with 0.1% Tween 20 (PBST) containing 3% BSA and incubated with the beads for 2 hours at room temperature. The absorbed sera were assayed for anti-SmD66-80 activity using ELISA.
Statistical analysis
Significance was determined using the two tailed Student’s t-test.
Results
TT lysate activates SmD66-80 cross-reactive T cells
We previously identified SmD66-80 as one of seven DR3 restricted SmD T cell epitopes using T-T hybridomas in our DR3 transgenic mouse model. Many of the T-T hybridomas have cross-reactivity to SmD66-80 and bacterial antigens (9). Experiments were designed to demonstrate cross-reactivity to SmD66-80 and bacterial antigens on a single T cell by the HLA-DR3 tetramer technology. First to confirm the specificity of the SmD66-80 DR3 tetramer, DR3 mice were immunized with SmD66-80 and SmD66-80 reactive T cells were detected using the SmD66-80 DR3 tetramer. DR3 mice immunized with SmD91-119 were used as controls. SmD91-119 lacks the DR3 restricted T cell epitopes. 6.23% SmD66-80 reactive CD4+ T cells were identified in the spleens of SmD66-80 immunized DR3 mice. In comparison, 1.24% CD4+ T cells were seen in the spleens of SmD91-119 immunized DR3 mice. The control DR3 tetramer loaded with non SmD peptides showed 0.27% and 0.11% staining in immunized mice, respectively (Supplementary Figure 1). These results showed the SmD66-80 DR3 tetramer specifically bound SmD66-80 reactive CD4+ T cells. After establishing the specificity of the SmD66-80 DR3 tetramer, it was used to stain PBMCs from one SLE patient. The PBMCs were stimulated with the autoantigen peptide SmD66-80, and TT lysate (a strong T cell dependent antigen) was used as a negative control. We showed in one DR3 positive SLE patient that 0.67% of the CD4+ T cells were stained with the SmD66-80 DR3 tetramer. Surprisingly, the TT lysate stimulation increased the SmD66-80 reactive T cells to 4.59% (Figure 1A). These results suggested that there are T cell epitopes in the TT lysate that are cross-reactive with SmD66-80. To seek further support of this thesis, we immunized humanized DR3 mice with SmD66-80 or TT, respectively. We found that the peptides from either SmD or TT were able to stimulate the splenic T cells of the other (Figure 1B). This showed that TT immunization activates SmD66-80 reactive T cells and vice versa. Furthermore, a DR3 restricted SmD66-80 reactive T cell hybridoma generated from SmD immunization of DR3 mice was activated by TT (Figure 1C). These results suggested one or more DR3 restricted T cell epitopes in TT lysate activated and expanded autoimmune SmD66-80 reactive CD4+ T cells.
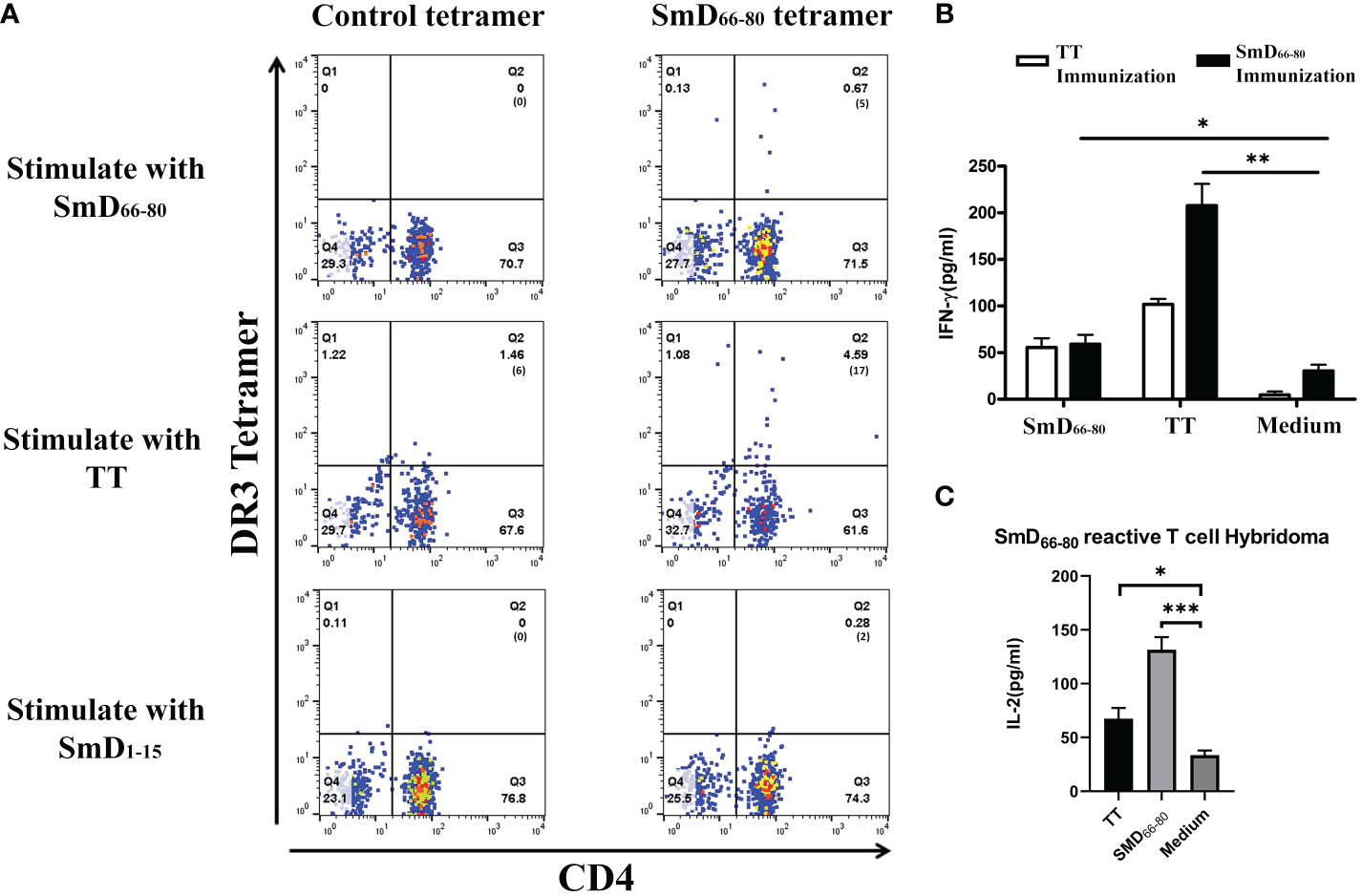
Figure 1 TT lysate activates autoimmune SmD66-80 reactive T cells. (A) The PBMCs from an SLE patient were stained with the SmD66-80 DR3 tetramer after stimulation with SmD66-80 or TT lysate. SmD1-15 was used as a negative control. The number of tetramer positive cells is shown in parentheses. (B) Humanized DR3 mice were immunized with TT (n=2) or SmD66-80 (n=2), respectively. The splenic T cell responses were recalled using SmD66-80 or TT. Supernatant IFN-γ was assayed for T cell activation. (C) T cell hybridoma F140 was previously shown to react to SmD66-80 (9). Its cross-reactivity to TT was assayed by stimulating it with TT. Supernatant IL-2 was assayed for hybridoma activation. *p < 0.05, **p < 0.01, ***p < 0.001 by Student’s two-tailed t-test. TT, Tetanus toxoid.
An epitope from our bacterial mimicry library shows both T and B cell cross-reactivity to the SmD66-80 epitope
As reported previously (9), a software program was developed to analyze bacterial protein sequences in order to create a library of mimic peptides that may stimulate SmD reactive T cells. From this library, we identified one epitope (the ABC247-261 Mimic) from the bacterium Clostridium tetani, to have cross-reactivity with SmD. It is of note that this bacterium produces TT. We showed this epitope could activate T cells from DR3 mice primed by SmD66-80 (Figure 2A). At the B cell level, the DR3 mice immunized with SmD66-80 produced anti-SmD antibodies with cross-reactivity to ABC247-261 Mimic. We showed anti-ABC247-261 Mimic antibodies could be generated by SmD66-80 immunization of DR3 mice and could be absorbed by SmD66-80 coated beads (Figure 2B). These results suggest at least one bacterial epitope from our mimicry library has both T and B cell cross-reactivity to the SmD66-80 autoimmune response.
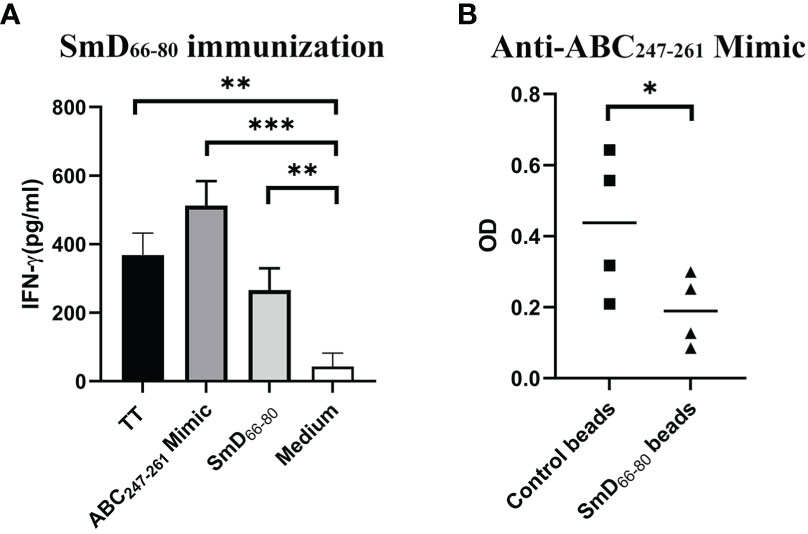
Figure 2 The ABC247-261 Mimic, one epitope from the bacterium Clostridium tetani, cross-reacts with SmD66-80 at both the T and B cell levels. (A) Splenic cells from two SmD66-80 immunized DR3 mice were stimulated with the ABC247-261 Mimic and SmD66-80 and TT lysate were used as positive controls. IFN-γ was assayed for T cell activation. (B) Sera from SmD66-80 immunized DR3 mice (n=4) were probed for ABC247-261 Mimic antibodies. SmD66-80 coated beads were used to absorb the cross-reactive antibodies between SmD66-80 and the ABC247-261 Mimic. *p < 0.05, **p < 0.01, ***p < 0.001 by Student’s two-tailed t-test. TT, Tetanus toxoid.
ABC247-261 Mimic and TT T cell epitopes induce cross-reactive T cells against SmD and TT
Seven DR3 restricted TT peptides have been described in the literatures (18–20). They are TT92-111, TT279-296, TT826-845, TT986-1005, TT1058-1077, TT1114-1133, and TT1272-1284 (Table 1). DR3 mice were immunized with individual TT peptides and ABC247-261 Mimic in incomplete Freund’s adjuvant (IFA). As a negative control, IFA was used (Figure 3I). Splenic T cells were cultured with the ABC247-261 Mimic, TT peptides and SmD peptides as recall antigens. The supernatants were analyzed for IFN-γ (Figures 3A-H). The ABC247-261 Mimic, TT279-296, TT826-845, TT986-1005, TT1058-1077, TT1114-1133, and TT1272-1284 epitopes efficiently activated T cells ex vivo. Among these peptides, the ABC247-261 Mimic is the most potent cross-reactive T cell epitope in that it cross-reacts with all tested TT epitopes except that of TT92-111 (Figures 3A-H). The chosen TT epitopes exhibit intramolecular cross-reactivity with one another and other TT epitopes, making TT a strong immunogen (9). Also, T cells from TT peptide-immunized DR3 mice showed modest T cell IFN-γ responses to SmD peptides (Figures 3B-H). Immunization with the ABC247-261 Mimic, TT826-845, and TT1058-1077 induced cross-reactive T cells to SmD46-75 or SmD61-90 which include the SmD66-80 epitope (Figures 3A-C). These three epitopes were chosen for further investigation based on their T cell cross-reactivity with SmD66-80.
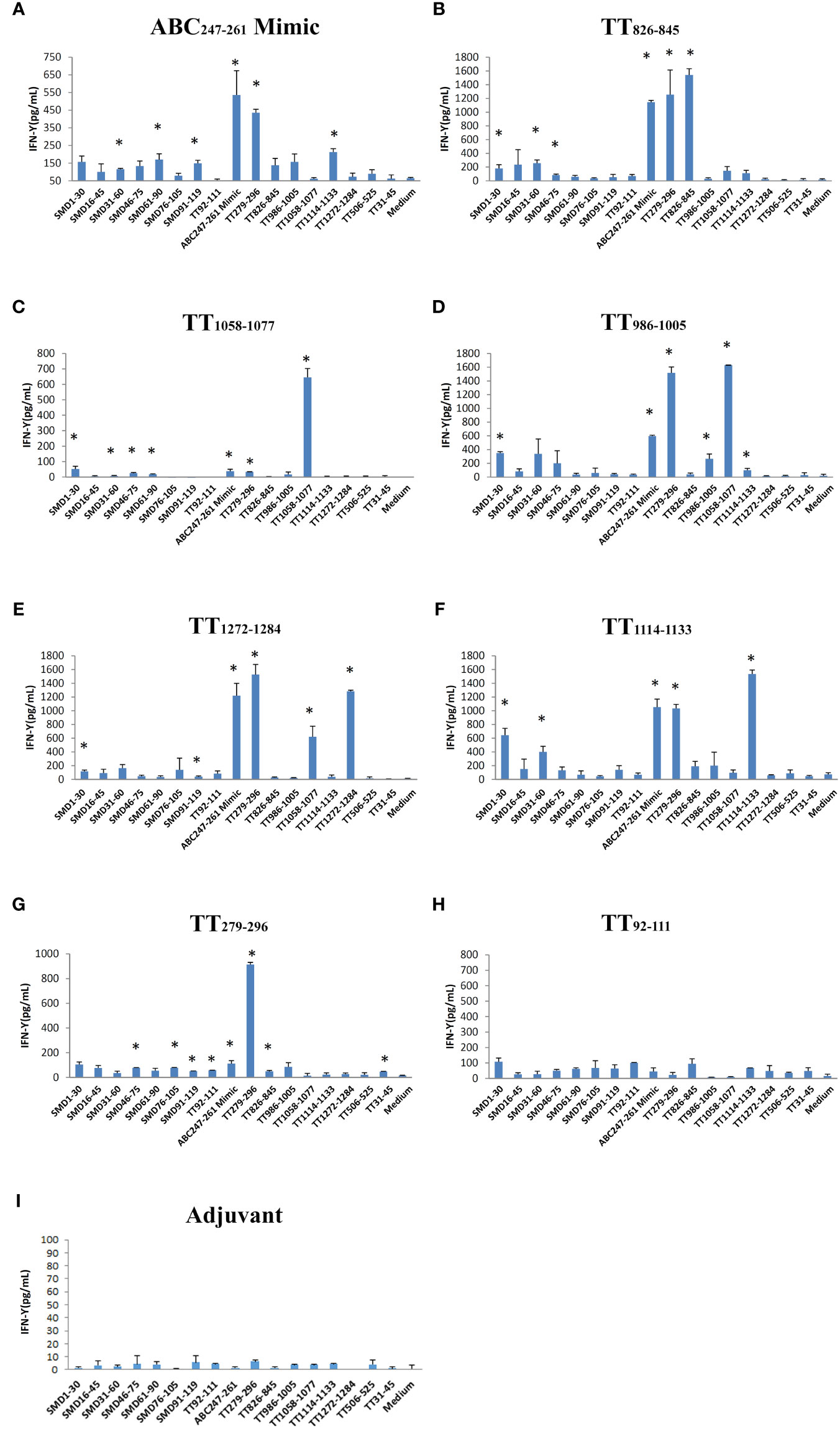
Figure 3 The ABC247-261 Mimic and single DR3 restricted TT epitopes induce cross-reactive T cells to SmD and TT epitopes. Peptides (A) the ABC247-261 Mimic, (B) TT826-845, (C) TT1058-1077, (D) TT986-1005, (E) TT1272-1284, (F) TT1114-1133, (G) TT279-296, and (H) TT92-111 were used to immunize DR3 mice. DR3 mice immunized with (I) adjuvant only were used as controls. Splenic cells from two immunized mice were stimulated with a panel of both SmD and TT epitope peptides. Supernatant IFN-γ was assayed for T cell activation. This experiment was replicated twice. * means the stimulating epitope peptide significantly increased the IFN-γ present in the supernatant compared to the medium only as indicated by Student’s two-tailed t-tests.
The SmD66-80 reactive CD4+ T cells induced after single epitope immunizations were assayed by SmD66-80 DR3 tetramer staining (Figure 4). The ABC247-261 Mimic, TT826-845, and TT1058-1077 induced SmD66-80 reactive CD4+ T cells. In the case of TT1058-1077, T cells from spleens of immunized mice showed staining by the control tetramer. We have no explanation for this staining. In this experiment, TT92-111 and TT279-296 induce SmD66-80 reactive T cells. This result differed from that in Figure 3, where TT92-111 and TT279-296 did not respond to SmD61-90 with IFN-γ secretion. This difference in results could be explained if the cross-reactive T cells induced by TT92-111 and TT279-296 are not of the Th1 subtype or do not initiate a Th1 immune response. The remaining three peptides, TT986-1005, TT1114-1133, and TT1272-1284 stimulation did not induce cross-reactive T cells to SmD66-80.
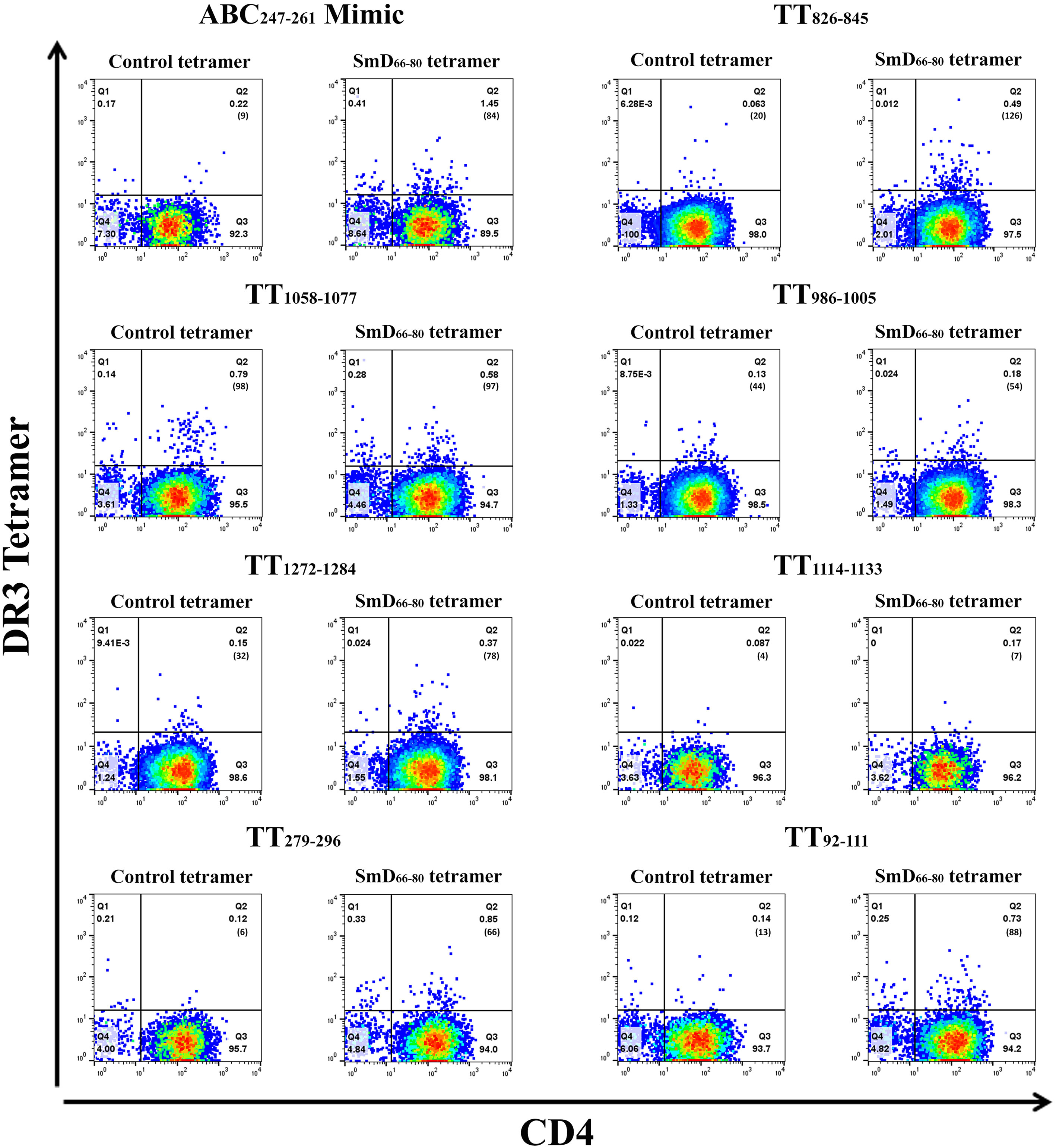
Figure 4 TT epitopes increase SmD66-80 reactive T cells in vivo. Peptides ABC247-261 Mimic, TT826-845, TT1058-1077, TT986-1005, TT1272-1284, TT1114-1133, TT279-296, and TT92-111 were used to immunize DR3 mice. At day 10 post immunization, the splenic CD4+ T cells from two or three mice were pooled together and stained with an SmD66-80 DR3 tetramer. The number of tetramer positive cells is shown in parentheses.
To identify the cross-reactive T cells in vivo due to TT exposure, we immunized DR3 mice with TT and detected the cross-reactive T cells using two DR3 tetramers with different fluorochromes. One was the SmD66-80 DR3 tetramer and the others were the ABC247-261 Mimic, TT826-845, and TT1058-1077 DR3 tetramers (Supplementary Figure 2). DR3 tetramer staining revealed 0.57% ABC247-261 Mimic cross-reactive T cells to SmD66-80 compared to 0.29% and 0.49% with the TT826-845 and TT1058-1077 peptides, respectively. The results showed cross-reactive T cells to SmD66-80 and the ABC247-261 Mimic are generated in DR3 mice after TT immunization as well as those cross-reactive to SmD66-80 and TT826-845 and TT1058-1077.
Immunization of DR3 mice with the ABC247-261 Mimic generates anti-SmD autoantibodies and leads to B cell epitope spreading to other lupus-related autoantigens
The ABC247-261 Mimic induced autoantibodies to SmD and other lupus-related autoantigens 42 days post immunization (Figure 5A). Compared to the ABC247-261 Mimic, peptides TT826-845 and TT1058-1077 failed to induce anti-SmD autoantibodies and induced much less B cell epitope spreading to other lupus-related autoantigens during the same time period (Figures 5D, G). Autoantibody titers remained detectable 58 days after DR3 mice immunizations with the ABC247-261 Mimic, the TT826-845 and the TT1058-1077 peptides, respectively (Figures 5B, E, H). The autoantibodies from the ABC247-261 Mimic immunizations started to decrease, while some autoantibodies from the TT826-845 and TT1058-1077 immunizations increased 90 days post immunization (Figures 5C, F, I). The adjuvant control showed no autoantibody production at any time point (Figures 5J–L).
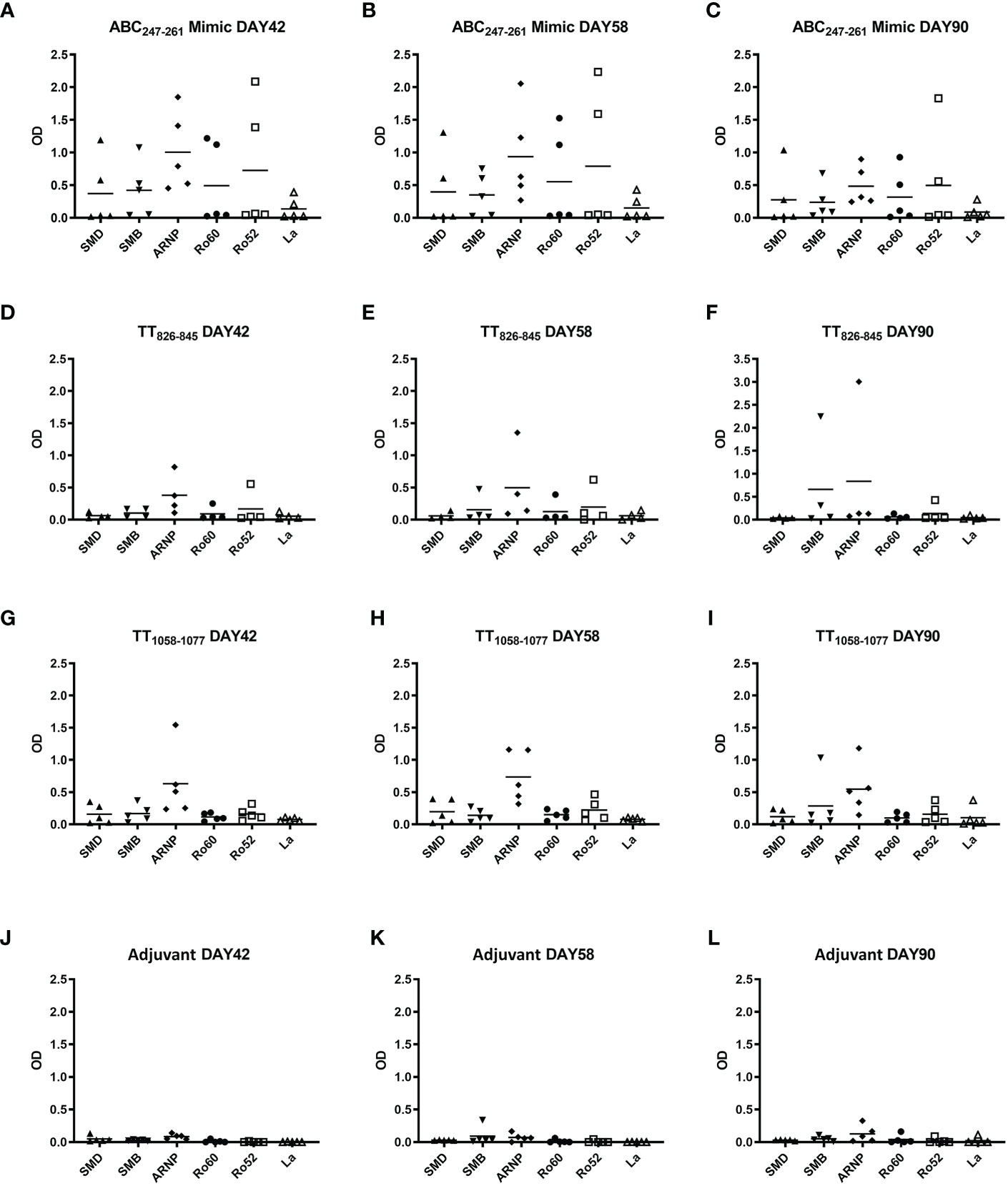
Figure 5 ABC247-261 Mimic, TT826-845, and TT1058-1077 induce autoantibodies to lupus-related autoantigens. Single T cell epitope peptides ABC247-261 Mimic (A–C), TT826-845 (D–F), and TT1058-1077 (G–I) were used to immunize DR3 mice. DR3 mice immunized with adjuvant only were used as controls (J–L). Autoantibodies to lupus-related autoantigens were assayed in mouse sera at day 42 (A, D, G, J), day 58 (B, E, H, K), and day 90 (C, F, I, L). Each dot indicates a single animal.
SLE patients have a greater percentage of ABC247-261 Mimic and TT826-845 cross-reactive T cells than healthy donors
To support the findings from our mouse studies, we evaluated the presence of SmD66-80, ABC247-261 Mimic, TT826-845 and TT1058-1077 mono- and cross-reactive T cells in DR3 SLE patients and healthy donors. The PBMCs from both groups were stimulated with TT lysate for 5-7 days and then stained with DR3 tetramers. Representative DR3 tetramer gating is shown for a single lupus patient and healthy donor (Figures 6A, B). SLE patients had a greater percentage of the ABC247-261 Mimic and the TT826-845 cross-reactive T cells to SmD66-80 compared to healthy donors (Figures 6C, D). Patients also had a greater percentage of SmD66-80 and ABC247-261 Mimic mono-reactive T cells than healthy donors (Figures 6F, G). However, there was no significant difference in the percentages of T cells cross-reactive with TT1058-1077 and SmD66-80 (Figure 6E). There were no significant differences of T cells reactive only to TT826-845 or TT1058-1077 between patients and healthy donors (Figures 6H, I).
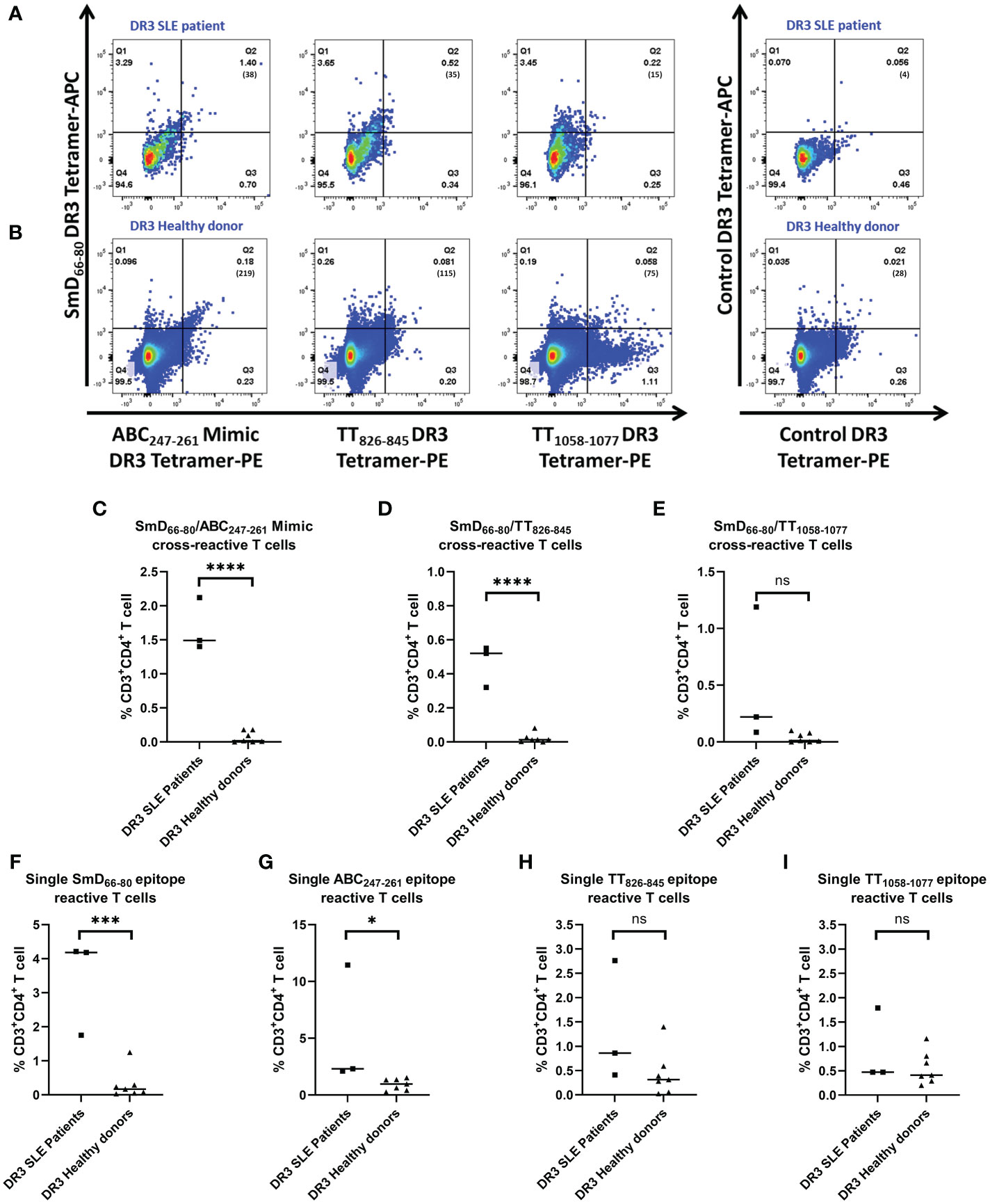
Figure 6 Percentages of ABC247-261 Mimic and TT826-845 cross-reactive and SmD66-80 and ABC247-261 Mimic single reactive T cells are greater in SLE patients. PBMCs were collected from DR3 SLE patients and healthy donors. They were stimulated with TT lysate for one week and stained with both SmD66-80 and one of three environmental epitope DR3 tetramers. Representative DR3 tetramer gating is shown for a single SLE patient (A) and healthy donor (B). The number of tetramer positive cells is shown in parentheses. (C–E) Percentages of the autoimmune, cross-reactive T cells detected in SLE patients and healthy donors. (F–I) Percentages of the single reactive T cells against SmD66-80, ABC247-261 Mimic, TT826-845, and TT1058-1077 present in patients and healthy donors. Each dot indicates a single individual. *p < 0.05, ***p < 0.001, ****p < 0.0001 by Student’s two-tailed t-test. ns, not significant.
Discussion
While analyzing T cells from a DR3 lupus patient, TT lysate was unexpectedly found to induce cross-reactive T cells against SmD66-80. This finding prompted us to examine TT epitopes and their abilities to drive autoimmune T cell responses and production of lupus-related autoantibodies. The possibility that vaccines may initiate autoimmune responses and even induce disease has been considered. There are case reports of lupus diagnoses occurring after TT immunization and lupus-related autoantibodies being generated after flu and hepatitis B virus (HBV) vaccines (21–23). These studies are limited because it is unclear whether the vaccines correlated with the production of autoantibodies and development of clinical symptoms, and they were unable to describe the details of the underlying mechanisms. To understand how TT T cell epitopes could drive autoimmune responses, we immunized humanized DR3 mice with single TT T cell epitopes. Interestingly, we found multiple intramolecular, cross-reactive T cell epitopes, a characteristic of self-antigens that makes them very antigenic (9). This explains the high level of antigenicity of TT. The ABC247-261 Mimic is one epitope from the same bacterium that produces TT. ABC247-261 Mimic reactive T cells from DR3 immunized mice are activated after priming with TT epitopes such as TT826-845, TT986-1005, TT1058-1077, TT1114-1133, and TT1272-1284. TT826-845 and TT1058-1077 induced autoantibodies as early as 10 days post immunization (data not shown). However, only the ABC247-261 Mimic induced anti-SmD autoantibodies and B cell epitope spreading to other lupus-related autoantigens months after immunization. These results suggest the ABC247-261 Mimic T cell epitope is a long-term driver of autoimmunity.
To analyze antigen-specific T cells in our studies, we used HLA tetramers: powerful tools to monitor T cells with specific epitope reactivity. Cross-reactive T cells that can be activated by both autoantigens and non-autoantigens have been detected in several autoimmune diseases in addition to lupus e.g. rheumatoid arthritis, antiphospholipid syndrome, celiac disease, autoimmune encephalomyelitis, multiple sclerosis and diabetes (24–33). In a mouse model of SLE, mouse H-2 tetramers were used to probe for U1-70131-150 (another lupus-related autoantigen epitope) reactive T cells. In the autoimmune-prone MRL/lpr mice, U1-70 reactive T cells increase with disease severity (34). However, the U1-70 study is limited by its use of mouse H-2 tetramers in a mouse system. In our experiments, we studied the SmD autoantigen epitope as well as the cross-reactive TT epitopes. Additionally, we employed humanized DR3 mice and human samples from DR3 lupus patients and healthy donors.
The phenotypes observed in mice are not directly attributable to humans because their immune systems are not the same (35). Thus, we also utilized human specimens in our studies to validate observations from our mouse model. Similar to our mouse studies, we detected T cells cross-reactive between SmD66-80 and Clostridium tetani epitopes in human PBMCs. Using the DR3 tetramers, it is not possible to rule out that within the population of cross-reactive T cells there are no dual T cell receptor (TCR) T cells present (36). However, given dual TCR T cells make up a small fraction of the entire population of T cells, it is more than likely that most of the T cells we identified are cross-reactive T cells. Further experimentation could be used to identify the specific TCRs of these cells. In addition, the percentages of cross-reactive T cells in our samples may be higher than what we detected due to competition between the two tetramers for binding to the cells (Supplementary Figure 3).
To our knowledge, there are no reports about TT epitopes that cross-react with self-antigens. In two studies of autoimmune diseases using tetramers, small percentages of cross-reactive T cells to microbial and self-epitopes were detected, similar to those detected in our study (30, 37).
Compared to healthy donors, lupus patients have greater percentages of SmD66-80 and ABC247-261 Mimic mono-reactive T cells, but not single TT826-845 and TT1058-1077 reactive T cells. This result is congruent with clinical findings that there is no difference in anti-TT titers in SLE patients and healthy donors after TT vaccination (38–40). But the differences in the percentages of cross-reactive T cells between lupus patients and healthy donors gives us insight into the activation of autoimmune T cells in these patients. As they encounter innumerable environmental mimics of self-antigens, autoimmune, cross-reactive T cells accumulate. Following accumulation, these cells proliferate and activate autoimmune, cross-reactive B cells, leading to autoantibody production and the breaking of self-tolerance. The autoimmune SmD66-80 T cells in lupus patients are not biased toward IL-17a expression (Supplementary Figure 4), indicating that these autoimmune CD4+ T cells may not only express IL-17a, but a mixture of other cytokines.
We are interested in investigating the abilities of environmental peptides to induce autoimmune T cells and autoantibody generation. Our hypothesis is that autoantibodies and autoreactive T cells are generated by the activation of T cells cross-reactive with autoantigens and environmental antigens (e.g. those present in bacteria, viruses, fungi, etc.) in an HLA-DR restricted manner. Molecular mimicry is the underlying mechanism of induction of autoantibodies by the ABC247-261 Mimic, TT826-845, and TT1058-1077 due to cross-reactivity to SmD66-80. Removing these autoimmune, cross-reactive T cell epitopes from vaccines may benefit individuals susceptible to developing lupus or other autoimmune diseases.
In this investigation, the importance of HLA-DR3 in the pathogenesis of SLE is emphasized. However, the genetics of lupus is very complex. The female mice of NZM2328.DR3.AE0 mice have more anti-SmD antibodies and more severe nephritis with early mortality (41). No anti-SmD antibodies were detected in SJL.DR3.AE0 mice immunized with ABC247-261 Mimic (Zhao, unpublished data), although significantly increased autoantibody to SmD was detected in the presented B10.DR3.AE0 immunization (Figures 5A-C). These data highlight the importance of non-major histocompatibility complex genes which may be protective and prevent autoimmunity. Thus, although TT and the ABC247-261 Mimic may induce autoantibodies, only in rare instances, diseases such as SLE and related disorders result.
Data availability statement
The original contributions presented in the study are included in the article/Supplementary Material. Further inquiries can be directed to the corresponding author.
Ethics statement
The animal study was reviewed and approved by the Animal Care and Use Committees at the University of Virginia.
Author contributions
ZZ, WWK, and SMF designed the research. ZZ, ANA, and CCK carried out the experiments. All the authors were involved in the analysis and interpretation of the data. ZZ, FG, ANA, and SMF wrote the manuscript. ZZ and SMF had full access to all the data and are responsible for the integrity and accuracy of the data analysis. All authors contributed to the article and approved the submitted version.
Funding
This work was supported in part by National Institutes of Health Grant R01 139673 (to SMF) and ANA was supported by training grants 5T32DK 072922 (PI: Mark Okusa) and 1TL1DK 132771 (PI: Portilla Didier).
Conflict of interest
The authors declare that the research was conducted in the absence of any commercial or financial relationships that could be construed as a potential conflict of interest.
Publisher’s note
All claims expressed in this article are solely those of the authors and do not necessarily represent those of their affiliated organizations, or those of the publisher, the editors and the reviewers. Any product that may be evaluated in this article, or claim that may be made by its manufacturer, is not guaranteed or endorsed by the publisher.
Supplementary material
The Supplementary Material for this article can be found online at: https://www.frontiersin.org/articles/10.3389/fimmu.2022.928374/full#supplementary-material
References
1. Tsokos GC. Systemic lupus erythematosus. New Engl J Med (2011) 365(22):2110–21. doi: 10.1056/nejmra1100359
2. Arbuckle MR, McClain MT, Rubertone MV, Scofield RH, Dennis GJ, James JA, et al. Development of autoantibodies before the clinical onset of systemic lupus erythematosus. N Engl J Med (2003) 349(16):1526–33. doi: 10.1056/NEJMoa021933
3. Murphy K, Weaver C. Chapter 15: Autoimmunity and transplantation. In: Janeway's immunobiology, 9th ed. New York, NY: Garland Science/Taylor & Francis Group, LLC (2016). p. 676.
4. Fu SM, Deshmukh US, Gaskin F. Pathogenesis of systemic lupus erythematosus revisited 2011: end organ resistance to damage, autoantibody initiation and diversification, and HLA-DR. J Autoimmun (2011) 37(2):104–12. doi: 10.1016/j.jaut.2011.05.004
5. Jiang C, Deshmukh US, Gaskin F, Bagavant H, Hanson J, David CS, et al. Differential responses to smith d autoantigen by mice with HLA-DR and HLA-DQ transgenes: dominant responses by HLA-DR3 transgenic mice with diversification of autoantibodies to small nuclear ribonucleoprotein, double-stranded DNA, and nuclear antigens. J Immunol (Baltimore Md 1950) (2010) 184(2):1085–91. doi: 10.4049/jimmunol.0902670
6. Cusick MF, Libbey JE, Fujinami RS. Molecular mimicry as a mechanism of autoimmune disease. Clin Rev Allergy Immunol (2012) 42(1):102–11. doi: 10.1007/s12016-011-8294-7
7. Deshmukh US, Sim DL, Dai C, Kannapell CJ, Gaskin F, Rajagopalan G, et al. HLA-DR3 restricted T cell epitope mimicry in induction of autoimmune response to lupus-associated antigen SmD. J Autoimmun (2011) 37(3):254–62. doi: 10.1016/j.jaut.2011.07.002
8. Szymula A, Rosenthal J, Szczerba BM, Bagavant H, Fu SM, Deshmukh US. T Cell epitope mimicry between sjögren's syndrome antigen a (SSA)/Ro60 and oral, gut, skin and vaginal bacteria. Clin Immunol (Orlando Fla) (2014) 152(1-2):1–9. doi: 10.1016/j.clim.2014.02.004
9. Zhao Z, Ren J, Dai C, Kannapell CC, Wang H, Gaskin F, et al. Nature of T cell epitopes in lupus antigens and HLA-DR determines autoantibody initiation and diversification. Ann Rheum Dis (2019) 78(3):380–90. doi: 10.1136/annrheumdis-2018-214125
10. Aringer M, Costenbader K, Daikh D, Brinks R, Mosca M, Ramsey-Goldman R, et al. 2019 European League against Rheumatism/American college of rheumatology classification criteria for systemic lupus erythematosus. Arthritis Rheumatol (2019) 71(9):1400–12. doi: 10.1002/art.40930
11. Bunce M. PCR-sequence-specific primer typing of HLA class I and class II alleles. Methods Mol Biol (2003) 210:143–71. doi: 10.1385/1-59259-291-0:143
12. Flynn J, Gorry P. Flow cytometry analysis to identify human CD4+ T cell subsets. New York: Springer (2019) p. 15–25.
13. Novak EJ, Liu AW, Nepom GT, Kwok WW. MHC class II tetramers identify peptide-specific human CD4+ T cells proliferating in response to influenza a antigen. J Clin Invest (1999) 104(12):R63–R7. doi: 10.1172/jci8476
14. Deshmukh US, Lewis JE, Gaskin F, Kannapell CC, Waters ST, Lou YH, et al. Immune responses to Ro60 and its peptides in mice. i. the nature of the immunogen and endogenous autoantigen determine the specificities of the induced autoantibodies. J Exp Med (1999) 189(3):531–40. doi: 10.1084/jem.189.3.531
15. Deshmukh US, Kannapell CC, Fu SM. Immune responses to small nuclear ribonucleoproteins: Antigen-dependent distinct b cell epitope spreading patterns in mice immunized with recombinant polypeptides of small nuclear ribonucleoproteins. J Immunol (2002) 168(10):5326–32. doi: 10.4049/jimmunol.168.10.5326
16. Kong YC, Lomo LC, Motte RW, Giraldo AA, Baisch J, Strauss G, et al. HLA-DRB1 polymorphism determines susceptibility to autoimmune thyroiditis in transgenic mice: definitive association with HLA-DRB1*0301 (DR3) gene. J Exp Med (1996) 184(3):1167–72. doi: 10.1084/jem.184.3.1167
17. Kruisbeek AM. Production of mouse T cell hybridomas. Curr Protoc Immunol (2001) 24:3.14.1–11. doi: 10.1002/0471142735.im0314s24. Chapter 3:Unit 3.14.
18. Da Silva Antunes R, Paul S, Sidney J, Weiskopf D, Dan JM, Phillips E, et al. Definition of human epitopes recognized in tetanus toxoid and development of an assay strategy to detect ex vivo tetanus CD4+ T cell responses. PloS One (2017) 12(1):e0169086. doi: 10.1371/journal.pone.0169086
19. James EA, Bui J, Berger D, Huston L, Roti M, Kwok WW. Tetramer-guided epitope mapping reveals broad, individualized repertoires of tetanus toxin-specific CD4+ T cells and suggests HLA-based differences in epitope recognition. Int Immunol (2007) 19(11):1291–301. doi: 10.1093/intimm/dxm099
20. Wienhold W, Malcherek G, Jung C, Stevanovic S, Jung G, Schild H, et al. An example of immunodominance: engagement of synonymous TCR by invariant CDR3β. Int Immunol (2000) 12(6):747–56. doi: 10.1093/intimm/12.6.747
21. Ruhrman-Shahar N, Torres-Ruiz J, Rotman-Pikielny P, Levy Y. Autoimmune reaction after anti-tetanus vaccination–description of four cases and review of the literature. Immunol Res (2017) 65(1):157–63. doi: 10.1007/s12026-016-8822-x
22. Więsik-Szewczyk E, Romanowska M, Mielnik P, Chwalińska-Sadowska H, Brydak LB, Olesińska M, et al. Anti-influenza vaccination in systemic lupus erythematosus patients: an analysis of specific humoral response and vaccination safety. Clin Rheumatol (2010) 29(6):605–13. doi: 10.1007/s10067-010-1373-y
23. Agmon-Levin N, Zafrir Y, Paz Z, Shilton T, Zandman-Goddard G, Shoenfeld Y. Ten cases of systemic lupus erythematosus related to hepatitis b vaccine. Lupus (2009) 18(13):1192–7. doi: 10.1177/0961203309345732
24. Gerstner C, Turcinov S, Hensvold AH, Chemin K, Uchtenhagen H, Ramwadhdoebe TH, et al. Multi-HLA class II tetramer analyses of citrulline-reactive T cells and early treatment response in rheumatoid arthritis. BMC Immunol (2020) 21(1):1–14. doi: 10.1186/s12865-020-00357-w
25. Ruff WE, Dehner C, Kim WJ, Pagovich O, Aguiar CL, Yu AT, et al. Pathogenic autoreactive T and b cells cross-react with mimotopes expressed by a common human gut commensal to trigger autoimmunity. Cell Host Microbe (2019) 26(1):100–13.e8. doi: 10.1016/j.chom.2019.05.003
26. Yang J, Jing L, James EA, Gebe JA, Koelle DM, Kwok WW. A novel approach of identifying immunodominant self and viral antigen cross-reactive T cells and defining the epitopes they recognize. Front Immunol (2018) 9:2811. doi: 10.3389/fimmu.2018.02811
27. Van Heemst J, Jansen DTSL, Polydorides S, Moustakas AK, Bax M, Feitsma AL, et al. Crossreactivity to vinculin and microbes provides a molecular basis for HLA-based protection against rheumatoid arthritis. Nat Commun (2015) 6(1):6681. doi: 10.1038/ncomms7681
28. Reynolds CJ, Sim MJW, Quigley KJ, Altmann DM, Boyton RJ. Autoantigen cross-reactive environmental antigen can trigger multiple sclerosis-like disease. J Neuroinflamm (2015) 12(1):91. doi: 10.1186/s12974-015-0313-9
29. Han A, Newell EW, Glanville J, Fernandez-Becker N, Khosla C, Chien YH, et al. Dietary gluten triggers concomitant activation of CD4+ and CD8+ αβ T cells and γδ T cells in celiac disease. Proc Natl Acad Sci USA (2013) 110(32):13073–8. doi: 10.1073/pnas.1311861110
30. Massilamany C, Steffen D, Reddy J. An epitope from acanthamoeba castellanii that cross-react with proteolipid protein 139-151-reactive T cells induces autoimmune encephalomyelitis in SJL mice. J Neuroimmunol (2010) 219(1-2):17–24. doi: 10.1016/j.jneuroim.2009.11.006
31. Ou D, Mitchell LA, Metzger DL, Gillam S, Tingle AJ. Cross-reactive rubella virus and glutamic acid decarboxylase (65 and 67) protein determinants recognised by T cells of patients with type I diabetes mellitus. Diabetologia (2000) 43(6):750–62. doi: 10.1007/s001250051373
32. Talbot PJ, Paquette J-SB, Ciurli C, Antel JP, Ouellet F. Myelin basic protein and human coronavirus 229E cross-reactive T cells in multiple sclerosis. Ann Neurol (1996) 39(2):233–40. doi: 10.1002/ana.410390213
33. Greiling TM, Dehner C, Chen X, Hughes K, Iñiguez AJ, Boccitto M, et al. Commensal orthologs of the human autoantigen Ro60 as triggers of autoimmunity in lupus. Sci Trans Med (2018) 10(434):eaan2306. doi: 10.1126/scitranslmed.aan2306
34. Kattah NH, Newell EW, Jarrell JA, Chu AD, Xie J, Kattah MG, et al. Tetramers reveal IL-17-secreting CD4+ T cells that are specific for U1-70 in lupus and mixed connective tissue disease. Proc Natl Acad Sci USA (2015) 112(10):3044–9. doi: 10.1073/pnas.1424796112
35. Richard ML, Gilkeson G. Mouse models of lupus: what they tell us and what they don't. Lupus Sci Med (2018) 5(1):e000199. doi: 10.1136/lupus-2016-000199
36. Schuldt NJ, Binstadt BA. Dual TCR T cells: Identity crisis or multitaskers? J Immunol (2019) 202(3):637–44. doi: 10.4049/jimmunol.1800904
37. Yang J, Danke NA, Berger D, Reichstetter S, Reijonen H, Greenbaum C, et al. Islet-specific glucose-6-Phosphatase catalytic subunit-related protein-reactive CD4+ T cells in human subjects. J Immunol (2006) 176(5):2781–9. doi: 10.4049/jimmunol.176.5.2781
38. Csuka D, Czirják L, Hóbor R, Illes Z, Bánáti M, Rajczy K, et al. Effective humoral immunity against diphtheria and tetanus in patients with systemic lupus erythematosus or myasthenia gravis. Mol Immunol (2013) 54(3-4):453–6. doi: 10.1016/j.molimm.2013.01.012
39. Kashef S, Ghazizadeh F, Derakhshan A, Farjadian S, Alyasin S. Antigen-specific antibody response in juvenile-onset SLE patients following routine immunization with tetanus toxoid. Iran J Immunol (2008) 5(3):181–4.
40. Devey ME, Bleasdale K, Isenberg DA. Antibody affinity and IgG subclass of responses to tetanus toxoid in patients with rheumatoid arthritis and systemic lupus erythematosus. Clin Exp Immunol (1987) 68(3):562–9.
Keywords: T cell epitope, HLA-DR3, environmental bacteria, molecular mimicry, autoantigen, Smith D (SmD), cross-reactive T cells, systemic lupus erythematosus (SLE)
Citation: Zhao Z, Anderson AN, Kannapell CC, Kwok WW, Gaskin F and Fu SM (2022) HLA-DR3 restricted environmental epitopes from the bacterium Clostridium tetani have T cell cross-reactivity to the SLE-related autoantigen SmD. Front. Immunol. 13:928374. doi: 10.3389/fimmu.2022.928374
Received: 25 April 2022; Accepted: 14 October 2022;
Published: 31 October 2022.
Edited by:
Diego Francisco Catalán, University of Chile, ChileReviewed by:
Ricardo Pujol Borrell, Universitat Autònoma de Barcelona, SpainKatina Schinnerling, University of Chile, Chile
Copyright © 2022 Zhao, Anderson, Kannapell, Kwok, Gaskin and Fu. This is an open-access article distributed under the terms of the Creative Commons Attribution License (CC BY). The use, distribution or reproduction in other forums is permitted, provided the original author(s) and the copyright owner(s) are credited and that the original publication in this journal is cited, in accordance with accepted academic practice. No use, distribution or reproduction is permitted which does not comply with these terms.
*Correspondence: Shu Man Fu, c2YyZUB2aXJnaW5pYS5lZHU=