- 1Translational Immunology and Nanotechnology Theme, School of Health and Biomedical Sciences, Royal Melbourne Institute of Technology (RMIT) University, Bundoora, VIC, Australia
- 2Integrated Photonics and Applications Centre (InPaC), School of Engineering, Royal Melbourne Institute of Technology (RMIT) University, Melbourne, VIC, Australia
High Tumor Necrosis Factor Receptor 2 (TNFR2) expression is characteristic of diverse malignant cells during tumorigenesis. The protein is also expressed by many immunosuppressive cells during cancer development, allowing cancer immune escape. A growing body of evidence further suggests a correlation between the circulating form of this protein and cancer development. Here we conducted a systematic meta-analysis of cancer studies published up until 1st October 2022, in which the circulating soluble TNFR2 (sTNFR2) concentrations in patients with cancers were recorded and their association with cancer risk was assessed. Of the 14,615 identified articles, 44 studies provided data on the correlation between cancer risk and the level of circulating sTNFR2. The pooled means comparison showed a consistently significant increase in the levels of sTNFR2 in diverse cancers when compared to healthy controls. These included colorectal cancer, ovarian cancer, breast cancer, non-Hodgkin’s lymphoma, Hodgkin’s lymphoma, lung cancer, hepatocarcinoma, and glioblastoma. In a random-effect meta-analysis, the cancer-specific odd ratios (OR) showed significant correlations between increased circulating sTNFR2 levels and the risk of colorectal cancer, non-Hodgkin’s lymphoma, and hepatocarcinoma at 1.59 (95% CI:1.20-2.11), 1.98 (95% CI:1.49-2.64) and 4.32 (95% CI:2.25-8.31) respectively. The overall result showed an association between circulating levels of sTNFR2 and the risk of developing cancer at 1.76 (95% CI:1.53-2.02). This meta-analysis supports sTNFR2 as a potential diagnostic biomarker for cancer, albeit with different predictive strengths for different cancer types. This is consistent with a potential key role for TNFR2 involvement in cancer development.
Introduction
Cancer remains one of the most lethal diseases and is currently the world’s second most common cause of death (1). According to the World Health Organization, approximately 9.6 million deaths occur annually because of cancer (1). Currently, the high mortality rate from cancers is mostly due to late diagnosis as many cancers can be efficiently treated if diagnosed early. The existing cancer diagnostics methods and techniques present various limitations. While diagnostic imaging techniques such as digital mammography, ultrasonography, computed tomography, and magnetic resonance imaging are non-invasive, they lack absolute sensitivity and specificity for the detection of different cancer types (2). Furthermore, these imaging techniques require expensive specialized equipment and highly trained medical personnel, which limits patient access due to high costs (3). On the other hand, while biopsy staining is useful for definitive cancer diagnosis, its invasive nature makes it unattractive for most patients and it is less sensitive to early-stage cancers. Thus, diagnostic biomarkers from a minimally invasive liquid biopsy such as blood that could identify the presence of specific cancers with high precision and at their early stage, are highly desired.
Tumor necrosis factor (TNF) is a cytokine implicated in inflammation and cancer development (4–6). In the tumor microenvironment, TNF via its receptor TNFR1 and TNFR2 plays a dual role to suppress or promote cancer proliferation and metastasis (7, 8). TNFR1 can be expressed by nearly all cells, while TNFR2 can be highly expressed by tumor cells (9–11). In malignant cells, TNFR2 promotes tumor cell proliferation and is increasingly being considered as an oncogene as it is overexpressed in more than 20 types of cancer, including multiple myeloma, human renal cell carcinoma, breast, oesophageal, myeloma, colon cancer, ovarian cancer, and cutaneous T-cell lymphomas, among others (9–11). The presence of TNFR2 at cancer sites has prompted research on utilizing TNFR2 as a target for therapeutic agents.
TNFR2 is also highly expressed in immune cells, which could be associated with tumorigenesis and tumor growth or conversely tumor controls (10, 12, 13). This protein however has been shown to be broadly expressed in the repertoire of immunosuppressive cells present on tumors and tumor microenvironments promoting pro-tumor activity (9). They include regulatory T cells (Tregs) (14, 15), natural killer cells (NK cells) (16), myeloid-derived suppressor cells (MDSCs) (17), mesenchymal stem cells (MSCs) (18, 19), endothelial progenitor cells (EPCs) (20), neural stem cells (NSCs) (21) and cancer-associated fibroblasts (CAFs) (22). The immunosuppressive cells are activated by the TNF-TNFR2 axis as well as the TNFR2 alone without its ligand, as TNFR2 can auto-associate in the absence of TNF and promote active signaling (23). The active immunosuppressive cells could then promote cancer immune evasion by suppressing the immune response against cancer. TNFR2 overexpression and TNF-TNFR2 signaling on Tregs results in their proliferation into a subpopulation of highly suppressive phenotype, promoting enhanced immunosuppressive activities within tumor microenvironments (24) which in turn promotes tumor cell proliferation (25). As an example, the expression of TNFR2 by Tregs in peripheral blood is strongly correlated with cancer development of the lymphatic system (lymph nodes), distant metastases, and advanced lung cancer disease (26). In NK cells, the TNF-TNFR2 axis acts as a checkpoint molecule, reducing NK cells’ tumoricidal activity (16). In MDSCs, TNFR2 boosts differentiation capacity and immunosuppressive activity of these immunosuppressive cells as well as promoting the activation of Tregs (27). TNFR2 promotes MDSC survival by inhibiting the apoptosis processes of the cells, which in turn contributes to tumorigenesis (12, 28). Similarly, in MSCs, EPCs, and NPCs, the TNF-TNFR2 axis promotes immunosuppression within the tumor microenvironment and induction of active Tregs (18, 20, 21, 29). In addition, the TNF-TNFR2 signaling on CAFs enhances the synthesis of immunosuppressive interleukin (IL)-33, which increases tumor cell migration and invasion (22). On the contrary, TNFR2 is an important costimulatory molecule to enhance the proliferation and activation of both CD4+ and CD8+ conventional effector T cells (Teffs) necessary to eliminate the neoplastic cells (14, 30, 31).
The levels of circulating form of TNFR2 (sTNFR2) has been shown to increase in chronic inflammatory conditions such as obesity and Type 2 diabetes (DM2), diabetic kidney disease characterized by increased albuminuria, and juvenile chronic arthritis (32–35), as well as in infectious diseases including severe malaria (36). The circulating sTNFR2 comes from membrane shedding or as a spliced variant, following immune cell activation (37–41). This soluble receptor is secreted in vivo by Tregs and consistently counteract the action of TNF (42), which in turn suppresses the active immune response exerted via TNFR1, and meanwhile, the membrane-bound TNFR2 on Tregs can independently promote immunosuppressive behavior of Tregs (14, 15). In vitro, some pathogens stimulate the secretion of sTNFR2 (43, 44). In vivo, TNFR2-overexpressing cancer cells promote the accumulation of TNFR2+ Tregs in the draining lymph nodes and increase the levels of sTNFR2 in the circulation (45). These studies suggest that elevated sTNFR2 could be an indicator of cancer (10, 12, 13). Therefore, the circulating sTNFR2 could potentially lend itself as a novel diagnostic biomarker to detect the presence of cancer. Thus, we conducted a systematic review and meta-analysis to test the utility of sTNFR2 as a diagnostic biomarker for cancer.
Materials and methods
Study design
The study was designed to evaluate the utility of sTNFR2 in plasma or serum as a diagnostic biomarker for various cancers and a prognostic biomarker to predict cancer outcome, by performing a meta-analysis on published studies. The literature research was conducted by the authors following the guideline set by the statements provided by “Preferred Reporting Items for Systemic Reviews and Meta-Analysis” (PRISMA) (46).
Search strategy
The literature was searched systematically in Medline, Embase, and Scopus databases, from inception to 1st October 2022, for studies investigating the associations between circulating sTNFR2 and cancer. The text word search included (TNFR2, TNFR2-p75, TNFR2p75, TNFRp75, TNFR-p75, sTNFR2 or CD120b) and (cancer, cancers, carcinoma, tumor, neoplasm, malignant, or malignancy). Duplicates were removed using EndNote20 software (Clarivate Analytics, Boston, USA), and this software was further used to select articles.
Inclusion and exclusion criteria
The selection of articles for studies was based on defined inclusion and exclusion criteria. Titles, abstracts, and full articles were first screened independently by three authors (AK, EC, MR). Case studies, conference papers, animal and in vitro studies were excluded. Secondary source articles such as meta-analyses and reviews that do not provide the original data of a study were excluded. No restriction to time or age was applied. Additional search by scanning the reference lists from other related articles was also performed. Relevant articles were then independently reviewed by the three authors (AK, EC, MR) and selected based on the content of the articles, which includes: 1. the study is on cancer, 2. the biomarker of interest is soluble TNFR2 in serum or plasma. The collected articles were sorted and recorded using the PRISMA flow diagram (46).
Quality assessment
The article’s quality was assured by noting the author, year, abstract, and number of citations. Importantly, the study fitness was assessed by two authors (AK, EC) in discussion, using the updated Strengthening the Reporting of Observational studies in Epidemiology (STROBE) checklist for diagnostic studies (47).
Data collection and extraction
Three reviewers (AK, EC, and MR) recorded data from all studies that met inclusion criteria using a standardized data collection procedure. Data collected was arranged in a table for all the studies (Supplementary Table 1). Study title, author’s name, name of journal, year, cancer type, country of study, the biological liquid used, population ethnicity, age, and gender were recorded. The number of patients and healthy controls participating in the study were recorded. Furthermore, the reported values related to sTNFR2 levels in serum or plasma were recorded. Additionally, the odds ratios presented in the studies were recorded or calculated based on the provided supporting data available in the studies.
Data analysis and statistics
The changes in the levels of sTNFR2 levels in various cancers were interrogated by determining the pooled mean values of sTNFR2 concentrations in serum/plasma from the controls and cancer patients. The pooled mean value is the mean of the weighted means from the selected studies, obtained by log-transforming the means as the sTNFR2 is expected to display log beta distribution and adjusting the means with their corresponding sample sizes (48). We also constructed the 95% confidence interval (CI) following log-transformed data. When median values were recorded, we estimated the mean values following Cochrane recommendations and the handbook (49)with the calculation described by Wan et al. (2014) (50). Then, we applied the Tukey test to assess the significant difference of sTNFR2 levels in cancer in comparison to controls. We then back-transformed the variables that have been log transformed for reporting purposes.
To evaluate the diagnostic utility of sTNFR2, we extracted odd ratio values from the selected studies, as this style of meta-analysis is frequently used to quantify disease occurrence in populations to answer concerns about disease risk (51). Since there is variability in study populations, especially with differs cancer types, the methodology of sample handling, as well as the methodology of sTNFR2 measurements, we utilized a random-effects meta-analysis model to evaluate the sTNFR2 correlation with the risk of cancer in our selected studies (52). We then generated the forest plot using Review Manager 5.4, London, UK, that calculated the overall odds ratios for each cancer type, and all cancers combined. An odds ratio of more than one with a P-value of <0.05 indicates that there is a likelihood of cancer occuring and a potential for sTNFR2 to indicate the presence of cancer. The between-study invariance in our random-effect meta-analysis was calculated using τ2, as the estimated standard deviation of underlying effects across included studies (49). The Chi2 and I2 tests measure the heterogeneity between studies. I2 above 50% could represent substantial heterogeneity depending on the magnitude and direction of the effects and the P-value of the Chi2 test (49). Publication bias was assessed based on the presence of data asymmetry on the Funnel plot (49).
Results
Study selection and characterization
A total of 18,502 relevant articles were identified from Medline, Embase and Scopus searches. Of the 18,502 articles, 3,887 were duplicates and removed, and 14,615 records were selected for screening. After excluding 2,787 animal studies, 4,089 in vitro studies, 2,642 secondary source articles, and 1,173 conference articles, 3,942 full-length articles were assessed for their eligibility. After applying the inclusion and exclusion criteria, 44 studies (53–97) were included in this meta-analysis. PRISMA Flow chart (Figure 1) shows the number of studies searched and selected. The full list of the selected studies is listed in Supplementary Table 1.
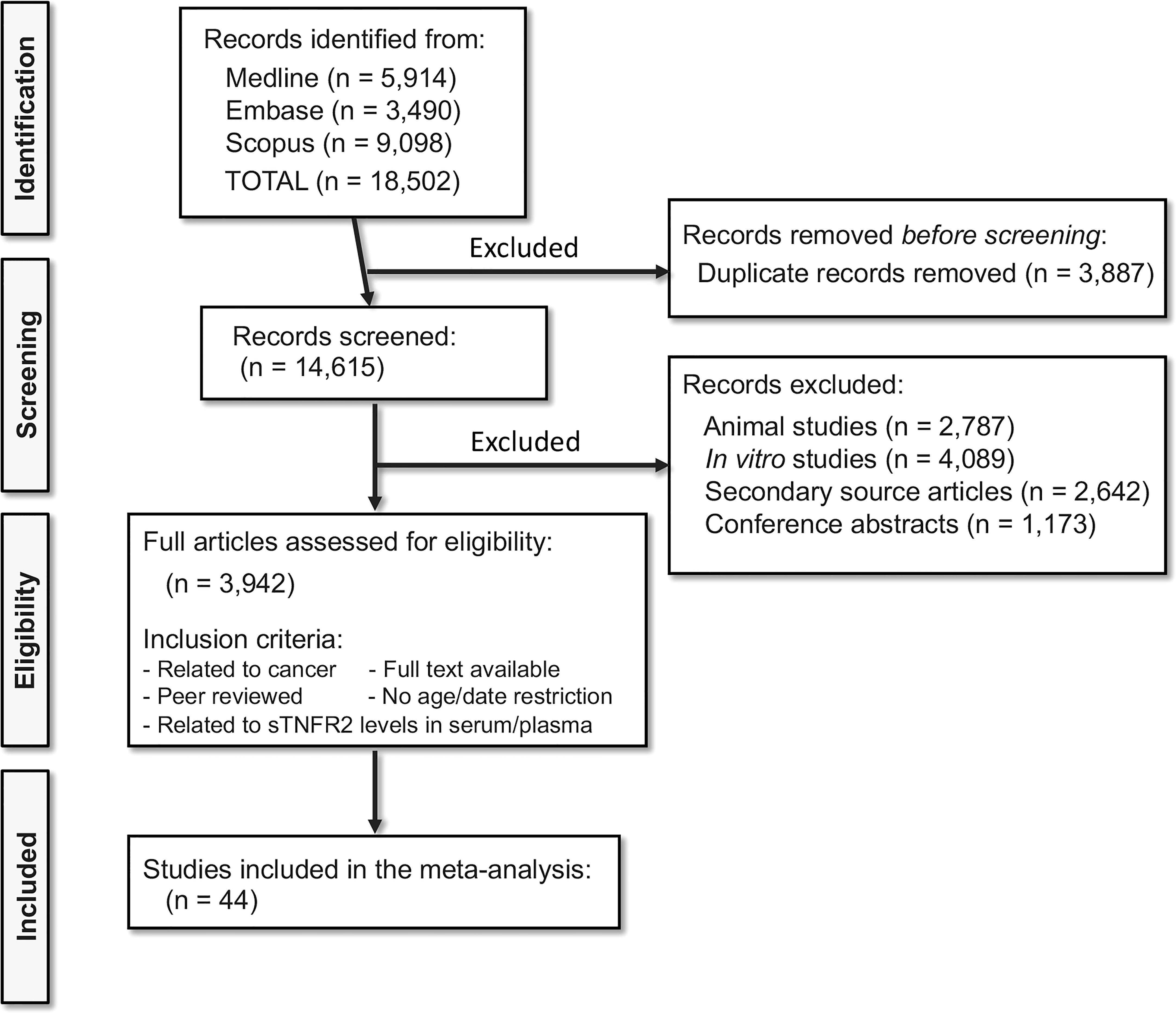
Figure 1 Selection of studies following PRISMA flowchart. Medline, Embase, and Scopus database searches resulted in the identification of 18,502 relevant articles. Following the exclusion and inclusion criteria, 44 articles were selected for the meta-analysis.
STROBE checklists
All the articles were assessed for their fitness following the STROBE checklist criteria. This includes meeting the criteria of participant selection, an introduction describing the background of the study, study design, the methods of sample handling, the results, and the outcome of the study. Each criterion scores one, and all the studies selected here were scored six and thus regarded as good quality studies for the purpose of this meta-analysis (Supplementary Table 1).
sTNFR2 levels in the circulation of cancer patients
We investigated the evidence for differences in sTNFR2 levels in serum/plasma between healthy subjects and cancer patients, to explore its potential as a diagnostic biomarker. Here, we analyzed the cancer types that are observed by at least two studies (Table 1). In total, we extracted data from 28 studies, encompassing 7520 healthy and 5981 cancer participants. The pooled mean values of the healthy controls did not differ significantly across different cancers. On the contrary, we observed that the pooled mean of sTNFR2 in patients with colorectal cancer at 2.69 ng/mL (95% CI:2.45-2.95) was significantly higher (P-value of the difference <0.001) than that of the healthy controls at 2.51 ng/mL (95% CI:2.36-2.68) in the same studies. Similarly, the pooled mean of sTNFR2 in patients with ovarian cancer at 3.23 ng/mL (95% CI:2.28-4.59) was also significantly higher than that of the healthy controls (P-value of the difference <0.05) at 2.27 ng/mL (95% CI:2.15-2.40) in the same studies (Table 1). The significant differences in the levels of sTNFR2 in the serum/plasma between controls and cancer participants were also observed in several other cancers including non-Hodgkin’s lymphoma, breast cancer, Hodgkin’s lymphoma, lung cancer, hepatocarcinoma, and glioblastoma (Figure 2, Table 1). This result suggests the potential involvement of circulating sTNFR2 concentrations in cancer and could prove its utility as a diagnostic biomarker for cancer.
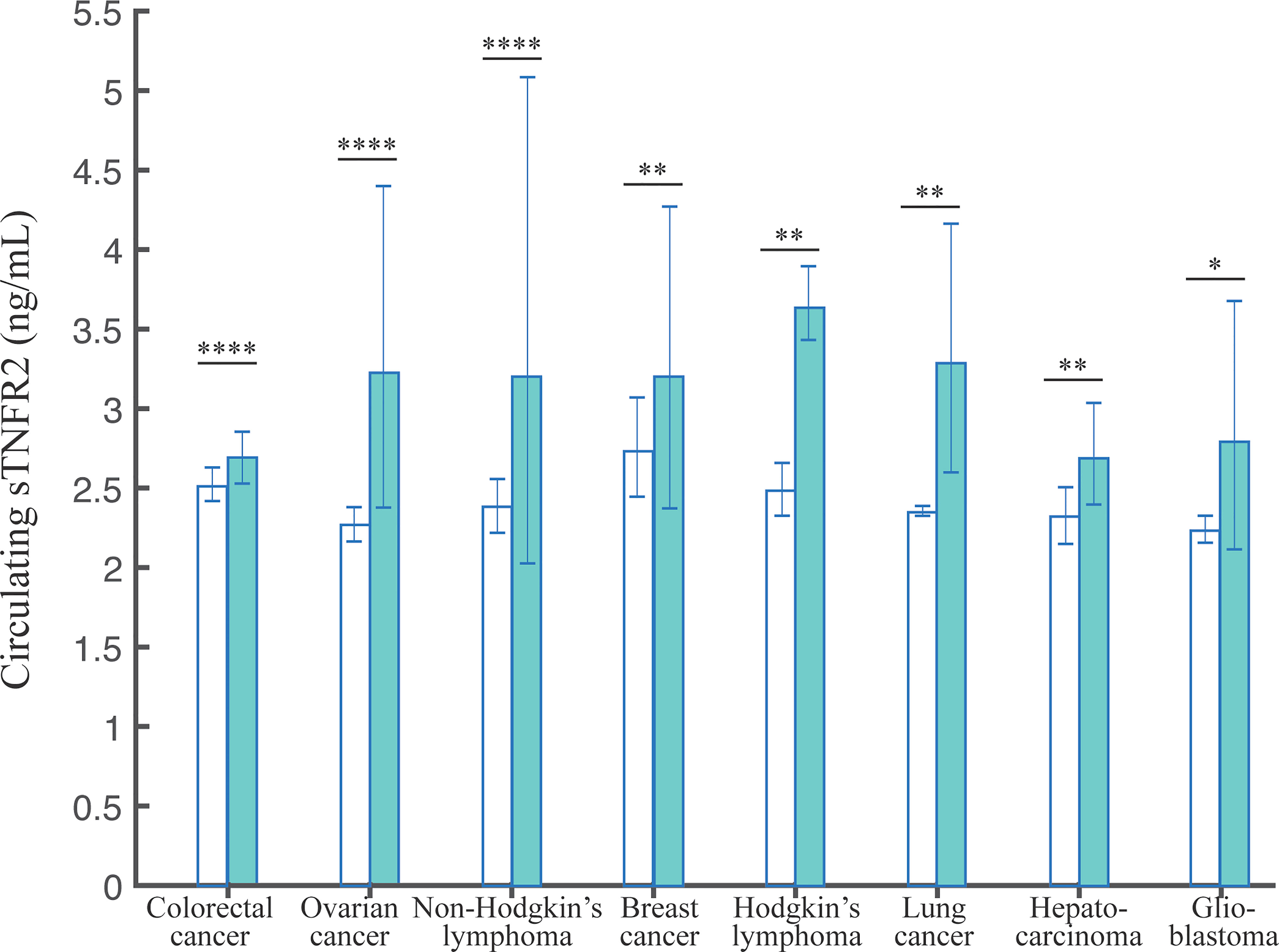
Figure 2 The pooled weighted means +/- 95% CI of sTNFR2 levels in serum/plasma from patients with the indicated cancers. The Tukey test was used to assess the significant difference of sTNFR2 levels, with *, **, and **** indicate P-values of <0.05, <0.01, <0.0001.
sTNFR2 association with the risk of developing cancer
Here, we extracted data from 34 eligible articles with sufficient data on the odd ratios (OR) for sTNFR2 and the risk of developing cancer (Figure 3). We divided the studies based on the cancer types that are being investigated and performed the random effect meta-analysis. Based on 8 studies, increased sTNFR2 levels showed significant association with colorectal cancer with pooled OR of 1.59 (95% CI:1.20-2.11), however significant heterogeneity of 66% between studies was also observed, although bias in heterogeneity could result from such a small number of studies (98). This heterogeneity result indicates that the strength of the association may differ between those studies. From 5 studies on non-Hodgkin’s lymphoma, increased sTNFR2 levels showed a significant association, with pooled OR of 1.98 (95% CI:1.49-2.64) and a non-significant heterogeneity of 38%. Additionally, 2 studies on hepatocarcinoma showed a strong significant association between sTNFR2 levels and this cancer, with pooled OR of 4.32 (95% CI:2.25-8.31) with a non-significant heterogeneity of 16%. On the other hand, studies with ovarian cancer, breast cancer, and glioblastoma did not show significant correlations between sTNFR levels and an increased risk in developing those cancers, with pooled OR of 1.19 (95% CI:0.95-1.49), 1.58 (95% CI:0.58-4.33), and 1.45 (95% CI:0.77-2.71) respectively. Overall pooled OR score from various cancers however showed a modest but significant correlation between sTNFR2 and an increased risk in cancer development, at 1.76 (95% CI:1.53-2.02), albeit with significant heterogeneity between studies of 54%. No publication bias was observed based on the funnel plot (Figure 4). These studies thus showed that sTNFR2 potentially increases the risk of some cancers to various extent, significantly in colorectal cancer, non-Hodgkin’s lymphoma, and hepatocarcinoma. This thus indicate sTNFR2 potential to be used as a circulating diagnostic biomarker for cancer.
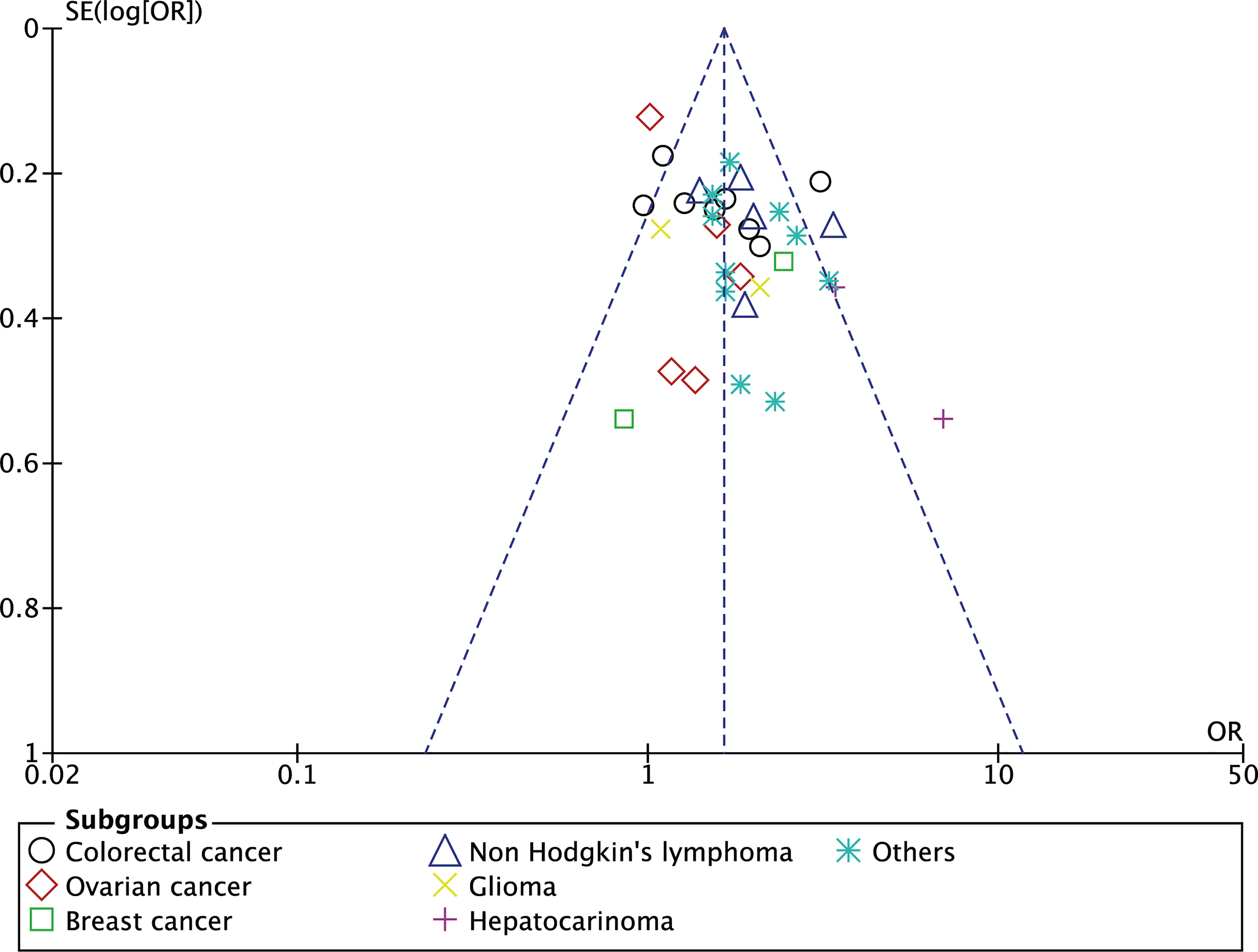
Figure 4 The funnel plot showing the OR of individuals studies against the standard error of the OR to detect bias and heterogeneity of between study.
We further tested our selected studies comprising various cancer types using a funnel plot, as this plot could indicate study heterogeneity and reporting bias. The funnel plot (Figure 4) shows a symmetrical feature which indicates the absence of reporting bias and that the random-effect model assumption used in this meta-analysis fits with the heterogeneity present in the selected studies (99).
Discussion
Overall, most cancers still present with a high mortality rate, due to late diagnosis. As such, there is a pressing need for the identification of reliable biomarkers facilitating early detection. It has been frequently proposed that inflammation, orchestrated by various cytokines may promote cancer formation and further its development (6, 48). Cancer and immune cells may secrete immune proteins to control inflammation, such as sTNFR2, that may also mark cancer formation. Thus, in this systematic review, we investigated the correlation between increased circulating sTNFR2 levels with the risk of developing cancer. Finding the utility of sTNFR2 as a diagnostic marker could be useful to improve the effectiveness of current cancer diagnosis and provide a convenient detection approach for patients, especially with various technologies have been developed recently to facilitate circulating cytokine detection (100).
Based on the calculated pooled mean values, circulating sTNFR2 levels were found to be consistently reported as significantly higher in various cancers in comparison to the healthy controls (Figure 5), suggesting sTNFR2 may be involved in cancer development. Using a random-effect OR meta-analysis, we observed significant correlations between sTNFR2 and several cancers, including colorectal cancers, non-Hodgkin’s lymphoma, and hepatocarcinoma. This indicates the potential of sTNFR2 as a diagnostic biomarker. Indeed, sTNFR2 levels are correlated with lung cancer development even 6 years before diagnosis (84). However, in other cancers, including ovarian, breast, and glioblastoma, the correlation was not significant, suggesting that sTNFR2 levels may not be sufficient as an independent diagnostic biomarker, especially for these cancers. It has been previously suggested that the circulating inflammatory biomarkers such as sTNFR2 which are highly correlated with cancer risks, could be combined with the circulating cancer-specific biomarkers that otherwise would have low sensitivity and specificity to indicate the presence of cancer (100). Furthermore, in agreement with our findings, a previous meta-analysis of prospective studies also found no significant association (of diagnostic value) between circulating levels of sTNFR2 and the risk of ovarian cancer (101). However, some studies show that high expression of TNFR2 at cancer sites is correlated with cancer size, metastasis and progression in epithelial ovarian cancer (90, 102), non-small lung carcinoma (103), anal carcinoma (104), and esophageal carcinoma (105, 106).
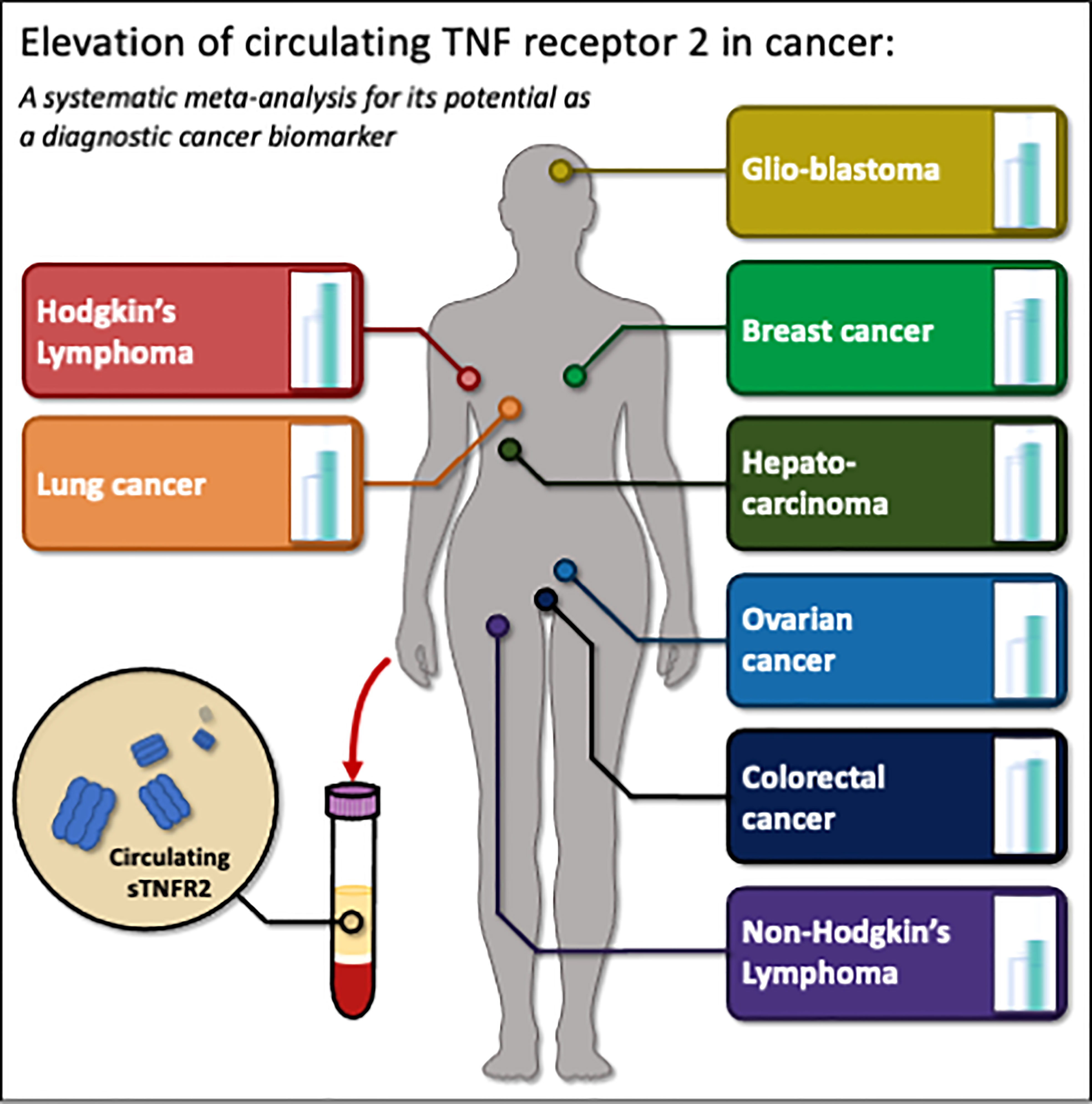
Figure 5 The summary schema of the elevated sTNFR2 levels in various cancers, and its potential as a biomarker for cancer detection. Figure was created using Microsoft power point.
The increased levels of circulating sTNFR2 do not only correlate with cancer risk but also cancer outcomes such as overall survival and progression-free survival, as has been shown by several studies of various cancers (56, 65–67, 78, 88, 107–113). In patients with ovarian cancer for example high levels of TNFR2+ Tregs have been associated with poor OS, while ovarian tissue with strong expression of TNFR2 was associated with longer PFS (14, 114). Additionally, a high pre-diagnosis plasma sTNFR2 level corelate with overall mortality in colorectal cancer patients (110). Moreover, several studies show that the effectiveness of anti-cancer drugs at reducing tumor size and improving survival is correlated with reduced levels of circulating sTNFR2 (115–119). This observation may thus extend the use of sTNFR2 not only as a minimally-invasive diagnostic biomarker to predict cancer outcome, but also to monitor therapy effectiveness during treatment (120).
In summary, our meta-analysis study confirms the correlation between increased circulating sTNFR2 levels and increased risk of cancers, albeit the extent of this association varies between different cancers. This indicates circulating sTNFR2 may have utility, perhaps combined with other blood accessible biomarkers, to aid in the diagnosis of cancer. We believe that the easy access to this biomarker through liquid biopsies makes it an ideal candidate to be used alone, or in combination with other markers, as a minimally-invasive cancer screening method, potentially accelerating the implementation of point-of-care devices (100) for cancer diagnosis in clinical settings outside of central laboratories.
Data availability statement
The raw data supporting the conclusions of this article will be made available by the authors, without undue reservation.
Author contributions
AK, MP conceptualized the study. AK designed and directed the study. AK, MR and EC performed literature research and analyzed the data. AK wrote the first draft of the manuscript. EC, MR, CS, CH, AM, MP wrote sections of the manuscript. All authors contributed to the article and approved the submitted version.
Funding
This research was funded by NHMRC No. 2011747.
Conflict of interest
The authors declare that the research was conducted in the absence of any commercial or financial relationships that could be construed as a potential conflict of interest.
Publisher’s note
All claims expressed in this article are solely those of the authors and do not necessarily represent those of their affiliated organizations, or those of the publisher, the editors and the reviewers. Any product that may be evaluated in this article, or claim that may be made by its manufacturer, is not guaranteed or endorsed by the publisher.
Supplementary material
The Supplementary Material for this article can be found online at: https://www.frontiersin.org/articles/10.3389/fimmu.2022.918254/full#supplementary-material
References
1. WHO. Cancer. Geneva, Switzerland: W.H.O (2022). Available at: https://www.who.int/health-topics/cancer.
2. García-Figueiras R, Baleato-González S, Padhani AR, Luna-Alcalá A, Vallejo-Casas JA, Sala E, et al. How clinical imaging can assess cancer biology. Insights into Imaging (2019) 10(1):28. doi: 10.1186/s13244-019-0703-0
3. Wu J, Dong M, Santos AR, Rigatto C, Liu Y, Lin F. Lab-on-a-Chip platforms for detection of cardiovascular disease and cancer biomarkers. Sensors (Basel Switzerland) (2017) 17(12):2934. doi: 10.3390/s17122934
4. Balkwill F. Tumour necrosis factor and cancer. Nat Rev Cancer (2009) 9(5):361–71. doi: 10.1038/nrc2628
5. Sethi G, Sung B, Aggarwal BB. TNF: A master switch for inflammation to cancer. Front Biosci (2008) 13:5094–107. doi: 10.2741/3066
6. Quinn KM, Kartikasari AE, Cooke RE, Koldej RM, Ritchie DS, Plebanski M. Impact of age-, cancer-, and treatment-driven inflammation on T cell function and immunotherapy. J Leukocyte Biol (2020) 108(3):953–65. doi: 10.1002/JLB.5MR0520-466R
7. Ham B, Fernandez MC, D'Costa Z, Brodt P. The diverse roles of the TNF axis in cancer progression and metastasis. Trends Cancer Raes (2016) 11(1):1–27.
8. Brenner D, Blaser H, Mak TW. Regulation of tumour necrosis factor signalling: live or let die. Nat Rev Immunol (2015) 15(6):362–74. doi: 10.1038/nri3834
9. Yang Y, Islam MS, Hu Y, Chen X. TNFR2: Role in cancer immunology and immunotherapy. ImmunoTargets Ther (2021) 10:103. doi: 10.2147/ITT.S255224
10. Li M, Zhang X, Bai X, Liang T. Targeting TNFR2: A novel breakthrough in the treatment of cancer. Front Oncol (2022) 12. doi: 10.3389/fonc.2022.862154
11. Vanamee É.S., Faustman DL. TNFR2: A novel target for cancer immunotherapy. Trends Mol Med (2017) 23(11):1037–46. doi: 10.1016/j.molmed.2017.09.007
12. Sheng Y, Li F, Qin Z. TNF receptor 2 makes tumor necrosis factor a friend of tumors. Front Immunol (2018) 9(1170). doi: 10.3389/fimmu.2018.01170
13. Bai J, Zhang Y, Ding B, Li H. Targeting TNFR2 in cancer: All roads lead to Rome. Front Immunol (2022) p:460. doi: 10.3389/fimmu.2022.844931
14. Kampan NC, Madondo MT, McNally OM, Stephens AN, Quinn MA, Plebanski M. Interleukin 6 present in inflammatory ascites from advanced epithelial ovarian cancer patients promotes tumor necrosis factor receptor 2-expressing regulatory T cells. Front Immunol (2017) 8(1482). doi: 10.3389/fimmu.2017.01482
15. Chen X, Wu X, Zhou Q, Howard OZ, Netea MG, Oppenheim JJ. TNFR2 is critical for the stabilization of the CD4+ Foxp3+ regulatory T cell phenotype in the inflammatory environment. J Immunol (2013) 190(3):1076–84. doi: 10.4049/jimmunol.1202659
16. Ivagnès A, Messaoudene M, Stoll G, Routy B, Fluckiger A, Yamazaki T, et al. TNFR2/BIRC3-TRAF1 signaling pathway as a novel NK cell immune checkpoint in cancer. Oncoimmunology (2018) 7(12):e1386826. doi: 10.1080/2162402X.2017.1386826
17. Hu X, Li B, Li X, Zhao X, Wan L, Lin G, et al. Transmembrane TNF-α promotes suppressive activities of myeloid-derived suppressor cells via TNFR2. J Immunol (2014) 192(3):1320–31. doi: 10.4049/jimmunol.1203195
18. Beldi G, Khosravi M, Abdelgawad ME, Salomon BL, Uzan G, Haouas H, et al. TNFα/TNFR2 signaling pathway: An active immune checkpoint for mesenchymal stem cell immunoregulatory function. Stem Cell Res Ther (2020) 11(1):1–15. doi: 10.1186/s13287-020-01740-5
19. Naserian S, Shamdani S, Arouche N, Uzan G. Regulatory T cell induction by mesenchymal stem cells depends on the expression of TNFR2 by T cells. Stem Cell Res Ther (2020) 11(1):1–7. doi: 10.1186/s13287-020-02057-z
20. Nouri Barkestani M, Shamdani S, Afshar Bakshloo M, Arouche N, Bambai B, Uzan G, et al. TNFα priming through its interaction with TNFR2 enhances endothelial progenitor cell immunosuppressive effect: new hope for their widespread clinical application. Cell Commun Signal (2021) 19(1):1–16. doi: 10.1186/s12964-020-00683-x
21. Shamdani S, Uzan G, Naserian S. TNFα-TNFR2 signaling pathway in control of the neural stem/progenitor cell immunosuppressive effect: different experimental approaches to assess this hypothetical mechanism behind their immunological function. Stem Cell Res Ther (2020) 11(1):1–5. doi: 10.1186/s13287-020-01816-2
22. Zhou Q, Wu X, Wang X, Yu Z, Pan T, Li Z, et al. The reciprocal interaction between tumor cells and activated fibroblasts mediated by TNF-α/IL-33/ST2L signaling promotes gastric cancer metastasis. Oncogene (2020) 39(7):1414–28. doi: 10.1038/s41388-019-1078-x
23. Chan FK-M, et al. A domain in TNF receptors that mediates ligand-independent receptor assembly and signaling. Science (2000) 288(5475):2351–4. doi: 10.1126/science.288.5475.2351
24. Chen X, Plebanski M. Editorial: The role of TNF-TNFR2 signal in immunosuppressive cells and its therapeutic implications. Front Immunol (2019) 10(2126). doi: 10.3389/fimmu.2019.02126
25. Ye L-L, Peng W-B, Niu Y-R, Xiang X, Wei X-S, Wang Z-H, et al. Accumulation of TNFR2-expressing regulatory T cells in malignant pleural effusion of lung cancer patients is associated with poor prognosis. Ann Trans Med (2020) 8(24):1647. doi: 10.21037/atm-20-7181
26. Yan F, Du R, Wei F, Zhao H, Yu J, Wang C, et al. Expression of TNFR2 by regulatory T cells in peripheral blood is correlated with clinical pathology of lung cancer patients. Cancer Immunol Immunother (2015) 64(11):1475–85. doi: 10.1007/s00262-015-1751-z
27. Polz J, Remke A, Weber S, Schmidt D, Weber-Steffens D, Pietryga-Krieger A, et al. Myeloid suppressor cells require membrane TNFR2 expression for suppressive activity. Immun Inflammation Dis (2014) 2(2):121–30. doi: 10.1002/iid3.19
28. Zhao X, Rong L, Zhao X, Li X, Liu X, Deng J, et al. TNF signaling drives myeloid-derived suppressor cell accumulation. J Clin Invest (2012) 122(11):4094–104. doi: 10.1172/JCI64115
29. Razazian M, Khosravi M, Bahiraii S, Uzan G, Shamdani S, Naserian S. Differences and similarities between mesenchymal stem cell and endothelial progenitor cell immunoregulatory properties against T cells. World J Stem Cells (2021) 13(8):971. doi: 10.4252/wjsc.v13.i8.971
30. Aspalter RM, Eibl MM, Wolf HM. Regulation of TCR-mediated T cell activation by TNF-RII. J leukocyte Biol (2003) 74(4):572–82. doi: 10.1189/jlb.0303112
31. Kim EY, Priatel JJ, Teh S-J, Teh H-S. TNF receptor type 2 (p75) functions as a costimulator for antigen-driven T cell responses in vivo. J Immunol (2006) 176(2):1026–35. doi: 10.4049/jimmunol.176.2.1026
32. Straczkowski M, Kowalska I, Nikolajuk A, Adamska A, Karolczuk-Zarachowicz M, Karczewska-Kupczewska M, et al. Plasma levels of soluble tumor necrosis factor-α receptors are related to total and LDL-cholesterol in lean, but not in obese subjects. Cardiovasc Diabetol (2006) 5(1):14. doi: 10.1186/1475-2840-5-14
33. Vendrell J, Broch M, Fernandez-Real JM, Gutiérrez C, Simón I, Megia A, et al. Tumour necrosis factor receptors (TNFRs) in type 2 diabetes. analysis of soluble plasma fractions and genetic variations of TNFR2 gene in a case-control study. Diabetes Med (2005) 22(4):387–92. doi: 10.1111/j.1464-5491.2004.01392.x
34. Murakoshi M, Gohda T, Suzuki Y. Circulating tumor necrosis factor receptors: A potential biomarker for the progression of diabetic kidney disease. Int J Mol Sci (2020) 21(6):1957. doi: 10.3390/ijms21061957
35. de Benedetti F, Pignatti P, Massa M, Sartirana P, Ravelli A, Cassani G, et al. Soluble tumour necrosis factor receptor levels reflect coagulation abnormalities in systemic juvenile chronic arthritis. Br J Rheumatol (1997) 36(5):581–8. doi: 10.1093/rheumatology/36.5.581
36. Dunning AJ, DiazGranados CA, Voloshen T, Hu B, Landolfi VA, Talbot HK. Correlates of protection against influenza in the elderly: results from an influenza vaccine efficacy trial. Clin Vaccine Immunol (2016) 23(3):228–35. doi: 10.1128/CVI.00604-15
37. Lainez B, Fernandez-Real JM, Romero X, Esplugues E, Cañete JD, Ricart W, et al. Identification and characterization of a novel spliced variant that encodes human soluble tumor necrosis factor receptor 2. Int Immunol (2004) 16(1):169–77. doi: 10.1093/intimm/dxh014
38. DeBerge MP, Ely KH, Wright PF, Thorp EB, Enelow RI. Shedding of TNF receptor 2 by effector CD8+ T cells by ADAM17 is important for regulating TNF-α availability during influenza infection. J Leukoc Biol (2015) 98(3):423–34. doi: 10.1189/jlb.3A0914-432RR
39. Bell JH, Herrera AH, Li Y, Walcheck B. Role of ADAM17 in the ectodomain shedding of TNF-alpha and its receptors by neutrophils and macrophages. J Leukoc Biol (2007) 82(1):173–6. doi: 10.1189/jlb.0307193
40. Philippe C, Roux-Lombard P, Fouqueray B, Perez J, Dayer J, Baud L. Membrane expression and shedding of tumour necrosis factor receptors during activation of human blood monocytes: regulation by desferrioxamine. Immunology (1993) 80(2):300.
41. Galve-de Rochemonteix B, Nicod LP, Dayer J-M. Tumor necrosis factor soluble receptor 75: the principal receptor form released by human alveolar macrophages and monocytes in the presence of interferon gamma. Am J Respir Cell Mol Biol (1996) 14(3):279–87. doi: 10.1165/ajrcmb.14.3.8845179
42. van Mierlo GJ, Scherer HU, Hameetman M, Morgan ME, Flierman R, Huizinga TW, et al. Cutting edge: TNFR-shedding by CD4+ CD25+ regulatory T cells inhibits the induction of inflammatory mediators. J Immunol (2008) 180(5):2747–51. doi: 10.4049/jimmunol.180.5.2747
43. Dri P, Gasparini C, Menegazzi R, Cramer R, Albéri L, Presani G, et al. TNF-induced shedding of TNF receptors in human polymorphonuclear leukocytes: role of the 55-kDa TNF receptor and involvement of a membrane-bound and non-matrix metalloproteinase. J Immunol (2000) 165(4):2165–72. doi: 10.4049/jimmunol.165.4.2165
44. Balcewicz-Sablinska MK, Keane J, Kornfeld H, Remold HG. Pathogenic mycobacterium tuberculosis evades apoptosis of host macrophages by release of TNF-R2, resulting in inactivation of TNF-α. J Immunol (1998) 161(5):2636–41.
45. Chen X, Li P, Yang X, Miao X, Luo H. Tumor necrosis factor receptor II (TNFR2) promotes the growth of mouse CT26 colon cancer. Am Assoc Immnol (2018) 200(1 Supplement):178.7.
46. Page MJ, McKenzie JE, Bossuyt PM, Boutron I, Hoffmann TC, Mulrow CD, et al. The PRISMA 2020 statement: An updated guideline for reporting systematic reviews. Bmj (2021) 372:n71. doi: 10.1136/bmj.n71
47. Cuschieri S. The STROBE guidelines. Saudi J Anaesth (2019) 13(Suppl 1):S31–s34. doi: 10.4103/sja.SJA_543_18
48. Amer H, Kartikasari AE, Plebanski M. Elevated interleukin-6 levels in the circulation and peritoneal fluid of patients with ovarian cancer as a potential diagnostic biomarker: A systematic review and meta-analysis. J Personal Med (2021) 11(12):1335. doi: 10.3390/jpm11121335
49. Thomas JHJ, Higgins JPT, Thomas J, Chandler J, Cumpston M, Li T, et al. Eds. Cochrane Handbook for Systematic Reviews of Interventions version 6.3 (updated February 2022). London, United Kingdom: Cochrane (2022).
50. Wan X, Wang W, Liu J, Tong T. Estimating the sample mean and standard deviation from the sample size, median, range and/or interquartile range. BMC Med Res Method (2014) 14(1):1–13. doi: 10.1186/1471-2288-14-135
51. Barker TH, Migliavaca CB, Stein C, Colpani V, Falavigna M, Aromataris E, et al. Conducting proportional meta-analysis in different types of systematic reviews: A guide for synthesisers of evidence. BMC Med Res Method (2021) 21(1):189. doi: 10.1186/s12874-021-01381-z
52. Borenstein M, Hedges LV, Higgins JP, Rothstein HR. A basic introduction to fixed-effect and random-effects models for meta-analysis. Res synthesis Methods (2010) 1(2):97–111. doi: 10.1002/jrsm.12
53. Aderka D, Englemann H, Hornik V, Skornick Y, Levo Y, Wallach D, et al. Increased serum levels of soluble receptors for tumor necrosis factor in cancer patients. Cancer Res (1991) 51(20):5602–7.
54. Viac J, Vincent C, Palacio S, Schmitt D, Claudy A. Tumour necrosis factor (TNF) soluble receptors in malignant melanoma: correlation with soluble ICAM-1 levels. Eur J Cancer (1996) 32a(3):447–9. doi: 10.1016/0959-8049(95)00541-2
55. Warzocha K, Bienvenu J, Ribeiro P, Moullet I, Dumontet C, Neidhardt-Berard E, et al. Plasma levels of tumour necrosis factor and its soluble receptors correlate with clinical features and outcome of hodgkin's disease patients. Br J Cancer (1998) 77(12):2357–62. doi: 10.1038/bjc.1998.391
56. Vinante F, Rigo A, Tecchio C, Morosato L, Nadali G, Ricetti MM, et al. Serum levels of p55 and p75 soluble TNF receptors in adult acute leukaemia at diagnosis: correlation with clinical and biological features and outcome. Br J Haematol (1998) 102(4):1025–34. doi: 10.1046/j.1365-2141.1998.00872.x
57. Tesarová P, Kvasnicka J, Umlaufová A, Homolková H, Jirsa M, Tesar V. Soluble TNF and IL-2 receptors in patients with breast cancer. Med Sci Monit (2000) 6(4):661–7.
58. Ammirati M, Rao S, Granger G. Detection of TNF inhibitors (soluble receptors) in the sera and tumor cyst fluid of patients with malignant astrocytomas of the brain. Front Biosci (2001) 6:B17–24. doi: 10.2741/ammirat
59. Alemán MR, Santolaria F, Batista N, de La Vega M, González-Reimers E, Milena A, et al. Leptin role in advanced lung cancer. a mediator of the acute phase response or a marker of the status of nutrition? Cytokine (2002) 19(1):21–6. doi: 10.1006/cyto.2002.1051
60. Krajcik RA, Massardo S, Orentreich N. No association between serum levels of tumor necrosis factor-alpha (TNF-alpha) or the soluble receptors sTNFR1 and sTNFR2 and breast cancer risk. Cancer Epidemiol Biomarkers Prev (2003) 12(9):945–6.
61. Melczer Z, Bánhidy F, Csömör S, Siklós P, Dworak O, Cseh K. ErbB-2/HER-2 protein expression, serum tumour necrosis factor-alpha (TFM-alpha) and soluble tumour necrosis factor receptor-2 (TNFR-2) concentrations in human carcinoma of the uterine cervix. Eur J Gynaecol Oncol (2003) 24(2):138–42.
62. Rzymski P, Opala T, Wilczak M, Woźniak J, Sajdak S. Serum tumor necrosis factor alpha receptors p55/p75 ratio and ovarian cancer detection. Int J Gynaecol Obstet (2005) 88(3):292–8. doi: 10.1016/j.ijgo.2004.12.015
63. Schmid I, Schmitt M, Streiter M, Meilbeck R, Haas RJ, Stachel DK. Effects of soluble TNF receptor II (sTNF-RII), IL-1 receptor antagonist (IL-1ra), tumor load and hypermetabolism on malnutrition in children with acute leukemia. Eur J Med Res (2005) 10(11):457–61.
64. Suruki RY, Mueller N, Hayashi K, Harn D, DeGruttola V, Raker CA, et al. Host immune status and incidence of hepatocellular carcinoma among subjects infected with hepatitis c virus: A nested case-control study in Japan. Cancer Epidemiol Biomarkers Prev (2006) 15(12):2521–5. doi: 10.1158/1055-9965.EPI-06-0485
65. Casasnovas RO, Mounier N, Brice P, Divine M, Morschhauser F, Gabarre J, et al. Plasma cytokine and soluble receptor signature predicts outcome of patients with classical hodgkin's lymphoma: A study from the groupe d'Etude des lymphomes de l'Adulte. J Clin Oncol (2007) 25(13):1732–40. doi: 10.1200/JCO.2006.08.1331
66. Gregorc V, Spreafico A, Floriani I, Colombo B, Ludovini V, Pistola L, et al. Prognostic value of circulating chromogranin a and soluble tumor necrosis factor receptors in advanced nonsmall cell lung cancer. Cancer (2007) 110(4):845–53. doi: 10.1002/cncr.22856
67. Dobrzycka B, Terlikowski SJ, Kowalczuk O, Kinalski M. Circulating levels of TNF-alpha and its soluble receptors in the plasma of patients with epithelial ovarian cancer. Eur Cytokine Netw (2009) 20(3):131–4. doi: 10.1684/ecn.2009.0161
68. Gu Y, Shore RE, Arslan AA, Koenig KL, Liu M, Ibrahim S, et al. Circulating cytokines and risk of b-cell non-Hodgkin lymphoma: A prospective study. Cancer Causes Control (2010) 21(8):1323–33. doi: 10.1007/s10552-010-9560-3
69. Chan AT, Ogino S, Giovannucci EL, Fuchs CS. Inflammatory markers are associated with risk of colorectal cancer and chemopreventive response to anti-inflammatory drugs. Gastroenterology (2011) 140(3):799–808, quiz e11. doi: 10.1053/j.gastro.2010.11.041
70. Dossus L, Becker S, Rinaldi S, Lukanova A, Tjønneland A, Olsen A, et al. Tumor necrosis factor (TNF)-α, soluble TNF receptors and endometrial cancer risk: the EPIC study. Int J Cancer (2011) 129(8):2032–7. doi: 10.1002/ijc.25840
71. Purdue MP, Lan Q, Bagni R, Hocking WG, Baris D, Reding DJ, et al. Prediagnostic serum levels of cytokines and other immune markers and risk of non-hodgkin lymphoma. Cancer Res (2011) 71(14):4898–907. doi: 10.1158/0008-5472.CAN-11-0165
72. Clendenen TV, Lundin E, Zeleniuch-Jacquotte A, Koenig KL, Berrino F, Lukanova A, et al. Circulating inflammation markers and risk of epithelial ovarian cancer. Cancer Epidemiol Biomarkers Prev (2011) 20(5):799–810. doi: 10.1158/1055-9965.EPI-10-1180
73. Grote VA, Kaaks R, Nieters A, Tjønneland A, Halkjær J, Overvad K, et al. Inflammation marker and risk of pancreatic cancer: A nested case-control study within the EPIC cohort. Br J Cancer (2012) 106(11):1866–74. doi: 10.1038/bjc.2012.172
74. Mielczarek-Palacz A, Kondera-Anasz Z, Sikora J. Higher serum levels of tumour necrosis factor and its soluble receptors are associated with ovarian tumours. Arch Med Sci (2012) 8(5):848–53. doi: 10.5114/aoms.2012.31384
75. Hosono K, Yamada E, Endo H, Takahashi H, Inamori M, Hippo Y, et al. Increased tumor necrosis factor receptor 1 expression in human colorectal adenomas. World J Gastroenterol (2012) 18(38):5360–8. doi: 10.3748/wjg.v18.i38.5360
76. Gross AL, Newschaffer CJ, Hoffman-Bolton J, Rifai N, Visvanathan K. Adipocytokines, inflammation, and breast cancer risk in postmenopausal women: A prospective study. Cancer Epidemiol Biomarkers Prev (2013) 22(7):1319–24. doi: 10.1158/1055-9965.EPI-12-1444
77. Poole EM, Lee IM, Ridker PM, Buring JE, Hankinson SE, Tworoger SS. A prospective study of circulating c-reactive protein, interleukin-6, and tumor necrosis factor α receptor 2 levels and risk of ovarian cancer. Am J Epidemiol (2013) 178(8):1256–64. doi: 10.1093/aje/kwt098
78. Nakamura N, Goto N, Tsurumi H, Takemura M, Kanemura N, Kasahara S, et al. Serum level of soluble tumor necrosis factor receptor 2 is associated with the outcome of patients with diffuse large b-cell lymphoma treated with the r-CHOP regimen. Eur J Haematol (2013) 91(4):322–31. doi: 10.1111/ejh.12139
79. Yeung CY, Tso AW, Xu A, Wang Y, Woo YC, Lam TH, et al. Pro-inflammatory adipokines as predictors of incident cancers in a Chinese cohort of low obesity prevalence in Hong Kong. PloS One (2013) 8(10):e78594. doi: 10.1371/journal.pone.0078594
80. Purdue MP, Hofmann JN, Kemp TJ, Chaturvedi AK, Lan Q, Park JH, et al. A prospective study of 67 serum immune and inflammation markers and risk of non-Hodgkin lymphoma. Blood (2013) 122(6):951–7. doi: 10.1182/blood-2013-01-481077
81. Song M, Wu K, Ogino S, Fuchs CS, Giovannucci EL, Chan AT. A prospective study of plasma inflammatory markers and risk of colorectal cancer in men. Br J Cancer (2013) 108(9):1891–8. doi: 10.1038/bjc.2013.172
82. Duvillard L, Ortega-Deballon P, Bourredjem A, Scherrer ML, Mantion G, Delhorme JB, et al. A case-control study of pre-operative levels of serum neutrophil gelatinase-associated lipocalin and other potential inflammatory markers in colorectal cancer. BMC Cancer (2014) 14:912. doi: 10.1186/1471-2407-14-912
83. Ho GY, Wang T, Zheng SL, Tinker L, Xu J, Rohan TE, et al. Circulating soluble cytokine receptors and colorectal cancer risk. Cancer Epidemiol Biomarkers Prev (2014) 23(1):179–88. doi: 10.1158/1055-9965.EPI-13-0545
84. Shiels MS, Katki HA, Hildesheim A, Pfeiffer RM, Engels EA, Williams M, et al. Circulating inflammation markers, risk of lung cancer, and utility for risk stratification. J Natl Cancer Inst (2015) 107(10):djv199. doi: 10.1093/jnci/djv199
85. Purdue MP, Lan Q, Kemp TJ, Hildesheim A, Weinstein SJ, Hofmann JN, et al. Elevated serum sCD23 and sCD30 up to two decades prior to diagnosis associated with increased risk of non-Hodgkin lymphoma. Leukemia (2015) 29(6):1429–31. doi: 10.1038/leu.2015.2
86. Kim C, Zhang X, Chan AT, Sesso HD, Rifai N, Stampfer MJ, et al. Inflammatory biomarkers, aspirin, and risk of colorectal cancer: Findings from the physicians' health study. Cancer Epidemiol (2016) 44:65–70. doi: 10.1016/j.canep.2016.07.012
87. Song M, Mehta RS, Wu K, Fuchs CS, Ogino S, Giovannucci El, et al. Plasma inflammatory markers and risk of advanced colorectal adenoma in women. Cancer Prev Res (Phila) (2016) 9(1):27–34. doi: 10.1158/1940-6207.CAPR-15-0307
88. Ahluwalia MS, Bou-Anak S, Burgett ME, Sarmey N, Khosla D, Dahiya S, et al. Correlation of higher levels of soluble TNF-R1 with a shorter survival, independent of age, in recurrent glioblastoma. J Neurooncol (2017) 131(3):449–58. doi: 10.1007/s11060-016-2319-2
89. Koshiol J, Gao YT, Corbel A, Kemp TJ, Shen MC, Hildesheim A, et al. Circulating inflammatory proteins and gallbladder cancer: Potential for risk stratification to improve prioritization for cholecystectomy in high-risk regions. Cancer Epidemiol (2018) 54:25–30. doi: 10.1016/j.canep.2018.03.004
90. Nomelini RS, Borges Júnior LE, de Lima CA, Chiovato AFC, Micheli DC, Tavares-Murta BM, et al. TNF-R2 in tumor microenvironment as prognostic factor in epithelial ovarian cancer. Clin Exp Med (2018) 18(4):547–54. doi: 10.1007/s10238-018-0508-3
91. Bastard JP, Fellahi S, Audureau É, Layese R, Roudot-Thoraval F, Cagnot C, et al. Elevated adiponectin and sTNFRII serum levels can predict progression to hepatocellular carcinoma in patients with compensated HCV1 cirrhosis. Eur Cytokine Netw (2018) 29(3):112–20. doi: 10.1684/ecn.2018.0413
92. Zhang T, Jiao J, Jiao X, Zhao L, Tian X, Zhang Q, et al. Aberrant frequency of TNFR2(+) treg and related cytokines in patients with CIN and cervical cancer. Oncotarget (2018) 9(4):5073–83. doi: 10.18632/oncotarget.23581
93. Cook MB, Barnett MJ, Bock CH, Cross AJ, Goodman PJ, Goodman GE, et al. Prediagnostic circulating markers of inflammation and risk of oesophageal adenocarcinoma: A study within the national cancer institute cohort consortium. Gut (2019) 68(6):960–8. doi: 10.1136/gutjnl-2018-316678
94. Guerrero CLH, Yamashita Y, Miyara M, Imaizumi N, Kato M, Sakihama S, et al. Proteomic profiling of HTLV-1 carriers and ATL patients reveals sTNFR2 as a novel diagnostic biomarker for acute ATL. Blood Adv (2020) 4(6):1062–71. doi: 10.1182/bloodadvances.2019001429
95. Kim J, Lee J, Oh JH, Chang HJ, Sohn DK, Shin A, et al. Plasma inflammatory biomarkers and modifiable lifestyle factors associated with colorectal cancer risk. Clin Nutr (2020) 39(9):2778–85. doi: 10.1016/j.clnu.2019.12.005
96. Marrone MT, Lu J, Visvanathan K, Joshu CE, Platz EA. Association between pre-diagnostic circulating adipokines and colorectal cancer and adenoma in the CLUE II cohort. Cancer Causes Control (2021) 32(8):871–81. doi: 10.1007/s10552-021-01441-1
97. Wu WY, Späth F, Wibom C, Björkblom B, Dahlin AM, Melin B. Pre-diagnostic levels of sVEGFR2, sTNFR2, sIL-2Rα and sIL-6R are associated with glioma risk: A nested case-control study of repeated samples. Cancer Med (2022) 11(4):1016–25. doi: 10.1002/cam4.4505
98. von Hippel PT. The heterogeneity statistic I(2) can be biased in small meta-analyses. BMC Med Res Methodol (2015) 15:35. doi: 10.1186/s12874-015-0024-z
99. Sterne JA, Sutton AJ, Ioannidis JP, Terrin N, Jones DR, Lau J, et al. Recommendations for examining and interpreting funnel plot asymmetry in meta-analyses of randomised controlled trials. Bmj (2011) 343:d4002. doi: 10.1136/bmj.d4002
100. Kartikasari AE, Huertas CS, Mitchell A, Plebanski M. Tumor-induced inflammatory cytokines and the emerging diagnostic devices for cancer detection and prognosis. Front Oncol (2021) 11. doi: 10.3389/fonc.2021.692142
101. Zeng F, Wei H, Yeoh E, Zhang Z, Ren ZF, Colditz GA, et al. Inflammatory markers of CRP, IL6, TNFα, and soluble TNFR2 and the risk of ovarian cancer: A meta-analysis of prospective studies. Cancer Epidemiol Biomarkers Prev (2016) 25(8):1231–9. doi: 10.1158/1055-9965.EPI-16-0120
102. Dobrzycka B, Terlikowski SJ, Garbowicz M, Niklińska W, Bernaczyk PS, Nikliński J, et al. Tumor necrosis factor-alpha and its receptors in epithelial ovarian cancer. Folia Histochem Cytobiol (2009) 47(4):609–13. doi: 10.2478/v10042-008-0117-1
103. Zhang YW, Chen QQ, Cao J, Xu LQ, Tang X, Wang J, et al. Expression of tumor necrosis factor receptor 2 in human non-small cell lung cancer and its role as a potential prognostic biomarker. Thorac Cancer (2019) 10(3):437–44. doi: 10.1111/1759-7714.12948
104. Haga T, Efird JT, Tugizov S, Palefsky JM. Increased TNF-alpha and sTNFR2 levels are associated with high-grade anal squamous intraepithelial lesions in HIV-positive patients with low CD4 level. Papillomavirus Res (2017) 3:1–6. doi: 10.1016/j.pvr.2016.11.003
105. Yang D, Li R, Wang H, Wang J, Li Y, Wang H, et al. Clinical significance of tumor necrosis factor receptor 2 in middle and lower thoracic esophageal squamous cell carcinoma. Oncol Lett (2018) 16(3):2971–8. doi: 10.3892/ol.2018.8998
106. Liu Z, Ma C, Ma G, Sun X, Liu J, Li S, et al. High expression of tumor necrosis factor receptor 2 in tissue is associated with progression and prognosis of esophageal squamous cell carcinoma. Hum Pathol (2018) 80:179–85. doi: 10.1016/j.humpath.2018.03.027
107. Goto N, Tsurumi H, Takemura M, Hara T, Sawada M, Kasahara S, et al. Serum-soluble tumor necrosis factor receptor 2 (sTNF-R2) level determines clinical outcome in patients with aggressive non-hodgkin's lymphoma. Eur J Haematol (2006) 77(3):217–25. doi: 10.1111/j.1600-0609.2006.00702.x
108. Heemann C, Kreuz M, Stoller I, Schoof N, von Bonin F, Ziepert M, et al. Circulating levels of TNF receptor II are prognostic for patients with peripheral T-cell non-Hodgkin lymphoma. Clin Cancer Res (2012) 18(13):3637–47. doi: 10.1158/1078-0432.CCR-11-3299
109. Tarhini AA, et al. A four-marker signature of TNF-RII, TGF-α, TIMP-1 and CRP is prognostic of worse survival in high-risk surgically resected melanoma. J Transl Med (2014) 12:19. doi: 10.1186/1479-5876-12-19
110. Babic A, Shah SM, Song M, Wu K, Meyerhardt JA, Ogino S, et al. Soluble tumour necrosis factor receptor type II and survival in colorectal cancer. Br J Cancer (2016) 114(9):995–1002. doi: 10.1038/bjc.2016.85
111. Hamaguchi T, Denda T, Kudo T, Sugimoto N, Ura T, Yamazaki K, et al. Exploration of potential prognostic biomarkers in aflibercept plus FOLFIRI in Japanese patients with metastatic colorectal cancer. Cancer Sci (2019) 110(11):3565–72. doi: 10.1111/cas.14198
112. Connor AE, Dibble KE, Boone SD, Baumgartner KB, Baumgartner RN. Systemic inflammation and risk of all-cause mortality after invasive breast cancer diagnosis among Hispanic and non-Hispanic white women from new Mexico. Cancer Epidemiol (2022) 76:102092. doi: 10.1016/j.canep.2021.102092
113. Bulska-Będkowska W, Czajka-Francuz P, Jurek-Cisoń S, Owczarek AJ, Francuz T, Chudek J. The predictive role of serum levels of soluble cell adhesion molecules (sCAMs) in the therapy of advanced breast cancer-a single-centre study. Medicina (Kaunas) (2022) 58(2):153. doi: 10.3390/medicina58020153
114. Silva Raju J, Abd Aziz NH, Atallah GA, Teik CK, Shafiee MN, Mohd Saleh MF, et al. Prognostic value of TNFR2 and STAT3 among high-grade serous ovarian cancer survivors according to platinum sensitivity. Diagnostics (2021) 11(3):526. doi: 10.3390/diagnostics11030526
115. Zeng Q, Gupta A, Xin L, Poon M, Schwarz H, et al. Plasma factors for the differentiation of hodgkin's lymphoma and diffused Large b cell lymphoma and for monitoring remission. J Hematol (2019) 8(2):47–54. doi: 10.14740/jh499
116. Wolny-Rokicka E, Petrasz P, Krajewski W, Sulimiera Michalak S, Tukiendorf A. Analysis of serum markers with regard to treatment procedures in advanced stage prostate cancer patients. Med Sci Monit (2020) 26:e925860. doi: 10.12659/MSM.925860
117. Zamaratskaia G, Mhd Omar NA, Brunius C, Hallmans G, Johansson JE, Andersson SO, et al. Consumption of whole grain/bran rye instead of refined wheat decrease concentrations of TNF-R2, e-selectin, and endostatin in an exploratory study in men with prostate cancer. Clin Nutr (2020) 39(1):159–65. doi: 10.1016/j.clnu.2019.01.007
118. Vladimirova LY, Frantsiyants EM, Samaneva NY, Storozhakova AE, Bandovkina VA, Cheryarina N, et al. Assessment of the risk of tumor progression in different subtypes of breast cancer. Am Soc Clin Oncol (2020), suppl.e12551. doi: 10.1200/JCO.2020.38.15_suppl.e12551
119. Noguchi G, Nakaigawa N, Umemoto S, Kobayashi K, Shibata Y, Tsutsumi S, et al. C-reactive protein at 1 month after treatment of nivolumab as a predictive marker of efficacy in advanced renal cell carcinoma. Cancer Chemother Pharmacol (2020) 86(1):75–85. doi: 10.1007/s00280-020-04088-y
Keywords: circulating biomarker, cancer, sTNFR2, diagnosis, prognosis
Citation: Kartikasari AER, Cassar E, Razqan MAM, Szydzik C, Huertas CS, Mitchell A and Plebanski M (2022) Elevation of circulating TNF receptor 2 in cancer: A systematic meta-analysis for its potential as a diagnostic cancer biomarker. Front. Immunol. 13:918254. doi: 10.3389/fimmu.2022.918254
Received: 12 April 2022; Accepted: 27 October 2022;
Published: 16 November 2022.
Edited by:
Harald Wajant, University Hospital Würzburg, GermanyReviewed by:
Sina Naserian, Hôpital Paul Brousse, FranceMohammad A. I. Al-Hatamleh, Universiti Sains Malaysia (USM), Malaysia
Copyright © 2022 Kartikasari, Cassar, Razqan, Szydzik, Huertas, Mitchell and Plebanski. This is an open-access article distributed under the terms of the Creative Commons Attribution License (CC BY). The use, distribution or reproduction in other forums is permitted, provided the original author(s) and the copyright owner(s) are credited and that the original publication in this journal is cited, in accordance with accepted academic practice. No use, distribution or reproduction is permitted which does not comply with these terms.
*Correspondence: Apriliana E. R. Kartikasari, april.kartikasari@rmit.edu.au; Magdalena Plebanski, magdalena.plebanski@rmit.edu.au