- 1Institute for Regenerative Medicine and Biotherapy (IRMB), Univ Montpellier, Institut national de la santé et de la recherche médicale (INSERM), Montpellier, France
- 2Institut du Cancer Avignon-Provence Sainte Catherine, Avignon, France
- 3Institute for Regenerative Medicine and Biotherapy, Univ Montpellier, Institut national de la santé et de la recherche médicale (INSERM), Centre national de la recherche scientifique (CNRS), Centre hospitalier universitaire (CHU) Montpellier, Montpellier, France
Antibody-dependent cell-mediated cytotoxicity (ADCC) is a potent cytotoxic mechanism that is mainly mediated in humans by natural killer (NK) cells. ADCC mediates the clinical benefit of several widely used cytolytic monoclonal antibodies (mAbs), and increasing its efficacy would improve cancer immunotherapy. CD16a is a receptor for the Fc portion of IgGs and is responsible to trigger NK cell-mediated ADCC. The knowledge of the mechanism of action of CD16a gave rise to several strategies to improve ADCC, by working on either the mAbs or the NK cell. In this review, we give an overview of CD16a biology and describe the latest strategies employed to improve antibody-dependent NK cell cytotoxicity.
Introduction
Immunoglobulins G (IgG) are essential for the immune system, and their characteristics have been harnessed to develop new innovative therapies, particularly in cancers and autoimmune diseases (1). They are composed of 4 chains, 2 heavy and 2 light, forming a Y-shape structure with distinct functions. The (Fab′)2 part is responsible for the specificity of the antibody to its target, and the second moiety, the fragment crystallizable (Fc), could be seen as a communication platform with the immune system. IgG and immune cell interaction take place through a family of receptors: the Fc receptors (FcR). In humans, the FcR family for IgG (FcγR) is composed of 6 receptors: FcγRI/CD64, FcγRIIa/CD32a, FcγRIIb/CD32b, FcγRIIc/CD32c, FcγRIIIa/CD16a, and FcγRIIIb/CD16b. Only CD64 is defined as a high-affinity receptor, while CD32b is the only inhibitory receptor (2, 3).
In humans, 4 subclasses of IgGs exist, IgG1, IgG2, IgG3, and IgG4. The different FcγRs bind with variable affinities to those subclasses (4). CD16a can interact with all of them, although IgG1 and IgG3 show the highest affinity (4).
Natural killer (NK) cells are innate lymphocytes that are very efficient in destroying stressed cells, such as virally infected or tumor-transformed cells (5, 6). Human NK cells mainly express CD16a, while CD16b is restricted to neutrophils (7). Of note, a subset of NK cells has been reported to express all CD32 variants (8). Human NK cells are divided into two main subsets: CD56bright and CD56dim. CD56bright NK cells are competent cytokine secretors but lack CD16a (9). CD56dim are highly cytotoxic and express CD16a (10, 11). Upon recognition of IgG-opsonized targets through CD16a, NK cells trigger a potent cytotoxic mechanism called antibody-dependent cell-mediated cytotoxicity (ADCC), leading to the death of the target cell. This mechanism relies on the formation of an immunological synapse and the degranulation of lytic granules containing perforin and granzymes (12). Besides degranulation, NK cells can also eliminate target cells by engaging target death receptors, e.g., DR4, DR5, or Fas, with their death receptors ligands, e.g., FasL and TRAIL (13).
Cellular therapies based on T lymphocytes, more precisely on Chimeric Antigen Receptor (CAR) T cells, recently became an important weapon in the anticancer arsenal, with good efficiency in hematological cancers. However, achieving success in solid cancers is more challenging (14). Moreover, CAR T-cell therapy could induce very serious adverse events, such as graft-versus-host disease (GvHD), neurotoxicity, or cytokine release syndrome (15). Interestingly, NK cells do not induce them (16). Nevertheless, there are still some limitations to their large-scale use in clinics (17), and hence, there is a need to improve their clinical efficiency, in particular on ADCC to release the full clinical capacity of monoclonal antibodies (mAbs).
Here, we first review the basic knowledge of CD16a, and second, we show how this fundamental knowledge helps increase ADCC activity and present promising advancements for improving immunotherapy.
CD16a Biology
Genetic
CD16a is encoded by FCGR3A gene, which is located on the long arm of chromosome 1, in the 1q23 region. Two functional polymorphisms of the CD16a have been described, 158 V/F (18) and 48L/H/R (19), sometimes called 176 V/F and L66/H/R, respectively, depending on when counted from the N-terminal of the mature protein or not. The first polymorphism is due to a nucleotide substitution T to G at position 559. The second is due to two possible different substitutions at position 230, either T to G or T to A. The 48L genotype was first described to have a lower binding to IgG than the 48R or 48H genotype (19). Later, it was shown that the differences are due to the 158 V/F genotype rather than the L48/H/R genotype (20, 21). However, there is a link between 158V/F and 48L/H/R genotypes, with 48L being preferentially expressed together with 158F and 48H or 48R with 158V (22). CD16a-bearing 158V phenotype shows a superior binding to IgG (20, 21). This increase in affinity has at least two consequences. First, CD16a 158V shows a better ADCC in vitro (23) and gives a better clinical response to rituximab therapy, most likely through ADCC (21, 24). Second, V/V patients display a faster clearance of rituximab during their treatment, possibly due to the better recognition by CD16a 158V (25).
It was believed that the 158V/F polymorphism could impact the amount of CD16a expressed by NK cells, and being 158V, the one expressing more CD16a (26). However, a subsequent study showed that depending on the clone of anti-CD16 used on cytometry, certain differences can arise (27). In conclusion, the amount of CD16a expressed by NK cells is probably not related to the 158V/F genotype.
Structure
CD16a is a transmembrane receptor with a short C-ter cytoplasmic tail and possesses two extracellular Ig-like domains (28). It does not possess any signaling component in its intracellular part. Thus, to transduce signals, it needs two immunoreceptor tyrosine-based activation motif (ITAM)-bearing signaling chains, as described later.
CD16a/IgG Interaction
CD16a interacts in a 1:1 stoichiometry fashion with the lower hinge/upper CH2 of IgG. The Fc N-glycan chain, linked to the N297 of the CH2, also plays a critical role in this interaction (29, 30). Thus, not only the amino acid (31) but also the glycan composition (32) can greatly influence the affinity of CD16a for the Fc and consequently the potency of ADCC.
Besides IgG Fc interaction with CD16a, recent data support that the Fab could be implicated in the IgG/CD16a interaction (33, 34). Moreover, mutations in the Fab seem to modulate ADCC, highlighting the potential interaction of Fab-CD16a during IgG-CD16a binding (35).
Glycosylation of CD16a
The Fc N297-linked glycan has a critical role in its interaction with CD16a (32). Less is known about the role of CD16a glycosylation and how it could impact the affinity for IgG. CD16a is decorated with 5 asparagine(N)-linked glycan at N38, N45, N74, N162, and N169 (7). The N-glycan composition of CD16a has been only recently solved, due to the difficulty to have enough material. Among the 3 donors studied, the N-glycans are composed of 23% of the high-mannose structure, 22% of hybrid type structure, and the remaining (55%) being complex type N-glycans (36). However, only the N162 N-glycan seems to directly impact CD16a affinity toward IgG, conversely with its position in the IgG-binding site (29, 30). Although the N45 N-glycan is not situated in the IgG-binding site, it also contributes to the binding to IgG (37) by stabilizing the CD16a structure (38, 39).
Finally, CD16a 48L/H polymorphism, which is close to the N45 glycan site, modulates the composition of its glycosylation (40) and potentially CD16a function. With the 158F/V polymorphism being also close to the N162 glycan, it is tempting to postulate that the same kind of relationship between amino acid sequence and N-glycosylation pattern could exist.
Signaling Pathway
NK cell cytotoxicity is regulated by a plethora of inhibitory or activating receptors. When NK cells interact with a potential target cell, they receive activating and inhibiting signals through those receptors. If the balance is in favor of inhibition, the target cell will survive; if not, the cell will be killed (41). It is worth noting that some inhibitory signals are very strong and need a large involvement of activating receptors to be overcome (42). CD16a probably provides the strongest signals and can overcome the inhibitory signals. The engaged CD16a forms clusters in lipid rafts (43), a cholesterol-rich lipidic microdomain structure, and hence, CD16a signaling takes place in them (44), enabling proper intracellular signal (45, 46).
As described previously, CD16a does not possess any ITAM domain in its cytoplasmic tail and thus needs the help of a tandem of two intracellular chains bearing ITAM domains, CD3ζ and FcϵRIγ (47–49), which are indistinctly used (50). Noteworthy, the two signaling chains are covalently associated between them, but not to CD16a (51). A unique feature of the intracytoplasmic tail of CD16a, compared to other FcγR, is the possibility to be phosphorylated by PKC (52). The downstream signaling pathway of phosphorylated CD16a favors cytokine production, while the unphosphorylated leads to stronger degranulation (52).
Upon CD16a engagement, a kinase belonging to the Src family, Lck, becomes activated (53) and phosphorylates the ITAM domains of CD3ζ and/or FcϵRIγ (54). The phosphorylated ITAMs allow the recruitment and the phosphorylation of kinases from the Syk family (55), such as Syk (56) and ZAP-70 (54), which are in turn responsible for the subsequent signaling. Among their substrates, PI3K is highly relevant (57, 58), because it converts PIP2 to PIP3, which is processed by PLC-γ (55) releasing IP3 and DAG (59). DAG activates the PKC family, which contributes to the triggering of degranulation. IP3 induces calcium release from the endoplasmic reticulum to the cytosol (59). This calcium influx is one of the major signals for ADCC triggering and also allows NFAT translocation in the nucleus, inducing transcription of its target genes (60). There are other pathways activated by CD16a engagement that contribute to ADCC, e.g., the ERK2 MAPK pathway.
Downregulation
After CD16a-dependent NK cell cytotoxicity, CD16a is rapidly downregulated. One of the mechanisms involved in the shedding of CD16a is mediated by a disintegrin and metalloprotease 17 (ADAM17) (61), which is expressed on NK cells. This metalloprotease cleaves the stalk region of CD16a between Ala195 and Val196 (62). The cleaving of CD16a by ADAM17 occurs in cis. This means that an ADAM17 expressing NK cell cannot induce CD16a shedding on another NK cell (62). Another mechanism of CD16a downregulation after activation is internalization, which occurs not only to CD16a but also to other intracellular signaling components such as CD3ζ, ZAP-70, and Syk. They are ubiquitinated, probably leading to their degradation (46, 62–64).
Improving Natural Killer Cell Antibody-Dependent Cell-Mediated Cytotoxicity
The strategies described in this section are summarized in Figure 1.
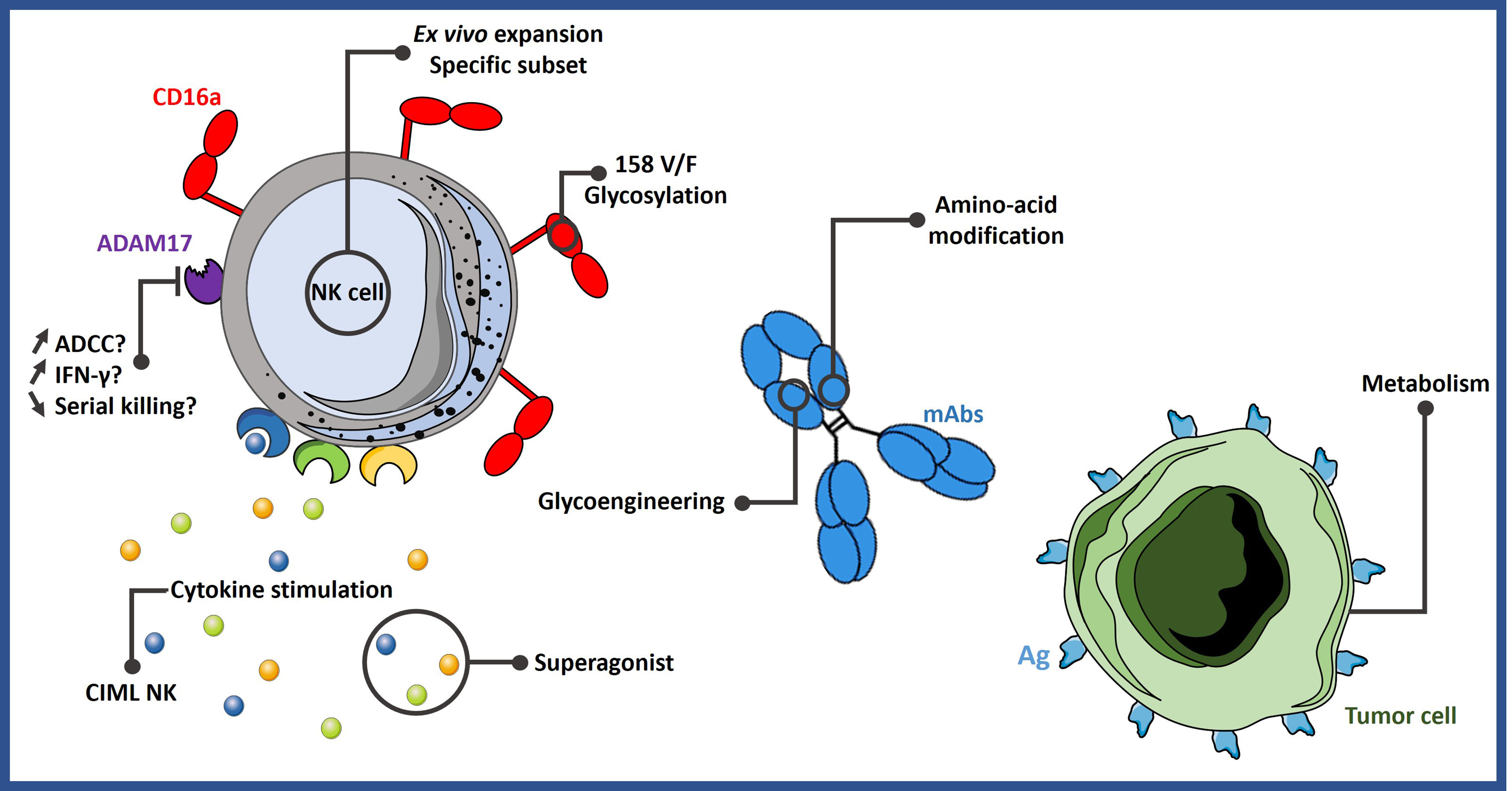
Figure 1 Different strategies to improve ADCC. NK cell-mediated ADCC can be enhanced by expanding them with specific protocols or by stimulating them with certain cytokine cocktails. It could be possible to select more potent NK cell subsets based on their phenotype or genotype. Engineering mAbs are also an efficient way to increase ADCC activity. Lastly, tumor cells can be sensitized to NK cell activity by modifying their metabolism. ADCC, antibody-dependent cell-mediated cytotoxicity; Ag, antigen; CIML NK, memory-like natural killer cells; mAbs, monoclonal antibodies.
Making Highly Active Natural Killer Cells for Therapy
NK cell engraftment has been successfully performed in several clinical assays, for example, with the infusion of cytokine-induced memory-like (CIML) NK cells (65, 66). The most utilized cocktail contains IL-12, IL-15, and IL-18 (67, 68). CIML NK cells express more IFN-γ than conventional NK and show superior cytotoxicity against leukemia cells and primary acute myeloid leukemia (AML) blasts (65). Moreover, haploidentical CIML NK transfused in relapsed or refractory AML patients exhibited anti-leukemia function (65). CIML NK transfusion for relapsed pediatric AML patients post-hematopoietic cell transplant achieved complete remission in 4 out of 8 patients without significant toxicities (66), highlighting the potential of this strategy to treat leukemia. However, a strong effort is currently done to further expand these cells ex vivo before infusion in allogeneic settings. Since NK cell expansion has recently been reviewed (69, 70), we shortly comment on it. Primary NK cells can be expanded from peripheral blood or umbilical cord blood, usually using feeder cells. The culture media is usually supplemented with various cytokines, including IL-15 and IL-2, and accessory cells. NK cells have also been generated from induced pluripotent stem cells (iPSCs). In general, the resulting expanded NK cells show high cytotoxic activities. For clinical purposes, i.e., to mediate ADCC, it is interesting to produce NK cells with high CD16a expression, such as the protocol that we have recently described (71), which produces cells with efficient ADCC against primary tumor cells (72, 73).
Recently a new subset of NK cells has been described in healthy individuals with prior exposure to human cytomegalovirus (HCMV). HCMV infection induces a peculiar subset of NK cells, which are mainly characterized by the absence of the FcϵRIγ (74–76), one of the two chains responsible for CD16a signaling, as described before. Although these cells express a lower amount of natural cytotoxic receptors and thus show weak responsiveness toward the K562 tumor cell line compared to FcϵRIγ+ NK cells (74), they display an increased activity through Ab-dependent stimulation (74) and ADCC (75). Recently, a method to ex vivo expand these so-called “g-NK” cells has been published (77). Although the expansion is only possible from previously HCMV-infected donors, the resulting cells possess some interesting features. Consistent with previous research, g-NK cells induce stronger ADCC than conventional NK cells. Also, whereas NK cells express relatively high CD38 levels, g-NK cells have the particularity to express low or no CD38. This ectoenzyme is the target for the clinical mAb daratumumab (DARZALEX) used in multiple myeloma treatment (78). Although daratumumab shows benefit in the clinic, the patient’s NK cell count rapidly decreases during treatment (79). Thus, the uncommon g-NK cells phenotype is an advantage for their use in these patients. Taken together, g-NK cells could be a powerful anticancer agent when utilized in combination with cytolytic mAbs.
Cytokine Priming
A simple way to improve ADCC activity is to stimulate NK cells with pro-inflammatory cytokines. IL-18 improves CD16a-induced IFN-γ production by NK cells after incubation on IgG-coated dishes. Moreover, IL-18-treated NK cells show increased ADCC with rituximab against the CD20+ Raji cell line, in vitro and in vivo (80).
IL-12 also increases IFN-γ and TNF-α production by NK cells after encountering trastuzumab-opsonized SKBR3 cells. Interestingly, a set of genes uniquely regulated by this co-stimulation have been identified. Among them, granzyme B was upregulated 10 times (81). IL-12 increases CD25 expression on NK cells (82). CD25 is the alpha subunit of the IL-2 receptor (IL-2Rα), which is composed of 3 subunits. The CD25/IL-2Rα is the subunit with the highest affinity for IL-2. The expression of CD25 by IL-12+ immobilized IgG-stimulated NK cells made them more sensitive to IL-2, particularly at low doses. In a phase I/II clinical trial, cetuximab was used along with IL-12 on patients suffering from unresectable or recurrent head and neck squamous cell carcinoma (83). Patients having experienced progression-free survival (PFS) longer than 100 days had their NK cells exerting more ADCC ex vivo as compared to patients with PFS shorter than 100 days. Thus, IL-12 priming of NK cells seems to increase their activity, particularly ADCC, even in treated patients. Remarkably, NK cells from these patients showed increased CD25 expression.
IL-15 is a cytokine essential for the survival and function of NK and CD8+ T cells (84). The IL-15 receptor is composed of 3 subunits, IL-15Rα (CD215), IL-15Rβ (CD122), and IL-15Rγ (CD132). The IL-15Rα is a high-affinity receptor for IL-15, although it is not expressed by NK cells but rather by antigen-presenting cells (APCs), such as monocytes and dendritic cells. APCs use the IL-15Rα to transpresent IL-15 to NK cells expressing the two other subunits (85). An IL-15 superagonist was described using this IL-15Rα subunit (86). This fusion protein between IL-15 and IL-15Rα displays stronger activity than the native IL-15 (86). Both native IL-15 and the IL-15/IL-15Rα construct have reached clinical trials. A 5-day IL-15 intravenous administration to various solid cancer patients leads to a 34-fold increase in NK cell counts (87). Treatment also increased NK cell-mediated ADCC ex vivo. This short-term IL-15 administration was well tolerated. Other recent clinical trials are using the fusion protein IL-15/IL-15Rα, such as NIZ985 in a phase I dose-escalating study as single-agent therapy in advanced solid cancers patients (88). Following treatment, NK cells and CD8+ T lymphocytes proliferated, and this was associated with increased plasma levels of IFN-γ, IL-18, and CXCL10. NKTR-255 is a polyethylene glycol-conjugated human IL-15, designed in an attempt to increase the pharmacokinetics (PK) of IL-15 while retaining the ability to interact with its receptor (89). In an in vivo model, NKTR-255 showed a better PK and an increase of granzyme B and CD107a positive NK cells compared to IL-15 or IL-15 complexed with IL-15Rα, as well as better survival in mice bearing Daudi lymphoma xenografts. NKTR-255 is currently investigated in a phase I trial as monotherapy or in combination with daratumumab or rituximab for the treatment of refractory multiple myeloma or non-Hodgkin’s lymphoma, respectively (NCT04136756). N-803, previously known as ALT-803, is a mutated N72D superagonist IL-15 bound to IL-15Rα and fused to an IgG1 Fc that showed enhanced NK cells activity in vitro and in vivo in preclinical models (90, 91). Recently, the PK and safety of N-803 have been assessed in healthy volunteers. It did not produce adverse events and persisted in circulation ~10-fold longer as compared to IL-15. Moreover, it increased NK and CD8 T-cell numbers (92). N-803 has been used instead of IL-2 to support cytokine-primed allogeneic NK cells transfer to AML patients in a relapse in two separate clinical trials (NCT03050216 and NCT01898793). Unexpectedly, the report stated that the use of N-803 instead of IL-2 led to reduced clinical activity (93). The authors showed that N-803 promotes recipient CD8+ T-cell activation, inducing a decrease in allogeneic NK cell persistence through rejection. Although IL-15-based immunotherapy could be seen as a promising strategy to support NK cell therapy, the best clinical protocol remains to be described.
ADAM17 Inhibition: A Double-Edged Sword?
As described above, ADAM17 is the main driver of CD16a downregulation after activation. One strategy to improve NK cell ADCC is to prevent CD16a shedding by targeting ADAM17. Some conflicting reports are published. A patient with a rare genetic deficiency resulting in an absence of expression of ADAM17 provided some insights into the relevance of this strategy (94). The patient’s NK cells showed a strong defect in cytokine secretion, while the response to CD16a engagement was similar to that of 2 healthy donors, in terms of IFN-γ production as well as ADCC efficiency. However, blocking ADAM17 activity in NK cells from healthy donors, by either a chemical or an antibody, increases IFN-γ production after antibody stimulation (61, 95). Similarly, others published that ADAM17 knock-out using CRISPR/Cas9 technology in purified NK cells from peripheral blood mononuclear cell (PBMC) shows better IFN-γ production and ADCC activity in vitro and in vivo (96). It is possible that the constitutive deficiency of ADAM17 in that patient-generated NK cells does not depend on this enzyme. Nevertheless, ADAM17 blocking by chemicals has been shown to reduce the survival of the NK cells as well as the CD16a-mediated serial killing, the cells being unable to detach from the target cells, impeding their motility and preventing them to go to another target (97).
In conclusion, the benefit of ADAM17 inhibition is still unclear. The success of this strategy will be unveiled by a clinical trial in process (NCT04023071) where the use of iPSC-derived NK cells bearing non-cleavable CD16a (98) will be assessed in AML and B-cell lymphoma.
Working on Monoclonal Antibodies
As a well-characterized protein, IgGs have been modified in their amino acid sequence to increase the Fc affinity to CD16a and, subsequently, improve ADCC (31, 99–101). Some mutations became “famous,” for example, the so-called GASDALIE, consisting of 4 substitutions in the Fc: G236A/S239D/A330L/I332E (102). This mutation shows a great increase of affinity to CD16a with almost no increase in CD32b affinity, which is the only inhibitory FcγR (103). Another mutation is referred to as Variant 18 (F243L/R292P/Y300L/V305I/P396L) and showed a remarkable increase in ADCC (104). These mutations have now reached the clinic as an anti-HER2 antibody, the margetuximab, and showed good results in phase I for the treatment of various HER2-positive carcinomas (105). Margetuximab is currently tested in breast cancer patients with chemotherapy versus trastuzumab plus chemotherapy (phase III SOPHIA trial). The preliminary results showed an increase in PFS for margetuximab compared to trastuzumab (106).
As stated previously, the N297 situated in the CH2 of the Fc region is linked to an N-glycan chain, which is extremely important for the interaction with all FcγRs, as aglycosylated IgGs cannot interact with them (107). Thus, glycoengineering the IgG Fc N-glycan holds great promise to increase affinity to CD16a (32). The most studied glycomodification is afucosylation, where the fucose attached to the first N-acetyl-glucosamine of the N-glycan chain is absent. This modification leads to an increased binding to CD16a and an improvement in ADCC (108). This has been used to generate the anti-CD20 antibody obinutuzumab (previously GA101), which displayed high efficacy in vitro and in vivo on monkeys (109). Moreover, afucosylation increases effector function through CD16a only if a certain amount of CD16a is expressed, such as on NK cells, in opposition with macrophages (110). It has also been described that afucosylated antibodies increase the IFN-γ secretion, as well as the serial killing (111). Finally, obinutuzumab reached the market under the brand name GAZYVARO in 2013 in the United States and in 2014 in Europe.
Natural Killer Cell Engagers
Apart from changes in the amino acid sequence and glycoengineering of Fc IgG, it is also possible to work on the format of the antibodies. Based on the success of the bispecific T-cell engager (BiTE) strategy, a similar format was developed for NK cells, the so-called bispecific Killer cell engager (BiKE). This format consists of two single-chain fragment variables (scFv) linked between them through a linker. One scFv targets CD16a, while the other targets a tumor antigen (112). The trispecific Killer cell engager (TRiKE) format also exists, in which 2 tumor antigens are targeted along with CD16a, even if others add IL-15 in place of the third scFv (112, 113). The use of such a construct allows a retargeting of NK cells to the tumor cells and leads to a strong ADCC against target cells. The majority of BiKE/TRiKE are developed for hematological cancers, such as the CD16xCD33 BiKE, which shows efficient ADCC against samples from AML patients and can reactivate patient NK cells (114). AFM13 is a tetravalent bispecific antibody targeting CD16a and CD30, which has shown interesting results in a phase Ib trial in combination with pembrolizumab for treatment of refractory or relapsed Hodgkin lymphoma (115). Four other clinical trials are scheduled for AFM13 (115). GTB-3550 is a TRiKE CD16/CD33/IL-15 that is currently being studied in a phase I/II trial for several types of leukemia (NCT03214666).
Researchers and clinicians are also trying to develop NK cell engagers for solid tumors. A TRiKE CAM1615HER2, which contains a VHH targeting CD16a, an scFv for HER2 and IL-15, shows an increase in NK cell proliferation and activation in vitro and tumor clearance in vivo (116). AFM24 is a tetravalent bispecific antibody, composed of one full-length IgG with two scFv linked on the C-terminal of the CH3, which targets CD16a and EGFR. It showed better activity than cetuximab in ADCC, regardless of the mutational status of the cell line. In monkeys, AFM24 seems to be harmless (117). Taken together, the NK cell engager/retargeting strategy seems promising by increasing NK cell activity, in particular regarding ADCC.
Increasing Natural Killer Cell Activity Through Metabolism: The Newest Field in Immunotherapy
It is known that metabolism dysregulation is a key driver of immunosuppression in the tumor microenvironment and also a cause of immunotherapy resistance (118). Many teams have shown that immune cell metabolism is critical for their function (119, 120). Recently, reports about phenotypic changes in leukemia cell lines through metabolic drugs show promising results regarding NK cell activity. Metformin, a drug originally used to treat type 1 diabetes, increases the expression of ligands, notably ICAM-1, which is recognized by lymphocyte function-associated antigen 1 (LFA-1). This is an integrin that also modulates lymphocyte intracellular signaling (121), including in NK cells (122, 123). Although metformin alone was not toxic for the cells, the combination with UCB-expanded NK cells (71) showed higher tumor cell clearance in vitro and in vivo (124). Another report shows that dichloroacetate (DCA), an inducer of oxidative phosphorylation (OXPHOS) metabolism, increases several stress ligands on leukemic cell lines, sensitizing them to NK natural cytotoxicity. However, this mechanism is linked to the p53 mutational status (125). Although these two reports highlight the potentiation of NK natural cytotoxicity on tumor cells by metabolic drugs, it is possible that this could increase as well ADCC. Further studies are needed to verify this.
Another strategy is to directly modulate NK cell metabolism. cMyc is a critical factor that regulates the metabolic machinery supporting glycolysis and OXPHOS in mouse NK cells. Lack of cMyc results in an impaired NK cell response (126). Glycogen synthase kinase-3 (GSK3) can mediate cMyc degradation in murine NK cells (126). Consistent with this, GSK3 overexpression has been detected in NK cells from AML patients, and this is linked to impaired cytotoxicity against AML cells. GSK3 inhibitors restore the NK cell activity in these AML patients against AML cell lines and primary AML cells. Moreover, expanded NK cells from donors treated with GSK3 inhibitors show superior activity in a mouse xenograft model of AML (127). Finally, NK cells treated with a GSK3 inhibitor during expansion resulted in enhanced tumor clearance in a xenograft mouse model of a human ovarian cancer cell line (128). As a promising approach, several clinical trials evaluated the efficacy of the GSK3 inhibitor LY2090314 in solid and hematological cancers (NCT01632306, NCT01287520, and NCT01214603). To our knowledge, the results are not published yet.
Conclusion
Immunotherapy has shown exciting results in cancer patients, particularly for hematological malignancies. However, further progresses are still needed to achieve further success in solid cancer. Even being a long-last studied FcγR, the CD16a has still some features that need to be unraveled. With the advancement in technology, new findings and subsequent clinical approaches are expected. This knowledge will allow us to select the most suitable NK cells bearing the most efficient CD16a to exploit the full potential of clinical mAbs.
Author Contributions
All authors were involved in preparing and writing the manuscript.
Funding
This work was supported by INCA/DGOS PRT-K program 2021 (MV; 2021-014) and from the 2021 AAP Companies On Campus by the MUSE (Montpellier Université d’Excellence (MV). LC is a recipient of a fellowship from MRT. This work was also supported by the “Investissements d’avenir” Grant LabEx MAbImprove: ANR-10-LABX-53 (MV).
Conflict of Interest
The authors declare that the research was conducted in the absence of any commercial or financial relationships that could be construed as a potential conflict of interest.
Publisher’s Note
All claims expressed in this article are solely those of the authors and do not necessarily represent those of their affiliated organizations, or those of the publisher, the editors and the reviewers. Any product that may be evaluated in this article, or claim that may be made by its manufacturer, is not guaranteed or endorsed by the publisher.
Acknowledgments
We acknowledge the imaging facility MRI, a member of the National Infrastructure France-BioImaging supported by the French National Research Agency (ANR-10-INBS-04, Investments for the future).
References
1. Lu R-M, Hwang Y-C, Liu I-J, Lee C-C, Tsai H-Z, Li H-J, et al. Development of Therapeutic Antibodies for the Treatment of Diseases. J BioMed Sci (2020) 27:1. doi: 10.1186/s12929-019-0592-z
2. Nimmerjahn F, Ravetch JV. Fcγ Receptors as Regulators of Immune Responses. Nat Rev Immunol (2008) 8:34–47. doi: 10.1038/nri2206
3. Bolland S, Ravetch JV. Inhibitory Pathways Triggered by ITIM-Containing Receptors. Adv Immunol (1999) 72:149–77. doi: 10.1016/s0065-2776(08)60019-x
4. Bruhns P, Iannascoli B, England P, Mancardi DA, Fernandez N, Jorieux S, et al. Specificity and Affinity of Human Fcgamma Receptors and Their Polymorphic Variants for Human IgG Subclasses. Blood (2009) 113:3716–25. doi: 10.1182/blood-2008-09-179754
5. Savoy SKA, Boudreau JE. The Evolutionary Arms Race Between Virus and NK Cells: Diversity Enables Population-Level Virus Control. Viruses (2019) 11:E959. doi: 10.3390/v11100959
6. Kim S, Iizuka K, Aguila HL, Weissman IL, Yokoyama WM. In Vivo Natural Killer Cell Activities Revealed by Natural Killer Cell-Deficient Mice. Proc Natl Acad Sci U.S.A. (2000) 97:2731–6. doi: 10.1073/pnas.050588297
7. Ravetch JV, Perussia B. Alternative Membrane Forms of Fc Gamma RIII(CD16) on Human Natural Killer Cells and Neutrophils. Cell Type-Specific Expression of Two Genes That Differ in Single Nucleotide Substitutions. J Exp Med (1989) 170:481–97. doi: 10.1084/jem.170.2.481
8. Dutertre C-A, Bonnin-Gélizé E, Pulford K, Bourel D, Fridman W-H, Teillaud J-L. A Novel Subset of NK Cells Expressing High Levels of Inhibitory FcgammaRIIB Modulating Antibody-Dependent Function. J Leukoc Biol (2008) 84:1511–20. doi: 10.1189/jlb.0608343
9. Cooper MA, Fehniger TA, Turner SC, Chen KS, Ghaheri BA, Ghayur T, et al. Human Natural Killer Cells: A Unique Innate Immunoregulatory Role for the CD56(bright) Subset. Blood (2001) 97:3146–51. doi: 10.1182/blood.v97.10.3146
10. Lanier LL, Le AM, Civin CI, Loken MR, Phillips JH. The Relationship of CD16 (Leu-11) and Leu-19 (NKH-1) Antigen Expression on Human Peripheral Blood NK Cells and Cytotoxic T Lymphocytes. J Immunol (1986) 136:4480–6.
11. Nagler A, Lanier LL, Cwirla S, Phillips JH. Comparative Studies of Human FcRIII-Positive and Negative Natural Killer Cells. J Immunol (1989) 143:3183–91.
12. Orange JS. Formation and Function of the Lytic NK-Cell Immunological Synapse. Nat Rev Immunol (2008) 8:713–25. doi: 10.1038/nri2381
13. Tuomela K, Ambrose AR, Davis DM. Escaping Death: How Cancer Cells and Infected Cells Resist Cell-Mediated Cytotoxicity. Front Immunol (2022) 13:867098. doi: 10.3389/fimmu.2022.867098
14. Dana H, Chalbatani GM, Jalali SA, Mirzaei HR, Grupp SA, Suarez ER, et al. CAR-T Cells: Early Successes in Blood Cancer and Challenges in Solid Tumors. Acta Pharm Sin B (2021) 11:1129–47. doi: 10.1016/j.apsb.2020.10.020
15. Neelapu SS, Tummala S, Kebriaei P, Wierda W, Gutierrez C, Locke FL, et al. Chimeric Antigen Receptor T-Cell Therapy - Assessment and Management of Toxicities. Nat Rev Clin Oncol (2018) 15:47–62. doi: 10.1038/nrclinonc.2017.148
16. Olson JA, Leveson-Gower DB, Gill S, Baker J, Beilhack A, Negrin RS. NK Cells Mediate Reduction of GVHD by Inhibiting Activated, Alloreactive T Cells While Retaining GVT Effects. Blood (2010) 115:4293–301. doi: 10.1182/blood-2009-05-222190
17. Villalba M, Alexia C, Bellin-Robert A, Fayd’herbe de Maudave A, Gitenay D. Non-Genetically Improving the Natural Cytotoxicity of Natural Killer (NK) Cells. Front Immunol (2019) 10:3026. doi: 10.3389/fimmu.2019.03026
18. Wu J, Edberg JC, Redecha PB, Bansal V, Guyre PM, Coleman K, et al. A Novel Polymorphism of FcgammaRIIIa (CD16) Alters Receptor Function and Predisposes to Autoimmune Disease. J Clin Invest (1997) 100:1059–70. doi: 10.1172/JCI119616
19. de Haas M, Koene HR, Kleijer M, de Vries E, Simsek S, van Tol MJ, et al. A Triallelic Fc Gamma Receptor Type IIIA Polymorphism Influences the Binding of Human IgG by NK Cell Fc Gamma RIIIa. J Immunol (1996) 156:2948–55.
20. Koene HR, Kleijer M, Algra J, Roos D, von dem Borne AE, de Haas M. Fc gammaRIIIa-158v/F Polymorphism Influences the Binding of IgG by Natural Killer Cell Fc gammaRIIIa, Independently of the Fc gammaRIIIa-48l/R/H Phenotype. Blood (1997) 90:1109–14. doi: 10.1182/blood.V90.3.1109
21. Treon SP, Hansen M, Branagan AR, Verselis S, Emmanouilides C, Kimby E, et al. Polymorphisms in FcgammaRIIIA (CD16) Receptor Expression are Associated With Clinical Response to Rituximab in Waldenström’s Macroglobulinemia. J Clin Oncol (2005) 23:474–81. doi: 10.1200/JCO.2005.06.059
22. Pérez-Romero CA, Sánchez IP, Naranjo-Piedrahita L, Orrego-Arango JC, Muskus-López CE, Rojas-Montoya W, et al. Frequency Analysis of the G.7081T>G/A and G.10872T>G Polymorphisms in the FCGR3A Gene (CD16A) Using Nested PCR and Their Functional Specific Effects. Genes Immun (2019) 20:39–45. doi: 10.1038/s41435-017-0001-0
23. Dall’Ozzo S, Tartas S, Paintaud G, Cartron G, Colombat P, Bardos P, et al. Rituximab-Dependent Cytotoxicity by Natural Killer Cells: Influence of FCGR3A Polymorphism on the Concentration-Effect Relationship. Cancer Res (2004) 64:4664–9. doi: 10.1158/0008-5472.CAN-03-2862
24. Cartron G, Dacheux L, Salles G, Solal-Celigny P, Bardos P, Colombat P, et al. Therapeutic Activity of Humanized Anti-CD20 Monoclonal Antibody and Polymorphism in IgG Fc Receptor FcgammaRIIIa Gene. Blood (2002) 99:754–8. doi: 10.1182/blood.v99.3.754
25. Tout M, Gagez A-L, Leprêtre S, Gouilleux-Gruart V, Azzopardi N, Delmer A, et al. Influence of FCGR3A-158v/F Genotype and Baseline CD20 Antigen Count on Target-Mediated Elimination of Rituximab in Patients With Chronic Lymphocytic Leukemia: A Study of FILO Group. Clin Pharmacokinet (2017) 56:635–47. doi: 10.1007/s40262-016-0470-8
26. Hatjiharissi E, Xu L, Santos DD, Hunter ZR, Ciccarelli BT, Verselis S, et al. Increased Natural Killer Cell Expression of CD16, Augmented Binding and ADCC Activity to Rituximab Among Individuals Expressing the Fc{gamma}RIIIa-158 V/V and V/F Polymorphism. Blood (2007) 110:2561–4. doi: 10.1182/blood-2007-01-070656
27. Congy-Jolivet N, Bolzec A, Ternant D, Ohresser M, Watier H, Thibault G. Fc Gamma RIIIa Expression Is Not Increased on Natural Killer Cells Expressing the Fc Gamma RIIIa-158V Allotype. Cancer Res (2008) 68:976–80. doi: 10.1158/0008-5472.CAN-07-6523
28. Woof JM, Burton DR. Human Antibody-Fc Receptor Interactions Illuminated by Crystal Structures. Nat Rev Immunol (2004) 4:89–99. doi: 10.1038/nri1266
29. Sondermann P, Huber R, Oosthuizen V, Jacob U. The 3.2-A Crystal Structure of the Human IgG1 Fc Fragment-Fc gammaRIII Complex. Nature (2000) 406:267–73. doi: 10.1038/35018508
30. Radaev S, Motyka S, Fridman WH, Sautes-Fridman C, Sun PD. The Structure of a Human Type III Fcgamma Receptor in Complex With Fc. J Biol Chem (2001) 276:16469–77. doi: 10.1074/jbc.M100350200
31. Shields RL, Namenuk AK, Hong K, Meng YG, Rae J, Briggs J, et al. And FcRn and Design of IgG1 Variants With Improved Binding to the Fc Gamma R. J Biol Chem (2001) 276:6591–604. doi: 10.1074/jbc.M009483200
32. Dekkers G, Treffers L, Plomp R, Bentlage AEH, de Boer M, Koeleman CAM, et al. Decoding the Human Immunoglobulin G-Glycan Repertoire Reveals a Spectrum of Fc-Receptor- and Complement-Mediated-Effector Activities. Front Immunol (2017) 8:877. doi: 10.3389/fimmu.2017.00877
33. Yogo R, Yamaguchi Y, Watanabe H, Yagi H, Satoh T, Nakanishi M, et al. The Fab Portion of Immunoglobulin G Contributes to its Binding to Fcγ Receptor III. Sci Rep (2019) 9:11957. doi: 10.1038/s41598-019-48323-w
34. Shi L, Liu T, Gross ML, Huang Y. Recognition of Human IgG1 by Fcγ Receptors: Structural Insights From Hydrogen-Deuterium Exchange and Fast Photochemical Oxidation of Proteins Coupled With Mass Spectrometry. Biochemistry (2019) 58:1074–80. doi: 10.1021/acs.biochem.8b01048
35. Sun Y, Izadi S, Callahan M, Deperalta G, Wecksler AT. Antibody-Receptor Interactions Mediate Antibody-Dependent Cellular Cytotoxicity. J Biol Chem (2021) 297:100826. doi: 10.1016/j.jbc.2021.100826
36. Patel KR, Roberts JT, Subedi GP, Barb AW. Restricted Processing of CD16a/Fc γ Receptor IIIa N-Glycans From Primary Human NK Cells Impacts Structure and Function. J Biol Chem (2018) 293:3477–89. doi: 10.1074/jbc.RA117.001207
37. Shibata-Koyama M, Iida S, Okazaki A, Mori K, Kitajima-Miyama K, Saitou S, et al. The N-Linked Oligosaccharide at Fc Gamma RIIIa Asn-45: An Inhibitory Element for High Fc Gamma RIIIa Binding Affinity to IgG Glycoforms Lacking Core Fucosylation. Glycobiology (2009) 19:126–34. doi: 10.1093/glycob/cwn110
38. Subedi GP, Falconer DJ, Barb AW. Carbohydrate-Polypeptide Contacts in the Antibody Receptor CD16A Identified Through Solution NMR Spectroscopy. Biochemistry (2017) 56:3174–7. doi: 10.1021/acs.biochem.7b00392
39. Subedi GP, Barb AW. CD16a With Oligomannose-Type N-Glycans is the Only “Low-Affinity” Fc γ Receptor That Binds the IgG Crystallizable Fragment With High Affinity. vitro. J Biol Chem (2018) 293:16842–50. doi: 10.1074/jbc.RA118.004998
40. Patel KR, Roberts JT, Barb AW. Allotype-Specific Processing of the CD16a N45-Glycan From Primary Human Natural Killer Cells and Monocytes. Glycobiology (2020) 30:427–32. doi: 10.1093/glycob/cwaa002
41. Chan CJ, Smyth MJ, Martinet L. Molecular Mechanisms of Natural Killer Cell Activation in Response to Cellular Stress. Cell Death Differ (2014) 21:5–14. doi: 10.1038/cdd.2013.26
42. Raulet DH, Vance RE. Self-Tolerance of Natural Killer Cells. Nat Rev Immunol (2006) 6:520–31. doi: 10.1038/nri1863
43. Zheng P, Bertolet G, Chen Y, Huang S, Liu D. Super-Resolution Imaging of the Natural Killer Cell Immunological Synapse on a Glass-Supported Planar Lipid Bilayer. J Vis Exp (2015) 11:52502. doi: 10.3791/52502
44. Galandrini R, Tassi I, Mattia G, Lenti L, Piccoli M, Frati L, et al. SH2-Containing Inositol Phosphatase (SHIP-1) Transiently Translocates to Raft Domains and Modulates CD16-Mediated Cytotoxicity in Human NK Cells. Blood (2002) 100:4581–9. doi: 10.1182/blood-2002-04-1058
45. Inoue H, Miyaji M, Kosugi A, Nagafuku M, Okazaki T, Mimori T, et al. Lipid Rafts as the Signaling Scaffold for NK Cell Activation: Tyrosine Phosphorylation and Association of LAT With Phosphatidylinositol 3-Kinase and Phospholipase C-Gamma Following CD2 Stimulation. Eur J Immunol (2002) 32:2188–98. doi: 10.1002/1521-4141(200208)32:8<2188::AID-IMMU2188>3.0.CO;2-T
46. Cecchetti S, Spadaro F, Lugini L, Podo F, Ramoni C. Functional Role of Phosphatidylcholine-Specific Phospholipase C in Regulating CD16 Membrane Expression in Natural Killer Cells. Eur J Immunol (2007) 37:2912–22. doi: 10.1002/eji.200737266
47. Lanier LL, Yu G, Phillips JH. Co-Association of CD3 Zeta With a Receptor (CD16) for IgG Fc on Human Natural Killer Cells. Nature (1989) 342:803–5. doi: 10.1038/342803a0
48. Wirthmueller U, Kurosaki T, Murakami MS, Ravetch JV. Signal Transduction by Fc Gamma RIII (CD16) Is Mediated Through the Gamma Chain. J Exp Med (1992) 175:1381–90. doi: 10.1084/jem.175.5.1381
49. Vivier E, Rochet N, Kochan JP, Presky DH, Schlossman SF, Anderson P. Structural Similarity Between Fc Receptors and T Cell Receptors. Expression of the Gamma-Subunit of Fc Epsilon RI in Human T Cells, Natural Killer Cells and Thymocytes. J Immunol (1991) 147:4263–70.
50. Blázquez-Moreno A, Park S, Im W, Call MJ, Call ME, Reyburn HT. Transmembrane Features Governing Fc Receptor CD16A Assembly With CD16A Signaling Adaptor Molecules. Proc Natl Acad Sci U.S.A. (2017) 114:E5645–54. doi: 10.1073/pnas.1706483114
51. Lanier LL, Yu G, Phillips JH. Analysis of Fc Gamma RIII (CD16) Membrane Expression and Association With CD3 Zeta and Fc Epsilon RI-Gamma by Site-Directed Mutation. J Immunol (1991) 146:1571–6.
52. Li X, Baskin JG, Mangan EK, Su K, Gibson AW, Ji C, et al. The Unique Cytoplasmic Domain of Human Fcγriiia Regulates Receptor-Mediated Function. J Immunol (2012) 189:4284–94. doi: 10.4049/jimmunol.1200704
53. Pignata C, Prasad KV, Robertson MJ, Levine H, Rudd CE, Ritz J. Fc Gamma RIIIA-Mediated Signaling Involves Src-Family Lck in Human Natural Killer Cells. J Immunol (1993) 151:6794–800.
54. Cone JC, Lu Y, Trevillyan JM, Bjorndahl JM, Phillips CA. Association of the P56lck Protein Tyrosine Kinase With the Fc Gamma RIIIA/CD16 Complex in Human Natural Killer Cells. Eur J Immunol (1993) 23:2488–97. doi: 10.1002/eji.1830231017
55. Ting AT, Dick CJ, Schoon RA, Karnitz LM, Abraham RT, Leibson PJ. Interaction Between Lck and Syk Family Tyrosine Kinases in Fc Gamma Receptor-Initiated Activation of Natural Killer Cells. J Biol Chem (1995) 270:16415–21. doi: 10.1074/jbc.270.27.16415
56. Ståhls A, Liwszyc GE, Couture C, Mustelin T, Andersson LC. Triggering of Human Natural Killer Cells Through CD16 Induces Tyrosine Phosphorylation of the P72syk Kinase. Eur J Immunol (1994) 24:2491–6. doi: 10.1002/eji.1830241035
57. Kanakaraj P, Duckworth B, Azzoni L, Kamoun M, Cantley LC, Perussia B. Phosphatidylinositol-3 Kinase Activation Induced Upon Fc Gamma RIIIA-Ligand Interaction. J Exp Med (1994) 179:551–8. doi: 10.1084/jem.179.2.551
58. Cerboni C, Gismondi A, Palmieri G, Piccoli M, Frati L, Santoni A. CD16-Mediated Activation of Phosphatidylinositol-3 Kinase (PI-3K) in Human NK Cells Involves Tyrosine Phosphorylation of Cbl and Its Association With Grb2, Shc, Pp36 and P85 PI-3K Subunit. Eur J Immunol (1998) 28:1005–15. doi: 10.1002/(SICI)1521-4141(199803)28:03<1005::AID-IMMU1005>3.0.CO;2-O
59. Cassatella MA, Anegón I, Cuturi MC, Griskey P, Trinchieri G, Perussia B. Fc Gamma R(CD16) Interaction With Ligand Induces Ca2+ Mobilization and Phosphoinositide Turnover in Human Natural Killer Cells. Role of Ca2+ in Fc Gamma R(CD16)-Induced Transcription and Expression of Lymphokine Genes. J Exp Med (1989) 169:549–67. doi: 10.1084/jem.169.2.549
60. Aramburu J, Azzoni L, Rao A, Perussia B. Activation and Expression of the Nuclear Factors of Activated T Cells, NFATp and NFATc, in Human Natural Killer Cells: Regulation Upon CD16 Ligand Binding. J Exp Med (1995) 182:801–10. doi: 10.1084/jem.182.3.801
61. Romee R, Foley B, Lenvik T, Wang Y, Zhang B, Ankarlo D, et al. NK Cell CD16 Surface Expression and Function is Regulated by a Disintegrin and Metalloprotease-17 (ADAM17). Blood (2013) 121:3599–608. doi: 10.1182/blood-2012-04-425397
62. Lajoie L, Congy-Jolivet N, Bolzec A, Gouilleux-Gruart V, Sicard E, Sung HC, et al. ADAM17-Mediated Shedding of Fcγriiia on Human NK Cells: Identification of the Cleavage Site and Relationship With Activation. J Immunol (2014) 192:741–51. doi: 10.4049/jimmunol.1301024
63. Paolini R, Serra A, Molfetta R, Piccoli M, Frati L, Santoni A. Tyrosine Kinase-Dependent Ubiquitination of CD16 Zeta Subunit in Human NK Cells Following Receptor Engagement. Eur J Immunol (1999) 29:3179–87. doi: 10.1002/(SICI)1521-4141(199910)29:10<3179::AID-IMMU3179>3.0.CO;2-9
64. Paolini R, Molfetta R, Piccoli M, Frati L, Santoni A. Ubiquitination and Degradation of Syk and ZAP-70 Protein Tyrosine Kinases in Human NK Cells Upon CD16 Engagement. Proc Natl Acad Sci U.S.A. (2001) 98:9611–6. doi: 10.1073/pnas.161298098
65. Romee R, Rosario M, Berrien-Elliott MM, Wagner JA, Jewell BA, Schappe T, et al. Cytokine-Induced Memory-Like Natural Killer Cells Exhibit Enhanced Responses Against Myeloid Leukemia. Sci Transl Med (2016) 8:357ra123. doi: 10.1126/scitranslmed.aaf2341
66. Bednarski JJ, Zimmerman C, Berrien-Elliott MM, Foltz JA, Becker-Hapak M, Neal CC, et al. Donor Memory-Like NK Cells Persist and Induce Remissions in Pediatric Patients With Relapsed AML After Transplant. Blood (2022) 139:1670–83. doi: 10.1182/blood.2021013972
67. Cooper MA, Elliott JM, Keyel PA, Yang L, Carrero JA, Yokoyama WM. Cytokine-Induced Memory-Like Natural Killer Cells. Proc Natl Acad Sci U.S.A. (2009) 106:1915–9. doi: 10.1073/pnas.0813192106
68. Romee R, Schneider SE, Leong JW, Chase JM, Keppel CR, Sullivan RP, et al. Cytokine Activation Induces Human Memory-Like NK Cells. Blood (2012) 120:4751–60. doi: 10.1182/blood-2012-04-419283
69. Kundu S, Gurney M, O’Dwyer M. Generating Natural Killer Cells for Adoptive Transfer: Expanding Horizons. Cytotherapy (2021) 23:559–66. doi: 10.1016/j.jcyt.2020.12.002
70. Gurney M, Kundu S, Pandey S, O’Dwyer M. Feeder Cells at the Interface of Natural Killer Cell Activation, Expansion and Gene Editing. Front Immunol (2022) 13:802906. doi: 10.3389/fimmu.2022.802906
71. Sanchez-Martinez D, Allende-Vega N, Orecchioni S, Talarico G, Cornillon A, Vo D-N, et al. Expansion of Allogeneic NK Cells With Efficient Antibody-Dependent Cell Cytotoxicity Against Multiple Tumors. Theranostics (2018) 8:3856–69. doi: 10.7150/thno.25149
72. Calvo T, Reina-Ortiz C, Giraldos D, Gascón M, Woods D, Asenjo J, et al. Expanded and Activated Allogeneic NK Cells Are Cytotoxic Against B-Chronic Lymphocytic Leukemia (B-CLL) Cells With Sporadic Cases of Resistance. Sci Rep (2020) 10:19398. doi: 10.1038/s41598-020-76051-z
73. Reina-Ortiz C, Constantinides M, Fayd-Herbe-de-Maudave A, Présumey J, Hernandez J, Cartron G, et al. Expanded NK Cells From Umbilical Cord Blood and Adult Peripheral Blood Combined With Daratumumab are Effective Against Tumor Cells From Multiple Myeloma Patients. Oncoimmunology (2020) 10:1853314. doi: 10.1080/2162402X.2020.1853314
74. Hwang I, Zhang T, Scott JM, Kim AR, Lee T, Kakarla T, et al. Identification of Human NK Cells That are Deficient for Signaling Adaptor Fcrγ and Specialized for Antibody-Dependent Immune Functions. Int Immunol (2012) 24:793–802. doi: 10.1093/intimm/dxs080
75. Zhang T, Scott JM, Hwang I, Kim S. Cutting Edge: Antibody-Dependent Memory-Like NK Cells Distinguished by Fcrγ Deficiency. J Immunol (2013) 190:1402–6. doi: 10.4049/jimmunol.1203034
76. Lee J, Zhang T, Hwang I, Kim A, Nitschke L, Kim M, et al. Epigenetic Modification and Antibody-Dependent Expansion of Memory-Like NK Cells in Human Cytomegalovirus-Infected Individuals. Immunity (2015) 42:431–42. doi: 10.1016/j.immuni.2015.02.013
77. Bigley AB, Spade S, Agha NH, Biswas S, Tang S, Malik MH, et al. Fcϵriγ-Negative NK Cells Persist In Vivo and Enhance Efficacy of Therapeutic Monoclonal Antibodies in Multiple Myeloma. Blood Adv (2021) 5:3021–31. doi: 10.1182/bloodadvances.2020002440
78. Reina-Ortiz C, Giraldos D, Azaceta G, Palomera L, Marzo I, Naval J, et al. Harnessing the Potential of NK Cell-Based Immunotherapies Against Multiple Myeloma. Cells (2022) 11:392. doi: 10.3390/cells11030392
79. Casneuf T, Xu XS, Adams HC, Axel AE, Chiu C, Khan I, et al. Effects of Daratumumab on Natural Killer Cells and Impact on Clinical Outcomes in Relapsed or Refractory Multiple Myeloma. Blood Adv (2017) 1:2105–14. doi: 10.1182/bloodadvances.2017006866
80. Srivastava S, Pelloso D, Feng H, Voiles L, Lewis D, Haskova Z, et al. Effects of Interleukin-18 on Natural Killer Cells: Costimulation of Activation Through Fc Receptors for Immunoglobulin. Cancer Immunol Immunother (2013) 62:1073–82. doi: 10.1007/s00262-013-1403-0
81. Campbell AR, Regan K, Bhave N, Pattanayak A, Parihar R, Stiff AR, et al. Gene Expression Profiling of the Human Natural Killer Cell Response to Fc Receptor Activation: Unique Enhancement in the Presence of Interleukin-12. BMC Med Genomics (2015) 8:66. doi: 10.1186/s12920-015-0142-9
82. Duggan MC, Campbell AR, McMichael EL, Opheim KS, Levine KM, Bhave N, et al. Co-Stimulation of the Fc Receptor and Interleukin-12 Receptor on Human Natural Killer Cells Leads to Increased Expression of Cd25. Oncoimmunology (2018) 7:e1381813. doi: 10.1080/2162402X.2017.1381813
83. McMichael EL, Benner B, Atwal LS, Courtney NB, Mo X, Davis ME, et al. A Phase I/II Trial of Cetuximab in Combination With Interleukin-12 Administered to Patients With Unresectable Primary or Recurrent Head and Neck Squamous Cell Carcinoma. Clin Cancer Res (2019) 25:4955–65. doi: 10.1158/1078-0432.CCR-18-2108
84. Kennedy MK, Glaccum M, Brown SN, Butz EA, Viney JL, Embers M, et al. Reversible Defects in Natural Killer and Memory CD8 T Cell Lineages in Interleukin 15-Deficient Mice. J Exp Med (2000) 191:771–80. doi: 10.1084/jem.191.5.771
85. Dubois S, Mariner J, Waldmann TA, Tagaya Y. IL-15Ralpha Recycles and Presents IL-15 In Trans to Neighboring Cells. Immunity (2002) 17:537–47. doi: 10.1016/s1074-7613(02)00429-6
86. Mortier E, Quéméner A, Vusio P, Lorenzen I, Boublik Y, Grötzinger J, et al. Soluble Interleukin-15 Receptor Alpha (IL-15R Alpha)-Sushi as a Selective and Potent Agonist of IL-15 Action Through IL-15R Beta/Gamma. Hyperagonist IL-15 X IL-15R Alpha Fusion Proteins. J Biol Chem (2006) 281:1612–9. doi: 10.1074/jbc.M508624200
87. Dubois SP, Miljkovic MD, Fleisher TA, Pittaluga S, Hsu-Albert J, Bryant BR, et al. Short-Course IL-15 Given as a Continuous Infusion Led to a Massive Expansion of Effective NK Cells: Implications for Combination Therapy With Antitumor Antibodies. J Immunother Cancer (2021) 9:e002193. doi: 10.1136/jitc-2020-002193
88. Conlon K, Watson DC, Waldmann TA, Valentin A, Bergamaschi C, Felber BK, et al. Phase I Study of Single Agent NIZ985, a Recombinant Heterodimeric IL-15 Agonist, in Adult Patients With Metastatic or Unresectable Solid Tumors. J Immunother Cancer (2021) 9:e003388. doi: 10.1136/jitc-2021-003388
89. Miyazaki T, Maiti M, Hennessy M, Chang T, Kuo P, Addepalli M, et al. NKTR-255, a Novel Polymer-Conjugated rhIL-15 With Potent Antitumor Efficacy. J Immunother Cancer (2021) 9:e002024. doi: 10.1136/jitc-2020-002024
90. Zhu X, Marcus WD, Xu W, Lee H, Han K, Egan JO, et al. Novel Human Interleukin-15 Agonists. J Immunol (2009) 183:3598–607. doi: 10.4049/jimmunol.0901244
91. Rosario M, Liu B, Kong L, Collins LI, Schneider SE, Chen X, et al. The IL-15-Based ALT-803 Complex Enhances Fcγriiia-Triggered NK Cell Responses and In Vivo Clearance of B Cell Lymphomas. Clin Cancer Res (2016) 22:596–608. doi: 10.1158/1078-0432.CCR-15-1419
92. Rubinstein MP, Williams C, Mart C, Beall J, MacPherson L, Azar J, et al. Phase I Trial Characterizing the Pharmacokinetic Profile of N-803, A Chimeric IL-15 Superagonist, in Healthy Volunteers. J Immunol (2022) 208:1362–70. doi: 10.4049/jimmunol.2100066
93. Berrien-Elliott MM, Becker-Hapak M, Cashen AF, Jacobs M, Wong P, Foster M, et al. Systemic IL-15 Promotes Allogeneic Cell Rejection in Patients Treated With Natural Killer Cell Adoptive Therapy. Blood (2022) 139:1177–83. doi: 10.1182/blood.2021011532
94. Tsukerman P, Eisenstein EM, Chavkin M, Schmiedel D, Wong E, Werner M, et al. Cytokine Secretion and NK Cell Activity in Human ADAM17 Deficiency. Oncotarget (2015) 6:44151–60. doi: 10.18632/oncotarget.6629
95. Mishra HK, Pore N, Michelotti EF, Walcheck B. Anti-ADAM17 Monoclonal Antibody MEDI3622 Increases Ifnγ Production by Human NK Cells in the Presence of Antibody-Bound Tumor Cells. Cancer Immunol Immunother (2018) 67:1407–16. doi: 10.1007/s00262-018-2193-1
96. Pomeroy EJ, Hunzeker JT, Kluesner MG, Lahr WS, Smeester BA, Crosby MR, et al. A Genetically Engineered Primary Human Natural Killer Cell Platform for Cancer Immunotherapy. Mol Ther (2020) 28:52–63. doi: 10.1016/j.ymthe.2019.10.009
97. Srpan K, Ambrose A, Karampatzakis A, Saeed M, Cartwright ANR, Guldevall K, et al. Shedding of CD16 Disassembles the NK Cell Immune Synapse and Boosts Serial Engagement of Target Cells. J Cell Biol (2018) 217:3267–83. doi: 10.1083/jcb.201712085
98. Zhu H, Blum RH, Bjordahl R, Gaidarova S, Rogers P, Lee TT, et al. Pluripotent Stem Cell-Derived NK Cells With High-Affinity Noncleavable CD16a Mediate Improved Antitumor Activity. Blood (2020) 135:399–410. doi: 10.1182/blood.2019000621
99. Lazar GA, Dang W, Karki S, Vafa O, Peng JS, Hyun L, et al. Engineered Antibody Fc Variants With Enhanced Effector Function. Proc Natl Acad Sci U.S.A. (2006) 103:4005–10. doi: 10.1073/pnas.0508123103
100. Moore GL, Chen H, Karki S, Lazar GA. Engineered Fc Variant Antibodies With Enhanced Ability to Recruit Complement and Mediate Effector Functions. MAbs (2010) 2:181–9. doi: 10.4161/mabs.2.2.11158
101. Richards JO, Karki S, Lazar GA, Chen H, Dang W, Desjarlais JR. Optimization of Antibody Binding to FcgammaRIIa Enhances Macrophage Phagocytosis of Tumor Cells. Mol Cancer Ther (2008) 7:2517–27. doi: 10.1158/1535-7163.MCT-08-0201
102. Smith P, DiLillo DJ, Bournazos S, Li F, Ravetch JV. Mouse Model Recapitulating Human Fcγ Receptor Structural and Functional Diversity. Proc Natl Acad Sci U.S.A. (2012) 109:6181–6. doi: 10.1073/pnas.1203954109
103. Ahmed AA, Keremane SR, Vielmetter J, Bjorkman PJ. Structural Characterization of GASDALIE Fc Bound to the Activating Fc Receptor Fcγriiia. J Struct Biol (2016) 194:78–89. doi: 10.1016/j.jsb.2016.02.001
104. Stavenhagen JB, Gorlatov S, Tuaillon N, Rankin CT, Li H, Burke S, et al. Fc Optimization of Therapeutic Antibodies Enhances Their Ability to Kill Tumor Cells In Vitro and Controls Tumor Expansion In Vivo via Low-Affinity Activating Fcgamma Receptors. Cancer Res (2007) 67:8882–90. doi: 10.1158/0008-5472.CAN-07-0696
105. Bang YJ, Giaccone G, Im SA, Oh DY, Bauer TM, Nordstrom JL, et al. First-In-Human Phase 1 Study of Margetuximab (MGAH22), an Fc-Modified Chimeric Monoclonal Antibody, in Patients With HER2-Positive Advanced Solid Tumors. Ann Oncol (2017) 28:855–61. doi: 10.1093/annonc/mdx002
106. Rugo HS, Im S-A, Cardoso F, Cortés J, Curigliano G, Musolino A, et al. Efficacy of Margetuximab vs Trastuzumab in Patients With Pretreated ERBB2-Positive Advanced Breast Cancer: A Phase 3 Randomized Clinical Trial. JAMA Oncol (2021) 7:573–84. doi: 10.1001/jamaoncol.2020.7932
107. Walker MR, Lund J, Thompson KM, Jefferis R. Aglycosylation of Human IgG1 and IgG3 Monoclonal Antibodies can Eliminate Recognition by Human Cells Expressing Fc Gamma RI and/or Fc Gamma RII Receptors. Biochem J (1989) 259:347–53. doi: 10.1042/bj2590347
108. Shields RL, Lai J, Keck R, O’Connell LY, Hong K, Meng YG, et al. Lack of Fucose on Human IgG1 N-Linked Oligosaccharide Improves Binding to Human Fcgamma RIII and Antibody-Dependent Cellular Toxicity. J Biol Chem (2002) 277:26733–40. doi: 10.1074/jbc.M202069200
109. Mössner E, Brünker P, Moser S, Püntener U, Schmidt C, Herter S, et al. Increasing the Efficacy of CD20 Antibody Therapy Through the Engineering of a New Type II Anti-CD20 Antibody With Enhanced Direct and Immune Effector Cell-Mediated B-Cell Cytotoxicity. Blood (2010) 115:4393–402. doi: 10.1182/blood-2009-06-225979
110. Bruggeman CW, Dekkers G, Bentlage AEH, Treffers LW, Nagelkerke SQ, Lissenberg-Thunnissen S, et al. Enhanced Effector Functions Due to Antibody Defucosylation Depend on the Effector Cell Fcγ Receptor Profile. J Immunol (2017) 199:204–11. doi: 10.4049/jimmunol.1700116
111. Karampatzakis A, Brož P, Rey C, Önfelt B, Cruz De Matos GDS, Rycroft D, et al. Antibody Afucosylation Augments CD16-Mediated Serial Killing and Ifnγ Secretion by Human Natural Killer Cells. Front Immunol (2021) 12:641521. doi: 10.3389/fimmu.2021.641521
112. Gleason MK, Verneris MR, Todhunter DA, Zhang B, McCullar V, Zhou SX, et al. Bispecific and Trispecific Killer Cell Engagers Directly Activate Human NK Cells Through CD16 Signaling and Induce Cytotoxicity and Cytokine Production. Mol Cancer Ther (2012) 11:2674–84. doi: 10.1158/1535-7163.MCT-12-0692
113. Vallera DA, Felices M, McElmurry R, McCullar V, Zhou X, Schmohl JU, et al. IL15 Trispecific Killer Engagers (TriKE) Make Natural Killer Cells Specific to CD33+ Targets While Also Inducing Persistence, In Vivo Expansion, and Enhanced Function. Clin Cancer Res (2016) 22:3440–50. doi: 10.1158/1078-0432.CCR-15-2710
114. Reusing SB, Vallera DA, Manser AR, Vatrin T, Bhatia S, Felices M, et al. CD16xCD33 Bispecific Killer Cell Engager (BiKE) as Potential Immunotherapeutic in Pediatric Patients With AML and Biphenotypic ALL. Cancer Immunol Immunother (2021) 70:3701–8. doi: 10.1007/s00262-021-03008-0
115. Bartlett NL, Herrera AF, Domingo-Domenech E, Mehta A, Forero-Torres A, Garcia-Sanz R, et al. A Phase 1b Study of AFM13 in Combination With Pembrolizumab in Patients With Relapsed or Refractory Hodgkin Lymphoma. Blood (2020) 136:2401–9. doi: 10.1182/blood.2019004701
116. Vallera DA, Oh F, Kodal B, Hinderlie P, Geller MA, Miller JS, et al. A HER2 Tri-Specific NK Cell Engager Mediates Efficient Targeting of Human Ovarian Cancer. Cancers (Basel) (2021) 13:3994. doi: 10.3390/cancers13163994
117. Wingert S, Reusch U, Knackmuss S, Kluge M, Damrat M, Pahl J, et al. Preclinical Evaluation of AFM24, A Novel CD16A-Specific Innate Immune Cell Engager Targeting EGFR-Positive Tumors. MAbs (2021) 13:1950264. doi: 10.1080/19420862.2021.1950264
118. Ramapriyan R, Caetano MS, Barsoumian HB, Mafra ACP, Zambalde EP, Menon H, et al. Altered Cancer Metabolism in Mechanisms of Immunotherapy Resistance. Pharmacol Ther (2019) 195:162–71. doi: 10.1016/j.pharmthera.2018.11.004
119. Oberholtzer N, Quinn KM, Chakraborty P, Mehrotra S. New Developments in T Cell Immunometabolism and Implications for Cancer Immunotherapy. Cells (2022) 11:708. doi: 10.3390/cells11040708
120. Traba J, Sack MN, Waldmann TA, Anton OM. Immunometabolism at the Nexus of Cancer Therapeutic Efficacy and Resistance. Front Immunol (2021) 12:657293. doi: 10.3389/fimmu.2021.657293
121. Verma NK, Kelleher D. Not Just an Adhesion Molecule: LFA-1 Contact Tunes the T Lymphocyte Program. J Immunol (2017) 199:1213–21. doi: 10.4049/jimmunol.1700495
122. Mace EM, Zhang J, Siminovitch KA, Takei F. Elucidation of the Integrin LFA-1-Mediated Signaling Pathway of Actin Polarization in Natural Killer Cells. Blood (2010) 116:1272–9. doi: 10.1182/blood-2009-12-261487
123. Barber DF, Faure M, Long EO. LFA-1 Contributes an Early Signal for NK Cell Cytotoxicity. J Immunol (2004) 173:3653–9. doi: 10.4049/jimmunol.173.6.3653
124. Allende-Vega N, Marco Brualla J, Falvo P, Alexia C, Constantinides M, de Maudave AF, et al. Metformin Sensitizes Leukemic Cells to Cytotoxic Lymphocytes by Increasing Expression of Intercellular Adhesion Molecule-1 (ICAM-1). Sci Rep (2022) 12:1341. doi: 10.1038/s41598-022-05470-x
125. Belkahla S, Brualla JM, Fayd’herbe de Maudave A, Falvo P, Allende-Vega N, Constantinides M, et al. The Metabolism of Cells Regulates Their Sensitivity to NK Cells Depending on P53 Status. Sci Rep (2022) 12:3234. doi: 10.1038/s41598-022-07281-6
126. Loftus RM, Assmann N, Kedia-Mehta N, O’Brien KL, Garcia A, Gillespie C, et al. Amino Acid-Dependent Cmyc Expression is Essential for NK Cell Metabolic and Functional Responses in Mice. Nat Commun (2018) 9:2341. doi: 10.1038/s41467-018-04719-2
127. Parameswaran R, Ramakrishnan P, Moreton SA, Xia Z, Hou Y, Lee DA, et al. Repression of GSK3 Restores NK Cell Cytotoxicity in AML Patients. Nat Commun (2016) 7:11154. doi: 10.1038/ncomms11154
Keywords: CD16a, NK cells, ADCC, monoclonal antibodies, cell therapy
Citation: Coënon L and Villalba M (2022) From CD16a Biology to Antibody-Dependent Cell-Mediated Cytotoxicity Improvement. Front. Immunol. 13:913215. doi: 10.3389/fimmu.2022.913215
Received: 05 April 2022; Accepted: 09 May 2022;
Published: 03 June 2022.
Edited by:
Ana Stojanovic, Heidelberg University, GermanyReviewed by:
Francisco Borrego, Biocruces Bizkaia Health Research Institute, SpainOscar Aguilar, University of California, San Francisco, United States
Copyright © 2022 Coënon and Villalba. This is an open-access article distributed under the terms of the Creative Commons Attribution License (CC BY). The use, distribution or reproduction in other forums is permitted, provided the original author(s) and the copyright owner(s) are credited and that the original publication in this journal is cited, in accordance with accepted academic practice. No use, distribution or reproduction is permitted which does not comply with these terms.
*Correspondence: Loïs Coënon, bG9pcy5jb2Vub25AaW5zZXJtLmZy