- Department of Pediatrics, The First Affiliated Hospital, Guangxi Medical University, Nanning, China
Immunoglobulin A vasculitis (IgAV) is the most common systemic small vessel vasculitis in childhood. Its clinical manifestations are non-thrombocytopenic purpura, accompanied by gastrointestinal tract, joint, kidney and other organ system involvement. The pathogenesis of IgAV has not been fully elucidated. It may be related to many factors including genetics, infection, environmental factors, and drugs. The most commonly accepted view is that galactose-deficient IgA1 and the deposition of IgA and complement C3 in small blood vessel walls are key contributors to the IgAV pathogenesis. Extensive neutrophil extracellular traps (NETs) in the peripheral circulation and skin, kidney, and gastrointestinal tissue of patients with IgAV has been identified in the past two years and is associated with disease activity. This mini-review provides a possible mechanism for NETs involvement in the pathogenesis of IgAV.
Introduction
Immunoglobulin A vasculitis (IgAV), also known as Henoch‐Schönlein purpura, is an inflammatory small vascular disease involving the capillaries, venules, or arterioles (1). The clinical manifestations of IgAV are non-thrombocytopenic purpura, mainly involving the skin, gastrointestinal tract, joint and kidneys, and deposition of IgA or IgA-immune complexes (IgA-ICs) in the vascular wall. The incidence of IgAV in children is 10-27/100,000 per year (2). The majority of cases occur between 2 and 10 years of age, with a peak onset between 4 and 7 years of age (3, 4). In most children, IgAV is self-limited and has a good prognosis, but a few cases have renal involvement and a recurrent or even prolonged course. IgAV nephritis (IgAVN), which is a major cause of mortality, is the cause of 1%–2% of pediatric end-stage renal disease cases (3). The pathogenesis of IgAV has not been fully elucidated. At present, it is believed to be caused by genetics, infection, environmental factors, drugs, and other factors (5). There is a wide range of immunological abnormalities in IgAV.
The Physiological Function of NETs and Their Role in Autoimmune Diseases
Neutrophils are the most abundant white blood cells in the human peripheral circulation. They play a key role in the innate immune system and constitute the body’s first line of defense against pathogens. Previous studies have shown that neutrophils phagocytose and kill bacteria directly through secretion of proteolytic enzymes, antibacterial proteins and reactive oxygen species (ROS), which are directly to kill bacteria (6, 7).A novel mechanism of neutrophil defense against infection through release of neutrophil extracellular traps (NETs) was reported in recent years (8). This process fundamentally differs from both cell death and necrotizing apoptosis and is called NETosis (9). At present, there are two mechanisms by which NETs are formed: suicide lytic NETosis and vital NETosis. In lytic NETosis, neutrophils release NETs through cell membrane lysis death, which depends on the Raf/MEK/ERK signaling pathway and the activation of NADPH oxidase. In lyticNETosis, the cell membrane breaks down and neutrophils are unable to secrete particles. In vital NETosis, DNA from the nucleus erupts in vesicles, passes through the cytoplasm and binds to the plasma membrane, transporting DNA outside the cell to formation of NETs without damaging the membrane and maintaining the integrity of neutrophil (10). The structure of NETs directly wraps around invading microorganisms and uses its highly concentrated antimicrobial peptides to degrade virulence factors and kill pathogenic microorganisms, preventing the spread and dissemination of infection, which plays an important role in infection defense (8). However, excessive formation of NETs and clearance of obstacles also has a toxic effect on the host. NETs related components, such as nucleic acids and proteins, were exposed as autoantigens in the inflammatory environment, which can stimulate the autoimmune response of susceptible individuals and promote various autoimmune diseases (11).
NETs Involved in the Pathogenesis of IgAV
In 2020 Bergqvist, C et al. (12) reported that NETs were significantly increase in skin tissues in the early stages of IC-mediated small vasculitis, such as allergic vasculitis and IgAV. Our previous study evaluated the level of NETs in the peripheral blood and gastrointestinal and renal tissues of children with IgAV at different periods. The study evaluated components of NETs, which included cell-free DNA (cf-DNA), myeloperoxidase-DNA (MPO-DNA), citrullinated-histone H3 (cit-H3), neutrophil elastase (NE), and cathelicidin antimicrobial peptides (CAMP, LL37). The level of NETs significantly increased in children with IgAV onset and active stage, while the level of NETs gradually returned to normal in children in the remission stage and drug withdrawal (13). In autoimmune diseases, excessive NETs are known to act as an exposed autoantigen in vivo, inducing the production of autoantibodies, thereby increasing the intensity of the inflammatory response. A continuous increase in NETs indicates a high inflammatory state, and reflects the imbalance between the formation and clearance of NETs in IgAV, leading to the accumulation of excessive NETs, which ultimately leads to autoimmune disorders, chronic inflammation and tissue damage. These processes have been associated with the development of autoimmune and inflammatory diseases (14). Several studies have shown that NETs are involved in the development and progression of autoimmune diseases such as ANCA-associated vasculitis, rheumatoid arthritis (RA), inflammatory bowel disease and systemic lupus erythematosus (SLE) (15–18). A recent study reported that MPO-DNA is significantly elevated in the circulation of patients with IgAV and positively correlates with IgA levels, which suggests that NETs are involved in the pathogenesis of IgAV (19). NETs may influence the activity or severity of IgAV (13, 19).
Mechanism of NETs in IgAV
Disordered Equilibrium Between NETs and DNase I
Our previous study (13) revealed that serum degradation of NETs significantly declines in children with IgAV onset and active IgAV. Children in drug withdrawal had a normal level of NETs degradation. The level of DNase I also decreases in children with IgAV onset and active IgAV. The reduced ability to degrade NETs is negatively correlated with the presence of DNase I, which is required to degrade NETs (20). The decreased activity of DNase I may be one of the reasons for the significant increase in NETs and thus may cause immune imbalance (21). In patients with SLE and eosinophilic granuloma, the ability of the extracellular and intracellular environment to degrade DNA is significantly reduced. This phenomenon seems to be a common characteristic of autoimmune diseases (22, 23). In addition, over-activation of complement system and over deposition of complement protein C1q also inhibit the production of DNase I, resulting in ineffective NETs degradation (24). Therefore, excessive NETs formation is related to deficient DNase I activity, which leads to disorders that promote immunological homeostasis dysregulation and tissue damage (25). Impaired self-degradation of NETs is associated with RA and lupus nephritis (26–29).The decreased activity of DNase I eventually leads to a reduced ability to degrade NETs, which is one of the reasons for the increase in NETs in IgAV.
Aberrant Glycosylation of IgA1 and IgA-ICs Induces NETs Formation in IgAV
Deposition of IgA on the vascular wall is characteristic of IgAV. IgA activate neutrophils and release NETs into tissues and the peripheral blood. Studies have shown that NETs are involved in various IC-mediated small vasculitis conditions, and that circulating NETs are related to the severity of vascular inflammation (12). In renal biopsies from patients with ANCA-associated vasculitis, the formation of NETs was found in the involved glomeruli and stroma lesions (30). IgA can induce neutrophils to release NETs via Fcα receptor I (FcaRI) (31). FcaRI is elevated in children with active IgAV (32). In idiopathic IgA nephropathy, proteinuria and leukocyte infiltration are more pronounced, and FcaRI activation leads to a more severe inflammatory response. It is believed that FcaRI promotes and aggravates tissue and kidney damage by activating the cascade reaction of cytokines and chemokines (33). NETs have been shown to induce an autoimmune response in other autoimmune diseases such as SLE and ANCA-associated vasculitis (34–36). In addition, in patients with RA, the level of circulating NETs is positively correlated with the severity of periodontitis (37).
NETs formation has been detected in tissue biopsies of patients in the early stages of IC-mediated vasculitis (14). These immobilized ICs induce human neutrophils to release NETs in vitro (38). The formation of NETs increases in the renal, gastric, and duodenal tissues of children during IgAV onset and active IgAV, which may be related to IgA-ICs deposition activating neutrophils to release NETs. In lupus nephritis, deposition of circulating ICs in the glomerular basement membrane is accompanied by the accumulation of NETs in the tissue, resulting in tissue damage (39). The deposition of IgA and C3 and the formation of NETs are common in the renal, gastric and duodenal tissues of children with IgAV. It is speculated that IgA-ICs and C3 deposition may be involved in the occurrence and development of IgAV through various mechanisms, such as complement activation, chemotaxis infiltration and aggregation of neutrophils to promote the release of NETs (Figure 1).
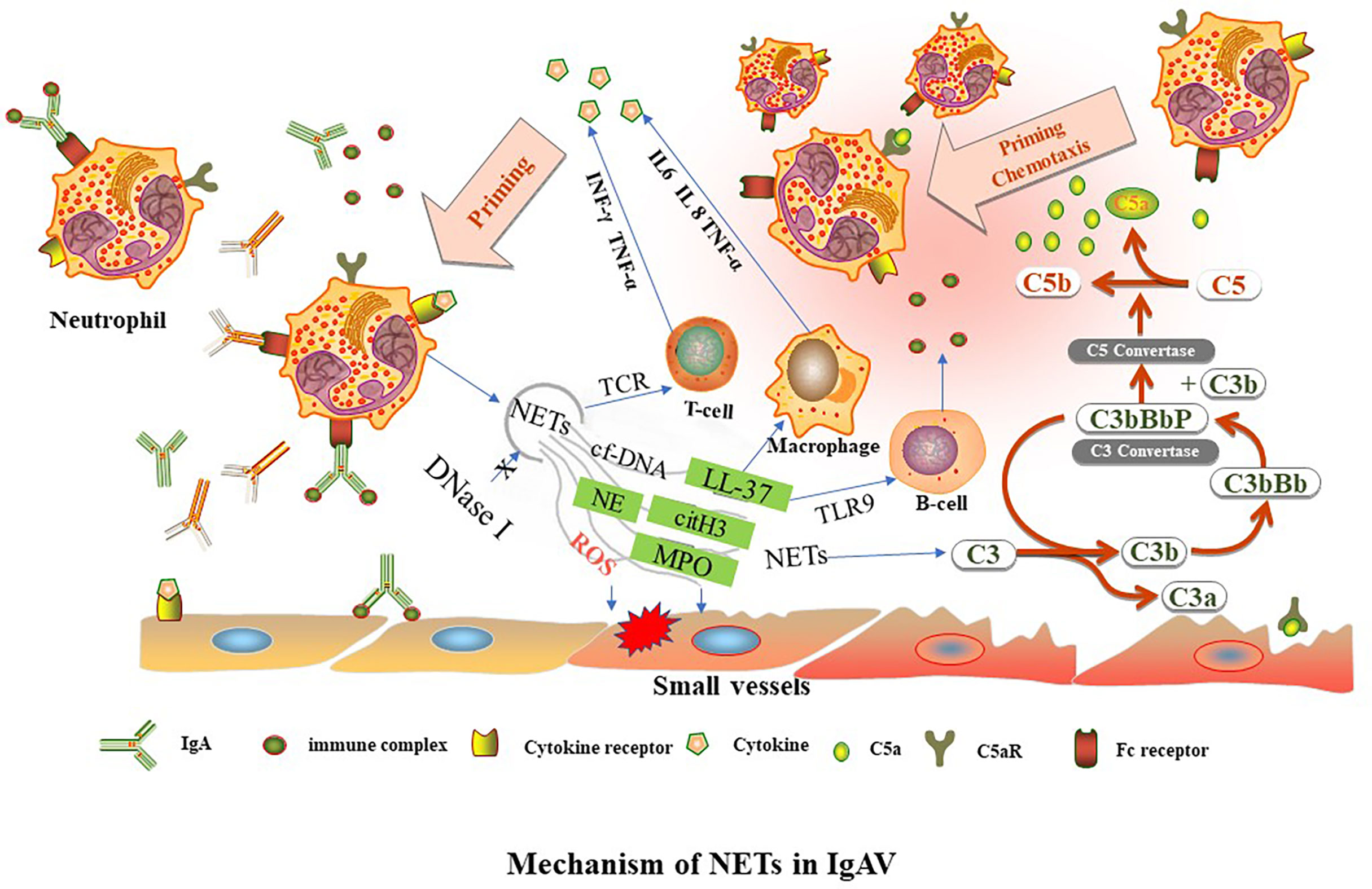
Figure 1 TNFα, tumor necrosis factor alpha. INF-γ, interferon gamma. NETs, neutrophil extracellular traps. LL37, cathelicidin antimicrobial peptides. C3, complement factor 3. IL, interleukin, MPO, myeloperoxidase. NE, neutrophil elastase. cit-H3, citrullinated-histone H3. cf-DNA, cell free DNA. TCR, T cell receptor. TLR9, toll-like receptor 9-dependent manner. Aberrant glycosylation of IgA1 and IgA immune complexes (IgA-ICs) induce NETs formation by binding to the Fc receptor of neutrophils. The level of DNase I decreases, leading to reduce of NETs degradation. NETs activate downstream target immune cells to release cytokines. NETs can activate immune-related cells, such as T lymphocytes (through TCR), B lymphocytes (through TLR9-dependent manner) and macrophages to release cytokines, such as IL6, IL 8, TNF-α, and INF-γ. NETs are involved in different complement bypass pathways.
NETs Activate Downstream Target Immune Cells to Release Cytokines
Neutrophils release related components of NETs such as MPO and protease, which further aggravates tissue damage. Similarly, IgA binds to the FcαRI junctions of neutrophils, releasing tumor necrosis factor α (TNF-α), leukotriene B4 (LTB4), etc. (40, 41). TNF-α can further stimulate endothelial cells to produce interleukin (IL)-8 (42). IgA may activate neutrophils to release IL-8 (1, 43). Studies show that LTB4 is significantly increased in children with IgAV (44). LTB4 can induce further neutrophil migration through a positive feedback pathway (40). Although LTB4 has no direct effect on microvascular injury of inflammatory tissues, it can make white blood cells adhere to vascular endothelial cells, resulting in increased vascular permeability and aggravating tissue injury (45). LTB4 plays an important role in inflammation, the immune system, and allergies. TNF-α is a pro-inflammatory factor involved in the occurrence and development of IgAV and is closely related to kidney damage. TNF-α can even reflect the degree of renal damage in IgAVN (46, 47). In addition, NETs-related components can activate immune-related cells such as B lymphocytes, T lymphocytes and antigen-presenting cells to release IL-6, IL-8, interferon γ (INF-γ), and TNF-α (14). In the interleukin family, IL-6, IL-8, IL-10, and IL-33 are all related to IgAV (46, 48–50). IL-2 is negatively correlated with the severity of the disease (51). IL-6 promotes the activation of B cells and the production of relevant antibodies, which are mainly deposited in the mesangial region of the kidney. Through the action of T cells, IL-6 stimulates the proliferation and fibrosis of mesangial tissues, aggravates kidney damage and leads to the occurrence and development of IgAVN (52). IL-10 plays a protective role by inhibiting the antigen presentation function of macrophages and indirectly inhibiting the function of natural killer cell (53). Under the stimulation of IL-8, an increase in the cytoplasmic Ca2+ of neutrophils mediates the release of hydrogen peroxide in a respiratory burst reaction, and lysosomal enzymes can be released through chemotaxis of neutrophils, leading to capillary destruction (46). IgA can activate the complement system through bypass and lectin pathways. The levels of C3a and C5a increase in the circulation of IgAV, and C3 and C5-C9 are deposited in the skin tissue and mesangial region of glomeruli (54, 55). These compounds can form membrane-attacking complexes that directly destroy the membranes of target cells, and deposition of C4d and C5B-9 in the kidney is associated with poor prognosis (56). NETs activate C3 and eventually convert it to C5a, which can induce chemotaxis and neutrophil aggregation, and stimulate endothelial cells to secrete IL8.
NETs May Be a Potential Biomarker to Assess Disease Activity in IgAV
NETs have been reported as a marker of disease activity in other diseases. The One predictor of inflammatory response and sepsis is cf-DNA (57, 58). Cit-H3 is a useful biomarker for early detection of liver dysfunction (59). NETs can be used as markers and therapeutic targets for ophthalmic diseases including dry eye, glaucoma, age-related macular degeneration, and diabetic retinopathy (60, 61). NETs and anti-NETs associated antibodies are indicators of SLE activity (11, 16). NETs significantly increase during IgAV onset and the active stage of IgAV but decrease in the remission and withdrawal stage of IgAV (13). Most patients in the active and relapse have IgAVN. It is speculated that changes in NETs levels may reflect disease activity of IgAV in children, especially those with IgAVN need corticosteroids or immune suppressive therapy.
There are no widely used biomarkers to predict disease activity or the prognosis of IgAVN. The combined indexes of blood examination, immunoglobulin, C-reactive protein, procalcitonin and trace elements have been used to predict the index (62–64). The detection of related metabolites in urine has also been considered. The soluble transferrin receptor concentration in urine increases significantly during the active stage of IgAVN, but the correlation coefficient is low (65). The ratio of urine (Fcα receptor × glutamine transferase)/urine protein and perforin 3 has also been used to predict disease activity, but clinical detection methods for these markers are limited (32, 66). The severity of IgAVN is correlated with alpha-smooth muscle actin (α-SMA) and C-Met, while IgAV with gastrointestinal involvement is correlated with fecal calprotectin, D-dimer and fibrin degradation products (67–69). Therefore, NETs may be a potential convenient biomarker and indicator of IgAVN disease activity, particularly in those patients who would have an ominous outcome.
NETs related components include cf-DNA, MPO-DNA, and NE. Peripheral blood cf-DNA is simple and convenient to measure, but Moss et al. (70) showed that cf-DNA can be released from a variety of cells other than neutrophils during inflammation. Whether cf-DNA alone can predict the level of NETs needs more research. Therefore, the use of NETs or their related components as biomarkers for disease still needs further study for confirmation.
Conclusion and Perspectives
In conclusion, IgA or IgA-ICs can activate neutrophils to release NETs. NETs-related components can directly damage tissues or secrete large amounts of cytokines by activating downstream target immune cells. Cytokines can aggravate tissue damage and cause neutrophil aggregation, forming a vicious cycle (Figure 1). NETs may be a potential biological indicator to assess disease activity in children with IgAV.
However, many unanswered questions about the mechanism of NETs in IgAV remain. The mechanism by which neutrophils mediate IgAV tissue damage is not completely clear. At present, the specific mechanism for NETs signaling pathway regulation and NETs related components in IgAV-induced tissue injury has not been elucidated. Which signaling pathway IgA/FcαR regulates the formation of NETs in neutrophil remains unclear.
Data Availability Statement
The raw data supporting the conclusions of this article will be made available by the authors.
Author Contributions
XQC were responsible for the conception, design and drafted the manuscript. QT and LH were responsible for design of the review. LT and YHQ revised the manuscript. All authors contributed to the article and approved the submitted version.
Funding
This study was supported by the Innovation Project of Guangxi Graduate Education (no. YCBZ2021047), the Research Basic Ability Enhancement Project for Young and Middle-aged Teachers in Guangxi Universities (no: 2021KY0096).
Conflict of Interest
The authors declare that the research was conducted in the absence of any commercial or financial relationships that could be construed as a potential conflict of interest.
Publisher’s Note
All claims expressed in this article are solely those of the authors and do not necessarily represent those of their affiliated organizations, or those of the publisher, the editors and the reviewers. Any product that may be evaluated in this article, or claim that may be made by its manufacturer, is not guaranteed or endorsed by the publisher.
References
1. Heineke MH, Ballering AV, Jamin A, Ben Mkaddem S, Monteiro RC, Van Egmond M. New Insights in the Pathogenesis of Immunoglobulin A Vasculitis (Henoch-Schonlein Purpura). Autoimmun Rev (2017) 16:1246–53. doi: 10.1016/j.autrev.2017.10.009
2. Piram M, Maldini C, Biscardi S, De Suremain N, Orzechowski C, Georget E, et al. Incidence of IgA Vasculitis in Children Estimated by Four-Source Capture-Recapture Analysis: A Population-Based Study. Rheumatol (Oxford) (2017) 56:1358–66. doi: 10.1093/rheumatology/kex158
3. Schnabel A, Hedrich CM. Childhood Vasculitis. Front Pediatr (2018) 6:421. doi: 10.3389/fped.2018.00421
4. Leung AKC, Barankin B, Leong KF. Henoch-Schonlein Purpura in Children: An Updated Review. Curr Pediatr Rev (2020) 16:265–76. doi: 10.2174/1573396316666200508104708
5. Oni L, Sampath S. Childhood IgA Vasculitis (Henoch Schonlein Purpura)-Advances and Knowledge Gaps. Front Pediatr (2019) 7:257. doi: 10.3389/fped.2019.00257
6. Dale DC, Boxer L, Liles WC. The Phagocytes: Neutrophils and Monocytes. Blood (2008) 112:935–45. doi: 10.1182/blood-2007-12-077917
7. Lacy P. Mechanisms of Degranulation in Neutrophils. Allergy Asthma Clin Immunol (2006) 2:98–108. doi: 10.1186/1710-1492-2-3-98
8. Brinkmann V, Reichard U, Goosmann C, Fauler B, Uhlemann Y, Weiss DS, et al. Neutrophil Extracellular Traps Kill Bacteria. Science (2004) 303:1532–5. doi: 10.1126/science.1092385
9. Guimaraes-Costa AB, Nascimento MT, Froment GS, Soares RP, Morgado FN, Conceicao-Silva F, et al. Leishmania Amazonensis Promastigotes Induce and are Killed by Neutrophil Extracellular Traps. Proc Natl Acad Sci USA (2009) 106:6748–53. doi: 10.1073/pnas.0900226106
10. Yipp BG, Kubes P. NETosis: How Vital is it? Blood (2013) 122:2784–94. doi: 10.1182/blood-2013-04-457671
11. Barnado A, Crofford LJ, Oates JC. At the Bedside: Neutrophil Extracellular Traps (NETs) as Targets for Biomarkers and Therapies in Autoimmune Diseases. J Leukoc Biol (2016) 99:265–78. doi: 10.1189/jlb.5BT0615-234R
12. Bergqvist C, Safi R, El Hasbani G, Abbas O, Kibbi A, Nassar D. Neutrophil Extracellular Traps are Present in Immune-Complex-Mediated Cutaneous Small Vessel Vasculitis and Correlate With the Production of Reactive Oxygen Species and the Severity of Vessel Damage. Acta Derm Venereol (2020) 100:adv00281. doi: 10.2340/00015555-3363
13. Chen XQ, Tu L, Zou JS, Zhu SQ, Zhao YJ, Qin YH. The Involvement of Neutrophil Extracellular Traps in Disease Activity Associated With IgA Vasculitis. Front Immunol (2021) 12:668974. doi: 10.3389/fimmu.2021.668974
14. Fousert E, Toes R, Desai J. Neutrophil Extracellular Traps (NETs) Take the Central Stage in Driving Autoimmune Responses. Cells (2020) 9:915. doi: 10.3390/cells9040915
15. Moore S, Juo HH, Nielsen CT, Tyden H, Bengtsson AA, Lood C. Role of Neutrophil Extracellular Traps Regarding Patients at Risk of Increased Disease Activity and Cardiovascular Comorbidity in Systemic Lupus Erythematosus. J Rheumatol (2020) 47:1652–60. doi: 10.3899/jrheum.190875
16. de Bont CM, Stokman MEM, Faas P, Thurlings RM, Boelens WC, Wright HL, et al. Autoantibodies to Neutrophil Extracellular Traps Represent a Potential Serological Biomarker in Rheumatoid Arthritis. J Autoimmun (2020) 113:102484. doi: 10.1016/j.jaut.2020.102484
17. Abreu-Velez AM, Smith JG Jr., Howard MS. Presence of Neutrophil Extracellular Traps and Antineutrophil Cytoplasmic Antibodies Associated With Vasculitides. N Am J Med Sci (2009) 1:309–13.
18. Liu Q, Yang Y, Ge S, Huo J, Wang D, Ma Y, et al. Serum Level of Advanced Oxidation Protein Products (AOPPs) in Patients With Henoch-Schonlein Purpura and its Relationship With Aberrant Glycosylation of IgA1 and Cosmc mRNA Expression. Int J Dermatol (2019) 58:1092–7. doi: 10.1111/ijd.14550
19. Takeuchi S, Kawakami T, Okano T, Shida H, Nakazawa D, Tomaru U, et al. Elevated Myeloperoxidase-DNA Complex Levels in Sera of Patients With IgA Vasculitis. Pathobiology (2022) 89:23–8. doi: 10.1159/000519869
20. Hakkim A, Furnrohr BG, Amann K, Laube B, Abed UA, Brinkmann V, et al. Impairment of Neutrophil Extracellular Trap Degradation is Associated With Lupus Nephritis. Proc Natl Acad Sci USA (2010) 107:9813–8. doi: 10.1073/pnas.0909927107
21. Malickova K, Duricova D, Bortlik M, Hruskova Z, Svobodova B, Machkova N, et al. Impaired Deoxyribonuclease I Activity in Patients With Inflammatory Bowel Diseases. Autoimmune Dis (2011) 2011:945861. doi: 10.4061/2011/945861
22. Pruchniak MP, Ostafin M, Wachowska M, Jakubaszek M, Kwiatkowska B, Olesinska M, et al. Neutrophil Extracellular Traps Generation and Degradation in Patients With Granulomatosis With Polyangiitis and Systemic Lupus Erythematosus. Autoimmunity (2019) 52:126–35. doi: 10.1080/08916934.2019.1631812
23. Masuda S, Nonokawa M, Futamata E, Nishibata Y, Iwasaki S, Tsuji T, et al. Formation and Disordered Degradation of Neutrophil Extracellular Traps in Necrotizing Lesions of Anti-Neutrophil Cytoplasmic Antibody-Associated Vasculitis. Am J Pathol (2019) 189:839–46. doi: 10.1016/j.ajpath.2019.01.007
24. Leffler J, Martin M, Gullstrand B, Tyden H, Lood C, Truedsson L, et al. Neutrophil Extracellular Traps That are Not Degraded in Systemic Lupus Erythematosus Activate Complement Exacerbating the Disease. J Immunol (2012) 188:3522–31. doi: 10.4049/jimmunol.1102404
25. Napirei M, Wulf S, Mannherz HG. Chromatin Breakdown During Necrosis by Serum Dnase1 and the Plasminogen System. Arthritis Rheum (2004) 50:1873–83. doi: 10.1002/art.20267
26. Bruschi M, Petretto A, Santucci L, Vaglio A, Pratesi F, Migliorini P, et al. Neutrophil Extracellular Traps Protein Composition is Specific for Patients With Lupus Nephritis and Includes Methyl-Oxidized Alphaenolase (Methionine Sulfoxide 93). Sci Rep (2019) 9:7934. doi: 10.1038/s41598-019-44379-w
27. Rother N, Pieterse E, Lubbers J, Hilbrands L, van der Vlag J. Acetylated Histones in Apoptotic Microparticles Drive the Formation of Neutrophil Extracellular Traps in Active Lupus Nephritis. Front Immunol (2017) 8:1136. doi: 10.3389/fimmu.2017.01136
28. Sur Chowdhury C, Giaglis S, Walker UA, Buser A, Hahn S, Hasler P. Enhanced Neutrophil Extracellular Trap Generation in Rheumatoid Arthritis: Analysis of Underlying Signal Transduction Pathways and Potential Diagnostic Utility. Arthritis Res Ther (2014) 16:R122. doi: 10.1186/ar4579
29. Aleyd E, Al M, Tuk CW, van der Laken CJ, van Egmond M. IgA Complexes in Plasma and Synovial Fluid of Patients With Rheumatoid Arthritis Induce Neutrophil Extracellular Traps via FcalphaRI. J Immunol (2016) 197:4552–9. doi: 10.4049/jimmunol.1502353
30. Kessenbrock K, Krumbholz M, Schonermarck U, Back W, Gross WL, Werb Z, et al. Netting Neutrophils in Autoimmune Small-Vessel Vasculitis. Nat Med (2009) 15:623–5. doi: 10.1038/nm.1959
31. Aleyd E, van Hout MW, Ganzevles SH, Hoeben KA, Everts V, Bakema JE, et al. IgA Enhances NETosis and Release of Neutrophil Extracellular Traps by Polymorphonuclear Cells via Fcalpha Receptor I. J Immunol (2014) 192:2374–83. doi: 10.4049/jimmunol.1300261
32. Moresco RN, Speeckaert MM, Zmonarski SC, Krajewska M, Komuda-Leszek E, Perkowska-Ptasinska A, et al. Urinary Myeloid IgA Fc Alpha Receptor (CD89) and Transglutaminase-2 as New Biomarkers for Active IgA Nephropathy and Henoch-Schonlein Purpura Nephritis. BBA Clin (2016) 5:79–84. doi: 10.1016/j.bbacli.2016.02.002
33. Kanamaru Y, Arcos-Fajardo M, Moura IC, Tsuge T, Cohen H, Essig M, et al. Fc Alpha Receptor I Activation Induces Leukocyte Recruitment and Promotes Aggravation of Glomerulonephritis Through the FcR Gamma Adaptor. Eur J Immunol (2007) 37:1116–28. doi: 10.1002/eji.200636826
34. Bruschi M, Bonanni A, Petretto A, Vaglio A, Pratesi F, Santucci L, et al. Neutrophil Extracellular Traps (NETs) Profiles in Patients With Incident SLE and Lupus Nephritis. J Rheumatol (2020) 47:181232. doi: 10.3899/jrheum.181232
35. O'Sullivan KM, Holdsworth SR. Neutrophil Extracellular Traps: A Potential Therapeutic Target in MPO-ANCA Associated Vasculitis? Front Immunol (2021) 12:635188. doi: 10.3389/fimmu.2021.635188
36. Lee KH, Kronbichler A, Park DD, Park Y, Moon H, Kim H, et al. Neutrophil Extracellular Traps (NETs) in Autoimmune Diseases: A Comprehensive Review. Autoimmun Rev (2017) 16:1160–73. doi: 10.1016/j.autrev.2017.09.012
37. Kaneko C, Kobayashi T, Ito S, Sugita N, Murasawa A, Nakazono K, et al. Circulating Levels of Carbamylated Protein and Neutrophil Extracellular Traps are Associated With Periodontitis Severity in Patients With Rheumatoid Arthritis: A Pilot Case-Control Study. PloS One (2018) 13:e0192365. doi: 10.1371/journal.pone.0192365
38. Granger V, Peyneau M, Chollet-Martin S, de Chaisemartin L. Neutrophil Extracellular Traps in Autoimmunity and Allergy: Immune Complexes at Work. Front Immunol (2019) 10:2824. doi: 10.3389/fimmu.2019.02824
39. Nishi H, Mayadas TN. Neutrophils in Lupus Nephritis. Curr Opin Rheumatol (2019) 31:193–200. doi: 10.1097/BOR.0000000000000577
40. van der Steen L, Tuk CW, Bakema JE, Kooij G, Reijerkerk A, Vidarsson G, et al. Immunoglobulin A: Fc(alpha)RI Interactions Induce Neutrophil Migration Through Release of Leukotriene B4. Gastroenterology (2009) 137:2018–29 e1-3. doi: 10.1053/j.gastro.2009.06.047
41. Anquetil F, Clavel C, Offer G, Serre G, Sebbag M. IgM and IgA Rheumatoid Factors Purified From Rheumatoid Arthritis Sera Boost the Fc Receptor- and Complement-Dependent Effector Functions of the Disease-Specific Anti-Citrullinated Protein Autoantibodies. J Immunol (2015) 194:3664–74. doi: 10.4049/jimmunol.1402334
42. Chen L, Liu X, Pan Z, Liu S, Han H, Zhao C, et al. The Role of IL-8/CXCR2 Signaling in Microcystin-LR Triggered Endothelial Cell Activation and Increased Vascular Permeability. Chemosphere (2018) 194:43–8. doi: 10.1016/j.chemosphere.2017.11.120
43. Nikolova EB. And Russell MW Dual Function of Human IgA Antibodies: Inhibition of Phagocytosis in Circulating Neutrophils and Enhancement of Responses in IL-8-Stimulated Cells. J Leukoc Biol (1995) 57:875–82. doi: 10.1002/jlb.57.6.875
44. Herlin T, Fogh K, Ternowitz T, Storm K, Bunger E. Chemotactic Leukotriene B4 Activity in Plasma From Children With Arthritis. Acta Pathol Microbiol Immunol Scand C (1987) 95:195–8. doi: 10.1111/j.1699-0463.1987.tb00030.x
45. Liao PY, Wu SH. [Serum Levels of IL-5 and LTB4 in Children With Henoch-Schonlein Purpura]. Zhongguo Dang Dai Er Ke Za Zhi (2006) 8:198–200. doi: 10.3969/j.issn.1008-8830.2006.03.008
46. Yuan L, Wang Q, Zhang S, Zhang L. Correlation Between Serum Inflammatory Factors TNF-Alpha, IL-8, IL-10 and Henoch-Schonlein Purpura With Renal Function Impairment. Exp Ther Med (2018) 15:3924–8. doi: 10.3892/etm.2018.5876
47. Pinheiro RR, Lencastre A. Henoch-Schonlein Purpura During Anti-TNFalpha Therapy: A Fortuitous Event or an Indication to Stop Therapy? Eur J Dermatol (2017) 27:304–5. doi: 10.1684/ejd.2017.2979
48. Yang B, Tan X, Xiong X, Wu D, Zhang G, Wang M, et al. Effect of CD40/CD40L Signaling on IL-10-Producing Regulatory B Cells in Chinese Children With Henoch-Schonlein Purpura Nephritis. Immunol Res (2017) 65:592–604. doi: 10.1007/s12026-016-8877-8
49. Xu H, Pan Y, Li W, Fu H, Zhang J, Shen H, et al. Association Between IL17A and IL17F Polymorphisms and Risk of Henoch-Schonlein Purpura in Chinese Children. Rheumatol Int (2016) 36:829–35. doi: 10.1007/s00296-016-3465-8
50. Wang F, Dong LL. [IL-33 and Sst2 Levels in Serum of Children With Henoch-SchOnlein Purpura and Their Clinical Significance]. Zhongguo Shi Yan Xue Ye Xue Za Zhi (2017) 25:517–21. doi: 10.7534/j.issn.1009-2137.2017.02.038
51. Zhao G, Fu R, Yang C, Luo C, Li F. [The Lymphocyte Subset and Blood Interleukin-2 Level in Children With Henoch-Schonlein Purpura Before and After Cimetidine Treatment]. Hua Xi Yi Ke Da Xue Xue Bao (2000) 31:93–4, 97.
52. Lin CY, Yang YH, Lee CC, Huang CL, Wang LC, Chiang BL. Thrombopoietin and Interleukin-6 Levels in Henoch-Schonlein Purpura. J Microbiol Immunol Infect (2006) 39:476–82.
53. Xu H, Jiang G, Shen H, Pan Y, Zhang J, Li W, et al. The Association Between Genetic Variation in Interleukin-10 Gene and Susceptibility to Henoch-Schonlein Purpura in Chinese Children. Clin Rheumatol (2017) 36:2761–7. doi: 10.1007/s10067-017-3852-x
54. Yang YH, Tsai IJ, Chang CJ, Chuang YH, Hsu HY, Chiang BL. The Interaction Between Circulating Complement Proteins and Cutaneous Microvascular Endothelial Cells in the Development of Childhood Henoch-Schonlein Purpura. PloS One (2015) 10:e0120411. doi: 10.1371/journal.pone.0120411
55. Gomez-Guerrero C, Alonso J, Lopez-Armada MJ, Ruiz-Ortega M, Gomez-Garre D, Alcazar R, et al. Potential Factors Governing Extracellular Matrix Production by Mesangial Cells: Their Relevance for the Pathogenesis of IgA Nephropathy. Contrib Nephrol (1995) 111:45–54. doi: 10.1159/000423876
56. Chua JS, Zandbergen M, Wolterbeek R, Baelde HJ, van Es LA, de Fijter JW, et al. Complement-Mediated Microangiopathy in IgA Nephropathy and IgA Vasculitis With Nephritis. Mod Pathol (2019) 32:1147–57. doi: 10.1038/s41379-019-0259-z
57. Dyer MR, Chen Q, Haldeman S, Yazdani H, Hoffman R, Loughran P, et al. Deep Vein Thrombosis in Mice is Regulated by Platelet HMGB1 Through Release of Neutrophil-Extracellular Traps and DNA. Sci Rep (2018) 8:2068. doi: 10.1038/s41598-018-20479-x
58. Margraf S, Logters T, Reipen J, Altrichter J, Scholz M, Windolf J. Neutrophil-Derived Circulating Free DNA (Cf-DNA/NETs): A Potential Prognostic Marker for Posttraumatic Development of Inflammatory Second Hit and Sepsis. Shock (2008) 30:352–8. doi: 10.1097/SHK.0b013e31816a6bb1
59. Nomura K, Miyashita T, Yamamoto Y, Munesue S, Harashima A, Takayama H, et al. Citrullinated Histone H3: Early Biomarker of Neutrophil Extracellular Traps in Septic Liver Damage. J Surg Res (2019) 234:132–8. doi: 10.1016/j.jss.2018.08.014
60. Wan W, Liu H, Long Y, Wan W, Li Q, Zhu W, et al. The Association Between Circulating Neutrophil Extracellular Trap Related Biomarkers and Retinal Vein Occlusion Incidence: A Case-Control Pilot Study. Exp Eye Res (2021) 210:108702. doi: 10.1016/j.exer.2021.108702
61. Martinez-Alberquilla I, Gasull X, Perez-Luna P, Seco-Mera R, Ruiz-Alcocer J, Crooke A. Neutrophils and Neutrophil Extracellular Trap Components: Emerging Biomarkers and Therapeutic Targets for Age-Related Eye Diseases. Ageing Res Rev (2021) 74:101553. doi: 10.1016/j.arr.2021.101553
62. Purevdorj N, Mu Y, Gu Y, Zheng F, Wang R, Yu J, et al. Clinical Significance of the Serum Biomarker Index Detection in Children With Henoch-Schonlein Purpura. Clin Biochem (2018) 52:167–70. doi: 10.1016/j.clinbiochem.2017.11.006
63. Deng Z, Yang Z, Ma X, Tian X, Bi L, Guo B, et al. Urinary Metal and Metalloid Biomarker Study of Henoch-Schonlein Purpura Nephritis Using Inductively Coupled Plasma Orthogonal Acceleration Time-of-Flight Mass Spectrometry. Talanta (2018) 178:728–35. doi: 10.1016/j.talanta.2017.10.013
64. Teng X, Wang Y, Lin N, Sun M, Wu J. Evaluation of Serum Procalcitonin and C-Reactive Protein Levels as Biomarkers of Henoch-Schonlein Purpura in Pediatric Patients. Clin Rheumatol (2016) 35:667–71. doi: 10.1007/s10067-014-2773-1
65. Delanghe SE, Speeckaert MM, Segers H, Desmet K, Vande Walle J, Laecke SV, et al. Soluble Transferrin Receptor in Urine, a New Biomarker for IgA Nephropathy and Henoch-Schonlein Purpura Nephritis. Clin Biochem (2013) 46:591–7. doi: 10.1016/j.clinbiochem.2013.01.017
66. Ge W, Wang HL, Sun RP. Pentraxin 3 as a Novel Early Biomarker for the Prediction of Henoch-Schonlein Purpura Nephritis in Children. Eur J Pediatr (2014) 173:213–8. doi: 10.1007/s00431-013-2150-0
67. Kanik A, Baran M, Ince FD, Cebeci O, Bozkurt M, Cavusoglu D, et al. Faecal Calprotectin Levels in Children With Henoch-Schonlein Purpura: Is This a New Marker for Gastrointestinal Involvement? Eur J Gastroenterol Hepatol (2015) 27:254–8. doi: 10.1097/MEG.0000000000000284
68. Hong J, Yang HR. Laboratory Markers Indicating Gastrointestinal Involvement of Henoch-Schonlein Purpura in Children. Pediatr Gastroenterol Hepatol Nutr (2015) 18:39–47. doi: 10.5223/pghn.2015.18.1.39
69. Zhang L, Han C, Sun C, Meng H, Ye F, Na S, et al. Serum Levels of Alpha-Smooth Muscle Actin and C-Met as Biomarkers of the Degree of Severity of Henoch-Schonlein Purpura Nephritis. Transl Res (2013) 161:26–36. doi: 10.1016/j.trsl.2012.09.001
Keywords: IgA vasculitis, neutrophil extracellular traps, pathogenesis, biomarker, neutrophils, IgA vasculitis nephritis
Citation: Chen XQ, Tu L, Tang Q, Huang L and Qin YH (2022) An Emerging Role for Neutrophil Extracellular Traps in IgA Vasculitis: A Mini-Review. Front. Immunol. 13:912929. doi: 10.3389/fimmu.2022.912929
Received: 05 April 2022; Accepted: 26 May 2022;
Published: 21 June 2022.
Edited by:
Raphaela Goldbach-Mansky, National Institutes of Health (NIH), United StatesReviewed by:
Loreto Massardo, Universidad de San Sebastián, ChileCopyright © 2022 Chen, Tu, Tang, Huang and Qin. This is an open-access article distributed under the terms of the Creative Commons Attribution License (CC BY). The use, distribution or reproduction in other forums is permitted, provided the original author(s) and the copyright owner(s) are credited and that the original publication in this journal is cited, in accordance with accepted academic practice. No use, distribution or reproduction is permitted which does not comply with these terms.
*Correspondence: Xiu-Qi Chen, Y2hlbnhpdXFpQGd4bXUuZWR1LmNu