- 1Division of Dermatology, Rush University Medical Center, Chicago, IL, United States
- 2Department of Dermatology, Venereology and Leprology, Postgraduate Institute of Medical Education and Research, Chandigarh, India
- 3Department of Dermatology, University Hospital Inselspital Bern, University of Bern, Bern, Switzerland
- 4Department of Internal Medicine, Rush University Medical Center, Chicago, IL, United States
Bullous pemphigoid is an autoimmune blistering disease caused by autoantibodies targeting BP180 and BP230. While deposits of IgG and/or complement along the epidermal basement membrane are typically seen suggesting complement -mediated pathogenesis, several recent lines of evidence point towards complement-independent pathways contributing to tissue damage and subepidermal blister formation. Notable pathways include macropinocytosis of IgG-BP180 complexes resulting in depletion of cellular BP180, direct induction of pro-inflammatory cytokines from keratinocytes, as well as IgE autoantibody- and eosinophil-mediated effects. We review these mechanisms which open new perspectives on novel targeted treatment modalities.
Introduction
Bullous pemphigoid (BP) is the most frequent autoimmune subepidermal blistering disease associated with an autoantibody response directed against the BP antigen 180 (BP180, BPAG2 or type XVII collagen) and the BP antigen 230 (BP230 or BPAG1-e). The latter are components of junctional adhesion complexes called hemidesmosomes that promote dermo-epidermal cohesion (1). Characteristically, BP is an intensely pruritic eruption with generalized blistering. However, in early stages or in atypical variants of the disease, only localized or generalized excoriated, eczematous, or urticarial lesions may be present. The disease, which has a chronic course, typically affects the older population after the age of 65 and has a significant impact on both the quality of life and life-expectancy (2). The one-year mortality ranges from 13% to 40%, while the mortality rate of patients with BP seems to be at least three times higher than that of age- and sex-matched subjects (3). The annual incidence has been estimated to be at least 6–13 new cases per million population with a striking increase after the age of 80 years (with more than 300 cases per million in individuals). Nonetheless, in the last two decades, there is evidence indicating a two to four-fold rise of the overall incidence of BP in the population, most likely due to the better recognition of atypical forms of BP and the increasing relative size of older age groups (4).
A recent consensus guideline on management of BP primarily recommends the use of high potency topical steroids and systemic corticosteroids as first-line therapeutic options (5). Immunomodulatory and immunosuppressive drugs may be considered in treatment-resistant cases or in cases at increased risk for steroid-related adverse events or in the presence of contraindications to systemic steroids. In recent years, a number of biologics have been used with promising results, such as omalizumab, dupilumab, interleukin-17, and IL-5Rα inhibitors (6, 7). In addition, a recently published phase 2a trial examined the use of nomacopan, a leukotriene B4 and C5 inhibitor, in BP patients. The drug appears to be well-tolerated by patients and has therapeutic potential for reducing acute BP flares (8). As BP is more common in the elderly, balancing management with patient comorbidities is almost invariably challenging. The efficacy of current treatments is limited and relatively unsatisfactory; patients’ unmet needs remain significant. Hopefully, several ongoing trials will allow more effective and better tolerated therapies to be validated in the near future. Such therapies should facilitate and improve the overall management of affected patients, which primarily consist of fragile and debilitated individuals.
Pathogenesis of Bullous Pemphigoid
There is ample evidence indicating that BP occurs due to a loss of immune tolerance leading to autoantibody formation against BP180 and BP230. BP180 is transmembrane protein with a large collagenous extra-cellular domain serving as an adhesion molecule. Its ectodomain binds to laminin 332 and type IV collagen, connecting the basal keratinocytes to the extracellular matrix of the epidermal basement membrane (9–11). BP230, the epithelial isoform of BPAG1, is a cytoplasmic protein of the plakin family of cytolinkers. It primarily connects the keratin intermediate filament system to hemidesmosomes at the basal keratinocyte cell membrane (1, 9, 12). Patients’ sera recognize multiple antigenic regions on both target antigens, although the NC16A domain, on the extracellular membrane of BP180, contains the immunodominant antigenic determinants (13, 14). The autoreactive B and T cell response in BP is primarily directed at this region of BP180 (15, 16). BP autoantibodies lead to an inflammatory response with a large number of eosinophils and, to a lesser degree, neutrophils, migrating to the dermis and degranulating. These cells contain and release upon activation dozens of cytokines, chemokines, hydrolytic degrading enzymes, including matrix metalloprotease 9 (MMP9) and neutrophil elastase, as well reactive oxygen species. This inflammatory cascade ultimately leads to tissue damage and subepidermal blister formation (17–21).
In vitro and in vivo studies have allowed the characterization of several pathways critically involved in BP pathogenesis that directly contribute to tissue damage. Among these, activation of the complement system with production of anaphylatoxins, and activation of the innate immune response with subsequent recruitment and activation of basophils, eosinophils, neutrophils, monocytes/macrophages, and mast cells, play a key role in BP (15, 19, 22–27). Complement components, including C1q, C3, C4, and the membrane attack complex (MAC) are usually found detected along the dermal-epidermal junction (DEJ) in the skin of both patients with BP and of mice with experimentally induced BP (28–31). Furthermore, complement proteins including anaphylatoxins are detectable in the blister fluid of BP patients (32). The presence of tissue-bound complement components and/or the ability of circulating autoantibodies to mediate complement activation are also likely to affect clinical and histopathological features, including overall disease activity, in affected patients (33). For example, the presence of tissue-bound C3 in the skin of BP positively correlates with the presence of circulating anti-BP180 antibodies targeting the NC16A domain (34). While the importance of complement was described as early as the 1970s (35), increasing evidence has emerged pointing to the presence of complement-independent pathways in mediating tissue damage and subepidermal formation in BP.
This review will seek to summarize the current understanding of complement-independent mechanisms in BP and provide a reference framework for future research aimed at further elucidating these processes. This new knowledge is expected to facilitate the development of new treatment modalities that should benefit the management of BP patients in the near future.
Complement-Independent Pathways in Tissue Damage and in Dermo-Epidermal Disadhesion
In the last decade, a number of laboratories have provided convincing evidence that complement-independent processes are implicated in the pathogenesis of BP, directly contributing to inflammation, tissue damage and dermo-epidermal separation. This idea is substantiated by a number of in vitro and in vivo experiments as well as clinical observations, including:
1) Serum derived anti-NC16A IgG antibodies and recombinant anti human NC16a IgG antibodies impair keratinocyte adhesion and deplete BP180 by induction of macropinocytosis (36–39); 2) Passive transfer of F(ab’)2 fragments of the human BP or IgG antibodies, against BP180, that cannot activate complement, are able to cause skin fragility in neonatal BP180-humanized mice (36); 3) C5-/- mice as well as C5ar1-/- mice injected with anti-NC15A antibodies, the murine analog to NC16A in humans, develop a BP-like phenotype, although its severity is milder when compared to that of wild type (WT) mice (40). In the latter study, pharmacologic inhibition of C5a receptor 1 fails to reduce clinical disease or neutrophil infiltration in mice with established cutaneous disease (40); Notably, this study demonstrates that inhibition of complement has therapeutic benefit, once again reaffirming the importance of complement in BP. However, C5ar-/- mice demonstrated a relatively increased extent of skin lesions following BP-IgG injection, raising the possibility of complement-independent induced blistering as well 4) Production of neutrophil reactive oxygen species (ROS), which contribute to tissue damage, does not differ between WT, C5ar1-/-, and C5ar2-/- mice (40), 5) Passive transfer of human BP-IgG into C3-/- BP180 humanized mice develop blisters (39); 6) Non-complement binding autoantibodies are able to cause blister formation in vivo (39, 41); 7) BP-IgG antibodies are able to induce IL-6, IL-8, and Hsp90 expression from cultured keratinocytes independent of complement (42–48); 8) IgG4 autoantibodies, which are the dominant IgG isotype in over 50% of BP patients, are able to induce blistering in cryosection assays (41, 49–54) 9) IgE autoantibodies and eosinophils contribute to blister formation by means of various mechanisms including secretion of proteases, eosinophil degranulation, and extracellular traps, as well as cytokine and chemokine release in a complement independent manner. However, IgE antibodies very rarely form in isolation of IgG. As such, while they are definitively pathogenic, their contribution relative to IgG is unclear. Finally, 10) in up to 20% of biopsy specimens obtained from BP patients, there is no evidence for complement deposition as assessed by direct immunofluorescence microscopy (DIF) (55). However, this study was limited by use of a single detection antibody against complement, specifically the C3c component. Sensitivity may increase with the use of multiple antibodies. Likewise, a case report of a patient developing BP despite C4 deficiency provides further support (56)
The recent Phase 2a trial results of the complement inhibiting drug nomacopan offer an important caveat to the prospect of physiologically significant complement-independent effects (8). In this study, 7 of 9 patients saw significant reductions in skin severity index scores by six weeks. However, the subjects who were non-responders to such complement inhibition raises the possibility of tissue damage and blister induction occurring outside of the complement system in these patients. Furthermore, randomized trials with much larger sample sizes are needed to truly assess the therapeutic efficacy of this drug.
Non-Immunologic Induction of Blistering by Anti-BP180 Antibodies on Keratinocytes
The direct non-immunologic, but complex biologic impact of anti-BP180 antibodies on basal keratinocytes represents an important means by which complement-independent mechanisms contribute to basement membrane disadhesion and dermo-epidermal blistering. Both ubiquitin- and proteasome-mediated degradation of BP180, as well as macropinocytic internalization of BP180 appear to be involved (57). Notably, this is seen with antibodies targeting the NC16a domain of BP180, but not the c-terminus, suggesting epitope dependent pathogenicity (58).
Early studies by Kitajima et al. first showed that the binding of anti-BP180 antibodies results in internalization of BP180 in cultured epidermal cells (59, 60). Further work by Iwata et al. confirmed that incubation of anti-BP-IgG autoantibodies causes internalization of BP180 from the cell membrane and provided evidence indicating that BP180 is depleted from the keratinocyte. In these experiments, BP-IgG treatment was able to reduce the amount of BP180 from cells by roughly 40% and 85% after two and six hours of respective incubation as assessed by immunoblotting. Notably, while BP180 was decreased, the amount of the α6β4 integrin, a key component of hemidesmosomes, remained unchanged, indicating that the effect on BP180 was specific. By semiquantitative analysis of BP180 content in BP patients’ skin by immunoblotting, the authors also found a reduction of ~40% of BP180 in BP patients’ skin when compared to that of control subjects. Finally, in vibration detaching assay using cultured keratinocytes, BP-IgG treatment caused a significant reduction of the adhesion of cells to the culture plate (61). Another study showed that both BP-IgG and BP-IgE are capable of directly binding to the surface of cultured human keratinocytes with subsequent loss of hemidesmosomes at the basement membrane zone (BMZ) (44). The studies mentioned above used in vitro models exclusively, and generalizations regarding this apparent BP180 internalization and subsequent loss of adhesion to living systems are limited.
In 2012, Natsuga et al. reported that rabbit antibodies raised against a distinct portion of the human NC16A region of BP180 (Arg522 to Gln545) decrease BP180 expression in cultured NHKs (36). More strikingly, the same group also found that the injection of the F(ab’)2 fragments of the rabbit anti-BP180-NC16A antibodies, thus lacking the complement-binding Fc domain, was able to cause dermal-epidermal splitting in neonatal BP180-humanized mice and also decreased expression of BP180 in murine skin by immunoblotting. Nevertheless, the observation that not all mice injected with the F(ab’)2 fragments displayed skin fragility implies that the anti-NC16A F(ab’)2 fragments have a less potent effect than BP-IgG (36). It is important to note that this study utilized neonatal mice. Skin fragility and neonatal immune response may not be predictive of human responses. Hiroyasu et al. subsequently confirmed the findings of Natsuga et al. using cultured 804G cells and normal human epidermal keratinocytes (NHEKs) as well as BP-IgG(Fab’)2 and BP-IgG Fab fragments (37). Hence, these observations strongly indicate that skin detachment in BP not only depends on a complement-mediated inflammatory cascade, but also involves a direct effect of BP antibodies on the adhesive function and cell expression of BP180, which directly impairs dermo-epidermal cohesion. It is unclear why previous mouse model studies failed to observe a direct effect of IgG(Fab’)2 fragments against BP180 on keratinocyte adhesions. These apparent discrepancies may be related to the variable experimental conditions of the used in vitro assays and in vivo models (22, 62, 63).
It has been speculated that blistering in BP first requires a weakening of the adhesive strength of keratinocytes, which is then accompanied by an inflammatory response that ultimately causes dermo-epidermal separation (37, 61). This model, which is not yet substantiated by experimental data, can however be applied on a subset of patients with pauci-inflammatory BP and other subepidermal bullous autoimmune diseases in which blistering primarily results from mechanical trauma and friction. This phenomenon is typically observed in patients with the mechano-bullous form of epidermolysis bullosa acquisita, with antibodies directed against type VII collagen.
BP-IgG induced internalization of BP180 occurs through the macropinocytosis pathway. In fluid uptake assays, the addition of macropinocytosis inhibitors are able to block the internalization of BP180 in both cultured 804G cells and NHEKs. This process seems to occur following a calcium-dependent phosphorylation of the intracellular domain of BP180 by the protein kinase C pathway (38). Inhibition of the macropinocytosis pathway is also able to block the negative effect of BP-IgG on the adhesive strength of cultured NHEKs following treatment (37). The entire BP180 molecule seems to be internalized as a complex bound with BP-IgG. The impact of this internalization of BP180 on hemidesmosome formation in vitro and potentially in vivo remains to be assessed (64).
Ujiie et al. also characterized the mechanisms by which BP-IgG induced BP180 depletion takes place (39), confirming that anti-BP180 BP-IgG can induce blistering in complement-deficient mice. Notably, these researchers have utilized a monoclonal antibody, mIgG2c (TS4-2) which has high complement activation activity, low ability to deplete BP180, and low affinity to hNC16A. The passive transfer of this mIgG2c anti-BP180 monoclonal antibody failed to cause blistering in most mice. Ujiie et al. also showed that BP180 is ubiquinated following treatment with BP-IgG. In fact, addition of a proteasome inhibitor, MG-132, prevents the depletion of BP180 in a dose-dependent manner. These results thus indicate that the ubiquitin/proteasome pathway is implicated in BP180 depletion. Noteworthy, in this latter experimental model, mice injected with the monoclonal antibody rhIgG4 against the human NC16A domain of BP180 (but unable to activate the complement) still developed blistering despite the administration of a proteasome inhibitor. This observation indicates that internalization of BP180 and adhesive weakening most likely precede the degradation of BP180 via proteasomes. In this context, it should be mentioned that proteins that enter cells via macropinocytosis are usually degraded by lysosomes. However, proteasomes may also be involved under certain circumstances, such as in relation to cross-presentation of antigens on the major histocompatibility complex I pathway (65). Hence, it remains unclear if BP180 is only degraded by proteasomal pathways or if lysosomes and/or other processes are also implicated (9).
Regulation of Inflammatory Responses by BP180 in Keratinocytes
In addition to the direct non-inflammatory effects of anti-BP180 autoantibodies, there is growing evidence for complement-independent inflammatory mechanisms by which BP antibodies can induce disease. Specifically, keratinocytes are able to secrete a variety of proinflammatory cytokines which appear to be pathogenetically relevant. Specifically, in 2000 Schmidt et al. found that treatment of cultured NHEKs with anti-NC16A BP-IgG, and not control IgG, results in an increased expression of IL-6 and IL-8 in a time- and concentration-dependent manner while IL-1α, IL-1-β, IL-10, and TNF-α were not detected and MCP-1 levels remained unchanged (46). Molecules such as IL-1β and TNF-α are known to upregulate complement factors (66). The upregulation of IL-6 and IL-8 was detected at both the mRNA and protein level. In addition, blocking the immunoreactivity of two distinct epitopes, NC16A and NC16A2, prevented the upsurge in IL-8. Also of note, since BP-IgG treatment of BP180-deficient keratinocytes did not cause an increased release of IL-6 or IL-8, these results suggest that the specific interaction between BP autoantibodies and BP180 ectodomain initiates an intracellular signal transduction pathway affecting transcription and translation with an increased release of keratinocyte-derived IL-6 and IL-8 (46).
Messingham and colleagues expanded on these results finding that not only IgG but also IgE anti-BP antibodies are capable of stimulating IL-6 and IL-8 production in cultured human keratinocytes and organ culture (44). Similar results were obtained following treatment with F(ab’)2 and Fab fragments prepared from IgE and IgG, confirming that these effects occur in an FcR-independent manner. Noteworthy, IgE appears to be a more potent stimulator of cytokine production compared to IgG (44). In this context, we recently identified a similar pro-inflammatory response from keratinocytes treated with IgG obtained from patients with laminin-332 pemphigoid, particularly with autoantibodies against the β3 subunit (67).
IL-8 derived from keratinocytes is chemotactic for neutrophils (68). The latter are critical for the formation of blisters in both animal and in vitro BP models (23, 69). Furthermore, Liu et al. showed that intradermal IL-8 injection into C-5 deficient mice can reverse their resistance to the pathogenic effects of rabbit-derived BP-IgG monoclonal antibodies (23). IL-6 is another pro-inflammatory cytokine. It is secreted by keratinocytes and can penetrate the BMZ (70). Its contribution in tissue damage is attested by the observation that IL-6-depleted mice do not develop blistering in a BP model (23). Finally, IL-6 and IL-8 levels are elevated in both the sera and blister fluid of BP patients (71, 72). Serum levels of these interleukins have also been correlated with disease activity in BP (73). Overall these observations indicate that keratinocytes, by releasing pro-inflammatory cytokines, also play a previously unrecognized role in the pathogenesis of pemphigoid diseases (67). Keratinocyte dependent complement-independent mechanisms are summarized in Figure 1.
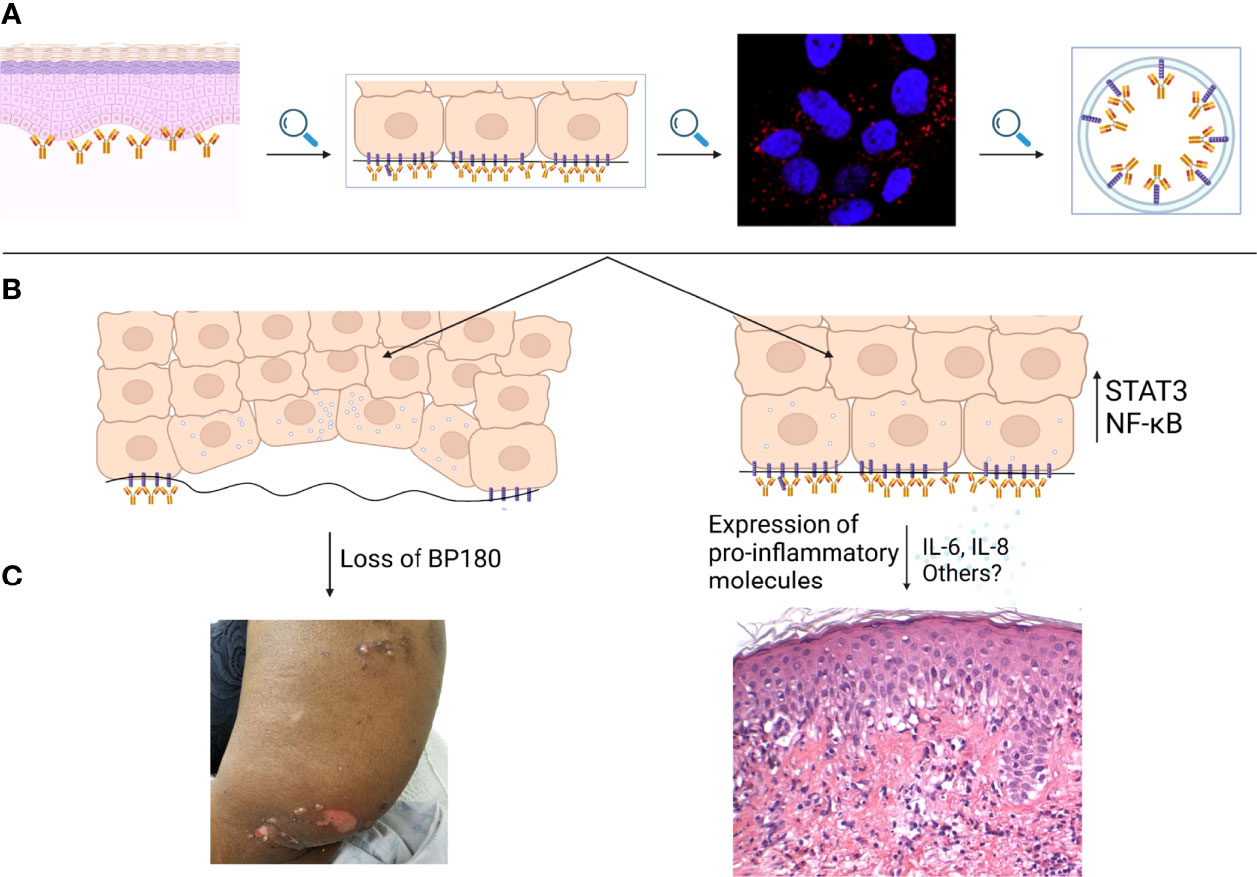
Figure 1 Keratinocyte mediated, complement-independent pathways in bullous pemphigoid. (A) IgG autoantibodies targeting BP180 bind to the epidermal basement membrane. IgG-BP180 complexes undergo macropinocytosis as shown by immunostain for EEA-1. (B) Internalized IgG-BP180 complexes lead to both loss of cellular BP180 and increased expression of proinflammatory mediators such as IL-6 and IL-8, resulting in both (C) pauci-inflammatory and pro-inflammatory complement-independent mechanisms.
Pharmacologic studies have also been useful to gain better insight into the inflammatory processes triggered by anti-BP antibodies (42, 45, 47). By ELISA, Tukaj et al. found that calcitriol decreased the BP-IgG-induced release of IL-6 and IL-8 in human keratinocytes in a dose- and time-dependent fashion (42). Moreover, this vitamin D metabolite reduced phosphorylation of STAT3 and suppressed NF-κβ activity in keratinocytes treated with BP-IgG, but did not affect levels of heat shock protein (Hsp) 70 or the vitamin D receptor (VDR), an observation implying that NF-κβ and STAT3 are both involved in mediating the pro-inflammatory effects. Dapsone, an anti-inflammatory agent used in BP management, was found to specifically suppress the release of IL-8, but not of IL-6, from NHEKs treated with BP-IgG antibodies isolated from both human and rabbit sera, in a dose-dependent manner (45). Evidence was further provided suggesting that the effect of dapsone occurs at the post-transcriptional level (45). Notably, dapsone can also exert a significant inhibitory effect on neutrophils (74). Therefore, conclusions drawn on the complement-independent mechanisms of BP from dapsone’s efficacy in the disease may be limited.
Hsp90 has been linked to the synthesis of various cytokines such as TNF-α, IL-1, IL-6, and IL-8 (48, 75–77). In human epidermal keratinocytes treated with BP-IgG, blockade of Hsp90 with 17-DMAG is able to suppress the IL-8, but not IL-6, release in a dose- and time-dependent manner (47). Blocking Hsp90 also impaired the NF-κβ p65 subunit activity in BP-IgG stimulated keratinocytes. These findings suggest that Hsp90 also exerts a regulatory role in BP-IgG-induced production of IL-8.
Van Den Bergh et al. sought to assess whether BP180 is directly involved in modulating this pro-inflammatory response (43). For this purpose, they measured IL-8 response under various inflammatory stimuli in both normal keratinocytes and in BP180-deficient keratinocytes derived from either a junctional epidermolysis bullosa patient or after shRNA-mediated knockdown of BP180. The BP180-deficient keratinocytes showed a dysregulated higher IL-8 response after treatment with lipopolysaccharide (LPS), ultraviolet-B radiation or tumor necrosis factor compared to normal human keratinocytes. Notably, inhibition of NF-κβ, but not p38MAPK, was able to normalize this response. The same group also found that LPS treatment of BP180-deficient keratinocytes increases the expression of an NF-κβ-driven reporter compared to normal cells. In LPS-treated cells, inhibition of NF-κβ activity in BP180-deficient keratinocytes normalized their IL-8 response. The results are in line with the idea that the effects of BP180 on IL-8 response are mediated by NF-κβ. Together, these results point toward BP180 serving as regulator of IL-8 involved inflammatory response of keratinocytes. It is as of yet unclear if autoantibodies to BP180 affect its interactions with other hemidesmosomal components, such as the α6β4 integrin and extracellular proteins, and if disturbance of this network has an impact on the inflammatory response. Importantly, many of the aforementioned studies looking at these inflammatory mechanisms and their regulation are limited by reliance on in vitro data. As such, drawing conclusions regarding the in vivo response must be done carefully.
In another study (78), genetically engineered mice which expressed a NC16A-truncated BP180 developed spontaneous inflammation of the skin and exhibited severe pruritus, compromised skin barrier, increased serum IgE, and immune cell infiltration. The pruritus was found to be independent of adaptive immunity or histamine, but was related to an increased expression of TSLP. This study suggests that dysfunction or structural alteration of BP180 is sufficient to trigger an inflammatory response similar to that seen in BP patients.
Finally, the effects of IgG4 autoantibodies provide evidence for other complement-independent inflammatory mechanisms in bullous pemphigoid. While IgG1 and IgG3 antibodies are known to fix complement, the IgG4 subclass does not (79). In 2007, Mihai et al. isolated both IgG1 and IgG4 BP antibodies from patient sera and introduced them into an ex vivo experimental model. Although the IgG4 antibodies did not activate complement, they were able to induce dermo-epidermal spitting and tissue damage via leukocyte recruitment and activation (79). While the pathogenic potential of the IgG4 autoantibodies was significantly less than that of IgG1 in this experiment, the study demonstrates the capability of IgG4 to induce a BP-like phenotype in the absence of complement, and introduces a novel role for IgG4 in this disease.
Eosinophil and IgE Mediated Blistering
Eosinophils can directly mediate dermo-epidermal separation in the presence of either IgG or IgE (80, 81). In both instances, complement is not required. Eosinophils, which are typically abundantly present in lesional skin of BP patients, play an important role in tissue damage by means of different mechanisms (82–86). Eosinophils are capable of secreting the matrix metalloproteinase 9 (MMP-9), which can degrade BP180 and thus contribute to dermo-epidermal separation, by cleaving the extracellular collagenous domain of BP180 and other proteins (21, 87–90).
Degranulated proteins from eosinophils can be detected in both the serum and blister fluid of BP patients (91–94). Eosinophil granules have also been found along the BMZ in patients with BP (94, 95). Release and deposition of eosinophil granules appear to be present even in the early stages of BP lesions (96, 97). We have demonstrated that the granule proteins eosinophil cationic protein (ECP) and eosinophil derived neurotoxin (EDN) induce keratinocyte expression of IL-5, eotaxin-1, and RANTES, as well as reactive oxygen species formation. ECP but not EDN is able to directly induce keratinocyte detachment (98).
Eosinophils can also produce extracellular traps (EETs) which are made up of granule proteins, DNA, and nuclear components in a network-like structure which can expand to be 15 times larger than the cell itself (99). EETs have been found to be present in BP. Based on ex vivo data obtained from experiments involving human skin and isolated eosinophils showing that dermo-epidermal separation is reduced with DNase affecting EETs, the latter may be directly involved in the amplification of the inflammatory response, although the exact mechanisms remain still unknown (100). It should be noted that neutrophil extracellular traps (NETs) may also play a role in BP. Using immunodetection of patient skin biopsies, NET formation has been shown to be associated with BP (101). Additionally, levels of NET biomarkers are correlated to BP disease activity (102). Thus, it is likely that these complexes play a role in the tissue damage involved in this disease. However, given the fact that neutrophils are likely recruited at least in part by complement (103), it is unclear whether these NET-related mechanisms may truly be complement-independent.
As described previously, eosinophils are involved in BP pathogenesis by mediating the effects of anti-BP180 IgE antibodies and contributing to dermo-epidermal separation (81). Anti-BP180 IgE autoantibodies are present in the majority of BP patients, and their levels are correlated with disease activity (104–107). In mice with grafted human skin, injection of anti-NC16A IgE resulted in inflammation with development of erythematous skin lesions and dermo-epidermal separation. Influx and degranulation of eosinophils have been here implicated (108). Similar results were obtained using human cryosection dermis in which IgE injection led to DEJ separation with associated eosinophil infiltration. The activation of eosinophils was mediated through the FCεRI receptor (109–111). Notably, the amount of anti-BP180 IgE and IgG was correlated to levels of circulating eosinophils in BP sera (111). Moreover, IgE autoantibodies against a component of the shed ectodomain of BPAG2 induce pruritus, erythema, eosinophil infiltration, and blistering when passively transferred (112).
Eosinophils also directly contribute to BP symptomatology by producing IL-31, a known pruritogen. Pruritus is a key feature of BP and can be a presenting symptom even in the absence of specific skin lesions (13). IL-31 activates endothelin-1 and causes subsequent upregulation of brain natriuretic peptide (BNP), an important mediator of pruritus (113, 114). IL-31 is known to be produced by eosinophils (107, 115), and increased levels of IL-31 have been found in both the lesional skin and serum of BP patients (116). Recent evidence even suggests that eosinophils are the central source of IL-31 in BP (117). Eosinophil-derived IL-31 certainly plays a role in BP itching, but it is still unclear whether this is the primary mediator of pruritus in BP or if other pathways are paramount.
The most compelling evidence for the role of eosinophils in mediating tissue injury in BP comes from Lin et al. who generated a transgenic mouse which expressed human hNC16A as well as the human FCεRI (118). In these mice, anti-NC16A IgE produced subepidermal splitting along with eosinophil infiltration and deposition of IgE along the epidermal BMZ. BP-IgE-induced blistering required the presence of eosinophils. In this model, the intensity of eosinophil infiltration also correlated with disease severity. Overall, these findings not only support the pathogenicity of anti-NC16A IgE antibodies in BP, but also show that eosinophils are the mediator in this process (118).
The work of Freire et al. further characterized the complex role of IgE antibodies in BP (119). Using ELISA and immunofluorescence to examine BP patient sera and skin respectively, they detected increased levels of both anti-BP180 and anti-BP230 IgE compared to healthy controls. The former were found to interact with the same NC16A region of BP180 known to be recognized by IgG. Furthermore, direct immunofluorescence studies showed the majority of BP patients (compared to none of the healthy controls) to have IgE+ cells in their skin. Surprisingly, IgE was rarely detected at the BMZ, and instead was primarily associated with mast cells and eosinophils in the dermis. The study also identified fragments of the extracellular domain of BP180 in the dermis and BMZ, often co-localized with IgE+ cells. These findings indicate that BP180 and IgE can form complexes on the same cells. Further degranulation assays revealed that these IgE-BP180 complexes are capable of cross-linking FCERI receptors and causing basophil degranulation. This process could conceivably lead to inflammation and tissue damage in BP skin. When taken overall, these findings provide strong evidence for an additional complement-independent, Th2-dependent, eosinophil-mediated pathway that contributes to tissue damage and clinical features in BP. A summary of IgE and eosinophil dependent pathomechanisms are shown in Figure 2.
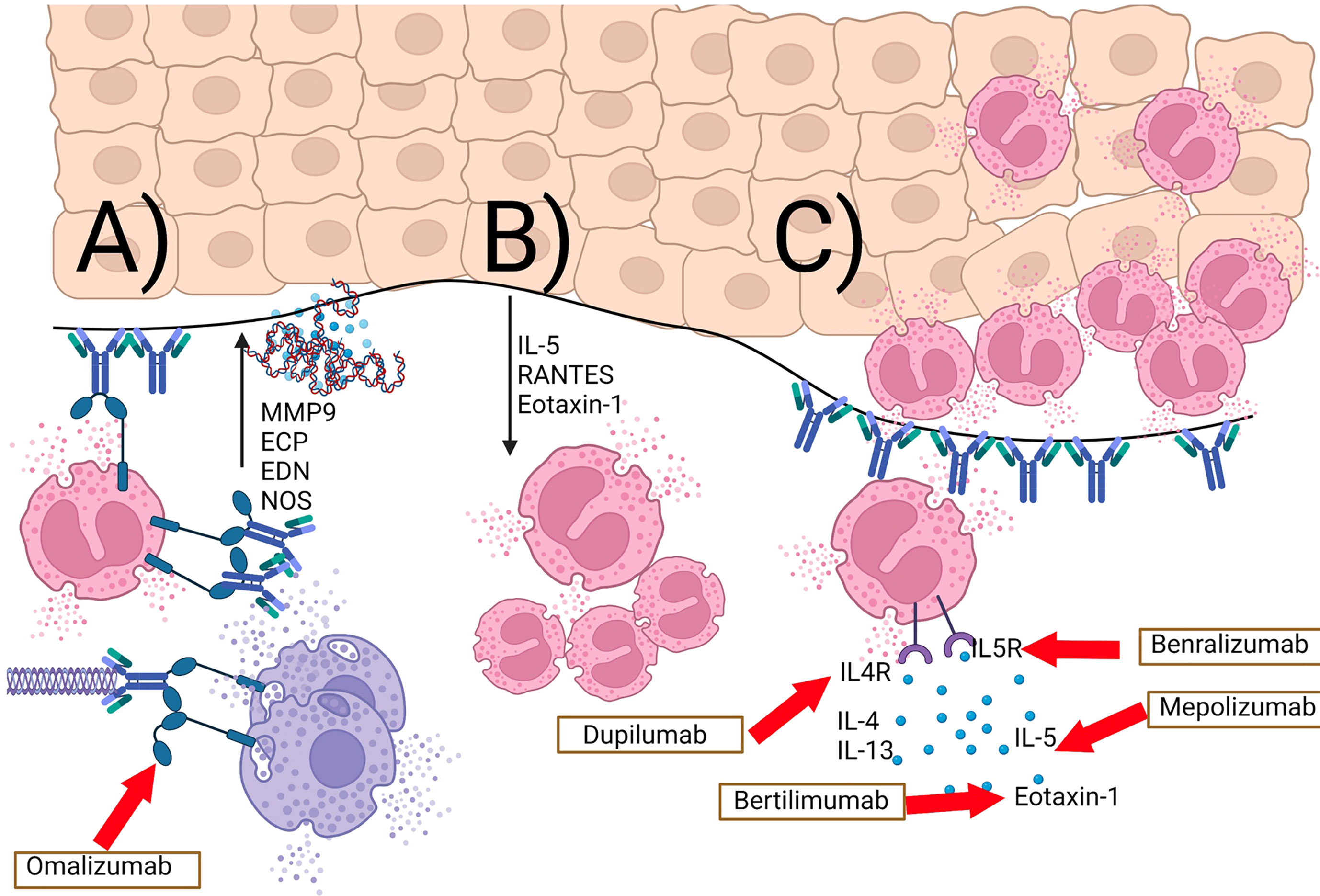
Figure 2 IgE- and eosinophil-mediated complement-independent pathways in bullous pemphigoid. (A) BP180 IgE autoantibodies and BP180-IgE complexes bind to the cutaneous basement membrane and the FcϵR1 on eosinophils as well as mast cells and basophils. This results in release of proteases (e.g. MMP9), eosinophil granule proteins (ECP, EDN), eosinophil extracellular traps, as well as reactive nitric oxide-derived oxidants (NOS). (B) Keratinocytes release IL-5, RANTES, and eotaxin-1 as a response to eosinophil granule proteins. (C) this positive feedback loop results in an increase in tissue eosinophilia and eosinophilic spongiosis. Inhibitory therapeutic antibodies are shown in boxes with red arrows leading to their downstream target.
Finally, investigation regarding IgM antibodies in pemphigoid also highlights the possibility of complement-independent mechanisms in these diseases. Cases of IgM bullous pemphigoid have been documented, with such patients exhibiting linear deposition of both IgM and C3 (120–125). In addition, several patients with only IgM deposition have been identified (126, 127). A recently published article from Boch et al. describes three patients with ‘non-bullous’ pemphigoid who presented with erythematous papules and plaques, two of whom displayed exclusive IgM deposition at the BMZ in the complete absence of complement. The other patient showed weak complement binding. Additionally all three patients demonstrated no serum complement activation capacity (128). Thus, complement-independent mechanisms may play a more prominent role in unique subtypes of AIBD such as this. However, as C3c deposition has been shown to be significantly decreased in non-bullous patients as compared to those with blisters (33, 55), these findings continue to reinforce the central importance of complement activation in actual blister formation as well.
Lessons From the Bedside and Targeted Therapies
The presence of complement-dependent and independent mechanisms in BP not only highlight the disease’s biologic complexity, but treatment challenges. Currently, several inhibitors of complement components are under investigation for the treatment of BP. Other than identifying the presence of complement on the epidermal BMZ which has significant limitations, it remains a challenge to stratify patients who may have significant contribution from complement-independent pathways. Nonetheless, biomarkers of complement independent pathways can be utilized to target these pathways.
Patients with BP often exhibit elevated serum IgE levels and circulating BP180- and BP230-specific IgE autoantibodies. These findings provide support to the idea that IgE has a role in BP pathogenesis (129, 130). In fact, it is thought that IgE autoantibodies directed against the ectodomain of BP180 are first bound to FcεRI on mast cells and eosinophils. This binding subsequently promotes degranulation and initiates an inflammatory reaction resulting in further tissue damage and blister formation (44, 97, 111, 131–133). In addition, binding of specific IgE autoantibodies to the ectodomain of BP180 on basal keratinocytes also triggers internalization of BP180 (see above) and thereby contributes to cell-substrate disadhesion (44, 119, 134). As a result, a humanized mAb that inhibits IgE binding to its high-affinity receptor (FcεRI), omalizumab (an approved treatment for severe asthma and chronic spontaneous urticaria), represents a logical alternative drug for BP. In 2009 Fairley et al. first reported the beneficial effect of omalizumab in a BP patient poorly controlled by oral corticosteroids, azathioprine, and minocycline. After 16 weeks of treatment, several patients showed a significant improvement despite discontinuation of corticosteroids. Since this first report, several case series have confirmed the value of omalizumab as either monotherapy or adjuvant therapy in patients with various forms of BP (105, 135–138). We have also successfully used omalizumab in a number of BP patients and found that omalizumab treatment results in a sharp decrease of FcεRI expression on circulating basophils and a strong reduction of FcεRI+ cells in the skin of treated patients (129). Our results are thus in line with the idea that omalizumab is able to sequester free IgE and prevent its binding to its high-affinity IgE receptor, FcεRI (139–141). This process has been proposed to then downregulate the expression of FcεRI on mast cells and basophils as well as antigen-presenting cells (139).
In addition to omalizumab, there are other biological targeted therapies in development for BP (142). Dupilumab, a human IgG4 monoclonal antibody binding the IL4-Rα inhibits IL-4 and IL-13. It is approved in atopic dermatitis and is being studied in BP, and has several reports of treatment success (7, 143–145). While a phase 2 study of mepolizumab, a humanized IgG1 monoclonal antibody targeting IL-5, was unsuccessful in BP (146), benralizumab, a human IgG1 monoclonal antibody targeting IL5-Rα that leads to apoptosis of eosinophils and basophils, is being studied.
Together, these observations corroborate the idea that complement-independent mechanisms, which play a role in BP pathogenesis, offer additional therapeutic targets beneficial for affected patients. The recent, promising results of the complement inhibitor nomacopan continue to reflect the well-established role of complement-dependent mechanisms as the primary driver in the pathogenesis of BP (8). As such, downregulation of the complement pathway should remain the priority for investigation of therapeutic targets in this disease. However, given the evidence laid out above for the existence of complement-independent mechanisms, it is reasonable to conclude that specific targeting of these pathways may offer additional benefit to patients in the future, or be a major treatment option for a subset of patients in which these mechanisms are predominant.
Conclusions
Increased insight into complement-independent mechanisms in BP has not only improved our understanding of BP pathogenesis but has also significant translational implication. The increasing knowledge gained from studies dissecting IgE- and eosinophil-dependent pathways have highlighted the importance of extending the therapeutic horizons beyond those predominantly focusing on complement-mediated pathways. Hence, several avenues remain to be therapeutically explored. For example, blocking the production of pro-inflammatory mediators released by keratinocytes represents a potential approach by which BP may be improved. Likewise, induction of BP180 expression in basal keratinocytes to compensate for BP-IgG induced BP180 loss could also have a beneficial effect.
The extent to which complement-dependent or -independent mechanisms contribute to phenotypic presentation remains to be determined. Dissecting contributory pathways has significant impact on personalized treatments. For example, autoantibodies to non-NC16a epitopes have been associated with a pauci-inflammatory phenotype (147). Could this be sufficiently explained by the lack of NC16a-mediated endocytosis and subsequent expression of pro-inflammatory molecules? Several other questions remain. Are anti-NC16a induced keratinocyte pro-inflammatory molecules sufficient to induce granulocyte infiltration in the absence of complement? Does anti-BP180 depletion on keratinocytes lead to skin fragility in patients or is this primarily protease driven? Do keratinocytes express other pro-inflammatory cytokines/chemokines that may account for the eosinophilia typically seen in BP? Is keratinocyte derived IL-8 the major inducer of neutrophil chemotaxis or complement? Can the presence or absence of complement fixing antibodies predict responses to different therapies?
While the whole-body application of topical corticosteroids may affect these pathways, their systemic absorption, local side effects, and their practical use in elderly patients constitute a therapeutic hurdle (148, 149). It is likely that in the near future, the possibility to more rapidly and easily obtain a comprehensive characterization of complement-independent pathways activated in lesional tissues obtained from BP patients, for example using gene expression profiling, transcriptomics and proteomics, will provide a means to better tailor the therapy plan to the individual affected by BP.
Author Contributions
All authors contributed to the writing and review of the manuscript. All authors approved of the final manuscript.
Funding
KA is supported in part by Office of Research Infrastructure Programs of the National Institute of Health (R21OD030057).
Conflict of Interest
KA has served as a consultant for AstraZeneca, Akari Therapeutics, Argenx, as well as received research support from AstraZeneca.
The remaining authors declare that the research was conducted in the absence of any commercial or financial relationships that could be construed as a potential conflict of interest.
Publisher’s Note
All claims expressed in this article are solely those of the authors and do not necessarily represent those of their affiliated organizations, or those of the publisher, the editors and the reviewers. Any product that may be evaluated in this article, or claim that may be made by its manufacturer, is not guaranteed or endorsed by the publisher.
Acknowledgments
Figures were created using BioRender.com
References
1. Borradori L, Sonnenberg A. Structure and Function of Hemidesmosomes: More Than Simple Adhesion Complexes. J Invest Dermatol (1999) 112(4):411–8. doi: 10.1046/j.1523-1747.1999.00546.x
2. Amber KT, Murrell DF, Schmidt E, Joly P, Borradori L. Autoimmune Subepidermal Bullous Diseases of the Skin and Mucosae: Clinical Features, Diagnosis, and Management. Clin Rev Allergy Immunol (2018) 54(1):26–51. doi: 10.1007/s12016-017-8633-4
3. Cortes B, Marazza G, Naldi L, Combescure C, Borradori L. Mortality of Bullous Pemphigoid in Switzerland: A Prospective Study. Br J Dermatol (2011) 165(2):368–74. doi: 10.1111/j.1365-2133.2011.10413.x
4. Joly P, Baricault S, Sparsa A, Bernard P, Bedane C, Duvert-Lehembre S, et al. Incidence and Mortality of Bullous Pemphigoid in France. J Invest Dermatol (2012) 132(8):1998–2004. doi: 10.1038/jid.2012.35
5. Schmidt E, et al. European Guidelines (S3) on Diagnosis and Management of Mucous Membrane Pemphigoid, Initiated by the European Academy of Dermatology and Venereology - Part II. J Eur Acad Dermatol Venereol (2021) 35(10):1926–48. doi: 10.1111/jdv.17395
6. Kremer N, Snast I, Cohen ES, Hodak E, Mimouni D, Lapidoth M, et al. Rituximab and Omalizumab for the Treatment of Bullous Pemphigoid: A Systematic Review of the Literature. Am J Clin Dermatol (2019) 20(2):209–16. doi: 10.1007/s40257-018-0401-6
7. Abdat R, Waldman RA, de Bedout V, Czernik A, McLeod M, King B, et al. Dupilumab as a Novel Therapy for Bullous Pemphigoid: A Multicenter Case Series. J Am Acad Dermatol (2020) 83(1):46–52. doi: 10.1016/j.jaad.2020.01.089
8. Sadik CD, Rashid H, Hammers CM, Diercks GFH, Weidinger A, Beissert S, et al. Evaluation of Nomacopan for Treatment of Bullous Pemphigoid: A Phase 2a Nonrandomized Controlled Trial. JAMA Dermatol (2022). doi: 10.1001/jamadermatol.2022.1156
9. Tuusa J, Kokkonen N, Tasanen K. BP180/Collagen XVII: A Molecular View. Int J Mol Sci (2021) 22(22):12233. doi: 10.3390/ijms222212233
10. Nishie W, Kiritsi D, Nystrom A, Hofmann SC, Bruckner-Tuderman L, et al. Dynamic Interactions of Epidermal Collagen XVII With the Extracellular Matrix: Laminin 332 as a Major Binding Partner. Am J Pathol (2011) 179(2):829–37. doi: 10.1016/j.ajpath.2011.04.019
11. Kamaguchi M, Iwata H, Nishie W, Toyonaga E, Ujiie H, Natsuga K, et al. The Direct Binding of Collagen XVII and Collagen IV Is Disrupted by Pemphigoid Autoantibodies. Lab Invest (2019) 99(1):48–57. doi: 10.1038/s41374-018-0113-9
12. Fontao L, Favre B, Riou S, Geerts D, Jaunin F, Saurat JH, et al. Interaction of the Bullous Pemphigoid Antigen 1 (BP230) and Desmoplakin With Intermediate Filaments Is Mediated by Distinct Sequences Within Their COOH Terminus. Mol Biol Cell (2003) 14(5):1978–92. doi: 10.1091/mbc.e02-08-0548
13. Schmidt T, Sitaru C, Amber K, Hertl M. BP180- and BP230-Specific IgG Autoantibodies in Pruritic Disorders of the Elderly: A Preclinical Stage of Bullous Pemphigoid? Br J Dermatol (2014) 171(2):212–9. doi: 10.1111/bjd.12936
14. Zidan A, Jones VA, Patel PM, Gibson FT, Cordova A, Amber K. The Role of Collagen XVII in Cancer: Squamous Cell Carcinoma and Beyond. J Anim Physiol Anim Nutr (Berl) (2020) 10:352.
15. Liu Z, Sui W, Zhao M, Li Z, Li N, Thresher R, et al. Subepidermal Blistering Induced by Human Autoantibodies to BP180 Requires Innate Immune Players in a Humanized Bullous Pemphigoid Mouse Model. J Autoimmun (2008) 31(4):331–8. doi: 10.1016/j.jaut.2008.08.009
16. Thoma-Uszynski S, Uter W, Schwietzke S, Schuler G, Borradori L, Hertl M, et al. Autoreactive T and B Cells From Bullous Pemphigoid (BP) Patients Recognize Epitopes Clustered in Distinct Regions of BP180 and BP230. J Immunol (2006) 176(3):2015–23. doi: 10.4049/jimmunol.176.3.2015
17. Cirillo N, Prime SS. A Scoping Review of the Role of Metalloproteinases in the Pathogenesis of Autoimmune Pemphigus and Pemphigoid. Biomolecules (2021) 11(10):1506. doi: 10.3390/biom11101506
18. de Graauw E, Sitaru C, Horn MP, Borradori L, Yousefi S, Simon D, et al. Monocytes Enhance Neutrophil-Induced Blister Formation in an Ex Vivo Model of Bullous Pemphigoid. Allergy (2018) 73(5):1119–30. doi: 10.1111/all.13376
19. Liu Z, Li N, Diaz LA, Shipley M, Senior RM, Werb Z, et al. Synergy Between a Plasminogen Cascade and MMP-9 in Autoimmune Disease. J Clin Invest (2005) 115(4):879–87. doi: 10.1172/JCI23977
20. Liu Z, Shipley JM, Vu TH, Zhou X, Diaz LA, Werb Z, et al. Gelatinase B-Deficient Mice Are Resistant to Experimental Bullous Pemphigoid. J Exp Med (1998) 188(3):475–82. doi: 10.1084/jem.188.3.475
21. Stahle-Backdahl M, Inoue M, Guidice GJ, Parks WC. 92-kD Gelatinase Is Produced by Eosinophils at the Site of Blister Formation in Bullous Pemphigoid and Cleaves the Extracellular Domain of Recombinant 180-kD Bullous Pemphigoid Autoantigen. J Clin Invest (1994) 93(5):2022–30. doi: 10.1172/JCI117196
22. Liu Z, Giudice GJ, Swartz SJ, Fairley JA, Till GO, Troy JL, et al. The Role of Complement in Experimental Bullous Pemphigoid. J Clin Invest (1995) 95(4):1539–44. doi: 10.1172/JCI117826
23. Liu Z, Giudice GJ, Zhou X, Swartz SJ, Troy JL, Fairley JA, et al. A Major Role for Neutrophils in Experimental Bullous Pemphigoid. J Clin Invest (1997) 100(5):1256–63. doi: 10.1172/JCI119639
24. Liu Z, Shapiro SD, Zhou X, Twining SS, Senior RM, Giudice GJ, et al. A Critical Role for Neutrophil Elastase in Experimental Bullous Pemphigoid. J Clin Invest (2000) 105(1):113–23. doi: 10.1172/JCI3693
25. Chen R, Ning G, Zhao ML, Fleming MG, Diaz LA, Werb Z, et al. Mast Cells Play a Key Role in Neutrophil Recruitment in Experimental Bullous Pemphigoid. J Clin Invest (2001) 108(8):1151–8. doi: 10.1172/JCI11494
26. Nelson KC, Zhao M, Schroeder PR, Li N, Wetsel RA, Diaz LA, et al. Role of Different Pathways of the Complement Cascade in Experimental Bullous Pemphigoid. J Clin Invest (2006) 116(11):2892–900. doi: 10.1172/JCI17891
27. Leighty L, Li N, Diaz LA, Liu Z. Experimental Models for the Autoimmune and Inflammatory Blistering Disease, Bullous Pemphigoid. Arch Dermatol Res (2007) 299(9):417–22. doi: 10.1007/s00403-007-0790-5
28. Li Q, Ujiie H, Shibaki A, Wang G, Moriuchi R, Qiao HJ, et al. Human IgG1 Monoclonal Antibody Against Human Collagen 17 Noncollagenous 16A Domain Induces Blisters via Complement Activation in Experimental Bullous Pemphigoid Model. J Immunol (2010) 185(12):7746–55. doi: 10.4049/jimmunol.1000667
29. Jordon RE. Complement Activation in Bullous Skin Diseases. J Invest Dermatol (1975) 65(1):162–9. doi: 10.1111/1523-1747.ep12598113
30. Provost TT, Tomasi TB Jr. Evidence for Complement Activation via the Alternate Pathway in Skin Diseases, I. Herpes Gestationis, Systemic Lupus Erythematosus, and Bullous Pemphigoid. J Clin Invest (1973) 52(7):1779–87. doi: 10.1172/JCI107359
31. Dahl MV, Falk RJ, Carpenter R, Michael A. Deposition of the Membrane Attack Complex of Complement in Bullous Pemphigoid. J Invest Dermatol (1984) 82(2):132–5. doi: 10.1111/1523-1747.ep12259679
32. Solimani F, Didona D, Li J, Bao L, Patel PM, Gasparini G, et al. Characterizing the Proteome of Bullous Pemphigoid Blister Fluid Utilizing Tandem Mass Tag Labeling Coupled With LC-Ms/MS. Arch Dermatol Res (2021). doi: 10.1007/s00403-021-02253-8
33. Chiorean RM, Baican A, Mustafa MB, Lischka A, Leucuta DC, Feldrihan V, et al. Complement-Activating Capacity of Autoantibodies Correlates With Disease Activity in Bullous Pemphigoid Patients. Front Immunol (2018) 9:2687. doi: 10.3389/fimmu.2018.02687
34. Stander S, Holtsche MM, Schmidt E, Hammers CM, Zillikens D, Ludwig RJ, et al. Presence of Cutaneous Complement Deposition Distinguishes Between Immunological and Histological Features of Bullous Pemphigoid-Insights From a Retrospective Cohort Study. J Clin Med (2020) 9(12):3928. doi: 10.3390/jcm9123928
35. Jordon RE, Day NK, Sams WM Jr.. The Complement System in Bullous Pemphigoid. I. Complement and Component Levels in Sera and Blister Fluids. J Clin Invest (1973) 52(5):1207–14. doi: 10.1172/JCI107288
36. Natsuga K, Nishie W, Shinkuma S, Ujiie H, Nishimura M, Sawamura D, et al. Antibodies to Pathogenic Epitopes on Type XVII Collagen Cause Skin Fragility in a Complement-Dependent and -Independent Manner. J Immunol (2012) 188(11):5792–9. doi: 10.4049/jimmunol.1003402
37. Hiroyasu S, Ozawa T, Kobayashi H, Ishii M, Aoyama Y, Kitajima Y, et al. Bullous Pemphigoid IgG Induces BP180 Internalization via a Macropinocytic Pathway. Am J Pathol (2013) 182(3):828–40. doi: 10.1016/j.ajpath.2012.11.029
38. Iwata H, Kamaguchi M, Ujiie H, Nishimura M, Izumi K, Natsuga K, et al. Macropinocytosis of Type XVII Collagen Induced by Bullous Pemphigoid IgG is Regulated via Protein Kinase C. Lab Invest (2016) 96(12):1301–10. doi: 10.1038/labinvest.2016.108
39. Ujiie H, Sasaoka T, Izumi K, Nishie W, Shinkuma S, Natsuga K, et al. Bullous Pemphigoid Autoantibodies Directly Induce Blister Formation Without Complement Activation. J Immunol (2014) 193(9):4415–28. doi: 10.4049/jimmunol.1400095
40. Karsten CM, Beckmann T, Holtsche MM, Tillmann J, Tofern S, Schulze FS, et al. Tissue Destruction in Bullous Pemphigoid Can Be Complement Independent and May Be Mitigated by C5ar2. Front Immunol (2018) 9:488. doi: 10.3389/fimmu.2018.00488
41. Dainichi T, Nishie W, Yamagami Y, Sonobe H, Ujiie H, Kaku Y, et al. Bullous Pemphigoid Suggestive of Complement-Independent Blister Formation With Anti-BP180 IgG4 Autoantibodies. Br J Dermatol (2016) 175(1):187–90. doi: 10.1111/bjd.14411
42. Tukaj S, Gruner D, Tukaj C, Zillikens D, Kasperkiewicz M, et al. Calcitriol Exerts Anti-Inflammatory Effects in Keratinocytes Treated With Autoantibodies From a Patient With Bullous Pemphigoid. J Eur Acad Dermatol Venereol (2016) 30(2):288–92. doi: 10.1111/jdv.12929
43. Van den Bergh F, Eliason SL, Burmeister BT, Giudice GJ. Collagen XVII (BP180) Modulates Keratinocyte Expression of the Proinflammatory Chemokine, IL-8. Exp Dermatol (2012) 21(8):605–11. doi: 10.1111/j.1600-0625.2012.01529.x
44. Messingham KN, Srikantha R, DeGueme AM, Fairley JA. FcR-Independent Effects of IgE and IgG Autoantibodies in Bullous Pemphigoid. J Immunol (2011) 187(1):553–60. doi: 10.4049/jimmunol.1001753
45. Schmidt E, Reimer S, Kruse N, Brocker EB, Zillikens D, et al. The IL-8 Release From Cultured Human Keratinocytes, Mediated by Antibodies to Bullous Pemphigoid Autoantigen 180, is Inhibited by Dapsone. Clin Exp Immunol (2001) 124(1):157–62.
46. Schmidt E, Reimer S, Kruse N, Jainta S, Brocker EB, Marinkovich MP, et al. Autoantibodies to BP180 Associated With Bullous Pemphigoid Release Interleukin-6 and Interleukin-8 From Cultured Human Keratinocytes. J Invest Dermatol (2000) 115(5):842–8. doi: 10.1046/j.1523-1747.2000.00141.x
47. Tukaj S, Gruner D, Zillikens D, Kasperkiewicz M. Hsp90 Blockade Modulates Bullous Pemphigoid IgG-Induced IL-8 Production by Keratinocytes. Cell Stress Chaperones (2014) 19(6):887–94. doi: 10.1007/s12192-014-0513-8
48. Tukaj S, Gruner D, Zillikens D, Kasperkiewicz M. Aberrant Expression and Secretion of Heat Shock Protein 90 in Patients With Bullous Pemphigoid. PloS One (2013) 8(7):e70496. doi: 10.1371/journal.pone.0070496
49. Dainichi T, Chow Z, Kabashima K. IgG4, Complement, and the Mechanisms of Blister Formation in Pemphigus and Bullous Pemphigoid. J Dermatol Sci (2017) 88(3):265–70. doi: 10.1016/j.jdermsci.2017.07.012
50. Zhou XP, Liu B, Xu Q, Yang Y, He CX, Zuo YG, et al. Serum Levels of Immunoglobulins G1 and G4 Targeting the Non-Collagenous 16A Domain of BP180 Reflect Bullous Pemphigoid Activity and Predict Bad Prognosis. J Dermatol (2016) 43(2):141–8. doi: 10.1111/1346-8138.13051
51. Lamb PM, Patton T, Deng JS. The Predominance of IgG4 in Prodromal Bullous Pemphigoid. Int J Dermatol (2008) 47(2):150–3. doi: 10.1111/j.1365-4632.2008.03361.x
52. Patton T, Plunkett RW, Beutner EH, Deng JS, Jukic DM, et al. IgG4 as the Predominant IgG Subclass in Pemphigoides Gestationis. J Cutan Pathol (2006) 33(4):299–302. doi: 10.1111/j.0303-6987.2006.00458.x
53. Buschman KE, Seraly M, Thong HY, Deng JS, Draviam RP, Abernethy J, et al. A Predominant IgG4 Subclass may be Responsible for False-Negative Direct Immunofluorescence in Bullous Pemphigoid. J Cutan Pathol (2002) 29(5):282–6. doi: 10.1034/j.1600-0560.2002.290504.x
54. Zuo Y, Evangelista F, Culton D, Guilabert A, Lin L, Li N, et al. IgG4 Autoantibodies Are Inhibitory in the Autoimmune Disease Bullous Pemphigoid. J Autoimmun (2016) 73:111–9. doi: 10.1016/j.jaut.2016.06.019
55. Romeijn TR, Jonkman MF, Knoppers C, Pas HH, Diercks GF. Complement in Bullous Pemphigoid: Results From a Large Observational Study. Br J Dermatol (2017) 176(2):517–9. doi: 10.1111/bjd.14822
56. Shiraishi S, Iio T, Shirakata Y, Sayama K, Nishimukai H, Miki Y. Bullous Pemphigoid in a Patient With a C4 Deficiency. Br J Dermatol (1991) 124(3):296–8. doi: 10.1111/j.1365-2133.1991.tb00579.x
57. Liu Y, Li L, Xia Y. BP180 Is Critical in the Autoimmunity of Bullous Pemphigoid. Front Immunol (2017) 8:1752. doi: 10.3389/fimmu.2017.01752
58. Wada M, Nishie W, Ujiie H, Izumi K, Iwata H, Natsuga K, et al. Epitope-Dependent Pathogenicity of Antibodies Targeting a Major Bullous Pemphigoid Autoantigen Collagen XVII/Bp180. J Invest Dermatol (2016) 136(5):938–46. doi: 10.1016/j.jid.2015.11.030
59. Kitajima Y, Hirako Y, Owaribe K, Mori S, Yaoita H, et al. Antibody-Binding to the 180-kD Bullous Pemphigoid Antigens at the Lateral Cell Surface Causes Their Internalization and Inhibits Their Assembly at the Basal Cell Surface in Cultured Keratinocytes. J Dermatol (1994) 21(11):838–46. doi: 10.1111/j.1346-8138.1994.tb03299.x
60. Kitajima Y, Nojiri M, Yamada T, Hirako Y, Owaribe K, et al. Internalization of the 180 kDa Bullous Pemphigoid Antigen as Immune Complexes in Basal Keratinocytes: An Important Early Event in Blister Formation in Bullous Pemphigoid. Br J Dermatol (1998) 138(1):71–6. doi: 10.1046/j.1365-2133.1998.02028.x
61. Iwata H, Kamio N, Aoyama Y, Yamamoto Y, Hirako Y, Owaribe K, et al. IgG From Patients With Bullous Pemphigoid Depletes Cultured Keratinocytes of the 180-kDa Bullous Pemphigoid Antigen (Type XVII Collagen) and Weakens Cell Attachment. J Invest Dermatol (2009) 129(4):919–26. doi: 10.1038/jid.2008.305
62. Sitaru C, Schmidt E, Petermann S, Munteanu LS, Brocker EB, Zillikens D, et al. Autoantibodies to Bullous Pemphigoid Antigen 180 Induce Dermal-Epidermal Separation in Cryosections of Human Skin. J Invest Dermatol (2002) 118(4):664–71. doi: 10.1046/j.1523-1747.2002.01720.x
63. Wang G, Ujiie H, Shibaki A, Nishie W, Tateishi Y, Kikuchi K, et al. Blockade of Autoantibody-Initiated Tissue Damage by Using Recombinant Fab Antibody Fragments Against Pathogenic Autoantigen. Am J Pathol (2010) 176(2):914–25. doi: 10.2353/ajpath.2010.090744
64. Iwata H, Kitajima Y. Bullous Pemphigoid: Role of Complement and Mechanisms for Blister Formation Within the Lamina Lucida. Exp Dermatol (2013) 22(6):381–5. doi: 10.1111/exd.12146
65. Zhou Y, Bosch ML, Salgaller ML. Current Methods for Loading Dendritic Cells With Tumor Antigen for the Induction of Antitumor Immunity. J Immunother (2002) 25(4):289–303. doi: 10.1097/00002371-200207000-00001
66. Hyc A, Osiecka-Iwan A, Strzelczyk P, Moskalewski S. Effect of IL-1beta, TNF-Alpha and IL-4 on Complement Regulatory Protein mRNA Expression in Human Articular Chondrocytes. Int J Mol Med (2003) 11(1):91–4.
67. Bao L, Li J, Solimani F, Didona D, Patel PM, Li X, et al. Subunit-Specific Reactivity of Autoantibodies Against Laminin-332 Reveals Direct Inflammatory Mechanisms on Keratinocytes. Front Immunol (2021) 12:775412. doi: 10.3389/fimmu.2021.775412
68. Barker JN, Jones ML, Mitra RS, Crockett-Torabe E, Fantone JC, Kunkel SL, et al. Modulation of Keratinocyte-Derived Interleukin-8 Which Is Chemotactic for Neutrophils and T Lymphocytes. Am J Pathol (1991) 139(4):869–76.
69. Gammon WR, Merritt CC, Lewis DM, Sams WM Jr., Carlo JR, Wheeler CE Jr, et al, et al. An In Vitro Model of Immune Complex-Mediated Basement Membrane Zone Separation Caused by Pemphigoid Antibodies, Leukocytes, and Complement. J Invest Dermatol (1982) 78(4):285–90. doi: 10.1111/1523-1747.ep12507222
70. Kondo S, Kooshesh F, Sauder DN. Penetration of Keratinocyte-Derived Cytokines Into Basement Membrane. J Cell Physiol (1997) 171(2):190–5. doi: 10.1002/(SICI)1097-4652(199705)171:2<190::AID-JCP9>3.0.CO;2-J
71. Kowalski EH, Kneibner D, Kridin K, Amber KT. Serum and Blister Fluid Levels of Cytokines and Chemokines in Pemphigus and Bullous Pemphigoid. Autoimmun Rev (2019) 18(5):526–34. doi: 10.1016/j.autrev.2019.03.009
72. Schmidt E, Ambach A, Bastian B, Brocker EB, Zillikens D. Elevated Levels of Interleukin-8 in Blister Fluid of Bullous Pemphigoid Compared With Suction Blisters of Healthy Control Subjects. J Am Acad Dermatol (1996) 34(2 Pt 1):310–2. doi: 10.1016/S0190-9622(96)80146-0
73. Inaoki M, Takehara K. Increased Serum Levels of Interleukin (IL)-5, IL-6 and IL-8 in Bullous Pemphigoid. J Dermatol Sci (1998) 16(2):152–7. doi: 10.1016/S0923-1811(97)00044-3
74. Ghaoui N, Hanna E, Abbas O, Kibbi AG, Kurban M, et al. Update on the Use of Dapsone in Dermatology. Int J Dermatol (2020) 59(7):787–95. doi: 10.1111/ijd.14761
75. Salminen A, Paimela T, Suuronen T, Kaarniranta K. Innate Immunity Meets With Cellular Stress at the IKK Complex: Regulation of the IKK Complex by HSP70 and HSP90. Immunol Lett (2008) 117(1):9–15. doi: 10.1016/j.imlet.2007.12.017
76. Taipale M, Jarosz DF, Lindquist S. HSP90 at the Hub of Protein Homeostasis: Emerging Mechanistic Insights. Nat Rev Mol Cell Biol (2010) 11(7):515–28. doi: 10.1038/nrm2918
77. Henderson B, Kaiser F. Do Reciprocal Interactions Between Cell Stress Proteins and Cytokines Create a New Intra-/Extra-Cellular Signalling Nexus? Cell Stress Chaperones (2013) 18(6):685–701. doi: 10.1007/s12192-013-0444-9
78. Zhang Y, Hwang BJ, Liu Z, Li N, Lough K, Williams SE, et al. BP180 Dysfunction Triggers Spontaneous Skin Inflammation in Mice. Proc Natl Acad Sci USA (2018) 115(25):6434–9. doi: 10.1073/pnas.1721805115
79. Mihai S, Chiriac MT, Herrero-Gonzalez JE, Goodall M, Jefferis R, Savage CO, et al. IgG4 Autoantibodies Induce Dermal-Epidermal Separation. J Cell Mol Med (2007) 11(5):1117–28. doi: 10.1111/j.1582-4934.2007.00081.x
80. de Graauw E, Sitaru C, Horn M, Borradori L, Yousefi S, Simon HU, et al. Evidence for a Role of Eosinophils in Blister Formation in Bullous Pemphigoid. Allergy (2017) 72(7):1105–13. doi: 10.1111/all.13131
81. Amber KT, Valdebran M, Kridin K, Grando SA. The Role of Eosinophils in Bullous Pemphigoid: A Developing Model of Eosinophil Pathogenicity in Mucocutaneous Disease. Front Med (Lausanne) (2018) 5:201. doi: 10.3389/fmed.2018.00201
82. Crotty C, Pittelkow M, Muller SA. Eosinophilic Spongiosis: A Clinicopathologic Review of Seventy-One Cases. J Am Acad Dermatol (1983) 8(3):337–43. doi: 10.1016/S0190-9622(83)70036-8
83. Nishioka K, Hashimoto K, Katayama I, Sarashi C, Kubo T, Sano S, et al. Eosinophilic Spongiosis in Bullous Pemphigoid. Arch Dermatol (1984) 120(9):1166–8. doi: 10.1001/archderm.1984.01650450048015
84. Bernard P, Venot J, Constant F, Bonnetblanc JM. Blood Eosinophilia as a Severity Marker for Bullous Pemphigoid. J Am Acad Dermatol (1987) 16(4):879–81. doi: 10.1016/S0190-9622(87)80227-X
85. van Beek N, Schulze FS, Zillikens D, Schmidt E. IgE-Mediated Mechanisms in Bullous Pemphigoid and Other Autoimmune Bullous Diseases. Expert Rev Clin Immunol (2016) 12(3):267–77. doi: 10.1586/1744666X.2016.1123092
86. Kridin K. Peripheral Eosinophilia in Bullous Pemphigoid: Prevalence and Influence on the Clinical Manifestation. Br J Dermatol (2018) 179(5):1141–7. doi: 10.1111/bjd.16679
87. Okada S, Kita H, George TJ, Gleich GJ, Leiferman KM, et al. Migration of Eosinophils Through Basement Membrane Components In Vitro: Role of Matrix Metalloproteinase-9. Am J Respir Cell Mol Biol (1997) 17(4):519–28. doi: 10.1165/ajrcmb.17.4.2877
88. Kelly EA, Liu LY, Esnault S, Quinchia Johnson BH, Jarjour NN, et al. Potent Synergistic Effect of IL-3 and TNF on Matrix Metalloproteinase 9 Generation by Human Eosinophils. Cytokine (2012) 58(2):199–206. doi: 10.1016/j.cyto.2012.01.009
89. Wiehler S, Cuvelier SL, Chakrabarti S, Patel KD. P38 MAP Kinase Regulates Rapid Matrix Metalloproteinase-9 Release From Eosinophils. Biochem Biophys Res Commun (2004) 315(2):463–70. doi: 10.1016/j.bbrc.2004.01.078
90. Verraes S, Hornebeck W, Polette M, Borradori L, Bernard P. Respective Contribution of Neutrophil Elastase and Matrix Metalloproteinase 9 in the Degradation of BP180 (Type XVII Collagen) in Human Bullous Pemphigoid. J Invest Dermatol (2001) 117(5):1091–6. doi: 10.1046/j.0022-202x.2001.01521.x
91. Giusti D, Gatouillat G, Le Jan S, Plee J, Bernard P, Antonicelli F, et al. Eosinophil Cationic Protein (ECP), a Predictive Marker of Bullous Pemphigoid Severity and Outcome. Sci Rep (2017) 7(1):4833. doi: 10.1038/s41598-017-04687-5
92. Czech W, Schaller J, Schopf E, Kapp A. Granulocyte Activation in Bullous Diseases: Release of Granular Proteins in Bullous Pemphigoid and Pemphigus Vulgaris. J Am Acad Dermatol (1993) 29(2 Pt 1):210–5. doi: 10.1016/0190-9622(93)70170-X
93. Caproni M, Palleschi GM, Falcos D, D'Agata A, Cappelli G, Fabbri P, et al. Serum Eosinophil Cationic Protein (ECP) in Bullous Pemphigoid. Int J Dermatol (1995) 34(3):177–80. doi: 10.1111/j.1365-4362.1995.tb01562.x
94. Tsuda S, Miyasato M, Iryo K, Nakama T, Kato K, Sasai Y, et al. Eosinophil Phenotypes in Bullous Pemphigoid. J Dermatol (1992) 19(5):270–9. doi: 10.1111/j.1346-8138.1992.tb03224.x
95. Dubertret L, Bertaux B, Fosse M, Touraine R. Cellular Events Leading to Blister Formation in Bullous Pemphigoid. Br J Dermatol (1980) 103(6):615–24. doi: 10.1111/j.1365-2133.1980.tb01683.x
96. Borrego L, Maynard B, Peterson EA, George T, Iglesias L, Peters MS, et al. Deposition of Eosinophil Granule Proteins Precedes Blister Formation in Bullous Pemphigoid. Comparison With Neutrophil and Mast Cell Granule Proteins. Am J Pathol (1996) 148(3):897–909.
97. Dvorak AM, Mihm MC Jr., Osage JE, Kwan TH, Austen KF, Wintroub BU, et al, et al. Bullous Pemphigoid, an Ultrastructural Study of the Inflammatory Response: Eosinophil, Basophil and Mast Cell Granule Changes in Multiple Biopsies From One Patient. J Invest Dermatol (1982) 78(2):91–101. doi: 10.1111/1523-1747.ep12505711
98. Amber KT, Chernyavsky A, Agnoletti AF, Cozzani E, Grando SA. Mechanisms of Pathogenic Effects of Eosinophil Cationic Protein and Eosinophil-Derived Neurotoxin on Human Keratinocytes. Exp Dermatol (2018) 27(12):1322–7. doi: 10.1111/exd.13782
99. Cortjens B, van Woensel JB, Bem RA. Neutrophil Extracellular Traps in Respiratory Disease: Guided Anti-Microbial Traps or Toxic Webs? Paediatr Respir Rev (2017) 21:54–61. doi: 10.1016/j.prrv.2016.03.007
100. Simon D, Hoesli S, Roth N, Staedler S, Yousefi S, Simon HU, et al. Eosinophil Extracellular DNA Traps in Skin Diseases. J Allergy Clin Immunol (2011) 127(1):194–9. doi: 10.1016/j.jaci.2010.11.002
101. Giusti D, Bini E, Terryn C, Didier K, Le Jan S, Gatouillat G, et al. NET Formation in Bullous Pemphigoid Patients With Relapse Is Modulated by IL-17 and IL-23 Interplay. Front Immunol (2019) 10:701. doi: 10.3389/fimmu.2019.00701
102. Fang H, Shao S, Xue K, Yuan X, Qiao P, Zhang J, et al. Neutrophil Extracellular Traps Contribute to Immune Dysregulation in Bullous Pemphigoid via Inducing B-Cell Differentiation and Antibody Production. FASEB J (2021) 35(7):e21746. doi: 10.1096/fj.202100145R
103. Petri B, Sanz MJ. Neutrophil Chemotaxis. Cell Tissue Res (2018) 371(3):425–36. doi: 10.1007/s00441-017-2776-8
104. Hashimoto T, Ohzono A, Teye K, Numata S, Hiroyasu S, Tsuruta D, et al. Detection of IgE Autoantibodies to BP180 and BP230 and Their Relationship to Clinical Features in Bullous Pemphigoid. Br J Dermatol (2017) 177(1):141–51. doi: 10.1111/bjd.15114
105. van Beek N, Luttmann N, Huebner F, Recke A, Karl I, Schulze FS, et al. Correlation of Serum Levels of IgE Autoantibodies Against BP180 With Bullous Pemphigoid Disease Activity. JAMA Dermatol (2017) 153(1):30–8. doi: 10.1001/jamadermatol.2016.3357
106. Bing L, Xiping Z, Li L, Jun P, Yi-Xia W, Min Y, et al. Levels of Anti-BP180 NC16A IgE do Not Correlate With Severity of Disease in the Early Stages of Bullous Pemphigoid. Arch Dermatol Res (2015) 307(9):849–54. doi: 10.1007/s00403-015-1598-3
107. Salz M, Haeberle S, Hoffmann J, Enk AH, Hadaschik EN. Elevated IL-31 Serum Levels in Bullous Pemphigoid Patients Correlate With Eosinophil Numbers and Are Associated With BP180-IgE. J Dermatol Sci (2017) 87(3):309–11. doi: 10.1016/j.jdermsci.2017.07.019
108. Fairley JA, Burnett CT, Fu CL, Larson DL, Fleming MG, Giudice GJ, et al. A Pathogenic Role for IgE in Autoimmunity: Bullous Pemphigoid IgE Reproduces the Early Phase of Lesion Development in Human Skin Grafted to Nu/Nu Mice. J Invest Dermatol (2007) 127(11):2605–11. doi: 10.1038/sj.jid.5700958
109. Hammers CM, Stanley JR. Mechanisms of Disease: Pemphigus and Bullous Pemphigoid. Annu Rev Pathol (2016) 11:175–97. doi: 10.1146/annurev-pathol-012615-044313
110. Messingham KN, Wang JW, Holahan HM, Srikantha R, Aust SC, Fairley JA. Eosinophil Localization to the Basement Membrane Zone Is Autoantibody- and Complement-Dependent in a Human Cryosection Model of Bullous Pemphigoid. Exp Dermatol (2016) 25(1):50–5. doi: 10.1111/exd.12883
111. Messingham KN, Wang JW, Holahan HM, Srikantha R, Aust SC, Fairley JA, et al. Human Eosinophils Express the High Affinity IgE Receptor, FcepsilonRI, in Bullous Pemphigoid. PloS One (2014) 9(9):e107725. doi: 10.1371/journal.pone.0107725
112. Zone JJ, Holahan HM, Frydman AS, Fullenkamp C, Srikantha R, Fairley JA. IgE Basement Membrane Zone Antibodies Induce Eosinophil Infiltration and Histological Blisters in Engrafted Human Skin on SCID Mice. J Invest Dermatol (2007) 127(5):1167–74. doi: 10.1038/sj.jid.5700681
113. Meng J, Moriyama M, Feld M, Buddenkotte J, Buhl T, Szollosi A, et al. New Mechanism Underlying IL-31-Induced Atopic Dermatitis. J Allergy Clin Immunol (2018) 141(5):1677–1689 e8. doi: 10.1016/j.jaci.2017.12.1002
114. Takamori A, Nambu A, Sato K, Yamaguchi S, Matsuda K, Numata T, et al. IL-31 is Crucial for Induction of Pruritus, But Not Inflammation, in Contact Hypersensitivity. Sci Rep (2018) 8(1):6639. doi: 10.1038/s41598-018-25094-4
115. Kunsleben N, Rudrich U, Gehring M, Novak N, Kapp A, Raap U. IL-31 Induces Chemotaxis, Calcium Mobilization, Release of Reactive Oxygen Species, and CCL26 in Eosinophils, Which Are Capable to Release IL-31. J Invest Dermatol (2015) 135(7):1908–11. doi: 10.1038/jid.2015.106
116. Bonciani D, Quintarelli L, Del Bianco E, Bianchi B, Caproni M. Serum Levels and Tissue Expression of Interleukin-31 in Dermatitis Herpetiformis and Bullous Pemphigoid. J Dermatol Sci (2017) 87(2):210–2. doi: 10.1016/j.jdermsci.2017.04.008
117. Rudrich U, Gehring M, Papakonstantinou E, Illerhaus A, Engmann J, Kapp A, et al. Eosinophils Are a Major Source of Interleukin-31 in Bullous Pemphigoid. Acta Derm Venereol (2018) 98(8):766–71. doi: 10.2340/00015555-2951
118. Lin L, Hwang BJ, Culton DA, Li N, Burette S, Koller BH, et al. Eosinophils Mediate Tissue Injury in the Autoimmune Skin Disease Bullous Pemphigoid. J Invest Dermatol (2018) 138(5):1032–43. doi: 10.1016/j.jid.2017.11.031
119. Freire PC, Munoz CH, Stingl G. IgE Autoreactivity in Bullous Pemphigoid: Eosinophils and Mast Cells as Major Targets of Pathogenic Immune Reactants. Br J Dermatol (2017) 177(6):1644–53. doi: 10.1111/bjd.15924
120. Baardman R, Horvath B, Bolling MC, Pas HH, Diercks GFH, et al. Immunoglobulin M Bullous Pemphigoid: An Enigma. JAAD Case Rep (2020) 6(6):518–20. doi: 10.1016/j.jdcr.2020.04.008
121. Braun-Falco O, Wolff HH, Ponce E. [Disseminated Cicatricial Pemphigoid]. Hautarzt (1981) 32(5):233–9.
122. Waisbourd-Zinman O, Ben-Amitai D, Cohen AD, Feinmesser M, Mimouni D, Adir-Shani A, et al. Bullous Pemphigoid in Infancy: Clinical and Epidemiologic Characteristics. J Am Acad Dermatol (2008) 58(1):41–8. doi: 10.1016/j.jaad.2007.08.010
123. Barth JH, Kelly SE, Wojnarowska F, Savin JA, Whittaker S, Cream JJ, et al. Pemphigoid and Ulcerative Colitis. J Am Acad Dermatol (1988) 19(2 Pt 1):303–8. doi: 10.1016/S0190-9622(88)70176-0
124. Motegi S, Abe M, Tamura A, Ishii N, Hashimoto T, Ishikawa O, et al. Childhood Bullous Pemphigoid Successfully Treated With Diaminodiphenyl Sulfone. J Dermatol (2005) 32(10):809–12. doi: 10.1111/j.1346-8138.2005.tb00849.x
125. Hashimoto T, Tsuruta D, Koga H, Fukuda S, Ohyama B, Komai A, et al. Summary of Results of Serological Tests and Diagnoses for 4774 Cases of Various Autoimmune Bullous Diseases Consulted to Kurume University. Br J Dermatol (2016) 175(5):953–65. doi: 10.1111/bjd.14692
126. Velthuis PJ, de Jong MC, Kruis MH. Is There a Linear IgM Dermatosis? Significance of Linear IgM Junctional Staining in Cutaneous Immunopathology. Acta Derm Venereol (1988) 68(1):8–14. doi: 10.2340/0001555568814
127. Helm TN, Valenzuela R. Continuous Dermoepidermal Junction IgM Detected by Direct Immunofluorescence: A Report of Nine Cases. J Am Acad Dermatol (1992) 26(2 Pt 1):203–6. doi: 10.1016/0190-9622(92)70027-D
128. Boch K, Hammers CM, Goletz S, Kamaguchi M, Ludwig RJ, Schneider SW, et al. Immunoglobulin M Pemphigoid. J Am Acad Dermatol (2021) 85(6):1486–92. doi: 10.1016/j.jaad.2021.01.017
129. Seyed Jafari SM, Gadaldi K, Feldmeyer L, Yawalkar N, Borradori L, Schlapbach , et al. Effects of Omalizumab on FcepsilonRI and IgE Expression in Lesional Skin of Bullous Pemphigoid. Front Immunol (2019) 10:1919. doi: 10.3389/fimmu.2019.01919
130. Messingham KN, Crowe TP, Fairley JA. The Intersection of IgE Autoantibodies and Eosinophilia in the Pathogenesis of Bullous Pemphigoid. Front Immunol (2019) 10:2331. doi: 10.3389/fimmu.2019.02331
131. Woodley DT. The Role of IgE Anti-Basement Membrane Zone Autoantibodies in Bullous Pemphigoid. Arch Dermatol (2007) 143(2):249–50. doi: 10.1001/archderm.143.2.249
132. Kaminer MS, Murphy GF, Zweiman B, Lavker RM. Connective Tissue Mast Cells Exhibit Time-Dependent Degranulation Heterogeneity. Clin Diagn Lab Immunol (1995) 2(3):297–301. doi: 10.1128/cdli.2.3.297-301.1995
133. Jones VA, Patel PM, Amber KT. Eosinophils in Bullous Pemphigoid. Panminerva Med (2021) 63(3):368–78. doi: 10.23736/S0031-0808.20.03997-X
134. Messingham KA, Holahan HM, Fairley JA. Unraveling the Significance of IgE Autoantibodies in Organ-Specific Autoimmunity: Lessons Learned From Bullous Pemphigoid. Immunol Res (2014) 59(1-3):273–8. doi: 10.1007/s12026-014-8547-7
135. Fairley JA, Baum CL, Brandt DS, Messingham KA. Pathogenicity of IgE in Autoimmunity: Successful Treatment of Bullous Pemphigoid With Omalizumab. J Allergy Clin Immunol (2009) 123(3):704–5. doi: 10.1016/j.jaci.2008.11.035
136. Dufour C, Souillet AL, Chaneliere C, Jouen F, Bodemer C, Jullien D, et al. Successful Management of Severe Infant Bullous Pemphigoid With Omalizumab. Br J Dermatol (2012) 166(5):1140–2. doi: 10.1111/j.1365-2133.2011.10748.x
137. London VA, Kim GH, Fairley JA, Woodley D. Successful Treatment of Bullous Pemphigoid With Omalizumab. Arch Dermatol (2012) 148(11):1241–3. doi: 10.1001/archdermatol.2012.1604
138. Balakirski G, Alkhateeb A, Merk HF, Leverkus M, Megahed M. Successful Treatment of Bullous Pemphigoid With Omalizumab as Corticosteroid-Sparing Agent: Report of Two Cases and Review of Literature. J Eur Acad Dermatol Venereol (2016) 30(10):1778–82. doi: 10.1111/jdv.13758
139. Liu J, Lester P, Builder S, Shire SJ. Characterization of Complex Formation by Humanized Anti-IgE Monoclonal Antibody and Monoclonal Human IgE. Biochemistry (1995) 34(33):10474–82. doi: 10.1021/bi00033a020
140. Beck LA, Marcotte GV, MacGlashan D, Togias A, Saini S. Omalizumab-Induced Reductions in Mast Cell Fce Psilon RI Expression and Function. J Allergy Clin Immunol (2004) 114(3):527–30. doi: 10.1016/j.jaci.2004.06.032
141. Chanez P, Contin-Bordes C, Garcia G, Verkindre C, Didier A, De Blay F. Omalizumab-Induced Decrease of FcxiRI Expression in Patients With Severe Allergic Asthma. Respir Med (2010) 104(11):1608–17. doi: 10.1016/j.rmed.2010.07.011
142. Kridin K, Kowalski EH, Kneiber D, Laufer-Britva R, Amber KT. From Bench to Bedside: Evolving Therapeutic Targets in Autoimmune Blistering Disease. J Eur Acad Dermatol Venereol (2019) 33(12):2239–52. doi: 10.1111/jdv.15816
143. Zhang Y, Xu Q, Chen L, Chen J, Zhang J, Zou Y, et al. Efficacy and Safety of Dupilumab in Moderate-To-Severe Bullous Pemphigoid. Front Immunol (2021) 12:738907. doi: 10.3389/fimmu.2021.738907
144. Seyed Jafari SM, Feldmeyer L, Bossart S, Simon D, Schlapbach C, Borradori L, et al. Case Report: Combination of Omalizumab and Dupilumab for Recalcitrant Bullous Pemphigoid. Front Immunol (2020) 11:611549. doi: 10.3389/fimmu.2020.611549
145. Kaye A, Gordon SC, Deverapalli SC, Her MJ, Rosmarin D. Dupilumab for the Treatment of Recalcitrant Bullous Pemphigoid. JAMA Dermatol (2018) 154(10):1225–6. doi: 10.1001/jamadermatol.2018.2526
146. Simon D, Yousefi S, Cazzaniga S, Burgler C, Radonjic S, Houriet C, et al. Mepolizumab Failed to Affect Bullous Pemphigoid: A Randomized, Placebo-Controlled, Double-Blind Phase 2 Pilot Study. Allergy (2020) 75(3):669–72. doi: 10.1111/all.13950
147. Nishie W. Dipeptidyl Peptidase IV Inhibitor-Associated Bullous Pemphigoid: A Recently Recognized Autoimmune Blistering Disease With Unique Clinical, Immunological and Genetic Characteristics. Immunol Med (2019) 42(1):22–8. doi: 10.1080/25785826.2019.1619233
148. Joly P, Roujeau JC, Benichou J, Delaporte E, D'Incan M, Dreno B, et al. A Comparison of Two Regimens of Topical Corticosteroids in the Treatment of Patients With Bullous Pemphigoid: A Multicenter Randomized Study. J Invest Dermatol (2009) 129(7):1681–7. doi: 10.1038/jid.2008.412
Keywords: bullous pemphigoid, complement - immunological terms, autoimmune blistering diseases, eosinophils – immunology, igE (Immunoglobulin E), BP180, BP230
Citation: Cole C, Vinay K, Borradori L and Amber KT (2022) Insights Into the Pathogenesis of Bullous Pemphigoid: The Role of Complement-Independent Mechanisms. Front. Immunol. 13:912876. doi: 10.3389/fimmu.2022.912876
Received: 05 April 2022; Accepted: 09 June 2022;
Published: 07 July 2022.
Edited by:
Enno Schmidt, University of Lübeck, GermanyReviewed by:
Cassian Sitaru, University of Freiburg Medical Center, GermanyHiroaki Iwata, Hokkaido University, Japan
Ning Li, University of North Carolina at Chapel Hill, United States
Copyright © 2022 Cole, Vinay, Borradori and Amber. This is an open-access article distributed under the terms of the Creative Commons Attribution License (CC BY). The use, distribution or reproduction in other forums is permitted, provided the original author(s) and the copyright owner(s) are credited and that the original publication in this journal is cited, in accordance with accepted academic practice. No use, distribution or reproduction is permitted which does not comply with these terms.
*Correspondence: Connor Cole, Y2pjb2xlMTI5MkBnbWFpbC5jb20=; Keshavamurthy Vinay, dmluYXkua2VzaGF2bXVydGh5QGdtYWlsLmNvbQ==; Luca Borradori, bHVjYS5ib3JyYWRvcmlAaW5zZWwuY2g=; Kyle T. Amber, a3lsZV9hbWJlckBydXNoLmVkdQ==