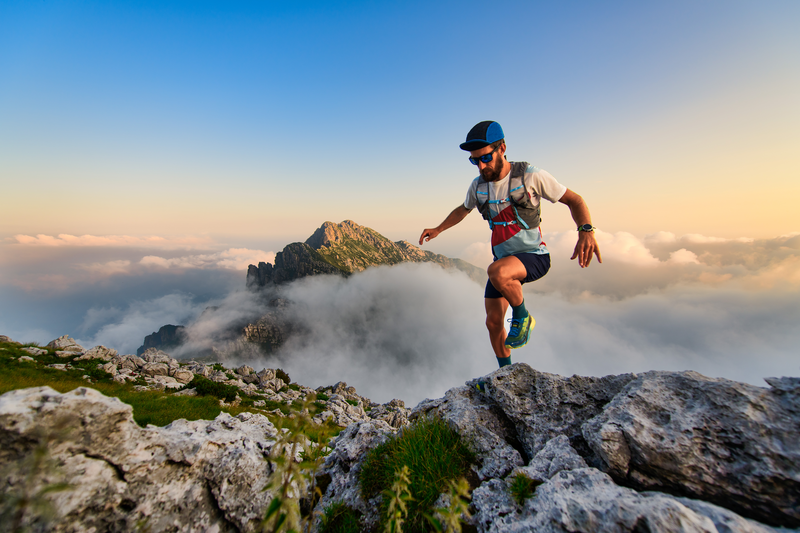
94% of researchers rate our articles as excellent or good
Learn more about the work of our research integrity team to safeguard the quality of each article we publish.
Find out more
REVIEW article
Front. Immunol. , 14 July 2022
Sec. T Cell Biology
Volume 13 - 2022 | https://doi.org/10.3389/fimmu.2022.911546
This article is part of the Research Topic Women in T Cell Biology View all 16 articles
T helper 22 (Th22) cells are a newly identified subset of CD4+ T cells that secrete the effector cytokine interleukin 22 (IL-22) upon specific antigen stimulation, barely with IFN-γ or IL-17. Increasing studies have demonstrated that Th22 cells and IL-22 play essential roles in skin barrier defense and skin disease pathogenesis since the IL-22 receptor is widely expressed in the skin, especially in keratinocytes. Herein, we reviewed the characterization, differentiation, and biological activities of Th22 cells and elucidated their roles in skin health and disease. We mainly focused on the intricate crosstalk between Th22 cells and keratinocytes and provided potential therapeutic strategies targeting the Th22/IL-22 signaling pathway.
The skin is the outmost sentinel barrier of the body and is continuously exposed to pathogens and the environment. The skin is composed of the epidermis, dermis, and subcutaneous tissues. Besides its mechanical barrier role, the skin acts as the first line of host immune defense. Keratinocytes are not only a component of the skin physical barrier but also have important immunoregulatory roles by secreting various cytokines and chemokines that work with cutaneous antigen-presenting cells (APC) such as Langerhans cells (LC), dendritic cells (DC), and macrophages, finally contributing to the innate immune system. To maintain skin immune homeostasis, innate and adaptive immune cells, especially T cells and tissue cells, interact finely with each other and constitute an intricate immune regulatory network (1, 2). As another leading actor of these crosstalk, dysregulated T cell immune responses can contribute to the pathogenesis of skin disorders. In the microenvironment and disease setting contexts, naive T cells can functionally differentiate into effector T helper (Th) cells. Furthermore, skin inflammatory diseases can be divided into four types based on the polarization of Th cells subsets. First, Th1-dominant skin diseases, such as lichen planus and vitiligo, share a lichenoid clinical phenotype. Atopic dermatitis (AD) is a clear example of Th2-specific skin diseases, characterized by autoantibody production and eosinophil-infiltrated eczematous patterns. Fibrogenesis is involved in regulatory T cells-polarized skin diseases, including systemic sclerosis. Finally, Th17 and Th22 cells, along with their cytokines IL-17 and IL-22, contribute to a neutrophil migration and wound-healing-like pattern, such as psoriasis (3–6).
The discovery of Th22 cells occurred much later than its defining cytokine, IL-22, which was initially regarded as a cytokine secreted by Th1 or Th17 cells. Nograles and Fujita proposed the possible existence of discrete Th22 cells, producing IL-22 without IFN-γ or IL-17 in the skin (7, 8). Then, Th22 cells were identified as a novel Th cell subset according to its particular transcriptional profile of aryl hydrocarbon receptor (AHR), chemokine receptors CCR4, CCR6, CCR10, as well as cytokines IL-22 instead of IFN-γ, IL-4, or IL-17 (9–11). Additionally, Th22 cells remain stable under inducible Th1, Th2, Th17, and Treg cells conditions, which reinforced that Th22 cells are a novel effector T subtype contributing to skin homeostasis and inflammation (10). Furthermore, results from an IL-17A fate-mapping reporter assay and a transcription profile supported Th22 cells as a distinct lineage from Th17 cells (12, 13).
Th22 cells have been proposed to play essential roles in autoimmune diseases including rheumatoid arthritis, inflammatory bowel diseases, asthma, multiple sclerosis, immune thrombocytopenia, nephropathy, hepatitis, thyroid diseases, and myasthenia gravis, as well as infectious diseases and tumors (14–22). Recently, emerging advances regarding the understanding of Th22 cells are allowing in-depth comprehension of the cutaneous immune system, which might promote the development of treatments targeting Th22 cells. Here, we review the current knowledge regarding Th22 cells and their main functional cytokine IL-22, especially their interaction with keratinocytes, in the pathogenesis of psoriasis, atopic dermatitis, lupus erythematosus, and other skin immune diseases. We also provided potential bases for treatments targeting the Th22/IL-22 signaling pathway.
Originally, Th22 cells were derived from the IL-22 study. IL-22 is the main effector cytokine of Th22 cells and belongs to the interleukin 10 (IL-10) family. IL-22 was first recognized as an IL-10-related T cell-derived inducible factor in 2000 (23, 24). Although IL-22 was primarily discovered that IL-22 was produced by Th1 and natural killer (NK) cells (25), IL-22 was usually viewed as a Th17 cytokine (26, 27) until the identification of Th22 cells (8–11). They were discovered as a new subset that only secreted IL-22, and barely produced IFN-γ, IL-4, or IL-17 (15, 20). Duhen estimated that about a half of IL-22 producing CD4+ memory T cells from healthy human peripheral blood secreted only IL-22, whereas around 30% and 13% separately co-expressed IFN-γ and IL-17, separately, with nearly 6% producing all three (9). Apart from its characteristic cytokine IL-22, Th22 cells can also produce IL-13 (10–12, 28), TNF-α (10, 11), IL-26 (11), and granzyme B (12). Additionally, Th22 cells orchestrate skin immunity and wound healing along with keratinocytes (10). Supporting the function of Th22 cells in the skin, they have been shown to possess skin-homing properties with CCR4, CCR6, and CCR10 expressions (9–11). Unsurprisingly, the frequency of cutaneous Th22 cells is higher in inflammatory skin than that in the blood, accounting for 30% of IL-22+ cells in the epidermis and dermis (10). Recently, Foster’s team generated IL17aeGFPIL22tdTom dual-reporter transgenic mice and proved that Th22 cells were not derived from Th17 cells as a distinct lineage in Th22-enriched culture conditions (12). Further, they validated this finding in vivo with triple-reporter mice in which Th22 cells seldom developed through the IL-17A-expressing stage during bacterial infection (13). Collectively, Th22 cells are regarded as a novel T cell subset. Considering the complexity of T cells subsets identification, flow cytometry appeared to be the most popular method to segregate Th22 cells by combining extracellular proteins (CCR4, CCR6, and CCR10), a transcription factor (AHR) and cytokine profiles (IL-22+, along with IFN-γ-, IL-4-, IL-9-, IL-10- and IL-17-) (29). Additionally, the dual cytokine-secretion assay allowes the isolation and purification of Th22 cells, which might provide possibilities for further characterizations (30).
The differentiation of T cells is primed by direct contact or the interaction with cytokines produced by APC and tissue cells and depends on complex lineage-defining transcriptional networks (31). Previous studies have demonstrated that TGF-β promotes IL-17 while differentially inhibits IL-22 during early Th cell differentiation (11, 32). Trifari has also found that human naive T cells co-cultured with IL-1β and IL-23 secreted IL-22 rather than IL-17 (11). Further, Yoon has revealed that tape stripping of mouse and human skin can lead to the activation of Toll-like receptors 4 (TLR4). Finally, the skin DC can be polarized by IL-23 and promote Th22 cell differentiation (33). On the other hand, Basu has shown that IL-6 alone can trigger the development of Th22 cells. (34). Although IL-23 can augment the polarization of Th22 cells primed by IL-6, IL-23 is dispensable since its blockage did not affect TCDD-induced IL-22 production. (34, 35). Moreover, Duhen has demonstrated that IL-6 and TNF-α contributed to Th22 cells production (9). Studies also suggested that IL21 or IL-26 enhanced Th22 differentiation (36, 37). Recently, Prostaglandin E2 (PGE2) has been proved to increase IL-22 under Th22-priming conditions and to stimulate IL-22 in vivo by binding to the EP4 receptor. (38). Prostaglandin I (2) analogs has been proved to augment IL-22 and IL-17 in vitro and expanded Th17 and TH22 cells as well (39). Jiao et al. have found that ICOS/ICOSL signaling increased the proliferation of CCR4+ CCR6+ Th22 cells (40). Besides various cytokines and molecules, APC could also regulate Th22 cells differentiation (Figure 1). Plasmacytoid DC (pDC) was more potent than conventional DC (cDC) to induce human Th22 cells via IL-6 secretion (9). For example, Wilkinson has shown that IL-6 is released by DC-polarized Th22 cells through classical, trans, and cluster signaling pathways. (41). Additionally, CD5+ LC and CD5+ DC were strong stimulators of Th22 cells (42). In another study, LC was more efficient than DC to induce Th22 polarization (8). Yang indicated that activated B cells also promoted Th22 cell formation (43). Although studies revealed that various factors regulated Th22 cells differentiation, there was no standard Th22 cells polarization protocol. Recently, the optimized Th22-skewing condition proposed by Plank was widely accepted, which was composed of IL-23, IL-1β, IL-6, the endogenous AHR ligand 6-Formylindolo[3,2-b]carbazole (FICZ), and the TGF-βR inhibitor Galunsertib (12). However, differentiation of Th22 cells in different context of disease settings and distinct immune microenvironments remains to be explored.
Figure 1 The characterization, differentiation of Th22 and the signaling pathway of Th22/IL-22. Native T cells induced by IL-6, IL-23, IL-1β and TNF-α, mediated by LC, DC or activated B cells could differentiate into Th22, while TGFβ could inhibit Th22 polarization. AHR, the master transcription factor of Th22, could be upregulated by ultraviolet B, microbiota, PGE2 and Notch signaling. Th22 secreted its effector cytokine IL-22, as well as IL-13, IL-26, TNF- α and granzyme B. Th22 might converted to Th1, Th2, Th17 under certain conditions. IL-22 could bind to receptor complex of IL-22R and IL-10R, activating TYK2 and JAK1, thus mediating multiple pathways. In contrast, the decoy receptor IL-22BP blocks the roles of IL-22. Th22, T helper 22 cell; LC, Langerhans cell; DC, dendritic cell; AHR, aryl hydrocarbon receptor; IL-22R, IL-22 receptor; IL-10R, IL-10 receptor; IL-22BP, IL-22 binding protein; PGE2, Prostaglandin E2. Created with Biorender.com.
The transcriptional mechanisms orchestrating the phenotypic identity of Th22 cells are not fully elucidated. Nevertheless, AHR is mainly considered as a characteristic transcription factor of Th22 cells. Studies have demonstrated that the AHR agonists β-naphthoflavone, FICZ, and dioxin upregulated not only induced the production of IL-22 but also of IL-17 (11, 35). Similarly, the AHR ligand VAF347 selectively promoted IL-22 by acting on monocytes and naive T cells (44). Conversely, inhibiting or silencing AHR in vitro, and knocking out AHR in vivo led to reduced IL-22 (11, 34, 45). Moreover, Alam et al. have identified a Notch-AHR axis using RBP-J deficient mice, where Notch1 and Notch2 compensated each other to control IL-22 expression. They have also discovered that Notch signaling can induce heat-labile molecules to stimulate AHR (45). Zeng has also shown that the Notch-Hes-1 axis facilitates the production of Th22 cells (46). Medroxyprogesterone acetate promoted Th22 cells through AHR and T-bet while inhibiting RORγt (47). Besides the essential roles of master regulatory, co-regulator, placeholder, and other epigenetic programs also cooperate together in a coordinated network to drive Th cells differentiation. For example, RORγt is a positive regulator of Th22 cell differentiation, (11, 12). combined with RUNX1, it promotes IL-22 expression through their enhancer, CNS-32 (48). Besides, HIF-1α and RUNX3 can also elevate the expression of IL-22 (49, 50). Notably, T-bet knock-out mice exhibited compromised IL-22 expression in Th22 cells without affecting IL-22 in Th17 cells (34). In contrast, Plank has described the inhibitory role of T-bet in vitro in Th22 cell cultures with potential minimal Th17 cell contamination (12). These controversial results motivated us to define Th22 cells accurately and explore the underlying mechanisms balancing their stability and plasticity.
Plasticity allows for heterogeneity, which facilitates Th cells to continuously adapt to the ever-changing surroundings and is crucial for immune homeostasis (31, 51). Studies have demonstrated that a proportion of Th22 cells can express IFN-γ (10, 12, 28, 32), IL-4 (10) IL-13 (12, 28), and IL-17 (10, 12, 13, 32), indicating a possible conversion from Th22 cells to Th1/2/17 cells. In turn, Th17 clones, derived from psoriatic skin, could be polarized to an IL-22-single-producing Th22 cell profile (52). Besides, T cells exposed to filaggrin-deficient skin equivalents shifted from a Th1/Th17 to a Th2/Th22 profile (53). Additionally, IL-22+ Th cells predominantly produce IFN-γ instead of IL-17 under Th0 and Th17 conditions, indicating co-regulation of IL-22 and IFN-γ (32). Shen et al. have described that in vitro generated mouse IL-22+ Th cells were relatively stable under Th1 or Th2 conditions in vitro, whilst acquired IL-17-expressing ability under Th17 conditions (54). However, Plank et al. have suggested that IL-17, IL-13, and IFN-γ expressions were augmented in Th22 cells under respective re-stimulated circumstances (12). The different polarization conditions that Shen used to differentiate Th22 cells with TGF-β while Plank induced Th22 differentiation with TGF-βR inhibitor Galunisertib with potential minimal Th17 contamination, which might account for the paradoxical results. The in vivo generated IL-22+ Th cells in IL-22-tdTomato mice lost IL-22 expression and converted to express IL-17 with higher T-bet. This was further confirmed by a colitis model in which transferred IL-22+T cells gained IFN-γ and IL-17 productions (54). Furthermore, IL-22+ Th cells generated in vivo using IL-22-tdTomato mice lost IL-22 expression and expressed IL-17 with higher T-bet, which was further confirmed by a colitis model in which the transferred IL-22+T cells started to produce IFN-γ and IL-17 (12). Human study proved that the plasticity of Th22 cells varied in different diseases with dysregulated Th-skewing. IL-4-secreting Th22 cells were only apparent in atopic eczema rather than allergic contact dermatitis or psoriasis, although IFN-γ and IL-17-producing Th22 cells could be found in all three (10). In summary, Th22 cells can acquire plasticity under certain influences including extrinsic factors like cytokine milieu or pathogen infection, which might play essential roles in the pathogenesis of skin immune diseases. Finally, uncovering the mechanisms that orchestrate the differentiation and plasticity of Th22 cells is crucial for therapeutic interventions.
As mentioned above, Th22 cells were initially discovered and play a vital role in the skin, particularly via its effector cytokine, IL-22. In addition to Th22 cells, cutaneous IL-22 is also expressed by innate and adaptive immune cells including NK cells, innate lymphoid cells (ILCs), lymphoid tissue inducer cells, NK T and γδT cells, DC, macrophages, mast cells, neutrophils, as well as Th1 cells, Th17 cells, and CD8+ T cells, and even fibroblasts (55–63) (Table S1). Generally, innate immune cells respond directly to local environment and quickly modulate surrounding epithelial host defense in the early state, while T cells induce strong and sustained immune responders in the late state. ILCs were supposed as the main producer of IL-22 in mucosa, while Th cells were believed as the primary sources of IL-22 in epithelial tissues (64). ILCs initiated inflammatory response rapidly after stimulation by IL-23 in psoriatic mice (65). Compared with ILCs targeting surface intestinal epithelial cells, Th22 cells have a nonredundant role in the intestinal crypts, revealing spatiotemporal differences between Th22 cells and ILC3 during infection (66). The differences of IL-22-producing ILCs and Th22 cells were not fully elucidated in the skin. ILCs were mostly clustered beneath the dermoepidermal junction and close to T cells (67), which indicated possible crosstalk between them. Strikingly, Mashiko proposed that mast cells were major IL-22 producers in psoriasis patients and atopic dermatitis individuals (68). The emergences of IL-22-expressing cancer-associated fibroblasts and immunofibroblats enhance the complexity of IL-22 production. The limited data requires further exploration of the different roles of various IL-22-expressing cells, both in physiological and pathological conditions. Questions such as whether IL-22-expressing innate immune cells respond earlier and result in acute inflammation while IL-22-producing T cells participate in later stages and cause chronic immune dysfunction, and what are the differences between Th17/Tc17 and Th22/Tc22, considering differentiation, regulation, and plasticity in specific pathological conditions remains unanswered. Also, it is important to consider the differences between murine and human skin when interpreting the underlying mechanism of skin disorders. For example, it is known that the interfollicular epidermis in humans is larger than that in mice. Moreover, the composition of their immune cells is different. For example, dendritic epidermal T cells are only present in the mouse skin. Here, we summarized published data and focused on the biological activities of Th22 cells and IL-22 in the skin.
IL-22 can be regulated by positive inducers including IL-6, IL-21, IL-23, IL-1β, IL-7, AHR, Notch and negative controllers such as TGF-β, IRF-4, IL-27, ICOS, and c-Maf (Figure 2). Interestingly, ultraviolet B irradiation of keratinocytes increased the productions of both AHR ligand (69, 70) and IL-22R1 (71), amplifying the roles of Th22 cells and IL-22 (Figure 1). Soluble IL-22 binding proteins (IL-22BP, or IL-22R2) serve as its natural inhibitor. Furthermore, a high concentration of IL-38 augmented IL-22 while a low concentration attenuated it (58, 60, 63, 72). IL-22 binds with a heterodimeric complex receptor composed of IL-22R1 and IL-10R2. Previously, it was determined that IL-22 bound to IL-22R1 first with high affinity, leading to a conformational adjustment which in turn promotes the combination with IL-10R. While IL-10R2 is widely expressed, IL-22R1 is restricted to non-hematopoietic epithelial cells such as keratinocytes and fibroblasts. IL-22 activates JAK1 and TYK2, transmitting the signal to downstream pathways, including STAT3, STAT1, STAT5, P38, MAPK, JNK and MEK, ERK, AKT, and mTOR (58–60, 63, 72, 73) (Figure 1). Considering the expressions of IL-22R1 and IL-22BP in keratinocytes, IL-22 functions to sustain the integrity and barrier functions of the skin. In contrast, IL-22 can also amplify inflammation cascade, and promote abnormal proliferation and differentiation in certain cases.
Figure 2 Cutaneous biological roles of Th22 cells and IL-22. Th22 cells exert biological functions through its effector cytokine IL-22 in the skin. Besides, IL-22 could also be secreted by ILC, NK cells, LTi, NKT, γδT, Th1/17, CD8+ T cell, DC, macrophage, mast cell, neutrophil and fibroblast in the skin. Both positive inducers and negative controllers could regulate the expression of IL-22. IL-22 plays a vital role in Th22-mediated host defense by communicating with keratinocytes, promoting antimicrobial peptides and keratinocytes proliferation, inhibiting keratinocyte differentiation, and regulating tissue remodeling as well as inflammation. Furthermore, IL-22 could amplify its biological activities through a positive feedback loop by inducing IL-20 production in keratinocytes, which has similar effects on keratinocytes. ILC, innate lymphoid cell; NKT, natural killer T cell; LTi, Lymphoid tissue inducer; γδT, gamma-delta T cell; DC, dendritic cell; Th, T helper cell; IL-22BP, IL-22 binding protein; MMP, matrix metalloproteinases; AHR, aryl hydrocarbon receptor. Created with Biorender.com.
IL-22 plays a vital role in Th22-mediated host defense by communicating with keratinocytes (Figure 2). The function of IL-22 on keratinocytes can be divided into five sections. First, IL-22 protects the host defense through promoting antimicrobial peptides (AMP), including psoriasin (S100A7), calgranulin A (S100A8), calgranulin B (S100A9), S100A12, S100A15, hBD2, and hBD3 (26, 74–77). Similarly, keratinocytes exposed to Th22 supernatants showed increased S100A7 (10). Mechanically, MSX2P1 promoted the progression and growth of IL-22-stimulated keratinocytes by targeting miR-6731-5p and activating S100A7 (78). Additionally, IL-22-induced SPRR2C has been reported to target the miR-330/STAT1/S100A7 axis in HaCaT cells (79). In contrast, CXCL8 and hBD2 were upregulated by IL-22 via STAT3-mediated Bcl-3 (77). Interestingly, IL-17 alone could not enhance S100A7 and S100A8, but, when combined with IL-22, upregulated S100A9 and hBD in primary keratinocytes (26). Unlike the described host defense role of IL-22 to induce Reg family proteins in intestinal epithelia, IL-22 alone could not induce Reg3α in keratinocytes (80). However, along with IL-17, IL-22 can elevate the levels of Reg3α, thereby promoting the proliferation of keratinocytes and inhibiting the terminal differentiation in feedback (81). Blocking either IL-17 or IL-22 increased the severity of Staphylococcus aureus infections, indicating complementary and nonredundant roles of IL-17 and IL-22 (82).
Second, IL-22 promotes keratinocytes proliferation. Among the IL-20 family, IL-22 induced the maximal level of proliferation both in monolayer keratinocytes and reconstituted human epithelial culture system (76). Yang proved that Nrf2 elevated keratin 6 (K6), K16, and K17 by regulating their antioxidant responsive element after IL-22 stimulation, finally promoting proliferation (83). By contrast, IL-22 inhibited apoptosis by increasing Bcl-xl and decreasing Bax (84). Recently, studies demonstrated that microRNAs (miRNAs) cooperated with IL-22 and regulated keratinocytes proliferation through miR-197/IL-22R, miR-122-5p/Sprouty2, miR-330/CTNNB1, miR-20a-3p/SFMBT1, miR-548a-3p/PPP3R1, MSX2P1/miR-6731-5p, miR-233/PTEN, NORAD/miR-26a, and miR-617/FOXO4 signaling pathways (78, 85–92). Furthermore, STAT3, Erk, Akt, MAPK, and JNK were demonstrated to be involved in the hyperproliferation of keratinocytes (93–97). Notably, IL-22 and IL-17 might induce keratinocytes stemness to promote regeneration (98).
Third, IL-22 can restrain the differentiation of keratinocytes and has essential impacts on epithelial architecture, leading to acanthosis, parakeratosis, and hypogranularity. Those differentiated proteins were inhibited by IL-22, including K1, K10, K7, kallikrein 7, profilaggrin/filaggrin, involucrin, loricrin, desmocollin 1, and CALM5 (75, 76, 99–103). Padhi further proved that IL-22 suppressed peptidylarginine deiminase-1 to hinder epithelial differentiation (104).
Fourth, IL-22 also contributes to tissue remodeling, particularly through matrix metalloproteinases (MMP) 1 and 3 in keratinocytes (75, 99, 105). Scratch wound healing and transwell assay further proved the mobility role of IL-22 (95, 103). Impaired skin showed a delayed healing process and reduced IL-22 (105, 106), while recombinant IL-22 alleviated skin damage in different wound-healing stages (103, 107). Additionally, IL-22 promoted collagen deposition in fibroblasts and accelerated wound healing (73, 108). Moreover, IL-22 activated TNF-induced keratinocytes, then promoted MMP1 in fibroblasts through increasing IL-22R (109). Recently, Li suggested that CD11c+ myeloid cells were important for the underlying mechanisms of wound healing via regulation of the IL-23/IL-22 axis (110).
Fifth, IL-22 can also regulates inflammation in keratinocytes, mainly through inducing granulocyte-attracting chemokines, such as CXCL1, CXCL5, and CXCL8 (76, 99, 111). Also, IL-22 can enhance IL-1, IL-8, and TNF-α (71, 112–114). Furthermore, IFNα increased IL-22R and amplified the pro-inflammatory role of IL-22 (112, 115). Interestingly, IL-22, IL-20, and IL-24 share the IL-22R1 subunit and have redundant roles in the skin (76, 100, 107). Moreover, IFNα increases IL-22R and amplifies the pro-inflammatory role of IL-22 (100).
Nowadays, increasing studies have proved that IL-22 exerted dual roles, both guardian and pathogenic, depending on the context (56, 63, 116). The dysregulated interplay between immune and epithelial cells can lead to the disruption of skin homeostasis. In contrast to its skin integrity protective function, excessive IL-22 can lead to skin disorders. There is emerging evidence that Th22 cells and IL-22 are involved in the development of skin diseases, particularly psoriasis and AD (55, 57, 62).
IL-22 is thought to multifactorial develop psoriatic lesions. Also, the pathological pattern of psoriasis can be viewed as an uncontrolled IL-22 physiological activity in all aspects. Gene-editing mice are widely used when exploring the mechanism of psoriasis-like models composed of hyperkeratosis, parakeratosis, acanthosis, and dermal inflammation. IL-22-transgenic mice showed neonatal mortality and psoriatic morphological changes (117). Reversely, IL-22-deficient mice were protected from IL-23- or imiquimod-induced psoriatic lesions (27, 118, 119). Similarly, neutralizing IL-22 significantly ameliorates murine psoriatic phenotype (27, 119, 120). Moreover, knocking down IL-22BP also elevates the production of Th22 and γδT cells, (121, 122). thereby deteriorating psoriasiform dermatitis. (121, 123). Zheng suggested that IL-23 induced IL-22 production and activated STAT3, while IL-6 was dispensable for IL-22 expression (27). Then, Lindroos verified that the expressions of IL-22R1 and IL-22BP were IL-6-dependent, although IL-6-/- T cells did not reduce IL-22 production (124). On the other hand, Chen has shown that K23/IL-22+/+ and K23/IL-22−/− mice are not different and hypothesized that IL-22 deficiency is not required for psoriasis development, although IL-22 aggravates psoriatic arthritis in K23 mice. (125). Hedrick demonstrated that IL-23 did not induce IL-22 in CCR6-/- mouse ears, while triggering IL-22 production in Rag-/- mice, implying that both T and non-T cell-derived IL-22 were involved in IL-23-induced ear swelling in murine psoriasis models (126). The previous studies were predominantly concentrated on the biological activities of IL-22 and simply considered it as a Th17 cytokine in the IL-23/IL-17/IL-22 axis in psoriasiform models without elucidating the sources of IL-22. Along with IL-22, IL-17, the primary effector of Th17 cells, also seemed necessary to induce IL-23-mediated murine psoriasis (118, 127). A 2D visualization of psoriasis RNA-seq datasets was unable to prove the relationship between IL-17A and IL-22, which indicated alternative sources of IL-22 such as Th22 or non-T cells rather than Th17 in psoriasis (128). Th22 and IL-22 producing CD8+ T cells are one of the primary sources of IL-22 in AD (129). For example, IL-22 mediates the proliferation of keratinocytes and epithelial thickness in AD-like models. (130). IL-22 mediated keratinocytes proliferation and epithelial thickness in AD-like models (131–133). Additionally, skin-specific IL-22 transgenic (K5-tTA-IL-22) mice exhibited chronic pruritic dermatitis with increased IL-4, IL-13, and gastrin-releasing peptides, along with elevated susceptibility to S. aureus infections (134). Epicutaneous-sensitized AD models promoted IL-22 expression as well as Th22 cell accumulation (33, 135–137). IL-23, triggered by endogenous TLR4, promoted DC-induced Th22 cell polarization after antigen application following tape stripping in mouse skin (33). Furthermore, Robb found that PGE2 markedly augmented IL-22 in both Th22 cells and ILCs (38, 138). Several studies proposed that ILCs and γδT cells were acknowledged as primary producers of IL-22 in psoriatic mouse models instead of Th cells (65, 119, 139–141).
Studies have revealed that Th22 cells play a regulatory role in the pathogenesis of varied immune disorders, infections, and tumors (14, 15, 21, 22, 142, 143). Here, we provided a brief summary of published research pertaining to the roles of Th22 cells in skin diseases.
Psoriasis is an inflammatory skin disease with high incidence, characterized by erythematous scaly patches or plaques usually on extensor locations, caused by immune-mediated hyperproliferation and chaotic differentiation of keratinocytes. Substantial progress has been made in relating skin lesions with systemic comorbidities such as psoriatic arthritis (PsA) and metabolic syndromes (144). Both circulation and skin lesion showed higher IL-22 expression in psoriasis patients, which was also associated with disease severity (84, 145–148). Although IL-22-producing Th22 cells were first discovered in psoriatic lesions, it is widely accepted that IL-17- and IL-22- expressing Th17 cells drive the inflammatory cascades and activate and recruit Th1 cells and Th22 cells, resulting in abnormal immune response and aberrant epidermal proliferation in psoriasis (149). Moreover, IL-22 enhanced the production of IL-17 in keratinocytes, promoting keratinocytes migration (150). IL-17 and IL-22 in synergism with each other induce the secretion of AMP, promote acanthosis, parakeratosis and inflammation. However, while IL-17 dominates inflammation by activating immune cells, IL-22 mainly regulates the proliferation and differentiation of keratinocytes.
IL-22 might be a genetic risk factor in psoriasis. Copy number variations (CNV) of IL-22 exon1 were significantly associated with psoriasis severity (151, 152). Pollock proposed IL-22 as a possible germ line risk locus for PsA (153) Furthermore, Nikamo found that a high-risk IL-22 promoter variant might lead to the onset of psoriasis before puberty (154). Similarly, Cordero discovered higher IL-22-expressing T cells in pediatric psoriasis patients rather than adult ones (155). Both studies indicated that pediatric psoriasis patients might benefit from anti-IL-22 therapy. Besides, differential IL-22 expression at different sites might result in diverse local manifestations of psoriasis. Serum IL-22 was elevated in PsA patients with entheseal and joint changes (148, 156). CD4+ IL-22+ T cells were lower in the synovial fluid of PsA (156), while the level of IL-22 was higher in the intestine of PsA patients (157). Moreover, Ahn found that scalp psoriasis had higher IL-22 derived from CD8+T cells (158). However, using GSEA and GSVA, Ruano has found a similar Th1/Th17 profile but less activation of Th22 cells in scalp psoriasis compared to skin psoriasis (159). Besides, these discordant expressions at different sites put forward a hypothesis that differential IL-22 expression might result in diverse local manifestations of psoriasis.
Collectively, both IL-22-producing Th17 cells and Th22 cells play important roles in psoriasis patients. Rather than dominant function of Th17 cells in psoriasis, Th22 cells, serve as immune response of Th17 cells, mostly act on keratinocytes leading to excessive proliferation and deviate differentiation in the skin. However, the emergence of IL-22-producing innate immune cells increase the complexity of immune activity in psoriasis. Future studies illustrating distinct roles among innate and adaptive IL-22-expressing immune cells are required.
AD is the most common pruritic inflammatory skin disease with recurrent eczematous dermatitis. AD affects all ages and ethnicities and can be classified as extrinsic and intrinsic phenotypes. Its pathophysiology is complicated and involves genetic predisposition and epidermal dysfunction, along with diverse T cell-related inflammation (160).
Numerous Studies demonstrated that increased expression of IL-22 was correlated with the Scoring of Atopic Dermatitis (SCORAD) (161–164). The elevated IL-22 was localized in skin lesions in mild AD (165), while affecting both local skin and circulation in severe AD (162). A comparative study revealed differential expression of chemokines in AD (CCL17/18/20) and psoriasis (CXCL1, IL-8, and CCL20), indicating that disease-specific microenvironments might be involved in Th differentiation (166) While psoriasis is Th17/Th22-skewing, AD tends to be Th2/Th22 polarized (129, 166, 167). The Th2-skewing microenvironment inhibited AMP, thus resulting in more vulnerable skin colonization with S. aureus in AD patients rather than in psoriasis individuals (162, 168).
The immune infiltration in AD is quite heterogeneous depending on the disease-specific manifestation-related contexts. Intrinsic AD presented higher skewing of Th17/TH22 than extrinsic phenotype (169), and the “Th2/Th22/PARC-dominant” cluster in four types of intrinsic AD patients showed the highest SCORAD (170). Besides, Th2/Th22 activation progressively intensified from acute to chronic while IL-17 was decreased from acute to chronic phase (168, 171). The immune cells infiltrations also vary among different AD ethnicities. African American AD patients (172), Tanzanian AD patients (173), and Asian AD patients (174, 175) had higher Th2/Th22 skewing than European Americans. Although common Th2/Th22 skewing can be found in different age groups (176), adult AD patients had elevated IL-22 frequencies than infants and adolescents (177, 178) but decreased with age in elderly AD patients especially those over 40 (179, 180). Future study with continuous monitoring in AD patients from infants to seniors might expand our knowledge in aged-related immune heterogeneity in AD. Moreover, gene predisposition influences the immune pathways in AD. AD children with FLG mutation demonstrated higher Th22 cell numbers without affecting the adaptive immunity composition (181).
Recently, laser capture micro-dissection has been used to detect IL-22 in the dermis (163). Compared to a skin biopsy, tape strip sampling and interstitial fluid from suction blistering are less invasive methods to identify similar inflammatory cytokines (182–184). However, the detection and qualification of cytokine profiles in interstitial fluid is unstable and sometimes might not detect IL-22 (185). Microneedle patch for ultrasensitive biomarkers quantification in interstitial fluids would facilitate point-of-care diagnosis and longitudinal monitoring and uncover the underlying mechanism of background immune activation in different ethnicities and age groups.
Lupus erythematosus is a heterogeneous autoimmune disease, ranging from skin lesions to nephritis, characterized by exaggerated B and T cell immune responses with impaired immune tolerance against self-antigens (186–188). Studies related with Th22 cells and IL-22 in lupus patients are quite controversial, possibly due to small sample sizes.
Some found decreased plasma IL-22 in inactive SLE individuals (189) and new-onset SLE patients (190). In contrast, other studies revealed elevated Th22/IL-22, implying its pathologic roles (191, 192). CNV of IL-22 and IL-17 were higher in SLE patients without synergistic contribution to SLE risks (193). Additionally, IL-22 gene rs2227513 polymorphism was associated with increased risk in renal SLE (194). The frequencies of Th22 cells vary among different lupus erythematosus subtypes, indicating a prognostic role of IL-22 in various types. Additionally, discoid erythematosus has higher levels of Th22 cells and IL-22 compared to subacute cutaneous lupus erythematosus in both skin lesions and circulation. (195, 196). Yang et al. have also found increased Th22 cells in sole skin involvement patients but decreased in sole nephritis ones (197). However, they later reported controversial results in which serum IL-22 levels in AD were higher compared to healthy controls, and anti-IL-22 monoclonal antibody treatment attenuated nephritis in MRL-lpr mice (198). Zhong found augmented CCR6+ Th22 cells in SLE patients with sole skin and/or renal impairments (199). In addition, Luk investigated and revealed higher IL-22 levels in the urinary sediment of SLE patients with proliferative nephritis than those with nonproliferative (200).
Systemic sclerosis (SSc) is an immune-mediated disease with T cell abnormalities, characterized by skin or/and organs fibrosis. SSc patients had increased Th22 cells in both circulation and lesional skin, indicating abnormal crosstalk between Th22 cells and fibroblasts (39, 108, 109, 201, 202). Hypertrophic scar and keloid caused by proliferative fibroblasts further confirmed the interaction between Th22 cells and fibroblasts with augmented Th22 numbers and IL-22 levels (195, 203, 204). Alopecia areata (AA) is a common non-scarring hair-loss immune disease with limited therapeutics (205). Some studies described elevated IL-22 in both AA lesions and serum (206), as well as expanded CLA+ Th22 cells in the blood (207). Meanwhile, other studies have suggested AA as Th1/Th2 skewing instead of Th17/Th22 (208). Larger cohort investigations are urgent to reveal underlying Th polarization in AA. The biology of Behcet’s disease (BD) is not fully understood, however, a recent study found that increased Th22 cells were associated with mucocutaneous BD (209). Hidradenitis suppurativa (HS) is a chronic inflammatory disorder with painful inflamed nodules, abscesses, sinus tracts, and fistulas in intertriginous skin, leading to severely decreased life quality (210). IL-22 deficiencies might participate in HS pathogenetic mechanisms (211, 212). In contrast, transcriptional profiles showed apparent Th22/IL-22-skewing in prurigo nodularis (213). Furthermore, Th22 cells were augmented in Graft-versus-host disease (GVHD), a complication following allogeneic hematopoietic-cell transplantation involving the skin (214, 215). IL-22 derived from donor T- cells alleviated cutaneous GVHD in mouse models (216, 217).
Taken together, Th22 cells and IL-22 play essential roles in in the pathogenesis of skin disorders. However, we found that most studies and clinical trials (Table S2) concentrate on the function of IL-22. As we know, IL-22 could be secreted by not only Th22 cells but other immune cells and tissue cells. Thus, future studies focused on the behaviors of Th22 cells n dermatological disorders are needed.
Over the past 20 years, biologics have remarkably updated our knowledge of dermatological treatments, especially in psoriasis and AD. Biologics are recommended as a first-line treatment of moderate to severe plaque psoriasis, including 11 approved inhibitors of TNF-α (infliximab, adalimumab, certolizumab pegol, golimumab, and etanercept), p19 of IL-23 (tildrakizumab, risankizumab, and guselkumab), p40 of IL-12/23 (ustekinumab), IL-17(secukinumab, ixekizumab, and brodalumab) (144, 218, 219). Another two drugs targeted IL-23 or IL-17, Mirikizumab (anti-p19 of IL-23) (220) or bimekizumab (anti-IL-17) (221), are in clinical development. Currently, three inhibitors are approved in AD, including dupilumab (anti-IL-4Rα), tralokinumab (anti-IL-13), baricitinib (JAK1/2 inhibitor). Antibodies of CCR4, CCL20, IL-6/IL-6R, along withother JAK inhibitors (detailed information see Table S2) are under clinical development. However, all of those biologics are not applicable in patients with active tuberculosis or hepatitis B virus infection, which might lead to deadly infection (218, 222, 223). Targeting the IL-22-IL-22R system might provide a new therapeutic with minimal side effects of infection due to the lack of IL-22R on hematopoietic cells (59).
Reich found that guselkumab had better long-term efficacy than secukinumab (224), which might because of broader spectrum of both IL-22 and IL-17 inhibiting roles of guselkumab than decrising Il-17 solely of sekinumab. Furthermore, Evidences of suppression of IL-22 expression were also discovered in infliximab (225), adalimumab (225–227), etanercept (228, 229), tildrakizumab (230), risankizumab (230), guselkumab (231, 232), ustekinumab (233–235), filgotinib (jak1 inhibitor) (236), upadacitinib (jak1 inhibitor) (230) and baricitinib (237), which is in consistent with the signaling pathway of Th22/IL-22. Besides, ruxolitinib exhibited extra role of inhibiting expression of IL-22R1 (238) (Table S3). In contrast, anakinra (recombinant IL-1Rα) increased production of IL-22 in Hidradenitis Suppurativa (239, 240), possibly via promoting the differentiation of Th22 cells. However, tapinarof (AHR agonist) increased the production of IL-22 in keratinocytes in vitro, while inhibited IL-22 in psoriasis mouse model (241). This discrepancy again reminds us of the complexity of IL-22 related immune responses with both innate and adaptive IL-22-producing immune cells, which urges us to clarify the different roles of IL-22-producing cells in human health and skin diseases.
Integrative biology techniques combing human transcriptome profiles with clinically relevant in vivo models have identified IL-22-targeting as a prospective intervention in psoriasis (242) Guselkumab (anti-IL-23) acted as a long-time effector by suppressing IL-17 and IL-22 after withdrawal in the treatment of psoriasis, indicating that IL-22 blockers might be effective in both active and maintenance phases (231). Surprisingly, clinical trials of anti-IL-22 monoclonal antibodies (mAb), ILV-094 (NCT00563524) and ILV-095 (NCT01010542), are discontinued because of no efficacy in psoriasis patients with PASI ≥ 11 and PGA ≥ 3 in active phases (Table 1). The negative results of both trials might be due to that IL-22 acts on keratinocytes and induces abnormal proliferation and differentiation of the epidermis rather than inflammation. Notably, after years of treatment with both infliximab and ustekinumab, IL-22-expressing CD4+ T cells-maintained secretion of IL-22 after stimulation in clinically-healed-lesions (248). Thus, targeting IL-22 might be used as a maintenance treatment to avoid psoriasis relapse. In some cases, patients do not respond to common biologics. For example, Fania reported that three psoriasis patients developed psoriasiform skin lesions with augmented expression of IL-22 after receiving adalimumab (249). Megna also discovered increased levels of IL-22 in psoriasis patients who switched to eczematous drug eruption after treatment of anti-IL-17 biologics (250). Thus, combined therapies of anti-TNF-α (or anti-IL-23/IL-17) and anti-IL-22 intervention might be effective in anti-TNF-α (or anti-IL-23/IL-17)-resistant psoriasis individuals. Another reason for the failure of anti-IL-22 therapy in psoriasis might be the overlapping activities of IL-22, IL-20 and IL-24 (218). Thus, inhibiting IL-22 or IL-20 (251) alone might not be enough for a strong clinical response. Targeting IL-22R might have better clinical improvements than IL-22 neutralization since it also inhibits IL-20 and IL-24 (Table 1). Moreover, Michiels proposed a specific noncanonical STAT3 activation targeting by IL-22R1 Y-independent pathways, which not only relieved psoriatic skin but also protected IL-22-dependent barrier defense function at mucosal sites (252). However, lower serum IL-22 was detected in psoriasis patients with metabolic syndromes than that in patients without systemic complications (253). As IL-22 proved beneficial effects in metabolic syndromes (254, 255), anti-IL-22 treatment might deteriorate metabolic changes in psoriasis patients.
Different from the negative results of anti-IL-22 in psoriasis trials, ILV-094 seems attractive in AD therapeutics, as it improved SCORAD and neutrophils infiltration of asthma in severe AD patients in a preliminary study (243, 244) (Table 1). Dupilumab could inhibit IL-4, IL-13 and IL-22 simultaneously (256). However, some refractory AD patients respond poorly to dupilumab. Interestingly, a mathematical model revealed that simultaneous inhibition of IL-13 and IL-22 would be a promising intervention for them (257). Conversely, recombinant IL-22, F-652 or UTTR1147A, might strengthen its protective role in IL-22-deficient diseases such as GVHD and ulcer (Table 1).
In summary, Th22 cells represent a novel Th cell subset. However, their differentiation and regulation mechanisms remain to be fully elucidated. Emerging evidences have suggested that Th22 cells and IL-22 can play either protective or pathogenetic roles in various skin disorders. The biological roles of IL-22 depend on the local environment and disease setting contexts. Further studies are required to elucidate the mechanisms of Th22 cells in the pathogenesis of different diseases, as well as explore therapeutics targeting the Th22/IL-22 signaling pathway in skin disorders. Non-invasive transdermal delivery systems, such as microneedles with cell-penetrating peptides (258), might expand our application of biologics in the future.
YP drafted and edited the manuscript, DD, LW and XW drafted and edited the figure and tables, GH and XJ revised the manuscript. All authors contributed to the article and approved the submitted version.
This work was supported by the National Natural Science Foundation of China (81872535, 22177084 and 82073473), the Fundamental Research Funds of Science & Technology Department of Sichuan Province (Grant Nos. 2022YFQ0054) and the 1.3.5 project for disciplines of excellence, West China Hospital, Sichuan University (ZYJC21036).
The authors declare that the research was conducted in the absence of any commercial or financial relationships that could be construed as a potential conflict of interest.
All claims expressed in this article are solely those of the authors and do not necessarily represent those of their affiliated organizations, or those of the publisher, the editors and the reviewers. Any product that may be evaluated in this article, or claim that may be made by its manufacturer, is not guaranteed or endorsed by the publisher.
We apologize to researchers in Th22 cells and skin immunity whose work we could not cite according to space constraints.
The Supplementary Material for this article can be found online at: https://www.frontiersin.org/articles/10.3389/fimmu.2022.911546/full#supplementary-material
1. Pasparakis M, Haase I, Nestle FO. Mechanisms Regulating Skin Immunity and Inflammation. Nat Rev Immunol (2014) 14(5):289–301. doi: 10.1038/nri3646
2. Kabashima K, Honda T, Ginhoux F, Egawa G. The Immunological Anatomy of the Skin. Nat Rev Immunol (2019) 19(1):19–30. doi: 10.1038/s41577-018-0084-5
3. Sabat R, Wolk K, Loyal L, Docke WD, Ghoreschi K. T Cell Pathology in Skin Inflammation. Semin Immunopathol (2019) 41(3):359–77. doi: 10.1007/s00281-019-00742-7
4. Gudjonsson JE, Kabashima K, Eyerich K. Mechanisms of Skin Autoimmunity: Cellular and Soluble Immune Components of the Skin. J Allergy Clin Immunol (2020) 146(1):8–16. doi: 10.1016/j.jaci.2020.05.009
5. Krohn IK, Aerts JL, Breckpot K, Goyvaerts C, Knol E, Van Wijk F, et al. T-Cell Subsets in the Skin and Their Role in Inflammatory Skin Disorders. Allergy (2022) 77(3):827–42. doi: 10.1111/all.15104
6. Seiringer P, Garzorz-Stark N, Eyerich K. T-CellMediated Autoimmunity: Mechanisms and Future Directions. J Invest Dermatol (2022) 142(3 PT B):804–10. doi: 10.1016/j.jid.2021.04.032
7. Nograles KE, Zaba LC, Guttman-Yassky E, Fuentes-Duculan J, Suárez-Fariñas M, Cardinale I, et al. Th17 Cytokines Interleukin (IL)-17 and IL-22 Modulate Distinct Inflammatory and Keratinocyte-Response Pathways. Br J Dermatol (2008) 159(5):1092–102. doi: 10.1111/j.1365-2133.2008.08769.x
8. Fujita H, Nograles KE, Kikuchi T, Gonzalez J, Carucci JA, Krueger JG. Human Langerhans Cells Induce Distinct IL-22-Producing CD4+ T Cells Lacking IL-17 Production. Proc Natl Acad Sci USA (2009) 106(51):21795–800. doi: 10.1073/pnas.0911472106
9. Duhen T, Geiger R, Jarrossay D, Lanzavecchia A, Sallusto F. Production of Interleukin 22 But Not Interleukin 17 by a Subset of Human Skin-Homing Memory T Cells. Nat Immunol (2009) 10(8):857–63. doi: 10.1038/ni.1767
10. Eyerich S, Eyerich K, Pennino D, Carbone T, Nasorri F, Pallotta S, et al. Th22 Cells Represent a Distinct Human T Cell Subset Involved in Epidermal Immunity and Remodeling. J Clin Invest (2009) 119(12):3573–85. doi: 10.1172/jci40202
11. Trifari S, Kaplan CD, Tran EH, Crellin NK, Spits H. Identification of a Human Helper T Cell Population That has Abundant Production of Interleukin 22 and is Distinct From T(H)-17, T(H)1 and T(H)2 Cells. Nat Immunol (2009) 10(8):864–71. doi: 10.1038/ni.1770
12. Plank MW, Kaiko GE, Maltby S, Weaver J, Tay HL, Shen W, et al. Th22 Cells Form a Distinct Th Lineage From Th17 Cells In Vitro With Unique Transcriptional Properties and Tbet-Dependent Th1 Plasticity. J Immunol (2017) 198(5):2182–90. doi: 10.4049/jimmunol.1601480
13. Barnes JL, Plank MW, Asquith K, Maltby S, Sabino LR, Kaiko GE, et al. T-Helper 22 Cells Develop as a Distinct Lineage From Th17 Cells During Bacterial Infection and Phenotypic Stability is Regulated by T-Bet. Mucosal Immunol (2021) 14(5):1077–87. doi: 10.1038/s41385-021-00414-6
14. Zhang N, Pan HF, Ye DQ. Th22 in Inflammatory and Autoimmune Disease: Prospects for Therapeutic Intervention. Mol Cell Biochem (2011) 353(1-2):41–6. doi: 10.1007/s11010-011-0772-y
15. Tian T, Yu S, Ma D. Th22 and Related Cytokines in Inflammatory and Autoimmune Diseases. Expert Opin Ther Targets (2013) 17(2):113–25. doi: 10.1517/14728222.2013.736497
16. Perusina Lanfranca M, Lin Y, Fang J, Zou W, Frankel T. Biological and Pathological Activities of Interleukin-22. J Mol Med (Berl) (2016) 94(5):523–34. doi: 10.1007/s00109-016-1391-6
17. Hou W, Wang B, Zhou Y, Xu K, Meng L, Zhu W, et al. IL22 Expression is Increased Variedly in the Initial Phase, Onset and Chronic Phase of a Pristaneinduced Arthritis Rat Model. Mol Med Rep (2017) 16(2):1109–16. doi: 10.3892/mmr.2017.6739
18. Wang B, Zhao P, Zhou Y, Meng L, Zhu W, Jiang C, et al. Increased Expression of Th17 Cytokines and Interleukin-22 Correlates With Disease Activity in Pristane-Induced Arthritis in Rats. PloS One (2017) 12(11):e0188199. doi: 10.1371/journal.pone.0188199
19. Tamasauskiene L, Sitkauskiene B. Role of Th22 and IL-22 in Pathogenesis of Allergic Airway Diseases: Pro-Inflammatory or Anti-Inflammatory Effect? Pediatr Neonatol (2018) 59(4):339–44. doi: 10.1016/j.pedneo.2017.11.020
20. Gong J, Zhan H, Liang Y, He Q, Cui D. Role of Th22 Cells in Human Viral Diseases. Front Med (Lausanne) (2021) 8:708140. doi: 10.3389/fmed.2021.708140
21. Hossein-Khannazer N, Zian Z, Bakkach J, Kamali AN, Hosseinzadeh R, Anka AU, et al. Features and Roles of T Helper 22 Cells in Immunological Diseases and Malignancies. Scand J Immunol (2021) 93(5):e13030. doi: 10.1111/sji.13030
22. Jiang Q, Yang G, Xiao F, Xie J, Wang S, Lu L, et al. Role of Th22 Cells in the Pathogenesis of Autoimmune Diseases. Front Immunol (2021) 12:688066. doi: 10.3389/fimmu.2021.688066
23. Dumoutier L, Louahed J, Renauld JC. Cloning and Characterization of IL-10-Related T Cell-Derived Inducible Factor (IL-TIF), a Novel Cytokine Structurally Related to IL-10 and Inducible by IL-9. J Immunol (2000) 164(4):1814–9. doi: 10.4049/jimmunol.164.4.1814
24. Xie MH, Aggarwal S, Ho WH, Foster J, Zhang Z, Stinson J, et al. Interleukin (IL)-22, a Novel Human Cytokine That Signals Through the Interferon Receptor-Related Proteins CRF2-4 and IL-22r. J Biol Chem (2000) 275(40):31335–9. doi: 10.1074/jbc.M005304200
25. Wolk K, Kunz S, Asadullah K, Sabat R. Cutting Edge: Immune Cells as Sources and Targets of the IL-10 Family Members? J Immunol (2002) 168(11):5397–402. doi: 10.4049/jimmunol.168.11.5397
26. Liang SC, Tan XY, Luxenberg DP, Karim R, Dunussi-Joannopoulos K, Collins M, et al. Interleukin (IL)-22 and IL-17 are Coexpressed by Th17 Cells and Cooperatively Enhance Expression of Antimicrobial Peptides. J Exp Med (2006) 203(10):2271–9. doi: 10.1084/jem.20061308
27. Zheng Y, Danilenko DM, Valdez P, Kasman I, Eastham-Anderson J, Wu J, et al. Interleukin-22, a T(H)17 Cytokine, Mediates IL-23-Induced Dermal Inflammation and Acanthosis. Nature (2007) 445(7128):648–51. doi: 10.1038/nature05505
28. Teraki Y, Sakurai A, Izaki S. IL-13/IL-22-Coproducing T Cells, a Novel Subset, are Increased in Atopic Dermatitis. J Allergy Clin Immunol (2013) 132(4):971–4. doi: 10.1016/j.jaci.2013.07.029
29. Mousset CM, Hobo W, Woestenenk R, Preijers F, Dolstra H, van der Waart AB. Comprehensive Phenotyping of T Cells Using Flow Cytometry. Cytom A (2019) 95(6):647–54. doi: 10.1002/cyto.a.23724
30. Wawrzyniak M, Ochsner U, Wirz O, Wawrzyniak P, van de Veen W, Akdis CA, et al. A Novel, Dual Cytokine-Secretion Assay for the Purification of Human Th22 Cells That do Not Co-Produce IL-17a. Allergy (2016) 71(1):47–57. doi: 10.1111/all.12768
31. Saravia J, Chapman NM, Chi H. Helper T Cell Differentiation. Cell Mol Immunol (2019) 16(7):634–43. doi: 10.1038/s41423-019-0220-6
32. Volpe E, Touzot M, Servant N, Marloie-Provost MA, Hupe P, Barillot E, et al. Multiparametric Analysis of Cytokine-Driven Human Th17 Differentiation Reveals a Differential Regulation of IL-17 and IL-22 Production. Blood (2009) 114(17):3610–4. doi: 10.1182/blood-2009-05-223768
33. Yoon J, Leyva-Castillo JM, Wang G, Galand C, Oyoshi MK, Kumar L, et al. IL-23 Induced in Keratinocytes by Endogenous TLR4 Ligands Polarizes Dendritic Cells to Drive IL-22 Responses to Skin Immunization. J Exp Med (2016) 213(10):2147–66. doi: 10.1084/jem.20150376
34. Basu R, O'Quinn DB, Silberger DJ, Schoeb TR, Fouser L, Ouyang W, et al. Th22 Cells are an Important Source of IL-22 for Host Protection Against Enteropathogenic Bacteria. Immunity (2012) 37(6):1061–75. doi: 10.1016/j.immuni.2012.08.024
35. Ramirez JM, Brembilla NC, Sorg O, Chicheportiche R, Matthes T, Dayer JM, et al. Activation of the Aryl Hydrocarbon Receptor Reveals Distinct Requirements for IL-22 and IL-17 Production by Human T Helper Cells. Eur J Immunol (2010) 40(9):2450–9. doi: 10.1002/eji.201040461
36. Yeste A, Mascanfroni ID, Nadeau M, Burns EJ, Tukpah AM, Santiago A, et al. IL-21 Induces IL-22 Production in CD4+ T Cells. Nat Commun (2014) 5, 3753:1–29. doi: 10.1038/ncomms4753
37. Niu Y, Ye L, Peng W, Wang Z, Wei X, Wang X, et al. IL-26 Promotes the Pathogenesis of Malignant Pleural Effusion by Enhancing CD4(+) IL-22(+) T-Cell Differentiation and Inhibiting CD8(+) T-Cell Cytotoxicity. J Leukoc Biol (2021) 110(1):39–52. doi: 10.1002/jlb.1ma0221-479rr
38. Robb CT, McSorley HJ, Lee J, Aoki T, Yu C, Crittenden S, et al. Prostaglandin E(2) Stimulates Adaptive IL-22 Production and Promotes Allergic Contact Dermatitis. J Allergy Clin Immunol (2018) 141(1):152–62. doi: 10.1016/j.jaci.2017.04.045
39. Truchetet ME, Allanore Y, Montanari E, Chizzolini C, Brembilla NC. Prostaglandin I(2) Analogues Enhance Already Exuberant Th17 Cell Responses in Systemic Sclerosis. Ann Rheum Dis (2012) 71(12):2044–50. doi: 10.1136/annrheumdis-2012-201400
40. Jiao Q, Qian Q, Liu C, Luo Y, Fang F, Wang M, et al. T Helper 22 Cells From Han Chinese Patients With Atopic Dermatitis Exhibit High Expression of Inducible T-Cell Costimulator. Br J Dermatol (2020) 182(3):648–57. doi: 10.1111/bjd.18040
41. Wilkinson AN, Chang K, Kuns RD, Henden AS, Minnie SA, Ensbey KS, et al. IL-6 Dysregulation Originates in Dendritic Cells and Mediates Graft-Versus-Host Disease via Classical Signaling. Blood (2019) 134(23):2092–106. doi: 10.1182/blood.2019000396
42. Korenfeld D, Gorvel L, Munk A, Man J, Schaffer A, Tung T, et al. A Type of Human Skin Dendritic Cell Marked by CD5 is Associated With the Development of Inflammatory Skin Disease. JCI Insight (2017) 2(18): e96101. doi: 10.1172/jci.insight.96101
43. Yang J, Yang X, Wang L, Li M. B Cells Control Lupus Autoimmunity by Inhibiting Th17 and Promoting Th22 Cells. Cell Death Dis (2020) 11(3):164. doi: 10.1038/s41419-020-2362-y
44. Baba N, Rubio M, Kenins L, Regairaz C, Woisetschlager M, Carballido JM, et al. The Aryl Hydrocarbon Receptor (AhR) Ligand VAF347 Selectively Acts on Monocytes and Naïve CD4(+) Th Cells to Promote the Development of IL-22-Secreting Th Cells. Hum Immunol (2012) 73(8):795–800. doi: 10.1016/j.humimm.2012.05.002
45. Alam MS, Maekawa Y, Kitamura A, Tanigaki K, Yoshimoto T, Kishihara K, et al. Notch Signaling Drives IL-22 Secretion in CD4+ T Cells by Stimulating the Aryl Hydrocarbon Receptor. Proc Natl Acad Sci USA (2010) 107(13):5943–8. doi: 10.1073/pnas.0911755107
46. Zeng C, Shao Z, Wei Z, Yao J, Wang W, Yin L, et al. The NOTCH-HES-1 Axis is Involved in Promoting Th22 Cell Differentiation. Cell Mol Biol Lett (2021) 26(1):7. doi: 10.1186/s11658-021-00249-w
47. Piccinni MP, Lombardelli L, Logiodice F, Kullolli O, Maggi E, Barkley MS. Medroxyprogesterone Acetate Decreases Th1, Th17, and Increases Th22 Responses via AHR Signaling Which Could Affect Susceptibility to Infections and Inflammatory Disease. Front Immunol (2019) 10:642. doi: 10.3389/fimmu.2019.00642
48. Sekimata M, Yoshida D, Araki A, Asao H, Iseki K, Murakami-Sekimata A. Runx1 and Rorγt Cooperate to Upregulate IL-22 Expression in Th Cells Through Its Distal Enhancer. J Immunol (2019) 202(11):3198–210. doi: 10.4049/jimmunol.1800672
49. Budda SA, Girton A, Henderson JG, Zenewicz LA. Transcription Factor HIF-1alpha Controls Expression of the Cytokine IL-22 in CD4 T Cells. J Immunol (2016) 197(7):2646–52. doi: 10.4049/jimmunol.1600250
50. Fu D, Song X, Hu H, Sun M, Li Z, Tian Z. Downregulation of RUNX3 Moderates the Frequency of Th17 and Th22 Cells in Patients With Psoriasis. Mol Med Rep (2016) 13(6):4606–12. doi: 10.3892/mmr.2016.5108
51. Bluestone JA, Mackay CR, O'Shea JJ, Stockinger B. The Functional Plasticity of T Cell Subsets. Nat Rev Immunol (2009) 9(11):811–6. doi: 10.1038/nri2654
52. Res PC, Piskin G, de Boer OJ, van der Loos CM, Teeling P, Bos JD, et al. Overrepresentation of IL-17A and IL-22 Producing CD8 T Cells in Lesional Skin Suggests Their Involvement in the Pathogenesis of Psoriasis. PloS One (2010) 5(11):e14108. doi: 10.1371/journal.pone.0014108
53. Wallmeyer L, Dietert K, Sochorová M, Gruber AD, Kleuser B, Vávrová K, et al. TSLP is a Direct Trigger for T Cell Migration in Filaggrin-Deficient Skin Equivalents. Sci Rep (2017) 7(1):774. doi: 10.1038/s41598-017-00670-2
54. Shen W, Hixon JA, McLean MH, Li WQ, Durum SK. IL-22-Expressing Murine Lymphocytes Display Plasticity and Pathogenicity in Reporter Mice. Front Immunol (2015) 6:662. doi: 10.3389/fimmu.2015.00662
55. Sabat R, Wolk K. Research in Practice: IL-22 and IL-20: Significance for Epithelial Homeostasis and Psoriasis Pathogenesis. J Dtsch Dermatol Ges (2011) 9(7):518–23. doi: 10.1111/j.1610-0387.2011.07611.x
56. Rutz S, Eidenschenk C, Ouyang W. IL-22, Not Simply a Th17 Cytokine. Immunol Rev (2013) 252(1):116–32. doi: 10.1111/imr.12027
57. Hao JQ. Targeting Interleukin-22 in Psoriasis. Inflammation (2014) 37(1):94–9. doi: 10.1007/s10753-013-9715-y
58. Rutz S, Wang X, Ouyang W. The IL-20 Subfamily of Cytokines–From Host Defence to Tissue Homeostasis. Nat Rev Immunol (2014) 14(12):783–95. doi: 10.1038/nri3766
59. Sabat R, Ouyang W, Wolk K. Therapeutic Opportunities of the IL-22-IL-22r1 System. Nat Rev Drug Discovery (2014) 13(1):21–38. doi: 10.1038/nrd4176
60. Dudakov JA, Hanash AM, van den Brink MR. Interleukin-22: Immunobiology and Pathology. Annu Rev Immunol (2015) 33:747–85. doi: 10.1146/annurev-immunol-032414-112123
61. Ueshima C, Kataoka TR, Hirata M, Koyanagi I, Honda T, Tsuruyama T, et al. NKp46 Regulates the Production of Serine Proteases and IL-22 in Human Mast Cells in Urticaria Pigmentosa. Exp Dermatol (2015) 24(9):675–9. doi: 10.1111/exd.12741
62. Jin M, Yoon J. From Bench to Clinic: The Potential of Therapeutic Targeting of the IL-22 Signaling Pathway in Atopic Dermatitis. Immune Netw (2018) 18(6):e42. doi: 10.4110/in.2018.18.e42
63. Arshad T, Mansur F, Palek R, Manzoor S, Liska V. A Double Edged Sword Role of Interleukin-22 in Wound Healing and Tissue Regeneration. Front Immunol (2020) 11:2148. doi: 10.3389/fimmu.2020.02148
64. Che Y, Su Z, Xia L. Effects of IL-22 on Cardiovascular Diseases. Int Immunopharmacol (2020) 81:106277. doi: 10.1016/j.intimp.2020.106277
65. Bielecki P, Riesenfeld SJ, Hütter JC, Torlai Triglia E, Kowalczyk MS, Ricardo-Gonzalez RR, et al. Skin-Resident Innate Lymphoid Cells Converge on a Pathogenic Effector State. Nature (2021) 592(7852):128–32. doi: 10.1038/s41586-021-03188-w
66. Zindl CL, Witte SJ, Laufer VA, Gao M, Yue Z, Janowski KM, et al. A Nonredundant Role for T Cell-Derived Interleukin 22 in Antibacterial Defense of Colonic Crypts. Immunity (2022) 55(3):494–511 e411. doi: 10.1016/j.immuni.2022.02.003
67. Bruggen MC, Bauer WM, Reininger B, Clim E, Captarencu C, Steiner GE, et al. In Situ Mapping of Innate Lymphoid Cells in Human Skin: Evidence for Remarkable Differences Between Normal and Inflamed Skin. J Invest Dermatol (2016) 136(12):2396–405. doi: 10.1016/j.jid.2016.07.017
68. Mashiko S, Bouguermouh S, Rubio M, Baba N, Bissonnette R, Sarfati M. Human Mast Cells are Major IL-22 Producers in Patients With Psoriasis and Atopic Dermatitis. J Allergy Clin Immunol (2015) 136(2):351–359.e351. doi: 10.1016/j.jaci.2015.01.033
69. Memari B, Nguyen-Yamamoto L, Salehi-Tabar R, Zago M, Fritz JH, Baglole CJ, et al. Endocrine Aryl Hydrocarbon Receptor Signaling is Induced by Moderate Cutaneous Exposure to Ultraviolet Light. Sci Rep (2019) 9(1):8486. doi: 10.1038/s41598-019-44862-4
70. Rolfes KM, Sondermann NC, Vogeley C, Dairou J, Gilardino V, Wirth R, et al. Inhibition of 6-Formylindolo[3,2-B]Carbazole Metabolism Sensitizes Keratinocytes to UVA-Induced Apoptosis: Implications for Vemurafenib-Induced Phototoxicity. Redox Biol (2021) 46:102110. doi: 10.1016/j.redox.2021.102110
71. Kim Y, Lee J, Kim J, Choi CW, Hwang YI, Kang JS, et al. The Pathogenic Role of Interleukin-22 and its Receptor During UVB-Induced Skin Inflammation. PloS One (2017) 12(5):e0178567. doi: 10.1371/journal.pone.0178567
72. Shabgah AG, Navashenaq JG, Shabgah OG, Mohammadi H, Sahebkar A. Interleukin-22 in Human Inflammatory Diseases and Viral Infections. Autoimmun Rev (2017) 16(12):1209–18. doi: 10.1016/j.autrev.2017.10.004
73. McGee HM, Schmidt BA, Booth CJ, Yancopoulos GD, Valenzuela DM, Murphy AJ, et al. IL-22 Promotes Fibroblast-Mediated Wound Repair in the Skin. J Invest Dermatol (2013) 133(5):1321–9. doi: 10.1038/jid.2012.463
74. Wolk K, Kunz S, Witte E, Friedrich M, Asadullah K, Sabat R. IL-22 Increases the Innate Immunity of Tissues. Immunity (2004) 21(2):241–54. doi: 10.1016/j.immuni.2004.07.007
75. Wolk K, Witte E, Wallace E, Döcke WD, Kunz S, Asadullah K, et al. IL-22 Regulates the Expression of Genes Responsible for Antimicrobial Defense, Cellular Differentiation, and Mobility in Keratinocytes: A Potential Role in Psoriasis. Eur J Immunol (2006) 36(5):1309–23. doi: 10.1002/eji.200535503
76. Sa SM, Valdez PA, Wu J, Jung K, Zhong F, Hall L, et al. The Effects of IL-20 Subfamily Cytokines on Reconstituted Human Epidermis Suggest Potential Roles in Cutaneous Innate Defense and Pathogenic Adaptive Immunity in Psoriasis. J Immunol (2007) 178(4):2229–40. doi: 10.4049/jimmunol.178.4.2229
77. Tohyama M, Shirakata Y, Hanakawa Y, Dai X, Shiraishi K, Murakami M, et al. Bcl-3 Induced by IL-22 via STAT3 Activation Acts as a Potentiator of Psoriasis-Related Gene Expression in Epidermal Keratinocytes. Eur J Immunol (2018) 48(1):168–79. doi: 10.1002/eji.201747017
78. Qiao M, Li R, Zhao X, Yan J, Sun Q. Up-Regulated lncRNA-MSX2P1 Promotes the Growth of IL-22-Stimulated Keratinocytes by Inhibiting miR-6731-5p and Activating S100a7. Exp Cell Res (2018) 363(2):243–54. doi: 10.1016/j.yexcr.2018.01.014
79. Luo M, Huang P, Pan Y, Zhu Z, Zhou R, Yang Z, et al. Weighted Gene Coexpression Network and Experimental Analyses Identify lncRNA SPRR2C as a Regulator of the IL-22-Stimulated HaCaT Cell Phenotype Through the miR-330/STAT1/S100A7 Axis. Cell Death Dis (2021) 12(1):86. doi: 10.1038/s41419-020-03305-z
80. Keir M, Yi Y, Lu T, Ghilardi N. The Role of IL-22 in Intestinal Health and Disease. J Exp Med (2020) 217(3):e20192195. doi: 10.1084/jem.20192195
81. Lai Y, Li D, Li C, Muehleisen B, Radek KA, Park HJ, et al. The Antimicrobial Protein REG3A Regulates Keratinocyte Proliferation and Differentiation After Skin Injury. Immunity (2012) 37(1):74–84. doi: 10.1016/j.immuni.2012.04.010
82. Chan LC, Chaili S, Filler SG, Barr K, Wang H, Kupferwasser D, et al. Nonredundant Roles of Interleukin-17a (IL-17A) and IL-22 in Murine Host Defense Against Cutaneous and Hematogenous Infection Due to Methicillin-Resistant Staphylococcus Aureus. Infect Immun (2015) 83(11):4427–37. doi: 10.1128/iai.01061-15
83. Yang L, Fan X, Cui T, Dang E, Wang G. Nrf2 Promotes Keratinocyte Proliferation in Psoriasis Through Up-Regulation of Keratin 6, Keratin 16, and Keratin 17. J Invest Dermatol (2017) 137(10):2168–76. doi: 10.1016/j.jid.2017.05.015
84. Wang B, Han D, Li F, Hou W, Wang L, Meng L, et al. Elevated IL-22 in Psoriasis Plays an Anti-Apoptotic Role in Keratinocytes Through Mediating Bcl-Xl/Bax. Apoptosis (2020) 25(9-10):663–73. doi: 10.1007/s10495-020-01623-3
85. Lerman G, Sharon M, Leibowitz-Amit R, Sidi Y, Avni D. The Crosstalk Between IL-22 Signaling and miR-197 in Human Keratinocytes. PloS One (2014) 9(9):e107467. doi: 10.1371/journal.pone.0107467
86. Jiang M, Ma W, Gao Y, Jia K, Zhang Y, Liu H, et al. IL-22-Induced miR-122-5p Promotes Keratinocyte Proliferation by Targeting Sprouty2. Exp Dermatol (2017) 26(4):368–74. doi: 10.1111/exd.13270
87. Shen H, Zeng B, Wang C, Tang X, Wang H, Liu W, et al. MiR-330 Inhibits IL-22-Induced Keratinocyte Proliferation Through Targeting Ctnnb1. BioMed Pharmacother (2017) 91:803–11. doi: 10.1016/j.biopha.2017.05.005
88. Li R, Qiao M, Zhao X, Yan J, Wang X, Sun Q. MiR-20a-3p Regulates TGF-β1/Survivin Pathway to Affect Keratinocytes Proliferation and Apoptosis by Targeting SFMBT1 In Vitro. Cell Signal (2018) 49:95–104. doi: 10.1016/j.cellsig.2018.06.003
89. Zhao X, Li R, Qiao M, Yan J, Sun Q. MiR-548a-3p Promotes Keratinocyte Proliferation Targeting PPP3R1 After Being Induced by IL-22. Inflammation (2018) 41(2):496–504. doi: 10.1007/s10753-017-0705-3
90. Wang R, Wang FF, Cao HW, Yang JY. MiR-223 Regulates Proliferation and Apoptosis of IL-22-Stimulated HaCat Human Keratinocyte Cell Lines via the PTEN/Akt Pathway. Life Sci (2019) 230:28–34. doi: 10.1016/j.lfs.2019.05.045
91. Li S, Zhu X, Zhang N, Cao R, Zhao L, Li X, et al. LncRNA NORAD Engages in Psoriasis by Binding to miR-26a to Regulate Keratinocyte Proliferation. Autoimmunity (2021) 54(3):129–37. doi: 10.1080/08916934.2021.1897976
92. Liu T, Feng X, Liao Y. miR-617 Promotes the Growth of IL-22-Stimulated Keratinocytes Through Regulating FOXO4 Expression. Biochem Genet (2021) 59(2):547–59. doi: 10.1007/s10528-020-09997-4
93. Sestito R, Madonna S, Scarponi C, Cianfarani F, Failla CM, Cavani A, et al. STAT3-Dependent Effects of IL-22 in Human Keratinocytes are Counterregulated by Sirtuin 1 Through a Direct Inhibition of STAT3 Acetylation. FASEB J (2011) 25(3):916–27. doi: 10.1096/fj.10-172288
94. Mitra A, Raychaudhuri SK, Raychaudhuri SP. IL-22 Induced Cell Proliferation is Regulated by PI3K/Akt/mTOR Signaling Cascade. Cytokine (2012) 60(1):38–42. doi: 10.1016/j.cyto.2012.06.316
95. Zhu X, Li Z, Pan W, Qin L, Zhu G, Ke Y, et al. Participation of Gab1 and Gab2 in IL-22-Mediated Keratinocyte Proliferation, Migration, and Differentiation. Mol Cell Biochem (2012) 369(1-2):255–66. doi: 10.1007/s11010-012-1389-5
96. Liang J, Chen P, Li C, Li D, Wang J, Xue R, et al. IL-22 Down-Regulates Cx43 Expression and Decreases Gap Junctional Intercellular Communication by Activating the JNK Pathway in Psoriasis. J Invest Dermatol (2019) 139(2):400–11. doi: 10.1016/j.jid.2018.07.032
97. Zhuang L, Ma W, Yan J, Zhong H. Evaluation of the Effects of IL−22 on the Proliferation and Differentiation of Keratinocytes In Vitro. Mol Med Rep (2020) 22(4):2715–22. doi: 10.3892/mmr.2020.11348
98. Ekman AK, Bivik Eding C, Rundquist I, Enerbäck C. IL-17 and IL-22 Promote Keratinocyte Stemness in the Germinative Compartment in Psoriasis. J Invest Dermatol (2019) 139(7):1564–1573.e1568. doi: 10.1016/j.jid.2019.01.014
99. Boniface K, Bernard FX, Garcia M, Gurney AL, Lecron JC, Morel F. IL-22 Inhibits Epidermal Differentiation and Induces Proinflammatory Gene Expression and Migration of Human Keratinocytes. J Immunol (2005) 174(6):3695–702. doi: 10.4049/jimmunol.174.6.3695
100. Wolk K, Witte E, Warszawska K, Schulze-Tanzil G, Witte K, Philipp S, et al. The Th17 Cytokine IL-22 Induces IL-20 Production in Keratinocytes: A Novel Immunological Cascade With Potential Relevance in Psoriasis. Eur J Immunol (2009) 39(12):3570–81. doi: 10.1002/eji.200939687
101. Gutowska-Owsiak D, Schaupp AL, Salimi M, Taylor S, Ogg GS. Interleukin-22 Downregulates Filaggrin Expression and Affects Expression of Profilaggrin Processing Enzymes. Br J Dermatol (2011) 165(3):492–8. doi: 10.1111/j.1365-2133.2011.10400.x
102. Zhang W, Dang E, Shi X, Jin L, Feng Z, Hu L, et al. The Pro-Inflammatory Cytokine IL-22 Up-Regulates Keratin 17 Expression in Keratinocytes via STAT3 and ERK1/2. PloS One (2012) 7(7):e40797. doi: 10.1371/journal.pone.0040797
103. Avitabile S, Odorisio T, Madonna S, Eyerich S, Guerra L, Eyerich K, et al. Interleukin-22 Promotes Wound Repair in Diabetes by Improving Keratinocyte Pro-Healing Functions. J Invest Dermatol (2015) 135(11):2862–70. doi: 10.1038/jid.2015.278
104. Padhi A, Srivastava A, Ramesh A, Ehrström M, Simon M, Sonkoly E, et al. IL-22 Downregulates Peptidylarginine Deiminase-1 in Human Keratinocytes: Adding Another Piece to the IL-22 Puzzle in Epidermal Barrier Formation. J Invest Dermatol (2022) 142(2):333–+. doi: 10.1016/j.jid.2021.07.155
105. Huang SM, Wu CS, Chao D, Wu CH, Li CC, Chen GS, et al. High-Glucose-Cultivated Peripheral Blood Mononuclear Cells Impaired Keratinocyte Function via Reduced IL-22 Expression: Implications on Impaired Diabetic Wound Healing. Exp Dermatol (2015) 24(8):639–41. doi: 10.1111/exd.12733
106. Sasaki JR, Zhang Q, Schwacha MG. Burn Induces a Th-17 Inflammatory Response at the Injury Site. Burns (2011) 37(4):646–51. doi: 10.1016/j.burns.2011.01.028
107. Kolumam G, Wu X, Lee WP, Hackney JA, Zavala-Solorio J, Gandham V, et al. IL-22r Ligands IL-20, IL-22, and IL-24 Promote Wound Healing in Diabetic Db/Db Mice. PloS One (2017) 12(1):e0170639. doi: 10.1371/journal.pone.0170639
108. Sawamura S, Jinnin M, Inoue K, Yamane K, Honda N, Kajihara I, et al. Regulatory Mechanisms of Collagen Expression by Interleukin-22 Signaling in Scleroderma Fibroblasts. J Dermatol Sci (2018) 90(1):52–9. doi: 10.1016/j.jdermsci.2017.12.017
109. Brembilla NC, Dufour AM, Alvarez M, Hugues S, Montanari E, Truchetet ME, et al. IL-22 Capacitates Dermal Fibroblast Responses to TNF in Scleroderma. Ann Rheum Dis (2016) 75(9):1697–705. doi: 10.1136/annrheumdis-2015-207477
110. Li Z, Lamb R, Coles MC, Bennett CL, Ambler CA. Inducible Ablation of CD11c(+) Cells to Determine Their Role in Skin Wound Repair. Immunology (2021) 163(1):105–11. doi: 10.1111/imm.13312
111. Bachmann M, Ulziibat S, Härdle L, Pfeilschifter J, Mühl H. Ifnα Converts IL-22 Into a Cytokine Efficiently Activating STAT1 and its Downstream Targets. Biochem Pharmacol (2013) 85(3):396–403. doi: 10.1016/j.bcp.2012.11.004
112. Carrier Y, Ma HL, Ramon HE, Napierata L, Small C, O'Toole M, et al. Inter-Regulation of Th17 Cytokines and the IL-36 Cytokines In Vitro and In Vivo: Implications in Psoriasis Pathogenesis. J Invest Dermatol (2011) 131(12):2428–37. doi: 10.1038/jid.2011.234
113. Cho KA, Kim JY, Woo SY, Park HJ, Lee KH, Pae CU. Interleukin-17 and Interleukin-22 Induced Proinflammatory Cytokine Production in Keratinocytes via Inhibitor of Nuclear Factor κb Kinase-α Expression. Ann Dermatol (2012) 24(4):398–405. doi: 10.5021/ad.2012.24.4.398
114. Cho KA, Suh JW, Lee KH, Kang JL, Woo SY. IL-17 and IL-22 Enhance Skin Inflammation by Stimulating the Secretion of IL-1β by Keratinocytes via the ROS-NLRP3-Caspase-1 Pathway. Int Immunol (2012) 24(3):147–58. doi: 10.1093/intimm/dxr110
115. Tohyama M, Yang L, Hanakawa Y, Dai X, Shirakata Y, Sayama K. IFN-Alpha Enhances IL-22 Receptor Expression in Keratinocytes: A Possible Role in the Development of Psoriasis. J Invest Dermatol (2012) 132(7):1933–5. doi: 10.1038/jid.2011.468
116. Alabbas SY, Begun J, Florin TH, Oancea I. The Role of IL-22 in the Resolution of Sterile and Nonsterile Inflammation. Clin Transl Immunol (2018) 7(4):e1017. doi: 10.1002/cti2.1017
117. Wolk K, Haugen HS, Xu W, Witte E, Waggie K, Anderson M, et al. IL-22 and IL-20 are Key Mediators of the Epidermal Alterations in Psoriasis While IL-17 and IFN-Gamma are Not. J Mol Med (Berl) (2009) 87(5):523–36. doi: 10.1007/s00109-009-0457-0
118. Rizzo HL, Kagami S, Phillips KG, Kurtz SE, Jacques SL, Blauvelt A. IL-23-Mediated Psoriasis-Like Epidermal Hyperplasia is Dependent on IL-17a. J Immunol (2011) 186(3):1495–502. doi: 10.4049/jimmunol.1001001
119. Van Belle AB, de Heusch M, Lemaire MM, Hendrickx E, Warnier G, Dunussi-Joannopoulos K, et al. IL-22 is Required for Imiquimod-Induced Psoriasiform Skin Inflammation in Mice. J Immunol (2012) 188(1):462–9. doi: 10.4049/jimmunol.1102224
120. Ma HL, Liang S, Li J, Napierata L, Brown T, Benoit S, et al. IL-22 is Required for Th17 Cell-Mediated Pathology in a Mouse Model of Psoriasis-Like Skin Inflammation. J Clin Invest (2008) 118(2):597–607. doi: 10.1172/jci33263
121. Martin JC, Wolk K, Bériou G, Abidi A, Witte-Händel E, Louvet C, et al. Limited Presence of IL-22 Binding Protein, a Natural IL-22 Inhibitor, Strengthens Psoriatic Skin Inflammation. J Immunol (2017) 198(9):3671–8. doi: 10.4049/jimmunol.1700021
122. Voglis S, Moos S, Kloos L, Wanke F, Zayoud M, Pelczar P, et al. Regulation of IL-22BP in Psoriasis. Sci Rep (2018) 8(5085):1–5. doi: 10.1038/s41598-018-23510-3
123. Fukaya T, Fukui T, Uto T, Takagi H, Nasu J, Miyanaga N, et al. Pivotal Role of IL-22 Binding Protein in the Epithelial Autoregulation of Interleukin-22 Signaling in the Control of Skin Inflammation. Front Immunol (2018) 9:1418. doi: 10.3389/fimmu.2018.01418
124. Lindroos J, Svensson L, Norsgaard H, Lovato P, Moller K, Hagedorn PH, et al. IL-23-Mediated Epidermal Hyperplasia is Dependent on IL-6. J Invest Dermatol (2011) 131(5):1110–8. doi: 10.1038/jid.2010.432
125. Chen L, Deshpande M, Grisotto M, Smaldini P, Garcia R, He Z, et al. Skin Expression of IL-23 Drives the Development of Psoriasis and Psoriatic Arthritis in Mice. Sci Rep (2020) 10(1):8259:1–11. doi: 10.1038/s41598-020-65269-6
126. Hedrick MN, Lonsdorf AS, Shirakawa AK, Richard Lee CC, Liao F, Singh SP, et al. CCR6 is Required for IL-23-Induced Psoriasis-Like Inflammation in Mice. J Clin Invest (2009) 119(8):2317–29. doi: 10.1172/jci37378
127. Tortola L, Rosenwald E, Abel B, Blumberg H, Schäfer M, Coyle AJ, et al. Psoriasiform Dermatitis is Driven by IL-36-Mediated DC-Keratinocyte Crosstalk. J Clin Invest (2012) 122(11):3965–76. doi: 10.1172/jci63451
128. Le ST, Merleev AA, Luxardi G, Shimoda M, Adamopoulos IE, Tsoi LC, et al. 2d Visualization of the Psoriasis Transcriptome Fails to Support the Existence of Dual-Secreting IL-17a/IL-22 Th17 T Cells. Front Immunol (2019) 10:589. doi: 10.3389/fimmu.2019.00589
129. Nograles KE, Zaba LC, Shemer A, Fuentes-Duculan J, Cardinale I, Kikuchi T, et al. IL-22-Producing "T22" T Cells Account for Upregulated IL-22 in Atopic Dermatitis Despite Reduced IL-17-Producing TH17 T Cells. J Allergy Clin Immunol (2009) 123(6):1244–52.e1242. doi: 10.1016/j.jaci.2009.03.041
130. Nakahara T, Kido-Nakahara M, Ohno F, Ulzii D, Chiba T, Tsuji G, et al. The Pruritogenic Mediator Endothelin-1 Shifts the Dendritic Cell-T-Cell Response Toward Th17/Th1 Polarization. Allergy (2018) 73(2):511–5. doi: 10.1111/all.13322
131. Nakagawa R, Yoshida H, Asakawa M, Tamiya T, Inoue N, Morita R, et al. Pyridone 6, a Pan-JAK Inhibitor, Ameliorates Allergic Skin Inflammation of NC/Nga Mice via Suppression of Th2 and Enhancement of Th17. J Immunol (2011) 187(9):4611–20. doi: 10.4049/jimmunol.1100649
132. Jang M, Kim H, Kim Y, Choi J, Jeon J, Hwang Y, et al. The Crucial Role of IL-22 and its Receptor in Thymus and Activation Regulated Chemokine Production and T-Cell Migration by House Dust Mite Extract. Exp Dermatol (2016) 25(8):598–603. doi: 10.1111/exd.12988
133. Lindahl H, Martini E, Brauner S, Nikamo P, Gallais Serezal I, Guerreiro-Cacais AO, et al. IL-22 Binding Protein Regulates Murine Skin Inflammation. Exp Dermatol (2017) 26(5):444–6. doi: 10.1111/exd.13225
134. Lou H, Lu J, Choi EB, Oh MH, Jeong M, Barmettler S, et al. Expression of IL-22 in the Skin Causes Th2-Biased Immunity, Epidermal Barrier Dysfunction, and Pruritus via Stimulating Epithelial Th2 Cytokines and the GRP Pathway. J Immunol (2017) 198(7):2543–55. doi: 10.4049/jimmunol.1600126
135. Olivry T, Mayhew D, Paps JS, Linder KE, Peredo C, Rajpal D, et al. Early Activation of Th2/Th22 Inflammatory and Pruritogenic Pathways in Acute Canine Atopic Dermatitis Skin Lesions. J Invest Dermatol (2016) 136(10):1961–9. doi: 10.1016/j.jid.2016.05.117
136. Glocova I, Brück J, Geisel J, Müller-Hermelink E, Widmaier K, Yazdi AS, et al. Induction of Skin-Pathogenic Th22 Cells by Epicutaneous Allergen Exposure. J Dermatol Sci (2017) 87(3):268–77. doi: 10.1016/j.jdermsci.2017.06.006
137. Leyva-Castillo JM, Yoon J, Geha RS. IL-22 Promotes Allergic Airway Inflammation in Epicutaneously Sensitized Mice. J Allergy Clin Immunol (2019) 143(2):619–630.e617. doi: 10.1016/j.jaci.2018.05.032
138. Duffin R, O'Connor RA, Crittenden S, Forster T, Yu C, Zheng X, et al. Prostaglandin E(2) Constrains Systemic Inflammation Through an Innate Lymphoid Cell-IL-22 Axis. Science (2016) 351(6279):1333–8. doi: 10.1126/science.aad9903
139. Pantelyushin S, Haak S, Ingold B, Kulig P, Heppner FL, Navarini AA, et al. Rorγt+ Innate Lymphocytes and γδ T Cells Initiate Psoriasiform Plaque Formation in Mice. J Clin Invest (2012) 122(6):2252–6. doi: 10.1172/jci61862
140. Zanvit P, Konkel JE, Jiao X, Kasagi S, Zhang D, Wu R, et al. Antibiotics in Neonatal Life Increase Murine Susceptibility to Experimental Psoriasis. Nat Commun (2015) 6:8424. doi: 10.1038/ncomms9424
141. Cibrian D, Saiz ML, de la Fuente H, Sánchez-Díaz R, Moreno-Gonzalo O, Jorge I, et al. CD69 Controls the Uptake of L-Tryptophan Through LAT1-CD98 and AhR-Dependent Secretion of IL-22 in Psoriasis. Nat Immunol (2016) 17(8):985–96. doi: 10.1038/ni.3504
142. Cui G. T(H)9, T(H)17, and T(H)22 Cell Subsets and Their Main Cytokine Products in the Pathogenesis of Colorectal Cancer. Front Oncol (2019) 9:1002. doi: 10.3389/fonc.2019.01002
143. Imperiale BR, Garcia A, Minotti A, Gonzalez Montaner P, Moracho L, Morcillo NS, et al. Th22 Response Induced by Mycobacterium Tuberculosis Strains is Closely Related to Severity of Pulmonary Lesions and Bacillary Load in Patients With Multi-Drug-Resistant Tuberculosis. Clin Exp Immunol (2021) 203(2):267–80. doi: 10.1111/cei.13544
144. Armstrong AW, Read C. Pathophysiology, Clinical Presentation, and Treatment of Psoriasis: A Review. JAMA (2020) 323(19):1945–60. doi: 10.1001/jama.2020.4006
145. Kagami S, Rizzo HL, Lee JJ, Koguchi Y, Blauvelt A. Circulating Th17, Th22, and Th1 Cells are Increased in Psoriasis. J Invest Dermatol (2010) 130(5):1373–83. doi: 10.1038/jid.2009.399
146. Michalak-Stoma A, Bartosińska J, Kowal M, Juszkiewicz-Borowiec M, Gerkowicz A, Chodorowska G. Serum Levels of Selected Th17 and Th22 Cytokines in Psoriatic Patients. Dis Markers (2013) 35(6):625–31. doi: 10.1155/2013/856056
147. Luan L, Ding Y, Han S, Zhang Z, Liu X. An Increased Proportion of Circulating Th22 and Tc22 Cells in Psoriasis. Cell Immunol (2014) 290(2):196–200. doi: 10.1016/j.cellimm.2014.06.007
148. Sokolova MV, Simon D, Nas K, Zaiss MM, Luo Y, Zhao Y, et al. A Set of Serum Markers Detecting Systemic Inflammation in Psoriatic Skin, Entheseal, and Joint Disease in the Absence of C-Reactive Protein and its Link to Clinical Disease Manifestations. Arthritis Res Ther (2020) 22(1):26. doi: 10.1186/s13075-020-2111-8
149. Schabitz A, Eyerich K, Garzorz-Stark N. So Close, and Yet So Far Away: The Dichotomy of the Specific Immune Response and Inflammation in Psoriasis and Atopic Dermatitis. J Intern Med (2021) 290(1):27–39. doi: 10.1111/joim.13235
150. Borowczyk J, Buerger C, Tadjrischi N, Drukala J, Wolnicki M, Wnuk D, et al. IL-17e (IL-25) and IL-17a Differentially Affect the Functions of Human Keratinocytes. J Invest Dermatol (2020) 140(7):1379–89.e1372. doi: 10.1016/j.jid.2019.12.013
151. Prans E, Kingo K, Traks T, Silm H, Vasar E, Kõks S. Copy Number Variations in IL22 Gene are Associated With Psoriasis Vulgaris. Hum Immunol (2013) 74(6):792–5. doi: 10.1016/j.humimm.2013.01.006
152. Zhou F, Shen C, Hsu YH, Gao J, Dou J, Ko R, et al. DNA Methylation-Based Subclassification of Psoriasis in the Chinese Han Population. Front Med (2018) 12(6):717–25. doi: 10.1007/s11684-017-0588-6
153. Pollock RA, Zaman L, Chandran V, Gladman DD. Epigenome-Wide Analysis of Sperm Cells Identifies IL22 as a Possible Germ Line Risk Locus for Psoriatic Arthritis. PloS One (2019) 14(2):e0212043. doi: 10.1371/journal.pone.0212043
154. Nikamo P, Cheuk S, Lysell J, Enerbäck C, Bergh K, Xu Landén N, et al. Genetic Variants of the IL22 Promoter Associate to Onset of Psoriasis Before Puberty and Increased IL-22 Production in T Cells. J Invest Dermatol (2014) 134(6):1535–41. doi: 10.1038/jid.2014.5
155. Cordoro KM, Hitraya-Low M, Taravati K, Sandoval PM, Kim E, Sugarman J, et al. Skin-Infiltrating, Interleukin-22-Producing T Cells Differentiate Pediatric Psoriasis From Adult Psoriasis. J Am Acad Dermatol (2017) 77(3):417–24. doi: 10.1016/j.jaad.2017.05.017
156. Benham H, Norris P, Goodall J, Wechalekar MD, FitzGerald O, Szentpetery A, et al. Th17 and Th22 Cells in Psoriatic Arthritis and Psoriasis. Arthritis Res Ther (2013) 15(5):R136. doi: 10.1186/ar4317
157. Ciccia F, Guggino G, Ferrante A, Raimondo S, Bignone R, Rodolico V, et al. Interleukin-9 Overexpression and Th9 Polarization Characterize the Inflamed Gut, the Synovial Tissue, and the Peripheral Blood of Patients With Psoriatic Arthritis. Arthritis Rheumatol (2016) 68(8):1922–31. doi: 10.1002/art.39649
158. Ahn R, Yan D, Chang HW, Lee K, Bhattarai S, Huang ZM, et al. RNA-Seq and Flow-Cytometry of Conventional, Scalp, and Palmoplantar Psoriasis Reveal Shared and Distinct Molecular Pathways. Sci Rep (2018) 8(1):11368. doi: 10.1038/s41598-018-29472-w
159. Ruano J, Suárez-Fariñas M, Shemer A, Oliva M, Guttman-Yassky E, Krueger JG. Molecular and Cellular Profiling of Scalp Psoriasis Reveals Differences and Similarities Compared to Skin Psoriasis. PloS One (2016) 11(2):e0148450. doi: 10.1371/journal.pone.0148450
160. Langan SM, Irvine AD, Weidinger S. Atopic Dermatitis. Lancet (2020) 396(10247):345–60. doi: 10.1016/S0140-6736(20)31286-1
161. Suarez-Farinas M, Tintle SJ, Shemer A, Chiricozzi A, Nograles K, Cardinale I, et al. Nonlesional Atopic Dermatitis Skin is Characterized by Broad Terminal Differentiation Defects and Variable Immune Abnormalities. J Allergy Clin Immunol (2011) 127(4):e951954–64. doi: 10.1016/j.jaci.2010.12.1124
162. Czarnowicki T, Gonzalez J, Shemer A, Malajian D, Xu H, Zheng X, et al. Severe Atopic Dermatitis is Characterized by Selective Expansion of Circulating TH2/TC2 and TH22/TC22, But Not TH17/TC17, Cells Within the Skin-Homing T-Cell Population. J Allergy Clin Immunol (2015) 136(1):104–115.e107. doi: 10.1016/j.jaci.2015.01.020
163. Esaki H, Ewald DA, Ungar B, Rozenblit M, Zheng X, Xu H, et al. Identification of Novel Immune and Barrier Genes in Atopic Dermatitis by Means of Laser Capture Microdissection. J Allergy Clin Immunol (2015) 135(1):153–63. doi: 10.1016/j.jaci.2014.10.037
164. Ungar B, Garcet S, Gonzalez J, Dhingra N, Correa da Rosa J, Shemer A, et al. An Integrated Model of Atopic Dermatitis Biomarkers Highlights the Systemic Nature of the Disease. J Invest Dermatol (2017) 137(3):603–13. doi: 10.1016/j.jid.2016.09.037
165. He H, Del Duca E, Diaz A, Kim HJ, Gay-Mimbrera J, Zhang N, et al. Mild Atopic Dermatitis Lacks Systemic Inflammation and Shows Reduced Nonlesional Skin Abnormalities. J Allergy Clin Immunol (2021) 147(4):1369–80. doi: 10.1016/j.jaci.2020.08.041
166. Fujita H, Shemer A, Suárez-Fariñas M, Johnson-Huang LM, Tintle S, Cardinale I, et al. Lesional Dendritic Cells in Patients With Chronic Atopic Dermatitis and Psoriasis Exhibit Parallel Ability to Activate T-Cell Subsets. J Allergy Clin Immunol (2011) 128(3):574–82.e571-512. doi: 10.1016/j.jaci.2011.05.016
167. Guttman-Yassky E, Nograles KE, Krueger JG. Contrasting Pathogenesis of Atopic Dermatitis and Psoriasis–Part II: Immune Cell Subsets and Therapeutic Concepts. J Allergy Clin Immunol (2011) 127(6):1420–32. doi: 10.1016/j.jaci.2011.01.054
168. Souwer Y, Szegedi K, Kapsenberg ML, de Jong EC. IL-17 and IL-22 in Atopic Allergic Disease. Curr Opin Immunol (2010) 22(6):821–6. doi: 10.1016/j.coi.2010.10.013
169. Suárez-Fariñas M, Dhingra N, Gittler J, Shemer A, Cardinale I, de Guzman Strong C, et al. Intrinsic Atopic Dermatitis Shows Similar TH2 and Higher TH17 Immune Activation Compared With Extrinsic Atopic Dermatitis. J Allergy Clin Immunol (2013) 132(2):361–70. doi: 10.1016/j.jaci.2013.04.046
170. Bakker DS, Nierkens S, Knol EF, Giovannone B, Delemarre EM, van der Schaft J, et al. Confirmation of Multiple Endotypes in Atopic Dermatitis Based on Serum Biomarkers. J Allergy Clin Immunol (2021) 147(1):189–98. doi: 10.1016/j.jaci.2020.04.062
171. Gittler JK, Shemer A, Suárez-Fariñas M, Fuentes-Duculan J, Gulewicz KJ, Wang CQ, et al. Progressive Activation of T(H)2/T(H)22 Cytokines and Selective Epidermal Proteins Characterizes Acute and Chronic Atopic Dermatitis. J Allergy Clin Immunol (2012) 130(6):1344–54. doi: 10.1016/j.jaci.2012.07.012
172. Sanyal RD, Pavel AB, Glickman J, Chan TC, Zheng X, Zhang N, et al. Atopic Dermatitis in African American Patients is T(H)2/T(H)22-Skewed With T(H)1/T(H)17 Attenuation. Ann Allergy Asthma Immunol (2019) 122(1):99–110.e116. doi: 10.1016/j.anai.2018.08.024
173. Lang CCV, Renert-Yuval Y, Del Duca E, Pavel AB, Wu J, Zhang N, et al. Immune and Barrier Characterization of Atopic Dermatitis Skin Phenotype in Tanzanian Patients. Ann Allergy Asthma Immunol (2021) 127(3):334–41. doi: 10.1016/j.anai.2021.04.023
174. Noda S, Suárez-Fariñas M, Ungar B, Kim SJ, de Guzman Strong C, Xu H, et al. The Asian Atopic Dermatitis Phenotype Combines Features of Atopic Dermatitis and Psoriasis With Increased TH17 Polarization. J Allergy Clin Immunol (2015) 136(5):1254–64. doi: 10.1016/j.jaci.2015.08.015
175. Wen HC, Czarnowicki T, Noda S, Malik K, Pavel AB, Nakajima S, et al. Serum From Asian Patients With Atopic Dermatitis is Characterized by TH2/TH22 Activation, Which is Highly Correlated With Nonlesional Skin Measures. J Allergy Clin Immunol (2018) 142(1):324–8.e311. doi: 10.1016/j.jaci.2018.02.047
176. Renert-Yuval Y, Del Duca E, Pavel AB, Fang M, Lefferdink R, Wu J, et al. The Molecular Features of Normal and Atopic Dermatitis Skin in Infants, Children, Adolescents, and Adults. J Allergy Clin Immunol (2021) 148(1):148–63. doi: 10.1016/j.jaci.2021.01.001
177. Czarnowicki T, Esaki H, Gonzalez J, Malajian D, Shemer A, Noda S, et al. Early Pediatric Atopic Dermatitis Shows Only a Cutaneous Lymphocyte Antigen (CLA)(+) TH2/TH1 Cell Imbalance, Whereas Adults Acquire CLA(+) TH22/TC22 Cell Subsets. J Allergy Clin Immunol (2015) 136(4):941–51.e943. doi: 10.1016/j.jaci.2015.05.049
178. Czarnowicki T, He H, Canter T, Han J, Lefferdink R, Erickson T, et al. Evolution of Pathologic T-Cell Subsets in Patients With Atopic Dermatitis From Infancy to Adulthood. J Allergy Clin Immunol (2020) 145(1):215–28. doi: 10.1016/j.jaci.2019.09.031
179. Zhou L, Leonard A, Pavel AB, Malik K, Raja A, Glickman J, et al. Age-Specific Changes in the Molecular Phenotype of Patients With Moderate-To-Severe Atopic Dermatitis. J Allergy Clin Immunol (2019) 144(1):144–56. doi: 10.1016/j.jaci.2019.01.015
180. Wang S, Zhu R, Gu C, Zou Y, Yin H, Xu J, et al. Distinct Clinical Features and Serum Cytokine Pattern of Elderly Atopic Dermatitis in China. J Eur Acad Dermatol Venereol (2020) 34(10):2346–52. doi: 10.1111/jdv.16346
181. Looman KIM, van Mierlo MMF, van Zelm MC, Hu C, Duijts L, de Jongste JC, et al. Increased Th22 Cell Numbers in a General Pediatric Population With Filaggrin Haploinsufficiency: The Generation R Study. Pediatr Allergy Immunol (2021) 32(6):1360–68. doi: 10.1111/pai.13502
182. Rojahn TB, Vorstandlechner V, Krausgruber T, Bauer WM, Alkon N, Bangert C, et al. Single-Cell Transcriptomics Combined With Interstitial Fluid Proteomics Defines Cell Type-Specific Immune Regulation in Atopic Dermatitis. J Allergy Clin Immunol (2020) 146(5):1056–69. doi: 10.1016/j.jaci.2020.03.041
183. Lyubchenko T, Collins HK, Goleva E, Leung DYM. Skin Tape Sampling Technique Identifies Proinflammatory Cytokines in Atopic Dermatitis Skin. Ann Allergy Asthma Immunol (2021) 126(1):46–53.e42. doi: 10.1016/j.anai.2020.08.397
184. Pavel AB, Renert-Yuval Y, Wu J, Del Duca E, Diaz A, Lefferdink R, et al. Tape Strips From Early-Onset Pediatric Atopic Dermatitis Highlight Disease Abnormalities in Nonlesional Skin. Allergy (2021) 76(1):314–25. doi: 10.1111/all.14490
185. Szegedi K, Lutter R, Res PC, Bos JD, Luiten RM, Kezic S, et al. Cytokine Profiles in Interstitial Fluid From Chronic Atopic Dermatitis Skin. J Eur Acad Dermatol Venereol (2015) 29(11):2136–44. doi: 10.1111/jdv.13160
186. Dorner T, Furie R. Novel Paradigms in Systemic Lupus Erythematosus. Lancet (2019) 393(10188):2344–58. doi: 10.1016/S0140-6736(19)30546-X
187. Wenzel J. Cutaneous Lupus Erythematosus: New Insights Into Pathogenesis and Therapeutic Strategies. Nat Rev Rheumatol (2019) 15(9):519–32. doi: 10.1038/s41584-019-0272-0
188. Anders HJ, Saxena R, Zhao MH, Parodis I, Salmon JE, Mohan C. Lupus Nephritis. Nat Rev Dis Primers (2020) 6(1):7. doi: 10.1038/s41572-019-0141-9
189. Cheng F, Guo Z, Xu H, Yan D, Li Q. Decreased Plasma IL22 Levels, But Not Increased IL17 and IL23 Levels, Correlate With Disease Activity in Patients With Systemic Lupus Erythematosus. Ann Rheum Dis (2009) 68(4):604–6. doi: 10.1136/ard.2008.097089
190. Lin J, Yue LH, Chen WQ. Decreased Plasma IL-22 Levels and Correlations With IL-22-Producing T Helper Cells in Patients With New-Onset Systemic Lupus Erythematosus. Scand J Immunol (2014) 79(2):131–6. doi: 10.1111/sji.12135
191. Zhao L, Jiang Z, Jiang Y, Ma N, Wang K, Zhang Y, et al. IL-22+CD4+ T-Cells in Patients With Active Systemic Lupus Erythematosus. Exp Biol Med (Maywood) (2013) 238(2):193–9. doi: 10.1177/1535370213477597
192. Zhao L, Ma H, Jiang Z, Jiang Y, Ma N. Immunoregulation Therapy Changes the Frequency of Interleukin (IL)-22+ CD4+ T Cells in Systemic Lupus Erythematosus Patients. Clin Exp Immunol (2014) 177(1):212–8. doi: 10.1111/cei.12330
193. Yu B, Guan M, Peng Y, Shao Y, Zhang C, Yue X, et al. Copy Number Variations of Interleukin-17f, Interleukin-21, and Interleukin-22 are Associated With Systemic Lupus Erythematosus. Arthritis Rheum (2011) 63(11):3487–92. doi: 10.1002/art.30595
194. Wang R, Zeng YL, Qin HM, Lu YL, Huang HT, Lei M, et al. Association of Interleukin 22 Gene Polymorphisms and Serum IL-22 Level With Risk of Systemic Lupus Erythematosus in a Chinese Population. Clin Exp Immunol (2018) 193(2):143–51. doi: 10.1111/cei.13133
195. Méndez-Flores S, Hernández-Molina G, Enríquez AB, Faz-Muñoz D, Esquivel Y, Pacheco-Molina C, et al. Cytokines and Effector/Regulatory Cells Characterization in the Physiopathology of Cutaneous Lupus Erythematous: A Cross-Sectional Study. Mediators Inflammation (2016) 2016:7074829. doi: 10.1155/2016/7074829
196. Méndez-Flores S, Hernández-Molina G, Azamar-Llamas D, Zúñiga J, Romero-Díaz J, Furuzawa-Carballeda J. Inflammatory Chemokine Profiles and Their Correlations With Effector CD4 T Cell and Regulatory Cell Subpopulations in Cutaneous Lupus Erythematosus. Cytokine (2019) 119:95–112. doi: 10.1016/j.cyto.2019.03.010
197. Yang XY, Wang HY, Zhao XY, Wang LJ, Lv QH, Wang QQ. Th22, But Not Th17 Might be a Good Index to Predict the Tissue Involvement of Systemic Lupus Erythematosus. J Clin Immunol (2013) 33(4):767–74. doi: 10.1007/s10875-013-9878-1
198. Yang X, Weng Q, Hu L, Yang L, Wang X, Xiang X, et al. Increased Interleukin-22 Levels in Lupus Nephritis and its Associated With Disease Severity: A Study in Both Patients and Lupus-Like Mice Model. Clin Exp Rheumatol (2019) 37(3):400–7.
199. Zhong W, Jiang Y, Ma H, Wu J, Jiang Z, Zhao L. Elevated Levels of CCR6(+) T Helper 22 Cells Correlate With Skin and Renal Impairment in Systemic Lupus Erythematosus. Sci Rep (2017) 7(1):12962. doi: 10.1038/s41598-017-13344-w
200. Luk CC, Tam LS, Kwan BC, Wong PC, Ma TK, Chow KM, et al. Intrarenal and Urinary Th9 and Th22 Cytokine Gene Expression in Lupus Nephritis. J Rheumatol (2015) 42(7):1150–5. doi: 10.3899/jrheum.140954
201. Truchetet ME, Brembilla NC, Montanari E, Allanore Y, Chizzolini C. Increased Frequency of Circulating Th22 in Addition to Th17 and Th2 Lymphocytes in Systemic Sclerosis: Association With Interstitial Lung Disease. Arthritis Res Ther (2011) 13(5):R166. doi: 10.1186/ar3486
202. Mathian A, Parizot C, Dorgham K, Trad S, Arnaud L, Larsen M, et al. Activated and Resting Regulatory T Cell Exhaustion Concurs With High Levels of Interleukin-22 Expression in Systemic Sclerosis Lesions. Ann Rheum Dis (2012) 71(7):1227–34. doi: 10.1136/annrheumdis-2011-200709
203. da Cunha Colombo Tiveron LR, da Silva IR, da Silva MV, Peixoto AB, Rodrigues DBR, Rodrigues V Jr. High in Situ mRNA Levels of IL-22, TFG-β, and ARG-1 in Keloid Scars. Immunobiology (2018) 223(12):812–7. doi: 10.1016/j.imbio.2018.08.010
204. Wu J, Del Duca E, Espino M, Gontzes A, Cueto I, Zhang N, et al. RNA Sequencing Keloid Transcriptome Associates Keloids With Th2, Th1, Th17/Th22, and JAK3-Skewing. Front Immunol (2020) 11:597741. doi: 10.3389/fimmu.2020.597741
205. Pratt CH, King LE Jr., Messenger AG, Christiano AM, Sundberg JP. Alopecia Areata. Nat Rev Dis Primers (2017) 3:17011. doi: 10.1038/nrdp.2017.11
206. Loh SH, Moon HN, Lew BL, Sim WY. Role of T Helper 17 Cells and T Regulatory Cells in Alopecia Areata: Comparison of Lesion and Serum Cytokine Between Controls and Patients. J Eur Acad Dermatol Venereol (2018) 32(6):1028–33. doi: 10.1111/jdv.14775
207. Czarnowicki T, He HY, Wen HC, Hashim PW, Nia JK, Malik K, et al. Alopecia Areata is Characterized by Expansion of Circulating Th2/Tc2/Th22, Within the Skin-Homing and Systemic T-Cell Populations. Allergy (2018) 73(3):713–23. doi: 10.1111/all.13346
208. Suarez-Farinas M, Ungar B, Noda S, Shroff A, Mansouri Y, Fuentes-Duculan J, et al. Alopecia Areata Profiling Shows TH1, TH2, and IL-23 Cytokine Activation Without Parallel TH17/TH22 Skewing. J Allergy Clin Immunol (2015) 136(5):1277–87. doi: 10.1016/j.jaci.2015.06.032
209. Aktas Cetin E, Cosan F, Cefle A, Deniz G. IL-22-Secreting Th22 and IFN-γ-Secreting Th17 Cells in Behçet's Disease. Mod Rheumatol (2014) 24(5):802–7. doi: 10.3109/14397595.2013.879414
210. Sabat R, Jemec GBE, Matusiak L, Kimball AB, Prens E, Wolk K. Hidradenitis Suppurativa. Nat Rev Dis Primers (2020) 6(1):18. doi: 10.1038/s41572-020-0149-1
211. Wolk K, Warszawska K, Hoeflich C, Witte E, Schneider-Burrus S, Witte K, et al. Deficiency of IL-22 Contributes to a Chronic Inflammatory Disease: Pathogenetic Mechanisms in Acne Inversa. J Immunol (2011) 186(2):1228–39. doi: 10.4049/jimmunol.0903907
212. Jones D, Banerjee A, Berger PZ, Gross A, McNish S, Amdur R, et al. Inherent Differences in Keratinocyte Function in Hidradenitis Suppurativa: Evidence for the Role of IL-22 in Disease Pathogenesis. Immunol Invest (2018) 47(1):57–70. doi: 10.1080/08820139.2017.1377227
213. Belzberg M, Alphonse MP, Brown I, Williams KA, Khanna R, Ho B, et al. Prurigo Nodularis Is Characterized by Systemic and Cutaneous T Helper 22 Immune Polarization. J Invest Dermatol (2021) 141(9):2208–2218.e2214. doi: 10.1016/j.jid.2021.02.749
214. Lamarthee B, Malard F, Saas P, Mohty M, Gaugler B. Interleukin-22 in Graft-Versus-Host Disease After Allogeneic Stem Cell Transplantation. Front Immunol (2016) 7:148. doi: 10.3389/fimmu.2016.00148
215. Zeiser R, Blazar BR. Pathophysiology of Chronic Graft-Versus-Host Disease and Therapeutic Targets. N Engl J Med (2017) 377(26):2565–79. doi: 10.1056/NEJMra1703472
216. Gartlan KH, Bommiasamy H, Paz K, Wilkinson AN, Owen M, Reichenbach DK, et al. A Critical Role for Donor-Derived IL-22 in Cutaneous Chronic GVHD. Am J Transplant (2018) 18(4):810–20. doi: 10.1111/ajt.14513
217. Pan B, Zhang F, Lu Z, Li L, Shang L, Xia F, et al. Donor T-Cell-Derived Interleukin-22 Promotes Thymus Regeneration and Alleviates Chronic Graft-Versus-Host Disease in Murine Allogeneic Hematopoietic Cell Transplant. Int Immunopharmacol (2019) 67:194–201. doi: 10.1016/j.intimp.2018.12.023
218. Ghoreschi K, Balato A, Enerback C, Sabat R. Therapeutics Targeting the IL-23 and IL-17 Pathway in Psoriasis. Lancet (2021) 397(10275):754–66. doi: 10.1016/S0140-6736(21)00184-7
219. Griffiths CEM, Armstrong AW, Gudjonsson JE, Barker J. Psoriasis. Lancet (2021) 397(10281):1301–15. doi: 10.1016/S0140-6736(20)32549-6
220. Reich K, Rich P, Maari C, Bissonnette R, Leonardi C, Menter A, et al. Efficacy and Safety of Mirikizumab (LY3074828) in the Treatment of Moderate-To-Severe Plaque Psoriasis: Results From a Randomized Phase II Study. Br J Dermatol (2019) 181(1):88–95. doi: 10.1111/bjd.17628
221. Blauvelt A, Papp KA, Merola JF, Gottlieb AB, Cross N, Madden C, et al. Bimekizumab for Patients With Moderate to Severe Plaque Psoriasis: 60-Week Results From BE ABLE 2, a Randomized, Double-Blinded, Placebo-Controlled, Phase 2b Extension Study. J Am Acad Dermatol (2020) 83(5):1367–74. doi: 10.1016/j.jaad.2020.05.105
222. Bellinato F, Gisondi P, Girolomoni G. Latest Advances for the Treatment of Chronic Plaque Psoriasis With Biologics and Oral Small Molecules. Biologics (2021) 15:247–53. doi: 10.2147/BTT.S290309
223. Thyssen JP, Thomsen SF. Treatment of Atopic Dermatitis With Biologics and Janus Kinase Inhibitors. Lancet (2021) 397(10290):2126–8. doi: 10.1016/S0140-6736(21)00717-0
224. Reich K, Armstrong AW, Langley RG, Flavin S, Randazzo B, Li S, et al. Guselkumab Versus Secukinumab for the Treatment of Moderate-To-Severe Psoriasis (ECLIPSE): Results From a Phase 3, Randomised Controlled Trial. Lancet (2019) 394(10201):831–9. doi: 10.1016/S0140-6736(19)31773-8
225. Olejniczak-Staruch I, Narbutt J, Bednarski I, Wozniacka A, Sieniawska J, Kraska-Gacka M, et al. Interleukin 22 and 6 Serum Concentrations Decrease Under Long-Term Biologic Therapy in Psoriasis. Postepy Dermatol Alergol (2020) 37(5):705–11. doi: 10.5114/ada.2020.100481
226. Goldminz AM, Suárez-Fariñas M, Wang AC, Dumont N, Krueger JG, Gottlieb AB. CCL20 and IL22 Messenger RNA Expression After Adalimumab vs Methotrexate Treatment of Psoriasis: A Randomized Clinical Trial. JAMA Dermatol (2015) 151(8):837–46. doi: 10.1001/jamadermatol.2015.0452
227. Luan L, Han S, Wang H, Liu X. Down-Regulation of the Th1, Th17, and Th22 Pathways Due to Anti-TNF-Alpha Treatment in Psoriasis. Int Immunopharmacol (2015) 29(2):278–84. doi: 10.1016/j.intimp.2015.11.005
228. Zaba LC, Cardinale I, Gilleaudeau P, Sullivan-Whalen M, Suárez-Fariñas M, Fuentes-Duculan J, et al. Amelioration of Epidermal Hyperplasia by TNF Inhibition is Associated With Reduced Th17 Responses. J Exp Med (2007) 204(13):3183–94. doi: 10.1084/jem.20071094
229. Caproni M, Antiga E, Melani L, Volpi W, Del Bianco E, Fabbri P. Serum Levels of IL-17 and IL-22 are Reduced by Etanercept, But Not by Acitretin, in Patients With Psoriasis: A Randomized-Controlled Trial. J Clin Immunol (2009) 29(2):210–4. doi: 10.1007/s10875-008-9233-0
230. Zhou L, Wang Y, Wan Q, Wu F, Barbon J, Dunstan R, et al. A non-Clinical Comparative Study of IL-23 Antibodies in Psoriasis. MAbs (2021) 13(1):1964420. doi: 10.1080/19420862.2021.1964420
231. Gordon KB, Armstrong AW, Foley P, Song M, Shen YK, Li S, et al. Guselkumab Efficacy After Withdrawal Is Associated With Suppression of Serum IL-23-Regulated IL-17 and IL-22 in Psoriasis: VOYAGE 2 Study. J Invest Dermatol (2019) 139(12):2437–2446.e2431. doi: 10.1016/j.jid.2019.05.016
232. Sweet K, Song Q, Loza MJ, McInnes IB, Ma K, Leander K, et al. Guselkumab Induces Robust Reduction in Acute Phase Proteins and Type 17 Effector Cytokines in Active Psoriatic Arthritis: Results From Phase 3 Trials. RMD Open (2021) 7(2):e001679. doi: 10.1136/rmdopen-2021-001679
233. Feldmeyer L, Mylonas A, Demaria O, Mennella A, Yawalkar N, Laffitte E, et al. Interleukin 23-Helper T Cell 17 Axis as a Treatment Target for Pityriasis Rubra Pilaris. JAMA Dermatol (2017) 153(4):304–8. doi: 10.1001/jamadermatol.2016.5384
234. Philipp S, Menter A, Nikkels AF, Barber K, Landells I, Eichenfield LF, et al. Ustekinumab for the Treatment of Moderate-To-Severe Plaque Psoriasis in Paediatric Patients (>/= 6 to < 12 Years of Age): Efficacy, Safety, Pharmacokinetic and Biomarker Results From the Open-Label CADMUS Jr Study. Br J Dermatol (2020) 183(4):664–72. doi: 10.1111/bjd.19018
235. Cesaroni M, Seridi L, Loza MJ, Schreiter J, Sweet K, Franks C, et al. Suppression of Serum Interferon-Gamma Levels as a Potential Measure of Response to Ustekinumab Treatment in Patients With Systemic Lupus Erythematosus. Arthritis Rheumatol (2021) 73(3):472–7. doi: 10.1002/art.41547
236. Roblin X, Serone A, Yoon OK, Zhuo L, Grant E, Woo J, et al. Effects of JAK1-Preferential Inhibitor Filgotinib on Circulating Biomarkers and Whole Blood Genes/Pathways of Patients With Moderately to Severely Active Crohn's Disease. Inflammation Bowel Dis (2021) 11(6):1–2. doi: 10.1093/ibd/izab253
237. Uchiyama A, Fujiwara C, Inoue Y, Ishikawa M, Motegi SI. Possible Suppressive Effects of Baricitinib on Serum IL-22 Levels in Atopic Dermatitis. J Dermatol Sci (2022) 106(3):189–92. doi: 10.1016/j.jdermsci.2022.04.006
238. Abikhair Burgo M, Roudiani N, Chen J, Santana AL, Doudican N, Proby C, et al. Ruxolitinib Inhibits Cyclosporine-Induced Proliferation of Cutaneous Squamous Cell Carcinoma. JCI Insight (2018) 3(17):e120750. doi: 10.1172/jci.insight.120750
239. Tzanetakou V, Kanni T, Giatrakou S, Katoulis A, Papadavid E, Netea MG, et al. Safety and Efficacy of Anakinra in Severe Hidradenitis Suppurativa: A Randomized Clinical Trial. JAMA Dermatol (2016) 152(1):52–9. doi: 10.1001/jamadermatol.2015.3903
240. Li J, Shouval DS, Doty AL, Snapper SB, Glover SC. Increased Mucosal IL-22 Production of an IL-10ra Mutation Patient Following Anakin Treatment Suggests Further Mechanism for Mucosal Healing. J Clin Immunol (2017) 37(2):104–7. doi: 10.1007/s10875-016-0365-3
241. Smith SH, Jayawickreme C, Rickard DJ, Nicodeme E, Bui T, Simmons C, et al. Tapinarof Is a Natural AhR Agonist That Resolves Skin Inflammation in Mice and Humans. J Invest Dermatol (2017) 137(10):2110–9. doi: 10.1016/j.jid.2017.05.004
242. Perera GK, Ainali C, Semenova E, Hundhausen C, Barinaga G, Kassen D, et al. Integrative Biology Approach Identifies Cytokine Targeting Strategies for Psoriasis. Sci Transl Med (2014) 6(223):223ra222. doi: 10.1126/scitranslmed.3007217
243. Guttman-Yassky E, Brunner PM, Neumann AU, Khattri S, Pavel AB, Malik K, et al. Efficacy and Safety of Fezakinumab (an IL-22 Monoclonal Antibody) in Adults With Moderate-To-Severe Atopic Dermatitis Inadequately Controlled by Conventional Treatments: A Randomized, Double-Blind, Phase 2a Trial. J Am Acad Dermatol (2018) 78(5):872–881 e876. doi: 10.1016/j.jaad.2018.01.016
244. Badi YE, Pavel AB, Pavlidis S, Riley JH, Bates S, Kermani NZ, et al. Mapping Atopic Dermatitis and Anti-IL-22 Response Signatures to Type 2-Low Severe Neutrophilic Asthma. J Allergy Clin Immunol (2021). doi: 10.1016/j.jaci.2021.04.010
245. Tang KY, Lickliter J, Huang ZH, Xian ZS, Chen HY, Huang C, et al. Safety, Pharmacokinetics, and Biomarkers of F-652, a Recombinant Human Interleukin-22 Dimer, in Healthy Subjects. Cell Mol Immunol (2019) 16(5):473–82. doi: 10.1038/s41423-018-0029-8
246. Arab JP, Sehrawat TS, Simonetto DA, Verma VK, Feng D, Tang T, et al. An Open-Label, Dose-Escalation Study to Assess the Safety and Efficacy of IL-22 Agonist F-652 in Patients With Alcohol-Associated Hepatitis. Hepatology (2020) 72(2):441–53. doi: 10.1002/hep.31046
247. Rothenberg ME, Wang Y, Lekkerkerker A, Danilenko DM, Maciuca R, Erickson R, et al. Randomized Phase I Healthy Volunteer Study of UTTR1147A (IL-22fc): A Potential Therapy for Epithelial Injury. Clin Pharmacol Ther (2019) 105(1):177–89. doi: 10.1002/cpt.1164
248. Cheuk S, Wikén M, Blomqvist L, Nylén S, Talme T, Ståhle M, et al. Epidermal Th22 and Tc17 Cells Form a Localized Disease Memory in Clinically Healed Psoriasis. J Immunol (2014) 192(7):3111–20. doi: 10.4049/jimmunol.1302313
249. Fania L, Morelli M, Scarponi C, Mercurio L, Scopelliti F, Cattani C, et al. Paradoxical Psoriasis Induced by TNF-α Blockade Shows Immunological Features Typical of the Early Phase of Psoriasis Development. J Pathol Clin Res (2020) 6(1):55–68. doi: 10.1002/cjp2.147
250. Megna M, Caiazzo G, Parisi M, Ruggiero A, Capasso G, Mascolo M, et al. Eczematous Drug Eruption in Patients With Psoriasis Under Anti-Interleukin-17a: Does Interleukin-22 Play a Key Role? Clin Exp Dermatol (2022) 47(5):918–25. doi: 10.1111/ced.15052
251. Gottlieb AB, Krueger JG, Sandberg Lundblad M, Göthberg M, Skolnick BE. First-In-Human, Phase 1, Randomized, Dose-Escalation Trial With Recombinant Anti-IL-20 Monoclonal Antibody in Patients With Psoriasis. PloS One (2015) 10(8):e0134703. doi: 10.1371/journal.pone.0134703
252. Michiels C, Puigdevall L, Cochez P, Achouri Y, Cheou P, Hendrickx E, et al. A Targetable, Noncanonical Signal Transducer and Activator of Transcription 3 Activation Induced by the Y-Less Region of IL-22 Receptor Orchestrates Imiquimod-Induced Psoriasis-Like Dermatitis in Mice. J Invest Dermatol (2021) 141(11):2668–+. doi: 10.1016/j.jid.2021.04.016
253. Brito-Luna MJ, Villanueva-Quintero DG, Sandoval-Talamantes AK, Fafutis-Morris M, Graciano-Machuca O, Sanchez-Hernandez PE, et al. Correlation of IL-12, IL-22, and IL-23 in Patients With Psoriasis and Metabolic Syndrome. Preliminary Report. Cytokine (2016) 85:130–6. doi: 10.1016/j.cyto.2016.06.020
254. Sabat R, Wolk K. Deciphering the Role of Interleukin-22 in Metabolic Alterations. Cell Biosci (2015) 5:68. doi: 10.1186/s13578-015-0060-8
255. Zou J, Chassaing B, Singh V, Pellizzon M, Ricci M, Fythe MD, et al. Fiber-Mediated Nourishment of Gut Microbiota Protects Against Diet-Induced Obesity by Restoring IL-22-Mediated Colonic Health. Cell Host Microbe (2018) 23(1): e44 41–53. doi: 10.1016/j.chom.2017.11.003
256. Bakker DS, van der Wal MM, Heeb LEM, Giovannone B, Asamoah M, Delemarre EM, et al. Early and Long-Term Effects of Dupilumab Treatment on Circulating T-Cell Functions in Patients With Moderate-To-Severe Atopic Dermatitis. J Invest Dermatol (2021) 141(8):1943–1953 e1913. doi: 10.1016/j.jid.2021.01.022
257. Miyano T, Irvine AD, Tanaka RJ. A Mathematical Model to Identify Optimal Combinations of Drug Targets for Dupilumab Poor Responders in Atopic Dermatitis. Allergy (2022) 77(2):582–94. doi: 10.1111/all.14870
Keywords: Th22 cells, IL-22, skin, inflammation, keratinocyte
Citation: Pan Y, Du D, Wang L, Wang X, He G and Jiang X (2022) The Role of T Helper 22 Cells in Dermatological Disorders. Front. Immunol. 13:911546. doi: 10.3389/fimmu.2022.911546
Received: 02 April 2022; Accepted: 14 June 2022;
Published: 14 July 2022.
Edited by:
Mariolina Salio, University of Oxford, United KingdomReviewed by:
Clare Hardman, University of Oxford, United KingdomCopyright © 2022 Pan, Du, Wang, Wang, He and Jiang. This is an open-access article distributed under the terms of the Creative Commons Attribution License (CC BY). The use, distribution or reproduction in other forums is permitted, provided the original author(s) and the copyright owner(s) are credited and that the original publication in this journal is cited, in accordance with accepted academic practice. No use, distribution or reproduction is permitted which does not comply with these terms.
*Correspondence: Gu He, aGVndUBzY3UuZWR1LmNu; Xian Jiang, amVubnl4aWFuakAxNjMuY29t
†These authors have contributed equally to this work
Disclaimer: All claims expressed in this article are solely those of the authors and do not necessarily represent those of their affiliated organizations, or those of the publisher, the editors and the reviewers. Any product that may be evaluated in this article or claim that may be made by its manufacturer is not guaranteed or endorsed by the publisher.
Research integrity at Frontiers
Learn more about the work of our research integrity team to safeguard the quality of each article we publish.