- 1Division of Maternal-Fetal Medicine, Department of Obstetrics, Gynecology, and Reproductive Sciences, University of California, San Francisco, San Francisco, CA, United States
- 2Department of Bioengineering and Therapeutic Sciences, University of California, San Francisco, San Francisco, CA, United States
- 3Institute for Human Genetics, University of California, San Francisco, San Francisco, CA, United States
- 4Department of Family Health Care Nursing, University of California, San Francisco, San Francisco, CA, United States
- 5Department of Pediatrics, University of California, San Francisco, San Francisco, CA, United States
- 6Division of Pediatric Infectious Diseases and Global Health, University of California, San Francisco, San Francisco, CA, United States
- 7Center for Reproductive Sciences, Department of Obstetrics, Gynecology, and Reproductive Sciences, University of California, San Francisco, San Francisco, CA, United States
Human milk contains three antibody classes that confer mucosal immunity to the breastfed infant: secretory IgA (SIgA), secretory IgM (SIgM), and IgG. Influenza and pertussis vaccines administered during pregnancy induce pathogen specific SIgA and IgG responses in human milk that have been shown to protect the breastfed infant from these respiratory illnesses. In addition, mRNA vaccines against the SARS-CoV-2 virus administered during pregnancy and lactation induce anti-SARS-CoV-2 IgG and IgA responses in human milk. This review summarizes the immunologic benefits of influenza, pertussis, and COVID-19 vaccines conferred by human milk. Additionally, future research direction in human milk immunity and public health needs to improve lactational support are discussed.
Introduction
Human milk has been shown to have numerous benefits for infants (1–5) as well as for breastfeeding mothers which experience short-term and long-term health benefits (5–8). The World Health Organization recommends exclusive breastfeeding for the first six months after birth, and up to two years with the introduction of complementary foods (9). Unfortunately, due to systemic and structural barriers such as racism, lack of workplace accommodations, and inequitable access to human milk feeding resources, breastfeeding disparities and inequities remain (10–12). In general, breastfeeding initiation and duration rates are higher among Asian and White mothers and lower among Black and Indigenous mothers in the U.S (13).
Vaccination during pregnancy and lactation not only has immune protection for the mother, but also provides immunologic benefits for their child through the transfer of immune factors in utero and through human milk. Pregnant women and those who have recently given birth may face increased vulnerability to infections and severe illness (14, 15). Thus, vaccines serve as a critical component of preventative healthcare for pregnant and lactating women and an important public health intervention (16, 17). However, inequities and disparities also extend to vaccinations. Presently, in the U.S., children, adolescents, and adults who are uninsured, living in rural communities, have lower levels of income, and identify as a person of color, experience lower rates of recommended vaccination (18–20). Given the benefits and significance of human milk, lactation, and vaccines across the life course, the barriers need to be addressed to make certain that all mothers and infants, especially those most marginalized, have access to critical resources and supports during the perinatal period.
In this review, we discuss the barriers that need to be addressed to improve equity, and summarize the literature regarding humoral immunity in the human milk after influenza and pertussis vaccinations, as well as the latest data on human milk immunity conferred by the mRNA-based COVID-19 vaccines.
Antibodies in Human Milk
For the first few months of life, the infant’s immune system is immature and they therefore rely on maternal passive immunity for protection and to distinguish pathogenic from commensal bacteria (21). During pregnancy, specific maternal IgG antibodies are transferred from the mother through the placenta to the fetal bloodstream to provide systemic immunity that confers protection for the first few months of infancy. Maternally-derived antibodies gradually decrease during the first year of life while the infant builds protective immune responses through vaccination and early life pathogen exposure (22). After birth, lactating mothers continue to transfer milk-derived antibodies to their newborn which provide passive mucosal immunity. Human milk contains protective immunologic components including immune cells, cytokines, glycoproteins (e.g. lactoferrin), human milk oligosaccharides, and antibodies such as maternal secretory IgA (SIgA), secretory IgM (SIgM), and IgG (21, 23, 24). In humans, mucosal barriers close shortly after birth, and therefore human milk antibodies are prevented from passing into the bloodstream due to decreasing permeability of the gut. As a result, milk antibodies predominately provide mucosal immunity (25, 26).
Serum IgA is a monomer, whereas mucosal IgA is a dimer. The IgA dimers in the mammary gland bind to polymeric immunoglobulin receptor (pIgR) on the basolateral surface of the epithelial cells and travel across the cell to the apical surface (27). There, the external domain of the pIgR bound to the dimeric mucosal IgA is cleaved, and the remaining compound is secreted into the human milk as SIgA (26). SIgA provides first line protection along mucosal surfaces including the respiratory and digestive tracts (27). It has also been shown to be protective against various diarrheal diseases as infants consuming human milk with higher SIgA levels were more likely to be asymptomatic for these diseases (24, 28–31). Pentameric secretory IgM usually produces the primary antibody response to an antigen and activates the complement cascade upon antigen binding. SIgM is delivered to human milk through the same mechanism as SIgA. IgG is the least prominent antibody in human milk. Monomeric IgG from maternal blood is delivered in human milk through binding of the neonatal Fc receptor (FcRn) on epithelial cells in the mammary gland (32–35). Human milk-derived maternal IgG binds intraluminal pathogens in the infant’s gut and helps protect against enteric infections (36, 37). Milk antibodies main functions are summarized in Table 1.
Antibody Composition in Human milk Post-Influenza and Pertussis Vaccination
Influenza Vaccination
Influenza (flu) viruses are RNA viruses (38), that can cause severe illness, particularly in pregnant people who are at high risk for infectious complications leading to hospitalization (39). Influenza vaccines are updated annually to optimize protection against circulating influenza viruses that are predicted to be the most common in the upcoming year (40). Currently in the U.S., inactivated virus quadrivalent vaccines are recommended for pregnant individuals, which protect against four different types of flu viruses. There is year-to-year variability in vaccine efficacy, due to a number of factors including antigenic mismatch, pre-existing immunity, and the limited ability to predict the dominant viruses each year. However, the efficiency of the flu vaccines typically ranges between 50-70% in pregnancy but may be less for other populations (41, 42).
Influenza vaccination during pregnancy leads to a 40% decreased risk of influenza-related hospitalization in pregnant women (43), as well as a significant increase in maternal and infant serum influenza IgG levels (44, 45). In addition, numerous studies have shown a decrease in the incidence of influenza in infants born to vaccinated mothers up to 6 months post-delivery (45–48). This protection is mostly attributed to transplacentally-derived IgG antibodies which are transferred during pregnancy, and wanes in the infants typically three to six months after delivery (44, 45, 49–51). However, in breastfed infants, human milk-derived antibodies may also provide additional layer of influenza protection in the infant during the breastfeeding period. A recent study evaluated longitudinal levels of anti-influenza IgA in human milk, samples were collected from lactating individuals after administering the trivalent inactivated influenza vaccine or a 23-valent pneumococcal polysaccharide vaccine (control) to pregnant women in the third trimester (52). Human milk anti-influenza IgA levels in milk were maintained at a significantly higher level in those who received the influenza vaccine for at least 6 months after delivery compared to controls (52). In addition to IgA, anti-influenza IgM and IgG are also present in milk but at lower levels (53). Human milk also contains varying levels of immune cells including innate cells, memory T cells, and plasma B cells (54–58), but limited data exists on the antigen-specificity of these cells and response to infection or vaccination. A prior study demonstrated influenza-specific CD8 T cells in human milk (57, 59). However, the degree of protection conferred by milk immune cells remains unknown. Breastfeeding exclusivity is associated with lower rate of infant febrile respiratory illness (52, 60) compared to non-exclusively breastfed infant. Additional studies are needed to understand the various factors in milk that confer this protection to infants.
Pertussis Vaccination
Pertussis (also known as whooping cough) is a childhood respiratory illness caused by the bacterium Bortadella pertussis. Pertussis (PT) booster immunization during the late second or third trimester of pregnancy is an important public health strategy to reduce the morbidity and mortality from whooping cough in neonates. Since 2010, the pertussis vaccine has been recommended for all pregnant people between 27- and 36-weeks of gestation in order to provide protective antibodies to the fetus for protection against pertussis, in the critical early months of the infant’s life when they are most at risk for serious disease (61, 62). In the U.S., this is typically administered through the combined Tdap vaccine, which also provides protection against tetanus, diphtheria, in addition to pertussis (63).
Studies have demonstrated that after maternal vaccination high levels of anti-PT IgG is present in newborn blood due to transplacental transfer from mother to infant (64). After delivery, pertussis-specific IgA as well as IgG are present in colostrum and mature human milk and are detected for at least 8 weeks postpartum after maternal vaccination during pregnancy (65, 66).
The effectiveness of maternal vaccination in infant protection against PT infection at the first months of life ranges from 88 to 93% (67–70). Further, infants with pertussis whose mothers received the TdaP vaccine had lower risks of hospitalization, ICU admission, and shorter hospital stays compared to mothers who were not vaccinated (71). In summary, vaccination with Tdap during or shortly after pregnancy greatly increases the level of anti-PT antibodies in human milk (64–66, 72, 73) and may contribute to the protection provided to the infant against pertussis infection.
Immune Responses in Human Milk Following COVID-19 Vaccination
BNT162b2 (BioNTech and Pfizer) and mRNA-1273 (Moderna) are mRNA-based vaccines approved by the Food and Drug Administration (FDA) to use against COVID-19 (74, 75). In addition, two vector-based vaccines AZD1222 (Oxford/AstraZeneca) and Ad26.COV2.S (Johnson & Johnson/Janssen) are widely used worldwide (76–80). However, due to the timing of vaccine approval, there is currently limited data on vector-based vaccines in pregnancy and lactation, and for purposes of this review we will focus on mRNA vaccines. BNT162b2 and mRNA-1273 vaccines contain the mRNA sequence of the SARS-CoV-2 Spike protein, coated by a lipid-nanoparticle envelope. Upon administration, the lipid nanoparticles are absorbed by cells, and the mRNA sequence is released into the cytoplasm, where it is translated into Spike protein that is presented on the cell surface of vaccinated cells. This Spike protein is recognized by immune cells to generate a robust and specific immune response against the Spike protein (81, 82). These vaccines have been found to be highly efficient in prevention of severe COVID-19 disease (83, 84) and to be safe for administration during pregnancy and lactation (85–95).
For mothers that were vaccinated while pregnant, their infants had detectable levels of anti-SARS-CoV-2 IgG antibodies in cord blood and in infant follow up blood samples, demonstrating transfer of these IgG antibodies via the placenta to the fetal bloodstream (96–98). Similar to influenza and pertussis vaccination during pregnancy (44, 46), SARS-CoV-2 vaccination during pregnancy reduced the risk of infant hospitalization for COVID-19 up to 4-6 months of age by 30-70% (99, 100). In contrast, infants born to mothers vaccinated after pregnancy did not have anti-SARS-CoV-2 IgG in their blood (25, 101). However, COVID-19 vaccination during pregnancy and lactation both elicited transfer of anti-SARS-CoV-2 antibodies to human milk (25, 96, 97, 102–105).
Since SARS-CoV-2 is a novel pathogen, the implementation of COVID-19 vaccines has provided a unique opportunity to understand primary immune responses in human milk to a novel antigen in lactating people. We have summarized multiple studies that have evaluated mRNA vaccination during lactation and human milk antibodies (Table 2). Most studies have found an initial increase of milk IgG 14-21 days after the first dose of vaccine, with further robust increased levels peaking at 7 days after the second dose and remaining elevated for at least 6 weeks (78, 96, 102–105). In most lactating people, 4-10 weeks after the second dose, anti-SARS-CoV-2 IgG levels in milk were still significantly higher compared to their levels before vaccination (25, 105). Additionally, IgA levels generally peak at 14-18 days after the first dose, increase slightly for one week after the second dose, but decrease thereafter (96, 102–105). In contrast to the significant increases in IgG levels after the second dose, studies have shown that IgA levels in milk do not rise further when measured > 18 days after the second dose (25, 101, 105).
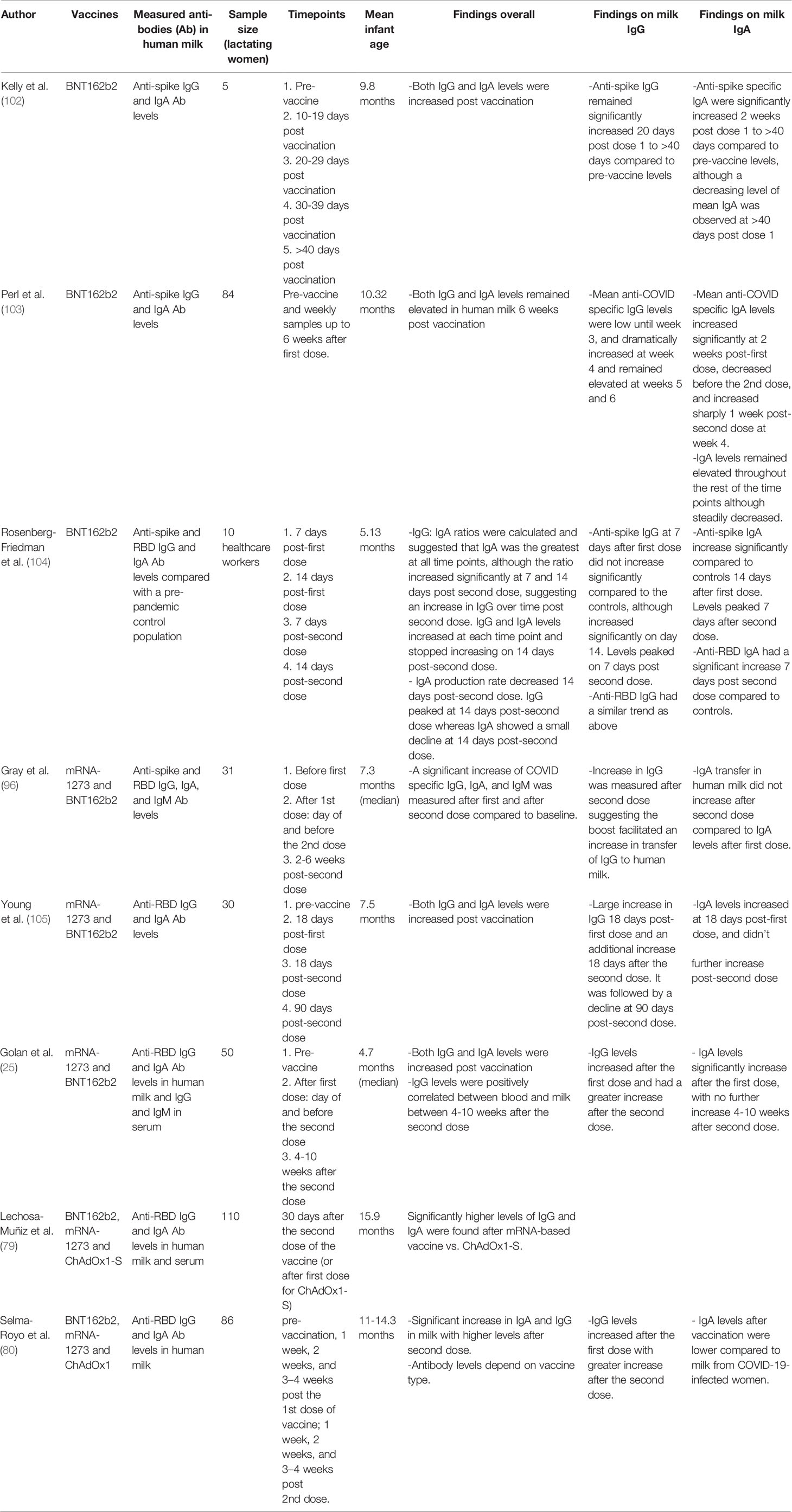
Table 2 Summary of various studies evaluating vaccination during lactation and human milk antibodies with regards to the mRNA-1273 and BNT162b2 vaccines including sample size of lactating women, timepoints measured, and mean infant age.
Studies on the association between blood and milk levels after SARS-CoV-2 vaccination during lactation have found a positive correlation between serum and human milk SARS-CoV-2 IgG levels measured at 4-10 weeks after second dose (25, 106). Interestingly, one study measured milk IgG and IgA in pregnant women who were vaccinated for both SARS-CoV-2 and TdaP during pregnancy and found similar levels between anti-Spike (SARS-CoV-2) antibodies and anti-tetanus toxoid (TT) antibodies (104). These findings further strengthen our knowledge about the mechanism and absolute level of transferred IgG antibodies from the serum to human milk via FcR transfer in the mammary gland (107).
Mothers who were infected with COVID-19 during pregnancy or lactation had a universally rapid anti-SARS-CoV-2 IgA secretion in human milk, lasting >90 days after diagnosis. In contrast, vaccination during pregnancy or lactation results in a robust anti-SARS-CoV-2 IgG secretion to milk with a less dominant IgA response (97, 105, 107–109). Though antibody functional responses may be similar after SARS-CoV-2 vaccination vs infection, as was demonstrated by comparable levels of neutralizing antibodies (97, 105). These findings suggest that exposure through natural infection leads to increased secretion of mucosal related IgA antibodies in mucosal organs, such as the mammary gland, which may be a distinct immune response than what is generated after mRNA-based vaccines. In animal models, additional intranasal vaccination induces mucosal boost immunity in addition to the systemic immunity that is induced after mRNA-based vaccines (110). Approaches boosting mucosal immunity may be useful to increase secretion of antibodies to human milk, however further research is needed in this area.
Similar to other vaccinations and infections, there is limited data on the presence SARS-CoV-2 antigen-specific human milk immune cells on infant protection against disease (111). Using animal models, it was shown that cells from milk can survive the digestive tract and can traffic into infant organs (112, 113). Interestingly two recent studies have demonstrated the presence of SARS-CoV-2 specific Spike-reactive T cells in human milk after vaccination (111, 114). However, it is unknown if human milk cells provide immune protection to the respiratory tract or gastrointestinal tract of human infants or if they are taken up in the infant gut into systemic circulation. The role of these antigen-specific immune cells in human milk in regard to infant protection requires further study.
Protection of Infants
Further studies are needed to evaluate the protective effects of breastfeeding and milk SARS-CoV-2 antibodies against COVID-19 infection in infants. Exclusively breastfed infants usually consume human milk every 1-3 hours, providing them with frequent doses of milk antibodies. Upon weaning, milk antibodies decay rapidly in the infant, and this mode of passive immunity ends. Neonates and infants with COVID-19 often present with gastrointestinal symptoms (115). However, there is limited information to date on whether SARS-CoV-2 achieves gastrointestinal viral invasion or whether SARS-CoV-2 causes bystander mucosal inflammation that contributes to these symptoms (116–118). Interestingly, anti-SARS-CoV-2 IgA and IgG have been detected in one-third of 24 infant stool samples after maternal vaccination (119). Further studies are needed to determine the impact of local mucosal protection by human milk derived SARS-CoV-2 antibodies in the infant gut.
COVID-19 Vaccines Safety During Lactation
COVID-19 vaccination for lactating women is recommended by the Centers for Disease Control and Prevention (CDC) to reduce the risk of complications from COVID-19, and the World Health Organization (WHO) recommends continuing of breastfeeding after vaccination (120, 121). Maternal vaccination during lactation protects the mother from severe COVID-19 disease and as discussed above may also protect the infant. A large survey-based study including over 10,000 lactating individuals found minimal disruption of lactation after vaccination (around 2% of the individuals), with 6% of individuals reporting decrease in milk supply (122). Reduction in milk supply was reported in 5-7% of the women, which was more common after the second dose. Most symptoms resolved within 24-72 hours after vaccination (25, 122, 123). Symptoms in the breastfed infant in the short term after maternal vaccination were reported in 2-7% the cases, with sleepiness and fussiness being the most common symptom (25, 122, 123). Other symptoms such as fever and gastrointestinal symptoms were reported in 1-2% of the infants (25, 122, 123). Few studies examined transfer of vaccine particles to human milk after vaccination (101, 124) and found minimal transfer of vaccine mRNA to human milk in less than 2% of the samples (out of 309 samples examined). In addition, a single study measured polyethylene glycol (PEG) which is present in the lipid nanoparticles of the mRNA-based vaccines in milk and found no significant increase in PEG in milk after vaccination (25). It is not clear whether the infant symptoms reported are specifically related to vaccine particle transfer, and further research is needed in this area. There is a lack of clinical trials that carefully examine infant side effects after vaccination in this vulnerable population of lactating dyads. Future trials should include these populations and outcomes. However, based on the data collected so far in multiple prospective studies, the benefits of vaccination outweigh the risk for mother and her infant.
COVID-19, Lactation, and Equity Issues
Despite the known maternal and infant health benefits of breastfeeding and vaccination, significant inequities persist among the most vulnerable groups that are presented with unique challenges to lactation support and vaccine access. There are a lack of studies examining barriers to breastfeeding during the COVID-19 pandemic. Access to commercial tele-lactation companies offering online lactation support is limited especially for those who have lost their jobs and may not be able to afford lactation or internet services (117). It is essential to provide resources to the communities and populations purposively marginalized. For instance, in certain parts of large cities with previous inequitable health care access, such as the South Side of Chicago, the COVID-19 pandemic has exacerbated the reduction of open hospitals (118). Hospitals are typically the primary source of breastfeeding education and in communities with already low rates of breastfeeding. Barriers of marginalized populations are being aggravated rather than reduced during the pandemic. There is also inadequate funding to lactation services in institutions and agencies. Addressing systemic and structural barriers and increasing funding to lower resourced communities can begin to reduce health care disparities by providing essential services, such as open hospitals and consistent breastfeeding education so that families understand the short- and long-term importance of vaccination and breastfeeding.
Discussion
Future Directions
The studies presented here have demonstrated the benefits of influenza, pertussis, and SARS-CoV-2 vaccination for pregnant and lactating individuals and the presence of anti-pathogens antibodies in human milk following vaccination. Further epidemiological studies are needed to determine the level of disease protection to infants against COVID-19 provided by maternal vaccination through human milk. Additionally, the quantity of human milk required to be ingested to confer a protective effect in an infant is unknown. To address this question, detailed study of infant feeding patterns is needed to distinguish between various quantities and patterns of human milk consumption. Current studies usually compare only exclusively breastfed to nonexclusively breastfed infants as a group. In addition, studies that measure the durability of milk antibodies in infant mucosal surfaces, such as the oropharynx, are necessary to better understand the protection of milk antibodies against pathogens that are transmitted via these organs.
Longitudinal studies to evaluate the persistence of human milk antibodies after vaccination, and the effect of a third and fourth mRNA-based vaccine doses on human milk are needed. Similarly, long-term follow up on infants of COVID-19 vaccinated mothers is needed as the pandemic evolves to provide more data on protection of these infants with continued breastfeeding.
In summary, while the immunologic benefits of breastfeeding have long been promoted, there is still much to learn regarding the dynamics of immune responses during lactation. More work is needed to understand the precise mechanisms of immune protection seen in breastfed infants. However, the potential benefits of breastfeeding and human milk are nullified if there is not equitable access and support for lactation, particularly in vulnerable communities. Research and financial support for qualitative studies and community-engaged programs are needed to improve advocacy for education and resources in lactating communities of color.
Author Contributions
SH and YG wrote the first draft. All authors revised the manuscript. All authors contributed to the article and approved the submitted version.
Funding
SLG- K08 AI141728/AI/NIAID NIH HHS/United States; IVA- K12 HD052163/HD/NICHD NIH HHS/United States; MP- K23 AI127886/AI/NIAID NIH HHS/United States; YG-Weizmann Institute of Science -National Postdoctoral Award Program for Advancing Women in Science and Human Frontier Science Program.
Conflict of Interest
The authors declare that the research was conducted in the absence of any commercial or financial relationships that could be construed as a potential conflict of interest.
Publisher’s Note
All claims expressed in this article are solely those of the authors and do not necessarily represent those of their affiliated organizations, or those of the publisher, the editors and the reviewers. Any product that may be evaluated in this article, or claim that may be made by its manufacturer, is not guaranteed or endorsed by the publisher.
References
1. Wright AL, Holberg CJ, Martinez FD, Morgan WJ, Taussig LM. Breast Feeding and Lower Respiratory Tract Illness in the First Year of Life. Group Health Medical Associates. BMJ (1989) 299:946–9. doi: 10.1136/bmj.299.6705.946
2. Pisacane A, Graziano L, Mazzarella G, Scarpellino B, Zona G. Breast-Feeding and Urinary Tract Infection. J Pediatr (1992) 120:87–9. doi: 10.1016/S0022-3476(05)80607-9
3. Howie PW, Forsyth JS, Ogston SA, Clark A, Florey CD. Protective Effect of Breast Feeding Against Infection. BMJ (1990) 300:11–6. doi: 10.1136/bmj.300.6716.11
4. Hanson LA. Breastfeeding Provides Passive and Likely Long-Lasting Active Immunity. Ann Allergy Asthma Immunol (1998) 81:523–33; quiz 533–4, 537. doi: 10.1016/S1081-1206(10)62704-4
5. Arthur I, Eidelman MD, Schanler MD, Margreete Johnston MD, Susan Landers MD, Larry Noble MD, Kinga Szucs MD, Laura Viehmann MD. Section on Breastfeeding. Breastfeeding and the Use of Human Milk. Pediatrics (2012) 129:e827–41. doi: 10.1542/peds.2011-3552
6. Dewey KG, Heinig MJ, Nommsen LA. Maternal Weight-Loss Patterns During Prolonged Lactation. Am J Clin Nutr (1993) 58:162–6. doi: 10.1093/ajcn/58.2.162
7. López-Olmedo N, Hernández-Cordero S, Neufeld LM, García-Guerra A, Mejía-Rodríguez F, Méndez Gómez-Humarán I. The Associations of Maternal Weight Change With Breastfeeding, Diet and Physical Activity During the Postpartum Period. Matern Child Health J (2016) 20:270–80. doi: 10.1007/s10995-015-1826-7
8. Victora CG, Bahl R, Barros AJD, França GVA, Horton S, Krasevec J, et al. Breastfeeding in the 21st Century: Epidemiology, Mechanisms, and Lifelong Effect. Lancet (2016) 387:475–90. doi: 10.1016/S0140-6736(15)01024-7
9. Breastfeeding . Available at: https://www.who.int/health-topics/breastfeeding (Accessed June 8, 2022).
10. An Official Position Statement of the Association of Women’s Health, Obstetric and Neonatal Nurses. Breastfeeding and the Use of Human Milk. J Obstet Gynecol Neonatal Nurs (2021) 50:e1–5. doi: 10.1016/j.jogn.2021.06.006
11. Asiodu IV, Bugg K, Palmquist AEL. Achieving Breastfeeding Equity and Justice in Black Communities: Past, Present, and Future. Breastfeed Med (2021) 16:447–51. doi: 10.1089/bfm.2020.0314
12. Bartick MC, Jegier BJ, Green BD, Schwarz EB, Reinhold AG, Stuebe AM. Disparities in Breastfeeding: Impact on Maternal and Child Health Outcomes and Costs. J Pediatr (2017) 181:49–55.e6. doi: 10.1016/j.jpeds.2016.10.028
13. Chiang KV, Li R, Anstey EH, Perrine CG. Racial and Ethnic Disparities in Breastfeeding Initiation ─ United States, 2019. MMWR Morb Mortal Wkly Rep (2021) 70:769–74. doi: 10.15585/mmwr.mm7021a1
14. CDC. Pregnant and Recently Pregnant People (2022). Centers for Disease Control and Prevention. Available at: https://www.cdc.gov/coronavirus/2019-ncov/need-extra-precautions/pregnant-people.html (Accessed June 8, 2022).
15. Kaur R, Callaghan T, Regan AK. Disparities in Maternal Influenza Immunization Among Women in Rural and Urban Areas of the United States. Prev Med (2021) 147:106531. doi: 10.1016/j.ypmed.2021.106531
16. Why Maternal Vaccines are Important (2021). Available at: https://www.cdc.gov/vaccines/pregnancy/hcp-toolkit/important-maternal-vaccines.html (Accessed June 8, 2022).
17. Office of Infectious Disease, HIV/AIDS Policy (OIDP). Vaccines for Pregnant Women. Hhs.Gov (2021). Available at: https://www.hhs.gov/immunization/who-and-when/pregnant/index.html (Accessed June 8, 2022).
18. Hill HA, Elam-Evans LD, Yankey D, Singleton JA, Kang Y. Vaccination Coverage Among Children Aged 19-35 Months - United States, 2016. MMWR Morb Mortal Wkly Rep (2017) 66:1171–7. doi: 10.15585/mmwr.mm6643a3
19. Kawai K, Kawai AT. Racial/ethnic and Socioeconomic Disparities in Adult Vaccination Coverage. Am J Prev Med (2021) 61:465–73. doi: 10.1016/j.amepre.2021.03.023
20. Tsai Y, Lindley MC, Zhou F, Stokley S. Urban-Rural Disparities in Vaccination Service Use Among Low-Income Adolescents. J Adolesc Health (2021) 69:114–20. doi: 10.1016/j.jadohealth.2020.10.021
21. Atyeo C, Alter G. The Multifaceted Roles of Breast Milk Antibodies. Cell (2021) 184:1486–99. doi: 10.1016/j.cell.2021.02.031
22. Mankarious S, Lee M, Fischer S, Pyun KH, Ochs HD, Oxelius VA, et al. The Half-Lives of IgG Subclasses and Specific Antibodies in Patients With Primary Immunodeficiency Who are Receiving Intravenously Administered Immunoglobulin. J Lab Clin Med (1988) 112:634–40.
23. Andreas NJ, Kampmann B, Mehring Le-Doare K. Human Breast Milk: A Review on its Composition and Bioactivity. Early Hum Dev (2015) 91:629–35. doi: 10.1016/j.earlhumdev.2015.08.013
24. Hanson LA, Korotkova M, Lundin S, Håversen L, Silfverdal S-A, Mattsby-Baltzer I, et al. The Transfer of Immunity From Mother to Child. Ann N Y Acad Sci (2003) 987:199–206. doi: 10.1111/j.1749-6632.2003.tb06049.x
25. Golan Y, Prahl M, Cassidy AG, Gay C, Wu AHB, Jigmeddagva U, et al. COVID-19 mRNA Vaccination in Lactation: Assessment of Adverse Events and Vaccine Related Antibodies in Mother-Infant Dyads. Front Immunol (2021) 12:777103. doi: 10.3389/fimmu.2021.777103
26. Van de Perre P. Transfer of Antibody via Mother’s Milk. Vaccine (2003) 21:3374–6. doi: 10.1016/S0264-410X(03)00336-0
27. Mostov KE. Transepithelial Transport of Immunoglobulins. Annu Rev Immunol (1994) 12:63–84. doi: 10.1146/annurev.iy.12.040194.000431
28. Hayani KC, Guerrero ML, Morrow AL, Gomez HF, Winsor DK, Ruiz-Palacios GM, et al. Concentration of Milk Secretory Immunoglobulin A Against Shigella Virulence Plasmid-Associated Antigens as a Predictor of Symptom Status in Shigella-Infected Breast-Fed Infants. J Pediatr (1992) 121:852–6. doi: 10.1016/S0022-3476(05)80327-0
29. Glass RI, Svennerholm AM, Stoll BJ, Khan MR, Hossain KM, Huq MI, et al. Protection Against Cholera in Breast-Fed Children by Antibodies in Breast Milk. N Engl J Med (1983) 308:1389–92. doi: 10.1056/NEJM198306093082304
30. Walterspiel JN, Morrow AL, Guerrero ML, Ruiz-Palacios GM, Pickering LK. Secretory Anti-Giardia Lamblia Antibodies in Human Milk: Protective Effect Against Diarrhea. Pediatrics (1994) 93:28–31. doi: 10.1542/peds.93.1.28
31. Cruz JR, Gil L, Cano F, Caceres P, Pareja G. Breast Milk Anti-Escherichia Coli Heat-Labile Toxin IgA Antibodies Protect Against Toxin-Induced Infantile Diarrhea. Acta Paediatr Scand (1988) 77:658–62. doi: 10.1111/j.1651-2227.1988.tb10726.x
32. Cianga P, Medesan C, Richardson JA, Ghetie V, Ward ES. Identification and Function of Neonatal Fc Receptor in Mammary Gland of Lactating Mice. Eur J Immunol (1999) 29:2515–23. doi: 10.1002/(SICI)1521-4141(199908)29:08<2515::AID-IMMU2515>3.0.CO;2-D
33. Adamski FM, King AT, Demmer J. Expression of the Fc Receptor in the Mammary Gland During Lactation in the Marsupial Trichosurus Vulpecula (Brushtail Possum). Mol Immunol (2000) 37:435–44. doi: 10.1016/S0161-5890(00)00065-1
34. Cianga P, Cianga C, Cozma L, Ward ES, Carasevici E. The MHC Class I Related Fc Receptor, FcRn, is Expressed in the Epithelial Cells of the Human Mammary Gland. Hum Immunol (2003) 64:1152–9. doi: 10.1016/j.humimm.2003.08.025
35. Mazanec MB, Kaetzel CS, Lamm ME, Fletcher D, Nedrud JG. Intracellular Neutralization of Virus by Immunoglobulin A Antibodies. Proc Natl Acad Sci U.S.A. (1992) 89:6901–5.
36. Caballero-Flores G, Sakamoto K, Zeng MY, Wang Y, Hakim J, Matus-Acuña V, et al. Maternal Immunization Confers Protection to the Offspring Against an Attaching and Effacing Pathogen Through Delivery of IgG in Breast Milk. Cell Host Microbe (2019) 25:313–323.e4. doi: 10.1016/j.chom.2018.12.015
37. Zheng W, Zhao W, Wu M, Song X, Caro F, Sun X, et al. Microbiota-Targeted Maternal Antibodies Protect Neonates From Enteric Infection. Nature (2020) 577:543–8. doi: 10.1038/s41586-019-1898-4
38. Hutchinson EC. Influenza Virus. Trends Microbiol (2018) 26:809–10. doi: 10.1016/j.tim.2018.05.013
39. Cuningham W, Geard N, Fielding JE, Braat S, Madhi SA, Nunes MC, et al. Optimal Timing of Influenza Vaccine During Pregnancy: A Systematic Review and Meta-Analysis. Influenza Other Respi Viruses (2019) 13:438–52. doi: 10.1111/irv.12649
40. CDC. Key Facts About Seasonal Flu Vaccine (2022). Centers for Disease Control and Prevention. Available at: https://www.cdc.gov/flu/prevent/keyfacts.htm (Accessed June 8, 2022).
41. Bansal A, Trieu M-C, Mohn KGI, Cox RJ. Safety, Immunogenicity, Efficacy and Effectiveness of Inactivated Influenza Vaccines in Healthy Pregnant Women and Children Under 5 Years: An Evidence-Based Clinical Review. Front Immunol (2021) 12:744774. doi: 10.3389/fimmu.2021.744774
42. Biswas A, Chakrabarti AK, Dutta S. Current Challenges: From the Path of “Original Antigenic Sin” Towards the Development of Universal Flu Vaccines. Int Rev Immunol (2020) 39:21–36. doi: 10.1080/08830185.2019.1685990
43. Thompson MG, Kwong JC, Regan AK, Katz MA, Drews SJ, Azziz-Baumgartner E, et al. Influenza Vaccine Effectiveness in Preventing Influenza-Associated Hospitalizations During Pregnancy: A Multi-Country Retrospective Test Negative Design Study, 2010-2016. Clin Infect Dis (2019) 68:1444–53. doi: 10.1093/cid/ciy737
44. Steinhoff MC, Omer SB, Roy E, Arifeen SE, Raqib R, Altaye M, et al. Influenza Immunization in Pregnancy–Antibody Responses in Mothers and Infants. N Engl J Med (2010) 362:1644–6. doi: 10.1056/NEJMc0912599
45. Eick AA, Uyeki TM, Klimov A, Hall H, Reid R, Santosham M, et al. Maternal Influenza Vaccination and Effect on Influenza Virus Infection in Young Infants. Arch Pediatr Adolesc Med (2011) 165:104–11. doi: 10.1001/archpediatrics.2010.192
46. Shakib JH, Korgenski K, Presson AP, Sheng X, Varner MW, Pavia AT, et al. Influenza in Infants Born to Women Vaccinated During Pregnancy. Pediatrics (2016) 137:e20152360. doi: 10.1542/peds.2015-2360
47. Regan AK, de Klerk N, Moore HC, Omer SB, Shellam G, Effler PV. Effect of Maternal Influenza Vaccination on Hospitalization for Respiratory Infections in Newborns: A Retrospective Cohort Study. Pediatr Infect Dis J (2016) 35:1097–103. doi: 10.1097/INF.0000000000001258
48. Tapia MD, Sow SO, Tamboura B, Tégueté I, Pasetti MF, Kodio M, et al. Maternal Immunisation With Trivalent Inactivated Influenza Vaccine for Prevention of Influenza in Infants in Mali: A Prospective, Active-Controlled, Observer-Blind, Randomised Phase 4 Trial. Lancet Infect Dis (2016) 16:1026–35. doi: 10.1016/S1473-3099(16)30054-8
49. Zaman K, Roy E, Arifeen SE, Rahman M, Raqib R, Wilson E, et al. Effectiveness of Maternal Influenza Immunization in Mothers and Infants. N Engl J Med (2008) 359:1555–64. doi: 10.1056/NEJMoa0708630
50. Benowitz I, Esposito DB, Gracey KD, Shapiro ED, Vázquez M. Influenza Vaccine Given to Pregnant Women Reduces Hospitalization Due to Influenza in Their Infants. Clin Infect Dis (2010) 51:1355–61. doi: 10.1086/657309
51. Poehling KA, Szilagyi PG, Staat MA, Snively BM, Payne DC, Bridges CB, et al. Impact of Maternal Immunization on Influenza Hospitalizations in Infants. Am J Obstet Gynecol (2011) 204:S141–8. doi: 10.1016/j.ajog.2011.02.042
52. Schlaudecker EP, Steinhoff MC, Omer SB, McNeal MM, Roy E, Arifeen SE, et al. IgA and Neutralizing Antibodies to Influenza a Virus in Human Milk: A Randomized Trial of Antenatal Influenza Immunization. PloS One (2013) 8:e70867. doi: 10.1371/journal.pone.0070867
53. Demers-Mathieu V, Huston RK, Markell AM, McCulley EA, Martin RL, Dallas DC. Antenatal Influenza A-Specific IgA, IgM, and IgG Antibodies in Mother’s Own Breast Milk and Donor Breast Milk, and Gastric Contents and Stools From Preterm Infants. Nutrients (2019) 11:1567. doi: 10.3390/nu11071567
54. Järvinen K-M, Suomalainen H. Leucocytes in Human Milk and Lymphocyte Subsets in Cow’s Milk-Allergic Infants. Pediatr Allergy Immunol (2002) 13:243–54. doi: 10.1034/j.1399-3038.2002.00087.x
55. Bertotto A, Gerli R, Fabietti G, Crupi S, Arcangeli C, Scalise F, et al. Human Breast Milk T Lymphocytes Display the Phenotype and Functional Characteristics of Memory T Cells. Eur J Immunol (1990) 20:1877–80. doi: 10.1002/eji.1830200838
56. Wirt DP, Adkins LT, Palkowetz KH, Schmalstieg FC, Goldman AS. Activated and Memory T Lymphocytes in Human Milk. Cytometry (1992) 13:282–90. doi: 10.1002/cyto.990130310
57. Sabbaj S, Ghosh MK, Edwards BH, Leeth R, Decker WD, Goepfert PA, et al. Breast Milk-Derived Antigen-Specific CD8+ T Cells: An Extralymphoid Effector Memory Cell Population in Humans. J Immunol (2005) 174:2951–6. doi: 10.4049/jimmunol.174.5.2951
58. Tuaillon E, Valea D, Becquart P, Al Tabaa Y, Meda N, Bollore K, et al. Human Milk-Derived B Cells: A Highly Activated Switched Memory Cell Population Primed to Secrete Antibodies. J Immunol (2009) 182:7155–62. doi: 10.4049/jimmunol.0803107
59. Demers-Mathieu V, DaPra C, Medo E. Influenza Vaccine Associated With the Gene Expression of T Cell Surface Markers in Human Milk. Breastfeed Med (2022) 17:218–25. doi: 10.1089/bfm.2021.0186
60. Henkle E, Steinhoff MC, Omer SB, Roy E, Arifeen SE, Raqib R, et al. The Effect of Exclusive Breast-Feeding on Respiratory Illness in Young Infants in a Maternal Immunization Trial in Bangladesh. Pediatr Infect Dis J (2013) 32:431–5. doi: 10.1097/INF.0b013e318281e34f
61. Tdap (Pertussis) Vaccine and Pregnancy (2021). Available at: https://www.cdc.gov/vaccines/pregnancy/hcp-toolkit/tdap-vaccine-pregnancy.html (Accessed June 8, 2022).
62. Winter K, Harriman K, Schechter R, Yamada E, Talarico J, Chavez G. Notes From the Field: Pertussis-California. MMWR Morb Mortal Wkly Rep (2010) 59(26):817.
63. About Diphtheria, Tetanus, and Pertussis Vaccination (2022). Available at: https://www.cdc.gov/vaccines/vpd/dtap-tdap-td/hcp/about-vaccine.html (Accessed June 8, 2022).
64. Vilajeliu A, Goncé A, López M, Costa J, Rocamora L, Ríos J, et al. Combined Tetanus-Diphtheria and Pertussis Vaccine During Pregnancy: Transfer of Maternal Pertussis Antibodies to the Newborn. Vaccine (2015) 33:1056–62. doi: 10.1016/j.vaccine.2014.12.062
65. Abu Raya B, Srugo I, Kessel A, Peterman M, Bader D, Peri R, et al. The Induction of Breast Milk Pertussis Specific Antibodies Following Gestational Tetanus-Diphtheria-Acellular Pertussis Vaccination. Vaccine (2014) 32:5632–7. doi: 10.1016/j.vaccine.2014.08.006
66. De Schutter S, Maertens K, Baerts L, De Meester I, Van Damme P, Leuridan E. Quantification of Vaccine-Induced Antipertussis Toxin Secretory IgA Antibodies in Breast Milk: Comparison of Different Vaccination Strategies in Women. Pediatr Infect Dis J (2015) 34:e149–52. doi: 10.1097/INF.0000000000000675
67. Amirthalingam G, Andrews N, Campbell H, Ribeiro S, Kara E, Donegan K, et al. Effectiveness of Maternal Pertussis Vaccination in England: An Observational Study. Lancet (2014) 384:1521–8. doi: 10.1016/S0140-6736(14)60686-3
68. Dabrera G, Amirthalingam G, Andrews N, Campbell H, Ribeiro S, Kara E, et al. A Case-Control Study to Estimate the Effectiveness of Maternal Pertussis Vaccination in Protecting Newborn Infants in England and Wales, 2012-2013. Clin Infect Dis (2015) 60:333–7. doi: 10.1093/cid/ciu821
69. Baxter R, Bartlett J, Fireman B, Lewis E, Klein NP. Effectiveness of Vaccination During Pregnancy to Prevent Infant Pertussis. Pediatrics (2017) 139:e20164091. doi: 10.1542/peds.2016-4091
70. Amirthalingam G, Campbell H, Ribeiro S, Fry NK, Ramsay M, Miller E, et al. Sustained Effectiveness of the Maternal Pertussis Immunization Program in England 3 Years Following Introduction. Clin Infect Dis (2016) 63:S236–43. doi: 10.1093/cid/ciw559
71. Winter K, Cherry JD, Harriman K. Effectiveness of Prenatal Tetanus, Diphtheria, and Acellular Pertussis Vaccination on Pertussis Severity in Infants. Clin Infect Dis (2017) 64:9–14. doi: 10.1093/cid/ciw633
72. Orije MRP, Larivière Y, Herzog SA, Mahieu LM, Van Damme P, Leuridan E, et al. Breast Milk Antibody Levels in Tdap-Vaccinated Women After Preterm Delivery. Clin Infect Dis (2021) 73:e1305–13. doi: 10.1093/cid/ciab260
73. Curtis CR, Baughman AL, DeBolt C, Goodykoontz S, Kenyon C, Watson B, et al. Risk Factors Associated With Bordetella Pertussis Among Infants ≤4 Months of Age in the Pre-Tdap Era: United States, 2002-2005. Pediatr Infect Dis J (2017) 36:726–35. doi: 10.1097/INF.0000000000001528
74. Office of the Commissioner. FDA Takes Key Action in Fight Against COVID-19 by Issuing Emergency Use Authorization for First COVID-19 Vaccine . US Food and Drug Administration. Available at: https://www.fda.gov/news-events/press-announcements/fda-takes-key-action-fight-against-covid-19-issuing-emergency-use-authorization-first-covid-19 (Accessed June 8, 2022).
75. Office of the Commissioner. FDA Takes Additional Action in Fight Against COVID-19 by Issuing Emergency Use Authorization for Second COVID-19 Vaccine . US Food and Drug Administration. Available at: https://www.fda.gov/news-events/press-announcements/fda-takes-additional-action-fight-against-covid-19-issuing-emergency-use-authorization-second-covid (Accessed June 8, 2022).
76. Voysey M, Clemens SAC, Madhi SA, Weckx LY, Folegatti PM, Aley PK, et al. Safety and Efficacy of the ChAdOx1 Ncov-19 Vaccine (AZD1222) Against SARS-CoV-2: An Interim Analysis of Four Randomised Controlled Trials in Brazil, South Africa, and the UK. Lancet (2021) 397:99–111. doi: 10.1016/S0140-6736(20)32661-1
77. Sadoff J, Gray G, Vandebosch A, Cárdenas V, Shukarev G, Grinsztejn B, et al. Safety and Efficacy of Single-Dose Ad26.COV2.S Vaccine Against Covid-19. N Engl J Med (2021) 384:2187–201. doi: 10.1056/NEJMoa2101544
78. Juncker HG, Mulleners SJ, Coenen ERM, van Goudoever JB, van Gils MJ, van Keulen BJ. Comparing Human Milk Antibody Response After 4 Different Vaccines for COVID-19. JAMA Pediatr (2022) 176:611–2. doi: 10.1001/jamapediatrics.2022.0084
79. Lechosa-Muñiz C, Paz-Zulueta M, Mendez-Legaza JM, Irure-Ventura J, Cuesta González R, Calvo Montes J, et al. Induction of SARS-CoV-2-Specific IgG and IgA in Serum and Milk With Different SARS-CoV-2 Vaccines in Breastfeeding Women: A Cross-Sectional Study in Northern Spain. Int J Environ Res Public Health (2021) 18:8831. doi: 10.3390/ijerph18168831
80. Selma-Royo M, Bäuerl C, Mena-Tudela D, Aguilar-Camprubí L, Pérez-Cano FJ, Parra-Llorca A, et al. Anti-SARS-CoV-2 IgA and IgG in Human Milk After Vaccination is Dependent on Vaccine Type and Previous SARS-CoV-2 Exposure: A Longitudinal Study. Genome Med (2022) 14:42. doi: 10.1186/s13073-022-01043-9
81. Polack FP, Thomas SJ, Kitchin N, Absalon J, Gurtman A, Lockhart S, et al. Safety and Efficacy of the BNT162b2 mRNA Covid-19 Vaccine. N Engl J Med (2020) 383:2603–15. doi: 10.1056/NEJMoa2034577
82. Baden LR, El Sahly HM, Essink B, Kotloff K, Frey S, Novak R, et al. Efficacy and Safety of the mRNA-1273 SARS-CoV-2 Vaccine. N Engl J Med (2021) 384:403–16. doi: 10.1056/NEJMoa2035389
83. Thomas SJ, Moreira ED Jr, Kitchin N, Absalon J, Gurtman A, Lockhart S, et al. Safety and Efficacy of the BNT162b2 mRNA Covid-19 Vaccine Through 6 Months. N Engl J Med (2021) 385:1761–73. doi: 10.1056/NEJMoa2110345
84. Dagan N, Barda N, Biron-Shental T, Makov-Assif M, Key C, Kohane IS, et al. Effectiveness of the BNT162b2 mRNA COVID-19 Vaccine in Pregnancy. Nat Med (2021) 27:1693–5. doi: 10.1038/s41591-021-01490-8
85. Male V. SARS-CoV-2 Infection and COVID-19 Vaccination in Pregnancy. Nat Rev Immunol (2022) 22:277–82. doi: 10.1038/s41577-022-00703-6
86. Muyldermans J, De Weerdt L, De Brabandere L, Maertens K, Tommelein E. The Effects of COVID-19 Vaccination on Lactating Women: A Systematic Review of the Literature. Front Immunol (2022) 13:852928. doi: 10.3389/fimmu.2022.852928
87. Zauche LH, Wallace B, Smoots AN, Olson CK, Oduyebo T, Kim SY, et al. Receipt of mRNA Covid-19 Vaccines and Risk of Spontaneous Abortion. N Engl J Med (2021) 385:1533–5. doi: 10.1056/NEJMc2113891
88. Magnus MC, Gjessing HK, Eide HN, Wilcox AJ, Fell DB, Håberg SE. Covid-19 Vaccination During Pregnancy and First-Trimester Miscarriage. N Engl J Med (2021) 385:2008–10. doi: 10.1056/NEJMc2114466
89. Lipkind HS, Vazquez-Benitez G, DeSilva M, Vesco KK, Ackerman-Banks C, Zhu J, et al. Receipt of COVID-19 Vaccine During Pregnancy and Preterm or Small-for-Gestational-Age at Birth - Eight Integrated Health Care Organizations, United States, December 15, 2020-July 22, 2021. MMWR Morb Mortal Wkly Rep (2022) 71:26–30. doi: 10.15585/mmwr.mm7101e1
90. Citu IM, Citu C, Gorun F, Sas I, Tomescu L, Neamtu R, et al. Immunogenicity Following Administration of BNT162b2 and Ad26.COV2.S COVID-19 Vaccines in the Pregnant Population During the Third Trimester. Viruses (2022) 14:307. doi: 10.3390/v14020307
91. Blakeway H, Prasad S, Kalafat E, Heath PT, Ladhani SN, Le Doare K, et al. COVID-19 Vaccination During Pregnancy: Coverage and Safety. Am J Obstet Gynecol (2022) 226:236. doi: 10.1016/j.ajog.2021.08.007
92. Shimabukuro TT, Kim SY, Myers TR, Moro PL, Oduyebo T, Panagiotakopoulos L, et al. Preliminary Findings of mRNA Covid-19 Vaccine Safety in Pregnant Persons. N Engl J Med (2021) 384:2273–82. doi: 10.1056/NEJMoa2104983
93. Olson C. COVID-19 Vaccine Safety in Pregnancy. In: Updates From the V-Safe COVID-19 Vaccine Pregnancy Registry.
94. Magnus MC, Örtqvist AK, Dahlqwist E, Ljung R, Skår F, Oakley L, et al. Association of SARS-CoV-2 Vaccination During Pregnancy With Pregnancy Outcomes. JAMA (2022) 327:1469–77. doi: 10.1001/jama.2022.3271
95. Fell DB, Dhinsa T, Alton GD, Török E, Dimanlig-Cruz S, Regan AK, et al. Association of COVID-19 Vaccination in Pregnancy With Adverse Peripartum Outcomes. JAMA (2022) 327:1478–87. doi: 10.1001/jama.2022.4255
96. Gray KJ, Bordt EA, Atyeo C, Deriso E, Akinwunmi B, Young N, et al. Coronavirus Disease 2019 Vaccine Response in Pregnant and Lactating Women: A Cohort Study. Am J Obstet Gynecol (2021) 225:303.e1–303.e17. doi: 10.1016/j.ajog.2021.03.023
97. Collier A-RY, McMahan K, Yu J, Tostanoski LH, Aguayo R, Ansel J, et al. Immunogenicity of COVID-19 mRNA Vaccines in Pregnant and Lactating Women. JAMA (2021) 325:2370–80. doi: 10.1001/jama.2021.7563
98. Prahl M, Golan Y, Cassidy AG, Matsui Y, Li L, Alvarenga B, et al. Evaluation of Transplacental Transfer of mRNA Vaccine Products and Functional Antibodies During Pregnancy and Early Infancy. medRxiv (2021). doi: 10.1101/2021.12.09.21267423
99. Halasa NB, Olson SM, Staat MA, Newhams MM, Price AM, Boom JA, et al. Effectiveness of Maternal Vaccination With mRNA COVID-19 Vaccine During Pregnancy Against COVID-19-Associated Hospitalization in Infants Aged <6 Months - 17 States, July 2021-January 2022. MMWR Morb Mortal Wkly Rep (2022) 71:264–70. doi: 10.15585/mmwr.mm7107e3
100. Carlsen EØ, Magnus MC, Oakley L, Fell DB, Greve-Isdahl M, Kinge JM, et al. Association of COVID-19 Vaccination During Pregnancy With Incidence of SARS-CoV-2 Infection in Infants. JAMA Intern Med (2022). doi: 10.1001/jamainternmed.2022.2442
101. Yeo KT, Chia WN, Tan CW, Ong C, Yeo JG, Zhang J, et al. Neutralizing Activity and SARS-CoV-2 Vaccine mRNA Persistence in Serum and Breastmilk After BNT162b2 Vaccination in Lactating Women. Front Immunol (2021) 12:783975. doi: 10.3389/fimmu.2021.783975
102. Kelly JC, Carter EB, Raghuraman N, Nolan LS, Gong Q, Lewis AN, et al. Anti-Severe Acute Respiratory Syndrome Coronavirus 2 Antibodies Induced in Breast Milk After Pfizer-BioNTech/BNT162b2 Vaccination. Am J Obstet Gynecol (2021) 225:101–3. doi: 10.1016/j.ajog.2021.03.031
103. Perl SH, Uzan-Yulzari A, Klainer H, Asiskovich L, Youngster M, Rinott E, et al. SARS-CoV-2-Specific Antibodies in Breast Milk After COVID-19 Vaccination of Breastfeeding Women. JAMA (2021) 325:2013–4. doi: 10.1001/jama.2021.5782
104. Rosenberg-Friedman M, Kigel A, Bahar Y, Werbner M, Alter J, Yogev Y, et al. BNT162b2 mRNA Vaccine Elicited Antibody Response in Blood and Milk of Breastfeeding Women. Nat Commun (2021) 12:6222. doi: 10.1038/s41467-021-26507-1
105. Young BE, Seppo AE, Diaz N, Rosen-Carole C, Nowak-Wegrzyn A, Cruz Vasquez JM, et al. Association of Human Milk Antibody Induction, Persistence, and Neutralizing Capacity With SARS-CoV-2 Infection vs mRNA Vaccination. JAMA Pediatr (2022) 176:159–68. doi: 10.1001/jamapediatrics.2021.4897
106. Esteve-Palau E, Gonzalez-Cuevas A, Guerrero ME, Garcia-Terol C, Alvarez MC, Casadevall D, et al. Quantification of Specific Antibodies Against SARS-CoV-2 in Breast Milk of Lactating Women Vaccinated With an mRNA Vaccine. JAMA Netw Open (2021) 4:e2120575. doi: 10.1001/jamanetworkopen.2021.20575
107. Atyeo C, DeRiso EA, Davis C, Bordt EA, De Guzman RM, Shook LL, et al. COVID-19 mRNA Vaccines Drive Differential Antibody Fc-Functional Profiles in Pregnant, Lactating, and Nonpregnant Women. Sci Transl Med (2021) 13:eabi8631. doi: 10.1126/scitranslmed.abi8631
108. Fox A, Marino J, Amanat F, Krammer F, Hahn-Holbrook J, Zolla-Pazner S, et al. Robust and Specific Secretory IgA Against SARS-CoV-2 Detected in Human Milk. iScience (2020) 23:101735. doi: 10.1016/j.isci.2020.101735
109. Shook LL, Atyeo CG, Yonker LM, Fasano A, Gray KJ, Alter G, et al. Durability of Anti-Spike Antibodies in Infants After Maternal COVID-19 Vaccination or Natural Infection. JAMA (2022) 327:1087–9. doi: 10.1001/jama.2022.1206
110. Lapuente D, Fuchs J, Willar J, Vieira Antão A, Eberlein V, Uhlig N, et al. Protective Mucosal Immunity Against SARS-CoV-2 After Heterologous Systemic Prime-Mucosal Boost Immunization. Nat Commun (2021) 12:6871. doi: 10.1038/s41467-021-27063-4
111. Armistead B, Jiang Y, Carlson M, Ford ES, Jani S, Houck J, et al. Mucosal Memory T Cells in Breastmilk are Modulated by SARS-CoV-2 mRNA Vaccination. bioRxiv (2021). doi: 10.1101/2021.12.03.21267036
112. Cabinian A, Sinsimer D, Tang M, Zumba O, Mehta H, Toma A, et al. Transfer of Maternal Immune Cells by Breastfeeding: Maternal Cytotoxic T Lymphocytes Present in Breast Milk Localize in the Peyer’s Patches of the Nursed Infant. PloS One (2016) 11:e0156762. doi: 10.1371/journal.pone.0156762
113. Camacho-Morales A, Caba M, García-Juárez M, Caba-Flores MD, Viveros-Contreras R, Martínez-Valenzuela C. Breastfeeding Contributes to Physiological Immune Programming in the Newborn. Front Pediatr (2021) 9:744104. doi: 10.3389/fped.2021.744104
114. Gonçalves J, Juliano AM, Charepe N, Alenquer M, Athayde D, Ferreira F, et al. Secretory IgA and T Cells Targeting SARS-CoV-2 Spike Protein are Transferred to the Breastmilk Upon mRNA Vaccination. Cell Rep Med (2021) 2:100468. doi: 10.1016/j.xcrm.2021.100468
115. Cao Q, Chen Y-C, Chen C-L, Chiu C-H. SARS-CoV-2 Infection in Children: Transmission Dynamics and Clinical Characteristics. J Formos Med Assoc (2020) 119:670–3. doi: 10.1016/j.jfma.2020.02.009
116. Yonker LM, Gilboa T, Ogata AF, Senussi Y, Lazarovits R, Boribong BP, et al. Multisystem Inflammatory Syndrome in Children is Driven by Zonulin-Dependent Loss of Gut Mucosal Barrier. J Clin Invest (2021) 131(14):e149633. doi: 10.1172/jci149633
117. Xiao F, Tang M, Zheng X, Liu Y, Li X, Shan H. Evidence for Gastrointestinal Infection of SARS-CoV-2. Gastroenterology (2020) 158:1831–1833.e3. doi: 10.1053/j.gastro.2020.02.055
118. Zhang Y, Geng X, Tan Y, Li Q, Xu C, Xu J, et al. New Understanding of the Damage of SARS-CoV-2 Infection Outside the Respiratory System. BioMed Pharmacother (2020) 127:110195. doi: 10.1016/j.biopha.2020.110195
119. Narayanaswamy V, Pentecost BT, Schoen CN, Alfandari D, Schneider SS, Baker R, et al. Neutralizing Antibodies and Cytokines in Breast Milk After Coronavirus Disease 2019 (COVID-19) mRNA Vaccination. Obstet Gynecol (2022) 139:181–91. doi: 10.1097/AOG.0000000000004661
120. CDC. COVID-19 Vaccines While Pregnant or Breastfeeding (2022). Centers for Disease Control and Prevention. Available at: https://www.cdc.gov/coronavirus/2019-ncov/vaccines/recommendations/pregnancy.html?s_cid=10484:covid (Accessed June 8, 2022).
121. WHO Recommends Continuing Breastfeeding During COVID-19 Infection and After Vaccination (2021). Available at: https://www.euro.who.int/en/media-centre/sections/press-releases/2021/who-recommends-continuing-breastfeeding-during-covid-19-infection-and-after-vaccination (Accessed June 8, 2022).
122. McLaurin-Jiang S, Garner CD, Krutsch K, Hale TW. Maternal and Child Symptoms Following COVID-19 Vaccination Among Breastfeeding Mothers. Breastfeed Med (2021) 16:702–9. doi: 10.1089/bfm.2021.0079
123. Kachikis A, Englund JA, Singleton M, Covelli I, Drake AL, Eckert LO. Short-Term Reactions Among Pregnant and Lactating Individuals in the First Wave of the COVID-19 Vaccine Rollout. JAMA Netw Open (2021) 4:e2121310. doi: 10.1001/jamanetworkopen.2021.21310
Keywords: human milk, COVID - 19, influenza, pertussis, vaccination, infant health, immunization
Citation: Hunagund S, Golan Y, Asiodu IV, Prahl M and Gaw SL (2022) Effects of Vaccination Against Influenza, Pertussis, and COVID-19 on Human Milk Antibodies: Current Evidence and Implications for Health Equity. Front. Immunol. 13:910383. doi: 10.3389/fimmu.2022.910383
Received: 01 April 2022; Accepted: 20 June 2022;
Published: 12 July 2022.
Edited by:
Johannes (Hans) Van Goudoever, Amsterdam University Medical Center, NetherlandsReviewed by:
Britt Van Keulen, Amsterdam University Medical Center, NetherlandsCopyright © 2022 Hunagund, Golan, Asiodu, Prahl and Gaw. This is an open-access article distributed under the terms of the Creative Commons Attribution License (CC BY). The use, distribution or reproduction in other forums is permitted, provided the original author(s) and the copyright owner(s) are credited and that the original publication in this journal is cited, in accordance with accepted academic practice. No use, distribution or reproduction is permitted which does not comply with these terms.
*Correspondence: Stephanie L. Gaw, Stephanie.Gaw@ucsf.edu
†These authors have contributed equally to this work