- 1Department of Medical Oncology, National Cancer Center/National Clinical Research Center for Cancer/Cancer Hospital, Chinese Academy of Medical Sciences and Peking Union Medical College, Beijing, China
- 2Department of Comprehensive Oncology, National Cancer Center/National Clinical Research Center for Cancer/Cancer Hospital, Chinese Academy of Medical Sciences and Peking Union Medical College, Beijing, China
Background: Synergistic anti-tumor effects were observed in vivo and in vitro when immune checkpoint inhibitors (ICIs) were combined with denosumab. However, the clinical benefit and safety of this synergy have not been adequately evaluated in non-small cell lung cancer (NSCLC).
Methods: Consecutive charts of NSCLC patients with bone metastases between December 2020 and December 2021 in the Chinese National Cancer Center were reviewed. The entire cohort was divided into one experimental group (denosumab + ICIs [DI]) and three control groups (denosumab + non-ICIs [DnI], phosphates + ICIs [PI], phosphates + non-ICIs [PnI]). Real-world objective response rates (ORRs), median progression-free survival (mPFS), skeletal-related events (SREs), and adverse events (AEs) were compared between groups.
Results: A total of 171/410 (41.7%) patients with advanced or recurrent NSCLC carrying bone metastases who received bone-targeted therapy were eligible for analysis. Although the DI group showed a better benefit trend, differences were not statistically significant concerning the therapeutic efficacy among the DI group (n = 40), PI group (n = 74), DnI group (n = 15), and PnI group (n = 42) (ORRs: 47.5%, 43.2%, 33.3%, and 40.5%, respectively, p = 0.799; and mPFS: 378, 190, 170, and 172 days, respectively, p = 0.115; SREs: 5%, 10.8%, 13.3%, and 11.9%, respectively, p = 0.733). Nevertheless, further analysis in the NON-DRIVER cohort revealed a greater benefit for the DI group (p = 0.045). Additionally, the AEs of the DI group were not significantly different from those of the PI, DnI, and PnI groups (AEs: 27.5%, 39.2%, 26.7%, and 28.6%, respectively, p = 0.742). Furthermore, the multivariate analysis revealed the independent prognostic role of DI treatment for PFS in the overall cohort. Within the DI group, we did not observe differences in benefit among different mutational subgroups (p = 0.814), but patients with single-site bone metastasis (p = 0.319) and high PD-L1 expression (p = 0.100) appeared to benefit more, though no significant differences were observed.
Conclusions: Denosumab exhibited synergistic antitumor efficacy without increasing toxicity when used concomitantly with ICIs in patients with advanced non-small cell lung cancer carrying bone metastases.
1. Introduction
In recent years, lung cancer incidence and mortality rates have remained high as the aging population has intensified, along with the effects of industrialization and air pollution (1). As the main body of lung cancer, the 5-year overall survival (OS) rate of metastatic non-small cell lung cancer (NSCLC) patients is only 5% (2). In the past 20 years, the treatment of lung cancer has undergone radical changes, especially with the in-depth development of the molecular pathology of lung cancer and the rise of immunotherapy, including monoclonal antibodies (mAbs) blocking programmed cell death 1 (PD-1)/PD1 ligand 1 (PD-L1) and the cytotoxic T-lymphocyte-associated antigen 4 (CTLA-4), known as immune checkpoint inhibitors (ICIs). In the renowned KEYNOTE-024 trial, pembrolizumab obtained a 5-year OS rate of 31.9%, which is granted as an effective first-line treatment option for NSCLC patients with PD-L1 TPS ≥50% by the FDA (3). However, ICI resistance is a challenge that we must embrace. To overcome the resistance and expand the population benefiting from ICIs, non-redundant mechanisms of tumor-induced immunosuppression need to be explored, and combinatory therapy is expected to be more effective (4).
Receptor activator of nuclear factorκB ligand (RANKL, also called TNFSF11) is a member of the tumor necrosis factor (TNF) superfamily and a ligand both for RANK (also called TNFRSF11A) and osteoprotegerin (OPG, also called TNFRSF11B) (5). The RANK–RANKL–OPG axis is essential for physiological bone resorption and destruction, and it also plays an important role in pathological states such as osteoporosis and bone destruction at the foci of bone metastases (2, 5–7). As the first fully human anti-RANKL mAb, denosumab was demonstrated to be non-inferior to zoledronic acid (ZA) in delaying time to the first on-study skeletal-related events (SREs) in a randomized, double-blind study enrolling multiple advanced cancer types (including lung cancer) (8) and has been approved by the FDA for preventing SREs in solid tumors. Unexpectedly, the exploratory analysis also revealed an OS benefit of denosumab over ZA in NSCLC patients with bone metastases (hazard ratio [HR] = 0.78, 9.5 vs. 8.0 months; p = 0.01) (9).
Increasing evidence indicates that the survival benefit may stem from the synergistic anti-tumor effects of the combination of ICIs and denosumab (10–14). Series studies conducted by Ahern et al. revealed via a mouse model, that the combination of RANKL inhibitor and ICIs significantly increased the number of infiltrating T cells and expression of anti-tumor cytokines (IFN-γ, etc.) in the tumor microenvironment (TME) compared to a single agent, and the combination therapy significantly reduced mouse tumor burden (11, 12).
Recently, several retrospective studies have suggested the feasibility of this combination regimen in advanced NSCLC patients with bone metastases (14–18). However, the findings of these studies need further confirmation due to the lack of suitable control groups and the presence of confounding factors. This study evaluated the efficacy and safety of the combination of ICIs and denosumab for advanced NSCLC patients with bone metastases in a real-world setting.
2. Methods
2.1. Study design and rationale
A retrospective, observational chart review was conducted on NSCLC patients with bone metastases who were enrolled in the Chinese National Cancer Center between December 2020 and December 2021. To fully assess the synergistic effects of denosumab and ICIs, based on the therapeutic pattern of systematic therapy and bone-targeted therapy (BTT), the entire cohort was divided into one experimental group (denosumab + ICIs [DI]) and three control groups (denosumab + non-ICIs [DnI], phosphates + ICIs [PI], phosphates + non-ICIs [PnI]). Real-world objective response rates (ORRs), median progression-free survival (mPFS), adverse events (AEs), and SREs were planned to be compared between groups. The DnI and PnI groups were set up to verify whether a difference in efficacy existed between denosumab and phosphates in the absence of ICIs (in the context of no synergistic condition existing), thus establishing a baseline for comparison between the DI and PI groups. On this basis, a synergistic effect of DI treatment would be confirmed if the efficacy of the DI group was better than that of the PI group (Figure 1).
2.2. Patient eligibility, grouping, and data collection
Patients diagnosed with NSCLC who have received chemotherapy either alone or along with ICI (pembrolizumab, nivolumab, atezolizumab, sintilimab, or camrelizumab) as well as concomitant BTT (phosphates [including zoledronic acid, pamidronate disodium, or ibandronate monosodium] or denosumab) were identified. Concomitant therapy was defined as receipt of BTT at any point before systematic therapy (chemotherapy combined with ICI or not) initiation, or no later than 30 days following systematic therapy initiated at least 4 months before the data cutoff (31 December 2021). Demographics, clinicopathological information, molecular features, and detailed treatment history data were extracted from electronic medical records. Patients with too much key clinical information missing were excluded.
Sub-cohorts were defined during the data analysis. The NON-DRIVER cohort included cases without EGFR, HER-2, ALK, ROS1, MET, RET, and BRAF mutations, except for KRAS mutations. The WILD-TYPE cohort included cases without EGFR, HER-2, ALK, ROS1, MET, RET, BRAF, or KRAS mutations.
All charts were reviewed by the primary author, the confidentiality of all patients was maintained by assigning each patient a study number, and all data were securely stored in the hospital. The study was conducted in accordance with the Declaration of Helsinki (as revised in 2013). Institutional Review Board approval of the study protocol was obtained (No.: NCC-008296) before study conduct and informed patient consent was waived as this was a retrospective study.
2.3. Treatment and efficacy/toxicity evaluation
In this real-world study, denosumab was administered subcutaneously at 120 mg approximately every 28 days, while phosphates were administered intravenously approximately every 21 days. The PD-1 or PD-L1 inhibitor was administered by intravenous injection approximately every 3 weeks, and the specific dosage was determined according to the specific drug instructions. Phosphates were generally administered within three days of the administration of ICIs. Patient compliance was confirmed from the electronic medical records.
Real-world tumor response was analyzed and produced by trained extractors following a pre-defined process, including an integrated assessment of radiologist reports and clinical documentation data. The frequency of imaging review to assess response was every 6–8 weeks in a real-world setting. The objective tumor response was determined according to the Response Evaluation Criteria in Solid Tumors (RECIST 1.1) guidelines (19). The objective response was divided into two categories: the objective response was divided into complete response (CR) and partial response (PR), while the disease control was divided into CR, PR, or stable disease (SD).
Toxicity was assessed according to the Common Terminology Criteria for Adverse Events (version 5.0). Acute phase AEs such as flu-like reactions, including fever, myalgia, and chills, were counted only as treatment-related if they occurred within 24 h of phosphate infusion; otherwise, they were not counted as AEs to BTT.
2.4. Statistical analysis
Categorical variables were reported as absolute numbers and percentages. The chi-square test was used for comparisons between different groups. The data cut-off date was 28 February 2022, when the disease status of the patients was confirmed. PFS was defined as the time from concomitant administration to disease progression or death from any cause. Patients who were lost to follow-up were judged to be censored and the last determinable time of survival was used as the time of termination of follow-up. The relationship between various variables and survival was evaluated using the Kaplan–Meier method. Differences between survival curves were tested for statistical significance using the Log-rank test. Significant prognostic predictors for patients identified by univariate analyses were further assessed by multivariate analyses using the Cox proportional hazards regression model. Statistical analyses were performed, and analytic graphs were created using GraphPad Prism 8 software (GraphPad Software, San Diego, CA, USA). An α value of 0.05 was used as the examination standard.
3. Results
3.1 Baseline characteristics
In total, 171/410 (41.7%) patients with NSCLC carrying bone metastases who were treated with BTT were enrolled at the Chinese National Cancer Center between December 2020 and December 2021 (Figure 2). Based on different treatment combinations of systematic therapy and BTT, the overall cohort was divided into 4 groups: DI (n = 40), PI (n = 74), DnI (n = 15), and PnI (n = 42). The baseline characteristics of the four groups are displayed in Table 1. A higher proportion of adenocarcinoma histology was observed in the DnI and PnI groups (p = 0.038), while a higher proportion of PD-L1 expression in the DI and PI groups (p = 0.010) was observed, and the highest proportion of KRAS mutation in the DI group (p = 0.145) was revealed, despite a significant difference being unreached. No statistically significant differences were observed for other baseline characteristics.
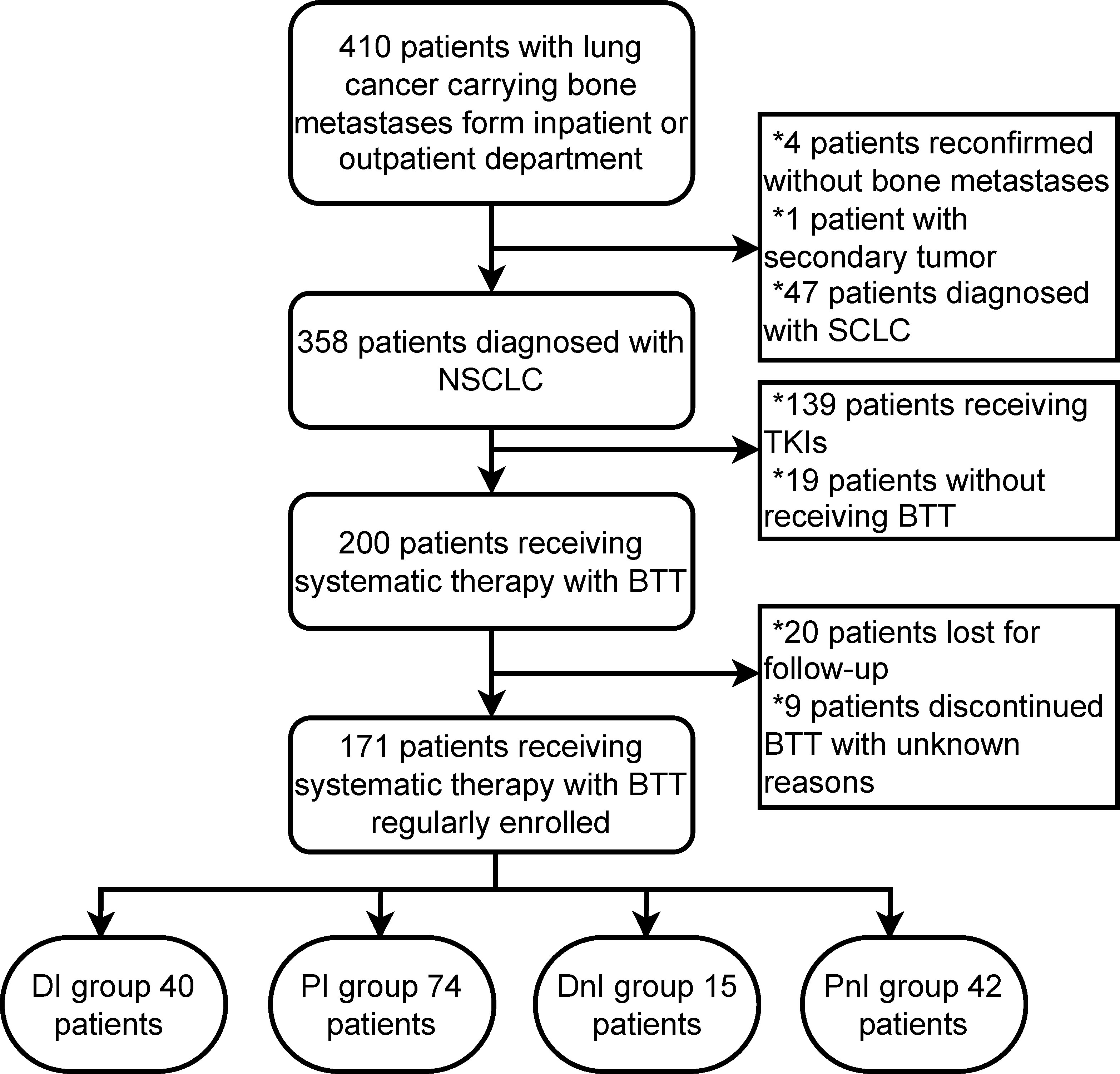
Figure 2 Flow chart of patient selection. NSCLC, non-small cell lung cancer; SCLC, small-cell lung cancer; BTT, bone-targeted therapy; TKIs, tyrosine-kinase inhibitors; DI, denosumab + ICIs; DnI, denosumab + non-ICIs; PI, phosphates + ICIs; PnI, phosphates + non-ICIs.
For the DI group, a predominant proportion of males (87.5%) was observed. More than half (60%) of patients were current smokers, and the majority of patients (77.5%) had adenocarcinoma histology. Non-driver patients (including KRAS-mutated and wild-type ones) account for most of the DI group. Nearly 70% of patients initiated BTT along with the first-line systematic therapy. Most patients had multiple bone metastases (85%) and a PS status of 1 (67.5%) (Table 1).
3.2 Efficacy evaluation
Among the 40 evaluable patients in the DI group, 19 (47.5%), 19 (47.5%), and two (5%) had PR, SD, and de novo resistance to DI treatment, respectively. The ORR was 47.5% and the disease control rate (DCR) was 95% (Figure 3). At the data cut-off date, the mPFS was 378 days (95% CI, 118.5–636.5 days), and the median follow-up duration was 198 days (95% CI, 181.6–214.4 days) in the DI group. The PFS was mature in 14 (35%) patients, and the tumors of 26 patients were still under control (Figure 4).
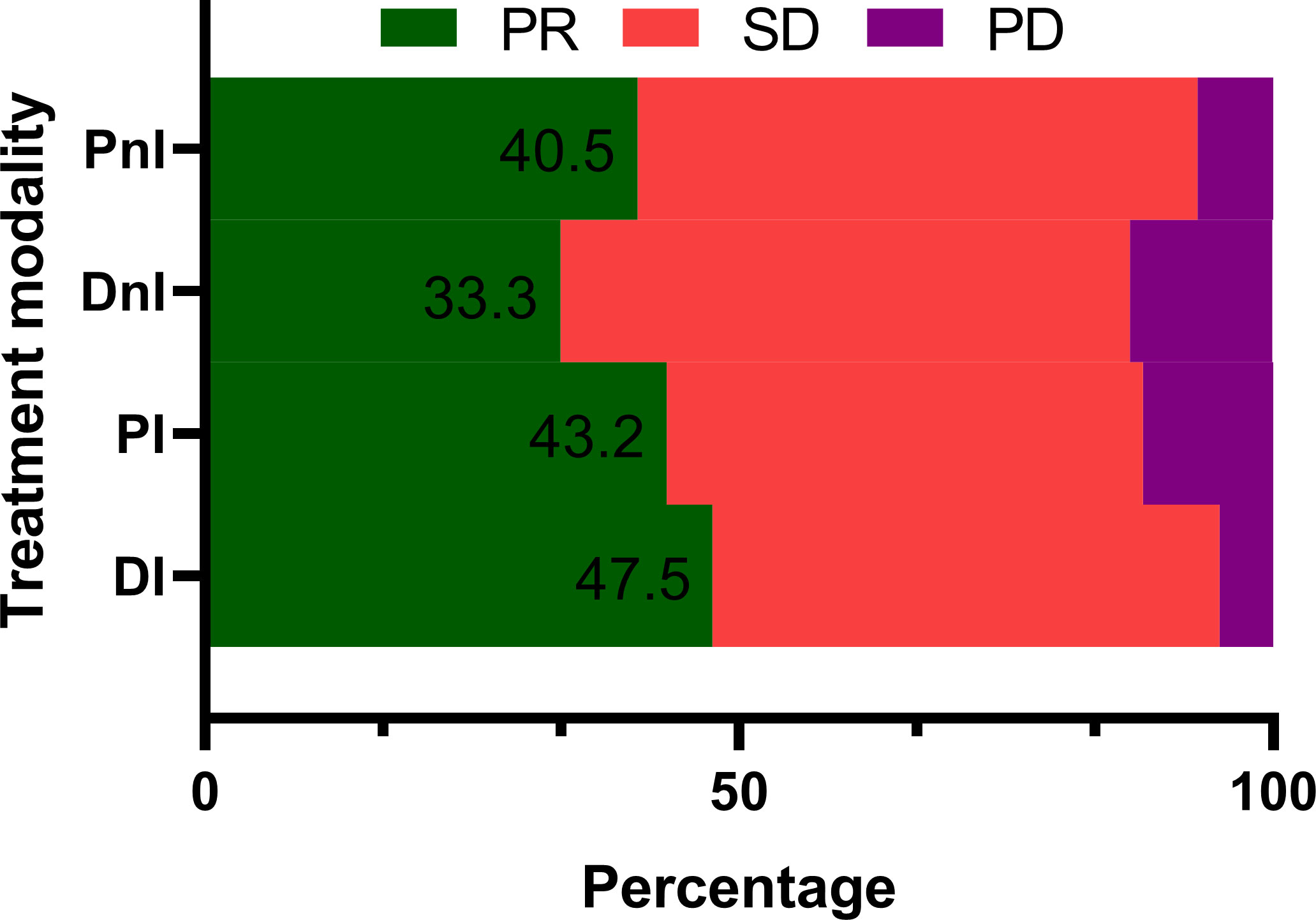
Figure 3 Treatment responses of different treatment modalities (n = 171). PR, partial response; SD, stable disease; PD, progressive disease; DI, denosumab + ICIs; DnI, denosumab + non-ICIs; PI, phosphates + ICIs; PnI, phosphates + non-ICIs.
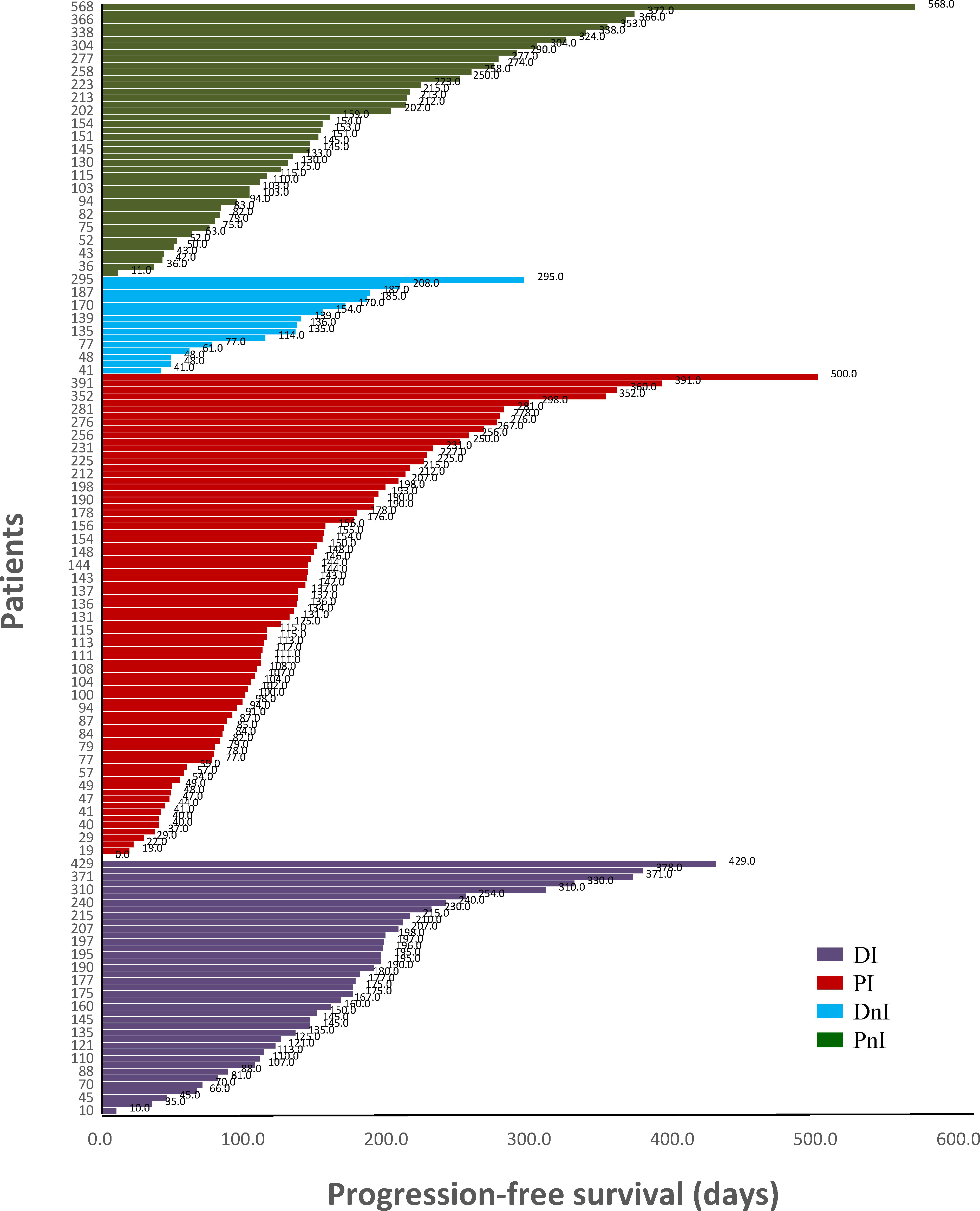
Figure 4 Swimming plot of different treatment modalities (n = 171). DI, denosumab + ICIs; DnI, denosumab + non-ICIs; PI, phosphates + ICIs; PnI, phosphates + non-ICIs.
By contrast, the DI group showed a trend for better ORR (Figure 3) and mPFS (Figure 5A) than those of the PI, DnI, and PnI groups (ORRs: 47.5%, 43.2%, 33.3%, and 40.5%, respectively, p = 0.799; and mPFS: 378, 190, 170, and 172 days, respectively, p = 0.115), though the differences were not statistically significant. To exclude the confounding effect of driver genes on efficacy, we extracted the NON-DRIVER (including KRAS-mutated and wild-type cases) cohort, WILD-TYPE cohort, and KRAS cohort from the overall cohort. Kaplan–Meier analysis in the NON-DRIVER cohort confirmed a statistically significant benefit for the DI group over the control groups (mPFS: NR, 225, 170, and 133 days, respectively, p = 0.045) (Figure 5B). In the WILD-TYPE cohort, a more pronounced benefit for the DI group appeared to be observed, but due to the reduced cohort scale, there was insufficient statistical power to demonstrate a statistically significant difference (p = 0.125) (Figure 5C). In the KRAS cohort, the DI group also showed a trend for better therapeutic efficacy than that of the control groups (mPFS: 230, 148, 170, and 133 days, respectively). Nevertheless, the advantage of the mPFS of the DI group was less obvious (p = 0.452) (Figure 5D).
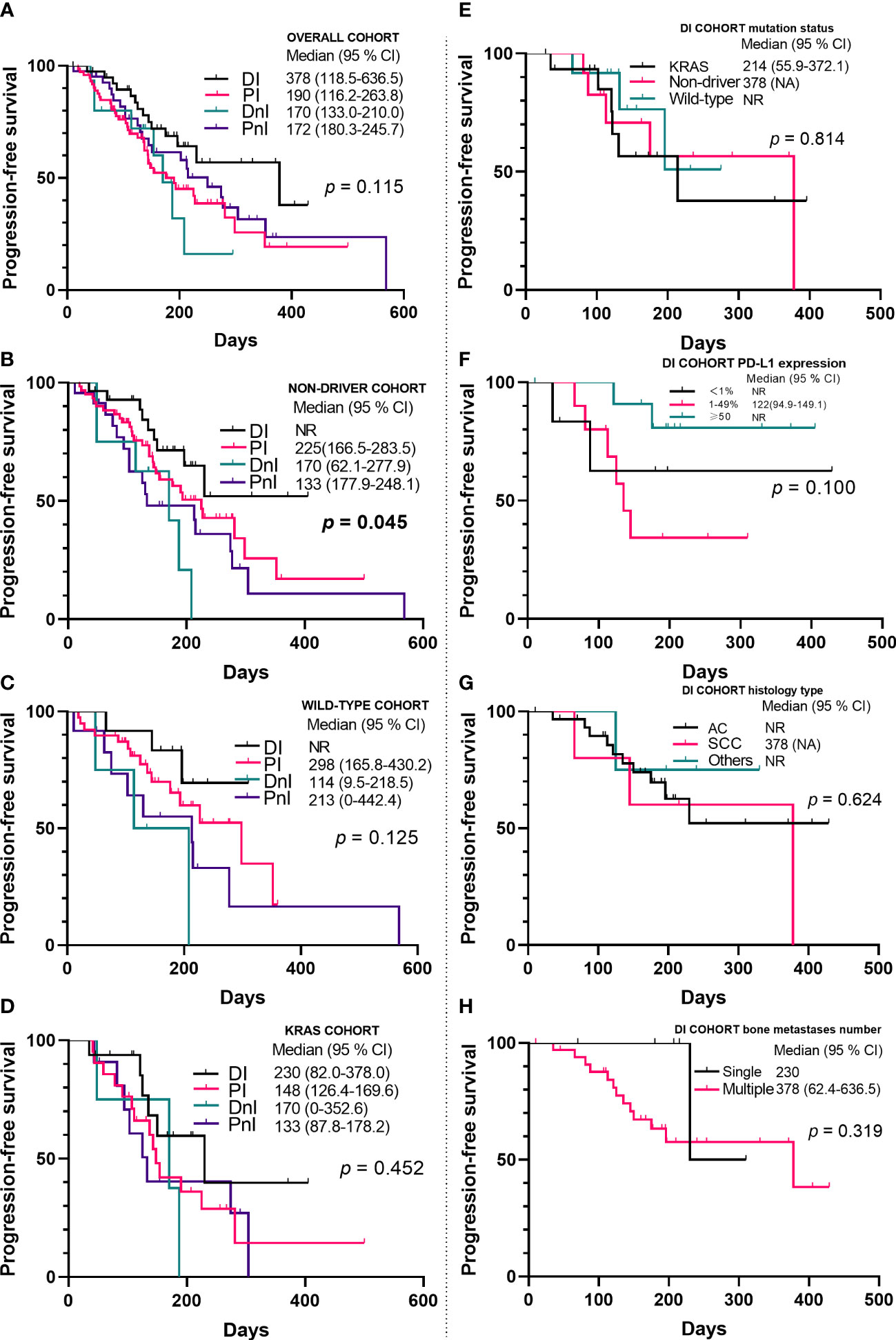
Figure 5 Kaplan–Meier analyses of progression-free survival in the overall cohort (A–D) (n = 171) and the DI cohort (E–H) (n = 40). NR, not reached; PD-L1, programmed death ligand-1; AC, adenocarcinoma; SCC, squamous cell carcinoma; DI, denosumab + ICIs; DnI, denosumab + non-ICIs; PI, phosphates + ICIs; PnI, phosphates + non-ICIs.
With regards to SRE prevention, the DI group demonstrated a trend for a lower SRE rate than that of the PI, DnI, and PnI groups (5%, 10.8%, 13.3%, and 11.9%, respectively), though a significant difference was not reached (p = 0.733) (Table 2).
3.3 Survival analysis
To determine the influence of different variates on prognosis, we conducted univariate and multivariate analyses (Table 3) for the whole cohort. In the univariate analysis, visceral metastases (p = 0.021), application line (p = 0.006), and Eastern Cooperative Oncology Group performance status (ECOG PS) (p = 0.001) were all statistically significant prognostic factors for PFS. In the multivariate analysis, mutation status (p = 0.043), PD-L1 expression level (p = 0.036), application line (p = 0.011), ECOG PS (p = 0.021), and treatment modality (p = 0.042) were independent predictors of PFS (Table 3). Specifically, we also examined the effect of different variables on the efficacy within the DI group (Figures 5E–H). We did not observe differences in benefit among different mutational subgroups (p = 0.814), but patients with single-site bone metastasis (p = 0.319) and high PD-L1 expression (p = 0.100) appeared to benefit more, though no significant differences were observed.
3.4 Toxicity evaluation
The most frequent AEs were pyrexia (12.3%) in the overall cohort, followed by fatigue (4.1%), arthralgia (3.5%), myalgia (3.5%), and renal failure (3.5%) (Table 4). The PI group showed a trend for higher overall AEs than those of the DI, DnI, and PnI groups (39.2%, 27.5%, 26.7%, and 28.6%, respectively), though a significant difference was not reached (p = 0.742). The DI group demonstrated relatively comparable levels of AEs to the PnI group but fewer AEs than the PI group regarding pyrexia, arthralgia, myalgia, and renal failure.
4. Discussion
Based on previous studies, we further explored and confirmed the synergistic effects and safety of ICIs and denosumab. Through setting three parallel comparative subgroups, we found that concomitant therapy in the DI group was associated with better PFS and with a good safety profile.
The RANK–RANKL pathway is best known for its essential role in the biological and pathological processes of bone. RANKL produced by osteoblasts and bone marrow mesenchymal cells can attract aggregation of RANK-expressing cancer cells and induce migration of cancer cells through specific signaling cascade activation (especially the MAPK pathway), thus leading to bone metastasis formation and bone destruction (2). In addition to its bone-derived role, evidence has shown that it plays an important role in promoting tumor growth in a variety of malignancies and is confirmed as a worse prognostic factor (2, 4–7). In a KRASG12D-driven lung cancer model, Rao et al. (20) found that RANK expression appeared in the early stages of highly plastic tumor development, suggesting that RANK was a driver of early tumor progression. Further studies revealed that the complex interaction of the RANK/RANKL pathway and mitochondrial respiratory metabolism ultimately directly stimulated the proliferation of KRASG12D mutant stem-like lung cancer cells through activation of the p38 and NF-κB pathways (20). Targeting RANKL seems to be a promising anti-tumor approach, but unfortunately, the randomized open-label phase III SPLENDOUR trial, which was designed to evaluate whether the addition of denosumab to standard first-line platinum-based doublet chemotherapy improved OS in advanced NSCLC, failed to demonstrate a clinical benefit (21).
However, besides cancer cells, RANK and RANKL are also expressed extensively in the TME, with RANK mainly on immature dendritic cells, immunosuppressive m2-type macrophages, and myeloid-derived suppressor cells, whereas RANKL mainly on CD8+ T cells (including regulatory T cells) (4). As a cytokine expressed on T cells, RANKL stimulates the survival of RANK-expressing dendritic cells (DCs) and enhances the ability of DCs to trigger the proliferation of naïve T cells (22). In the TME, RANKL interacts with RANK to coordinate various immunosuppressive processes through a variety of mechanisms (4). The 3LL lung adenocarcinoma mouse model constructed by Liede et al. showed that RANKL inhibitor combined with PD-1 mAb had a better therapeutic effect than RANKL inhibitor and PD-1 mAb alone (14). The study of Ahern et al. found that the combination of RANKL inhibitor and PD-1 mAb in a mouse model could further increase the number of infiltrating CD4+ and CD8+ T cells that can produce both IFN-γ and TNF in the TME, thus verifying the antitumor synergy effect of PD-1 mAb and RANKL inhibitor (12). However, in the early days, the immunomodulatory role of the RANK–RANKL pathway did not receive much attention or application until the development and application of denosumab.
Currently, as the first fully human anti-RANKL mAb, denosumab has been approved by the FDA for the prevention of SRE in solid tumors, including melanoma and lung cancer. For melanoma, in a retrospective study, Afzal and Shirai evaluated the synergistic effect of immune checkpoint inhibitors combined with denosumab in patients with metastatic melanoma, and the results showed that the PFS and OS of the group receiving the combination therapy were 11.6 months and 57 months, respectively, compared with 4.15 months and 22.8 months in the ICIs monotherapy group (10). For NSCLC, a retrospective clinical study that included 166 patients with advanced NSCLC by Liede et al. showed that continuous use of denosumab with ICIs significantly increased ORR (p <0.0001) and prolonged OS (p <0.0001) (14). Although the study of Liede et al. is very enlightening, due to the lack of an external control group, only patients with longer and shorter combination therapy within the study cohort were compared. This may lead to a reversal in deriving causality and consequent unfirm conclusion, because patients who were able to receive a longer duration of combination therapy (with better ORR and longer OS) may themselves be sensitive to immunotherapy, rather than as a result of the combination of denosumab. The same concern is also present in the study by Cao et al. (16). Bongiovanni et al. circumvented this by setting both the control group (immunotherapy monotherapy) and the experimental group (denosumab/ZA + immunotherapy). However, treatment with ZA was confounded in the study arm, so the synergistic effect of denosumab and immunotherapy could not be accurately assessed. Furthermore, it is possible that the additional prolongation of survival in the experimental group shown by the study results was due to the survival benefit of BTT by reducing SREs rather than synergy with immunotherapy (15). A summary of published data on the combination of denosumab and ICIs is demonstrated in Table 5.
Therefore, to exclude the effect of confounding factors and to enhance the persuasiveness of the study, we set up one experimental group and three control groups. Besides, we also explored the effect of mutation status on the efficacy of combination therapy. The results of the study suggested an improved PFS for the DI group compared with the PI group in the overall cohort (p = 0.115), which was more pronounced in the WILD-TYPE cohort (p = 0.125) and the NON-DRIVER cohort (p = 0.045). Meanwhile, we did not observe significant differences in PFS between the DnI and PnI groups. Hence, it is feasible to assume that the improved efficacy of denosumab over ZA originated from a synergistic effect with ICIs rather than from a difference in the ability to reduce SREs between denosumab and ZA. Our study results were partly supported by previous data from Bongiovanni et al. (15), whose work indicated a better mPFS (15.9 months; 95%CI, 5.1–not estimable) of patients receiving denosumab (n = 6) than those treated with ICIs alone or with ZA (p = 0.068).
Two prospective studies concerning the DI treatment of lung cancer are currently underway. The DENIVOS (NCT03669523) study aims to evaluate the combination of denosumab and nivolumab in the second line of NSCLC with bone metastases. The POPCORN study (ACTRN12618001121257) is designed to provide information about the activity and safety of the combination of denosumab and nivolumab compared with nivolumab alone in the preoperative treatment of resectable NSCLC (23). Hopefully, these studies will shed light on the future exploration and application of the combination of denosumab and ICIs in NSCLC.
This study has some inherent limitations. First, as this study was a single-center study, and the included patients were mainly from urban areas, there was a selection bias. Second, the number of patients in individual groups in the study cohort was too small, which may affect the statistical test validity. Third, there were confounding factors in the study, such as different brands of PD-1/PD-L1 inhibitors and phosphates. In addition, patients receiving denosumab may not have been randomly selected because some physicians may prefer denosumab to phosphates, and the relatively higher cost of denosumab may also be an important factor in deciding whether a patient will receive denosumab or not. Although these influencing factors were not assessed in this study, they may still have influenced the outcomes. Finally, potential efficacy biomarkers, including RANKL and RANK, were not investigated. All of these have the potential to affect the reliability of the study results. Therefore, the results of the study should be interpreted with caution.
In conclusion, our findings suggest a synergistic effect of denosumab in combination with ICIs in the treatment of NSCLC patients carrying bone metastases, and this combination has a good safety profile.
Data availability statement
The original data presented in the study are included in the article/supplementary material. Further inquiries can be directed to the corresponding authors.
Ethics statement
The studies involving human participants were reviewed and approved by the Ethics Committee of National Cancer Center/Cancer Hospital, Chinese Academy of Medical Sciences and Peking Union Medical College. The patients/participants provided their written informed consent to participate in this study.
Author contributions
Conception and design: H-SL, S-YL, H-YX, and YW. Administrative support: H-SL, JLL, PYX, XZH, HYX, and YW Provision of study materials or patients: J-LL, P-YX, X-ZH, HYX, and YW. Collection and assembly of data: H-SL and S-YL. Data analysis and interpretation: H-SL, S-YL, JLL, PYX, XZH, FX, and HYX Manuscript writing: All authors. Final approval of manuscript: All authors. All authors contributed to the article and approved the submitted version.
Funding
This work was supported by the National Natural Science Foundation of China (Grant No. 82072590) and the Beijing Health Promotion Association (Grant No. 2021-053-ZZ).
Acknowledgments
We would like to thank the support of all enrolled patients and their relatives, and we would also like to thank Editage (www.editage.cn) for English language editing.
Conflict of interest
The authors declare that the research was conducted in the absence of any commercial or financial relationships that could be construed as a potential conflict of interest.
Publisher’s note
All claims expressed in this article are solely those of the authors and do not necessarily represent those of their affiliated organizations, or those of the publisher, the editors and the reviewers. Any product that may be evaluated in this article, or claim that may be made by its manufacturer, is not guaranteed or endorsed by the publisher.
References
1. Barta JA, Powell CA, Wisnivesky JP. Global epidemiology of lung cancer. Ann Glob Health (2019) 85:1–16. doi: 10.5334/aogh.2419
2. Deligiorgi MV, Trafalis DT. Repurposing denosumab in lung cancer beyond counteracting the skeletal related events: an intriguing perspective. Expert Opin Biol Ther (2020) 20:1331–46. doi: 10.1080/14712598.2020.1790522
3. Reck M, Rodríguez-Abreu D, Robinson AG, Hui R, Csőszi T, Fülöp A, et al. Pembrolizumab versus chemotherapy for PD-L1-Positive non-Small-Cell lung cancer. N Engl J Med (2016) 375:1823–33. doi: 10.1056/NEJMoa1606774
4. Ahern E, Smyth MJ, Dougall WC, Teng MWL. Roles of the RANKL-RANK axis in antitumour immunity - implications for therapy. Nat Rev Clin Oncol (2018) 15:676–93. doi: 10.1038/s41571-018-0095-y
5. Peters S, Clézardin P, Márquez-Rodas I, Niepel D, Gedye C. The RANK-RANKL axis: an opportunity for drug repurposing in cancer? Clin Transl Oncol (2019) 21:977–91. doi: 10.1007/s12094-018-02023-5
6. Ming J, Cronin SJF, Penninger JM. Targeting the RANKL/RANK/OPG axis for cancer therapy. Front Oncol (2020) 10:1283. doi: 10.3389/fonc.2020.01283
7. Casimiro S, Vilhais G, Gomes I, Costa L. The roadmap of RANKL/RANK pathway in cancer. Cells (2021) 10(8):1978. doi: 10.3390/cells10081978
8. Henry DH, Costa L, Goldwasser F, Hirsh V, Hungria V, Prausova J, et al. Randomized, double-blind study of denosumab versus zoledronic acid in the treatment of bone metastases in patients with advanced cancer (excluding breast and prostate cancer) or multiple myeloma. J Clin Oncol Off J Am Soc Clin Oncol (2011) 29:1125–32. doi: 10.1200/jco.2010.31.3304
9. Scagliotti GV, Hirsh V, Siena S, Henry DH, Woll PJ, Manegold C, et al. Overall survival improvement in patients with lung cancer and bone metastases treated with denosumab versus zoledronic acid: subgroup analysis from a randomized phase 3 study. J Thorac Oncol (2012) 7:1823–9. doi: 10.1097/JTO.0b013e31826aec2b
10. Afzal MZ, Shirai K. Immune checkpoint inhibitor (anti-CTLA-4, anti-PD-1) therapy alone versus immune checkpoint inhibitor (anti-CTLA-4, anti-PD-1) therapy in combination with anti-RANKL denosumuab in malignant melanoma: a retrospective analysis at a tertiary care center. Melanoma Res (2018) 28:341–7. doi: 10.1097/cmr.0000000000000459
11. Ahern E, Harjunpää H, Barkauskas D, Allen S, Takeda K, Yagita H, et al. Co-Administration of RANKL and CTLA4 antibodies enhances lymphocyte-mediated antitumor immunity in mice. Clin Cancer Res Off J Am Assoc Cancer Res (2017) 23:5789–801. doi: 10.1158/1078-0432.Ccr-17-0606
12. Ahern E, Harjunpää H, O'Donnell JS, Allen S, Dougall WC, Teng MWL, et al. RANKL blockade improves efficacy of PD1-PD-L1 blockade or dual PD1-PD-L1 and CTLA4 blockade in mouse models of cancer. Oncoimmunology (2018) 7:e1431088. doi: 10.1080/2162402x.2018.1431088
13. Faget J, Contat C, Zangger N, Peters S, Meylan E. RANKL signaling sustains primary tumor growth in genetically engineered mouse models of lung adenocarcinoma. J Thorac Oncol (2018) 13:387–98. doi: 10.1016/j.jtho.2017.11.121
14. Liede A, Hernandez RK, Wade SW, Bo R, Nussbaum NC, Ahern E, et al. An observational study of concomitant immunotherapies and denosumab in patients with advanced melanoma or lung cancer. Oncoimmunology (2018) 7:e1480301. doi: 10.1080/2162402x.2018.1480301
15. Bongiovanni A, Foca F, Menis J, Stucci SL, Artioli F, Guadalupi V, et al. Immune checkpoint inhibitors with or without bone-targeted therapy in NSCLC patients with bone metastases and prognostic significance of neutrophil-to-Lymphocyte ratio. Front Immunol (2021) 12:697298. doi: 10.3389/fimmu.2021.697298
16. Cao Y, Afzal MZ, Shirai K. Does denosumab offer survival benefits? -our experience with denosumab in metastatic non-small cell lung cancer patients treated with immune-checkpoint inhibitors. J Thorac Dis (2021) 13:4668–77. doi: 10.21037/jtd-21-150
17. Manglaviti S, Galli G, Bini M, Labianca A, Zecca E, Brambilla M, et al. 184P bone-targeted agents (BTA) improve survival in advanced non-small cell lung cancer (aNSCLC) patients (pts) with high bone tumor burden (HBTB) treated with PD-(L)-1 inhibitors (ICIs). J Thorac Oncol (2021) 16:S797. doi: 10.1016/S1556-0864(21)02026-8
18. Asano Y, Yamamoto N, Demura S, Hayashi K, Takeuchi A, Kato S, et al. The therapeutic effect and clinical outcome of immune checkpoint inhibitors on bone metastasis in advanced non-Small-Cell lung cancer. Front Oncol (2022) 12:871675. doi: 10.3389/fonc.2022.871675
19. Therasse P, Arbuck SG, Eisenhauer A. New guidelines to evaluate the response to treatment in solid tumors (RECIST guidelines). J Natl Cancer Inst (2000) 92:201–16. doi: 10.1093/jnci/92.3.205
20. Rao S, Sigl V, Wimmer RA, Novatchkova M, Jais A, Wagner G, et al. RANK rewires energy homeostasis in lung cancer cells and drives primary lung cancer. Genes Dev (2017) 31:2099–112. doi: 10.1101/gad.304162.117
21. Peters S, Danson S, Hasan B, Dafni U, Reinmuth N, Majem M, et al. A randomized open-label phase III trial evaluating the addition of denosumab to standard first-line treatment in advanced NSCLC: The European thoracic oncology platform (ETOP) and European organisation for research and treatment of cancer (EORTC) SPLENDOUR trial. J Thorac Oncol (2020) 15:1647–56. doi: 10.1016/j.jtho.2020.06.011
22. Anderson DM, Maraskovsky E, Billingsley WL, Dougall WC, Tometsko ME, Roux ER, et al. A homologue of the TNF receptor and its ligand enhance T-cell growth and dendritic-cell function. Nature (1997) 390:175–9. doi: 10.1038/36593
23. Ahern E, Cubitt A, Ballard E, Teng MWL, Dougall WC, Smyth MJ, et al. Pharmacodynamics of pre-operative PD1 checkpoint blockade and receptor activator of NFkB ligand (RANKL) inhibition in non-small cell lung cancer (NSCLC): study protocol for a multicentre, open-label, phase 1B/2, translational trial (POPCORN). Trials (2019) 20:753. doi: 10.1186/s13063-019-3951-x
Keywords: immunotherapy, non-small cell lung cancer, bone metastases, efficacy, safety, denosumab, synergistic efficacy
Citation: Li H-S, Lei S-Y, Li J-L, Xing P-Y, Hao X-Z, Xu F, Xu H-Y and Wang Y (2022) Efficacy and safety of concomitant immunotherapy and denosumab in patients with advanced non-small cell lung cancer carrying bone metastases: A retrospective chart review. Front. Immunol. 13:908436. doi: 10.3389/fimmu.2022.908436
Received: 30 March 2022; Accepted: 05 August 2022;
Published: 29 August 2022.
Edited by:
Peter Brossart, University of Bonn, GermanyReviewed by:
Rong Jin, Peking University, ChinaMelina Elpi Marmarelis, University of Pennsylvania, United States
Roberto Ferrara, National Cancer Institute Foundation (IRCCS), Italy
Copyright © 2022 Li, Lei, Li, Xing, Hao, Xu, Xu and Wang. This is an open-access article distributed under the terms of the Creative Commons Attribution License (CC BY). The use, distribution or reproduction in other forums is permitted, provided the original author(s) and the copyright owner(s) are credited and that the original publication in this journal is cited, in accordance with accepted academic practice. No use, distribution or reproduction is permitted which does not comply with these terms.
*Correspondence: Yan Wang, d2FuZ3lhbnlpZnVAMTYzLmNvbQ==; Hai-Yan Xu, eHVoYWl5YW43NjA5QHNpbmEuY29t
†These authors have contributed equally to this work