- Institute of Pathogenic Biology, Hengyang Medical College, University of South China, Hunan Provincial Key Laboratory for Special Pathogens Prevention and Control, Hengyang, China
Respiratory diseases cause a high incidence and mortality worldwide. As a natural immunobiotic, Lactobacillus has excellent immunomodulatory ability. Administration of some Lactobacillus species can alleviate the symptoms of respiratory diseases such as respiratory tract infections, asthma, lung cancer and cystic fibrosis in animal studies and clinical trials. The beneficial effect of Lactobacillus on the respiratory tract is strain dependent. Moreover, the efficacy of Lactobacillus may be affected by many factors, such as bacteria dose, timing and host background. Here, we summarized the beneficial effect of administered Lactobacillus on common respiratory diseases with a focus on the mechanism and safety of Lactobacillus in regulating respiratory immunity.
1 Introduction
Probiotics are defined as “live microorganisms” and confer a health benefit on the host when properly administered (1). Many probiotics have been shown the beneficial properties, including Lactobacillus spp., Bifidobacterium spp., Enterococcus spp., Streptococcus spp., Propionibacterium spp., Bacillus cereus, Saccharomyces boulardii, and several specific strains of Escherichia coli (1, 2). Among probiotics, the most widely used are microorganisms of the genus Lactobacillus, which contains more than 200 species bacteria (3, 4). Lactobacillus spp. are gram-positive, facultative anaerobic bacterium that ferments carbohydrates to produce lactic acid (5), and has a high economic value due to the use in biotechnology, food as well as therapeutic application (4, 6, 7). In human hosts, Lactobacillus spp. exist in the gastrointestinal tract, vagina, oral cavity, respiratory tract and skin. They account for 6% and 95% of the total bacteria in the intestinal and vaginal tracts, respectively (8).
As natural immunobiotics, Lactobacilli have excellent immunomodulatory function, and their ability in improving gastrointestinal, oral and vaginal disorders is already well known (6, 9–12). Meanwhile, emerging evidence shows that Lactobacillus can also modulate respiratory immunity (13–16). Administration of Lactobacillus confer a beneficial role in respiratory diseases including respiratory tract infections (RTIs), asthma, lung cancer, cystic fibrosis (CF) and chronic obstructive pulmonary disease (COPD) (17–21). Administration of Lactobacillus may be an alternative strategy to alleviate respiratory diseases. In this review, we focus on the beneficial effect of probiotic Lactobacillus in promoting respiratory health and discuss its potential mechanism and safety.
2 Beneficial Role of Lactobacillus in Respiratory Diseases
2.1 Respiratory Tract Infections
RTIs are the major source of incidence and mortality in the world (22). Although many RTIs are of mild and self-limited nature, they caused 4 million deaths worldwide each year (23, 24). In 2017, influenza virus has infected 54.5 million people worldwide, resulting in about 145,000 deaths (25). Severe Acute Respiratory Syndrome Coronavirus-2 (SARS-CoV-2) is raging globally. Effective vaccines are not available for many respiratory pathogens, and the increase in drug-resistant microbe makes the effective treatment of RTIs extremely challenging. Therefore, it is important to find a safe and effective method to reduce the risk of RTIs. Recently, the probiotic Lactobacillus has been used to fight against a variety of RTIs, including virus and bacterial infection (Table 1). Oral administration of Lactobacillus can improve symptoms caused by poly(I:C) treatment (49). Many Lactobacillus species can prevent influenza virus infection, including L. rhamnosus GG, L. casei Shirota, L. plantarum DK119, L. paracasei MCC1849, L. gasseri SBT2055, L. fermentum CJl-112 and L. kunkeei YB38 (34, 50–55). Moreover, Lactobacilli also have excellent role as a vaccine or adjuvant in preventing influenza virus infection due to its safety and biotechnological advantage (56–58). It is worth pointing out that oral supplementation with L. johnsonii (strain not shown) in pregnant BALB/c mice can reduce Th2 type cytokines and lung inflammation in Respiratory Syncytial Virus (RSV)-infected newborn mice (59). It means Lactobacillus may have preventive effect for RTIs of offspring when administered to the mother. Notably, supplementation with live and inactivated bacteria of the same Lactobacillus strain (such as L. rhamnosus CRL1505) generally have similar effect (39, 40), suggesting that viability is not necessary for Lactobacillus achieve the protective immunoregulatory effect. However, nasally administration of,viable but not heat-killed L. rhamnosus CRL1506 can provide complete protection against RSV infection in mice (28). Although the reason needs further investigation, it shows that Lactobacilli efficacy is strain-dependent. Therefore, the selection of a Lactobacillus strain with potent immunomodulatory ability is crucial.
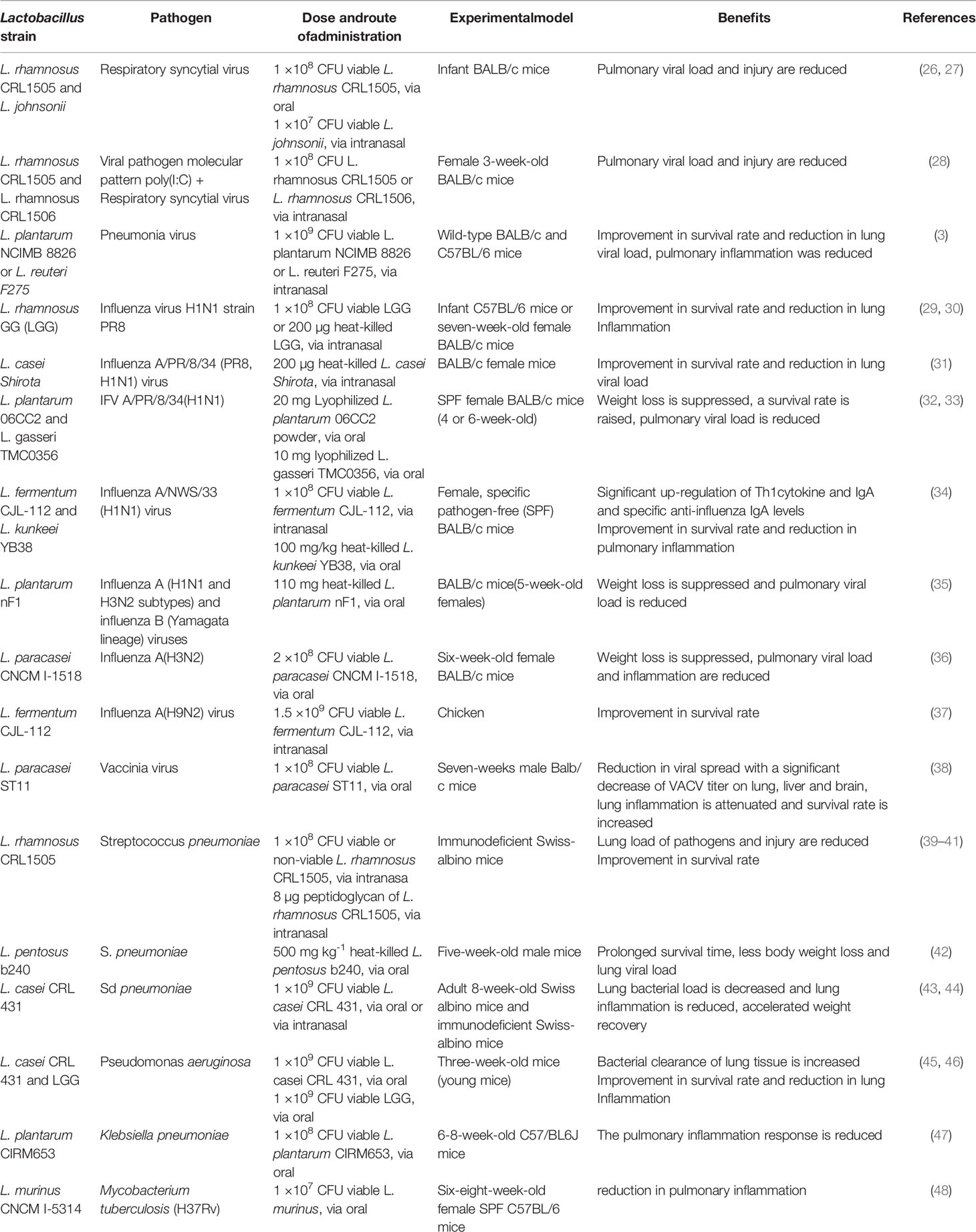
Table 1 Pre-clinical studies on the administration of the Lactobacillus for protection against bacterial and viral respiratory tract infections.
Although vaccines for SARS-CoV-2 are available, the rapidly SARS-CoV-2 mutating makes the effectiveness of these vaccines challenging (60). Notably, in vitro experiment has demonstrated that L. fermentum 90 TC-4 pretreatment increases the activity of SARS-CoV-2-infected Vero E6 cells (grass monkey kidney cells) (61). Pretreatment of L. plantarum MPL16 and CRL1506 can also inhibit the proliferation of SARS-CoV-2 in human lung epithelial cell line Calu-3 (62). In Central Europe, it has been reported that low death rate of COVID-19 patients is associated with the consumption of fermented vegetables containing many Lactobacilli species (63). Therefore, Lactobacilli may have the potential to become an adjuvant for treating SARS-CoV-2.
However, there are some limitations in the therapeutic effect of administering Lactobacillus. For example, neonatal C57BL/6 mice pre-treated with L. rhamnosus GG maintain 100% survival rate post influenza virus infection; however, the survival rate is only 10% when mice treated with L. rhamnosus GG at 48h post influenza virus infection (29). Similarly, the survival rate of BALB/c mice treated with L. plantarum at 24h post Pneumonia virus infection is 100%, but all mice die when L. plantarum administered at 72h post infection (3, 64). These results show that Lactobacillus administration post infection cannot exert an obvious protective immunoregulatory effect.
Lactobacilli can also provide resistance to respiratory bacterial infections. Administration of Lactobacilli such as L. rhamnosus CRL1505, L. casei CRL 431 and L. pentosus B240 increases resistance of mice to Streptococcus pneumoniae infection (41–43). Intranasal inoculation of L. rhamnosus CRL1505 is beneficial in S. pneumoniae infected-immunodeficient mice (39). Interestingly, peptidoglycan from L. rhamnosus CRL1505 shows a similar protective effect with the whole bacteria in preventing S. pneumoniae infection (40). Moreover, nasal administration of L. rhamnosus CRL1505 can also reduce pathogen load and lung damage of infant mice with RSV infection and secondary S. pneumoniae infection (65, 66).
In clinical trials, Lactobacilli are generally given in the form of tablets, capsules, powders, fermented yogurt or dairy products, and mainly used for preventive purposes (Table 2). For instance, oral L. rhamnosus GG in adults can reduce rhinovirus infection (83), and the combination of oral L. paracasei (strain not shown), L. casei CRL 431 and L. fermentium PCC also reduces rhinovirus-induced common and influenza-like infection (78). In addition, oral mixed probiotic (mainly Lactobacilli) can decrease the risk of respiratory failure in COVID-19 patients by 8-fold and reduce the rate of transfer to Intensive Care Unit and mortality (84). In patients with severe COVID-19, oral tablet (live B. longum, live L. bulgaricus and live S. thermophilus, strains not shown) also shortens the time to reach a negative nucleic acid test of SARS-CoV-2 and decreases blood C-reactive protein and procalcitonin (81). Moreover, clinically administration of many Lactobacillus species such as L. rhamnosus GG, L. paracasei N1115 and L. plantarum L-137 can decrease the total incidence as well as shorten the duration of RTIs (26, 27, 81). Therefore, the prospect of Lactobacillus clinical application in reducing the risk of RTIs is promising.
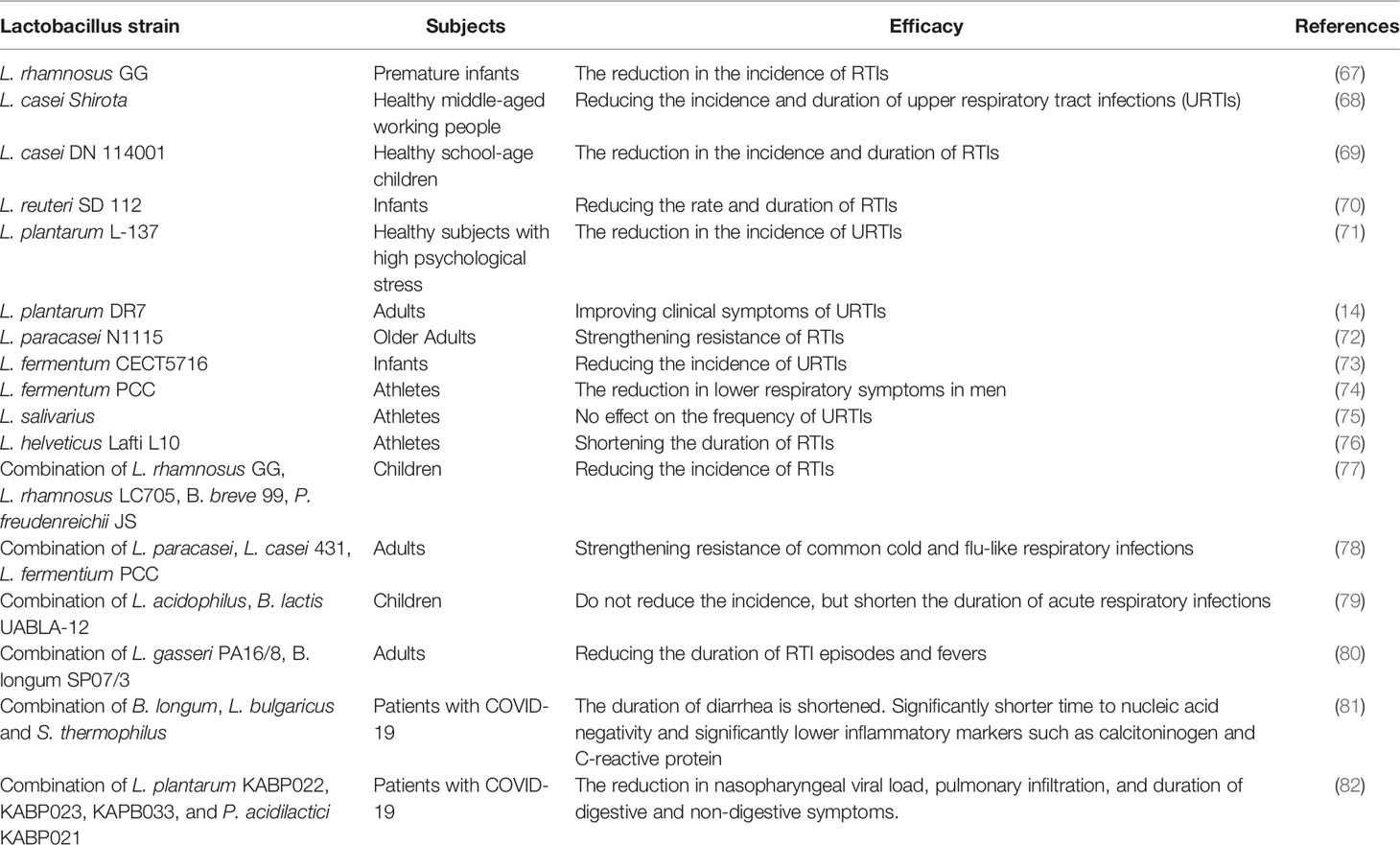
Table 2 Treatment effect of clinical trials regarding the application of Lactobacillus in improving symptoms of respiratory tract infections (RTIs).
2.2 Asthma
Asthma is a heterogeneous airway disease, which behaves as complex symptoms, including cough, intermittent wheezing, dyspnea, chest tightness, airway obstruction, and bronchial hyperresponsiveness. Asthma patients generally have a Th1/Th2 imbalance and are polarized toward Th2 type immune response, usually resulting in high level of allergen-specific IgE and eosinophilic airway inflammation (85). Although asthma can be treated by strategies include allergen avoidance and improvement of signs and symptoms by inhaled corticosteroids, anti-leukotrienes and β2 agonists, there is still no specific treatment for asthma and healthcare is expensive during exacerbation (86).
Emerging evidence suggests that respiratory asthma symptoms can be ameliorated when probiotic Lactobacillus is administered. Oral administration of many Lactobacillus species has shown an effective preventive role for asthma in animal studies, including L. rhamnosus GG, L. plantarum K37, L. reuteri (ATCC No. 23272), L. casei Shirota, L. paracasei HB89 and L. salivarius (strain not shown) (18, 87–91). Some Lactobacillus species such as L. rhamnosus GG, L. paracasei (strain not shown) and L. fermentum (strain not shown) are orally used in the clinical and have beneficial effect on asthma in children (92, 93). Interestingly, the acute asthma is closely associated with RTIs, especially rhinovirus infection (94). Consequently, the role of Lactobacillus administration in preventing RTIs may be useful in relieving exacerbation of acute asthma. Based on the “hygiene hypothesis,” exposure to specific microbial components early in life can decrease the susceptibility to asthma and allergic diseases (95). Therefore, oral administration of probiotic Lactobacillus in the early life may also play an important role in preventing asthma.
For the purpose of asthma prevention, the general route of administration of Lactobacillus species is oral delivery. However, a study indicates that although oral administration of L. paracasei NCC2461 could provide effective protection for female BALB/c mice with asthma, the efficacy of intranasal delivery is better (96). Nevertheless, two studies show that oral administration of L. rhamnosus GR-1 prevents the worsening of asthma in male BALB/c mice, but nasal administration has little effect on the improvement of asthma symptoms in male BALB/c mice (97, 98). This may be caused by the lower dose and duration of nasal administration. Interestingly, oral administration of L. paracasei NCC2461 in perinatal mice provides prevention for allergic airway inflammation in the offspring (99). Similarly, in clinical trials, oral L. rhamnosus GG has been shown to prevent atopic diseases in children in both prenatal and postnatal (100). Thus, Lactobacillus may have a preventive effect against offspring asthma when administered to the mother. Furthermore, supplementation with Lactobacillus GG also has a therapeutic rather than only a preventive effect on mice suffering from asthma (101). Additionally, animal studies have found that oral Lactobacillus can also reduce other airway allergic reactions such as allergic rhinitis. For example, oral L. rhamnosus GG, L. gasseri TMC0356, L. plantarum IM76, L. plantarum CJLP133 and CJLP243 can effectively improve the symptoms of allergic rhinitis (102–104). In clinical trials. L. gasseri KS-13, L. casei Shirota and L. acidophilus L-92 have been used to effectively prevent seasonal allergic rhinitis (105–107). Thus, the administration of Lactobacillus may have excellent preventive effect on airway allergic reaction.
2.3 Lung Cancer
Lung cancer is a malignant tumor with a high incidence and mortality rate in the world (108). In recent years, immunotherapy has sparked a new surge in tumor treatment. Although immune check inhibitors (ICIs) such as anti-PD-1/PD-L1 and anti-CTLA 4 antibodies has become the first-line clinical treatment for tumors, the application of ICIs increases T-cell activity and removes the “braking” of the immune system, and these drugs are likely been associated with immune-related adverse events, especially when used in combination (109). Therefore, the search for natural immunobiotics with immunomodulatory properties to assist in the treatment of cancer patients may be a direction. It has been reported that probiotics show the same degree of anti-tumor ability as PD-L1 inhibitors, while simultaneous combination therapy with PD-L1 inhibitors virtually eliminated tumor growth in mice (110). Moreover, in clinical trials, oral administration of yogurt with probiotics provides a potential protective effect against lung cancer (111). Lactobacillus is one of the most widely studied probiotic involved in the treatment of lung cancer. Administration of Lactobacillus can inhibit the metastasis of tumor cells to the lung. For example, nasal administration of L. rhamnosus GG can inhibit the metastasis of melanoma B16 to the lung in C57BL/6 mice (112). L. casei (strain not shown) has significant inhibitory effect on the proliferation of A549 lung cancer cells in vitro (113). Furthermore, intravenous and intradermal injection of L. casei YIT 9018 can increase the anti-tumor activity against Lewis lung carcinoma in C57BL/6 mice (114). In addition, oral administration of L. casei CRL 431 also reduces the side effects of chemotherapy (115), which may improve the prognosis of lung cancer patients. These suggest that some Lactobacillus species may have potential to become an effective adjuvant to treat lung cancer.
2.4 CF
CF is an autosomal recessive, monogenic disease with lesions affecting the lung, intestine, and other organ, but lung disease is the leading cause of morbidity and mortality in people with CF (116). CF is closely related to intestinal flora and significantly reduces intestinal flora diversity (117, 118). A study indicates that oral L. rhamnosus GG improves disorders of intestinal flora in children with CF (119). Moreover, clinical trial shows oral administration of L. rhamnosus GG reduces pulmonary exacerbation and hospitalization rate in CF patients (120). This suggests administration of L. rhamnosus GG may be able to alleviate the symptoms of CF. In another clinical trial, however, oral administration of L. rhamnosus GG did not promote respiratory health in patients of CF (121). The main reason may be due to different inclusion criteria for clinical trial subjects. The clinical efficacy of applying a single Lactobacillus strain on CF is unstable, and the combined administration of multiple probiotic Lactobacillus species may be more effective. For example, intestinal supplementation with probiotic capsules (consisting mainly of Lactobacillus species) significantly reduces the incidence of lung deterioration and improves the quality of life in patients with CF (19, 122). However, these are single-center clinical trials. Multicenter and expansion of the number of subjects are needed to further clarify the efficacy of Lactobacillus administration on CF in clinical trials.
2.5 Other Respiratory Diseases
Several studies have reported the potential of Lactobacillus in improving COPD. The commonly known causes of COPD are cigarettes and air pollutants (123). In vitro experiment has demonstrated that L. rhamnosus NutRes1 can reduce inflammatory mediators produced by cigarette-activated human macrophages (124). Moreover, oral feeding of L. rhamnosus (strain not shown) increases levels of IL-10 as well as SOCS3 and TIMP1/2, and attenuates lung injury of COPD induced by cigarette in C57BL/6 mice (21). Additionally, the development of COPD is associated with reduction of Lactobacillus spp. in lung (125).
In addition, a study indicates that a decrease in intestinal Lactobacillus may contribute to the development of pulmonary arterial hypertension (126). In clinical trial, intestinal supplementation with L. casei Shirota can reduce the incidence of Ventilator-Associated Pneumonia in hospitalized patients (127). Thus, Lactobacillus may also be beneficial in other respiratory diseases and need more studies.
3 Potential Mechanism of Probiotic Lactobacillus in Improving Respiratory Diseases
As mentioned above, many members of the genus Lactobacillus have immunomodulatory properties. Notably, not only the whole bacteria, but also their components can exert immunomodulatory function, including peptidoglycans, extracellular polysaccharides, surface proteins and metabolites (short-chain fatty acids, SCFAs) and inorganic polyphosphate liquids (40, 128–131). The mechanism of health-promoting effect of Lactobacillus on respiratory tract is complex. The same bacteria, when administered orally or nasally, or even administered live and inactivated bacteria, may not have the same effect on promoting respiratory health (49, 50, 52). The potential mechanisms include gut-lung axis and enhancement of the local mucosal immunity of the respiratory tract.
3.1 The Gut-Lung Axis
In recent years, many studies have reported that there is a crosstalk between gut and lung, and this connection described as gut-lung axis seems to be bidirectional (132, 133). Oral administration of some probiotics, especially Lactobacilli, could promote respiratory health via gut-lung axis (17, 30, 134). Although the exact mechanisms by which Lactobacillus enterica affects the lung immunity via gut-lung axis are not fully understood, there are three main aspects (Figure 1).
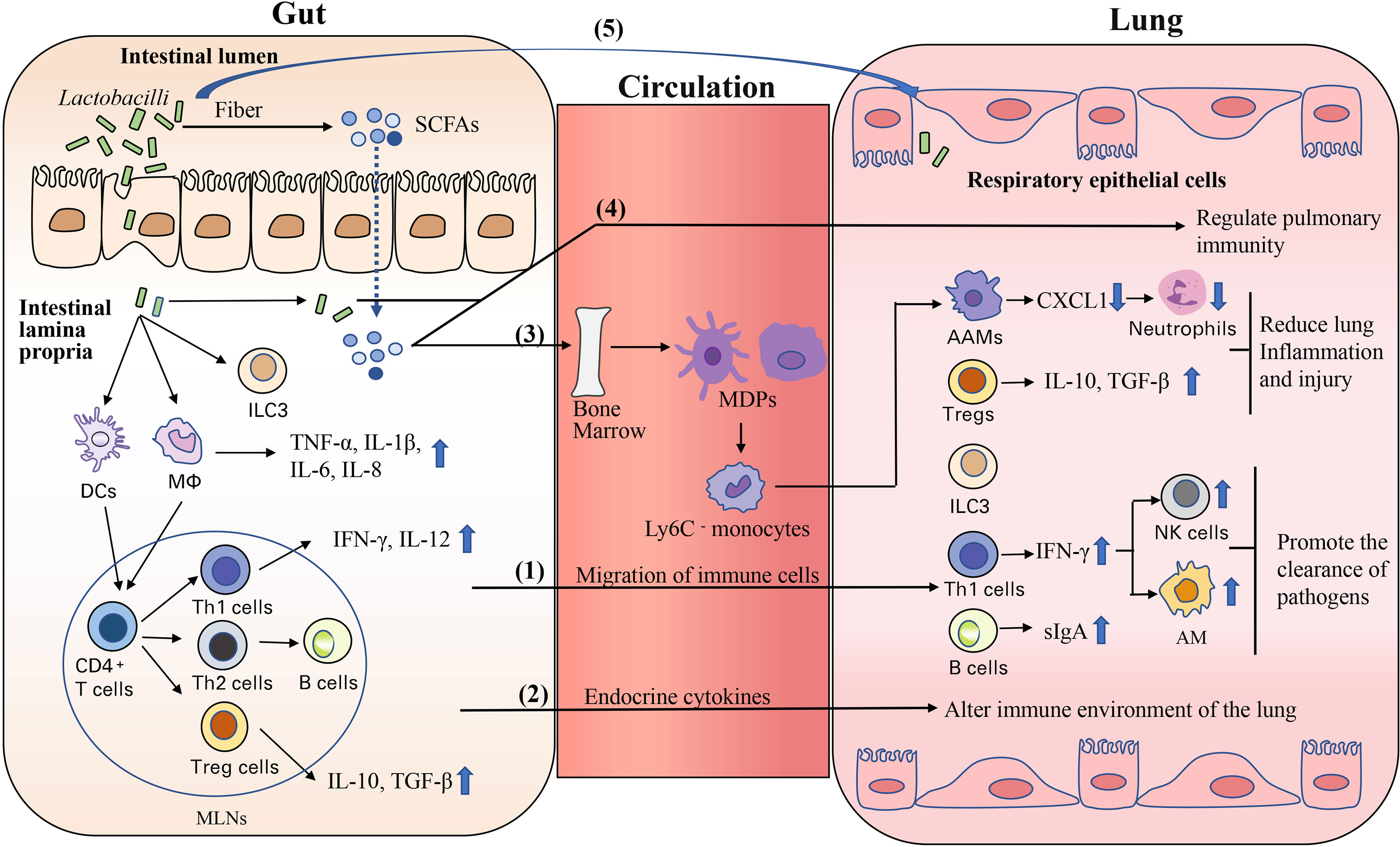
Figure 1 Potential mechanisms of Lactobacilli to modulate respiratory immunity via the gut-lung axis (1). Migration of activated immune cells and cytokines from mesenteric lymph nodes (MLNs) and intestinal lamina propria to the lung through the circulation (2). Some endocrine cytokines (such as TNF-α, IL-6) may migrate to the lung tissue through the circulation, and then alter immune environment of the lung (3). SCFAs affect bone marrow hematopoiesis and promote the conversion of macrophage and DC progenitors (MDPs) into Ly6C-monocytes, which reaches lung tissue and differentiates into anti-inflammatory alternatively activated macrophages (AAMs); AAMs inhibit chemokine CXCL1 production thus leading to reduced neutrophils recruitment in lung tissue (4). In the intestinal lumen, Lactobacilli or their components and production of metabolites (such as SCFAs) are taken up by intestinal epithelial cells and then enter to the lung via the circulation (5). Lactobacilli or their components from the intestinal lumen reach lung directly via microbreathing or esophageal reflux.
3.1.1 Direct Immigration of Immune Cells and Cytokines From the Intestine to the Respiratory Tract Through the Circulation
Recognition of Lactobacillus species or their components by pattern recognition receptors (PRRs, such as TLRs or NLRs, etc.) on immune cells in the intestinal mucosa can result in the activation of innate immune cells which could migrate to lung tissue via circulation. For instance, innate lymphoid group 3 cells (ILC3s) can migrate from the gut to the lung where IL-22+ILC3 exhibits resistance to pneumonia (135). Oral delivery of L. paracasei CNCM I-1518 also significantly increases ILC3 in lung tissue and enhances resistance to influenza virus (36). Intestinal supplements with L. paracasei MCC1849 can provide protection against influenza virus, which may be associated with an increased proportion of IgA+ B cells and follicular helper T cells in Peyer’s patches and significantly increases IgA secretion in lung (53). Villena suggests that Oral delivery of L. rhamnosus CRL1505 induces Th1 response in the gut-associated lymphoid tissue and promotes the migration of Th1 cell to the lung tissue where Th1 cells produces IFN-γ which induces activation of alveolar macrophages, natural killer cells, CD103+ and CD11bhigh dendritic cell (DC) (49). Additionally, a study indicates that intestinal supplementation with L. rhamnosus GG can inhibit pulmonary inflammation, which is associated with an increase in Tregs of the intestinal tract (136). Moreover, oral administration of L. murinus (strain not shown) to antibiotic-treated mice can also increase Tregs in lung (137). Tregs not only inhibit inflammation, but also inhibit Th2 type immune response (138). As controlling airway inflammation is crucial in preventing asthma progression (94), migration of Tregs to lung tissue may alleviate asthma symptoms.
Cytokines secreted in the mucosa of the gastrointestinal tract by Lactobacillus can reach the circulation and interact with other mucosal tissues, such as lung (139). Intestinal supplementation with L. rhamnosus CRL1505 can increase TNF-α, IFN-α, IFN-β, IFN-γ in bronchoalveolar lavage fluid which exert a significant antiviral effect in the respiratory tract, and these cytokines show a similar increased trend in the intestinal fluid (49).
3.1.2 Influence of Lactobacillus Metabolites on Pulmonary Immunity via the Circulation
In parallel to promoting the migration of intestinal immune cells and cytokines, members of Lactobacillus genus produce metabolites that can modulate host respiratory immunity. The most important intestinal bacteria metabolites affecting pulmonary immunity is SCFAs, such as propionate, butyrate and acetate (140). Studies show that intestinal supplementation with many Lactobacillus species can increase SCFAs in the intestine and blood (141–145). The unmetabolized SCFAs enter the circulation and may affect pulmonary immunity in two ways. The first way is that SCFAs enter the bone marrow through the circulation enhancing generation of macrophage and DC progenitors (MDPs) (146), and meanwhile SCFAs can increase the conversion of MDPs into Ly6C– monocytes (147, 148); then bone marrow Ly6C– monocytes migrate into lung tissue where they differentiate into alternatively activated macrophages (149). The second way is that SCFAs directly enter into the lung tissue where they may activate G protein-coupled receptors (GPCRs) or inhibit histone deacetylase (150–152). For example, butyrate can not only promote the generation of Tregs and IL-10 production by activating GPR109A (153), but also restore IL-10 in the lung by inhibiting histone deacetylase in mice (154). Another metabolite of Lactobacillus enterica, lithocholic acid, can also enhance Tregs function while inhibit Th17 response (155). Other Lactobacillus intestinal metabolites (such as lactic acids, polyamines and indole derivatives) with immunomodulatory properties are involved in intestinal homeostasis (156–158). Nevertheless, it remains to be studied whether these metabolites can impact respiratory health via the gut-lung axis. In summary, Lactobacillus metabolites, such as SCFAs (especially butyrate) and lithocholic acid have excellent anti-inflammatory capacity, which may contribute to alleviate the development of RTIs, asthma, COPD. In addition to anti-inflammatory effect, butyrate and propionate can induce apoptosis of lung cancer cells and inhibit proliferation of lung cancer cells in vitro (159–161). Therefore, oral administration of some lactobacilli may provide benefit for the treatment of lung cancer by producing butyrate and propionate.
3.1.3 Migration of Lactobacillus and Their Components to the Lung
There may be two main routes for transfer of intestinal bacteria to the lung tissue: the first is intestinal bacteria or bacterial components enter into the circulation through the mesenteric lymphatic system and then reach the lung tissue (162–164); the second is the migration of intestinal bacteria or bacterial components to the lung tissue through microbreathing and oropharyngeal reflux (165, 166). Therefore, Lactobacillus or components of Lactobacillus in the intestine may be directly transferred to lung tissue and thus modulate lung immunity.
3.2 Enhancement of the Mucosal Immunity of the Respiratory Tract
Intestinal supplementation with Lactobacillus has been shown to promote respiratory health, but direct action of immunobiotic Lactobacillus on the respiratory mucosa may modulate local immunity of the respiratory tract. It has been found that intranasal administration of Lactobacillus can induce better respiratory immune response than oral administration (3, 50, 167). Nasal administration of Lactobacillus does not generally produce SCFAs due to the absence of substrate. The potential mechanisms by which they regulate respiratory immunity mainly have two aspects.
The first one is that some components of Lactobacillus can be recognized by PRRs in the respiratory tract and then activate downstream pathways. For example, nasal priming with peptidoglycan from L. rhamnosus CRL1505 increases TNF-α and IL-10 levels of lung and upregulates TLR2 and TLR9 expression in alveolar macrophages, which is similar to intranasal administration of whole bacteria (40). Meanwhile, other studies have shown that nasal priming with peptidoglycan from L. rhamnosus CRL1505 can enhance the TLR3/RIG-I-triggered antiviral immune response by increasing IFN-γ and NK cell activity, thus contributing to higher viral clearance and reducing lung tissue damage (28, 65, 168). In addition, lung peptidoglycan can also be recognized by peptidoglycan recognition proteins (PGRPs), a type of PRRs, which mediates bactericidal effect (169). For instance, activated PGRP2 could promote neutrophil recruitment in lung tissue of S. pneumoniae infected mice (170). Of note, not all peptidoglycan of Lactobacillus species has the same protective effect. Nasal administration the peptidoglycan from L. rhamnosus CRL534 does not enhance resistance to S. pneumoniae infection in immunodeficient mice (41). This strongly suggests that the protective effect provided by Lactobacillus is strain-specific. Importantly, nasal administration of Lactobacillus may activate PRRs by multiple pathways. Even if one PRR is blocked, another pathway can be activated to provide protection in a compensatory manner. For example, studies have shown that only when both NOD2 and TLR2 are knocked out can L. plantarum BAA-793 lose its role in protecting against pneumonia virus infection (3, 64, 171). Therefore, components of Lactobacillus to activate the PRRs may be an important part of the protective role performed by intranasal Lactobacillus delivery.
The second one is that Lactobacillus can bind to host cells to antagonize adhesion or binding of pathogen. For the bacteria, experiments have demonstrated the ability of Lactobacillus to directly inhibit the adhesion of bacteria to respiratory epithelial cells. L. rhamnosus Kx151A1, L. reuteri PTA-5289, and L. salivarius LMG9477 can inhibit the adhesion of S. pyogenes to pharyngeal epithelial cells (172). Moreover, intranasal administration of L. murinus CNCM I-5314, a eubacterium of the murine lung, can provide a barrier function against the colonization of S. pneumoniae in the lung tissue (173). In the case of viruses, Lactobacillus binds competitively to viral receptor molecule to prevent viral entry into the host cell. For instance, lipopeptides released by L. curvatus, L. sakei and L. lactis (strains not shown) can bind to the receptor molecule (angiotensin-converting enzyme 2) of SARS-CoV-2 spike glycoprotein, and may prevent virus entry into host cells (174, 175). In addition to inhibiting the adhesion and binding of pathogenic bacteria, Lactobacillus directly displays antibacterial activity. Some Lactobacillus spp. exhibit antibacterial effect against group A Streptococcus in vitro (176). Similarly, L. rhamnosus Kx151A1 and L. reuteri PTA-5289 significantly inhibit hemolytic activity of S. pyogenes in vitro (172). Additionally, some proteins secreted by Lactobacillus have antimicrobial activity. For example, reuterin secreted by L. reuteri has broad-spectrum antibacterial effect (2). However, whether it will alter lung microbial composition and affect lung homeostasis remains further investigation.
4 Safety
As normal members of the human intestinal, vaginal, skin, oral and respiratory flora, Lactobacilli are low-toxicity commensal organisms and are mostly considered safe when taken as probiotics. In animal studies, long-term oral administration of L. plantarum PS128 had no bad side on the health in mice (177). In addition, nasal inoculation of L. reuteri F275 and L. rhamnosus GG, which are generally colonized in the intestinal tract. L. reuteri F275 is cleared in lung tissue less than 24 hours (178); live L. rhamnosus GG is detected on the nasal mucosa at 24 hours after intranasal administration, but not after 72 hours, and does not affect body weight or behavior in mice (97). In clinical trials, oral administration of some common Lactobacillus species such as L. reuteri DSM17938, L. casei Shirota and L. salivarius CECT5713 is safe in infants or children (2, 179–181). Moreover, with a long history of safe use, Lactobacilli are classified as GRAS (Generally Recognized as Safe) and QPS (Qualified Presumption of Safety) by the US Food and Drug Administration (FDA) and the European Food Safety Authority (EFSA, 2021), respectively (182). Therefore, administration of some Lactobacillus species is generally safe.
However, when Lactobacillus is given intranasally, it can colonize the respiratory tract for long periods of time, and whether this could interfere lung microbial homeostasis or induce more severe inflammation or even lead to bacteremia is not clear. Although Lactobacillus bacteremia is a rare disease, it has been found in the clinical that L. rhamnosus GG and L. casei (strain not shown), which are generally considered safe, can also cause bacteremia when in a state of immunosuppression, prolonged hospitalization or surgical intervention (183). Even non-pathogenic bacteria of L. salivarius (strain not shown) in the oral cavity have been found to cause bacteremia, septic chest and diabetic ketoacidosis due to respiratory failure in the clinical (184). Moreover, it has been reported that pneumonia and pleural abscess are caused by mixed Lactobacillus infection in elderly people with esophageal cancer (185). Although it occurs being rare and almost exclusively in infants, children and immune-compromised populations, this also suggests that live Lactobacillus is not absolutely safe, especially when administered intranasally. Further, the presence of antibiotic resistance genes and virulence genes in Lactobacillus and their possible transfer to other microorganisms is also a concern.
5 Concluding Remarks
The use of Lactobacillus is a promising strategy for the prevention and treatment of respiratory diseases and is generally safe. The mechanism of Lactobacillus in regulating respiratory immunity includes the gut-lung axis and activation of mucosal immunity. The beneficial role of Lactobacillus on the respiratory tract is strain-dependent, and may change in different species of Lactobacillus and even subspecies of each Lactobacillus (32). Therefore, for clinical applications, the selection of effective Lactobacillus strains is crucial. Additionally, a single Lactobacillus is not always effective for all respiratory diseases and efficacy may be inconsistent even if the same Lactobacillus is administered due to many factors such as subject, dose and time of administration in clinical trials. The uncertainty of the effectiveness of Lactobacilli is one of the main reasons limiting their application in the clinic. For this problem, co-administration of multiple probiotic Lactobacillus or co-administration of probiotic Lactobacillus and other probiotics such as Bifidobacterium can provide more stable and better efficacy, which is also the trend of probiotic application. Furthermore, clinical application of the main active ingredients of Lactobacillus or inactivated bacteria can provide more safer effect and stable efficacy relative to live bacteria. Besides, Lactobacillus has preventive effect for offspring respiratory disease when administered to the mother, and it can greatly reduce the occurrence and alleviate the symptoms of respiratory diseases in infants and children if the stability and safety of Lactobacillus efficacy is fully established. Additionally, the economic burden of clinical application of Lactobacillus is low (186). Overall, administration of Lactobacillus is beneficial in improving pulmonary health and its application in treating respiratory diseases needs more clinical studies.
Author Contributions
TD finished the original manuscript. NZ compiled table information. CZ and AL provided constructive comments and made critical revisions to the manuscript. All authors contributed to the article and approved the submitted version.
Funding
CZ was supported by National Natural Science Foundation of China (No. 31970177); AL was supported by the National Natural Science Foundation of Hunan Province (No. 2021JJ40475).
Conflict of Interest
The authors declare that the research was conducted in the absence of any commercial or financial relationships that could be construed as a potential conflict of interest.
Publisher’s Note
All claims expressed in this article are solely those of the authors and do not necessarily represent those of their affiliated organizations, or those of the publisher, the editors and the reviewers. Any product that may be evaluated in this article, or claim that may be made by its manufacturer, is not guaranteed or endorsed by the publisher.
Abbreviations
RTIs, Respiratory infections; CF, Cystic fibrosis; COPD, Chronic obstructive pulmonary disease; RSV, Respiratory Syncytial Virus; SARS-CoV-2, Severe Acute Respiratory Syndrome Coronavirus-2; SCFAs, Short-chain fatty acids; DC, Dendritic cell; BALF, Bronchoalveolar lavage fluid; PRR, Pattern recognition receptor; TLRs, Toll-like receptors; NLRs, NOD-like receptors; Tregs, Regulatory T cells.
References
2. Mu Q, Tavella VJ, Luo XM. Role of Lactobacillus Reuteri in Human Health and Diseases. Front Microbiol (2018) 9:757. doi: 10.3389/fmicb.2018.00757
3. Gabryszewski SJ, Bachar O, Dyer KD, Percopo CM, Killoran KE, Domachowske JB, et al. Lactobacillus-Mediated Priming of the Respiratory Mucosa Protects Against Lethal Pneumovirus Infection. J Immunol (2011) 186(2):1151–61. doi: 10.4049/jimmunol.1001751
4. Duar RM, Lin XB, Zheng J, Martino ME, Grenier T, Perez-Munoz ME, et al. Lifestyles in Transition: Evolution and Natural History of the Genus Lactobacillus. FEMS Microbiol Rev (2017) 41(Supp1):S27–48. doi: 10.1093/femsre/fux030
5. Goldstein EJ, Tyrrell KL, Citron DM. Lactobacillus Species: Taxonomic Complexity and Controversial Susceptibilities. Clin Infect Dis (2015) 60 Suppl 2:S98–107. doi: 10.1093/cid/civ072
6. Zhang Z, Lv J, Pan L, Zhang Y. Roles and Applications of Probiotic Lactobacillus Strains. Appl Microbiol Biotechnol (2018) 102(19):8135–43. doi: 10.1007/s00253-018-9217-9
7. O'Callaghan J, O'Toole PW. Lactobacillus: Host-Microbe Relationships. Curr Top Microbiol Immunol (2013) 358:119–54. doi: 10.1007/82_2011_187
8. Heeney DD, Gareau MG, Marco ML. Intestinal Lactobacillus in Health and Disease, a Driver or Just Along for the Ride? Curr Opin Biotechnol (2018) 49:140–7. doi: 10.1016/j.copbio.2017.08.004
9. Hojsak I. Probiotics in Functional Gastrointestinal Disorders. Adv Exp Med Biol (2019) 1125:121–37. doi: 10.1007/5584_2018_321
10. Margiotta G, Ferretti S, Graglia B, Gatto A, Capossela L, Bersani G, et al. Effect of Lactobacillus Reuteri Lre02-Lactobacillus Rhamnosus Lr04 Combination and Gastrointestinal Functional Disorders in an Emergency Department Pediatric Population. Eur Rev Med Pharmacol Sci (2021) 25(7):3097–104. doi: 10.26355/eurrev_202104_25564
11. Zheng N, Guo R, Wang J, Zhou W, Ling Z. Contribution of Lactobacillus Iners to Vaginal Health and Diseases: A Systematic Review. Front Cell Infect Microbiol (2021) 11:792787. doi: 10.3389/fcimb.2021.792787
12. Chee WJY, Chew SY, Than LTL. Vaginal Microbiota and the Potential of Lactobacillus Derivatives in Maintaining Vaginal Health. Microb Cell Fact (2020) 19(1):203. doi: 10.1186/s12934-020-01464-4
13. Gao F, Fang Z, Lu W. Regulation Divergences of Lactobacillus Fermentum Pcc and Lactobacillus Paracasei 431 on Penicillin-Induced Upper Respiratory Tract Microbial Dysbiosis in Balb/C Mice. Food Funct (2021) 12(23):11913–25. doi: 10.1039/d0fo02981e
14. Chong HX, Yusoff NAA, Hor YY, Lew LC, Jaafar MH, Choi SB, et al. Lactobacillus Plantarum Dr7 Improved Upper Respiratory Tract Infections Via Enhancing Immune and Inflammatory Parameters: A Randomized, Double-Blind, Placebo-Controlled Study. J Dairy Sci (2019) 102(6):4783–97. doi: 10.3168/jds.2018-16103
15. Strauss M, Micetic-Turk D, Pogacar MS, Fijan S. Probiotics for the Prevention of Acute Respiratory-Tract Infections in Older People: Systematic Review. Healthcare (Basel) (2021) 9(6):690. doi: 10.3390/healthcare9060690
16. Zelaya H, Laino J, Villena J, Marranzino G, Alvarez S, Aguero G. Lactobacillus Casei Crl431 Modulates Hemostatic Activation Induced by Protein Malnourishment and Pneumococcal Respiratory Infection. Appl Microbiol Biotechnol (2020) 104(24):10669–83. doi: 10.1007/s00253-020-10957-6
17. Percopo CM, Dyer KD, Garcia-Crespo KE, Gabryszewski SJ, Shaffer AL 3rd, Domachowske JB, et al. B Cells Are Not Essential for Lactobacillus-Mediated Protection Against Lethal Pneumovirus Infection. J Immunol (2014) 192(11):5265–72. doi: 10.4049/jimmunol.1400087
18. Forsythe P, Inman MD, Bienenstock J. Oral Treatment With Live Lactobacillus Reuteri Inhibits the Allergic Airway Response in Mice. Am J Respir Crit Care Med (2007) 175(6):561–9. doi: 10.1164/rccm.200606-821OC
19. Di Nardo G, Oliva S, Menichella A, Pistelli R, De Biase RV, Patriarchi F, et al. Lactobacillus Reuteri Atcc55730 in Cystic Fibrosis. J Pediatr Gastroenterol Nutr (2014) 58(1):81–6. doi: 10.1097/MPG.0000000000000187
20. Gui Q-F, Lu H-F, Zhang C-X, Xu Z-R, Yang Y-H. Well-Balanced Commensal Microbiota Contributes to Anti-Cancer Response in a Lung Cancer Mouse Model. Gen Mol Res (2015) 14(2):5642–51. doi: 10.4238/2015.May.25.16
21. Carvalho JL, Miranda M, Fialho AK, Castro-Faria-Neto H, Anatriello E, Keller AC, et al. Oral Feeding With Probiotic Lactobacillus Rhamnosus Attenuates Cigarette Smoke-Induced Copd in C57bl/6 Mice: Relevance to Inflammatory Markers in Human Bronchial Epithelial Cells. PloS One (2020) 15(4):e0225560. doi: 10.1371/journal.pone.0225560
22. Fendrick AM, Monto AS, Nightengale B, Sarnes M. The Economic Burden of Non-Influenza-Related Viral Respiratory Tract Infection in the United States. Arch Intern Med (2013) 163(4):487–94. doi: 10.1001/archinte.163.4.487
23. Ahmadi Badi S, Tarashi S, Fateh A, Rohani P, Masotti A, Siadat SD. From the Role of Microbiota in Gut-Lung Axis to Sars-Cov-2 Pathogenesis. Mediators Inflammation (2021) 2021:6611222. doi: 10.1155/2021/6611222
24. Ruopp M, Chiswell K, Thaden JT, Merchant K, Tsalik EL. Respiratory Tract Infection Clinical Trials From 2007-2012: A Systematic Review of Clinicaltrials.Gov. Ann Am Thorac Soc (2015) 12(12):1852–63. doi: 10.1513/AnnalsATS.201505-291OC
25. Macias AE, McElhaney JE, Chaves SS, Nealon J, Nunes MC, Samson SI, et al. The Disease Burden of Influenza Beyond Respiratory Illness. Vaccine (2021) 39 Suppl 1:A6–A14. doi: 10.1016/j.vaccine.2020.09.048
26. Garcia-Castillo V, Tomokiyo M, Raya Tonetti F, Islam MA, Takahashi H, Kitazawa H, et al. Alveolar Macrophages Are Key Players in the Modulation of the Respiratory Antiviral Immunity Induced by Orally Administered Lacticaseibacillus Rhamnosus Crl1505. Front Immunol (2020) 11:568636. doi: 10.3389/fimmu.2020.568636
27. Fonseca W, Lucey K, Jang S, Fujimura KE, Rasky A, Ting HA, et al. Lactobacillus Johnsonii Supplementation Attenuates Respiratory Viral Infection Via Metabolic Reprogramming and Immune Cell Modulation. Mucosal Immunol (2017) 10(6):1569–80. doi: 10.1038/mi.2017.13
28. Tomosada Y, Chiba E, Zelaya H, Takahashi T, Tsukida K, Kitazawa H, et al. Nasally Administered Lactobacillus Rhamnosus Strains Differentially Modulate Respiratory Antiviral Immune Responses and Induce Protection Against Respiratory Syncytial Virus Infection. BMC Immunol (2013) 14:40. doi: 10.1186/1471-2172-14-40
29. Kumova OK, Fike AJ, Thayer JL, Nguyen LT, Mell JC, Pascasio J, et al. Lung Transcriptional Unresponsiveness and Loss of Early Influenza Virus Control in Infected Neonates Is Prevented by Intranasal Lactobacillus Rhamnosus Gg. PloS Pathog (2019) 15(10):e1008072. doi: 10.1371/journal.ppat.1008072
30. Harata G, He F, Hiruta N, Kawase M, Kubota A, Hiramatsu M, et al. Intranasal Administration of Lactobacillus Rhamnosus Gg Protects Mice From H1n1 Influenza Virus Infection by Regulating Respiratory Immune Responses. Lett Appl Microbiol (2010) 50(6):597–602. doi: 10.1111/j.1472-765X.2010.02844.x
31. Hori T, Kiyoshima J, Shida K, Yasui H. Effect of Intranasal Administration of Lactobacillus Casei Shirota on Influenza Virus Infection of Upper Respiratory Tract in Mice. Clin Diagn Lab Immunol (2001) 8(3):593–7. doi: 10.1128/CDLI.8.3.593-597.2001
32. Takeda S, Takeshita M, Kikuchi Y, Dashnyam B, Kawahara S, Yoshida H, et al. Efficacy of Oral Administration of Heat-Killed Probiotics From Mongolian Dairy Products Against Influenza Infection in Mice: Alleviation of Influenza Infection by Its Immunomodulatory Activity Through Intestinal Immunity. Int Immunopharmacol (2011) 11(12):1976–83. doi: 10.1016/j.intimp.2011.08.007
33. Kawase M, He F, Kubota A, Yoda K, Miyazawa K, Hiramatsu M. Heat-Killed Lactobacillus Gasseri Tmc0356 Protects Mice Against Influenza Virus Infection by Stimulating Gut and Respiratory Immune Responses. FEMS Immunol Med Microbiol (2012) 64(2):280–8. doi: 10.1111/j.1574-695X.2011.00903.x
34. Yeo JM, Lee HJ, Kim JW, Lee JB, Park SY, Choi IS, et al. Lactobacillus Fermentum Cjl-112 Protects Mice Against Influenza Virus Infection by Activating T-Helper 1 and Eliciting a Protective Immune Response. Int Immunopharmacol (2014) 18(1):50–4. doi: 10.1016/j.intimp.2013.10.020
35. Park S, Kim JI, Bae JY, Yoo K, Kim H, Kim IH, et al. Effects of Heat-Killed Lactobacillus Plantarum Against Influenza Viruses in Mice. J Microbiol (2018) 56(2):145–9. doi: 10.1007/s12275-018-7411-1
36. Belkacem N, Serafini N, Wheeler R, Derrien M, Boucinha L, Couesnon A, et al. Lactobacillus Paracasei Feeding Improves Immune Control of Influenza Infection in Mice. PloS One (2017) 12(9):e0184976. doi: 10.1371/journal.pone.0184976
37. Youn HN, Lee YN, Lee DH, Park JK, Yuk SS, Lee HJ, et al. Effect of Intranasal Administration of Lactobacillus Fermentum Cjl-112 on Horizontal Transmission of Influenza Virus in Chickens. Poult Sci (2012) 91(10):2517–22. doi: 10.3382/ps.2012-02334
38. Dos Santos Pereira Andrade AC, Lima MT, Oliveira GP, Calixto RS, de Sales ESEL, da Gloria de Souza D, et al. Daily Ingestion of the Probiotic Lactobacillus Paracasei St11 Decreases Vaccinia Virus Dissemination and Lethality in a Mouse Model. Benef Microbes (2017) 8(1):73–80. doi: 10.3920/BM2016.0074
39. Barbieri N, Villena J, Herrera M, Salva S, Alvarez S. Nasally Administered Lactobacillus Rhamnosus Accelerate the Recovery of Humoral Immunity in B Lymphocyte-Deficient Malnourished Mice. J Nutr (2013) 143(2):227–35. doi: 10.3945/jn.112.165811
40. Kolling Y, Salva S, Villena J, Marranzino G, Alvarez S. Non-Viable Immunobiotic Lactobacillus Rhamnosus Crl1505 and Its Peptidoglycan Improve Systemic and Respiratory Innate Immune Response During Recovery of Immunocompromised-Malnourished Mice. Int Immunopharmacol (2015) 25(2):474–84. doi: 10.1016/j.intimp.2015.02.006
41. Kolling Y, Salva S, Villena J, Alvarez S. Are the Immunomodulatory Properties of Lactobacillus Rhamnosus Crl1505 Peptidoglycan Common for All Lactobacilli During Respiratory Infection in Malnourished Mice? PloS One (2018) 13(3):e0194034. doi: 10.1371/journal.pone.0194034
42. Tanaka A, Seki M, Yamahira S, Noguchi H, Kosai K, Toba M, et al. Lactobacillus Pentosus Strain B240 Suppresses Pneumonia Induced by Streptococcus Pneumoniae in Mice. Lett Appl Microbiol (2011) 53(1):35–43. doi: 10.1111/j.1472-765X.2011.03079.x
43. Zelaya H, Laino J, Haro C, Alvarez S, Aguero G. Modulation of the Immuno-Coagulative Response in a Pneumococcal Infection in Malnourished Mice Nasally Treated With Lactobacillus Casei. J Med Microbiol (2013) 62(Pt 1):145–54. doi: 10.1099/jmm.0.045526-0
44. Racedo S, Villena J, Medina M, Aguero G, Rodriguez V, Alvarez S. Lactobacillus Casei Administration Reduces Lung Injuries in a Streptococcus Pneumoniae Infection in Mice. Microbes Infect (2006) 8(9-10):2359–66. doi: 10.1016/j.micinf.2006.04.022
45. Khailova L, Baird CH, Rush AA, McNamee EN, Wischmeyer PE. Lactobacillus Rhamnosus Gg Improves Outcome in Experimental Pseudomonas Aeruginosa Pneumonia: Potential Role of Regulatory T Cells. Shock (2013) 40(6):496–503. doi: 10.1097/SHK.0000000000000066
46. Alvarez S, Herrero C, Bru E, Perdigon G. Effect of Lactobacillus Casei and Yogurt Administration on Prevention of Pseudomonas Aeruginosa Infection in Young Mi. J Food Prot (2001) 64(11):1768–74. doi: 10.4315/0362-028x-64.11.1768
47. Vareille-Delarbre M, Miquel S, Garcin S, Bertran T, Balestrino D, Evrard B, et al. Immunomodulatory Effects of Lactobacillus Plantarum on Inflammatory Response Induced by Klebsiella Pneumoniae. Infect Immun (2019) 87(11):e00570-19. doi: 10.1128/IAI.00570-19
48. Bernard-Raichon L, Colom A, Monard SC, Namouchi A, Cescato M, Garnier H, et al. A Pulmonary Lactobacillus Murinus Strain Induces Th17 and Rorgammat(+) Regulatory T Cells and Reduces Lung Inflammation in Tuberculosis. J Immunol (2021) 207(7):1857–70. doi: 10.4049/jimmunol.2001044
49. Villena J, Chiba E, Tomosada Y, Salva S, Marranzino G, Kitazawa H, et al. Orally Administered Lactobacillus Rhamnosus Modulates the Respiratory Immune Response Triggered by the Viral Pathogen-Associated Molecular Pattern Poly(I:C). BMC Immunol (2012) 13:53. doi: 10.1186/1471-2172-13-53
50. Youn HN, Lee DH, Lee YN, Park JK, Yuk SS, Yang SY, et al. Intranasal Administration of Live Lactobacillus Species Facilitates Protection Against Influenza Virus Infection in Mice. Antiviral Res (2012) 93(1):138–43. doi: 10.1016/j.antiviral.2011.11.004
51. Hori T, Kiyoshima J, Shida K, Yasui H. Augmentation of Cellular Immunity and Reduction of Influenza Virus Titer in Aged Mice Fed Lactobacillus Casei Strain Shirota. Clin Diagn Lab Immunol (2002) 9(1):105–8. doi: 10.1128/cdli.9.1.105-108.2002
52. Park MK, Ngo V, Kwon YM, Lee YT, Yoo S, Cho YH, et al. Lactobacillus Plantarum Dk119 as a Probiotic Confers Protection Against Influenza Virus by Modulating Innate Immunity. PloS One (2013) 8(10):e75368. doi: 10.1371/journal.pone.0075368
53. Arai S, Iwabuchi N, Takahashi S, Xiao JZ, Abe F, Hachimura S. Orally Administered Heat-Killed Lactobacillus Paracasei Mcc1849 Enhances Antigen-Specific Iga Secretion and Induces Follicular Helper T Cells in Mice. PloS One (2018) 13(6):e0199018. doi: 10.1371/journal.pone.0199018
54. Nakayama Y, Moriya T, Sakai F, Ikeda N, Shiozaki T, Hosoya T, et al. Oral Administration of Lactobacillus Gasseri Sbt2055 Is Effective for Preventing Influenza in Mice. Sci Rep (2014) 4:4638. doi: 10.1038/srep04638
55. Asama T, Uematsu T, Kobayashi N, Tatefuji T, Hashimoto K. Oral Administration of Heat-Killed Lactobacillus Kunkeei Yb38 Improves Murine Influenza Pneumonia by Enhancing Iga Production. Bioscience microbiota Food Health (2017) 36(1):1–9. doi: 10.12938/bmfh.16-010
56. Fonolla J, Gracian C, Maldonado-Lobon JA, Romero C, Bedmar A, Carrillo JC, et al. Effects of Lactobacillus Coryniformis K8 Cect5711 on the Immune Response to Influenza Vaccination and the Assessment of Common Respiratory Symptoms in Elderly Subjects: A Randomized Controlled Trial. Eur J Nutr (2019) 58(1):83–90. doi: 10.1007/s00394-017-1573-1
57. Yang WT, Yang GL, Yang X, Shonyela SM, Zhao L, Jiang YL, et al. Recombinant Lactobacillus Plantarum Expressing Ha2 Antigen Elicits Protective Immunity Against H9n2 Avian Influenza Virus in Chickens. Appl Microbiol Biotechnol (2017) 101(23-24):8475–84. doi: 10.1007/s00253-017-8600-2
58. Peiroten A, Landete JM. Natural and Engineered Promoters for Gene Expression in Lactobacillus Species. Appl Microbiol Biotechnol (2020) 104(9):3797–805. doi: 10.1007/s00253-020-10426-0
59. Fonseca W, Malinczak CA, Fujimura K, Li D, McCauley K, Li J, et al. Maternal Gut Microbiome Regulates Immunity to Rsv Infection in Offspring. J Exp Med (2021) 218(11):e20210235. doi: 10.1084/jem.20210235
60. Tao K, Tzou PL, Nouhin J, Gupta RK, de Oliveira T, Kosakovsky Pond SL, et al. The Biological and Clinical Significance of Emerging Sars-Cov-2 Variants. Nat Rev Genet (2021) 22(12):757–73. doi: 10.1038/s41576-021-00408-x
61. Soloveva IV, Ilyicheva TN, Marchenko VY, Pyankov OV, Tochilina AG, Belova IV, et al. Genome Features and in Vitro Activity Against Influenza a and Sars-Cov-2 Viruses of Six Probiotic Strains. BioMed Res Int (2021) 2021:6662027. doi: 10.1155/2021/6662027
62. Islam MA, Albarracin L, Tomokiyo M, Valdez JC, Sacur J, Vizoso-Pinto MG, et al. Immunobiotic Lactobacilli Improve Resistance of Respiratory Epithelial Cells to Sars-Cov-2 Infection. Pathogens (2021) 10(9):1197. doi: 10.3390/pathogens10091197
63. Bousquet J, Anto JM, Czarlewski W, Haahtela T, Fonseca SC, Iaccarino G, et al. Cabbage and Fermented Vegetables: From Death Rate Heterogeneity in Countries to Candidates for Mitigation Strategies of Severe Covid-19. Allergy (2021) 76(3):735–50. doi: 10.1111/all.14549
64. Percopo CM, Rice TA, Brenner TA, Dyer KD, Luo JL, Kanakabandi K, et al. Immunobiotic Lactobacillus Administered Post-Exposure Averts the Lethal Sequelae of Respiratory Virus Infection. Antiviral Res (2015) 121:109–19. doi: 10.1016/j.antiviral.2015.07.001
65. Clua P, Kanmani P, Zelaya H, Tada A, Kober AKMH, Salva S, et al. Peptidoglycan From Immunobiotic Lactobacillus Rhamnosus Improves Resistance of Infant Mice to Respiratory Syncytial Viral Infection and Secondary Pneumococcal Pneumonia. Front Immunol (2017) 8:948. doi: 10.3389/fimmu.2017.00948
66. Clua P, Tomokiyo M, Raya Tonetti F, Islam MA, García Castillo V, Marcial G, et al. The Role of Alveolar Macrophages in the Improved Protection Against Respiratory Syncytial Virus and Pneumococcal Superinfection Induced by the Peptidoglycan of Lactobacillus Rhamnosus Crl1505. Cells (2020) 9(7):1653. doi: 10.3390/cells9071653
67. Luoto R, Ruuskanen O, Waris M, Kalliomaki M, Salminen S, Isolauri E. Prebiotic and Probiotic Supplementation Prevents Rhinovirus Infections in Preterm Infants: A Randomized, Placebo-Controlled Trial. J Allergy Clin Immunol (2014) 133(2):405–13. doi: 10.1016/j.jaci.2013.08.020
68. Vaisberg M, Paixao V, Almeida EB, Santos JMB, Foster R, Rossi M, et al. Daily Intake of Fermented Milk Containing Lactobacillus Casei Shirota (Lcs) Modulates Systemic and Upper Airways Immune/Inflammatory Responses in Marathon Runners. Nutrients (2019) 11(7):1678. doi: 10.3390/nu11071678
69. Sanz JC, Mateos J, Conejo AM. Effect of Lactobacillus Casei on the Incidence of Infectious Conditions in Children. Nutrición hospitalaria (2006) 21(4):547–51.
70. Weizman Z, Asli G, Alsheikh A. Effect of a Probiotic Infant Formula on Infections in Child Care Centers: Comparison of Two Probiotic Agents. Pediatrics (2005) 115(1):5–9. doi: 10.1542/peds.2004-1815
71. Hirose Y, Yamamoto Y, Yoshikai Y, Murosaki S. Oral Intake of Heat-Killed Lactobacillus Plantarum L-137 Decreases the Incidence of Upper Respiratory Tract Infection in Healthy Subjects With High Levels of Psychological Stress. J Nutr Sci (2013) 2:e39. doi: 10.1017/jns.2013.35
72. Pu F, Guo Y, Li M, Zhu H, Wang S, Shen X, et al. Yogurt Supplemented With Probiotics Can Protect the Healthy Elderly From Respiratory Infections: A Randomized Controlled Open-Label Trial. Clin Interv Aging (2017) 12:1223–31. doi: 10.2147/CIA.S141518
73. Maldonado J, Canabate F, Sempere L, Vela F, Sanchez AR, Narbona E, et al. Human Milk Probiotic Lactobacillus Fermentum Cect5716 Reduces the Incidence of Gastrointestinal and Upper Respiratory Tract Infections in Infants. J Pediatr Gastroenterol Nutr (2012) 54(1):55–61. doi: 10.1097/MPG.0b013e3182333f18
74. West NP, Pyne DB, Cripps AW, Hopkins WG, Eskesen DC, Jairath A, et al. Lactobacillus Fermentum (Pcc(R)) Supplementation and Gastrointestinal and Respiratory-Tract Illness Symptoms: A Randomised Control Trial in Athletes. Nutr J (2011) 10:30. doi: 10.1186/1475-2891-10-30
75. Gleeson M, Bishop NC, Oliveira M, McCauley T, Tauler P, Lawrence C. Effects of a Lactobacillus Salivarius Probiotic Intervention on Infection, Cold Symptom Duration and Severity, and Mucosal Immunity in Endurance Athletes. Int J Sport Nutr Exerc Metab (2012) 12(4):235–42. doi: 10.1123/ijsnem.22.4.235
76. Michalickova D, Minic R, Dikic N, Andjelkovic M, Kostic-Vucicevic M, Stojmenovic T, et al. Lactobacillus Helveticus Lafti L10 Supplementation Reduces Respiratory Infection Duration in a Cohort of Elite Athletes: A Randomized, Double-Blind, Placebo-Controlled Trial. Appl Physiol Nutr Metab (2016) 41(7):782–9. doi: 10.1139/apnm-2015-0541
77. Hatakka K, Blomgren K, Pohjavuori S, Kaijalainen T, Poussa T, Leinonen M, et al. Treatment of Acute Otitis Media With Probiotics in Otitis-Prone Children-A Double-Blind, Placebo-Controlled Randomised Study. Clin Nutr (2007) 26(3):314–21. doi: 10.1016/j.clnu.2007.01.003
78. Zhang H, Yeh C, Jin Z, Ding L, Liu BY, Zhang L, et al. Prospective Study of Probiotic Supplementation Results in Immune Stimulation and Improvement of Upper Respiratory Infection Rate. Synth Syst Biotechnol (2018) 3(2):113–20. doi: 10.1016/j.synbio.2018.03.001
79. Gerasimov SV, Ivantsiv VA, Bobryk LM, Tsitsura OO, Dedyshin LP, Guta NV, et al. Role of Short-Term Use of L. Acidophilus Dds-1 and B. Lactis Uabla-12 in Acute Respiratory Infections in Children: A Randomized Controlled Trial. Eur J Clin Nutr (2016) 70(4):463–9. doi: 10.1038/ejcn.2015.171
80. de Vrese M, Winkler P, Rautenberg P, Harder T, Noah C, Laue C, et al. Effect of Lactobacillus Gasseri Pa 16/8, Bifidobacterium Longum Sp 07/3, B. Bifidum Mf 20/5 on Common Cold Episodes: A Double Blind, Randomized, Controlled Trial. Clin Nutr (2005) 24(4):481–91. doi: 10.1016/j.clnu.2005.02.006
81. Wang H, Wang Y, Lu C, Qiu L, Song X, Jia H, et al. The Efficacy of Probiotics in Patients With Severe Covid-19. Ann Palliat Med (2021) 10(12):12374–80. doi: 10.21037/apm-21-3373
82. Gutierrez-Castrellon P, Gandara-Marti T, Abreu YAAT, Nieto-Rufino CD, Lopez-Orduna E, Jimenez-Escobar I, et al. Probiotic Improves Symptomatic and Viral Clearance in Covid19 Outpatients: A Randomized, Quadruple-Blinded, Placebo-Controlled Trial. Gut Microbes (2022) 14(1):2018899. doi: 10.1080/19490976.2021.2018899
83. Tapiovaara L, Kumpu M, Makivuokko H, Waris M, Korpela R, Pitkaranta A, et al. Human Rhinovirus in Experimental Infection After Peroral Lactobacillus Rhamnosus Gg Consumption, a Pilot Study. Int Forum Allergy Rhinol (2016) 6(8):848–53. doi: 10.1002/alr.21748
84. d'Ettorre G, Ceccarelli G, Marazzato M, Campagna G, Pinacchio C, Alessandri F, et al. Challenges in the Management of Sars-Cov2 Infection: The Role of Oral Bacteriotherapy as Complementary Therapeutic Strategy to Avoid the Progression of Covid-19. Front Med (Lausanne) (2020) 7:389. doi: 10.3389/fmed.2020.00389
85. Khan AM, Shahzad M, Raza Asim MB, Imran M, Shabbir A. Zingiber Officinale Ameliorates Allergic Asthma Via Suppression of Th2-Mediated Immune Response. Pharm Biol (2015) 53(3):359–67. doi: 10.3109/13880209.2014.920396
86. Vézina K, Chauhan B, Ducharme F. Inhaled Anticholinergics and Short-Acting Beta2 -Agonists Versus Short-Acting Beta2-Agonists Alone for Children With Acute Asthma in Hospital (Review). Cochrane Database systematic Rev (2014) 31(7):CD010283. doi: 10.1002/14651858.CD010283.pub2
87. Wu CT, Lin FH, Lee YT, Ku MS, Lue KH. Effect of Lactobacillus Rhamnosus Gg Immunopathologic Changes in Chronic Mouse Asthma Model. J Microbiol Immunol Infect (2019) 52(6):911–9. doi: 10.1016/j.jmii.2019.03.002
88. Liu YW, Liao TW, Chen YH, Chiang YC, Tsai YC. Oral Administration of Heat-Inactivated Lactobacillus Plantarum K37 Modulated Airway Hyperresponsiveness in Ovalbumin-Sensitized Balb/C Mice. PloS One (2014) 9(6):e100105. doi: 10.1371/journal.pone.0100105
89. Lim LH, Li HY, Huang CH, Lee BW, Lee YK, Chua KY. The Effects of Heat-Killed Wild-Type Lactobacillus Casei Shirota on Allergic Immune Responses in an Allergy Mouse Model. Int Arch Allergy Immunol (2009) 148(4):297–304. doi: 10.1159/000170383
90. Lin CH, Tseng CY, Chao MW. Administration of Lactobacillus Paracasei Hb89 Mitigates Pm2.5-Induced Enhancement of Inflammation and Allergic Airway Response in Murine Asthma Model. PloS One (2020) 15(12):e0243062. doi: 10.1371/journal.pone.0243062
91. Yun X, Shang Y, Li M. Effect of Lactobacillus Salivarius on Th1/Th2 Cytokines and the Number of Spleen Cd4(+) Cd25(+) Foxp3(+) Treg in Asthma Balb/C Mouse. Int J Clin Exp Pathol (2015) 8(7):7661–74.
92. Du X, Wang L, Wu S, Yuan L, Tang S, Xiang Y, et al. Efficacy of Probiotic Supplementary Therapy for Asthma, Allergic Rhinitis, and Wheeze: A Meta-Analysis of Randomized Controlled Trials. Allergy Asthma Proc (2019) 40(4):250–60. doi: 10.2500/aap.2019.40.4227
93. Huang CF, Chie WC, Wang IJ. Efficacy of Lactobacillus Administration in School-Age Children With Asthma: A Randomized, Placebo-Controlled Trial. Nutrients (2018) 10(11):1678. doi: 10.3390/nu10111678
94. Ramsahai JM, Hansbro PM, Wark PAB. Mechanisms and Management of Asthma Exacerbations. Am J Respir Crit Care Med (2018) 199(4):423–32. doi: 10.1164/rccm.201810-1931CI
95. Daley D. The Evolution of the Hygiene Hypothesis: The Role of Early-Life Exposures to Viruses and Microbes and Their Relationship to Asthma and Allergic Diseases. Curr Opin Allergy Clin Immunol (2014) 14(5):390–6. doi: 10.1097/ACI.0000000000000101
96. Pellaton C, Nutten S, Thierry AC, Boudousquie C, Barbier N, Blanchard C, et al. Intragastric and Intranasal Administration of Lactobacillus Paracasei Ncc2461 Modulates Allergic Airway Inflammation in Mice. Int J Inflam (2012) 2012:686739. doi: 10.1155/2012/686739
97. Spacova I, Petrova MI, Fremau A, Pollaris L, Vanoirbeek J, Ceuppens JL, et al. Intranasal Administration of Probiotic Lactobacillus Rhamnosus Gg Prevents Birch Pollen-Induced Allergic Asthma in a Murine Model. Allergy (2019) 74(1):100–10. doi: 10.1111/all.13502
98. Spacova I, Van Beeck W, Seys S, Devos F, Vanoirbeek J, Vanderleyden J, et al. Lactobacillus Rhamnosus Probiotic Prevents Airway Function Deterioration and Promotes Gut Microbiome Resilience in a Murine Asthma Model. Gut Microbes (2020) 11(6):1729–44. doi: 10.1080/19490976.2020.1766345
99. Schabussova I, Hufnagl K, Tang ML, Hoflehner E, Wagner A, Loupal G, et al. Perinatal Maternal Administration of Lactobacillus Paracasei Ncc 2461 Prevents Allergic Inflammation in a Mouse Model of Birch Pollen Allergy. PloS One (2012) 7(7):e40271. doi: 10.1371/journal.pone.0040271
100. Kalliomäki M, Salminen S, Poussa T, Arvilommi H, Isolauri E. Probiotics and Prevention of Atopic Disease: 4-Year Follow-Up of a Randomised Placebo-Controlled Trial. Lancet (2003) 361(9372):1869–71. doi: 10.1016/s0140-6736(03)13490-3
101. Wu CT, Chen PJ, Lee YT, Ko JL, Lue KH. Effects of Immunomodulatory Supplementation With Lactobacillus Rhamnosus on Airway Inflammation in a Mouse Asthma Model. J Microbiol Immunol Infect (2016) 49(5):625–35. doi: 10.1016/j.jmii.2014.08.001
102. Kim WG, Kang GD, Kim HI, Han MJ, Kim DH. Bifidobacterium Longum Im55 and Lactobacillus Plantarum Im76 Alleviate Allergic Rhinitis in Mice by Restoring Th2/Treg Imbalance and Gut Microbiota Disturbance. Benef Microbes (2019) 10(1):55–67. doi: 10.3920/BM2017.0146
103. Choi SP, Oh HN, Choi CY, Ahn H, Yun HS, Chung YM, et al. Oral Administration of Lactobacillus Plantarum Cjlp133 and Cjlp243 Alleviates Birch Pollen-Induced Allergic Rhinitis in Mice. J Appl Microbiol (2018) 124(3):821–8. doi: 10.1111/jam.13635
104. Kawase M, He F, Kubota A, Hata JY, Kobayakawa S, Hiramatsu M. Inhibitory Effect of Lactobacillus Gasseri Tmc0356 and Lactobacillus Gg on Enhanced Vascular Permeability of Nasal Mucosa in Experimental Allergic Rhinitis of Rats. Biosci Biotechnol Biochem (2006) 70(12):3025–30. doi: 10.1271/bbb.60152
105. Ishida Y, Nakamura F, Kanzato H, Sawada D, Hirata H, Nishimura A, et al. Clinical Effects of Lactobacillus Acidophilus Strain L-92 on Perennial Allergic Rhinitis: A Double-Blind, Placebo-Controlled Study. J Dairy Sci (2005) 88(2):527–33. doi: 10.3168/jds.S0022-0302(05)72714-4
106. Dennis-Wall JC, Culpepper T, Nieves C Jr., Rowe CC, Burns AM, Rusch CT, et al. Probiotics (Lactobacillus Gasseri Ks-13, Bifidobacterium Bifidum G9-1, and Bifidobacterium Longum Mm-2) Improve Rhinoconjunctivitis-Specific Quality of Life in Individuals With Seasonal Allergies: A Double-Blind, Placebo-Controlled, Randomized Trial. Am J Clin Nutr (2017) 105(3):758–67. doi: 10.3945/ajcn.116.140012
107. Ivory K, Wilson AM, Sankaran P, Westwood M, McCarville J, Brockwell C, et al. Oral Delivery of a Probiotic Induced Changes at the Nasal Mucosa of Seasonal Allergic Rhinitis Subjects After Local Allergen Challenge: A Randomised Clinical Trial. PloS One (2013) 8(11):e78650. doi: 10.1371/journal.pone.0078650
108. Jones GS, Baldwin DR. Recent Advances in the Management of Lung Cancer. Clin Med (Northfield Il) (2018) 18(2):41–6. doi: 10.7861/clinmedicine.18-2-s41
109. Liu X, Cheng Y, Zang D, Zhang M, Li X, Liu D, et al. The Role of Gut Microbiota in Lung Cancer: From Carcinogenesis to Immunotherapy. Front Oncol (2021) 11:720842. doi: 10.3389/fonc.2021.720842
110. Sivan A, Corrales L, Hubert N, Williams JB, Keston A-M, Earley ZM, et al. Commensal Bifidobacterium Promotes Antitumor Immunity and Facilitates Anti– Pd-L1 Efficacy. Science (2015) 27(350):1084–9. doi: 10.1126/science.aac4255
111. Yang JJ, Yu D, Xiang YB, Blot W, White E, Robien K, et al. Association of Dietary Fiber and Yogurt Consumption With Lung Cancer Risk: A Pooled Analysis. JAMA Oncol (2020) 6(2):e194107. doi: 10.1001/jamaoncol.2019.4107
112. Le Noci V, Guglielmetti S, Arioli S, Camisaschi C, Bianchi F, Sommariva M, et al. Modulation of Pulmonary Microbiota by Antibiotic or Probiotic Aerosol Therapy: A Strategy to Promote Immunosurveillance Against Lung Metastases. Cell Rep (2018) 24(13):3528–38. doi: 10.1016/j.celrep.2018.08.090
113. Zhang M, Li M, Du L, Zeng J, Yao T, Jin Y. Paclitaxel-In-Liposome-In-Bacteria for Inhalation Treatment of Primary Lung Cancer. Int J Pharm (2020) 578:119177. doi: 10.1016/j.ijpharm.2020.119177
114. Matsuzaki T, Yokokura T, Azuma I. Anti-Tumour Activity of Lactobacillus Casei on Lewis Lung Carcinoma and Line-10 Hepatoma in Syngeneic Mice and Guinea Pigs. Cancer Immunol Immunother (1985) 20:18–22. doi: 10.1007/BF00199768
115. Mendez Utz VE, Perez Visnuk D, Perdigon G, de Moreno de LeBlanc A. Milk Fermented by Lactobacillus Casei Crl431 Administered as an Immune Adjuvant in Models of Breast Cancer and Metastasis Under Chemotherapy. Appl Microbiol Biotechnol (2021) 105(1):327–40. doi: 10.1007/s00253-020-11007-x
116. Turcios NL. Cystic Fibrosis Lung Disease: An Overview. Respir Care (2020) 65(2):233–51. doi: 10.4187/respcare.06697
117. Madan JC, Koestler DC, Stanton BA, Davidson L, Moulton LA, Housman ML, et al. Serial Analysis of the Gut and Respiratory Microbiome in Cystic Fibrosis in Infancy: Interaction Between Intestinal and Respiratory Tracts and Impact of Nutritional Exposures. mBio (2012) 3(4):e00251-12. doi: 10.1128/mBio.00251-12
118. Burke DG, Fouhy F, Harrison MJ, Rea MC, Cotter PD, O'Sullivan O, et al. The Altered Gut Microbiota in Adults With Cystic Fibrosis. BMC Microbiol (2017) 17(1):58. doi: 10.1186/s12866-017-0968-8
119. Bruzzese E, Callegari ML, Raia V, Viscovo S, Scotto R, Ferrari S, et al. Disrupted Intestinal Microbiota and Intestinal Inflammation in Children With Cystic Fibrosis and Its Restoration With Lactobacillus Gg: A Randomised Clinical Trial. PloS One (2014) 9(2):e87796. doi: 10.1371/journal.pone.0087796
120. Bruzzese E, Raia V, Spagnuolo MI, Volpicelli M, De Marco G, Maiuri L, et al. Effect of Lactobacillus Gg Supplementation on Pulmonary Exacerbations in Patients With Cystic Fibrosis: A Pilot Study. Clin Nutr (2007) 26(3):322–8. doi: 10.1016/j.clnu.2007.01.004
121. Bruzzese E, Raia V, Ruberto E, Scotto R, Giannattasio A, Bruzzese D, et al. Lack of Efficacy of Lactobacillus Gg in Reducing Pulmonary Exacerbations and Hospital Admissions in Children With Cystic Fibrosis: A Randomised Placebo Controlled Trial. J Cyst Fibros (2018) 17(3):375–82. doi: 10.1016/j.jcf.2017.10.014
122. Jafari S-A, Atieh M-H, Kianifar H-R, Hebrani P, Ahanchian H, Abbasnejad E. Effects of Probiotics on Quality of Life in Children With Cystic Fibrosis; a Randomized Controlled Trial. Iranian J Pediatr (2013) 23(6):669–74.
123. Nathell L, Nathell M, Malmberg P, Larsson K. Copd Diagnosis Related to Different Guidelines and Spirometry Techniques. Respir Res (2007) 8:89. doi: 10.1186/1465-9921-8-89
124. Mortaz E, Adcock IM, Ricciardolo FL, Varahram M, Jamaati H, Velayati AA, et al. Anti-Inflammatory Effects of Lactobacillus Rahmnosus and Bifidobacterium Breve on Cigarette Smoke Activated Human Macrophages. PloS One (2015) 10(8):e0136455. doi: 10.1371/journal.pone.0136455
125. Sze MA, Utokaparch S, Elliott WM, Hogg JC, Hegele RG. Loss of Gd1-Positive Lactobacillus Correlates With Inflammation in Human Lungs With Copd. BMJ Open (2015) 5(2):e006677. doi: 10.1136/bmjopen-2014-006677
126. Wedgwood S, Warford C, Agvatisiri SR, Thai PN, Chiamvimonvat N, Kalanetra KM, et al. The Developing Gut-Lung Axis: Postnatal Growth Restriction, Intestinal Dysbiosis, and Pulmonary Hypertension in a Rodent Model. Pediatr Res (2020) 87(3):472–9. doi: 10.1038/s41390-019-0578-2
127. Rongrungruang Y, Krajangwittaya D, Pholtawornkulchai K, Tiengrim S, Thamlikitkul V. Randomized Controlled Study of Probiotics Containing Lactobacillus Casei (Shirota Strain) for Prevention of Ventilator-Associated Pneumonia. J Med Assoc Thai (2015) 98(3):253–9.
128. Buda B, Dylus E, Sabina G-F, Brzozowska E, Gamian A. Biological Properties of Lactobacillus Surface Proteins. Postepy Higieny I Medycyny Doswiadczalnej (2013) 4(67):229–37. doi: 10.5604/17322693.1043393
129. Correa Deza MA, Rodriguez de Olmos A, Suarez NE, Font de Valdez G, Salva S, Gerez CL. Inorganic Polyphosphate From the Immunobiotic Lactobacillus Rhamnosus Crl1505 Prevents Inflammatory Response in the Respiratory Tract. Saudi J Biol Sci (2021) 28(10):5684–92. doi: 10.1016/j.sjbs.2021.06.010
130. Ksonzekova P, Bystricky P, Vlckova S, Patoprsty V, Pulzova L, Mudronova D, et al. Exopolysaccharides of Lactobacillus Reuteri: Their Influence on Adherence of E. Coli to Epithelial Cells and Inflammatory Response. Carbohydr Polym (2016) 141:10–9. doi: 10.1016/j.carbpol.2015.12.037
131. Wu T, Xu F, Su C, Li H, Lv N, Liu Y, et al. Alterations in the Gut Microbiome and Cecal Metabolome During Klebsiella Pneumoniae-Induced Pneumosepsis. Front Immunol (2020) 11:1331. doi: 10.3389/fimmu.2020.01331
132. Clarke TB. Early Innate Immunity to Bacterial Infection in the Lung Is Regulated Systemically by the Commensal Microbiota Via Nod-Like Receptor Ligands. Infect Immun (2014) 82(11):4596–606. doi: 10.1128/IAI.02212-14
133. Schuijt TJ, Lankelma JM, Scicluna BP, de Sousa e Melo F, Roelofs JJ, de Boer JD, et al. The Gut Microbiota Plays a Protective Role in the Host Defence Against Pneumococcal Pneumonia. Gut (2016) 65(4):575–83. doi: 10.1136/gutjnl-2015-309728
134. Mortaz E, Adcock IM, Folkerts G, Barnes PJ, Paul Vos A, Garssen J. Probiotics in the Management of Lung Diseases. Mediators Inflammation (2013) 2013:751068. doi: 10.1155/2013/751068
135. Gray J, Oehrle K, Worthen G, Alenghat T, Whitsett J, Deshmukh H. Intestinal Commensal Bacteria Mediate Lung Mucosal Immunity and Promote Resistance of Newborn Mice to Infection. Sci Transl Med (2017) 9(376):eaaf9412. doi: 10.1126/scitranslmed.aaf9412
136. Zhang J, Ma JY, Li QH, Su H, Sun X. Lactobacillus Rhamnosus Gg Induced Protective Effect on Allergic Airway Inflammation Is Associated With Gut Microbiota. Cell Immunol (2018) 332:77–84. doi: 10.1016/j.cellimm.2018.08.002
137. Han W, Tang C, Baba S, Hamada T, Shimazu T, Iwakura Y. Ovalbumin-Induced Airway Inflammation Is Ameliorated in Dectin-1-Deficient Mice, in Which Pulmonary Regulatory T Cells Are Expanded Through Modification of Intestinal Commensal Bacteria. J Immunol (2021) 206(9):1991–2000. doi: 10.4049/jimmunol.2001337
138. Karimi K, Inman MD, Bienenstock J, Forsythe P. Lactobacillus Reuteri-Induced Regulatory T Cells Protect Against an Allergic Airway Response in Mice. Am J Respir Crit Care Med (2009) 179(3):186–93. doi: 10.1164/rccm.200806-951OC
139. de Oliveira GLV, Oliveira CNS, Pinzan CF, de Salis LVV, Cardoso CRB. Microbiota Modulation of the Gut-Lung Axis in Covid-19. Front Immunol (2021) 12:635471. doi: 10.3389/fimmu.2021.635471
140. Espirito Santo C, Caseiro C, Martins MJ, Monteiro R, Brandao I. Gut Microbiota, in the Halfway Between Nutrition and Lung Function. Nutrients (2021) 13(5):1716. doi: 10.3390/nu13051716
141. Berni Canani R, De Filippis F, Nocerino R, Laiola M, Paparo L, Calignano A, et al. Specific Signatures of the Gut Microbiota and Increased Levels of Butyrate in Children Treated With Fermented Cow's Milk Containing Heat-Killed Lactobacillus Paracasei Cba L74. Appl Environ Microbiol (2017) 83(19):e01206-17. doi: 10.1128/AEM.01206-17
142. Li L, Fang Z, Lee YK, Zhao J, Zhang H, Lu W, et al. Prophylactic Effects of Oral Administration of Lactobacillus Casei on House Dust Mite-Induced Asthma in Mice. Food Funct (2020) 11(10):9272–84. doi: 10.1039/d0fo01363c
143. Li L, Fang Z, Liu X, Hu W, Lu W, Lee YK, et al. Lactobacillus Reuteri Attenuated Allergic Inflammation Induced by Hdm in the Mouse and Modulated Gut Microbes. PloS One (2020) 15(4):e0231865. doi: 10.1371/journal.pone.0231865
144. Chen L, Zhou X, Wang Y, Wang D, Ke Y, Zeng X. Propionate and Butyrate Produced by Gut Microbiota After Probiotic Supplementation Attenuate Lung Metastasis of Melanoma Cells in Mice. Mol Nutr Food Res (2021) 65(15):e2100096. doi: 10.1002/mnfr.202100096
145. Pham MT, Yang AJ, Kao MS, Gankhuyag U, Zayabaatar E, Jin SC, et al. Gut Probiotic Lactobacillus Rhamnosus Attenuates Pde4b-Mediated Interleukin-6 Induced by Sars-Cov-2 Membrane Glycoprotein. J Nutr Biochem (2021) 98:108821. doi: 10.1016/j.jnutbio.2021.108821
146. Trompette A, Gollwitzer ES, Yadava K, Sichelstiel AK, Sprenger N, Ngom-Bru C, et al. Gut Microbiota Metabolism of Dietary Fiber Influences Allergic Airway Disease and Hematopoiesis. Nat Med (2014) 20(2):159–66. doi: 10.1038/nm.3444
147. Trompette A, Gollwitzer ES, Pattaroni C, Lopez-Mejia IC, Riva E, Pernot J, et al. Dietary Fiber Confers Protection Against Flu by Shaping Ly6c– Patrolling Monocyte Hematopoiesis and Cd8+ T Cell Metabolism. Immunity (2018) 48(5):992–1005.e8. doi: 10.1016/j.immuni.2018.04.022
148. Dang AT, Marsland BJ. Microbes, Metabolites, and the Gut-Lung Axis. Mucosal Immunol (2019) 12(4):843–50. doi: 10.1038/s41385-019-0160-6
149. Landsman L, Jung S. Lung Macrophages Serve as Obligatory Intermediate Between Blood Monocytes and Alveolar Macrophages. J Immunol (2007) 179(6):3488–94. doi: 10.4049/jimmunol.179.6.3488
150. Gao H, Wang Y, Sun Y, Qu B, Wu Y, Pei G, et al. Identification of B-Arrestin2 as a G Protein-Coupled Receptor-Stimulated Regulator of Nf-Kb Pathways. Mol Cell (2004) 7(14):303–17. doi: 10.1016/s1097-2765(04)00216-3
151. Park BO, Kang JS, Paudel S, Park SG, Park BC, Han SB, et al. Novel Gpr43 Agonists Exert an Anti-Inflammatory Effect in a Colitis Model. Biomol Ther (Seoul) (2022) 30(1):48–54. doi: 10.4062/biomolther.2021.078
152. Koh A, De Vadder F, Kovatcheva-Datchary P, Backhed F. From Dietary Fiber to Host Physiology: Short-Chain Fatty Acids as Key Bacterial Metabolites. Cell (2016) 165(6):1332–45. doi: 10.1016/j.cell.2016.05.041
153. Singh N, Gurav A, Sivaprakasam S, Brady E, Padia R, Shi H, et al. Activation of Gpr109a, Receptor for Niacin and the Commensal Metabolite Butyrate, Suppresses Colonic Inflammation and Carcinogenesis. Immunity (2014) 40(1):128–39. doi: 10.1016/j.immuni.2013.12.007
154. Chakraborty K, Raundhal M, Chen BB, Morse C, Tyurina YY, Khare A, et al. The Mito-Damp Cardiolipin Blocks Il-10 Production Causing Persistent Inflammation During Bacterial Pneumonia. Nat Commun (2017) 8:13944. doi: 10.1038/ncomms13944
155. Hang S, Paik D, Yao L, Kim E, Trinath J, Lu J, et al. Bile Acid Metabolites Control Th17 and Treg Cell Differentiation. Nature (2019) 576(7785):143–8. doi: 10.1038/s41586-019-1785-z
156. Rishi P, Bharrhan S, Singh G, Kaur IP. Effect of Lactobacillus Plantarum and L-Arginine Against Endotoxin-Induced Liver Injury in a Rat Model. Life Sci (2011) 89(23-24):847–53. doi: 10.1016/j.lfs.2011.09.007
157. Orlando A, Messa C, Linsalata M, Cavallini A, Russo F. Effects of Lactobacillus Rhamnosus Gg on Proliferation and Polyamine Metabolism in Hgc-27 Human Gastric and Dld-1 Colonic Cancer Cell Lines. Immunopharmacol Immunotoxicol (2009) 31(1):108–16. doi: 10.1080/08923970802443631
158. Niu H, Zhou X, Gong P, Jiao Y, Zhang J, Wu Y, et al. Effect of Lactobacillus Rhamnosus Mn-431 Producing Indole Derivatives on Complementary Feeding-Induced Diarrhea Rat Pups Through the Enhancement of the Intestinal Barrier Function. Mol Nutr Food Res (2022) 66(2):e2100619. doi: 10.1002/mnfr.202100619
159. Xiao X, Cao Y, Chen H. Profiling and Characterization of Micrornas Responding to Sodium Butyrate Treatment in A549 Cells. J Cell Biochem (2018) 119(4):3563–73. doi: 10.1002/jcb.26547
160. Edmond V, Brambilla C, Brambilla E, Gazzeri S, Eymin B. Srsf2 Is Required for Sodium Butyrate-Mediated P21(Waf1) Induction and Premature Senescence in Human Lung Carcinoma Cell Lines. Cell Cycle (2011) 10(12):1968–77. doi: 10.4161/cc.10.12.15825
161. Kim K, Kwon O, Ryu TY, Jung CR, Kim J, Min JK, et al. Propionate of a Microbiota Metabolite Induces Cell Apoptosis and Cell Cycle Arrest in Lung Cancer. Mol Med Rep (2019) 20(2):1569–74. doi: 10.3892/mmr.2019.10431
162. Trivedi R, Barve K. Gut Microbiome a Promising Target for Management of Respiratory Diseases. Biochem J (2020) 477(14):2679–96. doi: 10.1042/BCJ20200426
163. Bingula R, Filaire M, Radosevic-Robin N, Bey M, Berthon JY, Bernalier-Donadille A, et al. Desired Turbulence? Gut-Lung Axis, Immunity, and Lung Cancer. J Oncol (2017) 2017:5035371. doi: 10.1155/2017/5035371
164. Shahbazi R, Yasavoli-Sharahi H, Alsadi N, Ismail N, Matar C. Probiotics in Treatment of Viral Respiratory Infections and Neuroinflammatory Disorders. Molecules (2020) 25(21):4891. doi: 10.3390/molecules25214891
165. Mathieu E, Escribano-Vazquez U, Descamps D, Cherbuy C, Langella P, Riffault S, et al. Paradigms of Lung Microbiota Functions in Health and Disease, Particularly, in Asthma. Front Physiol (2018) 9:1168. doi: 10.3389/fphys.2018.01168
166. Fangous MS, Lazzouni I, Alexandre Y, Gouriou S, Boisrame S, Vallet S, et al. Prevalence and Dynamics of Lactobacillus Sp. In the Lower Respiratory Tract of Patients With Cystic Fibrosis. Res Microbiol (2018) 169(4-5):222–6. doi: 10.1016/j.resmic.2018.03.005
167. Villena J, Barbieri N, Salva S, Herrera M, Alvarez S. Enhanced Immune Response to Pneumococcal Infection in Malnourished Mice Nasally Treated With Heat-Killed Lactobacillus Casei. Microbiol Immunol (2009) 53(11):636–46. doi: 10.1111/j.1348-0421.2009.00171.x
168. Zelaya H, Tada A, Vizoso-Pinto MG, Salva S, Kanmani P, Aguero G, et al. Nasal Priming With Immunobiotic Lactobacillus Rhamnosus Modulates Inflammation-Coagulation Interactions and Reduces Influenza Virus-Associated Pulmonary Damage. Inflammation Res (2015) 64(8):589–602. doi: 10.1007/s00011-015-0837-6
169. Dziarski R, Gupta D. Review: Mammalian Peptidoglycan Recognition Proteins (Pgrps) in Innate Immunity. Innate Immun (2010) 16(3):168–74. doi: 10.1177/1753425910366059
170. Dabrowski AN, Conrad C, Behrendt U, Shrivastav A, Baal N, Wienhold SM, et al. Peptidoglycan Recognition Protein 2 Regulates Neutrophil Recruitment Into the Lungs After Streptococcus Pneumoniae Infection. Front Microbiol (2019) 10:199. doi: 10.3389/fmicb.2019.00199
171. Rice TA, Brenner TA, Percopo CM, Ma M, Keicher JD, Domachowske JB, et al. Signaling Via Pattern Recognition Receptors Nod2 and Tlr2 Contributes to Immunomodulatory Control of Lethal Pneumovirus Infection. Antiviral Res (2016) 132:131–40. doi: 10.1016/j.antiviral.2016.06.002
172. Saroj SD, Maudsdotter L, Tavares R, Jonsson AB. Lactobacilli Interfere With Streptococcus Pyogenes Hemolytic Activity and Adherence to Host Epithelial Cells. Front Microbiol (2016) 7:1176. doi: 10.3389/fmicb.2016.01176
173. Yildiz S, Pereira Bonifacio Lopes JP, Berge M, Gonzalez-Ruiz V, Baud D, Kloehn J, et al. Respiratory Tissue-Associated Commensal Bacteria Offer Therapeutic Potential Against Pneumococcal Colonization. Elife (2020) 9:e53581. doi: 10.7554/eLife.53581
174. Li J, Zhao J, Wang X, Qayum A, Hussain MA, Liang G, et al. Novel Angiotensin-Converting Enzyme-Inhibitory Peptides From Fermented Bovine Milk Started by Lactobacillus Helveticus Klds.31 and Lactobacillus Casei Klds.105: Purification, Identification, and Interaction Mechanisms. Front Microbiol (2019) 10:2643. doi: 10.3389/fmicb.2019.02643
175. Manna S, Chowdhury T, Chakraborty R, Mandal SM. Probiotics-Derived Peptides and Their Immunomodulatory Molecules Can Play a Preventive Role Against Viral Diseases Including Covid-19. Probiotics Antimicrob Proteins (2021) 13(3):611–23. doi: 10.1007/s12602-020-09727-7
176. Wasfi R, Abd El-Rahman OA, Zafer MM, Ashour HM. Probiotic Lactobacillus Sp. Inhibit Growth, Biofilm Formation and Gene Expression of Caries-Inducing Streptococcus Mutans. J Cell Mol Med (2018) 22(3):1972–83. doi: 10.1111/jcmm.13496
177. Liu WH, Chuang HL, Huang YT, Wu CC, Chou GT, Wang S, et al. Alteration of Behavior and Monoamine Levels Attributable to Lactobacillus Plantarum Ps128 in Germ-Free Mice. Behav Brain Res (2016) 298(Pt B):202–9. doi: 10.1016/j.bbr.2015.10.046
178. Garcia-Crespo KE, Chan CC, Gabryszewski SJ, Percopo CM, Rigaux P, Dyer KD, et al. Lactobacillus Priming of the Respiratory Tract: Heterologous Immunity and Protection Against Lethal Pneumovirus Infection. Antiviral Res (2013) 97(3):270–9. doi: 10.1016/j.antiviral.2012.12.022
179. Saviano A, Brigida M, Migneco A, Gunawardena G, Zanza C, Candelli M, et al. Lactobacillus Reuteri Dsm 17938 (Limosilactobacillus Reuteri) in Diarrhea and Constipation: Two Sides of the Same Coin? Medicina (Kaunas) (2021), 57(7):643. doi: 10.3390/medicina57070643
180. Maldonado J, Lara-Villoslada F, Sierra S, Sempere L, Gomez M, Rodriguez JM, et al. Safety and Tolerance of the Human Milk Probiotic Strain Lactobacillus Salivarius Cect5713 in 6-Month-Old Children. Nutrition (2010) 26(11-12):1082–7. doi: 10.1016/j.nut.2009.08.023
181. Guo C, Lei M, Wang Y, Hua L, Xue S, Yu D, et al. Oral Administration of Probiotic Lactobacillus Casei Shirota Decreases Pneumonia and Increases Pulmonary Functions After Single Rib Fracture: A Randomized Double-Blind, Placebo-Controlled Clinical Trial. J Food Sci (2018) 83(8):2222–6. doi: 10.1111/1750-3841.14220
182. Colautti A, Arnoldi M, Comi G, Iacumin L. Antibiotic Resistance and Virulence Factors in Lactobacilli: Something to Carefully Consider. Food Microbiol (2022) 103:103934. doi: 10.1016/j.fm.2021.103934
183. Salminen MK, Rautelin H, Tynkkynen S, Poussa T, Saxelin M, Valtonen V, et al. Lactobacillus Bacteremia, Clinical Significance, and Patient Outcome, With Special Focus on Probiotic L. Rhamnosus Gg. Clin Infect Dis (2004) 38(1):62–9. doi: 10.1086/380455
184. Wang H-K, Teng L-J, Chen Y-C, Du S-H, Hsueh P-R. Lactobacillus Salivarius Empyema With Respiratory Failure. J Microbiol Immunol Infect (2017) 50(6):923–5. doi: 10.1016/j.jmii.2016.06.001
185. Chaini E, Chainis ND, Ioannidis A, Magana M, Nikolaou C, Papaparaskevas J, et al. Pneumonia and Pleural Empyema Due to a Mixed Lactobacillus Spp. Infection as a Possible Early Esophageal Carcinoma Signature. Front Med (Lausanne) (2016) 3:42. doi: 10.3389/fmed.2016.00042
Keywords: Lactobacillus, respiratory diseases, the gut-lung axis, mucosal immunity, safety
Citation: Du T, Lei A, Zhang N and Zhu C (2022) The Beneficial Role of Probiotic Lactobacillus in Respiratory Diseases. Front. Immunol. 13:908010. doi: 10.3389/fimmu.2022.908010
Received: 30 March 2022; Accepted: 02 May 2022;
Published: 31 May 2022.
Edited by:
Luigina Romani, University of Perugia, ItalyReviewed by:
María Fernanda Raya Tonetti, Consejo Nacional de Investigaciones Científicas y Técnicas (CONICET), ArgentinaZhihong Sun, Inner Mongolia Agricultural University, China
Paulraj Kanmani, Tohoku University, Japan
Copyright © 2022 Du, Lei, Zhang and Zhu. This is an open-access article distributed under the terms of the Creative Commons Attribution License (CC BY). The use, distribution or reproduction in other forums is permitted, provided the original author(s) and the copyright owner(s) are credited and that the original publication in this journal is cited, in accordance with accepted academic practice. No use, distribution or reproduction is permitted which does not comply with these terms.
*Correspondence: Cuiming Zhu, nhzhucuiming@usc.edu.cn