- 1Arthur D. Riggs Diabetes and Metabolism Research Institute, The Beckman Research Institute, City of Hope National Medical Center, Duarte, CA, Unites States
- 2Hematologic Malignancies and Stem Cell Transplantation Institute, City of Hope National Medical Center, Duarte, CA, Unites States
- 3Fujian Medical University Center of Translational Hematology, Fujian Institute of Hematology, and Fujian Medical University Union Hospital, Fuzhou, China
- 4Fred Hutchinson Cancer Research Center, University of Washington, Seattle, WA, United States
Allogeneic hematopoietic cell transplantation (Allo-HCT) is a curative therapy for hematological malignancies (i.e., leukemia and lymphoma) due to the graft-versus-leukemia (GVL) activity mediated by alloreactive T cells that can eliminate residual malignant cells and prevent relapse. However, the same alloreactive T cells can cause a serious side effect, known as graft-versus-host disease (GVHD). GVHD and GVL occur in distinct organ and tissues, with GVHD occurring in target organs (e.g., the gut, liver, lung, skin, etc.) and GVL in lympho-hematopoietic tissues where hematological cancer cells primarily reside. Currently used immunosuppressive drugs for the treatment of GVHD inhibit donor T cell activation and expansion, resulting in a decrease in both GVHD and GVL activity that is associated with cancer relapse. To prevent GVHD, it is important to allow full activation and expansion of alloreactive T cells in the lympho-hematopoietic tissues, as well as prevent donor T cells from migrating into the GVHD target tissues, and tolerize infiltrating T cells via protective mechanisms, such as PD-L1 interacting with PD-1, in the target tissues. In this review, we will summarize major approaches that prevent donor T cell migration into GVHD target tissues and approaches that augment tolerization of the infiltrating T cells in the GVHD target tissues while preserving strong GVL activity in the lympho-hematopoietic tissues.
Introduction
Allogeneic hematopoietic cell transplantation (Allo-HCT) provides curative therapy for hematological malignancies such as lymphoma and leukemia by relying on the graft-versus-leukemia/lymphoma (GVL) effects mediated by alloreactive T cells (1–6). However, the same alloreactive T cells also cause a severe side effect, graft-versus-host disease (GVHD). Prevention of GVHD while preserving GVL activity remains a long-sought and elusive goal.
Acute GVHD (aGVHD) is a dysregulated and exaggerated alloimmune response whose pathogenesis has been elegantly described in previous and recent reviews (7–11). Although GVHD and GVL activity are mediated by the same alloreactive T cells, they occur in different tissue compartments. GVHD target tissues include the skin, lung, liver and intestine (12), but leukemia/lymphoma cells reside mainly in lympho-hematopoietic tissues, including the bone marrow, spleen and lymph nodes (13). Current methods that suppress general alloreactive T cell activation and expansion such as immunosuppressants (i.e., tacrolimus and sirolimus) simultaneously reduce GVHD and GVL activity (14, 15). Blocking alloreactive T cell infiltration in GVHD target tissues while allowing full activation and expansion of alloreactive T cells that kill malignant cells in the lympho-hematopoietic compartment offers a better approach toward prevention of GVHD. In addition, cellular therapy such as infusion of Tr1 can prevent GVHD while exerting GVL activity in pre-clinical models (16–18) and can maintain alloreactive responses without causing GVHD in humans (19, 20). Moreover, CD8+ Tregs can enhance GVL activity while suppressing GVHD (21–23). In this review, we focus on the approaches that can maintain donor T cells in lymphohematopoietic tissue and augmentation of tissue PD-L1 mediating protection to prevent GVHD while preserving GVL activity. We summarize preclinical studies and clinical trials that have tested this compartmental approach for preventing GVHD while preserving GVL activity.
Inhibition of Tissue-Specific T Cell Homing and Chemokine Receptors
T cell migration into GVHD target tissues requires specific homing and chemokine receptor expression and release of the corresponding of chemokines in tissues. In general, T cell that express CXCR3, CCR9 or α4β7integrins interacting with their ligands CXCL9, CXCL10, CXCL11, CCL25 or MAdCAM-1 migrate into gut (24). T cell that express CCR5 or α4β1integrins interacting with its ligands CCL3, CCL4, CCL5 or VCAM-1 migrate into liver (24, 25). T cells that express CCR3, CCR4, or CCR6 interacting with their ligands CCL11, CCL17/CCL22 or CCL20 migrate into lung and skin (24, 26–28).
Various studies have tested the effects of targeting chemokine and chemokine receptors in experimental murine GVHD models. For example, a decrease in skin, liver and gut GVHD can be achieved through elimination of CXCR3+ T cells, or by neutralization of its ligands CXCL9-11 (29–31). Based on these results, several patent applications for CXCR3 antagonists have been filed, but no such antagonist has been approved for prevention of GVHD or treatment of other diseases in humans (32). Another novel approach involves the use of CXCR3-transfected regulatory T cells (Tregs) that migrate and accumulate in the liver, lung and gut, resulting in decreased GVHD severity (33). CCR5 is an important receptor that allows lymphocytes to migrate to the skin and causes the production of TNF-α, IL-2, and IFN-γ, leading to development of aGVHD in patients (34). A 32-nucleotide deletion of CCR5 (CCR5Δ32) in recipients significantly decreased the risk of GVHD (35). Moreover, in both recipient and donor cells, the CCR5Δ32 genotype exhibited the greatest protection (35). This protection may depend on the conditioning regimen (36). Maraviroc, which is an inhibitor of CCR5, has been approved for treatment of HIV infection. An initial report about Maraviroc for prevention of GVHD in human showed encouraging results (37), but a follow-up study did not (38).
CCR9 plays a critical role in the homing of lymphocytes into inflamed intestines, thereby contributing to the pathogenesis of colitis and Crohn’s disease (39). An orally bioactive inhibitor of CCR9, CCX282, has been developed and was well tolerated with encouraging results in clinical trials for Crohn’s disease (40), but no studies in treatment of GVHD has been reported so far. Surprisingly, in a murine GVHD model, lack of CCR9 expression by donor T cells did not ameliorate GVHD since CCR9 deficiency on donor T cells did not impact on inflammatory cytokine production and T cells accumulation in liver and intestine (41). These results suggested CCR9 seems to have a subordinate role for donor T cell homing in vivo during aGVHD.
The integrin α4β7 has a critical role in mediating aGVHD. Upregulation of α4β7 integrin expression by T cell subsets correlates with the development of gut aGVHD in humans (42, 43). In murine models, the severity of GVHD was lower with α4β7 deficient donor T cells than with wild-type (WT) T cells (41, 44). The α4β7 deficient donor T cells also showed intact graft-versus-tumor (GVT) activity or even enhanced activity (44). Similarly, MAdCAM-1 deficiency on recipients reduced GVHD in mice (41), moreover, administration of anti-MAdCAM-1 antibody reduced GVHD without impairing GVL effects in both nonirradiated recipients and in recipients treated with myeloablative conditioning (45). Recent study found intestinal stem cells were the primary target of alloreactive donor T cells (46). Furthermore, it was shown that this process relies on β7 integrin and MAdCAM-1 interactions, since the anti-MAdCAM-1 antibody reduced donor T cell invasion into the lower crypt regions of the mucosa resulting in less damage to the GI tract (46). Vedolizumab, a monoclonal antibody that binds to α4β7, has been approved for treatment of ulcerative colitis and Crohn’s disease and, more recently, has been examined as a treatment for steroid refractory gut GVHD with variable results (47–51), however there is no information about the use of Vedolizumab to prevent GVHD.
T cell tissue tropism and expression of chemokine receptors is imprinted by tissue CCR7+ dendric cells (DCs) in the draining lymph nodes (52–56). Consistently, anti-CD3-preconditioning effectively depletes CCR7+ DCs in mesenteric lymph nodes by inhibiting CCR7+ DC migration from gut tissue into MLN and inducing CCR7+ DC apoptosis in the MLN. Consequently, the expression of gut homing molecules α4β7 and CCR9, as well as skin homing molecules E-Lig, P-Lig, CCR4 and CCR10, is reduced in donor T cells. Additionally, anti-CD3 preconditioning reduced the expression of CCL17, CCL22, CCL27, and CCL28 in skin tissues. Anti-CD3 preconditioning also reduced gut, skin, and liver tissue expression of CCL3-5 and CXCL9-11, which decreased alloreactive donor T cell tissue tropism towards skin, liver, and gut. Therefore, anti-CD3 preconditioning allows fully activated donor T cells to be retained and mediate GVL activity in lympho-hematopoietic tissues where hematological cancer cells reside without causing GVHD (57, 58). Due to lack of depleting anti-human CD3 mAb, this approach has not yet been tested in humans.
Sequestering lymphocytes within secondary lymphoid organs offers an alternative to preventing GVHD by blocking migration of alloreactive donor lymphocytes to target tissues in the recipient. Exit of T cells from lymphoid tissues require their expression of sphingosine 1-phosphate receptors (S1PRs). The S1PRs agonist FTY720 retains alloreactive T cells in lymphoid tissues and prevents T cell infiltration of GVHD target tissues, thereby preventing GVHD while preserving GVL effects in both MHC mismatched and MHC-haploidentical murine GVHD models (59–62). On the other hand, FTY720 also reduced the numbers of host DCs in the recipient spleen before transplantation and slightly impaired GVL activity (63, 64). Fingolimod (FTY720), a first-in-class, orally bioavailable S1PR agonist has been approved in 2010 for treatment of relapsing forms of multiple sclerosis (MS). Published clinical testing for GVHD in humans, however, is limited to a single a 66-year-old patient with severe CNS GVHD who was treated successfully with fingolimod (65). Overall, few studies have evaluated pharmacological targeting of chemokines and chemokine receptors in clinical settings. This approach might be valid for treatment, but not for prevention of GVHD, if the inhibitor is given prior to the infusion of donor cells. In addition, the difficulty in controlling donor T cell migration may be promoted by pre-existing tissue resident T cells in the human GVHD target tissues (66).
Reduction of Target Tissue Inflammation
Tissue inflammation caused by pretransplant conditioning regimens triggers migration of alloreactive T cell into GVHD target tissues (67). Delayed lymphocyte infusion (DLI) of donor T cells after tissue inflammation has subsided reduced GVHD while augmenting GVL effect in murine models and humans (68–75). The DLI mediated GVL effect has been confirmed for chronic myeloid leukemia (CML) in numerous studies worldwide (76, 77), with up to 70–80% cytogenetic complete remissions (78). For patients with acute myeloid leukemia (AML) or myelodysplastic syndrome (MDS), the response rate to DLI is much lower (20–40%) and is lower still in those with acute lymphoid leukemia (ALL) (10–13%) (79).
According to most studies, conditioning regimens initiate aGVHD by triggering the production of cytokines (such as TNF-α, IFN-γ, IL-1, and IL-2) which, in turn, up-regulate the chemokine receptors and their ligands that drive the migration of T cells to GVHD target tissues (53, 55, 80, 81). Certain cytokines promote GVHD while also providing survival signals to leukemia cells. These include granulocyte-macrophage colony-stimulating factor (GM-CSF) (82, 83) and macrophage colony-stimulating factor 1 (CSF-1) (84) in AML, and Interleukin-6 (IL-6) in ALL (85) and multiple myeloma (MM) (86). Among these, the role of IL-6 has been demonstrated in the pathogenesis of GVHD in several murine GVHD models (87, 88). Expression of IL-6 and IL-6R is enhanced after allo-HCT, DCs are the principal source of IL-6 dysregulation after allo-HCT, and blockade of IL-6 signaling by in vivo administration of anti-IL-6R mAb attenuates GVHD with significant expansion of Tregs and reduction of inflammatory Th1 and Th17 cells (87, 88). Inhibition of classical signaling of IL-6R on donor T cells decreased the severity of Th17 and Th22-dependent GVHD without inhibiting GVL response against a primary blast crisis chronic myeloid leukemia cell line BCR-ABL/NUP98-HOXA9 (88). Similarly, tumor necrosis factor-alpha (TNF-α) blockade ameliorated GVHD mediated by both CD4+ and CD8+ T cells without blocking GVL activity (89). Another study, however, showed that recipients given TNF-α receptor deficient T cells had a significant impairment in donor GVL activity after HCT compared to recipients of WT T cells, indicating that TNF-α has an important role in GVL mediated by donor T cells (90). Neutralization of a single cytokine such as IL-6 and TNF has shown variable and conflicting results (89, 91, 92). A recent study, however, showed beneficial effects from dual blockade of both IL-6 and TNF in prevention of GVHD in both MHC-mismatched and minor antigen-mismatched aGVHD murine models and in sclerodermatous cGVHD murine models (93), while preserving GVL activity against A20 (B-cell lymphoma) and C1498 (acute myeloid leukemia) (93). In clinical trials, however, tocilizumab (TCZ), a monoclonal antibody against the interleukin-6 receptor, did not significantly reduce the incidence of grade 2-4 aGVHD, and did not improve long-term survival (94). Similarly, the clinical results of testing TNF blockade for prevention of GVHD have been disappointing (95, 96).
Infusion of Mesenchymal Stem Cells (MSCs)
MSCs are highly heterogeneous population of stem and progenitor cells that can be isolated and expanded from many tissues, such as bone marrow, placenta, umbilical cord (UC), adipose tissue (AT), and dental pulp (97–102). It has been demonstrated that MSC heterogeneity occurs within the same species, the same tissue preparations, and even on the same donor isolations (103–106). In general, MSCs inhibit the generation of cytotoxic T cells by secreting a soluble factor, but they do not interfere with CTL and NK cell lytic activity (107). Other studies have suggested that tolerance induction by MSCs may occur through inhibition of dendritic cell maturation and function (108–110), induction of myeloid-derived suppressor cells (MDSCs) (111), and suppression of B cells (112). Although it has been difficult to recover MSCs from BM of transplant recipients, MSCs can migrate to lymphoid organs and engraft at areas of tissue damage or tumor progression (113–115). These results indicate that MSCs are recruited mainly to tissues other than bone marrow for immune suppression in GVHD. The ability of MSCs to suppress infiltration of activated T cells into GVHD target tissues but not into the bone marrow contributes to the separation of GVL from GVHD. In addition, MSCs ameliorate GVHD through expansion of Tregs, especially the CD8+ Tregs (116–118). Unlike CD4+ Tregs, CD8+ Tregs suppress GVHD while preserving GVL activity (21–23). In clinic, third party, ex-vivo expanded, MSCs co-injection in a high risk, mismatched, unrelated-donor HCT reduced the severity of GVHD (119). Co-injection of MSCs and HSCs in HCT with HLA-identical sibling donors reduced the severity of aGVHD, but the incidence of relapse was significantly increased (120), and a comprehensive meta-analysis showed that co-administration of MSCs with allo-HCT has no demonstrable benefit regarding engraftment or prevention of aGVHD or cGVHD (121).
Exosomes are naturally occurring extracellular vesicles (EVs) that are released from many cell types and can be enriched from virtually all body fluids, including blood plasma, urine and saliva (122). Depending on their origin, some exosomes exert immune stimulatory or immune suppressive functions (122, 123). Since MSC exosomes represent a therapeutically active product of MSCs, it was suggested that EVs could have similar tissue repair capabilities as MSCs, making them a promising noncellular approach for GVHD prevention or treatment (124). In murine models bone marrow MSC derived EVs enhanced survival and reduced the severity of aGVHD (125), but MSC-EVs have not been tested for prevention of GVHD in humans.
Overall, the efficacy of MSCs treatment varies from study to study, possibly because MSCs are very heterogeneous, depending on their origin and the methods used to isolate and propagate them in vitro. Progress will require improved understanding of the mechanisms of MSCs and the development of methods that define the optimal source, in vitro culture methods, measurement of potency, cell dose, and the timing and frequency of administration.
Depletion of Naïve Donor T Cells Reduce Tissue Infiltration and Reduce GVHD While Preserving GVL Effect
Generally, T cells can be divided into two types: (1) naïve T cells (TN) which have not yet encountered their corresponding antigens, and (2) antigen-experienced T cells, which include memory and effector T cells (TM) composed primarily of clonal expansions of T cells specific to their respective antigens (126). Based on the phenotype, gene expression, metabolic profile, and function of these antigen-experienced T cells, they can further be subdivided into three main types: central memory T cells (TCM), effector memory T cells (TEM), and effector T cells (TE) (127). Most T cells in the blood of mice have a TN phenotype and both CD4+ and CD8+ T cells from peripheral blood mediated lethal GVHD in an MHC-mismatched HCT model (128). In contrast, bone marrow T cells are TM phenotype that failed to induce lethal GVHD but retained GVL activity and facilitated hematopoietic progenitor engraftment (128). This study indicated that preserving resident marrow TM cells but not blood TN cells in the transplant inoculum may result in the desirable outcome of GVL and facilitation of engraftment without causing GVHD.
In subsequent studies, several different groups evaluated TN and TM subsets for their ability to cause GVHD using various murine GVHD models (129–137). The models involved in these studies included different MHC disparity (e.g., MHC-mismatched, MHC-matched, and minor H antigen mismatched), distinct GVHD disease patterns (aGVHD and cGVHD), as well as CD8+ and CD4+ T cell-mediated models respectively. Consistently, TN caused severe GVHD, while TEM did not cause GVHD. Some studies showed that TCM can also cause intestinal damage that was less severe than with TN (131). More importantly, the CD8+ TM preserve GVL function in vivo (133, 135). Taken together, these preclinical murine studies indicated that TN consistently mediate GVHD, while TM either do not cause GVHD or cause only mild GVHD while still contributing to functional GVL effects.
Preclinical studies showed that human TN and TM have distinctly different fates after alloactivation in vitro (138). TM lost their function to recognize alloantigens, whereas the TN remained highly functional (138). These results suggested that TN-depletion was likely to reduce the expansion of alloreactive T cells after allo-HCT. Based on these discoveries, a single-arm clinical trial was designed to evaluate outcomes after CD45RA+ TN-depleted allo-HCT. Accordingly, 35 patients with high-risk leukemia received TN-depleted peripheral blood stem cell transplantation (PBSCT) from HLA-matched sibling donors after myeloablative conditioning (139). During the first three months after HCT, T cell immune reconstitution was comparable to that with unmanipulated bone marrow transplant (BMT) and was significantly better than with CD34+ selected pan-T cell depleted (TCD) PBSCT recipients, although the incidence of moderate (grade II-III) aGVHD was not reduced. GVHD in these patients, however, was uniformly responsive to corticosteroids, with a very low incidence of grade III-IV GVHD. The incidence of cGVHD was strikingly reduced compared to BMT. More importantly, the presence of TM in the graft contributed to rapid recovery of T cells and the transfer of protective virus-specific immunity. No excessive rates of infection or relapse was observed (139). Similar observation was reported recently on three prospective phase II clinical trials of 138 patients with acute leukemia and MDS received TN-depleted PBSCT from HLA matched related or unrelated donors, aGVHD was mild and corticosteroid-responsive; Strikingly, only 7% of patients developed cGVHD, which was also mostly mild and steroid-responsive. No apparent increase in relapse or fatal infections (140). TN-depletion of PBSCT is also being applied to the setting of HLA-haploidentical HCT (haplo HCT) (141–144). In a recent report summarizing preliminary data from the first 50 subjects enrolled in an ongoing clinical trial, the results indicated an increase in the 3-year overall survival (OS) and event-free survival (EFS) in non-chemo refractory recipients receiving TN-depleted grafts (78.9% and 77.7%, respectively) compared to historic T-cell depleted haplo HCT cohorts (46.7% and 42.7%, respectively; p = 0.004 and 0.003 respectively) (144). Based on these results, clinical trials are now in progress to compare TN-depleted PBSCT with standard unmanipulated allo-HCT along with other promising GVHD-reduction strategies (145, 146).
Collectively, the outcomes of TN-depleted allo-HCT are very encouraging, and the understanding obtained from various human studies generally correlate with the results of murine experiments. However, relapses still occur at a low incidence. The underlying mechanism by which TN and TM exert differential effects on alloreactivity remains unclear. It has been proposed that TN cause GVHD while TEM do not because they lack CD62L and CCR7, which are critical in directing TN toward to the sites of antigen presentation for GVHD initiation, such as lymph nodes (LN) and Peyer patches (PP). However, in murine GVHD model, Anderson et al. showed that CD62L and CCR7 were not required for TN-mediated GVHD, since CD62L-/- donor T cells still induced GVHD, and GVHD also developed in recipients that lacked LN and PP. Even when TEM constitutively express CD62L, they do not cause GVHD (147), indicating that targeting a single chemokine receptor alone on TN cells might not be an effective therapy. Therefore, to further clarify the mechanism in human, future studies are needed evaluate the difference between TN and TM related to the respective cell trafficking patterns, or whether pathogen-specific TM cross react with leukemia/lymphoma-associated antigens but with little cross-reactivity for alloantigen will need further investigation.
Host Tissue PD-L1 With Donor CD4+ and CD8+ T Cells
PD-L1 interact with receptors PD-1 and CD80 (148–152), and we proposed a general view that PD-L1 interactions with PD-1 and CD80 could differentially regulate GVHD and GVL, as summarized in our previous review (153). In the current review, we elaborate on how PD-L1 expressed by host parenchymal tissues or expressed by donor- and host-type lympho-hematopoietic cells regulates GVHD and GVL activity mediated by the same alloreactive T cells. Parenchymal tissue expression of PD-L1 can effectively tolerize infiltrating T cells by interaction with PD-1 on activated T cells and induction of T cell anergy, exhaustion, and apoptosis, together with expansion of FoxP3+ Treg cells and FoxP3-IL-10+ Tr1 cells (154–156). Nonetheless, upregulation of PD-L1 by host tissues did not effectively prevent aGVHD, although it can reduce the severity of aGVHD as indicated by exacerbation of aGVHD in PD-L1-/- recipients and with PD-1-/- donor T cells. In addition, transgenic expression of PD-L1 by hepatocytes via hydrodynamic injection of PD-L1 plasmid ameliorated aGVHD with expansion of FoxP3+CD4+ Treg cells (157). The lack of effective prevention of aGVHD by host-tissue PD-L1 may result from the cytokine environment, since GVHD target tissues express elevated levels of IL-2, IFN-γ, TNF-α, GM-CSF, and IL-6 (7, 158). Neutralizing TNF-α or IL-6 did not effectively prevent aGVHD, although the severity of GVHD was reduced in murine recipients and in human HCT recipients (87–89, 91, 92). Thus, other cytokines may regulate the effects of PD-L1/PD-1 pathway.
Host Tissue PD-L1 and Tolerogenic Anti-IL-2 mAb
Sorted donor CD4+ T cells can cause severe GVHD by expressing FASL and producing proinflammatory cytokines (e.g., IFN-γ and TNF-α) (159, 160), while sorted donor CD8+ T cells prevent graft rejection and mediate GVL effects by expressing perforin/granzyme, without causing aGVHD in animal models (161, 162). IL-2 produced by CD4+ T cells makes CD8+ T cells resistant to anergy and apoptosis induced by PD-1 signaling (163). We found that administration of tolerogenic anti-IL-2 mAb (JES6) that specifically blocks IL-2 interaction with IL-2Rβ effectively prevents aGVHD while preserving strong GVL effect in a host tissue PD-L1-depdent manner. In GVHD target tissues, blockade of IL-2β signaling increased inhibition of AKT-mTOR pathway mediated by PD-L1/PD-1 signaling, upregulated T cell expression of PD-1 and Blimp-1, and expanded IL-10+FoxP3-CD4+ Tr1 cells (156). In lymphoid tissues, donor CD8+ T cells expanded and had increased expression of granzyme B and generation of TCF-1+CD8+ memory progenitors that can give rise to cytotoxic effector cells, which contribute to strong GVL activity (156). Maintenance of donor CD8+ T cells in lymphoid tissues may result from the lack of host-type PD-L1 expression and lack of PD-L1/PD-1 signaling. Thus, administration of tolerogenic anti-IL-2 that specifically blocks IL-2Rβ signaling may represent a novel approach for preventing aGVHD while preserving strong GVL activity through the expansion of functional CD8+ T cells in lymphoid organs while inducing Tcon anergy/exhaustion in GVHD target tissues (Figure 1). An anti-human IL-2Rβ mAb has been developed (164) but has not been evaluated in clinical trials.
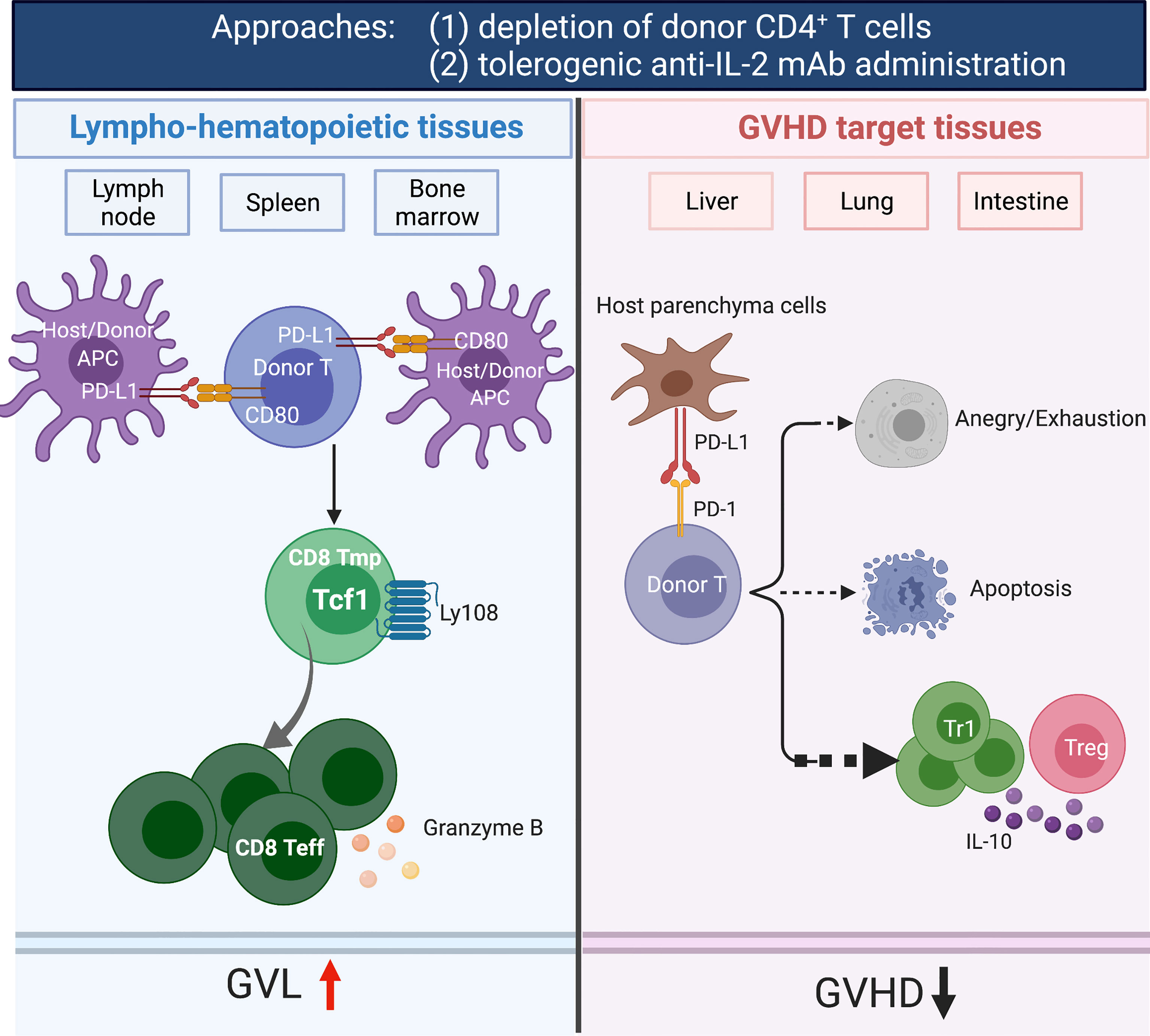
Figure 1 Depletion of donor CD4+ T cells and tolerogenic anti-IL-2 mAb (JES6-1) administration prevents acute GVHD while preserving GVL activity. The depletion of CD4+ T cells by anti-CD4 mAb prevented both acute and chronic GVHD while preserving GVL activity. In GVHD target tissues, depletion of CD4+ T cells allowed host tissue PD-L1 interaction with PD-1 expressed by donor CD8+ T cells to induce anergy, exhaustion and apoptosis. In lymphoid tissues, the treatment allowed PD-L1 and CD80 interactions among lymphocytes and DCs to augment expansion of CD8+ T cells that mediating GVL activity. Furthermore, CD4+ T cells help CD8+ T cells via their production of IL-2. Administration of tolerogenic anti-IL-2 mAb (JES6) expanded IL-10+FoxP3-CD4+ Tr1 cells in GVHD target tissues. In addition, increased expression of granzyme B and generation of TCF-1+CD8+ memory progenitors that can give rise to cytotoxic effector cells in lymphoid tissues, leading to effectively prevention of aGVHD while preserving GVL activity. Created with BioRender.com.
Administration of tolerogenic anti-IL-2 mAb that blocks IL-2 interaction with IL-2Rβ did not prevent cGVHD (156). Although the treatment was very effective at prevention of gut aGVHD, the recipients developed cGVHD with body weight loss (156). The lack of protection of thymus may result from autoreactive PD-1hiIFN-γ+IL-10+CD4+ T cell interactions with B cells. Our previous report showed that autoreactive IFN-γ+IL-10+CD4+ T cells can activate B cells to produce autoantibodies (165), and donor-type tissue-resident PD-1hiCD4+ T helper cells interact with B cells in the GVHD target tissues to produce autoantibodies that mediate thymus damage and cutaneous GVHD (166). Those PD-1hi T helper cells were derived from naïve CD4+ T cells in the graft (166), depletion of naïve T cells in the graft was recently reported to effectively prevent cGVHD (140).
Host Tissue PD-L1 and Depleting Anti-CD4 mAb
aGVHD is mediated by donor CD4+ and CD8+ T cells in the graft, and cGVHD is mediated mainly by CD4+ T cells from the graft and from T cells that are de novo generated from progenitors in the GVHD-damaged thymus (167, 168). With a murine model that reflects characteristic features of acute and chronic GVHD, we showed that sorted CD4+ T cells induce both acute and chronic GVHD. Sorted CD8+ T cells did not induce aGVHD but did induce cGVHD that depended on de novo generated CD4+ T cells (169). In follow-up studies, we administered a depleting anti-CD4 mAb weekly for 4 weeks after HCT to deplete donor CD4+ T cells derived from the graft and from de novo-regeneration early after HCT (163). Notably, the administration of anti-CD4 mAb did not affect bone marrow reconstitution and allowed full recovery of donor CD4+ T cells on day 100 after HCT (163). The depletion of CD4+ T cells by anti-CD4 mAb prevented both acute and chronic GVHD while preserving strong GVL activity in murine and humanized xeno-GVHD models (163). In GVHD target tissues, depletion of CD4+ T cells allowed host tissue PD-L1 interaction with PD-1 expressed by donor CD8+ T cells to induce anergy, exhaustion and apoptosis. In lymphoid tissues, however, the treatment allowed PD-L1/CD80 interactions to augment expansion of CD8+ T cells early after HCT, which contributed to strong GVL activity (163). CD4+ T cells help CD8+ T cells via their production of IL-2 that prevents T cell tolerance induced by PD-1 signaling (163). Deletion of CD4+ T cells not only removed the IL-2 effect on donor CD8+ T cells and augmented infiltrating CD8+ T tolerance but might also prevented formation of tissue resident CD4+ T cell helpers for B cells that mediate cGVHD. Administration of depleting anti-CD4 mAb may also deplete the pre-existing host-type tissue-resident CD4+ T cells in GVHD target tissues that augment induction of aGVHD (66). Therefore, administration of depleting anti-CD4 mAb early after HCT may represent one of the most effective approaches to prevent acute and chronic GVHD while preserving strong GVL activity (Figure 1).
Donor- and Host-Type Hematopoietic Cell Expression of PD-L1 Differentially Regulate Alloreactive T Cell Expansion and GVL Activity
PD-L1 is induced and constantly expressed by parenchymal cells in inflamed GVHD target tissues (163, 170). Host-type hematopoietic cells in the lympho-hematopoietic tissues are rapidly eliminated and replaced by donor-type cells early after HCT (171). Donor-type cells in lymphoid tissues expressed higher levels of PD-L1 and CD80 but lower levels of PD-1 as compared to those in the GVHD target tissues (163). Thus, PD-L1 interaction with CD80 in the lymphoid tissues is likely dominant in lymphoid tissues, while PD-L1 interaction with PD-1 is dominant in GVHD target tissues, although both interactions exist in the two compartments. Accordingly, PD-L1 interactions with PD-1 and CD80 differentially regulate GVHD and GVL activity (163).
Blazar’s group showed that while host-tissue PD-L1 ameliorated aGVHD, donor cell PD-L1 augmented T cell expansion and aGVHD (172). Since PD-L1 interaction with PD-1 always inhibits T cell expansion (173), the role of donor T cell PD-L1 on augmenting T cell expansion and GVHD must be through PD-L1 interaction with CD80 or other ligands. Consistently, we observed that PD-L1 or CD80 deficiency in donor T cells and specific blockade of PD-L1 interactions with CD80 by anti-PD-L1 mAb (43H12) given on day 0 before T cell activation in vivo reduced CD8+ T expansion (163). Blockade of PD-L1 interaction with CD80 after T cell activation on day 5 after HCT, however, augmented donor CD8+ T expansion (174). Although we assumed that PD-L1 interaction with CD80 occurred in trans (151, 157, 175), recent publications showed that PD-L1 interactions with CD80 do occur in cis and that PD-L1/CD80 interactions in cis on APCs reduced PD-L1 interaction with PD-1 and reduced CD80 interaction in trans with CTLA-4 on T cells, thereby augmenting CD8+ T cell expansion (176, 177). Our studies, however, showed that in vivo 43H12 blockade augmented expansion of CD44+CD62L-CD8+ memory/effector T cells in tumor draining lymph nodes (178). This effect is opposite from blocking cis PD-L1/CD80 interactions, suggesting that trans PD-L1/CD80 interaction occurs in vivo. Furthermore, these findings correlate with the observation that blockade at day 5 accelerated the expansion of donor CD8+ T cells in allogeneic recipients (174). Naïve T cell expressed low levels of PD-L1 and CD80, while activated T cells and APC express high levels of PD-L1 and CD80 (151). Thus, administration of 43H12 on day 0 predominantly blocks PD-L1/CD80 cis interactions on host APC (176, 177), while administration of 43H12 on day 5 primarily blocks trans PD-L1/CD80 interactions (153, 157).
Taken together, our studies suggest that when donor T cells interact with host APCs in lymphoid tissues early after HCT, the interaction of PD-L1 on donor T cells with CD80 on host-APCs augments donor T cell expansion. After donor T cells are fully activated and host APCs in the lymphoid tissues are eliminated, donor T cells interact with donor APCs, and the PD-L1/CD80 between donor T cells and APCs augment the tolerance effect of PD-1/PD-L1 interactions. This hypothesis is supported by our observation that blockade of PD-L1/CD80 interaction between activated donor CD4+ T cells and APCs in allogeneic recipients augmented the expansion of the CD4+ T cells, and the effect occurred only in WT donor T cells but not in PD-1-/- donor T cells (157).
Administration of anti-PD-1 or anti-PD-L1 mAb to the patients who with tumor relapsed after allo-HCT induced lethal aGVHD (179–182). Since blockade of PD-L1/CD80 interaction reduced but did not completely remove the inhibitory effect of PD-L1/PD-1 interactions, we expect that administration of antibodies to specifically block PD-L1/CD80 interaction will augment GVL effect in lymphoid tissues while maintaining the protective effect of PD-L1/PD-1 interactions in GVHD target tissues. Therefore, blockade of PD-L1/CD80 in patients with relapse could augment GVL activity with little GVHD.
Sequential Administration of Tolerogenic Anti-IL-2 and JAK Inhibitor
JAKs are intracellular signaling components that function as downstream signal mediators for many cytokines (183). The JAK family contains four members. Among these, JAK1, JAK2, and JAK3 may be important for the development of GVHD (184–188). JAKs regulate the function of immune cells that mediate GVHD, including APCs (189), T cells (190), and B cells (191). Thus, numerous studies have been conducted to investigate the role of JAKs inhibitors in regulating GVHD in preclinical models. John F. DiPersio’s group evaluated the effect of JAK1/JAK2 inhibitors in an MHC-mismatched murine model and showed that JAK1/JAK2 inhibitors inhibited IFNR and IL-6R signaling, which inhibited migration of alloreactive T cells to GVHD target organs by decreasing expression of CXCR3. JAK1/JAK2 inhibition also expanded Tregs, and the two effects effectively prevented GVHD (184, 192). Similarly, inhibition of JAK1/JAK3 inhibition also reduced aGVHD and enhanced survival (187). While significant evidence supports the role of multi-kinase inhibitors that target more than one JAK protein, selective JAK1, JAK2 or JAK3 inhibition is also effective in many GVHD models (188, 193, 194). The impact of JAK inhibitors on GVL activity, however, is variable. For example, Baricitinib (JAK1/JAK2 inhibitor) enhanced GVL effects by downregulating PD-L1 expression in tumors (192). While Ruxolitinib (JAK1/JAK2 inhibitor) impaired murine CTL activity against tumor cells in vitro, neither pacritinib (JAK2 inhibitor) nor ruxolitinib interfered with the GVL effect in vivo in MHC-mismatched murine models (186, 194). In xenograft models, however, ruxolitinib significantly impaired antitumor activity against U937 cells, while only pacritinib preserved CTL function (194). The success of many clinical studies evaluating the efficacy of JAK inhibitors for treatment of both SR-aGVHD and SR-cGVHD (185, 195–200), have prompted interest in testing JAK inhibitors for prevention of GVHD (201, 202). Since our previous study showed tolerogenic anti-IL-2 mAb effective prevent aGVHD and maintain GVL activity, but did not prevent cGVHD (156). It would also be of interest to test whether sequential administration of tolerogenic anti-IL-2 mAb and JAK inhibitors will effectively prevent both aGVHD and cGVHD while preserving GVL activity.
Summary
The cellular interactions that lead to GVHD occur in the skin, liver, gut and lung, while those that lead to GVL activity occur in lympho-hematopoietic tissues (12, 61). We summarized the approaches that GVHD could be prevented while preserving GVL activity in Figure 2. First, inhibiting alloreactive T migration and expansion in GVHD target tissues while allowing full activation and expansion of alloreactive T cells in lympho-hematopoietic tissues. Approaches that specifically prevent alloreactive T cell infiltration into GVHD target tissues include the following (Table 1): 1) targeting chemokine or chemokine receptors, such as CCR5, CXCR3, MAdCAM-1; 2) anti-CD3-preconditioning that depletes host DCs that imprint alloreactive T cell tissue tropism; 3) FTY720 that prevents alloreactive T cell egress from lymphoid tissues; and 4) neutralizing or blockade signaling pathways of inflammatory cytokines such as TNF-α and IL-6. Some of these approaches (i.e., FTY720, anti-TNF-α and anti-IL-6R) have been tested in the clinic, but the effect was minimal (94–96), while some (i.e., anti-CD3-preconditioning, anti-CCR5) have not been tested. Depletion of naïve T cells effectively prevents cGVHD and severe aGVHD. Evidence suggests that the high incidence of mild aGVHD in these patients helps to decrease the risk of relapse without increasing the risk of non-relapse mortality. Other promising approaches are to augment parenchymal tissue protective mechanisms mediated by PD-L1 interaction with PD-1 and CD80, including 1) administration of depleting anti-CD4 mAb that allows parenchymal tissue PD-L1/PD-1 interaction to tolerize infiltrating donor CD8+ T cells while allowing lymphoid tissue PD-L1 interaction with CD80 to augment expansion of donor CD8+ T cells that mediate GVL activity (163) (2); administration of tolerogenic anti-IL-2 mAb early after HCT that prevents aGVHD while preserving strong GVL activity (156), potentially in combination with JAK inhibitors to prevent cGVHD.
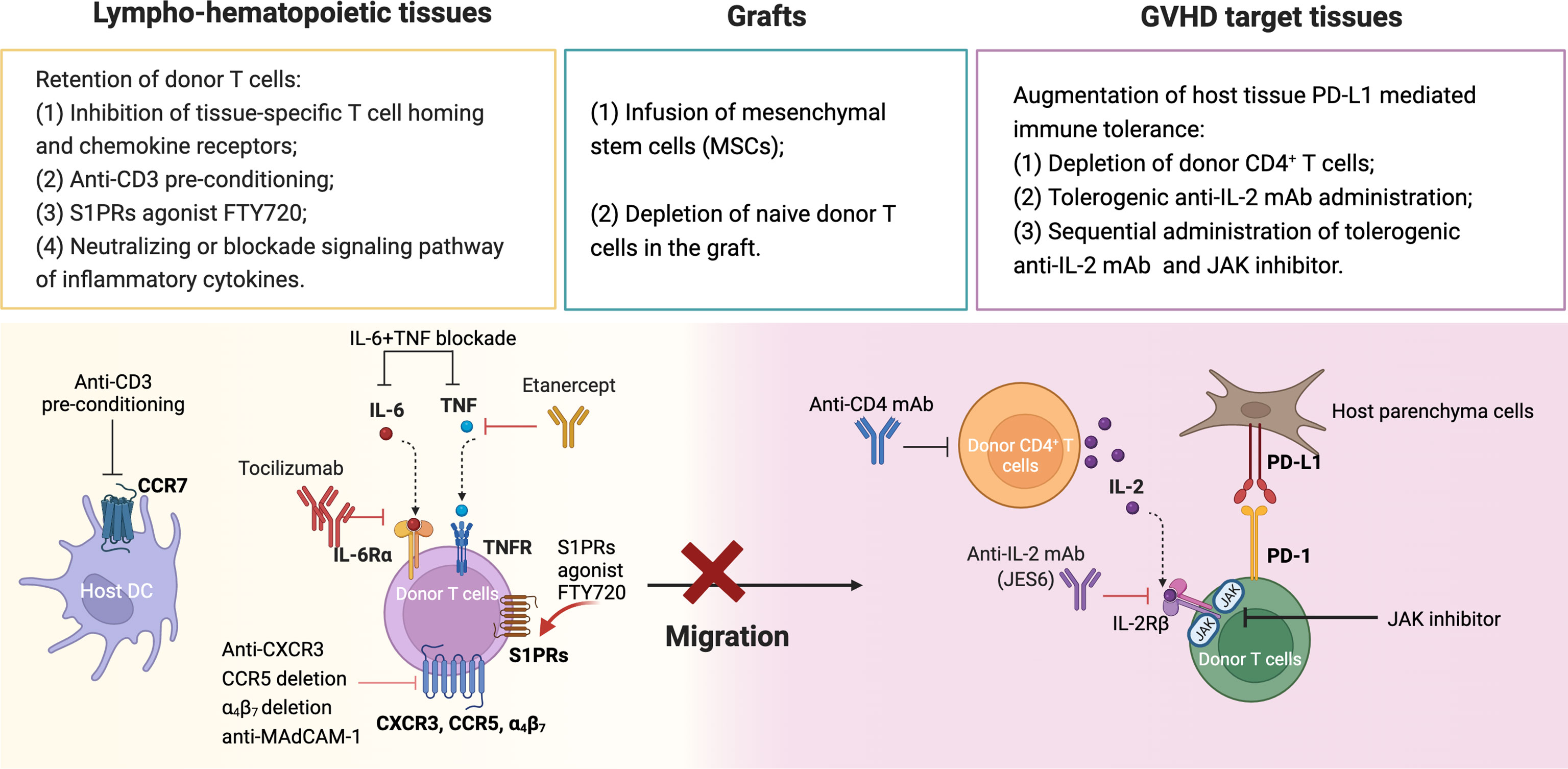
Figure 2 Summary of approaches for prevention of GVHD while preserving GVL activity. (1) Retention of donor T cells in lympho-hematopoietic tissue. (2) Depletion of naïve donor T cells and infusion of mesenchymal stem cells. (3) Augmentation of tissue PD-L1 mediated immune tolerance. Created with BioRender.com.
Author Contributions
QS wrote the review manuscript; UN edited the manuscript; RN, PM, and DZ critically reviewed and edited the manuscript. All authors contributed to the article and approved the submitted version.
Funding
This work was supported by National Institutes of Health Grant R01 CA228465 (to DZ) and National Natural Science Foundation of China (grant no. 82100226) to QS.
Conflict of Interest
The authors declare that the research was conducted in the absence of any commercial or financial relationships that could be construed as a potential conflict of interest.
Publisher’s Note
All claims expressed in this article are solely those of the authors and do not necessarily represent those of their affiliated organizations, or those of the publisher, the editors and the reviewers. Any product that may be evaluated in this article, or claim that may be made by its manufacturer, is not guaranteed or endorsed by the publisher.
References
1. Appelbaum FR. Haematopoietic Cell Transplantation as Immunotherapy. Nature (2001) 411(6835):385–9. doi: 10.1038/35077251
2. Reddy P, Maeda Y, Liu C, Krijanovski OI, Korngold R, Ferrara JLM. A Crucial Role for Antigen-Presenting Cells and Alloantigen Expression in Graft-Versus-Leukemia Responses. Nat Med (2005) 11(11):1244–9. doi: 10.1038/nm1309
3. Fefer A. Graft-Vs.-Tumor Responses. Thomas Hematopoietic Cell Transpl (2003) . p:369–79. doi: 10.1002/9780470987070.ch28
4. Riddell SR. The Graft-Versus-Leukemia Effect—Breaking the Black Box Open. Biol Blood Marrow Transplant (2008) 14(1):2–3. doi: 10.1016/j.bbmt.2007.10.004
5. Shlomchik WD. Graft-Versus-Host Disease. Nat Rev Immunol (2007) 7(5):340–52. doi: 10.1038/nri2000
6. Ito M, Shizuru JA. Graft-vs.-Lymphoma Effect in an Allogeneic Hematopoietic Stem Cell Transplantation Model. Biol Blood Marrow Transplant (1999) 5(6):357–68. doi: 10.1016/s1083-8791(99)70012-1
7. Hill GR, Koyama M. Cytokines and Costimulation in Acute Graft-Versus-Host Disease. Blood (2020) 136(4):418–28. doi: 10.1182/blood.2019000952
8. Zeiser R. Advances in Understanding the Pathogenesis of Graft-Versus-Host Disease. Br J Haematol (2019) 187(5):563–72. doi: 10.1111/bjh.16190
9. Betts BC, Yu X-Z. Editorial: Pathogenesis and Therapy of Graft-Versus-Host Disease. Front Immunol (2019) 10:1797(1797). doi: 10.3389/fimmu.2019.01797
10. Perkey E, Maillard I. New Insights Into Graft-Versus-Host Disease and Graft Rejection. Annu Rev Pathol: Mech Dis (2018) 13(1):219–45. doi: 10.1146/annurev-pathol-020117-043720
11. Ferrara JLM, Levine JE, Reddy P, Holler E. Graft-Versus-Host Disease. Lancet (2009) 373(9674):1550–61. doi: 10.1016/S0140-6736(09)60237-3
12. Zeiser R, Blazar BR. Acute Graft-Versus-Host Disease — Biologic Process, Prevention, and Therapy. N Engl J Med (2017) 377(22):2167–79. doi: 10.1056/nejmra1609337
13. Whiteley AE, Price TT, Cantelli G, Sipkins DA. Leukaemia: A Model Metastatic Disease. Nat Rev Cancer (2021) 21(7):461–75. doi: 10.1038/s41568-021-00355-z
14. Abouelnasr A, Roy J, Cohen S, Kiss T, Lachance S. Defining the Role of Sirolimus in the Management of Graft-Versus-Host Disease: From Prophylaxis to Treatment. Biol Blood Marrow Transplant (2013) 19(1):12–21. doi: 10.1016/j.bbmt.2012.06.020
15. Sharma N, Zhao Q, Ni B, Elder P, Puto M, Benson DM, et al. Effect of Early Post-Transplantation Tacrolimus Concentration on the Risk of Acute Graft-Versus-Host Disease in Allogenic Stem Cell Transplantation. Cancers (Basel) (2021) 13(4):613. doi: 10.3390/cancers13040613
16. Andolfi G, Fousteri G, Rossetti M, Magnani CF, Jofra T, Locafaro G, et al. Enforced Il-10 Expression Confers Type 1 Regulatory T Cell (Tr1) Phenotype and Function to Human Cd4+ T Cells. Mol Ther (2012) 20(9):1778–90. doi: 10.1038/mt.2012.71
17. Locafaro G, Andolfi G, Russo F, Cesana L, Spinelli A, Camisa B, et al. Il-10-Engineered Human Cd4 + Tr1 Cells Eliminate Myeloid Leukemia in an Hla Class I-Dependent Mechanism. Mol Ther (2017) 25(10):2254–69. doi: 10.1016/j.ymthe.2017.06.029
18. Cieniewicz B, Uyeda MJ, Chen P, Sayitoglu EC, Liu JM-H, Andolfi G, et al. Engineered Type 1 Regulatory T Cells Designed for Clinical Use Kill Primary Pediatric Acute Myeloid Leukemia Cells. Haematologica (2020) 106(10):2588–97. doi: 10.3324/haematol.2020.263129
19. Bacchetta R, Lucarelli B, Sartirana C, Gregori S, Lupo Stanghellini MT, Miqueu P, et al. Immunological Outcome in Haploidentical-Hsc Transplanted Patients Treated With Il-10-Anergized Donor T Cells. Front Immunol (2014) 5:16(16). doi: 10.3389/fimmu.2014.00016
20. Chen PP, Cepika A-M, Agarwal-Hashmi R, Saini G, Uyeda MJ, Louis DM, et al. Alloantigen-Specific Type 1 Regulatory T Cells Suppress Through Ctla-4 and Pd-1 Pathways and Persist Long-Term in Patients. Sci Trans Med (2021) 13(617):eabf5264. doi: 10.1126/scitranslmed.abf5264
21. Heinrichs J, Li J, Nguyen H, Wu Y, Bastian D, Daethanasanmak A, et al. Cd8(+) Tregs Promote Gvhd Prevention and Overcome the Impaired Gvl Effect Mediated by Cd4(+) Tregs in Mice. Oncoimmunology (2016) 5(6):e1146842. doi: 10.1080/2162402x.2016.1146842
22. Iamsawat S, Tian L, Daenthanasanmak A, Wu Y, Nguyen HD, Bastian D, et al. Vitamin C Stabilizes Cd8+ Itregs and Enhances Their Therapeutic Potential in Controlling Murine Gvhd and Leukemia Relapse. Blood Adv (2019) 3(24):4187–201. doi: 10.1182/bloodadvances.2019000531
23. Wang W, Hong T, Wang X, Wang R, Du Y, Gao Q, et al. Newly Found Peacekeeper: Potential of Cd8+ Tregs for Graft-Versus-Host Disease. Front Immunol (2021) 12:764786. doi: 10.3389/fimmu.2021.764786
24. Wysocki CA, Panoskaltsis-Mortari A, Blazar BR, Serody JS. Leukocyte Migration and Graft-Versus-Host Disease. Blood (2005) 105(11):4191–9. doi: 10.1182/blood-2004-12-4726
25. Murai M, Yoneyama H, Harada A, Yi Z, Vestergaard C, Guo B, et al. Active Participation of Ccr5(+)Cd8(+) T Lymphocytes in the Pathogenesis of Liver Injury in Graft-Versus-Host Disease. J Clin Invest (1999) 104(1):49–57. doi: 10.1172/jci6642
26. Yi T, Chen Y, Wang L, Du G, Huang D, Zhao D, et al. Reciprocal Differentiation and Tissue-Specific Pathogenesis of Th1, Th2, and Th17 Cells in Graft-Versus-Host Disease. Blood (2009) 114(14):3101–12. doi: 10.1182/blood-2009-05-219402
27. Lloyd CM, Delaney T, Nguyen T, Tian J, Martinez AC, Coyle AJ, et al. Cc Chemokine Receptor (Ccr)3/Eotaxin Is Followed by Ccr4/Monocyte-Derived Chemokine in Mediating Pulmonary T Helper Lymphocyte Type 2 Recruitment After Serial Antigen Challenge In Vivo. J Exp Med (2000) 191(2):265–74. doi: 10.1084/jem.191.2.265
28. Reiss Y, Proudfoot AE, Power CA, Campbell JJ, Butcher EC. Cc Chemokine Receptor (Ccr)4 and the Ccr10 Ligand Cutaneous T Cell-Attracting Chemokine (Ctack) in Lymphocyte Trafficking to Inflamed Skin. J Exp Med (2001) 194(10):1541–7. doi: 10.1084/jem.194.10.1541
29. Piper KP, Horlock C, Curnow SJ, Arrazi J, Nicholls S, Mahendra P, et al. Cxcl10-Cxcr3 Interactions Play an Important Role in the Pathogenesis of Acute Graft-Versus-Host Disease in the Skin Following Allogeneic Stem-Cell Transplantation. Blood (2007) 110(12):3827–32. doi: 10.1182/blood-2006-12-061408
30. He S, Cao Q, Qiu Y, Mi J, Zhang JZ, Jin M, et al. A New Approach to the Blocking of Alloreactive T Cell-Mediated Graft-Versus-Host Disease by in Vivo Administration of Anti-Cxcr3 Neutralizing Antibody. J Immunol (2008) 181(11):7581–92. doi: 10.4049/jimmunol.181.11.7581
31. Duffner U, Lu B, Hildebrandt GC, Teshima T, Williams DL, Reddy P, et al. Role of Cxcr3-Induced Donor T-Cell Migration in Acute Gvhd. Exp Hematol (2003) 31(10):897–902. doi: 10.1016/s0301-472x(03)00198-x
32. Pease JE, Horuk R. Chemokine Receptor Antagonists: Part 2. Expert Opin Ther Pat (2009) 19(2):199–221. doi: 10.1517/13543770802641353
33. Hasegawa H, Inoue A, Kohno M, Lei J, Miyazaki T, Yoshie O, et al. Therapeutic Effect of Cxcr3-Expressing Regulatory T Cells on Liver, Lung and Intestinal Damages in a Murine Acute Gvhd Model. Gene Ther (2008) 15:171–82. doi: 10.1038/sj.gt.3303051
34. Palmer LA, Sale GE, Balogun JI, Li D, Jones D, Molldrem JJ, et al. Chemokine Receptor Ccr5 Mediates Alloimmune Responses in Graft-Versus-Host Disease. Biol Blood Marrow Transplant (2010) 16(3):311–9. doi: 10.1016/j.bbmt.2009.12.002
35. Bogunia-Kubik K, Duda D, Suchnicki K, Lange A. Ccr5 Deletion Mutation and Its Association With the Risk of Developing Acute Graft-Versus-Host Disease After Allogeneic Hematopoietic Stem Cell Transplantation. Haematologica (2006) 91(12):1628–34.
36. Wysocki CA, Burkett SB, Panoskaltsis-Mortari A, Kirby SL, Luster AD, McKinnon K, et al. Differential Roles for Ccr5 Expression on Donor T Cells During Graft-Versus-Host Disease Based on Pretransplant Conditioning. J Immunol (2004) 173(2):845–54. doi: 10.4049/jimmunol.173.2.845
37. Reshef R, Luger SM, Hexner EO, Loren AW, Frey NV, Nasta SD, et al. Blockade of Lymphocyte Chemotaxis in Visceral Graft-Versus-Host Disease. N Engl J Med (2012) 367(2):135–45. doi: 10.1056/nejmoa1201248
38. Moy RH, Huffman AP, Richman LP, Crisalli L, Wang XK, Hoxie JA, et al. Clinical and Immunologic Impact of Ccr5 Blockade in Graft-Versus-Host Disease Prophylaxis. Blood (2017) 129(7):906–16. doi: 10.1182/blood-2016-08-735076
39. Saruta M, Yu QT, Avanesyan A, Fleshner PR, Targan SR, Papadakis KA. Phenotype and Effector Function of Cc Chemokine Receptor 9-Expressing Lymphocytes in Small Intestinal Crohn’s Disease. J Immunol (2007) 178(5):3293–300. doi: 10.4049/jimmunol.178.5.3293
40. Keshav S, Vaňásek T, Niv Y, Petryka R, Howaldt S, Bafutto M, et al. A Randomized Controlled Trial of the Efficacy and Safety of Ccx282-B, an Orally-Administered Blocker of Chemokine Receptor Ccr9, for Patients With Crohn’s Disease. PloS One (2013) 8(3):e60094. doi: 10.1371/journal.pone.0060094
41. Schreder A, Moschovakis GL, Halle S, Schlue J, Lee CW, Schippers A, et al. Differential Effects of Gut-Homing Molecules Cc Chemokine Receptor 9 and Integrin-β7 During Acute Graft-Versus-Host Disease of the Liver. Biol Blood Marrow Transplant (2015) 21(12):2069–78. doi: 10.1016/j.bbmt.2015.08.038
42. Chen YB, Kim HT, McDonough S, Odze RD, Yao X, Lazo-Kallanian S, et al. Up-Regulation of Alpha4beta7 Integrin on Peripheral T Cell Subsets Correlates With the Development of Acute Intestinal Graft-Versus-Host Disease Following Allogeneic Stem Cell Transplantation. Biol Blood Marrow Transplant (2009) 15(9):1066–76. doi: 10.1016/j.bbmt.2009.05.003
43. Chen YB, McDonough S, Chen H, Kennedy J, Illiano C, Attar EC, et al. Expression of α4β7 Integrin on Memory Cd8+ T Cells at the Presentation of Acute Intestinal Gvhd. Bone Marrow Transplant (2013) 48(4):598–603. doi: 10.1038/bmt.2012.191
44. Waldman E, Lu SX, Hubbard VM, Kochman AA, Eng JM, Terwey TH, et al. Absence of Beta7 Integrin Results in Less Graft-Versus-Host Disease Because of Decreased Homing of Alloreactive T Cells to Intestine. Blood (2006) 107 4:1703–11. doi: 10.1182/blood-2005-08-3445
45. Ueha S, Murai M, Yoneyama H, Kitabatake M, Imai T, Shimaoka T, et al. Intervention of Madcam 1 or Fractalkine Alleviates Graft Versuì Host Reaction Associated Intestinal Injury While Preserving Grafî Versuì Tumor Effects. J Leukocyte Biol (2007) 81(1):176–85. doi: 10.1189/jlb.0306231
46. Fu YY, Egorova A, Sobieski C, Kuttiyara J, Calafiore M, Takashima S, et al. T Cell Recruitment to the Intestinal Stem Cell Compartment Drives Immune-Mediated Intestinal Damage After Allogeneic Transplantation. Immunity (2019) 51(1):90–103.e3. doi: 10.1016/j.immuni.2019.06.003
47. Fløisand Y, Lazarevic VL, Maertens J, Mattsson J, Shah NN, Zachée P, et al. Safety and Effectiveness of Vedolizumab in Patients With Steroid-Refractory Gastrointestinal Acute Graft-Versus-Host Disease: A Retrospective Record Review. Biol Blood Marrow Transplant (2019) 25(4):720–7. doi: 10.1016/j.bbmt.2018.11.013
48. Coltoff A, Lancman G, Kim S, Steinberg A. Vedolizumab for Treatment of Steroid-Refractory Lower Gastrointestinal Acute Graft-Versus-Host Disease. Bone Marrow Transplant (2018) 53(7):900–4. doi: 10.1038/s41409-018-0094-8
49. Danylesko I, Bukauskas A, Paulson M, Peceliunas V, Gedde-Dahl DYT, Shimoni A, et al. Anti-α4β7 Integrin Monoclonal Antibody (Vedolizumab) for the Treatment of Steroid-Resistant Severe Intestinal Acute Graft-Versus-Host Disease. Bone Marrow Transplant (2019) 54(7):987–93. doi: 10.1038/s41409-018-0364-5
50. Mehta RS, Saliba RM, Jan A, Shigle TL, Wang E, Nieto Y, et al. Vedolizumab for Steroid Refractory Lower Gastrointestinal Tract Graft-Versus-Host Disease. Transplant Cell Ther (2021) 27(3):272.e1–.e5. doi: 10.1016/j.jtct.2020.12.011
51. Fløisand Y, Schroeder MA, Chevallier P, Selleslag D, Devine S, Renteria AS, et al. A Phase 2a Randomized Clinical Trial of Intravenous Vedolizumab for the Treatment of Steroid-Refractory Intestinal Acute Graft-Versus-Host Disease. Bone Marrow Transplant (2021) 56(10):2477–88. doi: 10.1038/s41409-021-01356-0
52. Randolph GJ, Ochando J, Partida-Sánchez S. Migration of Dendritic Cell Subsets and Their Precursors. Annu Rev Immunol (2008) 26(1):293–316. doi: 10.1146/annurev.immunol.26.021607.090254
53. He T, Tang C, Xu S, Moyana T, Xiang J. Interferon Gamma Stimulates Cellular Maturation of Dendritic Cell Line Dc2.4 Leading to Induction of Efficient Cytotoxic T Cell Responses and Antitumor Immunity. Cell Mol Immunol (2007) 4(2):105–11.
54. Kim TD, Terwey TH, Zakrzewski JL, Suh D, Kochman AA, Chen ME, et al. Organ-Derived Dendritic Cells Have Differential Effects on Alloreactive T Cells. Blood (2008) 111(5):2929–40. doi: 10.1182/blood-2007-06-096602
55. Sackstein R. A Revision of Billingham’s Tenets: The Central Role of Lymphocyte Migration in Acute Graft-Versus-Host Disease. Biol Blood Marrow Transplant (2006) 12(1 Suppl 1):2–8. doi: 10.1016/j.bbmt.2005.09.015
56. von Andrian UH, Mempel TR. Homing and Cellular Traffic in Lymph Nodes. Nat Rev Immunol (2003) 3(11):867–78. doi: 10.1038/nri1222
57. He W, Racine JJ, Johnston HF, Li X, Li N, Cassady K, et al. Depletion of Host Ccr7+ Dendritic Cells Prevented Donor T Cell Tissue Tropism in Anti-Cd3–Conditioned Recipients. Biol Blood Marrow Transplant (2014) 20(7):920–8. doi: 10.1016/j.bbmt.2014.03.029
58. Li N, Chen Y, He W, Yi T, Zhao D, Zhang C, et al. Anti-Cd3 Preconditioning Separates Gvl From Gvhd Via Modulating Host Dendritic Cell and Donor T-Cell Migration in Recipients Conditioned With Tbi. Blood (2009) 113(4):953–62. doi: 10.1182/blood-2008-06-165522
59. Huu DL, Matsushita T, Jin G, Hamaguchi Y, Hasegawa M, Takehara K, et al. Fty720 Ameliorates Murine Sclerodermatous Chronic Graft-Versus-Host Disease by Promoting Expansion of Splenic Regulatory Cells and Inhibiting Immune Cell Infiltration Into Skin. Arthritis Rheum (2013) 65(6):1624–35. doi: 10.1002/art.37933
60. Chiba K. Fty720, A New Class of Immunomodulator, Inhibits Lymphocyte Egress From Secondary Lymphoid Tissues and Thymus by Agonistic Activity at Sphingosine 1-Phosphate Receptors. Pharmacol Ther (2005) 108(3):308–19. doi: 10.1016/j.pharmthera.2005.05.002
61. Kim YM, Sachs T, Asavaroengchai W, Bronson R, Sykes M. Graft-Versus-Host Disease can Be Separated From Graft-Versus-Lymphoma Effects by Control of Lymphocyte Trafficking With Fty720. J Clin Invest (2003) 111(5):659–69. doi: 10.1172/jci16950
62. Hashimoto D, Asakura S, Matsuoka K, Sakoda Y, Koyama M, Aoyama K, et al. Fty720 Enhances the Activation-Induced Apoptosis of Donor T Cells and Modulates Graft-Versus-Host Disease. Eur J Immunol (2007) 37(1):271–81. doi: 10.1002/eji.200636123
63. Taylor PA, Ehrhardt MJ, Lees CJ, Tolar J, Weigel BJ, Panoskaltsis-Mortari A, et al. Insights Into the Mechanism of Fty720 and Compatibility With Regulatory T Cells for the Inhibition of Graft-Versus-Host Disease (Gvhd). Blood (2007) 110(9):3480–8. doi: 10.1182/blood-2007-05-087940
64. Yu X-Z, Anasetti C. Fty720 Prevents Gvhd: The Host Perspective. Blood (2007) 110(9):3094–. doi: 10.1182/blood-2007-08-102954
65. Gauthier J, Vermersch P, Chauvet P, Varlet P, Coiteux V, Magro L, et al. Successful Treatment With Fingolimod of Graft-Versus-Host Disease of the Central Nervous System. Blood Adv (2018) 2(1):10–3. doi: 10.1182/bloodadvances.2017011478
66. Divito SJ, Aasebø AT, Matos TR, Hsieh P-C, Collin M, Elco CP, et al. Peripheral Host T Cells Survive Hematopoietic Stem Cell Transplantation and Promote Graft-Versus-Host Disease. J Clin Invest (2020) 130(9):4624–36. doi: 10.1172/jci129965
67. Chakraverty R, Coêteí D, Buchli J, Cotter P, Hsu R, Zhao G, et al. An Inflammatory Checkpoint Regulates Recruitment of Graft-Versus-Host Reactive T Cells to Peripheral Tissues. J Exp Med (2006) 203(8):2021–31. doi: 10.1084/jem.20060376
68. Mapara MY, Kim Y-M, Wang S-P, Bronson R, Sachs DH, Sykes M. Donor Lymphocyte Infusions Mediate Superior Graft-Versus-Leukemia Effects in Mixed Compared to Fully Allogeneic Chimeras: A Critical Role for Host Antigen–Presenting Cells. Blood (2002) 100(5):1903–9. doi: 10.1182/blood-2002-01-0023
69. Mapara MY, Kim Y-M, Marx J, Sykes M. Donor Lymphocyte Infusion-Mediated Graft-Versus-Leukemia Effects in Mixed Chimeras Established With a Nonmyeloablative Conditioning Regimen: Extinction of Graft-Versus-Leukemia Effects After Conversion to Full Donor Chimerism1. Transplantation (2003) 76(2):297–305. doi: 10.1097/01.Tp.0000072014.83469.2d
70. Billiau AD, Fevery S, Rutgeerts O, Landuyt W, Waer M. Crucial Role of Timing of Donor Lymphocyte Infusion in Generating Dissociated Graft-Versus-Host and Graft-Versus-Leukemia Responses in Mice Receiving Allogeneic Bone Marrow Transplants. Blood (2002) 100(5):1894–902. doi: 10.1182/blood-2002-02-0419
71. Dey BR, McAfee S, Colby C, Sackstein R, Saidman S, Tarbell N, et al. Impact of Prophylactic Donor Leukocyte Infusions on Mixed Chimerism, Graft-Versus-Host Disease, and Antitumor Response in Patients With Advanced Hematologic Malignancies Treated With Nonmyeloablative Conditioning and Allogeneic Bone Marrow Transplantation. Biol Blood Marrow Transplant (2003) 9(5):320–9. doi: 10.1016/s1083-8791(03)00077-6
72. Spitzer TR, McAfee S, Sackstein R, Colby C, Toh HC, Multani P, et al. Intentional Induction of Mixed Chimerism and Achievement of Antitumor Responses After Nonmyeloablative Conditioning Therapy and Hla-Matched Donor Bone Marrow Transplantation for Refractory Hematologic Malignancies. Biol Blood Marrow Transplant (2000) 6(3a):309–20. doi: 10.1016/s1083-8791(00)70056-5
73. Mapara MY, Sykes M. Induction of Mixed vs Full Chimerism to Potentiate Gvl Effects After Bone-Marrow Transplantation. Methods Mol Med (2005) 109:469–74. doi: 10.1385/1-59259-862-5:469
74. Dazzi F, Szydlo RM, Cross NCP, Craddock C, Kaeda J, Kanfer E, et al. Durability of Responses Following Donor Lymphocyte Infusions for Patients Who Relapse After Allogeneic Stem Cell Transplantation for Chronic Myeloid Leukemia. Blood (2000) 96(8):2712–6. doi: 10.1182/blood.V96.8.2712
75. Frederik Falkenburg JH, Schmid C, Kolb HJ, Locatelli F, Kuball J. Delayed Transfer of Immune Cells or the Art of Donor Lymphocyte Infusion. In: Carreras E, Dufour C, Mohty M, Kröger N, editors. The Ebmt Handbook: Hematopoietic Stem Cell Transplantation and Cellular Therapies. Cham (CH: Springer Copyright 2019, EBMT and the Author(s (2019). p. 443–8.
76. Drobyski WR, Roth MS, Thibodeau SN, Gottschall JL. Molecular Remission Occurring After Donor Leukocyte Infusions for the Treatment of Relapsed Chronic Myelogenous Leukemia After Allogeneic Bone Marrow Transplantation. Bone Marrow Transplant (1992) 10(3):301–4.
77. Naparstek E, Or R, Nagler A, Cividalli G, Engelhard D, Aker M, et al. T-Cell-Depleted Allogeneic Bone Marrow Transplantation for Acute Leukaemia Using Campath-1 Antibodies and Post-Transplant Administration of Donor’s Peripheral Blood Lymphocytes for Prevention of Relapse. Br J Haematol (1995) 89(3):506–15. doi: 10.1111/j.1365-2141.1995.tb08356.x
78. Radujkovic A, Guglielmi C, Bergantini S, Iacobelli S, van Biezen A, Milojkovic D, et al. Donor Lymphocyte Infusions for Chronic Myeloid Leukemia Relapsing After Allogeneic Stem Cell Transplantation: May We Predict Graft-Versus-Leukemia Without Graft-Versus-Host Disease? Biol Blood Marrow Transplant (2015) 21(7):1230–6. doi: 10.1016/j.bbmt.2015.03.012
79. Zeiser R, Bertz H, Spyridonidis A, Houet L, Finke J. Donor Lymphocyte Infusions for Multiple Myeloma: Clinical Results and Novel Perspectives. Bone Marrow Transplant (2004) 34(11):923–8. doi: 10.1038/sj.bmt.1704670
80. Kittan NA, Hildebrandt GC. The Chemokine System: A Possible Therapeutic Target in Acute Graft Versus Host Disease. Curr Top Microbiol Immunol (2010) 341:97–120. doi: 10.1007/82_2010_23
81. Dutt S, Ermann J, Tseng D, Liu YP, George TI, Fathman CG, et al. L-Selectin and β7 Integrin on Donor Cd4 T Cells Are Required for the Early Migration to Host Mesenteric Lymph Nodes and Acute Colitis of Graft-Versus-Host Disease. Blood (2005) 106(12):4009–15. doi: 10.1182/blood-2005-06-2339
82. Gartlan KH, Koyama M, Lineburg KE, Chang K, Ensbey KS, Kuns RD, et al. Donor T-Cell–Derived Gm-Csf Drives Alloantigen Presentation by Dendritic Cells in the Gastrointestinal Tract. Blood Adv (2019) 3(19):2859–65. doi: 10.1182/bloodadvances.2019000053
83. Waller EK. A New Role for an Old Cytokine: Gm-Csf Amplifies Gvhd. Blood (2020) 135(8):520–1. doi: 10.1182/blood.2019004681
84. Alexander KA, Flynn R, Lineburg KE, Kuns RD, Teal BE, Olver SD, et al. Csf-1–Dependant Donor-Derived Macrophages Mediate Chronic Graft-Versus-Host Disease. J Clin Invest (2014) 124(10):4266–80. doi: 10.1172/jci75935
85. Bent EH, Millán-Barea LR, Zhuang I, Goulet DR, Fröse J, Hemann MT. Microenvironmental Il-6 Inhibits Anti-Cancer Immune Responses Generated by Cytotoxic Chemotherapy. Nat Commun (2021) 12(1):6218. doi: 10.1038/s41467-021-26407-4
86. Gadó K. Role of Interleukin-6 in the Pathogenesis of Multiple Myeloma. Cell Biol Int (2000) 24(4):195–209. doi: 10.1006/cbir.2000.0497
87. Chen X, Das R, Komorowski R, Beres A, Hessner MJ, Mihara M, et al. Blockade of Interleukin-6 Signaling Augments Regulatory T-Cell Reconstitution and Attenuates the Severity of Graft-Versus-Host Disease. Blood (2009) 114(4):891–900. doi: 10.1182/blood-2009-01-197178
88. Wilkinson AN, Chang K, Kuns RD, Henden AS, Minnie SA, Ensbey KS, et al. Il-6 Dysregulation Originates in Dendritic Cells and Mediates Graft-Versus-Host Disease Via Classical Signaling. Blood (2019) 134(23):2092–106. doi: 10.1182/blood.2019000396
89. Korngold R, Marini JC, de Baca ME, Murphy GF, Giles-Komar J. Role of Tumor Necrosis Factor-Alpha in Graft-Versus-Host Disease and Graft-Versus-Leukemia Responses. Biol Blood Marrow Transplant (2003) 9(5):292–303. doi: 10.1016/s1083-8791(03)00087-9
90. Hill GR, Teshima T, Gerbitz A, Pan L, Cooke KR, Brinson YS, et al. Differential Roles of Il-1 and Tnf-α on Graft-Versus-Host Disease and Graft Versus Leukemia. J Clin Invest (1999) 104(4):459–67. doi: 10.1172/jci6896
91. Markey KA, Burman AC, Banovic T, Kuns RD, Raffelt NC, Rowe V, et al. Soluble Lymphotoxin is an Important Effector Molecule in Gvhd and Gvl. Blood (2010) 115(1):122–32. doi: 10.1182/blood-2009-01-199927
92. Hill GR, Teshima T, Gerbitz A, Pan L, Cooke KR, Brinson YS, et al. Differential Roles of Il-1 and Tnf-Alpha on Graft-Versus-Host Disease and Graft Versus Leukemia. J Clin Invest (1999) 104(4):459–67. doi: 10.1172/jci6896
93. Khuat LT, Vick LV, Dunai C, Collins CP, More SK, Le CT, et al. Increased Efficacy of Dual Proinflammatory Cytokine Blockade on Acute Gvhd While Maintaining Gvt Effects. Blood (2021) 138(24):2583–8. doi: 10.1182/blood.2021011216
94. Kennedy GA, Tey S-K, Buizen L, Varelias A, Gartlan KH, Curley C, et al. A Phase 3 Double-Blind Study of the Addition of Tocilizumab vs Placebo to Cyclosporin/Methotrexate Gvhd Prophylaxis. Blood (2021) 137(14):1970–9. doi: 10.1182/blood.2020009050
95. Choi SW, Stiff P, Cooke K, Ferrara JL, Braun T, Kitko C, et al. Tnf-Inhibition With Etanercept for Graft-Versus-Host Disease Prevention in High-Risk Hct: Lower Tnfr1 Levels Correlate With Better Outcomes. Biol Blood Marrow Transplant (2012) 18(10):1525–32. doi: 10.1016/j.bbmt.2012.03.013
96. Kitko CL, Braun T, Couriel DR, Choi SW, Connelly J, Hoffmann S, et al. Combination Therapy for Graft-Versus-Host Disease Prophylaxis With Etanercept and Extracorporeal Photopheresis: Results of a Phase Ii Clinical Trial. Biol Blood Marrow Transplant (2016) 22(5):862–8. doi: 10.1016/j.bbmt.2015.11.002
97. Marigo I, Dazzi F. The Immunomodulatory Properties of Mesenchymal Stem Cells. Semin Immunopathol (2011) 33(6):593–602. doi: 10.1007/s00281-011-0267-7
98. Meirelles LDS, Chagastelles PC, Nardi NB. Mesenchymal Stem Cells Reside in Virtually All Post-Natal Organs and Tissues. J Cell Sci (2006) 119(11):2204–13. doi: 10.1242/jcs.02932
99. Igura K, Zhang X, Takahashi K, Mitsuru A, Yamaguchi S, Takahashi TA. Isolation and Characterization of Mesenchymal Progenitor Cells From Chorionic Villi of Human Placenta. Cytotherapy (2004) 6(6):543–53. doi: 10.1080/14653240410005366-1
100. Erices A, Conget P, Minguell JJ. Mesenchymal Progenitor Cells in Human Umbilical Cord Blood. Br J Haematol (2000) 109(1):235–42. doi: 10.1046/j.1365-2141.2000.01986.x
101. Zannettino ACW, Paton S, Arthur A, Khor F, Itescu S, Gimble JM, et al. Multipotential Human Adipose-Derived Stromal Stem Cells Exhibit a Perivascular Phenotype In Vitro and In Vivo. J Cell Physiol (2008) 214(2):413–21. doi: 10.1002/jcp.21210
102. Gronthos S, Mankani M, Brahim J, Robey PG, Shi S. Postnatal Human Dental Pulp Stem Cells (Dpscs) in Vitro and. In Vivo Proc Natl Acad Sci (2000) 97(25):13625–30. doi: 10.1073/pnas.240309797
103. Hass R, Kasper C, Böhm S, Jacobs R. Different Populations and Sources of Human Mesenchymal Stem Cells (Msc): A Comparison of Adult and Neonatal Tissue-Derived Msc. Cell Commun Signaling (2011) 9(1):12. doi: 10.1186/1478-811x-9-12
104. in 't Anker PS, Noort WA, Scherjon SA, Kleijburg-van der Keur C, Kruisselbrink AB, van Bezooijen RL, et al. Mesenchymal Stem Cells in Human Second-Trimester Bone Marrow, Liver, Lung, and Spleen Exhibit a Similar Immunophenotype But a Heterogeneous Multilineage Differentiation Potential. Haematologica (2003) 88(8):845–52.
105. Kern S, Eichler H, Stoeve J, Klüter H, Bieback K. Comparative Analysis of Mesenchymal Stem Cells From Bone Marrow, Umbilical Cord Blood, or Adipose Tissue. Stem Cells. (2006) 24(5):1294–301. doi: 10.1634/stemcells.2005-0342
106. Wang Z, Chai C, Wang R, Feng Y, Huang L, Zhang Y, et al. Single-Cell Transcriptome Atlas of Human Mesenchymal Stem Cells Exploring Cellular Heterogeneity. Clin Trans Med (2021) 11(12):e650. doi: 10.1002/ctm2.650
107. Rasmusson I, Ringdén O, Sundberg B, Le Blanc K. Mesenchymal Stem Cells Inhibit the Formation of Cytotoxic T Lymphocytes, But Not Activated Cytotoxic T Lymphocytes or Natural Killer Cells. Transplantation (2003) 76(8):1208–13. doi: 10.1097/01.Tp.0000082540.43730.80
108. Aggarwal S, Pittenger MF. Human Mesenchymal Stem Cells Modulate Allogeneic Immune Cell Responses. Blood (2005) 105(4):1815–22. doi: 10.1182/blood-2004-04-1559
109. Jiang X-X, Zhang Y, Liu B, Zhang S-X, Wu Y, Yu X-D, et al. Human Mesenchymal Stem Cells Inhibit Differentiation and Function of Monocyte-Derived Dendritic Cells. Blood (2005) 105(10):4120–6. doi: 10.1182/blood-2004-02-0586
110. Beyth S, Borovsky Z, Mevorach D, Liebergall M, Gazit Z, Aslan H, et al. Human Mesenchymal Stem Cells Alter Antigen-Presenting Cell Maturation and Induce T-Cell Unresponsiveness. Blood (2005) 105(5):2214–9. doi: 10.1182/blood-2004-07-2921
111. Wang R, Wang X, Yang S, Xiao Y, Jia Y, Zhong J, et al. Umbilical Cord-Derived Mesenchymal Stem Cells Promote Myeloid-Derived Suppressor Cell Enrichment by Secreting Cxcl1 to Prevent Graft-Versus-Host Disease After Hematopoietic Stem Cell Transplantation. Cytotherapy (2021) 23(11):996–1006. doi: 10.1016/j.jcyt.2021.07.009
112. Corcione A, Benvenuto F, Ferretti E, Giunti D, Cappiello V, Cazzanti F, et al. Human Mesenchymal Stem Cells Modulate B-Cell Functions. Blood (2006) 107(1):367–72. doi: 10.1182/blood-2005-07-2657
113. Zappia E, Casazza S, Pedemonte E, Benvenuto F, Bonanni I, Gerdoni E, et al. Mesenchymal Stem Cells Ameliorate Experimental Autoimmune Encephalomyelitis Inducing T-Cell Anergy. Blood (2005) 106(5):1755–61. doi: 10.1182/blood-2005-04-1496
114. Studeny M, Marini FC, Champlin RE, Zompetta C, Fidler IJ, Andreeff M. Bone Marrow-Derived Mesenchymal Stem Cells as Vehicles for Interferon-Beta Delivery Into Tumors. Cancer Res (2002) 62(13):3603–8.
115. Nauta AJ, Fibbe WE. Immunomodulatory Properties of Mesenchymal Stromal Cells. Blood (2007) 110(10):3499–506. doi: 10.1182/blood-2007-02-069716
116. Ni X, Xia Y, Zhou S, Peng H, Wu X, Lu H, et al. Reduction in Murine Acute Gvhd Severity by Human Gingival Tissue-Derived Mesenchymal Stem Cells Via the Cd39 Pathways. Cell Death Dis (2019) 10(1):13. doi: 10.1038/s41419-018-1273-7
117. Polchert D, Sobinsky J, Douglas G, Kidd M, Moadsiri A, Reina E, et al. Ifn-Γ Activation of Mesenchymal Stem Cells for Treatment and Prevention of Graft Versus Host Disease. Eur J Immunol (2008) 38(6):1745–55. doi: 10.1002/eji.200738129
118. Poggi A, Zocchi MR. Role of Bone Marrow Stromal Cells in the Generation of Human Cd8+ Regulatory T Cells. Hum Immunol (2008) 69(11):755–9. doi: 10.1016/j.humimm.2008.08.278
119. Baron F, Lechanteur C, Willems E, Bruck F, Baudoux E, Seidel L, et al. Cotransplantation of Mesenchymal Stem Cells Might Prevent Death From Graft-Versus-Host Disease (Gvhd) Without Abrogating Graft-Versus-Tumor Effects After Hla-Mismatched Allogeneic Transplantation Following Nonmyeloablative Conditioning. Biol Blood Marrow Transplant (2010) 16(6):838–47. doi: 10.1016/j.bbmt.2010.01.011
120. Ning H, Yang F, Jiang M, Hu L, Feng K, Zhang J, et al. The Correlation Between Cotransplantation of Mesenchymal Stem Cells and Higher Recurrence Rate in Hematologic Malignancy Patients: Outcome of a Pilot Clinical Study. Leukemia (2008) 22(3):593–9. doi: 10.1038/sj.leu.2405090
121. Kallekleiv M, Larun L, Bruserud Ø, Hatfield KJ. Co-Transplantation of Multipotent Mesenchymal Stromal Cells in Allogeneic Hematopoietic Stem Cell Transplantation: A Systematic Review and Meta-Analysis. Cytotherapy (2016) 18(2):172–85. doi: 10.1016/j.jcyt.2015.11.010
122. Ludwig A-K, Giebel B. Exosomes: Small Vesicles Participating in Intercellular Communication. Int J Biochem Cell Biol (2012) 44(1):11–5. doi: 10.1016/j.biocel.2011.10.005
123. Nguyen DG, Booth A, Gould SJ, Hildreth JEK. Evidence That Hiv Budding in Primary Macrophages Occurs Through the Exosome Release Pathway. J Biol Chem (2003) 278(52):52347–54. doi: 10.1074/jbc.m309009200
124. Lai P, Weng J, Guo L, Chen X, Du X. Novel Insights Into Msc-Evs Therapy for Immune Diseases. biomark Res (2019) 7(1):6. doi: 10.1186/s40364-019-0156-0
125. Fujii S, Miura Y, Fujishiro A, Shindo T, Shimazu Y, Hirai H, et al. Graft-Versus-Host Disease Amelioration by Human Bone Marrow Mesenchymal Stromal/Stem Cell-Derived Extracellular Vesicles Is Associated With Peripheral Preservation of Naive T Cell Populations. Stem Cells (2017) 36(3):434–45. doi: 10.1002/stem.2759
126. Lanzavecchia A, Sallusto F. Dynamics of T Lymphocyte Responses: Intermediates, Effectors, and Memory Cells. Science (2000) 290(5489):92–7. doi: 10.1126/science.290.5489.92
127. Farber DL, Yudanin NA, Restifo NP. Human Memory T Cells: Generation, Compartmentalization and Homeostasis. Nat Rev Immunol (2014) 14(1):24–35. doi: 10.1038/nri3567
128. Zeng D, Hoffmann P, Lan F, Huie P, Higgins J, Strober S. Unique Patterns of Surface Receptors, Cytokine Secretion, and Immune Functions Distinguish T Cells in the Bone Marrow From Those in the Periphery: Impact on Allogeneic Bone Marrow Transplantation. Blood (2002) 99(4):1449–57. doi: 10.1182/blood.V99.4.1449
129. Chen BJ, Cui X, Sempowski GD, Liu C, Chao NJ. Transfer of Allogeneic Cd62l– Memory T Cells Without Graft-Versus-Host Disease. Blood (2004) 103(4):1534–41. doi: 10.1182/blood-2003-08-2987
130. Zheng H, Matte-Martone C, Li H, Anderson BE, Venketesan S, Sheng Tan H, et al. Effector Memory Cd4+ T Cells Mediate Graft-Versus-Leukemia Without Inducing Graft-Versus-Host Disease. Blood (2008) 111(4):2476–84. doi: 10.1182/blood-2007-08-109678
131. Anderson BE, McNiff J, Yan J, Doyle H, Mamula M, Shlomchik MJ, et al. Memory Cd4+ T Cells Do Not Induce Graft-Versus-Host Disease. J Clin Invest (2003) 112(1):101–8. doi: 10.1172/jci17601
132. Anderson BE, Zheng H, Taylor PA, Matte-Martone C, McNiff JM, Jain D, et al. Memory T Cells in Gvhd and Gvl. Biol Blood Marrow Transplant (2008) 14(1 Suppl 1):19–20.
133. Zhang Y, Joe G, Zhu J, Carroll R, Levine B, Hexner E, et al. Dendritic Cell-Activated Cd44hicd8+ T Cells Are Defective in Mediating Acute Graft-Versus-Host Disease But Retain Graft-Versus-Leukemia Activity. Blood (2004) 103(10):3970–8. doi: 10.1182/blood-2003-09-3135
134. Dutt S, Tseng D, Ermann J, George TI, Liu YP, Davis CR, et al. Naive and Memory T Cells Induce Different Types of Graft-Versus-Host Disease. J Immunol (2007) 179(10):6547–54. doi: 10.4049/jimmunol.179.10.6547
135. Zheng H, Matte-Martone C, Jain D, McNiff J, Shlomchik WD. Central Memory Cd8+ T Cells Induce Graft-Versus-Host Disease and Mediate Graft-Versus-Leukemia. J Immunol (2009) 182(10):5938–48. doi: 10.4049/jimmunol.0802212
136. Li N, Matte-Martone C, Zheng H, Cui W, Venkatesan S, Tan HS, et al. Memory T Cells From Minor Histocompatibility Antigen–Vaccinated and Virus-Immune Donors Improve Gvl and Immune Reconstitution. Blood (2011) 118(22):5965–76. doi: 10.1182/blood-2011-07-367011
137. Huang W, Mo W, Jiang J, Chao NJ, Chen BJ. Donor Allospecific Cd44high Central Memory T Cells Have Decreased Ability to Mediate Graft-Vs.-Host Disease. Front Immunol (2019) 10:624(624). doi: 10.3389/fimmu.2019.00624
138. Bleakley M, Otterud BE, Richardt JL, Mollerup AD, Hudecek M, Nishida T, et al. Leukemia-Associated Minor Histocompatibility Antigen Discovery Using T-Cell Clones Isolated by in Vitro Stimulation of Naive Cd8+ T Cells. Blood (2010) 115(23):4923–33. doi: 10.1182/blood-2009-12-260539
139. Bleakley M, Heimfeld S, Loeb KR, Jones LA, Chaney C, Seropian S, et al. Outcomes of Acute Leukemia Patients Transplanted With Naive T Cell–Depleted Stem Cell Grafts. J Clin Invest (2015) 125(7):2677–89. doi: 10.1172/jci81229
140. Bleakley M, Sehgal A, Seropian S, Biernacki MA, Krakow EF, Dahlberg A, et al. Naive T-Cell Depletion to Prevent Chronic Graft-Versus-Host Disease. J Clin Oncol (2022) 40(11):1174–1185, Jco2101755. doi: 10.1200/jco.21.01755
141. Triplett BM, Shook DR, Eldridge P, Li Y, Kang G, Dallas M, et al. Rapid Memory T-Cell Reconstitution Recapitulating Cd45ra-Depleted Haploidentical Transplant Graft Content in Patients With Hematologic Malignancies. Bone Marrow Transplant (2015) 50(7):968–77. doi: 10.1038/bmt.2014.324
142. Shook DR, Triplett BM, Eldridge PW, Kang G, Srinivasan A, Leung W. Haploidentical Stem Cell Transplantation Augmented by Cd45ra Negative Lymphocytes Provides Rapid Engraftment and Excellent Tolerability. Pediatr Blood Cancer (2015) 62(4):666–73. doi: 10.1002/pbc.25352
143. Triplett BM, Muller B, Kang G, Li Y, Cross SJ, Moen J, et al. Selective T-Cell Depletion Targeting Cd45ra Reduces Viremia and Enhances Early T-Cell Recovery Compared With Cd3-Targeted T-Cell Depletion. Transplant Infect Dis (2018) 20(1):e12823. doi: 10.1111/tid.12823
144. Mamcarz E, Madden R, Qudeimat A, Srinivasan A, Talleur A, Sharma A, et al. Improved Survival Rate in T-Cell Depleted Haploidentical Hematopoietic Cell Transplantation Over the Last 15 Years at a Single Institution. Bone Marrow Transplant (2020) 55(5):929–38. doi: 10.1038/s41409-019-0750-7
145. Bleakley M, FHUoWCC. Multi-Center Phase Ii Randomized Controlled Trial of Naïve T Cell Depletion for Prevention of Chronic Graft-Versus-Host Disease in Children and Young Adults. (2019). Available at : https://www.clinicaltrials.gov/ct2/show/NCT03779854.
146. Bleakley M, FHUoWCC. A Phase Ii Randomized Controlled Trial Comparing Gvhd-Reduction Strategies for Allogeneic Peripheral Blood Transplantation (Pbsct) for Patients With Acute Leukemia: Selective Depletion of Cd45ra+ Naïve T Cells (Tnd) Vs. Post-Transplantation Cyclophosphamide (Ptcy) Vs. Tacrolimus and Methotrexate (Tacmtx) Pbsct. (2019). Available at : https://www.clinicaltrials.gov/ct2/show/NCT03970096.
147. Anderson BE, Taylor PA, McNiff JM, Jain D, Demetris AJ, Panoskaltsis-Mortari A, et al. Effects of Donor T-Cell Trafficking and Priming Site on Graft-Versus-Host Disease Induction by Naive and Memory Phenotype Cd4 T Cells. Blood (2008) 111(10):5242–51. doi: 10.1182/blood-2007-09-107953
148. Chen L, Flies DB. Molecular Mechanisms of T Cell Co-Stimulation and Co-Inhibition. Nat Rev Immunol (2013) 13(4):227–42. doi: 10.1038/nri3405
149. Okazaki T, Chikuma S, Iwai Y, Fagarasan S, Honjo T. A Rheostat for Immune Responses: The Unique Properties of Pd-1 and Their Advantages for Clinical Application. Nat Immunol (2013) 14(12):1212–8. doi: 10.1038/ni.2762
150. Sharpe AH, Pauken KE. The Diverse Functions of the Pd1 Inhibitory Pathway. Nat Rev Immunol (2018) 18(3):153–67. doi: 10.1038/nri.2017.108
151. Butte MJ, Keir ME, Phamduy TB, Sharpe AH, Freeman GJ. Programmed Death-1 Ligand 1 Interacts Specifically With the B7-1 Costimulatory Molecule to Inhibit T Cell Responses. Immunity (2007) 27(1):111–22. doi: 10.1016/j.immuni.2007.05.016
152. Butte MJ, Peña-Cruz V, Kim M-J, Freeman GJ, Sharpe AH. Interaction of Human Pd-L1 and B7-1. Mol Immunol (2008) 45(13):3567–72. doi: 10.1016/j.molimm.2008.05.014
153. Cassady K, Martin PJ, Zeng D. Regulation of Gvhd and Gvl Activity Via Pd-L1 Interaction With Pd-1 and Cd80. Front Immunol (2018) 9:3061. doi: 10.3389/fimmu.2018.03061
154. Keir ME, Liang SC, Guleria I, Latchman YE, Qipo A, Albacker LA, et al. Tissue Expression of Pd-L1 Mediates Peripheral T Cell Tolerance. J Exp Med (2006) 203(4):883–95. doi: 10.1084/jem.20051776
155. Francisco LM, Salinas VH, Brown KE, Vanguri VK, Freeman GJ, Kuchroo VK, et al. Pd-L1 Regulates the Development, Maintenance, and Function of Induced Regulatory T Cells. J Exp Med (2009) 206(13):3015–29. doi: 10.1084/jem.20090847
156. Song Q, Wang X, Wu X, Qin H, Li Y, Riggs AD, et al. Tolerogenic Anti–Il-2 Mab Prevents Graft-Versus-Host Disease While Preserving Strong Graft-Versus-Leukemia Activity. Blood (2021) 137(16):2243–55. doi: 10.1182/blood.2020006345
157. Deng R, Cassady K, Li X, Yao S, Zhang M, Racine J, et al. B7h1/Cd80 Interaction Augments Pd-1-Dependent T Cell Apoptosis and Ameliorates Graft-Versus-Host Disease. J Immunol (2015) 194(2):560–74. doi: 10.4049/jimmunol.1402157
158. Ju XP, Xu B, Xiao ZP, Li JY, Chen L, Lu SQ, et al. Cytokine Expression During Acute Graft-Versus-Host Disease After Allogeneic Peripheral Stem Cell Transplantation. Bone Marrow Transplant (2005) 35(12):1179–86. doi: 10.1038/sj.bmt.1704972
159. Yi T, Zhao D, Lin CL, Zhang C, Chen Y, Todorov I, et al. Absence of Donor Th17 Leads to Augmented Th1 Differentiation and Exacerbated Acute Graft-Versus-Host Disease. Blood (2008) 112(5):2101–10. doi: 10.1182/blood-2007-12-126987
160. Na IK, Lu SX, Yim NL, Goldberg GL, Tsai J, Rao U, et al. The Cytolytic Molecules Fas Ligand and Trail Are Required for Murine Thymic Graft-Versus-Host Disease. J Clin Invest (2010) 120(1):343–56. doi: 10.1172/jci39395
161. Schmaltz C, Alpdogan O, Horndasch KJ, Muriglan SJ, Kappel BJ, Teshima T, et al. Differential Use of Fas Ligand and Perforin Cytotoxic Pathways by Donor T Cells in Graft-Versus-Host Disease and Graft-Versus-Leukemia Effect. Blood (2001) 97(9):2886–95. doi: 10.1182/blood.v97.9.2886
162. Hsieh MH, Korngold R. Differential Use of Fasl- and Perforin-Mediated Cytolytic Mechanisms by T-Cell Subsets Involved in Graft-Versus-Myeloid Leukemia Responses. Blood (2000) 96(3):1047–55. doi: 10.1182/blood.V96.3.1047.015k36_1047_1055
163. Ni X, Song Q, Cassady K, Deng R, Jin H, Zhang M, et al. Pd-L1 Interacts With Cd80 to Regulate Graft-Versus-Leukemia Activity of Donor Cd8+ T Cells. J Clin Invest (2017) 127(5):1960–77. doi: 10.1172/jci91138
164. Trotta E, Bessette PH, Silveria SL, Ely LK, Jude KM, Le DT, et al. A Human Anti-Il-2 Antibody That Potentiates Regulatory T Cells by a Structure-Based Mechanism. Nat Med (2018) 24(7):1005–14. doi: 10.1038/s41591-018-0070-2
165. Zeng D, Dick M, Cheng L, Amano M, Dejbakhsh-Jones S, Huie P, et al. Subsets of Transgenic T Cells That Recognize Cd1 Induce or Prevent Murine Lupus: Role of Cytokines. J Exp Med (1998) 187(4):525–36. doi: 10.1084/jem.187.4.525
166. Kong X, Zeng D, Wu X, Wang B, Yang S, Song Q, et al. Tissue-Resident Psgl1locd4+ T Cells Promote B Cell Differentiation and Chronic Graft-Versus-Host Disease–Associated Autoimmunity. J Clin Invest (2021) 131(1):e135468. doi: 10.1172/jci135468
167. Zhang Y, Hexner E, Frank D, Emerson SG. Cd4+T Cells Generated De Novo From Donor Hemopoietic Stem Cells Mediate the Evolution From Acute to Chronic Graft-Versus-Host Disease. J Immunol (2007) 179(5):3305–14. doi: 10.4049/jimmunol.179.5.3305
168. Song Q, Kong X, Martin PJ, Zeng D. Murine Models Provide New Insights Into Pathogenesis of Chronic Graft-Versus-Host Disease in Humans. Front Immunol (2021) 12:700857. doi: 10.3389/fimmu.2021.700857
169. Wu T, Young JS, Johnston H, Ni X, Deng R, Racine J, et al. Thymic Damage, Impaired Negative Selection, and Development of Chronic Graft-Versus-Host Disease Caused by Donor Cd4+ and Cd8+ T Cells. J Immunol (2013) 191(1):488–99. doi: 10.4049/jimmunol.1300657
170. Li X, Deng R, He W, Liu C, Wang M, Young J, et al. Loss of B7-H1 Expression by Recipient Parenchymal Cells Leads to Expansion of Infiltrating Donor Cd8+ T Cells and Persistence of Graft-Versus-Host Disease. J Immunol (2012) 188(2):724–34. doi: 10.4049/jimmunol.1102630
171. Auffermann-Gretzinger S, Lossos IS, Vayntrub TA, Leong W, Grumet FC, Blume KG, et al. Rapid Establishment of Dendritic Cell Chimerism in Allogeneic Hematopoietic Cell Transplant Recipients. Blood (2002) 99(4):1442–8. doi: 10.1182/blood.v99.4.1442
172. Saha A, O’Connor RS, Thangavelu G, Lovitch SB, Dandamudi DB, Wilson CB, et al. Programmed Death Ligand-1 Expression on Donor T Cells Drives Graft-Versus-Host Disease Lethality. J Clin Invest (2016) 126(7):2642–60. doi: 10.1172/jci85796
173. Fife BT, Pauken KE, Eagar TN, Obu T, Wu J, Tang Q, et al. Interactions Between Pd-1 and Pd-L1 Promote Tolerance by Blocking the Tcr-Induced Stop Signal. Nat Immunol (2009) 10(11):1185–92. doi: 10.1038/ni.1790
174. Cassady KM, Zhou J, Riggs A, Zeng D. Pd-L1/Cd80 and Pd-L1/Pd-1 Signaling Reciprocally Regulate Alloreactive Cd8+ T Cell Glycolysis, Proliferation, Apoptosis and Gvhd-Inducing Capacity. Blood (2015) 126(23):4282–. doi: 10.1182/blood.V126.23.4282.4282
175. Park J-J, Omiya R, Matsumura Y, Sakoda Y, Kuramasu A, Augustine MM, et al. B7-H1/Cd80 Interaction is Required for the Induction and Maintenance of Peripheral T-Cell Tolerance. Blood (2010) 116(8):1291–8. doi: 10.1182/blood-2010-01-265975
176. Zhao Y, Lee CK, Lin CH, Gassen RB, Xu X, Huang Z, et al. Pd-L1:Cd80 Cis-Heterodimer Triggers the Co-Stimulatory Receptor Cd28 While Repressing the Inhibitory Pd-1 and Ctla-4 Pathways. Immunity (2019) 51(6):1059–73. doi: 10.1016/j.immuni.2019.11.003
177. Sugiura D, Maruhashi T, Okazaki IM, Shimizu K, Maeda TK, Takemoto T, et al. Restriction of Pd-1 Function by Cis-Pd-L1/Cd80 Interactions Is Required for Optimal T Cell Responses. Science (2019) 364(6440):558–66. doi: 10.1126/science.aav7062
178. Zhang Y, Song Q, Lee M, Tang H, Cassady K, Fu Y-X, et al. Abstract 3180: Blockade of Pd-L1 Interaction With Cd80 in Trans Augments Anti-Tumor Immunity by Increasing Nos2 in Tumor-Associated Macrophages. Cancer Res (2021) 81(13_Supplement):3180–. doi: 10.1158/1538-7445.Am2021-3180
179. Boekstegers AM, Blaeschke F, Schmid I, Wiebking V, Immler S, Hoffmann F, et al. Mrd Response in a Refractory Paediatric T-All Patient Through Anti-Programmed Cell Death 1 (Pd-1) Ab Treatment Associated With Induction of Fatal Gvhd. Bone Marrow Transplant (2017) 52(8):1221–4. doi: 10.1038/bmt.2017.107
180. Yao S, Jianlin C, Zhuoqing Q, Yuhang L, Jiangwei H, Guoliang H, et al. Case Report: Combination Therapy With Pd-1 Blockade for Acute Myeloid Leukemia After Allogeneic Hematopoietic Stem Cell Transplantation Resulted in Fatal Gvhd. Front Immunol (2021) 12:639217. doi: 10.3389/fimmu.2021.639217
181. Haverkos BM, Abbott D, Hamadani M, Armand P, Flowers ME, Merryman R, et al. Pd-1 Blockade for Relapsed Lymphoma Post-Allogeneic Hematopoietic Cell Transplant: High Response Rate But Frequent Gvhd. Blood (2017) 130(2):221–8. doi: 10.1182/blood-2017-01-761346
182. Hsiao M, Tatishchev S, Khedro T, Yaghmour B, O’Connell C, Yaghmour G. First Report of Severe Acute Graft-Versus-Host Disease After Allogeneic Stem Cell Transplant in a Patient With Myelodysplastic Syndrome Treated With Atezolizumab: Literature Review. World J Oncol (2020) 11(3):112–5. doi: 10.14740/wjon1263
183. Schwartz DM, Bonelli M, Gadina M, O’Shea JJ. Type I/Ii Cytokines, Jaks, and New Strategies for Treating Autoimmune Diseases. Nat Rev Rheumatol (2016) 12(1):25–36. doi: 10.1038/nrrheum.2015.167
184. Choi J, Ziga ED, Ritchey J, Collins L, Prior JL, Cooper ML, et al. Ifnγr Signaling Mediates Alloreactive T-Cell Trafficking and Gvhd. Blood (2012) 120(19):4093–103. doi: 10.1182/blood-2012-01-403196
185. Spoerl S, Mathew NR, Bscheider M, Schmitt-Graeff A, Chen S, Mueller T, et al. Activity of Therapeutic Jak 1/2 Blockade in Graft-Versus-Host Disease. Blood (2014) 123(24):3832–42. doi: 10.1182/blood-2013-12-543736
186. Carniti C, Gimondi S, Vendramin A, Recordati C, Confalonieri D, Bermema A, et al. Pharmacologic Inhibition of Jak1/Jak2 Signaling Reduces Experimental Murine Acute Gvhd While Preserving Gvt Effects. Clin Cancer Res (2015) 21(16):3740–9. doi: 10.1158/1078-0432.ccr-14-2758
187. Okiyama N, Furumoto Y, Villarroel VA, Linton JT, Tsai WL, Gutermuth J, et al. Reversal of Cd8 T-Cell–Mediated Mucocutaneous Graft-Versus-Host-Like Disease by the Jak Inhibitor Tofacitinib. J Invest Dermatol (2014) 134(4):992–1000. doi: 10.1038/jid.2013.476
188. Cetkovic-Cvrlje M, Roers BA, Waurzyniak B, Liu X-P, Uckun FM. Targeting Janus Kinase 3 to Attenuate the Severity of Acute Graft-Versus-Host Disease Across the Major Histocompatibility Barrier in Mice. Blood (2001) 98(5):1607–13. doi: 10.1182/blood.V98.5.1607
189. Heine A, Held SAE, Daecke SN, Wallner S, Yajnanarayana SP, Kurts C, et al. The Jak-Inhibitor Ruxolitinib Impairs Dendritic Cell Function in Vitro and In Vivo. Blood (2013) 122(7):1192–202. doi: 10.1182/blood-2013-03-484642
190. Sugimoto N, Nakahira M, Ahn H-J, Micallef M, Hamaoka T, Kurimoto M, et al. Differential Requirements for Jak2 and Tyk2 in T Cell Proliferation and Ifn-Γ Production Induced by Il-12 Alone or Together With Il-18. Eur J Immunol (2003) 33(1):243–51. doi: 10.1002/immu.200390027
191. Wang S-P, Iwata S, Nakayamada S, Sakata K, Yamaoka K, Tanaka Y. Tofacitinib, a Jak Inhibitor, Inhibits Human B Cell Activation In Vitro. Ann Rheumatic Dis (2014) 73(12):2213–5. doi: 10.1136/annrheumdis-2014-205615
192. Choi J, Cooper ML, Staser K, Ashami K, Vij KR, Wang B, et al. Baricitinib-Induced Blockade of Interferon Gamma Receptor and Interleukin-6 Receptor for the Prevention and Treatment of Graft-Versus-Host Disease. Leukemia (2018) 32(11):2483–94. doi: 10.1038/s41375-018-0123-z
193. Courtois J, Ritacco C, Dubois S, Canti L, Vandenhove B, Seidel L, et al. Itacitinib Prevents Xenogeneic Gvhd in Humanized Mice. Bone Marrow Transplant (2021) 56(11):2672–81. doi: 10.1038/s41409-021-01363-1
194. Betts BC, Bastian D, Iamsawat S, Nguyen H, Heinrichs JL, Wu Y, et al. Targeting Jak2 Reduces Gvhd and Xenograft Rejection Through Regulation of T Cell Differentiation. Proc Natl Acad Sci (2018) 115(7):1582–7. doi: 10.1073/pnas.1712452115
195. Zeiser R, Burchert A, Lengerke C, Verbeek M, Maas-Bauer K, Metzelder SK, et al. Ruxolitinib in Corticosteroid-Refractory Graft-Versus-Host Disease After Allogeneic Stem Cell Transplantation: A Multicenter Survey. Leukemia (2015) 29(10):2062–8. doi: 10.1038/leu.2015.212
196. Schroeder MA, Khoury HJ, Jagasia M, Ali H, Schiller GJ, Staser K, et al. A Phase 1 Trial of Itacitinib, a Selective Jak1 Inhibitor, in Patients With Acute Graft-Versus-Host Disease. Blood Adv (2020) 4(8):1656–69. doi: 10.1182/bloodadvances.2019001043
197. Jagasia M, Perales M-A, Schroeder MA, Ali H, Shah NN, Chen Y-B, et al. Ruxolitinib for the Treatment of Steroid-Refractory Acute Gvhd (Reach1): A Multicenter, Open-Label Phase 2 Trial. Blood (2020) 135(20):1739–49. doi: 10.1182/blood.2020004823
198. Holtzman NG, Im A, Ostojic A, Curtis LM, Parsons-Wandell L, Nashed J, et al. Efficacy and Safety of Baricitinib in Refractory Chronic Graft-Versus-Host Disease (Cgvhd): Preliminary Analysis Results of a Phase 1/2 Study. Blood (2020) 136(Supplement 1):1. doi: 10.1182/blood-2020-140392
199. Zeiser R, Von Bubnoff N, Butler J, Mohty M, Niederwieser D, Or R, et al. Ruxolitinib for Glucocorticoid-Refractory Acute Graft-Versus-Host Disease. N Engl J Med (2020) 382(19):1800–10. doi: 10.1056/nejmoa1917635
200. Zeiser R, Polverelli N, Ram R, Hashmi SK, Chakraverty R, Middeke JM, et al. Ruxolitinib for Glucocorticoid-Refractory Chronic Graft-Versus-Host Disease. N Engl J Med (2021) 385(3):228–38. doi: 10.1056/nejmoa2033122
201. Zhao Y, Shi J, Luo Y, Gao F, Tan Y, Lai X, et al. Calcineurin Inhibitors Replacement by Ruxolitinib as Graft-Versus-Host Disease Prophylaxis for Patients After Allogeneic Stem Cell Transplantation. Biol Blood Marrow Transplant (2020) 26(5):e128–e33. doi: 10.1016/j.bbmt.2020.01.012
202. Choe H, Shah NN, Chevallier P, Rubio MT, Schroeder MA, Hardy NM, et al. A Single-Arm, Open-Label Phase 1 Study of Itacitinib (Ita) With Calcineurin Inhibitor (Cni)-Based Interventions for Prophylaxis of Graft-Versus-Host Disease (Gvhd; Gravitas-119). Blood (2020) 136(Supplement 1):50–1. doi: 10.1182/blood-2020-140747
Keywords: graft versus leukaemia (GVL), graft versus host disease (GVHD), naive T cell depletion, PD-L1, chemokine and chemokine receptors, anti-IL-2
Citation: Song Q, Nasri U, Nakamura R, Martin PJ and Zeng D (2022) Retention of Donor T Cells in Lymphohematopoietic Tissue and Augmentation of Tissue PD-L1 Protection for Prevention of GVHD While Preserving GVL Activity. Front. Immunol. 13:907673. doi: 10.3389/fimmu.2022.907673
Received: 30 March 2022; Accepted: 26 April 2022;
Published: 23 May 2022.
Edited by:
Xi Zhang, Xinqiao Hospital, ChinaReviewed by:
Ying-Jun Chang, Peking University People’s Hospital, ChinaYongxia Wu, Medical College of Wisconsin, United States
Xiaoyu Zhu, The First Affiliated Hospital of University of Science and Technology of China Anhui Provincial Hospital, China
Jishi Wang, Affiliated Hospital of Guizhou Medical University, China
Copyright © 2022 Song, Nasri, Nakamura, Martin and Zeng. This is an open-access article distributed under the terms of the Creative Commons Attribution License (CC BY). The use, distribution or reproduction in other forums is permitted, provided the original author(s) and the copyright owner(s) are credited and that the original publication in this journal is cited, in accordance with accepted academic practice. No use, distribution or reproduction is permitted which does not comply with these terms.
*Correspondence: Qingxiao Song, cWluZ3hpYW9AY29oLm9yZw==; Defu Zeng, ZHplbmdAY29oLm9yZw==