- 1Department of Pediatrics, The First Affiliated Hospital of University of Science and Technology of China (USTC), Division of Life Sciences and Medicine, University of Science and Technology of China, Hefei, China
- 2Institute of Immunology, The Chinese Academy of Sciences (CAS) Key Laboratory of Innate Immunity and Chronic Disease, School of Basic Medical Sciences, Division of Life Sciences and Medicine, University of Science and Technology of China, Hefei, China
Food allergy is a growing concern due to its increasing world-wide incidence. Strict avoidance of allergens is a passive treatment strategy. Since the mechanisms responsible for the occurrence and development of food allergy have not yet been fully elucidated, effective individualized treatment options are lacking. In this review, we summarize the pathways through which food antigens enter the intestine and review the proposed mechanisms describing how the intestine acquires and tolerates food antigens. When oral tolerance is not established, food allergy occurs. In addition, we also discuss the contribution of commensal bacteria of the gut in shaping tolerance to food antigens in the intestinal tract. Finally, we propose that elucidating the mechanisms of intestinal uptake and tolerance of food antigens will provide additional clues for potential treatment options for food allergy.
Introduction
Globally, there is an increasing incidence of food allergy that affects the quality of life of those affected (1–3). Food allergies have been identified at all ages. Food allergy affects approximately 6% of children and 3 to 4% of adults (4). Allergies to certain foods that start in childhood can persist into adulthood, and new allergies can occur at any stage of life (5). For example, 40 to 60% of fish or shellfish allergies begin in adulthood (6). Peanut allergy affects nearly 5 million adults in the United States, and about one in six individuals with peanut allergy experience their first episode in adulthood (7).
Although food allergy has a wide incidence and is considered a substantial public health burden, its diagnosis and treatment are still inadequate due to the limitations of research into its pathogenesis (8) and the understanding of the mechanisms triggering food allergies (9). First, there are no clear and uniform diagnostic criteria. In addition, most symptoms of food allergy are not typical, such as cough, diarrhea, abdominal pain, and vomiting, which are similar to the symptoms of many other clinical diseases and are often ignored or misdiagnosed (10). Second, because of the lack of understanding of the pathogenic mechanisms of food allergy, there is a lack of safe and effective treatment options for individuals diagnosed with food allergy. The only safe and effective method for patients with a food allergy diagnosis is to strictly avoid the allergic antigens (11, 12). However, there is widespread concern that a strict and limited diet in patients with food allergies can lead to nutritional deficiencies and growth failure in children (13). Recently, allergen-specific immunotherapy has also emerged, and involves the administration of allergic antigens orally, sublingually, or epicutaneously to induce immune tolerance to allergens. Although allergen-specific immunotherapy has made considerable progress in the treatment of food allergies, during the course of clinical treatment, allergen-specific immunotherapy has obvious limitations in efficacy, safety, and durability (14). Thus, an in-depth exploration of the pathogenic mechanism of food allergy is necessary to further improve and optimize treatment plans.
It is well-known that the primary role of the intestinal mucosa is to act as a barrier to prevent harmful substances from entering the digestive system. Furthermore, as a selective filter, the intestinal mucosa allows the necessary dietary nutrients, water, and electrolytes to be diverted from the lumen into the blood circulation of the intestine (15). Although considered as foreign antigens, food antigens are selectively filtered into the circulation by the intestinal mucosa without triggering a defense immune attack response in the intestines but induces immune tolerance. Nevertheless, the disruption of this tolerance mechanism will lead to food allergies. Herein, we focus on how food antigens pass through the intestinal barrier and how they are acquired and tolerated by the intestinal immune system. We also discuss situations in which food allergy occurs when the intestinal immune regulation is disturbed. Finally, by summarizing and discussing these studies on food allergy, we hope to provide more clues to stimulate fundamental research and clinical applications in the field of food allergy.
How Do Food Antigens Cross the Intestinal Barrier?
Structurally, the intestinal barrier can be divided into three layers. The outer layer is the mucus layer that is symbiotic with intestinal microorganisms, the central layer is a specialized single cell layer consisting of epithelial cells, and the inner layer is the lamina propria (LP) composed of innate and adoptive immune cells. The mucus layer has a Sieve-like structure, among which mucins secreted by goblet/mucinous cells cover the intestinal epithelium (16). Mucins secreted in the mucus layer can protect intestinal epithelial cells from digestive enzymes and function as a defense barrier to prevent the invasion of foreign microorganisms (17–19). The central layer is made up of intestinal epithelial cells that are considered important components of the intestinal defense system and play a key role in the transport of substances into the intestinal tract. A variety of epithelial cell subsets, including absorptive enterocytes, goblet cells, Paneth cells (20, 21), tuft cells (22), enteroendocrine cells (23), microfold cells (M cells), and epithelial stem cells, have unique and specialized characteristics and functions, which cooperatively form a sophisticated epithelial layer against numerous antigens in the lumen (24). A set of highly organized intercellular junction complexes links these intestinal epithelial cells to form intestinal paracellular barriers. These junction complexes are in dynamic balance and are divided into three types: tight junctions (TJs), adherens junctions (AJs), and desmosomes (25). Paracellular barriers composed of these junction complexes function to maintain the integrity of the intestine and also mediate the regulation of nutrients that pass through the intestine through the paracellular pathway. Beyond the mucus layer and the epithelial layer, is the LP, which contains both innate and adaptive immune cells, such as dendritic cells (DCs), macrophages, T cells, and B cells. Immune cells in the LP are involved in both immune defense and immune regulation in the intestinal microenvironment (26). The intestinal barrier composed of these three layers can effectively prevent harmful substances from entering the body. However, this barrier is not completely impenetrable. The intestinal barrier allows foreign nutrients to enter the body to meet growth needs. We will first summarize the pathways through which nutrients from ingested food pass through the intestine.
Food is digested into peptides, amino acids, polysaccharides, monosaccharides, water, electrolytes, and other nutrients through chemical and mechanical activity in the digestive tract. These nutrients, including food antigens, enter the subepithelium through two main pathways, the paracellular pathway and the transcellular pathway.
Paracellular Pathway
As mentioned above, the intercellular junction complex located in intestinal epithelial cells is the main mediator that regulates the paracellular pathway. Among the three types of epithelia junction complexes, TJs are composed of transmembrane proteins (27), which interact with each other and with the intestinal immune system, making them the main rate-limiting step in controlling the permeability of the paracellular pathway (28, 29). The TJ proteins, such as zonula occludens (ZO) (30–32), occludins (33), and claudins (34) participate in the formation of TJs and control the permeability of paracellular pathways. Small molecules derived from food nutrients, such as solutes soluble in water, cross the intestinal barrier through paracellular pathways (Figure 1-(1)). Paracellular pathways have the selectivity of capacity, charge, and size for the substances they transport. This pathway is highly regulated by TJs to ensure that the transport of materials across the epithelial barrier is strictly controlled. However, when the epithelial barrier is damaged, such as following destruction of TJs, the paracellular pathway becomes nonrestrictive and allows the free passage of ions, water, macromolecules, and even bacteria or viruses. This increases the intestinal permeability and leads to pathological changes (Figure 1-(2)). For example, food cysteine proteases degrade the TJ protein occludin, thus increasing the permeability in the paracellular pathway, which can contribute to the sensitization process of food allergies (35). Furthermore, the paracellular pathway is disrupted in patients with inflammatory bowel disease (IBD) due to the reduced expression of TJs and AJs proteins in epithelial cells in the inflammatory zone, such as ZO-1, claudin, and E-cadherin (36). Thus, the paracellular pathway plays an indispensable role in mediating materials into the intestinal subepithelium, and this process is controlled by TJs between epithelial cells. Furthermore, damage to TJs disrupts the paracellular pathway, which leads to increased intestinal permeability and intestinal disorders such as food allergies and IBD.
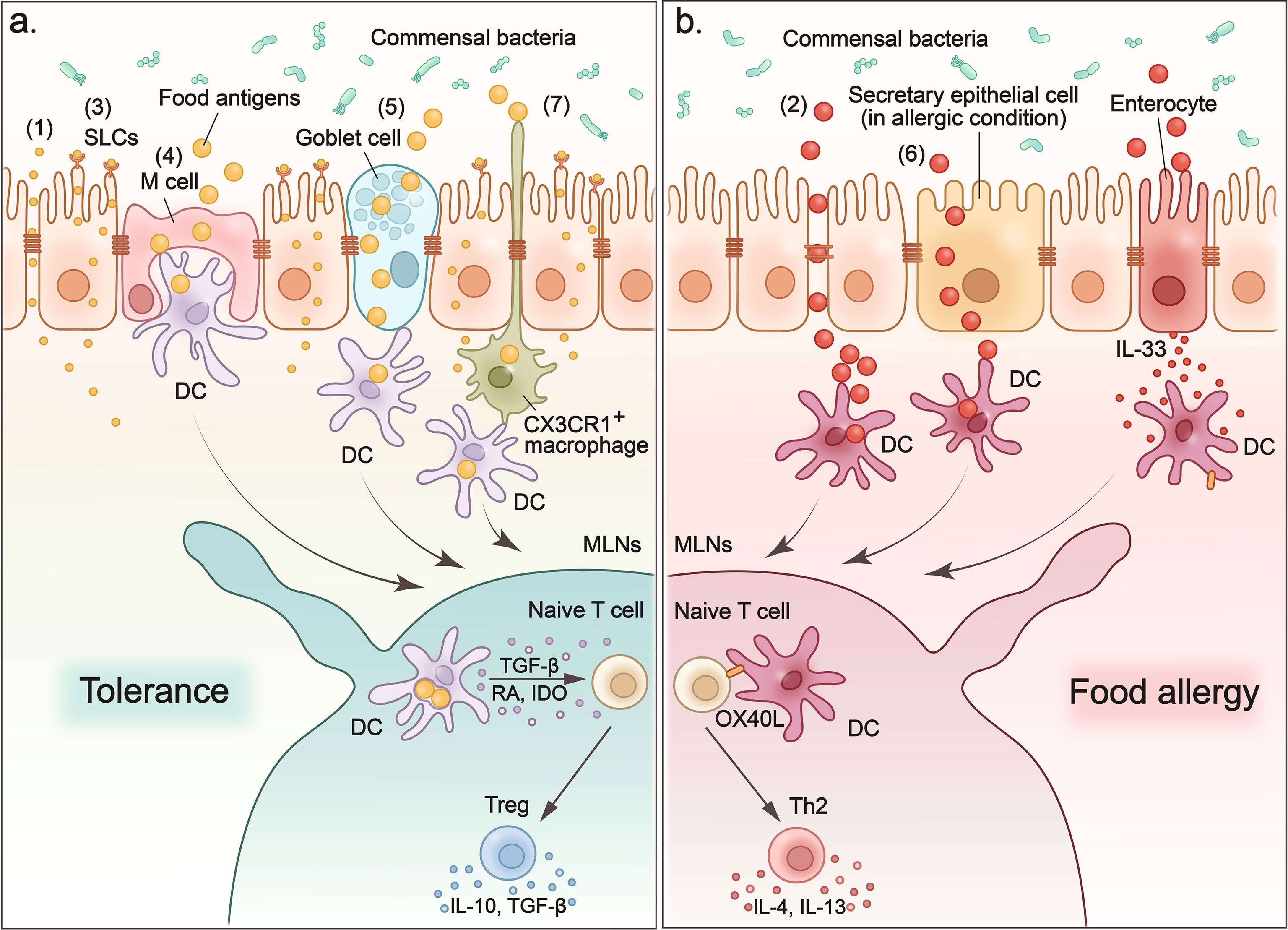
Figure 1 Food antigens cross the intestinal epithelium to induce tolerance or provoke allergy. (1) Small molecules such as electrolytes and water enter the sub-epithelium via paracellular pathways. (2) Degradation of tight junctions increases the permeability of the paracellular pathway, which leads to non-selective and uncontrolled entry of macromolecules into the intestinal sub-epithelium and contributes to the sensitization process of food allergies. (3) Small molecules such as amino acids and glucose that are transported across the epithelium by apical brush boundary transporters such as SLCs on enterocytes. (4) M cells sample food antigens, and then present them to dendritic cells. (5) Goblet cells form goblet-cell-associated antigen passage (GAP) to enclose luminal antigens (food antigens and microbial antigens) in internal sack-like vesicles, and then transport them across entire cells to dendritic cells. (6) In food allergy mouse models, secretory epithelial cells function as conduits to allow the transport of food antigens through the epithelium of the small intestine and induce a food-induced anaphylactic reaction. (7) CX3CR1+ macrophages rely on CX3CR1 expression to form dendrites to efficiently sample antigens from the intestinal lumen without disrupting the tight junctions between the cells. CX3CR1+ macrophages transfer antigens they captured to CD103+ DC. (A) When food antigens enter the intestinal sub-epithelium through above pathways, they are acquired and processed by dendritic cells. Then DCs migrate to MLNs where they present the processed food antigens and induce tolerance or food allergy. To induce oral tolerance, DCs secrete interleukin-10 (IL-10), transforming growth factor-β (TGF-β), and indoleamine 2,3-dioxygenase (IDO) to induce Tregs and promote their production of IL-10 and TGF-β to maintain tolerance. (B) In the case of allergy, oral antigens and adjuvants stimulate the expression of IL-33 in intestinal epithelial cells, which in turn upregulates the expression of the costimulatory molecule-OX40L on DCs. OX40L expression upregulates DCs to promote the TH2 response in the MLN and leads to more severe food allergies.
Transcellular Pathway
Enterocytes are the main type of intestinal epithelial cells and differ greatly in structure and composition between the small intestine and the colon. In the small intestine, enterocytes have villi that protrude into the lumen. The villi increase the surface area of the intestinal mucosa for better absorption of nutrients. These cells concentrate digestive enzymes (such as pancreatic proteolytic enzymes) on their apical surface, which are involved in the chemical digestion of lipids, carbohydrates and proteins, and absorb these digested nutrients through the apical brush boundary transporters (such as the SLC1A, SLC6A, and SLC7A families) (37). As we mentioned, small molecules such as water (38) and ions (39) can enter the intestinal subepithelium via paracellular pathways. They can also enter the intestinal cytoplasm from the apical membrane through epithelial transporters (integral membrane protein pumps or channels) and are discharged from the basolateral membrane (Figure 1-(3)). For example, the sodium-dependent transport of glucose (40), alanine (41), and glutamine (42) occurs on the surface of intestinal epithelial cells. However, macromolecules can only enter the cell through vesicles, which are formed by invagination and extrusion of the apical membrane. The vesicle transfer process is called endocytosis (43), which in intestinal cells is limited to pinocytosis (44). A variety of intestinal epithelial cells are involved in these intestinal epithelial-mediated transcellular pathways. Among them, M cells and goblet cells play a pivotal role in internalizing luminal antigens into gut-associated lymphoid tissues (GALTs) and in establishing intestinal tolerance or inducing intestinal immune responses to food antigens. They indiscriminately sample lumen contents, including food antigens, and transport the intact antigens to intestinal DCs to process and present these antigens (37).
M cells are specialized epithelial cells located in the follicle-associated epithelium overlying Peyer’s patches (PP) (45, 46). Their location facilitates M cells to transcytose a wide range of substances, such as food antigens and microbes, to underlying DCs for antigen processing and presentation (47) (Figure 1-(4)). In addition, M cells have specialized structures that lack the typical brushlike border and have thinner calyx glycosomes, making it easier to capture large particle antigens and transcytose them by pinocytosis in the fluid phase and by receptor-mediated endocytosis (48). Besides the delivery of intact antigens into the underlying lymphoid tissue of the GALT (49, 50), M cells also participate in Ag processing and presentation based on the observation that GALT M cells express MHC class II molecules and acidic endosomal-lysosomal compartments (51). Suzuki et al. developed an M cell-targeting Ag delivery system by combining antigens OVA with pσ1 protein that is known to bind to M cells in gut and nasal-associated lymphoid tissues (NALT) to investigate the role of M cells in oral tolerance (47, 52, 53). Using this M cell-targeting antigen delivery system, the authors determined that the recombinant protein OVA-pσ1 can induce mucosal unresponsiveness through two main mechanisms: clonal deletion of Ag-specific CD4+ T cells and the induction of acquired type Tregs cells (47). However, additional studies have also reported that oral tolerance could be established even in the absence of PP or the destruction of M cells to facilitate antigen transport to the PP (54, 55). Thus, the role of M cells in the induction of oral tolerance by transporting Ags from the lumen is controversial and further studies of the mechanism are needed.
Goblet cells are specialized mucous epithelial secretory cells. The secretory products of goblet cells, including mucins, trefoil factors, and other proteins, are essential for the integrity of the intestinal barrier and the prevention against the entry of harmful antigens (56). It should be noted that another important role of goblet cells is the formation of a goblet cell-associated antigen passage (GAP) to transport luminal antigens (food antigens and microbial antigens) to antigen-presenting cells (APC) in the LP (57, 58) (Figure 1-(5)). Preventing the entry of harmful antigens through mucus secretion and sampling luminal substances into the intestinal immune system through GAP formation are two divergent processes for goblet cells. Molecular mechanisms have revealed that a neurotransmitter called acetylcholine can trigger both mucus release and the GAP process in goblet cells in independent signaling pathways mediated by different receptors (59). This regulation of Ach allows goblet cells to accommodate the dynamically changing demands of the mucosal environment. Goblet cells can also deliver luminal substances, including food antigens and microbial antigens, and induce intestinal tolerance through the GAP process (60). First, goblet cells capture food antigens from the lumen, enclose them in internal sack-like vesicles, and then transport them across the entire cell. Then the APCs in the LP acquire luminal antigens to induce intestinal tolerance by maintaining pre-existing Tregs in the LP, and imprinting tolerogenic properties (60). However, Noah et al. reported that in food allergic mice, secretory epithelial cells, including goblet cells, enteroendocrine cells, and Paneth cells in the small intestine function as conduits to allow the transport of food antigens through the epithelium of the small intestine to the underlying immune cells and induce a food-induced anaphylactic reaction (61) (Figure 1-(6)). They also found that these secretory epithelial cell antigen passages (SAP) were induced by the Th2 cytokine-IL-13 in a CD38/cADPR-dependent manner (61). Additionally, blockade of this process reduced the passage of food antigens through the epithelium of the small intestine and alleviated the induction of the food allergic reaction in the intestine (61). Finally, they confirmed that SAP formation driven by IL-13 through the PI3K/CD38/cADPR pathway is conserved in the human intestine, indicating that blockade of this process, such as by inhibiting Th2 cytokines, might represent a potential therapeutic option for food allergy.
How Are Food Antigens Acquired and Tolerated By The Intestinal Immune System?
When food antigens enter the intestinal sub-epithelium through the above pathways, they are acquired and processed by APCs dispersed in the LP, PPs, and mesenteric lymph nodes (MLN). Oral tolerance to food antigens is often induced in MLNs (55) (Figure 1A). When the underlying APCs acquire the food antigens, they process them and present them to immune regulatory cells such as Treg cells in the MLN and induce tolerance (62).
Food Antigen Capture and Oral Tolerance Induction in the Intestine
As we mentioned above, food antigens can be internalized by M cells or acquired by goblet cell-associated passages. When the food antigens are captured by these specialized intestinal epithelial cells, they will be transferred to the migratory DCs in the intestinal PP (where the M cells deliver antigens to underlying DCs) (63) and LP (where the GAPs transport antigens to DCs) (57).
DCs and macrophages are the two main APCs for food antigens (64, 65). Rescigno et al. observed that DCs can penetrate the monolayers of the intestinal epithelium into the gut lumen by extending the transepithelial dendrites (66). This property provides DCs with access to antigens in the intestinal lumen. CX3CR1+ macrophages have also been reported to rely on CX3CR1 expression to form dendrites to efficiently sample antigens from the intestinal lumen (67). These antigen uptake macrophages quickly transfer food antigens to CD103+ DCs via connexin 43 in the gap junctions (67) (Figure 1-(7)).
In general, food antigens are collected directly by intestinal DCs or by CX3CR1+ macrophages or other epithelial cells (such as the M cells and goblet cells described earlier) and are delivered to DCs (68).
When loaded with food antigens, DCs migrate to the MLNs where they present the processed food antigens and induce tolerance (Figure 1A). Among various subsets of DCs, CD103+ DCs have been reported to play an important role in tolerance induction (67). CD103+ DCs are derived from circulating monocytes that express the gut homing marker-α4β7 integrin (69). They are located in the LP of the small and large intestine. When they acquire food antigens from the lumen, they migrate to MLNs to induce oral tolerance by activating Tregs. There are several mechanisms through which CD103+ DC induce Tregs. For example, intestinal CD103+ DCs have been reported to secrete retinoic acid (RA) and transforming growth factor-β (TGF-β) to promote the differentiation of Foxp3+Treg cells (70, 71). CD103+ DC also express indoleamine 2,3-dioxygenase (IDO), to sustain and differentiate Tregs, while inhibition of IDO in vivo has been reported to reduce Tregs specific to orally administered antigens and to impair the induction of oral tolerance (72).
Besides CD103+ DC, resident intestinal macrophages marked with high expression of the CX3C-chemokine receptor 1 (CX3CR1) also help to maintain Foxp3 expression in Tregs in the intestine by secreting IL-10 (73, 74). These intestinal resident macrophages have been proposed to provide additional survival signals for Tregs since they express high level of MHC class II, which enables them to undergo cognate interactions with specific Treg cells (75).
In addition to Treg-mediated oral tolerance, T cell clone anergy and/or deletion are also involved in oral tolerance (76, 77). The modality of oral tolerance induction depends on the dose of food antigens (77). Low dose of antigens induce Treg mediated immune suppression, while high doses of antigens lead to anergy and deletion of antigen-specific T cells (76–78).
Intestinal Commensal Bacteria Help Establish Oral Tolerance
Intestinal commensal bacteria also provide a large number of non-self-antigens that are tolerated by the intestinal immune system (73, 79, 80). Evidence indicates that the intestinal microbiota is crucial for the development and maturation of the intestinal immune system (81, 82). In particular, intestinal commensal bacteria could help shape intestinal tolerance. In germ-free (GF) mice, the frequency of Tregs and the levels of the anti-inflammatory cytokine IL-10 produced by Tregs are markedly reduced compared to mice free of specific pathogens (83–86). In one study, 17 strains of bacteria from the human gut microbiota were identified as Treg-cell-inducing bacterial strains. Treatment of these 17 Treg cell-inducing strains could alleviate intestinal inflammation, including allergic diarrhea (87). Furthermore, the observation that food allergen sensitization is enhanced in GF mice or mice that have been treated with antibiotics suggests that commensal bacteria are essential for the establishment of oral tolerance (88). Although the mechanisms by which the intestinal microbiota regulate allergic responses to food are not yet fully defined, studies have revealed that the composition of the gut microbiota, metabolites derived from intestinal bacteria and colonization of special functional bacteria are important factors that influence intestinal tolerance to food antigens.
Mechanistically, intestinal bacteria-derived metabolites, including inosine and short-chain fatty acids (SCFAs), are considered key factors promoting Treg differentiation and improve the production of regulatory cytokines such as IL-10 (89–91). In particular, SCFAs, which are produced during the bacterial fermentation of indigestible dietary fiber, have received much attention for their immunoregulatory activity. Butyrate, one of the most abundant SCFAs in the gut, has been reported to induce functional colonic Treg cells through its function to enhance histone H3 acetylation in the promoter and conserved non-coding sequence regions of the Foxp3 locus (92). In addition, it has been reported that SCFAs, particularly acetate and butyrate, could help establish oral tolerance and prevent food allergy by enhancing retinaldehyde dehydrogenase-2 (RALDH2) activity in CD103+ DC (93). RALDH2 converts vitamin A to retinoic acid, which promotes the differentiation of naive T cells into Treg cells and contributes to the establishment of oral tolerance (93, 94).
Stefka et al. reported that colonization of a Clostridia-containing microbiota can protect against sensitization to food allergens (88). Colonization of Clostridia induced early production of IL-22 by RORγt+ innate lymphoid cells (ILCs) and T cells in the intestine. This Clostridia-Induced IL-22 reduced the access of food allergen to the circulation (88).
In addition, dysbiosis of the gut microbiota leads to intolerance in the intestine. When the gut microbiota of infants allergic to milk protein was transplanted into GF mice, these recipient mice also showed an allergic response to milk-allergens (95).
Pathogenesis and Treatment of Food Allergy
The most important role of intestinal immune system is to distinguish innocuous food antigens and commensal microbes from pathogens. They initiate an immune response against pathogens and induce tolerance to food and commensal bacteria. However, a breakdown of the default oral tolerance to food leads to abnormal immune responses and results in food allergy (Figure 1B). Many factors including the genetic background, alteration of gut microbiota, food allergenicity and methods of food processing, may trigger a food allergy.
As we mentioned, the intestinal APCs, especially intestinal DCs play a pivotal role in the induction of tolerance. However, stimuli from food components or extrinsic adjuvants could activate DCs to trigger a food allergy. Although the detailed identification of stimuli and their reorganization are not very clear, it has been reported that glycoproteins from the allergenic foods could directly bind to C-type lectin receptors (CLR) on DCs to stimulate immune response to the food allergens (96). For instance, in the peanut induced allergy, the glycoprotein Ara h 1 was identified as the major peanut allergen able to bind to DC specific intercellular adhesion molecule-3-grabbing non-integrin (DC-SIGN), a C-type lectin receptor, on monocyte-derived DCs and subsequently activate DCs to induce allergic immune responses (97). Similarly, hazelnuts, walnuts, and egg whites have also been found to bind to DC-SIGN and related DC-SIGNR to activate DCs and contribute to the development of food allergies (98, 99).
In addition, alarmins such as IL-25, IL-33, and TSLP are also involved in the development of food allergies (100, 101). Among these allergenic alarmins, intestinal epithelial cell-derived IL-33 has been reported to act on different immune cells to expand the allergenic immune response in the intestine. In an allergenic mouse model, oral Ags and adjuvants stimulate the expression of IL-33 in intestinal epithelial cells, which in turn up-regulate the expression of the costimulatory molecule-OX40L in DCs. These DCs expressing upregulated OX40L promote the Th2 response in the MLN and lead to more severe food allergies (102, 103) (Figure 1B). In another study, IL-33 secreted by intestinal epithelial cells was found to act on type 2 ILC (ILC2) to enhance their expansion and induce their production of IL-4 (104). The IL-33 signal-stimulated production of IL-4 by ILC2 is indispensable for oral allergic sensitization and anaphylaxis (104, 105). Furthermore, IL-33 acts directly on mast cells to potentiate antigen-driven IgE-dependent degranulation of MC and promotes oral anaphylaxis after epicutaneous sensitization (106).
Based on current understanding of the mechanisms of oral tolerance and food allergy, there have been significant advances in treatment to food allergy, such as allergen specific immunotherapy, vaccines, and non-allergen specific therapies, which provide viable options for patients with food allergies.
For allergen specific immunotherapy, patients with food allergies are treated with their specific allergens to establish the tolerance to these allergens. This process is called desensitization. There are various approaches to treating patients with allergens including oral immunotherapy (OIT), sublingual immunotherapy (SLIT), and epicutaneous immunotherapy (EPIT). Specifically, patients with allergies are treated with their allergens in increasing amounts each time until a maintenance dose is reached, and then this dose is given periodically to patients (107). Compared to other allergen-specific immunotherapies, OIT has a higher efficiency, but also has a higher risk of systemic side effects, which may even require therapeutic intervention. Clinical trials have shown that OIT directed at milk, eggs, peanuts, and wheat allergens is therapeutically effective; however, OIT directed at these allergens generally caused significant adverse effects when the dose is increased (108–110). In addition to concerns about safety, there are many factors that limit the application of allergen-specific immunotherapy. There is a lack of standardization of clinical treatment, including the type of allergen used in the treatment, the administration method, the given dose, and frequency (111, 112). Further research is needed to promote and apply allergen-specific immunotherapy in the clinical treatment of food allergies.
Since the allergenic activity of natural allergen extracts is the most concerning side effect of allergen-specific immunotherapy, and broadly limits its applicability. However, recombinant allergens with genetic modifications that can reduce allergenic activity are produced to improve the safety of the immunotherapies (113). Clinical therapy trials suggest that recombinant allergens are effective for subcutaneous immunotherapy (114–116). Based on the promising results of these clinical trials, the first recombinant allergen-based vaccines will soon be registered and available for routine clinical use in patients with allergies.
Except for allergen-specific immunotherapy, nonallergen-specific therapies for food allergies have been developed, including immune antibody therapy and prebiotics treatment. As an immune antibody therapy, omalizumab has been tested in clinical trials as a monoclonal antibody against immunoglobulin E (IgE) (117). The combination of omalizumab and OIT has achieved promising results for the treatment of food allergies (118). In the future, with an in-depth understanding of the mechanism of food allergy and the identification of therapeutic targets, more targeted antibodies, such as antibodies against Th2 cytokines, will be developed and used in the treatment of food allergy.
Since intestinal microbiosis contributes significantly to allergic states in the intestine, the manipulation of intestinal microbes holds promise for the treatment of food allergy. Preclinical evidence has shown that prebiotics have a positive effect on remission of food allergy. For example, dietary supplementation with fructo-oligosaccharides, an immunomodulatory prebiotic, significantly improved allergic intestinal inflammation in OVA23-3 TCR-transgenic mice fed with an OVA-containing diet (119). Supplements consisting of Lactobacillus paracasei L9 reduced allergic responses in mice allergic to β-lactoglobulin (120). Oral administration of Lactobacillus murinus restored the deterioration of the intestinal flora in food-allergic mice and alleviated allergic reactions (121). Although prebiotic and probiotic trials are promising in food allergy treatment, there is currently no solid evidence to support the preventive or therapeutic effects of prebiotics and probiotics in relation to clinical food allergies. Therefore, future studies should uncover more specific details and mechanisms for the treatment of food allergies, while optimal functional probiotic strains should be selected and isolated for this approach.
Conclusion
The incidence of food allergy worldwide has increased progressively. When food allergies are diagnosed, there are limited treatment options for patients. Strictly avoiding allergens is one of the few safe and effective treatments in clinical application. However, such treatment is considered as a passive option with significant shortcomings. The limitations of clinical treatments for food allergies are largely attributable to unclear disease mechanisms. In recent years, significant progress has been made in elucidating the mechanisms involved in food antigen uptake and oral tolerance induction in the intestine. In this review, we summarized the pathways in which food antigens cross the intestinal epithelium and the processes through which they are transferred to the sub-epithelial compartment to induce tolerance or to provoke allergic reactions in the intestine. However, more mechanistic details need to be explored regarding processes associated with the promotion of clinical manifestations associated with food antigen-triggered anaphylaxis. More importantly, promising clinical strategies have been proposed, such as allergen-specific immunotherapy, vaccines, and non-allergen-specific therapies, which may provide additional viable options for patients with food allergies.
Author Contributions
HM and YX wrote the manuscript. GX provided important advice and suggestions. HM and MC supervised the writing. All authors contributed to the article and approved the submitted version.
Funding
This work was supported by the National Natural Science Foundation of China (#82171783, # 81871284).
Conflict of Interest
The authors declare that the research was conducted in the absence of any commercial or financial relationships that could be construed as a potential conflict of interest.
Publisher’s Note
All claims expressed in this article are solely those of the authors and do not necessarily represent those of their affiliated organizations, or those of the publisher, the editors and the reviewers. Any product that may be evaluated in this article, or claim that may be made by its manufacturer, is not guaranteed or endorsed by the publisher.
References
1. Dunlop JH, Keet CA. Epidemiology of Food Allergy. Immunol Allergy Clinics North Am (2018) 38(1):13. doi: 10.1016/j.iac.2017.09.002
2. Garkaby J, Epov L, Musallam N, Almog M, Bamberger E, Mandelberg A, et al. The Sesame-Peanut Conundrum in Israel: Reevaluation of Food Allergy Prevalence in Young Children. J Allergy Clin Immunol Pract (2021) 9(1):200–5. doi: 10.1016/j.jaip.2020.08.010
3. Vale LS, Lobb M, Netting JM, Murray K, Clifford R, Campbell DE, et al. A Systematic Review of Infant Feeding Food Allergy Prevention Guidelines-can We AGREE? World Allergy Organ J (2021) 14(6):100550. doi: 10.1016/j.waojou.2021.100550
4. Gunes Bayir A, Can B, Ekingen S. Food Allergy in Children. Bezmialem Sci (2021) 9(3):373–9. doi: 10.14235/bas.galenos.2020.4097
5. Mandavinia M. Food Allergy in Adults Presentations, Evaluation, and Treatment. Med Clinics North Am (2020) 104(1):145–55. doi: 10.1016/j.mcna.2019.08.008
6. Sicherer SH, Munoz-Furlong A, Sampson HA. Prevalence of Seafood Allergy in the United States Determined by a Random Telephone Survey. J Allergy Clin Immunol (2004) 114(1):159–65. doi: 10.1016/j.jaci.2004.04.018
7. Warren C, Lei D, Sicherer S, Schleimer R, Gupta R. Prevalence and Characteristics of Peanut Allergy in US Adults. J Allergy Clin Immunol (2021) 147(6):2263–70.e5. doi: 10.1016/j.jaci.2020.11.046
8. Suaini NHA, Wang YC, Soriano VX, Martino DJ, Allen KJ, Ellis JA, et al. Genetic Determinants of Paediatric Food Allergy: A Systematic Review. Allergy (2019) 74(9):1631–48. doi: 10.1111/all.13767
9. Luke AK, Flessner CA. Examining Differences in Parent Knowledge About Pediatric Food Allergies. J Pediatr Psychol (2020) 45(1):101–9. doi: 10.1093/jpepsy/jsz091
10. Ukleja-Sokolowska N, Kuzminski A, Tykwinska M, Bartuzi Z. In Vivo Studies in the Diagnosis of Food Allergy - Selected Practical Aspects. Alerg Astma Immunol (2021) 26(1):10–7.
11. Michelet M, Balbino B, Guilleminault L, Reber LL. IgE in the Pathophysiology and Therapy of Food Allergy. Eur J Immunol (2021) 51(3):531–43. doi: 10.1002/eji.202048833
12. Mayorga C, Palomares F, Canas JA, Perez-Sanchez N, Nunez R, Torres MJ, et al. New Insights in Therapy for Food Allergy. Foods (2021) 10(5):1037. doi: 10.3390/foods10051037
13. Pavic I, Kolacek S. Growth of Children With Food Allergy. Horm Res Paediatr (2017) 88(1):91–100. doi: 10.1159/000462973
14. Nicolaides RE, Parrish CP, Bird JA. Food Allergy Immunotherapy With Adjuvants. Immunol Allergy Clinics North Am (2020) 40(1):149–73. doi: 10.1016/j.iac.2019.09.004
15. Groschwitz KR, Hogan SP. Intestinal Barrier Function: Molecular Regulation and Disease Pathogenesis. J Allergy Clin Immunol (2009) 124(1):3–20. doi: 10.1016/j.jaci.2009.05.038
16. Bansil R, Turner BS. The Biology of Mucus: Composition, Synthesis and Organization. Adv Drug Deliv Rev (2018) 124:3–15. doi: 10.1016/j.addr.2017.09.023
17. Johansson MEV, Sjovall H, Hansson GC. The Gastrointestinal Mucus System in Health and Disease. Nat Rev Gastroenterol Hepatol (2013) 10(6):352–61. doi: 10.1038/nrgastro.2013.35
18. Johansson MEV, Hansson GC. Immunological Aspects of Intestinal Mucus and Mucins. Nat Rev Immunol (2016) 16(10):639–49. doi: 10.1038/nri.2016.88
19. Schroeder BO. Fight Them or Feed Them: How the Intestinal Mucus Layer Manages the Gut Microbiota. Gastroenterol Rep (Oxf) (2019) 7(1):3–12. doi: 10.1093/gastro/goy052
20. Bevins CL, Salzman NH. Paneth Cells, Antimicrobial Peptides and Maintenance of Intestinal Homeostasis. Nat Rev Microbiol (2011) 9(5):356–68. doi: 10.1038/nrmicro2546
21. Nakamura K, Sakuragi N, Takakuwa A, Ayabe T. Paneth Cell Alpha-Defensins and Enteric Microbiota in Health and Disease. Biosci Microb Food Health (2016) 35(2):57–67. doi: 10.12938/bmfh.2015-019
22. Gerbe F, Jay P. Intestinal Tuft Cells: Epithelial Sentinels Linking Luminal Cues to the Immune System. Mucosal Immunol (2016) 9(6):1353–9. doi: 10.1038/mi.2016.68
23. Worthington JJ. The Intestinal Immunoendocrine Axis: Novel Cross-Talk Between Enteroendocrine Cells and the Immune System During Infection and Inflammatory Disease. Biochem Soc Trans (2015) 43:727–33. doi: 10.1042/BST20150090
24. Salim SY, Soderholm JD. Importance of Disrupted Intestinal Barrier in Inflammatory Bowel Diseases. Inflamm Bowel Dis (2011) 17(1):362–81. doi: 10.1002/ibd.21403
25. Turner JR. Intestinal Mucosal Barrier Function in Health and Disease. Nat Rev Immunol (2009) 9(11):799–809. doi: 10.1038/nri2653
26. Vancamelbeke M, Vermeire S. The Intestinal Barrier: A Fundamental Role in Health and Disease. Expert Rev Gastroenterol Hepatol (2017) 11(9):821–34. doi: 10.1080/17474124.2017.1343143
27. Van Itallie CM, Anderson JM. Architecture of Tight Junctions and Principles of Molecular Composition. Semin Cell Dev Biol (2014) 36:157–65. doi: 10.1016/j.semcdb.2014.08.011
28. Lee B, Moon KM, Kim CY. Tight Junction in the Intestinal Epithelium: Its Association With Diseases and Regulation by Phytochemicals. J Immunol Res (2018) 2018:2645465. doi: 10.1155/2018/2645465
29. Zuo L, Kuo WT, Turner JR. Tight Junctions as Targets and Effectors of Mucosal Immune Homeostasis. Cell Mol Gastroenterol Hepatol (2020) 10(2):327–40. doi: 10.1016/j.jcmgh.2020.04.001
30. Stevenson BR, Siliciano JD, Mooseker MS, Goodenough DA. Identification OF ZO-1: A High-Molecular-Weight Polypeptide Associated With the Tight Junction (Zonula Occludens) in a Variety of Epithelia. J Cell Biol (1986) 103(3):755–66. doi: 10.1083/jcb.103.3.755
31. Jesaitis LA, Goodenough DA. Molecular Characterization and Tissue Distribution of Zo-2, a Tight Junction Protein Homologous to Zo-1 and the Drosophila Disks-Large Tumor-Suppressor Protein. J Cell Biol (1994) 124(6):949–61. doi: 10.1083/jcb.124.6.949
32. Haskins J, Gu LJ, Wittchen ES, Hibbard J, Stevenson BR. ZO-3, a Novel Member of the MAGUK Protein Family Found at the Tight Junction, Interacts With ZO-1 and Occludin. J Cell Biol (1998) 141(1):199–208. doi: 10.1083/jcb.141.1.199
33. Furuse M, Hirase T, Itoh M, Nagafuchi A, Yonemura S, Tsukita S, et al. Occludin - a Novel Integral Membrane-Protein Localizing at Tight Junctions. J Cell Biol (1993) 123(6):1777–88. doi: 10.1083/jcb.123.6.1777
34. Furuse M, Fujita K, Hiiragi T, Fujimoto K, Tsukita S. Claudin-1 and -2: Novel Integral Membrane Proteins Localizing at Tight Junctions With No Sequence Similarity to Occludin. J Cell Biol (1998) 141(7):1539–50. doi: 10.1083/jcb.141.7.1539
35. Grozdanovic MM, Cavic M, Nesic A, Andjelkovic U, Akbari P, Smit JJ, et al. Kiwifruit Cysteine Protease Actinidin Compromises the Intestinal Barrier by Disrupting Tight Junctions. Biochim Biophys Acta-General Subj (2016) 1860(3):516–26. doi: 10.1016/j.bbagen.2015.12.005
36. Fries W, Belvedere A, Vetrano S. Sealing the Broken Barrier in IBD: Intestinal Permeability, Epithelial Cells and Junctions. Curr Drug Targets (2013) 14(12):1460–70. doi: 10.2174/1389450111314120011
37. Allaire JM, Crowley SM, Law HT, Chang SY, Ko HJ, Vallance BA. The Intestinal Epithelium: Central Coordinator of Mucosal Immunity. Trends Immunol (2018) 39(9):677–96. doi: 10.1016/j.it.2018.04.002
38. Matsuzaki T, Susa T, Shimizu K, Sawai N, Suzuki T, Aoki T, et al. Function of the Membrane Water Channel Aquaporin-5 in the Salivary Gland. Acta Histochem Cytochem (2012) 45(5):251–9. doi: 10.1267/ahc.12018
39. Auchere D, Tardivel S, Gounelle JC, Drueke T, Lacour B. Role of Transcellular Pathway in Ileal Ca2+ Absorption: Stimulation by Low-Ca2+ Diet. Am J Physiol Gastrointest Liver Physiol (1998) 275(5):G951–G6. doi: 10.1152/ajpgi.1998.275.5.G951
40. Dyer J, Hosie KB, ShiraziBeechey SP. Nutrient Regulation of Human Intestinal Sugar Transporter (SGLT1) Expression. Gut (1997) 41(1):56–9. doi: 10.1136/gut.41.1.56
41. Christensen HN, Liang M, Archer EG. A Distinct NA+-Requiring Transport System for Alanine Serine Cysteine and Similar Amino Acids. J Biol Chem (1967) 242(22):5237–46. doi: 10.1016/S0021-9258(18)99417-2
42. Rose EM, Koo JCP, Antflick JE, Ahmed SM, Angers S, Hampson DR. Glutamate Transporter Coupling to Na,K-ATPase. J Neurosci (2009) 29(25):8143–55. doi: 10.1523/JNEUROSCI.1081-09.2009
43. Snoeck V, Goddeeris B, Cox E. The Role of Enterocytes in the Intestinal Barrier Function and Antigen Uptake. Microbes Infect (2005) 7(7-8):997–1004. doi: 10.1016/j.micinf.2005.04.003
44. Aderem A, Underhill DM. Mechanisms of Phagocytosis in Macrophages. Annu Rev Immunol (1999) 17:593–623. doi: 10.1146/annurev.immunol.17.1.593
45. Bockman DE, Cooper MD. Pinocytosis by Epithelium Associated With Lymphoid Follicles in Bursa of Fabricius, Appendix, and Peyers Patches - Electron-Microscopic Study. Am J Anat (1973) 136(4):455–77. doi: 10.1002/aja.1001360406
46. Jang MH, Kweon MN, Iwatani K, Yamamoto M, Terahara K, Sasakawa C, et al. Intestinal Villous M Cells: An Antigen Entry Site in the Mucosal Epithelium. Proc Natl Acad Sci USA (2004) 101(16):6110–5. doi: 10.1073/pnas.0400969101
47. Suzuki H, Sekine S, Kataoka K, Pascual DW, Maddaloni M, Kobayashi R, et al. Ovalbumin-Protein Sigma 1 M-Cell Targeting Facilitates Oral Tolerance With Reduction of Antigen-Specific CD4(+) T Cells. Gastroenterology (2008) 135(3):917–25. doi: 10.1053/j.gastro.2008.05.037
48. Sakhony OS, Rossy B, Gusti V, Pham AJ, Vu K, Lo DD. M Cell-Derived Vesicles Suggest a Unique Pathway for Trans-Epithelial Antigen Delivery. Tissue Barriers (2015) 3(1-2):e1004975. doi: 10.1080/21688370.2015.1004975
49. Neutra MR, Frey A, Kraehenbuhl JP. Epithelial M Cells: Gateways for Mucosal Infection and Immunization. Cell (1996) 86(3):345–8. doi: 10.1016/S0092-8674(00)80106-3
50. Gebert A, Rothkotter HJ, Pabst R. M Cells in Peyer's Patches of the Intestine. Int Rev Cytol (1996) 167:91–159. doi: 10.1016/s0074-7696(08)61346-7
51. Wolf JL, Bye WA. The Membranous Epithelial (M) Cell and the Mucosal Immune-System. Annu Rev Med (1984) 35:95–112. doi: 10.1146/annurev.me.35.020184.000523
52. Wu Y, Boysun MJ, Csencsits KL, Pascual DW. Gene Transfer Facilitated by a Cellular Targeting Molecule, Reovirus Protein Sigma 1. Gene Ther (2000) 7(1):61–9. doi: 10.1038/sj.gt.3301046
53. Wu YP, Wang XH, Csencsits KL, Haddad A, Walters N, Pascual DW. M Cell-Targeted DNA Vaccination. Proc Natl Acad Sci USA (2001) 98(16):9318–23. doi: 10.1073/pnas.161204098
54. Spahn TW, Fontana A, Faria AMC, Slavin AJ, Eugster HP, Zhang XM, et al. Induction of Oral Tolerance to Cellular Immune Responses in the Absence of Peyer's Patches. Eur J Immunol (2001) 31(4):1278–87. doi: 10.1002/1521-4141(200104)31:4<1278::AID-IMMU1278>3.0.CO;2-A
55. Spahn TW, Weiner HL, Rennert PD, Lugering N, Fontana A, Domschke W, et al. Mesenteric Lymph Nodes are Critical for the Induction of High-Dose Oral Tolerance in the Absence of Peyer's Patches. Eur J Immunol (2002) 32(4):1109–13. doi: 10.1002/1521-4141(200204)32:4<1109::AID-IMMU1109>3.0.CO;2-K
56. McCauley HA, Guasch G. Three Cheers for the Goblet Cell: Maintaining Homeostasis in Mucosal Epithelia. Trends Mol Med (2015) 21(8):492–503. doi: 10.1016/j.molmed.2015.06.003
57. McDole JR, Wheeler LW, McDonald KG, Wang BM, Konjufca V, Knoop KA, et al. Goblet Cells Deliver Luminal Antigen to CD103(+) Dendritic Cells in the Small Intestine. Nature (2012) 483(7389):345–U141. doi: 10.1038/nature10863
58. Knoop KA, Gustafsson JK, McDonald KG, Kulkarni DH, Kassel R, Newberry RD. Antibiotics Promote the Sampling of Luminal Antigens and Bacteria via Colonic Goblet Cell Associated Antigen Passages. Gut Microbes (2017) 8(4):400–11. doi: 10.1080/19490976.2017.1299846
59. Gustafsson JK, Davis JE, Rappai T, McDonald KG, Kulkarni DH, Knoop KA, et al. Intestinal Goblet Cells Sample and Deliver Lumenal Antigens by Regulated Endocytic Uptake and Transcytosis. Elife (2021) 10:e67292. doi: 10.7554/eLife.67292
60. Kulkarni DH, Gustafsson JK, Knoop KA, McDonald KG, Bidani SS, Davis JE, et al. Goblet Cell Associated Antigen Passages Support the Induction and Maintenance of Oral Tolerance. Mucosal Immunol (2020) 13(2):271–82. doi: 10.1038/s41385-019-0240-7
61. Noah TK, Knoop KA, McDonald KG, Gustafsson JK, Waggoner L, Vanoni S, et al. IL-13-Induced Intestinal Secretory Epithelial Cell Antigen Passages are Required for IgE-Mediated Food-Induced Anaphylaxis. J Allergy Clin Immunol (2019)144(4):1058–73.e3. doi: 10.1016/j.jaci.2019.04.030
62. Liu EG, Yin XY, Swaminathan A, Eisenbarth SC. Antigen-Presenting Cells in Food Tolerance and Allergy. Front Immunol (2021) 11. doi: 10.3389/fimmu.2020.616020
63. Lelouard H, Fallet M, de Bovis B, Meresse S, Gorvel JP. Peyer's Patch Dendritic Cells Sample Antigens by Extending Dendrites Through M Cell-Specific Transcellular Pores. Gastroenterology (2012) 142(3):592–601.e3. doi: 10.1053/j.gastro.2011.11.039
64. Kelsall B. Recent Progress in Understanding the Phenotype and Function of Intestinal Dendritic Cells and Macrophages. Mucosal Immunol (2008) 1(6):460–9. doi: 10.1038/mi.2008.61
65. Farache J, Zigmond E, Shakhar G, Jung S. Contributions of Dendritic Cells and Macrophages to Intestinal Homeostasis and Immune Defense. Immunol Cell Biol (2013) 91(3):232–9. doi: 10.1038/icb.2012.79
66. Rescigno M, Urbano M, Valzasina B, Francolini M, Rotta G, Bonasio R, et al. Dendritic Cells Express Tight Junction Proteins and Penetrate Gut Epithelial Monolayers to Sample Bacteria. Nat Immunol (2001) 2(4):361–7. doi: 10.1038/86373
67. Mazzini E, Massimiliano L, Penna G, Rescigno M. Oral Tolerance Can Be Established via Gap Junction Transfer of Fed Antigens From CX3CR1(+) Macrophages to CD103(+) Dendritic Cells. Immunity (2014) 40(2):248–61. doi: 10.1016/j.immuni.2013.12.012
68. Schulz O, Jaensson E, Persson EK, Liu XS, Worbs T, Agace WW, et al. Intestinal CD103(+), But Not CX3CR1(+), Antigen Sampling Cells Migrate in Lymph and Serve Classical Dendritic Cell Functions. J Exp Med (2009) 206(13):3101–14. doi: 10.1084/jem.20091925
69. Zeng R, Oderup C, Yuan R, Lee M, Habtezion A, Hadeiba H, et al. Retinoic Acid Regulates the Development of a Gut-Homing Precursor for Intestinal Dendritic Cells. Mucosal Immunol (2013) 6(4):847–56. doi: 10.1038/mi.2012.123
70. Coombes JL, Siddiqui KRR, Arancibia-Carcamo CV, Hall J, Sun CM, Belkaid Y, et al. A Functionally Specialized Population of Mucosal CD103(+) DCs Induces Foxp3(+) Regulatory T Cells via a TGF-Beta- and Retinoic Acid-Dependent Mechanism. J Exp Med (2007) 204(8):1757–64. doi: 10.1084/jem.20070590
71. Sun CM, Hall JA, Blank RB, Bouladoux N, Oukka M, Mora JR, et al. Small Intestine Lamina Propria Dendritic Cells Promote De Novo Generation of Foxp3 T Reg Cells via Retinoic Acid. J Exp Med (2007) 204(8):1775–85. doi: 10.1084/jem.20070602
72. Matteoli G, Mazzini E, Iliev ID, Mileti E, Fallarino F, Puccetti P, et al. Gut CD103(+) Dendritic Cells Express Indoleamine 2,3-Dioxygenase Which Influences T Regulatory/T Effector Cell Balance and Oral Tolerance Induction. Gut (2010) 59(5):595–604. doi: 10.1136/gut.2009.185108
73. Hadis U, Wahl B, Schulz O, Hardtke-Wolenski M, Schippers A, Wagner N, et al. Intestinal Tolerance Requires Gut Homing and Expansion of FoxP3(+) Regulatory T Cells in the Lamina Propria. Immunity (2011) 34(2):237–46. doi: 10.1016/j.immuni.2011.01.016
74. Murai M, Turovskaya O, Kim G, Madan R, Karp CL, Cheroutre H, et al. Interleukin 10 Acts on Regulatory T Cells to Maintain Expression of the Transcription Factor Foxp3 and Suppressive Function in Mice With Colitis. Nat Immunol (2009) 10(11):1178–U61. doi: 10.1038/ni.1791
75. Mowat AM. To Respond or Not to Respond - a Personal Perspective of Intestinal Tolerance. Nat Rev Immunol (2018) 18(6):405–15. doi: 10.1038/s41577-018-0002-x
76. Chen YH, Inobe J, Marks R, Gonnella P, Kuchroo VK, Weiner HL. Peripheral Deletion of Antigen-Reactive T-Cells in Oral Tolerance. Nature (1995) 376(6536):177–80. doi: 10.1038/376177a0
77. Friedman A, Weiner HL. Induction of Anergy or Active Suppression Following Oral Tolerance is Determined by Antigen Dosage. Proc Natl Acad Sci USA (1994) 91(14):6688–92. doi: 10.1073/pnas.91.14.6688
78. Chen Y, Kuchroo VK, Inobe J, Hafler DA, Weiner HL. Regulatory T Cell Clones Induced by Oral Tolerance: Suppression of Autoimmune Encephalomyelitis. Science (1994) 265(5176):1237–40. doi: 10.1126/science.7520605
79. Kim KS, Hong SW, Han D, Yi J, Jung J, Yang BG, et al. Dietary Antigens Limit Mucosal Immunity by Inducing Regulatory T Cells in the Small Intestine. Science (2016) 351(6275):858–63. doi: 10.1126/science.aac5560
80. Weiss JM, Bilate AM, Gobert M, Ding Y, de Lafaille MAC, Parkhurst CN, et al. Neuropilin 1 is Expressed on Thymus-Derived Natural Regulatory T Cells, But Not Mucosa- Generated Induced Foxp3(+) T Reg Cells. J Exp Med (2012) 209(10):1723–42. doi: 10.1084/jem.20120914
81. Cahenzli J, Koller Y, Wyss M, Geuking MB, McCoy KD. Intestinal Microbial Diversity During Early-Life Colonization Shapes Long-Term IgE Levels. Cell Host Microbe (2013) 14(5):559–70. doi: 10.1016/j.chom.2013.10.004
82. Sarkar A, Yoo JY, Dutra SVO, Morgan KH, Groer M. The Association Between Early-Life Gut Microbiota and Long-Term Health and Diseases. J Clin Med (2021) 10(3):459. doi: 10.3390/jcm10030459
83. Atarashi K, Tanoue T, Shima T, Imaoka A, Kuwahara T, Momose Y, et al. Induction of Colonic Regulatory T Cells by Indigenous Clostridium Species. Science (2011) 331(6015):337–41. doi: 10.1126/science.1198469
84. Geuking MB, Cahenzli J, Lawson MAE, Ng DCK, Slack E, Hapfelmeier S, et al. Intestinal Bacterial Colonization Induces Mutualistic Regulatory T Cell Responses. Immunity (2011) 34(5):794–806. doi: 10.1016/j.immuni.2011.03.021
85. Russell SL, Gold MJ, Hartmann M, Willing BP, Thorson L, Wlodarska M, et al. Early Life Antibiotic-Driven Changes in Microbiota Enhance Susceptibility to Allergic Asthma. EMBO Rep (2012) 13(5):440–7. doi: 10.1038/embor.2012.32
86. Wang YA, Brzozowska-Prechtl A, Karten HJ. Laminar and Columnar Auditory Cortex in Avian Brain. Proc Natl Acad Sci USA (2010) 107(28):12676–81. doi: 10.1073/pnas.1006645107
87. Atarashi K, Tanoue T, Oshima K, Suda W, Nagano Y, Nishikawa H, et al. T-Reg Induction by a Rationally Selected Mixture of Clostridia Strains From the Human Microbiota. Nature (2013) 500(7461):232–6. doi: 10.1038/nature12331
88. Stefka AT, Feehley T, Tripathi P, Qiu J, McCoy K, Mazmanian SK, et al. Commensal Bacteria Protect Against Food Allergen Sensitization. Proc Natl Acad Sci U S A (2014) 111(36):13145–50. doi: 10.1073/pnas.1412008111
89. Russler-Germain EV, Rengarajan S, Hsieh CS. Antigen-Specific Regulatory T-Cell Responses to Intestinal Microbiota. Mucosal Immunol (2017) 10(6):1375–86. doi: 10.1038/mi.2017.65
90. Mager LF, Burkhard R, Pett N, Cooke NCA, Brown K, Ramay H, et al. Microbiome-Derived Inosine Modulates Response to Checkpoint Inhibitor Immunotherapy. Science (2020) 369(6510):1481–9. doi: 10.1126/science.abc3421
91. Yang WJ, Yu TM, Huang XS, Bilotta AJ, Xu LQ, Lu Y, et al. Intestinal Microbiota-Derived Short-Chain Fatty Acids Regulation of Immune Cell IL-22 Production and Gut Immunity. Nat Commun (2020) 11(1):4457. doi: 10.1038/s41467-020-18262-6
92. Furusawa Y, Obata Y, Fukuda S, Endo TA, Nakato G, Takahashi D, et al. Commensal Microbe-Derived Butyrate Induces the Differentiation of Colonic Regulatory T Cells. Nature (2013) 504(7480):446–50. doi: 10.1038/nature12721
93. Tan J, McKenzie C, Vuillermin PJ, Goverse G, Vinuesa CG, Mebius RE, et al. Dietary Fiber and Bacterial SCFA Enhance Oral Tolerance and Protect Against Food Allergy Through Diverse Cellular Pathways. Cell Rep (2016) 15(12):2809–24. doi: 10.1016/j.celrep.2016.05.047
94. Jaensson E, Uronen-Hansson H, Pabst O, Eksteen B, Tian J, Coombes JL, et al. Small Intestinal CD103+ Dendritic Cells Display Unique Functional Properties That are Conserved Between Mice and Humans. J Exp Med (2008) 205(9):2139–49. doi: 10.1084/jem.20080414
95. Feehley T, Plunkett CH, Bao RY, Hong SMC, Culleen E, Belda-Ferre P, et al. Healthy Infants Harbor Intestinal Bacteria That Protect Against Food Allergy. Nat Med (2019) 25(3):448–53. doi: 10.1038/s41591-018-0324-z
96. Brown GD, Willment JA, Whitehead L. C-Type Lectins in Immunity and Homeostasis. Nat Rev Immunol (2018) 18(6):374–89. doi: 10.1038/s41577-018-0004-8
97. Shreffler WG, Castro RR, Kucuk ZY, Charlop-Powers Z, Grishina G, Yoo S, et al. The Major Glycoprotein Allergen From Arachis Hypogaea, Ara H 1, is a Ligand of Dendritic Cell-Specific ICAM-Grabbing Nonintegrin and Acts as a Th2 Adjuvant In Vitro. J Immunol (2006) 177(6):3677–85. doi: 10.4049/jimmunol.177.6.3677
98. Schnurr M, Then F, Galambos P, Scholz C, Siegmund B, Endres S, et al. Extracellular ATP and TNF-Alpha Synergize in the Activation and Maturation of Human Dendritic Cells. J Immunol (2000) 165(8):4704–9. doi: 10.4049/jimmunol.165.8.4704
99. Hsu S-C, Chen C-H, Tsai S-H, Kawasaki H, Hung C-H, Chu Y-T, et al. Functional Interaction of Common Allergens and a C-Type Lectin Receptor, Dendritic Cell-Specific ICAM3-Grabbing Non-Integrin (DC-SIGN), on Human Dendritic Cells. J Biol Chem (2010) 285(11):7903–10. doi: 10.1074/jbc.M109.058370
100. Li JN, Wang Y, Tang LH, de Villiers WJS, Cohen D, Woodward J, et al. Dietary Medium-Chain Triglycerides Promote Oral Allergic Sensitization and Orally Induced Anaphylaxis to Peanut Protein in Mice. J Allergy Clin Immunol (2013) 131(2):442–50. doi: 10.1016/j.jaci.2012.10.011
101. Khodoun MV, Tomar S, Tocker JE, Wang YH, Finkelman FD. Prevention of Food Allergy Development and Suppression of Established Food Allergy by Neutralization of Thymic Stromal Lymphopoietin, IL-25, and IL-33. J Allergy Clin Immunol (2018) 141(1):171–9.e1. doi: 10.1016/j.jaci.2017.02.046
102. Blazquez AB, Berin MC. Gastrointestinal Dendritic Cells Promote Th2 Skewing via OX40L. J Immunol (2008) 180(7):4441–50. doi: 10.4049/jimmunol.180.7.4441
103. Chu DK, Llop-Guevara A, Walker TD, Flader K, Goncharova S, Boudreau JE, et al. IL-33, But Not Thymic Stromal Lymphopoietin or IL-25, is Central to Mite and Peanut Allergic Sensitization. J Allergy Clin Immunol (2013) 131(1):187–U283. doi: 10.1016/j.jaci.2012.08.002
104. Rivas MN, Burton OT, Oettgen HC, Chatila T. IL-4 Production by Group 2 Innate Lymphoid Cells Promotes Food Allergy by Blocking Regulatory T-Cell Function. J Allergy Clin Immunol (2016) 138(3):801–11.e9. doi: 10.1016/j.jaci.2016.02.030
105. Hammad H, Lambrecht BN. Barrier Epithelial Cells and the Control of Type 2 Immunity. Immunity (2015) 43(1):29–40. doi: 10.1016/j.immuni.2015.07.007
106. Galand C, Leyva-Castillo JM, Juhan Y, Han A, Lee MS, McKenzie ANJ, et al. IL-33 Promotes Food Anaphylaxis in Epicutaneously Sensitized Mice by Targeting Mast Cells. J Allergy Clin Immunol (2016) 138(5):1356–66. doi: 10.1016/j.jaci.2016.03.056
107. Hamad A, Burks WA. Emerging Approaches to Food Desensitization in Children. Curr Allergy Asthma Rep (2017) 17(5):32. doi: 10.1007/s11882-017-0700-7
108. Wood RA. Food Allergen Immunotherapy: Current Status and Prospects for the Future. J Allergy Clin Immunol (2016) 137(4):973–82. doi: 10.1016/j.jaci.2016.01.001
109. Wang J, Sampson HA. Safety and Efficacy of Epicutaneous Immunotherapy for Food Allergy. Pediatr Allergy Immunol (2018) 29(4):341–9. doi: 10.1111/pai.12869
110. Scurlock AM. Oral and Sublingual Immunotherapy for Treatment of IgE-Mediated Food Allergy. Clin Rev Allergy Immunol (2018) 55(2):139–52. doi: 10.1007/s12016-018-8677-0
111. Gernez Y, Nowak-Wegrzyn A. Immunotherapy for Food Allergy: Are We There Yet? J Allergy Clin Immunol Pract (2017) 5(2):250–72. doi: 10.1016/j.jaip.2016.12.004
112. Sampath V, Sindher SB, Pinzon AMA, Nadeau KC. Can Food Allergy be Cured? What are the Future Prospects? Allergy (2020) 75(6):1316–26. doi: 10.1111/all.14116
113. Valenta R, Linhart B, Swoboda I, Niederberger V. Recombinant Allergens for Allergen-Specific Immunotherapy: 10 Years Anniversary of Immunotherapy With Recombinant Allergens. Allergy (2011) 66(6):775–83. doi: 10.1111/j.1398-9995.2011.02565.x
114. Jutel M, Jaeger L, Suck R, Meyer H, Fiebig H, Cromwell O. Allergen-Specific Immunotherapy With Recombinant Grass Pollen Allergens. J Allergy Clin Immunol (2005) 116(3):608–13. doi: 10.1016/j.jaci.2005.06.004
115. Pauli G, Larsen TH, Rak S, Horak F, Pastorello E, Valenta R, et al. Efficacy of Recombinant Birch Pollen Vaccine for the Treatment of Birch-Allergic Rhinoconjunctivitis. J Allergy Clin Immunol (2008) 122(5):951–60. doi: 10.1016/j.jaci.2008.09.017
116. Winther L, Poulsen LK, Robin B, Melac M, Malling H. Safety and Tolerability of Recombinant Bet V 1 (Rbet V 1) Tablets in Sublingual Immunotherapy (SLIT). J Allergy Clin Immunol (2009) 123(2):S215–S. doi: 10.1016/j.jaci.2008.12.822
117. Arasi S, Mennini M, Cafarotti A, Fiocchi A. Omalizumab as Monotherapy for Food Allergy. Curr Opin Allergy Clin Immunol (2021) 21(3):286–91. doi: 10.1097/ACI.0000000000000744
118. Michelet M, Reber LL, Guilleminault L. Focus on Omalizumab in Food Allergy. Rev Francaise D Allergol (2021) 61(3):170–6. doi: 10.1016/j.reval.2020.12.006
119. Tsuda M, Arakawa H, Ishii N, Ubukata C, Michimori M, Noda M, et al. Dietary Fructo-Oligosaccharides Attenuate Early Activation of CD4(+) T Cells Which Produce Both Th1 and Th2 Cytokines in the Intestinal Lymphoid Tissues of a Murine Food Allergy Model. Int Arch Allergy Immunol (2017) 174(3-4):121–32. doi: 10.1159/000481984
120. Yang J, Ren FZ, Zhang H, Jiang L, Hao YL, Luo XG. Induction of Regulatory Dendritic Cells by Lactobacillus Paracasei L9 Prevents Allergic Sensitization to Bovine Beta-Lactoglobulin in Mice. J Microbiol Biotechnol (2015) 25(10):1687–96. doi: 10.4014/jmb.1503.03022
Keywords: food antigens, food allergy, paracellular pathway, transcellular pathway, intestinal barrier, oral tolerance, intestinal immune system, gut microbiota
Citation: Xiong Y, Xu G, Chen M and Ma H (2022) Intestinal Uptake and Tolerance to Food Antigens. Front. Immunol. 13:906122. doi: 10.3389/fimmu.2022.906122
Received: 28 March 2022; Accepted: 16 May 2022;
Published: 10 June 2022.
Edited by:
Daming Zuo, Southern Medical University, ChinaReviewed by:
Xiaoshan Shi, Shenzhen Institutes of Advanced Technology (CAS), ChinaPeixiang Lan, Huazhong University of Science and Technology, China
Chuanfeng Wu, National Heart, Lung, and Blood Institute (NIH), United States
Copyright © 2022 Xiong, Xu, Chen and Ma. This is an open-access article distributed under the terms of the Creative Commons Attribution License (CC BY). The use, distribution or reproduction in other forums is permitted, provided the original author(s) and the copyright owner(s) are credited and that the original publication in this journal is cited, in accordance with accepted academic practice. No use, distribution or reproduction is permitted which does not comply with these terms.
*Correspondence: Hongdi Ma, bWFob25nZGlAdXN0Yy5lZHUuY24=; Mingwu Chen, Y2hlbm1pbmd3dTAxQDE2My5jb20=