- 1Biotherapy Center, Harbin Medical University Cancer Hospital, Harbin, China
- 2Department of Pharmacology (State-Province Key Laboratories of Biomedicine-Pharmaceutics of China, Key Laboratory of Cardiovascular Research, Ministry of Education), College of Pharmacy, Department of Pharmacology, Harbin Medical University, Harbin, China
Colorectal cancer (CRC) is the third most common cancer, with a high mortality rate and a serious impact on people’s life and health. In recent years, adoptive chimeric antigen receptor T (CAR-T) cells therapy has shown well efficacy in the treatment of hematological malignancies, but there are still many problems and challenges in solid tumors such as CRC. For example, the tumor immunosuppressive microenvironment, the low targeting of CAR-T cells, the short time of CAR-T cells in vivo, and the limited proliferation capacity of CAR-T cells, CAR-T cells can not effectively infiltrate into the tumor and so on. New approaches have been proposed to address these challenges in CRC, and this review provides a comprehensive overview of the current state of CAR-T cells therapy in CRC.
Introduction
CRC occurs worldwide, has a high mortality rate and is the third most common cancer (1, 2), which seriously affects human life and health. Due to the rarity of early diagnosis of CRC, existing treatment methods including surgery, chemotherapy and radiotherapy cannot completely inhibit the progression, metastasis and recurrence of CRC when cancer cells infiltrate or metastasize to surrounding tissues (3).
CAR-T cells have shown significant efficacy in immunotargeted therapy of hematologic tumors (4). The United States Food and Drug Administration has approved CAR-T cells for the treatment of hematologic tumors (5). In recent years, basic and clinical studies on CAR-T cells therapy for CRC have been published, and some studies have made encouraging progress (6). However, CAR-T cells face many challenges in the treatment of CRC, limiting their clinical application (7). This article reviews the progress of CAR-T cells therapy for CRC.
Extracellular region, hinge region, transmembrane region and intracellular signal region are the four components of CAR, and each plays an important role (8). The extracellular domain is usually Fab or single chain variable fragment (scFv) of monoclonal antibody, which has flexible splicing function and determines antigen specificity (9). The hinge domain consists of (Cluster of differentiation4)CD4、CD8、CD28 or IgG4, which connects the extracellular domain to the transmembrane domain (10). The transmembrane domain consists of CD8α, CD4, CD3 ζ, CD28 or ICOS, linking the extracellular domain to the intracellular domain and acting as an anchor of the cell membrane (11, 12). Intracellular signaling domains transmit stimuli into the cell (13). First-generation CAR, which consist of scFv and intracellular CD3ζ molecular signaling domain (14–16), have limited antitumor activity due to the lack of co-stimulation and interleukin signaling (17). The costimulatory domain of the second generation CAR consists of 4-1BB (CD137) or CD28, which mimics costimulatory signals during activation (18). The third generation of CAR has two costimulatory domains, further enhancing the function of CAR (19). The fourth generation CAR is based on the second generation CAR and secretes cytokines such as interleukin2(IL-2) and IL-12 (20, 21). A schematic diagram of the different generations of CARs is shown in Figure 1. Recently, researchers designed a combination of focused ultrasound (FUS) and CAR-T cells expressing heat-inducible genes (22). FUS activates heat-inducible genes by controlling local temperature in vivo (22). In animal experiments, CAR-T cells was injected into tumors in mice, and a small ultrasonic transducer was placed on the top of the skin of the tumor area (22). The tumor area was heated through the ultrasonic transducer in the FUS guided by magnetic resonance imaging. Only tumors exposed to ultrasound will be attacked by CAR-T, improving CAR-T targeting (22). This design is expected to be a promising CAR-T.
Basic experimental of CAR-T cells therapy for CRC
The genetic modification of peripherally derived T lymphocytes with CARs has achieved a remarkable effect in the treatment of hematologic malignancies (23, 24). CAR-T cells therapy for solid tumors still faces many challenges. Recently, there are some advances in CAR-T cells therapy for CRC. The targets of CAR-T cells therapy for CRC include carcino-embryonic antigen(CEA), Mesothelin (MSLN),Guanylyl cyclase C (GUCY2C), epithelial cell adhesion molecule(EpCAM), Human epidermal growth factor receptor-2 (HER2)、Doublecortin-like kinase 1 (DCLK1).
CEA is a glycoprotein formed by cells in the large intestine and a glycoprotein carcinoembryonic antigen, which has been considered as a sensitive marker of CRC (25). At present, there are many basic studies on CAR-T for CEA (26). CAR-T cells have excellent anti-tumor ability when dual targeting CEA and other targets such as CD30 antibody (27).The combination of CEA-CAR-T cells and recombinant human IL‐12(rhIL-12) significantly inhibited the growth of tumor xenografts (28).
MSLN is a cells surface glycoprotein, which is physiologically expressed in peritoneal, pleural and pericardial mesenchymal cells (29). Overexpression of MSLN can be detected in CRC (30). MSLN is an important CAR-T cells target in solid tumors (31, 32). In a recent study, the efficacy of MSLN-CAR-T cells on colon cancer xenografts was investigated. Compared with the control group, the mice in the MSLN-CAR-T cells group had more T lymphocytes in the peripheral blood and more granzyme B infiltrates in the tumor tissue (33). The experimental results showed that the MSLN-CAR-T cells group had a more significant anti-tumor effect (33).
GUCY2C is a binding receptor present in the enterocytes membranes that sustains balance by activating its hormone ligand guanosine or uridine to produce the second messenger cGMP (34). When GUCY2C signaling is blocked, it may lead to the pathogenesis of CRC. However, GUCY2C is expressed in both human primary and metastatic CRC, and GUCY2C is considered to be a tumor marker (35). GUCY2C is highly expressed in 95% of CRC metastasis (36). CAR-T cells targeting hGUCY2C mediated killing of CRC cells expressing hGUCY2C, and were nontoxic to intestinal epithelial cells expressing normal GUCY2C. Such CAR-T cells induce antigen-dependent T-cells activation and cytokine production, thereby enhancing antitumor efficacy (37).
EpCAM is one of the main surface tumor-associated antigens of CRC (38), which can promote the migration, proliferation and tumor growth of colon cancer cells (39). In the experimental treatment of CRC with EpCAM-CAR-T cells, compared with control T cells, EpCAM-CAR-T cells have greater lethality and specificity against cancer cells which express EpCAM (40).
HER2 is overexpressed in CRC (41), and is an important target for CAR-T cells therapy. HER2-CAR-T cells showed strong and particular cytotoxic capacity against colon cancer cells. In mouse models, HER2-CAR-T cells-treated mice showed significant tumor control, significantly improved overall survival, and suppressed distant metastasis of CRC to liver and lung (42).
DCLK1 is an enzyme that regulates epithelial mesenchymal transition (43). Mesenchymal DCLK1 labeling of tumor stem cells in a genetic mouse model of CRC (44). DCLK1-targeted CAR-T cells therapy inhibited xenograft tumor growth in mice without apparent toxicity (45).
Cbl-b is an E3 ubiquitin ligase that mediates ubiquitination, and removal of Cbl-b from CAR-T cells enhances the antitumor activity of CAR-T cells (46). Compared with the control group, Cbl-b -/-CAR-T cells significantly enhanced the killing ability of CAR-T cells against CRC cells, which was manifested by increased secretion of IFN-γ, TNF-α and granzyme B (47).
Challenges
Adoptive T cells therapy is a new option for tumor patients, but its efficacy is affected by various factors, it is imperative to find relevant strategies to solve the problem.
Immunosuppression in the tumor microenvironment
Hypoxia, acidic microenvironment and lack of substances necessary for the survival, proliferation and activation of T lymphocytes in tumor tissues will lead to immunosuppressive microenvironment, thereby weakening the killing effect of CAR-T cells on tumor cells (7). Tumor immunosuppressive microenvironment includes suppressive immune cells such as regulatory T cells (Treg), myeloid-derived suppressor cells (MDSC), immunosuppressive cytokines such as IL-10 and TGF-β, immunosuppressive molecules such as PD-1, and other molecules such as indoleamine dioxygenase 2-3 (IDO1) (7, 48). The immunosuppressive microenvironment promotes tumor immune escape (49). The occurrence and development of tumor are correlated strongly with immune escape (50), in which immune checkpoints play an important role (51). Programmed cell death protein 1 (PD-1) and its ligand PD-L1 are significant immune checkpoint proteins (52). PD-1 is an immune checkpoint receptor expressed in T lymphocytes, and PD-L1 is expressed mainly in the tumor microenvironment (53). When PD-1 encounters antigens, its expression is increased and binds to its ligand PD-L1, thereby inhibiting the immune response function of T cells and mediating immune suppression (54).
CAR-T cells does not effectively chemotaxis to tumor tissue
One of the challenges of CAR-T cells therapy for solid malignancies is the specific recognition of targeted antigens (55). Currently, the majority of tumor target antigens recognized by CAR-T cells are also expressed in normal cells, so when CAR-T cells are used to treat tumors, the therapeutic effect is ineffective (7). Meanwhile, CAR-T cells can also injury normal tissues and cause toxicity in vitro (7).
CAR-T cells can not proliferate and persist in the blood or tumor area
The persistence and proliferation of CAR-T cells in blood or tumor are important factors for the efficacy of CAR-T cells in cancer treatment (56). Firstly, different costimulatory molecules of CAR affect the survival and proliferation of CAR-T cells (57). Secondly, in the tumor microenvironment, there are a series of factors that affect the survival, proliferation and induce the failure of CAR-T cells. For example, when CAR-T cells are in chronic T helper 2 cells(Th2) inflammation state, their expansion ability is weakened and the number of apoptotic cells is increased (58). Thirdly, TGF-β and adenosine significantly inhibit the tumor cytotoxicity of CD8 + T cells by inhibiting the expression of granzyme (59, 60). In addition, the hypoxic acid microenvironment in the local tumor can cause damage to CAR-T cells, in which lactic acid accumulation can inhibit the production of IL-2, thereby affecting the proliferation and function of CAR-T cells (61). Further, the PD-1/PD-L1 axis affects the survival and function of CAR-T cells (62). Transcription factors T-bet and B lymphocyte-induced maturation protein 1 (Blimp1) regulate early CD8+T lymphocytes (63, 64). Forkhead box protein O1 (FoxO1) can regulate memory CD8+ T cell differentiation (65).
The level of CAR-T cells invasion in tumor tissue was low
When a CAR-T cells is used to treat a tumor, the CAR-T cells must reach the site of the tumor to perform their tumor-killing function (7). In solid tumors, CAR-T cells must overcome multiple obstacles to reach the tumor site, such as blood vessels and the tumor’s stroma (66, 67). Primarily, when intravenous infusion of CAR-T cells in the treatment of CRC, CAR-T cells must cross the vascular barrier and interstitial barrier to enter the tumor site to exert its efficacy (66). Intratumoral vascular beds and interstitial abnormalities are the key factors affecting the efficacy (66). Then, the inability of many T cells to reach the cancer cells may depend on the lack of surface-expressed chemokine receptor that match chemokine expressed in the tumor or tumor stroma (68). When the chemokines/chemokine receptors axis is mismatched, tumor cells secrete trace amounts of chemokines, resulting in the inability of T cells to reach the tumor tissues (68). For example, CXCL10 can make a variety of antitumor lymphocytes chemotactic to tumor tissues, such as CD8+ T cells, and is associated with T-lymphocytes infiltration in solid tumors (69).
Strategies
Develop drugs and measures that can improve the tumor microenvironment
In order to improve the tumor microenvironment to improve the anti-tumor efficacy and durability of CAR-T cells, there are currently the following methods.
It is essential that CAR-T cells secrete pro-inflammatory cytokines to protect them from the inhibitory tumor microenvironment. Studies have shown that secreted cytokines such as IL-7 and IL-12 CAR T cells can improve the immunosuppressive microenvironment (70, 71). Mesenchymal Stem Cells (MSCs) are the main components of tumor stroma and have the ability to actively migrate into tumor tissues (72, 73).By making MSCs capable of releasing IL-7 and IL-12 and combining CAR-T cells, researchers found that CAR-T cells could prolong the time of T cells attack on tumors and improve the tumor immunosuppressive microenvironment (74). IDO1 degrades tryptophan, an essential amino acid for T cells, which is required for T cells survival and immune responses (75). The expression of IDO1 is inhibited by miR-153 (76). When miR-153 was overexpressed in tumor cells, the tumor immunosuppressive environment was improved, CAR-T cells targeting epidermal growth factor receptor variant III(EGFRIII) were more effective in killing colon cancer cells overexpressing miR-153 (77). CD30 signaling can promote the differentiation of T cells to Th2, which has immunosuppressive function (78). In CRC, CAR-T cells dual targeting CD30 and CEA can produce a more significant proinflammatory response, manifested by higher granzyme B and perforin levels In T cells, which improves the ability of CAR-T cells to attack the tumor (27). IL-10 binds to its cognate receptor IL-10R to cause a wide range of immunosuppressive functions (79, 80). Recent studies have shown that CAR-T cells combined with IL-10 monoclonal antibody (mAb) can partially alleviate bone marrow cell-mediated immunosuppression by blocking IL-10 signaling, while promoting CAR-T cells expansion and enhancing killing effect, thereby increasing anti-tumor function (81).
Guo and his team demonstrated that intravenous injection of live attenuated Brucella in mice can promote the tumor microenvironment to a proinflammatory state, enhance the anti-tumor immunity of T cells, and reduce the resistance of tumors to CAR-T cells (82). Dopamine treatment can promote the differentiation of CD8+ T lymphocytes into CD103+ tissue-resident memory CD8+ T lymphocytes (TRM), and TRM can trigger stronger anti-tumor immunity. Moreover, dopamine treatment enhanced the anti-tumor function of CAR-T cells (83).
In addition, blockade of immune checkpoints can improve immunosuppression. Adding genes expressing PD-1 negative receptors to CAR-T cells can block intracellular immune checkpoints and enhance the lethality to target cells (84, 85). Investigators also used clustered regularly interspaced short palindromic repeats (CRISPR) and CRISPR-associated protein 9 (Cas9) (CRISPR-Cas9) systems to knock down the expression of PD-1 in CAR-T cells and achieved excellent preclinical efficacy by blocking PD-1/PD-L1 induced suppression of T-cell immune function (86–89).
Improve CAR-T cells targeting of tumors
Targeting multiple antigens and application of novel CAR can improve the targeting of CAR-T cells. Jiang and colleagues constructed a dual CAR system containing two extracellular domains, NKG2D and PD-1, and showed that such CAR-T cells effectively eliminated target cancer cells (90). CAR-T cells that dual target CD30 and CEA have a more specific ability to kill tumor cells, which is manifested by blocking the inhibition of cytotoxic T lymphocyte immune function induced by CD30 (27). In addition, when using the novel inhibitory CAR (iCAR) construct, the iCAR can trigger inhibitory signals when CAR-T cells are present in normal tissues, thereby inhibiting T cell function, avoiding the attack of normal tissues, and enhancing the targeting of tumor tissues (7). Additionally, switchable CAR T cells can increase their targeting, with the “switch” acting as a bridge between tumor cells and T cells, allowing T cells to specifically kill tumor cells (91). Besides, the combination of focused ultrasound (FUS) and CAR-T cells, in which only tumors exposed to ultrasound are attacked by CAR-T cells, also improves CAR-T cells targeting (22).
Amplification and long-term presence of CAR-T cells
How to maintain sustained expansion of CAR-T cells in vivo is a common consideration in the treatment of solid tumors with CAR-T cells. Cytokines such as IL-2, IL-7, IL-12 and IL-15 play an important role in T cells activation, proliferation and immune response (92–94). However, the content of immune stimulatory cytokines in the tumor microenvironment is very low. There are now several therapies for combining cytokines with CAR-T cells to treat tumors. CEA-CAR-T cells combined with rhIL-12 can increase the multiplication, persistence and cytokines release of CEA-CAR-T cells in vivo (28). When MSCs that can release IL-7 and IL-12 are used in combination with CAR-T cells, CAR-T cells survive longer and have better expansion ability in vivo, thereby improving the anti-tumor response (74). Li and his team demonstrated that inhibition of Wnt significantly inhibited TGF-β expression in tumor tissues and improved T cells infiltration (95). Moreover, after the inhibition of Wnt, the contents of T-bet and FoxO1 in the nucleus of CAR-T cells increased, and the expression of BLIMP1 increased, indicating that the inhibition of Wnt can make CAR-T cells early kill tumor function and differentiate into memory T lymphocytes (95). CD133 is expressed in cancer cells of various epithelial cell origins (96). A phase I trial of CAR-T cells targeting CD133 (CAR-T-133) in the treatment of advanced metastatic malignancies has found that CAR-T-133 cells can persist in vivo through multiple infusions and increase the content of immunostimulatory cytokines, which makes valid disease clearance and prevention of relapse possible (97). Previous studies have shown that increasing telomerase activity in CAR-T cells can enhance their proliferation ability and delay senescence (98). Other studies have shown that the costimulatory domain 4-1BB of CAR-T cells can improve the exhaustion of T cells and enhance their persistence in vivo (99).
Increased CAR-T cells invasion in tumors
Targeting tumor blood vessels and stroma and increasing the expression of chemokines are important methods to improve CAR-T cells infiltration into tumor tissues (100). Vascular blocker combretastatin A4 phosphate (CA4P) is a vascular interfering agent with high selectivity for tumor vascular system (101). Targeting CA4P can block the VE-cadherin signaling pathway, affect the stability of microtubule polymerization of tumor cell-related vascular endothelial cells, induce cell apoptosis, destroy the vascular system, reduce the blood supply in the tumor, and lead to tumor cell necrosis in the tumor tissue (101). CA4P combined with HER2-CAR-T cells therapy has a better antitumor effect than CA4P or HER2-CAR-T cell therapy alone, which can destroy tumor blood vessels, thereby promoting the infiltration of T cells into tumor tissues and enhancing the proliferation of CAR T cells (102). Vascular endothelial growth factor (VEGF)/VEGFR axis can promote the generation of vascular endothelial cells, which is a key signaling pathway of angiogenesis (103). VEGFR -targeting CAR T cells can disrupt vascular structures and obviously inhibit xenograft tumor growth, invasion, and metastasis (104). Cancer-associated fibroblasts (CAFs) are important components of tumor stroma (105). Fibroblast activating protein (FAP) is over expression in CAFs and suppresses tumor immune response by promoting the recruitment of immunosuppressive cells (106). At present, FAP-targeted CAR-T cells have achieved certain preclinical and clinical efficacy in solid tumors (107, 108). When the Wnt signaling pathway is blocked, it can up-regulate the expression of chemokine CXCL10, improve T cells tumor infiltration in cancer models, and improve the efficacy of CAR-T cells in CRC treatment (95, 109).
Clinical trials
In the past few years, immune cell therapy has been increasingly used in multicentre clinical trials. Multiple clinical trials targeting tumor antigens have been approved, including CEA, MSLN, EpCAM, HER2 and antigens, as well as NK group 2 member D ligands (NKG2DL), Mucin-1 (MUC1), B7-H3 (CD276), CD133, mesenchymal epithelial transfer factor(c-Met), which is overexpressed in colorectal cancer, can be used as a target for CAR-T cells. In Table 1, we summarized the clinical information available on ClinicalTrials.gov regarding CAR-T-cells therapy for CRC.
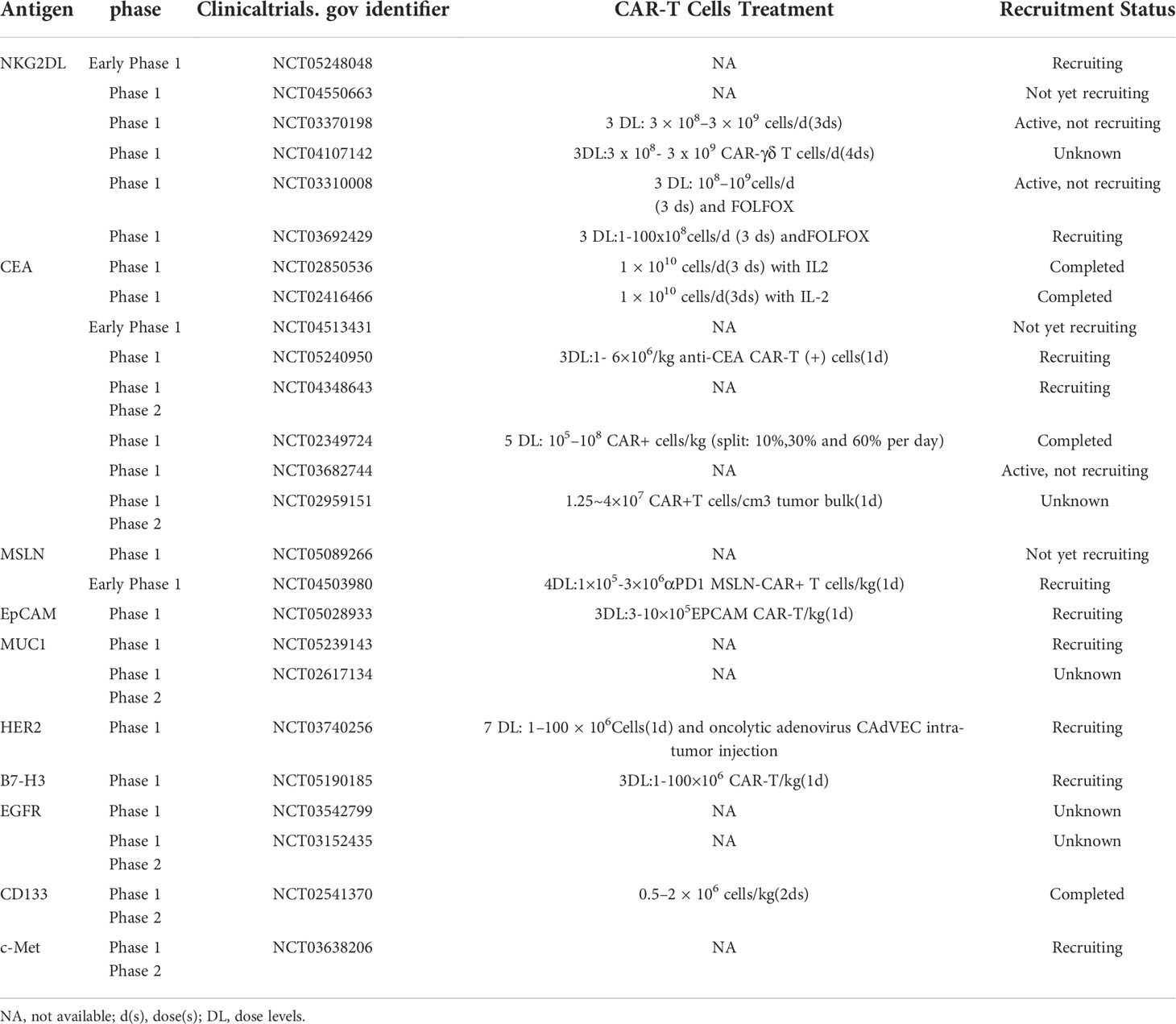
Table 1 Clinical trial of CAR-T cells in CRC(https://clinicaltrials.gov/).
In a phase I trial of CEA + CRC patients treated with CEA-CAR-T cells (NCT02349724), five dose-escalation CAR-T cells were administered to 10 patients with relapsed and refractory CRC metastases. No serious adverse events related to CAR-T cells therapy were observed in the trial (6). Among the 10 patients, 7 were stable after CAR-T cells therapy, of which 2 were stable for more than 30 weeks and 2 showed tumor shrinkage (6).
A phase 1B hepatic Immunotherapy for Metastases-selective internal irradiation therapy (HITM-SIR) trial was conducted in patients with liver metastases from CRC (NCT01373047). Six of them received anti-CEA CAR-T hepatic artery infusions (HAIs) and SIRT. Significant reductions in Granulocyte macrophage colony stimulating factor (GM-CSF), GM-CSF-R, IDO, and Programmed death ligand-1(PD-L1) were observed after HITM CAR-T HAI treatment, suggesting a reversal of immunosuppressed hepatic tumor microenvironment (TME). Subsequent increases in IL-2 and IL-6 in tumor biopsies after infusion further demonstrated pro-inflammatory liver TME. The median survival of patients in the trial was 8 months (110).
Conclusions
There are many approaches to CRC adoptive cell therapy, of which CAR-T cells are one of the most researched and promising, although clinical studies are still in the early stages of clinical trials. Many studies have demonstrated the efficacy and safety of CAR-T cells in the treatment of CRC. However, the therapy faces many challenges that limit its clinical application. In addition, CAR-T cells therapy can cause a number of toxic effects, the most common of which is cytokine release syndrome (CRS), which is a cytokine secretion response after CAR-T cells infusion (111). CRS has a series of non-characteristic manifestations, such as fever, nausea, decreased cardiac function, and hypotension (112). It can also cause other systemic toxicity, such as dyspnea, respiratory failure, arrhythmia, elevated myocardial enzymes, cardiac insufficiency, liver insufficiency, gastrointestinal reaction, coagulation dysfunction, muscle injury, neurotoxic allergy, etc (112). Only when these problems are effectively addressed can the efficacy of CAR-T cells therapy for CRC be improved and more patients receive effective treatment. In conclusion, CAR-T cells are a promising treatment for CRC and further research is needed.
Author contributions
CC and QL designed this manuscript. XQ wrote the main manuscript text. FW prepared figure and table. CC and QL revised the article. All authors contributed to the article and approved the submitted version.
Funding
This work was supported by the National Natural Science Foundation of China [grant number 81800242 and 81970382]; The Science foundation of Haiyan [grant number JJMS2022-16].
Conflict of interest
The authors declare that the research was conducted in the absence of any commercial or financial relationships that could be construed as a potential conflict of interest.
Publisher’s note
All claims expressed in this article are solely those of the authors and do not necessarily represent those of their affiliated organizations, or those of the publisher, the editors and the reviewers. Any product that may be evaluated in this article, or claim that may be made by its manufacturer, is not guaranteed or endorsed by the publisher.
References
1. Schrock AB, Ouyang C, Sandhu J, Sokol E, Jin D, Ross JS, et al. Tumor mutational burden is predictive of response to immune checkpoint inhibitors in MSI-high metastatic colorectal cancer. Ann Oncol (2019) 30(7):1096–103. doi: 10.1093/annonc/mdz134
2. Sung H, Ferlay J, Siegel RL, Laversanne M, Soerjomataram I, Jemal A, et al. Global cancer statistics 2020: GLOBOCAN estimates of incidence and mortality worldwide for 36 cancers in 185 countries. CA Cancer J Clin (2021) 71(3):209–49. doi: 10.3322/caac.21660
3. Sawicki T, Ruszkowska M, Danielewicz A, Niedźwiedzka E, Arłukowicz T, Przybyłowicz KE. A review of colorectal cancer in terms of epidemiology, risk factors, development, symptoms and diagnosis. Cancers (Basel) (2021) 13(9):2025. doi: 10.3390/cancers13092025
4. June CH, O'Connor RS, Kawalekar OU, Ghassemi S, Milone MC. CAR T cell immunotherapy for human cancer. Science (2018) 359(6382):1361–5. doi: 10.1126/science.aar6711
5. Fournier C, Martin F, Zitvogel L, Kroemer G, Galluzzi L, Apetoh L. Trial watch: Adoptively transferred cells for anticancer immunotherapy. Oncoimmunology (2017) 6(11):e1363139. doi: 10.1080/2162402X.2017.1363139
6. Zhang C, Wang Z, Yang Z, Wang M, Li S, Li Y, et al. Phase I escalating-dose trial of CAR-T therapy targeting CEA(+) metastatic colorectal cancers. Mol Ther (2017) 25(5):1248–58. doi: 10.1016/j.ymthe.2017.03.010
7. D’Aloia MM, Zizzari IG, Sacchetti B, Pierelli L, Alimandi M. CAR-T cells: The long and winding road to solid tumors. Cell Death Dis (2018) 9(3):282. doi: 10.1038/s41419-018-0278-6
8. Lipowska-Bhalla G, Gilham DE, Hawkins RE, Rothwell DG. Targeted immunotherapy of cancer with CAR T cells: Achievements and challenges. Cancer Immunol Immunother (2012) 61(7):953–62. doi: 10.1007/s00262-012-1254-0
9. Zhang G, Wang L, Cui H, Wang X, Zhang G, Ma J, et al. Anti-melanoma activity of T cells redirected with a TCR-like chimeric antigen receptor. Sci Rep (2014) 4:3571. doi: 10.1038/srep03571
10. Hudecek M, Sommermeyer D, Kosasih PL, Silva-Benedict A, Liu L, Rader C, et al. The nonsignaling extracellular spacer domain of chimeric antigen receptors is decisive for in vivo antitumor activity. Cancer Immunol Res (2015) 3(2):125–35. doi: 10.1158/2326-6066.CIR-14-0127
11. Bridgeman JS, Hawkins RE, Bagley S, Blaylock M, Holland M, Gilham DE. The optimal antigen response of chimeric antigen receptors harboring the CD3zeta transmembrane domain is dependent upon incorporation of the receptor into the endogenous TCR/CD3 complex. J Immunol (2010) 184(12):6938–49. doi: 10.4049/jimmunol.0901766
12. Guedan S, Posey AD Jr, Shaw C, Wing A, Da T, Patel PR, et al. Enhancing CAR T cell persistence through ICOS and 4-1BB costimulation. JCI Insight (2018) 3(1):e96976. doi: 10.1172/jci.insight.96976
13. Zhang C, Liu J, Zhong JF, Zhang X. Engineering CAR-T cells. biomark Res (2017) 5:22. doi: 10.1186/s40364-017-0102-y
14. Stancovski I, Schindler DG, Waks T, Yarden Y, Sela M, Eshhar Z. Targeting of T lymphocytes to Neu/HER2-expressing cells using chimeric single chain fv receptors. J Immunol (1993) 151(11):6577–82.
15. Hwu P, Shafer GE, Treisman J, Schindler DG, Gross G, Cowherd R, et al. Lysis of ovarian cancer cells by human lymphocytes redirected with a chimeric gene composed of an antibody variable region and the fc receptor gamma chain. J Exp Med (1993) 178(1):361–6. doi: 10.1084/jem.178.1.361
16. Hwu P, Yang JC, Cowherd R, Treisman J, Shafer GE, Eshhar Z, et al. In vivo antitumor activity of T cells redirected with chimeric antibody/T-cell receptor genes. Cancer Res (1995) 55(15):3369–73.
17. Thistlethwaite FC, Gilham DE, Guest RD, Rothwell DG, Pillai M, Burt DJ, et al. The clinical efficacy of first-generation carcinoembryonic antigen (CEACAM5)-specific CAR T cells is limited by poor persistence and transient pre-conditioning-dependent respiratory toxicity. Cancer Immunol Immunother (2017) 66(11):1425–36. doi: 10.1007/s00262-017-2034-7
18. Subklewe M, von Bergwelt-Baildon M, Humpe A. Chimeric antigen receptor T cells: A race to revolutionize cancer therapy. Transfus. Med Hemother. (2019) 46(1):15–24. doi: 10.1159/000496870
19. Huang R, Li X, He Y, Zhu W, Gao L, Liu Y, et al. Recent advances in CAR-T cell engineering. J Hematol Oncol (2020) 13(1):86. doi: 10.1186/s13045-020-00910-5
20. Tokarew N, Ogonek J, Endres S, von Bergwelt-Baildon M, Kobold S. Teaching an old dog new tricks: next-generation CAR T cells. Br J Cancer (2019) 120(1):26–37. doi: 10.1038/s41416-018-0325-1
21. Yeku OO, Brentjens RJ. Armored CAR T-cells: utilizing cytokines and pro-inflammatory ligands to enhance CAR T-cell anti-tumour efficacy. Biochem Soc Trans (2016) 44(2):412–8. doi: 10.1042/BST20150291
22. Wu Y, Liu Y, Huang Z, Wang X, Jin Z, Li J, et al. Control of the activity of CAR-T cells within tumours via focused ultrasound. Nat BioMed Eng (2021) 5(11):1336–47. doi: 10.1038/s41551-021-00779-w
23. Porter DL, Levine BL, Kalos M, Bagg A, June CH. Chimeric antigen receptor-modified T cells in chronic lymphoid leukemia. N Engl J Med (2011) 365(8):725–33. doi: 10.1056/NEJMoa1103849
24. Maude SL, Frey N, Shaw PA, Aplenc R, Barrett DM, Bunin NJ, et al. Chimeric antigen receptor T cells for sustained remissions in leukemia. N Engl J Med (2014) 371(16):1507–17. doi: 10.1056/NEJMoa1407222
25. Jelski W, Mroczko B. Biochemical markers of colorectal cancer - present and future. Cancer Manag. Res (2020) 12:4789–97. doi: 10.2147/CMAR.S253369
26. Fan J, Das JK, Xiong X, Chen H, Song J. Development of CAR-T cell persistence in adoptive immunotherapy of solid tumors. Front Oncol (2020) 10:574860. doi: 10.3389/fonc.2020.574860
27. Hombach AA, Rappl G, Abken H. Blocking CD30 on T cells by a dual specific CAR for CD30 and colon cancer antigens improves the CAR T cell response against CD30(-) tumors. Mol Ther (2019) 27(10):1825–35. doi: 10.1016/j.ymthe.2019.06.007
28. Chi X, Yang P, Zhang E, Gu J, Xu H, Li M, et al. Significantly increased anti-tumor activity of carcinoembryonic antigen-specific chimeric antigen receptor T cells in combination with recombinant human IL-12. Cancer Med (2019) 8(10):4753–65. doi: 10.1002/cam4.2361
29. Hassan R, Thomas A, Alewine C, Le DT, Jaffee EM, Pastan I. Mesothelin immunotherapy for cancer: Ready for prime time? J Clin Oncol (2016) 34(34):4171–9. doi: 10.1200/JCO.2016.68.3672
30. Morello A, Sadelain M, Adusumilli PS. Mesothelin-targeted CARs: Driving T cells to solid tumors. Cancer Discovery (2016) 6(2):133–46. doi: 10.1158/2159-8290.CD-15-0583
31. Liang Z, Dong J, Yang N, Li SD, Yang ZY, Huang R, et al. Tandem CAR-T cells targeting FOLR1 and MSLN enhance the antitumor effects in ovarian cancer. Int J Biol Sci (2021) 17(15):4365–76. doi: 10.7150/ijbs.63181
32. Schoutrop E, Renken S, Micallef Nilsson I, Hahn P, Poiret T, Kiessling R, et al. Trogocytosis and fratricide killing impede MSLN-directed CAR T cell functionality. Oncoimmunology (2022) 11(1):2093426. doi: 10.1080/2162402X.2022.2093426
33. Zhang Q, Liu G, Liu J, Yang M, Fu J, Liu G, et al. The antitumor capacity of mesothelin-CAR-T cells in targeting solid tumors in mice. Mol Ther Oncolytics (2021) 20:556–68. doi: 10.1016/j.omto.2021.02.013
34. Yarla NS, Gali H, Pathuri G, Smriti S, Farooqui M, Panneerselvam J, et al. Targeting the paracrine hormone-dependent guanylate cyclase/cGMP/phosphodiesterases signaling pathway for colorectal cancer prevention. Semin Cancer Biol (2019) 56:168–74. doi: 10.1016/j.semcancer.2018.08.011
35. Entezari AA, Snook AE, Waldman SA. Guanylyl cyclase 2C (GUCY2C) in gastrointestinal cancers: recent innovations and therapeutic potential. Expert Opin Ther Targets (2021) 25(5):335–46. doi: 10.1080/14728222.2021.1937124
36. Birbe R, Palazzo JP, Walters R, Weinberg D, Schulz S, Waldman SA. Guanylyl cyclase c is a marker of intestinal metaplasia, dysplasia, and adenocarcinoma of the gastrointestinal tract. Hum Pathol (2005) 36(2):170–9. doi: 10.1016/j.humpath.2004.12.002
37. Magee MS, Abraham TS, Baybutt TR, Flickinger JC Jr, Ridge NA, Marszalowicz GP, et al. Human GUCY2C-targeted chimeric antigen receptor (CAR)-expressing T cells eliminate colorectal cancer metastases. Cancer Immunol Res (2018) 6(5):509–16. doi: 10.1158/2326-6066.CIR-16-0362
38. Herlyn D, Herlyn M, Steplewski Z, Koprowski H. Monoclonal antibodies in cell-mediated cytotoxicity against human melanoma and colorectal carcinoma. Eur J Immunol (1979) 9(8):657–9. doi: 10.1002/eji.1830090817
39. Liang KH, Tso HC, Hung SH, Kuan II, Lai JK, Ke FY, et al. Extracellular domain of EpCAM enhances tumor progression through EGFR signaling in colon cancer cells. Cancer Lett (2018) 433:165–75. doi: 10.1016/j.canlet.2018.06.040
40. Feng M, Jin JQ, Xia L, Xiao T, Mei S, Wang X, et al. Pharmacological inhibition of β-catenin/BCL9 interaction overcomes resistance to immune checkpoint blockades by modulating t(reg) cells. Sci Adv (2019) 5(5):eaau5240. doi: 10.1126/sciadv.aau5240
41. La Salvia A, Lopez-Gomez V, Garcia-Carbonero R. HER2-targeted therapy: an emerging strategy in advanced colorectal cancer. Expert Opin Investig Drugs (2019) 28(1):29–38. doi: 10.1080/13543784.2019.1555583
42. Xu J, Meng Q, Sun H, Zhang X, Yun J, Li B, et al. HER2-specific chimeric antigen receptor-T cells for targeted therapy of metastatic colorectal cancer. Cell Death Dis (2021) 12(12):1109. doi: 10.1038/s41419-021-04100-0
43. Sureban SM, May R, Mondalek FG, Qu D, Ponnurangam S, Pantazis P, et al. Nanoparticle-based delivery of siDCAMKL-1 increases microRNA-144 and inhibits colorectal cancer tumor growth via a notch-1 dependent mechanism. J Nanobiotechno. (2011) 9:40. doi: 10.1186/1477-3155-9-40
44. Kim JH, Park SY, Jeon SE, Choi JH, Lee CJ, Jang TY, et al. DCLK1 promotes colorectal cancer stemness and aggressiveness via the XRCC5/COX2 axis. Theranostics (2022) 12(12):5258–71. doi: 10.7150/thno.72037
45. Sureban SM, Berahovich R, Zhou H, Xu S, Wu L, Ding K, et al. DCLK1 monoclonal antibody-based CAR-T cells as a novel treatment strategy against human colorectal cancers. Cancers (Basel) (2019) 12(1):54. doi: 10.3390/cancers12010054
46. Venuprasad K. Cbl-b and itch: Key regulators of peripheral T-cell tolerance. Cancer Res (2010) 70(8):3009–12. doi: 10.1158/0008-5472.CAN-09-4076
47. Kumar J, Kumar R, Kumar Singh A, Tsakem EL, Kathania M, Riese MJ, et al. Deletion of cbl-b inhibits CD8(+) T-cell exhaustion and promotes CAR T-cell function. J Immunother Cancer (2021) 9(1):e001688. doi: 10.1136/jitc-2020-001688
48. Klemm F, Joyce JA. Microenvironmental regulation of therapeutic response in cancer. Trends Cell Biol (2015) 25(4):198–213. doi: 10.1016/j.tcb.2014.11.006
49. Tang H, Qiao J, Fu YX. Immunotherapy and tumor microenvironment. Cancer Lett (2016) 370(1):85–90. doi: 10.1016/j.canlet.2015.10.009
50. Li F, Zhang R, Li S, Liu J. IDO1: An important immunotherapy target in cancer treatment. Int Immunopharmacol. (2017) 47:70–7. doi: 10.1016/j.intimp.2017.03.024
51. Pardoll DM. The blockade of immune checkpoints in cancer immunotherapy. Nat Rev Cancer (2012) 12(4):252–64. doi: 10.1038/nrc3239
52. Iwai Y, Ishida M, Tanaka Y, Okazaki T, Honjo T, Minato N. Involvement of PD-L1 on tumor cells in the escape from host immune system and tumor immunotherapy by PD-L1 blockade. Proc Natl Acad Sci U.S.A. (2002) 99(19):12293–7. doi: 10.1073/pnas.192461099
53. Okazaki T, Honjo T. PD-1 and PD-1 ligands: from discovery to clinical application. Int Immunol (2007) 19(7):813–24. doi: 10.1093/intimm/dxm057
54. Jiang X, Wang J, Deng X, Xiong F, Ge J, Xiang B, et al. Role of the tumor microenvironment in PD-L1/PD-1-mediated tumor immune escape. Mol Cancer (2019) 18(1):10. doi: 10.1186/s12943-018-0928-4
55. Ma S, Li X, Wang X, Cheng L, Li Z, Zhang C, et al. Current progress in CAR-T cell therapy for solid tumors. Int J Biol Sci (2019) 15(12):2548–60. doi: 10.7150/ijbs.34213
56. Ghorashian S, Kramer AM, Onuoha S, Wright G, Bartram J, Richardson R, et al. Enhanced CAR T cell expansion and prolonged persistence in pediatric patients with ALL treated with a low-affinity CD19 CAR. Nat Med (2019) 25(9):1408–14. doi: 10.1038/s41591-019-0549-5
57. Weinkove R, George P, Dasyam N, McLellan AD. Selecting costimulatory domains for chimeric antigen receptors: functional and clinical considerations. Clin Transl Immunol (2019) 8(5):e1049. doi: 10.1002/cti2.1049
58. Haabeth OA, Lorvik KB, Hammarström C, Donaldson IM, Haraldsen G, Bogen B, et al. Inflammation driven by tumour-specific Th1 cells protects against b-cell cancer. Nat Commun (2011) 2:240. doi: 10.1038/ncomms1239
59. Tang N, Cheng C, Zhang X, Qiao M, Li N, Mu W, et al. TGF-β inhibition via CRISPR promotes the long-term efficacy of CAR T cells against solid tumors. JCI Insight (2020) 5(4):e133977. doi: 10.1172/jci.insight.133977
60. Li N, Tang N, Cheng C, Hu T, Wei X, Han W, et al. Improving the anti-solid tumor efficacy of CAR-T cells by inhibiting adenosine signaling pathway. Oncoimmunology (2020) 9(1):1824643. doi: 10.1080/2162402X.2020.1824643
61. Calcinotto A, Filipazzi P, Grioni M, Iero M, De Milito A, Ricupito A, et al. Modulation of microenvironment acidity reverses anergy in human and murine tumor-infiltrating T lymphocytes. Cancer Res (2012) 72(11):2746–56. doi: 10.1158/0008-5472.CAN-11-1272
62. Lotfinejad P, Kazemi T, Mokhtarzadeh A, Shanehbandi D, Jadidi Niaragh F, Safaei S, et al. PD-1/PD-L1 axis importance and tumor microenvironment immune cells. Life Sci (2020) 259:118297. doi: 10.1016/j.lfs.2020.118297
63. Kallies A, Xin A, Belz GT, Nutt SL. Blimp-1 transcription factor is required for the differentiation of effector CD8(+) T cells and memory responses. Immunity (2009) 31(2):283–95. doi: 10.1016/j.immuni.2009.06.021
64. Joshi NS, Cui W, Chandele A, Lee HK, Urso DR, Hagman J, et al. Inflammation directs memory precursor and short-lived effector CD8(+) T cell fates via the graded expression of T-bet transcription factor. Immunity (2007) 27(2):281–95. doi: 10.1016/j.immuni.2007.07.010
65. Hess Michelini R, Doedens AL, Goldrath AW, Hedrick SM. Differentiation of CD8 memory T cells depends on Foxo1. J Exp Med (2013) 210(6):1189–200. doi: 10.1084/jem.20130392
66. Vignali D, Kallikourdis M. Improving homing in T cell therapy. Cytokine Growth Factor Rev (2017) 36:107–16. doi: 10.1016/j.cytogfr.2017.06.009
67. Hanahan D, Coussens LM. Accessories to the crime: functions of cells recruited to the tumor microenvironment. Cancer Cell (2012) 21(3):309–22. doi: 10.1016/j.ccr.2012.02.022
68. Moon EK, Wang LS, Bekdache K, Lynn RC, Lo A, Thorne SH, et al. Intra-tumoral delivery of CXCL11 via a vaccinia virus, but not by modified T cells, enhances the efficacy of adoptive T cell therapy and vaccines. Oncoimmunology (2018) 7(3):e1395997. doi: 10.1080/2162402X.2017.1395997
69. Romero JM, Grünwald B, Jang GH, Bavi PP, Jhaveri A, Masoomian M, et al. A four-chemokine signature is associated with a T-cell-Inflamed phenotype in primary and metastatic pancreatic cancer. Clin Cancer Res (2020) 26(8):1997–2010. doi: 10.1158/1078-0432.CCR-19-2803
70. Luo H, Su J, Sun R, Sun Y, Wang Y, Dong Y, et al. Coexpression of IL7 and CCL21 increases efficacy of CAR-T cells in solid tumors without requiring preconditioned lymphodepletion. Clin Cancer Res (2020) 26(20):5494–505. doi: 10.1158/1078-0432.CCR-20-0777
71. Kueberuwa G, Su J, Sun R, Sun Y, Wang Y, Dong Y, et al. CD19 CAR T cells expressing IL-12 eradicate lymphoma in fully lymphoreplete mice through induction of host immunity. Mol Ther Oncolytics (2018) 8:41–51. doi: 10.1016/j.omto.2017.12.003
72. Melzer C, Yang Y, Hass R. Interaction of MSC with tumor cells. Cell Commun Signal (2016) 14(1):20. doi: 10.1186/s12964-016-0143-0
73. Relation T, Yi T, Guess AJ, La Perle K, Otsuru S, Hasgur S, et al. Intratumoral delivery of interferonγ-secreting mesenchymal stromal cells repolarizes tumor-associated macrophages and suppresses neuroblastoma proliferation in vivo. Stem Cells (2018) 36(6):915–24. doi: 10.1002/stem.2801
74. Hombach AA, Geumann U, Günther C, Hermann FG, Abken H. IL7-IL12 engineered mesenchymal stem cells (MSCs) improve a CAR T cell attack against colorectal cancer cells. Cells (2020) 9(4):873. doi: 10.3390/cells9040873
75. Brandacher G, Perathoner A, Ladurner R, Schneeberger S, Obrist P, Winkler C, et al. Prognostic value of indoleamine 2,3-dioxygenase expression in colorectal cancer: effect on tumor-infiltrating T cells. Clin Cancer Res (2006) 12(4):1144–51. doi: 10.1158/1078-0432.CCR-05-1966
76. Zhang W, Mao S, Shi D, Zhang J, Zhang Z, Guo Y, et al. MicroRNA-153 decreases tryptophan catabolism and inhibits angiogenesis in bladder cancer by targeting indoleamine 2,3-dioxygenase 1. Front Oncol (2019) 9:619. doi: 10.3389/fonc.2019.00619
77. Huang Q, Xia J, Wang L, Wang X, Ma X, Deng Q, et al. miR-153 suppresses IDO1 expression and enhances CAR T cell immunotherapy. J Hematol Oncol (2018) 11(1):58. doi: 10.1186/s13045-018-0600-x
78. Del Prete G, De Carli M, D'Elios MM, Daniel KC, Almerigogna F, Alderson M, et al. CD30-mediated signaling promotes the development of human T helper type 2-like T cells. J Exp Med (1995) 182(6):1655–61. doi: 10.1084/jem.182.6.1655
79. Saraiva M, Vieira P, O’Garra A. Biology and therapeutic potential of interleukin-10. J Exp Med (2020) 217(1):e20190418. doi: 10.1084/jem.20190418
80. Ruffell B, Chang-Strachan D, Chan V, Rosenbusch A, Ho CM, Pryer N, et al. Macrophage IL-10 blocks CD8+ T cell-dependent responses to chemotherapy by suppressing IL-12 expression in intratumoral dendritic cells. Cancer Cell (2014) 26(5):623–37. doi: 10.1016/j.ccell.2014.09.006
81. Sullivan KM, Jiang X, Guha P, Lausted C, Carter JA, Hsu C, et al. Blockade of interleukin 10 potentiates antitumour immune function in human colorectal cancer liver metastases. Gut (2022) Jun 15:gutjnl-2021-325808. doi: 10.1136/gutjnl-2021-325808
82. Guo F, Das JK, Kobayashi KS, Qin QM, T AF, Alaniz RC, et al. Live attenuated bacterium limits cancer resistance to CAR-T therapy by remodeling the tumor microenvironment. J Immunother Cancer (2022) 10(1):e003760. doi: 10.1136/jitc-2021-003760
83. Chen Y, Yan SM, Pu Z, Feng J, Tan L, Li Y, et al. Dopamine signaling promotes tissue-resident memory differentiation of CD8+ T cells and antitumor immunity. Cancer Res (2022) 82(17):3130–42. doi: 10.1158/0008-5472.CAN-21-4084
84. Chen N, Morello A, Tano Z, Adusumilli PS. CAR T-cell intrinsic PD-1 checkpoint blockade: A two-in-one approach for solid tumor immunotherapy. Oncoimmunology (2017) 6(2):e1273302. doi: 10.1080/2162402X.2016.1273302
85. Chen C, Gu YM, Zhang F, Zhang ZC, Zhang YT, He YD, et al. Construction of PD1/CD28 chimeric-switch receptor enhances anti-tumor ability of c-met CAR-T in gastric cancer. Oncoimmunology (2021) 10(1):1901434. doi: 10.1080/2162402X.2021.1901434
86. Hu W, Zi Z, Jin Y, Li G, Shao K, Cai Q, et al. CRISPR/Cas9-mediated PD-1 disruption enhances human mesothelin-targeted CAR T cell effector functions. Cancer Immunol Immunother (2019) 68(3):365–77. doi: 10.1007/s00262-018-2281-2
87. Nakazawa T, Natsume A, Nishimura F, Morimoto T, Matsuda R, Nakamura M, et al. Effect of CRISPR/Cas9-mediated PD-1-Disrupted primary human third-generation CAR-T cells targeting EGFRvIII on in vitro human glioblastoma cell growth. Cells (2020) 9(4):998. doi: 10.3390/cells9040998
88. Choi BD, Yu X, Castano AP, Darr H, Henderson DB, Bouffard AA, et al. CRISPR-Cas9 disruption of PD-1 enhances activity of universal EGFRvIII CAR T cells in a preclinical model of human glioblastoma. J Immunother Cancer (2019) 7(1):304. doi: 10.1186/s40425-019-0806-7
89. Ren J, Zhao Y. Advancing chimeric antigen receptor T cell therapy with CRISPR/Cas9. Protein Cell (2017) 8(9):634–43. doi: 10.1007/s13238-017-0410-x
90. Jiang G, Ng YY, Tay JCK, Du Z, Xiao L, Wang S, et al. Dual CAR-T cells to treat cancers co-expressing NKG2D and PD1 ligands in xenograft models of peritoneal metastasis. Cancer Immunol Immunother (2022). doi: 10.1007/s00262-022-03247-9
91. Raj D, Yang MH, Rodgers D, Hampton EN, Begum J, Mustafa A, et al. Switchable CAR-T cells mediate remission in metastatic pancreatic ductal adenocarcinoma. Gut (2019) 68(6):1052–64. doi: 10.1136/gutjnl-2018-316595
92. Hernandez R, LaPorte KM, Hsiung S, Santos Savio A, Malek TR. High-dose IL-2/CD25 fusion protein amplifies vaccine-induced CD4(+) and CD8(+) neoantigen-specific T cells to promote antitumor immunity. J Immunother Cancer (2021) 9(9):e002865. doi: 10.1136/jitc-2021-002865
93. Lee JY, Nguyen B, Mukhopadhyay A, Han M, Zhang J, Gujar R, et al. Amplification of the CXCR3/CXCL9 axis via intratumoral electroporation of plasmid CXCL9 synergizes with plasmid IL-12 therapy to elicit robust anti-tumor immunity. Mol Ther Oncolytics (2022) 25:174–88. doi: 10.1016/j.omto.2022.04.005
94. Watkinson F, Nayar SK, Rani A, Sakellariou CA, Elhage O, Papaevangelou E, et al. IL-15 upregulates telomerase expression and potently increases proliferative capacity of NK, NKT-like, and CD8 T cells. Front Immunol (2020) 11:594620. doi: 10.3389/fimmu.2020.594620
95. Li W, Zhou Y, Wu Z, Shi Y, Tian E, Zhu Y, et al. Targeting wnt signaling in the tumor immune microenvironment to enhancing EpCAM CAR T-cell therapy. Front Pharmacol (2021) 12:724306. doi: 10.3389/fphar.2021.724306
96. Schmohl JU, Vallera DA. CD133, selectively targeting the root of cancer. Toxins (Basel) (2016) 8(6):165. doi: 10.3390/toxins8060165
97. Wang Y, Chen M, Wu Z, Tong C, Dai H, Guo Y, et al. CD133-directed CAR T cells for advanced metastasis malignancies: A phase I trial. Oncoimmunology (2018) 7(7):e1440169. doi: 10.1080/2162402X.2018.1440169
98. Bai Y, Kan S, Zhou S, Wang Y, Xu J, Cooke JP, et al. Enhancement of the in vivo persistence and antitumor efficacy of CD19 chimeric antigen receptor T cells through the delivery of modified TERT mRNA. Cell Discov (2015) 1:15040. doi: 10.1038/celldisc.2015.40
99. Long AH, Haso WM, Shern JF, Wanhainen KM, Murgai M, Ingaramo M, et al. 4-1BB costimulation ameliorates T cell exhaustion induced by tonic signaling of chimeric antigen receptors. Nat Med (2015) 21(6):581–90. doi: 10.1038/nm.3838
100. Zhang ZZ, Wang T, Wang XF, Zhang YQ, Song SX, Ma CQ. Improving the ability of CAR-T cells to hit solid tumors: Challenges and strategies. Pharmacol Res (2022) 175:106036. doi: 10.1016/j.phrs.2021.106036
101. Vincent L, Kermani P, Young LM, Cheng J, Zhang F, Shido K, et al. Combretastatin A4 phosphate induces rapid regression of tumor neovessels and growth through interference with vascular endothelial-cadherin signaling. J Clin Invest (2005) 115(11):2992–3006. doi: 10.1172/JCI24586
102. Deng C, Zhao J, Zhou S, Dong J, Cao J, Gao J, et al. The vascular disrupting agent CA4P improves the antitumor efficacy of CAR-T cells in preclinical models of solid human tumors. Mol Ther (2020) 28(1):75–88. doi: 10.1016/j.ymthe.2019.10.010
103. Ferrara N, Gerber HP, LeCouter J. The biology of VEGF and its receptors. Nat Med (2003) 9(6):669–76. doi: 10.1038/nm0603-669
104. Xing H, Yang X, Xu Y, Tang K, Tian Z, Chen Z, et al. Anti-tumor effects of vascular endothelial growth factor/vascular endothelial growth factor receptor binding domain-modified chimeric antigen receptor T cells. Cytotherapy (2021) 23(9):810–9. doi: 10.1016/j.jcyt.2021.05.008
105. Kalluri R, Zeisberg M. Fibroblasts in cancer. Nat Rev Cancer (2006) 6(5):392–401. doi: 10.1038/nrc1877
106. Yang X, Lin Y, Shi Y, Li B, Liu W, Yin W, et al. FAP promotes immunosuppression by cancer-associated fibroblasts in the tumor microenvironment via STAT3-CCL2 signaling. Cancer Res (2016) 76(14):4124–35. doi: 10.1158/0008-5472.CAN-15-2973
107. Ebert LM, Yu W, Gargett T, Toubia J, Kollis PM, Tea MN, et al. Endothelial, pericyte and tumor cell expression in glioblastoma identifies fibroblast activation protein (FAP) as an excellent target for immunotherapy. Clin Transl Immunol (2020) 9(10):e1191. doi: 10.1002/cti2.1191
108. Hiltbrunner S, Britschgi C, Schuberth P, Bankel L, Nguyen-Kim TDL, Gulati P, et al. Local delivery of CAR T cells targeting fibroblast activation protein is safe in patients with pleural mesothelioma: first report of FAPME, a phase I clinical trial. Ann Oncol (2021) 32(1):120–1. doi: 10.1016/j.annonc.2020.10.474
109. Grasso CS, Giannakis M, Wells DK, Hamada T, Mu XJ, Quist M, et al. Genetic mechanisms of immune evasion in colorectal cancer. Cancer Discovery (2018) 8(6):730–49. doi: 10.1158/2159-8290.CD-17-1327
110. Katz SC, Hardaway J, Prince E, Guha P, Cunetta M, Moody A, et al. HITM-SIR: phase ib trial of intraarterial chimeric antigen receptor T-cell therapy and selective internal radiation therapy for CEA(+) liver metastases. Cancer Gene Ther (2020) 27(5):341–55. doi: 10.1038/s41417-019-0104-z
111. Morris EC, Neelapu SS, Giavridis T, Sadelain M. Cytokine release syndrome and associated neurotoxicity in cancer immunotherapy. Nat Rev Immunol (2022) 22(2):85–96. doi: 10.1038/s41577-021-00547-6
Keywords: colorectal cancer, CAR-T cells, antigen, immunotherapy, cell therapy
Citation: Qin X, Wu F, Chen C and Li Q (2022) Recent advances in CAR-T cells therapy for colorectal cancer. Front. Immunol. 13:904137. doi: 10.3389/fimmu.2022.904137
Received: 25 March 2022; Accepted: 09 September 2022;
Published: 27 September 2022.
Edited by:
John Maher, King’s College London, United KingdomReviewed by:
Vita Golubovskaya, ProMab Biotechnologies, United StatesAndrei Havasi, Oncology Institute Prof. Dr. Ion Chiricuta, Romania
Copyright © 2022 Qin, Wu, Chen and Li. This is an open-access article distributed under the terms of the Creative Commons Attribution License (CC BY). The use, distribution or reproduction in other forums is permitted, provided the original author(s) and the copyright owner(s) are credited and that the original publication in this journal is cited, in accordance with accepted academic practice. No use, distribution or reproduction is permitted which does not comply with these terms.
*Correspondence: Qi Li, 529016822@qq.com; Chang Chen, chen213chang@163.com