- 1Department of Allergy and Rheumatology, Graduate School of Medicine, The University of Tokyo, Bunkyo-ku, Japan
- 2Department of Medicine and Rheumatology, Tokyo Metropolitan Geriatric Hospital, Itabashi-ku, Japan
- 3Department of Functional Genomics and Immunological Diseases, Graduate School of Medicine, The University of Tokyo, Bunkyo-ku, Japan
Systemic sclerosis (SSc) is an autoimmune disease that is characterized by vascular damage and fibrosis. Both clinical manifestations and immunological disturbances are diverse according to the disease duration. Particularly, changes in immunological processes are prominent in the early phase of SSc. The orchestration of several subsets of immune cells promotes autoimmune responses and inflammation, and eventually stimulates pro-fibrotic processes. Many reports have indicated that CD4+ T cells play pivotal roles in pathogenesis in the early phase of SSc. In particular, the pathogenic roles of regulatory T (Treg) cells have been investigated. Although the results were controversial, recent reports suggested an increase of Treg cells in the early phase of SSc patients. Treg cells secrete transforming growth factor-β (TGF-β), which promotes myofibroblast activation and fibrosis. In addition, the dysfunction of Treg cells in the early phase of SSc was reported, which results in the development of autoimmunity and inflammation. Notably, Treg cells have the plasticity to convert to T-helper17 (Th17) cells under pro-inflammatory conditions. Th17 cells secrete IL-17A, which could also promote myofibroblast transformation and fibrosis and contributes to vasculopathy, although the issue is still controversial. Our recent transcriptomic comparison between the early and late phases of SSc revealed a clear difference of gene expression patterns only in Treg cells. The gene signature of an activated Treg cell subpopulation was expanded in the early phase of SSc and the oxidative phosphorylation pathway was enhanced, which can promote Th17 differentiation. And this result was accompanied by the increase in Th17 cells frequency. Therefore, an imbalance between Treg and Th17 cells could also have an important role in the pathogenesis of the early phase of SSc. In this review, we outlined the roles of Treg cells in the early phase of SSc, summarizing the data of both human and mouse models. The contributions of Treg cells to autoimmunity, vasculopathy, and fibrosis were revealed, based on the dysfunction and imbalance of Treg cells. We also referred to the potential development in treatment strategies in SSc.
Introduction
Systemic sclerosis (SSc) is a connective tissue disease which is characterized by the combination of immunological disturbances, impaired peripheral circulation, and fibrotic features, such as skin and lung fibrosis (1). Advanced fibrosis causes lethal organ dysfunction, such as heart and respiratory failure. Various approaches have been taken for treatment, including immunosuppressive reagents, vasodilation, and antifibrotic therapy (2, 3). These approaches have gradually improved the survival rate of SSc patients. Despite the success of these approaches, the 5-year-survival of diffuse cutaneous SSc, which involves systemic organs, remains relatively low at 85.5%. Therefore, SSc is an intractable disease with a high lethality rate compared to other connective tissue diseases (4).
SSc patients present with various clinical manifestations depending on their disease stages. For example, Raynaud’s phenomenon, suggesting a peripheral circulation disorder and microangiopathy, is a relatively early clinical manifestation of SSc (1). The definition of “the early disease phase” varies among reports. Typically, less than five years at longest (5, 6), and less than three years of disease duration represents the early phase, especially in diffuse cutaneous SSc (7, 8). A majority of SSc patients experience peripheral circulation disorders in the early phase of their disease courses. In particular, Raynaud’s phenomenon (RP) and nailfold capillary abnormalities are characteristic features of peripheral circulation disorders in the early phase of SSc (1, 9, 10), and are caused by vascular damage and dysfunction. Nailfold videocapillaroscopy (NVC) abnormality is another feature of peripheral circulation disorders and is easily observed in the very early phase of SSc. Abnormalities of nailfold capillaries, such as enlargement, bleeding, and stenosis, suggest vascular dysfunction and damage. These features are associated with a vasculopathy that is characterized by endothelial dysfunction and fibrotic proliferation (11). To diagnose very early SSc, predictors of developing definite SSc have been proposed. An international multicenter study on the very early diagnosis of SSc (VEDOSS) determined that disease-specific autoantibody and puffy fingers were independent parameters predicting the development of definite SSc. They also showed that puffy fingers have a positive predictive value (12). Bellando-Randone et al. additionally reported that the presence of one or two VEDOSS criteria (systemic sclerosis-specific autoantibodies, nailfold capillaroscopy abnormalities, and puffy fingers) in patients with Raynaud’s phenomenon confers a progressively higher risk for SSc over time (13).
The dysregulated activation of the immune system is observed from the early stage of the disease prior to fibrosis (14, 15). Several immune cell subsets, such as T cells, B cells, and macrophages, play pivotal roles in the pathogenesis of SSc. In recent years, the importance of CD4+ T cells, particularly regulatory T (Treg) cells, in the pathophysiology of SSc has emerged as an important player both in immune disorders and in the fibrotic pathogenesis of SSc. This paper focuses on the immunological features in the early phase of SSc and outlines the possible role of Treg cells in the pathophysiology of the early phase of SSc.
Immunological Features of SSc
The main pathological features of the early phase of SSc are microvascular dysfunction and autoimmunity (16, 17).
Even though the precise mechanisms remain unknown, exposure to environmental risk factors, such as toxins, irritants, and viral infections, would trigger endothelial and epithelial damage (18, 19). These stimulants induce both innate and adaptive immune system activation, and inflammation. Indeed, the tissue infiltration of immune cells, predominantly around blood vessels, is prominent in the early phase of SSc. Although these immune cells mainly consist of CD4+ dominant T cells and macrophages (20), the orchestration of many types of immune cells promotes tissue inflammation, autoimmune responses, tissue damage, and eventually fibrosis.
Monocyte/macrophage subsets play crucial roles in innate immunity, tissue repair, and fibrosis (21). In a previous report, we performed bulk and single-cell RNA-sequencing (scRNA-seq) of peripheral blood immune cells from SSc and identified a SSc-related inflammatory gene module and a cluster of monocytes, similar to lung-infiltrating FCN1hi monocytes expressing IL1B. This study included SSc patients with relatively long disease durations, therefore we estimated that monocyte/macrophage subsets were important for SSc pathogenesis during the disease course (22). On the other hand, monocyte/macrophage features that are prominent in the early disease phase of SSc were reported many years ago. York et al. reported that the expression of Siglec-1, an IFN-regulated marker, in circulating monocytes from early SSc and the number of Siglec-1+ cells in skin samples from early SSc were increased compared with healthy controls (HC) (23). Recently, Slaug et al. reported that M1 and M2 macrophage signatures were higher in early SSc than in late SSc (8).
Dendritic cells (DCs) are broadly classified as myeloid DC (mDC) and plasmacytoid DC (pDC). pDC secrete not only IFN but also cytokines and chemokines including CXCL4, which is an early SSc marker (24).
B cells have variable immune functions including the production of antibodies, antigen presentation, and cytokine production. Therefore, much research has focused on the role of B cells in SSc pathogenesis. Genome-wide association study (GWAS) identified several B cell-related genes, such as BLK, CSK, and PTPN22, as SSc susceptibility genes (25). Sato et al. reported that transgenic mice that overexpressed CD19 generated spontaneous autoantibodies, and that the CD19 density on blood B cells from SSc patients was significantly higher compared with HC. They implied that gene expression changes in B cells contributed to autoimmunity in SSc (26). Studies using SSc tissue samples, including lung and skin samples, have also been published. As for histopathological studies, Lafyatis et al. showed that the number of CD20+ cells was higher in the pulmonary tissues from patients with SSc-associated interstitial lung disease compared to controls (27). Bosselo et al. reported that the number of CD20+ cells was higher in clinically involved skin than in uninvolved skin, and there was a greater number of CD20+ cells in patients with early SSc compared with patients with long-standing disease (28). As described above, many types of immune cells play important roles in the pathogenesis of SSc. We intensively discuss the roles of CD4+ T cells in a later section.
Genetics and Immunology in SSc
Genetic studies suggested the primary role of the immune system in the pathogenesis of SSc. A GWAS identified risk genes that are associated with innate and acquired immunity. For exaple, IRF5, one of the transcription factors involved in interferon (IFN) signaling, was the first interferon regulatory factor (IRF) gene reported to be associated with SSc (29). TNFAIP3 encodes a ubiquitin-editing enzyme involved in the TNF-mediated immune response, and one SSc-associated genetic variant of TNFAIP3 was strongly associated with the silica-induced profibrotic response of fibroblasts (30). PTPN22 encodes the protein tyrosine phosphatase lymphoid tyrosine phosphatase, which is a critical gatekeeper of T-cell receptor (TCR) signaling. The PTPN22 R620W polymorphism influences SSc genetic susceptibility (31). Notably, autoimmune diseases, such as systemic lupus erythematosus (SLE) and rheumatoid arthritis, share the same immune-related risk genes (32, 33). In addition, genes associated with fibrosis and vascular homeostasis were also identified as risk genes of SSc. For example, DDX6 is a regulator of vascular endothelial growth factor (34, 35). In this way, genetic analysis suggested that the pathogenesis of SSc is characterized by the combination of immune system dysregulation, vascular homeostasis, and fibrotic processes.
As described above, many immune cell subsets contribute to the pathogenesis of SSc, especially in the early disease phase. However, López-Isac et al. evaluated cell type-specific enrichment of SSc risk loci in representative epigenetic marks and reported that SSc-associated variants displayed significant enrichment in a histone mark of active genes in primary T cells and Natural Killer T cells (34). Moreover, in SSc, tissue-infiltrating T cells are predominantly CD4+ T cells, which express activation markers and show oligoclonal expansion (36). Taking these past reports into account, we focused on the role of CD4+ T cells in the immune system in early SSc.
CD4+ T Cells in Early SSc
Many reports on CD4+ T cells in early SSc were published in this decade (7, 8, 37–53). Using skin samples from early SSc, Maehara et al. reported that microscopy and quantitative image analysis showed prominent infiltration of CD4+ cytotoxic T cells and an accumulation of apoptotic cells in the skin (37). The authors speculated that cytotoxic T cells might induce the apoptotic death of endothelial cells and contribute to vasculopathy in SSc. Skaug et al. revealed that immune cells (including CD4+ T cells) and fibroblast signatures positively correlated with the skin thickness and progression rate in early SSc by using transcriptomic analysis of the skin samples (8, 38). They also performed immunohistochemical staining and showed that most early diffuse cutaneous SSc patients with high baseline T cell and macrophage numbers had declines in these numbers at follow-up (8). Zhou et al. reported that quantitative reverse transcription PCR (RT-qPCR), immunohistochemistry (IHC), and Western blotting analysis showed the overexpression of T-helper17 (Th17)-related cytokines, such as IL-17A, in early SSc skin lesions. They reported that protein expression of IL-17A was positively correlated with the modified Rodnan skin score of SSc (39).
Studies with peripheral blood have yielded controversial results. Many reports have examined Treg cells. For example, Shah et al. reported that fluorescence-activated cell sorting (FACS) analysis of peripheral blood showed reductions in activated total T cells, CD4+ T cells, and FOXP3+CD25+ Treg cells, and a greater bias towards an IL4+ T helper 2 (Th2)/T cytotoxic 2 (Tc2) phenotype based on the ratio of Th2/Th1 CD4+ and Tc2/Tc1 CD8+ T cells (41). Kataoka et al. reported that FACS analysis of peripheral blood showed that the proportion of Foxp3+ cells among the total CD4+ T cells decreased in patients with either early- or late-stage SSc compared with HC (45). The authors implied that the lower expression level of Runx1 mRNA in purified Tregs in SSc was related to the frequency of Tregs in SSc. Conversely, several reports on early SSc revealed an increased proportion of Treg or Treg subpopulations (42–44), and some reports also showed an increased proportion of Th17 cells (42, 44).
The published reports on Treg cell and Th17 subsets of early SSc were listed in Table 1 (7, 39, 40, 42–46, 54–59). The majority of the reports indicated that the frequencies of Treg cells in the blood or the skin were increased in early SSc in comparison with those in late SSc or HC (40, 42, 43). In addition, some reports showed the decreased regulatory capacity in Treg from SSc (43, 58). In the case of Th17 cells, a number of reports suggested the frequencies of Th17 or the levels of Th17-related cytokines were higher in the blood or the skin from early SSc (7, 40, 42, 44, 59), even though some articles reported that those were comparable between early and late SSc (57).
Taken together, evidence has accumulated to indicate that CD4+ T cell subsets contribute to the pathogenesis of early SSc. In particular, Treg subsets have been considered in many past reports on early SSc in the context of the balance of other subsets, especially Th17 cells.
Th17 Cells and Plasticity of Treg Cells
Th17 cells are characterized by secretion of IL-17A and their physiological role is the immunological defense against bacteria and yeast. In the context of the pathogenesis of SSc, IL-17A induces the production of pro-inflammatory cytokines, including chemokine (C-C motif) ligand 2 (CCL2), IL-6, and IL-8, by fibroblasts in human skin and lung (60, 61). IL-17A also affects vascular dysfunction through microangiopathy in SSc (60, 62, 63). IL-17A activates vascular smooth muscle cells via the ERK 1/2 pathway and increases collagen synthesis. Moreover, IL-17A promotes the migration of dermal vascular smooth cells. Therefore, Th17 cells could play variable roles in inflammation, fibrosis, and vascular dysfunction in SSc.
The heterogeneity of Th17 subsets is often discussed in view of the plasticity of Treg cells. It means that Treg cells have the capacity to convert into other Th cell subsets, including Th17 cells, in response to cytokine stimulation around their circumstances (64, 65). Recently, the plasticity of T cells was hypothesized as one of the causes of autoimmune diseases. For example, in collagen-induced arthritis mice, an animal model of rheumatoid arthritis (RA), CD25lo Foxp3+ CD4+ T cells convert to Th17 cells, mediated by synovial fibroblast-derived IL-6 (66). These “exFoxp3 Th17 cells” accumulate in inflamed joints and have a strong ability to induce osteoclasts. IL-17+ Foxp3+ T cells are also detected in the synovium of people with active RA. As described in inflammatory arthritis, the plasticity of Tregs cells can be thought to contribute to the pathogenesis of many other autoimmune-mediated diseases.
CD4 + Treg Cells
CD4+ Treg cells were first reported in 1995 as a cell population characterized by the surface expression of CD25 with immune suppressive function in mice (67). Thereafter, the transcription factor Forkhead box P3 (FOXP3) was reported as a critical master regulator for the differentiation and inhibitory ability of Treg cells (68). In humans, CD4+ CD25+ FOXP3+ Treg cells were also identified as an immune regulatory population (69). In addition, CD45RA expression defined the subpopulation of human Treg cells, that is, CD45RA-FOXP3highCD4+ Treg cells, as activated Treg cells with high regulatory activity (70). Transcriptomic analysis, especially single cell analysis, can determine detailed subpopulations of Treg cells. Zemmour et al. performed scRNA-seq of human and mouse Tregs and revealed the similarity of these species and the presence of subpopulations in each Treg subset (71).
Recently, the metabolic dynamism of Treg cells was unveiled. The conventional effector T cells including pathogenic Th17 have enhanced glycolysis, whereas Tregs are reliant on mitochondrial metabolism and oxidative phosphorylation rather than on glycolysis for energy production (72). In addition, oxidative phosphorylation in Tregs enforces Treg regulatory functions (73). On the other hand, under specific conditions, the metabolic status does not work to support Treg function. Shin et al. reported that oxidative phosphorylation facilitated TCR signaling and promoted Th17 cell-development, but not Treg development, under the existence of IL-6, TGF-β, and IL-23 (Th17 conditions) (74).
The association between the dysfunction of Treg cells and human immune-related diseases has been reported. Loss-of-function variants in the human FOXP3 gene are known to cause IPEX (immune dysregulation, polyendocrinopathy, enteropathy, and X-linked) syndrome, which causes multiple autoimmune disorders due to Treg cell deficiency or dysfunction (75). Recently, anti-PD-1 and anti-CTLA-4 antibodies, which are immune checkpoint inhibitors, have been applied as therapeutic options for progressed malignancies. Treg cells suppress anti-tumor immunity in malignant tumors, and it is known that people with a large amount of Treg cell infiltration in the tumor region, such as people with gastric cancer and ovarian cancer, have a poor prognosis (76, 77). These antibodies promote anti-cancer immunological attack by suppression of Treg cell functions. Importantly, autoimmune diseases termed immune-related adverse events (irAEs) can develop because of treatment with immune checkpoint inhibitors. In addition, the important roles of Treg cells in suppressing the onset and progression of autoimmune diseases, for example, SLE, and fibrotic-related diseases have been reported (78, 79).
Treg cells in the pathophysiology of SSc
Although it has not yet been thoroughly studied, the pathogenesis of SSc is based on immunological abnormalities as described above. The contribution of Treg cells to the pathophysiology of SSc has been explained by several mechanisms. First, the suppressive function of Treg cells in SSc patients is limited, causing the breakdown of the immune response, chronic inflammation, and fibrosis. Treg cells release some inhibitory cytokines, such as interleukin (IL)-10, TGF-β, and IL-35. These inhibitory cytokines function as immunosuppressive factors in various ways, such as suppressing the activation and maturation of immune cells (80). Several reports have pointed out the possibility of decreased inhibitory ability of Treg cells in SSc patients due to the decreased production of TGF-β and IL-10 (81). A second point is the promotion of fibrosis by pro-fibrotic cytokines produced by Treg cells. For example, TGF-β contributes to fibrotic pathology through the proliferation of fibroblasts. TGF-β promotes collagen production and extracellular matrix secretion, and also induces the epithelial-mesenchymal transition (EMT) (82). Some reports have suggested that Treg cells differentiate into Th2-like cells in SSc, and promote fibrosis through the production of cytokines, such as IL-4 and IL-13 (83). In this way, Treg cells are thought to be associated with several aspects of immune dysregulation and fibrosis during SSc pathogenesis.
In terms of TGF-β, there are three isoforms and TGF-β1 isoform is the major type. There remains unclear the respective roles of each TGF-β isotype in the pathogenesis of SSc. According to the studies reported so far, the sources of each TGF-β isotype are various including several immune cells and fibroblast/myofibroblast, and the role of each isotype is different depending on each cell type. For example, while many past reports referred to the relation of TGF-β1 and Treg or SSc (82), a recent report showed the epigenetic activation of TGFB2 enhancer in the skin fibroblast from SSc (84). Of note, the TGF-β-measuring methods were different according to the past studies. That is, some reports showed the results of measured active TGF-β, and the others measured latent protein of TGF-β (82). Since TGF-β is secreted as the large latent complex, the results could differ depending on which types of proteins are targeted. That makes an issue complicated and must be organized.
In animal models of fibrosis and SSc, the pathophysiological roles of Treg cells have been investigated in detail. The topoisomerase-immunized mouse is one of the representative mouse models of SSc, and these SSc model mice showed increased production of IL-6, TGF-β1, and IL-17, and decreased production of IL-10 (85). In these mice, Th1, Th2, Th17, and Treg cells were increased in the bronchoalveolar lavage fluid of the fibrotic lungs. In the other model of SSc, mice hetero-deficient in the transcription factor Friend leukemia virus integration 1 (Fli1), it has been reported that the induction rate of Treg cells in the skin at 1 week after the completion of bleomycin administration is lower than that in wild-type mice, which may contribute to the exacerbation of skin fibrosis (86). There were also several reports describing Treg cells in the classical model of fibrosis, the bleomycin-induced pulmonary fibrosis model. Birjandi et al. reported that an increase of CD4+CD25highFoxP3+ Treg cells in the lung resulted in a shift to a type 2 immune response and the exacerbation of lung fibrosis (87). On the other hand, Kamio et al. reported that lung fibrosis was reduced by Treg cell transfer 14 days after bleomycin administration (88). In addition, Boveda-Ruiz et al. demonstrated that the prognosis improved in a bleomycin-induced pulmonary fibrosis model when Treg cells were removed in an early phase by anti-CD25 antibody administration. They also reported that lung fibrosis was exacerbated when Treg cells were removed in a late phase of the disease. These reports suggested that the pathological roles of Treg cells in lung fibrosis may differ depending on the stage of disease (89).
Imbalance of Treg Cells and Th17 Cells in SSc
As mentioned in the section of “Th17 cells and plasticity of Treg cells”, the plasticity of Treg cells and the imbalance of Treg cells and other subsets, especially Th17 cells, have been focused on in the pathogenesis of SSc (90). For example, Th17 cells produce the inflammatory cytokine IL-17A, which promotes the proliferation and migration of dermal vascular smooth muscle cells and is involved in the vasculopathy of SSc (91). It was also suggested that IL-17A contributes to the transformation of myofibroblasts and promotes skin and lung fibrosis (92). In the case of SSc, several reports proposed that some Treg cell populations were transformed into Th17 or Th17-like cells in SSc. Liu et al. reported that CD4+ CD25+ FOXP3lowCD45RA-T cells were increased in the peripheral blood of people with SSc and that this population of cells produced high levels of IL-17, like Th17 cells (93). In our previous report on FACS analysis of peripheral blood, the frequency of Th17 cells was increased in the early phase of SSc patients compared to the late phase of SSc and HC (42). According to the reports on the skin lesions in SSc, Yang et al. performed immunohistochemistry using skin samples from people with active SSc and revealed the increased infiltration of IL-17+ cells in the skin (40). Based on FACS analysis, they also reported that elevated percentages of circulating Th17 cells and Th17-derived IL-17A were involved in fibroblast growth and collagen production in SSc. Truchetet, et al. also showed that the numbers of IL-17A producing cells correlated with the expression of CCR6 and indicated that these cells were prone to be recruited into the inflamed target tissue, for example, the skin and the lung in SSc (94).
The imbalance between Treg and Th17 cells could also contribute to gastrointestinal disorders in SSc. Gastrointestinal disorders are a very frequent problem in SSc patients (95). Any area of the gastrointestinal tract can be damaged, and the esophagus is particularly vulnerable (96). Nicola et al. investigated the concentrations of Treg, Th17, and Th1-related cytokines in the digestive juices of SSc patients (97). They showed higher concentrations of IL-17A and lower concentrations of IL-10 and TGF-β in SSc patients with esophageal involvement. Interestingly, IL-17A concentrations were increased in minor inflammatory gastritis compared to those in advanced atrophic gastritis. These results suggested that the imbalance between Treg and Th17 cells contributes to the early phase of gastrointestinal disorders in SSc. The authors also suggested that the overproduction of IL-17A contributed to gastrointestinal fibrosis, especially in the early stage of the disease. Thus, Th17 cells are a major player in the pathogenesis of SSc, and the imbalance between Treg and Th17 cells could promote the pathogenic course of gastrointestinal diseases.
In the context of IL-17 and fibrosis, the results were still controversial. Some past reports showed that the modified Rodman skin thickness score (MRSS) tended to be lower in patients with elevated IL-17A than those without (98, 99). Truchetet, et al. reported that the numbers of IL-17A+ cells were increased in SSc, and they inversely correlated with the MRSS (100). In addition, Nakashima, et al. reported that IL-17A signaling had an antifibrogenic effect via the upregulation of miR-129-5p and the downregulation of connective tissue growth factor and α1(I) collagen (99). Some other reports also showed the anti-fibrotic effects of Th17 or IL-17A (100–103).
Even though, considering the imbalance of Treg and Th17 cells could be one of the major pathogenic processes in SSc, targeting Th17 cells and/or IL-17 will be a promising treatment strategy for SSc. In terms of the mice models of SSc, Okamoto, et al. found that IL-17A deficiency reduced skin fibrosis in the bleomycin-induced mice and the TSK-1 model (104). Park, et al. also reported on the efficacy of targeting IL-17 in bleomycin-induced mice and chronic graft-versus-host disease models (105). Using Secukinumab, a monoclonal antibody against IL-17A, Karatas, et al. showed the amelioration of bleomycin-induced dermal fibrosis in mice (106). In humans, an active but not recruiting phase III trial of brodalumab (AMG 827), a human, anti-IL17 receptor monoclonal antibody, for SSc (Clinicaltrials.gov identifier: NCT03957681) is undergoing. Although further data accumulation is needed, correction of the imbalance of Treg and Th17 cells could be a promising therapeutic strategy for SSc.
Treg Cells in Early SSc Patients
There have been many reports about the frequencies and absolute numbers of Treg cells in the peripheral blood and tissues of SSc patients. However, the results were inconsistent between the studies. For example, using immunostaining, Antiga et al. determined that FOXP3+ Treg cells were less infiltrated in SSc skin tissues than HC (107). At the same time, they reported that serum TGF-β and IL-10 concentrations and the frequencies of peripheral blood CD4+ CD25brightFOXP3+ cells were decreased in SSc patients (107). In contrast, Yang et al. reported that the numbers of FOXP3+ Treg cells increased in the skin tissue of people in the early phase of SSc compared to people in the late phase of SSc and HC. The authors proposed that this increase of Treg cells suggested a regulatory feedback reaction for the exacerbation of immune response in the onset of SSc (40). The differences in the definitions of Treg cells, such as the surface markers, could cause these controversial results. Additionally, some populations of CD4+ CD25+ cells secret pro-inflammatory cytokines, which could cause the inconsistent results of the previous reports about the frequencies and functions of Treg cells in SSc. Different backgrounds of SSc patients in the studies could also contribute to the variation in the results. In recent years, a meta-analysis of peripheral blood Treg cell/CD4+ T cell ratios was reported by Deng et al. They reported that there was no statistically significant difference between the SSc patients and HC (108). However, in view of a variety of stages and clinical phenotypes in SSc, such a meta-analysis would not lead to a meaningful result. Indeed, Frants et al. reported that Treg cells tended to be higher in people in the early phase of SSc (81), and the frequencies of Treg cells would depend on the phase of the disease. In recent studies, some reports also showed the increase of Treg subsets or Treg subpopulations in early SSc (42–44, 58), although some studies reported the decrease of Treg subsets in early SSc (41, 45).
In addition, many reports suggested the functional inability of peripheral blood Treg cells to suppress the immune response in early SSc. Ugor et al. reported that the production of immunosuppressive cytokines by Tregs was diminished in early SSc (43). Radstake et al. showed a lower immunosuppressive ability of SSc patient-derived Treg cells in both early and late SSc (58). In this way, the frequencies and functions of Treg cells in SSc remain controversial. As they would depend on the phases and clinical phenotypes of SSc, these points should be considered in understanding the roles of Treg cells in SSc.
Our team recently published a catalogue of gene expression in the 28 immune cells from people with 10 autoimmune diseases, including SSc, called ImmuNexUT (Immune Cell Gene Expression Atlas from the University of Tokyo) (109). In this study, a cross-sectional analysis of multiple autoimmune disorders revealed that the expression of IFN-signature genes was high in autoimmune diseases such as SLE, and that IL-18 or IL-1β signature was high in autoinflammatory diseases such as Behcet’s disease. Notably, the gene expression of SSc patients varied greatly among individuals. Some showed a transcriptome similar to an autoimmune disease, while others had a transcriptome that similar to an autoinflammatory disease. These results suggested that SSc patients were immunologically heterogeneous, and this heterogeneity may cause the difficulty of obtaining consistent results in SSc.
Transcriptome Analysis of Treg Cells in Early SSc Patients
As described above, the clinical features differ between the early and the late stages of SSc (1). In the early phase of SSc, inflammation and autoimmune responses were prominent (14), whereas pro-fibrotic reactions were evident in the late phase of the disease. Therefore, the analysis of SSc-related immunological data for each stage of SSc could unveil stage-specific immunological disturbances. Therefore, our team performed FACS and RNA-seq analysis of peripheral blood immune cells in early SSc (defined as morbidity of less than 5 years) and late SSc (defined as morbidity of 5 years or more) to investigate the immunological characteristics of early SSc patients. As a result, in the comparison of early SSc vs. late SSc patients, the frequencies of Th17 cells were significantly higher in early SSc. In contrast, no significant difference was observed in the frequencies of the fraction II effector regulatory T cells (Fr. II eTreg) subset defined by CD4+CD25++ CD45RA- in FACS-based data. Next, we estimated the proportion of Treg subsets and subpopulations by deconvolution based on the previously reported single cell RNA-seq data. Interestingly, among the transcriptome of 24 cell subsets, differences in gene expression in Treg cells were only evident between early and late SSc. In addition, the proportion of “activated subpopulations” of Treg cells was significantly higher in early SSc, in association with a higher expression of activation markers. Pathway analysis suggested that oxidative phosphorylation was enhanced in “activated subpopulations” of Treg cells in early SSc. Treg cells rely on the oxidative phosphorylation pathway for most of their energy production (72). This result suggested that regulatory feedback for the activation of the immune system occurs in the early stage of SSc pathogenesis, or alternatively, a compensative feedback for the decrease in the immunosuppressive ability of Treg cells as reported previously (81). It was also reported that enhancement of the oxidative phosphorylation pathway promotes Th17 differentiation, and inhibition of the oxidative phosphorylation pathway induces differentiation into Treg cells (74). The enhanced oxidative phosphorylation pathway in early SSc Treg cells would represent a metabolic sign of transition of Treg to Th17 cells. In this study, we also performed differentially expressed gene (DEG) analysis and identified hub genes that regulate the expression of DEGs between early and late SSc in the gene network estimated with a non-knowledge-based method. This data-driven approach identified ARPC3 as a hub gene. ARPC3 is a crucial gene for immune synapse formation and T-cell receptor (TCR) signaling (110, 111). This result implied that the different gene expression patterns of Tregs between early SSc and late SSc were regulated by TCR signaling. This finding was consistent with previous reports suggesting that TCR signaling was essential in Tregs and was required for suppressive activity and differentiation to an activated phenotype (112, 113). In this study, the expression of ARPC3 was upregulated in early SSc. Therefore, it could enhance the sensitivity of TCR signaling and induce activation of Fr. II eTregs.
In previous reports, Treg cells in the peripheral blood of SSc patients were contradictory in both quantitative and qualitative evaluations. Our study focused on the subpopulation of activated Treg cells in early SSc patients. Therefore, we propose that meaningful results could be obtained by analyzing a “subpopulation” of Treg cells derived from homogeneous phases of disease and clinical features of SSc patients.
Discussion
We outlined the current understanding of the roles of Treg cells in SSc. It was suggested that Treg cells contribute to the pathophysiology of SSc via the decreased inhibitory ability and disruption of equilibrium with other helper T cell subsets (Figure 1). Here, our team demonstrated an increase in the Treg “activated subpopulation” accompanying an increase in oxidative phosphorylation in early SSc patients (42). In addition, an increase in Th17 cells was also observed in early SSc patients. These results suggested that the imbalance between Treg and Th17 plays an important role in the pathophysiology of early SSc. Importantly, some reports showed that targeting IL-17 was effective to improve the skin and lung fibrosis in SSc models (104–106). Even though it is still controversial and further studies will be needed (99), this imbalance between Treg and Th17 cells could be a therapeutic target of SSc, and control of abnormal Treg activation could be another therapeutic strategy for SSc. From the point of view of Treg plasticity, it could be effective to ameliorate inflammation using anti-cytokine agents, because the conversion of Treg into Th17 is prompted under the specific inflammatory condition (64). It was reported that tocilizumab, an anti-IL-6 receptor monoclonal antibody, was partially effective to preserve the lung function in SSc with interstitial lung diseases in the early disease phase (114). We suspected that tocilizumab could control the inflammatory environment and prevent Treg to Th17 conversion. Further studies of Treg cells in SSc will provide additional clues to solve the problem. Our study suggests that a detailed definition of Treg cell populations and a homogeneous stage and pathological background of the study target groups will be required to attain high quality and reliability of the results in SSc studies.
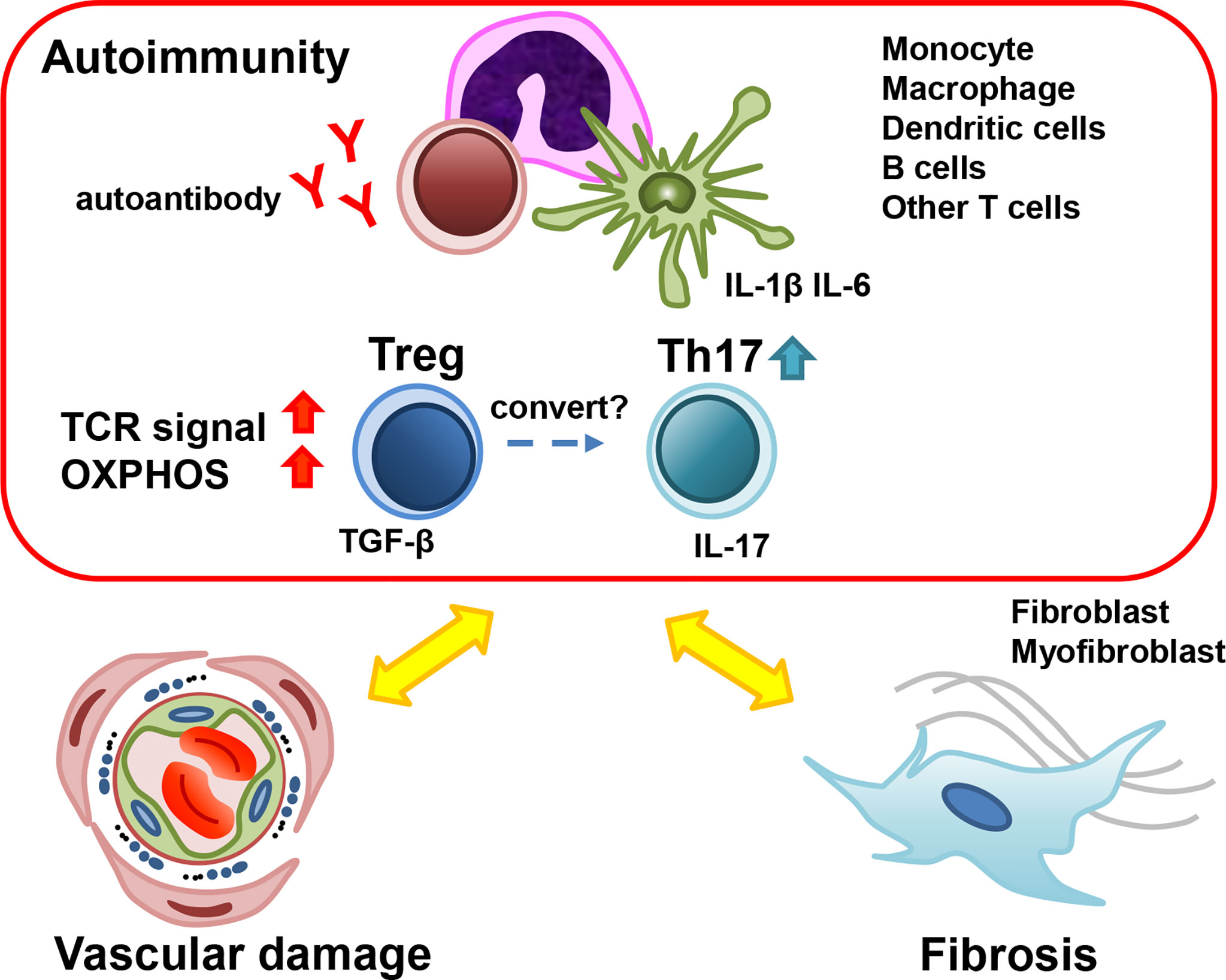
Figure 1 The role of Treg in the pathogenesis of early SSc. Many types of cells contribute to the pathogenesis of early SSc. Treg cells secrete TGF-β, which promotes myofibroblast activation and fibrosis. The dysfunction of Treg cells results in the development of autoimmunity and inflammation. Under the inflammatory condition, Treg could convert into Th17 and Th17 could promote inflammation and vasculopathy. SSc; systemic sclerosis, Treg; regulatory T cells, Th17; T-helper17 cells, TGF-β; transforming growth factor-β.
Author Contributions
SK, YN, HS, and KF designed the study and contributed to writing the manuscript. HS and KF supervised the study. All authors were involved in drafting the article or revising it critically for important intellectual content, and all authors approved the final version to be published.
Conflict of Interest
YN belongs to the Social Cooperation Program, Department of Functional Genomics and Immunological Diseases, supported by Chugai Pharmaceutical.
The remaining authors declare that the research was conducted in the absence of any commercial or financial relationships that could be construed as a potential conflict of interest.
Publisher’s Note
All claims expressed in this article are solely those of the authors and do not necessarily represent those of their affiliated organizations, or those of the publisher, the editors and the reviewers. Any product that may be evaluated in this article, or claim that may be made by its manufacturer, is not guaranteed or endorsed by the publisher.
References
1. Denton CP, Khanna D. Systemic Sclerosis. Lancet (2017) 390(10103):1685–99. doi: 10.1016/S0140-6736(17)30933-9
2. Kowal-Bielecka O, Fransen J, Avouac J, Becker M, Kulak A, Allanore Y, et al. Update of EULAR Recommendations for the Treatment of Systemic Sclerosis. Ann Rheum Dis (2017) 76(8):1327–39. doi: 10.1136/annrheumdis-2016-209909
3. Distler O, Highland KB, Gahlemann M, Azuma A, Fischer A, Mayes MD, et al. Nintedanib for Systemic Sclerosis-Associated Interstitial Lung Disease. N Engl J Med (2019) 380(26):2518–28. doi: 10.1056/NEJMoa1903076
4. Nihtyanova SI, Schreiber BE, Ong VH, Rosenberg D, Moinzadeh P, Coghlan JG, et al. Prediction of Pulmonary Complications and Long-Term Survival in Systemic Sclerosis. Arthritis Rheumatol (2014) 66(6):1625–35. doi: 10.1002/art.38390
5. Medsger TA Jr. Natural History of Systemic Sclerosis and the Assessment of Disease Activity, Severity, Functional Status, and Psychologic Well-Being. Rheum Dis Clin North Am (2003) 29(2):255–73, vi. doi: 10.1016/S0889-857X(03)00023-1
6. Hachulla E, Launay D, Mouthon L, Sitbon O, Berezne A, Guillevin L, et al. Is Pulmonary Arterial Hypertension Really a Late Complication of Systemic Sclerosis? Chest (2009) 136(5):1211–9. doi: 10.1378/chest.08-3042
7. Fox DA, Lundy SK, Whitfield ML, Berrocal V, Campbell P, Rasmussen S, et al. Lymphocyte Subset Abnormalities in Early Diffuse Cutaneous Systemic Sclerosis. Arthritis Res Ther (2021) 23(1):10. doi: 10.1186/s13075-020-02383-w
8. Skaug B, Lyons MA, Swindell WR, Salazar GA, Wu M, Tran TM, et al. Large-Scale Analysis of Longitudinal Skin Gene Expression in Systemic Sclerosis Reveals Relationships of Immune Cell and Fibroblast Activity With Skin Thickness and a Trend Towards Normalisation Over Time. Ann Rheum Dis (2021) 81(4):516–23. doi: 10.1136/annrheumdis-2021-221352
9. LeRoy EC, Medsger TA Jr. Criteria for the Classification of Early Systemic Sclerosis. J Rheumatol (2001) 28(7):1573–6.
10. Matucci-Cerinic M, Allanore Y, Czirják L, Tyndall A, Müller-Ladner U, Denton C, et al. The Challenge of Early Systemic Sclerosis for the EULAR Scleroderma Trial and Research Group (EUSTAR) Community. It is Time to Cut the Gordian Knot and Develop a Prevention or Rescue Strategy. Ann Rheum Dis (2009) 68(9):1377–80. doi: 10.1136/ard.2008.106302
11. Herrick AL, Cutolo M. Clinical Implications From Capillaroscopic Analysis in Patients With Raynaud's Phenomenon and Systemic Sclerosis. Arthritis Rheumatol (2010) 62(9):2595–604. doi: 10.1002/art.27543
12. Siqueira VS, Helbingen MFS, Medeiros-Ribeiro AC, Carriço da Silva H, Miossi R, Luppino-Assad AP, et al. Predictors of Progression to Systemic Sclerosis: Analysis of Very Early Disease of Systemic Sclerosis (VEDOSS) in a Large Single Cohort. Rheumatol (Oxf) (2022). doi: 10.1093/rheumatology/keac006
13. Bellando-Randone S, Galdo FD, Lepri G, Minier T, Huscher D, Furst DE, et al. Progression of Patients With Raynaud's Phenomenon to Systemic Sclerosis: A Five-Year Analysis of the European Scleroderma Trial and Research Group Multicentre, Longitudinal Registry Study for Very Early Diagnosis of Systemic Sclerosis (VEDOSS). Lancet Rheumatol (2021) 3:e834–4. doi: 10.1016/S2665-9913(21)00244-7
14. Zuber JP, Spertini F. Immunological Basis of Systemic Sclerosis. Rheumatol (Oxf) (2006) 45 Suppl 3:iii23–5. doi: 10.1093/rheumatology/kel285
15. Cossu M, van Bon L, Preti C, Rossato M, Beretta L, Radstake T. Earliest Phase of Systemic Sclerosis Typified by Increased Levels of Inflammatory Proteins in the Serum. Arthritis Rheumatol (2017) 69(12):2359–69. doi: 10.1002/art.40243
16. Gilbane AJ, Denton CP, Holmes AM. Scleroderma Pathogenesis: A Pivotal Role for Fibroblasts as Effector Cells. Arthritis Res Ther (2013) 15(3):215. doi: 10.1186/ar4230
17. Mahoney JM, Taroni J, Martyanov V, Wood TA, Greene CS, Pioli PA, et al. Systems Level Analysis of Systemic Sclerosis Shows a Network of Immune and Profibrotic Pathways Connected With Genetic Polymorphisms. PloS Comput Biol (2015) 11(1):e1004005. doi: 10.1371/journal.pcbi.1004005
18. Marie I, Gehanno JF, Bubenheim M, Duval-Modeste AB, Joly P, Dominique S, et al. Prospective Study to Evaluate the Association Between Systemic Sclerosis and Occupational Exposure and Review of the Literature. Autoimmun Rev (2014) 13(2):151–6. doi: 10.1016/j.autrev.2013.10.002
19. Randone SB, Guiducci S, Cerinic MM. Systemic Sclerosis and Infections. Autoimmun Rev (2008) 8(1):36–40. doi: 10.1016/j.autrev.2008.07.022
20. Roumm AD, Whiteside TL, Medsger TA Jr., Rodnan GP. Lymphocytes in the Skin of Patients With Progressive Systemic Sclerosis. Quantification, Subtyping, and Clinical Correlations. Arthritis Rheumatol (1984) 27(6):645–53. doi: 10.1002/art.1780270607
21. Murray PJ, Allen JE, Biswas SK, Fisher EA, Gilroy DW, Goerdt S, et al. Macrophage Activation and Polarization: Nomenclature and Experimental Guidelines. Immunity (2014) 41(1):14–20. doi: 10.1016/j.immuni.2014.06.008
22. Kobayashi S, Nagafuchi Y, Okubo M, Sugimori Y, Shirai H, Hatano H, et al. Integrated Bulk and Single-Cell RNA-Sequencing Identified Disease-Relevant Monocytes and a Gene Network Module Underlying Systemic Sclerosis. J Autoimmun (2021) 116:102547. doi: 10.1016/j.jaut.2020.102547
23. York MR, Nagai T, Mangini AJ, Lemaire R, van Seventer JM, Lafyatis R. A Macrophage Marker, Siglec-1, Is Increased on Circulating Monocytes in Patients With Systemic Sclerosis and Induced by Type I Interferons and Toll-Like Receptor Agonists. Arthritis Rheumatol (2007) 56(3):1010–20. doi: 10.1002/art.22382
24. van Bon L, Affandi AJ, Broen J, Christmann RB, Marijnissen RJ, Stawski L, et al. Proteome-Wide Analysis and CXCL4 as a Biomarker in Systemic Sclerosis. N Engl J Med (2014) 370(5):433–43. doi: 10.1056/NEJMoa1114576
25. Ota Y, Kuwana M. Updates on Genetics in Systemic Sclerosis. Inflammation Regen (2021) 41(1):17. doi: 10.1186/s41232-021-00167-6
26. Sato S, Hasegawa M, Fujimoto M, Tedder TF, Takehara K. Quantitative Genetic Variation in CD19 Expression Correlates With Autoimmunity. J Immunol (2000) 165(11):6635–43. doi: 10.4049/jimmunol.165.11.6635
27. Lafyatis R, O'Hara C, Feghali-Bostwick CA, Matteson E. B Cell Infiltration in Systemic Sclerosis-Associated Interstitial Lung Disease. Arthritis Rheumatol (2007) 56(9):3167–8. doi: 10.1002/art.22847
28. Bosello S, Angelucci C, Lama G, Alivernini S, Proietti G, Tolusso B, et al. Characterization of Inflammatory Cell Infiltrate of Scleroderma Skin: B Cells and Skin Score Progression. Arthritis Res Ther (2018) 20(1):75. doi: 10.1186/s13075-018-1569-0
29. Xu Y, Wang W, Tian Y, Liu J, Yang R. Polymorphisms in STAT4 and IRF5 Increase the Risk of Systemic Sclerosis: A Meta-Analysis. Int J Dermatol (2016) 55(4):408–16. doi: 10.1111/ijd.12839
30. Wei P, Yang Y, Guo X, Hei N, Lai S, Assassi S, et al. Identification of an Association of TNFAIP3 Polymorphisms With Matrix Metalloproteinase Expression in Fibroblasts in an Integrative Study of Systemic Sclerosis-Associated Genetic and Environmental Factors. Arthritis Rheumatol (2016) 68(3):749–60. doi: 10.1002/art.39476
31. Diaz-Gallo LM, Gourh P, Broen J, Simeon C, Fonollosa V, Ortego-Centeno N, et al. Analysis of the Influence of PTPN22 Gene Polymorphisms in Systemic Sclerosis. Ann Rheum Dis (2011) 70(3):454–62. doi: 10.1136/ard.2010.130138
32. Li D, Matta B, Song S, Nelson V, Diggins K, Simpfendorfer KR, et al. IRF5 Genetic Risk Variants Drive Myeloid-Specific IRF5 Hyperactivation and Presymptomatic SLE. JCI Insight (2020) 5(2). doi: 10.1172/jci.insight.124020
33. Abbasifard M, Imani D, Bagheri-Hosseinabadi Z. PTPN22 Gene Polymorphism and Susceptibility to Rheumatoid Arthritis (RA): Updated Systematic Review and Meta-Analysis. J Gene Med (2020) 22(9):e3204. doi: 10.1002/jgm.3204
34. López-Isac E, Acosta-Herrera M, Kerick M, Assassi S, Satpathy AT, Granja J, et al. GWAS for Systemic Sclerosis Identifies Multiple Risk Loci and Highlights Fibrotic and Vasculopathy Pathways. Nat Commun (2019) 10(1):4955. doi: 10.1038/s41467-019-12760-y
35. de Vries S, Naarmann-de Vries IS, Urlaub H, Lue H, Bernhagen J, Ostareck DH, et al. Identification of DEAD-Box RNA Helicase 6 (DDX6) as a Cellular Modulator of Vascular Endothelial Growth Factor Expression Under Hypoxia. J Biol Chem (2013) 288(8):5815–27. doi: 10.1074/jbc.M112.420711
36. Sakkas LI, Xu B, Artlett CM, Lu S, Jimenez SA, Platsoucas CD. Oligoclonal T Cell Expansion in the Skin of Patients With Systemic Sclerosis. J Immunol (2002) 168(7):3649–59. doi: 10.4049/jimmunol.168.7.3649
37. Maehara T, Kaneko N, Perugino CA, Mattoo H, Kers J, Allard-Chamard H, et al. Cytotoxic CD4+ T Lymphocytes may Induce Endothelial Cell Apoptosis in Systemic Sclerosis. J Clin Invest (2020) 130(5):2451–64. doi: 10.1172/JCI131700
38. Skaug B, Khanna D, Swindell WR, Hinchcliff ME, Frech TM, Steen VD, et al. Global Skin Gene Expression Analysis of Early Diffuse Cutaneous Systemic Sclerosis Shows a Prominent Innate and Adaptive Inflammatory Profile. Ann Rheum Dis (2020) 79(3):379–86. doi: 10.1136/annrheumdis-2019-215894
39. Zhou Y, Hou W, Xu K, Han D, Jiang C, Mou K, et al. The Elevated Expression of Th17-Related Cytokines and Receptors Is Associated With Skin Lesion Severity in Early Systemic Sclerosis. Hum Immunol (2015) 76(1):22–9. doi: 10.1016/j.humimm.2014.12.008
40. Yang X, Yang J, Xing X, Wan L, Li M. Increased Frequency of Th17 Cells in Systemic Sclerosis Is Related to Disease Activity and Collagen Overproduction. Arthritis Res Ther (2014) 16(1):R4. doi: 10.1186/ar4430
41. Shah A, Storek J, Woolson R, Pinckney A, Keyes-Elstein L, Wallace PK, et al. Lymphocyte Subset Abnormalities in Early Severe Scleroderma Favor a Th2 Phenotype and Are Not Altered by Prior Immunosuppressive Therapy. Rheumatol (Oxf) (2022). doi: 10.1093/rheumatology/keac015
42. Kobayashi S, Nagafuchi Y, Okubo M, Sugimori Y, Hatano H, Yamada S, et al. Dysregulation of the Gene Signature of Effector Regulatory T Cells in the Early Phase of Systemic Sclerosis. Rheumatol (Oxf) (2022). doi: 10.1093/rheumatology/keac031
43. Ugor E, Simon D, Almanzar G, Pap R, Najbauer J, Németh P, et al. Increased Proportions of Functionally Impaired Regulatory T Cell Subsets in Systemic Sclerosis. Clin Immunol (2017) 184:54–62. doi: 10.1016/j.clim.2017.05.013
44. Almanzar G, Klein M, Schmalzing M, Hilligardt D, El Hajj N, Kneitz H, et al. Disease Manifestation and Inflammatory Activity as Modulators of Th17/Treg Balance and RORC/FoxP3 Methylation in Systemic Sclerosis. Int Arch Allergy Immunol (2016) 171(2):141–54. doi: 10.1159/000450949
45. Kataoka H, Yasuda S, Fukaya S, Oku K, Horita T, Atsumi T, et al. Decreased Expression of Runx1 and Lowered Proportion of Foxp3+ CD25+ CD4+ Regulatory T Cells in Systemic Sclerosis. Mod Rheumatol (2015) 25(1):90–5. doi: 10.3109/14397595.2014.899736
46. Krasimirova E, Velikova T, Ivanova-Todorova E, Tumangelova-Yuzeir K, Kalinova D, Boyadzhieva V, et al. Treg/Th17 Cell Balance and Phytohaemagglutinin Activation of T Lymphocytes in Peripheral Blood of Systemic Sclerosis Patients. World J Exp Med (2017) 7(3):84–96. doi: 10.5493/wjem.v7.i3.84
47. Günther J, Kill A, Becker MO, Heidecke H, Rademacher J, Siegert E, et al. Angiotensin Receptor Type 1 and Endothelin Receptor Type A on Immune Cells Mediate Migration and the Expression of IL-8 and CCL18 When Stimulated by Autoantibodies From Systemic Sclerosis Patients. Arthritis Res Ther (2014) 16(2):R65. doi: 10.1186/ar4503
48. Vettori S, Cuomo G, Iudici M, D'Abrosca V, Giacco V, Barra G, et al. Early Systemic Sclerosis: Serum Profiling of Factors Involved in Endothelial, T-Cell, and Fibroblast Interplay Is Marked by Elevated Interleukin-33 Levels. J Clin Immunol (2014) 34(6):663–8. doi: 10.1007/s10875-014-0037-0
49. Chiba M, Yanaba K, Hayashi M, Yoshihara Y, Nakagawa H. Clinical Significance of Serum Soluble T-Cell Immunoglobulin and Mucin Domain 3 Levels in Systemic Sclerosis: Association With Disease Severity. J Dermatol (2017) 44(2):194–7. doi: 10.1111/1346-8138.13610
50. Wu M, Baron M, Pedroza C, Salazar GA, Ying J, Charles J, et al. CCL2 in the Circulation Predicts Long-Term Progression of Interstitial Lung Disease in Patients With Early Systemic Sclerosis: Data From Two Independent Cohorts. Arthritis Rheumatol (2017) 69(9):1871–8. doi: 10.1002/art.40171
51. Masui Y, Asano Y, Shibata S, Noda S, Akamata K, Aozasa N, et al. A Possible Contribution of Visfatin to the Resolution of Skin Sclerosis in Patients With Diffuse Cutaneous Systemic Sclerosis via a Direct Anti-Fibrotic Effect on Dermal Fibroblasts and Th1 Polarization of the Immune Response. Rheumatol (Oxf) (2013) 52(7):1239–44. doi: 10.1093/rheumatology/ket010
52. Yanaba K, Asano Y, Noda S, Akamata K, Aozasa N, Taniguchi T, et al. Increased Production of Soluble Inducible Costimulator in Patients With Diffuse Cutaneous Systemic Sclerosis. Arch Dermatol Res (2013) 305(1):17–23. doi: 10.1007/s00403-012-1292-7
53. Hasegawa M, Fujimoto M, Matsushita T, Hamaguchi Y, Takehara K. Augmented ICOS Expression in Patients With Early Diffuse Cutaneous Systemic Sclerosis. Rheumatol (Oxf) (2013) 52(2):242–51. doi: 10.1093/rheumatology/kes258
54. Kubo S, Nakayamada S, Miyazaki Y, Yoshikawa M, Yoshinari H, Satoh Y, et al. Distinctive Association of Peripheral Immune Cell Phenotypes With Capillaroscopic Microvascular Patterns in Systemic Sclerosis. Rheumatol (Oxf) (2019) 58(12):2273–83. doi: 10.1093/rheumatology/kez244
55. D'Amico F, Fiorito G, Skarmoutsou E, Granata M, Rossi GA, Trovato C, et al. FOXP3, ICOS and ICOSL Gene Polymorphisms in Systemic Sclerosis: FOXP3 Rs2294020 Is Associated With Disease Progression in a Female Italian Population. Immunobiology (2018) 223(1):112–7. doi: 10.1016/j.imbio.2017.10.004
56. Papp G, Barath S, Szegedi A, Szodoray P, Zeher M. The Effects of Extracorporeal Photochemotherapy on T Cell Activation and Regulatory Mechanisms in Patients With Systemic Sclerosis. Clin Rheumatol (2012) 31(9):1293–9. doi: 10.1007/s10067-012-2000-x
57. Mathian A, Parizot C, Dorgham K, Trad S, Arnaud L, Larsen M, et al. Activated and Resting Regulatory T Cell Exhaustion Concurs With High Levels of Interleukin-22 Expression in Systemic Sclerosis Lesions. Ann Rheum Dis (2012) 71(7):1227–34. doi: 10.1136/annrheumdis-2011-200709
58. Radstake TR, van Bon L, Broen J, Wenink M, Santegoets K, Deng Y, et al. Increased Frequency and Compromised Function of T Regulatory Cells in Systemic Sclerosis (SSc) Is Related to a Diminished CD69 and TGFbeta Expression. PloS One (2009) 4(6):e5981. doi: 10.1371/journal.pone.0005981
59. Radstake TR, van Bon L, Broen J, Hussiani A, Hesselstrand R, Wuttge DM, et al. The Pronounced Th17 Profile in Systemic Sclerosis (SSc) Together With Intracellular Expression of TGFbeta and IFNgamma Distinguishes SSc Phenotypes. PloS One (2009) 4(6):e5903. doi: 10.1371/journal.pone.0005903
60. Fossiez F, Djossou O, Chomarat P, Flores-Romo L, Ait-Yahia S, Maat C, et al. T Cell Interleukin-17 Induces Stromal Cells to Produce Proinflammatory and Hematopoietic Cytokines. J Exp Med (1996) 183(6):2593–603. doi: 10.1084/jem.183.6.2593
61. Yamamoto T, Eckes B, Hartmann K, Krieg T. Expression of Monocyte Chemoattractant Protein-1 in the Lesional Skin of Systemic Sclerosis. J Dermatol Sci (2001) 26(2):133–9. doi: 10.1016/S0923-1811(00)00169-9
62. Kurasawa K, Hirose K, Sano H, Endo H, Shinkai H, Nawata Y, et al. Increased Interleukin-17 Production in Patients With Systemic Sclerosis. Arthritis Rheumatol (2000) 43(11):2455–63. doi: 10.1002/1529-0131(200011)43:11<2455::AID-ANR12>3.0.CO;2-K
63. Yao Z, Painter SL, Fanslow WC, Ulrich D, Macduff BM, Spriggs MK, et al. Human IL-17: A Novel Cytokine Derived From T Cells. J Immunol (1995) 155(12):5483–6.
64. Hori S. Developmental Plasticity of Foxp3+ Regulatory T Cells. Curr Opin Immunol (2010) 22(5):575–82. doi: 10.1016/j.coi.2010.08.004
65. Koenen HJ, Smeets RL, Vink PM, van Rijssen E, Boots AM, Joosten I. Human CD25highFoxp3pos Regulatory T Cells Differentiate Into IL-17-Producing Cells. Blood (2008) 112(6):2340–52. doi: 10.1182/blood-2008-01-133967
66. Komatsu N, Okamoto K, Sawa S, Nakashima T, Oh-hora M, Kodama T, et al. Pathogenic Conversion of Foxp3+ T Cells Into TH17 Cells in Autoimmune Arthritis. Nat Med (2014) 20(1):62–8. doi: 10.1038/nm.3432
67. Sakaguchi S, Sakaguchi N, Asano M, Itoh M, Toda M. Immunologic Self-Tolerance Maintained by Activated T Cells Expressing IL-2 Receptor Alpha-Chains (CD25). Breakdown of a Single Mechanism of Self-Tolerance Causes Various Autoimmune Diseases. J Immunol (1995) 155(3):1151–64.
68. Hori S, Nomura T, Sakaguchi S. Control of Regulatory T Cell Development by the Transcription Factor Foxp3. Science (2003) 299(5609):1057–61. doi: 10.1126/science.1079490
69. Gavin MA, Torgerson TR, Houston E, DeRoos P, Ho WY, Stray-Pedersen A, et al. Single-Cell Analysis of Normal and FOXP3-Mutant Human T Cells: FOXP3 Expression Without Regulatory T Cell Development. Proc Natl Acad Sci USA (2006) 103(17):6659–64. doi: 10.1073/pnas.0509484103
70. Miyara M, Yoshioka Y, Kitoh A, Shima T, Wing K, Niwa A, et al. Functional Delineation and Differentiation Dynamics of Human CD4+ T Cells Expressing the FoxP3 Transcription Factor. Immunity (2009) 30(6):899–911. doi: 10.1016/j.immuni.2009.03.019
71. Zemmour D, Zilionis R, Kiner E, Klein AM, Mathis D, Benoist C. Single-Cell Gene Expression Reveals a Landscape of Regulatory T Cell Phenotypes Shaped by the TCR. Nat Immunol (2018) 19(3):291–301. doi: 10.1038/s41590-018-0051-0
72. Michalek RD, Gerriets VA, Jacobs SR, Macintyre AN, MacIver NJ, Mason EF, et al. Cutting Edge: Distinct Glycolytic and Lipid Oxidative Metabolic Programs are Essential for Effector and Regulatory CD4+ T Cell Subsets. J Immunol (2011) 186(6):3299–303. doi: 10.4049/jimmunol.1003613
73. Weinberg SE, Singer BD, Steinert EM, Martinez CA, Mehta MM, Martínez-Reyes I, et al. Mitochondrial Complex III Is Essential for Suppressive Function of Regulatory T Cells. Nature (2019) 565(7740):495–9. doi: 10.1038/s41586-018-0846-z
74. Shin B, Benavides GA, Geng J, Koralov SB, Hu H, Darley-Usmar VM, et al. Mitochondrial Oxidative Phosphorylation Regulates the Fate Decision Between Pathogenic Th17 and Regulatory T Cells. Cell Rep (2020) 30(6):1898–909.e4. doi: 10.1016/j.celrep.2020.01.022
75. Bennett CL, Christie J, Ramsdell F, Brunkow ME, Ferguson PJ, Whitesell L, et al. The Immune Dysregulation, Polyendocrinopathy, Enteropathy, X-Linked Syndrome (IPEX) Is Caused by Mutations of FOXP3. Nat Genet (2001) 27(1):20–1. doi: 10.1038/83713
76. Sasada T, Kimura M, Yoshida Y, Kanai M, Takabayashi A. CD4+CD25+ Regulatory T Cells in Patients With Gastrointestinal Malignancies: Possible Involvement of Regulatory T Cells in Disease Progression. Cancer (2003) 98(5):1089–99. doi: 10.1002/cncr.11618
77. Curiel TJ, Coukos G, Zou L, Alvarez X, Cheng P, Mottram P, et al. Specific Recruitment of Regulatory T Cells in Ovarian Carcinoma Fosters Immune Privilege and Predicts Reduced Survival. Nat Med (2004) 10(9):942–9. doi: 10.1038/nm1093
78. Kato H, Perl A. Blockade of Treg Cell Differentiation and Function by the Interleukin-21-Mechanistic Target of Rapamycin Axis Via Suppression of Autophagy in Patients With Systemic Lupus Erythematosus. Arthritis Rheumatol (2018) 70(3):427–38. doi: 10.1002/art.40380
79. Gieseck RL 3rd, Wilson MS, Wynn TA. Type 2 Immunity in Tissue Repair and Fibrosis. Nat Rev Immunol (2018) 18(1):62–76. doi: 10.1038/nri.2017.90
80. Vignali DA, Collison LW, Workman CJ. How Regulatory T Cells Work. Nat Rev Immunol (2008) 8(7):523–32. doi: 10.1038/nri2343
81. Frantz C, Auffray C, Avouac J, Allanore Y. Regulatory T Cells in Systemic Sclerosis. Front Immunol (2018) 9:2356. doi: 10.3389/fimmu.2018.02356
82. Lafyatis R. Transforming Growth Factor β–at the Centre of Systemic Sclerosis. Nat Rev Rheumatol (2014) 10(12):706–19. doi: 10.1038/nrrheum.2014.137
83. MacDonald KG, Dawson NA, Huang Q, Dunne JV, Levings MK, Broady R. Regulatory T Cells Produce Profibrotic Cytokines in the Skin of Patients With Systemic Sclerosis. J Allergy Clin Immunol (2015) 135(4):946–.e9. doi: 10.1016/j.jaci.2014.12.1932
84. Shin JY, Beckett JD, Bagirzadeh R, Creamer TJ, Shah AA, McMahan Z, et al. Epigenetic Activation and Memory at a TGFB2 Enhancer in Systemic Sclerosis. Sci Transl Med (2019) 11(497). doi: 10.1126/scitranslmed.aaw0790
85. Yoshizaki A, Yanaba K, Ogawa A, Asano Y, Kadono T, Sato S. Immunization With DNA Topoisomerase I and Freund's Complete Adjuvant Induces Skin and Lung Fibrosis and Autoimmunity via Interleukin-6 Signaling. Arthritis Rheumatol (2011) 63(11):3575–85. doi: 10.1002/art.30539
86. Miura S, Watanabe Y, Saigusa R, Yamashita T, Nakamura K, Hirabayashi M, et al. Fli1 Deficiency Suppresses RALDH1 Activity of Dermal Dendritic Cells and Related Induction of Regulatory T Cells: A Possible Role in Scleroderma. Arthritis Res Ther (2021) 23(1):137. doi: 10.1186/s13075-021-02520-z
87. Birjandi SZ, Palchevskiy V, Xue YY, Nunez S, Kern R, Weigt SS, et al. CD4(+)CD25(hi)Foxp3(+) Cells Exacerbate Bleomycin-Induced Pulmonary Fibrosis. Am J Pathol (2016) 186(8):2008–20. doi: 10.1016/j.ajpath.2016.03.020
88. Kamio K, Azuma A, Matsuda K, Usuki J, Inomata M, Morinaga A, et al. Resolution of Bleomycin-Induced Murine Pulmonary Fibrosis via a Splenic Lymphocyte Subpopulation. Respir Res (2018) 19(1):71. doi: 10.1186/s12931-018-0783-2
89. Boveda-Ruiz D, D'Alessandro-Gabazza CN, Toda M, Takagi T, Naito M, Matsushima Y, et al. Differential Role of Regulatory T Cells in Early and Late Stages of Pulmonary Fibrosis. Immunobiology (2013) 218(2):245–54. doi: 10.1016/j.imbio.2012.05.020
90. Mo C, Zeng Z, Deng Q, Ding Y, Xiao R. Imbalance Between T Helper 17 and Regulatory T Cell Subsets Plays a Significant Role in the Pathogenesis of Systemic Sclerosis. BioMed Pharmacother (2018) 108:177–83. doi: 10.1016/j.biopha.2018.09.037
91. Liu M, Yang J, Xing X, Cui X, Li M. Interleukin-17A Promotes Functional Activation of Systemic Sclerosis Patient-Derived Dermal Vascular Smooth Muscle Cells by Extracellular-Regulated Protein Kinases Signalling Pathway. Arthritis Res Ther (2014) 16(6):4223. doi: 10.1186/s13075-014-0512-2
92. Lei L, Zhong XN, Zhao C, Mi CD, Li JQ, Zeng JJ. [Expression and Significance of Th17 Cells and Related Cytokines in a Murine Model of Systemic Sclerosis]. Beijing Da Xue Xue Bao Yi Xue Ban (2012) 44(2):259–64.
93. Liu X, Gao N, Li M, Xu D, Hou Y, Wang Q, et al. Elevated Levels of CD4(+)CD25(+)FoxP3(+) T Cells in Systemic Sclerosis Patients Contribute to the Secretion of IL-17 and Immunosuppression Dysfunction. PloS One (2013) 8(6):e64531. doi: 10.1371/journal.pone.0064531
94. Truchetet ME, Brembilla NC, Montanari E, Allanore Y, Chizzolini C. Increased Frequency of Circulating Th22 in Addition to Th17 and Th2 Lymphocytes in Systemic Sclerosis: Association With Interstitial Lung Disease. Arthritis Res Ther (2011) 13(5):R166. doi: 10.1186/ar3486
95. Marie I, Antonietti M, Houivet E, Hachulla E, Maunoury V, Bienvenu B, et al. Gastrointestinal Mucosal Abnormalities Using Videocapsule Endoscopy in Systemic Sclerosis. Aliment Pharmacol Ther (2014) 40(2):189–99. doi: 10.1111/apt.12818
96. Sjogren RW. Gastrointestinal Motility Disorders in Scleroderma. Arthritis Rheumatol (1994) 37(9):1265–82. doi: 10.1002/art.1780370902
97. Nicola S, Rolla G, Bucca C, Geronazzo G, Ridolfi I, Ferraris A, et al. Gastric Juice Expression of Th-17 and T-Reg Related Cytokines in Scleroderma Esophageal Involvement. Cells (2020) 9(9). doi: 10.3390/cells9092106
98. Murata M, Fujimoto M, Matsushita T, Hamaguchi Y, Hasegawa M, Takehara K, et al. Clinical Association of Serum Interleukin-17 Levels in Systemic Sclerosis: Is Systemic Sclerosis a Th17 Disease? J Dermatol Sci (2008) 50:240–2. doi: 10.1016/j.jdermsci.2008.01.001
99. Nakashima T, Jinnin M, Yamane K, Honda N, Kajihara I, Makino T, et al. Impaired IL-17 Signaling Pathway Contributes to the Increased Collagen Expression in Scleroderma Fibroblasts. J Immunol (2012) 188(8):3573–83. doi: 10.4049/jimmunol.1100591
100. Truchetet ME, Brembilla NC, Montanari E, Lonati P, Raschi E, Zeni S, et al. Interleukin-17A+ Cell Counts are Increased in Systemic Sclerosis Skin and Their Number is Inversely Correlated With the Extent of Skin Involvement. Arthritis Rheumatol (2013) 65(5):1347–56. doi: 10.1002/art.37860
101. Vettori S, Barra G, Russo B, Borgia A, Pasquale G, Pellecchia L, et al. T-Cell Proapoptotic and Antifibrotic Activity Against Autologous Skin Fibroblasts In Vitro Is Associated With IL-17a Axis Upregulation in Systemic Sclerosis. Front Immunol (2020) 11:220. doi: 10.3389/fimmu.2020.00220
102. Dufour AM, Borowczyk-Michalowska J, Alvarez M, Truchetet ME, Modarressi A, Brembilla NC, et al. IL-17a Dissociates Inflammation From Fibrogenesis in Systemic Sclerosis. J Invest Dermatol (2020) 140(1):103–12.e8. doi: 10.1016/j.jid.2019.05.026
103. Brembilla NC, Montanari E, Truchetet ME, Raschi E, Meroni P, Chizzolini C. Th17 Cells Favor Inflammatory Responses While Inhibiting Type I Collagen Deposition by Dermal Fibroblasts: Differential Effects in Healthy and Systemic Sclerosis Fibroblasts. Arthritis Res Ther (2013) 15(5):R151. doi: 10.1186/ar4334
104. Okamoto Y, Hasegawa M, Matsushita T, Hamaguchi Y, Huu DL, Iwakura Y, et al. Potential Roles of Interleukin-17A in the Development of Skin Fibrosis in Mice. Arthritis Rheumatol (2012) 64(11):3726–35. doi: 10.1002/art.34643
105. Park MJ, Moon SJ, Lee EJ, Jung KA, Kim EK, Kim DS, et al. IL-1-IL-17 Signaling Axis Contributes to Fibrosis and Inflammation in Two Different Murine Models of Systemic Sclerosis. Front Immunol (2018) 9:1611. doi: 10.3389/fimmu.2018.01611
106. Karatas A, Celik C, Oz B, Akar ZA, Etem EO, Dagli AF, et al. Secukinumab and Metformin Ameliorate Dermal Fibrosis by Decreasing Tissue Interleukin-17 Levels in Bleomycin-Induced Dermal Fibrosis. Int J Rheum Dis (2021) 24(6):795–802. doi: 10.1111/1756-185X.14114
107. Antiga E, Quaglino P, Bellandi S, Volpi W, Del Bianco E, Comessatti A, et al. Regulatory T Cells in the Skin Lesions and Blood of Patients With Systemic Sclerosis and Morphoea. Br J Dermatol (2010) 162(5):1056–63. doi: 10.1111/j.1365-2133.2010.09633.x
108. Deng C, Li W, Chen S, Li Y. Meta-Analysis of the Changes of Peripheral Blood Regulatory T Cell to CD4(+) T Cell Ratio in Patients With Systemic Sclerosis. Sci Rep (2017) 7:43532. doi: 10.1038/srep43532
109. Ota M, Nagafuchi Y, Hatano H, Ishigaki K, Terao C, Takeshima Y, et al. Dynamic Landscape of Immune Cell-Specific Gene Regulation in Immune-Mediated Diseases. Cell (2021) 184(11):3006–21.e17. doi: 10.1016/j.cell.2021.03.056
110. Tsopoulidis N, Kaw S, Laketa V, Kutscheidt S, Baarlink C, Stolp B, et al. T Cell Receptor-Triggered Nuclear Actin Network Formation Drives CD4(+) T Cell Effector Functions. Sci Immunol (2019) 4(31). doi: 10.1126/sciimmunol.aav1987
111. Janssen E, Tohme M, Hedayat M, Leick M, Kumari S, Ramesh N, et al. A DOCK8-WIP-WASp Complex Links T Cell Receptors to the Actin Cytoskeleton. J Clin Invest (2016) 126(10):3837–51. doi: 10.1172/JCI85774
112. Levine AG, Arvey A, Jin W, Rudensky AY. Continuous Requirement for the TCR in Regulatory T Cell Function. Nat Immunol (2014) 15(11):1070–8. doi: 10.1038/ni.3004
113. Li MO, Rudensky AY. T Cell Receptor Signalling in the Control of Regulatory T Cell Differentiation and Function. Nat Rev Immunol (2016) 16(4):220–33. doi: 10.1038/nri.2016.26
Keywords: systemic sclerosis, the early disease phase, immune cells, regulatory T cells, transcriptome analysis
Citation: Kobayashi S, Nagafuchi Y, Shoda H and Fujio K (2022) The Pathophysiological Roles of Regulatory T Cells in the Early Phase of Systemic Sclerosis. Front. Immunol. 13:900638. doi: 10.3389/fimmu.2022.900638
Received: 21 March 2022; Accepted: 26 April 2022;
Published: 24 May 2022.
Edited by:
Vincent Sobanski, Université de Lille, CHU Lille, U1286 (INSERM), FranceReviewed by:
Minoru Hasegawa, Faculty of Medical Sciences, JapanCarlo Chizzolini, Université de Genève, Switzerland
Copyright © 2022 Kobayashi, Nagafuchi, Shoda and Fujio. This is an open-access article distributed under the terms of the Creative Commons Attribution License (CC BY). The use, distribution or reproduction in other forums is permitted, provided the original author(s) and the copyright owner(s) are credited and that the original publication in this journal is cited, in accordance with accepted academic practice. No use, distribution or reproduction is permitted which does not comply with these terms.
*Correspondence: Hirofumi Shoda, c2hvZGFoLWludEBoLnUtdG9reW8uYWMuanA=