- 1National Joint Engineering Research Center of Biodiagnostics and Biotherapy, Second Affiliated Hospital, Xi’an Jiaotong University, Xi’an, China
- 2Key Laboratory of Environment and Genes Related to Diseases, Xi’an Jiaotong University, Ministry of Education, Xi’an, China
- 3Institute of Molecular and Translational Medicine (IMTM), Xi’an Jiaotong University Health Science Center, Xi’an, China
- 4Department of Biochemistry and Molecular Biology, School of Basic Medical Sciences, Xi’an Jiaotong University Health Science Center, Xi’an, China
- 5National Regional Children’s Medical Center (Northwest), Key Laboratory of Precision Medicine to Pediatric Diseases of Shaanxi Province, Xi’an Key Laboratory of Children’s Health and Diseases, Shaanxi Institute for Pediatric Diseases, Xi’an Children’s Hospital, Affiliated Children’s Hospital of Xi’an Jiaotong University, Xi’an, China
Allergic asthma is a common chronic inflammation of the airways and causes airway remodeling eventually. For a long time, investigators have been focusing on the immunological mechanism of asthma. However, in recent years, the role of neuro-regulation in the occurrence of asthma has gradually attracted investigators’ attention. In this review, we firstly describe neuro-immune regulation in inflammation of allergic asthma from two aspects: innate immunity and adaptive immunity. Secondly, we introduce neuro-immune regulation in airway remodeling of asthma. Finally, we prospect the role of pulmonary neuroendocrine cells in the development of asthma. In general, the amount of researches is limited. Further researches on the neural regulation during the occurrence of asthma will help us clarify the mechanism of asthma more comprehensively and find more effective ways to prevent and control asthma.
Introduction
Allergic asthma is a chronic inflammation of the airways caused by repeated exposure to allergens such as dust, mites and pollens (1). It is a disease with high heterogeneity (2). The hallmark of asthma is airway hyperresponsiveness (AHR) with symptoms of cough, shortness of breath, wheeze and chest tightness. Asthma is a serious global problem with the prevalence rate of 5.8% in children under 18 and 8.4% in adults, and fatality rate of 1.3/100,000 (https://www.cdc.gov/nchs/fastats/asthma.htm [Accessed March 8, 2022]). Asthma can be divided into Type 2-High and Type 2-Low subtypes according to whether T helper 2 (Th2) cells are dominant (3). Type 2-High asthma, also known as allergic asthma, is characterized by large numbers of Th2 cells and group 2 innate lymphoid cells (ILC2s). By secreting type 2 cytokines, these two types of cells can initiate a series of signaling cascades (4–6). It starts with the trigger of allergen which is captured by antigen-presenting cells such as dendritic cells. Then Th2 cells in lymph nodes are induced and activated (7, 8) with an antigen specific manner. In addition, injured epithelial cells release cytokines such as IL-25, IL-33, and thymus stromal lymphopoietin (TSLP), which can directly recruit and activate Th2 cells and ILC2s in an antigen-nonspecific manner (9–11). These two types of cells produce a large number of type 2 cytokines and activate effector cells such as B cells, basophils and eosinophils, thus initiate the pulmonary inflammatory response (12, 13), causing pathological changes such as AHR, excess mucus secretion, and airway remodeling (14).
Although immune response plays a vital role in the occurrence and development of allergic asthma, neuro regulation, especially neuro-immune regulation in asthma has drawn more and more attention in recent years. Just as skin and intestine, the lung is an important organ that connects the body with the environment. Airway epithelial cells are exposed to all kinds of external irritants from air sources during breath. The lung is the first organ to sense and recognize dangers by alarming the body immediately and responding to these foreign invaders. During this process, nervous and immune system cooperate closely. They share many similarities, such as the same universal distribution almost all over the body, common transmitting mediators such as neurotransmitters and cytokines. In addition to exercising their basic innate defense function, the innate nervous/immune system of the body can also continuously promote adaptive regulation with the changes of external environment (15). Nervous and immune system interact with each other. Sensory nerve fibers express cytokine receptors, which can sense cytokines and send messages to the brain via the autonomic nervous system (15–17). Neurotransmitters can also regulate immune responses through neural receptors on the surface of immune cells (18, 19). This review focuses on neuro-immune regulation in inflammation and airway remodeling of allergic asthma.
Neuro-Immune Regulation in Inflammation of Allergic Asthma
The lung is a highly innerved organ. Nerves in the lung can be divided into sensory or afferent nervous system and motor or efferent nervous system according to the signal direction travelling within the nerve (20, 21). Sensory nerves from the airways relay stretch stimuli, mechanoreceptors, and chemical stimuli, chemoreceptors, along afferent sensory fibers or vagus nerves to the central nervous system (20). Sympathetic, parasympathetic, non-adrenergic and non-cholinergic parasympathetic nerves constitute the motor pathways of the lung. The parasympathetic nerve releases acetylcholine (ACh) and activates muscarinic M3 receptors on airway smooth muscle cells (ASMs), resulting in the effects of bronchial contraction (22, 23). The pulmonary sympathetic nerve innervates the blood vessels and submucosal glands of the bronchus and antagonizes parasympathetic action by noradrenaline (24). Non-adrenergic non-cholinergic parasympathetic nerves may mediate bronchiectasis through vasoactive intestinal peptides and nitric oxide (25).
Neuro-Immune Regulation in Innate Immune Cells
Like a guard, dendritic cells (DCs) are the first to sound the alarm when allergens invade. Allergen capture by antigen-presenting cells such as DC cells is the beginning of allergic asthma (7, 8). Nervous system regulates DCs by neuropeptides. Calcitonin gene-related peptide (CGRP) is a neuropeptide secreted by pulmonary neuroendocrine cells (PNECs) mainly in lung. It inhibits maturation of DCs by CGRP receptors expressed in DCs themselves, reducing the activation and proliferation of antigen-specific T cells (Figure 1). In mouse model of ovalbumin (OVA) induced asthma, CGRP-pretreated DCs alleviate inflammation of allergic asthma with reduced eosinophil numbers in bronchoalveolar lavage fluid (26). Interestingly, the nerve cells can also be a whistleblower to allergens thus giving instructions to DCs. A study using skin allergen exposure model found that allergens can directly activate transient receptor potential vanilloid 1 positive sensory neurons in skin, causing itch and pain behaviors. Activated neurons release neuropeptides such as substance P, which can induce the migration of CD301b+ DCs into the draining lymph nodes, where the differentiation of Th2 cells is initiated (27). Whether the nervous system is also the first responder of allergens during asthma, thereby triggering the accumulation of dendritic cells and subsequent immune responses needs further exploration. If so, inhibition of sensitization by the allergen to sensory nerve fibers could potentially reduce the activation of pulmonary inflammation during asthma. Besides, antigens can also lead to the variation of innervation, regulating inflammatory infiltration in tissues in an age-related manner. In neonatal mice, exposure to antigens could elevate neurotrophin 4 (NT4) levels of ASMs thus increase ASMs innervation through NT4/TrkB signaling. As a result, persistent AHR appears in adulthood (28) (Figure 1). Therefore, in addition to the familiar antigen-presentation process, the interaction between the nervous system and antigens might be more important during the initial phase of inflammation.
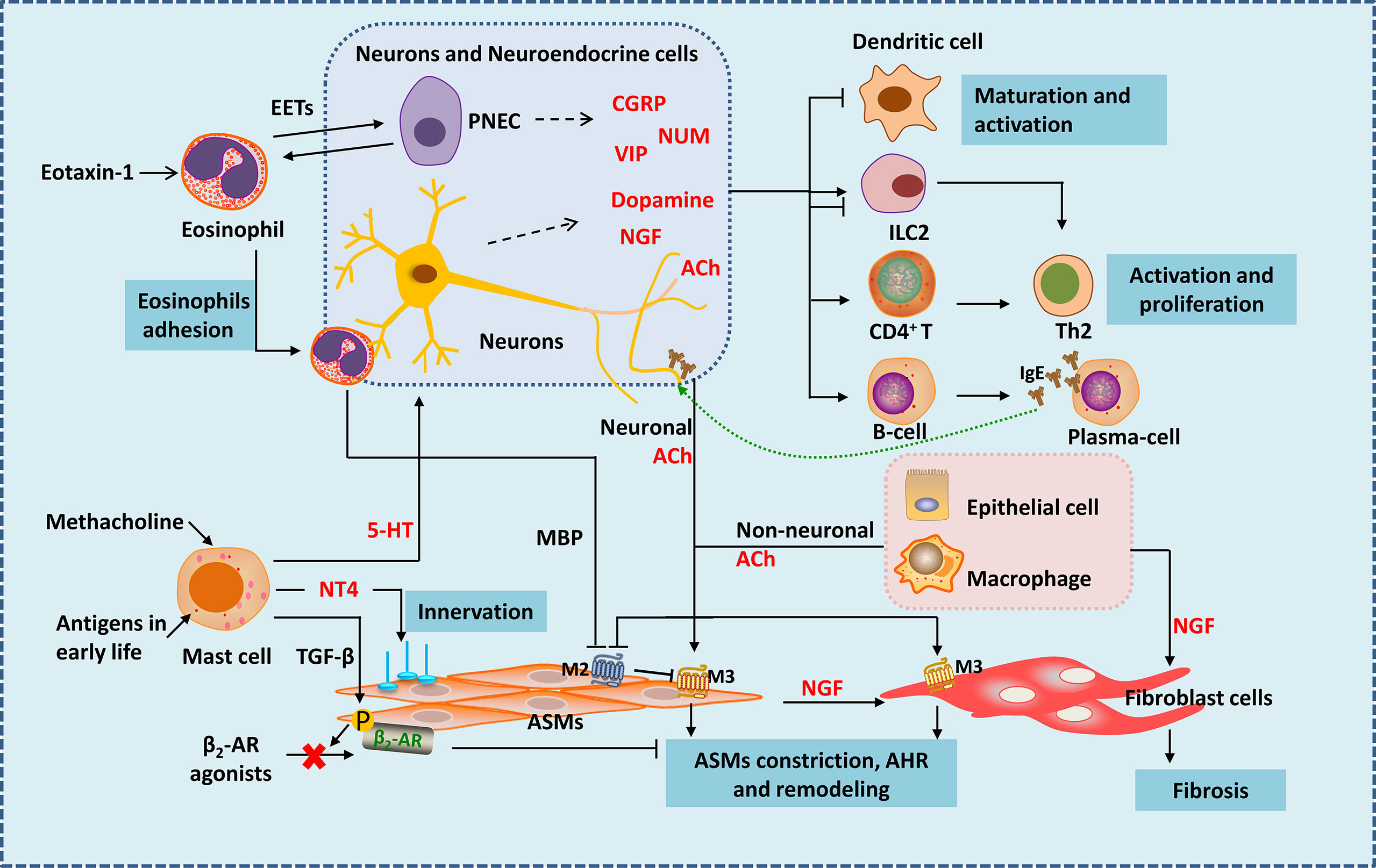
Figure 1 Neuro-immune interactions in inflammation and airway remodeling of allergic asthma. Eosinophils migrate to airway during inflammation via eotaxin-1. They release EETs surrounding and activating PNECs. Under the action of VLA-4 and CD11b, eosinophils adhere to VCAM-1 and ICAM-1 on parasympathetic fibers. Eosinophils are then activated and release MBP, which is an antagonist of M2 muscarinic receptor, thus enhancing parasympathetic mediated bronchoconstriction. Besides, eosinophils increase airway innervation. Under the stimulation of methacholine, mast cells release 5-HT, interacting with 5-HT2 receptors in parasympathetic nerves, thus release ACh (neuronal ACh), enhancing bronchoconstriction. Mast cells also produce NT4 following allergen exposure during early-life, increasing ASMs innervation through NT4/TrkB signaling, causing long-term airway dysfunction. Mast cells also produce TGF-β to induce β2-AR phosphorylation in ASMs, thereby causing β2-AR agonists resistance. Neuropeptides generate form neurons and neuroendocrine cells such as NMU and VIP activate ILC2s. CGRP generated mainly from PNECs inhibits maturation of DCs, it may have bidirectional effects on ILC2s. Besides neuronal ACh, non-neuronal ACh released from epithelia cells and macrophages mainly acted in small airways. ACh activate muscarinic 3 acetylcholine receptor on ASMs and fibroblast cells, causing airway contraction and airway remodeling. NGF released from neurons, epithelial cells, ASMs and other immune cells acts on fibroblasts leading to fibrosis. NGF can also activate Th2 cells and promote the differentiation of B cells into plasma cells. EETS, Eosinophil extracellular traps; PNEC, pulmonary neuroendocrine cell; MBP, major basic protein; 5-HT, 5-hydroxytryptamine; ACh, acetylcholine; NT4, neurotrophin 4; ASMs, airway smooth muscle cells; NGF, nerve growth factor.
In asthma, mast cells accumulate within or close to epithelium and smooth muscle under the stimulation of allergen (29). Mast cells could mediate acute inflammatory response of asthma by secreting a large number of pro-inflammatory and pro-airway constrictor mediators (29–32). Mast cell is now recognized to be involved in nerve regulation during the development of asthma—with muscarinic M3 receptors on its surface (33). Bronchial stimulation test by methacholine provocations is a classic method used for clinical diagnosis of asthma. Mast cells may play the key role in this response. Under the stimulation of methacholine, mast cells release 5-hydroxytryptamine (5-HT), interact with 5-HT2 receptors in parasympathetic nerves, thus release ACh (Figure 1). ACh, an endogenous neurotransmitter, enhances bronchoconstriction and AHR in house dust mite induced experimental asthma (33). Therefore, mast cells and parasympathetic neurons cooperate to form a vicious circle that amplifies the bronchial constriction of choline. Asthma gets worse as the result. Why are mast cells involved in parasympathetic regulation of ASMs? Why the parasympathetic nerve manipulates mast cells to enhance its control on ASMs? Is it the result of the body’s rapid response to foreign substances? Studies showed that the interference of mast cells in ASMs occur with the development of ASMs. Following allergen exposure during early-life, mast cells in lung can produce amount of NT4, which is essential for the innervation of ASMs during development (34), causing long-term airway dysfunction. Mast cells can also produce transforming growth factor β (TGF-β) to induce β2-adrenergic receptor (β2-AR) phosphorylation in ASMs, thereby reducing the airway dilation effect of β2-AR agonists (35) (Figure 1). Therefore, during the occurrence of allergic asthma, mast cells are activated by immunity and affect ASMs under nerve regulation, resulting in persistent airway contraction and AHR. Obviously, mast cells are closely related to the nervous system. Mast cells seem to control the distribution and function of nerves. Besides inflammatory factors, are mast cells also regulated by neurotransmitters? After all, signals travel much faster in nerves. More research is expected.
Eosinophil is accepted as an important kind of effector cells in the development of allergic asthma. Extensive eosinophil infiltration around the airway is considered a hallmark of allergic asthma (36–38). However, growing evidence suggests that eosinophils are inextricably linked to the nervous system during the development of asthma. Tropomyosin receptor kinase A is a high affinity receptor for nerve growth factor (NGF). In a tropomyosin receptor kinase A-knock-in mouse model, eosinophils are found to migrate to airway during inflammation via eotaxin-1 (39). Moreover, eosinophils are closely related to nerve fibers in asthma (40, 41). Under the action of VLA-4 and CD11b, eosinophils adhere to VCAM-1 and ICAM-1 on parasympathetic fibers (41) (Figure 1). It was found that in guinea pigs (42) and monkeys (43) during allergy, eosinophil binding to VCAM-1 or ICAM-1 is important in airway hyperreactivity. Eosinophils are then activated and release major basic protein (MBP), which is an antagonist of M2 muscarinic receptor in human, thus enhancing parasympathetic mediated bronchoconstriction (40) (Figure 1). Besides, airway and peripheral blood eosinophils are associated with increased airway innervation in asthma patients. Eosinophils increase airway sensory innervation in mice and humans, which is remarkable in moderate persistent asthma, thus leading to AHR (44). Strikingly, a novel study suggests that eosinophils play a much more interesting role in neuro-immune regulation than we previously have known. Eosinophil extracellular traps (EETs) are web-like DNA traps generated from eosinophils (Figure 1). In asthma tissues from human and mice, PNECs are surrounded by EETs and activated via the CCDC25–ILK–PKCα–CRTC1 pathway (45). It appears that PNECs are one type of the target cells of eosinophils, but previous studies have shown that eosinophils are influenced by cells such as PNECs (46). Such interactive network regulation is not unusual for sophisticated organisms like mammals, and suggests that we need to take a dialectical view of disease.
ILC2s are important players in both neural and immune regulation. They play a vital role in asthma by releasing type 2 cytokines. Besides respond to alarmins IL-25, IL-33 and TSLP which are released by epithelium, ILC2s could also be regulated by other stimuli. ILC2s are bidirectional regulated by the nervous system (Figure 1). In vitro, Nmur1 is highly expressed in ILC2s purified from mice, and neuropeptides neuromedin U (NMU) activate ILC2s by interacting with NMUR (18, 47, 48). In house dust induced airway inflammation model, NUM amplifies allergic inflammation with the combination of IL-25 (18). Vasoactive intestinal peptide (VIP) is another neuropeptide that belongs to non-adrenergic, non-cholinergic (NANC) system. VIP is one of the most potent endogenous bronchodilators and is proposed to have anti-inflammatory effects (49). However, recent study find it expressed at high levels in nodose ganglion neurons in the lung and has a positive stimulating effect on ILC2. Under the stimulation of IL-5 and OVA, the generation of VIP increase in nodose ganglion neurons both in vitro and in vivo. VIP may promote ILC2s activation partly by VIP-VPAC2 axis thus amplify the airway inflammatory (50). ILC2s are found resided in proximity to PNECs in naïve mice, and when cultured with CGRP and IL-33, ILC2s are activated with increased IL-5 production (46). However, another study analyzed single-cell RNA-seq atlas of lung ILCs and found that ILC2s express both CGRP and its encoding genes, and endogenous CGRP derived from neurons and neuroendocrine cells could negatively regulate IL-33 or IL-25 driven pulmonary ILC2s response, inhibit the production of ILC2-derived type 2 cytokines, thus reduce tissue damage under certain conditions (19). In a study of Nippostrongylus brasiliensis infection mouse model also showed that a subset of ILC2s in lung express CGRP receptor components, and CGRP limits ILC2s response and worm clearance (51). This suggests the complexity of CGRP function and ILC2s microenvironment in different disease models. Many neuro-derived factors can inhibit type 2 immune responses mediated by ILC2s. β2-AR is an important member of the adrenergic nervous system. β2-AR gene is detected in murine and human ILC2s. In N. brasiliensis and Alternaria alternata induced murine lung inflammation models, ILC-deficient mice exhibit increased levels of ILC2s in the lung after infection, which could be inhibited by β2-AR agonist treatment (52). β2-AR agonist is an important class of medicine in the treatment of asthma. It can reduce bronchial constriction by targeting β2-AR on ASMs. Whether β2-AR signaling also alleviate asthma symptoms by dampening ILC2 responses needs further study. α7-nicotinic acetylcholine receptor (α7nAChR) is thought to have anti-inflammatory effect in inflammatory diseases (53). α7nAChR is found expressed on murine ILC2s and α7nAChR agonist inhibited ILC2s function with decreased IL-5 and IL-13 production in vitro. By giving intranasal recombinant mouse IL-33 to Rag2-deficient mice (devoid of T and B cells), ILC2s are induced and a7nAChR agonist treatment could ameliorate ILC2s-mediated AHR by decreasing the expression of GATA-3, a key transcription factor of ILC2s (54). In conclusion, ILC2s express a variety of neuropeptide receptors. As the innate immune cells in the lung, ILC2s become the pivot of lung neuro-immune regulation.
We know that there are other types of innate immune cells, like macrophages, basophils and neutrophils. A special group of macrophages have been found near the large bronchi and airway nerves, named nerve-and airway-associated macrophages (55). This kind of macrophages can activate neurons to produce colony stimulating factor 1 (CSF1) by producing bone morphogenic protein 2, and CSF1 is the necessary signal to maintain its survival. So, nerve fibers provide nutrients for the survival of macrophages. But researches on their relationship with nervous system is still insufficient.
Neuro-Immune Regulation in Adaptive Immunocytes
As we mentioned above, large numbers of Th2 cells is a hallmark of Type 2-high asthma. Th2 cells, induced and activated in lymph nodes by DCs in an antigen specific manner, produce large amounts of type 2 cytokines thus activate further immune cascades. Stimulated by Th2 cells, B cells mature into plasma cells and secrete IgE in response to cytokines IL-4 and IL-13 (56). IgE interacts with cells that have receptors FcϵRI and CD23, such as mast cells, basophils, DCs and ASMs, causing acute allergic response and AHR (3, 57).
Differentiation of T cells into Th2 cells is a landmark in the development of allergic asthma, and the subsequent production of large amounts of type 2 cytokines cause persistent inflammatory changes. These are also important molecular indicators for determining asthma severity and evaluating the effectiveness of interventions in various studies. T cells are certainly an important battleground for the nervous system to participate in immune regulation. As a transient receptor potential ankyrin channel, transient receptor potential ankyrin 1 (TRPA1) is widely expressed in sensory neurons. It can be triggered by wide variety of stimuli and release amounts of inflammatory neuropeptides and neurotransmitters, facilitating the communication between the nervous system and the immune system (58). Furthermore, adaptive immune cells may directly participate in the battlefield of neuroimmune response through TRPA1. The expression of TRPA1 is increased in lung tissues and CD4+ T cells in an OVA-induced mouse model of asthma, accompanied with the development and exacerbation of asthma (59). It provides a complement to non-neural sources of TRPA1. As we have discussed before, the neurotransmitter VIP regulates the immune system by acting on its receptor VPAC2. With the help of VPAC2 expressed on T cells, VIP promotes the differentiation of CD4+ T cells into Th2 cells and increase the production of IL-5 and IL-13 correspondingly (50). NGF has been suggested to play an important role in neuro-immune regulation in airway inflammation by overwhelming studies (60–62). The aggravating role of NGF in lung inflammation can be explained partly by regulating T and B cells. NGF exacerbates inflammation and airway remodeling by enhancing Th2 in OVA sensitized asthma in rat model (63). Suppression of NGF could reduce Th2 immune response, decrease inflammatory infiltration and airway response in murine asthma model (64). The above effects in lung inflammation resulting from inhibition of NGF is also observed in mice infected with respiratory syncytial virus (RSV) (65). And RSV infection in infants is considered a risk factor for the development of asthma (66, 67). It seems that NGF is a broad and powerful molecule involved in neuroimmune inflammation. It is worth noting that the regulation of adaptive immunity by the nervous system may partly explain the higher incidence of allergic asthma in children. It is found that during postnatal development in mice and humans, sympathetic nerves in the lung in mice and humans undergo a transition from dopaminergic type to adrenergic type. In this process, dopamine is bound to a specific dopamine receptor, DRD4, then IL-2-STAT5 signaling is upregulated and histone trimethylation is induced at Th2 gene loci, as a result, differentiation of CD4+ T cell to Th2 cell is promoted (68). Thus, the dopamine-DRD4 pathway augments Th2 inflammation in the lung of young mice in allergen exposure models, which is not so evident in the lungs of adult mice dominated by the adrenergic type.
NGF can also affect the function of plasma cells in the development of asthma. In a mouse model of OVA induced allergic asthma, plasma cells from airways and spleen express different patterns of neurotrophins receptors, and TrkA is only expressed on pulmonary plasma cells. In vitro, NGF promotes survival of pulmonary plasma cells by increasing transcription factors in plasma cells (X-box binding protein 1 and NF-κB subunit RelA) responsible for production of immunoglobulins. Consistently, anti-NGF treatment reduce the number of pulmonary plasma cells and serum IgE (69). B cell activation and subsequent production of large amounts of antigen-specific IgE are known to be characteristic of allergic asthma. However, studies on neural regulation on B cells or plasma cells in asthma are handful and years in advance. Interestingly and excitingly, FcϵR1, a high-affinity IgE receptor, is found expressed on vagal nociceptor neurons in lung in an OVA sensitized mouse model. It means that this kind of neurons can sense invading allergens directly, inducing allergic inflammation (70). This finding is an important addition to the understanding of neuro-immune regulation in the development of asthma, and more research is expected.
Neuro-Immune Regulation in Airway Remodeling of Allergic Asthma
Airway remodeling, the ultimate pathological change in the development of many pulmonary diseases, is one of the most characteristic pathological features of persistent asthma and main reason for hospital care (3, 71). ASMs and fibroblasts play important roles in airway remodeling, and they are closely related to the nervous system.
ASMs express receptors for IgE and respond to IgE directly, causing airway obstruction in severe asthma (3, 72). ASMs is the main kind of effector cell of AHR and airway remodeling, especially in small airways (73). ASMs becomes innervated by parasympathetic fibers since embryogenesis, and its development partly rely on ACh secreted from parasympathetic nerves (74). In asthma, the airway tone is increased mainly because of ASMs contraction, induced by ACh (Figure 1). ACh is derived from two ways——neuronal (the classic) and non-neuronal way. The former is released from nerves like vagus and parasympathetic nerves, the latter comes from epithelial cells and macrophages, which is the main source in small airways (75). ACh activated muscarinic 3 acetylcholine receptor on ASMs and fibroblast cells, causing airway contraction and airway remodeling (75, 76). It is unclear whether the ACh from these two sources is different. Besides, just as we discussed, eosinophils increase parasympathetic ACh release by inhibiting M2 receptor function. An increase in airway eosinophils specifically enhances bronchial constriction in mice, independent of changes in muscarinic M3 receptors or 5-HT receptors in ASMs (77). Apparently, the body considers neuromodulation to be an efficient and economical way. Even without the involvement of nerve fibers, various cells of the body communicate with each other through neurotransmitters. During evolution for over tens of millions of years, human beings and other mammals have developed such elaborate structures. Making full use of the intrinsic transmitters and receptors not only enables us to respond quickly to the environment, but also is more cost-effective——far more efficient and economic than evolving one or more extra regulatory systems.
Excessive collagen deposition and subepithelial fibrosis is another striking feature of airway remodeling (78–81). Almost all these mechanisms are associated with the activation of fibroblasts and its trans-differentiation into myofibroblasts (82, 83). TGF-β1 is identified as one of the important factors in this process (84). However, more and more researches reveal that neural regulation also plays a vital role (Figure 1). Previous studies have shown that NGF was highly increased during allergic asthma, especially in chronic phase (85). And NGF induced the production of type III collagen in fibroblasts by activating p38 MAPK in a TGF-β1 independent way, thus promoting fibrosis (85). Interestingly, activated airway epithelium is a major source of NGF in allergic airway inflammation (86). And NGF can also be produced by ASMs and infiltrated immune cells including mast cells, T cells, eosinophils (87–90). It seems that when a man is going down-hill, everyone will give him a push.
Besides, macrophage may promote the development of pulmonary fibrosis (91) by affecting nerves in the lung. In a bleomycin inhaled mouse model, macrophage-derived neuronal guidance proteins such as netrin-1 is involved in pulmonary adrenergic nerve remodeling and promotes pulmonary fibrosis (92).
Regulation of PNECs in Allergic Asthma
PNECs are a rare and evolutionarily conserved type of lung epithelial cells, accounting for about 0.5% of airway epithelial cells (93), 0.01% of total lung cells (94). They were firstly described in 1954 (95). They scatter among lung epithelial cells, or exist in clusters, forming neuroepithelium bodies (NEBs) consisting of 5-20 PNEC cells (96–98). PNECs are located along the airway epithelium in the trachea and lung in humans and rodents, while NEBs are mainly distributed at the airway branch points (96), where inhalation particles gathered (99). PNECs originate from the endoderm or basal cells (100–102). PNECs are the first to differentiate in the lung (96). The differentiated PNECs gradually move towards the bronchial bifurcation to form NEBs. Nerve fibers then enter the NEBs to innervate them, and innate lung immune cells such as ILC2 settle around the NEBs (96). Although the population of PNECs is very limited, their function can be significant. PNECs have both neural and endocrine properties. They are the only innervated cells in the lung epithelium and the cytoplasm of PNECs is rich in core vesicles containing many kinds of substances like amines, amine metabolizing enzymes, purines, neuroendocrine markers, functional proteins and so on (103). In vitro, PNECs release their vesicle contents under the stimulation of oxygen, mechanical stretching and chemical spines, thereby inducing corresponding pathophysiological changes (104). Innervation of PNECs appear in rabbit embryos on the 16th day (105). The specific form of PNECs and nerve interaction varies in different species (96), which is still not well understood. Present study shows that the nerve innervating PNECs may be sensory nerve, and its cell body is located in the vagal ganglion or dorsal root ganglia (106). PNECs are considered as the most important “sense” cells in the airways. They can sense oxygen changes in the airways (107). The number of PNECs increases in hypoxic environment, and plays a protective role in the surrounding airway epithelial cells (100). Some members of the olfactory receptor family are found expressed in human PNECs, thus PNECs can sense a variety of chemical stimuli (108). Besides, they can also sense mechanical stimuli (109, 110). When the airway epithelium is damaged, PNECs act as progenitor cells to repair the damaged epithelium (97, 111, 112). PNECs are not the key cells to maintain lung development (46), but are necessary in many lung diseases including asthma (46, 113–115).
PNECs may serve as the center of neuro-immune regulation in the asthmatic lung (116). PNECs activate ILC2s by secreting neuropeptides thus amplify the allergen stimulation of immune cells in asthma (46). PNECs are the main source of gamma-aminobutyric acid (GABA) in the lung. GABA secreted by PNECs can promote the transformation of club cells around PNECs into goblet cells. In addition, GABA leads to excess mucus secretion in airway goblet cells by acting on GABA type α and GABA type β receptors (117) and worse symptoms in asthma. Adjacent to ILC2s, PNECs can directly stimulate ILC2s to produce IL5, IL-13 and other cytokines by secreting CGRP, and then trigger Th2 response (46) (Figure 2).
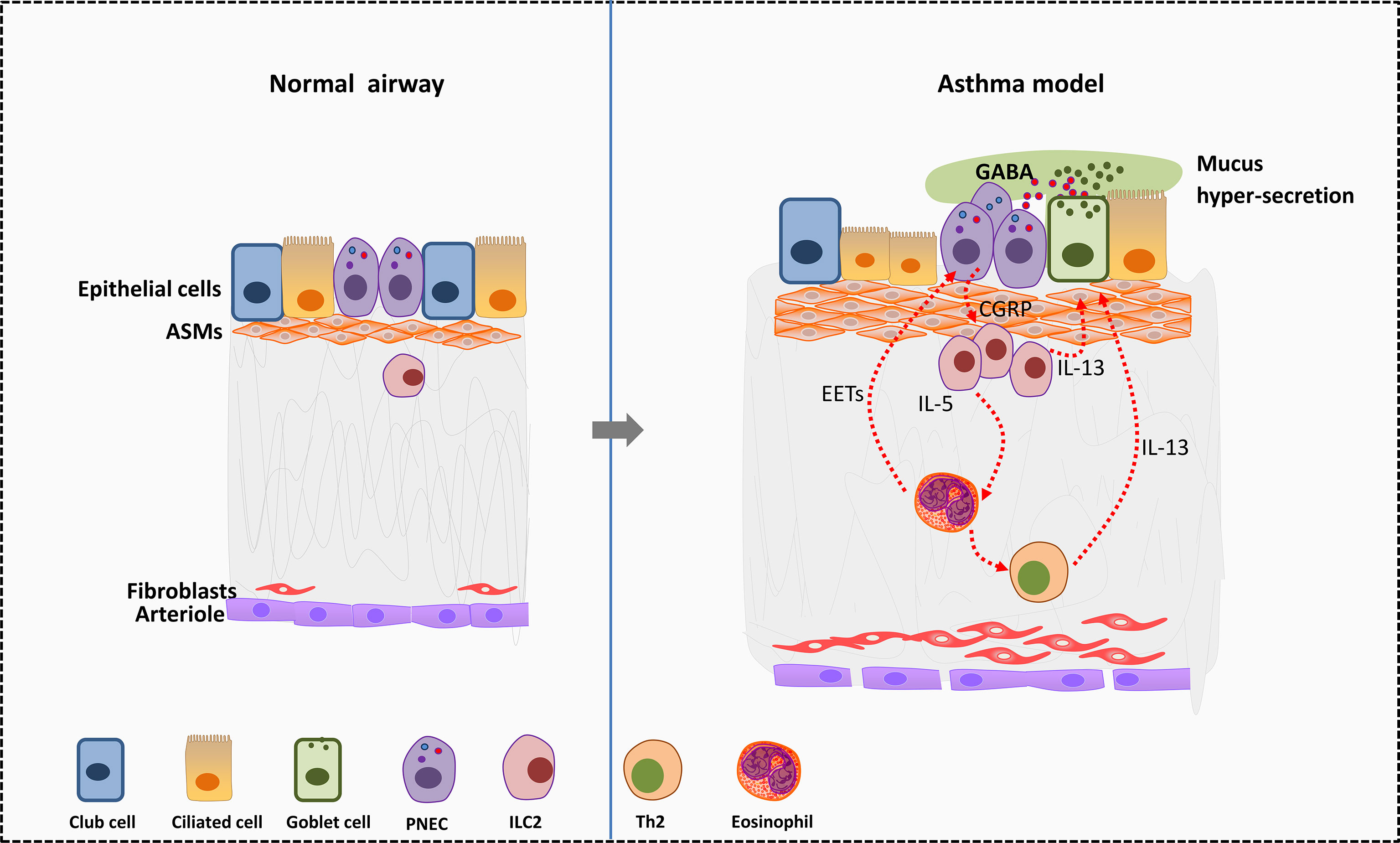
Figure 2 Effects of PNEC cells on surrounding cells in allergic asthma. In murine asthma model, PNECs secret CGRP to active ILC2s and further promote the differentiation of Th2 cells. Type 2 cytokines such as IL-5 and IL-13 secreted by ILC2s act on eosinophils and goblet cells. Eosinophils in turn activate PNECs by releasing EETs. Besides, PNECs secret GABA to promote transformation of club cells near PNECs into goblet cells. GABA leads to excess mucus secretion in airway goblet cells by acting on GABA type α and GABA type β receptors, thus worse symptoms in asthma. EETS, Eosinophil extracellular traps; PNECs, pulmonary neuroendocrine cells; GABA, gamma-aminobutyric acid; ASMs, airway smooth muscle cells.
Conclusion
The occurrence of asthma involves multi-factors such as heredity, environment and so on. Neuro-immune regulation is ubiquitous in the development of asthma and airway remodeling. The link between the nervous system and the immune system is intricate and interlocking. This review provides a new view for the study of the pathogenesis of allergic asthma and the search for effective treatment from the perspective of nerve regulation on immunity. The nervous system is closely related to the various cells of the immune system, but it is not clear whether neuro-immune regulation is the cause or the result for the development of asthma. With the discovery of PNECs, the function of neuro-regulation in the physiological and pathological aspects in asthma has been paid more attention gradually. However, related investigations are still very handful. There are still many questions to be answered. For example, most of the signaling pathways and key molecules that mediate neuro-immune regulation are not yet clear, and the function of some cells and molecules is controversial.
With the novel perspective of neuro-immune regulation, it is our hope to screen out the high-risk population of asthma in early childhood, help find a simpler and more specific monitoring method of asthma treatment, as well as develop new asthma treatments. While for now, more research is expected and this is definitely a field worth further exploration.
Author Contributions
Study design: CJ and SL. Original draft of manuscript: NZ. Manuscript preparation: NZ and JX. Financial support: CJ and SL. Finalized the manuscript: NZ, CJ, and SL. All authors contributed to the review article and approved the submitted version.
Funding
We are very grateful for the financial support from the National Natural Science Foundation of China (grant No. 81970029), Shaanxi Province Natural Science Foundation (Project No. 2021JQ-024) and fundamental research funds for the central universities (xjh012020026).
Conflict of Interest
The authors declare that the research was conducted in the absence of any commercial or financial relationships that could be construed as a potential conflict of interest.
Publisher’s Note
All claims expressed in this article are solely those of the authors and do not necessarily represent those of their affiliated organizations, or those of the publisher, the editors and the reviewers. Any product that may be evaluated in this article, or claim that may be made by its manufacturer, is not guaranteed or endorsed by the publisher.
Abbreviations
AHR, airway hyperresponsiveness; Th2, T helper 2; ILC2s, group 2 innate lymphoid cells; TSLP, thymus stromal lymphopoietin; ACh, acetylcholine; ASMs, airway smooth muscle cells; DCs, dendritic cells; CGRP, calcitonin gene-related peptide; PNECs, pulmonary neuroendocrine cells; NT4, neurotrophin 4; OVA, ovalbumin; 5-HT, 5-hydroxytryptamine; TGF-β, transforming growth factor β; β2-AR, β2-adrenergic receptor; NGF, nerve growth factor; EETs, eosinophil extracellular traps; NMU, neuromedin U; VIP, vasoactive intestinal peptide.
References
1. Lambrecht BN, Hammad H. The Immunology of Asthma. Nat Immunol (2015) 16:45–56. doi: 10.1038/ni.3049
2. Wenzel SE. Asthma Phenotypes: The Evolution From Clinical to Molecular Approaches. Nat Med (2012) 18:716–25. doi: 10.1038/nm.2678
3. Hammad H, Lambrecht BN. The Basic Immunology of Asthma. Cell (2021) 184:1469–85. doi: 10.1016/j.cell.2021.02.016
4. Fahy JV. Type 2 Inflammation in Asthma–Present in Most, Absent in Many. Nat Rev Immunol (2015) 15:57–65. doi: 10.1038/nri3786
5. Robinson D, Humbert M, Buhl R, Cruz AA, Inoue H, Korom S, et al. Revisiting Type 2-High and Type 2-Low Airway Inflammation in Asthma: Current Knowledge and Therapeutic Implications. Clin Exp Allergy (2017) 47:161–75. doi: 10.1111/cea.12880
6. Boonpiyathad T, Sozener ZC, Satitsuksanoa P, Akdis CA. Immunologic Mechanisms in Asthma. Semin Immunol (2019) 46:101333. doi: 10.1016/j.smim.2019.101333
7. Del Giacco SR, Bakirtas A, Bel E, Custovic A, Diamant Z, Hamelmann E, et al. Allergy in Severe Asthma. Allergy (2017) 72:207–20. doi: 10.1111/all.13072
8. Jirmo AC, Busse M, Happle C, Skuljec J, Daluge K, Habener A, et al. IL-17 Regulates DC Migration to the Peribronchial LNs and Allergen Presentation in Experimental Allergic Asthma. Eur J Immunol (2020) 50:1019–33. doi: 10.1002/eji.201948409
9. Tang W, Smith SG, Du W, Gugilla A, Du J, Oliveria JP, et al. Interleukin-25 and Eosinophils Progenitor Cell Mobilization in Allergic Asthma. Clin Transl Allergy (2018) 8:5. doi: 10.1186/s13601-018-0190-2
10. Wang W, Li Y, Lv Z, Chen Y, Li Y, Huang K, et al. Bronchial Allergen Challenge of Patients With Atopic Asthma Triggers an Alarmin (IL-33, TSLP, and IL-25) Response in the Airways Epithelium and Submucosa. J Immunol (2018) 201:2221–31. doi: 10.4049/jimmunol.1800709
11. Yi L, Cheng D, Zhang K, Huo X, Mo Y, Shi H, et al. Intelectin Contributes to Allergen-Induced IL-25, IL-33, and TSLP Expression and Type 2 Response in Asthma and Atopic Dermatitis. Mucosal Immunol (2017) 10:1491–503. doi: 10.1038/mi.2017.10
12. Toyama S, Okada N, Matsuda A, Morita H, Saito H, Fujisawa T, et al. Human Eosinophils Constitutively Express a Unique Serine Protease, PRSS33. Allergol Int (2017) 66:463–71. doi: 10.1016/j.alit.2017.01.001
13. Nakagome K, Nagata M. Involvement and Possible Role of Eosinophils in Asthma Exacerbation. Front Immunol (2018) 9:2220. doi: 10.3389/fimmu.2018.02220
14. Kuo CS, Pavlidis S, Zhu J, Loza M, Baribaud F, Rowe A, et al. Contribution of Airway Eosinophils in Airway Wall Remodeling in Asthma: Role of MMP-10 and MET. Allergy (2019) 74:1102–12. doi: 10.1111/all.13727
15. Salvador AF, de Lima KA, Kipnis J. Neuromodulation by the Immune System: A Focus on Cytokines. Nat Rev Immunol (2021) 21(8):526–41. doi: 10.1038/s41577-021-00508-z
16. Baral P, Udit S, Chiu IM. Pain and Immunity: Implications for Host Defence. Nat Rev Immunol (2019) 19:433–47. doi: 10.1038/s41577-019-0147-2
17. Da Mesquita S, Fu Z, Kipnis J. The Meningeal Lymphatic System: A New Player in Neurophysiology. Neuron (2018) 100:375–88. doi: 10.1016/j.neuron.2018.09.022
18. Wallrapp A, Riesenfeld SJ, Burkett PR, Abdulnour RE, Nyman J, Dionne D, et al. The Neuropeptide NMU Amplifies ILC2-Driven Allergic Lung Inflammation. Nature (2017) 549:351–6. doi: 10.1038/nature24029
19. Wallrapp A, Burkett PR, Riesenfeld SJ, Kim SJ, Christian E, Abdulnour RE, et al. Calcitonin Gene-Related Peptide Negatively Regulates Alarmin-Driven Type 2 Innate Lymphoid Cell Responses. Immunity (2019) 51:709–23.e6. doi: 10.1016/j.immuni.2019.09.005
20. Riches DWH, Martin TR. Overview of Innate Lung Immunity and Inflammation. Methods Mol Biol (2018) 1809:17–30. doi: 10.1007/978-1-4939-8570-8_2
21. Sun H, Lin AH, Ru F, Patil MJ, Meeker S, Lee LY, et al. KCNQ/M-Channels Regulate Mouse Vagal Bronchopulmonary C-Fiber Excitability and Cough Sensitivity. JCI Insight (2019) 4:e124467. doi: 10.1172/jci.insight.124467
22. Kocmalova M, Kollarik M, Canning BJ, Ru F, Adam Herbstsomer R, Meeker S, et al. Control of Neurotransmission by NaV1.7 in Human, Guinea Pig, and Mouse Airway Parasympathetic Nerves. J Pharmacol Exp Ther (2017) 361:172–80. doi: 10.1124/jpet.116.238469
23. Roth M. Airway and Lung Remodelling in Chronic Pulmonary Obstructive Disease: A Role for Muscarinic Receptor Antagonists? Drugs (2015) 75:1–8. doi: 10.1007/s40265-014-0319-0
24. Oh EJ, Mazzone SB, Canning BJ, Weinreich D. Reflex Regulation of Airway Sympathetic Nerves in Guinea-Pigs. J Physiol (2006) 573:549–64. doi: 10.1113/jphysiol.2005.104661
25. Balentova S, Conwell S, Myers AC. Neurotransmitters in Parasympathetic Ganglionic Neurons and Nerves in Mouse Lower Airway Smooth Muscle. Respir Physiol Neurobiol (2013) 189:195–202. doi: 10.1016/j.resp.2013.07.006
26. Rochlitzer S, Veres TZ, Kuhne K, Prenzler F, Pilzner C, Knothe S, et al. The Neuropeptide Calcitonin Gene-Related Peptide Affects Allergic Airway Inflammation by Modulating Dendritic Cell Function. Clin Exp Allergy (2011) 41:1609–21. doi: 10.1111/j.1365-2222.2011.03822.x
27. Perner C, Flayer CH, Zhu X, Aderhold PA, Dewan ZNA, Voisin T, et al. Substance P Release by Sensory Neurons Triggers Dendritic Cell Migration and Initiates the Type-2 Immune Response to Allergens. Immunity (2020) 53:1063–77.e7. doi: 10.1016/j.immuni.2020.10.001
28. Aven L, Paez-Cortez J, Achey R, Krishnan R, Ram-Mohan S, Cruikshank WW, et al. An NT4/TrkB-Dependent Increase in Innervation Links Early-Life Allergen Exposure to Persistent Airway Hyperreactivity. FASEB J (2014) 28:897–907. doi: 10.1096/fj.13-238212
29. Carter RJ, Bradding P. The Role of Mast Cells in the Structural Alterations of the Airways as a Potential Mechanism in the Pathogenesis of Severe Asthma. Curr Pharm Des (2011) 17:685–98. doi: 10.2174/138161211795428975
30. Mendez-Enriquez E, Hallgren J. Mast Cells and Their Progenitors in Allergic Asthma. Front Immunol (2019) 10:821. doi: 10.3389/fimmu.2019.00821
31. Elieh Ali Komi D, Bjermer L. Mast Cell-Mediated Orchestration of the Immune Responses in Human Allergic Asthma: Current Insights. Clin Rev Allergy Immunol (2019) 56:234–47. doi: 10.1007/s12016-018-8720-1
32. Yu M, Mukai K, Tsai M, Galli SJ. Thirdhand Smoke Component can Exacerbate a Mouse Asthma Model Through Mast Cells. J Allergy Clin Immunol (2018) 142:1618–27.e9. doi: 10.1016/j.jaci.2018.04.001
33. Mendez-Enriquez E, Alvarado-Vazquez PA, Abma W, Simonson OE, Rodin S, Feyerabend TB, et al. Mast Cell-Derived Serotonin Enhances Methacholine-Induced Airway Hyperresponsiveness in House Dust Mite-Induced Experimental Asthma. Allergy (2021) 76(7):2057–69. doi: 10.1183/13993003.congress-2021.PA862
34. Patel KR, Aven L, Shao F, Krishnamoorthy N, Duvall MG, Levy BD, et al. Mast Cell-Derived Neurotrophin 4 Mediates Allergen-Induced Airway Hyperinnervation in Early Life. Mucosal Immunol (2016) 9:1466–76. doi: 10.1038/mi.2016.11
35. Chachi L, Alzahrani A, Koziol-White C, Biddle M, Bagadood R, Panettieri RA Jr, et al. Increased Beta2-Adrenoceptor Phosphorylation in Airway Smooth Muscle in Severe Asthma: Possible Role of Mast Cell-Derived Growth Factors. Clin Exp Immunol (2018) 194:253–8. doi: 10.1111/cei.13191
36. Kanda A, Yasutaka Y, Van Bui D, Suzuki K, Sawada S, Kobayashi Y, et al. Multiple Biological Aspects of Eosinophils in Host Defense, Eosinophil-Associated Diseases, Immunoregulation, and Homeostasis: Is Their Role Beneficial, Detrimental, Regulator, or Bystander? Biol Pharm Bull (2020) 43:20–30. doi: 10.1248/bpb.b19-00892
37. Pope SM, Zimmermann N, Stringer KF, Karow ML, Rothenberg ME. The Eotaxin Chemokines and CCR3 Are Fundamental Regulators of Allergen-Induced Pulmonary Eosinophilia. J Immunol (2005) 175:5341–50. doi: 10.4049/jimmunol.175.8.5341
38. Ray A, Raundhal M, Oriss TB, Ray P, Wenzel SE. Current Concepts of Severe Asthma. J Clin Invest (2016) 126:2394–403. doi: 10.1172/JCI84144
39. Dileepan M, Ge XN, Bastan I, Greenberg YG, Liang Y, Sriramarao P, et al. Regulation of Eosinophil Recruitment and Allergic Airway Inflammation by Tropomyosin Receptor Kinase A. J Immunol (2020) 204:682–93. doi: 10.4049/jimmunol.1900786
40. Drake MG, Lebold KM, Roth-Carter QR, Pincus AB, Blum ED, Proskocil BJ, et al. Eosinophil and Airway Nerve Interactions in Asthma. J Leukoc Biol (2018) 104:61–7. doi: 10.1002/JLB.3MR1117-426R
41. Kingham PJ, Costello RW, McLean WG. Eosinophil and Airway Nerve Interactions. Pulm Pharmacol Ther (2003) 16:9–13. doi: 10.1016/S1094-5539(02)00093-7
42. Fryer AD, Costello RW, Yost BL, Lobb RR, Tedder TF, Steeber DA, et al. Antibody to VLA-4, But Not to L-Selectin, Protects Neuronal M2 Muscarinic Receptors in Antigen-Challenged Guinea Pig Airways. J Clin Invest (1997) 99:2036–44. doi: 10.1172/JCI119372
43. Wegner CD, Gundel RH, Reilly P, Haynes N, Letts LG, Rothlein R. Intercellular Adhesion Molecule-1 (ICAM-1) in the Pathogenesis of Asthma. Science (1990) 247:456–9. doi: 10.1126/science.1967851
44. Drake MG, Scott GD, Blum ED, Lebold KM, Nie Z, Lee JJ, et al. Eosinophils Increase Airway Sensory Nerve Density in Mice and in Human Asthma. Sci Transl Med (2018) 10:eaar8477. doi: 10.1126/scitranslmed.aar8477
45. Lu Y, Huang Y, Li J, Huang J, Zhang L, Feng J, et al. Eosinophil Extracellular Traps Drive Asthma Progression Through Neuro-Immune Signals. Nat Cell Biol (2021) 23:1060–72. doi: 10.1038/s41556-021-00762-2
46. Sui P, Wiesner DL, Xu J, Zhang Y, Lee J, Van Dyken S, et al. Pulmonary Neuroendocrine Cells Amplify Allergic Asthma Responses. Science (2018) 360:eaan8546. doi: 10.1126/science.aan8546
47. Klose CSN, Mahlakoiv T, Moeller JB, Rankin LC, Flamar AL, Kabata H, et al. The Neuropeptide Neuromedin U Stimulates Innate Lymphoid Cells and Type 2 Inflammation. Nature (2017) 549:282–6. doi: 10.1038/nature23676
48. Cardoso V, Chesne J, Ribeiro H, Garcia-Cassani B, Carvalho T, Bouchery T, et al. Neuronal Regulation of Type 2 Innate Lymphoid Cells via Neuromedin U. Nature (2017) 549:277–81. doi: 10.1038/nature23469
49. Misaka S, Aoki Y, Karaki S, Kuwahara A, Mizumoto T, Onoue S, et al. Inhalable Powder Formulation of a Stabilized Vasoactive Intestinal Peptide (VIP) Derivative: Anti-Inflammatory Effect in Experimental Asthmatic Rats. Peptides (2010) 31:72–8. doi: 10.1016/j.peptides.2009.09.032
50. Talbot S, Abdulnour RE, Burkett PR, Lee S, Cronin SJ, Pascal MA, et al. Silencing Nociceptor Neurons Reduces Allergic Airway Inflammation. Neuron (2015) 87:341–54. doi: 10.1016/j.neuron.2015.06.007
51. Nagashima H, Mahlakoiv T, Shih HY, Davis FP, Meylan F, Huang Y, et al. Neuropeptide CGRP Limits Group 2 Innate Lymphoid Cell Responses and Constrains Type 2 Inflammation. Immunity (2019) 51:682–95.e6. doi: 10.1016/j.immuni.2019.06.009
52. Moriyama S, Brestoff JR, Flamar AL, Moeller JB, Klose CSN, Rankin LC, et al. Beta2-Adrenergic Receptor-Mediated Negative Regulation of Group 2 Innate Lymphoid Cell Responses. Science (2018) 359:1056–61. doi: 10.1126/science.aan4829
53. Wang H, Yu M, Ochani M, Amella CA, Tanovic M, Susarla S, et al. Nicotinic Acetylcholine Receptor Alpha7 Subunit is an Essential Regulator of Inflammation. Nature (2003) 421:384–8. doi: 10.1038/nature01339
54. Galle-Treger L, Suzuki Y, Patel N, Sankaranarayanan I, Aron JL, Maazi H, et al. Nicotinic Acetylcholine Receptor Agonist Attenuates ILC2-Dependent Airway Hyperreactivity. Nat Commun (2016) 7:13202. doi: 10.1038/ncomms13202
55. Ural BB, Yeung ST, Damani-Yokota P, Devlin JC, de Vries M, Vera-Licona P, et al. Identification of a Nerve-Associated, Lung-Resident Interstitial Macrophage Subset With Distinct Localization and Immunoregulatory Properties. Sci Immunol (2020) 5:eaax8756. doi: 10.1126/sciimmunol.aax8756
56. Hoof I, Schulten V, Layhadi JA, Stranzl T, Christensen LH, Herrera de la Mata S, et al. Allergen-Specific IgG(+) Memory B Cells are Temporally Linked to IgE Memory Responses. J Allergy Clin Immunol (2020) 146:180–91. doi: 10.1016/j.jaci.2019.11.046
57. Redhu NS, Gounni AS. The High Affinity IgE Receptor (FcepsilonRI) Expression and Function in Airway Smooth Muscle. Pulm Pharmacol Ther (2013) 26:86–94. doi: 10.1016/j.pupt.2012.04.004
58. Talavera K, Startek JB, Alvarez-Collazo J, Boonen B, Alpizar YA, Sanchez A, et al. Mammalian Transient Receptor Potential TRPA1 Channels: From Structure to Disease. Physiol Rev (2020) 100:725–803. doi: 10.1152/physrev.00005.2019
59. Li M, Fan X, Yue Q, Hu F, Zhang Y, Zhu C. The Neuro-Immune Interaction in Airway Inflammation Through TRPA1 Expression in CD4+ T Cells of Asthmatic Mice. Int Immunopharmacol (2020) 86:106696. doi: 10.1016/j.intimp.2020.106696
60. Minnone G, De Benedetti F, Bracci-Laudiero L. NGF and Its Receptors in the Regulation of Inflammatory Response. Int J Mol Sci (2017) 18:1028. doi: 10.3390/ijms18051028
61. Prakash YS, Martin RJ. Brain-Derived Neurotrophic Factor in the Airways. Pharmacol Ther (2014) 143:74–86. doi: 10.1016/j.pharmthera.2014.02.006
62. Chen YL, Huang HY, Lee CC, Chiang BL. Small Interfering RNA Targeting Nerve Growth Factor Alleviates Allergic Airway Hyperresponsiveness. Mol Ther Nucleic Acids (2014) 3:e158. doi: 10.1038/mtna.2014.11
63. Yang YG, Tian WM, Zhang H, Li M, Shang YX. Nerve Growth Factor Exacerbates Allergic Lung Inflammation and Airway Remodeling in a Rat Model of Chronic Asthma. Exp Ther Med (2013) 6:1251–8. doi: 10.3892/etm.2013.1284
64. Shi Y, Jin Y, Guo W, Chen L, Liu C, Lv X. Blockage of Nerve Growth Factor Modulates T Cell Responses and Inhibits Allergic Inflammation in a Mouse Model of Asthma. Inflamm Res (2012) 61:1369–78. doi: 10.1007/s00011-012-0538-3
65. Wu X, Zhou X, Hu Y, Liu C, Wang J. Neutralization of Nerve Growth Factor (NGF) Inhibits the Th2 Response and Protects Against the Respiratory Syncytial Virus (RSV) Infection. Immunol Res (2017) 65:721–8. doi: 10.1007/s12026-017-8909-z
66. Jartti T, Gern JE. Role of Viral Infections in the Development and Exacerbation of Asthma in Children. J Allergy Clin Immunol (2017) 140:895–906. doi: 10.1016/j.jaci.2017.08.003
67. Raita Y, Perez-Losada M, Freishtat RJ, Harmon B, Mansbach JM, Piedra PA, et al. Integrated Omics Endotyping of Infants With Respiratory Syncytial Virus Bronchiolitis and Risk of Childhood Asthma. Nat Commun (2021) 12:3601. doi: 10.1038/s41467-021-23859-6
68. Wang W, Cohen JA, Wallrapp A, Trieu KG, Barrios J, Shao F, et al. Age-Related Dopaminergic Innervation Augments T Helper 2-Type Allergic Inflammation in the Postnatal Lung. Immunity (2019) 51:1102–18.e7. doi: 10.1016/j.immuni.2019.10.002
69. Abram M, Wegmann M, Fokuhl V, Sonar S, Luger EO, Kerzel S, et al. Nerve Growth Factor and Neurotrophin-3 Mediate Survival of Pulmonary Plasma Cells During the Allergic Airway Inflammation. J Immunol (2009) 182:4705–12. doi: 10.4049/jimmunol.0802814
70. Crosson T, Wang JC, Doyle B, Merrison H, Balood M, Parrin A, et al. FcepsilonR1-Expressing Nociceptors Trigger Allergic Airway Inflammation. J Allergy Clin Immunol (2021) 147:2330–42. doi: 10.1016/j.jaci.2020.12.644
71. Dunican EM, Elicker BM, Gierada DS, Nagle SK, Schiebler ML, Newell JD, et al. Mucus Plugs in Patients With Asthma Linked to Eosinophilia and Airflow Obstruction. J Clin Invest (2018) 128:997–1009. doi: 10.1172/JCI95693
72. Ferreira DS, Carvalho-Pinto RM, Gregorio MG, Annoni R, Teles AM, Buttignol M, et al. Airway Pathology in Severe Asthma is Related to Airflow Obstruction But Not Symptom Control. Allergy (2018) 73:635–43. doi: 10.1111/all.13323
73. Bonini M, Usmani OS. The Role of the Small Airways in the Pathophysiology of Asthma and Chronic Obstructive Pulmonary Disease. Ther Adv Respir Dis (2015) 9:281–93. doi: 10.1177/1753465815588064
74. Patel KR, Bai Y, Trieu KG, Barrios J, Ai X. Targeting Acetylcholine Receptor M3 Prevents the Progression of Airway Hyperreactivity in a Mouse Model of Childhood Asthma. FASEB J (2017) 31:4335–46. doi: 10.1096/fj.201700186R
75. Cazzola M, Calzetta L, Matera MG. Long-Acting Muscarinic Antagonists and Small Airways in Asthma: Which Link? Allergy (2021) 76(7):1990–2001. doi: 10.22541/au.160870966.64336849/v1
76. Matera MG, Page CP, Calzetta L, Rogliani P, Cazzola M. Pharmacology and Therapeutics of Bronchodilators Revisited. Pharmacol Rev (2020) 72:218–52. doi: 10.1124/pr.119.018150
77. Nie Z, Maung JN, Jacoby DB, Fryer AD. Lung Eosinophils Increase Vagus Nerve-Mediated Airway Reflex Bronchoconstriction in Mice. Am J Physiol Lung Cell Mol Physiol (2020) 318:L242–51. doi: 10.1152/ajplung.00040.2019
78. Fang L, Sun Q, Roth M. Immunologic and Non-Immunologic Mechanisms Leading to Airway Remodeling in Asthma. Int J Mol Sci (2020) 21:757. doi: 10.3390/ijms21030757
79. Fehrenbach H, Wagner C, Wegmann M. Airway Remodeling in Asthma: What Really Matters. Cell Tissue Res (2017) 367:551–69. doi: 10.1007/s00441-016-2566-8
80. Trejo Bittar HE, Yousem SA, Wenzel SE. Pathobiology of Severe Asthma. Annu Rev Pathol (2015) 10:511–45. doi: 10.1146/annurev-pathol-012414-040343
81. Hough KP, Curtiss ML, Blain TJ, Liu RM, Trevor J, Deshane JS, et al. Airway Remodeling in Asthma. Front Med (Lausanne) (2020) 7:191. doi: 10.3389/fmed.2020.00191
82. Wnuk D, Paw M, Ryczek K, Bochenek G, Sladek K, Madeja Z, et al. Enhanced Asthma-Related Fibroblast to Myofibroblast Transition is the Result of Profibrotic TGF-Beta/Smad2/3 Pathway Intensification and Antifibrotic TGF-Beta/Smad1/5/(8)9 Pathway Impairment. Sci Rep (2020) 10:16492. doi: 10.1038/s41598-020-73473-7
83. Ojiaku CA, Cao G, Zhu W, Yoo EJ, Shumyatcher M, Himes BE, et al. TGF-Beta1 Evokes Human Airway Smooth Muscle Cell Shortening and Hyperresponsiveness via Smad3. Am J Respir Cell Mol Biol (2018) 58:575–84. doi: 10.1165/rcmb.2017-0247OC
84. Saito A, Horie M, Nagase T. TGF-Beta Signaling in Lung Health and Disease. Int J Mol Sci (2018) 19:460. doi: 10.3390/ijms19082460
85. Kilic A, Sonar SS, Yildirim AO, Fehrenbach H, Nockher WA, Renz H. Nerve Growth Factor Induces Type III Collagen Production in Chronic Allergic Airway Inflammation. J Allergy Clin Immunol (2011) 128:1058–66.e1-4. doi: 10.1016/j.jaci.2011.06.017
86. Hahn C, Islamian AP, Renz H, Nockher WA. Airway Epithelial Cells Produce Neurotrophins and Promote the Survival of Eosinophils During Allergic Airway Inflammation. J Allergy Clin Immunol (2006) 117:787–94. doi: 10.1016/j.jaci.2005.12.1339
87. Kobayashi H, Gleich GJ, Butterfield JH, Kita H. Human Eosinophils Produce Neurotrophins and Secrete Nerve Growth Factor on Immunologic Stimuli. Blood (2002) 99:2214–20. doi: 10.1182/blood.V99.6.2214
88. Nassenstein C, Braun A, Erpenbeck VJ, Lommatzsch M, Schmidt S, Krug N, et al. The Neurotrophins Nerve Growth Factor, Brain-Derived Neurotrophic Factor, Neurotrophin-3, and Neurotrophin-4 Are Survival and Activation Factors for Eosinophils in Patients With Allergic Bronchial Asthma. J Exp Med (2003) 198:455–67. doi: 10.1084/jem.20010897
89. Freund V, Pons F, Joly V, Mathieu E, Martinet N, Frossard N. Upregulation of Nerve Growth Factor Expression by Human Airway Smooth Muscle Cells in Inflammatory Conditions. Eur Respir J (2002) 20:458–63. doi: 10.1183/09031936.02.00269202
90. Kemi C, Grunewald J, Eklund A, Hoglund CO. Differential Regulation of Neurotrophin Expression in Human Bronchial Smooth Muscle Cells. Respir Res (2006) 7:18. doi: 10.1186/1465-9921-7-18
91. Desai O, Winkler J, Minasyan M, Herzog EL. The Role of Immune and Inflammatory Cells in Idiopathic Pulmonary Fibrosis. Front Med (Lausanne) (2018) 5:43. doi: 10.3389/fmed.2018.00043
92. Gao R, Peng X, Perry C, Sun H, Ntokou A, Ryu C, et al. Macrophage-Derived Netrin-1 Drives Adrenergic Nerve-Associated Lung Fibrosis. J Clin Invest (2021) 131:e136542. doi: 10.1172/JCI136542
93. Boers JE, den Brok JL, Koudstaal J, Arends JW, Thunnissen FB. Number and Proliferation of Neuroendocrine Cells in Normal Human Airway Epithelium. Am J Respir Crit Care Med (1996) 154:758–63. doi: 10.1164/ajrccm.154.3.8810616
94. Travaglini KJ, Nabhan AN, Penland L, Sinha R, Gillich A, Sit RV, et al. A Molecular Cell Atlas of the Human Lung From Single-Cell RNA Sequencing. Nature (2020) 587:619–25. doi: 10.1038/s41586-020-2922-4
95. Feyrter F. [Argyrophilia of Bright Cell System in Bronchial Tree in Man]. Z Mikrosk Anat Forsch (1954) 61:73–81.
96. Kuo CS, Krasnow MA. Formation of a Neurosensory Organ by Epithelial Cell Slithering. Cell (2015) 163:394–405. doi: 10.1016/j.cell.2015.09.021
97. Song H, Yao E, Lin C, Gacayan R, Chen MH, Chuang PT. Functional Characterization of Pulmonary Neuroendocrine Cells in Lung Development, Injury, and Tumorigenesis. Proc Natl Acad Sci USA (2012) 109:17531–6. doi: 10.1073/pnas.1207238109
98. Weichselbaum M, Sparrow MP, Hamilton EJ, Thompson PJ, Knight DA. A Confocal Microscopic Study of Solitary Pulmonary Neuroendocrine Cells in Human Airway Epithelium. Respir Res (2005) 6:115. doi: 10.1186/1465-9921-6-115
99. Awadalla M, Miyawaki S, Abou Alaiwa MH, Adam RJ, Bouzek DC, Michalski AS, et al. Early Airway Structural Changes in Cystic Fibrosis Pigs as a Determinant of Particle Distribution and Deposition. Ann BioMed Eng (2014) 42:915–27. doi: 10.1007/s10439-013-0955-7
100. Shivaraju M, Chitta UK, Grange RMH, Jain IH, Capen D, Liao L, et al. Airway Stem Cells Sense Hypoxia and Differentiate Into Protective Solitary Neuroendocrine Cells. Science (2021) 371:52–7. doi: 10.1126/science.aba0629
101. Hor P, Punj V, Calvert BA, Castaldi A, Miller AJ, Carraro G, et al. Efficient Generation and Transcriptomic Profiling of Human iPSC-Derived Pulmonary Neuroendocrine Cells. iScience (2020) 23:101083. doi: 10.1016/j.isci.2020.101083
102. Mou H, Yang Y, Riehs MA, Barrios J, Shivaraju M, Haber AL, et al. Airway Basal Stem Cells Generate Distinct Subpopulations of PNECs. Cell Rep (2021) 35:109011. doi: 10.1016/j.celrep.2021.109011
103. Cutz E, Pan J, Yeger H, Domnik NJ, Fisher JT. Recent Advances and Contraversies on the Role of Pulmonary Neuroepithelial Bodies as Airway Sensors. Semin Cell Dev Biol (2013) 24:40–50. doi: 10.1016/j.semcdb.2012.09.003
104. Noguchi M, Furukawa KT, Morimoto M. Pulmonary Neuroendocrine Cells: Physiology, Tissue Homeostasis and Disease. Dis Model Mech (2020) 13:dmm046920. doi: 10.1242/dmm.046920
105. Pan J, Yeger H, Cutz E. Innervation of Pulmonary Neuroendocrine Cells and Neuroepithelial Bodies in Developing Rabbit Lung. J Histochem Cytochem (2004) 52:379–89. doi: 10.1177/002215540405200309
106. Chang RB, Strochlic DE, Williams EK, Umans BD, Liberles SD. Vagal Sensory Neuron Subtypes That Differentially Control Breathing. Cell (2015) 161:622–33. doi: 10.1016/j.cell.2015.03.022
107. Noguchi M, Sumiyama K, Morimoto M. Directed Migration of Pulmonary Neuroendocrine Cells Toward Airway Branches Organizes the Stereotypic Location of Neuroepithelial Bodies. Cell Rep (2015) 13:2679–86. doi: 10.1016/j.celrep.2015.11.058
108. Gu X, Karp PH, Brody SL, Pierce RA, Welsh MJ, Holtzman MJ, et al. Chemosensory Functions for Pulmonary Neuroendocrine Cells. Am J Respir Cell Mol Biol (2014) 50:637–46. doi: 10.1165/rcmb.2013-0199OC
109. Pan J, Copland I, Post M, Yeger H, Cutz E. Mechanical Stretch-Induced Serotonin Release From Pulmonary Neuroendocrine Cells: Implications for Lung Development. Am J Physiol Lung Cell Mol Physiol (2006) 290:L185–93. doi: 10.1152/ajplung.00167.2005
110. Garg A, Sui P, Verheyden JM, Young LR, Sun X. Consider the Lung as a Sensory Organ: A Tip From Pulmonary Neuroendocrine Cells. Curr Top Dev Biol (2019) 132:67–89. doi: 10.1016/bs.ctdb.2018.12.002
111. Reynolds SD, Giangreco A, Power JH, Stripp BR. Neuroepithelial Bodies of Pulmonary Airways Serve as a Reservoir of Progenitor Cells Capable of Epithelial Regeneration. Am J Pathol (2000) 156:269–78. doi: 10.1016/S0002-9440(10)64727-X
112. Guha A, Vasconcelos M, Cai Y, Yoneda M, Hinds A, Qian J, et al. Neuroepithelial Body Microenvironment is a Niche for a Distinct Subset of Clara-Like Precursors in the Developing Airways. Proc Natl Acad Sci USA (2012) 109:12592–7. doi: 10.1073/pnas.1204710109
113. Alshehri M, Cutz E, Banzhoff A, Canny G. Hyperplasia of Pulmonary Neuroendocrine Cells in a Case of Childhood Pulmonary Emphysema. Chest (1997) 112:553–6. doi: 10.1378/chest.112.2.553
114. Almquist DR, Sonbol MB, Ross HJ, Kosiorek H, Jaroszewski D, Halfdanarson T. Clinical Characteristics of Diffuse Idiopathic Pulmonary Neuroendocrine Cell Hyperplasia: A Retrospective Analysis. Chest (2021) 159:432–4. doi: 10.1016/j.chest.2020.08.012
115. Schaff DL, Singh S, Kim KB, Sutcliffe MD, Park KS, Janes KA. Fragmentation of Small-Cell Lung Cancer Regulatory States in Heterotypic Microenvironments. Cancer Res (2021) 81(7):18537–67. doi: 10.1101/2020.03.30.017210
116. Barrios J, Patel KR, Aven L, Achey R, Minns MS, Lee Y, et al. Early Life Allergen-Induced Mucus Overproduction Requires Augmented Neural Stimulation of Pulmonary Neuroendocrine Cell Secretion. FASEB J (2017) 31:4117–28. doi: 10.1096/fj.201700115R
Keywords: neuro-immune regulation, inflammation, airway remodeling, asthma, pulmonary neuroendocrine cells
Citation: Zhang N, Xu J, Jiang C and Lu S (2022) Neuro-Immune Regulation in Inflammation and Airway Remodeling of Allergic Asthma. Front. Immunol. 13:894047. doi: 10.3389/fimmu.2022.894047
Received: 22 March 2022; Accepted: 23 May 2022;
Published: 16 June 2022.
Edited by:
Ahmed Nadeem, King Saud University, Saudi ArabiaReviewed by:
Yusuke Murakami, Musashino University, JapanSumit Ghosh, The Research Institute at Nationwide Children’s Hospital, United States
Copyright © 2022 Zhang, Xu, Jiang and Lu. This is an open-access article distributed under the terms of the Creative Commons Attribution License (CC BY). The use, distribution or reproduction in other forums is permitted, provided the original author(s) and the copyright owner(s) are credited and that the original publication in this journal is cited, in accordance with accepted academic practice. No use, distribution or reproduction is permitted which does not comply with these terms.
*Correspondence: Congshan Jiang, amlhbmdjb25nc2hhbkB4anR1LmVkdS5jbg==; Shemin Lu, bHVzaGVtaW5AeGp0dS5lZHUuY24=