- Wellcome-Wolfson Institute for Experimental Medicine, Queen’s University Belfast, Belfast, United Kingdom
A review of our current knowledge of B cell antigen uptake mechanisms, the relevance of these processes to pathology, and outstanding questions in the field. Specific antigens induce B cell activation through the B cell receptor (BCR) which initiates downstream signaling and undergoes endocytosis. While extensive research has shed light on the signaling pathways in health and disease, the endocytic mechanisms remain largely uncharacterized. Given the importance of BCR-antigen internalization for antigen presentation in initiating adaptive immune responses and its role in autoimmunity and malignancy, understanding the molecular mechanisms represents critical, and largely untapped, potential therapeutics. In this review, we discuss recent advancements in our understanding of BCR endocytic mechanisms and the role of the actin cytoskeleton and post-translational modifications in regulating BCR uptake. We discuss dysregulated BCR endocytosis in the context of B cell malignancies and autoimmune disorders. Finally, we pose several outstanding mechanistic questions which will critically advance our understanding of the coordination between BCR endocytosis and B cell activation.
Introduction
The term endocytosis describes the production of intracellular membranes from the cell surface plasma membrane (PM), a phospholipid bilayer, to internalize extracellular components, such as cell surface receptors and soluble extracellular factors. Once internalized, the contents can be recycled back to the PM through recycling pathways or delivered to late endosomes and lysosomes for cellular degradation. The final destination of internalized cargo is dependent on post-translational modifications (PTM) and interactions with distinct trafficking adaptors (1). Endocytosis is a ubiquitous cellular process and cargo includes receptors for iron uptake, cytokine responses, growth factors, glucose, cholesterol, vitamins (2–4). As such, it plays a critical role in cell growth and metabolism, cell-cell interactions, adhesion, and migration.
In the immune system, multiple endocytic pathways, including phagocytosis, pinocytosis, receptor-mediated endocytosis and caveolae, allow the uptake of foreign antigens for processing and presentation, and subsequent initiation of immune responses. Different antigen presenting cells rely on distinct endocytic mechanisms; dendritic cells (DC) have the structural and membrane capacity for dramatic and energetically demanding forms of endocytosis such as macropinocytosis and phagocytosis of particulate antigen. In contrast, the size and membrane availability in B cells, together with their requirement to detect specific antigens, limits their antigen uptake capacity to receptor-mediated endocytosis. Soluble antigens encountered in the lymphatics or membrane-bound antigen displayed by antigen presenting cells (APC) (5), binds to the surface B cell receptor (BCR), which initiates downstream signaling cascades for B cell activation and rapid endocytosis to provide material for antigen presentation. In this review, we will discuss mechanisms of antigen-BCR endocytosis in B cells, their coordination with downstream BCR signaling and their dysregulation contributing to B cell malignancy and autoimmunity.
B Cell Activation
B cells promote clearance of invading pathogens and long-term immunity through two main cellular processes: antigen presentation on major histocompatibility complex class II (MHC-II) molecules to initiate T cell responses (6); and differentiation into memory or plasma cells for specific antibody production. Both processes require the coordination of signaling and endocytosis at the BCR.
The BCR is composed of surface immunoglobulin (Ig) and non-covalently associated Igα/β heterodimer. Igα and Igβ chains possess a cytoplasmic tail containing immunoreceptor tyrosine-based activation motifs (ITAMS), which allow signal transduction. Upon antigen binding, ITAMs can be phosphorylated to transmit downstream signals through the action of several enzymes (such as Src family kinase Lyn), reviewed in detail elsewhere (7).
In addition to signaling, antigen binding triggers BCR endocytosis and trafficking of the BCR-antigen complex into antigen-processing compartments, where antigenic peptides are loaded onto MHC-II. Presentation of antigen-loaded MHC-II molecules on the B cell surface promotes interaction with cognate CD4+ T cells, which stimulates B cell activation and transcriptional changes. Thus, BCR-mediated antigen internalization and processing determines the number and repertoire of antigen peptides presented, directly impacting B cell ability to compete for T cell help during the germinal center (GC) response (8).
Despite this interconnectivity, little is known about how BCR signaling and endocytosis are coordinated. Indeed, the two processes appear to be mutually exclusive for individual BCRs as the phosphorylation of ITAMs required to initiate signaling, is incompatible with binding of known endocytic adaptors (9, 10).
Molecular Mechanisms of BCR Internalization
Clathrin-Mediated Endocytosis
The best characterized mechanism of BCR internalization is clathrin-mediated endocytosis (CME) (11, 12). Upon antigen binding to the BCR, clathrin is recruited to BCR-antigen clusters via its essential adaptor protein-2 (AP-2), which binds ITAM residues in the cytoplasmic tails of Igα and Igβ (10). BCR-proximal Src family kinases mediate phosphorylation of clathrin heavy chain, promoting binding to clathrin light chain and polymerization to induce membrane-curvature and formation of clathrin-coated pits (CCP) (13–15). Clathrin heavy chain phosphorylation also promotes association of CCPs with the actin cytoskeleton (14, 16). BCR antigen binding also promotes tyrosine phosphorylation of several CCP-associated proteins, including Epsin15 and Intersectin2 (17, 18), further cementing the link between BCR signaling and CME. CCPs invaginate before scission from the PM mediated by the large GTPase dynamin. Dynamin forms a helical polymer around the constricted neck of the CCP and, upon hydrolysis of GTP, mediates membrane scission producing clathrin-coated vesicles (CCV). This mechanically demanding process requires actin polymerization which may be mediated by interaction between clathrin light chain and its actin-binding partner Hip1R (16, 19). The specific clathrin adaptors, redundancy, stoichiometry and dynamics may be altered depending on antigen concentration (20).
Experimental inhibition of CME has revealed significant plasticity in BCR internalization pathways (21). This may be particularly striking in GC B cells, which do not polarize several CME components (e.g. sorting nexins SNX9, SNX18) to the immunological synapse (22) and appear more reliant on clathrin-independent endocytosis (23). We discuss current knowledge of these alternative pathways below (and Figure 1).
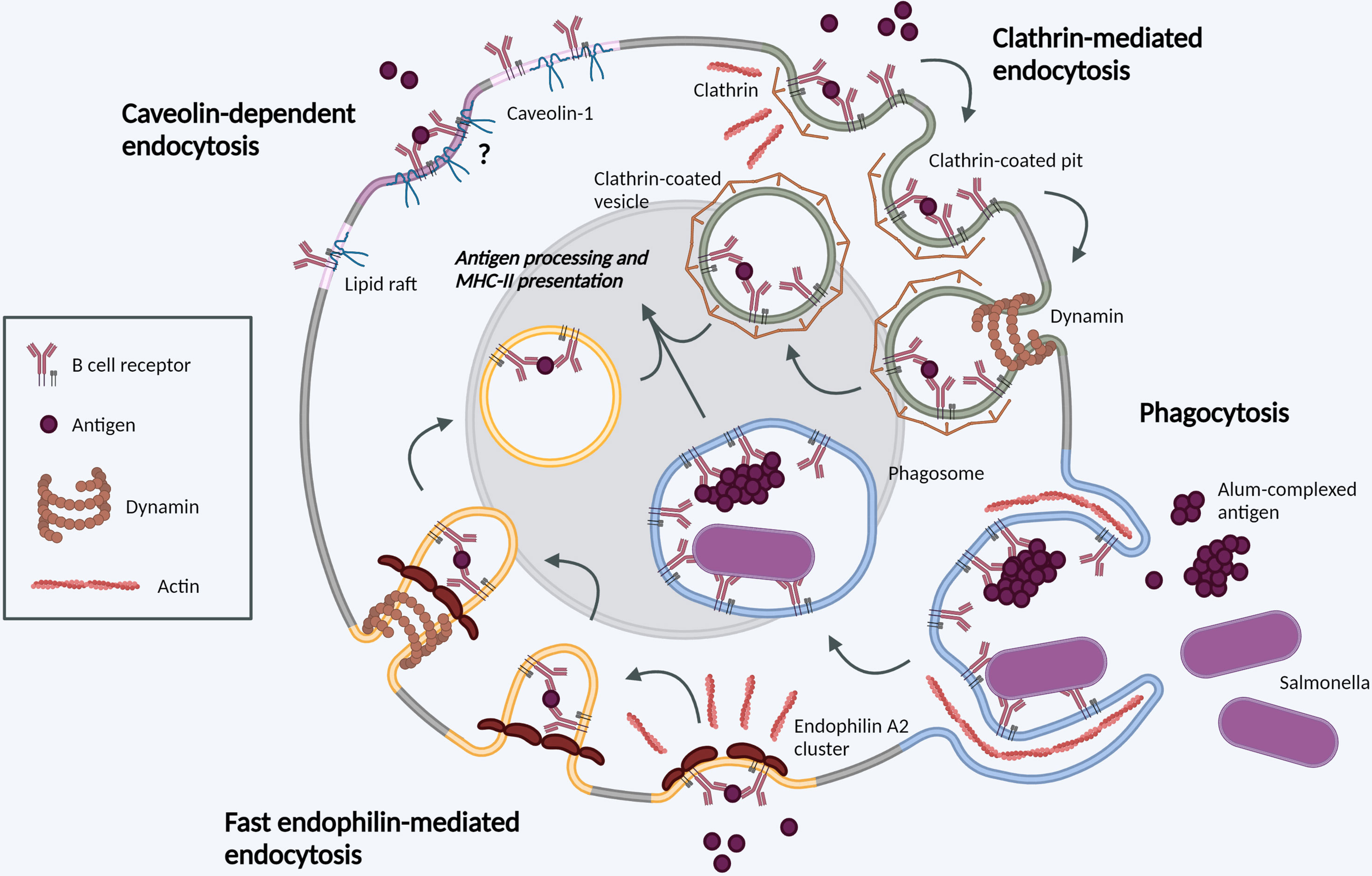
Figure 1 Molecular mechanisms of BCR internalization. Clathrin-mediated endocytosis (green). Following BCR antigen binding, clathrin is recruited to BCR-antigen clusters, binding ITAM residues in the cytoplasmic tails of Igα and Igβ. Clathrin polymerization induces membrane-curvature and formation of CCPs. CCPs invaginate before scission from the PM mediated by dynamin, forming a CCV. CCVs containing BCR-antigen complexes fuse with late endosomes for antigen processing and subsequent presentation. Phagocytosis (blue). BCR binding to large, particulate antigens can induce local actin rearrangements, driving membrane protrusion to form a large membrane invagination. Once internalized in phagosomes, the membrane vesicle undergoes fusion events in parallel with other endocytic processes. Fast endophilin-mediated endocytosis (yellow). Within endophilin-primed membrane patches, BCR antigen binding results in endophilin-mediated membrane curvature with subsequent dynamin scission to form an intracellular vesicle. This pathway is specific for ligand-triggered, signaling receptors. Caveolin-dependent endocytosis (pink). Caveolin-1 controls the distribution of surface BCR into lipid rafts. Its role in antigen-mediated BCR internalization requires further investigation. Created with BioRender.com.
Fast Endophilin-Mediated Endocytosis
FEME was described in 2014 as a fast-acting, tubulovesicular, clathrin-independent endocytic pathway. It mediates ligand-triggered uptake of several G-protein coupled receptors and receptor tyrosine kinases in a dynamin-dependent manner (24). FEME is mediated by BAR domain-containing endophilin proteins – Endophilin A1, A2 and A3. While Endophilin A1 and A3 are restricted to tissues such as brain, kidney, and testes, Endophilin A2 is ubiquitously expressed and importantly, the only endophilin family member expressed in B cells.
Endophilin A2 is present in PM patches, priming the membrane for FEME by sporadically forming and dissolving clusters. These clusters are highly dynamic, lasting 5-15s, and rely on production of PI (3, 4)P2 at the membrane (25). Within these clusters, productive receptor-ligand interactions result in cargo capture, endophilin-mediated membrane curvature and rapid endocytosis with subsequent dynamin-dependent scission. In contrast, in the absence of ligand binding, the membrane priming complex is dissembled and new endophilin clusters can form stochastically (26). The molecular mechanisms of FEME, and known or suspected cargo proteins in other cells have been extensively reviewed (25). Critically for B cells, unlike other pathways, FEME is non-constitutive ligand-triggered endocytosis.
Recent work identified the BCR as cargo for FEME. An unbiased whole-genome screen in a human B cell line identified a role for Endophilin A2 specifically in ligand-triggered BCR internalization. Although the stoichiometry and direct interactors remain to be discovered, recruitment of Endophilin A2 to the BCR was dependent on Grb2 and BLNK. Endophilin A2 deletion caused B cell-intrinsic defects resulting in decreased antigen-specific GC responses and reduced high-affinity IgG production (23).
Unlike CME, FEME critically requires Cdc42 activity and actin polymerization, concurrent with observations of BCR-antigen uptake in B cells. The use of FEME as an additional endocytic mechanism in B cell antigen internalization may guarantee internalization of phosphorylated BCRs, where machinery for signaling and clathrin-dependent endocytosis would normally compete for binding. In the context of other receptors, endophilin has also been described to interact with the CIN85-Cbl complex (27, 28), as well as ESCRT (endosomal sorting complexes required for transport) components ALIX and Tsg101 (29), which are highly expressed in germinal center B cells. Investigation of these interactions in B cells will advance our understanding of the coordination between BCR signaling and endocytosis. Further mechanistic insights will allow define the contribution of this pathway to antigen affinity discrimination, the dynamics and repertoire of antigen presentation, and its involvement in malignancy, given its specific requirement in Germinal Center B-cell like (GCB) and Burkitt lymphomas (23).
Caveolin-Dependent Endocytosis
Caveolin-1 is an integral membrane protein with a hairpin-like structure containing an internal intramembrane domain and both C- and N-termini facing the cytosol. Caveolin-1 promotes PM compartmentalization and the assembly of ordered lipid domains, such as caveolae - flask-shaped PM invaginations, with a diameter of 50-100nm, considered a form of lipid rafts. Early studies reported lack of expression of caveolin-1 in transformed B cells lines (30); however, subsequent work in primary cells has shown caveolin-1 expression in mouse and human B cells (31, 32). More recently, caveolin-1 was shown to control the distribution of IgM BCR clusters to ordered lipid domains on the B cell surface. Caveolin-1 function in BCR organization is dependent on Tyr14 phosphorylation by Src family kinases. In caveolin-1-deficient B cells, altered BCR nanoscale organization resulted in impaired BCR signaling. In immature B cells, loss of caveolin-1 resulted in defective central tolerance and expression of autoreactive BCRs leading to autoimmunity in mice (33). Interestingly, in these studies BCR internalization kinetics following soluble anti-IgM/IgD stimulation were unchanged in caveolin-1-deficient B cells. Questions remain regarding the mechanisms of caveolin-1 class-specific interactions with IgM or IgD BCR, and the potential role of caveolin-1 in cognate antigen uptake.
Phagocytosis
Phagocytosis is defined as the receptor-mediated ingestion of large (≥0.5-μm) particles through local rearrangements of the actin cytoskeleton. Irrespective of the surface receptor involved, this type of internalization results in a membrane-bound vacuole termed a phagosome, which matures through regulated fusion events, altering its composition and pH, in parallel with other endocytic pathways (34). Rho family GTPases, as master regulators of actin dynamics, play an essential role in PM and cytoskeleton remodeling during particle internalization (35, 36). The early paradigm dictated that phagocytosis was carried out by professional phagocytes, such as macrophages and DCs, providing APCs with antigen for presentation to lymphoid cells, and therefore playing a key role in the initiation of adaptive immune responses (36). B cells were thought to lack such phagocytic properties, due to the dramatic membrane and cytosolic rearrangements required, and have been used as negative controls in phagocytic assays (37). The discovery of macrophage-like B cell phagocytosis in a primitive vertebrate species transformed our understanding of the process (38). Subsequent studies identified BCR-mediated phagocytosis of bacteria in human B cells and murine innate-like B1 B cells, inducing robust antigen presentation and downstream B cell responses (39–41).
More recently, Martínez-Riaño et al. demonstrated that follicular B cell phagocytosis is BCR-driven and dependent on RhoG GTPase. This phagocytosis was important for development of a strong GC response and generation of high-affinity class-switched antibodies. Phagocytosis of antigen complexed to alum (common immunization adjuvant) provides a mechanism for initiating a humoral response against particulate antigens (42). The discovery that B cells phagocytose may play a pivotal role in vaccine design.
Trogocytosis
Trogocytosis is a process commonly described as cell gnawing, in which lymphocytes can internalize cellular material, including transfer of plasma membrane, from conjugated APCs. The process has been demonstrated in primary B cells and requires engagement of the BCR (43, 44) though may exhibit distinct signaling and cytoskeletal requirements from other cell types (45, 46). Most recently, trogocytosis was shown to mediate transfer of peptide-MHC II complexes from conventional DCs to marginal zone B cells in a complement receptor-dependent manner (47). This significantly expands the variety of antigens that marginal zone B cells can present to T cells, and opens further research avenues on the mechanisms and dynamics of peptide acquisition.
Endocytosis of Immobilized Antigens Following Proteolytic Extraction
A distinct mechanism of antigen endocytosis has been demonstrated for antigens presented on rigid substrates. In this model, B cell polarization towards the IS results in release of lysosomal content into the extracellular space (48, 49). Subsequent studies have revealed a role for Galectin8, a glycan-binding protein mediating extracellular matrix interactions, in B cell polarization, lysosome docking at the antigen contact site, and acidification of the IS space (50). Recent work provided further insight on lysosomal recruitment mechanisms. First, proteasome activity can promote actin clearance at the IS and lysosomal polarization towards immobilized antigens (51, 52). Second, interaction with surface-tethered high-affinity antigen induces B cell permeabilization and plasma membrane repair through calcium-induced lysosome exocytosis (53). Whether the specific endocytic mechanisms involved in recovery of proteolytically extracted antigen differ from those described above remains to be investigated.
The Role of Actin in BCR Endocytosis and Signaling
In B cells, actin plays a crucial role in antigen acquisition in several distinct stages. First, cortical actin reorganization coordinates B cell spreading and contraction at the immunological synapse (54–56). Second, actin modulates spatial patterning of the surface BCR, regulating the amplitude/strength and dynamics of clustering and signaling by the BCR (57–59). Third, actin interacts with endocytic processes for antigen extraction and internalization. The first two stages have been extensively reviewed elsewhere (60–62) and here we will focus on the interaction with endocytosis. Actin foci have been described at sites of antigen uptake in murine and human B cells (22, 63, 64) and generate the physical force required to extract membrane-bound antigen (15). Deletion of the actin motor protein myosin IIa significantly reduced antigen uptake in naïve B cells (65), further highlighting the requirement for mechanical force.
In CME, Lyn-mediated phosphorylation of clathrin heavy chain promotes interaction with actin (14, 16). Antigen-induced BCR signaling through Vav, Bam32 and Btk can also regulate actin polymerization and association with CCPs (66–68). In addition, several clathrin-interacting proteins (CYFIP1, Intersectin, Eps15) can promote actin polymerization through Wiskott-Aldrich Syndrome protein (WASp) family proteins (69) and B cell deficient in WASp exhibit reduced antigen uptake (70). Actin is crucially required for antigen extraction by GC B cells, which apply stronger physical forces and exhibit distinct synaptic architecture (22, 71, 72). Investigation of individual clathrin adaptors and actin regulators will likely reveal further molecular details and differential requirements; for example, deletion of Itsn2, a Cdc42 guanine nucleotide exchange factor (GEF), reduced germinal center formation but did not impact BCR-antigen uptake (73). This highlights the challenges in investigating direct and indirect effects in this process.
Actin polymerization is also required for all clathrin-independent pathways we describe in B cells. In FEME, actin polymerization drives local membrane deformation and formation of intracellular bud (25, 74). Molecular details remain to be investigated but FEME requires all three main small GTPases (RhoA, Rac1 and Cdc42), with Cdc42 required for priming and inhibition of RhoA or Rac1 inhibiting FEME entirely (24). Phagocytosis of larger antigens also requires intense remodeling of the actin cytoskeleton (42). Whether similar mechanisms are required for the poorly understood process of trogocytosis (45) remains to be investigated.
Although originally described to promote CME (14), lipid rafts (cholesterol-rich microdomains) have been shown to drive BCR internalization independently of clathrin (21). This uptake requires the immunoglobulin cytoplasmic domain (75) and is strongly dependent on intact actin remodeling (21). Caveolae are closely associated with actin filaments, through potential interactions with filaminA and Intersectin2L (76, 77). Dissociation of lipid rafts from the underlying cortical actin, through ezrin dephosphorylation and release, is critical for antigen-induced lipid raft coalescence and eventual uptake (78). Rapid cholesterol-mediated BCR uptake may explain uncoupled BCR signaling in anergic B cells (79). Whether this is related to reduced NFκB signaling observed in GC B cells (71) and, more broadly, the role of lipid rafts in GC antigen uptake requires further investigation. How lipid order in these microdomains directs protein interactions and whether rafts play a role in force-dependent affinity discrimination is unclear.
Post-Translational Modifications of BCR-Associated Endocytic Proteins
Common PTMs downstream of BCR engagement include phosphorylation and ubiquitination. As described above, phosphorylation by proximal kinases not only initiates downstream signaling cascades but is required for interaction with numerous endocytic regulators (70). Its involvement in several pathways is not well understood, for example, Endophilin A2 is itself phosphorylated at serine 288 and the level of modification is marginally increased upon antigen challenge (18), however, the significance of this for FEME or CME is unknown.
Activated BCRs have also been shown to associate with c-Cbl/Cbl-b E3-ubiquitin ligases, which results in the ubiquitination of Igα and Igβ (80, 81). This is likely to play a role in both BCR-antigen complex internalization and downstream intracellular trafficking (82). Cbl-mediated ubiquitination can promote BCR uptake in an actin-dependent mechanism (83), providing a potential mechanism to resolve the binding competition between signaling and endocytic regulators. The molecular mechanisms of ubiquitin-driven BCR endocytosis remain uncharacterized. Recent proteomic analysis by Satpathy et al. confirmed PTM changes in numerous CCP components and actin regulators following BCR ligation (PICALM, SWAP70, DOCK8, EPS15, EPN2, WASH complex) (18). Additionally, several endocytic accessory proteins, including epsins and Eps15R, contain ubiquitin-binding domains which may mediate interaction with ubiquitinated BCR. Depletion of CIN85 or Cbl, whose role in BCR signaling is clear (84, 85), also inhibits FEME (24) and reduces Endophilin A2 recruitment to the BCR (23).
Cbl and Cbl-b promote antigen uptake in naïve but not GC B cells, despite their high expression in the latter (86). This may reflect the requirement for naïve B cells to efficiently internalize antigen irrespective of its affinity for the BCR (71) and future insight into the molecular mechanisms will be exciting.
Altered BCR-Antigen Endocytosis in Malignant B Cells
The crucial interplay between BCR endocytosis and signaling is illustrated by the number of B cell malignancies that display altered antigen receptor endocytosis or trafficking (Figure 2). Igβ ITAM residues modulate ligand-induced signaling by regulating BCR internalization and are therefore essential for normal levels of cell surface BCR expression. Mutations in Igβ resulted in reduced BCR internalization and enhanced signaling in primary B cells, specifically Akt and Erk activation, which can promote cell survival and metabolic changes (87). Specific mutations in the first ITAM residue in Igβ have been observed in activated B cell-like (ABC) diffuse large B cell lymphoma (DLBCL). These increase surface BCR expression by attenuating Lyn kinase activity and reducing internalization, leading to chronic active BCR signaling as a pathogenic mechanism in ABC DLBCL (88).
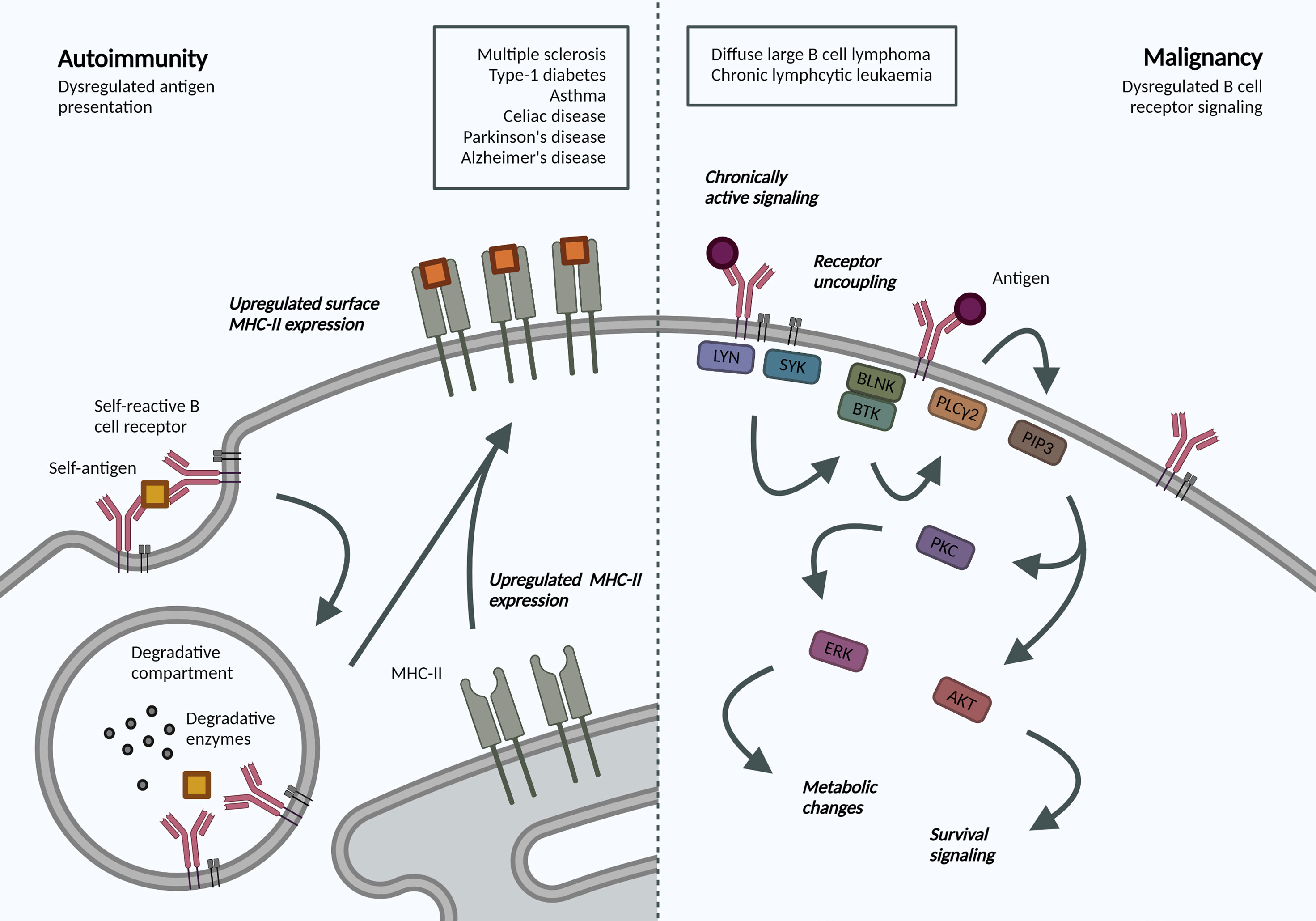
Figure 2 Altered BCR-antigen endocytosis, trafficking and presentation in B cell autoimmunity and malignancy. Autoimmunity. BCR-mediated self-antigen uptake can increase presentation of relevant peptides and drive disease progression. B cells in many autoimmune disorders exhibit increased surface MHC-II expression, though the repertoire of presented peptides has not been addressed. Malignancy. Following antigen binding to the BCR, a signaling cascade is initiated through phosphorylation of Igα/β ITAMs residues. Downstream activation of ERK and AKT mediates many metabolic changes and survival signaling, which are dysregulated in B cell malignancies due to localization and trafficking dynamics of the BCR. Created with BioRender.com.
Interestingly, in chronic lymphocytic leukemia (CLL), anergic cells exhibit reduced BCR signaling and increased internalization when compared to non-anergic counterparts (89). The specific signaling pathways and endocytic regulators were not addressed but the model supports mutually exclusive signaling and internalization functions and highlights changes in internalization dynamics in B cell malignancy. Understanding the dependency of different leukemias and lymphomas on distinct endocytic processes and how these may alter cell signaling can reveal novel disease mechanisms and therapeutic targets, specifically in our move towards precision medicine.
B Cell Antigen Trafficking and Presentation in Autoimmunity
Antigen presentation by B cells has been associated with the development and progression of various autoimmune disorders. An antigen presentation role for B cells, independent of autoantibody production, has been established in multiple sclerosis (MS) (90). Indeed, surface MHC-II expression was increased in B cells from relapsing MS patients (91), and this increase may be mechanistically linked to Epstein-Barr virus (EBV) infection (92). In animal models, B cell antigen presentation was sufficient to drive neuro-inflammation (93). B cell antigen presentation is also implicated in the development and progression of type-1 diabetes, irrespective of their ability to produce secreted antibodies (94), as well as asthma (95) and celiac disease (96). Most recently, single cell sequencing of B cells from patients with Parkinson’s disease also revealed increased expression of MHC-II genes (97); while transient B cell depletion significantly reduced Alzheimer’s disease progression in murine models (98). While these studies do not address the dynamics or specificity, they highlight an increased capacity for antigen-presentation in disease-associated B cells. Understanding antigen trafficking and presentation would provide exciting opportunities for B-cell targeted therapeutics in these pathologies.
Unanswered Questions and Future Milestones
How are BCR antigen internalization and signaling coordinated?
What is the fate of BCR-antigen complexes endocytosed by distinct mechanisms? How are these altered in malignancy, and can they promote progression?
How are actin and proteasome remodeling processes coordinated with endocytic mechanisms?
How are BCR uptake processes altered with ageing, and do they contribute to reduced B cell responses?
What is the role of ubiquitination in BCR antigen internalization? What is the relationship between different post-translational modifications and how do they interact with endocytic regulators?
Concluding Remarks
Antigen-induced B cell activation plays a key role in the adaptive immune response by regulating BCR signaling capacity and antigen availability for presentation. Many questions remain and the field will benefit from advances in imaging, genetic and proteomics techniques. A better understanding of these processes could provide the tools to modulate the process with direct implications for vaccine design, and potential for novel targeted therapeutics in B cell malignancies and autoimmune diseases.
Author Contributions
DM and ANMS wrote and edited the manuscript. All authors contributed to the article and approved the submitted version.
Funding
We would like to acknowledge funding from the NI Department for the Economy (ANMS), QUB Patrick G Johnston fellowship (DM), Royal Society (RGS\R2\212016) (DM) and Medical Research Council (MR/W025868/1) (DM).
Conflict of Interest
The authors declare that the research was conducted in the absence of any commercial or financial relationships that could be construed as a potential conflict of interest.
Publisher’s Note
All claims expressed in this article are solely those of the authors and do not necessarily represent those of their affiliated organizations, or those of the publisher, the editors and the reviewers. Any product that may be evaluated in this article, or claim that may be made by its manufacturer, is not guaranteed or endorsed by the publisher.
Glossary
References
1. Doherty GJ, McMahon HT. Mechanisms of Endocytosis. Annu Rev Biochem (2009) 78:857–902. doi: 10.1146/annurev.biochem.78.081307.110540
2. Wang J, Pantopoulos K. Regulation of Cellular Iron Metabolism. Biochem J (2011) 434(3):365–81. doi: 10.1042/BJ20101825
3. Foley K, Boguslavsky S, Klip A. Endocytosis, Recycling, and Regulated Exocytosis of Glucose Transporter 4. Biochemistry (2011) 50(15):3048–61. doi: 10.1021/bi2000356
4. Orth JD, Krueger EW, Weller SG, McNiven MA. A Novel Endocytic Mechanism of Epidermal Growth Factor Receptor Sequestration and Internalization. Cancer Res (2006) 66(7):3603–10. doi: 10.1158/0008-5472.CAN-05-2916
5. Carrasco YR, Batista FD. B Cells Acquire Particulate Antigen in a Macrophage-Rich Area at the Boundary Between the Follicle and the Subcapsular Sinus of the Lymph Node. Immunity (2007) 27(1):160–71. doi: 10.1016/j.immuni.2007.06.007
6. Lanzavecchia A. Receptor-Mediated Antigen Uptake and its Effect on Antigen Presentation to Class II-Restricted T Lymphocytes. Annu Rev Immunol (1990) 8(1):773–93. doi: 10.1146/annurev.iy.08.040190.004013
7. Rickert RC. New Insights Into Pre-BCR and BCR Signalling With Relevance to B Cell Malignancies. Nat Rev Immunol (2013) 13(8):578–91. doi: 10.1038/nri3487
8. Allen CDC, Okada T, Cyster JG. Germinal-Center Organization and Cellular Dynamics. Immunity (2007) 27(2):190–202. doi: 10.1016/j.immuni.2007.07.009
9. Hou P, Araujo E, Zhao T, Zhang M, Massenburg D, Veselits M, et al. B Cell Antigen Receptor Signaling and Internalization are Mutually Exclusive Events. PloS Biol (2006) 4(7):e200. doi: 10.1371/journal.pbio.0040200
10. Busman-Sahay K, Drake L, Sitaram A, Marks M, Drake JR. Cis and Trans Regulatory Mechanisms Control AP2-Mediated B Cell Receptor Endocytosis via Select Tyrosine-Based Motifs. PloS One (2013) 8(1):e54938. doi: 10.1371/journal.pone.0054938
11. Salisbury JL, Condeelis JS, Satir P. Role of Coated Vesicles, Microfilaments, and Calmodulin in Receptor-Mediated Endocytosis by Cultured B Lymphoblastoid Cells. J Cell Biol (1980) 87(1):132–41. doi: 10.1083/jcb.87.1.132
12. Guagliardi LE, Koppelman B, Blum JS, Marks MS, Cresswell P, Brodsky FM. Co-Localization of Molecules Involved in Antigen Processing and Presentation in an Early Endocytic Compartment. Nature (1990) 343(6254):133–9. doi: 10.1038/343133a0
13. Caballero A, Katkere B, Wen X-Y, Drake L, Nashar TO, Drake JR. Functional and Structural Requirements for the Internalization of Distinct BCR-Ligand Complexes. Eur J Immunol (2006) 36(12):3131–45. doi: 10.1002/eji.200636447
14. Stoddart A, Dykstra ML, Brown BK, Song W, Pierce SK, Brodsky FM. Lipid Rafts Unite Signaling Cascades With Clathrin to Regulate BCR Internalization. Immunity (2002) 17(4):451–62. doi: 10.1016/S1074-7613(02)00416-8
15. Natkanski E, Lee W-Y, Mistry B, Casal A, Molloy JE, Tolar P. B Cells Use Mechanical Energy to Discriminate Antigen Affinities. Sci (New York NY) (2013) 340(6140):1587–90. doi: 10.1126/science.1237572
16. Bonazzi M, Vasudevan L, Mallet A, Sachse M, Sartori A, Prevost M-C, et al. Clathrin Phosphorylation is Required for Actin Recruitment at Sites of Bacterial Adhesion and Internalization. J Cell Biol (2011) 195(3):525–36. doi: 10.1083/jcb.201105152
17. Matsumoto M, Oyamada K, Takahashi H, Sato T, Hatakeyama S, Nakayama KI. Large-Scale Proteomic Analysis of Tyrosine-Phosphorylation Induced by T-Cell Receptor or B-Cell Receptor Activation Reveals New Signaling Pathways. Proteomics (2009) 9(13):3549–63. doi: 10.1002/pmic.200900011
18. Satpathy S, Wagner SA, Beli P, Gupta R, Kristiansen TA, Malinova D, et al. Systems-Wide Analysis of BCR Signalosomes and Downstream Phosphorylation and Ubiquitylation. Mol Syst Biol (2015) 11(6):810. doi: 10.15252/msb.20145880
19. Chaturvedi A, Martz R, Dorward D, Waisberg M, Pierce SK. Endocytosed BCRs Sequentially Regulate MAPK and Akt Signaling Pathways From Intracellular Compartments. Nat Immunol (2011) 12(11):1119–26. doi: 10.1038/ni.2116
20. Roberts AD, Davenport TM, Dickey AM, Ahn R, Sochacki KA, Taraska JW. Structurally Distinct Endocytic Pathways for B Cell Receptors in B Lymphocytes. Mol Biol Cell (2020) 31(25):2826–40. doi: 10.1091/mbc.E20-08-0532
21. Stoddart A, Jackson AP, Brodsky FM. Plasticity of B Cell Receptor Internalization Upon Conditional Depletion of Clathrin. Mol Biol Cell (2005) 16(5):2339–48. doi: 10.1091/mbc.e05-01-0025
22. Kwak K, Quizon N, Sohn H, Saniee A, Manzella-Lapeira J, Holla P, et al. Intrinsic Properties of Human Germinal Center B Cells Set Antigen Affinity Thresholds. Sci Immunol (2018) 3(29):eaau6598. doi: 10.1126/sciimmunol.aau6598
23. Malinova D, Wasim L, Newman R, Martinez-Riano A, Engels N, Tolar P. Endophilin A2 Regulates B-Cell Endocytosis and is Required for Germinal Center and Humoral Responses. EMBO Rep (2021) 22:e51328. doi: 10.15252/embr.202051328
24. Boucrot E, Ferreira APA, Almeida-Souza L, Debard S, Vallis Y, Howard G, et al. Endophilin Marks and Controls a Clathrin-Independent Endocytic Pathway. Nature (2015) 517(7535):460–5. doi: 10.1038/nature14067
25. Casamento A, Boucrot E. Molecular Mechanism of Fast Endophilin-Mediated Endocytosis. Biochem J (2020) 477(12):2327–45. doi: 10.1042/BCJ20190342
26. Hak LCW, Khan S, Di Meglio I, Law AL, Hasler SLA, Quintaneiro LM, et al. FBP17 and CIP4 Recruit SHIP2 and Lamellipodin to Prime the Plasma Membrane for Fast Endophilin-Mediated Endocytosis. Nat Cell Biol (2018) 20(9):1023. doi: 10.1038/s41556-018-0146-8
27. Soubeyran P, Kowanetz K, Szymkiewicz I, Langdon WY, Dikic I. Cbl-CIN85-Endophilin Complex Mediates Ligand-Induced Downregulation of EGF Receptors. Nature (2002) 416(6877):183–7. doi: 10.1038/416183a
28. Petrelli A, Gilestro GF, Lanzardo S, Comoglio PM, Migone N, Giordano S. The Endophilin-CIN85-Cbl Complex Mediates Ligand-Dependent Downregulation of C-Met. Nature (2002) 416(6877):187–90. doi: 10.1038/416187a
29. Mercier V, Laporte MH, Destaing O, Blot B, Blouin CM, Pernet-Gallay K, et al. ALG-2 Interacting Protein-X (Alix) Is Essential for Clathrin-Independent Endocytosis and Signaling. Sci Rep (2016) 6(1):26986–15. doi: 10.1038/srep26986
30. Fra AM, Williamson E, Simons K, Parton RG. Detergent-Insoluble Glycolipid Microdomains in Lymphocytes in the Absence of Caveolae. J Biol Chem (1994) 269(49):30745–8. doi: 10.1016/S0021-9258(18)47340-1
31. Medina FA, Williams TM, Sotgia F, Tanowitz HB, Lisanti MP. A Novel Role for Caveolin-1 in B Lymphocyte Function and the Development of Thymus-Independent Immune Responses. Cell Cycle (2006) 5(16):1865–71. doi: 10.4161/cc.5.16.3132
32. Vargas L, Nore BF, Berglof A, Heinonen JE, Mattsson PT, Smith CIE, et al. Functional Interaction of Caveolin-1 With Bruton's Tyrosine Kinase and Bmx. J Biol Chem (2002) 277(11):9351–7. doi: 10.1074/jbc.M108537200
33. Minguet S, Kläsener K, Schaffer A-M, Fiala GJ, Osteso-Ibánez T, Raute K, et al. Caveolin-1-Dependent Nanoscale Organization of the BCR Regulates B Cell Tolerance. Nat Immunol (2017) 18(10):1150–9. doi: 10.1038/ni.3813
34. Desjardins M, Huber LA, Parton RG, Griffiths G. Biogenesis of Phagolysosomes Proceeds Through a Sequential Series of Interactions With the Endocytic Apparatus. J Cell Biol (1994) 124(5):677–88. doi: 10.1083/jcb.124.5.677
35. Chimini G, Chavrier P. Function of Rho Family Proteins in Actin Dynamics During Phagocytosis and Engulfment. Nat Cell Biol (2000) 2(10):E191–E6. doi: 10.1038/35036454
36. Flannagan RS, Jaumouille V, Grinstein S. The Cell Biology of Phagocytosis. Annu Rev Pathol-Mech (2012) 7:61–98. doi: 10.1146/annurev-pathol-011811-132445
37. Ochando JC, Homma C, Yang Y, Hidalgo A, Garin A, Tacke F, et al. Alloantigen-Presenting Plasmacytoid Dendritic Cells Mediate Tolerance to Vascularized Grafts. Nat Immunol (2006) 7(6):652–62. doi: 10.1038/ni1333
38. Li J, Barreda DR, Zhang YA, Boshra H, Gelman AE, LaPatra S, et al. B Lymphocytes From Early Vertebrates Have Potent Phagocytic and Microbicidal Abilities. Nat Immunol (2006) 7(10):1116–24. doi: 10.1038/ni1389
39. Souwer Y, Griekspoor A, Jorritsma T, de Wit J, Janssen H, Neefjes J, et al. B Cell Receptor-Mediated Internalization of Salmonella: A Novel Pathway for Autonomous B Cell Activation and Antibody Production. J Immunol (2009) 182(12):7473–81. doi: 10.4049/jimmunol.0802831
40. Gao JX, Ma XY, Gu WJ, Fu M, An JG, Xing Y, et al. Novel Functions of Murine B1 Cells: Active Phagocytic and Microbicidal Abilities. Eur J Immunol (2012) 42(4):982–92. doi: 10.1002/eji.201141519
41. Parra D, Rieger AM, Li J, Zhang YA, Randall LM, Hunter CA, et al. Pivotal Advance: Peritoneal Cavity B-1 B Cells Have Phagocytic and Microbicidal Capacities and Present Phagocytosed Antigen to CD4(+) T Cells. J Leukocyte Biol (2012) 91(4):525–36. doi: 10.1189/jlb.0711372
42. Martinez-Riano A, Bovolenta ER, Mendoza P, Oeste CL, Martin-Bermejo MJ, Bovolenta P, et al. Antigen Phagocytosis by B Cells is Required for a Potent Humoral Response. EMBO Rep (2018) 19(9):e46016. doi: 10.15252/embr.201846016
43. Puaux AL, Campanaud J, Salles A, Preville X, Timmerman B, Joly E, et al. A Very Rapid and Simple Assay Based on Trogocytosis to Detect and Measure Specific T and B Cell Reactivity by Flow Cytometry. Eur J Immunol (2006) 36(3):779–88. doi: 10.1002/eji.200535407
44. Hudrisier D, Aucher A, Puaux AL, Bordier C, Joly E. Capture of Target Cell Membrane Components via Trogocytosis is Triggered by a Selected Set of Surface Molecules on T or B Cells. J Immunol (2007) 178(6):3637–47. doi: 10.4049/jimmunol.178.6.3637
45. Aucher A, Magdeleine E, Joly E, Hudrisier D. Capture of Plasma Membrane Fragments From Target Cells by Trogocytosis Requires Signaling in T Cells But Not in B Cells. Blood (2008) 111(12):5621–8. doi: 10.1182/blood-2008-01-134155
46. Zhao S, Zhang L, Xiang S, Hu Y, Wu Z, Shen J. Gnawing Between Cells and Cells in the Immune System: Friend or Foe? A Rev Trogocytosis Front Immunol (2022) 13:791006. doi: 10.3389/fimmu.2022.791006
47. Schriek P, Ching AC, Moily NS, Moffat J, Beattie L, Steiner TM, et al. Marginal Zone B Cells Acquire Dendritic Cell Functions by Trogocytosis. Science (2022) 375(6581):eabf7470. doi: 10.1126/science.abf7470
48. Obino D, Diaz J, Sáez JJ, Ibañez-Vega J, Sáez PJ, Alamo M, et al. Vamp-7-Dependent Secretion at the Immune Synapse Regulates Antigen Extraction and Presentation in B-Lymphocytes. Mol Biol Cell (2017) 28(7):890–7. doi: 10.1091/mbc.e16-10-0722
49. Yuseff M-I, Reversat A, Lankar D, Diaz J, Fanget I, Pierobon P, et al. Polarized Secretion of Lysosomes at the B Cell Synapse Couples Antigen Extraction to Processing and Presentation. Immunity (2011) 35(3):361–74. doi: 10.1016/j.immuni.2011.07.008
50. Obino D, Fetler L, Soza A, Malbec O, Saez JJ, Labarca M, et al. Galectin-8 Favors the Presentation of Surface-Tethered Antigens by Stabilizing the B Cell Immune Synapse. Cell Rep (2018) 25(11):3110. doi: 10.1016/j.celrep.2018.11.052
51. Ibañez-Vega J, Del Valle Batalla F, Sáez JJ, Soza A, Yuseff M-I. Proteasome Dependent Actin Remodeling Facilitates Antigen Extraction at the Immune Synapse of B Cells. Front Immunol (2019) 10:225. doi: 10.3389/fimmu.2019.00225
52. Ibanez-Vega J, Del Valle F, Saez JJ, Guzman F, Diaz J, Soza A, et al. Ecm29-Dependent Proteasome Localization Regulates Cytoskeleton Remodeling at the Immune Synapse. Front Cell Dev Biol (2021) 9:650817. doi: 10.3389/fcell.2021.650817
53. Maeda FY, van Haaren JJH, Langley DB, Christ D, Andrews NW, Song WX. Surface-Associated Antigen Induces Permeabilization of Primary Mouse B-Cells and Lysosome Exocytosis Facilitating Antigen Uptake and Presentation to T-Cells. Elife (2021) 10:e66984. doi: 10.7554/eLife.66984
54. Fleire SJ, Goldman JP, Carrasco YR, Weber M, Bray D, Batista FD. B Cell Ligand Discrimination Through a Spreading and Contraction Response. Sci (New York NY) (2006) 312(5774):738–41. doi: 10.1126/science.1123940
55. Treanor B, Depoil D, Bruckbauer A, Batista FD. Dynamic Cortical Actin Remodeling by ERM Proteins Controls BCR Microcluster Organization and Integrity. J Exp Med (2011) 208(5):1055–68. doi: 10.1084/jem.20101125
56. Freeman SN, Lei V, Dang-Lawson M, Mizuno K, Roskelley CD, Gold MR. Cofilin-Mediated F-Actin Severing Is Regulated by the Rap GTPase and Controls the Cytoskeletal Dynamics That Drive Lymphocyte Spreading and BCR Microcluster Formation. J Immunol (2011) 187(11):5887–900. doi: 10.4049/jimmunol.1102233
57. Bolger-Munro M, Choi K, Scurll JM, Abraham L, Chappell RS, Sheen D, et al. Arp2/3 Complex-Driven Spatial Patterning of the BCR Enhances Immune Synapse Formation, BCR Signaling and B Cell Activation. Elife (2019) 8:e44574. doi: 10.7554/eLife.44574
58. Hao SL, August A. Actin Depolymerization Transduces the Strength of B-Cell Receptor Stimulation. Mol Biol Cell (2005) 16(5):2275–84. doi: 10.1091/mbc.e04-10-0881
59. Bolger-Munro M, Choi K, Cheung F, Liu YT, Dang-Lawson M, Deretic N, et al. The Wdr1-LIMK-Cofilin Axis Controls B Cell Antigen Receptor-Induced Actin Remodeling and Signaling at the Immune Synapse. Front Cell Dev Biol (2021) 9. doi: 10.3389/fcell.2021.649433
60. Harwood NE, Batista FD. The Cytoskeleton Coordinates the Early Events of B-Cell Activation. Cold Spring Harbor Perspect Biol (2011) 3(2):a002360. doi: 10.1101/cshperspect.a002360
61. Tolar P. Cytoskeletal Control of B Cell Responses to Antigens. Nat Rev Immunol (2017) 17(10):621–34. doi: 10.1038/nri.2017.67
62. Li JW, Yin W, Jing YK, Kang DQ, Yang L, Cheng JL, et al. The Coordination Between B Cell Receptor Signaling and the Actin Cytoskeleton During B Cell Activation. Front Immunol (2019) 9. doi: 10.3389/fimmu.2018.03096
63. Roper SI, Wasim L, Malinova D, Way M, Cox S, Tolar P. B Cells Extract Antigens at Arp2/3-Generated Actin Foci Interspersed With Linear Filaments. eLife (2019) 8:489. doi: 10.7554/eLife.48093
64. Kumari A, Pineau J, Saez PJ, Maurin M, Lankar D, San Roman M, et al. Actomyosin-Driven Force Patterning Controls Endocytosis at the Immune Synapse. Nat Commun (2019) 10(1):2870. doi: 10.1038/s41467-019-10751-7
65. Hoogeboom R, Natkanski EM, Nowosad CR, Malinova D, Menon RP, Casal A, et al. Myosin IIa Promotes Antibody Responses by Regulating B Cell Activation, Acquisition of Antigen, and Proliferation. Cell Rep (2018) 23(8):2342–53. doi: 10.1016/j.celrep.2018.04.087
66. Malhotra S, Kovats S, Zhang W, Coggeshall KM. B Cell Antigen Receptor Endocytosis and Antigen Presentation to T Cells Require Vav and Dynamin. J Biol Chem (2009) 284(36):24088–97. doi: 10.1074/jbc.M109.014209
67. Niiro H, Allam A, Stoddart A, Brodsky FM, Marshall AJ, Clark EA. The B Lymphocyte Adaptor Molecule of 32 Kilodaltons (Bam32) Regulates B Cell Antigen Receptor Internalization. J Immunol (2004) 173(9):5601–9. doi: 10.4049/jimmunol.173.9.5601
68. Sharma S, Orlowski G, Song W. Btk Regulates B Cell Receptor-Mediated Antigen Processing and Presentation by Controlling Actin Cytoskeleton Dynamics in B Cells. J Immunol (Baltimore Md 1950) (2009) 182(1):329–39. doi: 10.4049/jimmunol.182.1.329
69. Anitei M, Hoflack B. Bridging Membrane and Cytoskeleton Dynamics in the Secretory and Endocytic Pathways. Nat Cell Biol (2012) 14(1):11–9. doi: 10.1038/ncb2409
70. Onabajo OO, Seeley MK, Kale A, Qualmann B, Kessels M, Han J, et al. Actin-Binding Protein 1 Regulates B Cell Receptor-Mediated Antigen Processing and Presentation in Response to B Cell Receptor Activation. J Immunol (Baltimore Md 1950) (2008) 180(10):6685–95. doi: 10.4049/jimmunol.180.10.6685
71. Nowosad CR, Spillane KM, Tolar P. Germinal Center B Cells Recognize Antigen Through a Specialized Immune Synapse Architecture. Nat Immunol (2016) 17(7):870–7. doi: 10.1038/ni.3458
72. Li Y, Bhanja A, Upadhyaya A, Zhao X, Song W. WASp Is Crucial for the Unique Architecture of the Immunological Synapse in Germinal Center B-Cells. Front Cell Dev Biol (2021) 9:646077. doi: 10.3389/fcell.2021.646077
73. Burbage M, Gasparrini F, Aggarwal S, Gaya M, Arnold J, Nair U, et al. Tuning of In Vivo Cognate B-T Cell Interactions by Intersectin 2 is Required for Effective Anti-Viral B Cell Immunity. eLife (2018) 7:113. doi: 10.7554/eLife.26556
74. Hinze C, Boucrot E. Local Actin Polymerization During Endocytic Carrier Formation. Biochem Soc T (2018) 46:565–76. doi: 10.1042/BST20170355
75. Cheng PC, Dykstra ML, Mitchell RN, Pierce SK. A Role for Lipid Rafts in B Cell Antigen Receptor Signaling and Antigen Targeting. J Exp Med (1999) 190(11):1549–60. doi: 10.1084/jem.190.11.1549
76. Muriel O, Echarri A, Hellriegel C, Pavon DM, Beccari L, Del Pozo MA. Phosphorylated Filamin A Regulates Actin-Linked Caveolae Dynamics. J Cell Sci (2011) 124(16):2763–76. doi: 10.1242/jcs.080804
77. Klein IK, Predescu DN, Sharma T, Knezevic I, Malik AB, Predescu S. Intersectin-2l Regulates Caveola Endocytosis Secondary to Cdc42-Mediated Actin Polymerization. J Biol Chem (2009) 284(38):25953–61. doi: 10.1074/jbc.M109.035071
78. Gupta N, Wollscheid B, Watts JD, Scheer B, Aebersold R, DeFranco AL. Quantitative Proteomic Analysis of B Cell Lipid Rafts Reveals That Ezrin Regulates Antigen Receptor-Mediated Lipid Raft Dynamics. Nat Immunol (2006) 7(6):625–33. doi: 10.1038/ni1337
79. Blery M, Tze L, Miosge LA, Jun JE, Goodnow CC. Essential Role of Membrane Cholesterol in Accelerated BCR Internalization and Uncoupling From NF-Kappa B in B Cell Clonal Anergy. J Exp Med (2006) 203(7):1773–83. doi: 10.1084/jem.20060552
80. Zhang M, Veselits M, O’Neill S, Hou P, Reddi AL, Berlin I, et al. Ubiquitinylation of Ig Beta Dictates the Endocytic Fate of the B Cell Antigen Receptor. J Immunol (Baltimore Md 1950) (2007) 179(7):4435–43. doi: 10.4049/jimmunol.179.7.4435
81. Drake L, McGovern-Brindisi EM, Drake JR. BCR Ubiquitination Controls BCR-Mediated Antigen Processing and Presentation. Blood (2006) 108(13):4086–93. doi: 10.1182/blood-2006-05-025338
82. Drake JR. The Immunobiology of Ubiquitin-Dependent B Cell Receptor Functions. Mol Immunol (2018) 101:146–54. doi: 10.1016/j.molimm.2018.05.022
83. Jacob M, Todd L, Sampson MF, Pure E. Dual Role of Cbl Links Critical Events in BCR Endocytosis. Int Immunol (2008) 20(4):485–97. doi: 10.1093/intimm/dxn010
84. Niiro H, Jabbarzadeh-Tabrizi S, Kikushige Y, Shima T, Noda K, Ota S, et al. CIN85 is Required for Cbl-Mediated Regulation of Antigen Receptor Signaling in Human B Cells. Blood (2012) 119(10):2263–73. doi: 10.1182/blood-2011-04-351965
85. Katkere B, Rosa S, Drake JR. The Syk-Binding Ubiquitin Ligase C-Cbl Mediates Signaling-Dependent B Cell Receptor Ubiquitination and B Cell Receptor-Mediated Antigen Processing and Presentation. J Biol Chem (2012) 287(20):16636–44. doi: 10.1074/jbc.M112.357640
86. Li X, Gong L, Meli AP, Karo-Atar D, Sun W, Zou Y, et al. Cbl and Cbl-B Control the Germinal Center Reaction by Facilitating Naive B Cell Antigen Processing. J Exp Med (2020) 217(9):190. doi: 10.1084/jem.20191537
87. Gazumyan A, Reichlin A, Nussenzweig MC. Ig Beta Tyrosine Residues Contribute to the Control of B Cell Receptor Signaling by Regulating Receptor Internalization. J Exp Med (2006) 203(7):1785–94. doi: 10.1084/jem.20060221
88. Davis RE, Ngo VN, Lenz G, Tolar P, Young RM, Romesser PB, et al. Chronic Active B-Cell-Receptor Signalling in Diffuse Large B-Cell Lymphoma. Nature (2010) 463(7277):88–92. doi: 10.1038/nature08638
89. Coulter EM, Pepper A, Mele S, Nad F, Townsend W, Cuthill K, et al. In Vitro and In Vivo Evidence for Uncoupling of B-Cell Receptor Internalization and Signaling in Chronic Lymphocytic Leukemia. Haematologica (2018) 103(3):497–505. doi: 10.3324/haematol.2017.176164
90. Molnarfi N, Schulze-Topphoff U, Weber MS, Patarroyo JC, Prod’homme T, Varrin-Doyer M, et al. MHC Class II-Dependent B Cell APC Function is Required for Induction of CNS Autoimmunity Independent of Myelin-Specific Antibodies. J Exp Med (2013) 210(13):2921–37. doi: 10.1084/jem.20130699
91. Mathias A, Perriard G, Canales M, Soneson C, Delorenzi M, Schluep M, et al. Increased Ex Vivo Antigen Presentation Profile of B Cells in Multiple Sclerosis. Multiple sclerosis (Houndmills Basingstoke England) (2017) 23(6):802–9. doi: 10.1177/1352458516664210
92. Leffler J, Trend S, Ward N, Grau GE, Hawke S, Byrne SN, et al. Circulating Memory B Cells in Early Multiple Sclerosis Exhibit Increased IgA+ Cells, Globally Decreased BAFF-R Expression and an EBV-Related IgM+ Cell Signature. Front Immunol (2022) 13(812317). doi: 10.3389/fimmu.2022.812317
93. Parker C, Archambault A, Sim J, Ferris S, Mikesell R, Linington C, et al. B Cell Antigen Presentation is Sufficient to Drive Neuro-Inflammation in an Animal Model of Multiple Sclerosis. J Immunol (2015) 194(11):5077–84. doi: 10.4049/jimmunol.1402236
94. Wong FS, Wen L, Tang M, Ramanathan M, Visintin I, Daugherty J, et al. Investigation of the Role of B-Cells in Type 1 Diabetes in the NOD Mouse. Diabetes (2004) 53(10):2581–7. doi: 10.2337/diabetes.53.10.2581
95. Wypych TP, Marzi R, Wu GF, Lanzavecchia A, Sallusto F. Role of B Cells in TH Cell Responses in a Mouse Model of Asthma. J Allergy Clin Immunol (2018) 141(4):1395–410. doi: 10.1016/j.jaci.2017.09.001
96. Iversen R, Roy B, Stamnaes J, Hoydahl LS, Hnida K, Neumann RS, et al. Efficient T Cell-B Cell Collaboration Guides Autoantibody Epitope Bias and Onset of Celiac Disease. Proc Natl Acad Sci USA (2019) 116(30):15134–9. doi: 10.1073/pnas.1901561116
97. Wang P LM, Zhou W, Jin X, Xu Z, Yan S, Li Y, et al. Global Characterization of Peripheral B Cells in Parkinson’s Disease by Single-Cell RNA and BCR Sequencing. Front Immunol (2022) 13.13:814239. doi: 10.3389/fimmu.2022.814239
Keywords: B cell receptor (BCR), endocytosis, antigen uptake, antigen presentation, clathrin, endophilin A2, caveolae, phagocytosis
Citation: McShane AN and Malinova D (2022) The Ins and Outs of Antigen Uptake in B cells. Front. Immunol. 13:892169. doi: 10.3389/fimmu.2022.892169
Received: 08 March 2022; Accepted: 31 March 2022;
Published: 26 April 2022.
Edited by:
Robert Eisenberg, University of Pennsylvania, United StatesReviewed by:
Marko Radic, University of Tennessee College of Medicine, United StatesCopyright © 2022 McShane and Malinova. This is an open-access article distributed under the terms of the Creative Commons Attribution License (CC BY). The use, distribution or reproduction in other forums is permitted, provided the original author(s) and the copyright owner(s) are credited and that the original publication in this journal is cited, in accordance with accepted academic practice. No use, distribution or reproduction is permitted which does not comply with these terms.
*Correspondence: Dessislava Malinova, ZC5tYWxpbm92YUBxdWIuYWMudWs=